- 1MARE - Marine and Environmental Sciences Centre, Faculdade de Ciências, Universidade de Lisboa, Lisbon, Portugal
- 2Departamento de Biologia Animal, Faculdade de Ciências, Universidade de Lisboa, Lisbon, Portugal
Echinoid feeding biology is well known but their sluggish responses to chemical stimuli have turned them into inadequately worked in the field of chemoreception. Echinoid responses to chemical stimulation had allowed, so far, only qualitative analyses based on tube-feet activity, directional, or masticatory movements, and artificial agarose foods. Besides stimulation through plumes of dissolved organic compounds and response analysis based on tube-feet activity, we propose another method to chemically stimulate echinoids that allows for fast and unambiguous responses and thus, quantitative analyses. Small squared pieces of absorbent semi-synthetic cleaning cloths, soaked with specific chemical compounds (simulacra), such as water insoluble lipid oils, were deposited singly or concurrently with a blank on the aboral hemisphere of each sea urchin, allowing choice and eventual transport down to the mouth by tube feet and spines of one or both cloths. The responsiveness of Paracentrotus lividus was clearly dependent on its nutritional state. Well-fed sea urchins (maize whole grains) rarely responded, while the ones fed with less caloric rations (Kombu seaweed) responded faster and objectively. Stimulating sea urchin P. lividus with 41 different food-related compounds, such as carbohydrates, proteins, peptides and amino acids, oils and fatty acids, and purified chemicals related with some human basic tastes, it was possible to evidence a clear ability of this echinoid species to positively discriminate proteins, starches, and a very few oils. Perceived as incitants/stimulants we have only found among proteins gliadin (from wheat gluten) but not casein (from bovine milk), among polysaccharides starch but not laminarin (from kelp) or glycogen (from mussels), and among lipids only the fatty acid linolenic acid. Among tissues, Kombu alga flesh and mussel flesh were readily perceived as both incitant/stimulant but not Kombu and mussel extracts. Therefore, the combined results reported here provide evidence for P. lividus as an omnivorous species rather than a strictly herbivorous marine species. However, the restricted group of food-related compounds perceived by this species as incitants or suppressants and as stimulants or deterrents was shown to be remarkably related to other vertebrates whose kinship was confirmed by the sequencing of the genome of another plant-eater sea urchin.
Introduction
Sea urchin feeding ecology and preferences have been studied for many years, but their olfactory ability and relation to feeding behaviour still remain lesser-known subjects. Under laboratory conditions, there have been several observations of echinoderm capacity to identify and locate food items (Zafirou, 1972; Teruya et al., 2001). However, echinoid responses to chemical stimulation have allowed, so far, only qualitative analyses based on tube feet activity or directional and masticatory movements.
Few analytical studies have been conducted on echinoids using extracts and food-related chemicals such as amino acids, but very little has been done on the precise characterisation of the chemicals stimulatory to echinoids (Sloan and Campbell, 1982). Several authors have used complex natural food components to stimulate chemotactic behaviour (Hay et al., 1986). Hydrophobic volatile molecules have thus, been shown to have some attractive importance to Strongylocentrotus droebachiensis (Mann et al., 1984). Also, evidence has established carbohydrates (galactose) as phagostimulatory to Lytechinus variegatus (Klinger and Lawrence, 1984). In most echinoderms, compounds with low molecular weight, notably amino acids and amines, make up the primary feeding stimulants (Whittle and Blumer, 1970; Mann et al., 1984; McClintock et al., 1984). However, the precise characterisation of chemicals stimulatory to echinoids is still a wide-open field in which knowledge of chemical stimulants derived from algal food would be particularly useful (Sloan and Campbell, 1982).
According to Morris (1968), Chlorophyta: Chlorophyceae accumulate “true” starch as a reserve product, and cell walls invariably contain cellulose; Phaeophyta: Phaeophyceae include laminarin and mannitol as food storage products and alginic and fucinic acids as characteristic cell wall constituents; Rhodophyta: Rhodophyceae include floridean starch (which is somewhat similar to amylopectin) and galactoside floridoside as food storage products and polysulphate esters (agar-agar) as characteristic components of cell walls.
Based on those assumptions and as there are no known descriptions of stimulants specific for echinoids in general, we tested the capacity of P. lividus (Echinodermata: Echinoidea) to distinguish between dissolved food-related chemicals. Therefore, whenever available for acquisition, all those purified algal food storage products and cell wall components, as well as their respective constituent monomers, were tested to precise the chemicals stimulatory to this echinoid, generally acknowledged as a plant eater (Boudouresque and Verlaque, 2007). An animal polysaccharide, glycogen from mussels, was also tested and compared. Besides the emphasis put on carbohydrates, peptides, proteins, and their respective mixtures of amino acids resultant from hydrolysates, and fatty oils, both of plant and animal origins, were also put on test. Finally, purified chemicals related with human basic tastes (sweet, bitter, and acidic) were also examined given the acknowledged evolutionary relationship of modern echinoderms with vertebrates (Barnes, 1980).
So far, in studies on aquatic chemical perception, the common practice involves observing animals when exposed to diluted extracts and their component molecules. We also used this approach with several diluted chemicals and observed subsequent podia and spine motion, but their significance as a potential selective food intake could hardly be anticipated. In attempts to stimulate sea urchins with water insoluble lipid oils, we had to devise a different methodological procedure for presenting chemical stimuli. A method of sea urchin chemical stimulation, based on absorbent cleaning cloth material soaked with a specific stimulus (a simulacrum) and deposited on the aboral hemisphere of sea urchins, was exploited, and is reported here and compared with classical stimulation through diluted extracts.
The main objective was, therefore, to determine chemical perception toward several dissolved food-related organic compounds, such as proteins, peptides and amino acids, carbohydrates, and lipids, and tissues and tissue extracts of plant and animal origins, thus finding molecular evidence for the food preferences of the acknowledged plant-eater sea urchin Paracentrotus lividus and how they could affect feeding behaviour.
However, before such refined task became possible, given the novelty of the chemical stimulation procedure exploited here, some issues had to be inevitably and preliminarily addressed. Such issues were (1) distance food detection, ability to detect food or food-related molecules at a distance, and capability of moving toward the food target; (2) effect of the long term nutritional state, given the notorious sluggish responses of sea urchins to chemical stimuli; (3) selection of suitable absorbent-cleaning cloths with neutral features per se regarding themselves acting as potential feeding stimulants; (4) persistence of responses induced by chemical stimuli absorbed in cleaning cloths at longer periods of time; (5) concentration effectiveness of soaked chemical stimuli; and (6) effect of the presentation of soaked chemical stimuli through simulacra, singly or concurrently, with blanks.
Materials and Methods
Preliminary Studies
Distance Food Detection
Food Proximity
We intended to evaluate the capacity of distance food localization and orientation. Observations (n = 24 similar-sized sea urchins) on the direction of the sea urchins were carried out in the presence of selected food items currently used in the laboratory as raw feed to maintain the sea urchins, maize whole yellow grains, and fronds of Kombu seaweed Laminaria ochroleuca (Phaeophyceae), and fronds commonly found on the same sea urchin rocky shores, the seaweed Corallina elongata (Rhodophyceae) placed at a distance of.1 m from the sea urchins. This red seaweed was also selected for being referenced as a preference for this sea urchin (Boudouresque and Verlaque, 2007).
All the sea urchins were fed with each food item 2 days before the experiments and observed individually in a glass aquarium (1.5 × 0.4 × 0.25 m of water depth) integrated into the same seawater recirculation system where they were previously kept.
To reduce stressful conditions caused by illumination that could prevent motion, distance food detection assays were made in the absence of any light source, given that prior tests conducted before the experiments clearly showed the opposite motion of the sea urchins from light sources, and that motion velocity (escape response) positively correlated with light intensity. Sea urchin movements in darkness were followed using an infrared video camera (model VC2400; Vicon, Hauppauge, NY, United States) installed above the glass aquarium, which was illuminated on each longitudinal side by 300 infrared diodes (Siemens, Munich, Germany) mounted into two external rulers parallel to the water column.
Detection of Plumes of Dissolved Compounds
The sea urchins were stimulated in translucent 2 L cylindrical plastic beakers (0.13 m diameter; 0.18 m height) filled with 1 L of seawater to prevent immobilization in any corner, where it would be more difficult to observe its activity. All the tested sea urchins (n = 10 per tested stimulus) were kept under the same conditions of 20°C of seawater temperature and an attenuated fluorescent light illuminance of 16 lux on yjr beaker upper surface.
When the sea urchins were completely inactive and with most of the podia retracted or slightly distending, the stimulant and the control solutions were injected simultaneously in separate containers using turbulence traps, as shown in Figure 1, which allowed for a minimum disturbance that could be caused by the mechanical introduction of the solution for stimulation. The turbulence traps, consisting of small plastic cylinders, were connected using plastic tubing (0.5 cm diameter) to plastic syringes (model 22 Syringe Pump; Harvard Apparatus, Cambridge, MA, United States) that delivered the chemical stimuli at constant velocities of 0.005 L impulses with 60 s duration, which were separated by 60 s inter-impulse pauses. Each sea urchin was stimulated eight times in a total of 0.04 L of stimulant for a total time of 16 min. The control was tested simultaneously, so the disturbances caused by the presence of the observer or any residual mechanical stimulation could be immediately identified.
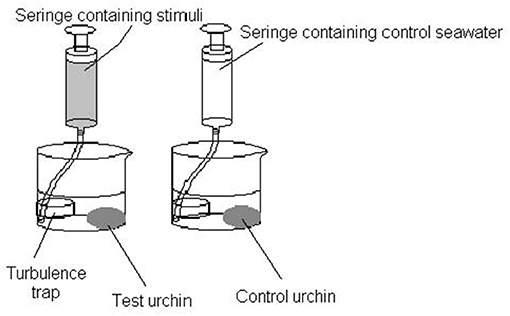
Figure 1. Side view of the stimulation apparatus used to introduce dissolved stimuli and control seawater in a controlled and non-turbulent manner.
Behavioural classifications were established according to the number of extended podia (<5, 5–20, or more than 20), the intensity of their movements (stationary, active, or very active), and their stretching based on exceeding or not spine length (slightly or fully extended). The grouping of the observed behaviours was arranged through parallelism with the observations of Ferner and Jumars (1999) in palps of polychaetes. The miniature size of the pedicelariae made their use impracticable in this study, despite their importance as chemoreceptive organs (Campbell and Laverack, 1968).
Dissolved Food-Related Compounds
The dissolved stimuli tested (25) for tube feet activity were extracts of the seaweed C. elongata, 22 single amino acids, soluble starch, and glucose. To prepare the seaweed extract, fronds of C. elongata, collected in the same place as the tested sea urchins, were wet-crushed and filtered up to 100 μm. This extract was used in 10% dilution. For the preparation of the amino acids and carbohydrate solutions, the seawater used was filtered until 1 μm and irradiated with UV. This seawater had the same source as the surrounding water of the sea urchins; thus, it was not necessary to make pH corrections. The 22 single amino acids were taurine (Sigma T-0625; Sigma Aldrich, St. Louis, MO, United States) and the 21 L- amino acids (kit LAA-21; Sigma Aldrich, St. Louis, MO, United States): alanine, arginine, asparagine, aspartate, cysteine, cystine, phenylalanine, glycine, glutamate, glutamine, histidine, isoleucine, leucine, lysine, methionine, proline, serine, threonine, tryptophan, tyrosine, and valine. Amino acid solutions were prepared in concentrations of 10 mM and the starch and glucose solutions in 10% concentration (0.01 kg of composition in 0.1 L seawater). The control consisted of seawater of the same origin as the one used for the preparation of the stimulants. The final concentrations in the test container after dilution resulted in 3.8 × 10−5 M for amino acids and 0.4% for the remaining stimulants. All the solutions were used at a temperature of 20°C.
