- 1Australian Institute of Marine Science, Indian Ocean Marine Research Centre, The University of Western Australia, Crawley, WA, Australia
- 2The University of Western Australia Oceans Institute and School of Biological Sciences, University of Western Australia, Crawley, WA, Australia
- 3Georgia Aquarium, Atlanta, GA, United States
- 4Department of Environment and Geography, University of York, Heslington, United Kingdom
Age and growth data are central to management or conservation strategies for any species. Circumstantial evidence suggests that male whale sharks (Rhincodon typus) grow to asymptotic sizes much smaller than those predicted by age and growth studies and consequently, there may be sex-specific size and growth patterns in the species. We tested this hypothesis by using stereo-video and photo-identification studies to estimate the growth rates of 54 whale sharks that were resighted over a period of up to a decade at Ningaloo Reef. We found that male growth patterns were consistent with an average asymptotic total length (TL) of approximately 8–9 m, a size similar to direct observations of size at maturity at aggregation sites world-wide and much smaller than the sizes predicted by earlier modeling studies. Females were predicted to grow to an average asymptotic length of around 14.5 m. Males had growth coefficients of K = 0.088 year–1, whereas limited resighting data suggested a growth coefficient of K = 0.035 year–1 for females. Other data including re-sightings of an individual male over two decades, records of sex-specific maximum sizes of individuals captured in fisheries and data from juveniles growing in aquaria were also consistent with the suggestion of sex-specific growth profiles for the species. We argue that selection for sex-specific growth patterns could explain many of the otherwise enigmatic patterns in the ecology of this species including the tendency of the species to form aggregations of juvenile males in coastal waters.
Introduction
Whale sharks are the world’s largest fish, growing to maximum known sizes of 18 m total length (TL) (McClain et al., 2015). The immense size of these animals implies slow growth rates and great longevity (Speakman, 2005), factors that are likely to promote low resilience to anthropogenic threats such as overfishing, warming oceans and ship strike. Indeed, declines in abundance have led to whale sharks recently being classified as Endangered by the IUCN Red List (Pierce and Norman, 2016).
Data on the growth patterns of whale sharks is very limited. It is mostly derived from age analysis of the vertebrae of a few individuals (n = 15) that have been stranded on shorelines in the western Indian Ocean (Wintner, 2000) and from sharks (n = 92) that were captured during a fishery (now closed) off the coast of Taiwan (Hsu et al., 2014). Interpretation of some of these data is complicated by problems in estimating ages from vertebrae (as is the case with other large sharks; Harry, 2018; Natanson et al., 2018). Furthermore, new research that has validated aging techniques using a bomb radiocarbon approach (Ong et al., 2020) shows that in analyses where biannual patterns in the deposition of bands growth has been assumed, growth rates were overestimated.
All growth rate studies of whale sharks, including a recent photo-identification approach by Perry et al. (2018), have mostly sampled juvenile males (3–7 m TL). Data from these animals has been used to extrapolate growth patterns of adults, with predicted asymptotic sizes sometimes exceeding the documented maximum size of 18 m for the species. This idea is difficult to reconcile with the direct observations of size at maturity of male sharks reported by several studies, as these show that (based on clasper morphology) males generally attain sexual maturity at around 8 m (Norman and Stevens, 2007). This implies that male size is more likely to asymptote at a much smaller size range, given well-established trade-offs between growth and sexual maturation in fishes (Roff, 1991; He and Stewart, 2001). Further support for asymptotic rather than indeterminate growth in whale sharks is provided by repeated observations of a 7.4 m TL mature male shark at Ningaloo Reef that has not changed in size in over two decades (Norman and Morgan, 2016). If male sharks do asymptote in size at <10 m, it is likely that only females grow to the much larger sizes observed in the field. Again, this suggestion is supported by direct observations where all the sharks that have been measured reliably to be larger than 13 m have been females (Hsu et al., 2012; Ramírez-Macias et al., 2012; McClain et al., 2015), with the exception of a single male from the equatorial Atlantic (Macena and Hazin, 2016). Conversely, in Qatar (Robinson et al., 2016) and Donsol in the Philippines (McCoy et al., 2018), where coastal aggregations of whale sharks often have high proportions of mature males, individuals over 10 m TL have not been recorded.
Sex-specific life history strategies are typical of many sharks, with numerous examples of species where females grow to larger sizes than males, including great hammerhead (Sphyrna mokarran; Piercy et al., 2010), bonnethead (Sphyrna tiburo; Carlson and Parsons, 1997), blacknose (Carcharhinus acronotus; Driggers et al., 2004), and shortfin mako (Isurus oxyrinchus; Semba et al., 2009) sharks. In these species, sexual dimorphism in size is accompanied by differences in growth rate, with males having higher growth coefficients than females so that they more rapidly approach a smaller asymptotic size. If this is the case for whale sharks, as predicted by direct observations and life history strategies in other sharks, we might thus expect males to grow faster than females, at least during the early juvenile phase. This might have important implications both for conservation strategies and for calculations of longevity for the species if male growth trajectories are extrapolated to maximum adult body sizes.
Here, we record the growth patterns of free-swimming whale sharks over a decade at an aggregation site in nearshore waters at Ningaloo Reef, Western Australia. This population, which is dominated by juvenile males of 3–7 m TL, has been the subject of long-term (10 years) research including photo-identification and stereo-video measurements of size (Meekan et al., 2006; Sequeira et al., 2016). We combine these data to identify repeat measurements of individual sharks over time and use them to estimate growth patterns, testing the hypothesis that male sharks show a clear pattern of rapid growth to a comparatively smaller asymptotic size. We review available evidence both from our study and from animals held in aquaria to examine the question whether female sharks have an initially slower growth trajectory than males but ultimately are likely to attain larger asymptotic sizes. We then examine the implications of sex-specific patterns in size and growth of whale sharks on the biology, ecology and conservation of the species.
Materials and Methods
Whale sharks have spot and stripe patterns on the body that are unique to individuals and photographs of these patterns taken by snorkelers or divers can be used as an identifying tag in mark-recapture studies (Meekan et al., 2006; Holmberg et al., 2009). We combined this photo-identification approach with stereo-video and photogrammetry (Sequeira et al., 2016) in order to calculate the body size of individuals through time. The use of this non-invasive approach to estimate growth rates is viable for whale sharks because they form predictable aggregations in shallow coastal waters in tropical and warm subtropical regions and display a high degree of site fidelity to these aggregations, with some recorded sporadically over periods of up to 20 years (Meekan et al., 2006; Norman and Morgan, 2016; Sequeira et al., 2016).
