- 1Hellenic Centre for Marine Research, Institute of Oceanography, Heraklion, Greece
- 2Hellenic Centre for Marine Research, Institute of Marine Biological Resources, Heraklion, Greece
- 3Department of Biology, University of Crete, Heraklion, Greece
Marine benthic ecosystems face well-documented changes as a result of human activities. Describing these changes is important for predicting ecosystem functioning. In this context, long-term changes in soft-bottom macrofaunal communities after a quarter of a century were studied in the south Aegean Sea with the purpose of investigating whether temporal changes in taxa diversity are accompanied by changes in functional diversity, and secondly to determine the main mechanisms driving these changes (i.e., deterministic versus stochastic processes). To achieve this, a large data set that included species abundance data collected in 1990 and 2014 from several sampling sites along a transect line was used. A biological trait analysis (BTA) was conducted to determine the species functional roles. The results revealed a decline in taxonomic alpha and beta diversity metrics between 1990 and 2014, a difference that was also reflected in functional richness, partially in functional redundancy, but not in functional composition. The stability of functional composition indicated that replacements of functionally similar taxa may occur, ensuring the resilience of the ecosystem to provide goods and services. Finally, the comparison of co-occurrence and functional networks for 1990 indicated a non-differentiation with the null model and, it was not possible to determine if the benthic community was structured due to stochastic processes (e.g., dispersal, natural phenomena) or an overlap of deterministic processes (e.g., niche-filtering, competition). In contrast, the comparison of networks for 2014 pointed out that environmental conditions have acted as a major filter on species distribution.
Introduction
Human activities are driving rapid changes in the marine environment. In the current times of global change, marine ecosystems are affected by many anthropogenic activities, and face unique threats such as ocean acidification and climate change (Halpern et al., 2008). The consequence of these stressors is a documented change in species richness, and composition (McCauley et al., 2015). Biodiversity change over time has two facets: temporal alpha diversity, which is an expression of change in the abundance and structure of assemblages, and temporal beta diversity which tracks changes attributed to species composition (Legendre, 2019; Magurran et al., 2019). The challenge in measuring beta diversity is to capture the ongoing temporal changes in a way that reflects the ecological processes, and the ecosystem functions, that are involved (Magurran et al., 2019). In this context, an increasing interest in the predictability in the function of marine ecosystems has been raised (Worm et al., 2006; Gogina et al., 2017). The identity of organisms in terms of their activity and function are known to influence both the magnitude, and the variability of ecosystem processes (Solan et al., 2008).
Considering the functional identity of organisms and grouping them by ecological equivalency (e.g., physiological, behavioral aspects) into functional groups is a widespread approach that has led to a better understanding of the role of species within a community and the relationship between ecological function, spatial distribution and environmental characteristics (Bolam et al., 2017). Trait-based indices provide a useful tool for the quantitative prediction of ecosystem processes as biological traits analysis (BTA) can provide a direct link to certain functional properties related to life history, behavioral or morphological characteristics of macrofaunal organisms that drive ecosystem functioning (Bremner, 2008).
Measuring functional diversity allows the assessment of the contributions of unique species to ecosystem functioning and the prediction of the impacts of species loss (Micheli and Halpern, 2005; Teichert et al., 2017). The order in which the species are lost as well as the trait similarity between species, determine the impact of biodiversity loss on ecosystem functioning (Ricotta et al., 2016; Teichert et al., 2017). Species with similar biological traits are functionally redundant and result in a community where a species loss may cause only minor impact in ecosystem functioning (Naeem, 1998). In contrast, species with little trait similarity support high functional diversity and a species loss may have a more severe impact in ecosystem functioning (Teichert et al., 2017).
Factors that could potentially induce long-term changes and shape benthic communities are either deterministic or stochastic (Connor and Simberloff, 1979; Drake, 1990). Deterministic factors may include environmental filtering and limiting similarity processes (i.e., competitive exclusion) (Macarthur and Levins, 1967; Somerfield et al., 2009; Vallée et al., 2019). In environmental filtering, environmental conditions act as the filter on species distribution (Colorado Zuluaga, 2015). A change in environmental constraints may drive community change. Environmental constraints or filters act by removing species that lack specific traits (Belyea and Lancaster, 1999). Thus, traits are filtered and, with them, species. In competitive exclusion, species with similar traits are negatively associated. Stochastic events may interrupt or weaken species interactions and niche filtering (Colorado Zuluaga, 2015). Under stochastic processes, it is expected that the distribution of species will not be different from a random pattern and no directional trends would occur (Gotelli and McGill, 2006).
One of the difficulties in correlating ecological shifts to driving factors is the relative absence of long-term time series (Clare et al., 2017). In the oligotrophic Eastern Mediterranean, long-term time series monitoring benthic macrofauna composition are rare (Salen-Picard and Arlhac, 2002). Nevertheless, comparison of current data with historical data has been already used in many cases and has been effective in inferring temporal changes (Cartes et al., 2009; Romero-Ramirez et al., 2016; Bonifácio et al., 2018; Tsikopoulou et al., 2018) but to the best of our knowledge, none of them investigate the changes in ecosystem functioning. In contrast, these studies are more common in the North Sea (Cartes et al., 2009; Meyer and Kröncke, 2019; Shojaei et al., 2021), the English Channel (Davoult et al., 1993, 1998; Dauvin and Pezy, 2013) and the Baltic Sea (Salo et al., 2020) and proved significant for the coastal management and the maintenance of the goods and services provided by marine ecosystems (Hinz et al., 2021; Shojaei et al., 2021).
In this context, long-term changes in soft-bottom macrofaunal communities between 1990 and 2014 were studied in the south Aegean Sea with the purpose of investigating whether temporal changes in species diversity are accompanied by changes in functional diversity. Specifically, the objectives were: (i) to test for temporal differences in taxonomic diversity and functional diversity of the benthic communities between 1990 and 2014, (ii) to estimate functional redundancy in benthic communities in 1990 and 2014, and (iii) to determine the main mechanisms that drove the potential differences in benthic communities between 1990 and 2014 by comparing the congruence between functional and co-occurrence networks. Our hypotheses were that: (1) there would be a difference in taxonomic diversity between 1990 and 2014, and (2) this difference would affect functional diversity.
Materials and Methods
Study Site and Sampling Strategy
The study was conducted along a transect line in Heraklion Bay, Crete (south Aegean Sea). The continental shelf along the north coast of Crete forms a relative open bay in front of Heraklion city and is considered as an oligotrophic environment (Psarra et al., 2000; Lampadariou and Eleftheriou, 2018). Heraklion Bay is under slight pressure mainly from winter rain related discharges of domestic wastes and nutrients from agriculture (Simboura et al., 2016). The construction of the Heraklion municipality wastewater treatment plant in 1996 further reduced the organic enrichment by Heraklion city waste discharges removing annually >160,000 kg of dry solids (Tsikopoulou et al., 2018). The annual average for historical and recent environmental conditions of the study area are presented in Table 1.
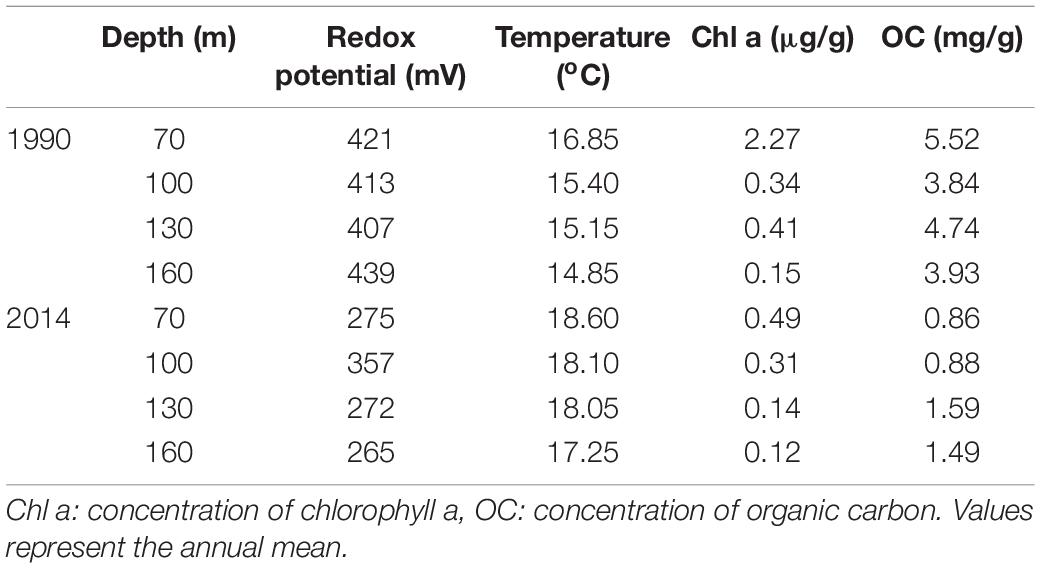
Table 1. Historical and recent data on the environmental conditions prevailed in the top layer of the sediment during the 1990 and 2014 samplings.
