- 1Massachusetts Division of Marine Fisheries, New Bedford, MA, United States
- 2Biology Department, University of Massachusetts Dartmouth, North Dartmouth, MA, United States
- 3State College of Florida, Bradenton, FL, United States
- 4School for Marine Science and Technology, University of Massachusetts Dartmouth, New Bedford, MA, United States
- 5Greater Atlantic Regional Fisheries Office, NOAA Fisheries, Gloucester, MA, United States
- 6Apex Predators Program, NOAA Fisheries, Narragansett, RI, United States
- 7School of Aquatic and Fishery Sciences, University of Washington, Seattle, WA, United States
- 8Biology Department, Woods Hole Oceanographic Institution, Woods Hole, MA, United States
The porbeagle (Lamna nasus) is a large, highly migratory endothermic shark broadly distributed in the higher latitudes of the Atlantic, South Pacific, and Indian Oceans. In the North Atlantic, the porbeagle has a long history of fisheries exploitation and current assessments indicate that this stock is severely overfished. Although much is known of the life history of this species, there is little fisheries-independent information about habitat preferences and ecology. To examine migratory routes, vertical behavior, and environmental associations in the western North Atlantic, we deployed pop-up satellite archival transmitting tags on 20 porbeagles in late November, 2006. The sharks, ten males and ten females ranging from 128 to 154 cm fork length, were tagged and released from a commercial longline fishing vessel on the northwestern edge of Georges Bank, about 150 km east of Cape Cod, MA. The tags were programmed to release in March (n = 7), July (n = 7), and November (n = 6) of 2007, and 17 (85%) successfully reported. Based on known and derived geopositions, the porbeagles exhibited broad seasonally-dependent horizontal and vertical movements ranging from minimum linear distances of 937 to 3,310 km and from the surface to 1,300 m, respectively. All of the sharks remained in the western North Atlantic from the Gulf of Maine, the Scotian Shelf, on George's Bank, and in the deep, oceanic waters off the continental shelf along the edge of, and within, the Gulf Stream. In general, the population appears to be shelf-oriented during the summer and early fall with more expansive offshore radiation in the winter and spring. Although sharks moved through temperatures ranging from 2 to 26°C, the bulk of their time (97%) was spent in 6-20°C. In the summer months, most of the sharks were associated with the continental shelf moving between the surface and the bottom and remaining < 200 m deep. In the late fall and winter months, the porbeagles moved into pelagic habitat and exhibited two behavioral patterns linked with the thermal features of the Gulf Stream: “non-divers” (n = 7) largely remained at epipelagic depths and “divers” (n = 10) made frequent dives into and remained at mesopelagic depths (200–1000 m). These data demonstrate that juvenile porbeagles are physiologically capable of exploiting the cool temperate waters of the western North Atlantic as well as the mesopelagic depths of the Gulf Stream, possibly allowing exploitation of prey not available to other predators.
Introduction
The porbeagle, Lamna nasus, is a large, highly migratory pelagic shark broadly distributed in the temperate latitudes of the Atlantic, South Pacific, and South Indian Oceans (Compagno, 2002). Historically, the species has been subjected to both commercial and recreational fisheries throughout its range (Curtis et al., 2016; Haugen, 2020). In the North Atlantic, the porbeagle is currently managed as two stocks (i.e., east and west) and the most recent stock assessment indicates that both are heavily overfished (ICCAT, 2009; Curtis et al., 2016). As a result, the IUCN Red List of Threatened Species assessed the species as Vulnerable in 2018 (Rigby et al., 2018). The porbeagle is also currently listed on Annex I of the United Nations Convention on Law of the Sea, Appendix II of the Convention for the Conservation of Migratory Species (CMS, 2008), and, since 2014, Appendix II of the Convention on International Trade in Endangered Species of Wild Fauna and Flora (www.cites.org; Curtis et al., 2016). In 2015, the International Commission for the Conservation of Atlantic Tunas (ICCAT) passed a recommendation requiring contracting and cooperating parties to release unharmed porbeagles caught in association with ICCAT fisheries. In 2016, the porbeagle was proposed to be listed for protection under the U.S. Endangered Species Act, but an evaluation of all available information determined that the listing was not warranted due to a low risk of extinction in the foreseeable future (Curtis et al., 2016). Although major harvesters of porbeagle have either stopped (European Union, Canada) or capped landings (USA) in an effort to allow for the rebuilding of stocks (Campana et al., 2015; reviewed by Haugen, 2020), this species occurs in the highest bycatch risk zone in the North Atlantic (Queiroz et al., 2019) and mortality associated with bycatch may be high (Haugen, 2020). The most optimistic projections predict that stock recovery on both sides of the Atlantic will require decades (ICCAT, 2009). Given the current status of porbeagle stocks, the Standing Committee on Research and Statistics in ICCAT proffered a suite of research recommendations that included a better understanding of stock structure, vertical and migratory movements, and habitat associations with oceanographic features (ICCAT, 2009). Such information on the temporal and spatial distribution of porbeagles in relation to environmental/ecosystem features would not only facilitate a better understanding of catch trends, but also spatial management to reduce potential fishing mortality (ICCAT, 2009).
Although the life history of the porbeagle has been well-studied (Jensen et al., 2002; Joyce et al., 2002; Natanson et al., 2019), much of what is known about stock structure, movements, habitat use, and spatial ecology has come from fisheries-dependent data (Campana and Joyce, 2004; Kohler and Turner, 2019). These studies (using conventional tags and commercial catch data) suggest that porbeagles exhibit seasonal shifts in abundance and are highly migratory in the western North Atlantic (WNA). However, relatively little is known about the three-dimensional movements and habitat use of these fish as well as the biotic and abiotic factors (e.g., ambient temperature, oceanic fronts, prey availability, and reproductive behaviors) that may influence their movements.
While fisheries-dependent data suggest that porbeagles do indeed have a wide thermal distribution (i.e., captured in waters with a sea surface temperature [SST] ranging from 2 to 23°C), most catches near the continental shelves are associated with the thermocline and with oceanographic frontal zones in a relatively narrow temperature range between 5 and 10°C (Campana and Joyce, 2004). Based on these data, Campana and Joyce (2004) suggested that the vertical distribution of the porbeagle in the WNA is relatively narrow (<200 m), although they did not rule out the possibility of vertical migrations to greater depths.
During the last two decades, the advent of electronic tagging technologies has allowed researchers to gain a more holistic, fisheries-independent understanding of the temporal and spatial movement patterns and stock structure of highly migratory fishes (reviewed by Costa et al., 2012). In addition, these new technologies allow for the correlation of movement patterns with oceanographic features (Braun et al., 2019) and, therefore, provide a more robust understanding of habitat use. The most commonly used technology to study the three-dimensional movement ecology in commercially important teleosts and elasmobranchs is the pop-up satellite archival transmitting (PSAT) tag (e.g., Block, 2005; Skomal et al., 2009, 2017; Campana et al., 2010; Galuardi et al., 2010; Musyl et al., 2011). To date, several studies have deployed PSAT tags to examine porbeagle movements in the Atlantic (Pade et al., 2009; Campana et al., 2010; Saunders et al., 2011; Biais et al., 2017). Work in the eastern North Atlantic (ENA) off the coast of the United Kingdom showed that porbeagles appear to exhibit deep (>300 m) and, in some cases, diel diving behavior and highly variable horizontal movements (Pade et al., 2009; Saunders et al., 2011). However, conclusions from those studies were based on relatively low sample sizes (total n = 7) and short track durations (22-122 days). In the WNA, the tracks of 21 porbeagles showed deep diving (up to 1,360 m) and broad horizontal movements as far as the Sargasso Sea (Campana et al., 2010). Although track durations spanned almost a year (60–348 days), the porbeagle sample in that study was dominated by large, sexually mature females. Based on those data, Campana et al. (2010) hypothesized that the Sargasso Sea may be an important parturition area for porbeagles in the WNA. Most recently, Biais et al. (2017) deployed PSAT tags on nine subadult/adult porbeagles in the Bay of Biscay for up to a year, and showed broad migrations to the Arctic Circle, Madeira, and the mid-Atlantic Ridge, as well as site fidelity to the tagging location. However, the lack of juveniles from both sexes in these studies limits the extent to which these findings are applicable to all age classes.
The porbeagle and other members of the family Lamnidae are unique among sharks in having the capacity to retain metabolically produced heat to significantly elevate tissue (i.e., eyes, brain, viscera and swimming muscle) temperatures above ambient (i.e., regional endothermy, Carey and Teal, 1969a; Goldman, 1997; Bernal et al., 2001; Goldman et al., 2004; Sepulveda et al., 2004). Among lamnids, the porbeagle and its congener the salmon shark (Lamna ditropis) are able to maintain their eyes, brain, viscera, and swimming muscles up to 20°C above ambient (Goldman et al., 2004; Bernal et al., 2005), resulting in a relatively stable elevated tissue operating temperature. This may allow lamnid sharks to make long duration or more frequent dives below the thermocline or to inhabit very cold, highly productive, subpolar waters (2–10°C) for prolonged periods (i.e., numerous months) of time without compromising locomotor, sensory, and digestive function. In the porbeagle, regional endothermy may allow them to expand their thermal niche to forage in cooler, more productive waters at greater depths and/or higher latitudes (Block and Carey, 1985; Carey et al., 1985; Bernal et al., 2001; Dickson and Graham, 2004). While the vertical movement patterns of large, sexually mature females support a wide thermal (i.e., depth and latitude) distribution (Campana et al., 2010), the vertical movement patterns of juvenile porbeagles remain unknown.
Information on porbeagle movement ecology, habitat use, and stock structure as it relates to juveniles is warranted given relatively little is known about the three-dimensional movements of these fish. As this stock is highly overexploited, fishery managers need more empirical data for the development of enhanced conservation measures to facilitate the restoration of porbeagle populations in the Atlantic. In this study, we used PSAT technology to examine long-term, three-dimensional movements and to characterize habitat use by juvenile porbeagles in the WNA.
