- 1Institute of Ecology and Evolutionary Biology, National Taiwan University, Taipei, Taiwan
- 2Department of Life Sciences, National Chung Hsing University, Taichung, Taiwan
- 3Department of Hydraulic and Ocean Engineering, National Cheng Kung University, Tainan, Taiwan
The Indo-Pacific humpback dolphin (Sousa chinensis) has been reported to prefer estuary habitats. This study explored the environmental factors affecting a critically endangered population off the coast of Yunlin, Taiwan. We measured dolphin sighting rates and estuary characteristics affected by the watershed, including seven physical factors (watershed rainfall, watershed runoff, estuarine turbidity, pH, salinity, temperature, and dissolved oxygen) and two biological factors (estuarine net primary production and chlorophyll a concentration), at the Hsinhuwei River estuary in Taiwan. Dolphin activity was measured by sighting rate and behavioral indices for feeding and traveling between 2017 and 2018. We observed that when the maximum net production increased alongside rising temperatures in spring, both the dolphin sighting rate and foraging activity increased. This trend was maintained until heavy rainfall or increased river runoff occurred during late summer, which resulted in high turbidity in autumn and winter. Turbidity was significantly negatively correlated with dolphin activity (sighting rate and foraging). Furthermore, we found that dolphin traveling positively correlated with the chlorophyll a concentration and maximum net production factors, which could attract dolphins expecting more abundant prey fish in the estuary supported by the high primary production. This study provides empirical evidence on how estuary characteristics affected by the watershed can affect the sighting rate and behavioral activities of Indo-Pacific humpback dolphins.
Introduction
Knowledge of the spatial distribution patterns of species is crucial for their conservation (Ferrier, 2002; Rushton et al., 2004) as it improves our understanding of the relationship between environmental factors and animal activities. Moreover, it elucidates habitat preferences. The Indo-Pacific humpback dolphin (Sousa chinensis) is found in the eastern Indian and the western Pacific Oceans, mainly in coastal and shallow waters at a depth of less than 20 m; they only rarely travel to waters deeper than 25 m (Jefferson, 2000; Folkens and Reeves, 2002; Shirihai et al., 2006). Since the habitat of S. chinensis typically overlaps with areas of human activity and industrial development, these dolphins have been experiencing direct or indirect negative effects, including environmental pollution, habitat destruction, ship collisions, fishing net entanglement, and noise pollution (Ross et al., 2010). The International Union for Conservation of Nature Red List of Threatened Species identifies the Taiwanese population found off the western coast as a subspecies (Sousa chinensis ssp. taiwanensis) and as critically endangered (Wang and Araujo-Wang, 2018). In Taiwan, the habitat of this dolphin is mainly concentrated around the central section of the west coast, ranging within a narrow strip from the Longfeng Harbor in Miaoli to the General Harbor of Tainan county, mainly within 3 km of land, in water at a depth of less than 20 m (Chou et al., 2014, 2018; Chou, 2018). In addition, its habitat has often been described as including estuarine areas (Dares et al., 2014; Chen et al., 2016; Karczmarski et al., 2016). Jefferson and Hung (2004) suggested that the range of the Indo-Pacific humpback dolphin is mainly dependent on the abundance of food resources. Generally, estuaries are recognized as fish aggregation areas, but not all estuaries are suitable for dolphins. For example, the distribution of dolphins in Taiwan is not consistent across all estuaries along the western coast (of which there are at least 20). Therefore, the association between estuary characteristics and dolphin activities requires further investigation.
The abundance of dolphins depends on the availability of the fish they prey on (Young and Cockcroft, 1994), which is influenced by primary productivity through both environmental factors (Young and Phillips, 2002) and rainfall (Lin et al., 2015; Sprogis et al., 2018). Among the ∼20 estuaries within the habitat range of S. chinensis in Taiwan, the Hsinhuwei estuary is a hotspot (Chou, 2018) for dolphin sightings. Lin et al. (2015) found that their activity pattern in the Hsinhuwei estuary significantly varied with the amount of rainfall. Moreover, they suggested that dolphin activity may be related to estuary productivity. Pan et al. (2016a) demonstrated that primary production in the Hsinhuwei estuary was higher than in two other nearby locations. This means that the area could support larger numbers of invertebrates and predatory fish, allowing for a larger population of Indo-Pacific humpback dolphins in the estuary. Many environmental factors and primary production in an estuary can be significantly affected by river runoff and the precipitation that falls within the drainage area (Cloern et al., 2014; Hosen et al., 2019). However, how watershed characteristics affect the activities of Indo-Pacific humpback dolphins in estuaries remains unclear. This study empirically explored the seasonal variation in and the association between environmental factors affected by the watershed and activity levels of Indo-Pacific humpback dolphins in the Hsinhuwei estuary.
Materials and Methods
Boat Surveys Near Hsinhuwei Estuary
From 2017 to 2018, boat surveys were conducted along two parallel transect lines, which ranged from the Zhuoshui River (23°52′N) to the south of Taizi village (23°34′N) in Yunlin county, approximately 1 NM from the land for the nearshore line (depth, ∼8 m) and 1.5 NM from the land for the offshore line (depth, ∼14 m). For this study, we selected sections near the Hsinhuwei River estuary (1 NM northward and southward from the middle of the estuary; Figure 1). A CT2-class fishing boat, approximately 11-m long, was utilized for surveys at speeds between 4 and 9 knots. At least four observers were on board, with three people using binoculars (8× and 10× magnification) and unaided eyes searching and observing at three positions (the bow and at a high position on both the left and right sides). The fourth person recorded the data and was provided with the opportunity to rest. The observers’ positions were shifted every 20 min. When a dolphin group was sighted, their GPS location, behavior types, and group size were recorded. The observers shot photos or videos of dolphins for later individual identification and behavioral confirmation.
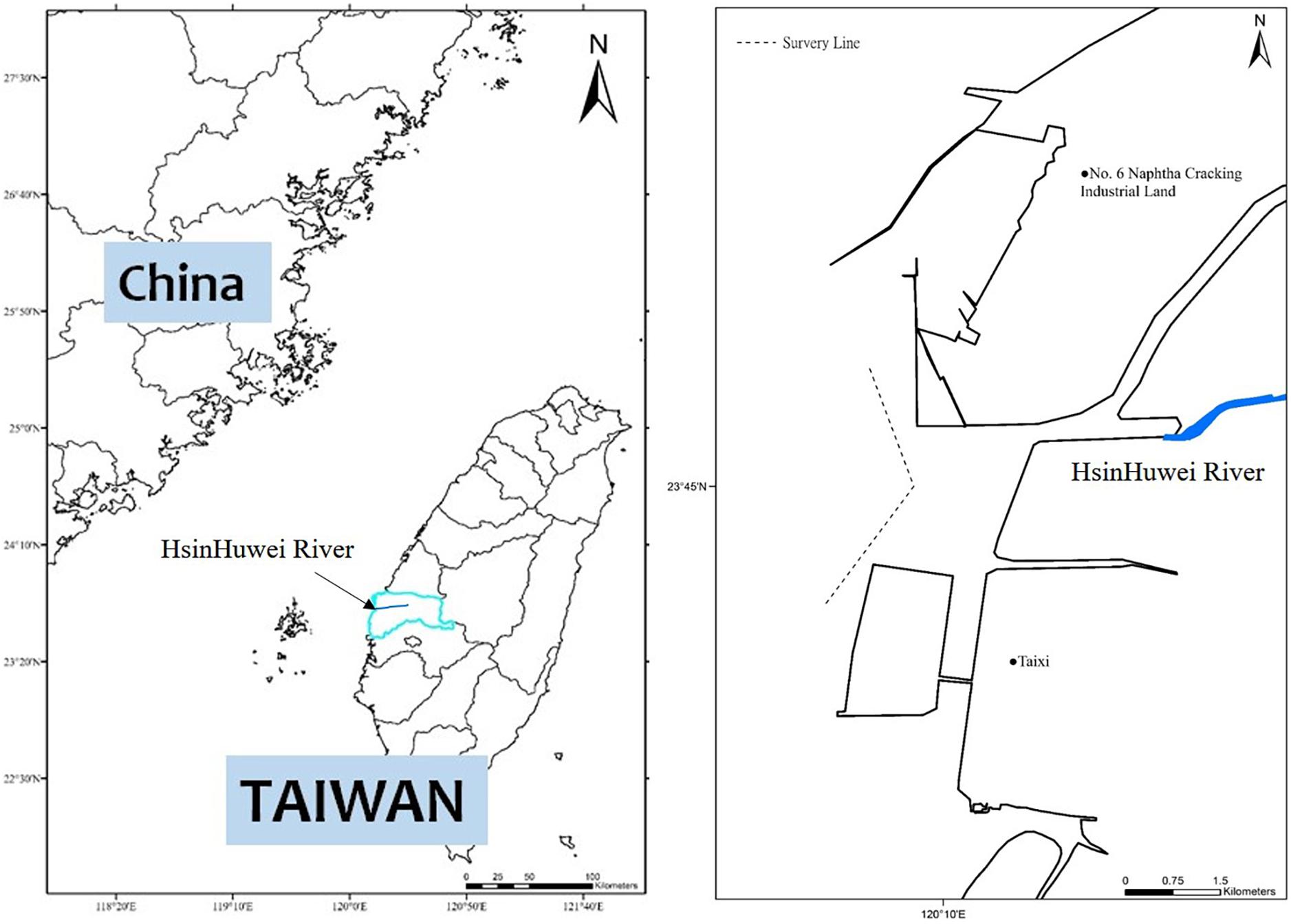
Figure 1. Survey area and the two parallel survey lines (solid and dashed “V”-shaped lines) near the Hsinhuwei River estuary.
