- 1Marine and Environmental Sciences Centre (MARE), Polytechnic Institute of Leiria, Peniche, Portugal
- 2Oceanic Observatory of Madeira, Regional Agency for the Development of Research, Technology and Innovation, Funchal, Portugal
- 3Interdisciplinary Centre of Marine and Environmental Research (CIIMAR), Matosinhos, Portugal
- 4Mariculture Centre of Calheta, Directorate of the Sea, Calheta, Portugal
- 5Centre of Marine Sciences (CCMAR), University of Algarve, Faro, Portugal
- 6LB3, Faculty of Science and Engineering, University of Madeira, Funchal, Portugal
- 7Instituto das Florestas e Conservação da Natureza – IP RAM, Funchal, Portugal
Sea urchins play an important role in island coastal ecosystems as keystone species and as potential resources for harvesting and aquaculture. In Southern Europe, the most valuable and harvested species is the European purple sea urchin Paracentrotus lividus, but there is a growing interest to explore other edible species like the blunt sea urchin Sphaerechinus granularis. The echinoderms biodiversity of Madeira archipelago was previously described, but their local habitat, population densities and size distribution, important to establish their harvest and aquaculture potential, are poorly known. The present study aimed to evaluate S. granularis population in the oligotrophic and narrow insular shelf of Madeira archipelago, mainly regarding its habitat, densities, reproduction season and roe marketable characteristics. Sphaerechinus granularis was found in low densities (0.01 ind./m2) from shallow to deep reefs (5–30 m). The highest densities occurred in shallow waters and breakwaters in association to encrusting algae, turf and erect brown macroalgae. Here, the low primary productivity ecosystem influenced the long reproductive season of the local population. This population showed a relatively high gonadosomatic index (maximum GI of 8), high gonad weight (5.00–12.19 g) with high proportion of bright yellow and orange gonads (37.5–60%) between February and November. Additionally, the high level of total carotenoids, the high PUFA (particularly EPA) content, and the ratio n-6/n-3 close to one support the high nutritional value of S. granularis roe. The trend of incremental GI between September and November and the increasing content of PUFA in autumn suggests this could be the preferential harvesting season. This information is key to evaluate a sustainable exploitation of this living marine resource.
Introduction
Sea urchins are major components of marine communities including 900 known species (Pearse, 2006). They are keystone species of the shallow coastal ecosystems (Steneck, 2013), playing a pivotal role in the community structure of subtidal bottoms, including seagrass meadows and rocky reefs in the islands of the Macaronesia biogeographic region (Hernández et al., 2008; Sangil et al., 2018). By grazing upon the seafloor, sea urchins are strong interactors and drivers of community structure controlling algal biomass (Pearse, 2006; Steneck, 2013).
Approximately 40 edible species are exploited worldwide for their gonads (or roe), a prized delicacy appreciated in many countries like Japan, Australia, New Zealand, United States, France, Italy and Spain (Hammer et al., 2013; Sun and Chiang, 2015). Globally, it is estimated that approximately 70,000 tons of sea urchins are harvested each year (Andrew et al., 2002; Sun and Chiang, 2015; Stefansson et al., 2017), and several species are over-exploited in many regions of the world (Andrew et al., 2002; González-Irusta et al., 2010). In the Northeast Atlantic coast, the most harvested and the most profitable species is the European purple sea urchin Paracentrotus lividus (Lamarck 1816), but other species such as Strongylocentrotus droebachiensis (Müller 1776), Psammechinus miliaris (Müller 1776) and Sphaerechinus granularis (Lamarck 1816) are also consumed (Andrew et al., 2002; Monfort, 2002; Martínez-Pita et al., 2008). This fishery is conducted both by professional and recreational fishermen collecting the sea urchins by hand with or without SCUBA diving gear. Due to the high level of illegal captures it is very difficult to quantify sea urchins’ captures in Europe (Pais et al., 2007, 2012; Bertocci et al., 2014). Nevertheless, the available data show that only in Spain approximately 700 tons are captured each year, with a market value of about one and a half million euros (González-Irusta et al., 2010; Fernández-Boán et al., 2012).
The blunt sea urchin S. granularis is a regular sea urchin characterized by a test covered by massive and short white or purple spikes with occasional white tips displayed regularly through the spikes (Guillou and Lumingas, 1998). The species is commonly found in the Mediterranean (Vafidis et al., 2020) and in the northeast Atlantic between the English Channel and the Gulf of Guinea (Guillou and Michel, 1993), including Madeira and other archipelagos of the Macaronesia biogeographic region (Alves et al., 2001; Riera et al., 2012; Madeira et al., 2019). Sphaerechinus granularis populations are characterized by low densities, although it can occur locally in high densities (e.g., in southern Brittany – Guillou and Michel, 1993, western Mediterranean – Sartoretto and Francour, 1997 and in the Bay of Algiers (Algeria) – Soualili et al., 1999). The species can be found from the intertidal zone to depths up to 130 m (Guillou and Michel, 1993), in both calm and exposed areas (Martínez-Pita et al., 2008) and is frequently associated with rhodoliths beds (aggregations of free-living coralline algae that cover extensive benthic areas of subtidal environments worldwide) (Guillou et al., 2002), although it may occur in sandy bottoms (Gaspar et al., 2001).
Sphaerechinus granularis is a grazer consuming mostly encrusting coralline algae (Sartoretto and Francour, 1997). However, in meadows of Posidonia oceanica, it consumes dead leaves (and their algal epiphytes), rhizomes and roots when they are accessible (Verlaque, 1981).
Studies on S. granularis growth conducted in the Bay of Brest (France) showed that this species has a fast growth rate during the first years of life (Lumingas and Guillou, 1994; Jordana et al., 1997). The longevity estimations conducted by Guillou and Michel (1993) showed that the S. granularis population of Brittany can attain 5 years old, with higher growth rates and shorter longevity when compared with P. lividus. Sphaerechinus granularis urchins eight to 10 months old can attain 15 mm of test diameter (TD) and 4 years old urchins have a mean TD of 100 mm (Guillou and Michel, 1993), whereas P. lividus only attains 50 mm TD in Northeast Atlantic coast and 45–65 mm in culture (Grosjean et al., 1998; Boudoresque and Verlaque, 2020).
Several studies showed that, concomitantly with other echinoids, the reproductive cycle of S. granularis follows a latitudinal gradient. The population inhabiting the English Channel presents a major spawning event in the early spring (Guillou and Michel, 1993), whereas in southern of Spain (western Mediterranean), the local population showed a single spawning event in summer months (Martínez-Pita et al., 2008). The recent study by Vafidis et al. (2020) corroborates the single annual reproductive season, with the S. granularis population of Aegean Sea (eastern Mediterranean) spawning during spring.
The unsustainable harvesting pressure on P. lividus in several areas of the Northeast Atlantic and Mediterranean (Farina et al., 2020), associated with its slow growth and low gonad yield have been driving the research for alternative species to supply the market demand on sea urchin roe and aquaculture production. Sphaerechinus granularis has been identified as an emergent species in Brittany (Guillou and Michel, 1993) and Mediterranean regions (Soualili et al., 1999; Martínez-Pita et al., 2010). However, the lack of knowledge on the biology (e.g., life cycle) and ecology of S. granularis has been referred to several regions (Guillou and Michel, 1993) and in Madeira archipelago that is no exception. There are no official records about the species harvesting, but anecdotal evidence indicates it is captured as an alternative to P. lividus.
The aim of the present work was to characterize the habitat, population density, size structure and reproductive biology of S. granularis under the oligotrophic, relatively stable seawater temperature, and narrow insular shelf ecosystem of Madeira archipelago. Additionally, it was conducted a first assessment of gonads’ nutritional quality and roe color characteristics along the year. This information will be useful to identify a potential harvesting season and to a for a future analysis of environmental and economic sustainability of this low-mobility resource in the archipelago.
Materials and Methods
Study Area
The Madeira archipelago is located in the eastern North Atlantic Ocean about 700 km west of Casablanca (Morocco) and comprises the islands of Madeira (742 km2), Porto Santo (43 km2), Desertas (14 km2) and Selvagens (3 km2) (Figure 1). The archipelago is surrounded by oligotrophic water masses (Campuzano et al., 2010), and stable seawater temperature year-round (average ranges from 18 to 24°C) (Caldeira et al., 2002). The major coastal marine environments are rocky shores characterized by pebble beaches, basalt platforms and areas armored by artificial breakwaters (Ribeiro, 2008). Madeira’s intertidal and subtidal domain comprises a rocky substrate (boulders, vertical walls, platforms, and outcroppings) down to depths of 15–20 m and sandy bottoms, which form a major part of this domain. The soft bottoms are formed by sediments ranging from coarse gravel to medium silt according to the classification of Wentworth (1922) (Instituto Hidrográfico, 2003, 2007, 2008).
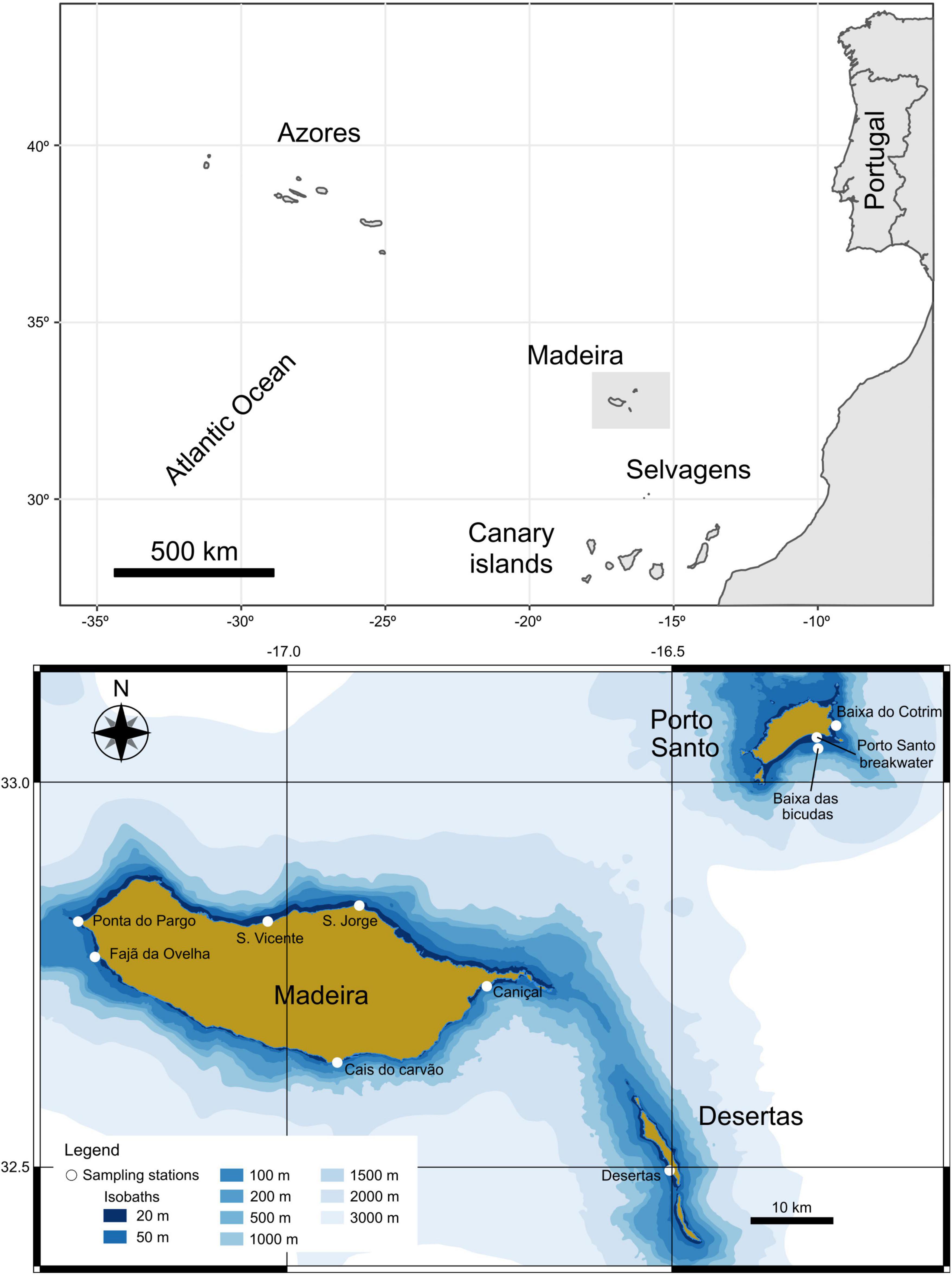
Figure 1. Regional context of study area and sampling locations evaluating Sphaerechinus granularis habitat, density and reproductive cycle in Madeira archipelago.