Materials and Methods
All methods associated with the capture and handling of sharks were conducted in accordance to standards from the Institutional Animal Care and Use Committee of the University of Massachusetts, Dartmouth (Protocol #05-07).
Tagging
During this study, 20 porbeagles were captured and tagged in the western North Atlantic Ocean east of Cape Cod, MA near Cultivator Shoal, George's Bank (41.844°N, 68.095°W) in November, 2006 aboard the F/V Eagle Eye II. Sharks were caught on pelagic longline fishing gear comprised of monofilament gangions (9.1 m; 180 kg test) with 18/0 circle hooks (Mustad Corp., Miami, FL, USA) baited with whole Atlantic mackerel (Scomber scombrus). Two longline sets of 210 and 315 hooks were allowed to soak for 3 and 5 h, respectively, before hauling. During each set, a temperature profile of the water column was obtained using a conductivity-temperature-depth meter (model SBE911, Sea-Bird Electronics, Bellevue, WA, USA).
All hooked sharks were brought alongside the vessel and inspected for physical trauma (e.g., hook damage); only healthy, actively swimming, mouth-hooked specimens that showed little or no bleeding were selected for this study. Each shark was guided by the monofilament leader into a mesh-lined cradle and quickly lifted to the deck for sex determination, accurate length (over the body fork length, FL) measurement, and PSAT tag attachment (n = 20, Model Mk10-PAT, Wildlife Computers Inc., Redmond, WA, USA). Each PSAT tag was tethered to an intramuscular nylon umbrella dart, which was implanted into the musculature and through the cartilaginous supports of the dorsal fin (Kohler and Turner, 2001). All PSAT tags were programmed to release if the shark showed no significant vertical movement (± 1 m) for 96 continuous hours.
Data Analyses
Tags were programmed to collect depth (minimum resolution: 0.5 m), ambient temperature (minimum resolution: 0.05°C), and light intensity (measured as irradiance at 550 nm wavelength) every 10 s for the duration of each deployment. Three subsets of deployed tags were programmed to detach from the sharks after 120 days (n = 7; T120; March, 2007), 240 days (n = 7; T240; July, 2007), and 360 days (n = 6; T360; November, 2007). Upon release, pre-processed binned data (summarized every 6 h [120 and 240 day deployments] or 12 h [360 day deployments]; proportion of time spent at depth [m]: <5, 10, 25, 50, 75, 100, 150, 200, 300, 400, 500, 600, 800, >800; proportion of time spent at ambient temperature: 2–26°C at 2°C intervals) were transmitted through the Argos satellite system over a period of 6–10 days. Each tag also transmitted a temperature-depth profile of the water column inhabited by the shark during each 6- or 12-h interval. This comprised minimum and maximum water temperatures in eight depth bins, ranging from the shallowest to the deepest swimming depths for that time interval. Finally, the tag processed the light level data onboard to correct for depth and subsequently estimated times of dawn, dusk, and midnight or midday.
Temperature profiles of the water column and each shark's possible association with a thermocline, were assessed by plotting depth vs. temperature for discrete periods of time when diving behavior indicated repeated use of a consistent depth range. Given our findings that porbeagles exhibited two distinct diving behaviors [i.e., epipelagic (0–200 m) and mesopelagic (200–1000 m)], we grouped them as “non-divers” and “divers,” respectively. This allowed the examination of depth associations, averaged across individuals within each behavior group, using contoured probability plots generated with the ffgrid package in MATLAB (version R2010a).
To estimate the horizontal movements of each shark, we then used the HMMoce package (Braun et al., 2018), which uses light-based position estimates (from the tag), sea surface temperature (SST), and depth-temperature profiles, to construct gridded likelihoods of occupancy using a state space hidden Markov model framework. Observation-based likelihoods were derived from remotely-sensed SST, light-based longitude, and depth-temperature profile data collected by the tags, using four separate likelihood calculations: (1) an SST likelihood was generated for tag-based SST values integrated according to an error term (+/- 1%) and compared to remotely-sensed SST from the Multi-scale Ultra-high Resolution (MUR) sea surface temperature analysis (MUR-SST, 0.01° resolution) (NASA JPL, 2015); (2) light-based longitudinal likelihood was derived using estimates of longitude from GPE2 software (Wildlife Computers, Inc., Redmond, WA, USA) that allowed visual checking of light curves; (3) depth-temperature profile summaries recorded by the tag were compared to daily reanalysis model depth-temperature products from the HYbrid Coordinate Ocean Model (Bleck, 2002; Chassignet et al., 2007; HYCOM, 0.08° resolution) at the standard depth levels available in the model and individual likelihood surfaces for each depth level were then multiplied together for an overall profile likelihood at that time point; and (4) Ocean Heat Content (OHC) was obtained by integrating the heat content of the water column above the minimum daily tag-measured temperature to the most shallow depth recorded by the PSAT and included in the HYCOM fields (Luo et al., 2015). Start and end locations were fixed in all model runs.
The resulting observation likelihoods, in all reasonable pairwise and triplicate combinations, were convolved with a diffusive movement kernel that allowed swim speeds up to 2 m/s (see Braun et al., 2018 for full details of the convolution, filtering, and smoothing components of the model). Model selection comparing different combinations of observation likelihoods used Akaike Information Criterion (AIC). Results from the final smoothing step of the selected model represent the posterior distribution of each state (position) over time. The spatial means of the daily posterior distributions were used to calculate a most probable track.
Residency distribution (RD) plots were generated using the posterior likelihood surfaces from the position estimates. RDs were determined by aggregating the daily posterior probabilities across all tagged sharks, seasonally. Gridded probabilities were calculated with a 0.1° resolution and seasons were based on the lunar calendar: fall, September 20–December 19; winter, December 20–March 19; spring, March 20–June 19; summer, June 20–September 19.
Results
A total of 88 juvenile porbeagles ranging from 90 to 174 cm FL were caught on two pelagic longline sets in November, 2006 on George's Bank (41°N, 68°W) with sea surface temperatures of 12.8–13.0°C and water depths of 90-140 m. A subset of 20 sharks (128–154 cm FL), 10 males (mean ± SE: 133 ± 2.5 cm FL) and 10 females (141 ± 2.3 cm FL) were selected for tagging (Table 1). We received data from 17 PSAT tags (7 males, 10 females, T120 n = 5, T240 n = 7, and T360 n = 5) resulting in 4,050 days of total tracking time (Table 1). Four tags released prior to their programmed date (T240 n = 2:185 and 221 days; T360 n = 2: 307 and 311 days) and three did not report (T120 n = 2 and T360 n = 1).
Horizontal Movements
The total minimum straight-line distance traveled by each of the tagged sharks ranged from 937 to 1,903 km for T120, 1,579 to 2,857 km for T240, and 2,517 to 3,310 km for T360 (Table 1). The porbeagles largely remained in the temperate WNA year-round, occurring in the Gulf of Maine, the Scotian Shelf, on George's Bank, and in the deep, oceanic waters off the continental shelf along the edge of, and within, the Gulf Stream (Figure 1). Geoposition estimates of latitude ranged from 35°N (off the Outer Banks, North Carolina, USA) to 45°N; longitude ranged from shelf waters east to 55°W (Figure 1). Residency distribution plots show that tagged porbeagles exhibited seasonal changes in distribution (Figure 2). During the fall, the sharks were broadly distributed from the Gulf of Maine to oceanic areas, but largely concentrated in deeper regions of the former. In the late fall and winter, porbeagles moved out of the Gulf and off the shelf into oceanic habitat along the northern edge of and within the Gulf Stream (Figure 2). In the spring, the porbeagles remained in oceanic habitat along the shelf edge prior to returning to the Gulf of Maine and Scotian Shelf in the summer (Figure 2).
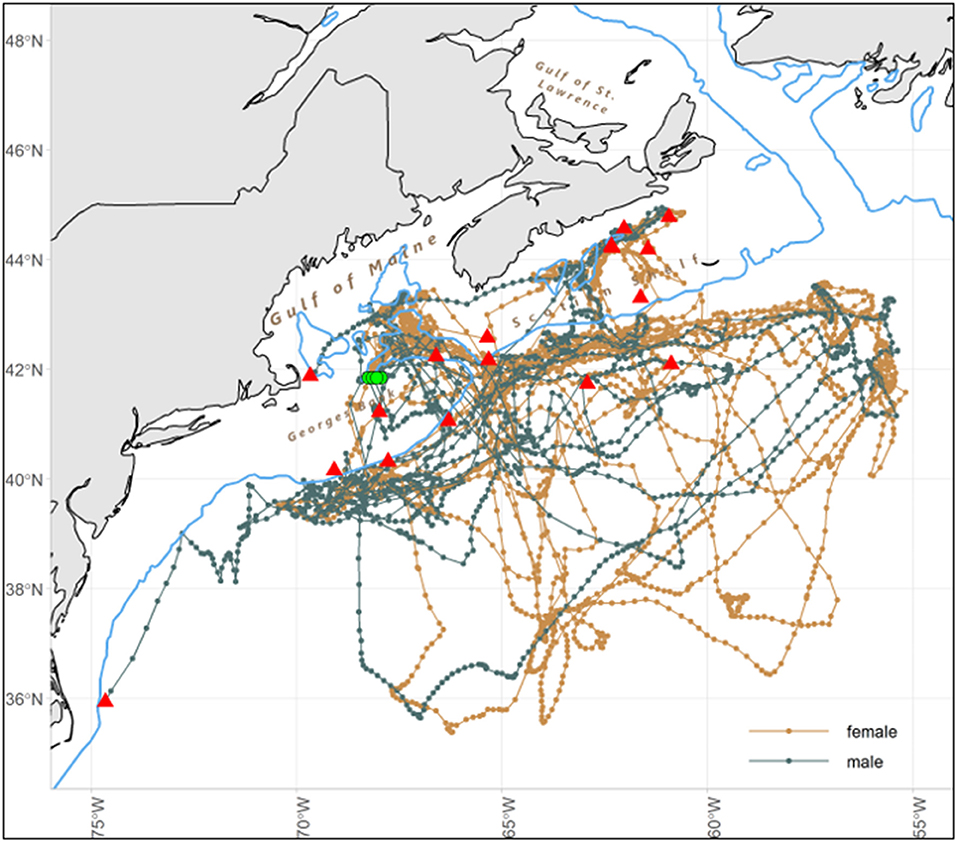
Figure 1. Most probable tracks for 17 juvenile porbeagle sharks, stratified by sex, tagged in the western North Atlantic with tagging (green points) and pop-up (red triangle) locations, and shelf edge (200 m isobath, blue line).
