- 1Departamento de Biología Animal, Universidad de Málaga, Málaga, Spain
- 2Centro Oceanográfico de Santander, Instituto Español de Oceanografía, Santander, Spain
- 3Centro Oceanográfico de Gijón, Instituto Español de Oceanografía, Gijón, Spain
This study analyzes the fauna composition of the community of brittle and sea stars associated with sponge aggregations located in Avilés Canyons System and El Cachucho, Marine Protected Area (MPA). Diverse sampling methods were used depending on bottom morphology, such as rock dredges and specific samplers for sedimentary bottoms, mainly beam trawl models. These banks are made up of sponge and coral species that build a very appropriate substrate for the proliferation of benthic species, which together create Vulnerable Marine Ecosystems that are highly relevant for management and conservation. Among these benthic species, echinoderms are of great interest due to their value as indicators of good habitat. In total, 1261 specimens were collected (934 brittle stars and 327 starfishes), belonging to 42 species (28 ophiuroids and 14 asteroids) from INDEMARES AVILÉS, ECOMARG, and SponGES project surveys. Specimens were distributed among four sponge aggregations (F: fields) that were considered according to the sponge records obtained in the same stations (36). These fields were defined and named based on the five most common sponge species: Aphrocallistes beatrix and Regadrella phoenix (F1: Avilés Canyon); Pheronema carpenteri (F2: Intraslope basin of Le Danois Bank); Asconema setubalense (F3: Le Danois Bank); and Neoschrammeniella aff. bowerbankii (F4: Corbiro Canyon). Faunistic results show that Ophiactis abyssicola (55.55% occurrence), Brisinga endecacnemos, Ophiolycus purpureus, and Peltaster placenta (33.33%) were the most frequently found species in F1; Psilaster andromeda (80%), Pseudarchaster parelii (60%), and Nymphaster arenatus (46.67%) in F2; Ophiura carnea (71.43%) and Ophiacantha smitti (42.86%) in F3; and Ophiacantha densa, and Henricia caudani (100%) in F4. The asteroid and ophiuroid species collected seem to be composed of four different communities that fit to areas with particular morphological and biological features, related to the presence of species specialized in the use of the resources they can find there. In general, the abiotic factor controlling this structure is depth. This assemblage structure, which favors the dissimilarity between the canyons and the bank, is not so clear, since the deepest stations are located on the intraslope basin of El Cachucho, Marine Protected Area (MPA), therefore, using it a priori could lead to misunderstandings. Once the structure of the echinoderm community was known, we compared the expected and obtained results to analyze evidence which should prove the existence of any association between echinoderms and sponges, which enabled us to refute the incongruous hypothesis.
Introduction
The benthic fauna of the Avilés Canyon System and the El Cachucho, Marine Protected Area (MPA) have been studied from a systematic point of view (mostly in Altuna, 2013; Altuna and Ríos, 2014; Manjón-Cabeza et al., 2014b; García-Guillén et al., 2018; Taboada et al., 2019), and under a general ecosystem approach (Sánchez et al., 2008, 2009, 2014a), providing a complete analysis of habitat scene for management and conservation. These studies were very valuable for these areas to be considered a vulnerable ecosystem and, as such, a protected area by the European Union (92/43/CEE) (Sánchez et al., 2014a,b, 2017; Punzón et al., 2016; Rodríguez-Basalo et al., 2019a). However, specific level studies are scarce, from the biological and ecological approach.
Echinoderms, together with sponges and corals, constitute the most important groups, in both biomass and/or abundance (Sánchez et al., 2008, 2009, 2014a, 2017; Manjón-Cabeza et al., 2014b; García-Guillén et al., 2018), as well as specific richness of the deep seabed. The importance of considering the association between these three groups is considerable, and all these previous studies provide us with a unique opportunity to carry out different kinds of analysis. Moreover, many of these bottoms are made up of sponge and coral species that build a very appropriate substrate for the proliferation of other benthic species. Among these benthic species, echinoderms are of high interest, mostly because of their inherent needs in order to survive (Murillo et al., 2012; Manjón-Cabeza et al., 2014a,b; Andrino-Abelaira, 2015; Gómez-Delgado, 2015; Murillo, 2015; Palma-Sevilla, 2015; Hurtado-García, 2016; Moya, 2016; Mah, 2020), or they are found to be associated to specific communities, leading them to be indicators of good habitat.
Recently, Ríos et al. (2020) studied the community composition and characterization of sponge aggregations in the Cantabrian Sea, showing that these aggregations constitute structuring habitats (3D communities) that function as support for different types of benthic communities (Peña-Cantero and Manjón-Cabeza, 2014, among others) and that certain benthic groups seem to have an intrinsic association with these sponge aggregations.
Based on these results, it should be possible to find an echinoderm reliance, preference, or association with these aggregations, but precise studies on the subject were not carried out, which is the main objective of the present study.
For this purpose, four sampling areas were chosen, two in the canyons and two on El Cachucho, Marine Protected Area (MPA), as well as two groups of echinoderms, starfishes, and brittle stars, because these echinoderm taxa tend to be indicators of different morphological and granulometry bottom features and of the assemblage of benthic communities.
According to this objective, to ascertain whether there exists any link between asteroids and ophiuroids and sponge aggregations on sea beds, we proposed the following hypotheses (Figure 1):
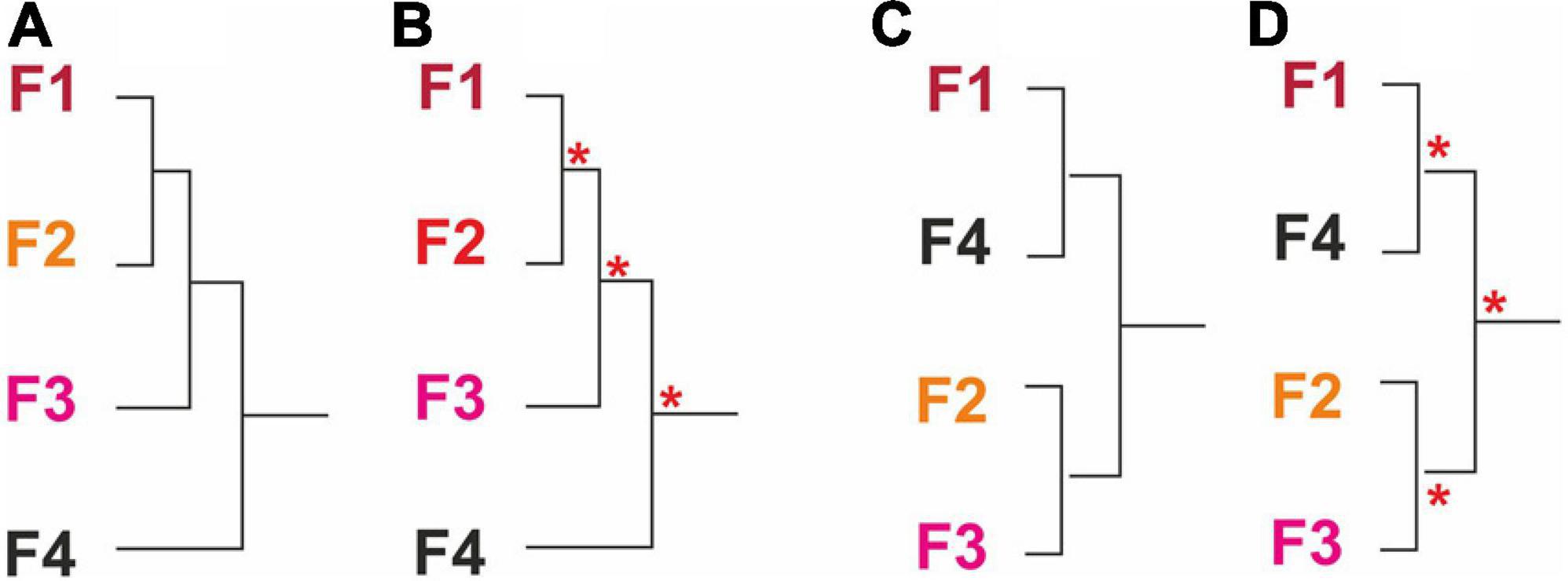
Figure 1. Hypothetical clusters of echinoderm community assemblages (A–D). Asterisks in red illustrates hypothetical strong boundaries segregating significant (P < 0.001) clusters (or groups) (B,D).
1 Although sponge aggregations present different specific compositions, there is not enough evidence to consider that the asteroid/ophiuroid community in canyons is different from on El Cachucho, Marine Protected Area (MPA) (Figures 1A,C).
2 These echinoderm species distributions will enable us to define four different echinoderm assemblages related to each sponge field (Figure 1B).
3 There are two different taxocoenosis, one in the canyons, and another on El Cachucho, Marine Protected Area (MPA) (Figure 1D).
Materials and Methods
Study Area and Description of Work Fields
The study area is located in the northern continental margin of the Iberian Peninsula in the Cantabrian Sea (Figure 2A), specifically the Avilés Canyon Systems (ACS: Figure 2B) and El Cachucho, Marine Protected Area (MPA) (LDB: Figure 2C), which present peculiar geomorphological and habitat features described in several previous papers (Ballesteros et al., 2006; Sánchez et al., 2008, 2009; Van Rooij et al., 2010). These features together with station locations were analyzed and georeferenced by ArcGIS 10.7.