To evaluate where S. granularis occur and estimate its abundance and density within the Madeira archipelago, several locations from Madeira, Desertas and Porto Santo islands were surveyed with SCUBA diving (Figure 1). These locations comprised the typical rocky reefs made of boulders (medium to big boulders), rock platforms, outcrops and rhodoliths beds. Only two locations at the South coast of Madeira (Cais do Carvão and Caniçal 1) were monitored seasonally (winter, spring, summer, and autumn) due to their easy access and protection from the prevailing weather conditions. Four locations—Caniçal 2, breakwater of port of Porto Santo Island (hereafter Porto Santo breakwater), Baixa das Bicudas and Baixa do Cotrim—were surveyed in two seasons (summer and autumn), whereas the remaining were only surveyed in summer, due to logistical limitations. For each location where S. granularis was detected, the diversity indices were calculated using Hill numbers according to the framework described by Jost (2006).
Density Data Collection
During the year 2017, surveys to estimate the density of S. granularis across the entire study area were conducted using underwater visual census (UVC’s) along strip transects of 25 m × 2 m, inside which all sea urchin specimens, and macrofaunal organisms that could be discerned as individuals (starfish, crabs, etc.) were counted. Moreover, to characterize the general habitat of the species in terms of sessile cover (macroalgae and macrofauna) 100 photographs were taken at each location, which were later analyzed to describe the habitat. All conspicuous sessile species observed were registered from imagery analysis and an abundance category approach used, with each species being classified according to DAFOR scale (D = Dominant: 51–100%; A = Abundant: 31–50%; F = Frequent: 16–30%; O = Occasional: 6–15%; R = Rare < 5%) (Tansley, 1993).
Biological Data Collection
Samples of sea urchins (n = 10) were collected monthly by SCUBA divers from subtidal rocky reef bottoms of Cais do Carvão (Figure 1). This sampling effort was not possible to be replicated in other locations during the study period, mostly due to logistical and team constraints. Therefore, additional samples (usually n = 10) were collected in other two locations, namely at Caniçal in every season and at Porto Santo in two seasons (summer and autumn). The specimens collected were transported in a cooler filled with seawater to the Funchal Marine Biology Station, where they were weighed to the nearest 0.01 g (body weight—BW) and the test diameter (TD) measured to the nearest 0.05 mm with a caliper. The individuals were then dissected by a circular incision around the peristomal membrane, and the gonads removed and weighed to the nearest 0.1 mg (gonad total weight—GTW). The gonad color was evaluated according to a semi-quantitative scale of four grades (Pearce et al., 2002): 1- bright yellow or orange; 2- yellow pale or orange (mustard); 3- brownish yellow, brownish orange, brownish red; 4- any other color. One gonad was fixed in 4% formalin and stored in ethanol 70% for histologic analysis of the maturation status and the remaining four were stored at −20°C to further biochemical analysis.
Histologic Analysis
The gonads of the urchins collected in Cais do Carvão, Caniçal and Porto Santo breakwater were dehydrated in baths of increasing ethanol concentration and xylol, embedded in paraffin and sectioned at 5 μm for histologic analysis. The sections were stained with haematoxylin periodic acid Schiff reagent and analyzed under a light microscope (Leica® DM 2000 LED) equipped with a Leica® MC170 5MP HD Camera and the LAS V4.4.0 software at magnification 50 × and 100 ×. Gonad microscopic maturity stages were assessed using the four stages scale defined by Walker et al. (2013) (Maturity scale description and Figure 1 in Supplementary Material).
Biological Indices
The sex ratio was determined by Equation 1, where Nmales and Nfemales are the number of S. granularis males and females collected, respectively:
The gonad index (GI) was determined individually as described in Equation 2. The GI monthly averages were determined by sex and by sampling site.
The maturity index (MI) was determined monthly as the mean of maturity stages frequencies through Equation 3, where nstg is the number of sea urchins assigned to each maturity stage at each month, stg is the numerical value assigned to each maturity stage (1—stage I; 2—stage II; 3—stage III and 4—stage IV), Nmonth is the number of sea urchins sampled monthly.
Chemical Analysis
The gonad samples were freeze-dried, pooled by sex and by season, ground, homogenized and stored at −20°C for further analyses. Prior to chemical analysis, residual moisture was determined using a Gibertini-Eurotherm dry weight balance, and the results were expressed in percentage of dry mater (% DM). All chemical analyses were conducted at least in duplicates.
Crude protein was assessed by the total nitrogen content obtained with an elemental analyzer TruSpec 630-200-200. The total nitrogen content was converted to total protein equivalents using the following relationship: percentage crude protein = percentage nitrogen × 6.25 (AOAC, 1990).
Total lipid content was determined gravimetrically. To 100 mg of freeze-dried sample was added 3 mL of a chloroform/methanol (1:2, v/v) mixture, containing 0.01% (w/v) BHT (butylated hydroxytoluene), and 400 μL of a saturated solution of potassium chloride. Then, 2 mL of chloroform and 2 mL of distilled water were added, and the mixture placed under agitation for 20 min. The organic phase was collected, and the solvent removed using a rotary evaporator at 40°C.
The fatty acids (FA) profile was analyzed by converting the FA in methyl esters (FAME) by adding methanol/acetyl chloride (19:1, v/v), during 1 h at 80°C, and adding n-heptane as extraction solvent (Fernandes et al., 2016). FAME were analyzed by gas chromatography-mass spectrometry following the conditions described in Nogueira et al. (2017) and identified by comparing the chromatographic retention times and mass spectra fragmentation with commercial standards (Supelco 37 component FAMEs and mix bacterial acid methyl esters CP mix) and by consulting the NIST spectral library. The identified FA were quantified by comparison with the retention area of Non-adecanoic acid (C19:0, 4 mg/g DM) used as internal standard due to its absence in the samples. Each sample was injected in duplicate, and the replicates had a variation lower than 5%.
Total carotenoid extraction in urchins gonads followed Rocha et al. (2019), where freeze-dried gonads (c.a. 0.5 g) were extracted with acetone (5:1, v/w). After centrifugation at 3,500 × G for 10 min, at 8°C, the supernatant was collected. The extraction procedure was repeated twice to obtain a colorless supernatant. The resulting supernatant was evaporated to dryness under a constant stream of nitrogen, re-dissolved in n-hexane and filtered with a 0.2 μm syringe filter for further analysis. Total carotenoid content was determined by UV-Visible spectrophotometry (Shimadzu UV-1603, Japan), using the absorption maximum (471–477 nm) in n-hexane and the extinction coefficient of 2,500 L/mol.cm.
Statistical Analysis
Mean density of the species S. granularis was calculated for each location surveyed. Differences among locations were tested between the five locations in which the species was present. Because the obtained data did not comply with the assumptions of normality and homogeneity of variances, the non-parametric Kruskal–Wallis test was selected. Whenever significant differences were found, a pairwise Wilcoxon rank sum test was performed between group levels with corrections for multiple testing.
A two-way ANOVA was applied to test for differences in species abundance among seasons (four levels) and location (two levels: Cais do carvão and Caniçal 1). As previously, violation of residuals normality occurred and therefore the Aligned Rank Transform for non-parametric factorial analyses (Wobbrock et al., 2011) was selected.
The biometric data used to determine the biological indices and reproductive biology was analyzed independently of sampling site. A two-way ANOVA was performed to assess the effect of the factors season and sex on the biometric parameters (TD and BW), reproductive variables (GTW, GI and MI), and protein (TP), lipid (TL), carotenoid (TC) and FA profile of sea urchin gonads. If ANOVA detected significant effects on single factors and/or factors’ interaction, individual means were compared using post hoc pairwise Tukey’s test. Whenever the assumptions of normality and homogeneity failed, a non-parametric factorial analysis was conducted using the aligned rank transform (ART) method. Whenever ART analysis showed significant differences, individual means were compared using the pairwise Wilcoxon test with a Bonferroni correction to the p-value. Differences in the maturity stages and color index distributions were analyzed by chi-square (χ2) test of independence. In all cases, significant differences were considered when p < 0.05. All statistical tests were performed in R environment (R Core Team, 2020).
Results
Sphaerechinus granularis Densities
The sea urchin S. granularis was found in five out of the eleven locations surveyed in this study: Cais do Carvão (five transects by season), Caniçal 1 (five transects by season, except in summer with only two), Caniçal 2 (18 transects in summer), Ponta do Pargo (six transects in summer) and Porto Santo breakwater (five transects in summer and six in autumn). In all these sampling locations, S. granularis occurred in low densities, with the maximum number of individuals (0.16 ind./m2) recorded at Cais do Carvão during spring (Figure 2). Regarding mean densities, the highest value was also recorded at Cais do Carvão in spring (0.064 ± 0.071 ind./m2). Porto Santo breakwater showed the second and the third higher density values in summer and autumn, respectively (Figure 2). The lowest values were recorded at Caniçal 1 (0 ind./m2) and Ponta do Pargo (0.007 ± 0.010 ind./m2) both in summer (Figure 2). Significant differences were observed in mean densities between locations (H4 = 1.45, p = 0.014). Pairwise comparisons showed significant differences only between Porto Santo breakwater and Caniçal 1 and between Porto Santo breakwater and Caniçal 2 (p = 0.022). The results from the two-way ANOVA indicated significant differences on the species densities between locations (F1 = 8.64, p = 0.0064), but no significant differences between seasons (F3 = 1.42, p = 0.26), nor for the factors interaction (F1,3 = 2.75, p = 0.06).
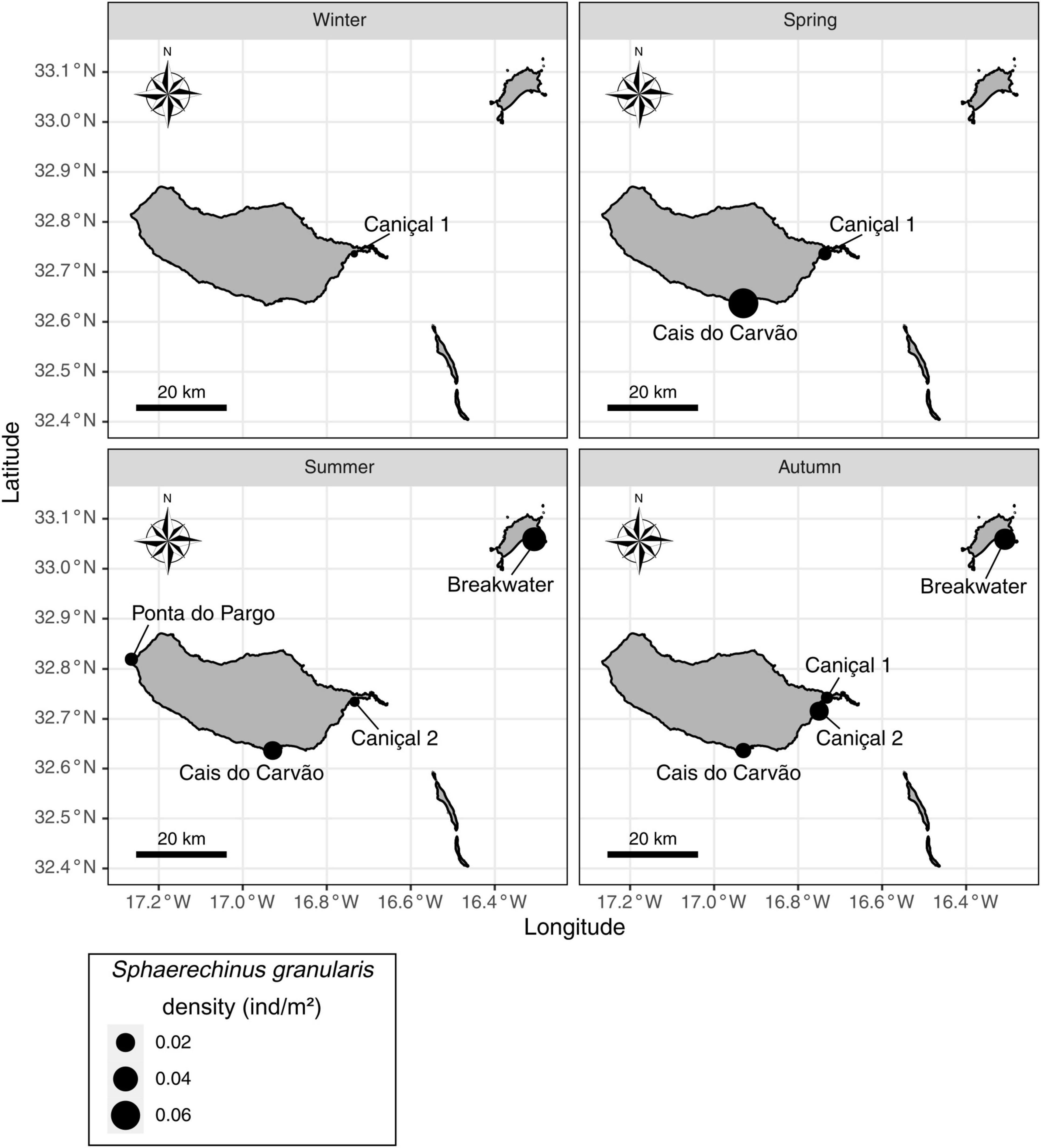
Figure 2. Spharaechinus granularis mean densities (ind./m2) by season in the five sampling locations where the species was recorded in Madeira archipelago.
