- 1The Food BioSciences Department, Teagasc Ashtown Food Research Centre, Dublin, Ireland
- 2Department of Marine Biotechnology, Nofima AS, The Norwegian Institute of Food, Fisheries and Aquaculture Research, Tromsø, Norway
- 3Department of Nutrition and Feed Technology, Nofima AS, Bergen, Norway
The abundance of fish and zooplankton (1 × 109 tons to 7 × 1010 tons) in the mesopelagic zone of the ocean is a source of novel raw materials that provides opportunities for sustainable new product development. The peculiar conditions of light and pressure in this ecological zone and the position of the specific organisms in the marine food chain results in diversity in their bio-composition. Mesopelagic fish are an underutilized resource, rich in proteins and omega-3 oils, and present opportunities to develop novel feed, food and functional food ingredients and products. However, there is also a need to ensure that this resource is not overfished and is processed to optimize the catch in line with sustainability goals. There is therefore a need to establish sustainable bioprocessing technologies to yield value added products from mesopelagic fish species. In the present study, various protein extracts from the mesopelagic fish Maurolicus muelleri (M) and Meganyctiphanes norvegica (Northern Krill) (K) and combinations of proteins from these species (C) were generated using hydrolysis methods. Protein Hydrolysates were generated using four different enzymes including Alcalase, endocut-01, endogenous M/K enzymes and FoodPro PNL. Hydrolysates were characterized and assessed for their ability to inhibit enzymes important in diseases associated with metabolic syndrome. The ability of generated Hydrolysates to inhibit enzymes including Angiotensin-1-converting enzyme (ACE-1; EC. 3.4.15.1) associated with blood pressure regulation, Acetylcholinesterase (AChE; EC 3.1.1.7) associated with maintenance of the nervous system, and Dipeptidyl peptidase IV (DPP-IV; EC 3.4.14.5) linked with development of type-2-diabetes, was determined. In a separate process, the same mesopelagic fish species were transformed into fishmeal, Hydrolysates, fish-silage, and aqueous extracts (AQ) and screened for bioactivities using the same bioassays. The Hydrolysates contained greater than 60% protein (dry weight basis) when analyzed using the DUMAS method. A hydrolysate from M. muelleri generated using FoodPro PNL (M1) inhibited the ACE-1 enzyme by 89.56% when assayed at a concentration of 1 mg/ml compared to the positive control Captopril©. Aqueous extract two (AQ2) inhibited ACE-1 by 95.28% when assayed at 1 mg/ml compared to the control. Sample M1 inhibited DPP-IV by 100% and aqueous extract one (AQ1) inhibited the same enzyme by 90.08% when assayed at a concentration of 1 mg/ml compared to Sitagliptin used as the positive control. All samples assayed did not significantly inhibit the enzyme AChE–fraction C3 (combined hydrolzate 3: Krill and M. muelleri) inhibited AChE by 27.48% only. Based on these results samples M1, C3, and AQ1 were selected for further characterization and the IC50 values for each were determined in relation to ACE-1 and DPP-IV inhibition as well as their amino acid composition. Glutamate and aspartate were the most abundant amino acids in the selected samples. IC50 values of <0.2 mg/ml and distinct terminal amino acids were identified in each of the three fractions. The study shows that targeting processing of mesopelagic fish have potential to generate Hydrolysates for use in the prevention of type-2-diabetes and hypertension.
Introduction
The mesopelagic zone refers to the zone of the ocean between 200 and 1,000 m below the surface–the intermediate zone of the ocean. Selected species of zooplankton including Krill are currently harvested and processed from this zone due to the content of omega-3 fatty acids (FAs) including eicosapentaenoic acid (EPA) and docosahexaenoic acid (DHA) that they contain. Indeed, Krill-derived omega-3 supplements are readily available in health stores and are recognized for their associated health benefits. Contrary to krill, the small size and bony structure of mesopelagic fish make them unsuitable for traditional culinary preparations but they may have potential as functional food ingredients. In addition, the global need for new, sustainable protein to feed the growing population makes these under-explored protein sources attractive for researchers to explore further in line with policy documents including those outlined by the UN. The main species of mesopelagic fish include Maurolicus muelleri (common name Silvery light fish), Northern Krill, Benthosema glaciale (Glacier lantern fish), and myctophids (lanternfish).
Some studies conducted to date demonstrate that these fish are a rich source of proteins (Alvheim et al., 2020), lipids (Wang et al., 2018), minerals and bioactives (Lauritano et al., 2020). An average protein content of 16.1 g/100 g fish was reported previously for the mesopelagic species Micromesistius poutassou (common name Blue whiting) while M. muelleri and B. glaciale were reported to contain average protein contents of 12.3 g/100 g of fish and 14 g/100 g fish, respectively (Alvheim et al., 2020). Total fat values were also reported previously and ranged from 17.8 g/100 g of fish for M. muelleri to 3.9 g/100 g fish for M. poutassou (Alvheim et al., 2020). However, fish hauls for mesopelagic species tend to contain mixed species and this poses challenges for processing. For example, fish hauls for mesopelagic species in open waters off the coast of Norway were examined previously and were found to contain mixtures of amphipods that caused the cadmium levels for the recovered biomass when processed into fish feed to exceed the maximum allowable level in feedstuffs (Olsen et al., 2020). Therefore, in addition to enhancing catch and processing methods, processing and refining of mesopelagic fish species for bioactive compounds such as peptides and amino acids may represent an economic and nutritionally sustainable approach to utilize this resource if the resource is managed correctly. Estimates suggest that 6–10 billion metric tons (MT) of mesopelagic fish are available globally (Irigoien et al., 2014). Several projects in the EU and globally continue to evaluate the biomass and estimation methods. Marine proteins have excellent nutritional value and protein quality due to the presence of essential amino acids including leucine, which is known for promoting muscle growth and has potential for use in the development of products to prevent sarcopenia (Gigliotti, 2007; Breen and Churchward-Venne, 2012). Enzymatic hydrolysis of fish and fisheries by-products with enzymes including pepsin, alcalase®, protamex®, and others is a known method to produce highly digestible and bioavailable proteins, peptides and amino acids (Valcarcel et al., 2020). The type of enzyme, rate of hydrolysis and initial raw material composition all have significant impacts on the resulting composition of the generated hydrolysate in terms of peptide and amino acid profiles (Gigliotti, 2007; Chalamaiah et al., 2012). Fish-derived bioactive peptide containing Hydrolysates are considered beneficial for prevention of ailments including high blood pressure (Hayes et al., 2016), type-2 diabetes (Slizyte et al., 2016) and other diseases associated with metabolic syndrome including high cholesterol (Pérez-Gálvez et al., 2015), inflammation and aging (Lees and Carson, 2020). Despite the abundance of mesopelagic fish species, few publications exist concerning the use of this resource for generation of protein-rich and bioactive Hydrolysates with potential to prevent common, human and animal diseases. One study carried out by Lauritano et al. (2020) identified the lanternfish Myctophum punctatum and the Mediterranean krill Meganyctiphanes norvegica as sources of anti-cancer and antimicrobial fractions rich in EPA, DHA, and eicosatetraenoic acid (ETA). The primary objective of this paper was to characterize mesopelagic protein Hydrolysates and extracts developed at Nofima, Norway using enzymatic hydrolysis, ensiling and aqueous extractions methods applied to mesopelagic fish species captured during different commercial trawls. Mesopelagic trawls resulted in the capture of mixed hauls of mesopelagic fish. The potential of mesopelagic Hydrolysates and aqueous fractions developed using this biomass to inhibit enzymes including Angiotensin converting enzyme (ACE-I; EC 3.4.15.1), Dipeptidyl peptidase IV (DPP-IV; 3.4.14.5), and Acetylcholinesterase (AChE; 3.1.1.7) was determined. Inhibition of these enzymes relates to prevention of high blood pressure, type-2-diabetes and nervous system issues, respectively.
