- Department of Zoology, Sidho-Kanho-Birsha University, Purulia, India
In vertebrates, melatonin (N-acetyl-5-methoxy-tryptamine, MEL) is synthesized from L-tryptophan (L-Trp), primarily in the pineal gland and additionally in several non-pineal tissues, including enterochromaffin cells of the gastrointestinal tract. The biosynthesis of MEL in the pinealocytes exhibits a daily rhythm with a nocturnal peak in synchronization with the dark phase of an environment. However, the temporal pattern of the gut melatoninergic system in fish is known to be synchronized with one or more non-photic external cue(s), of which most notable include the components of food and feeding time in a daily cycle. Experimental findings on the use of L-Trp (5-fold to −8-fold higher with respect to standard) as a fish food supplement suggest that it has a stimulatory influence on gut MEL synthesis that ultimately leads to elevated levels of plasma MEL. Several studies employing MEL as a fish feed additive report variable responses in relation to the physiological functions of the fish and its doses of application and modes of exposure. Oral administration of MEL (validated dose in general: 200 mg/kg fish food) causes an increase in gut MEL concentrations, food intake capacity, and micronutrient selection ability of fish, as well as enhanced activity of antioxidative enzymes and/or reduced levels of biological stress markers like plasma cortisol and lactate. The application of MEL with the doses of 50 and 250 mg/kg fish food to balanced diet results in precious maturation of gonads, high live sperm rate, and good quality seeds in catfish. A few studies on different fish groups administered with MEL through tank water reveal a significant increase in the fecundity rate, percentage of fertilization, and the number of hatched embryos. MEL injection through intraperitoneal or intramuscular routes also elicits variable responses in fish. Several studies suggest a major protective role of endogenous MEL against gastric ulcer induced by pathogenic bacterial infection. Taken together, MEL seems to be a multipotent physiological candidate involved in the regulation of a variety of body functions ranging from the synchronization of vital activities with environmental variables to the timing of reproduction in a changing system, from the acceleration of body growth to the determination of a pattern of development, and from the reduction of oxidative stress to the protection against microbial infections. Because aquaculture aims at substantially improving the quality and quantity of available aquatic resources employing convenient, consistent, and commercially exploitable measures, we find the reasons to suggest that the use of MEL or its precursor L-Trp as a fish food supplement may open up a new vista of aquaculture and, hence, present this review with an attempt to present the basic information on this area of interest to justify the hypothesis.
Introduction
Multiple natural resources are utilized for the production of aquatic animals and have become a primary factor on which the aquaculture relies, and capturing of wild fish has become a primary source of protein production from sea food sources (Boyd et al., 2020). Sustainable aquaculture has a primary goal of supplying farm-based aquatic nutrients continuously in bringing the sustainable benefit to human without hampering the existence of ecosystem or overutilization of natural resources to the extent from where it cannot be recovered. Nowadays, commonly practiced aquaculture management is exceedingly engaged with a continuous progress on the optimal usage of natural resources for the production of nutrients although it lacks provisions on the aspects of balanced fish growth and fishing mortality (Costello et al., 2020). Looking at the perspectives on the magnitude in general trend and growth in the categories of aquatic cultured animals are prerequisite for an understanding of the aquaculture diversity. According to the Food and Agriculture Organization, United Nations (Food Agriculture Organization, 2019), aquatic plants, mollusks, and freshwater fish encompass about 84% of the aquaculture, and the global aquaculture production for freshwater fish and for marine fish is 44.66 mt and is 3.10 mt, respectively.
Reproduction in most fish groups is seasonal, and an understanding of the underlying mechanisms used to synchronize this periodic event with the cyclic changes in environmental variables is an important aspect for sustainable aquaculture (Maitra, 2011). Such a synchronization is required for setting the spawning period at the most favorable time of the year for a better survival of the progeny, and vis-à-vis some predictive mechanisms are also developed in fish by using environmental cues and photoperiod for an anticipation of the initiation of gametogenesis at the proper time for the supply of mature healthy gametes at the time of spawning (Carrillo et al., 1993; Bromage et al., 2001). The supply of “good quality” gametes is an essential requirement for the sustainable culture of any species as “poor quality” may decrease the survival potential of hatched embryos (Tamura et al., 2008; Hasan et al., 2014). Specific environmental cues stimulate the pineal gland to secrete a hormone, melatonin (N-acetyl-5-methoxy-tryptamine, MEL), which plays a significant role in the regulation of diverse physiological functions, of which most important are seasonality of reproduction and antioxidative defense in a wide range of vertebrates, including fish (Falcón et al., 2010; Maitra et al., 2013). The identification of MEL-producing cells and subcellular localization of different enzymes regulating MEL synthesis are also well-documented in various tissues (Maitra et al., 2015). Apart from reproduction, several important physiological functions are associated with successful aquaculture. MEL, in addition to its housekeeping effects on rhythmic changes in different physiological variables, plays a key role in regulating several body functions in aquatic animals (Maitra, 2011). The impact of MEL on fish growth and development in association with digestive physiology are also evident from several studies (Falcón et al., 2003, 2007, 2010; Pal et al., 2016a; Pal and Maitra, 2018). Several lines of evidence suggest that MEL, along with its effect on enhancing the digestive enzyme activity in the gut, has a significant impact on the gut microbiota in fish as well as in other vertebrates (Yin et al., 2018, 2020; Wang et al., 2021; Zhang et al., 2021). A fish study by evincing a role of endogenous MEL in ameliorating microbial infection-induced pathogenesis implicates MEL in aquaculture against ulcer causative agents (Pal et al., 2016b). Environmental stressors and disease susceptibility are the two important aspects, which have major impacts on sustainable fish aquaculture. Several studies on fish also demonstrated the role of MEL in regulating the status and rhythmic functions of immune cells (Cuesta et al., 2007, 2008; Roy et al., 2008; Baekelandt et al., 2020). In the case of fish, external stressor-mediated MEL synthesis and the response of mealatonin to chronic stress are also known (Conde-Sieira et al., 2014; López-Patiño et al., 2014; Gesto et al., 2016). Thus, the significance of MEL as a multipotent physiological candidate involved in the regulation and maintenance of fish health is gaining momentum.
In the current state of knowledge, there are reasons to suggest that food composition, feeding habits, and feeding time may be the critical factors in aquaculture for the synchronization of MEL synthesis within the gastrointestinal tissues (Mukherjee and Maitra, 2018; Pal and Maitra, 2018; Yasmin et al., 2021). It is well-proclaimed that the poor quality of flesh and poor performance of fish growth are related to imbalanced food composition in aquaculture (Maitra, 2011). Thus, an interplay between food composition and the circadian axis in the regulation of growth, development, reproduction, and other physiological functions becomes an important facet of understanding for sustainable aquaculture (Campos-Mendoza et al., 2004). However, the emergence of a comprehensive idea depicting the potential use of MEL and/or its biosynthetic precursor as a component of fish food for application in aquaculture suffered a major setback mostly due to the lack of an effort to make the use of available data for a meaningful outcome. Hence, this review article aims to deal with existing literature studies on the unique features of MEL, gathered mostly from fish studies, and to highlight its hitherto neglected multipotent actions on fish health, which seems to be important for sustainability in the aquaculture industry.
Unique Features of Melatonin: Sources, Biosynthesis, and Regulation of Production
Melatonin is primarily synthesized within the pinealocytes of the pineal gland in a rhythmic manner, having extreme light sensitivity in its biosynthesis. The duration of the dark phase in a photoperiodic cycle or an external geophysical cycle is a key regulator of the nocturnal increase of MEL. This indole compound was first isolated, purified and characterized from the extract of bovine pineal gland (Lerner et al., 1958). Further studies revealed that MEL is also synthesized in several extra-pineal tissues, viz., retina (Iuvone and Besharse, 1983), gut (Bubenik, 1986; Maitra et al., 2015), placenta (Iwasaki et al., 2005), harderian gland (Menendez-Pelaez et al., 1987), and gonads (Itoh et al., 1999; Hasan et al., 2014). Thus, the distribution of MEL is ubiquitous. The major source of MEL is the pineal organ/gland or epiphysis cerebri, which is located on the roof of diencephalon. In the teleost fish, three distinct structures constitute the pineal gland: (1) The “end vesicle” is an elongated and a flattened vesicular body located close to the cranium. (2) The “pineal stalk” is a structural connection that connects the “end vesicle” to the brain. (3) “Dorsal sac” is a saccular structure, which encircles the “pineal stalk” at the cerebral end (Maitra et al., 2005). In fish, the pineal organ has both sensory and secretory functions; whereas in higher vertebrates (as in birds and mammals) it acts as an endocrine gland (Falcón et al., 2010).
Melatonin, in every tissue, is synthesized from the precursor molecule known as L-tryptophan (L-Trp) in a four-step pathway. The rhythmic process of MEL synthesis with respect to the environmental light-dark (LD) cycle is regulated primarily by the clock genes of the suprachiasmatic nucleus (SCN) of hypothalamus. Briefly, the entry of L-Trp into the site of synthesis from the blood stream and its conversion to 5-hydroxy-tryptophan (5-HTP) are also facilitated by the action of tryptophan hydroxylase, and the reaction takes place within the mitochondria (Lovenberg et al., 1967). Then, 5-HTP in the cytosol acts as a substrate for the enzyme 5-HTP decarboxylase, which forms 5-hydroxytryptamin (5-HT) or serotonin (Lovenberg et al., 1962) that is converted to N-acetyl serotonin by the action of arylalkylamine-N-acetyltransferase (AANAT) (Voisin et al., 1984), and finally MEL is synthesized by the enzyme hydroxyindole-O-methyltransferase (HIOMT), also known as acetylserotonin O-methyltransferase (ASMT) (Axelrod and Weissbach, 1960) (Figures 1A,B). AANAT is considered as the rate-limiting enzyme for MEL synthesis (Klein et al., 1997) and is intricately regulated by the environmental LD cycle (Collin et al., 1989). The oscillation in AANAT activity is congruent with the change in the MEL level and, for this reason, it is known as “the MEL rhythm generating enzyme” (Klein et al., 1997). MEL synthesis in carp, like other fish species, is dependent on an external LD cycle and is regulated through the circadian organization of melatoninergic system (Seth and Maitra, 2010). Endogenous clock of pineal-driven changes in the AANAT messenger RNA (mRNA) level is observed in zebra fish (Danio rerio) and sea bream (Sparus aurata), but not in rainbow trout (Oncorhynchus mykiss). Thus, it is apparent that the temporal organization and regulation of AANAT synthesis vary in different fish species (Bégay et al., 1998; Iigo et al., 2007).
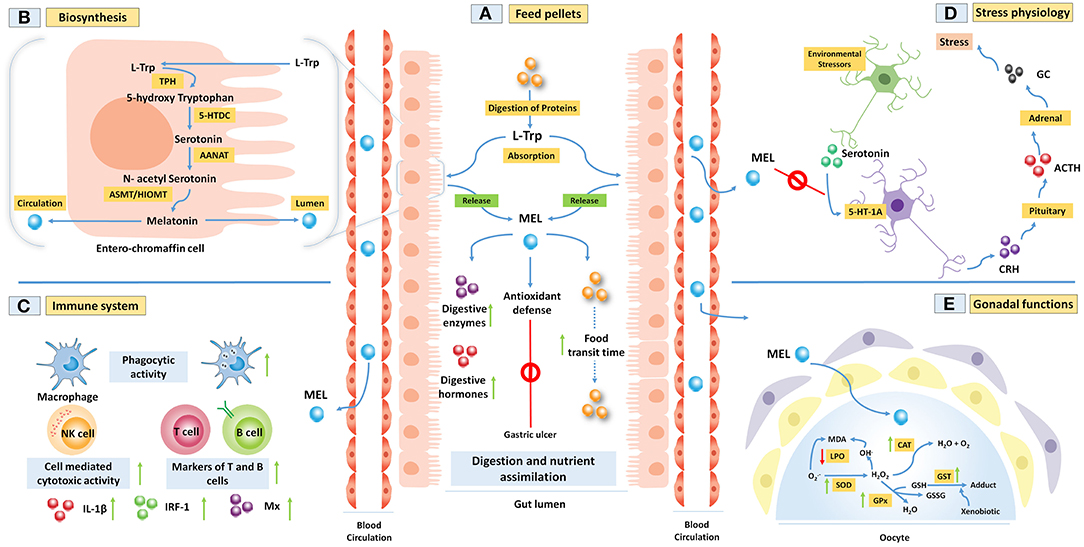
Figure 1. (A,B) Melatonin (MEL) is synthesized from amino acid L-tryptophan (L-Trp) within the fish gut epithelial cells. This process includes four steps of enzymatic reactions. Gut MEL increases the activity of digestive enzymes and helps in the assimilation of food materials. It also abrogates gastric ulcers by activating the antioxidant defense system. (C) MEL improves fish immune functions by increasing the phagocytic activity of the macrophages, cell-mediated cytotoxicity, markers of T and B cells, and several immune regulators like interleukin-1 beta (IL-1β), interferon-regulatory factor 1 (IRF-1), and Mx proteins. (D) Further, MEL downregulates the environmental stressor-induced release of glucocorticoid (GC) from the adrenal through the activation of 5-HT-1A receptor in fish. (E) MEL acts as a potent antioxidant through increasing the activity of antioxidative enzymes in carp ovary to regulate the spawning.
The environmental cues play a central role in the regulation of MEL production in the pineal organ and other peripheral tissues in fish (Maitra et al., 2015). Among various environmental factors, “duration of the solar day” or photoperiod is shown to be the most important component, which regulates the synthesis and release of pineal MEL (Falcón et al., 2010). However, light alone, or in addition to other environmental variables like temperature, rainfall, and humidity, seems to act as a synchronizer of MEL biosynthesis. These environmental signals are transduced by a pineal organ into a neuroendocrine signal in the form of MEL (Ekstrzm and Meissl, 1997). This indolamine hormone is synthesized in a rhythmic pattern, and this property is conserved in all vertebrate species (Migaud et al., 2010; Maitra et al., 2013). In fish, MEL formation at night and its termination during the day are strictly regulated by AANAT, which in the presence of light signal undergoes proteasomal proteolysis (Liu and Borjigin, 2005). The regulatory mechanism of circadian MEL production in various fish pineal organs is not identical. It may act as a light sensitive organ (e.g., rainbow trout O. mykiss) or a true circadian light-sensitive pacemaker (e.g., pike Esox lucius, zebra fish D. rerio, and sea bream S. aurata) in fish (Maitra et al., 2015). The nocturnal rhythmicity of MEL shows three types of profile, viz., types A, B, and C; which are identified in different vertebrates, including fish (Falcón et al., 2010; Migaud et al., 2010). In the cases of Atlantic cod (Gadus morhua) and haddock (Melanogrammus aeglefinus), the MEL level in blood shows a peak in the late dark phase and this is characterized as type A profile (Davie et al., 2007). Type B profile shows a peak in the MEL level at the mid-dark phase as observed in Nile tilapia, Oreochromis niloticus (Martinez-Chavez et al., 2008), whereas a rise in the MEL level just after the onset of dark phase is the character of type C profile, which is found in Atlantic salmon (Salmo salar), rainbow trout (O. mykiss), Atlantic halibut (Hippoglossus hippoglossus), and in most teleosts (Falcón et al., 2010; Migaud et al., 2010).
Ubiquitous Functions of Melatonin in Vertebrates
In the past few decades, classical physiological approaches are the commonly undertaking modes of investigation to reveal the role of the pineal gland and its secreted hormone MEL in the regulation of a wide range of physiological functions in diverse groups of animals, including fish. Many of such regulatory functions exhibit a pattern of circadian and/or seasonal rhythms, viz., food intake, growth, reproduction, migration, osmoregulation, pigmentation of skin, thermal inclination, plasma glucose, lipid and sterol contents, etc. (Falcón et al., 2007, 2010; Sköld et al., 2008). However, only those physiological functions of MEL are emphasized, the significance of them has been unequivocally demonstrated in most of the studied animals.