Habitat Characterization and Diversity Indices
A list of the dominant sessile macrobenthic flora and fauna is presented in Table 1. The substrate in the five locations where the sea urchin was found was mostly rocky reefs, formed by rock boulders (generally > 1 m)—Cais do Carvão, Caniçal 1, and Porto Santo breakwater, but also rock platform—Ponta do Pargo and rhodoliths beds—Caniçal 2. The subtidal reefs where S. granularis inhabits (5–30 m depth) are generally characterized by encrusting algal communities and macroalgae mainly formed by turf and erect algae (e.g., Asparagopsis taxiformis, Dasycladus vermicularis, Dictyota sp., Stypopodium zonale), whereas sessile macrofauna cover is mostly composed by sponges [e.g., Batzella inops, Callyspongia (Callyspongia) burtoni, Cliona sp., Ircinia sp., Phorbas fictitius], hydrozoans (Pennaria disticha and Macrorhynchia philippina) and serpulids. Intermediate and deeper rocks (10–30 m) are mainly covered by sessile invertebrates, primarily barnacles and bryozoans as Reptadonella violacea and Schizoporella dunkeri and sponges (e.g., Aplysina aerophoba, B. inops, Chondrosia reniformes, P. fictius, Petrosia ficiformes). Algae cover is mostly crustose coralline algae (Lithophyllum sp.) or rocks deprived of algae—barren rocks—where the long spine sea urchin Diadema africanum is usually abundant.
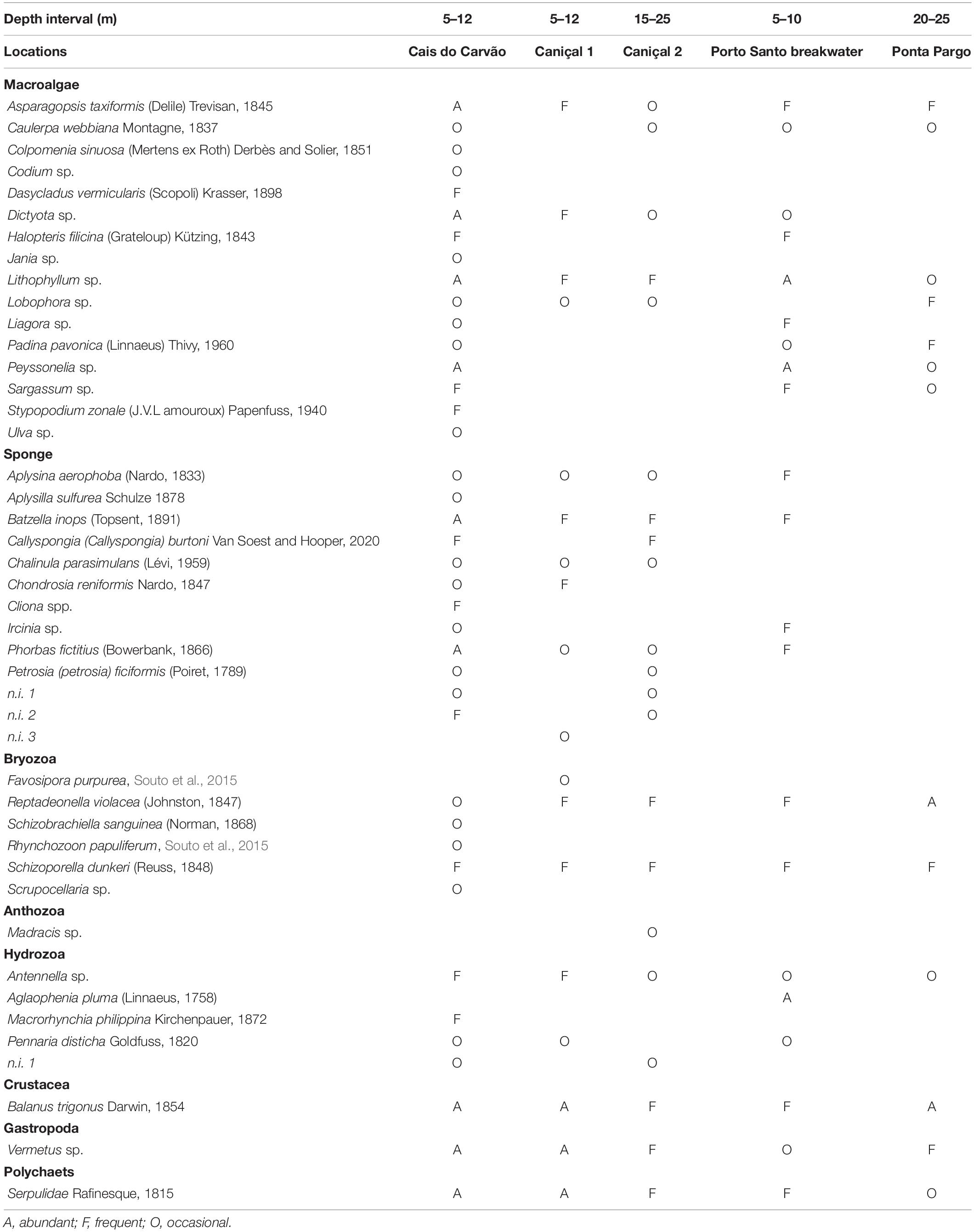
Table 1. List of the main sessile macrobenthic fauna and flora associated to the five locations where Sphaerechinus granularis was observed in Madeira archipelago.
Diversity indices for the mobile macrofauna associated to the S. granularis habitat at the five locations are presented in Table 2. The total species richness ranged between 10 and 16, the lowest value being recorded at Caniçal 1 and the highest at Cais do Carvão. For Shannon’s diversity index the highest value was recorded at Porto Santo breakwater and the minimum at Caniçal 1 (Table 2) and the same happening for the Simpson diversity index. Many of the macrofauna species detected inside locations and among locations showed low abundance and frequency of occurrence (e.g., the gastropods Bittium sp., Bursa sp., Felimare picta, Umbraculum umbraculum, and the echinoderms Ophidiaster ophidianus, Ophioderma longicauda), whereas the most abundant and frequently found species for most of the locations was the sea urchin D. africanum. nr most of the subtidaI effect, besides S. granularis, three more species of sea urchins were registered at these locations, namely Arbacia lixula, D. africanum and P. lividus (mean densities by season in Supplementary Table 1). The latter species was also recorded with low densities within most of the reefs surveyed, whereas A. lixula registered a total mean density three times higher than S. granularis in Porto Santo breakwater and Cais do Carvão reefs. D. africanum presented much higher densities for most of the locations, with a maximum of 5.6 ind./m2 recorded at Baixa das bicudas in autumn, a location where S. granularis was not detected.

Table 2. Diversity indices in each of the five locations where Sphaerechinus granularis was observed during the present study in Madeira archipelago.
Size Distribution and Reproduction Cycle
The TD and BW distributions of the collect urchins ranged between 40 and 90 mm (Figure 3A) and 40 g and 289 g (Figure 3B), respectively, indicate that only the adult component of the population was analyzed. The urchins mean TD was 65.82 ± 9.73 mm and mean BW 138.89 ± 52.56 g. It was observed a significant effect of season in the two parameters, with the urchins collected during spring being heavier (BW = 156.38 ± 54.83, N = 47) and larger (TD = 68.85 ± 8.89 mm) than those collected in winter (BW = 121.65 ± 52.37 g, TD = 62.39 ± 9.34 mm, N = 30) and in summer (BW = 122.72 ± 33.51, TD = 64.50 ± 5.93, N = 34). The mean BW and TD of the urchins collected in autumn was 149.94 ± 47.54 g and 67.41 ± 7.87 mm (N = 36). The two-away ANOVA output on the effect of season and sea urchin sex in the biometric parameters and biological indices is presented in Supplementary Table 2.
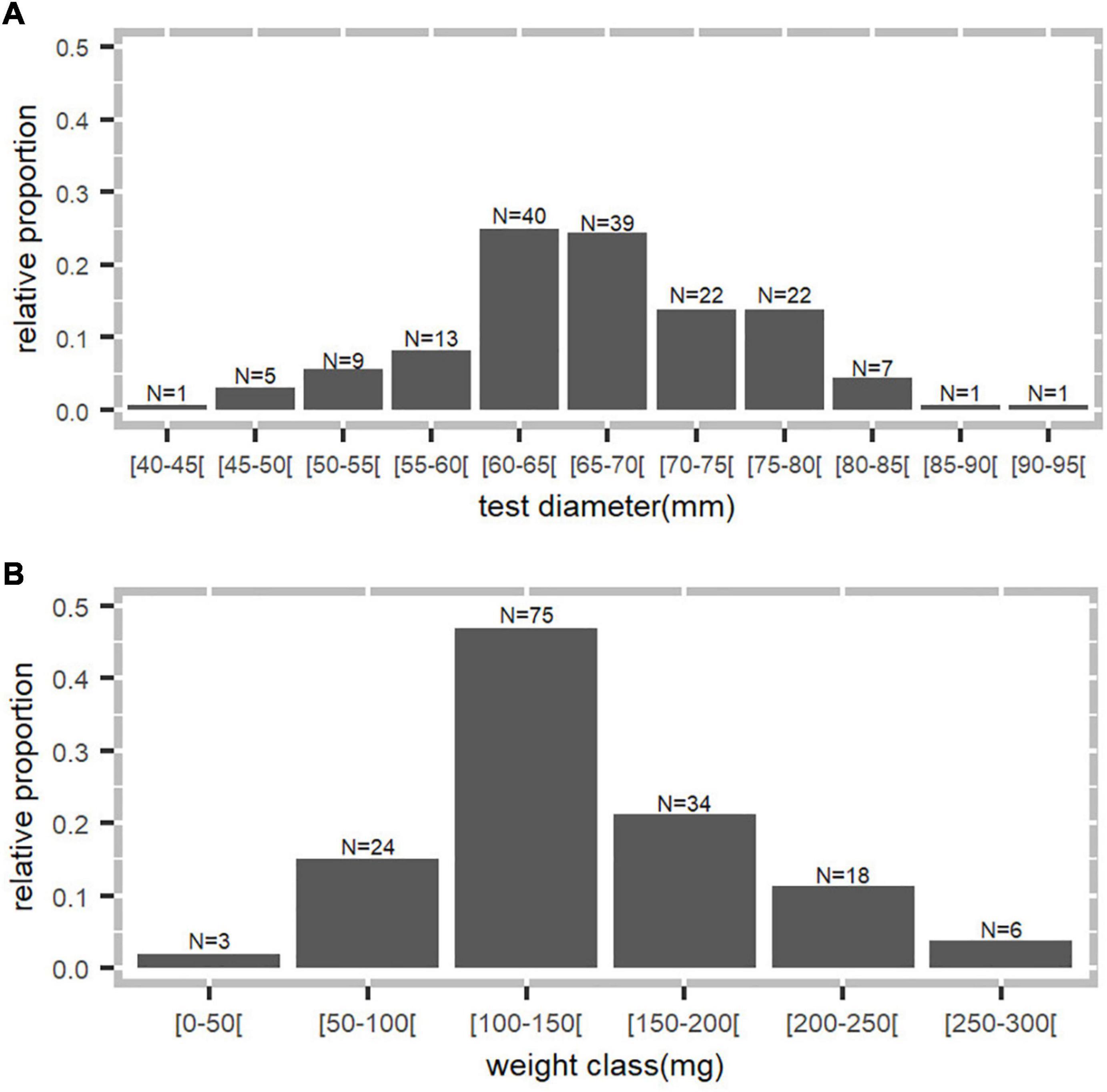
Figure 3. Test diameter distribution [TD, (A)], body weight distribution [BW, (B)] of Sphaerechinus granularis samples collected in Madeira archipelago.