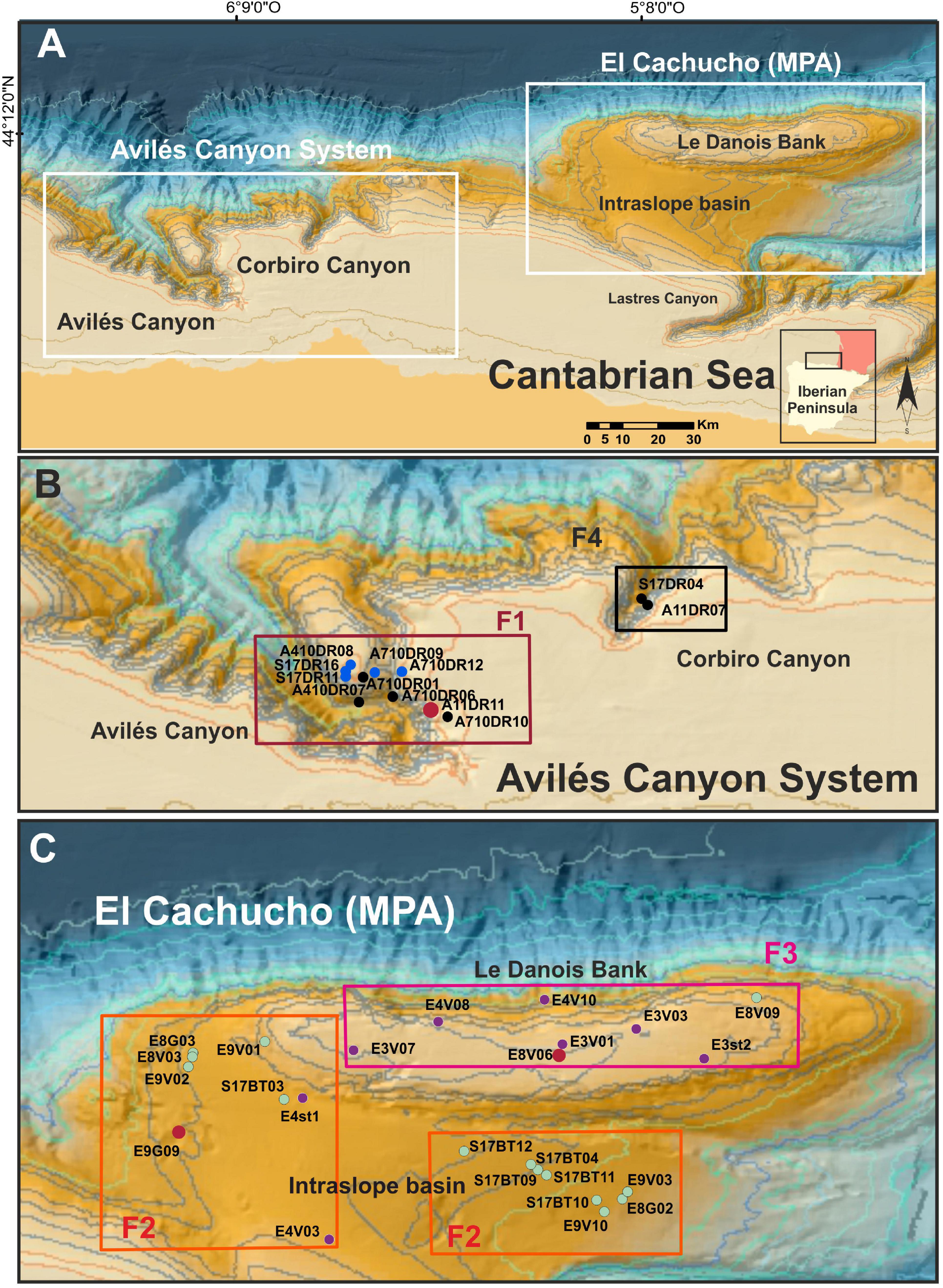
Figure 2. (A) Central Cantabrian Sea, sampling area. (B) Avilés Canyon System. Delimited field samling area described as F1: Avilés Canyon field; F4: Corbiro Canyon field. (C) El Cachucho, Marine Protected Area (MPA). Field sampling: F2: Intraslope basin and southern Bank Break; F3, Top of the Bank. Rectangle colors define the stations integrated in each sponge field, and dot colors are related to the cluster (Baroni-Urbani similarity coefficient) significant group, G1: purple; G2: black; G3: green; G4: blue (see Figure 7).
This work focuses on two canyon heads, Avilés Canyon (AC) and the Corbiro Canyon (CC) (Figure 2B), and Le Danois Bank and its intraslope basin (Figure 2C), based on sponge aggregation types of settlements described by different authors (Prado et al., 2019; Rodríguez-Basalo et al., 2019b; Ríos et al., 2020). Four sponge fields were established according to sponge species: F1 (AC), Aphrocallistes Beatrix Gray, 1858, and Regadrella phoenix Schmidt, 1880; F2 (intraslope basin of LDB), Pheronema carpenteri (Thomson, 1869); F3 (LBD), Asconema setubalense Kent, 1870; and F4 (CC), Neoschrammeniella aff. bowerbankii (Johnson, 1863; Figures 2B,C).
These four species were studied based on previous knowledge of the study area (García-Alegre et al., 2014; Sánchez et al., 2014a) and the criteria by which vulnerable marine ecosystems such as sponge grounds are considered (Hogg et al., 2010; Maldonado et al., 2016): they support high biodiversity of other species, are fragile and unlikely to recover from trawl damage, and are limited to discrete areas with suitable environmental conditions.
Sampling was carried out using different trawl gear and surveys, as described in previous studies (Sánchez et al., 2008; Rapp, 2019). A total of 36 stations (Table 1) meeting the afore-described criteria requirements were selected.
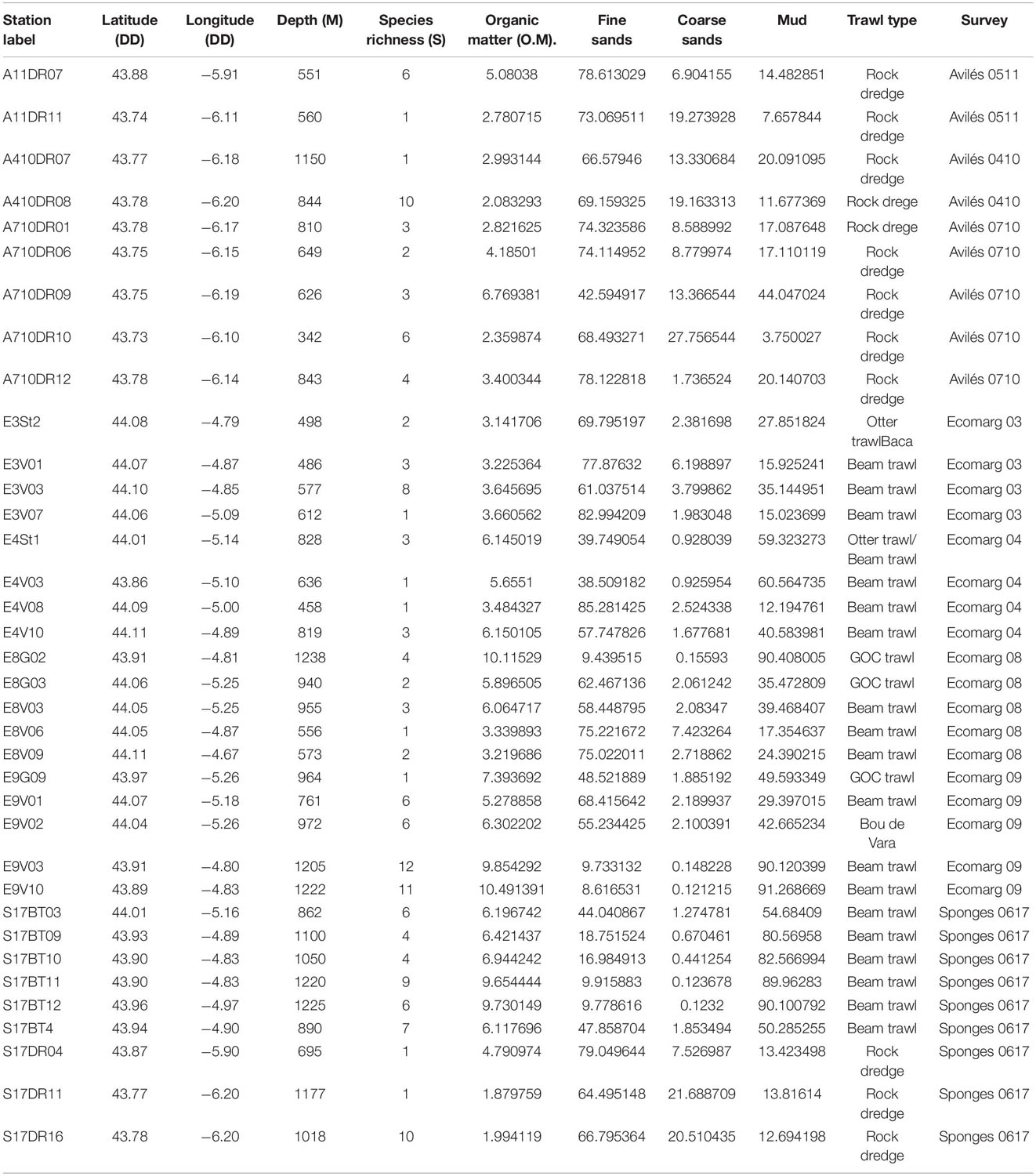
Table 1. Stations positions (DD: decimal degrees), trawl sampling methods, surveys per station included in the present study and depth (m: meters).
Material
The biological material consisted of 1261 specimens: 934 ophiuroids and 327 asteroids. Specimens were photographed and conserved in ethanol and identified based on their morphological characteristics using specialized literature (Mortensen, 1927, 1933; Lieberkind, 1935; Paterson, 1985; Clark and Downey, 1992; Southward and Campbell, 2006), and the appropriate protocols for their visualization (light microscopy or SEM).
Data Analysis
Echinoderm and sponge occurrence frequencies were calculated (to analyze the general faunal composition). Echinoderm species were classified into four categories according to their frequency across stations, which is a surrogate for evaluating their importance in the community: the most common species (50% of stations), very common species (between 25 and 50%), common species (between 25 and 10%), and rare or accidental species (<5%) (Mora, 1980; Manjón-Cabeza and García Raso, 1994; Manjón-Cabeza and Ramos, 2003, among others). To investigate the structure of echinoderms, assemblage similarities, related to sponge aggregations, were computed by a hierarchical cluster analysis (classification) using the UPGMA agglomerative algorithm (Sneath and Sokal, 1973; RMACOQUI ver. 1.0 software Olivero et al., 2011; RStudio Ver. 0.99.473) made on the similarity matrix of the Baroni-Urbani coefficients calculated from presence/absence data (Baroni-Urbani and Buser, 1976). The robustness of each cluster was supported by a test of biological significance of the boundaries between echinoderm assemblages. Strong and weak boundaries were defined between assemblages following (McCoy et al., 1986, P < 0.001). A strong boundary separates two significantly different clusters (red node number in Figure 7). A weak boundary (green asterisk in Figure 7) measures the homogeneity of species distribution between stations. When boundaries are not significant, it means that species are randomly distributed. Boundary analysis followed Olivero et al. (1998, 2011).