Dolphin Activity Indices
Dolphin activity was represented by three indices, namely, the sighting rate (SR), foraging behavioral index (BIF), and traveling index (BIT).
A standardized SR was calculated as follows:
where effective effort refers to the distance traveled on the fixed transect lines not including dolphin focal tracking (as opposed to ineffective effort, which refers to the distance traveled while observing dolphins, during boat failure, or any other unpredictable event that resulted in a deviation from the fixed route).
Dolphin behavior can be classified into five types: traveling, resting, maternal caring, foraging or feeding, and socializing or playing (Pryor and Norris, 1991). Only two types, foraging or feeding and traveling, were observed during the survey sections in this study. Traveling refers to the persistent movement of dolphins, either alone or in groups, in a constant direction. Foraging or feeding is when dolphins dive and chase after prey or even toss fish out of the water.
These two types of behavior were quantified in terms of frequency and duration. When a particular group of dolphins was tracked, the observers recorded their behavior types every 3–5 min (the mean interval was ∼4 min), which enabled the calculation of the behavior type frequency per 4-min interval. In terms of duration, the index for behavior x (BIx) was calculated as the proportion of time behavior x (bx) was observed during the total observation time period (T) during each survey as follows:
where bx denotes the length of time a dolphin group engaged in behavior x during each survey, and T denotes the total time a dolphin group was tracked.
Rainfall and Runoff Estimates in the River Basin of the Hsinhuwei River
The Hsinhuwei River flows 49.85 km and has a basin area of approximately 109.26 km2 (Water Resources Agency, Ministry of Economic Affairs, 2018). Land use in the river basin is mostly agricultural (78.4%), and residential and industrial areas account for 20.9 and 0.64%, respectively. Because the Hsinhuwei River basin has no streamflow station, the total daily runoff at the river mouth was estimated using the following steps. First, six rainfall stations, namely, Taixi, Lunbei, Erlun, Huwei, Wutong, and Douliu (Figure 2), were used to determine the average daily rainfall within the basin using Thiessen’s polygon method. Second, the daily direct runoff was calculated on the basis of average rainfall using Equation 1. Finally, the total daily runoff was calculated as the sum of the base flow (Chou et al., 2018) and the direct runoff:
where Q denotes the direct runoff (cm); C, the runoff coefficient (0.7 was determined considering the land use conditions of the Hsinhuwei River basin); i, the average rainfall (m day–1); and A, the drainage area (m2).
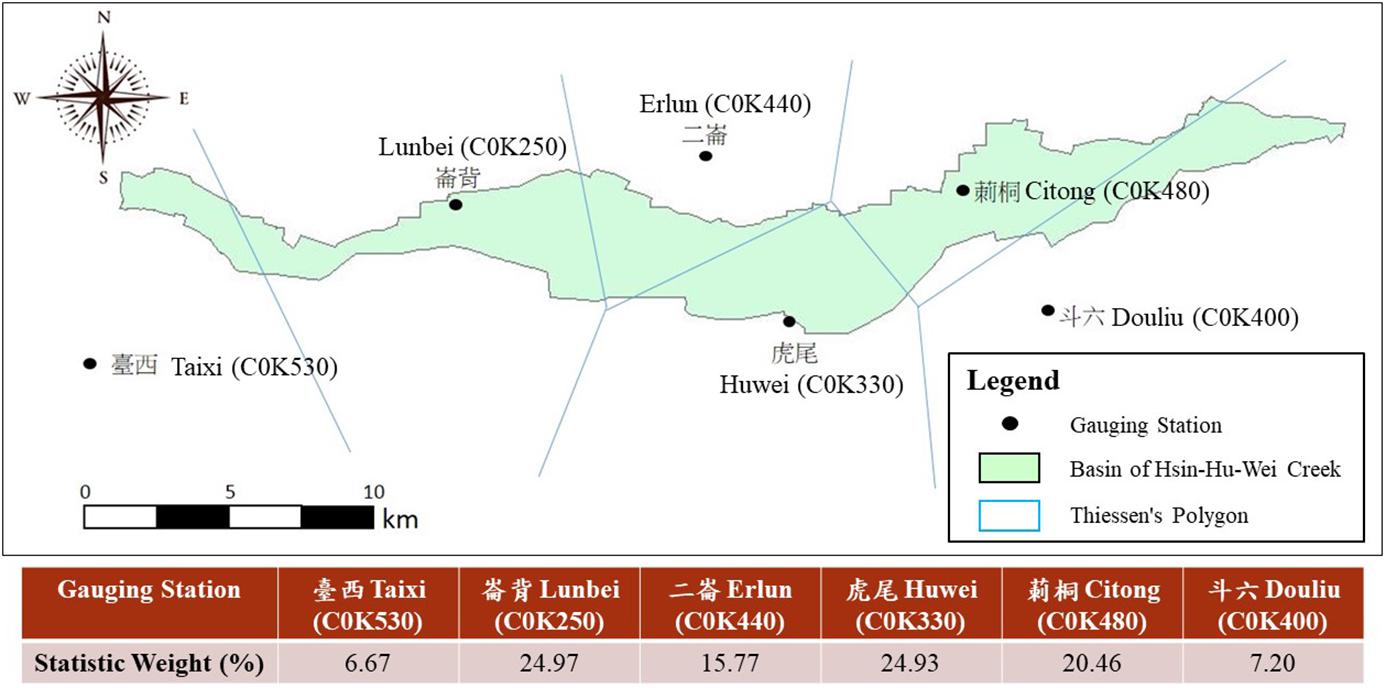
Figure 2. Division using Thiessen’s polygon method in the Hsinhuwei River basin for the estimation of rainfall and runoff variables. The full water flow range was 109 km2.
Measurement of Chlorophyll a Concentration and Primary Production
Seasonal water samples were collected at high tide from 05:00 to 07:30 on sunny days during the eight seasons of the study period. Data for the first season (winter 2017) was missing; as a substitute, we used measurements conducted during winter 2019.
