- 1Institute for Terrestrial and Aquatic Wildlife Research (ITAW), University of Veterinary Medicine Hannover Foundation, Büsum, Germany
- 2Center of Natural History (CeNak), University of Hamburg, Hamburg, Germany
- 3Fundación Macuáticos Colombia, Medellin, Colombia
The North Sea is one of the most heavily used shelf regions worldwide with a diversity of human impacts, including shipping, pollution, fisheries, and offshore constructions. These stressors on the environment can have consequences for marine organisms, such as our study species, the harbor porpoise (Phocoena phocoena), which is regarded as a sentinel species and hence has a high conservation priority in the European Union (EU). As EU member states are obliged to monitor the population status, the present study aims to estimate trends in absolute harbor porpoise abundance in the German North Sea based on almost two decades of aerial surveys (2002–2019) using line-transect methodology. Furthermore, we were interested in trends in three Natura2000 Special Areas of Conservation (SACs), which include the harbor porpoise as designated feature. Trends were estimated for each SAC and two seasons (spring and summer) as well as the complete area of the German North Sea. For the trend analysis we applied a Bayesian framework to a series of replicated visual surveys, allowing to propagate the error structure of the original abundance estimates to the final trend estimate and designed to deal with spatio-temporal heterogeneity and other sources of uncertainty. In general, harbor porpoise abundance decreased in northern areas and increased in the south, such as in the SAC Borkum Reef Ground. A particularly strong decline with a high probability (94.9%) was detected in the core area and main reproduction site in summer, the SAC Sylt Outer Reef (−3.79% per year). The overall trend for the German North Sea revealed a decrease in harbor porpoise abundance over the whole study period (−1.79% per year) with high probability (95.1%). The assessment of these trends in abundance based on systematic monitoring should now form the basis for adaptive management, especially in the SAC Sylt Outer Reef, where the underlying causes and drivers for the large decline remain unknown and deserve further investigation, also in a regional North Sea wide context.
Introduction
The harbor porpoise (Phocoena phocoena) is the smallest and by far most common cetacean species in the North Sea, including German waters (Benke et al., 1998; Siebert et al., 2006a; Hammond et al., 2013). Being described as a continental shelf species, it is distributed throughout the shallow North Sea, from coastal waters up to the Dogger Bank in the central North Sea (Gilles et al., 2009, 2011, 2016). Occasionally, harbor porpoises also enter estuaries and move upstream rivers (Wenger and Koschinski, 2012). In the North Sea, they are usually encountered solitary or in small groups (Benke et al., 1998; Gilles et al., 2009; Hammond et al., 2013). Females reach sexual maturity between 3 and 5 years (Sørensen and Kinze, 1994; Kesselring et al., 2017) and potentially give birth to a single calf every year thereafter (Sørensen and Kinze, 1994; Read and Hohn, 1995). However, the North-East Atlantic population which includes the North Sea, seems to have lower pregnancy rates (Pierce et al., 2008; Learmonth et al., 2014; Murphy et al., 2015).
Harbor porpoises feed on energy-rich fish (<30 cm) such as sandeels (Ammodytes spp.), gobies (Gobiidae), gadoids (e.g., Atlantic cod Gadus morhua and whiting Merlangius merlangus), clupeids (e.g., Atlantic herring Clupea harengus and sprat Sprattus sprattus), and flatfish (Pleuronectiformes) (Benke et al., 1998; Gilles, 2008; Leopold et al., 2015; Andreasen et al., 2017). As small, endotherm predators living in temperate waters, harbor porpoises have limited capacity to store energy (Koopman et al., 2002) and high metabolic rates (Spitz et al., 2012; Rojano-Doñate et al., 2018); also high feeding rates were reported for wild, mainly juvenile, harbor porpoises from the Baltic Sea (Wisniewska et al., 2016, 2018a). If such feeding rates were generally representative for harbor porpoises (but see Hoekendijk et al., 2018), this would imply that harbor porpoises have high energetic requirements and consequently are especially vulnerable to any anthropogenic disturbance.
In the Anthropocene, ongoing and accelerating industrialization leads to substantial changes in the ecosystem of the North Sea, known to be one of the most intensely used sea areas (Halpern et al., 2008, 2015; Emeis et al., 2015). A diversity of human activities introduces various stressors and pressures to the marine environment, such as pollution (including marine litter), overfishing and trawling, dredging, human-induced eutrophication, sand and gravel extraction, offshore construction and heavy ship traffic (Emeis et al., 2015). Besides being threatened by by-catch in fisheries (Vinther and Larsen, 2004; Read et al., 2006; Brownell et al., 2019) and chemical pollution (Siebert et al., 1999; Beineke et al., 2005; Law et al., 2012), harbor porpoises are particularly sensitive to underwater noise pollution caused by several human exploitations. A huge effort has been made to increase the extent of energy from renewables in the ocean, leading to an intensive construction of offshore wind farms (OWFs) (Berkenhagen et al., 2010; Esteban et al., 2011; Sun et al., 2012). The construction phase is accompanied by the introduction of impulsive noise of high energy when wind turbines are driven into the seabed, which has been found to affect harbor porpoise hearing (Kastelein et al., 2015, 2016) as well as behavior and distribution (Tougaard et al., 2009; Dähne et al., 2013; Brandt et al., 2018). Similar impulsive noise is generated during seismic surveys with air guns or clearance of unexploded World War ordnance, which can likewise affect hearing and distribution (Lucke et al., 2009; von Benda-Beckmann et al., 2015; Aarts et al., 2016; Merchant et al., 2020; Sarnocińska et al., 2020). As odontocetes, harbor porpoises rely on sound for orientation, predation and intraspecific communication (Clausen et al., 2010; Wisniewska et al., 2016; Sørensen et al., 2018), which is why they are particularly vulnerable to underwater noise and any hearing impairment should be prevented. Beside impulsive noise events, the global underwater soundscape is largely dominated by shipping noise proven to have negative effects on harbor porpoise behavior (Hermannsen et al., 2014; Dyndo et al., 2015; Akkaya Bas et al., 2017; Wisniewska et al., 2018b).
Another important anthropogenic stressor affecting harbor porpoises is fisheries. The fishing intensity in the North Sea is high, especially towards coastal waters (Pedersen et al., 2009a,b; Vespe et al., 2016; Eigaard et al., 2017), although fishing effort has decreased in recent years (ICES, 2019b; STECF, 2020). The use of mobile bottom-contacting gear (e.g., beam and otter trawls) is common (Pedersen et al., 2009a,b; Eigaard et al., 2017), with potential adverse effects on the diverse benthic communities. In the southern North Sea, the most important target species in terms of landing weight are sandeel, sprat, herring, mackerel (Scomber scombrus), and flatfish, such as plaice (Pleuronectes platessa), dab (Limanda limanda), common sole (Solea solea), and turbot (Scophthalmus maximus) (Pedersen et al., 2009b). These species are also important components of harbor porpoise diet in this region (Benke et al., 1998; Gilles, 2008; Leopold et al., 2015). Harbor porpoise distribution also overlaps spatio-temporally with specific fisheries (e.g., sandeel fisheries) in the North Sea (Herr et al., 2009). As several fish stocks in the North Sea, e.g., sandeel and cod, have plummeted over decades due to severe overfishing (ICES, 2019a,c), this raises concerns for marine predators which rely on the same resources.
The harbor porpoise has a high conservation priority in the European Union (EU). Among other legislative frameworks, it is protected under the EU Habitats Directive (EU HD, 92/43/EEC; in Annexes II and IV) and is also covered by the OSPAR Convention (OSPAR, 2008). EU Member States are required to establish national monitoring programs to report on abundance and distribution of harbor porpoises at national levels and assess trends every six years (Article 17, EU HD). As one of the first EU Member States, Germany initiated a dedicated visual monitoring program in 2002 to estimate harbor porpoise abundance and density, as well as to determine distributional patterns. Continuous monitoring efforts have now led to almost two decades of harbor porpoise abundance survey data that can be used for trend analyses.
In the North Sea, the overall abundance of harbor porpoises was estimated to be 345,000 individuals (CV = 0.18), based on the latest multi-national cetacean survey, SCANS-III, in 2016 (Hammond et al., 2017). The 2016 estimate is very similar to the estimates from previous large scale surveys in 2005 (355,000; CV = 0.22; revised from Hammond et al., 2013) and in 1994 (289,000; CV = 0.14; revised from Hammond et al., 2002, 2017). Although the North Sea-wide assessments found abundance to be similar, a notable southward shift in the distribution was detected with now highest porpoise densities in the south-western North Sea (Hammond et al., 2013, 2017). However, trend estimation is difficult when comparing only a few point estimates of abundance over a large area with their large uncertainty (Authier et al., 2020). While there are ways to derive trends between short and noisy time series from sporadic large scale survey estimates (see for example, Hammond et al., 2017), these rarely consider all sources of uncertainty and variability within the underlying data and more sophisticated approaches are needed (see for example, Authier et al., 2020). Statistical power to detect change in marine mammal population is crucial for policy making, but achieving statistical power is challenging and has long been debated in conservation practice (Taylor and Gerrodette, 1993; Wade, 2000; Taylor et al., 2007).
In this context, national monitoring programs are essential to gather dedicated survey data more frequently in higher temporal and spatial resolution and, ultimately, to infer on fine-scale trends at a national level to inform conservation and management needs. For instance, an increase in harbor porpoise densities with accompanied increased densities of porpoise calves in the southern German Bight between 2002 and 2013 was derived from subsets of the dataset used in the present study (Peschko et al., 2016). Moreover, fine-scale surveys are also helpful to improve our understanding of seasonal distribution patterns. It has been shown that harbor porpoises are most abundant in spring and summer in the German North Sea (Gilles et al., 2009, 2011). These findings of the early survey years led to adaptations of the present long-term monitoring scheme to focus efforts particularly on seasons with high density [as suggested e.g. by, Harvey (2008); Lindenmayer and Likens (2009), and Hammond (2010)].
Our aim of the present study was to determine trends in absolute abundance of harbor porpoises based on aerial surveys conducted in the German Exclusive Economic Zone (EEZ) in the North Sea. In addition, we were interested in the dynamics in the Special Areas of Conservation (SACs, as designated under the EU Natura2000 framework) “Borkum Reef Ground,” “Sylt Outer Reef,” and “Dogger Bank.” These sites were nominated in 2004 and officially approved by the European Commission in 2008. Only recently, in May 2020, Germany implemented management plans for these SACs that define conservation and restoration measures required to achieve the protection purposes. Amongst further Annex II qualifying species and habitats, these three sites were designated for harbor porpoises and specific conservation objectives of these sites demand a maintenance or restoration of a favorable conservation status of habitats and species. A trend analysis can be useful to detect changes in abundance and hence to inform management on the effectiveness of conservation efforts. As the assessment of the abundance of a wildlife population usually comes with an associated uncertainty, ideally, the anticipated analysis should propagate this uncertainty into the final trend estimate. Bayesian tools are particularly useful in that sense as they are a valid option for complex hierarchical problems such as trend estimation that require error propagation (Buckland et al., 2004; Newman et al., 2006; Humbert et al., 2009). Such tools are now also more easily applicable in user-friendly software packages (Hadfield, 2010; Johnson and Fritz, 2014). These allow researchers not only to quantify the magnitude of the trend, but also its uncertainty; i.e., all sources of uncertainty and intrinsic bias in the data will be propagated to a final estimate. In this study, we applied a Bayesian framework to a series of replicated visual surveys in the German North Sea to report trends in harbor porpoise abundance, based on 18 years of data from the regular national monitoring program for harbor porpoises.
Materials and Methods
Study Area
The study area encompassed the EEZ and the 12 nautical mile zone of the German North Sea, a shallow shelf sea region of the North East Atlantic (Figure 1). The coast is characterized by the shallow and tidally influenced Wadden Sea, with estuaries from the rivers Ems, Weser, Elbe and Eider (Becker et al., 1992). The deep, wedge-shaped post glacial valley of the river Elbe (>30 m water depth) extends from the Elbe estuary to the northwest and dominates the bathymetry of the study area. In the central part of the German North Sea, water depths lie between 40 and 60 m (Becker et al., 1992).
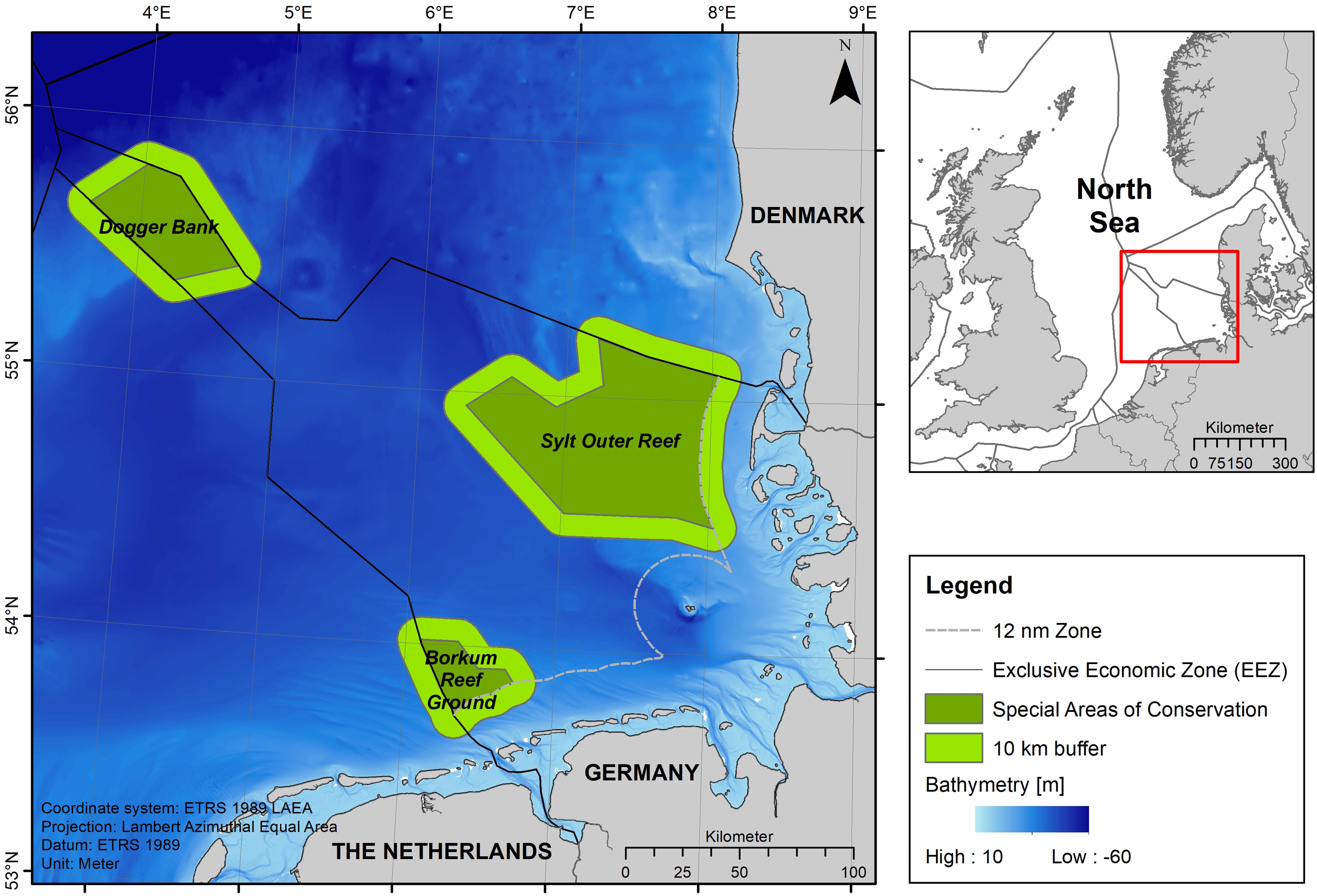
Figure 1. Overview map (top right) of the study area in the German North Sea marked by a red box. Dark green areas in the map on the left illustrate the three SACs located in the German North Sea EEZ: Dogger Bank, Sylt Outer Reef and Borkum Reef Ground. Light green areas around the SACs indicate the 10 km buffer zone used during the analysis stage.
