- 1College of Life Science, Qingdao Agricultural University, Qingdao, China
- 2Key Laboratory of Experimental Marine Biology, Institute of Oceanology, Chinese Academy of Sciences (IOCAS), Qingdao, China
- 3Laboratory for Marine Biology and Biotechnology, Pilot National Laboratory for Marine Science and Technology, Qingdao, China
- 4Center for Ocean Mega-Science, Chinese Academy of Sciences, Qingdao, China
The upregulation of heat shock protein 70 (hsp70) gene under high temperature stress is a common phenomenon. Although heat shock protein-mediated stress responses play an important role in intertidal Neopyropia yezoensis, the detailed regulatory mechanism of the hsp70 gene expression is still unclear. Here, a full-length sequence of the hsp70-2 gene was cloned and its’ expression regulation was analyzed. There was an activating transcription factors element (ATFE) of cAMP-dependent protein kinase (PKA) was found at the gene promoter region and a highly conserved deduced amino acid sequence with calmodulin-binding activity was detected. Reagents implicated in the induction of the hsp70 gene were then selected to treat the algal samples at 24°C, and the photosynthetic parameters, transcription and translation of this gene were determined. Results showed that quercetin inhibited the transcription of the hsp70-2 gene, significantly decreased the synthesis of the HSP70-2 protein, and lowered the photosynthetic activity of N. yezoensis under high temperature stress conditions. Although the addition of trifluoperazine (TFP), an inhibitor of calmodulin (CAM), downregulated the photosynthetic parameters, the transcription of the hsp70-2 gene was not influenced at high temperature treatment, implying that CAM was not involved in the transcription of the hsp70-2 gene but involved in the heat stress reponding pathways. 5′-aminoimidasole-4-carboxamide-1-β-d-ribofuranoside (AICAR) promoted the synthesis of hsp70-2 transcripts significantly and HSP70-2 protein slightly, which indicated that AMPK regulated the induction of the hsp70-2 gene in N. yezoensis. Forskolin also promoted the upregulation of the hsp70-2 gene. Thus, both AMPK and cAMP-dependent protein kinase (PKA) could phosphorylate HSF and activate the expression of the hsp70-2 gene in N. yezoensis. However, there was no strict correlation between transcripts of the hsp70-2 gene and HSP70-2 protein contents. It was proposed that the posttranscriptional mechanisms were involved in regulating the synthesis of the HSP70-2 protein. Which allowed it to adapt to the extremely variable intertidal environments with an instant responding manner, since the accumulated transcripts could be translated rapidly when needed.
Introduction
Temperature is one of the most important factors affecting plant metabolism and growth. High temperature can cause stress or even harm to plants, and in the long-term evolutionary process, plants have developed a series of mechanisms to sense and respond to high temperature stress (Larkindale and Vierling, 2008). Among them, the upregulation of heat shock protein (HSP) is considered as a common biological phenomenon in different organisms. When cells are stressed with heat, some proteins will lose their original folding state, resulting in loss of function, which leads to the induced expression of hsp genes. HSPs are usually accumulated at 30 minutes to 2 hours after stress (Sung et al., 2001). The most important function of induced HSP is as a molecular chaperone to participate in protein folding, advanced assembly and degradation of misfolding or aggregation protein, which contributes to the maintenance of intracellular homeostasis under appropriate and adverse conditions (Wang et al., 2004). hsp70 is one of the most conserved, abundant and widely studied HSPs in the HSP family (Cottin et al., 2015). And all hsp70 genes are also distinguished with constitutive and inducible in most organisms (Lindquist and Craig, 1988).
With the progress of research, more and more results showed that the expression of hsp70 gene was also induced by dry/water stress, cold, heavy metal ions, ultraviolet light and ABA (Sun et al., 2002), and the content of hsp70 in plants was significantly up-regulated. The increase of hsp70 synthesis significantly improves the stress tolerance of cell (Rizhsky et al., 2002; Cho and Hong, 2006; Cho and Choi, 2009; Zou et al., 2009). Murtha and Keller (2003) pointed out that hsp70 played a role in self-balance maintaining in cells against various physiological and environmental stresses. HSP protein can also play a function by inhibiting intracellular and extracellular signal pathways (Chatterjee and Burns, 2017), improve the tolerance of cells to environmental stress and restore cells or organisms from various stress states (Ellis and Hartl, 1996). Montero-Barrientos et al. (2010) transferred the hsp70 gene of Trichoderma harzianum into Arabidopsis, which effectively improved the tolerance of transgenic Arabidopsis to abiotic stresses such as high salt and high temperature.
Then, the heat shock protein transcription factor (HSF) has been proved to be a key molecule in induction of hsp70 gene expression. The HSF protein is relatively conservative, and its typical structural feature is the N-terminal highly structured DNA binding domain (DBD), which is responsible for identifying and locating heat stress elements in the target promoter (Scharf et al., 1990). Under normal conditions, HSF combines with HSP70 and is in an inactive dormant state. Under heat shock or other stress conditions, the content of misfolded proteins in cells increases, which caused dissociation of HSP70 from HSF and combined to the misfolded proteins. The released HSF is phosphorylated and combined to the heat shock element (HSE) to initiate the expression of hsp70 gene. Phosphorylated HSF can promote the transcription of hsp gene and the efficient translation of mRNA, producing a large number of HSPs (Schuetz et al., 1991). Sun et al. (2000) proposed another HSP signal transduction pathway model involving Ca2+-CAM. Under normal conditions, HSF remains inactivated by binding to cytoplasmic HSP70 (or HSP90). Heat stress leads to the increase of intracellular Ca2+ concentration, which directly activates CAM or promotes the expression of CaM. Increase of Ca2+-CAM concentration enhances the binding between CAM and cytoplasmic HSP70, resulting in the release of HSF from HSF-HSP70 complex. The binding of free HSF to HSE activates the transcription of hsp70 gene. There is evidence that the activated expression of HSP70 is related to the phosphorylation of HSF factor (Schuetz et al., 1991). Choi et al. (1991) found that the upregulation of reporter gene after heat shock protein promoter by using cAMP promoter of forskolin. It thus seems to be closely related to cAMP action and the expression of hsp70 gene. Researchers screened proteins that can interact with HSF in cells and found that HSF binds to the catalytic subunit of protein kinase A (PKA) and is then phosphorylated (Xu et al., 2007).
In recent years, there are lots of investigations on heat shock response in macroalgae. For example, high temperature can induce the up regulation of hsp70 gene in Ulva lactuca (Zhang et al., 2012). And the expression of Gracilaria lemaneiformis hsp70 is directly related to its ability to resist high temperature (Gu et al., 2012). High temperature stress and plant hormones can also induce the up-regulation of hsp70 gene expression (Lv et al., 2019). The hsp70 gene in umbilical laver was highly expressed under short-term high temperature stress and decreased slowly during subsequent recovery (Reith and Munholland, 1991). After 15 minutes of heat shock at 35°C, the expression of hsp70 gene increased 15 times in Porphyra haitanensis, and a heat shock response element was identified upstream of its promoter (Liu et al., 2012). Sun et al. (2015) screened a number of genes related to temperature stress response from N. yezoensis using digital gene expression profiling technology, and proposed that the high level of hsp70 gene mRNA in cells is one of the bases for its’ adaptation to the intertidal habitats. Zheng et al. (2020) found that under the conditions of inhibition of CAM or calcium channel, the photosynthetic activity of P. haitanensis was not affected at normal temperature, but decreased significantly under high temperature of 32 °C. The expression of hsp70 was also down regulated under the combination of high temperature and inhibitor of CAM or calcium. While, how does N. yezoensis perceives high temperature and activate the intracellular stress responding pathway? Does hsp70 gene also transcribe rapidly under heat shock conditions and then decrease slowly? Is the upregulation of hsp70 gene closely related to the calcium signaling and HSF phosphorylation? And what belongs to the specific kinase molecule involved in the activation of HSF? All above issues are the key details of hsp70 gene responding to heat shock stress in N. yezoensis.
N. yezoensis belongs to a large economic seaweed in the world. The annual total output value of it in China exceeds 5 billion yuan and the species is widely cultivated in the coastal areas of Jiangsu and Shandong Province (Lu et al., 2016). Under the conditions of global warming, the temperature in the cultivated sea area continues to rise, which affects the normal growth and metabolism of N. yezoensis, resulting in the decrease of both quantity and quality, even the occurrence of large-scale disease disaster. Here, different inhibitors implicated in the induction of hsp70 gene were used to treat N. yezoensis under high temperature. And the expression of hsp70-2 gene under different treatment times were determined. Then, the contents of HSP70-2 were detected. The molecular mechanism of induction of hsp70 gene was analyzed. This will provide a better understanding of the special role of HSP70-2 protein in intertidal algae and lay a solid foundation for the development of accurate molecular breeding strategies for the cultivation of high temperature resistant lines of N. yezoensis.
