- 1Marine Ecology, GEOMAR Helmholtz Centre for Ocean Research Kiel, Kiel, Germany
- 2The Alfred Wegener Institute, Helmholtz Centre for Polar and Marine Research, Bremerhaven, Germany
- 3British Antarctic Survey, Cambridge, United Kingdom
- 4Department of Oceanography, University of Hawaii at Mānoa, Honolulu, HI, United States
When pelagic organisms die and fall onto the deep-sea floor they create food falls, i.e., parcels of organic enrichment that subsidize deep benthic scavenging communities. The diversity and quantities of food falls remain unstudied for many ocean regions since they are stochastically deposited and rapidly scavenged. The Southern Ocean habitat supports large populations of megafauna but few food falls have been documented. To investigate the diversity and quantity of food falls in the northwestern Weddell Sea, we analyzed 8476 images from the deep seafloor that were captured during the expedition PS118 on RV Polarstern in 2019 by the camera system OFOBS (Ocean Floor Observation and Bathymetry System). OFOBS was towed 1.5 m above the seafloor along five transects (400 to 2200 m seafloor depth) east of the Antarctic Peninsula. We observed the carcasses of one baleen whale, one penguin, and four fish at depths of 647 m, 613 m, 647 m, 2136 m, 2165 m, and 2112 m, respectively, as well as associated scavenging fauna. To the best of our knowledge, we describe here the first in situ observations of deep-sea food falls for penguins and fish in the Southern Ocean. While the whale carcass seemed in an intermediate successional stage, both the penguin and the fish were likely recently deposited and three of the fish potentially resulted from fishery discards. Our relatively small data set suggests that a diverse array of food falls provide nutrients to the slopes of the Powell Basin.
Introduction
The deep seafloor is typically considered a food-depleted environment, where benthic fauna, other than chemosynthetic communities, primarily rely on the downward flux of organic matter produced in the overlying sunlit ocean layers (Dayton and Hessler, 1972; Gooday et al., 1990; Glover and Smith, 2003). This downward flux of particulate organic matter (POC), composed of fecal pellets, marine snow, and phytodetritus, is part of the “biological pump”, which results in the export of atmospheric carbon to the deep sea (Turner, 2015). In regional carbon budgets, the carbon consumption of deep benthic communities often exceeds the POC measured with sediment traps (Smith, 1987; Smith et al., 1992; Andersson et al., 2004). It is hypothesized that the discrepancy between measured and required energy in the deep sea in part results from selective collection by sediment traps (Smith and Kaufmann, 1999; Smith and Demopoulos, 2003; Robison et al., 2005) and an underestimation of the flux of carcasses of larger taxa such as macro- and megazooplankton and nekton, also known as food falls.
Food falls act as localized energy pulses to the deep seafloor (Stockton and DeLaca, 1982; Smith, 1985), with documented seafloor residence times ranging from hours to months depending on carcass size, depth, region, and composition. These food parcels constitute a much more labile source of organic matter than the more degraded smaller particles of the marine snow. Unlike marine snow, food falls can attract diverse scavenging megafauna which in turn can attract predators, also creating indirect feeding opportunities (Smith, 1985; Kemp et al., 2006). Despite increasing exploration of the deep-sea floor with in situ observations via ROVs (Remotely Operated Vehicles), AUVs (Automated Underwater Vehicles), and towed cameras, scientific reports of food falls are still rare. This is especially true for small- or medium-sized food falls (1-100 cm) (Hoving et al., 2017), which are rapidly consumed by scavengers (Soltwedel et al., 2003; Sweetman et al., 2014; Amon et al., 2017; Scheer et al., 2022). The rarity of such observations impedes estimations of their contribution to the biological carbon pump and assessments of their importance to scavengers.
Reports of natural medium-sized food falls, and their scavenging communities and rates, residence time, and estimated carbon contribution, at the moment of writing include carcasses of fish (Smith, 1985; Soltwedel et al., 2003; Yamamoto et al., 2009; Aguzzi et al., 2012; Higgs et al., 2014; Amon et al., 2017), cephalopods (Hoving et al., 2017), crustaceans (Christiansen and Boetius, 2000; Klages et al., 2001), jellyfish (Yamamoto et al., 2008; Sweetman and Chapman, 2011), and tunicates (Henschke et al., 2013; Stenvers et al., 2021). While for whale carcasses, the residence time, succession and consumption, scavenging communities and carbon contribution have been studied, both via naturally and experimentally deposited carcasses around the world (Fujiwara et al., 2007; Lundsten et al., 2010b; Lundsten et al., 2010a; Amon et al., 2013; Smith et al., 2014b; Smith et al., 2014a; Smith et al., 2015), descriptions of whale falls are still worthwhile to report. For example, it was relatively recently that a whale fall was documented for the first time in the southwestern Atlantic (Sumida et al., 2016). Residence time, scavenging communities and successional stages of food falls can depend on carcass size and composition (Smith, 1985; Rohlfer et al., 2022). Small- and medium-sized food falls are rapidly consumed, whereas large whale falls undergo a number of successional stages including mobile scavenger, enrichment opportunistic, and chemosynthetic stages, creating habitats for a diverse and unique community of organisms over longer time periods (Smith and Baco, 2003; Smith et al., 2015).
No reports of medium-sized food falls are yet published from the northwestern Weddell Sea or flanks of the Powell Basin. The pelagic community of the northwestern Weddell Sea is based on krill populations that supply feeding grounds for fish, cephalopods, seals, penguins and cetaceans (Atkinson et al., 2008; Trathan and Hill, 2016; Xavier et al., 2018). Additionally, gelatinous fauna such as the tunicate Salpa thompsoni, inhabit the pelagic realm of the region, and these taxa may become more important with ongoing warming in the Southern Ocean, which has been hypothesized to shift salp populations southwards (Słomska et al., 2021). Although the Southern Ocean attracts baleen whales from lower latitudes, only a few natural whale falls have been observed and studied, with the first of these discovered in 2012 (Amon et al., 2013; Smith et al., 2014b). The Antarctic Peninsula marine environment is affected by rapid regional warming and exploitation of fin-fish stocks (Vaughan et al., 2003; Ainley and Pauly, 2014). To better understand the food supply of deep benthic communities in a rapidly changing Antarctic ocean, we analyzed seafloor images from the northwestern Weddell Sea for the presence of food falls as well as their associated scavenging fauna.
Material and methods
Observations of the seafloor were collected during the PS118 expedition with RV Polarstern to the Weddell Sea in February-April 2019. The towed camera system ”OFOBS” (Ocean Floor Observation and Bathymetry System) was deployed seven times to assess seafloor habitats and fauna in areas recently cleared from permanent ice cover (Purser et al., 2021). A high-resolution camera system (iSiTEC, Canon EOS 5D Mark III) collected images with an interval of about 20 s. Additionally, the OFOBS operator could also trigger “hotkey” images at any timepoint. This resulted in 12,691 images. Data and power are both transmitted through optic/coaxial cable, connecting the subsea with the topside unit of OFOBS (Purser et al., 2019). The iXBlue Posidonia ultra-short base line (USBL) system was used to retrieve the positional data of the camera system to a likely accuracy of ca. 20 m. The OFOBS system was deployed at around 1.5-2 m above seafloor producing images capturing 4-6 m2 of the seafloor which was illuminated by four downward directed SeaLight Sphere 3150 LED lights and two iSiTEC UW_Blitz 250 strobe lights. The system was additionally equipped with three lasers, creating a triangle of laser points on each image with a fixed distance of 50 cm between the points.
