Deep-sea biology in undergraduate classrooms: Open access data from remotely operated vehicles provide impactful research experiences
- 1Biology Department, State University of New York at Geneseo, Geneseo, NY, United States
- 2Ocean Exploration, National Oceanic and Atmospheric Administration, U.S. Department of Commerce, Silver Spring, MD, United States
Opportunities for students to conduct research in courses increase feelings of belonging in science, retention in STEM majors, and sense of ownership in a student’s educational experience. However, many research fields are challenging to bring to students: for example, deep-sea biology often requires expensive expeditions, restricting participation and accessibility. Access to deep-sea systems has been expanded by programs such as the National Oceanic and Atmospheric Administration's Ocean Exploration (NOAA Ocean Exploration), which uses telepresence to bring deep-sea exploration to a global audience. Here, we present one example of how remotely operated vehicles can engage students in original research. Students in an undergraduate Marine Biology lab at at the State University of New York at Geneseo investigated the relationship between substrate rugosity and biodiversity of cold-water coral communities and associate fauna. The study site in the Pacific Remote Islands Marine National Monument was explored by NOAA Ocean Exploration using NOAA Ship Okeanos Explorer as part of the 2017 Mountains in the Deep Expedition, EX1705. Organism density was greater at higher rugosity levels, suggesting that complex substrates support higher abundances in deep-sea communities. This research experience enhanced student’s understanding of the scientific process, appreciation for deep-sea communities, and engagement in the course. Open access deep-sea data provide impactful opportunities for students to participate in original research, increasing the accessibility and reach of deep-sea science.
Introduction
While traditional undergraduate education focuses on answers—known facts from textbooks tested by recall on exams—science itself is a process driven by questions (e.g., Vale, 2013). Best practices in science, technology, engineering, and mathematics (STEM) pedagogy now call for students to be trained not only in content, but to experience the research process through discovery-based learning opportunities (e.g., Woodin et al., 2010). In 2010, the American Association for the Advancement of Science stated in their call to action in revising undergraduate biology education that, “Students should have opportunities to participate in authentic research experiences and learn how to evaluate complex biology problems from a variety of perspectives, not just recite facts and terminology” (Woodin et al., 2010). To engage undergraduates in research at the necessary scale requires not only internships, directed study, and research experiences for undergraduates (REU) opportunities, but for research to be brought directly into lab courses (e.g., Auchincloss et al., 2014; Spell et al., 2014).
Course-based research experiences1 offer students meaningful and accessible opportunities to engage in research as part of their science education (Auchincloss et al., 2014). During a laboratory course, for example, all students in a class work together to collect data and answer a scientific question. Because the research experience is built into an existing course, these programs increase access to research opportunities for students across identities (e.g., Woodin et al., 2010; Auchincloss et al., 2014; Shortlidge and Brownell, 2016). Research experiences also provide chances for active learning, which have been shown to lessen opportunity gaps between students from identity groups that have been overrepresented in the sciences and those who belong to historically excluded identity groups in STEM fields (Theobald et al., 2020). Bringing research into laboratory classes can reduce barriers for students—such as lack of awareness about research opportunities and financial barriers to volunteering in a lab—and be a valuable pathway to increase diversity in the sciences (Bangera and Brownell, 2014).
The benefits of course-based research experiences for undergraduates have been demonstrated across multiple types of institutions and projects (e.g., Mader et al., 2017) and are effective for students in both introductory courses (e.g., Kortz and Van Der Hoeven Kraft, 2016) and discipline-specific programs (e.g., Cooper and Brownell, 2018). Students see gains in multiple areas after course-based research opportunities, from understanding how research works and how scientists solve real problems to improving their abilities in analyzing data and understanding scientific papers (Mader et al., 2017). Students who participate in course-based research experiences tend to report increased feelings of belonging in science and a stronger personal science identity. These increases in science identity are stronger for students who collect their own data as part of the research experience than for those who analyze data collected by others. Students who collect and analyze their own data also feel greater emotional ownership of the project, increasing the impact of the course-based research experience (Cooper et al., 2020).
Certain fields are easier to adapt to course-based research experiences than others. Disciplines that require field work, rare samples, long time scales, and high levels of subject expertise can be challenging to bring to undergraduate classrooms. Further, the COVID-19 pandemic brought new barriers to engaging students in inquiry-based labs due to the shift to online learning. Online formats make student engagement especially important, due to the reduced opportunities to feel belonging and connect with peers and instructors (e.g. Martin and Bolliger, 2018). In both in-person and online courses, access to deep-sea science has been limited for undergraduates, due the expense and challenges of reaching deep habitats (Stephens et al., 2016). Yet, the deep seas represent the largest habitat on Earth (e.g., Ramirez-Llodra et al., 2010) and hold significant opportunities to engage students in original and impactful research in the classroom.
Effective course-based research experiences require five elements, according to established best practices. Throughout the research experience, students should: 1) use scientific techniques, 2) experience discovery, 3) work in broadly relevant and/or important fields, 4) collaborate with one another to develop both intellectual and communication skills, and 5) practice iteration, building on existing work and accumulating data over time (Auchincloss et al., 2014). These course-based research experiences have also been shown to be most effective when 1) the research component makes up the majority of the laboratory course, 2) when students contribute to study design, and 3) when results are original and unknown to both students and faculty when the project begins (Mader et al., 2017). Because course-based research experiences need to serve two objectives: those of the research and the pedagogical objectives, research-based courses require careful backward design to identify and meet learning outcomes (Cooper et al., 2017). These best practices of course-based research can be achieved studying deep-sea systems in the classroom, through the power of open access data. Here, we present an example of how students can conduct original research in deep-sea biology using publicly available videos from remotely operated vehicles (ROVs) and telepresence technology.
In recent years, telepresence-enabled vessels have expanded the accessibility of deep-sea habitats by broadcasting deep-sea exploration to global online audiences. Since the pioneering of ship-to-shore telepresence technology by Dr. Robert Ballard’s 1989 JASON Project, telepresence has been increasingly used for both education outreach and to broaden participation of a wider science party (Raineault et al., 2018). Today, programs by Ocean Exploration Trust’s EV Nautilus, Schmidt Ocean Institute’s RV Falkor, and National Oceanic and Atmospheric Administration (NOAA) Ocean Exploration using NOAA Ship Okeanos Explorer share deep-sea exploration through telepresence, allowing a global audience to engage with deep-sea habitats on unprecedented scales (Martinez and Keener-Chavis, 2006; Marlow et al., 2017; Raineault et al., 2018). Video from NOAA Ocean Exploration’s ROV dives are made publicly available through the Ocean Exploration Video Portal (https://www.ncei.noaa.gov/access/ocean-exploration/video/) and the platform SeaTube (Ocean Networks Canada, https://data.oceannetworks.ca/ExpeditionManagement). SeaTube also offers access to annotations of organisms made by various science parties and metadata such as latitude, longitude, and depth. Additional metadata including temperature, salinity, current speed, and oxygen concentration are also openly available through the NOAA Ocean Exploration data resources, including through the NOAA Ocean Exploration Data Atlas (https://www.ncei.noaa.gov/maps/ocean-exploration-data-atlas/). Other platforms also provide open-access deep-sea data, such as GenBank (genetic data, National Center for Biotechnology Information, https://www.ncbi.nlm.nih.gov/genbank/), MorphoSource (3D morphology data, Duke University, National Science Foundation, https://www.morphosource.org/), FathomNet (artificial intelligence processing of imagery data, MBARI, NOAA, National Geographic Society, Katija et al., 2022, https://fathomnet.org/), and the Deep-Sea ID App (taxonomic information, Natural History Museum, London, Glover et al., 2015, https://www.marinespecies.org/deepsea/app.php). These open access data provide unprecedented opportunities to bring deep-sea research experiences into undergraduate classrooms. Further, these telepresence-enabled expeditions often target diverse and captivating deep-sea habitats, such as hydrothermal vents, seeps, and deep-sea coral gardens with high-quality video that can engage students in deep-sea biology.
Coral reefs are some of the most diverse, captivating, economically important, and vulnerable marine habitats (e.g., Wagner et al., 2020). While shallow-water reef systems have received great attention from the fields of both biology and conservation, corals also inhabit the deep sea, to depths exceeding 4000 m (e.g., Fautin et al., 2009). These deep-sea corals live below the photic zone, relying on suspension feeding for nutrients, rather than on photosynthetic symbionts like their shallow-living counterparts (e.g., Maier et al., 2020). Deep-sea corals grow slowly, for some species on the order of 4–35 µm per year, reaching ages of over 4,000 years (Roark et al., 2009). Slow growth rates and long lifespans make deep-sea corals especially vulnerable to human impacts (e.g. Prouty et al., 2017). In addition to the corals themselves, deep-sea coral gardens create important habitat for associate fauna such as seastars, brittle stars, crabs, amphipods, acorn worms, barnacles, isopods, snails, and crinoids, among others (e.g., Freiwald and Roberts, 2005).
Most records of reef-building corals in international waters are known from habitats with steep topographic features such as seamounts (Wagner et al., 2020). While this association between deep-sea corals and large-scale features like ridges and seamounts and local peaks is well understood (e.g., Genin et al., 1986), the role that fine-scale changes in seafloor rugosity play in structuring deep-sea coral community biodiversity remains largely unknown. In an undergraduate Marine Biology lab at the State University of New York at Geneseo (SUNY Geneseo), we studied deep-sea benthic communities to understand how local changes in substrate roughness, or rugosity, influence biodiversity. In shallow water reef communities, substrate rugosity can enhance benthic biodiversity and habitat use (e.g., Yanovski et al., 2017; Mazzuco et al., 2020). We therefore hypothesized that deep-sea coral communities and associate fauna may also have higher biodiversity with increasing rugosity.
To study deep-sea coral community biodiversity in this course-based research experience, we used data from the Mountains in the Deep Expedition EX1705, which explored deep waters of the Pacific Remote Islands Marine National Monument (Cantwell et al., 2019). This expedition was part of NOAA’s CAPSTONE program—Campaign to Address Pacific monument Science, Technology, and Ocean Needs—a three-year effort that involved more than 250 scientists, student researchers, and managers (Leonardi et al., 2018). The CAPSTONE program conducted 187 dives with the ROV Deep Discoverer, mapped a seafloor area of almost 600,000 km2, and recorded more than 347,000 individual organisms (Kennedy et al., 2019). In this semester-long course, students studied the Jarvis Island dive (Dive 05, May 5th, 2017), between 300 and 800 meters to understand how local community diversity relates to substrate rugosity.
The goals of this manuscript are threefold. First, we present a model for how open access deep-sea video data may be used to create course-based research experiences in the classroom. Second, we report our findings regarding the relationship between deep-sea coral community biodiversity and substrate rugosity. Third, we assess the impacts of undergraduate research experiences based on NOAA Ocean Exploration video data in the classroom and in directed study research opportunities through student reflections and surveys. Together, these findings inform new understanding of the factors that structure community biodiversity in an important deep-sea habitat and share how undergraduate research experiences using deep-sea ROV footage can improve student engagement, identity as scientists, understanding of deep-sea habitats, and accessibility and inclusion in deep-sea biology.
Methods
Research experiences for undergraduate students using NOAA Ocean Exploration resources
Course-based research experience: Design and implementation
This undergraduate marine biology lab (SUNY Geneseo, BIOL 317, Fall 2020) met once per week online for two hours, during the period of remote instruction necessitated by the COVID-19 pandemic. During the first four labs of the semester, students completed more traditional lab activities, adapted to the Zoom format. These activities aimed to introduce the marine environment, practices of marine sciences, and tools for analyzing marine biological data using the statistical programming platform R (R Core Team, 2015). The remaining nine sessions of the laboratory course was structured around this course-based research experience.
