- 1Environmental Biochemistry, Institute for Chemistry and Biology of the Marine Environment (ICBM), Carl von Ossietzky Universität Oldenburg, Oldenburg, Germany
- 2BASF SE, Ludwigshafen am Rhein, Germany
- 3Product Stewardship & EHS Data Management, BASF Personal Care and Nutrition GmbH, Monheim am Rhein, Germany
- 4Helmholtz Institute for Functional Marine Biodiversity at the University of Oldenburg (HIFMB), Oldenburg, Germany
Coral reefs are highly endangered ecosystems. The identification and quantification of potential stress factors are essential to protect them. UV filters from sunscreens that are introduced to coral reef areas are considered as one of these stressors and their impact on corals needs to be further investigated. Even though UV filters are functionally similar, their structural features are very diverse. Their impact on limnic organisms have also been shown to be highly variable ranging from no or low to high toxicity. It is therefore to be expected that their effect on corals also differs significantly and that each compound has to be evaluated individually. The demand for conclusive benchmarks and guidelines from policy makers and the public over the past years shows the necessity for an objective literature review on the effects of various UV filters on scleractinian corals. Here, we review the present literature, summarize the data on the different UV filters and discuss the different approaches, advantages and limitations of the studies. However, the methods used in the latter studies vary greatly. They differ in many aspects such as species and life stage used, field and laboratory approaches, with exposure times ranging from hours to weeks. Some studies include analytics and measure the actual test concentration, others only provide nominal concentrations. The lack of standardized methods renders comparisons between studies futile. Additionally, most UV filters have only been investigated in a single or a few studies of different quality. Reliable thresholds are therefore impossible to draw on the basis of currently available studies. Nevertheless, certain UV filters repeatedly showed comparable toxicity in both freshwater and marine species tested. Yet, existing differences in results from coral tests emphasize the need for a standardized testing method comparable to those established for other aquatic organisms in order to allow for a more conclusive assessment. In this review, we describe what a scientifically sound testing proposal should include in order to obtain reliable and reproducible data, which ultimately should result in an internationally organized standardized ring test trial. Such standardized toxicity tests would enable validation of coral toxicity data related to UV filters, but also testing of other types of compounds that are known to be introduced and effect coral reefs, thus helping to identify significant stressors and enabling objective policy decisions.
Introduction
Scleractinian corals are the bioengineers of the most diverse marine ecosystem – coral reefs. Although coral reefs cover only 0.1% of the ocean floor (Spalding and Grenfell, 1997), roughly 25% of all known marine species depend on them as habitat or food source (Spalding et al., 2001). Coral reefs are also highly important for tropical coastal regions by providing irreplaceable economic and ecological functions including coastal protection, tourism and fisheries (Sebens, 1994; Sutton and VandenBelt, 1997; Coker et al., 2014). Despite growing knowledge of their socio-economic value by providing ecosystem services to around 10% of world’s population (Darling et al., 2019), coral reefs remain threatened mainly due to anthropogenic disturbances: the average loss of coral reefs within the last 40 years has been estimated at 20–50% and a further decline is expected for the rest of this century (Hughes et al., 2003, 2017b; Bruno and Selig, 2007; Ateweberhan et al., 2011; De’ath et al., 2012; Jackson et al., 2014).
The most relevant factor contributing to the global decline of coral reef habitats is climate change. Three previously unprecedented global bleaching events occurred in the years 1998, 2002, and 2016 (Hughes et al., 2017b), leading to widespread loss and degradation of vast coral reef areas throughout the tropics. In 2020, a large-scale bleaching event was observed again at the Great Barrier Reef [Australian Institute of Marine Science (AIMS), 2020]. Among all ecosystems coral reefs will be among the most affected by climate change, and the rising sea surface temperatures alone will already lead to a significant global decline (Hughes et al., 2017a).
However, on a local scale, more direct anthropogenic factors contribute to coral loss. For example, deforestation and construction operations can lead to coastal erosion onto coral reefs (Fabricius, 2005; Haapkylä et al., 2011; Fabricius et al., 2014). Dredging and other hydro-engineering activities can provoke increased sedimentation in reef habitats, causing intense smothering and loss of corals (Erftemeijer et al., 2012; Pollock et al., 2014; Gailani et al., 2016; Nelson et al., 2016). Furthermore, overfishing and destructive fishing methods are also problematic. Overfished reefs are less resilient against a variety of stressors (Hughes et al., 2007; Raymundo et al., 2009; Mumby and Harborne, 2010). Especially a reduction in herbivorous and spongivorous fish species can contribute substantially to phase shifts from coral dominated to algae or sponge dominated reefs (Edwards et al., 2013; Loh et al., 2015). The destructive potential of certain fishing methods such as dynamite and cyanide fishing is unrivaled and remains a threat even though being illegal in many countries (Fox et al., 2002; Mak et al., 2005).
Intensive agriculture and aquaculture, chemical industries and poorly treated wastewaters often cause influxes of increased levels of nutrients and other bioactive compounds onto coastal reefs (FAO, 2004; Crossland et al., 2005; Fabricius, 2005; UNEP/GPA, 2006; D’Angelo and Wiedenmann, 2014). Both natural and anthropogenic substances may impact the corals‘ health on a multitude of levels ranging from physiological responses in a subcellular to direct toxic effects on the organism level (Pastorok and Bilyard, 1985; Epstein et al., 2000; Negri et al., 2002; Shafir et al., 2007; Downs et al., 2016). However, the local dimension of the potential negative impact of chemical pollutants is not always fully understood.
In recent years, UV filters as well as the entire sunscreen products have gained both public and scientific attention as those cosmetic ingredients and products are likely to enter coastal areas indirectly via sewage treatment plant effluents or directly due to various leisure and recreational activities (e.g., swimming, snorkeling, and wave riding) (Giokas et al., 2007). Recent studies (Danovaro et al., 2008; Downs et al., 2014, 2016; McCoshum et al., 2016; Corinaldesi et al., 2018; Fel et al., 2019; He et al., 2019a, c; Stien et al., 2019, 2020; Sharifan, 2020) raised concern about the negative impact sunscreen products could have on coral reefs.
Danovaro et al. (2008) estimated an annual release of 4,000 to 6,000 metric tons of sunscreens in coral reef areas from swimmers with the potential to harm corals at local scales. Given their globally increasing usage and continuous release and dispersal in coastal waters, UV filters act as pseudo-persistent compounds (Pintado-Herrera and Lara Martín, 2020). Concentrations of UV filters in waters around coral reefs have mostly been documented in the range of ng L–1 (ppt) to low μg L–1 (ppb) (Tashiro and Kameda, 2013; Tsui et al., 2014a, b, 2017; Bargar et al., 2015; Sánchez Rodríguez et al., 2015; Downs et al., 2016; He et al., 2019a; Mitchelmore et al., 2019). Mitchelmore et al. (2021) recently reviewed field findings of organic UV-filters in sediments, water and coral tissue. In the wake of increased media attention to that topic, policymakers in Palau, United States virgin Islands and Hawaii decided to ban certain UV filter compounds that are commonly used in sunscreens probably based on these concerns (Hawaii State Legislature, 2018; PALAU, 2018).
Sunscreens and the UV filter systems within are designed to protect the human skin from sunburn, premature aging and skin cancer through absorption and reflection of deleterious UVA and UVB radiation (Chisvert and Salvador, 2007; Giokas et al., 2007; Osterwalder et al., 2014). Sun protection by the use of sunscreens is part of the world wide national skin cancer prevention strategy (Bundesanstalt für Arbeitsschutz und Arbeitsmedizin (BAuA), 2020; Centers for Disease Control and Prevention (CDC), 2020; HSE, 2020) and UV filter compositions are highly complex so that substituting one filter with another is very challenging and not always possible without changing the whole formulation (Narla and Lim, 2020; Pawlowski and Petersen-Thiery, 2020; Sohn et al., 2020).
Therefore, any new data generated require special attention and evaluation before any regulatory measure could be implemented. In other words, decisions to ban certain UV filters are controversial as there are no standardized or even validated toxicity tests on corals available and published data, using highly variable test protocols, are often not comparable or even scientifically sound.
Despite the need for a discussion on the regulatory framework, a growing number of consumers is already concerned about the potential effects of sunscreens to coral reefs. Several cosmetic labels already take these aspects into account by promoting products as being ‘reef friendly’ or ‘coral safe.’ However, such claims often lack a sound scientific basis (Levine, 2020). This controversy therefore needs a solid scientific basis. With this review we will provide an overview of the current publications on the effects of UV filters on corals with an emphasis on the strengths and limitations of the applied procedures. Furthermore, an outline for the urgent demand of high-quality coral toxicity studies along with the imminent need of standardized coral toxicity testing is given, which ultimately should lead to reliable, science-based regulatory decisions.
UV Filters
UV radiation is a significant threat to public health in terms of sunburn risk, photo-aging and skin cancer. On the electromagnetic spectrum, wavelengths of UV rays are shorter than visible light and transport more energy than electromagnetic waves of larger wavelengths. The solar UV range can be separated into UV-B (290–320 nm) and UV-A (320–400 nm) radiation, while UV-A can be further separated into UV-AI (340–400 nm) and UV-AII (320–340 nm). Its high energy load allows UV radiation to penetrate cells and cause harm on various levels, including permanent damages to DNA that stores genetic instructions for the development, functioning, growth and reproduction of all known macro- and microorganisms. UV-A rays lead to photo-oxidative damage down to the dermis and dermal capillary system (Elmets et al., 2014). Such photo-oxidative damage may alter the expression of certain genes, may lead to lipid oxidation as well as a depletion of enzymatic and non-enzymatic antioxidants in the stratum corneum (Schmid et al., 2004). UV-A radiation is responsible for premature skin aging, which results for example in wrinkling, skin dryness and pigment abnormalities (Fisher, 2005). UV-B rays, being the major cause of sunburn, mainly penetrate the epidermal basal cell layer of the skin and are more genotoxic than UV-A rays (Moore et al., 2013). UV radiation is the leading risk factor for melanoma and non-melanoma skin cancer (De Gruijl, 1999; Wang et al., 2001).
The human body’s protective response to UV exposure is rather limited and in fact does not reflect actual movement and activity behavior of humans (Pawlowski and Petersen-Thiery, 2020). In response to UV exposure, melanocytes produce melanin to protect the skin to a certain extent. However, the human body’s own protective mechanisms are not sufficient to avoid long-lasting damages through UV radiation (Brenner and Hearing, 2008). Avoidance of peak radiation and protective clothing are an essential way to prevent UV-damage (McCoshum et al., 2016). However, sunscreens are an additional and highly popular mean of protection against UV-A and UV-B radiation (Bernerd et al., 2000). UV filters in sunscreens are legislated by local and/or international agencies such as the European Union Cosmetics Regulation or the United States Food and Drug Administration (FDA) in order to ensure that adequate UV protection goes along with a safe use for humans (Tovar-Sánchez et al., 2013).
Every individual UV filter has its own peak absorption rate (DiNardo and Downs, 2018) at which it is most efficient and is classified either as a broad-spectrum protection, or for only UV-A or UV-B. UV filters in sunscreen formulations can be either organic (carbon based) or inorganic (mineral based). Inorganic UV filters reflect and absorb UV light (Egerton and Tooley, 2012; Gilbert et al., 2013). Zinc oxide (ZnO) and titanium dioxide (TiO2) are the two widely used inorganic UV filters (Schneider and Lim, 2019) and are mainly incorporated into sunscreen formulations as nanoparticles because the larger, non-nano size particles usually leave unpleasant white marks on the skin (Gilbert et al., 2013). Some of the mineral UV filters do get additional coatings such as alumina or incorporated manganese to minimize the formation of free radicals (Corinaldesi et al., 2018) or lipophilic coating with, e.g., triethoxycaprylylsilane to incorporate the filter in the oil phase of the sunscreen formulation.