Stations were identified using Canonical Correspondence Analysis (CCA) computed from the presence/absence matrix and based on the eigenvalues of χ2 distances between all data points (Ter Braak and Prentice, 1988; Hennebert and Lees, 1991; Legendre and Legendre, 1998), using PAST (paleontological statistics, ver. 3.25 computer program (Hammer et al., 2001).
Three analyses were performed: (CCA1) only with% sponge occurrence as the biotic variable; (CCA2) with all non-correlated abiotic variables, in this case only depth and granulometry (latitude and longitude were discarded) and biotic variable (% sponge occurrence); (CCA3) only abiotic variables (granulometry and depth). These were used to define ordination axes on which echinoderm data (with both stations and specimens) were plotted. Environmental variables were plotted as well as correlations with ordination axes.
Results
General Faunal Composition
The faunal composition of the study area presented 42 echinoderm species (28 ophiuroids and 14 starfishes) and 21 sponge species (Table 2 and Figures 3–6). Ophiolycus purpureus, Ophiophrixus spinosus, and Ophiotreta valenciennesi were new records for the area and as such will be included in the “Echinodermata Spanish Check List” (2020 in press, update of Ministerio de Agricultura y Pesca, Alimentación y Medio Ambiente, 2017).
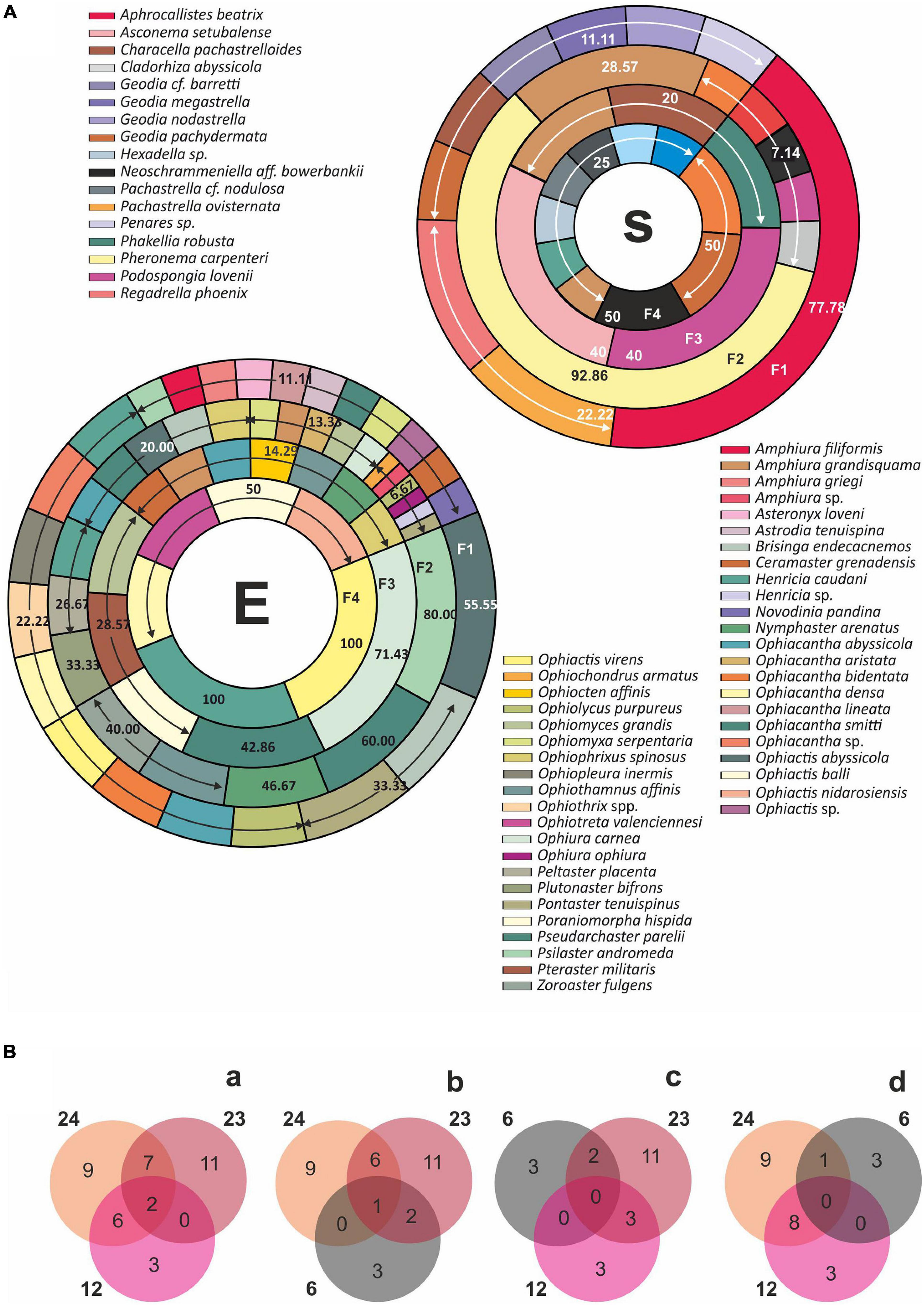
Figure 3. (A) Species richness by field. (B) Faunal composition. S: sponges; E: echinoderms, based on occurrence percentages related to the whole area of study.
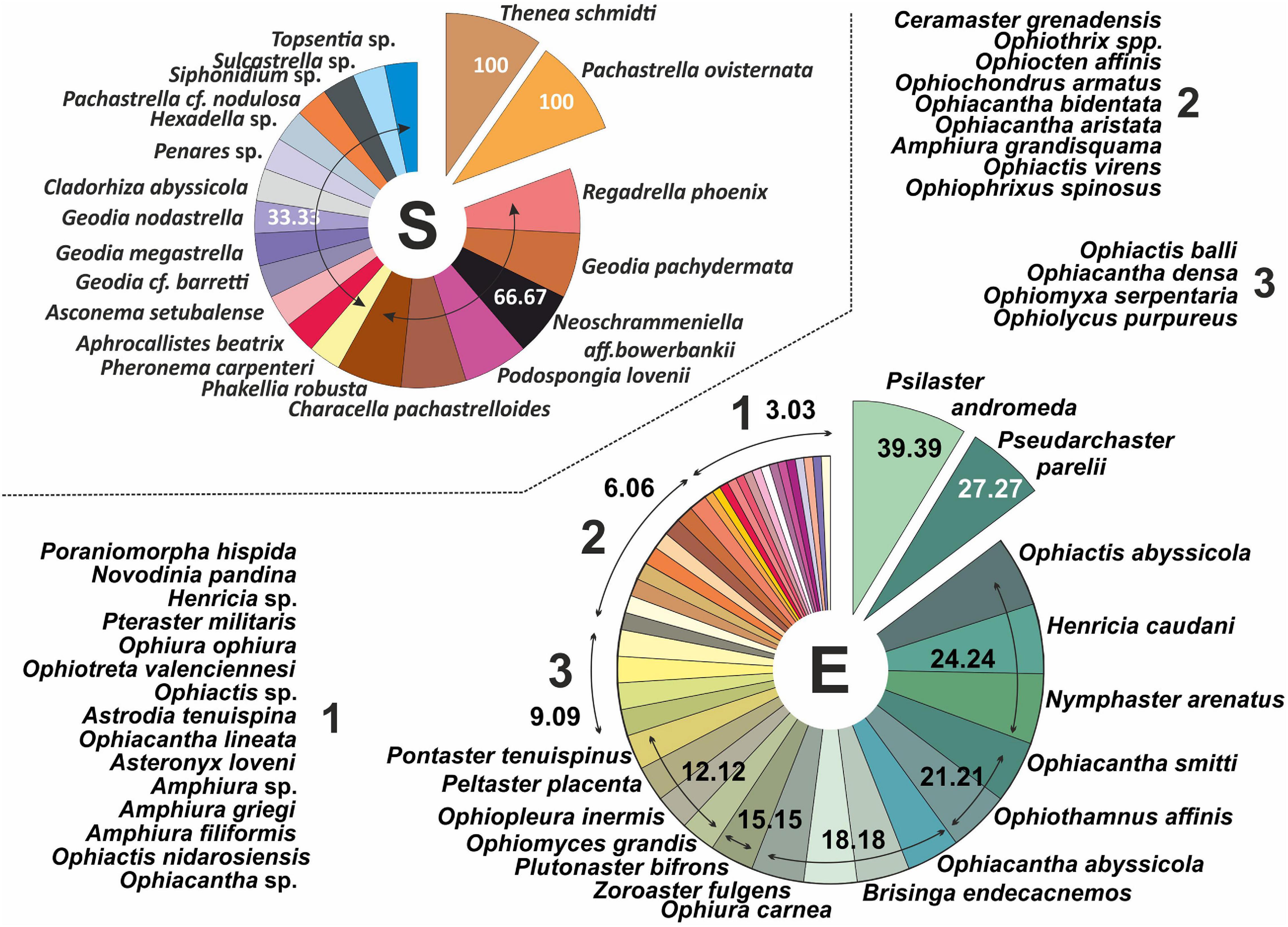
Figure 4. Faunal composition predefined sponge field (see “Materials and Methods” section). S: sponges; E: echinoderms, based on occurrence percentage related to the total number of stations included in each defined sponge field.
