- 1Aquarium La Rochelle, Centre d’Etudes et de Soins pour les Tortues Marines, La Rochelle, France
- 2MERCATOR-Ocean International (MOI), Toulouse, France
Unusual environmental events can push marine animals outside their physiological tolerances through changes in trophic and/or thermal conditions. Such events typically increase the risk of stranding. Rescue Centers offer a unique opportunity to report animals in distress and satellite track rehabilitated individuals to identify potential new habitats and support an effective conservation of these endangered species. By combining sightings (1988–2020) and tracking data (2008–2020) collected along the French Atlantic and English Channel coasts, our study assessed if the Bay of Biscay is an ecological trap or a favorable habitat for immature sea turtles. The largest tracked individuals migrated westward to pelagic waters, likely toward their natal beaches, while smaller individuals remained within the Bay of Biscay (BoB) and crossed colder (mean: 17.8 ± 3.0°C) but more productive waters. The turtles’ directions differed from the ones of ocean currents, excluding a passive advection to these unexpected habitats. Although the BoB might be thermally unsuitable in winter, the higher micronekton biomass predicted in this region could offer a productive foraging habitat for immature turtles. However, the majority of the sightings referred to individuals stranded alive (75%), suggesting this area could also act as an ecological trap for the smallest individuals that are mostly reported in winter suffering cold-stunning. Assumed to be outside the species range, our results reveal a potential foraging ground in the North-East Atlantic for these young turtles, confirming the crucial role of the rehabilitation centers and the need to continue prioritizing conservation of these endangered species, particularly vulnerable at this stage and at such temperate latitudes.
Introduction
Unusual environmental conditions can push marine animals outside their physiological tolerances through changes in trophic and/or thermal conditions. In sea turtles, such events are commonly caused by a drop in sea surface temperature (SST) below 10°C, making individuals lethargic and floating at the sea surface. The risk of stranding is inevitably increased during such cold-stunning events (George, 1997; Spotila et al., 1997). Rescue Centers offer a unique opportunity to satellite track the rehabilitated individuals to identify potential new habitats (assumed to be unfavorable due to extreme thermal conditions), and redefine Regional Management Units to ensure the conservation of these endangered species. Hypothermia of sea turtles induced by cold weather episodes have been reported in the North-West Atlantic (Burke et al., 1991; Still et al., 2005; Roberts et al., 2014; Griffin et al., 2019; Innis et al., 2019), Gulf of Mexico (Foley et al., 2007; McMichael et al., 2008; Avens et al., 2012; Shaver et al., 2017), Mexican coast (Koch et al., 2013; Salinas-Zavala et al., 2020) and Western Europe (Davenport, 1997; Witt et al., 2007; Bellido et al., 2008, 2010; Monzón-Argüello et al., 2012; Nicolau et al., 2016), affecting three main species, i.e., the green, the Kemp’s ridley and the loggerhead turtles.
The loggerhead turtle (Caretta caretta) is the most studied of the seven sea turtle species (Hays and Hawkes, 2018) and has a complex life cycle, being distributed in oceanic waters during its juvenile stage before recruiting to coastal habitats (Bolten, 2003). The movements and habitat use of immature individuals directly caught at-sea at their developmental habitats have been largely documented in the Mediterranean Sea (Cardona et al., 2005; Revelles et al., 2007; Casale et al., 2012; Abalo-Morla et al., 2018; Chimienti et al., 2020), Pacific (Polovina et al., 2001, 2004, 2006; Kobayashi et al., 2008; Briscoe et al., 2016b, 2021), Indian (Dalleau et al., 2014; Bousquet et al., 2020), and Atlantic Oceans (Mansfield et al., 2009, 2014; Varo-Cruz et al., 2016; Chambault et al., 2019). However, the spatial patterns of individuals after rehabilitation in unusual habitat (outside their geographical range-limit) remain undocumented in most regions, in particular in Western Europe.
Stranding events of sea turtles occur annually along the Western European coasts, including the Spanish (Bellido et al., 2010; Orós et al., 2016), Portuguese (Nicolau et al., 2016), British (Botterell et al., 2020) and French coasts (Witt et al., 2007; Morinière and Dell’Amico, 2011). Genetic analysis have demonstrated that immature loggerhead turtles stranded along the French Atlantic and English Channel coasts mostly originate from the North-East American coast (51%), and to a lesser extent to Cape Verde (26%) (Monzón-Argüello et al., 2012). Hatchlings emerging from Florida rookeries are known to perform a transatlantic oceanic migration toward several developmental habitats located in the Azores, Madeira, Cape Verde, and Canary Islands (Bolten et al., 1998). But despite the large number of stranding events observed each year (Witt et al., 2007; Bellido et al., 2010; Morinière and Dell’Amico, 2011; Nicolau et al., 2016; Orós et al., 2016; Botterell et al., 2020), no favorable developmental habitat has been identified yet along the continental European coast due to potentially unfavorable thermal conditions and the high latitudinal location of this areas, assumed to be outside the species usual range (Wallace et al., 2010). In addition to loggerhead turtles, green (Chelonia mydas) and Kemp’s ridley sea turtles (Lepidochelys kempii) originating from North-West Atlantic, Caribbean, and Gulf of Mexico (Manzella et al., 1988; Marquez, 2001; Wallace et al., 2010) are, two species rarely seen in European waters, annually gathering in lower proportions along the French Atlantic coast and English Channel coasts.
In 2010, Wallace et al. (2010) delineated 58 Regional Management Units (RMUs) based on published satellite tracks, tag returns and demographic and genetic data collected from nesting populations for the seven sea turtle species worldwide. However, these units have not incorporated some sites of immature turtle aggregation, in particular in the North-East Atlantic where immature loggerheads, greens and Kemp’s ridleys are frequently observed (Bellido et al., 2010; Morinière and Dell’Amico, 2011; Monzón-Argüello et al., 2012; Nicolau et al., 2016; Orós et al., 2016; Botterell et al., 2020). Satellite tracks and stranding reports collected from European waters therefore provide a unique source of data to update these RMUs and design more appropriate units of protection taking into account juvenile sea turtle habitats.
By combining sightings (1988–2020) and tracking data (2008–2020) collected by the CESTM (Center d’Études et de Soins pour les Tortues Marines) of the Aquarium La Rochelle along the French Atlantic and English Channel coasts, our study aims to assess if the Bay of Biscay could be a favorable habitat or rather an ecological trap for immature sea turtles. Depending on their size, we expect that the rehabilitated immature individuals will either: (i) remain in the North-East Atlantic ecoregion (smaller individuals) or (ii) migrate toward their natal beaches located along the North-Eastern American coast or Cape Verde islands (larger individuals). Our study provides the first evidence of new habitats for rehabilitated immature sea turtles across the North-East Atlantic. Our findings will support the use of sea turtles as bioindicators in the context of the European Marine Strategy Framework Directive (MSFD) and will help redesigning the Regional Management Units (Wallace et al., 2010) to prioritize conservation of these endangered species, particularly vulnerable at this stage.
Materials and Methods
Sightings
Sightings of sea turtles are reported throughout the year to the CESTM from the English Channel-North Sea to the Bay of Biscay-Iberian Coast (Figure 1A). This large dataset covering 33 years of sightings (1988–2020) complements the work of Witt et al. (2007) to investigate the temporal variability of the turtle size throughout the year, over a longer period. These sightings gather individuals stranded, bycaught in fishing nets or drifting at sea. Initiated in 1988 and compiling data from the four species found along Western Europe (leatherback, loggerhead, Kemp’s ridley and green), this dataset has been partly published (Witt et al., 2007; Morinière and Dell’Amico, 2011). To be consistent with the tracking analysis, we restricted this dataset to the three species satellite tracked in our study, i.e., the loggerhead, the Kemp’s ridley and the green turtle. The sightings located outside the three marine ecoregions defined by the MSFD were also discarded from the analysis.
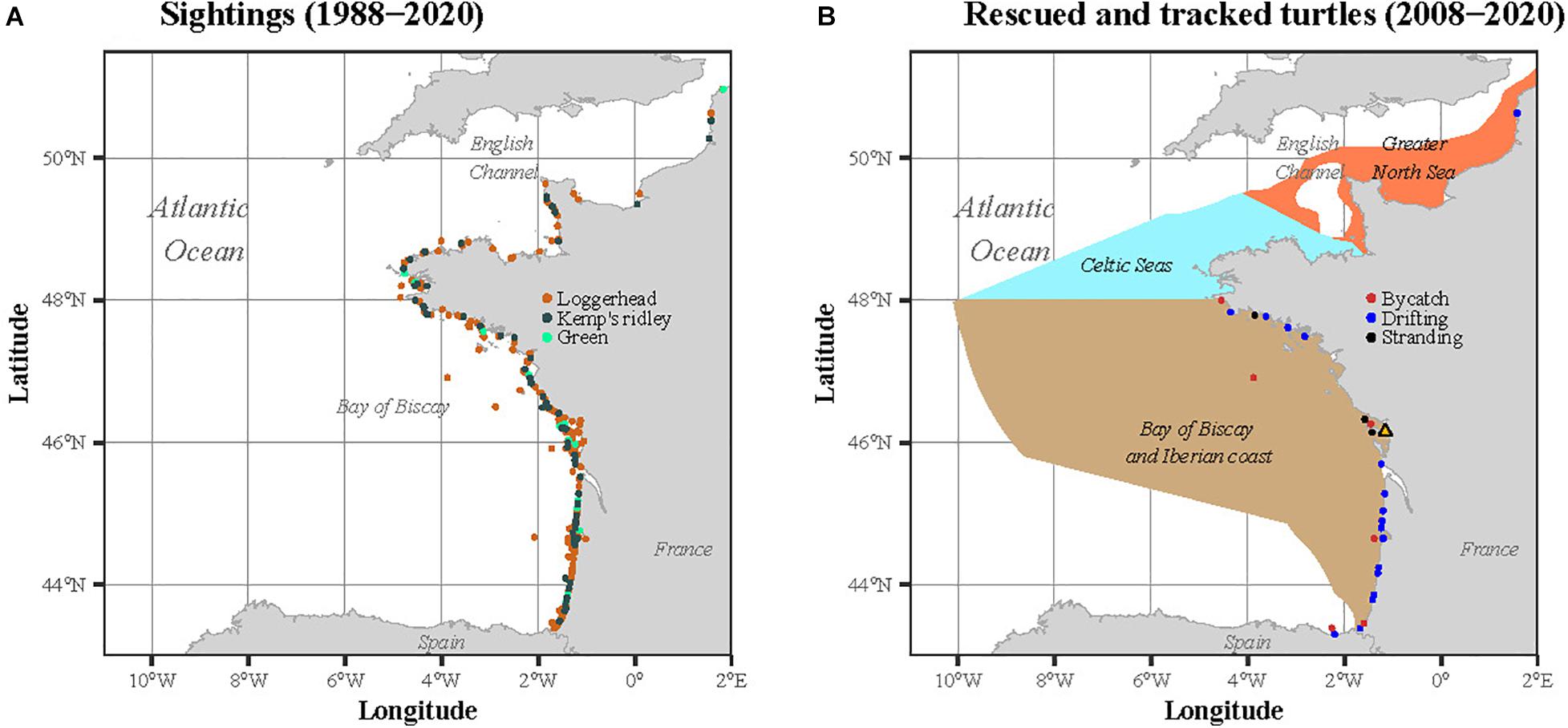
Figure 1. Locations of (A) the sightings recorded between 1988 and 2020 and (B) the immature sea turtles rescued and satellite tracked by the CESTM between 2008 and 2020. In (B), the three ecoregions as defined in the MSFD are represented in the background: the Greater North Sea, the Celtic Seas and the Bay of Biscay and Iberian coast.
Ethics Statements
This study meets the legal requirements of the country and the Rescue Center where this work was carried out, and follows all institutional guidelines. The Prefectoral Order N°2004-1104 approved the opening of the CESTM and delivered to Mrs. Florence Dell’Amico a certificate (n°2017 02173) to conduct care practices on non-domestic animals. The protocol was approved by the French “Regional environment, planning and housing” agency (DREAL, permit number: DREAL/56-2020), authorizing the transportation, tag deployment and release of endangered species. The Ministerial Order (30/12/2020) acts as an exception to the strict protection of species, authorizing the manipulation of protected species when found bycaught, drifting at sea or stranded.
Rescue and Rehabilitation
Between 2008 and 2020, 66 immature sea turtles were rescued and rehabilitated by the CESTM of the Aquarium La Rochelle. Among these 66 individuals, 28 were then equipped with satellite transmitters. The rescued individuals (one rescued twice) were found stranded (n = 17), bycaught (n = 8) or drifting at the sea surface (n = 3) within the three regions defined by the MSFD along the Atlantic French and English Channel coasts: the Greater North Sea, the Celtic Seas and the Bay of Biscay and Iberian coast. The majority of the rehabilitated individuals were loggerhead turtles (n = 23), but some were also Kemp’s ridley (n = 4) and green (n = 1) sea turtles. When rescued, each individual was measured (minimum Straight Carapace Length, hereafter SCLmin) and weighed. The same morphometric measures were also taken before attaching the tag, a few days before release.