Images from five of the seven PS118 OFOBS transects were used for this study (Figure 1). 1404, 3807, 2723, 158, and 384 images were checked for food falls from transects “PS118_6-9”, “PS118_39-1”, “PS118_69-1”,”PS118_77-1”, and “PS118_81-1”, respectively. This resulted in a dataset of 8476 analyzed images from water depths between 400 and 2200 m. A list of all analyzed images can be found in the Supplementary Materials. Approximately 1000 images were tagged as unusable because they were either taken from the water column or were too dark to identify possible food falls. Each image was inspected manually in Microsoft Photos with zoom and light adjustment functions applied if necessary. Images with possible food falls were extracted and the positional data of the images were mapped using the GIS software QGIS (v. 3.16.6-Hannover). Depths and coordinates of the food falls were extracted from the PANGAEA repository (Purser et al., 2020) and are summarized in the Supplementary Materials, together with the image IDs. For Figures 2-4, the original images were processed for illustrational reasons using Microsoft Photos and Adobe Photoshop (v. 23.4.2). Additionally, fishing effort data was downloaded from the “Global Fishing Watch” website to evaluate the influence of fishing discards (Global Fishing Watch Inc (2022). https://globalfishingwatch.org/data-download/datasets/public-fishing-effort [Accessed August 05, 2022].).
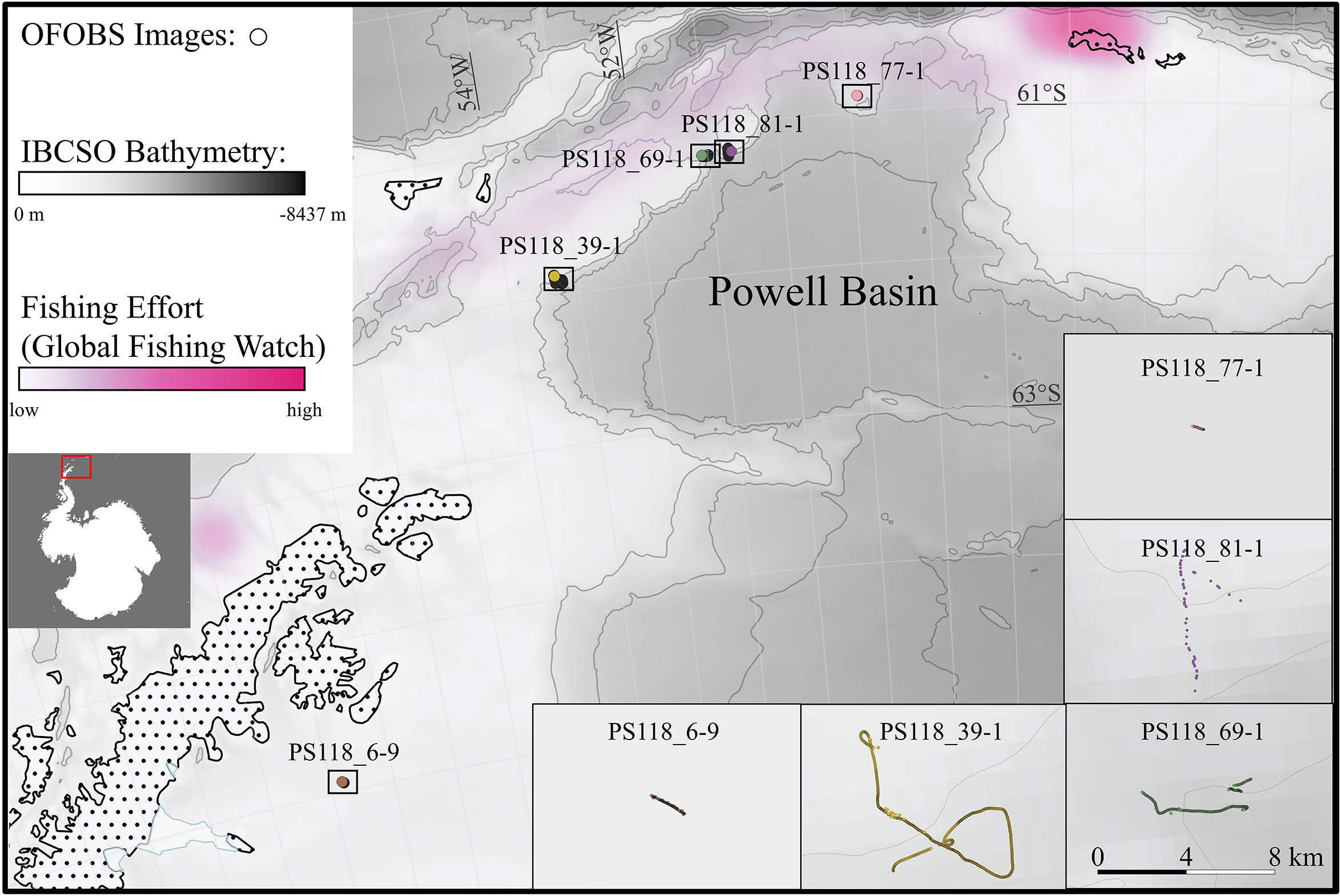
Figure 1 Location of the five OFOBS (Ocean Floor Observation and Bathymetry System) image transects during cruise PS118 from which images were analyzed to detect food falls. Analyzed images are depicted as points. For transect PS118_69-1 1073 and for transect PS118_81-1 335 images are not shown because of missing meta data. IBCSO bathymetry is represented as a grey scale with isobaths each 1000 m (Dorschel et al., 2022). Fishing effort data from globalfishingwatch.org is represented with a pink heatmap, indicating fishing effort from March 31 2019 when food falls “Fish_1”, “Fish_2”, and “Fish_3” were found. The Antarctic Polar Stereographic projection was used to display the map.