Organic UV filters, on the other hand, are the most commonly used type of UV filters and typically absorb UV rays through their chemical structure but some, similar to mineral filters, may also scatter and/or reflect UV rays (Herzog et al., 2004; Pawlowski and Petersen-Thiery, 2020). They are commonly used in combination with other types of organic and inorganic UV filters since single organic UV filters do not achieve sufficient UV protection (Gilbert et al., 2013). Furthermore, the percentage of individual UV-active compounds in sunscreen formulations is generally limited by the responsible legislative authority (Sohn et al., 2020). Owing to their photo-sensitivity, certain organic UV filters are also subject to decomposition from UV light exposure, making a composition of several UV filters necessary for photo-stability (Donglikar and Deore, 2016). In other words, any other possible alternative stabilizing agent requires the need for both sufficient UV-light absorbance and UV light stability during the application period.
Due to application needs, organic UV filters are typically rather lipophilic and show sufficient resilience against both, abiotic and biotic degradation (Pawlowski and Petersen-Thiery, 2020). Some authors have detected UV filters in various aquatic biota and concluded that some UV filters may be bioaccumulative (Balmer et al., 2005; Buser et al., 2006; Bachelot et al., 2012; Gomez et al., 2012; Gago-Ferrero et al., 2015; Peng et al., 2017; Cunha et al., 2018). Other studies even moved a step further, when they state that some UV filters have the potential to accumulate along the food chain (Gago-Ferrero et al., 2012; Tovar-Sánchez et al., 2013). However, a more recent evaluation of aquatic bioaccumulation data related to certain UV filters came to the conclusion that under standardized lab conditions reproducible bioconcentration factors (BCF) remain below any critical value and thus, accumulation of UV filters along the food chain is not likely to occur (Pawlowski et al., 2019).
Standardized Test Procedures and Environmental Risk Assessments Used in a Regulatory Context
Environmental scientific research is key for a rather flexible and unconventional investigation into recent topics of interest. It helps to identify scientific concerns. However, results from this type of investigation may not be considered as adequate to address regional or even global regulatory demands, if data is generated under non-standardized and non-validated test conditions. Standardization of testing protocols is mandatory to allow an equal assessment of substances such as UV filters within national chemical registration processes, but requires considerably more effort and time compared to typical scientific research. This validation process relies at first on a scientifically sound testing proposal, and second, on reproducible results obtained from internationally organized ring tests. Once being established within an approved test guideline framework, such as ISO, DIN, OPPT or OECD, studies are conducted under good laboratory practice (GLP) conditions allowing for the mutual acceptance of data amongst various regulatory bodies across the globe. Those data are considered the basis for the environmental fate and environmental toxicity profile of a substance and may, beyond this, allow for a comparable assessment of substances used in the same application (Pawlowski et al., 2020).
This evaluation procedure also includes the selection of suitable test organisms, as not all of them are globally available on a regular basis and can be kept with affordable efforts in enduring lab cultures. Furthermore, test organisms should be of suitable size, free from parasites and resilient to any potential inbreeding effects. Although wild caught species are in theory allowed, sustainability aspects should prevent the use of wild stock populations in laboratory testing, especially in light of the UN sustainable development goals (e.g., SDG 14 Conserve and sustainably use the oceans, seas and marine resources for sustainable development). All these aspects per se limit the choice of the test organism to be selected in standardized tests. For example, among the vast variety of fish species only 10 species are recommended for the use in acute fish toxicity tests according to OECD 203 (OECD, 2019b). One may conclude that the results from these single species tests may be limited in the applicability in environmental protection. However, for decades, this indeed existing uncertainty has been addressed by using accepted assessment factors (ECB, 2003). Following this concept, the safe use of chemicals has been demonstrated and approved within more than 10,000 chemical registrations across the globe (ECHA, 2020).
Taking the above-mentioned aspects into account, it is generally agreed that for the aquatic compartment, besides fish (secondary consumer), also algae (primary producers) and daphnids (invertebrate; primary consumer) are selected representatives of the aquatic trophic food chain. Other species (such as corals or mollusks) may be added as additional species, once validated and approved test protocols exist (ECB, 2003).
Toxicological Studies With Tropical Scleractinian Corals
Ecotoxicological studies on corals are challenging compared to many standardized tests with freshwater organisms due to the following reasons: all scleractinian corals are protected under appendix II or even I of Convention of International Trade of Endangered Species (CITES). Therefore, a variety of permits are needed to collect and transport them across borders to be used in laboratory experiments. A possible source for some coral species are aquarium shops as they have well established trade channels. However, newly imported corals are often stressed, slightly bleached, and need to recover for several weeks to regain symbionts and start growing normally (Petersen et al., 2004; Delbeek, 2008). If possible, corals obtained from ex situ fragmentation of long-term aquarium cultures should be preferred over wild collected specimens for sustainability reasons as well as for their better usability and standardization purposes. Excellent skills, knowledge and experience are needed to cultivate corals in an aquarium setup for a required longer period of time. The biology, chemistry (e.g., micronutrients) and complex technology (pumps, lighting etc.) in the aquarium system builds a fragile and complex ecological system that can easily be disturbed by inappropriate or even wrong handling due to inadequate knowhow or training (Borneman, 2008; Osinga et al., 2008; Craggs et al., 2017). Hence, reliable substance-related results can only be obtained when secondary unnoted stress factors do not impact the outcome of the study. Depending on the length of the experiment, different factors must be assured: at all times water parameters [i.e., pH, oxygen saturation, salinity (conductivity), and water temperature] must be of sufficient quality. Especially for those physiologically rather poorly understood organisms, already an imbalance of individual trace elements can greatly disturb a coral’s wellbeing. A reliable source of natural or artificial seawater of consistent quality is absolutely crucial. The best option is freshly obtained natural seawater from an intact coral reef, that is distant to possible influx from land (e.g., harbor, river, and sewage). This, however, is typically not possible for many laboratories, since local marine water quality likely differs from site to site and lab locations may even be far off from natural marine water resources. It is also possible to use natural seawater from temperate or other regions when conducting coral experiments. However, it should only be used with sufficient knowledge on water quality chemistry, since certain parameters potentially need to be adjusted. A suitable alternative is the use of high-quality artificial seawater which is commonly applied for reef aquaria in both zoological gardens and private households. Contamination, particularly with organic compounds, is rare and a consistent quality of synthetic salt mixtures is usually guaranteed by the manufacturer.
As scleractinian corals live mostly on nutrients provided by their symbiotic microalgae, adequate and sufficient lighting is key to successful coral farming. Only high-quality aquarium lamps are suitable for coral culture, maintenance and experiments. Output and quality must be tested and adjusted since the wrong spectrum, as well as too little or too much light can be stressful, even detrimental to the coral health. Furthermore, every coral has a rather narrow temperature range when considering optimal growth and health. While some corals have their optimum at 28°C, this temperature could be rather stressful to other species. Typically, temperature ranges from 25–28°C are suitable for coral experiments. Short time exposure to higher temperatures than those can lead to great stress and even coral bleaching. Short-term experiments in small water volumes, without water changes and water movement are possible with certain coral species and setups. However, when corals are supposed to be kept for more than a few days, adequate water movement provided by a circulation pump or an airlift is necessary. Bubble stones are not suited for this purpose as they often do not provide sufficient water movement and, more importantly, create very small air bubbles that can get stuck in the coral polyp and cause stress. When using airflow for water movement, bubbles should always be in the mm range.
Scleractinia is a very diverse order of 1631 valid names of living coral species (Hoeksema and Cairns, 2020). Even though most of them are found in typical coral reef environments, some have a very or slightly different ecology. Even within the tropical zooxanthellate shallow-water species there are differences in their preferred habitats. Some species are only found in very steady water conditions, others can be found on reef flats and tide pools where water parameters naturally change greatly throughout the day and year. Thus, some corals are more or less prone to environmental stressors. From our own experience, some species can be very tolerant to a variety of parameters but very sensitive to others. When selecting coral species for toxicological experiments one should aim for coral species that have successfully been established in the aquarium trade and the coral husbandry hobby. These species are commonly available, can be reproduced sustainably, and thus allow for a high replicability of the conducted experiments.
The adult stage of corals is only one of several highly distinctive stages of their life cycle. For a comprehensive and realistic risk assessment, other life stages should also be included. Most corals are broadcast spawners and release eggs and sperm into the water column in a highly synchronized yearly spawning event (Willis et al., 1985; Babcock et al., 1986, 1994; Baird et al., 2009). Fertilization, embryo development take place in the water column and the developed planula larvae eventually settle on the seafloor (Harrison and Wallace, 1990). Brooders, on the other hand, represent with roughly 20% (Kerr et al., 2011) a minority of coral species that release sperm into the water and the fertilization takes place inside the coral (Baird et al., 2009). After an internal embryo development, fully competent planula larvae are released into the water column. For both, spawning and brooding corals, settlement will usually take place within a week, however, larvae may survive up to several weeks in order to reach suitable habitats even far away from their parental colonies (Ayre and Hughes, 2000; Graham et al., 2013). Like other organisms with a distinct larvae or juvenile phase, also the fertilization, larval and coral larvae settlement stages might be considered in standardized lab tests as the different stages may react differently on the target test-compounds compared to adult corals (Richmond, 1997; Richmond et al., 2018). Figure 1 shows the different life stages with suitable assay types and end points.
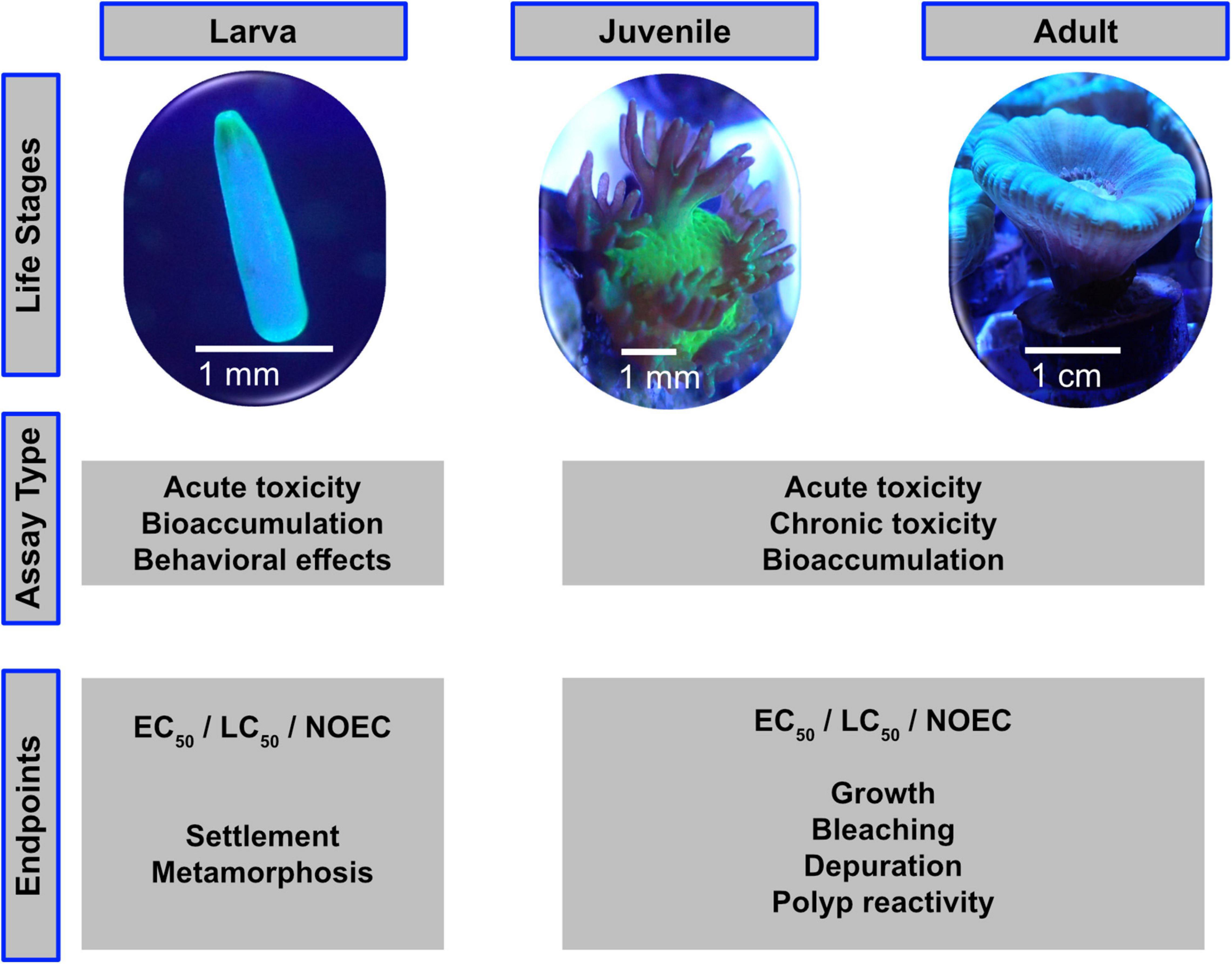
Figure 1. The life stages of scleractinian corals are suitable for different assay types and toxicity endpoints.
