- Department of Forestry and Natural Resources, Purdue University, West Lafayette, IN, United States
Integrated aquaponic food production systems are capable of producing more food on less land using less water than conventional food systems, and marine systems offer the potential of conserving freshwater resources. However, there have been few evaluations of species combinations or operational parameters in marine aquaponics. The goal of this experiment was evaluation of stocking density ratio of Pacific whiteleg shrimp (Litopenaeus vannamei) to three edible halophytes (Atriplex hortensis, Salsola komarovii, and Plantago coronopus) with two C/N ratios in a 3 × 2 factorial design. There were three stocking density ratios (shrimp: plant), 2:1, 3:1, and 5:1; and two C/N ratios, 12 and 15. The results indicated that stocking density ratio exerted a significant impact on shrimp growth. Shrimp reared in 2:1 and 3:1 treatments had better growth performance. In contrast, plants were affected by both stocking density ratio and C/N ratio. Halophytes grown in stocking density ratios of 3:1 and 5:1 with a C/N ratio of 15 had better growth performance and nutrient content. The concentrations of TAN and NO2– were below 0.2 mg/L throughout the experiment, including the higher stocking density ratio treatments. In conclusion, the stocking density ratio of 3:1 with a C/N ratio of 15 was suggested as the optimal condition for the operation of marine aquaponics in which whiteleg shrimp and the three halophytes are target crops.
Introduction
Integrated aquaponic food production systems are capable of producing more food, on less land, using less water and a lower environmental impact than conventional food production systems (Somerville et al., 2014; Alshrouf, 2017; Goddek et al., 2019). Adopting a salt-, or brackish water aquaponics system significantly increases the potential animal species that could be raised, and many of the potential species have good name recognition and demand in the marketplace. Further, marine aquaponics reduces the reliance on freshwater resources. Water usage in a marine recirculating aquaculture system can be as low as 16 L per kg of seafood produced, while usage in freshwater recirculating aquaculture system is approximately 50 L per kg of production (Klinger and Naylor, 2012). Marine systems rely on saltwater for initial fill and replacement of evaporative losses, given the system is near a natural source of saltwater (Klinger and Naylor, 2012). Freshwater would only be needed in these situations to adjust salinity to desired levels. Additionally, marine and/or brackish water species tend to have lower FCR (on average) and grow faster than freshwater species (Fry et al., 2018). Of the potential animal species, the Pacific whiteleg shrimp (Litopenaeus vannamei) appears to have potential in the short-term as global production was second highest among aquaculture industries in 2018 (FAO, 2020) (4966.2 thousand metric tons) and they display rapid growth (FAO, 2006), high market price (Ross et al., 2017) and strong global demand (FAO, 2020). Whiteleg shrimp are tolerant of a wide range of salinities (Gao et al., 2016; Ray and Lotz, 2017; Pinheiro et al., 2020; Chu and Brown, 2021) and stocking densities (Otoshi et al., 2007; Krummenauer et al., 2011; Araneda et al., 2020) (90 to 600 shrimp/m2) making them strong candidates for marine aquaponics. Further, shrimp might alleviate the “economic drain” of fish raised in freshwater aquaponic systems (Quagrainie et al., 2018). However, the complex interaction between marine aquaponic subsystems and taxa has received little attention in the scientific literature.
Halophytes (salt-tolerant plants) only represent 2% of terrestrial plant species and most of them are not common commodities; however, they have been used for many purposes such as food and forage crops, oilseeds, phytoremediation and medicinal purposes (Glenn et al., 1998, 1999; Ventura and Sagi, 2013; Panta et al., 2014; Panth et al., 2016; Kim et al., 2017). Red orache (Atriplex hortensis), okahijiki (Salsola komarovii), and minutina (Plantago coronopus) are edible halophytes that possess high nutrient concentrations (protein, amino acids, vitamins, and minerals) and have been successfully raised in marine aquaponics (Chu and Brown, 2021). However, the ratio of subsystem components, which will impact the flow of nutrients and health of subsystem taxa, has not been evaluated in marine aquaponic food production systems.
Several broad generalizations have been developed to help conceptualize the sizing and ratios of aquaponic subsystems. For example, current recommendations include 60 to 100 grams of fish feed/d/m2 of plant growing area, a 1:2 ratio of fish tank volume to hydroponic media, a 7.3:1 ratio of plant-bed surface to fish-tank surface area, and a 3:1 ratio of hydroponic tank volume to fish production tank volume (Rakocy, 2012; Somerville et al., 2014; Lam et al., 2015). Generalized ratios fail to acknowledge the biological variability associated with potential species combinations. For example, feed consumption varies between species of fish and crustacean, dietary formulations contain varying concentrations of crude protein and amino acids, nutrient needs of plants vary as a function of growth stage (vegetative vs. fruiting), plant nutrient uptake is influenced by environmental conditions (pH, temperature and the microbiome in the rhizosphere), and the recommendations assume the space and nutritional needs of the system microbiome are adequate. The ratio of specific animals to plants might be a more realistic view of the physiological interactions and flow of nutrients that must occur in an integrated system. Consequently, the ratio of animals to plants must be understood for economical and sustainable operation.
Biofloc technology (BFT) has been used for decades to manage and control the water quality at safe ranges for target organisms in high density aquaculture (Avnimelech, 1999). Biofloc technology is an agglomeration of diverse microbes, which includes heterotrophic and autotrophic bacteria, algae, zooplankton, fungi, and viruses. The major function of BFT is to assimilate and mineralize toxic metabolites such as total ammonia nitrogen (TAN) and nitrite (NO2–) in the water (Panigrahi et al., 2018; Luo et al., 2020). Biofloc technology requires the addition of organic carbon to manipulate the C/N ratio and accelerate the development of the microbial communities. A C/N ratio between 10 and 20 is recommended for aquaculture (Xu et al., 2016, 2018; Panigrahi et al., 2018), while the recommendation for aquaponic systems is lacking. Moreover, inoculating probiotics is a promising approach to ensure the dominant bacteria are beneficial organisms (Crab et al., 2012). While it is common to amend the growing environment for better plant nutrient uptake and a higher yield, there is little information about the optimal C/N ratio in nutrient solutions for plant cultivation (Rodríguez-Kábana, 1986; Schenck, 2001; White, 2012; Pyakurel et al., 2019; Li et al., 2020).
The goal of this project was to evaluate critical ratios of crop density in aquaculture and hydroponics subsystems and provide operational guidelines for marine aquaponics. Specific objectives were to evaluate stocking densities and the C/N ratio on growth and production of whiteleg shrimp and three halophytes.
Materials and Methods
Aquaponic System Design
Eighteen individual aquaponic systems were constructed at the Aquaculture Research Lab, Purdue University. The following are the components of each system; an aquaculture tank (113.6L), a hydroponic tank (102.2L), and a biofilter tank (18.9 L; Figure 1). To avoid shrimp escape and algal growth, plastic mesh, and lids were placed on the top of aquaculture tanks and hydroponic tanks, respectively. The lids on the top of hydroponic tanks were used to inhibit light into the tank, and also used as the floating rafts to support plants. Every biofilter tank was equipped with a 25-micron filter bag and bio-balls (surface area 98 ft2/ft3; Pentair Aquatic Eco-Systems, Inc., Apopka, FL, United States). Bio-balls in the biofilter tank were used to provide high surface area for attachment and colony expansion of microbes, and the filter bag was used to filter water from shrimp culture to prevent plant roots from clogging by biofloc. Air stones were installed in aquaculture tanks, hydroponic tanks, and biofilter tanks to maintain dissolved oxygen (DO) level above 6 mg/L. To maintain the water temperature within the optimal range 26–28°C for shrimp, submersible heaters (300w; Aqueon, Wisconsin, United States) were used in aquaculture tanks. Light source for plant growth was provided via LED light tubes (40w, 5000 lumens, 4000K daylight white; Kihung LED, Guangdong, China), that were suspended at a height of 16.5 cm over the plant growth bed. Quantum sensor (MQ-500 Full-Spectrum Quantum Meter; Apogee Instruments, Inc., UT, United States) was used to measure light intensity. The photosynthetically active radiation averaged 234 μmolm–2s–1. The photoperiod was 14 h light and 10 h dark.