In 1985, a comprehensive program (NATO funded GR-FISHECO) was initiated by the Institute of Marine Biology of Crete for the investigation of the structure and dynamics of benthic communities of the shelf ecosystem in the Cretan Sea. In 2014, the area was revisited twice (May and November) and the samples taken were compared to those taken in 1990 (May and November). Sampling took place in 4 sites at 70, 100, 130, and 160 m depth. Both sampling campaigns followed identical experimental designs and methodology in terms of sampling gear, techniques, sampling season and station coordinates.
Macrofaunal Analysis
At each site, 7 replicates were collected for macrofaunal analysis using a 0.1 m2 Smith-McIntyre grab. Benthic samples were sieved in situ over a 0.5 mm mesh, fixed with 5% buffered formalin, stained with Rose Bengal and stored for species identification. In the laboratory, the samples were sorted by hand and specimens were identified to the species or to the lowest possible taxonomic level and then counted. To address the issue of differences in taxonomic resolution between the surveys, and also the bias caused during the identification procedure and different taxonomic expertise between sampling periods, all analyses were performed on data aggregated at the genus level. Following the “taxonomic sufficiency” approach, aggregating to the genus level does not result in any loss of information (Mueller et al., 2013; Bolam et al., 2014; Bonifácio et al., 2018).
A BTA was conducted on the macrofaunal communities to determine their functional diversity. Ten biological traits (subdivided into 50 modalities) were chosen for the analysis reflecting species morphology, life history characteristics and behavior (Bolam et al., 2017) (Table 2). Individual taxa were coded for the modalities of each trait using a fuzzy-coding procedure, which allows assessment of the affinity of a taxon to multiple categories using a discrete score from 0 (no affinity) to 3 (total affinity). To avoid bias among the different modalities, the affinity scores for each trait were standardized so that the sum was equal to 1 (Liu et al., 2019). The taxa were classified into different functional categories based on information from a variety of literature sources (e.g., Queirós et al., 2013; Jumars et al., 2015; Renz et al., 2018; Wrede et al., 2018) and databases1 ,2. A table of stations by abundance-weighted traits was produced for 1990 and 2014. Specifically, trait scores of each taxon were multiplied by its abundance for every sample and then summed to provide the overall frequency of each trait modality per sample for the two sampling periods.
Data Analysis
To describe historical and recent taxonomic and functional diversity of the benthic communities, biodiversity and functional diversity indices were calculated for all sites in 1990 and 2014, respectively. These indices were richness (number of taxa per sample, S), total abundance per sample (N), and functional richness (FRic) and uniqueness, i.e., the functional contribution of single taxa to the overall redundancy of the whole assemblage (Ricotta et al., 2016). A two-way analysis of variance (ANOVA) was performed to identify significant differences (p < 0.05) in all indices between 1990 and 2014 for all depths. In cases of significant interaction between years and depths, simple effects were assessed (variance between years at each depth). Pairwise comparisons were performed using the Tukey post hoc test. Prior to the analysis, all datasets were examined for the assumptions of normality using the Shapiro-Wilk test and homogeneity of variance using Levine’s test. Seasonal fluctuations of the diversity indices were not the aim of this study and as a result, seasonal variation within each year was not presented here but can be found in Tsikopoulou et al. (2018).
Changes in functional composition through time were estimated using the temporal beta-diversity index (TBI) (Legendre, 2019) (Figure 1). First, TBI was computed using community trait data for each site, measuring the change in functional composition between the first (1990) and second sampling periods (2014). To calculate functional TBI, a Gower dissimilarity matrix was computed for the trait data (Bello et al., 2021). A principal coordinate analysis (PCoA) was applied to the square-rooted Gower dissimilarities and then, all PCoA axes were used as input matrices for the TBI analysis. Euclidean distance was the basis for computing the functional TBI (Legendre, 2019).
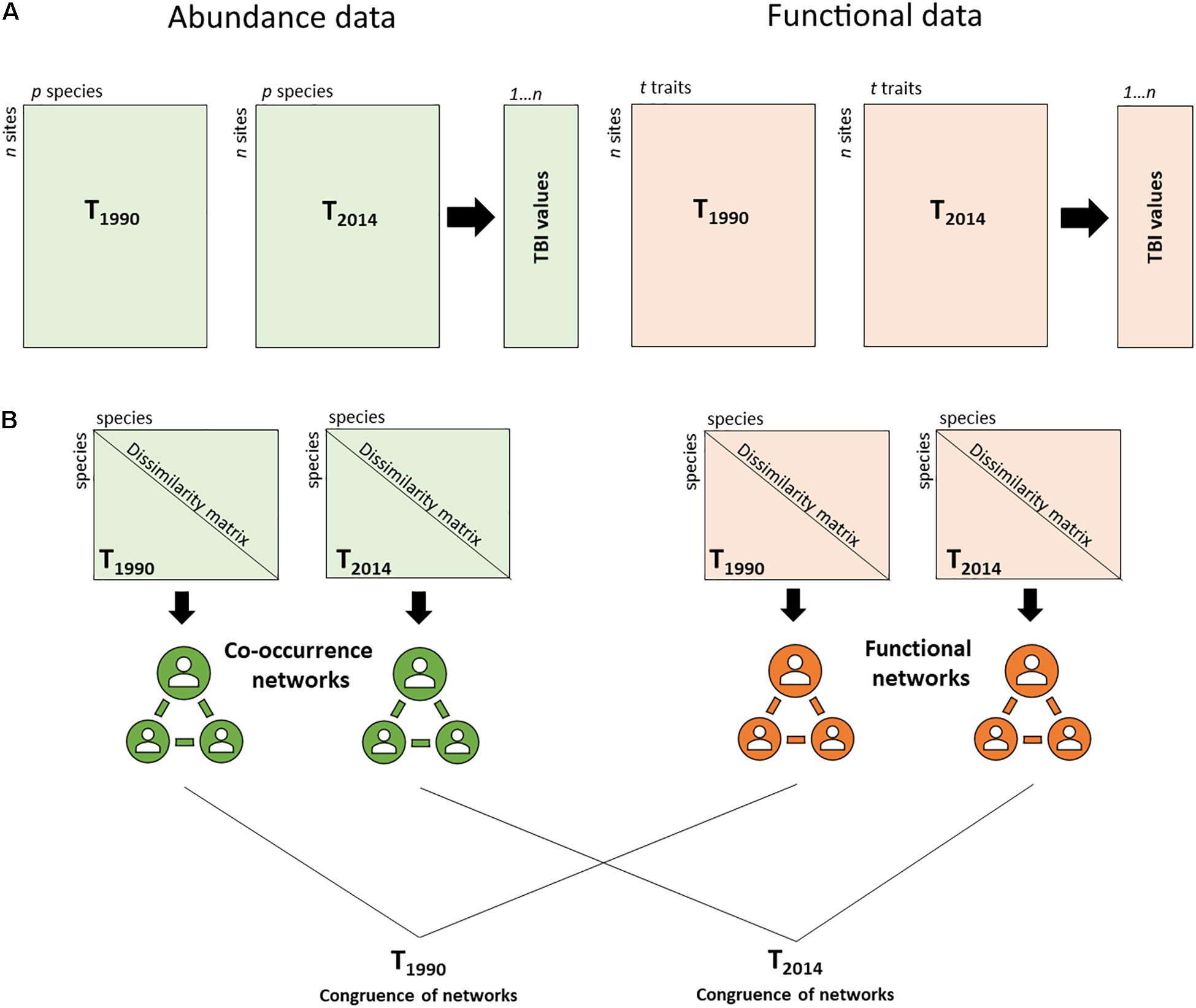
Figure 1. Methodological approach (A) comparing temporal taxonomic and functional beta diversity (TBI) for two time periods, 1990 and 2014, (B) assessing the congruence of the co-occurrence and functional networks to determine the main assembly rules of benthic communities for 1990 and 2014.
To better interpret possible changes in functional TBI, taxonomic TBI was also computed. Bray-Curtis dissimilarity was performed on log-transformed abundance data and used as input for computing taxonomic TBI. Taxonomic TBI was decomposed into β diversity explained by either species temporal losses or temporal gains. The significance of the processes (losses or gains) dominating and causing community composition changes between 1990 and 2014 was tested using parametric paired t-tests (Legendre, 2019).
