- 1State Key Laboratory of Marine Environmental Science, College of Ocean and Earth Sciences, Xiamen University, Xiamen, China
- 2Key Laboratory of Underwater Acoustic Communication and Marine Information Technology of the Ministry of Education, Xiamen University, Xiamen, China
- 3State Key Laboratory of Marine Environmental Science, College of the Environment and Ecology, Xiamen University, Xiamen, China
- 4South China Sea Fisheries Research Institute of the Chinese Academy of Fishery Sciences, Key Laboratory for Sustainable Utilization of Open-sea Fishery, Ministry of Agriculture and Rural Affairs, Guangzhou, China
Commercial fishing constitutes an important food source but induces undesirable bycatch on animals worldwide. This study extends bycatch research geographically to the Western Pacific Ocean, filling a knowledge gap regarding bycatch and mitigation measures in the region. Bycatch has caused many lethal consequences to the pantropical spotted dolphins (Stenella attenuata) during their interaction with fisheries in the research area. We provided a self-developed acoustic deterrent system and conducted field experiments, suggesting its effectiveness as a potential useful conservation tool to reduce bycatch. Dolphins departed the area and the number of dolphins in sight declined to zero after the deployment of the system. Additional evidence was reflected in acoustic recordings, showing the number of clicks emitted by dolphins decreased from 1,502 to 136 per minute after the ADS was activated. Meanwhile, click amplitude was reduced by 84%, indicating an increase in the distance between dolphins and the system. These combined results indicate that the system was effective in driving dolphins away to facilitate the conservation of the species by protecting them from potential bycatch.
1 Introduction
The acceleration of anthropogenic activities into the oceans has expanded since at least the 1950s, as a replenishment to the increasing scarcity of terrestrial resources and important food sources (Garcia and Rosenberg, 2010; Bostock et al., 2010; FAO, 2013). The resulting negative impact on marine mammals, including cetaceans, pinnipeds, sea otters, and sirenians, can be reflected in the threats imposed either directly or indirectly on the animals through fisheries, transportation, and oil and gas extraction (Berta et al., 1999; Committee on Taxonomy, 2016; Temple et al., 2021). This has raised an issue on how to reasonably develop an ocean-based economy while ensuring the conservation of marine mammals as 33 species have been currently classified as globally endangered (Avila et al., 2018).
Among the threats, fishery bycatch constitutes one of the primary factors that has led to population reductions of marine mammals since the 1970s (Mitchell, 1975; Hofman, 1995; Read et al., 2006; Hodgson et al., 2007; Read, 2008; Wilson et al., 2014; Liu et al., 2017; Moore et al., 2021; Wade et al., 2021). Bycatch results from the direct interactions between marine mammals and fishing gear when animals forage or prey spatiotemporally with anthropogenic fishing activities (Kiszka et al., 2008). Marine animals, even for odontocetes possessing an exceptional biosonar system, sometimes have a limited capability to detect fishing nets, especially at a long distance (Kastelein et al., 2000). Harbor porpoises (Phocoena phocoena) were unable to detect monofilament nets from distances greater than 3 to 6 m. This limitation in their biosonar system might mislead the animals to deliberately remove fish captured in gear and become entangled (Rabearisoa et al., 2015; Machernis et al., 2021). The fishery bycatch of marine mammals, imposed by the growing need for seafood consumption, has become a serious issue widely in the South Pacific, Indian, North Pacific, and Atlantic Oceans, involving dozens of cetaceans (Werner et al., 2015; Papageorgiou et al., 2022). Reeves et al. (2013) pointed out that the past two decades has witnessed a gillnet bycatch on at least 75% of odontocetes, 64% of mysticetes, 66% of pinnipeds, and all species of sirenians and marine mustelids. Interactions with fishing gear may result in incidental mortality or serious injury to individuals that led to infections, starvation, and a reduced ability to forage and can raise the possibility of being caught by predators (Machernis et al., 2021). The number of harbor porpoise carcasses found along the German Baltic Sea coast increased yearly, of which 47%–86% were confirmed to be caused by bycatch (Herr et al., 2009; Koschinski and Pfander, 2009).
Fishery bycatch was also found in the South China Sea, which is a vital habitat for more than one-third of extant cetacean species on Earth (Li et al., 2020). The high-intensity fisheries in this region have posed a great threat to the survival of cetaceans in the South China Sea (Li et al., 2020; Lin et al., 2021; Liu et al., 2022). A recent study showed a high overlap between humpback dolphin habitat and trammel-net fishing area in the northern Beibu Gulf, China (Wu et al., 2022). The fishery activities had a negative impact on dolphins, causing distinct injuries (Slooten et al., 2013; Chen et al., 2016). Liu et al. (2017) conducted a large-scale interview among local fishermen in Hainan Island, China, reporting a total number of 150 bycatch events involving more than 600 animals from 2000 to 2013 (Liu et al., 2017). Wang et al. (2015) summarized the bycatch data from 2000 to 2006 and found that a total of 66 bycatches and 30 injuries covering at least 18 Cetacean and Carnivora species were attributed to fishery bycatch.
Among the victims of fishery bycatch, the pantropical spotted dolphin (Stenella attenuata) has been recognized as one of the most affected, and the majority of deaths were attributed to fishing operations (Bernard and Hohn, 1989; Chivers and Myrick Jnr, 1993). Stenella attenuata is primarily distributed in tropical and subtropical waters (Perrin and Hohn, 1994; Perrin, 2009). Interactions between fisheries and this species in and around Hawaiian waters have induced its serious entanglements with fishing gear (Bradford and Lyman, 2015; Stack et al., 2019; Carretta et al., 2020). Sixteen pantropical spotted dolphins were caught by accident during fishery activities in the Yellow Sea, China (Wu et al., 2015). This also holds true in other sites where the bycatch of foraging spotted dolphins has frequently been documented in tuna fisheries and dolphins were found trapped in the fishing nets during their preys on tuna (Baird and Webster, 2020). The South China Sea is an important habitat for S. attenuata (Zhu et al., 2003; Gong et al., 2019; Liu et al., 2022). Though listed as a national protected animal, bycatch still constitutes a major concern for the conservation of the pantropical spotted dolphins inhabiting the South China Sea (Wang et al., 2021). Using field data and documentation from fishermen, researchers estimated bycatch-induced deaths of 20, 18, and 7 specimens in 2015, 2017, and early 2021, respectively (Wang et al., 2021). These aggregations demonstrate a serious status for the dolphins even though the real number of dolphin deaths induced by bycatch might have been underestimated as local fishermen stated that the dolphins occasionally get trapped and entangled by the fishing nets when trying to remove fish from nets (Figure 1). The fishery bycatch has significantly affected the conservation and recovery of the population in the South China Sea and caused serious loss of biomass catch for fishermen, calling for an urgent need to develop mitigation methods.
