- 1Chesapeake Biological Laboratory, University of Maryland Center for Environmental Science, Solomons, MD, United States
- 2Animal and Plant Health Directorate, Ministry for Primary Industries, Wellington, New Zealand
- 3Marine Invasive Species Program, California State Lands Commission, Sacramento, CA, United States
- 4Naval Research Laboratory, Washington, DC, United States
- 5Smithsonian Environmental Research Center, Edgewater, MD, United States
- 6U.S. Department of Transportation, Maritime Administration, Washington, DC, United States
Submerged ship surfaces are often inhabited by diverse sessile and sedentary marine organisms, which can directly impact vessel operations and increase the likelihood of non-indigenous species (NIS) establishment and impacts. Ship in-water cleaning (IWC) systems are now being incorporated into biofouling policy, and rigorous, transparent, and predictive verification testing is vital to regulatory success. Performance criteria for IWC approval should focus on environmental protection goals by including: qualified and independent testing; quantitative, robust, and statistically sound data, rather than qualitative observations; water sampling at all critical control points to characterize the release of harmful materials, including dissolved and particulate biocides; measurable and protective endpoints, rather than percent reductions; determinations of presence or absence of macro-organisms, irrespective of species origins or physiological state; and appropriately trained IWC operators.
Introduction
The colonization of submerged surfaces by sessile and sedentary organisms, including microbes, invertebrates, and macroalgae, has long been a significant challenge for coastal and ocean-going ships (Woods Hole Oceanographic Institute, 1952). Biofouling of the global shipping fleet, which is responsible for transporting approximately 80% of the world’s goods and materials (UNCTAD, 2018), can result in increased vessel corrosion rates, hydrodynamic drag, fuel consumption, and exhaust emissions (Townsin et al., 1981; Schultz, 2007; Li and Ning, 2019). Beyond the direct impacts on vessel operations, maintenance, and associated legal/contractual requirements (e.g., BIMCO, 2013, 2019; Altarriba and Halonen, 2019), which drive advances in biofouling prevention/management systems (Scianni and Georgiades, 2019), biofouling management is the focus of current and evolving environmental protection regulations.
Greenhouse gas (GHG) emissions from ships have been regulated since 2005 under Annex VI (Regulations for the Prevention of Air Pollution from Ships) of the International Convention for the Prevention of Pollution from Ships (MARPOL 73/78, 1997). Therefore, biofouling, which even at the biofilm (i.e., slime layer) level requires ships to increase fuel use to maintain speed (e.g., Schultz et al., 2011), will result in amplified GHG emissions that may exceed required limits.
The role of ship biofouling in the introduction, establishment, and subsequent spread of non-indigenous species (NIS) is also a significant concern (Hewitt and Campbell, 2010; Ruiz et al., 2015; Davidson et al., 2018; Bailey et al., 2020; Georgiades et al., 2021). Marine NIS have caused a range of impacts to various economic, ecological, societal, and cultural resources (Ruiz et al., 1997; Grosholz, 2002; Hewitt et al., 2004; Bell et al., 2011; Georgiades et al., 2021). Thus, guidelines and regulations to prevent NIS impacts via ship biofouling are beginning to emerge.
In response to ship operational needs, and to a lesser extent emissions and biosecurity regulations, a sophisticated antifouling system (AFS) industry continues to evolve to minimize biofouling accumulation. The primary AFS employed by ships is surface coatings, which inhibit macrofouling attachment (using biocides) or reduce adhesion (foul-release) to wetted surfaces (Dafforn et al., 2011). Biocidal coatings typically require regulatory approval for use to prevent unintended environmental degradation (Dafforn et al., 2011). Although some biocides are now banned [e.g., tributyl tin (TBT)] due to non-target environmental effects (e.g., Sonak et al., 2009), copper- and zinc-based biocidal AFS remain the most commonly applied on commercial ships (Scianni et al., 2021).
Despite continuous improvements in AFS efficacy and safety, coatings have a limited service life (reapplied at 5–10 year dry-docking intervals) and do not prevent biofouling accumulation on all ship surfaces (Davidson et al., 2016; Georgiades and Kluza, 2017), especially if ships have extended stationary/immobile periods (BIMCO, 2013; Davidson et al., 2020). Biofouling “hotspots” also exist that include areas on ships which are difficult to paint (e.g., dry-dock support strips) or sub-optimal for antifouling coating performance (e.g., niche areas such as rudders and sea chests) (Coutts and Taylor, 2004; Davidson et al., 2009, 2016). In-water removal of established macrofouling (i.e., individual organisms or colonies visible to the eye) is a common practice for many ships, primarily to increase fuel efficiency (e.g., Schultz et al., 2011).
Traditionally, ship in-water cleaning (IWC) has involved divers or remotely operated vehicles (ROV), which use scraping tools or cleaning carts to remove macrofouling from hull and propeller surfaces without capture of released debris (i.e., fouling organisms and coating material) (Jones, 1999; McClay et al., 2015; Morrisey and Woods, 2015). However, without debris capture, IWC of macrofouling can directly lead to discharges of NIS and harmful AFS biocides (Scianni and Georgiades, 2019; Tamburri et al., 2020a). Thus, IWC technology is rapidly developing to either (a) capture and process debris removed from ships or (b) conduct periodic proactive IWC (i.e., reduction/removal of biofilms to prevent or inhibit/limit macrofouling growth) (Tribou and Swain, 2010; Scianni and Georgiades, 2019; Tamburri et al., 2020a). Although proactive IWC is typically less abrasive than macrofouling removal, substantial amounts of microscopic material (biological and chemical) can be released into the environment. However, proactive IWC is viewed as a relatively low biosecurity risk because it may ultimately minimize the translocation of macrofouling species, including associated pathogens (where present) (Department of Agriculture [DOA] et al., 2015; Georgiades et al., 2021).