Materials and method
Algae materials
Fresh N. yezoensis thalli were collected from an algae culture farm in Jimo area (36° N, 120° E), Shandong Province, China. The ropes attached with algae were cut from the nets cultivated in the sea and put in the incubator, brought back to the laboratory as soon as possible and cultured in fresh seawater with PES medium at 15°C. The medium was constantly filled with air and replaced every day. During the temporary culture before treatments, the light intensity was maintained at 50 μmol photons m-2 s-1 in a 12-h light/12-h dark cycle.
Preparation of stock chemical solutions
The inhibitor of HSF, 1 ml Quercetin stock solution with a concentration of 150 μM was prepared with 45.3 mg quercetin powder and 1ml DMSO and stored at -20°C. The inhibitor of CAM stock solution was made by 3.26 mg trifluoperazine (TFP) powder and 1ml 75% ethanol and was stored at -20°C. Then, 1 mg powder pifithrin-μ was dissolved in 5.5 mL anhydrous ethanol to prepare a 1 μM stock inhibitor solution of HSP70. And the stock solution was stored at -80°C immediately. The promoter of PKA was prepared with 4 mg forskolin powder and 1.04 ml DMSO and the 10 mM stock solution was prepared. All the above stock solution was diluted 1000 times when it was used in the stress treatment experiment. A mount of 20 mg 5′-aminoimidasole-4-carboxamide-1-β-d-ribofuranoside (AICAR) powder was directly dissolved in 387.5 ml seawater to prepare the 0.2 mM working solution of AMP related phosphokinase (AMPK) promotor to initiate the experiment.
High temperature stress treatments under different chemicals
Firstly, the fresh seaweed samples were treated in 120‰ salinity seawater at 15°C for 0.5h. Then, the high salinity treated samples were recovered in normal seawater containing one of the inhibitors above mentioned and incubated for 2h at 15°C under 50 μmol photons m–2 s–1 of artificial light. The control group was just recovered in normal seawater. Then, the recovered N. yezoensis with the special inhibitor was put into the flasks filled with the corresponding chemical at 24°C and incubated for another 2h. During the intervals of 0.5h, 1h and 2h, the photosynthetic parameters of each treatment were determined and the corresponding sample was collected separately, immediately frozen with liquid nitrogen, and stored at -80°C.
Measurement of the photosynthetic parameters
The photosynthetic parameters of the algae under high temperature stress conditions containing the different chemicals were measured using a Dual-PAM-100 measuring system (Heinz Walz, Effeltrich, Germany). The determination procedure was set according to (Xie et al., 2020) and the actinic light at 57 µmol m–2 s–1 was employed. Maximal PS II quantum yield, Fv/Fm, the effective quantum yield Y(II), and the quantum yield of non-regulated energy dissipation in PS II [Y(NO)] were automatically calculated by the software provided. All samples were dark adapted for 10 min at room temperature before determining the chlorophyll fluorescence parameters and each sample was tested three replications.
Sequence analysis of the hsp70-2 gene
The DNA fragment of inducible hsp70 gene which was annotated as hsp70-2 was obtained from the previous transcriptome data, and its homology to other known sequences was analyzed using the BLAST program available at the NCBI website (http://www.ncbi.nlm.nih.gov/blast). Then, the full length of the gene and its’ regulatory sequence before the start codon were down loaded from NCBI and analyzed.
RNA extraction, cDNA synthesis and real-time quantitative PCR assay
The total RNA of each sample was extracted using RNAprep Pure Plant Kit (Tiangen Biotech Co., Beijing, China). The RNA integrity was checked by 1% agarose gel electrophoresis and the concentration and purity of RNA was detected by a NanoPhotometer NP80 Touch (Implen Gmbh, Munich, Germany). Then, the amount of 200 ng of obtained RNA of each sample was reverse transcribed by using Takara reverse transcriptase kit (Takara, Japan).
Based on the previous transcriptome data, a full-length sequence of hsp70-2 gene was obtained. According to the screen results of stable expressed genes under different conditions, 18S ribosomal RNA was used as the internal reference gene (Wang et al., 2014). Primers were designed with primer premier 5 (Premier Biosoft International, Palo Alto, CA, USA) and synthesized by Shenggong (Shanghai, China). After optimization of the amplification conditions by the traditional PCR, real-time quantitative PCR (qRT-PCR) experiment was carried out to detect the expression variations of hsp70-2 gene under high temperature conditions. Meanwhile, in order to investigate the effects of kinases on hsp70-2 expression, different protein kinase genes, including AMPK, PKA, and CaM gene and hsf gene were screened and the transcription analysis under different conditions were conducted. The determination was performed on a StepOne Plus Multicolor Real-Time PCR Detection System (ABI, Foster, CA, USA) using 2 × SYBR Green Master Mix (Roche, Germany). Three parallel replicates were set for each experimental sample. The relative gene expression value was calculated by the 2-ΔΔCt method (Livak and Schmittgen, 2001). The gene names and Primer sequences are shown in Table 1.
Immunoblotting of HSP70 in N. yezoensis
The algae treated with different chemicals were collected after 1h high temperature stress at 24°C and the total protein was prepared. Equal amounts of protein from different treatments, estimated by the BCA method, were separated on a 12% acrylamide gel. Then the proteins were transferred to a PVDF membrane and blocked with 5% fat free milk in TBST for 2h at room temperature. Washed with TBST for 3 times, the membrane was incubated in 1:1000 dilution anti-HSP70 antibody (ABclonal, Wuhan, China) in TBST overnight at 4°C. After washing with TBST, the membranes were incubated again with a 1:2000 dilution anti-rabbit Ig-horseradish peroxidase conjugate secondary antibodies for 2 h at RT. Then the western films were washed 3 times and the protein bands were visualized using 3,3’-diaminobenzidine reagent (Pierce, Rockford, IL, United States). Scanned with a GS-800 Calibrated Imaging Densitometer (Bio-Rad, Hercules, CA, United States), amounts of HSP70-2 in different treatments, defined as the optical density of the band multiplied with its area, were analyzed through Image analysis software Quantity One (Bio-Rad, version 4.6.9). According to the immunoblot band volume, the relative amounts of HSP70-2 protein in different treatments were obtained by the ratio of the values to that of the control.
Protein identification and quantitation by nano UHPLC-QTOF mass spectrometer
Equal amounts of proteins from different treatments as described above were separated using SDS-PAGE method, stained with Coomassie brilliant blue and the bands located near 72kDa in each lane were excised precisely. The proteins contained in those bands were digested respectively with trypsin according to Shevchenko et al. (2006). The prepared digested peptides samples were separated using C18 reverse phase column (Thermo peptide Map RSLC, 75 μm x 50 cm) and tested by an ESI QTOF (Bruker Daltonics, Compact II) system. During the process, linear acetonitrile gradient (from 5% to 35% for 120 min) with a flow rate of 300 nL/min was applied to elute the peptides and eluted peptides were detected in positive mode. The data collected was processed through Bruker Compass DataAnalysis software (v 4.4). Blast and identification of the peptides were performed against the NCBI red algae database (retrieved on 21 Jan, 2019) and the relative quantification of proteins were completed on a Spectrum Mill MS Proteomics Workbench (version A.03.03, Agilent). The variation of HSP70-2 in different samples was compared after normalization of the target protein by global intensity of each sample as described by Griffin et al. (2010). Based on the triplicate assays, the average variation of HSP70-2 in different samples was obtained.
Statistic analysis
All experimental data were derived from three independent measurements ( ± SD). Single factor analysis of variance (ANOVA) in SPSS 23.0 software was used to analyze the data, and significant difference was determined using Duncan’s test (p < 0.05).
Results
Changes in photosynthetic parameters under different chemical treatments
The results of photosynthetic parameters showed no significant variation in the control under high temperature stress from 15°C to 24°C for 2h. Except for the abnormal decrease in Fv/Fm in the sample treated with forskolin before high temperature stress, no significant changes in Y(II) and Y(NO) were found (P > 0.05) during the whole treatment. The fluctuation profiles of the photosynthetic parameters in the samples treated with AICAR and pifithrin-μ were consistent with those of the control. On adding quercetin, Y(II) and Fv/Fm decreased significantly after 0.5h of high temperature stress (P < 0.05) and showed a slight recovery after 1 and 2h, but were also significantly lower than those in control (Figures 1A, B). Similarly, Y(NO) increased to the maximum level, about twice that of the control, after 0.5h of high temperature stress and decreased slightly with the extension of stress time. No significant difference was found in photosynthetic activity between the samples treated with TFP and the control before high temperature treatment (Figure 1C). However, Y(II) and Fv/Fm decreased significantly, and Y(NO) presented a continuous rise during 0.5h to 2h high temperature stress at 24°C.
