- Borneo Marine Research Institute, Universiti Malaysia Sabah, Kota Kinabalu, Malaysia
Different types and inclusion levels of palm oil were incorporated in the enriched diets of L-type rotifer, Brachionus plicatilis, and fed to Asian seabass (Lates calcarifer) larvae. The dietary fish oil was replaced with either 50 or 75% of crude palm oil, CPO (CPO50, CPO75) and refined bleached deodorized palm olein, RPO (RPO50, RPO75). The enriched diet containing 100% fish oil (FO100) was used as the experimental control. Triplicate groups of the fish larvae of initial length 2.72 ± 0.14 mm were fed with enriched rotifer for 15 days. In general, palm oil-based enriched diets performed better than the control diet (FO100). Specifically, final mean body weight (31.3 ± 9.2 mg), final mean total length (11.5 ± 1.6 mm), SGR (29.0 ± 1.4%/day) and WG (7,769.4 ± 1,510.8%) of Asian seabass larvae fed RPO75 were significantly higher (P < 0.05) compared to those fed the other palm oil-based diet and FO100. The rotifer enriched with palm oil significantly affected the body proximate composition and fatty acid profiles of the fed larvae. The present study suggests that RPO and CPO can be considered as a good alternative dietary lipid for enrichment of rotifer to positively influence the nutritional requirements of the Asian seabass larvae and support their survival and growth.
Introduction
Asian seabass, Lates calcarifer, is a tropical marine fish that is widely farmed throughout Asia. Despite being an established aquaculture species, many obstacles remain in using an acceptable live feed in the first feeding of the larval rearing phase. Currently, rotifer (primarily the species, Brachionus plicatilis) is widely used as the first feed for Asian seabass larvae mainly because it is relatively easy to culture, highly digestible, rich in protein, and has been used as a vector for delivering compounds of diverse nutritional value to larval fish (Skalliaa and Robin, 2004; Das et al., 2012; Dey et al., 2015). It is well-documented that live feed should be enriched prior to providing it to the larvae, with many studies focusing on the benefits of live feed enrichment using highly unsaturated fatty acids, HUFA (Rainuzzo et al., 1994; Sargent et al., 1999; Benítez-Santana et al., 2007, Tocher, 2010, Radhakrishnan et al., 2020) such as docosahexaenoic (DHA, 22:6n−3), eicosapentaenoic acid (EPA, 20:5n−3), and arachidonic acid (ARA, 20:4n−6) as the main energy sources and to ensure proper larval development (Rainuzzo et al., 1997; Sargent et al., 1999; Benítez-Santana et al., 2007, Tocher, 2010). Major dietary lipid source used in larviculture is fish-oil based emulsion through live feed enrichment (Rainuzzo et al., 1997). With the growth of aquaculture industry the demand of fish oil for fish nutrition has increased (Alder et al., 2008; Nichols et al., 2010) resulting in shortages and threatening the sustainability of wild prey fish populations (Wassef et al., 2007). Fish oil substitution is, therefore, crucial to reducing the pressure on marine ecosystem while at the same time supporting the aquaculture industry (Tacon and Metian, 2008; Naylor et al., 2009; Alhazzaa, 2012). Developing aquafeeds by replacing fish oil and meal is an important area of research to pursue for reducing the pressure on forage fish. This will be among the major steps toward securing the sustainability of fed finfish aquaculture (Hua et al., 2019; Turchini et al., 2019).
Recent years have witnessed a heightened research interest in replacing fish oil with alternative lipid sources, mainly of plant origin. Palm oil is one of the vegetable oils that has received increasing attention in aquaculture feed research. It is abundantly available in several Asian countries. Apart from supplying dietary fat, palm oil is also a rich source of vitamin E, carotenoids, tocotrienols and natural antioxidants, and has a much cheaper price than the fish oil (Nasaretnam and Muhammad, 1993; Ng et al., 2007; Han et al., 2012; Singh et al., 2012). However, research on the use of palm oil as an alternative dietary fat source mostly involved juvenile and larger fish (Miller et al., 2008; Turchini et al., 2009; Torstensen and Tocher, 2010; Shapawi et al., 2011). In our earlier study based on juvenile stage of the Asian seabass (~5 g), the specimens fed diet based on RPO gained significantly higher (P < 0.05) weight than the other fish groups that were offered diets based on fish oil, soybean oil and canola oil at the end of feeding trials (Shapawi et al., 2011). Therefore, research is needed to investigate the potential of the abundantly available palm oil in the larval nutrition of Asian seabass to curtail the mortality and boost health and stamina of the most vulnerable phase of life of the fish. There is a glaring paucity of data on the application of edible plant-based oil enriched rotifer (Poh-Leong et al., 2012; Dhaneesh and Kumar, 2016; Campoverde and Eztevez, 2017), whereas the use of artemia has received a great deal of attention in nutrition of fish larvae (Villalta et al., 2007; Arulvasu and Munuswamy, 2009; Hafezieh et al., 2010). The present study reports the results of experimental trials carried out for testing the effectiveness of the two different types of palm oils (CPO and RPO) as the lipid source in the enriched diets for the rotifer on Asian seabass larvae. To the best of our knowledge this is the first report on the replacement of fish oil with palm oil in the enriched diets for rotifers in the Asian seabass larvae. Other attempts to use palm oil as a substitute of fish oil have focused on juvenile and grow-out stages. For example, Wan et al. (2013) demonstrated that complete dietary fish oil replacement with either palm oil or lipid from poultry waste in barramundi (Lates calcarifer) juveniles (~3.6 g initial body weight) did not affect growth or hepato-somatic index. In our previous study on humpback grouper (Cromileptes altivelis) juveniles of about 10 g initial body weight, CPO and RPO were shown to have successfully replaced fish oil at 50% replacement level in a fish meal-based formulated diets without any detrimental effects on growth and survival (Shapawi et al., 2008). Similarly, growth performance of hybrid grouper (Epinephelus fuscoguttatus x E. lancelatus) juveniles (~11 g initial body weight) fed CPO and RPO at 50% replacement level was slightly better than in those fed fish oil-based diet (Yong et al., 2019). Bell et al. (2002) have demonstrated that feeding diets containing palm oil (25 to 100% of added oil) to Atlantic Salmon (Salmo salar) juveniles (~55 g) had no significant effect on growth rate or feed conversion ratio, compared to fish fed 100% marine fish oil.