Tag Deployment
Different tags types were used between 2008 and 2020. They all recorded Argos locations. In 2008, a KiwiSat® Argos tag (SirTrack manufacturer) was deployed on the first individual after rehabilitation using the attachment procedure described in Balazs et al. (1996) for the biggest individuals. The 27 remaining tags (two SPLASH and 25 SPOT) deployed between 2009 and 2020 were provided by Wildlife Computers® and attached using the procedure developed by Seney et al. (2010) for the smallest individuals. This technique incorporating a neoprene layer takes into account the rapid growth of immature individuals to prevent the tags to detach too quickly, extending therefore the tracking duration. The complete list of deployed tags is given in Supplementary Table 1.
Data Prefiltering
Location data were obtained from the Argos Data Collection and Location System. The Least Square positioning algorithm was used for tags deployed before 2011 and the improved Kalman filtered positions were obtained for the other tags (Lopez et al., 2014). All statistical analyses were performed using R software version 4.0.2 (R Core Team, 2021). We restricted our dataset to positions associated with a travel speed lower than 10 km/h (Fossette et al., 2010). Locations on land and those associated with a location class Z were also discarded.
Movement Analysis
The individual tracks were then predicted daily using the foieGras package with a simple continuous-time random walk algorithm (Jonsen and Patterson, 2020; Jonsen et al., 2020). The algorithm accounted for the Argos quality to predict the daily locations using Argos error ellipses for the tags deployed after 2011, while the location classes (0, 1, 2, 3, A or B) were used for the remaining tags. Following Hays et al. (2021) and Hart et al. (2021), assessment of tag failure was conducted on the data available using the three following metrics: battery voltage, wet/dry switches and the number of Argos transmissions.
To identify turtle aggregation and hotspot habitats, we generated hexagonal maps by summing the number of predicted locations (from the random walk) in each grid cell of 0.5 and 0.1 degrees, respectively. To reduce tagging location and track length biases, the first week of tracking was removed for each individual.
Spatial patterns were visually identified based on the geographic difference between the last position recorded for each individual and the release site. Intrinsic (morphometric measurements) and extrinsic factors (environmental conditions encountered) were then compared according to the spatial pattern. The effect of rehabilitation time on the spatial pattern was tested using a Kruskal-Wallis test.
In order to assess if the behavior of the turtles was typical, we then compared the movement characteristics (tracking duration, swimming speed and distance traveled) of the injured individuals that were amputated (n = 4, Table 1, IDs with the asterisk) to the “not injured” turtles. The interaction between distance traveled and tracking duration was also tested. This was conducted using a Generalized Linear Model with a binomial distribution, and the state (injured vs. not injured) as a response variable.
Environmental Drivers
To assess the habitat used and selected by the turtles, a series of environmental variables were then extracted at each predicted daily location. Three oceanographic variables were extracted daily from E.U. Copernicus Marine Service Information1 at a resolution of 0.08 decimal degree: the zonal component of the surface currents (U, positive eastward), the meridional component of surface current (V, positive northward) and the Sea Surface Temperature (SST). Two products were used depending on the period: the Global Ocean Physics Reanalysis Glorys S2V4 (PHYS_001_024, after 2016) and the Global Ocean Physics Reanalysis Glorys12v1 (PHY_001_030, before 2016).
Predicted daily positions were used to compute the daily ground speeds of each turtle. The swimming speed of each individual was then calculated by subtracting the modeled ocean current velocity from the ground speed (Gaspar et al., 2006). Turtles and ocean currents headings were then estimated at each position. To assess if the habitats experienced by the turtles were productive, two low and mid-trophic level (LMTL) variables were also extracted daily from the Spatial Ecosystem and Populations Dynamics Model (SEAPODYM) at a 0.08 decimal degree (Lehodey et al., 2010): the mesozooplankton biomass (200 μm to 2 cm) and the epipelagic micronekton biomass (2–20 cm). Unlike primary productivity or chlorophyll a that are commonly used to predict the distribution of megafauna species, micronekton and zooplankton refer to mid-trophic levels encompassing the potential prey of sea turtles. Micronekton group gathers organisms of different taxa (e.g., fish, cephalopods, crustaceans) and these model outputs are made available through the CMEMS web portal (BGC_001_033). Due to the dive behavior of immature turtles of this size, we restricted our analysis to the epipelagic layer.
Habitat Modeling
The geographic space available to each animal was assessed by simulating a series of tracks for each individual using the availability package (Raymond et al., 2021), i.e., n = 50 simulated tracks per individual as suggested by Hindell et al. (2020). Using the mgcv package (Wood, 2017), the habitat selected by the turtles was then characterized using a Generalized Additive Model to relate the presence of the turtles to a series of environmental factors, i.e., SST, zooplankton biomass, micronekton biomass, ocean current velocity, zonal and meridional components of ocean currents and sea surface salinity. The turtle’s presence was used as a binary response variable (real track vs. simulated tracks). All numeric variables were first checked for collinearity using the VIF function from the usdm package (Naimi et al., 2014). To account for the inter-individual variability, turtle ID was added as a random factor. An autocorrelation term was also added to the model to account for the correlation structure in the data. A threefold cross-validation was used by partitioning the dataset into the training (2/3 of the data) and the validation dataset (1/3). Model evaluation was then done on the validation dataset using four performance metrics calculated for each model: the area under the curve (AUC), the sensitivity, the specificity and the true skill statistics (TSS).
To test the influence of the spatial pattern on the thermal and biological habitat used by the turtles, a generalized logistic model was performed using the mlogit package (Croissant, 2020) using the data collected along the entire route for each individual. The response variable was the spatial pattern with the four modalities, and the explanatory variables the environmental conditions encountered by the individuals: SST, zooplankton and micronekton biomasses.
Results
Sightings (1988–2020)
The sightings were reported throughout the year between 1988 and 2020 along the French Atlantic and English Channel coasts (Figure 1A). A total of 449 sightings of immature sea turtles were reported, including a majority of loggerhead turtles (n = 353), followed by Kemp’s ridleys (n = 75) and greens (n = 21). No green turtle was observed in spring and summer while a very few Kemp’s ridley were seen in April and late summer. The majority of the sightings referred to individuals stranded alive (65.5%), followed by stranded dead (23%), captured alive (6.9%), drifting alive (2.7%), captured dead (1.3%), drifting dead (0.4%) and observed in the water alive (0.2%). A larger proportion of sightings occurred during late autumn and winter, with a peak in February and March, representing 39% of the sightings (Figure 2A). The smallest individuals (≤32 cm) were mostly observed between January and April, while largest turtles (>40 cm) were found year-round (Figure 2B).
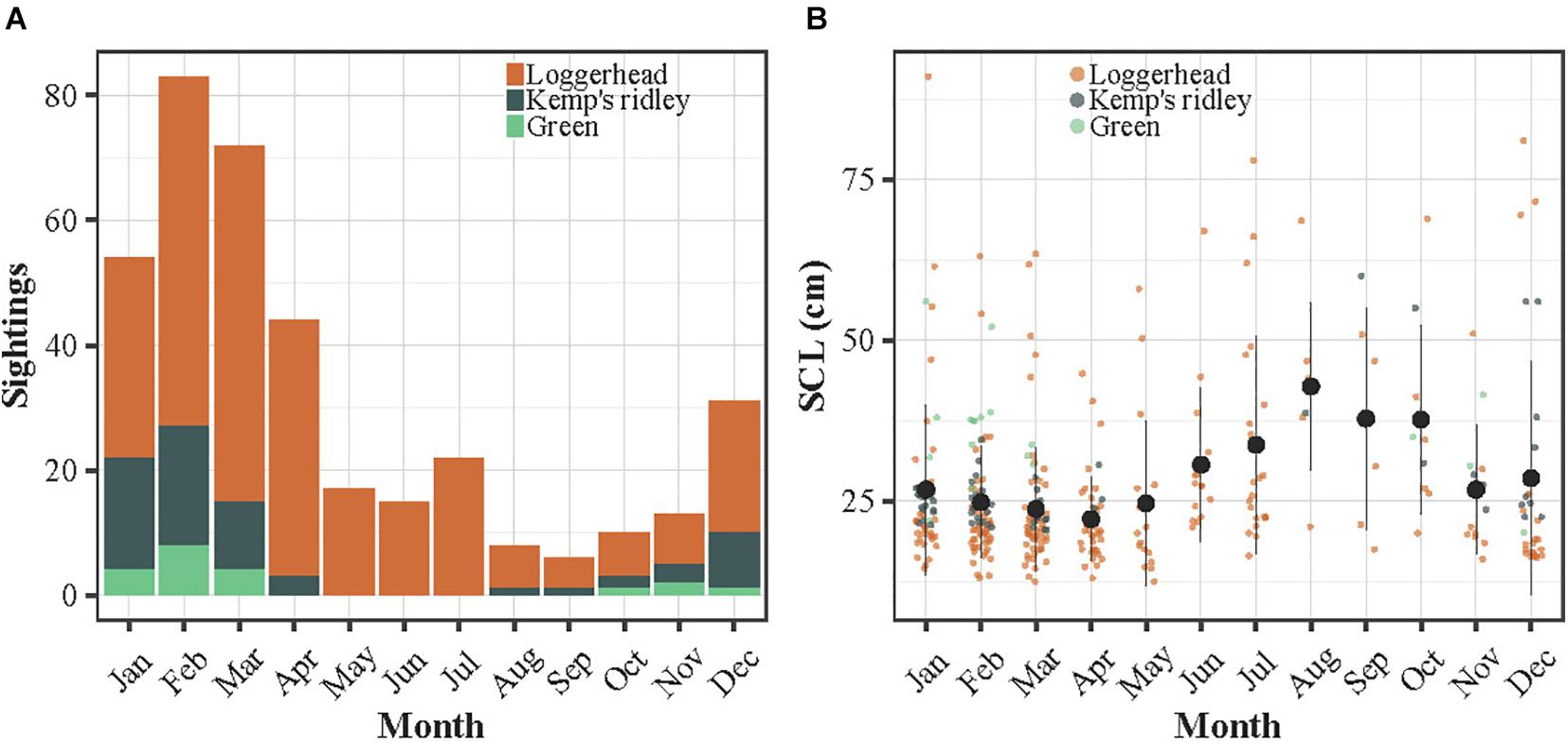
Figure 2. (A) Histogram of the number of sightings according to month and species. (B) Distribution of the SCL (SCLmin or SCL in cm) of the sightings over month. In (B), the dots refer to the monthly means and the bars to the standard errors.
Rehabilitation and Tracking Data (2008–2020)
Among the 28 satellite tracked turtles, one was removed from the analysis due to a very short tracking duration and only a few locations transmitted (Ino). This turtle was found stranded dead with the tag still attached only 10 days after release, so the end of transmission was not due to battery exhaustion (Supplementary Figure 1A). Among the 27 remaining turtles, four were amputated (Icare, Iodée, Kemen, and Kercambre). These injured individuals behaved similarly (in terms of swimming speed, tracking duration and distance traveled) compared to the “not injured” turtles (generalized linear model: all p > 0.05). Rehabilitation time had no effect on the spatial pattern (Kruskal-Wallis rank sum test, p > 0.05).
Sightings occurred throughout the year but there was a peak in fall and winter, representing 64% of the observations. The individuals were either found stranded (n = 17), bycaught (n = 7) or drifting at sea (n = 3) along the French Atlantic coast, from Bretagne to the Spanish border (Figure 1B). The duration between the observation and the release lasted between 32 (Karolina) to 4239 days (Francesca), and on average (± SD) 373 ± 771 days (Table 1). Most individuals were rehabilitated at the CESTM, but one turtle remained in another center for 12 years before being transferred to the CESTM for release with a satellite tag. The rehabilitation time spent at the CESTM varied between 30 and 551 days for an average of 190 ± 122 days. When released, the turtles measured between 21.5 and 87.5 cm in SCLmin (mean ± SD: 41.6 ± 16.6 cm), for a body mass ranging from 1.8 to 93.2 kg and a mean of 17.7 ± 21.6 kg.
Among the 27 satellite tracked turtles, one was found stranded again a few months after release (Antioche), and then equipped a second time in 2009 (Antioche 2). The average tracking duration was 145 ± 152 days, for an average traveled distance of 2,129 ± 2,409 km (range: 160–11,000 km) (Table 1). The time spent within the BoB varied between 5 and 319 days (mean ± SD: 73 ± 52 days). The total travel speed (including ocean currents) was 0.17 ± 0.12 m/s (max: 0.9 m/s). The haplotype was available for six individuals (Table 1) and have been previously published in Monzón-Argüello et al. (2012). The natal origin of five individuals could be determined and have already been published in Monzón-Argüello et al. (2012): one originates from Cape Verde, one from Dry Tortugas, Mexico or South Florida, and three could have multiple origins (e.g., Florida, Dry Tortugas, Mexico, Cape Verde).