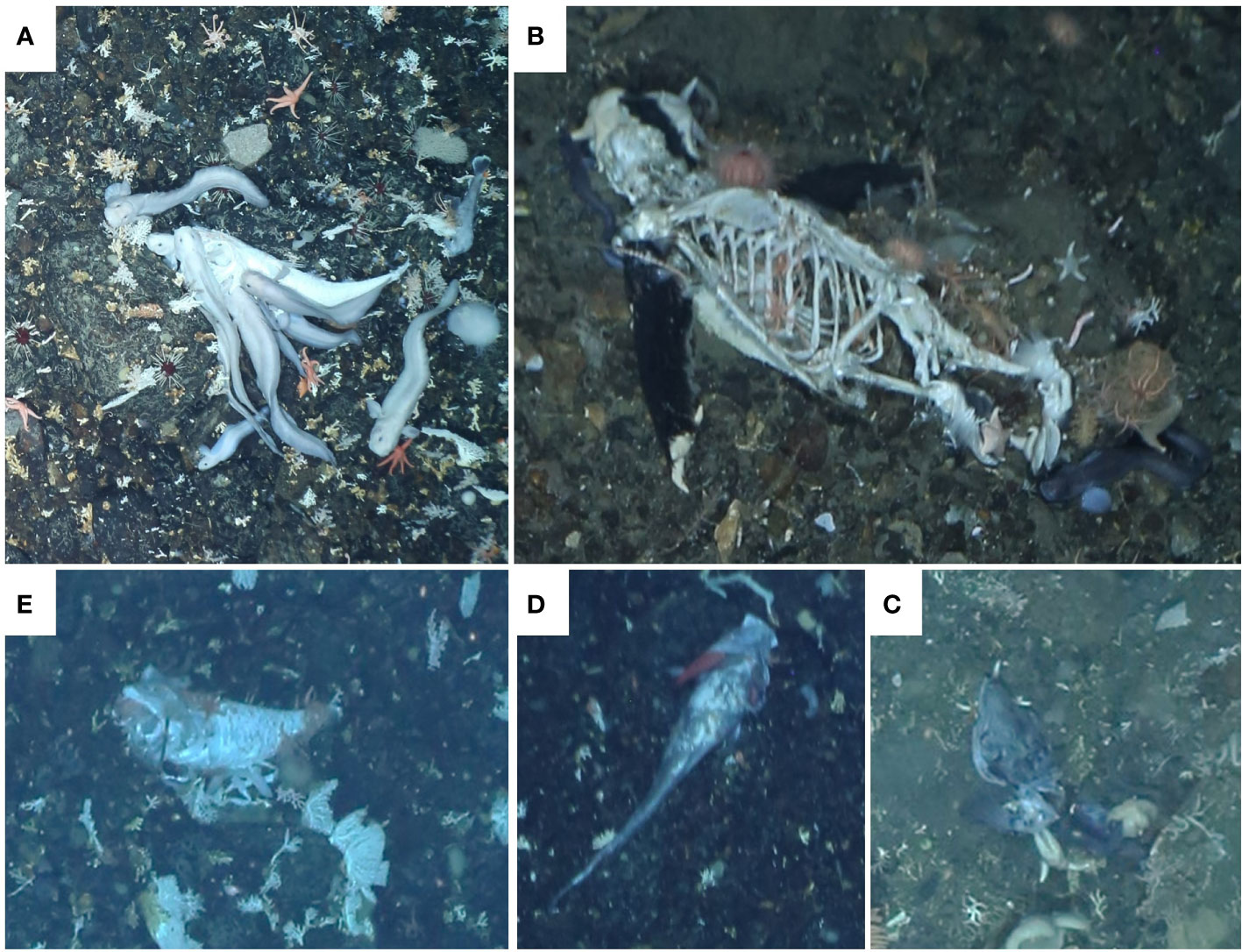
Figure 2 Medium-sized food falls and associated scavengers detected on the slopes of the Powell Basin during cruise PS118. (A) Fish carcass (”Fish_3”) at 2112 m scavenged by zoarcid fish. (B) Penguin carcass found at 613 m accompanied by echinoderms and zoarcid fish. (C) Fish carcass (“Fish_4”) at 647 m. (D) Fish remains (“Fish_2”) found at 2165 m with scavenging amphipod burrowed into its gill slit. (E) Fish carcass (“Fish_1”) from 2136 m. Images were cropped and light correction was applied if necessary.
Results
The two transects “PS118_69-1” and “PS118_39-1”, which covered seafloor areas of the slopes of the Powell Basin, yielded food falls (Figure 1). On transect “PS118_69-1” three fish carcasses (“Fish_1”, “Fish_2”, and “Fish_3”) were detected. And the transect “PS118_39-1” yielded one fish carcass (“Fish_4”), one dead penguin, one whale fall, and two bone assemblages.
The three fish carcasses (“Fish_1”, “Fish_2”, “Fish_3”) of “PS_118_69-1” detected at water depths of 2112 m, 2165 m, 2136 m (Figures 2A, D, E), and within vicinity of each other (distance between “Fish_1” and “Fish_3” was ca. 1200 m). The morphology of the three carcasses suggests that they all belong to Grenadier fish (Macrouridae) which were often observed alive close to the seafloor on other images. All three remains still had tissue connected to the skeletons suggesting recent deposition. Associated scavenger fauna included echinoderms, an amphipod, eelpouts, and unidentified fish. An amphipod was half burrowed into the gill slit of “Fish_2”. Eelpouts were visibly feeding on the carcass of “Fish_3”.
The fish carcass “Fish_4”, detected at 647 m water depth, was blueish and there appeared to be tissue left on the head of the carcass (Figure 2C). Judging by the head color and morphology (wide jaw), the remains could belong to an icefish (Channichthyidae) which were also observed alive on other images of the study area. There were at least seven eelpouts (Zoarcidae) present near the fish carcass. At least two starfish (Asteroidea) were attached to the fish remains and brittle stars (Ophiuroidea, likely Astrochlamys sol) were present near the carcass.
The penguin carcass was detected at a water depth of 613 m and was largely scavenged to the bones but still contained black tissue on its head and flippers (Figure 2B). Two eelpouts were observed close to the carcass. Various echinoderms including ophiuroids (likely Astrochlamys sol), at least two species of asteroids, and echinoids (Echinoidea, likely Sterechinus sp.) were on the carcass. Using the 50 cm laser points as size reference, the penguin carcass length was less than 30 cm which is too short for the penguins living around the Antarctic peninsula. An underestimation of the size may result from a sloped seafloor and because the carcass is not centered on the image.
There was no visible soft tissue left on the whale fall which was observed at a depth of 647 m and the vertebrae and bones were detached from one another (Figure 3). The skeleton was incomplete with limb bones and ribs missing or not visible. The skull morphology suggests that the remains belonged to a baleen whale. The images of the whale fall are underexposed and were taken from several meters above the seafloor and therefore appeared dark and of low resolution. This made it difficult to detect and identify scavenging organisms. The whale carcass too could not be properly measured because laser points were missing from the image due to the high relief of this region of the flank of the Powell Basin.