Materials and Methods
Enriched Diet Preparation
A 2 × 3 factorial design using two types of palm oil (CPO and RPO) and three different levels (0, 50, and 75%) was applied to substitute fish oil in the enrichment diets for the rotifer. Fish oil-based enriched diet (FO100) was used as a control treatment. The oil emulsion was prepared by mixing 0.5 g of soy-lecithin and 10 g of egg albumin (Watanabe et al., 1989). Other ingredients included 2.1 g vitamin premix and 1.0 g mineral premix. The emulsion weighing 100 g was used for respective concentrations (50%, 75% and 100%) of either palm oil or fish oil in addition to 35.4% filtered seawater as shown in Table 1. The oil emulsion was homogenized using IKA homogenizer (T-25 basic Ultra Turrax) at 14,000 rpm/minute.
Rotifer Culture and Enrichment Protocol
The L-type rotifer was obtained from Fish Hatchery of Borneo Marine Research Institute (BMRI), Universiti Malaysia Sabah (UMS). The rotifers were cultured in 150 L conical fiber reinforced plastic (FRP) tank with the seawater volume of 100L at the density of 600 rotifers/ml. The salinity and temperature of water were maintained at 30 ppt and 28.59 ± 0.16°C, respectively. Individual rotifer tanks were well-aerated (800 ml/second). Rotifers were enriched using fortified diets in a concentration of 0.5 g/L for 24 h. After this treatment, the enriched rotifers were harvested by using a 60 μm—nylon mesh screen, washed in de-chlorinated seawater and then used for larval feeding at the density of 20 individual/ml. A sample was stored for subsequent chemical analysis.
Proximate and Fatty Acid Analysis
The enriched diets, rotifer and larval whole-body proximate composition were analyzed by methods suggested by the Association of Official Analytical Chemists (AOAC, 1997). The enrichment diets and enriched rotifer were subjected to lipid extraction by using chloroform: methanol (1:1, v/v) following Bligh and Dyer (1959) method. The lipid extract was then fractionated by a short column filled with silica gel 60 F254 (Merck, Darmstadt, Germany) with a mesh size of 0.063–0.2 mm in a hexane: ethyl acetate solvent system (9:1, v/v). Methylation of the extract was carried out inside a reaction vessel for 2 h using sodium methylate. The extract was purified using the silica gel column system before the fatty acid methyl esters were analyzed in a gas chromatograph (Shimadzu GC-2010, Shimadzu Corporation, Kyoto, Japan) equipped with a flame ionization detector and an auto injector. The esters were separated using a capillary column (60 m × 0.25 mm ID; BPX70 column, SGE Australia Pty. Ltd., Ringwood, Vic., Australia) and chromatograph peaks were identified by comparing retention times with known standards (Supelco™ 37 Component FAME mix, Supelco Inc., Bellefonte, USA).
Experimental Design and Feeding Trial
Asian seabass larvae were obtained from the Fish Hatchery of BMRI (UMS). A total of 54,000 newly hatched larvae with an initial weight and length of 0.44 ± 0.11 mg and 2.72 ± 0.14 mm, respectively were observed for general health condition and held in the tanks for experimental trials. Triplicate groups of larvae were randomly distributed into 18 conical FRP tanks (150 liters) with initial stocking density of 30 individual per liter. The experimental tanks were randomly arranged in triplicate and in closed system, and supplied with continuous mild aeration (250 ml/second). Starting from 36 h after hatching (hAH), the respective batches of enriched rotifer were fed to the fish larvae at the density of 20 individual/ml. Nannochloropsis oculata was also provided in all the tanks at concentration of 0.5 × 106 cell/ml. There was no cleaning for up to 5 days to reduce the disturbance to the larvae. After 5 days of rearing, water was routinely changed (5%) to avoid accumulation of metabolic wastes. The density of enriched rotifer was monitored under a profile projector (Mitutoyo, PJ-A3000, Japan) twice daily at 0830 am and 1400 pm. The physiochemical parameters of water temperature, dissolved oxygen and pH throughout the experiment were 29.31 ± 0.80°C, 5.54 ± 0.11mg/L and 8.12 ± 0.04, respectively. The experimental duration was 15 days.
Performance Evaluation
Triplicate pooled sample of initial stock and 5 days after hatching (dAH)-larvae (n = 100) and 10 dAH and 15 dAH-larvae (n = 50) were measured using an analytical balance. At every 5 days interval up to 15 dAH, 10 larvae (n = 10) from each treatment were sampled, anesthetised with Transmore (alpha-methylquinoline) (Nika Trading, Puchong, Malaysia) and measured under a profile projector (Mitutoyo, PJ-A3000, Japan) for morphometric observations on total length (TL), standard length (SL), body height (BH), muscle height (MH), head length (HL), head depth (HD), gut height (GH), and eye diameter (ED). The larvae were examined under a light microscope (Nikon, Eclipse E600, Japan) for rotifer ingestion and gut observation. The condition factor and ratio of total length to body weight (CF) (Chatain, 1994) were determined at the end of the experiment.
Other parameters were calculated using the following formulas:
Specific growth rate (SGR, %/d) = [ln final weight, mg ln initial weight, mg)days] × 100
Survival (%) = [(Initial fish number final fish number) Initial fish number] × 100
Condition factor (CF) = [fish weight(total length)] × 100
Total length/ body weight (TL/W) ratio = [(total length, mm)(body weight, mg)]
Weight gain, WG (%) = [(final body weight, g − initial body weight, g)initial body weight, g] × 100.