While the North Sea is supplied with salty marine water in the north by the North Atlantic, salinity decreases toward the south due to river water input (Turrell et al., 1992; Prandle et al., 1997; Ducrotoy et al., 2000). There is an anti-clockwise current in the North Sea. Water masses enter the North Sea in the North between Scotland and Norway, travel along the east coast of Great Britain and mix with the waters of the English Channel in the south before continuing along the coastline of Belgium, the Netherlands, Germany, and Denmark. After entering the Skagerrak, water masses leave the North Sea along the Norwegian coast back into the North East Atlantic (Turrell et al., 1992; Ducrotoy et al., 2000). Strong west winds can reverse the direction of the current flow (Stanev et al., 2019).
The three SACs Borkum Reef Ground (EU Code: DE 2104-301), Sylt Outer Reef (DE 1209-301) and Dogger Bank (DE 1003-301) are located within the German EEZ (Figure 1). The SAC Borkum Reef Ground is the smallest of the three areas covering 625 km2 and is situated north of the East Frisian Wadden Sea islands Borkum and Juist, bordering Dutch waters (water depths: 18–33 m). It is characterized by a single submerged sandbank, including patches of reef, hosting a very diverse benthic community (Federal Agency for Nature Conservation, 2008a). The Sylt Outer Reef is the largest SAC in the German EEZ and is located to the west of the North Frisian Wadden Sea island of Sylt with a size of 5,314 km2 (water depths: 8–48 m). It consists of alternating sandbanks and reefs characterized by a rich benthic diversity and also provides an important habitat for many important fish species as well as endangered seabirds (Federal Agency for Nature Conservation, 2008b; Neumann et al., 2013, 2017). The SAC Sylt Outer Reef is considered as a core area for the population and as a main reproduction site for harbor porpoises in German waters with high numbers of calves (Sonntag et al., 1999; Siebert et al., 2006a; Gilles et al., 2009). The SAC Dogger Bank covers the part of the Dogger Bank (a large sandbank in the central North Sea) extending into the German EEZ; the SAC covers an area of 1,699 km2 (water depths: 29–40 m). The sediment mainly consists of fine sands and hosts a unique epi- and infauna community (Federal Agency for Nature Conservation, 2010; Neumann et al., 2013, 2017). The local hydrographic conditions also support a relatively high primary productivity and provide an ideal spawning ground for marine fishes attracting seabirds and marine mammals (Federal Agency for Nature Conservation, 2010).
Data on spatial extents of the SACs were retrieved from the EEA1.
Survey Methodology and Data Processing
Dedicated aerial line-transect distance sampling surveys (Buckland et al., 2001) were conducted in the German North Sea between 2002 and 2019 under the German monitoring program for harbor porpoises and smaller scale research projects (Figure 2). The study area was stratified into different survey blocks (i.e., strata) that could be covered on a single day. For each stratum, transects were designed to provide a systematic survey with even coverage of the survey region, following the principles described in Buckland et al. (2001) (Figure 2). If possible, strata boundaries and transect placement within strata were kept constant throughout the years. The study areas TF and BD were primarily designed to study the large scale effects of the construction of the OWFs “alpha ventus” and “Butendiek,” respectively, on harbor porpoise distribution (Dähne et al., 2013; Viquerat et al., 2015) (Figure 2D).
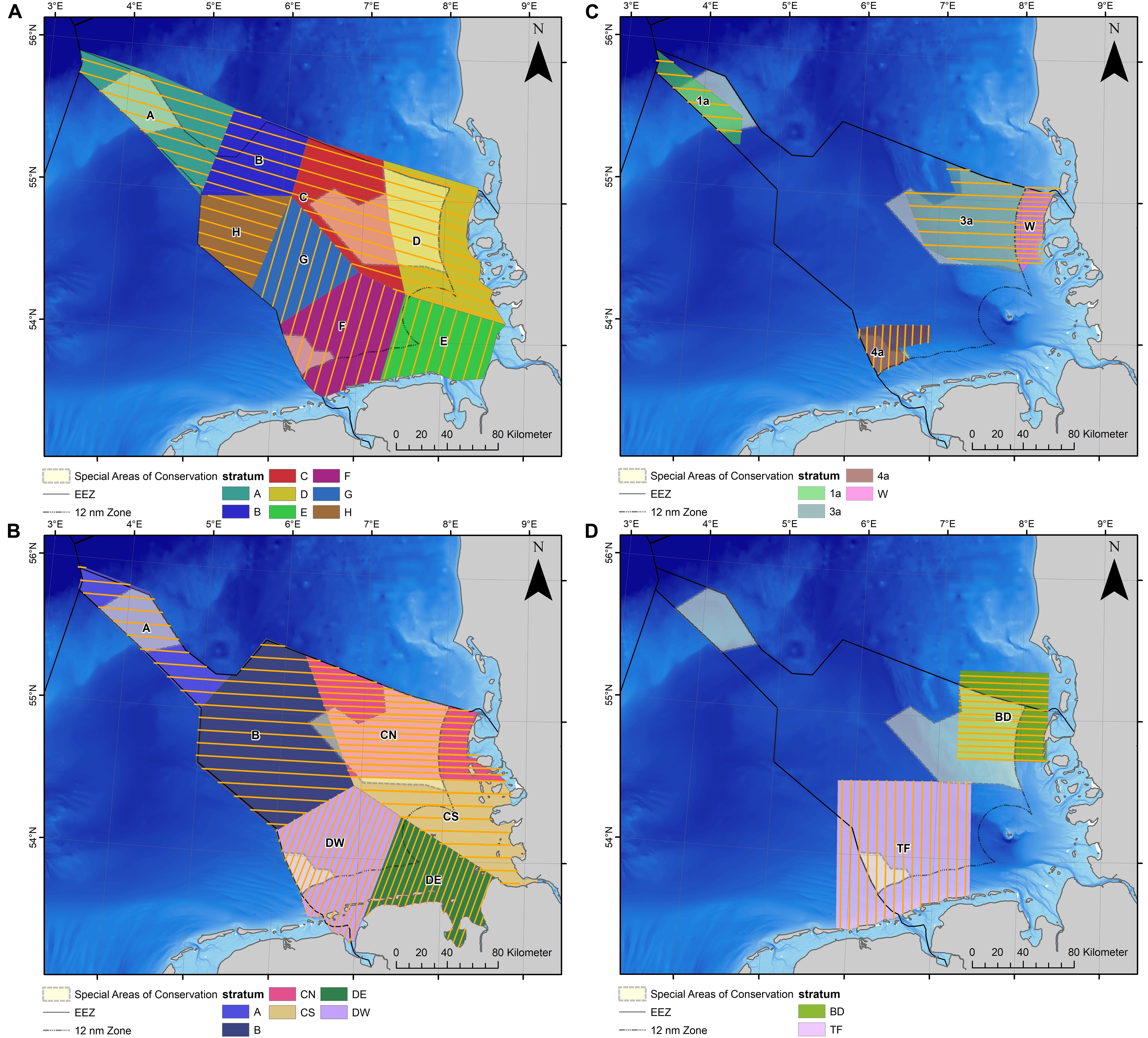
Figure 2. Survey and transect design of the German visual monitoring of harbor porpoises and related research projects: (A) TopMarine, since 2017; (B) MINOS and TopSpace, 2002–2016 (Gilles et al., 2009); (C) EMSON (1a, 3a, and 4a) and whale protection area (W), 2002–2003; (D) alpha ventus OWF test field 2008–2012 (TF; Dähne et al., 2013) and Butendiek 2014 (BD; Viquerat et al., 2015).
Field methods were identical during all surveys and detailed descriptions of aerial survey data collection protocols are given elsewhere (Scheidat et al., 2008; Gilles et al., 2009; Hammond et al., 2013). Briefly, all surveys were conducted using the same platform, a twin engine, high-wing aircraft (Partenavia P-68) equipped with two bubble windows. A constant altitude (600 ft) and speed over ground (90–100 km) were maintained while on the transect. Two observers, one at either side of the plane, monitored the area directly underneath the airplane and relayed all sightings with corresponding information via Intercom to a third person, the data recorder, operating a customized survey software running on a laptop connected to an external GPS device (Garmin GPSMAP 78S or GPS 72H). Surveys were only conducted when weather conditions were expected to be good (no low clouds, visibilities >5 km, Beaufort sea states 0–3); if conditions deteriorated during the flight, the survey was paused or canceled. All environmental conditions [sea state, glare (area obstructed by glare and glare strength), water turbidity, cloud cover] and corresponding changes were noted. Additionally, each observer reported on subjective sighting conditions (good, moderate, or poor), which reflect the likelihood of sighting a harbor porpoise given the above described environmental conditions on either side. Although this describes a subjective measure it is important to note that there was a consistent way of allocating these conditions based on prevailing environmental conditions. All observers were long-trained and highly experienced harbor porpoise observers, many of them also participating in the SCANS (Hammond et al., 2013, 2017) and T-NASS (Trans-North Atlantic Sightings Survey) aerial surveys (Gilles et al., 2020).
The probability of detecting an animal on the transect line (commonly known as g(0)), is often assumed to be 1 for marine mammals to provide minimum (uncorrected) estimates. However, due to diving and the often restricted visibility into the water column, we expect a substantial fraction of animals to be missed on the transect by the observers. This “false absence” is one of the main component of the availability bias, which modifies g(0) to below 1 (Laake et al., 1997). Another bias is introduced when the animal is indeed missed by the observer although it was actually visible, also called perception bias (Marsh and Sinclair, 1989). In order to estimate the fraction missed, in our surveys, the Hiby racetrack (or circle-back) data collection method (Hiby, 1999) was followed, where the survey aircraft circles back to resurvey a defined segment of transect (see details on field protocol on Scheidat et al., 2008). This presents a modification of the tandem formation method, as implemented, e.g., in SCANS 1994 (Hammond et al., 2002), where two survey aircraft would closely follow each other on the transect to determine leading/trailing pairs and consequently duplicates of porpoise sightings (Hiby and Lovell, 1998). The major advantage of this method is that it takes into account both availability and perception bias with the same data collected (Hiby and Lovell, 1998; Hiby, 1999). Per definition of the analytical approach, the Hiby racetrack method produces estimates of total effective strip width ESW (i.e., on both sides of the aircraft) that incorporates g(0). Here, ESWs were estimated separately for good and moderate conditions (poor conditions default to an ESW of 0). This provided a correction for missed animals on the transect line within varying sighting conditions that could also differ between observer sides (Scheidat et al., 2008; Hammond et al., 2013, 2017). The same (racetrack) data collection method and software, the same aircraft and a pool of the same experienced observers have been used in SCANS-II, SCANS-III and in harbor porpoise aerial surveys in the Netherlands (Scheidat et al., 2012; Hiby and Gilles, 2016). The estimate resulted in a total corrected ESW of 166 m (CV = 0.13) for good and of 83 m (CV = 0.13) for moderate porpoise sighting conditions, incorporating g(0) values of 0.42 and 0.21, respectively.
In the following, only surveys performed during spring (1 March–31 May) and summer (1 June–31 August) were considered, due to very low and fragmented survey coverage in other seasons (Gilles et al., 2009, 2011, 2016). Data were filtered to include only good and moderate survey conditions.
Stratum-Based Abundance Estimation
The data base for the trend analysis includes all survey data of the aircraft-based national monitoring campaigns from 2002 to 2019 in the areas of the German North Sea. Data were aggregated seasonally at stratum level to determine a total abundance and associated variance measures per study area per season (here, for spring and summer). For ease of calculation, all data were spatially aggregated (or “post-stratified”) based on the current stratum design (see Figure 2A). For the abundance estimation within the SACs, we post-stratified the data to the extent of the three SACs but added a 10 km buffer around each area (Figure 1) to enhance the data base for a more reliable estimation of density. According to distance sampling methodology, strata-based density estimates assume a uniform distribution of density within the strata and can thus be applied to smaller subsets of the strata to estimate abundances (Buckland et al., 2001). Thus, abundance was subsequently estimated based on the extent of the original SAC area without the 10 km buffer zone.
Prior to abundance estimation, the coverage of the post-stratified areas was assessed on two levels: transects and strata. First, all transects with effective transect lengths (i.e., effort in km under good or moderate conditions) shorter than 10 km were excluded [average length of all excluded transects: 2.9 ± 3.2 km (mean ± SD)]. Given the high survey speed (ca. 180 km/h) and encounter rates for harbor porpoises (ca. 0.1 porpoises/km in the full data set), these very short transects are very unlikely to provide reliable data due to the limited window of observation. Excluding these short transects led to an average transect length of 71.0 ± 50.4 km. Given the high effort in most strata (average effort per stratum is 790 ± 750 km) these short transects would not contribute to the overall estimate but rather inflate the uncertainty due to high randomness and false zero counts. Secondly, survey-specific coverage of strata was assessed, and only strata with sufficient realized area coverage and a representative spatial coverage were included. Conversely, strata were excluded if realized area coverage was deemed not be representative (e.g., missing a major part of the stratum due to closed air space or bad local weather conditions). Maps showing the coverage of the respective survey areas (German EEZ and the three SACs) are available in the Supplementary Material.
We then estimated abundances and their associated error structures for each stratum in a given season and year. As described above, the total ESW, i.e., on both sides of the transect, was estimated for good and moderate sighting conditions ( and , respectively) based on the racetrack method taking a detection probability of less than 1 on the transect into account. Abundance in stratum v was estimated as:
where Av is the stratum area in km2, Lv is the effective transect length in km in stratum v (i.e., effort under good or moderate conditions), ngv and nmv are the number of harbor porpoise group sightings made in good and moderate conditions in stratum v, respectively, and is the mean observed group size in stratum v in both good and moderate conditions combined. Absolute densities (individuals/km2) were estimated by dividing the abundance estimates by the area of the associated stratum.
Coefficients of variation (CVs) and 95% confidence intervals (CI) were estimated by non-parametric bootstrapping (999 replicates). More specific details on the abundance estimation method are provided in Scheidat et al. (2008) and Hammond et al. (2013).
Bayesian Trend Analysis of Harbor Porpoise Abundance
The data base from the previous step contains the abundance estimates and 95% CI per season, stratum and year for data from the national monitoring program from 2002 to 2019 within the areas of the German North Sea. In contrast to fitting a linear model to the design-based point estimates (i.e., in this case abundance estimates in one stratum in one season), we incorporated the structure of the uncertainty, i.e., the variability of the individual estimates and the associated errors over the course of the years. We take these assumptions:
1. The abundance and distribution of animals at any given point in time within any area is the sum of effects caused by observable drivers (either immediate such as seasonality or proxies such as spatial boundaries) and by an unknown number of interacting and unobservable (hidden) drivers (e.g., prey occurrence).
2. Our survey setup and effort is sufficient to detect a shift in abundance and distribution across large temporal scales (in our case between years, and between spring and summer) and is robust towards day to day fluctuations (within the same stratum). Furthermore, the survey frequency is sufficient to cover the observed within season, between season and between years variation of distribution and abundance. Thus, we assume that we have sufficient power to determine a trend based on the available data and that the coverage is representative in both space and time.
In order to assess a shift between years in our data, we assume a known stratum-based abundance and associated error structure (here, the standard error based on 95% CI) based on our survey efforts since 2002 as our a priori estimates for each stratum, season and year. The posteriori abundances are subsequently determined using a hierarchical Markov chain Monte Carlo model (MCMC).
We ran separate models for each data set (full data set of complete surveys of German EEZ, subsets for three individual SACs) for each season (spring, summer) across all available data between 2002 and 2019.
Equation 2 describes the log-normal model used for the abundance process for nij at site i = 1, …, I and time j = 1, …, J. L(μ,σ2) represents a log-normal distribution with location parameter μij (expected observed abundance) and scale parameter (in our case: standard error of observed abundance estimate). The model for the latent and missing true abundances Nij is given by Eq. 3.
with a vector of site and time specific covariates related to Nij, βi the site level covariates associated with abundance Ni, the variance of log(Nij) and ωij the parameters of a random walk process. Our true abundance estimates for site i at time j were drawn from the posterior distribution of Nij using Eq. 4.
where N is a vector of all Nij. φ is the vector of all parameters, [φ] is the prior distribution of these. s(i,j) is an indicator function that equals 1 if site I was surveyed at time j. We then considered the median of the posteriori distribution as the true abundance for site i and time j. We additionally chose 95% Bayesian credible intervals to illustrate the uncertainty and range of the posteriori distribution values.
