- 1Museu Oceanográfico “Prof. Eliézer de C. Rios”, Universidade Federal do Rio Grande-FURG, Rio Grande, Brazil
- 2Laboratório de Ecologia e Conservação da Megafauna Marinha (EcoMega), Instituto de Oceanografia, Universidade Federal do Rio Grande-FURG, Rio Grande, Brazil
- 3Kaosa, Rio Grande, Brazil
- 4Cetacean Ecology, Behaviour and Evolution Lab, College of Science and Engineering, Flinders University, Bedford Park, SA, Australia
- 5Molecular Ecology Laboratory, College of Science and Engineering, Flinders University, Bedford Park, SA, Australia
- 6Programa de Pós-graduação em Oceanografia Biológica, Universidade Federal do Rio Grande-FURG, Rio Grande, Brazil
Identifying threatened populations and quantifying their vulnerability is crucial for establishing priorities for conservation and providing robust information for decision-making. Lahille’s bottlenose dolphins have been long subjected to by-catch mortality in gillnet fisheries in coastal waters of southern Brazil, particularly in the Patos Lagoon estuary (PLE) and adjacent coastal waters, where dolphins from three populations (or Management Units) show overlapping home ranges. In this study we used a stage-classified matrix population model to conduct a demographic analysis of the PLE’s population with life-history data estimated through an 8 years mark-recapture study. A population viability analysis (PVA) was used to run a series of simulations where the risk was assessed under different by-catch scenarios, taking into account the effects of parameter uncertainty and stochasticity in the projections. In the absence of by-catch, we estimated that this dolphin population would growth at a rate of about 3% annually (95% CI: 1.2–5.8%). Under current by-catch rates, prognoses indicated high probabilities of viability over the next 60 years. These optimistic prognoses appear to be associated with the high survival of adult females. However, the eventual removal of very few mature females (one every year or two) would result in a prominent likelihood of decline from its current abundance at all pre-specified levels. The viability of the population would be substantially improved if the survival of juveniles/sub-adults could be increased. This may be achieved through the recently implemented dolphin protection area, which prohibits gillnet fisheries in the core area of this population. If the protection area reduces the entanglement rates of the most impacted life-stages (i.e., juvenile/sub-adult dolphins), there would be a substantial chance of the PLE’s dolphin population increasing above 20% of its current size, which is here proposed as conservation goal. If met, this goal has the potential to promote habitat quality, increase genetic diversity and connectivity with adjacent populations, enhancing the ability of bottlenose dolphins in southern Brazil to cope with environmental change and potential disease outbreaks.
Introduction
The central aim of conservation biology is to establish principles and tools for preserving biological diversity from unwarranted rates of extinction and the processes that sustain it (Soulé, 1985). Identifying threatened populations and quantifying their vulnerability is therefore crucial for establishing priorities for conservation and providing robust information to decide if an intervention is needed with minimal delay. Finding early warning signs before the severe decline of a population should have important implications for successful conservation attempts. However, objectively classifying populations according to their level of vulnerability is challenging (e.g., Ralls and Taylor, 1997).
Population viability analysis (PVA) is a framework widely used in conservation biology and management decision-making (e.g., Morris and Doak, 2003). PVA is a procedure that projects the population into the future based on models of population dynamics, allowing the incorporation of elements that can affect their likelihood of persistence, including environmental and demographic stochasticity, catastrophes, deterministic pressures (e.g., annual hunting quotas), uncertainties in parameter estimates, and control variables representing conservation strategies (e.g., Gilpin and Soulé, 1986; Possingham et al., 1993; Morris and Doak, 2003). Probably the most important uses of these models are for investigating the extinction or quasi-extinction probabilities of populations within pre-specified periods of time and under particular scenarios (e.g., Possingham et al., 1993), as well as for identifying the most important factors affecting the likelihood of extinction (Reed et al., 2002). This information can then be used to identify research priorities and guide conservation and management actions for protecting threatened populations (e.g., Possingham et al., 1993; Caswell, 2001).
In the Southwestern Atlantic Ocean, coastal bottlenose dolphins have a restricted distribution, ranging from southern Brazil to Central Argentina. They are regularly sighted along a narrow stretch of the coast in strong association with estuaries and river mouths (see Laporta et al., 2016 for a review). These costal dolphins were recently recognized as a different species (Lahille’s bottlenose dolphin, Tursiops gephyreus—Wickert et al., 2016; Hohl et al., 2020) of the abundant, worldwide-distributed common bottlenose dolphin (T. truncatus), although Marine Mammalogy’s Committee on Taxonomy argues that the lineages should be recognized at subspecies level 2018 (Costa et al., 2016, 2019; Marine Mammalogy’s Committee on Taxonomy, 2018). Due to the low number of individuals representing the entire lineage, and evidence of decline at least in part of its range due to bycatch and other unknown factors, the Lahille’s bottlenose dolphin was recently listed as Vulnerable under criterion D1of the IUCN (Vermeulen et al., 2019).
A population genetic study has suggested the existence of two Evolutionarily Significant Units for Lahille’s bottlenose dolphins: one ranging along central Argentina (Bahía San Antonio), and another ranging along southern Brazil and Uruguay (Southern Brazil-Uruguay) (Fruet et al., 2014). The Southern Brazil-Uruguay Evolutionarily Significant Unit is comprised of at least five Management Units (here after referred as populations) of dolphins with very low levels of genetic diversity, and restricted and asymmetrical dispersal between them (Fruet et al., 2014; Figure 1). There are no abundance estimates available for this entire Southern Brazil-Uruguay Evolutionarily Significant Unit, but mark-recapture studies conducted at several sites indicate that each local population is very small (<90 individuals), and some exhibit strong site fidelity and year-round residency to small embayments (e.g., Simões-Lopes and Fabian, 1999; Fruet et al., 2015a). By-catch in gillnets is recognized as the main threat for these dolphins, and it is known to occur throughout their range (Fruet et al., 2012, 2016a), but others agents such as coastal habitat degradation, chronic skin-diseases, boat strikes, chemical pollution and underwater noise also impact upon them in southern Brazil (e.g., Van Bressem et al., 2015; Fruet et al., 2016a; Righetti et al., 2019). The Patos Lagoon estuary (PLE) population is possibly the largest of all five within the Southern Brazil-Uruguay Evolutionarily Significant Unit, numbering approximately 90 individuals (Fruet et al., 2016b). By-catch of animals from this population used to occur sporadically and was not considered a reason for concern in the past (e.g., Pinedo, 1986). However, the increased number of observed resident dolphins with evidence of interaction with fisheries (piece of nets attached to the body and rostrum, mutilated appendices), and the significant increase in mortality in adjacent coastal beaches in the last decade, including some of the known resident PLE’s dolphins, raised concern about the viability of this population unit (Fruet et al., 2012, 2014). Lower survival rates of adult males and immature individuals compared to adult females from the PLE’s population (Fruet et al., 2015a) suggest these two age-sex classes may be more vulnerable to human-induced mortality, particularly in fisheries (see Fruet et al., 2012; Venuto et al., 2020).
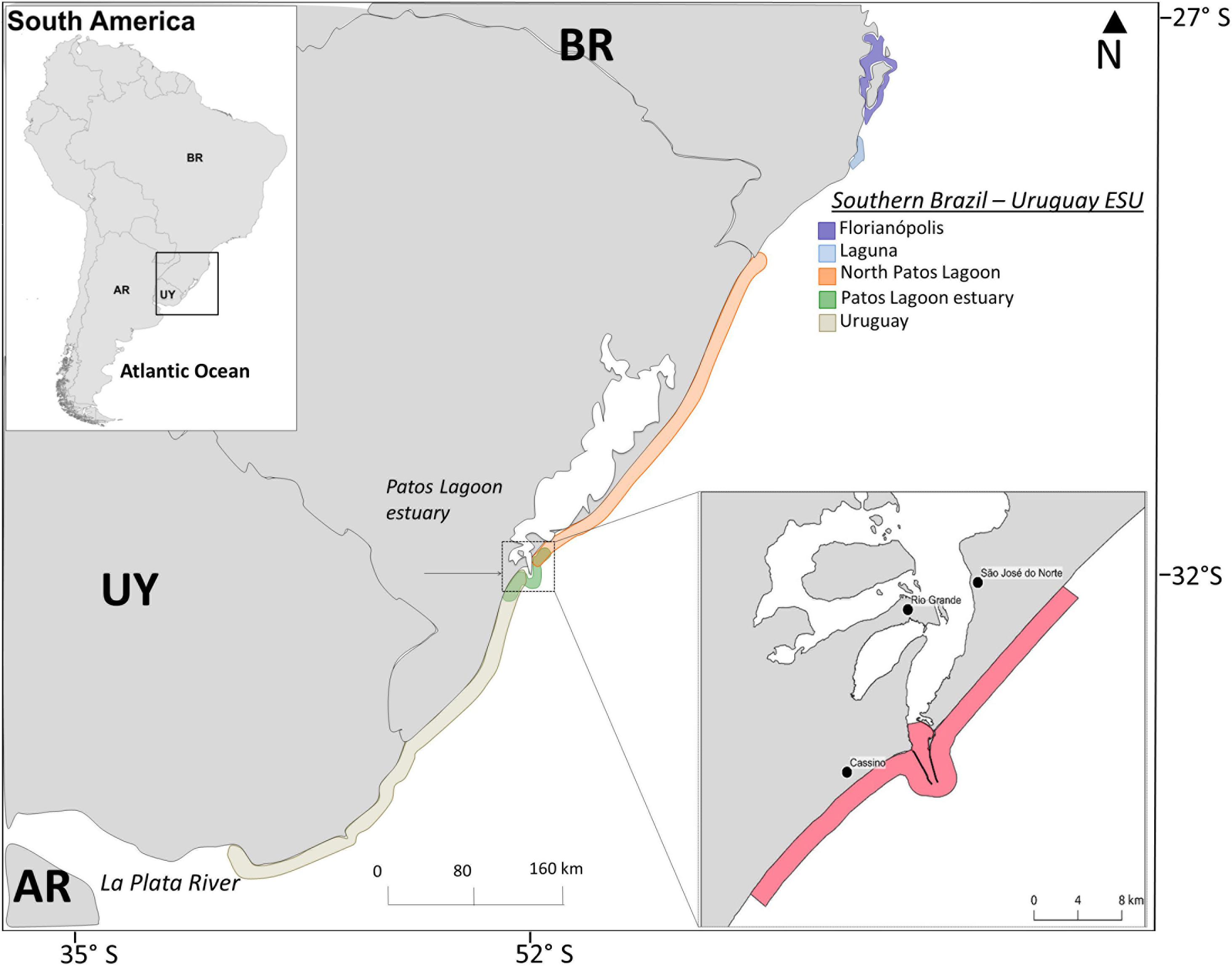
Figure 1. Map showing approximate geographic boundaries of the five bottlenose dolphin populations/Management Units (color contoured lines) identified along the Southern-Brazil/Uruguay Evolutionarily Significant Unit (SB-U ESU) (see Fruet et al., 2014 for details), and the recently implemented dolphin protection area in southern Brazil (denoted by dashed lines) where boat-based gillnet fisheries are banned (Ministério da Agricultura, Pecuária e Abastecimento, 2012).
