- 1Institute of Marine Research, Bergen, Norway
- 2Bjerknes Centre for Climate Research, Bergen, Norway
- 3Faroe Marine Research Institute, Tórshavn, Faroe Islands
- 4Marine and Freshwater Research Institute, Hafnarfjörður, Iceland
The ocean climate of the southern Norwegian Sea - the Norwegian Basin - is largely set by the relative amount of Atlantic Water in the eastern and Arctic Water in the western region. Here we utilized hydrographic data from repeated sections, together with annually gridded survey data of the upper 1000 m, to resolve the main hydrographic changes over the period 1995-2019. Based on integrated heat -and freshwater content, we divide into three periods. The first period 1995-2005, denoted Arctic, is characterized by relative fresh and cold Atlantic Water overlaying Arctic Intermediate Water that basically covers the whole Norwegian Basin. Differently, the conditions during the period 2006-2016, denoted Atlantic, are warmer and more saline, and the extent and thickness of Arctic Intermediate Water is greatly reduced. During the most recent period denoted Fresh, 2017-2019, there has been a major freshening of the Atlantic waters, the layer of Arctic Intermediate Water has not recovered, but instead a layer of warmer but relative fresh Arctic Water has expanded. We find that increased abundance of the Arctic zooplankton Calanus hyperboreus in the southern and eastern Norwegian Basin coincides with increased extent of Arctic Water. We also note that the overall mesozooplankton biomass in the Norwegian Basin is significantly higher during periods of relative high amount of Arctic Water. Furthermore, we show that both nitrate and silicate winter (pre-bloom) concentrations are significantly higher in the Arctic Water compared to Atlantic Water, and that there is a reduction in nutrients from the Arctic period compared subsequent Atlantic and Fresh periods. Since these nutrients can be interpreted as the potential for new production, changes in the influx of western Arctic waters are expected to have a bottom-up effect on the Norwegian Sea. Hence, this study indicates that the amount of Arctic waters and their concentration of nutrients and zooplankton are more important for the Norwegian Basin ecosystem functioning rather than the temperature of the Atlantic waters.
Introduction
The southern Norwegian Sea - the Norwegian Basin - is a confluence area of warm and saline Atlantic Water from the south and relative fresher and colder Arctic waters from the west (Helland-Hansen and Nansen, 1909; Figure 1). Over the last 60 years, there have been marked changes in the hydrographic conditions in the Norwegian Basin. During the 1960s to the 1990s, the Norwegian Basin experienced a cooling and freshening mainly because of increased supply of Arctic Water from the East Icelandic Current (EIC, Blindheim et al., 2000). The Norwegian Sea started to warm and become more saline from the mid-1990s due to advection of warmer inflowing Atlantic Water (Skagseth and Mork, 2012), associated with circulation changes in the North Atlantic (Häkkinen and Rhines, 2004; Hátún et al., 2005). The warming continued until mid-2000s when the Norwegian Basin remained in a relatively warm and saline state (e.g., Mork et al., 2019). During the recent years, a major freshening of the Norwegian Basin has been reported (Mork et al., 2019; Holliday et al., 2020). In this paper we investigate these major hydrographic changes and discuss some potential ecosystem effects in the Norwegian Basin over the period 1995-2019.
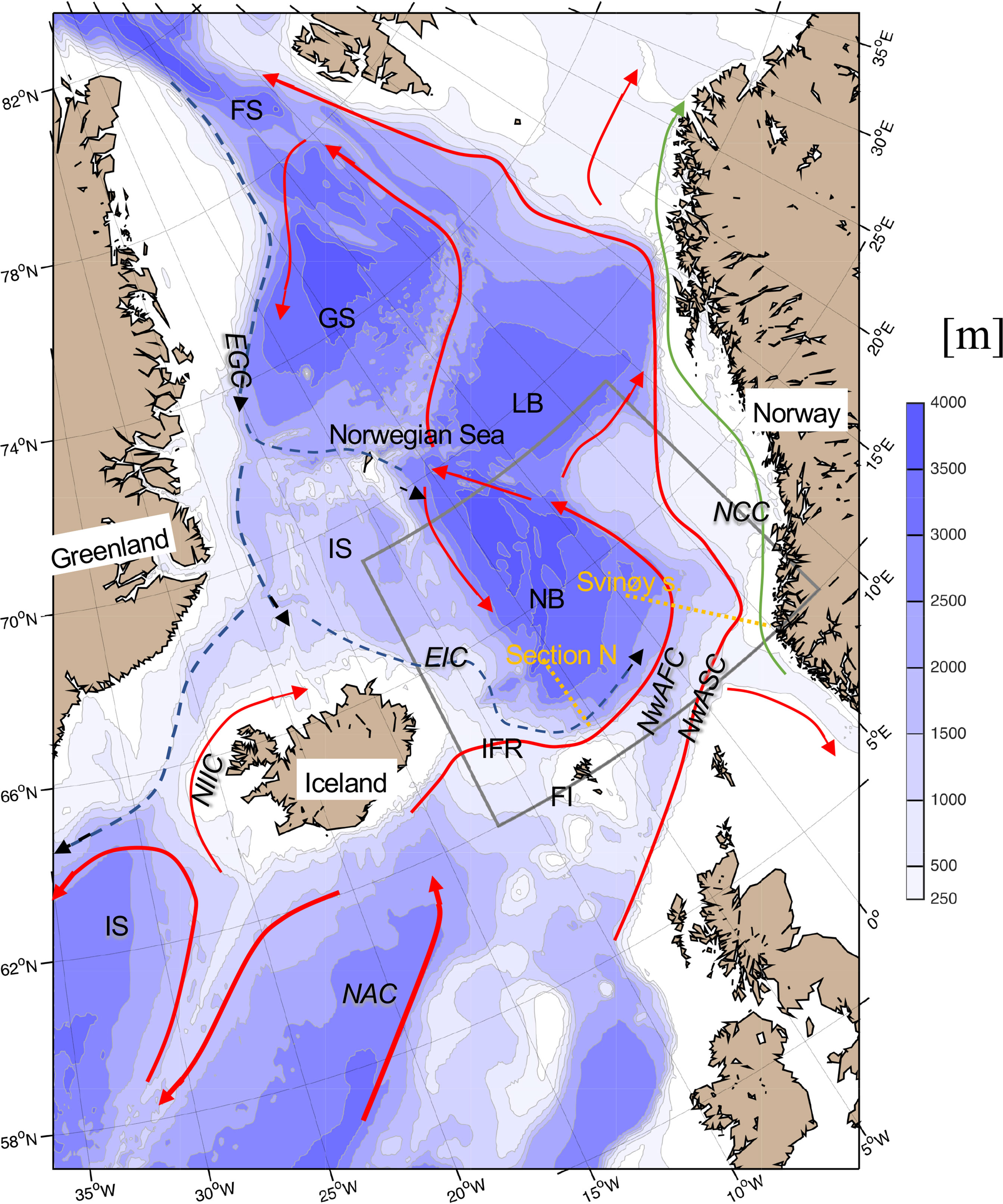
Figure 1 Map of the investigation area including schematic of the major currents associated with Atlantic Water in red, Arctic and Polar Water in black dashed, and freshwater boundary current in green. Yellow dashed lines show position of the repeated section used in this study; the Section N and the Svinøy section. The square shows the area used to calculate integrated quantities for the Norwegian Basin. North Atlantic Current, (NAC); Irminger Sea, (IS); North Icelandic Irminger Current, (NIIC); East Icelandic Current, (EIC); Iceland-Faroe Ridge, (IFR); Faroe Islands, (FI); Norwegian Atlantic Slope Current, (NwASC); Norwegian Atlantic Front Current, (NwAFC); Norwegian Coastal Current, (NCC); East Greenland Current, (EGC); Norwegian Basin, (NB); Lofoten Basin, (LB); Iceland Sea, (IS); Greenland Sea, (GS) and Fram Strait, (FS). Color show water depth in meters.
