- 1CEAAF Center for East Asian-Australasian Flyway Studies, Beijing Forestry University, Beijing, China
- 2School of Environment, Beijing Normal University, Beijing, China
- 3Groningen Institute for Evolutionary Life Sciences (GELIFES), University of Groningen, Groningen, Netherlands
- 4Department of Coastal Systems, NIOZ Royal Netherlands Institute for Sea Research, Texel, Netherlands
- 5Paulson Institute, Beijing, China
- 6College of Biological Science and Technology, Beijing Forestry University, Beijing, China
- 7Global Flyway Network, Nelson, New Zealand
Invasion by smooth cordgrass (Spartina alterniflora Loisel) has greatly impacted the intertidal ecosystems of China. Worldwide, chemical control is the most widely used method to control Spartina species, but it has not been widely implemented along the Chinese coast due to concerns about the potential impacts of herbicide residues on the environment and organisms. Macrobenthos, both natural and cultured on intertidal mudflats, is an important seafood resource, so human food safety is a particular concern. Here we tested the effectiveness of imazapyr (an imidazolinone herbicide inhibiting the synthesis of branched-chain amino acids) in controlling S. alterniflora from August 2020 to June 2021 on the Jiangsu Coast, an area severely impacted by S. alterniflora. We used two different concentrations of the herbicide and monitored the density of S. alterniflora seedlings and flower spikelets, the effects of herbicide use on macrobenthos, and residues in organisms and the environment at different times post-application. Ten months after application, imazapyr had killed all plants and within 30 days it inhibited the two reproductive processes of germination and flowering; there were no significant differences between the two concentrations used. Imazapyr residues were detected in the environment for up to 14 days post-application, but at very low concentrations and exponentially decreased with time. No residues were found in any macrobenthos. Imazapyr use did not result in a reduction of macrobenthos density. We conclude that the herbicide imazapyr effectively removes S. alterniflora with little collateral damage to other organisms and the environment. However, in view of the relatively small scale of our trials and the great extent of S. alterniflora in many sites in China, we recommend that larger scale field trails be conducted to assess any potential adverse effects when imazapyr is used at a landscape level.
1. Introduction
Human activities, both deliberate and accidental, have brought a long list of species from their places of origin to the far corners of the world. Some of them have become invasive, displacing local species and dominating communities (Seebens et al., 2015; Early et al., 2016). Cordgrasses (Spartina spp.) are perennial C4 grasses that are important ecosystem engineers in their native ecosystems, modifying, maintaining and/or creating habitats (Strong and Ayres, 2013). Because of their capacity to dissipate wave action, thus protecting dikes, reducing erosion and trapping sediments, various Spartina taxa have been introduced to coastal areas worldwide, frequently resulting in serious ecological problems (Li et al., 2009; Strong and Ayres, 2013; Meng et al., 2020). The world’s largest invasion of S. alterniflora is in China (Strong and Ayres, 2013), where it is now found in all coastal provinces; in 2019 it covered 67,532 hectares and is still increasing (Gu et al., 2021). The most severely impacted region is the coast of Jiangsu Province and Shanghai (Gu et al., 2021). In China S. alterniflora has resulted in serious negative impacts on local biodiversity, fisheries, aquaculture and ecosystem functioning (Chen et al., 2004; Chen et al., 2009; Ju et al., 2017; Zhang et al., 2019a; Jackson et al., 2021). As a result, effective treatments to control or remove S. alterniflora have been sought (Ju et al., 2017).
Worldwide, coastal managers have tried various methods to control or remove Spartina including physical removal, biological treatment and treatment with herbicides. These methods vary in terms of effectiveness, cost and potential adverse effects (Roberts and Pullin, 2008; Table 1). Physical removal includes burning, hand pulling, smothering by covering with plastic, mowing, waterlogging, and tilling, but always with limited success (Roberts and Pullin, 2008; Xie et al., 2019). Physical methods are costly and destroy the physical structure of underlying sediments and, when impoundments are constructed, result in the loss of intertidal habitat (Yuan et al., 2011; Xie et al., 2019). Biological control, e.g., using the plant hopper Prokelisia marginata (Grevstad et al., 2003), has yet to be demonstrated as an effective method of biological control of S. alterniflora and potentially may exacerbate problems by selecting for resistant genotypes (Garcia-Rossi et al., 2003). Herbicides are the most widely used method worldwide for controlling Spartina species (Roberts and Pullin 2006) because they are highly efficient, low cost, fast-acting, with small impacts on the physical characteristics of the habitat, and potentially with limited impacts on other organisms.
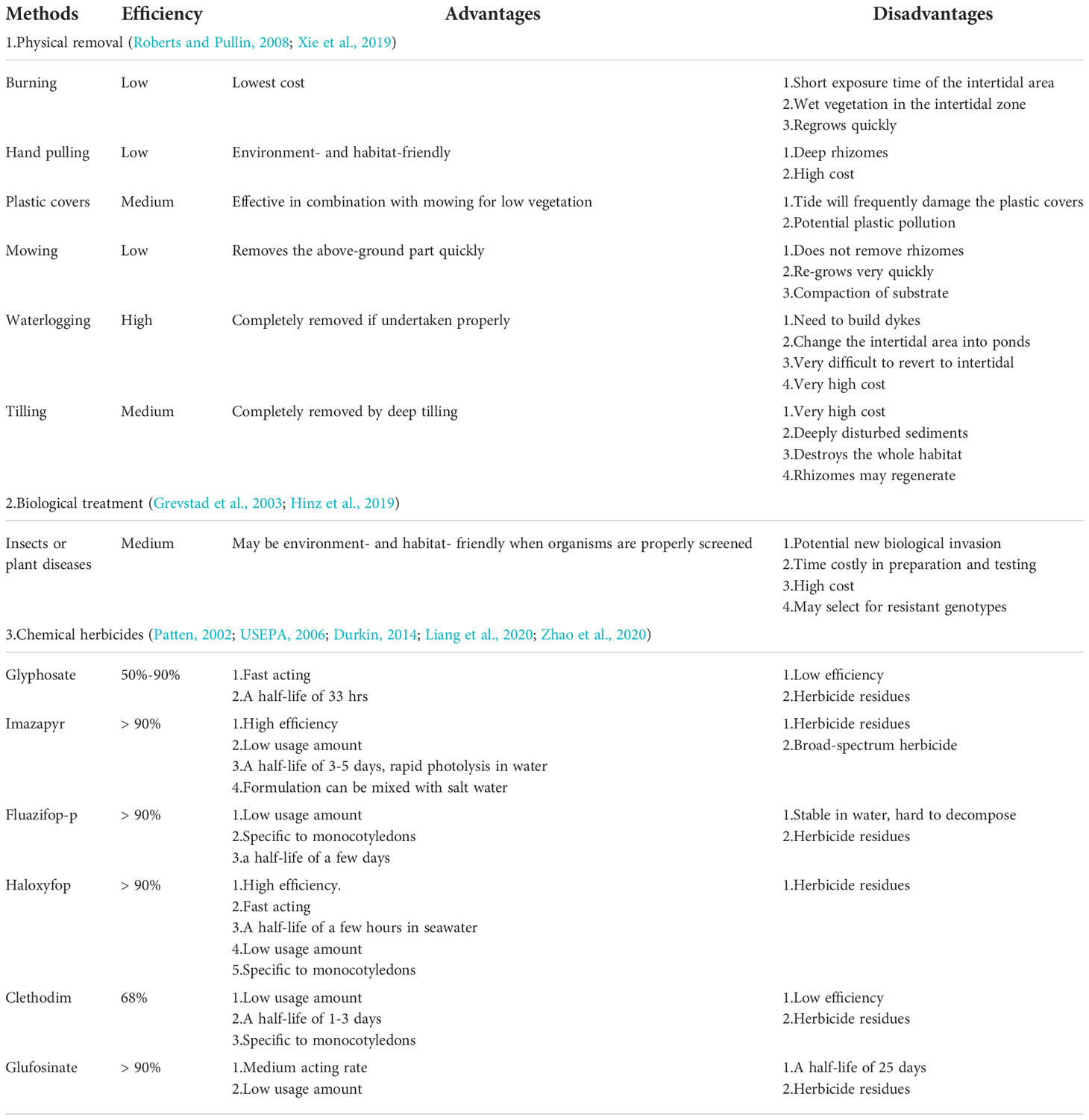
Table1 The efficiency, advantages and disadvantages of various methods of controlling or removing Spartina species.
Among the numerous herbicides used to control Spartina, glyphosate, imazapyr, fluazifop-p, haloxyfop, clethodim, and glufosinate have been used with success (Patten, 2002; Roberts and Pullin, 2008; Knott et al., 2013; Liang et al., 2020), imazapyr and haloxyfop are the most widely used (Roberts and Pullin 2006; Liang et al., 2020; Mo et al., 2021). Each of these herbicides has advantages and disadvantages (reviewed in Table 1). In China, haloxyfop has been used to control Spartina for more than 10 years, especially in Shanghai, which is one of the coastal areas most heavily invaded by Spartina (Liang et al., 2020). Imazapyr is among the most tested and used herbicides worldwide for Spartina control (Patten, 2002; Roberts and Pullin, 2008), however it has been used only once in China, but not in the most heavily invaded area, and no assessment of potential impacts on macrozoobenthos were made (Mo et al., 2021).
