- 1Departamento de Ecología y Biodiversidad, Facultad de Ciencias de la Vida, Universidad Andres Bello, Santiago, Chile
- 2Centro de Investigación Marina Quintay (CIMARQ), Facultad de Ciencias de la Vida, Universidad Andres Bello, Quintay, Chile
- 3Departamento de Ecología, Facultad de Ciencias, Universidad Católica de la Santísima Concepción, Concepción, Chile
- 4Centro de Investigación en Biodiversidad y Ambientes Sustentables (CIBAS), Universidad Católica de la Santísima Concepción, Concepción, Chile
- 5Universidad de Oriente, Cumaná, Venezuela
- 6Centro de Estudios Avanzados en Ambientes Marinos (CEAMAR), La Serena, Chile
- 7Instituto Milenio en Socio-Ecología Costera (SECOS), Santiago, Chile
- 8Departamento de Biología Marina, Universidad Católica del Norte, Coquimbo, Chile
- 9Centro de Investigación y Desarrollo Tecnológico en Algas y Otros Recursos Biológicos (CIDTA), Facultad de Ciencias del Mar, Universidad Católica del Norte, Coquimbo, Chile
- 10Centro de Investigación e Innovación para el Cambio Climático (CiiCC), Facultad de Ciencias, Universidad Santo Tomás, Santiago, Chile
- 11Institute of Ecology and Biodiversity (IEB), Santiago, Chile
- 12Doctorado en Conservación y Gestión de la Biodiversidad, Universidad Santo Tomás, Santiago, Chile
- 13Laboratorio de Estudios Ecosistémicos, Escuela de Ingeniería y Negocios, Universidad Viña del Mar, Viña del Mar, Chile
- 14Programa de Doctorado en Medicina de la Conservación, Universidad Andres Bello, Santiago, Chile
- 15Center of Applied Ecology and Sustainability (CAPES), Santiago, Chile
Several seaweed species are commercialized worldwide both due to high demand for food and feed and as a raw material for the extraction of phycocolloids such as agar, carrageenan, and alginates that are used broadly in the food, cosmetic, and pharmaceutical industries. Chile is the world’s leading marine seaweed biomass producer when it comes to the exploitation of natural kelp beds. This extraction pressure has persisted for decades and has resulted in a reduction in natural stocks along the benthic ecosystems of the Chilean coast. Over the last three decades, several strategies aimed at restoring seaweed stocks have been implemented (i.e., sexual and asexual reproduction, the use of spore-type propagules or fragments of thalli, and entire thallus transplants). Success rates have varied, but the biological feasibility of such strategies has been demonstrated for several species. However, technological improvements must be achieved to move from small-scale, pilot experiments to cost-effective restocking strategies that are easy to transfer to fisher communities and another end-user, scalable to marine field conditions, and socio-ecologically sustainable. Researchers in other geographic areas have explored similar pathways for developing kelp restocking strategies and have tackled the research gaps regarding its massification. This work summarizes the research activities carried out in recent decades in the search for sustainable strategies to restore algal stocks in Chile.
1. Introduction
Seaweeds are an important economic resource for coastal communities and have historic, cultural, and gastronomic value (Delaney et al., 2016). The Seaweed Manifesto (Giercksky and Doumeizel, 2020) has highlighted the contribution of these organisms to several of the Sustainable Development Goals due to their contributions as a source of quality food for human populations (food security) and their role in carbon capture and the mitigation of the consequences of ocean acidification (climate change mitigation). They are key organisms for the maintenance of coastal marine ecosystems and biodiversity conservation and are a relevant factor for facilitating coastal community resilience (Wernberg et al., 2019; Morris et al., 2020; Hynes et al., 2021; Cai et al., 2021a; Cuba et al., 2022; Earp et al., 2022).
There is a high worldwide demand for seaweeds biomass for food and feed and as a raw material for the extraction of phycocolloids which are used broadly in the food, cosmetic, and pharmaceutical industries (Chopin and Tacon, 2021). The value of this natural capital is currently estimated at over USD 13.3 billion, and the demand for it is expected to increase by more than 10% over the next decade, presenting an important challenge for countries that commercialize these resources (Cai et al., 2021a; Cai et al., 2021b; Chopin and Tacon, 2021). In 2019, the worldwide production of seaweed was 35.8 million tons, 97.1% of which was produced through seaweed aquaculture and only 2.9% (0.9 million tons) of which came from wild-harvested seaweed (Cai et al., 2021a; Cai et al., 2021b). The worldwide production of seaweeds is concentrated in eastern and south-eastern Asia, contributing 95% of production, whereas Europe and the Americas contribute about 1% each to global production (Cai et al., 2021a). It is precisely outside of the main production centers where seaweed collection is maintained as a productive practice. Chile presents an extractivist developmental model (Márquez and Vásquez, 2020) that involves the exploitation of natural seaweed populations and the export of raw materials with a low level of processing. Chile leads in the commercialization of non-cultivated seaweed biomass and has been the main producer of this type of biomass since 2015, harvesting over 340,000 tons annually (FAO, 2018).
Coastal ecosystems are threatened by factors such as ocean acidification, pollution, climatic events, and human intervention (a result of infrastructure development in coastal areas) leading to loss of natural habitats (e.g., Rangel-Buitrago et al., 2018; Cai et al., 2021b; Hynes et al., 2021). The consequences of the abovementioned phenomena on seaweeds are already noticeable, with clear evidence of a global decrease in algal populations (Krumhansl et al., 2016). Worldwide changes in the distribution and abundance of important species such as kelps have been recorded in different ecosystems owing to the direct or indirect effects of anthropogenic activities (Wernberg et al., 2019; Oyarzo-Miranda et al., 2020; Jara-Yáñez et al., 2021; Latorre-Padilla et al., 2021). However, the impact of these changes varies among geographical regions, indicating that local stressors and regional variations can modify the effects of global drivers of seaweed populations (Wernberg et al., 2019). For example, no great changes attributable to global drivers have been reported for kelp forest ecosystems located off the South American coast (Perú and Chile), probably owing to the effects of the Humboldt Current, which has prevented water warming over recent decades (Seabra et al., 2019; Smale, 2020). However, in this region, particularly along the Chilean coast, threats are associated with the extraction and potential direct overexploitation of large extensions of seaweeds along the entire coast, mainly kelp forest species as Lessonia trabeculata, Lessonia spicata, Lessonia berteroana and Macrocystis pyrifera (Vásquez et al., 2012; Vásquez et al., 2014). The consequences of this practice could be devastating for these ecosystems, and it should thus be regulated adequately to avoid their collapse (Márquez and Vásquez, 2020). In Chile, despite government efforts to regulate the exploitation of several seaweed species, there is clear evidence that species such as L. berteroana, L. spicata and L. trabeculata have been exploited beyond recovery in some locations (Vásquez, 2016; Westermeier et al., 2017; Bularz et al., 2022) due to the high pressure of extraction or the complete lack of management measures in some coastal areas. However, effective population replacement has been achieved at some locations and good extraction practices have been implemented.
Due to the above mentioned, one of the management measures that has gained support and that has been encouraged by the Chilean Undersecretariat for Fisheries and Aquaculture (SUBPESCA by its acronym in Spanish) is restocking. The restocking of different seaweed species aims to diversify, maintain, or increase the number of specimens that can be harvested, which supports the extractive activity, offering an alternative strategy to passive fishery management measures (e.g., limiting extraction participants). The Chilean law that regulates fisheries and aquaculture defines restocking as “sets of actions that have as their objective to increase or recover the population of a given hydrobiological species, through artificial or natural means, within its geographical distribution range”. SUBPESCA have promoted law No. 20.925, which involves a “Bonus for Restocking and Farming of Seaweeds” and have aimed to encourage changes in the productive matrix of the artisanal fishers’ organizations and a stimulus for the conservation of seaweed resources. However, this management tool, which provides subsidies for undertaking cultivation or repopulation actions, has remained exclusively focused on the cultivation of the red seaweed Gracilaria chilensis (Cárcamo et al., 2021). To date, it is not clear why seaweed restocking and/or farming actions in Chile are not being undertaken by end users such as fisher communities despite the incentives and evident need for them. Potential explanations for this lack of action could be related to biological, technological, administrative, or commercial limitations, or a combination of them.
Over the past 20 years, several scientific and technological pilot studies have been undertaken throughout Chile to develop and evaluate effective methods for restocking. However, many of these studies remain published only in the grey literature or have not been compared among different localities or approaches. In this study, we review scientific and project reports that have focused on red and brown seaweed restocking in Chile with an emphasis on the technological developments achieved, such as the propagation strategies adopted, and the strengths and limitations of the results obtained to date. We also compare these technological developments with actions undertaken in other areas of the world. This will allow us to propose strategies to identify knowledge and technological development gaps and to suggest possible solutions and recommendations.
2. Seaweed exploitation in Chile
The extraction of seaweed biomass for direct use or commercialization, along with strategies to recover or maintain the productivity of exploited algal populations, tends to take one of three main approaches: (a) collection/harvesting of thalli from natural populations, (b) fisheries management of natural populations, and (c) farming. The easiest approach is to collect stranded biomass, which are thalli that have been detached due to wave actions and displaced by bottom currents toward the beach, or to harvest from natural populations. To harvest kelp in subtidal environments, divers use crowbars to detach the holdfast from the substrate, whereas shore harvesters collect stranded biomass. It should be noted that the feasibility of collecting/harvesting thalli depends on the persistence of natural populations, biological and environmental factors that regulate such populations, and the extractive pressure that the fishery exerts. The systematic record of collected/harvested seaweed populations in Chile began with a red seaweed known as “pelillo” (G. chilensis) in 1960. However, there is archeological evidence of the use of “luche/nori” (Porphyra spp. and Pyropia spp.), “cochayuyo” (Durvillaea antarctica and Durvillaea incurvata), and “huiro” (Lessonia spp. and Macrocystis sp.) by several indigenous peoples along the South American Pacific coast (Dillehay et al., 2008). The collection/harvesting of thalli primarily requires basic knowledge, involving the identification of the target species, the local distribution of populations, the use of harvesting tools, and the level of humidity required for the direct use or commercialization of the biomass.
When the exploited seaweed populations are put at risk by harvesting pressure, the creation of consensual fishery management plans between communities and stakeholders emerges. Currently in northern Chile, brown seaweed management committees have been established in the regions of Arica and Parinacota (from 17°S to 19°S), Tarapacá (from 19°S to 21°S), Antofagasta (from 21°S to 25°S), Atacama (from 26°S to 29°S), and Coquimbo (from 29°S to 32°S). The “Bahía Chasco kelps Management Committee” (ca. 27°S) has also been established in the Atacama Region with a territorially delimited area of influence. These local or regional management plans encompass different dimensions (e.g., biological fisheries and environmental and socioeconomic factors) with different measures and management actions (SUBPESCA, n. d. a). A measure that is complementary to management plans is restocking, particularly for natural populations that have been subjected to high harvesting pressure. In protected marine areas, restocking can also be part of an ecological restoration strategy, particularly for seaweeds that function as foundational species or that are considered ecosystem engineers.
The third approach to reduce harvesting pressure on seaweed natural populations is seaweed farming, preferably through systems installed on infrastructures in the ocean that can be incorporated into management plans. However, seaweed cultivation requires complete knowledge of the life cycle and the physiological requirements of the species at different developmental stages.
Approximately 13 seaweed species are commercialized in Chile, with total landings mainly involving six brown and nine red seaweed species (SERNAPESCA, 2022) (Figure 1). Brown seaweed landings are represented by two “cochayuyo” species, namely, D. antarctica and D. incurvata, and four “huiro” species, namely, L. berteroana, L. spicata (“huiro negro” or “chascón”), L. trabeculata (“huiro palo”), and M. pyrifera (“huiro canutillo”), which together represent 60% of historical seaweed landings over the past decade. Conversely, red seaweed landings are more diverse in terms of species composition: Callophyllis variegata (“carola”), Gelidium spp. (“chasca”), Chondracanthus chamissoi (“chicoria de mar”), Gymnogongrus furcellatus (“líquen gomoso”), Porphyra spp. and Pyropia spp. (“luche”), Mazzaella laminarioides (“luga cuchara”), Sarcothalia crispata (“luga negra”), Sarcopeltis skottsbergii (formely Gigartina skottsbergii) (“luga roja”), and G. chilensis (“pelillo”). The latter three species are historically the most important in terms of annual landings (Figure 1).
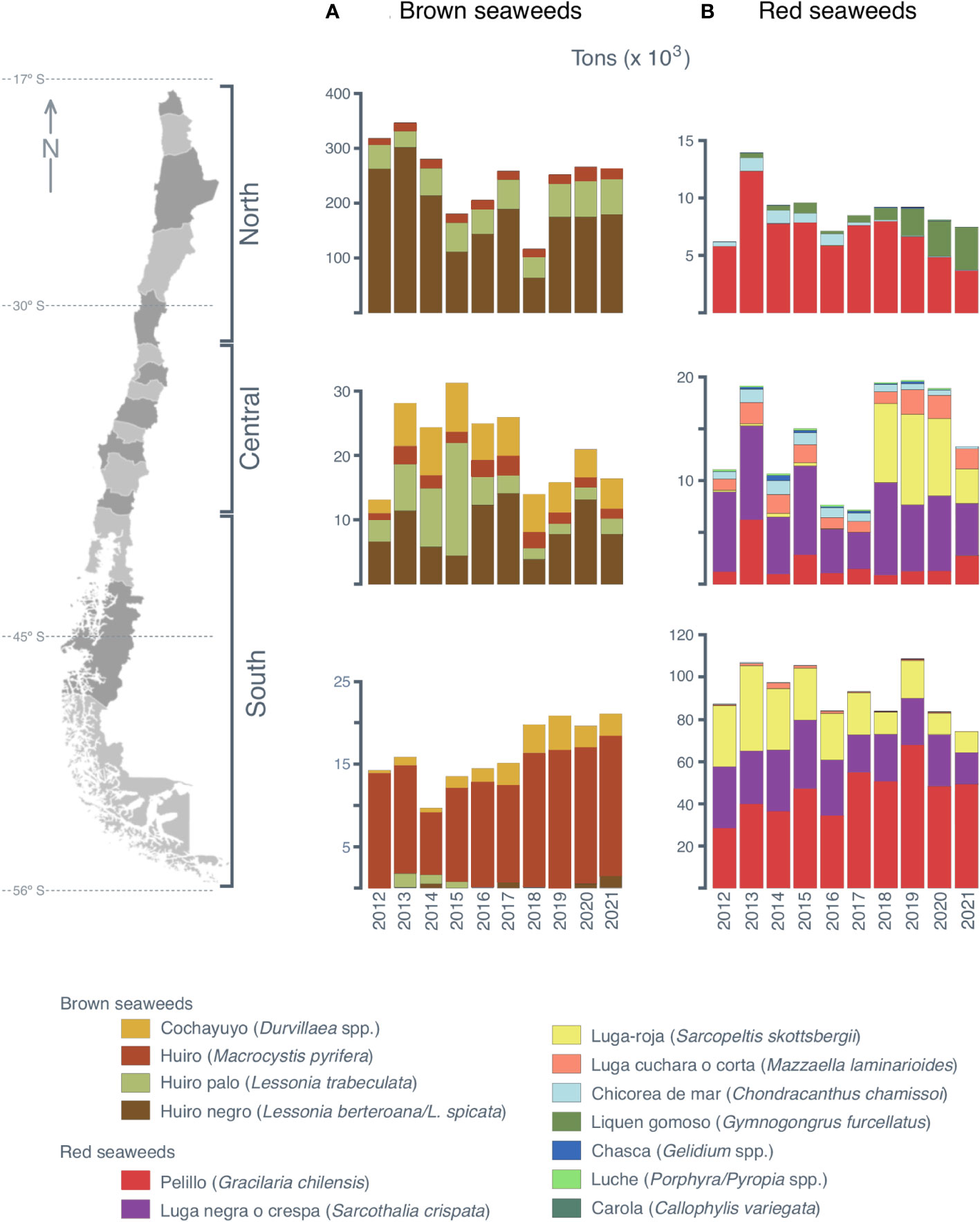
Figure 1 Annual landings (2012–2021; SERNAPESCA, 2022) of (A) brown seaweeds and (B) red seaweeds in three geopolitical areas along the coast of Chile.