To understand the causes of variability in the Norwegian Basin it is necessary to consider the relative contribution of Atlantic and Arctic waters as well as changes in the local ocean to atmosphere heat loss (Mork et al., 2019). Variability in the Atlantic inflow waters to the Norwegian Basin are related to atmospheric driven circulation changes in the North Atlantic sub-tropical and sub-polar gyres (Eden and Willebrand, 2001; Marshall et al., 2001; Häkkinen and Rhines, 2004). Numerous studies have reported that hydrographic anomalies propagate with the North Atlantic Current toward (Dickson et al., 1988; Krahman et al., 2001; Hátún et al., 2005; Holliday et al., 2008) and through the Norwegian Sea (Helland-Hansen and Nansen, 1909; Furevik, 2001; Chafik et al., 2015; Broome and Nilsson, 2018; Asbjørnsen et al., 2019). However, less focus has been on the Arctic flow from the west.
Arctic Water enters the Norwegian Basin from the Iceland and Greenland Seas. Mixing of Atlantic type water from the North Icelandic Irminger Current and waters from the East Greenland Current forms so-called North Icelandic Winter Water (Stefansson, 1962). This water type is a source to the EIC (Macrander et al., 2014). It is further transformed while flowing along the northern slope of the Iceland Faroes Ridge where it is denoted Modified East Icelandic Water (MEIW; Read and Pollard, 1992). MEIW contributes to the Arctic Intermediate Water (AIW) in the Norwegian Sea (Blindheim et al., 2000). However, the main sources of AIW are from further north in the Iceland Sea and from the Greenland Sea (Blindheim, 1990). These waters flow southward following the general cyclonic circulation along the western slope of the Norwegian Basin (Søiland et al., 2008; Voet et al., 2010) and contribute to the AIW in the Norwegian Sea (Blindheim, 1990; Blindheim et al., 2000; Mork and Blindheim, 2000; Jeansson et al., 2017).
The characteristics of the Atlantic and Arctic source waters also cause hydrographic variability in the Norwegian Basin. The most striking event is the “Great Salinity Anomaly” in the 1970s that propagated from the Arctic with the East Greenland Current into the North Atlantic and finally returned into the Norwegian Sea in the late 1970s (Dickson et al., 1988). Another example is the warmer and more saline Atlantic inflow after 2000 (Hátún et al., 2005; Hansen et al., 2015), which propagated north through the Nordic Seas and whereof a part thereafter recirculated in the Fram Strait. This increased the upper salinity in the Greenland and Iceland Seas, and hence changed the properties of the Arctic waters that subsequently enter the Norwegian Sea at depth (Lauvset et al., 2018). While such indirect changes may be more difficult to track, they are potentially more predictable due to their longer time scale.
In terms of integrated heat and freshwater content in the Norwegian Basin, there are three distinct multi–year periods during the years 1995 to 2019 (Mork et al., 2019; Figure 2). The first period, 1995-2005 (hereafter termed the Arctic period), is a transition period from cold and fresh “Arctic” condition to warmer and more saline “Atlantic” condition. This transition has been related to a switch from a relative strong to weak sub-polar gyre of the North Atlantic from 1995 to 1996 (Häkkinen and Rhines, 2004). Hátún et al. (2005) attributed the effect of this weakening gyre to more saline and warmer Atlantic Water around the Faroes, which is the southern entrance to the Norwegian Basin. In the second period from 2006-2016 (here termed the Atlantic period), the Norwegian Basin remained in a relatively warm and saline state. During this period the atmospheric forcing represented by the NAO-index did not show consistent deviation from the climatology. The third period includes the recent years 2017-2019 (termed the Fresh period), where there has been a notable freshening, but only minor reduction in heat content (Mork et al., 2019). This can partly be related to the “anomalous freshening” in the Northeast Atlantic in 2014-2015 that, with a time-lag of a few years, has propagated with the main circulation into the Norwegian Basin (Holliday et al., 2020), although with anomalous low ocean to atmosphere cooling over the Norwegian Basin (Mork et al., 2019).