Regulation of Reproduction
The role of MEL in the regulation of reproduction is one of the major areas of studies in different vertebrate groups that received serious attention (Reiter et al., 2009). MEL plays a central role in the timing of reproductive seasonality by acting as a neuroendocrine reflection of photoperiod. Reproductive events in a seasonal breeder are often synchronized with the periodic changes in one or more environmental components in such a way that reproduction occurs in the most favorable part of the year. Most teleosts, which breed at mid and high latitudes, and evenly a few species at low latitudes, use the information on the changes in the duration of the solar day or photoperiod, individually or in combination with water temperature or other component(s) of the environment, as a “driving force” to determine the temporal pattern of seasonal reproduction (Maitra et al., 2015). The processing of light information at the physiological level, thus seems to be the most important event that occurs in a neuroendocrine system to coordinate and/or synchronize different body functions associated with the seasonality of reproduction (Falcón et al., 2010). Here is the physiological significance of MEL, which follows the synthesis in a photosensitive organ, like pineal and retina, and is released through the circulation to reach the target organs and act on them through specific G-protein coupled receptors (Chattoraj et al., 2009). Obviously, the demonstration of MEL receptors in several tissues and organs, especially gonads in fish, has been a major breakthrough in understanding the role of MEL in the regulation of fish reproduction (Chattoraj et al., 2009; Moniruzzaman and Maitra, 2012). Nonetheless, one of the limitations of existing knowledge is that most of the studies dealing with the actions of MEL on the regulatory axis of reproduction have been confined to the circulatory profiles of endogenous MEL in relation to reproductive events in an annual cycle, or to the response mechanism at the target sites to exogenous MEL administration, leaving the endogenous source of MEL in circulation mostly undefined. Accordingly, we are compelled to discuss the role of MEL as a potent indolamine candidate in the regulation of reproduction using the data on the profiles of MEL in various organs and in circulation during the different phases of gonadal growth and development in an annual reproductive cycle, but more importantly, the data gathered from in vivo and in vitro studies on the mechanism of response in gonads and their regulatory axis to exogenous MEL in respective species (Maitra et al., 2013).
The mechanism of action of MEL in the regulation of reproduction has been extensively studied in fish and in other vertebrates. There is possibility that MEL interacts with the brain–pituitary–gonadal axis, or with one or more of a variety of peripheral and/or central sites, including the brain, the pituitary gland, and also on the gonads (Maitra et al., 2013). The hypothesis that emerged from such studies in common states that the actions of MEL on the reproductive axis are mediated by an interaction with the hypothalamic control of pituitary functions. The hypothalamo–hypophyseal system in periodic breeders in general plays a major role in the photoperiodic regulation of reproduction, where MEL provides temporal information related to day length and season (Popek et al., 1997). In fish, the preoptic area (POA) of hypothalamus is known to integrate the photoperiodic information from different sources as it receives neural inputs from the retina and the pineal organ, and hormonal (MEL) input from the pineal organ, and thereby occupies a pivotal position in the photoneuroendocrine regulation of reproduction (Maitra and Hasan, 2016). Neurons from the POA transmit monoaminergic (i.e., dopamine and 5-HT) and/or peptidergic information to the pituitary gland, which corresponds to the peptides that reach the neurohypophysis (e.g., isotocin, arginine, and vasotocin) or releasing factors that control the activity of the pituitary cells for the synthesis of a number of hormones associated with the seasonal growth and development of gonads. Thus, the effects of MEL on reproductive organs result from an interaction with the hypothalamic control of the pituitary function. Moreover, the detection of MEL receptors (both MT1 and MT2 subtypes) and the display of binding of 125IMel to tissue sections as well as to membrane preparations in pike and trout pituitary glands provide evidence that MEL might modulate neuroendocrine functions by targeting the pituitary gland itself (Falcón et al., 2003). Thus, it seems to be suggestive that variably influencing MEL under different experimental conditions and even in different parts of the reproductive cycle depends on the type of MEL receptors expressed in the pituitary glands of the concerned animals (Falcón et al., 2007). Obviously, MEL is per se neither anti-gonadotrophic nor pro-gonadotrophic, rather the changing duration of the nocturnal MEL message is a passive signal that provides the hypothalamic–pituitary–gonadal axis information as to the time of year (calendar information; Reiter et al., 2009). The reproductive axis uses the season-dependent MEL rhythm to adjust testicular and ovarian physiology accordingly. However, considering hypothalamus as the highest center of endocrine regulation, a more convincing explanation for the mechanism of action of MEL on this part of brain deserves special attention (Maitra et al., 2013).
Synchronization of Rhythmic Body Functions With the Environment
In all living organisms, MEL acts as an endogenous synchronizer either in stabilizing the rhythms (circadian) of body functions or in reinforcing them. Hence, MEL is called a “chronobiotic” molecule or “hormone of darkness” or “biological night” (Reiter et al., 2013). It has been demonstrated in mammalian species and human beings that the exogenous administration of MEL changes the timing of rhythms by increasing endogenous MEL and cortisol levels, and this phase shift of MEL depends upon its time of administration (Scheer and Czeisler, 2005). It phase advances the circadian clock when given in the evening and the first half of the night, whereas circadian rhythms in the second half of the night or at early daytime are phase delayed. The magnitude of phase advance or phase delay depends on the doses of MEL. Thus, a practical definition of MEL would be “a substance that adjusts the timing of internal biological rhythms” or more specifically “a substance that adjusts the timing of the central biological clock” as a chronological pacemaker or “zeitgeber” (time cue) as a calendar function though the appropriate zeitgeber circadian oscillators are found in every organ and indeed in every cell of the body (Arendt and Skene, 2005).
Regulation of Behavior and Brain Functions
The pineal gland promotes a homeostatic equilibrium through MEL and acts as a “tranquilizing organ” in stabilizing the electrical activity of the central nervous system. The classic endogenous or non-seasonal depression is characterized by sleep disorder, appetite suppression, weight loss, and an advanced onset of nocturnal MEL release, which starts in the spring and persists through the summer or through the winter during the period of light-phase shortening. Similar phenomena are associated with individuals having low nocturnal MEL levels and major neuronal disorders (Srinivasan et al., 2006). MEL secretion is shown to be wavelength dependent as the exposure to monochromatic light at 460 nm produced a 2-fold greater circadian phase delay. These results are further confirmed by measuring brain serotonin and tryptophan levels, which rise after MEL administration and are directly linked with an array of neuropsychiatric phenomena. Collectively, these findings suggest that appropriate exogenous MEL administration can restore neurological disorders with a direct impact on the general health of aged animals (Cardinali, 2019).
Scavenger of Free Radicals
Due to its lipophilic nature, melatonin crosses all morphological and physical barriers or a hematoencephalic barrier (blood–brain barrier (BBB), placenta) and reaches all tissues of the body within a very short period of time. As a result, MEL exhibits antioxidant effects and performs a very important receptor-independent metabolic function, i.e., a multifaceted scavenger of free radicals (Maitra and Hasan, 2016). The antioxidant effects of MEL have been well-described and included both direct and indirect effects with equal efficiency in multiple sites (nucleus, cytosol, and membranes) of the cell. It detoxifies a variety of free radicals and reactivates oxygen intermediates, including the hydroxyl radical/hydrogen peroxide, peroxy radicals, peroxynitrite anion, singlet oxygen, nitric oxide (NO), and lipid peroxidation (Tamura et al., 2008). Compared to vitamins C and E, MEL is a more potent antioxidant (Tan, 1993). The antioxidant property of MEL is shared by the two major metabolites of MEL, namely, N1-acetyl-N2-formyl-5-methoxykynuramine (AFMK) and with a considerably higher efficacy, N1-acetyl-5-methoxykynuramine (AMK). A broad-spectrum antioxidant activity of MEL also includes an indirect effect by upregulating several antioxidative enzymes and downregulating pro-oxidant enzymes in general, and 5- and 12-lipo-oxygenases, glutathione peroxidase (GPx), glutathione reductase, glucose-6-phospahte dehydogenase, superoxide dismutase (SOD), catalase (CAT), and NO synthases in particular (Reiter et al., 2013; Olcese, 2020).
Modulation of Immune Function
Melatonin is known to act as an immunomodulator in the regulation of the development, differentiation, and functions of lymphoid tissues. In rodents, postnatal pinealectomy suppresses immunity and thymic atrophy, whereas exogenous MEL treatment increases a cell-mediated immune function by increasing natural killer cell (NK cell) activity (Ma et al., 2020). The nocturnal rise in blood MEL levels is associated with the increased production of interleukin IL-1, IL-2, IL-6, and IL-12; thymosin 1a, thymulin, and tumor necrosis factor-alpha (Carrillo-Vico et al., 2006). MEL acts on immunocompetent cells (monocytes, B lymphocytes, natural killer lymphocytes, T-helper lymphocytes, and cytotoxic T lymphocytes) through MT1 and retinoid Z receptor (RZR)/RAR-related orphan receptor alpha (RORα) orphan nuclear receptor family and enhances cytokine production/secretions, cell proliferation, or oncostasis (Liu et al., 2001). In B lymphocytes, MEL binds to the orphan receptors to downregulate the gene expression of 5-lipoxygenase, which is an important enzyme in several inflammatory diseases. Thus, MEL and the immune system are linked by a complex bidirectional communication. The immune system, in turn, seems to reciprocally regulate pineal functions, mainly via cytokines produced by an activation of immune cells and depends on age, sex, and species (Carrillo-Vico et al., 2006). The exact mechanisms involved in autoimmune diseases are as yet largely unknown and need further study.
Implications of Melatonin on Fish in Aquaculture
Considering multipotent physiological actions of MEL, recent studies especially on different commercially important fish groups have opened up a new possibility for the application of most convenient and reliable measures for the manipulation of endogenous MEL levels, so as to elicit the desirable physiological response, which may be of considerable interest in aquaculture. Obviously, the manipulation of light conditions in the environment is considered as a classical tool for altering the circulating MEL milieu in the concerned fish. However, the employment of such measures has certain limitations in field studies. Therefore, a search for an alternative has been imperative for aquaculture purposes and, as a result, several attempts are made to augment endogenous MEL concentrations in the gut and thereto the circulation by using different dietary supplements.
Fish Feed Formulation for the Production of Endogenous Gut Melatonin
Two primarily important enzymes involved in a MEL biosynthetic pathway, namely AANAT and ASMT, are found in the gastrointestinal tissues of fish (Devi et al., 2016). The gene expression analysis revealed that aanat mRNA is detected in the gastrointestinal tissues of goldfish (Velarde et al., 2010), European sea bass (Paulin et al., 2015), tropical carp (Devi et al., 2016), rainbow trout (Muñoz-Pérez et al., 2016), and three-spined stickleback (Kulczykowska et al., 2017), and using the Western blot technique gut AANAT protein is also identified in freshwater carp (Mukherjee et al., 2014). Endogenous MEL synthesis from its precursor tryptophan in fish gut is well-established by various researchers. Dietary L-Trp supplementation in variable amounts with conventional fish meal has been shown to modulate the synthesis of MEL from digestive tissues (Yasmin et al., 2021). In the juvenile rainbow trout (O. mykiss), the administration of 3.57 g L-Trp/kg of dry feed paste (8-fold with respect to standard) for 7 days increased plasma MEL and plasma cortisol levels (stress marker) and is reduced after stress exposure (Lepage et al., 2005). In this experiment, in vitro incubation of digestive tissues of rainbow trout with graded concentration of L-Trp (0, 50, or 100 μg/ml of culture media; up to 24 h) also induces the synthesis of MEL from respective tissues at a higher dose (Lepage et al., 2005). This result is explained by an experiment on European sea bass after supplemented with two times of higher concentration of L-Trp, i.e., 5 g L-Trp/kg of mashed dry feed for 7 days (Herrero et al., 2007). Dietary supplementation of L-Trp at a dose of 4.2 g L-Trp/kg of dry feed (L-Trp is mixed with the final form of fish feed paste consisting of 35% fish meal, 28% mustard oil cake, 28% rice bran, 2% each sunflower and cod liver oil, and 5% carboxymethyl cellulose and multivitamin–multimineral tablets) in five times higher than standard for 7 days also heightened the synthesis of MEL from the digestive tissue in carp Catla catla (Mukherjee and Maitra, 2018). However, the increased levels of AANAT and MEL in the gut are sustained for about 12 h after the supply of an L-Trp-rich diet, and the highest amplitude of gut variables are found in the case of daytime food supply. Serum MEL concentration is also increased and sustained for about 12 h after the supply of an L-Trp-rich diet in a day, so this result clearly indicates that gut MEL has a positive correlation with the MEL pool in circulation (Mukherjee and Maitra, 2018). Interestingly, supplementing the fish with a high L-Trp-rich diet during night can cause a significant decrease in gut MEL synthesis as compared to day time due to a negative feedback loop regulated by food intake capacity (Mukherjee and Maitra, 2018). However, due to the lack of appropriate experimental studies, the question whether the gut MEL response to a particular dosage regimen of L-Trp-rich diet would vary with the feeding habit of the concerned fish, or more specifically among the carnivores, herbivores, and omnivores, remains unresolved and thereby warrants further study.
The application of L-Trp to fish diet is indeed a well-studied indirect method to increase MEL levels in gastric tissues. However, MEL is also used directly with fish meal as a feed additive (Conde-Sieira et al., 2014) to demonstrate its physiological impact. Apart from the composition of the fish food, feeding time and its frequency are also found to be major influencing factors on the diverse rhythmic pattern of MEL synthesis and AANAT expression in fish gut. Daily food supply at a fixed or randomly selected single time point can cause heightened MEL synthesis from the gut after 2–4 h of meal intake in freshwater carp (Mukherjee et al., 2014). In another study, carp C. catla starved (8 days) and refed (2–16 days) observed that, with the progression of starvation, the level of gut MEL and AANAT increases (higher mesor and amplitude) (Mukherjee and Maitra, 2015). However, refeeding is followed by a steady increase in the level of gut MEL up to 16 days after the first 2 days of experiment (Mukherjee and Maitra, 2015). Further, when fish received food at fixed two times in a day, a rhythmic pattern in the synthesis of gut MEL and the expression of AANAT are either disturbed (Devi et al., 2016) or peaked at night within a 24-h LD cycle (Choi et al., 2016). Rhythmicity in MEL synthesis and AANAT expression are unrecognizable when fish meals are given at fixed single time and kept unfed for several days and daily rhythmicity has been observed in case of prolonged starvation (Pal and Maitra, 2018). In zebra fish (D. rerio), altered timing of food supply (at night) for 30 days observed the rhythmic expression of core clock genes in the gut, irrespective of feeding time, which concludes that feeding cycle controls the gut MEL production (Mondal et al., 2021) (Table 1). Therefore, the timing of food supply and cycle of feeding have been shown to act as a zeitgeber (Sánchez-Vázquez et al., 1997) in the modulation of the rhythmicity of MEL synthesis from a digestive tract (Figure 1A). Existing information provide persuasive evidence that the supply of foods containing MEL or promoting its synthesis by influencing the availability of tryptophan, as well those containing vitamins and minerals, which generally acts as cofactors and activators in the synthesis of MEL, may modulate the diurnal profiles of MEL (Peuhkuri et al., 2012). Collectively, tryptophan-rich diet and the regulation in feeding time can be a factor to induce MEL synthesis from the gastrointestinal tissue, which is ultimately released into blood and contributes to the total pool of free MEL in the circulation (Pal et al., 2016a; Mukherjee and Maitra, 2018). However, it is not yet clear whether the effectiveness of MEL added to the diets would depend on the before- or post-challenge test and thus should remain as an interesting area of future research.