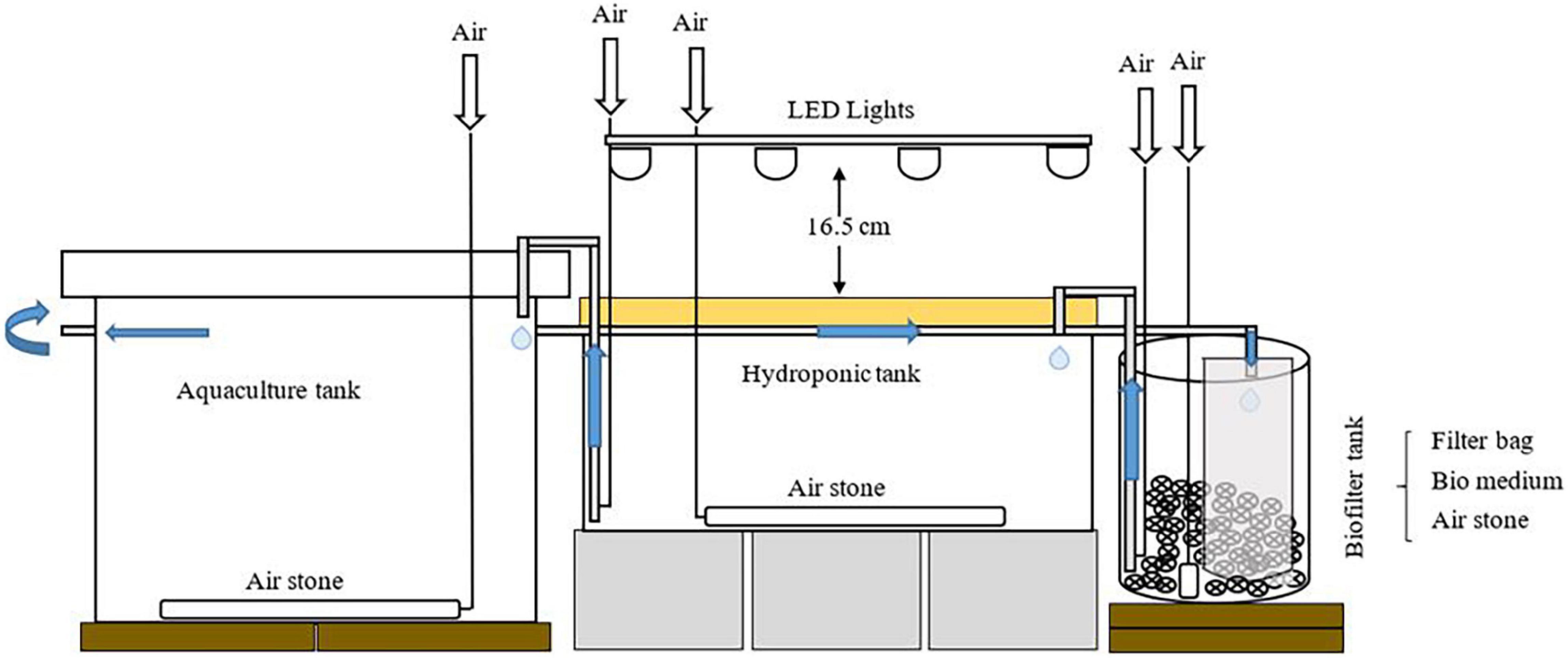
Figure 1. Schematic diagram of an aquaponics system unit (adapted from Chu and Brown (2021); https://creativecommons.org/licenses/by/4.0/).
Biological Material
Shrimp
Pacific whiteleg shrimp were purchased from a commercial shrimp farm (RDM Aquaculture, Fowler, IN, United States), and transported to the Aquaculture Research Lab. Water temperature during transport was 24°C and salinity was 15 ppt. Shrimp were separated into three 700 L tanks, and quarantined for 1 week before moving into aquaponic systems. During quarantine, shrimp were fed a commercial shrimp feed (Zeigler Brothers, Gardners, PA, United States) twice a day at 8 a.m. and 5 p.m., with a total daily amount of 3.0% of body weight divided into equal aliquots.
Plants
Seeds of red orache, okahijiki, and minutina, were purchased from a commercial source (Johnny’s Selected Seeds, Winslow, ME, United States) and sowed in horticubes, soilless foam medium (OASIS® Grower Solutions, Kent, OH, United States). Fresh water was used for plant irrigation in the first week of germination. To prevent osmotic shock on plants, salinity was increased at a rate of 2–3 ppt every 48 h from the second week until the desired salinity (15 ppt) was reached.
Experimental Design and System Management
A 3 × 2 experimental design was established in this study; 3 ratios of shrimp to plants (2:1, 3:1 or 5:1) and 2 C/N ratios (12 or 15). Treatments were designated 2:1–12 (stocking density ratio 2:1 with C/N ratio 12), 2:1–15, 3:1–12, 3:1–15, 5:1–12, and 5:1–15. Treatments were randomly assigned to three replicate experimental systems. The study was conducted for 4 weeks, from July 4 to August 1, 2020. Before the experiment started, all experimental systems were seeded with Bacillus spp. (EZ-Bio; Zeigler Brothers, Gardners, PA, United States), and inoculated water from established systems used in prior research. Sea salt (Instant Ocean®, Blacksburg, VA, United States) was used to adjust the salinity to 15 ppt. One week prior to the experiment, shrimp were weighed and placed in aquaculture tanks to produce nutrients for plants. The stocking density of shrimp was 200 shrimp/m2 (40 shrimp/tank), 300 shrimp/m2 (60 shrimp/tank), or 500 shrimp/m2 (100 shrimp/tank). The average weight of individual shrimp was 1.50 g. The stocking density of plants was 100 plants/m2, which was equivalent to 24 plants (8 plants per species) in each hydroponic tank. Commercial shrimp feed (Zeigler Brothers, Gardners, PA, United States) was provided twice a day at 8 a.m. and 5 p.m., with a total daily amount of 3.0% of body weight divided into equal aliquots. Guaranteed analysis of the feed was 35% protein, 7% fat, and a maximum of 2% fiber.
Probiotics (EZ-Bio; Zeigler Brothers, Gardners, PA, United States) were used to manage water quality and the microbial community within every system. EZ-bio (Bacillus spp.) was inoculated at 10 mg/L into every system once a week prior to starting the experiment. As soon as shrimp were moved into aquaculture tanks, additional doses of probiotics were added every other day in the first week, twice per week in the second week, and once per week beginning in the third week continuing until the end of the experiment (Crab et al., 2010; Chu, 2014). Molasses (Hawthorne Gardening Co., Vancouver, WA, United States) was added to aquaculture tank as an organic carbon source to adjust the C/N ratio in the water with the same frequency of the probiotic inoculations. The amount of molasses added was based on the carbon-nitrogen content of shrimp feed and the carbon content of the molasses to adjust the C/N ratio to 12 or 15 (Xu et al., 2016). Potassium bicarbonate was added to maintain the alkalinity above 60 mg/L, and 10% sulfuric acid was applied to keep the pH below 8. Throughout the 4-week experiment, there was no water discharged or exchanged except for replacement due to evaporation.
Measurement of Water Quality
During the experiment, dissolved oxygen, temperature (OxyGuard Handy Polaris DO meter, Farum, Denmark), and pH (pHTestr™ 10 Pocket pH Tester, Vernon Hills, IL, United States) were measured twice per day at 8 a.m. and 5 p.m. before feeding. Salinity (Vital Sine™ Salinity Refractometer, Pentair Aquatic Eosystems, Apopka, FL, United States) was measured once per day at 8 a.m. Water samples were collected twice per week from the aquaculture tank before feeding, to determine the concentrations of total ammonia nitrogen (TAN), nitrite-N (NO2–), nitrate-N (NO3–), phosphate (PO43–), and alkalinity using HACH reaction kits (HACH, Loveland, CO, United States). Total suspended solids (TSSs) and volatile suspended solids (VSSs) were measured once a week by United States EPA method 1684.
Growth Performance
Shrimp
Response parameters for shrimp included survival, weight gain, specific growth rate (SGR), and feed conversion ratio (FCR) The following formulae were used:
Plants
Only edible parts of individual plants were collected and weighed at the beginning and end of the experiment. Initial plant samples were obtained from the extra plant seedlings and chosen those that were similar to the seedlings transplanted into systems. Initial plant samples were weighed on the same day of the transplantation. Initial and final fresh weights were used to calculate relative growth rate (RGR). Dry weight was measured after plant samples were dried in an oven at 100 °C until constant weight. The water content (WC) in plants was determined through final fresh weights and final dry weights. Formulae used to calculate plant growth and water content are shown below. In addition, dried plant samples were ground and sieved (with a 10-mesh screen) and kept in 50 ml centrifuge tubes for nutrient analysis. Plant tissue analysis was conducted by the Midwest Laboratory (Omaha, NE, United States).
Statistical Analysis
Shrimp and plant growth performance, nutrient content in plants, and water quality parameters were analyzed using JMP v14.0 (SAS Institute Inc., Cary, NC, United States). Treatment means were compared by two-way analysis of variance (ANOVA). Statistical differences between means was determined by Tukey’s honestly significant difference test (HSD) at p ≤ 0.05.
Results
Shrimp Growth
The survival of shrimp in all treatments was above 95% and there were no significant (p > 0.05) differences among treatments. The growth of shrimp was significantly (p < 0.05) impacted by stocking density, but not by the C/N ratio or the interaction of factors (Table 1). Shrimp raised in 2:1–12 treatment had better growth performances (final weight, weight gain, and SGR) and a lower FCR. The mean values of final weight, weight gain, and SGR were significantly greater (p < 0.05) in the treatment 2:1–12, averaging 2.68g, 73.9%, and 1.97, respectively, compared to 5:1–12 and 5:1–15 treatments, yet, values were not significantly (p > 0.05) different from that of the 2:1–15, 3:1–12, and 3:1–15 treatments. Similarly, FCR was significantly lower (p < 0.05) in shrimp raised in 2:1–12 treatment compared to shrimp raised in 5:1–12 and 5:1–15 treatments, whereas it was not significantly (p > 0.05) different from the other treatments.