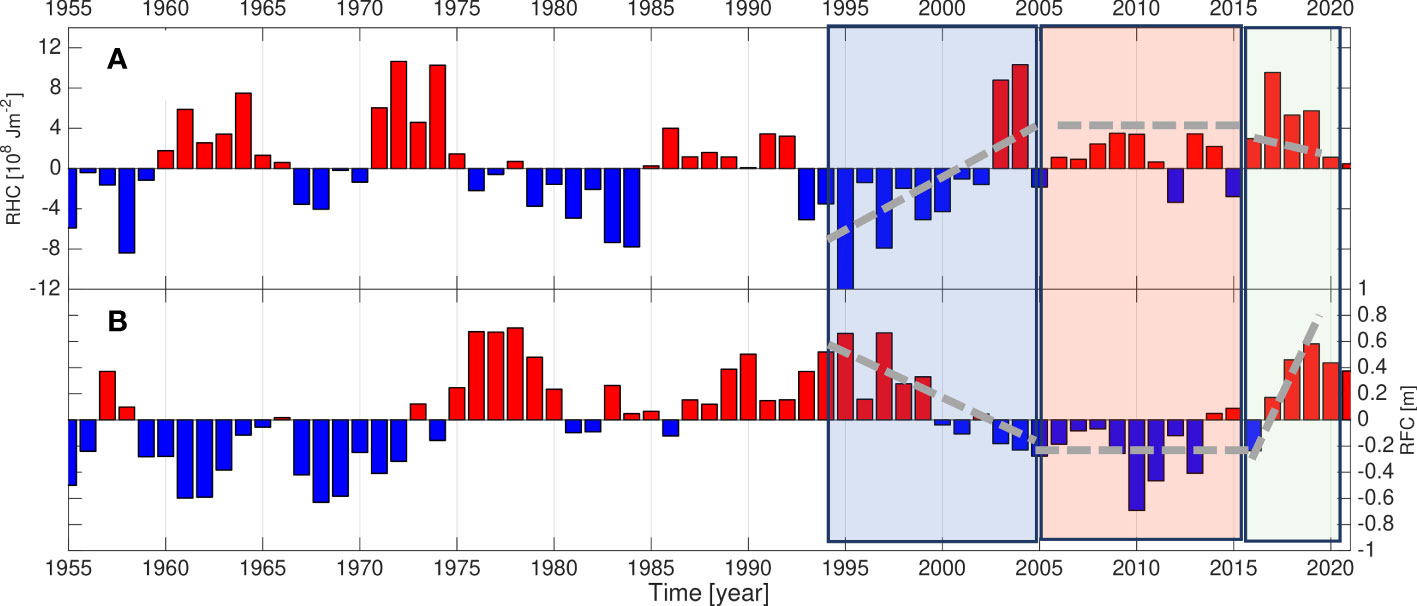
Figure 2 (A, B) Time series of Relative Heat Content (RHC) and Relative Freshwater Content (RFC) in the Norwegian Basin (after Mork et al., 2014).
In the Norwegian Basin, changes in the hydrographic conditions may affect the biological production in numerous ways (see Skjoldal, 2004 for a comprehensive review). It is a highly productive area and home of large commercial fish stocks that rely on zooplankton as prey during spring and summer. The seasonal cycle in light, and thus primary production, is pronounced and zooplankton represent the primary transfer of energy from nutrients and phytoplankton production to zooplankton and fish (Melle et al., 2014). As such, the overall abundance of zooplankton is important for the energy transfer across trophic levels.
The large arctic copepod, Calanus hyperboreus dominates the zooplankton biomass within the Iceland and Greenland Seas (Wiborg, 1954; Hirche, 1997; Astthorsson and Gislason, 2003; Gislason, 2018; Strand et al., 2020). The expatriate, C. hyperboreus, from the Iceland and Greenland Seas have been used as a tracer of Arctic Water variability in the southern Norwegian Basin (Wiborg, 1954; Kristiansen et al., 2019).
In this study, we explore the hydrographic variability within the Norwegian Basin from 1995 to 2019, with particular emphasis on the Arctic influx from the west and relate the contrasting hydrographic states to the pre-bloom (winter) nutrient concentrations and to the total zooplankton biomass in May. Furthermore, we investigate the abundance of the expatriate, C. hyperboreus, within the eastern Norwegian Basin to determine whether its traceability can also be detected within this region.
Data and Methods
Data
We utilize hydrographic data from several sources. To describe the variability in the cyclonic circulation in the southern and eastern Norwegian Basin, we use two repeated hydrographic sections (Figure 1). Extending northwards from the Faroes, the Section N was initiated in 1988 by the Faroe Marine Research Institute (FAMRI) (Larsen et al., 2012). Typically, it has been occupied four times a year on 14 fixed stations, with some modification since the work started. The Svinøy section extends northwestward from the Norwegian coast and has been regularly operated since the mid 1970s by the Institute of Marine Research (IMR), typically four to five times a year, with 17 fixed stations (Figure 1).
To describe the annual 3-D hydrographic variability from 1995 to 2019, we combined data sets from ICES (International Council for the Exploration of the Sea), PINRO (Polar Research Institute of Marine Fisheries and Oceanography, Russia), and the Argo Global Data Assembly Centre in France (Argo, 2000) (Mork et al., 2019). Data are from spring, between 15 April and 15 June, that coincide with the Northeast Atlantic Pelagic Ecosystem Surveys that include CTD stations every 60 nautical miles to a maximum depths of 1000 m. All hydrographic data are corrected for the climatological seasonal trend (Skagseth and Mork, 2012). The number of CTD profiles for the Norwegian Basin for each year varies from 50 to 150. For each year, at 5 m depth interval, the observations were interpolated using objective analysis on a grid with intervals of 0.5° and 0.25° in the zonal and meridional directions, respectively.
We used nutrient data collected on the repeated hydrographic surveys along Svinøy section by IMR (Gundersen et al., 2021). Here, we use nitrate and silicate data from standard depths 10, 30, 50, 70, 100, 200, 500, 800, 1000, 1500, 2000 m. Only winter data (January-March) are used, to avoid the effect of nutrient consumption by primary production (Baggøien et al., 2012). Here we contrast the depth-mean distributions for the Arctic (1995-2005), the Atlantic (2006-2016), and the Fresh (2017-2019) periods. Nutrient observations in the Section N started in 2013 and are therefore not included in this study.
The zooplankton biomass was sampled at the ICES co-ordinated IESNS (International Ecosystem Survey in Nordic Sea) ecosystem surveys by several countries and vessels, covering the Norwegian Sea and adjacent areas (ICES, 2021a; ICES, 2021b). In order to have comparable geographical coverage of the southern Norwegian Sea every year, the area reported in the present paper is bounded by the longitudes 8°W and 12°E and latitudes 62°N and 70°N. The zooplankton have been sampled as standard since 1995, and sampling was carried out from the end of April until the beginning of June. All zooplankton biomass samples were collected by vertical hauls with WP2 nets with 0.25m2 mouth area (Fraser, 1966) and mesh-size of 200 μm, except from the Norwegian vessels which used the modified mesh-size of 180 μm. The nets were hauled from 200 m to the surface or from the bottom whenever bottom depth is less than 200 m. The wet zooplankton samples were oven-dried at ~ 60°C for at least 24 h and data are presented as gram dry weight per m2. Due to avoidance of larger zooplankton when sampling with WP2, the dry weight presented is considered to represent the mesozooplankton biomass.