Statistical Analysis
IBM Statistical Package of Social Sciences Version 23.0 was used for all the statistical analyses. All the data in percentage were arcsine transformed before the analysis. The quantitative data was subjected to one-way analysis of variance (ANOVA) to determine the mean differences among the treatments at 0.05 significance level. The homogeneity of variance was tested using Levene's test and multiple comparisons among treatments were presented using a Duncan's multiple range test. Effects of oil types and levels, and their interactions were determined by two-way ANOVA.
Results
Growth Performance
Data on larval growth performance is presented in Table 2. Final mean body weight (31.3 ± 9.2 mg), final mean total length (11.5 ± 1.6 mm), SGR (29.0 ± 1.4%/day) and WG (7,769.4 ± 1,510.8%) of Asian seabass larvae fed RPO75 were significantly higher (P < 0.05) compared to those fed the other palm oil-based diet and FO100. The palm oil inclusion level significantly affected these parameters at the end of experiment. Table 3 and Figure 1 show the survival rate and variation (%) of the larvae in the 15 day experimental period. The different batches of enriched rotifer did not show any significant effect on 5 and 10 dAH. However, at the end of the experiment (15 dAH), the survival was significantly affected by palm oil level. CPO50 yielded higher survival (77.4 ± 9.4%) compared to FO100 (54.5 ± 2.0%) and RPO-based treatments (61.2 ± 8.7–67.8 ± 12.3%).
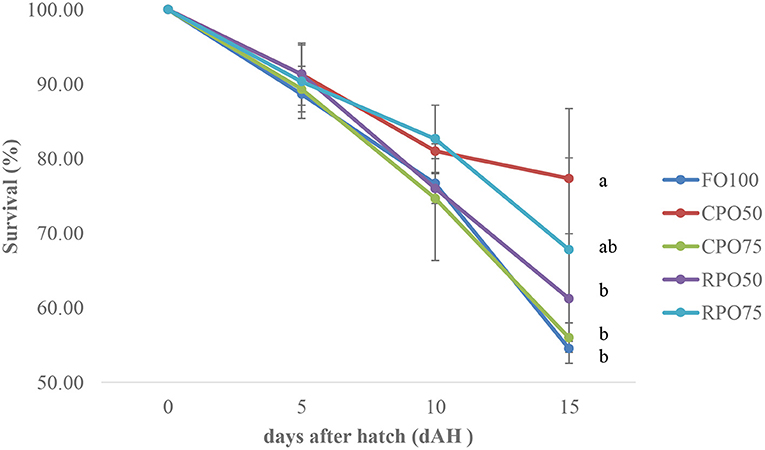
Figure 1. Survival variation (%) of Asian seabass larvae fed enriched rotifer. Mean with same superscripts in the same row are not significantly different (P > 0.05) from each treatment (Mean ± SD).
Morphometric Variables and Body Condition
The morphometric variables and body indices of Asian seabass at the end of the experiment are presented in Table 4. The morphometric value of each parameter was affected by palm oil level. RPO75 shows significantly higher (P < 0.05) SL, MH, ED (10.0 ± 1.0, 2.6 ± 0.5 and 1.0 ± 0.1, respectively). The lowest (P < 0.05) value of HL, HD and ED (2.5 ± 0.2, 1.9 ± 0.2 and 0.7 ± 0.1, respectively) was observed in fish fed FO100 compared to the other treatments. SL, BH, MH, GH (6.4 ± 0.8, 2.1 ± 0.2, 1.6 ± 0.1, 0.4 ± 0.1, respectively of FO100 treatment were significantly lower than the other ones, except RPO50. Meanwhile, the condition factor was not significantly affected by palm oil type and level. However, T:W was significantly influenced by oil type only. TL:W was significantly (P < 0.05) higher in FO100 and RPO50 compared to the other treatments.
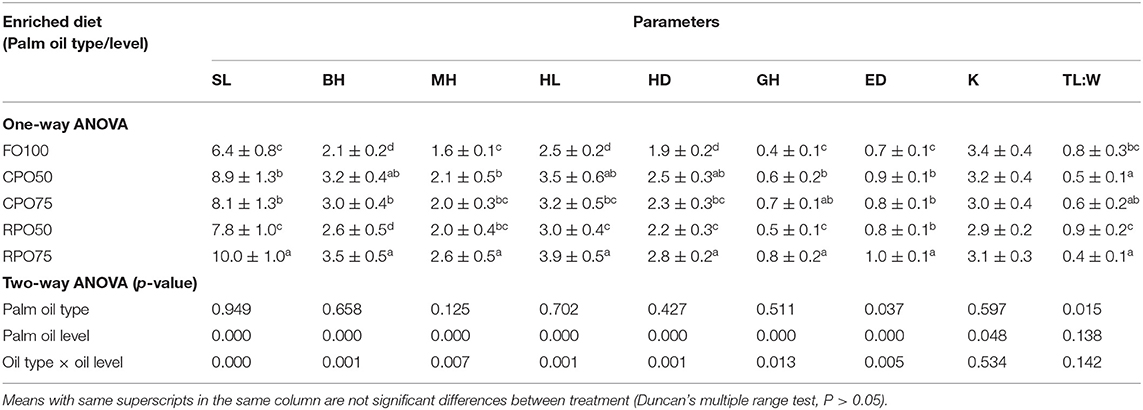
Table 4. Morphometric variables and body indices of Asian seabass larvae fed different types of enriched rotifers.
Proximate Composition of Enriched Rotifer and Larval Fish
Table 5 shows the proximate composition of enriched rotifer. The proximate composition of enriched rotifer was not significantly affected by palm oil type and level except for protein content that was markedly influenced by palm oil type (P = 0.005). RPO50-enriched rotifer contained significantly (P < 0.05) higher protein (55.2 ± 0.3%) compared to the other treatments. Meanwhile, the whole-body proximate composition of Asian seabass was not significantly affected by the enriched diet. Fish fed RPO-enriched diet resulted in slightly higher protein and lipid contents compared to the other treatments as shown in Table 6.
