- Global Coral Reef Alliance, Cambridge, MA, United States
Coral reefs are the fastest collapsing ecosystems from accelerating global warming, sea level, disease, and pollution. Urgent steps are essential to regenerate them, and their biodiversity and environmental services, if there is to be a sustainable “Blue Economy”. Methods that greatly increase settlement, growth, survival and resistance to extremely high temperature and pollutant stress are critically needed to regenerate coral reef and coastal ecosystems now and in the future. Safe Extremely Low Voltage (SELV) electrical fields generated by the Biorock electrolytic reef process have been documented for more than 35 years to greatly increase settlement, growth, healing, and survival of marine organisms in general under extreme stress conditions. The visibly observable benefits of electrical stress relief on marine organisms are summarized, and testable hypotheses are proposed to determine mechanisms and improve applications. Although these field observations strongly imply that electricity in the proper range improves stress resistance and health, the mechanisms have never been studied at cellular, biophysical, biochemical, molecular, or genetic levels. Electrical stimulation is the only method known to reverse the impacts of extreme stresses on corals and other marine organisms. Widespread regeneration of coral reef ecosystems by electrotherapy is urgently needed before they become functionally extinct.
Introduction
Coral stresses from pollution, climate change, and pathogens were negligible before the 1980s (Goreau et al., 1979; Hayes and Goreau, 1998) but have since then killed most corals in the world. Global warming passed the tipping point for mass coral bleaching in the 1980s (Hayes and Goreau, 1991; Goreau and Hayes, 1994; Goreau and Hayes, 2021), but governments failed to respond to the data with effective measures to save coral reefs.
Most studies of coral stress measure negative impacts of high levels of imposed stressors on coral health. This volume contains many state-of-the-art applications of recent advances in biological instrumentation and methods to quantify negative stress impacts. This chapter takes an alternative approach, using natural history observations under beneficial growth conditions instead of harmful ones. The effects of stress are only too well known; they are visible almost everywhere; dead and dying corals are now regarded as “normal” by most reef researchers with less than 50 years of experience. The Goreau coral reef photograph archive, the world’s largest from the 1940s, 1950s, and 1960s, which will be posted on line by the Global Coral Reef Alliance, shows no diseased or dying corals except those being smothered by dredging sediments.
Observations of Biorock electric reefs showed that all visible measures of good coral health are improved by the method (Goreau and Hilbertz, 2005). Nearly 35 years of field observations are systematically summarized here for the first time. The effects of Safe Extremely Low Voltage (SELV) direct current electrical stimulation of corals and all other marine organisms include significantly increased settlement, biodiversity, growth, healing, reproduction, survival, and resistance to lethal extreme environmental stresses. No adverse impacts have been seen. Testable hypotheses are proposed for research to elucidate the biophysical and biochemical mechanisms. The work of authors of other chapters of this volume will prove essential for testing these hypotheses and improving applications for coral reef regeneration.
The Biorock technique of ecosystem regeneration greatly increases coral growth under adverse water quality conditions and protects corals from severe bleaching mortality (Goreau and Hilbertz, 2005; Goreau and Trench, 2012), providing our most powerful tool to save coral reef ecosystems from functional extinction by global warming. Its discovery came from unexpected empirical field observations.
The use of electricity to grow solid limestone structures of any size or shape in the oceans was invented by architect Wolf Hilbertz in 1976 (Hilbertz, 1979), building on Michael Faraday’s original work on electrolysis of sea water (Goreau, 2012a). In 1987 I invited Wolf to Jamaica to collaborate applying the method to coral reef regeneration, and we worked around the globe until his death 20 years later (https://en.wikipedia.org/wiki/Wolf_Hilbertz ). Jamaican corals were being overgrown by harmful algae blooms caused by sewage (Goreau, 1992), so our first goal was to grow structures to revive dying coral populations above the slime smothering them. We quickly found that previously unsuspected biological benefits far outweighed purely physical ones. This chapter summarizes field observations from perhaps around a thousand Biorock reefs built by our students and us in 45 countries over more than four decades in a wide range of sizes, shapes, environments, and purposes. The process works over a wide range of electrical voltages and currents (Hilbertz, 1979) in the Safe Extremely Low Voltage (SELV) range that are insensible to humans, and a very wide range of power sources are used, including solar, wind, wave, ocean current, and conventional sources that span a wide range of conditions. As the electrical current depends on type of power source and size of the reef, most are probably run under sub-optimal conditions.
Low voltage direct current electrical stimulation of marine life is widely misunderstood due to fear of the well-known deadly effects of high voltage alternating currents. Demonstrated benefits of low voltage direct current are widely ridiculed by those who have never seen them and are unwilling to look for themselves. Nevertheless, a wide range of electro-therapy methods have been applied medically to wound healing and nervous system, bone, and muscle regeneration (Tai et al., 2018). These generally use alternating current at much higher voltages than the work described in this chapter, which deals only with Safe Extremely Low Voltage (SELV) direct current electrical fields.
All living organisms, from bacteria and archaea throughout the evolutionary tree of life, use proteins inherited from the last universal common ancestor, perhaps around 4 billion years ago (Weiss et al., 2018). These enzymes transform electrical currents flowing down electrical potential (voltage) differences across biological membranes of around a tenth of a volt to synthesize the universal biochemical energy currencies, ATP and NADP (O’Rourke et al., 2005).
All organisms lose a major part of their energy pumping ions, protons, and electrons back across the membrane to maintain the voltage gradient across it because if this collapses, they quickly run out of energy and die. Very low direct current electrical fields increase ATP, NADP, and protein synthesis in cell cultures up to an optimal voltage, with benefits then decreasing and turning negative at very high currents (Cheng et al., 1982).
Ambient electrical fields used in the Biorock process appear to reduce the energy organisms waste maintaining membrane voltage gradients, providing increased energy for growth, healing, reproduction, and general good health. We hypothesize that the Biorock process directly stimulates biochemical energy availability via increased end-use energy efficiency, providing all marine organisms with energy for superior health and stress resistance (Goreau, 2012b; Goreau, 2014). Despite overwhelming field evidence for its benefits, no studies of the biophysics and biochemistry of the process have yet been done. This chapter summarizes around 35 years of field observations on biological effects of electrical currents, and proposes many testable hypotheses for such future research studies relevant to field applications. A separate chapter by the author on the ecological effects of Biorock reef structures, Biorock electric reefs greatly enhance coral reef structure and function for climate change adaptation, has been submitted to a separate book, Corals - Habitat Formers From the Shallow to the Deep, Dr. Giovanni Chimienti, Editor.
Results: Visible Biological Health Benefits of Biorock
Growth Rates
Greatly accelerated coral growth rates were the first effect noted. On the first Biorock structure we built in Discovery Bay in the 1980s, I attached a few small loose fragments of Porites porites. This species had previously been very abundant but been almost entirely overgrown and killed by weedy algae overgrowth over-fertilized by sewage nutrients (Goreau, 1992). The first small pale fragments, a few centimeters across, grew astonishingly fast and tripled in size in three months, growing onto and over the Biorock structure, with polyp tentacles vigorously feeding and branching (Figures 1A, B). Thus we grew most Jamaican corals several times faster than documented record rates for those species in clean water, but in poor water quality where corals were dying (see photographs in Goreau and Hilbertz, 2012).
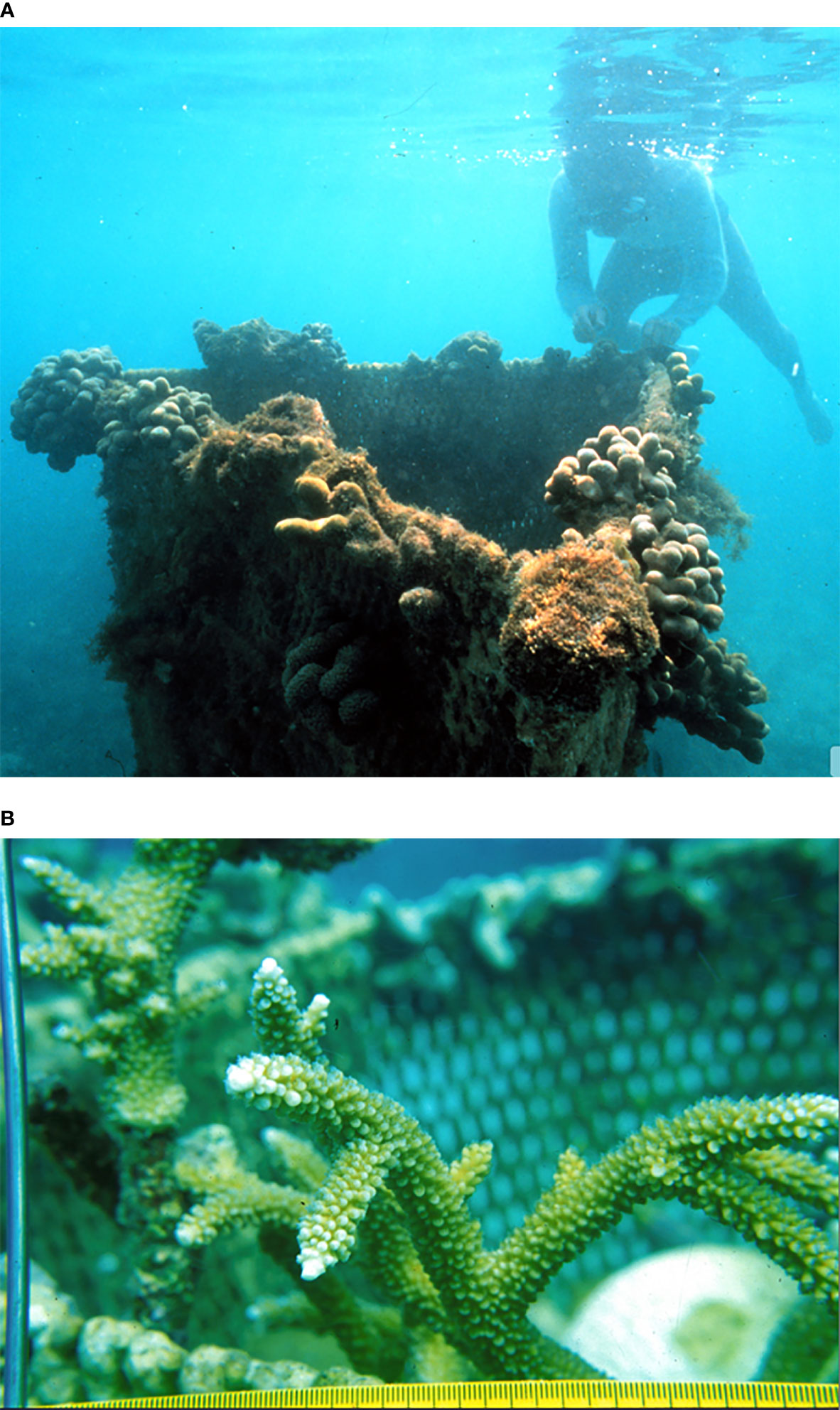
Figure 1 (A) The prolific growth of Porites porites on Biorock. Densely branching Porites porites colonies are interspersed with sand-producing red coralline algae that grew spontaneously (front corner of Biorock reef). Discovery Bay, Jamaica, 1989 photograph by Dr. Peter Goreau. (B) Acropora cervicornis grew 0.8 cm per week in an area of poor water quality. Negril, Jamaica, 1991 photograph by Wolf Hilbertz.
We first thought coral calcification was accelerated by high pH produced by water electrolysis. Although only coral fragments were placed on Biorock structures, all forms of marine life spontaneously settled on and around the Biorock structures, both with and without limestone skeletons. Greatly accelerated growth was seen in hard coral, soft corals, sponges, tunicates, oysters, mussels, giant clams, seagrasses, salt marsh grass, calcareous algae, and every marine animal and plant examined (See chapters in Goreau and Trench, 2012; reviewed in Goreau, 2014).
The growth rate increase compared to controls depends on precise electrical conditions and species. In general, most organisms measured grew 3-5 times faster than controls (Figure 2), but some cases were much higher, up to 6-20 times faster than controls (Figures 3A, B) (Bakti et al., 2012; Goreau, 2012b; Jompa et al., 2012; Munandar et al., 2018; Natasasmita et al., 2016; https://www.globalcoral.org/biorock-corals-grow-faster-longer-and-wider-depending-on-species-and-depth/). All organisms have been seen to benefit, but some proliferate faster than others. The effect is similar to throwing fertilizer on an empty field: the fastest growing weeds quickly dominate unless the lot is carefully weeded to produce roses or tomatoes.