C. hyperboreus was sampled at Section N and at the Svinøy section. The zooplankton time-series at Section N started in 1993, however no data are available for 1996, 1998 and 2006. The zooplankton time-series from the Svinøy secion started in 1995. Only the five northernmost stations along Section N, which are always north of the Iceland-Faroe Front (Kristiansen et al., 2016), and thus influenced by Arctic waters, are included in this paper. In the Svinøy section only the three westernmost stations are used and are thus in comparable waters as Section N. In Section N the data are during the period 10-27 May, in the Svinøy section from 17 April to 17 May. By using data from this period of the season, the analysis are refined to the overwintering generations, which makes the between-year abundances comparable. Zooplankton samples at both sections were collected by vertical hauls with WP2 nets with 0.25m2 mouth area (Fraser, 1966), and mesh-size 200 μm (Section N) and 180 μm (Svinøy section). Sampling was conducted from 50 m depth to the surface at the Section N, and from 200 m depth to the surface at the Svinøy section. All samples were preserved with 4% formaldehyde on board. In the laboratory, the samples were divided into subsamples with a plankton splitter, and an aliquot of around 200 to 300 animals from each sample was identified and counted. For the Svinøy section, C. hyperboreus has been identified to copepodite stages. The copepodite stage IV, V, and adult females and males were used in the present analysis and younger stages were omitted because these younger stages have great fluctuations in abundance during the growth season (Gislason, 2018). The older stages will in addition represent the overwintering stages (Hirche, 1997; Astthorsson and Gislason, 2003; Broms et al., 2009; Gislason, 2018). At Section N, C. hyperboreus has not been identified to copepodite stages and thus all stages are included. However, stages younger than CIII have very seldom been observed in the samples (pers.com. I. Kristiansen, Faroe Marine Research Institute) and we therefore interpret these data as overwintering stages. The data are presented as abundance per m3.
Methods
To show the focused period herein (1995-2019) in a longer time perspective updated series over the period 1955-2021 of heat and freshwater are presented (Mork et al., 2014). At each grid point from the gridded hydrography, the heat and freshwater contents were calculated above the depth of the specific volume anomaly equals to 2.1 × 10-7 m3 kg -1 (denoted here as s21). The surface s21 corresponds approximately to the surface σt = 27.9 that is close to the lower depth of the Atlantic layer in the Norwegian Basin (Mork and Blindheim, 2000; Rossby et al., 2009). Local time averaged temperature and salinity at the s21 surface were used as reference values. Relative heat and freshwater contents were calculated by removing the temporal averages before integrating over the Norwegian Basin (see Mork et al., 2014 for details). We do not have the full access to the Russian hydrographic data used in Mork et al. (2014) before 1995. However, a comparison of the estimated relative heat and freshwater contents using the s21 or the 1000 dbar surfaces as reference yield qualitatively similar results (not shown).
From the gridded hydrography we construct maps showing the depth-mean isohalines for two vertical layers 50-200 m and 300-800 for the three periods defined above (Figure 5). The volumetric census is made for Temperature-Salinity classes of 0.1°C by 0.01, respectively, (Figure 6) and confined to the area limited by 62-69°N and 12°W to 8°E and depth range 0-1000 m (see area in Figure 1). We use in-situ temperature throughout the manuscript. In the upper 1000 m considered here, the difference between temperature and potential temperature is less than 0.1°C. This would not affect the results. For salinity we use the traditional practical salinity unit to simplify comparison with earlier studies.
Nutrient concentrations are presented with median and 95% confidence interval (Figure 7). We contrast the depth-mean distributions for the Arctic, Atlantic and Fresh periods. We include mean estimates for three stations over the continental slope in Atlantic Water and for the three westernmost station representing Arctic Water (see Figure 3).
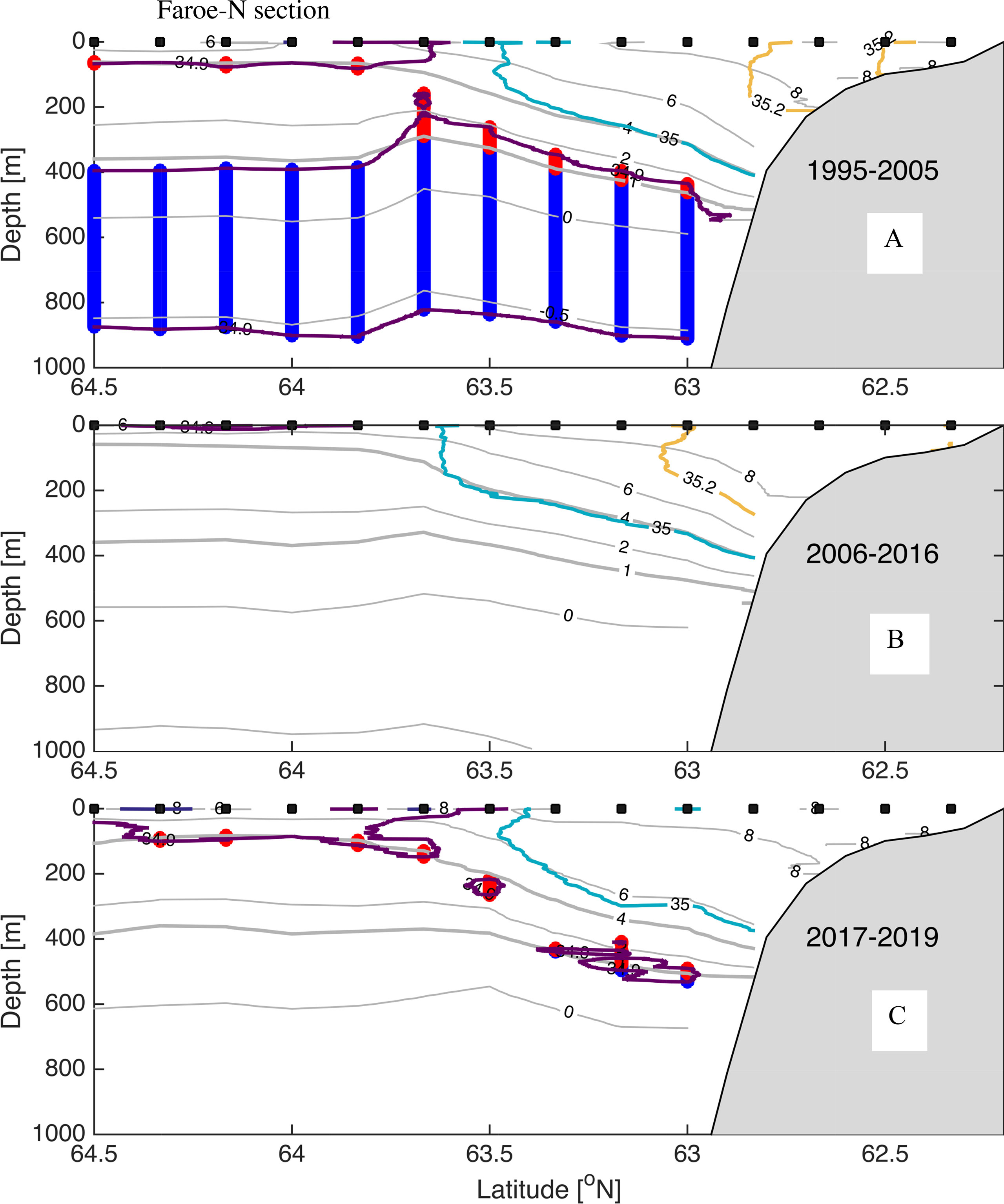
Figure 3 (A–C) Mean temperature and salinity for the Section N section for the periods (A) 1995-2005, (B) 2006-2016, and (C) 2017-2019. Colors are for salinity showing 34.9 (purple), 35.0 (light blue) and 35.2 (yellow) isohalines, and gray show isotherms -0.5, 0,1,.,8°C. Thick vertical lines indicate Arctic Intermediate Water (blue) and Modified East Icelandic Water (red). Black squares show positions of the stations.