Mature urchins were identified all year around, except for October (Figure 4). The proportion of immature and maturing individuals increased between May and September with a strong association between the immature individuals and spring (χ2 = 25.69, p = 0.002). Mature females appeared in similar proportions across all seasons, whereas mature males were strongly associated with winter (χ2 = 20.95, p = 0.013). The evolution of the maturity index through the year (Figure 5A) indicated the occurrence of two maturity peaks, one in April and another in November.
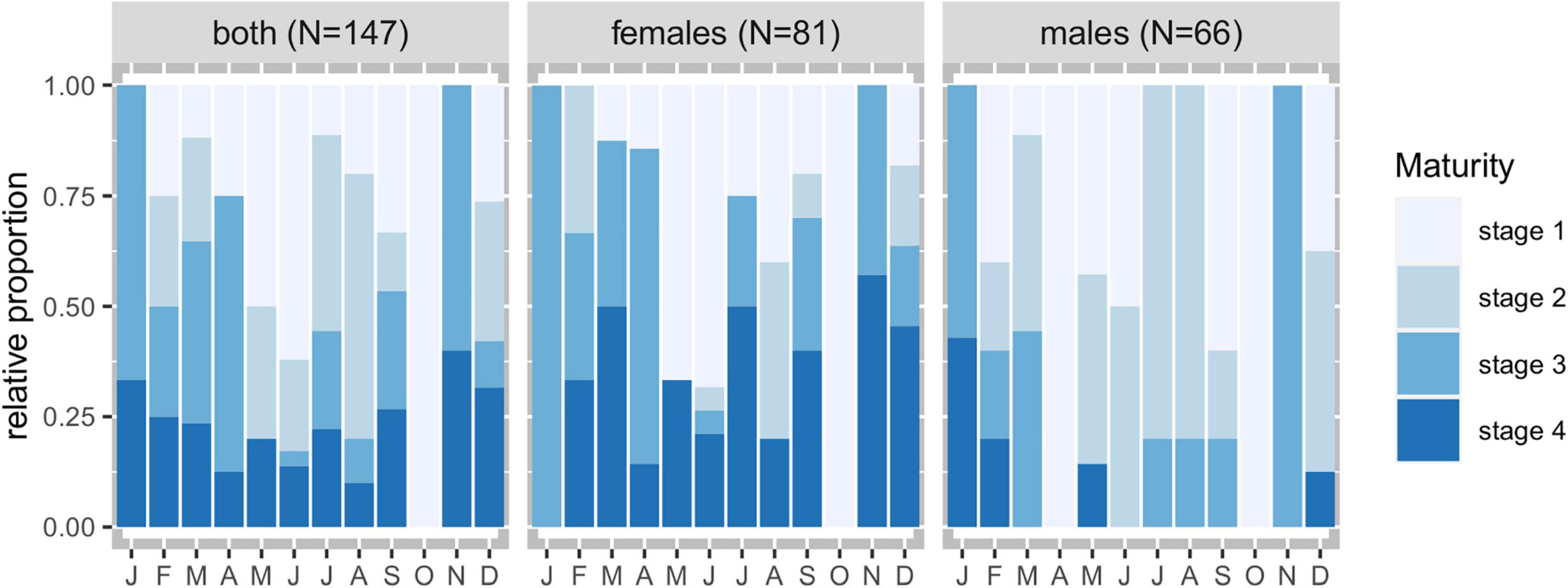
Figure 4. Maturity stages relative proportions by sex and by month of Sphaerechinus granularis in Madeira archipelago.
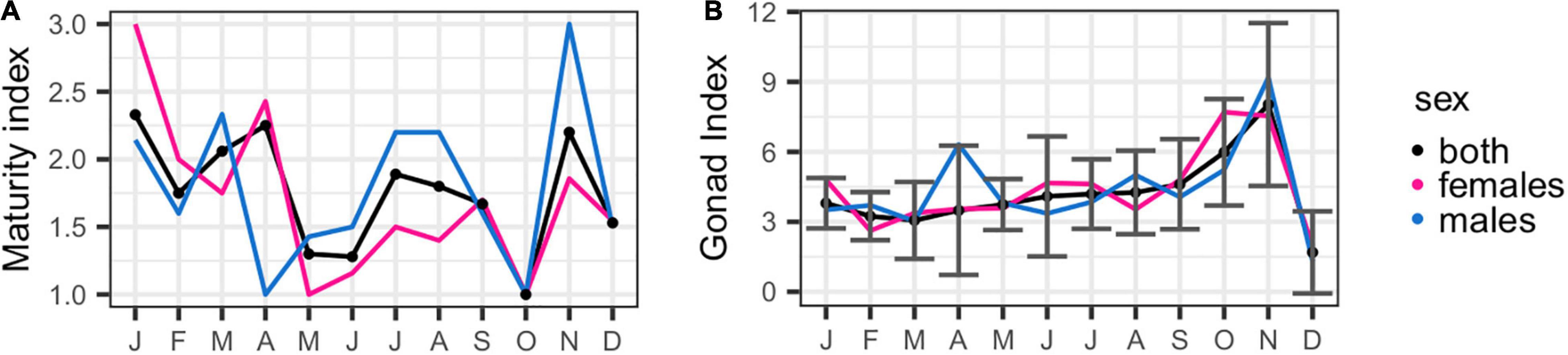
Figure 5. Monthly evolution of maturity index (A) and gonad index (B) of the Sphaerechinus granularis in Madeira archipelago.
Gonad Size, Color, and Nutritional Value
The average GI (Figure 5B) was 3.84 ± 2.54 and increased steadily from March to November, when attained its maximum (8.03 ± 3.49, N = 10), in synchrony with the maturation cycle. The GI decreased sharply between November and December, when it reached a minimum (1.69 ± 1.76, N = 19). Most of the sea urchins’ gonads were classified with a color grade between 1 and 2 (Figure 6). Gonad color distribution was influenced by season of capture (χ2 = 19.02, p = 0.025), with color grades 3 and 4 associated with autumn and winter. No statistically significant differences associated to the gonad sex were identified in the color grade distribution.
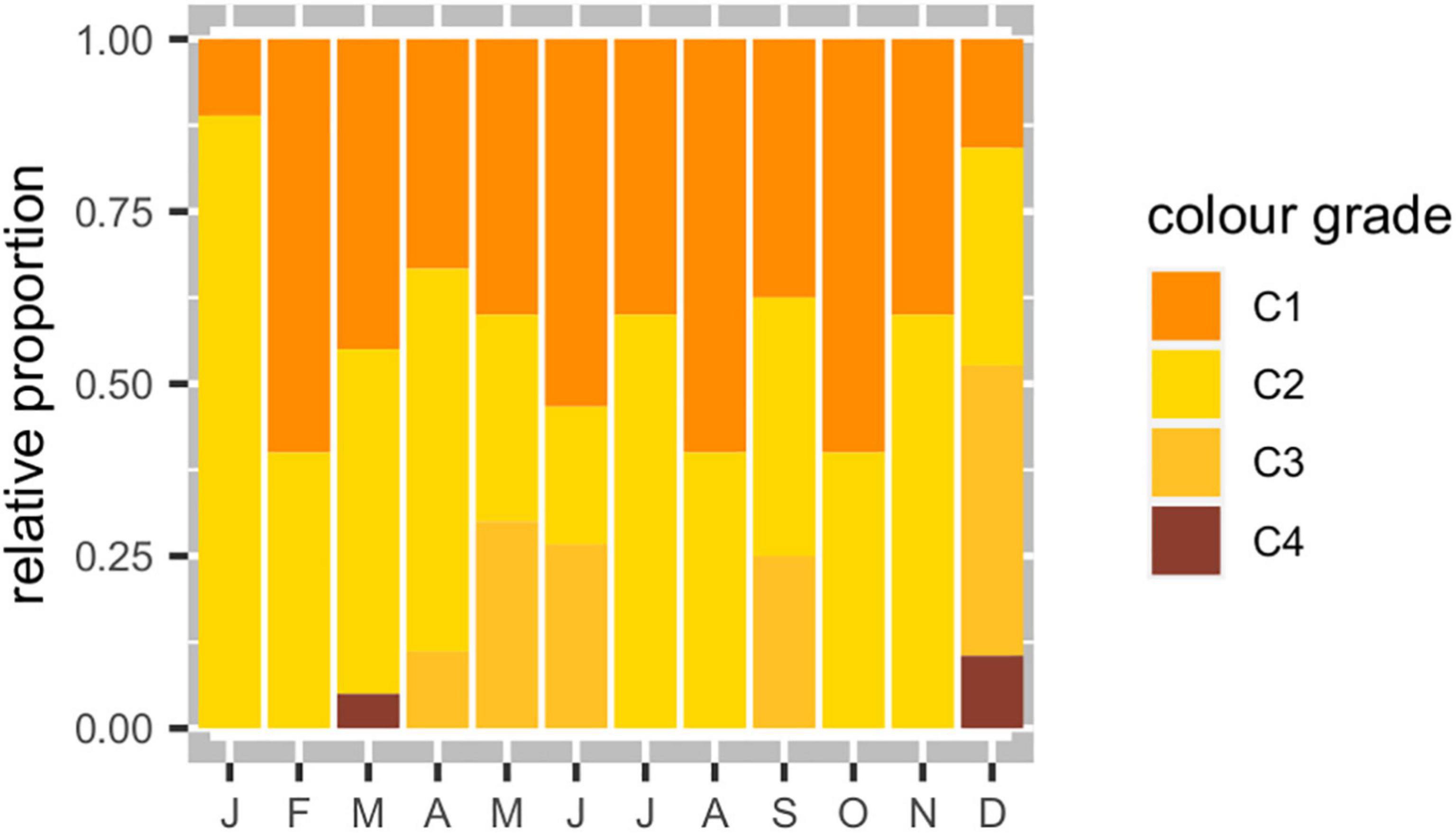
Figure 6. Gonad color distribution observed in the Sphaerechinus granularis population in Madeira archipelago.
The gonads of S. granularis presented a relatively high content of total carotenoids (average 296.36 ± 213.24 μg/g DM), significantly affected by the interaction of factors season and sex (F = 4.291, p = 0.012). The total carotenoid content was lower in summer (average 195.41 ± 34.15 μg/g DM) with identical concentration in both males and females (Figure 7A), followed by autumn (average 310.66 ± 52.41 μg/g DM). Differences in the total content of carotenoids between sexes were registered in spring and winter, being higher in females (Figure 7A).
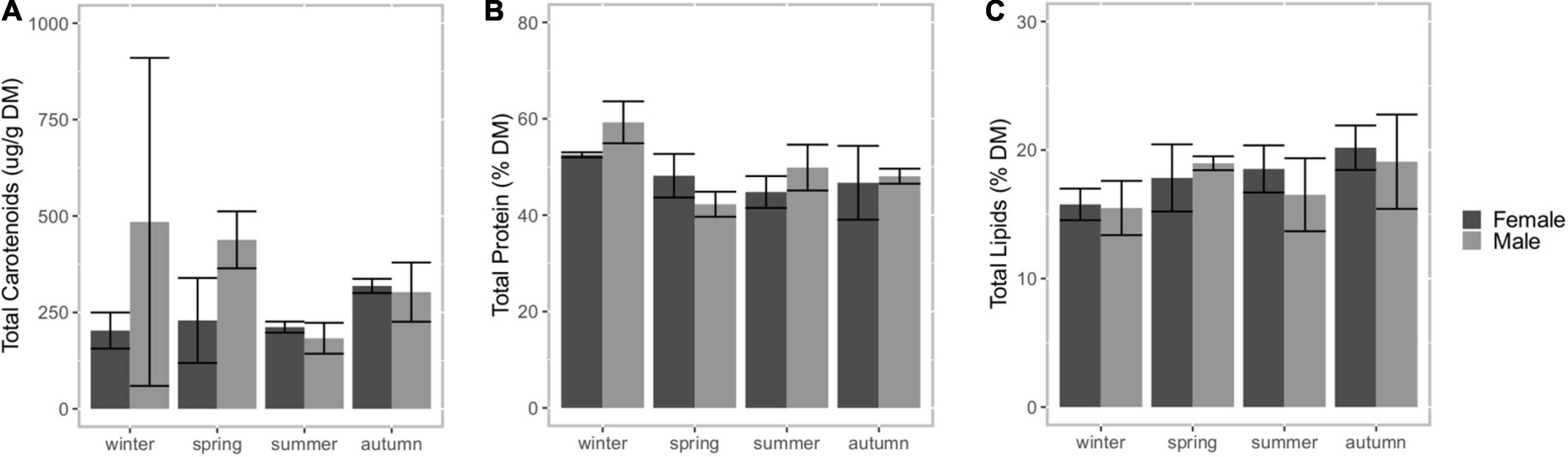
Figure 7. Total carotenoids [(A), mg/g dry matter DM], crude protein [(B), % DM] and total lipids [(C), % DM] present in female and male gonads of Sphaerechinus granularis by season in Madeira archipelago.