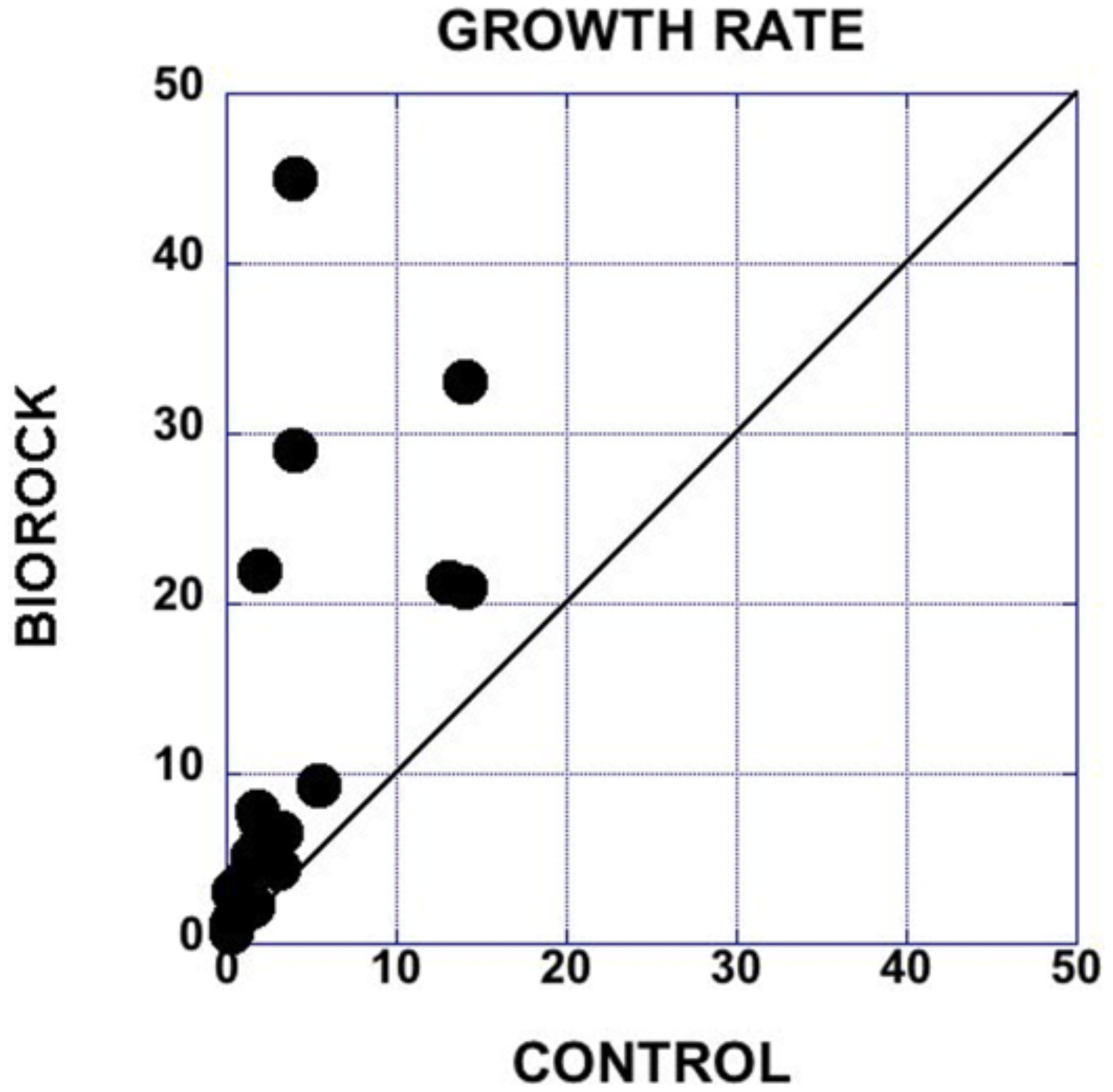
Figure 2 Comparisons in growth rates between various species on Biorock versus controls. Each dot is a different species on a different Biorock reef under different conditions, from Goreau (2014).
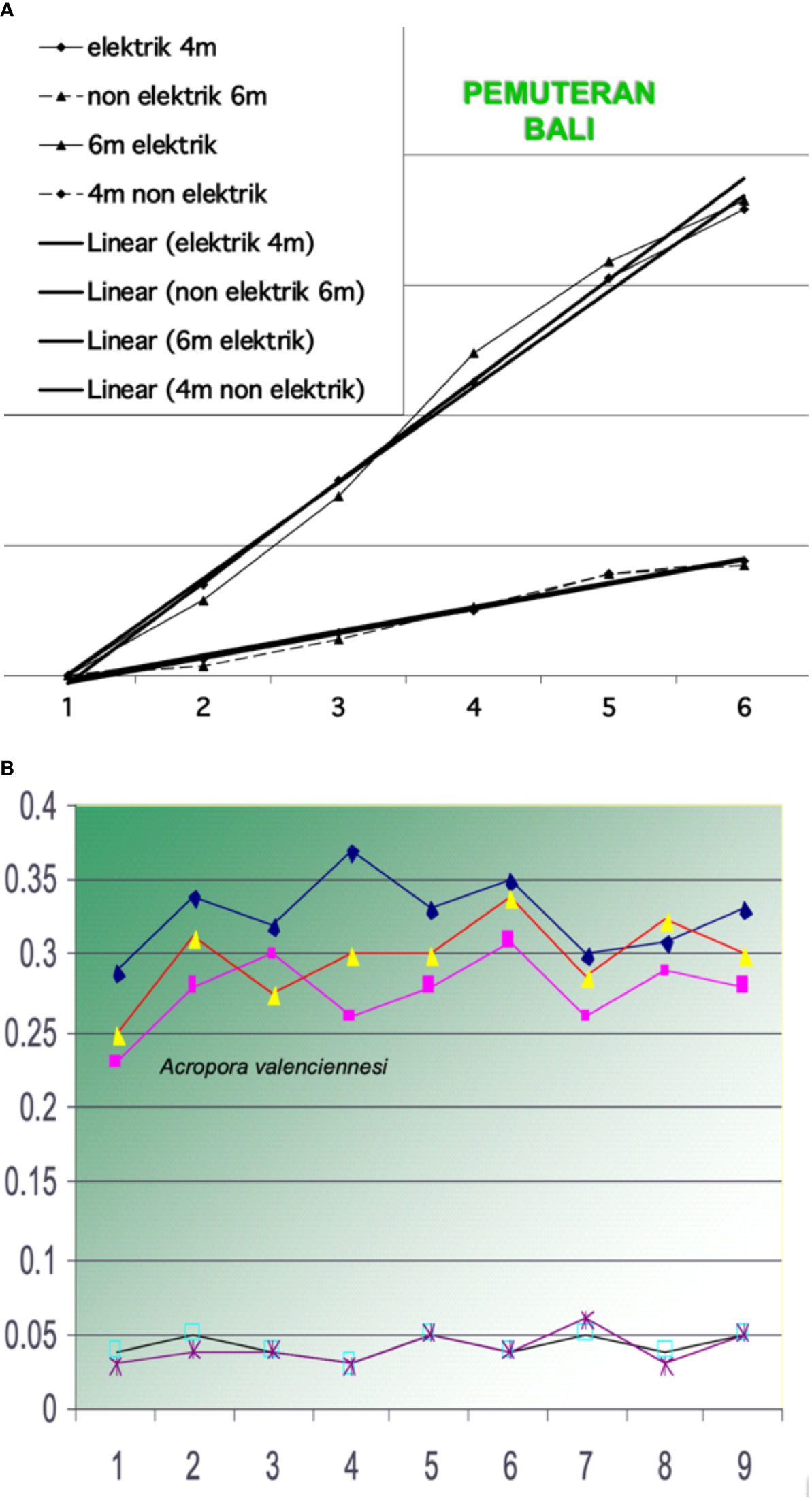
Figure 3 (A) The first measurements of coral growth in Pemuteran, Bali found Biorock corals (top two curves) grew 4.01 times faster than controls (bottom two curves, overlapping). However, it was later realized that this increase was an underestimate because the controls were also in the electrical field even though they were not directly connected to it. Their growth had also been stimulated more than if they had been farther away. The vertical axis is the coral length in centimeters, the horizontal axis is weeks, each data point is the average of 8 corals. Data by Putra Nyoman Dwija, Udayana University, from Jompa et al., 2012. (B) Enhanced growth rate with Biorock (Jompa et al., 2012). Colored symbols on the top three lines are Biorock, x symbols at the bottom are controls. Vertical axis growth rate in centimeters per week, horizontal axis weeks, from Bakti et al., 2012.
The effects of applied electrical currents on rapid settlement and growth of corals and all other forms of sessile reef organisms have long been familiar to commercial divers, who spend most of their time smashing living corals and other prolific growth off oil and gas rigs and other marine structures that are electrically protected from corrosion (Gabriel Despaigne & Paul Sammarco, personal communications). Unfortunately, no scientific papers have ever been written on this, nor a systematic comparison of settlement and growth on electrically-protected structures versus controls.
Healing From Physical Damage
Healing of damaged coral tissues by Biorock electrical fields was immediately noted. Coral fragments transplanted onto Biorock structures had been naturally broken and were almost all in poor condition when rescued. Many corals had been badly damaged from rolling on the bottom or been partially buried in sand and mud, with most tissue dead or rotting. These injured corals were releasing prolific mucus when collected and transported, which continues until they fully heal, typically days or weeks later, but corals recovered fully overnight after being placed in the electrical field and stopped releasing mucus.
The corals shown in the following photographs had been collected as damaged naturally broken fragments late afternoon the previous day in Gili Trawangan, Lombok, Indonesia. They looked terrible when they were placed lying loosely, not attached, on top of a Biorock mesh overnight, prior to transplantation onto Biorock reefs the next morning. Normally broken corals collected from the field like this release mucus from injured tissue for days afterward, a sign of stress (Hayes and Goreau, 1998), and most were slimy with mucus when put on the mesh, but they had no mucus the next morning. Figures 4A, B were taken early the next morning, after one night of exposure to Biorock electrical fields. All the corals that looked so sorry the day before regained vibrant colors overnight and their polyps were fully extended and actively feeding. In addition, orange fan tube worms in coral skeletons were fully expanded (lower right, Figure 4A). The transformation was so incredible we were astonished!
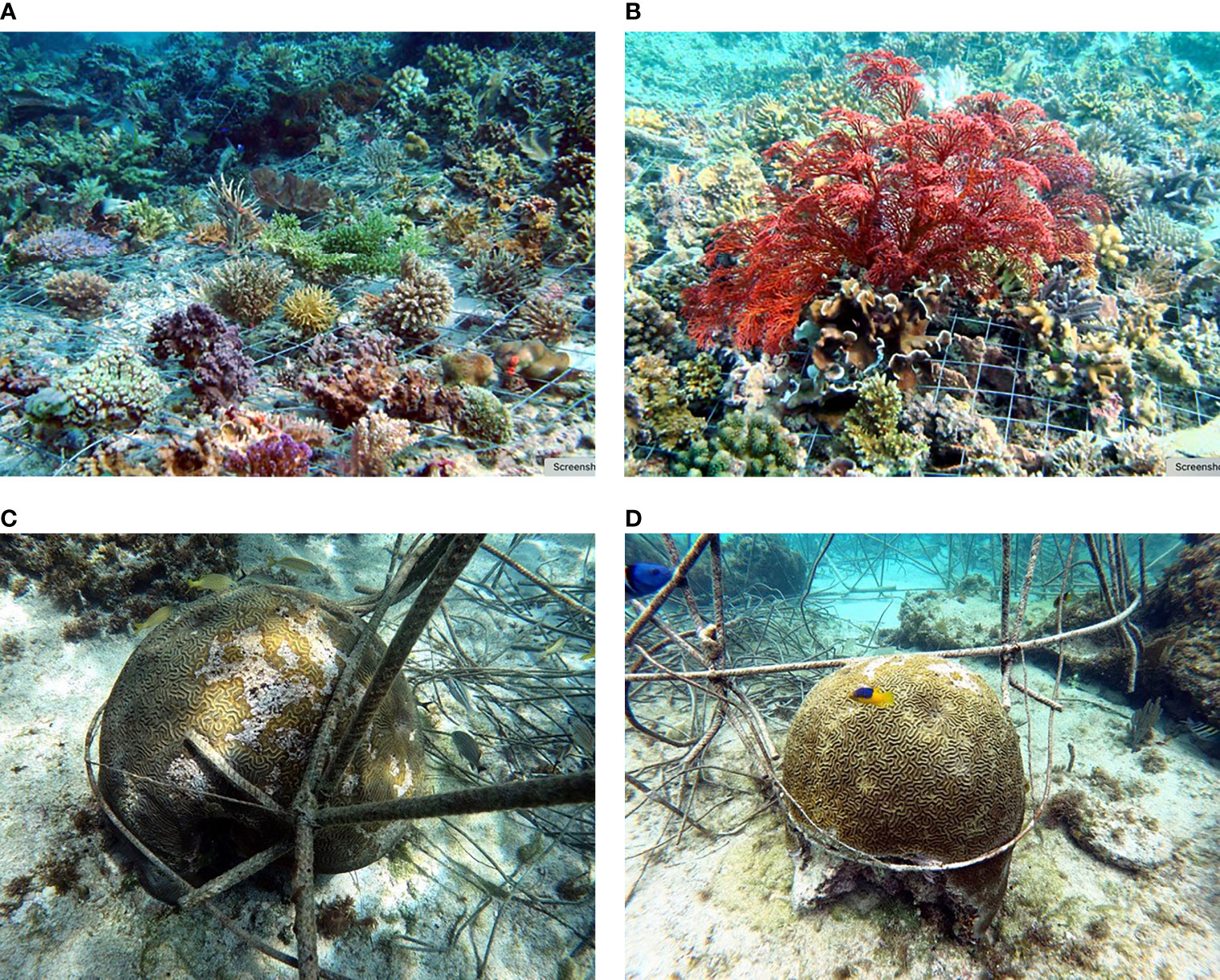
Figure 4 (A) Loose broken hard and soft corals, with bright colors and with polyps fully expanded, after one night of electrical stimulation. Gili Trawangan, Lombok, Indonesia. (B) The red soft coral had been broken across the base by a storm and had been completely limp when placed on the mesh. The next morning it had not only healed the broken stalk; it was fully expanded and feeding. Gili Trawangan, Lombok, Indonesia. (C) February 23 2021, after three months in the electrical field, the damaged areas have healed and are growing back as with dark rims of proliferating new tissue growing over the dead coral skeleton. Before being placed in the electrical field, the tissue next to them had been necrotic and dying back. (D) After eight months, on June 4 2021 this old coral was nearly completely healed from severe injuries. It had not been expected to survive before it was placed in the electrical field. Negril, Jamaica, photographs by Sharren Robinson.