A preliminary analysis of the sustainability of the PLE’s population was carried out by Fruet et al. (2012), using the Potential Biological Removal approach of Wade (1998). Previous results about Potential Biological Removal analysis suggested that by-catch levels recorded between 2002 and 2006 in coastal beaches adjacent to the PLE would be unsustainable, in the most optimistic scenario, if only individuals from the PLE’s population were removed (Fruet et al., 2012). In August 2012 the Brazilian Ministry of Fisheries and Aquaculture, and the Ministry of Environment, published a joint norm to regulate the gillnet fisheries in southeast and southern Brazil. Article 8 of this norm established a dolphin protection area that bans gillnetting in the core area of the PLE’s dolphin population (Ministério da Agricultura, Pecuária e Abastecimento, 2012). If effective, this fishery ban is expected to increase dolphin survival and the growth rate of this population.
The adoption of the Potential Biological Removal-based framework represented an important step for measuring the sustainability of the PLE population in the absence of enhanced biological information. However, a comprehensive demographic modeling analysis using life-history data estimated from the PLE population would provide a better baseline for understanding their population dynamics and conservation needs. The main advantage of structured models, such as matrix models, is that they are designed to capture the internal structure of a population, using a vector of numbers to represent the abundances for each of the different components of the population. These types of models yield more detailed forecasts and may lead to different predictions about the future of the population, but they often require considerably more data to parameterize. In cases where the age-composition of the population has been disturbed (e.g., because of differential mortality among classes due to a particular threat), structured models may be the best option to predict the consequence (e.g., Morris and Doak, 2003). By contrast, unstructured models, such as Potential Biological Removal, assume that variations among individuals make no difference to the overall dynamics of the population. However, there are often important dissimilarities among individuals, both with respect to reproductive output and survival rates, as well as, threat vulnerability, such as bycatch in fisheries.
In this study we used a stage-classified matrix population model (e.g., Caswell, 2001) for analyzing the demography of the PLE population of Lahille’s bottlenose dolphins, estimating population growth, projecting its potential trajectory into the future, and assessing the risk of decline based on current and population-specific life-history data. Effects of parameter uncertainty, demographic and environmental stochasticity, and by-catch mortality were also included in the analysis. Finally, we assessed the potential consequences of effectiveness or failure of the dolphin protection area established around PLE and adjacent coastal zone by modeling the expected effects it would have on dolphin survival rates as a result of reduction or increase in by-catch levels.
Materials and Methods
The Models
A stochastic stage-structured matrix population model developed to model the viability of the franciscana dolphin (Pontoporia blainvillei) and the Hector’s dolphin (Cephalorhynchus hectori) (Secchi, 2006) was adapted to depict the dynamics and estimate the viability of the PLE bottlenose dolphins. Since population growth rates in large non-monogamous mammals are mainly driven by female vital rates (e.g., Brault and Caswell, 1993; Burgman et al., 1993; Morris and Doak, 2003; Secchi and Fletcher, 2004), only females were included in the model. The model assumes that the population is closed to immigration and emigration processes. We used a stage-structured model because estimation of survival and fertility parameters are essentially stage-specific for the PLE’s bottlenose dolphins. We grouped individuals in three life-stages, following the classification used by Fruet et al. (2015a, b) to estimate the life history data: Stage 1 is represented by calves (0–2 years), stage 2 by juveniles/sub-adults (> 2 years and up to 8 years; all the immature non-calves), and stage 3 by adults (> 8 years; all the mature individuals) (Eq. 1). For details on the model structure and definitions refer to Caswell (2001).
where:
ni is the number of individuals in state class i;
Pi is the probability of surviving and staying in stage i;
Gi is the probability of surviving and moving to the stage i + 1; and
Fi is the number of female offspring at time t + 1 per adult female at time t.
Since the PLE’s population has a well-defined breeding season, with most births occurring during late spring and summer (Fruet et al., 2015b), we used a birth-pulse model. In this case the model will represent the post-birth census (see definitions in Caswell, 2001).
For a birth-pulse population,
Pi = σi(1-γi)
Gi = σiγi
Fi = Pimi + Gimi+1
where, for stage i:
σi is the stage-specific survival probability;
γi is the transition probability or growth probability; and
mi is the mean reproductive output of females (see Brault and Caswell, 1993; Caswell, 2001, p. 171–173).
Hence, for a three-stage birth-pulse population:
F2 = (1-γ2) σ2 m2 + γ2σ2 m3
F3 = σ3m3
G1 = σ1
G2 = γ2σ2
P1 = 0
P2 = (1-γ2) σ2
P3 = σ3
Finally, Eq. 1 can be rewritten as,
to represent the dynamic of a birth-pulse population.
To estimate the transition probability, we used a “variable stage duration” (Ti) (Caswell, 2001, p. 164).
where,
is the mean stage duration and V(Ti) is its variance.
Equation 3 depends on λ, the largest eigenvalue of the matrix A, whose entries are being estimated and it cannot be calculated before estimation is complete. However, it can be done through an iterative approach that requires an initial value of λ (for details see Caswell, 2001, p. 164).
Initial Models—Input Parameters and Uncertainty
Model input parameters were all obtained from PLE’s bottlenose dolphins throughout a long-term photo-identification and monitoring program (Fruet et al., 2015a, b). Uncertainty in parameter estimates was allowed using Monte Carlo methods (Manly, 1997) by running the model 1000 times, and randomly selecting parameter values from probability distributions representing parameters uncertainty. Whenever appropriate data (i.e., yielding robust parameter estimations) were available, they were used to estimate statistical distributions for the model parameters. When poor data (i.e., yielding low-quality parameter estimates due to little data, or only minimum and maximum observed values) were available, a uniform distribution was chosen to represent parameter uncertainty. With the exception of age at first reproduction and abundance, for which values were fixed (abundance estimates are precise and model output is not overly sensitive to variations in age at first reproduction and initial abundance), uncertainties were incorporated into all remaining model parameters.
Initial abundance was set as 44 females based on the last and more precise mark-recapture abundance estimation available (NT 2011 = 88 individuals, 95% CI = 82–94; Fruet et al., 2015a). Stage-specific survival rates were obtained from recent studies that have applied mark-recapture models to 8 years of photo-identification data (2005–2012) to estimate demographic parameters of the PLE’s population (Fruet et al., 2015a, b). For adults, female survival rates were used, but for juveniles and calves the available estimation was not sex-specific. It was assumed that survival rates of all immature dolphins were sex invariant. For survival rates, we chose a beta distribution for incorporating uncertainty into the estimates (White, 2000).
Female age at first reproduction varied from 8 to 10 years in PLE’s bottlenose dolphins (Fruet et al., 2015b). Thus, age at first reproduction was assumed to be 9 years. Fecundity was estimated as the number of female calves born per known mature female in the PLE’s population (Fruet et al., 2015b). Since the nature of the data analyzed may produce a downward bias in the estimation due to the potential of non-detected births before calves died, fecundity was also derived as the reciprocal of the average calving interval estimated by Fruet et al. (2015b), assuming an even sex ratio at birth. This estimate may bias fecundity upwards as longer calving intervals may not be detected due to the short-term nature of the data relative to the life expectancy in the wild of this long-lived species. These estimates were used to set the lower and upper bound values of fecundity to represent parameter uncertainty, which was expressed through a uniform distribution. The parameter estimates and the distributions that represented our level of uncertainty for different modeling scenarios are summarized in Table 1.