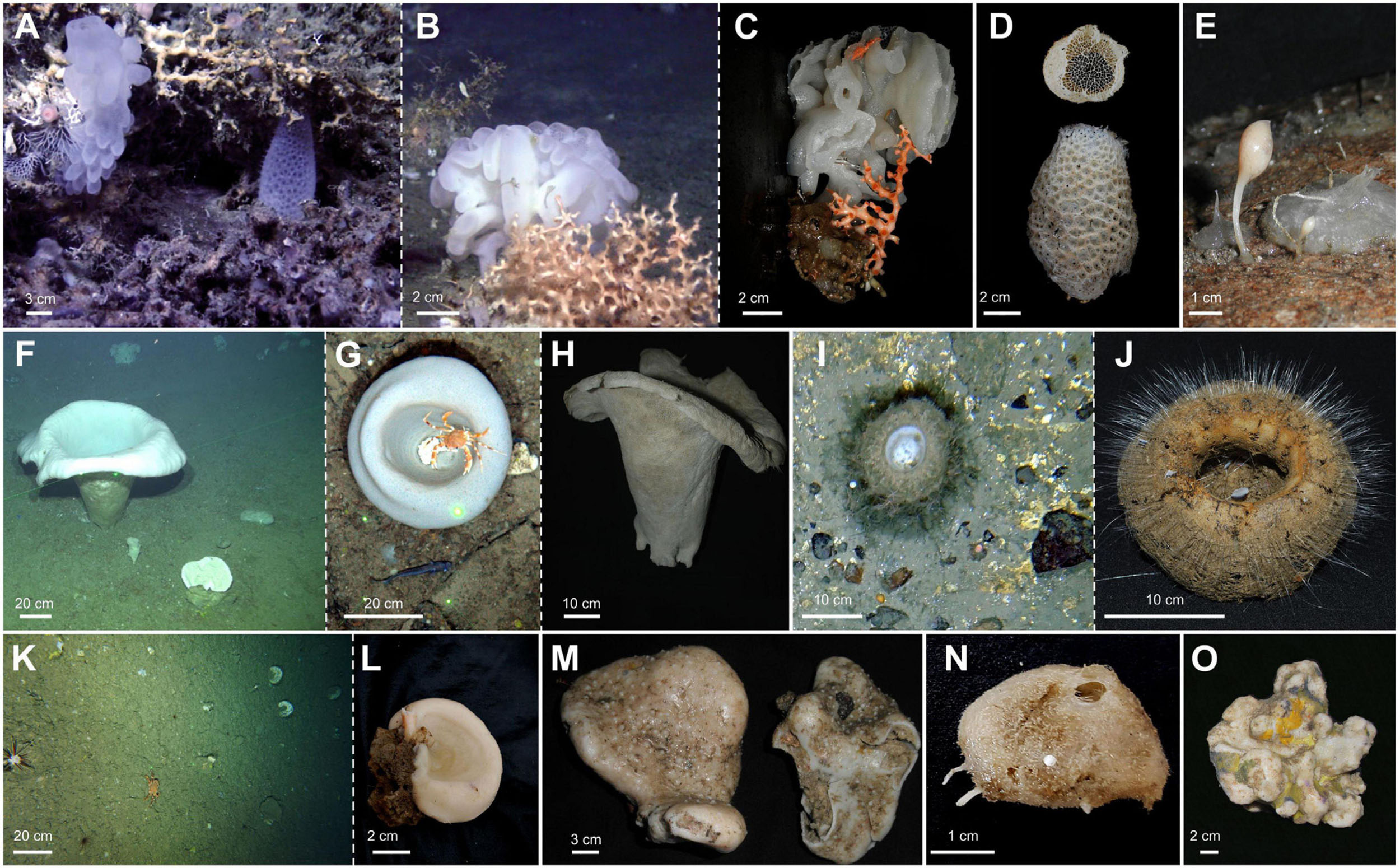
Figure 5. Habitats and species of 3D large sponge aggregations in the Cantabrian sea. (A) Aphrocallistes beatrix with Regadrella phoenix “in situ” Avilés Canyon System. Scale bar 3 cm. (B) Aphrocallistes beatrix “in situ” Avilés Canyon System. Scale bar 2 cm. (C) Aphrocallistes beatrix view of the same specimen. Scale bar 2 cm. (D) Regadrella phoenix. Scale bar 2 cm. (E) Podospongia lovenii. Scale bar 1 cm. (F,G) Asconema setubalense “in situ,” Le Danois Bank. Scale bar 20 cm. (H) Asconema setubalense. Scale bar 10 cm. (I) Pheronema carpenteri “in situ,” Le Danois Bank. Scale bar 10 cm. (J) Pheronema carpenteri. Scale bar 10 cm. (K) Neoschrameniella aff. bowerbankii “in situ” in El Corbiro Canyon. Scale bar 20 cm. (L) Neoschrameniella aff. bowerbankii. Scale bar 2 cm. (M) Geodia pachydermata.Scale bar 3 cm. (N) Thenea schmidti. Scale bar 1 cm. (O) Pachastrella ovisternata. Scale bar 2 cm.
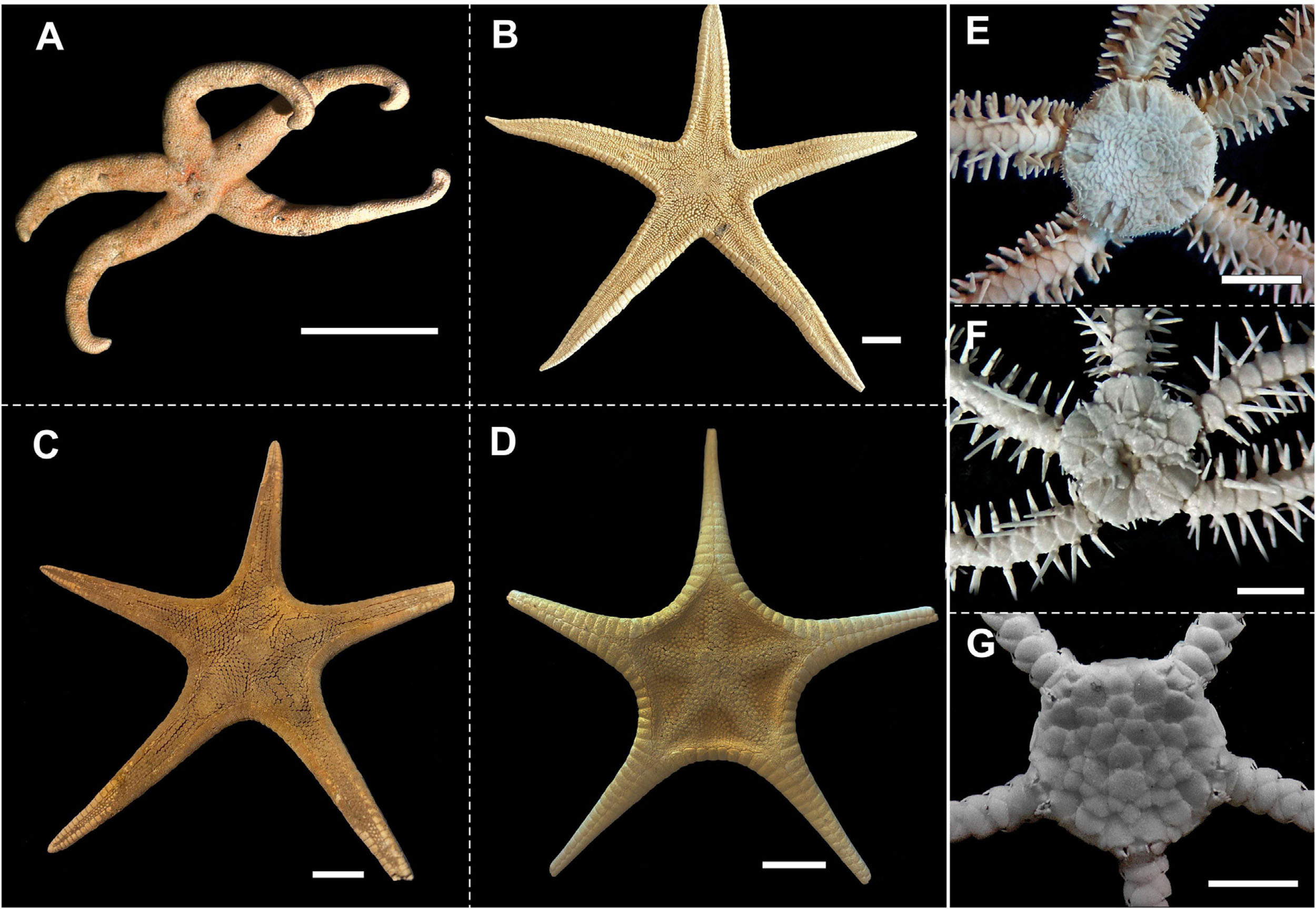
Figure 6. Images of characteristic echinoderm species from each field. (A) Henricia caudani. Scale bar 2 cm. (B) Psilaster andromeda. Scale bar 1 cm. (C) Pseudarchaster parelii. Scale bar 2 cm. (D) Nymphaster arenatus. Scale bar 2 cm. (E) Ophiactis balli. Scale bar 0.15 cm. (F) Ophiactis abyssicola. Scale bar 0.2 cm. (G) Ophiura carnea. Scale bar 0.2 cm.
Two sponge species, Thenea schmidtii and Pachastrella ovisternata, were the most common, while very common ones were: Regadrella phoenix, Geodia pachydermata, Neoschrammeniella aff. bowerbanki, Podospongia loveni, Characella pachastrelloides, and Phakellia robusta, present in more than half of occurrence of the stations (Figures 3, 5). In the case of echinoderm species, occurrence was more evenly spread between them. They were classified as: very common species (between 25 and 50%): Psilaster andromeda and Pseudarchaster parelii; common species (between 25 and 10%): Ophiactis abyssicola, Henricia caudani, Nymphaster arenatus, Ophiacantha smitti, Ophiothamnus affinis, Ophiacantha abyssicola, Brisinga endecacnemos, Ophiura carnea, Zoroaster fulgens, Plutonaster bifrons, Ophiomyces grandis, Ophiopleura inermis, Peltaster placenta, and Pontaster tenuispinus; and rare or accidental species (<5%): the rest (Figures 4, 6).
