- 1Department of Aquaculture, Faculty of Natural Sciences, Urmia University, Urmia, Iran
- 2Centro de San Carlos de la Rápita, Institut de Recerca i Tecnologia Agroalimentaries (IRTA), San Carlos de la Rápita, Tarragona, Spain
Larval rearing and ongrowing of meager have experienced a great advance during the last years and nowadays this species is considered as one of the most important new species in Mediterranean aquaculture. However, larval nutrition in meager still needs some improvements especially regarding live prey enrichment and fatty acid composition. In this study, a trial for larval rearing of meager (Argyrosomus regius) using different commercial products for rotifer enrichment (Multigain, Red Pepper and concentrated microalgae) with and without phospholipids (PL), and with a different fatty acid composition, especially regarding DHA, have been used to assess the growth and survival of the larvae and check the effect of PLs in larval growth. Lipid class composition was the same among the larvae whereas % DHA was always higher in larvae fed Multigain and Red Pepper enriched rotifers. The dominant fatty acids in the PL of the larvae and PL of the diets were well-correlated and the results suggested a close relationship between the composition of dominant PL fatty acids in the rotifer and in the larvae, especially in the case of DHA and 16:0 that show a high correlation.
Introduction
Nutrition and feeding during early development is one of the main important issues for marine fish larvae culture, especially for new species (Campoverde and Estévez, 2017). The success of larval rearing is influenced by the live prey selected for first feeding and their nutritional quality, being their content in lipids and their fatty acid profile the most important nutritional factors affecting larval growth and survival (Watanabe, 1993).
Marine lipids are rich in saturated and monounsaturated fatty acids, which are the main source of metabolic energy for the fast developing and growing fish larvae. Polyunsaturated fatty acids (PUFA) must also be provided in the first feeding diets of marine fish larvae, because they are considered essential fatty acids (EFA) since they cannot be biosynthesized. Three long chain PUFA (LC-PUFA), docosahexaenoic acid (DHA, 22:6n−3), eicosapentaenoic acid (EPA, 20:5n−3), and arachidonic acid (ARA, 20:4n−6) play several important roles in vital functions of fish. They are the main components of membranes and precursors of bioactive molecules such as eicosanoids which have hormone-like activity (Tocher, 2010). DHA also plays an important role during larval development as it is incorporated into nervous and retina tissue (Mourente and Tocher, 1992; Bell et al., 1996), and in the case that DHA is not provided to the larvae, poor growth, high mortality and several behavioral, physiological and morphological alterations have been observed (Lingenfelser et al., 1995; Tocher, 2010).
Phospholipids (PLs) are considered essential for fish larvae (Tocher et al., 2008; Cahu et al., 2009) due to their limited capacity of biosynthesis. Between 2 and 12% must be supplied in the diet for a normal growth and functional development as Tocher et al. (2008) suggested. Artemia and rotifers, the live preys used in aquaculture, have high PL content (Olsen, 2004) but their fatty acid composition, that is the absence of LC-PUFA, is not adequate for early larvae and they must be enriched before feeding them. On the other hand, copepods, the live prey that the larvae feed in the wild, are rich in phospholipids and in n−3 PUFA. Although the content of PL per dry weight (DW) of rotifers and Artemia cannot be manipulated, the fatty acid composition of PL can be modified in some degree (Guinot et al., 2013; Li et al., 2014; Rogacki et al., 2019). Supplementation of PL is usually carried out using commercial lecithin, either obtained from plants (soybean), chicken eggs, or pure phospholipids. Recently several marine phospholipids derived from krill, with a better fatty acid profile in terms of n−3 PUFA, are also available and have been used for Artemia enrichment (Guinot et al., 2013; Rogacki et al., 2019). One of the main problems in enriching the Artemia with DHA and PLs is the rapid metabolism of the metanauplii, that retroconvert DHA into EPA (Navarro et al., 1999), and when these LC-PUFA are delivered to Artemia as PLs, the metanauplii metabolize them into other lipid classes, particularly triacylglycerols (TAG), metabolizing EFA from PL fraction into the NL fraction (Guinot et al., 2013), being one of the most important handicaps for bioencapsulation of PLs and LC-PUFA in Artemia. On the other hand, in the case of rotifers Li et al. (2015) have shown that it is possible to enrich the DHA levels in PLs of rotifers to relatively high levels using short-term enrichment strategies.
Meager, Argyrosomus regius, is a new and valuable finfish species with increasing interest in European aquaculture due to its rapid growth during ongrowing and the quality of its meat with muscle low in fat content (Poli et al., 2003; Grigorakis et al., 2011). Protocols regarding spawning induction (Duncan et al., 2012), larval development (Jimenez et al., 2007; Cardeira et al., 2012) and larval rearing (Roo et al., 2010; Vallés and Estevez, 2013, 2015b; Campoverde et al., 2017) have already been published. Some information is also available about the nutritional requirements of the larva, including LC-PUFA, either using commercial enrichment products or microdiets for larval feeding (Vallés and Estevez, 2015a; Campoverde and Estévez, 2017; El Kertaoui et al., 2017). On the other hand, recent studies carried out with cod larvae have shown that DHA is better available to the larvae when it is incorporated into dietary PLs than in NLs (Olsen et al., 2014) for a better growth and organ and skeleton development.
Thus, the objective of the present study was to learn about the effect of different rotifer enrichment diets based on their content in LC-PUFA, and supplemented with or without marine phospholipids, on the growth, survival and fatty acid composition of meager larvae. The capacity of meager larvae to incorporate DHA from dietary PL in the early stages of feeding and growth was also examined.
Materials and Methods
Meager Larviculture and Sampling
Newly hatched meager (Argyrosomus regius) larvae were obtained from a commercial hatchery and transported by road to the Institut de Recerca i Tecnologia Agroalimentaries (IRTA, San Carles de la Rapita, Spain). Larvae were distributed into 18 40 L mesh-made basckets provided with air-lifts, placed in triplicates in 200 L tanks at a density of 50 larvae L−1. The tanks were connected to a recirculation unit (IRTAmarTM) and water temperature was controlled every day and maintained at 20.0 ± 1.2°C, salinity at 35.82 ± 0.33 ppt, dissolved oxygen at 7.2 ± 1.0 mg L−1 whereas pH (7.97 ± 0.06) nitrites (0.02 ± 0.02 mg L−1) and ammonia (0.1 ± 0.05 mg L−1) were checked 2 times per week (Hach Colorimeter DR/890, USA). Photoperiod was kept at 16 h light: 8 h darkness and light intensity was maintained at 500 lux at water surface following the recommendations of Vallés and Estevez (2013). At mouth opening, 2 days post-hatching (dph), larvae started being fed enriched rotifers until 14 dph twice daily (09:00 and 17:00 h) at a density of 10 rotifers mL−1.
Samples of 10 larvae were collected from each bascket at the beginning and at the end of the trial (15 dph) and killed with an overdose of anesthetic MS222 (1,000 mg L−1). Standard length (SL) was measured using a dissecting microscope and an image analyzer (Analysis, SIS Gmbh, Germany). The upper jaw length (Shirota, 1978) was measured in order to analyze larvae species gape size (GS) and the mouth opening at 90° calculated (Shirota, 1970). The same larvae were then washed on a mesh with distilled water, dry blotted to remove excess water, and pooled onto pre-weighted coverslips. They were then oven-dried at 60°C for 24 h and weighted to determine dry weight (DW) on a Mettler A-20 microbalance (Mettler Toledo, Columbus, OH, USA) to the nearest ±1 μg. Specific growth rate (SGR) was calculated at the end of the trials using the formula:
Where lnWf = the natural logarithm of the final weight; lnWi = the natural logarithm of the initial weight; and t = time (days) between lnWf and lnWi.