The SIMPER analysis (Clarke and Gorley, 2015) was performed in Primer 7 software to identify the 90% of the taxa that contributed mostly to dissimilarities in community composition between 1990 and 2014. Afterward, to determine the main drivers that influenced the structure of the communities between 1990 and 2014, the taxa co-occurrence networks (based on abundances) were compared to the functional networks (based on traits) for the two periods, following a methodological approach based on the network theory and modularity concept described in Legras et al. (2019) (Figure 1). Briefly, the methodology included three steps: First, the functional and the taxa co-occurrence networks were defined. The functional network was derived from Gower dissimilarities computed from the trait matrix (1 – Gower index). The values of this matrix were used to weight edges between genera in the functional network. For the co-occurrence network, the values of Bray-Curtis similarity matrix (1 – Bray-Curtis dissimilarity index) were used to weight the edges between taxa. Modularity (using Louvain’s optimization methodology) was used to define groups of taxa in the functional and co-occurrence networks (Legras et al., 2019). In the next step, an index of module diversity was calculated for each functional group. This index allows one to assess if taxa from a specific functional group in the functional network tend to be also in the same co-occurrence group. In the final step, the mean of all module diversity indices (DgM) was compared with DgM obtained from null models. DgM should be lower than 5% of the null distribution if environmental filtering dominates and higher than 95% of the null distribution if limiting similarity and competition is dominant. In contrast, if there is a dominance of stochastic processes or a mix of both deterministic and stochastic processes the observed DgM is expected to range between 5 and 95% of the null distribution.
All the aforementioned statistical analyses were carried out with “FD” (Laliberté and Legendre, 2010), “gawdis” (Bello et al., 2021), “adespatial” (Dray et al., 2012), “ade4” (Dray et al., 2007), “modMax” (Schelling and Hui, 2015) and “ecodist” (Goslee and Urban, 2007) packages in the R program (version 4.0.2).
Results
Alpha Diversity Differences Between 1990 and 2014
Changes in the univariate biodiversity and functional diversity indices among the factors, year and depth, and their statistical significance are shown in Figure 2 and Table 3, respectively. Specifically, significant decreases in richness (S) and total abundance (N) were recorded between 1990 and 2014 (Table 3). Functional richness was also significantly lower in 2014 than in 1990 for all depths (Tukey tests at 70 m q = 10.644, p < 0.01; at 100 m q = 6.034, p < 0.01; at 130 m q = 5.358, p < 0.01) except for the station at 160 m depth where no differences were recorded. Uniqueness was significantly different between 1990 and 2014 only for the stations at 100 m (Tukey test; q = 3.402, p = 0.019) and 130 m (Tukey test; q = 8.461, p < 0.001) depth.
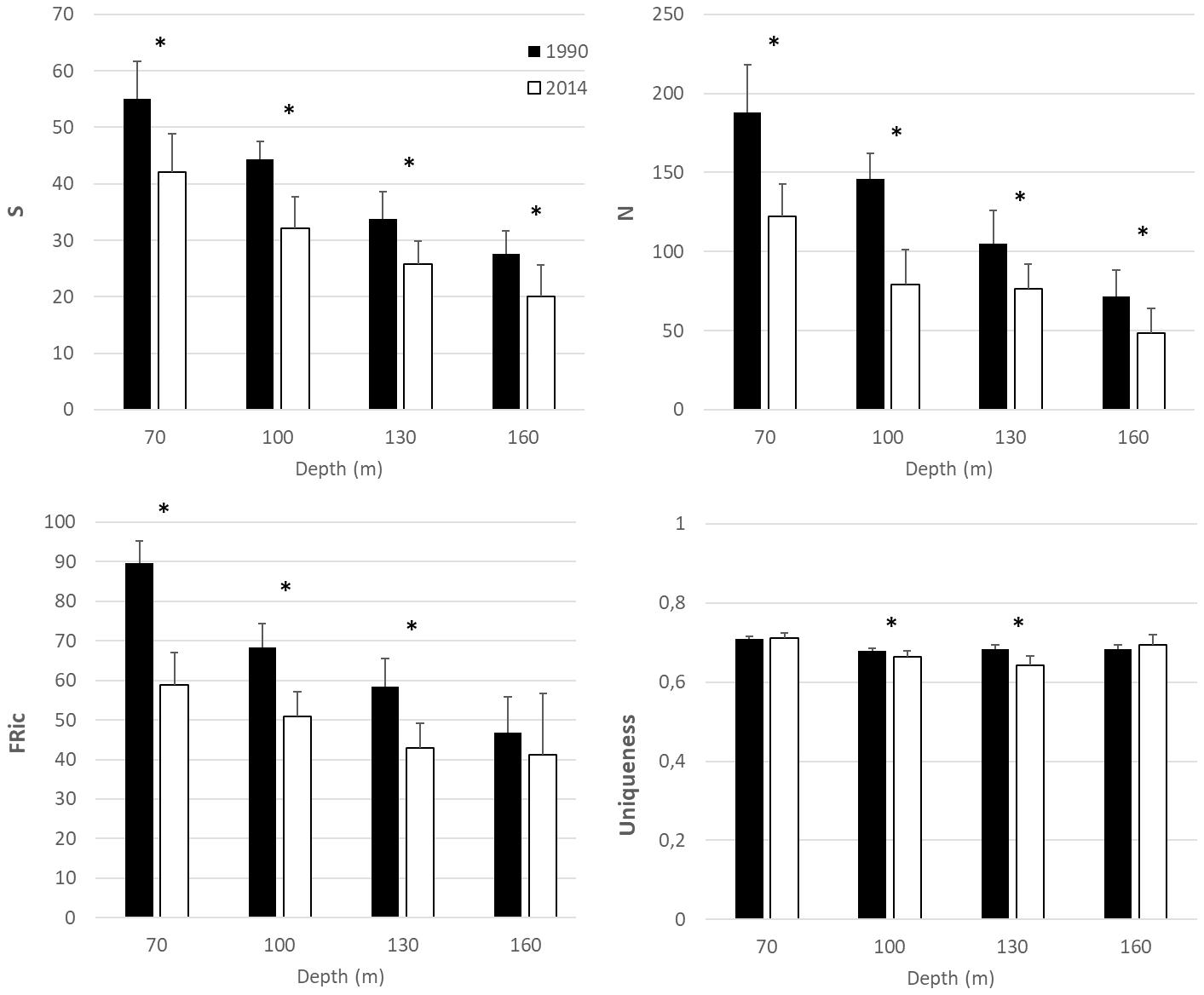
Figure 2. Temporal change in alpha diversity indices (S: richness, N: total abundance, FRic: Functional richness and Uniqueness) between 1990 and 2014. Black bars indicate samples collected in 1990 and white bars in 2014. Error bars indicate standard deviation. Asterisks indicate statistically significant differences at p < 0.05.

Table 3. Two-way ANOVA results for the temporal comparisons of the diversity metrics (S: richness, N: total abundance, FRic: Functional richness and Uniqueness).
Beta Diversity Differences Between 1990 and 2014
The taxonomic TBI dissimilarities in benthic communities of Heraklion Bay showed that beta diversity was significantly different between 1990 and 2014 (mean D = 0.698, p = 0.0001) (Table 4). Detailed analysis of the species losses and gains from TBI analysis indicated that the changes mostly consisted of taxa losses (Table 4 and Figure 3). The SIMPER analysis indicated that 126 taxa contributed to 90% of the dissimilarity between 1990 and 2014. The top 20 genera and their functional characteristics are presented in Table 5.
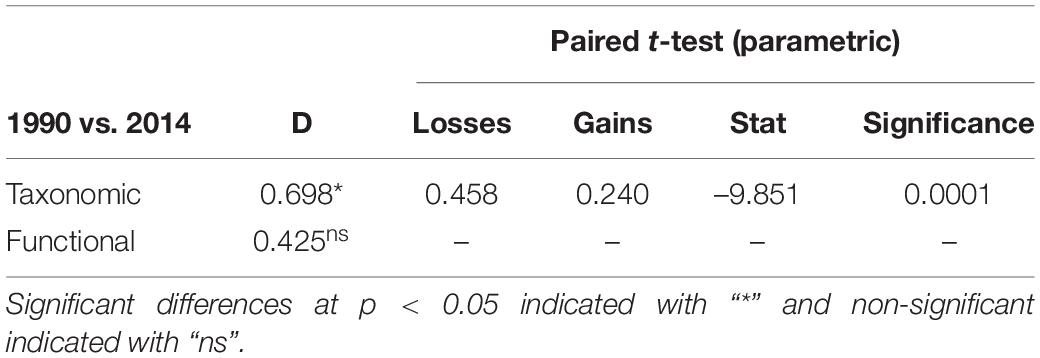
Table 4. TBI results for taxonomic and functional temporal beta diversity differences and results of the paired t-test of significance of the processes (losses or gains) dominated and caused taxa composition changes between 1990 and 2014.