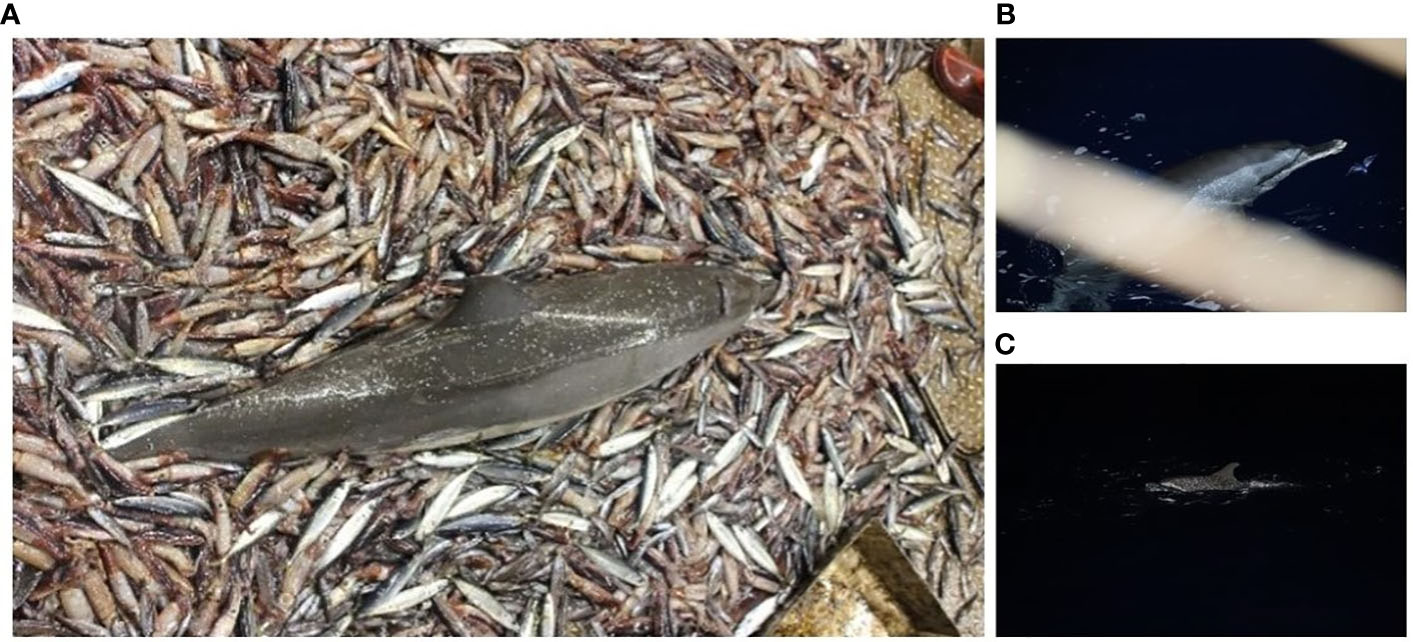
Figure 1 (A) A dead specimen in fishery bycatch. (B) Sea observations of a pantropical spotted dolphin showing the white beak tip. (C) A dolphin wandering around the vessel during a fishing activity.
Countermeasures to fishery bycatch have been proposed accordingly (Bjørge et al., 2013; Geijer and Read, 2013; Senko et al., 2013; Senko et al., 2013; Dunn et al., 2014; Hamer et al., 2015; Passadore et al., 2015; Thode et al., 2015; Werner et al., 2015; Prado et al., 2021). Establishing nature reserves and restricting fishing activities in the reserves have been useful to revive the animal populations (Zhou et al., 2007; Guinet et al., 2015). Changing the operation mode of fishing by using a mesh with larger size purse seines was also effective in reducing the bycatch of dolphins which chased after the same fish runs (Yang, 2005). Using barium sulfate-enhanced material to fabricate nets can acoustically raise its reflectivity and create a greater detection distance for animals to potentially reduce entanglement and bycatch (Mooney et al., 2004; Mooney et al., 2007).
However, these means cannot force the animals to actively swim away from fishing sites. Using sounds sensitive to the potential bycatch victims was useful to exclude marine mammals, e.g., odontocetes from fishery regions by increasing their minimum distance to the threat, mitigating bycatch situations (Au, 1993; Barlow and Cameron, 2003; Kastelein et al., 2006; Nachtigall and Supin, 2008; Graham et al., 2009; Mooney et al., 2009; Schakner and Blumstein, 2013), as has been demonstrated in studies previously conducted on porpoises and beaked whales (Culik et al., 2001; Koschinski et al., 2006; Carretta et al., 2008; Carlström et al., 2009; Papageorgiou et al., 2022). Porpoises were excluded hundreds of meters away from the fishing sites, and bycatch cases of beaked whales dropped to zero after the acoustic deterrent device was deployed to work. Apparently, acoustic deterrence constitutes an appropriate method to reduce fishery bycatch with respect to feasibility and cost, as well as acceptability for fishermen. In this paper, we developed an acoustic deterrent system (ADS) for the pantropical spotted dolphins (S. attenuata) and carried out field experiments in the South China Sea.
2 Materials and methods
2.1 Development of the ADS
The ADS was integrated into a waterproof portable box, including a high-capacity lithium battery power module, providing power to the entire system; a signal generator; a digital-to-analog signal transform module; a power amplifier module; and a transducer (Figure 2A). Signal generation was completed by feeding LabView (National Instruments, Austin, USA)-based codes to the system to generate the source signal in demand, which was further transformed to an analog signal through a digital-to-analog signal transform module (DAQ Card USB-6216, National Instruments, Austin, USA) at a sampling rate of 400 kS/s. The output was then fed into the customized power amplifier module to boost the signal energy, reaching up to 120 W. The signal was transmitted into the water through a non-directional underwater transducer (WBT 30, China Shipbuilding Industry Group Co., Ltd., Hangzhou, China) with a centroid frequency of 30 kHz.
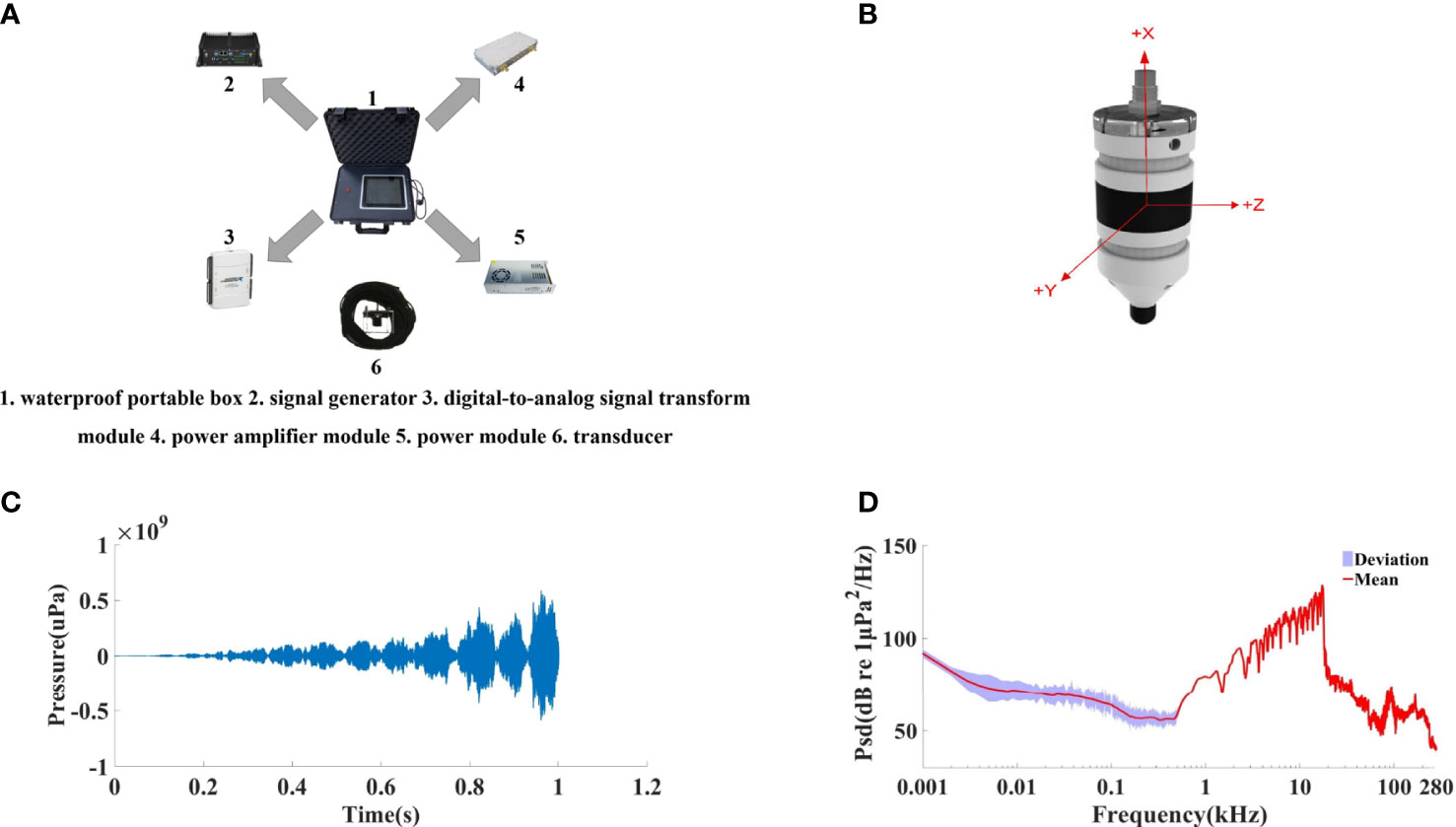
Figure 2 (A) Components of the self-developed acoustic deterrent system. (B) Outlook of the acoustic recording SoundTrap 300 HF. (C) Waveform and (D) the mean power spectrum density level of the deterring signal measured in a laboratory condition.