Macrobenthic animals play core roles in coastal wetland food webs both as primary and secondary consumers and as food for higher trophic level consumers such as fish and birds (Herman et al., 1999; Rakhimberdiev et al., 2018; Zhang et al., 2019b). Macrobenthos also influence the physicochemical processes in sediments through bioturbation (van der Zee et al., 2012; Adámek and Maršálek, 2013; Donadi et al., 2013; Donadi et al., 2014). Because of their generally limited mobility and sensitivity to environmental changes, macrobenthic species can serve as environmental indicator organisms (Bianchelli et al., 2018). Along China’s coasts, macrobenthos (especially bivalves) are important economic products; most intertidal flats along the Chinese coast are occupied by mollusc aquaculture, and cultured species dominate the macrobenthic communities (Peng et al., 2021) affecting the survival of other organisms in the ecosystem (Piersma et al., 2016). Since macrobenthos is important as seafood for humans (Li et al., 2011), smooth cordgrass in China usually has been subject to physical control rather than the use of herbicides (Ju et al., 2017) due to concerns about possible contamination. However, the extent of Spartina invasion in China is now such that herbicides are the only effective method of control in most areas, but there is a need to ensure they have no adverse ecological effects.
Imazapyr is an imidazolinone herbicide with excellent herbicidal activity against a broad range of plants, including terrestrial annual and perennial grasses and broadleaved herbs, woody species, and riparian and emergent aquatic species (USEPA, 2006). Its mechanism of action is to inhibit the synthesis of branched-chain amino acids (WSSA, 2014). Imazapyr possesses a low sorption capacity, high water solubility, and high persistence in soils (WSSA, 2014), and has been proved to present no risk to or toxic effects on local aquatic organisms, birds and insects (Fisher et al, 2003; USEPA, 2006). This study was designed to test the effectiveness of, and assess the impact on macrobenthos of, imazapyr used to control smooth cordgrass. To explore the effectiveness of imazapyr for the removal of smooth cordgrass, and to study the effects of herbicide residues in the environment and macrobenthos, we used controlled field experiments and monitored the residues of herbicides in macrobenthos, sediment and water.
2. Methods and materials
2.1. Study area
This study was conducted at Dongtai Estuary (Figure 1), within the ‘experimental zone’ of the Yancheng National Rare Birds Nature Reserve (YNRBNR), located on the coast of Jiangsu Province, China (N 32.95°, E 120.92°) (Ma et al., 2009). On the border between the northern subtropical zone and the southern warm temperate zone, it has four distinct seasons with cold winters and hot summers, and light but abundant precipitation (average precipitation is 1000 mm, Huang et al., 2015). YNRBNR is a key staging site for hundreds of thousands of waterbirds along the East Asian-Australasian Flyway (EAAF), and was listed as a World Heritage Site in 2019 (UNESCO, 2019).
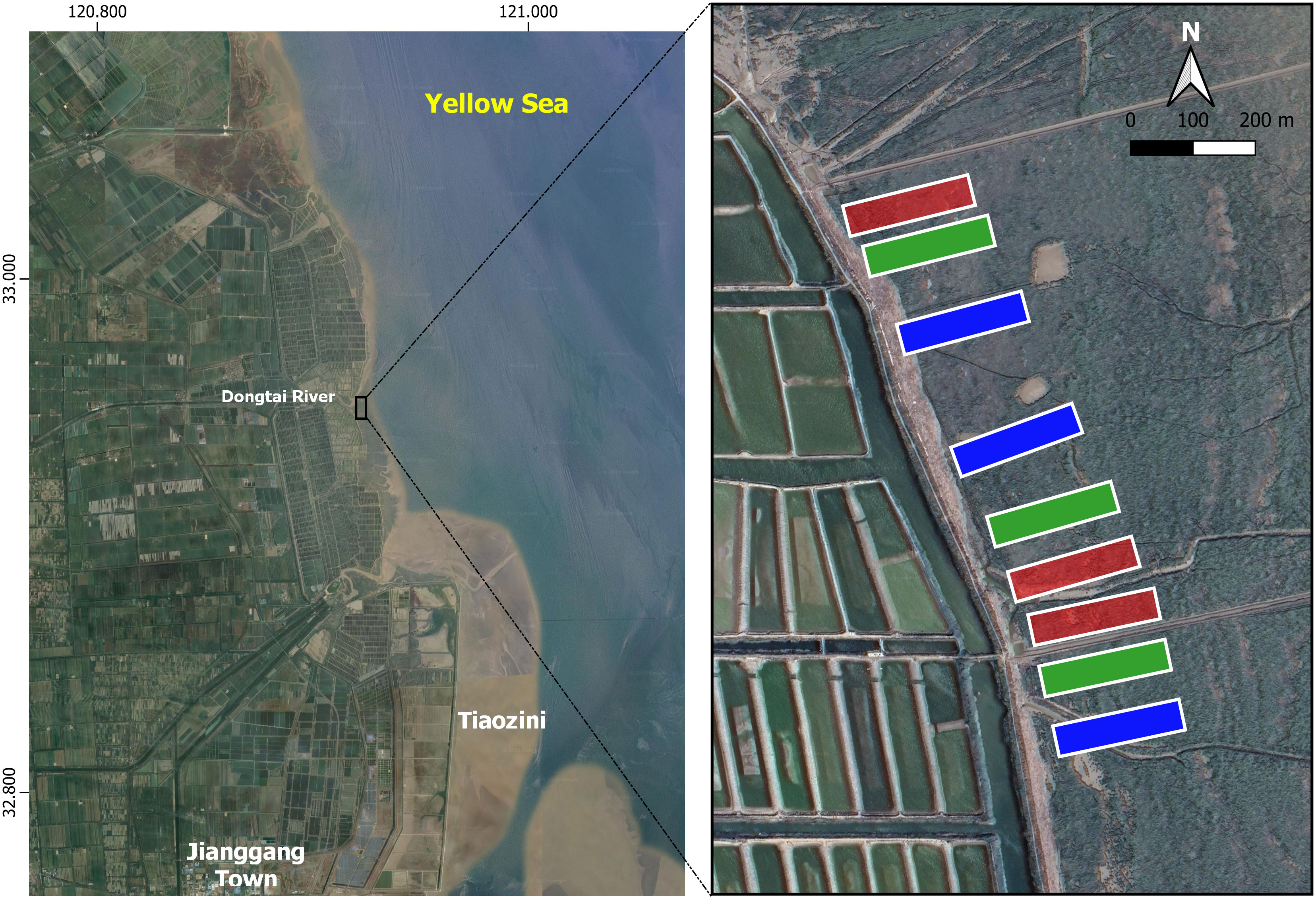
Figure 1 Study area in Jiangsu Province, China. Red plots are those treated with a high concentration of imazapyr, green plots are those treated with a low concentration of imazapyr, and blue plots show the untreated control plots.
Spartina alterniflora was first planted in Jiangsu in 1982 (Chung, 2006), since when it has rapidly invaded open mudflats (Gu et al., 2021), resulting in the loss of habitat for migrating birds (Ju et al., 2017). The experiments took place in the southern area of YNRBNR, where S. alterniflora has taken over all of the shoreline and extends up to 1.5 km or more offshore.
2.2. Plot setting and experiment treatment
Nine plots adjacent to the seawall were selected, each being 50 m × 200 m in size (1 ha in area). The tides submerged the plots for one (neap tide) to four (spring tide) hours during the high tide almost every day in August, but in September the plots did not submerge during the neap tide.
The plots received three treatments of imazapyr herbicide (Polaris, Nufarm Americas Inc.) (Table 2): high concentration group (10%), low concentration group (5%), and the control group which was not sprayed, with three replicates for each treatment (Figure 1). The minimum distance between sprayed plots was 20 m, and the minimum distance between the control and sprayed groups was 50 m to minimize the potential effect of spray drift on the control group. We used the same dose of Polaris (7L/ha) in both the high and low concentration plots, which was recommended by the herbicide manufacturer and previous researchers in the United States (Patten, 2002), the difference in concentration being due to the amount of water mixed; the application rate was twice as much using the low concentration mix (Table 2). We did this to test whether the application of more spray volume may lead to increased runoff (loss) of Polaris to the ground/water, which may have an impact on uptake by S. alterniflora.
The spraying of imazapyr was conducted on 13 August 2020 (Table 3); a sunny day with a neap tide, and wind speed < 4 m/s; water only submerged the lower parts of the stems of plants in our plots for 1 hour over a single tide which occurred 10 hours after spraying, thus ensuring that neither tide nor rain washed off the imazapyr shortly after spraying. Agricultural drones (DJI Agras T20) were used for spraying. To ensure effective spraying and prevent wastage, we set fixed computerized GPS tracks, flying at a height of 2.5 - 4 m above ground level (~1.5m above S. alterniflora) with a spraying range (the width of the area sprayed by each drone track) of 3.6 m.
