- 1Marine Turtle Biology and Assessment Program, Pacific Islands Fisheries Science Center, NOAA Fisheries, Honolulu, HI, United States
- 2Hawai‘i Island Hawksbill Project, Hawai‘i Volcanoes National Park, HI, United States
- 3Hawai‘i Wildlife Fund, Maui, HI, United States
- 4Hawaiian Hawksbill Conservation, Maui, HI, United States
- 5Pacific Islands Fish and Wildlife Office, US Fish and Wildlife Service, Honolulu, HI, United States
- 6Pacific Islands Regional Office, NOAA Fisheries, Honolulu, HI, United States
- 7Marine Mammal and Turtle Division, Southwest Fisheries Science Center, NOAA Fisheries, La Jolla, CA, United States
- 8Golden Honu Services of Oceania, Honolulu, HI, United States
- 9Nicholas School of the Environment, Duke University, Durham, NC, United States
- 10Fisheries Research and Monitoring Division, Pacific Islands Fisheries Science Center, NOAA Fisheries, Honolulu, HI, United States
Evaluating wildlife population trends is necessary for the development of effective management strategies, which are particularly relevant for highly threatened species. Hawksbill marine turtles (Eretmochelys imbricata) are considered endangered globally and are rare in Hawai‘i. Remnant hawksbill nesting beaches were identified in Hawai‘i in the late 1980s and the primary sites have been monitored since that time. In this study we summarize all available hawksbill nesting activity around the Hawaiian Islands between 1988 and 2018, highlighting relevant demographic and geographic data for the species. Because monitoring effort varied substantially across space and time, we implemented a predictive modeling approach that accounted for varying effort to explore potential trends in annual number of nesting females and nests over time. Field monitoring efforts documented an annual average of 14 ± 4.3 (range: 5–26) nesting females and 48 ± 19.0 (range: 12–93) nests, with a cumulative total of 178 individual nesting females and 1,280 nests recorded across all years. Nesting has been documented on four Hawaiian Islands, with the overwhelming majority of nesting females (78.4%) and nests (86.5%) recorded at four beaches along the southern coast of Hawai‘i Island. Recent monitoring (2018) at a beach on Moloka‘i Island revealed numbers similar to the most important beaches on Hawai‘i Island. Despite difficulty discerning obvious trends when looking solely at the raw tabulated numbers from field monitoring, our analysis suggests both the number of nesting females and nests have been positively trending since 2006, and this is supported by a higher percentage (57.1% of annual cohorts) of neophyte (vs. remigrant) nesters over the second half of the monitoring timeframe. The masking of obvious trends in the tabulated numbers is likely due to decreased overall monitoring effort as a result of reduced funding in recent years, coupled with a shift in focal monitoring effort from the historical primary nesting site of Kamehame, to the more recently established nesting site of Pōhue. Although the positive trend is encouraging, our findings highlight the precarious state of hawksbills in Hawai‘i and the need to enhance monitoring across all sites to support more robust population assessments and management decision making.
Introduction
Evaluating the size and dynamics of wildlife populations is a core underpinning of conservation ecology (Gaillard et al., 1998), and information generated from population monitoring represents a fundamental component of species status assessments and management decision making (Thompson, 2004). Collecting population information is particularly relevant for highly threatened species as doing so can help determine the levels of protection necessary (Clark et al., 2002).
Marine turtles are a taxon of global conservation concern (Pritchard, 1996; Avise, 2007), and although several techniques are available for evaluating populations (e.g., capture-recapture, tagging, photo-ID), monitoring the number of nesting females that come ashore and the number of nests deposited on beaches is often the most logistically and economically feasible method (NRC, 2010; Willson et al., 2020). Hawksbill turtles (Eretmochelys imbricata) are one of the rarest of the seven extant species of marine turtles and their scarcity has been recognized by the government of the United States (US) and other nations, as well as by international resource management institutions (e.g., International Union for the Conservation of Nature or IUCN; Mortimer and Donnelly, 2008). Hawksbills are listed as Endangered under the US Endangered Species Act (ESA) and as Critically Endangered by the IUCN, the highest level of protection under both of these management frameworks. Notwithstanding nation-wide and global classifications, the status of individual populations can vary dramatically, with some populations increasing (e.g., Richardson et al., 2006; Beggs et al., 2007; Allen et al., 2010) and others facing major recovery challenges (e.g., Gaos et al., 2010, 2017a; Liles et al., 2011; Bell et al., 2020).
The Hawaiian archipelago is a chain of isolated volcanic islands that collectively represent the largest landmass in the central North Pacific Ocean (Neall and Trewick, 2008). Although the islands host a robust population of green turtles (Chelonia mydas; honu in Hawaiian) (Balazs and Chaloupka, 2004; Seminoff et al., 2015), hawksbill turtles (honu‘ea or just ‘ea in Hawaiian) are rare in the region (Van Houtan et al., 2012; Becker et al., 2019; Gaos et al., 2020; Brunson et al., in press). Hawksbill nesting was first documented in the State of Hawai‘i in the late 1960s (Ernst and Barbour, 1972), and monitoring and conservation were initiated at several beaches in the late 1980s (Seitz et al., 2012). Despite ongoing monitoring at the primary nesting beaches and a limited number of technical governmental reports and conference proceedings (e.g., King et al., 2007; Seitz et al., 2012; Roberson et al., 2016; HHTN, 2018), comprehensive peer-reviewed publications of hawksbill nesting ecology on the Hawaiian archipelago remain scarce.
In the US, marine turtles are jointly managed by federal agencies (i.e., National Marine Fisheries Service and US Fish and Wildlife Service) in collaboration with state and territory governments. Nongovernmental organizations (NGOs) and other stakeholder groups also often play important roles in monitoring, conservation, and management activities (Jacobson et al., 2010). Hawksbills were listed as endangered under the ESA in 1970 (Witzell, 1983) and official US Recovery Plans were subsequently developed for the species in both the Pacific and Atlantic Oceans (NMFS and USFWS, 1993, 1998). When the US Recovery Plan for Pacific hawksbill populations was developed in 1998, limited information existed on hawksbills in Hawai‘i and thus received limited recognition (NMFS and USFWS, 1998). Notwithstanding this perspective, since the early 1990s, a consortium of federal, state, and NGO partners, collectively referred to as the Hawaiian Hawksbill Turtle Network, have collaboratively worked to identify and implement a diverse suite of hawksbill research and conservation activities in Hawai‘i (HHTN, 2018). The initiative has also led to the publication of several relevant papers on Hawaiian hawksbills in recent years, including topics such as movement behavior (Parker et al., 2009), age-to-maturity (Snover et al., 2012; Van Houtan K.D. et al., 2016), threats (Brunson et al., in press), and genetic structure (Gaos et al., 2020). Reviews are periodically undertaken to reevaluate the status of ESA listed species and update recovery goals and recommendations, providing important opportunities to incorporate these and other newly generated data (Plotkin, 1995; NMFS and USFWS, 2007, 2013).
In this study we summarize all available information on hawksbill nesting around the Hawaiian archipelago between 1988 and 2018. By identifying the primary nesting beaches, summarizing nesting female and nest abundance, exploring population trends, and providing other key demographic information, we contribute fundamental information needed to aid population assessments and the development of management strategies, while also establishing a baseline for future monitoring efforts. Given the current research and management context, this study is both timely and relevant.
Materials and Methods
Study Sites and Monitoring Background
The main Hawaiian Islands (MHI) are part of a remote archipelago and consist of eight islands situated in the central North Pacific, approximately 4,000 km west of mainland United States and 6,000 km east of Japan. The State of Hawai‘i includes the MHI, as well as the Northwestern Hawaiian Islands (NWHI), the latter representing an isolated and uninhabited chain of small islands and atolls stretching approximately 2,000 km to the northwest of the MHI. These are collectively protected (and culturally revered) through the Papahānaumokuākea Marine National Monument (with the exception of Midway Atoll, which is a US Territory and not a part of the State of Hawai‘i). This study identifies all confirmed hawksbill nesting beaches in the MHI (Figure 1), as no hawksbill nesting has been documented in the NWHI to date. Individual beaches were classified according to whether coastlines were interrupted by natural barriers (e.g., cliffs, rock outcrops) or if they had previously been given distinct local nomenclature (Liles et al., 2011; Gaos et al., 2017a). We recognized a site as a nesting beach if it was confirmed to receive at least one nest during any given year during the course of this study. Due to the geographic clustering of three sets of beaches on Hawai‘i Island, as well as high female nesting site fidelity to these areas (see Results), we further recognized the existence of three nesting beach complexes, which were named after the primary nesting beach in each complex (Figure 1).
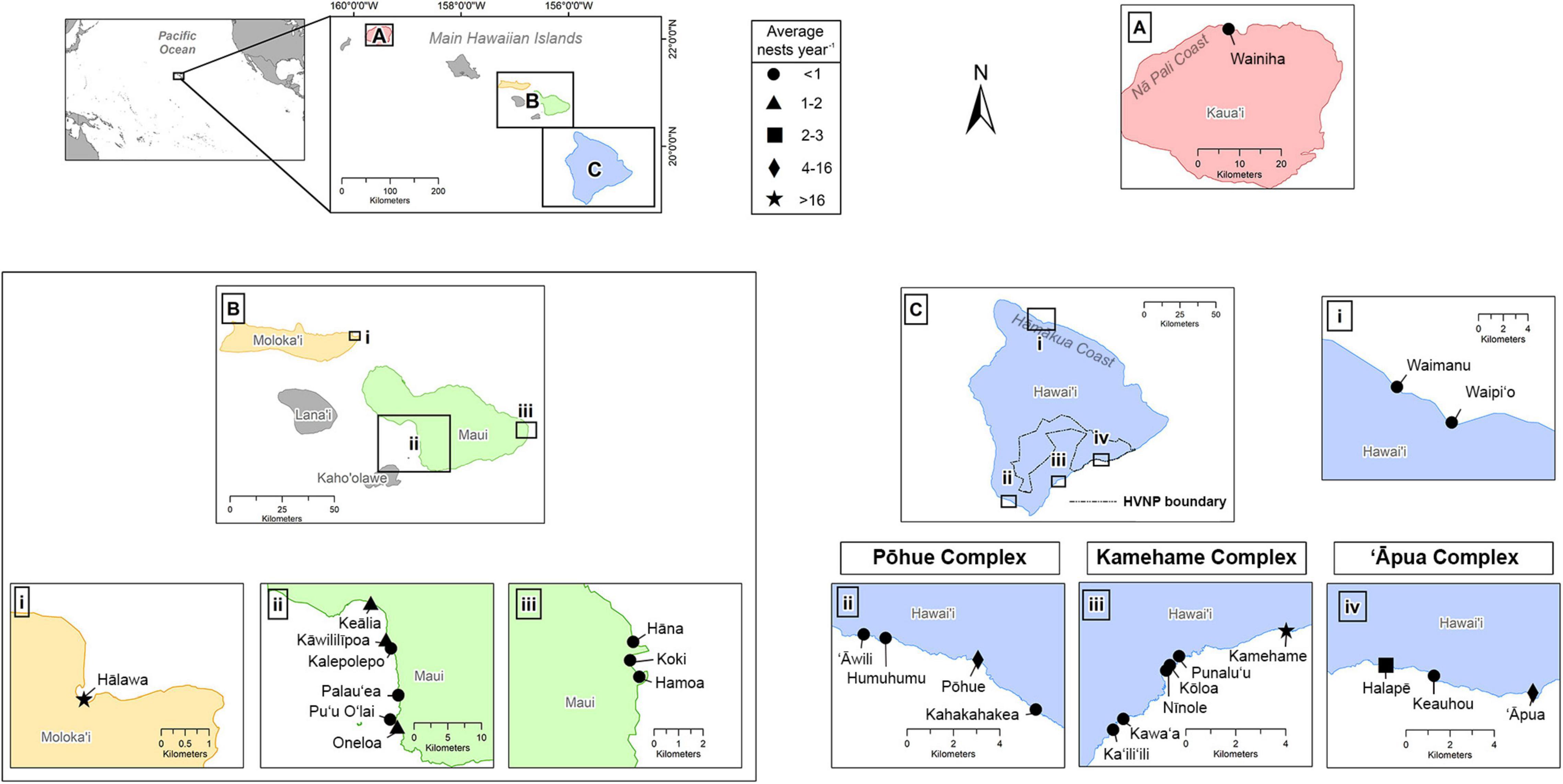
Figure 1. Confirmed hawksbill nesting beaches on the Hawaiian Islands, including panels for (A) Kaua’i, (B) Maui Nui, and (C) Hawai’i Island. Symbols correspond to the average number of nests per year based on years consistently monitored. The three primary nesting complexes on Hawai’i Island (Pōhue, Kamehame, and ‘Āpua) and the boundary for Hawai’i Volcanoes National Park (HVNP) shown in (C) for reference.