The zooplankton biomass estimates (Figure 8) are based on a bootstrapping method. For each year, we draw from the observations series of length equal to the number of samples with replacement. This procedure is repeated 1000 times from which median and the 95% confidence interval is presented. The main assumption behind this method is statistical stationarity within the area.
Results
Three Contrasting Periods
As a starting point, we investigate the integrated heat and freshwater content (Mork et al., 2014) in the Norwegian Basin (Figure 2). Three periods are defined: i) 1995-2005, Arctic - associated with a transition from cold/fresh to warm and saline, ii) 2006-2016, Atlantic - a generally consistent warm and saline period, and iii) 2017-2019, Fresh - a period that show record strong freshening while temperatures remained high.
Variable Water Mass Composition – Hydrographic Sections
We utilize the Section N (Figure 3) and the Svinøy section (Figure 4) to illustrate the variable distribution of water masses in the inflow region to the Norwegian Basin. During the Arctic period there was a several hundred-meter-thick layer of AIW at both sections. Common for both sections, there have been large changes in the Arctic Intermediate layer, with an almost total loss of AIW (S < 34.9 and -0.5°C < T< 1.0°C) from the Arctic period (Figures 4A, 5A) to the subsequent Atlantic period (Figures 4B, 5B). AIW, defined by the above TS criteria, has not re-appeared at either of these sections. At Section N section there are some minor traces of low salinity Arctic water MEIW/AIW (S < 34.9 and 1.0°C < T <4°C) during the Fresh period 2017-2019, but this is not observed in the Svinøy section (Figures 4C, 5C). A curious observation, at both sections, is a remarkable close resemblance between the 35 isohaline and the 4°C isotherm in the Atlantic inflow domain during the first two decades (1995-2005 and 2006-2016, Figures 3A, B and 4A, B). However, during the Fresh period the 35 isohaline is closer to the 6°C isotherm in the Section N (Figure 3C), and this is also observed downstream in the Svinøy section, but only for the Norwegian Atlantic Front Current west of 1.25°E (Figure 4C). At the eastern part of the section, the 35 isohaline still resembles the 4°C isotherm fairly well.
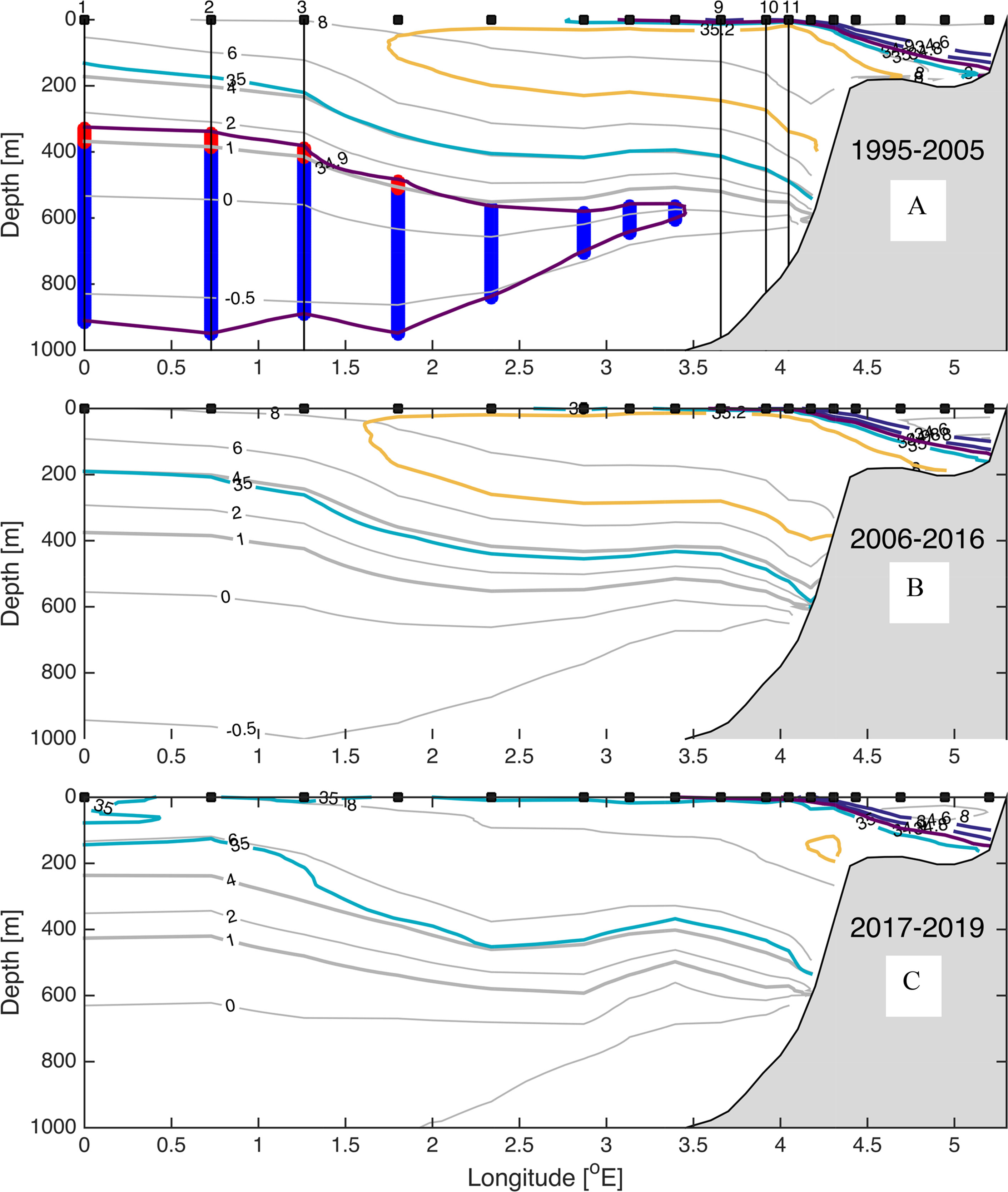
Figure 4 (A–C) Mean temperature and salinity for the Svinøy section for the periods (A) 1995-2005, (B) 2006-2016, and (C) 2017-2019. Colors are for salinity showing 34.9 (purple), 35.0 (light blue) and 35.2 (yellow) isohalines, and gray show isotherms -0.5, 0, 1,., 8°C. Vertical lines show nutrient data used in Figures 8, 9 stations 1-3 represent the western edge of the Norwegian Atlantic Front Current, and stations 9-11 represent the “core” Atlantic water. Thick vertical lines indicate Arctic Intermediate Water (blue) and Modified East Icelandic Water (red). Black squares show positions of the stations.