The large Jamaican head coral shown in Figures 4C, D was broken off by hurricane waves in November 2020, and rolled around upside down on rocks and sand in heavy waves for nearly a month before we could rescue it and place it in the Biorock electrical field. By that time most living tissue on top had been scraped off, and the skeleton surface was abraded and damaged. Within months healthy dark yellow-brown new coral tissue grew back over dead areas, and nearly completely healed over the damaged areas (Figures 4C, D). Such healing is now exceptional in Jamaica; severely damaged corals rarely recover like they used to in the old days unless they are in extremely clean water. Now we usually observe damaged coral tissues to be slimy, develop infectious diseases, and die, with tissue necrosis often expanding from damaged areas to kill the entire coral (Goreau et al., 1998).
Settlement
Rapid spontaneous settlement of larval corals and other species was quickly noticed on Biorock reefs (Figure 5). Tiny newly settled juvenile corals, about a millimeter across, were seen on Biorock at densities near one per square centimeter, orders of magnitudes higher than reported in natural coral reefs (Goreau, 2014).
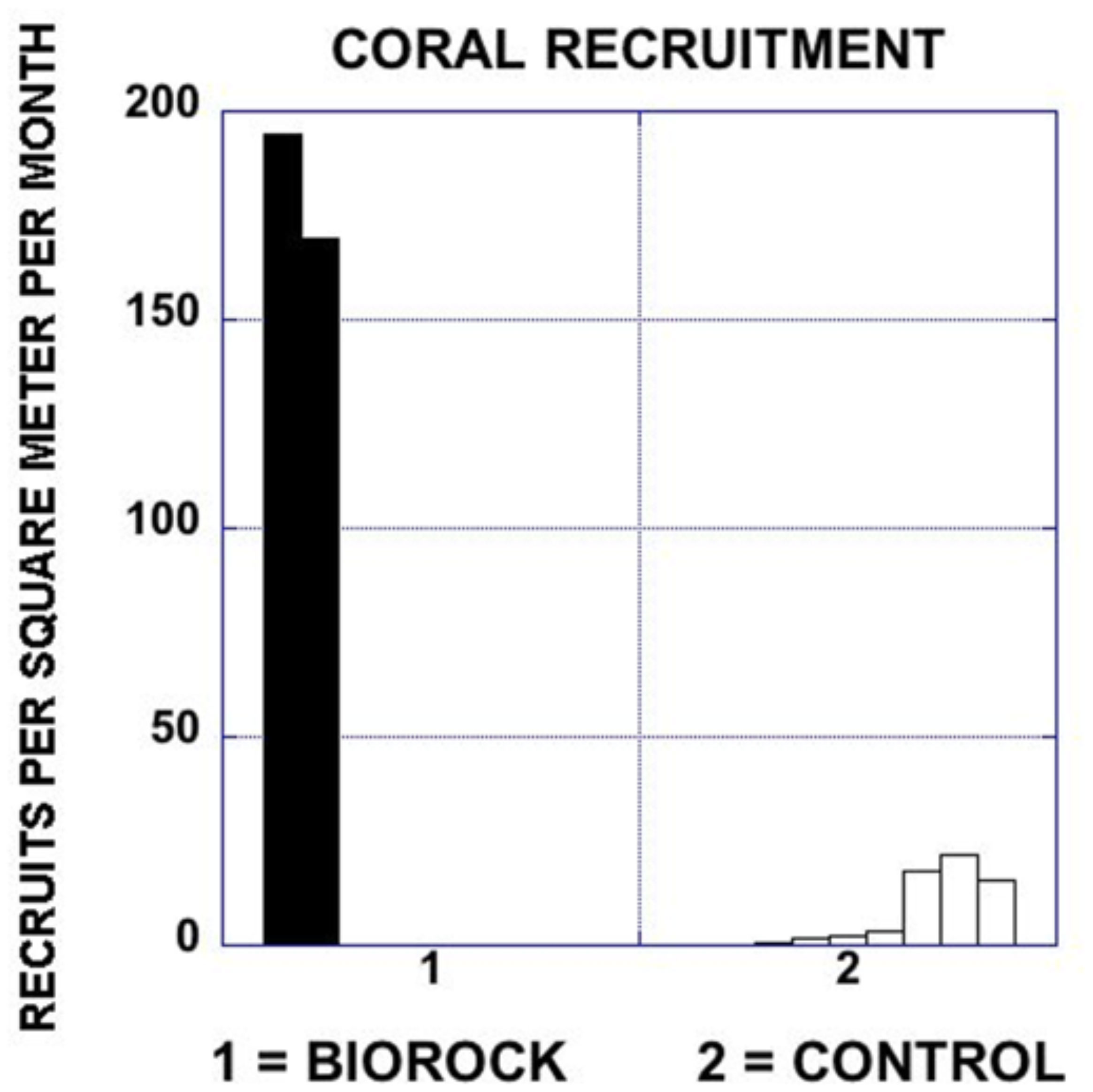
Figure 5 Coral recruitment, from Goreau, 2014.
After Hurricane Gilbert in 1989 I found rusty chicken wire fencing scraps in the bushes, wired them to a Biorock reef, and two years later found hundreds of young Favia fragum and Agaricia agaricites corals had settled all over the biorock coated wire mesh and grown several centimeters across (Figures 6A, B). Unfortunately, this reef had little or no natural coral recruitment for years since dead coral was smothered by weedy fleshy algae (Goreau and Hilbertz, 2012).
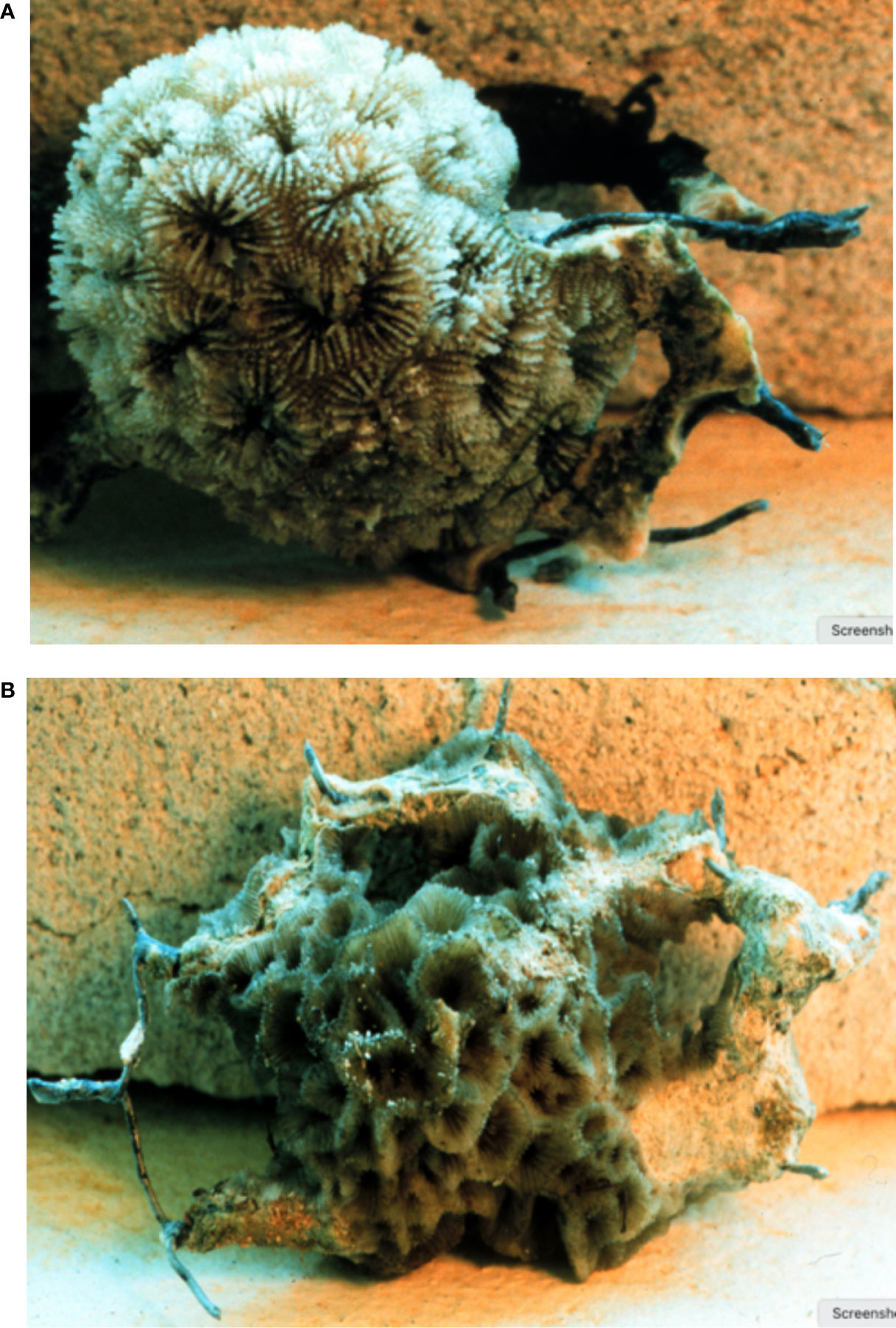
Figure 6 (A, B) Favia fragum (above) and Agaricia agaricites (below) spontaneously settle on one inch chicken wire mesh, about 1-2 years old. Photographs by T. J. F. Goreau, 1990, Discovery Bay, Jamaica.
We have since seen many Biorock structures around the world spontaneously overgrown by corals and other marine organisms such as sponges, tunicates, oysters, mussels, and barnacles, and entire reefs spring up spontaneously all around them (Figures 7, 8), and see video from Indonesia at https://www.globalcoral.org).
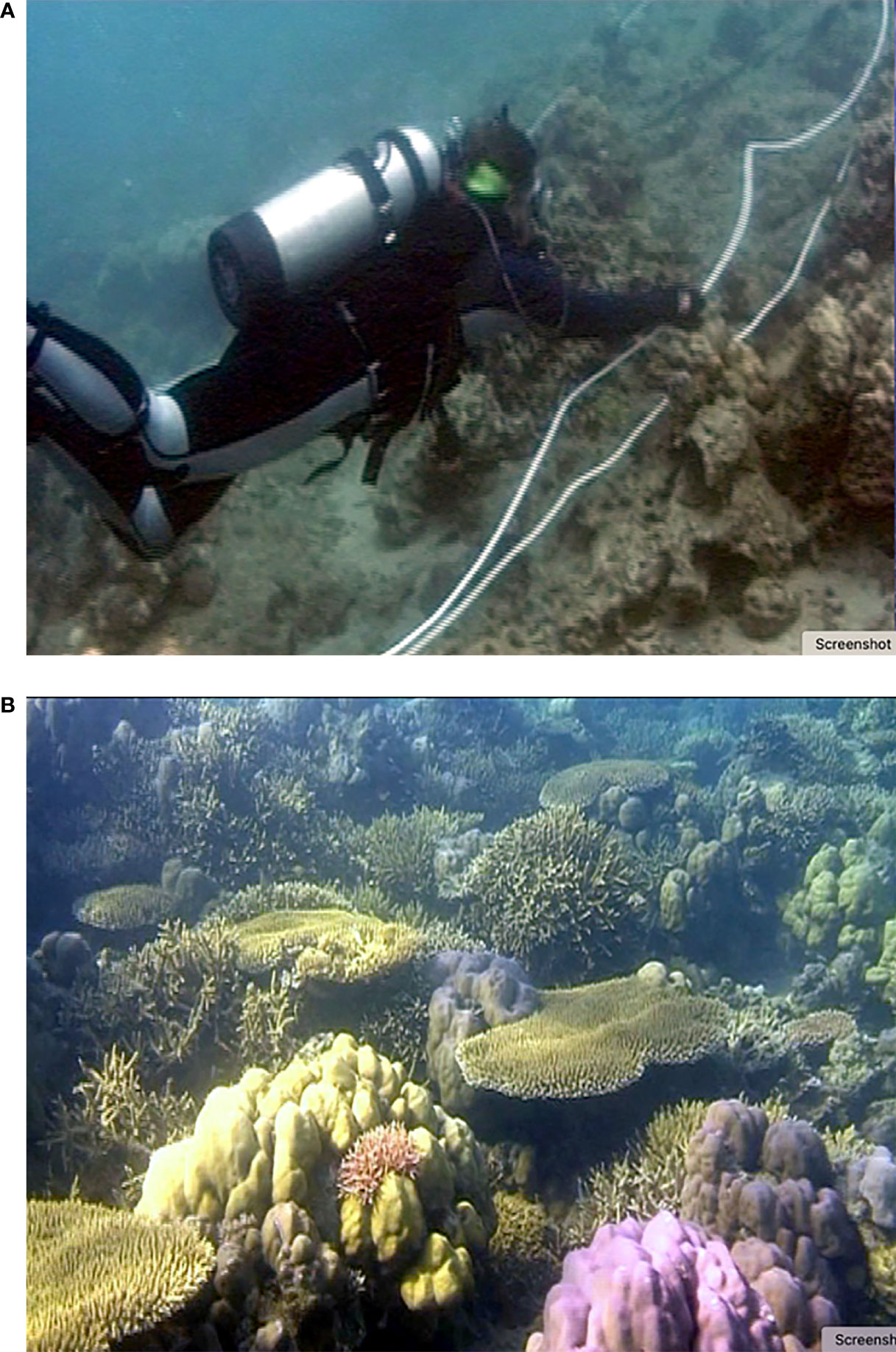
Figure 7 (A) Before Biorock the site looked like a barren moonscape. The project started two years after almost all the corals had bleached and died in 1998. Pemuteran, Bali, Photograph by Rani Morrow-Wuigk. (B) Same site ten years later. Seemingly dead Porites heads recovered, and large amounts of spontaneous Acropora recruitment took place on top of the dead reef corals. The cable is invisible because it was buried under up to a meter of coral growth. Photograph by Rani Morrow-Wuigk.