The species composition and landing volume of brown and red seaweeds over the past decade has varied among the geopolitical zones of Chile. In the northern region of the country, brown seaweed landings have ranged from 100,000 to 300,000 tons per year (dry weight), with a greater representation of “huiro negro” L. berteroana/L. spicata. Red seaweed landings have ranged from 5000 to 15,000 annual tons and have been represented mainly by “pelillo” (G. chilensis) (Figure 1).
In the central region of Chile, brown seaweed landings have been lower than in the northern zone in the historical series, ranging from 10,000 to 30,000 annual tons, and include “cochayuyo” (D. incurvata and D. antarctica). Red seaweed landings in this region have ranged from 5000 to 20,000 annual tons, with a historical series represented mainly by “luga negra” (S. crispata) and “luga roja” (S. skottsbergii) over the past three years (Figure 1).
In the southern region of the country, brown seaweed landings have ranged from 7000 to 15,000 annual tons, with a marked presence of “huiro canutillo” (M. pyrifera) in the historical series. In addition, there has been a considerable increase in red seaweed landings, which have ranged from 80,000 to 110,000 annual tons. The most abundant red seaweed species in this area have been “luga negra”, “luga roja”, and “pelillo” (Figure 1).
Artisanal fisheries have enormous social and economic importance in Chile, with over 71,000 registered shore gatherers, seaweed collectors, or free divers on the Artisanal Fishery Register (AFR), of whom 71% are men and 29% are women (SERNAPESCA, 2022). Another 11,000 users, mostly men, are registered as hookah divers. To estimate the potential capture pressure of this operating fishery force along the Chilean coast, the fisheries authority has performed periodic direct and indirect evaluations to estimate the total biomass of natural seaweed populations since the end of the past century. In addition, data on the fraction of available biomass (standing stock) and harvestable biomass (standing crop) have been collected to establish the exploitation state of these natural seaweed populations.
In general, the results of direct evaluations of brown seaweed over time indicate high harvesting pressure in northern Chile (ca. 18 to 32°S), which is reflected in populations characterized by a substantial fraction of juveniles and recruits and a low representation of adult plants (ABIMAR, 2017; UCN, 2018; ECOS, 2020). This has also negatively affected the biomass collected in natural stranding areas (Vega et al., 2014). For this reason, exploitation has been strongly regulated, and all species involved are under a general access regime, which has resulted in user rights being allocated to small-scale fisheries organized by fishery unions or syndicates (Ríos and Gelcich, 2017). Based on the region and seaweed species, other complementary measures have been undertaken. For example, management plans in northern Chile include regulatory measures such as quotas, bans, extraction limits, areas of operation, and others (SUBPESCA, n. d. a). Accordingly, in addition to actions and management measures for the conservation of brown seaweed resources, restocking strategies should be developed and areas fit for restocking should be selected Vásquez et al. (2010).
No equivalent management plans have been implemented for red seaweeds in southern Chile. However, proposed management measures for “luga roja” (S. skottsbergii) populations in the region of Magallanes (ca. 48°S to 56°S) include an annual extractive ban (May–September), a minimum capture size of 20 cm with a 10% tolerance for specimens below the minimum size, and fishing area rotations (IFOP, 2004). Similar management measures can also be applied to “luga negra” (S. crispata). The management plan for the Bay of Ancud in the region of Los Lagos (ca. 41°S) also established a biological ban, in this case from May to December for “luga negra” and “luga roja”, to facilitate the natural restocking of populations. However, the level of historical exploitation of “luga” species in southern Chile suggests the need to implement restocking strategies in addition to the established management measures (Otaíza and Cáceres, 2015a; Otaíza and Cáceres, 2015b).
3. Seaweed restocking in Chile
For this section, a review of the scientific and grey literature about restocking was done according to the adaptation of the PRISMA methodology (Moher et al., 2009). Specifically, we performed the four steps of systematic analysis.
(1) Identification of a topic and the research question, which was: why do we, in Chile, still have gaps in knowledge about decreasing seaweed populations, including economic and social problems?
(2) Screening information, which limited to texts in English and Spanish, and searched for the following key words: “seaweed” or “algae” combined with “restocking”, “repopulation”, “recovery”, “rehabilitation” and “restocking and Chile” in Scopus, Web of Science, and Springer Link, plus the repository of the National Research and Development Agency (known in Chile as ANID). A search on the web using Google as a search engine, to access articles such as published manuals and procedures, among others, was also done.
A total of 105 studies were initially identified in the bibliographic search, which was reduced to 81 studies after eliminating duplicate records.
(3) Eligibility was established with the following criteria: first, studies carried out in Chile using seaweeds as biological model, second, empirical studies of restocking, either “in vitro or in situ”, and third, studies being written either in Spanish or English. 55 studies were discarded, leaving only 26 for a full text reading and information extraction.
(4) Inclusion, where the 26 studies were submitted to qualitative analysis and ordination of information by title, authors and year of publication, seaweed species and phylum used as model for the restocking technique, and the region of the country and habitat where the activities or restocking technique were applied with the specific reproduction and propagation strategy. This information is described and ordered in the following text, tables and figures.
3.1. General aspects
In response to the need for seaweed biomass, efforts to develop restocking strategies in Chile have focused on red (Rhodophyta) and brown (Phaophyceae) seaweeds, as these two groups are the most commercialized in the country. More publications refer to red (54%; 14 reports) than to brown seaweeds (46%, 12 reports), and most of this research has been financed through government funds. These reports suggest that more restocking studies have been done in the northern region (41%), followed by the central (37%) and southern (22%) regions (Table 1).
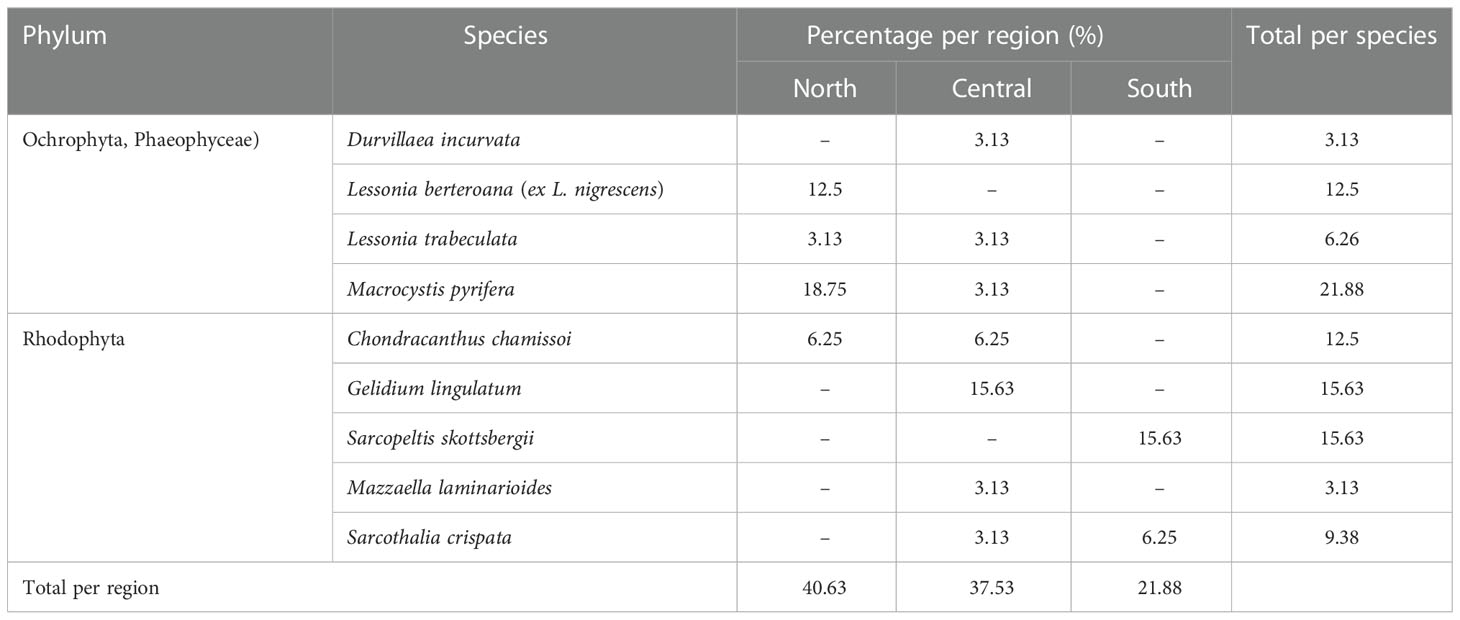
Table 1 Restocking studies in Chile. Information obtained and adapted from Díaz (2020). The symbol “–” indicates no availability of information on restocking studies for this species in the area.
The reproduction and propagation strategies employed for restocking natural populations vary among and within species. Most of the evaluated strategies use asexual propagation, except for D. incurvata and M. pyrifera, for which the sexual production of juveniles has been used. The juveniles of these species are produced in laboratory conditions and then seeded in intertidal or subtidal environments (Table 2). For species in which restocking has been attempted through asexual propagation (i.e., C. chamissoi and G. lingulatum), the most common strategy has been the seeding of multicellular propagules obtained through thallus fragmentation (Table 2). The studies have, on occasions, used more than one propagation strategy for the same species; for example, both the seeding of spores on natural substrates and seeding by spore dispersion have been used with S. skottsbergii and both transplantation and disk fragmentation have been used for L. berteroana (Westermeier et al., 2016; Ávila et al., 2017) (Table 2).
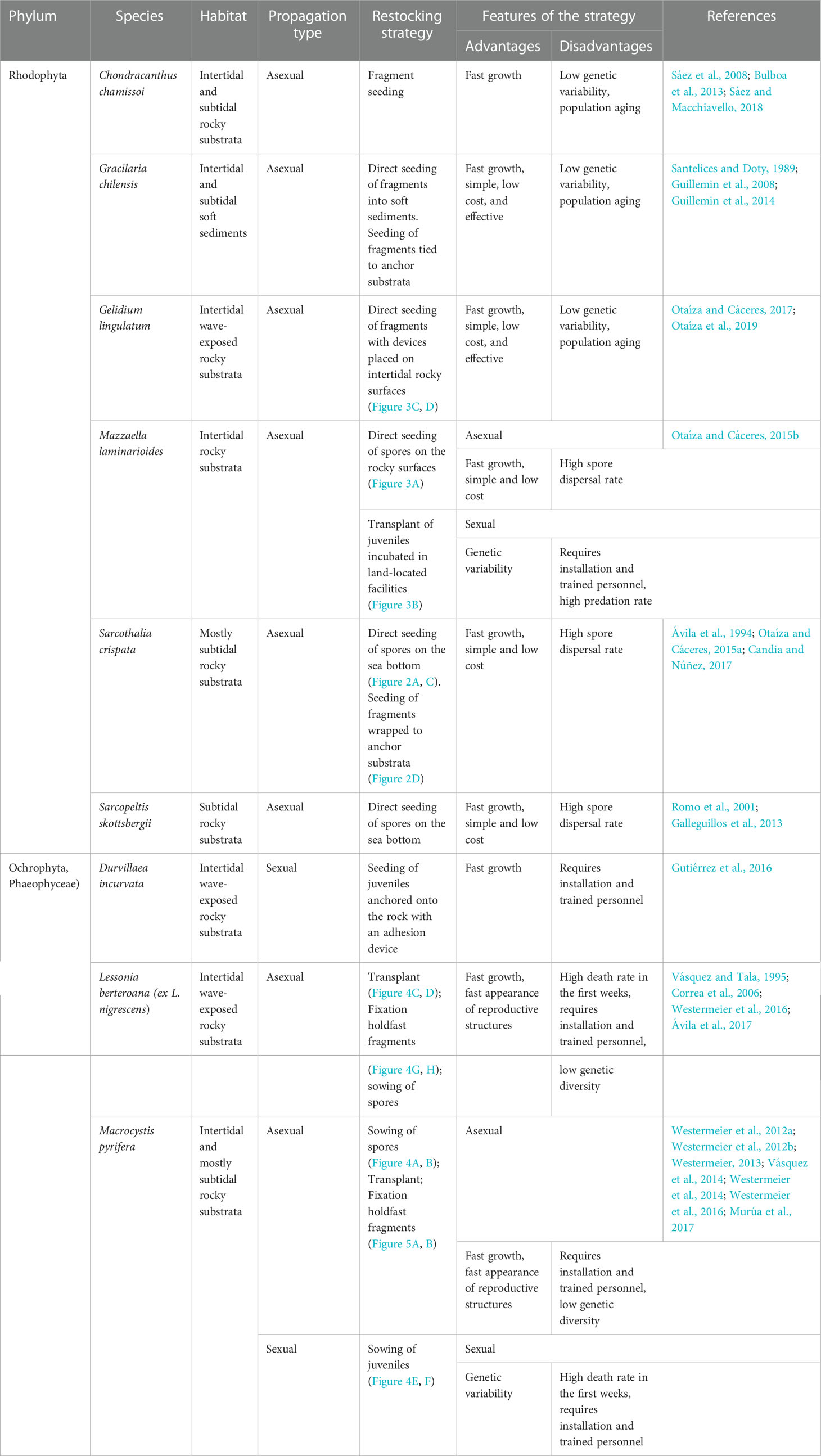
Table 2 Summary of main restocking strategies used in Chile, considering species; sexual or asexual propagation; and biological, technical, or economic advantages/disadvantages in each case.
Between 2017 and 2019, a Chilean government initiative implemented through SUBPESCA aimed to provide economic support to small aquaculture farmers, fishers, and/or artisanal fisher's organizations by funding up to 70% of the total costs for projects linked to the restocking and cultivation of seaweeds. The program financed 426 projects involving a total of 199 individuals or groups who applied, with an approximate value of USD 2 million (Table 3). Regrettably, only three restocking projects were approved, representing only 1% of this program’s budget. The main reason for the rejection of restocking projects was failure to comply with the monitoring program necessary to apply for this benefit (SUBPESCA, n. d. b). The total area used for this state-funded restocking project is uncertain. However, numerous investigations have been carried out in pursuit of seaweed restocking. A recent systematic study by Eger et al. (2022) analyzes the global efforts of kelp forest restoration. In the study they gathered data from different countries, Chile among them, with a total of 18 journal manuscripts reporting a total of 329.6 m2 of kelp restocking. This number does not include efforts made privately by fishing communities.
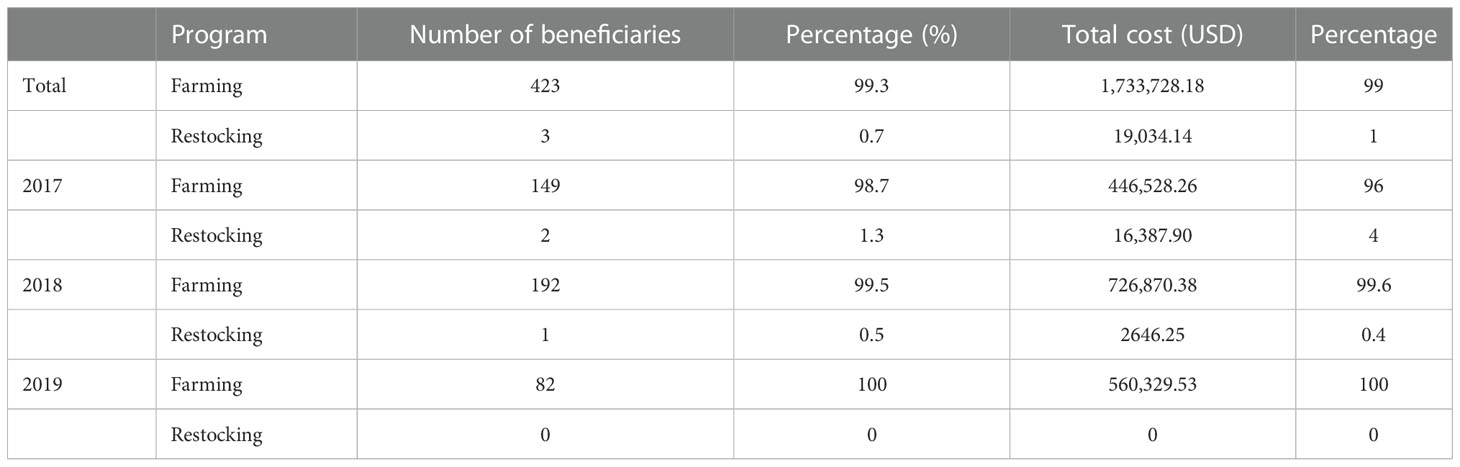
Table 3 Total number of approved projects by program duration in years and cost (USD) related to seaweed cultivation and restocking in Chile (SUBPESCA, 2017; SUBPESCA, 2018a; SUBPESCA, 2018b; SUBPESCA, 2018c; SUBPESCA, 2019).