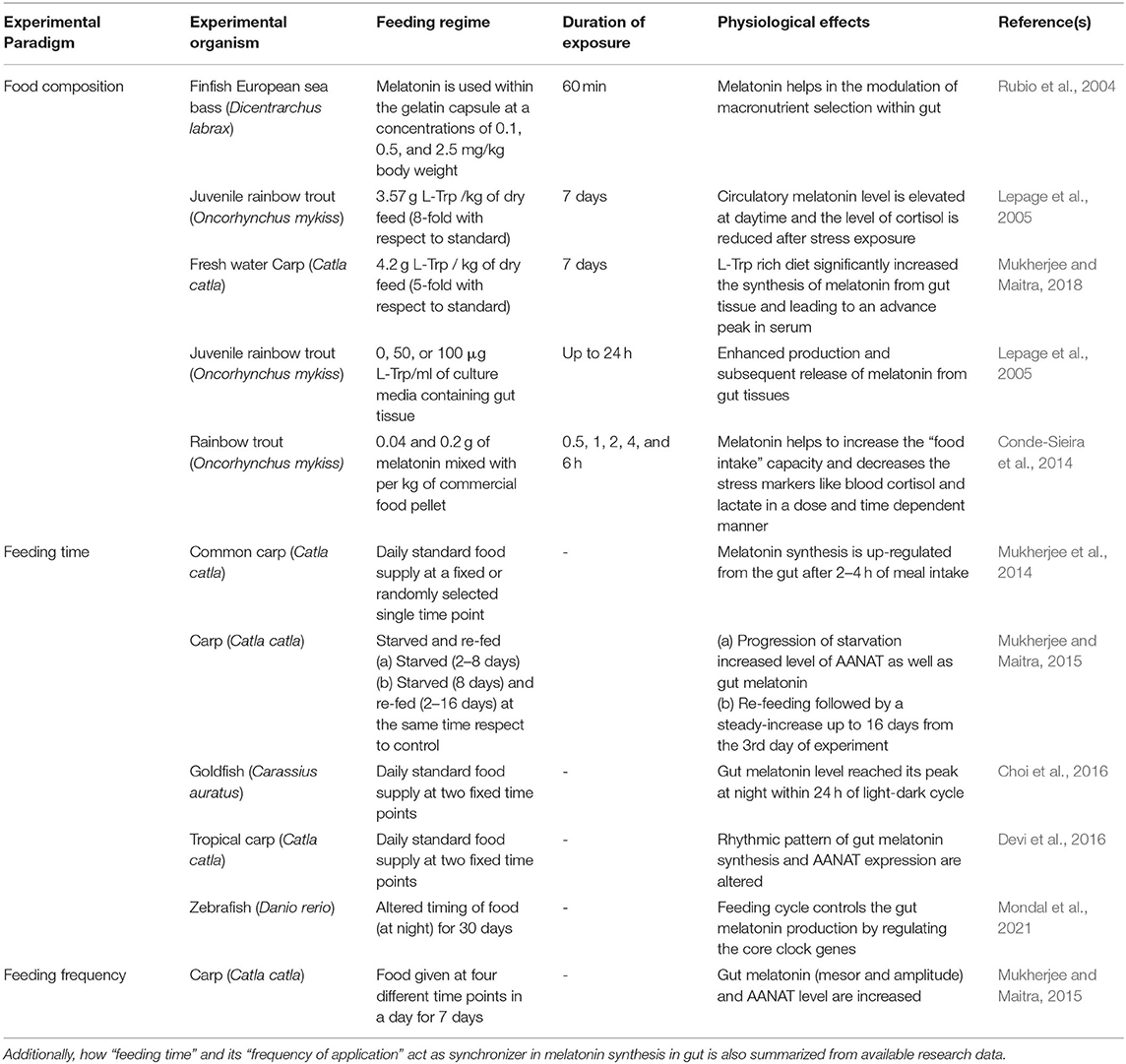
Table 1. Use of melatonin (directly or its precursor L-Trp) as fish feed additive in different experimental model animals and its effects on various physiological functions are well-described.
Application of Melatonin in the Regulation of Fish Growth
Photoperiod and food availability act synergistically in the feeding behavior of various organisms. Various studies demonstrated that the seasonal pattern of these variables on which fish growth rely varies in accordance with the day length (Boeuf and Falcon, 2001). Normal growth and development of fish larvae require light intensity at a minimal threshold. Furthermore, in salmonoid and other marine fish species, photoperiodic manipulations, like long days in diurnal fish, stimulate growth process. Food intake, pattern of digestion, and processes of growth are associated with the specific rhythm of behavior, and certainly pineal hormone MEL might have a key role on this aspect (Porter et al., 1999; Mayer, 2000; Zhdanova et al., 2001; Piccinetti et al., 2013). Both short photoperiod and intraperitoneal MEL administration (25 and 50 μg/fish) for 21 and 34 (or 38) days accelerate postexposure growth and weight in Carassius auratus (de Vlaming, 1980). Additionally, in vitro culture of trout pituitary gland with physiologically relevant concentration of MEL, viz., 10−12, 10−10, and 10−8 M for 12 h increase the release of growth hormone and sustainably inhibit prolactin release (Falcón et al., 2003). Thus, it is evident that the antagonistic interaction of these two hormones promotes fish growth. In rainbow trout, MEL at different concentrations (0.04 and 0.2 g/kg food) is mixed with the final form of commercial food paste, then dried at 37°C for 24 h, and applied as fish feed for 0.5, 1, 2, 4, and 6 h (Conde-Sieira et al., 2014). Such a study clearly demonstrates that MEL increases “food intake capacity,” an indication of fish growth and reduces the stress markers like cortisol and lactate in plasma in a dose- and time-dependent manner. In another experiment, MEL was used at the doses of 0.1, 0.5, and 2.5 mg/kg body weight within the gelatin capsule with either carbohydrate, or protein, or fat and supplied in the tank as food of finfish European sea bass for 60 min (Dicentrarchus labrax) (Rubio et al., 2004); which significantly modulates the pattern of macronutrient selection (Table 1). Collectively, exogenous- and/or tryptophan-derived endogenous gut MEL may have some impacts on growth and developmental processes by modulating pituitary hormones (Table 2).
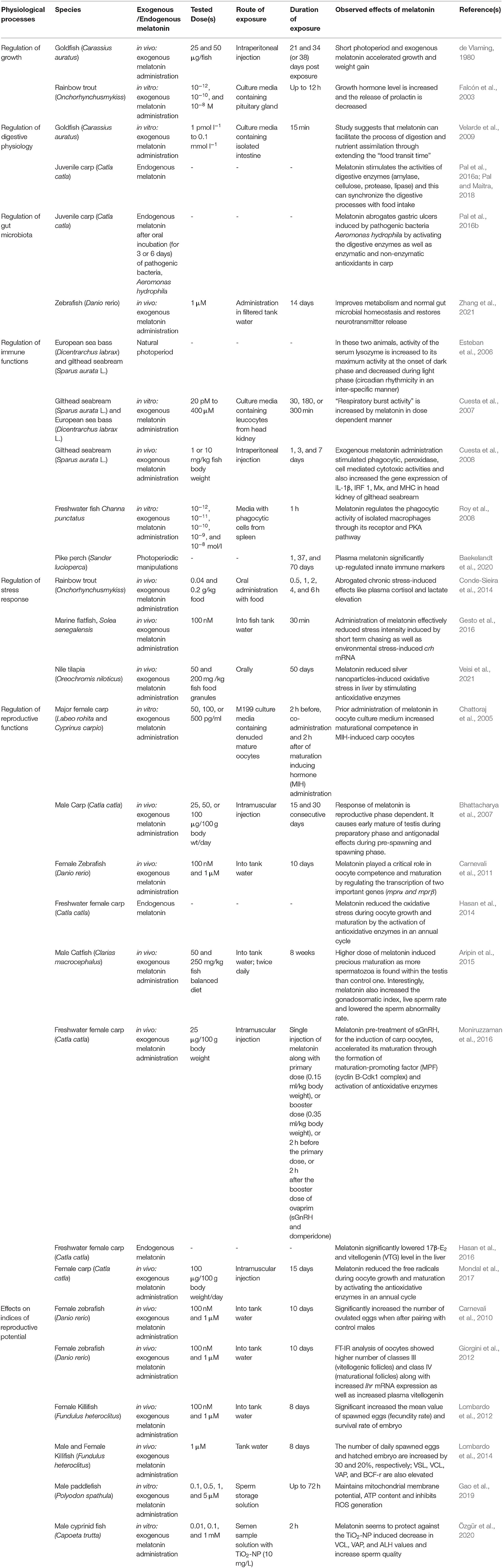
Table 2. Some critical findings on melatonin in the regulation of various physiological functions of both freshwater and marinewater fish at a glance: why melatonin can be incorporated in fish diet in sustainable aquaculture?
Influences of Melatonin on the Physiology of Digestion in Fish
Intestinal MEL receptors have been reported in the gut of various animals, including mice and humans (Bubenik et al., 1993), and MEL is thought to exert luminal, as well as, endocrine and/or, autocrine/paracrine mode of actions in the gut epithelium (Bubenik, 2008; Pal and Maitra, 2018). In fish gut cells, elevation in the postprandial MEL synthesis is made to occur by increased AANAT activity and intracellular signaling molecules (Pal et al., 2016b) which in turn regulates the transportation of ions and electrolytes through the adjustment of water content in the fecal matter (Bubenik and Pang, 1994; Lee et al., 1995). MEL can also have an impact on the relaxation of the smooth muscle of digestive tract as it inhibits the contraction of smooth muscles through preprandial and postprandial motility of the stomach, ileum, and colon in isolated rat intestine (Bubenik, 1986), and also by specifically inhibiting nicotinic channels or serotonin actions in gastric fundus of rat (Storr et al., 2000). A study suggests that MEL supplementation (1 pmol l−1 to 0.1 mmol l−1) in culture media containing the isolated intestine of goldfish (C. auratus) for 15 min can facilitate the process of digestion and nutrient assimilation through extending the “food transit time” (Velarde et al., 2009) by lowering the power of gut motility through the AANAT-mediated acetylation of potent motility factors, serotonin, and dopamine (Nisembaum et al., 2013). The acetylated forms are non-functional in inducing the motility of the gut (Figure 1A). MEL also stimulates the activities of digestive enzymes and can also synchronize the digestive process with food intake in carp (Pal et al., 2016a; Pal and Maitra, 2018). Apart from digestive enzymes, MEL also facilitates the synthesis of hormones and peptides related to gastrointestinal physiology, viz., leptin, ghrelin, and peptide YY (Aydin et al., 2008; Taheri et al., 2019). MEL-mediated regulation of appetite and hydrochloric acid secretion from parietal cells are operated by mucosal cell-derived bicarbonate, which can lessen the ulcerative complications in the gut (Sjöblom and Flemström, 2003).
Impact of Melatonin on Microbiota in Fish Gut
Experimental studies on the influences of MEL on the gut microbial dynamics have so far been limited mostly to mammalian species, and the reports from piscine studies are scanty. Until recently, a direct impact of MEL-formulated fish feed on the gut microbial dynamics and their physiological implications remained unknown. Just like the supplementary information, it may be noted that in zebra fish (D. rerio) larvae, the infection of Lactobacillus rhamnosus enhances the transcription of melatonin receptor 1 (MT1R) ba and bb, as well as the expression of MT1R protein, and these effects are the same due to the influences of continuous darkness on the same fish, suggesting a possible involvement of MEL in such a process (Lutfi et al., 2021). A relation between the LD cycle and gut microbial dynamics has been sought in a piscine representative tambaqui (Colossoma macropomum) (Fortes-Silva et al., 2016). In this study, lower nocturnal percentage of Gram-positive (24%) and Gram-negative cocci (38%) as well as Gram-positive (42%) and Gram-negative (44%) Bacillus are observed relative to their respective diurnal values. In glyphosate-treated Chinese mitten crab (Eriocheir sinensis), the supply of MEL (80 mg/kg food) as a supplement to the standard feed for 14 days significantly increases the relative abundance and Shannon index of Proteobacteria and Bacteroidete along with an enhanced activity of amylase, lipase, and trypsin in the gut (Song et al., 2020). Further, in zebra fish (D. rerio), 1 μM MEL administration in filtered tank water for 14 days improves metabolism and normal gut microbial homeostasis, and restores neurotransmitter release (Zhang et al., 2021). Bacillus, Lactobacillus, and Escherichia coli constitute the major microbial populations in fish gut and significantly improve various physiological activities during the rearing of fish in aquaculture practice (Carnevali et al., 2004; Hasan and Banerjee, 2020). However, substantial evidences in favor of a functional relationship between the profiles of MEL and these microbes in the fish gut are still not available in the literature studies, and thereby, the role of MEL in the regulation of gastrointestinal microbe populations in fish remains as an important area of future research.
In freshwater carp (C. catla), the involvement of gut melatoninergic system on pathophysiological alterations induced by oral incubation of pathogenic bacteria Aeromonas hydrophila (consecutively for 3 or 6 days) is reported (Pal et al., 2016b). Infection arbitrated morphological alterations includes the degeneration of lamina propria and tunica mucosa, associated with the disintegration of epithelial cells, and reduced goblet cell population and concomitant reduced activity of digestive enzymes are also observed under the progression of the infection (Table 2). This type of intestinal damage can lead to reduced growth performance as observed in a different study on the replacement of fishmeal with soybean meal, leading to the development of damaged intestinal mucosa in the orange-spotted group (Epinephelus coioides; Wang et al., 2017). Altered gut morphology and heightened gut inflammation (increased level of pro-inflammatory cytokines) are also found to be associated with reduced gut functionality and digestivity in case of Japanese sea bass (Lateolabrax japonicas; Zhang et al., 2018). Interestingly, with the progression of microbial infection and increasing gut damage, serum, and the gut MEL level as well as the gut AANAT level are found to be higher (Pal et al., 2016a). Moreover, the increased level of gut MEL with the development of infection is well-corroborated with the amplified antioxidative enzyme activity (SOD, CAT, and GPx) in the said experiment (Figure 2). Gastric ulcers are primarily induced by the pathogen containing ingested food particles and generated oxidative stress within the luminal epithelium, and MEL abrogates these effects by activating the antioxidative defense system (Konturek et al., 2011; Pal et al., 2016a). Very recent evidence in mammals also supported the results obtained from different experiments on fish that exogenous MEL improves the digestive physiology after microbial infection (Yin et al., 2020). However, there is a scarcity of detailed information regarding the relationships between MEL and microbial infection in case of fish gut.
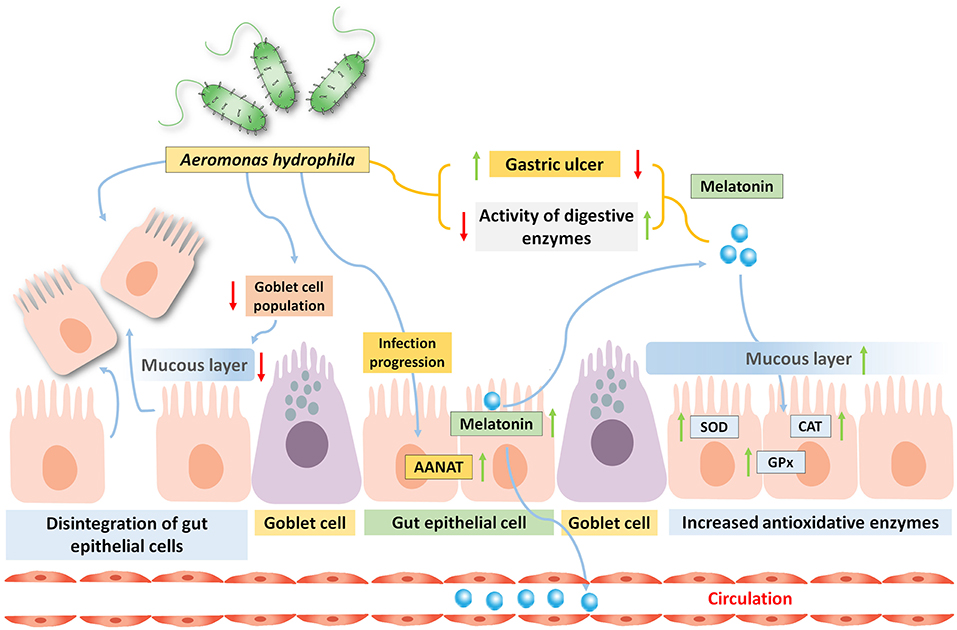
Figure 2. Oral administration of bacteria Aeromonas hydrophila in carp Catla catla induces gastric ulcer, and reduces the activities of several digestive enzymes. By the progression of the infection, the gut MEL level is significantly increased due to high arylalkylamine-N-acetyltransferase (AANAT) enzyme activity and this is related to enhance circulatory MEL pool. Moreover, the activity of antioxidative enzyme is also elevated by the gut MEL.