Fatty Acid Composition of Enriched diets, Rotifer, and Larval Fish
In general, the replacement of fish oil with palm oil (CPO and RPO) increased the C:16 (palmitic acid), C18:1n9 (oleic acid) and reduced total PUFA and n-3HUFA, especially DHA content of the enriched diets. The total saturates, monoenes and total PUFA were affected by palm oil level and n-3 and n-3/n-6 were affected by the palm oil type and level (Table 7). The total saturated fatty acids in palm oil-based enriched rotifer were significantly (P < 0.05) higher than in the control diet, ranging from 39.0 ± 0.1% to 40.9 ± 0.1% (Table 8). The replacement of fish oil with dietary palm oil had significantly affected the level of saturates and monoenes of the enriched rotifers. The total PUFA, n-3 PUFA, n-3/n-6, EPA and DHA levels were significantly higher in FO100 than in other groups. The total fatty acids of enriched rotifer were significantly affected by palm oil type and level. Table 9 shows the whole body fatty acid composition of Asian seabass at the end of experiment. Total fatty acids of Asian seabass body composition yielded the same pattern as in the enriched diet and rotifer. The palm oil-based diets contained significantly (P < 0.05) higher level of C:16 and C18:1n9 compared to FO100. Overall, the total fatty acids of palm oil enriched diets, rotifers and Asian seabass larvae were significantly affected by the palm oil type and level except total PUFA and n-3 which showed no significant effect by the palm oil type.
Discussion
Lipid is the main energy source for marine fish, especially in the early stage of its life. Short-term enrichment of rotifer using fish oil emulsion results in lipid-encapsulated rotifers rich in EPA and DHA. Generally, this is a nutritionally stable composition and makes the diet palatable which is important especially for fish larvae (Dhert et al., 2001). In the present study, Asian seabass larvae achieved faster growth by ingesting the palm-oil enriched rotifer, indicating the ability of the larvae to utilize the available lipid for energy consumption and growth. Fish fed palm oil-based enriched rotifer showed highest growth performance compared to control diet. It is well-documented that palm oil is characterized by high level of ß-carotene and is a rich source of antioxidants. These components are known to be excellent natural free radical acceptors and may exert beneficial effects on growth when fish are fed high levels of palm oil (Lim et al., 2001; Ng et al., 2003). A study by Jalali et al. (2008) emphasized that supplementation of antioxidants is necessary when high levels of n-3 PUFA are incorporated in larval diets to avoid adverse effects and to improve larval performance on beluga (Huso huso) larvae. Investigations conducted by Tocher et al. (2003) have shown that supplementation of α-tocopherol which is present in palm oil improved the growth and reduced the lipid peroxidation in sea bream and turbot (Psetta maxima) tissues. Betancor et al. (2013) observed that the elevation of dietary vitamin E levels in the palm oil markedly reduced the incidence of these symptoms and increased the tissue content of several PUFA, and improved the growth in European sea bass. Espe et al. (2007) stated that feeding lower level of fish oil than plant oil improved n-3 HUFA retention efficiency. However, inclusion of high level of fish oil in the enriched diet resulted in decline in growth of Asian seabass larvae, mainly due to the poor retention efficiency of fatty acids (Bell et al., 2002; Espe et al., 2007). Lipid oxidation impairs the nutritional value of animal products due to high proportion of unsaturated fatty acids. This is consistent with the findings reported by Jalali et al. (2010) and Babalola et al. (2013). Studies on the use of palm oil in enriching the live feed, particularly rotifer, are limited and the information is scarce on marine fish larvae. Poh-Leong et al. (2012) used palm oil mill effluent (POME)- enriched bacteria as food for rotifer and fed it to marble goby, Oxyeoleotris marmorata. The study reported that the POME significantly enhanced the rotifer production, and improved growth and survival. Concordant findings were also obtained on other plant oil-based enriched live foods for different species (Smith et al., 2004; Menoyo et al., 2007; Huanng et al., 2008; Hafezieh et al., 2010; Tehrani et al., 2014; Kazemi et al., 2016).
The morphological features (phenotypic characters), CF and TL/W ratio that are normally used as indicators of nutritional status of fish larvae showed that the Asian seabass larvae were developing normally based on the consistent pattern in all the treatment groups. During the larval phase, survival appeared to be strongly influenced by the energy status of the larvae. Thus, the high levels of fatty acids in the live prey are essential to satisfy the elevated energy demand and to promote growth (Dhaneesh and Kumar, 2016). Current study shows that the survival is affected by palm oil level and fatty acid composition particularly DHA and EPA. Rich amounts of these substances in the control treatment significantly reduced the survival. A study conducted by Planas and Cunha (1999) also suggested that higher levels of DHA or n-3 HUFA reduced the survival of marine fish larvae. Izquierdo et al. (1992) demonstrated that in the larvae of Japanese, P. olivaceus, DHA content in Artemia metanauplii did not affect the survival rate but influenced the size when the larvae were fed with artemia containing high level of DHA. Thus, the requirement of dietary DHA level of marine finfish larvae is species dependant as highlighted by Sargent et al. (1999).
The fatty acid composition of enriched rotifer and Asian seabass larvae reflected the fatty acid profile in the enriched diets. Earlier studies have demonstrated that fatty acid composition of enriched rotifer is a reflection of its enrichment and, therefore, it depends on the culture condition and presence of nutrients (Kotani et al., 2013; Hamre, 2016). Specifically, the fish oil replacement at 50% with CPO and 75% with RPO performed better than the other palm oil treatments and fish oil-based enriched diet in terms of the level of EPA and DHA that were significantly reduced in the rotifer fed palm oil-based enriched diets (EPA ranged from 5.12 to 7.93% and DHA ranged from 6.43 to 10.61%). It is evident from these findings that the n3:n9 ratio and variable fatty acid composition, particularly palmitic (C16:0) and oleic acid (C18:1n9), in the enriched rotifer promoted better growth of Asian seabass. Analysis of the growth performance of the palm oil-enriched rotifer in the present study suggested that the essential fatty acids in the enriched rotifer were sufficient to support the normal growth of the larvae. This investigation also revealed that the RPO75, CPO50, and RPO50 containing n3:n9 were 1.1, 1.4, and 2.1, respectively were effective and were within the recommended ratio of 0.58–2.24:1 as suggested by Watanabe et al. (1989) and Williams and Barlow (1999). However, fish fed RPO75 enriched rotifer showed a significant correlation between the significantly high growth performance and DHA:EPA ratio (1.4) compared to other palm-oil based treatments. Current study further showed that high dietary DHA level did not result in any significant growth benefit to the larvae. However, the nutritional requirements for DHA and EPA have been found to be both species-specific as well as specific to the developmental stage of the larvae (Copeman et al., 2002; Villalta et al., 2005).