Assessment of tag failure was conducted over the 27 individuals, and for only four turtles, the drop in battery voltage below 3.0 V was indicative of battery exhaustion at the end of the tracking (Supplementary Figure 1A). Data on the wet/dry switches was available for only 13 individuals. Except for Antioche 2, the clear difference between “dry” and “wet” states suggested biofouling was not the reason for the cessation of transmissions (Supplementary Figure 1B).
Spatial Patterns
The 26 turtles dispersed largely from the European west coast to Bermuda, passing near the Azores and Canary Islands (Figure 3). Among the four Kemp’s ridleys, two migrated northward while the last two remained within the BoB. The only green turtle satellite tracked (Flot) remained within the BoB during the entire tracking duration (36 days). According to the last position recorded for each individual compared to the release site, four different spatial patterns were identified: Bay of Biscay, North, South and West (Figure 3). The Bay of Biscay pattern refers to 11 resident individuals that remained within this region along the French Atlantic coast. The North pattern includes three individuals that migrated north toward United Kingdom, the Netherlands and Norway, crossing the English Channel and the North Sea. Among the three North individuals, two were Kemp’s ridley turtles. The South group (n = 3) headed toward Portugal, the Mediterranean Sea and Western Sahara, while the West group (n = 10) migrated westward within the North Atlantic in the open ocean, targeting the Azores, the Canary Islands or even Bermuda.
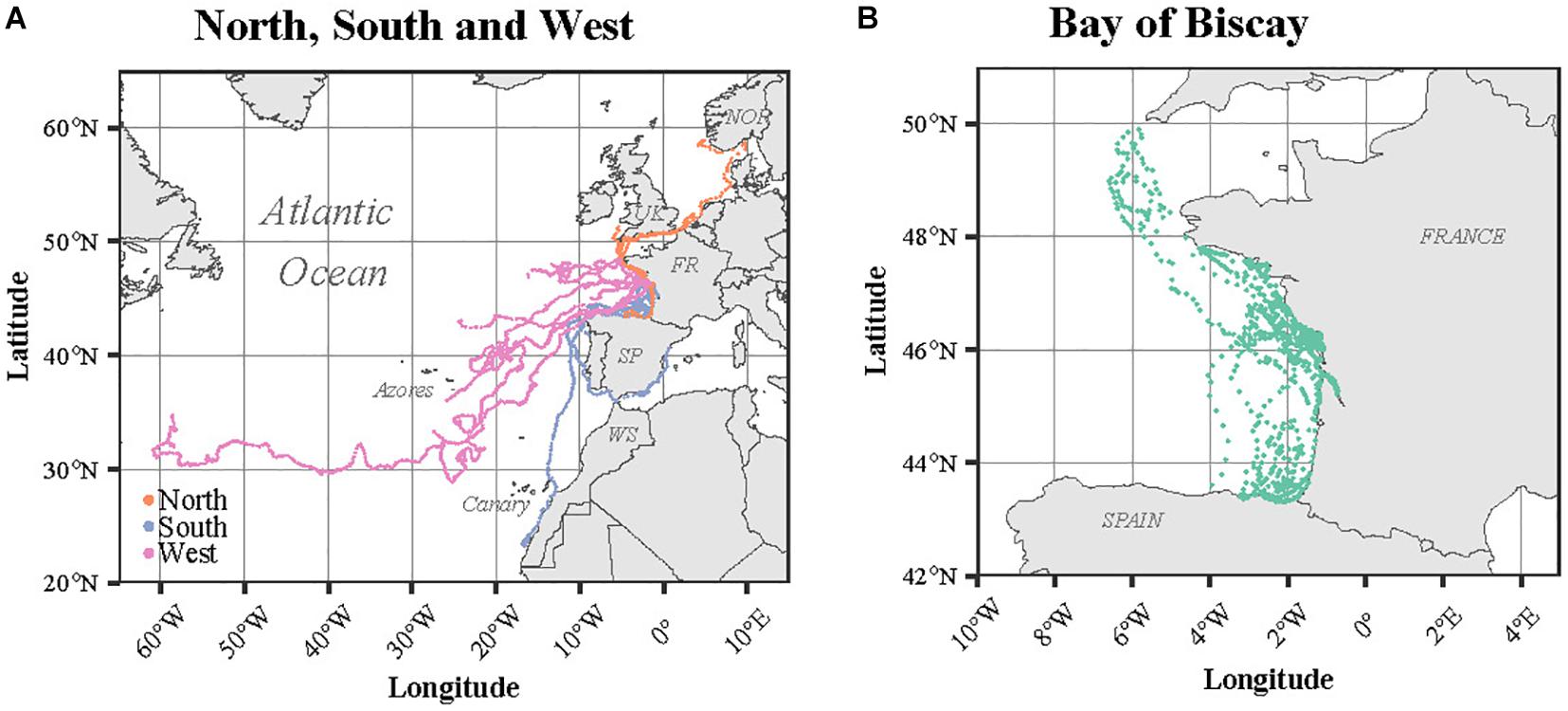
Figure 3. Satellite tracks of the immature turtles according to their spatial pattern: (A) North (n = 3), South (n = 3) and West (n = 10) and (B) Bay of Biscay (n = 11). The names of the countries are indicated in capital letters: NOR (Norway), UK (United Kingdom), FR (France), SP (Spain), and WS (Western Sahara).
Figure 4A shows a heatmap over the entire study region, highlighting the habitats of interest located mainly in the Bay of Biscay. This map confirms that the individuals crossed both international waters and numerous Exclusive Economic Zones (16 ZEEs), e.g., Spain, France, Portugal, Western Sahara. At a finer scale, the heatmap in Figure 4B confirms that the area of turtle aggregation is located close to the shoreline near the release site, and is included into the Gironde estuary and sea of Pertuis Marine Natural Park.
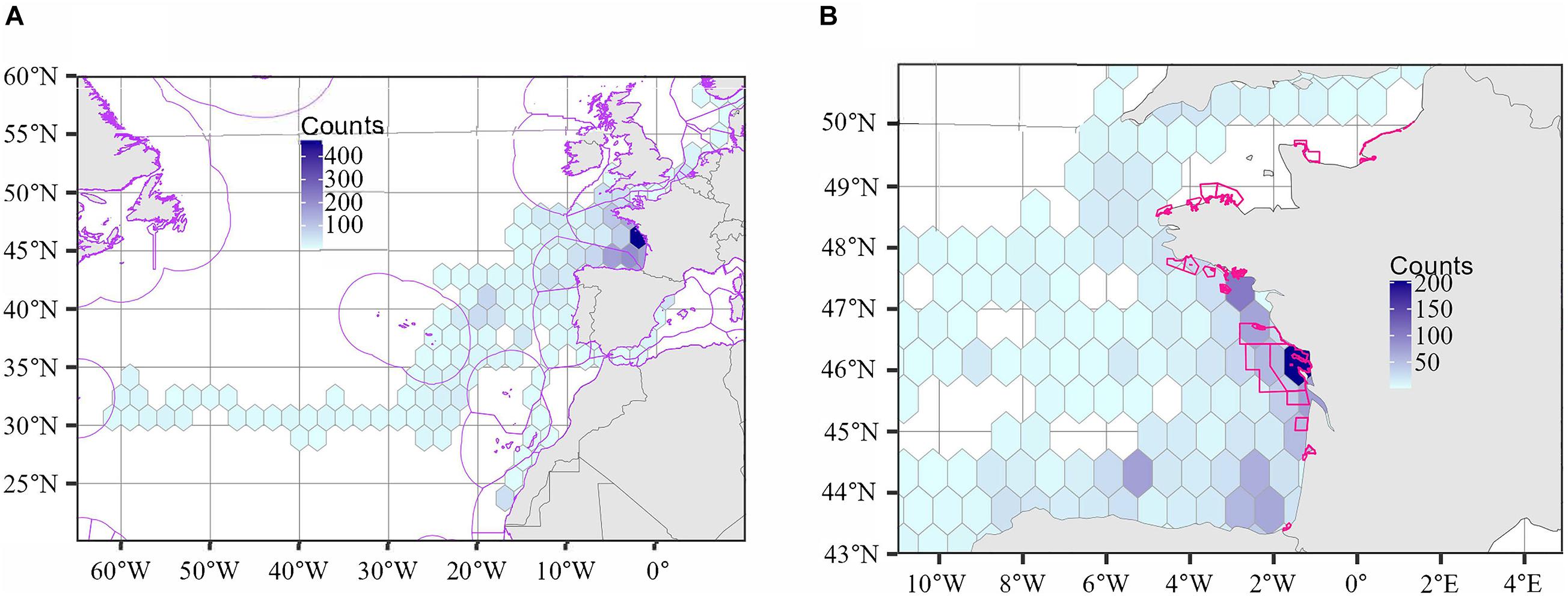
Figure 4. Density plots of the 26 sea turtles (A) over the entire study region and (B) a focus over the French Atlantic coast and English Channel. The counts refer to the total number of locations recorded in each grid cell. The first week of tracking was removed to discard the potential effect of the release site and atypical behavior after release. Exclusive Economic Zones were overlaid (in purple, left) and Marine Protected Areas (in pink, right) in (A,B), respectively.
The SCLmin and body mass varied significantly according to the spatial pattern (Figure 5). The SCLmin was significantly larger for individuals migrating westwards (mean ± SD: 53.1 ± 14.9 cm) and northwards (mean ± SD: 61.9 ± 13.5 cm), and minimum for the resident turtles that remained in the Bay of Biscay (BoB, mean ± SD: 32.7 ± 9.3 cm) and the South group (mean ± SD: 44.9 ± 10.1 cm, Kruskal-Wallis rank sum test, p < 0.001). Similarly, body mass differed significantly with the spatial pattern (Kruskal-Wallis rank sum test, p < 0.001). Body mass was maximum for the North (mean ± SD: 39.4 ± 19.9 kg) and West (mean ± SD: 31.3 ± 19.8 kg) groups and minimum for the BoB (mean ± SD: 7.7 ± 6.5 kg) and South (mean ± SD: 17.7 ± 16.0 kg) groups, being below the average values (mean ± SD: 41.6 ± 16.6 cm and 17.7 ± 21.6 kg).
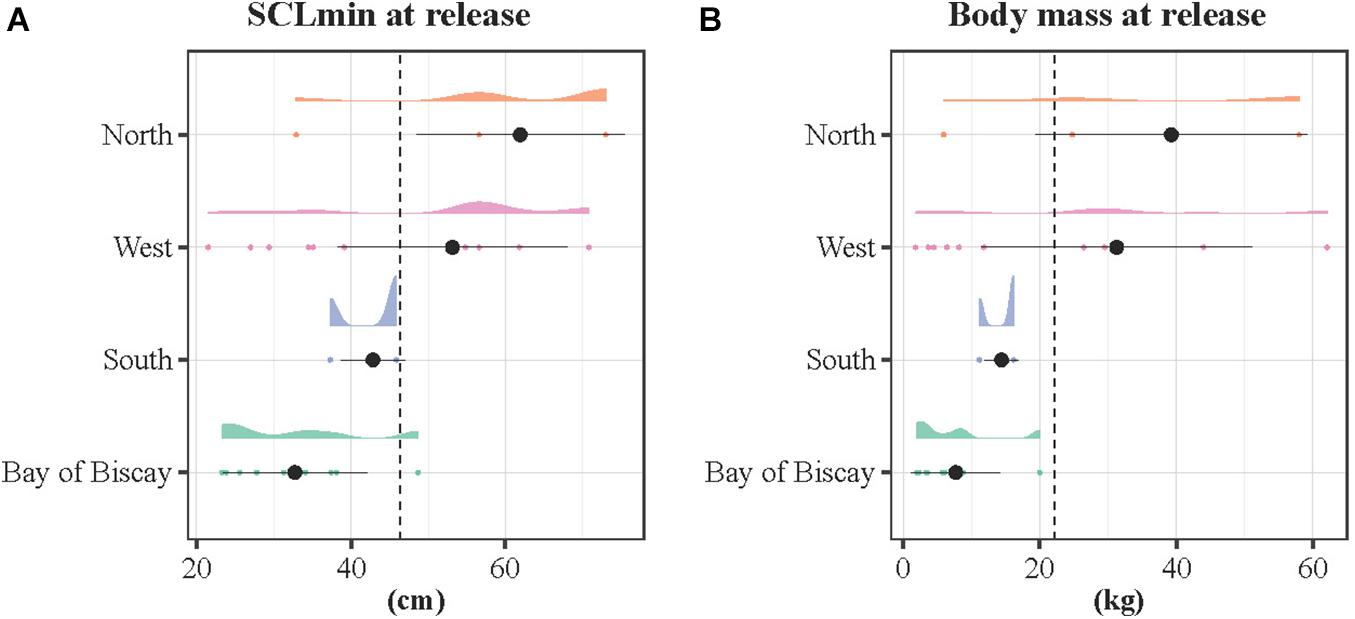
Figure 5. Density distributions of the (A) SCLmin and (B) body mass at release according to the spatial pattern. The density distributions are color-coded by spatial pattern, with a cloud of points below. The dotted lines refer to the average SCLmin and body mass considering the 26 tracked individuals (Francesca was removed because unrepresentative of the sample size). The black dots stand for the mean and the bars the standard deviation for each group.
