- 1Tianjin Key Laboratory of Environmental Technology for Complex Trans-Media Pollution, Tianjin International Joint Research Center for Environmental Biogeochemical Technology, College of Environmental Science and Engineering, Nankai University, Tianjin, China
- 2Changchun Bureau of Ecology and Environment, Changchun, China
- 3Centre for Biogeochemistry in the Anthropocene, Department of Chemistry, University of Oslo, Oslo, Norway
Environmental effects of microplastic are rather due to their adsorption capacity of contaminants than themselves. Aging is a key factor influencing adsorption properties of environmental microplastics. In order to clarify this influence, polyethylene (PE), polypropylene (PP), and polyethylene terephthalate (PET) microplastics with particle sizes of <0.9 mm, 0.9–2 mm, and 2–5 mm were artificially aged in seawater for 12 months. This enabled an assessment of the change in Cu(II) adsorption capacity to the microplastics particles under aging. According to the FTIR spectra, fresh microplastics were oxidized during the UV induced aging process. The adsorption capacities of microplastic were positively correlated with their aging time. After 12-months aging, the amount of Cu(II) adsorbed to the aged microplastics was 1.45–2.92 times higher than on the fresh microplastic particles. For PP and PET, the aging effect increased with decreasing size of the microplastic particles. In the case of PE, particles with the medium particle size (0.9–2 mm) had the strongest aging effect.
Introduction
Plastics bring great convenience to daily life, but poses also potential risks to the environment. Annual global production of plastic in 2018 was 360 million tons (Plastic Europe, 2019). Only 9% of the plastic waste is recycled. Most of it is thus dumped into landfills or just disposed of. Since plastic can take between 50 to more than 200 years to decompose, it eventually accumulates in the environment (Barnes et al., 2009; Jambeck et al., 2015; Geyer et al., 2017). It was estimated that about 4.8–12.7 million tons of plastic waste flows into the marine environment each year (Jambeck et al., 2015). If the current practice continues the weight of plastics in the ocean will be greater than the weight of fish by 2050 (World Economic Forum, 2020). Plastic waste accounts for 60–80% of all marine debris (Wang et al., 2018), and about 92% of all plastic fragments in the marine environment are in the form of microparticles (Wang et al., 2019).
Microplastics may adsorb pollutants due to their small size, large specific surface area and high hydrophobicity. Although microplastics are likely not toxic in themselves, this adsorption of contaminants to the microplastic particles may cause them to serve as a carrier or transport vector of toxic compounds in the environment. Considering the magnitude of microplastic particles in the marine environment this may significantly alter the fate and effect of pollutants in the environment (Wang et al., 2017). Heavy metals on microplastics collected from river sediments are found to have originated from the surrounding environment (Deheyn and Latz, 2006). Adsorption properties of microplastics will change over time in the environmental mainly due to exposure to solar radiation and physical weathering by e.g., wind or wave chopping. This aging causes modifications to the physicochemical properties of microplastic surfaces. Aged microplastics are found to adsorb more heavy metals than fresh microplastics (Deheyn and Latz, 2006; DeForest et al., 2007; Holmes et al., 2014; Turner and Holmes, 2015). For example, Holmes et al. (2014) reported that the adsorption capacity of beached plastic pellets was at least an order of magnitude greater than that of virgin pellets (Holmes et al., 2014). Photo-oxidation through UV exposure changes the physical morphology as well as the functional groups on the plastic polymer surfaces. Despite the potentially important role of microplastics in governing fate and effect of contaminants in the environment, there are few detailed studies on the photolytic aging process and how this aging affects metal adsorption capacity of microplastics with time in seawater.
In this article the UV aging effect on polyethylene (PE), polypropylene (PP), and polyethylene terephthalate (PET), the three most consumed types of plastics in the world1, 2, 3, are studied in laboratory experiments. Copper (Cu), which may cause toxic effects on humans and represent an ecological risk (Fu et al., 2017), is selected as target contaminant to explore the heavy metal adsorption capacity of microplastics with different degree of aging. The effect of particle size of the microplastics on the Cu adsorption capacity is also assessed.