Contact Food Detection
Animals and Conditions
Adult sea urchins, with test diameters ranging from 40 to 50 mm (60–70 mm including spines), were collected in September in Cabo Raso, Cascais (distance: 30 km west from Lisbon, Portugal). Those without evident signs of deterioration (no loss of spines and firmly attached to the substrate) were carried to Guia Marine Laboratory (Cascais) and kept until they were used for experiments in a natural seawater recirculation system made up of eight black fibreglass tanks (400 L each, 80 m diameter and 0.8 m water column). Water quality was maintained in the recirculation system through biological and protein skimmer filtration. Chemical and physical parameters (pH, dissolved salts, and gases) were regularly measured and showed to always fall within recommended values compatible with the water quality of a well-balanced aquarium system (Wickins and Helm, 1981). The temperature has been kept at 19°C, salinity at 35 psu, and pH at 7.8–8. The quality of the water was assured, since no sea urchin died or lost spines during the period in captivity, except in the first week following collection.
All the sea urchins were acclimatized to light:dark cycles of 14:10 h. Indirect light (diffused) from the laboratory was provided by ceiling fluorescent daylight tubes, reaching the surface of the perforated rectangular dark blue plastic containers where the sea urchins were assayed with a measured illuminance of 350 lux (Gossen Lunasix, Gossen, Germany). The need for direct and immediate observations of large numbers of simultaneously tested sea urchins prevented the assays to be carried out in total darkness and infrared illumination.
Food excess and/or excrements were removed by water siphoning once a week when the tanks were cleaned, and 10% of the volume of the recirculation system was replaced by stored natural seawater pumped directly from the sea adjacent to the laboratory and mechanically filtered through sand gravel.
The sea urchins, whatever their diet, were fed only two times a week (Tuesdays and Fridays) and when applicable, only after assays, given that it is well-known that recently fed sea urchins, in general, are less responsive to foods (Lawrence et al., 2007). Between the assays, the pool of sea urchins (150) was kept at the bottom of one of the 400-L fibreglass tanks of the recirculation system (equivalent to 300 sea urchins m−2 of tank bottom area), but to prevent them from fully adhering to the bare bottom, they were placed within a container made of a semi-rigid plastic mesh (2.5 cm) moulded to the shape of the rigid tank. This would facilitate the removal of sea urchins and lessen the impact on adhesion podia caused by frequent and periodic recollection for assays.
Methodology to Soak Up Food-Related Compounds
As a convenient way to absorb organic compounds, such as fats, and other water-insoluble molecules, we tested absorbent cleaning cloths (~20 × 20 cm) available on the market. Based on the partial incorporation of synthetic fibres, in the beginning, we tentatively selected one brand (Lever Fabergé) manufactured from natural cellulose (60%) and synthetic fibres (40%). Squared portions (2 × 2 cm) were cut out from the original 20 × 20 cm yellow cloth, so that each absorbent portion resembled a piece of drift material on the seashore (simulacrum).
Tested sea urchins (4 batches of 8 sea urchins, thus a total of 32 sea urchins for each assay) were placed in four perforated rectangular dark blue plastic containers (60 × 39 × 15 cm deep), each one partially immersed in one of the available four cylindrical 400-L tanks of the natural seawater recirculation system in the laboratory. The containers were divided by a plastic mesh in eight sections (18 × 11 × 12 cm of water depth) in which a group of eight sea urchins, with diameters ranging from 6 to 7 cm including spines, could be tested simultaneously and independently. The sea urchins were allowed to adapt until quietness for a period of 60 min before the assays were performed.
The chemical stimulation in this method consisted of placing vertically either one or simultaneously two simulacra with similar shape and size between the spines of the aboral hemisphere, as shown in Figure 2, one soaked with a chemical stimulus and the other soaked with just background seawater (blank). Thirty-two pieces of absorbent cleaning cloths were simultaneously soaked in each of the tested stimulus solutions (including blanks) for 10 min before the assays in order to allow for the adsorption of the stimulant. The soluble food-related compounds were freshly prepared by continuous magnetic bar agitation. Pure oils and other insoluble compounds were rendered temporarily emulsified using a hand-held blender (450 W; Braun, Kronberg, Germany). To avoid the eventual floatation of the simulacra due to air retention, each set of cloths was squeezed/released three times with an empty smaller-volume glass beaker pressed against the slightly larger bottom of the cloth container beaker. Each assay took place during a period of 30 min until the full inactivity of every sea urchin, which concurred with the time taken by most sea urchins to carry adopted standard macroalgae flesh squared portions to the oral region.
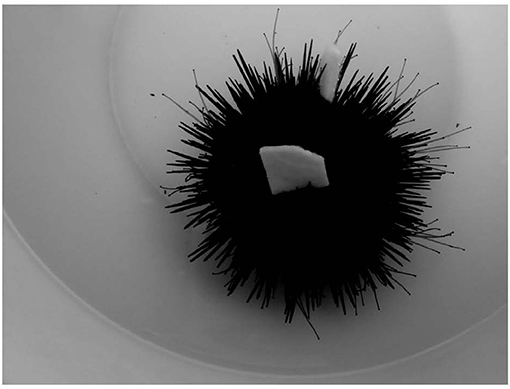
Figure 2. Contact chemical stimulation of the sea urchins with absorbent cleaning cloths (simulacra). The one in the centre of the image was soaked with just background seawater (blank) and the other in the foreground, already transported to the oral region, was soaked with a chemical stimulus.
All the assays were carried out during the daytime (from 10 to 17 h), and no sea urchin was tested two times in the same day. After each assay, every rectangular dark blue plastic container was immediately scoured with an abrasive sponge to remove completely adhered locomotory podia (footprints) left by the removal of tested sea urchins that could eventually interfere with the next batch of sea urchins.
The behavioural classification of responses to soaked chemical compounds was straightforward and reduced to very few categories based on the transportation of simulacra to the oral hemisphere (phagostimulatory responses), their discarding (phagodepressant responses), and unresponsiveness. These responses were further reclassified as simulacra carried to the mouth and bitten (CMB) and simulacra carried to the mouth but not bitten (CMNB), whose summation made the total simulacra carried to the mouth (CM). The discarded simulacra (D) were so classified whenever a simulacrum was actively rejected and dropped to the bottom of the container. Based on these resultant behavioural responses and according to the feeding model of Lee and Meyers (1997), we classified each chemical stimulus into the classes of suppressant (D), incitant (CM), stimulant (CMB), and deterrent (CMNB). As a behavioural response characteristic of sea urchins, unresponsiveness, or simulacra held on the aboral region all the time, was classified as the category of cover (H). To each of these categories of behavioural responses, the major sensory structures of sea urchins could be associated (Barnes, 1980). Aboral tube feet (away from the mouth) can capture and hold drift material and are thus, capable of generating Incitant (CM), Suppressant (D), or Hold (H) response. Peristomial tube feet (arising from the peristomial membrane, surrounding the mouth) are probably chemo-sensitive participating in feeding and thus, capable of generating the Stimulant (CMB) or the Deterrent (CMNB) response.
Nutritional State and Life History Effects
Patterns of feeding vary throughout the life cycle of aquatic invertebrates. Thus, chemoattractants and feeding stimulants to which they respond should change with time. The nutritional state of captive sea urchins must certainly condition the responses to feeding stimulants. Before any assay on the perception of food-related compounds could be carried out on sea urchins, the influence of their nutritional state on responses to feeding stimulants had to be preliminarily evaluated to select a standard diet that could provide fast behavioural responses without the inconveniences either of a fasting regime (too responsive) or a high energy regime (insufficiently responsive).
The maturation stage of sea urchins could also interfere. However, evidence from a previous study (Luis et al., 2005) suggested that feeding P. lividus in captivity with the brown seaweed Kombu (L. ochroleuca) resulted in poor gonad maturation throughout the year.
Captive groups of sea urchins were subjected to 8 months of three defined diets fed two times a week: whole yellow maize grains, sectioned pieces of commercially available dehydrated fronds of the brown seaweed L. ochroleuca (Alga Kombu, Algamar, Galiza, Espanha), and the resultant combined diet by alternating maize and kombu weekly. Each chemical stimulus, glucose 10% (Merck, Darmstadt, Germany), soluble starch 10% (Darmstadt, Germany), sucrose 10% (cane sugar), olive oil 10%, and a 22-amino acid 1 mM mixture, was tested on 30 sea urchins from each of the three diets as well as on an equal number of wild sea urchins recollected 15 days prior to testing and were kept unfed.
Selection of Suitable Absorbent Cleaning Cloths
The results of the preliminary tests using the cleaning cloth made of natural cellulose (60%) and synthetic fibres (40%) showed similar chances for the blank simulacra to be carried to the oral region as the simulacra containing chemical stimuli, which suggested that the natural cellulose component could also contribute to the response and induce undesired behavioural responses. Thus, alternative cleaning cloths had to be evaluated in order to select the few with the most desired attribute for a blank simulacrum, i.e., poor incitant response or the least carried to the mouth.
Absorbent cleaning cloths, available on the market and made of different combinations of natural and artificial fibres (either synthetic or semi-synthetic), reduced to strips of 20 × 2 cm and then cut out into squared pieces of 2 × 2 cm, were tested on the sea urchins: (1) 100% viscose (artificial cellulose fibre); (2) 70% viscose; 15% polypropylene; 15% polyester (Vileda brand); (3) 70% viscose; 15% polypropylene; 15% polyester (Ballerina brand); (4) 100% microfibres: [80% polyester + 20% polyamide]; (5) 60% latex; 28% viscose; 12% polyester; (6) 35% cotton; 65% cellulose; (7) 85% cotton; 15% cellulose; control: similar size pieces of Kombu seaweed.
A total of 32 sea urchins (four batches of 8 sea urchins) for each of the eight assays were allocated and tested as already described.
Persistence of Responses Mediated by Absorbent Cleaning Cloths at Longer Periods of Time
As stated, each assay with soaked chemical stimuli took place during a period of 30 min that concurred with the time taken by most sea urchins to carry adopted standard kombu seaweed flesh (L. ochroleuca), sectioned in squared portions, to the oral region immediately followed by biting. It was also assumed that longer periods of stimulation would result in a more complete sea urchin response. If the initial 30 min response allowed the transportation of a food simulacrum or a piece of kombu seaweed to the mouth followed by biting, then longer periods of time (3–4 days) would allow for a more thorough consumption of the food items (including the artificial absorbent cloths), eventually up to full ingestion by the sea urchin. On the other hand, if whatever food item was initially discarded, then more time would not revert the response. If the final outcomes were similar for natural foods and food simulacra items, then we could more plainly accept absorbent cloths (food simulacra) as trusted vehicles to soak up any kind of chemical stimuli.