The hierarchical modeling framework was provided by “agTrend” (Johnson and Fritz, 2014) in R 3.4.4 (R Core Team, 2018). The “agTrend” package was designed to provide a high level framework for Bayesian trend and abundance estimation from sites with uneven sample schemes over time, while accounting for variability due to survey replication (Johnson and Fritz, 2014). For this analysis, we extracted the posterior distribution of true abundances from agTrend but used our own metric to assess trends between points in time.
agTrend offers additional model hierarchy for availability bias and covariates on site level and all levels of observation. Since we used the racetrack method to estimate g(0) and have been following standardized protocols throughout the data acquisition, we assumed availability and survey related covariates to be 1. Site specific variables were considered irrelevant over the last 20 years and were assumed to be 1 as well.
In order to identify the relative change in abundance between two points in time, we decided to use the coefficient of a linear model that links the distribution of true abundances at year a with the distribution of true abundances at year b (assuming but neglecting an intercept). We then consider the model coefficient (including variance) ma,b as trend for that given time period:
with Na the distribution of true abundances at year a and Nb the distribution of true abundances at year b. ma,b is the coefficient for the linear model and is considered the relative trend between year a and year b. The empirical cumulative distribution function (ecdf) of the resulting distribution of relative trend estimates was used to quantify the probability that a trend between two given years was negative (p less than 0) or positive (p greater than 0), indicating a decline in true abundance or incline, respectively.
For the SAC Borkum Reef Ground the first three surveys (2002, 2003, and 2005) in summer were removed from the analyses since the stratum-based abundance estimates were either zero or very close to zero due a lack of sightings (see Supplementary Table 3). This led to computational issues in the estimation of trends, as abundances in “agTrend” are modeled using a log-normal distribution (Johnson and Fritz, 2014). The logarithm of zero is not defined. To overcome the problem, we have arbitrarily added the value 1 to the estimate and error structure, which solves the computational issue but is not advised (O’Hara and Kotze, 2010). The resulting model also revealed that the posterior distributions were clearly centered on the original abundance point estimates, as the low estimate with a narrow variance indicates a very precise prior value as a starting point. When removing the first three survey years, the model was deemed more realistic and anticipates very low abundances in the first years in this area and season.
Results
Survey Effort and Harbor Porpoise Sightings
A total effort of 122,344 km was realized under good or moderate conditions during 247 individual aerial survey flights between 2002 and 2019 in spring and summer (Figure 3). A combined total of 12,942 harbor porpoise groups (15,910 individuals) were observed, resulting in an overall mean observed group size of 1.2.
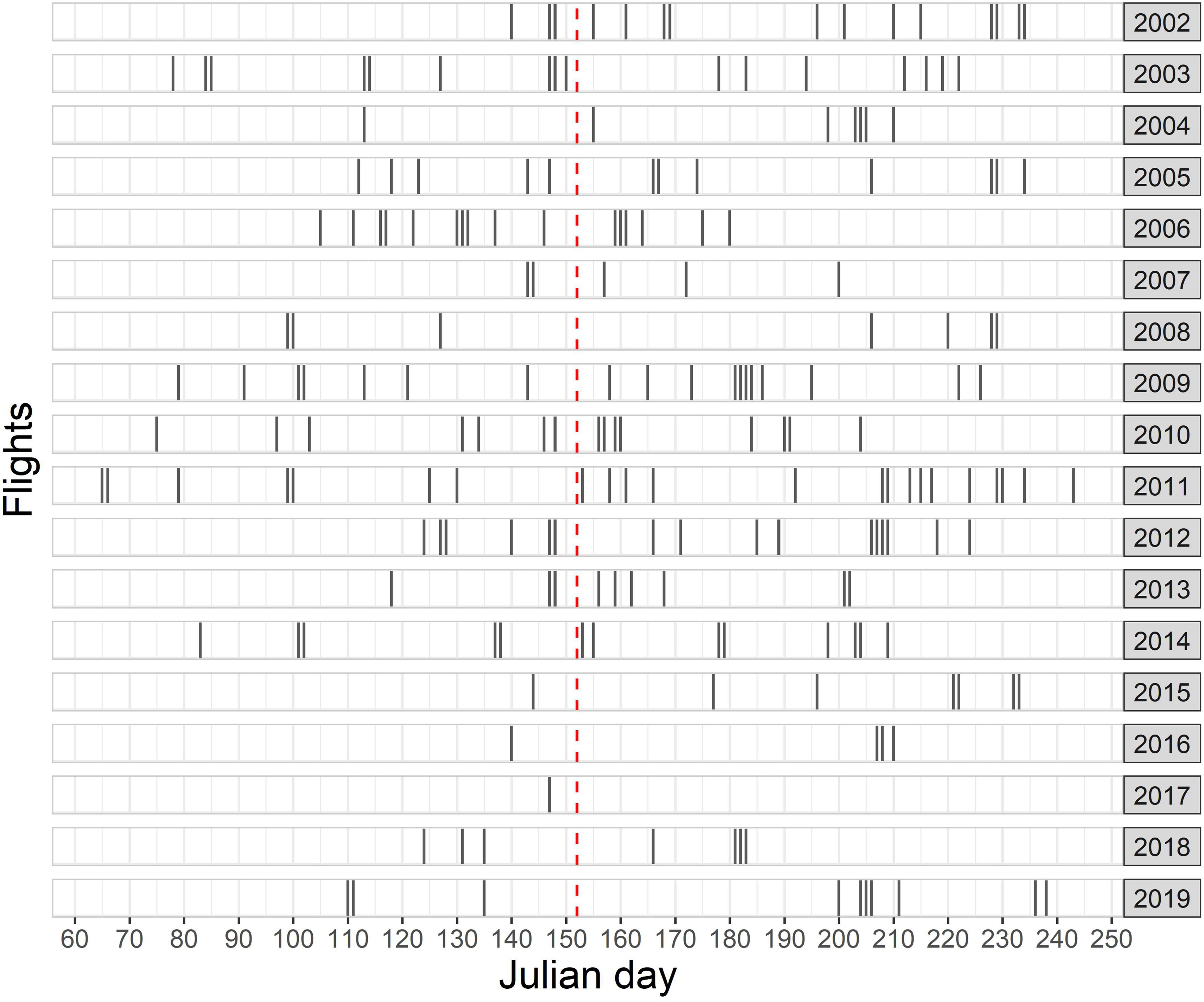
Figure 3. Total number of aerial survey flights conducted in the frame of the national visual monitoring and related research projects between 2002 and 2019 in the German North Sea. Timing of each flight is shown with respect to the Julian day. The red dashed line shows the transition from spring to summer.
The German North Sea EEZ (excluding the Dogger Bank stratum A) was surveyed completely in summer in 7 of 18 years (Figure 4A). The total effective survey effort (i.e., under good or moderate conditions) summed up to 27,633 km. A total of 2,614 harbor porpoise groups were recorded (Supplementary Table 1).
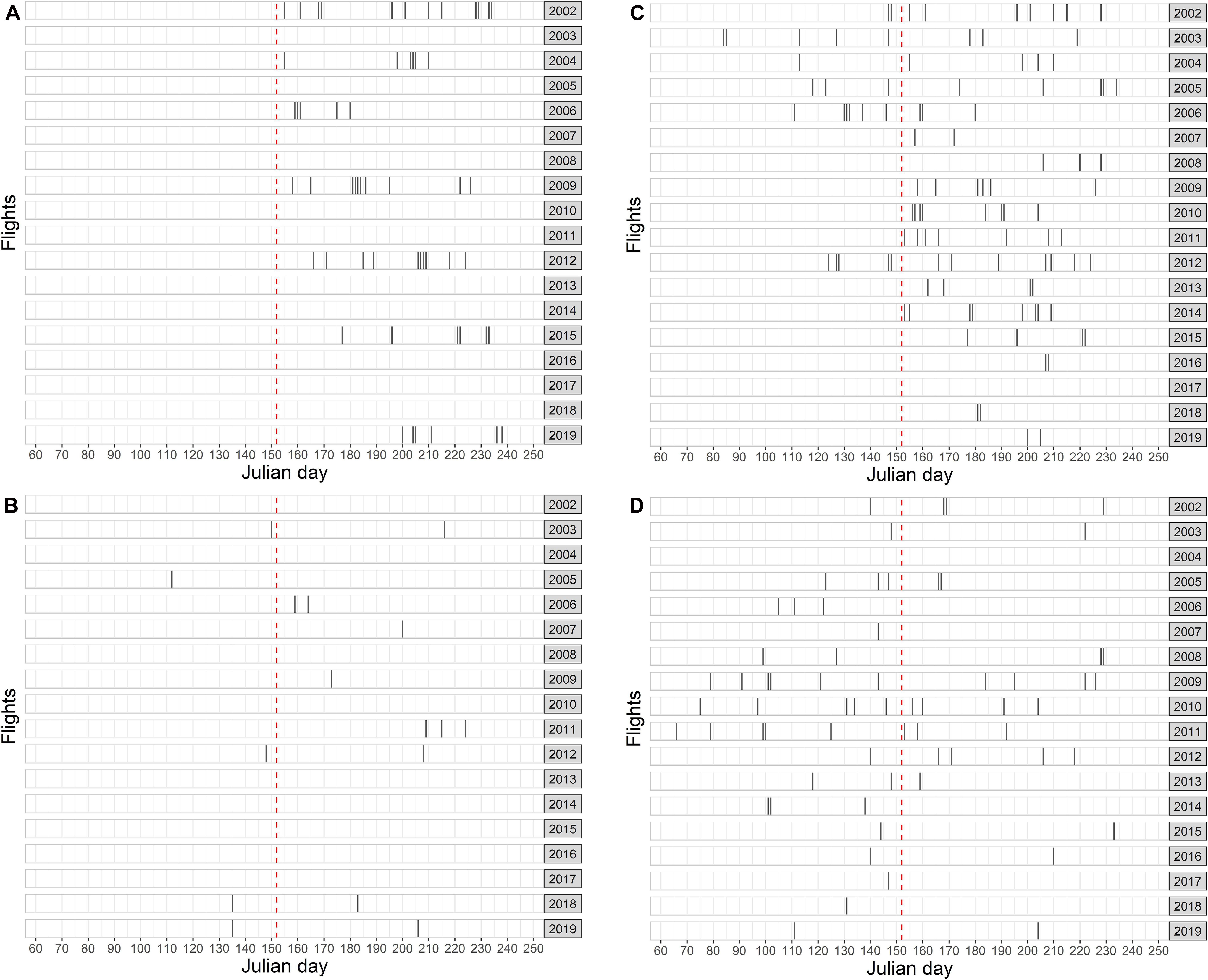
Figure 4. Aerial survey flights in the different study areas: (A) German North Sea EEZ, (B) SAC Dogger Bank, (C) SAC Sylt Outer Reef, (D) SAC Borkum Reef Ground. Timing of each flight is shown with respect to the Julian day. The red dashed line shows the transition from spring to summer.
In spring, the SAC Dogger Bank was surveyed in 5 of 18 years with a total effective effort (i.e., under good or moderate conditions) of 1,263 km, while in summer months 1,975 km were covered in 8 years (Figure 4B). In spring, a total of 195 harbor porpoise groups was recorded, while in summer 233 porpoise group sightings were recorded (Supplementary Tables 2, 3).
The SAC Sylt Outer Reef was covered in 5 of 18 years in spring, whereas it was surveyed in 17 years in summer (Figure 4C). The effective effort summed up to 5,213 km in spring and 25,747 km in summer. During spring surveys, a total of 1,322 harbor porpoise groups were sighted. In summer, 3,992 groups were observed (Supplementary Tables 2, 3).
In spring, the SAC Borkum Reef Ground was surveyed in 17 of 18 years with a total effective effort of 5,748 km, while during summer 3,563 km were covered in 12 years (Figure 4D). In spring, a total of 868 harbor porpoise groups were observed, while in summer 553 group sightings were recorded (Supplementary Tables 2, 3).
German North Sea EEZ: Trends of Harbor Porpoise Abundance
A visual inspection of the model-estimated harbor porpoise abundance in the German North Sea EEZ suggests decreasing numbers between 2002 and 2019 (Figure 5A). After an initial slight increase until 2006, the abundance declined sharply before leveling off toward the end of the study period. The probability that the trend was indeed negative (i.e., less than 0% per year) is 95.1% (Figure 6). The median relative change in abundance per year was −1.79% with a narrow credibility interval (95% credibility interval: −3.15 – −0.01%).
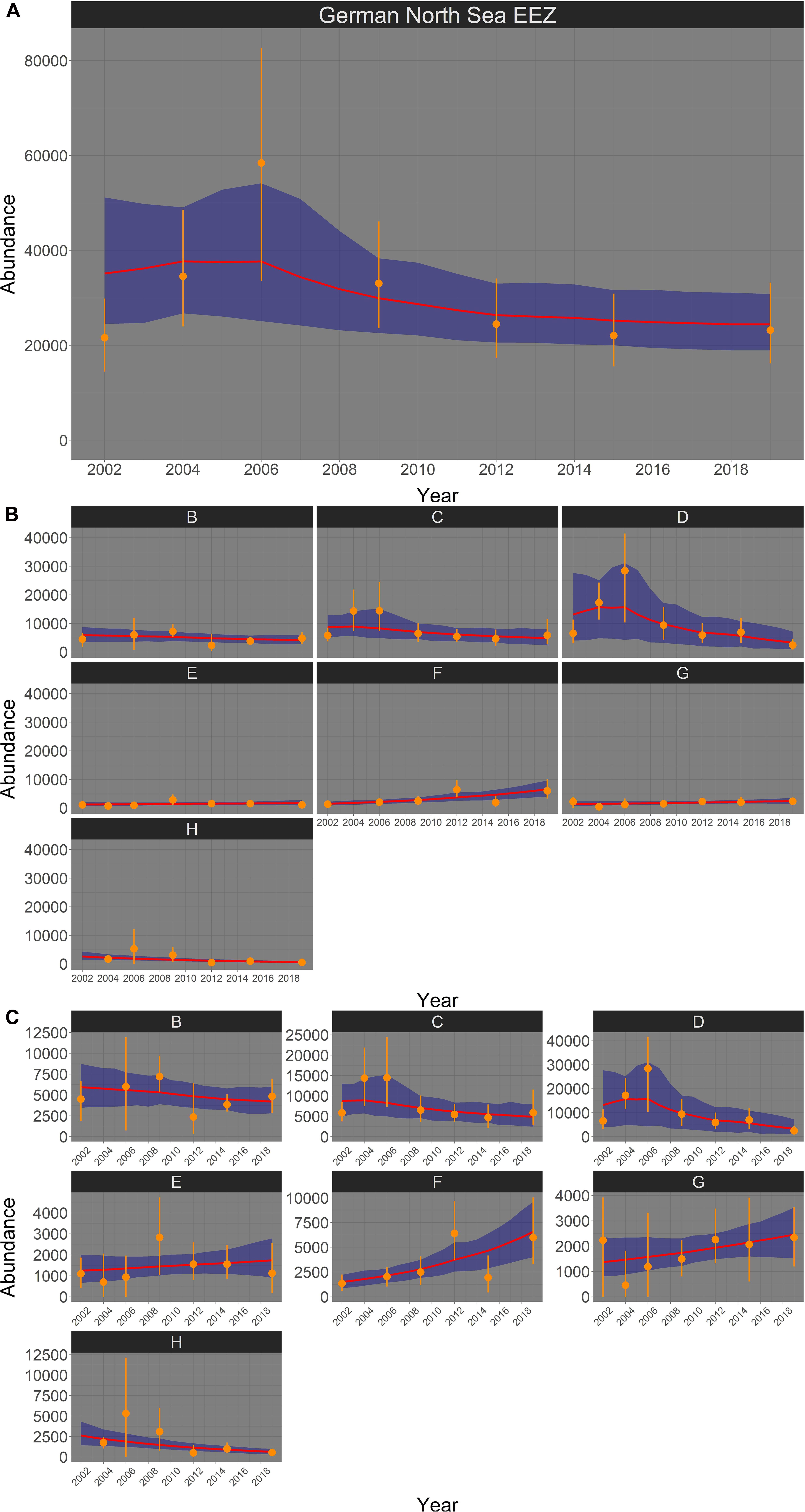
Figure 5. Bayesian abundance trend analysis for harbor porpoises in the German North Sea EEZ (aggregated trend) (A) and for each stratum separately (B,C) based on post-stratified aerial survey data in summer, between 2002 and 2019. Only years are included when the complete study area was covered (excluding Dogger Bank stratum A). Orange dots illustrate stratum-based abundance estimates and orange lines represent the associated 95% CI. The red line shows the median of the posteriori distribution of the calculated “true” abundances from the Bayesian model, the blue shaded area displays the corresponding 95% credibility intervals. Please note that all sub-figures in (B) share a common y-axis, while the y-axis is free in (C).