3.2. Red seaweed restocking technologies
3.2.1. Restocking by spore seeding
Spores (haploid tetraspores and diploid carpospores) are the main asexual reproductive cells of red algae. These propagules can be produced in large quantities for some species of interest and are the only reproduction and dispersion mechanism of some species.
Preliminary experiments and pilot studies have been undertaken in Chile to seed spores of commercially important red seaweeds directly onto the seafloor. Based on the idea of a brown seaweed “spore bag” (Ohno and Critchley, 1993), subtidal blades of red seaweeds in an advanced maturity stage have been suspended a short distance above the seafloor while natural spore release occurs. Ávila et al. (1994) undertook direct spore seeding experiments using S. crispata blades intertwined with ropes kept vertically, using a buoy and a weight as an anchor. Galleguillos et al. (2013) used mesh “curtains” with S. crispata and S. skottsbergii in the region of Los Lagos (from 40°S to 44°S), whereas Otaíza and Cáceres (2015a) used “curtains” with S. crispata blades in the region of Biobío (from 36°S to 38°S). In the latter case, the curtains were made from vertical polypropylene ropes connected transversally at the upper and lower ends, and suspended from buoys, using weights as anchors (Figure 2A, B). In all cases, the seeding units could be relocated along the area to be seeded and the blades could be replaced when necessary. In order to decrease spore dispersion and favor settlement in the selected areas, seeding units can also be placed horizontally, a short distance from the substrate (Figure 2C). Using a different strategy, Ávila et al. (1994) wrapped mature S. crispata fronds over boulders on the seafloor (Figure 2D, E). In this way, spore dispersion was minimized and settlement in groups was favored, facilitating the coalescence of juveniles, which could reach greater sizes in less time than individuals generated from individual spores (Santelices and Aedo, 2006; Santelices et al., 2011). For red seaweeds on intertidal rocks, such as Mazzaella laminarioides, devices that keep pieces of reproductive tissue in contact with the substrate can be installed to allow spore release directly to the surrounding areas (Otaíza and Cáceres, 2015b) (Figure 3A).
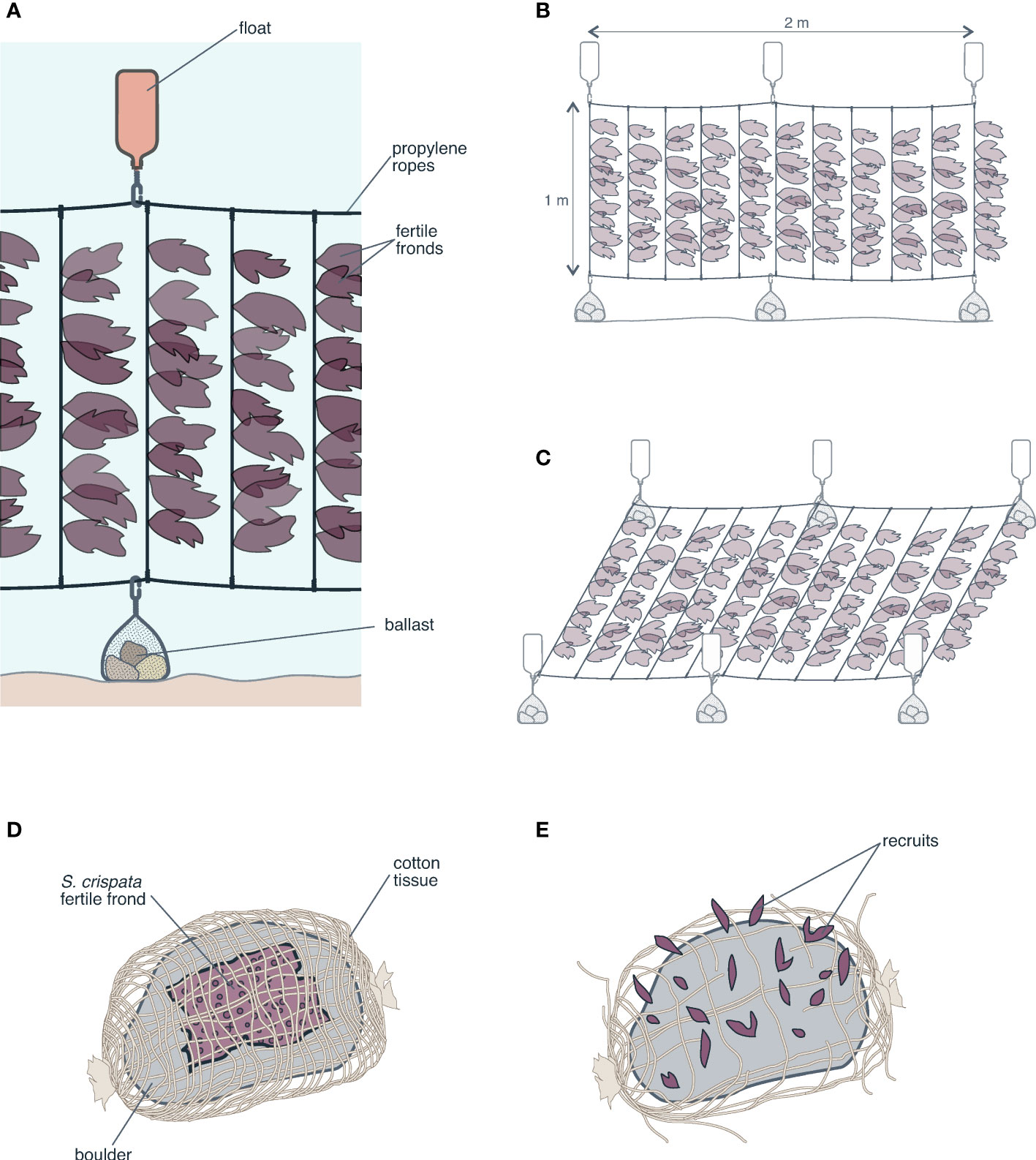
Figure 2 Illustration of some techniques to increase spore release from Sarcothalia crispata into the surrounding area. “Curtains” with blades of the seaweed are made of polypropylene ropes kept vertically between floats and weights, which are easy to move and reinstall elsewhere. The curtains can be installed vertically (A, B) or horizontally (C). The latter brings the reproductive tissue closer to the substrate, reducing the excess dispersion of spores. Fragments of reproductive tissue wrapped on boulders with cotton mesh (D, E) will favor the inoculation of spores.
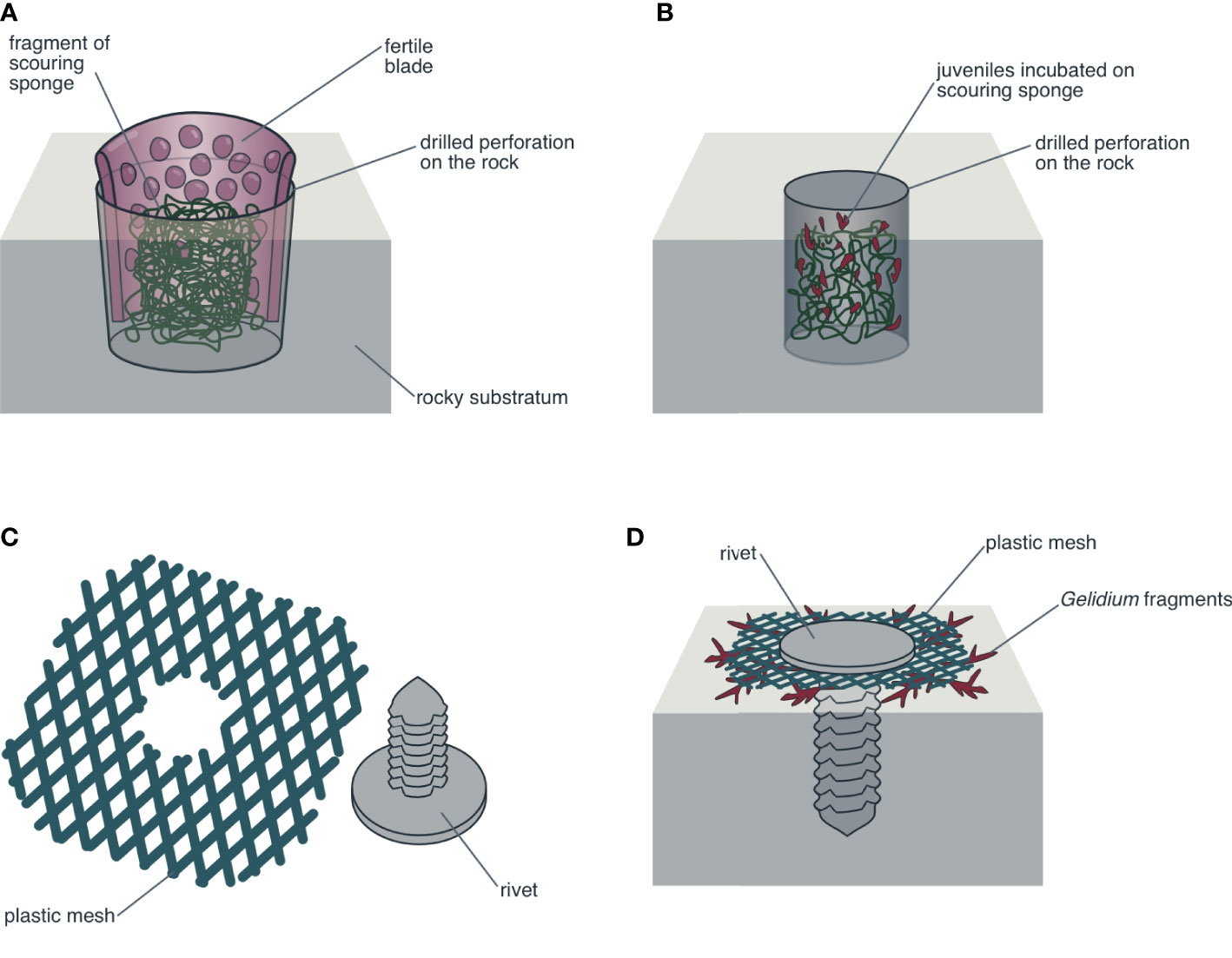
Figure 3 Mechanisms to produce new individuals on the rocks. (A) Fragment of reproductive blade of Mazzaella laminarioides trapped in position with a small fragment of abrasive sponge inside a perforation previously drilled in the substrate (intertidal rocky area), favoring the release of groups of spores in the area surrounding the perforation. (B) Fragment of abrasive sponge that has been previously inoculated with spores of M. laminarioides and incubated in the laboratory and after 4–6 weeks transplanted into holes previously drilled in the rocks. (C, D) Rivet and plastic mesh “washer” used to secure vegetative fragments of Gelidium lingulatum pressed against the substratum until secondary attachment structures are formed and the fragments regrow, generating new patches of this species.
An alternative procedure to direct spore inoculation in the natural environment is spore inoculation on natural or artificial substrates under controlled conditions (e.g., in a laboratory or in tanks in greenhouse or outdoor conditions) until the juveniles are sufficiently developed to be transplanted to the natural environment. Otaíza and Cáceres (2015b) successfully transplanted M. laminarioides juveniles incubated in the laboratory to holes previously drilled in intertidal rocks (Figure 3B). However, the initial inoculation and incubation of substrata with spores requires installations on land and trained personnel. This strategy could be an alternative for population restoration projects for this or similar species.
3.2.2. Restocking by planting fragments
Several red seaweeds exhibit fragmentation and reattachment of benthic thalli. This asexual reproduction via fragments, or vegetative reproduction, is a natural mechanism for producing new individuals. In addition, entire reproductive structures, like papillae, can break off (e.g., cystocarps; Oróstica et al., 2012) and act as dispersing structures. Additionally, the formation of “unattached juveniles” from spores that germinate and start to develop without attaching to a substrate has been observed in some species, resulting in small drifting multicellular spheres (Otaíza and Carrasco, 2002; Barrientos and Otaíza, 2014; Tiang et al., 2017). Multicellular propagules that can reattach to the substrate and fragments might be preferable alternatives to spores in restocking strategies because, unlike spores, they are multicellular entities whose size, structure, and organization may favor survival.
The most successful and emblematic case of red seaweed restocking in Chile through the planting of fragments is that of G. chilensis (Santelices and Doty, 1989). In this case, part of each fragment was buried directly, or indirectly with the aid of a tool, into a soft substrate. Alternatively, the thalli of G. chilensis can be tied to different anchoring systems (e.g., plastic sleeves filled with sand, stakes, or boulders) and laid on the sea bottom so that, with time, new algal patches develop. This is the only species for which a restocking technique has been applied extensively in different regions of Chile with considerable production results.
For other red seaweed species, fragment seeding has been conducted at the experimental level or in pilot studies. Fragments of C. chamissoi have been seeded by wrapping blade fragments together with a substrate (e.g., boulders or walkway bricks, see Figure 2D) using plastic or cotton mesh to secure the thalli (Otaíza and Cáceres, 2015c). In species like C. chamissoi, a blade can produce many secondary attachment discs (Sáez et al., 2008), which persist for at least a year and produce new blades (Bulboa et al., 2013; Otaíza, R., Fonseca, F., Barrientos, E., unpublished data). Planting fragment of Gelidium lingulatum has been carried out with devices that keep the fragments in close contact with the rocky surface in the wave-exposed, lower intertidal zone (Figure 3C, D). The application of this technique produced small patches of G. lingulatum in a few months, which could expand through vegetative propagation (Otaíza and Cáceres, 2017; Otaíza et al., 2019).
3.2.3. Restocking through transplants of adult thalli
An alternative to fragment planting is the removal of entire individuals from natural substrates and relocating them to new sites. These methods have been used in population restoration studies or in experimental ecological studies, almost exclusively with subtidal brown seaweeds. Most of these studies employed the direct transplantation of juveniles, adults, or previously inoculated substrates with propagules and incubation under laboratory conditions (Hernández-Carmona et al., 2000; Terawaki et al., 2003; Carney et al., 2005; for a review see Jacob et al., 2018). We found no reports of transplants of individuals aiming at the repopulation of commercially important red seaweeds in Chile. These methods could be laborious to implement, but the techniques could be used as restoration strategies to repopulate intertidal rocky shorelines after anthropogenic or natural disasters. In this way, patches of spore releasing individuals could be transplanted to favor the recruitment of new individuals around them.
3.3. Brown seaweed restocking
3.3.1. Restocking from spores
The first mention of an attempt at brown seaweed restocking in Chile from spores can be found in a study by Vásquez and Tala (1995), in which spores were directly seeded onto rocks. The authors concluded that the experiment was successful based on an increase of five juvenile seaweeds in the seeded area in comparison with the unseeded areas (zero juveniles) after two months.
The second attempt using this technique was undertaken several years later with M. pyrifera and involved attaching reproductive fronds (with sori) to boulders using cotton mesh (Westermeier et al., 2012a) (Figure 4A). The authors reported a 44% success rate with this technique (Figure 4B); however, there were high mortality rates associated with substrate problems and spore viability, which were highly variable.