The total protein content of S. granularis gonads was, on average, 49.76% DM. The season (F = 12.51, p = 0.001) and its interaction with sea urchin sex (F = 4.472, p = 0.009) significantly affected the total protein content of gonads. During winter, males were richer in protein than females, and females presented a significantly higher protein content in spring and summer. In autumn no significant differences were recorded between sexes, with the mean protein content of the gonads being 47.40 ± 5.18% DM (Figure 7B).
The mean lipidic content of S. granularis gonads was 17.51 ± 2.64% DM. The lipidic content was significantly affected by season (F = 0.92, p = 0.003) but not affected by sex (F = 1.87, p = 0.179) nor by factors’ interaction (F = 0.92, p = 0.442). Sea urchin gonads collected during spring and autumn presented higher lipidic content (spring average 18.29 ± 2.06% DM; autumn average 19.63 ± 2.72% DM) followed by the gonads collected in summer (17.38 ± 2.58%) (Figure 7C).
Both saturated fatty acids (SFA) and polyunsaturated fatty acids (PUFA) were equally abundant in the gonads of S. granularis (Table 3), with their content being mainly affected by season (SFA: F = 3.44, p = 0.030; PUFA: F = 5.98, p = 0.002; Table 3). The most abundant SFA was the palmitic acid (C16:0), whose content was unaffected by season and sex. The most abundant PUFA in the gonads was the eicosapentaenoid acid (EPA, C20:5n3), which was affected by season (F = 4.69, p = 0.007; Table 3) and the highest content observed in the autumn (Table 3). The second most abundant PUFA was the arachidonic acid (ARA, C20:4n6) and its content was independent of season and sex (Table 3). The most abundant monounsatured fatty acids (MUFA) were the oleic acid (C18:1n9) and the gondoic acid (C20:1n9). The content of C18:1n9 in the gonads was also independent of season and sex, whereas C20:1n9 changed with season, being higher during autumn (Table 3). The content of other FA was affected by sex. For example, the content of myristic acid (C14:0) was higher in females during winter, spring and summer (Table 3). The FA pentadecanoic acid (C15:0), stearic acid (C18:0) and 10-non-adecenoic acid (C19:1n9) were more abundant in males, and these differences were more evident during spring (Table 3). The dihomo-linoleic acid (C20:2n6) was more abundant in males across all sampling seasons (Table 3).
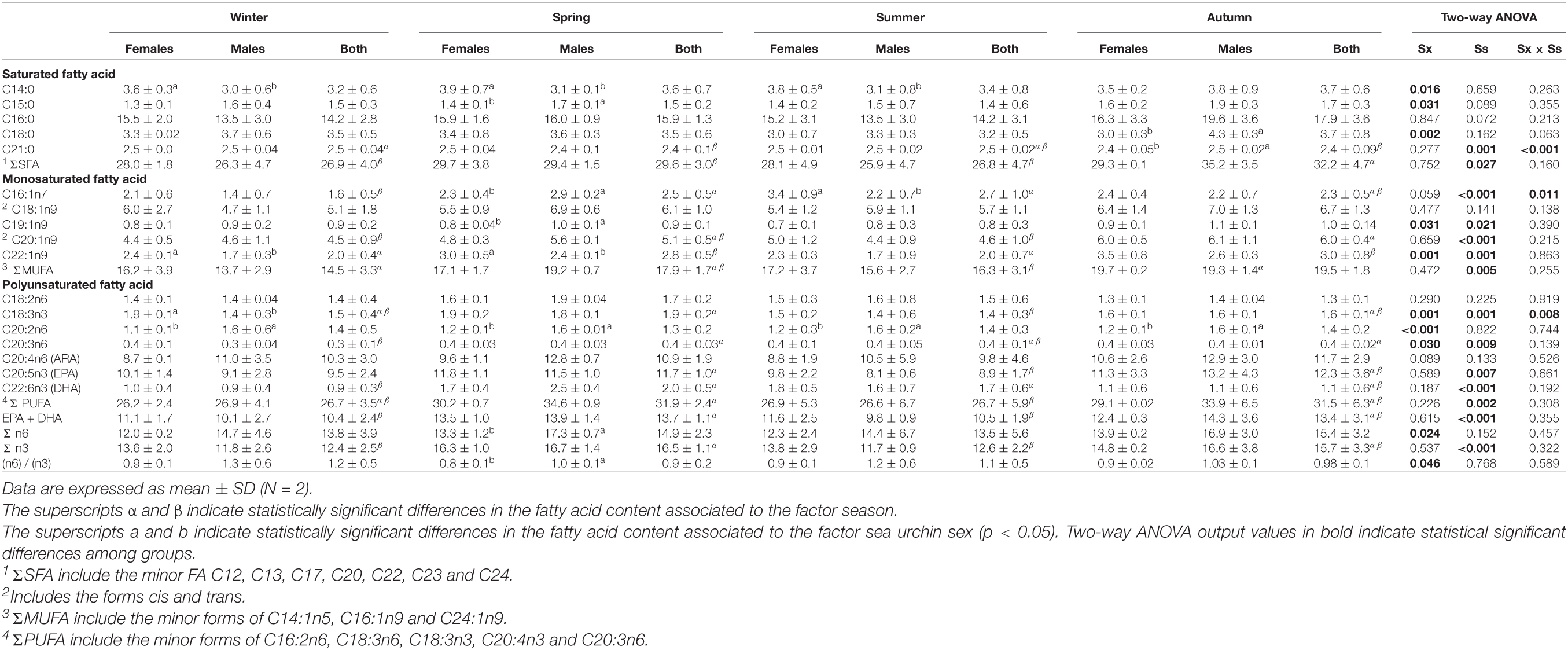
Table 3. Fatty acids (mg/g DM) profile and ANOVA results of Sphaerechinus granularis from Madeira archipelago.
Discussion
For most of the subtidal bottoms surveyed in Madeira archipelago, S. granularis occurred at low densities (average 0.01 ind./m2), from shallow to deep reefs (5–30 m). In effect the densities obtained were in average lower that those reported by Alves et al. (2001) who already referred average densities lower than 2 ± 0.6 ind./m2. The present results were much lower than those reported for the northwestern (< 30 ind./m2 to < 2 ind./m2 in Marseille Bay– Sartoretto and Francour, 1997) and for the southwestern (1.4–4.9 ind./m2 in Algeria—Soualili et al., 1999) Mediterranean coast. Both studies indicated that the species density was affected by sea urchin size, depth, and season, with higher densities found during summer and autumn in shallower grounds and composed by relatively small animals (diameter < 58 mm) (Sartoretto and Francour, 1997). These observations can explain the high densities indicated for the region of southern Brittany (50 ind./m2—Guillou and Michel, 1993). In fact, the low densities of S. granularis registered within the subtidal habitats of Madeira archipelago can be driven by several factors. Among these, is the high abundance of the sea urchin, D. africanum (Alves et al., 2001; Ribeiro and Neves, 2020). In highly abundant aggregations, D. africanum displays an aggressive behavior competing for food or even predating other echinoids (Shulman, 1990), which can cause detrimental effects in the S. granularis population within most of the locations sampled. Additionally, the typical oligotrophic conditions that characterize Madeira archipelago (Longhurst et al., 1995), comprising nutrient-poor waters and low chlorophyll concentrations (Falkowski et al., 1998) can also contribute to S. granularis low densities. A similar situation was reported in the oligotrophic southern part of the Aegean Sea (Vafidis et al., 2020). Yet, studies addressing this issue are needed for the study region.
The habitat of this species consists of a subtidal rocky reefs’ ecosystem described for these oceanic islands (e.g., Bianchi et al., 1998; Alves et al., 2001, 2019; Friedlander et al., 2017; Sangil et al., 2018; Neves and Ribeiro, 2019; Ribeiro and Neves, 2020). The five locations where the species was observed revealed similarity in species composition for macroflora and macrofauna, especially among the most abundant species (Table 1), nonetheless the highest densities of S. granularis occurred in locations presenting higher diversity indices (Table 2). Recently, Neves et al. (2019) referred that S. granularis inhabits the “Frondose algal communities” (EUNIS code MB1.15/A3.15) and “Faunal communities on moderate energy infralittoral rock” (EUNIS code MB1.24/A3.24). Our findings showed that among the major groups of macrobenthic mobile fauna associated with the surveyed subtidal communities are the echinoderms, with the sea urchin D. africanum as the most abundant as mentioned previously. In fact, this species has been pointed to effectively reduce algal abundance and determine their community structure within Madeira archipelago rocky reefs (Alves et al., 2003; Tuya et al., 2004) and responsible for the variability pattern of those benthic communities (Alves et al., 2019).
Sphaerechinus granularis is frequently associated to rhodoliths beds (Guillou et al., 2002). Nonetheless, according to this study and other surveys performed in rhodoliths beds within Madeira archipelago (Neves et al., 2021) the species is not abundant within this habitat, particularly when compared to rocky reefs. Data obtained from an ongoing habitat-mapping project revealed high abundance of the sea urchin D. africanum within rhodoliths beds as in rocky reefs (a maximum of 13.4 ind./m2 was recorded in intermediate and deep strata) (Ribeiro and Neves, 2020). As previously mentioned, the D. africanum voracious and aggressive behavior can restrict S. granularis to the vicinity of rhodoliths beds and deeper reefs, as shown in the neighbor Canaries archipelago (Tuya et al., 2001, 2007). Moreover, observations made by the authors (CR and PN) in ongoing monitoring surveys (Neves et al., 2018, 2019; Ribeiro and Neves, 2020), suggests this habitat shift of S. granularis to shallow reefs, especially in some natural rock breakwaters (e.g., breakwater of marina of Quinta do Lorde—Caniçal, of Porto Santo harbor and Fortaleza de Santiago- Funchal). Our results and additional observations allow to hypothesize that increasing load of nutrients from terrestrial sources/activities in these locations of higher anthropogenic pressure can provide more trophic resources (e.g., increasing macroalgal coverage) to the species, as have been shown elsewhere (Jordana et al., 1997).
Most of S. granularis urchins were collected from 5 to 12 m depth stratum of Cais do Carvão, Porto Santo breakwater and Caniçal. The diameter (average 65 mm) and weight distribution (average 139 g) were unimodal and showed that these depths are occupied mainly by adults, using as reference the presence of mature gonads for urchins with mean diameter > 40 mm (Guillou and Michel, 1993). The seasonal differences found in the size and weight distributions are related with the contribution of sample collected in Porto Santo breakwater which included urchins significantly larger and heavier than those collected in Cais do Carvão and Caniçal locations.