Active Swimming or Passive Advection?
We found differences between directions of ocean currents and those of the turtles across the four patterns (Figure 6). For the Bay of Biscay group, ocean currents flowed mainly south-eastwards while the individuals headed north-west in the opposite direction (Figures 6A,B). For the South and the West group, ocean currents flowed south-west and south-east, while the turtles swam mainly west for both groups (Figures 6C–F). The North individuals headed northwest and northeast and the ocean currents mainly west and north-east (Figures 6G,H). The swim speeds of the turtles were significantly faster (mean ± SD: 0.17 ± 0.11 m/s) than the speed of ocean currents (mean ± SD: 0.10 ± 0.08 m/s, Mann-Whitney U-test, p < 0.001). The turtle swimming speed was positively correlated to the velocity of ocean currents and to the zonal component of the currents U (LMM, p < 0.001).
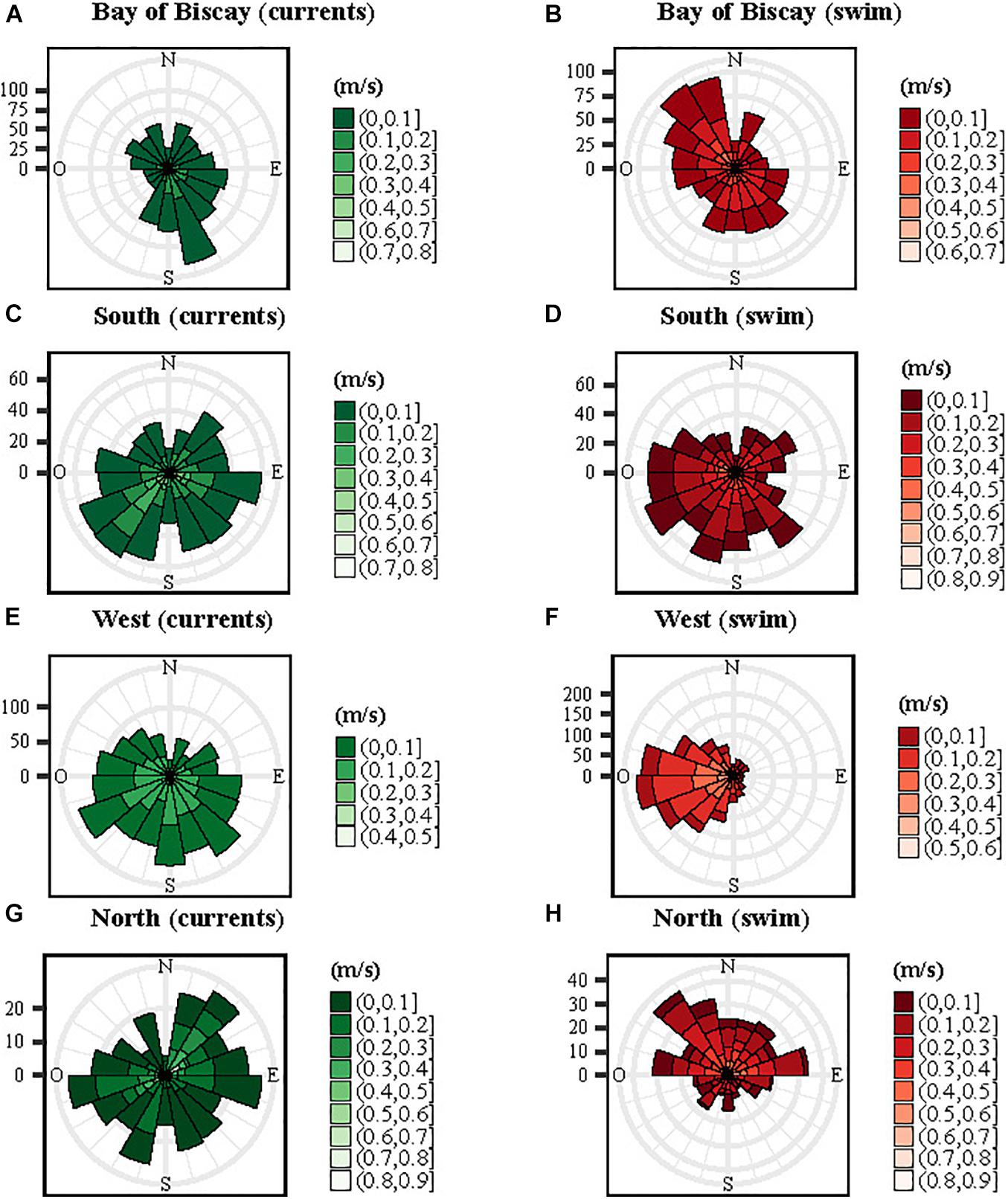
Figure 6. Rose diagram frequency distributions of ocean currents (in green) and turtles (in red) orientation and velocity (in m/s) for the four spatial patterns: Bay of Biscay (A,B), South (C,D), West (E,F) and North (G,H). The currents were extracted at each turtle location.
Habitat Selection
The explained deviances derived from the GAMs ranged between 23.1 and 23.4%, and the seven predictors were highly significant (p < 0.001). The performance of the models was high and negligible variability was noticed across the models: 0.270 < R2 < 0.273, 0.79 < AUC < 0.80, 0.68 < sensitivity < 0.69, 0.740 < specificity < 0.741 and 0.42 < TSS < 0.43. Current velocity and SSS had a negative relationship with the turtle’s presence, while micronekton and zooplankton biomasses increased with the probability to find a turtle (Figure 7). Turtle’s presence was at its highest for low and high values of U and V currents. The GAM indicated an optimum SST around 17–18°C, showing lower probabilities of turtle’s presence at both low and high sea temperatures. The SST extracted at turtle’s locations varied between 8 and 27.6°C for an average temperature of 18.4 ± 3.3°C (Supplementary Table 2). The SST varied significantly according to the spatial pattern, being minimum for the North group (16.1 ± 3.8°C, logistic model estimate: −0.28, p < 0.001) and maximum for the West group (19.8 ± 2.7°C) (see Figure 8A, logistic model estimate for BoB = −0.30, p < 0.001 and south = −0.16, p < 0.001).
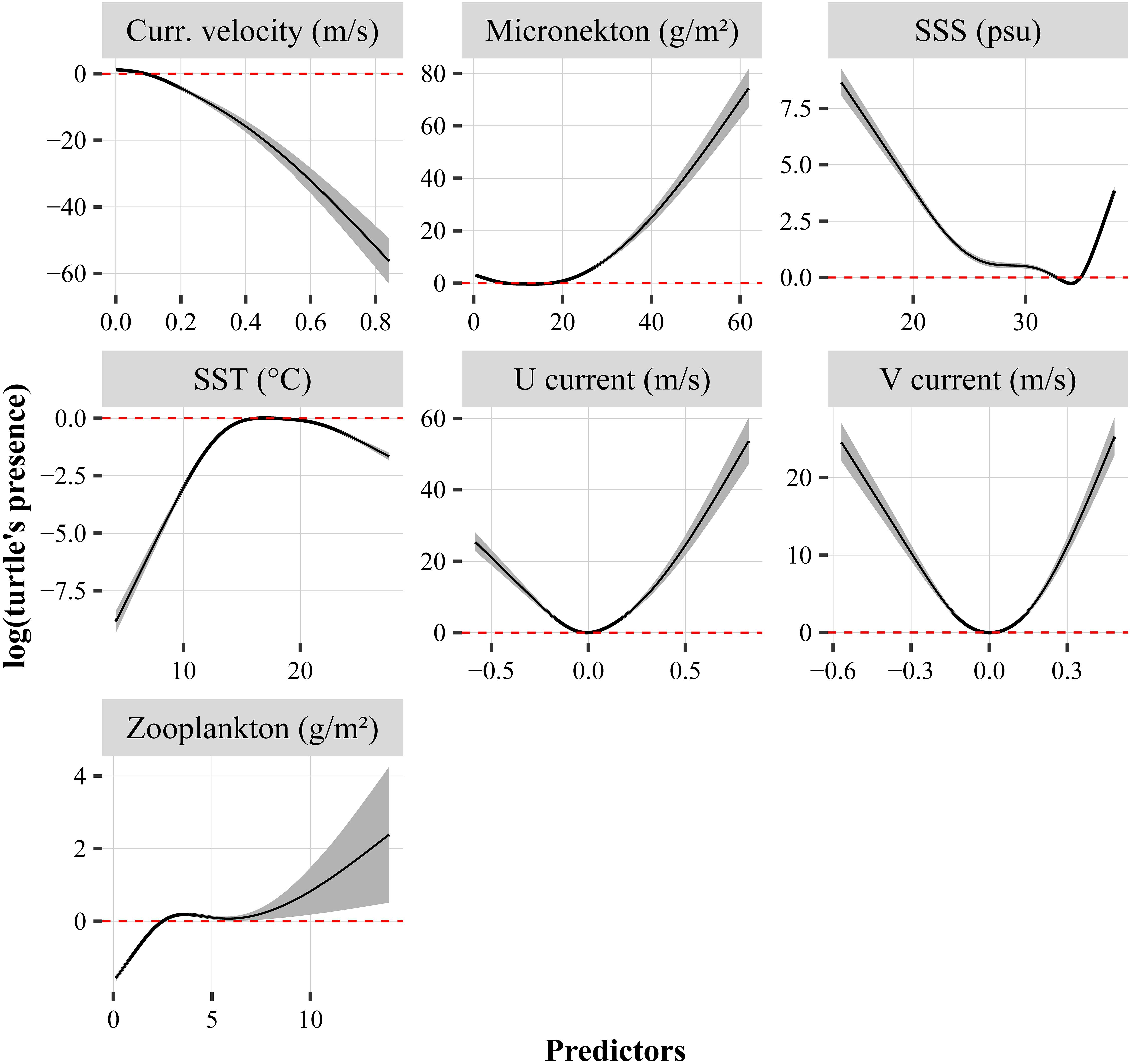
Figure 7. Relationships between turtle’s presence (y-axis) and their associated environmental variables obtained from the GAMs. The solid black line in each plot is the smooth function estimate and the shaded regions refer to the approximate 95% confidence intervals. The y-axis represents the response variable expressed in log scale. Positive values on the y- axis indicate a high probably of turtle’s presence, and conversely. The horizontal dotted lines indicate the probability of turtle presence is not significantly positively or negatively influenced by the predictor. SSS refers to Sea Surface Salinity.
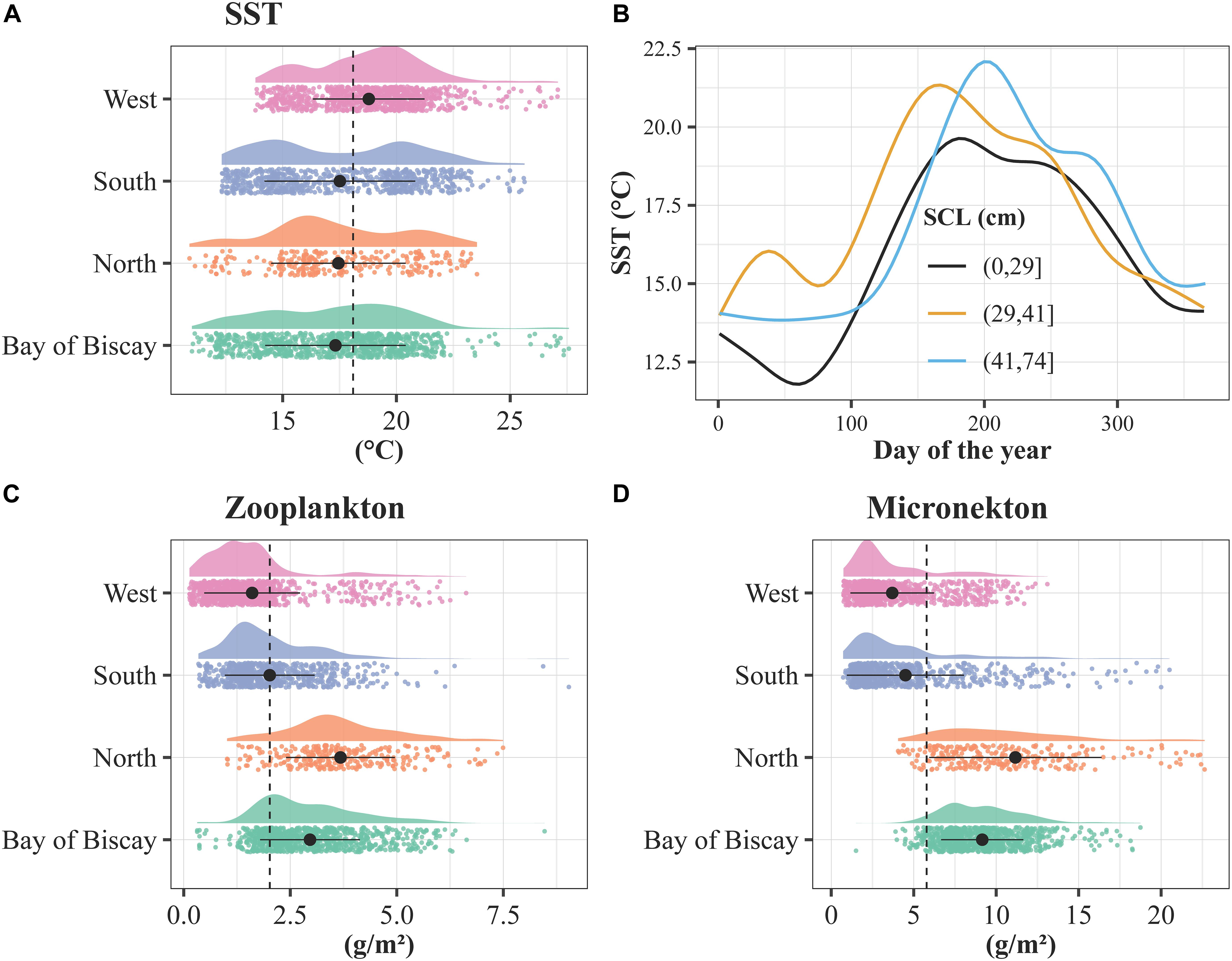
Figure 8. (A) Smooth lines of the SST at turtle’s locations according to days of the year and SCLmin classes (in cm). Density distributions of the (B) SST, (C) zooplankton biomass and (D) micronekton biomass extracted at the turtle’s locations according to the spatial pattern. The density distributions are color-coded by spatial pattern, with a cloud of points below. The dotted lines refer to the average zooplankton and micronekton biomasses considering the 26 tracked individuals. The black dots stand for the mean and the bars the standard deviation for each group.