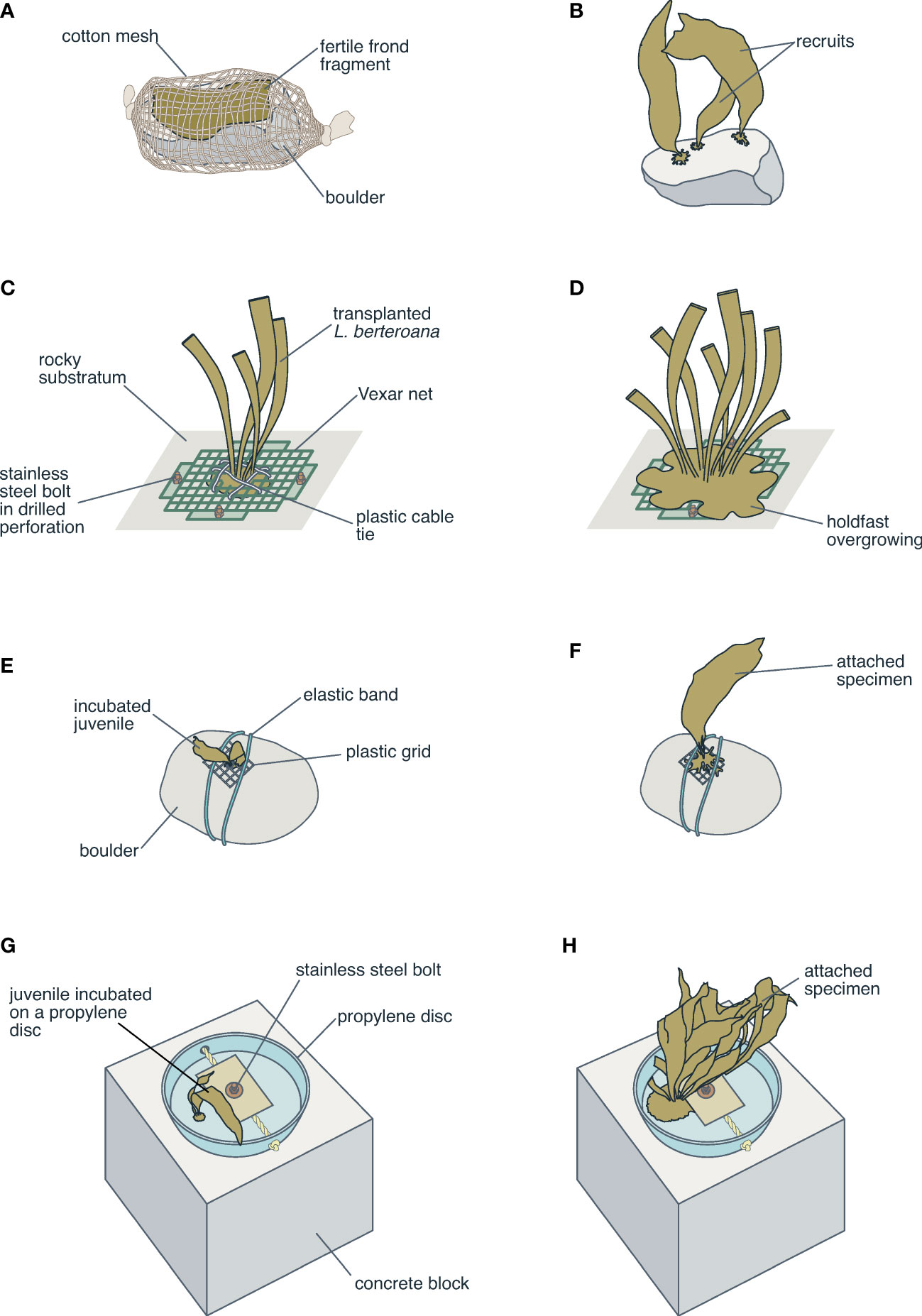
Figure 4 Illustration representing restocking techniques used for brown seaweeds in Chile. (A, B) Boulder with cotton mesh and fertile frond of M. pyrifera for sowing spores (Westermeier et al., 2012a; Westermeier et al., 2012b). (C, D) Anchoring system of L. berteroana sporophytes on rocks (Correa et al., 2006). (E, F) Seeding juvenile of M. pyrifera on boulders with elastic band and plastic grids (Westermeier et al., 2012a). (G, H) Disk-anchoring system requiring incrustation into the rock for L. berteroana sporophytes (Ávila et al., 2017).
3.3.2. Restocking from seeding or transplants of juvenile sporophytes
The study by Vásquez and Tala (1995) reported transplantation of reproductive adult L. berteroana individuals using concrete nails and cable ties to fasten them to rocks. Although there was 50% mortality in the first two weeks after transplantation, in the following months 38% of individuals had reproductive structures. Ten years later, Correa et al. (2006) published a second study on restocking in Chile, also using the transplant of adult individuals of L. berteroana (Figure 4C, D). A transplantation system was designed using materials such as mesh, cable ties, and bolts that were later anchored to the rock using a drill. Although the results indicated that adult individuals are effectively capable of surviving and growing after being transplanted (with 70% survival three months after the transplants), the authors recognized the complexity of the anchoring system and the difficulties of utilizing this strategy on a large scale in environments with high exposure to wave action.
Westermeier et al. (2012b) published results of experiments using M. pyrifera that evaluated different strategies and substrates. The techniques used for the transplant of juveniles included planting seedlings on different substrates, such as boulders, pottery, or mesh, and fastening them with elastic bands (Figure 4E, F) or glue (cyanoacrylate) to later place them in subtidal environments (Westermeier, 2013; Westermeier et al., 2014). Vásquez et al. (2014) also used M. pyrifera seedlings on three different substrates: pottery, tuffy pads, and clam shells; laboratory and field experiments showed that a greater biomass of seedlings attached to clam shells (60% more biomass than other substrates), and they concluded that the installation of substrate units with seaweed seedlings could be an option for the recovery of exploited M. pyrifera subtidal kelp forests.
The only study on the genus Durvillaea (i. e. “cochayuyo,”) was published by Gutiérrez et al. (2016), who reported transplanting D. incurvata seedlings by anchoring them directly onto the rock with an adhesion device similar to that used by Correa et al. (2006).
One of the most recent publications was a study by Ávila et al. (2017), who developed a technique using 3 cm L. berteroana seedlings on a plate made with nonbiodegradable material. Although this anchoring device supports wave simulation, which is positive for seaweed development, it requires incrustation into the rock, which increases both the logistical and replication difficulty (Figures 4G, H).
3.3.3. Restocking from holdfast fragment planting
The attachment of holdfast fragments of M. pyrifera to boulders with elastic bands (Figure 5A), boulders with cyanoacrylate glue, and rock platforms with cyanoacrylate glue was evaluated by Westermeier et al. (2016) and Murúa et al. (2017). The results showed that new thalli increased in size and developed reproductive structures (Figure 5B), which suggest good potential for use in restoration strategies (Murúa et al., 2017). In addition, seedling recovery and rapid holdfast growth were observed (Westermeier et al., 2016). Additionally, it is well known that M. pyrifera grows under specific laboratory conditions where haploid zoospores are seeded on ropes, from where diploid juvenile grow (Figure 5C). These ropes are arranged in longline structures in the sea (Figure 5D). The Final objective is that the juveniles will grow and develop reproductive structures that will help the population recover progressively (Figure 5E).
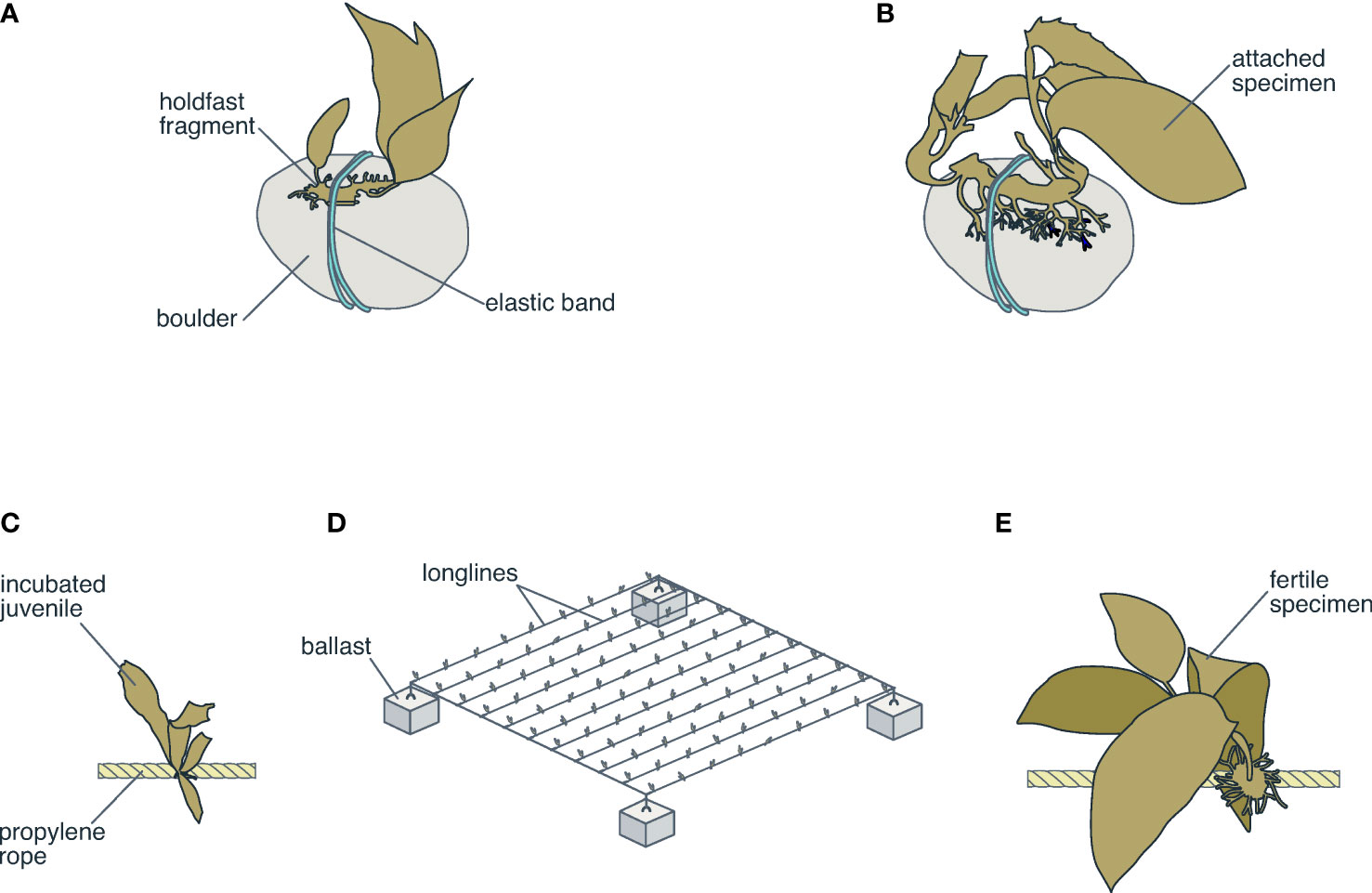
Figure 5 (A, B) Illustration of restocking technology for adults of M. pyrifera using holdfast fragments onto boulders (Murúa et al., 2017). (C–E) The most common culture system for M. pyrifera based on longline structures.
It should be noted that there has been considerable progress in Chile with respect to the knowledge of the biology and domestication of brown seaweed species. However, although 27 years have passed since the first report of an attempt to restock, technological developments have neither improved nor been widely applied to solve the practical difficulties of these strategies.
3.4. Seaweed restocking experience: Fisher communities and territorial users rights for fisheries
In Chile, benthic fisheries management is based on Territorial Users Rights for Fisheries (TURF). These are spatial user rights that grant exclusive access to fishers to manage benthic resources in a sustainable manner within a given geographic area (González et al., 2006; San Martín et al., 2010). This system assumes that the success or failure of management depends on users taking care of the management area and its resources, avoiding overexploitation.
A series of restocking experiments on benthic resources have been undertaken within TURF, although mostly without assessing reliable indicators of success (Cárcamo et al., 2021). The implementation of restocking efforts in management areas over large scales remains very low. For example, in the 803 active TURF along the Chilean coast, 109 restocking actions in 82 TURF were officially reported between 2010 and 2018. Of these, 41 corresponded to seaweed restocking, most frequently involving the two red species S. crispata and G. chilensis and the brown seaweed L. berteroana. Seaweed-restocking efforts within TURF respond directly to the importance that each specific organization gives to the species in their management plan (Figure 6).
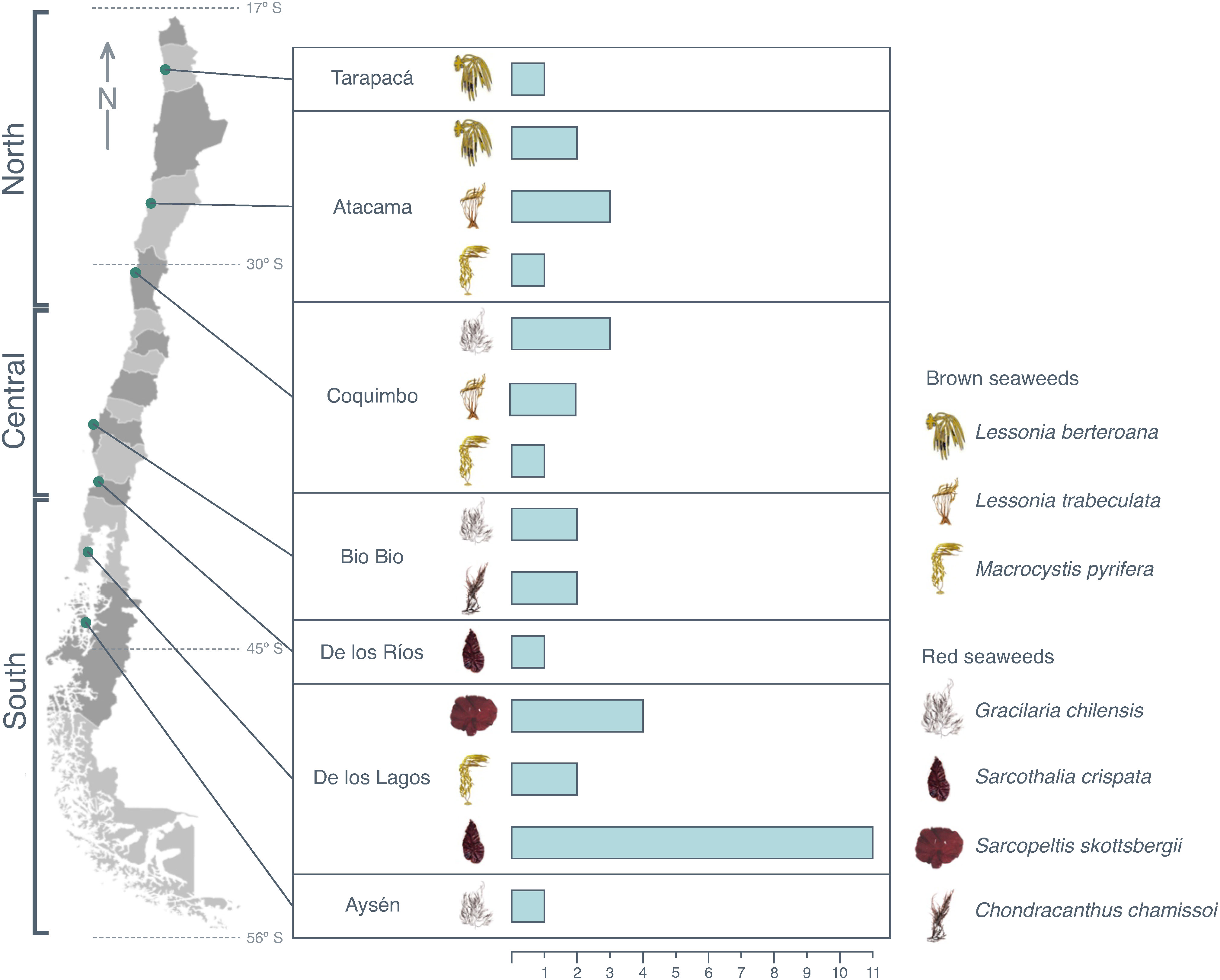
Figure 6 Number of seaweed-restocking activities along the Territorial Users Rights for Fisheries (TURF) in Chile by region and species for the period 2010–2018.
In northern Chile (i.e., Tarapacá, Atacama, and Coquimbo, see Figure 6), activities have focused mainly on the restocking of brown seaweeds (Lessonia spp. and M. pyrifera), which support the constantly growing fisheries in the area, with effects that are socially and ecologically relevant. On the other hand, in the central and southern regions of Chile, red seaweeds are more relevant in terms of exploitation due to an increase in their biomass (Figure 6). No restocking efforts have been reported for Patagonian ecosystems (Figure 6), which represent a relevant research gap for kelps distributed along the southern Chile region. It should be noted that the Magallanes benthic management committee recently agreed to implement an extractive ban on brown seaweeds (i.e., M. pyrifera) that focusses on conserving the ecosystem services that kelp forests provide in Patagonia (Vega et al., 2021).
Restocking activities have focused mainly on seedling transplantation using two techniques: direct seeding and the installation of seedlings with anchoring systems. The most common techniques, widely used with red seaweeds, are direct seeding using net bags with boulders (Figure 7A) or the use of a line or rope system (Figure 7B). For brown seaweeds, restocking systems have focused on the installation of seedlings with anchoring systems (Figure 7C) and the experimental use of cyanoacrylate as a glue (Figure 7D). In both cases, the seedlings are protected from herbivores such as the sea urchins Tetrapygus niger and Tegula atra (Cárcamo et al., 2021).