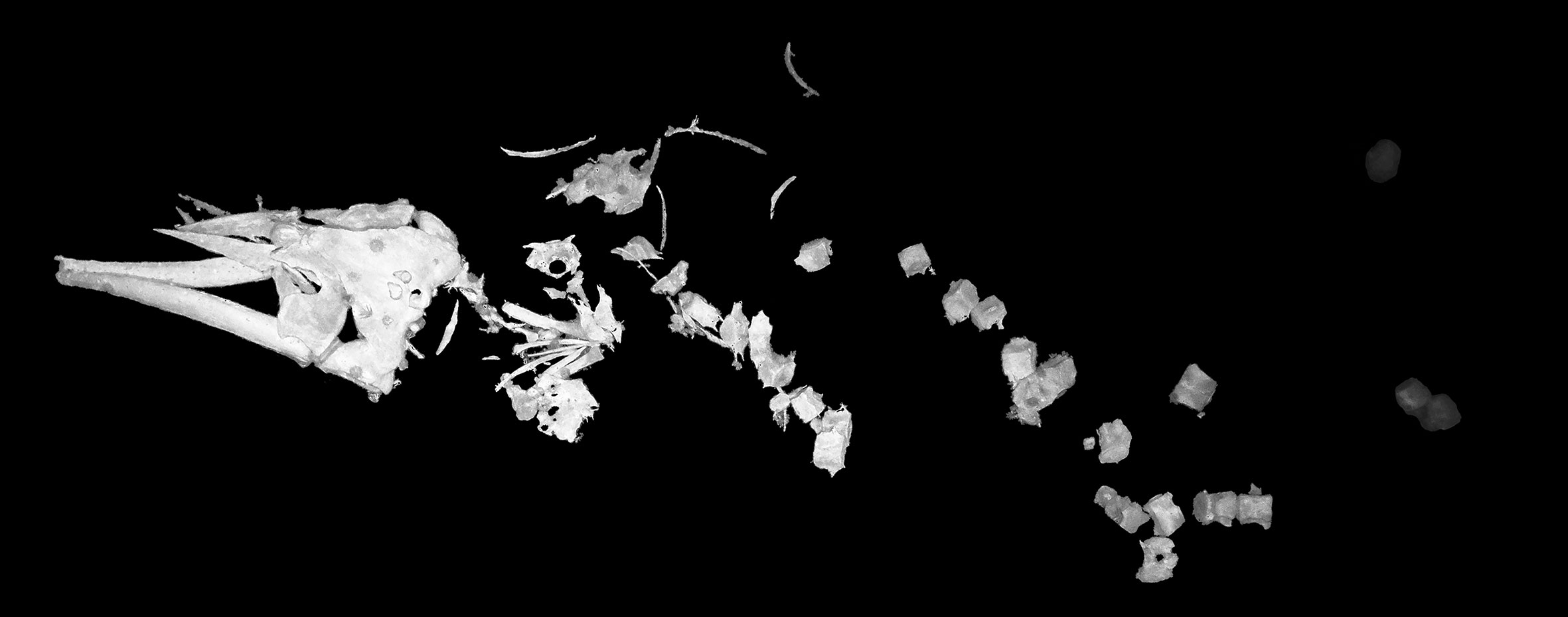
Figure 3 Whale fall of a baleen whale as observed on the slopes of the Powell Basin during the cruise PS118. For better visibility, the skeleton was cropped from its image, dropped on a black background and a black-and-white filter was applied.
Two bone assemblages were found (Figure 4). There was no visible tissue left on the bones nor were they arranged in a way that would allow identification of their origin. The bone assemblage found at 1330 m (“Bones_1”) consisted of around eight individual bones. The second Bone assemblage (“Bones_2”) consisted of just one bone at a water depth of 1328 m. The sizes of the bones suggest that they belonged to mammals.
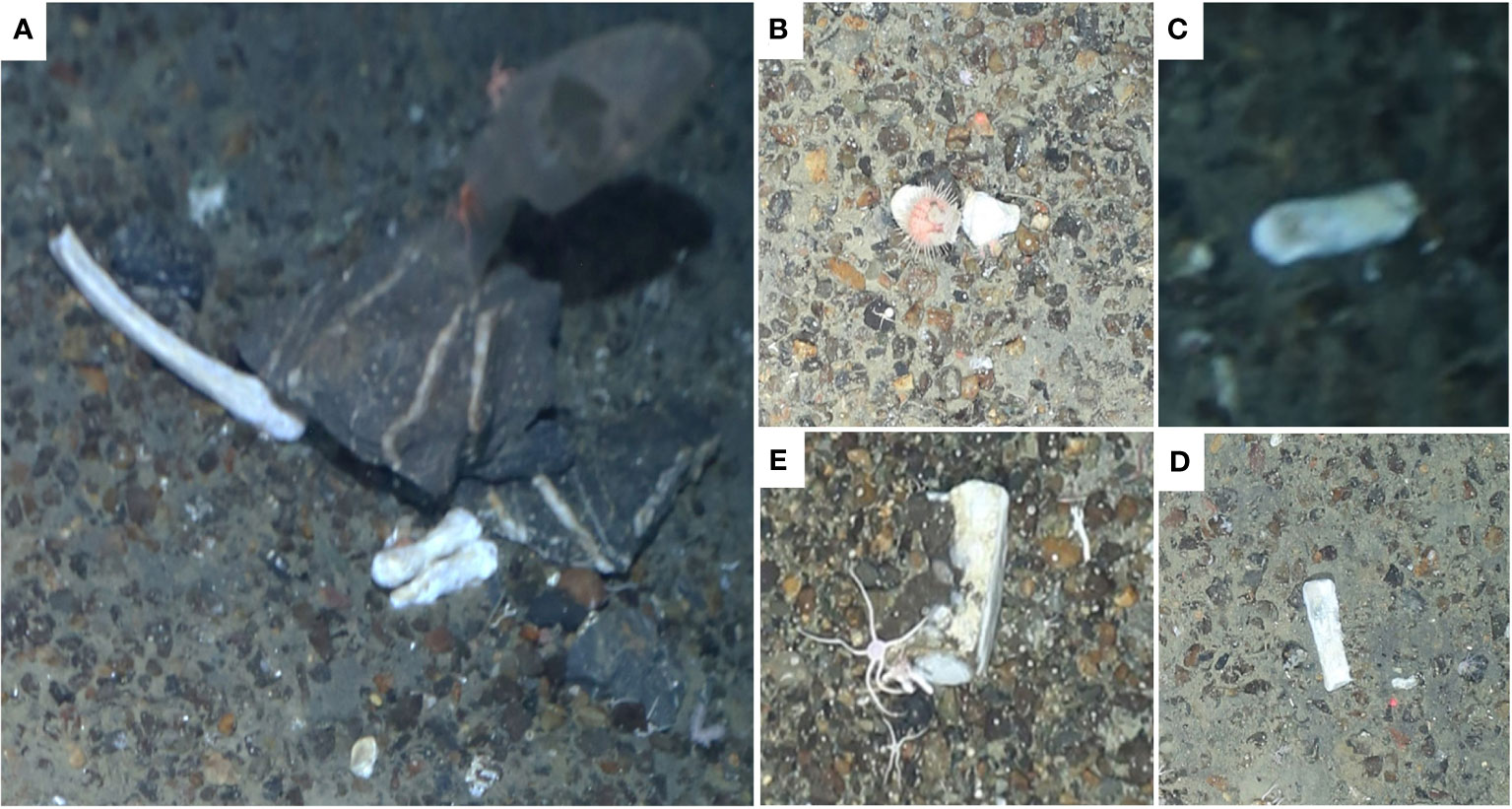
Figure 4 Loose bone assemblages found on the slopes of the Powell Basin during the cruise PS118. Bones (A-D) were found on three consecutive images at a water depth of around 1330 m (“Bones_1”). Bone (E) was found at a water depth of 1328 m (“Bones_2”). Images were cropped and light correction was applied if necessary.
Discussion
Despite the small sample size and the limited image resolution, we here document among the first food fall observations in the deep Weddell Sea. Because of the uneven bathymetry of the study region and other methodological limitations, we were unable to calculate exact size and densities of food falls. However, our unique observations allowed preliminary insights in the role of nekton in the coupling between the Antarctic pelagic and benthic habitats.