Considering the two coral reproductive strategies, spawners rely on field sampling and are basically available only once a year, whereas the brooders are considered to be constantly available in laboratory cultures. Therefore, the use of brooders is generally recommended for larvae testing. Nevertheless, it is valuable to compare relative sensitivity of larvae from brooders versus those from spawning corals.
It is considerably simple to make fragments of most adult small-polyped coral species using hole drills, bone cutters or other sharp tools to avoid squeezing of coral tissue. After the latter invasive treatment, the new coral fragments must be left to heal from the wounds before using them in any experimental setup to avoid a stress response by the coral (i.e., infections), which would consequently impact or even invalidate study results. The minimum healing time depends on the wound to healthy tissue ratio, the coral species, and the aquarium system they are left to heal in. An ideal fragment ready to be used in standardized tests shows visible new growth and no lesions.
A number of endpoints can be looked at in coral experiments ranging from physiological (DNA, RNA, enzymes) to morphological changes such as coral bleaching to the death of the coral. Like for many other standardized acute toxicity tests, a distinct endpoint like 50% mortality or related measure (e.g., bleaching) is most appropriate. On a chronic test level, sublethal endpoints such as growth, bleaching or other population relevant aspects may be suitable additional measurements. However, it should be taken into account, that the observation of effect levels might be easier at the larval compared to the adult coral stage (colony). Since bleaching is an easily recognizable coral stress response and a great variety of techniques are readily available to assess the extent of bleaching, past studies often used bleaching as a common endpoint to evaluate the effect of different chemical compounds (Danovaro et al., 2008; Downs et al., 2014, 2016; Corinaldesi et al., 2018; Fel et al., 2019; He et al., 2019b, c; Wijgerde et al., 2020). Furthermore, already minor variation in the color of the coral can be correlated to bleaching and thus can be evaluated (Siebeck et al., 2006). For example, smaller changes in symbiont photochemical efficiency can be measured with pulse amplitude modulated (PAM) fluorometry (Berkelmans and van Oppen, 2006; Schreiber, 2016; Nielsen et al., 2018; Fel et al., 2019). When determining symbiont densities, the coral tissue can be sampled and Symbiodiniaceae content counted under a microscope (Chen et al., 2005; Bourne et al., 2008; Cunning and Baker, 2013). Counting numbers of expelled symbionts is not ideal as expelled symbionts may decompose fast and healthy corals expel Symbiodiniaceae to different degrees (Titlyanov et al., 1996; Jones and Yellowlees, 1997; Dimond and Carrington, 2008). Other possible endpoints for short-term coral experiments could be: gene expression, metabolomics, respiration rate and polyp retraction. For longer-term experiments, skeletal growth, tissue growth (e.g., biomass, tissue thickness) and energy reserves (total lipid, protein, or carbohydrate content) can additionally be observed.
Depending on the compound tested, it must always be ensured that the basic water parameters are not changed by the addition of (1) the target compound or (2) the potential carrier substance in which the compound of interest might be dissolved. The latter is of particular interest for smaller water volumes, since this might indirectly lead to a toxicological effect. Furthermore, acute toxicity tests, which run over a longer period of time (weeks), may experience a substantial loss of evaporation, which can also lead to stressful conditions.
Likewise, to other well established aquatic OECD tests, water parameters like pH, major nutrient concentrations, oxygen saturation, salinity (conductivity) and temperature are critical during the whole testing period and should therefore be measured on a regular basis (at least daily) or even continuously during the entire exposure period. Any carrier-solvent [i.e., organic solvents such as methanol, ethanol, or dimethyl sulfoxide (DMSO)] that may be required to introduce particularly poor water-soluble substances should be checked beforehand for any negative impact on coral health. Basically, the use of solvents should be avoided whenever possible (OECD, 2019a). In addition to health impacts, some solvents, i.e., DMSO are known to interact with biological membranes which modify the bioavailability of the test compounds and thus should not be used at all (Gironi et al., 2020). When using a solvent, it is mandatory to run a separate solvent control, along with the treatment groups and the negative control (medium) (OECD, 2019a). The solvent concentration in each replicate and treatment (including the solvent control) should be at the same level to avoid any biased impact on the test results (e.g., lower concentrations in a treatment group may result to precipitation of the test material and potential physical effects).
Effect concentrations such as LCx/ECx [concentration at which x-% of the test organisms died (lethal concentration-LC) or were affected (effective concentration-EC)], NOEC (No Observed Effect Concentration) can either be related to nominal (e.g., loading rate) or mean measured test concentrations. However, whereas the first option also applies to mixtures of unknown composition, the latter one is considered to be mandatory if well-defined substances with available analytical methods are tested. Especially for poorly water-soluble substances analytical monitoring is required to demonstrate that (1) maximum test concentrations are close to the solubility limit under test conditions and not above, (2) that the test substance concentrations are consistent and stable during the entire testing period (3) to exactly determine actual effect concentrations (nominal values are not representative for such substances) (OECD, 2019a).
Figure 2 summarizes the main points that should be considered when planning a standardized coral toxicity test design.
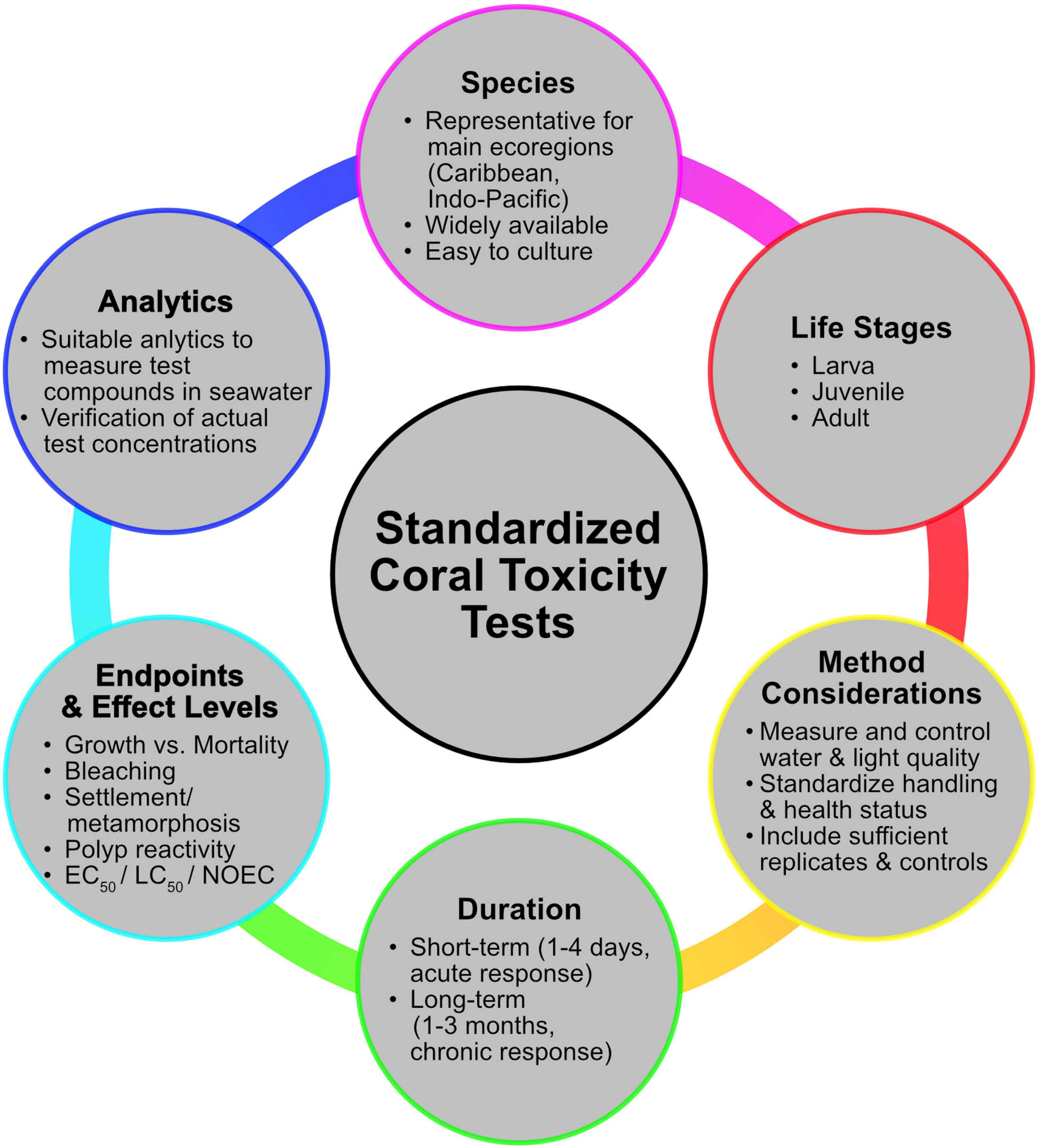
Figure 2. The main aspects that need to be considered when planning standardized toxicity testes with scleractinian corals.
Monitoring
UV filters mainly enter coastal waters either directly through recreational activities such as swimming, or indirectly through municipal and industrial wastewater effluents (rather low concentrations) as well as storm and surface water runoff (Giokas et al., 2007). Coastal areas located particularly close to coral reefs often lack sufficient wastewater treatment to remove anthropogenic substances including UV filters from the effluent stream (Burke et al., 2011). Local concentrations of filters in the marine compartment may vary due to specific topology and local currents. In addition it is still unclear which environmental concentrations impact coral health, because of the limited availability of monitoring and specifically of coral health effect data (Mitchelmore et al., 2019). Nevertheless, adequate monitoring data can help to address environmental concerns of potential hazardous substances (e.g., substances that have shown detrimental effects on test organisms) being released into the marine area.
However, measuring the presence of low environmental concentrations of these lipophilic compounds is challenging due to the following reasons: lipophilic substances tend to adsorb to each kind of surface, like for example particles in the water column of marine environments or the glass surface in an experimental set-up, but –of course– also to the surface of the corals. According to current guidelines, this fraction is not considered to be bioavailable anymore (OECD, 2019a). Thus, the bioavailable fraction in whole water samples is lower than that of the total fraction. In order to have a high bioavailable fraction – ideally corresponding to the applied test substance concentration, the sampling flasks should be of inert material (e.g., brown glass flasks), pre-saturated with sampling water, adequately transported and stored, otherwise a “loss” of substance prior to analysis may occur. Thus, in addition to the above- mentioned measures, also the spiking of the samples with an internal standard right after sampling will account for any substance losses during the period of sample preparation until chemical analysis. The overall results will be more reliable and reproducible. Analytical methods should be validated and sensitive enough to also quantify very low substance concentrations. The use of passive samplers as a surrogate to determine actual concentrations in the water should be taken with caution, as this method may be more applicable for the determination of a substance concentration in biota rather than in the water phase, as the sample concentration is related to the exposure time and thus is considered time dependent.
For the analysis of both sediment and tissue samples, in addition suitable extraction techniques (e.g., for sediments) and/or lipid normalization of data (for tissue concentrations) are required.
To date, several studies have aimed to qualify and quantify UV filters in the marine environment (Tashiro and Kameda, 2013; Bargar et al., 2015; Sánchez Rodríguez et al., 2015; Downs et al., 2016; He et al., 2017, 2019a; Tsui et al., 2017, 2014a, 2014b; Mitchelmore et al., 2019; Labille et al., 2020).