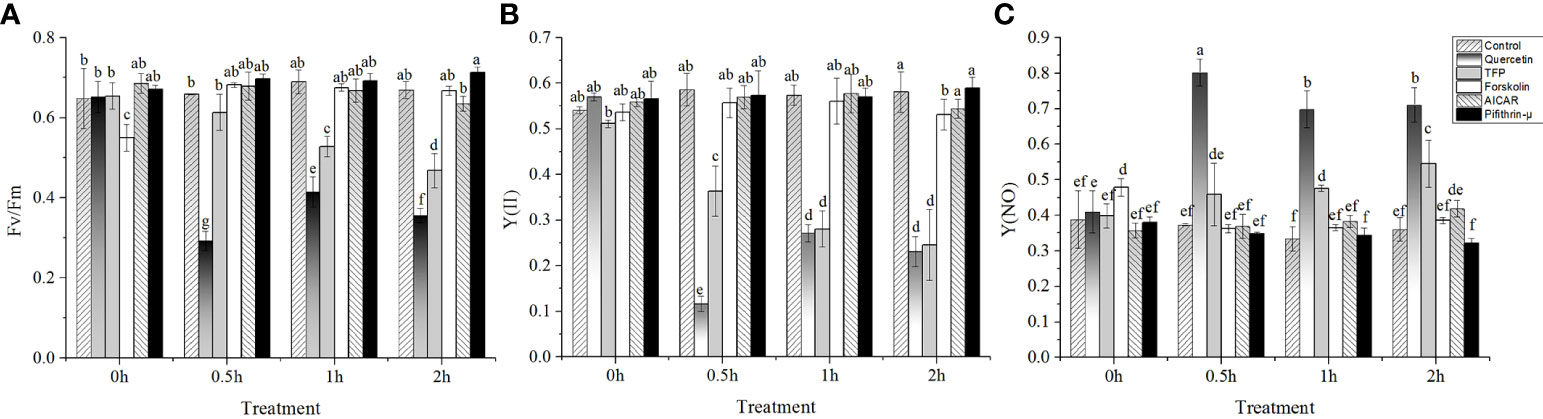
Figure 1 Variations of photosynthetic parameters of N. yezoensis treated with different chemicals under high-temperature stress. (A), the maximal PS II quantum yield (Fv/Fm), (B), the quantum yields of PS II (YII), (C), the quantum yield of non-regulated energy dissipation in PS II [Y(NO)]. The abscissa represented the different sampling time under high temperature (24°C) stress conditions. Each data point was the mean value ± standard deviation (SD) (n=3). Different letters indicated significant differences (P<0.05).
Sequence analysis of inducible hsp70 gene in N. yezoensis
We cloned an hsp70 gene according to the previous transcriptome data. The full-length transcript comprised 2,007 nucleotides and encoded a protein of 668 amino acids. The putative molecular weight was 72.1 kDa, and the protein possessed heat shock 70-kDa protein conserved structure revealed by the basic local alignment search tool (BLAST) through NCBI online analysis (https://www.ncbi.nlm.nih.gov/Structure/cdd/wrpsb.cgi). Moreover, a highly conserved special amino acid sequence of PRSQRRLRTACERAKRTLSSA was detected. It was extremely similar to the LTS sequence (KRAVRRLRTACERAKRTLSSS) with CAM-binding activity in mouse blood cells except the difference at positions 1, 3, 4, and 21. This strongly implied that HSP70 interacted with CAM under certain conditions. The analysis of the 5´-flanking sequence of the hsp70 gene of N. yezoensis showed an ATF-like element located at -231 to -223 relative to the start site. This sequence (-231)GTTACGCA(-223) was quite similar to the 5´-GT(G/T)ACG(T/A)CA sequence, which conferred cAMP inducibility, except for an absence of T/A at the third site counting backward. Thus, the induction of hsp70-2 gene was most likely related to PKA activation.
Effects of different chemicals on hsp70 gene expression
Under 24°C high temperature stress conditions, the expression of the hsp70 gene increased obviously in 1h, which was four times that of the control, and then decreased after 2h. Although the expression of the hsp70 gene in the samples treated with quercetin also showed the same trend as that in control, but the expression was significantly lower than that in control (P < 0.05). It should be noted that the expression of the hsp70 gene was significantly upregulated before high temperature stress (five and two times that of the control, respectively) in the samples treated with AICAR and TFP. Under the following high temperature stress conditions, the expression profiles of the hsp70 gene were consistent with those of the control. Moreover, the extent of the hsp70 gene upregulation was significantly higher than that of the control. Except for the sudden upregulation under 0.5h high temperature stress, the addition of cAMP promoter forskolin did not significantly enhance the expression of the hsp70 gene at other stress durations. The level of hsp70 transcripts increased continuously during the high temperature treatments in the presence of pifithrin-μ, although the level was equivalent to that of the control in the first an hour of high temperature stress. Also, no falling-back trend as that in control was found after 2h stress at 24°C. The mRNA of the hsp70 gene accumulated after adding pifithrin-μ and was 31 times that in the nonstressed algae (15°C without pretreatment with chemicals) (Figure 2).
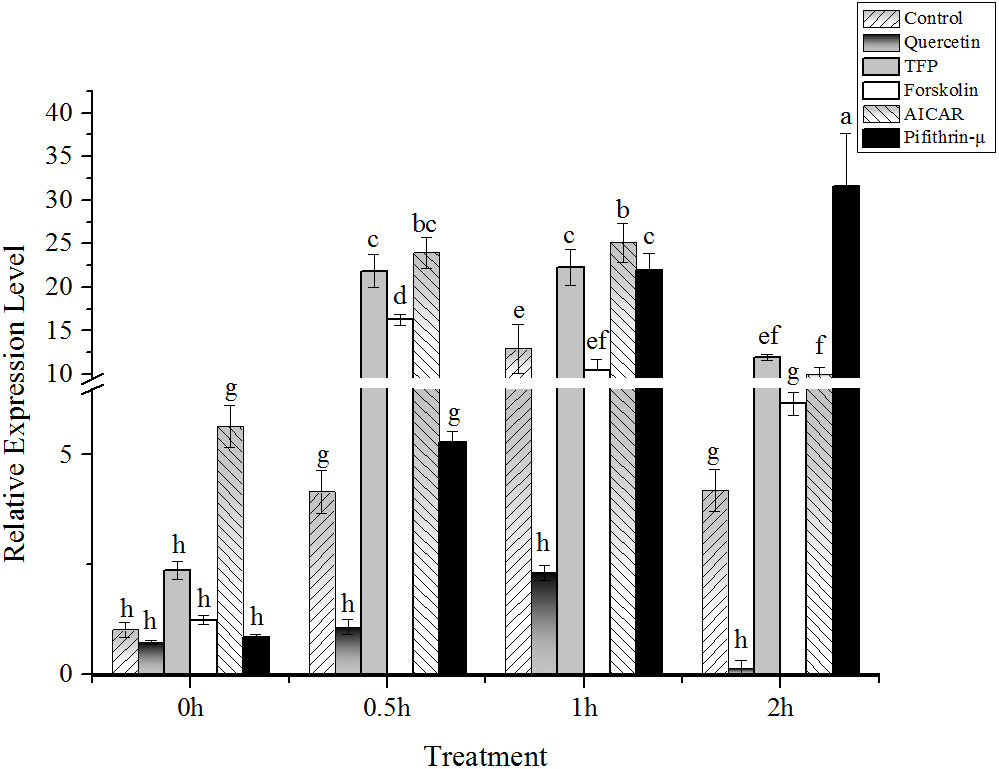
Figure 2 Relative expression of hsp70-2 gene under high-temperature stress with different chemicals. The abscissa represented the different sampling time under high temperature (24°C) stress conditions. Data were Mean ± SD (n=3). Different letters indicate significant differences (P<0.05).
Effects of different chemicals on the potential regulators involved in hsp70-2 expression
The results showed that under high temperature stress, the expression of AMPK and PKA was significantly upregulated at 24°C (Figures 3A, B). Interestingly, the expression of AMPK and PKA presented an upward trend after addition of AICAR before implementing heat stress (Figures 3A, B). Moreover, the profiles of both AMPK and PKA expression after treatment with AICAR were consistent with the control without chemical treatment. However, it seemed that the transcription of these two kinases was suppressed a little bit by forskolin except in the case of PKA under heat stress after 1h (Figures 3A, B). On the contrary, PKA was strongly induced by TFP during half an hour of heat stress, and pifithrin-μ also upregulated its expression compared with the control. Regardless of high temperature, quercetin and pifithrin-μ showed a suppressive effect on the expression of AMPK compared with the control. The variation in CaM gene expression showed that it was downregulated under high temperature stress (Figure 3C). The addition of quercetin, pifithrin-μ, forskolin, and TFP increased CaM expression before heat stress treatment, but it decreased rapidly under high temperature conditions and this downward trend continued with the duration of the stress applied.