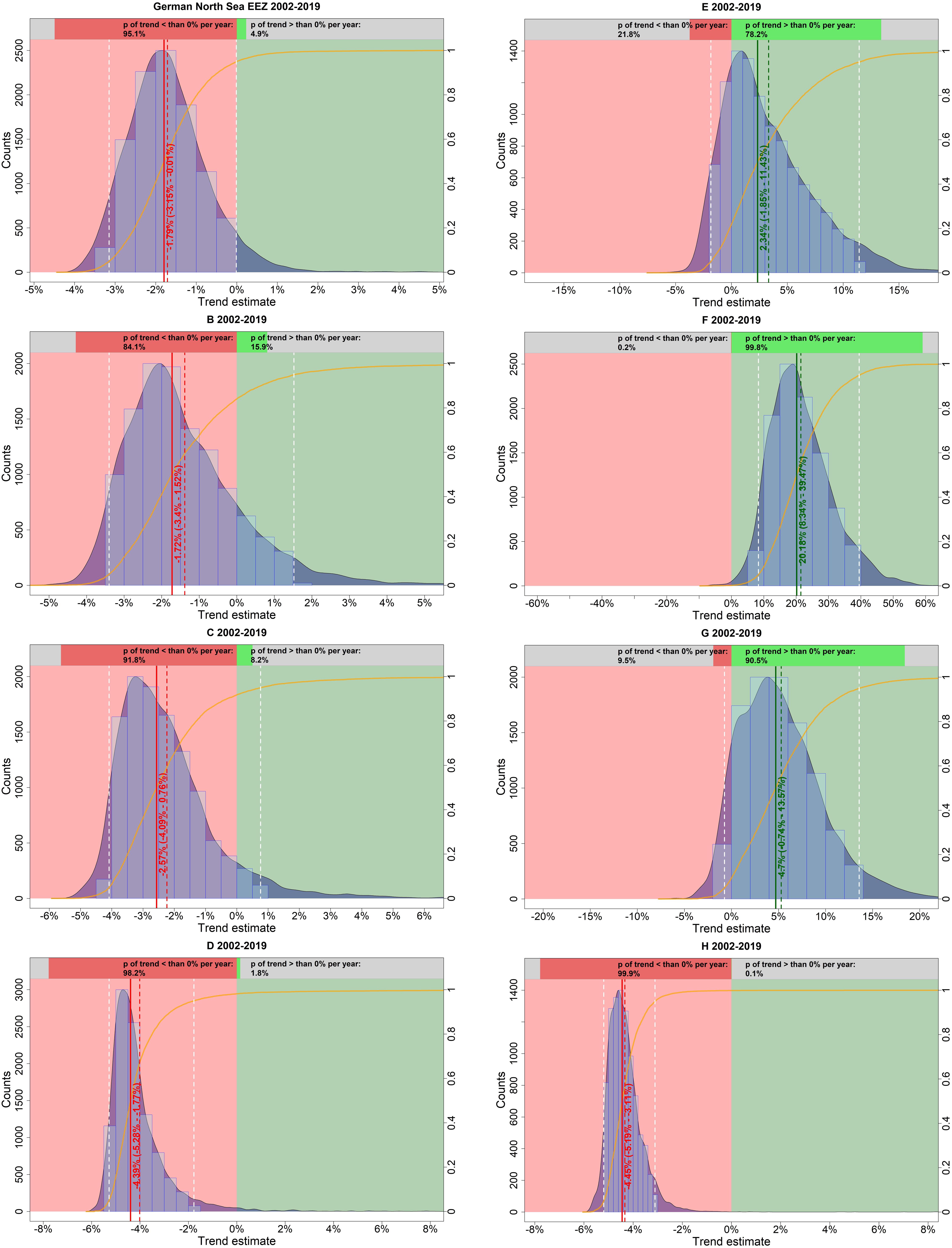
Figure 6. Estimated trends in harbor porpoise abundance in the German North Sea EEZ (but excluding Dogger Bank) and in each strata (B–H) separately in summer, between 2002 and 2019. The light blue histogram and the blue shaded density curve show the distribution of the trend estimates (relative change in abundance) between 2002 and 2019 (x-axis); the median is indicated by the solid red vertical line. The mean is shown by the vertical red dashed line, while the white dashed lines represent the upper and lower 95% credibility intervals of the trend estimate. The red colored area corresponds to an area with a negative trend, whereas the green colored area represents a positive trend. The orange solid line illustrates the empirical cumulative distribution function of the trend estimates, giving the probability of a trend estimate at a specified value (e.g., 0%). The upper bar chart hence indicates how likely it is that the trend is either negative (i.e., p < 0%; red) or positive (i.e., p > 0%; green).
Trends in harbor porpoise abundance were also calculated for each site (i.e., stratum) within the whole region, contributing to the aggregated trend. Abundances differed between strata and also the trend varied between the sites with some areas showing increases and others declines (Figures 5B,C). Generally, strata in the north (e.g., B, C, and D) were apparently exhibiting negative trends, whereas southern areas (e.g., E and F) showed positive trends (Figure 6).
SACs: Trends of Harbor Porpoise Abundance in Spring
In spring, harbor porpoise abundance in the SAC Dogger Bank seemed to be stable between 2002 and 2019 (Figure 7A). The probability that the trend was negative (i.e., less than 0% per year) is 58.4% (Figure 8A). The median change in abundance per year was −0.62%, but there was also a large credibility interval (95% credibility interval: −4.00% – +15.17%). The trend analysis suggests a decline of harbor porpoises in the SAC Sylt Outer Reef in the first years until reaching a certain level (Figure 7B). However, this apparently stable trend in the years 2006 until 2019 is mainly caused due to a lack of surveys (only one estimate in 2012), resulting in large credibility intervals. The probability that the trend was indeed negative between 2002 and 2019 is 70.0% (Figure 8B). The median change in harbor porpoise abundance per year was −1.56%, with a large uncertainty (95% credibility interval: −3.86% – +4.41%). Harbor porpoise abundance in the SAC Borkum Reef Ground exhibited high fluctuations over the study period (Figure 7C). The Bayesian trend analysis indicates an increase until 2005, relatively constant abundance until 2007 before dropping and increasing again until 2012. After 2012, the posteriori abundances declined steadily and remained rather low until 2019. Nevertheless, when assessing the trend from 2002 until 2019, the trend was likely to be positive with 86.0% probability (Figure 8C). Harbor porpoise abundances increased by 10.47% per year (95% credibility interval: −3.45% – +48.89%).
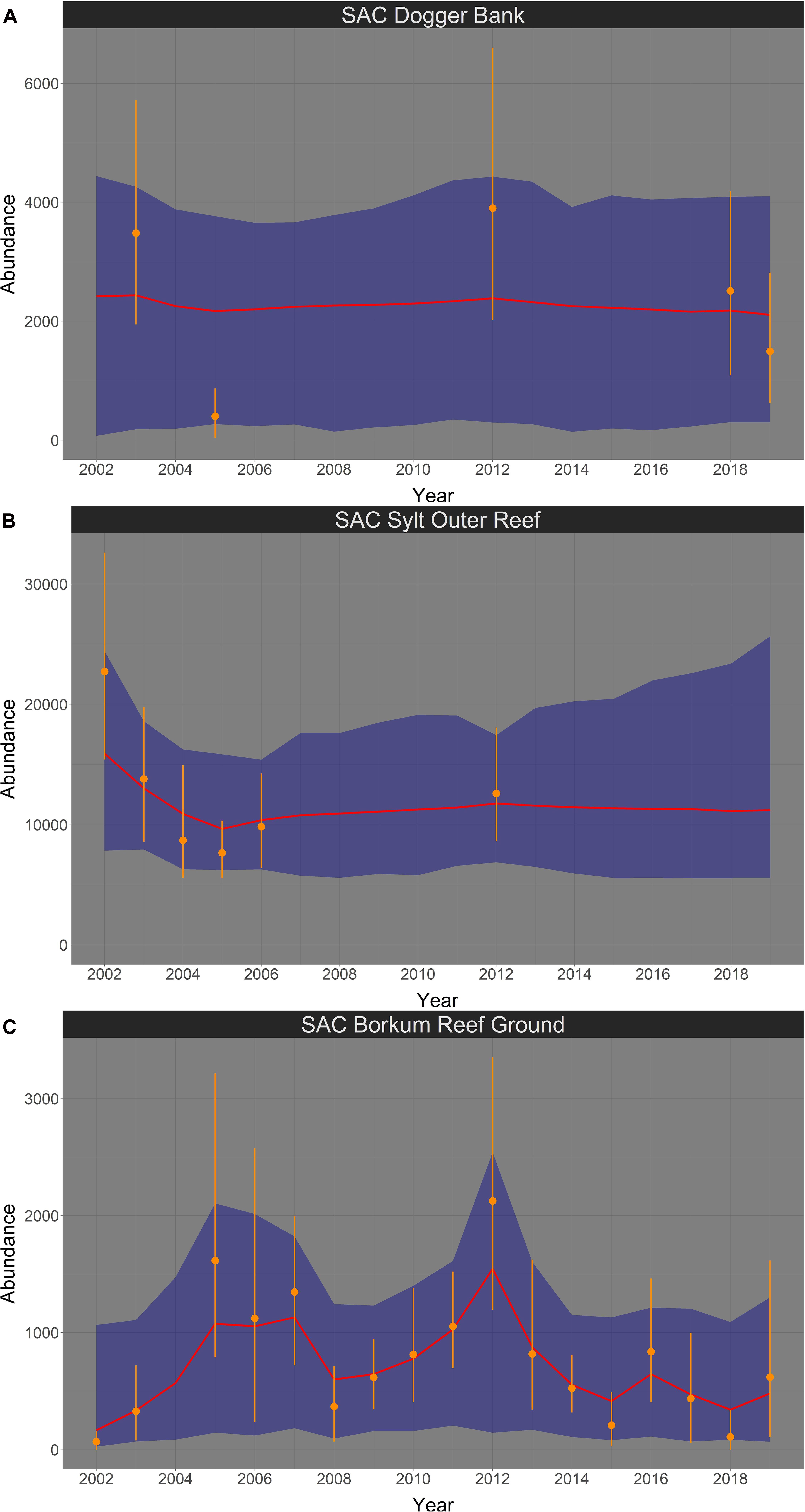
Figure 7. Bayesian abundance trend analysis for harbour porpoises in the three SACs Dogger Bank (A), Sylt Outer Reef (B), and Borkum Reef Ground (C), based on post-stratified aerial survey data in spring, between 2002 and 2019. Orange dots illustrate stratum-based abundance estimates and orange lines the associated 95% CI. The red line shows the median of the posteriori distribution of the calculated ‘true’ abundances from the Bayesian model, the blue shaded area displays the corresponding 95% credibility intervals. Please note in all figures the y-axis is free.
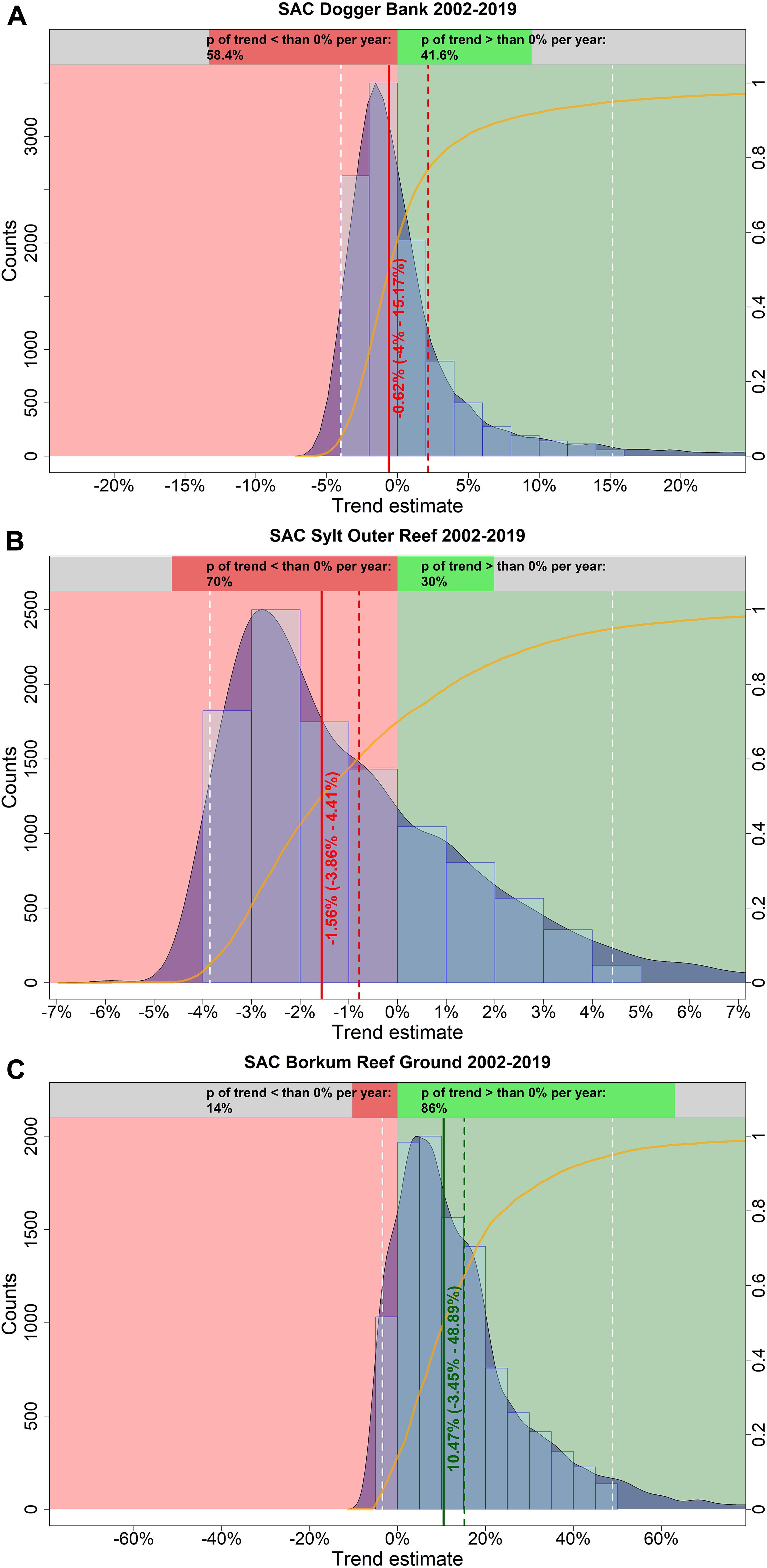
Figure 8. Estimated trends in harbour porpoise abundance in the three SACs Dogger Bank (A), Sylt Outer Reef (B), and Borkum Reef Ground (C) in spring, between 2002 and 2019. The light blue histogram and the blue shaded density curve show the distribution of the trend estimates (relative change in abundance) between 2002 and 2019 (x-axis); the median is indicated by the solid red vertical line. The mean is shown by the vertical red dashed line, while the white dashed lines represent the upper and lower 95% credibility intervals of the trend estimate. The red coloured area corresponds to an area with a negative trend, whereas the green coloured area represents a positive trend. The orange solid line illustrates the empirical cumulative distribution function of the trend estimates, giving the probability of a trend estimate at a specified value (e.g. 0%). The upper bar chart hence indicates how likely it is that the trend is either negative (i.e., p < 0%; red) or positive (i.e., p > 0%; green).
SACs: Trends of Harbor Porpoise Abundance in Summer
In summer, harbor porpoise abundance seemed to increase in the SAC Dogger Bank from 2002 until 2019 (Figure 9A). The probability for a positive trend was 85.6% (Figure 10A). The median increase in abundance was 6.61% per year (95% credibility interval: −2.33% – +36.11%). The Bayesian model suggests an almost linear decline of harbor porpoise abundance in the SAC Sylt Outer Reef over the study period from 2002 until 2019 (Figure 9B). The trend was highly likely to be negative with a probability of 94.9% (Figure 10B). The change in abundance between 2002 and 2019 was −3.79% per year (95% credibility interval: −5.16% – +0.03%). In contrast, harbor porpoise abundances seemed to increase in the SAC Borkum Reef Ground over the study period (Figure 9C). The probability that the trend in SAC Borkum Reef Ground was positive is 88.6% (Figure 10C). The posteriori abundance increased strongly with a median of 12.43% per year (95% credibility interval: −2.09% – +59.44%).