Some studies did not only include water analytics, but also sediment and coral tissue samples in their analyzes (Tsui et al., 2017; Mitchelmore et al., 2019). The latter may help to understand whether the bioaccumulation behavior of substances in corals differs significantly from those of standard OECD fish bioaccumulation studies (OECD, 2012). However, as environmental concentrations often vary much depending on current, tide, season, or precipitation, resulting tissue sample monitoring data bear a great uncertainty and can typically not be adequately used for regulatory purposes (Tsui et al., 2014a; Pawlowski et al., 2019). Instead, tissue analysis from standardized lab tests with constant exposure concentrations will certainly help to solve this merit.
Literature Review
An overview table of the different UV-filters and their published effects on corals can be found in the Supplementary Table 1.
Effects on Coral Larvae
In a first study it was concluded that coral larvae and recruits are thought to be more sensitive to a variety of substances compared to adults of the same species (Kushmaro et al., 1997).
Downs et al. (2016) investigated the toxicological effects of BP-3 on coral larvae of the scleractinian Stylophora pistillata. In the aforementioned study, larvae were exposed to different BP-3 concentrations and light regimes for up to 24 h, and examined with histopathology and cellular pathology, planula morphology, bleaching, DNA damage and planula mortality. The concentrations were not analytically verified but the LC50 under light conditions was described as 103.8 μg L–1 based on nominal concentrations, and the EC50 which led to deformations of the larvae was calculated to be 17 μg L–1 nominal concentration. EC50 and LC50 were higher during dark conditions and shorter exposure time. The same authors concluded that BP-3 causes immediate damage to the symbiotic zooxanthellae under light conditions. The observed bleaching under dark conditions, on the other hand, is the result of symbiophagy, which describes the consumption of the symbiont by the gastrodermal cells of the coral host (Downs et al., 2014). While EC50 represents the planulae deformation, Downs et al. (2016) also pointed out that BP-3 acts as a skeletal endocrine disruptor, a currently not defined term, which leads to ossification of the planula, eventually encasing the entire planula in its own skeleton. They also examined the DNA abasic lesions in planulae and showed that increasing concentrations of BP-3 cause significantly higher levels of DNA abasic lesions in the planulae which were exposed to both, light and dark conditions. Downs et al. (2016) also tested the effect of BP-3 on in vitro cell cultures of 7 different coral species and found a LC50 range from 8 to 340 μg L–1 (nominal concentrations). However, the quality of the study results is limited by the lack of analytical data to obtain the actual concentration of BP-3.
Downs et al. (2014) carried out a similar study with Stylophora pistillata larvae and in vitro cell cultures where they examined the impacts of the organic UV filter benzophenone-2 (BP-2), which can be found as additive in various non-cosmetic products. The results showed that BP-2 also induces different pathologies in both, light and dark experiments. The latter authors claim that BP-2, while exposed to complete darkness, caused coral bleaching because of symbiophagy. During light exposure, on the other hand, bleaching is caused through the direct damage to Symbiodiniaceae. Downs et al. (2014) concluded that this light-driven effect is based on the subcellular pathomorphology of chloroplasts and thylakoids resulting from photo-oxidative stress to the membrane structures. BP-2 appears to be a genotoxic to corals which is shown by the significant increase in DNA AP sites of planulae exposed to BP-2, similar to the BP-3 experiments from 2016. Even though the study showed effects on the coral symbionts, the lack of analytical data significantly limits the expressiveness of the obtained data, similar to Downs et al. (2016).
Effects on Adult Corals
Danovaro et al. (2008) exposed adult corals of the genus Acropora to several organic UV filters, namely butyl methoxy dibenzoyl methane (BMDBM), BP-3, ethylhexyl methoxycinnamate (EHMC), ethylhexyl salicylate (EHS), 4-methyl benzylidene camphor (MBC) and octocrylene (OCR) as well as various sunscreen formulations. Danovaro et al. (2008) carried out in situ experiments at different reef sites located in Indonesia, Mexico, Thailand and Egypt. The number of replicates per treatment ranged from 3 to 15, and measured water temperatures varied from 24 to 30°C, depending on the investigated sites. However, salinity (e.g., conductivity), pH and other relevant water quality parameters (e.g., ammonia and associated nitrogen-derivates, TOC) were not measured. During the entire 96 h-exposure period, no analytically concentration control analysis was carried out and thus it remains unclear whether any degradation of the test material occurred during the course of the experiment, which may also have an impact on relevant water quality parameters.
Danovaro et al. (2008) chose to carry out the experiments in the field with fragments of Acropora spp. that were immersed in polyethylene whirl-pack bags before placing them onto the reef (Danovaro et al., 2008). The subsequent release of Symbiodiniaceae under exposure to all tested sunscreen formulations and individual filters MBC, EHS, EHMC, and BP-3, led to the conclusion that UV filters induce a lytic viral cycle that leads to coral bleaching. However, the study is limited by the lack of analytical data as well as flaws in the experimental setup. For example, the enclosed whirl pack system with stagnant water, including the added organic material, is not an ideal experimental setup, particularly for scleractinian corals that need adequate water circulation. Also, large amounts of organic material in an enclosed containment can be a breeding ground for bacteria. The fact that similar effects were documented for all sunscreen brands but not all UV filters shows that other ingredients in the formulations might contribute to the detrimental effects on the corals. Such ingredients are known to be readily biodegradable and quickly broken down by bacteria affecting likely the water quality and are at least partially responsible for the negative effects on the corals. It would have been helpful to measure basic water parameters like O2 concentration, pH and maybe oxidation-reduction potential (ORP) at the end of the experiments to exclude indirect effects due to bacterial blooms.
He et al. (2019b, c) published two studies looking at the impact of organic UV filters on marine organisms. In the ex situ experiment (He et al., 2019c), the effects of four benzophenone analogs, namely BP-1, BP-3, BP-4, and BP-8 were investigated on the brooding scleractinian corals Pocillopora damicornis and Seriatopora caliendrum. In the other study, the effects of both EHMC and OCR were examined on the same two species (He et al., 2019b). The exposure phase for adults and coral larvae was 7 and 14 days, respectively. The larvae showed an overall higher sensitivity than the adult corals. The results in terms of toxicity of the single compounds was categorized in the following order: BP-8 > BP-1 > BP-3 > EHMC > BP-4 = OCR which implicates that BP-8 is most toxic to the tested coral species while BP-4 is the compound with the least toxicity. In addition, they inspected the bioaccumulation potential of the single compounds in both species and came to the following result: EHMC > OCR > BP-8 > BP-1 = BP-3 > BP-4. BP-8 has the highest bioaccumulation potential of the four benzophenones with the tested species while BP-4 shows the least. The LC50 in the larvae were >1,000 μg L–1 for all four benzophenone compounds, while for adult fragments of S. caliendrum (BP-1 and BP-8) and P. damicornis (BP-8) LC50s of <1,000 μg L–1 were derived. The lowest observed effect concentrations (LOECs) in S. caliendrum planulae were 250 and 500 μg L–1 for coral bleaching and death, respectively. The authors discovered that settled larvae bleach more rapidly (occurred after three days) compared to swimming larvae of the same species. Based on their experiments, they concluded that P. damicornis larvae and fragments are in general more tolerant toward the tested compounds than S. caliendrum. He et al. also suggested that the mode of action for BP’s toxicity to be the induction of oxidative stress and/or genotoxicity in the tested corals. They also documented that a total polyp retraction occurs before coral bleaching takes place, which is considered a typical behavior of stressed/unhealthy corals (He et al., 2019c). The fact that He and colleagues tested several individual UV filters and used precise analytics to determine the tested concentrations makes their study results one of the most significant in the field since their study allows an assessment of adequate effect values under the given experimental conditions.
Stien et al. (2019, 2020) published two studies with similar methods. In the first study (Stien et al., 2019), fragments of P. damicornis were exposed to different nominal OCR concentrations over the course of seven days. These experiments led to the observation that OCR transforms into conjugated fatty acids which then may accumulate in the corals tissue. They also found elevated acylcarnitine levels suggesting a mitochondrial disfunction. In the second study Stien et al. (2020) conducted a metabolomic profiling with different UV filters and found elevated concentrations of certain steroids under higher nominal concentrations of OCR, EHS, and BP-3. A metabolomic stress response could be verified within the range of test concentrations for bis-ethylhexyloxyphenol methoxyphenyl triazine (BEMT), BMDBM, diethylhexyl butamido triazone (DBT), diethylamino hydroxybenzoyl hexyl benzoate (DHHB), ethylhexyl triazone (EHT), homomenthyl salicylate (HMS), and methylene bis-benzotriazolyl tetramethylbutylphenol (MBBT). However, both the studies present only nominal concentrations of the assays and give little information about other physiological responses or even physical effects. Furthermore, screening for metabolomic profiles in corals will not allow to conclude on any adverse effect within the organism.
Fel et al. (2019) is currently one of the very few studies that include both, longer exposure times and a follow-up chemical analysis of the compound of interest. They exposed fragments of Stylophora pistillata to various UV filters, namely terephthalylidene dicamphor sulfonic acid (TDSA), drometrizole trisiloxane (DTS), DMDBM, EHT and OCR (all organic) as well as the inorganic nanoparticle ZnO. Coral fragments were exposed to the test substance (dissolved in methanol) under semi-static conditions (5-day renewal period) at 25°C and a 12/12 h day-night cycle (150 μmol photons m–2 s–1). The tested corals were fed once a week with life Artemia salina nauplii. Exposure was carried out in 15 L full glass aquaria and stirred using a pump having a current speed of 4 mL min–1. Additional data regarding the water quality parameters were not provided. The test setup included a control, a solvent control (e.g., 67 μL L–1 methanol) and up to four treatment concentration ranging from 10 to 5000 μg L–1. Considering that several of the organic UV filters were rather poorly soluble in water, the stated maximum tested concentrations were far above known water solubility limits. For example, despite the use of a solvent (here methanol), the solubility limits for some organic UV absorbers were reached to an extent (e.g.,> 5000 μg L–1 for EHT), which may bear the risk of compound precipitation, leading to a physical effect rather than a substance intrinsic effect and could lead to confounding results. Furthermore, linking measured test concentrations to nominal values could implicate an environmental fate of the substance during the exposure phase which may be due to adsorption and precipitation rather than abiotic or biotic degradation processes. Nevertheless, in this study, it can be concluded that once exposed to all organic UV filters no adverse effects occur in corals up to the known solubility limit in water. For ZnO, however, a negative impact on the photosystem II of the algae (the functionality was reduced by 38% compared to the controls) was observed after exposure to 90 μg L–1 for 35 days.
Wijgerde et al. (2020) explored the additive effect of BP-3 and elevated water temperatures over 6 weeks. Corals (i.e., Stylophora pistillata and Acropora tenuis) were exposed to a nominal concentration of 1 μg L–1 of BP-3 (measured 0.05–0.06 μg L–1) or control water (both with 5 replicates each) in 20 flow-through 12 L aquaria for two weeks at 26°C. After that time, 10 tanks were subjected to a heat wave at 33°C, whereas the others remained at 26°C. Survival, growth rate, photosynthesis efficiency, zooxanthellae density (only for S. pistillata) were monitored and in addition, 16S rRNA gene sequencing was carried out. Salinity, alkalinity, calcium, nitrate and phosphate were measured weekly and remained mostly stable with salinity rising slightly throughout the experiment. In both species, elevated temperatures were the main cause for reduced survival, growth and zooxanthellae density. A small additive effect of both stressors (e.g., temperature and BP-3) is suggested but not explicit from the provided results on survival, and zooxanthellae health. Oxybenzone without heat stress only resulted in a slight reduction in photosynthetic efficiency (4–5% in the BP-3 groups cultivated at 26°C, compared to 12–33% in the heated tanks with BP-3). An additive effect of both stressors was found for the microbial community; however, the relevance of those shifts remains unclear. Over the testing period of 6 weeks the survival of controls in S. pistillata microcolonies was 100% and of A. tenuis microcolonies 67%, just below the 70% required as an acceptable control survival from standardized OECD test guidelines (e.g., OECD 211). This is the first laboratory study aiming for an additive effects on UV-filter and temperature, indicating although the UV filter has an intrinsic hazard potential, at the low (environmental) concentrations tested, temperature remains the driving force for mortality, growth reduction and zooxanthellae density (Wijgerde et al., 2020).