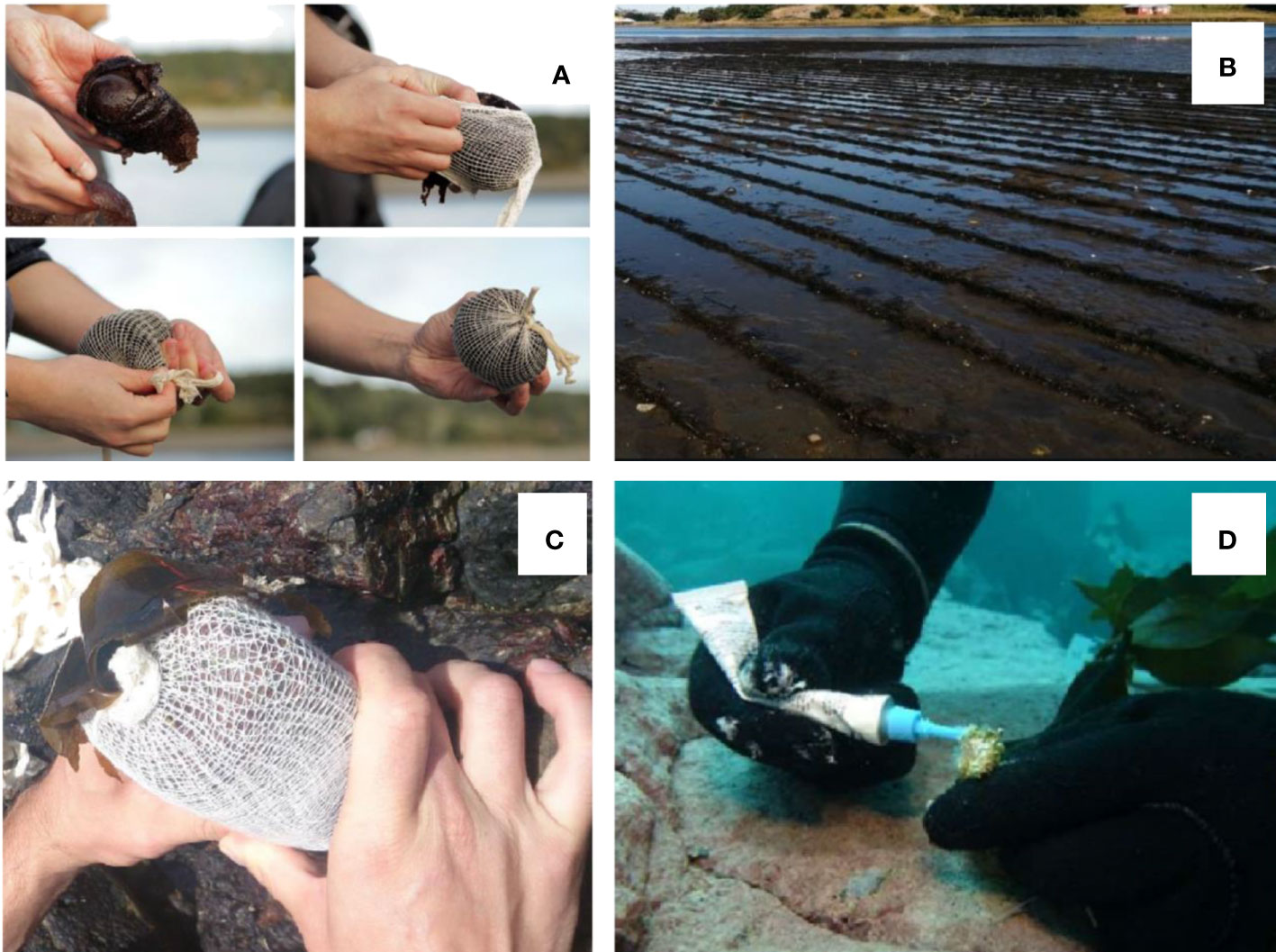
Figure 7 Photographs of algal restocking systems reported in the monitored TURF. (A) Repopulation system for Sarcothalia crispata reported in the Bajos de Lami by Ecos Consultores (2015). (B) Gracilaria chilensis restocking system recorded in Caulin, Chiloé in August 2020. (C) Repopulation system reported for Lessonia trabeculata in the Pajonales TURF by Bitecma Consultores (2015). (D) Experiment involving transplanting Lessonia trabeculata seedlings to a direct substrate with cyanoacrylate glue in the TURF of Chungungo by the Instituto de Fomento Pesquero (Henríquez-Antipa et al., 2019).
Ninety percent of these activities have been financed through either central or local government subsidies, whereas only 10% have been financed through competitive bidding for private funds. Restocking activities in TURF have ranged from a few units to a maximum of almost 50,000 seedlings (this was reported for L. trabeculata in Torres del Inca in the region of Atacama, ca. 26°S). However, there are exceptions, as was reported for G. chilensis in Playa Changa (ca. 29°S) in the region of Coquimbo (central Chile, Figure 6), where up to 90 tons of biological material were used.
These restocking efforts have focused mainly on the recovery of areas where target species have been previously overexploited or that have suffered from environmental/oceanographic changes created by large-scale events such as the El Niño Southern Oscillation (ENSO; Camus, 1994; Martínez et al., 2003; Vega et al., 2005). It is not yet known whether these activities have been successful, and for most, there are no long-term evaluations to assess their efficacy in terms of population recovery.
4. Recent proposals of restocking techniques elsewhere
Several restocking strategies have been described worldwide, which use similar biological principles and transplantation strategies as those mentioned for Chile. In this section, recently publications in other countries are described as examples about restocking in red and brown seaweeds that might be considered for Chile.
4.1. Red seaweeds
Globally, there are still few studies on the impact on coastal communities of the loss of economically important red seaweed biomass due to environmental forcing or overexploitation or the corresponding restoration efforts. Although they are smaller than large Laminaria and Fucus species (Phaeophyceae)), they provide similar ecosystem functions over their spatial scale, such as CO2 capture, provision of refuge areas for small invertebrates, and substrate stabilization. Several red seaweeds are part of the understory stratum or turf assemblages (Pessarrodona et al., 2021). There are few existing studies that have used transplantation techniques with different devices to evaluate, for example, ecophysiological responses to environmental variability such as solar radiation (e.g., Quintano et al., 2019) or wave exposure (Shaugnessy and DeWreede, 2001) or ecological relationships in seaweed–animal interactions (Enríquez et al., 2009). The effects of wave exposure in relation to the morphology of the red seaweeds Mazzaella splendens and Mazzaella linearis have been evaluated through intertidal transplantation with a device consisting of a base of vinyl-covered metal mesh attached to a rock with screws inserted using a pneumatic hammer drill that supports seaweeds inserted with a polypropylene rope attached to the metal mesh with wire cable ties (Shaughnessy and DeWreede, 2001). The interaction between the red alga Jania pedunculata var. adhaerens (formerly Jania adhaerens) and the sponge Haliclona caerulea was evaluated using artificial substrates tied to heavy concrete blocks (Enríquez et al., 2009). Individuals were induced to reattach to the artificial substrate (10 × 10 × 0.5 cm CaCO3 squares) by fastening them with cable ties, which allowed the development of their anchoring systems. Experimental transplantation of Gelidium corneum in the subtidal area used several stainless-steel elements, with a frame attached to the rock with rapid-drying concrete, a platform, and screwed cubical containers (Quintano et al., 2019). The devices described in these experiments could be considered prototypes that should be evaluated in future red seaweed repopulation/restoration studies.
4.2. Brown seaweeds
Recently Eger et al. (2022) reviewed the history about the kelp restoration projects highlighting the increase in attempts made around the word, using 10 different technologies and 17 kelp genera, becoming the group of brown algae that has received the most attention. The restoration of seaweed forests worldwide has typically followed two general strategies: assisted recovery and active restoration (Wood et al., 2019; Layton et al., 2020). Techniques range from bulk nursery-raised propagules to the cultivation of propagules with more intensive care in individual units. In general, propagules are maintained for several months until they reach the juvenile stage, following which they are transplanted to the sea (Fredriksen et al., 2020; Vanderklift et al., 2020).
A recently described technique is called “green gravel,” in which the general procedure involves collecting fertile Saccharina latissima individuals to obtain spores, which are then inoculated on rocks (2–5 cm in diameter) in a laboratory. The rocks are kept in tanks until seedlings are visible and can be transplanted to the sea, and the substrate is seeded directly onto the seafloor (Figure 8A) (Fredriksen et al., 2020). Although this technique can be effective, it is labor-intensive and requires upstream cultivation steps for the maintenance and growth of the seedlings. This methodology also has been evaluated in Ecklonia radiata (Alsuwaiyan et al., 2022) under indoor conditions.
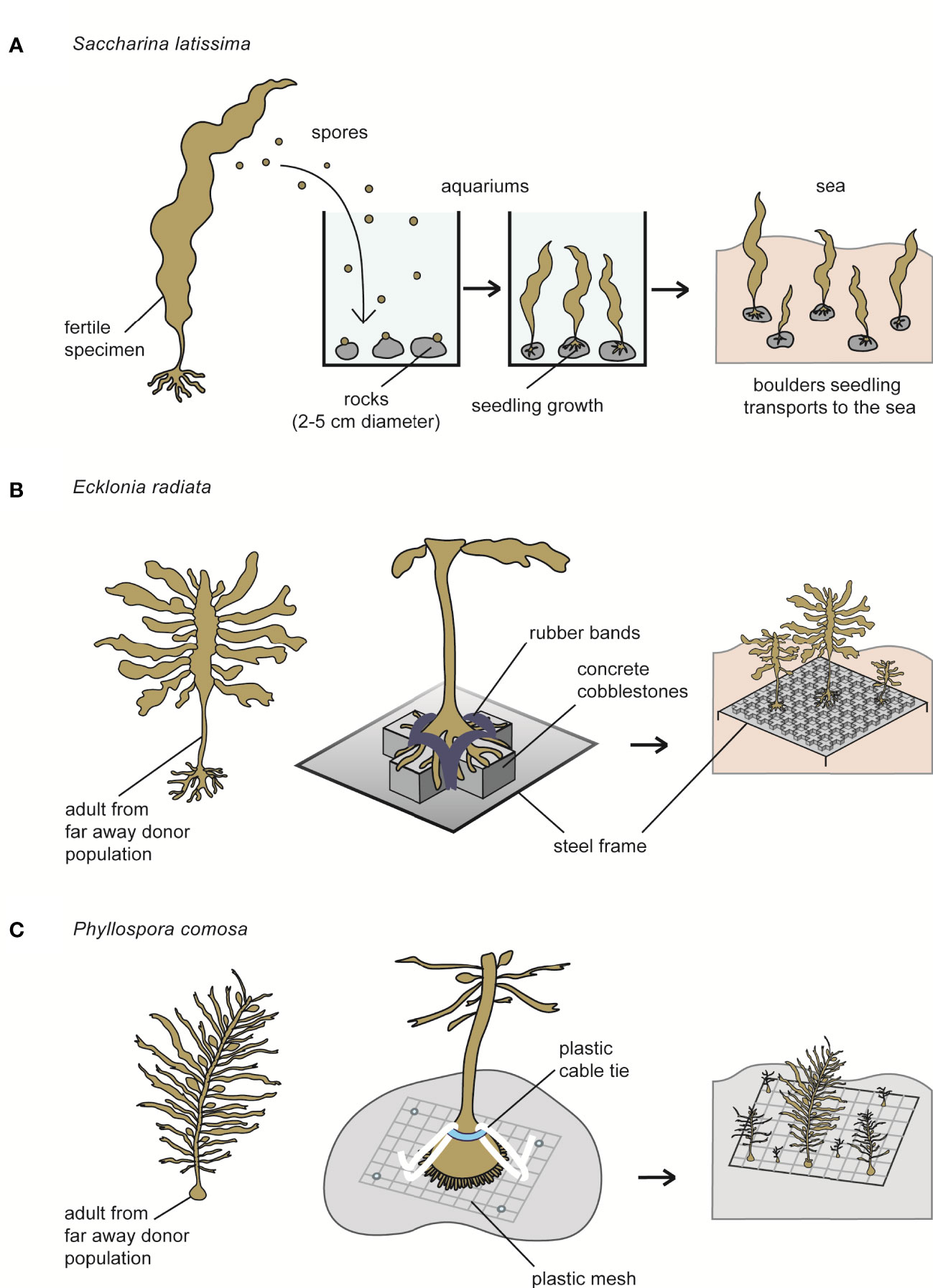
Figure 8 Some illustrations about restocking strategies used for brown seaweeds worldwide. (A) Saccharina latissima. (B) Ecklonia radiata. (C) Phyllospora comosa.
Another evaluated method is the transplantation of adult Ecklonia radiata individuals using concrete cobblestones attached to a steel frame while anchoring the holdfasts with rubber bands (Figure 8B) (Layton et al., 2021). The reattachment of individuals to the surface occurs within 3–6 weeks, with survival rates of 75%. According to the authors, this technology is adequate; however, the effort required to transport large cobblestones to the transplantation areas and the use of individuals collected from nature for restocking are inconvenient. It is important to note that the transplantation is based on harvesting individuals from a source/donor population, and thus the technique produces a decrease in the abundance of the source population, at least over the short term. Moreover, the success of the method would also depend on the health of the donor population, its context, its genetic variability, and its capacity to tolerate environmental stressors that could occur at the new sites.
Several restocking strategies have been employed in Australia, including deploying artificial substrates to which algae recruit naturally, replanting sporophytes (i.e., kelp reproductive fronds), and transplanting ropes seeded with small (5 mm) juvenile seaweed previously cultivated in the laboratory. A repopulation strategy for the kelp Phyllospora comosa was successful. It was based on transport from a distant donor population to the repopulation site to establish a population sufficiently mature and reproductive to create juveniles. Specifically, the seaweeds were harvested carefully, including the holdfast, which was attached to the transplantation site using cable ties on 0.25 m2 plastic meshes (Figure 7C) (Campbell et al., 2014). The efficacity of this approach was demonstrated by the observation of multiple new generations of this species spreading thousands of meters from the initial repopulation sites. Repopulation also reduces the risks of habitat diversity loss for the epifauna and its consumers (Marzinelli et al., 2016). Although this strategy has been effective, it is not always viable for all kelp due to the differences in their growth rates and types of natural substrate. There is also a genetic risk when translocating seaweeds from one place to another, in addition to the risk of the transportation of their associated epibionts with a genetic risk to the epibionts’ populations (Bonthond et al., 2020). This strategy also uses plastic material, and thus more sustainable technologies would be preferred. For example, biodegradable mesh drilled into the ocean floor is currently being used (http://www.operationcrayweed.com).
5. Discussion
5.1. Restocking technologies
Chilean official fisheries statistics indicate that commercial landings have involved more than ten seaweed resource species (some of which may include two or three related biological species) in the last 20 years (SERNAPESCA, 2022). A few of these species have been landed only occasionally, but for the remaining crops the harvesting pressure on natural populations has been persistent over time and along most of their Chilean geographic distribution. Although farming methods for commercially important seaweed species have been developed at the experimental or pilot stage, only one red seaweed, Gracilaria chilensis, is commercially cultivated in Chile. For other red seaweed resources, attempts have only recently been made to design techniques that can increase their abundance or replenish areas affected by natural disasters or anthropogenic activities (Table 2), but more extensive and longer-term restocking trials are needed. In the case of brown seaweeds, experimental restocking has been carried out for few species due to the difficulties of working over a large scale in environments that are highly impacted by wave action.
Some basic studies have been carried out on the biological features of most of these species, which have been successfully cultivated in laboratory conditions at an experimental scale (reviewed in Saavedra et al., 2019). Nonetheless, there are few reports of attempts to apply this knowledge to install new individuals in the field in restocking efforts. Increased awareness of increasing seaweed harvests in Chile, together with the recognition of the environmental services that they provide, have motivated research aimed at exploring seeding techniques that can be integrated into the management practices of six Rhodophyta and three Phaeophyceae) species (Table 2).
In other parts of the world, the situation for restocking activities is similar, although a great effort is being made to address the impact of climate change (e.g., heat waves) on populations of kelp forests due to their ecosystem value. For economically important seaweeds that are constantly harvested without an adequate management program, a lack of appropriate restocking practices may lead to over-extraction, affecting the seaweed populations and the products obtained from them (e.g., Callaway, 2005). Reports of the installation of new individuals in the field mainly involve the transplantation of individuals for experimental purposes (e.g., Enríquez et al., 2009; Quintano et al., 2019), and scale-up for mass implementation would be costly and unfeasible. On the other hand, the attributes of the seaweed resources present on other coasts, together with technological and implementation capabilities and the environmental conditions of those coasts, have allowed for the cultivation of seaweed rather than restocking in some cases (e.g., Eucheuma). Overall, large-scale population restocking techniques for the purpose of biomass production, conservation, and recovery of seaweed ecosystem functions are still lacking.