2.3. Monitoring methods
The monitoring was conducted from 11 August 2020 to 9 June 2021. S. alterniflora and macrobenthos were measured both before and after spraying. Imazapyr residues were measured 5 times within 31 days after spraying (Table 2).
2.3.1. Plant
In each plot, 5 subplots (1m x 1m each) selected randomly at a distance of 20 m from adjacent subplots were sampled. We cut off all plants above the ground in each subplot and checked whether they were dead or alive, and counted the number of living S. alterniflora stems. No other plant species were found in our study plots. We randomly selected ten S. alterniflora plants in each subplot and measured the height (measured from the base of the plant (above ground) to the tip of the stems), leaf length and leaf width (of the longest leaf of the selected plant), stem diameter (at the thickest part of the selected plant), and the length of the flower spikelet.
S. alterniflora propagates sexually via seeds and also asexually by tillers and rhizomes (Patten, 2002). To understand the effect of imazapyr on the reproduction of S. alterniflora, we monitored seedlings and spikelets to assess the effects of imazapyr on reproduction. We identified all S. alterniflora plants which were <5 cm tall as ‘seedlings’ (although these could include new growth from tillers and rhizomes), taller shoots were noted as ‘stems’. Spikelet length was only measured after the plant was eared. Plant monitoring was carried out 2 days before treatment, and 31, 61, and 300 days after treatment (Table 3).
2.3.2. Macrozoobenthos
In each plot, three sampling sites were randomly selected with a distance of at least 50m from each other, and a sediment core (surface area 0.019 m2) was taken to a depth of 20 cm and washed over a 0.5 mm sieve (Peng et al., 2021). The sieved macrozoobenthos were stored frozen (-18°C) prior to analysis. In the laboratory, macrozoobenthos were identified to species level using a dissecting microscope and counted (Peng et al., 2021). Macrozoobenthos monitoring was carried out 2 days before treatment, and 15, 31, 300 days after treatment (Table 2).
2.3.3. Residues of imazapyr
Samples for testing imazapyr residues were collected from 6 treated plots (3 high and 3 low concentration plots) and one untreated plot. In each plot, four sampling sites were selected with three being in vegetated areas and one in bare ground (with a size > 4m2). At each vegetated sample site, three benthic species (the gastropods Cerithideopsis largillierti and Assiminea latericea, and the crab Helice latimera) were collected by hand from the surface. Because these three species live on cordgrass or on the soil surface, they are more likely to be exposed to herbicides; furthermore, all three species feed on S. alterniflora, which makes them more likely to accumulate herbicides. At least 30g wet weight flesh for each species was collected at each sampling site, to enable sufficient flesh to be sampled for analysis. In areas of bare ground only one species, Cerithideopsis largillierti, was found. Approximately 10 g of soil was collected using a small shovel from the surface and at a depth of 5 cm, respectively and stored in sealed ziplock bags for measuring herbicide residues. Additionally, two 50ml water samples were collected from channels or shallows in the plots and saved in 50-ml centrifuge tube. All benthos, soil, and water samples were stored in a freezer (-18°C) within 12 hours of collection. Residue sampling was carried out on 1, 7, 14, 21, 30 days after treatment (Table 3).
2.4. Determination of the imazapyr residues in sediments and benthic organisms
2.4.1. Pretreatment of samples
For sediment samples, 1g of sediment was weighed and transferred into a 5.0-ml brown centrifuge tube, and then 2.0 ml of ammonium acetate solution (2.0 mM) was added and vortexed for 5 min, after which the mixture was treated with ultrasonic power of 100W for 15 min on an ice bath. Then, the samples were centrifuged at 10000 g for 10 min to collect the supernatant, followed by filtration through 0.45 μm hydrophilic filter. For benthic organisms, 1g of flesh pooled from several animals was put into a 10-ml tube, followed by the addition of 2.0 ml ammonium acetate solution (2.0 mM). Then, the samples were fully homogenized using a hand-held tissue homogenizer, after which 1.0 ml of the homogenate was transferred into a 4.0-ml centrifuge tube, and 2.0 ml of trichloroacetic acid (12.0%, w/w) was added to precipitate proteins in the solution. After vortex treatment for 5 minutes, the mixture was subjected to ultrasonic treatment for 15 minutes on an ice bath, followed by centrifugation (10000 g) for 10 minutes at 4°C. Finally, the supernatant was collected for determination after filtration through 0.45 μm hydrophilic filter.
2.4.2. UPLC-ESI-MSn determination of imazapyr
UPLC-ESI-MSn analyses were carried out using an Agilent 1260 Infinity II LC system coupled with a QTRAP® 5500 system (AB, USA). Analytical separation was carried out at 30°C on a ZORBAX RRHD SB-C18 column (2.1 mm id × 50 mm, 1.8 μm). Isocratic gradient elution was performed with a mixture of 94% ammonium acetate solution and 6% acetonitrile. The flow rate was kept at 0.3 mL/min, and 3.0 μL of each sample solution was injected in each run. Multiple reaction monitoring (MRM) was used for detecting transitions in a positive ionization mode. The operating parameters were set as follows: electrospray source; spray voltage, 4000 V; source temperature, 350°C; nebulizing gas, 15 psi; heating gas, 55 psi; curtain gas, 12 psi; gas flow rate 11L/min. The quantitative ion-pair was selected as 262.1/117.1, and external calibration curves of imazapyr were established with ten concentrations ranging from 0.0144 to 144 ng/mL. The calibration curve was linear over the entire concentration range and the Pearson’s correlation coefficient (r) was more than 0.99. The limit of detection (LOD) and limit of quantification (LOQ) of the imazapyr ranged from 0.0014 to 0.042 ng/mL. Analyst 1.6.1 software (Applied Biosystems, MDS Sciex, Canada) was used to run the above analysis.
2.5. Data analysis
Tukey’s HSD test was used to compare the difference among the groups at different times, including the density of plant, plant height, leaf length, leaf width, stem diameter, the density of seedlings and spikelet, and length of spikelet. Tukey’s HSD test was used to compare the difference in the density and number of species of macrobenthos among the groups at different times. To compare the residues of imazapyr in the surface soil, deep soil, water and macrobenthos among groups in different stages, Tukey’s HSD test was used separately. For the density of macrozoobenthos in June 2021, because the snail A. latericea falls to the ground when S. alterniflora dies, we compared the density of A. latericea in three treated groups by paired t-test; we further compared the density of all macrozoobenthos excluding A. latericea to remove the effect of their falling down with the dead plants. p < 0.05 indicates a significant difference. All statistical analyses were made using RStudio 1.4 for Windows.
3. Results
3.1. Plants
Only S. alterniflora was recorded in the nine plots we monitored; no other plants were found. Before treatment, the overall density of S. alterniflora stems across the plots was 113 ± 27 m-2 (n = 45), and there were no differences among the plots (p>0.05 in all cases. Figure 2A). Plant densities measured 31 days and 61 days after treatment did not change significantly for each of the three groups (Figure 2A, all p > 0.05). After 300 days post-treatment (the second growing season of our study), stem density of the untreated group increased significantly to 180 ± 19 stems m-2 (n = 15), but all plants in the high and low concentration treatment groups were dead (Figure 2A). Before treatment, the height of S. alterniflora was 140.4 ± 31.0 cm (n=448), with no differences among the three groups (p>0.05 in all cases. Figure 2D). After treatment, the heights of S. alterniflora measured on Day 31 and Day 61 did not change in either the high (p=0.55) or low concentration group (p=0.18), and all plants in these two groups were dead after 300 days (Figure 2D). In contrast, the plant height increased significantly in the untreated control group during the first two months after treatment (p<0.001), but decreased to the lowest level measured 300 days after treatment, the second growing season of our study as the stems died naturally over winter (Figure 2D).