Semi-consistent hawksbill monitoring efforts were initiated on several beaches on Hawai‘i Island in 1989, and flipper tagging of nesting females began in 1991. More intense monitoring efforts began on Hawai‘i Island and Maui in 1993 and 1996, respectively. Data on identified nesting female turtles and nest demographics are derived solely from these two islands. Reports of hawksbill nesting on other beaches of the MHI have largely been the result of opportunistic encounters of nesting females, their tracks, or emerging hatchlings, with the exception of Hālawa on Moloka‘i, where morning beach surveys were conducted consistently to document evidence of nesting throughout the 2018 nesting season.
Data Collection
Monitoring effort was only tracked on Hawai‘i Island and was recorded as either night camps or morning day checks. Night camps consisted of overnight stays at nesting beaches during which all-night monitoring was conducted to encounter nesting females and observe nest oviposition. Day checks consisted of visits to beaches in the early morning hours to document tracks of nesting females (nesting females almost exclusively emerge at night to lay eggs) and potential nest areas from the previous night(s). Combined, these efforts enable us to document hawksbill nesting events across the season, which we define as any evidence of a hawksbill emerging to nest, whether successful or not. We considered nesting events as successful if we could confirm nest presence, either by directly observing egg deposition or via post-hatching nest excavations, while unconfirmed nests were recorded as possible nests and not included in our analysis or results. When hawksbills were observed, we applied Inconel flipper tags (National Band & Tag, Newport, KY, United States) to front and/or rear flippers, allowing for the identification of nesting females across seasons and years. In 2016, we also began consistently applying internal passive integrated transponder (PIT) tags (Biomark, Boise, ID, United States) to the front or rear flippers of nesting hawksbills to maximize tag retention and ongoing identification. Measurements of curved (CCL) and straight (SCL) carapace length (from the nuchal notch to posterior-most tip of marginal scutes), as well as location, date, and time of the emergence event were recorded. Turtles observed across years were classified as remigrants, with remigration intervals representing the number of years between (observed) successive nesting events. Internesting intervals were calculated by the number of days between (observed) successive nests by an individual turtle within a season. Neophyte (i.e., turtles with no tags) and remigrant turtles were identified each year. Clutch frequency (i.e., the number of clutches laid per season) was calculated by averaging the total number of nests for known (i.e., observed/tagged) individual turtles for each season.
Nests were left in-situ with the exception of those deemed to be at risk of tidal inundation which were relocated to areas above the high tide line. Nest locations were monitored throughout the incubation period and when feasible, nest contents were excavated by hand 24–72 h following hatchling emergence. We estimated the number of hatchlings that successfully emerged from each nest by counting empty egg shells (consisting of > 50% of the shell) and subtracting any dead hatchlings. Whole eggs, or eggs that were perforated (and contained yolk/hatchling remnants), were considered unsuccessful. Successful and unsuccessful eggs were summed to get the total number of eggs laid. The hatching success rate for each nest was calculated by dividing the number of successful eggs by the total number of eggs laid. We took measurements (cm) from level ground to the top and bottom of the egg chamber whenever possible. We calculated the average annual number of nesting females and hatchlings using data from 1993 to 2018. The average annual number of nests for each beach was calculated for years during which each beach received monitoring, omitting beaches where nests were only observed opportunistically (e.g., via reports by the public).
Statistical Analysis
A generalized additive modeling (GAM) approach was used to evaluate trends of both nesting females and nests on Hawai‘i Island, where we had a constant record of monitoring effort. Models were fitted using the gam function in the mgcv R package (Wood, 2011; R Core Team, 2021). The monthly number of observed individual nesting turtles and confirmed nests were used as the response variables (y) in a hierarchical GAM model (Pedersen et al., 2019). The fitted model structure for month m, site s, in year t was
where campm,s,t is the number of night camps, daym,s,t is the number of morning day checks, αs is a site-level random effect and f(…) denotes a penalized GAM (Wood, 2003) effect. The GAM effects are:
• fs (t) is a penalized site-specific factor thin-plate regression spline,
• f (m) is a cyclic cubic spline constrained so the f (1) ≈ f (12),
• f (camp m,s,t) and f (daym,s,t) are effort main effect thin-plate regression splines
• f (campm,s,t, t) and f (daym,s,t, t) are 2-dimensional tensor-product smooths that represent effort year interactions (Wood, 2017), and
• f (campm,s,t, rs) and f (daym,s,t, rs) are 2-dimensional tensor product smooths that represent effort and region (rs = region of site s) interaction effects. The marginal smooth of rs is an independent random effect.
The interaction terms in the model allow the effect of a given effort to vary by year and region, e.g., if nest searchers become more efficient over time, then for a comparable amount of effort, more nests will be seen in later years. The selection method of Marra and Wood (2011) was used to automatically eliminate terms that were not useful in predicting counts. In a preliminary analysis a Tweedie distribution was used to model overdispersion and zero-inflation (Wood and Fasiolo, 2017), but it was determined that this was not necessary because the fitted Tweedie parameters indicated the distribution was very close to Poisson (i.e., ϕ ≈ 1, p ≈ 1). Therefore, a Poisson response distribution was used in further modeling.
After fitting the model, we used the approach of Johnson and Fritz (2014) to remove the effects of varying survey effort and missing data to allow aggregation over sites and estimation of yearly growth trends in total nesting female and nest counts. First, the effort corrected counts were estimated from the fitted model using
where and are fixed effort values for each site and month. Here we used the 85th percentile for each site and month over all years to estimate what the count would be if a consistently “good” survey effort was conducted at each site in each month over the entirety of the study. Other values could be chosen for this, e.g., 50th percentile would adjust all counts to a “typical” effort at each site and month. Because the overall goal is to examine trends over time, the adjustment makes relatively little difference as the overall fit is simply adjusted up or down uniformly. Thus, we chose the 85th percentile to give a closer estimate to the true count when maximal effort is expended. However, we did not want outlier effort values to overestimate the maximal count. Once predictions are normalized for survey effort, the predicted annual count by site for effort c*m,s and can be estimated using
Finally, yearly growth (% form) can be calculated as
for site s and for the aggregated population, respectively. To estimate average growth over a given time span, a linear model is fitted to log for all t in the time span. The slope, β, is then transformed to percent growth via the transform 100*[exp (β) − 1]. To accurately propagate uncertainty in the estimates, a parametric bootstrap was used to draw an empirical Bayesian posterior sample (size = 200,000) from the GAM coefficients and their estimated covariance matrix. With each draw, the predictions and growth trends were calculated, forming an approximate posterior sample for those derived parameters.
Data generated via field monitoring activities were also tabulated, analyzed, and graphed using Microsoft Excel v.14.16.2. Mean and mode values, standard deviations (SD), and ranges are reported throughout the present paper.
Results
Monitoring Outcomes
Monitoring effort varied spatially and temporarily, but was particularly limited prior to 1993, coinciding with limited monitoring results (Figure 2 and Supplementary Table 1). Overall monitoring effort (i.e., night camps and day checks) steadily increased over the first two decades of the study timeframe, reaching a peak in 2007, before subsequently decreasing. The average number of annual night camps across all beaches on Hawai‘i Island was 409.6 (±212.5) and day checks was 398.7 (±280.4) between 1989 and 2018 (Supplementary Table 1). The lowest average annual night camps (78.8 ± 60.4) and day checks (21.3 ± 13.7) occurred between 1989 and 1992, while the highest average annual night camps (704.0 ± 46.2) and day checks (785.3 ± 135.7) occurred between 2005 and 2010 (Figure 2 and Supplementary Table 1). No monitoring effort was recorded during 1988.
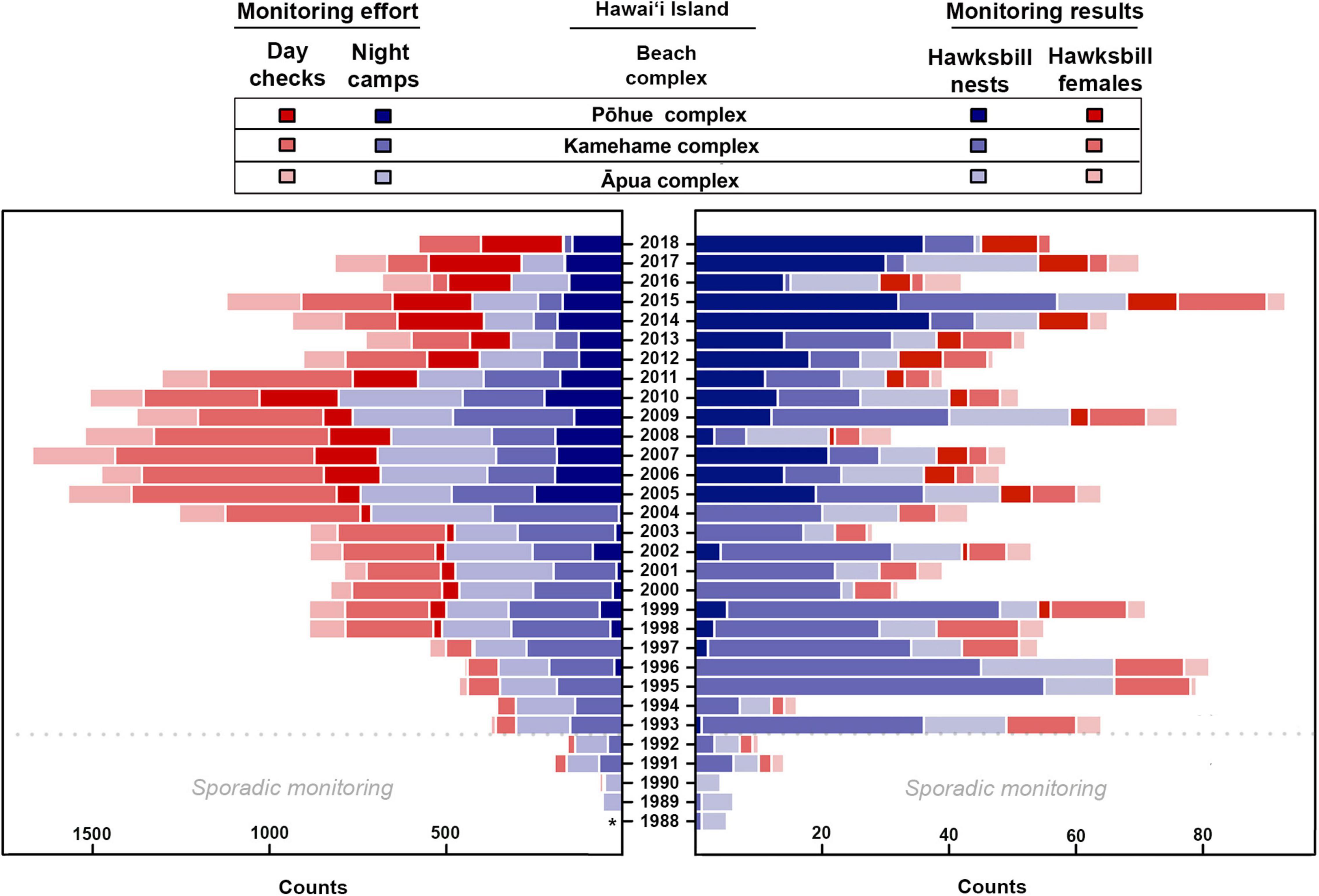
Figure 2. Monitoring effort, including day checks and night camps (red bars and purple bars, respectively, on left), as well as monitoring results, including confirmed hawksbill nests and observed hawksbill females (purple bars and red bars, respectively, on right), documented annually between 1988 and 2018 at the three main nesting complexes on Hawai‘i Island. Asterisk indicates no data available. Note that no female turtles were tagged prior to 1991 and only sporadic monitoring was carried out at all sites prior to 1993.