Influences of Melatonin in Modulation of Fish Immunity
Innovative technology based on improved knowledge regarding the biological aspects of farmed fish allowed the development of species-specific fish feed, which results into large-scale fish production and ultimately the whole process to be affected by the exposure to stressors, deteriorating environmental conditions, and spreading of disease (Balcázar et al., 2006). This has become a major challenge in large-scale fish production to control the spreading of diseases among the cultured fish, thus, in present aquaculture practices, the elucidation of fish immune system and modulators that can improve the immune defense mechanisms of fish are the important factors that are to be considered the most.
In vertebrates, endocrine and nervous systems interact synergistically with the immune system, and unarguably immune responses also involve rhythmic patterns (circadian and circannual). In birds and mammals, it is evident that MEL interacts with immune system due to a correlation in circadian and seasonal alterations in an immune function with MEL production and is significantly altered by pinealectomy (Esteban et al., 2013). In case of the exposure of two major marine finfish, European sea bass (D. labrax) and gilthead sea bream (S. aurata L.), to constant LD photoperiod and temperature, immunological parameters shows circadian rhythmicity, which is correlated with the rhythmic pattern of daily MEL level (Esteban et al., 2006). In these two species, the activity of the serum lysozyme also showed circadian rhythmicity in an interspecific manner, increased to its maximum level of activity at the onset of the dark phase, and decreased during the light phase. Complement system activation status is found to be relatively similar in these two species, shows higher during light hours, and shows a lower level of activation during dark hours. There is a potential interaction of environmental stress producers with the function of immune system, size of lymphatic organ, and occurrence of disease (Nelson and Demas, 1996), and the seasonality of the stressor also affects the competence and response of the immune system and disease prevalence in vertebrates (Bowden et al., 2007). Immune defense is considered as an effective factor for promoting better physiological conditions and improved survival rate in fish (Nelson and Demas, 1996). Further studies revealed that circadian rhythmicity in immune function is modulated by photoperiod and by MEL (Esteban et al., 2013). The organization of the immune system in fish is strikingly interesting as it is devoid of bone marrow and lymph nodes (Manning, 1998). In fish, the primary lymphoid tissues for the production and development of B lymphocytes is the head kidney (pronephros) also known as lympho-haematopoietic tissue, whereas T lymphocytes are developed and matured in the thymus (Baekelandt et al., 2020). Spleen is considered as the secondary lymphoid organ in fish, and the scattered leucocyte populations in gills [gill-associated lymphoid tissue (GIALT)], skin [skin-associated lymphoid tissue (SALT)], and gut [gut-associated lymphoid tissue (GALT)] constitute the mucosal-associated lymphoid tissue (MALT) (Salinas, 2015; Baekelandt et al., 2020). There is a scarcity of information regarding the interaction of MEL with cellular and humoral factors of the fish immune system. In vivo MEL exposure (1 or 10 mg/kg fish body weight) intraperitoneally to gilthead sea bream (S. aurata L.) for 1, 3, and 7 days significantly heightened phagocytic, peroxidase, cell-mediated cytotoxic activities and upregulated interleukin-1 beta (IL-1β), interferon-regulatory factor 1 (IRF-1), Mx, and major histocompatibility complex (MHC) genes as well as the marker proteins of B and T lymphocytes in head kidney cells in a dose- and time-dependent manner up to 7 days postexposure (Cuesta et al., 2008) (Figure 1C). Immune cell populations like lymphocytes, macrophages, and other granulocytes perform a cytotoxic activity analogous to the more specialized cells like NK cells performing the same function as in mammals under the innate immune system. However, the isolated leucocytes from the head kidney of Gilthead sea bream (S. aurata L.) and European sea bass (D. labrax L.) are incubated with physiological and pharmacological doses (low and high doses, respectively) of MEL (20 pM to 400 μM for 30, 180, or 300 min) and showed that, at the physiological level, MEL did not alter the response of an innate immune system but a higher pharmacological dose increased respiratory burst activity in sea bass (Cuesta et al., 2007). These observations provide the information regarding the interaction of MEL with an innate immune system of finfish, and further studies need to be performed regarding this particular aspect in a mechanistic approach. Phagocytic activity of the spleen of a freshwater fish Channa punctatus showed diurnal rhythmicity with the highest activity during the light phase, and interestingly exogenous MEL can inhibit this rhythmic pattern (Roy et al., 2008). This study also revealed that the administration of luzindol, which is a MEL receptor (cell surface) antagonist, altered the effect of MEL (10−12, 10−11, 10−10, 10−9, and 10−8 mol/l) in the inhibition of phagocytic activity of the isolated macrophages from the spleen (Roy et al., 2008). This inhibitory effect of MEL is operated through a pathway that involves adenylate cyclase (AC) and protein kinase A (PKA) by which MEL causes the inhibition of the phagocytic activity in C. punctatus (Roy et al., 2008). Very recently, in the case of pike perch (Sander lucioperca), a significant correlation between plasma MEL with innate immune markers has been explored (Baekelandt et al., 2020) (Table 2). Thus, the interpretations from different studies collectively supported that MEL can be used to modulate and improve fish immunity and resistance to certain pathogens in aquaculture.
Melatonin in the Physiological Regulation of Stress in Fish
Various environmental stress factors have a major impact on fish physiology and are mainly related to temperature elevation, organic or inorganic pollutants, etc. In aquaculture, routine husbandry includes some other important factors, sometimes comes from human interferences like feeding, handling, social interactions, and stocking conditions (Deane and Woo, 2009; Sánchez et al., 2009). Stress factors can influence the melatoninergic pathway in the pineal gland, and different studies have also reported on these aspects in both in vivo and in vitro conditions (Ceinos et al., 2008; Kulczykowska et al., 2018). In case of fish, pineal MEL shows sensitivity toward various environmental stressors in a stress and species-specific manner. The increased level of MEL at night in the pineal gland and blood plasma are reported in case of rainbow trout after transferring in isosmotic and hyperosmotic conditions from their initial freshwater adaptive condition both in short- and long-term treatment (López-Patiño et al., 2011). This result is well-corroborated with the increased amount of pineal aanat2 mRNA and AANAT2 enzyme activity in hypertonic conditions and suggested that external salinity induces MEL synthesis in rainbow trout (López-Patiño et al., 2014). High stocking density and chasing disrupts MEL rhythm by reducing serotonin level, aanat2 mRNA and AANAT2 enzyme activity in pineal at night (López-Patiño et al., 2014). Stress response in fish is physiologically mediated by the synergistic actions of hypothalamus-pituitary-interrenal (HPI) tissues and brain (hypothalamus)-sympathetic-chromaffin (HSC) tissues in response to stressors (Barton, 2002). A few studies indicated that in the case of alleviated stress response, MEL might have some crucial roles in teleosts, which involves two neuroendocrine axes mentioned earlier. In case of rainbow trout, oral MEL administration through food (0.04 and 0.2 g/kg mashed food) or through dissolving in water (10 μM) abrogates chronic stress-induced effects like plasma cortisol and lactate elevation, reduced food intake, and decreased activity of digestive enzymes (Conde-Sieira et al., 2014). Low dose (100 nM) administration of MEL effectively reduced stress intensity induced by short-term chasing in Solea senegalensis (Gesto et al., 2016). A very recent study by Veisi et al. (2021) demonstrated that the application of MEL (50 and 200 mg /kg dry food) through fish food granules for 50 days significantly reduced silver nanoparticles-induced oxidative stress in the liver by stimulating the redox sensitive enzymes in Nile tilapia (O. niloticus) (Veisi et al., 2021). In fish, the response to acute and chronic stress as well as social stress, neuroendocrine responses are supposed to be activated by the brain serotonergic system (Gesto et al., 2015; Backström and Winberg, 2017). Immediately after acquaintance to the stressor, serotonergic function is increased, which affects hypothalamus and telencephalon through serotonergic neuronal endings (Gesto et al., 2015). The stimulation of HPI axis by serotonin occurs at hypothalamus-POA through an increasing in the release of corticotropin releasing hormone (CRH), which in turn activates downstream glucocorticoid (GC) stress response (Barton, 2002; Backström and Winberg, 2017) (Table 2). It is found that environmental stress-induced increased crh mRNA expression is declined by MEL administration, suggesting a possible cross talk between MEL with CRH secreting neurons present in POA of hypothalamus in marine flatfish S. senegalensis (Gesto et al., 2016). The scarcity of information regarding the underlying mechanism of action behind the interaction of MEL and serotonin level in the brain but it is evident in case of Gulf toadfish that, in response to crowding stress, increases in crh mRNA and ACTH involve 5-HT1A-like receptors (Medeiros et al., 2014; Sánchez-Vázquez et al., 2019) and this receptor is also involved in the modulation of a HPI axis in Arctic charr (Salvelinus alpinus; Höglund et al., 2002) (Figure 1D). However, further studies are required for the elucidation of the mechanisms by which dietary tryptophan-derived gut MEL modulates the physiology of stress induced by environmental stressors in fish.
Melatonin as a Pivotal Candidate in the Regulation of Fish Reproduction
Reproduction in fish is primarily under the intricate regulation of neuroendocrine and endocrine axis as well as autocrine and/or paracrine actions of some growth factors, and this endogenous circuit is further regulated by the environmental factors (Maitra et al., 2015) like temperature, photoperiod, rainfall to synchronize oocyte development, and timely release of mature gametes to ensure maximal rate of external fertilization. In these respects, a large number of studies on diverse groups of fish have contributed largely to provide persuasive evidence suggesting that MEL indeed plays a pivotal role in the regulation of reproduction in fish.
Role of Endogenous Melatonin in the Regulation of Female Reproduction
The pineal organ, the lateral eyes and the SCNs act as circadian oscillators as well as sensors to regulate the synchronization between environment and annual reproductive cycle (Reiter et al., 2013), which is also evident in fish (García-Allegue et al., 2001; Iigo et al., 2006). Evidence on the interaction of MEL with the reproductive axis of an animal is insufficient although a few carefully controlled studies in recent past provide the information on the direct or indirect interaction of MEL with the reproductive physiology as it can regulate reproduction through a hypothalamo-pituitary axis, through modulating the action of sex steroids on meiotic resumption and more interestingly, it can have also a role on ovarian pathophysiology over a reproductive cycle by acting as a free radical scavenger (Tamura et al., 2008). The study on ovarian MEL rhythmicity and its action on seasonal reproduction is a major concern in aquaculture. Research on ovarian MEL was restricted only in mammalian ovary (Rönnberg et al., 1990) but in the case of carp, it has been evident recently and its temporal pattern during a reproductive cycle is also enlightened for the first time (Hasan et al., 2014).
Interestingly, in carp, diurnal rhythmicity of MEL varies in relation to the annual reproductive phase, i.e., it shows type A profile in the preparatory phase, and the rest of the phases in an annual cycle show type B profile (Maitra et al., 2005). This rhythmic change of plasma MEL is closely correlated with the different female sex hormones in fish (Maitra et al., 2013). A recent study showed that in carp C. calta, the peak value of seasonal serum MEL is negative correlated with the peak value of plasma 17β-E2 and 17α, 20β-diOH-progesterone [maturation-inducing steroid (MIS)] (Hasan et al., 2016). The result clearly indicated a functional interplay between MEL and ovarian steroids during the temporal regulation of oocyte growth in an annual reproductive cycle. In fish, gonadal growth and development throughout an annual breeding cycle occur sequentially and are further subdivided into different phases having distinct ovarian and germ cell profiles: (a) the preparatory phase, (b) the pre-spawning phase, (c) the spawning phase, and (d) the post-spawning phase (Maitra et al., 2005). Previous studies showed that pinealectomy results into various observations on reproductive effects: the sexual maturation is accelerated in Heteropneustes fossilis (Joy and Agha, 1989) and C. punctatus (Joy and Khan, 1991). Pinealectomy during the growth phase (more specifically, vitellogenesis) causes ovarian steroidogenic dysfunction and decreased plasma 17β-E2 level (Popek et al., 1997). In Gasterosteus aculeatus, Astyanax mexicanus, Fundulus heteroclitus, and C. auratus, pinealectomy did not show any such effects (Lal and Singh, 1995) (Table 2). Further observations were made through pinealectomy in relation to environmental photothermal conditions. It showed that pinealectomy stimulates gonadal development in fish exposed to short photoperiod, whereas the opposite effect is observed in case of long-day exposed fish. Likewise, in catfish, pinealectomy in the preparatory phase accelerated ovarian recrudescence under a long photoperiod, was ineffective under a short photoperiod and was also ineffecive during pre-spawning and post-spawning under either of the photoperiodic condition (long or short).
Role of Exogenous Melatonin in the Regulation of Female Reproduction
Effects on Ovarian Growth and Development
Melatonin when administered exogenously showed pro-gonadal, anti-gonadal, or no effects, that depends upon the annual reproductive cycle of a fish. Daily injection of exogenous MEL (for 15 or 30 days) caused the appearance of yolky stage-II oocytes in the ovary of fish during the preparatory phase of an annual reproductive cycle (precocious growth of the ovary and thus may be considered as the pro-gonadal effect of MEL; Maitra et al., 2005). In C. catla, exogenously administered MEL accelerated oocyte growth during the preparatory phase in spite of a decrease in the number of stage-II and stage-III oocytes during the pre-spawning and spawning phase, respectively, and further, no significant changes were observed during the post-spawning phase (Maitra et al., 2005). Pro-gonadal effects of exogenous MEL are observed in catfish (Singh and Lal, 1994), whereas the studies on some tropical fish, H. fossilis (Sundararaj and Keshavanath, 1976; Joy and Agha, 1991), Mystus tengara (Saxena and Anand, 1977), and Clarias batrachus (Singh and Lal, 1994) showed that MEL reduced 17α-hydroxyprogesterone level and vitellogenesis with increased follicular atresia, and these effects depend upon the photoperiod, reproductive phase, dose, and duration of the treatment. Further studies demonstrated that fish hepatic MEL concentration is negatively correlated with 17β-E2 as well as vitellogenin (VTG) protein in both the serum and liver of tropical carp C. catla (Hasan et al., 2016) (Figure 3).