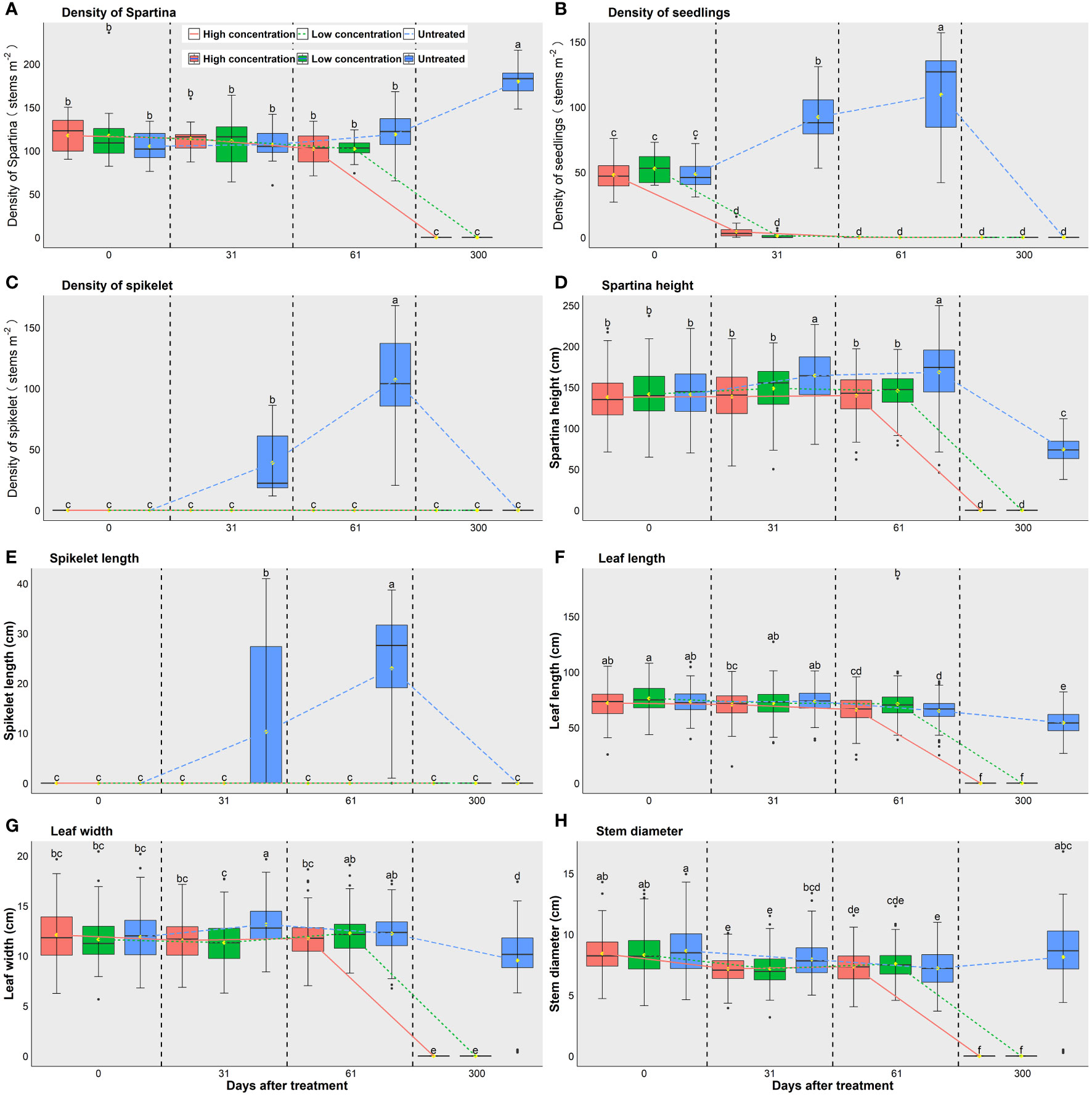
Figure 2 Plant densities and features of S. alterniflora in different treatment groups at different times. (A) Density of S. alterniflora. (B) Density of seedlings. (C) Density of flower spikelet. (D) Height of S. alterniflora. (E) Length of flower spikelet. (F) Length of leaves. (G) Width of leaves. (H) Diameter of stem. The different lowercase letters indicate significant differences between groups (p < 0.05). Red symbols and lines show plots treated with a high concentration of imazapyr, green symbols and lines show low concentration plots, blue symbols and lines show untreated control plots.
The average length of leaves was 74.0 ± 12.7 cm (n=448) before treatment, with no differences among groups (p<0.05 in all cases. Figure 2F). After treatment, the leaf length in both high and low concentration plots decreased slightly by Day 31, but significantly by Day 61, and no leaf was found on Day 300 when all plants were dead (Figure 2F). In contrast, the leaf length in the untreated plots did not change much after 31 days, but had decreased greatly after 300 days (p<0.001. Figure 2F) as the stems died naturally overwinter. The width of leaves was on average 11.9 ± 2.4 mm (n=448) before treatment and there was no difference between groups (p<0.05 in all cases. Figure 2G). In high concentration plots, the leaf width did not change by Day 31 and Day 61 (p=0.20), and no leaves were left by Day 300 when all plants were dead. In low concentration plots, the leaf width did not change by Day 31, but increased by Day 61 with an overall increasing trend during this period (p=0.02, Figure 2G). In untreated plots, the leaf width increased as measured on Day 31, and then decreased to the lowest level as measured on Day 300 in the next year (p<0.001). The stem diameter was 7.9 ± 1.5 mm (n=146) before treatment and no differences were detected between groups (p>0.05 in all cases. Figure 2H). The stem diameters in both high and low concentration plots decreased within 61 days after spraying (p<0.001). In untreated plots, the stem diameter also decreased within 61 days after spraying (p<0.001), but it increased to a similar level after 300 days to that measured at Day 0 (Figure 2H).
The density of seedlings of S. alterniflora was 50 ± 12 stems m-2 (n=45) before treatment, and not significantly different among the three groups (p>0.05 in all cases). After treatment, the densities of seedlings decreased to a very low level on Day 31 and even to zero on Day 61 in both high (p<0.001) and low concentration plots (p<0.001) (Figure 2B). In contrast, the seedling density in untreated plots increased significantly on Day 31 and Day 61 (p<0.001), but was recorded as ‘zero’ on Day 300 in the following June because all stems were taller than 5 cm. Because the experiment started before flowering, no spikelet was recorded in all three groups before the treatment. After treatment, no spikelet was found in either the high or the low concentration plots during the whole experiment. However, the density and length of the spikelet in untreated plots increased significantly after 31 days and 61 days (p<0.001. Figures 2C, E), and was zero 300 days later in the following June before the next flowering season.
3.2. Macrobenthos
Nine benthic species were found from 2020 to 2021 in the study plots (Figure 3A). The most abundant species were the gastropods Cerithideopsis largillierti (average density 131 individuals m-2), followed by Assiminea latericea (96 individuals m-2), the nereid polychaete Tylorrhynchus heterochaetus (39 individuals m-2), the crab Chiromantes haematocheir (14 individuals m-2), the bivalve Glauconome chinensis (10 individuals m-2), the crab Ilyoplax pingi (1 individual m-2), the gastropod Nerita yoldii (1 individual m-2), and the crab Uca arcuata (1 individual m-2). Before treatment, the mean number of species of macrobenthos was 2.6 ± 0.7 species/plot (n = 27); there were no differences between high concentration, low concentration and untreated plots (p > 0.05, Figure 3B).
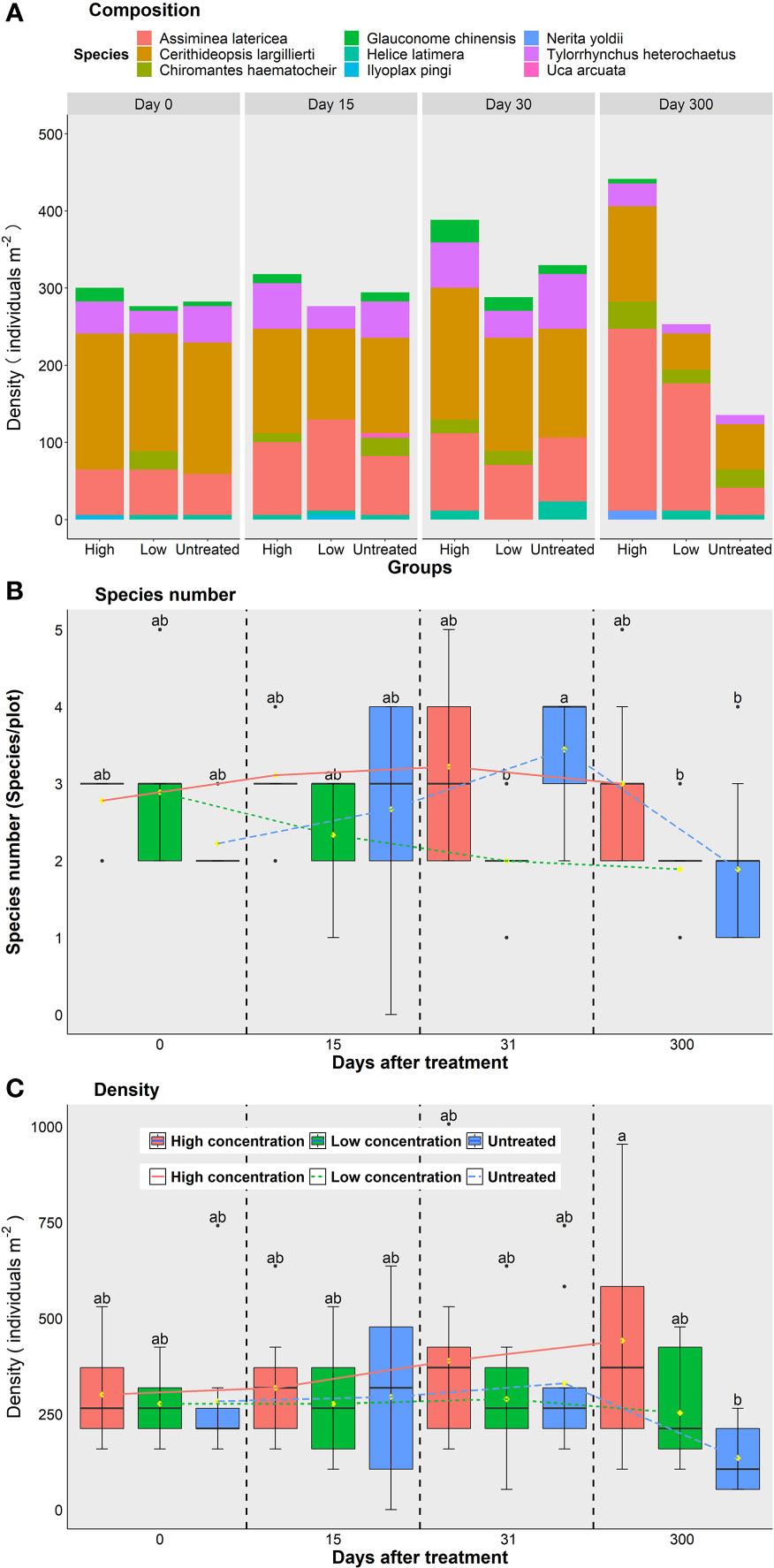
Figure 3 Species number and density of macrobenthos under different treatments at different times. (A) Overview of the composition of macrobenthos, different colors show the different species, the length of bar indicates the density of each species. (B) Species number and (C) density of macrobenthos in different treatments at different times, the different lowercase letters indicate significant differences between groups (p < 0.05). Red symbols and lines show high concentration plots, green symbols and lines show low concentration plots, blue symbols and lines show untreated control plots.