Essential aspects that must be considered when scaling up restocking techniques include the genetic diversity of the population that is being sampled and its genetic relatedness to the population at the site that is being repopulated. The genetic information is relevant to evaluate the potential impact of introducing new individuals on the genetic diversity of the natural populations (Ottewell et al., 2016). The health of the donor population, its context, its genetic variability, and its capacity to tolerate several environmental stressors that could occur at the new repopulation sites can determine the success of restocking (Reynolds et al., 2012; Wernberg et al., 2018; González et al., 2020; Chemello et al., 2022). Depending on the restocking strategy used, a loss of genetic diversity can lead to situations such as those described for G. chilensis because of domestication through clonal translation to management and cultivation areas (Guillemin et al., 2008; Guillemin et al., 2014).
In addition, an effort should be made to ensure that devices that are permanently deployed in the field are environmentally friendly, e.g., trying to replace plastic pieces with equivalent ones made of biodegradable materials. The chemical stability of plastic materials used in different techniques implies serious environmental problems and human health implications (Li et al., 2020; Gola et al., 2021; Wu et al., 2022), which require evaluation, especially for those practices with potential massive use. Restocking strategies require the use of biodegradable polymers. A possible transformation could involve the use of biopolymers such as polylactic acid (PLA), which has provided an important boost in biomedicine due to its properties such as biocompatibility, water solubility, handling properties, well-established production technologies (Casalini et al., 2019), and 3D printing possibilities (Singh et al., 2019). New techniques exist that could facilitate the anchoring or attachment of kelps to a rocky habitat, such as submersible hammer drills, which have been successfully used for perforation (Wainwright et al., 2020), or the use of organic resins that have strong underwater adhesive capacity (Yan et al., 2022), many of which have been inspired by the adhesion capacity of mollusks (Priemel et al., 2021) and other marine organisms (Fan and Gong, 2022).
The economic evaluation of ecosystem services and functions to the coastal environment of the new seaweeds should be considered as a relevant input during the decision-making process when considering implementing a large-scale restocking program (Cuba et al., 2022). The proper implementation of success indicators of restocking activities, from the individual to the ecosystem level, must include local/geographical considerations in relation to abiotic and biotic factors, the demographics of the populations to be impacted, and the timing for achieving changes in coastal ecosystems.
5.2. Social implications of seaweeds restocking
The management of benthic resources takes place in a socio-ecological system with social, political, and cultural characteristics that must be considered (Reyers et al., 2018). To begin the implementation of a large-scale restocking effort, it is necessary to evaluate whether the socio-ecological system presents the necessary enabling conditions for the implementation of such a strategy (Cárcamo et al., 2021). This raises important challenges in the Chilean case. First, artisanal fishers’ organizations must have the organizational maturity and social capacities to successfully implement technological and stocking projects (Abelson et al., 2016; Chen et al., 2020). In this sense, there is a lack of knowledge in Chile that necessitates the prioritization of organizations that have the necessary organizational maturity to implement restocking projects. Secondly, artisanal fishing in Chile and in particular the TURF system is highly vulnerable to illegal fishing (Gelcich et al., 2017; Oyanedel et al., 2018). Recent studies have shown that benthic resources are particularly exposed to poaching and illegal extraction (Romero et al., 2022). Restocking projects may generate a greater abundance of resources, and therefore greater incentives for illegal extraction (Cárcamo et al., 2021). The implementation of monitoring and surveillance systems emerges as a central element for the success of restocking projects in Chile. These systems should consider enforcement efforts by government agencies and the capacity for self-monitoring of artisanal fisher's organizations that manage TURF areas. Self-monitoring has been recognized as a central element in the sustainability of the Chilean TURF system (Estévez and Gelcich, 2020). Thirdly, it is necessary to evaluate the willingness of fisher’s organizations to participate in restocking initiatives that may not represent direct economic benefits in the short term (Blount et al., 2017). Therefore, given the number and size of the restocking initiatives carried out by TURF, it is necessary to consider which management tools could encourage restocking initiatives: possibilities of participation in organizations in the development of projects (Garaway et al., 2006), risk perception of the viability of the resource or the generation of other parallel financing instruments such as blue carbon markets. Although the willingness to participate in restocking programs has not been evaluated in Chile, the TURF system offers an opportunity to develop projects within the framework of a co-management model.
In Chile, restocking activities have been developed through management actions in an annual TURF plan or through the application for a bonus in accordance with Law NO. 20.925. The enactment of Law No. 20.925 “Bonus for the Repopulation and Farming of Seaweeds” in 2016 sought to diversify and increase the coverage of commercially important algae. This bonus is potentially a great opportunity for the artisanal fishing and small-scale aquaculture sector from an ecological and economic point of view. However, an a priori analysis indicates that the law has not achieved its objective of diversifying and protecting resources. On the one hand, administrative procedures and enforcement conditions by artisanal fisher's organizations make it difficult to obtain permits for these activities. On the other hand, this subsidy has concentrated on the cultivation of the red algae G. chilensis without promoting the restocking of a diversity of species (Quiroga, 2018; Carcamo et al., 2021). Thus, the true impact of the law on the resource, the increase in its coverage, the organizations, and the environment is unknown.
To strengthen the system and reduce the failure of restocking experiences, it is necessary to redesign some elements of the subsidy. At present, incentives are assigned at the end of the activity and after positive evidence and productive results are provided. This can be considered a weakness in the law given that it ignores the high variability and instability of the marine environment, in addition to externalities such as pollution, tsunamis, and climate change, which may affect the development of actions aimed at repopulation and/or cultivation. Alternatively, subsidies should be associated with the generation of early incentives that support the implementation of these practices, with a follow-up of their effectiveness over time, as well as assistance regarding the commercialization and innovation needed to create added value (Henríquez-Antipa and Cárcamo, 2019). In addition, some of the additional challenges remaining are: (1) to promote fishery diversification by incorporating other algae into productive activities, (2) to scale up restocking efforts, (3) to generate science-based technological entities that are in charge of supplying seeds and seedlings for seaweed production, (4) to develop appropriate infrastructure in the coastal areas for developing these technologies, and (5) to garner substantial support from the state for small-scale activities.
6. Conclusions
The information compiled on seaweed restocking in Chile underlines a need to accelerate the processes of increasing research on new restocking methods in a greater number of species and scaling up those methods for the benefit of the environment and the communities that depend on these activities. Efforts must be participatory, and actions should be interdisciplinary. It is important that governments and public administration, together with private seaweed companies, actively participate in seaweed restocking initiatives. A restocking program will be associated with a continuous effective restoration exercise that will allow structural/compositional replication, ecosystemic functional success and durability while considering its socioecosystemic and dynamic nature. Thus, restocking interventions advance initiatives related to ecological restoration, reclamation, and rehabilitation by impacting ecosystem structure (e.g., species diversity and structural complexity) and ecosystem functions (e.g., C sequestration, nutrient recycling, productivity), moving a degraded ecosystem to an intermediate or original state.
From a biological point of view, the genetic component of the entities to be restocked must be considered, and efforts should be made to increase genetic diversity and/or select genotypes that are resilient to future scenarios of change. Therefore, it would be appropriate to explicitly include the verification of the genetic variability of the thalli to be used in the repopulation programs to ensure not only a greater probability of success of settlement, but also the viability and ecosystemic health of the seaweed assemblages. Additionally, the impact of these techniques must involve caution regarding their effect on other species and the interaction of the target algae with them. Furthermore, seaweed restocking efforts must consider the technological complexity of the activity, of using species with complex life cycles that require in most cases infrastructure for the development of laboratory and hatchery stages, and of the working requirements and the use of structures that can withstand underwater conditions in areas exposed to waves and storm surges. In this task, of course, there are technological gaps, whose overcoming would imply a more effective approach between scientists and the technical and professional areas of the execution of these practices, as well as governmental entities and regional technical-logistical support institutions, such as hatcheries and monitoring laboratories. The implementation of these technologies would enable the continuous flow of the replenishment of biomass appropriate to the nature of the populations and ensure the permanence of a minimum population size. Therefore, although in Chile and the world, there are multisectoral experiences and capacities in seaweed restocking strategies, these must be strengthened and focused with clear environmental and social recovery objectives. See Figure 9 for conceptual scheme summarizing the challenges and recommendations for scaling-up restocking efforts.
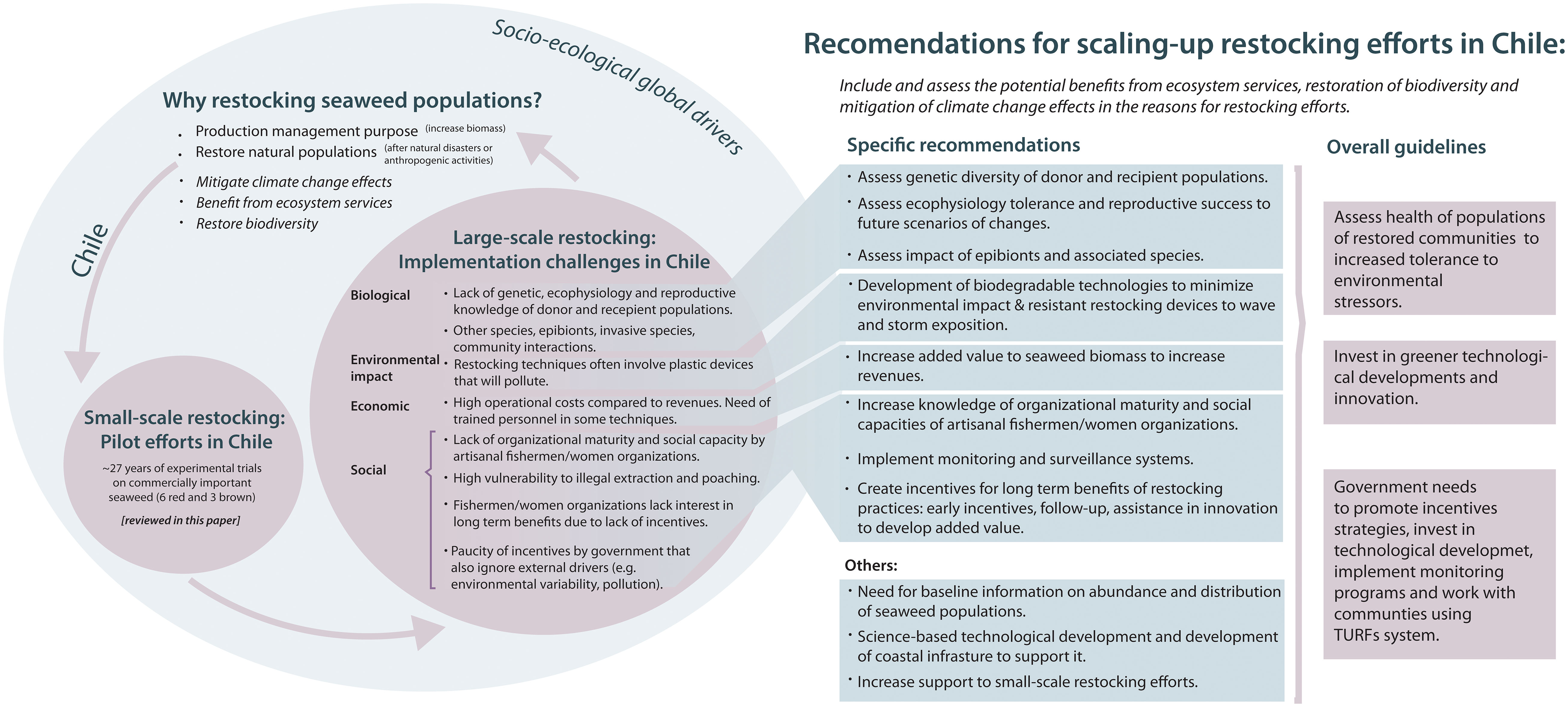
Figure 9 Conceptual figure summarizing the challenges and recommendations for scaling-up restocking efforts for seaweed populations in Chile.
Author contributions
CO-M, CB and LC-P conceived the paper. All authors contributed to the article and approved the submitted version. Then each author has had a part of the paper to write: Introduction by CB, LC-P, RO and FT. Seaweed restocking in Chile by all the authors. Principal restocking techniques used worldwide by all the authors. Discussion and Conclusions by all the authors.
Funding
This research was funded by the ANID Millennium Science Initiative Program ICN 2019_015, ANID PIA/BASAL FB0002, ANID/BASAL FB210006 and the CNRS International Research Network Diversity and Biotechnology of Marine Algae (contract number 00022).
Acknowledgments
The authors would like to thank Renata Garretón for her contribution to the elaboration of Figures 1 and 8 of this work.
Conflict of interest
The authors declare that the research was conducted in the absence of any commercial or financial relationships that could be construed as a potential conflict of interest.
Publisher’s note
All claims expressed in this article are solely those of the authors and do not necessarily represent those of their affiliated organizations, or those of the publisher, the editors and the reviewers. Any product that may be evaluated in this article, or claim that may be made by its manufacturer, is not guaranteed or endorsed by the publisher.
References
Abelson A., Halpern B. S., Reed D. C., Orth R. J., Kendrick J. A., Beck M. W., et al. (2016). Upgrading marine ecosystem restoration using ecological-social concepts. BioScience 66, 156–163. doi: 10.1093/biosci/biv171
ABIMAR (2017). Evaluación directa de macroalgas impacto de la extracción sobre la comunidad bentónica, IV Región. Proyecto FIPA 2014-18. Final report (Santiago: Instituto de fomento pesquero).
Alsuwaiyan N. A., Filbee-Dexter K., Vranken S., Burkholz C., Cambridge M., Coleman M. A., et al. (2022). Green gravel as a vector of dispersal for kelp restoration. Front. Mar. Sci. 9. doi: 10.3389/fmars.2022.910417
Ávila M., Merino C., Piel M. I., Riquelme R., Guissen K., Pizarro P., et al. (2017). Manual para el cultivo y repoblamiento del huiro negro (Lessonia berteroana) en la Región de Antofagasta. Serie programa educativo participativo para la pesca artesanal. III cultivo y repoblamiento de huiro negro (Iquique: Universidad Arturo Prat).
Ávila M., Otaíza R., Norambuena R., Núñez M., Candia A., Poblete A. (1994). Desarrollo de tecnología de cultivo y repoblación de luga negra en la X Región. Final report, Proyecto CORFO-IFOP (Santiago: Instituto de fomento pequero).
Barrientos E., Otaíza R. (2014). Juveniles generados a partir de esporas no asentadas de Chondracanthus chamissoi (Rhodophyta, Gigartinales) presentan capacidad de adhesión al sustrato. Rev. Biol. Mar. Oceanogr. 49, 135–140. doi: 10.4067/S0718-19572014000100015
Blount C., O’Donnell P., Reeds K., Taylor M. D., Boyd S., Van Derwalt B., et al. (2017). Tools and criteria for ensuring estuarine stock enhancement programs maximise benefits and minimise impacts. Fish. Res. 186, 413–425. doi: 10.1016/j.fishres.2016.08.019
Bonthond G., Bayer T., Krueger-Hadfield S. A., Barboza F. R., Nakaoka M., Valero M. (2020). How do microbiota associated with an invasive seaweed vary across scales? Mol. Ecol. 29, 2094–2108. doi: 10.1111/mec.15470
Bularz B., Fernández M., Subida M. D., Wieters E. A., Pérez-Matus A. (2022). Effects of harvesting on subtidal kelp forests (Lessonia trabeculata) in central Chile. Ecosphere 13, 1–14. doi: 10.1002/ecs2.3958
Bulboa C., Véliz K., Sáez F., Sepúlveda C., Vega L., Macchiavello J. (2013). A new method for cultivation of the carragenophyte and edible red seaweed Chondracanthus chamissoi based on secondary attachment disc: Development in outdoor tanks. Aquaculture 410, 86–94. doi: 10.1016/j.aquaculture.2013.06.018
Cai J., Lovatelli A., Aguilar-Manjarrez J., Cornish L., Dabbadie L., Desrochers A., et al. (2021a). Seaweeds and microalgae: An overview for unlocking their potential in global aquaculture development. FAO fisheries and aquaculture circular no. 1229 (FAO: Rome). doi: 10.4060/cb5670en
Cai J., Lovatelli S., Stankus A., Zhou X. (2021b). Seaweed revolution: Where is the next milestone? FAO Aquaculture Newslett. 63, 13–16. Available at: http://www.fao.org/3/cb4850en/cb4850en.pdf#page=13
Callaway E. (2015). Lab Staple agar runs low: dwindling seaweed harvest imperils reagent essential for culturing microbes. Nature 528, 171–112. doi: 10.1038/528171a
Campbell A. H., Marzinelli E. M., Vergés A., Coleman M. A., Steinberg P. D. (2014). Towards restoration of missing underwater forests. PloS One 9 (1), e84106. doi: 10.1371/journal.pone.0084106
Camus P. A. (1994). Dinámica geográfica en las poblaciones de Lessonia nigrescens Bory (Pheophyta) en el norte de Chile: importancia de la extinción local durante eventos El niño de gran intensidad. Rev. Investig. Cient. Tecnol. Ser. Cienc. Mar. 3, 58–70.