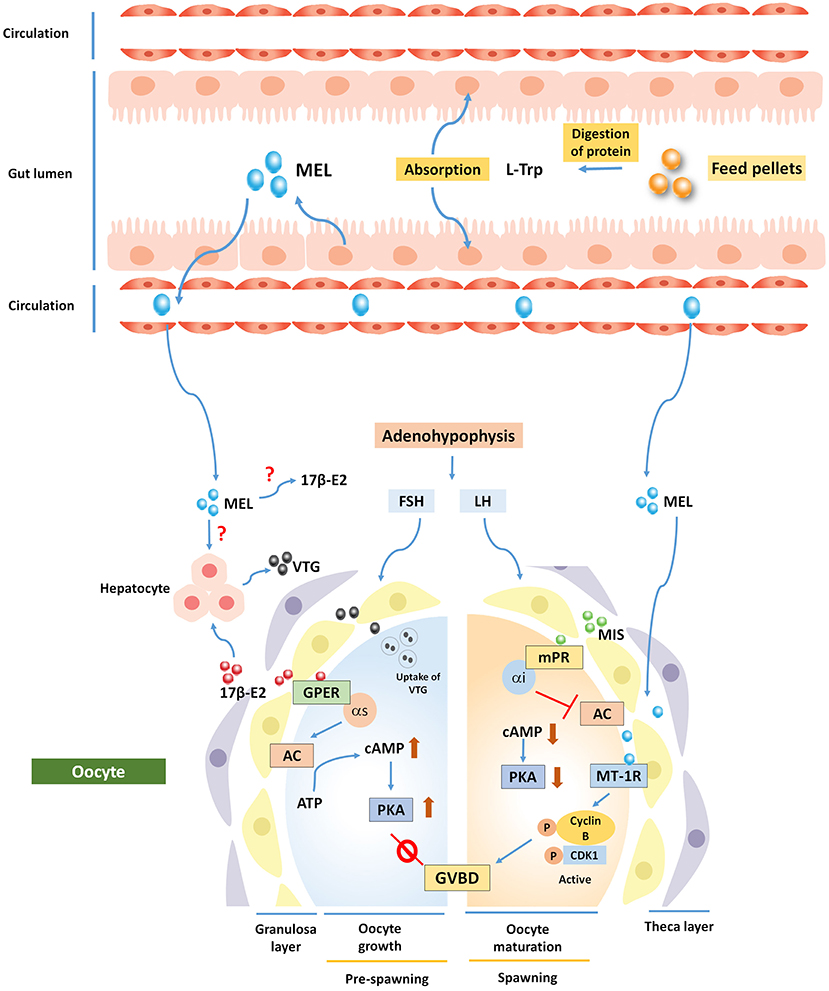
Figure 3. Potential involvement of MEL in the regulation of fish oogenesis. Ovarian follicles synthesize 17β-E2, which acts via membrane bound G-protein-coupled estrogen receptor 1 (GPER) and increases intra-oocyte cAMP level to inhibit oocyte maturation. A shift in steroidogenesis is governed by maturation-inducing steroid (MIS), which acts on oocyte via Membrane progestin receptor (mPR) to regulate germinal vesicle breakdown (GVBD). MEL is thought to exert its action through melatonin receptor 1 (MT1R) to regulate oocyte maturation. MEL has also a negative role in synthesizing vitellogenin (VTG) protein from carp hepatocyte, but direct actions on 17β-E2 and VTG are still speculative.
Effects on Oocyte Maturation
An in vitro study demonstrated for the first time that MEL locally acts on the ovary and accelerates the action of MIS-induced oocyte maturation through the formation of a maturation-promoting factor (MPF). This extra-hypothalamic action of MEL has become established after the localization and dynamics of a 37- kDa melatonin receptor protein (MT1R) in fish oocytes (Chattoraj et al., 2009) (Figure 3). In vitro administration of exogenous MEL at the doses of 50, 100, or 500 pg/ml in an oocyte incubation medium at 2 h before, co-administration, and 2 h after the administration of MIS can increase maturational competence in MIS-induced carp oocyte (Chattoraj et al., 2005). In zebra fish, it is reported that MEL (100 nM and 1 μM) in tank water for 10 days plays a critical role in seasonal reproduction through the activation of kisspeptin, which induces the production of a gonadotropin-releasing hormone (GnRH), and by acting directly on the gonads that regulate the transcription of two important genes (mprα and mprβ) whose proteins are involved in oocyte competence and maturation (Carnevali et al., 2011). Likewise, a study on killifish (Lombardo et al., 2012) provides evidence that endogenously synthesized or exogenously added MEL acts on steroidogenesis by regulating steroidogenic enzyme activities in theca and granulosa cells of the ovary (Hasan et al., 2016; Mondal et al., 2017).
Effects of Melatonin on Synthetic GnRH-Induced Oocyte Maturation
Observations on the amelioration potential of MEL pre-treatment on the induction of synthetic GnRH along with domperidone- (ovaprim-) mediated oocyte maturation in carp C. catla has been made in a study to evaluate MEL concentration and activity of different antioxidative enzymes in the ovary and to attempt conforming the documentation of the same observation during the spawning phase under natural photothermal conditions (Moniruzzaman et al., 2016). In this study on carp, the pre-treatment (2 h before) of MEL (25 μg/100 g body weight) before ovaprim administration leads to MPF formation [complex of cyclin B and cyclin-dependent kinase 1 (Cdk1)] and ensued the shortest latency and a higher rate of ovulation. However, the administration of exogenous MEL significantly reduced intraovarian oxidative stress by elevating antioxidative enzyme activity as well as non-enzymatic antioxidant level in the ovary regardless of the schedule of administration. Nevertheless, pre-treatment with MT1R blocker, luzindol, does not induce these effects, which clearly indicates that MEL pre-treatment augments the ovaprim action on oocyte maturation as well as improving antioxidative milieu in preovulatory oocyte, leading to the formation of improved quality of oocytes (Moniruzzaman et al., 2016) (Table 2). Thus, it seems rational to suggest that dietary MEL supplement may have an impact on final oocyte maturation and high-quality oocyte formation under natural photothermal condition, so it can be practiced as a hormonal tool in case of induced breeding.
Role of Melatonin in the Regulation of Male Reproduction
Existing literature studies on the influence of MEL in the regulation of annual gonadal events (male and female) are primarily based on the observations regarding the response of the gonad after pinealectomy and/or exogenously administered MEL and are often fragmentary studies with certain contradictions. In vertebrates, the expression of genes involved in MEL biosynthesis and further activities (the synthesis of proteins) are well-studied in the ovary (Coelho et al., 2015; He et al., 2016) and a daily variation in ovarian MEL level has also been observed (Khan et al., 2016). Nonetheless, information regarding the gene expression pattern of testicular MEL synthesizing enzymes as well as its daily and/or seasonal variation is scarce. In a very recent study, testicular MEL synthesizing parameters like mRNA expression and tissue-specific immunohistochemical localization of AANAT and ASMT along with serum and testicular MEL concentration has well-documented in the case of zebra fish in a 24-h LD cycle (Devi et al., 2021). In this experiment, end-point PCR, agarose gel electrophoresis showed a gene specific to single band, and quantitative reverse transcription- (RT-) PCR showed a gene-specific single peak, collectively indicating that MEL biosynthesizing genes are expressed in zebra fish testis and immunohistochemical study further confirmed the testicular distribution of AANAT and ASMT proteins. Interestingly, the mRNA expression pattern of Aanat1, Aanat2, and Tph1 showed rhythmicity while arrhythmic pattern was observed for Asmt. At noon, there is higher concentration of MEL observed in testis compared to serum MEL but both the concentration are similar at midnight. These data collectively revealed that there is a potential involvement of possible existence of MEL to regulate the testicular function in an autocrine and/or a paracrine manner (Devi et al., 2021). Intramuscular injection of MEL at graded doses (25, 50, or 100 μg/100 g body wt/day) for 15 and 30 consecutive days determines the influence of MEL in the regulation of annual events in a testicular cycle in carp C. catla (Bhattacharya et al., 2007). The study also includes the functional involvement of endogenous MEL in the regulation of annual testicular events by manipulating normal light/dark cycle (continuous darkness, DD; or continuous light, LL) for 30 days, and the experimental paradigm is kept constant in different reproductive phases. In this study, an identical testicular response is observed for exogenous MEL administration (at a dose of 100 μg for 30 days) and continuous darkness (for 30 days) in each reproductive period, however, remarkably, in these two groups precociously mature testis is found during the preparatory phase; in contrast to pre-spawning and spawning phases where the testicular function is reduced. This is the first ever study providing information regarding the role of MEL in the regulation of annual testicular events in a surface dweller, subtropical fish C. catla (Bhattacharya et al., 2007). Dietary MEL supplement at doses of 50 or 250 mg/kg balanced fish food (final form) mix to male brood stock (first puberty stage) for 8 weeks is used in a study to investigate the impact of exogenously supplied MEL on testis maturational study (gonadosomatic index, histological analysis and microscopic study of testis, live sperm rate, concentration and kinetic parameters, sperm abnormality, and level of testosterone) in walking catfish, Clarias macrocephalus (Aripin et al., 2015). The study revealed that mean gonadosomatic index, live sperm rate, and progressive motility are increased at the dose of 250 mg/kg fish food of MEL. Additionally, the results also showed that mature spermatozoa are more prevalent than immature spermatogonium due to the administration of MEL (higher dose) than the control group of fish. Reduced sperm abnormality with enhanced testicular maturation by MEL treatment in male brood stock indicated that MEL improves the quality of male seeds (Aripin et al., 2015).
Mechanism of Actions of Melatonin in the Regulation of Reproduction
Receptor-Mediated Action of Melatonin as a Hormone
In mammalian reproductive organs, the identification of MEL binding sites (Woo et al., 2001) raised the possibility of the receptor-mediated action of this hormone on the gonads. Radiolabeled techniques demonstrated the presence of three different types of MEL receptors, namely, MT1, MT2, and Mel1c (Dubocovich et al., 2000). A previous study on fish suggested that MEL treatment and pinealectomy regulate the process of vitellogenesis in the liver through its receptors followed by its active incorporation into the developing oocytes (Mazurais et al., 2000). In teleosts, the detection of MT1 (Mel1a) receptor protein on both the membrane and cytosolic fractions of the isolated oocytes supported the conjecture that MEL may have a direct action on oocyte maturation (Chattoraj et al., 2009; Maitra et al., 2013). Another study on the same carp also revealed that photoperiodic manipulation regulates ovarian functions using the interactions between the plasma profiles of MEL and its MT1 and MT2 receptors in the ovary (Moniruzzaman and Maitra, 2012).
Receptor-Independent Actions of Melatonin as an Antioxidant
An elevation in oxidative stress is observed during oocyte maturation, and ovulation as free radicals is generated in a large amount (Tamura et al., 2008). The development of oocyte requires the synthesis of steroids actively, which is accompanied by the generation of free radicals, e.g., reactive oxygen species (ROS) and reactive nitrogen species (RNS) (Valenzuela et al., 2015) that can lead to the acceleration of oocyte aging and production of low-quality seeds (Olcese, 2020). However, MEL acts as a scavenger of free radicals (Tan, 1993), which reduces free radical-induced damages and improves the quality of oocytes (Reiter et al., 2013). MEL can also enhance the activity of antioxidative enzymes in ovarian follicles, like SOD, CAT, and GPx, and non-enzymatic antioxidants are also enhanced by the action of MEL (Maitra and Hasan, 2016). In carp, it has been reported that the ovarian malondialdehyde (MDA) (a marker of intracellular oxidative stress) level exhibited a seasonal variation such as very high during the post-spawning season and low during the spawning phase in an annual cycle, and this pattern has a negative correlation with the level of ovarian MEL. This data suggested that MEL reduces the generated oxidative stress in the ovary by enhancing the activities of SOD, CAT, and GPx during oocyte growth and maturation (Hasan et al., 2014) (Figure 1E). A very recent study on carp also demonstrated that hepatic MEL acts as a potent antioxidant by increasing the antioxidative enzymes and glutathione level for the reduction of ROS in an annual ovarian cycle (Hasan et al., 2020). As MEL is a lipophilic molecule, so the possibility of MEL incorporation within the ovary from the gut through circulation after L-Trp metabolism may not be ruled out as AANAT protein is not detected in carp ovary (Hasan et al., 2014; Pal et al., 2016a) to regulate oocyte growth and maturation.
Efficacy of Melatonin on Egg and Sperm Quality, Fertilization Potential, and Fecundity Indices
This section summarizes the role of MEL on fertility, fecundity, and sperm quality in fish. In zebra fish (D. rerio), the effects of MEL on female fecundity is assessed by taking the two different concentrations 100 nM and 1 μM for 10 days in a semi-static condition (Carnevali et al., 2010). Fecundity is assessed in this study by measuring the number of ovulated eggs in both the treatment. It is observed that in both the treatment groups, MEL significantly increased the number of ovulated eggs in females after pairing with control males. Moreover, the expression of Cox2A gene (in class IIIb oocytes) is positively correlated with the increased rate of fecundity in both the treatment groups (Carnevali et al., 2010). Fourier-transform infrared spectroscopy (FT-IR) analysis of oocytes from MEL treated (100 nM and 1 μM for 10 days) zebra fish (D. rerio) results into a higher number of classes III (vitellogenic follicles) and IV (maturational follicles), increased lhr mRNA expression and increased plasma VTG (Giorgini et al., 2012), and this provides a molecular basis on the influence of MEL on zebra fish fecundity. In killifish or mummichog, F. heteroclitus, the two doses of MEL (100 nM and 1 μM) are used to study the impact of this treatment on fecundity, rate of hatching, embryo survival through biochemical, molecular, and vibrational studies (Lombardo et al., 2012). After 8 days of treatment, a significant increase in the mean value of spawned eggs (fecundity rate) and the survival rate of embryo are found to be higher in fish treated with 1 μM MEL, whereas embryo hatching rate is not significantly improved after either of the MEL treatment (Lombardo et al., 2012). In vitro maturational study on postvitellogenic prematurational follicles reveals MEL (100 pg/ml) co-administration with maturational steroid has the highest maturation potential compared to control (Lombardo et al., 2012). Further, MEL-mediated modulation of neuroendocrine axis in the regulation of reproduction in killifish, F. heteroclitus, is revealed by a 30% increase in the number of daily spawned eggs and a 20% increase in the number of hatched embryo in case of MEL treated group with respect to the untreated group (Lombardo et al., 2014). Exogenous MEL administration also improved the oocyte quality in carp C. catla by reducing the oxidative stress (Moniruzzaman et al., 2016; Mondal et al., 2017). Further analysis reveals that the administration of MEL to male killifish increased the percentage of total spermatozoa (motile) and rapid spermatozoa. The level of straight-line (VSL), curvilinear (VCL), and average path velocities (VAP), and beat cross frequency (BCF-r) are found to be significantly higher, whereas the unaltered amplitude of lateral head displacement in case of rapid sperm (ALH-r) is observed in the MEL treated group (Lombardo et al., 2014), further indicating the efficacy of MEL to produce a high-quality sperm. The protective effects of MEL are also evaluated against titanium dioxide nanoparticles (TiO2-NP) toxicity in wild Capoeta trutta (male) (Özgür et al., 2020). TiO2-NP (10 mg/l) treatment decreases 79% VSL value with that of control, and MEL (0.1 mM) supplementation along with TiO2-NP increase this value by 77%. Moreover, 1 mM MEL improves 72% of VCL value with respect to the group treated with TiO2-NP. MEL seems to protect against the TiO2-NP-induced decrease in VAP and ALH values, and thereby argue in favor of a positive impact of MEL on the quality of sperm (Özgür et al., 2020). MEL supplement (0.5 μM) is also found to improve sperm quality in paddlefish (Polyodon spathula) during storage as it maintains mitochondrial membrane potential, ATP content, and inhibits ROS generation (Gao et al., 2019).
Conclusion
The gathered information so far indicates that food composition is one of the most vital environmental cues, which regulate MEL synthesis in the gastrointestinal tract. Thus, worldwide contemporary research is now mainly focused on the role of MEL as a fish food additive on various physiological aspects dealing with the improvement of growth and reproduction to act as a critical synchronizer in aquaculture. Several studies clearly suggested that the dietary intake of MEL or its precursor L-Trp results in an increase in the gut MEL production in rainbow trout and European sea bass. Because the increased level of MEL within the gut, resulting either from the administration of MEL or an addition of L-Trp to the food, is transported to all central and peripheral organs, the possibility of its actions in an endocrine fashion in regulating various body functions earns consideration. Recent studies also reveal that the application of MEL orally improves gut microbial homeostasis in zebra fish. Endogenous MEL in fresh water carp is known to protect the gut from pathogenic bacterial infection related to disease, which is a major issue in aquaculture. Most importantly, exogenous MEL treatment improves the reproductive performance by stimulating the fecundity rates, percentage of fertilization, and improving the quality of male and female seeds in fish. Accordingly, the use of MEL or L-Trp as an additive to fish food trends to be a common practice for achieving MEL-induced response in fish. In conclusion, the current state of knowledge clearly provides reasonable indications that considering multipotent functions of MEL, the use of this hormone, or its precursor as a fish food additive may be an effective tool for attaining better fish growth and a higher rate of reproduction in a sustainable aquaculture system.
