- 1NORCE Norwegian Research Centre, Bergen, Norway
- 2Department of Chemistry, University of Bergen, Bergen, Norway
- 3Department of Geography, University of Bergen, Bergen, Norway
- 4Department of Biological Sciences, University of Bergen, Bergen Norway
- 5Faculty of Mathematics and Natural Sciences, University of Bergen, Bergen, Norway
The Norwegian Coastal Current transports natural debris and plastic waste along the Norwegian coastline. Deposition occurs in so-called wreck-bays and includes floating debris, such as seaweed, driftwood and volcanic pumice, and increasing amounts of plastics during the last decades. Deposition in these bays is controlled by ocean currents, tidal movements, prevailing winds and coastal morphology. We have compared soil profiles, analyzed the vegetation and inspected aerial photos back to 1950 in wreck-bays and defined three zones in the wreck-bays, where accumulation follows distinct physical processes. Zone 1 includes the foreshore deposition and consists of recent deposits that are frequently reworked by high tides and wave erosion. Thus, there is no accumulation in Zone 1. Zone 2 is situated above the high tide mark and includes storm embankments. Here, there is an archive of accumulated debris potentially deposited decades ago. Zone 3 starts above the storm embankments. The debris of Zone 3 is transported by wind from Zone 1 and Zone 2, and the zone continues onshore until the debris meets natural obstacles. Plastic accumulation seems to escalate soil formation as plastic is entangled within the organic debris Mapping and characterizing the soil layers indicates that deep soils have been formed by 50 or more years’ accumulation, while the pre-plastic soil layers are thin. The plastic soil forms dams in rivers and wetlands, changing the shape and properties of the coastal landscape, also altering the microhabitat for plants. This case-study describes an ongoing landscape and vegetation change, evidently co-occurring with the onset of plastic accumulation. Such processes are not limited to the Norwegian coastline but are likely to occur wherever there is accumulation of plastic and organic materials. If this is allowed to continue, we may witness a continued and escalating change in the shape and function of coastal landscapes and ecosystems globally.
Introduction
Plastics are lightweight, durable materials made of synthetic polymers, and have become an integrated part of modern society. Since mass-production of plastics began in the 1950s, the production has increased rapidly and has now reached over 360 million metric tons per year (Plastics Europe.com). Due to poor waste management, plastics are now omnipresent in the marine environment and have become of increasing scientific and public concern. The occurrence of plastic in the oceans and consequences for marine life have been reported since industrial production commenced in the 1960s (e.g., Heyerdahl, 1971; Kartar et al., 1973; Laist, 1987; Thompson et al., 2004, Thompson et al., 2009; Pham et al., 2014). Plastic in beach sediments was first reported by Merrell (1980) who observed the vast amount of fishery related waste along a section of the Alaskan coast from 1972 to 1974 and remarked how the plastic was encapsulated into the beach sediments and thereby covered by vegetation. Now, approximately 70 years after the onset of industrial plastic production, waste management is still unable to prevent plastic from entering the marine environment (Thompson et al., 2004; Ivar et al., 2009; Jambeck et al., 2015; Lebreton et al., 2017; Borrelle et al., 2020; Napper and Thompson, 2020).
The Norwegian Coastal Current transports natural debris and plastic waste from the North Sea region and Norwegian waters northward along the Norwegian coastline (OSPAR, 2007; Thiel et al., 2011; Cózar et al., 2017). Deposition of floating debris typically occurs in so-called “wreck bays” (Eriksson et al., 2013). These bays are largely unchanged over centuries, controlled by ocean currents, prevailing winds, tidal movements and coastal morphology. Originally, the floating debris included seaweed, kelp, driftwood and volcanic pumice. Nowadays, plastic is a substantial part of the debris. Plastic, as opposed to organic materials, does not decompose and can therefore accumulate where it is deposited. Thus, plastic accumulates along shorelines that are not regularly cleaned, such as in remote and uninhabited regions. The Norwegian coastline is more than 100 000 km long and is sparsely populated, with the implication that cleaning operations along the Norwegian coast until recently have focused on inhabited areas, such as near settlements, in recreational areas and near infrastructure. However, less accessible distal coves and bays at uninhabited islands and on the mainland are rarely cleaned and have accumulated debris for several decades.
We recently mapped a 70 km stretch of sparsely inhabited coastline in southwestern Norway and found plastic in over 800 accumulation sites (Bastesen et al., 2020), suggesting that remote areas along the southwestern coast of Norway are heavily polluted with plastic. Here, we argue that plastic accumulation is not only a concern for the affected wildlife that risk entanglement and death by contact with macroplastic, or the generation of microplastic that enters the ecosystem (Barnes et al., 2009). Based on our observations, the plastic accumulation also changes the physical properties of the soil and landscape, and thereby may alter the physicochemical properties and the function of the coastal ecosystems.
In this study, we focus on two locations that illustrate how landscape changes may occur because of long-term accumulation of marine plastic litter. This study aims to increase our understanding of the ongoing and potential landscape changes resulting from at least 50 years of plastic accumulation in coastal regions. Our investigation of the soil and vegetation aims to elucidate and describe the processes that started at the onset of plastic production and accumulation. If the accumulation continues, effects on soil and vegetation will be irreversible without a severe and costly human effort. The cleaning process itself may also be detrimental to the stability and resilience of the ecosystem. In order to mitigate detrimental effects of plastic accumulation, we need to understand the processes and speed at which they operate and their biological effects (e.g., Laist, 1987).
Our investigation of the plastic accumulation and coastal morphological changes encompassed three primary tasks. First to document the geomorphic character of two wreck bays of different physical properties; second to qualitatively map the distribution of plastic litter on the surface, in the soil and sediments according to a suggested accumulation/deposition zone framework. And third, to describe and qualitatively analyze the effects of plastic accumulation on the landscape development.
Materials and Methods
Physical Factors Controlling Plastic Accumulation in Western Norway
The coastline along Western Norway can be referred to as rocky (Storlazzi and Field, 2000; Trenhaile, 2016) and consists of glacially eroded crystalline rocks forming a rugged landscape with a vast number of bays, coves and beaches. Sediments are mostly glacial deposits and are under erosion (Mangerud et al., 2011).
Onshore accumulation of marine plastic is controlled by ocean currents, tidal movements, wind and coastal shape. Important geographical properties that control deposition include shape and orientation of the bay, shoreline slope and substratum (Haarr et al., 2019). Plastic accumulation in western Norway is localized into bays and coves (Bastesen et al., 2020). Most plastic waste accumulates in bays that are oriented toward the sea and prevailing wind and current directions, and commonly above the high tide mark. This is often a result of winter storm events. Historical wind data from the period 2005-2018 (see1) shows that, there were on average more than 25 events with moderate gale (> 15 m/s), 8 events with severe gale (21–24 m/s), and 1.5 storm events (> 25 m/s) annually. Winds are mostly from the SW, W or NW direction.
Case Study of Two Wreck Bays in Western Norway
Two sites (Figure 1) representing so called hot spots for accumulation of marine waste were selected for the case study. Case 1 is facing the open sea, whereas case 2 is facing a large fjord basin that is open toward the outer coastline. The two sites are somewhat different in terms of the energy-level that directs the physical processes; however, both represent examples of exposed and semi-exposed locations where plastic waste accumulates.
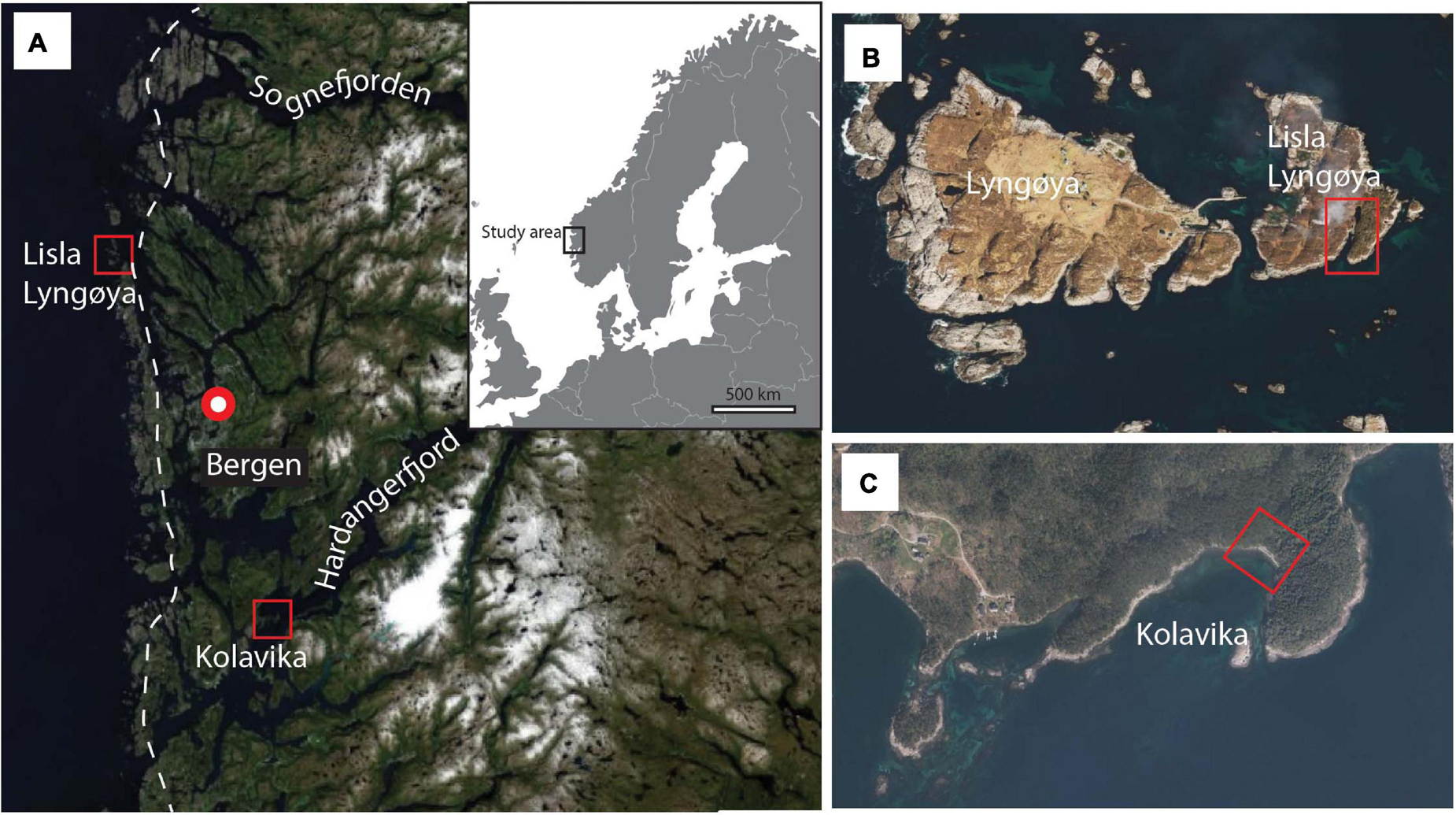
Figure 1. (A) Location of the two study sites in the NW part of the North Sea basin. (B) Lisle Lyngøyna aerial photo from (Norway in pictures). (C) Kolavika (aerial photo from Norway in pictures).
Case 1: Lisle Lyngøyna Island
Lisle Lyngøyna is an island located at the northern extent of Øygarden municipality at the southwestern exterior coast of Norway facing the North Sea (Figure 1). The area of the island is 0.3 km2 (0.5 × 0.5 km). It has a narrow cove with a lateral length of 100 m and width of 30 m facing the sea to the S-SW. A freshwater pond is in the N-NE continuation of the cove. The bedrock in the archipelago consists of felsic gneiss (acidic). The vegetation is classified as coastal heathland (Fremstad et al., 1991; Hjelle et al., 2010), consisting of a mosaic of heather (Calluna vulgaris) dominated vegetation, nutrient poor peatbogs and areas of barren rocks. In some areas there is regrowth with shrubs and trees. Coastal heathlands are cultural landscapes created and managed through regular burning and mowing of heather, and by grazing and browsing by sheep throughout the year. Historically, the area has also been used for peat extraction (until the 1950s), and remnants of peat carvings and irrigation channels are still visible in the landscape.