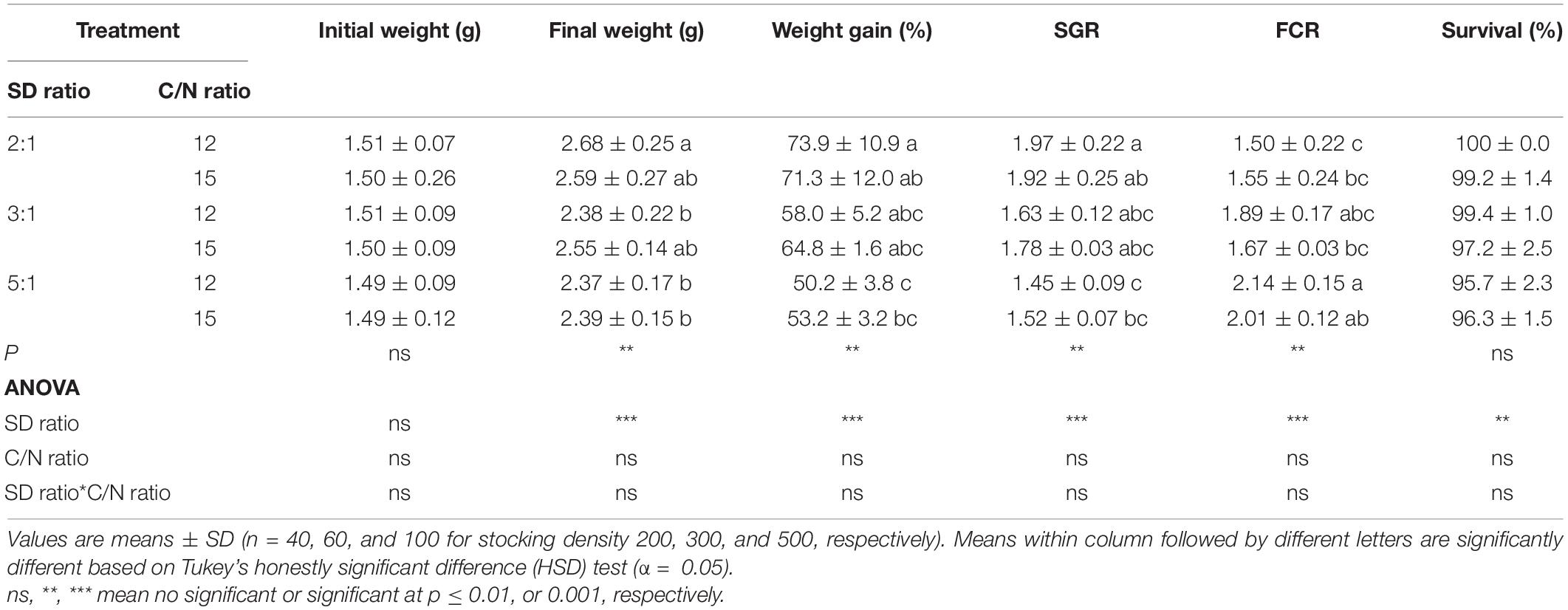
Table 1. Response of shrimp in marine aquaponics at three stocking density ratios (SD ratio) of shrimp to plant and two C/N ratios for 4 weeks.
Plants
Survival of red orache, okahijiki, and minutina was 100% in all treatments. Stocking density ratio significantly (p < 0.05) affected the growth of all plants, and C/N ratio significantly (p < 0.05) affected water content (WC) of red orache (Table 2), the final fresh weight (FFW), final dry weight (FDW), RGR and WC of okahijiki (Table 3), and the FFW and RGR of minutina (Table 4). The interaction of the two factors exerted no significant (p > 0.05) effect on growth of plants.
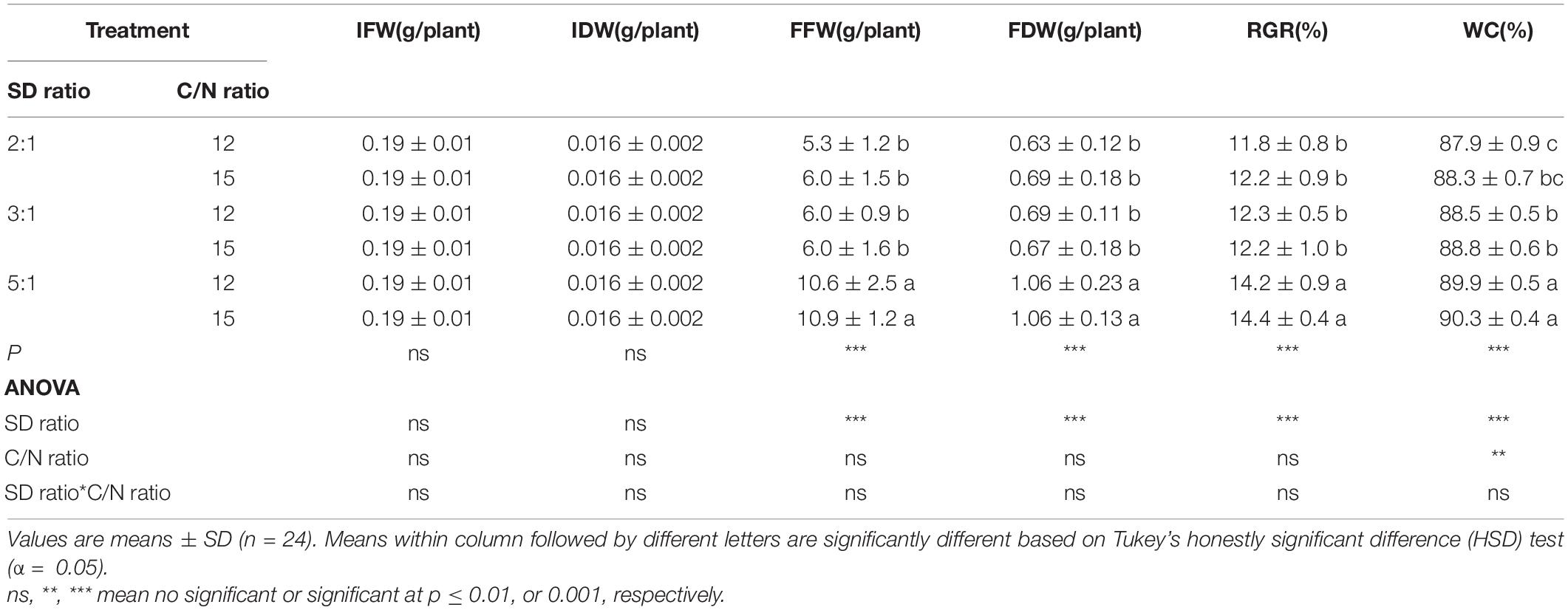
Table 2. Initial fresh weight (IFW), initial dry weight (IDW), final fresh weight (FFW), final dry weight (FDW), relative growth rate (RGR), and water content (WC) of red orache cultivated in marine aquaponics at three stocking density ratios (SD ratio) of shrimp to plant and two C/N ratios for 4 weeks.
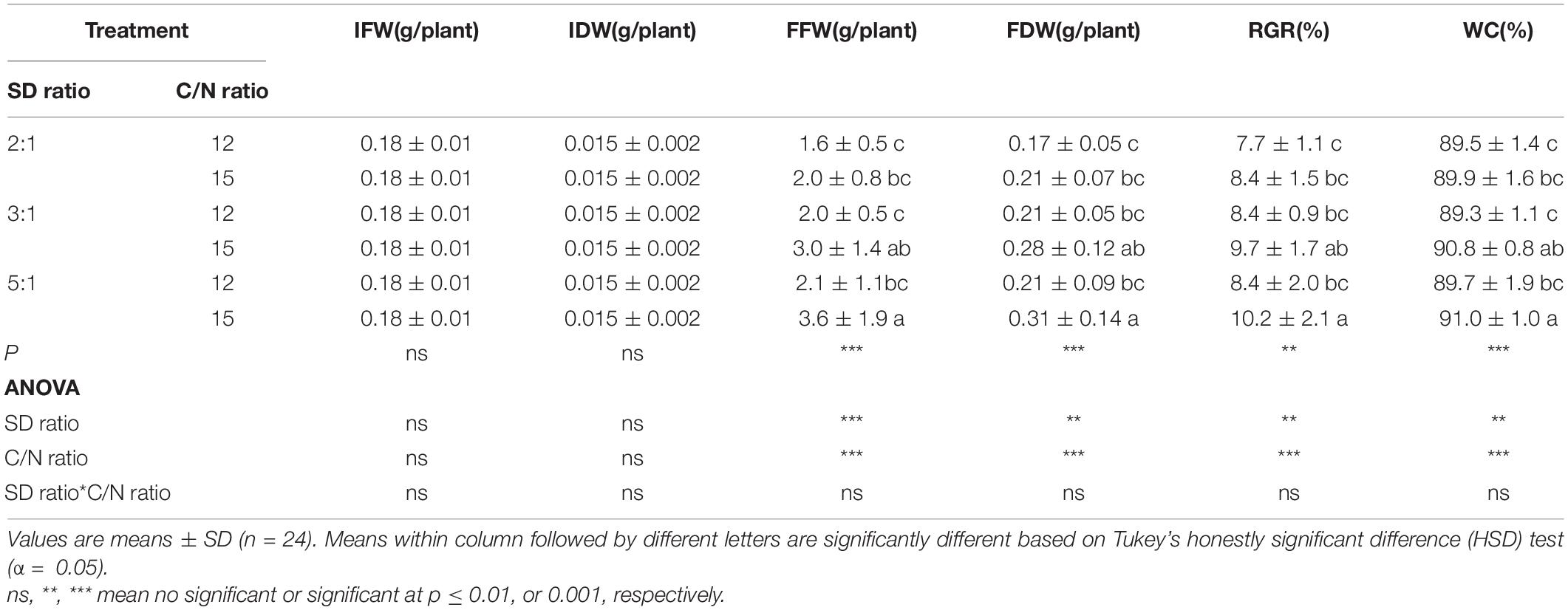
Table 3. Initial fresh weight (IFW), initial dry weight (IDW), final fresh weight (FFW), final dry weight (FDW), relative growth rate (RGR), and water content (WC) of okahijiki cultivated in marine aquaponics at three stocking density ratios (SD ratio) of shrimp to plant and two C/N ratios for 4 weeks.