When all individuals and the four patterns were pooled together, the seasonal trend was also observed and differed according to the size of the turtles with the smallest individuals (SCLmin ≤ 29 cm) exploiting waters generally colder (17.2 ± 2.6°C) than the largest individuals (SCLmin ≥ 41 cm, 18.9 ± 3.7°C) (see Figure 8B). Nearly half of the locations recorded (49%) were associated with waters between 15 and 20°C, and only 18% below 15°C (Supplementary Figure 2).
The zooplankton biomass varied between 0.10 and 9.0 g.m–2 for an average biomass of 2.3 ± 1.4 g.m–2 (Figure 8C and Supplementary Table 2). The zooplankton biomass varied significantly according to the spatial pattern (logistic model estimates: BoB = 0.32, north = 0.60, south = 0.23, p < 0.001), being minimum for the West group (1.4 ± 1.1 g.m–2) and maximum for the North group (3.7 ± 1.5 g.m–2). The micronekton biomass varied between 0.48 and 49.9 g.m–2 for an average biomass of 6.4 ± 4.9 g.m–2. The micronekton biomass varied significantly according to the spatial pattern (logistic model estimates: BoB = 0.60, north = 0.66, south = 0.12, p < 0.001), being minimum for the West group (3.3 ± 2.5 g.m–2) and maximum for the North group (12.9 ± 7.4 g.m–2) (see Figure 8D).
Discussion
By compiling the sighting data from three species together with the first dataset on rehabilitated individuals satellite tracked from the French Atlantic coast, our study sheds light on contrasting spatial patterns driven by individual size, and provided evidence that the Bay of Biscay might act as an ecological trap for the smallest individuals in winter due to low sea temperatures, but also as a potential foraging habitat in summer and autumn.
Spatial Pattern Driven by Turtle Size
The four spatial patterns identified seemed mainly driven by the size of the individuals, with the smallest turtles remaining in close proximity to the release site in the Bay of Biscay, while the largest turtles migrated either northwards or performed long westward migration in pelagic waters. The majority (51%) of the immature loggerhead turtles frequently observed along the French Atlantic coast originate from Florida (Monzón-Argüello et al., 2012), suggesting that the largest turtles heading westward in our study were targeting their natal beach as observed for other sea turtles species in the Indian Ocean (Dalleau et al., 2014) and the Caribbean (Chambault et al., 2018). The size of mature individuals in loggerhead turtles (80–90 cm, Wyneken et al., 2013) is larger than any in the West group of oceanic turtles tracked in our study (SCLmin: 52.2 ± 14.6 cm). However, these immature individuals might well initiate their return journey early, possibly stopping at an intermediate foraging site to develop and reach sexual maturity. Similar to some immature loggerhead turtles satellite tracked from the North-East American coast (Mansfield et al., 2014), one of our individuals reached Bermuda where the tag stopped emitting. Located 1,600 km from Florida, these oceanic islands could be a stopover before reaching the nesting beach in Florida or a permanent foraging ground, as it has been evidenced in immature loggerhead turtles originating from Florida (Mansfield et al., 2009). The clear westward heading of the turtles in the West group, independently of the current direction also confirms that these turtles are willingly migrating westwards. Furthermore, the haplotypes of five of the 26 satellite tracked turtles were available, showing that these turtles mainly originated from Florida, Dry Tortugas or Mexico. Among the satellite tracked turtles, only Beline originated from Cape Verde. Genetic samples were collected from all the satellite tracked individuals, allowing in the near future the comparison between their natal origin and their trajectory to confirm this natal homing hypothesis (Meylan et al., 1990).
Among the largest individuals tracked in our study (SCLmin > 45 cm), three turtles headed northwards in critically unsuitable habitats due to a strong thermal constraint in winter (SST < 8°C). It is worth noting that among these three turtles, two were Kemp’s ridley turtles, a species originating from the Gulf of Mexico and the Atlantic coast of the United States (Manzella et al., 1988; Marquez, 2001). The reasons for this surprising northward migration of this species remain unclear but could be due to either a disorientation or unusually productive and/or warm waters masses, channeling turtles out of their common range (Griffin et al., 2019). The higher micronekton and zooplankton biomasses found along the tracks of these three individuals compared to the three other spatial patterns reinforce the assumption that they were actually targeting productive waters. The low bathymetry and the narrow strip characterizing the English Channel and North Sea could, however, be responsible for the underestimation of the coastal circulation in this region in the framework of the SEAPODYM model, possibly resulting in an overestimation of the primary productivity by remote sensing. Consequently, zooplankton and micronekton biomasses might be slightly overestimated in this area (Conchon, personal communication). More Kemp’s ridley turtles need to be satellite tracked from the French Atlantic waters in order to elucidate these unexpected movements toward higher latitudes.
The coastal behavior of the smallest individuals was surprising and against the main hypothesis that the rehabilitated turtles would migrate back to the open ocean after release. The majority of immature loggerhead and green turtles are known to spend many years in the open ocean to grow and avoid predators, before swimming back to coastal habitats (Bolten, 2003). Coastal migrations have also been observed in some juveniles loggerheads (Mansfield et al., 2009), but only in much larger individuals (SCL: 64.8 ± 10.9 cm) than the ones remaining in European coastal waters (SCL: 32.9 ± 9.6 cm). The location and the date of the release might partly influence the individuals’ movements. Similar to a recent study conducted in Australia (Robson et al., 2017), future work should focus on simulating the active dispersal of turtles (size of the rehabilitated individuals) to confirm that the location and date of release are optimal for the rescued individuals, but also to determine if this coastal behavior is the result of environmental variability.
Resident Behavior in European Coastal Waters
Forty-one percent of the satellite tracked individuals exhibited a coastal behavior in the French Atlantic waters during the entire tracking duration, with a strong aggregation close to the release site off La Rochelle that could be partly attributed to the release location. Release sites are voluntarily located in close proximity to the CESTM (< 1 h) to prevent a stress induced by a long transportation of the rehabilitated turtles to be released (Hunt et al., 2019). The timing of the release is also strongly based on tidal cycles (during ebb tides, strong coefficients) to help the individuals swimming away from the shore. Despite a similar behavior has been observed in other taxa such as birds (Giunchi et al., 2003; Wallraff and Wallraff, 2005), this aggregation close to the release site is probably more intentional and indicative of a productive and favorable area, likely due to the presence of several river plumes acting as nursery grounds for a wide variety of fish (Yamashita et al., 2000; Le Pape et al., 2003). Alternatively, these small individuals might not be strong enough to swim away from the shore and migrate back to oceanic waters. But given their good condition after rehabilitation and the relatively small size of several turtles migrating westward to the open ocean, this hypothesis is unlikely. Among the 11 turtles that used the BoB the entire tracking duration, tag failure could be assessed for three of them, and evidence of battery exhaustion was only demonstrated for one turtle (Indigo). For two other individuals, biofouling was not indicative of tag failure, but the decrease in swimming speed might suggest that the turtles could have died due to cold-stunning in winter. The rapid growth of immature loggerhead turtles could also explain a premature tag detachment (Hays et al., 2021), leading to a shorter tag life (mean: 145 ± 152 days, range: 18–679 days) compared to other studies based on adult individuals, e.g., loggerheads retain 50% of their tags for 584 days (Hart et al., 2021). For future deployments, we recommend a careful battery management based on a severe duty cycling to extend tag life in immature individuals (Christiansen et al., 2016).
Although these results are in agreement with a previous study based on aerial surveys (Darmon et al., 2017), this finding is surprising because our individuals were relatively small (SCL: 32.9 ± 9.6 cm), a range of size at which they are thought to be fully pelagic (Bolten, 2003). Also, a recent study has demonstrated that European waters were not a suitable habitat for immature loggerhead turtles (Harrison et al., 2021). The authors restricted the model simulations to a passive dispersal of neonate turtles during the first year at sea, rather than simulating the active movements of immature turtles, probably explaining why the BoB was not identified as a suitable habitat for this species. New simulations using recent active dispersal models (Gaspar and Lalire, 2017; Lalire and Gaspar, 2019), should be performed to verify if loggerheads born in Florida might reach the BoB and find there suitable habitats, at least during part of the year.
Tidal currents are indeed known to play an important role in the coastal circulation of the BoB (Karagiorgos et al., 2020). A higher resolution regional ocean reanalysis including tidal forcing (IBI: Atlantic-Iberian Biscay Irish Ocean Physics Reanalysis, Sotillo et al., 2015) is available from the Copernicus Marine Service, but was not used as this reanalysis does not cover our entire tracking period at the finest resolution (1/36° decimal degree). Further work with the fraction of our tracking data covered by the IBI reanalysis should be conducted in the near future to more precisely investigate the role of tidal currents on turtles’ movements within the BoB.
The BoB is a highly dynamic ecosystem characterized by several currents flowing in opposite directions. There is also a seasonal inversion, with the main slope current flowing northwards in winter while toward the equator in summer and fall (Michel et al., 2009), making this region highly variable in terms of oceanic circulation. Together with the main oceanic circulation in fall, the Iberian shoreline might also act as a physical barrier, preventing the southward migration of these individuals at this period. That might explain why no seasonal north-south pattern was observed for the resident turtles, unlike their conspecifics in the Pacific (Polovina et al., 2001; Briscoe et al., 2016b) and Atlantic (Mansfield et al., 2009). The active swimming in young turtles has recently been detected by numerous studies (Gaspar et al., 2012; Briscoe et al., 2016a; Putman et al., 2016). Chambault et al. (2019) observed that large immature loggerheads (SCL: 36.3–61.1 cm) tracked around the Azores were clearly active. Our results confirmed that active swimming behavior is already present in the smaller individuals tracked in this study. Our findings therefore exclude the hypothesis that the turtles remained in their habitats due to ocean currents transport after release, but rather suggest a real habitat use (at least during summer and autumn), indicating that the Bay of Biscay might provide a suitable habitat in terms of both thermal and trophic conditions. This hypothesis was supported by two turtles from the BoB group which transmitted data until the next winter and even the next spring.
Trophic and Thermal Constraints
Mid-trophic level models (zooplankton and micronekton) confirmed the high productivity of the BoB, suggesting that coastal turtles could be feeding during the tracking period. Indeed, the BoB hosts all-year-round a wide variety of marine megafauna species, from seabirds to cetaceans (Lambert et al., 2017). The highest zooplankton and micronekton biomasses found in this area compared to the three other spatial patterns confirmed that this presumably unsuitable habitat could be used as an important feeding ground for immature sea turtles. The lack of seasonality for micronekton biomass also suggests this area is a favorable foraging ground for these species throughout the year. The analysis of stomach contents from necropsied individuals found along the French Atlantic coast also confirms that some turtles were feeding in proximity to their stranding site (Dell’Amico, personal communication). The numerous river plumes along the French Atlantic coast (Gironde, Charente, Loire) could also contribute to the high productivity of this area, and constitute a critical habitat for many species (Lambert et al., 2018). Unlike other marine species that use the shelf edge and the abyssal margin of the BoB, the tracked turtles remained in close proximity to the shore (75% of the locations < 100 m isobaths) and occasionally exploited river plumes, with two individuals using the Gironde Estuary. The importance of the BoB as a critical habitat or an ecological trap should be further investigated using longer tracking durations, additional individuals and model simulations. But so far, the 449 sightings collected over 33 years by the CESTM tend to show that immature individuals from the three species use these waters year-round, including the smallest ones mostly in winter, probably due to cold-stunning.