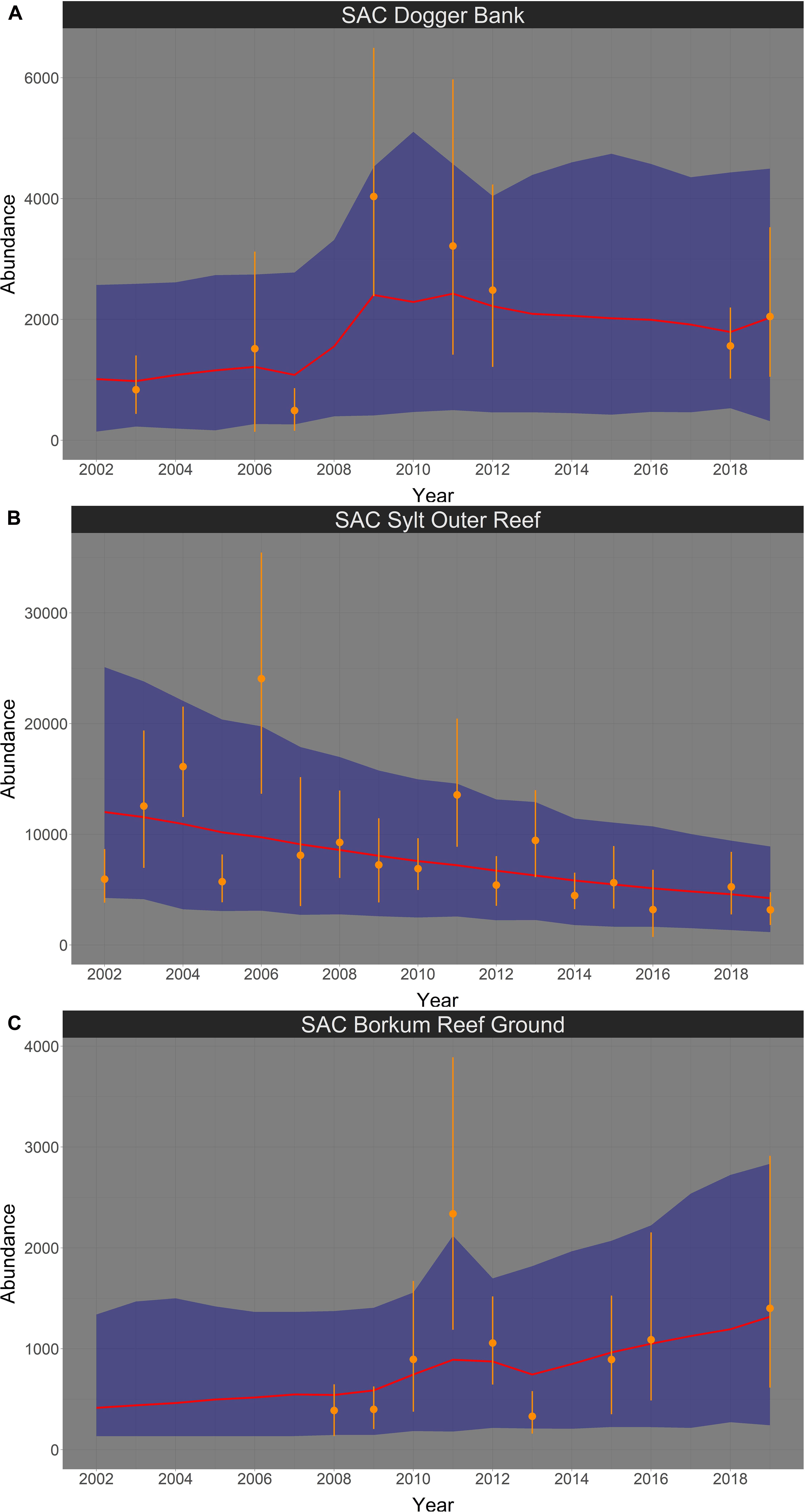
Figure 9. Bayesian abundance trend analysis for harbour porpoises in the three SACs Dogger Bank (A), Sylt Outer Reef (B), and Borkum Reef Ground (C), based on post-stratified aerial survey data in summer, between 2002 and 2019. Orange dots illustrate stratum-based abundance estimates and orange lines the associated 95% CI. The red line shows the median of the posteriori distribution of the calculated ‘true’ abundances from the Bayesian model, the blue shaded area displays the corresponding 95% credibility intervals. Please note in all figures the y-axis is free.
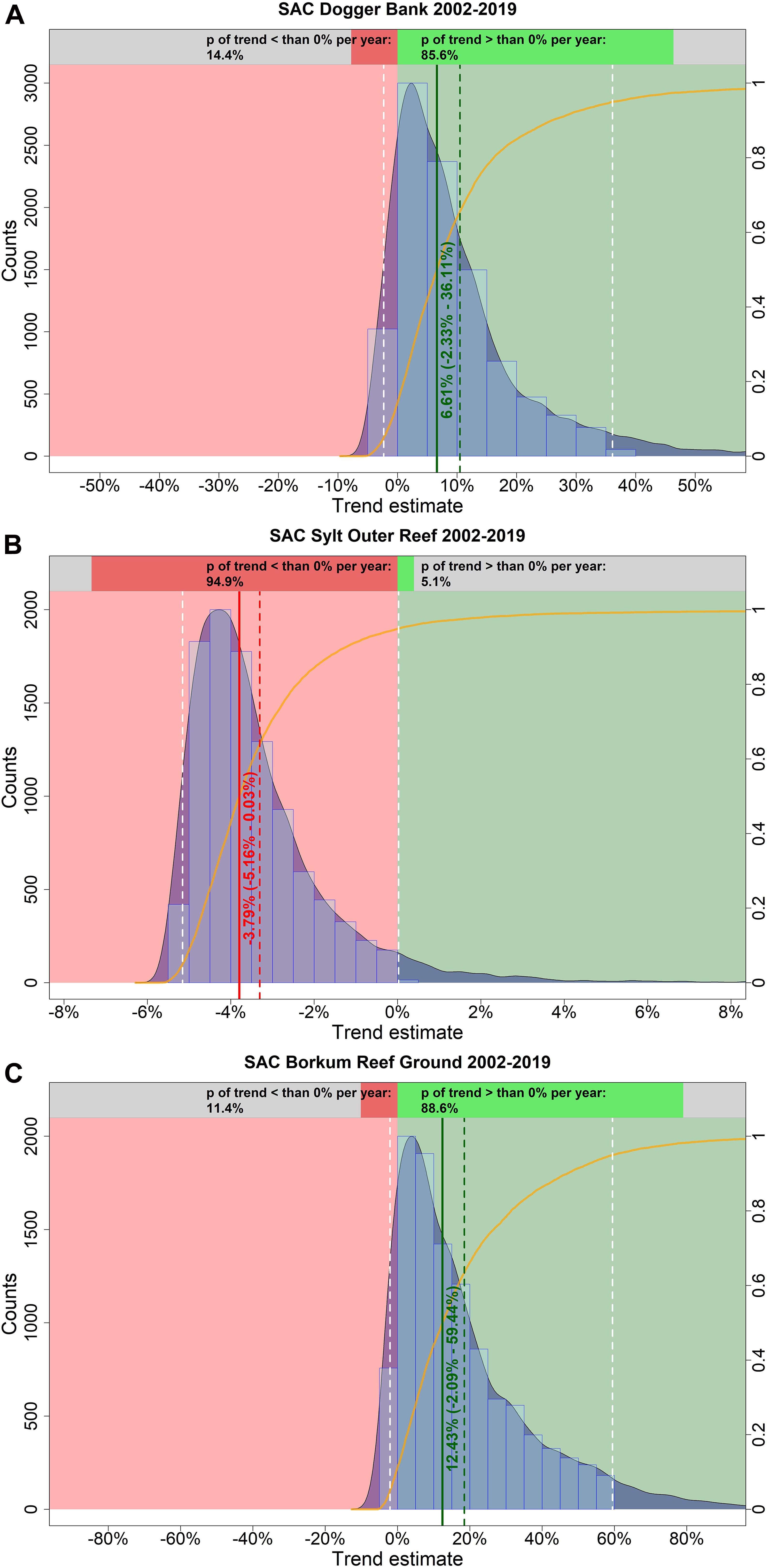
Figure 10. Estimated trends in harbour porpoise abundance in the three SACs Dogger Bank (A), Sylt Outer Reef (B), and Borkum Reef Ground (C) in summer, between 2002 and 2019. The light blue histogram and the blue shaded density curve show the distribution of the trend estimates (relative change in abundance) between 2002 and 2019 (x-axis); the median is indicated by the solid red vertical line. The mean is shown by the vertical red dashed line, while the white dashed lines represent the upper and lower 95% credibility intervals of the trend estimate. The red coloured area corresponds to an area with a negative trend, whereas the green coloured area represents a positive trend. The orange solid line illustrates the empirical cumulative distribution function of the trend estimates, giving the probability of a trend estimate at a specified value (e.g. 0%). The upper bar chart hence indicates how likely it is that the trend is either negative (i.e., p < 0%; red) or positive (i.e., p > 0%; green).
Discussion
In this study, unbiased abundance estimates of harbor porpoises obtained from aerial surveys in the German North Sea over 18 years were used to estimate trends in abundance by applying a Bayesian framework, considering error propagation. Most of the areas were covered continuously over the study period with a comparable survey frequency. We focused the trend analyses on spring and summer, as survey effort was highest in these seasons. Autumn and winter had a lower coverage due to low densities of harbor porpoises during these times (Gilles et al., 2009). Distance sampling requires a certain minimum amount of sightings to provide accurate abundance estimates, which is why monitoring efforts and resources were concentrated on seasons with sufficiently high densities and encounter rates. This hampers a seasonal comparison in some respect but ensures a good comparison between years.
Data presented here were collected as part of an ongoing national long-term monitoring of harbor porpoise status in German waters. Long-term monitoring is necessary to better understand population dynamics, to determine changes in population size and, ultimately, to advise conservation efforts and management (Marsh and Trenham, 2008; Magurran et al., 2010). Also, long time-series are needed to achieve sufficient statistical power to detect trends, in particular if changes are relatively subtle (Forney et al., 1991; White, 2019), whereas short time series can be underpowered and, thus, misleading. At least 10 years of monitoring is usually required to achieve a high level of statistical power to infer with confidence on population trends (White, 2019). Our evaluation presented here can be regarded reliable in the sense of (i) being based on adequate data collected over a sufficiently long-time frame and (ii) resulting from an analysis designed to deal with spatio-temporal heterogeneity and other sources of uncertainty (Johnson and Fritz, 2014). Furthermore, we have developed a methodological approach whose graphical output is clearly transferable to stakeholders and policy as the interpretation of the probability of change can be quickly grasped using the color codes.
SACs in the German North Sea
Our analyses revealed obvious trends in specific areas and seasons. Harbor porpoise abundance overall increased in SAC Borkum Reef Ground in both spring and summer, however, there were fluctuations over the course of the whole study period. The overall increase in harbor porpoise abundances in the south-western German Bight, where SAC Borkum Reef Ground is located, was also described in Peschko et al. (2016) for earlier years (2002–2013), including a subset of data used in this study. These results are in line with findings from neighboring countries in the southern North Sea. Harbor porpoise sighting rates have increased substantially in Dutch coastal waters, especially from the early 1990s onward (Camphuysen, 2004, 2011). Similar results were also reported from Belgian and French waters, including a steep increase in harbor porpoise strandings (Haelters et al., 2011, 2018; Peltier et al., 2013; Ijsseldijk et al., 2020). This sharp increase in sighting rates and strandings has been attributed to a southward shift of the North Sea harbor porpoise population as detected between the large scale surveys SCANS in 1994 and SCANS II in 2005 (Hammond et al., 2002, 2013). It has been hypothesized that the shift was caused by a change in distribution of principal prey species (Hammond et al., 2013). National monitoring in the southern North Sea showed indeed that the seasonal pattern of occurrence has changed. For instance, in the Netherlands harbor porpoises showed a higher abundance in winter and spring and lower abundances in summer (Camphuysen, 2011; Scheidat et al., 2012). Recently, this pattern has changed (2012–2017): harbor porpoise abundance increased markedly in summer and abundance and density are now comparable to spring (Geelhoed and Scheidat, 2018). Additionally, mother-calf pairs are now regularly sighted (Geelhoed et al., 2013; Geelhoed and Scheidat, 2018). The increasing abundances reported in the Netherlands corroborate the increasing trends in the southern German Bight, including SAC Borkum Reef Ground, in spring and summer as seen in this study.
Although being an overall positive trend, it is noteworthy that harbor porpoise abundance decreased after 2012 following the initial increase in SAC Borkum Reef Ground in spring. This is not congruent with the patterns described above for neighboring areas. Also, in summer the abundances in SAC Borkum Reef Ground continue to increase. In general, the SAC Borkum Reef Ground is the smallest of the three SACs examined here. As harbor porpoises are highly mobile species and their distribution can be variable, small protected areas may not adequately reflect their spatial requirements and, conversely, may not be effective. The variability of the abundance estimates and the associated confidence intervals in this area could be an indication of a rather variable distribution and small-scale distribution shifts in spring. Since 2007, harbor porpoises have been observed periodically entering the rivers Ems, Weser and Elbe following anadromous fish shoals in spring (Wenger and Koschinski, 2012; Wenger et al., 2016; Weel et al., 2018). It is noteworthy that the occurrence of harbor porpoises in the estuaries and rivers is subject to annual fluctuations, with some years of absence and other years of high usage. Since these estuaries are located close to the SAC Borkum Reef Ground, this could be a further explanation for the variable abundances and a reduced usage of this area in the last years.
In SAC Sylt Outer Reef, a highly likely and strong decline was observed in summer. The decline is continuous and almost linear from 2002 until 2019. The trend for spring also suggests a decline, however, data are much sparser in this season, in particular toward the end of the study period. Further monitoring efforts should concentrate on gathering more data in spring to get a better insight into seasonal developments in the SAC Sylt Outer Reef. Being identified as a core area of harbor porpoises in the German North Sea, with regular high densities, and its high relevance as a calving ground, beyond national borders (Sonntag et al., 1999; Gilles et al., 2009, 2011, 2016), the SAC Sylt Outer Reef was specifically designated for the protection of harbor porpoises. As mentioned before, the North Sea is characterized by a diversity of human exploitations affecting harbor porpoises on different levels. Although management plans of all three SACs are now in place since May 2020, fisheries are not yet specifically managed within the areas. There is high fishing pressure from mobile bottom contacting gear, e.g. beam and otter trawls, in SAC Sylt Outer Reef (Pedersen et al., 2009a,b), which may negatively impact the delicate reef communities. One of the main target species in SAC Sylt Outer Reef are sandeels (Ammodytes spp.) (Pedersen et al., 2009b), which constitute a generally important part of the harbor porpoise diet (Gilles, 2008; Leopold et al., 2015). However, sandeel biomass in this management unit has been repeatedly below the reference value according to the precautionary approach since 2004 due to the high fishing pressure (ICES, 2019c; Otto et al., 2019). In this context, the estimated negative trends of harbor porpoises in the core area SAC Sylt Outer Reef could be an indicator of fishing-related habitat degradation and a competition with fisheries (Herr et al., 2009), which is particularly relevant for a species depending on continuous food intake as shown for harbor porpoises in the Baltic Sea (Wisniewska et al., 2016). A recent joint recommendation for fisheries management measures in the two SACs Sylt Outer Reef and Borkum Reef Ground was rejected by the European Commission as the proposed measures were insufficient, in particular with respect to the conservation of harbor porpoises, endangered seabirds and important habitat types (STECF, 2019). Appropriate measures to reduce fishing activities within the SAC Sylt Outer Reef are needed and should be implemented soon to protect harbor porpoises and other sensitive marine biota.