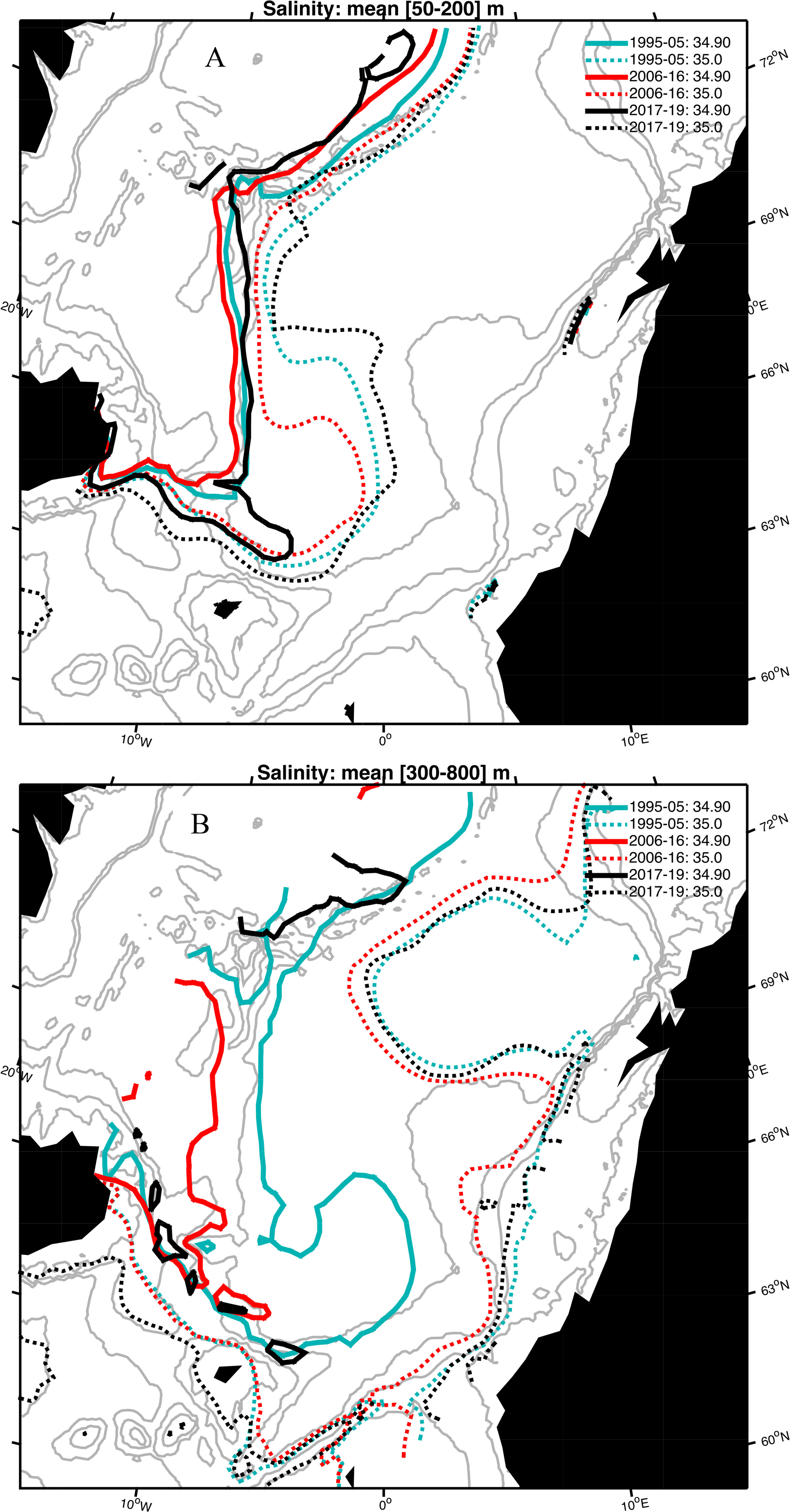
Figure 5 (A, B) Depth-mean salinity over the depth range (A) 50-200m, and (B) 300-800m for the three periods 1995-2005, 2006-2016, and 2017-2019. Isohalines included are 34.9 (solid) and 35.0 (dashed).
Horizontal Maps of Salinity
The depth-mean salinity maps illustrate some marked differences between the upper layer (50-200 m) that include the MEIW and intermediate layer (300-800 m) that contain the AIW (Figure 5). For the 50-200 m layer, the 34.9 isohaline typically align with the shelf slope bordering the Iceland Sea and the Norwegian Basin (Figure 5A). However, during the Fresh period a tongue of the 34.9 isohaline extends into the southern Norwegian Basin. For the 35 isohaline we find that this extends farthest west for the Atlantic period, and farthest east for the Fresh period, and with the largest differences in the interior Norwegian Basin. For the 300-800 m layer the substantial eastward extent of the 34.9 isohaline into the Norwegian is limited to the Arctic period (Figure 5B). The 35 isohaline is confined to the eastern shelf break of the Norwegian Basin, slightly more westward during the Atlantic period, before turning west and encircling the deep Atlantic Water in the Lofoten Basin.