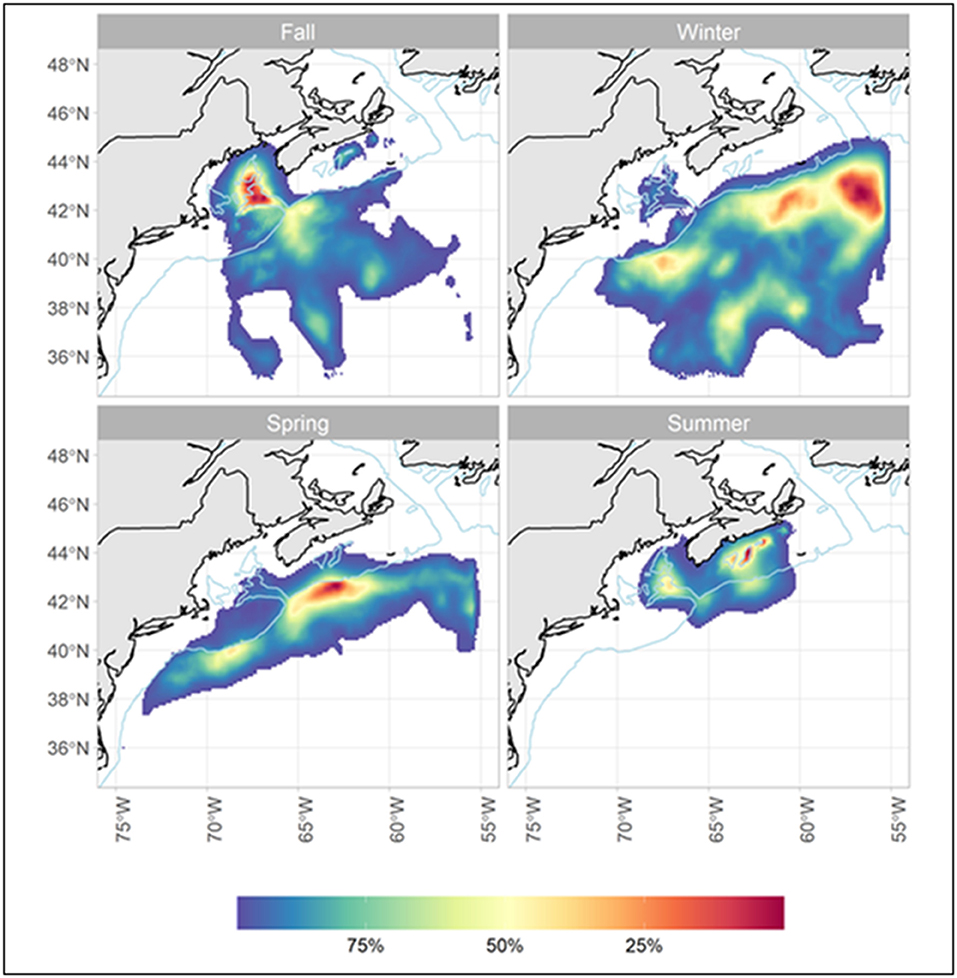
Figure 2. Residency density plots showing seasonal distribution of juvenile porbeagles tagged in the western North Atlantic; seasons are based on the lunar calendar (mo/d: Fall, 9/20–12/19; Winter, 12/20–3/19; Spring, 3/20–6/19; Summer, 6/20–9/19).
Vertical Movements
For all sharks combined, ambient water temperature and swimming depth ranged from 2 to 24°C and from the surface to 1,304 m, respectively. During the late fall and winter months, two general patterns of vertical behavior were observed: (1) “non-divers” (2 females and 5 males) largely remained at shallow depths and rarely penetrated depths >600 m (Figure 3); and (2) “divers” (8 females and 2 males) made frequent dives into and remained at mesopelagic depths (200-1000 m) for 38–109 days (average 71 ± 26 days from fall into winter), rarely moving into the upper epipelagic zone (Figure 4). Overall, these two distinct dive patterns did not appear to be influenced by the broad-scale horizontal (coastal to offshore) movements of these fish as there were no significant differences in total minimum straight-line distances traveled between divers (2,450 ± 659 km) and non-divers (2,288 ± 715 km; p > 0.05, Student's paired T-test). When stratified by dive behavior, the estimated geopositions of the porbeagles during the late fall and winter indicate that non-divers were almost exclusively associated with the cold side of the northern edge of the Gulf Stream, while divers primarily occupied the warm side of this edge, within the Gulf Stream, or in warm water south of the Gulf Stream (Figure 5).
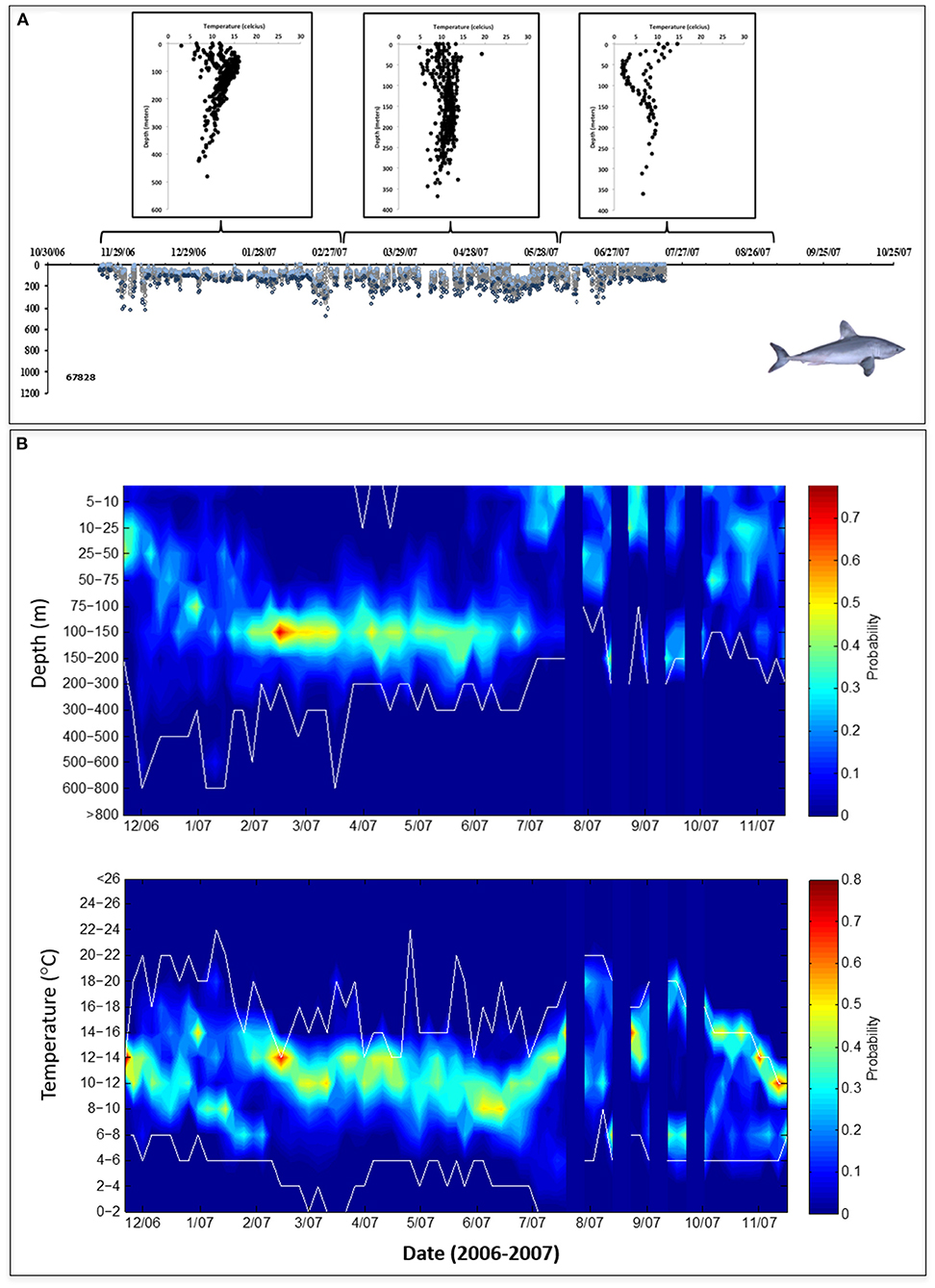
Figure 3. (A) Vertical behavior of a representative “non-diver” porbeagle (Shark 4, PTT 67828) showing depth-temperature profiles at 3-month intervals (upper) corresponding to daily depth observations (lower) comprised of minimum (light blue circles), maximum (dark blue circles), and intermediate (gray circles) depths. (B) Contoured probability plots of all depth (upper) and water temperature (lower) data collected from porbeagles classified as non-divers (n = 7); white lines indicate upper and lower limits of the data and blue vertical bars indicate areas of missing data.