Materials and Methods
Chemicals and Microplastic Materials
Cu(NO3)2 was obtained from Shanghai Aladdin Bio-Chem Technology Corporation (Shanghai, China). HNO3 was purchased from Bohua Chemical Reagent Corporation (Tianjin, China). NaCl, Na2SO4, KCl, NaHCO3, KBr, H3BO3, NaF, MgCl2⋅6H2O, CaCl2⋅2H2O, and SrCl2⋅6H2O, which are all used to prepare artificial seawater, were acquired from Macklin Bio-Chem Technology Corporation (Shanghai, China). All chemicals were analytical grade or higher purity. PE, PP, and PET pellets were procured from Yousuo Chemical Technology Corporation (Shandong, China).
Microplastic Aging Experiment
The aging experiment of microplastics was carried out in the laboratory using UV radiation and artificial seawater. PE, PP, and PET microplastics with particle sizes of 5 mm were crushed using a high-speed crusher. The crushed microplastic particles were then sequentially sieved through 20-, 10-, and 4- mesh screens in order to separate the particle sizes <0.9 mm, 0.9–2 mm, and 2–5 mm. The prepared size fractions of microplastics were submerged by artificial seawater in petri dishes with a diameter of 20 cm. The petri dishes were placed about 3 cm under two parallel placed UV-340 mm lamps with irradiation intensity of 29.80 J s–1⋅m–2. Artificial seawater was prepared according to the method by Kester et al. (1967). The simulated aging time was estimated by the following equation:
In which n is the ratio of radiation intensity between the experiment and ambient conditions, E0 (J⋅s–1⋅m–2) is irradiation intensity of UV lamps, and E1 (J m–2) is annual outdoor ultraviolet (UV) radiation. In the Tianjin area, which is the location of the experiment, the yearly UV radiation is 178.85 × 106 J m–2 (Li, 1999), yielding an n value of 5.2545. The microplastics were taken out after being exposed to UV radiation for 556, 1,112, and 1,668 h, which are equivalent to 4, 8, and 12 months of natural aging, respectively. The artificially aged microplastics were rinsed several times with distilled water and dried before storage in the dark until characterized or used for the adsorption experiments.
Characterization by Fourier Transformed Infrared
Functional groups on the surface of PE, PP, and PET microplastics before and after aging were characterized by attenuated total reflection Fourier transformed infrared spectroscopy (ATR-FTIR, Bruker Tensor II, Germany). The ATR-FTIR is a single beam, percent transmission technique that runs 40 scans per sample at a resolution of 0.4 cm–1 over the wavelength range from 4,000 to 350 cm–1.
Adsorption Experiments
The fresh and aged PP, PE, and PET microplastics with particle size of <0.9 mm, 0.9–2 mm, and 2–5 mm were used to conduct the Cu(II) adsorption experiments. The experiments were carried out at room temperature in centrifuge tubes, each containing 0.5 g microplastics and 50 mL solutions of Cu(II) with concentration of 5 mg L–1. The adsorption time was 240 h, which was the time required to assure equilibrium based on a pre-experiment. All experiments were conducted in duplicate. After adsorption, the microplastics were separated from the solution by filtration using filter paper with a pore size of 15–20 μm. The microplastics on the filter were dried and transferred to 10 mL centrifuge tubes. Then, 5 mL 2% HNO3 was added to the tube and sonicated using ultrasound for 10 min to desorb the Cu(II) metal ions from the microplastic surfaces. The sonicated mixtures were subsequently filtered through 0.45 μm syringe filters transferring the filtered solutions to clean PP centrifuge tubes for measuring the Cu(II) concentration using an inductively coupled plasma mass spectrometry (ICP-MS, Elan drc-e, United States).