Data Collection
We collected yearly length measurements of whale sharks at Ningaloo Reef, Western Australia (22°41′39″ S, 113°37′54″ E), from 2009 to 2019, during the peak of the annual whale shark aggregation (Taylor, 1996), typically in the first week of May each year (Supplementary Figure 1). Upon locating sharks (usually by aerial survey), snorkelers entered the water and (i) took high-resolution identification (ID) photos of the flank above each pectoral fin from the fifth gill slit to the posterior point of the pectoral fin on both sides (Speed et al., 2007), (ii) assessed sex by examining the presence or absence of claspers, and (iii) recorded full-body video sequences using a diver-operated stereo-video system (DOVs)1 to conduct measurements of body length. All observations of whale sharks received an individual identification code. We used the ID photos to identify repeated sighting and measurements of individual whale sharks within and between years, based on distinguishing patterns of spots or stripes (Meekan et al., 2006). The DOVs contained two Canon HFG25 (25 frames per second, 1920 × 1080 pixel resolution, wide field of view) or GoPro Hero 4 Black cameras (30 frames per second, 1920 × 1080 pixel resolution, medium field of view) mounted ∼0.85 to 1 m apart at an inwardly converged angle (∼4°), and set in a custom housing designed to maintain calibration stability. The separation distance between the cameras used here was larger than conventional systems designed for finfish as the larger separation distance allows for more accurate measurements of targets likely to be further away (Boutros et al., 2015). Before each field trip, camera calibrations were conducted on a large calibration square (∼2 × 2 m) set on the bottom of a pool and measured at a distance of >5 m to reflect the likely ranges of targets in the field. Calibrations were conducted using the CAL software (see “Footnote 1”) and calibration accuracy was verified by measuring known lengths on a scale bar post-calibration in the swimming pool. We measured the fork length (FL; 2009–2019) and TL (2009–2011) of individual whale sharks using the EventMeasure software (see “Footnote 1”). We found that FL was a more consistent and reliable measurement, as TL was more prone to bias from tail flexion. However, to enhance comparability with other published studies on whale sharks, we converted estimates of all resighted individuals to TL using the derived relationship between FL-TL (calculated using 2009–2011 paired estimates across individuals fit with a linear model; Figure 1).
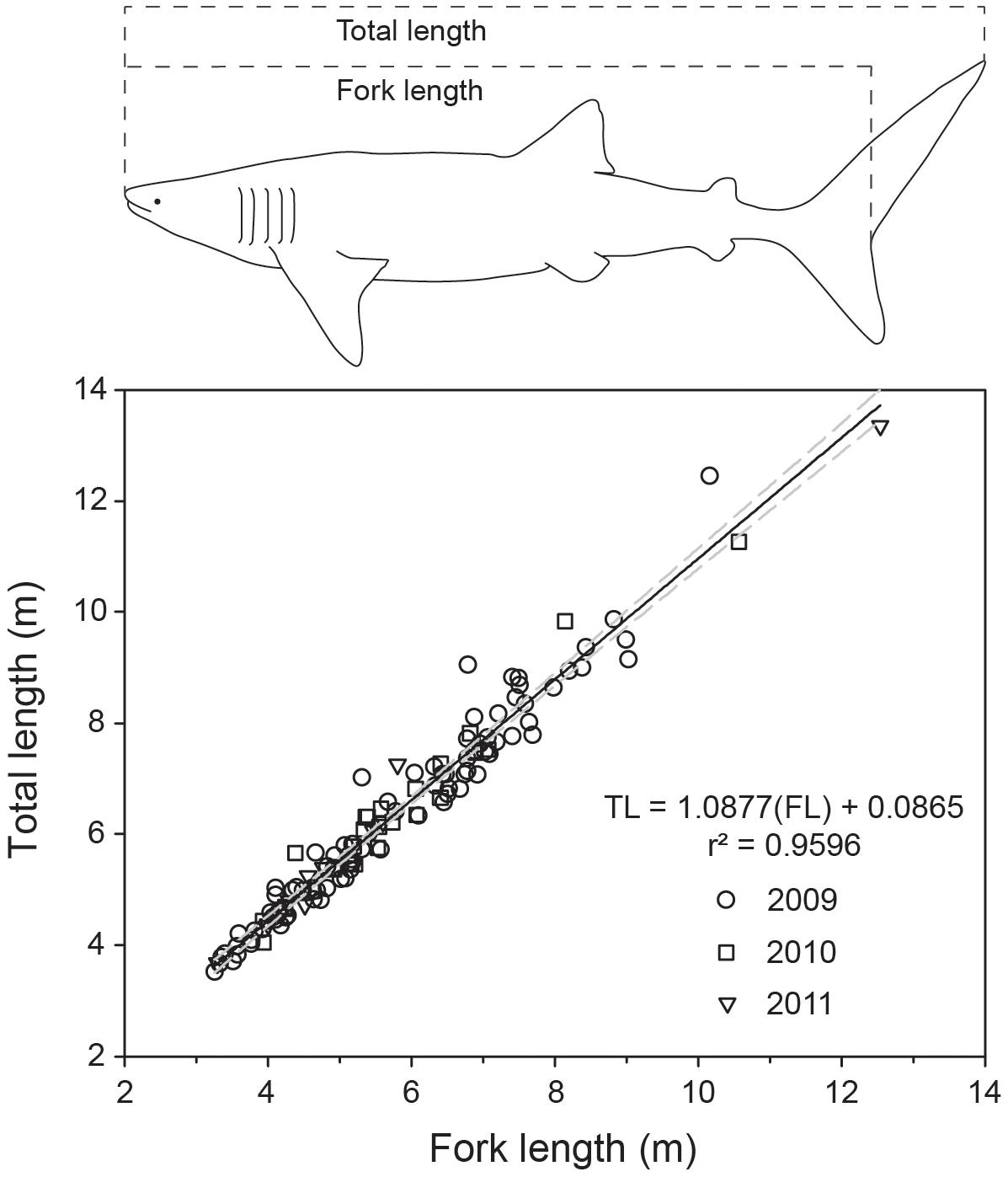
Figure 1. Linear relationship predicting total length from fork length for whale sharks, based on 3 years of sampling and 124 paired shark measurements. Whale shark outline redrawn from Rohner et al. (2011).
Analysis of Growth
We estimated sex-specific growth profiles for whale sharks using size differences between resightings by fitting the von Bertalanffy growth model (VBGM) to estimate the parameters L∞ (the mean asymptotic TL in m) and the growth coefficient K (which describes the curvature of growth toward L∞; in units year–1). We used the VBGM formulation arranged for tagging data (Fabens, 1965; Francis, 1988):
where L1 is the TL at first sighting, ΔL is the difference in TL between first and final sighting, and Δt is the time at liberty (in decimal years) between first and final sightings for an individual. We solved variables K and L∞ for each equation using non-linear least-squares estimation facilitated through the nls() function in R (Baty et al., 2015). Plots of growth models were constrained (y-intercept) to 0.6 m TL to reflect estimates of size at birth (Joung et al., 1996) and growth parameters were estimated for males and females separately because the numerical dominance of males precludes the interpretation of the result as a combined growth profile. Models were fitted with parameter ranges fixed at 0.0–0.5 year–1 for K and 6–25 m for L∞. Confidence intervals were determined through 10,000 iterations of bootstrap resampling. To examine whether differences in sex-specific growth were simply caused by the low number and range of female observations, we repeated this model fitting for males while including only individuals that matched the initial length range (4–7 m TL) and time at liberty (1–5 years) of female observations.