Jovanović and Guzmán (2014) tested the effect of TiO2 nanoparticle suspensions (nominal 0.1 and 10 mg L–1) on the Caribbean coral Orbicella faveolata (formerly Montastrea). Coral fragments (N = 54) were obtained from free living coral stocks located at different sites, transferred and distributed equally among three outside tanks (210 L each) and left for 3 days prior to the start of the experiment. Two tanks filled with reef water served as treatment groups and the third one as control. The tanks were equipped with a water pump to provide a constant water circulation. The experiment was run for 17 days under semi-static conditions (50% water renewal every 4 days) in these acclimatization tanks. Water temperature ranged between 25.2 and 28.9°C and dissolved oxygen was measured daily, although no data on oxygen levels were provided. Salinity (ca. 33.6 g L–1) was constant and the temperature difference among all three tanks was less than 0.4°C at any time of temperature measurement. Although no mortality was observed, symbiotic algae were released from the host at both TiO2 concentrations. In addition, the expression of heat shock proteins (potential stress indicators) was observed after 48 h of the nanoparticle exposure, however, this was also down-regulated afterwards. Whereas the latter response was regarded as a recovery of the corals from apparent stress (increase in particles), no recovery was observed for release of Symbiodiniaceae (Jovanović and Guzmán, 2014). However, as particle suspension was tested, it remains unclear whether the observed effect relates to intrinsic (chemical) properties, a nano-specific or a sole particle effect of the test material, since additional control groups such as inert quartz sand nanoparticle and larger, μm-scale TiO2 particles were missing.
Corinaldesi et al. (2018) tested nominal concentrations of 6.3 mg L–1 of uncoated ZnO nano-particles and two coated forms of TiO2 nanoparticles (Eusolex®- T2000 and OptisolTM) on Acropora spp. in a 48 h short-term experiment. The experiments were performed in triplicates per treatment in 2 L closed systems without steady water movement, and the water temperature was kept constant by a water bath at in situ conditions (28°C). The uncoated ZnO induced severe coral bleaching, whereas both forms of TiO2 did not cause any bleaching at the concentration tested. Controls with inert particles were missing, however, the difference between the 3 tested particular substances indicated a direct effect of the uncoated ZnO particles.
Tang et al. (2017) investigated the effect of ZnO nanoparticles on cell membranes in Seriatopora caliendrum in a 24 h short-term experiment. The experiment was conducted in aerated 1.8 L bottles with 7 replicated per treatment. Salinity, oxygen and pH was tested before, during and after the experiment and remained almost constant throughout the experiment. They found changes in lipid profiles through glycerophosphocholine (GPC) profiling and concluded a mechanical disturbance due to the presence of ZnO nanoparticles (Tang et al., 2017). However, like Jovanović and Guzmán (2014) and Corinaldesi et al. (2018) this study does not include an inert particle control and observed effects are therefore hard to evaluate. In addition, results rely on subcellular responses and does therefore not conclude on any possible adversity on the entire organism.
Chronic Toxicity Tests on Corals
Currently there are few tests with adult corals that could be considered as chronic test (35 days: Fel et al., 2019, 6 weeks: Wijgerde et al., 2020). There are no chronic toxicity tests with other coral live-stages publicly available. These types of tests are typically a follow-up (level 2) based on existing acute toxicity tests (level 1) of the same species (ECB, 2002; ECHA, 2017).
Discussion
Coral reefs, which are often termed the tropical rainforest of the oceans, harbor 25% of the marine biodiversity and provide ecosystem services to over 500 million people. In the last decades, coral reefs have been increasingly suffering from severe degradation due to the combined effect of anthropogenic perturbations and global change (Hughes et al., 2018). Their current global decrease provides reasons for serious concerns about their future existence. Thus, it is crucial to identify recent threats and focus on measures helping to protect extant reef areas. UV filters used in sunscreens are amongst those candidates that may harm corals and impact coral survival. Monitoring data confirmed that UV filters are already present in the marine aquatic environment, which is not a surprise considering the known exposure scenario into account (i.e., direct release into marine waters through recreational watersport activities). However, environmental concentrations are very difficult to evaluate as concentrations in water are highly variable due to daily and seasonal changes, environmental fate and adsorptive behavior of UV filters, current and tidal activities, etc., Furthermore, timely limited sampling, variation in processing of analytical water samples (filtered versus unfiltered) and the analytical methods used will lead to differences in measured concentrations. This certainly requires more frequent and continuous monitoring of relevant sites and a standardization of sampling techniques and analytical procedures.
Estimates of exposure levels based on production volumes may not be adequate, since local use and release for UV filters into the aquatic environment will differ strongly from site to site and in addition not all applied UV filters will end up in coastal ocean waters (Proiger et al., 2001; Poiger et al., 2004; Balmer et al., 2005). An application-based risk assessment, like those implemented for plant protection products or biocides registered within the EU regulatory framework, would provide a suitable alternative, although the framework for such a marine scenario is still needed to be defined.
Nevertheless, environmental concentrations (either predicted or measured) are mandatory to identify a potential risk of hazardous UV filters in the marine compartment. How hazardous a particular UV filter is will be concluded from ecotoxicity tests relevant for the respective environmental compartment (i.e., marine water or marine sediment), and thus it will be independent from the presence corresponding tests on corals. In other words, corals can be considered as a member of organisms, that contribute to the marine aquatic compartment along with results from other invertebrate, vertebrate and even plant organisms. In addition to other taxonomic groups, the toxicity of UV filters has recently been tested on corals. The toxicity data that are currently available for corals, however, do not allow for a scientifically sound assessment and conclusion. Variability in the test design, treatment of the test material and test organisms have even led to confounding results and are therefore not considered suitable for regulatory decision-making processes. To allow for a profound assessment of the intrinsic hazard profile of substances (including UV filters), a standardized and validated test design is required. This, however, requires additional efforts to develop test protocols built to cover various coral life stages (i.e., larvae, juveniles, and adults) on a short-term (acute tests) and long-term (chronic tests) exposure level. This encompasses a selection of specific test species, adequate culture and test conditions. Guidance can be provided by existing aquatic toxicity tests validated within nationally or even internationally acceptable frameworks such as DIN, OPPTS, ISO, or OECD. By applying such test protocols one can expect to generate results that are comparable independently from the laboratory, person, and time the test was carried out.
UV filters are substances which are very difficult to handle in aquatic test systems. In many cases low water solubility corresponds to a high adsorption potential, which makes a reliable chemical control analysis difficult, considering that the maximum solubility may be significantly different in saltwater, compared to freshwater or even to distilled water. Although available OECD guidance documents, i.e., OECD 23 (OECD, 2019b), provide adequate recommendations on how to treat such “difficult substances,” setting up test concentrations at the limit of maximum practical water solubility requires sufficient expertise and potentially time-intense resources. Therefore, one has to make sure, that any observed effect must be connected to the dissolved fraction of the test material only. This would identify the intrinsic hazard property of a substance rather than physical effects (OECD, 2019a; Pawlowski and Petersen-Thiery, 2020). In order to better understand, whether the bioaccumulation potential (BCF) of UV filters differs significantly from known BCFs using standardized fish bioaccumulation studies, it is also recommended to develop a standardized coral bioaccumulation test. This is of special importance since data derived from field measurements always bear a high uncertainty related to fluctuations in exposure concentrations, feeding conditions, actual stress, etc. (Pawlowski et al., 2019).
Combining both, a variable non-standardized test system with (1) an inadequate treatment of the test material or (2) the lack of adequate control groups will increase the uncertainty in the obtained results. These aspects are of special interest in tests with nano-sized test material aiming to identify a so-called “nano-effect” of the tested material. Corals are known to be sensitive to high particle loads which are not untypical even in the natural habitat due to sedimentation processes (Moeller et al., 2017). Taking this into account, besides a blank control, additional control groups (i.e., non-nano-sized test materials and nanoparticles composed of inert quartz sand) may be required to allow for discrimination into a substance-specific nano-effect.
Testing of biodegradable substances or even mixtures, as found in sunscreens, in static exposure systems without a regularly control of water quality parameters, may lead to results caused by secondary effects (i.e., drop in water quality, increased bacteria growth) that could not be linked to the toxicity of the test substance as such.
For some UV filters like BP-3, EHMC, OCR, and ZnO, results from standardized laboratory experiments have already demonstrated that they are acute and/or chronically toxic to aquatic organisms other than corals (ECHA, 2006a, b, c, d). Hence an apparent toxicity in other non-standard aquatic organisms such as corals can be expected at a similar compound concentration. Especially if algae belonging to the same taxonomic class as the coral symbionts (i.e., Symbiodiniaceae) turned out to be the most sensitive species in standard tests (OECD, 2011). On the other hand, other UV filters have been shown to be non-toxic to aquatic organisms. Therefore, one can conclude that, although functionality of UV filters in sunscreens is rather similar, structural features and the known ecotoxicological hazard profile of each filter differ significantly (Pawlowski et al., 2020). For this reason, care should be taken when UV filters are grouped together or considered as equally hazardous to the environment, because this will certainly lead to misinterpretation and false conclusions. This may result in the unwarranted exclusion and ban of certain UV filters from the sunscreen market, which will also negatively impact the options for high quality sunscreen formulation aiming to protect humans from sunburn and skin cancer (Sohn et al., 2020).
Taking all latter aspects into account, it can be concluded that most of the currently available toxicity data on corals linked to UV filters used in sunscreens, may be regarded as preliminary. Since corals are culture sensitive and non-standard laboratory test organisms, it is of special importance to develop standardized coral-based test systems to allow for a profound assessment of substances potentially affecting coral health.
Once testing protocols become available, additional validation and standardization processes including a ring-test trial would provide an excellent platform for the development of test systems that will comply with internationally acceptable standards ready to be used for decision making.
Author Contributions
MM, SP, MP-T, and PJS planned the manuscript. MM, SP, MP-T, IM, SN, and YH-S wrote the first draft of the manuscript. All authors read and contributed to the final manuscript.
Funding
MM, IM, and PJS acknowledge support by BASF SE grant “Toxicity tests with corals and coral reef communities”. The authors declare that this study received funding from BASF SE. The funder was not involved in the study design, collection, analysis, interpretation of data, the writing of this article or the decision to submit it for publication.
Conflict of Interest
MP-T and SP are employed by BASF SE.
The remaining authors declare that the research was conducted in the absence of any commercial or financial relationships that could be construed as a potential conflict of interest.
Supplementary Material
The Supplementary Material for this article can be found online at: https://www.frontiersin.org/articles/10.3389/fmars.2021.665548/full#supplementary-material
References
Ateweberhan, M., Mcclanahan, T. R., Graham, N. A. J., and Sheppard, C. R. C. (2011). Episodic heterogeneous decline and recovery of coral cover in the indian ocean. Coral Reefs 30, 739–752. doi: 10.1007/s00338-011-0775-x
Australian Institute of Marine Science (AIMS) (2020). Annual Summary Report on coral reef condition for 2019/2020. Initial recovery of the Great Barrier Reef threatened by the third mass bleaching event in five years. Townsvile: Australian Institute of Marine Science.