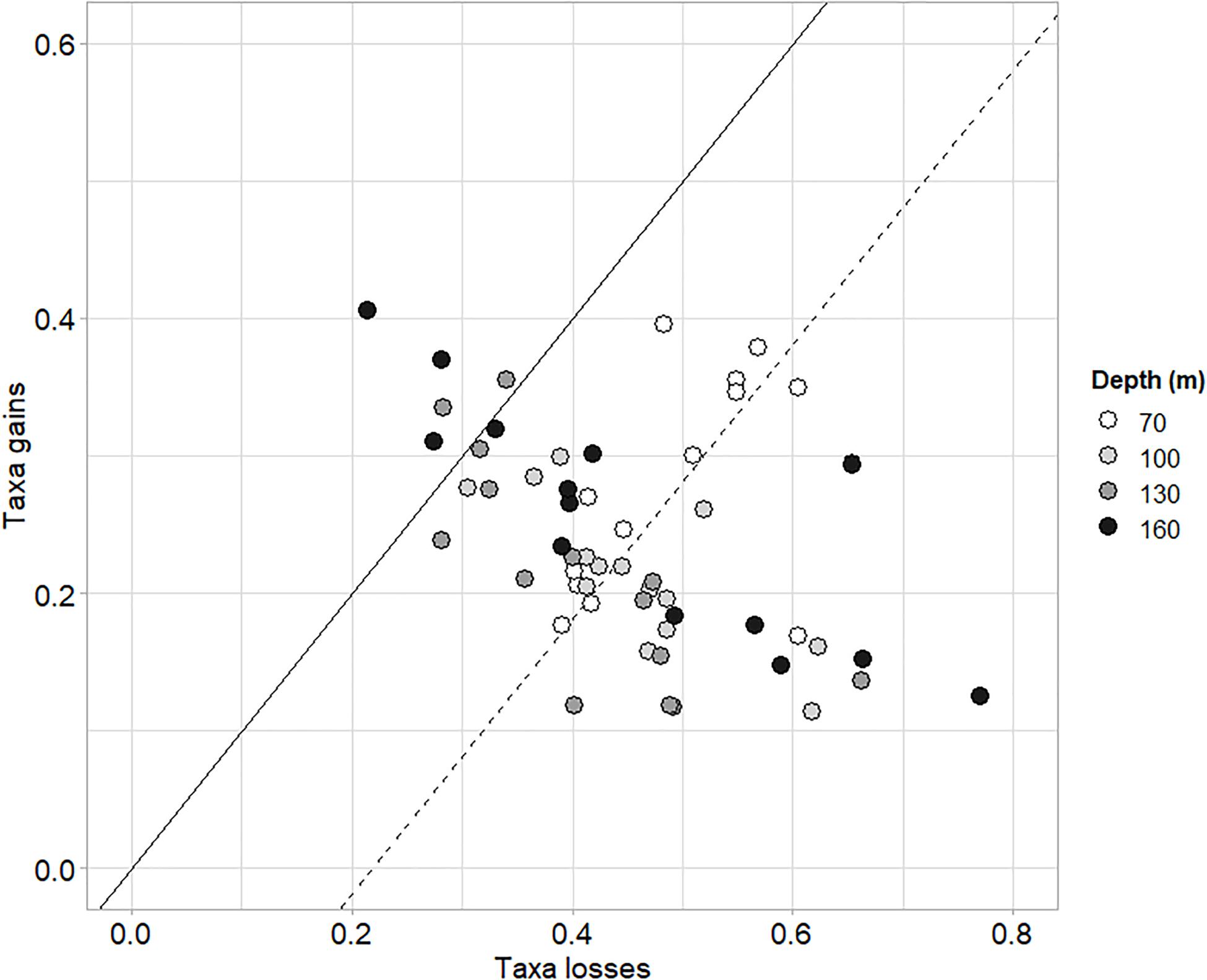
Figure 3. Losses and gains of taxa between the samplings of 1990 and 2014. Gray shades indicate stations depth. The solid line represents the theoretical positions of samples where gains equal losses. The dashed line parallel to the black line passes through the centroid of all samples. Its position below the solid line indicates that, on average, taxa losses dominated gains from 1990 to 2014.
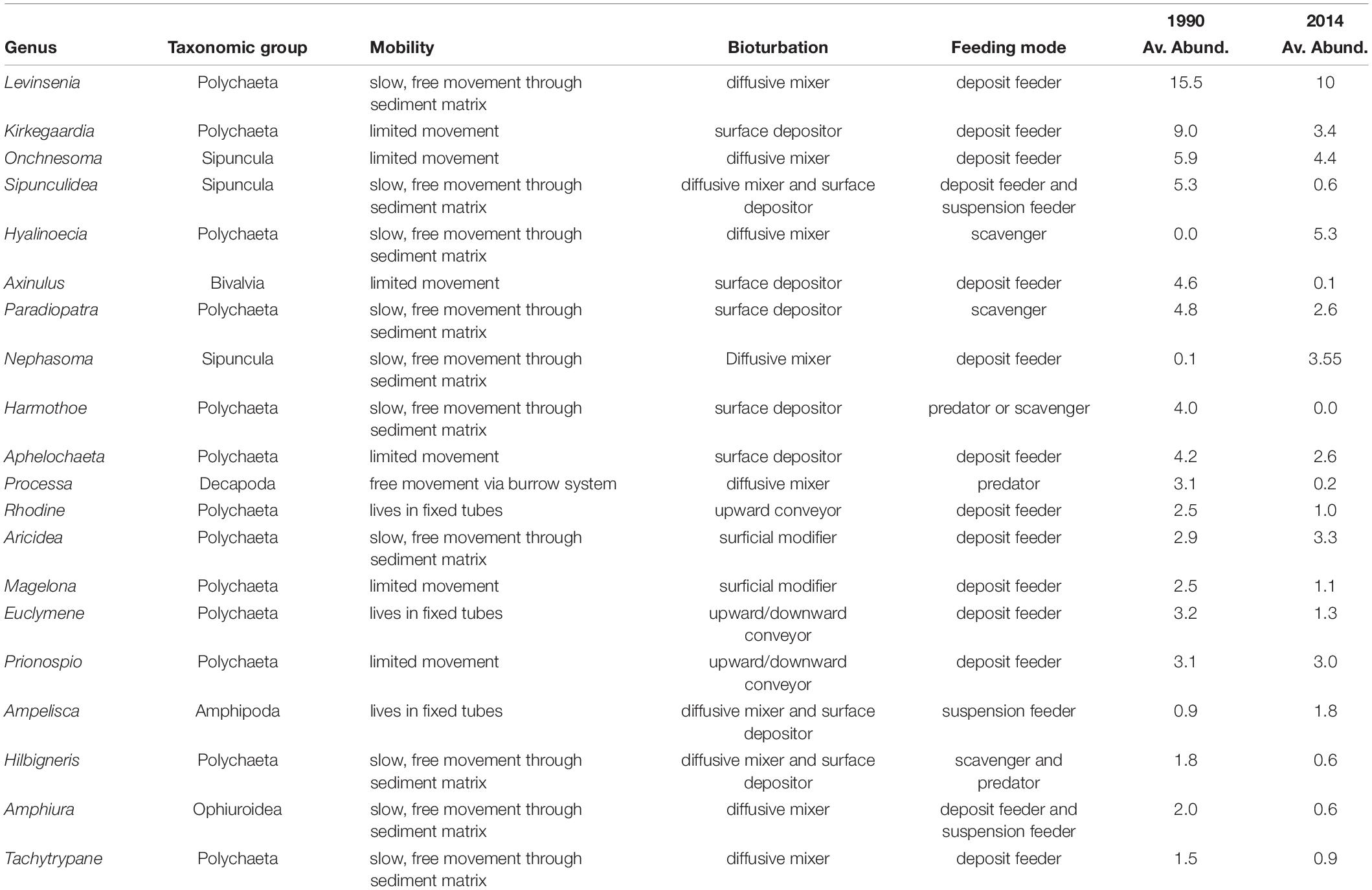
Table 5. Average abundance per sample and primary functional characteristics of top 20 taxa contributed most to dissimilarities between 1990 and 2014 according to the SIMPER analysis.
In spite of the differences in taxonomic beta diversity, TBI analysis of trait data revealed that functional beta diversity did not change significantly between 1990 and 2014 (mean D = 0.425, p > 0.05) (Table 4).
The abundance-weighted means of trait modalities were plotted to visualize the trait composition of all depths in 1990 and 2014 (Figure 4). Benthic communities at all stations were dominated mainly by infaunal polychaetes (0–5 cm) with medium and small body size (11–100 mm) and short longevity (1–3 years). The mobility traits free-living, sessile or low mobility were dominant at all stations, while the most common feeding mode was surface or subsurface deposit feeding. At 70 m, surface depositors were dominant in both 1990 and 2014, whereas diffusive mixing was the most common bioturbation mode at the deeper stations in both years. Regarding life strategy traits, at 70 m and 100 m, dominant reproduction mode was sexual and the reproductive traits included eggs that are released in the water column and grown to lecithotrophic or planktotrophic larvae. At the deeper stations, the dominant reproduction mode was also sexual but species brood eggs with no intermediate larval stages (direct development). It is also worth noting that differences in trait composition between 1990 and 2014 for all depths were rather quantitative than qualitative (Figure 4).