A total of 32 sea urchins (four batches of 8 sea urchins) for each of the five assays were allocated and tested as already described. The assays involved testing blank food simulacra (85% cotton and 15% cellulose) for 3 and 4 days, crushed fresh mussel (3 days), and a previously known phagostimulant, food simulacra soaked with a 10% starch solution (3 days). Also, identical-sized squared portions of kombu seaweed served as an experimental control for a 3-day period.
Concentration Effectiveness of Soaked Chemical Stimuli
During previous experiments, solutions of chemical stimuli at 10% concentration were generally used, except for single or mixtures of amino acids that were used only at 1% concentration. Nevertheless, either dissolved in plumes or soaked in artificial food simulacra, amino acids were apparently well-detected by sea urchins. Therefore, evidence was necessary to be gathered to clarify the issue of the concentration effectiveness of selected chemical stimuli.
A total of 32 sea urchins (four batches of eight sea urchins) for each of the 20 assays were allocated and tested as already described. The assays involved testing 20 diverse chemical stimuli as follows:
Carbohydrates: agar-agar; alginate (sodium); alginic acid; cellulose (alpha); glucose; glycogen; mannitol; saccharose; starch (unmodified), and starch (soluble). Proteins and peptides: Amicase; casein; gliadin and HyPEP. Fats: Cod liver oil; corn oil; linseed oil and olive oil. Tissue extracts: Kombu seaweed extract and mussel extract.
Effect of Presentation of Soaked Chemical Stimuli: Singly or Concurrently With Blanks
When chemical stimuli soaked in simulacra were chosen by sea urchins, i.e., transported from the aboral to the adoral side of the body and bitten, we wanted to have the assurance that they were chosen based on purpose and not on chance. A total of 41 chemical stimuli (fully described below) at 1% concentration soaked in simulacra were tested either singly or against concurrent blanks. Therefore, for the experimental design, an ANOVA with two factors was performed. A total of 16 observations of batches of eight sea urchins were carried out involving eight observations with single chemical-carrying simulacra and another eight observations of chemical-carrying simulacra concurrently with blank simulacra.
Refined Experiments
Once all the issues addressed in the preliminary studies were settled, the experiments then proceeded to finally determine which food-related compounds were stimulatory to sea urchin P. lividus.
Stripes from refills of mop heads made of an absorbent yellow cloth material (85% cotton and 15% semi-synthetic cellulosic fibres) were used throughout the entire refined experiments for testing the responses of the selected 41 food-related chemical compounds. The chemical stimulants derived from algal food were the first priority to be put under test. Thus, starch, unmodified (S5127; Sigma Aldrich, St. Louis, MO, United States), starch, soluble, (33615; Riedel-de-Haen, Seelze, Germany), and alpha-cellulose (C8002; Sigma Aldrich, St. Louis, MO, United States) were selected as stimulants from the Chlorophyta; Laminarin (from Laminaria digitata, L9634; Sigma Aldrich, St. Louis, MO, United States), D-mannitol (M4125; Sigma Aldrich, St. Louis, MO, United States), and alginic acid (A7003; Sigma Aldrich, St. Louis, MO, United States) were selected as stimulants from the Phaeophyta; agar-agar (powder, José M. Vaz Pereira) and D-(+)-galactose (G0750; Sigma Aldrich, St. Louis, MO, United States) were selected as stimulants from the Rhodophyta; glucose (8337; Merck, Darmstadt, Germany), saccharose (7651; Merck, Darmstadt, Germany), fructose (Cem Porcento; Ignoramus, Santarem, Portugal), glycogen (104202; Merck, Darmstadt, Germany), alginate, sodium (Fagron Iberica, Barcelona, Spain), and cotton (85%, isolated blanks) were selected as other carbohydrates. Among the proteins, peptides and free amino acids, we selected some of the few available on the market: casein (purified powder from bovine milk, C-5890; Sigma Aldrich, St. Louis, MO, United States), Amicase (casein acid hydrolysate mixture of free amino acids without peptides, A2427; Sigma Aldrich, St. Louis, MO, United States), gliadin (from wheat gluten, G3375; Sigma Aldrich, St. Louis, MO, United States), gluten from wheat also available from Sigma Aldrich (St. Louis, MO, United States) (G5004) but given that it was not pure protein (80 % protein, 7% fat) it could not be elected for testing, HyPEP 4601 (protein hydrolysate from wheat gluten, rich source of L-glutamine, H6784; Sigma Aldrich, St. Louis, MO, United States), peptone (bacteriological peptone powder, from pancreatic digested animal proteins; Oxoid L37, Basingstoke, United Kingdom) , glutamate, monosodium (flavour enhancer; Ajinomoto, Tokyo, Japan), L-glutamic acid (G-1251; Sigma Aldrich, St. Louis, MO, United States), L-glutamine (G-3126; Sigma Aldrich, St. Louis, MO, United States), L-tryptophan (T-0254; Sigma Aldrich, St. Louis, MO, United States), L-cystine (C-8755; Sigma Aldrich, St. Louis, MO, United States), L-cysteine (C-7755; Sigma Aldrich, St. Louis, MO, United States), L-valine (V-0500; Sigma Aldrich, St. Louis, MO, United States), L-threonine (T-8625; Sigma Aldrich, St. Louis, MO, United States), L-leucine (L-8000; Sigma Aldrich, St. Louis, MO, United States), and L-tyrosine (T-3754; Sigma Aldrich, St. Louis, MO, United States). The single L-amino acids, except L-glutamine, were restricted only to those eight that had previously generated a significant tube feet activity. New single amino acid solutions at 1% concentration were prepared fresh and used immediately. Among the fats and fatty acids, we selected some of the most common oils (and respective most abundant purified fatty acid) used for human consumption: linseed oil [55% linolenic acid; Emile Noel (Huilerie) France], linolenic acid (C18:3n-3, L2376; Sigma Aldrich, St. Louis, MO, United States), corn oil [linoleic acid > 45%; linolenic acid <2%; Solmil (Lisbon, Portugal)], linoleic acid (C18:2n-6 L1376; Sigma Aldrich, St. Louis, MO, United States), olive oil (acidity 1.5°; Galo), oleic acid (C18:1n-9, O1008; Sigma Aldrich, St. Louis, MO, United States), and Cod liver oil (61018; José M. Vaz Pereira, Santarem, Portugal). Among the bitter compounds, the alkaloid caffeine (white powder, BDH, Biochemical 213390) was selected. Tissues and tissue extracts were obtained from two sources, one from a plant (year-round commercially available dehydrated fronds of kombu brown seaweed L. ochroleuca) and the other from an animal (year-round commercially available fresh mussel Mytilus galloprovincialis): Kombu flesh, Kombu extract, mussel flesh, mussel extract; Kombu flesh against mussel flesh and Kombu seaweed extract against mussel extract.
The blank solution (control) was, in every case, made up of recirculated tank natural seawater.
Each complete stimulus assay consisted of eight observations on 64 sea urchins (eight batches of eight sea urchins) stimulated only with a single-stimulus carrying simulacra.
As the laboratory pool of sea urchins could only be tested a maximum of two times a week, the assays took almost a year to complete. To avoid life history interferences, each complete assay was divided in half, four observations (batches) on 32 sea urchins. Each one of the half sets was assayed with at least a 6-month interval.
Statistical Analysis
The data from stimulation through soaked chemical compounds were analysed according to phagostimulatory or phagodepressant responses. In the first case, only behaviours involving the transportation of simulacra to the mouth were compared, the remaining ones were considered collectively as non-responsive. In the second case, only behaviours involving the discard of one of the simulacra were analysed, the remaining ones were collectively considered as nonresponsive. As results are reported under a nominal form, non-parametric statistics were used (Siegel, 1975). Responsiveness to each chemical stimulus was analysed by the χ2 tests, and within responses, preference for one or the other simulacrum was compared using binomial tests. The null hypothesis was an equal probability for the occurrence of any of the responses. Response comparisons (phagostimulatory or phagodepressant) between diets were also analysed by χ2 tests.
The “Statistica for Windows” software package was used for statistical analyses. Differences between two data means were analysed by Student's t-distribution for independent values (Bailey, 1959). Multiple averages were compared by one-way analysis of variance (ANOVA). Levene's statistic test was performed to test for the homogeneity of variances for all data. Data with homogeneous variances were analysed by one-way ANOVA with Tukey's multiple comparisons to determine differences among the independent factors. Data with heterogeneous variances were analysed using by the Kruskal–Wallis statistic test followed by multiple comparisons of mean ranks for all the groups (Zar, 1999). The significance level used was P < 0.05.
Results
Preliminary Studies
Distance Food Detection
Food Proximity
Whenever the sea urchins were placed in the centre of the aquarium, regardless of the type of illumination (including darkness), they immediately initiated displacement with an apparently erratic course with several changes in direction that generally came to a halt when any of the corners of the aquarium was reached and then they stayed put for days without any further motion. The study, conducted under appropriate conditions of illumination (darkness), showed that food proximity (0.1 m) could not incite the sea urchins to displace or change directions while displacing. The occurrences in which newly placed sea urchins reached food were rare (8%), thus suggesting random encounters more than the presence of food as a cause (χ2: P > 0.2, df = 1 by comparison with similar situations of darkness but no food present). The results showed that this method is not convenient to chemically stimulate sea urchin P. lividus.
Tube Feet Activity
Tube feet responses were compared on the same sea urchins when exposed to food-related stimuli and control stimulus. The behavioural responses clearly varied with stimuli (P < 0.001). The sea urchins showed no behavioural differences relative to the control for 15 out of the total 25 tested stimuli. These were aspartate, alanine, arginine, asparagine, glutamine, glycine, histidine, isoleucine, lysine, methionine, phenylalanine, proline, serine, taurine, and glucose (P > 0.05).
However, for the 10 remaining tested stimuli, significant differences relative to the control were observed for the red seaweed extract of C. elongata (P < 0.001), the amino acids cystine, cysteine, leucine, tryptophan, tyrosine, and valine (P < 0.01), glutamate and threonine (P < 0.05), as well as starch (P < 0.01). The more frequent response observed was the full stretching of more than 20 tube feet but without agitation. Displacement was observed on 2% of all the tested sea urchins but was restricted only to those exposed to the red seaweed extract of C. elongata. Among the behaviours not directly related to tube feet activity, spine activity showed no variation when the sea urchins were exposed to stimuli.
The true meaning of these responses regarding the feeding behaviour of sea urchins remained unclear. All we could conclude was that any of the 10 chemical stimuli were recognized by sea urchins, but nothing could be drawn regarding the power of each stimulus as phagostimulant or phagodepressant.
Contact Food Detection
Long-Term Nutritional State Effects
The frequency of feeding responses is independent of the kind of chemical stimulus presented (P > 0.05, df = 3), but is clearly dependent on the nutritional state of the sea urchins (P < 0.001, df = 3; Figure 3). When fed with seaweed fronds exclusively, the sea urchins showed a greater response frequency toward simulacra (either test and/or blank) than those fed with any of the other diets tested or even the unfed ones (P < 0.001, df = 1). The sea urchins fed with maize, either exclusively or in combination with seaweed fronds, showed the least response frequency (P > 0.05, df = 1).