At the end of the experiment, all the larvae remaining in the tanks were anesthetized with MS-222, concentrated in a mesh, and counted to calculate the survival rate. All the larvae from the three replicates were washed with distilled water before freezing at −20°C for later analysis of lipids, lipid class composition, and fatty acids.
Rotifer Enrichment
Rotifers (Brachionus sp. Cayman) were cultivated in batch culture in 100 l conical fiberglass tanks at a salinity of 26 ppt, water temperature of 26°C, and 8 mg l−1 dissolved oxygen. The rotifer culture was aerated and daily fed microalgae (Tetraselmis chuii) at 4 × 105 cell ml−1 and yeast (Mauripan, Spain) at 0.7 g/million rotifers. The daily ration of rotifers was calculated after counting the rotifers, carefully harvested and divided into six oxygenated 10 l containers filled with UV filtered seawater and enriched for 12 h with the commercial enrichers Larviva Multigain (MG, a powder product, Biomar, Denmark), Red Pepper (RP, liquid product, Bernaqua, Belgium) and concentrated Nannochloropsis (NC, concentrated microalgae 6.25 × 109 cels ml−1, Blueclownfish, Spain) at a density of 500 rotifers ml−1 and 28 ± 1°C. The enrichment products were administered to the rotifers in 2 doses of 0.6 g l−1 of MG, 2 doses of 0.9 g l−1 of RP, and 2 doses of 2.5 g l−1 of NC following the recommendations of the products, doses were distributed at the beginning (0 h) and after 6 h enrichment. After 12 h the rotifers were gently filtered and washed using a 40 μm mesh with UV filtered seawater and disinfected by further rinsing for 1 min with freshwater, before feeding to the larvae. The same dose of rotifers, previously counted, enrichments, and treatments were used for the rotifers enriched with phospholipids, but in that case, a short-term enrichment, also known as boosting, was carried out for 3 h adding 0.6 g l−1 of LC60 (phospholipids extracted from krill, Phosphotech, France). The rotifers were then concentrated, rinsed, and washed with freshwater before feeding the larvae.
Samples of enriched rotifers for biochemical analyses were collected during the trial (days 2, 8, and 14), the samples were concentrated, washed with distilled water, and freezed at −20°C until analysis. All the analyses were carried out in duplicates.
Rotifers were chosen as the only live prey due to the difficulties in enriching Artemia with phospholipids and/or n−3 PUFA. Most studies of DHA enrichment of PL in Artemia showed that this fatty acid ended up in other lipid classes, mostly triacylglycerols (TAG), and very little was incorporated into PL, as Guinot et al. (2013), Monroig et al. (2006), and Sargent et al. (1999) have already established. Li and Olsen (2015) using a long-term rotifer enrichment protocol for cod larval feeding obtained high DHA levels in the PL fraction of rotifers showing a better capacity of this live prey to be enriched in PLs.
Commercial enrichment products Multigain and Red Pepper were selected due to its high use in European hatcheries and their particularities, Multigain is a powder product with 90% NL and 10% PL whereas Red Pepper is a liquid composed only by NLs.
Phospholipid enrichment of rotifers was carried out for 3 h to assure enough quantity of DHA in the PL of the rotifers (E. Almansa, pers.com., Mylonas et al., 2016).
Lipid Content, Lipid Class Composition and Fatty Acid Analysis
Total lipids were extracted in chloroform:methanol (2:1, v:v) using the method of Folch et al. (1957) and quantified gravimetrically after evaporation of the solvent under a nitrogen flow followed by vacuum desiccation overnight. Total lipids were stored in chloroform:methanol (2:1, 20 mg ml−1) containing 0.01% butylated hydroxytoluene (BHT) at −20°C prior to analysis. Acid catalyzed transmethylation was carried out using the method of Christie (1982). Methyl esters were extracted twice using isohexane diethyl ether (1:1, v:v), purified on TLC plates (Silica gel 60, VWR, Lutterworth, UK) and analyzed by gas–liquid chromatography on a Thermo TraceGC (Thermo Fisher, Spain) fitted with a BPX70 capillary column (30 m × 0.25 mm id; SGE, UK), using a two-stage thermal gradient from 50°C (injection temperature) to 150°C after ramping at 40°C min−1 and holding at 250°C after ramping at 2°Cmin−1. Helium (1.2 ml min−1 constant flow rate) as the carrier gas and on-column injection and flame ionization detection at 250°C were used. Peaks of each fatty acid were identified by comparison with known standards (Supelco Inc., Spain) and a well-characterized fish oil, and quantified by means of the response factor to the internal standard, 21:0 fatty acid, added prior to transmethylation, using a Chrom-card for Windows (TraceGC, Thermo Fisher, Spain).
Lipid class composition was determined by high-performance thin-layer chromatography (HPTLC). Approximately 10 μg of lipid was applied as a 2 mm streak and the plate developed to two-thirds distance with methyl acetate/isopropanol/chloroform/methanol/0.25% aqueous KCl (25:25:25:10:9, by vol.), to separate polar lipid classes, and then fully developed with isohexane/diethyl ether/acetic acid (85:15:1, by vol.). Lipid classes were visualized by charring at 160°C for 15 min after spraying with 3% (w/v) aqueous cupric acetate containing 8% (v/v) phosphoric acid and quantified by densitometry using a GS-800 Densitometer (Bio-Rad Laboratories, Spain) following Olsen and Henderson (1989). The identities of individual lipid classes were confirmed by comparison with authentic standards.
In order to analyze the fatty acid profile of the PL and NL separatedly, an additional HPTLC analysis was carried out to separate the two fractions. The HPTLC plates were developed in the same solvents but in this case the neutral and polar lipids marked, the bands scrapped off and lipids extracted and methylated as described above.
All the analyses were carried out in duplicates.
Statistics
Results in terms of larval DW, SL, mouth size, and survival of the larvae; as well as lipid classes and fatty acid profile of the larvae and the live prey were compared by one way analysis of variance (ANOVA). Percentage values were arcsine transformed and the assumption of homogeneity of variances was checked using the Shapiro–Wilk test. Data was analyzed at a significance level of 0.05. When significant differences were found, the Tukey HSD multiple range test was performed, using SigmaPlot 12.0 software. All data are given as mean values and standard deviations (±SD). A regression analysis was also performed with the results of the fatty acid composition of PL fraction in rotifers and larvae in order to assess the relationship between them. All data were analyzed using SigmaPlot 12.0 (Systat Software Inc., USA).
Results
Tables 1, 2 show the total lipid, lipid classes, and fatty acid composition of the enrichment products (Table 1) used for live prey enrichment and the enriched rotifers (Table 2). Statistically significant differences in the composition of enrichment products was observed not only in total lipid content, that was significantly lower in NC and higher in LC60, but also in the total amount of PL (significantly higher in LC60), NL (significantly higher in RP), total saturated fatty acids (SAT, significantly lower in NC), monounsaturated fatty acids (MUFA, significantly higher in NC), n−6 PUFA content (lower in NC and LC60), and in the content of EPA (higher in NC and lower in MG) and DHA (lower in NC) as well as in total PUFA (higher in RP and MG) and in the ratios n−3/n−6 (higher in LC60) and DHA/EPA (higher in MG).
