- Wageningen Marine Research, Wageningen University & Research, Den Helder, Netherlands
In the Space@Sea project a conceptual study is performed to develop standardized cost efficient floating modular islands with low environmental impact. As these floating modular islands are introduced for a purpose which is likely to determine the environmental impact, possible applications were considered: living, aquaculture, ports and logistics, and energy hub. The aim of this study is to develop a structured approach for Environmental Impact Assessments (EIAs) of floating modular islands and their applications as these are considered within the Space@Sea project. To contribute to the efficiency and sustainability of future floating island developments, early awareness of the likely environmental consequences is important and requires a solid knowledge base. To that end we recommend a screening approach to identify the main threats to the marine ecosystem and their potential impacts at the earliest (conceptual) stage of development. For each Space@Sea type of application, this screening approach should identify the main threats through an EIA that links critical pressures with sensitive ecosystem components. While conventional impact assessments only consider negative impacts, we also consider potential environmental benefits of floating islands. This not only to enhance more environmental-friendly designs but also to provide a balanced perspective which considers not only threats but also opportunities in future developments and implementation of floating islands and their applications.
Introduction
With increasing human population and rising sea level there is a scarcity for space on land for the people to live, grow food and harvest renewable energy. In search for solutions to this emerging threat, initiatives for spatial developments are shifting to the offshore environment, e.g., (Buck and Langan(eds), 2017; Wang et al., 2019, 2020; Ang et al., 2020; Elliott et al., 2020; Ruzzo et al., 2021). The Space@Sea project is such an initiative, involving a conceptual study to develop a standardized floating solution providing offshore space (Flikkema and Waals, 2019). Four applications focusing on energy hub, living, aquaculture, and maritime transport are described and for two locations a business case of a combination of these applications (i.e., multi-use) will be examined (Flikkema and Waals, 2019). The Space@Sea project aims to support the development of a standardized cost efficient floating modular island with low environmental impact. A state of the art review of multidisciplinary analyses of multi-use platforms shows that most of the research has been focused on the technological aspects, followed by the socio-economic aspects, and then the environmental impact aspects (Abhinav et al., 2020). This study is part of the Space@Sea project and aims to assess environmental impacts and guide the development of a knowledge base for such initiatives. To that end this study developed a structured approach to identify (potential) impacts to the marine environment of multi-use floating islands.
An environmental assessment is required to predict the environmental impacts of proposed initiatives before they are allowed to be carried out. There are many types of environmental assessments, e.g., “environmental impact assessment” (EIA), “strategic environmental assessment” (SEA), “cumulative effect assessment” (CEA), “cumulative impact assessment” (CIA), and “environmental (or ecological) risk assessment” (ERA). Although many different approaches exist, serving different purposes in various contexts under different names, they all share the common goal of assessing the effect, impact, or risk of human activities on the ecosystem (Tamis et al., 2016). These approaches therefore all have in common that they require a framework building on the principle that activities cause pressures that may lead to adverse effects on the ecosystem (Knights et al., 2013, 2015; Tamis et al., 2016). Such a framework falls within the concept of a mental or conceptual model, which is used across a diverse spectrum of academic disciplines to think and communicate about human-environment interactions (Lynam et al., 2012). The use of an appropriate environmental assessment methodology should provide a solid, broadly applicable and transferable basis to guide the (further) development of an ecological knowledge base for offshore floating island initiatives.
The European Commission has recognized that the environmental effects of ocean energy installations have not yet been identified, nor how environmental legislation in the different phases of projects should be applied (Martínez Pérez, 2017). For decades, EIAs have been one of the primary policy instruments for environmental management and have become a globally consistent approach in managing impacts of human activities in all kind of environments, including the coastal and marine environment (Gibbs and Browman, 2015). The purpose of an EIA is to inform and/or influence planning decisions for individual projects, such as the installation of an offshore construction, often as part of a permit application. In most cases, the development and design process has been conducted prior to the EIA, so many design and development decisions have already been made. Thus to make the best use of the environmental assessments, it should start on a high strategic level when alternative options for development that may benefit conservation are still open and should be explicitly considered to guide decision-making (Partidário, 2000). SEA, a structured proactive process to strengthen the role of environmental issues in strategic decision making (Verheem and Tonk, 2012), is intended for such purpose. This results in early environmental awareness and consequently more informed, efficient, and focused project-level assessments and decisions (Noble et al., 2013) and reduces the emphasis on compliance-oriented EIA (Morgan, 2012; Guerra et al., 2015).
Several European research projects have already considered the environmental impacts of (floating) marine constructions or, in general, the development of an EIA. The EU projects ODEMM (Knights et al., 2013; Knights et al., 2015) and AQUACROSS (Borgwardt et al., 2019) developed generic EIA approaches that included a broad range of marine ecosystem components, pressures, activities and their relationships. These provided a robust basis to develop a comprehensive framework as part of this study for the potential environmental impacts of floating island applications. In addition, the EU projects TROPOS (TROPOS, 2014) and MERMAID (Airoldi et al., 2016) provided specific information regarding environmental impacts of floating islands and their applications. These sources provided both broad and specific information which we here combined in a comprehensive EIA framework for floating island applications.
Here we develop and apply an EIA approach for Space@Sea floating island multi-use applications (energy hub, living, aquaculture, and maritime transport). Potential environmental impacts are identified and discussed together with potential benefits, allowing for more balanced decision-making.
Methodology
Space@Sea floating islands are designed to enable multi-use applications, e.g., combining transport activities with living facilities and aquaculture (Flikkema and Waals, 2019). These different applications comprise multiple activities taking place within the same space and time dimensions. Besides assessing each application individually, the methodology should therefore also allow to assess their combined or cumulative impacts. A generic approach to EIA is followed, that is also suited for CIA (Tamis et al., 2016). Two main steps can be identified: (1) scoping and (2) assessment.
Scoping
This scoping step determines what should be covered in the EIA specified for floating island applications. The scoping step results in a conceptual model of the ecological system (i.e., a linkage framework of activities, pressures, and ecosystem components) at an appropriate level of detail. Scoping should cover the following aspects:
Spatial Scale
The spatial scale of the EIA needs to be identified, both in terms of detail and spatial extent (João, 2002). The cases are situated in the North Sea and Mediterranean (Flikkema and Waals, 2019) and are addressed at a regional level. This means that the spatial detail is at the level of the North Sea and Mediterranean Sea, without site-specific information such as species distribution within these areas.
Temporal Scale
An indication of the time frame, ranging from the present situation (current, ongoing activities, and pressures) to inclusion of past and future activities and pressures. All Space@Sea activities are in the planning phase and may be realized in future.
Information Requirements
The decision-making level and the availability of appropriate information determines the EIA methodology, ranging from data-rich (e.g., monitoring data allowing quantitative approaches) to data-poor (e.g., expert judgment based qualitative approaches). For high-level strategic advice such as required for e.g., SEA but also EIA, as identified for this Space@Sea study, data-poor approaches may suffice (Tamis et al., 2016) and can be used to guide the further elaboration of the knowledge base toward future application of more quantitative and information-heavy approaches. In addition, several methodological issues need to be explicitly considered (Piet et al., 2017). For other applications such as in case of a license application this will require a site-specific, project-level assessment which generally come with high information requirements.