Case 2: Kolavika Bay
Kolavika is a SW facing bay located at the northern shores of the outer Hardangerfjord system. The bay is a pebble beach that is 50 m wide and consists of reworked moraine material. The sediment depth is unknown, and basement rocks crop out in the middle of the bay. The valley is bordered by small hills and has two small streams running toward the sea. Kolavika is part of a nature reserve2, and is protected because of its characteristic geology, nature types and biodiversity. The vegetation in the area is coastal forest; both rich deciduous and pine forest types are found there. They bay is surrounded by these vegetation types but was itself until recently a plantation forest of Picea sitchensis. Kolavika (kol = coal) was historically a location for charcoal production from pine. Remnants of the coal production can be observed in the substratum as a coal layer.
Equipment and Sampling
Pictures and Historical Images to Investigate Landscape Changes
A DJI Phantom 4 pro drone was used to collect high resolution images from flight heights of 20 m and 70 m using pre-programed flight routes (DroneDeploy version 2018). Images was collected during clear days in May and November 2018 for the Lisle Lyngøy site and in November 2019 for the Kolavika site. Images were processed by photogrammetric methods (Agisoft Photoscan 1.6.2 (Metashape); Westoby et al., 2012). Detailed orthomosaics and digital terrain models were subsequently generated and used in detailed mapping of the bays using the 3D visualization software Lime (version 2.2.2) (Buckley et al., 2019), for detecting plastic polluted areas, mapping of vegetation and analyzing plastic-modified terrain morphology. In order to analyze the evolution of the landscape, aerial images pre-dating the onset of the plastic pollution was compared with images from the present. This analysis was only performed at the Lisle Lyngøyna since historical images was not accessible over the Kolavika location. Aerial photos were provided by Norway digital and included the series, Midthordland 2019 (orthophoto resolution 0.08 m); Midthordland 2004 (orthophoto resolution 0.08 m) and Sotra-Fedje 1962 (orthophoto resolution 0.2 m) (©Kartverket, Geovekst, Øygarden; Tysnes). The georeferenced images were interpreted in ArcGIS (Esri version 10.1) by comparing the coastline, and the shoreline of ponds and other recognizable features. Observed landscape changes, such as increase in soil thickness due to plastic accumulation were quantified by area and volumetric measurement of the plastic waste using the 3D visualization software. An estimate of litter types and the amount of litter on surface was given mostly within per cent ranges based on inspections of drone photos. To quantify volumes of the plastic contaminated soil in field, measurements such as average depth of soil was multiplied with the surface area.
Sediment and Soil Stratigraphy
The soil layers were investigated first by using a metal rod for measuring the thickness of the soil down to the bedrock or sediment substrate. To characterize the 3D distribution of plastic, and to elucidate the formation process of the plastic soils, we retrieved full-length cores from the soil where possible. It was challenging to retrieve cores from this substratum as the plastic items and ropes were entangled and layered within the soil. We attempted to core using a Russian Peat Corer and cylinders with sharpened ends without success. Instead, we dug small transects (soil profiles of ∼1 × 1 m) with shovels and knives to describe the composition and layering of the substrate. Soil cores (Tables 1, 2) were retrieved using serrated knives (Figure 2E). The cores were wrapped in aluminum foil and transported to the laboratory for detailed investigation of plastic fragments. The bottom sediments in the pond at Lisle Lyngøyna were sampled by a hand-held van Veen grab, deployed from a small rubber boat. Also, we snorkeled the pond to describe plastic distribution and littering at the bottom. At Lisle Lyngøyna 10 locations were studied whereas 6 samples were collected for laboratory studies (Table 1). At Kolavika 5 locations were studied and 2 full cores were successfully retrieved for laboratory analysis (Table 2).
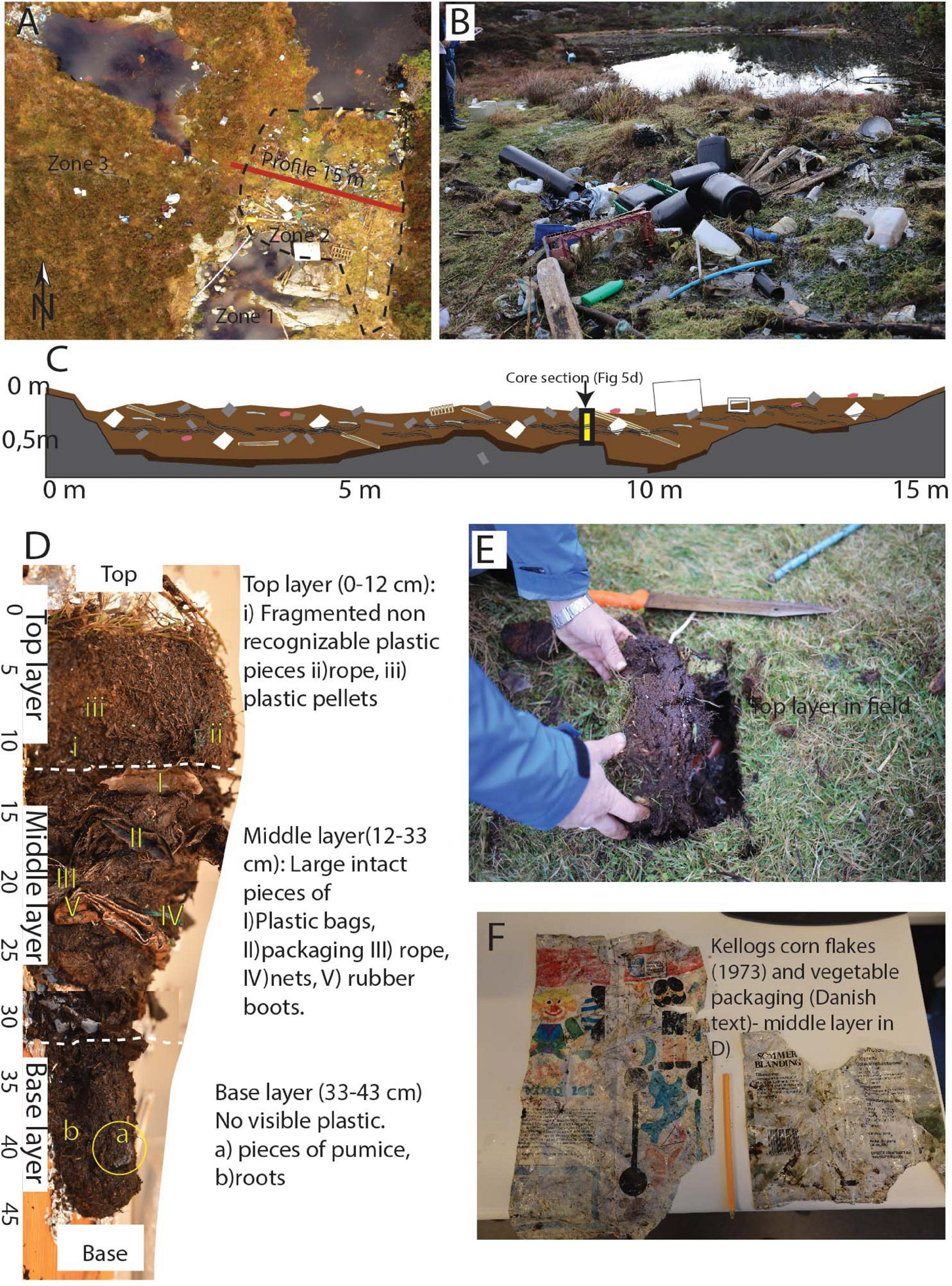
Figure 2. (A) Drone photo of the Zone 2 embankment that limits the pond from Zone 1. The profile that was used for measuring depth to basement rocks is denoted. (B) Close up image showing the type and amount of marine litter on the surface. Note the water saturated surface and water protruding from the pond in Zone 3. (C) Illustration of the depth profile through the embankment. Colored items illustrate plastic fragments of various sizes and types. (D) Photo of core taken in central part of the storm embankment. Various items observed on the surface are denoted. Upper part comprises small fragmented plastic pieces embedded in black organic rich soil, middle part consists of large and preserved marine litter, lower part consists of a base soil layer free from plastic. Colored pieces represent pumice that may be of volcanic origin. (E) Coring technique by using a serrated knife. (F) Pieces of preserved packaging plastic found in the middle part of the core. The Kellogg’s cornflakes packaging is dated 1973.
Vegetation Types
Mapping of vegetation in zones 2 and 3 was done in field with a GPS-receiver in combination with aerial imagery. Dominant and characteristic species were noted within each vegetation type. We counted annual growth rings (dendrochronology) of ash trees that grew in the plastic infused soil on the beach in Kolavika. The age of the oldest trees can be an indicator of when the plastic accumulation started.
Results
General Deposition Zones in the Studied Beaches
Based on our qualitative analysis and on the results of Bastesen et al. (2020) and Haarr et al. (2019), we suggest a general division of deposition zones at beaches (Figure 3). We adhere to this division when describing our results. We here define three zones that can be recognized in wreck-bays, based on the natural processes that impact the accumulation and deposition of debris. These zones, although of varying shape and size among locations, can be used to describe and understand their physical properties.
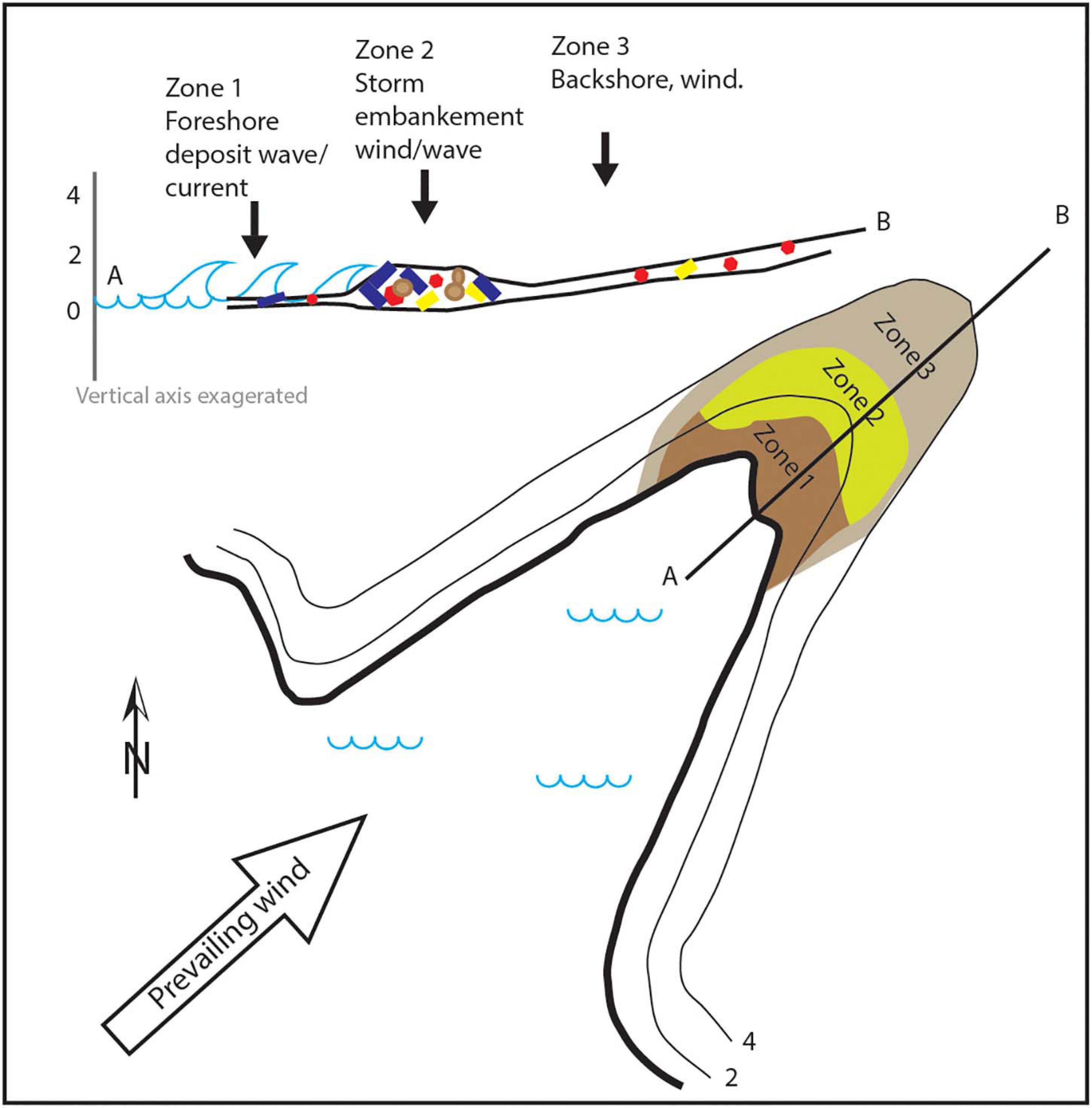
Figure 3. Conceptual sketch of wreck bays based on observation in the two localities. Zone 1 foreshore deposition. Zone 2 backshore/storm embankment, Zone 3 area of dominantly wind deposited plastic.