Results and Discussion
Fourier Transformed Infrared Characterization of Microplastics With Different Degree of Aging
Attenuated total reflection Fourier transformed infrared spectra of the PE, PP, and PET microplastics surfaces with different exposure times to UV irradiation are shown in Figure 1. The spectra show that new functional groups are generated on the surface of PE and PP microplastic particles. For PE, characteristic peaks for –OH, –COOH, and –C–O– were evolved at wave numbers of 3,360 cm–1 (Figure 1A-1), 1,610 cm–1 (Figure 1A-2), and 1,150 cm–1 (Figure 1A-3), indicating that alcohol, carboxylic acid and fatty ether functional groups were formed by the photolytic oxidation of the surfaces. The peak observed at 850 cm–1 is due to the adsorption by –CCl3. This indicates that a substitution reaction occurred during the aging process, in which the hydrogen in the carbon polymer was replaced by chlorine in the artificial seawater. For aged PP, only a peak at 3,360 cm–1 appeared (Figure 1B), indicating that PP microplastics were oxidized to less extent than PE during the aging process by UV irradiation in seawater environment. The peak is attributed to the adsorption of –OH, implying that alcohol functional groups were produced. For PET, there was no significant change in the surface functional groups based on the FTIR spectra (Figure 1C). Its greater resistance toward degradation is also documented by other studies (Chamas et al., 2020). It was worthy to note that no obvious peaks detected for 12-month-aged 2∼5 mm PE and 12-month-aged 0.9∼2 mm PP. Because ATR-FTIR has a rigorous demand for sample surface flatness, the uneven protrusions found on the surface of two particles might lead to the peak lack.
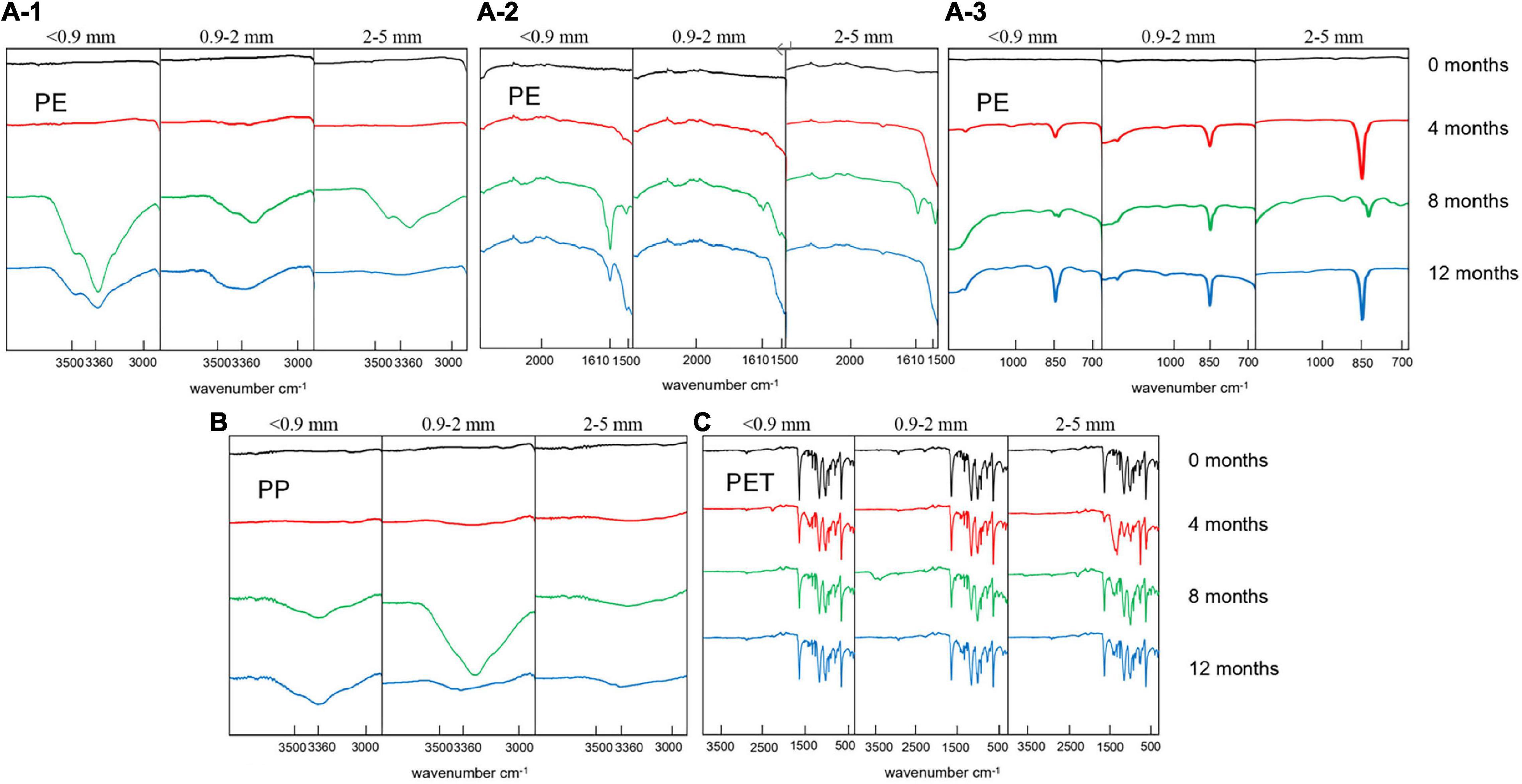
Figure 1. Selection of FTIR spectra of PE, PP, and PET microplastics surfaces with different aging time.
Aging Effect of Microplastics on Cu(II) Adsorption
As shown in Figure 2, the sequence of Cu(II) adsorption capacity for the three virgin microplastic materials is PE > PET > PP. Smaller microplastic particle sizes have, as could be expected due to greater surface area, higher Cu(II) adsorption capacity. For PE the capacity increases from 0.3 μg/g, for particle sizes between 0.9 and 5 mm, to 0.6 μg/g in particles smaller than 0.9 mm. This is less than expected considering that the surface area