Activities
Identification of the different activities related to Space@Sea floating island applications, e.g., construction, operation, and decommissioning (Piet et al., in press) likely to cause a different range of pressures. Here, the Space@Sea applications energy hub, living, aquaculture and ports (Flikkema and Waals, 2019) represent such different activities. For the assessment we include incidents that are part of regular operation, such as small operational spills, but not calamities.
Pressures
Identification of the pressures, i.e., the mechanism through which the activities may impact the ecosystem and its components (Tamis et al., 2016). A standard list of pressures is available from the Marine Strategy Framework Directive (MSFD) (European Commission, 2017a, b). Here, the results of different EU projects (Knights et al., 2013, 2015; TROPOS, 2014; Airoldi et al., 2016; Borgwardt et al., 2019), which also address the MSFD, were used as the basis for the pressures considered relevant to the Space@Sea applications.
Ecosystem Components
Ecosystem components are the basis for the assessment of Good Environmental Status (GES) as required in the MSFD and can be described at different levels of detail. For example at the lowest level three broadscale habitats are distinguished, i.e., benthic, pelagic, and ice habitats, and five species groups, i.e., birds, mammals, reptiles, fish, and cephalopods, but these can be subdivided into smaller habitat types or species groups. Ultimately specific species can be distinguished. For the broadscale habitats applies that these encompass both the physical habitat as well as its associated community such as the benthic communities in case of seabed habitats or planktonic communities in case of water column habitats. The MSFD broadscale habitats are aligned with the European Nature Information System (EUNIS) Habitat Classification, which is the main comprehensive pan-European hierarchical classification of habitats covering both the marine and terrestrial realms (Moss, 2008; Chytrı et al., 2020). The EUNIS was also used by Borgwardt et al. (2019) to identify 82 ecosystem components across seven European case studies and is accessible through the website portal http://eunis.eea.europa.eu/habitats.jsp (Moss, 2008).
Linkages
Establishing the links between activities, their pressures and ecosystem components, also referred to as impact chains (Knights et al., 2015). A wide range of human activities in the marine environment have already been linked to potential pressures and ecosystem components (Knights et al., 2013, 2015; Borgwardt et al., 2019), which was used as a basis for this study. These interactions only include direct effects. For reasons of simplicity, indirect effects and interactions between elements (e.g., food web interactions) were disregarded.
Assessment
The assessment estimates the (cumulative) effects of the activities and their pressures and how these may impact the ecosystem. Methodologies used for assessing (and integrating) the relationships are available for different levels of detail (Tamis et al., 2016):
• (semiquantitative) weighting of relationships using available information and/or expert judgment and classification schemes, which is most suitable for a broad scale, low-detailed assessment on a high process (strategic) level and thus used for this study;
• quantitative assessment of intensities of pressures and sensitivities of ecosystem components, which is most suitable for a focused, high-detailed assessment based on functional relationships or;
• a combination of the above, depending on the above mentioned suitability and data availability.
Impacts are identified for each Space@Sea application by using specific information regarding environmental impacts of floating islands and their applications as provided by the EU projects TROPOS (TROPOS, 2014; Papandroulakis et al., 2017) and MERMAID (Airoldi et al., 2016).
Results
Framework
The framework for floating island applications identifies the activities (Table 1) causing pressures (Table 2) that may lead to adverse effects on the ecosystem (Table 3) and is schematically presented in Figure 1. The tables list the activities, pressures and ecosystem components including their coverage by the EU projects AQUACROSS (Borgwardt et al., 2019), TROPOS (TROPOS, 2014; Papandroulakis et al., 2017), and MERMAID (Airoldi et al., 2016), which were used as information sources for development of the EIA and its application (next section). In addition, it is indicated in each table whether the activities, pressures and ecosystem components are included in Annex III of the MSFD (the indicative lists of ecosystem elements, anthropogenic pressures and human activities relevant to the marine waters) (European Commission, 2017b).
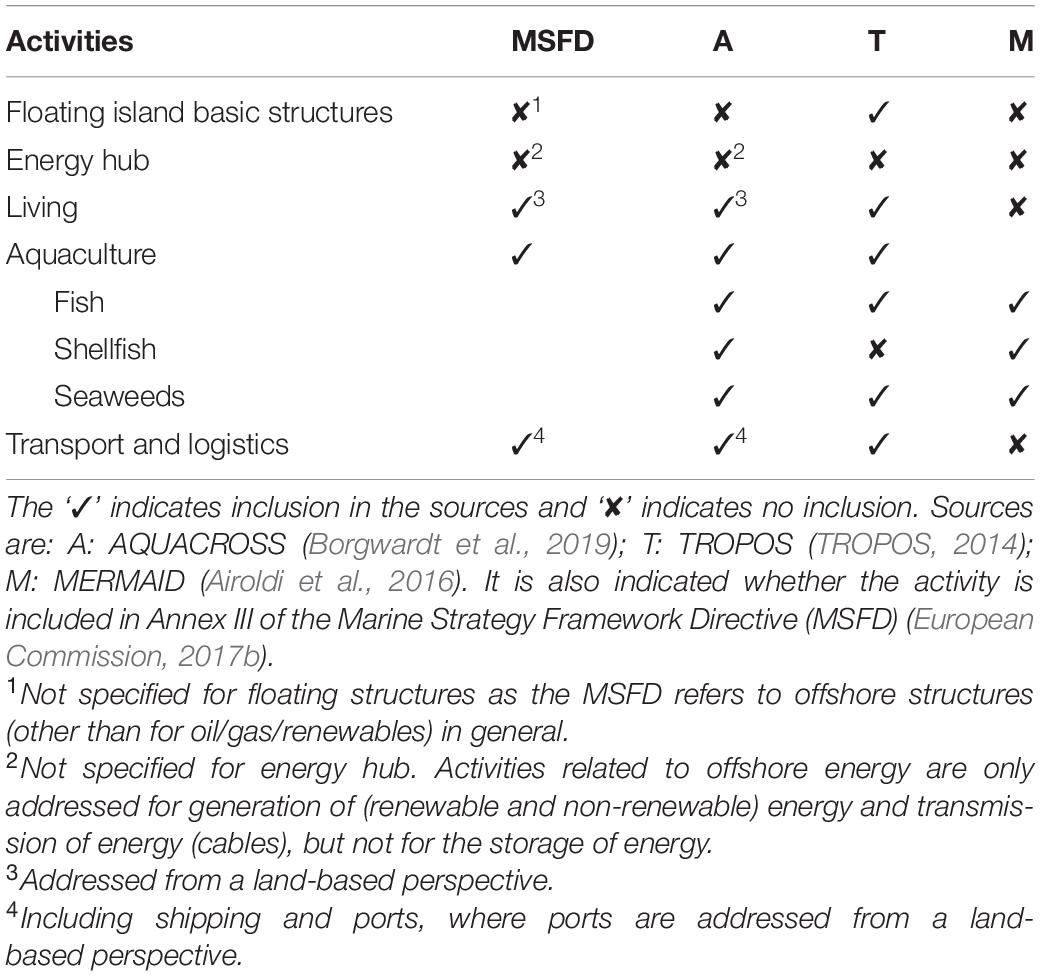
Table 1. List of activities identified for Space@Sea, representing the applications as described by Flikkema and Waals (2019).