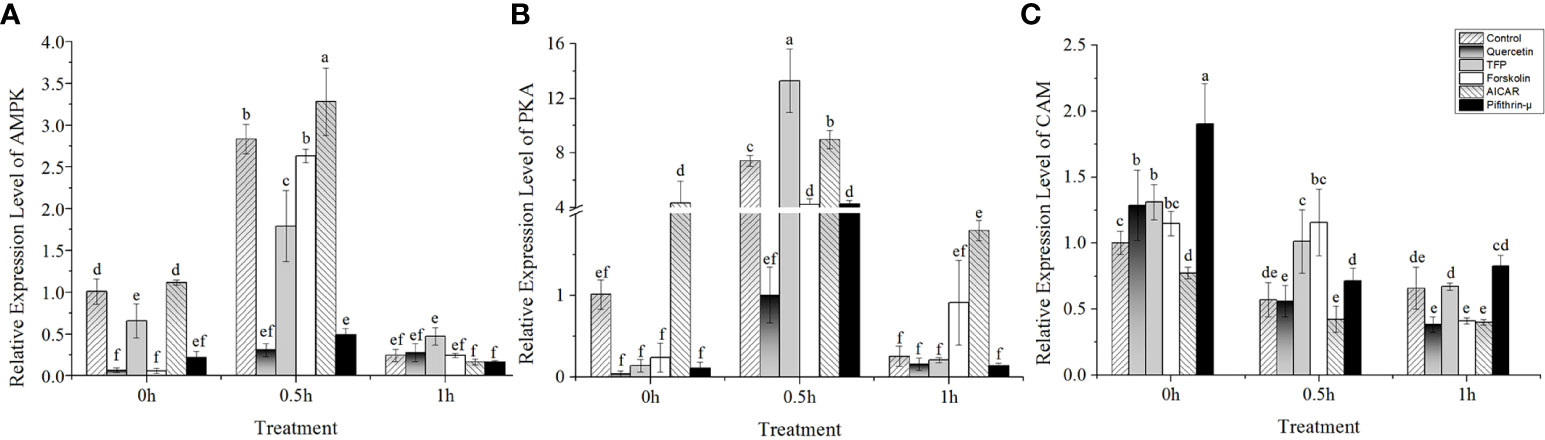
Figure 3 Relative expression of potential regulators involved in hsp70-2 transcription under high-temperature stress with different chemicals. (A) AMP-dependent protein kinase gene (AMPK), (B) cAMP-dependent protein kinase gene (PKA), and (C) calmodulin gene (CaM). The abscissa represented the different sampling time under high temperature (24°C) stress conditions. Data were Mean ± SD (n=3). Different letters indicate significant differences (P<0.05).
Changes in HSP70-2 protein in N. yezoensis determined by western blotting
The immunoblotting results showed that a HSP70 was located near the molecular weight of 72 kDa (Figure 4). However, except for the slight upregulation in the samples treated only with high temperature and those in the AICAR group, the HSP70 level remained relatively stable under other chemical treatments (Figure 4). However, no significant variation in HSP70 proteins was found after treatment with quercetin and TFP. Hence, it was reasonable to believe that the bands indicated by immunoblotting were not only the target HSP70-2 protein but also other fractions of HSP70.

Figure 4 Synthesis of HSP70-2 protein in samples treated with different chemicals under high-temperature stress. Western blot results of HSP70-2 protein under different treatments. The relative content of HSP70-2 protein derived from the normalization of western blot band intensity by that of the blank. All the samples were collected at 1h after different treatments and equal proteins were separated.
LC-MS/MS analysis
Considering the complexity of HSP70 proteins in cells, the content of the target HSP70-2 was investigated and analyzed using LC-MS/MS. Results showed that the HSP70-2 protein was upregulated to 1.08 times of the control under high temperature stress. Obviously, it was only 0.76 times of the control in the samples treated with quercetin. However, the level of the HSP70-2 protein under the treatments with other chemicals was not significantly different from the control (Figure 5).
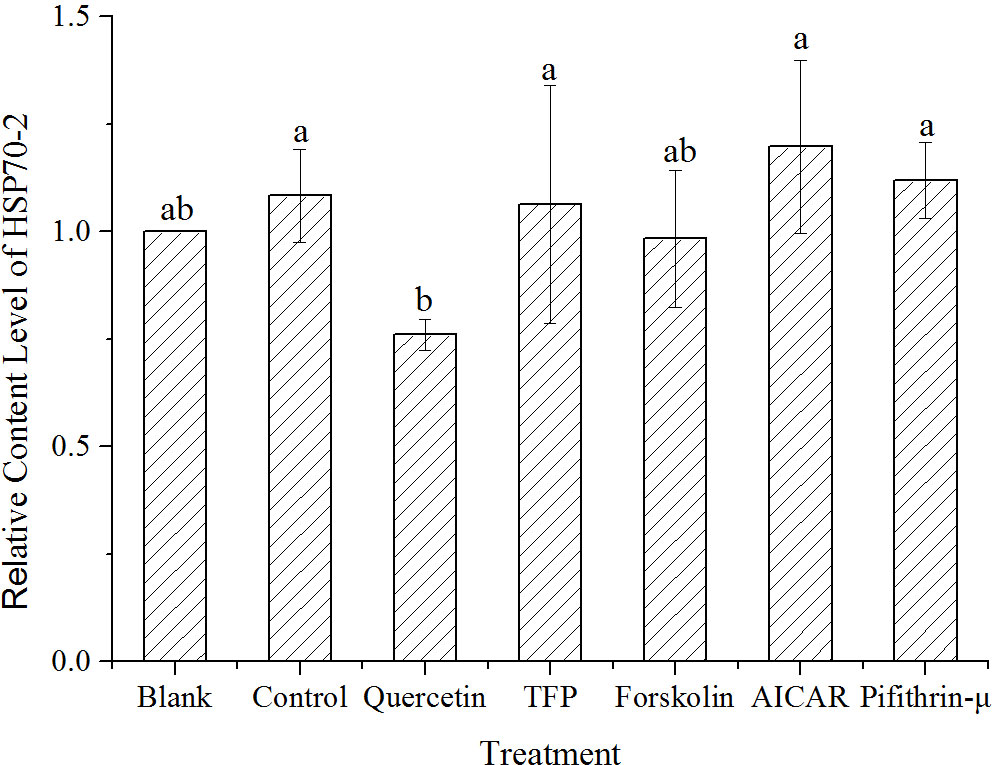
Figure 5 Quantification of HSP70-2 protein by LC-MS/MS in samples treated with different chemicals after 1h high-temperature stress. The translational changes of HSP70-2 derived from the ratio of normalized target protein intensity by that of the control. Blank, incubated at 15°C, Control, stressed at 24°C for 1h, Quercetin, TFP, Foskolin, AICAR and pifithrin-μ represented the corresponding treatment at 24°C for 1h. Data were Mean ± SD (n=3). Different letters indicate significant differences (P<0.05).
Besides the HSP70-2, there were HSP70-1 and a chloroplast genome encoding HSP70 were identified by LC-MS/MS. The results showed that the total contents composed of the three different HSP70 proteins remained stable. However, HSP70-1 and chloroplast genome encoded HSP70 showed a slight decrease under high temperature stress at 1h. And quercetin showed a tendency to decrease the concentration of HSP70, while AICAR showed a slight increase of HSP70 content. Other chemicals had no obvious effect on the synthesis of HSP70. The variation of total content of all detectable HSP70s and the various HSP70 protein were summarized in Supplementary Figures 1-3.
Discussion
High temperature induced the upregulation of the hsp70-2 gene in N. yezoensis
Ambient temperature affects almost all physiological processes in organisms, and extremely high temperatures cause severe cellular injury within minutes (Wahid et al., 2007). In Pyropia, high temperature makes itprone to diseases, and even leading to a reduction in yield (Yan et al., 2009; Xu et al., 2014). A transient elevation of 10–15°C above ambient temperature is considered as heat stress in general (Wahid et al., 2007). Heat stress can cause an increase in the amounts of misfolded proteins, and the primary response of cells is the synthesis of HSPs (Shatilina et al., 2011). Among these, the upregulation of hsp70 genes is one of the most studied molecular processes. The most characteristic feature of hsp70 synthesis is the rapid induction, usually in 30 min to 2h after exposure to heat stress (Sung et al., 2001). Five different hsp70 genes were identified in Pseudo-nitzschia seriata, and the one located in the cytoplasm showed obvious upregulation under thermal stimulation conditions (Choi et al., 2015). The online sequence alignment analysis showed that the hsp70-2 gene obtained here in N. yezoensis showed a high similarity to the Phhsp70-2 gene of N. haitanensis, which was located in the cytoplasm and strongly induced by high temperature (Ji et al., 2015). Thus, it was reasonable to believe that the hsp70-2 gene in N. yezoensis was alsoinducible expression in the cytoplasm and played an important protective role in heat stress responses.