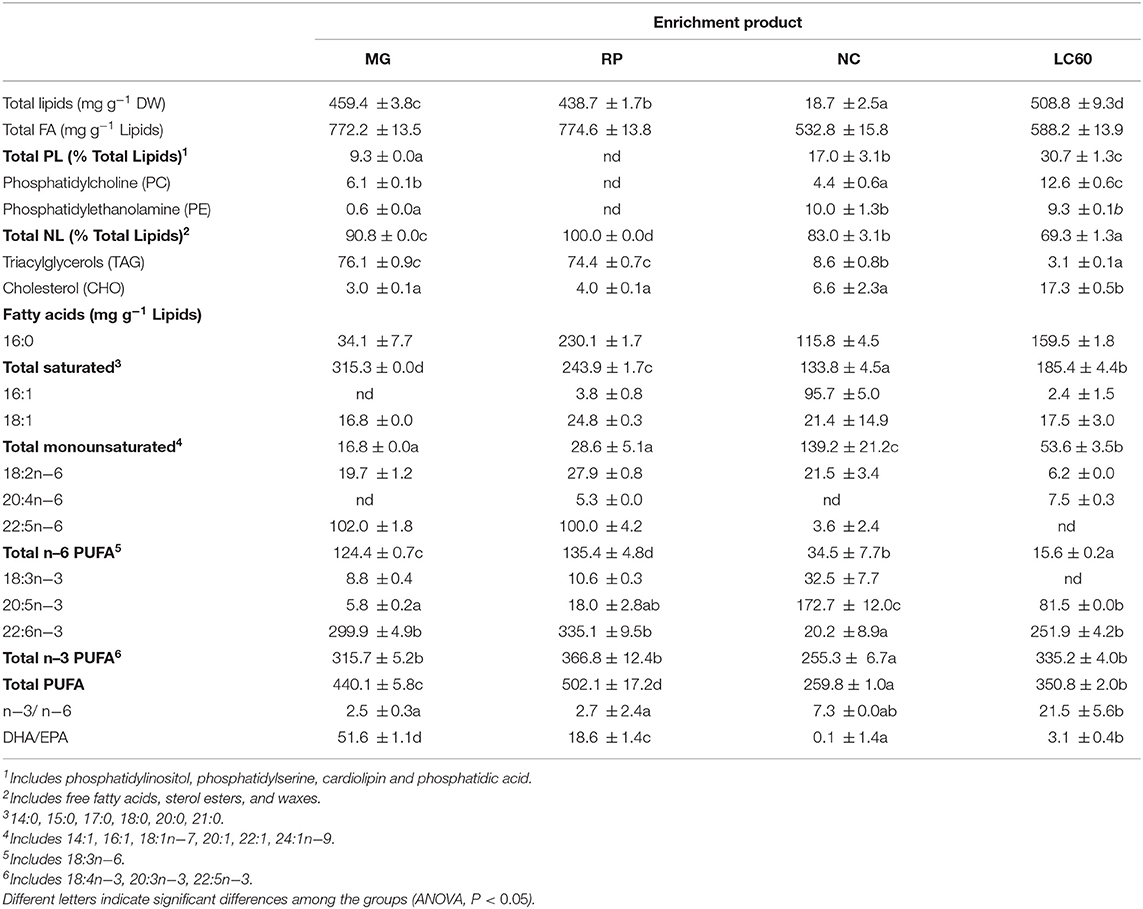
Table 1. Total lipid and main lipid class content and fatty acid composition of the commercial products used for rotifer enrichment: Larviva Multigain (MG), Red Pepper (RP), Concentrated Nannochloropsis (NC), and marine phospholipids (LC60).
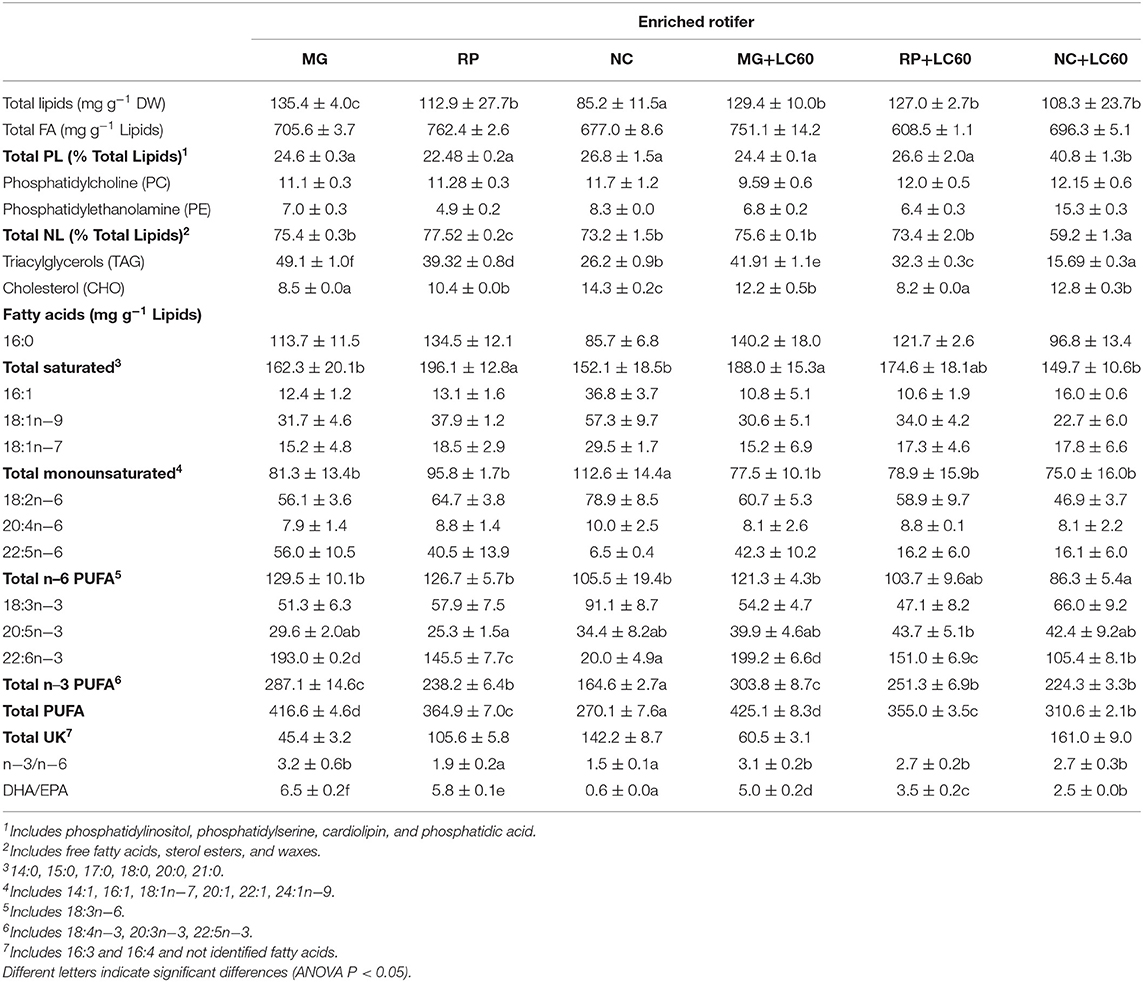
Table 2. Total lipid and main lipid class content and fatty acid composition of the enriched rotifers used for larval feeding: Larviva Multigain (MG), Red Pepper (RP), Concentrated Nannochloropsis (NC), and marine phospholipids (LC60).