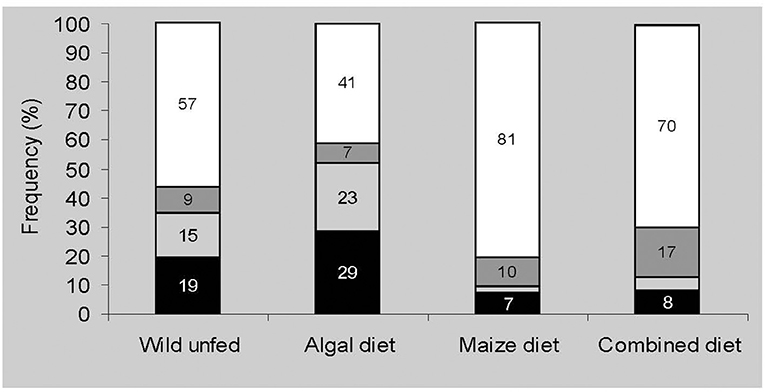
Figure 3. Nutritional state effects of sea urchins on the response toward food-related compounds soaked in simulacra. Black bars represent frequencies of carried simulacra-containing stimulus, light grey bars represent carried blank simulacra, dark grey bars represent discarded simulacra, and white bars represent unresponsiveness (N = 150 sea urchins under each dietary regime). For every diet, five food-related compounds were tested [glucose, starch, sucrose, olive oil, amino acid (AA) mixture] and expressed as their pooled responses.
Whenever simulacra were placed over the individual sea urchins, spine reactions were always observed, although subsequent tube feet responses did not always result in phagostimulation or phagodepression. In many instances, the sea urchins did not convey any of the presented simulacra to the mouth. For most of the tested chemical stimuli, the sea urchins choose indistinguishably either the test simulacrum or the control (blank) simulacrum. This was the case for the 22-amino acid mixture, olive oil, sucrose, and glucose (P > 0.05). However, regardless of diet, when soluble starch was the tested stimulus, the sea urchins clearly showed a preference for the respective simulacrum relative to the blank one (P < 0.05).
Blank simulacra, irrespective of sea urchin diet, were chosen as frequently as stimulus-carrying simulacra, except when this was starch. The natural cellulose component (60%) of the cloth simulacra must certainly have been responsible for the observed sea urchin feeding behaviour, thus suggesting its sensitivity to this complex carbohydrate.
The discarding of simulacra was less frequent than the frequency of carried simulacra responses but were again dependent on sea urchin nutritional state (P < 0.001, df = 4). The sea urchins fed on the combined diet of rejected simulacra more frequently than those fed with seaweed fronds or the unfed ones (algal diet vs. combined diet: P < 0.02, df = 1; combined diet vs. wild unfed: P < 0.05, df = 1). Differences among the other tested diets were found to be not significant (P > 0.05, df = 1). The sea urchins showed equal chances to discard either the test or the blank simulacrum as revealed by binomial analysis (P > 0.05). The discard frequency of simulacra was not correlated with the kind of tested stimulus (P > .05, df = 1) being observed in <5 cases for each tested diet.
Selection of Suitable Absorbent Cleaning Cloths
The simulacra carried to the oral region (CM), which summarizes both the least desired attributes for a blank simulacrum, carried to the mouth and bitten (CMB) and carried to the mouth but not bitten (CMNB), as shown in Table 1, showed the lowest values for 85% cotton, 35% cotton, and 100% microfibres (non-significant differences, Fisher's LSD test, P > 0.05). Given that the 85% cotton cleaning cloth was available already in stripes from mop head refills, it was selected for convenience for the following refined experiments.
Persistence of Responses Mediated by Absorbent Cleaning Cloths at Longer Periods of Time
The results shown in Table 2 seem to confirm the early assumption that sea urchins must not be kept unfed beyond 3 days, as on the 4th day the number of blank simulacra is clearly more carried to the mouth and bitten (CMB). On the other hand, the results also confirm that the selected absorbent cleaning cloth, once soaked with an appropriate food-related stimulus, seems to behave like real food given that 50% of the cloths soaked in starch were carried to the mouth and even ingested (CMB's half or more consumed).
The capacity of the selected cleaning cloths to retain chemical stimulus even when kept immersed for three consecutive days in seawater was evidenced when all the pieces of cloths previously soaked in the solution of starch stayed glued to filter paper after air drying. The same did not happen with the cloths used as blanks.
Concentration Effectiveness of Soaked Chemical Stimuli
Only 4 out of the 20 tested chemical stimuli, 20% of the tested comparisons between 1 and 10% stimuli concentrations, were shown to be significantly different (p < 0.05) when analysed according to the more relevant response concerning food-related compounds, the simulacra carried to the mouth (CM and incitants), which included either the simulacra carried to the mouth and bitten (CMB, incitants, and stimulants) and/or the carried ones to the mouth but not bitten (CMNB, incitants, and deterrents). The tested 1% chemical stimulus concentration seems to be more effective than the alternative 10% concentrations, as shown in Table 3, where all the incitant responses (CM) tested significantly different are related with the former concentration.
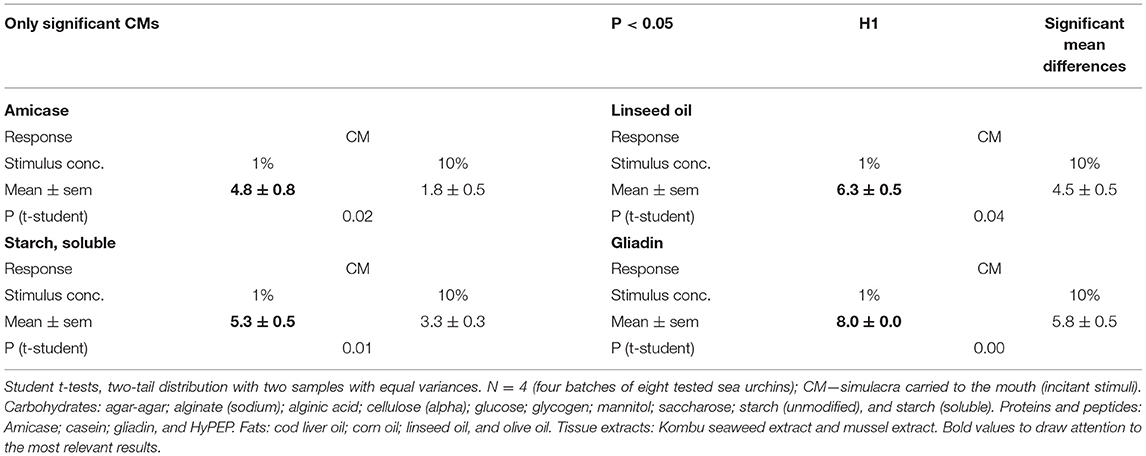
Table 3. Comparisons of 20 chemical stimuli at 1 and 10% concentration presented as single simulacra, i.e., without concurrent blank simulacra.
Therefore, given that 80% of the mean concentration differences between 1 and 10% were insignificant (P > 0.05) and that all the remaining 20% significantly different (P > 0.05) showed higher responses to the 1% concentration of chemical stimulus, we adopted this later condition for all subsequent experiments.
Effect of Presentation of Soaked Chemical Stimuli: Singly or Concurrently With Blanks
As shown in Table 4, whenever simulacra soaked with chemical stimuli are carried to mouth (CM), either bitten (CMB), or not bitten (CMNB), their averages were always greater than the averages of blank simulacra. As expected, the reverse was true only for the discarded (D) and held (H) simulacra. This suggests that, in general terms, the adopted simulacra of an artificial drift material allowed sea urchin P. lividus to clearly discriminate chemical-carrying simulacra from blank simulacra. As also shown in Table 4, the isolated blanks tend to be more ingested (CMB) than the blanks presented concurrent with stimulus (P < 0.001), thus attesting to the very low incidence of ingestion of blank simulacra throughout all the assays.
If each one of the 40 stimuli paired means with and without blanks (all the 41 chemical stimuli except the cotton blank stimulus) are compared by Student's “T-tests” (N = 16); then, most of the observed differences between means for any of the registered responses are not significant (P > 0.05, df = 14). Depending on the behavioural response, only 5–10% (2–4 out of 40) of the stimuli means were shown to be significantly different (P < 0.05) with no clear dominance for any of the treatments. Likewise, none of the global pooled means with and without blanks analysed by each behavioural response (D, CM, CMB, CMNB, and H) were shown to be significantly different (P > 0.05, df = 78).
However, if both factors are considered simultaneously (ANOVA, two factors), stimuli presented singly (N = 8 batches) and stimuli presented concurrently with a blank (N= 8 batches) for all the 40 tested stimuli, then, as shown in Table 5, some of the sea urchin behavioural responses are affected by the presence of a blank simulacrum. Behavioural responses D (suppressant) and CMB (incitant/stimulant) to stimuli either with a concurrent blank or without a blank (single stimulus) showed no differences between the respective response means or interferences from the concurrent blank simulacra, but the CMNB responses (incitant/deterrent) and consequently the CM responses (incitant) clearly showed the presence of concurrent blank simulacra as having interference with the final sea urchin choice.
Thus, as shown in Table 6, the final refined experiments for the identification and classification of food-related chemical stimuli are carried out based on simulacra presented singly to sea urchins but weighted by the results observed when the same chemical stimuli were presented concurrently with blanks. Nine of the latter 41 tested stimuli resulted in responses like the concurrent blanks (t-test, P > 0.05), and they were: cotton (blanks), alginic acid, saccharose, glutamate mNa, glutamic acid, glutamine, valine, leucine, and caffeine. Twenty-one (51.2%) of the 41 stimuli highlighted in Table 6 are coincidentally classified by both methods of stimuli presentation (with or without blanks). However, the 20 other tested stimuli resulted in some divergence in chemical stimuli classifications, although most of them (36.6%) were related with just discrimination from incitant, incitant/stimulant, and incitant /deterrent, or deterrent and incitant/deterrent classes. Only six of the tested stimuli (14.6%), namely, alginate sodium, glycogen, saccharose, fructose, amicase, and cod liver oil, showed no relationship between the two modes of chemical stimuli presentation and had to be classified as indeterminables.
