- 1College of Marine Sciences, South China Agricultural University, Guangzhou, China
- 2Pearl River Fisheries Research Institute, Chinese Academy of Fishery Sciences, Guangzhou, China
Despite the commercial importance of the sea cucumber Holothuria leucospilota increasing in recent years, little is known of its spatial distribution and ecology in the South China Sea. We investigate the distribution, abundance, and recruitment of H. leucospilota from 2017 to 2020 at two sites (S1 and S2) in Daya Bay, a typical subtropical bay which is strongly influenced by human activities in the South China Sea. We report hypoxia to drive H. leucospilota from deeper into shallower waters with higher DO concentrations at the mouth of Dapeng Cove in Daya Bay (S1), particularly during summer. Population size at S1 decreased by 90% from 2017 levels, before this area was opened to the public in 2018; recruitment was not observed by August (summer) of 2020. In contrast, in summers of 2017 and 2020, H. leucospilota abundance at S2, a protected open-water area, increased by 84%, and the proportion of small-sized (recently recruited) sea cucumber in the population increased by 20%. Severe summer hypoxia at S1 could negatively influence H. leucospilota spawning and larval settlement, which combined with depletion of broodstock because of fishing pressure and/or hypoxia-induced mortality, could inhibit recruitment. In contrast, higher DO concentrations and abundant broodstock during summer (the breeding season) favoured recruitment of H. leucospilota at S2. Overall, hypoxia and anthropogenic disturbance impede recovery of H. leucospilota at S1, while at the protected S2, larvae may be released to settle in nearby areas. It is imperative to develop a better understanding of the biology, ecology and conservation of tropical sea cucumbers in China.
Introduction
The sea cucumber Holothuria leucospilota occurs widely in shallow tropical and subtropical Indo-Pacific Ocean waters, where it is usually found on seagrass beds, and sandy and muddy substrata with rubble or coral reefs (Bonham and Held, 1963; Liao, 1997; Purcell et al., 2012; Yu et al., 2013). Being edible, H. leucospilota is an important component of traditional subsistence fisheries for some Pacific Island nations (Drumm et al., 1999; Dzeroski and Drumm, 2003; Drumm and Loneragan, 2005; Purcell et al., 2012; Huang et al., 2018). It is also a major source of bioactive substances such as polysaccharides and saponins for biotechnology and medicine, which potentially increase its market value (Yu et al., 2013; Huang et al., 2018).
Holothuria leucospilota plays an important ecological role in nearshore marine systems by moving large amounts of sand, selectively feeding on organically rich components within the sediment, increasing nutrient and dissolved oxygen exchange between water and sediments, and altering sediment biota and carbonate dynamics (Bonham and Held, 1963; Sloan, 1979; Che, 1990; Zamani et al., 2018). Additionally, it is a proposed bioremediator of organic polluted environments; its co-culture in aquaculture systems mitigates organic pollution and improves environmental quality, rendering it a good candidate for integrated multi-trophic aquaculture (IMTA) systems (Yu et al., 2012, 2013).
Sea cucumbers are considered a delicacy and medicinal food by Chinese and other Asian peoples. Nowadays most tropical sea cucumber species have been overexploited, especially in China, because of increased demand for their product. This overexploitation could indirectly, deleteriously affect marine ecosystems (Purcell et al., 2012; Harith et al., 2018; Huang et al., 2018; Ramírez-González et al., 2020). While H. leucospilota is an abundant species in tropical and subtropical waters, and not in danger of extinction like other commercially fished species (e.g., Stichopus horrens), it is severely threatened by overexploitation and environmental degradation (Purcell et al., 2012; Harith et al., 2018). Throughout Asia this species is fished in China, Malaysia, Thailand, Indonesia, the Philippines, and Viet Nam, and where it is fished there are few or no regulations pertaining to its harvesting (Purcell et al., 2012). Increased market demand drove the price of dried H. leucospilota in the Philippines from USD 1.83 kg–1 in 1998 to USD 4.89 kg–1 in 2000 (Akamine, 2001), and it has acquired more commercial value in recent years [current prices in Guangzhou market range USD 20–40 kg–1 (unpublished data)]. Overfishing and a lack of fishing regulations in Malaysia could put H. leucospilota at risk of local extinction (Harith et al., 2018).
The distribution and abundance of H. leucospilota is well studied in many countries such as Malaysia, Indonesia, Japan, and the Marshall and Cook islands (Bonham and Held, 1963; Drumm et al., 1999; Dzeroski and Drumm, 2003; Harith et al., 2018; Setyastuti et al., 2018; Tanita and Yamada, 2019). Although this species was reported to be widely distributed in the South China Sea (Liao, 1997; Yu et al., 2013), little is known of its spatial distribution and ecology in these waters. The present study was aimed at investigating the distribution and abundance of this species, and how environmental and anthropogenic disturbances affect them, in Daya Bay, a typical subtropical bay in the South China Sea. These results will be of value in stock assessment surveys, and sea cucumber sustainable management in China’s waters.
Materials and Methods
Study Site
Sampling was performed around the mouth of Dapeng Cove, a body of water about 4.5 km (N–S) by 5 km (E–W) (Tang et al., 2003; Wang et al., 2008) in southwest Daya Bay, a semi-enclosed water mass about 15 km wide and 30 km long (about 600 km2) in the South China Sea. Daya Bay is influenced by the East Guangdong upwelling and a thermocline from June to August; water column stratification (temperature and salinity) in this bay begins to develop in June, and is strongest from July to September (Wang et al., 2008). H. leucospilota is the most abundant holothurian in Daya Bay (Yu et al., 2013; Huang et al., 2018).
Fish and oyster aquaculture in Dapeng Cove has thrived since the 1990s. In this region fish farms have increased to 30 ha, with an annual production of 450 metric tons, and oyster farms to 200 ha, with an annual production of 1.8 × 104 metric tons. Although mariculture activities within Dapeng Cove contribute greatly to local economy, environmental quality in the bay has deteriorated because of organic and nutrient contamination, and aquaculture waste decomposition, which depletes oxygen and exacerbates problems with hypoxia on the seabed during summer (Yu et al., 2013, 2014, 2017). Thermal pollution from discharged coolant water from two nearby nuclear power plants on the north shore of Dapeng Cove – the Daya Bay Nuclear Power Plant, operating since 1993, and Lingao Nuclear Power Plant, since 2003 – has also profoundly affected the ecology of Dapeng Cove. The thermal plume from these power plants affects water column vertical mixing during winter and stratification in summer. An obvious decline in biomass and richness of benthic taxa has occurred near these power plants between 1991 and 2004 (Tang et al., 2003; Wang et al., 2008). Local fishermen also report that about 30 years prior to mariculture and nuclear power plant development in this region that there were many mangroves, corals, Sargassum and sea cucumbers, which have since almost vanished. Large numbers of H. leucospilota now occur only around the mouth of this bay in an area that has been closed to the public for more than 10 years, from 2009.
We investigate the abundance and distribution of H. leucospilota at two areas – one open to the public (S1) and another that is protected (S2). S1 is located at the mouth of Dapeng Cove (22°34′16″N, 114°32′46″E), about 2.5 km south of the two nuclear power plants. The area around this site was formerly closed and used for sea urchin (Anthocidaris crassispina) ranching until 2017, after which these ranching activities were banned by the government and the sea area was opened to public for fisheries and tourism. No regulations were enacted to protect wildlife, and large numbers of sea cucumbers and sea urchins were immediately collected by local fishermen and tourists, especially during summer 2018 when this area was first opened to the public. S2 is located at breakwaters constructed in the mid-1990s outside a yacht harbour in open water (22°33′36″N, 114°33′12″E). The sea area around S2 has been closed to the public since 2009 in preparation for the 26th Summer Universiade in Shenzhen in 2011, fisheries, SCUBA diving and snorkelling tourism are forbidden (Figure 1).