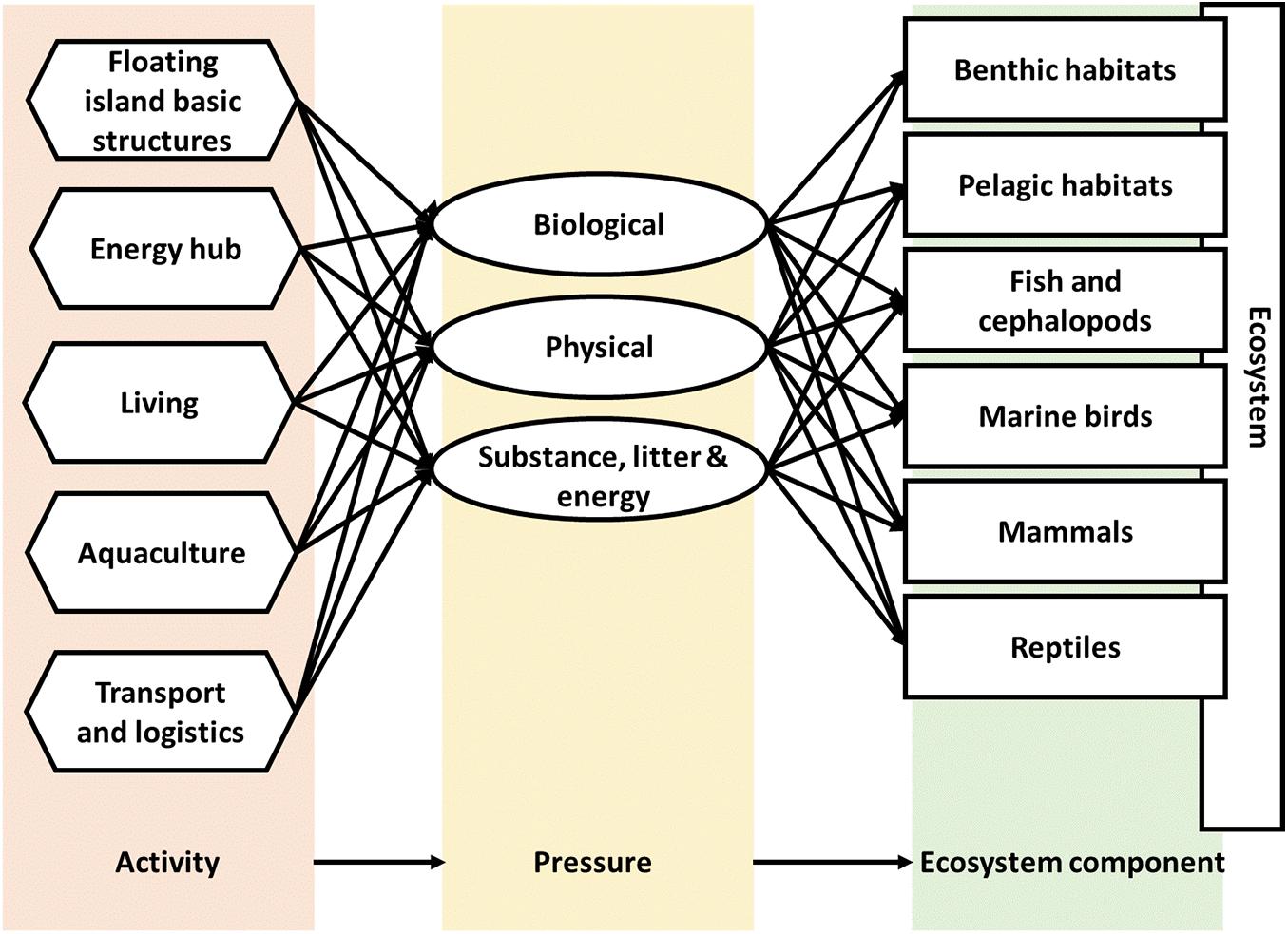
Figure 1. Framework for floating island applications showing relationships between activities (left), pressures (middle), and ecosystem components (right). Note that pressure are aggregated and presented by MSFD pressure theme (European Commission, 2017b).
The floating island applications, as described by Flikkema and Waals (2019), represent the activities (Table 1). Each activity is addressed by one or more sources of information, providing the relevant potential pressures caused by these activities that may lead to adverse effects on the ecosystem.
The pressure list (Table 2) was collated from various sources, starting from MSFD Annex III but complemented with additional pressures from other sources, e.g., AQUACROSS (Artificialization of habitat; Barrier to species movement; and Change of habitat structure/morphology), TROPOS (Attraction of wild animals; Escape of fish; Shading; Emissions to atmosphere; and Risk of accident and unplanned event), and MERMAID (N&P Depletion). The pressure Risk of accident and unplanned event is not included in the pressure list (Table 2) because calamities are outside the scope of this study (see section “Methodology”). Moreover, accidents are in fact the cause of pressures and not a pressure as such. For example, an explosion and subsequent fire on board of a floating island involves emissions of light and noise and may lead to emissions of hazardous substances and litter when facilities and storages are damaged.
Ecosystem components (Table 3) are defined differently by the sources: the components represent groups of species and habitats which may differ between the sources. Ecosystem components are not systematically included within MERMAID, but some ecosystem components are mentioned. Within MERMAID, potential impacts are described as activity-pressure combinations (which we consider threats) and, depending on the effect, ecosystem components are included ad hoc.
TROPOS includes microorganisms as separate group (not shown in Table 3), which are here assumed to be part of the benthic and pelagic habitats. In its ecosystem status assessment the MSFD includes in addition to the structural aspects of the ecosystem also its functioning, comprising of physical and hydrological characteristics, chemical characteristics, biological characteristics, functions and processes (not shown in Table 3). Here we only consider the structural elements and assume that this adequately covers the functional aspects. Furthermore, TROPOS includes humans as receptor, which is not considered an ecosystem component in the current study.
Assessment
The results in Table 4 show the potential impacts of the various applications that are considered in Space@Sea, based on the identified potential impacts in former studies, i.e., TROPOS, MERMAID, and AQUACROSS. The specific design of the Space@Sea applications was not taken into account in the assessment but will be considered in the “Discussion” section. Note that importance of impacts is based on TROPOS results only. Although AQUACROSS also includes a semi-quantification of impacts, AQUACROSS has not been used as literature source to determine the importance of impacts for this study. Instead it has been used only for the identification of potential links. The scope of AQUACROSS (sector activities on a regional sea scale) is not suited to assess the impact of the project scale activities which are the subject of this paper. MERMAID only includes a qualitative assessment and could therefore not be used as a source to determine importance of impact.