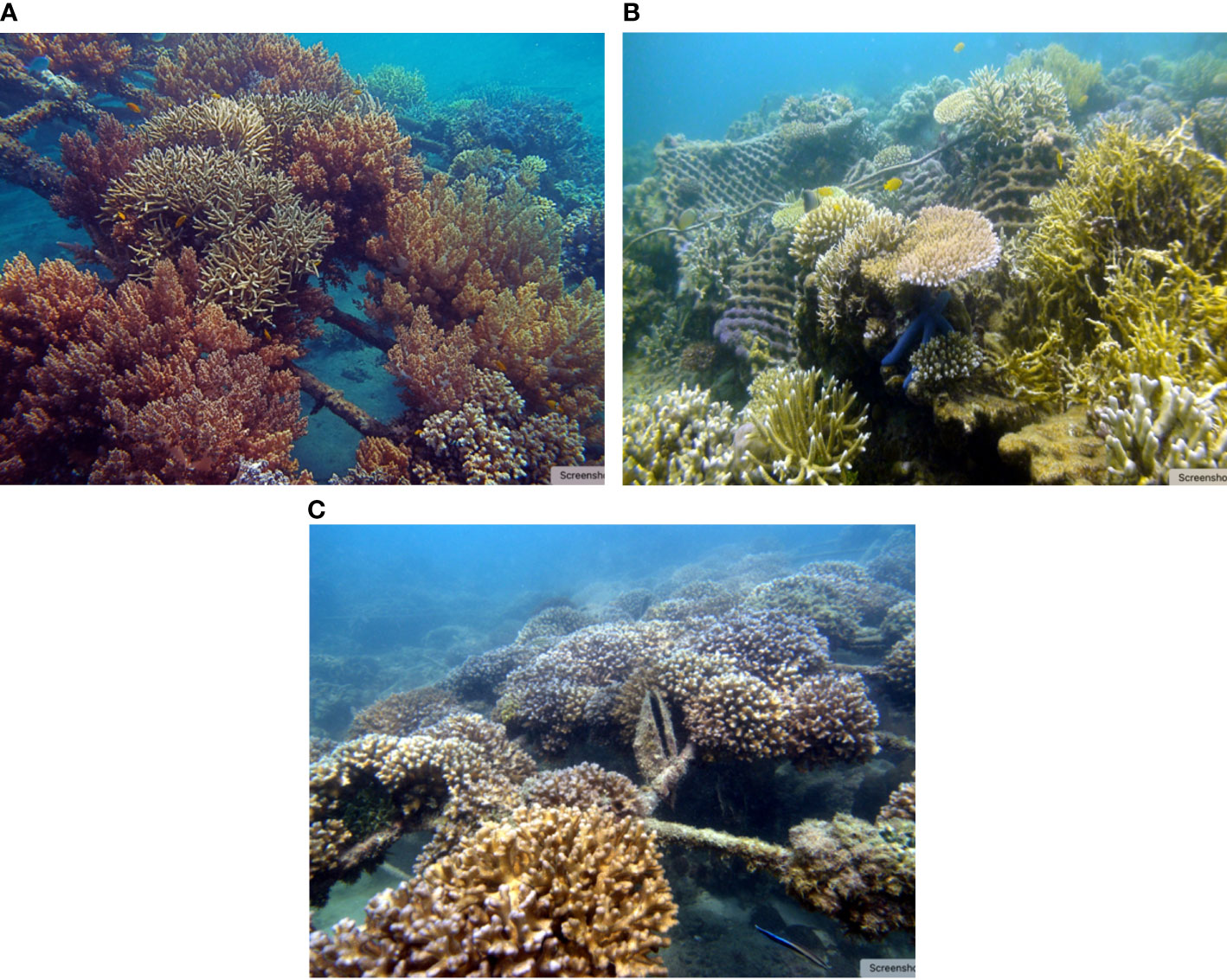
Figure 8 (A) Most hard corals seen here were started from small fragments, but soft corals spontaneously settled over this Biorock reef in Pemuteran, Bali. Photograph by Rani Morrow Wuigk. (B) Corals quickly overgrow Biorock mesh that stabilizes dead coral rubble. Pemuteran, Bali. Many corals settled on the underside of the mesh and grew up through it. Photograph by Wolf Hilbertz. (C) Spontaneous settlement of corals and oysters on a two year old Biorock reef, Ko Samui, Thailand, photograph by Thomas Sarkisian.
Biodiversity
Rapid Biodiversity increase on Biorock structures was noted, giving them the name “Biorock Coral Reef Arks”. Only small, loose, naturally broken coral fragments found on the bottom below the reef were transplanted onto Biorock reefs, but all other components of coral reef biodiversity spontaneously followed. A year old Jamaican Biorock reef about 1.5 square meters in the area had more than a hundred species living on it (Goreau and Hilbertz, 2012). A wide range of sessile organisms, such as corals, settled spontaneously onto the structure as larvae, and mobile marine organisms, such as juvenile reef fishes, crabs, sea urchins, and others migrated to it.
An astonishing local increase in biodiversity has been observed on Biorock reefs on sandy eutrophic rubble devoid of corals and fishes worldwide. In many places the biodiversity of the Biorock reefs is now considerably more remarkable than the reefs had been before, for example the corals and fishes in Pemuteran, Bali (Figures 9A, B). Fish densities and diversities are visibly higher on Biorock than surrounding areas, often by astonishing amounts, but few measurements have been made.
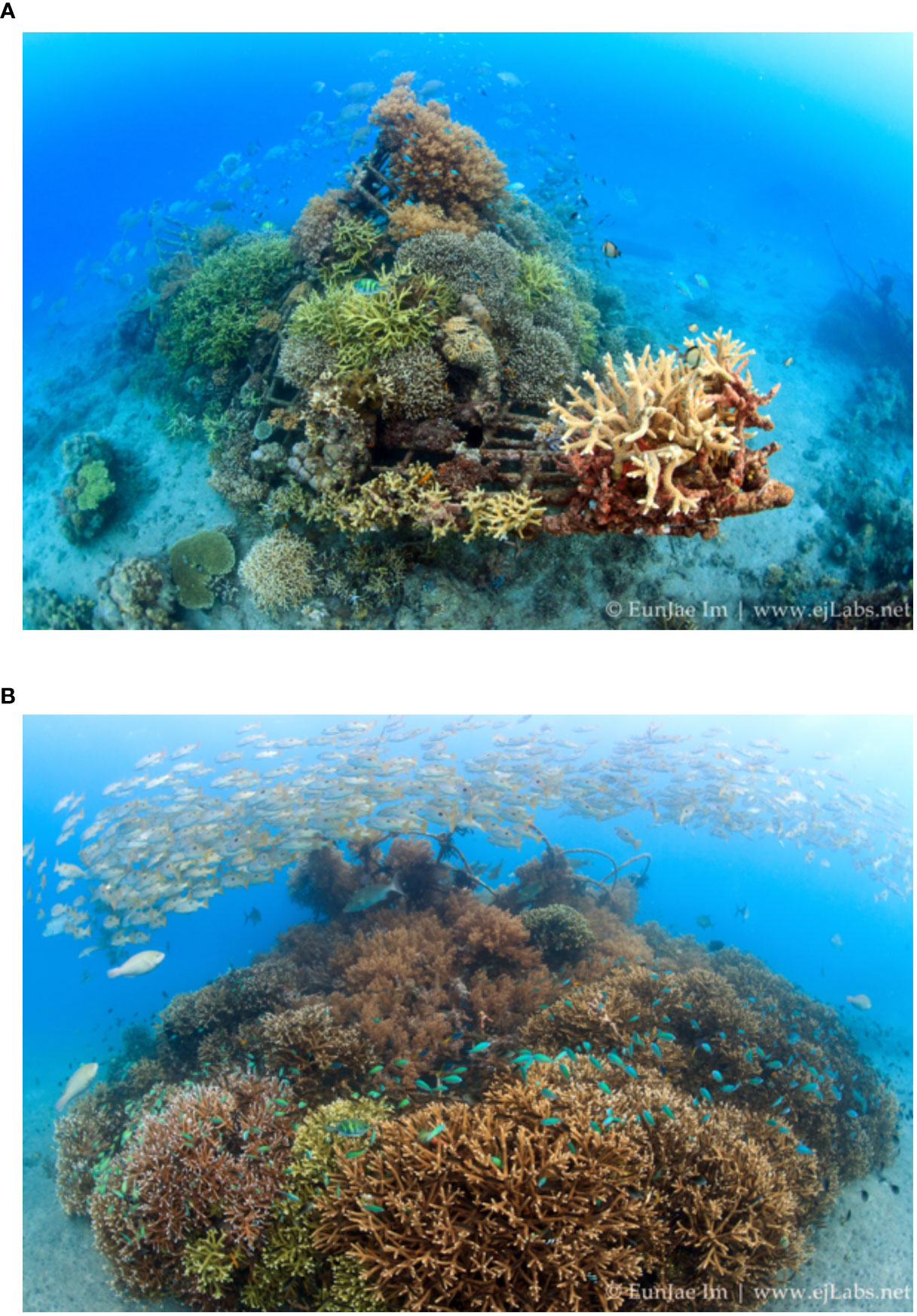
Figure 9 (A) 4 Year old Biorock reef in Pemuteran, Bali. Photograph by Eun Jae Im. (B) High fish diversities and densities are found in four year-old Biorock reefs over sand and rubble previously barren of corals and fishes. Photograph Pemuteran, Bali, by Eun Jae Im.
Different shaped Biorock structures side by side are dominated by different species of fish. The abundance of juvenile reef fish around Biorock reefs also corresponds to electrical currents, decreasing when power is turned off and rapidly increasing when restored. Biorock lobster habitats in Jamaica and Mexico had dozens to hundreds of lobsters crowded into around 2 square meters. This opens the possibilities of sustainable mariculture of entire ecosystems that grow their own food without external input and designed to the specific needs of desired species (Goreau, 2018).
Biodiversity increase in Biorock reefs needs to be measured by environmental DNA (eDNA). This method reveals nearly complete biodiversity of the habitat sampled, not just that which is visible. eDNA measurements reveals large amounts of cryptic biodiversity, identifying species playing a role in the ecosystem that are not observed by short term observers. The new species identified are especially noticeable in Indonesia, the center of marine biodiversity (Gelis et al., 2021; Maduppa et al., 2021). Therefore, Biorock reefs should be compared for eDNA of all taxa in comparison to reefs on either side.
Fluorescence
Biorock corals were noted to have more fluorescence than controls. The corals became brighter while under electrical power, but when power was turned off, they became notably paler over several days, and when power was restored, they visibly brightened within hours (Figure 10A). Observations first made in Jamaica were later confirmed in Maldives, Seychelles, Antigua, and Indonesia. Coral fluorescence is thought to be a measure of coral health, but there is little data.