Variations of photosynthetic parameters in heat-stressed N. yezoensis under different chemical treatments
The photosynthetic parameters of algae treated with different chemicals under high temperature conditions were studied to detect the physiological importance of HSP70. Fv/Fm is the maximum photochemical efficiency of photosystem II (PSII), which indicates the primary light energy conversion efficiency of PSII reaction center. Under normal conditions, Fv/Fm generally changes slightly. When Fv/Fm decreases significantly, it means that the organism has been stressed by environmental conditions (Choi et al., 2015). We found that quercetin and the CAM antagonist TFP led to the downregulation of Fv/Fm in N. yezoensis under high temperature stress, but Fv/Fm remained stable at ambient temperature (Figure 1B). On the other hands, the Y(NO) value increased, indicating the damage to the algal photosynthetic system (Figure 1C). Therefore, it was inferred that the response of N. yezoensis to high temperature stress is affected by quercetin and TFP. As mentioned above, quercetin repressed the transcription of hsp70-2 gene. Thus, it was speculated that the expression of hsp70-2 gene was involved in the protection of the photosynthetic system of N. yezoensis. And the heat stress response of photosystem was closely related to CAM since the addition of antagonist to CAM decreased the photosynthetic activities. As one of the most delicate and complex apparatus, the macromolecules related to photosynthesis are easily affected by different environmental factors (Alpert et al., 2006) and most environmental stress factors inevitably cause oxidative damage to cells (Kaur and Pati, 2016). Whether HSP70 protein participates in the regulation of antioxidant enzyme gene expression and the specific mechanism needs further research.
Effects of different chemicals on hsp70-2 gene transcription and translation
Under high temperature stress, the expression of the hsp70-2 gene in N. yezoensis increased first and then decreased with the extension of stress time (Figure 2), which was consistent with other research results on umbilical laver (Reith and Munholland, 1991). A similar trend of hsp70-2 gene expression was observed in the presence of CAM inhibitor of TFP (Figure 2). Therefore, CAM seemed to have no correlation with the expression of the hsp70 gene. At the same time, the addition of kinase promoters, no matter AICAR or forskolin, made the hsp70-2 gene expression profiles similar to that of the control under high temperature, except that the variations were earlier than that of the control. Thus, it indicated that the kinase promoter indeed accelerated the response of the hsp70-2 gene to high temperature stress, and this effect was more obvious in the presence of AICAR (Figure 2). As an effective HSP70 inhibitor, pifithrin-μ selectively interacted with HSP70 and interfered with its physical association with co-chaperones (Leu et al., 2009). Under its action, the expression of the hsp70 gene was supposed to be declined. However, the results here showed that the expression was upregulated continuously with the extension of stress time. It was reported that pifithrin-μ increased ROS production and caused cytotoxicity, leading to the oligomerization and aggregation of many cellular proteins to influence diverse biological processes and signaling pathways in a time- and dose-dependent manner (Leu et al., 2009). The deleterious consequences of this compound caused the accumulation of denatured proteins (Anathan et al., 1986), which showed a competitive combination with the HSP70 protein, resulting in the release of HSF and induction of hsp gene expression. This seemed to be the reason that hsp70 transcription was still upregulated in the presence of pifithrin-μ.
The subsequent protein quantification (Figures 4 and 5) revealed the induction of the hsp70-2 gene under high temperature treatments, and this induction was restrained by the addition of quercetin. Based on the LC-MS/MS analysis, no group showed the significant upregulation of HSP70-2 among the different chemical treatments compared with the blank (Figure 5). Therefore, the synthesis of the HSP70-2 protein was significantly different from its transcription. It was proposed that posttranscriptional regulation existed in N. yezoensis. As for the possible kinase molecule involved in the activation of hsp70-2 expression, evidence from both protein quantification and qRT-PCR results supported the possible phosphorylation of HSF from AMPK.
AMPK might regulate the induction of the hsp70-2 gene in N. yezoensis
A cellular stress response is a highly conserved phenomenon in eukaryotes, which is usually monitored by the induction of “stress” or “heat shock” proteins. Increasing evidence shows that heat stress can change the metabolisms of cells and regulate the utilization of energy. When stress response occurs, ATP is largely decomposed into ADP and AMP, which can activate AMPK to close the biosynthetic pathways of energy consumption (Corton et al., 1994). The specific process can be summarized as follows: the increase in AMP promotes the phosphorylation and activation of AMPK through special signaling pathways in cells, and then the activated AMPK phosphorylates downstream factors, promotes intracellular catabolism, inhibits anabolism, and completes the signal transmission process (Kahn et al., 2005; Zhang et al., 2013). It seems that ATP deficiency always occurred under stress conditions. The depletion of ATP and the activation of AMPK are therefore considered as the trigger of cell stress response (Ritossa, 1964).
At the molecular level, the primary response of cells to temperature elevation is the synthesis of HSPs (Shatilina et al., 2011). The HSF has been proven to be a key phosphorylated molecule that plays a role in inducing hsp gene expression when the cells experience stress (Koizumi et al., 2013). Studies illustrated that the phosphorylation of HSF was extremely important for the transcriptional activation of the hsp70 gene. The activation of HSF was divided into two steps. First, the conformational changes in HSF occurred under heat induction. The second step involved the phosphorylation of the HSF protein by certain kinases (Schuetz et al., 1991). Several AMPK genes were found from the transcriptome data of N. yezoensis under high-salinity stress. Obviously, it seemed likely that the activation of these molecules might be closely related to the adaptation of N. yezoensis to the environments in the intertidal zone. As shown in Figure 2, the addition of AICAR, an AMPK-specific activator, led to the upregulation of the hsp70-2 gene compared with the control, no matter under high temperature stress or normal conditions. This implied that the phosphorylation activation of the HSF factor might be mediated by AMPK. Moreover, the results of immunoblotting and LC-MS/MS also supported the possibility of the involvement of AMPK in the induction of the HSP70 protein, although the upregulation was quite weak (Figures 4 and 5).
PKA was also involved in the induction of the hsp70-2 gene in N. yezoensis
It seemed unreasonable to propose that the upregulation of the hsp70 gene in N. yezoensis was only mediated by AMPK, since the addition of forskolin also promoted the upregulated transcription of the hsp70 gene, although the degree was much lower than that by AICAR (Figure 2). However, the expression of PKA gene measured was not promoted by the treatment of forskolin under 24°C, except the case of 1h (Figure 3B). These results prompted us to suspect whether PKA might regulate the expression of the hsp70 gene. Studies on the expression of hsps provided evidence of a complex pattern of regulation (Choi et al., 1991). For example, other studies reported that both the basal and the heat-induced expression of the human hsp70 promoter-driven reporter gene could be inhibited by the specific protein inhibitor of PKA but was stimulated by the cAMP-elevating agents such as forskolin (10μM) and 8-Br-cAMP (1mM) (Choi et al., 1991). The analysis of the 5’-flanking sequence of the hsp70-2 gene of N. yezoensis showed an ATF-like element (GTTACGCA) located at -231 to -223 relative to the start site, showing similarity to the 5’-GT(G/T)ACG(T/A)CA sequence, which conferred cAMP inducibility. The presence of the ATF-like element prompted us to consider that the expression of hsp70-2 in N. yezoensis might also be regulated by PKA (Lin and Green, 1988; Choi et al., 1991). The notion that the ATF-like element was also involved in the stimulation of the hsp70 gene was supported by a report on humans (Choi et al., 1991). Although the recognition sequence of GTGAGTAA in humans was different from the consensus GTGACGTCA due to the absence of a cytosine residue, it showed cross-reactivity to the transcription factor (Lin and Green, 1988) and was considered to be involved in regulating hsp70 gene expression (Choi et al., 1991).
In summary, the upregulation of hsp70-2 in N. yezoensis was regulated by not only AMPK but also PKA. More importantly, the expression of PKA might be also regulated by AICAR and since it showed upregulation under the treatment of AICAR (Figure 3B). This made the induction of hsp70-2 gene expression was upregulated in the samples without heat shock treatment (Figure 2). The co-existence of multiple signaling pathways might be a significant feature of stress response in N. yezoensis. We proposed that the synergistic mechanism of different molecular pathways regarding the same metabolic process should be outcomes of the long adaptive evolution process. This was extremely important for the algae to survive under the extremely variable environmental conditions in the intertidal zone. However, how AICAR affects the transcription of PKA requires more detailed studies in the future.