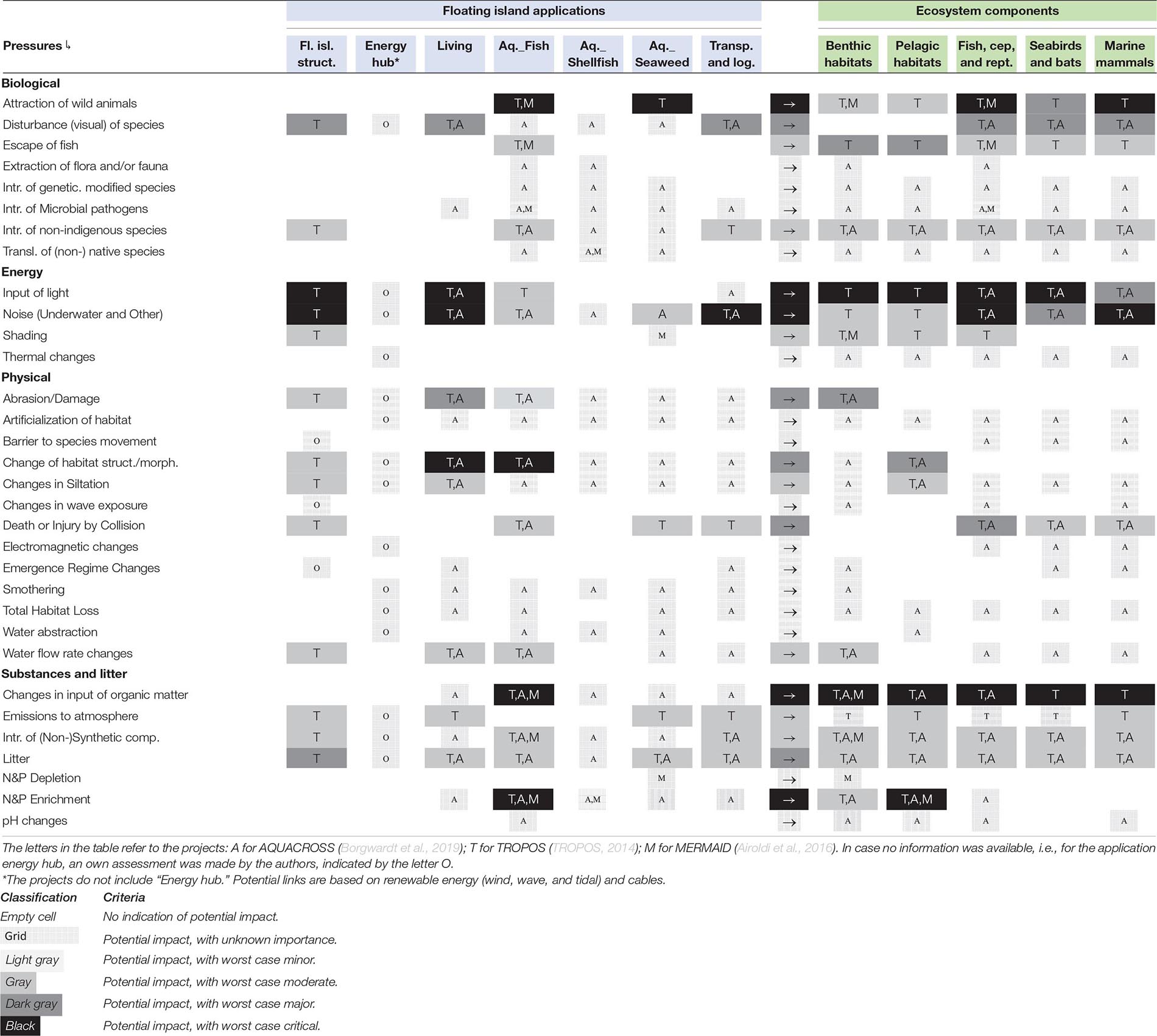
Table 4. Potential impacts of the various applications that are considered in Space@sea, based on a compilation of results from different projects.
Not all pressures identified in the pressure list (Table 2) are included in the framework of potential impacts of floating island applications (Table 4). Three pressures were not associated with either of the floating island applications: selective extraction of non-living resources (substrate e.g., gravel); introduction of radionuclides; and salinity changes.
Some elaboration was needed to define the ecosystem components, as the different sources defined them differently (Table 3). For the integration of results, the ecosystem components were harmonized resulting in a slightly different representation of the ecosystem components in Table 4.
There are considerable differences between the various Space@Sea applications in terms of the pressures they cause, both in terms of type, amount of pressures and their relative importance (Table 4). For the floating island structures and for living, two pressures are considered potentially critical (input of light and noise). For the energy hub the importance of impact could not be assessed because of limited information. For fish culture, three pressures could be critical (attraction of wild animals, input of organic matter, N&P enrichment). Because of limited information the importance of impacts resulting from mussel culture is not included, but the number of pressures caused by mussel culture is less than fish culture (17, as opposed to 24 for fish culture). Seaweed culture (macro algae) may cause a total of 23 pressures, but only one impact may be critical (attraction of wild animals). For transport and logistics one pressure may be critical (noise).
The importance of impacts resulting from a pressure may vary depending on the ecosystem components (Table 4). For seafloor habitats, the main threat is input of light and organic matter. For pelagic habitats, critical impact may be caused by input of light, organic matter and N&P enrichment. For fish, cephalopods and reptiles, critical impacts may be caused by attraction of wild animals, input of light, noise and organic matter. For seabirds, only changes of input of organic matter may cause critical impact. For marine mammals, critical impacts may be caused by attraction of wild animals, noise and organic matter.
Discussion
Methodology and (Future) Application
In this paper, a structured approach is developed and applied to assess the potential environmental impacts of floating islands and their offshore applications. This was based on earlier studies covering the environmental assessment of various specific designs and applications of floating structures in different environments (TROPOS, 2014; Airoldi et al., 2016) thereby providing a comprehensive overview of potential pathways through which sectoral activities may affect ecosystem components (Borgwardt et al., 2019). Here we combined this knowledge and developed a generic approach appropriate to assess the risk that these activities compromise the achievement of GES in EU waters according to the MSFD. This exercise revealed some gaps in the pressures from activities at offshore locations, that were previously not considered, such as shading.
The framework (i.e., the activities, pressures, ecosystem components, and their relationships) has a broad scope with low information requirements, which is most suitable for more strategic advice (Tamis et al., 2016). Consequently, the activities and ecosystem components are included at a highly aggregated level. Application of the framework resulted in a prioritization of the major threats or potential impacts and the activities and pressures causing them. This methodology is consistent with risk-based approaches, which are considered most suited for assessing (cumulative) impacts on the marine environment (GESAMP, 2008; Knights et al., 2015; Piet et al., 2015, 2019; Stelzenmüller et al., 2018; Culhane et al., 2019). Clearly further elaboration of this approach is possible in the future, in terms of detail but this comes with additional information requirements. Using this approach to guide this process has the advantage of utilizing existing knowledge and avoiding spending unnecessarily much time and space on irrelevant issues. For instance, a quantitative outcome might be required to assess whether potential effects of the proposed development are within boundaries set by environmental policies and legislations.