Ayre, D. J., and Hughes, T. P. (2000). Genotypic diversity and gene flow in brooding and spawning corals along the great barrier reef, australia. Evolution 54, 1590–1605. doi: 10.1111/j.0014-3820.2000.tb00704.x
Babcock, R. C., Bull, G. D., Harrison, P. L., Heyward, A. J., Oliver, J. K., Wallace, C. C., et al. (1986). Synchronous spawnings of 105 scleractinian coral species on the great barrier reef. Mar. Biol. 90, 379–394. doi: 10.1007/BF00428562
Babcock, R. C., Wills, B. L., and Simpson, C. J. (1994). Mass spawning of corals on a high latitude coral reef. Coral Reefs 13, 161–169. doi: 10.1007/BF00301193
Bachelot, M., Li, Z., Munaron, D., Le Gall, P., Casellas, C., Fenet, H., et al. (2012). Organic UV filter concentrations in marine mussels from french coastal regions. Sci. Total Environ. 420, 273–279. doi: 10.1016/j.scitotenv.2011.12.051
Baird, A. H., Guest, J. R., and Willis, B. L. (2009). Systematic and biogeographical patterns in the reproductive biology of scleractinian corals. Annu. Rev. Ecol. Evol. Syst. 40, 551–571. doi: 10.1146/annurev.ecolsys.110308.120220
Balmer, M. E., Buser, H. R., Müller, M. D., and Poiger, T. (2005). Occurrence of some organic UV filters in wastewater, in surface waters, and in fish from swiss lakes. Environ. Sci. Technol. 39, 953–962. doi: 10.1021/es040055r
Bargar, T. A., Alvarez, D. A., and Garrison, V. H. (2015). Synthetic ultraviolet light filtering chemical contamination of coastal waters of virgin Islands national park, St. John, U.S. virgin islands. Mar. Pollut. Bull. 101, 193–199. doi: 10.1016/j.marpolbul.2015.10.077
Bundesanstalt für Arbeitsschutz und Arbeitsmedizin (BAuA) (2020). Protection against UV radiation of the sun – Natural UV radiation of the sun poses an increased risk of cancer for employees who work outdoors. Available online at: https://www.baua.de/EN/Topics/Work-design/Physical-agents-and-work-environment/Optical-radiation/UV-radiation-of-the-sun.html. (accessed December 22, 2020).
Berkelmans, R., and van Oppen, M. J. H. (2006). The role of zooxanthellae in the thermal tolerance of corals: a “nugget of hope” for coral reefs in an era of climate change. Proc. Biol. Sci. 273, 2305–2312. doi: 10.1098/rspb.2006.3567
Bernerd, F., Vioux, C., and Asselineau, D. (2000). Evaluation of the protective effect of sunscreens on in vitro reconstructed human skin exposed to UVB or UVA irradiation. Photochem. Photobiol. 71:314. doi: 10.1562/0031-865520000710314EOTPEO2.0.CO2
Borneman, E. (2008). “Introduction to the husbandry of corals in aquariums: A review,” in Adv. coral Husb. public aquariums, eds R. J. Leewis and M. Janse (Arnhem, Netherlands: University of Houston), 3–14.
Bourne, D., Iida, Y., Uthicke, S., and Smith-Keune, C. (2008). Changes in coral-associated microbial communities during a bleaching event. ISME J. 2, 350–363. doi: 10.1038/ismej.2007.112
Brenner, M., and Hearing, V. J. (2008). The protective role of melanin against UV damage in human skin. Photochem. Photobiol. 84, 539–549. doi: 10.1111/j.1751-1097.2007.00226.x
Bruno, J. F., and Selig, E. R. (2007). Regional decline of coral cover in the indo-pacific: timing, extent, and subregional comparisons. PLoS One 2:e711. doi: 10.1371/journal.pone.0000711
Burke, L., Reytar, K., Spalding, M., and Perry, A. (2011). Reefs at risk revisited. Washington, DC: World Resources Institute.
Buser, H. R., Balmer, M. E., Schmid, P., and Kohler, M. (2006). Occurrence of UV filters 4-methylbenzylidene camphor and octocrylene in fish from various Swiss rivers with inputs from wastewater treatment plants. Environ. Sci. Technol. 40, 1427–1431. doi: 10.1021/es052088s
Centers for Disease Control and Prevention (CDC). (2020). Sun Safety Tips for Employers. In: Ski. Cancer. Available Online at: https://www.cdc.gov/cancer/skin/basic_info/sun-safety-tips-employers.htm. Available online at: https://www.cdc.gov/cancer/skin/basic_info/sun-safety-tips-employers.htm (accessed December 5, 2020).
Chen, C. A., Wang, J. T., Fang, L. S., and Yang, Y. W. (2005). Fluctuating algal symbiont communities in acropora palifera (scleractinia: acroporidae) from taiwan. Mar. Ecol. Prog. Ser. 295, 113–121. doi: 10.3354/meps295113
Chisvert, A., and Salvador, A. (2007). “UV Filters in Sunscreens and other Cosmetics. Regulatory Aspects and Analytical Methods,” in Analysis of Cosmetic Products, eds A. Salvador and A. Chisvert (Amsterdam: Elsevier), 83–120. doi: 10.1016/B978-044452260-3/50028-0
Coker, D. J., Wilson, S. K., and Pratchett, M. S. (2014). Importance of live coral habitat for reef fishes. Rev. Fish Biol. Fish. 24, 89–126. doi: 10.1007/s11160-013-9319-5
Corinaldesi, C., Marcellini, F., Nepote, E., Damiani, E., and Danovaro, R. (2018). Impact of inorganic UV filters contained in sunscreen products on tropical stony corals (acropora spp.). Sci. Total Environ. 637–638, 1279–1285. doi: 10.1016/j.scitotenv.2018.05.108
Craggs, J., Guest, J. R., Davis, M., Simmons, J., Dashti, E., and Sweet, M. (2017). Inducing broadcast coral spawning ex situ: closed system mesocosm design and husbandry protocol. Ecol. Evol. 7, 11066–11078. doi: 10.1002/ece3.3538
Crossland, C. J., Kremer, H. H., Lindeboom, H., Marshall Crossland, J. I., and Le Tissier, M. D. (2005). Coastal fluxes in the anthropocene: the land-ocean interactions in the Coastal Zone Project of the International Geosphere-Biosphere Programme. Berlin: Springer-Verlag.
Cunha, S. C., Trabalón, L., Jacobs, S., Castro, M., Fernandez-Tejedor, M., Granby, K., et al. (2018). UV-filters and musk fragrances in seafood commercialized in europe union: occurrence, risk and exposure assessment. Environ. Res. 161, 399–408. doi: 10.1016/j.envres.2017.11.015
Cunning, R., and Baker, A. C. (2013). Excess algal symbionts increase the susceptibility of reef corals to bleaching. Nat. Clim. Chang. 3, 259–262. doi: 10.1038/nclimate1711
D’Angelo, C., and Wiedenmann, J. (2014). Impacts of nutrient enrichment on coral reefs: new perspectives and implications for coastal management and reef survival. Curr. Opin. Environ. Sustain. 7, 82–93. doi: 10.1016/j.cosust.2013.11.029
Danovaro, R., Bongiorni, L., Corinaldesi, C., Giovannelli, D., Damiani, E., Astolfi, P., et al. (2008). Sunscreens cause coral bleaching by promoting viral infections. Environ. Health Perspect. 116, 441–447. doi: 10.1289/ehp.10966
Darling, E. S., Mcclanahan, T. R., Maina, J., and Gurney, G. G. (2019). Social–environmental drivers inform strategic management of coral reefs in the Anthropocene | Nature Ecology & Evolution. Available Online at: https://www.nature.com/articles/s41559-019-0953-8
De Gruijl, F. R. (1999). Skin cancer and solar UV radiation. Eur. J. Cancer 35, 2003–2009. doi: 10.1016/S0959-8049(99)00283-X
De’ath, G., Fabricius, K. E., Sweatman, H., and Puotinen, M. (2012). The 27-year decline of coral cover on the great barrier reef and its causes. Proc. Natl. Acad. Sci. 109, 17995–17999. doi: 10.1073/pnas.1208909109
Delbeek, J. C. (2008). “Collecting and shipping live coral: techniques, tips and headaches”, Public aquarium husbandry series. Advances in coral husbandry in public aquarium, eds R. J. Leewis and M. Janse (Arnhem: Public Aquarium Husbandry Series. Burgers’ Zoo), 363–373.
Dimond, J., and Carrington, E. (2008). Symbiosis regulation in a facultatively symbiotic temperate coral: zooxanthellae division and expulsion. Coral Reefs 27, 601–604. doi: 10.1007/s00338-008-0363-x
DiNardo, J. C., and Downs, C. A. (2018). Dermatological and environmental toxicological impact of the sunscreen ingredient oxybenzone/benzophenone-3. J. Cosmet. Dermatol. 17, 15–19. doi: 10.1111/jocd.12449
Donglikar, M. M., and Deore, S. L. (2016). Sunscreens: a review. Pharmacogn. J. 8, 171–179. doi: 10.5530/pj.2016.3.1
Downs, C. A., Kramarsky-Winter, E., Fauth, J. E., Segal, R., Bronstein, O., Jeger, R., et al. (2014). Toxicological effects of the sunscreen UV filter, benzophenone-2, on planulae and in vitro cells of the coral, stylophora pistillata. Ecotoxicology 23, 175–191. doi: 10.1007/s10646-013-1161-y
Downs, C. A., Kramarsky-Winter, E., Segal, R., Fauth, J., Knutson, S., Bronstein, O., et al. (2016). Toxicopathological effects of the sunscreen UV Filter, oxybenzone (benzophenone-3), on coral planulae and cultured primary cells and its environmental contamination in hawaii and the U.S. virgin islands. Arch. Environ. Contam. Toxicol. 70, 265–288. doi: 10.1007/s00244-015-0227-7
ECB. (2002). Technical Guidance Document on Risk Assessment. Ispra, Italy: European Chemicals Bureau.
ECB. (2003). Technical Guidance Document on Risk Assessment Part II. Ispra, Italy: European Chemicals Bureau, 337.
ECHA. (2006b). EHMC: 2-Ethylhexyl trans-4-methoxycinnamate - Registration Dossier. 15876. Helsinki: European Chemicals Agency.
ECHA. (2017). Guidance on Information Requirements and Chemical Safety Assessment Chapter R.7b: Endpoint specific guidance. Helsinki: European Chemicals Agency.
Edwards, C. B., Friedlander, A. M., Green, A. G., Hardt, M. J., Sala, E., Sweatman, H. P., et al. (2013). Global assessment of the status of coral reef herbivorous fishes: evidence for fishing effects. Proc. R. Soc. B Biol. Sci. 281, 7–11. doi: 10.1098/rspb.2013.1835
Egerton, T. A., and Tooley, I. R. (2012). UV absorption and scattering properties of inorganic-based sunscreens. Int. J. Cosmet. Sci. 34, 117–122. doi: 10.1111/j.1468-2494.2011.00689.x
Elmets, C. A., Cala, C. M., and Xu, H. (2014). Photoimmunology. Dermatol. Clin. 32, 277–290. doi: 10.1016/j.det.2014.03.005
Epstein, N., Bak, R. P. M., and Rinkevich, B. (2000). Toxicity of third generation dispersants and dispersed egyptian crude oil on red sea coral larvae. Mar. Pollut. Bull. 40, 497–503. doi: 10.1016/S0025-326X(99)00232-5
Erftemeijer, P. L. A., Riegl, B., Hoeksema, B. W., and Todd, P. A. (2012). Environmental impacts of dredging and other sediment disturbances on corals: a review. Mar. Pollut. Bull. 64, 1737–1765. doi: 10.1016/j.marpolbul.2012.05.008
Fabricius, K. E. (2005). Effects of terrestrial runoff on the ecology of corals and coral reefs: review and synthesis. Mar. Pollut. Bull. 50, 125–146. doi: 10.1016/j.marpolbul.2004.11.028
Fabricius, K. E., Logan, M., Weeks, S., and Brodie, J. (2014). The effects of river run-off on water clarity across the central great barrier reef. Mar. Pollut. Bull. 84, 191–200. doi: 10.1016/j.marpolbul.2014.05.012
FAO. (2004). Fertilizer requirements in 2015 and 2030 revisited. Rome, Italy: Food and Agriculture Organization.