While a suitable habitat should be productive, it also needs to be thermally optimal or at least acceptable for the species to survive. Similarly to other ectotherm species, ambient temperature plays a crucial role in reptile development and survival (Angilletta et al., 2002), driving the at-sea distribution of sea turtles. For this reason, immature loggerhead and green turtles during their pelagic phase generally target the 17–18°C isotherms (Polovina et al., 2000; Mansfield et al., 2014, 2021; Patel et al., 2021), and are rarely seen in habitats colder than 14°C (Robinson et al., 2020). Ten percent of our dataset was, however, associated with such cold waters, including 26% of the individuals remaining in these unsuitable areas for weeks or even months (range: 5–128 days, mean: 53 days). Similar to other loggerheads populations, smaller individuals experienced colder waters compared to larger ones (Abecassis et al., 2013), which could be related to increasing diving capacities with increased size. The larger turtles could target deeper, richer but colder layers, and therefore need to rewarm at the sea surface. Similar thermoregulation behaviors have been evidenced in other marine ectotherms (Di Santo and Bennett, 2011). The colder temperature range experienced by the smaller individuals could also partly explain why five of these small turtles (range: 3.3–8.7 kg) were found dead (n = 4) or stranded again alive (n = 1) during the following winter after release in European waters, possibly due to cold-stunning. This phenomenon is not uncommon as in Australia, 8.6% of the rehabilitated turtles were recaptured (Flint et al., 2017). This raises a serious concern regarding the thermal favorability of the BoB, where satellite-derived SSTs range between 10 and 20°C (Huret et al., 2018). Water temperatures can even drop below 10°C in very coastal areas used by the resident turtles. All the rescued turtles were in distress when found, suggesting that the BoB might act as an ecological trap for these young individuals in winter.
It is worth mentioning that the European waters are also commonly used by sub-adult and adult leatherback turtles (Witt et al., 2007; Nicolau et al., 2016), especially in summer, likely due to large aggregations of jellyfish (Houghton et al., 2006). Unlike the hard-shelled species (loggerhead, Kemp’s ridley and green turtle), leatherback strandings occur mainly during fall when weather conditions favor carcasses to drift to the shore before being stranded. Although the cause of mortality is in most cases difficult to determine, due to the level of decomposition, the endothermic ability of this gigantotherm species should make them less vulnerable to cold temperatures in such temperate habitats. But in response to global warming, these temperate habitats might become more and more thermally suitable as SST is expected to increase in the future, e.g., 0.23°C/decade in the Western English Channel coastal waters (L’Hévéder et al., 2017). As it has already been predicted for many species (Walther et al., 2002; Parmesan and Yohe, 2003), immature sea turtles might therefore experience a northern shift toward new habitats in response to global warming (Patel et al., 2021). However, the rise of temperature could be associated with a decrease in ocean productivity, leading to a trade-off between a more thermally favorable habitat but less abundant resources.
Conservation Implications
The identification of a species geographical range is crucial to implement effective management of endangered species, reinforcing the interest of our findings. All sea turtle species inhabiting the North Atlantic are listed on the IUCN Red list, with status vulnerable (loggerhead turtle), endangered (green turtle) and critically endangered (Kemp’s ridley turtle, Wibbels and Bevan, 2019). The French East Atlantic sea turtle network (RTMAE), coordinated by the CESTM of Aquarium La Rochelle, therefore provides an unprecedented dataset to confirm the turtle presence in the BoB. It is worth mentioning that all the satellite tracked turtles were found in distress (stranded, bycaught or drifting at the sea surface), supporting the idea that without the rescue center and rehabilitation, these turtles may have likely died. Given the number of rescued individuals per year is relatively low, it is hard to estimate the real proportion of immature sea turtles inhabiting the European waters, especially in winter. Future work should therefore be dedicated to genetic analysis and model simulations to assess if the BoB is either an ecological trap or an important habitat used by these immature sea turtles year-round. Such results will undoubtedly support conservation measures, especially the update of the RMUs by potentially including the BoB as an important habitat for loggerhead turtles. As RMUs are population specific, the dataset of the two other species (Kemp’s ridley and green turtles) need to be first augmented to draw reliable conclusions regarding their distributions. Alternative approaches such as aerial surveys are available to asses marine megafauna species distribution in the BoB and English Channel (Darmon et al., 2017; Lambert et al., 2018), but such observations generally miss small individuals like immature sea turtles, preventing the identification at the species level, and inevitably leading to an underestimation of the population. Our findings also confirm the importance of the Marine Protected Areas, including the small MPA located off the CESTM (Gironde estuary and the Sea of Pertuis Marine Natural Park), where a large proportion of the tracked individuals aggregated.
Although some individuals were found in bad condition (four were amputated), they showed no sign of atypical behavior after release, which is in agreement with other immature turtles satellite tracked from North-East America (Robinson et al., 2020). Among these four injured individuals, the longest tracking duration was even recorded for one of them (679 days), a sub-adult loggerhead turtle describing a typical trajectory despite being amputated from the left pectoral flipper, probably due to vessel collision or entrapment in fishing gear. Even though sea turtles are not the main marine megafauna species bycaught in the North East Atlantic (Bonanomi et al., 2019; Peltier et al., 2021), 29% of our rehabilitated and tracked individuals were found bycaught dead or alive (n = 3), stranded alive (n = 1) or stranded dead (n = 4) only few weeks after release. Indeed, the BoB hosts large populations of heavily small pelagic fishes (anchovy, sardine), in particular along the Spanish Atlantic coast (Ruiz et al., 2021), where several turtles aggregated. This clearly increases the entrapment risk in fishing nets.
In addition to bycatch, sea turtles face many other threats at sea such as marine debris (entrapment or ingestion) and organic pollutants, which are two of the 11 Descriptors listed in the Annex I of the European Marine Strategy Framework Directive (MSFD, 2008/56/EC), aiming to determine the Good Environmental Status (GES) of the EU’s waters by 2020. Among other marine megafauna species (e.g., cetaceans, seabirds), sea turtles are used as bioindicators of the ocean health to reach the good environmental status. The work carried out by Rescue Centers such as the CESTM of Aquarium La Rochelle is therefore critical and should be supported since they offer the unique possibility to collect a wide variety of data from both rescued and dead animals, e.g., satellite tracking for movement analysis (Descriptor 1), tissue sample for genetics (Descriptor 1), pollutants analyses (Descriptor 8) and interactions with marine litter from necropsies (Descriptor 10).
Data Availability Statement
The datasets presented in this article are not readily available because the dataset concerns endangered species and cannot be communicated. Requests to access the datasets should be directed to FD’A, dG9ydHVlc0BhcXVhcml1bS1sYXJvY2hlbGxlLmNvbQ==.
Ethics Statement
The animal study was reviewed and approved by the Prefectoral Order N°2004-1104 DREAL permit number: DREAL/56-2020 Ministerial Order (30/12/2020).
Author Contributions
FD’A: designing experiment and data collection. PC: data analysis and writing. FD’A, PC, and PG: interpretation of the results. All authors contributed to the article and approved the submitted version.
Funding
This study was financed by the Aquarium La Rochelle and supported by the French National Centre for Space Studies (CNES) and the French Agency for Biodiversity (Office français de la biodiversité). This study was achieved by the support of the volunteers of the French East Atlantic sea turtle network [authorized by French Ministry of the Ecological Transition (Ministère de la Transition écologique)] and fishermen involved in recovering sea turtles from bycatch. The authors also appreciate the support of Mercator Océan International.
Conflict of Interest
The authors declare that the research was conducted in the absence of any commercial or financial relationships that could be construed as a potential conflict of interest.
Publisher’s Note
All claims expressed in this article are solely those of the authors and do not necessarily represent those of their affiliated organizations, or those of the publisher, the editors and the reviewers. Any product that may be evaluated in this article, or claim that may be made by its manufacturer, is not guaranteed or endorsed by the publisher.
Acknowledgments
We would like to thank Julien Temple-Boyer for running complementary analyses, Gaëlle Darmon for the data analysis on stomach content and Anna Conchon and Maxime Lalire for their valuable comments on the paper.
Supplementary Material
The Supplementary Material for this article can be found online at: https://www.frontiersin.org/articles/10.3389/fmars.2021.736604/full#supplementary-material
Supplementary Figure 1 | Metrics used to assess tag failure: (A) battery voltage, (B) Wet-dry switches (dry state in red and wet state in blue) and (C) the total number of Argos transmissions over time. For (A), the red lines (3.0 V) refer to the threshold below which there was a drop in battery voltage indicative of battery exhaustion. In (B), no data regarding wet-dry states was available for 15 individuals. In (C), the red lines refer to the threshold before battery exhaustion (26,688 transmissions) calculated from Horia based on the battery voltage graphic.
Supplementary Figure 2 | Individual tracks colored by the SST for the (A) North, (B) South and (C) Bay of Biscay groups. The black triangles refer to the last location recorded.
Supplementary Table 1 | Summary of the types of tag deployed on the 28 immature turtles.
Supplementary Table 2 | Characteristics of the habitat used (thermal and trophic) for each individual. The column SST refers to the mean ± SD and the numbers in parentheses to min and max in °C. Zooplankton and micronekton biomasses are expressed in g.m–2.
Footnotes
References
Abalo-Morla, S., Marco, A., Tomás, J., Revuelta, O., Abella, E., Marco, V., et al. (2018). Survival and dispersal routes of head-started loggerhead sea turtle (Caretta caretta) post-hatchlings in the Mediterranean Sea. Mar. Biol. 165:51. doi: 10.1007/s00227-018-3306-2
Abecassis, M., Senina, I., Lehodey, P., Gaspar, P., Parker, D., Balazs, G., et al. (2013). A model of loggerhead sea turtle (Caretta caretta) habitat and movement in the oceanic North Pacific. PLoS One 8:e73274. doi: 10.1371/journal.pone.0073274
Angilletta, M. J., Niewiarowski, P. H., and Navas, C. A. (2002). The evolution of thermal physiology in ectotherms. J. Therm. Biol. 27, 249–268. doi: 10.1016/S0306-4565(01)00094-8
Avens, L., Goshe, L. R., Harms, C. A., Anderson, E. T., Hall, A. G., Cluse, W. M., et al. (2012). Population characteristics, age structure, and growth dynamics of neritic juvenile green turtles in the northeastern Gulf of Mexico. Mar. Ecol. Prog. Ser. 458, 213–229. doi: 10.3354/meps09720
Balazs, G. H., Miya, R., and Beavers, S. (1996). “Procedures to attach a satellite transmitter to the carapace of an adult green turtle, Chelonia mydas,” in Proceedings of the Fifteenth Annual Symposiumon Sea Turtle Biology and Conservation, February 20-25, 1995, eds J. A. Keinath, D. E. Barnard, J. A. Musick, and B. A. Bell (HiltonHead, SC: U.S. Dep. Commer. NOAA Tech), 21–26.
Bellido, J. J., Báez, J. C., Castillo, J. J., Pinto, F., Martín, J. J., Mons, J. L., et al. (2010). Loggerhead strandings and captures along the southern spanish coast: body size–based differences in natural versus anthropogenic injury. Chelonian Conserv. Biol. 9, 276–282. doi: 10.2744/CCB-0802.1
Bellido, J. J., Báez, J. C., Sanchez, R. F., Castillo, J. J., Martín, J. J., Mons, J. L., et al. (2008). Mass strandings of cold-stunned loggerhead turtles in the south Iberian Peninsula: ethological implications. Ethol. Ecol. Evol. 20, 401–405. doi: 10.1080/08927014.2008.9522520
Bolten, A. B. (2003). “Variation in sea turtle life history patterns: neritic vs. oceanic developmental stages,” in The Biology of Sea Turtles, eds P. L. Lutz, J. A. Musick, and J. Wyneken (Boca Raton, FL: CRC Press), 243–257.
Bolten, A. B., Bjorndal, K. A., Martins, H. R., Dellinger, T., Biscoito, M. J., Encalada, S. E., et al. (1998). Transatlantic developmental migrations of loggerhead sea turtles demonstrated by mtdna sequence analysis. Ecol. Appl. 8, 1–7.