Since 1993, an average of 14.0 (±4.3) unique female hawksbills have been recorded nesting annually in the MHI, with a minimum of five females in 1994 and a maximum of 26 females in 2015 (Table 1 and Figure 2). In total, 178 individual nesting female hawksbills have been tagged; 167 (93.8%) on Hawai‘i Island and 11 (6.2%) on Maui. The overwhelming majority (81.5%) of these turtles were first observed and tagged on three beaches on Hawai‘i Island, with remaining nesting females (18.5%) observed and tagged across a total of 11 additional beaches (Figure 3). Only 15 (8.4%) of the 178 nesting females were documented nesting on more than one beach, and on Hawai‘i Island, only two (1.1%) turtles were documented using beaches across nesting complexes (Figure 1), exemplifying high nesting site fidelity. Individual nesting females were observed on 351 occasions, of which 178 (50.7%) events corresponded to neophyte nesters and 173 (49.3%) to remigrant nesters (Figure 4). Neophyte nesters were less common (42.9% of annual cohorts) than remigrant nesters between 1991 and 2005, then more common (57.1% of annual cohorts) between 2006 and 2018.
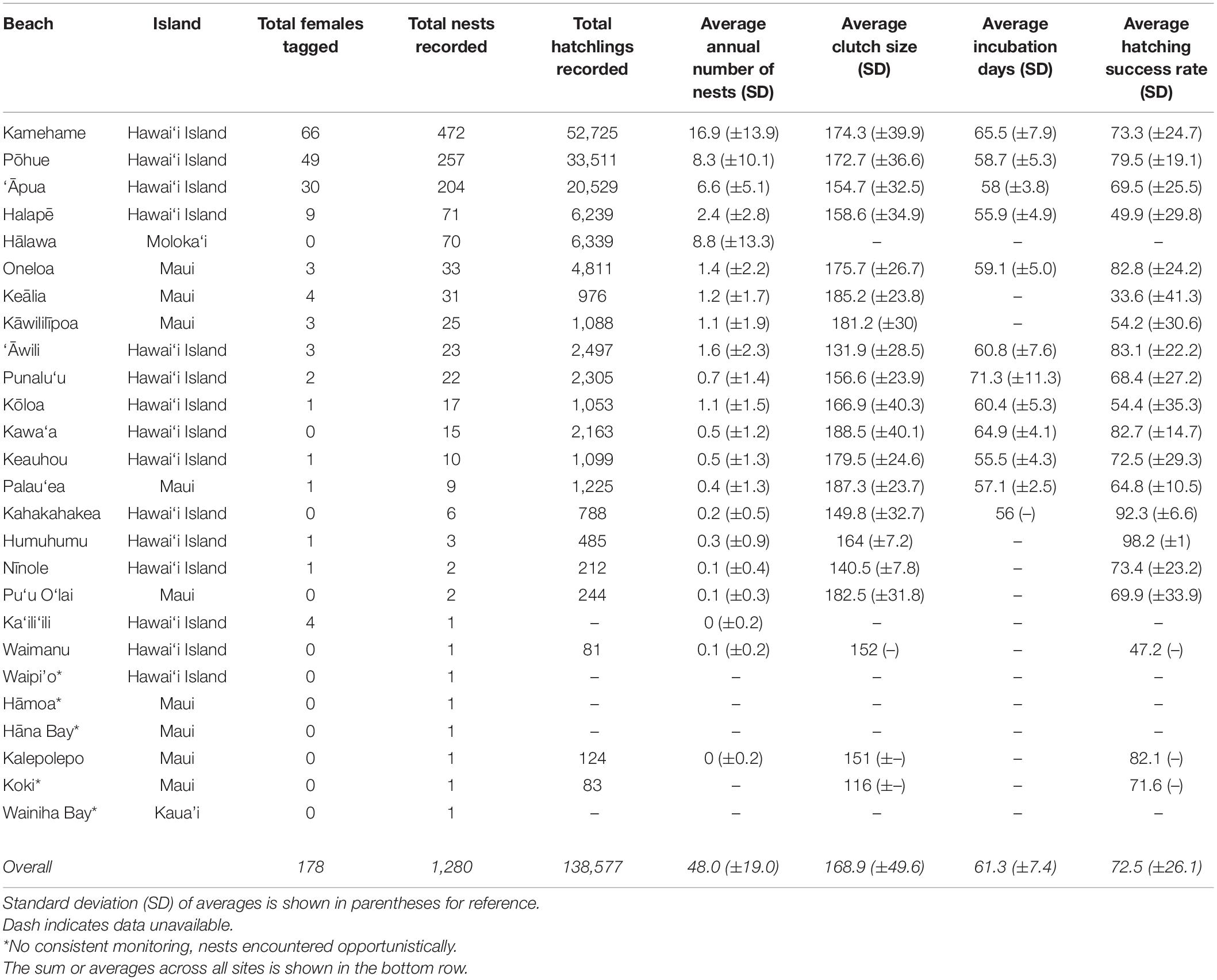
Table 1. Information on 26 beaches confirmed to host nesting hawksbills, including beach name, island, total number of females identified, nests, and hatchlings recorded between 1988 and 2018, as well as average annual number of nests, average clutch size, average incubation days, and average hatching success rate (1993–2018) on the main Hawaiian Islands.
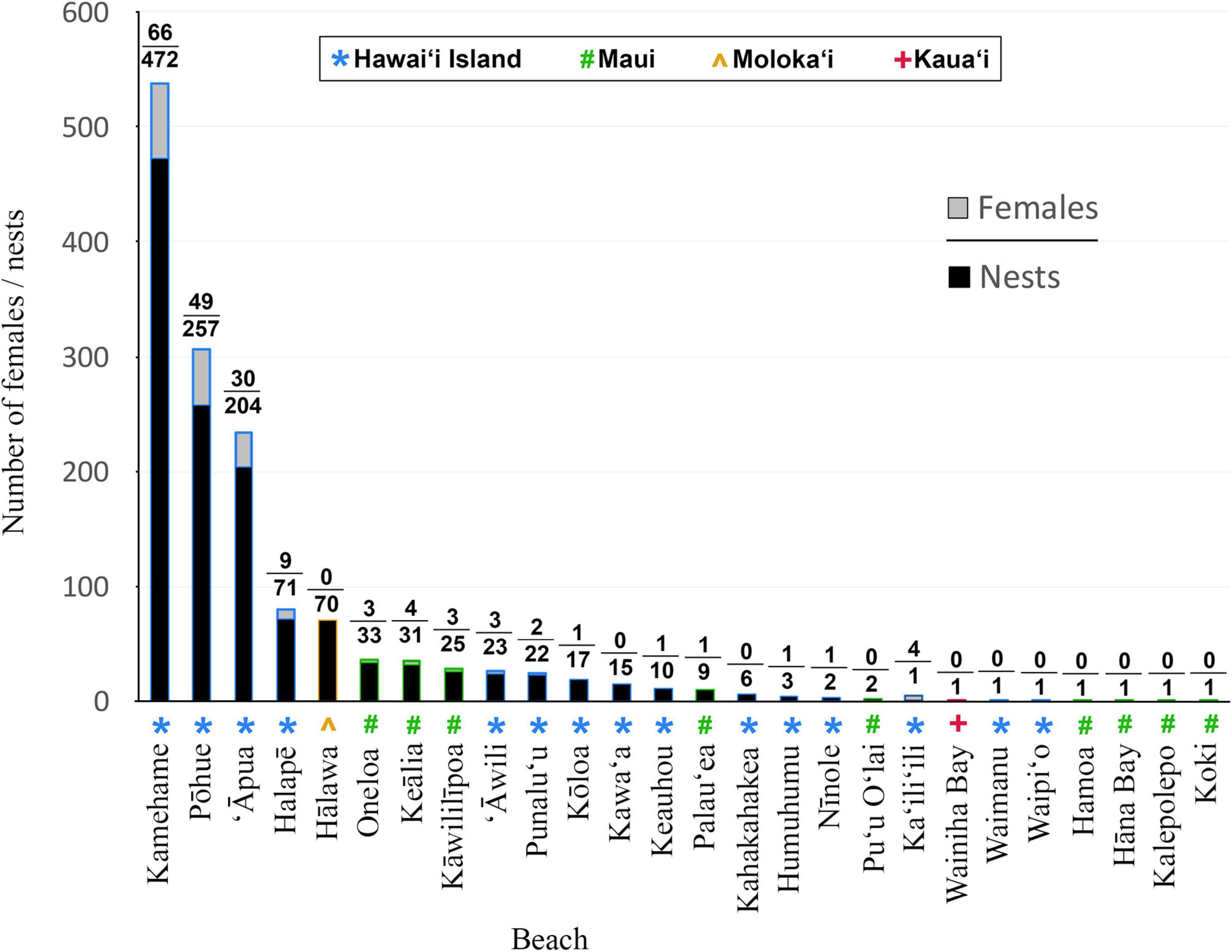
Figure 3. Total number of hawksbill females and nests recorded at beaches and islands around the main Hawaiian Islands between 1988 and 2018. Note that only sporadic monitoring was carried out prior to 1993.