Materials and Methods
Chemical and Materials
Mesopelagic samples prepared by Nofima, supplied to Teagasc as either freeze-dried powders or liquid samples, and subsequently stored at −80°C until further use. The ACE-1 (EC 3.4.15.1) inhibition of mesopelagic Hydrolysates and samples was determined using the ACE-1 inhibition colorimetric assay kit supplied by Dojindo Laboratories (Dojindo Laboratories, Kumamoto, Japan). Captopril© was supplied by Sigma Aldrich (Steinheim, Germany). A Fluorometric AChE (EC 3.1.1.7) assay kit purchased from Abcam Chemicals (Cambridge, United Kingdom) was used to study inhibition of AChE activity by mesopelagic samples. Acotiamide dihydrochloride (98% purity) was purchased from Sigma-Aldrich (Dublin, Ireland) and was used as positive control/standard AChE inhibitor. Cambridge Biosciences (Cambridge, England, United Kingdom) supplied the DPP-IV inhibitor screening assay kit, containing the DPP-IV inhibitor and positive control Sitagliptin. All other chemicals supplied were chemical grade and supplied by Sigma-Aldrich (Dublin, Ireland).
Silage, Fishmeal, Hydrolysates, and Aqueous Extract Generation From Mesopelagic Hauls
Silage Preparation
In the preparation of the silage biomass, 2.5 g of Grindox 1032 (tocopherol blend) and 25 g of 99% formic acid were added to 1,000 g of ground raw material. The mixture was stirred at 22°C for 47 h and then heated to 90°C in a microwave oven and kept at 90°C for 10 min. After centrifugation at 20,000 × g for 30 min, the liquid phase was decanted into a separatory funnel, and the oil and water phases were separated.
Fishmeal Process
To 1,000 g of ground raw material were added 2.5 g of Grindox 1032 and 500 g of water. The mixture was heated with stirring to 85°C over 56 min, kept at 85°C for 10 min, then pressed in a tincture press. The liquid phase was then centrifuged and poured into a separatory funnel where the oil phase and the water phase were separated. The sediment after centrifugation was mixed with the press cake and homogenized.
Hydrolysis
To 1,000 g of ground raw material were added 2.5 g of Grindox 1032 and 500 g of water. The mixture was heated with stirring to 55°C over 20 min. 1.1 g of Promod 439L enzyme solution was added and the mixture was stirred at 55°C for 60 min. It was then heated to 90°C over 20 min and kept at that temperature for 10 min. The mixture was then centrifuged at 20,000 × g, 40°C for 20 min. The liquid phase was decanted into a separatory funnel, and the oil and water phases were separated.
Mesopelagic protein Hydrolysates generated using proteolytic enzymes and aqueous extracts (AQ) were generated using processes developed at Nofima in Tromsø, Bergen and Fyllingsdalen, Norway. Briefly, enzymatic hydrolysis was performed using the three commercial enzymes Alkalse 2.4L (Al2.4), Endocut 01-L (01-L), Foodpro PNL (PNL) at optimum temperatures ranging from 50 to 55°C. Additionally, endogenous enzymes (i.e., No additional enzymes at reaction start, abbreviation Endo) were used at 30°C. All enzyme-reactions had identical setups: 1:1 w:v mix with water (∼500 g of each), 1 h hydrolysis time and 15 min deactivation at 90°C. After deactivation, the Hydrolysates were separated by centrifugation (30 min, 7,000 g) into an oil-phase, hydrolysate, and sediment fraction. Weight measurements were performed on all the separate fractions. In a separate method, biomass was processed for fishmeal, Hydrolysates and silage. Aqueous extracts were separated from the solid and lipid fraction of each preparation, freeze-dried and supplied to Teagasc, Ireland for bioactivity assessments in vitro.
The mesopelagic Hydrolysates were abbreviated as Krill (K), M. muelleri (M) and a combination of Krill and M. muelleri (C) and 1–4 based on the enzyme used for hydrolysis i.e., Alcalase, endocut-01, endogenous M/K enzymes, and FoodPro PNL. Further AQ 1–8 were generated. The first three aqueous samples (AQ1–AQ3) were aqueous phase from fishmeal process derived from H4, H6, and H7 trawls, AQ4–AQ6 was aqueous phase from enzymatic process derived from H4, H6, and H7 trawls and AQ7–AQ9 was aqueous phase from Silage process obtained from H4, H6, and H7 trawls.
Proximate Composition of Hydrolysates and Aqueous Extracts
The total protein content of the mesopelagic Hydrolysates and AQ was estimated using two different methods. Initially protein was determined using a LECO FP628 Protein analyzer (LECO Corp., St. Joseph, MI, United States) based on the Dumas method and according to the Association of Official Analytical Chemists (AOAC) method 955.04 (Association of Official Analytical (AOAC), 2000). A nitrogen conversion factor of 6.25 was used to calculate protein content in the samples. The Bicinchoninic Acid (BCA) method was also used in accordance with the manufacturers’ instructions (Sigma-Aldrich, Dublin, Ireland) and according to the method of Walker (1996). The lipid content of samples was quantified using AOAC Method 2008.06 with an Oracle rapid NMR fat analyzer. Samples were prepared according to standard procedures used for these analyses as described previously (Association of Official Analytical (AOAC), 1998).
ACE-1 Inhibition Assay–Determination of Potential Heart Health Effects
The ACE-1 inhibition bioassay of mesopelagic protein Hydrolysates and AQ was carried out as described previously (Naik and Hayes, 2019) using the ACE-1 inhibition assay kit from WST, Dojindo Laboratories, Kumamoto, Japan.1 In brief, 20 μL of each test sample aqueous solution at a concentration of 1 mg/ml was added to 20 μL of substrate and 20 μL of enzyme working solution in triplicate. Captopril was used as a positive control at a concentration of 0.05 μM as recommended by the manufacturer’s. Samples were incubated at 37°C for 1 h. A 200 μL volume of indicator working solution was then added to each well, and subsequent incubation at room temperature was carried out for 10 min. Absorbance at 450 nm was read using a FLUOstar Omega microplate reader (BMG LABTECH GmbH, Offenburg, Germany). The percentage of inhibition was calculated using the following equation:
where, A0 is the substrate absorbance at 450 nm in the presence of ACE-I and absence of inhibitor, and AI is the substrate absorbance at 450 nm in the presence of ACE-I and the inhibitor or the positive control Captopril©. Activity was expressed as mean IC50 value (inhibitory concentration that inhibits ACE-1 by 50%) ± SD from three independent replicates (n = 3).
AChE Inhibition by Mesopelagic Samples–Determination of Anticholinesterase Activity
The AChE Fluorometric assay kit from Abcam, Cambridge, United Kingdom,2 was used to study inhibition of AChE activity by mesopelagic samples to assess their potential to impact positively on mental health. The assays was carried out in accordance with the manufacturers’ instructions. Acotiamide dihydrochloride was used as the standard, positive control AChE inhibitor. Fluorescence was recorded at an excitation and emissions wavelength of 490/520 nm. Inhibition percentage for all the samples were calculated from these fluorescence values as described previously (Naik et al., 2020). Activity was expressed as mean IC50 value (inhibitory concentration that inhibits AChE by 50%) ± SD from three independent replicates (n = 3).