Volumetric TS Plots
Finally, the volumetric TS-plots illustrate the major changes in the water masses during the three defined periods in the Norwegian Basin (Figure 6). In the climatology, all water classes during the period 1995-2019 are included. The most prominent feature is the broad distribution in TS-space ranging from warm and saline Atlantic Water (35.2-35.3, 6-8°C) that is cooled and freshened in the Norwegian Sea and Arctic, to finally Norwegian Sea Deep Water (NSDW) with salinity of 34.91 and T below 0°C (Figure 6A). AIW and MEIW are characterized by lower salinities due to Polar (or Arctic) influence. The surface water, located in a band of temperature about 8°C and salinties from 34.4 to 35 is remnants of the Norwegian Coastal Current.
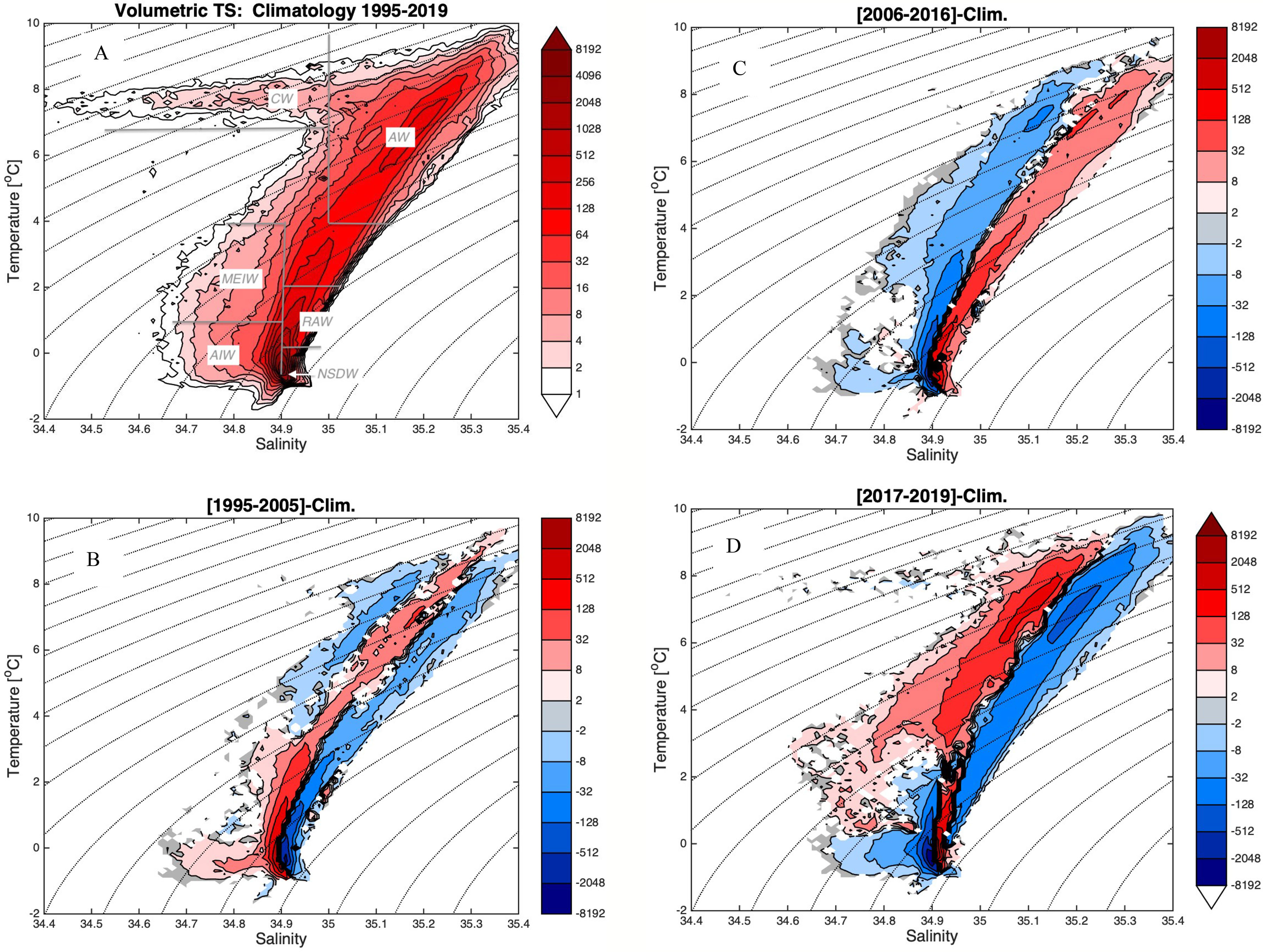
Figure 6 (A–D) Volumetric TS-plots for the Norwegian Basin. (A) Climatology 1995-2019 including schematic distribution of water masses discussed, (B) difference mean 1995-2005 and the climatology, (C) difference mean 2006-2016 and climatology, and (D) difference mean 2017-2019 and climatology. The values represent number of grid points 0.5° longitude x 0.25° latitude over 5 m depth. The color scale is log 2. In difference plots b-d red colors are positive and blue colors are negative. Data include the depth layers 0-1000m and the area is limited by 62 and 69°N and 12°W and 8°E (see area Figure 1). Dashed lines are isopycnal lines. Abbreviations for water masses includes Atlantic Water (AW), Return Atlantic Water (RAW), Artic Intermediate Water (AIW), Modified East Icelandic Water (MEIW), Norwegian Sea Deep Water (NSDW) and Coastal Water (CW).
Compared to the climatology the distribution during the sub-periods discussed here is more confined (compare Figure 6A and Figures 6B–D). As such, the period 1995-2005 distributes as a relative confined anomalous positive band of Atlantic Water TS-classes (Figure 6B) seen as a red band (positive) between blue bands (negative) both on the saline and fresh side. Another marked feature is the positive anomaly of the relative cold and fresh AIW (-0.5 < T < 1°C , S<34.9). During the following period 2006-2016 the Atlantic Water classes are anomalously warm and especially saline, and the AIW is greatly reduced (Figure 6C). During 2017-2019 large changes have occurred, which are different from the two previous decades (Figure 6D). First, the Atlantic Waters have become anomalous fresh exceeding by far the two previous decades. Second, the AIW indicate a further decrease, and instead the fresher and warmer MEIW (T in the range [1-4] °C and S < 34.9) has again become more dominant.