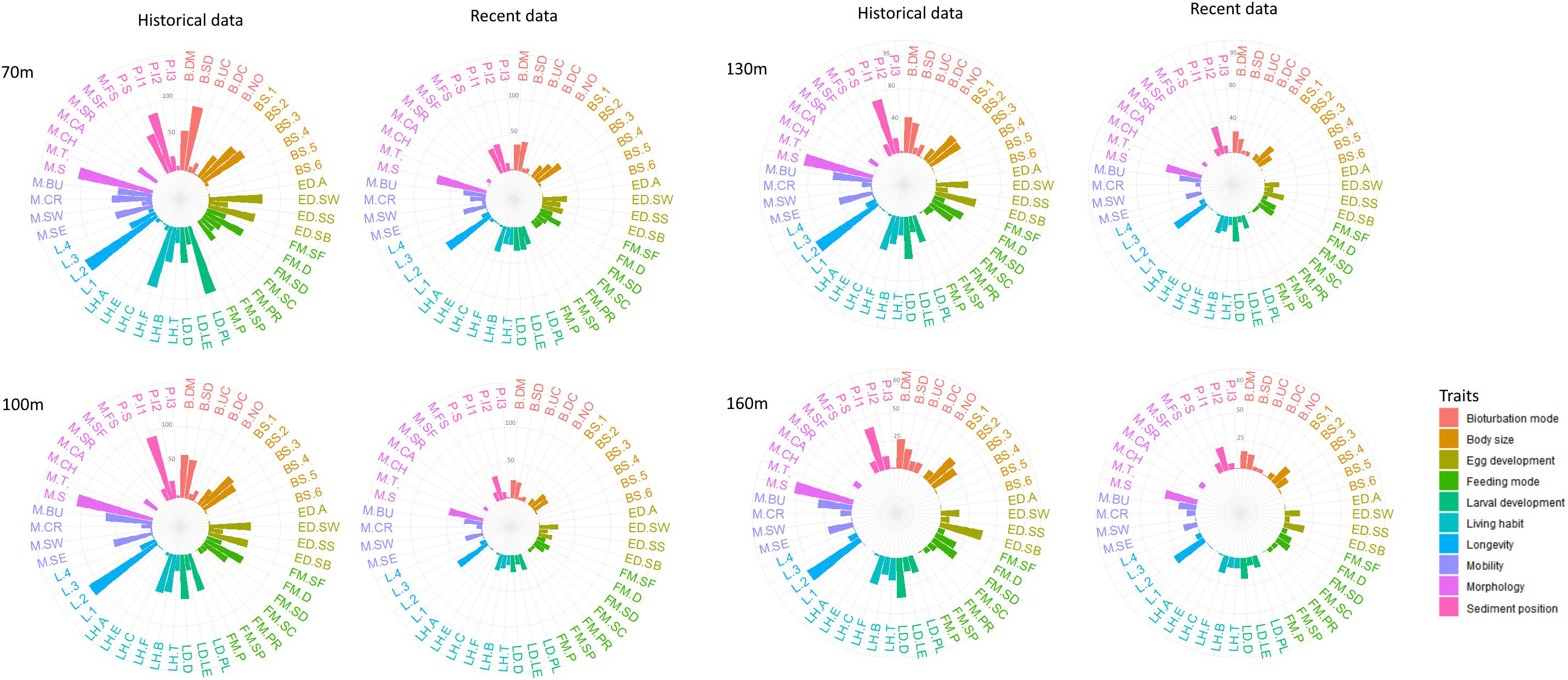
Figure 4. Abundance-weighted means of trait modalities at different sampling depths (i.e., 70, 100, 130, 160 m) for 1990 (historical data) and 2014 (recent data). Colors represent different traits; individual bars represent the trait modalities. For trait modalities codes see Table 2.
Congruence of Taxa Co-occurrence and Functional Networks
In order to determine the mechanisms that drove the differences in benthic communities between 1990 and 2014, the species co-occurrence networks were compared to the functional networks for the two periods. In 1990, both the functional and the co-occurrence networks consisted of 4 groups, while in 2014, taxa were classified in 6 functional groups and in 3 co-occurrence groups (Figure 5). In addition, the DgM index in 1990 was 0.74 showing that the distribution of taxa within networks was not different from a random pattern of distribution (Table 6). In 1990, the observed DgM (p = 0.19) ranged between 5 and 95% of the null distribution of the DgM indicating that stochastic processes were the main factors structuring the community or that there was not a clear dominance of deterministic processes, i.e., environmental filtering or competition. In contrast, the DgM index in 2014 was 0.79 and it was slightly lower than 5% of values obtained with null model (p = 0.047), indicating that environmental filtering structured the benthic community in 2014 (Table 6).
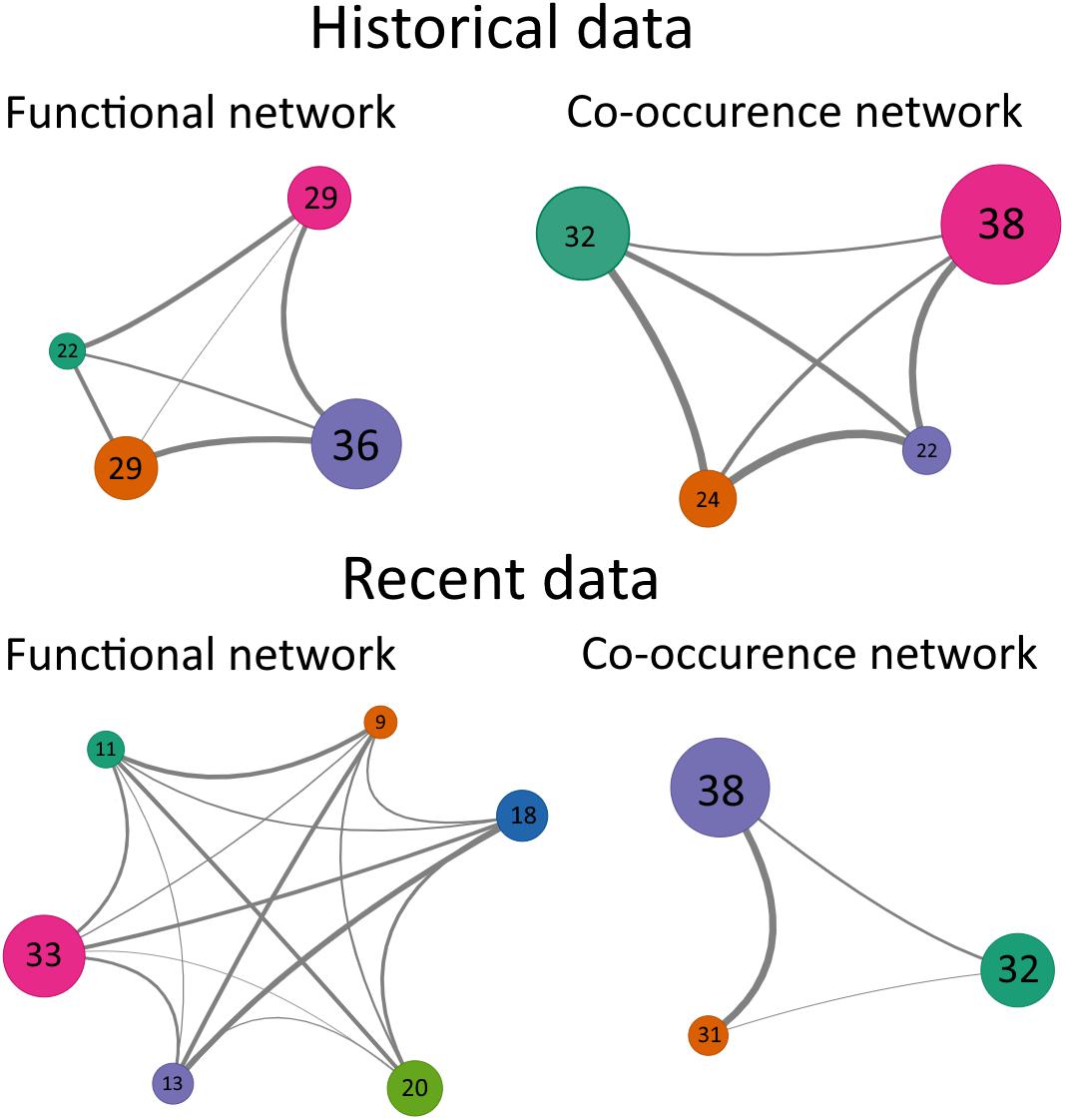
Figure 5. Schematic representation of networks obtained for benthic communities in 1990 (historical data) and 2014 (recent data). The numbers inside the circles correspond to the number of taxa composing each node and the width of edges is proportional to the adjacency between the nodes. The networks obtained from the trait data (functional networks) are on the left side and the network obtained from the taxa abundance data (co-occurrence networks) are on the right side.