The removal or prevention of macrofouling through reactive IWC with capture or proactive IWC, respectively, may represent rare win-win solutions for both the shipping industry and the environment. However, it is critical to ensure these practices do not result in unintended consequences, including: (a) increased biosecurity risk, (b) increased discharge of AFS biocides, and (c) diminished coating condition that reduces AFS performance or service life (Scianni and Georgiades, 2019; Tamburri et al., 2020a). Therefore, as various authorities develop new biofouling policies that include the use of approved ship IWC systems, comprehensive, evidence-based consideration of system efficacy and environmental safety is paramount.
This policy brief assesses the methods proposed for evaluating IWC systems, describes the challenges associated with quantifying IWC system performance and environmental safety, and proposes a series of practical and feasible recommendations for the verification testing and approval of ship IWC systems.
Policy Options and Implications
Ship biofouling management guidelines (IMO, 2011) and regulations (California Code of Regulations, 2017; MPI, 2018) have been developed at international, national, and regional levels (Georgiades et al., 2020; Scianni et al., 2021) to minimize biosecurity risks. Importantly, these guidelines and regulations identify vessel IWC or treatment as important tools for ship maintenance (IMO, 2011; Department of Agriculture [DOA] et al., 2015; Scianni et al., 2017; Georgiades et al., 2018), renewing the interest in appropriate and proven technologies.
The Australian and New Zealand antifouling and IWC guidelines were drafted in 2011 (Ministry of Agriculture and Forestry, 2011). This action was coincident with the development of IMO biofouling guidelines and movement toward biofouling management regulations in Australia, New Zealand, and California, and followed the 2001 International Convention on the Control of Harmful Anti-fouling Systems on Ships that banned TBT internationally (IMO, 2001). The intent of these guidelines was to provide a risk-based framework for approving biofouling management practices by considering both chemical contamination and biosecurity risks. These guidelines were published in 2013 and were updated by Australia in 2015 (Department of Agriculture [DOA] et al., 2015).
New Zealand’s Craft Risk Management Standard for Biofouling on Vessels Arriving to New Zealand (CRMS-BIOFOUL) requires ships to take preventive measures to maintain a “clean hull” prior to arrival (MPI, 2018). Compliance is through the presentation of documentary evidence showing that one of the following measures has been undertaken: (a) continual maintenance following best practice, or (b) cleaned within 30 days prior to arrival in New Zealand, or (c) scheduled arrangement with an MPI-approved provider for cleaning or treatment within 24 h of arrival. While there are no MPI-approved providers for cleaning or treatment of international ships within New Zealand territorial waters, technical advice for evaluation of IWC systems has been produced for external hulls and niche areas (Morrisey et al., 2015) and internal niche areas (Growcott et al., 2019), with the former being recently tested (Jones and McClary, 2021).
In the United States, IWC is regulated under the National Pollutant Discharge Elimination System (NPDES) section of the Clean Water Act (33 U.S.C. 1342). IWC without capture is regulated as a discharge incidental to the normal operation of a vessel under the 2013 Vessel General Permit (USEPA, 2013), whereas IWC with capture requires a separate NPDES permit typically issued by a state or regional water quality regulatory entity. This arrangement will change with the adoption of regulations developed by the USEPA and U.S. Coast Guard under the authority of the 2018 Vessel Incidental Discharge Act (VIDA, 33 U.S.C. 1322). Final rules have not been published, and there remains uncertainty as to how these activities will be regulated once they are in place.
In 2021, the Baltic and International Maritime Council (BIMCO) and International Chamber of Shipping (ICS) proposed an industry standard for IWC with capture (BIMCO/ICS, 2021a,b). The intent of this standard is to help “ensure that the in-water cleaning of a ship’s hull, and niche areas, including the propeller, can be carried out safely, efficiently and in an environmentally sustainable way.” However, several components of the proposed standard and approval regime may limit the latter goal. These include, among others: (a) allowance of 10% of macrofouling to remain on the hull following cleaning, (b) reliance on percent reduction for removal of material from the effluent that allows for proportional increases of material release with increasing vessel size and fouling extent, (c) use of untested systems on substantial portions of vessels (up to 5%), and (d) option to not include measurements for AFS biocide release in evaluations/approvals.
The Australian Government consulted on their draft IWC standard in 2021 (DAWE, 2021). The draft standard includes water quality (biocide) testing, captured debris effluent filtration to 10 μm (or treatment to render organisms non-viable), and relies on a percentage cut-off for capture akin to (but a higher level than) BIMCO/ICS. Similarly, Transport Canada released draft voluntary guidance for relevant authorities on ship IWC for consultation (Transport Canada, 2021). Their draft guidelines introduce secondary treatment of captured debris, in addition to particle separation (10 μm post-2023), and also require the discharge to meet all legal requirements within the jurisdiction of the activity. Importantly, while independent testing is fundamental to both drafts, detailed guidance regarding quantitative IWC system evaluation is not provided.