of a sphere increases by a factor of 25 when its size increases from 0.5 to 2.5 mm. The adsorbed Cu(II) increased with increasing aging time on all plastic materials and particle sizes. After 12 months aging, the amount of adsorbed Cu(II) on the aged microplastic particles were between 1.45 and 2.92 times greater than that on the fresh microplastic particles. Such increased Cu (II) adsorption in this study was also observed for aged beach plastic pellets (Holmes et al., 2014). However, the aging time was unclear in the study of Holmes et al. (2014).
Increased Cu(II) adsorption on aged microplastic particles can be attributed to that the surface of the microplastic materials are oxidized during the aging process. The oxygen-containing functional groups that are produced, provide sorption sites and thus enhanced adsorption capacity of metal ions. Moreover, the UV light alter the surface morphology of microplastics by generating a rougher surface, which increase the specific surface area and thereby the adsorption capacity of metal ions (Wang et al., 2020). The increase in metal adsorption by aged PE and PP microplastics may thus be due to both the chemical reaction and morphological change taking place in the aging process. However, for PET microplastics, due to the lack of chemical changes, the increased adsorption capacity can only be explained by the physical morphological change caused by the aging process.
The aging-derived increased adsorption capacity for Cu(II) on microplastics (Figure 3) is quantitatively evaluated by the linear regression slopes of adsorbed Cu(II) on microplastics against aging time (Figure 2). Generally, the aging-derived increases in adsorption capacity for PE and PET were much higher than that for PP. This implies that PE and PET microplastics have higher potential to act as vectors for heavy metal transport than PP. Moreover, for PP and PET, the increase rate in Cu(II) adsorption capacity exhibited an increasing trend with the decrease of microplastic particle size. In case of PE, the increase in capacity for smaller size particles (<0.9 mm and 0.9–2 mm) are similarly higher than that for the large size fraction (2–5 mm). This implies that the aging of smaller microplastics might have greater potential for impacting the fate and effect of heavy metals in the environment than the larger microplastic particles.