Discussion
Describing how communities change over time is important to better understand and predict ecosystem functioning (Dirzo et al., 2014; Barbier, 2017). Changes in biodiversity usually result in changes in functional diversity, however, the relationship between them is not always straightforward (Naeem, 1998; Hiddink et al., 2009; Clare et al., 2015). The order in which the taxa are lost, as well as the trait similarity between them determine the impact of biodiversity loss on ecosystems functioning. In this study, the impact of temporal changes in taxonomic diversity on ecosystem functions was assessed in an oligotrophic environment in the Eastern Mediterranean. Our working hypothesis that the temporal differences in taxonomic diversity would affect the functionality of the benthic communities was partially rejected. Specifically, the results revealed a decline in taxonomic alpha and beta diversity metrics of the benthic community in Heraklion Bay between 1990 and 2014. This difference was also reflected in functional richness but less in uniqueness (i.e., functional redundancy) and none in functional beta diversity. Trait composition was similar in 1990 and 2014 at all depths. The stability of functional composition indicated that replacements of functionally similar taxa may occur, ensuring the resilience of the ecosystem to provide goods and services (Shojaei et al., 2021).
The decline in taxonomic diversity metrics recorded in 2014 was higher for the shallower stations than the deeper ones. Shallow stations are more susceptible to disturbance because they are more exposed to human activities in the coastal zone (Tsikopoulou et al., 2018), and also to weather and current driven resuspension events (Labrune et al., 2007). Functional richness followed the same pattern of variation with richness. Functional richness was defined as the amount of functional space occupied by a species assemblage and it is expected to be higher if the range of traits within a community is high, and lower if the range is low (Villéger et al., 2008). Maintaining FRic in ecosystems is important because reduced functional space occupied by a community could, for instance, allow alien species to use free niche space formerly occupied by indigenous taxa (Taupp and Wetzel, 2019).
The temporal differences in taxonomic alpha diversity were accompanied by temporal differences in community composition (taxonomic beta diversity). Partitioning of the temporal beta diversity revealed that taxa loss was the dominant pattern in most of the sites between 1990 and 2014. The local extinction and/or population decline of certain taxa, mainly deposit feeders, with slow or limited movement ability was responsible for changes in community composition between the sampling years. In addition, the dominance of the polychaete Hyalinoecia and the flourishing of sipunculans in 2014 could be indicative of a reduction in food availability in the study area. Hyalinoecia species are described both as scavengers and surface deposit feeders (Jumars et al., 2015) that inhabit nutrient-poor soft bottoms, while Sipuncula have competitive advantages to obtain food deeper in the sediment (Karakassis and Eleftheriou, 1997). The establishment of the wastewater treatment plant that occurred between the sampling periods and the reduction in the organic load in the area (Table 1) could be responsible for the dominance of these taxa (Tsikopoulou et al., 2018).
Besides food availability, temperature is a limiting factor in structuring macrofaunal communities, by regulating and setting limits for recruitment and mortality (Hale et al., 2011; Valentine and Jablonski, 2015). Recurrent water mass exchanges between the Aegean Sea and the Eastern Mediterranean result in the appearance of low salinity and temperature, nutrient-rich water in the Cretan Sea (Kassis et al., 2015). Such an event was recorded in 1987–1995 (Velaoras et al., 2015) and found to impact ecological processes in the area (Psarra et al., 2000; Tselepides et al., 2000). This event was probably responsible for the lower temperature and higher organic matter recorded at 1990 than 2014 in the study area which in turn, may have caused the observed temporal variation in community composition. In addition, Rivetti et al. (2014) reviewed the mass mortality events of benthic invertebrates in the Mediterranean over the period 1945–2011 and linked them to positive thermal anomalies at regional scales highlighting the impact of temperature on species survivance.
Despite the changes in taxa composition and functional richness, uniqueness was stable through the years in two of the four study sites indicating that unique trait combinations were maintained in the benthic community. Uniqueness is an index that describes both functional redundancy and vulnerability by taking into account not only taxa trait dissimilarities but also abundances (Ricotta et al., 2016). In this context, it could be concluded that not only rare but also common taxa make unique contributions to functional diversity (Chapman et al., 2018).
The high functional redundancy of the benthic community was also indicated by the insignificant change in the functional temporal beta diversity. Our results are consistent with other studies that have applied BTA to assess temporal variability in the functioning of benthic communities and found that, despite shifts in taxonomic composition, trait composition either persisted or changed and recovered after a short period (Clare et al., 2015). For example, Shojaei et al. (2021) showed that a positive power function explained the relationship between taxonomic and functional diversity of benthic assemblages in the North Sea indicating that in species-rich communities, functional redundancy among the species would allow for only minor changes in functionality even at considerable variations in taxonomic diversity.
To detect the mechanisms that determined the taxa occurrence and community assembly in 1990 and 2014, the taxa co-occurrence networks were compared to the functional networks for the two periods. The result of this comparison showed that in 1990, the distribution of taxa within the networks was not different from a random pattern of distribution, indicating either that stochastic processes were the main factors structuring the community or that there was not a clear dominance of deterministic processes - environmental filtering or competition (Legras et al., 2019). The coastal zone is exposed to changes, natural or human induced. In such habitats, disturbances may blur assembly patterns (Cornwell and Ackerly, 2009).
Conversely, in 2014, environmental filtering had the strongest influence on structuring the benthic community. The environmental filtering hypothesis assumes that co-existing species are likely to share most similar ecological and functional characteristics, as they respond to the same environmental filters. Thus, when species are facing similar environmental constraints, functional diversity is expected to be relatively low and assemblages are composed of functionally closely related species, thereby increasing the number of different species that exhibit similar ecological functions (Mouillot et al., 2007; Craven et al., 2018; Boet et al., 2020). Although, data availability did not allow us to determine which environmental constraint played the leading role in structuring the benthic community in 2014, it could be assumed that both sediment temperature and food availability affected benthic community composition.
Conclusion
The results of the present study suggest that in slightly disturbed coastal benthic habitats such this in Heraklion Bay, where assemblages are experiencing temporal changes in species composition, a high functional overlap among species would imply a considerable capacity of the ecosystem to maintain key processes and functions in response to environmental changes.
Data Availability Statement
The raw data supporting the conclusions of this article will be made available by the authors, without undue reservation.
Author Contributions
IT: conceptualization, methodology, formal analysis, investigation, writing – original draft, visualization, and supervision. PD: investigation and writing – review and editing. IK and CS: resources, methodology, and writing – review and editing. NL and NP: resources and writing – review and editing. All authors contributed to the article and approved the submitted version.
Funding
This research was funded in the context of the project “Sources of variability in functional diversity of the benthic ecosystem: functional redundancy as an indicator of seafloor integrity” (MIS 5049084) under the call for proposals “Research Grant for Researcher Support with emphasis on Young Scientists (second call)” (EDBM103). The project is co-financed by Greece and the European Union (European Social Fund-ESF) by the Operational Programme Human Resources Development, Education and Lifelong Learning 2014–2020.
Conflict of Interest
The authors declare that the research was conducted in the absence of any commercial or financial relationships that could be construed as a potential conflict of interest.
Publisher’s Note
All claims expressed in this article are solely those of the authors and do not necessarily represent those of their affiliated organizations, or those of the publisher, the editors and the reviewers. Any product that may be evaluated in this article, or claim that may be made by its manufacturer, is not guaranteed or endorsed by the publisher.
Acknowledgments
The sampling and analyses in 1989–90 were carried out in the framework of the FISHECO project, funded by the NATO-SFS programme. Thanks are due to all collaborators within the FISHECO project. The second sampling period was made possible due to ship time provided by Manolis Tsapakis and the HCMR team of Anastasia Tsiola, Ioanna Kalantzi, Eleni Rousselaki, and Santi Diliberto. Helpful comments by the reviewers are gratefully acknowledged.
Footnotes
References
Barbier, E. B. (2017). Marine ecosystem services. Curr. Biol. 27, R507–R510. doi: 10.1016/j.cub.2017.03.020
Bello, F., Botta-Dukat, Z., Leps, J., and Fibich, P. (2021). Towards a more balanced combination of multiple traits when computing functional differences between species. Methods Ecol. Evol. 12, 443–448. doi: 10.1111/2041-210X.13537
Belyea, L. R., and Lancaster, J. (1999). Assembly rules within a contingent ecology. Oikos 86, 402–416.