A more detailed framework involves dividing the applications into specific activities and stages (i.e., installation, production, and decommissioning) and transferring the ecosystem components into a lower aggregation level. For example, the EUNIS is here included at level 1 involving three different habitats (benthic habitats, pelagic habitats, ice associated habitats) of which two (benthic- and pelagic habitats) were included in the framework. Including the EUNIS at level 2 comprises 42 benthic habitat types and 10 pelagic habitat types (Moss, 2008). The biotic groups of fish, birds and mammals could also be subdivided into e.g., demersal and pelagic fish, waterbirds and seabirds, pinnipeds and cetaceans, or even be implemented at a species level. Clearly increased detail of the ecosystem components comes with increased requirements on information availability. This should be reconciled already in the scoping step. Criteria for the selection of relevant ecosystem components could be policy and legislation concerning species and/or habitat protection in force, stakeholders involvement and dependency on species and/or habitats, ecological important species and/or habitats (e.g., a relatively large part of the population inhabiting the study area or vital processes, species and/or habitats, such as coral), species and habitats that are (specially) sensitive to the pressures that are caused by the proposed activities.
The outcome of the EIA may be influenced by several uncertainties such as emerging from the (often unknown) sensitivity of ecosystem components for pressures. Addressing uncertainty is important when assessing cause-effect relationships (Stelzenmüller et al., 2018). Our assessment does not take uncertainty into account as the potential impacts are all based on worst case assumptions. For example, the impact of artificial lighting on birds and bats is assessed as moderately negative but in fact can be negative, positive, or none at all (TROPOS, 2014). Incorporating uncertainty into the framework could be part of future developments. The actual impacts then need to be validated through monitoring (see e.g., FAO (2009) regarding EIA and monitoring in aquaculture), which could be part of permit conditions.
Potential Impact and Mitigation
Among the seven applications included in this impact assessment, the main threat appears to be caused by the application of floating islands for fish culture. The potential impacts identified for aquaculture are primarily caused through the pressures such as attraction of wild animals, changes in input of organic matter and N&P Enrichment, all related to discharges of food and feces. Regarding environmental impacts from aquaculture a large knowledge base is available, including practical guidelines from the Food and Agriculture Organization of the United Nations (FAO). Buck and Langan(eds) (2017) already emphasized that multi-use systems that incorporate aquaculture development need to be guided by international policy instruments such as the FAO Code of Conduct for Responsible Fisheries (FAO, 2011) and the FAO Guidelines for an Ecosystems Approach to Aquaculture (FAO, 2010). Recent literature suggests well-balanced and properly managed marine aquaculture operations should not significantly alter the environment and should be the standard for aquaculture development (Casadevall et al., 2021). Designing the Space@Sea aquaculture plans as closed systems should ensure zero discharge of substances to the marine environment thereby alleviating the main threat caused by these pressures. The use of closed cages for fish farming in floating structures may have many other benefits, as described by Olsen (2020).
The location of the floating island is important for the environmental impact because of spatial differences in the local abiotic environmental conditions and the density of sensitive ecosystem components. Local abiotic environmental conditions, e.g., the depth and currents, determine whether pressures related to the input of substances may lead to environmental concentrations above threshold values causing detrimental effects. This needs to be considered within the selection of suitable areas for marine aquaculture in order to minimize environmental impact (Yucel-Gier et al., 2019; Casadevall et al., 2021). In addition, the abundance and vulnerability of ecosystem components may vary among locations and may consequently lead to different detrimental effect levels. For example, TROPOS assessed the potential impacts of floating island applications at two locations and concluded that the same species groups show higher vulnerability in Gran Canaria as compared to Crete (TROPOS, 2014). It is mentioned that the location near Crete is not expected to have very critical detrimental effects on the environment, because the existing environment is not of extremely high sensitivity and it is very deep there. However, the waters are phosphorous-limited and the deposits and remains from the fish aquaculture may result in a boost of local primary production due to phosphorous input (TROPOS, 2014). For most pressures, negative environmental impacts can be prevented or mitigated by site selection based on suitable abiotic and biotic conditions with regard to nutrient availability, current speed, near-bed currents, shear from waves, seabed characteristics, dilution and nutrient absorption rate, and sensitive nature values (e.g., natural populations of seaweed or seagrass, protected areas) (Airoldi et al., 2016). For example, negative impacts of suspended mussel/oyster farming on seabed can be reduced if farms are located in exposed areas where near-bed currents and shear from waves regularly erode, resuspend, and disperse waste (Airoldi et al., 2016). Location may also be of importance regarding impact of shading. Shading, or light deficit, caused by offshore floating platforms may affect primary production and/or vulnerable species such as coral reefs and seagrass (Benham et al., 2016; Karpouzoglou et al., 2020). Karpouzoglou et al. (2020) studied the effects of floating solar installations on the hydrodynamics and the ecodynamics of the North Sea, with a physical-biogeochemical model. The model showed that for up to 20% coverage of the water surface with floating platforms, the changes in net primary production were less than 10%. For higher percentages of coverage, primary production decreased substantially with strong dependence on the characteristics of the location. The impact of light deficit was relatively high under stratified conditions, whereas at well-mixed locations, hydrodynamic effects resulted in partial compensation for effects of light deficit (Karpouzoglou et al., 2020). Furthermore, the tidal amplitude at the location may determine the degree of seafloor abrasion. Any dragging of the platform mooring cables as the floating structure moves with the wind and waves will cause scour. Tidal changes in water depths may result in extended periods during which slack mooring cables interact with the seabed (Hooper et al., 2020).
Other aspects to consider for selecting a suitable location for floating islands are the presence of other human activities in the area, in order to minimize conflicts with other activities and major environmental impacts, which falls under the field of Marine Spatial Planning (Stelzenmüller et al., 2013; Swedish Agency for Marine and Water Management, 2017; Schmidtbauer Crona et al., 2017; Rodriguez, 2017). The framework developed here can be used to structure such evaluation (Stelzenmüller et al., 2013).
Positive Effects
Where impacts are generally considered as negative aspects resulting from activities, there may also be positive impact, i.e., benefits. For example, impacts from artificial structures may increase local biodiversity through the design of the structures (O’Shaughnessy et al., 2020). These positive impacts are generally not taken into account in EIAs. The physical structure provides a habitat for hard substrate organisms attached to floating structures and attract higher trophic levels such as fish, turtles and marine mammals that find food or a living space. From the TROPOS study it was further concluded that the shading effects of an island may be beneficial to fish and turtles in the water by providing shelter from predators (TROPOS, 2014).
Whether the impact of artificial light on birds and bats has a positive, negative or no effect remains to be seen because the responses to artificial light are expected to differ between species and may involve disorientation, attraction, or repulsion (Wiese et al., 2001; Poot et al., 2008; Marquenie et al., 2013; Debrot, 2014; Stone et al., 2015; May et al., 2017). For marine aquaculture, it has been suggested that currently available scientific observations cannot clearly identify the positive or negative effects of artificial light on wildlife (Casadevall et al., 2021).
Underwater structures may have a positive effect on benthos because structures may provide additional settling ground for sessile benthic organisms. In addition, an indirect positive effect can be expected from excluding commercial fisheries. By excluding fisheries, these structures can act as important refuges for a variety of taxa (Frumkes, 2002; Claisse et al., 2014; van Elden et al., 2019). Particularly bottom trawl fishing, probably the biggest threat to the seabed habitats (Halpern et al., 2008; Knights et al., 2015; Piet et al., 2019). The so-called reef effects of solid structures in some environments add to biodiversity. However introduction the structures may facilitate non-indigenous species (Glasby et al., 2007) and therewith promote the spread of invasive species using structures as “stepping stones” (Afonso et al., 2020).