Following the best practices laid out by Shortlidge and Brownell (2016) and the call for instructors to use backward design in creating course-based research experiences (Cooper et al., 2017), learning outcomes for this deep-sea research project were defined prior to the beginning of the course. These general learning outcomes were as follows. Throughout this course-based research experience students would: 1) conduct independent research in marine biology, experiencing the scientific method from hypothesis formation through to dissemination of results; 2) work collaboratively, developing strategies for effective communication and teamwork, vital to scientific success, and 3) develop an in-depth understanding of an important and vulnerable marine environment.
During the Fall 2020 semester, students investigated the relationship between substrate rugosity and deep-sea community biodiversity off Jarvis Island in the Pacific Remote Islands Marine National Monument using open access NOAA Ocean Exploration data (https://oceanexplorer.noaa.gov/data/access/access.html). To conduct this research, students worked in small teams of four to five people in Zoom breakout rooms, with a total of 20 students in the course (BIOL 317, 4 credits, Lecture and Lab). Each team elected a group leader who was responsible for promoting team time management, communicating with the class about their group’s efforts, and facilitating the group in meeting the standards they agreed upon in a group collaboration plan at the beginning of the semester. The project was conducted over a total of nine lab sessions, beginning with an introduction to the project, followed by data collection, data analysis, a writing workshop, and the writing of a final paper. Specific goals, expectations, and detailed instructions were provided at each stage of the project, with opportunities for student autonomy, particularly in the hypothesis design and testing components of this course-based research experience. At the beginning of the project, the course instructor provided the overarching research question: how does substrate rugosity impact community biodiversity? Each group then developed and tested hypotheses related to the overarching research question and their own original questions, for example, related to specific substrate and organism types.
Students were assessed on the research portion of the lab course in three ways. First, they submitted a reflection detailing their contribution to the project, what they had learned, and how they worked as a collaborator. They also designed figures to analyze and present their data, building on work we had done early in the semester learning the statistical programming platform R (R Core Team, 2015). Finally, students were assessed on a writing contribution. There were certain integral sections of the final paper that all groups wrote, and other sections that were divided between groups. Basic teaching materials including assignment instructions are provided in the Supplementary Materials with this article. Additional teaching materials such as R code and rubrics are available upon written request to the corresponding author.
Undergraduate directed study experiences
Another advantage of the course-based research experience is that interested students can easily transition into independent directed study research, continuing or expanding the project they worked on in class in following semesters. Four students from the Fall 2020 Marine Biology Lab continued work on this project in the Spring of 2021 as part of the Gerringer Lab at SUNY Geneseo. To assess the impacts of research experiences more directly, we also include surveys from eight students who completed 1–2 credit directed study research in the Spring of 2022. Seven of these students worked with NOAA Ocean Exploration video data, while one designed their own project involving local lake ecosystems. Six students worked together to assess the fish community biodiversity in the deep waters of Puerto Rico using NOAA Ocean Exploration video data. One student assessed fish swimming speed and kinematics with increasing habitat depth using NOAA Ocean Exploration videos and data collected as part of a summer REU program. Scientific results of these studies will be presented elsewhere, but the impacts of these research experiences are reported here.
Assessing the impact of research experiences for students
Students who participated in the course-based research experience (Fall 2020, n=20, SUNY Geneseo BIOL 317) wrote a self-reflection about the project and what they had learned as part of the course. To assess overall student experiences in this course-based research program, we anonymized these reflections and report key themes. To assess the impact of research experiences through 1–2 credit directed study courses, eight students were surveyed anonymously about their understanding of and attitudes toward science and their own science identities at the beginning and end of the semester (Spring 2022, SUNY Geneseo BIOL 299, BIOL 396, BIOL 399), using the Survey of Undergraduate Research Experiences (Lopatto, 2009). The end-of-semester survey also allowed students to assess potential gains during the research experience from a standardized list and a place for open comments (Lopatto, 2009). Survey questions are provided in the Supplementary Material. We compared self-reported rankings in confidence in abilities at the beginning of the semester to those reported at the beginning of the semester to those reported after the research experience and use these results to assess the impacts of this research experience.
Concerns about the efficacy of using self-reported gains in evaluating undergraduate research experiences have been raised (e.g., Linn et al., 2015). However, true control groups for independent assessments were not available for the present study. Because the Fall 2020 course operated online and during the numerous external stressors brought on the pandemic, we chose not to compare test scores or course grades to previous years’ Marine Biology classes due to the high number of uncontrolled variables that could influence students’ academic experience. Instead, we interpret the assessment results with the limitations of self-reporting in mind.
Research methods used in this course-based lab research program
Video collection: NOAA Ship Okeanos Explorer EX1705
In 2017 (April 27–May 19), NOAA Ocean Exploration conducted the Mountains in the Deep Expedition (EX1705). The expedition targeted dive sites in the National Marine Sanctuary of American Samoa, the Cook Islands’ Marae Moana marine park, and the Pacific Remote Islands Marine National Monument. Using the two-body ROV platform Deep Discoverer and Seirios, the program conducted 12 dives spanning a depth range of 230–4573 m (Cantwell et al., 2019). The ROV is equipped with high-definition video cameras that record in PRORES 1080i format and two lasers, 10 cm apart, allowing for the measurement of in situ features (e.g., Kennedy et al., 2019). We used video and still frame grabs from the video camera for this research. Additional details about the ROV survey approach employed on this expedition are provided by Kennedy et al. (2019). Environmental data such as depth, temperature, salinity, and oxygen concentration were collected via a CTD with dissolved oxygen sensors (Sea-Bird, SBE 9) and processed using software version Seasave V 7.23.2 (Kennedy et al., 2019).
To investigate the differences in community biodiversity across substrate rugosity at a fine scale, we examined Dive 5 Jarvis Island, which reached a maximum depth of 819.3 m and left the seafloor at a depth of 356.8 m after 7 hours and 6 minutes of bottom time. The dive site began at 00°23.977’S, 159°57.967’W and the ROV left the seafloor at the end of the dive at 00°23.463’S, 159°57.951’W (Cantwell et al., 2019). This dive was chosen for this course-based research experience due to the high abundance and diversity of organisms observed and the varied local topography. This dive also offered especially effective scenes for engaging students, including a predation event at 433 m, where ophiuroid brittle stars caught a squid (c.f. Abralia) from the water and an eroded carbonate tower crowded with sponges, corals, and other taxa at 375 m (Figure 1, Cantwell et al., 2019). The Jarvis Island dive was annotated for organism identifications by expedition participants live during the telecast and quality assurance, quality control for these annotations was provided by the France Lab (University of Louisiana at Lafayette).
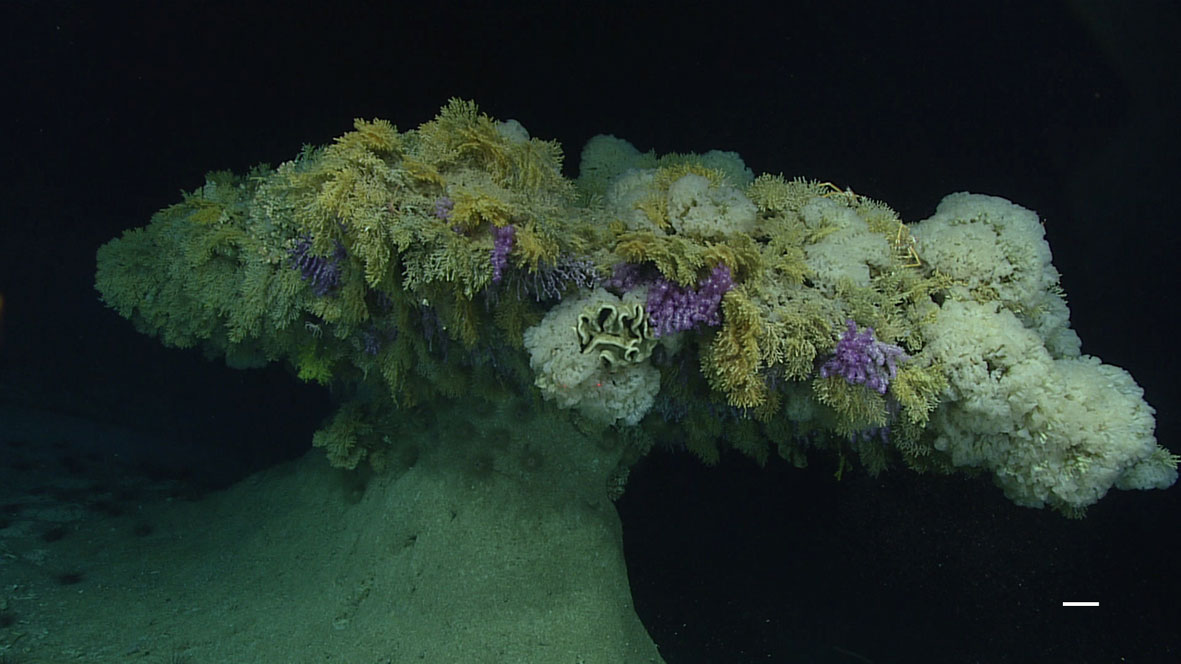
Figure 1 Deep-sea rock with a variety of sponges, corals, and other organisms. Red lasers on the center of the rock are 10 cm apart, and the white scale bar is 10 cm long (EX1705 Dive 5 Jarvis Island, 375 m). Morphotypes found on this feature include glass sponges, crabs, octocorals, scorpionfish, thin eels, sea urchins, snails, squat lobsters, and zoanthids. Photo credit: NOAA Ocean Exploration.
In-class data collection
Students accessed dive video through the NOAA Ocean Exploration Video Portal (National Centers for Environmental Information https://www.ncei.noaa.gov/access/ocean-exploration/video). Annotations and location metadata were accessed in SeaTube (Ocean Networks Canada, https://data.oceannetworks.ca/SeaTube). Future users can access SeaTube data through the new platform at https://data.oceannetworks.ca/ExpeditionManagement. Organisms that were greater than 1 cm in width and/or length that were within clear view of the ROV were counted and identified to the greatest taxonomic resolution available, using the NOAA Benthic Animal ID Guide (https://oceanexplorer.noaa.gov/okeanos/animal_guide/animal_guide.html). Taxonomic hierarchies and spellings were verified using the World Register of Marine Species (WoRMS Editorial Board, 2022). Common names of organisms were also recorded. Students measured organism sizes when laser scale marks (10 cm apart) were available using ImageJ (Schneider et al., 2012). ImageJ was also used to count highly abundant organisms from still images taken at ~20–30 second intervals, while the ROV was transiting. Still images were taken to cover the total area observed by the ROV, with smaller intervals between stills when the ROV traveled faster. Fishes were classified as benthic, pelagic, or demersal, while all other taxa were classified as benthic or pelagic. Organisms were also classified as either sessile or mobile. Associations between organisms such as predation events, one organism being perched on another, or large external parasites were also recorded. Students recorded approximate height of topographic features that organisms were found on and the approximate height of organisms off the seafloor. Rugosity was classified on a qualitative scale of 1–4, with 1 representing flat seafloor, 2 corresponding to flat seafloor punctuated with smaller rocks <50 cm in height, 3 associated with medium-sized rocks up to 1.5 m in height, and 4 representing complex rocky cliff habitat (Figure 2). The dive was divided into tracks according to the observed substrate. A new track began each time substrate rugosity changed. The linear distance of each track was calculated using the latitude and longitude of the first and last observations along that track (Table 1).