Actionable Recommendations
Comprehensive, consistent, and rigorous performance verification of any technology, and its subsequent approval for use, is often a complicated process that requires: (a) careful consideration of policy goals, (b) clear communication of technology performance requirements, (c) availability of accepted/validated verification test methods and approaches, (d) defensible, independent test results, and (e) post-approval compliance and enforcement processes (e.g., Bedson and Sargent, 1996; USEPA ETV, 2010; Tamburri et al., 2020b). This complexity is particularly true for the diverse suite of novel IWC systems designed for use on various types of ships (e.g., numerous designs, coatings, ages, and operational profiles) and biofouling (e.g., assorted stages, types, coverages, and locations), and under diverse environmental conditions (e.g., variable visibilities, swells, currents, and ambient water qualities) (Scianni and Georgiades, 2019; Tamburri et al., 2020a). This section provides advice to help avoid pitfalls in verification testing and approval of IWC systems, which can undermine the success of emerging environmental regulations.
The likelihood of NIS introduction and establishment from ship biofouling is driven by a variety of factors (e.g., volume of traffic; vessel operational profiles; antifouling measures employed; time since dry-docking; fouling type, extent, and maturity; and environmental conditions of fouling origin and recipient locations) (Coutts et al., 2010; Inglis et al., 2010; Davidson et al., 2020). While it is unrealistic to expect ships to remain completely free of all biofouling at all times, minimizing the species richness, abundance, and maturity of macrofouling lowers the biosecurity risks (Georgiades and Kluza, 2017; Georgiades et al., 2021). As different authorities consider acceptable measures for minimizing ship biofouling and acceptable biofouling levels, statistical comparisons of biofouling on various areas of ships (i.e., hull and niche areas) before and after IWC, including treated (cleaned) and control (not cleaned) areas, remains key to protective and robust IWC system approval.
Detailed methods for quantitative assessments of ship biofouling for NIS inspections, and in association with both reactive and proactive IWC performance testing, are available (e.g., Morrisey et al., 2015; ACT/MERC, 2019, ACT/MERC, 2020; Growcott et al., 2019; Georgiades and Kluza, 2020; Tamburri et al., 2020a). While these approaches vary to some extent, they all require a sufficient level of biofouling sampling and analysis that will produce the statistically sound and predictive data needed for meaningful IWC system assessment. While a balance between testing practicability (in particular, time and cost) and comprehensive data for IWC approval is needed, the use of qualitative estimates of biofouling (e.g., inconsistent, non-numerical video assessments) as part of IWC system approval is ineffective and discouraged.
Ship biofouling is an open system, with live organisms and coating biocides directly exposed to local waters. Nevertheless, IWC activities can exacerbate the release of harmful material to the environment through: (a) the lack of, or ineffective, capture of debris at the cleaning unit/vessel interface, (b) cleaning operations related disturbances (e.g., support boat movement, diver or ROV movement, umbilical or hose management), and (c) incomplete/ineffective processing and disposal of captured debris (if attempted) (Figure 1). Therefore, to identify measurable environmental impacts, if any, verification testing of IWC systems should include appropriate water sampling and analysis at all points critical for potential harmful material release, and for statistical comparisons to be made against ambient water not influenced by IWC (e.g., ACT/MERC, 2019,ACT/MERC, 2020; Tamburri et al., 2020a; Jones and McClary, 2021).