The fatty acid composition of the diets was clearly reflected in the enriched rotifer and body fatty acid composition of Asian seabass larvae. However, n-3 HUFA level in fish body fed RPO75 and CPO50 showed inconsistent profile where n-3 HUFA levels were higher (9.9 and 11.4%, respectively) which contrasted with the supplied enriched rotifer (6.5 and 8.1%, respectively). Findings of Tocher et al. (2003) and Mohd-Yusof et al. (2010) have revealed that Asian seabass was able to maintain levels of DHA above the dietary level and attributed it to the selective retention of these nutrients in the palm oil linked to the conversion of EPA to DHA. This study demonstrated that the nutritional value of rotifer was not influenced by the enrichment diets except the protein level. RPO-enriched rotifer showed higher protein level compared to the other treatments. The mean protein level in rotifers varied in the range of 45.9–55.2%. Generally, protein level in rotifers are relatively stable at ~34–52% as reported by Caric et al. (1993) and Hamre et al. (2013); Hamre (2016). Slight changes in lipid level in rotifers have been observed to affect the dry matter protein content (Srivastava et al., 2006). Variable protein level in rotifers in the present study may have been influenced by palm oil type that affects the ingestion rate of rotifer with 24 h of the enrichment process. The acceptability of rotifer might be due to the preference of available food as a consequence of the response of rotifers upon encountering the supplied feed (Wallace, 1980; Hotos, 2003). Evidently, the enriched diet did not significantly affect the whole-body proximate composition which suggested that Asian seabass larvae efficiently utilized the enriched rotifer to support survival and growth.
Conclusion
Palm oil in the form of CPO and RPO can be successfully used as a dietary lipid source in the enriched diets for rotifer and is able to support good survival and growth of Asian seabass larvae. Replacing 75% fish oil with RPO performed better than the other palm oil-based enriched diets and the control. A successful replacement of fish oil with palm oil in the enriched diets for rotifer will be able to reduce larval rearing production cost of Asian seabass. It is also a significant finding in larval nutrition research that can contribute to sparing the natural populations of forage fish from unsustainable exploitation. Rotifer represents a suitable live prey for enrichment with the ingredients that positively influence the nutritional requirements of the early larval stages of the fish widely used in aquaculture. Further research toward optimizing the alternative sources of protein and lipid from plants for live feeds will help in shaping the aquaculture industry consistent with the criteria of sustainable development.
Data Availability Statement
The raw data supporting the conclusions of this article will be made available by the authors, without undue reservation.
Ethics Statement
The study was conducted following the Researcher's guidelines on code of practice for the care and use of animals for scientific purposes (Universiti Malaysia Sabah).
Author Contributions
NS conducted the larval rearing experiment, raw data collection and analysis, and draft writing. RS leader of research project, designed the experimental layout, analysis of results, and manuscript writing. SM manuscript reviewing and improving presentation of data. FC involved in larval rearing design and execution. All authors contributed to the article and approved the submitted version.
Conflict of Interest
The authors declare that the research was conducted in the absence of any commercial or financial relationships that could be construed as a potential conflict of interest.
Acknowledgments
This study was partially supported by a research grant (FRG0503-1/2019) awarded by Ministry of Higher Learning Malaysia.
References
Alder, J., Campbell, B., Karpouzi, V., Kaschner, K., and Pauly, D. (2008). Forage fish: from ecosystems to markets. Annu. Rev. Environ. Resour. 33, 153–166. doi: 10.1146/annurev.environ.33.020807.143204
Alhazzaa, R. (2012). Alternative sources of omega-3 oils for barramundi, Lates calcarifer, aquaculture [Ph.D. thesis]. University of Tasmania, Hobart, TAS, Australia.
AOAC (1997). Association of Official Analytical Chemists International Official Methods of Analysis, 16th Edn. Arlington, VA: AOAC.
Arulvasu, C., and Munuswamy, N. (2009). Survival, growth and composition of Poecilia latipinna fry fed enriched Artemia nauplii. Curr. Sci. 96, 114–119.
Babalola, T. O., Adejumo, I. O., and Bolu, S. A. (2013). Fish oil replacement with palm oil and soybean oil in the diet of Heterobranchus longifilis affects liver biochemical compositions. Asian J. Agric. Food Sci. 1, 2321–1571.
Bell, J. G., Henderson, R. J., Tocher, D. R., McGhee, F., Dick, J. R., Porter, A., et al. (2002). Substituting fish oil with crude palm oil in the diet of Atlantic salmon (Salmo salar) affects tissue fatty acid compositions and hepatic fatty acid metabolism. J. Nutr. 132, 222–230. doi: 10.1093/jn/132.2.222
Benítez-Santana, T., Masuda, R., Juárez-Carrillo, E., Ganuza, E., Valencia, A., Hernández-Cruz, C. M., et al. (2007). Dietary n-3 HUFA deficiency induces a reduced visual response in gilthead seabream Sparus aurata larvae. Aquaculture 264, 408–417. doi: 10.1016/j.aquaculture.2006.10.024
Betancor, M. B., Caballero, M. J., and Izquerdo, M. S. (2013). Oxidative stress in sea bass (Dicentrarchus labrax) larvae fed on high DHA microdiets. Involvement of several antioxidant nutrients. Commun. Agric. Appl. Biol. Sci. 78, 41–42.