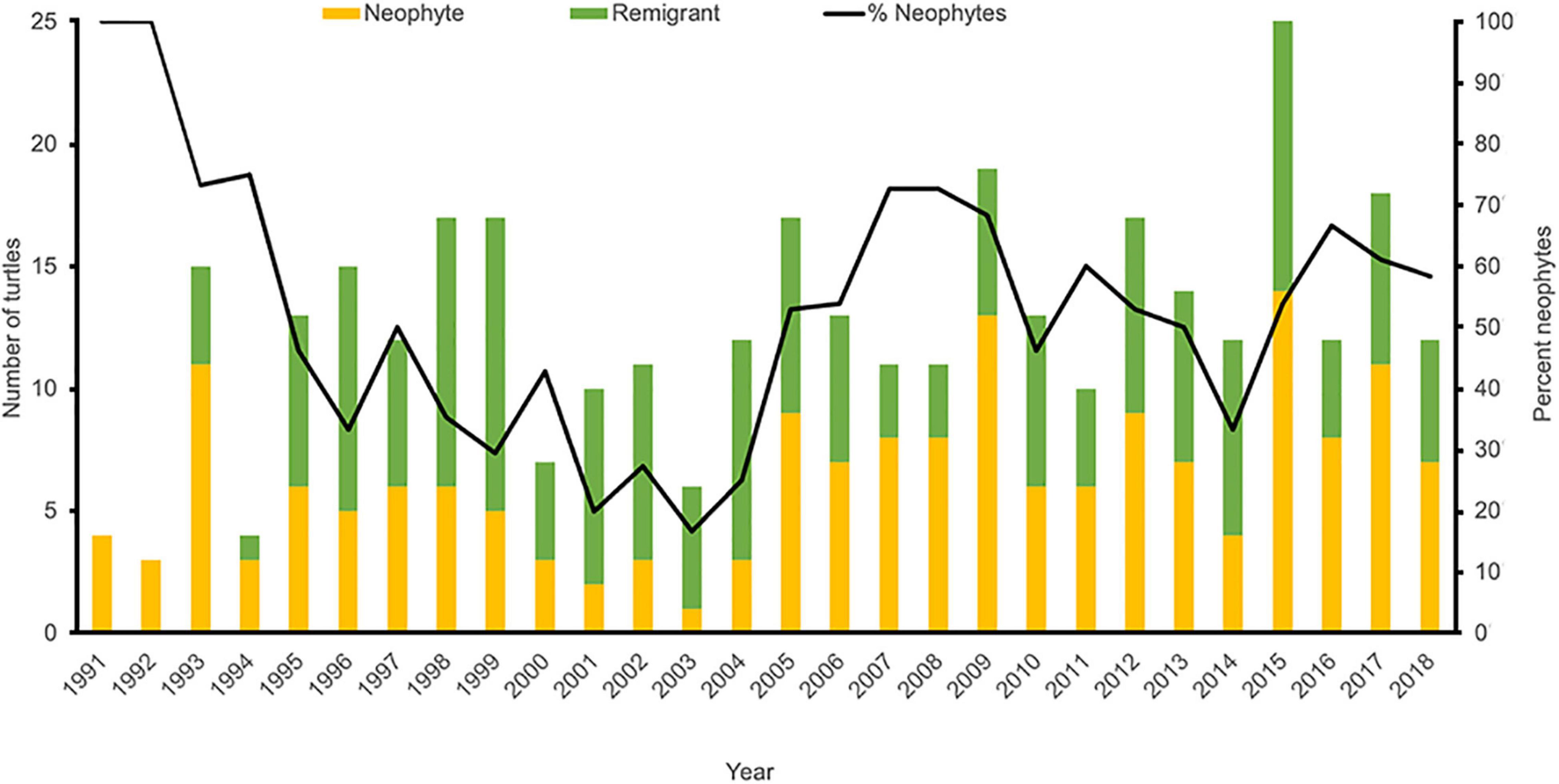
Figure 4. Proportion of nesting events corresponding to neophyte (orange bars) and remigrant (green bars) turtles, as well as percent neophytes (black line), between 1991 and 2018. Note that no female turtles were tagged prior to 1991 and only sporadic monitoring was carried out prior to 1993.
The mean internesting interval for hawksbills (n = 517) was 23.3 (±9.9) days, but the modal internesting interval was 18 days (13.9%) and 20 days (13.9%), followed by 19 days (12.4%), 17 days (10.6%), and 21 days (9.5%) (Supplementary Figure 1A). The mean remigration interval for hawksbills seen across years (n = 178) was 4.3 (±2.7) years, but the modal remigration interval was 3 years (32.6%), followed by 2 years (23.0%), 4 years (12.4%), and 5 years (12.4%), with a range of 2–17 years (Supplementary Figure 1B). Nesting activity occurred primarily between the months of April and December each year, with peak nesting occurring during the months of July (33.5%) and August (30.1%) (Supplementary Figure 1C).
Average hawksbill CCL (n = 73) was 86.6 cm ± 6.0 (range 71.0–104.0 cm), SCL (n = 155) was 81.8 cm ± 4.4 (range 68.0–99.0 cm), clutch size (n = 1,083) was 168.9 eggs ± 37.1 (range 34–299 eggs), and clutch frequency (n = 290) was 2.7 ± 1.3 clutches (range 1–6 clutches). In total, we recorded 3,231 nesting events (including successful and unsuccessful events), 1,997 (61.8%) during which the turtle was observed and 1,234 (38.2%) during which only tracks or signs of nesting were encountered.
We calculated an annual average of 48.0 (±19.0) hawksbill nests in the MHI between 1993 and 2018, and a grand total of 1,280 nests between 1988 and 2018 (Table 1). Nests were recorded on four of the eight MHI, including Hawai‘i Island (86.4%), Maui (8.1%), Moloka‘i (5.5%), and Kaua‘i (0.1%). The overwhelming majority (83.9%, n = 1,074) were documented at five beaches, including four beaches on Hawai‘i Island [Kamehame (16.9 ± 13.9 nests yr–1), Pōhue (8.3 ± 10.1 nests yr–1), ‘Āpua (6.6 ± 5.1 nests yr–1), and Halapē (2.4 ± 2.8 yr–1)] and one beach on Moloka‘i [Hālawa (25.5 ± 21.9 nests yr–1)] (Table 1 and Figure 3). The only other beaches that averaged ≥1.0 nest per season were ‘Āwili (1.6 ± 2.3 nests yr–1) and Kōloa (1.1 ± 1.5 nests yr–1) on Hawai‘i, and Kāwililīpoa (1.1 ± 1.9 nests yr–1), Keālia (1.2 ± 1.7 nests yr–1), and Oneloa (1.4 ± 2.2 nests yr–1) on Maui. The remaining (6.0%) nests were deposited across 16 different beaches, each averaging less than a single nest yr–1 over the study timeframe (Table 1 and Figure 1). The average depth to the top of the egg chamber (n = 888) was 28.7 cm (±10.8 cm) and to the bottom of the egg chamber (n = 895) was 45.9 cm (± 29.4 cm).
We recorded an average of 5,025.3 (± 1,923.9.4) hatchlings per year between 1993 and 2018, and a total of 138,577 hatchlings across all years (Table 1). The majority (77.0%) of hatchlings were documented at three sites on Hawai‘i Island, including Kamehame (38.0%, n = 52,725), Pōhue (24.2%, n = 33,511), and ‘Āpua (14.8.0%, n = 20,529). The average incubation period (n = 561) across all sites and years was 61.3 ± 7.4 days (range 49–91 days). The average hatching success rate (n = 1,064) across all sites and years was 72.5% (±26.1). Considering only beaches that received >10 nests, hatching success rates were <50% at two sites, including Keālia (Maui; 33.6% ± 41.3) and Halapē (Hawai‘i Island; 49.9% ± 29.8), and >80% at three sites, including Kawa‘a (Hawai‘i Island; 82.7% ± 14.7), Oneloa (Maui; 82.8% ± 24.2), and ‘Āwili (Hawai‘i Island; 83.8% ± 23.1). Nest excavation data was attained for 1,064 (83.1%) of the nests recorded across years. Due to deficiencies in data capture, it is unclear what percentage of the remaining 216 nests (16.9%) were predated or simply not relocated (i.e., excavations not possible).
Model Output
Using the constant 85th percentile effort schedule across years, the GAM models predicted a mean of 27.1 (±13.3) nesting females and 92.5 (±32.1) nests on Hawai‘i Island each year (Supplementary Table 1). The estimated annual number of nesting females and nests declined each consecutive year between 1989 and 2006, followed by consecutive annual increases after 2006 (Figure 5 and see Supplementary Figures 2, 3 for individual site results). Similarly, growth rates of the number of nesting females and nests were negative from 1989 to 2005, then began trending positive in 2006, with average annual growth rates of 8.2% (CI: 3.1–13.8) and 7.2% (CI: 4.2–10.6) since that time, respectively.
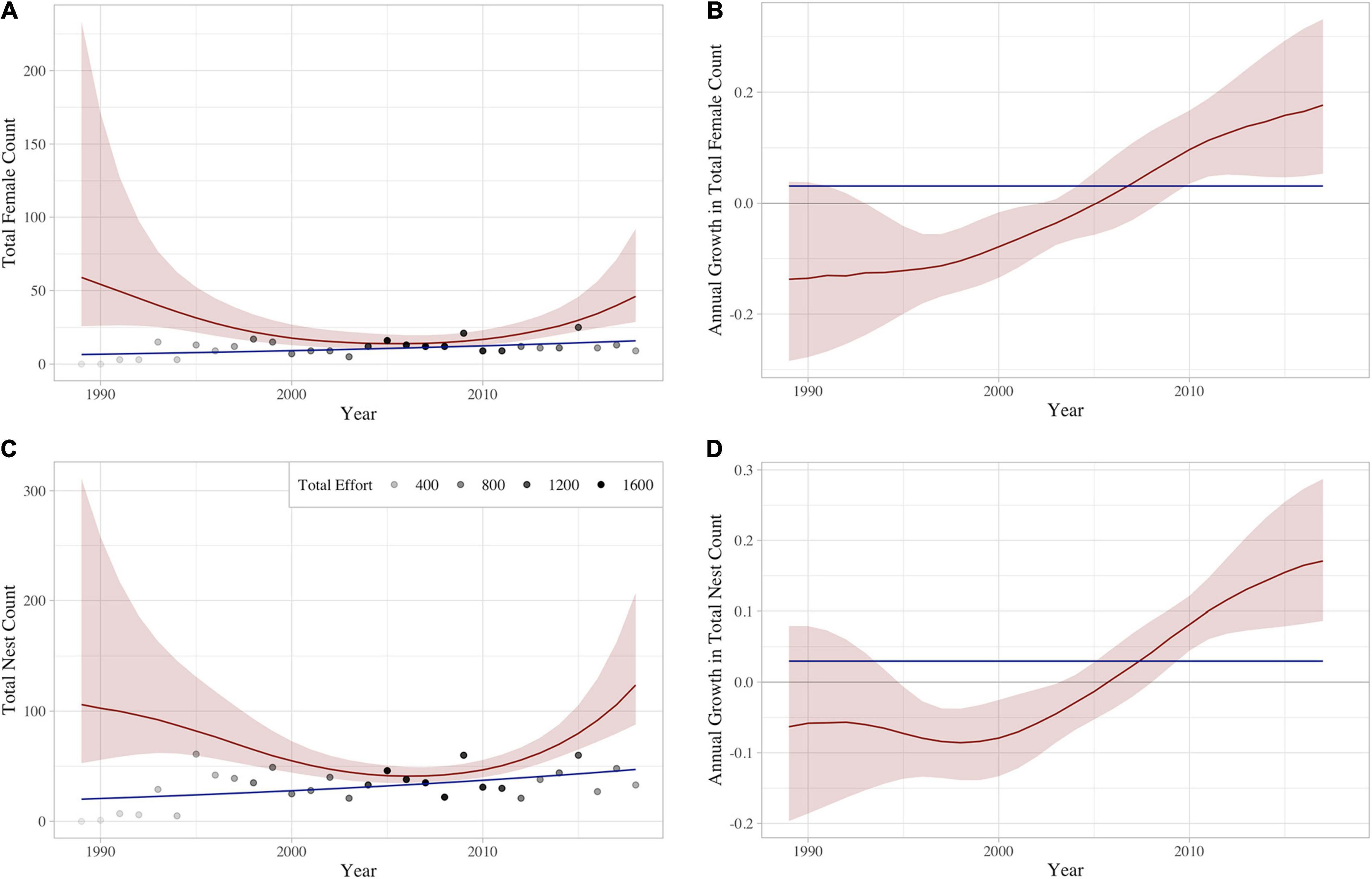
Figure 5. Yearly aggregated prediction of (A) females and (C) nests, as well as associated annual growth rates for (B) females and (D) nests. Solid red curves correspond to the empirical Bayes posterior mean, and the estimated 95% credible bands are shaded in red. Effort levels (shaded dots) are shown in panels (A,C) for reference. Total effort is the sum of all night camps and days surveys throughout the year. The blue line represents the constant slope fit for the raw tabulated numbers of (A) females and (C) nests, as well as the associated constant yearly growth for tabulated (B) females and (D) nests.
Discussion
Results of our field monitoring and GAM model predictions indicate a limited number of hawksbill nesting females and nests occur in Hawai‘i each year, demonstrating the precarious nature of the population. Notwithstanding low annual and overall numbers, our GAM analysis suggests that the hawksbill nesting population in Hawai‘i has been increasing since 2006 (Figure 5).