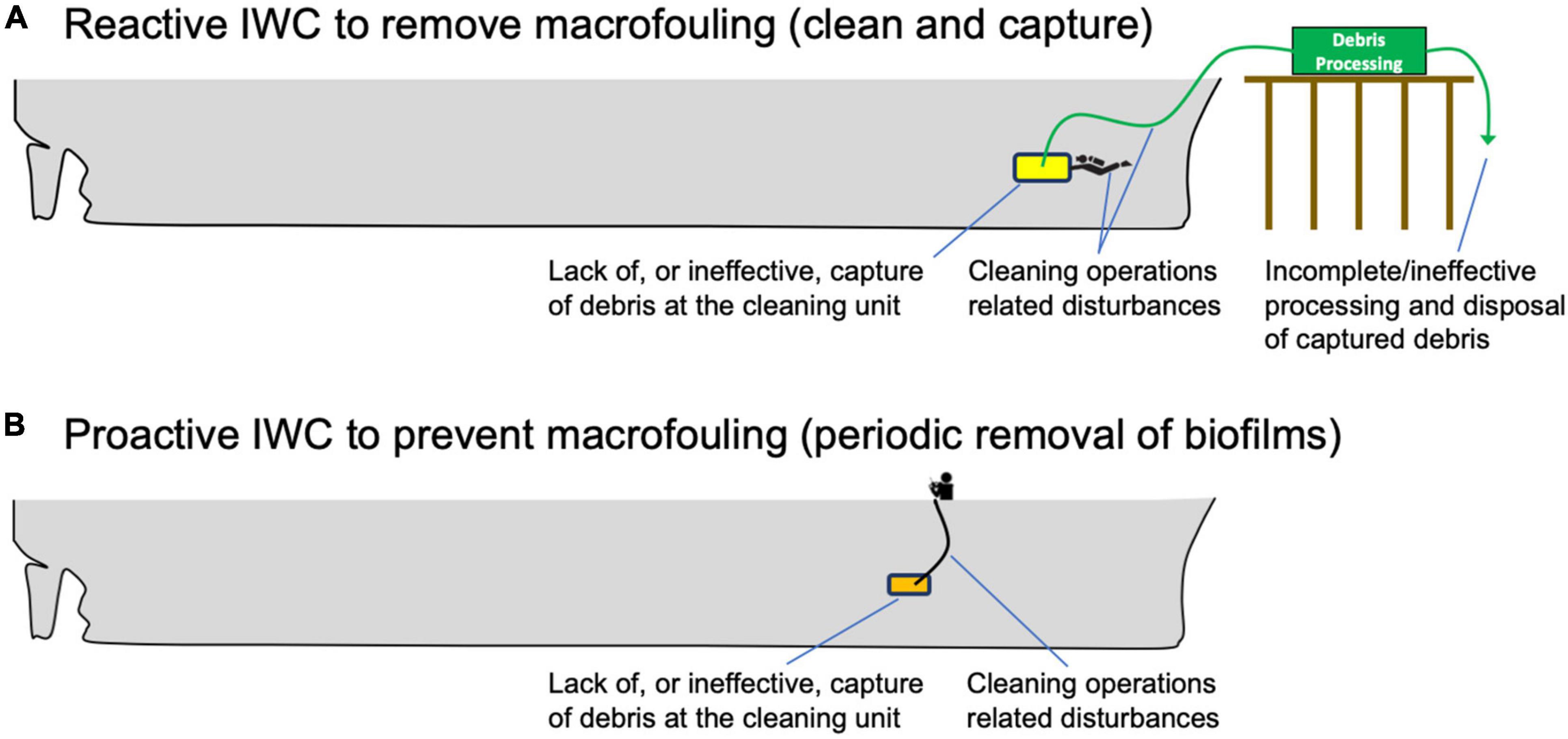
Figure 1. Possible sources for the release of harmful material during ship (A) reactive and (B) proactive in-water cleaning (IWC).
It has been demonstrated that water quality sampling and analyses targeting both chemical contaminants of concern (e.g., copper and/or zinc when cleaning a biocidal coating) and total suspended solids (TSS, as a proxy for particulate debris, including macrofouling organisms) is a feasible and effective approach (e.g., Tamburri et al., 2020a). Importantly, both the particulate and dissolved forms of coating biocides should be assessed to enable a complete understanding of environmental impacts (e.g., Tamburri et al., 2020a; Jones and McClary, 2021). Examination of possible chemical contamination during IWC should only be an optional consideration for non-biocidal coatings.
Visual approaches to detect the release of harmful material (e.g., visible plumes) during IWC are also under consideration. While such observations can help to identify areas of concern, they are subjective, qualitative estimates that are highly influenced by environmental conditions (e.g., sea state, water clarity/visibility, amount and angle of light, distance of observations). Visual observations of debris capture or release should, therefore, only be considered supplementary information, and not as approval criteria.
Determination of measurable environmental impacts as a result of IWC system use avoids the drawbacks associated with requiring a percent reduction in levels of potentially harmful materials. IWC performance standards based on percent reductions (whether in biofouling removal or in debris capture and disposal) are inappropriate because quantitative, mass balance measures of: (a) what is removed from a ship’s submerged surface, (b) what is captured, and (c) what is released over the entire cleaning process (e.g., from cleaning unit to shore processing waste disposal and discharge) would be required but not currently (nor ever likely to be) practical or feasible. Furthermore, while arbitrary criteria of 90, 95, or 99% reductions would clearly decrease risks, if initial biofouling levels or debris loads are high, particularly in the case of larger vessels, even > 99% capture and removal can lead to considerable discharges of harmful material, which may significantly impact local waters (Figure 2).
The physiological state of dislodged organisms, and their origins, are also being considered as part of biofouling policy and IWC approval. Ideally, effective reactive IWC would release very few, if any, live macrofouling organisms (and their propagules or associated pathogens) into the environment. However, because no technology (especially new and complex technologies) is perfect, interest in requiring the release of only dead, non-viable or local/native organisms would appear reasonable. The problem remains that methods for appropriate levels of species identification, and for definitive determination of live vs. dead and/or viable vs. non-viable (including propagules and tissue fragments but excluding residual baseplates or basal shell material remaining after reactive IWC) are in dispute and often challenging and error-prone (e.g., Zaiko et al., 2016; Blatchley et al., 2018). However, where IWC systems are required to minimize discharges of the dissolved and particulate components of biocidal coatings to appropriate levels, or to treat/disinfect debris to prevent the release of possible pathogens, this secondary processing of debris will likely have a similar effect on macrofouling organisms and their propagules (Scianni and Georgiades, 2019; Georgiades et al., 2021).