DPP-IV Inhibition by Mesopelagic Samples–Determination of Potential Anti-Diabetic Activity
This assay was carried out using a DPP-IV inhibitor screening assay kit in accordance with the manufacturer’s instructions.3 Fluorescence intensity was recorded with a FLUOstar Omega microplate reader (BMG Labtech, Ortenburg, Germany) using an excitation wavelength of 355 nm and an emission wavelength of 460 nm. Sitagliptin was used as a positive control and it has a known IC50 value of 19 mM (Thomas et al., 2008). DPP-IV inhibition was defined as the percentage of DPP-IV activity inhibited by a given concentration of peptide compared to the control. Activity was expressed as mean IC50 value (inhibitory concentration that inhibits DPP-IV by 50%) ± SD from three independent replicates (n = 3). 10 μL of each sample inhibitor (at a concentration of 1 mg/ml assay buffer) was added to 30 μL diluted assay buffer followed by 10 μL diluted DPP-IV, and 50 μL substrate solution (n = 3). Samples were incubated at 37°C for 30 min. Fluorescence was read with excitation wavelengths of 355 nm and emission wavelengths of 460 nm using a FLUOstar Omega microplate reader (BMG LABTECH GmbH, Offenburg, Germany). The percentage inhibition was calculated using the following equation:
Amino Acid Composition of the Bioactive Peptides
Determination of the total amino acid composition of the Hydrolysates was done by further hydrolyzing Hydrolysates using 6 M HCL at 100°C for 24 h (Fountoulakis and Lahm, 1998). The samples were then de-proteinized by mixing equal volumes of 24% (w/v) tri-chloroacetic acid and sample. These were allowed to stand for 10 min at room temperature before centrifugation at 14,400 × g for 10 min. The supernatants were removed and diluted with 0.2 M sodium citrate buffer, pH 2.2 to give approximately 250 nmol of each amino acid residue. Samples were then diluted 1:2 with the internal standard nor-leucine to give a final concentration of 125 nm/ml. Amino acids were quantified using a UHPLC-DAD, Agilent 1290. Two different System Suitability Tests (SSTs) were measured along with the samples to verify the calibration. Every sample was measured in duplicate (n = 2).
Statistical Analysis
All assays were performed in triplicate (n = 3). Excel was used for determination of the standard deviation values. Graphpad prism was used to perform one way ANOVA using Tukey’s multiple comparison test at α = 0.05.
Results and Discussion
Proximate Composition
All mesopelagic Hydrolysates had protein contents greater than 70% based on dry weight (Table 1). The protein content of the Hydrolysates varied between 72.34 ± 0.05 (K3) and 77.76 ± 0.19 (M1) g/100 g hydrolysate on dry weight basis. The ash content of the Hydrolysates varied between 13.66 ± 1.9 (M1) and 19.44 ± 0.66 (M3) while the lipid content was low at <0.8% for all the samples analyzed (Figure 1). The AQ were analyzed for protein and these varied between 61.59 ± 1.17 g protein/100 g of sample for K1 to 75.41 ± 0.70 g/100 g for aqueous extract M4 on a dry weight basis. Thus, the M. muelleri Hydrolysates and AQ had a greater percentage protein content than the krill samples. Fish protein Hydrolysates (FPH) are amorphous powders that are hygroscopic and usually contain 81–93% protein, <5% fat and 3–8% ash, and 1–8% moisture (Venugopal, 2016). The protein values obtained in this work are less than what is required for an ideal FPH and ash content is greater than 8%, which would limit applications in food. However, as protein content for all Hydrolysates of the studied mesopelagic species was greater than 70% they could be a valuable source for marine protein with high nutritional value.
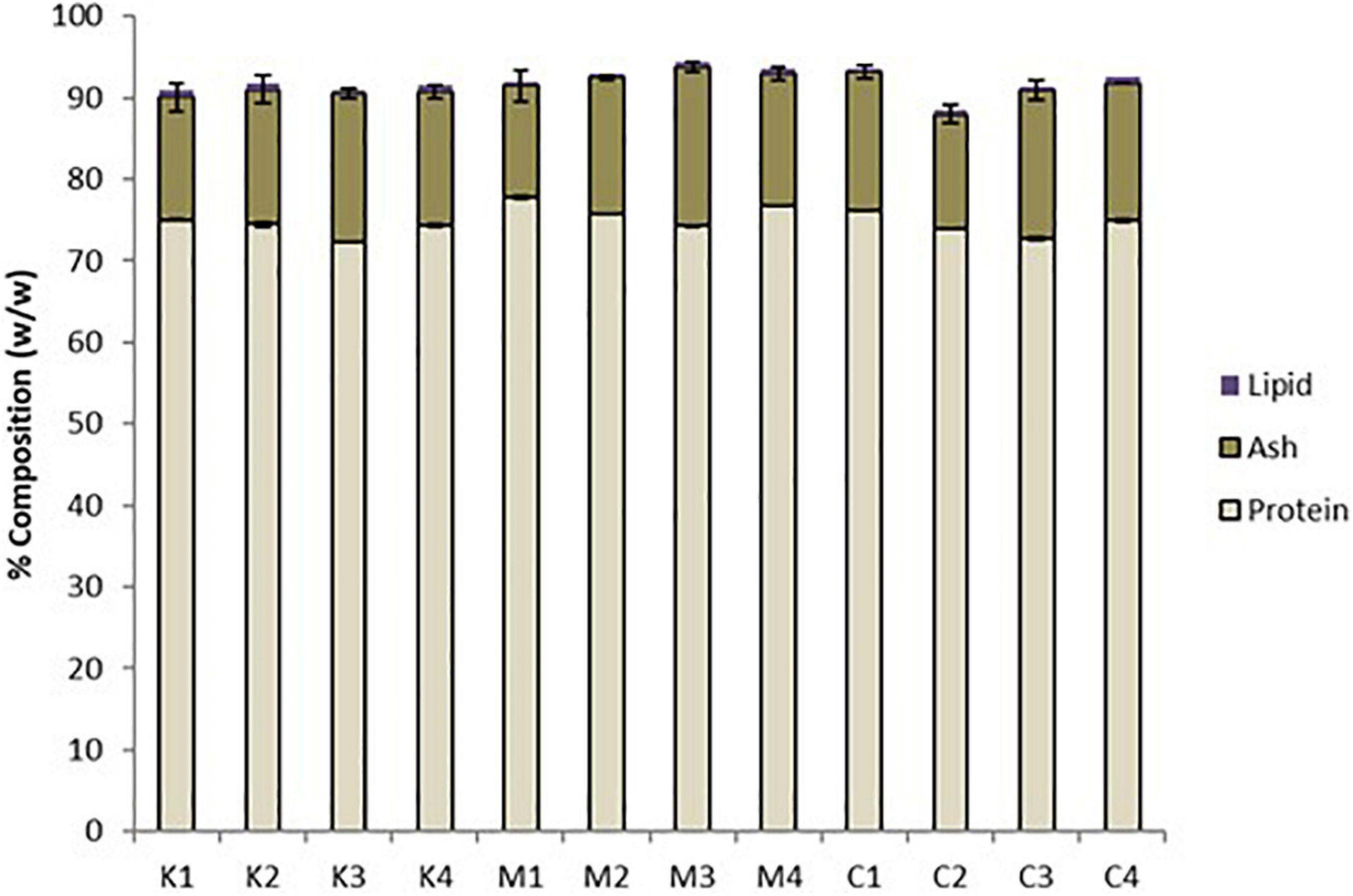
Figure 1. Proximate composition of mesopelagic Hydrolysates derived from Krill (K), M. muelleri (M), and a combination of Krill and M. muelleri (C) by method 955.04 (Association of Official Analytical (AOAC), 2000; n = 3). One way ANOVA using Tukey’s multiple comparison test at α = 0.05 showed no significant differences between protein, ash, and lipid content of the 12 mesopelagic Hydrolysates.