The ADS was first tested in a laboratory pool with a size of 27 m × 12 m × 3 m, prior to its application in the field. The system had a stable performance during the tests, and the actual deterring signal transmitted was recorded by a self-contained, calibrated professional underwater acoustic recorder, SoundTrap 300 HF (Ocean Instruments, Auckland, New Zealand) (Figure 2B), which was placed at 1 m from the transducer. Both the ADS and SoundTrap were hung in the water column, 1.5 m below the water surface. The received signal had a peak frequency of 17.3 kHz, sound energy was mainly distributed from 1 to 18 kHz, and the −20-dB frequency bandwidth equaled 12.5 kHz, ranging from 5.49 to 17.94 kHz (Figure 2D). The amplitude dropped rapidly over 18 kHz. The test was repeated 20 times. Peak-to-peak sound source level was 181.59 dB (re 1 μPa) (Figure 2C).
2.2 Field applications
The deployment of the ADS followed a protocol in which dolphins were sighted and faced potential bycatch during fishing (Figure 3B). Two separate field surveys were conducted in 2019 and 2021.
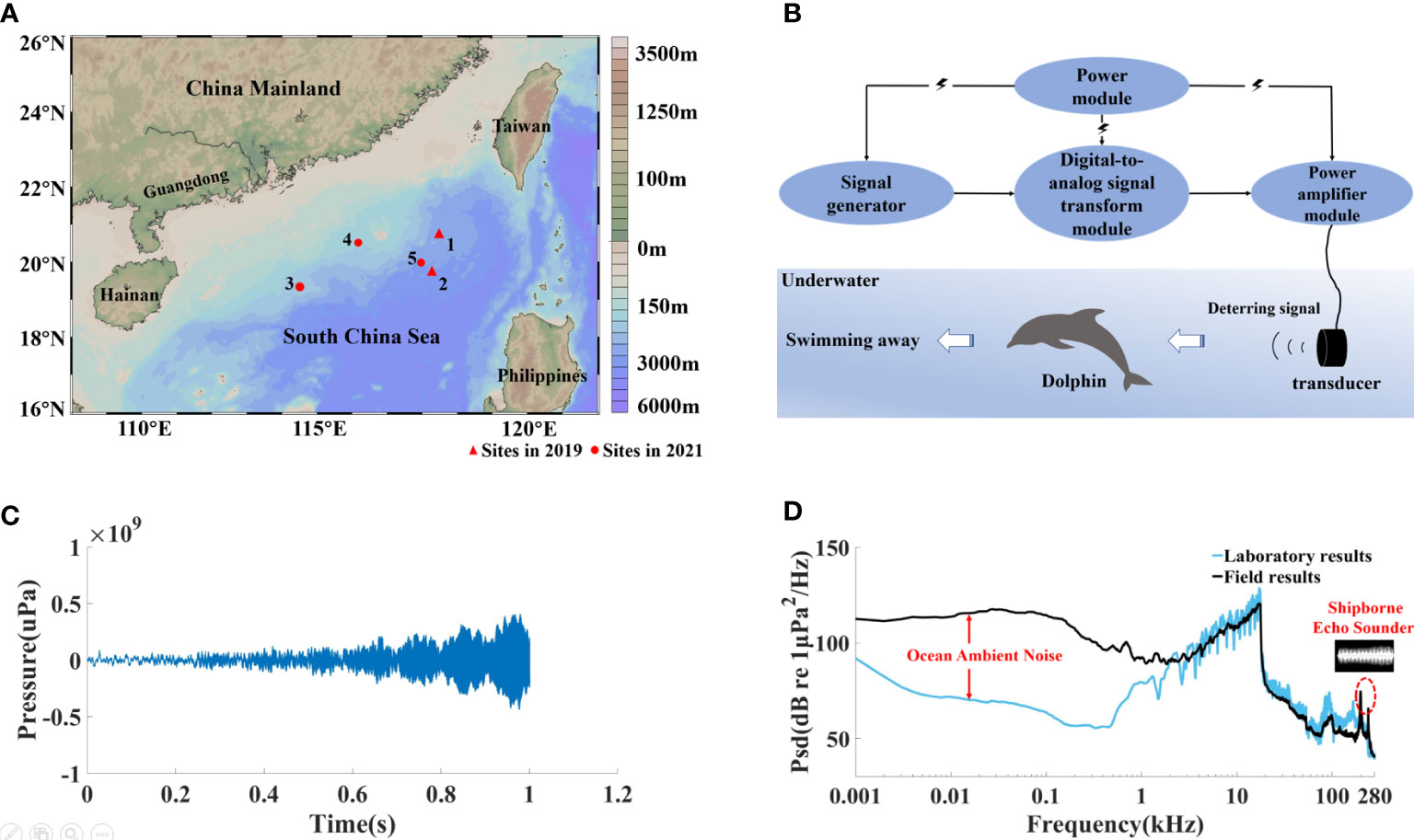
Figure 3 (A) Sites of the field tests conducted in the South China Sea. (B) Illustration of procedure workflow of the acoustic deterrent system. (C) Waveform and (D) the mean power spectrum density level of the deterring signals measured in the field, which was compared to signals measured in the laboratory condition.
We took advantage of the annual fishery resource survey voyage organized by the South China Sea Fisheries Research Institute of the Chinese Academy of Fishery Sciences to deploy the ADS during encounters with the pantropical spotted dolphins when fishing (Figure 3A). The marine fishery survey vessel, China Fishery 301, had a size of 42.8 m in length, 8 m in width, and 5.2 m in height, with a gross tonnage of 398 allowing for a continuous survey of 30 days. The actual deterring signal transmitted in field tests was recorded by SoundTrap 300 HF (Figure 3C), which was compared with the lab-based measurements (Figure 3D).
During the survey, the vessel sailed during the day and conducted light falling-net fishing at night. Before releasing the fishing net, all the lights on the vessel were turned on to attract fish to aggregate around the vessel. This huge aggregation of biomass, including various fishes and squids, attracted the pantropical spotted dolphins to the operating area to prey, consistent with the observations in a previous study (Qin et al., 2009). The ADS system was deployed, by putting the sound transducer into the water, which was suspended 3 m underwater by a rope with an iron block tied at the end as ballast. The deterring signal was transmitted by pressing the starting button on the ADS. The surfacing positions and movements of dolphins as well as the number of the total animals were documented through visual observation both before and after the deployment of the ADS. A fish-detecting sonar, Simrad EY60 (Kongsberg Maritime, Kongsberg, Norway), was equipped laterally at the vessel to specifically help determine the relative position of dolphins to the vessel, and another fishing sonar, and Simrad EY60 (Kongsberg Maritime, Kongsberg, Norway), was deployed at the bottom of the vessel to monitor fish biomass. The same SoundTrap 300 HF that was used in the lab was used in the field in 2021 to record the clicks emitted by dolphins. Results from visualizations, fish sonar images, and acoustic analysis were combined to quantitatively evaluate the efficiency of the ADS.
2.2.1 Trials in 2019
During the 30-day survey voyage in 2019, we opportunistically encountered dolphins in two of our fishing events on May 19 and 28 (Figure 3A). We visually observed the movements of dolphins in addition to the deployment of the EY60 in the horizontal direction, and the EK60 was used to monitor the abundance of fish in the vertical direction. Calibrations of the two sonars were done in the waters off Wanshan Island, Zhuhai, before the field experiments (Foote et al., 1987).
The acoustic images of sonars in the horizontal plane were used to monitor the distance between dolphins and the vessel, serving as an important reference to examine whether the dolphins swam away after the ADS was set to work. Those in the vertical plane were processed to calculate the nautical area scattering coefficient (NASC) within the beam range of sonar, to estimate the biomass of fishes (Zhang et al., 2017; Kang et al., 2020).
The image data were processed through the software Echoview (v6.1, Echoview Software Pty. Ltd., Australia), to reconstruct the tomography of an area (6 to 120 m in the horizontal direction and 10 to 50 m in the vertical direction) by inputting the depths of the sonars and the operation area. The acoustic images were reconstructed before the ADS started to work to compare with those selected 20 min after the deployment of the ADS. Data were then filtered using multiple filter variables to eliminate noise for further processing (Tarling et al., 2009).