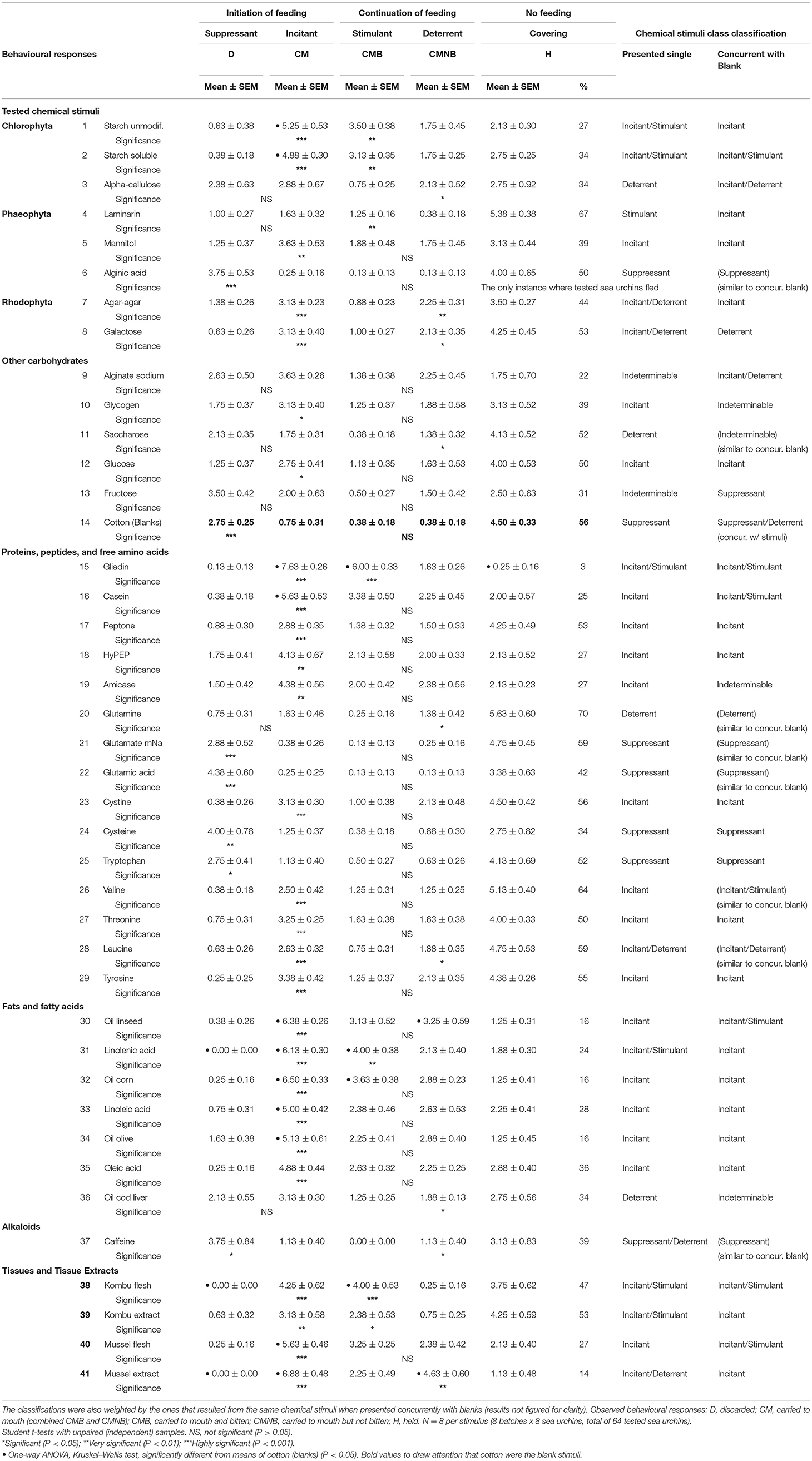
Table 6. Sea urchin behavioural responses to 41 singly presented chemical stimuli as solutions at 1% concentration soaked in cleaning cloths or as tissues, and resultant classifications of the chemical stimuli.
Refined Experiments: Stimuli Classification
The identification and most probable classification of the selected 41 food-related chemical stimuli according to their behavioural sea urchin responses are summarized in Table 6. Each individual chemical stimulus was first classified as an incitant if the average number of simulacra carried to the mouth was significantly greater (Student t-test, independent samples, P < 0.05) than the average number of simulacra dropped to the bottom of the container, away from the sea urchins. The reverse resulted in the chemical stimulus being classified as a suppressant. Afterwards, the same stimulus (incitant) was classified further as a stimulant if the average number of simulacra carried to the mouth and bitten was significantly greater (Student t-test, independent samples, P < 0.05) than the average number of simulacra carried to the mouth but not bitten. The reverse resulted in the chemical stimulus (incitant) being classified further as a deterrent. If no response was observed at all (no feeding), then the resultant behaviour was just classified as the so-called covering-feeding behaviour (de Ridder and Lawrence, 1982; Verlaque and Nedelec, 1983).
Independent of the chemical stimuli class classification shown in Table 6 and based only on the observed averages of the simulacra-carrying chemical stimuli, when all the means of the tested chemical stimuli (except the eight ones resulting in responses similar to cotton blanks) are compared by each behavioural response (one-way ANOVA and Kruskal-Wallis tests), we could only observe three means (Mussel extract, Kombu flesh, and linolenic acid) significantly less discarded (D) than the cotton blanks (P < 0.05); 11 means (gliadin, mussel extract, corn oil, linseed oil, linolenic acid, casein, mussel flesh, unmodified starch, olive oil, linoleic acid, and starch soluble) were more significantly carried to the mouth (CM) than the cotton blanks (P < 0.05); 4 means (gliadin, linolenic acid, Kombu flesh, and corn oil) were more significantly carried to the mouth and bitten (CMB) than the cotton blanks (P < 0.05); 2 means (mussel extract and linseed oil) were more significantly carried to the mouth but not bitten (CMNB) than the cotton blanks (P < 0.05); and finally only a mean (gliadin) was significantly less used as covering (H) than the cotton blanks (P < 0.05).
Merging behavioural responses and class classifications, six stimuli clearly emerged as the ones most perceived as food-related compounds: gliadin, linolenic acid, Kombu flesh, Kombu extract, starch unmodified, and starch soluble. All of the six were carried to the mouth and bitten (CMB), and as such, classified as incitants/stimulants. The majority (three stimuli) were complex molecules (gliadin, a protein, and starches, complex carbohydrates). Only one of the six stimuli was a simple molecule, linolenic acid, a fatty acid of plant origin. The remaining two were the intact structure of a section from a frond of a brown macroalgae or their resultant tissue extracts.
The most retained simulacra as covering behaviour (H), although not significantly different from the cotton blanks (56%), were amino acids (glutamine, 70%; valine, 64%; and leucine, 59%), laminarin (67%) and glutamate monosodium (59%) (ANOVA, -one-way, non-parametric, Kruskal–Wallis test, P > 0.05). On the opposite, the only and least retained one was the protein gliadin (ANOVA—one-way, parametric, Tukey HSD test, P < 0.05) followed by the mussel extract (14%) and the complex oils, each with 16% of the occurrences (linseed, corn, and olive oils).
The next tables, Tables 6.1–6.4, are continuations of Table 6, each arranged by selected chemical stimuli based on constituents or derivatives to be submitted to further statistical comparisons.
In Table 6.1, concerning carbohydrates, several specific and general comparisons involving 14 chemical stimuli are made evident. Starch was well-perceived as food whatever its chemical structure, unmodified (insoluble) or soluble. Although cotton is 91% cellulose (blanks: 85% cotton, 15% cellulose) it is much less perceived as food than alpha-cellulose (a polymer of glucose), and even glucose. The suppressant alginic acid, but not alginate sodium, was the only instance that induced the sea urchins (around 50%) to run away from the allocated sites of deposition during the testing period. However, 50% of the sea urchins retained (H) the alginic acid-carrying simulacra against the 22% that retained the alginate sodium-carrying simulacra. Agar-agar (agarose as its main constituent) is as well-perceived as a food incitant as galactose (contained in agarose and an isomer of glucose). Saccharose and its two constituents, glucose and fructose, as well as mannitol, a derivative from fructose all showed low incitant food response. In what concerns algae storage products, starch showed a clear larger perception related to food than mannitol or even laminarin, a polysaccharide from fronds of the macroalgae L. digitata. However, if cell wall constituents were concerned, then neither alpha-cellulose nor alginic acid showed any food-related response, except agar-agar. None of the tested monosaccharides, glucose, galactose, and fructose, showed a clear higher incitant food power. If all the polysaccharides, either the ones based in glucose or all the tested set, are taken into consideration, then again, only starch shows a clear high food-related response.
In Table 6.2, concerning lipids, several specific and general comparisons involving seven chemical stimuli are made evident. Most of the fats, except for cod liver oil of animal origin, and fatty acids showed to be clearly incitants except for linolenic acid, which showed a clear high food-related response (incitant/stimulant). Differences between each fat and its respective main constituent fatty acid are negligible.
In Table 6.3, concerning proteins, several specific and general comparisons involving 15 chemical stimuli are made evident. In general terms, more complex molecules, proteins, and peptides were better perceived as food-related compounds than simpler molecules such as amino acids. In absolute terms, only gliadin, a protein of plant origin, clearly showed the best result as a food-related stimulus, incitant/stimulant, while casein, an animal protein, could also show a classification as incitant but not simultaneously as stimulant. Gliadins are monomeric proteins rich in cysteine or proline and glutamine; nevertheless, these amino acids showed poor perception as food-related stimuli (suppressants or deterrents). Peptides either of animal origin, peptone, or peptone of plant origin (wheat gluten), HyPEP, induced similar sea urchin responses as incitants. Proteins, both of plant and animal origins, showed better responses than the peptides of plant and animal origins. If casein, a protein from an animal source, is compared with amicase, a free amino acid mixture from casein, responses showed little differences. However, if a peptide of plant origin, HyPEP (rich source of glutamine), is compared with glutamine, a pure amino acid, then food-related responses are clearly in favour of the peptide. Cystine, the oxidized form of the amino acid cysteine, showed a higher food-related response than cysteine. Grouping the amino acids by their classification into suppressants or incitants showed their coherence given insignificant differences in the food-related responses. Grouping the amino acids by their essentiality (essential amino acids, EAA), however, showed significant food-related responses. Despite their essentiality, which assumed all responses at least as incitants, the comparisons showed a variety of food-related responses, either positive (incitant) or negative (suppressant): tryptophan, an aromatic, cyclical derivative of alanine—suppressant; threonine, a cyclical derivative of alpha-amino butyric acid and most abundant FAA in sea urchin gonads (together with glutamic acid)—incitant; valine and leucine, the only branched-chain aliphatic amino acids known to occur in nature—incitant of the former, incitant/deterrent of the latter. Finally, trying to find any correlation of the classification of food-related responses with the polarity of the nine amino acids, there are no associations found between side chain polarity and response, e.g., incitant and incitant/deterrent.
In Table 6.4, concerning tastes, several specific and general comparisons involving 11 chemical stimuli are made evident. As expected, caffeine, an alkaloid of plant origin, showed clearly the worst result as a food-related stimulus, generating a suppressant/deterrent response while the other tested bitterness stimuli, three amino acids, showed a classification more in accordance with incitant stimuli. In what concerns sweetness, the five tested stimuli showed results in accordance with incitant responses. Finally, the umami-related stimuli, glutamic acid and glutamate monosodium, both showed similar suppressant responses.
The methods of sea urchin chemical stimulation developed in this study also allowed to explore the question about their food preferences, i.e., is P. lividus a plant or meat eater? As shown in Table 7, flesh tissues, either Kombu or mussel, are clearly preferred (incitant/stimulant) over extracts (incitant), but mussel extract is clearly preferred over Kombu extract when tested concurrently. However, it must be noted that the response facing mussels (either flesh or extract) is more immediate when compared with the response to Kombu (either flesh or extract). Whenever the tested alga is involved, a larger fraction of the resultant responses (70% as flesh or 64% as extract) was recorded as covering behaviour (H). These results obviously suggest P. lividus as an omnivorous species instead of a strictly herbivorous marine species.