Zone 1 includes the upper littoral zone or foreshore, placed at or immediately above the high tide mark; an area affected by high energy, such as waves and tidal movements. The zone comprises mostly non-vegetated to sparsely vegetated beach or bare rock faces. The materials that deposit include seaweed, driftwood and plastic debris. Characteristic for these zones is that materials are only temporarily placed there and may be washed away or blown to another location during storms or high tides.
Zone 2 is situated above the high tide mark and includes more permanent deposits, or storm embankments. Deposits may often comprise large items that only can be transported and deposited by severe storms and storm surges. These embankments are common morphological features in coastal bays of Norway and may be referred to as so-called drift embankments (Carlsen and Bär, 2016). During calm periods, such as the summer seasons, the vegetation thrives on the nutritious substratum (decomposed seaweed and kelp). The vegetation cover inhibit erosion during storm seasons. This annual cycle of winter storm deposition and summertime vegetation may form growth of deposits accumulated over several decades. Soil profiles of Zone 2 may therefore constitute a chronological archive of accumulation, which can be used for dating and stratigraphic investigation of long-term plastic accumulation.
Zone 3 is defined as the zone above (and around) Zone 2. Zone 3 accumulates wind-transported debris, and continues onshore until the debris meets natural obstacles, such as trees or boulders. This debris represents a scattered selection of materials and is to a lesser degree a chronological and layered archive of accumulation. The extent of Zone 3 depends on the morphology and physical properties of the area, such as ponds, vegetation, rocks, coves or other shapes that either facilitate or prevent wind transportation.
Case 1 – The Lisle Lyngøyna Island
At Lisle Lyngøyna, plastic litter covered a large area from the inner part of the cove to approximately 120 m inland (Figure 4). Large amounts of plastic items of considerable size were also found in and along the shores of the pond, demonstrating the high energy events that occur here and lead to the deposition of plastic waste. Evidence of long-term plastic accumulation was observed, and in some places the entire edge of the pond was comprised of plastic entangled into or overgrown with vegetation. In the following, landscape characteristics and sediment stratigraphy are described in each of the accumulation zones.
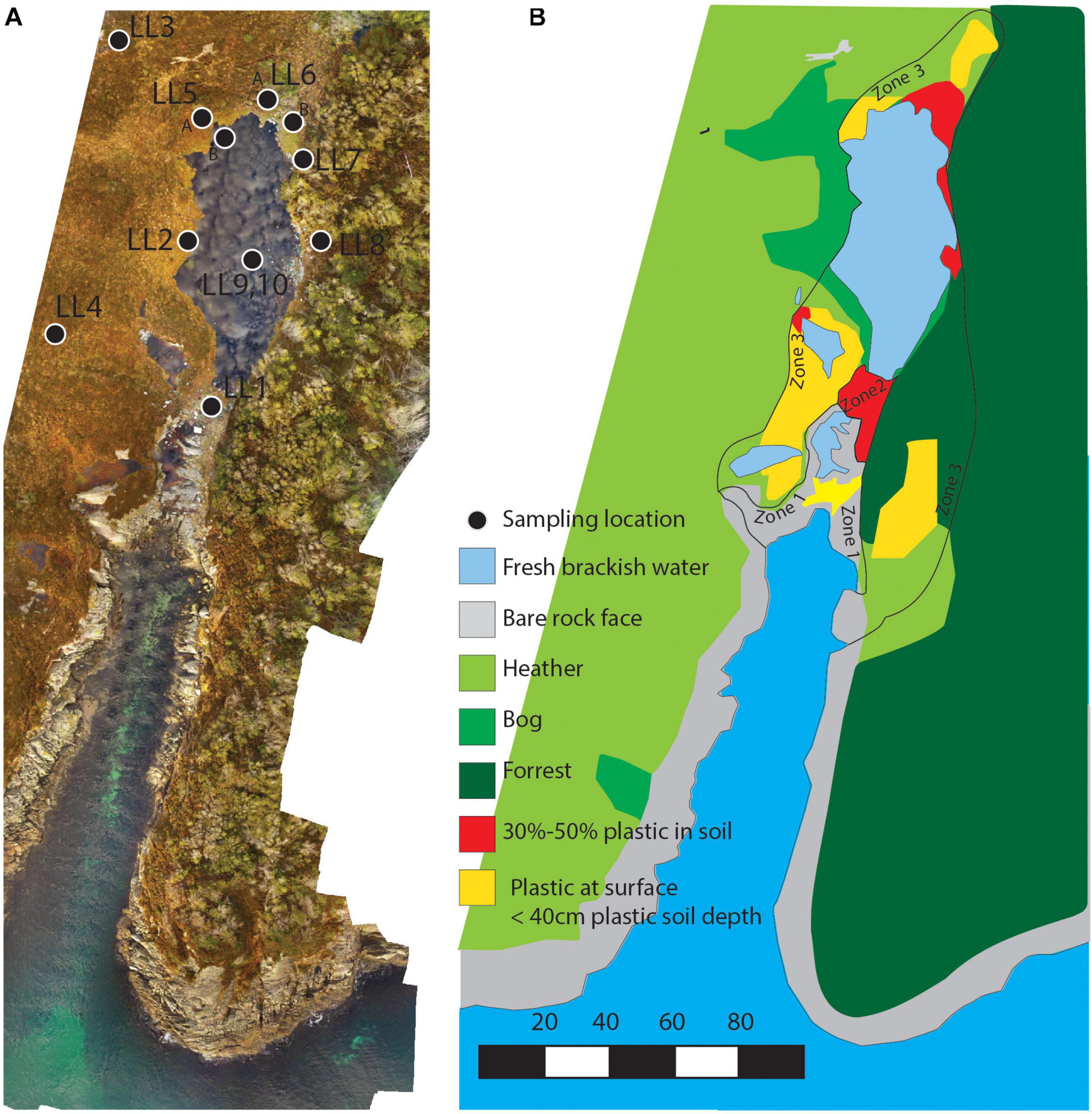
Figure 4. Map showing the plastic pollution in Lisle Lyngøyna and typical vegetation types. (A) Drone orthomosaic. (B) Map based on field work and image interpretation.
Lisle Lyngøyna Zone 1
Zone 1 was comprised of the shore and inner part of the cove up to about 20 m inland from the high tide mark (Figure 5). The area consisted mostly of barren rock faces, small tidal ponds and small patches of grass (Figure 5). Plastic intermixed with seaweed was accumulated into small depressions within Zone 1. The two drone images in Figure 5 illustrate the dynamics of these depositions. The images were retrieved at different times, May (Figure 5A) and November (Figure 5B) 2018. In November the near shore part of Zone 1 was filled with plastic items dominated by bottles. In May earlier the same year this same spot was completely cleared. This gives an indication of the accumulation rate and it also shows the dynamics of the lower foreshore area and that more plastic is observed at winter season compared to summer season. Plastic covered below 10% of the surface. Several lumps of tar originating from bitumen/oil, were attached to the rocks immediately above the high tide mark. In some cases, plastic pellets were embedded in the tar. The thin layer of soil beneath the scattered grass patches (Figure 5A), were investigated by digging, revealing only a thin soil layer without plastic.
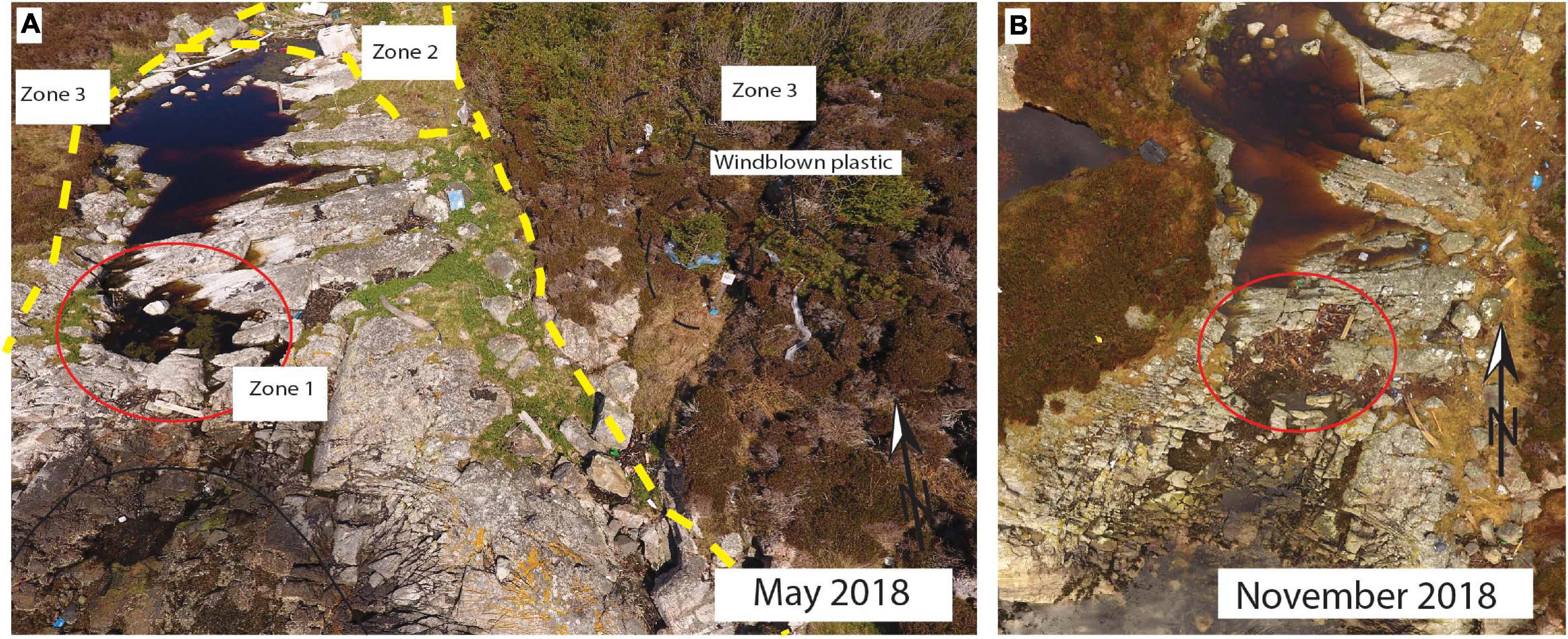
Figure 5. Zone 1 Drone photo of the cove area representative of Zone 1. (A) Photo taken in May 2018, note the clear area (red circle) (B) drone photo acquired in November (winter) with a substantial increase in deposited waste. No cleaning had been performed in the area.