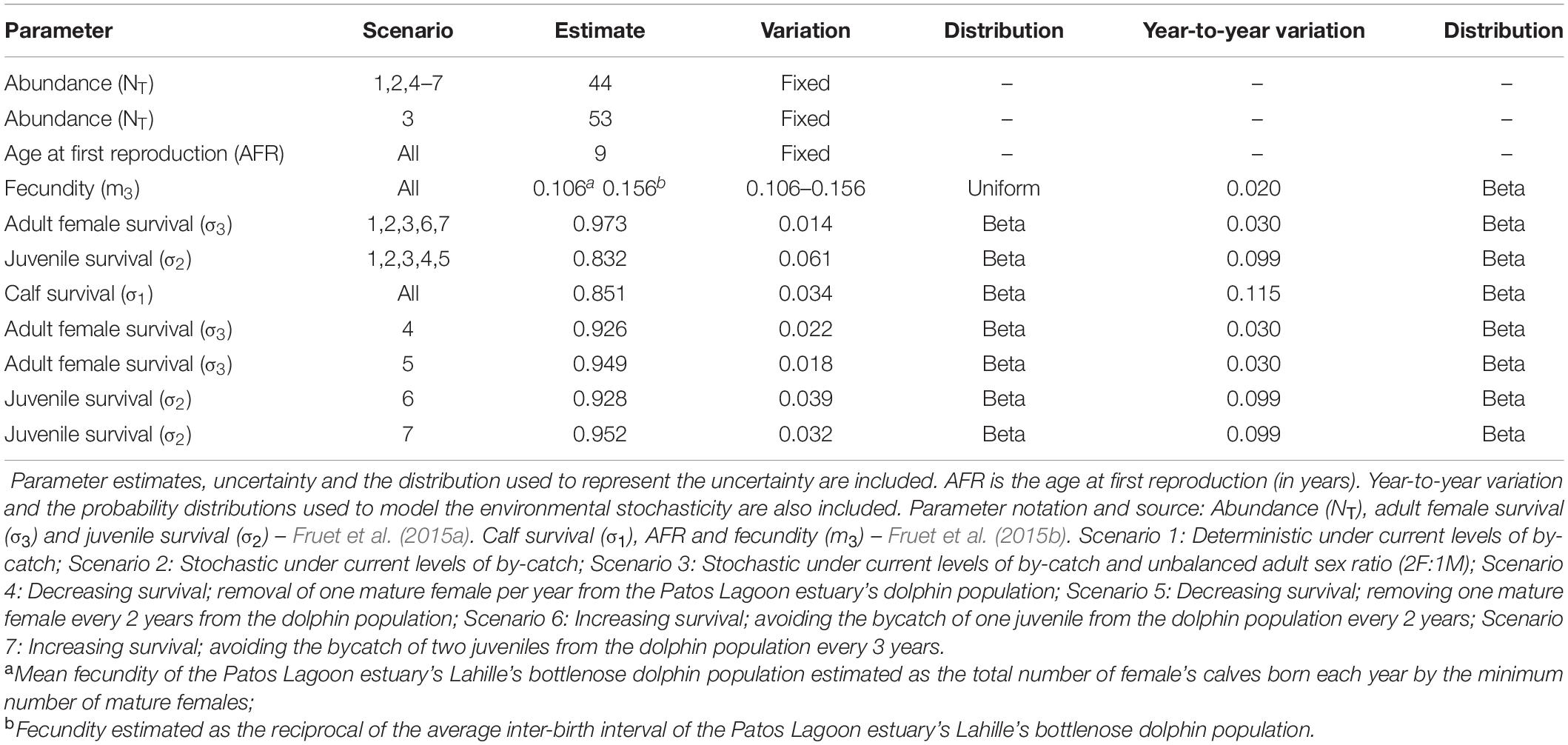
Table 1. Input parameters for all scenarios used to model the dynamics and viability of the Patos Lagoon estuary’s Lahille’s bottlenose dolphin population.
Incorporating Effects of Stochasticity
Year-to-year variation in survival rates, expressed as the standard deviation for each life-stage estimate, was obtained using the sampling variance component analysis in Program Mark (White and Burnham, 1999). For fecundity, the standard deviation value was obtained from the year-to-year variation for the estimate of this parameter (Fruet et al., 2015b). Demographic stochasticity (i.e., the variation in the average chance of a population being made up of a finite, integer number of individuals) was modeled using a binomial distribution from which we drew a random number for each individual. If the random number exceeded the stage-specific survival rate for that year, the individual died; otherwise the individual remained alive. Likewise, the number of newborns each year was a random variable from a binomial distribution including the reproductive rate and the number of breeding females.
All projections were run for 60 years, corresponding to about three generation-periods (one generation1 is estimated to be 21.1 years for common bottlenose dolphins in a stable population—see Taylor et al., 2007). This is probably a reasonable length of time for management purposes. The parameters were selected according to the following steps to represent parameter uncertainty and/or stochasticity (adapted from Secchi, 2006):
1. For one 3-generation run, select a mean survival rate for each stage class and a mean reproductive rate from distributions that represent parameter uncertainty.
2. Select the value of each parameter in each year by selecting at random from a distribution representing temporal environmental stochasticity for that parameter. The mean of the distribution is that chosen in step 1. The standard deviation is fixed at a pre-specified estimated value. For one scenario (see below), the model was set to be deterministic, in which case the standard deviations were set to zero.
3. Incorporate demographic stochasticity using a binomial distribution, involving (i) a survival rate and the relevant number of individuals from the previous year, or (ii) the reproductive rate and the number of breeding females.
4. Repeat steps 1–3 1000 times.
The model and simulations were written in Matlab.
Simulation Scenarios for Demography and Management Goals
The model-scenarios we considered for PLE’s dolphin population are specified below:
Scenario 1: Deterministic under current levels of by-catch;
Scenario 2: Stochastic under current levels of by-catch;
Scenario 3: Stochastic under current levels of by-catch and unbalanced adult sex ratio;
Scenario 4: Decreasing survival; removal of one mature female per year from the population;
Scenario 5: Decreasing survival; removing one mature female every 2 years from the population;
Scenario 6: Increasing survival; avoiding the bycatch of one juvenile every 2 years from the population;
Scenario 7: Increasing survival; avoiding the bycatch of two juveniles every 3 years from the population.
In scenarios 1 and 2 we used the best up to date stage-specific estimates of survival rates for estimating the population growth rate and risk of extinction and for exploring the effects of stochasticity in projections under the current fishery impact. This is because the mark-recapture survival rates estimation contains both natural and non-natural dolphin mortality (Fruet et al., 2015a), with by-catch as the main source of human-induced mortality for this dolphin population (Fruet et al., 2012; Prado et al., 2016; Venuto et al., 2020). In scenario 3 we modeled the effects of the unbalanced adult sex ratio reported for the PLE dolphin population (2F:1M) (see Fruet et al., 2015a). We fixed the number of calves and juveniles estimated in scenario 2 (calculated assuming a stable age-structure distribution and initial abundance of 44 females), and used the number of mature females known to be alive in the PLE’s dolphin population (N = 37) in 2011 as the adult female abundance. This resulted in an initial population size of 53 as opposed to 44 females used in scenarios 1 and 2, which assumed a 1:1 sex ratio overall for the population. The effects of potential changes in by-catch rates were simulated by artificially increasing or decreasing entanglement, which would affect survival rates (scenarios 4–7). We simulated the increase in survival of immature animals because it is possible that the by-catch mortality of this most affected life-stage will be reduced if the dolphin protection area turns out to be effective. However, as some types of artisanal and recreational gillnetting are still allowed in the vicinities of the protection area, and are known to catch and kill bottlenose dolphins (Fruet et al., 2018), simulating a slightly increase in by-catch of adult females due to increasing fishery effort is justifiable to allow for variations in fishing effort.
The effects of changes in survival rates was simulated by manipulating the fate of known individuals directly in the mark-recapture matrix used by Fruet et al. (2015b) for estimating the current survival rates of the PLE’s dolphins. For example, the effects of increased by-catch was assessed by simulating a decrease in the survival rate of adult females (scenarios 4 and 5), through manually setting the last 2 years of the survival history at zero for some individuals that had survived until the end of the study period (2005–2012). By contrast, for scenarios simulating the effectiveness of the dolphin protection area (scenarios 6 and 7), some immature dolphins were artificially “resurrected” through setting the capture history of some individuals that were already dead to one until the end of the period. In scenario 6, three individuals were added back to the population. This represents the minimum number of juvenile resident dolphins know to have been caught during the period of the mark-recapture study (2005–2012). In scenario 7, four dolphins were ‘resurrected’ as it is likely that some incidentally killed individuals do not show clear signs of fishing-related mortality. Thus, the survival rates estimated from these simulations potentially provide a reasonable approximation of the expected juvenile survival in the absence of by-catch (i.e., after the implementation of the dolphin protection area). All mark-recapture simulations were run in Program MARK (White and Burnham, 1999) using the most parsimonious model of the Robust Design approach as reported in Fruet et al. (2015a).