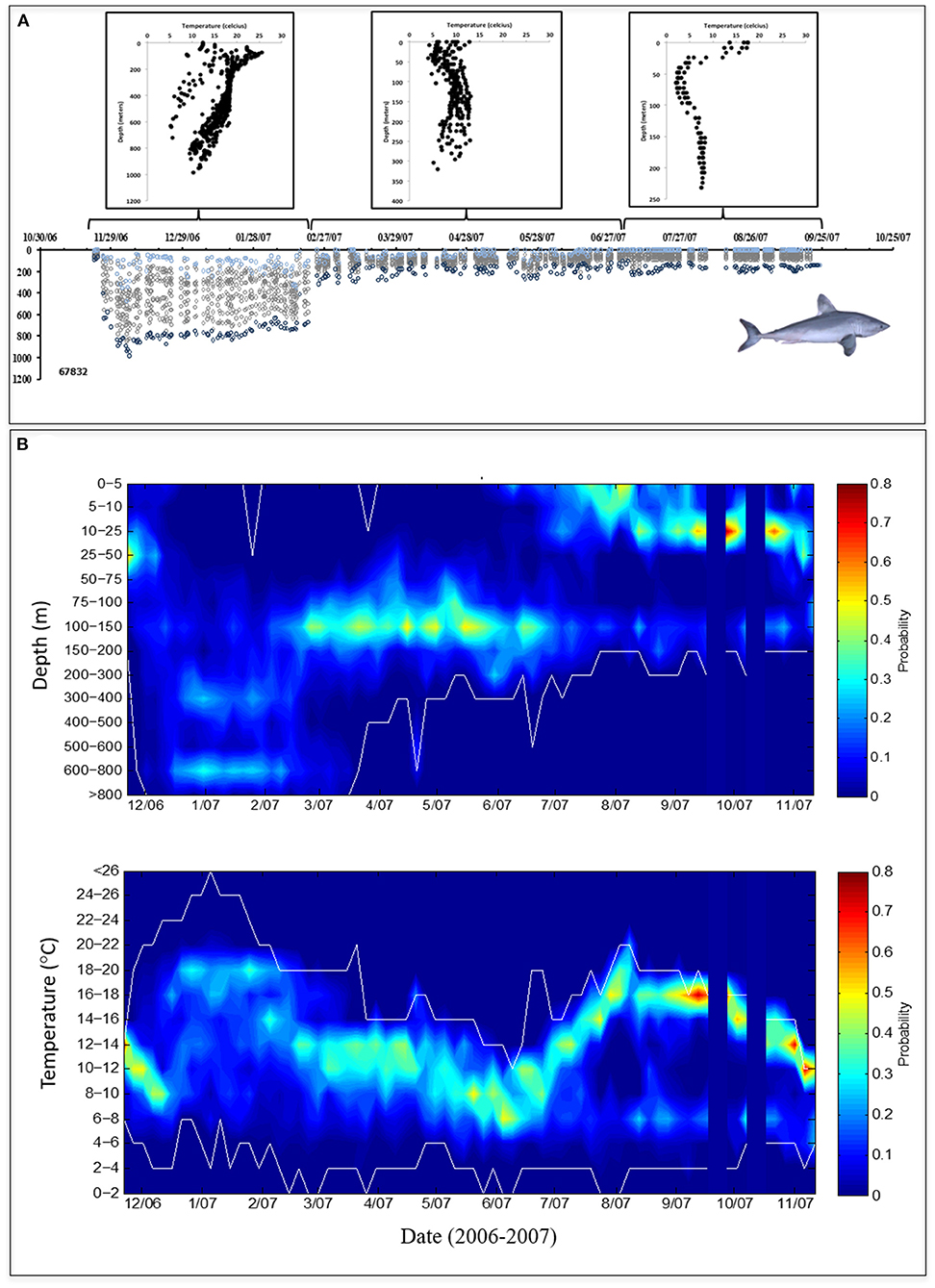
Figure 4. (A) Vertical behavior of a representative “diver” porbeagle (Shark 9, PTT 67832) showing depth-temperature profiles at 3-month intervals (upper) corresponding to daily depth observations (lower) comprised of minimum (light blue circles), maximum (dark blue circles), and intermediate (gray circles) depths. (B) Contoured probability plots of all depth (upper) and water temperature (lower) data collected from porbeagles classified as divers (n = 10); white lines indicate upper and lower limits of the data and blue vertical bars indicate areas of missing data.
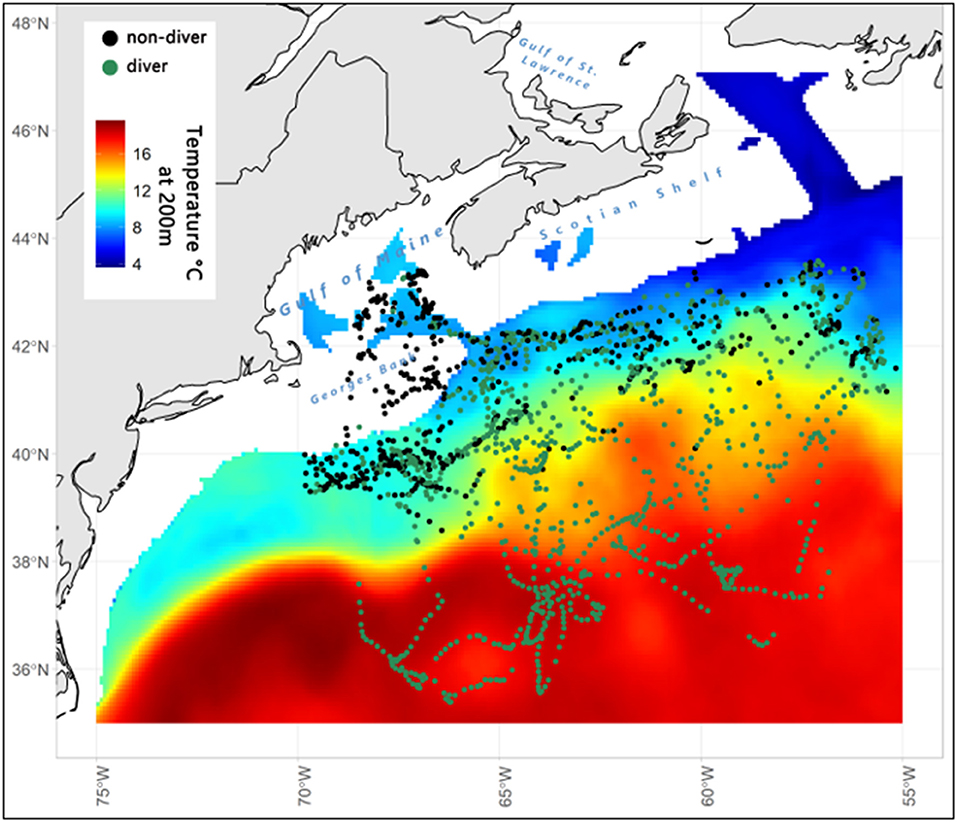
Figure 5. Estimated geopositions for porbeagle divers and non-divers, overlaid on a composite of temperature (°C) at 200 m (HYCOM, 0.08° resolution) for December 2006–March 2007.
When analyzed together, all fish, regardless of season, spent 75% and 50% of their time at depths of <200 m and 75-200 m, respectively, and 97% of their time in temperatures of 6–20°C. In general, porbeagles occupied similar depth and temperature distributions, but divers and non-divers diverged during the fall and winter months as they moved off the shelf (Figures 6, 7). In the fall, divers occupied a broad range of depths from 5 to 800 meters, spending 31% of their time >200 m (Figure 6), and 68% of the time in water temperatures ranging from 8 to 14°C (Figure 7). In contrast, non-divers did not move frequently to mesopelagic depths in the fall spending only 11% of the time >200 m, but in a similar temperature range (79% at 8–14°C) when compared to divers. During the winter months, divers exhibited a bimodal depth and temperature distribution comprised of a shallow (40% at 75–200 m), warm (17% at 18–20°C) component and a deep (43% at 300–800 m), cool (22% at 12–14°C) component (Figures 6, 7). However, non-divers remained at shallow depths in the winter (89% <200 m), yet occupied a similar temperature range to that of the divers (Figure 7). In addition, these fish were not associated with the warmer (18–20°C) waters encountered by the divers during the same time period. In the spring, both groups returned to epipelagic depths along the edge of the continental shelf with 70% and 92% of the time between 75 and 200 m and in an ambient temperature range of 6–14°C, respectively (Figures 3, 4, 6, 7). In the summer, all fish were <200 m (99% of the time) experiencing a wider range of ambient temperatures (from 4 to 18°C) for 97% of the time, but showed a bimodal depth distribution with shallow (59% <25 m) and deeper (25% at 100–200 m) components (Figures 3, 4, 6, 7).