Other potential positive, but indirect, effects of the floating island structures involve the exclusion of shipping traffic (except for platform related service and maintenance) from the entire area for many years (e.g., 25 years), which could have a positive effect on air and water quality, microorganisms, benthic flora and fauna, fish and turtles and marine mammals because shipping is related to many environmental impacts (Erbe et al., 2020).
Future Floating Structure Designs
Two business case studies are evaluated in Space@Sea demonstrating their multi-use application (Flikkema and Waals, 2019). In the North Sea case study, transport and logistic activities are combined with living facilities and aquaculture. In the Mediterranean case study an energy hub will be placed from where wind farms can be serviced, combined with aquaculture and the generation of wave energy. The structured approach to EIA presented here, enables to address the cumulative impacts of these multiple-use applications. By assigning numerical scores to weight each impact chain, the relative impact risks can be assessed, aggregated and ranked to identify activities and/or pressures causing highest impacts and/or ecosystem components receiving highest impact, see e.g., (Knights et al., 2015; Borgwardt et al., 2019). Thus this approach is suited for both EIA and CIA.
For floating structures there is the opportunity to ensure, from the outset, that designs maximize positive environmental outcomes (Hooper et al., 2020). For other offshore sectors, i.e., offshore wind energy, nature-inclusive designs have been formalized within the development process: in the Netherlands, the permit holder must make demonstrable efforts to design and build the wind farm in such a way that it actively enhances the sea’s ecosystem, helping to foster conservation efforts and goals relating to sustainable use of naturally occurring species and habitats (Hermans et al., 2020). However, to date there is not enough experience with nature-inclusive designs in the offshore domain to understand which design option will result in the highest ecological benefits (Hermans et al., 2020). More knowledge is required to improve the understanding of the ecological consequences of floating engineered structures to support decision-making by developers, regulators and planners committed to sustainable oceans (Sheehan et al., 2020). Based on the available structured knowledge on environmental impacts from floating structure applications presented in this study, some suggestions for mitigation are made (section “Potential Impact and Mitigation”), for example the use of closed cages for fish farming.
Concluding Remarks
The approach presented here is intended to provide a basis and initial guidelines for future EIAs for floating island and platform applications. Also, the results provide a first indication of the likely threats to the ecosystem and its components that may emerge from these activities and their pressures. As such, it provides a basis to adapt design, implementation and operation of the platforms to mitigate the identified negative impacts (or possibly increase the benefits) on the environment. Site selection was identified as an important consideration for which the EIA can provide guidance if adequate spatial information is available of the main sensitive ecosystem components. If cumulative impacts cannot be excluded, an appropriate monitoring strategy and effective management plan can be implemented to minimize long-term negative effects on the environment. This EIA can be used to identify the ecosystem components that primarily need to be covered by such a monitoring strategy or management plan.
Data Availability Statement
The original contributions presented in the study are included in the article, further inquiries can be directed to the corresponding author/s.
Author Contributions
All authors listed have made a substantial, direct and intellectual contribution to the work, and approved it for publication.
Funding
Space@Sea partners received a total of €6,766,793.02 funding from the European Commission as part of the Horizon 2020 research program under grant number 774253. Space@Sea was funded under Research and Innovation call H2020-BG-04-2017. Space@Sea partner Wageningen University & Research contributed to this paper.
Disclaimer
The opinions in this document reflect only the author’s view and in no way reflect the European Commission’s opinions. The European Commission is not responsible for any use that may be made of the information it contains.
Conflict of Interest
The authors declare that the research was conducted in the absence of any commercial or financial relationships that could be construed as a potential conflict of interest.
Acknowledgments
Space@Sea is a partnership between the following partners: MARIN, DeltaSync, DST, NEMOS, TU Delft, MOCEAN, TU Hamburg Harburg, Bluewater, University of Rostock, GICON, Wageningen University & Research, University of Duisburg-Essen, TU Graz, Waterstudio, Icepronav, VAL FoU, and DEME.
References
Abhinav, K. A., Collu, M., Benjamins, S., Cai, H., Hughes, A., Jiang, B., et al. (2020). Offshore multi-purpose platforms for a Blue Growth: A technological, environmental and socio-economic review. Sci. Total Environ. 734:138256. doi: 10.1016/j.scitotenv.2020.138256
Afonso, I., Berecibar, E., Castro, N., Costa, J. L., Frias, P., Henriques, F., et al. (2020). Assessment of the colonization and dispersal success of non-indigenous species introduced in recreational marinas along the estuarine gradient. Ecol. Indic. 113:106147. doi: 10.1016/j.ecolind.2020.106147
Airoldi, L., Møhlenberg, F., Evriviadou, M., Jimenez, C., Hansen, B., Dávila, O. G., et al. (2016). “EIA Manual for MUOP (Multi Use Offshore Platforms),” in Mermaid Project Report ID 3.5 to the European Commission, (Belgium: European Commission).
Ang, K. K., Dai, J., Hellan, O., Watn, A., and Si, M. B. I. (2020). “Design and Potential Applications of Floating Structures in Singapore,” in Lecture Notes in Civil Engineering, (Berlin: Springer), 135–154. doi: 10.1007/978-981-13-8743-2_7
Benham, C. F., Beavis, S. G., Hendry, R. A., and Jackson, E. L. (2016). Growth effects of shading and sedimentation in two tropical seagrass species: Implications for port management and impact assessment. Mar. Pollut. Bull. 109, 461–470. doi: 10.1016/j.marpolbul.2016.05.027
Borgwardt, F., Robinson, L., Trauner, D., Teixeira, H., Nogueira, A. J. A., Lillebø, A. I., et al. (2019). Exploring variability in environmental impact risk from human activities across aquatic ecosystems. Sci. Total Environ. 652, 1396–1408. doi: 10.1016/j.scitotenv.2018.10.339
Buck, B. H., and Langan, R. (eds) (2017). in Aquaculture Perspective of Multi-Use Sites in the Open Ocean: The Untapped Potential for Marine Resources in the Anthropocene, eds B. H. Buck and R. Langan (Cham: Springer Nature), doi: 10.1007/978-3-319-51159-7_7
Casadevall, M., Rodríguez-prieto, C., Torres, J., and Eira, C. (2021). Editorial?: Marine Aquaculture Impacts on Marine Biota. Front. Mar. Sci. 8:615267. doi: 10.3389/fmars.2021.615267
Chytrı, M., Tichı, L., Hennekens, S. M., Knollová, I., Janssen, J. A. M., Rodwell, J. S., et al. (2020). EUNIS Habitat Classification: Expert system, characteristic species combinations and distribution maps of European habitats. Appl. Veg. Sci. 12519. doi: 10.1111/avsc.12519
Claisse, J. T., Pondella, D. J., Love, M., Zahn, L. A., Williams, C. M., Williams, J. P., et al. (2014). Oil platforms off California are among the most productive marine fish habitats globally. Proc. Natl. Acad. Sci. U S A. 111, 15462–15467. doi: 10.1073/pnas.1411477111
Culhane, F., Teixeira, H., Nogueira, A. J. A., Borgwardt, F., Trauner, D., Lillebø, A., et al. (2019). Risk to the supply of ecosystem services across aquatic ecosystems. Sci. Total Environ. 660, 611–621. doi: 10.1016/j.scitotenv.2018.12.346
Debrot, A. O. (2014). Nocturnal foraging by artificial light in three Caribbean bird species. J. Carib. Ornithol. 27, 40–41.