Induction of the hsp70-2 gene was independent of Ca2+-CAM signaling in heat-stressed N. yezoensis
The notion that PKA stimulated the hsp70 promoter through ATF binding was conceptually simple. It has been reported that the content of CAM increased significantly during heat shock treatments (Gong et al., 1997; Gong et al., 1998). CAM has been confirmed to be one of the major intercellular signaling molecules. The Ca2+-CAM signal system regulates a series of intracellular physiological processes and has attracted increasing attention in the study of plant signal transduction. However, the role of CAM in the regulation of hsp gene expression in N. yezoensis under heat stress is still unclear. It therefore proposed a model of the hsp70 gene transduction pathway involving Ca2+-CAM, suggesting that the HSP70 protein released HSF after binding with CAM, resulting in the upregulation of the hsp70 gene due to the association of released HSF with the regulatory site of the hsp70 gene (Sun et al., 2000). However, the variation in the hsp70-2 gene treated with TFP, the inhibitor of CAM, showed a similar upregulation as that of the control after high temperature treatment in N. yezoensis (Figure 2). Thus, it implied that there was no corresponding relationship existed between CAM and hsp70-2 gene expression in N. yezoensis. This conflicted with the results reported in N. haitanensis thalli that the inhibition of the Ca2+ influx under high temperature stress conditions led to the downregulation of CaM expression, CAM abundance levels, and photosynthetic activities (Zheng et al., 2020). Therefore, it seemed that the mechanism of hsp70 upregulation in Neopyropia was diverse, which was a complicate regulatory process worthy of further exploration.
In addition, it is believed that there are other elements may also regulate the phosphorylation of HSF and upregulate the expression of the hsp70 gene. In wheat leaves, the contents of intracellular calcium and CAM increased significantly during heat shock treatment (Liu et al., 2001). A large number of changes in the Ca2+ concentration mediated the kinase activity and thus activated the HSF to promote the synthesis of hsp genes (Sangster and Queitsch, 2005). Calcium-activated protein kinases, such as calmodulin-regulated kinases II/IV (CAMKII/IV), may be key factors for activating hsp70 gene transcription (Price and Calderwood, 1991). However, the expression of the CaM gene in N. yezoensis showed continuous downregulation under heat shock treatments (Figure 3B) while the hsp70-2 expression showed an increasing trend in the presence of TFP (Figure 2). The opposite transcription between CaM and hsp70-2 indicated that the expression of the hsp70 gene showed no association with the synthesis of CAM. Moreover, the Ca2+-CAM-dependent protein kinases were absent from the genome of Porphyra (Brawley et al., 2017). In addition, the LC-MS/MS results also did not show that the level of the HSP70 protein reduced under treatment with TFP (Figure 5). Therefore, it was speculated that the Ca2+-CAM signaling was not involved in the upregulation of the hsp70 gene in N. yezoensis.
Posttranscriptional mechanisms of the hsp70-2 gene in N. yezoensis
It was puzzling that no corresponding relationship existed between the variation in HSP70-2 protein quantification and the qRT-PCR result of the samples. For example, the expression of the hsp70-2 gene was strongly inhibited by quercetin, but with no strictly corresponding downregulation of immunoblotting intensity was detected. Moreover, the results of Western blotting and LC-MS/MS analysis showed no significant variation in the HSP70-2 protein level compared with the control. Therefore, the transcription of the hsp70-2 gene was promoted under the action of kinase promoters, but its translation remained strictly restricted. It was proposed that the posttranscriptional mechanisms might be involved in regulating of the HSP70-2 protein in N. yezoensis.
Nowadays, the consensus notion about synthesis of HSPs was that these proteins were not necessarily newly synthesized, but rather normally synthesized at a basal rate and whose synthesis was greatly increased when subjected to heat or other stress conditions (Tanguay, 1983). However, the situation in N. yezoensis was much complex. Our results indicated that the intracellular HSP70-2 content indeed increased to a certain extent under certain stress conditions. And it was more remarkable that the synthesis of HSP70-2 protein was suppressed when quercetin was applied, while the HSP70-2 protein was relatively stable in the presence of a kinase promoter, although the transcription was significantly upregulated. It demonstrated that the translational rather than the transcriptional control operated during heat shock response in N. yezoensis. And the transcription of the hsp70-2 gene laid the foundation for rapid translation when needed. A similar study also reported that in Xenopus oocytes (Bienz and Gurdon, 1982). But the detailed mechanism was still obscure. Perhaps it was essential to compensate for the transcriptional block of RNA polymerase II under heat stress (Findly and Pederson, 1981).
The drastic upregulation of hsp70-2 transcription might be due to two aspects: one was due to the newly transcription, and the other was due to the inhibition of the degradation of mRNAs. The ability to stabilize hsp70 mRNA under heat stress was proved in many species (Moseley et al., 1993). Under the circumstances, the hsp70 mRNA level increased rapidly and was maintained through blocking of certain mRNA degrading mechanism pre-exists. Otherwise, the hsp mRNAs were gradually degraded during the cell recovery from heat shock (Didomenico et al., 1982). Several lines of evidence also suggested that HSPs themselves might be involved in the repression of their mRNAs (Didomenico et al., 1982). If the cell was pretreated with a mild heat shock that caused HSP70 protein synthesis, the following severe heat shock would repress the translation of hsp70 mRNAs (Petersen and Lindquist, 1989). Natural N. yezoensis is rooted in the intertidal zone and often encounters different environmental stresses. This leads to a relatively high level of HSP70 protein in N. yezoensis cells due to the frequent stress response. The immunoblotting results of the present study also showed that the HSP70-2 levels were high even in control. Therefore, we speculated that the transcripts of hsp70-2 were rapidly synthesized but the translation was repressed even under the action of kinase promoters in N. yezoensis samples incubated at 24°C. This conferred well to the extreme variable intertidal environments since the accumulated transcripts could easily be translated to proteins when needed.
Data availability statement
The data presented in the study are deposited in the Oceanographic Data Center repository, accession number PAPER2022090001-01, http://dx.doi.org/10.12157/IOCAS.20220915.002
Author contributions
JN and GW conceived and designed research. ZS, WG, ZF, and JN conducted the experiments. JN, ZS, YF and WG analyzed the data. JN and ZS wrote the manuscript. GW checked and revised the manuscript. JN and GW serve as the authors who were responsible for contact and ensuring communication. All authors contributed to the article and approved the submitted version.
Funding
This study was funded by the National Key R&D Program of China (2018YFD0901500), China Agriculture Research System of MOF and MARA, the Key Deployment Project of the Centre for Ocean Mega-Research of Science, the Chinese Academy of Sciences (COMS2019Q02), and the Major Scientific and Technological Innovation Project of Shandong Provincial Key Research and Development Program (2019JZZY010815).
Conflict of interest
The authors declare that the research was conducted in the absence of any commercial or financial relationships that could be construed as a potential conflict of interest.
Publisher’s note
All claims expressed in this article are solely those of the authors and do not necessarily represent those of their affiliated organizations, or those of the publisher, the editors and the reviewers. Any product that may be evaluated in this article, or claim that may be made by its manufacturer, is not guaranteed or endorsed by the publisher.
Supplementary material
The Supplementary Material for this article can be found online at: https://www.frontiersin.org/articles/10.3389/fmars.2022.1004600/full#supplementary-material
Supplementary Figure 1 | Quantification of HSP70-1 protein by LC-MS/MS in samples treated with different chemicals after 1h high-temperature stress. The translational changes of HSP70-2 derived from the ratio of normalized target protein intensity by that of the control. Blank, incubated at 15°C, Control, stressed at 24°C for 1h, Quercetin, TFP, Foskolin, AICAR and pifithrin-μ represented the corresponding treatment at 24°C for 1h. Data were Mean ± SD (n=3). Different letters indicate significant differences (P<0.05).
Supplementary Figure 2 | Quantification of chloroplast genome encoded HSP70 protein by LC-MS/MS in samples treated with different chemicals after 1h high-temperature stress. The translational changes of HSP70-2 derived from the ratio of normalized target protein intensity by that of the control. Blank, incubated at 15°C, Control, stressed at 24°C for 1h, Quercetin, TFP, Foskolin, AICAR and pifithrin-μ represented the corresponding treatment at 24°C for 1h. Data were Mean ± SD (n=3). Different letters indicate significant differences (P<0.05).
Supplementary Figure 3 | Quantification of the total contents of the three different HSP70 proteins by LC-MS/MS in samples treated with different chemicals after 1h high-temperature stress. The translational changes derived from the ratio of normalized target protein intensity by that of the control. Blank, incubated at 15°C, Control, stressed at 24°C for 1h, Quercetin, TFP, Foskolin, AICAR and pifithrin-μ represented the corresponding treatment at 24°C for 1h. Data were Mean ± SD (n=3). Different letters indicate significant differences (P<0.05).