The productivity of phytoplankton was measured in the field close to the estuary of the Hsinhuwei River using the dissolved oxygen (DO) method (Marshall, 2005). Local water was collected and maintained in biological oxygen demand (BOD) bottles (300 mL) in triplicate. Each group of BOD bottles was shaded at 0, 30, 50, 70, and 100% by covering them with screens of different mesh sizes on sunny days under ambient light conditions around noon (10:00 to 14:00) when irradiance was at the saturation level for photosynthesis. A DO sensor (YSI 52, YSI Inc., OH, United States) was used to measure the DO concentration in water (mg L–1) before and after culturing. The net production of phytoplankton was derived from the change in the DO concentration in the BOD bottles using Equation 2:
where NP denotes the net production per unit time and volume (mg C m–3 h–1), [O2]start and [O2]end, the start and end of DO concentrations (mg L–1) of the 0% shaded BOD bottle, respectively; tp, the culture time (h); 32, the molecular weight of O2; and 1.2, the molar ratio of produced oxygen and fixed carbon dioxide through phytoplankton photosynthesis (Hatcher et al., 1977). The net production of phytoplankton as a function of incident irradiance was described by the modified equation of Jassby and Platt (1976):
where NPm denotes the maximum NP (mg C m–2 h–1) in the absence of photoinhibition under optimal irradiance; α, the initial slope of the line under low irradiance when photosynthesis is assumed to be proportional to photon density; and E, the irradiance measured as photosynthetically active radiation in μmol photons m–2 s–1. Photosynthesis–irradiance (P–I) curves were plotted using SigmaPlot (version 12.5; Systat Software Inc., San Jose, CA, United States) for nonlinear regression fitting, and the results were regarded as acceptable when the fit was significant (p < 0.05) and the power of the conducted test was > 0.80. In this study, the maximum NP was used to represent the potential net primary production for the food web in the estuary.
Measurement of Environmental Factors
A YSI 600XLM (YSI, United States) device was utilized to measure the environmental factors (water temperature, DO, salinity, and pH value) at each station. A Lamotte 2020e Turbidity Meter (LaMotte, United States) was used to measure the turbidity of the surface water. Each environmental factor was measured five times at each station.
Definition of Seasons
Four seasons were defined according to monthly average seawater temperature, and 20 and 28°C were used as cutoff points. Winter (December–March) temperatures ranged between 14 and 20°C, and summer (June–September) temperatures ranged between 28 and 32°C. In spring (April–May) and fall (October–November), the temperatures ranged between 20 and 28°C. All weather data were obtained from the Fenzi Central Weather Bureau station in Yunlin county.
Data Analysis
Comprehensive environmental and biological factors and habitat use indicated by SR and behavioral indices (BIT and BIF) were analyzed by determining the correlations between the two sets of data. To obtain independent variables, principal component analysis (PCA) was conducted on nine environmental and biological factors, which could then be used for correlation analysis with dolphin SR as well as BIT and BIF.
Results
In total, 45 boat surveys were conducted between 2017 and 2018. The surveys were unevenly distributed among the seasons as unfavorable sea conditions during winter frequently prevented fieldwork. A total of 20 single-species (S. chinensis) dolphin groups were sighted on the nearshore transect line, with no sightings on the offshore line. The group size ranged from 1 group to 13 groups, with a mean size (standard deviation; SD) of 6.25 ± 4 (n = 8; Table 1). The mean SR for the nearshore transect line was 2.98 (SD = 1.08, n = 8). The dolphin tracking duration for each sighting ranged from 6 to 56 min per group (mean 31 ± 15 min). The total frequency and duration, respectively, were 106 counts and 419 min for foraging behavior and 50 counts and 201 min for traveling behavior (Table 2). Foraging behavior accounted for 68% of the total counts and duration.
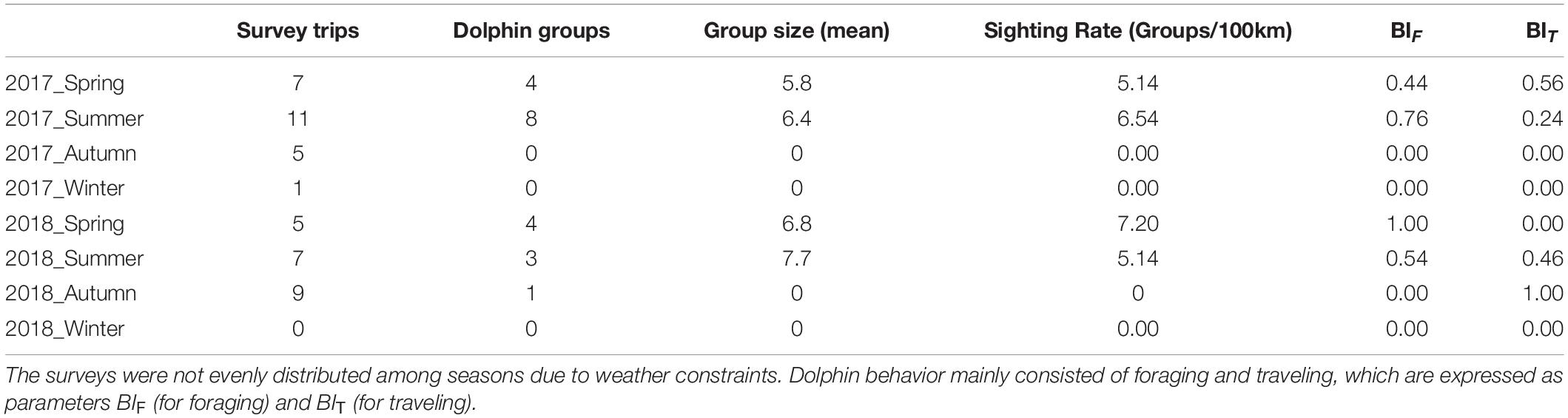
Table 1. Data for 45 dolphin surveys conducted during eight seasons between 2017 and 2018 off the coast of Yunlin county, Taiwan.
The mean chlorophyll a concentration was 2.49 ± 0.93 (mg m–3), with the highest values observed in the spring of 2017 and summer of 2018. The maximum mean NP was 3.75 ± 3.59 (mg C m–3 h–1), peaking at 15.35 (mg C m–3 h–1) during spring and bottoming at -13.53 (mg C m–3 h–1) in the autumn of both years.
Of the seven physical environmental factors investigated, only pH and salinity did not exhibit a clear seasonal variation. The temperature ranged between 17.8 and 29.9°C, reflecting typical seasonal temperature changes. The average rainfall and runoff in the basin were 136.4 ± 62.2 mm and 295.3 ± 68.7 m3 s–1 day–1, respectively, but the values widely varied between seasons; for example, high values in summer (342.76–484.66 mm and 539.34–662.49 m3 s–1 day–1, respectively) and considerably lower values (18.64 mm and 142.56 m3 s–1 day–1, respectively) in autumn. Turbidity exhibited smaller seasonal variation, ranging from 5.72 NTU to 38.57 NTU; whereas, DO ranged from 4.74 to 9.34. The values of these two factors were lower in spring and summer and higher in autumn and winter. Salinity and pH did not exhibit obvious seasonal variations; their ranges were 26.45–33.38 ppt and 7.4–8.23, respectively (Table 3).
The results of the PCA highlighted two major independent factors that explained 61 and 21% of the variance observed in the environmental changes in the Hsinhuwei estuary (Table 4). Factor 1 was significantly loaded by temperature, DO, chlorophyll a concentration, and turbidity (with loading coefficients greater than 0.86 or lower than −0.87). Factor 2 was mainly loaded by pH, with a coefficient of −0.88.
The layout of the environmental characteristic sets of the seasons according to PCA Factor 1 and Factor 2 demonstrated a significant seasonal–cyclic change among three clusters. The autumn and winter seasons formed cluster 1, whereas spring and summer formed clusters 2 and 3, respectively. When the temperature increased in spring and summer, the reductions in turbidity and DO was accompanied by increases in chlorophyll a concentration and maximum net production, which facilitated the transformation of cluster 1 into cluster 2. When rainfall and runoff significantly increased in summer, cluster 2 shifted to cluster 3 (Figures 3, 4). Correlation analysis of the environmental factors revealed the following: maximum net production increased with high chlorophyll a concentration in spring; river runoff was positively correlated with rainfall and affected the salinity in the estuary; and an increase in temperature led to a decrease in DO. These results suggest that the seasonal changes in the environmental factors of the Hsinhuwei River estuary were significantly affected by rainfall and runoff in the basin (Table 5).