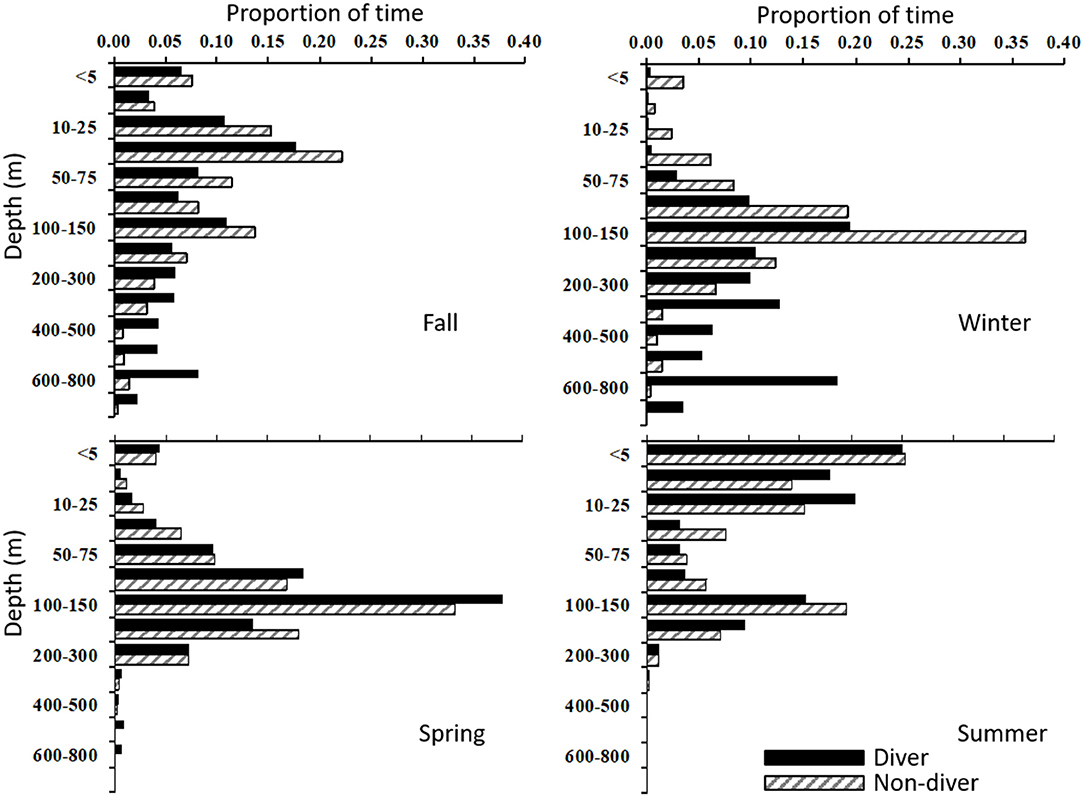
Figure 6. Seasonal time-at-depth histograms for porbeagle divers (solid bar; n = 10) and non-divers (hatched bar, n = 7) tagged in the western North Atlantic.
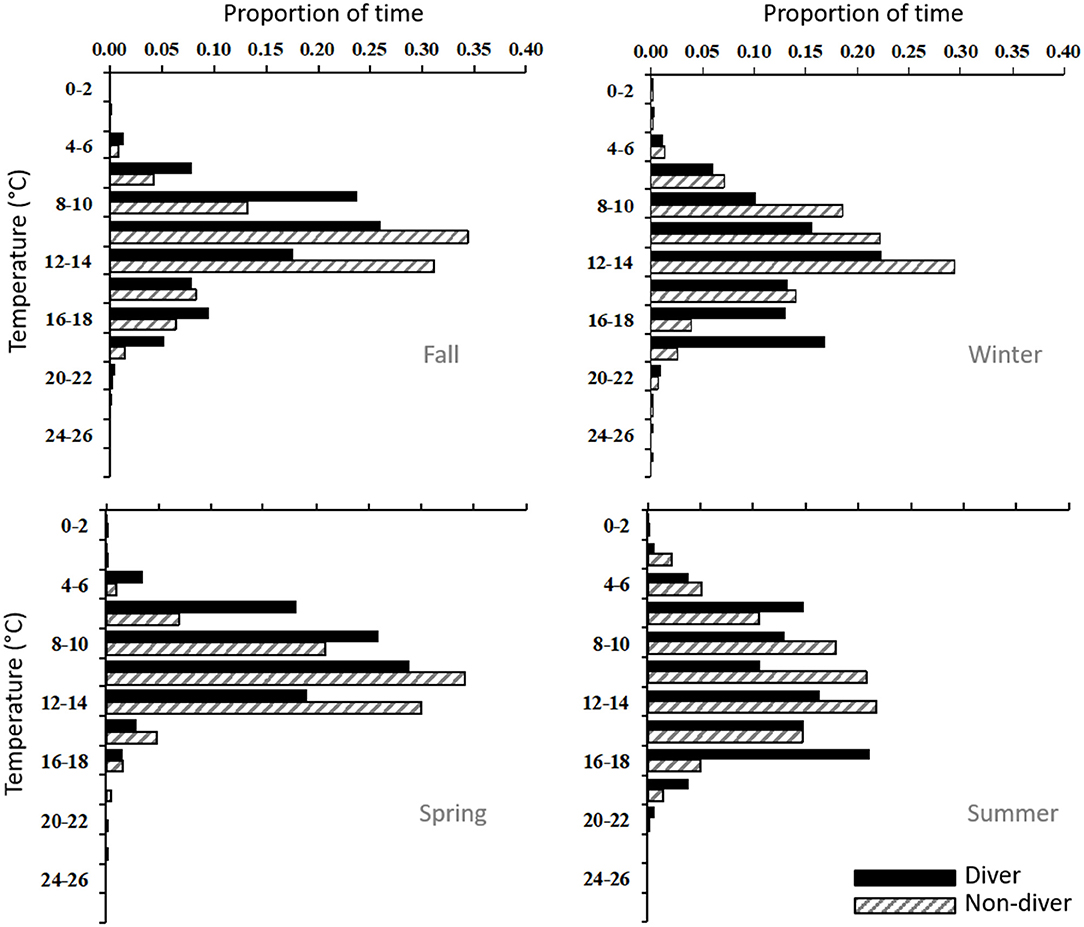
Figure 7. Seasonal time-at-temperature histograms for porbeagle divers (solid bar; n = 10) and non-divers (hatched bar, n = 7) tagged in the western North Atlantic.
Discussion
Based on fisheries-independent data derived from 17 PSAT tags, we show that juvenile porbeagles tagged in the western North Atlantic exhibited broad, seasonally-dependent horizontal and vertical movements, including movement into the Gulf Stream. In the fall, juvenile porbeagles were associated with shelf waters in the Gulf of Maine and George's Bank (Figure 2). The sharks tagged in this study were captured on the northern side of Georges Bank in 90–140 m of water as part of 88 juveniles hooked on only two longline sets in this area—this was clearly indicative of high abundance in the area. As fall transitions to winter, juvenile porbeagles moved out of the Gulf of Maine, off the shelf, and into the oceanic waters of the WNA for the winter months, during which some of the sharks moved into the Gulf Stream and exhibited mesopelagic residence. As northeastern shelf waters warm during the spring months, these sharks transitioned back to the shelf where they spent the summer in the Gulf of Maine and on the Scotian Shelf moving between the surface and the bottom. Although some of these results are consistent with historical fisheries-dependent conventional tagging data (Kohler and Turner, 2019), this study provided evidence of more expansive offshore movement in the WNA. All the porbeagles tagged in this study remained in temperate waters north of 35°N, which differs from other pelagic shark species that seasonally move into more southerly latitudes, including the blue shark (Prionace glauca; Kohler and Turner, 2019), the basking shark (Cetorhinus maximus; Skomal et al., 2009), and endothermic lamnid sharks (e.g., white shark Carcharodon carcharias, Skomal et al., 2017; shortfin mako, Isurus oxyrinchus; Vaudo et al., 2017; Kohler and Turner, 2019).
These results are in sharp contrast to previous findings for juvenile porbeagles tagged with this technology in the WNA. Campana et al. (2010) concluded that males and juvenile sharks of both sexes remained primarily in cool temperate waters on the continental shelf, always north of latitude 38°N, for periods of up to 348 days after tagging. However, their findings are likely linked to sample size and composition. Of the 21 sharks tagged in that study, 52% were adult females, two were adult males, and the remaining eight were juvenile females. Most of the tracks of the latter were relatively short (<76 days) and did not span the winter months during which our tagged juveniles moved offshore. Campana et al. (2010) did show that adult females move into the Gulf Stream and as far as the Sargasso Sea. Our findings demonstrate that juveniles also move into and south of the Gulf Stream, but not as far south as adult females (~20°N).
To date, three PSAT tagging studies have been conducted on porbeagles in the ENA: Pade et al. (2009; n = 4; UK), Saunders et al. (2011; n = 3; Ireland), and Biais et al. (2017; n = 9; Bay of Biscay). Although samples sizes were relatively low, collectively these studies show that porbeagles are associated with relatively shallow shelf waters during the spring and summer months and move to offshore, deeper waters in the fall and winter months. Most recently, Biais et al. (2017) found that porbeagles tagged in the Bay of Biscay moved extensively (up to 2,000 km) in the ENA as far as north as the Arctic Circle, west to the mid-Atlantic Ridge, and south to Madeira in late summer and returned to the Bay of Biscay in spring of the following year. However, like those tagged by Campana et al. (2010), these fish were primarily sub-adult and adult females, which appear to migrate more extensively than juveniles.
Diving Behavior and Water Temperature
As porbeagles migrated seasonally from shelf to offshore habitat, the juveniles tracked during this study also exhibited seasonal changes in their vertical and thermal distribution (Figures 6, 7). Not surprisingly, other pelagic fish have been shown to exhibit similar seasonal shifts in their depth distribution, which are associated with foraging behavior. For example, the movement of blue sharks from shallow to deep habitat is thought to be correlated with prey availability (Queiroz et al., 2010). Similarly, the movements of juvenile porbeagles that we observed are likely linked to foraging behavior mediated by temperature.
In the WNA, Joyce et al. (2002) examined the stomach contents of 1,022 porbeagles and concluded that this species is an opportunistic feeder on a diverse assemblage of pelagic, epipelagic, and benthic fish species, as well as cephalopods, depending on availability. In juveniles (<150 cm), they found that a variety of fishes and cephalopods constituted major portions of the diet. They also observed a strong seasonal diet shift linked to spatial changes in shark distribution, although they grouped the stomach samples coarsely into spring (January-June) and fall (July-December) “seasons” and, hence, do not coincide with true seasons. Regardless, there are some indications that the movements observed in our study are associated with feeding behavior. In stomachs sampled during the months of January to June, Joyce et al. (2002) found a high proportion of cephalopods and pelagic teleosts (e.g., lancetfish, Alepisaurus ferox; Atlantic herring, Clupea harengus). In our study, this roughly coincides with offshore foraging behavior at epipelagic and mesopelagic depths during the winter. With progression into the autumn months, juvenile porbeagles transitioned off the shelf (Figure 2), expanding their vertical behavior. During the late fall and winter, they were broadly distributed along the shelf edge and in offshore regions of the WNA, including the Gulf Stream. Those that we qualify as non-divers remained north of the Gulf Stream or along its northern edge and moved through epipelagic depths (<200 m), spending 84% of the time in a temperature range of 8–16°C. Those juveniles that migrated further offshore into the warm waters of the Gulf Stream and beyond (i.e., divers, Figure 5) shifted from epipelagic to mesopelagic habitat and exhibited a bimodal temperature distribution reflective of warm, shallow water and cooler waters at depth. This suggests that, in a manner similar to blue sharks (Carey et al., 1990; Braun et al., 2019) and white sharks (Gaube et al., 2018), these juvenile sharks may potentially dive deep within the Gulf Stream and associated features (i.e., fronts, eddies) in order to exploit more abundant food resources, including pelagic teleosts and cephalopods.