Discussion
Based on several previous echinoderm observations on food perception and capture, we expected chemotactic behaviour to occur in the presence of food as well as the ensuing arrival at food and feeding behaviour (e.g., Teruya et al., 2001). Nevertheless, we observed a low arrival frequency. Such a result suggests that sea urchins are incapable of distance food perception. The individual diversity that was observed, as evidenced by the erratic trajectory of the sea urchins, was also verified by Dance (1987).
The incapacity of the sea urchin P. lividus for distance food perception was observed and attested by a low arrival frequency in the presence of food, a trait not exclusive to this species. The apparent lack of chemotaxis has been shown to occur in several other echinoderm species, which besides sea urchins, such as L. variegatus (Klinger and Lawrence, 1985), Strongylocentrotus spp. (Laur et al., 1986), and Evechinus chloroticus (Andrew and Stocker, 1986), also include some starfish (Dayton et al., 1977). The observed trait seems to be in accordance with the habitat selection of this species; places of high-water turbulence where chemical cues are much diffused and where food comes more as a drift material rather than as a searched item. Subnutrition, however, seems to be capable of inducing oriented feeding responses, as are the known cases of some sea urchins such as Strongylocentrotus franciscanus (Mattison et al., 1977) and L. variegatus (Klinger and Lawrence, 1985). However, whether subnutrition could induce chemotaxis on P. lividus remains unclear, as the condition is not addressed in this study.
In spite of an apparent incapacity for distance food location, sea urchins certainly have chemoreceptors that allow them to detect and react when stimulated by food-related molecules (Klinger and Lawrence, 1984; Mann et al., 1984; Hay et al., 1986; Prince and LeBlanc, 1992). Displacement was a rare event even when large tube-feet activity was recorded in response to food-related chemical stimulation, a result in general agreement with most other marine invertebrates that also rarely react to similar chemical stimulation by displaying whole-body responses (Laverack, 1988).
Given the sluggish responses of sea urchins to chemical stimuli, we had to make use of two parallel stimulation methodologies in this study, odorant plumes and stimuli soaked in simulacra.
The responses obtained with the presentation of stimulants in the form of odorant plumes allowed for the suggestion of the existence of specific receptors. The increasing activity of tube feet was evident when sea urchins were placed in the presence of preferred algae (C. elongata). Tube feet, along with pedicellariae, are most likely responsible for the collection of food and consequent transport to the mouth (Campbell and Laverack, 1968; Barnes, 1980; Sloan and Campbell, 1982; Flammang et al., 1998), so its observed high reactivity will not be surprising.
Although the approaches used in this study had not identified specific receptors responsible for the detection of algae extract, the results suggested the presence of specific receptors for 8 out of the 22 amino acids (cystine, cysteine, glutamate, leucine, threonine, tryptophan, tyrosine, and valine) and further, a carbohydrate (starch), which was found to generate increased activity of the tube feet. Except for glutamate, all the remaining amino acids that generated positive responses were composed of neutral side chains (Zubay et al., 1995). It was found that the reactivity raised by these stimuli was not as marked as in the case of stimulation with a complex mixture of molecules formed by seaweed extracts, similarly to what happens in crustaceans (Carr et al., 1984). It could not be neglected in this study that the poor response to most of the 22 amino acids tested may have been related to the low concentration used (in the order of 10−5 M), although it has been found that in aquatic environments, sea urchins are exposed to external concentrations in the order of 10−6 M (Pavillon and Rault, 1990).
Although the methodology used is the most common in early studies on chemoreception (Laverack, 1988), the limitation in this type of procedure is the interpretation that can be made from the recorded behaviours. Stirring and distension of tube feet may be part of sea urchin activities set to select and carry stimuli to the receptor cells (Derby and Atema, 1988), but not necessarily food stimuli.
The second method based on the well-known sea urchin behaviour of carrying drift material by tube feet and spines to the mouth, where feeding is initiated if the food is acceptable to the gustatory senses, generated fast responses that could be clearly related with feeding behaviour. Testing the responses of sea urchins to lipid compounds, unless they are soaked and adsorbed in a solid material, is difficult to accomplish with the first method. The unavoidable initial mechanical stimulation caused by the placement of simulacra between the aboral spines certainly sped up responses by imitating the natural deposition of organic suspended material, thus rendering displacement irrelevant for food gathering.
Initial spongy cloth blank simulacra, whose high natural cellulose content was associated with its undesirable sensitivity to this complex carbohydrate turning it into a main limitation, was solved by the study concerning the selection of more suitable absorbent cleaning cloths, which showed cotton-based blank simulacra as appropriate for this assignment.
Depending on the sea urchin diet, there were differences in the feeding responses. Sea urchins that received the diet exclusively from Laminaria alga were those that had a higher frequency of food response. This seaweed, although often described as suitable for the growth and maintenance of sea urchins (Hagen, 1998), has a much lower energy content than the alternative corn diet (Crampton and Harris, 1969; Saa, 2002). In animals subjected to a combined diet, there was a feeding frequency response intermediate between the other two, which suggests that the frequency response of food is correlated with the nutritional status of sea urchins and the energy obtained from food. Lawrence et al. (2003) found a relationship between the energy absorbed from food and feed rate in the sea urchin L. variegatus.
In sea urchins fasted for 15 days, there was a food frequency response lower than that observed in sea urchins subjected to the Laminaria diet. This suggests that 15 days is not enough for sea urchins to exhaust all their energy reserves.
It is a recurrent observation that the rate of food consumption increases with a reduction in the content of organic matter (Gago et al., 2003; Vaietilingon et al., 2003). This is consistent with the finding that the nutritional status of sea urchins is very important in stimuli eliciting feeding activity. Thus, the responsiveness of P. lividus was clearly dependent on its nutritional state. Well-fed sea urchins (maize whole grains) rarely responded, while the ones fed with less caloric rations (Kombu seaweed) responded faster and objectively.
Gliadins and glutenins are the two main components of the gluten protein fraction of wheat. Gluten is split about evenly between the gliadins and glutenins, although there are variations found in different sources. There are three main types of gliadin (α, γ, and ω). γ-gliadin is an ancestral form of cysteine-rich gliadin, with only inter-chain disulfide bridges, that makes gliadin unable to form polymers in the cell (Markgren et al., 2020). Gliadin is the water-insoluble component of gluten, and glutenin is water-soluble.
A gene coding for gamma-gliadin was recently found in the genome of the purple sea urchin Strongylocentrotus purpuratus (NCBI Strongylocentrotus purpuratus Annotation Release 102, 2019)1. This may suggest an explanation for the clearest food-related response of P. lividus toward the gliadin stimulus, as specific receptors for gliadin may exist in sea urchins.
Some of the eight amino acids that give rise to the increased activity of tube feet could, with this new approach, tentatively be identified as effective indicators as phagostimulants (incitants) for sea urchins, valine, threonine, tyrosine, and cystine, as revealed by observations with mop stripes soaked with dissolved amino acid-related stimuli. However, the four other amino acids, leucine, tryptophan, glutamate, and cysteine, although also generating increased tube feet activity, were found to be responsible for phagodepressants (suppressants)-related responses.
The development of a positive response related with the presence of valine and threonine amino acids (both incitants) could not be explained by its abundance in natural food (Crampton and Harris, 1969; Saa, 2002). Nitrogen content, which is often assumed to be positively correlated with the choice of food (Boudouresque and Verlaque, 2007), is not decisive in the choice of some amino acids over the others. With regards to the chemical constitution of molecules, both possess a similar side chain, differing only in the replacement of a neutral group (-CH3) by a hydroxyl group (-OH) on threonine (Zubay et al., 1995).
After finding that the amino acids tryptophan, glutamate, and cysteine raise the activity of tube feet, it was found that the same amino acids, when embedded in simulacra, were rejected by sea urchins (suppressants). The attractive activity of glutamate, cysteine, and cystine to the predator starfish Luidia clathrata (McClintock et al., 1984) is known. Tryptophan is also an important stimulant for some species of predatory fish (Carr et al., 1996). One possible explanation for the negative feedback of sea urchins to these compounds may be related with the indication of predator proximity (Hagen et al., 2002); in this case, the agitation of the tube feet verified served as preparation for escape and the rejection of simulacrum as withdrawal from the stimulus source. This study noted that in none but one (Alginic acid) of the cases sea urchin flight had occurred, having just checked rejection of the simulacra, which suggests that the sole chemical stimulus is not enough to raise this behaviour, being necessary, for example, that the stimulus reaches sea urchin via a water current (Hagen et al., 2002). However, the case of alginic acid, which induced almost half of the tested sea urchins to run away, ruled out this explanation.
The amino acids tyrosine and leucine increased the activity of tube feet and were apparently responsible for the preferential transport of the simulacra to the mouth or of their rejection. A possible explanation for these behaviours can be related to their chemical constitution that is similar to that of other amino acids—tyrosine (incitant) has an aromatic ring similar to that of tryptophan (suppressant), and leucine (incitant/deterrent) has a side chain similar to that of valine (incitant), differing only in the number of carbon atoms (Zubay et al., 1995)—so the agitation of the tube feet may indicate the detection of amino acids. The apparent lack of distinction of the simulacra embedded in leucine in relation to the control suggests that they do not contain enough information for the sea urchins to feed or not to feed, but responses showed a capacity of sea urchins to transport simulacra to the mouth and then reject them.
However, evidence for and knowledge of chemoreceptors responsible for the observed reactivity to the eight amino acids, and more extensive studies are required to relate food or flight responses with the respective electrophysiological responses to stimuli.
For the remaining organic compounds associated with simulacra that were presented to sea urchins, regardless of whether the animals were subjected to different diets, starch appears as one of the tested compounds that potentiate distinction between targeted food responses to the blank control simulacrum and the embedded chemical stimuli simulacra, with higher frequency response to the simulacra soaked with starch. This trend was also observed when the activity of the tube feet was increased. In Strongylocentrotus sp., the importance of carbohydrates as attractants was also verified (Mann et al., 1984).
Based on the evidence of the importance of the constituents of natural food in the stimulation of sea urchins, it was expected that the main constituents of the natural diet of sea urchins might prove most stimulatory, as happens in other taxa (Robertson et al., 1981; Laverack, 1988; Riordan and Lindsay, 2002). Several studies show that P. lividus diet is dominated by plant foods (Maggiore et al., 1987; San Martin, 1987; Boudouresque and Verlaque, 2007), despite the variety of diets depending on the habitat they occupy (Boudouresque and Verlaque, 2007). The cells of plant tissues of algae are rich in starch, which is stored in the form of grains (Raven et al., 1999) and can be easily liberated in considerable amounts when the plant undergoes decomposition. Thus, the detection and preference for starch revealed in this study strongly support the numerous observations of herbivory in this species. The preference for foods with high starch content does not occur in sea urchin L. variegatus (Klinger and Lawrence, 1984), while simple carbohydrates function as phagostimulant molecules. Although also feeding on algae, this species is more often described as omnivorous (Watts et al., 2001), feeding on an equal proportion of plants and animals (McClintock et al., 1982).