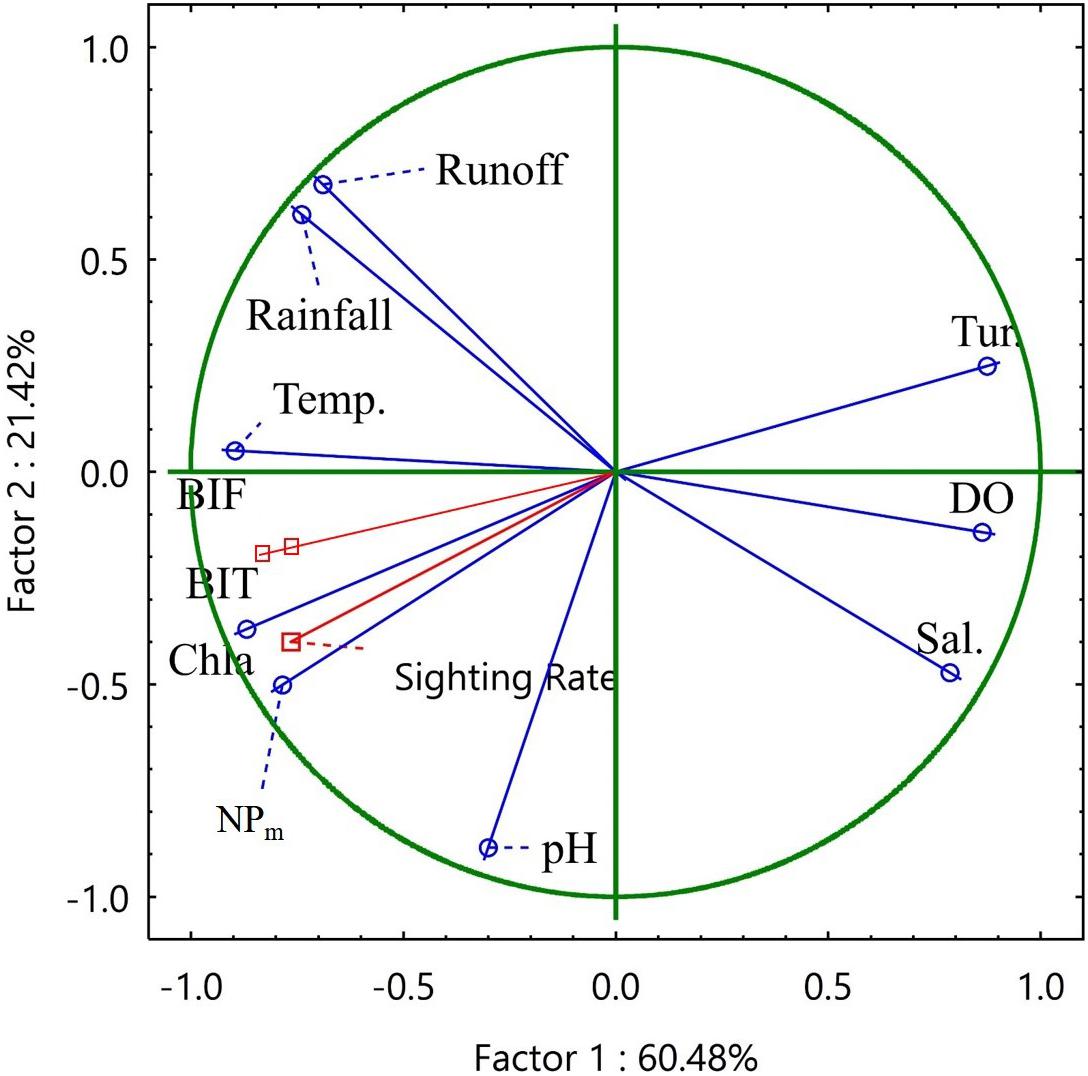
Figure 3. Principal component analysis of the environmental factors. The analysis revealed that Factor 1 was mainly loaded by rainfall, runoff, temperature, and chlorophyll a concentration, whereas Factor 2 was only influenced by pH. Dolphin activities, as indicated by the sighting rate, foraging, and traveling activity, were significantly related to Factor 1.
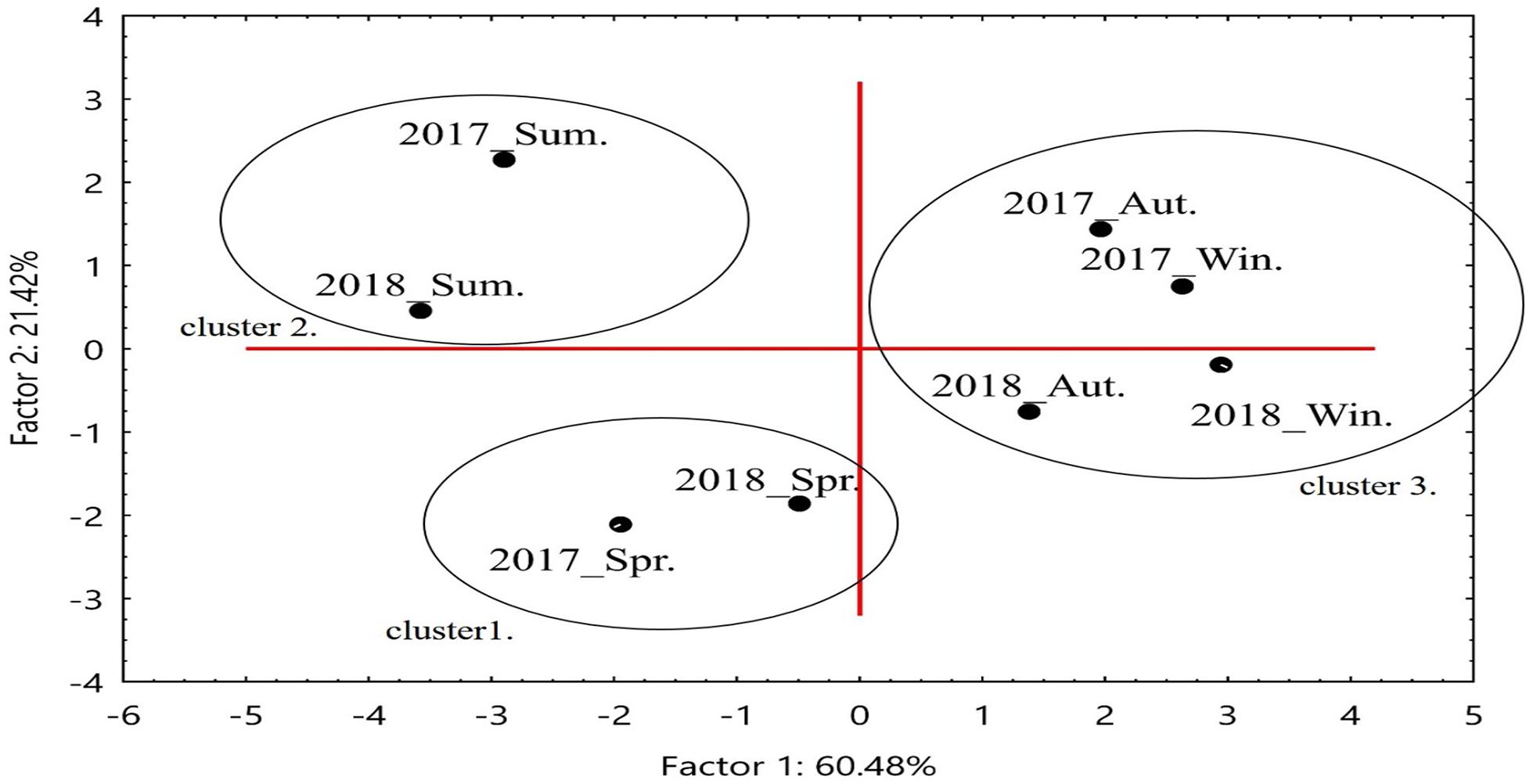
Figure 4. Seasonal variation of the environmental factors expressed by Factors 1 and 2. Data revealed an obvious cycle, starting with high maximum net production in spring and proceeding to high rainfall and runoff in summer as a result of the rainy season and typhoons, which led to significant changes in dissolved oxygen, turbidity, and salinity in the estuary area.