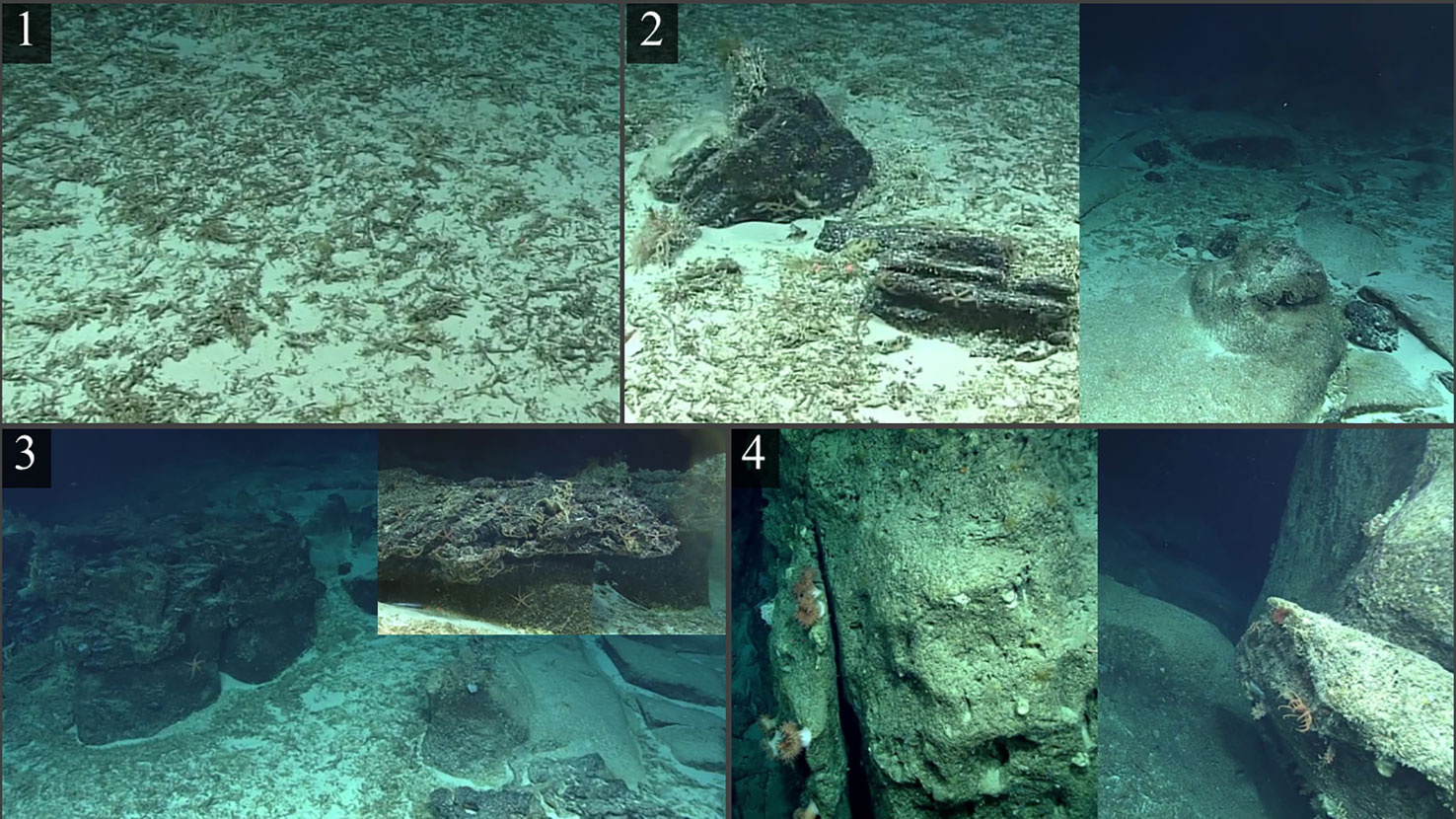
Figure 2 Qualitative classifications of substrate rugosity used to assess the relationship between habitat and biodiversity in deep-sea communities (1–4, EX1705 Dive 05, Jarvis Island). Rock feature height and abundance were used to classify rugosity. Two examples of each rugosity classification are shown for levels 2–4. Photo credit: NOAA Ocean Exploration.
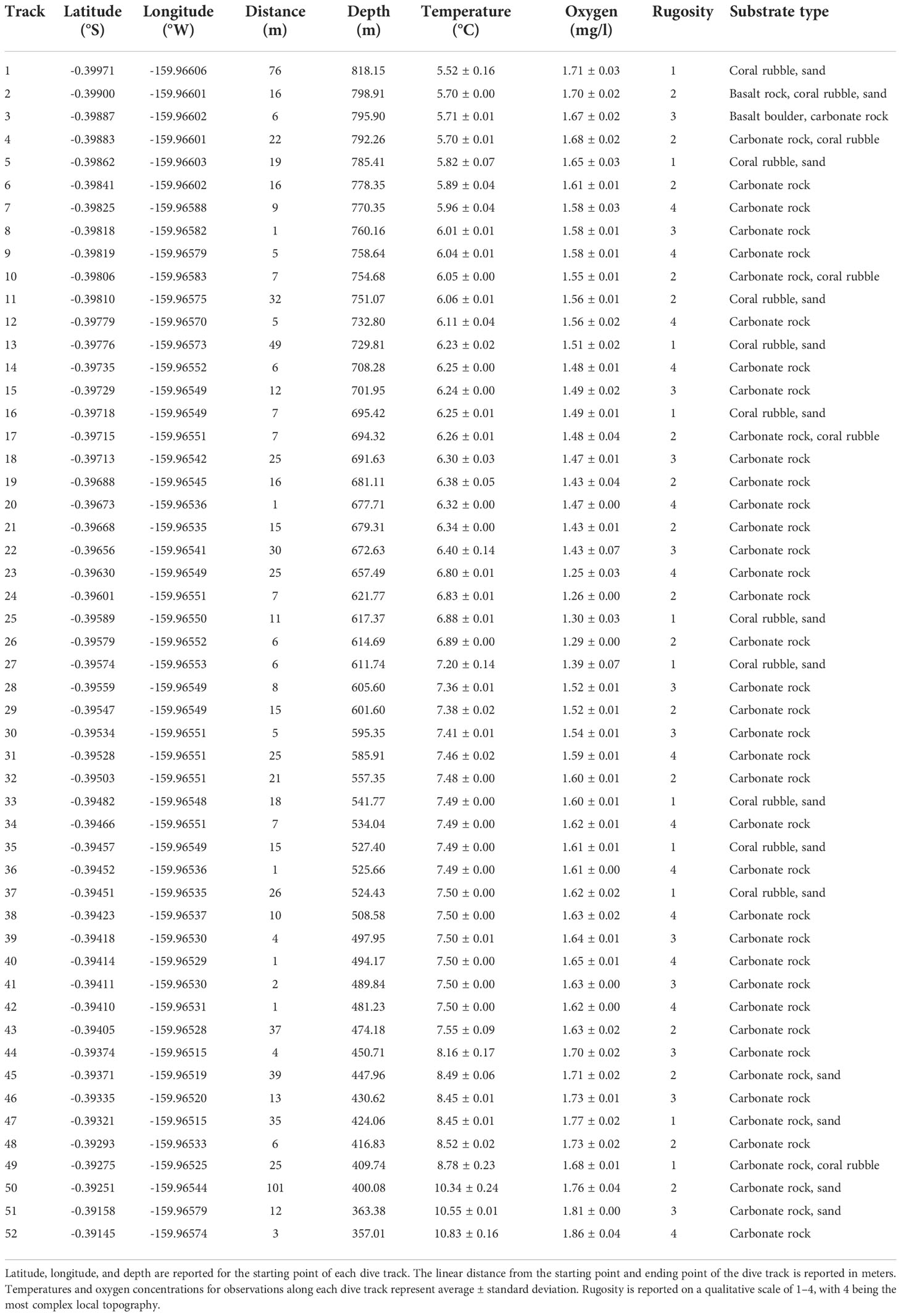
Table 1 Dive tracks of consistent rugosities identified on Dive 05 Jarvis Island, NOAA Ocean Exploration, EX1705.
All annotations were checked by a second group member to support both data quality and to incorporate the iterative and collaborative processes of science that feature in effective course-based research design (Auchincloss et al., 2014). Prior to data analysis and publication, data were checked by the instructor (MG) to ensure consistencies between rugosity classification and to check for accuracy of organism classifications.
Data analysis
Data were analyzed using the statistical programming platform R (R Core Team, 2015). Species accumulation curves were used to assess sampling efforts using the package vegan, with observations defined dive tracks of consistent rugosities, as described above (Oksanen et al., 2016). During dives, the ROV does not complete straight transects, rather it follows organisms and geology of interest to feature in video. To compare abundances of organisms at different rugosities, we divided the total number of organisms seen on a dive track by the linear distance between the location of the first and last observation. Results are reported as number of observations per linear meter. Because these abundances are not taken from straight transects, we use these values only for internal comparison within this dive site and we do not compare these values to other studies that use more precise methods to calculate densities e.g., (Price et al., 2019; Rowden et al., 2020). Diversity observed on each dive track was calculated using Shannon Diversity Index and evenness was calculated using the Shannon Equitability Index. These indices are reported at the phylum and morphotype level. For this analysis, we defined morphotypes as organisms with distinct common names, which often translated to class or family level distinctions. To compare community structure between rugosities, we used analysis of similarity (ANOSIM) tests, which analyzed Bray-Curtis Dissimilarity with 999 random permutations. Figures were created using ggplot2 (Wickam, 2009).
Results
Local variation in biodiversity of deep-sea coral communities
To assess the impact of local topography on community structure in deep-sea coral ecosystems, we identified and quantified organisms living on different substrate types and rugosities using open access ROV video from Jarvis Island (NOAA Ocean Exploration, EX1705, Dive 05). A total of 52 dive tracks of consistent rugosities were identified (Table 1). These ranged from flat seafloor, classified as rugosity level 1, to steep, cliff-like topography, classified as rugosity level 4. The calculated total distance of the dive across these 52 tracks was 867 m. Environmental conditions were also collected throughout the dive, from bottom depths 821 to 342 meters. Salinity ranged from 34.54–34.79 PSU (average 34.60 PSU) during the bottom time of the dive. Temperatures rose steadily throughout the dive, beginning at 5.32°C at deeper depths and reaching up to 11.03°C at the shallowest parts of the dive (average 7.38°C, Figure 3A). Oxygen concentrations ranged from 1.72 mg/L to 2.71 mg/L (52.26–82.46 µmol/kg) with an average of 2.27 mg/L (68.95 µmol/kg) during the bottom time of the dive. The lowest oxygen concentrations were encountered between 600 and 700 meters (Figure 3B).