Population Status
The lack of an obvious trend in the number of nesting females or nests when looking solely at raw tabulated numbers contrasts with the recent positive trend suggested by our GAM analysis (Figure 5), and this can most likely be attributed to recent changes in monitoring effort. Although Kamehame received the overwhelming majority of nesting over the 30-year study period (Figure 3), consistent monitoring has been limited since 2012 (Figure 2) due to staff safety concerns. Coincident with the suspension of consistent monitoring at Kamehame, hawksbill nesting activity increased at Pōhue –a beach that had not historically received much nesting activity (Figure 2)– and program staff largely redirected monitoring efforts to this site (Supplementary Figures 2, 3). This shift in effort and the ongoing lack of simultaneous, consistent monitoring at what represent the two most important hawksbill nesting sites in Hawai‘i, are likely the primary reasons for the discrepancy between the tabulated numbers and the GAM analysis. Increased funding to support more robust field monitoring, coupled with the potential implementation of novel survey tools such as uncrewed aerial systems to facilitate the evaluation of isolated beaches, will be important in quantifying nesting activity more accurately.
Our GAM analysis also suggests that current tabulated numbers of nesting females and nests are likely underestimates, which is supported by the number of untagged adult females observed in-water and via strandings (King and McLeish, 2019; Brunson et al., in press), as well as by the number of neophyte turtles that have been documented during recent years. As expected, during the initial years of monitoring the overwhelming majority of turtles were neophyte nesters, with an increase in the number of observed remigrants in subsequent years (Figure 4). However, although it was expected that the number of remigrants would continuously increase over time as monitoring efforts advanced toward tag saturation of the population, the percentage of neophyte nesters was actually higher during the latter half of the study, compared to the first half (Figure 4). Overall, approximately 50% of annual nesting cohorts have consisted of neophyte nesters, which potentially represent new recruits to the nesting population.
Hawksbill nesting at Hālawa was only systematically monitored (i.e., day checks) for the first time in 2018 (i.e., the final year included in this study). That effort led to the documentation of 41 nests, which represents 44.1% of the total nests documented in the State of Hawai‘i during 2018, although monitoring on Hawai‘i Island was limited that year due to logistical complications associated with the eruption of Kīlauea Volcano. Sporadic reports of hawksbill nesting at Hālawa have been recorded over the years (e.g., Ernst and Barbour, 1972; Balazs, 1978; NMFS and USFWS, 1998), suggesting that hawksbills have been nesting at the site for decades. Whether nesting levels at the site have changed over time remains unclear. Notwithstanding this perspective, the findings at Hālawa highlight the importance of the site for hawksbills, while underscoring the need to establish consistent monitoring efforts to support future population evaluations. Combined, the recent positive trend in the number of nesting females and nests, the shift in monitoring at two key nesting sites, and the documentation of a large proportion of nests at the newly monitored nesting beach of Hālawa, bode well for the future of the population.
The positive trend we report here coincides with recent findings that many sea turtle populations are increasing globally (Mazaris et al., 2017). Despite these encouraging findings, the average annual number of hawksbills nesting in Hawai‘i based on both our monitoring (∼14 year–1) and model output (∼27 year–1) is orders of magnitude smaller than those reported for several major nesting populations in the Indian, Atlantic, and Pacific Oceans (e.g., Richardson et al., 2006; Allen et al., 2010; Santos et al., 2013; Gaos et al., 2017a; Pilcher, 2021). The small population, coupled with recent genetic findings that Hawaiian hawksbills represent a closed population (Gaos et al., 2020), highlight the vulnerability of the population as major distrubances could impact both nesting and foraging grounds simultaneously.
Although it is possible that the Hawaiian hawksbill population is reduced compared to historical numbers (Van Houtan et al., 2012; Van Houtan K.S. et al., 2016), the lack of historical information makes it difficult to put the current state of the population into context. It is possible the species has persisted at relatively low levels in the region for centuries (see references in Van Houtan et al., 2012). The low number of nesting hawksbills around Hawai‘i contrasts sharply with findings for green turtles (Becker et al., 2019), with an average of 265 green turtles nesting annually between 1973 and 2018 at their primary nesting site on East Island, French Frigate Shoals (PIFSC unpublished data). Nonetheless, our results suggest the nesting populations of both species in Hawai‘i have been increasing over the last several years (this study; Balazs and Chaloupka, 2006; Seminoff et al., 2015). Combined, these findings suggest the conservation actions and legislative protections afforded both species starting in the 1970s appear to be working (Aguirre et al., 1998; Balazs and Chaloupka, 2004). The discrepancy in the approximate population size of these two species around Hawai‘i remains unclear, but studies in the western and eastern Pacific Ocean have also found that hawksbills are much less abundant than their green or olive ridley (Lepidochelys olivacea) turtle counterparts (Márquez et al., 2002; Eguchi et al., 2007; Gaos et al., 2017a; Becker et al., 2019).
Hawksbills face a myriad of threats in the Pacific, as do other sea turtle species whose populations remain abundant; therefore, attributing limited hawksbill populations solely to anthropogenic causes would not seem prudent. Hawksbills often forage in coral reef ecosystems and these habitats face a litany of threats and are thought to have declined around Hawai‘i during the past half century (Friedlander et al., 2005; Rodgers et al., 2017). This may have reduced dietary resource availability for hawksbills, thus limiting population expansion. Furthermore, as is common with coral reefs under environmental stress, some of these habitats may have undergone regime shifts to substrates dominated by macroalgae (Folke et al., 2004), which represent dietary items typical of green turtles. This could support the population expansion of greens over hawksbills. Broad-scale environmental forcing factors that are not easily detected could also be differentially impacting these species (Suryan et al., 2009).
Monitoring Outcomes
There were differences in the monitoring effort at the known hawksbill nesting beaches, with some sites only receiving sporadic visits (e.g., every few days) or strictly morning surveys to search for evidence of nesting (i.e., tracks), vs. other sites that received consistent night patrols in search of nesting females (Supplementary Figures 2, 3). As a result, it was often difficult to confirm whether nests were successfully laid (vs. unsuccessful nesting events), and some nesting events likely went undocumented each year. This is supported by our GAM analysis, which suggests more nesting females and nests occur on Hawai‘i each year than are recorded during field monitoring. Furthermore, a large proportion (38.2%) of nesting activity records consisted of events during which the actual turtle was not observed, making it difficult to confidently assess several parameters for nesting turtles.
For example, the mean internesting and remigration interval for nesting females was 23.3 (±9.9) days and 4.3 (±2.7) years, respectively, and both values are considerably larger than those reported for conspecifics in other ocean regions (Van Buskirk and Crowder, 1994; Spotila, 2004; Richardson et al., 2006; Gaos et al., 2017a). The averages for Hawai‘i are likely skewed by turtles that had internesting intervals of >40 days (n = 37) and remigration intervals of >5 years (n = 35). Although it is possible that these turtles did not nest during these timeframes, it is more likely that they went undetected between two or more observation events due to limited monitoring effort. Indeed, the mode of internesting interval for all hawksbills was 17–21 days, accounting for 60.8% of all internesting interval records (Supplementary Figure 1A). Similarly, the most common (55.9%) remigration interval for all hawksbills was 3 years (32.4% of observations), followed by 2 years (23.5% of observations) (Supplementary Figure 1B). These values are similar to intervals reported for the species by other studies (e.g., Bjorndal et al., 1985; Van Buskirk and Crowder, 1994; Spotila, 2004; Gaos et al., 2017a). Although annual remigration intervals are common (10.7%) for hawksbills in the eastern Pacific Ocean (Gaos et al., 2017a), annual remigrations have never been recorded for hawksbills in Hawai‘i. Whereas, hawksbills in Hawai‘i primarily use habitats consisting of coral and rocky reef substrates, in the eastern Pacific they commonly use mangrove estuaries (Gaos et al., 2012a,b). The differences in remigration intervals could be tied to resource quality differences between these two foraging habitats, with mangrove estuaries being particularly resource-rich (see references in Gaos et al., 2017a) and facilitating more frequent nesting.
Low observation rates may also contribute to the fact that approximately 28.1% (n = 50) of the 178 identified hawksbills have not been observed in the past 10 years. However, considering remigration records of >10 years are rare (4.5% of all remigration observations), it is reasonable to assume that many of these nesting females no longer constitute part of the reproductive population.
Sporadic monitoring and low observation rates are a result of several factors, including the diffuse and less colonial nesting habits exhibited by hawksbills (Witzell, 1983; Liles et al., 2011), as well as the isolated and inaccessible nature of many of the known nesting beaches around Hawai‘i, which complicate monitoring. Hawaiian hawksbills also often traverse extensive lava fields or large boulders and nest on beaches composed of crushed coral substrates, which can complicate the detection of tracks that would help identify nesting activity and potential nesting beaches. When coupled with the low number of hawksbills in Hawai‘i, these factors make it difficult to detect, monitor, and quantify nesting hawksbills and their nesting habitats. Diminished funding for monitoring beginning in 2012 has also led to reduced effort in recent years (Figure 2).
There are also some beaches around the MHI that appear to host adequate hawksbill nesting habitat, but have never been monitored because they are even more isolated and difficult to access than known nesting sites, making monitoring logistically and financially impractical. For example, the Nā Pali Coast of Kaua‘i (Figure 1) hosts a handful of potential beaches, but a lack of vehicular access presents major challenges to monitoring. Similar access limitations prohibit monitoring along the isolated Hāmākua Coast on Hawai‘i Island, although opportunistic nesting has been documented on two occasions in the area (i.e., Waimanu and Waipi‘o; Figure 1). There has been speculation that occasional hawksbill nesting may even occur on the windward (eastern) coast of O‘ahu as a total of 40 hawksbill hatchlings have been found stranded in the area between 1986 and 2016, yet no nests have been documented to date (Brunson et al., in press). However, a drifter satellite tag deployed offshore of Hālawa on Moloka‘i was encountered approximately 3 weeks later along windward O‘ahu, suggesting the stranded hatchlings may be originating from this nesting site (PIFSC unpublished data). The location of Hālawa in relation to O‘ahu (Figure 1), combined with the prevailing easterly Trade Winds typical of Hawai‘i (Juvik et al., 1999), would facilitate this hatchling dispersal pattern. Although the identification of “missing” nesting beaches is needed to better understand the status and management needs of hawksbills in the region, even if they are found, they would likely be limited in number and would not change the general conclusion that the Hawaiian hawksbill population is very small.
Management Policy and Framework
Various biodiversity measurement, risk assessment, and conservation priority schemes exist for marine turtles at both national and international scales (Seminoff and Shanker, 2008). The primary policy in the US is the ESA and as previously mentioned, hawksbills are considered endangered under this policy. Distinct Population Segments (DPS) are currently being developed under the ESA framework to identify sea turtle populations that are geographically and demographically discrete, and significant in relation to the species as a whole (Karl and Bowen, 1999). A conservation status (e.g., threatened or endangered) is subsequently assigned to each population that can facilitate region-specific tailoring of research and conservation activities (USFWS and NMFS, 1996). The results of DPS designations strongly influence marine turtle policy and management actions within the US and abroad, and DPS evaluations have been completed for green, loggerhead (Caretta caretta), and leatherback (Dermochelys coriacea) marine turtles (Conant et al., 2009; Seminoff et al., 2015; NMFS and USFWS, 2020). In 2013, the National Marine Fisheries Service (NMFS) and the US Fish and Wildlife Service (FWS) completed a 5-year review for the hawksbill and recommended an in-depth analysis be carried out to determine the application of the DPS framework to the species in the future (NMFS and USFWS, 2013). This study, combined with recent hawksbill genetic (Gaos et al., 2016, 2017b,2020, 2020; Vargas et al., 2016; Banerjee et al., 2019) and movement research (Parker et al., 2009; Gaos et al., 2012a,b, c; Hamilton et al., 2021) across the Pacific Ocean, should support future DPS evaluations.