In the correlation analysis between Factor 1 and Factor 2 with dolphin SR, only Factor 1 demonstrated a significant association (p < 0.05; Figure 5). When the four major loaded variables of Factor 1 were individually applied to correlation tests with dolphin activity variables, we found that SR was significantly negatively correlated with turbidity and DO (p < 0.01 and p < 0.05, respectively) but significantly positively correlated with temperature (p < 0.05; Figure 6). With regard to behavioral activity, the BIF coefficient exhibited a similar trend, being significantly negatively correlated with turbidity but significantly positively correlated with temperature (p < 0.05; Figure 7). However, while BIT was not significantly correlated with turbidity, it was significantly positively correlated with chlorophyll a concentration and maximum net production (p < 0.01 and p < 0.05, respectively; Figure 8). It is clear that seasonal variations in the occurrence and behavioral activities of S. chinensis in the Hsinhuwei estuary corresponded well with the seasonal changes in rainfall and runoff in the basin and the resulting estuary characteristics.
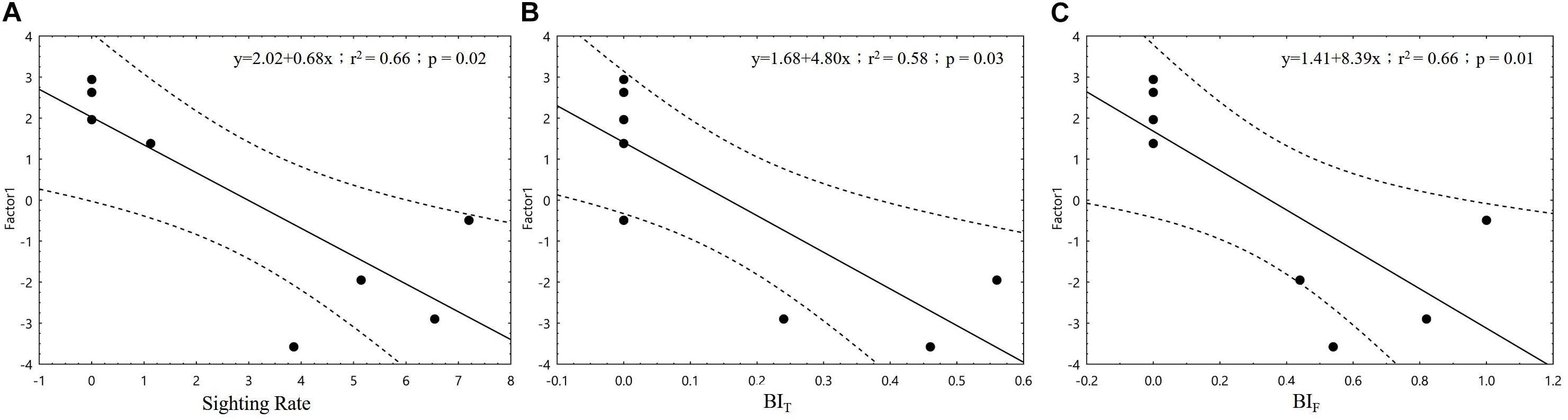
Figure 5. There are negative correlations between three dolphin activity indices [sighting rate (A), foraging (B), and traveling (C)] and Factor 1.
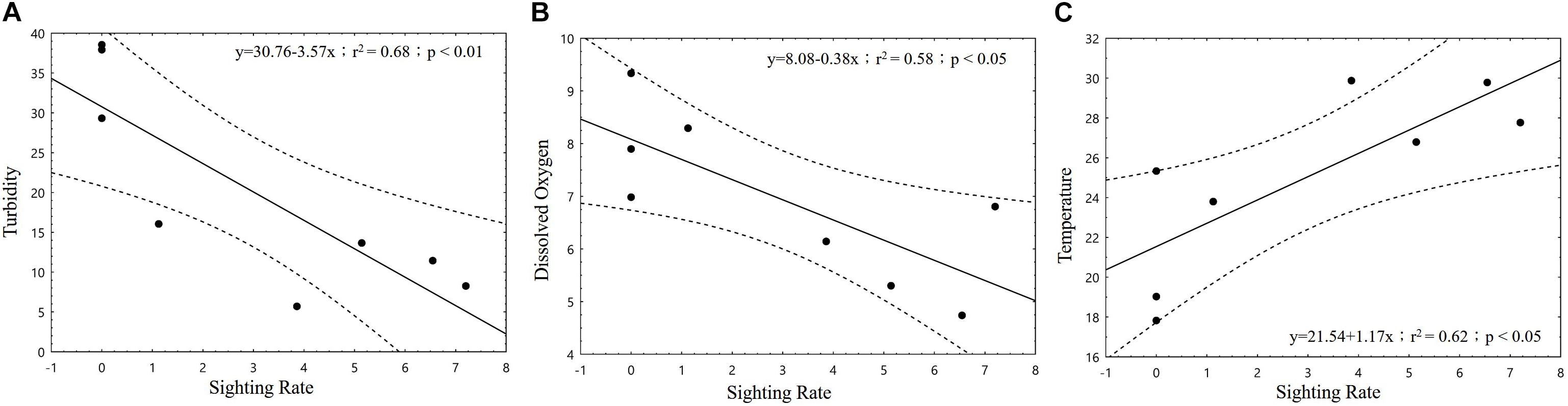
Figure 6. Correlations between dolphin sighting rate (SR) and environmental factors. Both turbidity (A) and dissolved oxygen (B) exhibited significantly negative correlations with SR (p < 0.01 and p < 0.05, respectively), whereas temperature (C) revealed a significant positive correlation with SR (p < 0.05).
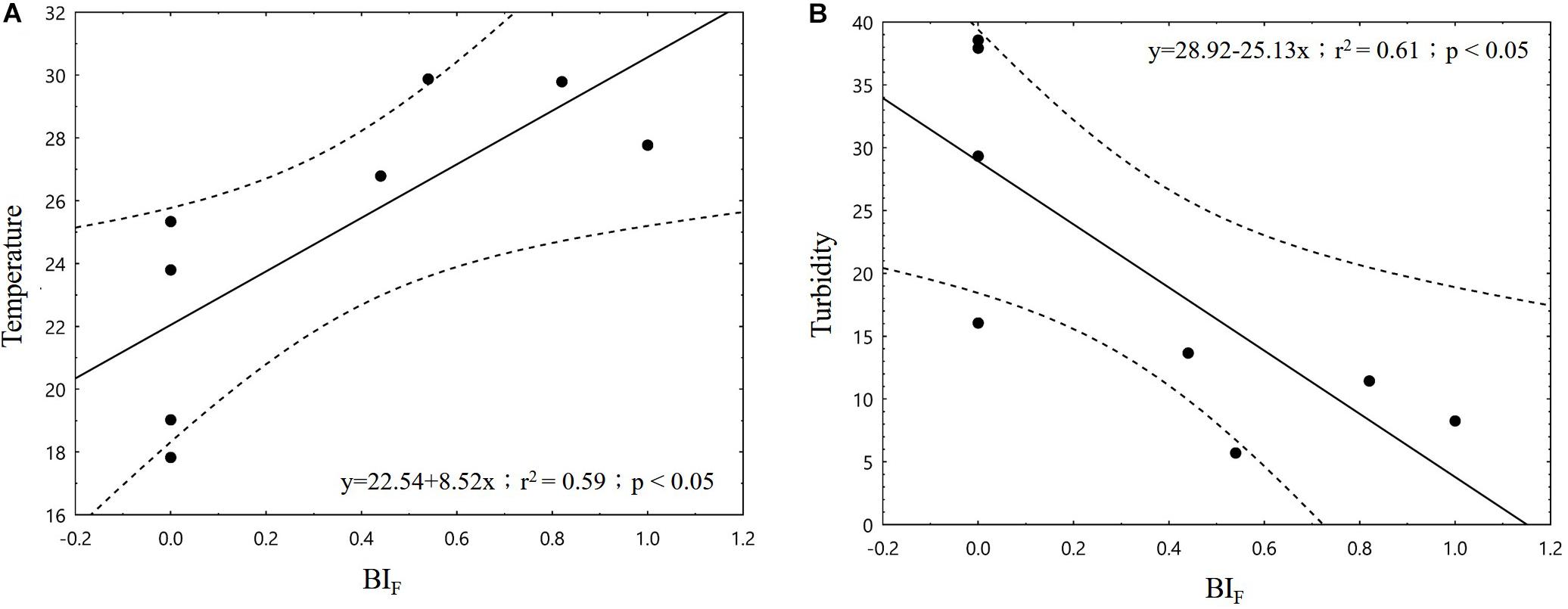
Figure 7. Foraging behavioral index was significantly positively correlated with temperature (A) but negatively correlated with turbidity (B) (p < 0.05). BIF, foraging behavioral index.