The apparent indifference verified with the stimulation with glucose (incitant) and saccharose (deterrent) associated with simulacra in this study suggests that carbohydrates, in general, do not raise food responses, but only those that are in large proportion in natural food of sea urchins. Simple carbohydrates (sugars) do not seem to arouse great interest from marine animals (Laverack, 1988). This hypothesis was also corroborated in the present study by other tested complex carbohydrates, such as glycogen (incitant) that most urchins use as energy reserve and laminarin (incitant) from seaweed Phaeophyta (Raven et al., 1999). The selectivity of foods based on the composition of their reserves can explain the food preferences of sea urchins for certain algae, as many studies on food preferences registered difficulty in relating favourite foods with their energy content or protein content (Vadas, 1977; Larson et al., 1980; Beddingfield and McClintock, 1998).
In several published studies dealing with the direct contact of source with chemoreceptors, Klinger and Lawrence (1984), using artificial agarose [3,6 anydro-galactose + D(+) galactose] foods containing starch, glycogen, alginate, casein, or peptone, also at a concentration of 1% (dry weight), reported these chemical stimuli as not phagostimulatory to sea urchin Lytechynus variegatus, but galactose and the amino acid L-phenylalanine were found to be phagostimulatory. However, all the artificial agarose food items were initially moved to the mouth and fed upon, rendering them as too responsive to be used as neutral simulacra. Agar disks or artificial agarose foods used as a methodology for studying feeding stimulation, as shown in this study, seem to be inappropriate given their intrinsic incitant or incitant/deterrent quality and not the suppressant quality shown by the cotton-based absorbent cleaning cloth used in this study.
Boudouresque and Verlaque (2007), based on gut content and non-limiting macrophyte resources, classified P. lividus as an herbivorous species and clearly elected brown algae (Fucophyceae) as the strongly “preferred” food of large individuals. Although macroalgae and seagrasses constitute the main feeding resources of P. lividus in situ, this species appears to be an opportunistic generalist able to exploit any kind of food resource including other marine invertebrates, especially under conditions of limited resources.
The restricted group of food-related compounds perceived by this species as incitants or suppressants and as stimulants or deterrents was shown to be remarkably related to other vertebrates whose kinship was confirmed by the sequencing of the genome of another plant-eater, the purple sea urchin S. purpuratus (Sea Urchin Genome Sequencing Consortium, 2006). This was the non-chordate deuterostome marine animal of any kind to be sequenced. Its genome, with 23,500 genes, includes about 979 genes for proteins expressly designed to sense light or odours, a number on par with what vertebrates have and more than in the invertebrates studied to date. The genome of the sea urchin S. purpuratus has revealed a large family of chemoreceptor genes, with some expressed in tube feet or pedicellariae, indicating a complex chemosensory system. Over 600 genes encode putative G-protein-coupled chemoreceptors. Many of these genes encode amino acid motifs that are a characteristic of vertebrate chemosensory and odorant receptors. The response of sea urchin P. lividus to the bitter caffeine stimulus was well-illustrative of its kinship to other vertebrates including humans.
Concerning tastes, caffeine, an alkaloid of plant origin, clearly showed the worst result as a food-related stimulus, generating a suppressant/deterrent response while the other tested bitterness stimuli, three amino acids (Osaku et al., 2007), showed a classification more in accordance with incitant stimuli and not, as expected, for bitter stimuli, at least suppressants. In what concerns sweetness, the five tested stimuli (Osaku et al., 2007) show results that are in accordance with incitant responses, but it remains unclear if sea urchins do really sense the sweet quality of a food. Finally, the umami-related stimuli, glutamic acid and glutamate monosodium, both showed similar suppressant responses, suggesting a poor sense for umami taste.
Concerning the non-feeding response (H), covering, it has been considered to be a protection against light, but it occurs both in the presence and absence of light. It has also been considered a protection against predation. de Ridder and Lawrence (1982) and Verlaque and Nedelec (1983) coined the term covering-feeding behaviour, suggesting that the covering process may play a role in feeding, allowing drift algae and sea grass leaves to be caught and held for later consumption.
Conclusion
The feeding behaviour of the tested sea urchins was clearly affected by their previous nutritional state; therefore, energetically poor diets are recommended to study echinoid chemical perception.
The proposed methodology to stimulate sea urchins based on simulacra seems to be valuable in terms of response objectivity and quantification, as it is possible to attribute connotation to food for any chemical stimulus and get bivariate binary responses (treatment or control). However, at least immediately after stimulation, a great percentage of the tested sea urchins did not show any kind of response at all, which implied that a considerable number of replicates should be submitted for statistical analysis.
Stimulating sea urchin P. lividus with 41 different food-related compounds such as carbohydrates, proteins, peptides and amino acids, oils and fatty acids, and purified chemicals related with some human basic tastes, it was possible to evidence a clear ability of this echinoid species to positively discriminate for proteins, starches, and very few oils. Perceived as incitants/stimulants, we have only found, among proteins, gliadin (from wheat gluten) but not casein (from bovine milk), among polysaccharides, starch but not laminarin (from kelp) or glycogen (from mussels), and among lipids, only the fatty acid linolenic acid. Among tissues, the flesh of either Kombu or mussel was clearly preferred (incitant/stimulant) over extracts (incitant).
The phagostimulants (incitants/stimulants) that were found, when presented alone, were, by order of magnitude, gliadin, linolenic acid, Kombu flesh, starch unmodified, starch soluble, and Kombu extract. If stimuli were presented concurrently with blanks, then seven phagostimulants (incitants/stimulants) were found by order of magnitude gliadin, Kombu flesh, linseed oil, casein, mussel flesh, starch soluble, and valine. As the classification incitant/stimulant (CMB) was not affected by the presence of blanks, eight such stimuli could be forwarded: gliadin, Kombu flesh, linolenic acid, casein, mussel flesh, starch soluble, starch unmodified, and Kombu extract. Being cautionary, we can, thus, suggest that under any circumstance gliadin, Kombu flesh, and starch were the only stimuli we can classify clearly as phagostimulants for sea urchin P. lividus. The lipids, linseed oil or its main constituent fatty acid linolenic acid, follow closely.
On the contrary, we found as phagodepressors (suppressants and suppressants/deterrents) eight stimuli by order of magnitude glutamate mNa, caffeine, cysteine, fructose, glutamic acid, tryptophan, cotton, and alginic acid.
Data Availability Statement
The raw data supporting the conclusions of this article will be made available by the authors, without undue reservation.
Author Contributions
Both authors listed have made a substantial, direct and intellectual contribution to the work, and approved it for publication.
Conflict of Interest
The authors declare that the research was conducted in the absence of any commercial or financial relationships that could be construed as a potential conflict of interest.
Publisher's Note
All claims expressed in this article are solely those of the authors and do not necessarily represent those of their affiliated organizations, or those of the publisher, the editors and the reviewers. Any product that may be evaluated in this article, or claim that may be made by its manufacturer, is not guaranteed or endorsed by the publisher.
Acknowledgments
We would like to express gratitude to Laboratorio Maritimo da Guia (Guia Marine Laboratory, Cascais, Portugal) for all the facilities conceded during all the long process of preparation and collection of data. This publication was financed by Portuguese national funds through FCT - Fundaa̧ão IP under project reference UIDB/04292/2020, and by the European Union's Horizon 2020 Research and Innovation Programme under grant agreement N810139: Project Portugal Twinning for Innovation and Excellence in Marine Science and Earth Observation - PORTWIMS.
Footnotes
1. ^NCBI Strongylocentrotus purpuratus Annotation Release 102. LOC575734 gamma-gliadin [Strongylocentrotus purpuratus (purple sea urchin)] 2019.
References
Andrew, N. L., and Stocker, L. J. (1986). Dispersion and phagokinesis in the echinoid Evechinus chloroticus (Val.). J. Exp. Mar. Biol. Ecol. 100, 11–23. doi: 10.1016/0022-0981(86)90152-8
Beddingfield, S. D., and McClintock, J. B. (1998). Differential survivorship, reproduction, growth and nutrient allocation in the regular echinoid Lytechinus variegatus (Lamarck) fed natural diets. J. Exp. Mar. Biol. Ecol. 226, 195–215. doi: 10.1016/S0022-0981(97)00247-5
Boudouresque, C. F., and Verlaque, M. (2007). “Ecology of Paracentrotus lividus,” in Edible Sea Urchins: Biology and Ecology, ed J. M. Lawrence (Amsterdam: Elsevier), 243–285. doi: 10.1016/S0167-9309(07)80077-9
Campbell, A. C., and Laverack, M. S. (1968). The responses of pedicellariae from Echinus esculentus (L.). J. Exp. Mar. Biol. Ecol. 2, 191–214. doi: 10.1016/0022-0981(68)90016-6
Carr, W. E. S., Netherton, J. C. III., Gleeson, R. A., and Derby, C. D. (1996). Stimulants of feeding behavior in fish: analyses of tissues of diverse marine organisms. Biol. Bull. 190, 149–160. doi: 10.2307/1542535
Carr, W. E. S., Netherton, J. C. III., and Milstead, M. L. (1984). Chemoattractants of the shrimp, Palaemonetes pugio: variability in responsiveness and the stimulatory capacity of mixtures containing amino acids, quaternary ammonium compounds, purines and other substances. Comp. Biochem. Physiol. 77A, 469–474. doi: 10.1016/0300-9629(84)90213-5
Crampton, E. F., and Harris, L. E. (1969). Applied Animal Nutrition—The Use of Feedstuffs in the Formulation of Livestock Rations, 2nd Edn. San Francisco, CA: Freeman
Dance, C. (1987). Patterns of activity of the sea urchin Paracentrotus lividus in the bay of Port-Cros (Var, France, Mediterranean). Mar. Ecol. 8, 131–142. doi: 10.1111/j.1439-0485.1987.tb00179.x
Dayton, P. K., Rosenthal, R. J., Mahen, L. C., and Antezana, T. (1977). Population structure and foraging biology of the predaceous Chilean asteroid Meyenaster gelatinosus and the escape biology of its prey. Mar. Biol. 39, 361–337. doi: 10.1007/BF00391939
de Ridder, C., and Lawrence, J. M. (1982). “Food and feeding mechanisms: echinoides,” in Echinoderm Nutrition, eds M. Jangoux and J. M. Lawrence (Rotterdam: A.A.Balkema), 57–115.
Derby, D., and Atema, J. (1988). “Chemoreceptor cells in aquatic invertebrates: peripheral mechanisms of chemical signal processing in decapod crustaceans,” in Sensory Biology of Aquatic Animals, eds J. Atema, R. Fay, N. A. Popper, and W. N. Tavolga (New York, NY: Springer-Verlag), 365–385. doi: 10.1007/978-1-4612-3714-3_14
Ferner, M. C., and Jumars, P. A. (1999). Responses of deposit-feeding spionid polychaetes to dissolved chemical cues. J. Exp. Mar. Biol. Ecol. 236, 89–106. doi: 10.1016/S0022-0981(98)00196-8
Flammang, P., Michel, A., Cauwenberge, A. V., Alexandre, H., and Jangoux, M. (1998). A study of the temporary adhesion of the podia in the sea star Asterias rubens (Echinodermata: Asteroidea) through their footprints. J. Exp. Biol. 201, 2383–2395. doi: 10.1242/jeb.201.16.2383
Gago, J., Range, P., and Luís, O. (2003). “Growth, reproductive biology and habitat selection of the sea urchin Paracentrotus lividus in the coastal waters of Cascais, Portugal,” in Echinoderm Research 2001, eds J. P. Féral and B. David (Lisse: Swets and Zeitlinger), 269–275.