The importance of cephalopods in the diets of large pelagic fishes is well documented in the world's oceans (Smale, 1996). Off the coast of New Zealand, cephalopods were found to be a major component of the porbeagle's diet (Horn et al., 2013). In the offshore waters of the Gulf Stream and Central North Atlantic, Logan et al. (2013) examined stomach samples from nine species of large pelagic tunas, swordfish, and sharks and found that ommastrephid squids were the most common prey group across predator species. Other important prey included octopod, histioteuthid, and architeuthid cephalopods, as well as mesopelagic fishes. Although porbeagle sharks were not sampled by that study, the predominance and abundance of cephalopod species in the region, and reflected in the diets of other pelagic predators, make them a probable component of the porbeagle's diet. During the late fall and winter months, some of the juvenile porbeagles tagged in this study moved into these offshore areas (Figure 5), which are dominated by cephalopod prey (Logan et al., 2013). Thus, the temporal and spatial distribution of these key prey species may be driving the seasonal differences in depth distribution observed in juvenile porbeagle sharks in this study.
During the spring months, when the porbeagles moved back to the shelf edge and George's Bank (Figure 2), they all inhabited epipelagic depths and a predominant temperature range of 6–14°C. During the summer, the porbeagles were shelf-oriented (Figure 2), spending all of their time <200 m and 59% of the time in the top 25 m (Figure 6). Moving from the surface to the bottom through a well-stratified water column, these sharks spent 97% of the time in the temperature range of 4–18°C (Figures 3A, 4A). In those fish sampled from July to December, Joyce et al. (2002) found a greater proportion of benthic teleosts (groundfish) and fewer pelagics in their stomachs. This reflects summer movement onto the Northeast Shelf Ecosystem, which includes the Gulf of Maine and Georges Bank, and is historically one of the most productive ecosystems in the world, supporting large numbers of fish, invertebrate, and cetacean species (Bigelow and Schroeder, 1953: Kenney and Winn, 1986; German, 1987; Sherman et al., 1988; Fogarty and Murawski, 1998).
The seasonal depth distribution that we observed in porbeagle sharks differs markedly from those previously described in the WNA based on fisheries-dependent catch data (Campana and Joyce, 2004) and PSAT tags (Campana et al., 2010), which suggested that juvenile sharks do not commonly dive >200 m. However, such behavior was observed in the large adult females tagged by Campana et al. (2010). In a pattern remarkably similar to what we observed in our “divers,” these mature females encountered the Gulf Stream between 22 December and 9 March, which resulted in an “almost instantaneous initiation of deep diving behavior (daily maximum depth of <248 m before entry and a mean of 845 m after entry)” (Campana et al., 2010). These authors suggested that the porbeagles were migrating beneath the main flow of the Gulf Stream to maximize their net swimming speed, to minimize their ambient temperature, and to ultimately reach the Sargasso Sea for parturition (Campana et al., 2010). Our findings that juvenile porbeagles also exhibit this behavior suggest that foraging, and not reproduction, is likely a key driver of this behavior. As noted by Pade et al. (2009), it is becoming increasingly apparent that the porbeagle exhibits considerable plasticity in horizontal and vertical habitat use and the variable behaviors likely reflect the need for different search strategies depending on habitat and prey types encountered.
Collectively, the results presented in this and previous studies indicate that while porbeagles have broad thermal tolerance, their vertical and horizontal movements are driven by an optimal temperature range. Although the juvenile porbeagles tagged in this study exhibited extensive seasonal shifts in habitat use, both vertically and horizontally, and occupied a broad temperature range of 2–24°C, they spent 97% of the time at 6–20°C. The lower limits reported herein are colder than previous findings using PSAT technology in the WNA (8.0°C, Campana et al., 2010), ENA (9°C, Saunders et al., 2011), and Southern Hemisphere (4.6°C, Francis et al., 2015), but it should be noted that our tagged fish spent only 2% of the time in water <6°C. The sharks in this study also moved into water that was warmer than the maximum reported in the ENA (18.5°C, Pade et al., 2009), but similar to that reported by Campana et al. (2010; 25.4°C). This is associated with movement into the Gulf Stream, yet temperatures in excess of 20°C constituted <1% of the observations. Campana et al. (2010) suggested that the deep diving behavior observed in adult females while in Gulf Stream and Sargasso Sea was driven by water temperature because the surface waters (>22°C) were uninhabitable. Our findings also suggest that the surface waters (<200 m) of the Gulf Stream and temperatures in excess of 20°C represent a thermal barrier that influence the vertical behavior of juvenile porbeagles.
It is important to consider the extent to which, if any, the depth and temperature distribution of porbeagles are influenced (or limited) by their unique thermal physiology. While the body temperature of most sharks closely matches ambient water temperature (i.e., ectothermy) due to loss of all metabolically produced heat via conductive and convective transfer to the surrounding water (Brill et al., 1994), a few sharks (i.e., lamnids and one alopiid) have evolved a suite of morphological adaptations that allow certain regions of their body (i.e., eye, brain, viscera, aerobic swimming muscles) to be maintained warmer relative to ambient temperature (i.e., regional endothermy; Carey and Teal, 1966, 1969a,b; Carey et al., 1971, 1985; Block and Carey, 1985). Regional endothermy may allow these sharks to maintain a more thermally stable internal operating environment during their forays across thermal fronts (Bernal et al., 2001, 2018). Thus, the selective advantages of regional endothermy may have allowed these species, including porbeagles, to expand their thermal niche and make accessible the additional food resources of the cooler, more productive waters at both a greater depth and at higher latitudes (Block and Carey, 1985; Carey et al., 1985; Bernal et al., 2001; Dickson and Graham, 2004).
Carey et al. (1985) compiled in vivo muscle and visceral temperatures and compared a suite of anatomical attributes associated with metabolic heat production and conservation (e.g., relative heart size, the amount and distribution of red muscle, the number of vessels in the lateral cutaneous rete) in lamnid sharks and ranked each species by its capacity to elevate its temperature. These endothermic rankings of Carey et al. (1985) are now being validated by PSAT technology, which is generating new horizontal and vertical data about habitat use by these species. For example, it has now been demonstrated that the Pacific salmon shark, ranked first, occupies subarctic winter habitat and demonstrates the greatest tolerance of cold waters, spending 68% of its time in waters cooler than 10°C (Weng et al., 2005). The endothermic capacity of the porbeagle, ranked second by Carey et al. (1985) likely allows this species to remain in northern latitudes year-round in the North Atlantic, spending 46% of the time in waters <12°C based on our findings. In sharp contrast, the white shark (ranked third by Carey et al., 1985) migrates seasonally into temperate latitudes of the WNA, including the Gulf of Maine and the Scotian Shelf, but returns south as water temperatures cool in the late fall; it spends 95% of its time at temperatures >12°C (Skomal et al., 2017). Similarly, the shortfin mako (ranked fifth by Carey et al., 1985), migrates seasonally to northeastern latitudes (59°N) in the WNA (Vaudo et al., 2016; Kohler and Turner, 2019), but habitat use is strongly associated with warmer features and water temperatures between 10 and 15°C represent the lower limit for this species, thereby constraining them horizontally and vertically over the course of the year (Vaudo et al., 2016).
Management
Although juvenile, subadult, and adult porbeagles have been PSAT-tagged on both sides of the Atlantic, none, including the juveniles tagged in this study, have provided evidence of trans-Atlantic movement. The juvenile porbeagle sharks tagged in this study demonstrated an inshore-offshore movement pattern consistent with fidelity to the WNA. Similarly, Biais et al. (2017) found evidence for porbeagle site fidelity and consistent return migrations to and from the Bay of Biscay in the ENA. These porbeagles exhibited migrations as far west as the Mid-Atlantic Ridge, with subsequent movements back to the Bay of Biscay, thereby supporting the idea of a separate ENA stock (Biais et al., 2017). Collectively, these findings support the two stock hypotheses put forth by Pade et al. (2009) and Saunders et al. (2011), and are consistent with conventional tagging data (Kohler and Turner, 2019), in which there is only one documented instance of an immature female porbeagle moving 4,260 km during a trans-Atlantic crossing from Ireland to Canada (Cameron et al., 2018). Nonetheless, molecular analyses have found that porbeagles sampled in the WNA and ENA are not genetically distinct, suggesting some level of migration and mixing (Testerman, 2014). Most recently, Haugen (2020) conducted an interdisciplinary review of all available information (i.e., life history, genetics, and movement) to evaluate North Atlantic stock structure in this species and concluded that geographic variation in life-history traits and movement patterns indicate one phenotypic stock in the WNA and another in the ENA, despite genetic analyses indicating gene flow and genetic homogeneity between the two regions. Based on our findings, coupled with this information, management of the porbeagle should be based on two stocks.