Emphasis on proactive IWC and “clean-before-you-leave” approaches may eliminate many of the organism-based concerns, compared to reactive IWC (Department of Agriculture [DOA] et al., 2015; Scianni and Georgiades, 2019; Georgiades et al., 2021). However, to date, no proactive IWC system has been independently assessed for efficacy and environmental safety. The applicability of clean-before-you-leave is also dependent on: (a) the vessel’s prior itinerary, (b) NIS and pathogen status of areas previously visited since last dry-docking or cleaning event and the recipient area, and (c) proximity to high-value areas (Department of Agriculture [DOA] et al., 2015; Georgiades et al., 2021). While not insurmountable, it requires authorities to determine the boundaries for what would be considered “local/regional” biofouling (Department of Agriculture [DOA] et al., 2015; DAWE, 2021) noting that the larger the area, the less protective it will be in terms of NIS spread (Outinen et al., 2021). This determination should be underpinned by suitable knowledge of the presence/absence of macro-organisms and pathogens (e.g., surveillance programs; Sim-Smith and Diggles, 2019; Woods et al., 2019), while also recognizing that NIS continue to occur and their distributions constantly shift (Bailey et al., 2020). Further, authorities should continue to be mindful of the potential for chemical release associated with all IWC activities and potential for AFS damage (Scianni and Georgiades, 2019; Tamburri et al., 2020a).
Finally, all current IWC operations are conducted by divers or ROV pilots (with support staff). Therefore, any IWC approval should also explicitly consider both operator safety and proficiency. It is recommended that, at minimum, standards be developed for required levels of diver and ROV pilot training, expertise, and experience to ensure consistent and effective IWC.
Conclusion
Independent, transparent, and predictive verification testing of IWC systems is fundamental to regulatory success of emerging biofouling policies. Performance criteria required for IWC approval should focus on the most environmentally protective variables, including presence or absence of macro-organisms (irrespective of species origins or physiological state), and measurable impacts to local water quality (as opposed to percent reductions in the release of debris). Equally important is the need to measure the selected performance criteria with scientifically and statistically sound, quantitative methods that provide regulatory agencies, approval bodies, ship owners/operators, and the public with the confidence needed that IWC of ship biofouling is safe and effective.
Author Contributions
MT led the overall project design, execution, and drafting of this manuscript. EG, CS, MF, GR, and CJ contributed to information, expertise and insight, and assisted in drafting various sections of the publication. All authors contributed to the article and approved the submitted version.
Funding
This effort was funded by the US Department of Transportation Maritime Administration and Maryland Department of Transportation’s Port Administration, through the University of Maryland Center for Environmental Science, as a precursor to an ACT/MERC international workshop on developing science-based, IWC system verification guidelines.
Author Disclaimer
The information contained within the manuscript reflects the views of the authors only.
Conflict of Interest
The authors declare that the research was conducted in the absence of any commercial or financial relationships that could be construed as a potential conflict of interest.
Publisher’s Note
All claims expressed in this article are solely those of the authors and do not necessarily represent those of their affiliated organizations, or those of the publisher, the editors and the reviewers. Any product that may be evaluated in this article, or claim that may be made by its manufacturer, is not guaranteed or endorsed by the publisher.
References
ACT/MERC (2019). Evaluation of Subsea Global Solutions in-Water Cleaning and Capture Technology for Ships. Baltimore, MD: ACT/MERC, ACT/MERC IWCC Evaluation Report ER01-19. Available online at: www.maritime-enviro.org/Downloads/Reports/Other_Publications/ACT_MERC_SGS_IWCC _Evaluation_Report.pdf (accessed September, 2021).
ACT/MERC (2020). Test Plan for the Evaluations of the Jotun HullSkater: A Proactive Biofouling in-Water Cleaning Solution. ACT/MERC Test Plan EP01-20. Available online at: https://www.maritime-enviro.org/Downloads/ Other_Publications/ACT_MERC_Jotun_Proactive_IWC_Test_Plan_ 5Aug2020_signed.pdf (accessed September, 2021).
Altarriba, E., and Halonen, J. (2019). “Onboard measurements to verify biofouling effect on ship performance,” in Proceedings of the 18th International Congress of the Maritime Association of the Mediterranean (IMAM 2019), eds P. Georgiev and C. Guedes Soares (Varna: CRC Press), doi: 10.1201/9780367810085
Bailey, S. A., Brown, L., Campbell, M. L., Canning-Clode, J., Carlton, J. T., Castro, N., et al. (2020). Trends in the detection of aquatic non-indigenous species across global marine, estuarine and freshwater ecosystems: a 50-year perspective. Divers. Distrib. 26, 1780–1797. doi: 10.1111/ddi.13167
Bedson, P., and Sargent, M. (1996). The development and application of guidance on equipment qualification of analytical instruments. Accredit. Qual. Assur. 1, 265–274. doi: 10.1007/s007690050083
Bell, A., Philipps, S., Denny, C., Georgiades, E., and Kluza, D. (2011). Risk Analysis: Vessel Biofouling. Wellington: Ministry of Agriculture and Forestry Biosecurity.
BIMCO (2013). Hull Fouling Clause for Time Charter Parties 2013. Available online at: https://www.bimco.org/contracts-and-clauses/bimco-clauses/earlier/hull_fouling_clause_for_time_charter_parties_2013 (accessed November, 2021).
BIMCO (2019). Hull Fouling Clause for Time Charter Parties 2019. Available online at: https://www.bimco.org/contracts-and-clauses/bimco-clauses/current/hull_fouling_clause_for_time_charter_parties_2019 (accessed November, 2021).
BIMCO/ICS (2021a). Industry Standard on in-Water Cleaning With Capture. V1.01. Available online at: www.bimco.org/-/media/bimco/ships-ports-and-voyage-planning/environment-protection/biofouling/2021-industry-standard-in-water-cleaning-final.ashx?rev=7e4ea382b7864d59951bf53f6595e4b2 (accessed September, 2021).