The rotifers enriched for 12 h with the commercial enrichers MG, RP and NC show a total lipid content that reflects the content of the enrichment product used. Similarly, the highest amounts of PL were observed in NC group, as in the case of the product used for enrichment. The use of 3 h post-enrichment with marine phospholipids LC60 showed a positive effect and all the enriched rotifers slightly increased their levels of PLs, although not showing statistically significant differences, with the exception of the rotifers enriched with the microalga (NC+LC60) that had a 40.8% of PLs. Rotifers enriched with Red Pepper (RP) showed the highest amounts of NL, reflecting the composition of the commercial product. Statistically significant differences were found in SAT and MUFA, and in both cases, the 3 h post-enrichment with LC60 induced a reduction in their levels, except in MG+LC60 enriched rotifers that showed an increase in SAT. N−6 PUFA content was very similar in all the enriched rotifers and the addition of LC60 induced a reduction due to the lower content of linoleic acid (LA, 18:2n−6). Total n−3 PUFA was always significantly higher in the rotifer enriched with MG, and the addition of LC60 contributed to increase EPA (20:5n−3) and DHA (22:6n−3) content and reduce the alpha linolenic acid (ALA, 18:3n−3). NC enriched rotifers also showed a statistically significant increase in EPA and DHA being 1.25 and 5.3 times higher in NC+LC60 group, respectively.
Larval growth (standard length, dry weight, and SGR results), size of the mouth, and survival rate are presented in Table 3. The use of rotifers enriched with LC60 contributed to a significantly higher growth in length and dry weight with no significant differences in the size of the mouth and low survival rate in all the groups. This low survival can be a consequence of feeding the larvae only with rotifers, considering that the standard protocol for meager larval rearing includes feeding with Artemia nauplii from day 8 post-hatch, the use of only rotifers may have had an effect in larval survival due to cannibalism, although as explained previously the trial was designed to use only rotifers for their easy way to enrich them with phospholipids, not that easy in the case of Artemia.
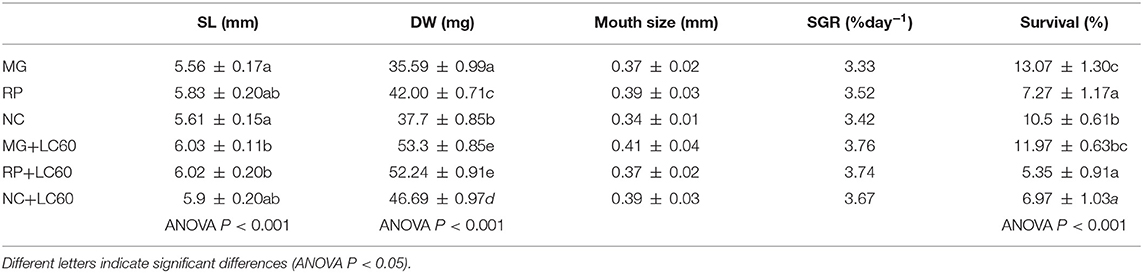
Table 3. Standard length (SL), dry weight (DW), and specific growth (SGR) and survival rates of meager larvae fed enriched rotifers with and without marine phospholipids: Larviva Multigain (MG), Red Pepper (RP), Concentrated Nannochloropsis (NC), and marine phospholipids (LC60).
Total lipid and lipid class content and fatty acid composition of the larvae at the end of the trial is shown in Table 4. The content of total lipids were significantly higher in 15 dph larvae fed the rotifers boosted with marine phospholipids in contrast with those fed standard enriched rotifers, except for RP group. On the contrary, total fatty acids were very similar between newly hatched and 15 dph larvae fed the different diets (P > 0.05). Total PL and NL were clearly different having the newly hatched larvae a higher content of NL probably due to the presence of the yolk sac and lipid droplet. Fatty acid composition of the newly hatched larvae also showed a higher content of MUFA (mainly 18:1n−9) and EPA and a lower content of ARA (20:4n−6) and 22:5n−6 that is quite high in the enrichment products and in the 15 dph larvae fed enriched rotifers. The most important differences found among the 15 dph larvae was the lower amount of lipids in MG group, the significantly higher content of SAT in MG and MG+LC60 larvae, and the significantly higher content in MUFA, EPA and lower content of DHA in larvae fed NC and NC+LC60 enriched rotifers. Consequently, DHA/EPA ratios were significantly lower in NC and NC+LC60 fed larvae and higher in MG fed groups. Similar amounts of total PL and total NL and in the fatty acid composition were observed in all the groups. A positive, although not significant, correlation (r = 0.352) was found between the DHA content in the rotifers and the final DW of the larvae that suggested a possible relationship between both parameters. Thus, to check which could have been the effect of the addition of marine PLs (LC60) in rotifer enrichment on larval growth, a further analysis of the fatty acid composition of PL and NL was carried out separately and the results of the fatty acid profile of PL in enriched rotifers and larvae are presented in Table 5 and Figure 1. Due to the small amount of sample analyzed no replicates and consequently no statistical analysis could be performed. The composition of the dominant fatty acids in PL of the rotifers was reflected in 15 dph larvae which show the highest content of DHA both in the rotifers and in MG fed larvae, and the lowest in NC enriched rotifers and fed larvae, in this case, the addition of marine phospholipids (LC60) contribute to a slight increase in the levels of DHA in MG, RP and NC larvae. On the contrary 16:0 and total SAT was increased in the rotifers enriched with MG and MG+LC60 and the larvae that fed on them, whereas they were reduced in NC+LC60 fed rotifers and larvae. Figure 1 shows a summary of these results. In MG and MG+LC60 fed larvae an increase of DHA (7%), EPA (31%), ARA (10%), and 18:1 (5%) fatty acids was observed whereas the content in 16:0 showed a 35% increase produced by the addition of LC60. RP and RP+LC60 larvae also showed similar amounts of 18:1n−9, but in this case the addition of marine phospholipids induced a reduction in EPA content (−42.8%), and a higher accumulation of DHA (18.4%), ARA (17.9%), and 16:0 (10.4%) fatty acids. In the case of NC and NC+LC60 larvae all the fatty acids, ARA (100%), DHA (31%), 18:1n−9 (13.7%), EPA (4.9%), and 16:0 (12.7%) increased with the addition of LC60.