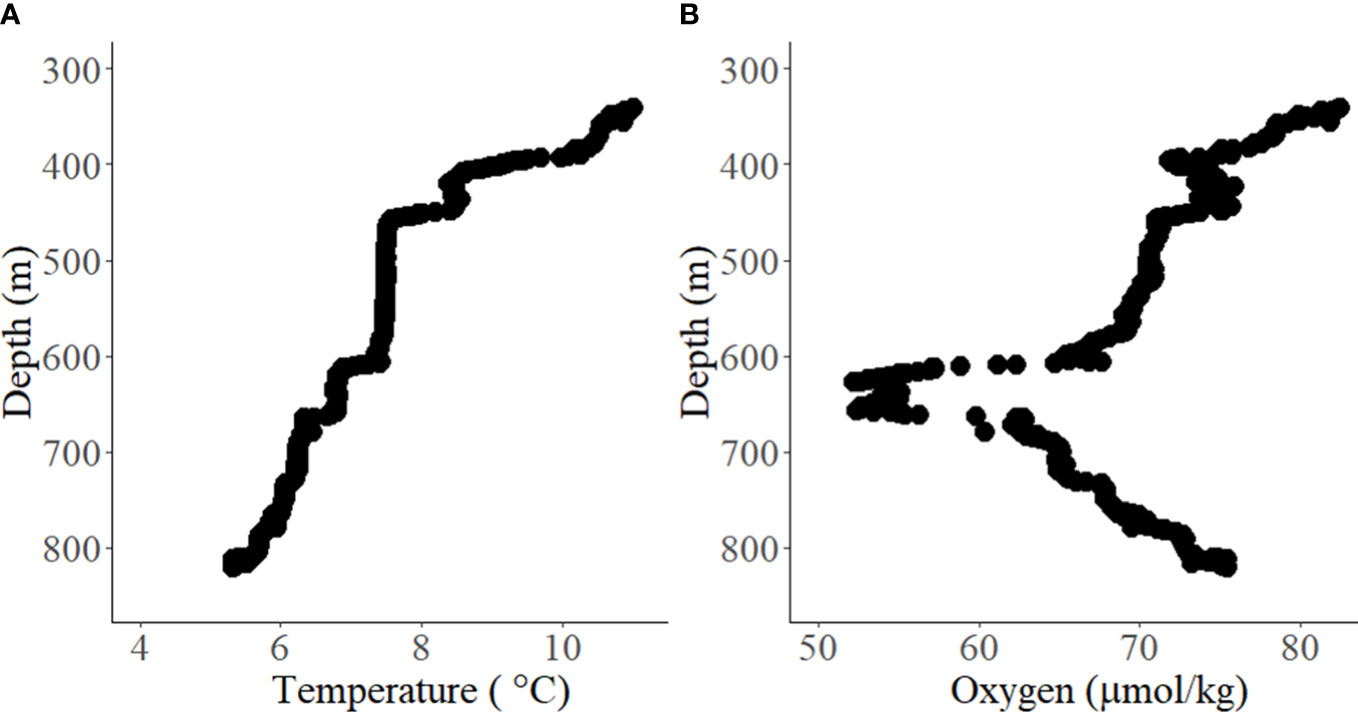
Figure 3 Environmental conditions near the seafloor throughout Dive 5, Jarvis Island (NOAA Ship Okeanos Explorer, EX1705). (A) Temperature (°C) recorded the two-bodied ROV system Deep Discoverer and Seirios by depth (m). (B) Oxygen concentration in µmol/kg by depth recorded throughout the dive.
Throughout the dive, we recorded a total of 13,660 individual organisms that could be identified to seven phyla. The most abundant phylum observed was the Echinodermata (70.4% of observed individuals), followed by the Cnidaria (18.4%), Porifera (5.9%), Annelida (2.7%), Chordata (1.5%), Arthropoda (0.8%), and Mollusca (0.3%). At the phylum level, our modeled species accumulation curve shows that the system was well sampled, with 7 phyla observed and 7.06 phyla predicted (Supplementary Figure 1, Lomolino model). Seventy-one unique morphotypes, classified by organismal common name (e.g., squat lobster, brittle star, rattail) were identified throughout the dive. The most abundant of these were sea cucumbers (24.7% of observations), primarily in the benthic genus Psolus (class Holothuroidea, family Psolidae). Other abundant taxa included brittle stars (12.3%), miscellaneous corals (12.0%), sea urchins (10.4%), crinoids (10.2%), octocorals (9.5%), and sponges (4.9%). At the morphotype level, which we delineated by unique common names, our species accumulation curve predicted 112.07 types of organisms are likely present in the community, more than our observed 71 (Figure 4, Lomolino model).
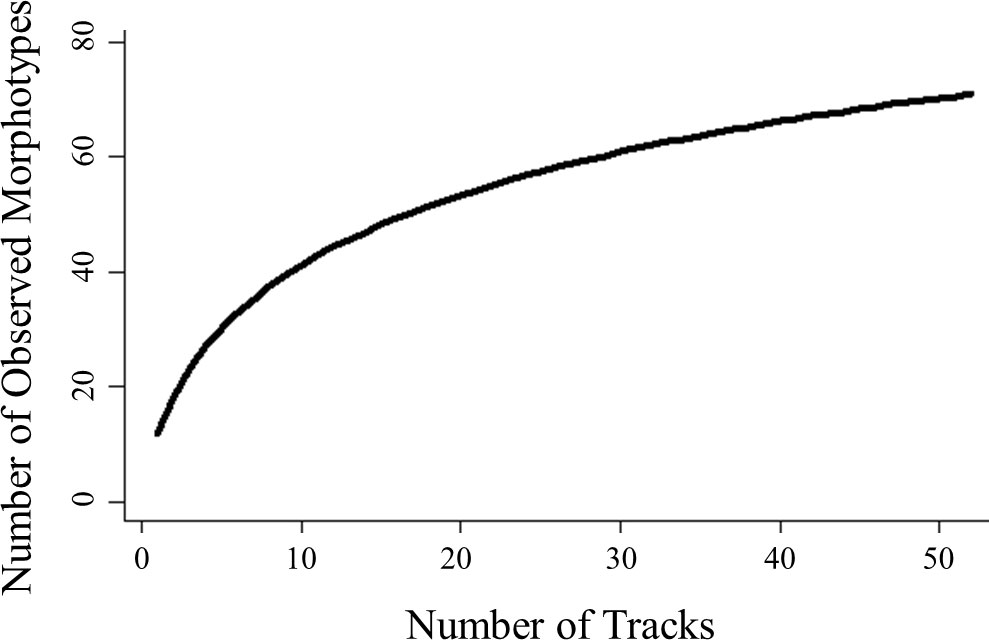
Figure 4 Species accumulation curve modeling the number of morphotypes likely to be observed with increasing sampling effort. Tracks represent dive sections of distinct rugosities. Morphotypes are classified by common name (e.g., shrimp, octopus, gold coral). The model predicts 112.07 types of organisms present in this community (Lomolino).
Environmental factors of temperature, oxygen, substrate type, and habitat depth correlated with some, but not all, aspects of community structure. At the phylum level, temperature was not significantly correlated with diversity (Shannon Diversity Index, ANOVA, F1,50 = 2.029, p=0.161), evenness (Shannon Equitability Index, ANOVA, F1,50 = 2.808, p=0.1), or the number of organisms observed per linear meter traveled by the ROV (ANOVA, F1,50 = 0.839, p=0.364). However, at the morphotype level, both community diversity and evenness decreased with increasing temperature (Shannon Diversity Index, ANOVA, α=0.01, F1,50 = 3.378, p=0.072; Shannon Equitability Index, ANOVA, α=0.05, F1,49 = 5.931, p=0.0186). Organismal abundance and community structure did correlate with oxygen concentration. Evenness decreased with increasing oxygen concentration at both the phylum and morphotype levels (ANOVA, α=0.05, phylum: F1,50 = 6.779, p=0.0121; morphotype: F1,49 = 7.195, p=0.00994). Biodiversity of observed megafauna decreased with increasing oxygen concentrations at the phylum level (ANOVA, α=0.1, F1,50 = 3.411, p=0.0707), but not at the morphotype level (F1,50 = 0.631, p=0.431). The number of organisms observed per meter traveled did not correlate with environmental oxygen concentration (ANOVA, F1,50 = 1.291, p=0.261). Substrate type did not significantly correlate with the number of organisms observed per meter, biodiversity, or evenness (ANOVA, F5,46 = 0.617, 0.412, 0.471, p>0.1). Habitat depth, which would include other environmental factors such as light and food availability, most strongly correlated with community composition. At deeper depths, community diversity was higher (Figure 5, Shannon Diversity Index, ANOVA, α=0.01, phylum level: F1,50 = 7.226, p=0.00973; morphotype level: F1,50 = 7.206, p=0.00983). Evenness was also higher at greater habitat depths along the dive (Figure 5, Shannon Equitability Index, ANOVA, α=0.01, phylum level: F1,50 = 7.958, p=0.00685; morphotype level: F1,49 = 9.023, p=0.00419). Despite these trends, diversity and evenness data showed a high degree of scatter across depths (Figure 5). The number of organisms observed per linear meter was higher at the shallower depths of the dive, although this trend was less strong than the relationships between evenness and diversity and habitat depth (ANOVA, α=0.1, F1,50 = 3.548, p=0.0654).
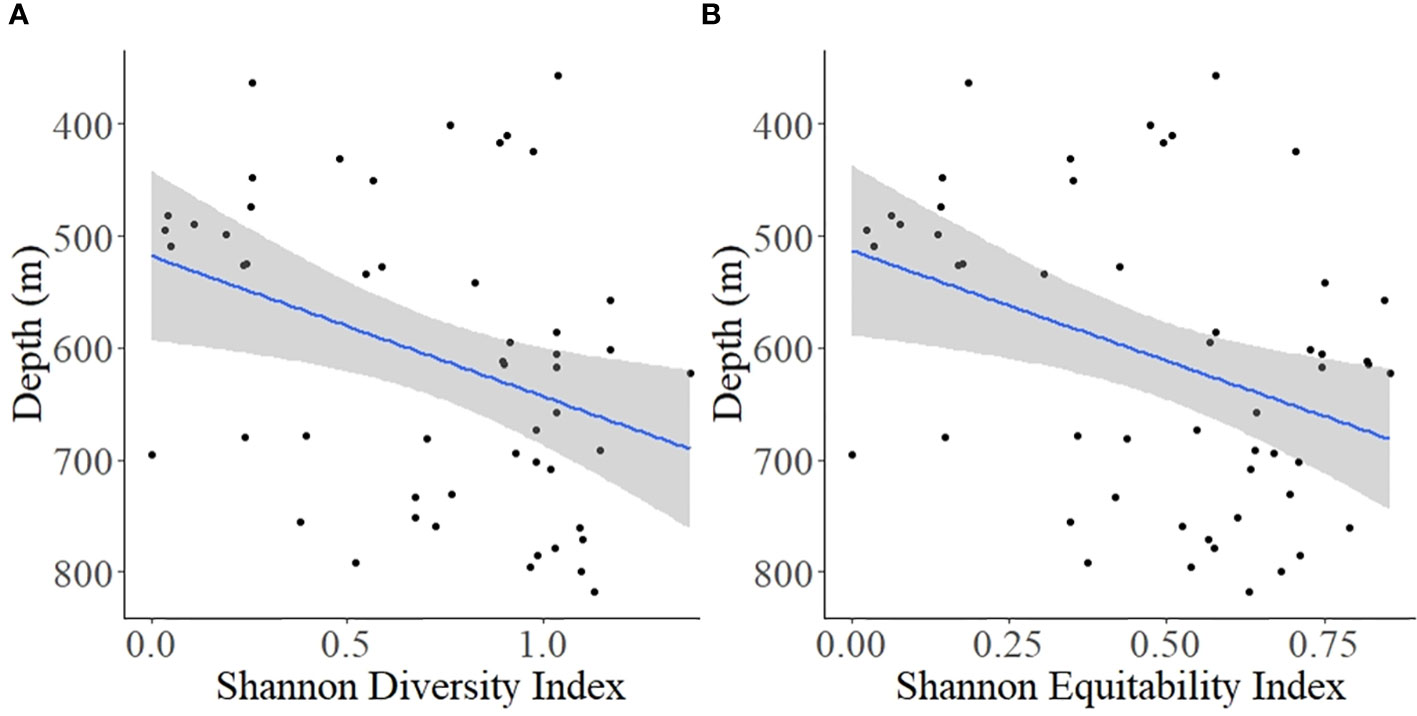
Figure 5 Diversity and evenness of megafaunal communities off Jarvis Island by habitat depth (m). Shannon Diversity Index (A) and Shannon Equitability Index (B) of dive tracks at consistent rugosities by depth are shown.