Elliott, M., Borja, A., and Cormier, R. (2020). Activity-footprints, pressures-footprints and effects-footprints – Walking the pathway to determining and managing human impacts in the sea. Mar. Pollut. Bull. 155:111201. doi: 10.1016/j.marpolbul.2020.111201
Erbe, C., Smith, J. N., Redfern, J. V., and Peel, D. (2020). Editorial: Impacts of Shipping on Marine Fauna. Front. Mar. Sci. 7:637. doi: 10.3389/fmars.2020.00637
European Commission (2017a). COMMISSION DECISION (EU) 2017/848 - of 17 May 2017 - laying down criteria and methodological standards on good environmental status of marine waters and specifications and standardised methods for monitoring and assessment, and repealing Decision 2010/4. Belgium: European Commission.
European Commission (2017b). COMMISSION DIRECTIVE (EU) 2017/845 - of 17 May 2017 - amending Directive 2008/56/EC of the European Parliament and of the Council as regards the indicative lists of elements to be taken into account for the preparation of marine strategies. Belgium: European Commission.
FAO (2009). Environmental impact assessment and monitoring in aquaculture. Requirements, practices, effectiveness and improvements. Rome: FAO.
Flikkema, M., and Waals, O. (2019). Space@Sea the Floating Solution. Front. Mar. Sci. 6:553. doi: 10.3389/fmars.2019.00553
Frumkes, D. (2002). The status of the California Rigs-to-Reefs Programme and the need to limit consumptive fishing activities. ICES J. Mar. Sci. 59, S272–S276. doi: 10.1006/jmsc.2002.1289
GESAMP (2008). Assessment and communication of environmental risks in coastal aquaculture. Rome: FAO.
Gibbs, M. T., and Browman, H. I. (2015). Introduction: Risk assessment and risk management: A primer for marine scientists. ICES J. Mar. Sci. 72, 992–996. doi: 10.1093/icesjms/fsu232
Glasby, T. M., Connell, S. D., Holloway, M. G., and Hewitt, C. L. (2007). Nonindigenous biota on artificial structures: Could habitat creation facilitate biological invasions? Mar. Biol. 151, 887–895. doi: 10.1007/s00227-006-0552-5
Guerra, F., Grilo, C., Pedroso, N. M., and Cabral, H. (2015). Environmental Impact Assessment in the marine environment: A comparison of legal frameworks. Environ. Impact Assess. Rev. 55, 182–194. doi: 10.1016/j.eiar.2015.08.003
Halpern, B. S., Walbridge, S., Selkoe, K. A., Kappel, K. V., Micheli, F., D’Agrosa, C., et al. (2008). A global map of human impact on marine ecosystems. Science 319, 948–952. doi: 10.1126/science.1149345
Hermans, A., Bos, O. G., and Prusina, I. (2020). Nature-Inclusive Design: a catalogue for offshore wind infrastructure?: Technical report. Wageningen: Wageningen University & Research.
Hooper, T., Armstrong, A., and Vlaswinkel, B. (2020). Environmental impacts and benefits of marine floating solar. Sol. Energy 2020:10. doi: 10.1016/j.solener.2020.10.010
João, E. (2002). How scale affects environmental impact assessment. Environ. Impact Assess. Rev. 22, 289–310. doi: 10.1016/S0195-9255(02)00016-1
Karpouzoglou, T., Vlaswinkel, B., and Van Der Molen, J. (2020). Effects of large-scale floating (solar photovoltaic) platforms on hydrodynamics and primary production in a coastal sea from a water column model. Ocean Sci. 16, 195–208. doi: 10.5194/os-16-195-2020
Knights, A. M., Koss, R. S., and Robinson, L. A. (2013). Identifying common pressure pathways from a complex network of human activities to support ecosystem-based management. Ecol. Appl. 23, 755–765. doi: 10.1890/12-1137.1
Knights, A. M., Piet, G. J., Jongbloed, R. H., Tamis, J. E., White, L., Akoglu, E., et al. (2015). An exposure-effect approach for evaluating ecosystem-wide risks from human activities. ICES J. Mar. Sci. 72, 1105–1115. doi: 10.1093/icesjms/fsu245
Lynam, T., Mathevet, R., Etienne, M., Stone-Jovicich, S., Leitch, A., Jones, N., et al. (2012). Waypoints on a journey of discovery: Mental models in human- environment interactions. Ecol. Soc. 17:170323. doi: 10.5751/ES-05118-170323
Marquenie, J., Donners, M., Poot, H., Steckel, W., and De Wit, B. (2013). Bird-friendly light sources: Adapting the spectral composition of artificial lighting. IEEE Ind. Appl. Mag. 19, 56–62. doi: 10.1109/MIAS.2012.2215991
Martínez Pérez, E. J. (2017). “The Environmental Legal Framework for the Development of Blue Energy in Europe,” in The Future of the Law of the Sea: Bridging Gaps Between National, Individual and Common Interests, ed. G. Andreone (Cham: Springer), 1–269. doi: 10.1007/978-3-319-51274-7
May, R., Åström, J., and Hamre, Ø, and Dahl, E. L. (2017). Do birds in flight respond to (ultra)violet lighting? Avian Res. 8:33. doi: 10.1186/s40657-017-0092-3
Morgan, R. K. (2012). Environmental impact assessment: The state of the art. Impact Assess. Proj. Apprais. 30, 5–14. doi: 10.1080/14615517.2012.661557
Moss, D. (2008). EUNIS habitat classification users guide v2. Copenhagen: European Environment Agency.