In the North Atlantic, major harvesters of porbeagle have either ceased landing (European Union, Canada) or capped landings (USA) in an effort to allow for the rebuilding of stocks (Campana et al., 2015; reviewed by Haugen, 2020). However, porbeagle bycatch occurs in several fisheries including pelagic longlines, gillnets, and trawls (Curtis et al., 2016; Haugen, 2020). In this study, we found that juvenile porbeagles ranged from shelf waters to the Gulf Stream and are, therefore, susceptible to these fisheries. A recent analysis of the overlap of shark distribution and pelagic longline fishing effort concluded that porbeagle occur in the highest-risk zone in the North Atlantic with high potential for mortality as a result of incidental bycatch (Queiroz et al., 2019). Although the ICCAT (2009) assessment concluded that longline bycatch on the high seas is minor and does not pose a significant threat to the species, at-vessel and post-release mortality is thought to be high (average = 47%; reviewed by Curtis et al., 2016). In the current study, we captured 88 juvenile porbeagles on two pelagic longline sets (525 hooks total) with relatively short soak times (3–5 h) and 21 (24%) sharks were dead at haulback. This not only indicates that porbeagle catch rates can be high on pelagic longlines when these sharks are associated with the shelf, but could lead to significant at-vessel mortality.
In addition to pelagic longline gear, porbeagles are also taken by trawl and gillnet fisheries while in shelf waters. A recent analysis of observer data collected from US gillnet and trawl fisheries indicated that annual bycatch and discards of porbeagles can be on the order of several hundred metric tons in recent years (Haugen, 2020). Unfortunately, these estimates have not been incorporated into stock assessments for this species. Clearly, bycatch mortality might be more substantial than previously thought and all sources need to be taken into consideration in future stock assessments. Our findings show a relatively high risk for juveniles in multiple fisheries across the distribution of this species and highlight the need for continued protection for stock rebuilding.
In this study, we demonstrate that juvenile porbeagles are more broadly distributed in the WNA using near-coastal, shelf habitat during the warm months and migrating to pelagic habitat from the shelf edge to the Gulf Stream during the winter. When in the latter, these sharks exhibited mesopelagic foraging behavior likely driven by cephalopod prey abundance and water temperature. The residency of juvenile porbeagles in temperate latitudes throughout the year is likely facilitated by its endothermic capacity. Additional studies that link these movements with the foraging ecology and physiology of this species are warranted.
Data Availability Statement
The raw data supporting the conclusions of this article will be made available by the authors, without undue reservation.
Ethics Statement
All methods associated with the capture and handling of sharks were reviewed and approved by Institutional Animal Care and Use Committee of the University of Massachusetts, Dartmouth (Protocol #05-07).
Author Contributions
GS and DB conceived the study. GS, HM, and LN performed the field work. GS, HM, BG, and CB conducted the data analyses. GS, HM, and DB wrote the initial draft and all authors provided edits and approval. All authors contributed to the article and approved the submitted version.
Funding
This research was funded by the Large Pelagics Research Center (Grant 06-125).
Conflict of Interest
The authors declare that the research was conducted in the absence of any commercial or financial relationships that could be construed as a potential conflict of interest.
Acknowledgments
This research was carried out under Exempted Fishing Permit SHK-EFP-06-05 issued by the NMFS Highly Migratory Species Management Division. Special thanks to the crew of F/V Eagle Eye II for working with us to find and tag porbeagles. This research was funded by the Large Pelagics Research Center (Grant 06-125) and Federal Aid in Sport Fish Restoration. This is Massachusetts Division of Marine Fisheries Contribution No. 118.
References
Bernal, D., Dickson, K. A., Shadwick, R. E., and Graham, J. B. (2001). Review: analysis of the evolutionary convergence for high performance swimming in lamnid sharks and tunas. Comp. Biochem. Physiol. A 129, 695–726. doi: 10.1016/S1095-6433(01)00333-6
Bernal, D., Donley, J. M., Shadwick, R. E., and Syme, D. A. (2005). Mammal-like muscles power swimming in a cold-water shark. Nature 437, 1349–1352. doi: 10.1038/nature04007
Bernal, D., Reid, J. P., Roessig, J. M., Matsumoto, S., Sepulveda, C. A., Cech, J. J., et al. (2018). Temperature effects on the blood oxygen affinity in sharks. Fish Physiol. Biochem. 44, 949–967. doi: 10.1007/s10695-018-0484-2
Biais, G., Coupeau, Y., Séret, B., Calmettes, B., Lopez, R., Hetherington, S., et al. (2017). Return migration patterns of porbeagle shark (Lamna nasus) in the Northeast Atlantic: implications for stock range and structure. ICES J. Mar. Sci. 74, 1268–1276. doi: 10.1093/icesjms/fsw233
Bigelow, H. B., and Schroeder, W. C. (1953). Fishes of the Gulf of Maine (No. 592). Washington, DC: US Government Printing Office.
Bleck, R. (2002). An oceanic general circulation model framed in hybrid isopycnic-Cartesian coordinates. Ocean Model 4, 55–88. doi: 10.1016/S1463-5003(01)00012-9
Block, B. A. (2005). Physiological ecology in the 21st century: Advancements in biologging science. Integr. Comp. Biol. 45, 305–320. doi: 10.1093/icb/45.2.305
Block, B. A., and Carey, F. G. (1985). Warm brain and eye temperatures in sharks. J. Comp. Physiol. B 156, 229–236. doi: 10.1007/BF00695777
Braun, C. D., Galuardi, B., and Thorrold, S. R. (2018). HMMoce: An R package for improved geolocation of archival-tagged fishes using a hidden Markov method. Meth. Ecol. Evol. 9, 1212–1220. doi: 10.1111/2041-210X.12959
Braun, C. D., Gaube, P., Sinclair-Taylor, T. H., Skomal, G. B., and Thorrold, S. R. (2019). Mesoscale eddies release pelagic sharks from thermal constraints to foraging in the ocean twilight zone. Proc. Natl. Acad. Sci. U.S.A. 116, 17187–17192. doi: 10.1073/pnas.1903067116
Brill, R. W., Dewar, H., and Graham, J. B. (1994). Basic concepts relevant to heat transfer in fishes, and their use in measuring the physiological thermoregulatory abilities of tunas. Environ. Biol. Fish. 40, 109–124. doi: 10.1007/BF00002538
Cameron, L. W. J., Roche, W., Green, P., Houghton, J. D. R., and Mensink, P. J. (2018). Transatlantic movement in porbeagle sharks (Lamna nasus). Fish. Res. 207, 25–27. doi: 10.1016/j.fishres.2018.05.014
Campana, S. E., Fowler, M., Houlihan, D., Joyce, W. N., Showell, M., Simpson, M., et al. (2015). Recovery potential assessment for porbeagle (Lamna nasus) in Atlantic Canada. DFO Can. Sci. Advis. Sec. Res. Doc. 41:45.
Campana, S. E., Joyce, W., and Fowler, M. (2010). Subtropical pupping ground for a cold-water shark. Can. J. Fish. Aquat. Sci. 67, 769–773. doi: 10.1139/F10-020
Campana, S. E., and Joyce, W. N. (2004). Temperature and depth associations of the porbeagle shark (Lamna nasus) in the northwest Atlantic. Fish. Oceanog. 13, 52–64. doi: 10.1111/j.1365-2419.2004.00236.x
Carey, F. G., Casey, J. G., Pratt, H., Urquhart, D., and McCosker, J. E. (1985). Temperature, heat production, and heat exchange in lamnid sharks. Mem. S. Cal. Acad. Sci. 9, 92–108.
Carey, F. G., Scharold, J. V., and Kalmijn, A. J. (1990). Movements of blue sharks (Prionace glauca) in depth and course. Mar. Biol. 106, 329–342. doi: 10.1007/BF01344309
Carey, F. G., and Teal, J. M. (1966). Heat conservation in tuna fish muscle. Proc.Nat. Acad. Sci. U.S.A. 56:1464. doi: 10.1073/pnas.56.5.1464
Carey, F. G., and Teal, J. M. (1969a). Regulation of body temperature by the bluefin tuna. Comp. Biochem. Physiol. 28, 205–213. doi: 10.1016/0010-406X(69)91336-X
Carey, F. G., and Teal, J. M. (1969b). Mako and porbeagle: warm-bodied sharks. Comp. Biochem. Physiol. 28, 199–204. doi: 10.1016/0010-406X(69)91335-8
Carey, F. G., Teal, J. M., Kanwisher, J. W., Lawson, K. D., and Beckett, J. S. (1971). Warm-bodied fish. Amer. Zool. 11, 137–143. doi: 10.1093/icb/11.1.137
Chassignet, E. P., Hurlburt, H. E., Smedstad, O. M., Halliwell, G. R., Hogan, P. J., Wallcraft, A. J., et al. (2007). The HYCOM (HYbrid Coordinate Ocean Model) data assimilative system. J. Mar. Syst. 65, 60–83. doi: 10.1016/j.jmarsys.2005.09.016
CMS (2008). Proposal for Inclusion of Species on the Appendices of the Convention on the Conservation of Migratory Species of Wild Animals. Proposal II /10/Rev.1. 16pp.
Compagno, L. J. V. (2002). FAO Species Catalogues. Sharks of the World. An Annotated and Illustrated Catalogue of the Shark Species Known to Date, parts 1 and 2. Rome: FAO Fish.
Costa, D. P., Breed, G. A., and Robinson, P. W. (2012). New insights into pelagic migrations: implications for ecology and conservation. Annu. Rev. Ecol. Evol. Syst. 43, 73–96. doi: 10.1146/annurev-ecolsys-102710-145045
Curtis, T. H., Laporte, S., Cortes, E., DuBeck, G., and McCandless, C. (2016). Status Review Report: Porbeagle Shark (Lamna nasus). Final Report to National Marine Fisheries Service, Office of Protected Resources.