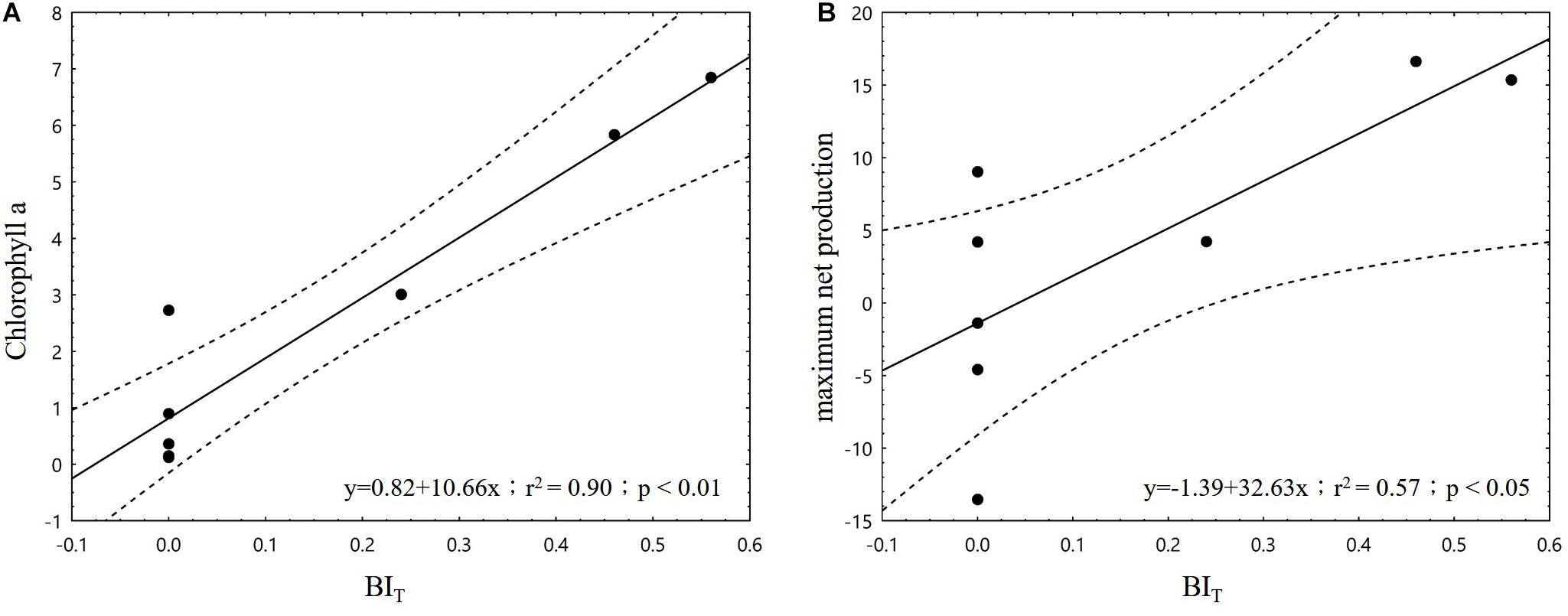
Figure 8. Correlations between the traveling behavioral index and biological factors. Significantly positive correlations were observed between traveling behavioral index and both chlorophyll a concentration (A) and maximum net production (B) (p < 0.05). BIT, traveling behavioral index.
Discussion
This study revealed not only that dolphin activities exhibit seasonal changes but also that the environmental factors of the Hsinhuwei estuary have obvious cyclic changes between seasons. Although the sample size in this study was small, similar results in terms of changes in dolphin sightings were observed through long-term acoustic monitoring at the same estuary (Lin et al., 2015). Seasonal variations in the acoustic dolphin detection rate were correlated with estuary runoff, positively in the dry season with lower levels of runoff (<5 mm) but negatively in the wet season with much higher levels of runoff (>5 mm; Lee, 2016). Seasonal variations in humpback dolphin sightings indicate an outward shift from the estuary during the rainy season and after periods of heavy rainfall resulting from typhoons (Lin, 2013). This finding is consistent with those from studies on bottlenose dolphin activity conducted by Gibson et al. (1996) and Hampel et al. (2003). They reported that seasonal changes affected the movement pattern of bottlenose dolphins, with a higher frequency of sightings during spring flooding. They claimed that dolphin movement patterns were related to the influence of tidal changes on fish movement patterns in the estuary area (Gibson et al., 1996; Hampel et al., 2003). Thus, we posit that the large volume of river runoff during the wet season, especially during the typhoon period, increases water turbidity, which in turn alters the abundance of prey near the river mouth. These changes lead to the seasonal distribution gradient of the humpback dolphin in Taiwan.
The abundance of the fish, on which dolphins prey, is a major factor that could explain the variation in dolphin activity. Although the exact diet of the Taiwanese population of S. chinensis remains unclear due to the dearth of data from stomach samples, isotope analysis of muscle samples from one such dolphin beached on the west coast of Taiwan revealed that they are likely pure fish eaters (Liu et al., 2015). Studies of another population of the same species in Hong Kong and a closely related species in northern Australia (Sousa sahulensis) indicated that their prey mainly consisted of benthic fish and sea bream but also included fish from the mesopelagic zone, such as carp (Barros et al., 2004; Krishnan et al., 2008; Parra and Jedensjö, 2009). These fish feed mainly on zooplankton and benthic animals (Xue et al., 2005). Areas with high primary production are frequently major fishing grounds (Nixon, 1988; Cloern, 2001); thus, they are likely to experience dolphin aggregation. Jefferson and Hung (2004) reported that the extent of dolphin activity is mainly related to the availability of food resources, and primary production is one of the crucial factors influencing various taxa in the food web. However, primary production can be influenced or controlled by numerous environmental factors.
In the current study, the nine environmental and biological factors can be categorized into four groups. Group 1 includes temperature and DO, associated with cyclic changes between seasons. Warmer seasons lead to a high growth rate and a rich food web reflected in high chlorophyll a concentration and maximum net production (group 2), which are negatively influenced by heavy rainfall and runoff in the basin (group 3). The high turbidity and low salinity arising in group 3 produces conditions for group 4. Only high turbidity, and not salinity, significantly inhibits the maximum net production (group 2), which leads to a decline in dolphin activity. In conclusion, we found that the maximum net production (group 2) and turbidity (group 4) are the main factors influencing dolphin distribution.