Conclusion
Microplastic particles of PE, PP, and PET polymers in a seawater environment were aged to different degrees by UV irradiation in order to explore the effect of aging on their adsorption capacity of Cu(II).
The adsorption capacity of Cu(II) differs between microplastic polymer types. PE has the highest adsorption capacity to Cu(II), while PP has the lowest. The adsorption capacity of Cu(II) increased with the degree of aging. Microplastics with smaller particle size have a higher adsorption capacity. The adsorption capacity of microplastics with particle size less than 0.9 mm is about twice as large as for particle size between 2 and 5 mm. From this we can deduce that microplastics with smaller particle size have higher potential to have a significant effect on the fate and effect of heavy metals in the marine environment.
Data Availability Statement
The original contributions presented in the study are included in the article/supplementary material, further inquiries can be directed to the corresponding author/s.
Author Contributions
XH: experiment, data curation, and writing-original draft preparation. RV: conceptualization and writing-review and editing. JZ, BZ, and XY: experiment. JF: supervision, writing-review and editing, and resources. XL: conceptualization, supervision, writing-review and editing, and resources. All authors contributed to the article and approved the submitted version.
Funding
This work was financially supported by the projects from the National Key Research and Development Program of China (2018YFC1406403 and 2020YFC1909500).
Conflict of Interest
The authors declare that the research was conducted in the absence of any commercial or financial relationships that could be construed as a potential conflict of interest.
Publisher’s Note
All claims expressed in this article are solely those of the authors and do not necessarily represent those of their affiliated organizations, or those of the publisher, the editors and the reviewers. Any product that may be evaluated in this article, or claim that may be made by its manufacturer, is not guaranteed or endorsed by the publisher.
Footnotes
- ^ The Essential Chemical Industry (ECI): Poly(ethene) (Polyethylene) [OL]. [2018-09-06]. http://www.essentialchemicalindustry.org/polymers/polyethene.html.
- ^ The Essential Chemical Industry (ECI): Poly(propene) (Polypropylene) [OL]. [2018-09-06]. http://www.essentialchemicalindustry.org/polymers/polypropene.html.
- ^ The Essential Chemical Industry (ECI): Polyesters [OL]. [2018-09-06]. http://www.essentialchemicalindustry.org/polymers/polyesters.html.
References
Barnes, D. K. A., Galgani, F., Thompson, R. C., and Barlaz, M. (2009). Accumulation and fragmentation of plastic debris in global environments. Philos. Trans. R. Soc. Lond. B Biol. Sci. 364, 1985–1998. doi: 10.1098/rstb.2008.0205
Chamas, A., Moon, H., Zheng, J., Qiu, Y., Tabassum, T., Jang, J. H., et al. (2020). Degradation rates of plastics in the Environment. ACS Sustainable Chem. Eng. 8, 3494–3511. doi: 10.1021/acssuschemeng.9b06635
DeForest, D. K., Brix, K. V., and Adams, W. J. (2007). Assessing metal bioaccumulation in aquatic environments: the inverse relationship between bioaccumulation factors, trophic transfer factors and exposure concentration. Aquat. Toxicol. 84, 236–246. doi: 10.1016/j.aquatox.2007.02.022
Deheyn, D. D., and Latz, M. I. (2006). Bioavailability of metals along a contamination gradient in San Diego Bay (California, USA). Chemosphere 63, 818–834. doi: 10.1016/j.chemosphere.2005.07.066
Fu, Z., Guo, W., Dang, Z., Hu, Q., Wu, F., Feng, C., et al. (2017). Refocusing on nonpriority toxic metals in the aquatic environment in China. Environ. Sci. Technol. 51, 3117–3118. doi: 10.1021/acs.est.7b00223
Geyer, R., Jambeck, J. R., and Law, K. L. (2017). Production, use, and fate of all plastics ever made. Sci. Adv. 3:e1700782. doi: 10.1126/sciadv.1700782
Holmes, L. A., Turner, A., and Thompson, R. C. (2014). Interactions between trace metals and plastic production pellets under estuarine conditions. Mar. Chem. 167, 25–32. doi: 10.1016/j.marchem.2014.06.001
Jambeck, J. R., Geyer, R., Wilcox, C., Siegler, T. R., Perryman, M., Andrady, A., et al. (2015). Plastic waste inputs from land into the ocean. Science 347, 768–771. doi: 10.1126/science.1260352
Kester, D. R., Duedall, I. W., Connors, D. N., and Pytkowicz, R. M. (1967). Preparation of Artificial Seawater. Limnol. Oceanogr. 12, 176–179.