We compared our data set of individual growth trajectories with published estimates of whale shark growth profiles (Wintner, 2000; Hsu et al., 2014; Perry et al., 2018; Ong et al., 2020). For those studies providing growth parameter estimates, we plotted the individual shark growth trajectories from Ningaloo as line segments overlaid on respective von Bertalanffy growth curves, whereby length and age combinations for initial observations were presumed to fall on the estimated age-at-length predicted by the growth model. We performed a similar exercise to examine the concordance between our growth parameter estimates and observed growth trajectories from whale sharks in captivity (National Museum of Marine Biology and Aquarium, Taiwan; Okinawa Expo Aquarium and Osaka Aquarium Kaiyuken, Japan) using published data with growth intervals ranging from <1 year to nearly two decades (five males, two females, and one unsexed individual, ranging from 0.6 to 4.9 m TL at initial size; Chang et al., 1997; Uchida et al., 2000; Wintner, 2000; Nishida, 2001; Matsumoto et al., 2019) and also new data from the Georgia Aquarium (two males, two females - ranging from 4.1 to 4.7 m TL at initial size and observed for over a decade).
Results
A total of 54 (6 females, 48 males) whale sharks were resighted and successfully measured across the 10-year sampling period. Males were more commonly encountered and resighted (n = 48), ranging in TL (considering both initial and final sightings) from 3.65 to 8.9 m, and in time at liberty from 1 to 10 years (Supplementary Figure 2). Only six females were remeasured across years, and these ranged in TL from 4.00 to 8.04 m, with times at liberty spanning 1–5 years.
Model fitting using least squares estimation provided good model fits for males (Figure 2). Model validity for females was more difficult to determine given the low number of observations. Male and female growth profiles differed substantially with males having slightly faster growth in the first decade but slowing to reflect asymptotic growth in the later stages of the life span (von Bertalanffy growth parameters: K = 0.088 year–1 [0.057–0.125 95% CI]; L∞ = 8.45 m TL [7.91–9.53 95% CI]; Figure 3). Conversely, our growth model suggests females display slower initial growth rates that are sustained to a degree throughout their life span, resulting in a more indeterminate growth pattern with larger size-at-age past approximately 20 years (von Bertalanffy growth parameters: K = 0.035 year–1 [0.017–0.084 95% CI]; L∞ = 14.55 m TL [9.65–23.26 95% CI]; Figure 3). Despite having only six females, bivariate data clouds from bootstrap re-estimation of growth parameter pairs demonstrated that male and female estimate distributions barely overlapped (Figure 3B), indicating that growth profiles differed considerably between the sexes. This discrepancy between sexes was sustained when growth parameters were modeled with the limited set of male observations that matched the ranges of initial lengths and times at liberty of females (male parameters K = 0.191 year–1; L∞ = 7.17 m TL).
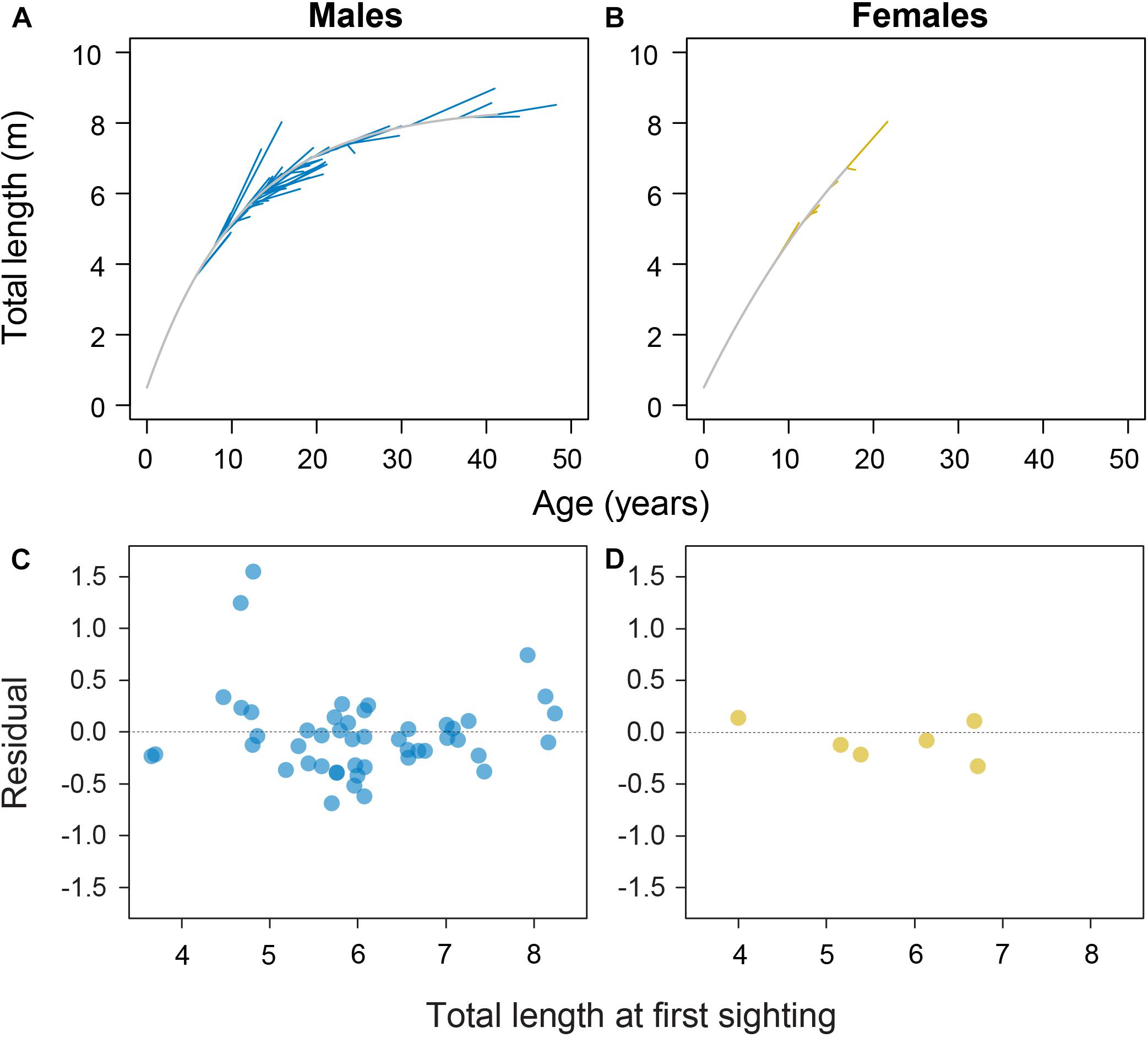
Figure 2. Sex-specific growth profiles (in gray) with individual shark growth trajectories plotted in blue for 48 male (A) and yellow for 6 female whale sharks (B) with associated residual plots (C,D) from the von Bertalanffy growth model fitted to whale shark resighting data.