The density of observed organisms per linear meter traveled by the ROV was greater at higher rugosities (Figure 6, ANOVA, α=0.01, F1,50 = 9.441, p=0.00343). Rugosity did not significantly correlate with community composition at the phylum level (ANOSIM, n=52, R=0.01561, p=0.284, Figure 7) or at the morphotype level, classified by unique common names (Figure 8, ANOSIM, n=52, R=0.08831, p=0.012). At the morphotype level, community evenness decreased at higher rugosities (Shannon Equitability Index, ANOVA, α=0.05, F3,47 = 2.905, p=0.0445). Rugosity did not significantly correlate with community diversity at the morphotype level (Shannon Diversity Index, ANOVA, F3,48 = 0987, p=0.407). The abundance of sponges found on each dive track increased with greater substrate complexity, being highest at rugosity level 4 (ANOVA, α=0.05, F3,48 = 4.146, p=0.0108). The abundance of other phyla did not significantly vary by rugosity (Figure 7). In terms of relative abundance, Echinodermata was the most commonly observed phylum across rugosities, followed by Cnidaria. At rugosities 2 and 3, Annelida were third most abundant, while at rugosities 1 and 4, Annelida were the least abundant phyla. Chordata were third most abundant at rugosity 1 and Porifera were third most abundant at rugosity 4 (Figure 7). The diversity of the echinoderms drove the observed trends in community composition at the morphotype level (Figure 8), with some tracks being dominated by sea cucumbers, while others had more abundant brittle stars, or crinoids.
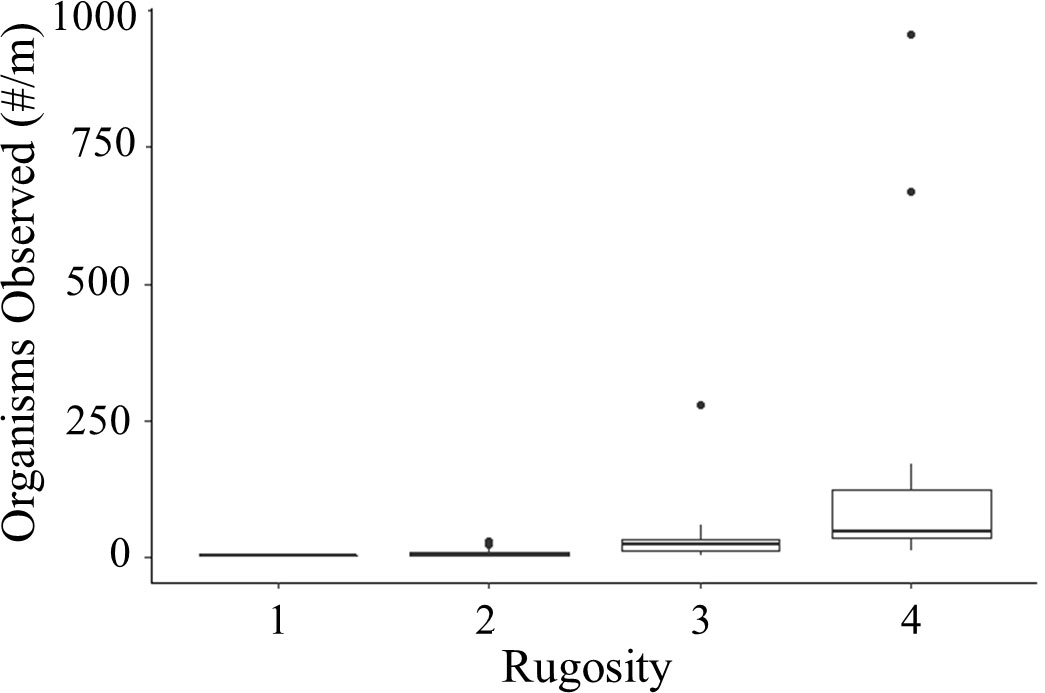
Figure 6 Density of observed organisms (number of observations per linear distance traveled by the ROV along each dive track) at different rugosities in deep waters off Jarvis Island, with 1 being the smoothest seafloor, 2 flat seafloor with rocks <50 cm, 3 medium-sized rocks 0.5–1.5 m, and 4 being the most complex substrate with rocks >1.5 m.
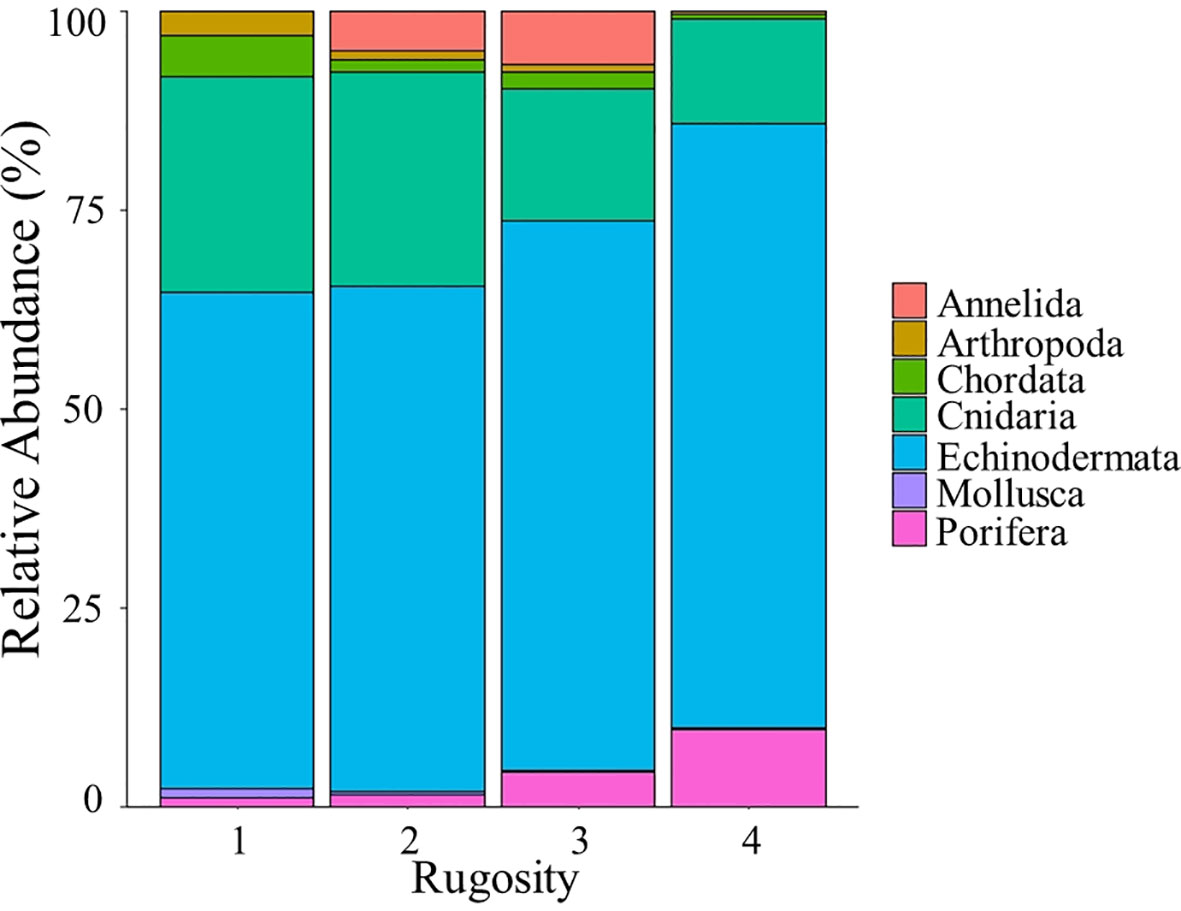
Figure 7 Relative abundances of different phyla by substrate rugosity in deep waters off Jarvis Island. Phyla are represented by color and appear in alphabetical order from the top to the bottom of each bar.
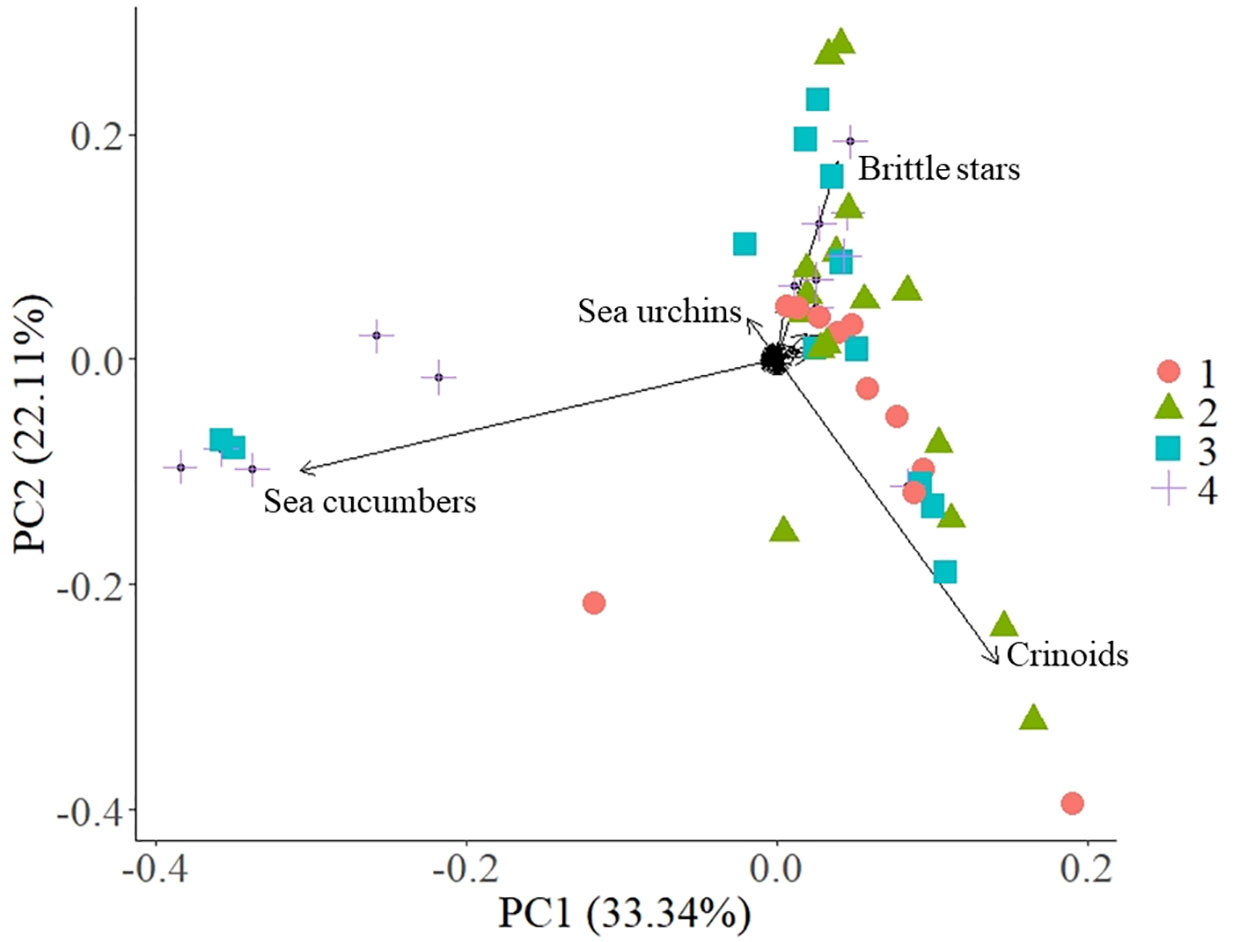
Figure 8 Principal components analysis comparing community composition by morphotype (common name) at different rugosities in waters off Jarvis Island (NOAA Ocean Exploration EX1705), with 1 being the smoothest seafloor and 4 being the most structurally complex topography. Loadings show the contributions of taxa that most strongly drive community differences between rugosities.