Li, C. (1999). Observation of the intensity of solar UV rradiation in tianjin area. J. Clin. Dermatol. 28, 29–30.
Plastic Europe (2019). An Analysis of European Plastics Production, Demand and Waste. Data. Plastics – The Facts 2019. Brussels: Plastic Europe.
Turner, A., and Holmes, L. A. (2015). Adsorption of trace metals by microplastic pellets in fresh water. Environ. Chem. 12:600. doi: 10.1071/EN14143
Wang, F., Wong, C. S., Chen, D., Lu, X., Wang, F., and Zeng, E. Y. (2018). Interaction of toxic chemicals with microplastics: a critical review. Water Res. 139, 208–219. doi: 10.1016/j.watres.2018.04.003
Wang, J., Peng, J., Tan, Z., Gao, Y., Zhan, Z., Chen, Q., et al. (2017). Microplastics in the surface sediments from the Beijiang River littoral zone: composition, abundance, surface textures and interaction with heavy metals. Chemosphere 171, 248–258. doi: 10.1016/j.chemosphere.2016.12.074
Wang, Q., Zhang, Y., Wangjin, X., Wang, Y., Meng, G., and Chen, Y. (2020). The adsorption behavior of metals in aqueous solution by microplastics effected by UV radiation. J. Environ. Sci. 87, 272–280. doi: 10.1016/j.jes.2019.07.006
Wang, W., Gao, H., Jin, S., Li, R., and Na, G. (2019). The ecotoxicological effects of microplastics on aquatic food web, from primary producer to human: a review. Ecotoxicol. Environ. Saf. 173, 110–117. doi: 10.1016/j.ecoenv.2019.01.113
Keywords: microplastics, aging effect, adsorption, Cu(II), particle sizes
Citation: Han X, Vogt RD, Zhou J, Zheng B, Yu X, Feng J and Lu X (2021) Increased Cu(II) Adsorption Onto UV-Aged Polyethylene, Polypropylene, and Polyethylene Terephthalate Microplastic Particles in Seawater. Front. Mar. Sci. 8:770606. doi: 10.3389/fmars.2021.770606
Received: 04 September 2021; Accepted: 24 September 2021;
Published: 26 October 2021.
Edited by:
Xiaoshan Zhu, Tsinghua University, ChinaReviewed by:
Chun Ciara Chen, Shenzhen University, ChinaZhenghua Duan, Tianjin University of Technology, China
Copyright © 2021 Han, Vogt, Zhou, Zheng, Yu, Feng and Lu. This is an open-access article distributed under the terms of the Creative Commons Attribution License (CC BY). The use, distribution or reproduction in other forums is permitted, provided the original author(s) and the copyright owner(s) are credited and that the original publication in this journal is cited, in accordance with accepted academic practice. No use, distribution or reproduction is permitted which does not comply with these terms.
*Correspondence: Xueqiang Lu, bHV4cUBuYW5rYWkuZWR1LmNu