In comparison to two nearby sites, the Zhuoshui River estuary (Ez) and an offshore control site (Dm), dolphin sightings were much higher in the Hsinhuwei River estuary than at the Ez or Dm sites. Pan et al. (2016b) explored the mechanism underlying this distinct distribution by constructing Ecopath trophic models of the three sites to compare energy flows within the food web. The trophic model of the Hsinhuwei River estuary suggested that the relatively high phytoplankton production resulted in more abundant benthic invertebrates and fish, especially large and small benthic-feeding fish, which could be the main prey for S. chinensis. The greater density of this dolphin species in the Hsinhuwei River estuary can be attributed to higher phytoplankton production and greater biomasses of benthic invertebrates and fish prey than those found in the other two areas.
In the Beibu Gulf (Gulf of Tonkin), bathymetry and chlorophyll a concentration are major variables contributing to S. chinensis habitat configuration, which is characterized by a shallow water depth and a high primary production (Huang et al., 2019). The current study indicated that the occurrence of S. chinensis in the Hsinhuwei River estuary was negatively correlated with DO and turbidity. S. chinensis forage more in low-turbidity water and travel more in areas with high net production and chlorophyll a concentration. Furthermore, turbidity was negatively correlated with the maximum net production and chlorophyll a concentration. A similar study in the Brunei Bay, Malaysia, revealed that salinity and turbidity affect the behavior of Irrawaddy dolphins; the amount of traveling was negatively correlated with surface water salinity and positively correlated with turbidity (Mahmud et al., 2018). Consequently, we argue that turbidity plays a significant role in an estuary. Estuaries are biologically productive systems that support many cetacean populations and serve as crucial nursery grounds for their prey; they are also highly dynamic systems with fine-scale environmental gradients and microhabitats. Thus, the identification of dolphin occurrence and behavioral patterns in an estuary is challenging.
In conclusion, we demonstrated significant seasonal changes in estuary environmental factors, affected by the rainfall and runoff in the basin, which lead to seasonal variations in the occurrence and behavioral activities of S. chinensis in the Hsinhuwei estuary. During spring and summer, rising temperatures (accompanied by low DO and turbidity) increased chlorophyll a concentration and primary production, which benefited fish and invertebrates in the estuary. The abundance of prey then attracted more S. chinensis to the area. However, heavy rainfall and runoff (typically associated with typhoons) in the basin in the late summer led to high turbidity, which limited primary production and may have led to a diminished food web in the estuary; dolphin occurrence and activity then declined. To the best of our knowledge, this is the first study to explore the integrated effect of biological and environmental factors influenced by the watershed on the activity of Indo-Pacific humpback dolphins in a single estuary. Whether the significant seasonal–cyclic change in dolphin–environment association we have demonstrated is present at other estuaries (at least 20 estuaries are within the home range of this dolphin population) requires further investigation. Future research could elucidate to what extent S. chinensis depends on estuary characteristics, including physical and biological factors.
Data Availability Statement
The raw data supporting the conclusions of this article will be made available by the authors, without undue reservation.
Ethics Statement
Ethical review and approval was not required for the animal study because During the investigation, only an appropriate distance was observed, and no invasive experiments were performed.
Author Contributions
H-JL provided the data of primary productivity. J-PS calculated of runoff and rainfall in river watershed. C-HL and L-SC performed the integration analysis of dolphin behavior and estuarine factors. All authors contributed to the article and approved the submitted version.
Funding
This article was subsidized by Ministry of Science and Technology and National Taiwan University (NTU), Taiwan. We would like to thank the sponsors of the Forestry Bureau and Formosa Plastics Group. The authors declare this study received funding from Formosa Plastics Group. The funder was not involved in the study design, collection, analysis, interpretation of data, the writing of this article or the decision to submit it for publication.
Conflict of Interest
The authors declare that the research was conducted in the absence of any commercial or financial relationships that could be construed as a potential conflict of interest.
Publisher’s Note
All claims expressed in this article are solely those of the authors and do not necessarily represent those of their affiliated organizations, or those of the publisher, the editors and the reviewers. Any product that may be evaluated in this article, or claim that may be made by its manufacturer, is not guaranteed or endorsed by the publisher.
References
Barros, N. B., Jefferson, T. A., and Parsons, E. C. M. (2004). Feeding habitat of Indo-Pacific humpback dolphins (Sousa chinensis) stranded in Hong Kong. Aquat. Mamm. 30, 179–188. doi: 10.1578/AM.30.1.2004.179
Chen, B., Xu, X., Jefferson, T. A., Olson, P. A., Qin, Q., Zhang, H., et al. (2016). Chapter five. conservation status of the Indo-Pacific humpback dolphin (Sousa chinensis) in the Northern Beibu Gulf, China. Adv. Mar. Biol. 73, 119–139.
Chou, L. S., Linand, H. J., and Chen, M. H. (2014). The Project of the Correlation Between the Indo-Pacific Humpback Dolphin (Sousa Chinensis) and the Estuarine Ecosystem Along the Coast of Yunlin, Taiwan. Taoyuan City: Formosa Plastics Group. (Unpublish).
Chou, L. S. (2018). The Project of the Monitoring of Indo-Pacific Humpback Dolphin (Sousa chinensis) Populations Along the Coast of Yunlin, Taiwan. Taoyuan City: Formosa Plastics Group. (Unpublish).
Chou, L. S., Lin, H. J., and Suen, J. P. (2018). The Habitat Monitoring of the Indo-Pacific Humpback Dolphin (Sousa chinensis) Population Ecology and Estuarine, A Research Project Subsidized by the Forestry Bureau of the Agricultural Committee of the Executive Yuan. Available online at: https://conservation.forest.gov.tw/0002026
Cloern, J. E. (2001). Our evolving conceptual model of the coastal eutrophication problem. Mar. Ecol. Progr. Ser. 210, 223–253. doi: 10.3354/meps210223
Cloern, J. E., Foster, S. Q., and Kleckner, A. E. (2014). Phytoplankton primary production in the world’s Estuarine-coastal ecosystems. Biogeosciences 11, 2477–2501. doi: 10.5194/bg-11-2477-2014
Dares, L. E., Hoffman, J. M., Yang, S. C., and Wang, J. Y. (2014). Short note: habitat characteristics of the critically endangered Taiwanese humpback dolphins (Sousa chinensis) of the Eastern Taiwan Strait. Aquat. Mamm. 40, 368–374. doi: 10.1578/AM.40.4.2014.368
Ferrier, S. (2002). Mapping spatial pattern in biodiversity for regional conservation planning: where to from here? Syst. Biol. 51, 331–363. doi: 10.1080/10635150252899806
Folkens, P., and Reeves, R. (2002). Guide to Marine Mammals of the World. New York, NY: Alfred A. Knopf, Inc.
Gibson, R. N., Robb, L., Burrows, M. T., and Ansell, A. D. (1996). Tidal, diel and longer term changes in the distribution of fishes on a Scottish Sandy Beach. Mar. Ecol. Progr. Ser. 130, 1–17. doi: 10.3354/meps130001
Hampel, H., Cattrijsse, A., and Vincx, M. (2003). Tidal, diel and semi lunar changes in the faunal assemblage of an intertidal salt-marsh Creek. Estuar. Coast. Shelf Sci. 56, 795–805. doi: 10.1016/S0272-7714(02)00296-2
Hatcher, B. G., Chapman, A. R. O., and Mann, K. H. (1977). An annual carbon budget for the kelp Laminaria longicruris. Mar. Biol. 44, 85–96. doi: 10.1007/BF00386909
Hosen, J. D., Aho, K. S., Appling, A. P., Creech, E. C., Fair, J. H., Hall, R. O. Jr., et al. (2019). Enhancement of primary production during drought in a temperate watershed is greater in larger rivers than headwater streams. Limnol. Oceanogr. 64, 1458–1472. doi: 10.1002/lno.11127
Huang, S. L., Peng, C., Chen, M., Wang, X., Jefferson, T. A., Xu, Y., et al. (2019). Habitat configuration for an obligate shallow-water delphinid: the Indo-Pacific humpback dolphin, Sousa chinensis, in the Beibu Gulf (Gulf of Tonkin). Aquat. Conserv. Mar. Freshw. Ecosyst. 29, 472–485. doi: 10.1002/aqc.3000
Jassby, A. D., and Platt, T. (1976). Mathematical formulation of the relationship between photosynthesis and light for phytoplankton. Limnol. Oceanogr. 21, 540–547. doi: 10.4319/lo.1976.21.4.0540
Jefferson, T. (2000). Wildlife monographs. population biology of the Indo-Pacific hump-backed dolphin in Hong Kong waters. Wildl. Monogr. 144, 1–65.