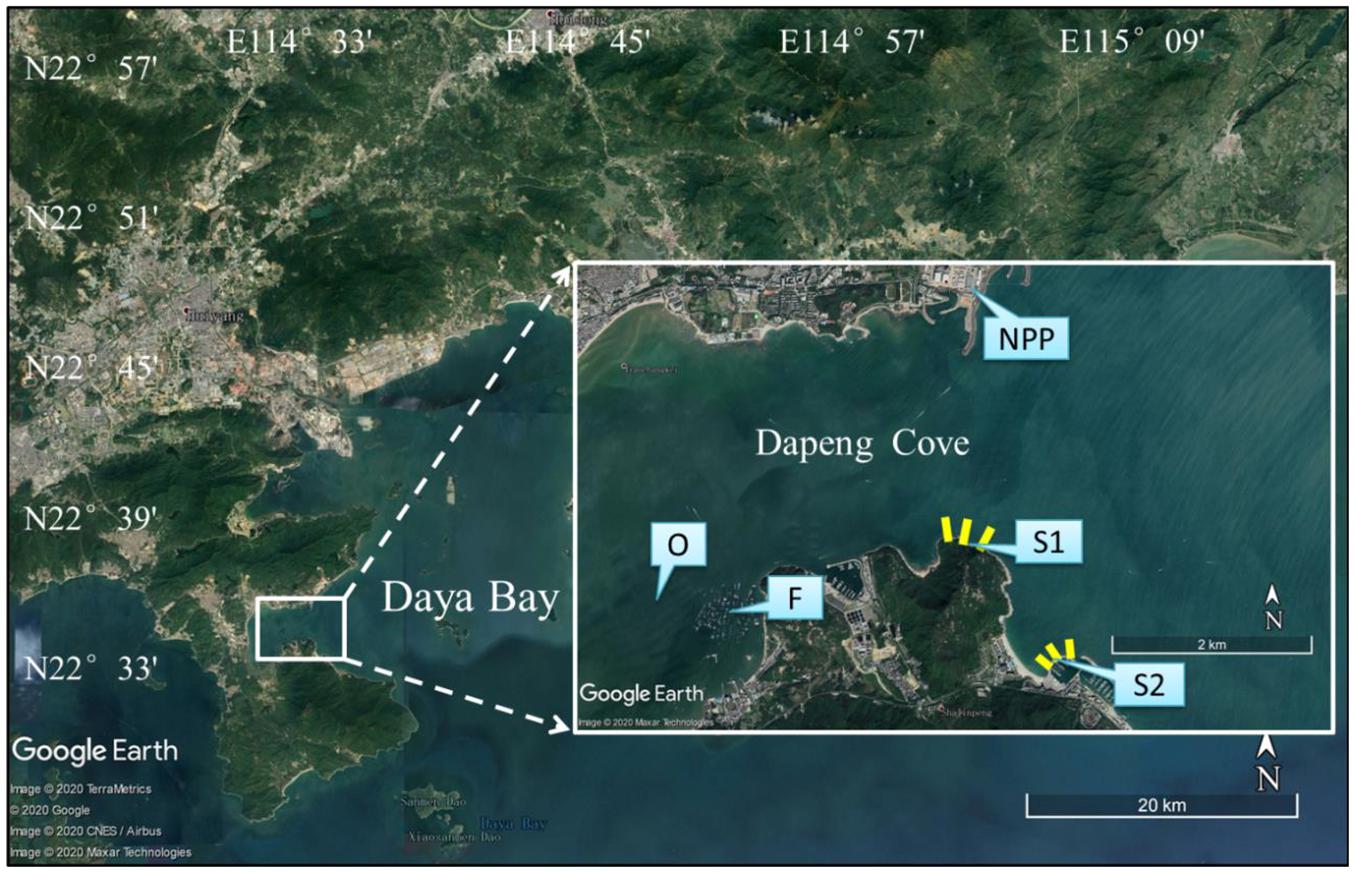
Figure 1. Map of study sites, with inset depicting the open area (S1) at the mouth of Dapeng Cove, and S2, the protected area in open water; yellow bars indicate transect lines. NPP, nuclear power plants; O, oyster farm; F, fish farm.
Distribution and Abundance of H. leucospilota
From February 28, 2017 to August 24, 2020, line transects were used to determine the distribution and abundance of H. leucospilota. Three permanent 50-m line transects were established at each site (the 50-m length was chosen because the H. leucospilota was found to occur mainly within 50 m of the shore in the pilot study). Transect lines began on shore at the water’s edge, and ran perpendicular to the shore, spaced about 50 m apart. Surveys along transects were performed on SCUBA at both sites during the day around high tide on February 28 (winter), May 23 (spring), August 26 (summer), and November 21 (autumn) in 2017, and August 24 (summer) in 2020; an additional survey occurred at S1 on November 11, 2019.
All H. leucospilota encountered within 1–2 m (distance determined by visibility) either side of the transect line were recorded along 10-m increments (i.e., 0–10, 10–20, 20–30, 30–40, and 40–50 m). Density along each transect and 10-m increment was calculated by dividing the total number of individuals by area surveyed and expressing this as individuals per square meter. Body length of animals in summer was measured (dorsal distance from mouth to anus) to the nearest 1 cm using a standard ruler; individuals were also photographed in situ with an Olympus Tough TG4 camera (to verify measurements); substrate type, depth, and distance from shore (DS) were simultaneously recorded.
No hand contact was made with sea cucumbers when measuring them, or their length was measured immediately after removal from the shelter (before they contracted); no sea cucumber along the transect was removed during any survey. In any survey, an area of 100–200 m2 per transect and 300–600 m2 per site was investigated.
Because H. leucospilota is active during the day, does not completely burrow into the sediment, and extends its highly mobile anterior end outward to feed, it is easily observed and surveyed (Massin and Doumen, 1986; Tanita and Yamada, 2019). We believe that any underestimation of its density during surveys (because of possible cryptic behaviour) would have been negligible.
Population Structure of H. leucospilota
During summer of 2017, 35 sea cucumbers from S2 (about 50 m outside the transect) were collected, measured (as above), and their wet weight determined (to the nearest 1 g) by portable handheld electronic scale after being drained on sterile gauze for 1 min (to constant weight). All animals were returned to the ocean after measurement. Wet weight and body length relationships were evaluated by linear regression, from which wet weights of sea cucumbers within transects were subsequently derived.
To evaluate annual changes in reproductive potential, the sizes of sea cucumbers surveyed during the summers of 2017 and 2020 were classified into three groups according to wet weight: small (≤90 g), medium (90–180 g), and large (≥180 g). This season and these sizes were chosen because the gonad index of H. leucospilota is greatest during August in Daya Bay (Huang et al., 2018), and wet weights > 180 g represent mature individuals in the breeding season (Gaudron et al., 2008; Purcell et al., 2012).
Environmental Characteristics
Substratum type, depth, and DS at locations where sea cucumbers occurred were recorded simultaneously. Bottom type at each 10-m increment along each transect was visually classified into sand (<2 mm), rubble (2–63 mm), rock (>63 mm) size fractions, or shell (primarily empty bivalves) (Zhou and Shirley, 1996; Tanita and Yamada, 2019).
Water parameters were monitored along transects at distances of 5, 15, 25, 35, and 45 m from the shore, with seafloor temperature (°C), dissolved oxygen (mg L–1), salinity, and pH measured using an In-Situ smarTROLL Multiparameter Handheld instrument (In-Situ Inc., Fort Collins, CO, United States).
Statistical Analysis
Analyses were performed using IBM SPSS Statistics for Windows, version 19.0 (IBM Corp., Armonk, NY, United States). A linear regression was used to determine relationships between sea cucumber wet weight and body length for summer of 2017. Separate two-way ANOVAs were conducted to assess the effects of sampling time and DS on sea cucumber density within each 10-m increment along each transect, at both sites. Average sea cucumber abundance at each site at different sampling times was analysed by one-way ANOVA, followed by a comparison of mean values using the Student–Newman–Keuls (SNK) test. Differences in average sea cucumber abundance at the two sites on common sampling occasions were analysed by Student’s t-test. For all data sets, normality was evaluated using the Kolmogorov–Smirnov test, and homogeneity was evaluated by Levene’s test. Density data for 10-m increments along each transect were log-transformed [log (x + 1)] to improve normality and homogeneity of variances, but we present results in an untransformed state. Statistical significance is set at P < 0.05.
Results
Environmental Characteristics
Water depth varied with DS at both sites (Figure 2). Depth at S1 increased with DS along the transect, while the seabed plateaued at S2 at DS > 15 m. Depths (at high tide) at DS of 5, 15, 25, 35, and 45 m at S1 were about 1.8, 3.0, 3.7, 4.6, and 5.7 m, respectively, while those for S2 were about 1.6, 4.7, 5.2, 5.2, and 5.3 m, respectively.
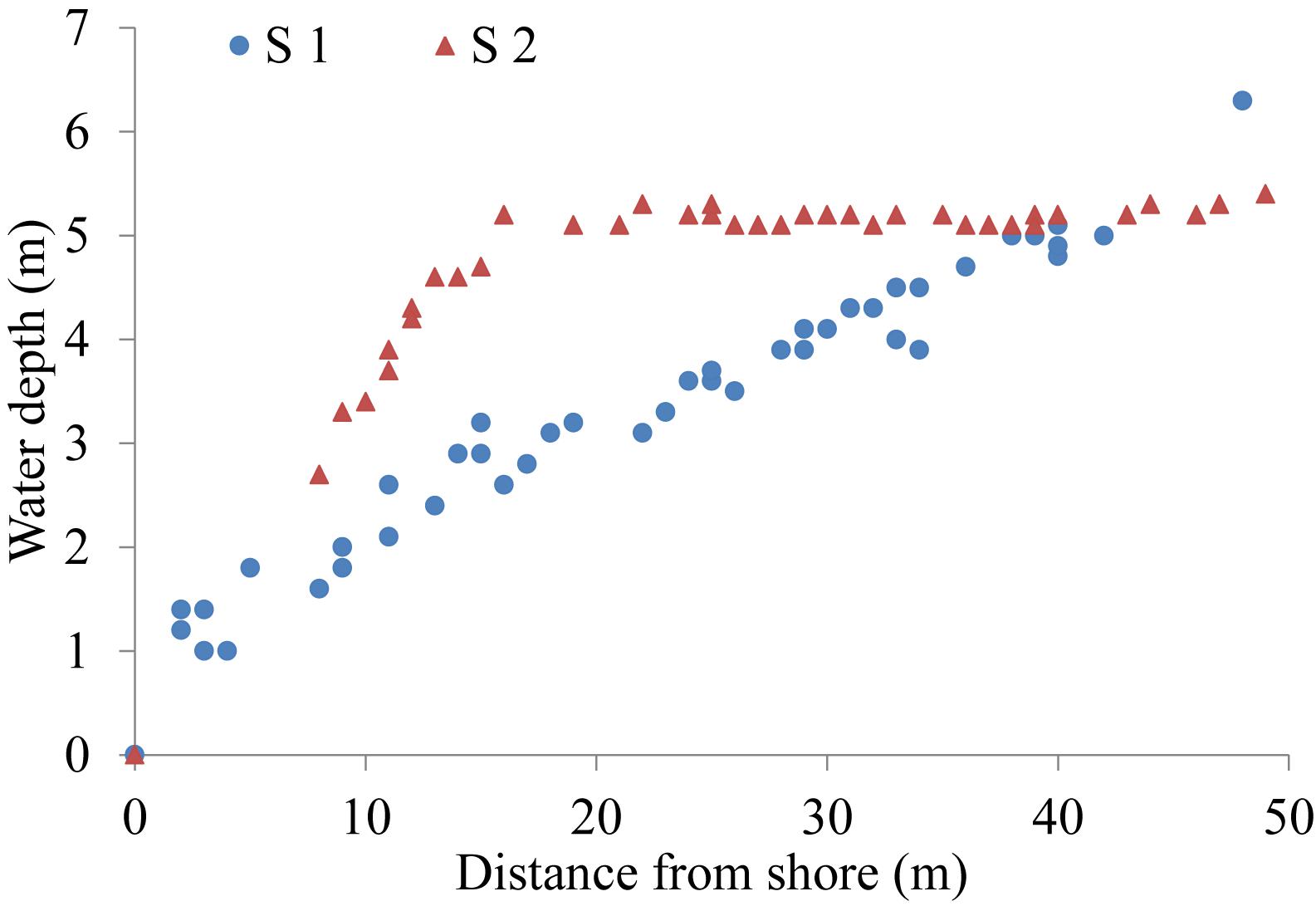
Figure 2. Water depth by distance from shore at each site (data collected on SCUBA at high tide, February 28, 2017).