BIMCO/ICS (2021b). Approval Procedure for in-Water Cleaning Companies. Available online at: www.bimco.org/-/media/bimco/ships-ports-and-voyage-planning/environment-protection/biofouling/2021-approval-procedure-in-water-cleaning-final.ashx?rev=8656cf3a826149ccb3c72f9e06649076 (accessed September, 2021).
Blatchley, E. R. III, Cullen, J. J., Petri, B., Bircher, K., and Welschmeyer, N. (2018). The biological basis for ballast water performance standards: “Viable/Non-viable” or “Live/Dead”. Environ. Sci. Technol. 52, 8075–8086. doi: 10.1021/acs.est.8b00341
California Code of Regulations (2017). Biofouling Management to Minimize the Transfer of Nonindigenous Species from Vessels Arriving at California Ports. California, CA: California Code of Regulations.
Coutts, A. D., and Taylor, M. D. (2004). A preliminary investigation of biosecurity risks associated with biofouling on merchant vessels in New Zealand. N. Z. J. Mar. Freshwater Res. 38, 215–229.
Coutts, A. D. M., Piola, R. F., Hewitt, C. L., Connell, S. D., and Gardner, J. P. A. (2010). Effect of vessel voyage speed on survival of biofouling organisms: implications for translocation of non-indigenous marine species. Biofouling 26, 1–13. doi: 10.1080/08927010903174599
Dafforn, K. A., Lewis, J. A., and Johnston, E. L. (2011). Antifouling strategies: history and regulation, ecological impacts and mitigation. Mar. Pollut. Bull. 62, 453–465. doi: 10.1016/j.marpolbul.2011.01.012
Davidson, I., Scianni, C., Hewitt, C., Everett, R., Holm, E., Tamburri, M., et al. (2016). Mini-review: assessing the drivers of ship biofouling management–aligning industry and biosecurity goals. Biofouling 32, 411–428. doi: 10.1080/08927014.2016.1149572
Davidson, I. C., Brown, C. W., Sytsma, M. D., and Ruiz, G. M. (2009). The role of containerships as transfer mechanisms of marine biofouling species. Biofouling 25, 645–655. doi: 10.1080/08927010903046268
Davidson, I. C., Scianni, C., Minton, M. S., and Ruiz, G. M. (2018). A history of ship specialization and consequences for marine invasions, management, and policy. J. Appl. Ecol. 55, 1799–1811. doi: 10.1111/1365-2664.13114
Davidson, I. C., Smith, G., Ashton, G. V., Ruiz, G. M., and Scianni, C. (2020). An experimental test of stationary lay-up periods and simulated transit on biofouling accumulation and transfer on ships. Biofouling 36, 455–466. doi: 10.1080/08927014.2020.1769612
DAWE (2021). Australian in-Water Cleaning Standards: Consultation Draft. Canberra, ACT: Department of Agriculture, Water and the Environment.
Department of Agriculture [DOA], Department of the Environment [DOE], and New Zealand Ministry for Primary Industries [MPI] (2015). Antifouling and in-Water Cleaning Guidelines. Canberra, ACT: Department of Agriculture.
Georgiades, E., Growcott, A., and Kluza, D. (2018). Technical Guidance on Biofouling Management for Vessels Arriving to New Zealand. MPI Technical Paper No: 2018/07. Wellington: Ministry for Primary Industries.
Georgiades, E., Kluza, D., Bates, T., Lubarsky, K., Brunton, J., Growcott, A., et al. (2020). Regulating vessel biofouling to support New Zealand’s marine biosecurity system - Ű A blue print for evidence-based decision making. Front. Mar. Sci. 7:390. doi: 10.3389/fmars.2020.00390
Georgiades, E., and Kluza, D. (2017). Evidence-based decision making to underpin the thresholds in New Zealand’s CRMS: biofouling on vessels arriving to New Zealand. J. Mar. Sci. Technol. 51, 76–88. doi: 10.4031/mtsj.51.2.5
Georgiades, E., and Kluza, D. (2020). Technical Advice: Conduct of In-Water Biofouling Surveys for Domestic Vessels. Biosecurity New Zealand Technical Paper No: 2020/04. Wellington: Ministry for Primary Industries.
Georgiades, E., Scianni, C., Davidson, I., Tamburri, M. N., First, M. R., Ruiz, G., et al. (2021). The role of vessel biofouling in the translocation of marine pathogens: management considerations and challenges. Front. Mar. Sci. 8:660125. doi: 10.3389/fmars.2021.660125
Grosholz, E. (2002). Ecological and evolutionary consequences of coastal invasions. Trends Ecol. Evol. 17, 22–27. doi: 10.1016/50169-5347(01)02358-8
Growcott, A., Kluza, D., and Georgiades, E. (2019). Technical Advice: Evaluation of in-Water Systems to Reactively Treat or Remove Biofouling within Vessel Internal Niche Areas. MPI Technical Paper No: 2019/02. Wellington: Ministry for Primary Industries.
Hewitt, C. L., and Campbell, M. L. (2010). The Relative Contribution of Vectors to the Introduction and Translocation of Invasive Marine Species. Canberra ACT: Commissioned by the Department of Agriculture, Fisheries and Forestry.