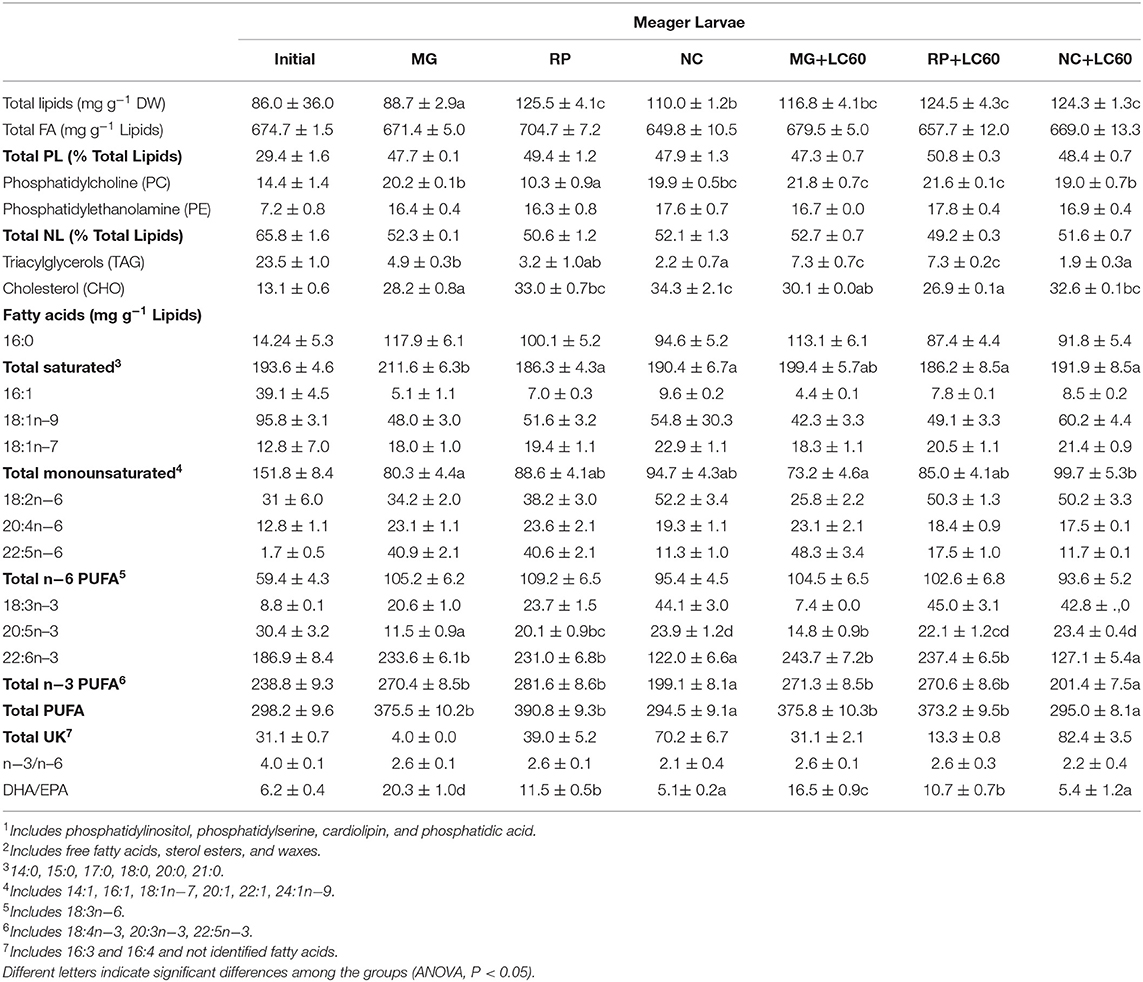
Table 4. Total lipid and main lipid class content and fatty acid composition of 1 dph and 15 dph meager larvae fed rotifers enriched with Larviva Multigain (MG), Red Pepper (RP), Concentrated Nannochloropsis (NC), and marine phospholipids (LC60).
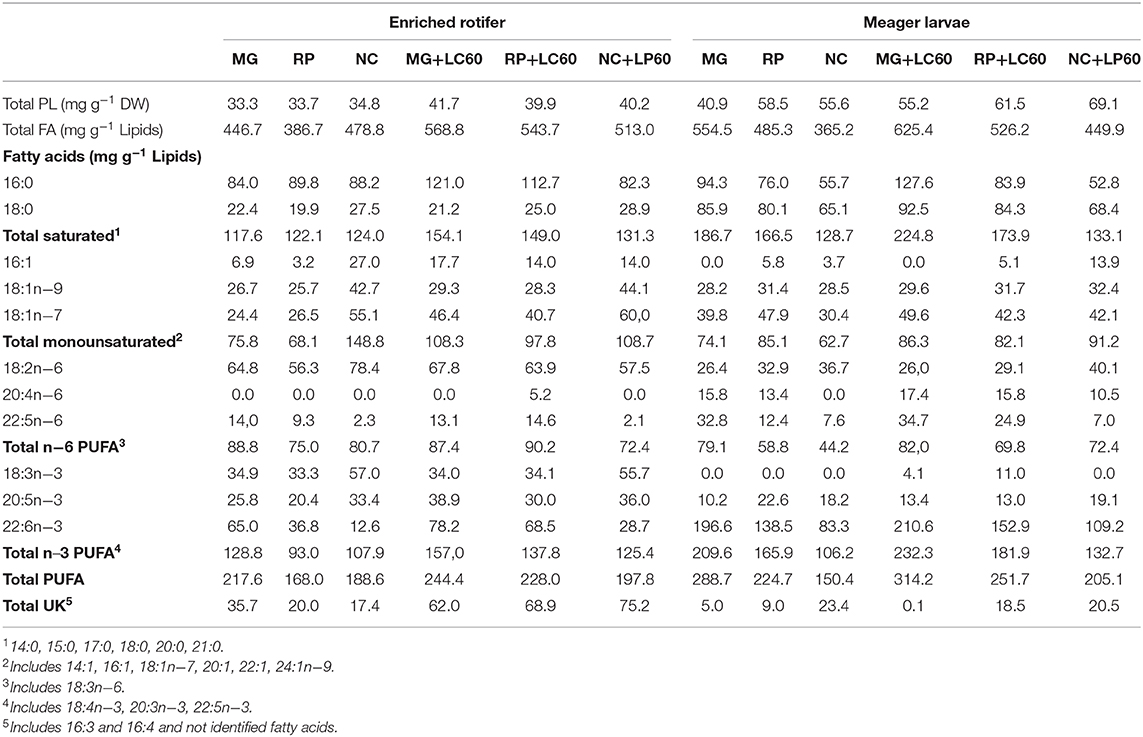
Table 5. Total PL content and fatty acid profile of the PL fraction of enriched rotifers and meager larvae.
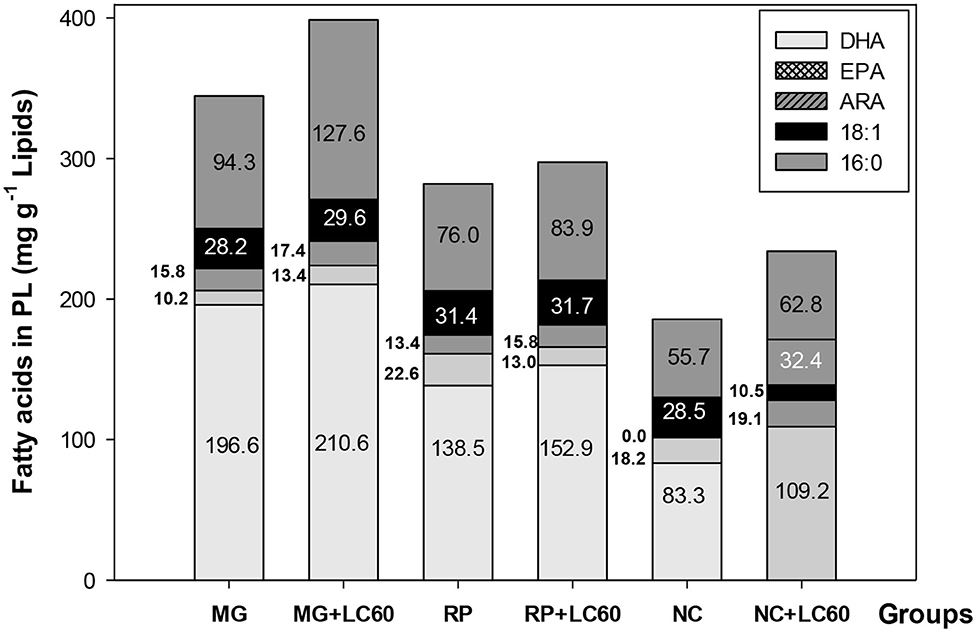
Figure 1. Main fatty acid content (mg) in PL of meager larvae fed different enriched rotifers until 15 dph.