After treatment, the species number of macrobenthos remained relatively stable in the high (p>0.05) and low concentration (p>0.05) plots across all study stages Figure 3B. The species number of macrobenthos in the untreated plots did not change significantly after 31 and 61 days (p>0.05), but it had decreased to 1.9 ± 1.1 species/plot (n = 9) when measured on Day 300 in June 2021 Figure 3B. The average density of macrobenthos across all 9 plots was 287 ± 126 individuals m-2 (n=27) before treatment, and there were no differences among the three groups (p>0.05). After treatment, the densities of macrobenthos in all three groups were similar when measured on Day 31 (p>0.05) and Day 61 (p>0.05), but the density in the high concentration group was significantly higher than those in the untreated plots when measured on Day 300 (p<0.05, Figure 3C). This is because the density difference of A. latericea among groups, where densities of A. latericea in untreated plots were significantly lower than treated plots (all p < 0.05). When excluding A. latericea, the densities of macrozoobenthos among the three treatments showed no differences on Day 300 (all p > 0.05).
3.3. Imazapyr residues
Samples from four kinds of environmental materials (i.e., surface soil, deep soil, water and macrobenthos) were tested for imazapyr residues. No imazapyr residues were detected in any macrobenthos sample collected from the treated and untreated plots (Figure 4). Imazapyr residues were detected in the surface and deep (5cm) soil samples from both the high concentration and low concentration plots, however the concentration of residues decreased exponentially with time until Day 21 after which no residue was detected in any soil sample (Figure 4).
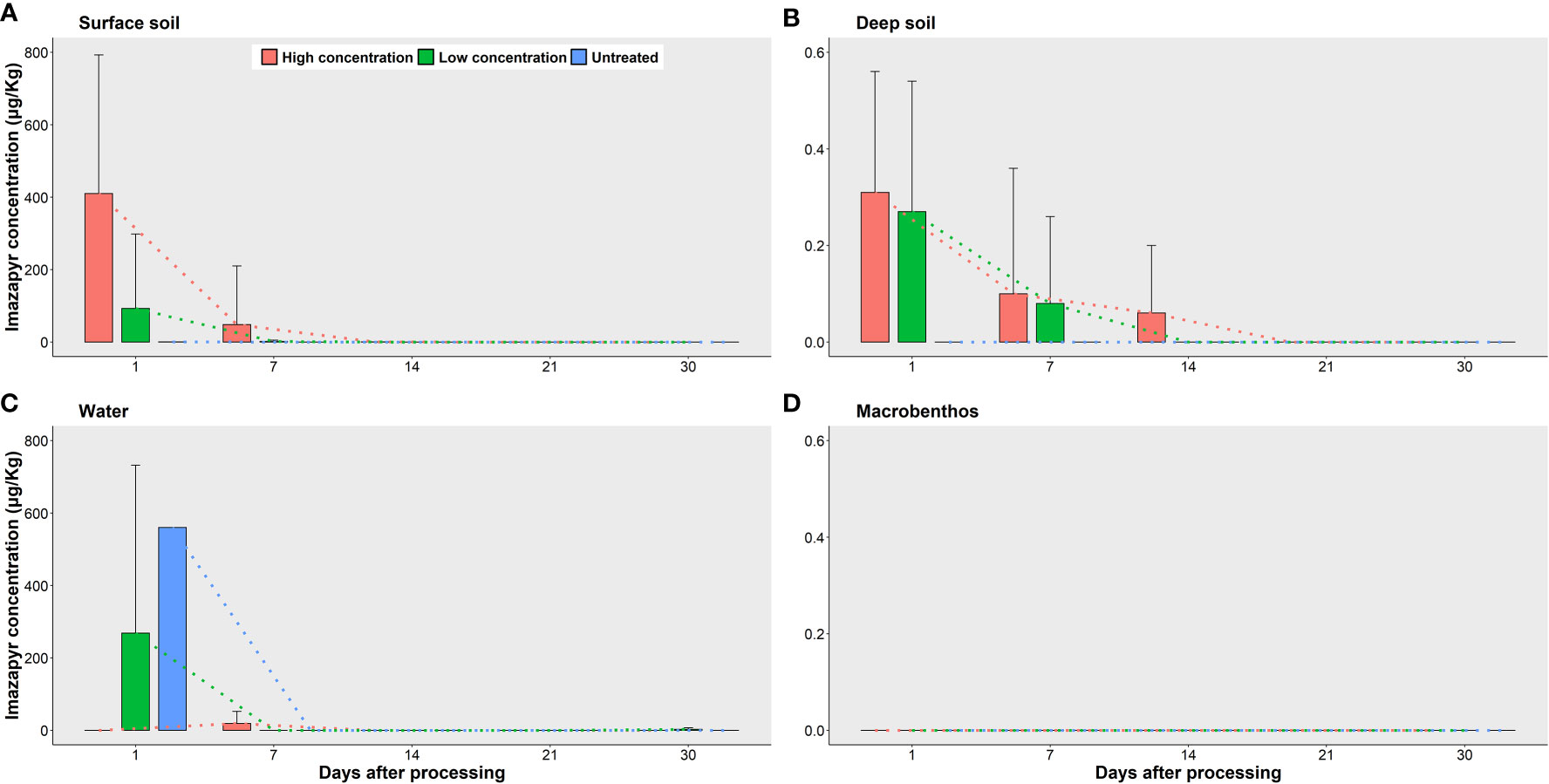
Figure 4 Changes of the concentration of Imazapyr acid residues in different environmental substances of different treated groups at different times. (A) Surface soil. (B) Deep soil (5cm). (C) Water. (D) Macrobenthos. Red symbols and lines show high concentration plots, green symbols and lines show low concentration plots, blue symbols and lines show untreated plots.
The concentration of the residues was higher in the surface soil than in the deep soil as measured at the same time for both high concentration and low concentration plots, but the residues remained in the soil longer in the high concentration plots than in the low concentration plots (Figure 4). No residue was detected in the soil beyond Day 14 in the low concentration plots, but beyond Day 21 in the high concentration plots, although at very low concentrations (0.08± 0.21 μg/kg for surface soil and 0.06 ± 0.14 μg/kg for deep soil). For the untreated plots, the residue was found in one out of three surface samples at an average concentration of 0.11 ± 0.18 μg/kg (n = 3) on Day 1 only, and not detected after Day 7 (Figure 4A).
No imazapyr residue was detected in the water samples from the high concentration plots on Day 1, but was detected from one out of three samples at an average concentration of 19.01 ± 32.93 μg/Kg (n = 3) on Day 7, and no imazapyr residue was found on or beyond Day 14. For the low concentration plots, imazapyr residues were found in two out of three samples at 268.37 ± 464.29 μg/kg (n=3) on Day 1, but was not recorded in the following sampling. The residues were detected in the water samples from the untreated plots at 560.57 μg/kg (n = 1) on Day 1, but thereafter was not detected (Figure 4C).
4. Discussion
4.1. The efficiency of imazapyr herbicide in killing Spartina alterniflora
All S. alterniflora was dead within 10 months of spraying in both high and low concentration plots. In addition, both asexual and sexual reproduction were effectively prevented in the treatment groups, and no new plants were produced in the following summer. The high control efficiency was consistent with other reported results (Roberts and Pullin, 2008; Mo et al., 2021), confirming that imazapyr can effectively inhibit the tillering and flowering of S. alterniflora, as well as the growth of plants, and eventually kill all the plants.