Population Viability Analysis (PVA)
Following the IUCN Red List categories and criteria (criterion A3) (IUCN, 2012) the risk was measured as the probability of the PLE’s dolphin population declining below a set of pre-specified levels. Considering the relative stability in abundance in the last three decades (Castello and Pinedo, 1977; Fruet et al., 2015a), the population will be classified as Critically Endangered (CR), Endangered (EN), and Vulnerable (VU) if > 50% of model runs predicted the population to decline 80, 50 and 30% of its current abundance, respectively. The conservation goal should ensure the long-term viability and ecological function of bottlenose dolphins in their environments. Thus, the management goal of the protected area assumed here was to promote a 20% increase in abundance of the PLE’s dolphin population over 60 years. This seems to be a satisfactory assumption from both biological and management perspectives. First, because it symbolizes a reasonable increase over such long-term period and because this population potentially is operating slightly below of it carrying capacity. Second, if achieved, this is expected to allow to increase the chances of the population to expand its range and intermixing at higher rates with the adjacent local populations (Management Units), thus enhancing genetic variability and adaptation potential. The goal of the dolphin protection area will be considered achieved only if at least 90% of the runs predicted the population to be at a level 20% higher than its current size.
Results
The effects of stochasticity and skewed adult female sex ratio in the PLE’s dolphin population had low impact in estimates of growth rate (r) under current by-catch rates and uncertainties in parameter estimates (Table 2 and Figure 2). Current by-catch rates appear to have a modest effect in the population dynamics. All simulations that took into account current by-catch rates indicated a growing rate of approximately 1.2–1.3% per year, and projected low probabilities of decline and high likelihood of an increase 20% above their current size over three generations (i.e., approximately 60 years) (Table 2). Therefore, under current conditions, the PLE population would not be classified as endangered under criterion A3 of the IUCN Red List categories and criteria (IUCN, 2012). However, the removal of a few adult females would have severe consequences for its dynamics and likelihood of future persistence. In the face of the current bycatch rates, model runs indicated that if one adult female was captured every year or every 2 years, the PLE’s dolphin population would experience very high probabilities of decline at all pre-specified levels. According to these scenarios, this population would decline at a rate of 2.3 and 0.5% per year, respectively, suggesting that such by-catch rates would be unsustainable.
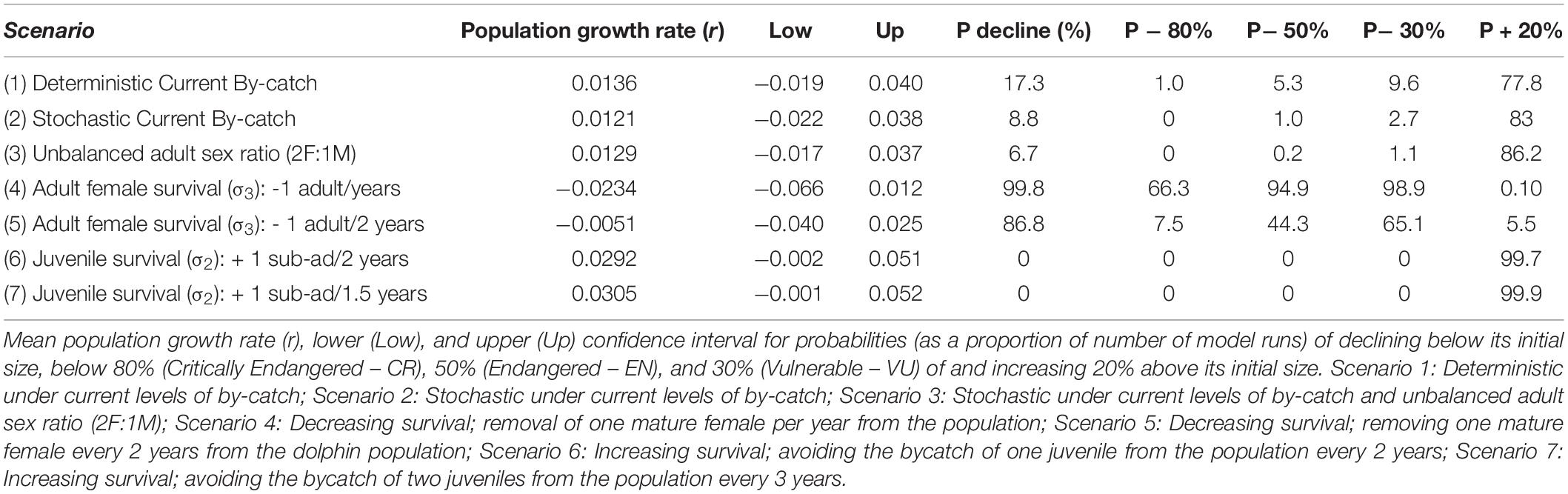
Table 2. Results of simulations used to estimate the growth rates and viability of the Patos Lagoon estuary’s Lahille’s bottlenose dolphin population under several scenarios of by-catch.
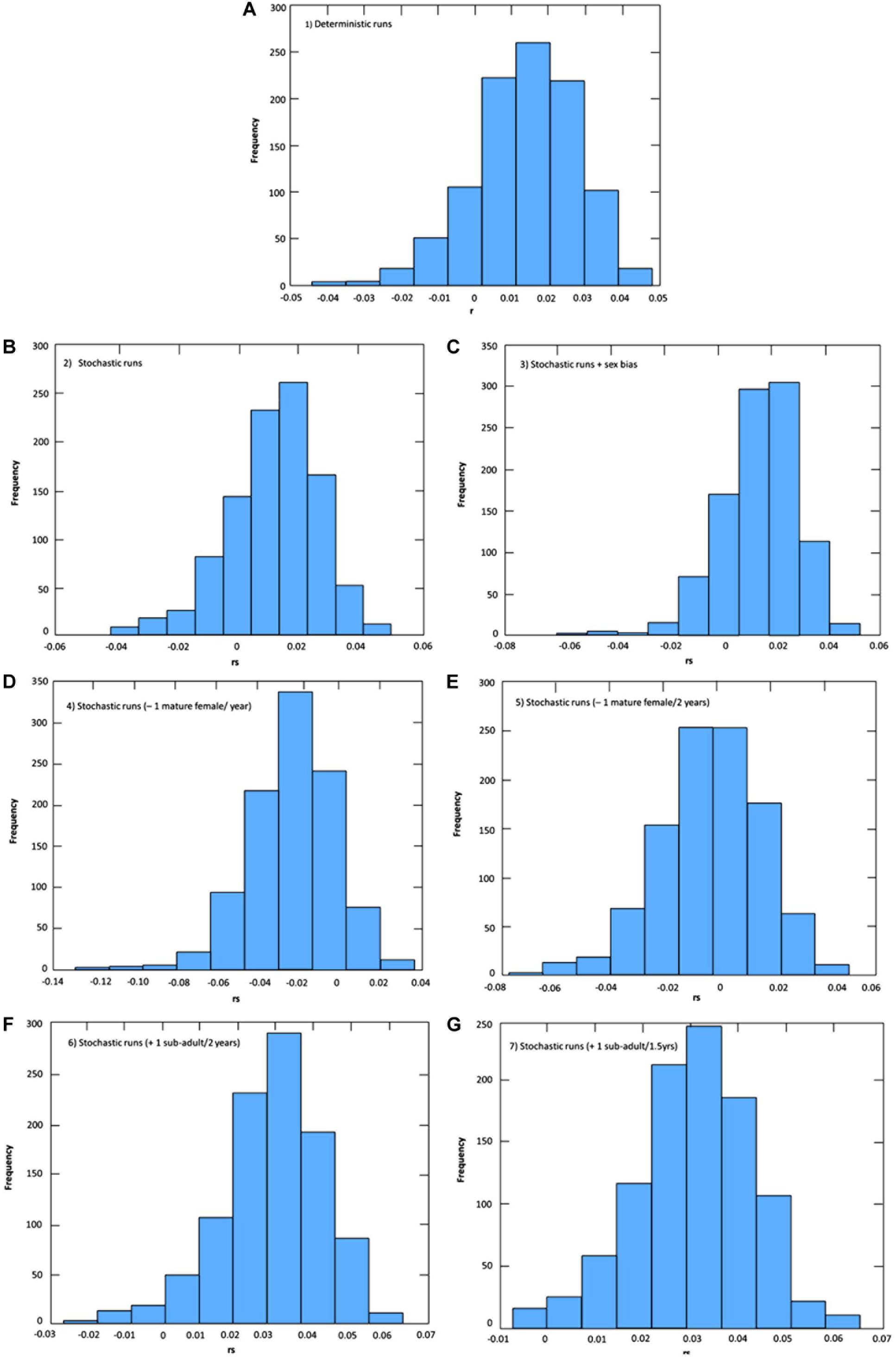
Figure 2. Uncertainties in population growth rates (r: deterministic; rs: stochastic) estimations for the Lahille’s bottlenose dolphin population of the Patos Lagoon estuary under different levels of by-catch and initial number of females (scenario 3). (A) Scenario 1: Deterministic under current levels of by-catch; (B) Scenario 2: Stochastic under current levels of by-catch; (C) Scenario 3: Stochastic under current levels of by-catch and unbalanced adult sex ratio; (D) Scenario 4: Decreasing survival; removal of one mature female per year from the Patos Lagoon estuary’s dolphin population; (E) Scenario 5: Decreasing survival; removing one mature female every 2 years from the dolphin population; (F) Scenario 6: Increasing survival; avoiding the bycatch of one juvenile from the dolphin population every 2 years; Scenario (G) 7: Increasing survival; avoiding the bycatch of two juveniles from the dolphin population every 3 years.