Boet, O., Arnan, X., and Retana, J. (2020). The role of environmental vs. Biotic filtering in the structure of European ant communities: a matter of trait type and spatial scale. PLoS One 15:228625. doi: 10.1371/journal.pone.0228625
Bolam, S. G., Coggan, R. C., Eggleton, J., Diesing, M., and Stephens, D. (2014). Sensitivity of macrobenthic secondary production to trawling in the English sector of the Greater North Sea: A biological trait approach. J. Sea Res. 85, 162–177. doi: 10.1016/j.seares.2013.05.003
Bolam, S. G., Garcia, C., Eggleton, J., Kenny, A. J., Buhl-Mortensen, L., Gonzalez-Mirelis, G., et al. (2017). Differences in biological traits composition of benthic assemblages between unimpacted habitats. Mar. Environ. Res. 126, 1–13. doi: 10.1016/j.marenvres.2017.01.004
Bonifácio, P., Grémare, A., Gauthier, O., Romero-Ramirez, A., Bichon, S., Amouroux, J. M., et al. (2018). Long-term (1998 vs. 2010) large-scale comparison of soft-bottom benthic macrofauna composition in the Gulf of Lions, NW Mediterranean Sea. J. Sea Res. 131, 32–45. doi: 10.1016/j.seares.2017.08.013
Bremner, J. (2008). Species traits and ecological functioning in marine conservation and management. J. Exp. Mar. Bio. Ecol. 366, 37–47. doi: 10.1016/j.jembe.2008.07.007
Cartes, J. E., Maynou, F., Fanelli, E., Papiol, V., and Lloris, D. (2009). Long-term changes in the composition and diversity of deep-slope megabenthos and trophic webs off Catalonia (western Mediterranean): Are trends related to climatic oscillations? Prog. Oceanogr. 82, 32–46. doi: 10.1016/j.pocean.2009.03.003
Chapman, A. S. A., Tunnicliffe, V., and Bates, A. E. (2018). Both rare and common species make unique contributions to functional diversity in an ecosystem unaffected by human activities. Divers. Distrib. 24, 568–578. doi: 10.1111/ddi.12712
Clare, D. S., Robinson, L. A., and Frid, C. L. J. (2015). Community variability and ecological functioning: 40 years of change in the North Sea benthos. Mar. Environ. Res. 107, 24–34. doi: 10.1016/J.MARENVRES.2015.03.012
Clare, D. S., Spencer, M., Robinson, L. A., and Frid, C. L. J. (2017). Explaining ecological shifts: the roles of temperature and primary production in the long-term dynamics of benthic faunal composition. Oikos 126, 1123–1133. doi: 10.1111/oik.03661
Clarke, K. R., and Gorley, R. N. (2015). PRIMER v7: User Manual/Tutorial PRIMER-E: Plymouth. Auckland: PRIMER-E Ltd.
Colorado Zuluaga, G. (2015). How ecological communities are structured: A review on ecological assembly rules. Rev. EIA/English version 12, 27–53. doi: 10.24050/reia.v12i24.873
Connor, E. F., and Simberloff, D. (1979). The Assembly of Species Communities: Chance or Competition? Ecology 60:1132. doi: 10.2307/1936961
Cornwell, W. K., and Ackerly, D. D. (2009). Community assembly and shifts in plant trait distributions across an environmental gradient in coastal California. Ecol. Monogr. 79, 109–126. doi: 10.1890/07-1134.1
Craven, D., Hall, J. S., Berlyn, G. P., Ashton, M. S., and Breugel, M. V. (2018). Environmental filtering limits functional diversity during succession in a seasonally wet tropical secondary forest. J. Veg. Sci. 29, 511–520. doi: 10.1111/jvs.12632
Dauvin, J. C., and Pezy, J. P. (2013). Long-term changes of the Seine estuary suprabenthos (1996–2012). J. Exp. Mar. Bio. Ecol. 448, 93–103. doi: 10.1016/J.JEMBE.2013.06.019
Davoult, D., Dewarumez, J. M., and Frontier, S. (1993). Long-term changes (1979–1990) in three benthic communities (eastern English Channel): Use of factor analysis and rank-frequency diagrams for studying structural developments. Netherlands J. Aquat. Ecol. 27, 415–426. doi: 10.1007/BF02334803
Davoult, D., Dewarumez, J. M., and Migneacute, A. (1998). Long-term changes (1979–1994) in two coastal benthic communities (English Channel): analysis of structural developments. Oceanol. Acta 21, 609–617. doi: 10.1016/S0399-1784(98)80043-1
Dirzo, R., Young, H. S., Galetti, M., Ceballos, G., Isaac, N. J. B., and Collen, B. (2014). Defaunation in the Anthropocene. Science 345, 401–406. doi: 10.1126/science.1251817
Drake, J. A. (1990). Communities as assembled structures: Do rules govern pattern? Trends Ecol. Evol. 5, 159–164. doi: 10.1016/0169-5347(90)90223-Z
Dray, S., Dufour, A.-B., and Chessel, D. (2007). The ade4 package - II: Two-table and K-table methods. R News 7, 47–52.
Dray, S., Pelissier, R., Couteron, P., Fortin, M. J., Legendre, P., Peres-Neto, P. R., et al. (2012). Community ecology in the age of multivariate multiscale spatial analysis. Ecol. Monogr. 82, 257–275. doi: 10.1890/11-1183.1
Gogina, M., Morys, C., Forster, S., Grawe, U., Friedland, R., and Zettler, M. L. (2017). Towards benthic ecosystem functioning maps: Quantifying bioturbation potential in the German part of the Baltic Sea. Ecol. Indic. 73, 574–588. doi: 10.1016/j.ecolind.2016.10.025
Goslee, S. C., and Urban, D. L. (2007). The ecodist package for dissimilarity-based analysis of ecological data. J. Stat. Softw. 22, 1–19. doi: 10.18637/jss.v022.i07
Gotelli, N. J., and McGill, B. J. (2006). Null versus neutral models: what’s the difference? Ecography 29, 793–800. doi: 10.1111/j.2006.0906-7590.04714.x
Hale, R., Calosi, P., Mcneill, L., Mieszkowska, N., and Widdicombe, S. (2011). Predicted levels of future ocean acidification and temperature rise could alter community structure and biodiversity in marine benthic communities. Oikos 120, 661–674. doi: 10.1111/j.1600-0706.2010.19469.x
Halpern, B. S., Walbridge, S., Selkoe, K. A., Kappel, C. V., Micheli, F., DAgrosa, C., et al. (2008). A global map of human impact on marine ecosystems. Science 319, 948–952. doi: 10.1126/science.1149345
Hiddink, J. G., Wynter Davies, T., Perkins, M., MacHairopoulou, M., and Neill, S. P. (2009). Context dependency of relationships between biodiversity and ecosystem functioning is different for multiple ecosystem functions. Oikos 118, 1892–1900. doi: 10.1111/j.1600-0706.2009.17556.x
Hinz, H., Tornroos, A., and de Juan, S. (2021). Trait-based indices to assess benthic vulnerability to trawling and model loss of ecosystem functions. Ecol. Indic. 126:107692. doi: 10.1016/j.ecolind.2021.107692
Jumars, P. A., Dorgan, K. M., and Lindsay, S. M. (2015). Diet of worms emended: an update of polychaete feeding guilds. Ann. Rev. Mar. Sci. 7, 497–520. doi: 10.1146/annurev-marine-010814-020007
Karakassis, I., and Eleftheriou, A. (1997). The continental shelf of Crete: Structure of macrobenthic communities. Mar. Ecol. Prog. Ser. 160, 185–196. doi: 10.3354/meps160185
Kassis, D., Korres, G., Petihakis, G., and Perivoliotis, L. (2015). Hydrodynamic variability of the Cretan Sea derived from Argo float profiles and multi-parametric buoy measurements during 2010–2012. Ocean Dyn. 65, 1585–1601. doi: 10.1007/s10236-015-0892-0
Labrune, C., Gremare, A., Guizien, K., and Amouroux, J. M. (2007). Long-term comparison of soft bottom macrobenthos in the Bay of Banyuls-sur-Mer (north-western Mediterranean Sea): A reappraisal. J. Sea Res. 58, 125–143. doi: 10.1016/j.seares.2007.02.006
Laliberté, E., and Legendre, P. (2010). A distance-based framework for measuring functional diversity from multiple traits. Ecology 91, 299–305. doi: 10.1890/08-2244.1
Lampadariou, N., and Eleftheriou, A. (2018). Seasonal dynamics of meiofauna from the oligotrophic continental shelf of Crete (Aegean Sea, eastern Mediterranean). J. Exp. Mar. Bio. Ecol. 502, 91–104. doi: 10.1016/j.jembe.2017.12.014
Legendre, P. (2019). A temporal beta-diversity index to identify sites that have changed in exceptional ways in space–time surveys. Ecol. Evol. 9, 3500–3514. doi: 10.1002/ece3.4984
Legras, G., Loiseau, N., Gaertner, J.-C., Poggiale, J. C., Ienco, D., Mazouni, N., et al. (2019). Assessment of congruence between co-occurrence and functional networks: A new framework for revealing community assembly rules. Sci. Rep. 9:19996. doi: 10.1038/s41598-019-56515-7
Liu, K., Lin, H., He, X., Huang, Y., Li, Z., Lin, J., et al. (2019). Functional trait composition and diversity patterns of marine macrobenthos across the Arctic Bering Sea. Ecol. Indic. 102, 673–685. doi: 10.1016/j.ecolind.2019.03.029
Macarthur, R., and Levins, R. (1967). The Limiting Similarity, Convergence, and Divergence of Coexisting Species. Am. Soc. Nat. 101, 377–385.