For the images gained in the horizontal plane, the “single target detection” was used to extract the acoustic image of dolphins by setting specific target strength thresholds. To process an acoustic image in the vertical plane, data were divided into 2-min × 10-m integration units to calculate their respective NASC values, which were averaged as the final output. The Kolmogorov–Smirnov (K-S) and Kruskal–Wallis (K-W) tests were conducted, respectively, to compare the average NASC values gained before and after the ADS was set to work at a significance level of 0.05.
2.2.2 Trials in 2021
Another set of trials occurred on June 2, 4, and 10 during the 30-day survey in 2021 (Figure 3A). We deployed the SoundTrap 300 HF at the same depth of 3 m underwater as that of the ADS on June 4, to record clicks of dolphins to provide additional assessments on the efficiency of the ADS in addition to visual observations. Analyses were conducted to estimate the relative number and amplitude of the clicks recorded before and after the use of the ADS, respectively. Visual data were analyzed to determine the relative distance between the dolphins and the transducer as well as the change in the number of dolphins within eyesight after the ADS was set to operate. To analyze the clicks, we used customized MATLAB (MathWorks, USA) codes to extract clicks from background noise and the deterring sound of ADS. To begin, a threshold was determined to isolate the clicks from the surrounding background noise. Thus, the time location of each click can be roughly determined, which was then used to extract clicks.
To separate the principal part from each click, we selected a certain number of neighboring data points around the location of the amplitude peak to calculate their power spectral density. The number of clicks per minute and the amplitude were compared between the data sets recorded before and after the application of ADS (Carlström et al., 2009). The background noise and deterrent sound were filtered for the data set obtained after the use of ADS because the amplitude of the clicks was lower than the deterring signals.
3 Results
3.1 Visual observation and fish sonar imaging
The pantropical spotted dolphins swam away from the fishing vessel shortly after the deployment of the ADS, and all animals disappeared beyond visual sight 20 min later. The summary of the alerting experiments suggested that 20 min after the trigger of the ADS, dolphins were out of sight (Table 1, Figure 4). In a control experiment conducted on 28 May 2019, the treatments were presented by periodically turning on/off the ADS. Dolphins were gone 20 min after the ADS was turned on and gathered around the fish school near the survey vessel again about 30 min after the ADS was turned off. The application of the ADS reduced the number of dolphins by 70, 26, and 20 during field trials conducted on June 2, 4, and 10 (2021), respectively.

Table 1 Results of the number and distances of dolphins 20 min before and after activation of the deterrent system in 2021.
Additional evidence can be found in the images from the fish-detecting sonars. The images of the pantropical spotted dolphins were extracted by setting the target strength thresholds of −25, −30, and −35 dB, referring to previous studies (Au et al., 2007; Zhang et al., 2010). After thresholding and extraction, the output can be regarded as an individual dolphin within the sonar beam and preset detection range, which was further examined by manually examining its shape (Figure 5). The analysis can help to determine the distance between the animals and the fish sonar. The target strengths of the pantropical spotted dolphins and their location were examined by analyzing the images within a 20-min window before the ADS was set to work, which were compared with those in the same 20-min window after the deployment of the ADS (Table 2). The number of pixels representing the dolphin was reduced, and the average distance of the dolphins increased after the ADS worked, suggesting that the use of the deterring signal drove the pantropical spotted dolphins away from the fishing site. On May 28, the image numbers all reduced to 0 after the ADS worked, indicating that dolphins were “driven” beyond the sonar detection range (120 m). At the same time, we found that after the use of the ADS, the average NASC of integration units increased from 19.44 to 36.70 (P < 0.05) and from 18.58 to 32.41 (P < 0.05) in the experiments conducted, respectively, on 19 and 28 May 2019, indicating that the biomass of fish increased after the ADS was activated.
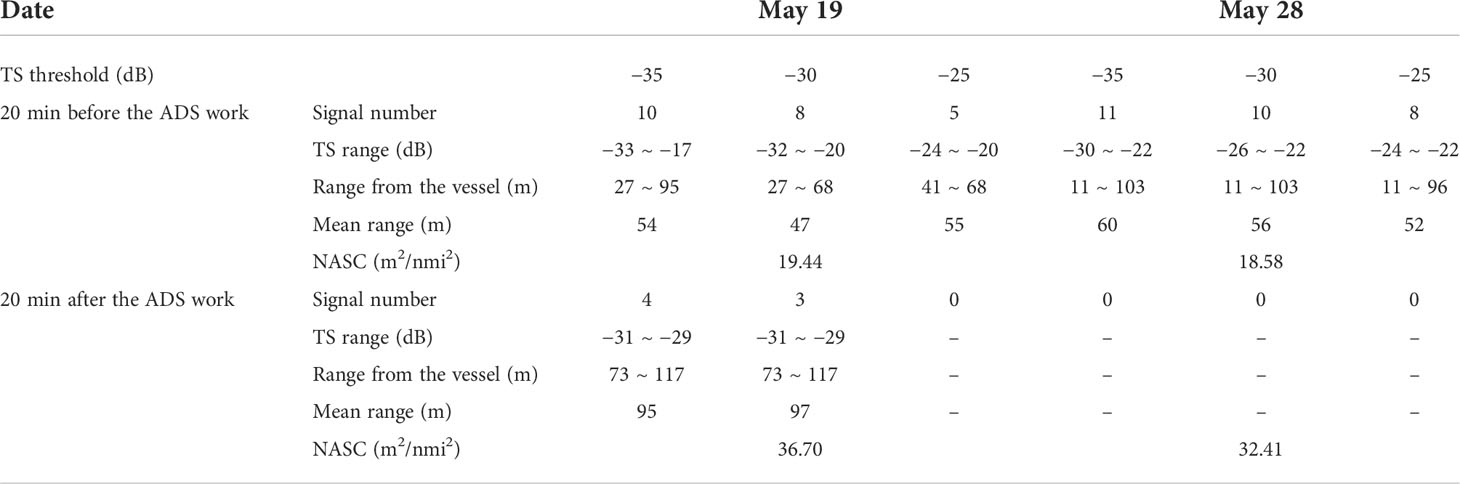
Table 2 Comparisons of the number and average distance of the acoustic images of the pantropical spotted dolphins, and the average NASC of integration units, 20 min before and after the trigger of ADS in the trails conducted on 19 and 28 May 2019.
3.2 Passive acoustic monitoring
The deterring signals were recorded by the SoundTrap 300 HF in the field experiments conducted on 4 June 2021 (Figure 3C). The mean power spectrum density of 20 deterring pulses was compared with those tested in the laboratory (Figure 3D). The ambient noise in the field data was added to the low-frequency (below 2 kHz) components, which can be ascribed to biological sources, e.g., fishes and shrimps as well as wave fluctuations. Two extra peaks were found in the field data at 200 and 240 kHz, respectively, corresponding to the sounds emitted by the shipborne depthometers.
Clicks recorded by the SoundTrap recorder served as an extra examination of the efficiency of the ADS. We extracted the clicks of the pantropical spotted dolphins (Figures 6A, B) recorded on 4 June 2021 and found that the number of clicks per minute decreased from 1,502 to 136, dropping by 90.9%, and the average amplitude dropped by 84.0% after the ADS was set to work (Figure 6C). These drops in both the number and amplitude demonstrated an increase in the distance between the animals and the survey vessel as the sound energy attenuated with the propagation range. Overall, the results suggested that the number of dolphins around the survey vessel was reduced and dolphins actively swam away under the deterrence of the ADS.