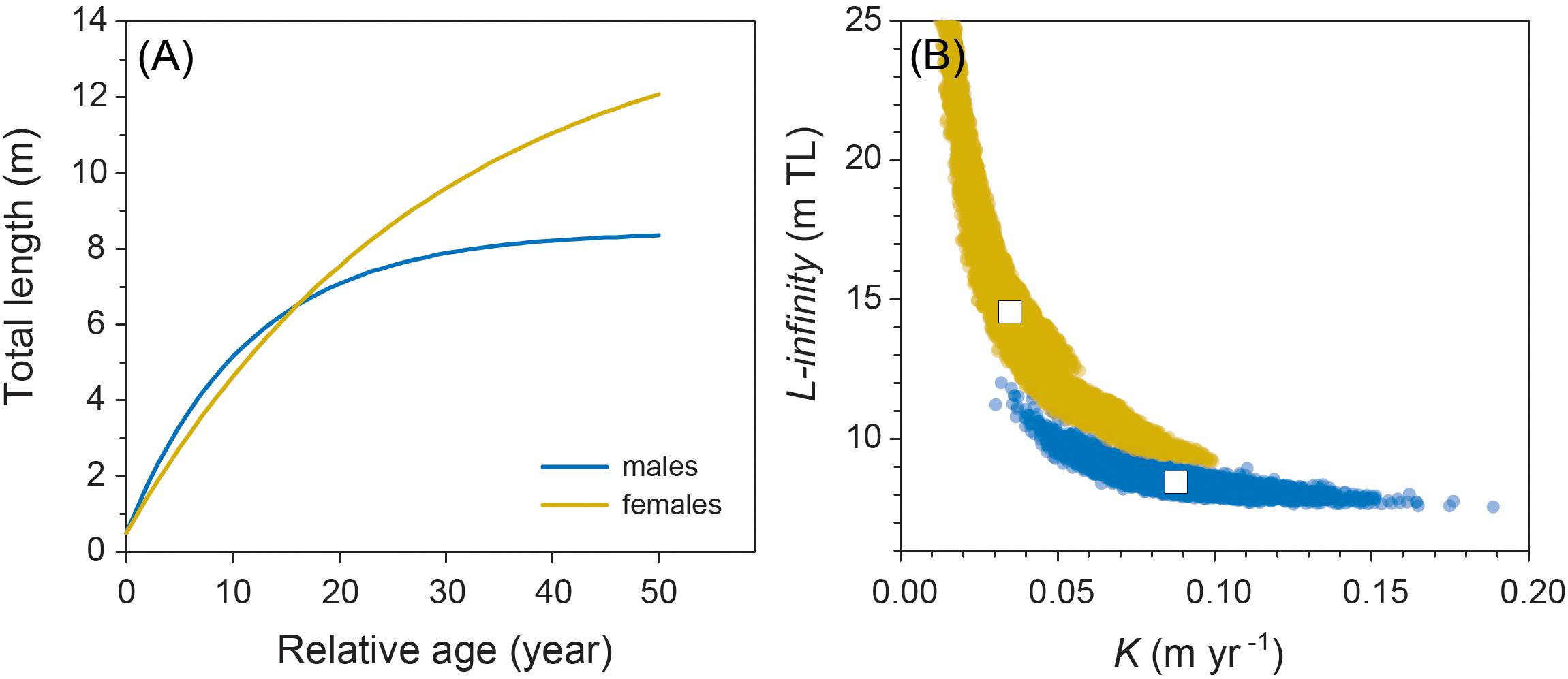
Figure 3. (A) Male and female growth profiles from the von Bertalanffy growth model fitted to resighting data from 48 male and 6 female whale sharks. (B) Bivariate confidence data clouds of paired estimates of von Bertalanffy growth parameters K and L∞ based on 200 bootstrapped re-estimates. White boxes represent coordinates of mean parameter estimates.
Two males were observed to have anomalously high growth from initially small sizes (Figure 2A). These observations were double-checked and confirmed as appropriately paired identifications through time with stereo-video measurements of high precision. Nevertheless, we reran the growth model with these two individuals omitted to see what influence they had on the growth model; growth parameters changed to L∞ = 8.91 m TL and K = 0.058 year–1, demonstrating a considerable influence of these two individuals on growth estimates.
Observations of growth of whale sharks kept in aquaria were largely congruent with our sex-specific growth models (Figure 4). Where male and female growth trajectories overlapped at approximately 5–6 m TL, male growth rates declined faster than those of females. However, the growth trajectories of individuals we observed in the field at Ningaloo Reef did not conform to growth profiles derived from other studies, all of which displayed patterns that were much more indeterminate (Supplementary Figure 4).
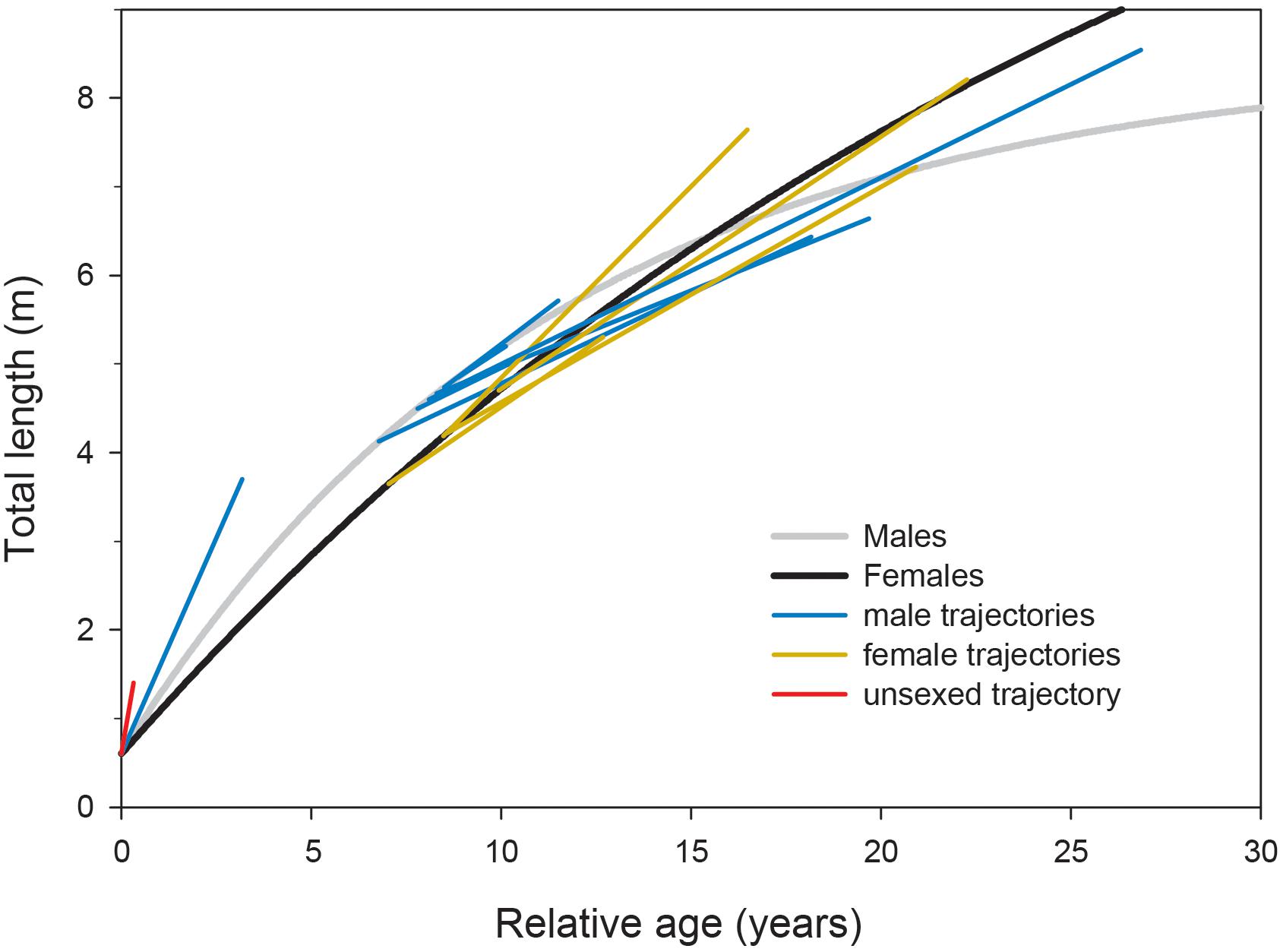
Figure 4. Growth trajectories for whale sharks kept in captivity overlaid onto growth profiles modeled from Ningaloo Reef, Western Australia, with the initial age predicted by sex-specific growth curve parameters. Four observations (two males, two females; unpublished data) are from the Georgia Aquarium (United States), each spanning over a decade; two observations (one male, one female; Wintner, 2000) are from the Osaka Aquarium Kaiyukan (Japan) spanning 3–8 years; five observations (four males, one female; Uchida et al., 2000; Nishida, 2001; Matsumoto et al., 2019) are from the Okinawa Churaumi Aquarium (Japan) spanning approximately 1–18 years; and one observation (unsexed; Chang et al., 1997) is from the National Museum of Marine Biology and Aquarium (Taiwan) spanning 120 days.