Four main substratum types occurred along transects (Table 1). At S1 where high numbers of bivalve shells occurred, rock and shell substrata characterised the seabed at DS < 10 m; sand and shell substrata prevailed elsewhere. At S2, rock prevailed at DS < 10 m; sand and rubble prevailed elsewhere.
Sample site water parameters are summarised in Table 2. Lowest water temperatures at S1 occurred in winter 2017; higher values occurred in summer; temperature decreased with DS in summer, and a thermocline was present at about 4 m depth (from DS 25 to 35 m); values at different DS were similar during other seasons. DO decreased gradually with DS in spring, but dramatically in summer. Hypoxia (DO < 2.0 mg L–1) (Vaquer-Sunyer and Duarte, 2008) occurred in all near-bottom waters in summer of 2017, with the exception of 5 m DS (2.31 mg L–1). Although hypoxic conditions were not apparent along all S1 transects in summer 2020, DO decreased seaward, with values at 5 and 15 m DS 2–3 × those at 45 m (2.75 mg L–1) DS. At S1, salinity was high and relatively constant with DS on any given sampling event, but values at 5 and 15 m DS were lower than those elsewhere in summer 2020, mainly because of rainfall which preceded sampling; pH was very similar at different DS on any given sampling event, except for summer, when values decreased with DS; pH was low (<8.00) in summer 2017, but more alkaline (>8.09) in summer 2020. Similar trends occurred in most cases for water parameters at S2. While the DO at 35 and 45 m DS was low (1.61 and 1.45 mg L–1, respectively), it was 1.6× and 2.3× that at corresponding DS at S1 in summer 2017. Fairly stable water parameters at different DS in winter and autumn indicated strong seasonal vertical water column mixing.
Population Characteristics of H. leucospilota
Distribution
A two-way ANOVA revealed the distribution of H. leucospilota to vary significantly over time and DS at both sites, with an obvious interaction between sampling time and DS at S1 (Table 3). The distribution of H. leucospilota at S1 is shown in Figure 3A. During the 2017 winter, H. leucospilota occurred along all 10-m increments of transects, with densities at DS 20–30 m (0.23 ± 0.03 ind m–2) and 30–40 m (0.35 ± 0.05 ind m–2) higher than those elsewhere. During spring, comparatively high densities occurred at DS < 30 m, with values at 10–20 m (0.51 ± 0.17 ind m–2) more than 2 × those found at DS 0–10 m or 20–30 m; no or very few individuals occurred at DS > 30 m. During summer, the highest sea cucumber density occurred at DS 0–10 m (0.51 ± 0.02 ind m–2), almost 2× that from 10 to 20 m (0.24 ± 0.08 ind m–2); no live animal occurred at DS > 30 m, where the water column was stratified and the DO < 1 mg L–1. Although sea cucumbers occurred along all 10-m transect increments in autumn 2017, their densities at DS > 30 m were lower than winter values in that same year. Sea cucumbers also occurred along all 10-m increments along transects in autumn 2019, but densities at DS < 40 m were lower than those at comparable DS in autumn 2017. The density of sea cucumbers at DS of 10–20 m in summer 2020 (0.12 ± 0.03 ind m–2) was less than one-quarter that at this DS in summer of 2017. Unlike summer 2017, when many sea cucumbers gathered at 0–10 m DS, none could be found at the same DS in summer 2020. From this we conclude that H. leucospilota at S1 tended to aggregate in nearshore shallow areas from winter to summer but dispersed deeper in autumn.

Table 3. Results of two-way ANOVA on effects of sampling time (time) and distance from shore (distance) on Holothuria leucospilota density at 10-m increments along transects.
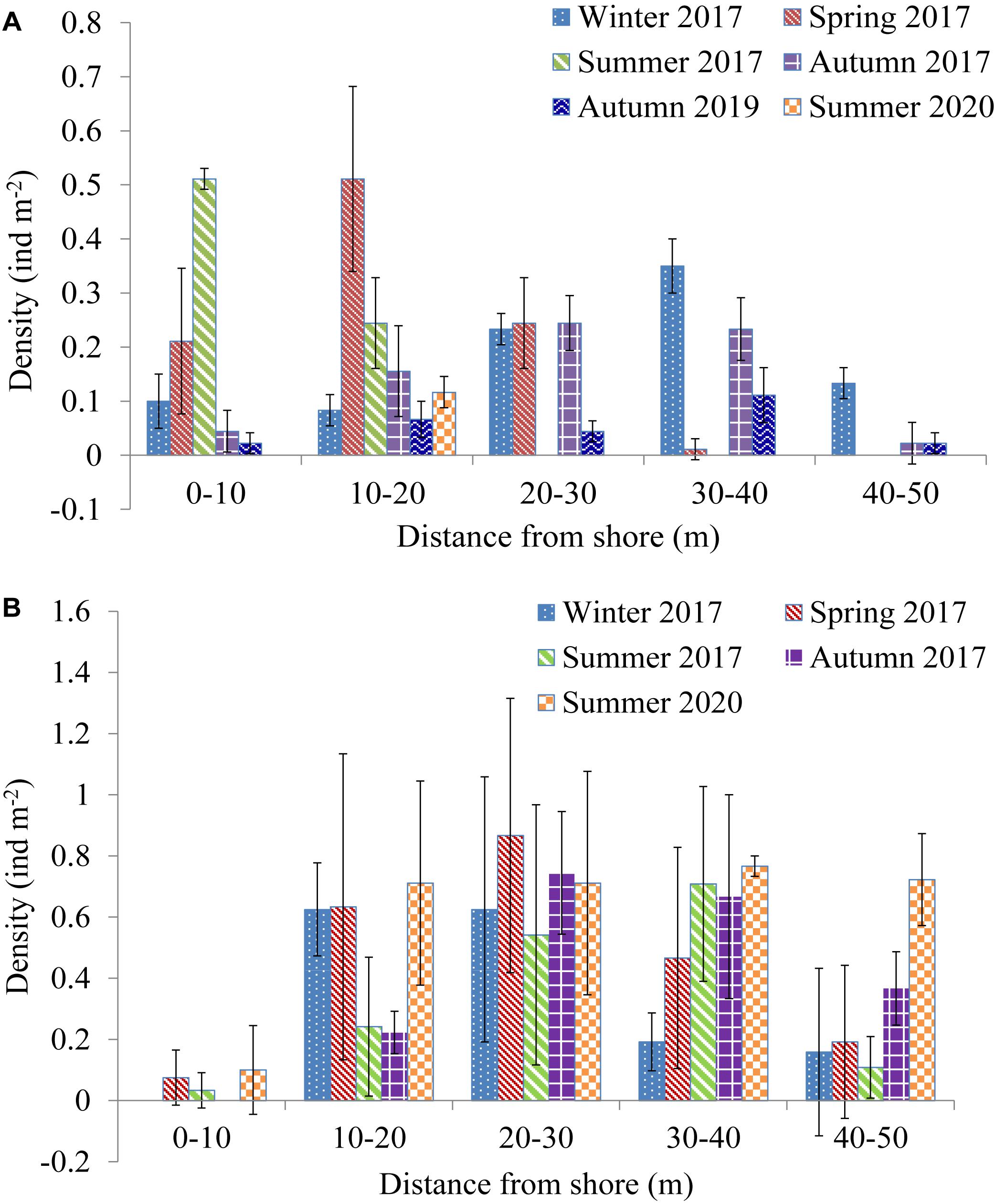
Figure 3. Temporal and spatial distribution of Holothuria leucospilota at 10-m increments along S1 (A) and S2 (B).
The distribution of H. leucospilota at S2 is shown in Figure 3B. Sea cucumbers occurred along all 10-m transect increments in spring and summer, but none occurred from 0 to 10 m DS in winter and autumn. For 2017, high densities occurred at 10–20 m and 20–30 m DS in winter and spring, at densities generally 2–3× those found at 30–40 m and 40–50 m DS at the same sampling time. Most sea cucumbers aggregated at 20–30 m and 30–40 m DS in summer and autumn, with high densities also occurring at 40–50 m DS in autumn (0.37 ± 0.12 ind m–2). Densities were low at 0–10 m DS (0.10 ± 0.15 ind m–2) in summer 2020, but values were high and generally similar along all other 10-m transect increments at DS > 10 m (0.71–0.76 ind m–2). Unalike 2017, high densities of sea cucumber still occurred at > 40 m DS in summer 2020.