Fel, J. P., Lacherez, C., Bensetra, A., Mezzache, S., Béraud, E., Léonard, M., et al. (2019). Photochemical response of the scleractinian coral Stylophora pistillata to some sunscreen ingredients. Coral Reefs 38, 109–122. doi: 10.1007/s00338-018-01759-4
Fox, H. E., Pet, J. S., Dahuri, R., and Caldwell, R. L. (2002). Coral reef restoration after blast fishing in indonesia. Proc. Ninth Int. Coral Reef Symp. Bali, Indones. 2, 969–975. doi: 10.1111/rec.12866
Gago-Ferrero, P., Díaz-Cruz, M. S., and Barceló, D. (2012). An overview of UV-absorbing compounds (organic UV filters) in aquatic biota. Anal. Bioanal. Chem. 404, 2597–2610. doi: 10.1007/s00216-012-6067-7
Gago-Ferrero, P., Díaz-Cruz, M. S., and Barceló, D. (2015). UV filters bioaccumulation in fish from iberian river basins. Sci. Total Environ. 51, 518–525. doi: 10.1016/j.scitotenv.2015.03.026
Gailani, J. Z., Lackey, T. C., King, D. B., Bryant, D., Kim, S. C., and Shafer, D. J. (2016). Predicting dredging-associated effects to coral reefs in apra harbor, guam - Part 1: sediment exposure modeling. J. Environ. Manage. 168, 16–26. doi: 10.1016/j.jenvman.2015.10.027
Gilbert, E., Pirot, F., Bertholle, V., Roussel, L., Falson, F., and Padois, K. (2013). Commonly used UV filter toxicity on biological functions: review of last decade studies. Int. J. Cosmet. Sci. 35, 208–219. doi: 10.1111/ics.12030
Giokas, D. L., Salvador, A., and Chisvert, A. (2007). UV filters: from sunscreens to human body and the environment. TrAC Trends Anal. Chem. 26, 360–374. doi: 10.1016/j.trac.2007.02.012
Gironi, B., Kahveci, Z., McGill, B., Lechner, B. D., Pagliara, S., Metz, J., et al. (2020). Effect of DMSO on the mechanical and structural properties of model and biological membranes. Biophys. J. 119, 274–286. doi: 10.1016/j.bpj.2020.05.037
Gomez, E., Bachelot, M., Boillot, C., Munaron, D., Chiron, S., Casellas, C., et al. (2012). Bioconcentration of two pharmaceuticals (benzodiazepines) and two personal care products (UV filters) in marine mussels (Mytilus galloprovincialis) under controlled laboratory conditions. Environ. Sci. Pollut. Res. 19, 2561–2569. doi: 10.1007/s11356-012-0964-3
Graham, E. M., Baird, A. H., Willis, B. L., and Connolly, S. R. (2013). Effects of delayed settlement on post-settlement growth and survival of scleractinian coral larvae. Oecologia 173, 431–438. doi: 10.1007/s00442-013-2635-6
Haapkylä, J., Unsworth, R. K. F., Flavell, M., Bourne, D. G., Schaffelke, B., and Willis, B. L. (2011). Seasonal rainfall and runoff promote coral disease on an inshore reef. PLoS One 6:0016893. doi: 10.1371/journal.pone.0016893
Harrison, P. L., and Wallace, C. C. (1990). “Reproduction, dispersal and recruitment of scleractinian corals,” in Ecosystems of the World, 25. Coral Reefs, ed. Z. Dubinsky (Amsterdam: Elsevier Science), 132–207.
Hawaii State Legislature. (2018). Hawaii State Legislature, The Senate Twenty-ninth legislature, 2018, State of Hawaii, S.B. NO. 2571, A Bill for an Act. Available Online at: https://www.capitol.hawaii.gov/session2018/bills/SB2571_.HTM
He, K., Hain, E., Timm, A., Tarnowski, M., and Blaney, L. (2019a). Occurrence of antibiotics, estrogenic hormones, and UV-filters in water, sediment, and oyster tissue from the chesapeake bay. Sci. Total Environ. 650, 3101–3109. doi: 10.1016/j.scitotenv.2018.10.021
He, T., Tsui, M. M. P., Tan, C. J., Ma, C. Y., Yiu, S. K. F., Wang, L. H., et al. (2019b). Toxicological effects of two organic ultraviolet filters and a related commercial sunscreen product in adult corals. Environ. Pollut. 245, 462–471. doi: 10.1016/j.envpol.2018.11.029
He, T., Tsui, M. M. P., Tan, C. J., Ng, K. Y., Guo, F. W., Wang, L. H., et al. (2019c). Comparative toxicities of four benzophenone ultraviolet filters to two life stages of two coral species. Sci. Total Environ. 651, 2391–2399. doi: 10.1016/j.scitotenv.2018.10.148
He, K., Timm, A., and Blaney, L. (2017). Simultaneous determination of UV-filters and estrogens in aquatic invertebrates by modified quick, easy, cheap, effective, rugged, and safe extraction and liquid chromatography tandem mass spectrometry. J. Chromatogr. A 1509, 91–101. doi: 10.1016/j.chroma.2017.06.039
Herzog, B., Quass, K., Schmidt, E., Müller, S., and Luther, H. (2004). Physical properties of organic particulate UV absorbers used in sunscreens: II. UV-attenuating efficiency as function of particle size. J. Colloid Interface Sci. 276, 354–363. doi: 10.1016/j.jcis.2004.04.009
Hoeksema, B. W., and Cairns, S. (2020). World List of Scleractinia. Available Online at http://www.marinespecies.org/scleractinia on 2020-08-22
HSE. (2020). Skin at work: Outdoor workers and sun exposure. Merseyside: Health and Savety Executive.
Hughes, T. P., Baird, A. H., Bellwood, D. R., Card, M., Connolly, S. R., Folke, C., et al. (2003). Climate change, human impacts, and the resilience of coral reefs. Science 301, 929–934. doi: 10.1126/science.1085046
Hughes, T. P., Barnes, M. L., Bellwood, D. R., Cinner, J. E., Cumming, G. S., Jackson, J. B. C., et al. (2017a). Coral reefs in the anthropocene. Nature 546, 82–90. doi: 10.1038/nature22901
Hughes, T. P., Kerry, J. T., Álvarez-Noriega, M., Álvarez-Romero, J. G., Anderson, K. D., Baird, A. H., et al. (2017b). Global warming and recurrent mass bleaching of corals. Nature 543, 373–377. doi: 10.1038/nature21707
Hughes, T. P., Bellwood, D. R., Folke, C. S., McCook, L. J., and Pandolfi, J. M. (2007). No-take areas, herbivory and coral reef resilience. Trends Ecol. Evol. 22, 1–3. doi: 10.1016/j.tree.2006.10.009
Hughes, T. P., Connolly, S. R., Eakin, C. M., Dietzel, A., McWilliam, M. J., Hoey, A. S., et al. (2018). Global warming transforms coral reef assemblages. Nature 556, 492–496. doi: 10.1038/s41586-018-0041-2
Jackson, J. B. C., Donovan, M. K., Cramer, K. L., and Lam, V. V. (2014). Status and Trends of Caribbean Coral Reefs: 1970-2012. Gland, Switzerland: Global Coral Reef Monitoring Network.
Jones, R. J., and Yellowlees, D. (1997). Regulation and control of intracellular algae (= zooxanthellae) in hard corals. Philos. Trans. R. Soc. B Biol. Sci. 352, 457–468. doi: 10.1098/rstb.1997.0033
Jovanović, B., and Guzmán, H. M. (2014). Effects of titanium dioxide (TiO2) nanoparticles on caribbean reef-building coral (montastraea faveolata). Environ. Toxicol. Chem. 33, 1346–1353. doi: 10.1002/etc.2560
Kerr, A. M., Baird, A. H., and Hughes, T. P. (2011). Correlated evolution of sex and reproductive mode in corals (anthozoa: scleractinia). Proc. Biol. Sci. 278, 75–81. doi: 10.1098/rspb.2010.1196
Kushmaro, A., Rosenberg, E., Fine, M., and Loya, Y. (1997). Bleaching of the coral oculina patagonica by vibrio AK- 1. Mar. Ecol. Prog. Ser. 147, 159–165. doi: 10.3354/meps171131
Labille, J., Slomberg, D., Catalano, R., Robert, S., Apers-Tremelo, M. L., Boudenne, J. L., et al. (2020). Assessing UV filter inputs into beach waters during recreational activity: a field study of three french mediterranean beaches from consumer survey to water analysis. Sci. Total Environ. 706:136010. doi: 10.1016/j.scitotenv.2019.136010
Levine, A. (2020). Sunscreen use and awareness of chemical toxicity among beach goers in hawaii prior to a ban on the sale of sunscreens containing ingredients found to be toxic to coral reef ecosystems. Mar. Policy 117:103875. doi: 10.1016/j.marpol.2020.103875
Loh, T.-L., McMurray, S. E., Henkel, T. P., Vicente, J., and Pawlik, J. R. (2015). Indirect effects of overfishing on caribbean reefs: sponges overgrow reef-building corals. PeerJ 3:e901. doi: 10.7717/peerj.901
Mak, K. K. W., Yanase, H., and Renneberg, R. (2005). Cyanide fishing and cyanide detection in coral reef fish using chemical tests and biosensors. Biosens. Bioelec. 20, 2581–2593. doi: 10.1016/j.bios.2004.09.015
McCoshum, S. M., Schlarb, A. M., and Baum, K. A. (2016). Direct and indirect effects of sunscreen exposure for reef biota. Hydrobiologia 776, 139–146. doi: 10.1007/s10750-016-2746-2
Mitchelmore, C. L., Burns, E. E., Conway, A., Heyes, A., and Davies, I. A. (2021). A critical review of organic ultraviolet filter exposure, hazard, and risk to corals. Environ. Toxicol. Chem. 2021, 1–22. doi: 10.1002/etc.4948
Mitchelmore, C. L., He, K., Gonsior, M., Hain, E., Heyes, A., Clark, C., et al. (2019). Occurrence and distribution of UV-filters and other anthropogenic contaminants in coastal surface water, sediment, and coral tissue from Hawaii. Sci. Total Environ. 670, 398–410. doi: 10.1016/j.scitotenv.2019.03.034
Moeller, M., Nietzer, S., Schils, T., and Schupp, P. J. (2017). Low sediment loads affect survival of coral recruits: the first weeks are crucial. Coral Reefs 36, 39–49. doi: 10.1007/s00338-016-1513-1
Moore, C., Cevikbas, F., Pasolli, H. A., Chen, Y., Kong, W., Kempkes, C., et al. (2013). Erratum: UVB radiation generates sunburn pain and affects skin by activating epidermal TRPV4 ion channels and triggering endothelin-1 signaling. Proc. Natl. Acad. Sci. U. S. A. 110:15502. doi: 10.1073/pnas.1315603110
Mumby, P. J., and Harborne, A. R. (2010). Marine reserves enhance the recovery of corals on caribbean reefs. PLoS One 5:0008657. doi: 10.1371/journal.pone.0008657
Narla, S., and Lim, H. W. (2020). Sunscreen: FDA regulation, and environmental and health impact. Photochem. Photobiol. Sci. 19, 66–70. doi: 10.1039/c9pp00366e
Negri, A. P., Smith, L. D., Webster, N. S., and Heyward, A. J. (2002). Understanding ship-grounding impacts on a coral reef: potential effects of anti-foulant paint contamination on coral recruitment. Mar. Pollut. Bull. 44, 111–117. doi: 10.1016/S0025-326X(01)00128-X
Nelson, D. S., McManus, J., Richmond, R. H., King, D. B., Gailani, J. Z., Lackey, T. C., et al. (2016). Predicting dredging-associated effects to coral reefs in apra harbor, guam - part 2: potential coral effects. J. Environ. Manage. 168, 111–122. doi: 10.1016/j.jenvman.2015.10.025
Nielsen, D. A., Petrou, K., and Gates, R. D. (2018). Coral bleaching from a single cell perspective. ISME J. 12, 1558–1567. doi: 10.1038/s41396-018-0080-6
OECD. (2011). Test No. 201: Freshwater Alga and Cyanobacteria, Growth Inhibition Test, OECD Guidelines for the Testing of Chemicals, Section 2. Paris: OECD Publishing.
OECD. (2012). Test No. 316: Phototransformation of Chemicals in Water – Direct Photolysis, Section 3. Paris: OECD Publishing, 1–72.