Lisle Lyngøyna Zone 2
Zone 2 was adjacent to Zone 1, covering the area until the beginning of the pond located approximately 30 meters from the high tide mark. It was comprised of an 11 × 11 m (approx. 50–60 m3) storm embankment (Figures 2, 4), The embankment surface was on average elevated 1.9–2.3 m above mean sea level and had a characteristic flat surface.
Zone 2 typically had more and larger plastic items than Zone 1, such as buckets, containers, bottles, fishnets, ropes, pipes and plastic films (Figure 2B). Compared to zone 1, drone images and ground investigations shows that in places more than 50% of the surface are covered by visible plastic (Figure 2A). Plastics were also embedded deeper in the soil and fully or partly overgrown by vegetation, thus plastics were in fact covering close to 100% of the surface, although not visible from aerial photos. In places this resulted in a lumpy surface. We documented plants, such as Hydrocotyle vulgaris, Scutellaria galericulata, Lychnis flos-cuculi, and more nutrient-demanding species such as Iris pseudocarus, Galeopsis sp., and Argentina anserine. The soil was between 0.3 to 0.7 m thick down to bedrock. Transects revealed an irregular bedrock topography (Figure 2C). The embankment thus smooths out depressions in the landscape. The water level of the pond was at the same level as the embankment. There was no visible outlet/stream from the pond, however channels protruding from the pond extend into the embankment (Figure 2A). During excavations of the embankment, the ditches that were dug were filled rapidly with water.
Excavations, soil coring and steel probe profiling showed that the soil from the embankment consisted of three main layers (Soil core LL1, Figures 2D,E). The upper 5 cm consisted of organic rich soil intermixed with macro plastic items that were partly buried into the ground. The soil immediately underneath (5–35 cm depth) was composed of dark brown soil with roots and mostly fragmented plastics, small rope pieces and pellets. In this section the soil to plastic ratio was around 80/20. Immediately under this layer was a thick layer of water-saturated, soft mass comprised of large plastic items and organic rich dark brown soil. Litter included fishnets, ropes, plastic bags and packaging, bottles, glass and pieces of wood, as well as smaller fragments of plastic, pellets and expanded polystyrene beads. Characteristic for this layer is the large amount of well-preserved plastic items, such as food packaging from thick plastic film, containers of detergents and personal care products. The packaging design could be recognized on the items, indicating the production-period (Figure 2F). Most of the items found had a typical design belonging to the 1970-1990 period. This layer also contained half-burned and melted plastics and some pumice rocks. This layer consisted of a soil to plastic ratio of 30/70. Throughout Zone 2 this layer had thicknesses of 30–70 cm. The base-layer of the embankment consisted of a black soil layer about 10 cm thick, densely packed with roots, driftwood and pumice rocks. This layer rests immediately on the basement rocks. In cores and in samples investigated in field there was no plastic observed in this layer (Figure 2D). This may be the original surface layer before the onset of plastic accumulation that formed the embankment.
Lisle Lyngøyna Zone 3
Zone 3 was comprised of areas covered by windblown plastic. The zone included the freshwater pond, its northern shores, the bog surrounding the pond and the hills to the east and west of the pond and bay, where windblown plastic items were found in and among the heather and trees (Figures 4–6). The bog in Zone 3 is a nutrient poor type and was dominated by various peat mosses (Sphagnum sp.) and Trichophorum cespitosum, Calluna vulgaris, Erica tetralix, Vaccinum uligonosum, Oxycoccus palustris, Chamaepericlymenum suecicum and Potentilla erecta.
The drone photo in Figure 6a illustrates that the distribution of plastic at the surface of the bog in the northern shore varies considerably. From west to east there was a visible increase in surface plastic debris. From 0-5% cover at the western side up to 50–100% cover on the eastern side. The litter was accumulated in shelters/bays/edges facing SW, typically controlled by the prevailing wind directions from the SW (Figure 6b). Items were for example 10–50 L industrial containers, fish boxes, pieces and objects of expanded polystyrene, baskets, bottles, and a litterbin with labeling showing that it was of United Kingdom origin (long transported). Larger pieces of plastic were also scattered on the bottom of the pond.
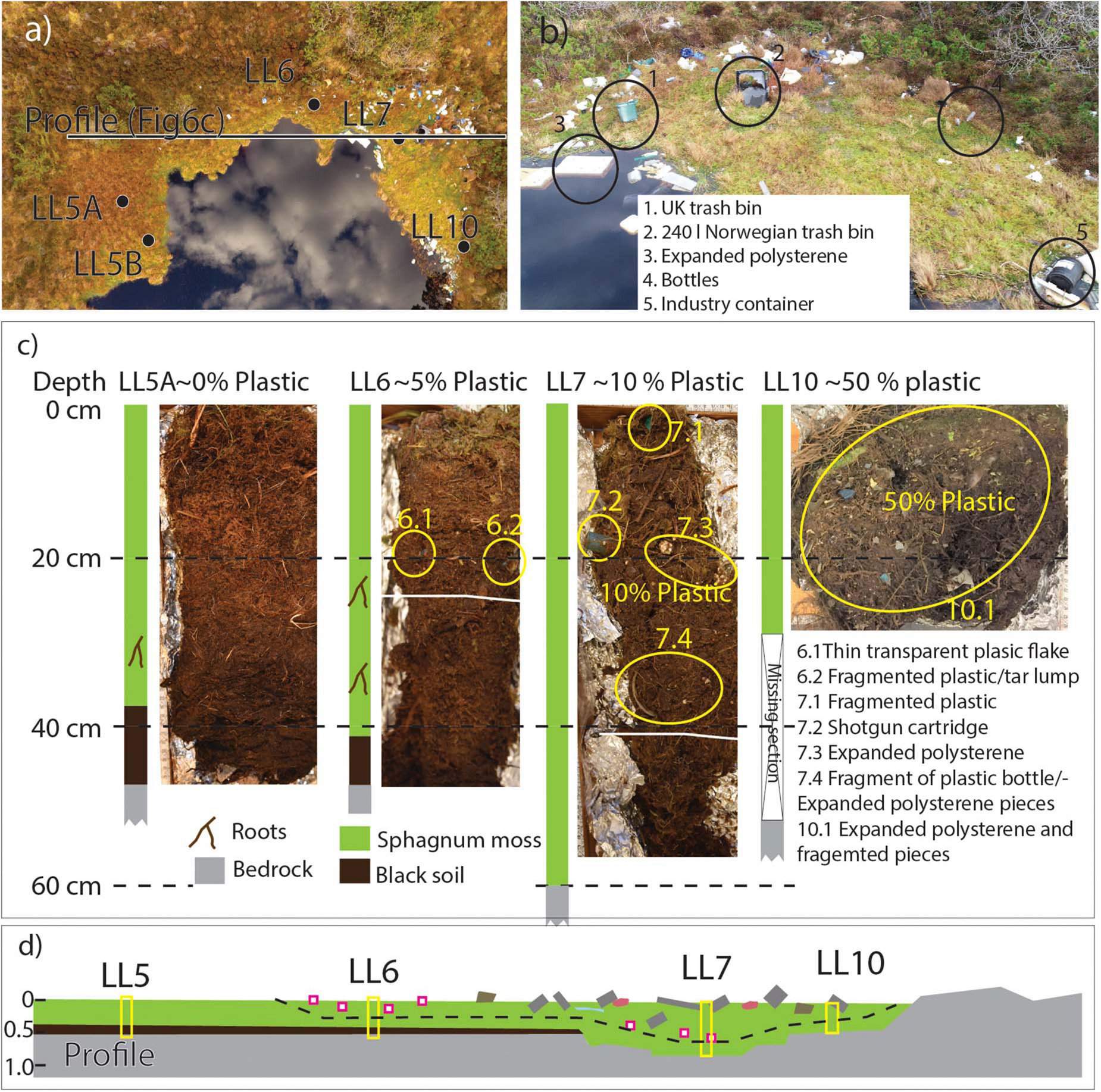
Figure 6. Samples and field work in northern part of pond Zone 3. (a) Drone photo of a bog in the northern part of the pond. Note decreasing amount of surface litter from east to west (the image is oriented NNW-SSE), subsurface soil core sample sites and profile (c and d) are denoted. (b) Close up drone photo of the NE part of pond displaying the high amount of litter observed on surface. (c) Cores LL5, LL6, LL7 and LL10. Samples display clearly how plastic is incorporated into the soil. (d) Profile based on the soil profiles and steel probe measurement.
Soil cores (Figure 6c) that were retrieved at the shores of the pond revealed a large amount of plastic found deep into the bog at the northern and eastern shores of the pond (LL6 and LL7), whereas there was little to no plastic found in the deep section at the western shore of the pond (LL5 A and B). The organic content of the soil was dominated by peat moss (sphagnum), on average about 40–50 cm thick and resting on a flat bedrock surface (Figure 6d). The bedrock surface deepened at the NE part of the pond to a depth of 70–100 cm.
The profiles from soil cores around the lake indicate that plastics are present in the substratum whenever plastic is also present on the surface. Cores retrieved at the western side of the pond, LL5 A and B, were dominated by a thick layer of peat and only 1 small visible plastic fragment was observed in LL5B. With prevailing winds from the W-SW, no plastic litter had accumulated on the western side of the pond but instead accumulated at NE side of the pond. The LL5A core was 50 cm deep from surface to bedrock and consisted of three peat layers, where the upper 20 cm consisted of green sphagnum. The middle part consisted of a lighter green, more large leaved sphagnum. The lower part consisted of a 10 cm dark brown soil with roots of wooden plants (heather). At the bottom, pieces of angular pebble sized rock fragments were found. LL5B was retrieved 1 m from the edge of the pond and 5 m from LL5A. The core was 48 cm deep and included green sphagnum in the upper 10 cm. At 10–30 cm below the surface there was a darker moss-like sphagnum with abundant wooden roots throughout (heather). Presence of such roots indicates that this site may previously have been drier and covered by heather. The lower 10 cm consisted of dark peat.
LL6 and LL7 were both collected near the NE part of the shore (Figure 6c) where there was a high amount of plastic on the surface. Sample LL6 (Figures 6c,d) was retrieved in an area with mostly smaller plastic particles on the surface. The LL6 core had a 5 cm layer of sphagnum at the top followed by a 15 cm layer of brown moss and heather roots (Figure 6c). Plastics in the top 5 cm consisted of small pieces (< 1 cm) including nylon threads, colored hard plastic, clear plastic foil and pellets. A tar lump was observed at 15 cm from the top of core. The subsequent layer was dark brown moss (17 cm to 38 cm in the core) with green sphagnum and no plastic. The bottom 10 cm layer consisted of dark soil without plastic.
The sampling site LL7 was at the deepest part of the bog where there was a depression in the bedrock, and the core sampled was 60 cm deep. Plastic was found in the upper 50 cm in a matrix of large-leaved moss with a well-preserved green color. The deeper layers of moss had no visible plastics. The base layer consisted of a 10 cm thick layer of dark soil with the presence of pumice rocks.