Enzyme Inhibition Bioassays
ACE-1 Inhibition
The mesopelagic Hydrolysates and the AQ all inhibited ACE-1 when tested at a concentration of 1 mg/ml. Inhibition of ACE-1 varied from 76.75 ± 4.7% to 95.28 ± 0.88% (Figures 2A,B). Sample M4 (the hydrolysate generated from M. muelleri using FoodPro PNL) inhibited ACE-1 by 89.56%. Second to this in terms of ACE-I inhibition was the hydrolysate generated from Krill using Alcalase (K1) and the combined sample generated from Krill and M. muelleri (C3; using endogenous enzymes). The aqueous extract AQ2 inhibited ACE-1 by 95.28% when assayed at a concentration of 1 mg/ml compared to the positive control Captopril. ACE-1 regulates peripheral blood pressure mainly through the renin–angiotensin and Kinin-nitric oxide systems. It also inactivates the vasodilator, bradykinin (Li et al., 2017). Inhibition of ACE-1 is a target for prevention of hypertension; these results demonstrate that mesopelagic extracts and Hydrolysates have potential for further development as anti-hypertensive agents in functional foods or supplements. However, further characterization of the active peptides and in vivo trials would be required for commercialization. The IC50 values obtained for all fractions tested for ACE-1 inhibition were all less than 0.2 mg/ml. This value compares favorably with other studies that have investigated ACE-1 inhibitors from fish hydrolysate sources (Salampessy et al., 2017). The low IC50 values may be due to synergetic/cumulative effect of various active peptides present in each hydrolysate or aqueous extract, and highlights the need for further identification and characterization of peptides.
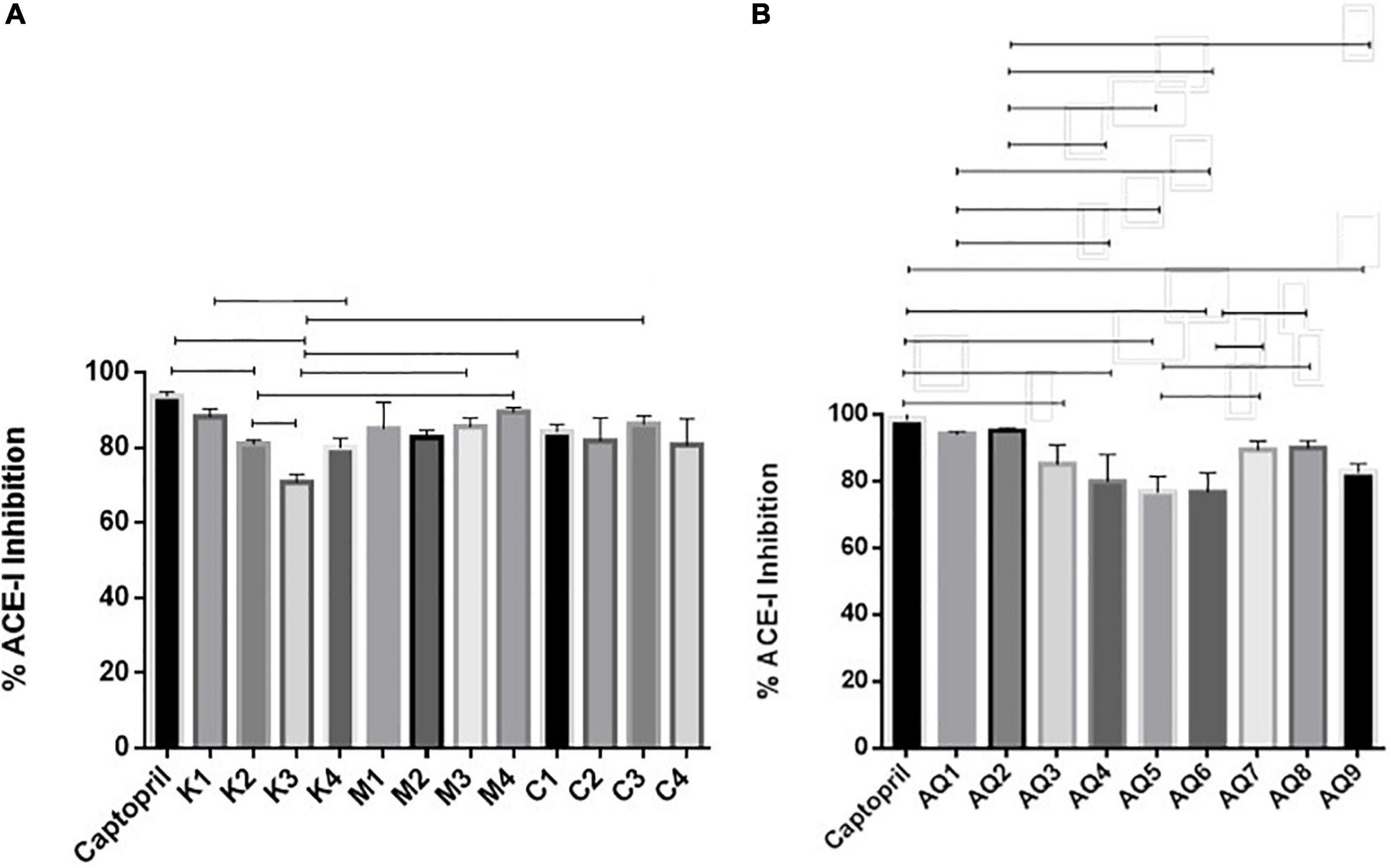
Figure 2. Angiotensin converting enzyme (ACE-1) inhibition by mesopelagic hydrolysates (A) and aqueous extracts (B) derived from Krill (K), M. muelleri (M) and a combination of both (C). AQ, aqueous extracts; (n = 3). One way ANOVA was done using Tukey’s multiple comparison test at α = 0.05.
DPP-IV Inhibition
Samples M1 and AQ1 were found to inhibit DPP-IV by 100 and 90.08%, respectively, when assayed at a concentration of 1 mg/ml, and compared to the commercial, positive control and drug Sitagliptin (Figures 3A,B). DPP-IV breaks down the incretins–glucagon-like peptide-1 and the glucose-dependent insulinotropic polypeptide. These hormones can increase insulin secretion during the post-prandial phase and inhibition of DPP-IV can prevent the degradation of incretins and improve serum glucose regulation (Sarteshnizi et al., 2021). As discussed recently by Sarteshnizi et al. (2021) and previously by Lacroix and Li-Chan (2012) Hydrolysates with ability to inhibit DPP-IV often contain hydrophobic amino acids and/or proline or alanine amino acids as part of their peptide sequences. As shown in Table 2, all samples tested for inhibition of DPP-IV contained 2–3.71 g proline/100 g sample and 3.22–3.86 g alanine/100 g sample. The DPP-IV values observed in this study compare favorably with previously published work where dairy proteins were assessed for ability to inhibit DPP-IV (Lacroix and Li-Chan, 2012).