Discussion
Our analysis of the growth patterns of 54 whale sharks that were resighted for up to a decade at Ningaloo Reef suggested that males in this population attained average asymptotic (total) lengths of approximately 8.5–9.0 m. This size range is much smaller than estimates based on prior vertebral analyses (Wintner, 2000; Hsu et al., 2014) and resighting data (Perry et al., 2018) (summarized in Table 1). For example, Perry et al. (2018) estimated male asymptotic size to be over 18 m, whereas Hsu et al. (2014) calculated sizes of 18 and close to 20 m depending on the rate of band deposition in the vertebrae. Wintner (2000) estimated a size (15 m) that was closer to our estimates for females when theoretical data points were added to the calculation of the VBGM, but a size of almost 20 m when the relationship was unconstrained by these points. Similarly, Ong et al. (2020) predicted asymptotic sizes of 22 m for whale sharks off the coast of Taiwan using a von Bertalanffy model of growth (but only 11 m using a logistic growth model). As these researchers noted, these asymptotic sizes of males are likely to largely reflect the selective sampling of whale shark populations in each location. All data sets for growth analyses (including the present study) have been dominated by juvenile males, with few adults to constrain the upper portion of the curve. No growth study to date has included sharks over ∼10 m (Supplementary Figure 3). When the data sets lack such individuals, very large asymptotic sizes may be an analytical by-product of the relatively fast growth rates of the smallest sharks; indeed, the analysis by Wintner (2000) shows how the addition of larger (theoretical) individuals to a data set has a major impact that reduces estimates of asymptotic size. Unlike the aggregation in the Maldives, which was documented by Perry et al. (2018), the aggregation at Ningaloo Reef is not completely composed of juveniles, but also includes some adult males. These adults serve to anchor the top end of the growth curve data, so that our estimates of asymptotic length correlate well with reported sizes at first maturity for males both at this (Norman and Stevens, 2007) and other (Ramírez-Macias et al., 2012; Rohner et al., 2015) aggregation sites. Importantly, observations of a mature male resighted over decades at Ningaloo Reef also supports our much smaller estimates of asymptotic length. This individual did not continue to grow through time but plateaued in size at around 7.4 m for at least 20 years (Norman and Morgan, 2016).
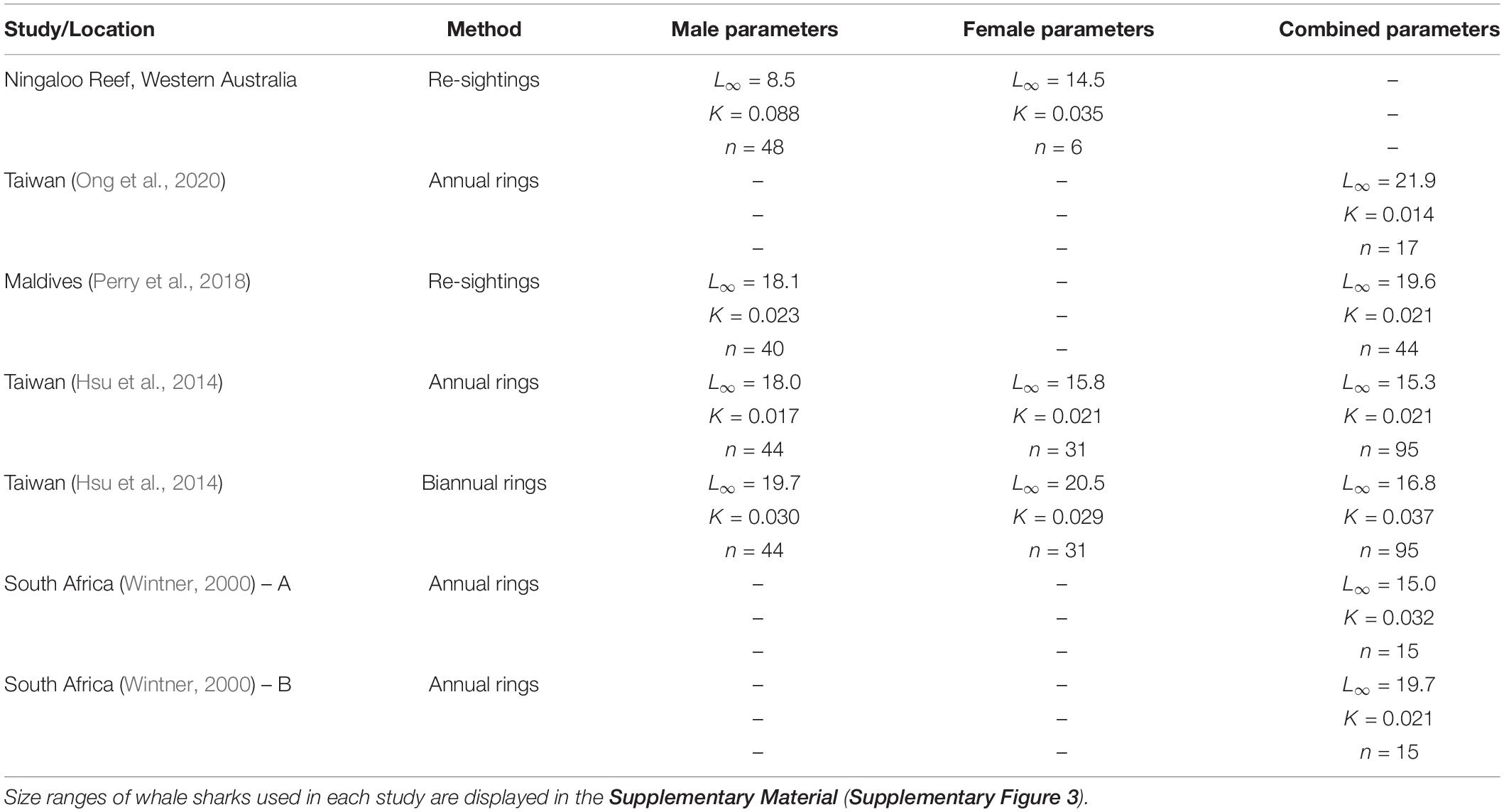
Table 1. von Bertalanffy growth parameter estimates from different studies and regions based on resighting or vertebral data.