Bligh, E. G., and Dyer, W. J. (1959). A rapid method for total lipid extraction and purification. Can. J. Biochem. Physiol. 37, 911–917. doi: 10.1139/o59-099
Campoverde, C., and Eztevez, A. (2017). The effect of live food enrichment with docosahexaenoic acid (22:6n:3) rich emulsions on growth, survival and fatty acid composition of meagre (Argyrosomus regius) larvae. Aquaculture 478, 16–24. doi: 10.1016/j.aquaculture.2017.05.012
Caric, M., Sanko-Njire, J., and Skaramuca, B. (1993). Dietary effects of different feeds on thebiochemical composition of the rotifer (Brachionus plicatilis). Aquaculture 110,141–150. doi: 10.1016/0044-8486(93)90268-4
Chatain, B. (1994). “Quality assessment of marine fish larvae and juveniles,” in Measures for Success, eds P. Kestemont, J. Muir, F. Sevila, and P. Williot (CEMAGREF), 181–183.
Copeman, L. A., Parrish, C. C., Harel, M., and Brown, J. A. (2002). Effects of docosahexaenoic, eicosapentaenoic, and arachidonic acids on the early growth, survival, lipid composition and pigmentation of yellowtail flounder (Limanda ferruginea): a live food enrichment experiment. Aquaculture 210, 285–304. doi: 10.1016/S0044-8486(01)00849-3
Das, P., Mandal, S. C., Bhagabati, S. K., Akhtar, M. S., and Singh, S. K. (2012). “Important live food organisms and their role in aquaculture,” in Frontiers in Aquaculture, ed M. Sukham (New Delhi: Narendra Publishing House), 69–86.
Dey, A., Ghosh, K., and Hazra, N. (2015). An overview on bioencapsulation of live food organisms with probiotics for better growth and survival of freshwater fish juveniles. Int. J. Fish. Aquac. 5, 74–83.
Dhaneesh, K. V., and Kumar, T. T. A. (2016). Oil extraction from microalgae for live prey enrichment and larviculture of clownfish Amphiprion percula. J. Mar. Biol. Assoc. 97, 1–16. doi: 10.1017/S0025315415002167
Dhert, P., Rombaut, G., Suantika, G., and Sorgeloos, P. (2001). Advancement of rotifer culture and manipulation techniques in Europe. Aquaculture 200, 129–146. doi: 10.1016/S0044-8486(01)00697-4
Espe, M., Lemme, A., Petri, A., and El-Mowafi, A. (2007). Assessment of lysine requirement for maximal protein accretion in Atlantic salmon using plant protein diets. Aquaculture 263, 168–178. doi: 10.1016/j.aquaculture.2006.10.018
Hafezieh, M., Kamarudin, M. S., Kamal, A. S., Agh, M., Valinasab, N., Sharifian, M., et al. (2010). Effect of enriched Artemia urmiana on growth, survival and fatty acid composition of the Persian sturgeon larvae (Acipenser persicus). Iran. J. Fish. Sci. 9, 61–72.
Hamre, K. (2016). Nutrient profiles of rotifers (Brachionus sp.) and rotifer diets from four different marine fish hatcheries. Aquaculture 450, 136–142. doi: 10.1016/j.aquaculture.2015.07.016
Hamre, K., Yúferam, M., Rønnestad, I., Boglione, C., Conceiçáo, L. E. C., and Izquierdo, M. (2013). Fish larval nutrition and feed formulation: knowledge gaps and bottlenecks for advances in larval rearing. Rev. Aquacult. 5, 26–58. doi: 10.1111/j.1753-5131.2012.01086.x
Han, Y. Z., Ren, T. J., Jiang, Z. Q., Jiang, B. Q., Gao, J., Koshio, S., et al. (2012). Effects of palm oil blended with oxidized fish oil on growth performances, hematology and several immune parameters in juvenile Japanese seabass, Lateolabrax japonicas. Fish Physiol. Biochem. 38, 1785–1794. doi: 10.1007/s10695-012-9675-4
Hotos, G. N. (2003). Growth, filtration and ingestion rate of the rotifer Brachionus plicatilis fed with large (Asteromonasgracilis) and small (Chlorella sp.) celled algal species. Aquacult. Res. 34, 793–802. doi: 10.1046/j.1365-2109.2003.00868.x
Hua, K., Cobcroft, J. M., Cole, A., Condon, K., Jerry, D. R., Mangott, A., et al. (2019). The future of aquatic protein: implications for protein sources in aquaculture diets. One Earth 1, 316–329. doi: 10.1016/j.oneear.2019.10.018
Huanng, S. S. Y., Fu, C. H. L., Higgs, D. A., Balfry, S. K., Schulte, P. M., and Brauner, C. J. (2008). Effects of dietary canola oil level on growth performance, fatty acid composition and immuonoregulatory development of spring Chinook salmon parr, Oncorhynchus tshawytscha. Aquaculture 274, 109–117. doi: 10.1016/j.aquaculture.2007.11.011
Izquierdo, M. S., Arakawa, T., Takeuchi, T., Haroun, R., and Watanabe, T. (1992). Effect of n-3 HUFA levels in Artemia on growth of larval Japanese flounder (Paralichthys olivaceus). Aquaculture 105, 73–82. doi: 10.1016/0044-8486(92)90163-F
Jalali, M. A., Hosseini, S. A., and Imanpour, M. R. (2008). Effect of vitamin E and highly unsaturated fatty acid-enriched Artemia urmiana on growth performance, survival and stress resistance of Beluga (Huso huso) larvae. Aquac. Res. 39, 1286–1291. doi: 10.1111/j.1365-2109.2008.01992.x
Jalali, H. A., Mahboobi-Soofiani, N., Sadeghi, A. A., Chamani, M., and Riazi, G. (2010). Effects of supplemental dietary Lcarnitine and ractopamine on the performance of juvenile rainbow trout (Oncorhynchus mykiss). Aqua. Res. 41, 1582–1591. doi: 10.1111/j.1365-2109.2009.02462.x
Kazemi, E., Agh, N., and Malekzadeh, V. R. (2016). Potential of plant oils as alternative to fish oil for live food enrichment: effects on growth, survival, body compositions and resistance against environmental stresses in rainbow trout, Oncorhynchus mykiss. Iran. J. Fish. Sci. 15, 1–15.