In the samples from LL10 (Figures 6c,d) on the eastern shore, plastic was observed in the entire core, except for a gravel layer at the base. The gravel was not possible to retrieve for lab inspection. Several other shorter cores in the surrounding 5–10 m radius had top layers containing plastic embedded in the moss, and lower layers without plastic. However, it was difficult to retrieve the cores from the bog due to the water content, depth and abundant plastic litter. Most of these cores included plastic down to 30–40 cm depth and had an underlying layer of black soil or gravel with pumice rocks. Large macroplastic items were found down to 40 cm depths at several places.
The Pond in Zone 3
The prevailing south westerly winds have over time caused aggregates of plastic in the inner northwestern part and at the western side of the pond (Figures 6, 7). On the pond surface, plastics were concentrated at the eastern and northern bank, whereas on the western and northwestern bank, no plastic was seen on the surface. A gradual increase in surface plastic concentration was observed toward the east, where plastics were gradually entangled and incorporated into the macrophytes and peat moss, fully or in part covering the floating items (Figure 7B). These “Green Plastic islands” behave as buoyant floats of vegetation and plastic, and are clearly not attached to the ground, but are thick and were in some cases stable enough to carry an adult human.
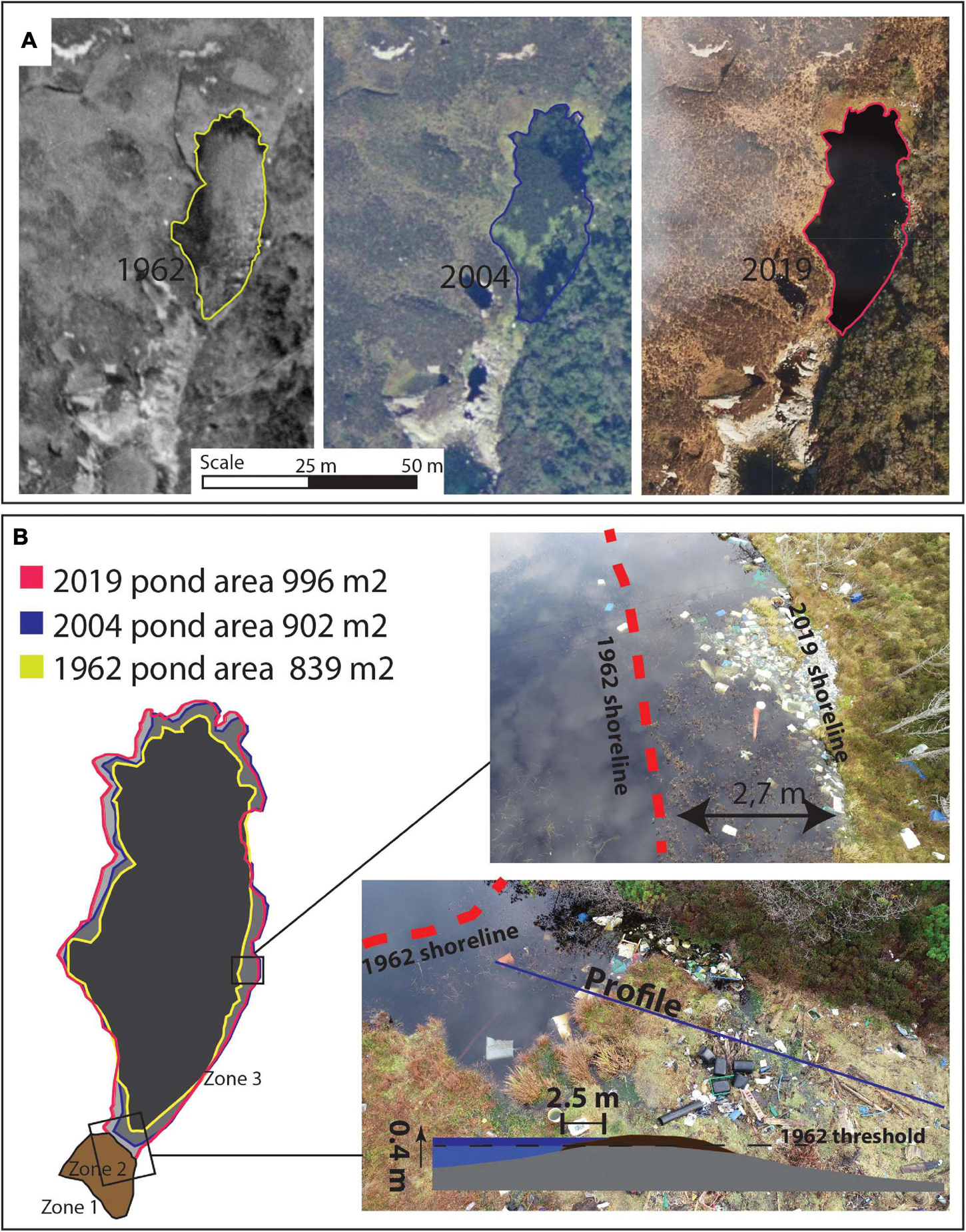
Figure 7. (A) Comparing landscape features from historical images from 1962 and 2004 to current situation in 2019. (B) Shoreline comparison shows a pond increase from 1962 to today. The increase is observed as 2–3 meter horizontal extension? along the eastern and northern shores. At western shores the horizontal increase is less due to steep shore gradient. In Zone 2 where the pond is dammed by the embankment, shoreline has moved toward pond, indicating that the embankment has increased in size since 1962.
The bottom sediments of the pond consisted of mineral clay and coarse sand, probably a result of surface weathering and marine deposits (sample LL9, Table 1). No plastic fragments could be observed, and no large plastic items were caught in the grab. The sediment layer was thin and was not sampled for further core description. Divers observed large pieces of well-preserved plastic sheets and tarpaulins on the bottom of the pond, mainly in areas of macrophytic vegetation that trapped the plastic among the stems. The species recorded in the pond included: Menyanthes trifoliata, Comarum palustre, Sparganium angustifolium, Hipporus vulgaris, Juncus articulatis, Nymphaea alba, and Myriophyllum alterniflorum. Most of these species grow in nutrient poor lakes, but the latter two also grow under nutrient-rich conditions.
Evidence of Landscape Changes
At Lisle Lyngøyna, aerial images show plastic debris on the island dating back to 2004. The comparison of pond size and outline on imagery from 1962 with later imagery (2004 and 2019) showed a change in pond shape and size from 1962 to 2004 and 2019 (Figure 7A). The 1962 image is black and white, which challenge the discriminations between vegetated areas, bedrocks and reflection from the sun. The resolution is too low to identify marine litter/debris from 1962 (which normally would be mainly driftwood). The shoreline of the pond was however clearly detectable in the images, facilitating a detailed interpretation. These images also indicated that a substantial part of the soil/peat was extracted at the location north of the pond, this is seen as sharp cuttings in the landscape (see Figure 7A). In the 2004 and 2019 images it is possible to observe plastic in the images, even though it is vague in the 2004 images due to the resolution. The shoreline trace of the three images shows an increase in pond size from 1962 to 2004 and 2019. The increase is largest at the flat bogs at the north and eastern shores showing a move of the shoreline by 2.5–3 meters (Figure 7B). There is also an apparent change in Zone 2 from 1962 to modern time. Although the image quality in the 1962 images is not sufficient to conclude, there seems to be a barren rock surface where the storm deposit (Zone 2) is placed today.
Case 2 - Kolavika Bay
Kolavika Zone 1
The gravel and pebble beach (1–10 cm pebble diameter) in Kolavika made up Zone 1 (Figures 8, 9). It was dominated by a belt of fresh seaweed at the high tide mark. The seaweed was mixed with plastic items of recent date, such as plastic tobacco (snus) containers, ropes, lids, plastic films, corks and smaller items, such as packaging film. As is typical for Zone 1, the sediment is mobile and shifts from season to season similar to what was observed in Lisle Lyngøyna.
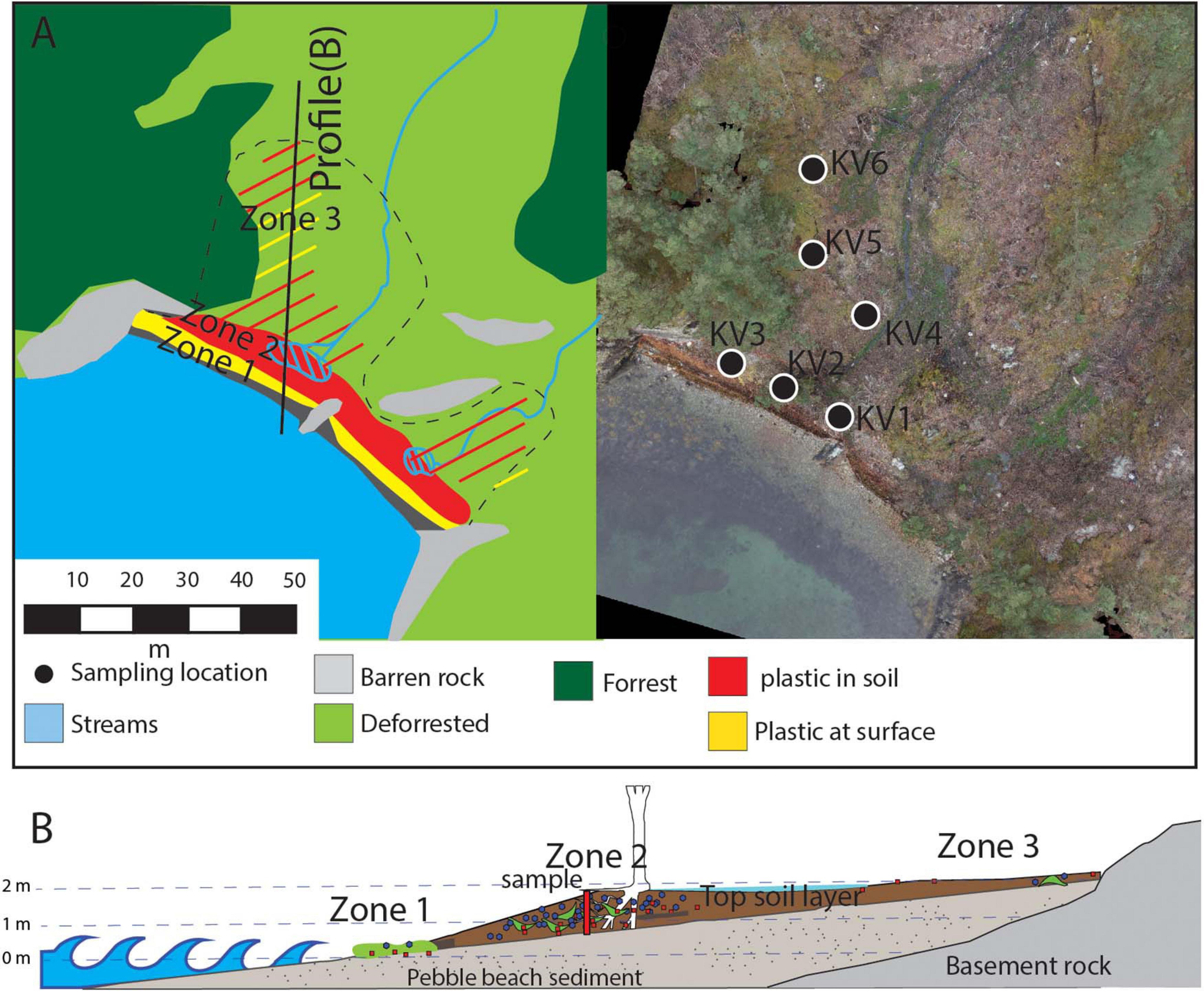
Figure 8. (A) Map of the Kolavika location from high resolution drone images acquired in autumn of 2019. The map illustrates the distribution of deposits in zones 1, 2 and 3 (B) Profile from the shoreline and 50 meters onshore illustrating the soil thickness and plastic litter distribution in the substratum.