In contrast to our results and direct observations, earlier studies have depicted lifetime profiles of growth of whale sharks that are largely indeterminate; that is, growth rate changes minimally across size and age classes, leading to predicted sizes often in excess of the largest individuals ever recorded. Our suggestion that male whale sharks exhibit more asymptotic (or determinate) growth profiles than previously reported stems from a more representative range of size observations between juvenile and mature stages (Supplementary Figure 3) coupled with the clear change in individual growth trajectories observed with increasing shark size (Figure 2). Acknowledging the potential for regional variation in growth rates and profiles, the pairing of Ningaloo growth trajectories with predicted growth profiles from other studies shows a consistent pattern for males, whereby observed trajectories switch from higher to lower than predicted as shark size increases (Supplementary Figure 4). Using a back-calculation approach on vertebral increments, Wintner (2000) demonstrated that the earliest growth trajectories may be J-shaped, implying a slow initial start that results in a growth profile that conforms better to a logistic rather than von Bertalanffy model. Indeed, use of a logistic model in Ong et al. (2020) produced estimates of asymptotic length (10.7 m) more similar to our estimates for males, and a slower initial growth rate in general might account for the differences in predicted age-specific growth rates between our study and others. Although there are very few published observations of growth rates for whale shark pups, a full-term embryo (sex not provided) raised for 120 days in Taiwan (taken from a commercially harvested mother; Chang et al., 1997) and a male embryo raised for 1157 days in the Okinawa Churaumi Aquarium (Nishida, 2001) displayed linear growth trajectories in excess of rates predicted even by the present study (Figure 4). This may not be surprising given that aquarium-kept animals often have greater growth rates than wild conspecifics because of the constant availability of energy-rich food in a situation where there are minimal metabolic costs for prey acquisition. However, these early growth trajectories did reasonably reflect early growth patterns predicted by our models, whereas the observed age-at-size for the older juvenile (terminal age of 3.2 years) was approximately 6–13 years lower than ages predicted by vertebral counts for similar-sized whale sharks in other studies. In contrast, observations of the growth of much larger juveniles kept in aquaria, some for more than a decade (six males and four females; Uchida et al., 2000; Wintner, 2000; Matsumoto et al., 2019; data from Georgia Aquarium) conformed very well to our predicted sex-specific growth profiles (Figure 4) constructed from the wild population at Ningaloo.
Although based on very limited data for females, due to the male bias in sex ratios of sharks in the aggregation at Ningaloo (as occurs in most other aggregations worldwide), we predicted that female whale sharks grew to an average asymptotic length of around 14.5 m. Females also had lower growth coefficients than males (K = 0.035 year–1 vs. K = 0.088 year–1, respectively) implying they approach asymptotic size more slowly, but keep growing over a much longer time period than males. This pattern is consistent with observations of other sharks that display sex-specific asymptotic sizes (Carlson and Parsons, 1997; Driggers et al., 2004; Semba et al., 2009; Piercy et al., 2010). Our contention that males do not grow as large as females and have average asymptotic sizes of 8.5–9 m is also supported by records of the largest sharks either observed in situ or captured by fisheries. In nearly all these cases, individuals over 12 m in TL have been females, including the largest individuals ever recorded estimated to be 18 m in length (McClain et al., 2015). This is further circumstantial evidence that our estimates of K and asymptotic length for males are biologically realistic. In this context, it is important to note that the parameter L∞ represents average values of asymptotic length for both males and females; this parameter is sometimes confused with maximum size. Therefore, in species with determinate growth patterns, this implies that approximately half of all individuals will be larger than this value, and thus occasional observations of very large males are entirely consistent with the model, although attainment of such large sizes will likely require a very long life span. Indeed, our bootstrapped estimates of asymptotic size for males and females (Figure 3B) show that a wide range of values is possible and for females, could easily include individuals of the largest sizes (18 m TL) observed in the wild by other studies. It is notable, however, that inclusion of the four observations of female growth trajectories in aquaria to our original growth model yields an even smaller predicted asymptotic length of 12.1 m TL.
Differences in growth and size between sexes might explain several otherwise enigmatic patterns in the biology of whale sharks. For example, the need for males to grow faster than females could account for the sex-specific distributions of whale sharks, with coastal aggregations dominated by juvenile males despite evidence for equal sex ratios of males and females at birth (Joung et al., 1996). These aggregations form in areas where there are predictable bursts of planktonic productivity and thus probably assist males to maintain higher growth coefficients (Meekan et al., 2015). Such high rates are likely to be more difficult to achieve in the open ocean where diving during the day to access food in the cool waters of the deep scattering layer is accompanied by the need to bask in surface waters to maintain body temperatures (Thums et al., 2013; Meekan et al., 2015). Vertical movements in the warm, well-mixed water column on the shelf of Ningaloo Reef do not impose this metabolic cost. In contrast, the slower growth rates of females may be maintained irrespective of the cost of feeding in the deep scattering layer during the day, without the need to inhabit coastal waters where there may be a heightened risk of predation (Fitzpatrick et al., 2006; Lester et al., 2020) and/or harassment of immature females by larger males for mating. For females, very large maximum body sizes could be advantageous given the potential need to provision and brood a multitude of pups–up to 300 at one time (Joung et al., 1996).
In summary, we found good evidence that male whale sharks attain asymptotic sizes of around 8.5–9 m at Ningaloo Reef, which suggests that females are more likely to reach the largest sizes (>12 m) observed in the species. This idea is consistent with direct observations of male and female size by other studies and with the growth rates of juvenile males and females held in aquaria for more than a decade. We cannot exclude the possibility that a sampling bias toward slow-growing sharks occurs in our study, if fast-growing individuals attain maturity more quickly and depart the aggregation, so are less likely to be re-sighted. Similarly, data from a fishery may also be biased toward smaller, slower-growing sharks because a rapid reduction in average sizes of individuals is a characteristic feature of fisheries that have targeted the species (Rowat and Brooks, 2012; Pierce and Norman, 2016). However, as noted above, our models are consistent with independent observations of the growth rates of sharks both in aquaria and in the field at Ningaloo and with sizes at maturity recorded across a range of localities worldwide.