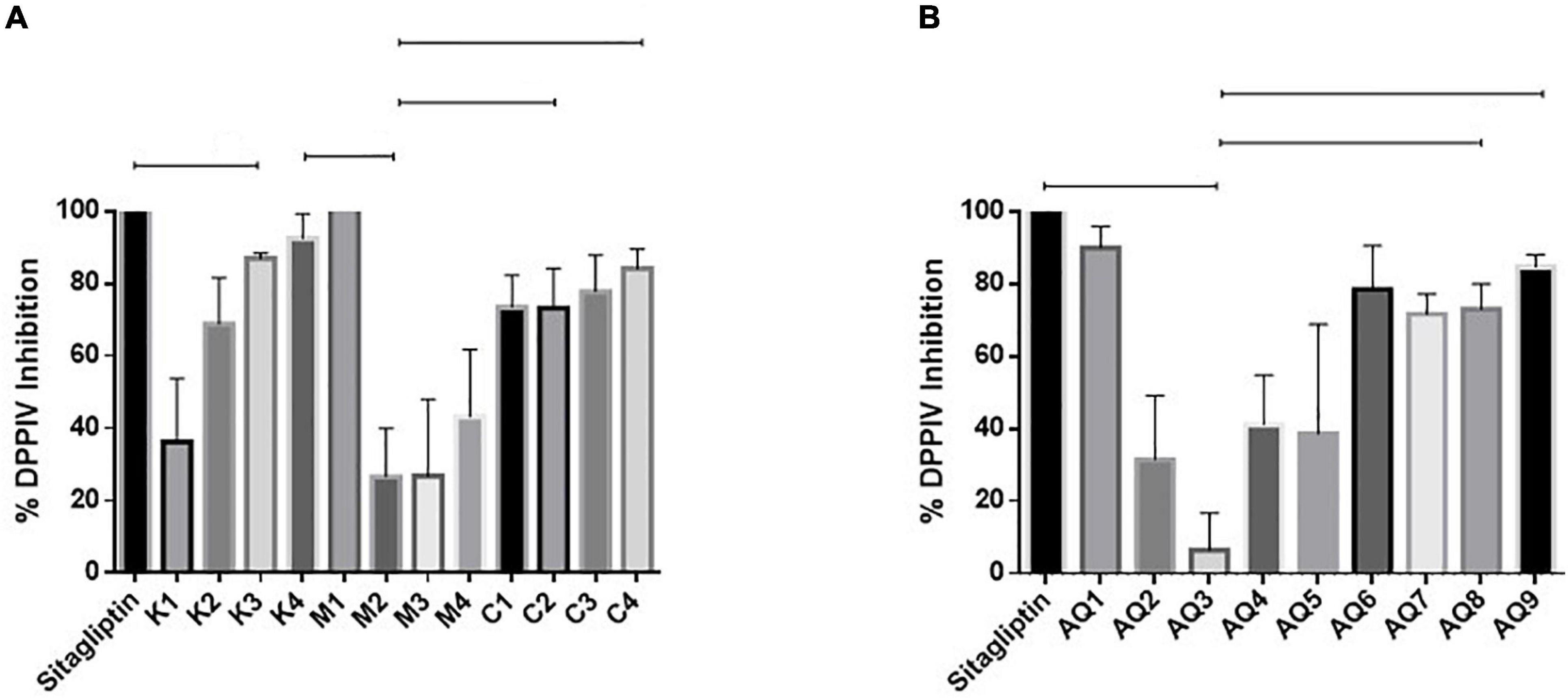
Figure 3. (A,B) Dipeptidyl peptidase enzyme (DPP-IV) inhibition by mesopelagic Hydrolysates generated from Krill (K), M. muelleri (M), and a combination of both (C). Aqueous extracts (AQ). (A) One way ANOVA was done using Tukey’s multiple comparison test at α = 0.05.
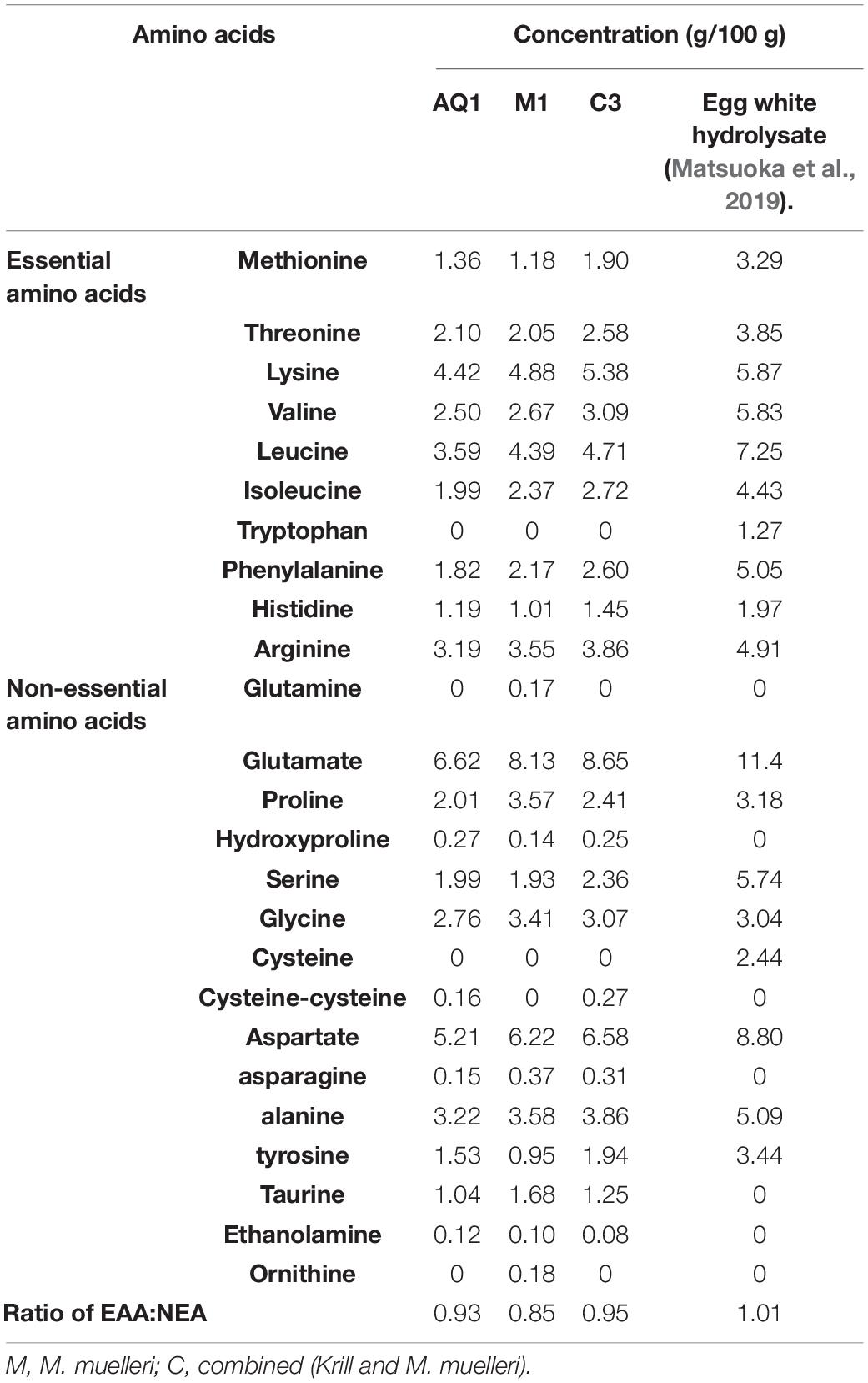
Table 2. Amino acid composition (mM) of mesopelagic Hydrolysates M1 and C3 and aqueous extract AQ1 compared to egg white hydrolysate.