It may take years or even decades before full DPS designations are completed for all species of marine turtle (Conant et al., 2009; Seminoff et al., 2015), but it is important that region-specific needs are considered for any recovery actions. Fortunately, the Hawaiian Hawksbill Turtle Network has been collaboratively working to identify hawksbill research and management needs in Hawai‘i since the early 1990s (Balazs et al., 1992; HHTN, 2018), and the group continues to meet regularly to outline priority actions. These efforts have been essential for guiding local research and management activities in the absence of region-specific designations (e.g., DPS) and will likely remain so even if hawksbills in Hawai‘i are formally recognized as a DPS in the future.
Management Implications
Approximately 85% of all confirmed hawksbills nests in the State of Hawai‘i have been recorded in the district of Ka‘ū, on the southern coast of Hawai‘i Island (Figures 1, 3), highlighting the importance of beaches in this area for the population. Development or proposed development near several of the primary nesting beaches were stopped, including a large resort and housing development near Punalu‘u in 2007 (Bambico, 2009: Hawai‘i resolution 169-07) and a space industry launch site near Pōhue in 2018 (Ancheta, 2018), yet threats from development are ongoing. Fortunately, several important nesting beaches, including ‘Āpua, Halapē, and Oneloa are located in national or state parks [e.g., Hawai‘i Volcanoes National Park (HVNP; Figure 1) and Mākena State Park] and benefit from permanent protection against major coastal development. However, even in these areas nesting habitat can be impacted by recreational activities (e.g., camping, driving on beaches, light pollution) that can negatively affect both nesting females and hatchlings (HHTN, 2018), thus stronger controls might be warranted.
Additionally, shorelines along much of the MHI, including sites located within HVNP, are highly susceptible to sea level rise and associated increased beach erosion (Anderson et al., 2018). The projected escalation in storm frequency and intensity has the potential to increase nest inundation events and embryonic mortality (Foley et al., 2006; Fuentes et al., 2010). Many coastlines around Hawai‘i have already lost significant nesting area to erosion (Baker et al., 2006; HHTN, 2018), and the reduction of areas available for nesting is expected to continue under future climate scenarios (Patrício et al., 2021). Such reductions could amplify density-dependent issues (Girondot et al., 2002; Tiwari et al., 2010), which are already a problem at the small hawksbill nesting beaches in Hawai‘i. For instance, although Kamehame is approximately 135 m long, the overwhelming majority of nesting actually occurs along a stretch of beach that is only 35 m wide, and as a result, many nests deposited early in the season are dug up by subsequent nesters. Although hawksbill often nest under naupaka (Scaevola taccada) on Hawai‘i and elsewhere (e.g., Mortimer et al., 2020), patches on the primary nesting beaches outlined on this study can grow so dense that they become impenetrable, further limited nesting areas available to turtles.
Although it has been suggested that marine turtles may be able to shift nesting to more suitable areas (Hamann et al., 2013; Abella Perez et al., 2016; Mainwaring et al., 2017), the high nesting site fidelity exhibited by Hawaiian hawksbills may inhibit their ability to do so. Furthermore, whether additional beaches with adequate habitat for hawksbills exist around Hawai‘i also remains unclear.
Mongoose (Herpestes javanicus), rat (Rattus spp.), feral cat (Felis catus), and feral pig (Sus scrofa) predation of hawksbill eggs and hatchlings is a major issue in Hawai‘i (HHTN, 2018). Temporary protective enclosures (Addison, 1997) have been placed around nests in some areas experiencing high predation, and efforts have been undertaken to reduce non-native predators at many sites since monitoring was initiated (Seitz et al., 2012). These approaches have been efficient at reducing predation, but require ongoing temporal and financial investments and are never 100% effective. Non-native vegetation is also an issue as the dense root systems of several species, particularly coconut (Cocos nucifera) and pepper (Schinus terebinthifolia) trees, as well as buffel grass (Cenchrus ciliaris), inhibit nest chamber excavation by females and can even trap hatchlings as they emerge (King et al., 2007; Seitz et al., 2012). Coastal lighting has been found to misdirect nesting females and hatchlings as they attempt to orient to the ocean, particularly at some of the more developed areas on Maui (King et al., 2007). Other threats to hawksbills around Hawai‘i include mortality in nearshore hook-and-line fisheries, geographic entrapment (e.g., in volcanic fissures), vessel strikes, vehicular impacts, and marine debris entanglement and ingestion (Van Houtan K.S. et al., 2016; Lynch, 2018; Brunson et al., in press). Ongoing conservation efforts as well as local outreach and awareness activities are essential in minimizing threats to hawksbills throughout the MHI.
Data Availability Statement
The raw data supporting the conclusions of this article will be made available by the authors, without undue reservation.
Ethics Statement
The animal study was reviewed and approved by Institutional Animal Care and Use Committee.
Author Contributions
AG drafted the manuscript. LK, HB, CK, KD, JB, EN, IK, GB, KV, TJ, and SM helped to develop the manuscript. DJ, TE, SM, and AG conducted the analyses. LK, HB, LS, KD, and CK collected the field data with support from AG, KV, GB, and EN. All authors approved the final article.
Funding
The hawksbill projects were largely supported via financial contributions from the NOAA Pacific Islands Regional Office under grant awards NA19NMF4540045, NA15NMF4540116, NA15NMF4540113, NA14NMF4540135, NA13NMF4540072, NA12NMF4540208, NA10NMF4540137, NA09NMF4540158, NA08NMF4540524, and NA07NMF4540171, from the USFWS under agreements 12200-2-G001 and F19AC00570 and from the National Park Service under agreements P12AC15875, P17AC01306, P21AC10410, CA8048-AO-001, H8080040012, and J8306072005.
Conflict of Interest
The authors declare that the research was conducted in the absence of any commercial or financial relationships that could be construed as a potential conflict of interest.
Publisher’s Note
All claims expressed in this article are solely those of the authors and do not necessarily represent those of their affiliated organizations, or those of the publisher, the editors and the reviewers. Any product that may be evaluated in this article, or claim that may be made by its manufacturer, is not guaranteed or endorsed by the publisher.
Acknowledgments
We recognize the Hawai‘i Island Hawksbill Project, World Turtle Trust, and NPS Hawai‘i Volcanoes National Park for monitoring activities carried out on Hawai‘i Island, as well as Hawai‘i Wildlife Fund and Hawaiian Hawksbill Conservation for work carried out on Maui. We also thank Pūlama Lāna‘i, Maui Ocean Center Marine Institute, Hawai‘i Pacific Parks Association, University of Hawai‘i at Mānoa–Pacific Cooperative Studies Unit, University of Hawai‘i at Hilo, the Three Mountain Alliance and ‘Imi Pono no ka ‘Āina, Yamanaka Enterprises Inc., Nani Kahuku ‘Āina LLC, the Nature Conservancy of Hawai‘i, Ka‘ū High School, the Trust for Public Land, Hawai‘i County, and the Hawai‘i State Department of Land and Natural Resources. We are indebted to Lawrence Katahira, Will Seitz, Kyle Kagimoto, Barbara Luehrs, Skippy Hau, Bill Gilmartin, Joan Yoshioka, Rhonda Loh, Sierra McDaniel, Kelly Peebles, Megan Lamson, Ingrid Yañez, Christi Feeter, Meghan Soukup, and the thousands of dedicated volunteers that have participated in hawksbill monitoring over the years. All hawksbill handling procedures were approved (SWPI_2013-05R) by the Institutional Animal Care and Use Committee (IACUC) in accordance with the requirements pertaining to animal subjects protections within the Public Health Service Policy and USDA Animal Welfare Regulations. Research was carried out under USFWS permits # TE739923, TE829250, TE739350, and TE72088A and State of Hawai‘i Department of Land and Natural Resources Special Activity Permits.
Supplementary Material
The Supplementary Material for this article can be found online at: https://www.frontiersin.org/articles/10.3389/fmars.2021.770424/full#supplementary-material
Supplementary Figure 1 | (A) Internesting intervals (days) and (B) Remigration intervals (years) of identified hawksbills, as well as (C) percentage of nesting activity by month between 1989 and 2018 in the state of Hawai‘i.
Supplementary Figure 2 | Yearly aggregated prediction of female counts by sites on Hawai‘i Island (A), and associated annual growth rates (B). Solid red curves correspond to the empirical Bayes posterior mean, and the estimated 95% credible bands are shaded in red. Effort levels (shaded dots) are shown in panels (A) for reference and represent the total of all night camps and day surveys.
Supplementary Figure 3 | Yearly aggregated prediction of nest counts by sites on Hawai‘i Island (A), and associated annual growth rates (B). Solid red curves correspond to the empirical Bayes posterior mean, and the estimated 95% credible bands are shaded in red. Effort levels (shaded dots) are shown in panels (A) for reference and represent the total of all night camps and day surveys.
Supplementary Table 1 | Results (±95% credible intervals in parentheses) from the Bayesian GAM analysis for the annual number of hawksbill nesting females and nests, as well as the number of night camps, day checks, and total monitoring effort (i.e., night camps + day checks), between 1989 and 2018 on Hawai‘i Island.
References
Abella Perez, E., Marco, A., Martins, S., and Hawkes, L. A. (2016). Is this what a climate change-resilient population of marine turtles looks like? Biol. Conserv. 193, 124–132. doi: 10.1016/j.biocon.2015.11.023
Addison, D. S. (1997). Galvanized wire cages can prevent nest depredation. Mar. Turt. Newsl. 76, 8–11.
Aguirre, A. A., Spraker, T. R., Balazs, G. H., and Zimmerman, B. (1998). Spirorchidiasis and fibropapillomatosis in green turtles of the hawaiian Islands. J. Wildl. Dis. 34, 91–98. doi: 10.7589/0090-3558-34.1.91
Allen, Z. C., Shah, N. J., Alastair, G., Gilles-David, D., and Bell, D. (2010). Hawksbill turtle monitoring in Cousin Island special reserve, Seychelles: an eight-fold increase in annual nesting numbers. Endanger. Species Res. 11, 195–200. doi: 10.3354/esr00281
Ancheta, D. (2018). Big Island’s South Point Won’t be Home to Satellite-Launching Catapult. Hawaii News Now. Available online at: http://www.hawaiinewsnow.com/story/37853764/big-islands-south-point-wont-be-home-to-satellite-launching-catapult (accessed March 31, 2018)
Anderson, T. R., Fletcher, C. H., Barbee, M. M., Romine, B. M., Lemmo, S., and Delevaux, J. M. S. (2018). Modeling multiple sea level rise stresses reveals up to twice the land at risk compared to strictly passive flooding methods. Sci. Rep. 8:14484. doi: 10.1038/s42598-018-32658-x
Avise, J. C. (2007). “Conservation genetics of marine turtles – ten years later,” in Frontiers in Wildlife Science: Linking Ecological Theory and Management Applications, eds D. Hewitt and T. Fulbright (Boca Raton, FL: CRC Press), 295–314. doi: 10.1201/9781420007619.ch17
Balazs, G. H., and Chaloupka, M. (2006). Recovery trend over 32 years at the Hawaiian green turtle rookery of French frigate shoals. Atoll Res. Bull. 543, 147–158.
Balazs, G. H., and Chaloupka, M. (2004). Thirty-year recovery trend in the once depleted Hawaiian green turtle stock. Biol. Conserv. 117, 491–498. doi: 10.1016/j.biocon.2003.08.008
Balazs, G. H., Hirth, H. F., Kawamoto, P. Y., Nitta, E. T., Ogren, L. H., Wass, R. C., et al. (1992). Interim Recovery Plan for Hawaiian Sea Turtles. Administrative Report H-92-01 to NOAA/NMFS-SWFSC. Honolulu, HI: Honolulu Laboratory, 83.
Balazs, G. H. (1978). Terrestrial critical habitat for sea turtles under United States jurisdiction in the Pacific region. Elepaio J. Hawaii Audubon Soc. 39, 37l–34l.