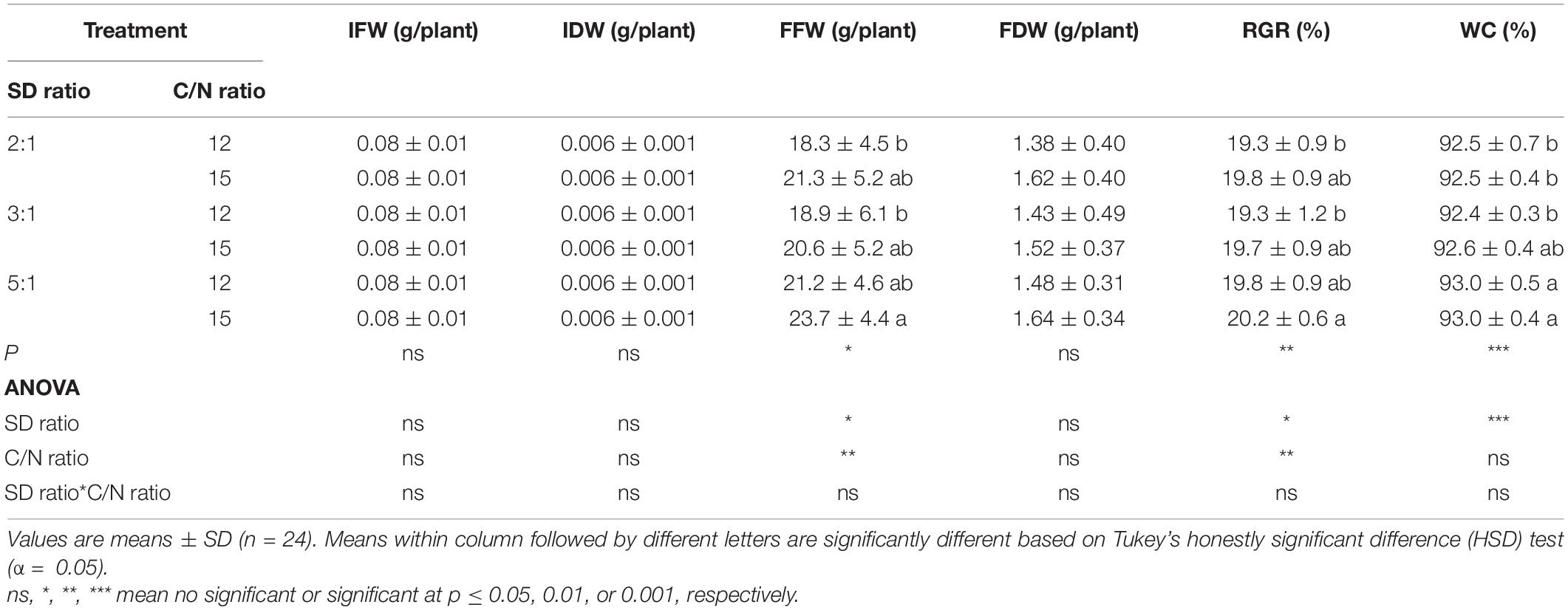
Table 4. Initial fresh weight (IFW), initial dry weight (IDW), final fresh weight (FFW), final dry weight (FDW), relative growth rate (RGR), and water content (WC) of minutina cultivated in marine aquaponics at three stocking density ratios (SD ratio) of shrimp to plant and two C/N ratios for 4 weeks.
Yield and Mineral Nutrient Content
According to the result of two-way ANOVA (Table 5), the yield, and the concentration of N, P, K, Mg, Ca, and S were significantly (p < 0.05) affected by plant species and stocking density ratio. The C/N ratio significantly (p < 0.05) affected the yield and the concentration of Mg. The interactions between plant species and stocking density ratio significantly (p < 0.05) affected the results of yield and the concentration of N, P, K, Mg, and Ca.
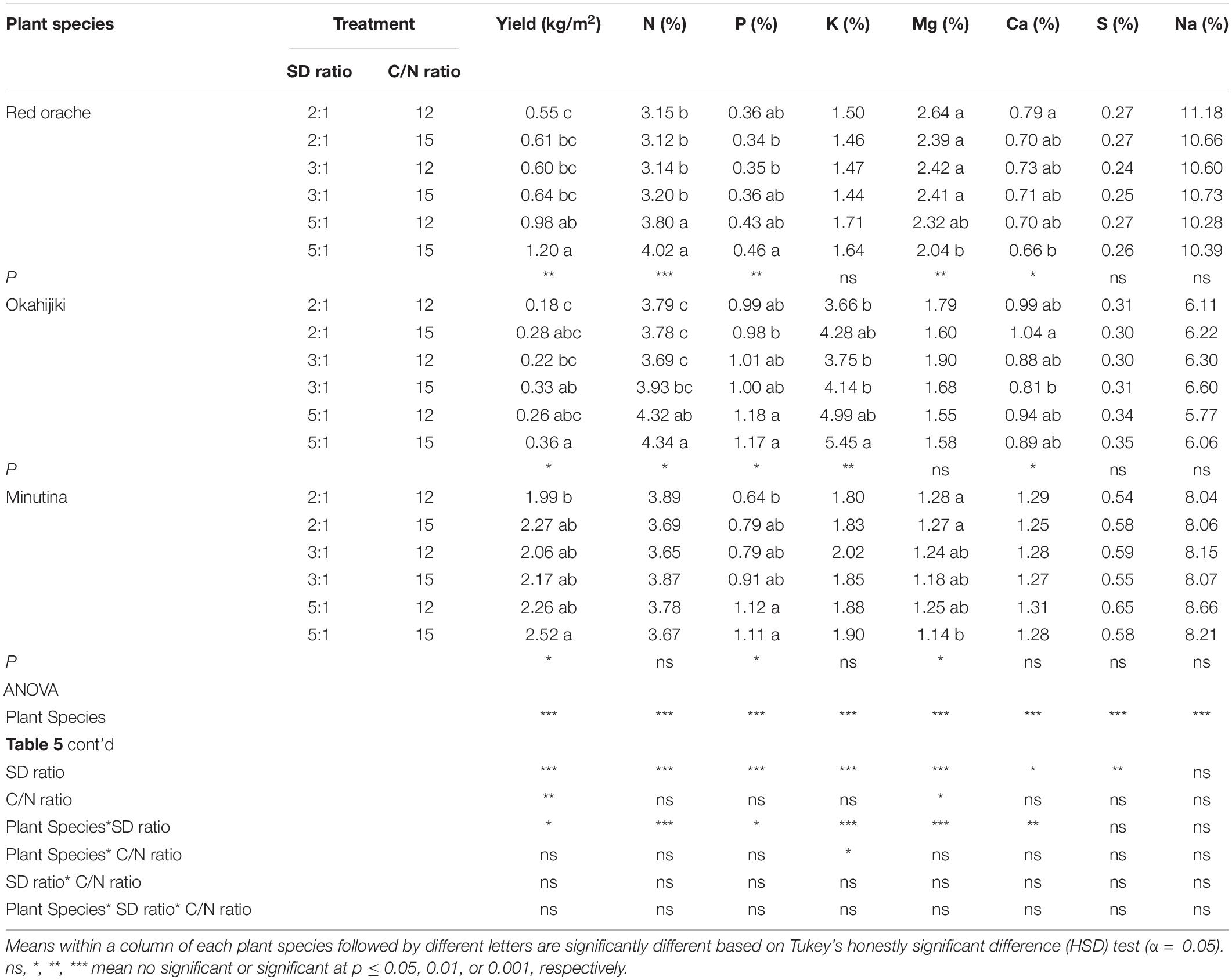
Table 5. Average yield and mineral nutrient concentrations of the three halophytic plants cultivated in marine aquaponics at three stocking density ratios (SD ratio) of shrimp to plant and two C/N ratios for 4 weeks.
The 5:1–15 treatment had better plant production among treatments (Table 5). Red orache and okahijiki had significantly higher (p < 0.05) yield in 5:1–15 treatment. The yield was about twice that of red orache, while around 1.5 to 2 times higher than 2:1–12 and 3:1–12 treatments in okahijiki. Minutina had a better yield among the three species. Its production was also higher in 5:1–15 treatment, whereas, the production in the 5:1–15 treatment was only higher than that of 2:1–12 treatment.
In general, the concentrations of N, P, K in plants were increased with the increasing stocking density and C/N ratio (Table 5). In contrast, the concentration of Mg and Ca displayed an opposite trend. The treatment with lower stocking density ratio and C/N ratio had a higher concentration of Mg and Ca, while the concentrations of S and Na in plants were not significantly (p > 0.05) different among treatments.
Water Quality
During the experiment, temperature and dissolved oxygen (DO) were maintained at 28–29°C and 6.1–7.3 mg/L in all treatments, respectively. The salinity was monitored and controlled every day to maintain at the desired level, 15 ppt. The alkalinity in all treatments was not significantly different (p > 0.05) among each other. There were no significant differences (p > 0.05) found in the concentrations of total suspended solids (TSS) or volatile suspended solids (VSS) (Table 6). The pH was affected by the stocking density ratio, the lower stocking density ratio tended to have a higher pH than the higher stocking density ratio. Starting on day 9, the value was significantly higher (p < 0.05) in the stocking ratio of 2:1–12 and 2:1–15 treatment than that of 5:1–12 and 5:1–15 (Figure 2).