Acetylcholinesterase Inhibition
AChE-targeted therapy may assist in prevention of memory degeneration (Zhao et al., 2017). Samples C4 and AQ4 were found to inhibit AChE by 27.48 and 25.43% when assayed at a concentration of 1 mg/ml, respectively, compared to the positive control Acotiamide dihydrochloride (Figures 4A,B). Zhao et al. (2017) previously observed the anti-acetylcholinesterase activities of anchovy (Coilia mystus) protein Hydrolysates. An AChE IC50 value of 159.76 ± 0.03 mg ml–1 was observed in this study and was attributed to the content of lysine in the hydrolysate. Values observed in this study did not warrant further investigation into the AChE IC50 value of the Hydrolysates and AQ and percentage inhibition values were all less than 50% when samples were assayed at a concentration of 1 mg/ml (Figure 5). Based on the obtained bioactivity results, samples M1, C3, and AQ1 were characterized further for their amino acid composition.
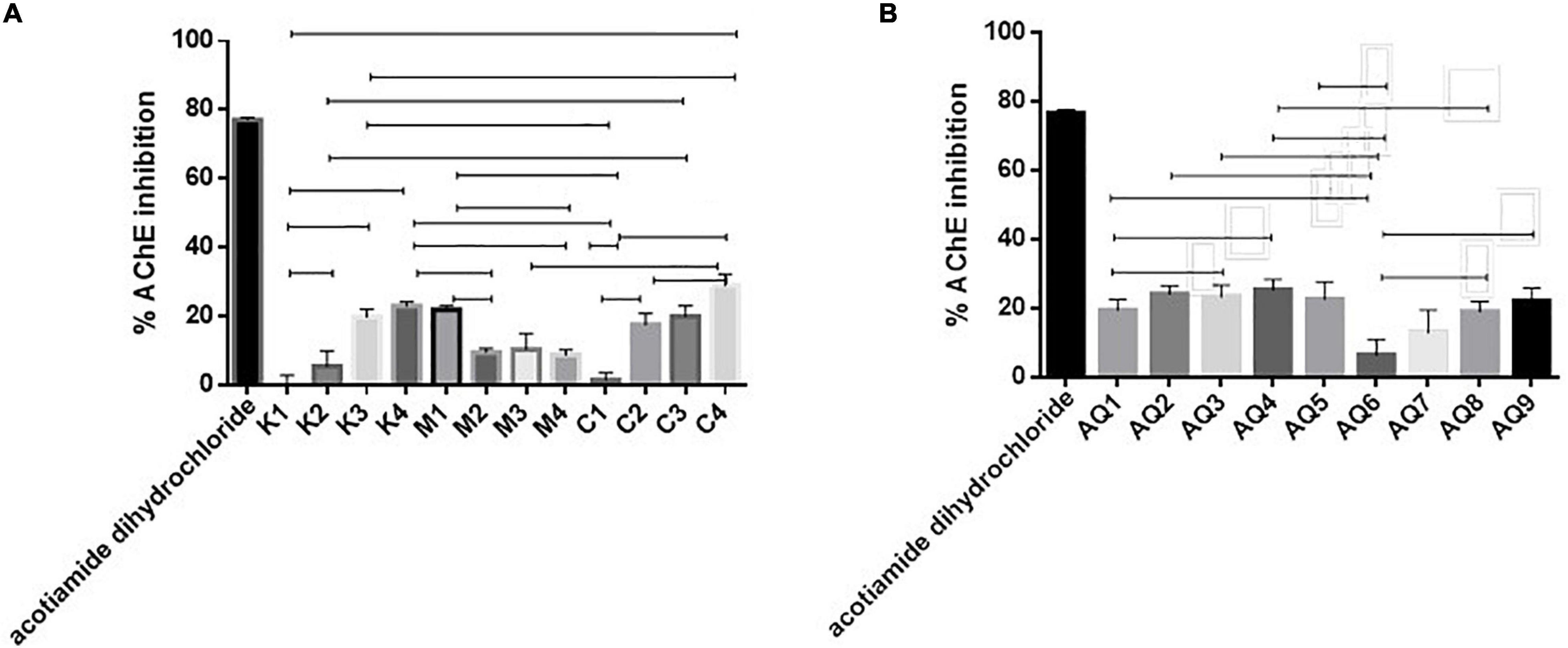
Figure 4. (A,B) Acetylcholine-esterase enzyme (AChE) inhibition by mesopelagic Hydrolysates generated from Krill (K), M. muelleri (M), and a combination of both and aqueous extracts (AQ) (n = 3). One way ANOVA was done using Tukey’s multiple comparison test at α = 0.05, where all the aqueous extracts (AQ) gave significantly less inhibition when compared to the positive control inhibitor acotiamide dihydrochloride. Amongst the Hydrolysates inhibition differences have been included on the graph.
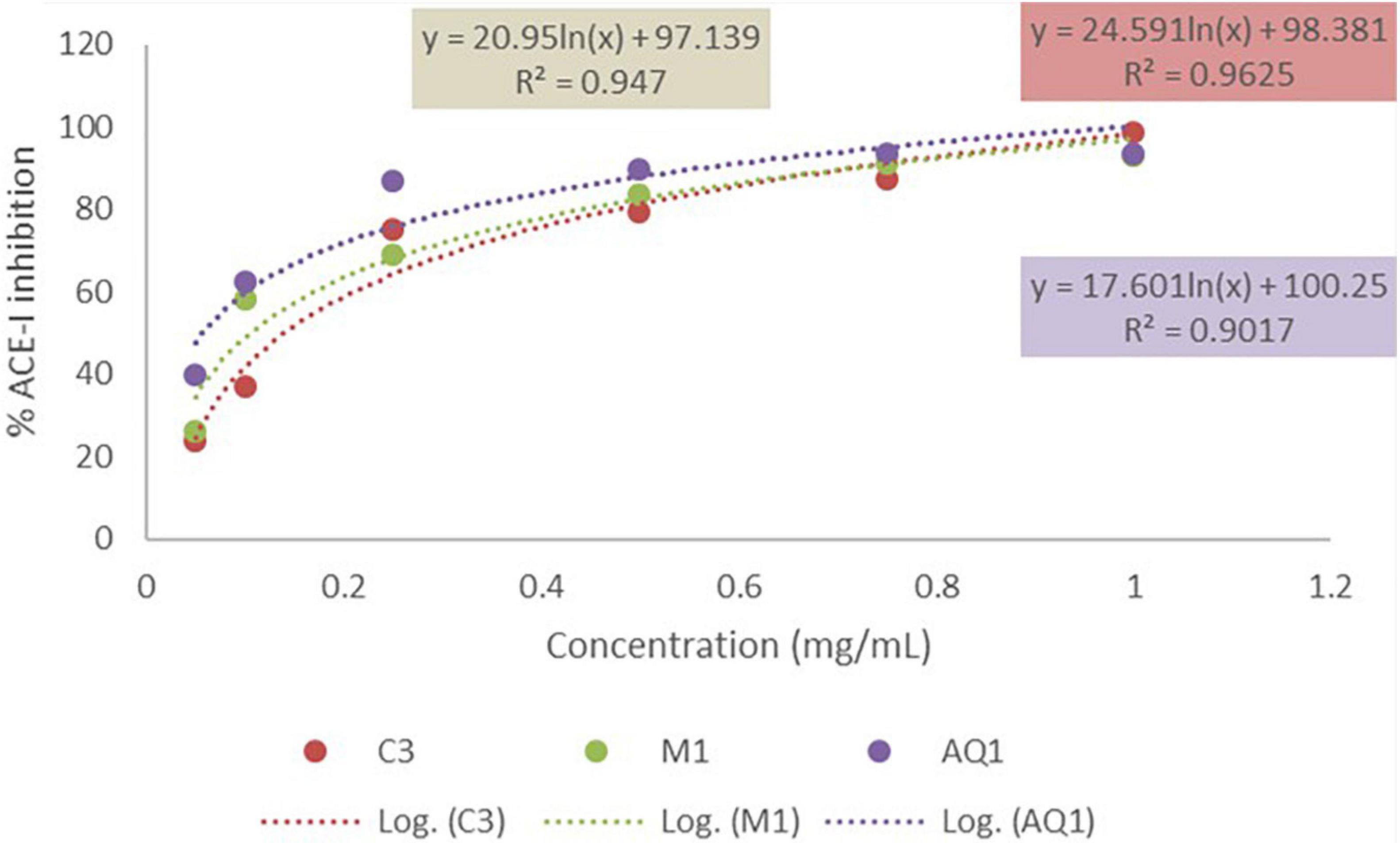
Figure 5. IC50 valus of mesopelagic hydrolysates MI and C3 and aqueous extract AQ1 for Angiotensin-1-converting enzyme (ACE-1) (n = 3). C, combined (Krill and M. muelleri).