Noble, B., Ketilson, S., Aitken, A., and Poelzer, G. (2013). Strategic environmental assessment opportunities and risks for Arctic offshore energy planning and development. Mar. Policy 39, 296–302. doi: 10.1016/j.marpol.2012.12.011
O’Shaughnessy, K. A., Hawkins, S. J., Evans, A. J., Hanley, M. E., Lunt, P., Thompson, R. C., et al. (2020). Design catalogue for eco-engineering of coastal artificial structures: a multifunctional approach for stakeholders and end-users. Urban Ecosyst. 23, 431–443. doi: 10.1007/s11252-019-00924-z
Olsen, T. O. (2020). “Fish Farming in Floating Structures,” in WCFS2019. Lecture Notes in Civil Engineering, Vol. 41, eds C. Wang, S. Lim, and Z. Tay (Singapore: Springer), doi: 10.1007/978-981-13-8743-2_10
Papandroulakis, N., Thomsen, C., Mintenbeck, K., Mayorga, P., and Hernández-Brito, J. J. (2017). “The EU-project ‘TROPOS,” in Aquaculture Perspective of Multi-Use Sites in the Open Ocean: The Untapped Potential for Marine Resources in the Anthropocene, eds R. Langan and B. H. Buck (Cham: Springer International Publishing), 355–374. doi: 10.1007/978-3-319-51159-7_12
Partidário, M. R. (2000). Elements of an SEA framework - Improving the added-value of SEA. Environ. Impact Assess. Rev. 20, 647–663. doi: 10.1016/S0195-9255(00)00069-X
Piet, G. J., Jongbloed, R. H., Knights, A. M., Tamis, J. E., Paijmans, A. J., van der Sluis, M. T., et al. (2015). Evaluation of ecosystem-based marine management strategies based on risk assessment. Biol. Conserv. 186, 158–166. doi: 10.1016/j.biocon.2015.03.011
Piet, G. J., Knights, A. M., Jongbloed, R. H., Tamis, J. E., de Vries, P., and Robinson, L. A. (2017). Ecological risk assessments to guide decision-making: Methodology matters. Environ. Sci. Policy 68, 1–9. doi: 10.1016/J.ENVSCI.2016.11.009
Piet, G. J., Tamis, J. E., Volwater, J., de Vries, P., van der Wal J. T., and Jongbloed, R. H. (in press). A roadmap towards quantitative cumulative impact assessments: every step of the way. Sci. Total Environ.
Piet, G., Culhane, F., Jongbloed, R., Robinson, L., Rumes, B., and Tamis, J. (2019). An integrated risk-based assessment of the North Sea to guide ecosystem-based management. Sci. Total Environ. 654, 694–704. doi: 10.1016/j.scitotenv.2018.11.001
Poot, H., Ens, B. J., de Vries, H., Donners, M. A. H., Wernand, M. R., and Marquenie, J. M. (2008). Green light for nocturnally migrating birds. Ecol. Soc. 13:130247. doi: 10.5751/ES-02720-130247
Rodriguez, N. (2017). A comparative analysis of holistic marine management regimes and ecosystem approach in marine spatial planning in developed countries. Ocean Coast. Manag. 137:23. doi: 10.1016/j.ocecoaman.2016.12.023
Ruzzo, C., Muggiasca, S., Malara, G., Taruffi, F., Belloli, M., Collu, M., et al. (2021). Scaling strategies for multi-purpose floating structures physical modeling: state of art and new perspectives. Appl. Ocean Res. 108:102487. doi: 10.1016/j.apor.2020.102487
Schmidtbauer Crona, J., Ruskule, A., Kopti, M., Käppeler, B., Dael, S., and Wesolowska, M. (eds) (2017). The Ecosystem Approach in Maritime Spatial Planning - A Checklist Toolbox. Sweden: Balticscope. Available online at: http://www.balticscope.eu/
Sheehan, E. V., Bridger, D., Nancollas, S. J., and Pittman, S. J. (2020). PelagiCam: a novel underwater imaging system with computer vision for semi-automated monitoring of mobile marine fauna at offshore structures. Environ. Monit. Assess. 192, 1–13. doi: 10.1007/s10661-019-7980-4
Stelzenmüller, V., Breen, P., Stamford, T., Thomsen, F., Badalamenti, F., and Borja, Á, et al. (2013). Monitoring and evaluation of spatially managed areas: A generic framework for implementation of ecosystem based marine management and its application. Mar. Policy 37, 149–164. doi: 10.1016/j.marpol.2012.04.012
Stelzenmüller, V., Coll, M., Mazaris, A. D., Giakoumi, S., Katsanevakis, S., Portman, M. E., et al. (2018). A risk-based approach to cumulative effect assessments for marine management. Sci. Total Environ. 612, 1132–1140. doi: 10.1016/j.scitotenv.2017.08.289
Stone, E. L., Harris, S., and Jones, G. (2015). Impacts of artificial lighting on bats: A review of challenges and solutions. Mamm. Biol. 80, 213–219. doi: 10.1016/j.mambio.2015.02.004
Swedish Agency for Marine and Water Management (2017). Symphony – a tool for ecosystem-based marine spatial planning. Sweden: Swedish Agency for Marine and Water Management.
Tamis, J. E., de Vries, P., Jongbloed, R. H., Lagerveld, S., Jak, R. G., Karman, C. C., et al. (2016). Toward a harmonized approach for environmental assessment of human activities in the marine environment. Integr. Environ. Assess. Manag. 12, 632–642. doi: 10.1002/ieam.1736
TROPOS (2014). Report on Environmental Impact Assessment and Mitigation Strategies. Richland, WA: PNNL.
van Elden, S., Meeuwig, J. J., Hobbs, R. J., and Hemmi, J. M. (2019). Offshore Oil and Gas Platforms as Novel Ecosystems: A Global Perspective. Front. Mar. Sci. 6:548. doi: 10.3389/fmars.2019.00548
Verheem, R. A. A., and Tonk, J. A. M. N. (2012). Impact Assessment and Project Appraisal Strategic environmental assessment: one concept, multiple forms. Impact Assess. Proj. Apprais. 18, 177–182. doi: 10.3152/147154600781767411
Wang, G., Drimer, N., and Goldfeld, Y. (2020). Modular floating structures (MFS) for offshore dwelling a hydrodynamic analysis in the frequency domain. Ocean Eng. 216:107996. doi: 10.1016/j.oceaneng.2020.107996
Wang, G., Goldfeld, Y., and Drimer, N. (2019). Expanding coastal cities – Proof of feasibility for modular floating structures (MFS). J. Clean. Prod. 222, 520–538. doi: 10.1016/j.jclepro.2019.03.007
Wiese, F. K., Montevecchi, W. A., Davoren, G. K., Huettmann, F., Diamond, A. W., and Linke, J. (2001). Seabirds at risk around offshore oil platforms in the North-west Atlantic. Mar. Pollut. Bull. 42, 1285–1290. doi: 10.1016/S0025-326X(01)00096-0
Keywords: environmental impact assessment, floating modular island, marine ecosystem, cumulative effect assessment, cumulative impact assessment, Space@Sea, multi-use, multi-purpose
Citation: Tamis JE, Jongbloed RH, Piet GJ and Jak RG (2021) Developing an Environmental Impact Assessment for Floating Island Applications. Front. Mar. Sci. 8:664055. doi: 10.3389/fmars.2021.664055
Received: 04 February 2021; Accepted: 22 March 2021;
Published: 22 April 2021.
Edited by:
Frank Adam, University of Rostock, GermanyReviewed by:
Bela H. Buck, Alfred Wegener Institute Helmholtz Center for Polar and Marine Research (AWI), GermanyDoris Soto Benavides, University of Concepcion, Chile
Copyright © 2021 Tamis, Jongbloed, Piet and Jak. This is an open-access article distributed under the terms of the Creative Commons Attribution License (CC BY). The use, distribution or reproduction in other forums is permitted, provided the original author(s) and the copyright owner(s) are credited and that the original publication in this journal is cited, in accordance with accepted academic practice. No use, distribution or reproduction is permitted which does not comply with these terms.
*Correspondence: Jacqueline E. Tamis, SmFjcXVlbGluZS50YW1pc0B3dXIubmw=