Reducing by-catch of juveniles/sub-adults dolphins (scenarios 6 and 7) would result in remarkable chances of an increase of 20% above the current size in 60 years, with the conservation goal achieved. Since scenario 6 used survival rates obtained from the simulation of the survival of three by-caught resident juvenile dolphins, this scenario is probably the most realistic for representing the expected “natural” juvenile survival. Under this scenario, the PLE’s dolphin population would have a mean annual potential intrinsic growth rate of 2.9%, with its maximum estimated at 5.1%.
Discussion
In this study the first comprehensive risk assessment of an estuarine, resident population of the endangered Lahille’s bottlenose dolphin is provided. We used stage-structured matrix population model to depict the dynamics and to assess the viability of the PLE’s dolphin population (Management Unit). Projections and estimates of declining probability were explored under different bycatch scenarios. Despite uncertainties in parameter estimates, all deterministic and stochastic projections indicate high chances of persistence of the local population in the next 60 years under current conditions. The results, however, suggest substantial vulnerability of this population to even a slight decrease in the survival rates of adult females. Nevertheless, this study has also shown the high probability of meeting the management goal of increasing the current population size by 20% if the proposed conservation strategy takes place.
The robustness of a population viability analysis results relies on the synergy of three central points: appropriate design of the model structure, parameters of interest that represents well the population dynamics and threats, and the input parameter values (e.g., Beissinger and Westphal, 1998). Several studies and approaches have been used to estimate the potential intrinsic growth rate of cetacean species and to model population viability (e.g., Reilly and Barlow, 1986; Caswell et al., 1998; Secchi, 2006; Slooten, 2007; Vermeulen and Bräger, 2015; Manlik et al., 2016), but many of the demographic analyses have been conducted with limited data or overly simplistic model structure. Stage and sex-specific survival rates, which is the parameter that population growth rate is most sensitive to in long-lived and slow-reproducing species (e.g., Caswell, 2001), is lacking for most cetacean species. Therefore, for cetaceans and several other threatened species, this information is generally obtained by constructing life-table models using survival curves from species with similar life histories (e.g., Caswell et al., 1998; Secchi, 2006; Hashimoto et al., 2013). Although valid, these models are constructed under a series of assumptions and their accuracy depends on the appropriate selection of model species and the time scaling procedures. This is particularly relevant when using structured models because the intrinsic population growth parameter (λ) is influenced disproportionally by variations in demographic traits of each life-stage (Caswell, 2001; Morris and Doak, 2003). Despite uncertainties, our structured model was tailored and used the best and most up to date stage-specific parameters estimated through a systematic mark-recapture study of the population unit under study.
Potential Intrinsic Growth Rates of the Patos Lagoon Estuary (PLE) Bottlenose Dolphins
Our results suggested that the PLE’s dolphin population would growth about 3% annually if by-catch of juveniles could be reduced to what we predicted to approximate their natural survival (scenarios 6 and 7). The maximum estimated potential annual growth rate was 5.1%. Despite parameter uncertainties, this estimate is consistent with other cetacean studies (Taylor et al., 2007). In general, the intrinsic growth rate of small cetaceans is likely to be approximately 4% (Reilly and Barlow, 1986; Taylor et al., 2007), with multiple populations of the franciscana dolphin (Pontoporia blainvillei) exhibiting an annual growth rate between 0.8 and 3.8% (Secchi, 2006), while the intrinsic growth rate of finless porpoises (Neophocoena asiaorientalis) has been estimated at between 4.1 and 5.6% (Hashimoto et al., 2013). For bottlenose dolphins, the only available potential intrinsic growth rate of 4.7% per year, was estimated for the population inhabiting the Indian River Lagoon (Florida, United States), based on a demographic analysis and age-at-death life-table data (Stolen and Barlow, 2003). This is in between our estimates of 3 and 5.1%.
Effects of Stochasticity
Stochastic effects and initial number of adult females appear to have little or no influence on the population growth rate and viability of PLE’s dolphins. Stochasticity arises from intrinsic or extrinsic forces that alter individual’s fitness and lead to variations in population size, which is independent of the average growth rate of the population (Burgman et al., 1993). In our study, stochasticity had minimal effects in the projections of long-term viability. Projections were similar for both stochastic and deterministic models. These results suggest that year-to-year fluctuations in the environmental conditions of the PLE during the study period did not significantly affect the reproduction and survival rates of individual females, as it would be expected if environmental variability affects prey availability, for example. Our data from the PLE’s dolphin population showed that the number of births, fecundity and survival rates were relatively constant over the last 8 years (Fruet et al., 2015a, b). This does not mean that the population is invulnerable to stochastic effects. The time-scale used to obtain year-to-year variation in reproduction and survival rates probably was not long enough to allow for the detection the effects of extreme weather events such as strong La Niña or El Niño, which could have severely impacted upon these parameters (e.g., Trillmich and Ono, 1991; Leaper et al., 2006; Seyboth et al., 2016). Given the low abundance of the PLE’s dolphin population, vulnerability to environmental and demographic stochasticity over the long-term should be further explored (e.g., Lande, 1988).
Viability of the Patos Lagoon Estuary’s (PLE) Dolphin Population
An early study used a simple logistic model to determine the Potential Biological Removal (sensu Wade, 1998) for the PLE’s dolphin population (Fruet et al., 2012). Simulations indicated this small, resident dolphin population would potentially decline if by-catch levels reported after 2002 remained at those levels (Fruet et al., 2012). However, the results of the present study suggest that entanglements have a potential lower effect on the demographic processes than previously thought (scenarios 1–3). The mean annual growth rate estimated in our study, considering current levels of by-catch, was low but positive (1.2% per year), with a probability of decline < 10%.
The discrepancies between these two studies are possibly due to the Potential Biological Removal approach being overly conservative (Hall and Donovan, 2001). The Potential Biological Removal model did not include the sex and life-stage of the by-caught individuals, and the number of dolphins killed in the fishery used in the simulations was likely overestimated (Fruet et al., 2012). Any dolphin found dead along the open coast near the PLE was assumed to belong to the PLE’s dolphin population (Fruet et al., 2012). Although reasonable based on knowledge at that time, we now know that there are two aditional bottlenose dolphin populations transiting (Genoves et al., 2018, 2020), and being captured in fishing nets near this area (Ecomega, unpubl. data). Hence animals stranded in the open coast area may belong to any of the three dolphin populations (Management Units) (Fruet et al., 2014; Genoves et al., 2018, 2020). In addition, Potential Biological Removal considers minimum abundance estimates (i.e., the 20th percentile of a log-normal distribution based on the absolute estimate of the number of animals in the population—see Wade, 1998), and that the population cannot grow more than half of the default maximum population growth of 4%, which was suggested for impacted small cetacean species (Reilly and Barlow, 1986). This approach also uses a recovery factor for populations lacking good demographic data (see Wade, 1998). In contrast, our stage-structured model used specific life history parameters estimated from mark-recapture data of photo-identified resident individuals from the dolphin population of interest. Hence, effects of fishing related mortality were implicitly considered as a component of survival rates. In fact, the results found in the present study were similar to the mark-recapture estimates of yearly changes in abundance (). Between 2005 and 2012 the abundance remained relatively stable, with no clear trends, with an average estimated at 0.00 (ranging from −1 to 7%) (Fruet et al., 2015a).
Different stage-specific vulnerability to entanglements is likely to maintain the PLE’s dolphin population relatively stable and with a high likelihood of growing if current levels and the structure of by-catch is maintained or reduced. While immature dolphins are particularly susceptible to entanglements, fishing-related mortality of adult females appears to be a rare event (Fruet et al., 2012). Previous studies using sensitivity and elasticity analyses comparing life histories across several taxa have suggested that adult survival has greater influence on fitness of long-lived and slow-reproducing species than other parameters (e.g., Caswell, 2001; Morris and Doak, 2003). Therefore, the high adult female survival rate of PLE’s bottlenose dolphins (Fruet et al., 2015a) appears to buffer the current, lower survival of juvenile dolphins.
The Bottlenose Dolphin Protection Area and the Future of the Patos Lagoon Estuary’s (PLE) Population
Although the abundance of dolphins is likely to increase under current by-catch rates that is affecting mostly juvenile individuals, there is no guarantee that this resident population can persist over the long-term under the current state. Predictions based on Population Viability Analyses (PVA) are generally optimistic unless all potential threats are included in the model (e.g., Lacy, 1993; Young, 1994; Ralls and Taylor, 1997). Thus, the PLE’s dolphin population would persist only if its habitat and other potential threats that could affect their vital rates remains the same during the projected time (i.e., no substantial changes in habitat quality or impacts that would cause additional deaths or reproductive failure). Such scenario is uncertain though very unlikely in a long-term, given accelerated environmental degradation and climate changes. Increased frequency of extreme climate events, such as El Nino, is expected as a consequence of global warming (Cai et al., 2015). In addition, other important factors with high potential to affect the dynamics of this small dolphin population (e.g., infectious disease outbreaks, genetic factor) were not taken into account in this PVA.