Jefferson, T. A., and Hung, S. K. (2004). A review of the status of the Indo-Pacific humpback dolphin (Sousa chinensis) in Chinese Waters. Aquat. Mamm. 30, 149–158. doi: 10.1578/AM.30.1.2004.149
Karczmarski, L., Huang, S. L., Or, C. K., Gui, D., Chan, S. C., Lin, W., et al. (2016). Humpback dolphins in Hong Kong and the Pearl River Delta: status, threats and conservation challenges. Adv. Mar. Biol. 73, 27–64. doi: 10.1016/bs.amb.2015.09.003
Krishnan, A. A., Yousuf, K. S., Kumaran, P. L., Harish, N., Anoop, B., Afsal, V. V., et al. (2008). Stomach contents of cetaceans incidentally caught along Mangalore and Chennai coasts of India. Estuar. Coast. Shelf Sci. 76, 909–913. doi: 10.1016/j.ecss.2007.08.004
Lee, C. Y. (2016). Passive Acoustic Monitoring on Activity Patterns of Indo-Pacific Humpback Dolphins (Sousa chinensis) in an Estuary. Ph.D thesis. Taiwan: National Taiwan University.
Lin, T. H. (2013). The Application of Passive Acoustic Monitoring for Studying Indo-Pacific Humpback Dolphin Behavior and Habitat Use off Western Taiwan. Ph. D. dissertation. Taiwan: National Taiwan University.
Lin, T. H., Akamatsu, T., and Chou, L. S. (2015). Seasonal distribution of Indo-Pacific humpback dolphins at an Estuarine habitat: influences of upstream rainfall. Estuar. Coast. 38, 1376–1384. doi: 10.1007/s12237-014-9886-2
Liu, J. Y., Chou, L. S., and Chen, M. H. (2015). Investigation of trophic level and niche partitioning of 7 Cetacean species by stable isotopes, and cadmium and arsenic tissue concentrations in the Western Pacific Ocean. Mar. Pollut. Bull. 93, 270–277. doi: 10.1016/j.marpolbul.2015.01.012
Mahmud, A. I., Jaaman, S. A., Muda, A. M., Muhamad, H. M., Zhang, X., and Scapini, F. (2018). Factors influencing the behaviour of Irrawaddy dolphins Orcaella brevirostris (Owen in Gray, 1866) in Brunei Bay, Malaysia. J. Ethol. 36, 169–180. doi: 10.1007/s10164-018-0549-9
Marshall, H. G. (2005). “Metabolic rate measurements,” in Standard Methods for the Examination of Water and Wastewater, eds A. D. Eaton and M. A. H. Franson (Washington, DC: American Public Health Association).
Nixon, S. W. (1988). Physical energy inputs and the comparative ecology of lake and marine ecosystems. Limnol. Oceanogr. 33, 1005–1025. doi: 10.4319/lo.1988.33.4_part_2.1005
Pan, C.-W., Chen, M.-H., Chou, L.-S., and Lin, H.-J. (2016a). The trophic significance of the Indo-Pacific humpback dolphin, Sousa chinensis, in Western Taiwan. PLoS One 11:e0165283. doi: 10.1371/journal.pone.0165283
Pan, C.-W., Chuang, Y.-L., Chou, L.-S., Chen, M.-H., and Lin, H.-J. (2016b). Factors governing phytoplankton biomass and production in tropical estuaries of Western Taiwan. Cont. Shelf Res. 118, 88–99. doi: 10.1016/j.csr.2016.02.015
Parra, G. J., and Jedensjö, M. (2009). Feeding Habits of Australian Snubfin (Orcaella heinsohni) and Indo-Pacific Humpback Dolphins (Sousa chinensis). Cairns, QLD: Reef and Rainforest Research Centre Limited.
Pryor, K., and Norris, K. S. (Eds.) (1991). Dolphin Societies: Discoveries and Puzzles. Berkeley, CA: University of California Press.
Ross, P. S., Dungan, S. Z., Hung, S. K., Jefferson, T. A., Macfarquhar, C., Perrin, W. F., et al. (2010). Averting the baiji syndrome: conserving habitat for critically endangered dolphins in Eastern Taiwan Strait. Aquat. Conserv. Mar. Freshw. Ecosyst. 20, 685–694. doi: 10.1002/aqc.1141
Rushton, S. P., Ormerod, S. J., and Kerby, G. (2004). New paradigms for modelling species distributions? J. Appl. Ecol. 41, 193–200. doi: 10.1111/j.0021-8901.2004.00903.x
Shirihai, H., Jarrett, B., and Kirwan, G. (2006). Whales, Dolphins, and Other Marine Mammals of the World. Princeton, NJ: Princeton University Press.
Sprogis, K. R., Christiansen, F., Wandres, M., and Bejder, L. (2018). El Niño Southern oscillation influences the abundance and movements of a marine top predator in coastal waters. Glob. Change Biol. 24, 1085–1096. doi: 10.1111/gcb.13892
Wang, J. Y., and Araujo-Wang, C. (2018). Sousa chinensis ssp. Taiwanensis (Amended Version of 2017 Assessment). IUCN Red List of Threatened Species 2018: e. T133710A122515524. Available online at: https://dx.doi.org/10.2305/IUCN.UK.2017-3.RLTS.T133710A122515524.en.
Water Resources Agency, and Ministry of Economic Affairs. (2018). Hydrological Year Book of Taiwan Republic of China. ISSN 0257.5442. Available online at: https://gweb.wra.gov.tw/wrhygis/ (accessed June, 2018).
Xue, Y., Jin, X., Zhang, B., and Liang, Z. (2005). Seasonal, diel and ontogenetic variation in feeding patterns of small yellow croaker in the Central Yellow Sea. J. Fish Biol. 67, 33–50. doi: 10.1111/j.0022-1112.2005.00677.x
Young, D. D., and Cockcroft, V. G. (1994). Diet of common dolphins (Delphinus delphis) off the South-East Coast of Southern Africa: opportunism or specialization? J. Zool. 234, 41–53. doi: 10.1111/j.1469-7998.1994.tb06055.x
Keywords: Indo-Pacific humpback dolphin, turbidity, chlorophyll a concentration, net primary production, watershed
Citation: Lin C-h, Lin H-J, Suen J-P and Chou L-S (2021) Association Between Estuary Characteristics and Activities of the Critically Endangered Indo-Pacific Humpback Dolphin (Sousa chinensis). Front. Mar. Sci. 8:577976. doi: 10.3389/fmars.2021.577976
Received: 30 June 2020; Accepted: 06 July 2021;
Published: 09 August 2021.
Edited by:
Randall William Davis, Texas A&M University at Galveston, United StatesReviewed by:
Gail Schofield, Queen Mary University of London, United KingdomStephanie Plön, Bayworld Centre for Research and Education, South Africa
Carol Palmer, Charles Darwin University, Australia
Simon David Berrow, Galway-Mayo Institute of Technology, Ireland
Copyright © 2021 Lin, Lin, Suen and Chou. This is an open-access article distributed under the terms of the Creative Commons Attribution License (CC BY). The use, distribution or reproduction in other forums is permitted, provided the original author(s) and the copyright owner(s) are credited and that the original publication in this journal is cited, in accordance with accepted academic practice. No use, distribution or reproduction is permitted which does not comply with these terms.
*Correspondence: Lien-Siang Chou, Y2hvdWxzQG50dS5lZHUudHc=