Amino Acid Composition
Mesopelagic Hydrolysates and aqueous extracts M1, C3, and AQ1 were characterized in terms of their total amino acid composition (TAA, Table 2). All known essential amino acids with the exception of tryptophan was detected. The TAA g/100 g values were lower than the values observed for an egg white hydrolysate (Matsuoka et al., 2019) and the overall protein content of the Hydrolysates were also less than that observed for egg white hydrolysate. Previous studies where Hydrolysates were generated from herring by-products found that the essential amino acids Asp and Glu (cationic amino acids) were present in the greatest quantities and Arg (anionic amino acids), previously (Durand et al., 2020). Anti-inflammatory activities were observed in cell studies with these Hydrolysates. The ratio of essential to non-essential amino acid was highest for the C3 hydrolysate (0.95), followed by AQ1 extract (0.93), and lowest for M1 hydrolysate at 0.85 as seen in Table 2. At 0.95 the ratio was close to the ideal ratio of 1 found in egg and milk protein and was better than plant sources like soy, pea, hemp protein and even some marine proteins (Matsuoka et al., 2019). Lysine was the most abundant essential amino acid in all three samples, and was highest in hydrolysate C3 at 5.38 g/100 g hydrolysate. Thus, sample C3 showed a favorable amino acid profile in comparison to the other two samples. Glutamate, often associated with umami taste of food products, were found to be the most abundant amino acid in the samples analyzed followed by aspartate, which was found in all the Hydrolysates. Specific amino acids such as L-histidine are known to be bitter-tasting, L-glutamate, a non-essential amino acid is a source of umami taste and L-threonine (essential amino acid) contributes to the sweet profile of foods in which it is found (Bachmanov et al., 2016). The sensory acceptability of the final product would thus depend on the prevalence of these individual amino acids.
Conclusion
Mesopelagic fish are an underutilized marine resource that show potential for sustainable exploitation as biomass for functional food ingredient development. The mesopelagic Hydrolysates and AQ generated in this work displayed an ability to inhibit ACE-1, DPP-V, and AChE and hold potential for further, functional food/supplement development. In addition, the results show that, without modification, the mesopelagic fish Hydrolysates and AQ have nutritive value due to their protein contents (all >70%) and total amino acid compositions, which are comparable to egg white protein. Further characterization work is required if the three Hydrolysates and aquatic extracts are to be developed as alternatives to anti-hypertensive drugs such as Captopril© and anti-diabetic drugs such as Sitagliptin including mass spectrometry characterization of the peptides and in vivo trials in animals and humans. However, this work is valuable as it is the first report detailing the development of mesopelagic fish as Hydrolysates with bioactivities to potentially improve human health.
Data Availability Statement
The raw data supporting the conclusions of this article will be made available by the authors, without undue reservation.
Author Contributions
ASN: data curation, formal analysis, investigation, methodology, visualization, validation, and writing—original draft. MH: conceptualization, writing, funding acquisition, project administration, supervision, review, and editing. All authors contributed to the article and approved the submitted version.
Funding
This manuscript and described work have been financed by the EU H2020 MEESO Project “Ecologically and Economically Sustainable Mesopelagic Fisheries” Grant Agreement No. 817669.
Conflict of Interest
The authors declare that the research was conducted in the absence of any commercial or financial relationships that could be construed as a potential conflict of interest.
Publisher’s Note
All claims expressed in this article are solely those of the authors and do not necessarily represent those of their affiliated organizations, or those of the publisher, the editors and the reviewers. Any product that may be evaluated in this article, or claim that may be made by its manufacturer, is not guaranteed or endorsed by the publisher.
Footnotes
- ^ https://www.dojindo.eu.com/TechnicalManual/Manual_A502.pdf
- ^ https://www.abcam.com/acetylcholinesterase-assay-kit-fluorometric-green-ab138872.html
- ^ https://www.abcam.com/dipeptidyl-peptidase-iv-dpp4-inhibitor-screening-assay-kit-ab133081.html
References
Alvheim, A. R., Kjellevold, M., Strand, E., Sanden, M., and Wiech, M. (2020). Mesopelagic species and their potential contribution to food and feed security-a case study from Norway. Foods 9, 344–363. doi: 10.3390/foods9030344
Association of Official Analytical (AOAC) (1998). Official Methods of Analysis of AOAC International. Maryland: AOAC International.
Association of Official Analytical (AOAC) (2000). Official Methods of Analysis. The Association of Official Analytical Chemists, 17th Edn, Gaithersburg, MD: AOAC.
Bachmanov, A. A., Bosak, N. P., Glendinning, J. I., Inoue, M., Li, X., Manita, S., et al. (2016). Genetics of amino acid taste and appetite. Adv. Nutr. 7, 806S–822S.
Breen, L., and Churchward-Venne, T. A. (2012). Leucine: a nutrient ‘trigger’ for muscle anabolism, but what more? J. Physiol. 590, 2065–2066. doi: 10.1113/jphysiol.2012.230631
Chalamaiah, M., Kumar, D. B., Hemalatha, R., and Jyothirmayi, T. (2012). Fish protein hydrolysates: proximate composition, amino acid composition, antioxidant activities and applications: a review. Food Chem. 135, 3020–3038. doi: 10.1016/j.foodchem.2012.06.100
Durand, R., Pellerin, G., Thibodeau, H., Fraboulet, E., Marette, A., and Bazinet, L. (2020). Screening for metabolic syndrome applications of a herring by-product hydrolysate after its separation by electrodialysis with ultrafiltration membranes and identification of novel anti-inflammatory peptides. Separat. Purif. Technol. 235:116205. doi: 10.1016/j.seppur.2019.116205
Fountoulakis, M., and Lahm, H. W. (1998). Hydrolysis and amino acid composition of proteins. J. Chromatogr. A 826, 109–134. doi: 10.1016/s0021-9673(98)00721-3
Gigliotti, J. C. (2007). “Determination of the Nutritional Value, Protein Quality and Safety of Krill Protein Concentrate Isolated Using an Isolelectric Solubilization/Precipitation Technique” Graduate Theses, Dissertations, and Problem Reports. 2555. Available online at: https://researchrepository.wvu.edu/etd/2555 (accessed February 24, 2021).