References
Alpert D. R., Mandl L. A., Erkan D., Salmon J. E. (2006). Anti-heparin/platelet factor 4 antibodies in SLE patients with and without antiphospholipid antibodies. Arthritis Rheumatism 54 (12), 4078–4079. doi: 10.1136/ard.2007.074476
Anathan J., Goldberg A. L., Voellmy R. (1986). Abnormal proteins serve as eucaryotic stress signals and trigger the activation of heat shock genes. Science 232, 522–524. doi: 10.1126/science.3083508
Bienz M., Gurdon J. B. (1982). The heat-shock response in xenopus oocytes is controlled at the translational level. Cell 29 (3), 811–819. doi: 10.1016/0092-8674(82)90443-3
Brawley S. H., Blouin N. A., Ficko-Blean E., Wheeler G. L., Lohr M., Goodson H. V., et al. (2017). Insights into the red algae and eukaryotic evolution from the genome of Porphyra umbilicalis (Bangiophyceae, rhodophyta). Proc. Natl. Acad. Sci. U.S.A. 114 (31), E6361–E6370. doi: 10.1073/pnas.1703088114
Chatterjee S., Burns T. F. (2017). Targeting heat shock proteins in cancer: A promising therapeutic approach. Int. J. Mol. Sci. 18 (9), 1–39. doi: 10.3390/ijms18091978
Cho E. K., Choi Y. J. (2009). A nuclear-localized HSP70 confers thermoprotective activity and drought-stress tolerance on plants. Biotechnol. Lett. 31 (4), 597–606. doi: 10.1007/s10529-008-9880-5
Cho E. K., Hong C. B. (2006). Over-expression of tobacco NtHSP70-1 contributes to drought-stress tolerance in plants. Plant Cell Rep. 25 (4), 349–358. doi: 10.1007/s00299-005-0093-2
Choi S., Kim S. H., Im. S. O., Jeong W. J., Hwang M. S., Park E. J., et al. (2015). The heat shock protein 70a from Pyropia seriata increases heat tolerance in chlamydomonas. Plant Omics J. 8(4), 323–334.
Choi H. S., Li B. S., Lin Z., Huang L. E., Liu A. Y. C. (1991). Camp and camp-dependent protein-kinase regulate the human heat-shock protein-70 gene promoter activity. J. Biol. Chem. 266 (18), 11858–11865. doi: 10.1016/s0021-9258(18)99036-8
Corton J. M., Gillespie J. G., Hardie D. G. (1994). Role of the amp-activated protein-kinase in the cellular stress-response. Curr. Biol. 4 (4), 315–324. doi: 10.1016/S0960-9822(00)00070-1
Cottin D., Foucreau N., Hervant F., Piscart C. (2015). Differential regulation of hsp70 genes in the freshwater key species Gammarus pulex (Crustacea, amphipoda) exposed to thermal stress: effects of latitude and ontogeny. J. Comp. Physiol. B. 185 (3), 303–313. doi: 10.1007/s00360-014-0885-1
Didomenico B. J., Bugaisky G. E., Lindquist S. (1982). The heat shock response is self regulated at both the transcriptional and post transcriptional levels. Cell 3 Pt 2), 593–603. doi: 10.1016/0092-8674(82)90315-4
Ellis R. J., Hartl F. U. (1996). Protein folding in the cell: Competing models of chaperonin function. FASEB J. 10 (1), 20–26. doi: 10.1096/fasebj.10.1.8566542
Findly R. C., Pederson T. (1981). Regulated transcription of the genes for actin and heat-shock proteins in cultured drosophila cells. J. Cell Biol. 88 (2), 323–328. doi: 10.1083/jcb.88.2.323
Gong M., Li Y. J., Dai X., Tian M., Li Z. G. (1997). Involvement of calcium and calmodulin in the acquisition of heat-shock induced thermotolerance in maize seedlings. J. Plant Physiol. 150 (5), 615–621. doi: 10.1016/S0176-1617(97)80328-8
Gong M., van der Luit A. H., Knight M. R., Trewavas A. J. (1998). Heat-shock-induced changes in intracellular Ca2+ level in tobacco seedlings in relation to thermotolerance. Plant Physiol. 116 (1), 429–437. doi: 10.1104/pp.116.1.429
Griffin N. M., Yu J., Long F., Oh P., Shore S., Li Y., et al. (2010). Label-free, normalized quantification of complex mass spectrometry data for proteomic analysis. Nat. Biotechnol. 28 (1), 83–89. doi: 10.1038/nbt.1592
Gu Y., Zhang X., Lu N., Zang X., Zhang X., Li G. (2012). Cloning and transcription analysis of hsp70-1 and hsp70-2 of gracilaria lemaneiformis under heat shock. Aquacult. 358-359, 284–291. doi: 10.1016/j.aquaculture.2012.05.040
Ji D. H., Li B., Xu Y., Chen C. S., Xie C. T. (2015). Cloning and quantitative analysis of five heat shock protein 70 genes from Porphyra haitanensis. J. Appl. Phycol. 27 (1), 499–509. doi: 10.1007/s10811-014-0314-6
Kahn B. B., Alquier T., Carling D., Hardie D. G. (2005). AMP-activated protein kinase: Ancient energy gauge provides clues to modern understanding of metabolism. Cell Metab. 1 (1), 15–25. doi: 10.1016/j.cmet.2004.12.003
Kaur G., Pati P. K. (2016). Analysis of cis-acting regulatory elements of respiratory burst oxidase homolog (Rboh) gene families in Arabidopsis and rice provides clues for their diverse functions. Comput. Biol. Chem. 62, 104–118. doi: 10.1016/j.compbiolchem.2016.04.002
Koizumi S., Suzuki K., Yamaguchi S. (2013). Heavy metal response of the heat shock protein 70 gene is mediated by duplicated heat shock elements and heat shock factor 1. Gene 522 (2), 184–191. doi: 10.1016/j.gene.2013.03.090
Larkindale J., Vierling E. (2008). Core Genome Responses Involved in Acclimation to High Temperature. Plant Physiology 146, 748–761
Leu J. I. J., Pimkina J., Frank A., Murphy M. E., George D. L. (2009). A small molecule inhibitor of inducible heat shock protein 70. Mol. Cell 36 (1), 15–27. doi: 10.1016/j.molcel.2009.09.023
Lindquist S., Craig E. A. (1988). The heat-shock proteins. Annu. Rev. Genet. 22, 631–677. doi: 10.1146/annurev.ge.22.120188.003215
Lin Y. S., Green M. R. (1988). Interaction of a common cellular transcription factor, ATF, with regulatory elements in both E1a- and cyclic AMP-inducible promoters. Proc. Natl. Acad. Sci. U.S.A. 85 (10), 3396–3400. doi: 10.1073/pnas.85.10.3396
Liu H. T., Zhao H., Li B., Sun D.Y., Zhou R. G. (2001). Involvement of calciumcalmodulin in the expression of hsp26 gene in wheat. Acta Botanica Sinica (in Chinese) 43, 766. doi: 10.3321/j.issn:1672-9072.2001.07.018
Liu W., Yang Y., Xu L. N., Zhang X. L., Wang Y. J., Sun X.. (2012). Cloning and Expression of hsp70 for Porphyra haitanensis. J. Ningbo. Univ. (in Chinese) 25, 17–25. doi: 10.3969/j.issn.1001-5132.2012.02.004
Livak K. J., Schmittgen T. D. (2001). Analysis of relative gene expression data using real-time quantitative PCR and the 2(T)(-delta delta c) method. Methods 25 (4), 402–408. doi: 10.1006/meth.2001.1262
Lu Q. Q., Zhou W., Deng Y. Y. (2016). Overview of economic and ecological benefits of laver cultivation. Aquacult. Fisheries1(in Chinese) 1, 53–54. doi: 10.3969/j.issn.1002-6681.2016.01.026
Lv Y., Wang J. F., Lin C. L., Xu N. J., Lu K. X., Sun x. (2019). Response of RBCL and HSP70 in asparagus to high temperature and phytohormones. Aquacult. Fish. (in Chinese) 43, 886–894. doi: 10.11964/jfc.20170910965
Montero-Barrientos M., Hermosa R., Cardoza R. E., Gutierrez S., Nicolas C., Monte E. (2010). Transgenic expression of the Trichoderma harzianum hsp70 gene increases Arabidopsis resistance to heat and other abiotic stresses. J. Plant Physiol. 167 (8), 659–665. doi: 10.1016/j.jplph.2009.11.012
Moseley P. L., Wallen E. S., Mccafferty J. D., Flanagan S., Kern J. A. (1993). Heat-stress regulates the human 70-kda heat-shock gene through the 3'-untranslated region. Am. J. Physiol. 264 (6), L533–L537. doi: 10.1152/ajplung.1993.264.6.L533