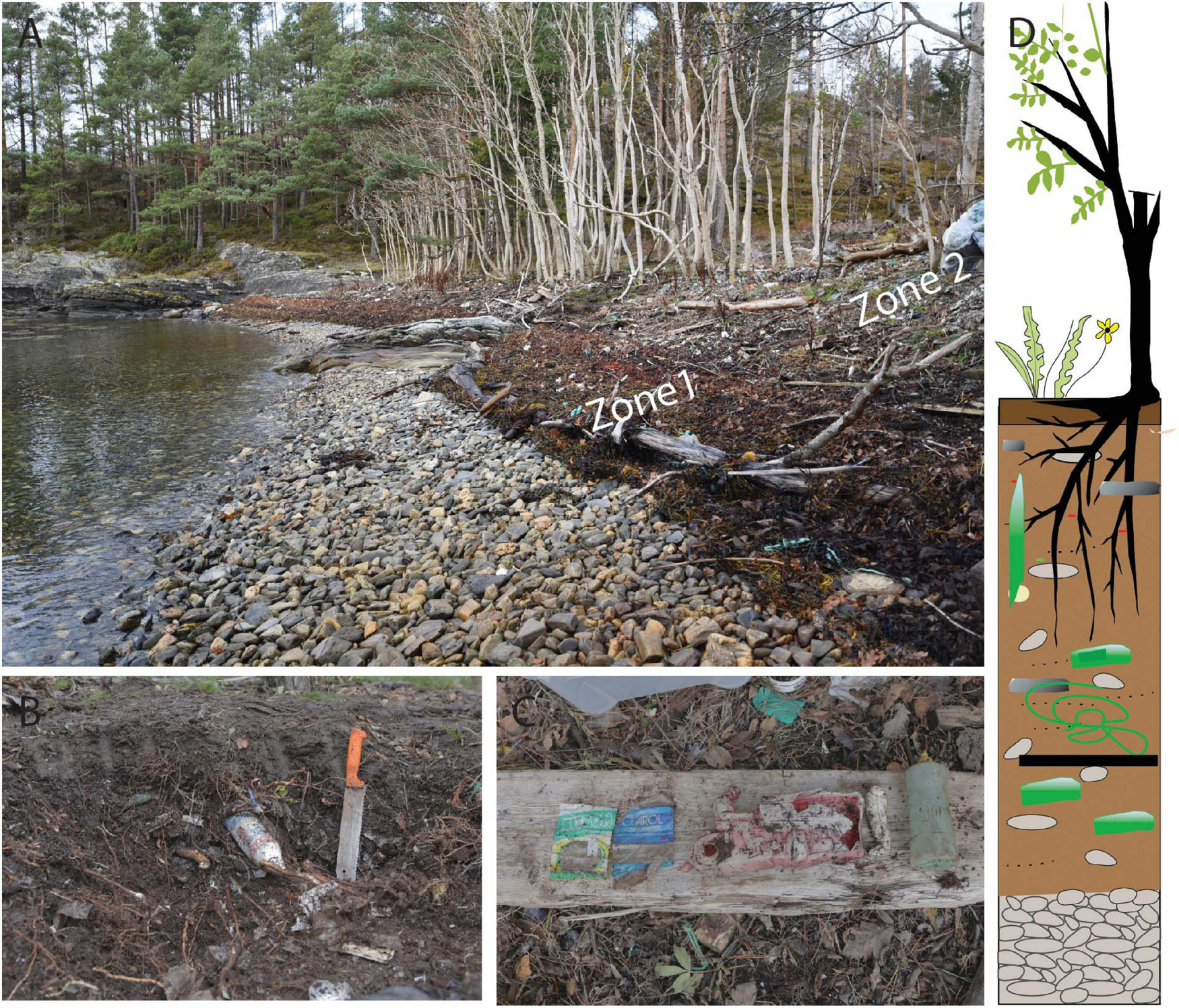
Figure 9. (A) Field photo of pebble beach and Zone 1 and Zone 2 deposits at Kolavika location. (B) profile section (sample KV 2), (C) typical well-preserved items found in the storm embankment (Zone 2), (D) Sketch displaying the soil in Zone 2 with an ash tree growing in the plastic polluted soil.
Kolavika Zone 2
This zone was made up of a 0.5 m high (on average), 48 m long and 2 to 8 m wide embankment consisting of large quantities of plastic, soil and driftwood that lined the entire width of the bay (Figures 8, 9). The embankment was terrace shaped with a flat top and steeper (20°) slope down toward the sea. The upper layer was comprised of organic matter with a 5 cm layer of sand and clay, mixed with pieces of bark on top. The sediment composition of this layer was similar to the composition of sediments in the stream, which indicates that it was deposited by the stream during a recent flood event. The middle layer consisted of soil rich in roots, driftwood, beach pebbles (rip-up clasts) and plastic fragments. Plastic was present down to 90 cm depth in the embankment. We estimated that the storm embankment consisted of 50/50 soil to plastic ratio by volume. The pieces of plastic were often well preserved and could be recognized in terms of purpose of use, labels and prints (Figure 9B). In the bottom layer at 40-60 cm below the surface, there were brittle, but well-preserved plastic toys with a typical 1960’s design (Figure 9C), plastic perfume containers, bottles for liquid dishwashing detergent and candy wrappings with a 1960-1970 design.
The vegetation was dominated by a group of young European ash trees (Fraxinus excelsior) (Figure 9A), a few specimens of Alnus glutinosa in the eastern part and Plantago lanceolata, Lotus corniculatus, Rubus nemoralis and Geranium robertianum in the field layer. The moister parts of the upper, flat embankment were dominated by Juncus bufonius, Cardamine amara and Triglochin maritima.
The ash trees grew in the plastic soil, with roots surrounding and penetrating plastic fragments. The tree diameter at breast height varied between 17 and 57 cm. Based on tree ring counts, many were under 20 years old and one was 55 years old. These observations indicate that the trees started growing in the plastic infused substrate after the onset of plastic accumulation.
Kolavika, Zone 3
Zone 3 in Kolavika started behind the storm embankment (Zone 2) and extended inland toward the NE to about 60 m from the high tide mark (Figure 8). In general, a 30-50 cm thick layer of soil covered a base layer of beach pebbles. The post glacial rebound after the last ice age caused the beach zone to have gradually been uplifted. The shoreline has consequently moved gradually toward the SW, and beach sediments can therefore be found under the soil some distance from the present shoreline. The map in Figure 8 (dashed black line) illustrates where we can assume to find beach sediments under the soil layer, based on the assumption that areas with a consistent 5–6° slope gradient are remnant beaches. Zone 3 consisted mainly of a recently clear-cut area. It was dominated by different species of herbs and moss, such as Veronica officinalis, Mercurialis perennis, Hylocomium splendens and Pleurozium schreberi on the drier parts and Juncus bufonius, Cardamine amara and Triglochin maritima on the moister parts and along the stream. Pieces of plastic were scattered on the surface in Zone 3. Soil samples (sample KL 5 and 6) contained about 90/10 soil plastic ratio. Plastic was also found at a land-tongue of about 35 m in length and 20 meter in width in a NE direction. The soil profile in Zone 3, 25 m from the high tide mark indicated that the upper 10 cm mostly consisted of organic matter and included also clear and colored plastic flakes from plastic bags, and expanded polystyrene beads, all of which could easily be windblown to this position. Fragmented hard plastics were also observed and could stem from larger pieces that could be windblown, and later break up or degrade into smaller pieces. Below this upper vegetated layer pieces of plastic were found within the thin soil down to 20 cm above the base layer of pebbles and gravel.
Discussion
The sites described in this paper include two accumulation sites for marine debris that have not been cleaned for several decades. A general mapping of parts of the Norwegian archipelago (Bastesen et al., 2020) indicates that such sites are common along the outer archipelago, and likely all along the Norwegian coastline where conditions are favorable for accumulation of debris. Similar observations are also reported elsewhere in the world (Merrell, 1980; Andrady, 2003), and likely share some common features. To our knowledge no other studies have investigated the potential long-term effect of plastic accumulation on the development of the coastal landscape, soil and vegetation, and the corresponding consequences for coastal ecosystems.
Signs of Landscape Changes Due to Plastic Accumulation
Table 3 summarizes the quantifiable landscape changes observed at the two localities based on estimation from the field work and from 3D model interpretation. Accumulation of plastic soil is evident at both study sites. The accumulation has had a profound impact on the surrounding soil generation, vegetation and landscape, although with somewhat different consequences for the landscape development at the two sites. At Lisle Lyngøyna the historical images show that the pond increased in size, which may coincide with the increased accumulation of plastic in the storm embankment (Zone 2). At Kolavika, increased plastic pollution caused the formation of an oversized drift embankment. We estimated an increase in soil volume of 100–125 m3 at Lisle Lyngøyna and about 75 m3 at Kolavika (Table 3). Both places can be described as typical hot spots where ocean current, wind and landscape shapes are favorable for accumulation of marine debris.
Landscape Changes in Lisle Lyngøyna
Based on the observations, a two staged evolutionary model of the landscape changes at Lisle Lyngøyna can be sketched (Figure 10).
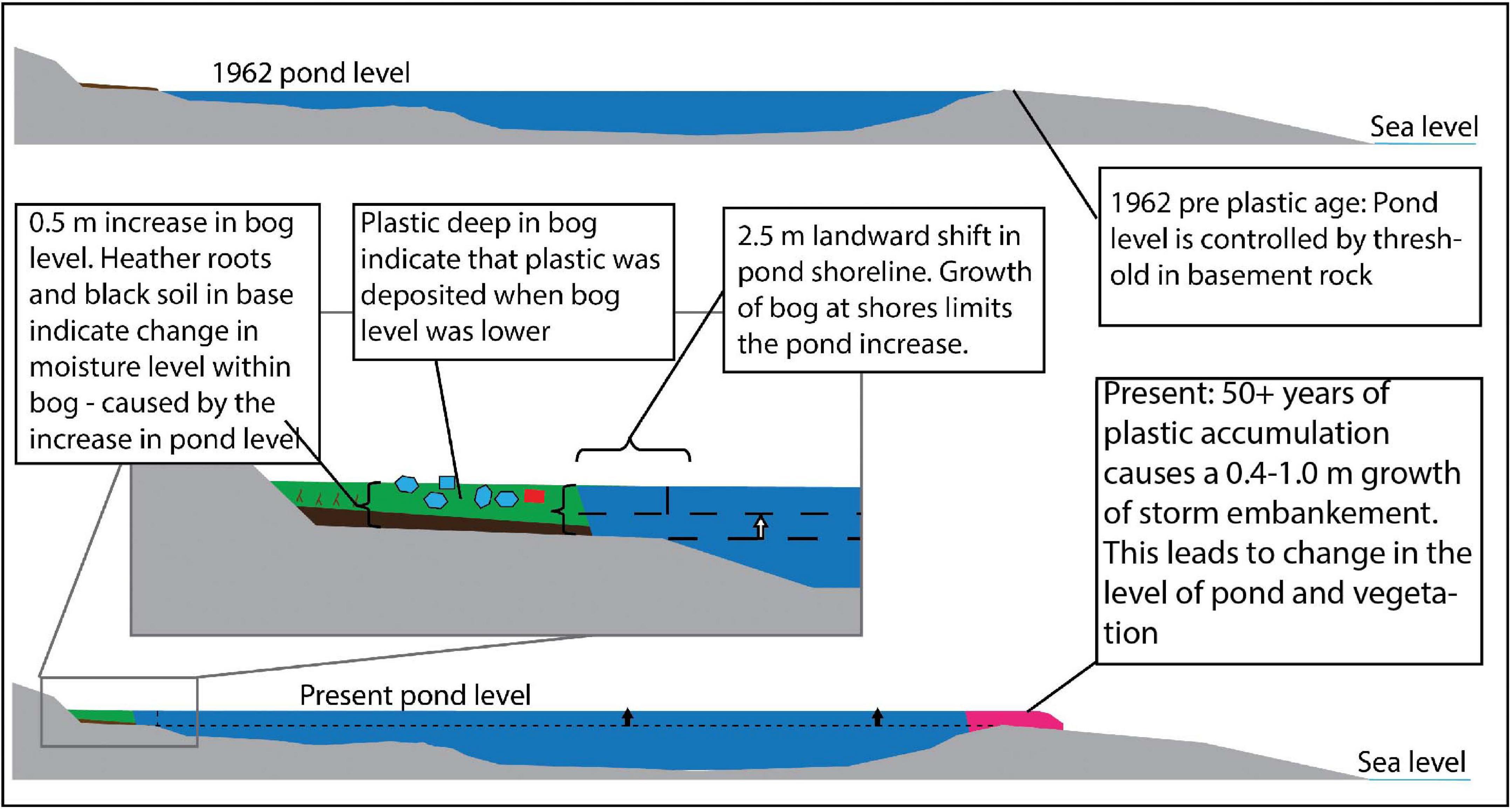
Figure 10. Model displaying the evolution of the Lisle Lyngøyna from the onset of plastic in the 1960s. See insets and text for explanation.