Work Field Faunal Features
Sponge (S) and echinoderm (E) composition of each field are shown in Figure 3. F1 (Aphrocallistes and Regadrella aggregation) presented nine sponge species, the most frequent of which were Aphrocallistes beatrix (77.78%) followed by Regadrella phoenix (22.22%) and Pachastrella ovisternata (22.22%) (Figures 3A–S). Twenty-three echinoderm species were recorded, 12 of which were exclusive (Figure 3B): the most commonly occurring echinoderm species was Ophiactis abyssicola (55.5%) (Figures 4A–E). F2 (Pheronema aggregation) was represented by Pheronema carpenteri with 92% of sponge species occurrence (Figure 4A-S), while the echinoderm community consisted of 24 species, nine of which were only present in F2 (Figure 3B). In this case the most common species were Psilaster andromeda with 75% and Pseudarchaster parelii with 56.25% occurrence, respectively. F3 (Asconema aggregation): Two sponges, Asconema setubalense, Podospongia loveni, established a new field with 40% occurrence. Regarding echinoderms, 12 species were distinguished, three of which were exclusive. Echinoderm composition was 14 species, 10 of which were starfish, and four of which were very rare brittle stars (in terms of occurrence). The species featured were Ophiura carnea with 55.56% and Ophiacantha smitti with 33.33%. F4 (Neoschrammeniella aggregation): the most frequent sponges (50%) were Neoschrammeniella aff. bowerbankii, Pachastrella ovisternata, and Geodia pachydermata. Six echinoderm species made up this field, three of which were exclusive. Ophiacantha densa and Henricia caudani, were recorded in all stations of this field.
Structure of Echinoderm Assemblages
Classification Analysis
Presence-absence species matrix, and occurrence percentage used for data analysis (Table 3). Cluster results display a clear discontinuity between different station groups (Figure 7, see dot colors), revealing the existence of four distinctive assemblages (G1, G2, G3, and G4), divided by strong boundaries (red nodes), while three stations did not match up with any other.
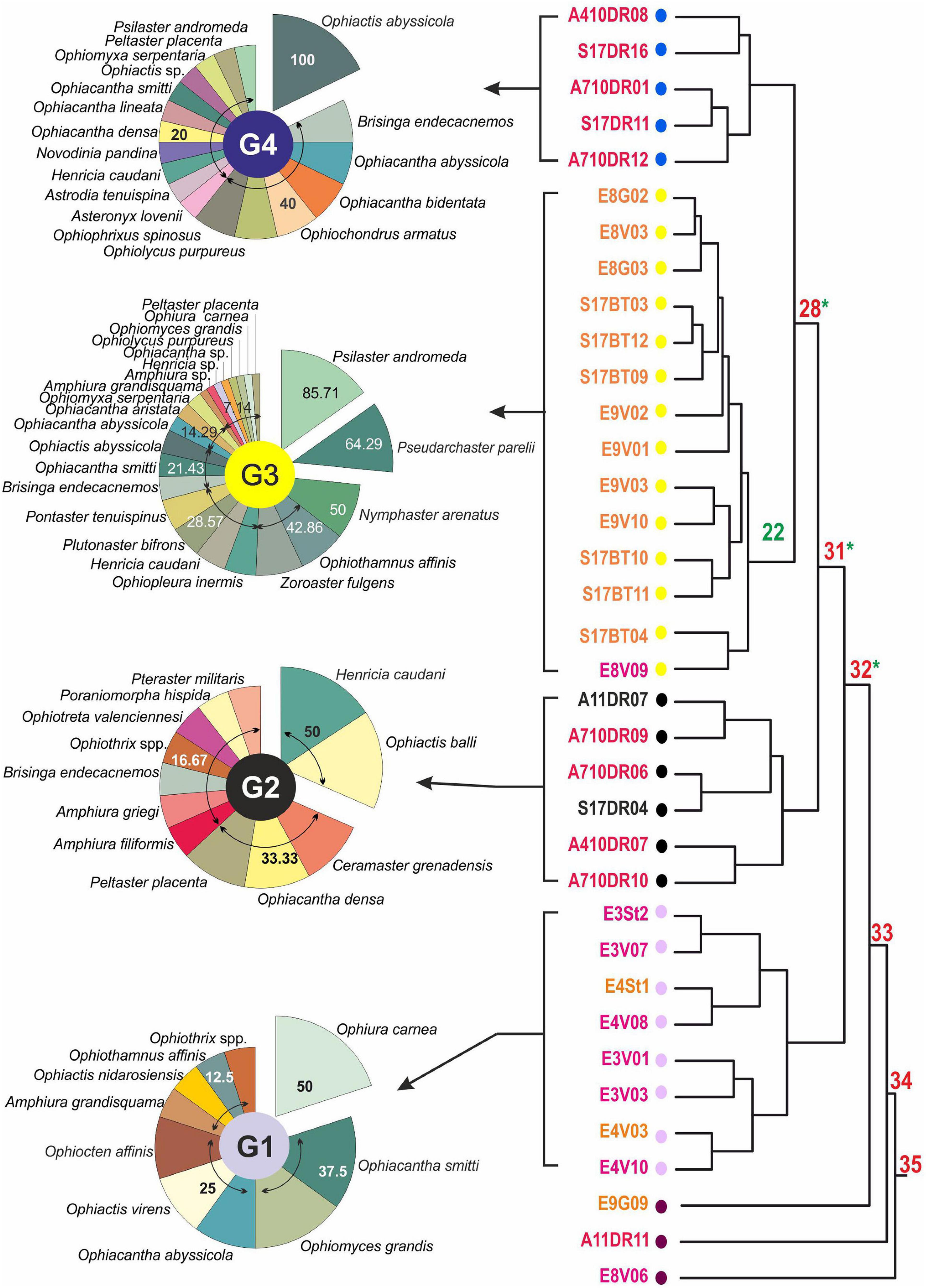
Figure 7. Cluster resulting from Echinoderm species classification (Baroni-Urbani index). Noted group color and dot colors. They are related to the cluster (Baroni-Urbani similarity coefficient) significant group, G1: purple; G2: black; G3: green; G4: blue. Node number in red illustrates strong boundaries segregating significant (P < 0.001) clusters (or groups), whereas green asterisks denote where weak boundaries (P < 0.001) were found, measuring the homogeneity of species distribution between stations included in these clusters or group. No node number shows non-significant boundaries (P > 0.001), in these cases species are randomly distributed (following Olivero et al., 1998, 2011). Sector diagrams show species occurrence percentage related to each significant cluster.
However, G3 and G4 represent very homogeneous groups because of the weak boundary found on nodes 22 and 28 (green nodes). G3 was composed of stations from work field F2, except E8V09 which belongs to F3. The most frequent species in this group were starfishes, such as Psilaster andromeda (85.71%), Pseudarchaster parelii (64.29%), Nymphaster arenatus (50%), and Zoroaster fulgens (42.85%). Only one brittle star should be mentioned, Ophiothamnus affinis (42.86%). G4, on the same cluster branch as G3, consists of stations exclusively from F1. In these cases, the most commonly occurring were Ophiactis abyssicola, (100%), followed by Brisinga endecacnemos, Ophiacantha abyssicola, Ophiacantha bidentata, Ophiochondrus armatus, Ophiolycus purpureus, and Ophiophrixus spinosus, present in 40% of stations.
G2 contains four stations from F1 and two stations from F4. In this case there was no evidence of homogeneity, but it was a consolidated group (node 31; P < 0.00001) as well as G1. This group was mainly composed of Henricia caudani and Ophiactis balli (Figure 6E) (both 50% occurrence), followed by Ceramaster grenadensis, Ophiacantha densa, and Peltaster placenta (33.33%).
G1 also presents a mix of stations from F2 (2) and F3 (6). It was made up of a group with a high dissimilarity with the rest, consisting exclusively of ophiuroid species. The most frequent species was Ophiura carnea.
Reliability of Setting to Preset Fields
Percentage fit of the different cluster groups to the working fields are: G1, 75% of F3 stations; G2, 33.33% of F4 (considering G2 is the only group with F4 stations); G3, 94.33% of F2; and G4 representing 100% of F1 stations.
Ordination Analysis
Results from CCA analysis are shown in Table 4 (Figures 8, 9). CCA1 was carried out only with sponge frequency as a biotic variable and echinoderms were ordered according to these, which was not significant (Table 4). However, when granulometry was taken into account (CCA2) (Figure 8), the CCA results became significant despite the very low% explanation. In the case of CCA3, only granulometry and depth significance were taken into account, showing the highest significance (Table 4 and Figure 9).