Despite the limitations related to the small sample size (N ≈ 10 urchins per month), the analysis of the maturity stages distribution throughout the year pointed to a single annual gametogenic cycle for S. granularis in line with the observations made by Spirlet et al. (1998) for other echinoid species. Our data indicate that, in Madeira archipelago, the reproductive season occurred between November and April with a notable synchrony between females and males. The evolution of the GI, increasing from March until a maximum in November, supports this extended reproductive cycle. The maturity index indicated two spawning peaks, one in April (preceded by relatively high values since January) and another stronger peak in November. These results show a delay of the reproductive season in relation to other regions, indicating that S. granularis could be a winter spawner. In the Mediterranean Sea, the study conducted by Martínez-Pita et al. (2008) indicate that reproduction season occurred during summer-autumn, starting with a spawning peak in June in southern location of the study area and ending with another and longer spawning peak in November in the northern site of the study area. Vafidis et al. (2020) defined a shorter reproductive season with a single spawning event during spring for the S. granularis population of the Aegean Sea (eastern region of the Mediterranean Sea) in line with the studies conducted by Guillou and Michel (1993) and Guillou and Lumingas (1998). These differences in the energy allocation for reproduction may be related with population densities, local productivity, and hydrodynamics. Larger populations within regions of high primary productivity tend to show relatively lower investment in reproduction (Lozano et al., 1995). In the sea urchins, as in other invertebrates, gametogenesis and spawning occur in synchrony with the best environmental conditions in terms of seawater temperature and local primary productivity (Soualili and Guillou, 2009; Amri et al., 2017). In fact, a winter reproduction season is also observed in the local populations of other sea urchin species as P. lividus (Lourenço, unpublished data) and other intertidal species as the gastropod Patella candei (Henriques et al., 2012) and P. aspera (Sousa et al., 2017). Both echinoderms and gastropods have in common a planktotrophic larval phase whose feeding success depends on phytoplankton blooms that in Madeira archipelago occurring from late autumn to early spring (Caldeira et al., 2002; Kaufmann et al., 2015).
Most sea urchin species are harvested for their gonads, or roe as commercially referred. High quality roe usually consists of large golden orange to yellow gonads with a distinct sweet ocean taste commercially identified as uni (Sun and Chiang, 2015). Sphaerechinus granularis adults attained particularly large body diameter and gonads when compared with P. lividus, a species of high commercial interest and the most harvested sharing the same habitat. The average GI (3.84 ± 2.54) of the local population of S. granularis is within the range observed by Guillou and Lumingas (1999) for the Bay of Brest (Brittany) and by Martínez-Pita et al. (2008) in the western Mediterranean Sea and is higher than those observed in the eastern Mediterranean (Aegean Sea), where the GI averaged approximately 2.0 (Vafidis et al., 2020). This difference between the Madeira archipelago and the eastern Mediterranean populations could be related to differences in the seawater temperature regimes. During the survey period, the local seawater temperature (measured in Funchal harbor) ranged between 18 °C (in March) and 24 °C (in September), whereas sea-bottom temperature recorded in the Aegen Sea ranged between 13°C in March and 27°C in July. The relative stability in seawater temperature at Madeira among seasons could benefit the gonadal growth. Nevertheless, the effect of local temperature trends in sea urchins GI is not clear (González-Irusta et al., 2010; Machado et al., 2019). Additionally, higher roe production yield could be obtained between September and November when gonads weight and GI attain its maximum (GI = 8.02 ± 3.49; GTW = 11.34 ± 7.34 g).
Most of the gonads observed were classified with color grade I (bright yellow or orange) and II (pale yellow or orange), ranging between 11 and 60% and between 20 and 89%, respectively, and independently of season. This intense orange color was consistent with the higher content of total carotenoids in their gonads. In fact, S. granularis gonads presented a particularly high carotenoid content, comparable to S. droebachiensis from Norway (Hagen et al., 2008). Both populations present higher carotenoid content in relation to those of P. lividus from the northwestern Portuguese coast (Rocha et al., 2019), S. droebachiensis from Newfoundland (Liyana-Pathirana et al., 2002), and P. miliaris from the west coast of Scotland (Symonds et al., 2007). The relatively high content of carotenoids in the S. granularis gonads may be related with the dominance of brown and red macroalgae rich in ß-carotene (e.g., Dictyota sp., Halopteris filicina, Peyssonelia sp. and Sargassum sp. in Table 1) in the diet of local population that use this molecule to synthesize the echinenone, the predominant carotenoid of sea urchin gonads (Symonds et al., 2007). The total carotenoid content varied significantly with season, being lower during summer, following the GI trend, when gonads are at their lowest level of stored reserves and start to recover from spawning. The high variability of total carotenoid content in male gonads may be related with the pooled samples as they included different maturity stages with various levels of stored carotenoids. According to Hagen et al. (2008) male and female urchins exhibit different patterns of carotenoid variation. Throughout the annual reproductive cycle, female carotenoid levels were generally lower and less variable than male carotenoids levels. Males do not loose carotenoids during spawning, and their concentration of carotenoids vary widely as a passive result of seasonal variation of gonad size and structure.
To our knowledge, this study constitutes the first analysis of the nutritional characteristics of S. granularis gonads. Both lipidic and protein content were in line to studies conducted in species partially sharing the same subtidal rocky habitat, namely P. lividus, in Madeira archipelago and in other regions like the Mediterranean Sea (Siliani et al., 2016) and the Northeast Atlantic coast (Montero-Torreiro and Garcia-Martinez, 2003). This consistency within the content of the major macronutrients in the sea urchin gonads between species and across regions could be related with the fact that sea urchins feed intake is mainly controlled by the dietary protein level. In sea urchins, the feed intake increases when the dietary protein level is low and decreases when the dietary protein level is high based in a dietary protein intake threshold (Heflin et al., 2016; Lourenço et al., 2020). On the other hand, the ratio between gonad lipidic and protein content is influenced by season as a response to the reproductive cycle. The lipidic content of the local population of S. granularis was on average 17.51 ± 2.64% DM independently of the sea urchins’ sex, but with variations across seasons. Sphaerechinus granularis gonads collected in winter presented lower lipidic content. The pattern of seasonal variation of the gonad lipidic content is complementary with the gonad protein content. Particularly in males, the lower lipidic content in winter matched with the highest protein content. This high protein content of males gonads is associated with the relatively high carotenoid content of their gonads, due to the binding relationship between carotenoids and protein in the sea urchins storage tissues (Pilbrow et al., 2014).
The FA profile of the S. granularis was highly influenced by sex, but also by season, as a proxy of seasonal changes in the gonad structure, and seasonal variations of diet. Particularly, the content of SFA C14:0, C15:0 and C18:0 in the gonad were affected by sex, in line with the results obtained by Martínez-Pita et al. (2010) for P. lividus. Females were richer in C14:0 while males were richer in C15:0 and C18:0. In contrast, the content of most abundant FA (C16:0) was not affected by season nor by sex, indicating the strong herbivorous behavior of this species (Kelly et al., 2008) as reported for other species such as P. lividus, A. lixula (Martínez-Pita et al., 2010), Spatangus purpureus and Echinus melo (Angioni and Addis, 2014). The MUFAs content was affected by season, particularly the C16:1n7 and the C20:1n9 suggesting that these two fatty acids are associated to the seasonal variation of food sources. The most abundant MUFA was C20:1n9 in line with the profile presented for the S. granularis of Sardinian Sea (Mediterranean basin) (Angioni and Addis, 2014). Both C19:1n9 (males with higher content) and C22:1n9 (females with higher content) were affected by the sea urchin sex suggesting it could be associated to gonads structural differences. The structural role of C22:1n9 in the sea urchins gonads is also evident in P. lividus, though with males presenting higher content of this fatty acid (Martínez-Pita et al., 2010). The seasonal and sex related variation of C22:1n9 was already reported in other studies (Rocha et al., 2019). The C18:3n3 and C20:3n6 were both affected by sex and season, also revealing a structural role in the gonad. The C18:2n6 and particularly ARA are very abundant in the gonads of S. granularis independently of season or gonad sex. The C18:2n6 and C18:3n3 are both involved in the “Δ8 metabolic pathway” that leads to the bio-synthesis of ARA and EPA, respectively (Kabeya et al., 2017). EPA influences many physiological processes such as reproduction, immunity efficiency and osmoregulation (Bret and Müller-Navarra, 1997). The absence of the PUFA C18:4n3 and C16:4 from the FAs profile in the gonads of the local population of S. granularis reveals that green algae and seagrasses do not have a predominant role in the diet of the species local population (Hughes et al., 2005) unlike the evidences observed by Angioni and Addis (2014) in the population of Sardinia Sea (Western Mediterranean). Moreover, the low gonadal content of C22:6n3 (DHA) reveals that the carnivory level of this species was also very low. The C22:6n3 (DHA) is an indicator of carnivory, as it is associated to filter feeding preys that in their turn feed on dinoflagellates (Cook et al., 2000; Hughes et al., 2005). The low level of DHA together with the absence of C18:4n3 and C16:4 indicates that S. granularis population of Madeira archipelago is closed associated with brown algae coverage, probably Dictiota sp., the most abundant brown macroalgae in the region (Graeve et al., 2002).
Despite the significant differences among females and males, the ratio n-6/n-3 was in all cases close to 1, denoting the balance between the more abundant PUFA, EPA, ARA and LA.
Conclusion
In the Madeira archipelago, the blunt sea urchin, S. granularis occurred in low densities in shallow subtidal rocky reefs and in breakwaters. In these ecosystems, the species is associated with encrusting algae and turf and erect macroalgae communities, on which the species grazes upon, mainly over brown macroalgae. Here, the S. granularis presents a long reproductive season occurring from late autumn to early spring, being considered a winter spawner. This reproductive strategy is associated with the southern limit of species distribution and with the low level of primary productivity and stable seawater temperature of this oceanic insular ecosystem. The species presents a relatively high gonad yield and proportion of sea urchins of bright orange color in line with roe market demand. Additionally, the high level of total carotenoids, the high PUFA (particularly EPA) content, and ratio n-6/n-3 close to one supports the high nutritional value of S. granularis roe.
The increasing interest in sea urchin roe in many regions of Mediterranean and southern Europe (James et al., 2016), the willingness of the local population to harvest and consume seafood and the growing interest of the local touristic sector for endogenous resources, foresees the increasing pressure to explore S. granularis and other edible species (e.g., P. lividus) directly to market or to sea urchin gonad enhancement programs. The consistency of high proportions of gonads with highly marketable color and the single annual GI peak, indicates that S. granularis has potential to be included in the edible sea urchins. Nevertheless, the species reduced densities demand for a precautionary approach when projecting any future exploitation of their roe. Further studies on the use of S. granularis as marine resource should focus in its full-cycle aquaculture in parallel with studies on the potential market for sea urchin roe at the regional level.
Data Availability Statement
The raw data supporting the conclusions of this article will be made available by the authors upon request.
Author Contributions
SL contributed to this study with conceptualization, methodology, formal analysis, original draft writing, review, and editing. RJ contributed with methodology, data collection and curation, original draft review, and editing. PN contributed with methodology, data collection and curation, formal analysis, original draft writing, review, and editing. AG and NC collected and analyzed chemical data and original draft writing, review, and editing. CA contributed with resources allocation, funding acquisition, and original draft review and editing. CR contributed to this study with methodology selection, data collection and curation, formal analysis, original draft writing, review, and editing. All authors contributed significantly to the content of this manuscript and the work that supported and agreed with its final content before submission.
Funding
The chemical analyses were supported by ISLANDAP project (INTERREG MAC/1.1a/207). RJ, CR, and PN were financially supported by the Oceanic Observatory of Madeira Project (M1420-01-0145-FEDER-000001-Observatório Oceanico da Madeira-OOM). SL was financially supported by a post-doctoral grant form ARDITI (Regional Agency for Development of Research, Technology, and Innovation of Madeira), Project M1420-09-5369-FSE-000001.
Conflict of Interest
The authors declare that the research was conducted in the absence of any commercial or financial relationships that could be construed as a potential conflict of interest.
Publisher’s Note
All claims expressed in this article are solely those of the authors and do not necessarily represent those of their affiliated organizations, or those of the publisher, the editors and the reviewers. Any product that may be evaluated in this article, or claim that may be made by its manufacturer, is not guaranteed or endorsed by the publisher.
Acknowledgments
We would like to thank Margarida Costa for the support on the sea urchin gonads histologic sectioning suggestions that significantly improved this manuscript.
Supplementary Material
The Supplementary Material for this article can be found online at: https://www.frontiersin.org/articles/10.3389/fmars.2021.699942/full#supplementary-material
References
Alves, F., Canning-Clode, J., Ribeiro, C., Gestoso, I., and Kaufmann, M. (2019). Local benthic assemblages in shallow rocky reefs find refuge in a marine protected area at Madeira Island. J. Coast. Conserv. 23, 373–383. doi: 10.1007/s11852-018-0669-y
Alves, F., Chícharo, L. M., Serrao, E., and Abreu, A. D. (2001). Algal cover and sea urchin spatial distribution at Madeira Island (NE Atlantic). Sci. Mar. 65, 383–392. doi: 10.3989/scimar.2001.65n4383
Alves, F., Chícharo, L. M., Serrão, E., and Abreu, A. D. (2003). Grazing by Diadema antillarum (Philippi) upon algal communities on rocky substrates. Sci. Mar. 67, 307–311. doi: 10.3989/scimar.2003.67n3307
Amri, S., Bensouilah, M., and Ouali, K. (2017). Variation of the condition index and sex-ratio of the sea urchin Paracentrotus lividus in the Southeast Mediterranean. J. Biol. Sci. 17, 76–83. doi: 10.3923/jbs.2017.76.83
Andrew, N., Agatsuma, Y., Ballesteros, E., Bazhin, A., Creaser, E., Barnes, D. K. A., et al. (2002). Status and management of world sea urchin fisheries. Oceanogr. Mar. Biol. 40, 343–425. doi: 10.1201/9780203180594.ch7
Angioni, A., and Addis, P. (2014). Characterization of the lipid fraction of wild sea urchin from the Sardinian Sea (Western Mediterranean). J. Food Sci. 79, C155–C162. doi: 10.1111/1750-3841.12330
AOAC (1990). Official Methods of Analysis of the Association of Official Analytical Chemists, 15th Edn. Arlington, VA: Association of Official Analytical Chemists, Inc.