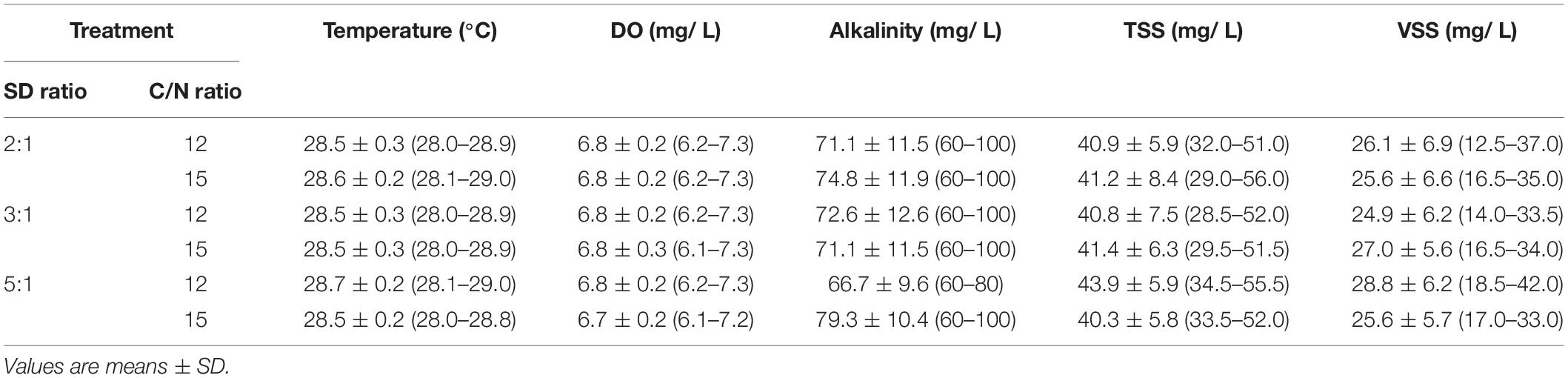
Table 6. Mean water quality values (range) for marine aquaponics at three stocking density ratios (SD ratio) of shrimp to plant and two C/N ratios for 4 weeks.
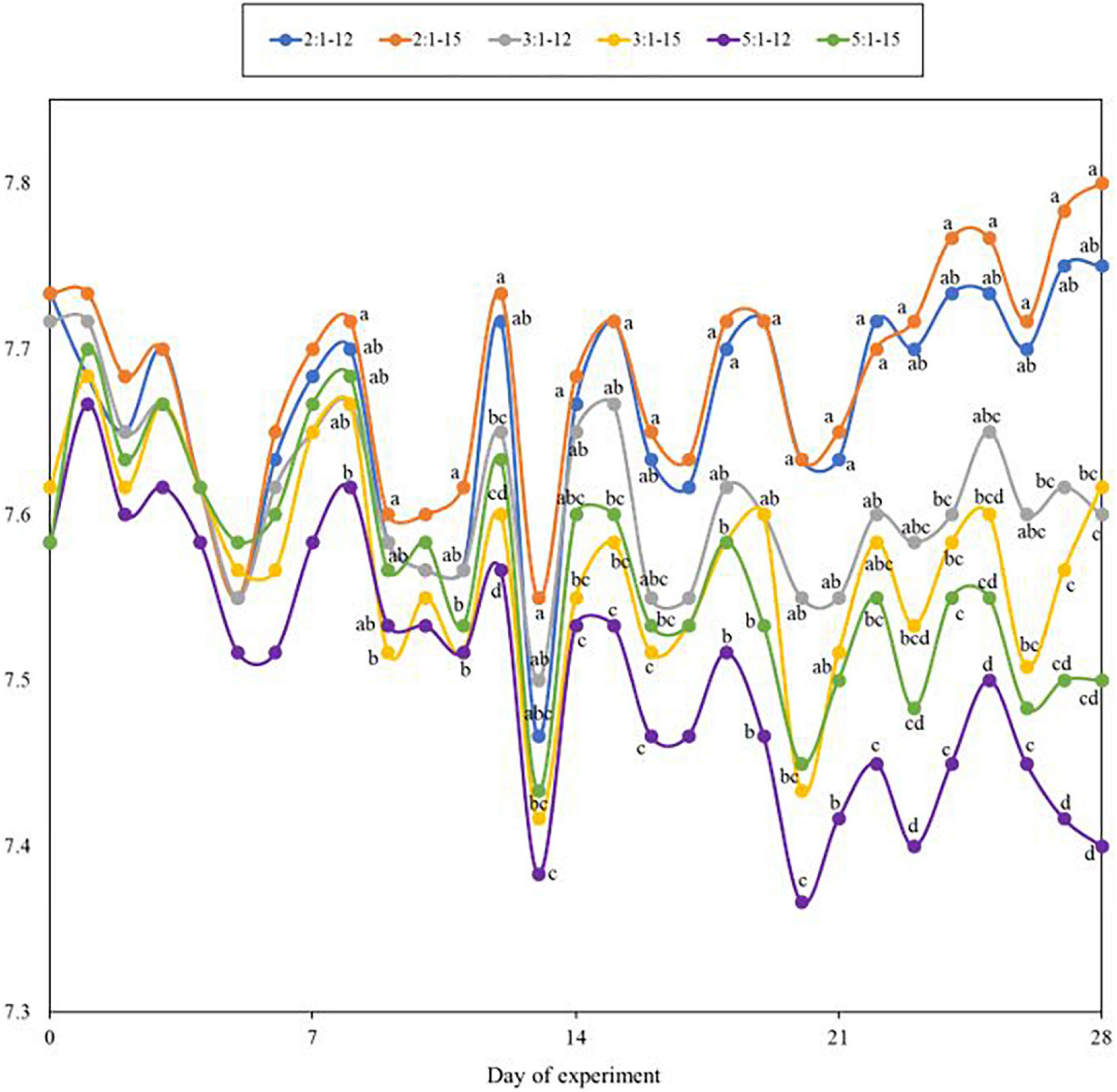
Figure 2. Dynamic change of pH measured during the 4-week experiment. Each point represents the means of 3 replicates, and lower-case alphabet letters represent significant differences, followed by one-way ANOVA and Tukey’s HSD test (α = 0.05).
The concentrations of TAN, and NO2–, which are toxic to aquatic animals and plants, remained at safe and low concentrations (both were below 0.2 mg/ L) throughout the experiment in all treatments, although the treatments with higher stocking density ratio, 5:1–12 and 5:1–15, had significantly higher (p < 0.05) concentrations than that of lower stocking density ratio, 2:1–12 and 2:1–15 (Figures 3A,B). On the other hand, concentrations of NO3– and PO43– continued increasing throughout the experiment. In general, 5:1–12 and 5:1–15 treatments had significantly higher (p < 0.05) concentrations of NO3– and PO43– than 2:1–12 and 2:1–15 treatments (Figures 3C,D).
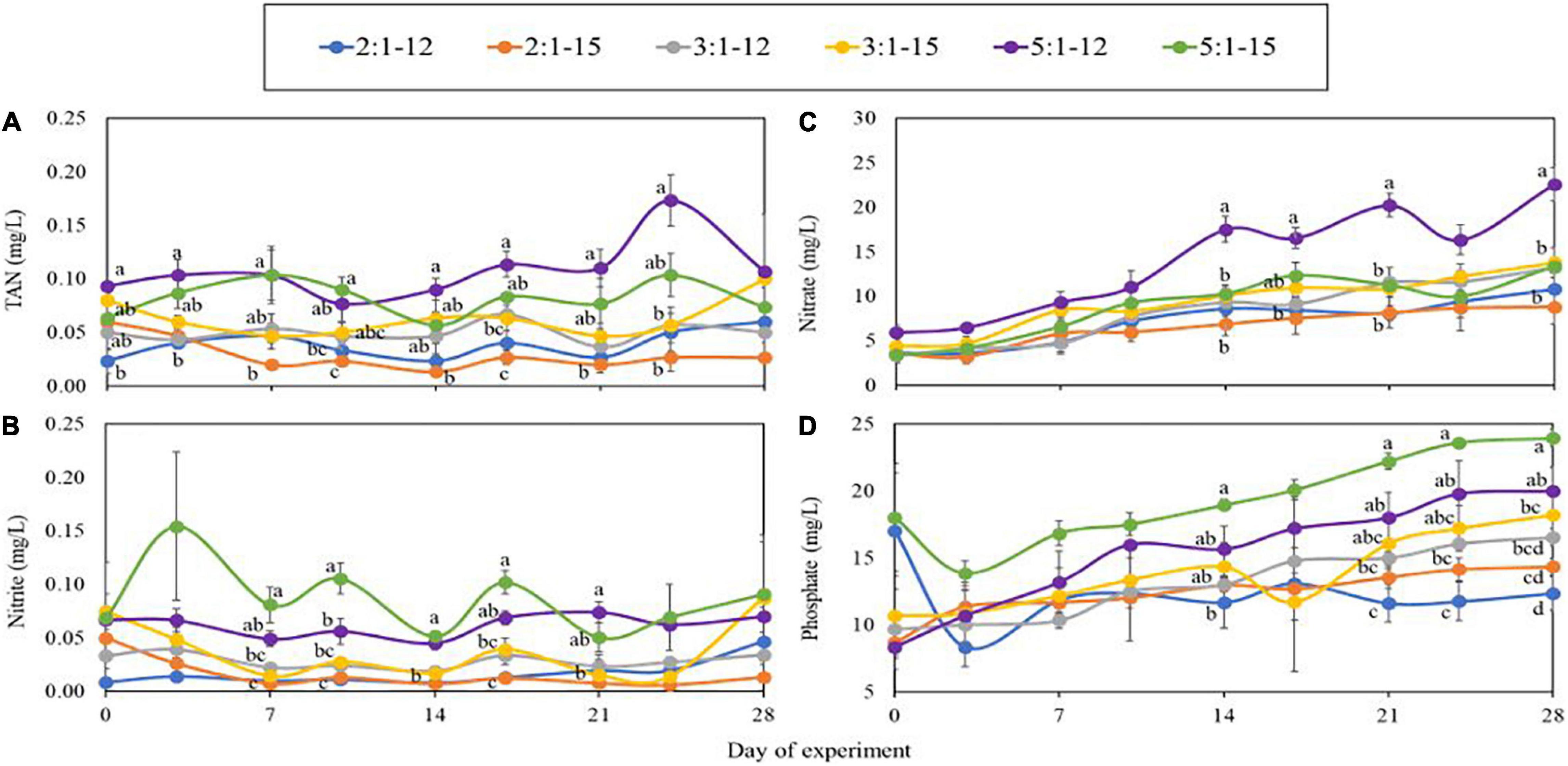
Figure 3. Concentrations of TAN (A), nitrite (B), nitrate (C), and phosphate (D) measured during the 4-week experiment. Each point represents the means of 3 replicates ± standard error. Lower-case alphabet letters represent significant differences, followed by one-way ANOVA and Tukey’s HSD test (α = 0.05).