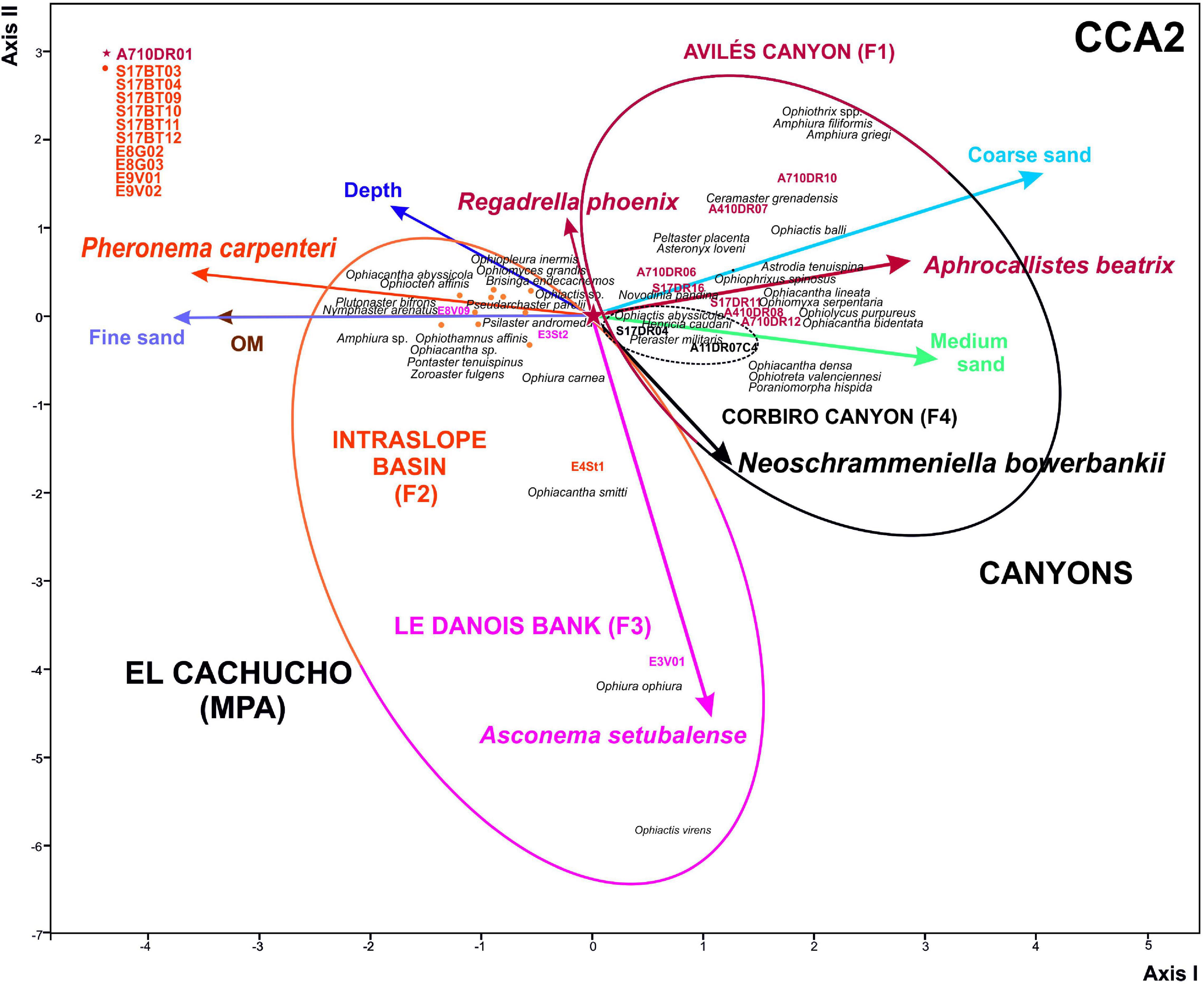
Figure 8. Ordination studies. Canonical Correspondence Analysis (CCA2). Physical environmental variables and biotic variable (most relevant sponges): Granulometry characteristics and depth. F1, 2, 3, 4 described in Figure 2. Statistical parameters and null hypothesis shown in Table 4.
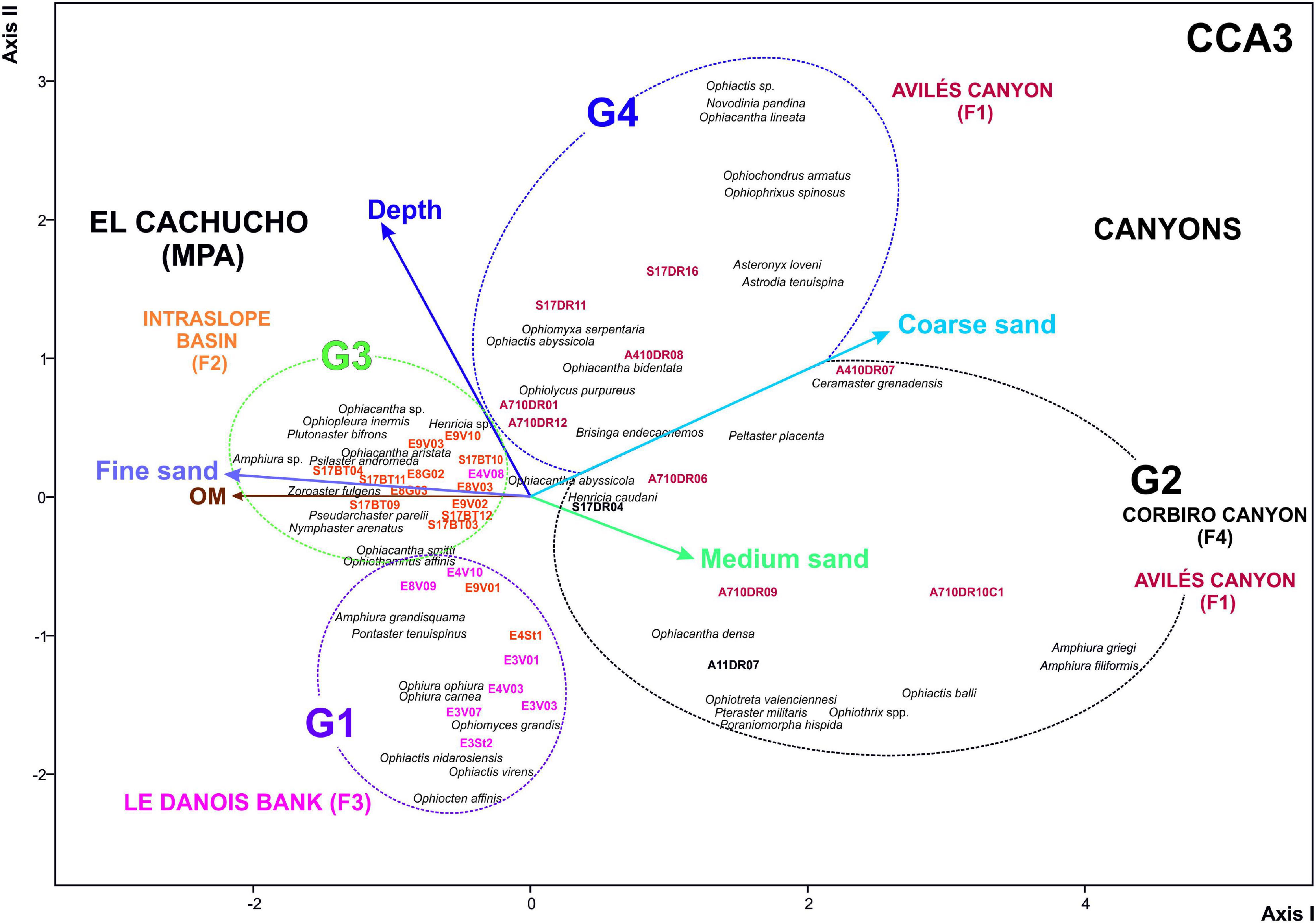
Figure 9. Ordination studies. Canonical Correspondence Analysis (CCA3). Physical environmental variables and granulometry characteristics and depth. F1, F2, F3, F4 described in Figure 2. CCA3: variables: sponge occurrence%, depth and granulometry. Statistical parameters and null hypothesis shown in Table 4.
Discussion
Echinoderm Assemblages and Control of Their Environmental Variables
The echinoderm community seems to be composed of four different communities that fit to areas with particular morphological and biological features, related to the presence of species specialized in the use of the resources they can find there (Sánchez et al., 2008; Ríos et al., 2020).
In general, the abiotic factor that mainly controls this community structure is depth. In fact, it is very frequent in echinoderm assemblage studies (Manjón-Cabeza and Ramos, 2003; Moya, 2016). This assemblage structure, which favors the dissimilarity between the canyons and El Cachucho, Marine Protected Area (MPA), is not so clear, since the deepest stations are located on the intraslope basin of the bank, therefore, its use a priori could lead to misunderstandings (Figures 8, 9).
Group G4 would represent a community associated to deep, hard bottoms covered by coarse sands. The stations fit perfectly at the head of the Avilés Canyon (Figures 2, 7). The taxa making up this community are suspension feeder species, such as brisingid species like Brisinga endecacnemos and Novodina pandina (Downey, 1986; Clark and Downey, 1992), which take advantage of the pedestals offered by the rock outcrops, or coral patches of Madrepora oculata Linnaeus, 1758; and Desmophyllum pertusum; Linnaeus, 1758 (Sánchez et al., 2014b). Coral aggregations are used as support by some ophiacanthids such as Ophiochondrus armatus, or species of the Genus Ophiacantha. However, Ophiactis abyssicola, like the rest of species in the Ophiactis genus, lives associated with bottoms that have cavities available, such as oscula sponges (Schejter et al., 2012; Sivadas et al., 2014; Çinar et al., 2019), little holes in stones or associated with dead corals or rest of calcareous algae (rhodoliths), which provides them with shelter (Gofas et al., 2014; Manjón-Cabeza et al., 2014c; Palma-Sevilla, 2015).
The community closest to G4 is G3, which is found on the intraslope basin of the Le Danois bank. This affinity is mainly due to species richness (Figures 3, 7). Depth seems to be the abiotic environmental factor controlling these two communities (Figures 7, 8), although other variables should be taken into account, such as the slope. In fact, steep areas could favor the settlement of structuring species (3D) such as Pheronema carpenteri, which would determine the echinoderm community (Cristobo et al., 2010; Sánchez et al., 2010, 2014a). In contrast with the rest of the communities studied, the species making up this one are mainly Asteroids, like Psilaster andromeda, Pseudarchaster parelii, Nymphaster arenatus, and Zoroaster fulgens, and others of lesser occurrence such as Plutonaster bifrons and Pontaster tenuispinus. This community is the most homogeneous one, and the one with the greatest specific richness. In this case, the abiotic factor mainly affecting species composition is the presence of fine sands with a high content of organic matter, preferred by sand burrow species such as those belonging to the Genus Amphiura (Sánchez et al., 2008).
On the other hand, G1 is represented by stations located mostly on Le Danois Bank which has fine sand bottoms with Asconema setubalense. These features are very well defined in previous publications where the characteristic habitats of the area are described, and a large occurrence of Callogorgia veticillata (Pallás, 1766) is attributed to the upper zone of the bank (Sánchez et al., 2008, 2017). These features, indeed, explain the presence of species as diverse as Ophiura carnea, Ophiomyces grandis, Ophiocten affinis, and Ophiothamnus affinis, which live on sandy bottoms; and species of the Genus Ophiacantha and Ophiothrix (Granja-Fernández et al., 2014) that have a preference for corals, especially gorgonians.