Kotani, T., Fushimi, H., Ohta, Y., Miyashima, A., Sudoh, K., Hayashi, M., et al. (2013). Effect of graded levels of dietary DHA included in rotifers Brachionus plicatilis on larviculture performance of red sea bream Pagrus major. Aquacult. Sci. 61, 321–330. doi: 10.11233/aquaculturesci.61.321
Lim, P. K., Boey, P. L., and Ng, W. K. (2001). Dietary palm oil level affects growth performance, protein retention and tissue vitamin E concentration of African catfish, Clarias gariepinus. Aquaculture 202, 101–112. doi: 10.1016/S0044-8486(01)00563-4
Menoyo, D., Lopez, C., Diez, A., and Obach, A. (2007). Impact of n-3 fatty acid chain length and n-3/ n-6 ratio in Atlantic salmon (Salmo salar) diets. Aquaculture 267, 248–259. doi: 10.1016/j.aquaculture.2007.02.031
Miller, M. R., Nichols, P. D., and Carter, C. G. (2008). n-3 Oil sources for use in aquaculture –alternatives to the unsustainable harvest of wild fish. Nutr. Res. Rev. 21, 85–96. doi: 10.1017/S0954422408102414
Mohd-Yusof, N. Y., Monroig, O., Mohd-Adnan, A., Wan, K. L., and Tocher, D. R. (2010). Investigation of highly unsaturated fatty acid metabolism in the Asian seabass, Lates calcarifer. Fish Physiol. Biochem. 36, 827–843. doi: 10.1007/s10695-010-9409-4
Nasaretnam, K., and Muhammad, B. (1993). “Nutritional properties of palm oil,” in Selected Readings on the Palm Oil and Its Uses, eds Organizing Committee of 1993 Palm Oil Familiarization Programme (Kuala Lumpur: Palm Oil Research Institute of Malaysia), 57–67.
Naylor, R. L., Hardy, R. W., Bureau, D. P., Chiu, A., Elliott, M., Farrell, A. P., et al. (2009). Feeding aquaculture in an era of finite resources. Proc.Natl. Acad. Sci. U.S.A. 106, 15103–15110. doi: 10.1073/pnas.0905235106
Ng, W. K., Lim, P. K., and Boey, P. L. (2003). Dietary lipid and palm oil source affects growth, fatty acid composition and muscle α-tocopherol concentration of African catfish, Clarias gariepinus. Aquaculture 215, 229–243. doi: 10.1016/S0044-8486(02)00067-4
Ng, W. K., Tocher, D. R., and Bell, J. G. (2007). The use of palm oil in aquaculture feeds for salmonid species. Eur. J. Lipid Sci. Technol. 109, 394–399. doi: 10.1002/ejlt.200600209
Nichols, P. D., Petrie, J., and Singh, S. (2010). Long-chain omega-3 oils–an update on sustainable sources. Nutrients 2, 572–585. doi: 10.3390/nu2060572
Planas, M., and Cunha, I. (1999). Larviculture of marine fish: problems and perspectives. Aquaculture 177, 171–190. doi: 10.1016/S0044-8486(99)00079-4
Poh-Leong, L., Ving-Ching, C., and Sabaratnam, V. (2012). Production of live food from palm oil mill effluent (POME) for culture of marble goby. J. Oil Palm Res. 24, 1566–1572.
Radhakrishnan, D. K., Akbarali, I., Schmidt, B. V., John, E. M., Sivanpillai, S., and Vasunambesan, S. T. (2020). Improvement of nutritional quality of live feed for aquaculture: an overview. Aqua. Res. 51, 1–17. doi: 10.1111/are.14357
Rainuzzo, J. R., Reitan, K. I., and Olsen, Y. (1994). Effect of short- and long-term lipid enrichment on total lipids, lipid class and fatty acid composition in rotifers. Aqua. Int. 2, 19–32. doi: 10.1007/BF00118530
Rainuzzo, J. R., Reitan, K. I., and Olsen, Y. (1997). The significance of lipids at early stages of marine fish: a review. Aquaculture 155, 103–115. doi: 10.1016/S0044-8486(97)00121-X
Sargent, J. R., McEvoy, L., Estevez, A., Bell, G., Bell, M., Henderson, J., et al. (1999). Lipid nutrition of marine fish during early development: current status and future directions. Aquaculture 179, 217–229. doi: 10.1016/S0044-8486(99)00191-X
Shapawi, R., Mustafa, S., and Ng, W. K. (2008). Effects of dietary fish oil replacement with vegetable oils on growth and tissue fatty acid composition of humpback grouper, Cromileptes altivelis (Valenciennes). Aquac. Res. 39, 315–323. doi: 10.1111/j.1365-2109.2007.01882.x
Shapawi, R., Mustafa, S., and Ng, W. K. (2011). A comparison of the growth performance and body composition of the humpback grouper, Cromileptes altivelis fed on farm-made feeds, commercial feeds and trash fish. J. Fish. Aquat. Sci. 6, 523–534. doi: 10.3923/jfas.2011.523.534
Singh, S. K., Rather, M. A., Mandal, S. C., Das, P., Pawar, N., Singh, Y. J., et al. (2012). Effects of dietary fish oil substitution with palm oil on growth, survival, and muscle proximate composition of Cirrhinus mrigala (Hamilton, 1822). Isr. J. Aquac. 64, 1–5. doi: 10.46989/001c.20607
Skalliaa, A., and Robin, J. H. (2004). Requirement of n-3 long chain polyunsaturated fatty acids for European sea bass (Dicentrarchus labrax) juveniles: growth and fatty acid composition. Aquaculture 240, 399–415. doi: 10.1016/j.aquaculture.2004.06.036
Smith, D. M., Hunter, B. J., Allen, G. L., Roberts, D. C. K., Booth, M. A., and Glencross, B. D. (2004). Essential fatty acids in diet on silver perch, Bidyanus bidyanus: effect of linolenic and linoleic acid on growth and survival. Aquaculture 236, 377–390. doi: 10.1016/j.aquaculture.2003.10.021
Srivastava, A., Hamre, K., Stoss, J., Chakrabarti, R., and Tonheim, S. K. (2006). Protein content and amino acid composition of the live feed rotifer (Brachionus plicatilis): with emphasis on the water-soluble fraction. Aquaculture 254, 534–543. doi: 10.1016/j.aquaculture.2005.11.014
Tacon, A. G. J., and Metian, M. (2008). Global overview on the use of fish meal and fish oil in industrially compounded aquafeeds: trends and future prospects. Aquaculture 285, 146–158. doi: 10.1016/j.aquaculture.2008.08.015
Tehrani, J. M., Dorche, E. E., Goli, S. A. H., and Akbary, P. (2014). Enrichment of Artemia (Leach) nauplii with canola oil: effect on Heros severus (Heckel) larvae performance and environmental stress. Adv. Microbiol. 4, 1242–1249. doi: 10.4236/aim.2014.416134
Tocher, D. R. (2010). Fatty acid requirements in ontogeny of marine and freshwater fish. Aqua. Res. 41, 717–732. doi: 10.1111/j.1365-2109.2008.02150.x
Tocher, D. R., Mourente, G., Van, A., Evjemo, J. O., Diaz, E., Wille, M., et al. (2003). Comparative study of antioxidant defence mechanisms in marine fish fed variable levels of oxidized oil and vitamin E. Aqua. Int. 11, 195–216. doi: 10.1023/A:1024127003997
Torstensen, B. E., and Tocher, D. R. (2010). “The effects of fish oil replacement on lipid metabolism of fish,” in Fish Oil Replacement and Alternative Lipid Sources in Aquaculture Feeds, eds G. M. Turchini, W. K. Ng, and D. R. Tocher (Boca Raton, Fl: CRC Press), 405–437.