Discussion
Shrimp Growth
The results of this study indicate that an increase in stocking density ratio was a negative factor on shrimp growth. In addition, the FCR increased with the increasing stocking density, which increases the cost of production. Similar results were observed in other studies that investigated the effect of stocking density on shrimp production (Moss and Moss, 2004; Esparza-Leal et al., 2010; Neal et al., 2010; Krummenauer et al., 2011; Sookying et al., 2011; Façanha et al., 2016; Araneda et al., 2020; Fleckenstein et al., 2020). However, survival of shrimp in our experiment was above 95%, while other researchers reported that survival decreased with increasing density (Neal et al., 2010; Krummenauer et al., 2011; Araneda et al., 2020).
The reduced survival and growth of shrimp at higher stocking densities can be related to several factors including the availability of space for growth, competition for feed, cannibalism, and increased waste excretion leading to degraded water quality (Arnold et al., 2006; Wang and Gu, 2010; Ferreira et al., 2020; Luo et al., 2020). The high survival at high stocking density in this study might be attributed to the application of probiotics and molasses, because probiotics improve the resistance to adverse environments and the ability to tolerate stress (Martínez Cruz et al., 2012; Buruianǎ et al., 2014; Nemutanzhela et al., 2014; Olmos et al., 2020). The use of molasses increased the C/N ratio in the environment, which provided a beneficial environment for probiotics to assimilate nitrogenous waste (Avnimelech, 1999; Crab, 2010; Crab et al., 2012) and compete with pathogens, such as Vibrio spp. (Panigrahi et al., 2018). The increased C/N ratio also led to the generation of biofloc, which can be a supplementary food for shrimp (Avnimelech, 1999; Browdy et al., 2012; Crab et al., 2012). Moreover, the application of molasses improved the ability of plants to uptake nutrients from the water column (White, 2012). The combined effect from the application of probiotics and molasses maintained the water quality and led to higher survival in the present study, albeit, with reduced growth. It is unclear if the increased density and potential increases in marketable shrimp would offset the slower growth and increased feed costs associated with super-high-density culture, but this possibility needs to be explored.
Plants
In general, the growth performance of the three halophytes was better with the increasing stocking ratio and C/N ratio. The nutrient balance is one of the key factors to the success of aquaponics; too few animals (inadequate nitrogen) is limiting to plants, and excessive animal density will result in nitrogen excretion in excess of the plants’ ability to uptake compounds, and may lead to chronic or even acute toxicity to both animals and plants (Somerville et al., 2014).
In our study, plants were benefiting from a higher shrimp stocking density, in which more nutrients are provided for plants. All plants had greater yield in treatment 5:1 (Table 5). A similar trend was also reported by Shete et al. (2015); the plant production was higher in fish to plant ratio of 1:1, followed by1:2 and then 1:3. Furthermore, the concentration of macronutrients (N, P, and K) in plant tissues were also higher in both 5:1 treatments than the other stocking density ratio treatments. The higher concentrations of TAN, NO2–, NO3–, and PO43– found in the 5:1 treatment was likely the result of the higher feed inputs into those treatments. The steady increase in NO3–, and PO43– in all treatments may indicate the saturation of plant’s assimilation limits. Further research is needed to evaluate a longer culture duration or a different strategy on plant harvest (sequential stocking) since the concentration of all N- and P-compounds in the water will likely increase after every harvest (Yang and Kim, 2020b), which might be a concern for shrimp culture.
While not as pronounced as the effect of shrimp stocking density, the C/N ratio also exerted an impact on plant production characteristics. Additional carbon for amending the growing environment improves nutrient uptake by plants, increases crop yield, and alleviates phytotoxicity, caused by trace metals, salinity, pesticides, phytotoxins, or allelochemicals (Rodríguez-Kábana, 1986; Schenck, 2001; White, 2012; Pyakurel et al., 2019; Li et al., 2020). Overall, the effect of C/N ratio on plant growth, yield, and mineral nutrient concentrations in plants was relatively minor compared to stocking density.
Water Quality
To manage the water quality well, a robust microbial community is indispensable in aquaponics. Promoting the establishment of microbial flora by inoculation of probiotics or inoculations from mature water, used-biomedia, or biofloc from stabilized systems to new systems are efficient practices (Otoshi et al., 2011; Xu and Pan, 2012; Pinheiro et al., 2020; Chu and Brown, 2021). Xu and Pan (2012) inoculated bioflocs, characterized by Bacillus sp. as the predominant bacteria, into experimental tanks before their study. The concentrations of toxic nitrogenous waste, TAN and NO2–, in their study were maintained below 0.51 mg/L and 1.25 mg/L, respectively. In the present study, the concentration of TAN and NO2– remained lower than 0.2 mg/L throughout the experiment, even in the high stocking density treatments. Additional research needs to be conducted examining the frequency of probiotic application and varying harvest scenarios, as after every plant harvest, the TAN and NO2– will likely increase (Yang and Kim, 2020b).
Concentrations of TAN and NO2– were higher in the higher C/N treatments while the NO3– concentrations were lower. Similar results were also reported in other studies (Xu et al., 2016, 2018). The additional organic carbon facilitates growth of heterotrophic bacteria, which compete for nutrients and space inside the biofilm or biomedia with the nitrifying bacteria (NB), which are composed of ammonia-oxidizing bacteria (AOB) and nitrite-oxidizing bacteria (NOB). The rate of reproduction of heterotrophic bacteria is much faster than NB, while the reproduction rate of AOB is faster than NOB (Hu et al., 2015). Due to the competition with heterotrophic bacteria, and the slower growth rate of AOB and NOB, the efficiency of nitrification decreases with the increasing C/N ratio, resulting in a decrease in TAN removal rate and NO3– productivity (Zhu and Chen, 2001; Michaud et al., 2006; Luo et al., 2020). However, in our study, all nitrogenous waste products remained below concentrations considered toxic.
The concentrations of PO43– continued to accumulate throughout the experiment, similar to previous results (Boxman et al., 2018; Yang and Kim, 2019, 2020a,b; Chu and Brown, 2021; Huang et al., 2021), and was higher in the treatments with the higher stocking densities and C/N ratio, likely due to higher feed inputs. Another explanation could be the higher C/N ratios resulted in bioflocs dominated by heterotrophic bacteria and less algae (Xu et al., 2016). Moreover, the accumulation of PO43– suggests the saturation of plant’s assimilation ability. PO43–, one of the compounds that causes eutrophication, is an issue for aquaponics; hence, more research is required to improve the management of PO43– in aquaponics and determine how to improve plant’s ability to assimilate PO43–.
In aquaponics, pH is another vital parameter that can be affected by nitrification, nutrient assimilation by plants and heterotrophic bacteria, and CO2 excretion by aquatic animals, as well as other factors (Yang and Kim, 2019; Li et al., 2020). The process of nitrification and nitrogenous waste assimilation by bacteria, and CO2 released through the respiration of aquatic animals and microorganisms tends to decrease the pH. Conversely, CO2 removal and nutrient assimilation by plant tends to raise the pH (Ebeling et al., 2006; White, 2012; Somerville et al., 2014). This may explain why the pH level was lowest at the stocking density of 5:1, followed by 3:1, then 2:1. Also, the lower pH can be another possible reason for a better plant growth in higher stocking density treatments, because nutrient availability increases with the decreasing pH (Somerville et al., 2014).
Conclusion
The stocking density ratio and C/N ratio exerted significant impacts on the performance of shrimp and plants in marine aquaponics. Shrimp performed better in the stocking density of 2:1 and 3:1, with no impact from the C/N ratio. Conversely, plants performed better in the stocking density of 3:1 and 5:1 with the C/N ratio at 15. Therefore, a stocking density ratio of 3:1 with a C/N ratio at 15 is suggested as the optimal condition for shrimp and the three halophytes in an indoor marine aquaponic food production system. Inoculating the water with biofloc and applying probiotics regularly can enhance the management of water quality and the health of shrimp and plants in aquaponics. Although water quality was maintained at safe levels for shrimp and halophytes during the experiment, more studies with a longer period of cultivation are needed for a better understanding of marine aquaponics using these species.
Data Availability Statement
The original contributions presented in the study are included in the article/supplementary material, further inquiries can be directed to the corresponding author.
Author Contributions
Y-TC: conceptualization, methodology, investigation, validation, formal analysis, data curation, and writing – original draft preparation and editing. PB: resources, supervision, project administration, funding acquisition, and writing – review and editing. Both authors have read and agreed to the published version of the manuscript.