Bonanomi, S., Clarke, M. W., Couperus, B., Dorrien, C., von Evans, P., Fernandez, R., et al. (2019). Working group on bycatch of protected species (WGBYC). ICES Sci. Rep. 1:163. doi: 10.17895/ices.pub.5563
Botterell, Z. L. R., Penrose, R., Witt, M. J., and Godley, B. J. (2020). Long-term insights into marine turtle sightings, strandings and captures around the UK and Ireland (1910–2018). J. Mar. Biol. Assoc. U.K. 100, 869–877. doi: 10.1017/S0025315420000843
Bousquet, O., Dalleau, M., Bocquet, M., Gaspar, P., Bielli, S., Ciccione, S., et al. (2020). Sea turtles for ocean research and monitoring: overview and initial results of the STORM project in the Southwest Indian Ocean. Front. Mar. Sci. 7:859. doi: 10.3389/fmars.2020.594080
Briscoe, D. K., Parker, D. M., Bograd, S., Hazen, E., Scales, K., Balazs, G. H., et al. (2016b). Multi-year tracking reveals extensive pelagic phase of juvenile loggerhead sea turtles in the North Pacific. Mov. Ecol. 4:23. doi: 10.1186/s40462-016-0087-4
Briscoe, D. K., Parker, D. M., Balazs, G. H., Kurita, M., Saito, T., Okamoto, H., et al. (2016a). Active dispersal in loggerhead sea turtles (Caretta caretta) during the ‘lost years’. Proc. R. Soc. B 283:20160690. doi: 10.1098/rspb.2016.0690
Briscoe, D. K., Turner Tomaszewicz, C. N., Seminoff, J. A., Parker, D. M., Balazs, G. H., Polovina, J. J., et al. (2021). Dynamic thermal corridor may connect endangered loggerhead sea turtles across the Pacific Ocean. Front. Mar. Sci 8:630590. doi: 10.3389/fmars.2021.630590
Burke, V. J., Standora, E. A., and Morreale, S. J. (1991). Factors affecting strandings of cold-stunned juvenile kemp’s ridley and loggerhead sea turtles in Long Island, New York. Copeia 1991, 1136–1138. doi: 10.2307/1446115
Cardona, L., Revelles, M., Carreras, C., Félix, M. S., Gazo, M., and Aguilar, A. (2005). Western mediterranean immature loggerhead turtles: habitat use in spring and summer assessed through satellite tracking and aerial surveys. Mar. Biol. 147, 583–591. doi: 10.1007/s00227-005-1578-9
Casale, P., Affronte, M., Scaravelli, D., Lazar, B., Vallini, C., and Luschi, P. (2012). Foraging grounds, movement patterns and habitat connectivity of juvenile loggerhead turtles (Caretta caretta) tracked from the Adriatic Sea. Mar. Biol. 159, 1527–1535. doi: 10.1007/s00227-012-1937-2
Chambault, P., Baudena, A., Bjorndal, K. A., Santos, M. A. R., Bolten, A. B., and Vandeperre, F. (2019). Swirling in the ocean: immature loggerhead turtles seasonally target old anticyclonic eddies at the fringe of the North Atlantic gyre. Prog. Oceanogr. 175, 345–358. doi: 10.1016/j.pocean.2019.05.005
Chambault, P., Thoisy, B., de Huguin, M., Martin, J., Bonola, M., Etienne, D., et al. (2018). Connecting paths between juvenile and adult habitats in the Atlantic green turtle using genetics and satellite tracking. Ecol. Evol. 8, 12790–12802. doi: 10.1002/ece3.4708
Chimienti, M., Blasi, M. F., and Hochscheid, S. (2020). Movement patterns of large juvenile loggerhead turtles in the Mediterranean Sea: ontogenetic space use in a small ocean basin. Ecol. Evol. 10, 6978–6992. doi: 10.1002/ece3.6370
Christiansen, F., Putman, N. F., Farman, R., Parker, D. M., Rice, M. R., Polovina, J. J., et al. (2016). Spatial variation in directional swimming enables juvenile sea turtles to reach and remain in productive waters. Mar. Ecol. Prog. Ser. 557, 247–259. doi: 10.3354/meps11874
Croissant, Y. (2020). Mlogit: Multinomial Logit Models. Available online at: https://CRAN.R-project.org/package=mlogit (accessed September 8, 2021).
Dalleau, M., Benhamou, S., Sudre, J., Ciccione, S., and Bourjea, J. (2014). The spatial ecology of juvenile loggerhead turtles (Caretta caretta) in the Indian Ocean sheds light on the “lost years” mystery. Mar. Biol. 161, 1835–1849. doi: 10.1007/s00227-014-2465-z
Darmon, G., Miaud, C., Claro, F., Doremus, G., and Galgani, F. (2017). Risk assessment reveals high exposure of sea turtles to marine debris in French Mediterranean and metropolitan Atlantic waters. Deep Sea Res. 2 Top. Stud. Oceanogr. 141, 319–328. doi: 10.1016/j.dsr2.2016.07.005
Davenport, J. (1997). Temperature and the life-history strategies of sea turtles. J. Therm. Biol. 22, 479–488. doi: 10.1016/S0306-4565(97)00066-1
Di Santo, V., and Bennett, W. A. (2011). Effect of rapid temperature change on resting routine metabolic rates of two benthic elasmobranchs. Fish Physiol. Biochem. 37, 929–934. doi: 10.1007/s10695-011-9490-3
Flint, J., Flint, M., Limpus, C. J., and Mills, P. (2017). Status of marine turtle rehabilitation in Queensland. PeerJ 5:e3132. doi: 10.7717/peerj.3132
Foley, A., Singel, K., Dutton, P., Summers, T., Redlow, A., and Lessman, J. (2007). Characteristics of a green turtle (Chelonia mydas) assemblage in northwestern Florida determined during a hypothermic stunning event. Gulf Mex. Sci. 25, 131–143. doi: 10.18785/goms.2502.04
Fossette, S., Hobson, V. J., Girard, C., Calmettes, B., Gaspar, P., Georges, J.-Y., et al. (2010). Spatio-temporal foraging patterns of a giant zooplanktivore, the leatherback turtle. J. Mar. Syst. 81, 225–234. doi: 10.1016/j.jmarsys.2009.12.002
Gaspar, P., and Lalire, M. (2017). A model for simulating the active dispersal of juvenile sea turtles with a case study on western Pacific leatherback turtles. PLoS One 12:e0181595. doi: 10.1371/journal.pone.0181595
Gaspar, P., Benson, S. R., Dutton, P. H., Réveillère, A., Jacob, G., Meetoo, C., et al. (2012). Oceanic dispersal of juvenile leatherback turtles: going beyond passive drift modeling. Mar. Ecol. Prog. Ser. 457, 265–284. doi: 10.3354/meps09689
Gaspar, P., Georges, J.-Y., Fossette, S., Lenoble, A., Ferraroli, S., and Maho, Y. L. (2006). Marine animal behaviour: neglecting ocean currents can lead us up the wrong track. Proc. R. Soc. B Biol. Sci. 273, 2697–2702. doi: 10.1098/rspb.2006.3623
George, R. (1997). “Health problems and diseases of sea Turtles,” in The Biology of Sea Turtles Vol. 1, eds, P. Lutz and J.A. Musick (Boca Raton, FL: CRC Press), 363–385.
Giunchi, D., Pollonara, E., and Baldaccini, N. E. (2003). The influence of transport conditions on the initial orientation of sand martins (Riparia riparia). Ethol. Ecol. Evol. 15, 83–97. doi: 10.1080/08927014.2003.9522693
Griffin, L. P., Griffin, C. R., Finn, J. T., Prescott, R. L., Faherty, M., Still, B. M., et al. (2019). Warming seas increase cold-stunning events for Kemp’s ridley sea turtles in the northwest Atlantic. PLoS One 14:e0211503. doi: 10.1371/journal.pone.0211503
Harrison, C. S., Luo, J. Y., Putman, N. F., Li, Q., Sheevam, P., Krumhardt, K., et al. (2021). Identifying global favourable habitat for early juvenile loggerhead sea turtles. J. R. Soc. Interface 18:20200799. doi: 10.1098/rsif.2020.0799
Hart, K. M., Guzy, J. C., and Smith, B. J. (2021). Drivers of realized satellite tracking duration in marine turtles. Mov. Ecol. 9:1. doi: 10.1186/s40462-020-00237-3
Hays, G. C., and Hawkes, L. A. (2018). Satellite tracking sea turtles: opportunities and challenges to address key questions. Front. Mar. Sci 5, 1–12. doi: 10.3389/fmars.2018.00432
Hays, G. C., Laloë, J.-O., Rattray, A., and Esteban, N. (2021). Why do Argos satellite tags stop relaying data? Ecol. Evol. 11, 7093–7101. doi: 10.1002/ece3.7558
Hindell, M. A., Reisinger, R. R., Ropert-Coudert, Y., Hückstädt, L. A., Trathan, P. N., Bornemann, H., et al. (2020). Tracking of marine predators to protect Southern Ocean ecosystems. Nature 580, 87–92. doi: 10.1038/s41586-020-2126-y
Houghton, J. D. R., Doyle, T. K., Wilson, M. W., Davenport, J., and Hays, G. C. (2006). Jellyfish aggregations and leatherback turtle foraging patterns in a temperate coastal environment. Ecology 87, 1967–1972.
Hunt, K. E., Innis, C., Merigo, C., Burgess, E. A., Norton, T., Davis, D., et al. (2019). Ameliorating transport-related stress in endangered Kemp’s ridley sea turtles (Lepidochelys kempii) with a recovery period in saltwater pools. Conserv. Physiol. 7:coy065 doi: 10.1093/conphys/coy065
Huret, M., Bourriau, P., Doray, M., Gohin, F., and Petitgas, P. (2018). Survey timing vs. ecosystem scheduling: degree-days to underpin observed interannual variability in marine ecosystems. Prog. Oceanogr. 166, 30–40. doi: 10.1016/j.pocean.2017.07.007
Innis, C. J., McGowan, J. P., and Burgess, E. A. (2019). Cold-Stunned loggerhead sea turtles (Caretta caretta): initial vs. convalescent physiologic status and physiologic findings associated with death. J. Herpetol. Med. Surg. 29, 105–112. doi: 10.5818/19-06-204.1
Jonsen, I. D., Patterson, T. A., Costa, D. P., Doherty, P. D., Godley, B. J., Grecian, W. J., et al. (2020). A continuous-time state-space model for rapid quality control of argos locations from animal-borne tags. Mov. Ecol. 8:31. doi: 10.1186/s40462-020-00217-7
Jonsen, I., and Patterson, T. (2020). Foiegras: Fit Latent Variable Movement Models To Animal Tracking Data For Location Quality Control And Behavioural Inference Zenodo. doi: 10.5281/zenodo.3899972
Karagiorgos, J., Vervatis, V., and Sofianos, S. (2020). The impact of tides on the bay of biscay dynamics. J. Mar. Sci. Eng. 8:617. doi: 10.3390/jmse8080617
Kobayashi, D. R., Polovina, J. J., Parker, D. M., Kamezaki, N., Cheng, I.-J., Uchida, I., et al. (2008). Pelagic habitat characterization of loggerhead sea turtles, Caretta caretta, in the North Pacific Ocean (1997–2006): insights from satellite tag tracking and remotely sensed data. J. Exp. Mar. Biol. Ecol. 356, 96–114. doi: 10.1016/j.jembe.2007.12.019
Koch, V., Peckham, H., Mancini, A., and Eguchi, T. (2013). Estimating at-sea mortality of marine turtles from stranding frequencies and drifter experiments. PLoS One 8:e56776. doi: 10.1371/journal.pone.0056776
L’Hévéder, B., Speich, S., Ragueneau, O., Gohin, F., and Bryère, P. (2017). Observed and projected sea surface temperature seasonal changes in the Western English Channel from satellite data and CMIP5 multi-model ensemble. Int. J. Climatol. 37, 2831–2849. doi: 10.1002/joc.4882
Lalire, M., and Gaspar, P. (2019). Modeling the active dispersal of juvenile leatherback turtles in the North Atlantic Ocean. Mov. Ecol. 7:7.
Lambert, C., Authier, M., Doray, M., Dorémus, G., Spitz, J., and Ridoux, V. (2018). Decadal stability in top predator habitat preferences in the Bay of Biscay. Prog. Oceanogr. 166, 109–120. doi: 10.1016/j.pocean.2018.03.007
Lambert, C., Pettex, E., Dorémus, G., Laran, S., Stéphan, E., Canneyt, O. V., et al. (2017). How does ocean seasonality drive habitat preferences of highly mobile top predators? Part II: the eastern North-Atlantic. Deep Sea Res. 2 Top. Stud. Oceanogr. 141, 133–154. doi: 10.1016/j.dsr2.2016.06.011
Le Pape, O., Chauvet, F., Mahévas, S., Lazure, P., Guérault, D., and Désaunay, Y. (2003). Quantitative description of habitat suitability for the juvenile common sole (Solea solea. L.) in the Bay of Biscay (France) and the contribution of different habitats to the adult population. J. Sea Res. 50, 139–149. doi: 10.1016/S1385-1101(03)00059-5
Lehodey, P., Murtugudde, R., and Senina, I. (2010). Bridging the gap from ocean models to population dynamics of large marine predators: a model of mid-trophic functional groups. Prog. Oceanogr. 84, 69–84. doi: 10.1016/j.pocean.2009.09.008
Lopez, R., Malarde, J.-P., Royer, F., and Gaspar, P. (2014). Improving argos doppler location using multiple-model kalman filtering. IEEE Trans. Geosci. Remote Sens. 52, 4744–4755. doi: 10.1109/TGRS.2013.2284293
Mansfield, K. L., Saba, V. S., Keinath, J. A., and Musick, J. A. (2009). Satellite tracking reveals a dichotomy in migration strategies among juvenile loggerhead turtles in the Northwest Atlantic. Mar. Biol. 156, 2555–2570. doi: 10.1007/s00227-009-1279-x
Mansfield, K. L., Wyneken, J., and Luo, J. (2021). First Atlantic satellite tracks of ‘lost years’ green turtles support the importance of the Sargasso Sea as a sea turtle nursery. Proc. R. Soc. B Biol. Sci. 288:20210057. doi: 10.1098/rspb.2021.0057
Mansfield, K. L., Wyneken, J., Porter, W. P., and Luo, J. (2014). First satellite tracks of neonate sea turtles redefine the ‘lost years’ oceanic niche. Proc. R. Soc. Lond. B Biol. Sci. 281:20133039. doi: 10.1098/rspb.2013.3039
Manzella, S., Caillouet, C. W., and Fontaine, C. (1988). Kemp’s ridley. Lepidochelys kempi, sea turtle head start tag recoveries: distribution, habitat, and method of recovery. Mar. Fish. Rev. 50, 24–32.