The relationship between the content of the dominant fatty acids in the live feed vs. those of the corresponding 15 dph larvae is presented in Figure 2. The results suggested a close relationship between the composition of dominant PL fatty acids in the rotifer and in the larvae, especially in the case of DHA and 16:0 that show a high correlation (r2 = 0.872, P = 0.006 and r2 = 0.546, P = 0.093, respectively).
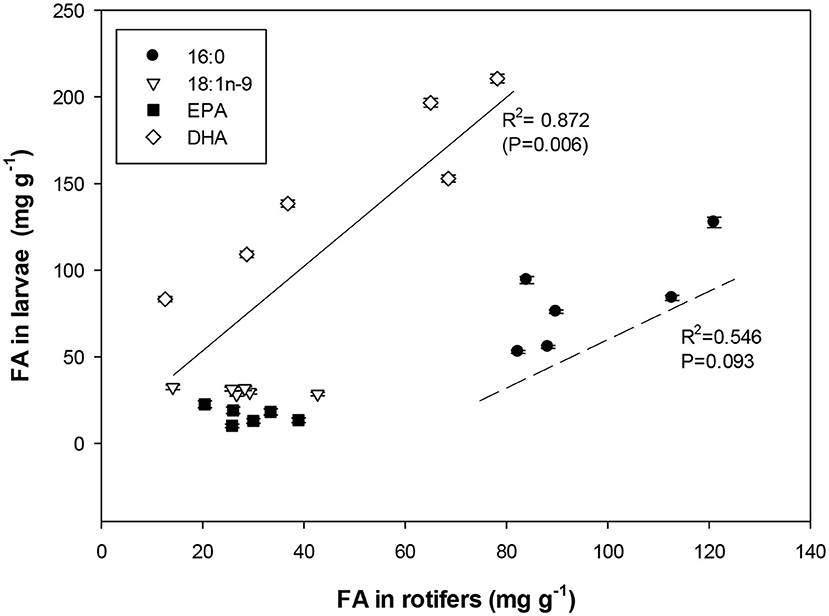
Figure 2. Linear regressions found between the most important fatty acids in PL in 15 dph meager larvae as a function of the respective fatty acid composition in the PL of enriched rotifers.
Discussion
The fatty acid composition of enriched live prey and larvae reflected the profile obtained in the products used for enrichment, as already published in previous reports (Vallés and Estevez, 2015b; Campoverde and Estévez, 2017). There is a clear effect of the DHA level in the live prey on larval fatty acid composition (see Tables 3, 4, regression coefficient r2 = 0.79, P = 0.062) and growth (regression coefficient between DHA content in the enriched rotifer and final weight r = 0.379, P = 0.459) although in this case not as high as expected. DHA levels found in this study,193 mg g−1 lipids (27% of total fatty acids) for MG enriched rotifers are similar to those reported by Vallés and Estevez (2015b) using commercial enrichers (24.9% DHA in MG enriched rotifers) and considered the most adequate for the proper growth and development of meager larvae. The authors concluded that DHA levels around 12–15% TFA in live prey, that correspond to around 40–50% of TFA in the enrichment product, might be considered as the required levels for this species. Similar levels of DHA are cited for other marine fish larvae, such as gilthead sea bream (Salhi et al., 1994) and red porgy (Roo et al., 2010), for growth and to prevent skeletal deformations. Similar amounts of DHA were used in the present study, except for diets NC and NC+LC60.
There is a clear effect of DHA content in the rotifers as a result of boosting rotifers with marine phospholipids (LC60) on larval growth. However, no effects on survival rate could be detected mainly due to the use of rotifer as the only live prey during larval rearing, inducing cannibalism of the fast growing larvae on their slow growing counterparts, as already have been observed in this species (Campoverde and Estévez, 2017). Similar results regarding DHA or fatty acid levels having no effect on larval survival have been cited for other species such as cod (Park et al., 2006; Garcia et al., 2008; Copeman and Laurel, 2010) or striped trumpeter (Bransden et al., 2005). These authors observed a clear relationship between the length and dry weight of the larvae at the end of the experiment with dietary fatty acids, whereas survival rate was not influenced by them. Other studies carried out with other species of marine fish larvae showed that high levels of DHA (or n−3 PUFA) reduce larval survival (Planas and Cunha, 1999). In the case of Japanese flounder (Paralichthys olivaceus) larvae, Izquierdo et al. (1992) showed that either low or high DHA content in live prey did not affect survival rate, being the larvae significantly larger when fed Artemia containing a high percentage of DHA (up to 3.5%). All these results with different species confirmed what Sargent et al. (1999) suggested, that the requirement of dietary DHA levels of marine finfish larvae is species dependent.
Another question addressed in this trial was to study if meager larvae can incorporate DHA into their tissues more efficiently if they are provided by dietary PLs and not TAG in the early stages of first feeding, and if the larvae can grow and perform better. We have found that meager larvae fed on rotifers enriched with different commercial enrichment diets rich or not in PLs showed a positive relationship between the percentage of DHA and 16:0 (or total SAT) in meager larval PL and the same percentage in rotifer PL (Figure 2, r2 = 0.872 for DHA and 0.546 for 16:0). A high percentage of DHA in larval tissues and PLs coincided with the higher larval growth rate at 15 dph (r = 0.352). Thus, the results indicate that the availability of fatty acids in general and LC-PUFA in particular might be different if they are provided as PLs or TAG, being those provided from PLs more accessible and the main source for larval PL synthesis and growth.
In this study one of the main challenges was to incorporate PLs in the rotifers and manipulate their fatty acid composition. The use of commercial enrichers for 12 h followed by the addition of marine phospholipids (LC60) using a short-term enrichment of 3 h was effective for that in the case of rotifers, as it has been shown by other authors (Olsen et al., 2014; Li and Olsen, 2015; Mylonas et al., 2016). In the case of Artemia a recent paper published by Rogacki et al. (2019) showed that a certain amount of PLs can be obtained using Multigain as enricher and LC60 as the phospholipid source, similarly to our results, and open the possibility of using this PL-enriched Artemia in the future.
Olsen et al. (2014) and Li et al. (2014) obtained a level of 9.4% of DHA (in % total fatty acids) in the PL fraction of the rotifers enriched with Marol E and suggested that this low level might be genetically or metabolically constrained. In the present study, DHA was present between 3.3% (NC group) and 13.7% (MG+LC60 group) of total fatty acids in the PLs of the rotifers, indicating that higher levels can be obtained using a different approach, either in the enrichment process or products used. Other result that is very interesting and needs more research is the positive correlation between the content of 16:0 in rotifer PLs and its content in the larvae. Li and Olsen (2015) also found high levels of 16:0 in MG enriched rotifers that may act as a stimulant of PL biosynthesis not only in the rotifers but also in the larvae, as the authors suggested. In the case of humans, it has been shown that 16:0 facilitates the incorporation of dietary DHA into plasma PC (Subbaiah et al., 1993) and the 16:0-DHA-PC is preferably retained in the plasma membrane (Williams et al., 1999). It seems that in meager larvae something similar might occur and need more research.