G2 group does not make much biological sense and its stations seem to be a consequence of the scarcity of stations from F4 in the Corbiro Canyon (only two). Another reason that could explain this artifact would be due to stations from F1, associated would have a similar sponge species contents, and Aphrocallistes beatrix were not as frequent as in the rest of stations from Avilés Canyon or in the other way round, Neoschrammeniella aff. bowerbankii, does not represent any echinoderm association. Therefore, the sea star and brittle star community of Corbiro Canyon should be more profusely studied in the near future.
Does There Exist a Real Association of Echinoderms With Sponge Aggregations?
Once the structure of the echinoderm community was known, we were able to compare the expected and obtained results in order to analyze the evidence which should prove the existence of any association between echinoderms and sponges, which enable us to refute the incongruous hypothesis.
In this way, the results obtained do not conform to any of the proposed hypotheses (Figures 1, 7), and the reasons that would explain this issue are developed below.
(1) Although station fit is quite high in G1, G3, and G4 clusters, the G2 cluster has a very low percentage station affiliation.
(2) Asteroid/ophiuroid community assemblages do not fit the sponge species composition.
Given the high percentage of adjustment that some of the fields present, it is possible that these small imbalances can be explained, since fields were delimited on the basis of main sponge species, although this occurrence may vary between stations (Figures 4, 8), and then some of them did not fit the field as we expected.
On the other hand, CCA using only an environmental variable set is more significant than using it in combination with occurrence of sponges. There are two ways to address this question: (1) sponges are not a very good biotic factor to control the echinoderm community; (2) echinoderms depend more on other bottom types (for instance related to granulometry, Figure 9), so the variable data set should be improved.
Finally, these results enable us to infer that the association of asteroids and ophiuroids with sponge aggregations is conditioned to environmental factors, like granulometry, which control fields such as habitat. Sponge species composition, or the structure they provide, would not be the main reason for explaining echinoderm assemblage structure.
Data Availability Statement
The raw data supporting the conclusions of this article will be made available by the authors, without undue reservation.
Author Contributions
MM-C and PR conceived and designed the study and wrote the manuscript. PR, TI, AR-B, FS, and JC collected the specimens and the pre-identification major taxa. PR and JC identified the sponges. MM-C, PR, AM-R, and LG-G identified the echinoderms, analyzed the data, prepared the figures and tables, reviewed drafts of the manuscript, and helped to writing the manuscript. MM-C, FS, and JC acquired the funding. All authors contributed to the article and approved the submitted version.
Conflict of Interest
The authors declare that the research was conducted in the absence of any commercial or financial relationships that could be construed as a potential conflict of interest.
Funding
This research has been performed in the scope of the SponGES and INTEMARES projects. SponGES received funding from the European Union’s Horizon 2020, Research and Innovation Programme under grant agreement no. 679849. The LIFE IP INTEMARES project, is coordinated by the Biodiversity Foundation of the Ministry for the Ecological Transition and the Demographic Challenge. It receives financial support from the European Union’s LIFE programme (LIFE15 IPE ES 012). Also, the Spanish Ministry of Environment through a management agreement with the Spanish Institute of Oceanography (IEO) has funded the scientific monitoring of the El Cachucho MPA (ECOMARG Project). This study was partially funded by the Universidad de Málaga/Instituto Español de Oceanografía agreement (contract n°: 2013/0032460 – 80625/47.6006).
Acknowledgments
This study was made possible thanks to the invaluable work of all the participants in the eight surveys involved and the crews of the R/Vs. Vizconde de Eza (SGPM), Ramón Margalef (IEO), and Ángeles Alvariño (IEO). We would like to thank Shani Jones and Meryl Jones (from Wyn Academy), American native English professional teachers for the revision of the manuscript.
References
Altuna, A. (2013). Scleractinia (cnidaria: anthozoa) from ECOMARG 2003, 2008 and 2009 expeditions to bathyal waters off north and northwest spain (northeast atlantic). Zootaxa 3641, 101–128. doi: 10.11646/zootaxa.3641.2.1
Altuna, A., and Ríos, P. (2014). Scleractinia (cnidaria: anthozoa) from INDEMARES 2010–2012 expeditions to the avilés canyon system (bay of biscay, spain, northeast atlantic). Helgol. Mar. Res. 68, 399–430.
Andrino-Abelaira, J. (2015). Estructura de la taxocenosis de Holoturoideos de la Plataforma y cañones submarinos de Patagonia. Spain: Universidad de Málaga, 48.
Ballesteros, M., Acosta, J., Muñoz, A., Rivera, J., and Sánchez, F. (2006). 5a Asamblea Hispano-Portuguesa de Geodesia y Geofísica, Estudio morfoestructural de “El Cachucho” (Le Danolis Bank) basado en batimetría multihaz. Proyecto ECOMARG, Spain: Universidad de Málaga.
Çinar, M.E., Bakir, K., Doǧan, A., Açik, S., Kurt, G., and Kataǧan, T. et al. (2019). Macro-benthic invertebrates associated with the black sponge Sarcotragus foetidus (Porifera) in the levantine and aegean seas, with special emphasis on alien species. Estuar. Coast. Shelf Sci. 22:106306. doi: 10.1016/j.ecss.2019.106306
Clark, A.M., and Downey, M.E. (1992). Starfishes of the Atlantic. Chapman & Hall. Identification Guides, 3, London: Chapman & Hall, 794.
Cristobo, J., Ríos, P., Sánchez, F., Muñoz, A., Polonio, V., González, D., et al. (2010). Diversity of sponges in bathyal coral reefs of a subsidiary canyon of Aviles Canyon complex (Cantabrian Sea). VIII World Sponge Conference, Spain: Instituto Español de Oceanografía.
Downey, M.E. (1986). Revision of the atlantic brisingida (echinodermata: asteroidea), with description of a new genus and family. Smithson. Contr. Zool. 435:57.
García-Alegre, A., Sánchez, F., Gómez-Ballesteros, M., Hinz, H., Serrano, A., and Parra, S. et al. (2014). Modeling and mapping the local distribution of relevant species on the le danois bank, el cachucho marine protected area (cantabrian sea). Deep-Sea Res. II 106, 151–164.
García-Guillén, L.M., Rios, P., and Manjón-Cabeza, M.E. (2018). Asteroideos del mar Cantábrico. Resultados faunísticos de los proyectos LIFE+INDERMARES del norte de España. I Congreso de jóvenes investigadores del Mar. Spain: Universidad de Málaga
Gofas, S., Goutayer, J., Luque, A., Salas, C., and Templado, J. (2014). “espacio marino de alborán. proyecto life+ indemares,” in del Ministerio de Agricultura, Alimentación y Medio Ambiente, ed. Fundación Biodiversidad, Spain: Agricultura, Alimentación y Medio Ambiente en España, 129.
Gómez-Delgado, A. I. (2015). Ofiuroideos de la Plataforma Patagónica Argentina. Trabajo Fin de Máster. Spain: Universidad de Málaga, 46.
Granja-Fernández, R., Herrero-Pérezrul, M. D., López-Pérez, R.A., Hernández, L., Rodríguez-Zaragoza, F. A., Jones, R. W., et al. (2014). Ophiuroidea (echinodermata) from coral reefs in the mexican pacific. ZooKeys 406, 101–145. doi: 10.3897/zookeys.406.6306
Hammer, Ø., Harper, D.A.T., and Rya, P. D. (2001). PAST: paleontological statistics software package for education and data analysis. Palaeontol. Electron. 4, 1–9.
Hennebert, M., and Lees, A. (1991). Environmental gradients in carbonate sediments and rocks detected by correspondence analysis: examples from the recent of norway and the dinantian of southwest england. Sedimentology 38, 623–642.
Hogg, M.M., Tendal, O.S., Conway, K.W., Pomponi, S.A., van Soest, R.W.M., and Gutt, J. et al. (2010). Deep-sea Sponge Grounds: Reservoirs of Biodiversity. UNEP-WCMC Biodiversity Series No. 32, Cambridge, UK: UNEP-WCMC.
Hurtado-García, J. (2016). Asteroideos (Asteroidea: Echinodermata) de la Plataforma Patagónica Argentina. Trabajo Fin de Máster, Spain: Universidad de Málaga, 52.
Mah, C. (2020). New species, occurrence records and observations of predation by deep-sea asteroidea (echinodermata) from the north atlantic by NOAA ship okeanos explorer. Zootaxa 4766, 201–260.
Maldonado, M., Aguilar, R., Bannister, R.J., Bell, J. J., Conway, K.W., Dayton, P.K., et al. (2016). “Sponge grounds as key marine habitats: a synthetic review of types, structure, functional roles, and conservation concerns”. In: Marine Animal Forests: The Ecology of Benthic Biodiversity Hotspots, eds S. Rossi, L. Bramanti, A. Gori, and C. Orejas, (Berlín: Springer International Publishing). 145–183.
Manjón-Cabeza, M.E., and García Raso, J.E. (1994). Structure and evolution of a decapod crustacean community from the coastal detritic bottoms of barbate (cadiz, southern spain). J. Nat. Hist. 32, 1619–1630.
Manjón-Cabeza, M. E., and Ramos, A. (2003). Ophiuroid community structure of the south shetlands islands and antarctic peninsula region. Polar Biol. 26, 691–699.
Manjón-Cabeza, M. E., Gómez-Delgado, A. I., and Moya, F. (2014a). Ofiuroideos Del Mar De Bellingshausen. Xviii Simposio Ibérico De Estudios De Biología Marina. Spain: Agricultura, Alimentación y Medio Ambiente en España.
Manjón-Cabeza, M. E., Palma-Sevilla, N., Gómez-Delgado, A. I., Andrino-Abelaira, J., and Ríos, P. (2014b). “Echinoderms assemblages of Aviles canyons (preliminary results) (Biscay Bay) (INDEMARES+LIFE Project),” in XVIII Simposio Ibérico de Estudios de BiologíaMarina. Libro De Resúmenes. eds P. Ríos, L. A. Suárez, and J. Cristobo, (Gijón: Centro Oceanográfico de Gijón).