Hewitt, C. L., Campbell, M. L., Thresher, R. E., Martin, R. B., Boyd, S., Cohen, B. F., et al. (2004). Introduced and cryptogenic species in Port Phillip Bay, Victoria, Australia. Mar. Biol. 144, 183–202. doi: 10.1007/s00227-003-1173-x
IMO (2001). International Convention on the Control of harmful Anti-fouling Systems on Ships. London: International Maritime Organization.
IMO (2011). Guidelines for the Control and Management of Ships’ Biofouling to Minimize the Transfer of Invasive Aquatic Species. London: International Maritime Organization.
Inglis, G. J., Floerl, O., Ahyong, S., Cox, S., Unwin, M., Ponder-Sutton, A., et al. (2010). The Biosecurity Risks Associated with Biofouling on International Vessels Arriving in New Zealand: Summary of the Patterns and Predictors of Fouling. Prepared for Ministry of Agriculture Biosecurity New Zealand. Wellington: Ministry of Agriculture Biosecurity New Zealand.
Jones, D. F. (1999). Controlling Marine Fouling with Antifouling Paints and Underwater Hull Cleaning. PCE November 1999. Pittsburgh, PA: Technology Publishing Company.
Jones, E., and McClary, D. (2021). Summary – Testing of Reactive In-Water Cleaning Systems for Removal of Vessel Biofouling. Biosecurity New Zealand Technical Paper No: 2021/11. Wellington: Biosecurity New Zealand.
Lewis, J. A. (2013). In-Water Hull Cleaning and Filtration System: In-Water Cleaning Trials – 26–28 November 2012. Available online at: www.fish.wa.gov.au/Documents/occasional_publications/fop114.pdf (accessed September, 2021).
Li, Y., and Ning, C. (2019). Latest research progress of marine microbiological corrosion and bio-fouling, and new approaches of marine anti-corrosion and anti-fouling. Bioact. Mater. 4, 189–195. doi: 10.1016/j.bioactmat.2019.04.003
MARPOL 73/78 (1997). Annex IV, Prevention of Air Pollution from Ships. International Convention for the Prevention of Pollution from Ships (MARPOL). London: International Maritime Organization.
McClay, T., Zabin, C., Davidson, I., Young, R., and Elam, D. (2015). Vessel Biofouling Prevention and Management Options Report. Available online at: https://apps.dtic.mil/dtic/tr/fulltext/u2/a626612.pdf (accessed September, 2021).
Ministry of Agriculture and Forestry (2011). Draft Anti-Fouling and in-Water Cleaning Guidelines. MAF Discussion Paper no.: 2011/13. Wellington: Ministry for Agriculture and Forestry.
Morrisey, D., Gadd, J., Page, M., Lewis, J., Bell, A., and Georgiades, E. (2013). In-Water Cleaning of Vessels: Biosecurity and Chemical Contamination Risks. New Zealand Ministry for Primary Industries Technical Paper No. 2013/11. Wellington: Ministry for Primary Industries.
Morrisey, D., Inglis, G., Tait, L., Woods, C., Lewis, J., and Georgiades, E. (2015). Procedures for Evaluating in-Water Systems to Remove or Treat Vessel Biofouling. MPI Technical Paper No: 2015/39. Wellington: Ministry for Primary Industries.
Morrisey, D., and Woods, C. (2015). In-Water Cleaning Technologies: Review of Information. MPI Technical Paper No: 2015/38. Wellington: Ministry for Primary Industries.
Moser, C. S., Wier, T. P., First, M. R., Grant, J. F., Riley, S. C., Robbins-Wamsley, S. H., et al. (2017). Quantifying the extent of niche areas in the global fleet of commercial ships: the potential for “super-hot spots” of biofouling. Biol. Invasion 19, 1745–1759. doi: 10.1007/s10530-017-1386-4
MPI (2018). Craft Risk Management Standard: Biofouling on Vessels Arriving to New Zealand. CRMS-BIOFOUL. Wellington: Ministry for Primary Industries.
Outinen, O., Baily, S. A., Broeg, K., Chasse, J., Clarke, S., Daigle, R. M., et al. (2021). Exceptions and exemptions under the Ballast Water Management Convention: sustainable alternatives for ballast water management? J. Environ. Manag. 293:112832. doi: 10.1016/j.jenvman.2021.112823
Ruiz, G. M., Carlton, J. T., Grosholz, E. D., and Hines, A. H. (1997). Global invasions of marine and estuarine habitats by nonindigenous species: mechanisms, extent, and consequences. Am. Zool. 37, 621–632. doi: 10.1093/icb/37.6.621
Ruiz, G. M., Fofonoff, P. W., Steves, B. P., and Carlton, J. T. (2015). Invasion history and vector dynamics in coastal marine ecosystems: a North American perspective. Aquat. Ecosyst. Health Manag. 18, 299–311. doi: 10.1080/14634988.2015.1027534
Schultz, M. P. (2007). Effects of coating roughness and biofouling on ship resistance and powering. Biofouling 23, 331–341. doi: 10.1080/08927010701461974
Schultz, M. P., Bendick, J. A., Holm, E. R., and Hertel, W. M. (2011). Economic impact of biofouling on a naval surface ship. Biofouling 27, 87–98. doi: 10.1080/08927014.2010.542809
Scianni, C., Falkner, M., and DeBruyckere, L. (2017). Biofouling in the U.S. Pacific States and British Columbia. Coastal Commission of the Western Regional Panel on Aquatic Nuisance Species. Available online at: http://westernregionalpanel.org/wp-content/uploads/2019/11/Final-CC-Biofouling-White-Paper-11Apr17.pdf (accessed September, 2021).