This study showed that there was no significant change in the density of living S. alterniflora (average height of 1.5 m) as measured within 61 days after spraying. In contrast, more than 95% of ‘seedlings’ (<5 cm in our study) were dead by 30 days post-spraying, and all were dead after 61 days. In a study in Fujian Province, southern China, half of the S. alterniflora was dead 30 days after spraying, and more than 90% of the plants were dead by 60 days (Mo et al., 2021), even though the concentration (5.7%) of active ingredient (imazapyr) was lower than the high concentration treatment in our study (10%). Although S. alterniflora on the Jiangsu Coast took longer to die completely than elsewhere (Patten, 2002; Mo et al., 2021), the final efficiency using imazapyr was nearly 100% in our sampling subsamples, and more than 95% in the entire treated plots (Figure 5). Imazapyr effectively inhibits both sexual and asexual reproduction of S. alterniflora, so the plants are unable to complete generation turnover, and also can kill the mature, tall plant, so it is possible to use imazapyr to completely remove all the invasive S. alterniflora.
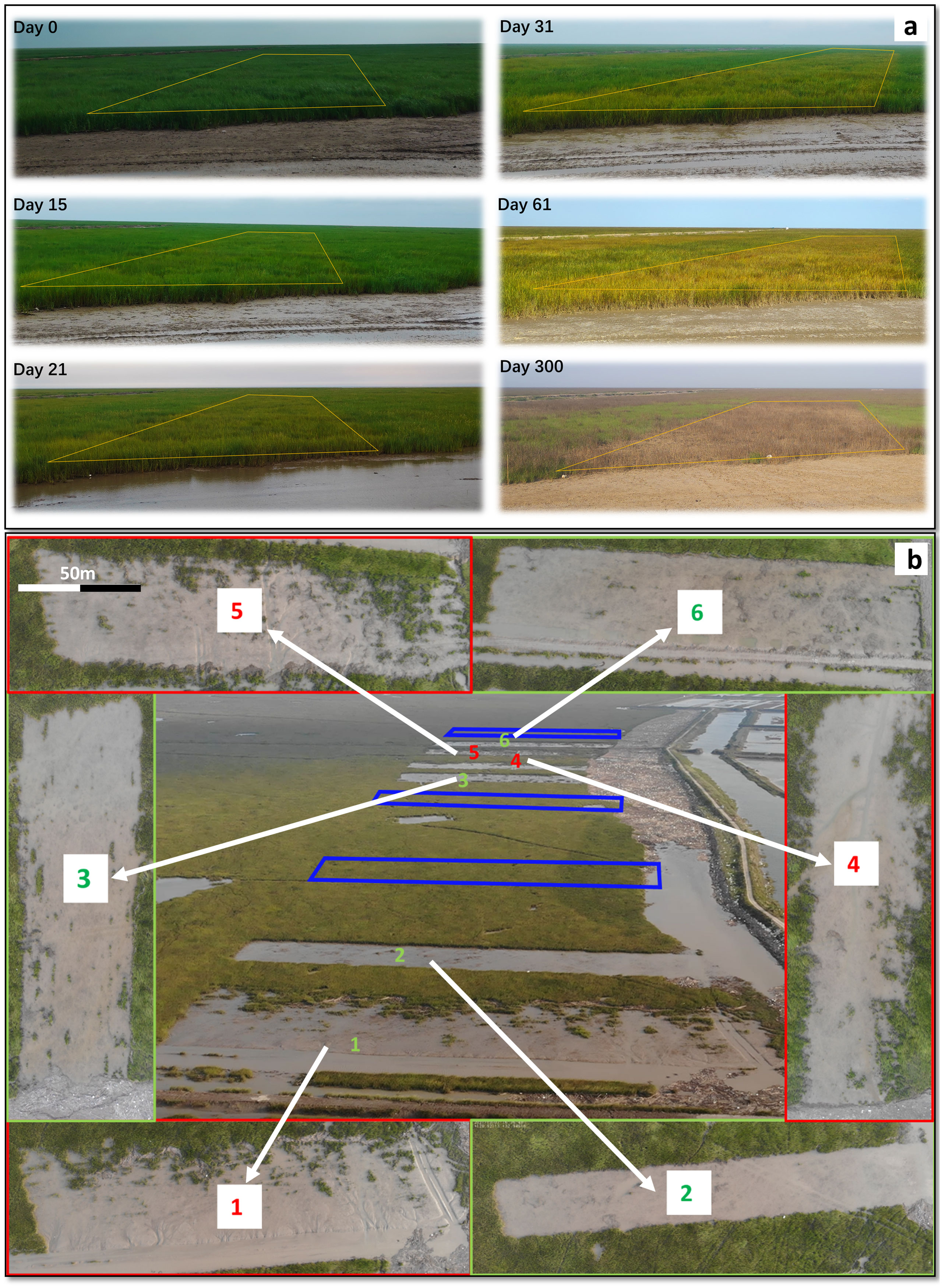
Figure 5 The effectiveness of imazapyr herbicide on S. alterniflora. (A) The changes of S. alterniflora in one treated plot (plot 2 in b) from day 0 to day 300 after treatment. (B) The overview of treatment effectiveness, photos were taken after treatment for 14 months, a typhoon had cleared away the dead vegetation. Red letters and plots show high concentrations plots, green letters and plots show low concentrations, and blue plots show the untreated plots.
When using similar or higher doses of imazapyr, it took longer to kill S. alterniflora in Jiangsu than in Fujian. This may be related to the fact that the stems of S. alterniflora along the Jiangsu coast are 3 times higher than those in Fujian; furthermore, we sprayed at a time when the plants were nearing flowering (August), whereas in Fujian, Mo et al. (2021) sprayed during the active growing period (May), and it is recognized that a higher-concentration of herbicide and longer-acting time is required to kill mature plants (DNR, 2012).
We found no differences in any of the metrics of S. alterniflora that we monitored between the high and low concentration treatment groups across the study period, and there were also no significant differences in macrobenthos density and imazapyr residues. This suggests that mixing different amounts of water does not affect the absorption of imazapyr by S. alterniflora and the environmental impacts of imazapyr. As a result, we recommend using the high concentration of imazapyr (10%) to remove S. alterniflora, because this reduces the amount of drone flying time needed to apply it, which will therefor reduce costs.
4.2. Residues of imazapyr in the environment
The highest herbicide residues were detected in water samples from channels and shallows. Surprisingly, these herbicide residues were found in the untreated plot on Day 1 after spraying. However, on Day 7, no imazapyr residues were found in the water of the low concentration and untreated plots, while only a very low amount was detected in the high concentration plots where none was detected on Day 1. No residues were detected two weeks later, or subsequently. All plots were subject to tidal inundation for 1-4 h every day in August, which will have washed imazapyr from the plants while the high tidal range ~5m (Kang et al., 2015) will have resulted in strong tidal flushing. Since the half-life of imazapyr in water averages 3-5 days (USEPA, 2006), it is likely that any imazapyr flowing elsewhere decomposed quickly. It is possible that the detection of imazapyr residue in water samples of the untreated plots on Day 1 resulted from spray-drift, but we think that this is unlikely as spraying was done on a calm day and there was no evidence of S. alterniflora mortality in the control plots.
We found imazapyr residues in the sediments (both surface soil and deep (5cm) soil) of the treatment plots, with higher residues in the high concentration plots than in the low concentration plots; none was found in the control plots. The concentration of residues decreased exponentially with time until only a very low level was detected in high concentration plots and none in low concentration plots in the second week, and no residues were detected in the third week or thereafter. In view of the fact that imazapyr is stable to hydrolysis, aerobic and anaerobic soil degradation (USEPA, 2006), this suggests that residues were washed from the sediment by tidal movement. Thus, imazapyr residues did not accumulate in the sediments of the intertidal area. For these reasons, we also recommend that spraying should be done during the neap tides such that the tide only submerges the plants and tidalflats for a short period, thereby ensuring that plants have enough time to absorb the herbicide, while also allowing tidal flushing of herbicide residues.
The imazapyr residues found in the deep soil may be due to the effects of tidal water. The plots were covered by tides one (neap tide) to four (spring tide) hours per day in August, and the water might carry the residues from the surface layer to the deeper layers. It is also possible that the residues flowed into the deeper soils with the water during our sampling.
4.3. Effects of imazapyr use on macrozoobenthos
Organisms other than the target plants have been the most important indicators to assess the safety of herbicides (Rani et al., 2021). A previous study using herbicides to control S. alterniflora along the Chinese coast suggested that the use of haloxyfop-r-methyl or glyphosate herbicide may lead to a short-term decline in crab density (Qiao et al., 2019), but the authors did not investigate whether this may have resulted from direct effects or indirect effects such as the death of S. alterniflora affecting habitat structure and/or food resources. Whilst this could be taken as suggesting that herbicides may affect the biomass of benthic fauna and thus potentially the yield of the human seafood, the U.S. Environmental Protection Agency determined that for imazapyr ‘there are no risks of concern to terrestrial birds, mammals, and bees, or to aquatic invertebrates and fish’ (USEPA, 2006), and Sheng et al. (2014) using haloxyfop, and Zhao et al. (2020) using haloxyfop, detected no adverse effects on macrozoobenthos and meiofauna respectively. We did not detect imazapyr residues in any macrobenthos samples throughout the study period, whether snails or crabs, the most abundant species in the study area. These two groups of benthic animals are active in plants and on the surface soil, feeding on plant debris and the plants themselves (Wang et al., 2014), and thus may be more vulnerable to exposure to herbicides than macrobenthos in the mudflats, however our results suggest that imazapyr did not accumulate in macrobenthos.