Sex-specific life-history patterns in whale sharks could provide plausible explanations for many of the features of the ecology of the species, such as the tendency to form aggregations of juvenile males in shallow coastal waters of the tropics. Ultimately, these may be linked to the remarkable biology of the species that involves gigantothermy and large litters of pups.
Data Availability Statement
All datasets generated for this study (resighting data from Ningaloo Reef and whale shark growth in aquaria) are included in the Supplementary Material.
Ethics Statement
This study was carried out with approval by the University of Western Australia Animal Ethics Office.
Author Contributions
MM conceived and funded the study. MM, MT, EL, LF, AS, AD, MB, and KB collected the data. AA, BT, MB, and KB performed video analysis. BT analyzed the data. MM and BT wrote the manuscript with critical input from all authors.
Funding
This work has been funded by Santos, the Australian Institute of Marine Science, the Save Our Seas Foundation, and the Georgia Aquarium.
Conflict of Interest
The authors declare that the research was conducted in the absence of any commercial or financial relationships that could be construed as a potential conflict of interest.
Acknowledgments
We are grateful to the many staff and volunteers who assisted with field data collection over the years and to Terry Maxwell, Kelly Woodvine, Dani Rob, Emily Wilson, and Peter Barnes for supporting our work.
Supplementary Material
The Supplementary Material for this article can be found online at: https://www.frontiersin.org/articles/10.3389/fmars.2020.575683/full#supplementary-material
Footnotes
References
Baty, F., Ritz, C., Charles, S., Brutsche, M., Flandrois, J.-P., and Delignette-Muller, M.-L. (2015). A toolbox for nonlinear regression in R: the package nlstools. J. Stat. Softw. 66, 1–21.
Boutros, N., Shortis, M. R., and Harvey, E. S. (2015). A comparison of calibration methods and system configurations of underwater stereo-vdeo systems for applications in marine ecology. Limnol. Oceanogr. Meth. 13, 224–236. doi: 10.1002/lom3.10020
Carlson, J. K., and Parsons, G. R. (1997). Age and growth of the bonnethead shark, Sphyrna tiburo, from northwest Florida, with comments on clinal variation. Environ. Biol. Fishes 50, 331–341.
Chang, W.-B., Leu, M.-Y., and Fang, L.-S. (1997). Embryos of the whale shark, Rhincodon typus: early growth and size distribution. Copeia 2, 444–446. doi: 10.2307/1447769
Driggers, W. B., Carlson, J. K., Cullum, B., Dean, J. M., Oakley, D., and Ulrich, G. (2004). Age and growth of the blacknose shark, Carcharhinus acronotus, in the western North Atlantic Ocean with comments on regional variation in growth rates. Environ. Biol. Fishes 71, 171–178. doi: 10.1007/s10641-004-0105-z
Fabens, A. J. (1965). Properties and fitting of the von Bertalanffy growth curve. Growth 29, 265–289.
Fitzpatrick, B., Meekan, M., and Richards, A. (2006). Shark attacks on a whale shark (Rhincodon typus) at Ningaloo Reef, Western Australia. Bull. Mar. Sci. 78, 397–402.
Francis, R. I. C. C. (1988). Maximum likelihood estimation of growth and growth variability from tagging data. New Zeal. J. Mar. Fresh. 22, 42–51.
Harry, A. V. (2018). Evidence for systemic age underestimation in shark and ray ageing studies. Fish Fish. 19, 185–200. doi: 10.1111/faf.12243
He, J. X., and Stewart, D. J. (2001). Age and size at first reproduction of fishes: predictive models based only on growth trajectories. Ecology 82, 784–791. doi: 10.1890/0012-9658(2001)082[0784:aasafr]2.0.co;2
Holmberg, J., Norman, B., and Arzoumanian, Z. (2009). Estimating population size, structure, and residency time for whale sharks Rhincodon typus through collaborative photo-identification. Endanger. Species Res. 7, 39–53. doi: 10.3354/esr00186
Hsu, H. H., Joung, S. J., Hueter, R. E., and Liu, K. M. (2014). Age and growth of the whale shark (Rhincodon typus) in the north-western Pacific. Mar. Freshw. Res. 65, 1145–1154. doi: 10.1071/mf13330
Hsu, H. H., Joung, S. J., and Liu, K. M. (2012). Fisheries, management and conservation of the whale shark Rhincodon typus in Taiwan. J. Fish Biol. 80, 1595–1607. doi: 10.1111/j.1095-8649.2012.03234.x
Joung, S. J., Chen, C. T., Clarck, E., Uchida, S., and Huang, W. Y. P. (1996). The whale shark, Rhincodon typus, is a livebearer: 300 embryos found in one ‘megamamma’ supreme. Environ. Biol. Fishes 46, 219–223. doi: 10.1007/bf00004997
Lester, E., Meekan, M. G., Barnes, P., Raudino, H., Rob, D., Waples, K., et al. (2020). Multi-year patterns in scarring, survival and residency of whale sharks in Ningaloo Marine Park, Western Australia. Mar. Ecol. Prog. Ser. 634, 115–125. doi: 10.3354/meps13173
Macena, B. C. L., and Hazin, F. H. (2016). Whale shark (Rhincodon typus) seasonal occurrence, abundance and demographic structure in the mid-equatorial Atlantic Ocean. PLoS One 11:e0164440. doi: 10.1371/journal.pone.0164440
Matsumoto, R., Matsumoto, Y., Ueda, K., Suzuki, M., Asahina, K., and Sato, K. (2019). Sexual maturation in a male whale shark (Rhincodon typus) based on observations made over 20 years of captivity. Fish. Bull. 117, 78–86. doi: 10.7755/fb.117.1-2.9
McClain, C. R., Balk, M. A., Benfield, M. C., Branch, T. A., Chen, C., Cosgrove, J., et al. (2015). Sizing ocean giants: patterns of intraspecific size variation in marine megafauna. PeerJ 3:e715. doi: 10.7717/peerj.715
McCoy, E., Burce, R., David, D., Aca, E. Q., Hardy, J., Labaja, J., et al. (2018). Long-term photo-identification reveals the population dynamics and strong site fidelity of adult whale sharks to the coastal waters of Donsol, Philippines. Front. Mar. Sci. 5:271. doi: 10.3389/fmars.2018.00271
Meekan, M. G., Bradshaw, C. J. A., Press, M., McLean, C., Richards, A., Quasnichka, S., et al. (2006). Population size and structure of whale sharks Rhincodon typus at Ningaloo Reef, Western Australia. Mar. Ecol. Prog. Ser. 319, 275–285. doi: 10.3354/meps319275
Meekan, M. G., Fuiman, L. A., Davis, R., Berger, Y., and Thums, M. (2015). Swimming strategy and body plan of the world’s largest fish: implications for foraging efficiency and thermoregulation. Front. Mar. Sci. 2:64. doi: 10.3389/fmars.2015.00064
Natanson, L. J., Skomal, G. B., Hoffman, S. L., Porter, M. E., Goldman, K. J., and Serra, D. (2018). Age and growth of sharks: do vertebral band pairs record age? Mar. Freshw. Res. 69, 1440–1452. doi: 10.1071/mf17279
Nishida, K. (2001). “Whale shark – the worlds largest fish,” in Fishes of the Kuroshio Current, Japan, ISBN4-931418-04-XC0645, eds T. Kakabo, Y. Machida, K. Yamaoka, and K. Nishida (Osaka: Osaka Aquarium Kaiyukan), 20–35.