The three fish carcasses found on transect “PS118_69-1” were all in relatively close proximity to each other (ca. 1200 m). Carcasses of 1-100 cm are scavenged very fast. Food fall experiments with Mackerel (Scomber scombrus) showed scavenging rates of 31.6 g h-1 between 1360 and 1440 m in the southern Norwegian Sea (Scheer et al., 2022) and 57.8 g h-1 at 1250 m in a Norwegian fjord (Sweetman et al., 2014) with bait residence times of approximately 9 h and 7 h respectively. Another experiment at 1300 m in the northeast Pacific with fish baits of 1, 2, 4, and 40 kg showed 90% of the baits were scavenged within 7 h to three weeks (Smith, 1985). Soltwedel and colleagues observed a zoarcid fish that was attacked and eaten by scavenging amphipods in one of their baited traps deployed at the HAUSGARTEN Observatory in the Fram Strait (Soltwedel et al., 2003). The 45 cm long zoarcid fish, with an estimated wet weight of ca. 700 g, was skeletonised within 11 h after entering the trap. Because the three carcasses were detected in a similar stage of decomposition and because carcasses of this size are scavenged rapidly, we can say that the three fish remains were deposited within a short time period of each other and potentially under 12 h. Their proximity, the fact that all three remains seemed to belong to the same taxonomic group (Macrouridae) and their similar decomposition stage, further suggests that the fish originated from a common source. One possibility is that they were fishery discards. Grenadier fish (Macrouridae) are common by-catch in Southern Ocean longline fishing for toothfish (Dissostichus spp.) (Morley et al., 2004; Petrov and Gordeev, 2015). The transect where the three remains were found lies in the fishing subarea 48.1, an area that was closed for fin-fishing in 1990 to protect fish stocks after intense depletion (Ainley and Pauly, 2014). For the 2018/19 season, fishing for toothfish and other fin-fish was still prohibited in subarea 48.1 with exceptions for research purposes (CCAMLR, 2018). Subarea 48.1 at time of writing was mainly used for krill (Euphausiacea) fisheries via midwater trawling, where macrourids are not common by-catch (Watters, 1996; Arana and Rolleri, 2020). Although, the three carcasses are unlikely to originate from krill fishery by-catch, it is possible that they resulted from fisheries, in particular since fishing vessels were present close to the study location on the day the carcasses were found (Figure 1).
The extraction of carbon in the form of large fish by fisheries could possibly limit carbon sequestration, since fishing prevents the natural death of these fish and subsequently their contribution to the biological carbon pump as food falls (Mariani et al., 2020). On the other hand, we can argue that on smaller scales, fisheries can also stimulate carbon sequestration through fisheries discards. Almost 10% of the global annual marine catch is discarded (Zeller et al., 2018), and although only a fraction of these discards will probably be sequestered in the deep sea, these discards may still act as localized pulses of labile carbon, stimulating the biological carbon pump regionally. The connection between fisheries discards, stimulation of food fall deposition and the biological carbon pump needs consideration in future research.
The fourth fish carcass, found on transect “PS118_39-1”, likely belonged to the icefish family (Channichthyidae). Icefish are endemic to the Southern Ocean and were also observed alive on our image transects. Icefish build nests on the seafloor. Huge assemblies of these nests have been documented in the eastern Weddell Sea between depths of 420 m and 535 m (Purser et al., 2022) and were also observed occasionally on the image transects analyzed in this study. The cause of death of this specimen is unknown.
We observed a baleen whale fall at 647 m. Smith and Baco (2003) suggested that deep-sea whale falls go through four stages of decomposition, with each stage exhibiting its own characteristics. These different stages are 1) the mobile-scavenger stage, 2) the enrichment-opportunist stage, 3) the sulphophilic stage, and 4) the reef stage. The first Southern Ocean natural whale fall was detected in the South Sandwich Arc at water depths between 1444 and 1447 m in 2012 (Amon et al., 2013). The skeleton belonged to an Antarctic minke whale (Balaenoptera bonaerensis) and was in an advanced stage of decomposition with no tissue left, no mobile scavengers present and blackened anoxic sediment below the bones, indicating that the whale fall was in the enrichment-opportunist or sulfophilic stage. Another Southern Ocean whale fall was previously reported from the slope of Anvers Island at a depth of 1430 m in 2013 (Smith et al., 2014b). Because of its size and location, it was assumed that the carcass also belonged to an Antarctic minke whale. The remains were found to be in the mobile scavenger stage with tissue still covering the skeleton and with zoarcids, Grenadier fish, and crustaceans beside and on the carcass. When comparing the images of these two published whale falls with the one presented here, it seems that the PS118 whale fall was found at an intermediate stage between the two other Southern Ocean whale falls. Although no tissue was left and mobile scavenging fauna were not visible, the bones did not appear to be as degraded as the bones of the whale fall discovered in the South Sandwich Arc. Unfortunately, the images from the PS118 whale fall are overall too dark to allow to distinguish sediment darkness under the bones. So, we assume that the whale fall of this report was found during the enrichment-opportunist stage or early sulphophilic stage. Because there are no time-series studies of intact whale falls at bathyal depths in the Southern Ocean, it is very difficult to estimate how long this whale carcass has been at the seafloor. It does appear to have passed through the mobile scavenger stage, which for similar depths in the northeast Pacific, would suggest it has been at the seafloor for at least 4-5 months (Smith and Baco, 2003; Lundsten et al., 2010b). Scavenging rates may be slower in the Weddell Sea, which lacks some of the principle bathyal scavengers of the northeast Pacific, in particular sharks and hagfish. Thus, we hypothesize that this carcass has been at the seafloor for a minimum of five months, and potentially substantially longer. Due to the advanced state of decomposition, it was not possible to determine the source organisms of the two found bone assemblages.
The penguin fall represents another example of coupling between the pelagic zone and deep sea. To our knowledge, this is the first in situ observation of a penguin carcass found in the deep sea. Tissue was still visible on the carcass and zoarcid fish as mobile scavengers were present, indicating recent deposition of the carcass. The nearest (ca. 80 km) penguin colonies to the penguin fall site is Clarence Island, which is home to chinstrap penguins (Pygoscelis antarcticus) and macaroni penguins (Eudyptes chrysolophus) (Croxall and Kirkwood, 1979; Harris et al., 2015). Since foraging ranges of macaroni penguins on South Georgia can exceed 400 km (Horswill et al., 2016), the penguin fall site is within reach (80 km) of the colonies on Clarence Island. Furthermore, the penguin fall site lies within foraging areas of Adélie penguins (Pygoscelis adeliae) from King George Island and long-distance migration routes of chinstrap penguins (Trivelpiece et al., 2007; Hinke et al., 2015; Oosthuizen et al., 2022). Major predators of penguins include leopard seals (Hydrurga leptonyx), which are known to discard penguin carcasses, creating a possible source for penguin food falls (Hall-Aspland and Rogers, 2004). Because of the high abundance of penguins in the area of the Antarctic Peninsula (over 50,000 breeding pairs on Elephant Island alone (Strycker et al., 2021)), penguin falls could represent a significant food input for deep benthic communities of the Weddell Sea. Indeed, toothfish of the genus Dissostichus scavenge penguin carcasses and were also observed on the images analyzed here (Fenaughty et al., 2003; Roberts et al., 2011). Roberts et al. (2011) found several penguin remains in toothfish (Dissostichus sp.) stomachs captured from the South Sandwich Islands at over 900 m depth. One of these included the almost complete remains of a chinstrap penguin, providing support for short residence times of medium-sized food falls and emphasizing the difficulty to observe them.