Candia A., Núñez M. (2017). Manual de técnicas de repoblamiento y cultivo de “luga negra” (Sarcothalia crispata). Proyecto HUAM-FONDED AQ 08I1031 (Santiago: Instituto de Fomento Pesquero).
Cárcamo P., Henríquez-Antipa L., Galleguillos F., Figueroa-Fabrega L., Taylor M. (2021). Marine stocking in Chile: A review of past progress and future opportunities for enhancing marine artisanal fisheries. Bull. Mar. Scie. 97, 729–748. doi: 10.5343/bms.2020.0052
Carney L. T., Waaland J. R., Klinger T., Ewing K. (2005). Restoration of the bull kelp Nereocystis luetkeana in nearshore rocky habitats. Mar. Ecol. Prog. Ser. 302, 49–61. doi: 10.3354/meps302049
Casalini T., Rossi F., Castrovinci A., Perale G. (2019). A perspective on polylactic acid-based polymers use for nanoparticles synthesis and applications. Front. Bioeng. Biotechnol. 7. doi: 10.3389/fbioe.2019.00259
Chemello S., Signa G., Mazzola A., Ribeiro Pereira T., Sousa Pinto I., Vizzini S. (2022). Limited stress response to transplantation in the Mediterranean macroalga Ericaria amentacea, a key species for marine forest restoration. Int. J. Environ. Res. Public Health 19, 12253. doi: 10.3390/ijerph191912253
Chen J. L., Hsu K., Chuang C. T. (2020). How do fishery resources enhance the development of coastal fishing communities: Lessons learned from a community-based sea farming project in Taiwan. Ocean. Coast. Manage. 184, 105015. doi: 10.1016/j.ocecoaman.2019.105015
Chopin T., Tacon A. G. (2021). Importance of seaweeds and extractive species in global aquaculture production. Rev. Fish. Sci. Aquac. 29, 139–148. doi: 10.1080/23308249.2020.1810626
Correa J., Lagos N., Medina M., Castilla J., Cerda M., Ramírez M., et al. (2006). Experimental transplants of the large kelp Lessonia nigrescens (Phaeophyceae) in high-energy wave exposed rocky intertidal habitats of northern Chile: Experimental, restoration and management applications. J. Exp. Mar. Biol. Ecol. 335, 13–18. doi: 10.1016/j.jembe.2006.02.010
Cuba D., Guardia-Luzon K., Cevallos B., Ramos-Larico S., Neira E., Pons A., et al. (2022). Ecosystem services provided by kelp forests of the Humboldt current system: A comprehensive review. Coasts 2, 259–277. doi: 10.3390/coasts2040013
Delaney A., Frangoudes K., Ii S. A. (2016). “Society and seaweed: Understanding the past and present,” in Seaweed in health and disease prevention. Eds. Fleurence J., Levine I. (London: Academic Press), 7–40. doi: 10.1016/C2014-0-02206-X
Díaz B. E. (2020). Análisis del desarrollo biotecnológico para implementar acciones de repoblamiento de algas marinas en Chile: una revisión sistemática [dissertation/Ungraduate thesis] (Santiago, Chile: Universidad Andrés Bello).
Dillehay T. D., Ramírez C., Pino M., Collins M. B., Rossen J., Pino-Navarro J. D. (2008). Monte Verde: Seaweed, food, medicine and the peopling of south America. Science 320, 784–786. doi: 10.1126/science.1156533
Earp H. S., Smale D. A., Pérez-Matus A., Gouraguine A., Shaw P. W., Moore P. J. (2022). A quantitative synthesis of approaches, biases, successes, and failures in marine forest restoration, with considerations for future work. Aquat. Conserv: Mar. Freshw. Ecosyst. 32, 1–15. doi: 10.1002/aqc.3880
ECOS (2020). Evaluación de biomasa y análisis del estado de explotación de las praderas naturales de algas pardas (huiro negro, huiro palo y huiro flotador) en las áreas de libre acceso de la Región de Atacama y Coquimbo. Proyecto FIPA 2017-53. Final report (Santiago: Subsecretaria de Pesca y Acuicultura).
Ecos Consultores (2015). Expediente de Repoblamiento Recursos Luga negra (Sarcothalia crispata) (Calbuco, X Región: Área de Manejo Bajos de Lami), 20 pp.
Eger A. M., Marzinelli E. M., Christie H., Fagerli C. W., Fujita D., Gonzales A. P., et al. (2022). Global kelp forest restoration: Past lessons, present status, and future directions. Biol. Rev. 97, 1449–1475. doi: 10.1111/brv.12850
Enríquez S., Ávila E., Carballo J. L. (2009). Phenotypic plasticity induced in transplant experiments in a mutualistic association between the red alga Jania adhaerens (Rhodophyta, Corallinales) and the sponge Haliclona caerulea (Porifera: Haplosclerida): morphological responses of the alga. J. Phycol. 45, 81–90. doi: 10.1111/j.1529-8817.2008.00640.x
Estévez R., Gelcich S. (2020). “Collective action spaces and transformations in the governance of fisheries resources towards democratic and deliberative management,” in Marine and fisheries policies in Latin America: A comparison of selected countries. Eds. Muller M., Oyanedel R., Monteferri B. B. (London, UK: Routledge), 138–148.
Fan H. L., Gong J. P. (2022). Bioinspired underwater adhesives. Adv. Mater. 33, 2102983. doi: 10.1002/adma.202102983
Fredriksen S., Filbee-Dexter K., Norderhaug K. M., Steen H., Bodvin T., Coleman M. A., et al. (2020). Green gravel: A novel restoration tool to combat kelp forest decline. Sci. Rep. 10, 3983. doi: 10.1038/s41598-020-60553-x
Galleguillos F., Aroca G., Saavedra S. (2013). Protocolo de repoblación: luga negra, luga roja. Proyecto HUAM AQ08I1031 (Santiago: Instituto de Fomento Pesquero).
Garaway C. J., Arthur R. I., Chamsingh B., Homekingkeo P., Lorenzen K., Saengvilaikham B., et al. (2006). A social science perspective on stock enhancement outcomes: lessons learned from inland fisheries in southern lao PDR. Fis. Res. 80, 37–45. doi: 10.1016/j.fishres.2006.03.012
Gelcich S., Cinner J., Donlan C. J., Tapia-Lewin S., Godoy N., Castilla J. C. (2017). Fisher’ perception on the Chilean coastal TURF system after two decades: Problems, benefits, and emerging needs. Bull. Mar. Sci. 93, 53–67. doi: 10.5343/bms.2015.1082
Giercksky E., Doumeizel V. (2020). Seaweed revolution, a manifesto for a sustainable future. United Nations (London: Loyd’s Register Foundation).
Gola D., Tyagi P. K., Arya A., Chauhan N., Agarwal M., Singh S. K., et al. (2021). The impact of microplastics on marine environment: A review. Environ. Nanotechnol. Monit. Manage. 16, 100552. doi: 10.1016/j.enmm.2021.100552
González J., Stotz W., Garrido J., Orensanz J. M., Parma A. M., Tapía C., et al. (2006). The Chilean TURF system: how is it performing in the case of the loco fishery? Bull. Mar. Sci. 78, 499–527.
González A., Santelices B., Vásquez J., Tala F. (2020). Production of macroalgae chimera. Patent no CL WO2019010588 (Santiago: Instituto Nacional de Propiedad Industrial (INAPI)).
Guillemin M. L., Faugeron S., Destombe C., Viard F., Correa J. A., Valero M. (2008). Domestication and distribution of genetic variation in wild and cultivated populations of the haploid diploid red alga Gracilaria chilensis: how a traditional framing practice favour asexual reproduction and heterozygocity? Evolution 62, 1500–1519. doi: 10.1111/j.1558-5646.2008.00373.x
Guillemin M. L., Valero M., Faugeron S., Nelson W., Destombe C. (2014). Tracing the trans-pacific evolutionary history of a domesticated seaweed (Gracilaria chilensis) with archaeological and genetic data. PloS One 9 (12), 114039–114039. doi: 10.1371/journal.pone.0114039
Gutiérrez A., Varela D., Zúñiga A., Paredes J., Villarroel A., Ruiz A., et al. (2016). Desarrollo de tecnologías de cultivo y repoblamiento de Durvillaea antarctica, “cochayuyo”: Implicancias para la diversificación de la acuicultura y manejo de poblaciones naturales. VD primer semestre (Puerto Mont: Universidad de los Lagos).
Henríquez-Antipa L., Cárcamo F. (2019). Stakeholder’s multidimensional perceptions on policy implementation gaps regarding the current status of Chilean small-scale seaweed aquaculture. Mar. Pol. 103, 138–147. doi: 10.1016/j.marpol.2019.02.042
Henríquez-Antipa L. A., Galleguillos Foix F., Saavedra Muñoz S., Torres AAvilés D., Cook Alvarado S., Cárcamo VVargas F., et al (2019). Informe Final Convenio de Desempeño 2018: Programa integral de desarrollo de acuicultura de algas para pescadores artesanales. Etapa 2 Instituto de Fomento Pesquero. 237 pp.
Hernández-Carmona G., García O., Robledo D., Foster M. (2000). Restoration techniques for Macrocystis pyrifera (Phaeophyceae) populations at the southern limit of their distribution in méxico. Bot. Mar. 43, 273–284. doi: 10.1515/BOT.2000.029
Hynes S., Chen W., Vondolia K., Armstrong C., O’Connor E. (2021). Valuing the ecosystem service benefits from kelp forest restoration: A choice experiment from Norway. Ecol. Econ. 179, 106833. doi: 10.1016/j.ecolecon.2020.106833
IFOP (2004). Investigación y manejo de praderas de luga roja en la XII Región. Proyecto FIP 2002-27 (Santiago: Subsecretaria de Pesca y Acuicultura).
Jacob C., Buffard A., Pioch S., Thorin S. (2018). Marine ecosystem restoration and biodiversity. Ecol. Eng. 120, 585–594. doi: 10.1016/j.ecoleng.2017.09.007
Jara-Yáñez R., Meynard A., Acosta G., Latorre-Padilla N., Oyarzo-Miranda C., Castañeda F., et al. (2021). Negative consequences on the growth, morphometry, and community structure of the kelp Macrocystis pyrifera (Phaeophyceae, Ochrophyta) by a short pollution pulse of heavy metals and PAHs. Toxics 9, 190. doi: 10.3390/toxics9080190
Krumhansl K. A., Okamoto D. K., Rassweiler A., Novak M., Bolton J. J., Cavanaugh K. C., et al. (2016). Global patterns of kelp forest change over the past half-century. Proc. Natl. Acad. Sci. 113, 13785–13790. doi: 10.1073/pnas.1606102113
Latorre-Padilla N., Meynard A., Rivas J., Contreras-Porcia L. (2021). Transfer of pollutants from Macrocystis pyrifera to Tetrapygus niger in a highly impacted coastal zone from Chile. Toxics 9 (10), 244. doi: 10.3390/toxics9100244
Layton C., Cameron M. J., Shelamoff V., Tatsumi M., Wright J. T., Johnson J. R. (2021). A successful method of transplanting adult Ecklonia radiata kelp, and relevance to other habitat-forming macroalgae. Restor. Ecol. 29, e13412. doi: 10.1111/rec.13412
Layton C., Coleman M. A., Marzinelli E. M., Steinberg P. D., Swearer S. E., Vergés A., et al. (2020). Kelp forest restoration in Australia. Front. Mar. Sci. 7. doi: 10.3389/fmars.2020.00074
Li Q., Feng Z., Zhang T., Ma C., Shi H. (2020). Microplastics in the commercial seaweed nori. J. Hazard. Mater. 388, 122060. doi: 10.1016/j.jhazmat.2020.122060
Márquez R., Vásquez J. (2020). El Extractivismo de las algas pardas en el norte de Chile. Rev. Eur. Estud. Latinoam. Caribe. 110, 101–121. doi: 10.32992/erlacs.10590
Martínez E. A., Cárdenas L., Pinto R. (2003). Recovery and genetic diversity of the intertidal kelp Lessonia nigrescens (Phaeophyceae) 20 years after El niño 1982/831. J. Phycol. 39, 504–508. doi: 10.1046/j.1529-8817.2003.02191.x
Marzinelli E. M., Leong M. R., Campbell A. H., Steinberg P. D., Vergés A. (2016). Does restoration of a habitat-forming seaweed restore associated faunal diversity? Restor. Ecol. 24, 81–90. doi: 10.1111/rec.12292
Moher D., Liberati A., Tetzlaff J., Altman D. G., Altman D., Antes G., et al. (2009). Preferred reporting items for systematic reviews and meta-analyses: the PRISMA statement (Chinese edition). Chin. J. Integr. Med. 7 (9), 889–896. doi: 10.3736/jcim20090918
Morris R. L., Hale R., Strain E. M., Reeves S. E., Vergés A., Marzinelli E. M., et al. (2020). Key principles for managing recovery of kelp forests through restoration. BioScience 70 (8), 688–698. doi: 10.1093/biosci/biaa058
Murúa P., Müller D. G., Patiño D. J., Westermeier R. (2017). Giant kelp vegetative propagation: Adventitious holdfast elements rejuvenate senescent individuals of the Macrocystis pyrifera “integrifolia” ecomorph. J. Phycol. 53, 230–234. doi: 10.1111/jpy.12493
Ohno M., Critchley A. T. (1993). Seaweed cultivation and marine ranching (Yokouka: Kamagawa international Fisheries Training Center).
Oróstica M. H., Otaíza R. D., Neill P. E. (2012). Blades and papillae as likely dispersing propagules in Chilean populations of Mastocarpus sp. (Rhodophyta, Gigartinales). Rev. Biol. Mar. Oceanog. 47, 109–119. doi: 10.4067/S0718-19572012000100010
Otaíza R. D., Cáceres J. (2015a). Manual de una técnica para el repoblamiento de la luga negra, Sarcothalia crispata (Bory) Leister (Rhodophyta, Gigartinales), en praderas naturales, Región del Biobío. Proyecto FONDEF-HUAM AQ12I0004 (Santiago: Universidad Santo Tomas), 44 pp.
Otaíza R. D., Cáceres J. (2015b). Manual de una técnica para el repoblamiento de la luga corta, Mazzaella laminarioides (Bory) Fredericq (Rhodophyta, Gigartinales), en roqueríos intermareales, Región del Biobío. Proyecto FONDEF-HUAM AQ12I0004 (Santiago: Universidad Santo Tomas), 40 pp.
Otaíza R. D., Cáceres J. (2015c). Manual de una técnica para el repoblamiento de chicoria de mar, Chondracanthus chamissoi (C. agardh) Kützing (Rhodophyta, Gigartinales), en praderas naturales, Región del Biobío. Proyecto FONDEF-HUAM AQ12I0004 (Santiago: Universidad Santo Tomas), 40 pp.
Otaíza R. D., Cáceres J. (2017). Manual de una técnica para el repoblamiento de la chasca delgada, Gelidium lingulatum Kützing (Rhodophyta, gelidiales), en roqueríos intermareales, Región del Biobío. Proyecto FONDEF-REGIONAL D13R20031 (Santiago: Universidad Santo Tomas), 40 pp.
Otaíza R. D., Cáceres J. H., Rodríguez C. Y., Sanhueza Á.G. (2019). Seeding of fragments of the agarophyte Gelidium lingulatum (Rhodophyta, Gelidiales) for the repopulation of lower levels of exposed rocky shores. J. Appl. Phycol. 31, 2133–2143. doi: 10.1007/s10811-018-1695-8
Otaíza R. D., Carrasco L. (2002). Juveniles no adheridos como propágulos de Gigartinales (Rhodophyta). [Dissertation] (Chile: XXII Congreso de Ciencias del Mar; Valdivia).