Examples of benthic invertebrates sighted at S1 in summer 2017 are shown in Figure 4. Large numbers of decomposing Charybdis japonica and Portunus pelagicus crabs (Figures 4A,B), and tests of the sea urchin Echinocardium spp. and shells of the bivalve Asaphis violascens (Figures 4C,D) were observed from 30 to 50 m DS. No survived sea cucumber remained at DS > 20 m, and three H. leucospilota corpses with expelled Cuvierian tubules were observed from 20 to 40 m DS (Figures 4E,F). These decaying corpses indicate severe hypoxia to anoxia had occurred at DS > 30 m before sampling in summer. In contrast, sea cucumbers from 0 to 10 m DS had normal postures and continued to feed on surface sediments (Figures 4G,H). Few sea cucumbers occurred in 2020 summer sampling; no mass mortality of benthic invertebrates was observed along any 10-m transect increment.
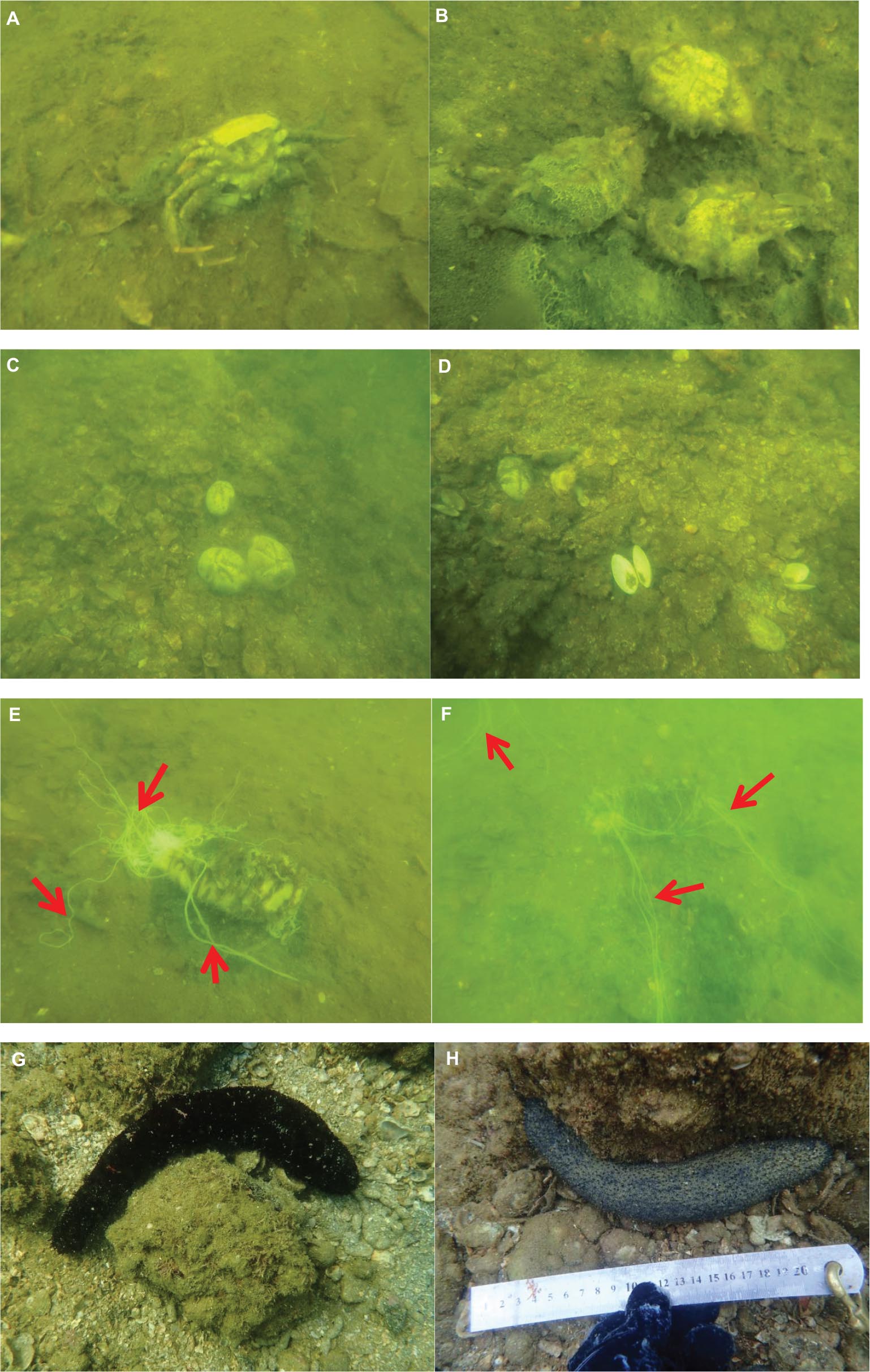
Figure 4. Representative benthic invertebrates at S1 in summer 2017. Decaying corpses of crabs Charybdis japonica (A) and Portunus pelagicus (B) at DS 30–50 m; tests of the heart urchin Echinocardium spp. (C) and shells of the bivalve Asaphis violascens (D) at DS 30–50 m; dead Holothuria leucospilota at DS 20–40 m (E,F), with arrows indicating Cuvierian tubules; live H. leucospilota at DS 0–10 m (G,H).
No H. leucospilota corpses or other dead benthic invertebrates occurred along S2 transects in summer 2017. All observed sea cucumbers were extending their anterior ends to feed on surface sediments, and most at >30 m DS had their anus (at the posterior end of body) oriented upward (Figures 5A–D). In contrast, individuals observed <20 m DS usually had normal posture (Figures 5E,F). All sea cucumbers had normal posture in 2020 summer sampling, and no benthic invertebrate corpses were found along any 10 m transect increment at either site.
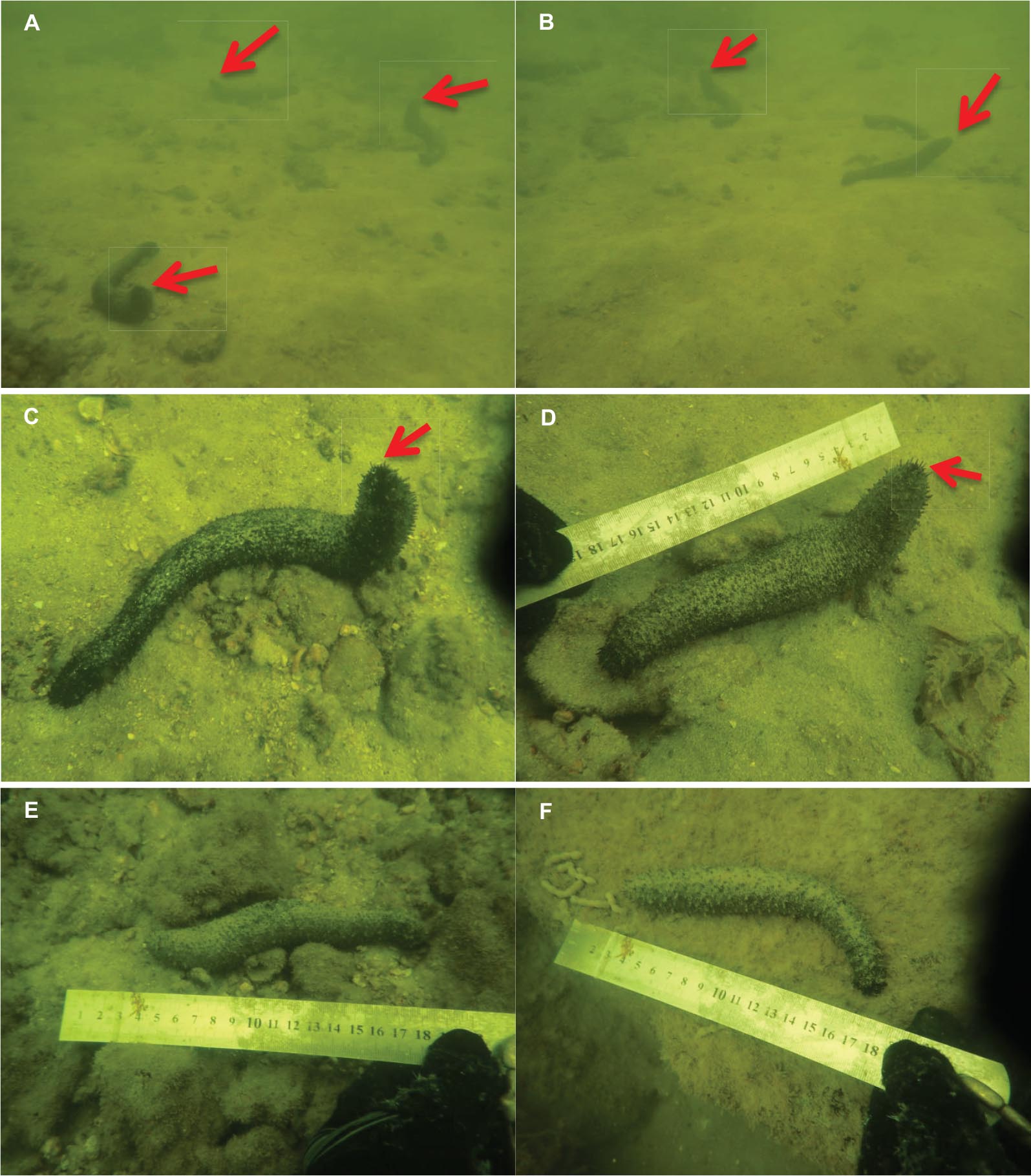
Figure 5. Sea cucumber Holothuria leucospilota at S2 in summer 2017: (A–D) DS 30–50 m, with arrows indicating anus; (E,F) DS 0–20 m.