Bell, I. P., Meager, J. J., Eguchi, T., Dobbs, K. A., Miller, J. D., and Madden Hof, C. A. (2020). Twenty-eight years of decline: nesting population demographics and trajectory of the north-east Queensland endangered hawksbill turtle (Eretmochelys imbricata). Biol. Conserv. 241:108376. doi: 10.1016/j.biocon.2019.108376
Banerjee, S. M., Komoroske, L. M., Frey, M., Hancock-Hanser, B., Morin, P. A., Archer, F. I., et al. (2019). Single nucleotide polymorphism markers for genotyping hawksbill turtles (Eretmochelys imbricata). Conserv. Genet. Resour. 12, 353–356. doi: 10.1007/s12686-019-01112-z
Baker, J. D., Littnan, C. L., and Johnston, D. W. (2006). Potential effects of sea level rise on the terrestrial habitats of endangered and endemic megafauna in the Northwestern Hawaiian islands. Endanger. Species Res. 2, 21–30. doi: 10.3354/esr002021
Becker, S. L., Brainard, R. E., and Van Houtan, K. S. (2019). Densities and drivers of sea turtle populations across Pacific coral reef systems. PLoS One 14:e0214972. doi: 10.1371/journal.pone.0214972
Beggs, J. A., Horrocks, J. A., and Krueger, B. H. (2007). Increase in hawksbill sea turtle Eretmochelys imbricata nesting in Barbados, West Indies. Endanger. Species Res. 3, 159–168. doi: 10.3354/esr003159
Bjorndal, K. A., Carr, A., Meylan, A. B., and Morimter, J. A. (1985). Reproductive Biology of the Hawksbill Eretmochelys imbricata at Tortuguero, Costa Rica, with notes on the ecology of the species in the Caribbean. Biol. Conserv. 34, 353–368. doi: 10.1016/0006-3207(85)90040-0
Clark, A. J., Hoekstra, J. M., Borersma, P. D., and Kareiva, P. (2002). Improving U.S. endangered species act recovery plans: key findings and recommendations of the SCB recovery plan project. Conserv. Biol. 16, 1510–1519. doi: 10.1046/j.1523-1739.2002.01376.x
Conant, T. A., Dutton, P. H., Eguchi, T., Epperly, S. P., Fahy, C. C., Godfrey, M. H., et al. (2009). Loggerhead Sea Turtle (Caretta caretta) 2009 Status Review Under the U.S. Endangered Species Act. Report of the Biological Review Team to the National Marine Fisheries Service, 222. Avilable online at: https://www.biologicaldiversity.org/species/reptiles/loggerhead_sea_turtle/pdfs/2009-Loggerhead-Status-Review.pdf
Eguchi, T., Gerrodette, T., Pitman, R. L., Seminoff, J. A., and Dutton, P. H. (2007). At-sea density and abundance estimates of olive ridley turtle in the eastern tropical Pacific. Endanger. Species Res. 3, 191–203. doi: 10.3354/esr003191
Ernst, C. H., and Barbour, R. W. (1972). “Cheloniidae: marine turtles,” in Turtles of the United States, ed. C. John (Lexington: The University Press of Kentucky), 69.
Foley, A. M., Peck, S. A., and Harman, G. R. (2006). Effects of sand characteristics and inundation on the hatchling success of loggerhead sea turtle (Caretta caretta) clutches on low-relief mangrove islands in Southwest Florida. Chelonian Conserv. Biol. 5, 32–41. doi: 10.2744/1071-8443(2006)5[32:eoscai]2.0.co;2
Folke, C., Carpenter, S., Walker, B., Sheffer, M., Elmqvist, T., Gunderson, L., et al. (2004). Regime shifts, resilience, and biodiversity in ecosystem management. Annu. Rev. Ecol. Evol. Syst. 35, 557–581. doi: 10.1146/annurev.ecolsys.35.021103.105711
Friedlander, A., Aeby, G., Brown, E., Clark, A., Coles, S., Dollar, S., et al. (2005). “The state of coral reef ecosystems of the main Hawaiian Islands,” in The State of Coral Reef Ecosystems of the United States and Paci‘c Freely Associated States: 2005. NOAA Technical Memorandum NOS NCCOS 11 ed. J. Waddell (Silver Spring, MD: NOAA/NCCOS Center for Coastal Monitoring and Assessment’s Biogeography Team), 222–269.
Fuentes, M. M. P. B., Limpus, C. J., Hamann, M., and Dawson, J. (2010). Potential impacts of projected sea-level rise on sea turtle rookeries. Aquat. Conserv Mar. Freshw. Ecosyst. 20, 132–139. doi: 10.1002/aqc.1088
Gaillard, J. M., Festa-Bianchet, M., and Yoccoz, N. G. (1998). Population dynamics of large herbivores: variable recruitment with constant adult survival. Tree 13, 58–63. doi: 10.1016/s0169-5347(97)01237-8
Gaos, A. R., LaCasella, E. L., Kurpita, L., Balazs, G., Hargrove, S., King, C., et al. (2020). Hawaiian hawksbills: a distinct and isolated nesting colony in the Central North Pacific ocean revealed by mitochondrial DNA. Conserv. Genet. 21, 771–783. doi: 10.1007/s10592-020-01287-1
Gaos, A. R., Lewison, R. L., Liles, M. J., Gadea, V., Altamirano, E., Henrquez, A. V., et al. (2016). Hawksbill turtle terra incognita: conservation genetics of eastern Pacific rookeries. Ecol. Evol. 6, 1251–1264. doi: 10.1002/ece3.1897
Gaos, A. R., Lewison, R., Jensen, M., Liles, M., Henriquez, A., Chavarria, S., et al. (2018). Rookery contributions, movements and conservation needs of hawksbill turtles at foraging grounds in the eastern Pacific Ocean. Mar. Ecol. Prog. Ser. 586, 203–216. doi: 10.3354/meps12391
Gaos, A. R., Liles, M., Gadea, V., Peña de Niz, A., Vallejo, F., Miranda, C., et al. (2017a). Living on the edge; hawksbill turtle nesting and conservation along the Eastern Pacific rim. Lat. Am. J. Aquat. Res. 45, 572–584. doi: 10.3856/vol45-issue3-fulltext-7
Gaos, A. R., Lewison, R. L., Jensen, M. P., Liles, M. J., Henriquez, A., Chavarria, S., et al. (2017b). Natal foraging philopatry of hawksbill turtles in the eastern Pacific Ocean. R. Soc. Open Sci. 4:170153. doi: 10.1098/rsos.170153
Gaos, A. R., Lewison, R. L., Wallace, B., Yañez, I. L., Liles, M., Baquero, A., et al. (2012c). Dive behaviour of adult hawksbills (Eretmochelys imbricata, Linnaeus 1766) in the eastern Pacific Ocean highlights shallow depth-use by the species. J. Exp. Mar. Biol. Ecol. 432-433, 171–178. doi: 10.1016/j.jembe.2012.07.006
Gaos, A. R., Lewison, R. L., Yañez, I. L., Wallace, B. P., Liles, M. J., Nichols, W. J., et al. (2012a). Shifting the life-history paradigm: discovery of novel habitat use by hawksbill turtles. Biol. Lett. 8, 54–56. doi: 10.1098/rsbl.2011.0603
Gaos, A. R., Lewison, R. L., Wallace, B. P., Yañez, I. L., Liles, M. J., Nichols, W. J., et al. (2012b). Spatial ecology of critically endangered hawksbill turtles Eretmochelys imbricata: implications for management and conservation. Mar. Ecol. Prog. Ser. 450, 181–194. doi: 10.3354/meps09591
Gaos, A. R., Abreu-Grobois, F. A., Alfaro-Shigueto, J., Amorocho, D., Arauz, R., Baquero, A., et al. (2010). Signs of hope in the eastern Pacific: international collaboration reveals encouraging status for a severely depleted population of hawksbill turtles Eretmochelys imbricata. Oryx 44, 595–601. doi: 10.1017/S0030605310000773
Girondot, M., Tucker, A. D., Rivalan, P., Godfrey, M. H., and Chevalier, J. (2002). Density-dependent nest destruction and population fluctuations of Guianan leatherback turtles. J. Anim. Conserv. 5, 75–84. doi: 10.1017/S1367943002001099
Hamann, M., Fuentes, M. M. P. B., Ban, N. C., and Mocellin, V. J. L. (2013). “Climate change and marine turtles,” in The Biology of Sea Turtles, Book 3, eds J. Wyneken, K. J. Lohmann, and J. A. Musick (Boca Raton, FL: CRC Press), 353–378.
Hamilton, R. J., Desbiens, A., Pita, J., Brown, C. J., Vuto, S., Atu, W., et al. (2021). Satellite tracking improves conservation outcomes for nesting hawksbill turtles in Solomon Islands. Biol. Conserv. 261:109240. doi: 10.1016/j.biocon.2021.109240
HHTN (2018). Action Plan for Research and Management of Hawksbill Sea Turtles (Eretmochelys imbricata) in Hawai‘i: 2018–2022. Pacific Islands Region Technical Report. Washington, DC: U.S. Fish and Wildlife Service, 37.
Jacobson, C. A., Organ, J. F., Decker, D. J., Batcheller, G. R., and Carpenter, L. (2010). A conservation institution for the 21st century: implications for state wildlife agencies. J. Wildl. Manage. 74, 203–209. doi: 10.2193/2008-485
Juvik, S., Juvik, J., and Thomas, R. (1999). Atlas of Hawai‘i. Honolulu, HI: University Press of Hawai‘i.
Johnson, D. S., and Fritz, L. W. (2014). agTrend: a Bayesian approach for estimating trends of aggregated abundance. Methods Ecol. Evol 5, 1110–1115. doi: 10.1371/journal.pone.0222232
Karl, S. A., and Bowen, B. B. (1999). Evolutionary significant units versus geopolitical taxonomy: molecular systematics of an endangered sea turtle (genus Chelonia). Soc. Cons. Biol. 13, 990–999. doi: 10.1046/j.1523-1739.1999.97352.x
King, C. S., and McLeish, D. A. (2019). “Through the camera lens: a photo-identification catalog (1998–2015) that superbly captures the lives of critically endangered Hawaiian hawksbill sea turtles,” in Proceedings of the 36th Annual Symposium on Sea Turtle Biology and Conservation, eds J. C. Mangel, A. Rees, M. Pajuelo, F. Córdova, and N. Acuña (Lima: U.S. Dept. of Commerce NOAA Tech Mem NMFS-SEFSC).
King, C. S., Gilmartin, W. G., Hau, S., Bernard, H. J., Canja, S. M., Nakai, G., et al. (2007). “Nesting Hawksbill Turtles (Eretmochelys imbricata) on the island of Maui, Hawai‘i from 1996-2003,” in Proceedings of the 24th Annual Symposium on Sea Turtle Biology and Conservation, eds R. B. Mast, B. J. Hutchinson, and A. H. Hutchinson (San Jose, CA: NOAA), 134–135.
Liles, M. J., Jandres, M. V., Lopez, W. A., Mariona, G. I., Hasbun, C. R., and Seminoff, J. A. (2011). Hawksbill turtles Eretmochelys imbricata in El Salvador: nesting distribution and mortality at the largest remaining nesting aggregation in the eastern Pacific Ocean. Endanger. Species Res. 14, 23–30. doi: 10.3354/esr00338
Lynch, J. M. (2018). Quantities of marine debris ingested by sea turtles: global meta-analysis highlights need for standardized data reporting methods and reveals relative risk. Environ. Sci. Technol. 52, 12026–12038. doi: 10.1021/acs.est.8b02848
Mainwaring, M. C., Barber, I., Deeming, D. C., Pike, D. A., Roznik, E. A., and Hartley, I. R. (2017). Climate change and nesting behaviour in vertebrates: a review of the ecological threats and potential for adaptive responses. Biol. Rev. Camb. Philos. Soc. 92, 1991–2002. doi: 10.1111/brv.12317
Marra, G., and Wood, S. N. (2011). Practical variable selection for generalized additive models. Comput. Stat. Data Anal. 55, 2372–2387. doi: 10.1016/j.csda.2011.02.004
Márquez, M. R., Carrasco, M. A., and Jiménez, M. C. (2002). “The marine turtles of Mexico: an update,” in Proceedings of the Western Pacific Sea Turtle Cooperative Research and Management Workshop, Hawaii, ed. I. Kinan (Honolulu, HI: Western Pacific Regional Fishery Management Council), 281–285.