Author Contributions
KNH and AA have developed the idea. All authors contributed to this review and approved the submitted version.
Funding
This work was funded by University Grants Commission (UGC) [F.30-448/2018(BSR)] to KNH and DBT-Boost Program Govt. of West Bengal, India (118/14/BT(Estt)/1P-4/2013).
Conflict of Interest
The authors declare that the research was conducted in the absence of any commercial or financial relationships that could be construed as a potential conflict of interest.
Publisher's Note
All claims expressed in this article are solely those of the authors and do not necessarily represent those of their affiliated organizations, or those of the publisher, the editors and the reviewers. Any product that may be evaluated in this article, or claim that may be made by its manufacturer, is not guaranteed or endorsed by the publisher.
Acknowledgments
We are very much thankful to Department of Zoology, Sidho-Kanho-Birsha University, Purulia, India for providing necessary support. The authors express their deep sense of gratitude to Prof. Saumen Kumar Maitra, Visva-Bharati, and Santiniketan, for his continuous motivation, academic encouragement, and unconditional support. The award of Junior Research Fellow (JRF) [F. No. 09/1156(0006)/2018-EMR-I] to AA from the Council of Scientific and Industrial Research (CSIR), Govt. of India, is appreciatively acknowledged. The authors are grateful to the editor, associate editor, and the three reviewers for their critique and invaluable comments on the previous version of the article.
References
Arendt, J., and Skene, D. J. (2005). Melatonin as a chronobiotic. Sleep Med. Rev. 9, 25–39. doi: 10.1016/j.smrv.2004.05.002
Aripin, S. A., Jintasataporn, O., and Yoonpundh, R. (2015). Effects of exogenous melatonin in Clarias macrocephalus male broodstock first puberty stage. J. Aquac. Res. Development 6:307. doi: 10.4172/2155-9546.1000307
Axelrod, J., and Weissbach, H. (1960). Enzymatic O-methylation of N-acetylserotonin to melatonin. Science 131, 1312–1312. doi: 10.1126/science.131.3409.1312
Aydin, M., Canpolat, S., Kuloglu, T., Yasar, A., Colakoglu, N., and Kelestimur, H. (2008). Effects of pinealectomy and exogenous melatonin on ghrelin and peptide YY in gastrointestinal system and neuropeptide Y in hypothalamic arcuate nucleus: immunohistochemical studies in male rats. Regul. Pept. 146, 197–203. doi: 10.1016/j.regpep.2007.09.009
Backström, T., and Winberg, S. (2017). Serotonin coordinates responses to social stress-what we can learn from fish. Front. Neurosci. 11:595. doi: 10.3389/fnins.2017.00595
Baekelandt, S., Milla, S., Cornet, V., Flamion, E., Ledor,é, Y., Redivo, B., et al. (2020). Seasonal simulated photoperiods influence melatonin release and immune markers of pike perch Sander lucioperca. Sci. Rep. 10, 1–10. doi: 10.1038/s41598-020-59568-1
Balcázar, J. L., De Blas, I., Ruiz-Zarzuela, I., Cunningham, D., Vendrell, D., and Múzquiz, J. L. (2006). The role of probiotics in aquaculture. Vet. Microbiol. 114, 173–186. doi: 10.1016/j.vetmic.2006.01.009
Barton, B. A. (2002). Stress in fishes: a diversity of responses with particular reference to changes in circulating corticosteroids. Integr. Comp. Biol. 42, 517–525. doi: 10.1093/icb/42.3.517
Bégay, V., Falcón, J., Cahill, G. M., Klein, D. C., and Coon, S. L. (1998). Transcripts encoding two melatonin synthesis enzymes in the teleost pineal organ: circadian regulation in pike and zebrafish, but not in trout. Endocrinology 139, 905–912. doi: 10.1210/endo.139.3.5790
Bhattacharya, S., Chattoraj, A., and Maitra, S. K. (2007). Melatonin in the regulation of annual testicular events in carp Catla catla: evidence from the studies on the effects of exogenous melatonin, continuous light, and continuous darkness. Chronobiol. Int. 24, 629–650. doi: 10.1080/07420520701534665
Bowden, T. J., Thompson, K. D., Morgan, A. L., Gratacap, R. M. L., and Nikoskelainen, S. (2007). Seasonal variation and the immune response: a fish perspective. Fish Shellfish Immunol. 22, 695–706. doi: 10.1016/j.fsi.2006.08.016
Boyd, C. E., D'Abramo, L. R., Glencross, B. D., Huyben, D. C., Juarez, L. M., Lockwood, G. S., et al. (2020). Achieving sustainable aquaculture: historical and current perspectives and future needs and challenges. J. World Aquac. Soc. 51, 578–633. doi: 10.1111/jwas.12714
Bromage, N., Porter, M., and Randall, C. (2001). The environmental regulation of maturation in farmed finfish with special reference to the role of photoperiod and melatonin. Aquaculture 197, 63–98. doi: 10.1016/S0044-8486(01)00583-X
Bubenik, G. A. (1986). The effect of Serotonin, N-acetylserotonin, and Melatonin on spontaneous contractions of isolated rat intestine. J. Pineal Res. 3, 41–54. doi: 10.1111/j.1600-079X.1986.tb00725.x
Bubenik, G. A., Niles, L. P., Pang, S. F., and Pentney, P. J. (1993). Diurnal variation and binding characteristics of melatonin in the mouse brain and gastrointestinal tissues. Comp. Biochem. Physiol. C Comp. 104, 221–224. doi: 10.1016/0742-8413(93)90027-I
Bubenik, G. A., and Pang, S. F. (1994). The role of serotonin and melatonin in gastrointestinal physiology: ontogeny, regulation of food intake, and mutual serotonin-melatonin feedback. J. Pineal Res. 16, 91–99. doi: 10.1111/j.1600-079X.1994.tb00088.x
Campos-Mendoza, A., McAndrew, B. J., Coward, K., and Bromage, N. (2004). Reproductive response of Nile tilapia (Oreochromis niloticus) to photoperiodic manipulation: effects on spawning periodicity, fecundity and egg size. Aquaculture 231, 299–314. doi: 10.1016/j.aquaculture.2003.10.023
Cardinali, D. P. (2019). Melatonin: clinical perspectives in neurodegeneration. Front. Endocrinol. 10:480. doi: 10.3389/fendo.2019.00480
Carnevali, O., Gioacchini, G., Maradonna, F., Olivotto, I., and Migliarini, B. (2011). Melatonin induces follicle maturation in Danio rerio. PLoS ONE 6:e19978. doi: 10.1371/journal.pone.0019978
Carnevali, O., Gioacchini, G., Piccinetti, C. C., Maradonna, F., Lombardo, F., Giorgini, E., et al. (2010). Melatonin control of oogenesis and metabolic resources in Zebrafish. J. Appl. Ichthyol. 26, 826–830. doi: 10.1111/j.1439-0426.2010.01549.x
Carnevali, O., Zamponi, M. C., Sulpizio, R., Rollo, A., Nardi, M., Orpianesi, C., et al. (2004). Administration of probiotic strain to improve sea bream wellness during development. Aquac. Int. 12, 377–386. doi: 10.1023/B:AQUI.0000042141.85977.bb
Carrillo, M., Zanuy, S., Prat, F., Serrano, R., and Bromage, N. (1993). “Environmental and hormonal control of reproduction in sea bass,” in Recent Advances in Aquaculture, eds J. F. Muir, R. J. Roberts (Oxford: Blackwell Scientific Publications; Institute of Aquaculture), 43–54.
Carrillo-Vico, A., Reiter, R. J., Lardone, P. J., Herrera, J. L., Fernández-Montesinos, R., Guerrero, J. M., et al. (2006). The modulatory role of melatonin on immune responsiveness. Curr. Opin. Investig. Drugs. 7, 423–431.
Ceinos, R. M., Polakof, S., Illamola, A. R., Soengas, J. L., and Míguez, J. M. (2008). Food deprivation and refeeding effects on pineal indoles metabolism and melatonin synthesis in the rainbow trout Oncorhynchus mykiss. Gen. Comp. Endocrinol. 156, 410–417. doi: 10.1016/j.ygcen.2008.01.003
Chattoraj, A., Bhattacharya, S., Basu, D., Bhattacharya, S., Bhattacharya, S., and Maitra, S. K. (2005). Melatonin accelerates maturation inducing hormone (MIH): induced oocyte maturation in Carps. Gen. Comp. Endocrinol. 140, 145–155. doi: 10.1016/j.ygcen.2004.10.013
Chattoraj, A., Seth, M., and Maitra, S. K. (2009). Localization and dynamics of Mel1a melatonin receptor in the ovary of carp Catla catla in relation to serum melatonin levels. Comp. Biochem. Physiol. A Mol. Integr. Physiol. 152, 327–333. doi: 10.1016/j.cbpa.2008.11.010
Choi, J. Y., Kim, N. N., Choi, Y. J., Park, M. S., and Choi, C. Y. (2016). Differential daily rhythms of melatonin in the pineal gland and gut of goldfish Carassius auratus in response to light. Biol. Rhythm Res. 47, 145–161. doi: 10.1080/09291016.2015.1094964
Coelho, L. A., Peres, R., Amaral, F. G., Reiter, R. J., and Cipolla-Neto, J. (2015). Daily differential expression of melatonin-related genes and clock genes in rat cumulus-oocyte complex: changes after pinealectomy. J. Pineal Res. 58, 490–499. doi: 10.1111/jpi.12234
Collin, J. P., Voisil, P., Falcón, J., Faure, J. P., Brisson, P., and Defaye, J. R. (1989). Pineal transducers in the course of evolution: molecular organization, rhythmic metabolic activity and role. Arch. Histol. Cytol. 52, 441–449. doi: 10.1679/aohc.52.Suppl_441
Conde-Sieira, M., Muñoz, J. L. P., López-Patiño, M. A., Gesto, M., Soengas, J. L., and Míguez, J. M. (2014). Oral administration of melatonin counteracts several of the effects of chronic stress in rainbow trout. Domest. Anim. Endocrinol. 46, 26–36. doi: 10.1016/j.domaniend.2013.10.001
Costello, C., Cao, L., Gelcich, S., Cisneros-Mata, M., Free, C. M., Froehlich, H. E., et al. (2020). The future of food from the sea. Nature 588, 95–100. doi: 10.1038/s41586-020-2616-y
Cuesta, A., Cerezuela, R., Esteban, M. Á., and Meseguer, J. (2008). In vivo actions of melatonin on the innate immune parameters in the teleost fish gilthead seabream. J. Pineal Res. 45, 70–78. doi: 10.1111/j.1600-079X.2008.00557.x
Cuesta, A., Rodríguez, A., Calderón, M. V., Meseguer, J., and Esteban, M. Á. (2007). Effect of the pineal hormone melatonin on teleost fish phagocyte innate immune responses after in vitro treatment. J. Exp. Zool. A Ecol. Genet. Physiol. 307, 509–515. doi: 10.1002/jez.404
Davie, A., Mazorra de Quero, C., Bromage, N., Treasurer, J., and Migaud, H. (2007). Inhibition of sexual maturation in tank reared haddock (Melanogrammus aeglefinus) through the use of constant light photoperiods. Aquaculture 270, 379–389. doi: 10.1016/j.aquaculture.2007.04.052
de Vlaming, V. (1980). Effects of pinealectomy and melatonin treatment on growth in the goldfish, Carassius auratus. Gen. Comp. Endocrinol. 40, 245–250. doi: 10.1016/0016-6480(80)90130-6
Deane, E. E., and Woo, N. Y. S. (2009). Modulation of fish growth hormone levels by salinity, temperature, pollutants and aquaculture related stress: a review. Rev. Fish Biol. Fish. 19, 97–120. doi: 10.1007/s11160-008-9091-0
Devi, H. S., Rajiv, C., Mondal, G., Khan, Z. A., Dharmajyoti Devi, S., Yumnamcha, T., et al. (2016). Melatonin bio-synthesizing enzyme genes (Tph1, Aanat1, Aanat2, and Hiomt) and their temporal pattern of expression in brain and gut of a tropical carp in natural environmental conditions. Cogent Biol. 2:1230337. doi: 10.1080/23312025.2016.1230337
Devi, S. D., Mondal, G., Khan, Z. A., Sarma, H. K., and Chattoraj, A. (2021). Differential gene expression and immunohistochemical localization of the key melatonin biosynthesizing enzymes in the testis of zebrafish (Danio rerio). Biol. Rhythm Res. 2021, 1–17. doi: 10.1080/09291016.2021.1926078
Dubocovich, M. L., Cardinali, D. P., Delagrange, P., Krause, D. N., Strosberg, D., Sugden, D., et al. (2000). “Melatonin receptors,” in The IUPHAR Compendium of Receptor Characterization and Classification, ed D. Girdlestone (London: IUPHAR Media), 271-277.
Ekstrzm, P., and Meissl, H. (1997). The pineal organ of teleost fishes. Rev. Fish Biol. Fisheries 7, 199–284. doi: 10.1023/A:1018483627058
Esteban, M. Á., Cuesta, A., Chaves-Pozo, E., and Meseguer, J. (2013). Influence of melatonin on the immune system of fish: a review. Int. J. Mol. Sci. 14, 7979–7999. doi: 10.3390/ijms14047979
Esteban, M. Á., Cuesta, A., Rodríguez, A., and Meseguer, J. (2006). Effect of photoperiod on the fish innate immune system: a link between fish pineal gland and the immune system. J. Pineal Res. 41, 261–266. doi: 10.1111/j.1600-079X.2006.00362.x
Falcón, J., Besseau, L., Fazzari, D., Attia, J., Gaildrat, P., Beauchaud, M., et al. (2003). Melatonin modulates secretion of growth hormone and prolactin by trout pituitary glands and cells in culture. Endocrinol. 144, 4648–4658. doi: 10.1210/en.2003-0707
Falcón, J., Besseau, L., Sauzet, S., and Boeuf, G. (2007). Melatonin effects on the hypothalamo-pituitary axis in fish. Trends Endocrinol. Metab. 18, 81–88. doi: 10.1016/j.tem.2007.01.002
Falcón, J., Migaud, H., Muñoz-Cueto, J. A., and Carrillo, M. (2010). Current knowledge on the melatonin system in teleost fish. Gen. Comp. Endocrinol. 165, 469–482. doi: 10.1016/j.ygcen.2009.04.026
Food Agriculture Organization (2019). Fishery and Aquaculture Statistics. Global Aquaculture Production 1950–2017 (FishstatJ). Rome: FAO Fisheries and Aquaculture Department. Available online at: www.fao.org/fishery/statistics/software/fishstatj/en (accessed April 21, 2021).