OECD. (2019a). Guidance document on aquatic toxicity testing of difficult substances and mixtures. series on testing and assessment No. 23 (2nd edition). Organ. Econ. Co-operation Dev. 23, 1–81. doi: 10.1787/0ed2f88e-en
OECD. (2019b). Test No. 203: Fish, Acute Toxicity Test, OECD Guidelines for the Testing of Chemicals, Section 2. Paris: OECD Publishing.
Osinga, R., Janssen, M., and Janse, M. (2008). The role of light in coral physiology and its implications of coral husbandry. Adv. coral Husb. Public Aquariums 2, 173–183.
Osterwalder, U., Sohn, M., and Herzog, B. (2014). Global state of sunscreens. Photodermatol. Photoimmunol. Photomed. 30, 62–80. doi: 10.1111/phpp.12112
PALAU. (2018). Responsible Tourism Education Act 2018. Available Online at: https://www.palaugov.pw/wp-content/uploads/2018/08/Proposed-Legislation-re.-Responsible-Tourism-Education-Act-of-2018.pdf
Pastorok, R., and Bilyard, G. (1985). Effects of sewage pollution on coral-reef communities. Mar. Ecol. Prog. Ser. 21, 175–189. doi: 10.3354/meps021175
Pawlowski, S., Herzog, B., Sohn, M., Petersen−Thiery, M., and Acker, S. (2020). Eco sun pass: a tool to evaluate the ecofriendliness of UV filters used in sunscreen products. Int. J. Cosmet. Sci. 2020, 1–10. doi: 10.1111/ics.12681
Pawlowski, S., Lanzinger, A. C., Dolich, T., Füßl, S., Salinas, E. R., Zok, S., et al. (2019). Evaluation of the bioaccumulation of octocrylene after dietary and aqueous exposure. Sci. Total Environ. 672, 669–679. doi: 10.1016/j.scitotenv.2019.03.237
Pawlowski, S., and Petersen-Thiery, M. (2020). Sustainable sunscreens: a challenge between performance, animal testing ban, and human and environmental safety. Handb. Environ. Chem. 94, 185–207. doi: 10.1007/698_2019_444
Peng, X., Fan, Y., Jin, J., Xiong, S., Liu, J., and Tang, C. (2017). Bioaccumulation and biomagnification of ultraviolet absorbents in marine wildlife of the pearl river estuarine, south china sea. Environ. Pollut. 225, 55–65. doi: 10.1016/j.envpol.2017.03.035
Petersen, D., Laterveer, M., Van Bergen, D., and Kuenen, M. (2004). Transportation techniques for massive scleractinian corals. Zoo Biol. 23, 165–176. doi: 10.1002/zoo.10127
Pintado-Herrera, M. G., and Lara Martín, P. A. (2020). “Fate and Behavior of UV Filters in the Marine Environment,” in Handbook of Environmental Chemistry. Berlin Heidelberg: Springer, 1–12.
Poiger, T., Buser, H. R., Balmer, M. E., Bergqvist, P. A., and Müller, M. D. (2004). Occurrence of UV filter compounds from sunscreens in surface waters: regional mass balance in two swiss lakes. Chemosphere 55, 951–963. doi: 10.1016/j.chemosphere.2004.01.012
Pollock, F. J., Lamb, J. B., Field, S. N., Heron, S. F., Schaffelke, B., Shedrawi, G., et al. (2014). Sediment and turbidity associated with offshore dredging increase coral disease prevalence on nearby reefs. PLoS One 9:0102498. doi: 10.1371/journal.pone.0102498
Proiger, T., Buser, H., and Müller, M. D. (2001). Verbrauch, Vorkommen in Oberflächengewässern und Verhalten in der Umwelt von Substanzen, die als UV Filter in Sonnenschutzmitteln eingesetzt werden. Abschlussarbeit. Wädenswil: Federal Research Institution.
Raymundo, L. J., Halford, A. R., Maypa, A. P., and Kerr, A. M. (2009). Functionally diverse reef-fish communities ameliorate coral disease. Proc. Natl. Acad. Sci. 106:nas–0900365106. doi: 10.1073/pnas.0913116107
Richmond, R. (1997). Reproduction and recruitment in corals: Critical links in the persistence of Reefs. Life and Death of Coral Reefs. London, UK: CHAPMAN & HALL.
Richmond, R. H., Tisthammer, K. H., and Spies, N. P. (2018). The effects of anthropogenic stressors on reproduction and recruitment of corals and reef organisms. Front. Mar. Sci. 5:226. doi: 10.3389/fmars.2018.00226
Sánchez Rodríguez, A., Rodrigo Sanz, M., and Betancort Rodríguez, J. R. (2015). Occurrence of eight UV filters in beaches of gran canaria (canary islands). an approach to environmental risk assessment. Chemosphere 131, 85–90. doi: 10.1016/j.chemosphere.2015.02.054
Schmid, D., Cornelia, S., and Fred, Z. (2004). UV-A sunscreen from red algae for protection against premature skin aging. In: Cosmetics and Toiletries Manufacture Worldwide. Buchs: Mibelle Group, 139–143.
Schneider, S. L., and Lim, H. W. (2019). A review of inorganic UV filters zinc oxide and titanium dioxide. Photodermatol. Photoimmunol. Photomed. 35, 442–446. doi: 10.1111/phpp.12439
Schreiber, U. (2016). Pulse-amplitude-modulation (PAM) fluorometry and saturation pulse method: an overview. Chlorophyll a Fluoresc. a Signat. Photosynth. 1367, 137–147. doi: 10.1007/978-1-4939-3130-9_11
Sebens, K. P. (1994). Biodiversity of coral reefs: what are we losing and why? Integr. Comp. Biol. 34, 115–133. doi: 10.1093/icb/34.1.115
Shafir, S., Van Rijn, J., and Rinkevich, B. (2007). Short and long term toxicity of crude oil and oil dispersants to two representative coral species. Environ. Sci. Technol. 41, 5571–5574. doi: 10.1021/es0704582
Sharifan, H. (2020). Alarming the impacts of the organic and inorganic UV blockers on endangered coral’s species in the persian gulf: a scientific concern for coral protection. Sustain. Futur. 2:100017. doi: 10.1016/j.sftr.2020.100017
Siebeck, U. E., Marshall, N. J., Klüter, A., and Hoegh-Guldberg, O. (2006). Monitoring coral bleaching using a colour reference card. Coral Reefs 25, 453–460. doi: 10.1007/s00338-006-0123-8
Sohn, M., Krus, S., Schnyder, M., Acker, S., Petersen, M., Pawlowski, S., et al. (2020). How to overcome the new challenges in sun care. Sofw 146, 2–10.
Spalding, M., Ravilious, C., and Green, E. (2001). World Atlas of Coral Reefs. California: University of California Press.
Spalding, M. D., and Grenfell, A. M. (1997). New estimates of global and regional coral reef areas. Coral Reefs 16, 225–230. doi: 10.1007/s003380050078
Stien, D., Clergeaud, F., Rodrigues, A. M. S., Lebaron, K., Pillot, R., Romans, P., et al. (2019). Metabolomics reveal that octocrylene accumulates in pocillopora damicornis tissues as fatty acid conjugates and triggers coral cell mitochondrial dysfunction. Anal. Chem. 91, 990–995. doi: 10.1021/acs.analchem.8b04187
Stien, D., Suzuki, M., Rodrigues, A. M. S., Yvin, M., Clergeaud, F., Thorel, E., et al. (2020). A unique approach to monitor stress in coral exposed to emerging pollutants. Sci. Rep. 10, 1–12. doi: 10.1038/s41598-020-66117-3
Sutton, P., and VandenBelt, M. (1997). The value of the world’s ecosystem services and natural capital. Nature 387, 253–260. doi: 10.1038/387253a0
Tang, C. H., Lin, C. Y., Lee, S. H., and Wang, W. H. (2017). Membrane lipid profiles of coral responded to zinc oxide nanoparticle-induced perturbations on the cellular membrane. Aquat. Toxicol. 187, 72–81. doi: 10.1016/j.aquatox.2017.03.021
Tashiro, Y., and Kameda, Y. (2013). Concentration of organic sun-blocking agents in seawater of beaches and coral reefs of okinawa island. Japan. Mar. Pollut. Bull. 77, 333–340. doi: 10.1016/j.marpolbul.2013.09.013
Titlyanov, E. A., Titlyanova, T. V., Leletkin, V. A., Tsukahara, J., Van Woesik, R., and Yamazato, K. (1996). Degradation of zooxanthellae and regulation of their density in hermatypic corals. Mar. Ecol. Prog. Ser. 139, 167–178. doi: 10.3354/meps139167
Tovar-Sánchez, A., Sánchez-Quiles, D., Basterretxea, G., Benedé, J. L., Chisvert, A., Salvador, A., et al. (2013). Sunscreen products as emerging pollutants to coastal waters. PLoS One 8:0065451. doi: 10.1371/journal.pone.0065451
Tsui, M. M. P., Lam, J. C. W., Ng, T. Y., Ang, P. O., Murphy, M. B., and Lam, P. K. S. (2017). Occurrence, distribution, and fate of organic UV filters in coral communities. Environ. Sci. Technol. 51, 4182–4190. doi: 10.1021/acs.est.6b05211
Tsui, M. M. P., Leung, H. W., Lam, P. K. S., and Murphy, M. B. (2014a). Seasonal occurrence, removal efficiencies and preliminary risk assessment of multiple classes of organic UV filters in wastewater treatment plants. Water Res. 53, 58–67. doi: 10.1016/j.watres.2014.01.014
Tsui, M. M. P., Leung, H. W., Wai, T. C., Yamashita, N., Taniyasu, S., Liu, W., et al. (2014b). Occurrence, distribution and ecological risk assessment of multiple classes of UV filters in surface waters from different countries. Water Res. 67, 55–65. doi: 10.1016/j.watres.2014.09.013
UNEP/GPA. (2006). The State of the Marine Environment: Trends and Processes. Nairobi, Kenya: UNEP/Earthprint.
Wang, S. Q., Setlow, R., Berwick, M., Polsky, D., Marghoob, A. A., Kopf, A. W., et al. (2001). Ultraviolet a and melanoma: a review. J. Am. Acad. Dermatol. 44, 837–846. doi: 10.1067/mjd.2001.114594
Wijgerde, T., van Ballegooijen, M., Nijland, R., van der Loos, L., Kwadijk, C., Osinga, R., et al. (2020). Adding insult to injury: effects of chronic oxybenzone exposure and elevated temperature on two reef-building corals. Sci. Total Environ. 733:139030. doi: 10.1016/j.scitotenv.2020.139030
Keywords: UV filters, corals, toxicology, sunscreen, standardization
Citation: Moeller M, Pawlowski S, Petersen-Thiery M, Miller IB, Nietzer S, Heisel-Sure Y, Kellermann MY and Schupp PJ (2021) Challenges in Current Coral Reef Protection – Possible Impacts of UV Filters Used in Sunscreens, a Critical Review. Front. Mar. Sci. 8:665548. doi: 10.3389/fmars.2021.665548
Received: 08 February 2021; Accepted: 22 March 2021;
Published: 14 April 2021.
Edited by:
Noga Stambler, Bar-Ilan University, IsraelReviewed by:
Esti Kramarsky-Winter, Ben-Gurion University of the Negev, IsraelTinka Murk, Wageningen University & Research, Netherlands
Copyright © 2021 Moeller, Pawlowski, Petersen-Thiery, Miller, Nietzer, Heisel-Sure, Kellermann and Schupp. This is an open-access article distributed under the terms of the Creative Commons Attribution License (CC BY). The use, distribution or reproduction in other forums is permitted, provided the original author(s) and the copyright owner(s) are credited and that the original publication in this journal is cited, in accordance with accepted academic practice. No use, distribution or reproduction is permitted which does not comply with these terms.
*Correspondence: Mareen Moeller, bWFyZWVuLm1vZWxsZXJAdW5pLW9sZGVuYnVyZy5kZQ==; bWFyZWVuLm1vZWxsZXJAdW9sLmRl; Peter J. Schupp, cGV0ZXIuc2NodXBwQHVuaS1vbGRlbmJ1cmcuZGU=