Dickson, K. A., and Graham, J. B. (2004). Evolution and consequences of endothermy in fishes. Physiol. Biochem. Zool. 77, 998–1018. doi: 10.1086/423743
Fogarty, M. J., and Murawski., S. A. (1998). Large-scale disturbance and the structure of marine systems: fishery impacts on Georges Bank. Ecol. Appl. 8, S6–S22. doi: 10.1890/1051-0761(1998)8[S6:LDATSO]2.0.CO;2
Francis, M. P., Holdsworth, J. C., and Block, B. A. (2015). Life in the open ocean: seasonal migration and diel diving behaviour of Southern Hemisphere porbeagle sharks (Lamna nasus). Mar. Biol. 162, 2305–2323. doi: 10.1007/s00227-015-2756-z
Galuardi, B., Royer, F., Golet, W., Logan, J., Nielson, J., and Lutcavage, M. (2010). Complex migration routes of Atlantic Bluefin tuna question current population structure paradigm. Can J. Fish. Aquat. Sci. 67, 966–976. doi: 10.1139/F10-033
Gaube, P., Braun, C. D., Lawson, G. L., McGillicuddy, D. J., Penna, A., Della, S.komal, G. B., et al. (2018). Mesoscale eddies influence the movements of mature female white sharks in the Gulf Stream and Sargasso Sea. Sci. Rep. 8:7363. doi: 10.1038/s41598-018-25565-8
German, A. W. (1987). “History of the early fisheries: 1720–1930,” Georges Bank, R. Backus ed (Cambridge, MA, Massachusetts Institute of Technology), 409–424.
Goldman, K. J. (1997). Regulation of body temperature in the white shark, Carcharadon carcharias. J. Comp. Physiol. B 167, 423–429. doi: 10.1007/s003600050092
Goldman, K. J., Anderson, S. D., Latour, R. J., and Musick, J. A. (2004). Homeothermy in adult salmon sharks, Lamna ditropis. Env. Biol. Fish. 71, 403–411. doi: 10.1007/s10641-004-6588-9
Haugen, J. B. (2020). Fishery Management, Conservation, and Bycatch of North Atlantic Porbeagle. [dissertation] University of Massachusetts Dartmouth, Dartmouth, MA, United States.
Horn, P. L., Ballara, S. L., Sutton, P., and Griggs, L. H. (2013). Evaluation of the Diets of Highly Migratory Species in New Zealand waters. Wellington: Ministry for Primary Industries.
Jensen, C. F., Natanson, L. J., Pratt, Jr, H. L., Kohler, N. E., and Campana, S. E. (2002). The reproductive biology of the porbeagle shark (Lamna nasus) in the western North Atlantic Ocean. Fish. Bull. 100, 727–738.
Joyce, W. N., Campana, S. E., Natanson, L. J., Kohler, N. E., Pratt, Jr, H. L., and Jensen, C. F. (2002). Analysis of stomach contents of the porbeagle shark (Lamna nasus Bonaterre) in the northwest Atlantic. ICES J. Mar. Sci. 59, 1263–1269. doi: 10.1006/jmsc.2002.1286
Kenney, R. D., and Winn, H. E. (1986). Cetacean high-use habitats of the northeast United States continental shelf. Fish. Bull. 84, 345–357.
Kohler, N. E., and Turner, P. A. (2001). Shark tagging: a review of conventional methods and studies. Env. Biol. Fish. 60, 191–223. doi: 10.1007/978-94-017-3245-1_12
Kohler, N. E., and Turner, P. A. (2019). Distributions and movements of Atlantic shark species: a 52-year retrospective atlas of mark and recapture data. Mar. Fish. Rev. 81, 1–93. doi: 10.7755/MFR.81.2.1
Logan, J. M., Toppin, R., Smith, S., Galuardi, B., Porter, J., and M., Lutcavage (2013). Contribution of cephalopod prey to the diet of large pelagic fish predators in the central North Atlantic Ocean. Deep Sea Res. II 95, 74–82. doi: 10.1016/j.dsr2.2012.06.003
Luo, J., Ault, J. S., Shay, L. K., Hoolihan, J. P., Prince, E. D., Brown, C., et al. (2015). Ocean heat content reveals secrets of fish migrations. PLoS ONE 10:e0141101. doi: 10.1371/journal.pone.0141101
Musyl, M., Brill, R., McNaughton, L., Swimmer, Y., Domeier, M., Nasby-Lucas, N., et al. (2011). Performance of pop-up satellite archival tags. Fish. Ocean. 433, 1–28. doi: 10.3354/meps09202
NASA JPL (2015). GHRSST Level 4 MUR Global Foundation Sea Surface Temperature Analysis (v4.1). Pasadena.
Natanson, L. J., Deacy, B. M., Joyce, W., and Sulikowski, J. (2019). Presence of a resting population of female porbeagles (Lamna nasus), indicating a biennial reproductive cycle, in the western North Atlantic Ocean. Fish. Bull. 117:8. doi: 10.7755/FB.117.1-2.8s
Pade, N. G., Queiroz, N., Humphries, N. E., Witt, M. J., Jones, C. S., Noble, L. R., et al. (2009). First results from satellite-linked archival tagging of porbeagle shark, Lamna nasus: area fidelity, wiser-scale movements and plasticity in diel depth changes. J. Exp. Mar. Biol. Ecol. 370, 64–74. doi: 10.1016/j.jembe.2008.12.002
Queiroz, N., Humphries, N. E., Couto, A., Vedor, M., Da Costa, I., Sequeira, A., et al. (2019). Global spatial risk assessment of sharks under the footprint of fisheries. Nature 572, 461–466. doi: 10.1038/s41586-019-1444-4
Queiroz, N., Humphries, N. E., Noble, L. R., Santos, A. M., and Sims, D. W. (2010). Short-term movements and diving behavior of satellite-tracked blue sharks Prionace glauca in the northeastern Atlantic Ocean. Mar. Ecol. Prog. Ser. 406, 265–279. doi: 10.3354/meps08500
Rigby, C. L., Barreto, R., Carlson, J., Fernando, D., Fordham, S. V., Francis, M. P., et al. (2018). Lamna nasus: The IUCN Red List of Threatened Species 2019 Global. International Union for Conservation of Nature. Available online at: https://www.iucnredlist.org/species/11200/500969 (accessed 17 February 2020).
Saunders, R. A., Royer, F., and Clarke, M. W. (2011). Winter migration and diving behaviour of porbeagle shark, Lamna nasus, in the Northeast Atlantic. ICES J. Mar. Sci. 68, 166–174. doi: 10.1093/icesjms/fsq145
Sepulveda, C. A., Kohin, S., Chan, C., Vetter, R., and Graham, J. B. (2004). Movement patterns, depth preferences, and stomach temperatures of free-swimming juvenile mako sharks, Isurus oxyrinchus, in the Southern California Bight. Mar. Biol. 145, 191–199. doi: 10.1007/s00227-004-1356-0
Sherman, K., Grosslein, M., Mountain, D., Busch, D., O'Reilly, J., and Theroux, R. (1988). “The continental shelf ecosystem off the northeast coast of the United States,” in Ecosystems of the World 27: Continental Shelves, H. Postema, and J. J. Zijlstra, eds (Amsterdam: Elsevier Press), 279–337.
Skomal, G. B., Braun, C. D., Chisholm, J. H., and Thorrold, S. R. (2017). Movements of the white shark Carcharodon carcharias in the North Atlantic Ocean. Mar. Ecol. Prog. Ser. 580, 1–16. doi: 10.3354/meps12306
Skomal, G. B., Zeeman, S. I., Chisholm, J. H., Summers, E. L., Walsh, H. J., McMahon, K. W., et al. (2009). Transequatorial migrations by basking sharks in the western Atlantic Ocean. Cur. Biol. 19, 1019–1022. doi: 10.1016/j.cub.2009.04.019
Smale, M. J. (1996). Cephalopods as prey. IV. Fishes. Philos. Trans. R. Soc. London B Biol. Sci. 351, 1067–1081. doi: 10.1098/rstb.1996.0094
Testerman, C. B. (2014). Molecular Ecology of Globally Distributed Sharks. [dissertation] Nova Southeastern University, Fort Lauderdale, FL, United States.
Vaudo, J. J., Byrne, M. E., Wetherbee, B. M., Harvey, G. M., and Shivji, M. S. (2017). Long-term satellite tracking reveals region-specific movements of a large pelagic predator, the shortfin mako shark, in the western North Atlantic Ocean. J. App. Ecol. 54, 1765–1775. doi: 10.1111/1365-2664.12852
Vaudo, J. J., Wetherbee, B. M., Wood, A. D., Weng, K., Howey-Jordan, L. A., Harvey, G. M., et al. (2016). Vertical movements of shortfin mako sharks Isurus oxyrinchus in the western North Atlantic Ocean are strongly influenced by temperature. Mar. Ecol. Prog. Ser. 547, 163–175. doi: 10.3354/meps11646
Keywords: porbeagle movements, diving behavior, Western North Atlantic Ocean, Gulf Stream, endothermy
Citation: Skomal G, Marshall H, Galuardi B, Natanson L, Braun CD and Bernal D (2021) Horizontal and Vertical Movement Patterns and Habitat Use of Juvenile Porbeagles (Lamna nasus) in the Western North Atlantic. Front. Mar. Sci. 8:624158. doi: 10.3389/fmars.2021.624158
Received: 30 October 2020; Accepted: 06 January 2021;
Published: 01 February 2021.
Edited by:
David Wells, Texas A&M University at Galveston, United StatesReviewed by:
Brittany Finucci, National Institute of Water and Atmospheric Research (NIWA), New ZealandNuno Queiroz, Centro de Investigacao em Biodiversidade e Recursos Geneticos (CIBIO-InBIO), Portugal
Copyright © 2021 Skomal, Marshall, Galuardi, Natanson, Braun and Bernal. This is an open-access article distributed under the terms of the Creative Commons Attribution License (CC BY). The use, distribution or reproduction in other forums is permitted, provided the original author(s) and the copyright owner(s) are credited and that the original publication in this journal is cited, in accordance with accepted academic practice. No use, distribution or reproduction is permitted which does not comply with these terms.
*Correspondence: Gregory Skomal, Z3JlZ29yeS5za29tYWxAbWFzcy5nb3Y=