Our simulations indicated that a minimal reduction in current by-catch would maximize the chances (>99%) of the PLE’s dolphin population increasing 20% above its current size in three generations. Increasing abundance could potentially increase gene flow among the three adjacent populations, which are genetically differentiated and depauperated (Fruet et al., 2014). In turn, this could lead to an increase of genetic diversity, and enhanced resilience to environmental changes (e.g., Frankham, 1995; Reed and Frankham, 2003). Excluding gillnet fisheries from the dolphin protection area may also promote an increase in habitat quality (e.g., by increasing prey densities), as estuarine waters provide critical habitat for various fish and crustacean species (e.g., Costa et al., 1997; Haimovici et al., 2006; Vieira et al., 2010).
If enforcement fails, fishing effort could increase, shift to other areas or to other fishing types, such as beach fixed trammel gillnetting, which is still allowed within the protection area (Ministério da Agricultura, Pecuária e Abastecimento, 2012). The consequences of such changes cannot be foreseen but could lead to higher risk of entanglements to both coastal and estuarine dolphins. These potential changes are a source of concern because these dolphin populations are small and with localized ranges, and its viability highly susceptible to non-natural mortality or catastrophic events. As shown here, if only a few mature females were removed from the PLE’s dolphin population, the effect on its viability would be severe (Table 2 and Figures 2, 3). Therefore, continued systematic monitoring of these three dolphin populations around PLE is highly recommended to evaluate the effectiveness of the dolphin protection area for each population’s long-term viability. Monitoring schemes should be designed according to the ecological characteristics of each dolphin population and consider their home-ranges, movements, habitat use and residency patterns.
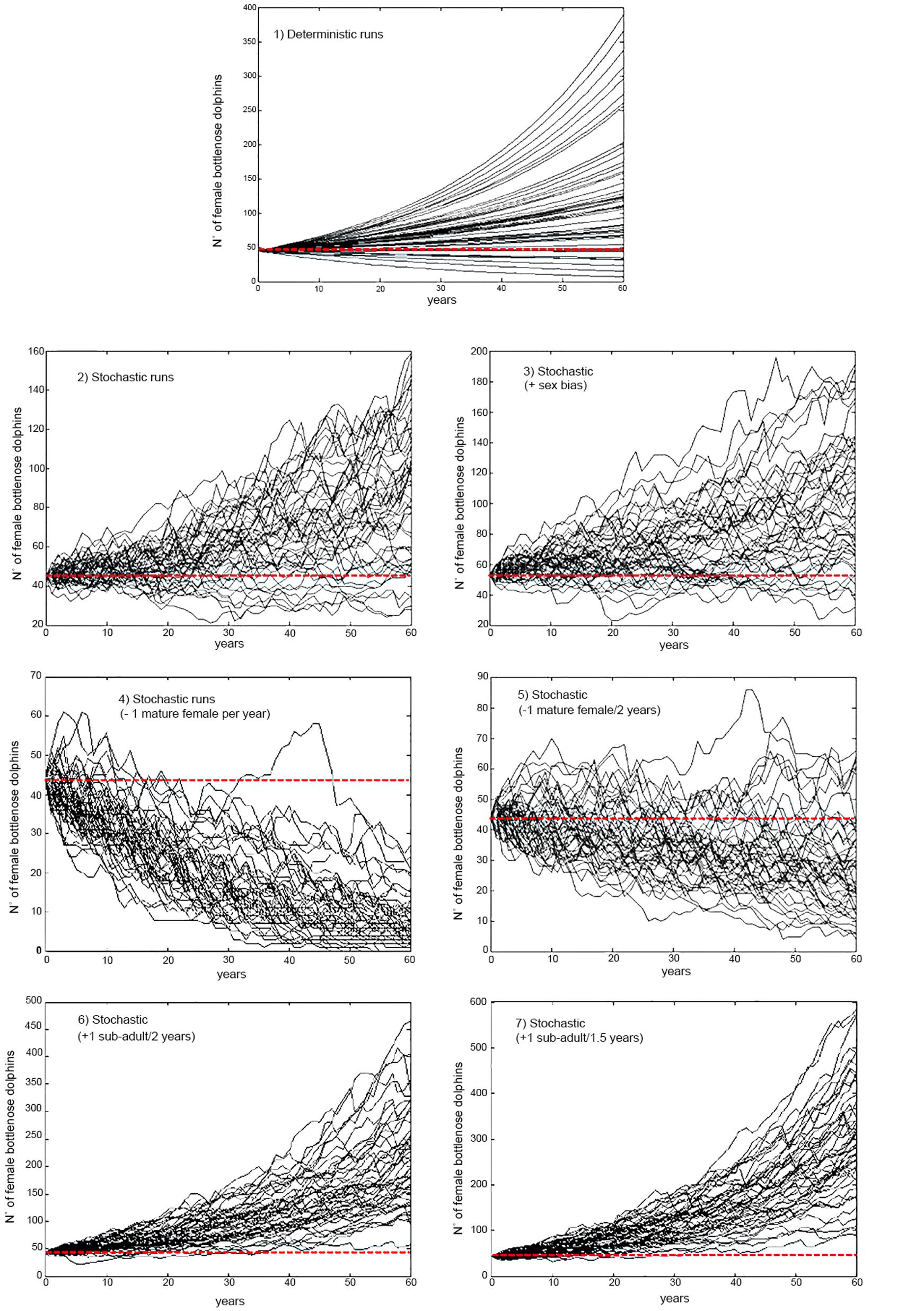
Figure 3. Samples (n = 50) from 1000 projections of the size of the resident population of bottlenose dolphin of the Patos Lagoon estuary for a period of three generations under different scenarios of by-catch and initial size considering parameter uncertainty in the estimates of stochastic population growth rate. Red dotted line highlights the initial female population size. Scenario 1: Deterministic under current levels of by-catch; Scenario 2: Stochastic under current levels of by-catch; Scenario 3: Stochastic under current levels of by-catch and unbalanced adult sex ratio; Scenario 4: Decreasing survival; removal of one mature female per year from the Patos Lagoon estuary’s dolphin population; Scenario 5: Decreasing survival; removing one mature female every 2 years from the dolphin population; Scenario 6: Increasing survival; avoiding the bycatch of one juvenile from the dolphin population every 2 years; Scenario 7: Increasing survival; avoiding the bycatch of two juveniles from the dolphin population every 3 years.
It is recommended that the potential effects of other human-induced impacting factors and genetics to be considered in future PVAs under the metapopulation framework. These dolphins are exposed to cumulative effects of a variety of human activities in coastal areas throughout their range and hold remarkably low genetic variability (Fruet et al., 2014; Costa et al., 2019). Finally, we advocate for managers to consider set increase 20% of the current abundance of PLE dolphin population over the next 60 years as one reasonable and attainable management goal. If met, this goal has the potential to promote habitat quality, increase genetic diversity and connectivity with adjacent populations, enhancing the ability of bottlenose dolphins in southern Brazil to cope with extreme environmental events and outbreaks (as the recent unprecedent morbillivirus mass mortality among Guiana dolphins, Sotalia guianensis, in Rio de Janeiro, Southeast Brazil—Groch et al., 2018; Flach et al., 2019).
Data Availability Statement
The original contributions presented in the study are included in the article/supplementary material, further inquiries can be directed to the corresponding author/s.
Ethics Statement
Ethical review and approval was not required for the animal study because this study does not involve any kind of data acquisition. It is purely mathematical.
Author Contributions
PF contributed to the conception, data analysis, interpretation, and drafting the manuscript. ES contributed to the conception and interpretation of the results, the PVA model, and revised drafts of the manuscript for its intellectual content. LM contributed to interpretation of the results, drafting of the manuscript, and revising its intellectual content. All authors contributed to the article and approved the submitted version.
Funding
This study was made possible by the financial support of the Organization for the Conservation of South American Aquatic Mammals—YAQU PACHA e.V., Nuremberg Zoo, Rancho Texas de Lancerote, Fundação O Boticário de Proteção à Natureza, Refinaria de Petróleo Riograndense, and Porto do Rio Grande RS. This study was partially funded by the Brazilian Long-Term Ecological Research Program (PELD) from CNPq (Proc.441492/2016-9), and the Fundação de Amparo à Pesquisa do Estado do Rio Grande do Sul (Proc. 16/2551-0000102-2). The Coordination for the Improvement of Higher Education Personnel (CAPES) provided access to the Portal de Periódicos and financial support through Programa de Excelência Acadêmica—PROEX. CNPq also provided a fellowship to ES (PDE 229334/20113-0 and PQ 310597/2018-8) and an international fellowship to PF (SWE 201567/2011-3). Flinders University of South Australia provided a fee waiver as part of PF’s Ph.D. cotutelle agreement between this university and Universidade Federal do Rio Grande (FURG). This agreement is within the scope of the Capes PrInt Program (public notice 041/2017).
Conflict of Interest
The authors declare that the research was conducted in the absence of any commercial or financial relationships that could be construed as a potential conflict of interest.