Murtha J. M., Keller E. T. (2003). Characterization of the heat shock response in mature zebrafish (Danio rerio). Exp. Gerontol. 38 (6), 683–691. doi: 10.1016/S0531-5565(03)00067-6
Petersen R. B., Lindquist S. (1989). Regulation of Hsp70 synthesis by messenger-rna degradation. Cell Regul. 1 (1), 135–149. doi: 10.1091/mbc.1.1.135
Price B. D., Calderwood S. K. (1991). Ca2+ is essential for multistep activation of the heat-shock factor in permeabilized cells. Mol. Cell. Biol. 11 (6), 3365–3368. doi: 10.1128/Mcb.11.6.3365
Reith M., Munholland J. (1991). An hsp70 homolog is encoded on the plastid genome of the red alga, Porphyra umbilicalis. FEBS Letters 294 (1–2), 116–120. doi: 10.1016/0014-5793(91)81355-c
Ritossa F. (1964). Experimental activation of specifific loci in polytene chromosomes of drosophila. Exp. Cell Res. 35 (3), 601–607. doi: 10.1016/0014-4827(64)90147-8
Rizhsky L., Liang H. J., Mittler R. (2002). The combined effect of drought stress and heat shock on gene expression in tobacco. Plant Physiol. 130 (3), 1143–1151. doi: 10.1104/pp.006858
Sangster T. A., Queitsch C. (2005). The HSP90 chaperone complex, an emerging force in plant development and phenotypic plasticity. Curr. Opin. Plant Biol. 8 (1), 86–92. doi: 10.1016/j.pbi.2004.11.012
Scharf K. D., Rose S., Zott W., Schoffl F., Nover L. (1990). Three tomato genes code for heat stress transcription factors with a region of remarkable homology to the DNA-binding domain of. EMBO J. 9 (13), 4495–4501. doi: 10.1002/j.1460-2075.1990.tb07900.x
Schuetz T. J., Gallo G. J., Sheldon L., Tempst p., Kingston R. E. (1991). “Isolation of a cDNA for HSF2: evidence for two heat shock factor genes in humans,” in Proceedings of the National Academy of Sciences, United States of America, Vol. 88. 6911–6915. doi: 10.1073/pnas.88.16.6911
Shatilina Z. M., Wolfgang Riss H., Protopopova M. V., Trippe M., Meyer E. I., Pavlichenko V. V., et al. (2011). The role of the heat shock proteins (HSP70 and sHSP) in the thermotolerance of freshwater amphipods from contrasting habitats. J. Thermal. Biol. 36 (2), 142–149. doi: 10.1016/j.jtherbio.2010.12.008
Shevchenko A., Tomas H., Havlis J., Olsen J. V., Mann M. (2006). In-gel digestion for mass spectrometric characterization of proteins and proteomes. Nat. Protoc. 1 (6), 2856–2860. doi: 10.1038/nprot.2006.468
Sung D. Y., Kaplan F., Guy C. L. (2001). Plant HSP70 molecular chaperones: protein structure, gene family, expression and function. Physiologia Plantarum. 113, 443–451. doi: 10.1034/j.1399-3054.2001.1130402.x
Sun X. T., Li B., Zhou G. M., Tang W. Q., Bai J., Sun D. Y., et al. (2000). Binding of the maize cytosolic Hsp70 to calmodulin, and identification of calmodulin-binding site in Hsp70. Plant Cell Physiol. 41 (6), 804–810. doi: 10.1093/pcp/41.6.804
Sun P., Mao Y., Li G., Cao M., Kong F., Wang L., et al. (2015). Comparative transcriptome profiling of Pyropia yezoensis (Ueda) M.S. hwang & H.G. choi in response to temperature stresses. BMC Genomics 16, 463. doi: 10.1186/s12864-015-1586-1
Sun W. N., Van Montagu M., Verbruggen N. (2002). Small heat shock proteins and stress tolerance in plants. Biochim. Et Biophys. Acta-Gene Structure Expression 1577 (1), 1–9. doi: 10.1016/S0167-4781(02)00417-7
Tanguay R. M. (1983). Genetic-regulation during heat-shock and function of heat-shock proteins - A review. Can. J. Biochem. Cell Biol. 61 (6), 387–394. doi: 10.1139/o83-053
Wahid A., Gelani S., Ashraf M., Foolad M. R. (2007). Heat tolerance in plants: An overview. Environ. Exp. Bot. 61 (3), 199–223. doi: 10.1016/j.envexpbot.2007.05.011
Wang W. X., Vinocur B., Shoseyov O., Altman A. (2004). Role of plant heat-shock proteins and molecular chaperones in the abiotic stress response. Trends Plant Sci. 9 (5), 244–252. doi: 10.1016/j.tplants.2004.03.006
Wang W., Wu X., Wang C., Jia Z., He L. W., Wei Y. F., et al. (2014). Exploring valid internal-control genes in Porphyra yezoensis (Bangiaceae) during stress response conditions. Chin. J. Oceanol. Limnol. 32 (4), 783–791. doi: 10.1007/s00343-014-3245-9
Xie X. J., Lu X. P., Wang L. P., He L. W., Wang G. C. (2020). High light intensity increases the concentrations of j3-carotene and zeaxanthin in marine red macroalgae. Algal. Research-Biomass Biofuels Bioproducts 47, 101852. doi: 10.1016/j.algal.2020.101852
Xu Y., Chen C. S., Ji D. H., Hang N., Xie C. T. (2014). Proteomic profile analysis of Porphyra haitanensis in response to high-temperature stress. J. Appl. Phycol. 26 (1), 607–618. doi: 10.1007/s10811-013-0066-8
Xu W., Kasper L. H., Lerach S., Jeevan T., Brindle P. K. (2007). Individual CREB-target genes dictate usage of distinct cAMP-responsive coactivation mechanisms. EMBO J. 26 (12), 2890–2903. doi: 10.1038/sj.emboj.7601734
Yan X. H., Lv F., Liu C. J., Zheng Y. F. (2009). Selection and characterization of a high-temperature tolerant strain of Porphyra haitanensis Chang et zheng (Bangiales, rhodophyta). J. Appl. Phycol. 22 (4), 511–516. doi: 10.1007/s10811-009-9486-x
Zhang Y. L., Guo H. L., Zhang C. S., Lin S. Y., Yin Z. Y., Peng Y. Y., et al. (2013). AMP as a low-energy charge signal autonomously initiates assembly of AXIN-AMPK-LKB1 complex for AMPK activation. Cell Metab. 18 (4), 546–555. doi: 10.1016/j.cmet.2013.09.005
Zhang H. N., Li W., Li J. J., Fu W. D., Yao J. T., Duan D. L. (2012). Characterization and expression analysis of hsp70 gene from ulva prolifera j. agardh (Chlorophycophyta, chlorophyceae). Mar. Genomics 5, 53–58. doi: 10.1016/j.margen.2011.10.001
Zheng H. Y., Wang W. L., Xu K., Xu Y., Ji D. H., Chen C. S., et al. (2020). Ca2+ influences heat shock signal transduction in Porphyra haitanensis. Aquaculture 516, 734618. doi: 10.1016/j.aquaculture.2019.734618
Keywords: heat shock protein 70, high-temperature stress, AMP-activated protein kinase, cAMP-dependent protein kinase, Neopyropia yezoensis
Citation: Sun Z, Gu W, Feng Z, Fan Y, Niu J and Wang G (2022) Both AMP-activated and cAMP-dependent protein kinase regulate the expression of heat shock protein 70-2 gene in Neopyropia yezoensis. Front. Mar. Sci. 9:1004600. doi: 10.3389/fmars.2022.1004600
Received: 28 July 2022; Accepted: 29 August 2022;
Published: 26 September 2022.
Edited by:
Xiaotong Wang, Ludong University, ChinaReviewed by:
Yuehuan Zhang, South China Sea Institute of Oceanology (CAS), ChinaZhengquan Gao, Shandong University of Technology, China
Wenjun Wang, Yellow Sea Fisheries Research Institute (CAFS), China
Copyright © 2022 Sun, Gu, Feng, Fan, Niu and Wang. This is an open-access article distributed under the terms of the Creative Commons Attribution License (CC BY). The use, distribution or reproduction in other forums is permitted, provided the original author(s) and the copyright owner(s) are credited and that the original publication in this journal is cited, in accordance with accepted academic practice. No use, distribution or reproduction is permitted which does not comply with these terms.
*Correspondence: Jianfeng Niu, jf_niu@qdio.ac.cn; Guangce Wang, gcwang@qdio.ac.cn