Bertocci, I., Dominguez, R., Machado, I., Freitas, C., Godino, J., Sousa-Pinto, I., et al. (2014). Multiple effects of harvesting on populations of the purple sea urchin Paracentrotus lividus in north Portugal. Fish. Res. 150, 60–65. doi: 10.1016/j.fishres.2013.10.010
Bianchi, N. C., Morri, C., Sartoni, G., and Wirtz, P. (1998). Sublittoral epibenthic communities around Funchal (Ilha da Madeira, NE Atlantic). Bol. Museu Munic. Funchal (Hist. Nat.) 5, 59–80.
Boudoresque, C. F., and Verlaque, M. (2020). “Paracentrotus lividus,” in Sea Urchins: Biology and Ecology, 4th Edn, Vol. 43, ed. J. M. Lawrence (Amsterdam: Elsevier), 447–485.
Bret, M. T., and Müller-Navarra, D. C. (1997). The role of highly unsaturated fatty acids in aquatic food web processes. Freshw. Biol 13, 1055–1063. doi: 10.1046/j.1365-2427.1997.00220.x
Caldeira, R. M. A., Groom, S., Miller, P., Pilgrim, D., and Nezlin, N. P. (2002). Sea-surface signatures of the island mass effect phenomena around Madeira Island, Northeast Atlantic. Remote Sens. Environ. 80, 336–360. doi: 10.1016/S0034-4257(01)00316-9
Campuzano, F., Nunes, S., Malhadas, M., and Neves, R. (2010). Modelling hydrodynamics and water quality of Madeira Island (Portugal). GLOBEC International Newsletter. 16, 40–42.
Cook, E. J., Bell, M. V., Black, K. D., and Kelly, M. S. (2000). Fatty acid compositions of gonadal material and diets of the sea urchin, Psammechinus miliaris: trophic and nutritional implications. J. Exp. Mar. Biol. Ecol. 255, 261–274. doi: 10.1016/S0022-0981(00)00301-4
Falkowski, P. G., Barber, R. T., and Smetacek, V. (1998). Biogeochemical controls and feedbacks on ocean primary production. Science 281, 200–206.
Farina, S., Baroli, M., Brundu, R., Conforti, A., Cucco, A., De Falco, G., et al. (2020). The challenge of managing the commercial harvesting of the sea urchin Paracentrotus lividus: advanced approaches are required. Peer J 8:e10093. doi: 10.7717/peerj.10093
Fernandes, T., Fernandes, I., Andrade, C. A. P., and Cordeiro, N. (2016). Changes in fatty acid biosynthesis in marine microalgae as a response to medium nutrient availability. Algal Res. 18, 314–320. doi: 10.1016/j.algal.2016.07.005
Fernández-Boán, M., Fernández, L., and Freire, J. (2012). History and management strategies of the sea urchin Paracentrotus lividus fishery in Galicia (NW Spain). Ocean Coast. Manag. 69, 265–272. doi: 10.1016/j.ocecoaman.2012.07.032
Friedlander, A. M., Ballesteros, E., Clemente, S., Gonçalves, E. J., Estep, A., Rose, P., et al. (2017). Contrasts in the marine ecosystem of two Macaronesian islands: a comparison between the remote Selvagens Reserve and Madeira Island. PLoS One 12:e0187935. doi: 10.1371/journal.pone.0187935
Gaspar, M. B., Dias, M. D., Campos, A., Monteiro, C. C., Santos, M. N., Chicharo, A., et al. (2001). The influence of dredge design on the catch of Callista chione (Linnaeus, 1758). Hydrobiologia 465, 153–167. doi: 10.1023/A:1014587212528
González-Irusta, J. M., Goñi de Cerio, F., and Canteras, J. C. (2010). Reproductive cycle of the sea urchin Paracentrotus lividus in the Cantabrian Sea (northern Spain): environmental effects. J. Mar. Biol. Assoc. U.K. 90, 699–709. doi: 10.1017/S002531540999110X
Graeve, M., Kattner, G., Wiencke, C., and Karsten, U. (2002). Fatty acid composition of Arctic and Antarctic macroalgae: indicator of phylogenetic and trophic relationships. Mar. Ecol. Prog. Ser. 231, 67–74. doi: 10.3354/meps231067
Grosjean, P., Spirlet, C., Gosselin, P., Vaïtilingon, D., and Jangoux, M. (1998). Land-based, closed-cycle echinoculture of Paracentrotus lividus (Lamarck) 8Echinoidea: Echinodermata): a long-term experiment at a pilot scale. J. Shellfish Res. 17, 1523–1531.
Guillou, M., and Lumingas, L. J. L. (1998). The reproductive cycle of the ‘blunt’ sea urchin. Aquacult. Int. 6, 147–160. doi: 10.1023/A:1009290307840
Guillou, M., and Lumingas, L. J. L. (1999). Variation in the reproductive strategy of the sea urchin Sphaerechinus granularis (Echinodermata: Echinoidea) related to food availability. J. Mar. Biol. Assoc. U.K. 79, 131–136.
Guillou, M., and Michel, C. (1993). Reproduction and growth of Sphaerechinus granularis (echinodermata: Echinoidea) in southern Brittany. J. Mar. Biol. Assoc. U.K. 73, 179–192. doi: 10.1017/S0025315400032719
Guillou, M., Grall, J., and Connan, S. (2002). Can low sea urchin densities control macro-epiphytic biomass in a north-east Atlantic mäerl bed ecosystem (Bay of Brest, Brittany, France)? J. Mar. Biol. Assoc. U.K. 82, 867–876. doi: 10.1017/S0025315402006276
Hagen, N. T., Jørgensen, I., and Egeland, E. S. (2008). Sex-specific seasonal variation in the carotenoid content of sea urchin gonads. Aquat. Biol. 3, 227–235. doi: 10.3354/ab00084
Hammer, H., Powell, M., and Watts, S. (2013). Species Profile: Sea Urchins Of The Southern Region. SRAC Publication no 7211, 1-6, USDA-NIFA. Stoneville, MS: Southern Regional Aquaculture Center Publication.
Heflin, L. E., Raubenheimer, D., Simpson, S. J., and Watts, S. A. (2016). Balancing macronutrient intake in cultured Lytechinus variegatus. Aquaculture 450, 295–300. doi: 10.1016/j.aquaculture.2015.08.001
Henriques, P., Sousa, R., Pinto, A. R., Delgado, J., Faria, G., Alves, A., et al. (2012). Life history traits of the exploited limpet Patella candei (Mollusca: Patellogastropoda) of the north-eastern Atlantic. J. Mar. Biol. Assoc. U.K. 92, 1379–1387. doi: 10.1017/S0025315411001068
Hernández, J. C., Clemente, S., Sangil, C., and Brito, A. (2008). The key role of the sea urchin Diadema aff. Antillarum in controlling macroalgae assemblages throughout the Canary Islands (eastern Subtropical Atlantic): a spatio-temporal approach. Mar. Environ. Res. 66, 259–270.
Hughes, A. D., Catarino, A. I., Kelly, M. S., Barnes, D. K. A., and Black, K. D. (2005). Gonad fatty acids and trophic interactions of the echinoid Psammechinus miliaris. Mar. Ecol. Prog. Ser. 305, 101–111. doi: 10.3354/meps305101
Instituto Hidrográfico (2003). Dinâmica Sedimentar Da Costa Sul Da Ilha Da Madeira. Lisboa: Instituto Hidrográfico.
Instituto Hidrográfico (2007). Dinâmica Sedimentar Da Costa Sul Da Ilha Da Madeira (Cabo Girão À Ponta De São Lourenço). Lisboa: Instituto Hidrográfico.
Instituto Hidrográfico (2008). Caracterização Dos Depósitos Sedimentares Da Plataforma Insular Sul da Ilha do Porto Santo. (Project GM 52OP02). Lisboa: Instituto Hidrográfico (Portuguese Navy).
James, P., Noble, C., Hannon, C., Stefánsson, G., Fiórarinsdóttir, G., Ziemer, N., et al. (2016). Sea Urchin Fisheries, Management And Policy Review (Activity A4.2.1 of the URCHIN project). Nofima Rapportserie 1–18. Available online at: http://hdl.handle.net/11250/2390039 (accessed April 23, 2021)
Jordana, E., Guillou, M., and Lumingas, L. J. L. (1997). Age and growth of the sea urchin Sphaerechinus granularis in southern Brittany. J. Mar. Biol. Assoc. U.K. 77, 1199–1212. doi: 10.1017/s0025315400038716
Kabeya, N., Sanz-Jorquera, A., Carboni, S., Davie, A., Oboh, A., and Monroig, O. (2017). Biosynthesis of polyunsaturated fatty acids in sea urchins: molecular and functional characterisation of three fatty acyl desaturases from Paracentrotus lividus (Lamark 1816). PLoS One 12:e0169374. doi: 10.1371/journal.pone.0169374
Kaufmann, M., Santos, F., and Maranhão, M. (2015). Checklist of nanno – and microphytoplankton off Madeira island (Northeast Atlantic) with some historical notes. Nova Hedwigia 101, 205–232.
Kelly, J. R., Scheibling, R. E., Iverson, S. J., and Gagnon, P. (2008). Fatty acid profiles in the gonads of the sea urchin Strongylocentrotus droebachiensis on natural algal diets. Mar. Ecol. Prog. Ser. 373, 1–9. doi: 10.3354/meps07746
Liyana-Pathirana, C., Shahidi, F., Whittick, A., and Hooper, R. (2002). Lipid and lipid soluble components of gonads f green sea urchin (Strongylocentrotus Droebachiensis). Food Lipids 9, 105–126. doi: 10.1111/j.1745-4522.2002.tb00213.x
Longhurst, A., Sathyendranath, S., Platt, T., and Caverhill, C. (1995). An estimate of global primary production in the ocean from satellite radiometer data. J. Plankton Res. 17, 1245–1271.
Lourenço, S., José, R., Andrade, C., and Valente, L. M. P. (2020). Growth performance and gonad yield of sea urchin Paracentrotus lividus (Lamarck, 1816) fed with diets of increasing protein: energy ratios. Anim. Feed Sci. Technol. 270:114690. doi: 10.1016/j.anifeedsci.2020.114690
Lozano, J., Galera, J., Lopez, S., Turon, X., Palacin, C., and Morera, G. (1995). Biological cycles and recruitment of Paracentrotus lividus (Echinodermata: Echinoidea) in two contrasting habitats. Mar. Ecol. Prog. Ser. 122, 179–192.
Lumingas, L. J. L., and Guillou, M. (1994). Growth zones and back-calculation for the sea urchin, Sphaerechinus granularis, from the bay of Brest, France. J. Mar. Biol. Assoc. U.K. 74, 671–686. doi: 10.1017/S0025315400047731
Machado, I., Moura, P., Pereira, F., Vasconcelos, P., and Gaspar, M. B. (2019). Reproductive cycle of the commercially harvested sea urchin (Paracentrotus lividus) along the western coast of Portugal. Invertebr. Biol. 138, 40–54. doi: 10.1111/ivb.12238
Madeira, P., Kroh, A., Cordeiro, R., Frias, A. M., and Ávila, S. P. (2019). The echinoderm fauna of Azores (NE Atlantic Ocean). Zootaxa 4639, 1–231.