Moreover, several OWFs have been built in the vicinity of the SAC Sylt Outer Reef since 2013 and in 2014 the OWF “Butendiek” was constructed within the SAC (Viquerat et al., 2015; Brandt et al., 2018). During the construction phase a large scale disturbance of harbor porpoises has been reported for those and other OWFs in the North Sea (Dähne et al., 2013; Viquerat et al., 2015; Brandt et al., 2018). The construction close to and within the SAC Sylt Outer Reef took place during the peak breeding and calving season of harbor porpoises in June and July (Hasselmeier et al., 2004; Kesselring et al., 2019). It has been predicted that an almost continuous construction of OWFs over several years close to important core areas, such as foraging grounds, would lead to a high degree of disturbance and a substantial population decline (Nabe-Nielsen et al., 2018). The predicted scenario is comparable to the situation seen in the SAC Sylt Outer Reef. Although the decline in harbor porpoise abundance had started already before the first OWFs were built, the construction of OWFs added another level of disturbance to the already existing list of stressors acting on the population and could hence be an additional confounding factor for the decline.
Similar to SAC Sylt Outer Reef, a decreasing trend in harbor porpoise abundance has also been observed in neighboring areas to the north, in Danish waters, including the SAC “Southern North Sea”. Aerial surveys were conducted yearly in summer between 2011 and 2018 with the same methodology as in this study, and results suggest a decline over the study period (Sveegaard et al., 2019). This further supports the negative trends seen in the adjacent SAC Sylt Outer Reef. It also highlights the importance of aligned and joint national monitoring programs, as demanded by the EU Marine Strategy Framework Directive (MSFD), to gather information on fine-scale changes in distribution and abundance in between large scale, quasi-decadal surveys such as SCANS. Aggregated national monitoring data can also be put in a larger, North Sea wide context by applying spatial modeling (see e.g., Gilles et al., 2016).
German North Sea EEZ
Obviously, there are clear differences in trends between strata and seasons – however, it is also important to have a holistic view on the entire German North Sea EEZ since the motivation of the monitoring program is to assess the status of key marine top predators. Germany has to meet the protection obligations under international and European law and is i.a. obliged to determine trends and assess conservation status of Annex II species populations in their marine waters for incorporation in HD Art. 17 assessments and to report on progress toward Good Environmental Status for marine mammals under Art. 8 of MSFD. For OSPAR, the status of cetaceans in the Northeast Atlantic needs to be assessed and especially the status of the harbor porpoise, as occurring on the OSPAR List of Threatened and/or Declining Species and Habitats (OSPAR, 2008). The key indicator for MSFD Descriptor 1 is population size, and to assess trends with confidence. State-of- the-art methods fitting to MSFD requirements and concepts should be prioritized (Palialexis et al., 2019) and our approach outlines a fitting approach to this concept.
Overall, the abundance in the German North Sea EEZ shows a pronounced decline over the years. The decline is mainly driven by the strong decrease in absolute numbers of porpoises in the SAC Sylt Outer Reef, which make up the majority of all harbor porpoises in the German North Sea. When looking at an even larger scale, such as the entire North Sea, there is no indication for a decline of harbor porpoises based on the SCANS III survey in 2016 but high densities in the south and south-west and low densities in the east (Hammond et al., 2017). A joint analysis of stranding records from North Sea neighboring countries from 1990 to 2017 showed a steep increase in strandings in the southern North Sea since 2005 (Ijsseldijk et al., 2020). Harbor porpoises were almost absent in the English Channel two decades ago but are now regularly seen in this region (Hammond et al., 2013, 2017; Bouveroux et al., 2020). The further southward population shift implies that harbor porpoises are now more exposed to human high-use areas, which might represent a less optimal habitat. The southern North Sea is characterized by high shipping traffic, a high fishing intensity and a high density of OWFs that will likely increase strongly in the near future (Pedersen et al., 2009a; Berkenhagen et al., 2010; Emeis et al., 2015; Vespe et al., 2016) leading to an environment-user conflict. This is of conservation concern, also in view of the ongoing industrialization of the North Sea as forced by the EUs Blue Growth strategy (European Commission, 2020). In this respect there is a large knowledge gap regarding exposure limits for marine mammals.
Considering the variety and wealth of anthropogenic stressors, the long-term reproductive success of harbor porpoises has been questioned in the heavily used North Sea. Female harbor porpoises from the German North Sea were found to be sexually mature at an age of 4.95 (±0.6) years, while the average age of death was determined at 5.7 (±0.27) years, especially due to a high mortality of young animals which may not had the chance to reproduce (Kesselring et al., 2017). Similarly, there is an exceptionally high mortality of neonates along the German and Danish North Sea coast compared to other North Sea regions (Ijsseldijk et al., 2020). This again underlines the importance of the SAC Sylt Outer Reef area as a calving habitat but also raises concerns about the high neonate mortality. A high mortality of young individuals of a population would be expected, however, it was shown recently that juvenile harbor porpoises carry a higher load of neurotoxic substances than adults - probably due to a selective maternal transfer of these substances through lactation (Williams et al., 2020). In general, harbor porpoises in the North Sea exhibit a longer calving interval, lower pregnancy rates, a higher incidence of severe lesions (especially of the respiratory tract), and higher pollutant burdens by PCBs, DDT, Hg and PBDEs than in areas with less human impacts, such as in the Arctic (Siebert et al., 1999, 2001, 2006b, 2009; Wunschmann et al., 2001; Beineke et al., 2005; Lehnert et al., 2005; Murphy et al., 2015). Given the high contaminant load, high mortality of young harbor porpoises and the potential lower reproductive output, the relevance of a calving ground such as the SAC Sylt Outer Reef could diminish over time affecting the whole population.
It has been argued that porpoises have a high movement potential. For instance, porpoises from the Danish Baltic Sea and Skagerrak disperse over a large area and can cover vast distances (Teilmann et al., 2007; Sveegaard et al., 2011). This is also known from tagging studies of porpoises in Greenland (Nielsen et al., 2018); however, these individuals also exhibit a high degree of site fidelity, with many individuals returning to the tagging site after their seasonal migration. Other harbor porpoise populations show a remarkably high residency and limited large-scale movements, such as on the US East and West Coast (Read and Westgate, 1997; Johnston et al., 2005; Forney et al., 2017; Elliser et al., 2018). There are also indications that harbor porpoises from the North Sea show site fidelity and high residency over weeks and months, based on offshore observations in the Netherlands (Camphuysen and Krop, 2011) and satellite tracking studies in the Danish Wadden Sea (van Beest et al., 2018; Stalder et al., 2020). In particular, recent telemetry results from the Wadden Sea suggest limited dispersal of harbor porpoises throughout the whole year (Stalder et al., 2020). More information on the (seasonal) movements of harbor porpoises in the North Sea by means of satellite tracking studies are needed to improve our interpretation of observed changes in distribution and population trends.
Considering the predominant anthropogenic pressures and our presented trends in harbor porpoise abundance, it is imperative to better understand the underlying drivers and causalities, also in a regional North Sea wide context. Habitat-based modeling, including proxies for environmental dynamics that aggregate prey, are valuable tools to identify important predictors influencing distribution and changes therein (e.g., Hammond et al., 2013; Gilles et al., 2016). However, our understanding of anthropogenic drivers and impacts could be improved if data with high temporal and spatial resolution on pressures such as ship traffic, fishing effort (and bycatch rates) and noise propagation of major anthropogenic activities would be included in such models and integrated in a Bayesian hierarchical framework (see, e.g., Forney et al., 2020). Such data could also feed into agent-based population models to predict population dynamics (Nabe-Nielsen et al., 2018; Pirotta et al., 2018). Yet, these data are not readily available at the needed temporal and spatial resolution but will be vital to ensure a better understanding of harbor porpoise ecology and the impact of anthropogenic disturbances on the population level as well as exposure limits, ultimately leading to effective management and conservation.
Conclusion
Overall, harbor porpoise abundance in summer has decreased in the German EEZ between 2002 and 2019. Within the German North Sea, local and seasonal differences in trends of abundance were noted: Harbor porpoises have decreased in the north-east and increased in the south, such as the southern German Bight, including SAC Borkum Reef Ground. A particularly strong decline was found in the SAC Sylt Outer Reef in summer. Due to its significance as a core area and main reproduction site beyond national borders, the decline is of conservation concern and needs to be further investigated. Further, monitoring efforts should be enlarged to survey more frequently in spring to get a better insight into seasonal developments of harbor porpoises in the SAC Sylt Outer Reef. The underlying causes for the observed trends are unknown and probably due to cumulative effects of numerous stressors, where of most we lack adequate data on. If the trends were driven by anthropogenic stressors, these should rapidly be identified, and appropriate management measures developed and implemented. The necessary next step will be to put the here seen trends in a North Sea-wide context reflecting the range and distribution of this population.
Data Availability Statement
Publicly available datasets were analyzed in this study. This data can be found here: https://geodienste.bfn.de/schweinswalmonitoring?lang=en.
Ethics Statement
Ethical review and approval was not required for the animal study because of no invasive monitoring.
Author Contributions
AG, SV, and US conceptualized the study and acquired funding. SV, AG, BU, DN, and NR-M collected the data and processed the survey data. DN and SV conducted the analysis and generated the figures. DN, SV, and AG prepared the manuscript. All authors provided comments on the manuscript and approved the final version.
Funding
The surveys in Germany were funded by the Federal Ministry for the Environment, Nature Conservation and Nuclear Safety (BMU; projects MINOS, MINOSplus, and StUKplus; project ref. no. 0327520, 0329946/B/C/D, and 0327689A), the Federal Agency for Nature Conservation [BfN; projects EMSON, “Development of a harbor porpoise monitoring concept to fulfil the EU Habitats Directive reporting requirements,” “Monitoring of marine mammals in the framework of NATURA 2000,” “Erforschung der Raumnutzung und Raum-Zeit-Muster von Seevögeln und marinen Säugetieren in Nord- und Ostsee sowie Weiterentwicklung und Anpassung der Erfassungsmethoden (TopSpace; project ref. no. Z1.2 161453202-2014/11),” and “Erfassung Mariner Topprädatoren in Nord- und Ostsee als Grundlage für Trends, Indikatoren und Bewertungen (TopMarine; project ref. no. 3518532213)”] and the Federal Ministry of Food and Agriculture (BMEL; 514-33.29/03HS059).
Conflict of Interest
The authors declare that the research was conducted in the absence of any commercial or financial relationships that could be construed as a potential conflict of interest.
Acknowledgments
We thank all observers and navigators for their dedication and hard work and aircraft pilots for safe flights. We are grateful to Devin Johnson for helpful advice on the use of agTrend. We would also like to thank Tobias Schaffeld, Stephanie Gross, Matthieu Authier, Karin Forney, and the two reviewers for their valuable comments, which improved earlier versions of this manuscript.
Supplementary Material
The Supplementary Material for this article can be found online at: https://www.frontiersin.org/articles/10.3389/fmars.2020.606609/full#supplementary-material
Footnotes
References
Aarts, G., Von Benda-Beckmann, A. M., Lucke, K., Sertlek, H. Ö, Van Bemmelen, R., Geelhoed, S. C. V., et al. (2016). Harbour porpoise movement strategy affects cumulative number of animals acoustically exposed to underwater explosions. Mar. Ecol. Prog. Ser. 557, 261–275. doi: 10.3354/meps11829
Akkaya Bas, A., Christiansen, F., Amaha, Öztürk, A., Öztürk, B., and McIntosh, C. (2017). The effects of marine traffic on the behaviour of Black Sea harbour porpoises (Phocoena phocoena relicta) within the Istanbul Strait, Turkey. PLoS One 12:e0172970. doi: 10.1371/journal.pone.0172970
Andreasen, H., Ross, S. D., Siebert, U., Andersen, N. G., Ronnenberg, K., and Gilles, A. (2017). Diet composition and food consumption rate of harbor porpoises (Phocoena phocoena) in the western Baltic Sea. Mar. Mammal. Sci. 33, 1053–1079. doi: 10.1111/mms.12421
Authier, M., Galatius, A., Gilles, A., and Spitz, J. (2020). Of power and despair in cetacean conservation: estimation and detection of trend in abundance with noisy and short time-series. PeerJ 8:e9436. doi: 10.7717/peerj.9436
Becker, G., Dick, S., and Dippner, J. (1992). Hydrography of the German Bight. Mar. Ecol. Prog. Ser. 91, 9–18. doi: 10.3354/meps091009
Beineke, A., Siebert, U., McLachlan, M., Bruhn, R., Thron, K., Failing, K., et al. (2005). Investigations of the potential influence of environmental contaminants on the thymus and spleen of harbor porpoises (Phocoena phocoena). Environ. Sci. Technol. 39, 3933–3938. doi: 10.1021/es048709j
Benke, H., Siebert, U., Lick, R., Bandomir, B., and Weiss, R. (1998). The current status of harbour porpoises (Phocoena phocoena) in German waters. Arch. Fish. Mar. Res. 46, 97–123.
Berkenhagen, J., Döring, R., Fock, H. O., Kloppmann, M. H. F., Pedersen, S. A., and Schulze, T. (2010). Decision bias in marine spatial planning of offshore wind farms: problems of singular versus cumulative assessments of economic impacts on fisheries. Mar. Policy 34, 733–736. doi: 10.1016/j.marpol.2009.12.004
Bouveroux, T., Waggitt, J. J., Belhadjer, A., Cazenave, P. W., Evans, P. G. H., and Kiszka, J. J. (2020). Modelling fine-scale distribution and relative abundance of harbour porpoises in the Southern Bight of the North Sea using platform-of-opportunity data. J. Mar. Biol. Assoc. United Kingdom 100, 481–489. doi: 10.1017/s0025315420000326
Brandt, M. J., Dragon, A. C., Diederichs, A., Bellmann, M. A., Wahl, V., Piper, W., et al. (2018). Disturbance of harbour porpoises during construction of the first seven offshore wind farms in Germany. Mar. Ecol. Prog. Ser. 596, 213–232. doi: 10.3354/meps12560
Brownell, R. Jr., Reeves, R., Read, A., Smith, B., Thomas, P., Ralls, K., et al. (2019). Bycatch in gillnet fisheries threatens critically endangered small cetaceans and other aquatic megafauna. Endanger. Species Res. 40, 285–296. doi: 10.3354/esr00994
Buckland, S. T., Borchers, D. L., and Thomas, L. (2001). Introduction to Distance Sampling. Estimating Abundance of Biological Populations. New York, NY: Oxford University Press.
Buckland, S. T., Newman, K. B., Thomas, L., and Koesters, N. B. (2004). State-space models for the dynamics of wild animal populations. Ecol. Modell. 171, 157–175. doi: 10.1016/j.ecolmodel.2003.08.002
Camphuysen, C. J. (2004). The return of the harbour porpoise (Phocoena phocoena) in Dutch coastal waters. Lutra 47, 135–144.
Camphuysen, K. C. J. (2011). Recent trends and spatial patterns in nearshore sightings of harbour porpoises (Phocoena phocoena) in the Netherlands (Southern Bight, North Sea), 1990-2010. Lutra 54, 39–47.
Camphuysen, K. C. J., and Krop, A. (2011). Maternal care, calf-training and site fidelity in a wild harbour porpoise in the North Sea. Lutra 54, 123–126.
Clausen, K. T., Wahlberg, M., Beedholm, K., DeRuiter, S., and Madsen, P. T. (2010). Click communication in harbour porpoises Phocoena phocoena. Bioacoustics 20, 1–28. doi: 10.1080/09524622.2011.9753630
Dähne, M., Gilles, A., Lucke, K., Peschko, V., Adler, S., Krügel, K., et al. (2013). Effects of pile-driving on harbour porpoises (Phocoena phocoena) at the first offshore wind farm in Germany. Environ. Res. Lett. 8:025002. doi: 10.1088/1748-9326/8/2/025002
Ducrotoy, J.-P., Elliott, M., and de Jonge, V. N. (2000). The North Sea. Mar. Pollut. Bull. 41, 5–23. doi: 10.1016/S0025-326X(00)00099-0
Dyndo, M., Wiśniewska, D. M., Rojano-Doñate, L., and Madsen, P. T. (2015). Harbour porpoises react to low levels of high frequency vessel noise. Sci. Rep. 5:11083. doi: 10.1038/srep11083
Eigaard, O. R., Bastardie, F., Hintzen, N. T., Buhl-Mortensen, L., Buhl-Mortensen, P., Catarino, R., et al. (2017). The footprint of bottom trawling in European waters: distribution, intensity, and seabed integrity. ICES J. Mar. Sci. 74, 847–865. doi: 10.1093/icesjms/fsw194
Elliser, C. R., MacIver, K. H., and Green, M. (2018). Group characteristics, site fidelity, and photo-identification of harbor porpoises, Phocoena phocoena, in Burrows Pass, Fidalgo Island, Washington. Mar. Mammal Sci. 34, 365–384. doi: 10.1111/mms.12459
Emeis, K.-C., van Beusekom, J., Callies, U., Ebinghaus, R., Kannen, A., Kraus, G., et al. (2015). The North Sea — a shelf sea in the Anthropocene. J. Mar. Syst. 141, 18–33. doi: 10.1016/j.jmarsys.2014.03.012
Esteban, M. D., Diez, J. J., López, J. S., and Negro, V. (2011). Why offshore wind energy? Renew. Energy 36, 444–450. doi: 10.1016/j.renene.2010.07.009
Federal Agency for Nature Conservation (2008a). Conservation Objectives for the Borkum Reef Ground SCI (DE 2104-301) in the German North Sea EEZ. German: Federal Agency for Nature Conservation.