Marquez, M. (2001). Status and Distribution of the Kemp’s Ridley Turtle, Lepidochelys kempii, in the Wider Caribbean Region. Santo Domingo: UNEP-CEP, 46–51.
McMichael, E., Seminoff, J., and Carthy, R. (2008). Growth rates of wild green turtles, Chelonia mydas, at a temperate foraging habitat in the northern Gulf of Mexico: assessing short-term effects of cold-stunning on growth. J. Nat. Hist. 42, 2793–2807. doi: 10.1080/00222930802357335
Meylan, A., Bowen, B., and Avise, J. (1990). A genetic test of the natal homing versus social facilitation models for green turtle migration. Science 248, 724–727. doi: 10.1126/science.2333522
Michel, S., Treguier, A.-M., and Vandermeirsch, F. (2009). Temperature variability in the Bay of Biscay during the past 40 years, from an in situ analysis and a 3D global simulation. Cont. Shelf Res. 29, 1070–1087. doi: 10.1016/j.csr.2008.11.019
Monzón-Argüello, C., Dell’Amico, F., Morinière, P., Marco, A., López-Jurado, L. F., Hays, G. C., et al. (2012). Lost at sea: genetic, oceanographic and meteorological evidence for storm-forced dispersal. J. R. Soc. Interface 9, 1725–1732. doi: 10.1098/rsif.2011.0788
Morinière, P., and Dell’Amico, F. (2011). Synthèse des observations de tortues marines sur la façade Manche-Atlantique de 1988 à 2008. Bull. Soc. Herp. Fr. 139–140, 131–141.
Naimi, B., Hamm, N. A. S., Groen, T. A., Skidmore, A. K., and Toxopeus, A. G. (2014). Where is positional uncertainty a problem for species distribution modelling? Ecography 37, 191–203. doi: 10.1111/j.1600-0587.2013.00205.x
Nicolau, L., Ferreira, M., Santos, J., Araújo, H., Sequeira, M., Vingada, J., et al. (2016). Sea turtle strandings along the Portuguese mainland coast: spatio-temporal occurrence and main threats. Mar. Biol. 163:21. doi: 10.1007/s00227-015-2783-9
Orós, J., Montesdeoca, N., Camacho, M., Arencibia, A., and Calabuig, P. (2016). Causes of stranding and mortality, and final disposition of loggerhead sea turtles (Caretta caretta) admitted to a wildlife rehabilitation center in gran canaria island, spain (1998-2014): a long-term retrospective study. PLoS One 11:e0149398. doi: 10.1371/journal.pone.0149398
Parmesan, C., and Yohe, G. (2003). A globally coherent fingerprint of climate change impacts across natural systems. Nature 421, 37–42. doi: 10.1038/nature01286
Patel, S. H., Winton, M. V., Hatch, J. M., Haas, H. L., Saba, V. S., Fay, G., et al. (2021). Projected shifts in loggerhead sea turtle thermal habitat in the Northwest Atlantic Ocean due to climate change. Sci. Rep. 11:8850. doi: 10.1038/s41598-021-88290-9
Peltier, H., Authier, M., Caurant, F., Dabin, W., Daniel, P., Dars, C., et al. (2021). In the wrong place at the wrong time: identifying spatiotemporal co-occurrence of bycaught common dolphins and fisheries in the bay of biscay (ne atlantic) from 2010 to 2019. Front. Mar. Sci 8:617342. doi: 10.3389/fmars.2021.617342
Polovina, J. J., Balazs, G. H., Howell, E. A., Parker, D. M., Seki, M. P., and Dutton, P. H. (2004). Forage and migration habitat of loggerhead (Caretta caretta) and olive ridley (Lepidochelys olivacea) sea turtles in the central North Pacific Ocean. Fish. Oceanogr. 13, 36–51. doi: 10.1046/j.1365-2419.2003.00270.x
Polovina, J. J., Howell, E., Kobayashi, D. R., and Seki, M. P. (2001). The transition zone chlorophyll front, a dynamic global feature defining migration and forage habitat for marine resources. Prog. Oceanogr. 49, 469–483. doi: 10.1016/S0079-6611(01)00036-2
Polovina, J. J., Kobayashi, D. R., Parker, D. M., Seki, M. P., and Balazs, G. H. (2000). Turtles on the edge: movement of loggerhead turtles (Caretta caretta) along oceanic fronts, spanning longline fishing grounds in the central North Pacific, 1997–1998. Fish. Oceanogr. 9, 71–82. doi: 10.1046/j.1365-2419.2000.00123.x
Polovina, J., Uchida, I., Balazs, G., Howell, E. A., Parker, D., and Dutton, P. (2006). The kuroshio extension bifurcation region: a pelagic hotspot for juvenile loggerhead sea turtles. Deep Sea Res. 2 Top. Stud. Oceanogr. 53, 326–339. doi: 10.1016/j.dsr2.2006.01.006
Putman, N. F., Lumpkin, R., Sacco, A. E., and Mansfield, K. L. (2016). Passive drift or active swimming in marine organisms? Proc. R. Soc. B Biol. Sci. 283:20161689. doi: 10.1098/rspb.2016.1689
R Core Team. (2021). R: A Language and Environment for Statistical Computing. R Foundation for Statistical Computing, Vienna, Austria.
Raymond, B., Wotherspoon, S., Jonsen, I., and Reisinger, R. R. (2021). Availability: Estimating Geographic Space Available to Animals Based on Telemetry Data. Available online at: https://github.com/AustralianAntarcticDivision/availability (accessed August 9, 2021).
Revelles, M., Isern-Fontanet, J., Cardona, L., San Félix, M., Carreras, C., and Aguilar, A. (2007). Mesoscale eddies, surface circulation and the scale of habitat selection by immature loggerhead sea turtles. J. Exp. Mar. Biol. Ecol. 347, 41–57. doi: 10.1016/j.jembe.2007.03.013
Roberts, K., Collins, J., Paxton, C. H., Hardy, R., and Downs, J. (2014). Weather patterns associated with green turtle hypothermic stunning events in St. Joseph Bay and Mosquito Lagoon, Florida. Phys. Geogr. 35, 134–150. doi: 10.1080/02723646.2014.898573
Robinson, N. J., Deguzman, K., Bonacci-Sullivan, L. Jr, DiGiovanni, R. A., and Pinou, T. (2020). Rehabilitated sea turtles tend to resume typical migratory behaviors: satellite tracking juvenile loggerhead, green, and kemp’s ridley turtles in the northeastern USA. Endanger. Species Res. 43, 133–143. doi: 10.3354/esr01065
Robson, N. A., Hetzel, Y., Whiting, S., Wijeratne, S., Pattiaratchi, C. B., Withers, P., et al. (2017). Use of particle tracking to determine optimal release dates and locations for rehabilitated neonate sea turtles. Front. Mar. Sci 4:173. doi: 10.3389/fmars.2017.00173
Ruiz, J., Louzao, M., Oyarzabal, I., Arregi, L., Mugerza, E., and Uriarte, A. (2021). The Spanish purse-seine fishery targeting small pelagic species in the Bay of Biscay: landings, discards and interactions with protected species. Fish. Res. 239:105951. doi: 10.1016/j.fishres.2021.105951
Salinas-Zavala, C. A., Morales-Zárate, M. V., Martínez-Rincón, R. O., Salinas-Zavala, C. A., Morales-Zárate, M. V., and Martínez-Rincón, R. O. (2020). An empirical relationship between sea surface temperature and massive stranding of the loggerhead turtle (Caretta caretta) in the Gulf of Ulloa. Mexico. Lat. Am. J. Aquat. Res. 48, 214–225. doi: 10.3856/vol48-issue2-fulltext-2348
Seney, E. E., Higgins, B. M., and Landry, A. M. (2010). Satellite transmitter attachment techniques for small juvenile sea turtles. J. Exp. Mar. Biol. Ecol. 384, 61–67. doi: 10.1016/j.jembe.2010.01.002
Shaver, D. J., Tissot, P. E., Streich, M. M., Walker, J. S., Rubio, C., Amos, A. F., et al. (2017). Hypothermic stunning of green sea turtles in a western Gulf of Mexico foraging habitat. PLoS One 12:e0173920. doi: 10.1371/journal.pone.0173920
Sotillo, M. G., Cailleau, S., Lorente, P., Levier, B., Aznar, R., Reffray, G., et al. (2015). The MyOcean IBI ocean forecast and reanalysis systems: operational products and roadmap to the future copernicus service. J. Oper. Oceanogr. 8, 63–79. doi: 10.1080/1755876X.2015.1014663
Spotila, J. R., O’Connor, M. P., and Paladino, F. V. (1997). “Thermal biology,” in The Biology Of Sea Turtles, Vol. 1, eds J. A. Musick and P. L. Lutz (Boca Raton, FL: CRC Press), 297.
Still, B., Griffin, C. R., and Prescott, R. (2005). Climatic and oceanographic factors affecting daily patterns of juvenile sea turtle cold-stunning in Cape Cod Bay, Massachusetts. Chelonian Conserv. Biol. 4, 883–890.
Varo-Cruz, N., Bermejo, J. A., Calabuig, P., Cejudo, D., Godley, B. J., López-Jurado, L. F., et al. (2016). New findings about the spatial and temporal use of the Eastern Atlantic Ocean by large juvenile loggerhead turtles. Divers. Distrib. 22, 481–492. doi: 10.1111/ddi.12413
Wallace, B., DiMatteo, A., Hurley, B., Finkbeiner, E., Bolten, A., Chaloupka, M., et al. (2010). Regional management units for marine turtles: a novel framework for prioritizing conservation and research across multiple scales. PLoS One 5:e15465. doi: 10.1371/journal.pone.0015465
Wallraff, H. G., and Wallraff, H. G. (2005). Avian Navigation: Pigeon Homing as a Paradigm. New York, NY: Springer Science & Business Media.
Walther, G.-R., Post, E., Convey, P., Menzel, A., Parmesan, C., Beebee, T. J. C., et al. (2002). Ecological responses to recent climate change. Nature 416:389. doi: 10.1038/416389a
Wibbels, T., and Bevan, E. (2019). Lepidochelys kempii. The IUCN Red List of Threatened Species 2019: e.T11533A155057916. Gland: IUCN.
Witt, M. J., Penrose, R., and Godley, B. J. (2007). Spatio-temporal patterns of juvenile marine turtle occurrence in waters of the European continental shelf. Mar. Biol. 151, 873–885. doi: 10.1007/s00227-006-0532-9
Wood, S. N. (2017). Generalized Additive Models: An Introduction with R, 2nd Edn. Boca Raton, FL: CRC Press.
Wyneken, J., Lohmann, K. J., and Musick, J. A. (2013). The Biology of Sea Turtles. Boca Raton, FL: CRC Press.
Keywords: Bay of Biscay, loggerhead turtle, green turtle, micronekton, sea surface temperature, Kemp’s ridley turtle
Citation: Chambault P, Gaspar P and Dell’Amico F (2021) Ecological Trap or Favorable Habitat? First Evidence That Immature Sea Turtles May Survive at Their Range-Limits in the North-East Atlantic. Front. Mar. Sci. 8:736604. doi: 10.3389/fmars.2021.736604
Received: 05 July 2021; Accepted: 30 September 2021;
Published: 26 October 2021.
Edited by:
Jorge M. Pereira, University of Coimbra, PortugalReviewed by:
Graeme Clive Hays, Deakin University, AustraliaAutumn Iverson, University of California, Davis, United States
Copyright © 2021 Chambault, Gaspar and Dell’Amico. This is an open-access article distributed under the terms of the Creative Commons Attribution License (CC BY). The use, distribution or reproduction in other forums is permitted, provided the original author(s) and the copyright owner(s) are credited and that the original publication in this journal is cited, in accordance with accepted academic practice. No use, distribution or reproduction is permitted which does not comply with these terms.
*Correspondence: Philippine Chambault, UGhpbGlwcGluZS5DaGFtYmF1bHRAZ21haWwuY29t