Ottewell K. M., Bickerton D. C., Byrne M., Lowe A. J. (2016). Bridging the gap: A genetic assessment framework for population-level threatened plant conservation prioritization and decision-making. Divers. Distrib. 22, 174–188. doi: 10.1111/ddi.12387
Oyanedel R., Keim A., Castilla J. C., Gelcich S. (2018). Illegal fishing and territorial user rights in Chile. Conser. Biol. 32, 619–627. doi: 10.1111/cobi.13048
Oyarzo-Miranda C., Latorre N., Meynard A., Rivas J., Bulboa C., Contreras-Porcia L. (2020). Coastal pollution from the industrial park Quintero bay of central Chile: Effects on abundance, morphology, and development of the kelp Lessonia spicata (Phaeophyceae). PlosOne 15 (10), e0240581. doi: 10.1371/journal.pone.0240581
Pessarrodona A., Filbee-Dexter K., Krumhansl K. A., Moore P. J., Wernberg T. (2021). A global dataset of seaweed net primary productivity. Sci. Data. 9, 484. doi: 10.1038/s41597-022-01554-5
Priemel T., Palia G., Förste F., Jehle F., Sviben S., Mantouvalou I., et al. (2021). Microfluidic-like fabrication of metal ion-cured bioadhesives by mussels. Science 374, 206–211. doi: 10.1126/ciencia.abi9702
Quintano E., Celis-Plá P. S. M., Martínez B., Díez I., Muguerza N., Figueroa F. L., et al. (2019). Ecophysiological responses of a threatened red alga to increased irradiance in an in-situ transplant experiment. Mar. Environ. Res. 144, 166–177. doi: 10.1016/j.marenvres.2019.01.008
Quiroga M. J. (2018). Determinar el beneficio potencial de la implementación de la ley de bonificación de algas, y las acciones necesarias para su logro, en los beneficiarios de las Regiones de Biobío y Los Lagos. Proyecto FIPA n° 2016-57. Final report (Santiago: Subsecretaria de Pesca y Acuicultura).
Rangel-Buitrago N., Contreras-López M., Martínez C., Williams A. (2018). Can coastal scenery be managed? the Valparaíso Region, Chile as a case study. Ocean. Coast. Manage. 163, 383–400. doi: 10.1016/j.ocecoaman.2018.07.016
Reyers B., Folke C., Moore M. L., Biggs R., Galaz V. (2018). Social-ecological systems insights for navigating the dynamics of the anthropocene. Annu. Rev. Environ. Res. 43, 267–289. doi: 10.1146/annurev-environ-110615-085349
Reynolds L. K., McGlathery K. J., Waycott M. (2012). Genetic diversity enhances restoration success by augmenting ecosystem services. PLoSONE 7 (6), e38397. doi: 10.1371/journal.pone.0038397
Ríos M., Gelcich S. (2017). Los derechos de pesca en Chile: Asimetrías entre armadores artesanales e industriales (Santiago: Center of Applied Ecology and Sustainability, Pontificia Universidad Católica de Chile). Available at: https://www.sociedadpoliticaspublicas.cl/archivos/noveno/Energia_Rios_Monica.pdf.
Romero P., Estévez R. A., Romero P., Gelcich S. (2022). Artisanal fisher association leaders’ estimates of poaching in their exclusive access management areas. Front. Mar. Sci. 8. doi: 10.3389/fmars.2021.796518
Romo H., Ávila M., Candia A. (2001). Manual de técnicas de cultivo y repoblamiento de “luga roja” (Gigartina skottsbergii). Publicación técnica, Proyecto FONDEF D97 I1064, IFOP (Santiago: Universidad de Concepción).
Saavedra S., Henríquez L., Leal P., Galleguillos F., Cook S., Cárcamo F. (2019). Cultivo de macroalgas: Diversificación de la acuicultura de pequeña escala en Chile (Santiago: Instituto de Fomento Pesquero).
Sáez F., Macchiavello J. (2018). Secondary attachment discs: A new alternative for restoring populations of Chondracanthus chamissoi (Gigartinales, Rhodophyta). Lat. Am. J. Aquat. 46, 140–146. doi: 10.3856/vol46-issue1-fulltext-14
Sáez F., Macchiavello J., Fonck E., Bulboa C. (2008). The role of the secondary attachment discs in the vegetative propagation of Chondracanthus chamissoi (Gigartinales: Rhodophyta). Aqua. Bot. 89, 63–65. doi: 10.1016/j.aquabot.2008.01.004
San Martín G., Parma A. M., Orensanz J. L. (2010). “The Chilean experience with territorial use rights in fisheries,” in Handbook of marine fisheries conservation and management. Eds. Grafton Q., Hilborn R., Squires D., Tait M., Williams M. (Mississippi, US: Oxford University Press), 324–337.
Santelices B., Aedo D. (2006). Group recruitment and early survival of Mazzaella laminarioides. J. Appl. Phycol. 18, 583–589. doi: 10.1007/s10811-006-9067-1
Santelices B., Alvarado J., Chianale C., Flores V. (2011). The effects of coalescence on survival and development of Mazzaella laminarioides (Rhodophyta, Gigartinales). J. Appl. Phycol. 23, 395–400. doi: 10.1007/s10811-010-9566-y
Santelices B., Doty M. S. (1989). A review of Gracilaria farming. Aquaculture. 78, 95–133. doi: 10.1016/0044-8486(89)90026-4
Seabra R., Varela R., Santos A. M., Gomez-Gesteira M., Meneghesso C., Wethey D. S., et al. (2019). Reduced nearshore warming associated with eastern boundary upwelling systems. Front. Mar. Sci. 6. doi: 10.3389/fmars.2019.00104
SERNAPESCA (2022). Anuarios estadísticos de pesca y acuicultura (Santiago: Ministerio de Economía, Fomento y Turismo).
Shaughnessy F. J., DeWreede R. E. (2001). Size, survival and the potential for reproduction in transplants of Mazzaella splendens and M. linearis (Rhodophyta). Mar. Ecol. Prog. Ser. 222, 109–118. doi: 10.3354/meps222109
Singh S., Prakash C., Singh M., Mann G. S., Gupta M. K., Singh R., et al. (2019). “Poly-lactic-Acid: Potential material for bio-printing applications,” in Biomanufacturing. Eds. Prakash C., Singh S., Songh R., Ramakrishna S., Sanjeev Puri B. S. P., et al (Cham: Springer). doi: 10.1007/978-3-030-13951-3_3
Smale D. A. (2020). Impacts of ocean warming on kelp forest ecosystems. New. Phytol. 225 (4), 1447–1454. doi: 10.1111/nph.16107
SUBPESCA Comités de manejo. Available at: https://www.subpesca.cl/portal/616/w3-propertyvalue-38010.html (Accessed in June 2022).
SUBPESCA Programa de bonificación al repoblamiento y cultivo de algas. Available at: https://www.subpesca.cl/portal/617/w3-propertyvalue-63108.html (Accessed in June 2022).
SUBPESCA (2017). Resolución exenta: Fija nómina de beneficiarios y de rechazados que indica, en el marco del programa de bonificación para el repoblamiento y cultivo de algas año 2017, subprograma nacional (Santiago: Subsecretaría de Pesca).
SUBPESCA (2018a). Resolución exenta: Fija nómina de beneficiarios que indica, en el marco del programa de bonificación para el repoblamiento y cultivo de algas 2018, subprograma nacional (Santiago: Subsecretaria de Pesca).
SUBPESCA (2018b). Resolución exenta: Fija nómina de beneficiarios que indica, en el marco del programa de bonificación para el repoblamiento y cultivo de algas año 2018, subprograma cultivo suspendido (Santiago: Subsecretaría de Pesca).
SUBPESCA (2018c). Resolución exenta: Fija nómina de beneficiarios que indica, en el marco del programa de bonificación para el repoblamiento y cultivo de algas año 2018, subprograma de repoblamiento (Santiago: Subsecretaría de Pesca).
SUBPESCA (2019). Fija nómina de beneficiarios que indica, en el marco del programa de bonificación al cultivo y repoblamiento de algas año 2019, subprograma cultivo de fondo (Santiago: Subsecretaría de Pesca).
Terawaki T., Yoshikawa K., Yoshida G., Uchimura M., Iseki K. (2003). Ecology and restoration techniques for Sargassum beds in the seto inland Sea, Japan. Mar. Pollut. Bull. 47, 198–201. doi: 10.1016/S0025-326X(03)00054-7
Tiang Y., Liu Y., Qi S., Zhang X. (2017). Early development and life history of Mazzaella japonica (MIkami) hommersand. J. Appl. Phycol. 30, 1933–1941. doi: 10.1007/s10811-017-1356-3
UCN (2018). Evaluación de biomasa y análisis del estado de explotación de las praderas naturales de algas pardas (huiro negro, huiro palo y huiro flotador) en las áreas de libre acceso de la Región de Arica y Parinacota, Atacama y Antofagasta. Proyecto FIPA 2017-52. Final report (Coquimbo: Universidad Católica del Norte).
Vanderklift M. A., Doropoulos C., Gorman D., Leal I., Minne A. J. P., Statton J., et al. (2020). Using propagules to restore coastal marine ecosystems. Front. Mar. Sci. 7. doi: 10.3389/fmars.2020.00724
Vásquez J. A. (2016). “The fishery of brown seaweed in Chile,” in Fisheries and aquaculture in the modern world. Ed. Mikkola H. (Croatia: InTechOpen). doi: 10.5772/62876
Vásquez X., Gutiérrez A., Buschmann A., Flores R., Farías D., Leal P. (2014). Evaluation of repopulation techniques for the giant kelp Macrocystis pyrifera (Laminariales). Bot. Mar. 5, 123–130. doi: 10.1515/bot-2014-0005
Vásquez J. A., Piaget N., Tala F., Vega J. M. A. (2010). Evaluación de la biomasa de praderas naturales y prospección de potenciales lugares de repoblamiento de algas pardas en la costa de XV, I y II regiones. Proyecto FIPA 2008-38. Final report (Coquimbo: Universidad Católica del Norte).
Vásquez J. A., Piaget N., Vega J. M. A. (2012). The Lessonia nigrescens fishery in northern Chile: How you harvest is more important than how much you harvest. J. Appl. Phycol. 24, 417–426. doi: 10.1007/s10811-012-9794-4
Vásquez J. A., Tala F. (1995). Experimental repopulation of Lessonia nigrescens (Phaeophyta, Laminariales) in intertidal areas of northern Chile. J. Appl. Phycol. 7, 347–349. doi: 10.1007/BF00003791
Vega J. M. A., Olivares C., Piaget N., Jorquera L., Núñez I., Díaz M., et al. (2014). Seguimiento biológico pesquero y evaluación económica, como insumo para plan de manejo de la pesquería de algas pardas. III Región 2013-2014. Final report (Santiago: Subsecretaría de Pesca y Acuicultura).
Vega J. M. A., Pérez E., Tapia C., Chávez J. (2021). Contribución al plan de adaptación en pesca y acuicultura, mediante la conservación y uso sustentable de los ecosistemas de algas pardas y estudio de su aporte al carbono azul. Proyecto SdP CHL/SDP/040/2021 –MMA/Subpesca/PNUD. Final report (Santiago: Programa de las Naciones Unidas para el Desarrollo).
Vega J. M. A., Vásquez J. A., Buchmann A. H. (2005). Biología poblacional de huirales submareales de Macrocystis integrifolia y Lessonia trabeculata (Laminariales, Phaeophyceae) en un ecosistema de surgencia del norte de Chile: variabilidad interanual y El niño 1997-1998. Rev. Chil. Hist. Nat. 78, 33–50. doi: 10.4067/S0716-078X2005000100004
Wainwright B. J., Zahn G. L., Afiq-Rosli L., Tanzil J. T., Huang D. (2020). Host age is not a consistent predictor of microbial diversity in the coral Porites lutea. Sci. Rep. 10, 14376. doi: 10.1038/s41598-020-71117-4
Wernberg T., Coleman M. A., Bennett S., Thomsen M. S., Tuya F., Kelaher B. P. (2018). Genetic diversity and kelp forest vulnerability to climatic stress. Sci. Rep. 8, 1851. doi: 10.1038/s41598-018-20009-9
Wernberg T., Krumhansl K., Filbee-Dexter K., Pedersen M. F. (2019). “Status and trends for the world’s kelp forests,” in World seas: An environmental evaluation. Ed. Sheppard C. (London: Academic Press), 57–78. doi: 10.1016/B978-0-12-805052-1.00003-6
Westermeier R. (2013). Repoblamiento de Macrocystis integrifolia en la Región de Atacama. Fase II. Final report (Valdivia: Universidad Austral de Chile).
Westermeier R., Murúa P., Patiño D. J., Müller D. G. (2017). Population biology and long-term mariculture studies in the brown alga Lessonia trabeculata in Atacama, Chile. J. Appl. Phycol. 29 (5), 2267–2275. doi: 10.1007/s10811-016-1019-9
Westermeier R., Murúa P., Patiño D., Muñoz L., Atero C., Müller D. (2014). Repopulation techniques for Macrocystis integrifolia (Phaeophyceae: Laminariales) in Atacama, Chile. J. Appl. Phycol. 26, 511–518. doi: 10.1007/s10811-013-0069-5
Westermeier R., Murúa P., Patiño J., Muñoz L., Müller D. (2016). Holdfast fragmentation of Macrocystis pyrifera (integrifolia morph) and Lessonia berteroana in Atacama (Chile): A novel approach for kelp bed restoration. J. Appl. Phycol. 28, 2969–2977. doi: 10.1007/s10811-016-0827-2
Westermeier R., Murúa P., Patiño D., Muñoz L., Ruiz A., Atero C., et al. (2012b). Utilization of holdfast fragments for vegetative propagation of Macrocystis integrifolia in Atacama, northern Chile. J. Appl. Phycol. 25, 639–642. doi: 10.1007/s10811-012-9898-x
Westermeier R., Patiño D., Murúa P., Muñoz L., Ruíz A., Atero C. (2012a). Repoblamiento de Macrocystis integrifolia en la Región de Atacama. Final report (Valdivia: Universidad Austral de Chile).
Wood G., Marzinelli E. M., Coleman M. A., Campbell A. H., Santini N. S., Kajlich L., et al. (2019). Restoring subtidal marine macrophytes in the anthropocene: trajectories and future-proofing. Mar. Freshw. Res. 70, 936–951. doi: 10.1071/MF18226
Wu P., Li S., Cao G., Wu J., Jin H., Wang C., et al. (2022). Absorption, distribution, metabolism, excretion and toxicity of microplastics in the human body and health implications. J. Hazard. Mater. 437, 129361. doi: 10.1016/j.jhazmat.2022.129361
Keywords: fishers, management, marine conservation, restoration, restocking
Citation: Oyarzo-Miranda C, Otaíza R, Bellorín A, Vega JMA, Tala F, Lagos NA, Oyarzún FX, Estévez RA, Latorre-Padilla N, Mora Tapia AM, Figueroa-Fábrega L, Jara-Yáñez R, Bulboa C and Contreras-Porcia L (2023) Seaweed restocking along the Chilean coast: History, present, and inspiring recommendations for sustainability. Front. Mar. Sci. 9:1062481. doi: 10.3389/fmars.2022.1062481
Received: 06 October 2022; Accepted: 12 December 2022;
Published: 09 January 2023.
Edited by:
Renato A. Quiñones, University of Concepción, ChileReviewed by:
Alejandro H. Buschmann, University of Los Lagos, ChileTrine Bekkby, Norwegian Institute for Water Research (NIVA), Norway
Leonel Pereira, University of Coimbra, Portugal
Copyright © 2023 Oyarzo-Miranda, Otaíza, Bellorín, Vega, Tala, Lagos, Oyarzún, Estévez, Latorre-Padilla, Mora Tapia, Figueroa-Fábrega, Jara-Yáñez, Bulboa and Contreras-Porcia. This is an open-access article distributed under the terms of the Creative Commons Attribution License (CC BY). The use, distribution or reproduction in other forums is permitted, provided the original author(s) and the copyright owner(s) are credited and that the original publication in this journal is cited, in accordance with accepted academic practice. No use, distribution or reproduction is permitted which does not comply with these terms.
*Correspondence: Cristian Bulboa, Y2J1bGJvYUB1bmFiLmNs; Loretto Contreras-Porcia, bG9yZXR0b2NvbnRyZXJhc0B1bmFiLmNs