Federal Agency for Nature Conservation (2008b). Conservation Objectives for the Sylt Outer Reef SCI (DE 1209-301) in the German North Sea EEZ. German: Federal Agency for Nature Conservation.
Federal Agency for Nature Conservation (2010). Conservation Objectives for the Dogger Bank SCI (DE 1003-301) in the German North Sea EEZ. German: Federal Agency for Nature Conservation.
Forney, K., Southall, B., Slooten, E., Dawson, S., Read, A., Baird, R., et al. (2017). Nowhere to go: noise impact assessments for marine mammal populations with high site fidelity. Endanger. Species Res. 32, 391–413. doi: 10.3354/esr00820
Forney, K. A., Hanan, D. A., and Barlow, J. (1991). Detecting trends in harbor porpoise abundance from aerial surveys using analysis of covariance. Fish. Bull. 89, 367–377.
Forney, K. A., Moore, J. E., Barlow, J., Carretta, J. V., and Benson, S. R. (2020). A multidecadal Bayesian trend analysis of harbor porpoise (Phocoena phocoena) populations off California relative to past fishery bycatch. Mar. Mammal Sci. 1–15. doi: 10.1111/mms.12764
Geelhoed, S. C. V., and Scheidat, M. (2018). Abundance of harbour porpoises (Phocoena phocoena) on the Dutch Continental Shelf, aerial surveys 2012 - 2017. Lutra 61, 127–136.
Geelhoed, S. C. V., Scheidat, M., van Bemmelen, R. S. A., and Aarts, G. (2013). Abundance of harbour porpoises (Phocoena phocoena) on the Dutch Continental Shelf, aerial surveys in July 2010-March 2011. Lutra 56, 45–57.
Gilles, A. (2008). Characterisation of Harbour Porpoise (Phocoena phocoena) Habitat in German Waters. Ph.D. thesis, Christian-Albrechts-Universität zu Kiel, Kiel. 1–151. Available online at: https://macau.uni-kiel.de/receive/diss_mods_00003429
Gilles, A., Adler, S., Kaschner, K., Scheidat, M., and Siebert, U. (2011). Modelling harbour porpoise seasonal density as a function of the German Bight environment: implications for management. Endanger. Species Res. 14, 157–169. doi: 10.3354/esr00344
Gilles, A., Gunnlaugsson, T., Mikkelsen, B., Pike, D. G., and Víkingsson, G. A. (2020). Summer abundance of harbour porpoises (Phocoena phocoena) in the coastal waters of Iceland and the Faroe Islands. NAMMCO Sci. Publ. 11, 1–11. doi: 10.7557/3.4939
Gilles, A., Scheidat, M., and Siebert, U. (2009). Seasonal distribution of harbour porpoises and possible interference of offshore wind farms in the German North Sea. Mar. Ecol. Prog. Ser. 383, 295–307. doi: 10.3354/meps08020
Gilles, A., Viquerat, S., Becker, E. A., Forney, K. A., Geelhoed, S. C. V., Haelters, J., et al. (2016). Seasonal habitat-based density models for a marine top predator, the harbor porpoise, in a dynamic environment. Ecosphere 7:e01367. doi: 10.1002/ecs2.1367
Hadfield, J. D. (2010). MCMCglmm: MCMC methods for multi-response GLMMs in R. J. Stat. Softw. 33, 1–22. doi: 10.1002/ana.22635
Haelters, J., Kerckhof, F., Jacques, T. G., and Degraer, S. (2011). The harbour porpoise Phocoena phocoena in the Belgian part of the North Sea: trends in abundance and distribution. Belgian J. Zool. 141, 75–84.
Haelters, J., Kerckhof, F., and Jauniaux, T. (2018). Strandings of cetaceans in Belgium from 1995 to 2017. Lutra 61, 107–126.
Halpern, B. S., Frazier, M., Potapenko, J., Casey, K. S., Koenig, K., Longo, C., et al. (2015). Spatial and temporal changes in cumulative human impacts on the world’s ocean. Nat. Commun. 6:7615. doi: 10.1038/ncomms8615
Halpern, B. S., Lbridge, S., Selkoe, K. A., Kappel, C. V., Micheli, F., D’Agrosa, C., et al. (2008). A global map of human impact on marine ecosystems. Science 319, 948–952. doi: 10.1126/science.1149345
Hammond, P. S. (2010). “Estimating the abundance of marine mammals,” in Marine Mammal Ecology and Conservation: A Handbook of Techniques, eds I. L. Boyd, W. D. Bowen, and S. J. Iverson (New York, NY: Oxford University Press), 42–67.
Hammond, P. S., Berggren, P., Benke, H., Borchers, D. L., Collet, A., Heide-Jørgensen, M. P., et al. (2002). Abundance of harbour porpoise and other cetaceans in the North Sea and adjacent waters. J. Appl. Ecol. 39, 361–376. doi: 10.1046/j.1365-2664.2002.00713.x
Hammond, P. S., Lacey, C., Gilles, A., Viquerat, S., Börjesson, P., Herr, H., et al. (2017). Estimates of Cetacean Abundance in European Atlantic Waters in Summer 2016 From the SCANS-III Aerial and Shipboard Surveys. Yerseke: Wageningen Marine Research.
Hammond, P. S., Macleod, K., Berggren, P., Borchers, D. L., Burt, L., Cañadas, A., et al. (2013). Cetacean abundance and distribution in European Atlantic shelf waters to inform conservation and management. Biol. Conserv. 164, 107–122. doi: 10.1016/j.biocon.2013.04.010
Harvey, J. T. (2008). ““Abundance,”,” in Encyclopedia of Ecology, ed. S. E. Jorgensen (Amsterdam: Elsevier B.V), 4–10.
Hasselmeier, I., Abt, K. F., Adelung, D., and Siebert, U. (2004). Stranding patterns of harbour porpoises (Phocoena phocoena) in the German North and Baltic Seas: when does the birth period occur. J. Cetacean Res. Manag. 6, 259–263.
Hermannsen, L., Beedholm, K., Tougaard, J., and Madsen, P. T. (2014). High frequency components of ship noise in shallow water with a discussion of implications for harbor porpoises (Phocoena phocoena). J. Acoust. Soc. Am. 136, 1640–1653. doi: 10.1121/1.4893908
Herr, H., Fock, H. O., and Siebert, U. (2009). Spatio-temporal associations between harbour porpoise Phocoena phocoena and specific fisheries in the German Bight. Biol. Conserv. 142, 2962–2972. doi: 10.1016/j.biocon.2009.07.025
Hiby, L., and Gilles, A. (2016). Estimating effective strip width in SCANS-III aerial surveys. Int. SCANS-III Project Rep. 1–16.
Hiby, L., and Lovell, P. (1998). Using aircraft in tandem formation to estimate abundance of harbour porpoise. Biometrics 54:1280. doi: 10.2307/2533658
Hiby, L. R. (1999). “The objective identification of duplicate sightings in aerial survey for porpoise,” in Marine Mammal Survey and Assessment Methods, eds G. W. Garner, S. C. Amstrup, J. L. Laake, B. F. Manly, L. L. McDonald, and D. G. Robertson (Rotterdam: Balkema), 179–189.
Hoekendijk, J. P. A., Spitz, J., Read, A. J., Leopold, M. F., and Fontaine, M. C. (2018). Resilience of harbor porpoises to anthropogenic disturbance: must they really feed continuously? Mar. Mammal. Sci. 34, 258–264. doi: 10.1111/mms.12446
Humbert, J. Y., Mills, L. S., Horne, J. S., and Dennis, B. (2009). A better way to estimate population trends. Oikos 118, 1940–1946. doi: 10.1111/j.1600-0706.2009.17839.x
ICES (2019a). Cod (Gadus morhua) in Subarea 4, Division 7.d, and Subdivision 20 (North Sea, Eastern English Channel, Skagerrak). Copenhagen: ICES.
ICES (2019c). Sandeel (Ammodytes spp.) in Divisions 4.b–c and Subdivision 20, Sandeel Area 2r (Central and Southern North Sea). Copenhagen: ICES.
Ijsseldijk, L. L., ten Doeschate, M. T. I., Brownlow, A., Davison, N. J., Deaville, R., Galatius, A., et al. (2020). Spatiotemporal mortality and demographic trends in a small cetacean: strandings to inform conservation management. Biol. Conserv. 249:108733. doi: 10.1016/j.biocon.2020.108733
Johnson, D. S., and Fritz, L. (2014). agTrend: a bayesian approach for estimating trends of aggregated abundance. Methods Ecol. Evol. 5, 1110–1115. doi: 10.1111/2041-210X.12231
Johnston, D. W., Westgate, A. J., and Read, A. J. (2005). Effects of fine-scale oceanographic features on the distribution and movements of harbour porpoises Phocoena phocoena in the Bay of Fundy. Mar. Ecol. Prog. Ser. 295, 279–293. doi: 10.3354/meps295279
Kastelein, R. A., Gransier, R., Marijt, M. A. T., and Hoek, L. (2015). Hearing frequency thresholds of harbor porpoises (Phocoena phocoena) temporarily affected by played back offshore pile driving sounds. J. Acoust. Soc. Am. 137, 556–564. doi: 10.1121/1.4906261
Kastelein, R. A., Helder-Hoek, L., Covi, J., and Gransier, R. (2016). Pile driving playback sounds and temporary threshold shift in harbor porpoises (Phocoena phocoena): effect of exposure duration. J. Acoust. Soc. Am. 139, 2842–2851. doi: 10.1121/1.4948571
Kesselring, T., Viquerat, S., Brehm, R., and Siebert, U. (2017). Coming of age: - Do female harbour porpoises (Phocoena phocoena) from the North Sea and Baltic Sea have sufficient time to reproduce in a human influenced environment? PLoS One 12:e0186951. doi: 10.1371/journal.pone.0186951
Kesselring, T., Viquerat, S., IJsseldijk, L. L., Langeheine, M., Wohlsein, P., Gröne, A., et al. (2019). Testicular morphology and spermatogenesis in harbour porpoises (Phocoena phocoena). Theriogenology 126, 177–186. doi: 10.1016/j.theriogenology.2018.11.031
Koopman, H. N., Pabst, D. A., McLellan, W. A., Dillaman, R. M., and Read, A. J. (2002). Changes in blubber distribution and morphology associated with starvation in the harbor porpoise (Phocoena phocoena): evidence for regional differences in blubber structure and function. Physiol. Biochem. Zool. 75, 498–512. doi: 10.1086/342799
Laake, J. L., Calambodikis, J., Osmek, S. D., and Rugh, D. J. (1997). Probability of detecting harbor porpoise from aerial surveys: estimating g(0). J. Wildl. Manage. 61:63. doi: 10.2307/3802415
Law, R. J., Barry, J., Barber, J. L., Bersuder, P., Deaville, R., Reid, R. J., et al. (2012). Contaminants in cetaceans from UK waters: status as assessed within the cetacean strandings investigation programme from 1990 to 2008. Mar. Pollut. Bull. 64, 1485–1494. doi: 10.1016/j.marpolbul.2012.05.024
Learmonth, J. A., Murphy, S., Luque, P. L., Reid, R. J., Patterson, I. A. P., Brownlow, A., et al. (2014). Life history of harbor porpoises (Phocoena phocoena) in Scottish (UK) waters. Mar. Mammal. Sci. 30, 1427–1455. doi: 10.1111/mms.12130
Lehnert, K., Raga, J., and Siebert, U. (2005). Macroparasites in stranded and bycaught harbour porpoises from German and Norwegian waters. Dis. Aquat. Organ. 64, 265–269. doi: 10.3354/dao064265
Leopold, M. F., Begeman, L., Heße, E., van der Hiele, J., Hiemstra, S., Keijl, G., et al. (2015). Porpoises: from predators to prey. J. Sea Res. 97, 14–23. doi: 10.1016/j.seares.2014.12.005
Lindenmayer, D. B., and Likens, G. E. (2009). Adaptive monitoring: a new paradigm for long-term research and monitoring. Trends Ecol. Evol. 24, 482–486. doi: 10.1016/j.tree.2009.03.005
Lucke, K., Siebert, U., Lepper, P. A., and Blanchet, M.-A. (2009). Temporary shift in masked hearing thresholds in a harbor porpoise (Phocoena phocoena) after exposure to seismic airgun stimuli. J. Acoust. Soc. Am. 125, 4060–4070. doi: 10.1121/1.3117443
Magurran, A. E., Baillie, S. R., Buckland, S. T., Dick, J. M. P., Elston, D. A., Scott, E. M., et al. (2010). Long-term datasets in biodiversity research and monitoring: assessing change in ecological communities through time. Trends Ecol. Evol. 25, 574–582. doi: 10.1016/j.tree.2010.06.016
Marsh, D. M., and Trenham, P. C. (2008). Current trends in plant and animal population monitoring. Conserv. Biol. 22, 647–655. doi: 10.1111/j.1523-1739.2008.00927.x
Marsh, H., and Sinclair, D. F. (1989). Correcting for visibility bias in strip transect aerial surveys of aquatic Fauna. J. Wildl. Manage. 53, 1017–1024. doi: 10.2307/3809604
Merchant, N. D., Andersson, M. H., Box, T., Le Courtois, F., Cronin, D., Holdsworth, N., et al. (2020). Impulsive noise pollution in the Northeast Atlantic: reported activity during 2015–2017. Mar. Pollut. Bull. 152:110951. doi: 10.1016/j.marpolbul.2020.110951
Murphy, S., Barber, J. L., Learmonth, J. A., Read, F. L., Deaville, R., Perkins, M. W., et al. (2015). Reproductive failure in UK harbour porpoises Phocoena phocoena: legacy of pollutant exposure? PLoS One 10:e0131085. doi: 10.1371/journal.pone.0131085
Nabe-Nielsen, J., van Beest, F. M., Grimm, V., Sibly, R. M., Teilmann, J., and Thompson, P. M. (2018). Predicting the impacts of anthropogenic disturbances on marine populations. Conserv. Lett. 11:e12563. doi: 10.1111/conl.12563
Neumann, H., Diekmann, R., Emeis, K.-C., Kleeberg, U., Moll, A., and Kröncke, I. (2017). Full-coverage spatial distribution of epibenthic communities in the south-eastern North Sea in relation to habitat characteristics and fishing effort. Mar. Environ. Res. 130, 1–11. doi: 10.1016/j.marenvres.2017.07.010
Neumann, H., Reiss, H., Ehrich, S., Sell, A., Panten, K., Kloppmann, M., et al. (2013). Benthos and demersal fish habitats in the German Exclusive Economic Zone (EEZ) of the North Sea. Helgol. Mar. Res. 67, 445–459. doi: 10.1007/s10152-012-0334-z
Newman, K. B., Buckland, S. T., Lindley, S. T., Thomas, L., and Fernández, C. (2006). Hidden process models for animal population dynamics. Ecol. Appl. 16, 74–86. doi: 10.1890/04-0592
Nielsen, N., Teilmann, J., Sveegaard, S., Hansen, R., Sinding, M., Dietz, R., et al. (2018). Oceanic movements, site fidelity and deep diving in harbour porpoises from Greenland show limited similarities to animals from the North Sea. Mar. Ecol. Prog. Ser. 597, 259–272. doi: 10.3354/meps12588
O’Hara, R. B., and Kotze, D. J. (2010). Do not log-transform count data. Methods Ecol. Evol. 1, 118–122. doi: 10.1111/j.2041-210X.2010.00021.x
OSPAR (2008). OSPAR List of Threatened and/or Declining Species and Habitats. OSPAR Agreement 2008-6. London: OSPAR.