Turchini, G. M., Torstensen, B. E., and Ng, W. K. (2009). Fish oil replacement in finfish nutrition. Rev. Aquac. 1, 10–57. doi: 10.1111/j.1753-5131.2008.01001.x
Turchini, G. M., Trushenski, J. T., and Glencross, B. D. (2019). Thoughts for the future of aquaculture nutrition. Realigning perspectives to reflect contemporary issues related to judicious use of marine resources in aquafeeds. North Am. J. Aquacult. 81, 13–39. doi: 10.1002/naaq.10067
Villalta, M., Estèvez, A., Bransden, M. P., and Bell, J. G. (2007). Effects of dietary eicosapentaenoic acid on growth, survival, pigmentation and fatty acid composition in Senegal sole (Solea senegalensis) larvae during the Artemia feeding period. Aquac. Nutr. 14, 232–241. doi: 10.1111/j.1365-2095.2007.00522.x
Villalta, M., Estevez, A., Bransden, M. P., and Bell, J. G. B. (2005). The effect of graded concentrations of dietary DHA on growth, survival and tissue fatty ac id profile of Senegal sole (Solea senegalensis) larvae during the Artemia feeding period. Aquaculture 249, 353–365. doi: 10.1016/j.aquaculture.2005.03.037
Wallace, R. L. (1980). Ecology of sessile rotifers. Hydrobiologia 73, 181–193. doi: 10.1007/BF00019445
Wan, A. R., Stone, D. A. J., and Schuller, K. A. (2013). Dietary fish oil replacement with palm or poultry oil increases fillet oxidative stability and decreases liver glutathione peroxidase activity in barramundi (Lates calcarifer). Fish Physiol. Biochem. 39, 1631–1640. doi: 10.1007/s10695-013-9815-5
Wassef, E. A., Wahby, O. M., and Sakr, E. M. (2007). Effect of dietary vegetable oils on health and liver histology of gilthead seabream (Sparus aurata) growers. Aquac. Res. 38, 852–861. doi: 10.1111/j.1365-2109.2007.01738.x
Watanabe, T., Izquierdo, M. S., Takeuchi, T., Satoh, S. Y., and Kitajima, C. (1989). Comparison between eicosapentaenoic and docosahexaenoic acids in terms of essential fatty acid efficacy in larval red seabream. Bull. Japan. Soc. Scien. Fish 55, 1635–1640. doi: 10.2331/suisan.55.1635
Williams, K. C., and Barlow, C. G. (1999). Dietary Requirement and Optimal Feeding Practices for Barramundi Lates calcarifer). Project 92/63, Final Report to Fisheries RandD Corporation, Canberra, ACT, Australia.
Yong, A. S. K., Mubarak, N. S. S., and Shapawi, R. (2019). Effects of partial replacement of fish oil with different vegetable oils on growth, feed utilization and fatty acid profile of hybrid grouper juvenile (Epinephelus fuscoguttatus × Epinephelus lanceolatus). J. Oil Palm Res. 31, 110–121. doi: 10.21894/jopr.2019.0003
Keywords: Brachionus plicatilis, palm oil, fish oil replacement, live feed, Asian seabass
Citation: Safiin NSZ, Mustafa S, Ching FF and Shapawi R (2021) Palm Oil-Based Enriched Diets for the Rotifer, Brachionus plicatilis, Improved the Growth of Asian Seabass (Lates calcarifer) Larvae. Front. Mar. Sci. 8:613312. doi: 10.3389/fmars.2021.613312
Received: 02 October 2020; Accepted: 24 March 2020;
Published: 27 April 2021.
Edited by:
Sami Souissi, Lille University of Science and Technology, FranceReviewed by:
Yun-Zhang Sun, Jimei University, ChinaGhasem Rashidian, Tarbiat Modares University, Iran
Copyright © 2021 Safiin, Mustafa, Ching and Shapawi. This is an open-access article distributed under the terms of the Creative Commons Attribution License (CC BY). The use, distribution or reproduction in other forums is permitted, provided the original author(s) and the copyright owner(s) are credited and that the original publication in this journal is cited, in accordance with accepted academic practice. No use, distribution or reproduction is permitted which does not comply with these terms.
*Correspondence: Rossita Shapawi, cm9zc2l0YUB1bXMuZWR1Lm15