Fortes-Silva, R., Oliveira, I. E., Vieira, V. P., Winkaler, E. U., Guerra-Santos, B., and Cerqueira, R. B. (2016). Daily rhythms of locomotor activity and the influence of a light and dark cycle on gut microbiota species in tambaqui (Colossoma macropomum). Biol. Rhythm Res. 47, 183–190. doi: 10.1080/09291016.2015.1094972
Gao, Y., Yang, C., Huang, C., Zhang, G., Ji, H., and Dong, W. (2019). The effects of melatonin supplement on paddlefish (Polyodon spathula) sperm quality and ATP content in sperm during in vitro storage. Aquaculture 503, 475–482. doi: 10.1016/j.aquaculture.2019.01.010
García-Allegue, R., Madrid, J. A., and Sánchez-Vázquez, F. J. (2001). Melatonin rhythms in European sea bass plasma and eye: influence of seasonal photoperiod and water temperature. J. Pineal Res. 31, 68–75. doi: 10.1034/j.1600-079X.2001.310110.x
Gesto, M., Álvarez-Otero, R., Conde-Sieira, M., Otero-Rodiño, C., Usandizaga, S., Soengas, J. L., et al. (2016). A simple melatonin treatment protocol attenuates the response to acute stress in the sole Solea senegalensis. Aquaculture 452, 272–282. doi: 10.1016/j.aquaculture.2015.11.006
Gesto, M., López-Patiño, M. A., Hernández, J., Soengas, J. L., and Míguez, J. M. (2015). Gradation of the stress response in rainbow trout exposed to stressors of different severity: the role of brain serotonergic and dopaminergic systems. J. Neuroendocrinol. 27, 131–141. doi: 10.1111/jne.12248
Giorgini, E., Gioacchini, G., Conti, C., Ferraris, P., Sabbatini, S., Tosi, G., et al. (2012). The role of melatonin on zebrafish follicle development: an FT-IR imaging approach. Vib. Spectrosc. 62, 279–285. doi: 10.1016/j.vibspec.2012.06.009
Hasan, K. N., and Banerjee, G. (2020). Recent studies on probiotics as beneficial mediator in aquaculture: a review. J. Basic Applied Zool. 81:53. doi: 10.1186/s41936-020-00190-y
Hasan, K. N., Moniruzzaman, M., and Maitra, S. K. (2014). Melatonin concentrations in relation to oxidative status and oocyte dynamics in the ovary during different reproductive phases of an annual cycle in carp Catla catla. Theriogenology 82, 1173–1185. doi: 10.1016/j.theriogenology.2014.08.001
Hasan, K. N., Pal, P. K., and Maitra, S. K. (2016). Diurnal and seasonal profiles of melatonin in the liver and their correlates of ovarian functions under natural photo-thermal conditions in adult carp Catla catla. Biol. Rhythm Res. 47, 947–965. doi: 10.1080/09291016.2016.1212535
Hasan, K. N., Pal, P. K., and Maitra, S. K. (2020). Temporal relationship between the levels of melatonin and different antioxidants in the liver of a surface feeding carp Catla catla. Biol. Rhythm Res. 51, 373–391. doi: 10.1080/09291016.2018.1533728
He, C., Wang, J., Zhang, Z., Yang, M., Li, Y., Tian, X., et al. (2016). Mitochondria synthesize melatonin to ameliorate its function and improve mice oocyte's quality under in vitro conditions. Int. J. Mol. Sci. 17:939. doi: 10.3390/ijms17060939
Herrero, M. J., Martínez, F. J., Míguez, J. M., and Madrid, J. A. (2007). Response of plasma and gastrointestinal melatonin, plasma cortisol and activity rhythms of European sea bass (Dicentrarchus labrax) to dietary supplementation with tryptophan and melatonin. J. Comp. Physiol. B Biochem. Syst. Environ. Physiol. 177, 319–326. doi: 10.1007/s00360-006-0131-6
Höglund, E., Blam, P. H. M., and Winberg, S. (2002). Stimulatory and inhibitory effects of 5-HT1A receptors on ACTH and cortisol secretion in a teleost fish, the Arctic charr (Salvelinus alpinus). Neurosci. Lett. 324, 193–196. doi: 10.1016/S0304-3940(02)00200-8
Iigo, M., Abe, T., Kambayashi, S., Oikawa, K., Masuda, T., Mizusawa, K., et al. (2007). Lack of circadian regulation of in vitro melatonin release from the pineal organ of salmonid teleosts. Gen. Comp. Endocrinol. 154, 91–97. doi: 10.1016/j.ygcen.2007.06.013
Iigo, M., Ikeda, E., Sato, M., Kawasaki, S., Noguchi, F., and Nishi, G. (2006). Circadian rhythms of ocular melatonin in the wrasse Halichoeres tenuispinnis, a labrid teleost. Gen. Comp. Endocrinol. 145, 32–38. doi: 10.1016/j.ygcen.2005.06.010
Itoh, M. T., Ishizuka, B., Kuribayashi, Y., Amemiya, A., and Sumi, Y. (1999). Melatonin, its precursors, and synthesizing enzyme activities in the human ovary. Mol. Hum. Reprod. 5, 402–408. doi: 10.1093/molehr/5.5.402
Iuvone, P. M., and Besharse, J. C. (1983). Regulation of indoleamine N-Acetyltransferase activity in the retina: effects of light and dark, protein synthesis inhibitors and cyclic nucleotide analogs. Brain Res. 273, 111–119. doi: 10.1016/0006-8993(83)91099-5
Iwasaki, S., Nakazawa, K., Sakai, J., Kometani, K., Iwashita, M., Yoshimura, Y., et al. (2005). Melatonin as a local regulator of human placental function. J. Pineal Res. 39, 261–265. doi: 10.1111/j.1600-079X.2005.00244.x
Joy, K. P., and Agha, A. K. (1989). Seasonal effects of pinealectomy on the ovary of the catfish Heteropneustes fossilis (Bloch) under normal photothermal conditions. J. Pineal Res. 7, 281–289. doi: 10.1111/j.1600-079X.1989.tb00451.x
Joy, K. P., and Agha, A. K. (1991). Seasonal effects of administration of melatonin and 5-methoxytryptophol on ovarian activity in the catfish Heteropneustes fossilis (Bloch). J. Pineal Res. 10, 65–70. doi: 10.1111/j.1600-079X.1991.tb00012.x
Joy, K. P., and Khan, I. A. (1991). Pineal-gonadal relationship in the teleost Channa punctatus (Bloch): evidence for possible involvement of hypothalamic serotonergic system. J. Pineal Res. 11, 12–22. doi: 10.1111/j.1600-079X.1991.tb00821.x
Khan, Z. A., Yumnamcha, T., Rajiv, C., Sanjita Devi, H., Mondal, G., Devi, S. D., et al. (2016). Melatonin biosynthesizing enzyme genes and clock genes in ovary and whole brain of zebrafish (Danio rerio): differential expression and a possible interplay. Gen. Comp. Endocrinol. 233, 16–31. doi: 10.1016/j.ygcen.2016.05.014
Klein, D. C., Coon, S. L., Roseboom, P. H., Weller, J. L., Bernard, M., Gastel, J. A., et al. (1997). The melatonin rhythm-generating enzyme: molecular regulation of serotonin N-acetyltransferase in the pineal gland. Recent Prog. Horm. Res. 52, 307–357.
Konturek, P. C., Brzozowski, T., and Konturek, S. J. (2011). Stress and the gut: pathophysiology, clinical consequences, diagnostic approach and treatment options. J. Physiol. Pharmacol. 62, 591–599.
Kulczykowska, E., Kalamarz-Kubiak, H., Gozdowska, M., and Sokołowska, E. (2018). Cortisol and melatonin in the cutaneous stress response system of fish. Comp. Biochem. Physiol. A Mol. Integr. Physiol. 218, 1–7. doi: 10.1016/j.cbpa.2018.01.003
Kulczykowska, E., Kleszczyńska, A., Gozdowska, M., and Sokołowska, E. (2017). The time enzyme in melatonin biosynthesis in fish: day/night expressions of three aralkylamine N-acetyltransferase genes in three-spined stickleback. Comp. Biochem. Physiol. A Mol. Integr. Physiol. 208, 46–53. doi: 10.1016/j.cbpa.2017.03.005
Lal, B., and Singh, T. P. (1995). Role of pineal in timing of photothermo-sexual responses in fish. Pineal Gland Mol. Sign. 1, 77–84.
Lee, P. P. N., Shiu, S. Y. W., Chow, P. H., and Pang, S. F. (1995). Regional and diurnal studies of melatonin and melatonin binding sites in the duck gastro-intestinal tract. Biol. Signals. 4, 212–224. doi: 10.1159/000109445
Lepage, O., Larson, E. T., Mayer, I., and Winberg, S. (2005). Tryptophan affects both gastrointestinal melatonin production and interrenal activity in stressed and non-stressed rainbow trout. J. Pineal Res. 38, 264–271. doi: 10.1111/j.1600-079X.2004.00201.x
Lerner, A. B., Case, J. D., Takahashi, Y., Lee, T. H., and Mori, W. (1958). Isolation of melatonin, the pineal gland factor that lightens melanocytes. J. Am. Chem. Soc. 80, 2587–2587. doi: 10.1021/ja01543a060
Liu, F., Ng, T. B., and Fung, M. C. (2001). Pineal indoles stimulate the gene expression of immunomodulating cytokines. J. Neural Transm. 108, 397–405. doi: 10.1007/s007020170061
Liu, T., and Borjigin, J. (2005). N-acetyltransferase is not the rate-limiting enzyme of melatonin synthesis at night. J. Pineal Res. 39, 91–96. doi: 10.1111/j.1600-079X.2005.00223.x
Lombardo, F., Gioacchini, G., Fabbrocini, A., Candelma, M., D'Adamo, R., Giorgini, E., et al. (2014). Melatonin-mediated effects on killifish reproductive axis. Comp. Biochem. Physiol. A Mol. Integr. Physiol. 172, 31–38. doi: 10.1016/j.cbpa.2014.02.008
Lombardo, F., Giorgini, E., Gioacchini, G., Maradonna, F., Ferraris, P., and Carnevali, O. (2012). Melatonin effects on Fundulus heteroclitus reproduction. Reprod. Fertil. Dev. 24, 794–803. doi: 10.1071/RD11267
López-Patiño, M. A., Gesto, M., Conde-Sieira, M., Soengas, J. L., and Míguez, J. M. (2014). Stress inhibition of melatonin synthesis in the pineal organ of rainbow trout (Oncorhynchus mykiss) is mediated by cortisol. J. Exp. Biol. 217, 1407–1416. doi: 10.1242/jeb.087916
López-Patiño, M. A., Rodríguez-Illamola, A., Gesto, M., Soengas, J. L., and Míguez, J. M. (2011). Changes in plasma melatonin levels and pineal organ melatonin synthesis following acclimation of rainbow trout (Oncorhynchus mykiss) to different water salinities. J. Exp. Biol. 214, 928–936. doi: 10.1242/jeb.051516
Lovenberg, W., Jequier, E., and Sjoerdsma, A. (1967). Tryptophan hydroxylation: measurement in pineal gland, brain stem and carcinoid tumor. Science 155, 217–219. doi: 10.1126/science.155.3759.217
Lovenberg, W. E., Weissbach, H., and Udenfriend, S. (1962). Aromatic L-amino acid decarboxylase. J. Biol. Chem. 237, 89–93. doi: 10.1016/S0021-9258(18)81366-7
Lutfi, E., Basili, D., Falcinelli, S., Morillas, L., Carnevali, O., Capilla, E., et al. (2021). The probiotic Lactobacillus rhamnosus mimics the dark-driven regulation of appetite markers and melatonin receptors' expression in zebrafish (Danio rerio) larvae: understanding the role of the gut microbiome. Comp. Biochem. Physiol. B Biochem. Mol. Biol. 2021:110634. doi: 10.1016/j.cbpb.2021.110634
Ma, N., Zhang, J., Reiter, R. J., and Ma, X. (2020). Melatonin mediates mucosal immune cells, microbial metabolism, and rhythm crosstalk: a therapeutic target to reduce intestinal inflammation. Med. Res. Rev. 40, 606–632. doi: 10.1002/med.21628
Maitra, S. K. (2011). “Strategic management of photoperiodic schedule and melatonin profile in the regulation of carp reproduction,” in Carp: Habitat, Management and Diseases, eds J. D. Sanders, S. B. Peterson (New York, NY: Nova Science Publishers, Inc.), 1–20.
Maitra, S. K., Chattoraj, A., and Bhattacharyya, S. (2005). Implication of melatonin in oocyte maturation in Indian major carp Catla catla. Fish Physiol. Biochem. 31, 201–207. doi: 10.1007/s10695-006-0025-2
Maitra, S. K., Chattoraj, A., Mukherjee, S., and Moniruzzaman, M. (2013). Melatonin: a potent candidate in the regulation of fish oocyte growth and maturation. Gen. Comp. Endocrinol. 181, 215–222. doi: 10.1016/j.ygcen.2012.09.015
Maitra, S. K., and Hasan, K. N. (2016). The role of melatonin as a hormone and an antioxidant in the control of fish reproduction. Front. Endocrinol. 7:38. doi: 10.3389/fendo.2016.00038
Maitra, S. K., Mukherjee, S., and Hasan, K. N. (2015). “Melatonin: endogenous sources and role in the regulation of fish reproduction,” in Indoleamines: Sources, Role in Biological Processes and Health Effects, ed A. Catalá (New York, NY: Nova Science Publishers, Inc.), 43–77.
Manning, M. J. (1998). “Immune defence systems,” in Biology of Farmed Fish, eds K. D. Black, A. D. Pickering (Sheffield: Sheffield Academic Press), 180–221.