Stage 1 before1960: the landscape was managed heathland (Fremstad et al., 1991). Peat was extracted from the northern shores of the pond leaving a thin soil layer above the bedrock. The pond level was below the flat bedrock surface leaving this area mostly dry. The storm embankment at the cove (Zone 2) consisted of driftwood and other organic materials. The deposit level was about 5–10 cm thick and was kept stable by a balance between deposition through storms, erosion and biodegradation. Furthermore, driftwood may also have been harvested by local farmers for firewood, reducing the amount of accumulation.
Stage 2 1960 to the present: A visible growth of the storm embankment took place, and our hypothesis is that this was due to an increasing proportion of plastic waste in the marine debris. Plastic waste accumulated in the storm embankment, in the pond, and at the shores of the pond. The plastic within the embankment was not subjected to extensive fragmentation or degradation and protected the organic material from erosion. Consequently, there was an escalating growth of the storm embankment. Deposition of plastic debris on high grounds took place during major storms. An increased height of the embankment caused an elevation of the threshold at the outlet of the pond and consequently the pond level rose.
The cores and profiles at the northern shore (LL5, LL6, LL7, Figure 6) of the pond showed that plastic is entangled into the vegetation down to 40 cm below the surface. These plastic fragments were found below the present pond water level (down to 40 cm). Some items, such as pellets and tar were found at 20–30 cm depth (Figure 6), these materials that are buoyant and compact are less likely to be deposited by wind and more likely deposited by water. Hence, the material must have been deposited at the water surface of the pond. Since it is now present 20-30 cm below surface it indicates that the water level has risen gradually during the period with accumulation of plastic. The assumed bare land north of the pond was gradually flooded and overgrown with peat moss (Figures 7, 10). The increased area of the pond is opposite to a natural succession where ponds grow in with vegetation and over time develop into bogs and finally forest.
The lack of aerial images from 1970 until 2000 makes some uncertainties to the model, however observation of items in the soil profile with the deepest items dating back to the 1970’s, support the idea that soil has been formed at least from this time onward.
Landscape Changes in Kolavika
In Kolavika, the storm embankment consists of plastic, soil and vegetation, and has formed a semi-impermeable plastic filled barrier for the small streams running through the area toward the sea. Although the streams percolated in part through the pebbles at the base of the embankment, the area behind the embankment was still saturated with water. This area experiences heavy rains throughout all seasons, and poor drainage will therefore easily lead to an increased water saturation. Plastic is causing an increased barrier to ground water flow.
Soil Formation/Microhabitats
Observations of the deposits in the two locations also support the impression that plastic debris increases soil formation by forming a scaffold that binds the organic material and protects the decomposing seaweed from being washed away during the subsequent winter storms. The plastic will also prevent evaporation and retain moisture in the soil and reduce the exchange of gases through impermeable layers of plastic. Together this may alter or change soil formation and soil properties compared to plastic free conditions.
At Lisle Lyngøyna the soil layer is thicker in areas with plastic than in areas without plastic. Moreover, the organic rich and humid plastic soil may provide a favorable microhabitat for some plants. Experiments have shown that microplastic alters the structure and biophysical properties of soils with various effects on plant growth, e.g., biomass above and below ground, and changed growth (Boots et al., 2019; Rillig et al., 2019; Rillig and Lehmann, 2020). Changing growth conditions in plastic polluted soils can lead to change in competition among plant species and eventually in their composition (Lozano and Rillig, 2020). In the vicinity of the Lisle Lyngøyna storm embankment, we found some nutrient demanding species in an otherwise nutrient poor environment (Figure 2). However, we cannot exclude that their presence is due to natural nutrient rich conditions caused by the accumulation of seaweeds in this zone. In Kolavika, a similar build-up of a plastic-soil embankment seems to be the main reason that ash trees have managed to establish in an environment that otherwise would have been unstable (Figure 9). The group of ash trees growing on the storm embankments are evidently all younger than 55 years, indicating that the trees started growing in the plastic infused substrate after the onset of plastic accumulation. In this case plastic contamination can have facilitated growth of trees that normally would not have survived due to lack of soil for nutrients and root attachment. In other words, seedlings and saplings growing in a more permeable embankment on the pebble beach would be vulnerable to droughts and erosion. We hypothesize that the plastic accumulation has created sufficient stable, humid soil masses and protective shelter for seeds to germinate and permanently establish in the storm embankment.
Trends of waste in the soil profiles may reflect the amounts of plastic and the historical development of plastic use and waste generation. Investigation of the plastic layers may reveal sources, as well as consumer patterns from the time of accumulation. In this study we have tried to keep the location untouched and we have therefore not removed enough to conclude if there is a stratigraphic deposition following the principles of the law of superposition. This law states that within a sequence of sediments the oldest layer is at the base and the layers are progressively younger upward in the sequence. At the storm embankments a base layer pre-dates the plastic deposit should be found in the base and gradually younger plastic pieces should be found upward. Some trends can however be observed. At Lisle Lyngøyna as well as in Kolavika, base layer of the storm deposit (zone 2) seems to be plastic free, marine debris is wooden pieces and pumice fragments. The latter would be the most persistent to degradation. Volcanic pumice from nearby sources (Iceland) is however Holocene age (older than 4000 years), pumice fragments of this age is found scattered along the entire north Atlantic (Newton, 2000; Larsen et al., 2014). It will therefore be of great importance to geochemically compare the pumice found in and below the plastic deposits, are these pumices redeposited Holocene pumices or do they represent modern pumice from a far distance source for instance the South Sandwich Island eruption in 1962 (Risso et al., 2002)? Other similarities were the common debris from the fishing and boat industry are represented by ropes, fish nets, containers, aquaculture feed-tubes and fish crates. The plastic industry is also represented, as plastic resin pellets are commonly observed in all soil layers.
Physical fragmentation of the plastic litter is a severe problem for the environment as this process generates microplastic and such sites could therefore be considered sources of microplastic (Thompson et al., 2004; Barnes et al., 2009). In some areas of the field sites fragmented plastic is quite common, typically in Zone 3 (sample LL7 Figure 6). After deposition, the plastic will be exposed to UV-light and frost degradation at the surface, that speeds up the degradation rate (Andrady et al., 2003). Some items will be degraded quickly into smaller fragments, especially thinner plastic items, such as plastic from bags or foil. In Zone 2 a substantial part of the plastic observed in the soil was nevertheless well preserved (Figures 2, 9). For example, the mid layer down to 60 cm in the soil in the storm deposit at Lisle Lyngøyna and Kolavika was composed of well-preserved plastic pieces from the 1970s and 1980s (Figures 2, 9). The good preservation could be due to the polymer types used in plastic materials in the 1970’s and 1980’s, or that the thicker plastic we find is more resilient to weathering in the low intensity sunlight of Norway, while the thinner plastics from the same time may already have fragmented. The fast accumulation and burial in these zones may also protect these plastic items from further physical degradation processes.
Man-Made Landscape Changes and Restoration
It is important to consider the changes that have occurred over the last decades in the coastal cultural landscape and how humans use the coast. The coastal areas have been cultivated and populated for centuries, and the resources offered by the areas were valuable for the coastal population. It was common to exploit driftwood for firewood and seaweed for fertilizer or food for livestock. Peat was cut from bogs and burned for heating (see example from Lisle Lyngøyna in Figure 7). We can assume that Lisle Lyngøyna, Kolavika and many other wreck bays were cleaned regularly to exploit these resources. This may have influenced the way the storm deposits evolved, as some bays may have been cleared annually for most loose material. After 1960 modernization caused a change in the way people lived and these resources from the sea were left behind and allowed to accumulate or degrade.
Cleaning operations are rarely complete when it comes to the plastic buried in the soil after decades of accumulation as it would require intensive efforts and resource. So far, we lack knowledge about the effects of the plastic as well as from disturbances caused by cleaning operations. Removing all the plastic soil to restore the landscape to its “natural” state may potentially cause more damage to the aquatic life in the pond, the vegetation and inhabitants at the plastic contaminated sites, and cause more immediate harm than if we were to leave it and perform regular clean-ups to gradually remove emerging debris and allow the changes to take place slowly. In cases when the plastic has negligible impact on the ecosystem, we can remove visible debris on the surface and restore recreational value for humans. However, we should not automatically assume that the plastic in the soil profile is of pressing concern to conservation of ecological integrity. Decisions on abatement measures that are not knowledge-based can lead to a waste of conservation resources and in the worst case be harmful for the ecosystem, e.g., by remobilization of buried contaminants or by disturbing the ecosystem.
Conclusion
We have made the following observations that support the hypotheses of landscape changes due to plastic accumulation:
(1) Plastic accumulation accelerates the formation of a thick storm embankment by acting as a scaffolding for organic deposits. This observation is supported by transects in the deposition zone showing a 5–10 cm bottom layer of compact and plastic-free soil with dense roots under a 40–70 cm layer of organic rich and wet soil mixed with plastic items. The soil and plastic items are distributed throughout the soil, indicating that the soil was generated alongside the accumulation of plastic.
(2) Aerial photos of Lisle Lyngøyna, indicate that the pond has increased in size from 1960 to 2015, as opposed to the natural succession where ponds grow in with vegetation and over time develop into bogs and finally forest over time. This suggests that plastic deposits and newly generated plastic-rich soil can form dams and prevent natural drainage of near shore wetlands and ponds.
(3) Our observation indicates that plastic accumulation caused changes in the vegetation. This is supported by the soil profiles around the ponds and bogs including a base layer with peat moss and heather overlain by a layer of peat moss with incorporation of plastic fragments. This finding indicates that the wet conditions leading to growth of peat moss instead of heather co-occurred with the deposition of plastic fragments. In support of this, we also see that plastic fragments float in the pond before they are trapped and deposited in the vegetation around the pond. The current bog vegetation grows around the plastic fragments, and over time, peat soil will overlay the plastic.
The observed accumulation and distribution patterns of marine plastic debris and long-term changes to the structure of soils, and the associated morphological development/changes of the landscape should not be assumed to occur only along the Norwegian coastline. This is likely a global phenomenon. Wherever there is debris, tidal movement, wind and a potential for accumulation it is likely that similar processes occur, potentially leading to large changes to costal ecosystems around the globe. The remedy is, first and foremost, to prevent and mitigate plastic waste accumulation by better waste management systems and frequent beach cleans. Such measures are likely far more realistic and desirable than the complete cessation of the use and release of plastic, although it is an enormous cleaning job that will go on for decades, long after the input of plastic to the ocean has stopped. Simply exchanging non-biodegradable plastic with biodegradable polymers is not recommended by the recent SAPEA report (Science Advice for Policy by European Academies (SAPEA), 2020) due to risk of continuation of the waste problem. However, biodegradable plastics may be a solution for some applications with high risk of loss to the open environment and where it is difficult or expensive to remove it from the environment. Sound application of biodegradable plastics designed for industrial composting is dependent on a working waste management system, in the same manner as conventional plastics.