Mazaris, A. D., Schofield, G., Gkazinou, C., Almpanidou, V., and Hays, G. C. (2017). Global sea turtle conservation successes. Sci. Adv. 3:e1600730. doi: 10.1126/sciadv.1600730
Mortimer, J., Esteban, N., Guzman, A., and Hays, G. (2020). Estimates of marine turtle nesting populations in the south-west Indian Ocean indicate the importance of the Chagos Archipelago. Oryx 54, 1–12. doi: 10.1017/s0030605319001108
Mortimer, J. A., and Donnelly, M. (2008). Eretmochelys imbricata. The IUCN Red List of Threatened Species. Available online at: http://www.iucnredlist.org (accessed May 15, 2018)
Neall, V. E., and Trewick, S. A. (2008). The age and origin of the Pacific islands: a geological overview. Phil. Trans. R. Soc. B. 363, 3293–3308. doi: 10.1098/rstb.2008.0119
NMFS, and USFWS (2020). Endangered Species Act status review of the leatherback turtle (Dermochelys coriacea). Report to the National Marine Fisheries Service Office of Protected Resources and U.S. Fish and Wildlife Service. Silver Spring, MD: NMFS.
NMFS, and USFWS (2013). Hawksbill Sea Turtle (Eretmochelys imbricata) 5-year Review: Summary and Evaluation. Silver Spring, MD: NMFS, 89.
NMFS, and USFWS (2007). Hawksbill Sea Turtle (Eretmochelys imbricata) 5-year review: summary and evaluation. National Marine Fisheries Service Office of Protected Resources. Silver Spring, MD: NMFS.
NMFS, and USFWS (1998). Recovery Plan for U.S. Pacific Populations of the Hawksbill Turtle (Eretmochelys imbricata) National Marine Fisheries Service. Silver Spring, MD: NMFS.
NMFS, and USFWS (1993). Recovery Plan for Hawksbill Turtle (Eretmochelys imbricata) in the U.S. Caribbean, Atlantic, and Gulf of Mexico. St. Petersburg, FL: National Marine Fisheries Service.
NRC (2010). Assessment of Sea-Turtle Status and Trends: Integrating Demography and Abundance. Washington, DC: National Academies Press.
Parker, D. M., Balazs, G. H., King, C. S., Katahira, L., and Gilmartin, W. (2009). Short-range movements of hawksbill turtles (Eretmochelys imbricata) from nesting to foraging areas within the Hawaiian Islands. Pac. Sci. 63, 371–382. doi: 10.2984/049.063.0306
Patrício, A. R., Hawkes, L. A., Monsinjon, J. R., Godley, B. J., and Fuentes, M. M. P. B. (2021). Climate change and marine turtles: recent advances and future directions. Endanger Species Res. 44, 363–395. doi: 10.3354/ESR01110
Pedersen, E. J., Miller, D. D., Simpson, G. L., and Ross, N. (2019). Hierarchical generalized additive models in ecology: an introduction with mgcv. Peer J. 7:e6876. doi: 10.7717/peerj.6876
Pilcher, N. J. (2021). Status of Sea Turtles in the Pacific Ocean – Literature Review. Apia: Secretariat of the Pacific Regional Environment Programme, 132.
Plotkin, P. T. (1995). National Marine Fisheries Service and U. S. Fish and Wildlife Service Status Reviews for Sea Turtles Listed under the Endangered Species Act of 1973. Silver Spring, MD: National Marine Fisheries Service.
Pritchard, P. C. H. (1996). “Evolution, phylogeny, and current status,” in The Biology of Sea Turtles, eds P. L. Lutz and J. A. Musick (Boca Raton, FL: CRC Press), 1–28. doi: 10.1525/california/9780520250925.003.0001
R Core Team (2021). R: A Language and Environment for Statistical Computing. Vienna: R Foundation for Statistical Computing.
Richardson, J., Hall, D., Mason, P., Andrews, K., Bjorkland, R., Cai, Y., et al. (2006). Eighteen years of saturation tagging data reveal a significant increase in nesting hawksbill sea turtles (Eretmochelys imbricata) on Long Island. Antigua. Anim. Conserv. 9, 302–307. doi: 10.1111/j.1469-1795.2006.00036.x
Roberson, K., Kendall, M. S., Parker, D., and Murakawa, S. (2016). Sea turtles. in Marine Biogeographic Assessment of the Main Hawaiian Islands. eds Costa B. M. and Kendall M. S. (Washington, D.C: Bureau of Ocean Energy Management and National Oceanic and Atmospheric Administration).
Rodgers, K. S., Keisha, D. B., Jokiel, P. L., and Doná, A. R. (2017). Patterns of bleaching and mortality following widespread warming events in 2014 and 2015 at the Hanauma Bay Nature Preserve, Hawai‘i. PeerJ. 5:e3355. doi: 10.7717/peerj.3355
Santos, A. J. B., Bellini, C., Vieira, D. H. G., Neto, L. D., and Corso, G. (2013). Northeast Brazil shows highest hawksbill turtle nesting density in the South Atlantic. Endanger Species Res. 21, 25–32. doi: 10.3354/esr00505
Seitz, W. A., Kagimoto, K. M., Luehrs, B., and Katahira, L. (2012). Twenty Years of Conservation and Research Findings of the Hawai’i Island Hawksbill Turtle Recovery Project, 1989 to 2009. Pacific Cooperative Studies Unit Technical Report University of Hawaii-Manoa. Honolulu, HI: Department of Botany.
Seminoff, J. A., Allen, C. D., Balazs, G. H., Dutton, P. H., Eguchi, T., Haas, H. L., et al. (2015). Status Review of the Green Turtle (Chelonia mydas) Under the U.S. Endangered Species Act. Hawaii, HI: NOAA Technical Memorandum.
Seminoff, J. A., and Shanker, K. (2008). Marine turtles and IUCN red listing: a review of the process, the pitfalls, and novel assessment approaches. J. Exp. Mar. Biol. Ecol. 356, 52–68. doi: 10.1016/J.JEMBE.2007.12.007
Snover, M. L., Balazs, G. H., Murakawa, S. K. K., Hargrove, S. K., Rice, M. R., and Seitz, W. A. (2012). Age and growth rates of Hawaiian hawksbill turtles using skeletochronology. Mar. Biol. 160, 37–46. doi: 10.1007/s00227-012-2058-7
Spotila, J. R. (2004). Sea Turtles: A Complete Guide to Their Biology, Behavior, and Conservation. Baltimore, MD: The Johns Hopkins University Press.
Suryan, R. M., Saba, V. S., Wallace, B. P., Hatch, S. A., Frederiksen, M., and Wanless, S. (2009). Environmental forcing on life history strategies: evidence for multi-trophic level responses at ocean basin scales. Prog. Oceanogr. 81, 214–222. doi: 10.1016/j.pocean.2009.04.012
Thompson, W. L. (2004). Sampling Rare or Elusive Species: Concepts, Designs, and Techniques for Estimating Population Parameters. Washington, DC: Island Press.
Tiwari, M., Balazs, G. H., and Hargrove, S. (2010). Estimating carrying capacity at the green turtle nesting beach of East Island, French Frigate Shoals. Mar. Ecol. Prog. Ser. 419, 289–294. doi: 10.3354/meps08833
USFWS, and NMFS (1996). Policy regarding the recognition of distinct vertebrate population segments under the endangered species act. Fed. Regist. 61, 4722–4725.
Van Buskirk, J., and Crowder, L. (1994). Life-history variation in marine turtles. Copeia 1994, 66–81. doi: 10.2307/1446672
Van Houtan, K. D., Andrews, A. H., Jones, T. T., Murakawa, S. K. K., and Hagemann, M. E. (2016). Time in tortoiseshell: a bomb radiocarbon-validated chronology in sea turtle scutes. Proc. R. Soc. B. 283:20152220. doi: 10.1098/rspb.2015.2220
Van Houtan, K. S., Francke, D. L., Alessi, S., Jones, T. T., Martin, S. L., Kurpita, L., et al. (2016). The developmental biogeography of hawksbill sea turtles in the North Pacific. Ecol. Evol. 6, 2378–2398. doi: 10.1002/ece3.2034
Van Houtan, K. S., Kittinger, J. N., Lawrence, A. L., Yoshinaga, C., Ray Born, V., and Fox, A. (2012). Hawksbill sea turtles in the Northwestern Hawaiian Islands. Chelonian. Conserv. Biol. 11, 117–121. doi: 10.2744/CCB-0984.1
Vargas, S. M., Jensen, M. P., Ho, S. Y. W., Mobaraki, A., Broderick, D., Mortimer, J. A., et al. (2016). Phylogeography, genetic diversity, and management units of hawksbill turtles in the Indo-Pacific. J. Hered. 107, 199–213. doi: 10.1093/jhered/esv091
Willson, A., Witherington, B., Baldwin, R., Tiwari, M., Al Sariri, T., Al Kiyumi, A., et al. (2020). Evaluating the long-term trend and management of a globally important loggerhead population nesting on masirah island, sultanate of oman. Front. Mar. Sci. 7:666. doi: 10.3389/fmars.2020.00666
Witzell, W. (1983). Synopsis of Biological Data on the Hawksbill Turtle, Eretmochelys Imbricata (Linnaeus, 1766). FAO Fisheries Synopsis. Miami, FL: Southeast Fisheries Center.
Wood, S. N. (2017). P-splines with derivative based penalties and tensor product smoothing of unevenly distributed data. Stat. Comput. 27, 985–989. doi: 10.1007/s11222-016-9666-x
Wood, S. N., and Fasiolo, M. (2017). A generalized fellner-schall method for smoothing parameter optimization with application to tweedie location, scale and shape models. Biometrics 73, 1071–1081. doi: 10.1111/biom.12666
Wood, S. N. (2011). Fast stable restricted maximum likelihood and marginal likelihood estimation of semiparametric generalized linear models. J. R. Stat. Soc. Series B. 73, 3–36. doi: 10.2307/41057423
Keywords: population trend, management, endangered, hawksbill turtle, Hawai‘i, honu‘ea, reproductive biology
Citation: Gaos AR, Kurpita L, Bernard H, Sundquist L, King CS, Browning JH, Naboa E, Kelly IK, Downs K, Eguchi T, Balazs G, Van Houtan K, Johnson D, Jones TT and Martin SL (2021) Hawksbill Nesting in Hawai‘i: 30-Year Dataset Reveals Recent Positive Trend for a Small, Yet Vital Population. Front. Mar. Sci. 8:770424. doi: 10.3389/fmars.2021.770424
Received: 03 September 2021; Accepted: 28 October 2021;
Published: 01 December 2021.
Edited by:
Graeme Clive Hays, Deakin University, AustraliaReviewed by:
Gail Schofield, Queen Mary University of London, United KingdomAntonios D. Mazaris, Aristotle University of Thessaloniki, Greece
Nicole Esteban, Swansea University, United Kingdom
Copyright © 2021 Gaos, Kurpita, Bernard, Sundquist, King, Browning, Naboa, Kelly, Downs, Eguchi, Balazs, Van Houtan, Johnson, Jones and Martin. This is an open-access article distributed under the terms of the Creative Commons Attribution License (CC BY). The use, distribution or reproduction in other forums is permitted, provided the original author(s) and the copyright owner(s) are credited and that the original publication in this journal is cited, in accordance with accepted academic practice. No use, distribution or reproduction is permitted which does not comply with these terms.
*Correspondence: Alexander R. Gaos, alexander.gaos@noaa.gov