Otto, T., Opitz, S., and Froese, R. (2019). How Does Sandeel Fishery Impact the Marine Ecosystem in the Southern North Sea and the Achievement of the Conservation Objectives of the Marine Protected Areas in the German EEZ of the North Sea?. Kiel: Helmholtz-Centre for Ocean Research.
Palialexis, A., Connor, D., Damalas, D., Gonzalvo, J., Micu, D., Mitchel, I., et al. (2019). Indicators for Status Assessment of Species, Relevant to MSFD Biodiversity Descriptor. EUR 29820 EN. Luxembourg: Publications Office of the European Union.
Pedersen, S. A., Fock, H., Krause, J., Pusch, C., Sell, A. L., Böttcher, U., et al. (2009a). Natura 2000 sites and fisheries in German offshore waters. ICES J. Mar. Sci. 66, 155–169. doi: 10.1093/icesjms/fsn193
Pedersen, S. A., Fock, H. O., and Sell, A. F. (2009b). Mapping fisheries in the German exclusive economic zone with special reference to offshore Natura 2000 sites. Mar. Policy 33, 571–590. doi: 10.1016/j.marpol.2008.12.007
Peltier, H., Baagøe, H. J., Camphuysen, K. C. J., Czeck, R., Dabin, W., Daniel, P., et al. (2013). The stranding anomaly as population indicator: the case of harbour porpoise Phocoena phocoena in North-Western Europe. PLoS One 8:e62180. doi: 10.1371/journal.pone.0062180
Peschko, V., Ronnenberg, K., Siebert, U., and Gilles, A. (2016). Trends of harbour porpoise (Phocoena phocoena) density in the southern North Sea. Ecol. Indic. 60, 174–183. doi: 10.1016/j.ecolind.2015.06.030
Pierce, G. J., Santos, M. B., Murphy, S., Learmonth, J. A., Zuur, A. F., Rogan, E., et al. (2008). Bioaccumulation of persistent organic pollutants in female common dolphins (Delphinus delphis) and harbour porpoises (Phocoena phocoena) from western European seas: geographical trends, causal factors and effects on reproduction and mortalit. Environ. Pollut. 153, 401–415. doi: 10.1016/j.envpol.2007.08.019
Pirotta, E., Booth, C. G., Costa, D. P., Fleishman, E., Kraus, S. D., Lusseau, D., et al. (2018). Understanding the population consequences of disturbance. Ecol. Evol. 8, 9934–9946. doi: 10.1002/ece3.4458
Prandle, D., Hydes, D. J., Jarvis, J., and McManus, J. (1997). The seasonal cycles of temperature, salinity, nutrients and suspended sediment in the Southern North Sea in 1988 and 1989. Estuar. Coast. Shelf Sci. 45, 669–680. doi: 10.1006/ecss.1996.0227
R Core Team (2018). R: A Language and Environment for Statistical Computing. Vienna: R Foundation for Statistical Computing.
Read, A. J., Drinker, P., and Northridge, S. (2006). Bycatch of marine mammals in U.S. and Global Fisheries. Conserv. Biol. 20, 163–169. doi: 10.1111/j.1523-1739.2006.00338.x
Read, A. J., and Hohn, A. A. (1995). Life in the fast lane: the life history of harbor porpoises from the Gulf of Maine. Mar. Mammal. Sci. 11, 423–440. doi: 10.1111/j.1748-7692.1995.tb00667.x
Read, A. J., and Westgate, A. J. (1997). Monitoring the movements of harbour porpoises (Phocoena phocoena) with satellite telemetry. Mar. Biol. 130, 315–322. doi: 10.1007/s002270050251
Rojano-Doñate, L., McDonald, B. I., Wisniewska, D. M., Johnson, M., Teilmann, J., Wahlberg, M., et al. (2018). High field metabolic rates of wild harbour porpoises. J. Exp. Biol. 221:jeb185827. doi: 10.1242/jeb.185827
Sarnocińska, J., Teilmann, J., Balle, J. D., van Beest, F. M., Delefosse, M., and Tougaard, J. (2020). Harbor porpoise (Phocoena phocoena) reaction to a 3D seismic airgun survey in the North Sea. Front. Mar. Sci. 6:824. doi: 10.3389/fmars.2019.00824
Scheidat, M., Gilles, A., Kock, K., and Siebert, U. (2008). Harbour porpoise Phocoena phocoena abundance in the southwestern Baltic Sea. Endanger. Species Res. 5, 215–223. doi: 10.3354/esr00161
Scheidat, M., Verdaat, H., and Aarts, G. (2012). Using aerial surveys to estimate density and distribution of harbour porpoises in Dutch waters. J. Sea Res. 69, 1–7. doi: 10.1016/j.seares.2011.12.004
Siebert, U., Gilles, A., Lucke, K., Ludwig, M., Benke, H., Kock, K.-H., et al. (2006a). A decade of harbour porpoise occurrence in German waters - analyses of aerial surveys, incidental sightings and strandings. J. Sea Res. 56, 65–80. doi: 10.1016/j.seares.2006.01.003
Siebert, U., Tolley, K., Vikingsson, G. A., Ólafsdóttir, D., Lehnert, K., Weiss, R., et al. (2006b). Pathological findings in harbour porpoises (Phocoena phocoena) from Norwegian and Icelandic waters. J. Comp. Pathol. 134, 134–142. doi: 10.1016/j.jcpa.2005.09.002
Siebert, U., Joiris, C., Holsbeek, L., Benke, H., Failing, K., Frese, K., et al. (1999). Potential relation between mercury concentrations and necropsy findings in cetaceans from German Waters of the North and Baltic Seas. Mar. Pollut. Bull. 38, 285–295. doi: 10.1016/S0025-326X(98)00147-7
Siebert, U., Prenger-Berninghoff, E., and Weiss, R. (2009). Regional differences in bacterial flora in harbour porpoises from the North Atlantic: environmental effects? J. Appl. Microbiol. 106, 329–337. doi: 10.1111/j.1365-2672.2008.04006.x
Siebert, U., Wünschmann, A., Weiss, R., Frank, H., Benke, H., and Frese, K. (2001). Post-mortem findings in harbour porpoises (Phocoena phocoena) from the German North and Baltic Seas. J. Comp. Pathol. 124, 102–114. doi: 10.1053/jcpa.2000.0436
Sonntag, R., Benke, H., Hiby, A. R., Lick, R., and Adelung, D. (1999). Identification of the first harbour porpoise (Phocoena phocoena) calving ground in the North Sea. J. Sea Res. 41, 225–232. doi: 10.1016/S1385-1101(98)00050-1
Sørensen, P. M., Wisniewska, D. M., Jensen, F. H., Johnson, M., Teilmann, J., and Madsen, P. T. (2018). Click communication in wild harbour porpoises (Phocoena phocoena). Sci. Rep. 8:9702. doi: 10.1038/s41598-018-28022-8
Sørensen, T. B., and Kinze, C. C. (1994). Reproduction and reproductive seasonality in Danish harbour porpoises, Phocoena phocoena. Ophelia 39, 159–174. doi: 10.1080/00785326.1994.10429541
Spitz, J., Trites, A. W., Becquet, V., Brind’Amour, A., Cherel, Y., Galois, R., et al. (2012). Cost of living dictates what whales, dolphins and porpoises eat: the importance of prey quality on predator foraging strategies. PLoS One 7:e50096. doi: 10.1371/journal.pone.0050096
Stalder, D., van Beest, F., Sveegaard, S., Dietz, R., Teilmann, J., and Nabe-Nielsen, J. (2020). Influence of environmental variability on harbour porpoise movement. Mar. Ecol. Prog. Ser. 648, 207–219. doi: 10.3354/meps13412
Stanev, E. V., Badewien, T. H., Freund, H., Grayek, S., Hahner, F., Meyerjürgens, J., et al. (2019). Extreme westward surface drift in the North Sea: public reports of stranded drifters and Lagrangian tracking. Cont. Shelf Res. 177, 24–32. doi: 10.1016/j.csr.2019.03.003
STECF (2019). Scientific, Technical and Economic Committee for Fisheries (STECF): 60th Plenary Meeting Report (PLEN-19-01). Luxembourg: STECF.
STECF (2020). Scientific, Technical and Economic Committee for Fisheries (STECF) – Monitoring the Performance of the Common Fisheries Policy (STECF-Adhoc-20-01). Luxembourg: STECF.
Sun, X., Huang, D., and Wu, G. (2012). The current state of offshore wind energy technology development. Energy 41, 298–312. doi: 10.1016/j.energy.2012.02.054
Sveegaard, S., Galatius, A., Kyhn, L. A., and Teilmann, J. (2019). “Havpattedyr - sæler og marsvin,” in Marine områder 2018. NOVANA, eds J. W. Hansen and S. Høgslund (Aarhus: Aarhus University, DCE – Danish Centre for Environment and Energy), 87–99.
Sveegaard, S., Teilmann, J., Tougaard, J., Dietz, R., Mouritsen, K. N., Desportes, G., et al. (2011). High-density areas for harbor porpoises (Phocoena phocoena) identified by satellite tracking. Mar. Mammal. Sci. 27, 230–246. doi: 10.1111/j.1748-7692.2010.00379.x
Taylor, B. L., and Gerrodette, T. (1993). The uses of statistical power in conservation biology: the vaquita and Northern Spotted Owl. Conserv. Biol. 7, 489–500. doi: 10.1046/j.1523-1739.1993.07030489.x
Taylor, B. L., Martinez, M., Gerrodette, T., Barlow, J., and Hrovat, Y. N. (2007). Lessons from monitoring trends in abundance of marine mammals. Mar. Mammal Sci. 23, 157–175. doi: 10.1111/j.1748-7692.2006.00092.x
Teilmann, J., Larsen, F., and Desportes, G. (2007). Time allocation and diving behaviour of harbour porpoises (Phocoena phocoena) in Danish and adjacent waters. J. Cetacean Res. Manag. 9, 201–210.
Tougaard, J., Carstensen, J., Teilmann, J., Skov, H., and Rasmussen, P. (2009). Pile driving zone of responsiveness extends beyond 20 km for harbor porpoises (Phocoena phocoena (L.)). J. Acoust. Soc. Am. 126, 11–14. doi: 10.1121/1.3132523
Turrell, W. R., Henderson, E. W., Slesser, G., Payne, R., and Adams, R. D. (1992). Seasonal changes in the circulation of the northern North Sea. Cont. Shelf Res. 12, 257–286. doi: 10.1016/0278-4343(92)90032-F
van Beest, F. M., Teilmann, J., Dietz, R., Galatius, A., Mikkelsen, L., Stalder, D., et al. (2018). Environmental drivers of harbour porpoise fine-scale movements. Mar. Biol. 165:95. doi: 10.1007/s00227-018-3346-7
Vespe, M., Gibin, M., Alessandrini, A., Natale, F., Mazzarella, F., and Osio, G. C. (2016). Mapping EU fishing activities using ship tracking data. J. Maps 12, 520–525. doi: 10.1080/17445647.2016.1195299
Vinther, M., and Larsen, F. (2004). Updated estimates of harbour porpoise (Phocoena phocoena) bycatch in the Danish North Sea bottom-set gillnet fishery. J. Cetacean Res. Manag. 6, 19–24.
Viquerat, S., Gilles, A., Herr, H., Siebert, U., Gallus, A., Krügel, K., et al. (2015). Monitoring Von Marinen Säugetieren 2014 in der Deutschen Nord- und Ostsee. Visuelle Erfassung von Schweinswalen. Endbericht für das Bundesamt für Naturschutz. Insel Vilm: Bundesamt für Naturschutz.
von Benda-Beckmann, A. M., Aarts, G., Sertlek, H. Ö, Lucke, K., Verboom, W. C., Kastelein, R. A., et al. (2015). Assessing the impact of underwater clearance of unexploded ordnance on harbour porpoises (Phocoena phocoena) in the Southern North Sea. Aquat. Mamm. 41, 503–523. doi: 10.1578/AM.41.4.2015.503
Wade, P. R. (2000). Bayesian methods in conservation biology. Conserv. Biol. 14, 1308–1316. doi: 10.1046/j.1523-1739.2000.99415.x
Weel, S. M. H., Geelhoed, S. C. V., Tulp, I., and Scheidat, M. (2018). Feeding behaviour of harbour porpoises (Phocoena phocoena) in the Ems estuary. Lutra 61, 137–152.
Wenger, D., and Koschinski, S. (2012). Harbour porpoise (Phocoena phocoena Linnaeus, 1758) entering the Weser river after decades of absence. Mar. Biol. Res. 8, 737–745. doi: 10.1080/17451000.2012.676184
Wenger, D., Siebert, U., and Hennig, V. (2016). “The return of harbour porpoises (Phocoena phocoena) to the lower Elbe and Weser rivers, following anadromous fish shoals, foraging in Hamburg harbour,” Poster Presented at the Conference of the European Cetacean Society, Madeira.
White, E. R. (2019). Minimum time required to detect population trends: the need for long-term monitoring programs. Bioscience 69, 26–39. doi: 10.1093/biosci/biy144
Williams, R. S., Curnick, D. J., Barber, J. L., Brownlow, A., Davison, N. J., Deaville, R., et al. (2020). Juvenile harbor porpoises in the UK are exposed to a more neurotoxic mixture of polychlorinated biphenyls than adults. Sci. Total Environ. 708:134835. doi: 10.1016/j.scitotenv.2019.134835
Wisniewska, D. M., Johnson, M., Teilmann, J., Rojano-Doñate, L., Shearer, J., Sveegaard, S., et al. (2018a). Response to “Resilience of harbor porpoises to anthropogenic disturbance: must they really feed continuously?”. Mar. Mammal. Sci. 34, 265–270. doi: 10.1111/mms.12463
Wisniewska, D. M., Johnson, M., Teilmann, J., Siebert, U., Galatius, A., Dietz, R., et al. (2018b). High rates of vessel noise disrupt foraging in wild harbour porpoises (Phocoena phocoena). Proc. R. Soc. B Biol. Sci. 285:20172314. doi: 10.1098/rspb.2017.2314
Wisniewska, D. M. M., Johnson, M., Teilmann, J., Rojano-Doñate, L., Shearer, J., Sveegaard, S., et al. (2016). Ultra-high foraging rates of harbor porpoises make them vulnerable to anthropogenic disturbance. Curr. Biol. 26, 1441–1446. doi: 10.1016/j.cub.2016.03.069
Wunschmann, A., Siebert, U., Frese, K., Weiss, R., Lockyer, C., Heide-Jørgensen, M. P., et al. (2001). Evidence of infectious diseases in harbour porpoises (Phocoena phocoena) hunted in the waters of Greenland and by-caught in the German North Sea and Baltic Sea. Vet. Rec. 148, 715–720. doi: 10.1136/vr.148.23.715
Keywords: harbor porpoise, North Sea, monitoring, Bayesian trend analysis, marine mammal conservation, biodiversity indicators, marine protected areas
Citation: Nachtsheim DA, Viquerat S, Ramírez-Martínez NC, Unger B, Siebert U and Gilles A (2021) Small Cetacean in a Human High-Use Area: Trends in Harbor Porpoise Abundance in the North Sea Over Two Decades. Front. Mar. Sci. 7:606609. doi: 10.3389/fmars.2020.606609
Received: 15 September 2020; Accepted: 01 December 2020;
Published: 07 January 2021.
Edited by:
Jeremy Kiszka, Florida International University, United StatesReviewed by:
Philip Hammond, University of St Andrews, United KingdomPatrick Halpin, Duke University, United States
Copyright © 2021 Nachtsheim, Viquerat, Ramírez-Martínez, Unger, Siebert and Gilles. This is an open-access article distributed under the terms of the Creative Commons Attribution License (CC BY). The use, distribution or reproduction in other forums is permitted, provided the original author(s) and the copyright owner(s) are credited and that the original publication in this journal is cited, in accordance with accepted academic practice. No use, distribution or reproduction is permitted which does not comply with these terms.
*Correspondence: Anita Gilles, YW5pdGEuZ2lsbGVzQHRpaG8taGFubm92ZXIuZGU=