Abundance
Average sea cucumber abundance at S1 varied with time (one-way ANOVA, P = 0.000) (Figure 6). Abundance increased from winter to spring in 2017, but then decreased over time, with abundances in autumn 2019 (0.06 ± 0.00 ind m–2) and summer 2020 (0.02 ± 0.01 ind m–2) significantly lower than those during four sampling events in 2017 (0.18 ± 0.02, 0.20 ± 0.04, 0.15 ± 0.01, and 0.14 ± 0.03 ind m–2). The rate at which abundance decreased accelerated over time, by 61% from autumn 2017 to autumn 2019, and 58% from autumn 2019 to summer 2020. Average sea cucumber abundance in 2020 was about 10% of 2017 values.
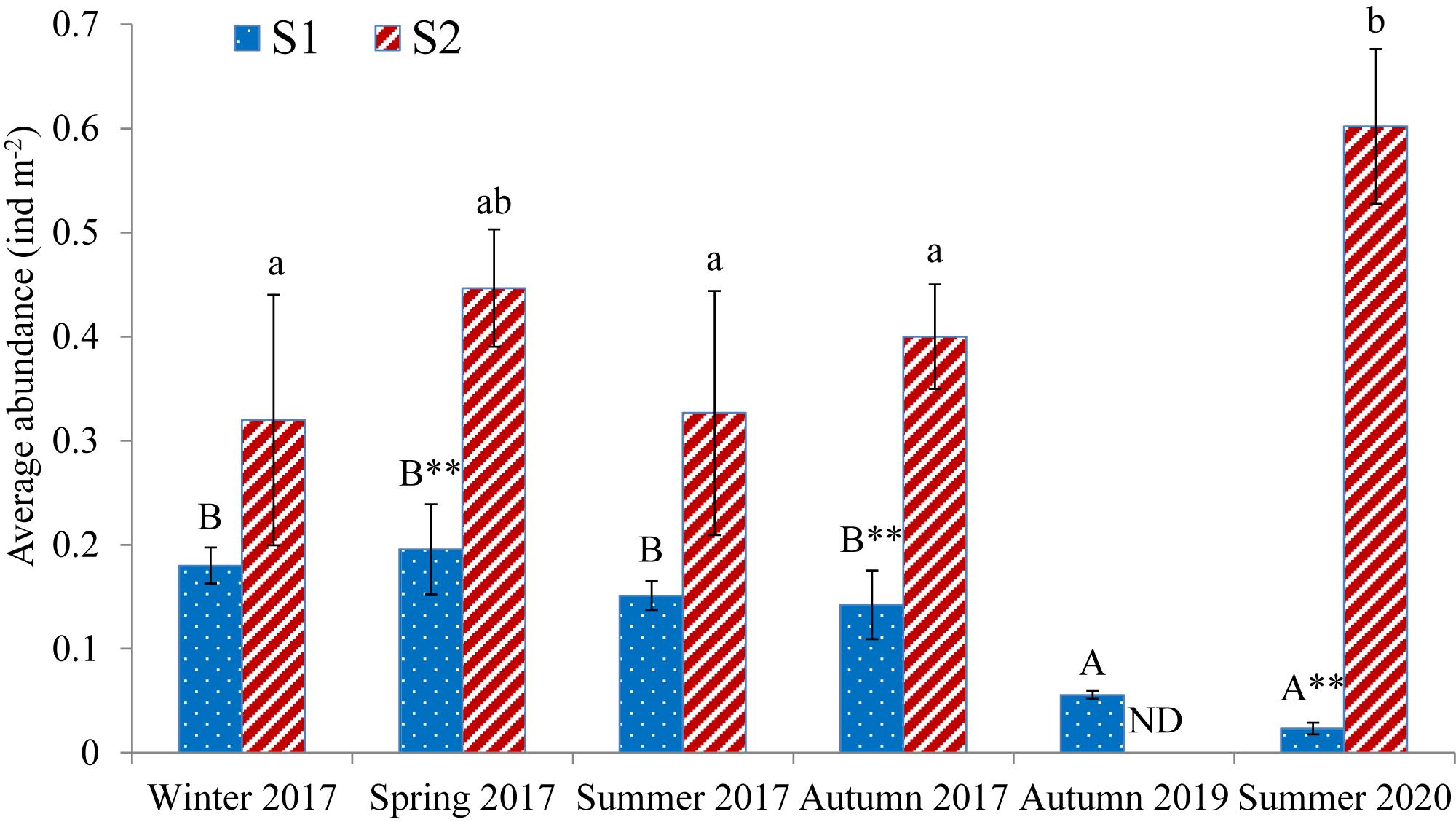
Figure 6. Temporal variation in average abundance of Holothuria leucospilota [mean ± SD (n = 3); ND = no data]. Different superscript letters indicate significant differences in time within a site; asterisks (∗) indicate significant differences between sites at the same time (∗∗P < 0.01).
Average sea cucumber abundance at S2 varied with time (one-way ANOVA, P = 0.019) (Figure 6), and increased by 84% from summer 2017 (0.33 ± 0.12 ind m–2) to summer 2020 (0.60 ± 0.07 ind m–2). Average sea cucumber abundance at S1 was significantly lower than it was at S2 in spring and autumn 2017, and summer 2020 (t-test, P = 0.004, P = 0.002, and P = 0.005, respectively). Average abundances at S2 were 1.8–2.8× those at S1 in 2017, and 25.8× those of S1 in summer 2020.
Population Structure
Summertime sea cucumber body lengths at S1 ranged 11–34 cm in 2017 and 16–30 cm in 2020; at S2 these values were 10–40 cm in 2017 and 7–36 cm in 2020. Relationships between sea cucumber wet weight (W, g) and body length (L, cm) for summer 2017 are described by a positive linear correlation: W = 10.715L − 65.421 (n = 35, R2 = 0.618, P = 0.000). We derive wet weights of sea cucumbers along each transect during summers of 2017 and 2020 using this equation.
Wet-weight population structure at each site in summer of 2017 and 2020 is shown in Figure 7. Sea cucumbers of medium size were prevalent at both sites, excepting S2 in summer 2017, where large individuals were proportionally more abundant (67.86%). The percentage of small-sized sea cucumbers at S1 decreased from 10.29% in 2017 to 0% in 2020, while values at S2 increased from 4.59 to 24.72%. Proportions of large-sized individuals at S1 decreased from 39.71% in 2017 to 28.57% in 2020, while values at S2 decreased from 67.86 to 33.58%.
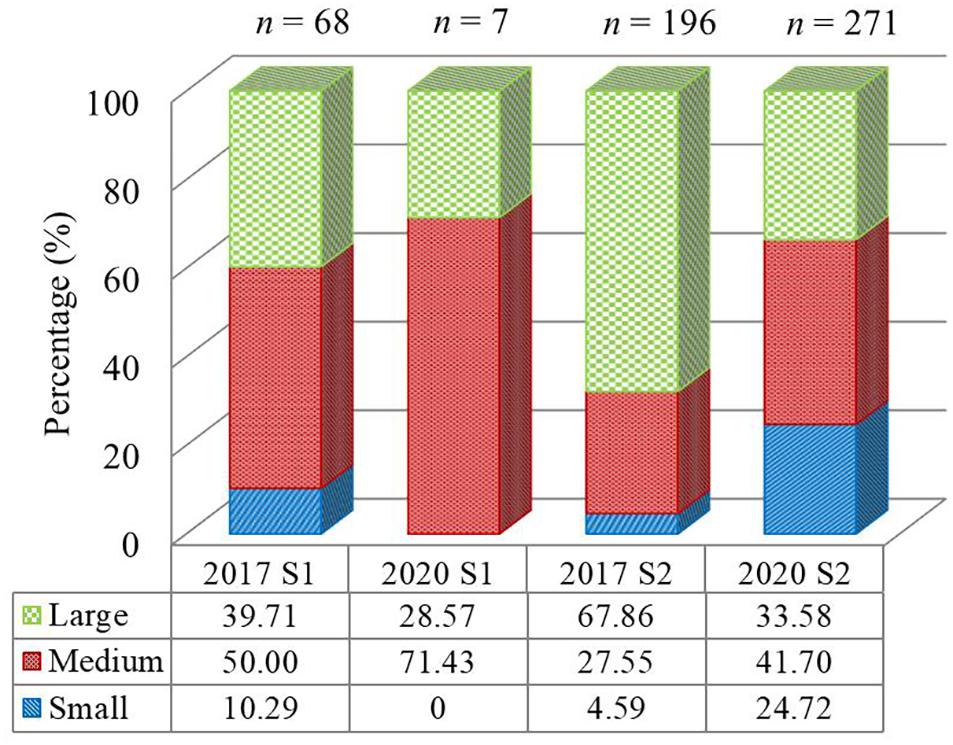
Figure 7. Population structure of the Holothuria leucospilota in summer 2017 and 2020. Size classified by wet weight: small (≤90 g), medium (90–180 g), and large (≥180 g). n = 7–271.