Funding
Funding for this project was provided by the Purdue University Department of Forestry and Natural Resources, the College of Agriculture, and the US Department of Agriculture.
Conflict of Interest
The authors declare that the research was conducted in the absence of any commercial or financial relationships that could be construed as a potential conflict of interest.
Publisher’s Note
All claims expressed in this article are solely those of the authors and do not necessarily represent those of their affiliated organizations, or those of the publisher, the editors and the reviewers. Any product that may be evaluated in this article, or claim that may be made by its manufacturer, is not guaranteed or endorsed by the publisher.
Acknowledgments
We would like to thank Zeigler Bros., Inc for their donation of commercial shrimp feed and EZ-bio. We would like to thank the manager, Robert Rode, of the Aquaculture Research Lab for his help and support for the study.
References
Alshrouf, A. (2017). Hydroponics, aeroponic and aquaponic as compared with conventional farming. Am. Sci. Res. J. Eng. Technol. Sci. 27, 247–255.
Araneda, M., Gasca-Leyva, E., Vela, M. A., and Domínguez-May, R. (2020). Effects of temperature and stocking density on intensive culture of Pacific white shrimp in freshwater. J. Therm. Biol. 94:102756. doi: 10.1016/j.jtherbio.2020.102756
Arnold, S. J., Sellars, M. J., Crocos, P. J., and Coman, G. J. (2006). Intensive production of juvenile tiger shrimp Penaeus monodon: an evaluation of stocking density and artificial substrates. Aquaculture 261, 890–896. doi: 10.1016/j.aquaculture.2006.07.036
Avnimelech, Y. (1999). Carbon/nitrogen ratio as a control element in aquaculture systems. Aquaculture 176, 227–235. doi: 10.1016/S0044-8486(99)00085-X
Boxman, S. E., Nystrom, M., Ergas, S. J., Main, K. L., and Trotz, M. A. (2018). Evaluation of water treatment capacity, nutrient cycling, and biomass production in a marine aquaponic system. Ecol. Eng. 120, 299–310. doi: 10.1016/j.ecoleng.2018.06.003
Browdy, C. L., Ray, A. J., Leffler, J. W., and Avnimelech, Y. (2012). “Biofloc-based aquaculture systems,” in Aquaculture Production Systems, ed. J. Tidwell (Oxford: Johy Wiley & Sons, Inc), 278–307. doi: 10.1002/9781118250105.ch12
Buruianǎ, C. T., Profir, A. G., and Vizireanu, C. (2014). Effects of probiotic Bacillus species in aquaculture – an overview. Ann. Univ. Dunarea Jos Galati Fascicle VI Food Technol. 38, 9–17. doi: 10.1016/j.fsi.2021.07.007
Chu, Y.-T. (2014). Effects of Different Probiotics on Water Qualities and Growth in Close Culture System of Litpenaeus vannamei. Master’s Thesis. Keelung: National Taiwan Ocean University.
Chu, Y.-T., and Brown, P. B. (2021). Evaluation of Pacific whiteleg shrimp and three halophytic plants in marine aquaponic systems under three salinities. Sustainability 13:269. doi: 10.3390/su13010269
Crab, R. (2010). Bioflocs Technology: An Integrated System for the Removal of Nutrients and Simultaneous Production of Feed in Aquaculture. Ph.D. Thesis. Brusseis: Ghen Unversity.
Crab, R., Chielens, B., Wille, M., Bossier, P., and Verstraete, W. (2010). The effect of different carbon sources on the nutritional value of bioflocs, a feed for Macrobrachium rosenbergii postlarvae. Aquac. Res. 41, 559–567. doi: 10.1111/j.1365-2109.2009.02353.x
Crab, R., Defoirdt, T., Bossier, P., and Verstraete, W. (2012). Biofloc technology in aquaculture: beneficial effects and future challenges. Aquaculture 35, 351–356. doi: 10.1016/j.aquaculture.2012.04.046
Ebeling, J. M., Timmons, M. B., and Bisogni, J. J. (2006). Engineering analysis of the stoichiometry of photoautotrophic, autotrophic, and heterotrophic removal of ammonia-nitrogen in aquaculture systems. Aquaculture 257, 346–358. doi: 10.1016/j.aquaculture.2006.03.019
Esparza-Leal, H. M., Ponce-Palafox, J. T., Aragón-Noriega, E. A., Arredondo-Figueroa, J. L., García-Ulloa Gómez, M., and Valenzuela-Quiñonez, W. (2010). Growth and performance of the whiteleg shrimp Penaeus vannamei (boone) cultured in low-salinity water with different stocking densities and acclimation times. Aquac. Res. 41, 878–883. doi: 10.1111/j.1365-2109.2009.02367.x
Façanha, F. N., Oliveira-Neto, A. R., Figueiredo-Silva, C., and Nunes, A. J. P. (2016). Effect of shrimp stocking density and graded levels of dietary methionine over the growth performance of Litopenaeus vannamei reared in a green-water system. Aquaculture 463, 16–21. doi: 10.1016/j.aquaculture.2016.05.024
FAO (2020). The State of World Fisheries and Aquaculture 2020. Sustainability in Action., Nature and Resources. Rome: FAO.
Ferreira, G. S., Silva, V. F., Martins, M. A., da Silva, A. C. C. P., Machado, C., Seiffert, W. Q., et al. (2020). Strategies for ammonium and nitrite control in Litopenaeus vannamei nursery systems with bioflocs. Aquac. Eng. 88:102040. doi: 10.1016/j.aquaeng.2019.102040
Fleckenstein, L. J., Kring, N. A., Tierney, T. W., Fisk, J. C., Lawson, B. C., and Ray, A. J. (2020). The effects of artificial substrate and stocking density on Pacific white shrimp (Litopenaeus vannamei) performance and water quality dynamics in high tunnel-based biofloc systems. Aquac. Eng. 90:102093. doi: 10.1016/j.aquaeng.2020.102093
Fry, J. P., Mailloux, N. A., Love, D. C., Milli, M. C., and Cao, L. (2018). Feed conversion efficiency in aquaculture: do we measure it correctly? Environ. Res. Lett. 13:024017. doi: 10.1088/1748-9326/aaa273
Gao, W., Tian, L., Huang, T., Yao, M., Hu, W., and Xu, Q. (2016). Effect of salinity on the growth performance, osmolarity and metabolism-related gene expression in white shrimp Litopenaeus vannamei. Aquac. Reports 4, 125–129. doi: 10.1016/j.aqrep.2016.09.001
Glenn, E. P., Brown, J. J., and Blumwald, E. (1999). Salt tolerance and crop potential of halophytes. CRC. Crit. Rev. Plant Sci. 18, 227–255. doi: 10.1080/07352689991309207
Glenn, E. P., Brown, J. J., and Leary, J. W. O. (1998). Irrigating crops with seawater. Sci. Am. 279, 76–81. doi: 10.1038/scientificamerican0898-76
Goddek, S., Joyce, A., Kotzen, B., and Butnell, G. M. (2019). “Nutrient cycling in aquaponics systems,” in Aquaponics Food Production Systems, 1st Edn, eds S. Goddek, A. Joyce, B. Kotzen, and G. M. Butnell (Cham: Springer), doi: 10.1007/978-3-030-15943-6_9
Hu, Z., Lee, J. W., Chandran, K., Kim, S., Brotto, A. C., and Khanal, S. K. (2015). Effect of plant species on nitrogen recovery in aquaponics. Bioresour. Technol. 188, 92–98. doi: 10.1016/j.biortech.2015.01.013
Huang, C. C., Lu, H. L., Chang, Y. H., and Hsu, T. H. (2021). Evaluation of the water quality and farming growth benefits of an intelligence aquaponics system. Sustain. 13, 1–15. doi: 10.3390/su13084210
Kim, J. H., Suk, S., Jang, W. J., Lee, C. H., Kim, J. E., Park, J. K., et al. (2017). Salicornia extract ameliorates salt-induced aggravation of nonalcoholic fatty liver disease in obese mice fed a high-fat diet. J. Food Sci. 82, 1765–1774. doi: 10.1111/1750-3841.13777
Klinger, D., and Naylor, R. (2012). Searching for solutions in aquaculture: charting a sustainable course. Annu. Rev. Environ. Resour. 37, 247–276. doi: 10.1146/annurev-environ-021111-161531
Krummenauer, D., Peixoto, S., Cavalli, R. O., Poersch, L. H., and Wasielesky, W. (2011). Superintensive culture of white shrimp, Litopenaeus vannamei, in a biofloc technology system in Southern Brazil at different stocking densities. J. World Aquac. Soc. 42, 726–733. doi: 10.1111/j.1749-7345.2011.00507.x
Lam, S. S., Ma, N. L., Jusoh, A., and Ambak, M. A. (2015). Biological nutrient removal by recirculating aquaponic system: optimization of the dimension ratio between the hydroponic & rearing tank components. Int. Biodeterior. Biodegrad. 102, 107–115. doi: 10.1016/j.ibiod.2015.03.012
Li, S., Zhao, X., Ye, X., Zhang, L., Shi, L., Xu, F., et al. (2020). The effects of condensed molasses soluble on the growth and development of rapeseed through seed germination, hydroponics and field trials. Agriculture 10, 1–20. doi: 10.3390/agriculture10070260
Luo, G., Xu, J., and Meng, H. (2020). Nitrate accumulation in biofloc aquaculture systems. Aquaculture 520:734675. doi: 10.1016/j.aquaculture.2019.734675
Martínez Cruz, P., Ibáñez, A. L., Monroy Hermosillo, O. A., and Ramírez Saad, H. C. (2012). Use of probiotics in aquaculture. ISRN Microbiol. 2012, 1–13. doi: 10.5402/2012/916845
Michaud, L., Blancheton, J. P., Bruni, V., and Piedrahita, R. (2006). Effect of particulate organic carbon on heterotrophic bacterial populations and nitrification efficiency in biological filters. Aquac. Eng. 34, 224–233. doi: 10.1016/j.aquaeng.2005.07.005
Moss, K. R. K., and Moss, S. M. (2004). Effects of artificial substrate and stocking density on the nursery production of Pacific white shrimp Litopenaeus vannamei. J. World Aquac. Soc. 35, 537–542. doi: 10.1111/j.1749-7345.2004.tb00121.x
Neal, R. S., Coyle, S. D., Tidwell, J. H., and Boudreau, B. M. (2010). Evaluation of stocking density and light level on the growth and survival of the pacific white shrimp, Litopenaeus vannamei, reared in zero-exchange systems. J. World Aquac. Soc. 41, 533–544. doi: 10.1111/j.1749-7345.2010.00393.x
Nemutanzhela, M. E., Roets, Y., Gardiner, N., and Lalloo, R. (2014). “The use and benefits of Bacillus based biological agents in aquaculture,” in Sustainable Aquaculture Techniques, eds M. Hernandez-Vergara and C. Perez-Rostro (Rijeka: Intech Publishing), 1–34. doi: 10.1016/j.colsurfa.2011.12.014
Olmos, J., Acosta, M., Mendoza, G., and Pitones, V. (2020). Bacillus subtilis, an ideal probiotic bacterium to shrimp and fish aquaculture that increase feed digestibility, prevent microbial diseases, and avoid water pollution. Arch. Microbiol. 202, 427–435. doi: 10.1007/s00203-019-01757-2
Otoshi, C. A., Naguwa, S. S., Falesch, F. C., and Moss, S. M. (2007). Shrimp behavior may affect culture performance at super-intensive stocking densities. Glob. Aquac. Advocate 2, 67–69.