Undergraduate reflections on classroom research experiences
Students reported high buy-in to this course-based research experience. In qualitative written self-reflections at the end of the semester, the twenty students who participated in this research described what they felt they had learned during the lab course and evaluated their efforts as a scientific collaborator. The largest challenges that students described related to learning the mechanisms of data collection, working with new tools, and the challenges of collaborating in the online format of the lab course. Students reflected on how they met these challenges and focused primarily on the benefits of the research experience, with overwhelmingly positive comments.
Three major themes about student gains emerged from these reflections. First, students expanded their view of the oceans and of what lives in the deep sea. Many described having thought of the deep ocean floor as “lifeless” or “barren” prior to taking the course. After participating in data collection, they had a stronger grasp of deep-sea environments and used terms like “diverse population,” “fantastic sites,” and “full of life” when describing this deep-water habitat. Second, another major theme of these self-reflections was that students felt like scientists and were excited to engage in some of “the work actual biologists do.” They reported having a stronger understanding of the steps and time that go into both data collection and data analysis and the importance of attention to detail in the scientific process. Students shared that the format of the NOAA Ocean Exploration videos, which were described as “fascinating,” “interesting,” and “mesmerizing,” allowed them to feel like they were part of the dive team and to feel an authentic sense of exploration. Third, many students reported gains in their understanding of science as a collaborative process. These improvements in collaboration came from both working in small groups with peers during the research experience and from listening to the collaborations of scientists on the dive itself. Students cited gains in their own abilities to effectively communicate and how they learned to work together as a group as the weeks of research progressed. Student researchers also reflected on the way that the participating scientists interacted and collaborated throughout the dive. They reported learning that it is acceptable to not know an answer offhand and to seek additional resources and insights from other experts, highlighting the important theme that “it is ok to ask questions.” They described feeling reassured by cases where one of the narrating scientists’ identifications was initially incorrect and how they were willing to admit if they had said something wrong. Students reflected on how these examples and helped them become more comfortable asking questions and increased both their science identities and abilities to collaborate effectively.
Survey of undergraduate research experiences results
There were six fourth year students, one third year student and one first year student who participated in the research experience and surveys. Five of the surveyed students self-identified as female, while three identified as male. All students were Biology majors. Four students had no previous research experience. One student identified as Black/African, one Hispanic, one Greek, and five as White. All surveyed students reported that it was not difficult to balance research and their coursework. No students reported difficulties finding sufficient time with their research mentor to discuss the project and all agreed that it was easy to plan and schedule work with their research team. Seven students stated that research was more interesting than coursework, with one neutral, and five reported that they learned more from their research experience than from courses (three neutral).
After the directed study experience, 75% of students rated their understanding of what it means to be a career scientist with higher confidence than they did at the beginning of the semester (Table 2). Students rated their perceptions of their math and social skills the same at the beginning and end of the semester, but some reported increased perceptions of writing skills (25%), public speaking skills (12.5%), and computer skills (12.5%). One student reported lower confidence in computer skills at the end of the semester than they did at the beginning of the semester and a different student reported a decrease in perceptions of social skills at the end of the semester than at the beginning. Most students stated that the research experience was much better than they expected (75%; 12.5% a little better than expectations; 12.5% met expectations). Students felt that their research supervisor was an outstanding teacher and mentor (100%). Students reported satisfaction with their research experience (12.5% mildly satisfied, 87.5% very satisfied) and were likely or very likely to choose another research experience (100% of 7 responses, 1 student preferred not to answer that question). Seven students reported that working with other students was one of the best parts of the research experience. One student who worked remotely, rather than in person with the group reported that working with other students moderately enhanced their research experience. Students reported multiple gains from their research experience across areas, including in the ability to analyze data, working independently, understanding the scientific process, and becoming part of a learning community (Table 2).
Discussion
Small-scale habitat heterogeneity influences deep-sea benthic communities
In more complex local topography, we observed greater abundances of organisms per meter traveled by the ROV. Our results from the Pacific Remote Islands Marine National Monument support previous findings from the Atlantic Ocean that local substrate complexity plays a role in structuring deep-water communities, with more organisms being observed on more complex terrain (Price et al., 2019). The present results suggest that substrate complexity, in addition to substrate type, influenced the number of organisms present, as most dive tracks were dominated by hard substrate. However, unlike in Explorer Canyon in the NE Atlantic (Price et al., 2019), total community diversity did not significantly vary across rugosity in the deep waters off Jarvis Island in the central Pacific in our study. This discrepancy could be due to observation gaps in our study, which used 2D video identifications instead of high-resolution 3D photogrammetry. It is also possible that while total community diversity did not significantly vary with changing rugosities, community assemblages were varying at a finer scale. A similar phenomenon has been shown in megafaunal biodiversity on seamounts in the Northeast Pacific Ocean, where total biodiversity does not vary with habitat depth, but the composition of species assemblages across the seamount did change considerably (McClain et al., 2010). Our species accumulation curves suggest that all megafaunal phyla present were likely sampled (Supplementary Figure 1), while ~63% of predicted morphotypes were seen (Figure 4), suggesting that hidden diversity is likely present in the ecosystem. Investigating rugosity effects on community structure in other deep-sea benthic habitats would be an important future direction to resolve this question. Community evenness was lower at higher rugosities, reflecting the dominance of certain organisms like the benthic holothurian Psolus on vertical rock faces. These benthic holothurians were only present on the most complex habitat structures, where they were observed in high abundances. Sponge abundance also increased with increasing rugosity, likely reflecting both a preference for hard substrate and an optimum habitat for filter feeding due to current flow over and around large rock structures at high rugosities. Although other phyla were not shown to be more abundant at higher rugosities in our dataset, the abundance of sponges could contribute to increased diversity and abundance of other megafauna on a long-term scale, as seen in deep Atlantic ecosystems (Beazley et al., 2013).
Community structure was also influenced by environmental factors, even on the small scale covered by this single dive. At greater dive depths (~800 m), community diversity was higher and more even than at shallower dive depths (~300 m). Organismal abundance was slightly higher at the shallower depths of the dive. The variable of depth includes multiple changes in biologically relevant parameters, including light levels, nutrient input, temperature, oxygen, and pressure. At higher temperatures, community diversity was lower, accounting for some, but not all, of the depth-related differences observed. These findings are consistent with the literature, which shows that depth plays a significant role in structuring deep-sea coral communities, particularly due to changes in temperature (e.g., Auscavitch et al., 2020). Relationships between oxygen concentration and biodiversity were less strong. Community diversity was lower at higher oxygen concentrations at the phylum level, but not at the morphotype level and communities were more even at lower oxygen concentrations. Organismal abundance did not correlate with oxygen level. The exact threshold of oxygen minimum zones remains hard to define, but oxygen concentrations from 60–120 µmol/kg can by hypoxic for and physiologically constrain many marine organisms (e.g., Gilly et al., 2013). It is therefore likely that the entirety of the dive site, which ranged from 52.26 to 82.46 µmol/kg oxygen concentration is oxygen limited and that the observed trends may reflect variable tolerance to low-oxygen conditions across taxa.
In addition to live coral, dead coral have been shown to provide habitat for associate organisms in deep-sea systems (Mortensen et al., 1995; Rowden et al., 2020). At rugosity level 1, the smoothest surfaces identified in this study, there were often fields of coral rubble, skeleton pieces of 5–20 cm in length scattered across the seafloor. While larger organisms such as brittle stars and crinoids were visible on these rubble fields, abundances were lower than on the rocky features seen on the same dive (Figure 6). However, when the ROV zoomed in to examine these rubble fields at a fine scale, many small organisms such as hydroids, polychaetes, snails, and crustaceans were visible. These organisms were below the size threshold of this study (<1 cm in length) and not counted, but their presence reveals that another, smaller scale of substrate complexity could also be influencing deep-sea community structure. Future research should continue to consider community diversity and heterogeneity across scales to best characterize deep-sea habitats and inform conservation and management measures.
The factors that structure deep-sea coral community biodiversity are known to be complex, and include influences such as depth, overlying water mass (Auscavitch et al., 2020), and prevailing deep-water currents (Bashah et al., 2020). While broad-scale trends in community diversity and biogeography with depth have received the most attention (e.g., Costello and Chaudhary, 2017), our results suggest that the complex drivers of deep-sea community abundance and structure also extend to fine-scale differences in substrate rugosity. These findings support those of previous studies that demonstrate the influence of physical structural complexity and community diversity across habitats, including in shallow (e.g., Richardson et al., 2017; Yanovski et al., 2017), submarine canyon (McClain and Barry, 2010), and deep coral reefs (Price et al., 2019). Management and conservation efforts should consider these small-scale differences in community abundance and structure to protect these vulnerable and diverse habitats (e.g., Rowden et al., 2020; Wagner et al., 2020).
Open access data from the deep ocean provide valuable opportunities for undergraduate research experiences
Our quantitative results from surveys of students who conducted independent directed study research using NOAA Ocean Exploration data demonstrate the benefits of undergraduate research experiences, adding to a growing literature in favor of undergraduate research opportunities (e.g., Lopatto, 2009; Bangera and Brownell, 2014; Linn et al., 2015; Kortz and Van Der Hoeven Kraft, 2016; Pallant et al., 2016; Cooper et al., 2020). Students reported gains in clarifying their career path, confidence in their potential to become teachers of science, and their tolerance for facing obstacles in research. At the end of the semester, they presented results at an undergraduate research conference at SUNY Geneseo (one individual oral presentation, one group presentation, and one poster presentation). Surveyed students cited especially strong gains in giving effective oral presentations (Table 2). Students reported feeling strong improvements in understanding of the scientific process and of data analysis, highlighting that research experiences provide not only content knowledge in a specific field, but development of skills including critical thinking.
The collaborative component of both the course-based research experience and the directed study research experiences stood out as being especially impactful for students. In quantitative survey results, students identified the largest gains in becoming part of a learning community. The positive collaborative elements of the research greatly enhanced student experiences. In qualitative reflections, students identified the value of listening to scientific discourse and how scientists collaborated to answer questions throughout the dive. This benefit to telepresence-enabled undergraduate research experiences was also seen by Stephens et al. (2016), who evaluated the experiences of seven students who participated in a two-week ROV cruise via telepresence in the Transforming Remotely Conducted Research through Ethnography, Education, and Rapidly Evolving Technologies (TREET) Educational Program (Pallant et al., 2016). Here, we show that the benefits can come from listening to recorded dives and collaborating with other students in data collection, in addition to participating directly in telepresence-enabled research cruises. The ability for students to gain this benefit of research from open access video, rather than only from live participation, greatly expands accessibility for undergraduates to experience effective course-based research.
Successfully implementing course-based research experiences requires significant investment of time, both in terms of instructor preparation and in the class time devoted to research. Indeed, the greater the amount of time students invest in the research project, the greater the reported gains and more positive the attitudes toward the experience (Shaffer et al., 2014; Mader et al., 2017). This time investment helps students to shift from the role of passive observer of scientific information to active contributor in the scientific method (Shaffer et al., 2014). Although implementing course-based research programs takes time, instructors can also benefit from creating stronger connections between their research and teaching (Cooper and Brownell, 2018). Faculty who teach research-based courses have also reported enjoying the lab courses more than traditional labs and that developing course-based research opportunities contributed positively to tenure and promotion (Shortlidge et al., 2016). Course-based research experiences have also been identified as a pathway to recruiting students into faculty research programs (Shortlidge et al., 2016). Multiple students continued research in future semesters from the course-based research example presented in this manuscript, positively contributing to the research lab. We argue that the benefits of these course-based research experiences—which include making deep-sea science more inclusive and accessible and increasing student belonging, performance, and retention in science majors—make these efforts well worth the investment. Platforms like Ocean Networks Canada’s SeaTube and programs such as NOAA Ocean Exploration and Ocean Exploration Trust’s EV Nautilus bring powerful opportunities to increase accessibility and reach of deep-sea science, including by bringing deep-sea research directly to students in the classroom. The power of open access data calls for ROV video to be made openly available through platforms such as SeaTube after quality control, providing valuable opportunities not only for access to deep-sea research experiences, but to enhance our understanding of deep-sea communities.