Conclusions
Rotifers can be enriched with phospholipids using marine phospholipids derived products (LC60) and short-term enrichment (3 h boosting) procedures especially when the products used for enrichment are deficient in PLs (Red Pepper or concentrated microalgae).
The use of phospholipid enriched rotifers contributed to an increase in growth rate and a relationship was found between the DHA content in the rotifer and the final body weight of the larvae.
A clear relationship was found between the 16:0 and DHA content in the PLs of the rotifers and the larvae. More research is needed to understand the role of 16:0 in PL synthesis and larval growth.
Data Availability Statement
The raw data supporting the conclusions of this article will be made available by the authors, without undue reservation.
Ethics Statement
The animal study was reviewed and approved by Generalitat de Cataluña.
Author Contributions
AE designed the trial, gave instructions about enrichment and sampling, help with the biochemical analyses, and wrote the manuscript. SG performed the trial with larvae, collected the samples, helped in the biochemical analyses, and contributed in writing the manuscript. All authors contributed to the article and approved the submitted version.
Conflict of Interest
The authors declare that the research was conducted in the absence of any commercial or financial relationships that could be construed as a potential conflict of interest.
Acknowledgments
Authors are grateful to S. Molas and M. Sastre for their technical assistance in fish rearing and biochemical analyses, respectively. SG thanks the Iranian Ministry of Science for her scholarship to carry out this experiment in IRTA facilities in Spain.
References
Bell, M. V., McEvoy, L. A., and Navarro, J. C. (1996). Deficit of dicosahexanoyl phospholipid in eyes of larval sea bass fed an essential fatty acid deficient diet. J. Fish Biol. 49, 941–952. doi: 10.1111/j.1095-8649.1996.tb00091.x
Bransden, M. P., Battaglene, S. C., Morehead, D. T., Dunstan, G. A., and Nichols, P. D. (2005). Effect of dietary 22:6n-3 on growth, survival and tissue fatty acid profile of striped trumpeter (Latris lineata) larvae fed enriched Artemia. Aquaculture 243, 331–344. doi: 10.1016/j.aquaculture.2004.11.002
Cahu, C. L., Gisbert, E., Villeneuve, L. A. N., Morais, S., Hamza, N., Wold, P. A., et al. (2009). Influence of dietary phospholipids on early ontogenesis of fish. Aquac. Res. 40, 989–999 doi: 10.1111/j.1365-2109.2009.02190.x
Campoverde, C., and Estévez, A. (2017). The effect of live food enrichment with docosahexaenoic acid (22:6n-3) rich emulsions on growth, survival and fatty acid composition of meagre (Argyrosomus regius) larvae. Aquaculture 478, 16–24. doi: 10.1016/j.aquaculture.2017.05.012
Campoverde, C., Rodriguez, C., Perez, J., Gisbert, E., and Estévez, A. (2017). Early weaning in meagre Argyrosomus regius: effects on growth, survival, digestión and skeletal deformities. Aquac. Res. 48, 5289–5299. doi: 10.1111/are.13342
Cardeira, J., Vallés, R., Dionísio, G., Estévez, A., Gisbert, E., Pousao-Ferreira, P., et al. (2012). Osteology of the axial and appendicular skeletons of the meagre Argyrosomus regius (Sciaenidae) and early skeletal development at two rearing facilities. J. Appl. Ichthyol. 28, 464–470. doi: 10.1111/j.1439-0426.2012.01979.x
Copeman, L. A., and Laurel, B. (2010). Experimental evidence of fatty acid limited growth and survival in Pacific cod larvae. Mar. Ecol. Prog. Ser. 412, 259–272. doi: 10.3354/meps08661
Duncan, N., Estévez, A., Porta, J., Carazo, I., Norambuena, F., Aguilera, C., et al. (2012). Reproductive development, GnRHa-induced spawning and egg quality of wild meagre (Argyrosomus regius) acclimatized to captivity. Fish Physiol. Biochem. 38, 1273–1286. doi: 10.1007/s10695-012-9615-3
El Kertaoui, N., Hernandez-Cruz, C. M., Montero, D., Caballero, M. J., Saleh, R., Afonso, J. M., et al. (2017). The importance of dietary HUFA for meagre larvae (Argyrosomus regius Asso, 1801). and its relation with antioxidant vitamins E and C. Aquac. Res. 48, 419–433. doi: 10.1111/are.12890
Folch, J., Lees, M., and Sloane-Stanley, G. H. (1957). A simple method for the isolation and purification of total lipids from animal tissues. J. Biol. Chem. 22, 497–509.
Garcia, A. S., Parrish, C. C., and Brown, J. A. (2008). A comparison among differently enriched rotifers (Brachionus plicatilis) and their effect on Atlantic cod (Gadus morhua) larvae early growth, survival and lipid composition. Aquac. Nutr. 14, 14–30. doi: 10.1111/j.1365-2095.2007.00500.x
Grigorakis, K., Fountoulaki, E., Vasilaki, A., Mittakos, I., and Nathanailides, C. (2011). Lipid quality and filleting yield of reared meagre (Argyrosomus regius). Food Sci. Technol. 46, 711–716. doi: 10.1111/j.1365-2621.2010.02537.x
Guinot, D., Monroig, O., Hontoria, F., Amat, F., Varó, I., and Navarro, J. C. (2013). Enriched on-grown Artemia metanauplii actively metabolise unsaturated fattu acid-rich phospholipids. Aquaculture 412–413, 173–178. doi: 10.1016/j.aquaculture.2013.07.030
Izquierdo, M. S., Arakawa, T., Takeuchi, T., Haroun, R., and Watanabe, T. (1992). Effect of n-3 HUFA levels in Artemia on growth of larval Japanese flounder (Paralichthys olivaceus). Aquaculture 105, 73–82. doi: 10.1016/0044-8486(92)90163-F
Jimenez, M. T., Rodriguez de la Rúa, A., Sanchez, R., and Cárdenas, S. (2007). Atlas del Desarrollo de la Corvina Argyrosomus regius (Piseces:Sciaenidae) Durante su Primer Mes de Vida. REDVET. Available online at: http://www.veterinaria.org/revistas/redvet/n010107.html
Li, K., Kjorsvik, E., Bergvik, M., and Olsen, Y. (2014). Manipulation of the fatty acid composition of phosphatidylcholine (PC) and phospatidylethanolamine (PE) in rotifers Brachionus Nevada and Brachionus Cayman. Aquac. Nutr. 21, 85–97. doi: 10.1111/anu.12140
Li, K., and Olsen, Y. (2015). Effect of enrichment time and dietary DHA and non-highly unsaturated fatty acid composition on the efficiency of DHA enrichment in phospholipid of rotifer (Brachionus Cayman). Aquaculture 446, 310–317. doi: 10.1016/j.aquaculture.2015.05.005
Lingenfelser, J. T., Blazer, V. S., and Gay, J. (1995). Influence of fish oil in production catfish feeds on selecteddisease resistance factors. J. Appl. Aquac. 5, 37–48. doi: 10.1300/J028v05n02_04
Monroig, O., Navarro, J. C., Amat, F., Gonzalez, P., Bermejo-Nogales, A., and Hontoria, F. (2006). Enrichment of Artemia nauplii in essential fatty acids with different types of liposomes and their use in the rearing of gilthead sea bream (Sparus aurata) larvae. Aquaculture 251, 491–508. doi: 10.1016/j.aquaculture.2005.06.026
Mourente, G., and Tocher, D. R. (1992). Effects of weaning onto a pelleted diet on docosahexaenoic acid (22:6 n-3) levels in brain of developing turbot (Scophthalmus maximus L.). Aquaculture 105, 363–377. doi: 10.1016/0044-8486(92)90100-Y
Mylonas, C. C., Katharios, P., Grigorakis, K., Papandroulakis, N., Robles, R., Fernandez-Palacios, H., et al. (2016). Advances in greater amberjack (Seriola dumerilii) research: the Diversify Project. Aquac. Europe 41, 12–19.