There were no differences in macrobenthos density when S. alterniflora density was similar during the first 61 days following spraying among the three treatments, but by June 2021 (300 days post-spraying) A. latericea was significantly more abundant in the treatment plots where all plants had died. Because A. latericea prefers to attach itself to the stems and leaves of S. alterniflora (Wang et al., 2014), it may be that A. latericea scattered to the ground surface after the dead S. alterniflora collapsed. We sampled the macrobenthos mainly from the surface to 20 cm deep sediment, and did not survey the macrobenthos on the plants, which may have caused the density of A. latericea to be significantly higher in treated plots than in untreated plots on Day 300.
The densities of macrozoobenthos 300 days post-spraying did not differ significantly among the three treated groups when A. latericea was excluded the from the analysis. This supports the contention that the herbicide itself may not affect the survival of macrobenthos directly, because it does not accumulate in macrobenthos.
Previous toxicological studies have proven that imazapyr has little toxicity effect on mammals, birds, fish and aquatic invertebrates and it is considered to be non-toxic to them when it is used as instructed (USEPA, 2006). Additionally, the short half-life of imazapyr in water (3-5 days) (USEPA, 2006) and strong tidal flushing along much of China’s Yellow Sea coast, indicate that the toxicity risk of imazapyr to benthic animals in the intertidal area is very low. In view of the importance of China’s intertidal areas for seafood production future studies should further assess imazapyr levels in commercially important species to ensure their safety for consumption – currently the USA has a safety level for imazapyr of >1.0 ppm for fish and > 0.1 ppm for shellfish (Department of Health and Human Services, 2022) and Germany has a limit of 0.1 ppm (De Witte et al., 2022).
5. Conclusion
The present study demonstrates that the herbicide imazapyr results in a high mortality of S. alterniflora with low ecological risk if applied strictly in accordance with the product instructions, however further study may still be needed in the future to confirm its safety with respect to commercial seafood. The application of imazapyr thus seems a rather benign option for the control of S. alterniflora in China and the use of plant protection drones provides a practical method for future large-scale operations. However, as imazapyr is a broad-spectrum herbicide, it needs to be used with caution in coastal wetlands where native salt marsh plants and mangroves also occur. Indeed, as S. alterniflora now occupies more than 60,000 ha in China, the amount of herbicides required for eradication will be very high. We recommend that larger scale field tests be conducted to examine the environmental impacts of the wider application of imazapyr.
Data availability statement
The original contributions presented in the study are included in the article/supplementary material. Further inquiries can be directed to the corresponding authors.
Author contributions
H-BP, JS, and XG designed and performed the experiments. H-BP drafted the manuscript. H-BP took samples. JZ and CM measured the imazapyr residues. H-BP performed the statistical analysis of the original data. JS, TP and DM revised the manuscript. All authors contributed to the article and approved the submitted version.
Funding
This project was supported by the Paulson Institute and Heren Charitable Foundation.
Acknowledgments
We thank Shou-Dong Zhang, Wanjuan Ke, Yan Gao for their help in the sampling and measurement. We thank Shou-Dong Zhang for the help in processing macrobenthic samples. We thank Jinjin Du for his help in coordinating the fieldwork.
Conflict of interest
The authors declare that the research was conducted in the absence of any commercial or financial relationships that could be construed as a potential conflict of interest.
Publisher’s note
All claims expressed in this article are solely those of the authors and do not necessarily represent those of their affiliated organizations, or those of the publisher, the editors and the reviewers. Any product that may be evaluated in this article, or claim that may be made by its manufacturer, is not guaranteed or endorsed by the publisher.
References
Adámek Z., Maršálek B. (2013). Bioturbation of sediments by benthic macroinvertebrates and fish and its implication for pond ecosystems: a review. Aquacult. Int. 21, 1–17. doi: 10.1007/s10499-012-9527-3
Bianchelli S., Buschi E., Danovaro R., Pusceddu A. (2018). Nematode biodiversity and benthic trophic state are simple tools for the assessment of the environmental quality in coastal marine ecosystems. Ecol. Indic. 95, 270–287. doi: 10.1016/j.ecolind.2018.07.032
Chen Z., Guo L., Jin B., Wu J., Zheng G. (2009). Effect of the exotic plant spartina alterniflora on macrobenthos communities in salt marshes of the Yangtze river estuary, China. Estuar Coast. Shelf S. 82, 265–272. doi: 10.1016/j.ecss.2009.01.014
Chen Z., Li B., Zhong Y., Chen J. (2004). Local competitive effects of introduced spartina alterniflora on scirpus mariqueter at dongtan of chongming island, the Yangtze river estuary and their potential ecological consequences. Hydrobiologia 528, 99–106. doi: 10.1007/s10750-004-1888-9
Chung C. H. (2006). Forty years of ecological engineering with Spartina plantations in China. Ecol. Eng. 27, 49–57. doi: 10.1016/j.ecoleng.2005.09.012
Food and Drug Administration (FDA) (2011). Fish and fishery products hazards and controls guidance, 4th Edn. (Washington, DC: Department of Health and Human Services, Food and Drug Administration, Center for Food Safety and Applied Nutrition).
De Witte B., Coleman B., Bekaert K., Boitsov S., Botelho M. J., Castro-Jiménez J., et al. (2022). Threshold values on environmental chemical contaminants in seafood in the European economic area. Food Control 138, 108978. doi: 10.1016/j.foodcont.2022.108978
DNR (2012) Imazapyr chemical fact sheet. Available at: https://dnr.wisconsin.gov/sites/default/files/topic/Lakes/ImazapyrFactsheet.pdf.
Donadi S., van der Zee E. M., van der Heide T., Weerman E. J., Piersma T., van de Koppel. J., et al. (2014). The bivalve loop: Intra- specific facilitation in burrowing cockles through habitat modification. J. Exp. Mar. Biol. Ecol. 461, 44–52. doi: 10.1016/j.jembe.2014.07.019
Donadi S., Westra J., Weerman E. J., van der Heide T., van der Zee E. M., van de Koppel J., et al. (2013). Non-trophic interactions control benthic producers on intertidal flats. Ecosystems 16, 1325–1335. doi: 10.1007/s10021-013-9686-8
Durkin P. R. (2013). Scoping/Screening level risk assessment on fluazifop-p-butyl (Syracuse Environmental Research Associates Inc., New York, NY, USA), 293. Available online: https://www.fs.fed.us/foresthealth/pesticide/pdfs/Fluazifop-P-butyl.pdf (accessed on 7 July 2019).
Early R., Bradley B. A., Dukes J. S., Lawler J. J., Olden J. D., Blumenthal D. M., et al. (2016). Global threats from invasive alien species in the twenty-first century and national response capacities. Nat. Commun. 7, 12485. doi: 10.1038/ncomms12485
Fisher J.P., Mavros B., Walker D., Heller M., Suedel B., Gillespie B., Slocomb J. (2003). Ecological risk assessment of the proposed use of the herbicide imazapyr to control invasive cordgrass (Spartina spp.) in estuarine habitat of Washington State. (Prepared for the Washington State Department of Agriculture by ENTRIX, INC., Olympia, Washington), pp 163.
Garcia-Rossi D., Rank N., Strong D. R. (2003). Potential for self-defeating biological control? variation in herbivore vulnerability among invasive Spartina genotypes. Ecol. Appl. 13, 1640–1649. doi: 10.1890/01-5301
Grevstad F. S., Strong D. R., Garcia-Rossi D., Switzer R. W., Wecker M. S. (2003). Biological control of spartina alterniflora in willapa bay, Washington using the planthopper prokelisia marginata: agent specificity and early results. Biol. Control 27, 32–42. doi: 10.1016/S1049-9644(02)00181-0
Gu J., Jin R., Chen G., Ye Z., Li Q., Wang H., et al. (2021). Areal extent, species composition, and spatial distribution of coastal saltmarshes in China. IEEE J-STARS. 14, 7085–7094. doi: 10.1109/JSTARS.2021.3093673
Herman P. M. J., Middelburg J. J., Van de Koppel J., Heip C. H. R. (1999). Ecology of estuarine macrobenthos. Adv. Ecol. Res. 29, 195–240. doi: 10.1016/S0065-2504(08)60194-4
Hinz H. L., Winston R. L., Schwarzländer M. (2019). How safe is weed biological control? a global review of direct nontarget attack. Q. Rev. Biol. 94, 1–27. doi: 10.1086/702340
Huang L., Zhang Y., Shi Y., Liu Y., Wang L., Yan N. (2015). Comparison of phosphorus fractions and phosphatase activities in coastal wetland soils along vegetation zones of yancheng national nature reserve, China. Estuar Coast. Shelf S. 157, 93–98. doi: 10.1016/j.ecss.2014.09.027
Jackson M. V., Fuller R. A., Gan X., Li J., Mao D., Melville D. S., et al. (2021). Dual threat of tidal flat loss and invasive spartina alterniflora endanger important shorebird habitat in coastal mainland China. J. Environ. Manage. 278, 111549. doi: 10.1016/j.jenvman.2020.111549
Ju R., Li H., Shang L., Qiu S., Li J., Nie M., et al. (2017). “Saltmarsh cordgrass spartina alterniflora loisel,” in Biological invasions and its management in China (Singapore: Springer), 187–198. doi: 10.1007/978-981-10-3427-5_14
Kang Y. Y., Ding X. R., Zhang C. K. (2015). Maximum tidal range analysis of radial sand ridges in the southern yellow Sea. Indian J. Geo-Marine Sci. 44, 971–976.