Manjón-Cabeza, M.E., Palma-Sevilla, N., and Salas, C. (2014c). Los Equinodermos De La Isla De Alborán. XVIII Simposio Lbérico de Estudios de Biología Marina. Spain: Agricultura, Alimentación y Medio Ambiente en España.
McCoy, E.D., Bell, S. S., and Walters, K. (1986). Identifying biotic boundaries along environmental gradients. Ecology 68, 749–759.
Ministerio de Agricultura y Pesca, Alimentación y Medio Ambiente, (2017). Boletín Oficial del Estado. 16648–16649. Available Online at: https://www.boe.es/diario_boe/txt.php?id=BOE-A-2017-2401.
Mora, J. (1980). Poblaciones bénticas de la Ría de Arosa. Ph.D. Thesis. Spain: Universidad de Santiago de Compostela, 335.
Mortensen, T. (1927). Handbook of the Echinoderms of the British Isles. Tomo: Oxford University press, 146-254.
Moya, F. (2016). Equinodermos del mar de Bellingshausen. Ph.D. Thesis. Málaga: Universidad de Málaga, 313.
Murillo, F. J., Durán Muñoz, P., Cristobo, J., Ríos, P., González, C., Kenchington, E., et al. (2012). Deep-sea sponge grounds of the flemish cap, flemish pass and the grand banks of newfoundland (northwest atlantic ocean): distribution and species composition. Mar. Biol. Res. 8, 842–854. doi: 10.1080/17451000.2012.682583
Murillo, F.J. (2015). Estudio de los ecosistemas epibentónicos del Atlántico Noroccidental: Gran Banco de Terranova y Flemish CAP. PhD Thesis. Spain: Universidad de Santiago de Compostela.
Olivero, J., Real, R., and Márquez, A. L. (2011). Fuzzy chorotypes as a conceptual tool to improve insight into biogeographic patterns. Systematic Biol. 60, 645–660. doi: 10.1093/sysbio/syr026
Olivero, J., Real, R., and Vargas, J. M. (1998). Distribution of breeding, wintering, and resident waterbirds in europe: biotic regions and the macroclimate. Ornis Fennica 75, 153–175.
Palma-Sevilla, N. (2015). Equinodermos del mar de Alborán. Proyecto LIFE + INDEMARES. Trabajo Fin de Máster. Málaga: Universidad de Málaga.
Paterson, G. L. J. (1985). The deep-sea ophiuroidea of the north atlantic ocean. Bull. Br. Mus. Zool. 49, 1–162.
Peña-Cantero, Á.L., and Manjón-Cabeza, M.E. (2014). Hydroid assemblages from the bellingshausen sea (antarctica): environmental factors behind their spatial distribution. Polar Biol. 37, 1733–1740. doi: 10.1007/s00300-014-1557-z
Prado, E., Sánchez, F., Ríos, P., Rodríguez-Basalo, A., and Cristobo, J. (2019). In-situ growth rate assessment of hexactinellid Asconema setubalense using 3D photogrammetric reconstruction in El cachucho marine protected area (le danois bank, cantabrian sea). Front. Mar. Sci. 6:163. doi: 10.3389/conf.fmars.2019.08.00163
Punzón, A., Arronte, J.C., Sánchez, F., and García-Alegre, A. (2016). Spatial characterization of the fisheries in the avilés canyon system (cantabrian sea, spain). Ciencias Mar. 42, 237–260.
Rapp, H.T. (2019). Deliverable D1.3 Report On The 2nd Set Of Sampling Cruises. Sponges Project. Bergan: University of Bergen.
Ríos, P., Prado, E., Carvalho, F. C., Sánchez, F., Rodríguez-Basalo, A., Xavier, J. R., et al. (2020). Community composition and habitat characterization of a rock sponge aggregation (porifera, corallistidae) in the cantabrian sea. Front. Mar. Sci. 7:578. doi: 10.3389/fmars.2020.00578
Rodríguez-Basalo, A., Delgado, F., Ortega, E., Ríos, P., and Sánchez, F. (2019a). High resolution spatial distribution for the hexactinellid sponges Asconema setubalense and Pheronema carpenteri in the central cantabrian sea. Front. Mar. Sci. 6:29. doi: 10.3389/conf.fmars.2019.08.00029
Rodríguez-Basalo, A., Sánchez, F., Punzón, A., and Gómez-Ballesteros, M. (2019b). Updating the master management plan for el cachucho mpa (cantabrian sea) using a spatial planning approach. Cont. Shelf Res. 19:10. doi: 10.1016/j.csr.2019.06.010
Sánchez, F. M., Serrano, A. G., and Ballesteros, M. G. (2009). Photogrammetric quantitative study of habitat and benthic communities of deep cantabrian sea hard grounds. Contin. Shelf Res. 29, 1174–1188. doi: 10.1016/j.csr.2009.01.004
Sánchez, F., Gómez-Ballesteros, M., González-Pola, C., Punzón-Merino, A.M., García-Alegre, A., and Druet, M. et al. (2014a). “Sistema de cañones submarinos de Avilés. Proyecto LIFE +INDEMARES,” in del Ministerio de Agricultura, Alimentación y Medio Ambiente, Ed. Fundación Biodiversidad, (Madrid: Universidad de Málaga), 114.
Sánchez, F., González-Pola, C., Druet, M., García-Alegre, A., Acosta, J., Cristobo, J., et al. (2014b). Habitat characterization of deep-water coral reefs in La gaviera canyon (avilés canyon system, cantabrian sea). Deep Sea Res. Part II. 106, 118–140. doi: 10.1016/j.dsr2.2013.12.014
Sánchez, F., Muñoz, A., Cristobo, J., Serrano, A., Druet, M., and Parra, S. et al. (2010a). “Caracterización Bionómica Y Geomorfológica De Un Profundo Cañón Submarino Situado En La Zona De Influencia Del Cañón De Avilés (Mar Cantábrico),” in XVI Simposio Ibérico de Estudios de Biología Marina. (Málaga: Universidad de Málaga).
Sánchez, F., Rodríguez-Basalo, A., García-Alegre, A., and Gómez-Ballesteros, M. (2017). Hard-bottom bathyal habitats and keystone epibenthic species on Le danois bank (cantabrian sea). J. Sea Res. 130, 134–153. doi: 10.1016/j.seares.2017.09.005
Sánchez, F., Serrano, A., Cartes, J.E., Preciado, I., Arronte, J.C., and Parra, S. et al. (2010b). “Estimating The Mpa Management Effects On Le Danois Bank Deep-Sea Ecosystem (El Cachucho) Using Trophodynamic Modelization,” in Abstract book of 12 Symposium of Oceanography of the Bay of Biscay. France: Universidad de Málaga).
Sánchez, F., Serrano, A., Parra, S., Ballesteros, M., and Cartes, J. E. (2008). Habitat characteristics as determinant of the structure and spatial distribution of epibenthic and demersal communities of Le danois bank (cantabrian sea, n. spain). J. Mar. Syst. 72, 64–86. doi: 10.1016/j.jmarsys.2007.04.008
Schejter, L., Chiesa, I., Doti, B., and Bremec, C. (2012). Mycale (Aegogropila) magellanica (Porifera, Demospongiae) in the south west atlantic ocean: endobiotic fauna and new distributional information. Sci. Marina. 76, 753–761. doi: 10.3989/scimar.03490.21A
Sivadas, S., Redij, A., Sagare, P., Thakur, N., and Ingole, B. (2014). Temporal variation in macroinvertebrates associated with intertidal sponge Ircinia fusca (carter 1880) from ratnagiri, west coast, india. Indian J. Geo-Mar. Sci. 43, 921–926.
Sneath, P. H. A., and Sokal, R. R. (1973). Numerical Taxonomy. The Principles And Practices Of Numerical Classification. San Francisco: Freeman, 573.
Southward, E.C., and Campbell, A.C. (2006). “Echinoderms. synopses of the british fauna (new series),” in Linnean Society of London, ed. Crothers J.H., & Hayward P.J, (Avon: The Bath Press), 272.
Taboada, S., Altuna, A., Sánchez, F., and Junoy, J. (2019). A new species of Astacilla (crustacea: isopoda: valvifera) from the le danois bank (n spain, ne atlantic), living on Placogorgia sp. (cnidaria: octocorallia) with notes on the cnidarian host. Deep Sea Res. 148, 108–122. doi: 10.1016/j.dsr.2019.04.016
Ter Braak, C.J.F., and Prentice, I. C. (1988). A theory of gradient analysis. Adv. Ecol. Res. 18, 271–317.
Keywords: Asteroidea, asteroids, Ophiuroidea, ophiuroids, environmental control, sponge aggregations, VMEs
Citation: Manjón-Cabeza ME, Ríos P, García-Guillén LM, Macías-Ramírez A, Sánchez F, Rodríguez-Básalo A, Ibarrola TP and Cristobo J (2021) Asteroids and Ophiuroids Associated With Sponge Aggregations as a Key to Marine Habitats. A Comparative Analysis Between Avilés Canyons System and El Cachucho, Marine Protected Area. Front. Mar. Sci. 7:606749. doi: 10.3389/fmars.2020.606749
Received: 15 September 2020; Accepted: 04 December 2020;
Published: 08 January 2021.
Edited by:
Shirley A. Pomponi, Florida Atlantic University, United StatesReviewed by:
Lorenzo Angeletti, National Research Council (CNR), ItalyChris Mah, National Museum of Natural History (SI), United States
Copyright © 2021 Manjón-Cabeza, Ríos, García-Guillén, Macías-Ramírez, Sánchez, Rodríguez-Básalo, Ibarrola and Cristobo. This is an open-access article distributed under the terms of the Creative Commons Attribution License (CC BY). The use, distribution or reproduction in other forums is permitted, provided the original author(s) and the copyright owner(s) are credited and that the original publication in this journal is cited, in accordance with accepted academic practice. No use, distribution or reproduction is permitted which does not comply with these terms.
*Correspondence: Maria Eugenia Manjón-Cabeza, bWVjbG91dGVAdW1hLmVz; Pilar Ríos, cGlsYXIucmlvc0BpZW8uZXM=