Our results support that we have entered the Anthropocene/Plastocene period whereupon layers of mismanaged plastic debris is influencing the landscape, the vegetation and geological processes and one day will be evident in sedimentary records of the future.
Data Availability Statement
The original contributions presented in the study are included in the article/supplementary material, further inquiries can be directed to the corresponding author/s.
Author Contributions
EB did the idea and project leader and field work and manuscript writing. MH did the field work and initiative to the study. GA did the field work on bothanics. GV did the Field work biology and lake and contributed with ideas. GB did the field work biology. CK did the field work. All authors contributed to the article and approved the submitted version.
Conflict of Interest
The authors declare that the research was conducted in the absence of any commercial or financial relationships that could be construed as a potential conflict of interest.
Acknowledgments
Special thanks to Rune Gaasø (Clean Shores Global) and Kenneth Bruvik (Norwegian Hunter- and Anglers’ Association) for making us aware of the plastic accumulation at Lisle Lyngøyna and for their engagement in developing the Lisle Lyngøyna project into a scientific study. Cato Lyngøy, representative of the landowners, is thanked for letting us work at the location and for providing valuable historical input. Sveinung Toppe and Gudrun Fatland from Bergen Recreational Council (Bergen Omland Friluftsråd) for financial support, aiding in boat transport to the Kolavika bay and for fruitful discussions regarding plastic accumulation in western Norway. The Retailers’ Environment Fund (Handelens Miljøfond) for supporting the project “Plastrent Tysnes.” Bergen and Øygarden municipalities for financing several small projects regarding plastic mapping leading up to this project. Christoph Postler, Benjamin Dolva, Tobias Kurz, and Simon Buckley are thanked for photogrammetric work and drone image collection. Jan Tveranger is thanked for providing knowledge and discussion around coastal sedimentary systems. Emily Lyng is thanked for proofreading and language correction. Two reviewers are thanked for comments and suggestions that significantly improved the manuscript.
Footnotes
- ^ https://klimaservicesenter.no/observations/
- ^ https://lovdata.no/dokument/LF/forskrift/2014-12-12-1669
References
Andrady, A. L., Hamid, H. S., and Torikai, A. (2003). Effects of climate change and UV-B on materials. Photochem. Photobiol. Sci. 2, 68–72.
Barnes, D. K., Galgani, F., Thompson, R. C., and Barlaz, M. (2009). Accumulation and fragmentation of plastic debris in global environments. Philos. Trans. R. Soc. Lond. B Biol. Sci. 364, 1985–1998. doi: 10.1098/rstb.2008.0205
Bastesen, E., Haave, M., Avlesen, H., and Neby, S. (2020). Utvikling av Kartleggingsmetoder og Estimat for Oppryddingskostnader for Makroplast i Strandsonen. NORCE Report nr 4, (Bergen, BC: NORCE Research), doi: 10.1098/rstb.2008.0205
Boots, B., Russell, C. W., and Green, D. S. (2019). Effects of microplastics in soil ecosystems: above and below ground. Environ. Sci. Technol. 53, 11496–11506. doi: 10.1021/acs.est.9b03304
Borrelle, S. B., Ringma, J., Law, K. L., Monnahan, C. C., Lebreton, L., and McGivern, A., et al., (2020). Predicted growth in plastic waste exceeds efforts to mitigate plastic pollution. Science 369, 1515–1518. doi: 10.1126/science.aba3656
Buckley, S. J., Ringdal, K., Naumann, N., Dolva, B., Kurz, T. H., and Howell, J. A., et al., (2019). LIME: software for 3-D visualization, interpretation, and communication of virtual geoscience models. Geosphere 15, 222–235. doi: 10.1130/ges02002.1
Carlsen, T., and Bär, A. (2016). Skjøtselsplan for beiteområde for beiteområde på Bliksvær i Bodø kommune, Nordland. Nord-Trøndelag: NIBIO.
Cózar, A., Martí, E., Duarte, C. M., García-de-Lomas, J., Van Sebille, E., and Ballatore, T. J., et al., (2017). The Arctic Ocean as a dead end for floating plastics in the North Atlantic branch of the Thermohaline circulation. Sci. Adv. 3:e1600582. doi: 10.1126/sciadv.1600582
Eriksson, C., Burton, H., Fitch, S., Schulz, M., and van den Hoff, J. (2013). Daily accumulation rates of marine debris on sub-Antarctic island beaches. Mar. Pollut. Bull. 66, 199–208. doi: 10.1016/j.marpolbul.2012.08.026
Fremstad, E., Aarrestad, P. A., and Skogen, A. (1991). Kystlynghei på Vestlandet og i Trøndelag: Naturtype og Vegetasjon i Fare, Vol. 029. Trondheim: Norsk institutt for naturforskning.
Haarr, M. L., Westerveld, L., Fabres, J., Iversen, K. R., and Busch, K. E. T. (2019). A novel GIS-based tool for predicting coastal litter accumulation and optimising coastal cleanup actions. Mar. Pollut. Bull. 139, 117–126. doi: 10.1016/j.marpolbul.2018.12.025
Heyerdahl, T. (1971). Atlantic Ocean pollution and biota observed by the “Ra” expeditions. Biol. Conserv. 3, 164–167. doi: 10.1016/0006-3207(71)90158-3
Hjelle, K. L., Halvorsen, L. S., and Overland, A. (2010). Heathland development and relationship between humans and environment along the coast of western Norway through time. Quat. Int. 220, 133–146. doi: 10.1016/j.quaint.2009.09.023
Ivar, D. S. J., and Spengler, Â, and Costa, M. F. (2009). Here, there and everywhere. small plastic fragments and pellets on beaches of Fernando de Noronha (equatorial western Atlantic). Mar. Pollut. Bull. 58:1236. doi: 10.1016/j.marpolbul.2009.05.004
Jambeck, J. R., Geyer, R., Wilcox, C., Siegler, T. R., Perryman, M., and Andrady, A., et al., (2015). Marine pollution. plastic waste inputs from land into the ocean. Science 347, 768–771. doi: 10.1126/science.1260352
Kartar, S., Milne, R. A., and Sainsbury, M. (1973). Polystyrene waste in the Severn Estuary. Mar. Pollut. Bull. 4:144. doi: 10.1016/0025-326x(73)90010-6
Laist, D. W. (1987). Overview of the biological effects of lost and discarded plastic debris in the marine environment. Mar. Pollut. Bull. 18, 319–326. doi: 10.1016/s0025-326x(87)80019-x
Larsen, G., Eiríksson, J., and Gudmundsdóttir, E. R. (2014). Last millennium dispersal of air-fall tephra and ocean-rafted pumice towards the north Icelandic shelf and the Nordic seas. Geol. Soc. Lond. Spec. Publ. 398, 113–140. doi: 10.1144/sp398.4
Lebreton, L. C. M., van der Zwet, J., Damsteeg, J. W., Slat, B., Andrady, A., and Reisser, J. (2017). River plastic emissions to the world’s oceans. Nat. Commun. 8:15611.
Lozano, Y. M., and Rillig, M. C. (2020). Effects of microplastic fibers and drought on plant communities. Environ. Sci. Technol. 54, 6166–6173. doi: 10.1021/acs.est.0c01051
Mangerud, J., Gyllencreutz, R., and Lohne, Ø, and Svendsen, J. I. (2011). “Glacial history of Norway. Dev. Quat. Sci. 15, 279–298.
Merrell, T. R. Jr. (1980). Accumulation of plastic litter on beaches of Amchitka Island. Alaska Mar. Environ. Res. 3, 171–184. doi: 10.1016/0141-1136(80)90025-2
Napper, I. E., and Thompson, R. C. (2020). Plastic debris in the marine environment: history and future challenges. Glob. Chall. 4:1900081. doi: 10.1002/gch2.201900081
Newton, A. (2000). Ocean-Transported Pumice in the North Atlantic. Doctoral dissertation, University of Edinburgh, Edinburgh.
OSPAR (2007). OSPAR Pilot Project on Monitoring Marine Beach Litter – Monitoring of Marine Litter in the OSPAR Region. OSPAR Commission (2007). London: OSPAR
Pham, C. K., Ramirez-Llodra, E., Alt, C. H. S., Amaro, T., Bergmann, M., and Canals, M., et al., (2014). Marine litter distribution and density in European Seas, from the shelves to deep basins. PLoS One 9:e95839. doi: 10.1371/journal.pone.0095839
Rillig, M. C., and Lehmann, A. (2020). Microplastic in terrestrial ecosystems. Science 368, 1430–1431. doi: 10.1126/science.abb5979
Rillig, M. C., Lehmann, A., de Souza Machado, A. A., and Yang, G. (2019). Microplastic effects on plants. New Phytol. 223, 1066–1070. doi: 10.1111/nph.15794
Risso, C., Scasso, R. A., and Aparicio, A. (2002). Presence of large pumice blocks on Tierra del Fuego and South Shetland Islands shorelines, from 1962 South Sandwich Islands eruption. Mar. Geol. 186, 413–422. doi: 10.1016/s0025-3227(02)00190-1
Science Advice for Policy by European Academies (SAPEA) (2020). Biodegradability of Plastics in the Open Environment. Berlin: SAPEA. doi: 10.26356/biodegradabilityplastics
Storlazzi, C. D., and Field, M. E. (2000). Sediment distribution and transport along a rocky, embayed coast: Monterey Peninsula and Carmel Bay. Calif. Mar. Geol. 170, 289–316. doi: 10.1016/s0025-3227(00)00100-6
Thiel, M., Hinojosa, I. A., Joschko, T., and Gutow, L. (2011). Spatio-temporal distribution of floating objects in the German Bight (North Sea). J. Sea Res. 65, 368–379. doi: 10.1016/j.seares.2011.03.002
Thompson, R. C., Olsen, Y., Mitchell, R. P., Davis, A., Rowland, S. J., and John, A. W., et al., (2004). Lost at sea: where is all the plastic? Science 304:838. doi: 10.1126/science.1094559
Thompson, R. C., Swan, S. H., Moore, C. J., and Vom Saal, F. S. (2009). Our plastic age. Philos. Trans. R. Soc. Lond. B Biol. Sci. 364, 1973–1976. doi: 10.1098/rstb.2009.0054
Trenhaile, A. (2016). Rocky coasts—their role as depositional environments. Earth Sci. Rev. 159, 1–13.
Keywords: macroplastic, Norwegian coastline, landscape changes, wreck bays, sea current
Citation: Bastesen E, Haave M, Andersen GL, Velle G, Bødtker G and Krafft CG (2021) Rapid Landscape Changes in Plastic Bays Along the Norwegian Coastline. Front. Mar. Sci. 8:579913. doi: 10.3389/fmars.2021.579913
Received: 03 July 2020; Accepted: 09 February 2021;
Published: 05 March 2021.
Edited by:
Montserrat Filella, Université de Genève, SwitzerlandReviewed by:
Christian Joshua Sanders, Southern Cross University, AustraliaConrad Sparks, Cape Peninsula University of Technology, South Africa
Copyright © 2021 Bastesen, Haave, Andersen, Velle, Bødtker and Krafft. This is an open-access article distributed under the terms of the Creative Commons Attribution License (CC BY). The use, distribution or reproduction in other forums is permitted, provided the original author(s) and the copyright owner(s) are credited and that the original publication in this journal is cited, in accordance with accepted academic practice. No use, distribution or reproduction is permitted which does not comply with these terms.
*Correspondence: Eivind Bastesen, RWliYUBub3JjZXJlc2VhcmNoLm5v