Otoshi, C. A., Rodriguez, N., and Moss, S. M. (2011). Establishing nitrifying bacteria in super-intensive biofloc shrimp production. Glob. Aquac. Advocate 14, 24–26.
Panigrahi, A., Saranya, C., Sundaram, M., Vinoth Kannan, S. R., Das, R. R., Satish Kumar, R., et al. (2018). Carbon: nitrogen (C:N) ratio level variation influences microbial community of the system and growth as well as immunity of shrimp (Litopenaeus vannamei) in biofloc based culture system. Fish Shellfish Immunol. 81, 329–337. doi: 10.1016/j.fsi.2018.07.035
Panta, S., Flowers, T., Lane, P., Doyle, R., Haros, G., and Shabala, S. (2014). Halophyte agriculture: success stories. Environ. Exp. Bot. 107, 71–83. doi: 10.1016/j.envexpbot.2014.05.006
Panth, N., Park, S. H., Kim, H. J., Kim, D. H., and Oak, M. H. (2016). Protective effect of Salicornia europaea extracts on high salt intake-induced vascular dysfunction and hypertension. Int. J. Mol. Sci. 17:1176. doi: 10.3390/ijms17071176
Pinheiro, I., Carneiro, R. F. S., Vieira, F., do, N., Gonzaga, L. V., Fett, R., et al. (2020). Aquaponic production of Sarcocornia ambigua and Pacific white shrimp in biofloc system at different salinities. Aquaculture 519, 1–9. doi: 10.1016/j.aquaculture.2019.734918
Pyakurel, A., Dahal, B. R., and Rijal, S. (2019). Effect of molasses and organic fertilizer in soil fertility and yield of Spinach in Khotang. Nepal. Int. J. Appl. Sci. Biotechnol. 7, 49–53. doi: 10.3126/ijasbt.v7i1.23301
Quagrainie, K. K., Flores, R. M. V., Kim, H. J., and McClain, V. (2018). Economic analysis of aquaponics and hydroponics production in the U.S. Midwest. J. Appl. Aquac. 30, 1–14. doi: 10.1080/10454438.2017.1414009
Rakocy, J. E. (2012). “Aquaponics — Integrating fish and plant culture,” in Aquaculture Production Systems, ed. J. Tidwell (Oxford: Johy Wiley & Sons, Inc), 343–386. doi: 10.1002/9781118250105.ch14
Ray, A. J., and Lotz, J. M. (2017). Comparing salinities of 10, 20, and 30‰ in intensive, commercial-scale biofloc shrimp (Litopenaeus vannamei) production systems. Aquaculture 476, 29–36. doi: 10.1016/j.aquaculture.2017.03.047
Rodríguez-Kábana, R. (1986). Organic and inorganic nitrogen amendments to soil as nematode suppressants. J. Nematol. 18, 129–134.
Ross, W., Gallaudet, R. T., and Oliver, C. (2017). Fisheries of the United States, 2017. U.S. Department of Commerce, NOAA Current Fishery Statistics No. 2017. Washington, DC: NOAA.
Schenck, S. (2001). Molasses Soil Amendment for Crop Improvement and Nematode Management. Honolulu, HI: Hawaii Agriculture. Reserch. Center, 1–7.
Shete, A. P., Verma, A. K., Chadha, N. K., Prakash, C., and Chandrakant, M. H. (2015). A comparative study on fish to plant component ratio in recirculating aquaponic system with common carp and mint. J. Env. Bio-Sci 29, 323–329.
Somerville, C., Cohen, M., Pantanella, E., Stankus, A., and Lovatelli, A. (2014). Small-Scale Aquaponic Food Production. Integrated fish and Plant Farming. Fisheries and Aquaculture Technical Paper, No 589. Rome: FAO, 262.
Sookying, D., Silva, F. S. D., Davis, D. A., and Hanson, T. R. (2011). Effects of stocking density on the performance of Pacific white shrimp Litopenaeus vannamei cultured under pond and outdoor tank conditions using a high soybean meal diet. Aquaculture 319, 232–239. doi: 10.1016/j.aquaculture.2011.06.014
Ventura, Y., and Sagi, M. (2013). Halophyte crop cultivation: the case for salicornia and sarcocornia. Environ. Exp. Bot. 92, 144–153. doi: 10.1016/j.envexpbot.2012.07.010
Wang, Y., and Gu, Q. (2010). Effect of probiotics on white shrimp (Penaeus vannamei) growth performance and immune response. Mar. Biol. Res. 6, 327–332. doi: 10.1080/17451000903300893
White, P. J. (2012). “Ion uptake mechanisms of individual cells and roots: short-distance transport,” in Marschner’s Mineral Nutrition of Higher Plants, ed. P. Marschner (Cambridge, MA: Academic Press), 7–47. doi: 10.1016/B978-0-12-384905-2.00002-9
Xu, W. J., Morris, T. C., and Samocha, T. M. (2016). Effects of C/N ratio on biofloc development, water quality, and performance of Litopenaeus vannamei juveniles in a biofloc-based, high-density, zero-exchange, outdoor tank system. Aquaculture 453, 169–175. doi: 10.1016/j.aquaculture.2015.11.021
Xu, W. J., Morris, T. C., and Samocha, T. M. (2018). Effects of two commercial feeds for semi-intensive and hyper-intensive culture and four C/N ratios on water quality and performance of Litopenaeus vannamei juveniles at high density in biofloc-based, zero-exchange outdoor tanks. Aquaculture 490, 194–202. doi: 10.1016/j.aquaculture.2018.02.028
Xu, W. J., and Pan, L. Q. (2012). Effects of bioflocs on growth performance, digestive enzyme activity and body composition of juvenile Litopenaeus vannamei in zero-water exchange tanks manipulating C/N ratio in feed. Aquaculture 356–357, 147–152. doi: 10.1016/j.aquaculture.2012.05.022
Yang, T., and Kim, H. J. (2019). Nutrient management regime affects water quality, crop growth, and nitrogen use efficiency of aquaponic systems. Sci. Hortic. 256:108619. doi: 10.1016/j.scienta.2019.108619
Yang, T., and Kim, H. J. (2020b). Comparisons of nitrogen and phosphorus mass balance for tomato-, basil-, and lettuce-based aquaponic and hydroponic systems. J. Clean. Prod. 274:122619. doi: 10.1016/j.jclepro.2020.122619
Yang, T., and Kim, H. J. (2020a). Characterizing nutrient composition and concentration in tomato-, basil-, and lettuce-based aquaponic and hydroponic systems. Water 12:1259. doi: 10.3390/W12051259
Keywords: marine aquaponics, shrimp to plant ratio, C/N ratio, Litopenaeus vannamei, halophytic plants, biofloc, sustainable food production, water pumpless system design
Citation: Chu Y-T and Brown PB (2021) Sustainable Marine Aquaponics: Effects of Shrimp to Plant Ratios and C/N Ratios. Front. Mar. Sci. 8:771630. doi: 10.3389/fmars.2021.771630
Received: 06 September 2021; Accepted: 15 November 2021;
Published: 07 December 2021.
Edited by:
Xiangli Tian, Ocean University of China, ChinaReviewed by:
Nathalie Rose Le François, Montreal Biodome, CanadaLi Li, Ocean University of China, China
Copyright © 2021 Chu and Brown. This is an open-access article distributed under the terms of the Creative Commons Attribution License (CC BY). The use, distribution or reproduction in other forums is permitted, provided the original author(s) and the copyright owner(s) are credited and that the original publication in this journal is cited, in accordance with accepted academic practice. No use, distribution or reproduction is permitted which does not comply with these terms.
*Correspondence: Paul B. Brown, cGJAcHVyZHVlLmVkdQ==