Magurran, A. E., Dornelas, M., Moyes, F., and Henderson, P. A. (2019). Temporal β diversity—A macroecological perspective. Glob. Ecol. Biogeogr. 28, 1949–1960. doi: 10.1111/geb.13026
McCauley, D. J., Pinsky, M. L., Palumbi, S. R., Estes, J. A., Joyce, F. H., and Warner, R. R. (2015). Marine defaunation: Animal loss in the global ocean. Science 347:1255641. doi: 10.1126/science.1255641
Meyer, J., and Kröncke, I. (2019). Shifts in trait-based and taxonomic macrofauna community structure along a 27-year time-series in the south-eastern North Sea. PLoS One 14:226410. doi: 10.1371/journal.pone.0226410
Micheli, F., and Halpern, B. S. (2005). Low functional redundancy in coastal marine assemblages. Ecol. Lett. 8, 391–400. doi: 10.1111/j.1461-0248.2005.00731.x
Mouillot, D., Dumay, O., and Tomasini, J. A. (2007). Limiting similarity, niche filtering and functional diversity in coastal lagoon fish communities. Estuar. Coast. Shelf. Sci. 71, 443–456. doi: 10.1016/j.ecss.2006.08.022
Mueller, M., Pander, J., and Geist, J. (2013). Taxonomic sufficiency in freshwater ecosystems: Effects of taxonomic resolution, functional traits, and data transformation. Freshw. Sci. 32, 762–778. doi: 10.1899/12-212.1
Naeem, S. (1998). Species redundancy and ecosystem reliability. Conserv. Biol. 12, 39–45. doi: 10.1046/j.1523-1739.1998.96379.x
Psarra, S., Tselepides, A., and Ignatiades, L. (2000). Primary productivity in the oligotrophic Cretan Sea (NE Mediterranean): Seasonal and interannual variability. Prog. Oceanogr. 46, 187–204. doi: 10.1016/S0079-6611(00)00018-5
Queirós, A. M., Birchenough, S. N. R., Bremner, J., Godbold, J. A., Parker, R. E., Romero-Ramirez, A., et al. (2013). A bioturbation classification of European marine infaunal invertebrates. Ecol. Evol. 3, 3958–3985. doi: 10.1002/ece3.769
Renz, J. R., Powilleit, M., Gogina, M., Zettler, M. L., Morys, C., and Forster, S. (2018). Community bioirrigation potential (BIPc), an index to quantify the potential for solute exchange at the sediment-water interface. Mar. Environ. Res. 141, 214–224. doi: 10.1016/j.marenvres.2018.09.013
Ricotta, C., de Bello, F., Moretti, M., Caccianiga, M., Cerabolini, B. E. L., and Pavoine, S. (2016). Measuring the functional redundancy of biological communities: a quantitative guide. Methods Ecol. Evol. 7, 1386–1395. doi: 10.1111/2041-210X.12604
Rivetti, I., Fraschetti, S., Lionello, P., Zambianchi, E., and Boero, F. (2014). Global warming and mass mortalities of benthic invertebrates in the Mediterranean Sea. PLoS One 9:115655. doi: 10.1371/journal.pone.0115655
Romero-Ramirez, A., Bonifacio, P., Labrune, C., Sardá, R., Amouroux, J. M., Bellan, G., et al. (2016). Long-term (1998-2010) large-scale comparison of the ecological quality status of gulf of Lion (NW Mediterranean) benthic habitats. Mar. Pollut. Bull. 102, 102–113. doi: 10.1016/j.marpolbul.2015.11.052
Salen-Picard, C., and Arlhac, D. (2002). Long-term changes in a Mediterranean benthic community: Relationships between the polychaete assemblages and hydrological variations of the Rhône River. Estuaries 25, 1121–1130. doi: 10.1007/BF02692209
Salo, T., Mattila, J., and Eklof, J. (2020). Long−term warming affects ecosystem functioning through species turnover and intraspecific trait variation. Oikos 129, 283–295. doi: 10.1111/oik.06698
Schelling, M., and Hui, C. (2015). modMax: Community Structure Detection via Modularity Maximization. 1–19. Available online at: https://cran.r-project.org/package=modMax (accessed July 24, 2015).
Shojaei, M. G., Gutow, L., Dannheim, J., Schroder, A., and Brey, T. (2021). Long-term changes in ecological functioning of temperate shelf sea benthic communities. Estuar. Coast. Shelf Sci. 249:107097. doi: 10.1016/J.ECSS.2020.107097
Simboura, N., Pavlidou, A., Bald, J., Tsapakis, M., Pagou, K., Zeri, C., et al. (2016). Response of ecological indices to nutrient and chemical contaminant stress factors in Eastern Mediterranean coastal waters. Ecol. Indic. 70, 89–105. doi: 10.1016/j.ecolind.2016.05.018
Solan, M., Batty, P., Bulling, M. T., and Godbold, J. A. (2008). How biodiversity affects ecosystem processes: Implications for ecological revolutions and benthic ecosystem function. Aquat. Biol. 2, 289–301. doi: 10.3354/ab00058
Somerfield, P. J., Arvanitidis, C., Faulwetter, S., Chatzigeorgiou, G., Vasileiadou, A., Amouroux, J. M., et al. (2009). Assessing evidence for random assembly of marine benthic communities from regional species pools. Mar. Ecol. Prog. Ser. 382, 279–286. doi: 10.3354/meps07934
Taupp, T., and Wetzel, M. A. (2019). Functionally similar but taxonomically different: Benthic communities in 1889 and 2006 in an industrialized estuary. Estuar. Coast. Shelf Sci. 217, 292–300. doi: 10.1016/j.ecss.2018.11.012
Teichert, N., Lepage, M., Sagouis, A., Borja, A., Chust, G., Ferreira, M. T., et al. (2017). Functional redundancy and sensitivity of fish assemblages in European rivers, lakes and estuarine ecosystems. Sci. Rep. 7, 1–11. doi: 10.1038/s41598-017-17975-x
Tselepides, A., Zervakis, V., Polychronaki, T., Danovaro, R., and Chronis, G. (2000). Distribution of nutrients and particulate organic matter in relation to the prevailing hydrographic features of the Cretan Sea (NE Mediterranean). Prog. Oceanogr. 46, 113–142. doi: 10.1016/S0079-6611(00)00015-X
Tsikopoulou, I., Moraitis, M. L., Geropoulos, A., Papadopoulou, K. N., Papageorgiou, N., Plaiti, W., et al. (2018). Long-term changes in the structure of benthic communities: Revisiting a sampling transect in Crete after 24 years. Mar. Environ. Res. 2018:8. doi: 10.1016/j.marenvres.2018.11.008
Valentine, J. W., and Jablonski, D. (2015). A twofold role for global energy gradients in marine biodiversity trends. J. Biogeogr. 42, 997–1005. doi: 10.1111/jbi.12515
Vallée, V., Villanueva, M. C., and Blanchard, F. (2019). Functional richness and turnover patterns reveal assembly rules structuring marine fish communities on the continental shelf of French Guiana. Mar. Ecol. Prog. Ser. 630, 183–195. doi: 10.3354/meps13129
Velaoras, D., Krokos, G., and Theocharis, A. (2015). Recurrent intrusions of transitional waters of Eastern Mediterranean origin in the Cretan Sea as a tracer of Aegean Sea dense water formation events. Prog. Oceanogr. 135, 113–124. doi: 10.1016/j.pocean.2015.04.010
Villéger, S., Mason, N. W. H., and Mouillot, D. (2008). New multidimensional functional diversity indices for a multifaceted framework in functional ecology. Ecology 89, 2290–2301. doi: 10.1890/07-1206.1
Worm, B., Barbier, E. B., Beaumont, N., Duffy, J. E., Folke, C., Halpern, B. S., et al. (2006). Impacts of biodiversity loss on ocean ecosystem services. Science 314, 787–790. doi: 10.1126/science.1132294
Keywords: biological trait analysis (BTA), benthic ecology, macrofauna, temporal beta diversity, ecosystem functioning, networks comparison, long-term comparison, uniqueness
Citation: Tsikopoulou I, Dimitriou PD, Karakassis I, Lampadariou N, Papadopoulou N and Smith CJ (2021) Temporal Variation in the Ecological Functioning of Benthic Communities After 20 Years in the Eastern Mediterranean. Front. Mar. Sci. 8:768051. doi: 10.3389/fmars.2021.768051
Received: 31 August 2021; Accepted: 24 September 2021;
Published: 12 October 2021.
Edited by:
Stefano Aliani, National Research Council (CNR), ItalyReviewed by:
Anabella Covazzi Harriague, Università degli Studi di Genova, ItalyJuan Moreira Da Rocha, Autonomous University of Madrid, Spain
Copyright © 2021 Tsikopoulou, Dimitriou, Karakassis, Lampadariou, Papadopoulou and Smith. This is an open-access article distributed under the terms of the Creative Commons Attribution License (CC BY). The use, distribution or reproduction in other forums is permitted, provided the original author(s) and the copyright owner(s) are credited and that the original publication in this journal is cited, in accordance with accepted academic practice. No use, distribution or reproduction is permitted which does not comply with these terms.
*Correspondence: Irini Tsikopoulou, aXRzaWtvcG91bG91QGhjbXIuZ3I=