Hayes, M., Mora, L., Hussey, K., and Aluko, R. E. (2016). Boarfish protein recovery using the pH-shift process and generation of protein hydrolysates with ACE-I and antihypertensive bioactivities in spontaneously hypertensive rats. Innov. Food Sci. Emerg. Technol. 37, 253–260. doi: 10.1016/j.ifset.2016.03.014
Irigoien, X., Klevjer, T. A., Røstad, A., Martinez, A., Boyra, G., Acuna, J. L., et al. (2014). Large mesopelagic fishes biomass and trophic efficiency in the open ocean. Nat. Commun. 5, 3271–3282.
Lacroix, I. M. E., and Li-Chan, E. C. Y. (2012). Dipeptidyl peptidase-IV inhibitory activity of dairy protein hydrolysates. Intern. Dairy J. 25, 97–102. doi: 10.1016/j.idairyj.2012.01.003
Lauritano, C., Martínez, K. A., Battaglia, P., Granata, A., de la Cruz, M., Cautain, B., et al. (2020). First evidence of anticancer and antimicrobial activity in Mediterranean mesopelagic species. Sci. Rep. 10:4929. doi: 10.1038/s41598-020-61515-z
Lees, M. J., and Carson, B. P. (2020). The potential role of fish-derived protein hydrolysates on metabolic health, skeletal muscle mass and function in ageing. Nutrients 12, 2434–2440. doi: 10.3390/nu12082434
Li, X., Zhang, C., and Zhuo, J. L. (2017). The vasoprotective axes of the renin-angiotensin system: physiological relevance and therapeutic implications in cardiovascular, hypertensive and kidney diseases. Pharmacol. Res. 125, 21–38. doi: 10.1016/j.phrs.2017.06.005
Matsuoka, R., Kurihara, H., Nishijima, N., Oda, Y., and Handa, A. (2019). Egg white hydrolysate retains the nutritional value of proteins and is quickly absorbed in rats. Sci. World J. 27, 1–6. doi: 10.1155/2019/5475302
Naik, A. S., and Hayes, M. (2019). Bioprocessing of mussel by-products for value added ingredients. Trends Food Sci. Technol. 92:111–121. doi: 10.1016/j.tifs.2019.08.013
Naik, A. S., Mora, L., and Hayes, M. (2020). Characterisation of seasonal Mytilus edulis By-products and generation of bioactive hydrolysates. Appl. Sci. 10, 6892–6899. doi: 10.3390/app10196892
Olsen, R. E., Strand, E., Melle, W., Nørstebø, J. T., Lall, S. P., Ringø, E., et al. (2020). Can mesopelagic mixed layers be used as feed sources for salmon aquaculture? Deep Sea Res. Part II Top. Stud. Oceanogr. 180, 104722–104732. doi: 10.1016/j.dsr2.2019.104722
Pérez-Gálvez, R., García-Moreno, P. J., Morales-Medina, R., Guadix, A., and Guadix, E. M. (2015). Bile acid binding capacity of fish protein hydrolysates from discard species of the West Mediterranean Sea. Food Funct. 6, 1261–1267. doi: 10.1039/c4fo01171f
Salampessy, J., Reddy, N., Phillips, M., and Kailasapathy, K. (2017). Isolation and characterisation of nutraceutically potential ACE-1 inhibitory peptides from leatherjacket (Meuchenia sp.) protein hydrolysates. LWT 80, 430–436. doi: 10.1016/j.lwt.2017.03.004
Sarteshnizi, R. A., Ali Sahari, M., Gavlighi, H. A., Regenstein, J. M., Nikoo, M., and Udenigwe, C. C. (2021). Influence of fish protein hydrolysate-pistachio green hull extract interactions on antioxidant activity and inhibition of α-glucosidase, α-amylase, and DPP-IV enzymes. LWT 142:111019. doi: 10.1016/j.lwt.2021.111019
Slizyte, R., Rommi, K., Mozuraityte, R., Eck, P., Five, K., and Rustad, T. (2016). Bioactivities of fish protein hydrolysates from defatted salmon backbones. Biotechnol. Rep. 11, 99–109. doi: 10.1016/j.btre.2016.08.003
Thomas, L., Eckhardt, M., Langkopf, E., Tadayyon, M., Himmelsbach, F., and Mark, M. (2008). (R)-8-(3-amino-piperidin-1-yl)-7-but-2-ynyl-3-methyl-1-(4-methyl-quinazolin-2-ylmethyl)-3,7-dihydro-purine-2,6-dione (BI 1356), a novel xanthine-based dipeptidyl peptidase 4 inhibitor, has a superior potency and longer duration of action compared with other dipeptidyl peptidase-4 inhibitors. J. Pharmacol. Exp. Ther. 325, 175–182. doi: 10.1124/jpet.107.135723
Valcarcel, J., Sanz, N., and Vazquez, J. A. (2020). Optimization of the enzymatic protein hydrolysis of by-products from Seabream (Sparus aurata) and Seabass (Dicentrarchus labrax), chemical and functional characterization. Foods 9:1503. doi: 10.3390/foods9101503
Venugopal, V. (2016). Marine Enzymes Biotechnology: Production and Industrial Applications, Part 1 – Production of Enzymes (Advances in Food and Nutrition Research). Cambridge, MA: Academic Press.
Walker, J. M. (1996). “The Bicinchoninic Acid (BCA) assay for protein quantitation,” in The Protein Protocols Handbook. Springer Protocols Handbooks, ed. J. M. Walker (Totowa, NJ: Humana Press), doi: 10.1007/978-1-60327-259-9_3
Wang, F., Wu, Y., Cui, Y., Chen, Z., Li, Z., Zhang, J., et al. (2018). Factors affecting the fatty acid composition of mesopelagic fish of the continental slope in the South China Sea. PeerJ [Preprints] 6:e26498v1. doi: 10.7287/peerj.preprints.26498v1
Keywords: mesopelagic fish, Maurolicus muelleri, Northern Krill, ACE-1 inhibition, DPP-IV, type-2-diabetes
Citation: Naik AS, Whitaker RD, Albrektsen S, Solstad RG, Thoresen L and Hayes M (2021) Mesopelagic Fish Protein Hydrolysates and Extracts: A Source of Novel Anti-Hypertensive and Anti-Diabetic Peptides. Front. Mar. Sci. 8:719608. doi: 10.3389/fmars.2021.719608
Received: 02 June 2021; Accepted: 29 July 2021;
Published: 03 September 2021.
Edited by:
Wolfram Brück, University of Applied Sciences and Arts of Western Switzerland, SwitzerlandReviewed by:
Gaurav Kumar Pal, Kriti Nutrients Limited, IndiaJiajun Wu, City University of Hong Kong, Hong Kong, SAR China
Copyright © 2021 Naik, Whitaker, Albrektsen, Solstad, Thoresen and Hayes. This is an open-access article distributed under the terms of the Creative Commons Attribution License (CC BY). The use, distribution or reproduction in other forums is permitted, provided the original author(s) and the copyright owner(s) are credited and that the original publication in this journal is cited, in accordance with accepted academic practice. No use, distribution or reproduction is permitted which does not comply with these terms.
*Correspondence: Maria Hayes, bWFyaWEuaGF5ZXNAdGVhZ2FzYy5pZQ==