Norman, B. M., and Morgan, D. L. (2016). The return of “Stumpy” the whale shark: two decades and counting. Front. Ecol. Environ. 14, 449–450. doi: 10.1002/fee.1418
Norman, B. M., and Stevens, J. D. (2007). Size and maturity status of the whale shark (Rhincodon typus) at Ningaloo Reef in Western Australia. Fish. Res. 84, 81–86. doi: 10.1016/j.fishres.2006.11.015
Ong, J. J. L., Meekan, M. G., Hsu, H. H., Fanning, L. P., and Campana, S. E. (2020). Annual bands in vertebrae validated by bomb radiocarbon assays provide estimates of age and growth of whale sharks. Front. Mar. Sci. 7:188. doi: 10.3389/fmars.2020.00188
Perry, C. T., Figeiredo, J., Vaudo, J. J., Hancock, J., Rees, R., and Shivji, M. (2018). Comparing length-measurement methods and estimating growth parameters of free-swimming whale sharks (Rhincodon typus) near the South Ari Atoll, Maldives. Mar. Freshw. Res. 69, 1487–1495. doi: 10.1071/mf17393
Pierce, S. J., and Norman, B. (2016). Rhincodon typus. IUCN Red List Threat. Spec. 2016:eT19488A2365291. doi: 10.2305/IUCN.UK.2016-1.RLTS.T19488A2365291.en
Piercy, A. N., Carlson, J. K., and Passerotti, M. S. (2010). Age and growth of the great hammerhead shark, Sphyrna mokarran, in the north-western Atlantic Ocean and Gulf of Mexico. Mar. Freshw. Res. 61, 992–998. doi: 10.1071/mf09227
Ramírez-Macias, D., Vázquez-Haikin, A., and Vázquez-Juárez, R. (2012). Whale shark Rhincodon typus populations along the west coast of the Gulf of California and implications for management. Endanger. Species Res. 18, 115–128. doi: 10.3354/esr00437
Robinson, D. P., Jaidah, M. Y., Bach, S., Lee, K., Jabado, R. W., Rohner, C. A., et al. (2016). Population structure, abundance and movement of whale sharks in the Arabian Gulf and the Gulf of Oman. PLoS one 11:e0158593. doi: 10.1371/journal.pone.0158593
Roff, D. A. (1991). The evolution of life-history variation in fishes, with particular reference to flatfishes. Netherl. J. Sea Res. 27, 197–207. doi: 10.1016/0077-7579(91)90024-u
Rohner, C. A., Richardson, A. J., Marshall, A. D., Weeks, S. J., and Pierce, S. J. (2011). How large is the world’s largest fish? Measuring whale sharks Rhincodon typus with laser photogrammetry. J. Fish Biol. 78, 378–385. doi: 10.1111/j.1095-8649.2010.02861.x
Rohner, C. A., Richardson, A. J., Prebble, C. E. M., Marshall, A. D., Bennett, M. B., Weeks, S. J., et al. (2015). Laser photogrammetry improves size and demographic estimates for whale sharks. PeerJ 3:e886. doi: 10.7717/peerj.886
Rowat, D., and Brooks, K. S. (2012). A review of the biology, fisheries and conservation of the whale shark Rhincodon typus. J. Fish Biol. 80, 1019–1056. doi: 10.1111/j.1095-8649.2012.03252.x
Semba, Y., Nakano, H., and Aoki, I. (2009). Age and growth analysis of the shortfin mako, Isurus oxyrinchus, in the western and central North Pacific Ocean. Environ. Biol. Fishes 84, 377–391. doi: 10.1007/s10641-009-9447-x
Sequeira, A. M. M., Thums, M., Brooks, K., and Meekan, M. G. (2016). Error and bias in size estimates of whale sharks: implications for understanding demography. R. Soc. Open Sci. 3:150668. doi: 10.1098/rsos.150668
Speakman, J. R. (2005). Body size, energy metabolism and lifespan. J. Exp. Biol. 208, 1717–1730. doi: 10.1242/jeb.01556
Speed, C. W., Meekan, M. G., and Bradshaw, C. J. A. (2007). Spot the match – wildlife photo-identification using information theory. Front. Zool. 4:2. doi: 10.1186/1742-9994-4-2
Taylor, J. G. (1996). Seasonal occurrence, distribution and movements of the whale shark, Rhincodon typus, at Ningaloo Reef, Western Australia. Mar. Freshw. Res. 47, 637–642. doi: 10.1071/mf9960637
Thums, M., Meekan, M. G., Stevens, J., Wilson, S., and Polovina, J. (2013). Evidence for behavioural thermoregulation by the world’s largest fish. J. R. Soc. Interface 10:20120477. doi: 10.1098/rsif.2012.0477
Uchida, S., Toda, M., Kamei, Y., and Teruya, H. (2000). “The husbandry of 16 whale sharks Rhincodon typus, from 1980 to 1998 at the Okinawa expo aquarium,” in Proceedings of the AES Whale Shark Meeting, La Paz.
Keywords: asymptotic size, sexual dimorphism, Ningaloo, Rhincodon typus, photo-identification
Citation: Meekan MG, Taylor BM, Lester E, Ferreira LC, Sequeira AMM, Dove ADM, Birt MJ, Aspinall A, Brooks K and Thums M (2020) Asymptotic Growth of Whale Sharks Suggests Sex-Specific Life-History Strategies. Front. Mar. Sci. 7:575683. doi: 10.3389/fmars.2020.575683
Received: 24 June 2020; Accepted: 25 August 2020;
Published: 16 September 2020.
Edited by:
Lars Bejder, University of Hawai‘i at Mānoa, United StatesReviewed by:
Alessandro Ponzo, Large Marine Vertebrates Research Institute Philippines (LAMAVE), PhilippinesSimon J. Pierce, Marine Megafauna Foundation, United States
Copyright © 2020 Meekan, Taylor, Lester, Ferreira, Sequeira, Dove, Birt, Aspinall, Brooks and Thums. This is an open-access article distributed under the terms of the Creative Commons Attribution License (CC BY). The use, distribution or reproduction in other forums is permitted, provided the original author(s) and the copyright owner(s) are credited and that the original publication in this journal is cited, in accordance with accepted academic practice. No use, distribution or reproduction is permitted which does not comply with these terms.
*Correspondence: Mark G. Meekan, bS5tZWVrYW5AYWltcy5nb3YuYXU=