More than a decade of deep-sea exploration and over forty ROV cruises by NOAA Ocean Exploration provide ample opportunities for future course-based research experiences. To further enhance the scope of the research question and the collaborative nature of the course-based research experience, future projects could combine data from multiple class years. For example, each year could investigate a research question in one dive and compare how their findings relate to previous classes. This multi-year model would reinforce the iterative nature of science, an important aspect of successful course-based research experiences (Auchincloss et al., 2014). In advanced courses, students could also design their own research projects after an introduction to using NOAA Ocean Exploration resources, providing further opportunity for autonomy and ownership. Future efforts could also include building a discipline-based education research experience into a pedagogy course (Cooper and Brownell, 2018) to specifically assess which aspects of deep-sea science best translate to course-based research experiences. One important future direction for deep-sea pedagogy research would be to assess the scalability of course-based research experiences to determine, for example, how a program could best be implemented to serve larger numbers of students.
Studying vulnerable marine habitats in the classroom can also inspire students to pursue conservation and management careers that focus on lesser-known biodiversity hotspots. While many students who enroll in undergraduate marine biology courses have strong interests in conservation, most are not aware of either the diversity of deep-sea habitats or how human activities impact deep-sea systems. Although home to high biodiversity and species that are especially vulnerable to human impacts, more than three quarters of recorded reef-building corals in international waters reside in unprotected waters (Wagner et al., 2020). Students who explore these important, but oft unseen deep-water coral communities in the classroom may be more likely to consider these habitats in future conservation efforts. Broader awareness of deep-sea habitats like cold-water corals is a critical step in effective deep-ocean conservation (e.g., Ankamah-Yeboah et al., 2020). Course-based research experiences with open access deep-sea video data offer students an awareness of these vulnerable habitats and a better understanding of gaps in the knowledge needed to inform management decisions about these habitats. The increased content knowledge and autonomy that course-based research experiences provide may empower these young scientists to take meaningful action toward protecting these important habitats.
Conclusions
In conclusion, open access data such as the ROV videos collected by NOAA Ocean Exploration offer students valuable opportunities to conduct original research. The user-friendly data platforms such as SeaTube provide accessible ways to bring deep-sea biology into undergraduate laboratory courses. Students identified three major gains of this course-based research experience: improved understanding of deep-sea systems, increased feelings of belonging in science, and enhanced collaboration skills. For successful implementation of course-based deep-sea research, we recommend a backward design approach, sufficient instructional time, clear communications of expectations and progress, and opportunities for student autonomy in the research design process. This study adds to a growing body of evidence about the benefits of open access data and demonstrates that NOAA Ocean Exploration resources can be successfully used in classrooms to increase accessibility of deep-sea science.
Data availability statement
The original contributions presented in the study are included in the article Supplementary Material. Further inquiries can be directed to the corresponding author.
Ethics statement
The studies involving human participants were reviewed and approved by Institutional Review Board, SUNY Geneseo, IRB #202122034. The participants provided their written informed consent to participate in this study.
Author contributions
MG devised the study, served as the course instructor of record for BIOL 317 Marine Biology and research mentor in the Spring of 2022, managed and implemented the course-based research experience, curated and checked data, and wrote the main manuscript text. KC oversaw the execution of the expedition and data collection on EX1705. YI, KC, AC, FG, RB, JC, AF, LF, HN, HL, JQ, NS, and BW collected and analyzed data, contributed to the writing of the manuscript, drafted figures, and revised the manuscript text. All authors contributed to the article and approved the submitted version.
Funding
The authors thank their funding sources: National Marine Sanctuaries Foundation and NOAA Ocean Exploration Education Mini-Grants program (Award #22-01-B-417 to MG), the State University of New York at Geneseo, the Geneseo Research Foundation, and the SUNY PRODiG program.
Acknowledgments
We thank the captains and crews of the NOAA Ship Okeanos Explorer and all participants of the EX1705 Mountains in the Deep Expedition for their work collecting the video used in this work and to NOAA’s Office of Ocean Exploration and Research for sharing their footage open access. The authors acknowledge the Ocean Networks Canada and the SeaTube teams for curating these video data. We thank Scott France and the France Lab (University of Louisiana at Lafayette) for their work annotating and quality checking the dive used in this research project. Thanks to Scott France (University of Louisiana at Lafayette), and Kimberly Galvez (NOAA Ocean Exploration, University of Miami) for consultation in project planning. Thanks to all the scientists who participated on the EX1705 Dive 5: Alain Murphy (Cook Islands), Amanda Netburn (NOAA Ocean Exploration), Amber Hale (McNeese State University), Asako Matsumoto (Planetary Exploration Research Center, Chiba Institute of Technology), Astrid Leitner (University of Hawaiʻi at Mānoa) Bruce Mundy (NOAA NMFS Pacific Islands Fisheries Science Center), Charlie Wilkins (NOAA Ship Okeanos Explorer), Chris Mah (Department of Invertebrate Zoology, NMNH Smithsonian Institution), Christopher Kelley (University of Hawaiʻi), Craig Smith (University of Hawaiʻi), Danielle Dodge (FAU Harbor Branch), Deborah Glickson (National Academies of Sciences, Engineering, and Medicine), Del Bohnenstiehl (North Carolina State University), Diva Amon (University of Hawaiʻi at Mānoa), Erin Easton (Ecology and Sustainable Management of Oceanic Islands), Heidi Hirsch (NOAA NMFS PIRO Marine National Monuments Program), Jaymes Awbrey (University of Louisiana, Lafayette), Kevin Jerram (University of New Hampshire), Les Watling (University of Hawaiʻi at Mānoa), Mashkoor Malik (NOAA Ocean Exploration), Natalie Summers (University of Hawaiʻi at Mānoa), Neah Baechler (NOAA Contractor), Shirley Pomponi (HBOI-FAU CIOERT), Sonia Rowley (University of Hawaiʻi at Mānoa), Timothy Shank (Woods Hole Oceanographic Institution), and Tina Molodtsova (P.P. Shirshov Institute of Oceanology RAS). These dedicated teams make research experiences like these possible, and we are grateful for the time and work of these many contributors. The authors are grateful to the other students in the State University of New York at Geneseo’s BIOL 317 Marine Biology Fall 2020 class for actively participating in this course-based research project: X. Delcid, J.L. Donaldson, G. Kaur, J. M. Son, J.A. Stern, M. Tetreault, and S.A. Virginia. We are also grateful to the survey participants who completed directed study research in the Spring of 2022. Thanks to SUNY Geneseo, The Geneseo Research Foundation, and the SUNY PRODiG (Promoting Recruitment, Opportunity, Diversity, Inclusion, and Growth) program for funding to present this work and Sheila McCart, Suzanna Rubright, Anne Baldwin, and Betsy Colón for administrative and grant submission support. We thank the reviewers and editor for their time and comments to improve this manuscript. The authors also thank the National Marine Sanctuaries Foundation, NOAA Ocean Exploration, and the Geneseo Research Foundation.
Conflict of interest
The authors declare that the research was conducted in the absence of any commercial or financial relationships that could be construed as a potential conflict of interest.
Any use of trade, product, or company names is for descriptive purposes of the methodology used only and does not imply endorsement by NOAA or the US Government.
Publisher’s note
All claims expressed in this article are solely those of the authors and do not necessarily represent those of their affiliated organizations, or those of the publisher, the editors and the reviewers. Any product that may be evaluated in this article, or claim that may be made by its manufacturer, is not guaranteed or endorsed by the publisher.
Supplementary material
The Supplementary Material for this article can be found online at: https://www.frontiersin.org/articles/10.3389/fmars.2022.1033274/full#supplementary-material
Footnotes
- ^ These research education programs are also known throughout the literature as course-based undergraduate research experiences (CURES) and course-based research experiences (CREs). Here, we choose to avoid the acronyms to increase the accessibility of this manuscript.
References
Ankamah-Yeboah I., Xuan B. B., Hynes S., Armstrong C. W. (2020). Public perceptions of deep-sea environment: Evidence from Scotland and Norway. Front. Mar. Sci. 7 (March). doi: 10.3389/fmars.2020.00137
Auchincloss L. C., Laursen S. L., Branchaw J. L., Eagan K., Graham M., Hanauer D. I., et al. (2014). Assessment of course-based undergraduate reserach experiences: A meeting report. CBE-Life Sci. Educ. 13, 29–40. doi: 10.1187/cbe.14-01-0004
Auscavitch S. R., Deere M. C., Keller A. G., Rotjan R. D., Shank T. M., Cordes E. E., et al. (2020). Oceanographic drivers of deep-sea coral species distribution and community assembly on seamounts, islands, atolls, and reefs within the Phoenix Islands Protected Area. Front. Mar. Sci. 7. doi: 10.3389/fmars.2020.00042
Bangera G., Brownell S. E. (2014). Course-based undergraduate research experiences can make scientific research more inclusive. CBE-Life Sci. Educ. 13, 602–606. doi: 10.1187/cbe.14-06-0099
Bashah S., Galvez K. C., Eberli G. P., Cantwell K. (2020). Control of deep currents on sediment and cold-water coral distribution on the northern manihiki plateau. Front. Mar. Sci. 7. doi: 10.3389/fmars.2020.00288
Beazley L. I., Kenchington E. L., Murillo F. J., Sacau M. (2013). Deep-sea sponge grounds enhance diversity and abundance of epibenthic megafauna in the Northwest Atlantic. ICES J. Mar. Sci. 70 (7), 1471–1490. doi: 10.1093/icesjms/fst124
Cantwell K., Bohnenstiehl D., France S. C., White M. P., Netburn A. N., Bowman A. (2019). Cruise report: EX1705, mountains in the deep- exploring the central Pacific basin (ROV and mapping). (MD, United States: NOAA Office of Ocean Exploration and Research Silver Spring). doi: 10.25923/ykmv-b048
Cooper K. M., Brownell S. E. (2018). Developing course-based research experiences in discipline-based education research: Lessons learned and recommendations. J. Microbiol. Biol. Educ. 19 (2), 1–6. doi: 10.1128/jmbe.v19i2.1567
Cooper K. M., Knope M. L., Munstermann M. J., Brownell S. E. (2020). Students who analyze their own data in a course-based undergraduate research experience (CURE) show gains in scientific identity and emotional ownership of research. J. Microbiol. Biol. Educ. 21 (3), 1–11. doi: 10.1128/jmbe.v21i3.2157
Cooper K. M., Soneral P. A., Brownell S. E. (2017). Define your goals before you design a CURE: A call to use backward design in planning course-based undergraduate research experiences. J. Microbiol. Biol. Educ. 18 (2), 1–7. doi: 10.1128/jmbe.v18i2.1287
Costello M. J., Chaudhary C. (2017). Marine biodiversity, biogeography, deep-sea gradients, and conservation. Curr. Biol. 27 (11), R511–R527. doi: 10.1016/j.cub.2017.04.060
Fautin D. G., Guinotte J. M., Orr J. C. (2009). Comparative depth distribution of corallimorpharians and scleractinians (Cnidaria: Anthozoa). Mar. Ecol. Prog. Ser. 397, 63–70. doi: 10.3354/meps08271
Freiwald A., Roberts J. M. (Eds.) (2005). Cold-water corals and ecosystems (1st ed.) (Berlin, Heidelberg: Springer). doi: 10.1007/3-540-27673-4
Genin A., Dayton P. K., Lonsdale P. F., Spiess F. N. (1986). Corals on seamount peaks provide evidence of current acceleration over deep-sea topography. Nature 322, 59–61. doi: 10.1038/322059a0
Gilly W., Beman J., Litvin S., Robison B. (2013). Oceanographic and biological effects of shoaling of the oxygen minimum zone. Annu. Rev. Mar. Sci. 5, 393–420. doi: 10.1146/annurev-marine-120710-100849
Glover A. G., Higgs N. D., Horton T., Porrer A. (2015) ). deep Sea ID v.1.2 a field guide to the marine life of the deep sea. Available at: http://www.marinespecies.org/deepsea.