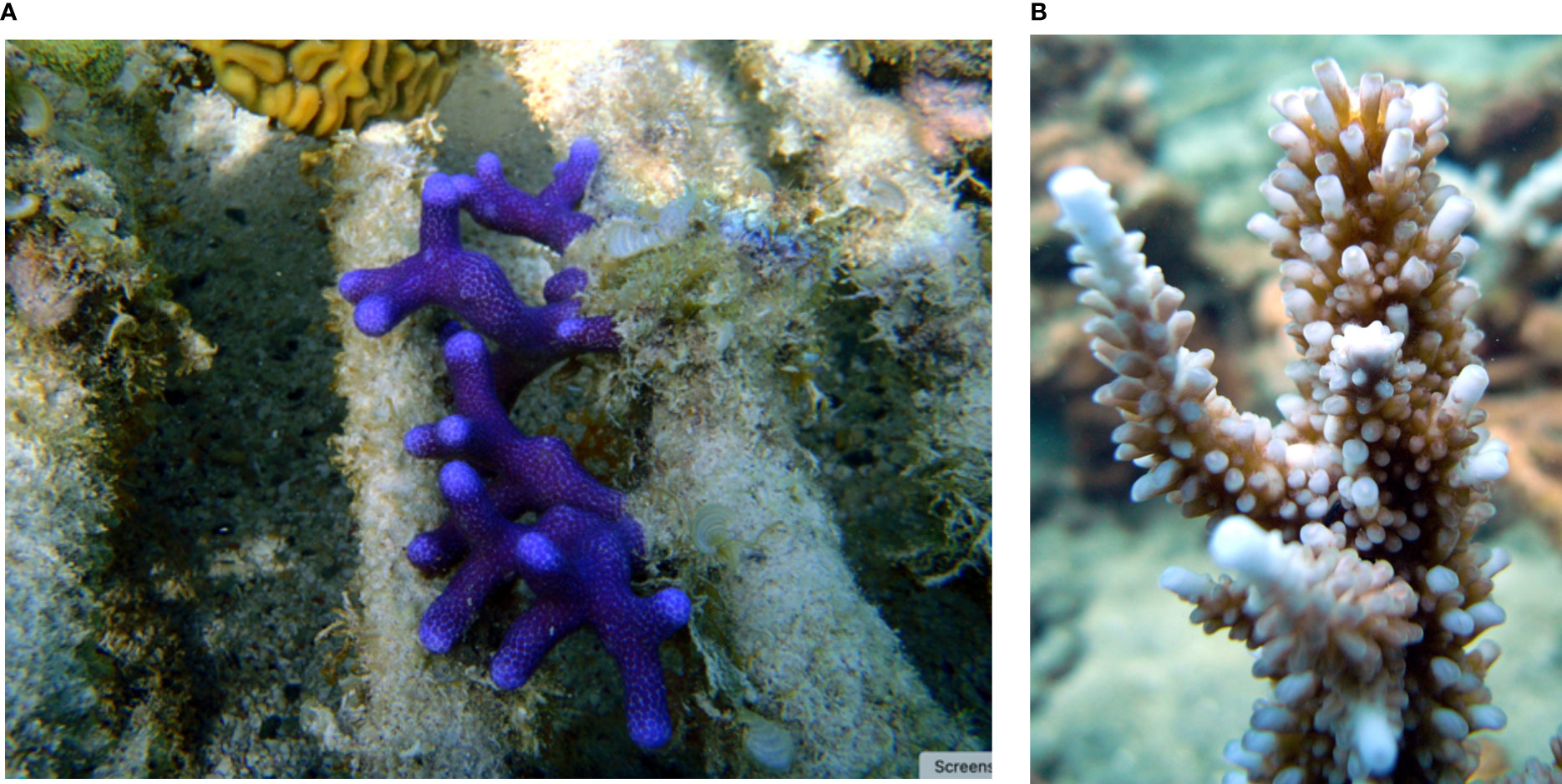
Figure 10 (A) The intense glowing purple color of these Biorock corals fades when power is off for a few days and darkens in hours when power is restored. Antigua, photograph by Martha Watkins Gilkes. (B) Biorock corals have more densely packed and more brightly colored polyp tips than controls. Pemuteran, Bali. Closeup photograph by Wolf Hilbertz.
Budding and Branching
Coral budding on Biorock showed very high polyp densities and dense formation of new growing tips (Figure 10B). Branching patterns were denser and better developed for all coral forms, including branching corals, foliose corals, head corals, and encrusting corals. Corals growing on Biorock show exceptional perfection in shape, with dense branching and bright colors compared to genetically identical corals growing away from the electrical field. This strongly suggests the Biorock process stimulates tissue growth more than calcification, with increased skeletal growth a secondary consequence of improved tissue health.
Survival
Survival of all organisms on Biorock, hard corals, soft corals, oysters, seagrass, salt marsh, etc. has been found to be higher on Biorock than nearby controls in the same habitat, typically 3-5 times greater, but often infinitely higher after extreme stress events when all the controls die and most growing on Biorock survive (Figure 11, from Goreau, 2014).
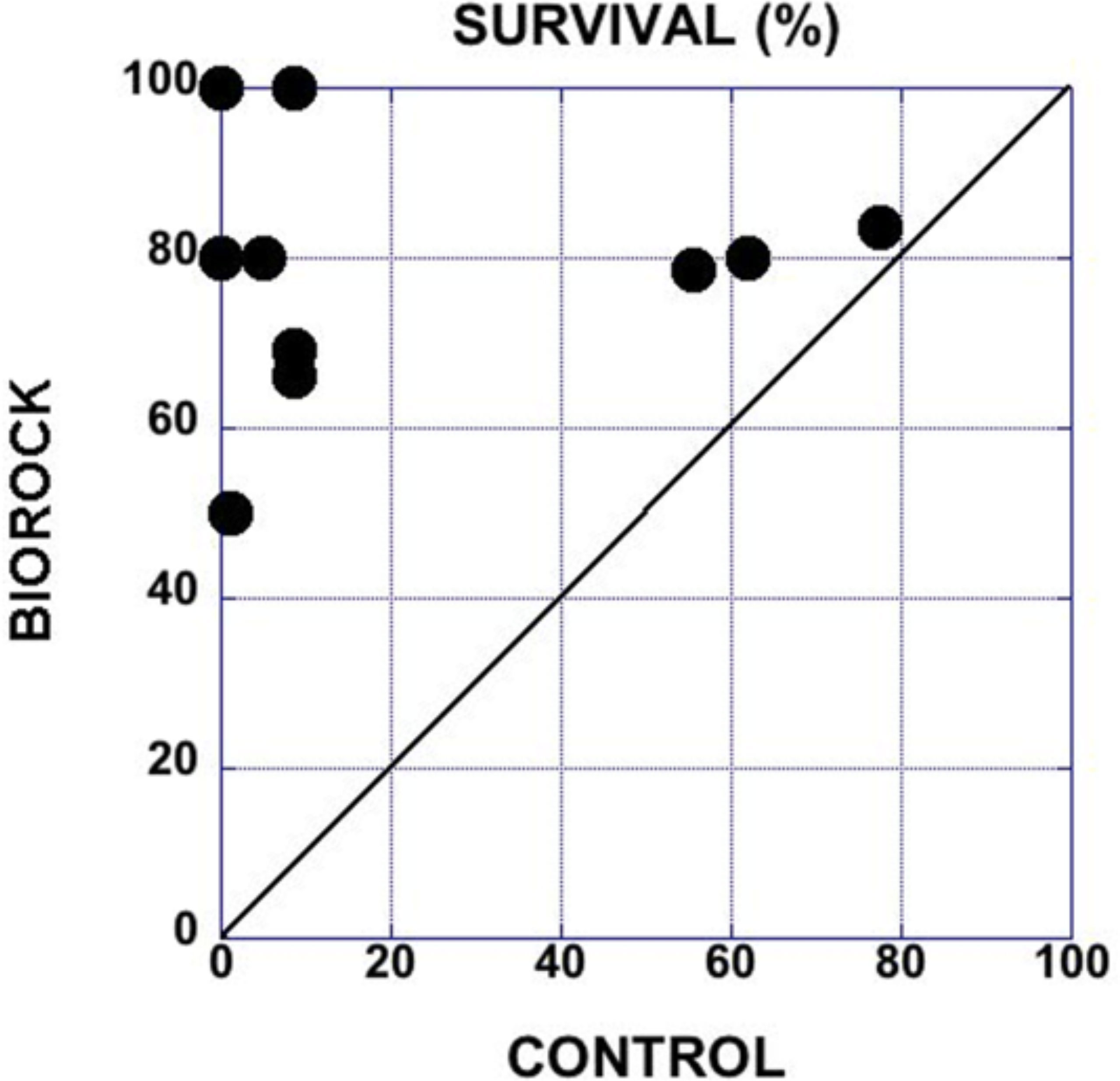
Figure 11 Survival on Biorock versus controls for hard corals, soft corals, oysters, and other marine organisms. Each dot represents comparisons of Biorock versus controls for a different species on a different project under different conditions, from Goreau (2014).
Resistance to Mud and Nutrient Stress
Resistance of corals on Biorock reefs to extremely high mud and nutrient stress was seen in Jamaica, Panama, Dominican Republic, Thailand, Indonesia, the Philippines, Dubai, and India. We have been able to grow mud-intolerant species, such as Acropora cervicornis and Acropora palmata, in places with poor water quality where they normally could not survive. We grew dense coralline sand-producing algae around Biorock reefs, replacing weedy algae that smother hard bottoms and prevent natural regeneration. We grew beautiful, healthy Acropora at the Jumeirah Palm in Dubai in the Persian Gulf. The Biorock corals survived near-opaque turbidity from dredging, but we could not photograph them because the visibility of the water was only 1-2 cm, less than the minimum focusing distance of the camera.
Recovery From Coral Bleaching
Biorock is the only method known to greatly increase coral survival from extreme bleaching events that imminently threaten to wipe out the world’s remaining coral reefs (Goreau and Hayes, 1994; Goreau and Hayes, 2021). Survival of Biorock corals from severe bleaching was first observed during the 1998 Maldives mass bleaching event. I had been filming natural reefs as controls to compare their coral growth to Biorock corals, as well as thousands of coral fragments that had been previously cemented onto concrete and coral blocks by Azeez Hakeem, which had grown well for years. Around 95-99% of the corals on the natural reef died in a few weeks, along with every single one (100%) of the thousands of previously transplanted control corals, while most corals on Biorock survived the extremely high temperatures (Goreau and Hilbertz, 2005). Many surviving corals had been killed on their tops by high temperature and light, but portions of surviving tissue underneath then overgrew the dead coral areas above. These surviving corals may have been a Noah’s Ark that helped reseed Maldivian reefs, which lost most of their reproducing corals so reef recovery had to wait for new recruits from Indonesia during the East monsoon to reach reproductive age (Goreau, 2022). Biorock kept reefs alive with very little coral mortality and complete recovery of fully bleached corals following repeated severe bleaching events that killed 90-99% of corals on nearby reefs in the Maldives, Thailand, and Indonesia. Less bleaching and much faster recovery were noted (Goreau, 2012b, See Figure 12). Photographs and video of Biorock reefs recovered from severe bleaching in Indonesia can be seen at: https://www.globalcoral.org.
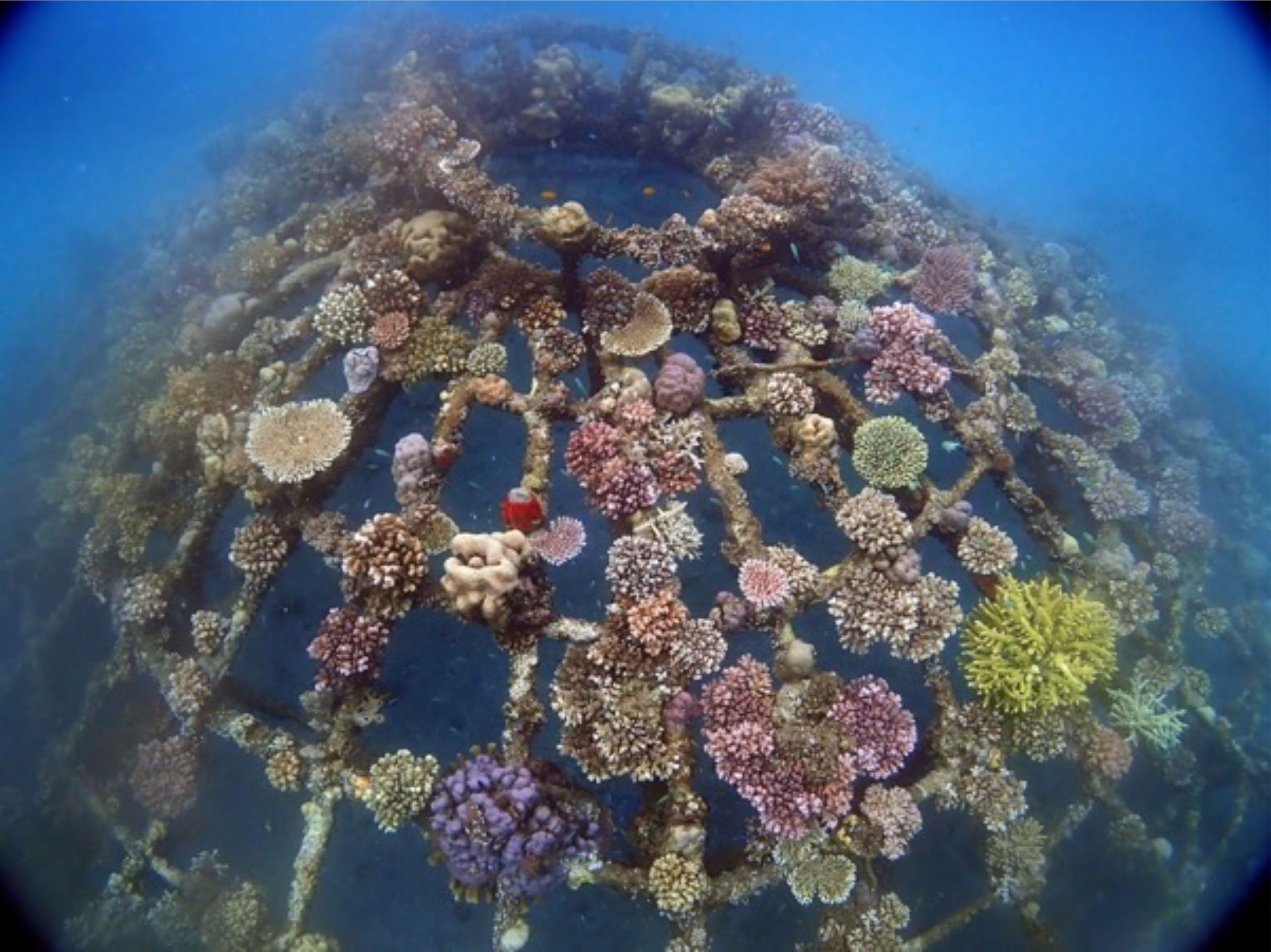
Figure 12 Twenty year-old Biorock reef in Bali, Indonesia that has survived two severe bleaching events. Photographed August 5 2021 by Komang Astika.
Symbiosis
Six different genera of corals were found to have higher symbiotic algae densities on Biorock than controls, along with higher division rates of these algae, but had generally lower chlorophyll per algal cell (Goreau et al., 2004). Reminiscent of light shock adaptation of corals exposed to sudden light increases suppressing algae photosynthesis. This suggests Biorock methods may help experimentally decouple symbiotic interactions and provide insight into their regulation under stress (Goreau, 2021).