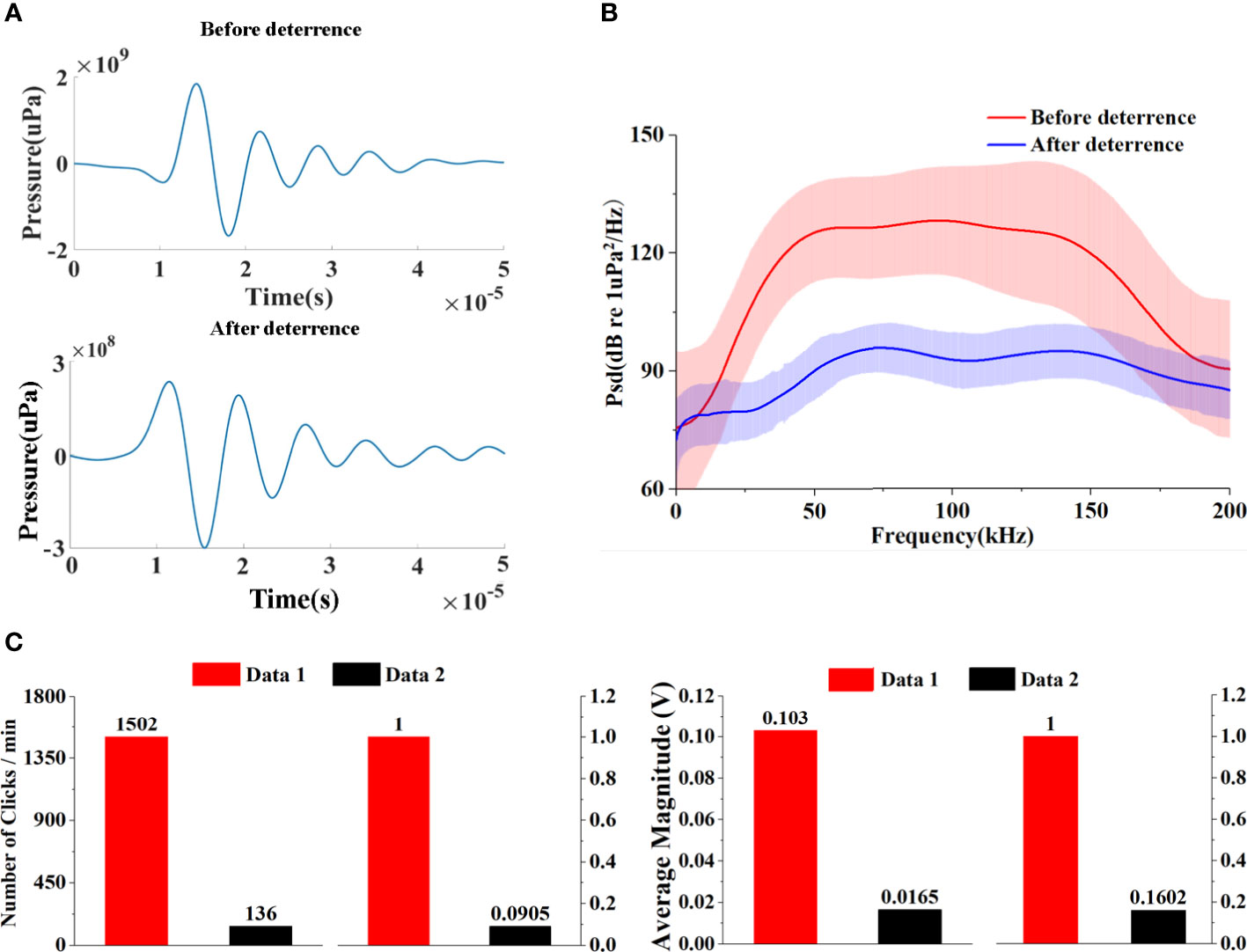
Figure 6 (A) Example of clicks before and after the ADS deterrence. (B) Mean power spectrum density level of dolphin clicks recorded in the field experiments before and after the ADS deterrence. (C) Comparison of the number and average magnitude of clicks before (data 1) and after deterrence (data 2).
4 Discussion
The interaction between fishery activities and cetaceans has led to serious bycatch worldwide (Werner et al., 2015), raising an important issue on how to enhance the conservation on animals under serious threat. The victims include the pantropical spotted dolphins across the Pacific and Atlantic Oceans (Bradford and Lyman, 2015; Zhao et al., 2017; Stack et al., 2019; Carretta et al., 2020). The self-developed ADS system showed its potential to serve as a conservation tool for the pantropical spotted dolphins. Meanwhile, the deterrence did not negatively affect fishing because the fish biomass increased after the ADS worked (Table 2). Gazo et al. (2008) found a decrease in the fish catch after the deterrence on bottlenose dolphins. It may be that the animals caught in our fishing spots have different sensitivities to those in Gazo et al. (2008), and it is challenging to determine this inconsistency.
The self-developed ADS had a source level of 181.59 dB re 1 μPa, higher than that of a previous system (Dawson et al., 2013). In some studies, previously conducted on acoustic deterrence, many odontocetes including porpoises and killer whales (Orcinus orca) get habituated to the deterring signals after a prolongation of the acoustic stimulation (Cox et al., 2001; Carlström et al., 2002; Schakner and Blumstein, 2013; Tixier et al., 2014; Guinet et al., 2015). Porpoises were fully adapted to the deterring signals on day 11 after the initial deployment of the deterring system (Cox et al., 2001). When first exposed to the deterring device, killer whales moved away from the vessel. However, the deterring effect disappeared after successive stimulation exposures (Tixier et al., 2014; Guinet et al., 2015). The long-term efficiency of our ADS has not been tested, and how pantropical spotted dolphins react to exposure to this deterring signal in the South China Sea needs to be further examined. The potential for habituation requires developing a next-generation deterrent system incorporating multiple channels to irregularly change deterring signals from different channels. This improvement may accommodate the need to drive different species away because the sensitivity may vary among species.
The application of the ADS will not significantly influence the distribution of the odontocetes as fishery cannot fully cover their habits simultaneously (Larsen and Eigaard, 2014; Northridge et al., 2017). Larsen and Eigaard (2014) found that gillnet fishery in the North Sea of Denmark covered <1% of the harbor porpoise habitat. Northridge et al. (2017) suggested that even if all gillnet fisheries in southwest England used acoustic deterrent devices, the habitat of the dolphins was affected by <1%, and the range it imposed on was ≤2 km. Thus, the impact of deterrence on animals’ habitats was negligible. The pantropical spotted dolphins inhabit a wide range in the South China Sea, and it is reasonable to assume that the ADS has little impact on the general habitat of this population, but it can help to reduce bycatch.
There is a controversy over the possibility of potential hearing damage caused by acoustic deterrent devices on cetaceans (Ace-Hopkins, 2002). The concern about the negative impacts of ADS on animal hearing capability can neither be proven nor dismissed (Gordon and Northridge, 2002). Despite a potential risk that has not been quantitatively examined, the application of the ADS has to date been one of the most efficient ways to protect the odontocetes from fishery bycatch to enhance conservation. Fishing operations were the biggest source causing the death of the pantropical spotted dolphins (Bernard and Hohn, 1989; Chivers and Myrick Jnr, 1993). The ADS proves effective to prevent animals from fishing sites and enhance population conservation. This application of the ADS can also be extended to other scenarios to prevent animals from negative influence resulting from anthropogenic zones, prior to the onset of pile driving, ammunition blasting, etc. Though the development of the ADS and its application was effective as a tool to conserve the pantropical spotted dolphins and facilitate fishery events in the South China Sea, the ADS should be improved to be handy for fishers to operate and inexpensive for user expansion, which can further enhance animal protection and fishery activities.
Data availability statement
The raw data supporting the conclusions of this article will be made available by the authors, without undue reservation.
Ethics statement
Ethical review and approval was not required for the animal study because the acoustic deterring system deployed in this study meets NMFS’s evaluation criteria and all the tests were conducted in the open sea, with animals free to move without any restrictions. There was no negative effect on the animals.
Author contributions
Conceptualization: YZ, PZ, ZS, and TW. Methodology: ZS, WF, ZG, and JL. Software: WF and ZG. Validation: YZ and ZS. Formal analysis: WF, ZG, JL, and TW. Investigation: PZ, JL, TW, and WF. Resources: YZ, PZ, ZS, and TW. Data curation: WF, ZG, and JL. Writing --original draft: ZS and WF. Writing --review and editing: ZS, YZ, PZ, and TW. Visualization: WF, ZG, and JL. Supervision: ZS, YZ, and TW. Project administration: YZ and PZ. Funding acquisition: YZ, PZ, ZS, and TW. All authors contributed to manuscript revision and read and approved the submitted version.