Katija K., Orenstein E., Schlining B., Lundsten L., Barnard K., Sainz G., et al. (2022). FathomNet: A global image database for enabling artificial intelligence in the ocean. Sci. Rep. 12, 15914. doi: 10.1038/s41598-022-19939-2
Kennedy B. R. C., Cantwell K., Malik M., Kelley C., Potter J., Elliott K., et al. (2019). The unknown and the unexplored: Insights into the Pacific deep-sea following NOAA CAPSTONE expeditions. Front. Mar. Sci. 6. doi: 10.3389/fmars.2019.00480
Kortz K. M., Van Der Hoeven Kraft K. J. (2016). Geoscience education research project: Student benefits and effective design of a course-based undergraduate research experience. J. Geosci. Educ. 64 (1), 24–36. doi: 10.5408/15-11.1
Leonardi A., Armor J., Montanio P., Werner C. (2018). CAPSTONE: NOAA’s campaign to address Pacific monument science, technology, and ocean needs. Oceanography 29, 48–52. Available at: https://www.fisheries.noaa.gov/inport/item/52222.
Linn M. C., Palmer E., Baranger A., Gerard E., Stone E. (2015). Undergraduate research experiences: Impacts and opportunities. Science 347, 6222. doi: 10.1126/science.1261757
Lopatto D. (2009). Science in solution: The impact of undergraduate reserach on student learning (First edit) (Tucson, AZ, United State: Research Corporation for Science Advancement).
Mader C. M., Beck C. W., Grillo W. H., Hollowell G. P., Hennington B. S., Staub N. L., et al. (2017). Multi-institutional, multidisciplinary study of the impact of course-based research experiences. J. Microbiol. Biol. Educ. 18 (2), 1–11. doi: 10.1128/jmbe.v18i2.1317
Maier S. R., Kutti T., Bannister R. J., Fang J. K., Van Breugel P., Van Rijswijk P., et al. (2020). Recycling pathways in cold- water coral reefs: Use of dissolved organic matter and bacteria by key suspension feeding taxa. Nat. Sci. Rep. 10, 9942. doi: 10.1038/s41598-020-66463-2
Marlow J., Borrelli C., Jungbluth S. P., Hoffman C., Marlow J., Girguis P. R., et al. (2017). Telepresence is a potentially transformative tool for field science. Proc. Natl. Acad. Sci. 114 (19), 4841–4844. doi: 10.1073/pnas.1703514114
Martin F., Bolliger D. U. (2018). Engagement matters: Student perceptions on the importance of engagement strategies in the online learning environment. Online Learn. J. 22 (1), 205–222. doi: 10.24059/olj.v22i1.1092
Martinez C., Keener-Chavis P. (2006). NOAA Ship Okeanos Explorer: Telepresence in the Service of Science, Education and Outreach. OCEANS pp. 1–5. doi: 10.1109/OCEANS.2006.306993
Mazzuco A. C. D. A., Stelzer P. S., Bernardino A. F. (2020). Substrate rugosity and temperature matters: Patterns of benthic diversity at tropical intertidal reefs in the SW Atlantic. PeerJ 8, e8289. doi: 10.7717/peerj.8289
McClain C. R., Barry J. P. (2010). Habitat heterogeneity, disturbance, and productivity work in concert to regulate biodiversity in deep submarine canyons. Ecology 91 (4), 964–976. doi: 10.1890/09-0087.1
McClain C. R., Lundsten L., Barry J., DeVogelaere A. (2010). Assemblage structure, but not diversity or density, change with depth on a northeast Pacific seamount. Mar. Ecol. 31 (s1), 14–25. doi: 10.1111/j.1439-0485.2010.00367.x
Mortensen P. B., Hovland M., Brattegard T., Farestveit R. (1995). Deep water bioherms of the scleractinian coral Lophelia pertusa (L.) at 64° N on the Norwegian shelf: Structure and associated megafauna. Sarsia 80 (2), 145–158. doi: 10.1080/00364827.1995.10413586
Oksanen J., Blanchet F. G., Kind R., Legendre P., Minchin P. R., O’Hara R. B., et al. (2016). Vegan: Community ecology package (R package version 2.3-4; p (R package version 2), 3–4. Available at: https://cran.r-project.org/package=vegan.
Pallant A., Mcintyre C., Stephens A. L. (2016). Transforming undergraduate research opportunities using telepresence. J. Geosci. Educ. 64 (2), 138–146. doi: 10.5408/15-118.1
Price D. M., Robert K., Callaway A., Lo C., Hall R. A., Huvenne V. A. I. (2019). Using 3D photogrammetry from ROV video to quantify cold- water coral reef structural complexity and investigate its influence on biodiversity and community assemblage. Coral Reefs 38, 1007–1021. doi: 10.1007/s00338-019-01827-3
Prouty N., Roark E., Andrews A., Robinson L., Hill T., Sherwood O., et al. (2017). Age, growth rates, and paleoclimate studies in deep-sea corals of the United States. CA, United States: NOAA Pacific Coastal and Marine Science Center Santa Cruz.
Raineault N. A., Bell K. L., Girguis P. (2018). Advancing ocean science and exploration through telepresence. Deep Sea Res. Part II: Topical Stud. Oceanography 150, 1–3. doi: 10.1016/j.dsr2.2018.05.008
Ramirez-Llodra E., Brandt A., Danovaro R., De Mol B., Escobar E., German C. R., et al. (2010). Deep, diverse and definitely different: Unique attributes of the world’s largest ecosystem. Biogeosciences 7 (9), 2851–2899. doi: 10.5194/bg-7-2851-2010
R Core Team. (2015). R: A language and environment for statistical computing (Vienna, Au: R Foundation for Statistical Computing).
Richardson L. E., Graham N. A. J., Hoey A. S. (2017). Cross-scale habitat structure driven by coral species composition on tropical reefs. Sci. Rep. 7, 7557. doi: 10.1038/s41598-017-08109-4
Roark E. B., Guilderson T. P., Dunbar R. B., Fallon S. J., Mucciarone D. A. (2009). Extreme longevity in proteinaceous deep-sea corals. Procedings Natl. Acad. Sci. 106 (13), 5204–5208. doi: 10.1073/pnas.0810875106
Rowden A. A., Pearman T. R. R., Bowden D. A., Anderson O. F., Clark M. R. (2020). Determining coral density thresholds for identifying structurally complex vulnerable marine ecosystems in the deep sea. Front. Mar. Sci. 7. doi: 10.3389/fmars.2020.00095
Schneider C. A., Rasband W. S., Eliceiri K. W. (2012). NIH Image to ImageJ: 25 years of image analysis. Nat. Methods 9 (7), 671–675. doi: 10.1038/nmeth.2089
Shaffer C. D., Alvarez C. J., Bednarski A. E., Dunbar D., Goodman A. L., Reinke C., et al. (2014). A course-based research experience: How benefits change with increased investment in instructional time. CBE-Life Sci. Educ. 13, 111–130. doi: 10.1187/cbe-13-08-0152
Shortlidge E. E., Bangera G., Brownell S. E. (2016). Faculty perspectives on developing and teaching course-based undergraduate research experiences. BioScience 66 (1), 54–62. doi: 10.1093/biosci/biv167
Shortlidge E. E., Brownell S. E. (2016). How to assess your CURE: A practical guide for instructors of course-based undergraduate research experiences. J. Microbiol. Biol. Educ. 17 (3), 399–408. doi: 10.1128/jmbe.v17i3.1103
Spell R. M., Guinan J. A., Miller K. R., Beck C. W. (2014). Redefining authentic research experiences in introductory biology laboratories and barriers to their implementation. CBE-Life Sci. Educ. 13, 102–110. doi: 10.1187/cbe.13-08-0169
Stephens A. L., Pallant A., McIntyre C. (2016). Telepresence-enabled remote fieldwork: Undergraduate reserach in the deep sea. Int. J. Sci. Educ. 38 (13), 2096–2113. doi: 10.1080/09500693.2016.1228128
Theobald E. J., Hill M. J., Tran E., Agrawal S., Arroyo E. N., Behling S., et al. (2020). Active learning narrows achievement gaps for underrepresented students in undergraduate science, technology, engineering, and math. Procedings Natl. Acad. Sci. 117 (12), 6476–6483. doi: 10.1073/pnas.1916903117
Vale R. D. (2013). The value of asking questions. Mol. Biol. Cell 24 (6), 680–682. doi: 10.1091/mbc.E12-09-0660
Wagner D., Friedlander A. M., Pyle R. L., Brooks C. M., Gjerde K. M., Wilhelm T. A. (2020). Coral reefs of the high seas: Hidden biodiversity hotspots in need of protection. Front. Mar. Sci. 7. doi: 10.3389/fmars.2020.567428
Wickam H. (2009). ggplot2: elegant graphics for data analysis. New York, United States: Springer Verlag.
Woodin T., Carter V. C., Fletcher L. (2010). Vision and change in biology undergraduate education, a call for action-initial responses. CBE-Life Sci. Educ. 9, 71–73. doi: 10.1187/cbe.10
WoRMS Editorial Board (2022). World register of marine species. Available from https://www.marinespecies.org at VLIZ. Accessed 2020-2022. doi: 10.14284/170
Keywords: telepresence, CURES, cold-water corals, rugosity, biodiversity, NOAA Ocean Exploration
Citation: Gerringer ME, Ismail Y, Cannon KA, Camilo Hernández A, Gonzales Peralta F, Bohen R, Cartwright JC, Feasley A, Fregosi L, Lehman H, Niles H, Quay J, Sherpa N, Woodworth BH and Cantwell K (2023) Deep-sea biology in undergraduate classrooms: Open access data from remotely operated vehicles provide impactful research experiences. Front. Mar. Sci. 9:1033274. doi: 10.3389/fmars.2022.1033274
Received: 31 August 2022; Accepted: 22 November 2022;
Published: 10 January 2023.
Edited by:
Erik Cordes, Temple University, United StatesReviewed by:
Anja Schulze, Texas A&M University at Galveston, United StatesKatrin Linse, British Antarctic Survey (BAS), United Kingdom
Copyright © 2023 Gerringer, Ismail, Cannon, Camilo Hernández, Gonzales Peralta, Bohen, Cartwright, Feasley, Fregosi, Lehman, Niles, Quay, Sherpa, Woodworth and Cantwell. This is an open-access article distributed under the terms of the Creative Commons Attribution License (CC BY). The use, distribution or reproduction in other forums is permitted, provided the original author(s) and the copyright owner(s) are credited and that the original publication in this journal is cited, in accordance with accepted academic practice. No use, distribution or reproduction is permitted which does not comply with these terms.
*Correspondence: Mackenzie E. Gerringer, gerringer@geneseo.edu