Increased Electro-taxis and Cellular Development
At the Woods Hole Marine Biological Laboratory course on marine algae physiology in the 1970s I learned larvae of algae and all marine organisms are electrically polarized. The examples demonstrated were the green alga Ulva, but were claimed to be general for marine organisms. One end of the larva is positively charged, the other negative, and they migrate toward the negative terminal along an imposed electrical field like Biorock electrolysis (electro-taxis). A calcium ion current flows down the central axis of marine larvae, cueing cellular differentiation of the embryo into the head-tail axis. Then a diffuse return electrical current flows back through the water to complete the electrical circuit.
The axial electrical current cues tissue development, segmentation, cell differentiation, and organism development (Whited and Levin, 2019). Responses of cells to mechanical forces are mediated by ATP generated by calcium electrical currents (Fukui et al., 2021). Electro-taxis is known to be important in wound healing; electrical currents in one direction can close wounds, while those in the opposite direction open them. Although electro-taxis is long known to be a universal feature of all marine larvae, both animals and plants, no research appears to have been done for a generation on marine organisms larval electro-taxis. Although Biorock negative terminals show dense settlement of all types of marine organisms, none are attracted to the other terminal, creating a small barren area that is vastly smaller in size. Ecological implications of electro-taxis and Biorock are discussed below.
High densities of zooplankton were noted at night in the electrical field, but were not present further away (Dr. Nathalie Ledee, personal communication), indicating that electro-taxis could also be providing increased food supplies for Biorock filter-feeding organisms. Further studies are needed on the response of zooplankton and marine larvae to electrical fields.
Growth Throughout Dormant Periods
Oysters grown at the New York City Superfund toxic waste site grew about 7.67 times faster in length on Biorock than controls (Shorr et al., 2012); and also in width and height, so 451 times greater in volume, over the summer growth season (Figure 13A). Biorock oysters grew all winter continuously with zero mortality and without any normal winter dormant period. At the same time, 93% of control oysters died over winter, and the 7% surviving control oysters shrank in size due to shell dissolution during winter dormancy (Figure 13B, after data in Shorr et al., 2012). Biorock oyster shells were growing, shiny, and bright instead of chalky and crumbling like control oysters.
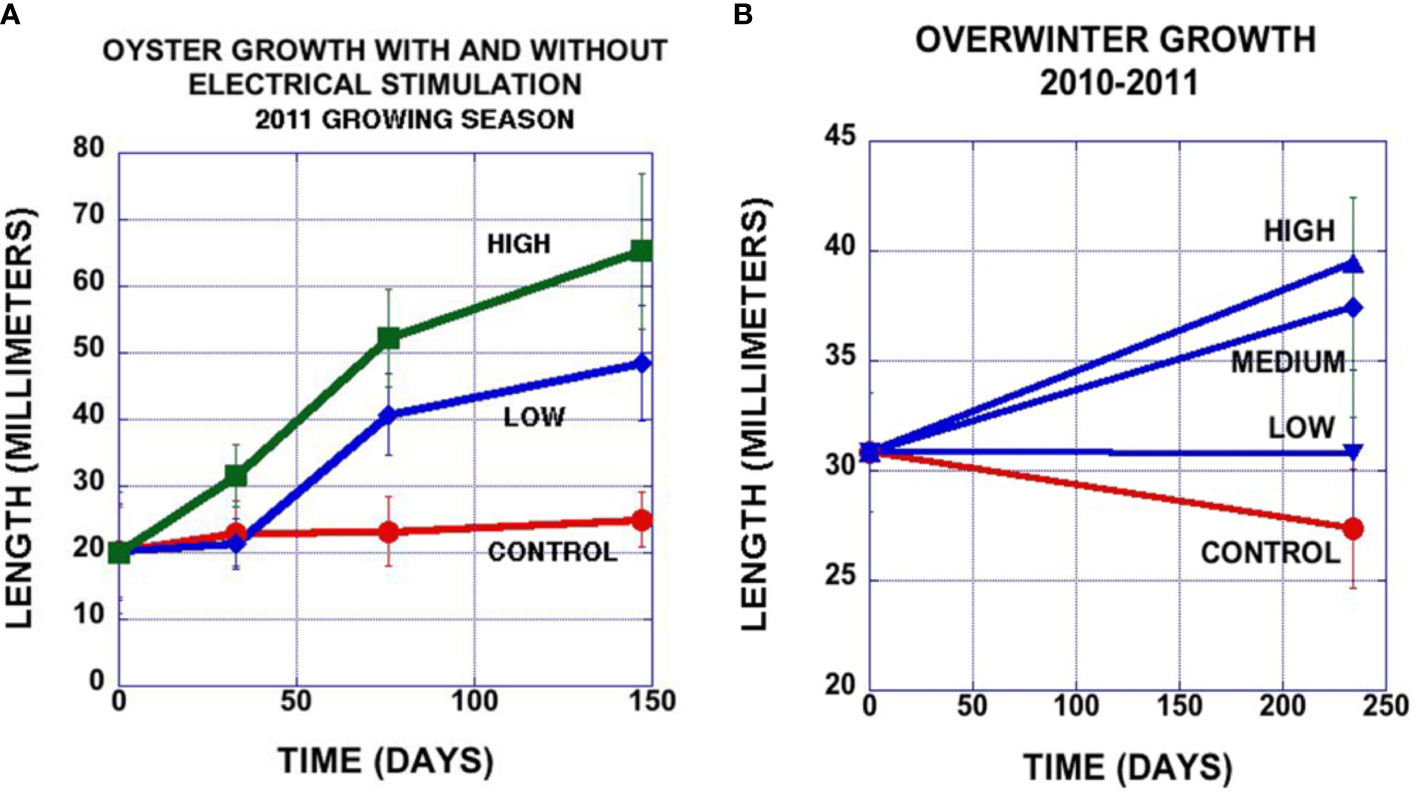
Figure 13 (A) Summer growth of oysters with high, low, and no electricity. Data from Shorr et al., 2012. (B) Growth of oysters over winter with high, medium, low, and no electrical current. All high current Biorock oysters survived and kept growing all winter long. 93% of control oysters died, and the survivors all went dormant and shrank in size as their shells dissolved in cold acidic water. Data from Shorr et al., 2012.
Underground Plant Growth
All species of seagrass have been seen to proliferate around Biorock reefs in Indonesia, Panama, and the Bahamas, as well as sand-producing coralline algae. In Italy, we grew the seagrass Posidonia oceanica on Biorock placed on bare limestone rock. This had been thought impossible because seagrass roots usually require 5-10 centimeters of sand or mud to grow in. However, Biorock sea grass roots grew so prolifically that they anchored themselves to bedrock and were quickly overgrown by mussels and oysters, with rich crab, shrimp, and juvenile fish populations (Figures 14A–C and 15A) (Vaccarella and Goreau, 2012). The controls without electricity all died.
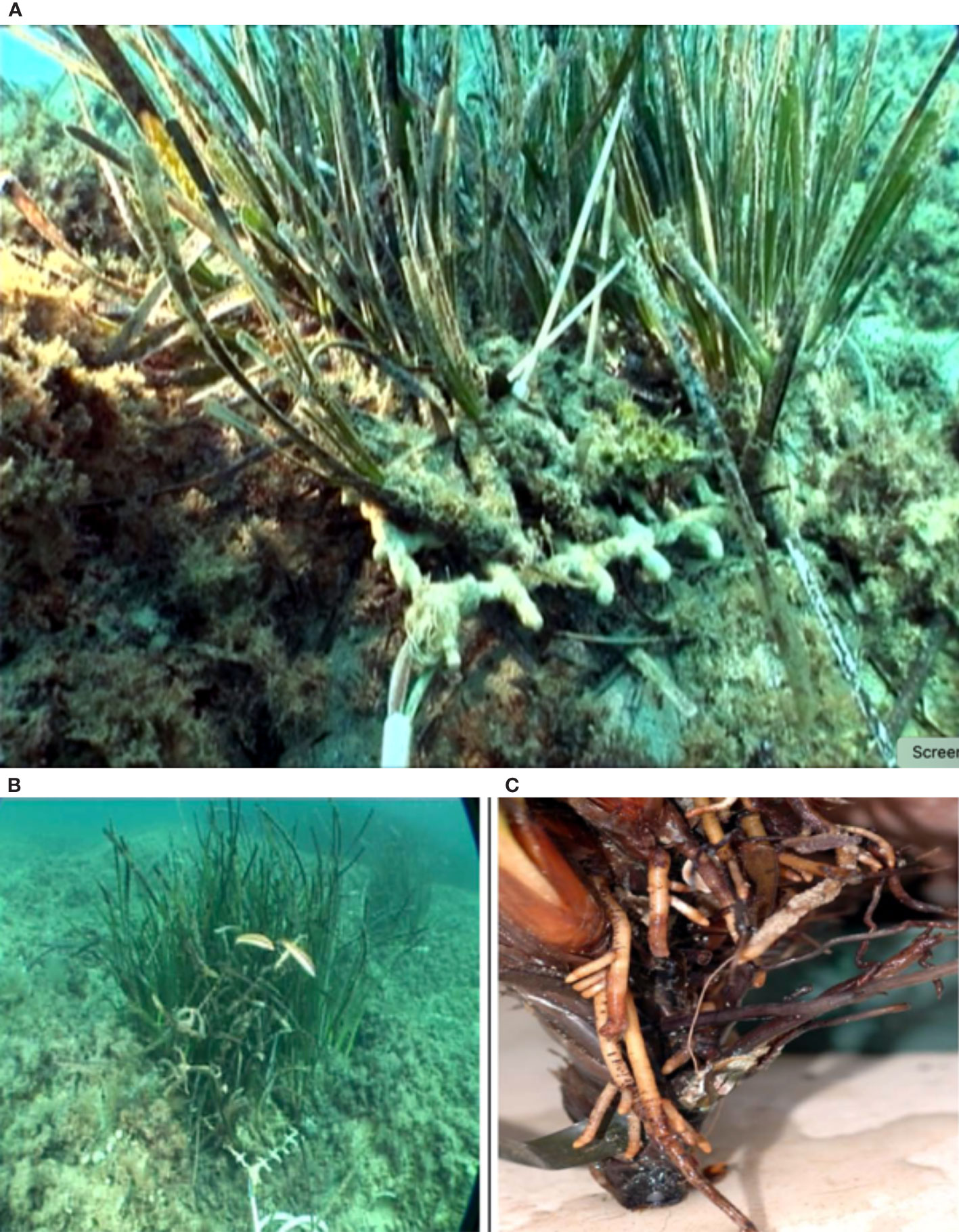
Figure 14 (A–C) Rapid growth of seagrass roots attached to limestone rock and produced dense and diverse sea grass communities. Photographs Torre de Guaceto, Puglia, Italy, Raffaele Vaccarella.
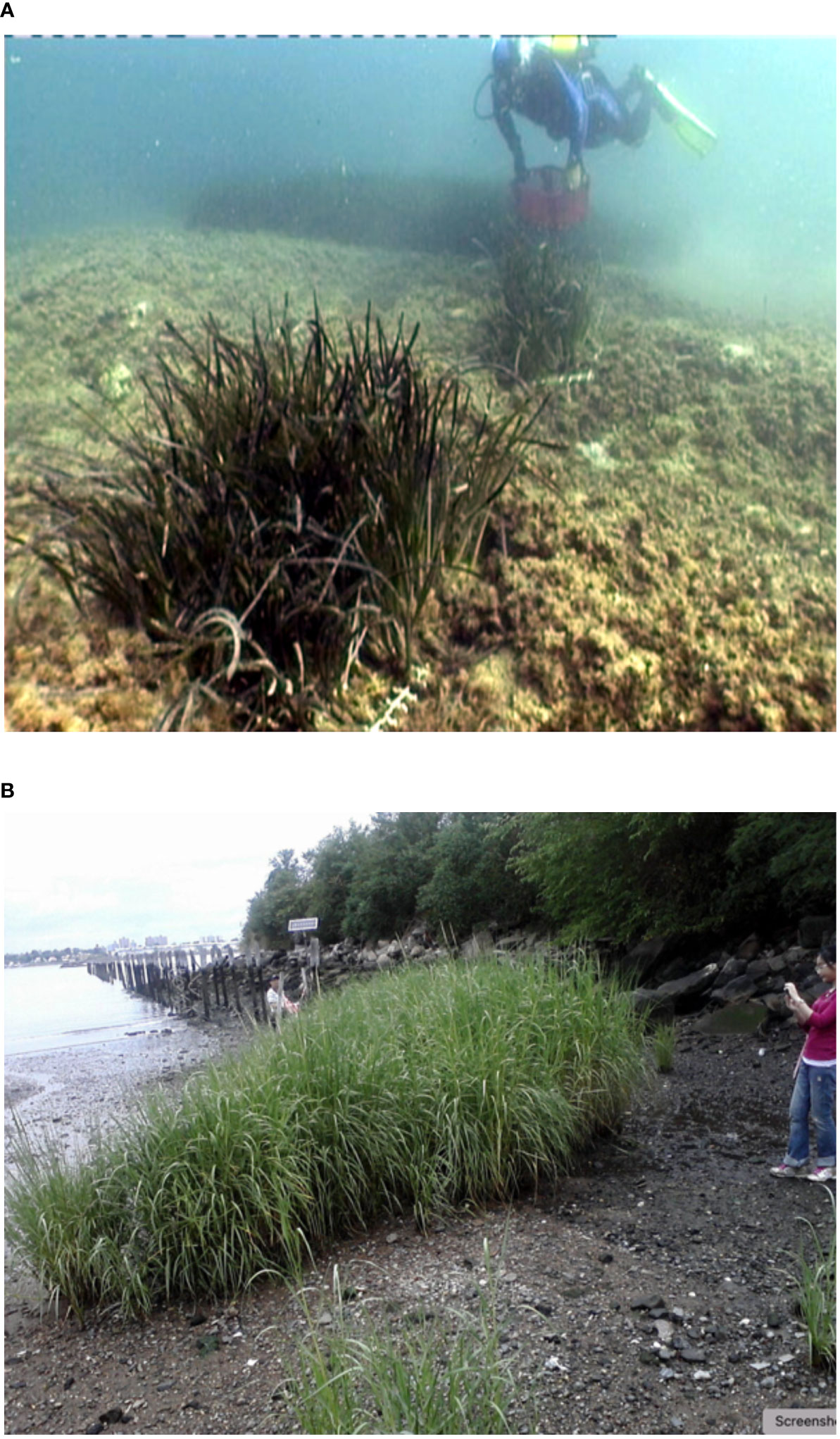
Figure 15 (A) Two patches of Biorock seagrass growth on limestone bedrock in the Adriatic Sea, Italy. Controls all died. Photograph by Raffaele Vaccarella. (B) Saltmarsh grass grows higher and spreads underground, powered by a solar panel. Biorock salt marsh grass at right, controls at left and in front. Biorock salt marsh survives winters and expands seaward, while controls die and wash away. College Point, New York City, photograph by James Cervino.