Discussion
Most tropical sea cucumber species in China are intensely fished to meet growing market demand for their products (Purcell et al., 2012; Harith et al., 2018; Huang et al., 2018). Of these, H. leucospilota occurs widely throughout tropical and subtropical oceans, but limited information existed regarding natural stocks of this species in the South China Sea. We report the tendency of one population in an area open to the public (S1) to aggregate in shallow water from winter to summer, but to disperse to deeper water in autumn. From a fishery perspective, the rate of decline in abundance of this species increased from 2017, no small-sized animals were found in 2020, and we consider that the local extinction of this species is an eventual possibility. In contrast, H. leucospilota abundance in a protected area (S2) increased by 84% from summer 2017 to summer 2020, and the percentages of small-sized, recently recruited sea cucumber increased by 20% over this same period.
Distribution
The distribution of sea cucumbers is influenced by various environmental (e.g., water temperature, salinity, substratum, depth, hydrodynamics) and biological (e.g., reproduction, predators, and even the presence of other holothurian species) factors (Massin and Doumen, 1986; Zhou and Shirley, 1996; Drumm et al., 1999; Mercier et al., 2000; Woodby et al., 2000; Harith et al., 2018; Tanita and Yamada, 2019). Relationships between sea cucumbers and their environment also vary geographically, such as the presence of juvenile H. leucospilota being positively correlated with seagrass cover at Pai Island, Indonesia (Setyastuti et al., 2018), but being absent from seagrass beds at Laing Island, Papua New Guinea (Massin and Doumen, 1986). The distribution of coral reef-dwelling H. leucospilota in tropical oceans can be also regulated by physicochemical parameters, substratum type, and hydrodynamics (Massin and Doumen, 1986; Harith et al., 2018; Setyastuti et al., 2018). No comparable information is available for H. leucospilota in coastal waters of China. We report sediment types to comprise relatively homogeneous sands and shell/rubbles from 10 to 50 m DS at each sampling site, and for sea cucumber density to not be very high, especially at S1. Therefore, substratum type and/or food availability might not be key factors regulating the distribution of this species at DS > 10 m at either site. Similarly, no relationship was observed between sea cucumber density and sediment type in the coastal waters of Ishigaki Island (Tanita and Yamada, 2019).
Coastal hypoxia and anoxia represent major stresses for benthic marine species. Mobile fauna can actively avoid hypoxia by migrating from these conditions to more oxygenated waters, with migrations and avoidance behaviour generally starting at DO < 3.0 mg L–1 (Wu, 2002; Riedel et al., 2012, 2014; Cosme and Hauschild, 2016). Echinoderms are aerobic animals with only moderate tolerance to environmental anoxia (Gray et al., 2002; Levin et al., 2009; Riedel et al., 2014; Cosme and Hauschild, 2016), and their threshold for escape responses to hypoxic conditions varies: e.g., the ophiuroid Amphiura filiformis abandons its burrow at DO < 1.2 mg L–1, but A. chiajei emerges from sediments at 0.8 mg L–1 (Rosenberg et al., 1991); and the sea urchin Psammechinus microtuberculatus begins to discard its camouflage and move from beneath bioherms at DO < 2.8 mg L–1, while the infaunal Schizaster canaliferus begins to emerge onto the sediment surface at DO < 1.4 mg L–1 (Riedel et al., 2014).
We report DO levels at S1 to decrease with DS during spring and summer (Table 2). Although we did not directly observe sea cucumber escape behaviours during sampling, hypoxia stress should have already forced H. leucospilota to migrate from deeper into shallower waters (with higher DO), particularly in summer, when specimens aggregated at DS < 20 m (Figure 3A). Decreased pH can also significantly influence benthic macrofaunal fitness and survival (Riedel et al., 2012). We report low pH in summer 2017, especially at S1, which possibly also influenced the distribution of H. leucospilota.
At S2 the seabed was largely flat, lacking refuge atop elevated substrata at >15 m DS (Figure 2). Additionally, S2 bottom waters had higher DO levels, possibly exceeding concentrations that would initiate escape behaviour for H. leucospilota, with no mass migration or avoidance reaction triggered during spring or summer. Sea cucumbers obtain most of their oxygen via their respiratory trees – blind-ended evaginations of the posterior digestive system – which rhythmically inflate with seawater that is drawn in through the anus located atop the posterior end of the body (Conand et al., 1997; Jaeckle and Strathmann, 2013; Weinrauch and Blewett, 2019). Most sea cucumbers at DS > 30 m may have elevated their anus to source oxygenated water from higher in the water column during periods of summer hypoxia (Figure 5). Sea cucumbers were absent from 0 to 10 m DS at S2, where rock prevailed during winter and autumn. This distribution is consistent with (Massin and Doumen, 1986), who reported H. leucospilota preferred non-rocky substrata. However, a few individuals could be observed on rocks in shallow water during spring and summers. The occurrence of H. leucospilota on nearshore rocks might also be explained by their seeking shallow water refuges when DO was low during spring and summer. While the onset and extent of hypoxia disturbance is difficult to forecast and study in the field, animal behaviour can be used to assess hypoxia stress in marine ecosystems (Riedel et al., 2014). The typical “escape” and/or “anus-lifted” behaviours of H. leucospilota may serve as early warning signals of hypoxic conditions.
Abundance
The sea cucumber is a common and conspicuous animal in tropical and subtropical coastal areas. Comparatively high abundances of H. leucospilota can be observed on tropical reefs: e.g., 0.5–1.2 ind m–2 at Reunion Island, Indian Ocean (Conand et al., 1997; Gaudron et al., 2008), 0.8 ind m–2 at One Tree Reef, Great Barrier Reef, Australia (Schneider et al., 2011), and 2.6 ind m–2 at Satang Besar Island, Sarawak, Malaysia (Harith et al., 2018). The abundance of this species appears much lower in subtropical areas, where, for example, on a reef fringing Ishigaki Island, southern Japan, it reaches only 0.31 ind m–2 (Tanita and Yamada, 2019). We report average abundances at S1 from 0.14 to 0.20 ind m–2 in 2017 in an area closed to the public (deemed “virgin abundance”), but for densities at this site to decrease to 0.02 ind m–2 (about 10% of 2017 levels) by summer 2020 after the area was opened to public for fisheries and tourism in 2018. This decrease suggests that this population has been severely over-exploited (a stock between 10 and 30% of virgin abundance) (Friedman et al., 2011; Purcell et al., 2013). In contrast, abundance at S2 (protected from both fisheries and tourism) increased by 84% from 2017 to 2020, and reached 0.60 ind m–2 by summer 2020.
Hypoxia can seriously affect marine life and the ecological balance of coastal waters, mortality of benthic fauna occurs at DO concentrations <2.0–0.5 mg L–1 (Gray et al., 2002; Vaquer-Sunyer and Duarte, 2008). In Gullmarfjord, Sweden, all macrofauna died at DO < 1.4 mg L–1 (Josefson and Widbom, 1988). Benthic macrofauna most resistant to hypoxia include molluscs, followed by polychaetes, echinoderms, and crustaceans (Gray et al., 2002; Levin et al., 2009). Sublethal hypoxia thresholds for echinoderms range 0.7–1.7 mg L–1 (Chu and Tunnicliffe, 2015). For H. leucospilota, survival rate decreases by 18% after 4 d hypoxia exposure (2 mg L–1), and 80% after 22 d exposure (Zamani et al., 2018). We previously reported bottom-cultured H. leucospilota and Apostichopus japonicus to be able to endure high levels of organic and sulphide pollution beneath a fish farm in Dapeng Cove, but not sustained summer hypoxia, and for all individuals to have died after late July when DO concentrations in bottom water dropped below 2.5 mg L–1 (Yu et al., 2013, 2014).
Field studies have shown that DO < 1.0 mg L–1 prevailing for days to weeks can significantly affect benthic community structure (Levin et al., 2009). We report DO < 1.04 mg L–1 at DS > 20 m in summer 2017 (Table 2), and concomitant mass mortality of benthic invertebrates (e.g., crabs, sea cucumbers, sea urchins, bivalves) (Figure 4). While short-term hypoxia does not induce evisceration in sea cucumbers, long-term hypoxia can, and the H. leucospilota can reject the Cuvierian tubules when confront prolonged hypoxia stress (Zamani et al., 2018; Weinrauch and Blewett, 2019). We report dead H. leucospilota at S1 with expelled Cuvierian tubules, indicating long-term exposure to hypoxia. While Cuvierian tubule expulsion could indicate severe hypoxia and the collapse of benthic ecosystems, summer hypoxia-induced mortality may not be the main cause of sea cucumber population decline at S1. Because bottom water oxygen depletion is typically a slow and gradual process, and sea cucumbers at S1 tended to aggregate in shallow water from winter to summer (Figure 3A), most of them could have found shelter from hypoxia at DS < 20 m before severe hypoxia or anoxia occurred in summer 2017. No apparent decline in H. leucospilota abundance occurred in 2017, prior to S1 being opened to the public, but it did decline afterward, with few individuals being found in summer of 2020 when DO levels were relatively high, and there had been no apparent mass mortality of benthic fauna.