Funding
This work was financially supported by the National Natural Science Foundation of China (Grant Nos. 42106181, 12074323, 62231011); the Development of a novel high performance platform for deep-sea fish farming and research of its key performances for Science and Technology Major Project of Fujian Province (Grant No. 2021NZ033016); the Guangzhou Science and Technology Foundation (No. 202102020901); the Financial Fund of the Ministry of Agriculture and Rural Affairs, P.R. China (No. NFZX2021); the Central Public-Interest Scientific Institution Basal Research Fund, South China Sea Fisheries Research Institute, CAFS (2021SD18); the China National Postdoctoral Program for Innovative Talents (Grant No. BX2021168); and the Outstanding Postdoctoral Scholarship, State Key Laboratory of Marine Environmental Science at Xiamen University.
Acknowledgments
The authors are grateful to Xiaohui Xu and Zhen Xiao for their assistance in conducting this study.
Conflict of interest
The authors declare that the research was conducted in the absence of any commercial or financial relationships that could be construed as a potential conflict of interest.
Publisher’s note
All claims expressed in this article are solely those of the authors and do not necessarily represent those of their affiliated organizations, or those of the publisher, the editors and the reviewers. Any product that may be evaluated in this article, or claim that may be made by its manufacturer, is not guaranteed or endorsed by the publisher.
Abbreviations
ADS, acoustic deterrent system; NASC, nautical area scattering coefficient.
References
Ace-Hopkins J. (2002). “Humane predator control: the case for acoustics,” in Ace aquatec company report AA-01-044. (Dundee: Ace Aquatec Ltd).
Au W. W. L., Houser D. S., Dankiewicz L. A. (2007). Acoustic backscatter from a diving dolphin. J. Acoust. Soc Am. 121, 3106. doi: 10.1121/1.4808554
Avila I. C., Kaschner K., Dormann C. F. (2018). Current global risks to marine mammals: Taking stock of the threats. Biol. Conserv. 221, 44–58. doi: 10.1016/j.biocon.2018.02.021
Baird R. W., Webster D. L. (2020). Using dolphins to catch tuna: Assessment of associations between pantropical spotted dolphins and yellowfin tuna hook and line fisheries in Hawaii. Fish. Res. 230, 105652. doi: 10.1016/j.fishres.2020.105652
Barlow J., Cameron G. A. (2003). Field experiment show that acoustic pingers reduce marine mammal bycatch in the California drift gill net fishery. Mar. Mammal Sci. 19, 265–283. doi: 10.1111/J.1748-7692.2003.TB01108.X
Bernard H. J., Hohn A. A. (1989). Differences in feeding habits between pregnant and lactating spotted dolphins (Stenella attenuata). J. Mammol. 70, 211–215. doi: 10.2307/1381693
Berta A., Sumich J. L., Kovacs K. M. (1999). Marine mammals evolutionary biology (San Diego: Academic Press).
Bjørge A., Skern-Mauritzen M., Rossman M. C. (2013). Estimated bycatch of harbour porpoise (Phocoena phocoena) in two coastal gillnet fisheries in Norway 2006–2008. mitigation and implications for conservation. Biol. Conserv. 161, 164–173. doi: 10.1016/j.biocon.2013.03.009
Bostock J., McAndrew B., Richards R., Jauncey K., Telfer T., Lorenzen K., et al. (2010). Aquaculture: global status and trends. Philos. T. R. Soc B. 365, 2897–2912. doi: 10.1098/rstb.2010.0170
Bradford A. L., Lyman E. G. (2015). Injury determinations for humpback whales and other cetaceans reported to NOAA response networks in the Hawaiian islands during 2007-2012. U.S. Dep. Commer., NOAA Tech. Memo., NOAA-TM-NMFS-PIFSC-45, 29p. (Honolulu: Pacific Islands Fisheries Science Center). doi: 10.7289/V5TX3CB1
Carlström J., Berggren P., Dinnétz F., Börjesson P. (2002). A field experiment using acoustic alarms (pingers) to reduce harbour porpoise by-catch in bottom-set gillnets. ICES J. Mar. Sci. 59, 816–824. doi: 10.1006/jmsc.2002.1214
Carlström J., Berggren P., Tregenza N. J. C. (2009). Spatial and temporal impact of pingers on porpoises. Can. J. Fish. Aquat. Sci. 66, 72–82. doi: 10.1139/F08-186
Carretta J. V., Barlow J., Enriquez L. (2008). Acoustic pingers eliminate beaked whale bycatch in a gill net fishery. Mar. Mammal Sci. 24, 956–961. doi: 10.1111/j.1748-7692.2008.00218.x
Carretta J. V., Forney K. A., Oleson E. M., Weller D. W., Lang A. R., Baker J., et al. (2020) U.S. pacific marine mammal stock assessments: 2019 (U.S. Department of Commerce, National Oceanic and Atmospheric Administration). doi.org/10.25923/trxr-z635 (Accessed November 25, 2021).
Chen B. Y., Xu X. R., Jefferson T. A., Olson P. A., Qin Q. R., Zhang H. K., et al. (2016). Conservation Status of the Indo-Pacific Humpback Dolphin (Sousa chinensis) in the Northern Beibu Gulf, China. Adv. Mar. Biol. 73, 119–139. doi: 10.1016/bs.amb.2015.10.001
Chivers S. J., Myrick Jnr A. C. (1993). Comparison of age at sexual maturity and other reproductive parameters for two stocks of spotted dolphin, Stenella attenuata. Fish. Bull. 91, 611–618.
Committee on Taxonomy (2016)List of marine mammal species and subspecies. In: Society for marine mammalogy. Available at: https://www.marinemammalscience.org/species-information/list-marine-mammal-species-subspecies/ (Accessed November 18, 2021).
Cox T. M., Read A. J., Solow A., Tregenza N. (2001). Will harbor porpoises (Phocoena phocoena) habituate to pingers? J. Cetacean Res. Manage. 3, 81–96.
Culik B. M., Koschinski S., Tregenza N., Ellis G. M. (2001). Reactions of harbor porpoises phocoena phocoena and herring clupea harengus to acoustic alarms. Mar. Ecol. Prog. Ser. 211, 255–260. doi: 10.3354/meps211255
Dawson S. M., Northridge S., Waples D., Read A. (2013). To ping or not to ping: The use of active acoustic devices in mitigating interactions between small cetaceans and gillnet fisheries. Endanger. Species. Res. 19, 201–221. doi: 10.3354/esr00464
Dunn D. C., Boustany A. M., Roberts J. J., Brazer E., Sanderson M., Gardner B., et al. (2014). Empirical move-on rules to inform fishing strategies: a new England case study. Fish. Fish. 15, 359–375. doi: 10.1111/faf.12019
FAO (2013). The state of world fisheries and aquaculture 2012 (Rome: Food and Agriculture Organization of the United Nations).
Foote K. G., Knudsen H. P., Vestnes G., MacLennan D. N., Simmonds J. E. (1987). Calibration of acoustic instruments for fish density estimation: A practical guide. ICES Coop. Res. Rep. 144, 1–69. doi: 10.1121/1.396130
Garcia S. M., Rosenberg A. A. (2010). Food security and marine capture fisheries: characteristics, trends, drivers and future perspectives. Phil. Trans. R. Soc B 365, 2869–2880. doi: 10.1098/rstb.2010.0171
Gazo M., Gonzalvo J., Aguilar A. (2008). Pingers as deterrents of bottlenose dolphins interacting with trammel nets. Fish. Res. 92, 70–75. doi: 10.1016/j.fishres.2007.12.016
Geijer C. K. A., Read A. J. (2013). Mitigation of marine mammal bycatch in U.S. fisheries since 1994. Biol. Conserv. 159, 54–60. doi: 10.1016/j.biocon.2012.11.009
Gong Z. N., Dong L. J., Caruso F., Lin M. L., Liu M. M., Dong J. C., et al. (2019). Echolocation signals of free-ranging pantropical spotted dolphins (Stenella attenuata) in the south China Sea. J. Acoust. Soc Am. 145, 3480. doi: 10.1121/1.5111742
Gordon J., Northridge S. (2002)Potential impacts of acoustic deterrent devices on Scottish marine wildlife. In: Scottish Natural heritage commissioned report F01AA404. Available at: http://ecologicuk.co.uk/SNHADDReview.pdf (Accessed November 12, 2021).