The salt marsh grass Spartina alterniflora grown with Biorock in construction debris at a New York City Superfund toxic waste site, grew twice as fast in height as controls, and was also darker green, had many more stems per clump (Figure 15B), flowered earlier and more, and attracted denser mussel populations around the roots that raised the height of the bottom by 5-10 centimeters around the plants. Saltmarsh grass was grown with Biorock in water normally too deep to grow without the roots being washed out. As a result the Biorock plants proliferated from only five root clumps to hundreds of plants, springing back more abundantly each spring after winter die-back. Control plantings had poor summer growth, died in winter, and failed to grow back in the spring (Cervino et al., 2012).
Shore Regeneration and Adaptation to Sea-Level Rise
Most large planting projects of seagrass, salt marsh, and mangroves fail because waves washed away plants before their roots can grow. Biorock greatly increases below-ground and above-ground productivity. It is the best hope of extending wave protecting marine plant ecosystems seaward along shores where they are now retreating landward due to global sea-level rise.
Biorock structures and their associated coral, oyster, mussel, and seagrass ecosystems greatly reduce wave energy at the shoreline, regenerating severely eroded beaches at record rates (Goreau and Prong, 2017). Furthermore, applied to seagrass, salt marsh, and mangrove regeneration, they can greatly increase underground root biomass and peat carbon production. The Biorock process itself also creates geochemical conditions leading to increased carbon preservation and storage (see below).
Naturally High Electrical Environments
Biorock electrical signals attract larvae at high levels. Because seawater is an electrical conductor, electrical currents are induced whenever water flows through the Earth’s magnetic field (Xu et al., 2018; Dommermuth, 2020). These natural electrical currents are strongest where waves break on hard reef surfaces. Reef-building organisms seek high wave energy environments. Marine larvae are hypothesized here to use electro-taxis to the electrical fields naturally generated by breaking waves to find high-energy conditions they need. Corals in high wave energy sites are also seen grow faster and are more brightly colored, as if they can tap the electrical field to produce more biochemical energy more than corals in low wave energy sites, another hypothesis needing testing. It is hypothesized that organisms living in very low energy muddy environments, like benthic deposit feeders, might exhibit negative electro-taxis. Experimental tests are required.
Settlement of hard corals, soft corals, tunicates, sponges, and all other reef fauna, including fishes, are very prominent around Biorock structures, typically orders of magnitude higher than further away, creating lush oases of life (video of spectacular settlement and growth on these reefs can be seen at https://www.globalcoral.org). Much more field and laboratory research on electro-taxis and their ecological effects is needed to understand how to use them to most rapidly regenerate biodiversity. However, it is already clear that the largest variety of structural shapes and interior passages give the fastest results.
Reproduction
Several observations suggest the profound effects of Biorock on reproduction of corals and other species although systematic studies on the impact remain to be done. Porites porites on the first Biorock projects in Jamaica in the 1980s quickly showed pink polyp colors associated with coral egg and sperm reproduction on a lunar calendar cycle. Branches of Acropora cervicornis on Biorock projects in Curaçao were observed to release coral planulae by Michael Duss of Biorock Curaçao. There is strong reason to hypothesize that Biorock corals will have much higher reproductive output due to their generally healthier conditions. Still no studies of reproduction of corals on Biorock have yet been made. If corals are found to be more fecund in electrical fields, Biorock Coral Arks will become essential to efforts to maintain reproducing populations and coral genetic diversity despite the extinction emergency from global warming and global sea level rise. Experimental work on coral fecundity on Biorock is badly needed.
Disease
Corals in several Biorock projects in the Caribbean and Indonesia have been affected by deadly coral diseases at times when waves of disease pass through local reefs, so it appears that Biorock does not prevent attack by pathogens that land on corals. However, in several cases, we have noticed virulent coral diseases on Biorock corals that would normally kill the entire colony, suddenly stop spreading, recover, and new coral tissue from the surviving areas to eventually regrow the dead areas, something we now rarely see in the reef. This included White Plague on table Acroporas in Indonesia and the deadly White Plague variant now widely mis-named Stony Coral Tissue Loss Disease on Acropora palmata in Saint Barthelemy (Nathalie Ledee, personal communication). Experimental work is needed to see if Biorock corals are more resistant to disease.
Hurricanes
The first Biorock reefs built in Jamaica in the 1980s have had no power for more than 30 years and have survived every hurricane that has hit since. Biorock reefs survived direct wave impacts on reef crests from Category 5 hurricanes that destroyed almost all the buildings on the land in the Turks and Caicos Islands with little or no coral damage (Wells et al., 2010), and Saint Barthelemy (Nathalie Ledee, personal communication, see images in https://www.youtube.com/watch?v=cv2h8Uv-MEs).
Algae
A fine surface fuzz of brownish-green micro-filamentous algae coating the structures is avidly grazed by fishes, whose bite marks down to limestone indicate the entire crop is harvested and converted into fish biomass. No measurements have yet been made of the importance of this food source. Still the sizes of the herbivorous fish populations indicate it is ecologically and energetically significant for local fisheries’ food chains, and studies are badly needed. If protected from herbivores, Biorock might be able to increase the growth of algae in mariculture (Goreau, 2018).
Weedy fleshy algae have never been a problem on Biorock reefs, even when local benthic harmful algae blooms are caused by seasonal nutrient inputs or eutrophication. This is because of intense grazing by herbivorous fish that move into Biorock reefs. Structures defended from herbivores by damselfish develop fleshy algae populations not seen on Biorock reefs free of damselfish. The vast majority of Biorock reefs have few weedy algae, and damselfish have established themselves in only around 4 or 5 out of more than 150 Biorock structures at Pemuteran. These may be structures too small to build up resident grouper populations to control damselfish.
Instead of weedy fleshy algae, what proliferates around Biorock reefs are sand-producing coralline algae like Halimeda and Amphiroa. Their limestone skeletons noticeably whiten the sediment under and around the structures and have piled up to regenerate an entire beach in Pemuteran (Goreau and Prong, 2017).
Microbiology
The Biorock process creates intensely localized sources of alkalinity and hydrogen at some sites and acidity and oxygen (plus a bit of chlorine) at others, stimulating the growth of microbes with extreme requirements, in particular hydrogen-oxidizing bacteria and nitrogen-fixing bacteria that consume hydrogen and make fixed nitrogen. Microbial nitrogen fixation is the only new source of ecosystem nitrogen from external sources (other than that internally recycled) and plays a key role limiting productivity and biodiversity. The Biorock reefs are hypothesized to be a significant source of new nitrogen driving the food chain. This can be measured using stable isotopes. No isotopic studies have yet been made, nor have any of the Biorock microbial community. Still it is hypothesized that environmental DNA measurements will reveal essential and unexpected components of the microbial flora.
Higher Vertebrate Attractant
Fish of all kinds swarm around Biorock reefs, and higher vertebrates appear to seek them out too. A friendly turtle has been sleeping blissfully on the same Biorock reef in Indonesia every night for around ten years, and really seems to get a charge from it! We have done no Biorock projects in places with marine mammals, so we have no information on their responses.
Humans snorkeling on Biorock reefs are observed smiling blissfully on exiting because of the numbers and diversity of fishes and corals (Figures 12). Entire Indonesian villages and islands live from tourists who come to snorkel in the Biorock reef gardens. Local people are smiling and happy because Biorock charges their economy too: people come from all over the world to their villages to see the underwater beauty they nourish (see figures above).
Shark Attack Preventer
All marine animals seen seem to be attracted except predatory sharks, which seem to avoid Biorock as we never see them! They are inhibited from biting by Biorock because the Biorock electrical field confuses the electrical sense organs used in the final bite: they avoid biting fish they know are in front of their mouth and want to eat (Uchoa et al., 2017). Biorock’s ability to reduce shark-human interaction is a positive benefit for both sharks and humans, making Biorock reefs exceptionally safe for ecotourism. On the other hand, nurse sharks (Panama) and sting rays (Maldives) have freely chosen to sleep in Biorock reefs at night, but they are bottom feeders who don’t use electrical senses like predatory sharks do and are minor threat to humans except through acts of stupidity.
Negative Impacts of Biorock
In contrast to more than 22 different visible biological benefits described above, no adverse biological effects of Biorock have been observed. Negative effects of acidity and chlorine at the positive electrode are limited by design and conditions and are very limited in range: fish swim past it without avoidance, and if the positive electrode is placed directly on living coral, tissue growth is inhibited in the immediate contact zone only but has no effect on coral tissue beyond 1-2 millimeters distance.
Conclusions: Coral Reef Electrotherapy Research and Development Needs
By directly stimulating the health of marine organisms, Biorock provides unique advantages for marine ecosystem service preservation, regeneration, and adaptation to extreme stress events, which will be increasingly needed as global warming, pollution, and storm strength increase. Biorock methods are ideally suited for keeping marine ecosystems alive during lethal stress events from excessive temperatures, mud and nutrient pollution, and hurricanes and for rapidly regenerating entire complex ecosystems in places where there has been no natural recovery.
The work in this paper is based purely on observational natural history, as we had no funding for laboratories or research equipment. Our results were entirely unexpected. Testing the hypotheses raised in this paper with modern research methods will provide valuable insights for marine biology and marine resource management.
Since there has never been funding for Biorock scientific research, laboratories, and equipment from any funding agency we worked for decades in the field with local groups in developing countries to build more than a thousand Biorock reef structures in some 45 countries, each different. There is a tremendous need for systematic experimental research to measure the degree of stimulation of different species to determine their optimal values, which may be different for each species, and how varying power levels can affect competitive outcomes.
Why does Biorock work so well by every single visually observable biological measure of good health? The Biorock process appears to directly stimulate natural membrane electrical potentials and the fundamental mechanism of biochemical energy formation for all forms of marine life, fortifying natural health promoting mechanisms. Unlike conventional mariculture of mono-specific populations, Biorock significantly increases all components of biodiversity, growing complete ecosystems that generate their own food without additional external feeds (Goreau, 2018). Yet despite the profound effects observed for 35 years, there has been no funding for systematic work on how the biophysical effects of low voltage electricity affect biochemical energy and the health of marine organisms. Specific tests of these hypotheses are mentioned here, but the process involves all aspects of science. To build local research capacity where it is most needed, most of this work should be done by students from coral reef countries.
With coral reef ecosystems threatened by extinction from global warming, it is critically urgent that work be done to understand the mechanisms by which Biorock works and how to manage Biorock marine ecosystem regeneration to maximize the natural healing power of electrotherapy. The electricity needed to grow back a hundred meters of severely eroded beach in front of a hotel might be one or two air conditioner’s worth of electricity, a small amount compared to the benefits of a beach, with a living coral reef and healthy fisheries, and could save the hotel itself from hurricanes and sea level rise, and fishing villages from starving.
Electrotherapy is no shocking execution; it accentuates natural energy production with a life-giving, healing electro-trickle charge that organisms respond to visibly as if electro-tickled orgasmically! This book chapter is the first to document 35 years of systematic observations of remarkable large-scale healing properties of low-voltage direct current on marine organisms. Suppose these findings are acted on the scale and urgency needed, in that case they can help rescue dying marine ecosystems and fisheries, reverse the worst impacts of global warming and sea level rise, and create sustainable Blue Economies in now collapsing coastal ecosystems. Governments of rich countries have ignored inconvenient warnings about their energy use impacts and knowingly let corals die for more than 30 years. For poor countries, regenerating reefs is a matter of survival until global warming and global sea-level rise are reversed. Coral reef countries have been the first and worst victims of climate change, sacrificed by fossil fuel-addicted countries’ politically-motivated inaction on global warming. An immediate crash Biorock Coral Ark program is needed if coral reefs are to survive coming years. At time of submission of this chapter a prolonged La Niña is ending and the next El Niño is just starting, which will cause severe bleaching in many places. Coral reef countries urgently need to be prepared to regenerate all the coastal reefs they can by recharging their reefs with Biorock Coral Arks to save their biodiversity, biomass, and ecosystem services.