Although macrobenthic species biomass and richness in the lower portion of Daya Bay, particularly Dapeng Cove, was negatively influenced by coolant water (heated) discharged from nuclear power plants (Wang et al., 2008), it is unlikely that the abundance of H. leucospilota was directly affected by discharge plumes in recent years. It is more likely that the decrease in abundance of H. leucospilota at S1 was due to other anthropogenic disturbances, particularly fishing pressure from fishermen and tourists, with summer being the tourist season, and with swimming and snorkelling being popular aquatic sports in Daya Bay. Many fishermen and tourists visited S1 in summer from 2018, when H. leucospilota would have migrated to shallow waters within the range of snorkellers. These individuals would be easily captured, possibly explaining why no individual was observed at DS < 10 m (with high DO), and few individuals were found at DS 10–20 m (greater depth along a transect would afford increased protection from fishing) in summer 2020. During summer of 2017, the highest sea cucumber densities occurred at DS < 10 m in waters with increased DO. Concurrently, H. leucospilota abundance increased at S2, which was closed to the public and protected from fisheries and tourism, over this same period.
Wild sea cucumber stocks are easily affected by fisheries, and marine reserves can assist in conserving their declining populations (Uthicke and Benzie, 2000; Anderson et al., 2011; Purcell et al., 2012, 2013; Wolfe and Byrne, 2017; Ramírez-González et al., 2020). In Ngardmau State, Palau, a decline in sea cucumber abundance occurred in one area open to fishing, while recovery occurred within a marine reserve (Rehm et al., 2014). On the Great Barrier Reef, densities of the sea cucumber Holothuria nobilis on reefs protected from fishing were about four times those on reefs open to fishing (Uthicke and Benzie, 2000). We consider S2 functions to an extent as a reserve for sea cucumbers in Daya Bay.
Population Recruitment
Hypoxia can negatively affect reproduction, larval supply, settlement and recruitment of benthic invertebrates: e.g., the brittle star A. filiformis spawning is affected by low DO (2.7 and 1.8 mg L–1) (Nilsson and Skold, 1996), and larvae of the spionid polychaete Paraprionospio pinnata do not settle until DO exceeds 2.0 mg L–1 (Powers et al., 2001). H. leucospilota breed in Daya Bay in summer (Huang et al., 2018), at which time the water column is stratified and pollution (organic enrichment from fish and oyster aquaculture inside the bay, and coolant water from nuclear power plants) could exacerbate hypoxia in Dapeng Cove (Tang et al., 2003; Wang et al., 2008; Yu et al., 2013, 2014). Severe summer hypoxia at S1 might negatively affect spawning and subsequent settlement of larval H. leucospilota, which, combined with broodstock depletion because of fishing pressure and/or hypoxia-induced mortality, might limit recruitment. Abundance declined by 90%, and the proportion of small H. leucospilota decreased from 10.29% in 2017 to 0% (no recruitment) in 2020 at S1.
Sea cucumbers might experience an Allee effect – a correlation between population size or density and mean individual fitness – induced by large reductions in abundance, resulting in slow and negligible recovery, and even population collapse (Uthicke and Benzie, 2000; Uthicke et al., 2009; Anderson et al., 2011; Wolfe and Byrne, 2017). As a traditional fishery target species, H. leucospilota in the open area of Daya Bay will continue to be exploited heavily by local fishermen and tourists. Overfishing and a lack of fisheries and tourism regulations at S1 are typical of the situation throughout China with regard to tropical sea cucumber resources. Recovery of H. leucospilota in this area is unlikely, unless fisheries and tourism desist. In contrast, higher DO concentrations and abundant sea cucumber broodstock in the breeding season at S2 favour recruitment of H. leucospilota, with abundance at this site tending to increase between 2017 and 2020. Site S2 might function as a nursery ground for sea cucumbers, and provide planktonic larvae for recruitment into nearby areas, although it is unknown if these larvae will disperse to areas that are fished. An improved understanding of the biology, ecology, and conservation of tropical sea cucumbers in China is required.
Conclusion
Our results demonstrated that the summer hypoxia at the mouth of a subtropical bay (S1) could force sea cucumber H. leucospilota to aggregate in shallower waters, where they were easily captured by fishermen and tourists after this area was opened to the public. The hypoxia at S1 could negatively influence H. leucospilota spawning and larval settlement, which combined with depletion of broodstock because of fishing pressure and/or hypoxia-induced mortality, could inhibit recruitment. In contrast, higher DO concentrations and abundant broodstock during summer favoured recruitment of H. leucospilota at the protected open-water area (S2). Overall, hypoxia and anthropogenic disturbance impede recovery of H. leucospilota at S1, while the protected S2 might function as a nursery ground for sea cucumbers of the nearby area.
Data Availability Statement
The original contributions presented in the study are included in the article/supplementary material, further inquiries can be directed to the corresponding author.
Author Contributions
ZY: conceptualisation, funding acquisition, investigation, data curation, writing – original draft, review, and editing. YZ: data curation, writing – original draft, review, and editing. LZ and HS: investigation. All authors contributed to the article and approved the submitted version.
Funding
This research was supported by the National Key R&D Program of China (2019YFD0900800 and 2020YFD0901104) and the National Natural Science Foundation of China (41676162).
Conflict of Interest
The authors declare that the research was conducted in the absence of any commercial or financial relationships that could be construed as a potential conflict of interest.
Publisher’s Note
All claims expressed in this article are solely those of the authors and do not necessarily represent those of their affiliated organizations, or those of the publisher, the editors and the reviewers. Any product that may be evaluated in this article, or claim that may be made by its manufacturer, is not guaranteed or endorsed by the publisher.
Acknowledgments
We are grateful to Manhong Wang for his assistance in field investigations.
References
Akamine, J. (2001). Holothurian exploitation in the Philippines: continuities and discontinuities. Tropics 10, 591–607. doi: 10.3759/tropics.10.591
Anderson, S. C., Flemming, J. M., Watson, R., and Lotze, H. K. (2011). Serial exploitation of global sea cucumber fisheries. Fish Fish. 12, 317–339. doi: 10.1111/j.1467-2979.2010.00397.x
Bonham, K., and Held, E. E. (1963). Ecological observations on the sea cucumbers Holothuria atra and H. leucospilota at Rongelap Atoll. Marshall Islands. Pac. Sci. 17, 305–314.
Che, R. G. O. (1990). Aspects of feeding biology of Holothuria leucospiolota brandt (Echinodermata: Holothuroidea) in Hong Kong. Asian Mar. Biol. 7, 133–146.
Chu, J. W. F., and Tunnicliffe, V. (2015). Oxygen limitations on marine animal distributions and the collapse of epibenthic community structure during shoaling hypoxia. Glob. Change Biol. 21, 2989–3004. doi: 10.1111/gcb.12898
Conand, C., Morel, C., and Mussard, R. (1997). A new case of asexual reproduction in holothurians: fission in Holothuria leucospilota populations on Reunion island in the Indian Ocean. SPC Beche-de-mer Inf. Bull. 9, 5–11.
Cosme, N., and Hauschild, M. Z. (2016). Effect factors for marine eutrophication in LCIA based on species sensitivity to hypoxia. Ecol. Indic. 69, 453–462. doi: 10.1016/j.ecolind.2016.04.006
Drumm, D. J., and Loneragan, N. R. (2005). Reproductive biology of Holothuria leucospilota in the Cook Islands and the implications of traditional fishing of gonads on the population. N. Zealand J. Mar. Fresh. 39, 141–156. doi: 10.1080/00288330.2005.9517297
Drumm, D., Purvis, M., and Zhou, Q. (1999). “Spatial ecology and artificial neural networks: modelling the habitat preference of the sea cucumber (Holothuria leucospilota) on Rarotonga, cook Islands,” in Proceedings of The 11th Annual Colloquium of the Spatial Information Research Centre. Dunedin, New Zealand: University of Otago.
Dzeroski, S., and Drumm, D. (2003). Using regression trees to identify the habitat preference of the sea cucumber (Holothuria leucospilota) on Rarotonga. Cook Islands. Ecol. Model. 170, 219–226. doi: 10.1016/s0304-3800(03)00229-1
Friedman, K., Eriksson, H., Tardy, E., and Pakoa, K. (2011). Management of sea cucumber stocks: patterns of vulnerability and recovery of sea cucumber stocks impacted by fishing. Fish Fish. 12, 75–93. doi: 10.1111/j.1467-2979.2010.00384.x
Gaudron, S. M., Kohler, S. A., and Conand, C. (2008). Reproduction of the sea cucumber Holothuria leucospilota in the Western Indian Ocean: biological and ecological aspects. Invertebr. Reprod. Dev. 51, 19–31. doi: 10.1080/07924259.2008.9652253
Gray, J. S., Wu, R. S. S., and Or, Y. Y. (2002). Effects of hypoxia and organic enrichment on the coastal marine environment. Mar. Ecol. Prog. Ser. 238, 249–279. doi: 10.3354/meps238249
Harith, M. N., Md Desa, M. H. I., and Ilias, Z. (2018). Holothuria leucospilota population in Satang Besar Island. Sarawak, Malaysia. Int. J. Zool. 2018:8047029.
Huang, W., Huo, D., Yu, Z., Ren, C., Jiang, X., Luo, P., et al. (2018). Spawning, larval development and juvenile growth of the tropical sea cucumber Holothuria leucospilota. Aquaculture 488, 22–29. doi: 10.1016/j.aquaculture.2018.01.013
Jaeckle, W. B., and Strathmann, R. R. (2013). The anus as a second mouth: anal suspension feeding by an oral deposit-feeding sea cucumber. Invertebr. Biol. 132, 62–68. doi: 10.1111/ivb.12009
Josefson, A. B., and Widbom, B. (1988). Differential response of benthic macrofauna and meiofauna to hypoxia in the Gullmar Fjord basin. Mar. Biol. 100, 31–40. doi: 10.1007/bf00392952
Levin, L. A., Ekau, W., Gooday, A. J., Jorissen, F., Middelburg, J. J., Naqvi, S. W. A., et al. (2009). Effects of natural and human-induced hypoxia on coastal benthos. Biogeosciences 6, 2063–2098. doi: 10.5194/bg-6-2063-2009
Liao, Y. (1997). Fauna Sincia: Phylum Echinodermata Class Holothuroidea. Beijing, China: Science Press.