Martinez-Chavez, C. C., Minghetti, M., and Migaud, H. (2008). GPR54 and rGnRH I gene expression during the onset of puberty in Nile tilapia. Gen. Comp. Endocrinol. 156, 224–233. doi: 10.1016/j.ygcen.2008.01.019
Mayer, I. (2000). Effect of long-term pinealectomy on growth and precocious maturationin Atlantic salmon, Salmo salar parr. Aquat. Living Resour. 13, 139–144. doi: 10.1016/S0990-7440(00)01053-6
Mazurais, D., Porter, M., Lethimonier, C., Le Dréan, G., Le Goff, P., Randall, C., et al. (2000). Effects of melatonin on liver estrogen receptor and vitellogenin expression in rainbow trout: an in vitro and in vivo study. Gen. Comp. Endocrinol. 118, 344–353. doi: 10.1006/gcen.2000.7472
Medeiros, L. R., Cartolano, M. C., and McDonald, M. D. (2014). Crowding stress inhibits serotonin 1A receptor-mediated increases in corticotropin-releasing factor mRNA expression and adrenocorticotropin hormone secretion in the Gulf toadfish. J. Comp. Physiol. B Biochem. Syst. Environ. Physiol. 184, 259–271. doi: 10.1007/s00360-013-0793-9
Menendez-Pelaez, A., Howes, K. A., Gonzalez-Brito, A., and Reiter, R. J. (1987). N-Acetyltransferase activity, hydroxyindole-O-methyltransferase activity, and melatonin levels in the Harderian glands of the female Syrian hamster: changes during the light: dark cycle and the effect of 6-parachlorophenylalanine administration. Biochem. Biophys. Res. Commun. 145, 1231–1238. doi: 10.1016/0006-291X(87)91569-5
Migaud, H., Davie, A., and Taylor, J. F. (2010). Current knowledge on the photoneuroendocrine regulation of reproduction in temperate fish species. J. Fish Biol. 76, 27–68. doi: 10.1111/j.1095-8649.2009.02500.x
Mondal, G., Dharmajyoti Devi, S., Khan, Z. A., Yumnamcha, T., Rajiv, C., Sanjita Devi, H., et al. (2021). The influence of feeding on the daily rhythm of mRNA expression on melatonin bio-synthesizing enzyme genes and clock associated genes in the zebrafish (Danio rerio) gut. Biol. Rhythm Res. 2021, 1–18. doi: 10.1080/09291016.2021.1905989
Mondal, P., Hasan, K. N., Pal, P. K., and Maitra, S. K. (2017). Influences of exogenous melatonin on the oocyte growth and oxidative status of ovary during different reproductive phases of an annual cycle in carp Catla catla. Theriogenology 87, 349–359. doi: 10.1016/j.theriogenology.2016.09.021
Moniruzzaman, M., Hasan, K. N., and Maitra, S. K. (2016). Melatonin actions on ovaprim (synthetic GnRH and domperidone)-induced oocyte maturation in carp. Reproduction 151, 285–296. doi: 10.1530/REP-15-0391
Moniruzzaman, M., and Maitra, S. K. (2012). Influence of altered photoperiods on serum melatonin and its receptors (MT1 and MT2) in the brain, retina, and ovary in carp Catla catla. Chronobiol. Int. 29, 175–188. doi: 10.3109/07420528.2011.645753
Mukherjee, S., and Maitra, S. K. (2015). Effects of starvation, re-feeding and timing of food supply on daily rhythm features of gut melatonin in carp (Catla catla). Chronobiol. Int. 32, 1264–1277. doi: 10.3109/07420528.2015.1087020
Mukherjee, S., and Maitra, S. K. (2018). Daily profiles of serum and gastrointestinal melatonin in response to daytime or night-time supply of tryptophan-rich diet in carp (Catla catla). Biol. Rhythm Res. 49, 315–327. doi: 10.1080/09291016.2017.1361157
Mukherjee, S., Moniruzzaman, M., and Maitra, S. K. (2014). Daily and seasonal profiles of gut melatonin and their temporal relationship with pineal and serum melatonin in carp Catlacatla under natural photo-thermal conditions. Biol. Rhythm Res. 45, 301–315. doi: 10.1080/09291016.2013.817139
Muñoz-Pérez, J. L., López-Patiño, M. A., Álvarez-Otero, R., Gesto, M., Soengas, J. L., and Míguez, J. M. (2016). Characterization of melatonin synthesis in the gastrointestinal tract of rainbow trout (Oncorhynchus mykiss): distribution, relation with serotonin, daily rhythms and photoperiod regulation. J. Comp. Physiol. B Biochem. Syst. Environ. Physiol. 186, 471–484. doi: 10.1007/s00360-016-0966-4
Nelson, R. J., and Demas, G. E. (1996). Seasonal changes in immune function. Q. Rev. Biol. 71, 511–548. doi: 10.1086/419555
Nisembaum, L. G., Tinoco, A. B., Moure, A. L., Alonso Gómez, A. L., Delgado, M. J., and Valenciano, A. I. (2013). The arylalkylamine-N-acetyltransferase (AANAT) acetylates dopamine in the digestive tract of goldfish: a role in intestinal motility. Neurochem. Int. 62, 873–880. doi: 10.1016/j.neuint.2013.02.023
Olcese, J. M. (2020). Melatonin and female reproduction: an expanding universe. Front. Endocrinol. 11:85. doi: 10.3389/fendo.2020.00085
Özgür, M. E., Ulu, A., Noma, S. A. A., Özcan, I., Balcioglu, S., Ateş, B., et al. (2020). Melatonin protects sperm cells of Capoeta trutta from toxicity of titanium dioxide nanoparticles. Environ. Sci. Pollut. Res. 27, 17843–17853. doi: 10.1007/s11356-020-08273-7
Pal, P. K., Hasan, K. N., and Maitra, S. K. (2016a). Temporal relationship between the daily profiles of gut melatonin, oxidative status and major digestive enzymes in carp Catlacatla. Biol. Rhythm Res. 47, 755–771. doi: 10.1080/09291016.2016.1191697
Pal, P. K., Hasan, K. N., and Maitra, S. K. (2016b). Gut melatonin response to microbial infection in carp Catla catla. Fish Physiol. Biochem. 42, 579–592. doi: 10.1007/s10695-015-0161-7
Pal, P. K., and Maitra, S. K. (2018). Response of gastrointestinal melatonin, antioxidants, and digestive enzymes to altered feeding conditions in carp (Catla catla). Fish Physiol. Biochem. 44, 1061–1073. doi: 10.1007/s10695-018-0494-0
Paulin, C. H., Cazaméa-Catalan, D., Zilberman-Peled, B., Herrera-Perez, P., Sauzet, S., Magnanou, E., et al. (2015). Subfunctionalization of arylalkylamine N-acetyltransferases in the sea bass Dicentrarchus labrax: two-ones for one two. J. Pineal Res. 59, 354–364. doi: 10.1111/jpi.12266
Peuhkuri, K., Sihvola, N., and Korpela, R. (2012). Dietary factors and fluctuating levels of melatonin. Food Nutr. Res. 56, 17252. doi: 10.3402/fnr.v56i0.17252
Piccinetti, C. C., Migliarini, B., Olivotto, I., Simoniello, M. P., Giorgini, E., and Carnevali, O. (2013). Melatonin and peripheral circuitries: insights on appetite and metabolism in Danio rerio. Zebrafish 10, 275–282. doi: 10.1089/zeb.2012.0844
Popek, W., Epler, P., Bieniarz, K., and Sokołowska-Mikołajczyk, M. (1997). Contribution of factors regulating melatonin release from pineal gland of carp (Cyprinus carpio L.) in normal and in polluted environments. Fish Aquatic. Sci. 5, 59–75.
Porter, M. J. R., Duncan, N. J., Mitchell, D., and Bromagea, N. R. (1999). The use of cage lighting to reduce plasma melatonin in Atlantic salmon (Salmo salar) and its effects on the inhibition of grilsing. Aquaculture 176, 237–244. doi: 10.1016/S0044-8486(99)00113-1
Reiter, R. J., Rosales-Corral, S. A., Manchester, L. C., and Tan, D. X. (2013). Peripheral reproductive organ health and melatonin: ready for prime time. Int. J. Mol. Sci. 14, 7231–7272. doi: 10.3390/ijms14047231
Reiter, R. J., Tan, D. X., Manchester, L. C., Paredes, S. D., Mayo, J. C., and Sainz, R. M. (2009). Melatonin and reproduction revisited. Biol. Reprod. 81, 445–456. doi: 10.1095/biolreprod.108.075655
Rönnberg, L., Kauppila, A., Leppäluoto, J., Martikainen, H., and Vakkuri, O. (1990). Circadian and seasonal variation in human preovulatory follicular fluid melatonin concentration. J. Clin. Endocrinol. Metab. 71, 493–496. doi: 10.1210/jcem-71-2-493
Roy, B., Singh, R., Kumar, S., and Rai, U. (2008). Diurnal variation in phagocytic activity of splenic phagocytes in freshwater teleost Channa punctatus: melatonin and its signaling mechanism. J. Endocrinol. 199, 471. doi: 10.1677/JOE-08-0270
Rubio, V. C., Sánchez-Vázquez, F. J., and Madrid, J. A. (2004). Oral administration of melatonin reduces food intake and modifies macronutrient selection in European sea bass (Dicentrarchus labrax, L.). J. Pineal Res. 37, 42–47. doi: 10.1111/j.1600-079X.2004.00134.x
Salinas, I. (2015). The mucosal immune system of teleost fish. Biology 4, 525–539. doi: 10.3390/biology4030525
Sánchez, J. A., López-Olmeda, J. F., Blanco-Vives, B., and Sánchez-Vázquez, F. J. (2009). Effects of feeding schedule on locomotor activity rhythms and stress response in sea bream. Physiol. Behav. 98, 125–129. doi: 10.1016/j.physbeh.2009.04.020
Sánchez-Vázquez, F. J., López-Olmeda, J. F., Vera, L. M., Migaud, H., López-Patiño, M. A., and Míguez, J. M. (2019). Environmental cycles, melatonin, and circadian control of stress response in fish. Front. Endocrinol. 10:279. doi: 10.3389/fendo.2019.00279
Sánchez-Vázquez, F. J., Madrid, J. A., Zamora, S., and Tabata, M. (1997). Feeding entrainment of locomotor activity rhythms in the goldfish is mediated by a feeding-entrainable circadian oscillator. J. Comp. Physiol. A Sensory, Neural, Behav. Physiol. 181, 121–132. doi: 10.1007/s003590050099
Saxena, P. K., and Anand, K. (1977). A comparison of ovarian recrudescence in the catfish, Mystus tengara (Ham.), exposed to short photoperiods, to long photoperiods, and to melatonin. Gen. Comp. Endocrinol. 33, 506–511. doi: 10.1016/0016-6480(77)90109-5
Scheer, F. A., and Czeisler, C. A. (2005). Melatonin, sleep, and circadian rhythms. Sleep Med. Rev. 9, 5–9. doi: 10.1016/j.smrv.2004.11.004
Seth, M., and Maitra, S. K. (2010). Importance of light in temporal organization of photoreceptor proteins and melatonin-producing system in the pineal of carp Catla catla. Chronobiol. Int. 27, 463–486. doi: 10.3109/07420521003666416
Singh, T. P., and Lal, P. (1994). “Endocrine physiology of reproduction in Indian catfish,” in Advances in Fish Biology, ed H. R. (Delhi: Singh Hindustan Publishing Corporation), 147–154.
Sjöblom, M., and Flemström, G. (2003). Melatonin in the duodenal lumen is a potent stimulant of mucosal bicarbonate secretion. J. Pineal Res. 34, 288–293. doi: 10.1034/j.1600-079X.2003.00044.x
Sköld, H. N., Amundsen, T., Svensson, P. A., Mayer, I., Bjelvenmark, J., and Forsgren, E. (2008). Hormonal regulation of female nuptial coloration in a fish. Horm. Behav. 54, 549–556. doi: 10.1016/j.yhbeh.2008.05.018
Song, Y., Song, X., Wu, M., Pang, Y., Shi, A., Shi, X., et al. (2020). The protective effects of melatonin on survival, immune response, digestive enzymes activities and intestinal microbiota diversity in Chinese mitten crab (Eriocheir sinensis) exposed to glyphosate. Comp. Biochem. Physiol. C Toxicol. Pharmacol. 238:108845. doi: 10.1016/j.cbpc.2020.108845
Srinivasan, V., Smits, M., Spence, W., Lowe, A., Kayumov, L., Pandi-Perumal, S., et al. (2006). Melatonin in mood disorders. World J. Biol. Psychiatry. 7, 138–151. doi: 10.1080/15622970600571822
Storr, M., Schusdziarra, V., and Allescher, H. D. (2000). Inhibition of small conductance K+-channels attenuated melatonin-induced relaxation of serotonin-contracted rat gastric fundus. Can. J. Physiol. Pharmacol. 78, 799–806. doi: 10.1139/y00-059
Sundararaj, B. I., and Keshavanath, P. (1976). Effects of melatonin and prolactin treatment on the hypophysial-ovarian system in the catfish, Heteropneustes fossilis (Bloch). Gen. Comp. Endocrinol. 29, 84–96. doi: 10.1016/0016-6480(76)90010-1
Taheri, P., Mogheiseh, A., Shojaee Tabrizi, A., Nazifi, S., Salavati, S., and Koohi, F. (2019). Changes in thyroid hormones, leptin, ghrelin and, galanin following oral melatonin administration in intact and castrated dogs: a preliminary study. BMC Vet. Res. 15, 1–13. doi: 10.1186/s12917-019-1894-9
Tamura, H., Takasaki, A., Miwa, I., Taniguchi, K., Maekawa, R., Asada, H., et al. (2008). Oxidative stress impairs oocyte quality and melatonin protects oocytes from free radical damage and improves fertilization rate. J. Pineal Res. 44, 280–287. doi: 10.1111/j.1600-079X.2007.00524.x
Valenzuela, F. J., Vera, J., Venegas, C., Pino, F., and Lagunas, C. (2015). Circadian system and melatonin hormone: risk factors for complications during pregnancy. Obstet. Gynecol. Int. 2015:825802. doi: 10.1155/2015/825802
Veisi, S., Sarkheil, M., Johari, S. A., and Safari, O. (2021). Dietary supplementation with melatonin: influence on growth performance, oxidative stress status, and amelioration of silver nanoparticles-induced toxicity in Nile tilapia (Oreochromis niloticus). Trop. Anim. Health Prod. 53:314. doi: 10.1007/s11250-021-02760-w
Velarde, E., Alonso-Gómez, A. L., Azpeleta, C., Isorna, E., and Delgado, M. J. (2009). Melatonin attenuates the acetylcholine-induced contraction in isolated intestine of a teleost fish. J. Comp. Physiol. B 179, 951–959. doi: 10.1007/s00360-009-0373-1
Velarde, E., Cerdá-Reverter, J. M., Alonso-Gómez, A. L., Sánchez, E., Isorna, E., and Delgado, M. J. (2010). Melatonin-synthesizing enzymes in pineal, retina, liver, and gut of the goldfish (Carassius): mRNA expression pattern and regulation of daily rhythms by lighting conditions. Chronobiol. Int. 27, 1178–1201. doi: 10.3109/07420528.2010.496911
Voisin, P., Namboodiri, M. A. A., and Klein, D. C. (1984). Arylamine N-acetyltransferase and arylalkylamine N-acetyltransferase in the mammalian pineal gland. J. Biol. Chem. 259, 10913–10918. doi: 10.1016/S0021-9258(18)90600-9
Wang, B., Zhu, S., Liu, Z., Wei, H., Zhang, L., He, M., et al. (2021). Increased expression of colonic mucosal melatonin in patients with irritable bowel syndrome correlated with gut dysbiosis. Genom. Proteom. Bioinforma. 2021:S1672–022900018-8. doi: 10.1101/2020.03.03.20030635
Wang, Y. R., Wang, L., Zhang, C., and xiao, Song, K. (2017). Effects of substituting fishmeal with soybean meal on growth performance and intestinal morphology in orange-spotted grouper (Epinephelus coioides). Aquac. Reports. 5, 52–57. doi: 10.1016/j.aqrep.2016.12.005
Woo, M. M. M., Tai, C. J., Kang, S. K., Nathwani, P. S., Pang, S. F., and Leung, P. C. K. (2001). Direct action of melatonin in human granulosa-luteal cells. J. Clin. Endocrinol. Metab. 86, 4789–4797. doi: 10.1210/jcem.86.10.7912
Yasmin, F., Sutradhar, S., Das, P., and Mukherjee, S. (2021). Gut melatonin: a potent candidate in the diversified journey of melatonin research. Gen. Comp. Endocrinol. 303:113693. doi: 10.1016/j.ygcen.2020.113693
Yin, J., Li, Y., Han, H., Chen, S., Gao, J., Liu, G., et al. (2018). Melatonin reprogramming of gut microbiota improves lipid dysmetabolism in high-fat diet-fed mice. J. Pineal Res. 65:e12524. doi: 10.1111/jpi.12524
Yin, J., Li, Y., Han, H., Ma, J., Liu, G., Wu, X., et al. (2020). Administration of exogenous melatonin improves the diurnal rhythms of the gut microbiota in mice fed a high-fat diet. mSystems 5, e00002–20. doi: 10.1128/mSystems.00002-20
Zhang, C., Rahimnejad, S., Wang, Y. R., Lu, K., Song, K., Wang, L., et al. (2018). Substituting fish meal with soybean meal in diets for Japanese seabass (Lateolabrax japonicus): effects on growth, digestive enzymes activity, gut histology, and expression of gut inflammatory and transporter genes. Aquaculture 483, 173–182. doi: 10.1016/j.aquaculture.2017.10.029
Zhang, Z., Peng, Q., Huo, D., Jiang, S., Ma, C., Chang, H., et al. (2021). Melatonin regulates the neurotransmitter secretion disorder induced by caffeine through the microbiota-gut-brain axis in zebrafish (Danio rerio). Front. Cell Dev. Biol. 9:678190. doi: 10.3389/fcell.2021.678190
Keywords: aquaculture, melatonin, gut bacteria, tryptophan, reproduction, stress
Citation: Acharyya A, Das J and Hasan KN (2021) Melatonin as a Multipotent Component of Fish Feed: Basic Information for Its Potential Application in Aquaculture. Front. Mar. Sci. 8:734066. doi: 10.3389/fmars.2021.734066
Received: 30 June 2021; Accepted: 20 August 2021;
Published: 17 September 2021.
Edited by:
Samad Rahimnejad, University of South Bohemia in Ceské Budějovice, CzechiaReviewed by:
Francisco Javier Moyano, University of Almeria, SpainOmid Safari, Ferdowsi University of Mashhad, Iran
Amalia Pérez-Jiménez, University of Granada, Spain
Copyright © 2021 Acharyya, Das and Hasan. This is an open-access article distributed under the terms of the Creative Commons Attribution License (CC BY). The use, distribution or reproduction in other forums is permitted, provided the original author(s) and the copyright owner(s) are credited and that the original publication in this journal is cited, in accordance with accepted academic practice. No use, distribution or reproduction is permitted which does not comply with these terms.
*Correspondence: Kazi Nurul Hasan, a2F6aWhhc2FuNUBnbWFpbC5jb20=