Graham I. M., Harris R. N., Denny B., Fowden D., Pullan D. (2009). Testing the effectiveness of an acoustic deterrent device for excluding seals from Atlantic salmon rivers in Scotland. ICES J. Mar. Sci. 66, 860–864. doi: 10.1093/icesjms/fsp111
Guinet C., Tixier P., Gasco N., Duhamel G. (2015). Long-term studies of crozet island killer whales are fundamental to understanding the economic and demographic consequences of their depredation behaviour on the Patagonian toothfish fishery. ICES J. Mar. Sci. 72, 1587–1597. doi: 10.1093/icesjms/fsu221
Hamer D. J., Childerhouse S. J., McKinlay J. P., Double M. C., Gales N. J. (2015). Two devices for mitigating odonotcete bycatch and depredation at the hook in tropical pelagic longline fisheries. ICES J. Mar. Sci. 72, 1691–1705. doi: 10.1093/icesjms/fsv013
Herr H., Siebert U., Benke H. (2009). “Stranding numbers and bycatch implications of harbour porpoises along the German Baltic Sea coast,” in 16th ASCOBANS advisory committee meeting (Brugge, Belgium), 3. Document AC16/Doc.62. (Bonn: ASCOBANS Secretariat).
Hodgson A. J., Marsh H., Delean S., Marcus L. (2007). Is attempting to change marine mammal behaviour a generic solution to the bycatch problem? A dugong case study. Anim. Conserv. 10, 263–273. doi: 10.1111/j.1469-1795.2007.00104.x
Hofman R. J. (1995). The changing focus of marine mammal conservation. Trends Ecol. Evol. 10, 462–465. doi: 10.1016/S0169-5347(00)89184-3
Kang M., Fajaryanti R., Son W. J., Kim J.-H., La H. S. (2020). Acoustic detection of krill scattering layer in the Terra Nova bay polynya, Antarctica. Front. Mar. Sci. 7. doi: 10.3389/fmars.2020.584550
Kastelein R. A., Au W. W. L., Haan D. D. (2000). Detection distances of bottom-set gillnets by harbour porpoises (Phocoena phocoena) and bottlenose dolphins (Tursiops truncatus). Mar. Environ. Res. 49, 359–375. doi: 10.1016/S0141-1136(99)00081-1
Kastelein R. A., Jennings N., Verboom W. C., de Haan D., Schooneman N. M. (2006). Differences in the response of a striped dolphin (Stenella coeruleoalba) and a harbour porpoise (Phocoena phocoena) to an acoustic alarm. Mar. Environ. Res. 61, 363–378. doi: 10.1016/j.marenvres.2005.11.005
Kiszka J., Pelourdeau D., Ridoux V. (2008). Body scars and dorsal fin disfigurements as indicators of interaction between small cetaceans and fisheries around the Mozambique channel island of Mayotte. Western Indian Ocean J. Mar. Sci. 7, 185–193. doi: 10.4314/wiojms.v7i2.48276
Koschinski S., Culik B. M., Trippel E. A., Ginzkey L. (2006). Behavioral reactions of free-ranging harbor porpoises Phocoena phocoena encountering standard nylon and BaSO4 mesh gillnets and warning sound. Mar. Ecol. Prog. Ser. 313, 285–294. doi: 10.3354/meps313285
Koschinski S., Pfander A. (2009). “By-catch of harbour porpoises (Phocoena phocoena) in the Baltic coastal waters of angeln and schwansen (Schleswig-Holstein, Germany),” in 16th ASCOBANS advisory committee meeting(Brugge, Belgium), 5. Document AC16/Doc.60 (P). (Bonn: ASCOBANS Secretariat).
Larsen F., Eigaard O. R. (2014). Acoustic alarms reduce bycatch of harbour porpoises in Danish north Sea gillnet fisheries. Fish. Res. 153, 108–112. doi: 10.1016/j.fishres.2014.01.010
Li S. H., Lin M. L., Caruso F., Dong L. J., Lin W. Z., Rosso M., et al. (2020). Cetaceans under threat in south China Sea. Science 368, 1074–1075. doi: 10.1126/science.abc7557
Lin M. L., Liu M. M., Caruso F., Rosso M., Tang X. M., Dong L. J., et al. (2021). A pioneering survey of deep-diving and off-shore cetaceans in the northern south China Sea. Integr. Zool. 16, 440–450. doi: 10.1111/1749-4877.12508
Liu M. M., Lin M. L., Li S. H. (2022). Species diversity and spatiotemporal patterns based on cetacean stranding records in China 1950–2018. Sci. Total Environ. 822, 153651. doi: 10.1016/j.scitotenv.2022.153651
Liu M. M., Lin M. L., Turvey S. T., Li S. H. (2017). Fishers’ knowledge as an information source to investigate bycatch of marine mammals in the south China Sea. Anim. Conserv. 20, 182–192. doi: 10.1111/acv.12304
Machernis A. F., Stack S. H., Olson G. L., Sullivan F. A., Currie J. J. (2021). External scarring as an indicator of fisheries interactions with bottlenose (Tursiops truncatus) and pantropical spotted (Stenella attenuata) dolphins in Maui nui, hawai’i. Aquat. Mamm. 47, 482–498. doi: 10.1578/AM.47.5.2021.482
Mitchell E. (1975). Review of biology and fisheries for smaller cetaceans. J. Fish. Res. Board Can. 32, 887–983. doi: 10.1139/f75-114
Mooney T. A., Au W. W. L., Nachtigall P. E., Trippel E. A. (2007). Acoustic and stiffness properties of gillnets as they relate to small cetacean bycatch. ICES J. Mar. Sci. 64, 1324–1332. doi: 10.1093/ICESJMS/FSM135
Mooney T. A., Nachtigall P. E., Au W. W. L. (2004). Target strength of a nylon monofilament and an acoustically enhanced gillnet: predictions of biosonar detection ranges. Aquat. Mamm. 30, 220–226. doi: 10.1578/AM.30.2.2004.220
Mooney T. A., Pacini A. F., Nachtigall P. E. (2009). False killer whale (Pseudorca crassidens) echolocation and acoustic disruption: implications for longline bycatch and depredation. Can. J. Zool. 87, 726–733. doi: 10.1139/Z09-061
Moore J. E., Heinemann D., Francis T. B., Hammond P. S., Long K. J., Punt A. E., et al. (2021). Estimating bycatch mortality for marine mammals: Concepts and best practices. Front. Mar. Sci. 8, 752356. doi: 10.3389/fmars.2021.752356
Nachtigall P. E., Supin A. Y. (2008). A false killer whale adjusts its hearing when it echolocates. J. Exp. Biol. 211, 1714–1718. doi: 10.1242/jeb.013862
Northridge S., Coram A., Kingston A., Crawford R. (2017). Disentangling the causes of protected-species bycatch in gillnet fisheries. Conserv. Biol. 31, 686–695. doi: 10.1111/cobi.12741
Papageorgiou M., Hadjioannou L., Jimenez C., Georgiou A., Petrou A. (2022). Understanding the interactions between cetaceans and other megafauna with the albacore tuna fishery: A case study from the cyprus’ pelagic longline fishery. Front. Mar. Sci. 9, 868464. doi: 10.3389/fmars.2022.868464
Passadore C., Domingo A., Secchi E. R. (2015). Analysis of marine mammal bycatch in the Uruguayan pelagic longline fishery operating in the southwestern Atlantic ocean. ICES J. Mar. Sci. 72, 1637–1652. doi: 10.1093/icesjms/fsu250
Perrin W. F. (2009). “Pantropical spotted dolphin stenella attenuata”, in Encyclopedia of marine mammals (Second edition). Eds. Schlitzer W. F., Würsig B., Thewissen. J. G. M. (Cambridge: Academic Press), 819–821.
Perrin W. F., Hohn A. A. (1994). “Pantropical spotted dolphin stenella attenuata,” in Handbook of marine mammals, Vol.5: The first book of dolphins (Cambridge: Academic Press), 71–98.