We found carcasses of various species in a relatively small data set on the slopes of the Powell Basin at depths between approximately 600 and 2200 m. The number of food falls in our seafloor transects suggests a potentially important role of carcasses in nourishing the deep-sea benthos of the northwestern Weddell Sea. The food falls resulted from a diverse pelagic macro- and megafauna in the productive Weddell Sea and this flux could be further increased by fisheries discards, an unnatural coupling that should be considered when measuring benthic fluxes in the region.
Data availability statement
The original contributions presented in the study are included in the article/Supplementary Material. Analyzed images and meta data can be found on PANGAEA (https://doi.org/10.1594/PANGAEA.911904). Further inquiries can be directed to the corresponding author.
Author contributions
HJH provided the initial idea. AP was responsible for organizing and running the OFOBS data collection during PS118 and provided the imagery data that was analyzed by JS with the help of HJH. HG assisted the OFOBS team during PS118, operating the camera, as well as aiding in identification of fauna in images, with references to samples he collected with epibenthic sledding during the expedition. CS aided with the interpretation of food falls. The manuscript was assembled by JS with the contribution of all co-authors.
Funding
HJH and JS are funded by the DFG (Deutsche Forschungsgemeinschaft) under grant HO 5569/2-1 (Emmy Noether Junior Research Group) and HO 5569/3-1. The Ocean Floor Observation and Bathymetry System (OFOBS) was partially funded by the FRAM project. HG was funded through NERC core funding to the British Antarctic Survey Biodiversity, Evolution and Adaptation Team. We acknowledge financial support by Land Schleswig-Holstein within the funding programme Open Access Publikationsfonds.
Acknowledgments
We would like to thank the captain and the crew of RV Polarstern as well as Laura Hehemann for their support with the OFOBS deployments during the PS118 expedition.
Conflict of interest
The authors declare that the research was conducted in the absence of any commercial or financial relationships that could be construed as a potential conflict of interest.
Publisher’s note
All claims expressed in this article are solely those of the authors and do not necessarily represent those of their affiliated organizations, or those of the publisher, the editors and the reviewers. Any product that may be evaluated in this article, or claim that may be made by its manufacturer, is not guaranteed or endorsed by the publisher.
Supplementary material
The Supplementary Material for this article can be found online at: https://www.frontiersin.org/articles/10.3389/fmars.2022.1055318/full#supplementary-material
References
Aguzzi J., Jamieson A. J., Fujii T., Sbragaglia V., Costa C., Menesatti P., et al. (2012). Shifting feeding behaviour of deep-sea buccinid gastropods at natural and simulated food falls. Mar. Ecol. Prog. Ser. 458, 247–253. doi: 10.3354/meps09758
Ainley D. G., Pauly D. (2014). Fishing down the food web of the Antarctic continental shelf and slope. Polar Rec. 50, 92–107. doi: 10.1017/S0032247412000757
Amon D. J., Glover A. G., Wiklund H., Marsh L., Linse K., Rogers A. D., et al. (2013). The discovery of a natural whale fall in the Antarctic deep sea. Deep Sea Res. Part II: Topical Stud. Oceanogr. 92, 87–96. doi: 10.1016/j.dsr2.2013.01.028
Amon D. J., Hilario A., Arbizu P. M., Smith C. R. (2017). Observations of organic falls from the abyssal clarion-clipperton zone in the tropical eastern pacific ocean. Mar. Biodiver. 47, 311–321. doi: 10.1007/s12526-016-0572-4
Andersson J. H., Wijsman J. W. M., Herman P. M. J., Middelburg J. J., Soetaert K., Heip C. (2004). Respiration patterns in the deep ocean. Geophys. Res. Lett. 31, L03304. doi: 10.1029/2003GL018756
Arana P., Rolleri R. (2020). Incidental catch of marine organisms registered in the Chilean Antarctic krill fishery, years 2012-2016. Lat Am. J. Aquat. Res. 48, 429–439. doi: 10.3856/vol48-issue3-fulltext-2434
Atkinson A., Siegel V., Pakhomov E. A., Rothery P., Loeb V., Ross R. M., et al. (2008). Oceanic circumpolar habitats of Antarctic krill. Mar. Ecol. Prog. Ser. 362, 1–23. doi: 10.3354/meps07498
CCAMLR (2018) Schedule of conservation measures in force 2018/19. Available at: https://www.ccamlr.org/en/document/publications/schedule-conservation-measures-force-2018/19 (Accessed August 22, 2022).
Christiansen B., Boetius A. (2000). Mass sedimentation of the swimming crab Charybdis smithii (Crustacea: Decapoda) in the deep Arabian Sea. Deep Sea Res. Part II: Topical Stud. Oceanogr. 47, 2673–2685. doi: 10.1016/S0967-0645(00)00044-8
Croxall J. P., Kirkwood E. D. (1979). The distribution of penguins on the Antarctic Peninsula and islands of the Scotia Sea. (Cambridge: British Antarctic Survey). Available at: http://nora.nerc.ac.uk/id/eprint/525143/.
Dayton P. K., Hessler R. R. (1972). Role of biological disturbance in maintaining diversity in the deep sea. Deep Sea Res. Oceanogr. Abstracts 19, 199–208. doi: 10.1016/0011-7471(72)90031-9
Dorschel B., Hehemann L., Viquerat S., Warnke F., Dreutter S., Tenberge Y. S., et al. (2022). The international bathymetric chart of the southern ocean version 2. Sci. Data 9, 275. doi: 10.1038/s41597-022-01366-7
Fenaughty J. M., Stevens D. W., Hanchet S. M. (2003). Diet of the antarctic toothfish (Dissostichus mawsoni) from the Ross Sea, Antarctica (Subarea 88.1). CCAMLR Sci. 10, 113–123. Available at: https://www.ccamlr.org/en/publications/science_journal/ccamlr-science-volume-10/ccamlr-science-volume-10113-123.
Fujiwara Y., Kawato M., Yamamoto T., Yamanaka T., Sato-Okoshi W., Noda C., et al. (2007). Three-year investigations into sperm whale-fall ecosystems in Japan. Mar. Ecol. 28, 219–232. doi: 10.1111/j.1439-0485.2007.00150.x
Global Fishing Watch Inc (2022). Available at: https://globalfishingwatch.org/data-download/datasets/public-fishing-effort (Accessed August 05, 2022).
Glover A. G., Smith C. R. (2003). The deep-sea floor ecosystem: current status and prospects of anthropogenic change by the year 2025. Environ. Conserv. 30, 219–241. doi: 10.1017/S0376892903000225
Gooday A. J., Turley C. M., Allen J. A., Charnock H., Edmond J. M., McCave I. N., et al. (1990). Responses by benthic organisms to inputs of organic material to the ocean floor: a review. Philos. Trans. R. Soc. London Ser. A Math. Phys. Sci. 331, 119–138. doi: 10.1098/rsta.1990.0060
Hall-Aspland S. A., Rogers T. L. (2004). Summer diet of leopard seals (Hydrurga leptonyx) in prydz bay, Eastern Antarctica. Polar Biol. 27, 729–734. doi: 10.1007/s00300-004-0662-9
Harris C. M., Lorenz K., Fishpool L. D. C., Lascelles B., Cooper J., Coria N. R., et al. (2015). Important Bird Areas in Antarctica. Cambridge: BirdLife International and Environmental Research & Assessment Ltd.