Massin, C., and Doumen, C. (1986). Distribution and feeding of epibenthic holothuroids on the reef flat of Laing Island (Papua New Guinea). Mar. Ecol. Prog. Ser. 31, 185–195. doi: 10.3354/meps031185
Mercier, A., Battaglene, S. C., and Hamel, J. F. (2000). Periodic movement, recruitment and size-related distribution of the sea cucumber Holothuria scabra in Solomon Islands. Hydrobiologia 440, 81–100. doi: 10.1007/978-94-017-1982-7_8
Nilsson, H. C., and Skold, M. (1996). Arm regeneration and spawning in the brittle star Amphiura filiformis (O.F. Muller) during hypoxia. J. Exp. Mar. Biol. Ecol. 199, 193–206. doi: 10.1016/0022-0981(95)00188-3
Powers, S. P., Harper, D. E., and Rabalais, N. N. (2001). “Effect of hypoxia/anoxia on the supply and settlement of benthic invertebrate larvae,” in Coastal and Estuarine Sciences: Coastal Hypoxia: Consequences for Living Resources and Ecosystems, Vol 58, ed. N. N. Rabalais. Washington, DC: AGU.
Purcell, S. W., Mercier, A., Conand, C., Hamel, J.-F., Toral-Granda, M. V., Lovatelli, A., et al. (2013). Sea cucumber fisheries: global analysis of stocks, management measures and drivers of overfishing. Fish Fish. 14, 34–59. doi: 10.1111/j.1467-2979.2011.00443.x
Purcell, S. W., Samyn, Y., and Conand, C. (2012). Commercially Important Sea Cucumbers of the World. Rome: FAO.
Ramírez-González, J., Moity, N., Andrade-Vera, S., and Reyes, H. (2020). Overexploitation and more than a decade of failed management leads to no recovery of the galápagos sea cucumber fishery. Front. Mar. Sci. 7:554314.
Rehm, L., Koshiba, S., Mereb, G., Olsudong, D., Seksei, F., and Remeliik, K. (2014). Status of Sea Cucumber Populations Inside and Outside a Marine Protected Area in Ngardmau State, Palau, PICRC Technical Report. Koror: Palau International Coral Reef Center
Riedel, B., Pados, T., Pretterebner, K., Schiemer, L., Steckbauer, A., Haselmair, A., et al. (2014). Effect of hypoxia and anoxia on invertebrate behaviour: ecological perspectives from species to community level. Biogeosciences 11, 1491–1518. doi: 10.5194/bg-11-1491-2014
Riedel, B., Zuschin, M., and Stachowitsch, M. (2012). Tolerance of benthic macrofauna to hypoxia and anoxia in shallow coastal seas: a realistic scenario. Mar. Ecol. Prog. Ser. 458, 39–52. doi: 10.3354/meps09724
Rosenberg, R., Hellman, B., and Johansson, B. (1991). Hypoxic tolerance of marine benthic fauna. Mar. Ecol. Prog. Ser. 79, 127–131. doi: 10.3354/meps079127
Schneider, K., Silverman, J., Woolsey, E., Eriksson, H., Byrne, M., and Caldeira, K. (2011). Potential influence of sea cucumbers on coral reef CaCO3 budget: a case study at one tree reef. J. Geophys. Res. 116:G04032.
Setyastuti, A., Dharmawan, I. W., Hafizt, M., Vimono, I., and Aji, L. (2018). Discovery of Holothuria leucospilota juveniles on Pai Island, Biak-Papua, and an overview of sea cucumber nursery grounds in Indonesia. SPC Beche-de-mer Inf. Bull. 38, 29–36.
Sloan, N. A. (1979). Microhabitat and resource utilization in cryptic rocky inter-tidal echinoderms at Aldabra Atoll, Seychelles. Mar. Biol. 54, 269–279. doi: 10.1007/bf00395789
Tang, D. L., Kester, D. R., Wang, Z. D., Lian, J. S., and Kawamura, H. (2003). AVHRR satellite remote sensing and shipboard measurements of the thermal plume from the Daya Bay, nuclear power station, China. Remote Sens. Environ. 84, 506–515. doi: 10.1016/s0034-4257(02)00149-9
Tanita, I., and Yamada, H. (2019). Distribution of sea cucumbers in relation to sediment characteristics in coral reef lagoons and adjacent waters around Ishigaki Island, southern Japan. Mar. Ecol. 40:e12564.
Uthicke, S., and Benzie, J. (2000). Effect of bêche-de-mer fishing on densities and size structure of Holothuria nobilis (Echinodermata: Holothuroidea) populations on the Great Barrier Reef. Coral Reefs 19, 271–276. doi: 10.1007/s003380000118
Uthicke, S., Schaffelke, B., and Byrne, M. (2009). A boom–bust phylum? ecological and evolutionary consequences of density variations in echinoderms. Ecol. Monogr. 79, 3–24. doi: 10.1890/07-2136.1
Vaquer-Sunyer, R., and Duarte, C. M. (2008). Thresholds of hypoxia for marine biodiversity. Proc. Natl. Acad. Sci. USA 105, 15452–15457. doi: 10.1073/pnas.0803833105
Wang, Y. S., Lou, Z. P., Sun, C. C., and Sun, S. (2008). Ecological environment changes in Daya Bay, China, from 1982 to 2004. Mar. Pollut. Bull. 56, 1871–1879. doi: 10.1016/j.marpolbul.2008.07.017
Weinrauch, A. M., and Blewett, T. A. (2019). Anoxia tolerance in the sea cucumbers Parastichopus californicus and Cucumaria miniata reflects habitat use. J. Exp. Mar. Biol. Ecol. 520:151203. doi: 10.1016/j.jembe.2019.151203
Wolfe, K., and Byrne, M. (2017). Population biology and recruitment of a vulnerable sea cucumber, Stichopus herrmanni, on a protected reef. Mar. Ecol. 38:e12397. doi: 10.1111/maec.12397
Woodby, D., Smiley, S., and Larson, R. (2000). Depth and habitat distribution of Parastichopus californicus near sitka, Alaska. Alaska Fish. Res. Bull. 7, 22–32.
Wu, R. S. S. (2002). Hypoxia: from molecular responses to ecosystem responses. Mar. Pollut. Bull. 45, 35–45. doi: 10.1016/s0025-326x(02)00061-9
Yu, Z., Hu, C., Qi, Z., Jiang, H., Ren, C., and Luo, P. (2012). Co-culture of sea cucumber Holothuria leucospilota with the Pacific white shrimp Litopenaeus vannamei. J. Fish. China 36, 1081–1087. doi: 10.3724/sp.j.1231.2012.27864
Yu, Z., Hu, C., Zhou, Y., Li, H., and Peng, P. (2013). Survival and growth of the sea cucumber Holothuria leucospilota Brandt: a comparison between suspended and bottom cultures in a subtropical fish farm during summer. Aquac. Res. 44, 114–124. doi: 10.1111/j.1365-2109.2011.03016.x
Yu, Z., Zhou, Y., Qi, Z., Luo, P., and Hu, C. (2017). Temporal dynamics in clearance rate of the Portuguese oyster Crassostrea angulata cultivated in Dapeng Cove, southern China. Aquaculture 479, 824–828. doi: 10.1016/j.aquaculture.2017.07.030
Yu, Z., Zhou, Y., Yang, H., Ma, Y., and Hu, C. (2014). Survival, growth, food availability and assimilation efficiency of the sea cucumber Apostichopus japonicus bottom-cultured under a fish farm in southern China. Aquaculture 426, 238–248. doi: 10.1016/j.aquaculture.2014.02.013
Zamani, N., Assidqi, K., and Madduppa, H. (2018). Study of the tolerance of black sea cucumber Holothuria leucospilota to hypoxia stress. Pertanika J. Trop. Agr. Sci. 41, 1511–1521.
Keywords: sea cucumber, summer hypoxia, overexploitation, fishing pressure, recruitment, benthic invertebrates, escape behaviour, mass mortality
Citation: Yu Z, Zhang Y, Zhou L and Sun H (2021) The Effects of Hypoxia and Anthropogenic Disturbance on the Distribution, Abundance and Recruitment of the Sea Cucumber Holothuria leucospilota at the Mouth of a Subtropical Bay, China. Front. Mar. Sci. 8:739657. doi: 10.3389/fmars.2021.739657
Received: 11 July 2021; Accepted: 23 August 2021;
Published: 09 September 2021.
Edited by:
Huang Wei, Ministry of Natural Resources, ChinaReviewed by:
Xiutang Yuan, Yantai Institute of Coastal Zone Research, Chinese Academy of Sciences (CAS), ChinaMalcolm Beveridge, Retired, Crieff, United Kingdom
Copyright © 2021 Yu, Zhang, Zhou and Sun. This is an open-access article distributed under the terms of the Creative Commons Attribution License (CC BY). The use, distribution or reproduction in other forums is permitted, provided the original author(s) and the copyright owner(s) are credited and that the original publication in this journal is cited, in accordance with accepted academic practice. No use, distribution or reproduction is permitted which does not comply with these terms.
*Correspondence: Zonghe Yu, eXV6aEBzY2F1LmVkdS5jbg==