Acknowledgments
We are very grateful to all field assistants and researchers from Ecomega for helping with data collection over the years, especially Rodrigo C. Genoves and Juliana C. Di Tullio. We also thank Lauro Barcellos, director of the Museu Oceanográfico “Prof Eliezer C. Rios” at FURG for providing logistical support to the fieldwork. We are in debt with Yaqu Pacha EV for their long-term support. Paul Kinas, Luciano Dalla Rosa, and Alexandre Azevedo provided useful advice in the early drafts of the manuscript. Dimas Gianuca, Mauricio Aresso, and Christian Göbel kindly helped with figures editing. We are especially indebted to David Fletcher (University of Otago, New Zealand) for advising during the model construction. This article is part of PF’s Ph.D. thesis in Biological Oceanography under the supervision of ES (Universidade Federal do Rio Grande, FURG) and LM (Flinders University) and it is a contribution of the Research Group Ecologia e Conservação da Megafauna Marinha – EcoMega –FURG/CNPq.
Footnotes
- ^ Generation length is the average age of parents of the current cohort (i.e., newborn individuals in the population), reflecting the turnover rate of breeding individuals in a population (Taylor et al., 2007).
References
Beissinger, S. R., and Westphal, M. I. (1998). On the use of demographic models of population viability in endangered species management. J. Wildlife Manage. 62, 821–841. doi: 10.2307/3802534
Brault, S., and Caswell, H. (1993). Pod-specific demography of Killer whales (Orcinus orca). Ecology 74, 1444–1454. doi: 10.2307/1940073
Burgman, M. A., Ferson, S., and Akçakaya, H. R. (1993). Risk Assessment in Conservation Biology. New York, NY: Chapman and Hall.
Cai, W., Borlace, S., Lengaigne, M., van Rensch, P., Collins, M., Vecchi, G. A., et al. (2015). Increasing frequency of extreme El Niño events due to greenhouse warming. Nat. Clim. Chang. 4, 111–116.
Caswell, H. (2001). Matrix Population Models: Construction, Analysis and Interpretation, 2nd Edn. Sunderland: Sinauer Associates.
Caswell, H., Brault, S., Read, A. J., and Smith, T. D. (1998). Harbor porpoise and fisheries: an uncertainty analysis of incidental mortality. Ecol. Appl. 8, 1226–1238. doi: 10.1890/1051-0761(1998)008[1226:HPAFAU]2.0.CO;2
Costa, A. P. B., Fruet, P. F., Secchi, E. R., Daura-Jorge, F. G., Simões-Lopes, P. C., Di Tullio, J. C., et al. (2019). Ecological divergence and speciation in common bottlenose dolphins in the western South Atlantic. J. Evol. Biol. doi: 10.1111/jeb.13575 [Online print of ahead]
Costa, A. P. B., Rosel, P. E., Daura-Jorge, F. G., and Simões-Lopes, P. C. (2016). Offshore and coastal common bottlenose dolphins of the western South Atlantic face-to-face: what the skull and the spine can tell us. Mar. Mam. Sci. 32, 1433–1457. doi: 10.1111/mms.12342
Costa, C. S. B., Seeliger, U., Oliveira, C. P. L., and Mazo, A. M. M. (1997). Distribuição, funções e valores das marismas e pradarias submersas no estuário da Lagoa dos Patos (RS, Brasil). Atlântica 19, 65–83.
Flach, L., Alonso, M. B., Marinho, T., Van Waerebeek, K., and Van Bressem, M. F. (2019). Clinical signs in free-ranging Guiana dolphins Sotalia guianensis during a morbillivirus epidemic: case study in Sepetiba Bay Brazil. Dis. Aquat. Organ. 133, 175–180. doi: 10.3354/dao03343
Fruet, P. F., Daura-Jorge, F. G., Möller, L. M., Genoves, R. C., and Secchi, E. R. (2015a). Abundance and demography of bottlenose dolphins inhabiting a subtropical estuary in the Southwestern Atlantic Ocean. J. Mammal 96, 332–343. doi: 10.1093/jmammal/gyv035
Fruet, P. F., Genoves, R. C., Möller, L. M., Botta, S., and Secchi, E. R. (2015b). Using mark-recapture and stranding data to estimate reproductive traits in female bottlenose dolphins (Tursiops truncatus) of the Southwestern Atlantic Ocean. Mar. Biol. 162, 661–673. doi: 10.1007/s00227-015-2613-0
Fruet, P. F., Kinas, P. G., Silva, K. G., Di Tullio, J. C., Monteiro, D. S., Dalla Rosa, L., et al. (2012). Temporal trends in mortality and effects of by-catch on common bottlenose dolphins, Tursiops truncatus, in southern Brazil. J. Mar. Biolog. Assoc. U.K. 92, 1865–1876. doi: 10.1017/s0025315410001888
Fruet, P. F., Laporta, P., and Flores, P. A. (2016b). Report of the working group on population parameters and demography of Tursiops truncatus in the southwest Atlantic Ocean. Lat. Am. J. Aquatic Mam. 11, 71–78.
Fruet, P. F., Prado, J., Genoves, R. C., Di Tullio, J. C., and Secchi, E. R. (2018). Preliminary Evidences Suggest that the Establishment of a Bottlenose Dolphin Protection Area in Southern Brazil is Failing Against the Reduction of Bycatch. Cambridge: International Whaling Commission.
Fruet, P. F., Secchi, E. R., Daura-Jorge, F. G., Vermeulen, E., Flores, P. A. C., Simões-Lopes, P. C., et al. (2014). Remarkably low genetic diversity and strong population structure in common bottlenose dolphins (Tursiops truncatus) from coastal waters of the Southwestern Atlantic Ocean. Conserve. Genet. 15, 879–895. doi: 10.1007/s10592-014-0586-z
Fruet, P. F., Zappes, C. A., Bisi, T. L., Simões-Lopes, P. C., Laporta, P., Loureiro, J. D., et al. (2016a). Report of the working group on interactions between humans and tursiops truncatus in the southwest atlantic ocean. Lat. Am. J. Aquat. Mam. 11, 79–98. doi: 10.5597/lajam00218
Genoves, R. C., Fruet, P. F., Botta, S., Beheregaray, L. B., Moüller, L. M., and Secchi, E. R. (2020). Fine-scale genetic structure in Lahille’s bottlenose dolphins (Tursiops truncatus gephyreus) is associated with social structure and feeding ecology. Mar. Biol. 167:34. doi: 10.1007/s00227-019-3638-6
Genoves, R. C., Fruet, P. F., Di Tullio, J. C., Moüller, L. M., and Secchi, E. R. (2018). Spatiotemporal use predicts social partitioning of bottlenose dolphins with strong home range overlap. Ecol. Evol. 8, 12597–12614. doi: 10.1002/ece3.4681
Gilpin, M. E., and Soulé, M. E. (1986). “Minimum viable populations: process of species extinction,” in Conservation Biology: The Science of Scarcity and Diversity, ed. M. E. Soulé (Sunderland: Sinauer Associates), 19–34.
Groch, K. R., Santos-Neto, E. B., Díaz-Delgado, J., Ikeda, J. M. P., Carvalho, R. R., Oliveira, R. B., et al. (2018). Guiana Dolphin unusual mortality event and link to cetacean morbillivirus, Brazil. Emerg. Infect. Dis. 24, 1349–1354. doi: 10.3201/eid2407.180139
Haimovici, M., Rossi-Wongtschowski, C. L. D. B., Cergole, M. C., Madureira, L. S., Bernardes, R. A., and Ávila-Da-Silva, A. O. (2006). “Recursos Pesqueiros da região Sudeste-Sul,” in Programa REVIZEE: Avaliação do Potencial Sustentável de Recursos Vivos da Zona Econômica Exclusiva. Relatório Executivo. (Brasília: Ministério do Meio Ambiente), 207–242.
Hall, M. A., and Donovan, G. P. (2001). “Environmentalists, fishermen, cetaceans and fish: is there a balance and can science help to find it?,” in Marine Mammals: Biology and Conservation, eds P. G. H. Evans and J. A. Raga (New York, NY: Kluwer Academic), 491–521. doi: 10.1007/978-1-4615-0529-7_14
Hashimoto, M., Shirakihara, K., Shirakihara, M., and Hiramatsu, K. (2013). Estimating the rate of increase for the finless porpoise with special attention to predictions for the Inland Sea population in Japan. Popul. Ecol. 55, 441–449. doi: 10.1007/s10144-013-0374-5
Hohl, L. S. L., Sicuro, F. L., Wickert, J. C., Moreno, I. B., Rocha-Barbosa, O., and Barreto, A. S. (2020). Skull morphology of bottlenose dolphins from different ocean populations with emphasis on South America. J. Morphol. 281, 564–577. doi: 10.1002/jmor.21121
Lacy, R. C. (1993). VORTEX: a computer simulation model for population viability analysis. Wildlife Res. 20, 45–65. doi: 10.1071/wr9930045
Lande, R. (1988). Demographic models of the northern spotted owl (Strix occidentalis caurina). Oecologia 75, 601–607. doi: 10.1007/bf00776426
Laporta, P., Martins, C. C. A., Lodi, L., Domit, C., Vermeulen, E., and Di Tullio, J. C. (2016). Report of the working group on habitat use of Tursiops truncatus in the Southwest Atlantic Ocean. Lat. Am. J. Aquat. Mamm. 11, 47–61. doi: 10.5597/lajam00215
Leaper, R., Cooke, J., Trathan, P., Reid, K., Rowntree, V., and Payne, R. (2006). Global climate drives southern right whale (Eubalaena australis) population dynamics. Biol. Lett. 2, 289–292. doi: 10.1098/rsbl.2005.0431
Manlik, O., McDonald, J. A., Mann, J., Raudino, H. C., Bejder, L., Krutzen, M., et al. (2016). The relative importance of reproduction and survival for the conservation of two dolphin populations. Ecol. Evol. 6, 3496–3612. doi: 10.1002/ece3.2130
Manly, B. F. J. (1997). Randomization, bootstrap and Monte Carlo Methods in Biology, 2nd Edn. London: Chapman and Hall.