Navarro, J. C., Henderson, R. J., McEvoy, L. A., Bell, M. V., and Amat, F. (1999). Lipid conversions during enrichment of Artemia. Aquaculture 174, 155–166. doi: 10.1016/S0044-8486(99)00004-6
Olsen, R. E., and Henderson, R. J. (1989). The rapid analysis of neutral and polar marine lipids using double-development HPTLC scanning densitometry. J. Exp. Mar. Biol. Ecol. 129, 189–197. doi: 10.1016/0022-0981(89)90056-7
Olsen, Y. (2004). “Live food technology of cold-water marine fish larvae,” in Culture of Cold-Water Marine Fish pages, eds E. Moksness, E. Kjørsvik, and Y. Olsen (Oxford: Blackwell Publishing Ltd.), 73–128.
Olsen, Y., Evjemo, J. O., Kjorsvik, E., Larssen, H., Li, K., Overrein, I., et al. (2014). DHA content in dietary phospholipids affects DHA content in phospholipids of cod larvae and larval performance. Aquaculture 428–429, 203–214. doi: 10.1016/j.aquaculture.2014.03.002
Park, H. G., Puvanendran, V., Kellett, A., Parrish, C. C., and Brown, J. A. (2006). Effect of enriched rotifers on growth, survival, and composition of larval Atlantic cod (Gadus morhua). ICES J. Mar. Sci. 63, 285–295. doi: 10.1016/j.icesjms.2005.10.011
Planas, M., and Cunha, I. (1999). Larviculture of marine fish: problems and perspectives. Aquaculture 177, 171–190 doi: 10.1016/S0044-8486(99)00079-4
Poli, B. M., Parisi, G., Zampacavallo, G., Iurzan, F., Mecatti, M., Lupi, P., et al. (2003). Preliminary results on quality and quality changes in reared meagre(Argyrosomus regius): body and fillet traits and freshness changes in refrigerated commercial-size fish. Aquac. Int. 11, 301–311. doi: 10.1023/A:1024840804303
Rogacki, T. C., Davie, A., King, E., Esnault, S., Migaud, H., and Monroig, O. (2019). Short-term lecithin enrichments can enhance the phospholipid and DHA contents of the polar lipid fraction of Artemia nauplii. Aquaculture 510, 122–130. doi: 10.1016/j.aquaculture.2019.05.041
Roo, J., Hernández-Cruz, C. M., Borrero, C., Schuchardt, D., and Fernandez-Palacios, H. (2010). Effect of larval density and feeding sequence on meagre ( Argyrosomus regius, Asso, 1801) larval rearing. Aquaculture 302, 82–88. doi: 10.1016/j.aquaculture.2010.02.015
Salhi, M., Hernandez-Cruz, C. M., Bessonart, M., Izquierdo, M. S., and Fernandez-Palacios, H. (1994). Effect of different dietary polar lipid level and different n-3 HUFA content in polar lipids on gut and liver histological structure of gilthead seabream (Sparus aurata) larvae. Aquaculture 124, 275–282 doi: 10.1016/0044-8486(94)90389-1
Sargent, J., Mc Evoy, L. A., Estevez, A., Bell, G. J., Bell, M. V., Henderson, J., et al. (1999). Lipid nutrition of marine fish during early development: current status and future directions. Aquaculture 179, 217–229. doi: 10.1016/S0044-8486(99)00191-X
Shirota, A. (1970). Studies on the gape size of fish larvae. Bull. Jpn. Soc. Sci. Fish. 36, 353–368. doi: 10.2331/suisan.36.353
Shirota, A. (1978). Studies on the gape size of fish larvae. II: specific characteristics of the upper jaw length. Bull. Jpn. Soc. Sci. Fish. 44, 1171–1177. doi: 10.2331/suisan.44.1171
Subbaiah, P. V., Kaufman, D., and Bagdade, J. D. (1993). Incorporation of dietary n-3 fatty acids into molecular species of phosphatidylcholine and cholesteryl ester in normal human plasma. Am. J. Clin. Nutr. 58, 360–368. doi: 10.1093/ajcn/58.3.360
Tocher, D. R. (2010). Fatty acid requirements in ontogeny of marine and freshwater fish. Aquac. Res. 41, 717–732. doi: 10.1111/j.1365-2109.2008.02150.x
Tocher, D. R., Bendiksen, E. A., Campbell, P. J., and Bell, J. G. (2008). The role of phospholipids in nutrition and metabolism of teleost fish. Aquaculture 280, 21–34. doi: 10.1016/j.aquaculture.2008.04.034
Vallés, R., and Estevez, A. (2013). Light conditions for larval rearing of meagre (Argyrosomus regius). Aquaculture 376–379, 15–19. doi: 10.1016/j.aquaculture.2012.11.011
Vallés, R., and Estevez, A. (2015a). Use of Ox-Aquaculture for disinfection of live prey and meagre larvae, Argyrosomus regius (Asso, 1801). Aquac. Res. 43, 413–419. doi: 10.1111/are.12187
Vallés, R., and Estevez, A. (2015b). Effect of different enrichment products rich in docosahexaenoic acid on growth and survival of meagre, Argyrosomus regius (Asso, 1801). J. World Aquac. Soc. 42, 191–200. doi: 10.1111/jwas.12175
Watanabe, T. (1993). Importance of docosahexaenoic acid in marine larval fish. J. World Aquac. Soc. 24, 152–161. doi: 10.1111/j.1749-7345.1993.tb00004.x
Williams, E. E., May, B. D., Stillwell, W., and Jenski, L. J. (1999). Docosahexaenoic acid (DHA) alters the phospholipid molecular species composition of membranous vesicles exfoliated from the surface of a murine leukemia cell line. Biochem. Biophys. Acta 1418, 185–196. doi: 10.1016/S0005-2736(99)00032-2
Keywords: survival, growth, marine phospholipids, rotifers (Brachionus plicatilis), meager (Argyrosomus regius), fatty acid (composition)
Citation: Ghaderpour S and Estevez A (2020) Effect of Short-Term Rotifer Enrichment With Marine Phospholipids on Growth, Survival, and Composition of Meager (Argyrosomus regius) Larvae. Front. Mar. Sci. 7:579002. doi: 10.3389/fmars.2020.579002
Received: 01 July 2020; Accepted: 17 November 2020;
Published: 09 December 2020.
Edited by:
Per Meyer Jepsen, Roskilde University, DenmarkReviewed by:
Takeshi Yamamoto, Japan Fisheries Research and Education Agency (FRA), JapanS.S.S. Sarma, National Autonomous University of Mexico, Mexico
Copyright © 2020 Ghaderpour and Estevez. This is an open-access article distributed under the terms of the Creative Commons Attribution License (CC BY). The use, distribution or reproduction in other forums is permitted, provided the original author(s) and the copyright owner(s) are credited and that the original publication in this journal is cited, in accordance with accepted academic practice. No use, distribution or reproduction is permitted which does not comply with these terms.
*Correspondence: Alicia Estevez, YWxpY2lhLmVzdGV2ZXpAaXJ0YS5lcw==