Henschke N., Bowden D. A., Everett J. D., Holmes S. P., Kloser R. J., Lee R. W., et al. (2013). Salp-falls in the Tasman Sea: a major food input to deep-sea benthos. Mar. Ecol. Prog. Ser. 491, 165–175. doi: 10.3354/meps10450
Higgs N. D., Gates A. R., Jones D. O. B. (2014). Fish food in the deep Sea: Revisiting the role of Large food-falls. PloS One 9, 1–9. doi: 10.1371/journal.pone.0096016
Hinke J. T., Polito M. J., Goebel M. E., Jarvis S., Reiss C. S., Thorrold S. R., et al. (2015). Spatial and isotopic niche partitioning during winter in chinstrap and adélie penguins from the south Shetland islands. Ecosphere 6, art125. doi: 10.1890/ES14-00287.1
Horswill C., Matthiopoulos J., Ratcliffe N., Green J. A., Trathan P. N., McGill R. A. R., et al. (2016). Drivers of intrapopulation variation in resource use in a generalist predator, the macaroni penguin. Mar. Ecol. Prog. Ser. 548, 233–247. doi: 10.3354/meps11626
Hoving H. J. T., Bush S. L., Haddock S. H. D., Robison B. H. (2017). Bathyal feasting: Post-spawning squid as a source of carbon for deep-sea benthic communities. Proc. R. Soc. B: Biol. Sci. 284, 10–12. doi: 10.1098/rspb.2017.2096
Kemp K. M., Jamieson A. J., Bagley P. M., McGrath H., Bailey D. M., Collins M. A., et al. (2006). Consumption of large bathyal food fall, a six month study in the NE Atlantic. Mar. Ecol. Prog. Ser. 310, 65–76. doi: 10.3354/meps310065
Klages M., Vopel K., Bluhm H., Brey T., Soltwedel T., Arntz W. E. (2001). Deep-sea food falls: first observation of a natural event in the Arctic ocean. Polar Biol. 24, 292–295. doi: 10.1007/s003000000199
Lundsten L., Paull C. K., Schlining K. L., McGann M., Ussler W. (2010a). Biological characterization of a whale-fall near Vancouver island, British Columbia, Canada. Deep Sea Res. Part I: Oceanogr. Res. Pap. 57, 918–922. doi: 10.1016/j.dsr.2010.04.006
Lundsten L., Schlining K. L., Frasier K., Johnson S. B., Kuhnz L. A., Harvey J. B. J., et al. (2010b). Time-series analysis of six whale-fall communities in Monterey canyon, California, USA. Deep Sea Res. Part I: Oceanogr. Res. Pap. 57, 1573–1584. doi: 10.1016/j.dsr.2010.09.003
Mariani G., Cheung W. W. L., Lyet A., Sala E., Mayorga J., Velez L., et al. (2020). Let more big fish sink: Fisheries prevent blue carbon sequestration–half in unprofitable areas. Sci. Adv. 6, eabb4848. doi: 10.1126/sciadv.abb4848
Morley S. A., Mulvey T., Dickson J., Belchier M. (2004). The biology of the bigeye grenadier at south Georgia. J. Fish. Biol. 64, 1514–1529. doi: 10.1111/j.0022-1112.2004.00405.x
Oosthuizen W. C., Pistorius P. A., Korczak-Abshire M., Hinke J. T., Santos M., Lowther A. D. (2022). The foraging behavior of nonbreeding adélie penguins in the western Antarctic peninsula during the breeding season. Ecosphere 13, e4090. doi: 10.1002/ecs2.4090
Petrov A. F., Gordeev I. I. (2015). Distribution and biological characteristics of Antarctic toothfish Dissostichus mawsoni in the weddell Sea. J. Ichthyol. 55, 210–216. doi: 10.1134/S0032945215020137
Purser A., Dreutter S., Griffiths H., Hehemann L., Jerosch K., Nordhausen A., et al. (2021). Seabed video and still images from the northern weddell Sea and the western flanks of the Powell basin. Earth Syst. Sci. Data 13, 609–615. doi: 10.5194/essd-13-609-2021
Purser A., Hehemann L., Boehringer L., Tippenhauer S., Wege M., Bornemann H., et al. (2022). A vast icefish breeding colony discovered in the Antarctic. Curr. Biol. 32, 842–850.e4. doi: 10.1016/j.cub.2021.12.022
Purser A., Hehemann L., Dreutter S., Dorschel B., Nordhausen A. (2020). OFOBS seafloor images from the Antarctic peninsula and Powell basin, collected during RV POLARSTERN cruise PS118. Alfred Wegener Institute, Helmholtz Centre for Polar and Marine Research, Bremerhaven, PANGAEA. doi: 10.1594/PANGAEA.911904
Purser A., Marcon Y., Dreutter S., Hoge U., Sablotny B., Hehemann L., et al. (2019). Ocean floor observation and bathymetry system (OFOBS): A new towed Camera/Sonar system for deep-Sea habitat surveys. IEEE J. Oceanic Eng. 44, 87–99. doi: 10.1109/JOE.2018.2794095
Roberts J., Xavier J. C., Agnew D. J. (2011). The diet of toothfish species Dissostichus eleginoides and Dissostichus mawsoni with overlapping distributions. J. Fish. Biol. 79, 138–154. doi: 10.1111/j.1095-8649.2011.03005.x
Robison B. H., Reisenbichler K. R., Sherlock R. E. (2005). Giant larvacean houses: Rapid carbon transport to the deep Sea floor. Sci. (1979) 308, 1609–1611. doi: 10.1126/science.1109104
Rohlfer E. K., Scheer S. L., Bergmann M., Sweetman A. K., Hoving H. J. T. (2022). Contrasting residence time and scavenging communities of experimental invertebrate food falls in the Arctic deep sea. Deep Sea Res. Part I: Oceanogr. Res. Pap. 189, 103832. doi: 10.1016/j.dsr.2022.103832
Scheer S. L., Sweetman A. K., Piatkowski U., Rohlfer E. K., Hoving H. J.T. (2022). Food fall-specific scavenging response to experimental medium-sized carcasses in the deep sea. Mar. Ecol. Prog. Ser. 685, 31–48. doi: 10.3354/meps13973
Słomska A. W., Panasiuk A., Weydmann-Zwolicka A., Wawrzynek-Borejko J., Konik M., Siegel V. (2021). Historical abundance and distributions of Salpa thompsoni hot spots in the southern ocean and projections for further ocean warming. Aquat. Conserv. 31, 2095–2102. doi: 10.1002/aqc.3443
Smith C. R. (1985). Food for the deep sea: utilization, dispersal, and flux of nekton falls at the Santa catalina basin floor. Deep Sea Res. Part A. Oceanogr. Res. Pap. 32, 417–442. doi: 10.1016/0198-0149(85)90089-5
Smith C. R., Baco A. R. (2003). Ecology of whale falls at the deep-sea floor. Oceanogr. Mar. Biol. - an Annu. Rev. 41, 311–354. doi: 10.1201/9780203180570
Smith C. R., Bernardino A. F., Baco A., Hannides A., Altamira I. (2014a). Seven-year enrichment: macrofaunal succession in deep-sea sediments around a 30 tonne whale fall in the northeast pacific. Mar. Ecol. Prog. Ser. 515, 133–149. doi: 10.3354/meps10955
Smith C. R., Demopoulos A. W. J. (2003). “Ecology of the deep pacific ocean floor,” in Ecosystems of the world volume 28: Ecosystems of the deep ocean. Ed. Tyler P. A. (Amsterdam: Elsevier), 179–218.