Marine Mammalogy’s Committee on Taxonomy (2018). List of Marine Mammal Species and Sub- Species. Available online at: https://www.marinemammalscience.org (accessed November 16, 2020).
Ministério da Agricultura, Pecuária e Abastecimento (2012). Instrução Normativa Interministerial MPA/MMA número 12 de 22 de agosto de 2012. Dispõe sobre critérios e padrões para o ordenamento da pesca praticada com o emprego de redes de emalhe nas águas jurisdicionais brasileiras das regiões Sudeste e Sul. Diário Oficial da União de 24/08/2012, n°165, Seção 1. Brasília: Ministério da Agricultura, Pecuária e Abastecimento.
Morris, W. F., and Doak, D. F. (2003). Quantitative Conservation Biology: Theory and Practice of Population Viability Analysis. Sunderland: Sinauer Associates.
Pinedo, M. C. (1986). “Mortalidade de Pontoporia blainvillei, Tursiops gephyreus, Otaria flavescens e Arctocephalus australis na costa do Rio Grande do Sul, Brasil, 1976–1983,” in Proceedings of Primeira Reunión de Trabajo de Expertos em Mamíferos Acuáticos de América del Sur, August, 25–29 (Buenos Aires), 187–199.
Possingham, H. P., Lindenmayer, D. B., and Norton, T. W. (1993). A framework for improved management of threatened species based on population viability analysis (PVA). Pac. Conserv. Biol. 1, 39–45. doi: 10.1071/pc930039
Prado, J. H. F., Mattos, P. H., Silva, K. G., and Secchi, E. R. (2016). Long-term seasonal and interannual patterns of marine mammal strandings in subtropical Western South Atlantic. PLoS One 11:e0146339. doi: 10.1371/journal.pone.0146339
Ralls, K., and Taylor, B. L. (1997). “How viable is population viability analysis?,” in The Ecological Basis of Conservation, eds T. A. Pickett, R. S. Ostfeld, M. Shachak, and G. E. Likens (New York, NY: Chapman and Hall), 228–235. doi: 10.1007/978-1-4615-6003-6_23
Reed, D. H., and Frankham, R. (2003). Correlation between fitness and genetic diversity. Conserv. Biol. 17, 230–237. doi: 10.1046/j.1523-1739.2003.01236.x
Reed, J. M., Mill, L. S., Dunning, J. B. Jr., Menges, E. S., McKelvey, K. S., et al. (2002). Emerging issues in population viability analysis. Conserv. Biol. 16, 7–19.
Reilly, S. B., and Barlow, J. (1986). Rates of increase in dolphin population size. Fish. Bull. 84, 527–533.
Righetti, B. P. H., Mattos, J. J., Siebert, M. N., Daura-Jorge, F. G., Bezamat, C., Fruet, P. F., et al. (2019). Biochemical and molecular biomarkers in integument biopsies of free- ranging coastal bottlenose dolphins from southern Brazil. Chemosphere 225, 139–149. doi: 10.1016/j.chemosphere.2019.02.179
Secchi, E. R. (2006). Modelling the Population Dynamics and Viability Analysis of Franciscana (Pontoporia blainvillei) and Hector’s Dolphins (Cephalorhynchus hectori) Under the Effects of Bycatch in Fisheries, Parameter Uncertainty and Stochasticity. Dunedin, NZ: University of Otago. PhD Thesis.
Secchi, E. R., and Fletcher, D. (2004). Estimating Survival Rates of Franciscana by Fitting the Siler Model to Data on Age-at Death of Beachcast and Bycatch and by a Modeling Approach Using Life Tables of Similar Species: a comparison. Cambridge: International Whaling Commission.
Seyboth, E., Groch, K., Dalla Rosa, L., Reid, K., Flores, P. A. C., and Secchi, E. R. (2016). Southern right whale (Eubalaena australis) reproductive success is influenced by krill (Euphausia superba) density and climate. Sci. Rep. 6:28205. doi: 10.1038/srep28205
Simões-Lopes, P. C., and Fabian, M. E. (1999). Residence patterns and site fidelity in bottlenose dolphins, Tursiops truncatus (Montagu) (Cetacea, Delphinidae) off Southern Brazil. Zoologia 16, 1017–1024. doi: 10.1590/s0101-81751999000400012
Slooten, E. (2007). Conservation management in the face of uncertainity: effectiveness of four options for managing Hector’s dolphin bycatch. Endanger. Species Res. 3, 169–179. doi: 10.3354/esr003169
Stolen, M. K., and Barlow, J. (2003). A model life table for bottlenose dolphins (Tursiops truncatus) from the Indian River Lagoon System, Florida, USA. Mar. Mamm. Sci. 19, 630–649. doi: 10.1111/j.1748-7692.2003.tb01121.x
Taylor, B. L., Chivers, S. J., Larese, J., and Perrin, W. F. (2007). Generation Length and Percent Mature Estimates for IUCN Assessments of Cetaceans. Administrative report LJ-07-01. La Jolla, CL: Southwest Fisheries Science Center.
Trillmich, F., and Ono, K. A. (1991). Pinnipeds and El Niño: Responses to Environmental Stress. Berlin: Springer-Verlag.
Van Bressem, M. F., Simões-Lopes, P. C., Félix, F., Kiszka, J. J., Daura-Jorge, F. G., Avila, I. C., et al. (2015). Epidemiology of lobomycosis-like disease in bottlenose dolphins Tursiops spp. from South America and southern Africa. Dis. Aquat. Organ. 117, 59–75. doi: 10.3354/dao02932
Venuto, R., Botta, S., Barreto, A. S., Secchi, E. R., and Fruet, P. F. (2020). Age structure of strandings and growth of Lahille’s bottlenose dolphin (Tursiops truncatus gephyreus). Mar. Mamm. Sci. 36:e12683. doi: 10.1111/mms.12683
Vermeulen, E., and Bräger, S. (2015). Demographics of the disappearing bottlenose dolphin in Argentina: a common species on its way out? PLoS One 10:e0119182. doi: 10.1371/journal.pone.0119182
Vermeulen, E., Fruet, P. F., Costa, A., Coscarella, M., and Laporta, P. (2019). Tursiops truncatus ssp. gephyreus. The IUCN Red List of Threatened Species 2019. doi: 10.2305/IUCN.UK.2019-3.RLTS.T134822416A135190824.en
Vieira, J. P., Garcia, A. M., and Moraes, L. E. (2010). “Assembléia de Peixes,” in O Estuário da Lagoa dos Patos: um século de transformações, eds U. Seeliger and C. Odebrecht (Rio Grande, RS: Editora da FURG), 79–90.
Wade, P. R. (1998). Calculating limits to the allowable human caused mortality of cetaceans and pinnipeds. Mar. Mamm. Sci. 14, 1–37. doi: 10.1111/j.1748-7692.1998.tb00688.x
White, G. C. (2000). “Population viability analysis: data requirements and essential analyses,” in Research Techniques in Animal Ecology: Controversies and Consequences, eds L. Boitani and T. K. Fuller (New York, NY: Columbia University Press), 288–331.
White, G. C., and Burnham, K. P. (1999). Program Mark: survival estimation from populations of marked animals. Bird Study 46, 120–138.
Wickert, J. C., von Eye, S. M., Oliveira, L. R., and Moreno, I. B. (2016). Revalidation of Tursiops gephyreus Lahille, 1908 (Cetartiodactyla: Delphinidae) from the southwestern Atlantic Ocean. J. Mammal. 97, 1728–1737. doi: 10.1093/jmammal/gyw139
Keywords: conservation, management, population viability analysis, by-catch, bottlenose dolphins, growth rates, protected areas, survival
Citation: Fruet PF, Möller LM and Secchi ER (2021) Dynamics and Viability of a Small, Estuarine-Resident Population of Lahille’s Bottlenose Dolphins From Southern Brazil. Front. Mar. Sci. 7:593474. doi: 10.3389/fmars.2020.593474
Received: 10 August 2020; Accepted: 07 December 2020;
Published: 12 January 2021.
Edited by:
Rob Harcourt, Macquarie University, AustraliaReviewed by:
Alana Grech, ARC Centre of Excellence for Coral Reef Studies, AustraliaKatrina Joan Davis, University of Oxford, United Kingdom
Copyright © 2021 Fruet, Möller and Secchi. This is an open-access article distributed under the terms of the Creative Commons Attribution License (CC BY). The use, distribution or reproduction in other forums is permitted, provided the original author(s) and the copyright owner(s) are credited and that the original publication in this journal is cited, in accordance with accepted academic practice. No use, distribution or reproduction is permitted which does not comply with these terms.
*Correspondence: Pedro F. Fruet, cGZydWV0QGdtYWlsLmNvbQ==; Eduardo R. Secchi, ZWR1LnNlY2NoaUBmdXJnLmJy