Martínez-Pita, I., García, F. J., and Pita, M. L. (2010). Males and females gonad fatty acids of the sea urchins Paracentrotus lividus and Arbacia lixula (Echinodermata). Helgol. Mar. Res. 64, 135–142. doi: 10.1007/s10152-009-0174-7
Martínez-Pita, I., Sànchez-Espana, A. I., and García, F. J. (2008). Gonadal growth and reproduction in the sea urchin Sphaerechinus granularis (Lamarck 1816) (Echinodermata: Echinoidea) in southern Spain. Sci. Mar. 72, 603–611. doi: 10.3989/scimar.2008.72n3603
Montero-Torreiro, M. F., and Garcia-Martinez, P. (2003). Seasonal changes in the biochemical composition of body components of sea urchin, Paracentrotus lividus, in Lorbé (Galicia, north-western Spain). J. Mar Biol. Ass. U.K. 83, 575–581.
Neves, P., and Ribeiro, C. (2019). Resultados Do Programa De Monitorização Dos Recifes Artificiais CORDECA e CORCEIRA. funchal: CIIMAR-Madeira, 69.
Neves, P., Braga-Henriques, A., and Ribeiro, C. (2019). Reference State Characterization For Marine Habitats And Biocenosis Of Madeira – Final Report. Funchal: CIIMAR-Madeira, 183.
Neves, P., Kaufmann, M., and Ribeiro, C. (2018). Resultados Do Programa De Monitorização Da Biodiversidade Marinha Dos Habitats Naturais E Artificiais Subtidais Na Ilha Do Porto Santo. funchal: CIIMAR-Madeira.
Neves, P., Silva, J., Peña, V., and Ribeiro, C. (2021). Pink round stones – maërl beds: an overlooked habitat in Madeira archipelago. Biodivers. Conserv. 30, 3359–3383. doi: 10.1007/s10531-021-02251-2
Nogueira, N., Fernandes, I., Fernandes, T., and Cordeiro, N. (2017). A comparative analysis of lipid content and fatty acid composition in muscle, live rand gonads of Seriola fasciata Bloch 1793 based on gender and maturation stage. J. Food Comp. Anal. 59, 68–73. doi: 10.1016/j.jfca.2016.11.005
Pais, A., Chessa, L. A., Serra, S., Ruiu, A., Meloni, G., and Donno, Y. (2007). The impact of commercial and recreational harvesting for Paracentrotus lividus on shallow rocky reef sea urchin communities in North-western Sardinia, Italy. Estuar. Coast. Shelf Sci. 73, 589–597. doi: 10.1016/j.ecss.2007.02.011
Pais, A., Serra, S., Meloni, G., Saba, S., Ceccherelli, G., Pais, A., et al. (2012). Harvesting effects on Paracentrotus lividus population structure: a case study from northwestern Sardinia, Italy, before and after the fishing season. J. Coast. Res. 28:570. doi: 10.2112/jcoastres-d-10-00119.1
Pearce, C. M., Daggett, T. L., and Robinson, S. M. C. (2002). Effect of urchin size and diet on gonad yield and quality in the green sea urchin (Strongylocentrotus droebachiensis). Aquaculture 233, 337–367. doi: 10.1016/j.aquaculture.2003.09.027
Pearse, J. S. (2006). Ecological role of purple sea urchins. Science 314, 940–941. doi: 10.1126/science.1131888
Pilbrow, J., Sabherwal, M., Garama, D., and Carne, A. (2014). A novel fatty acid-binding protein-like carotenoid- Binding protein from the gonad of the New Zealand sea urchin Evechinus chloroticus. PLoS One 9:e106465. doi: 10.1371/journal.pone.0106465
R Core Team (2020). R: A Language And Environment For Statistical Computing. Vienna: R Foundation for Statistical Computing.
Ribeiro, C. C. (2008). Comparison of rocky reef fish communities among protected, unprotected and artificial habitats in Madeira island coastal waters using underwater visual techniques. PhD Thesis. Faculdade de Ciências da Universidade de Lisboa, Lisboa. Available online at: http://repositorio.ul.pt/bitstream/10451/1541/1/16395_Tese_Claudia%2520Ribeiro.pdf (accessed December 8, 2013).
Ribeiro, C., and Neves, P. (2020). Habitat mapping of cabo girão marine park (Madeira island): a tool for conservation and management. J. Coast. Conserv. 24, 1–16. doi: 10.1007/s11852-019-00724-9
Riera, R., Delgado, J. D., Rodríguez, M., and Monterroso, Ó, and Ramos, E. (2012). Macrofaunal communities of threatened subtidal maerl seabeds on Tenerife (Canary Islands, north-east Atlantic Ocean) in summer. Acta Oceanol. Sin. 31, 98–105. doi: 10.1007/s13131-012-0181-4
Rocha, F., Baião, L. F., Moutinho, S., Reis, B., Oliveira, A., Arenas, F., et al. (2019). The effect of sex, season and gametogenic cycle on gonad yield, biochemical composition and quality traits of Paracentrotus lividus along the north Atlantic coast of Portugal. Sci. Rep. 9:2994. doi: 10.1038/s41598-019-39912-w
Sangil, C., Martins, G. M., Hernández, J. C., Alves, F., Neto, A. I., Ribeiro, C., et al. (2018). Shallow subtidal macroalgae in the North-eastern Atlantic archipelagos (Macaronesian region): a spatial approach to community structure. Eur. J. Phycol 53, 83–98. doi: 10.1080/09670262.2017.1385098
Sartoretto, S., and Francour, P. (1997). Quantification of bioerosion by Sphaerechinus granularis on ‘coralugène’ concretions of the western mediterranean. J. Mar. Biol. Assoc. U.K. 77, 565–568. doi: 10.1017/S0025315400071885
Shulman, M. J. (1990). Aggression among sea urchins on caribbean coral reefs. J. Exp. Mar. Biol. Ecol. 140, 197–207.
Siliani, S., Melis, R., Loi, B., Guala, I., Baroli, M., Sanna, R., et al. (2016). Influence of seasonal and environmental patterns on the lipid content and fatty acid profiles in gonads of the edible sea urchin Paracentrotus lividus from Sardinia. Mar. Environ. Res. 113, 124–133. doi: 10.1016/j.marenvres.2015.12.001
Souto, J., Kaufmann, M. J., and Canning-Clode, J. (2015). New species and new records of bryozoans from shallow waters of Madeira Island. Zootaxa 3925, 581–593. doi: 10.11646/zootaxa.3925.4.7
Soualili, D. L., Guillou, M., and Semroud, R. (1999). Age and growth of the echinoid Sphaerechinus granularis from the Algerian coast. J. Mar. Biol. Assoc. U.K. 79, 1139–1140. doi: 10.1017/S0025315499001460
Soualili, D., and Guillou, M. (2009). Variation in the reproductive cycle of the sea urchin Paracentrotus lividus in three differently polluted locations near Algiers (Algeria). Mar. Biodivers. Rec. 2:e100. doi: 10.1017/s175526720900092x
Sousa, R., Delgado, J., Pinto, A. R., and Henriques, P. (2017). Growth and reproduction of the north-eastern Atlantic keystone species Patella aspera (Mollusca: Patellogastropoda). Helgol. Mar. Res. 71:8. doi: 10.1186/s10152-017-0488-9
Spirlet, C., Grosjean, P., and Jangoux, M. (1998). Reproductive cycle of the echinoid Paracentrotus lividus: analysis by means of the maturity index. Invertebr. Reprod. Dev. 34, 69–81. doi: 10.1080/07924259.1998.9652355
Stefansson, G., Kristinsson, H., Ziemer, N., Hannon, C., and James, P. (2017). Markets For Sea Urchins: A Review of Global Supply and Markets. Matís - Food Research, Innovation & Safety, Iceland, 45. doi: 10.13140/RG.2.2.12657.99683
Steneck, R. S. (2013). “Sea urchins as drivers of shallow benthic marine community structure,” in Sea Urchins: Biology and Ecology, 3rd Edn, ed. J. M. Lawrence (Amsterdam: Elsevier Science), 195–212.
Sun, J., and Chiang, F.-S. (2015). “Use and exploitation of sea urchins,” in Echinoderms Aquaculture, 1st Edn, eds N. P. Brown and S. D. Eddy (Hoboken, NJ: Wiley-Blackwell), 25–46. doi: 10.1002/9781119005810.ch2
Symonds, R. C., Kelly, M. S., Caris-Veyrat, C., and Young, A. J. (2007). Carotenoids in the sea urchin Paracentrotus lividus: occurrence of 9′-cis-echinenone as the dominant carotenoid in gonad colour determination. Comp. Biochem. Physiol. B Biochem. Mol. Biol. 148, 432–444. doi: 10.1016/j.cbpb.2007.07.012
Tansley, A. G. (1993). An introduction To Plant Ecology. New Delhi: Discovery Publishing House, 228.
Tuya, F., Boyra, A., Sanchez-Jerez, P., Barbera, C., and Haroun, R. J. (2004). Relationships between rocky-reef fish assemblages, the sea urchin Diadema antillarum and macroalgae throughout the Canaria Archipelago. Mar. Ecol. Prog. Ser. 278, 157–169.
Tuya, F., Cisneros-Aguirre, J., Ortega-Borges, L., and Haroun, R. J. (2007). Bathymetric segregation of sea urchins on reefs of the canarian archipelago: role of flow-induced forces. Estuar. Coast. Shelf Sci. 73, 481–488. doi: 10.1016/j.ecss.2007.02.007
Tuya, F., Martín, J. A., Reuss, G. M., and Luque, A. (2001). Food preferences of the sea urchin Diadema antillarum in Gran Canaria (Canary Islands, central-east Atlantic Ocean). J. Mar. Biol. Assoc. U.K. 81, 845–849. doi: 10.1017/S0025315401004672
Vafidis, D., Antoniadou, C., and Ioannidi, V. (2020). Population density, size structure, and reproductive cycle of the comestible sea urchin Sphaerechinus granularis (Echinodermata: Echinoidea) in the pagasitikos gulf (aegean sea). Animals 10, 1–12. doi: 10.3390/ani10091
Verlaque, M. (1981). Preliminary data on some Posidonia feeders. Rapp. PV Réun. Commiss. Internatl Explor. Sci. Méditerr. (CIESM) 27, 201–202.
Walker, C., Lesser, M. P., and Unuma, T. (2013). “Sea urchin Gametogenesis – structural, functional and molecular/genomic biology,” in Sea Urchins: Biology and Ecology, 3rd Edn, ed. J. M. Lawrence (Amsterdam: Elsevier), 26–42.
Wentworth, C. K. (1922). A scale of grade and class terms for clastic sediments, J. Geol. 30, 377–392.
Wobbrock, J. O., Findlater, L., Gergle, D., and Higgins, J. J. (2011). “The aligned rank transform for nonparametric factorial analyses using only ANOVA procedures,” in Proceedings of the 2011 Annual Conference on Human Factors in Computing Systems – CHI’11: Presented At The The 2011 Annual Conference, (Vancouver, BC: ACM Press), 143. doi: 10.1145/1978942.1978963
Keywords: Macaronesia region, gonad yield, gonad color, carotenoids, fatty acids, Sphaerechinus granularis, Madeira archipelago
Citation: Lourenço S, José R, Neves P, Góis A, Cordeiro N, Andrade C and Ribeiro C (2022) Population Density, Reproduction Cycle and Nutritional Value of Sphaerechinus granularis (Echinodermata: Echinoidea) in an Oceanic Insular Ecosystem. Front. Mar. Sci. 8:699942. doi: 10.3389/fmars.2021.699942
Received: 24 April 2021; Accepted: 16 December 2021;
Published: 27 January 2022.
Edited by:
Mhd Ikhwanuddin, University of Malaysia Terengganu, MalaysiaReviewed by:
Carlos Vergara-Chen, Technological University of Panama, PanamaDaniele Grech, International Marine Centre Foundation, Italy
Copyright © 2022 Lourenço, José, Neves, Góis, Cordeiro, Andrade and Ribeiro. This is an open-access article distributed under the terms of the Creative Commons Attribution License (CC BY). The use, distribution or reproduction in other forums is permitted, provided the original author(s) and the copyright owner(s) are credited and that the original publication in this journal is cited, in accordance with accepted academic practice. No use, distribution or reproduction is permitted which does not comply with these terms.
*Correspondence: Sílvia Lourenço, c2xvdXJlbmNvMkBnbWFpbC5jb20=
†ORCID: Sílvia Lourenço, orcid.org/0000-0003-4426-0894; Pedro Neves, orcid.org/0000-0001-6659-0398; Nereida Cordeiro, orcid.org/0000-0001-6006-3415; Cláudia Ribeiro, orcid.org/0000-0002-0703-8795