Knott C. A., Webster E. P., Nabukalu P. (2013). Control of smooth cordgrass (Spartina alterniflora) seedlings with four herbicides. J. Aquat. Plant Manage. 51, 132–135.
Liang Q., Yan Z., Li X. (2020). Influence of the herbicide haloxyfop-r-methyl on bacterial diversity in rhizosphere soil of spartina alterniflora. Ecotox. Environ. Safe. 194, 110366. doi: 10.1016/j.ecoenv.2020.110366
Li B., Liao C.-H., Zhang X.-D., Chen H.-L., Wang Q., Chen Z.-Y., et al. (2009). Spartina alterniflora invasions in the Yangtze river estuary, China: an overview of current status and ecosystem effects. Ecol. Eng. 35, 511–520. doi: 10.1016/j.ecoleng.2008.05.013
Li X., Li J., Wang Y., Fu L., Fu Y., Li B., et al. (2011). Aquaculture industry in China: current state, challenges, and outlook. Rev. Fisheries Sci. 19, 187–200. doi: 10.1080/10641262.2011.573597
Ma Z., Li B., Li W., Han N., Chen J., Watkinson A. R. (2009). Conflicts between biodiversity conservation and development in a biosphere reserve. J. Appl. Ecol. 46, 527–535. doi: 10.1111/j.1365-2664.2008.01528.x
Meng W., Feagin R. A., Innocenti R. A., Hu B., He M., Li H. (2020). Invasion and ecological effects of exotic smooth cordgrass spartina alterniflora in China. Ecol. Eng. 143, 105670. doi: 10.1016/j.ecoleng.2019.105670
Mo X., Dong P., Xie L., Xiu Y., Wang Y., Wu B., et al. (2021). Effects of imazapyr on spartina alterniflora and soil bacterial communities in a mangrove wetland. Water 13, 3277. doi: 10.3390/w13223277
Patten K. (2002). Smooth cordgrass (Spartina alterniflora) control with imazapyr. Weed Technol. 16, 826–832. doi: 10.1614/0890-037X(2002)016[0826:SCSACW]2.0.CO;2
Peng H.-B., Chan Y.-C., Compton T. J., Cheng X. F., Melville D. S., Zhang S. D., et al. (2021). Mollusc aquaculture homogenizes intertidal soft-sediment communities along the 18,400 km long coastline of China. Diver. Distr. 27, 1553–1567. doi: 10.1111/ddi.13302
Piersma T., Lok T., Chen Y., Hassell C. J., Yang H.-Y., Boyle A., et al. (2016). Simultaneous declines in summer survival of three shorebird species signals a flyway at risk. J. Appl. Ecol. 53, 479–490. doi: 10.1111/1365-2664.12582
Qiao P., Wang A., Xie B., Wang L., Han G., Mei B., et al. (2019). Effects of herbicides on invasive spartina alterniflora in the yellow river delta. Acta Ecologica Sin. 39, 5627–5634.
Rakhimberdiev E., Duijns S., Karagicheva J., Camphuysen C. J., Dekinga A., Dekker R., et al. (2018). Fuelling conditions at staging sites can mitigate Arctic warming effects in a migratory bird. Nat. Commun. 9, 1–10. doi: 10.1038/s41467-018-06673-5
Rani L., Thapa K., Kanojia N., Sharma N., Singh S., Grewal A. S., et al. (2021). An extensive review on the consequences of chemical pesticides on human health and environment. J. Clean. Prod. 283, 124657. doi: 10.1016/j.jclepro.2020.124657
Roberts P. D., Pullin A. S. (2008). The effectiveness of management interventions for the control of spartina species: A systematic review and meta-analysis. Aquat. Conserv. 18, 592–618. doi: 10.1002/aqc.889
Seebens H., Essl F., Dawson W., Fuentes N., Moser D., Pergl J., et al. (2015). Global trade will accelerate plant invasions in emerging economies under climate change. Glob. Change Biol. 21, 4128–4140. doi: 10.1111/gcb.13021
Sheng Q., Huang M., Tang C., Niu D., Ma Q., Wu J. (2014). Effects of different eradication measures for controlling spartina alterniflora on plants and macrobenthic invertebrates. Acta Hydrobiologica Sin. 38, 279–290.
Strong D. R., Ayres D. R. (2013). Ecological and evolutionary misadventures of spartina. Annu. Rev. Ecol. Evol. S. 44, 389–410. doi: 10.1146/annurev-ecolsys-110512-135803
UNESCO (2019) Migratory bird sanctuaries along the coast of yellow Sea-bohai gulf of China (Phase I). Available at: https://whc.unesco.org/en/list/1606/.
USEPA (2006). Reregistration eligibility decision (RED) for imazapyr (Washington, DC, USA: United States Environmental Protection Agency).
van der Zee E. M., van der Heide T., Donadi S., Eklöf J. S., Eriksson B. K., Olff H., et al. (2012). Spatially extended habitat modification by intertidal reef- building bivalves has implications for consumer-resource interactions. Ecosystems 15, 664–673. doi: 10.1007/s10021-012-9538-y
Wang S. K., Chu T. J., Huang D. Q., Li B., Wu J. H. (2014). Incorporation of exotic spartina alterniflora into diet of deposit-feeding snails in the Yangtze river estuary salt marsh: stable isotope and fatty acid analyses. Ecosystems 17, 567–577. doi: 10.1007/s10021-013-9743-3
WSSA (2014). “Imazapyr,” in Herbicide handbook, 10th ed. Ed. Shaner D. L. (Lawrence, KS, USA: Weed Science Society of America), 258–259.
Xie B., Han G., Qiao P., Mei B., Wang Q., Zhou Y., et al. (2019). Effects of mechanical and chemical control on invasive spartina alterniflora in the yellow river delta, China. PeerJ 7, e7655. doi: 10.7717/peerj.7655
Yuan L., Zhang L., Xiao D., Huang H. (2011). The application of cutting plus waterlogging to control spartina alterniflora on saltmarshes in the Yangtze estuary, China. Estuar. Coast. Shelf S. 92, 103–110. doi: 10.1016/j.ecss.2010.12.019
Zhang S.-D., Ma Z., Choi C.-Y., Peng H.-B., Melville D. S., Zhao T. T., et al. (2019b). Morphological and digestive adjustments buffer performance: How staging shorebirds cope with severe food declines. Ecol. Evol. 9, 3868–3878. doi: 10.1002/ece3.5013
Zhang Y., Pennings S. C., Li B., Wu J. (2019a). Biotic homogenization of wetland nematode communities by exotic spartina alterniflora in China. Ecology 100, e02596. doi: 10.1002/ecy.2596
Keywords: Spartina alterniflora, imazapyr, macrobenthos, Jiangsu coast, intertidal mudflats, biological invasion
Citation: Peng H-B, Shi J, Gan X, Zhang J, Ma C, Piersma T and Melville DS (2022) Efficient removal of Spartina alterniflora with low negative environmental impacts using imazapyr. Front. Mar. Sci. 9:1054402. doi: 10.3389/fmars.2022.1054402
Received: 26 September 2022; Accepted: 30 November 2022;
Published: 15 December 2022.
Edited by:
Serena Moseman-Valtierra, University of Rhode Island, United StatesReviewed by:
Sikai Wang, Chinese Academy of Fishery Sciences, ChinaGuanglong Qiu, Guangxi Academy of Sciences, China
Copyright © 2022 Peng, Shi, Gan, Zhang, Ma, Piersma and Melville. This is an open-access article distributed under the terms of the Creative Commons Attribution License (CC BY). The use, distribution or reproduction in other forums is permitted, provided the original author(s) and the copyright owner(s) are credited and that the original publication in this journal is cited, in accordance with accepted academic practice. No use, distribution or reproduction is permitted which does not comply with these terms.
*Correspondence: Jianbin Shi, amJzaGlAYm51LmVkdS5jbg==; He-Bo Peng, aGViby5wZW5nQG91dGxvb2suY29t
†These authors have contributed equally to this work