We strongly urge island nations to make their own decisions to take full advantage of breakthrough endogenous island technologies like Biorock instead of funding mass coral breaking and killing methods of coral reef “restoration” used as foreign scientific tourism and volunteers in place of developing endogenous management capacity of coastal populations. The standard fragmentation methods greatly increase coral tissue stress instead of healing it as Biorock does. Their corals grow only until the first extreme high temperature, mud, pollution, hurricane, or disease event kills them (Foo and Asner, 2020), conditions Biorock reefs can survive. They mean well, but in the long run, they are just killing corals. Biorock is the only method of reef regeneration with a hope of working until CO2 is reduced to safe pre-industrial levels for coral reefs (Goreau, 2014). The fundamental limitation of Biorock is being able to provide direct current at the site, so it can only protect sites where that is cost effective, which will not be the case for remote sites. But if we don’t save all that we can, what will we have left?
Dedication
This paper is dedicated to Wolf Hilbertz, inventor of Biorock, my inspired collaborator for 20 years, and my wise coral teachers: Maurice Yonge, Thomas F. Goreau, Nora I. Goreau, and Robert K. Trench.
Author Contributions
All authors listed have made a substantial, direct, and intellectual contribution to the work and approved it for publication.
Conflict of Interest
The author invented the word Biorock in the 1980s as a better synonym for what had previously been called Mineral Accretion, Seacrete, and Seament. Biorock technology is open source and the copyright on Biorock® is held by Blue Regeneration, a company for which the author is Chief Scientist. As President of the Global Coral Reef Alliance, a non-profitable organization, he received no financial benefit from 35 years of work described in this paper, but wishes he had, because he and the late Wolf Hilbertz exhausted their personal resources (and their families’). Only occasional small donations from kind individuals kept them marching onward through the fog.
Publisher’s Note
All claims expressed in this article are solely those of the authors and do not necessarily represent those of their affiliated organizations, or those of the publisher, the editors and the reviewers. Any product that may be evaluated in this article, or claim that may be made by its manufacturer, is not guaranteed or endorsed by the publisher.
Acknowledgments
I thank all of the hundreds of great people who have worked with us on all the Biorock projects over the decades around the world. You know who you are, and you will be all be listed as partners when we write the chronicle of the projects. Thanks go to constructive suggestions by a reviewer. Special thanks go to all those who preserved these projects with the beautiful images shown, they are individually identified in the captions with their photographs.
References
Bakti L. A. A., Virgota A., Damayanti L. P. A., Radiman T. H. U., Retnowulan A., Sabil A., et al. (2012). “Biorock Reef Restoration in Gili Trawangan, North Lombok, Indonesia,” in Innovative Methods of Marine Ecosystem Restoration. Eds. Goreau T. J., Trench R. K. (Boca Raton: CRC Press), 59–80. doi: 10.1201/b14314-7
Cervino J., Gjoza D., Lin C., Weeks R., Goreau T. J. (2012). “Electrical Fields Increase Salt Marsh Survival and Growth and Speed Restoration in Adverse Conditions,” in Innovative Technologies for Marine Ecosystem Restoration. Eds. Goreau T. J., Trench R. K. (Boca Raton: CRC Press).
Cheng N., Van Hoof H., Bockx E., Hoogmartens M. J., Mulier J. C., De Ducker F. J., et al. (1982). The Effects of Electric Currents on ATP Generation, Protein Synthesis, and Membrane Transport in Rat Skin. Clin. Orthopaedics Related Res. 171, 264–272. doi: 10.1097/00003086-198211000-00045
Dommermuth D. G. (2020). “Magnetic Induction Due to the Effects of Breaking Ocean Waves,” in Breaking Wave Analysis Technical Report. (San Diego) vol. 11, 1–7.
Foo S. A., Asner G. P. (2020). Sea Surface Temperature in Coral Reef Restoration Outcomes. Environ. Res. Lett. 15, 074045. doi: 10.1088/1748-9326/ab7dfa
Fukui H., Chow R. W. Y., Xie J., Foo Y., Yap C. H., Minc N., et al. (2021). Bioelectric Signaling, and the Control of Cardiac Cell Identity in Response to Mechanical Forces. Science 374, 351–354. doi: 10.1126/science.abc6229
Gelis E. R. E., Kamal M. M., Subhan B., Bachtiar I., Sani L. M. I., Madduppa H. (2021). Environmental Biomonitoring of Reef Fish Community Structure With eDNA Metabarcoding in the Coral Triangle. Environ. Biol. Fish 104, 887–903. doi: 10.1007/s10641-021-01118-3
Goreau T. J. (1992). Bleaching and Reef Community Change in Jamaica: 1951-1991. In Symposium On Long Term Dynamics Of Coral Reefs. Am. Zoologist 32, 683–695. doi: 10.1093/icb/32.6.683
Goreau T. J. (2012a). “Marine Electrolysis for Building Materials and Environmental Restoration,” in Electrolysis. Eds. Kleperis J., Linkov V. (Rijeka, Croatia: InTech Publishing), 273–290.
Goreau T. J. (2012b). “Marine Ecosystem Electrotherapy: Practice and Theory,” in Innovative Technologies for Marine Ecosystem Restoration. Eds. Goreau T. J., Trench R. K. (Boca Raton: CRC Press).
Goreau T. J. (2014). Electrical Stimulation Greatly Increases Settlement, Growth, Survival, and Stress Resistance of Marine Organisms. Natural Resources 5, 527–537. doi: 10.4236/nr.2014.510048
Goreau T. (2014). “Global Biogeochemical Restoration to Stabilize CO2 at Safe Levels in Time to Avoid Severe Climate Change Impacts to Earth’s Life Support Systems: Implications for the United Nations Framework Convention on Climate Change,” in Geotherapy: Innovative Technologies for Soil Fertility Restoration, Carbon Sequestration, and Reversing Atmospheric CO2 Increase. Eds. Goreau T. J., Larson R. G., Campe J. A. (Boca Raton: CRC Press).
Goreau T. J. F. (2018). “Biorock Technology: A Novel Tool for Large-Scale Whole-Ecosystem Sustainable Mariculture Using Direct Biophysical Stimulation of Marine Organism’s Biochemical Energy Metabolism,” in Proceedings of the International Summit on Fisheries and Aquaculture (Amsterdam: Scientific Federation), 24.
Goreau T. J. F. (2021). “Robert Kent Trench: In Memoriam,” in Global Coral Reef Alliance (Cambridge, MA). Available at: https://www.globalcoral.org/robert-kent-trench-in-memoriam-2/.
Goreau T. J. F. (2022). “Maldives Biorock: Past Results and Future Applications,” in Global Coral Reef Alliance White Paper (Cambridge, MA). Available at: https://www.globalcoral.org/maldives-biorock-past-results-future-applications/.
Goreau T. J., Cervino J., Goreau M., Hayes R., Hayes M., Richardson L., et al. (1998). Rapid Spread of Diseases in Caribbean Coral Reefs. Rev. Biol. Tropical 46 Supl5, 157–171.
Goreau T., Cervino J., Polina R. (2004). Increased Zooxanthellae Numbers and Mitotic Index in Electrically Stimulated Corals. Symbiosis 37, 107–120.
Goreau T. F., Goreau N. I., Goreau T. J. (1979). Corals and Coral Reefs. Sci. American 241, 124–136. doi: 10.1038/scientificamerican0879-124
Goreau T. J. F., Hayes R. L. (2021). Global Warming Triggers Coral Reef Bleaching Tipping Point. Ambio 50, 1137–1140. doi: 10.1007/s13280-021-01512-2
Goreau T. J., Hilbertz W. (2005). Marine Ecosystem Restoration: Costs and Benefits for Coral Reefs. World Resource Rev. 17, 375–409.
Goreau T. J., Hilbertz W. (2012). “Reef Restoration Using Seawater Electrolysis in Jamaica,” in Innovative Technologies for Marine Ecosystem Restoration. Eds. Goreau T. J., Trench R. K. (Boca Raton: CRC Press).
Goreau T. J. F., Prong P. (2017). Biorock Reefs Grow Back Severely Eroded Beaches in Months. In T. Wahl, J. E. O. Nilsen, I. Haigh, & S. Brown (Eds.). P. 243-263 Coastal Sea Levels, Impacts, and Adaptation. J. Mar. Sci. Eng. 5 (4), 48. doi: 10.3390/jmse5040048
Goreau T. J., Trench R. K. (Eds.) (2012). Innovative Technologies for Marine Ecosystem Restoration (Boca Raton: CRC Press).
Hayes R. L., Goreau T. J. (1991). The Tropical Coral Reef Ecosystem as a Harbinger of Global Warming. Proc 2nd International Conference on Global Warming. World Resour. Rev. 3, 306–322.
Hayes R. L., Goreau N. I. (1998). The Significance of Emerging Diseases in the Tropical Coral Reef Ecosystem. Rev. Biol. Trop. 46 Supp5, 173–185.
Hilbertz W. H. (1979). Electrodeposition of Minerals in Sea Water: Experiments and Applications. IEEE J. Oceanic Eng. OE4, 94–113. doi: 10.1109/JOE.1979.1145428
Jompa J., Suharto J., Anpusyahnur E. M., Dwija P. N., Subagio J., Alimin I., et al. (2012). “Electrically Stimulated Corals in Indonesia Reef Restoration Projects Show Greatly Accelerated Growth Rates,” in Innovative Methods of Marine Ecosystem Restoration. Eds. Goreau T. J., Trench R. K. (Boca Raton: CRC Press), 47–58. doi: 10.1201/b14314-6
Maduppa H., Cahyani N. K. D., Anggoro A. W., Subhan B., Jefri E., Sani L. M. I., et al. (2021). eDNA Metabarcoding Illuminates Species Diversity and Composition of Three Phyla (Chordata, Mollusca and Echinodermata) Across Indonesian Coral Reefs. Biodiversity Conserv. 30, 3087–3114. doi: 10.1007/s10531-021-02237-0
Munandar M., Rizal M., Rani C., Faizal A. (2018). The Escalation of Coral Growth by Biorock Technology Applied in Sabang Marine Ecotourism. AACL Bioflux 11 (5), 1633–1647.
Natasasmita D., Wijayanti D. P., Suryono C. A. (2016). The Effects of Electrical Voltage Differences and Initial Fragment Size on Growth Performance and Survival Rate of Coral Acropora Cerealis in Biorock Method. J. Aquaculture Marine Biol. 4 (4), 00086. doi: 10.15406/jamb.2016.04.00086
O’Rourke B., Cortassa S., Aon M. A. (2005). Mitochondrial Ion Channels: Gatekeepers of Life and Death. Physiology 20, 303–315. doi: 10.1152/physiol.00020.2005
Shorr J., Cervino J., Lin C., Weeks R., Goreau T. J. (2012). “Electrical Stimulation Increases Oyster Growth and Survival in Restoration ProjectsIn,” in Innovative Technologies for Marine Ecosystem Restoration. Eds. Goreau T. J., Trench R. K. (Boca Raton: CRC Press).
Tai G., Tai M., Zhao M. (2018). Electrically Stimulated Cell Migration and its Contribution to Wound Healing. Burns Trauma 6, 20. doi: 10.1186/s41038-018-0123-2
Uchoa M. P., O’Connell C. C., Goreau T. J. (2017). The Effects of Biorock-Associated Electric Fields on the Caribbean Reef Shark (Carcharhinus Perezi) and the Bull Shark (Carcharhinus Leucas). Anim. Biol. 67, 191–208. doi: 10.1163/15707563-00002531
Vaccarella R., Goreau T. J. (2012). “Restoration of Seagrass Mats (Posidonia Oceanica) With Electrical Stimulation,” in Innovative Technologies for Marine Ecosystem Restoration. Eds. Goreau T. J., Trench R. K. (Boca Raton: CRC Press).
Weiss M. C., Preiner M., Xavier J. C., Zimorski V., Martin W. F. (2018). The Last Universal Common Ancestor Between Ancient Earth Chemistry and the Onset of Genetics. PloS Genet. 14, e1007518. doi: 10.1371/journal.pgen.1007518
Wells L., Perez F., Hibbert M., Clervaux L., Johnson J., Goreau T. (2010). Effect of Severe Hurricanes on Biorock Coral Reef Restoration Projects in Grand Turk, Turks and Caicos Islands. Rev. Biol. Tropical 58, 141–149.
Whited J. L., Levin M. (2019). Bioelectrical Controls on Morphogenesis: From Ancient Mechanisms of Cell Coordination to Biomedical Opportunities. Curr. Opin. Genet. Dev. 57, 61–69. doi: 10.1016/j.gde.2019.06.014
Keywords: coral reefs, electricity, healing, growth, stimulation, regeneration
Citation: Goreau TJF (2022) Coral Reef Electrotherapy: Field Observations. Front. Mar. Sci. 9:805113. doi: 10.3389/fmars.2022.805113
Received: 29 October 2021; Accepted: 31 March 2022;
Published: 12 May 2022.
Edited by:
Ranjeet Bhagooli, University of Mauritius, MauritiusReviewed by:
Ricardo Tapilatu, Ricardo F. Tapilatu, IndonesiaMunasik Munasik, Diponegoro University, Indonesia
Copyright © 2022 Goreau. This is an open-access article distributed under the terms of the Creative Commons Attribution License (CC BY). The use, distribution or reproduction in other forums is permitted, provided the original author(s) and the copyright owner(s) are credited and that the original publication in this journal is cited, in accordance with accepted academic practice. No use, distribution or reproduction is permitted which does not comply with these terms.
*Correspondence: Thomas J. F. Goreau, Z29yZWF1QGdsb2JhbGNvcmFsLm9yZw==