Smith C. R., Glover A. G., Treude T., Higgs N. D., Amon D. J. (2015). Whale-fall ecosystems: Recent insights into ecology, paleoecology, and evolution. Ann. Rev. Mar. Sci. 7, 571–596. doi: 10.1146/annurev-marine-010213-135144
Smith K. E., Thatje S., Singh H., Amsler M. O., Vos S. C., McClintock J. B., et al. (2014b). Discovery of a recent, natural whale fall on the continental slope off anvers island, western Antarctic peninsula. Deep Sea Res. Part I: Oceanogr. Res. Pap. 90, 76–80. doi: 10.1016/j.dsr.2014.04.013
Smith K. L., Kaufmann R. S. (1999). Long-term discrepancy between food supply and demand in the deep Eastern north pacific. Sci. (1979) 284, 1174–1177. doi: 10.1126/science.284.5417.1174
Smith K. L., Baldwin R. J., Williams P. M. (1992). Reconciling particulate organic carbon flux and sediment community oxygen consumption in the deep north pacific. Nature 359, 313–316. doi: 10.1038/359313a0
Smith K. L. Jr. (1987). Food energy supply and demand: A discrepancy between particulate organic carbon flux and sediment community oxygen consumption in the deep ocean. Limnol. Oceanogr. 32, 201–220. doi: 10.4319/lo.1987.32.1.0201
Soltwedel T., von Juterzenka K., Premke K., Klages M. (2003). What a lucky shot! photographic evidence for a medium-sized natural food-fall at the deep seafloor. Oceanol. Acta 26, 623–628. doi: 10.1016/S0399-1784(03)00060-4
Stenvers V. I., Hauss H., Osborn K. J., Neitzel P., Merten V., Scheer S., et al. (2021). Distribution, associations and role in the biological carbon pump of Pyrosoma atlanticum (Tunicata, thaliacea) off cabo Verde, NE Atlantic. Sci. Rep. 11, 9231. doi: 10.1038/s41598-021-88208-5
Stockton W. L., DeLaca T. E. (1982). Food falls in the deep sea: occurrence, quality, and significance. Deep Sea Res. Part A. Oceanogr. Res. Pap. 29, 157–169. doi: 10.1016/0198-0149(82)90106-6
Strycker N., Borowicz A., Wethington M., Forrest S., Shah V., Liu Y., et al. (2021). Fifty-year change in penguin abundance on elephant island, south Shetland islands, Antarctica: results of the 2019–20 census. Polar Biol. 44, 45–56. doi: 10.1007/s00300-020-02774-4
Sumida P. Y. G., Alfaro-Lucas J. M., Shimabukuro M., Kitazato H., Perez J. A. A., Soares-Gomes A., et al. (2016). Deep-sea whale fall fauna from the Atlantic resembles that of the pacific ocean. Sci. Rep. 6, 22139. doi: 10.1038/srep22139
Sweetman A. K., Chapman A. (2011). First observations of jelly-falls at the seafloor in a deep-sea fjord. Deep Sea Res. Part I: Oceanogr. Res. Pap. 58, 1206–1211. doi: 10.1016/j.dsr.2011.08.006
Sweetman A. K., Smith C. R., Dale T., Jones D. O. B. (2014). Rapid scavenging of jellyfish carcasses reveals the importance of gelatinous material to deep-sea food webs. Proc. R. Soc. B: Biol. Sci. 281, 20142210. doi: 10.1098/rspb.2014.2210
Trathan P. N., Hill S. L. (2016). “The importance of krill predation in the southern ocean,” in Biology and ecology of Antarctic krill. Ed. Siegel V. (Cham: Springer International Publishing), 321–350. doi: 10.1007/978-3-319-29279-3_9
Trivelpiece W. Z., Buckelew S., Reiss C., Trivelpiece S. G. (2007). The winter distribution of chinstrap penguins from two breeding sites in the south Shetland islands of Antarctica. Polar Biol. 30, 1231–1237. doi: 10.1007/s00300-007-0283-1
Turner J. T. (2015). Zooplankton fecal pellets, marine snow, phytodetritus and the ocean’s biological pump. Prog. Oceanogr. 130, 205–248. doi: 10.1016/j.pocean.2014.08.005
Vaughan D. G., Marshall G. J., Connolley W. M., Parkinson C., Mulvaney R., Hodgson D. A., et al. (2003). Recent rapid regional climate warming on the Antarctic peninsula. Clim. Change 60, 243–274. doi: 10.1023/A:1026021217991
Watters G. (1996). By-catch of fishes captured by the krill fishing vessel chiyo maru no. 2 in statistical area 58 (January to march 1995). CCAMLR Sci. 3, 111–123.Available at: https://www.ccamlr.org/en/publications/science_journal/ccamlr-science-volume-3/ccamlr-science-volume-3111-123.
Xavier J. C., Cherel Y., Allcock L., Rosa R., Sabirov R. M., Blicher M. E., et al. (2018). A review on the biodiversity, distribution and trophic role of cephalopods in the Arctic and Antarctic marine ecosystems under a changing ocean. Mar. Biol. 165, 93. doi: 10.1007/s00227-018-3352-9
Yamamoto J., Hirose M., Ohtani T., Sugimoto K., Hirase K., Shimamoto N., et al. (2008). Transportation of organic matter to the sea floor by carrion falls of the giant jellyfish Nemopilema nomurai in the Sea of Japan. Mar. Biol. 153, 311–317. doi: 10.1007/s00227-007-0807-9
Yamamoto J., Nobetsu T., Iwamori T., Sakurai Y. (2009). Observations of food falls off the shiretoko peninsula, Japan, using a remotely operated vehicle. Fish. Sci. 75, 513–515. doi: 10.1007/s12562-008-0055-z
Keywords: scavenging, penguin, Antarctic Peninsula, Powell Basin, OFOBS, fisheries discards, carbon pump, baleen whale
Citation: Stauffer JB, Purser A, Griffiths HJ, Smith CR and Hoving H-JT (2022) Food falls in the deep northwestern Weddell Sea. Front. Mar. Sci. 9:1055318. doi: 10.3389/fmars.2022.1055318
Received: 27 September 2022; Accepted: 01 November 2022;
Published: 17 November 2022.
Edited by:
Eleonora Puccinelli, Université de Bretagne Occidentale, FranceReviewed by:
Mauricio Shimabukuro, Universidade Federal do Rio Grande, BrazilEva Ehrnsten, Stockholm University, Sweden
Andrzej Kaim, Institute of Paleobiology, Polish Academy of Sciences, Poland
Copyright © 2022 Stauffer, Purser, Griffiths, Smith and Hoving. This is an open-access article distributed under the terms of the Creative Commons Attribution License (CC BY). The use, distribution or reproduction in other forums is permitted, provided the original author(s) and the copyright owner(s) are credited and that the original publication in this journal is cited, in accordance with accepted academic practice. No use, distribution or reproduction is permitted which does not comply with these terms.
*Correspondence: Julian B. Stauffer, anN0YXVmZmVyQGdlb21hci5kZQ==