Prado J. H., Kinas P. G., Pennino M. G., Seyboth E., Silveira F. R. G., Ferreira E. C., et al. (2021). Definition of no-fishing zones and fishing effort limits to reduce franciscana bycatch to sustainable levels in southern Brazil. Anim. Conserv. 24, 770–782. doi: 10.1111/acv.12679
Qin C. Y., Fang Z. Q., Zhou H. Y., An D. (2009). Contents and evaluation of heavy metals in pantropical spotted dolphin from beibu bay in south China Sea. J. Oceanogr. Taiwan Strait 28, 192–198. doi: 10.3969/j.issn.1000-8160.2009.02.007
Rabearisoa N., Bach P., Marsac F. (2015). Assessing interactions between dolphins and small pelagic fish on branchline to design a depredation mitigation device in pelagic longline fisheries. ICES J. Mar. Sci. 72, 1682–1690. doi: 10.1093/icesjms/fsu252
Read A. J. (2008). The looming crisis: interactions between marine mammals and fisheries. J. Mammal. 89, 541–548. doi: 10.1644/07-MAMM-S-315R1.1
Read A. J., Drinker P., Northridge S. (2006). Bycatch of marine mammals in U.S. and global fisheries. Conserv. Biol. 20, 163–169. doi: 10.1111/j.1523-1739.2006.00338.x
Reeves R. R., McClellan K., Werner T. B. (2013). Marine mammal bycatch in gillnet and other entangling net fisheries 1990 to 2011. Endanger. Species Res. 20, 71–97. doi: 10.3354/esr00481
Schakner Z. A., Blumstein D. T. (2013). Behavioral biology of marine mammal deterrents: A review and prospectus. Biol. Conserv. 167, 380–389. doi: 10.1016/j.biocon.2013.08.024
Schlitzer R. (2021) Ocean data view. Available at: https://odv.awi.de.
Senko J., White E. R., Heppell S. S., Gerber L. R. (2013). Comparing bycatch mitigation strategies for vulnerable marine megafauna. Anim. Conserv. 17, 5–18. doi: 10.1111/acv.12051
Slooten E., Wang J. Y., Dungan S. Z., Forney K. A., Hung S. K., Jefferson T. A., et al. (2013). Impacts of fisheries on the critically endangered humpback dolphin Sousa chinensis population in the eastern Taiwan strait. Endang. Species. Res. 22, 99–114. doi: 10.3354/esr00518
Stack S. H., Currie J. J., McCordic J. A., Olson G. L. (2019). Incidence of odontocetes with dorsal fin collapse in Maui nui, Hawaii. Aquat. Mamm. 45, 257–269. doi: 10.1578/AM.45.3.2019
Tarling G. A., Klevjer T., Fielding S., Watkins J., Atkinson A., Murphy E., et al. (2009). Variability and predictability of Antarctic krill swarm structure. Deep Sea Res. Part I 56, 1994–2012. doi: 10.1016/j.dsr.2009.07.004
Temple A. J., Westmerland E., Berggren P. (2021). By-catch risk for toothed whales in global small-scale fisheries. Fish. Fish. 22, 1155–1159. doi: 10.1111/faf.12581
Thode A., Mathias D., Straley J., O’Connell V., Behnken L., Falvey D., et al. (2015). Cues, creaks, and decoys: using passive acoustic monitoring as a tool for studying sperm whale depredation. ICES J. Mar. Sci. 72, 1621–1636. doi: 10.1093/icesjms/fsv024
Tixier P., Gasco N., Duhamel G., Guinet C. (2014). Habituation to an acoustic harassment device (AHD) by killer whales depredating demersal longlines. ICES J. Mar. Sci. 72, 1673–1681. doi: 10.1093/icesjms/fsu166
Wade P. R., Long K. J., Francis T. B., Punt A. E., Hammond P. S., Dennis H., et al. (2021). Best practices for assessing and managing bycatch of marine mammals. Front. Mar. Sci. 8, 757330. doi: 10.3389/fmars.2021.757330
Wang Y. M., Li W., Van Waerebeek K. (2015). Strandings, bycatches and injuries of aquatic mammals in china 2000 –2006, as reviewed from official documents: A compelling argument for a nationwide strandings programme. Mar. Policy 51, 242–250. doi: 10.1016/j.marpol.2014.07.016
Wang T., Zhang P., li J., Fu W. J., Xie B., Yan L., et al. (2021). Acoustic deterrent of pantropical spotted dolphins (Stenella attenuata) from light-falling net fisheries in the south China Sea. J. Fish. Sci. China 28, 348–354. doi: 10.12264/jfsc2020-0106
Werner T. B., Northridge S., Press K. M., Young N. (2015). Mitigating bycatch and depredation of marine mammals in longline fisheries. ICES J. Mar. Sci. 72, 1576–1586. doi: 10.1093/icesjms/fsv092
Wilson S. M., Raby G. D., Burnett N. J., Hinch S. G., Cooke S. J. (2014). Looking beyond the mortality of bycatch: sublethal effects of incidental capture on marine animals. Biol. Conserv. 171, 61–72. doi: 10.1016/j.biocon.2014.01.020
Wu H. P., Li Q. H., Wang C. Y., Wu Q. W., Peng C. W., Jefferson T. A., et al. (2022). Bycatch mitigation requires livelihood solutions, not just fishing bans: A case study of the trammel-net fishery in the northern beibu gulf, China. Mar. Policy 139, 105018. doi: 10.1016/j.marpol.2022.105018
Wu F. X., Wang X. Y., Zhang Q. X., Miao X., Zhang T., Zhu Q. (2015). First record of pantropical spotted dolphins stenella attenuata in the yellow Sea, China. Chin. J. Ocean. Limnol. 33, 869–877. doi: 10.1007/s00343-015-4163-1
Yang L. (2005). Selected fishing technology to reduce bycatch. Modern Fish. Inf. 20, 6-8, 12. doi: 10.3969/j.issn.1004-8340.2005.04.002
Zhang J., Jiang Y. E., Chen Z. Z., Gong Y. Y., Chen G. B. (2017). Preliminary study on the nautical area scattering coefficient and distribution of mesopelagic fish species in the central-southern part of the south China Sea. J. Fish. Sci. China 24, 120. doi: 10.3724/SP.J.1118.2017.16095
Zhang B., Ma Z. C., Liu W. Z. (2010). Theoretical and experimental studies of the scattering characteristic of dolphin. Appl. Acoust. 29, 122–128. doi: 10.1360/972010-1292
Zhao L. Y., Zhu Q., Miao X., Min X., Wu. F. X., Dai Y. F., et al. (2017). An overview of cetacean strandings, bycatches and rescues along the western coast of the Taiwan strait, China: 2010–2015. Acta Oceanol. Sin. 36, 31–36. doi: 10.1007/s13131-017-1100-5
Zhou B., Zhang J. Z., Zhou R. (2007). Study on influence of tonggu channel channel construction project on zhujiang estuary Chinese white dolphin nature reserve. Ocean Technol. 26, 38–41. doi: 10.3969/j.issn.1003-2029.2007.02.011
Keywords: pantropical spotted dolphins (Stenella attenuata), bycatch mitigation, acoustic deterrent system, fishery interaction, acoustic monitoring
Citation: Fu W, Song Z, Wang T, Gao Z, Li J, Zhang P and Zhang Y (2022) Acoustic deterrence to facilitate the conservation of pantropical spotted dolphins (Stenella attenuata) in the Western Pacific Ocean. Front. Mar. Sci. 9:1023860. doi: 10.3389/fmars.2022.1023860
Received: 20 August 2022; Accepted: 05 October 2022;
Published: 24 October 2022.
Edited by:
Jeffrey C Mangel, Prodelphinus, PeruReviewed by:
Salvatore Siciliano, Fundação Oswaldo Cruz (Fiocruz), BrazilDenise Lore Herzing, Florida Atlantic University, United States
Copyright © 2022 Fu, Song, Wang, Gao, Li, Zhang and Zhang. This is an open-access article distributed under the terms of the Creative Commons Attribution License (CC BY). The use, distribution or reproduction in other forums is permitted, provided the original author(s) and the copyright owner(s) are credited and that the original publication in this journal is cited, in accordance with accepted academic practice. No use, distribution or reproduction is permitted which does not comply with these terms.
*Correspondence: Zhongchang Song, c29uZ3pob25nY2hhbmdAZm94bWFpbC5jb20=; Teng Wang, dHdhbmdzaGFuZ2hhb0AxNjMuY29t; Yu Zhang, eXV6aGFuZ0B4bXUuZWR1LmNu