Scianni, C., and Georgiades, E. (2019). Vessel in-water cleaning or treatment: identification of environmental risks and science needs for evidence-based decision making. Front. Mar. Sci. 6:467. doi: 10.3389/fmars.2019.00467
Scianni, C., Lubarsky, K., Ceballos-Osuna, L., and Bates, T. (2021). Yes, we CANZ: initial compliance and lessons learned from regulating vessel biofouling management in California and New Zealand. Manag. Biol. Invasions 12, 727–746. doi: 10.3391/mbi.2021.12.3.14
Sim-Smith, C., and Diggles, B. (2019). Review of International Aquatic Health Inspection and Surveillance Programmes. MPI Technical Paper No: 2019/05. Prepared for Ministry for Primary Industries, New Zealand. Available online at: www.mpi.govt.nz/dmsdocument/33600-Review-of-International-Aquatic-Health-Inspection-and-Surveillance-Programmes (accessed September, 2021)
Sonak, S., Pangam, P., Giriyan, A., and Hawaldar, K. (2009). Implications of the ban on organotins for protection of global coastal and marine ecology. J. Environ. Manag. 90, 96–108. doi: 10.1016/j.envman.2008.08.017
Tamburri, M. N., Bailey, S. A., Everett, R. A., First, M. R., Gollasch, S., Outinen, O., et al. (2020b). Protocol for the verification of ballast water compliance monitoring devices. ICES techniques in marine. Environ. Sci. 63:13. doi: 10.17895/ices.pub.5465
Tamburri, M. N., Davidson, I. C., First, M. R., Scianni, C., Newcomer, K., Inglis, G. J., et al. (2020a). In-water cleaning and capture to remove ship biofouling: an initial evaluation of efficacy and environmental safety. Front. Mar. Sci. 7:437. doi: 10.3389/fmars.2020.00437
Townsin, R. L., Byrne, D., Svensen, T. E., and Milne, A. (1981). Estimating the technical and economicpenalties of hull and propeller roughness. Trans. SNAME 89, 295–318. doi: 10.1080/08927014.2021.1890044
Transport Canada (2021). Draft Voluntary Guidance for Relevant Authorities on In-water Cleaning of Vessels for Consultation. Available online at: https://tc.canada.ca/en/corporate-services/consultations/consultation-draft-voluntary-guidance-relevant-authorities-water-cleaning-vessels (accessed September, 2021).
Tribou, M., and Swain, G. (2010). The use of proactive in-water grooming to improve the performance of ship hull antifouling coatings. Biofouling 26, 47–56. doi: 10.1080/08927010903290973
UNCTAD (2018). 50 Years of Review of Maritime Transportation, 1968-2018: Reflecting on the Past, Exploring the Future. United Nations Transport and Trade Facilitation Series No.11. Geneva: UNCTAD.
USEPA (2013). Vessel General Permit for Discharges Incidental to the Normal Operation of a Vessel. Available online at: www3.epa.gov/npdes/pubs/vgp_permit2013.pdf (accessed September, 2021).
USEPA ETV (2010). Generic Protocol for the Verification of Ballast Water Treatment Technology, Version 5.1, EPA/600/R-10/146. Washington, DC: US Environmental Protection Agency.
Woods, C., Seaward, K., Pryor Rodgers, L., Buckthought, D., Carter, M., Lyon, W., et al. (2019). Marine High Risk Site Surveillance. Annual Synopsis Report for All High Risk Sites 2018-2019. Biosecurity New Zealand Technical Paper No. 2019/31. Wellington: Ministry for Primary Industries.
Woods Hole Oceanographic Institute (1952). Marine Fouling and its Prevention. Contribution No. 580 from Woods Hole Oceanographic Institute. Annapolis, MD: United States Naval Institute.
Keywords: ship biofouling, in-water cleaning, non-indigenous species, environmental regulations, verification testing
Citation: Tamburri MN, Georgiades ET, Scianni C, First MR, Ruiz GM and Junemann CE (2021) Technical Considerations for Development of Policy and Approvals for In-Water Cleaning of Ship Biofouling. Front. Mar. Sci. 8:804766. doi: 10.3389/fmars.2021.804766
Received: 01 November 2021; Accepted: 22 November 2021;
Published: 15 December 2021.
Edited by:
Wei-Bo Chen, National Science and Technology Center for Disaster Reduction (NCDR), TaiwanReviewed by:
Lena Granhag, Chalmers University of Technology, SwedenLida Teneva, Independent Researcher, Sacramento, CA, United States
Copyright © 2021 Tamburri, Georgiades, Scianni, First, Ruiz and Junemann. This is an open-access article distributed under the terms of the Creative Commons Attribution License (CC BY). The use, distribution or reproduction in other forums is permitted, provided the original author(s) and the copyright owner(s) are credited and that the original publication in this journal is cited, in accordance with accepted academic practice. No use, distribution or reproduction is permitted which does not comply with these terms.
*Correspondence: Mario N. Tamburri, dGFtYnVycmlAdW1jZXMuZWR1