Hagen, N. T. (1998). Effect of food availability and body size on out-of-season gonad yield in the green sea urchin, Strongylocentrotus droebachiensis. J. Shellfish Res. 17, 1533–1539.
Hagen, N. T., Andersen, A., and Stabell, O. B. (2002). Alarm responses of the green sea urchin, Strongylocentrotus droebachiensis, induced by chemically labelled durophagous predators and simulated acts of predation. Mar. Biol. 140, 365–374. doi: 10.1007/s002270100694
Hay, M. E., Lee, R. R. J., Guieb, R. A., and Bennett, M. M. (1986). Food preference and chemotaxis in the sea urchin Arbacia punctulata (Lamarck) Philippi. J. Exp. Mar. Biol. Ecol. 96, 147–153. doi: 10.1016/0022-0981(86)90239-X
Klinger, T. S., and Lawrence, J. M. (1984). Phagostimulation of Lytechinus variegatus (Lamarck) (Echinodermata: Echinoidea). Mar. Behav. Physiol. 11, 49–67. doi: 10.1080/10236248409387034
Klinger, T. S., and Lawrence, J. M. (1985). Distance perception of food and the effect of food quantity on feeding behaviour of Lytechinus variegatus (Lamarck) (Echinodermata: Echinoidea). Mar. Behav. Physiol. 11, 327–344. doi: 10.1080/10236248509387057
Larson, B. R., Vadas, R. L., and Keser, M. (1980). Feeding and nutritional ecology of the sea urchin Strongylocentrotus drobachiensis in Maine, USA. Mar. Biol. 59, 49–62. doi: 10.1007/BF00396982
Laur, D. R., Ebeling, A. W., and Reed, D. C. (1986). Experimental evaluations of substrate types as barriers to sea urchin (Strongylocentrotus spp.) movement. Mar. Biol. 93, 209–215. doi: 10.1007/BF00508258
Laverack, M. S. (1988). “The diversity of chemoreceptors,” in Sensory Biology of Aquatic Animals, eds J. Atema, R. Fay, N. A. Popper, W. N. Tavolga (New York, NY: Springer-Verlag), 287–312. doi: 10.1007/978-1-4612-3714-3_11
Lawrence, J. M., Lawrence, A. L., and Watts, S. A. (2007). “Feeding, digestion, and digestibility,” in Edible Sea Urchins: Biology and Ecology, ed J. M Lawrence (Amsterdam: Elsevier), 135–158. doi: 10.1016/S0167-9309(07)80071-8
Lawrence, J. M., Plank, L. R., and Lawrence, A. L. (2003). The effect of feeding frequency on consumption of food, absorption efficiency, and gonad production in the sea urchin Lytechinus variegatus. Comp. Biochem. Physiol. Part A 134, 69–75 doi: 10.1016/S1095-6433(02)00222-2
Lee, P. G., and Meyers, S. P. (1997). “Chemoattraction and feeding stimulation,” in Crustacean Nutrition, eds L. R. D'Abramo, D. E. Conklin, and D. M. Akiyama (Baton Rouge, LA: World Aquaculture Society), 292–352.
Luis, O., Delgado, F., and Gago, J. (2005). Year-round captive spawning performance of the sea urchin Paracentrotus lividus: relevance for the use of its larvae as live feed. Aquat. Living Resour. 18, 45–54. doi: 10.1051/alr:2005004
Maggiore, F., Berthon, J. F., Boudouresque, C. F., and Lawrence, J. (1987). “Donnes preliminaires sur les relations entre Paracentrotus lividus, Arbacia lixula et le phytobenthos dans la baie de Port-Cros (Var, France, Mediterranee),” in Colloque International sur Paracentrotus Lividus et les Oursins Comestibles, ed C. F. Boudouresque (Marseille: Gis Posidonie Publications), 65–82.
Mann, K. H., Wright, J. L. C., Welsford, B. E., and Hatfield, E. (1984). Responses of the sea urchin Strongylocentrotus droebachiensis (O. F. Muller) to water-borne stimuli from potential predators and potential food algae. J. Exp. Mar. Biol. Ecol. 79, 233–244. doi: 10.1016/0022-0981(84)90197-7
Markgren, J., Hedenqvist, M., Rasheed, F., Skep,ö, M, and Johansson, E. (2020). Glutenin and gliadin, a piece in the puzzle of their structural properties in the cell described through monte carlo simulations. Biomolecules 10:1095. doi: 10.3390/biom10081095
Mattison, J. E., Trent, J. D., Shanks, A. L., Akin, T. B., and Pearse, J. S. (1977). Movement and feeding activity of red sea urchins (Strongylocentrotus franciscanus) adjacent to a kelp forest. Mar. Biol. 39, 25–30. doi: 10.1007/BF00395589
McClintock, J. B., Klinger, T. S., and Lawrence, J. M. (1982). Feeding preferences of echinoids for plant and animal food models. Bull. Mar. Sci. 32, 365–369.
McClintock, J. B., Klinger, T. S., and Lawrence, J. M. (1984). Chemoreception in Luidia clathrata (Echinodermata: Asteroidea): qualitative and quantitative aspects of chemotactic responses to low molecular weight compounds. Mar. Biol. 84, 47–52. doi: 10.1007/BF00394526
Osaku, K., Fujii, A., Ruttanapornvareesakul, Y., Nagano, N., Kuwahara, K., and Okamoto, A. (2007). Differences in free amino acid composition between testis and ovary of sea urchin Anthocidaris crassispina during gonadal development. Fish. Sci. 73, 660–667. doi: 10.1111/j.1444-2906.2007.01379.x
Pavillon, J. F., and Rault, P. (1990). Variations mensuelles des acides aminés libres dissous (AALD) présents en un point de la rade de Villefranche-sur-Mer: utilisation par les larves de Paracentrotus lividus et de Sphaerechinus granularis. Aquat. Living Resour. 3, 147–150 doi: 10.1051/alr:1990015
Prince, J. S., and LeBlanc, W. G. (1992). Comparative feeding preference of Strongylocentrotus droebachiensis (Echinoidea) for the invasive seaweed Codium fragile ssp. tomentosoides (Chlorophyceae) and four other seaweeds. Mar. Biol. 113, 159–163. doi: 10.1007/BF00367649
Raven, P. H., Evert, R. E., and Eichhorn, S. E. (1999). Biology of Plants, 6th Edn. New York, NY: Freeman.
Riordan, T. J., and Lindsay, S. M. (2002). Feeding responses to particle-bound cues by a deposit-feeding spionid polychaete, Dipolydora quadrilobata (Jacobi 1883). J. Exp. Mar. Biol. Ecol. 277, 79–95. doi: 10.1016/S0022-0981(02)00292-7
Robertson, J. R., Fudge, J. A., and Vermeer, G. K. (1981). Chemical and live feeding stimulants of the sand fiddler crab, Uca pugilator (Bosc.). J. Exp. Mar. Biol. Ecol. 53, 47–64. doi: 10.1016/0022-0981(81)90083-6
Saa, C. F. (2002). Algas de Galicia, Alimento y Salud. Las verduras del Oceano Atlantico. Pontevedra: Algamar.
San Martin, G. A. (1987). “Comportement alimentaire de Paracentrotus lividus (Lmk) (Echinodermata: echinoidea) dans l'Étang de Thau (Herault, France),” in Colloque international sur Paracentrotus lividus et les Oursins Comestibles, ed C. F. Boudouresque (Marseille: Gis Posidonie Publicatons), 37–57.
Sea Urchin Genome Sequencing Consortium (2006). The genome of the sea urchin Strongylocentrotus purpuratus. Science 314, 941–952. doi: 10.1126/science.1133609
Sloan, N. A., and Campbell, A. C. (1982). “Perception of food,” in Echinoderm Nutrition, eds M. Jangoux and J. M. Lawrence (Rotterdam: A.A.Balkema), 3–23. doi: 10.1201/9781003078920-2
Teruya, T., Suenaga, K., Koyama, T., Nakano, Y., and Uemura, D. (2001). Arachidonic acid and α-linolelic acid, feeding attractants for the crown-of-thorns sea star Acanthaster planci, from the sea urchin Toxopneustes pileolus. J. Exp. Mar. Biol. Ecol. 266, 123–134. doi: 10.1016/S0022-0981(01)00337-9
Vadas, R. L. (1977). Preferential feeding: an optimization strategy in sea urchins. Ecol. Monogr. 47, 337–371. doi: 10.2307/1942173
Vaietilingon, D., Rasolofonirina, R., and Jangoux, M. (2003). Feeding preferences, seasonal gut repletion indices, and diel feeding patterns of the sea urchin Tripneustes gratilla (Echinodermata: Echinoidea) on a coastal habitat off Toliara (Madagascar). Mar. Biol. 143, 451–458. doi: 10.1007/s00227-003-1111-y
Verlaque, M. Q., and Nedelec, H. (1983). Biology of Paracentrotus lividus (Lamarck) on rocky bottoms in Corsica (Mediterranean, France): diet of adults. Vie Milieu 33, 191–201.
Watts, S. A., McClintock, J. B., and Lawrence, J. M. (2001). “The ecology of Lytechinus variegates,” in Edible Sea Urchins: Biology and Ecology, ed J. M. Lawrence (Amsterdam: Elsevier), 375–394. doi: 10.1016/S0167-9309(01)80023-5
Whittle, K. J., and Blumer, M. (1970). “Interactions between organisms and dissolved organic substances in the sea: chemical attraction of the starfish Asterias vulgaris to oysters,” in Organic Matter in Natural Waters, ed D. W. Hood (Alaska: Institute of Marine Science University of Alaska) 495–507.
Wickins, J. F., and Helm, M. M. (1981). “Seawater treatment,” in Aquarium Systems, ed A. D. Hawkins (London: Academic Press), 63–128.
Keywords: sea urchins, chemical perception, food related stimuli, simulacra, seaweed extracts
Citation: Luis OJ and Gago JM (2021) Perception of Dissolved Food-Related Compounds by the Sea Urchin Paracentrotus lividus (Echinodermata: Echinoidea). Front. Mar. Sci. 8:719670. doi: 10.3389/fmars.2021.719670
Received: 02 June 2021; Accepted: 23 August 2021;
Published: 04 October 2021.
Edited by:
Janine Barbara Adams, Nelson Mandela University, South AfricaReviewed by:
Rodrigo Riera, University of Las Palmas de Gran Canaria, SpainNadia Ruocco, Anton Dohrn Zoological Station, Italy
Copyright © 2021 Luis and Gago. This is an open-access article distributed under the terms of the Creative Commons Attribution License (CC BY). The use, distribution or reproduction in other forums is permitted, provided the original author(s) and the copyright owner(s) are credited and that the original publication in this journal is cited, in accordance with accepted academic practice. No use, distribution or reproduction is permitted which does not comply with these terms.
*Correspondence: Orlando J. Luis, b2psdWlzQGZjLnVsLnB0