Pre-Bloom (Winter) Nutrients
Given the stark changes especially in the AIW, but also the MEIW, it is of interest to investigate if these changes also are associated with changes in the nutrient concentrations. We used pre-bloom (winter) data (Jan-Mar) from the Svinøy section and from the same periods hitherto discussed: 1995-2005, 2006-2016, and 2017-2019. Near-surface nutrient concentrations in the north-western part of the section (Figures 7C, D) west of the Norwegian Atlantic Front Current (Figure 1) are generally higher than in the eastern part of the section (Figures 7A, B) in the Norwegian Atlantic Slope Current. Binned over the defined periods, both nitrate and silicate concentrations were higher during the Arctic compared to the subsequent Atlantic and Fresh periods (Figure 7). The decline is most apparent in near-surface waters (0-200/500m) and most pronounced for silicate concentrations. In deeper waters (2000-2500 m) there is little difference between the two periods.
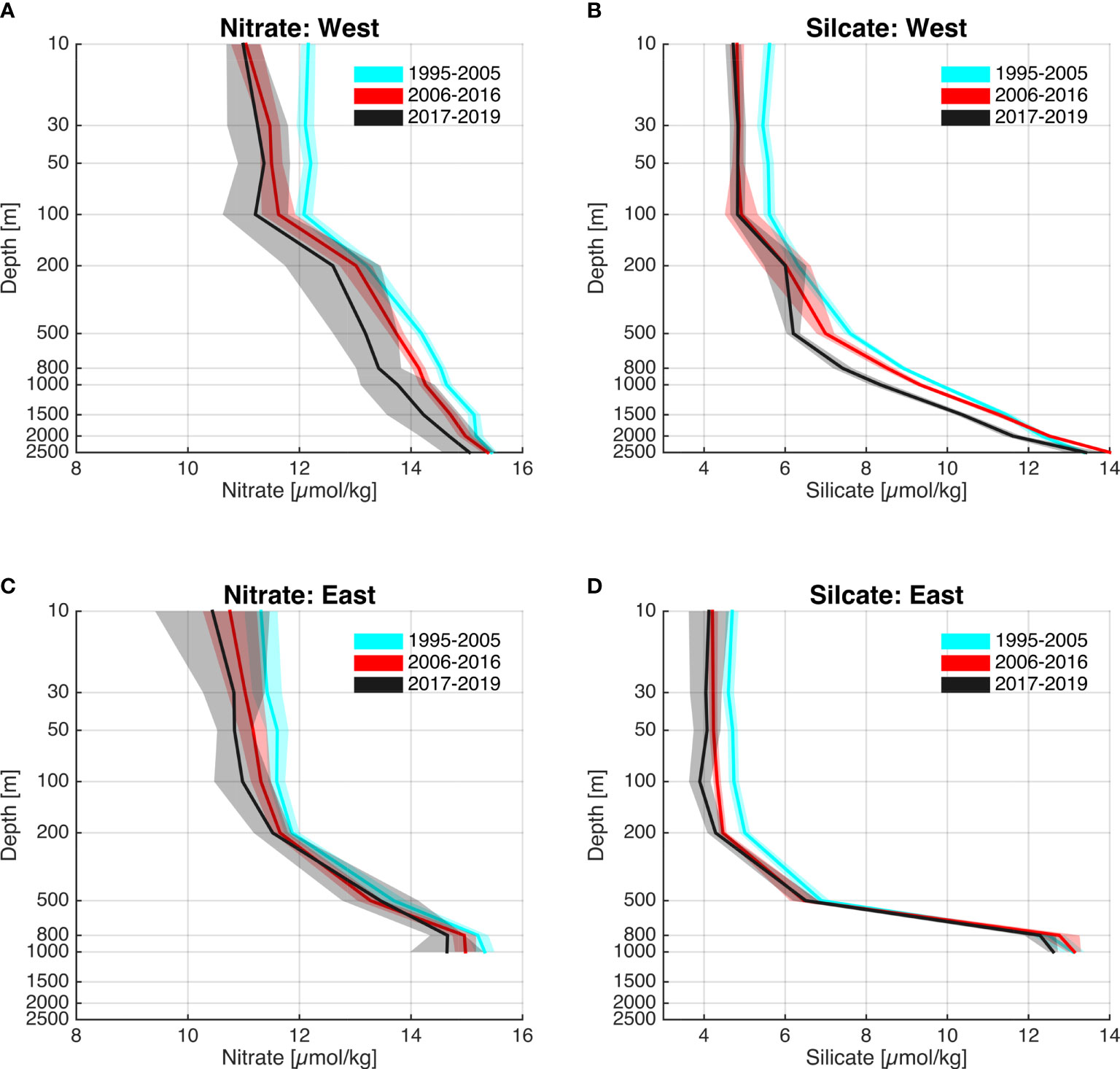
Figure 7 (A–D) Time-mean depth profiles of nutrients in the Svinøy section showing (A) nitrate, and (B) silicate from the stations 1-3, and (C) nitrate and (D) silicate from the stations 9-11. The legend shows the periods 1995-2005 (magenta), 2006-2016 (red), and 2017-2019 (black). Data are from months January-March. The shaded areas show the 95% confidence interval. The positions of the stations are indicated in Figure 4A.
Zooplankton
The large hydrographic changes are likely to impact the zooplankton abundances and species composition through nutrient driven influence on primary production or by direct lateral influxes of animals from the west (Kristiansen et al., 2019). To explore such ecosystem effects, we investigate the coinciding changes in mesozooplankton biomass in the upper 200 meters of the Norwegian Basin, sampled in May (Figure 8). The mesozooplankton biomass index represents a larger area covering the southern Norwegian Sea, including the Norwegian Basin and adjacent areas (Figure 1). The biomass is mainly represented by Calanus finmarchicus within the Norwegian Sea in the productive season, and the seasonal time-window of sampling will mainly represent the overwintering generation of C. finmarchicus (Skjoldal et al., 2004; Broms and Melle, 2007; Broms et al., 2009; Bagøien et al., 2012; Strand et al., 2020). The biomass showed high values in the first part of the time-series, from 1995 to mid-2000s. Thereafter, the biomass was largely reduced and reached a minimum around 2010. The period after mid-2000s can be regarded as a period with lower biomass, however an increase towards the end of the time-series is observed. When relating the mesozooplankton biomass to the three identified time-periods, the Arctic period has a mean of 10 g m-2, the Atlantic period has a mean of 6.4 g m-2, and the Fresh period have a mean of 9.5 g m-2.
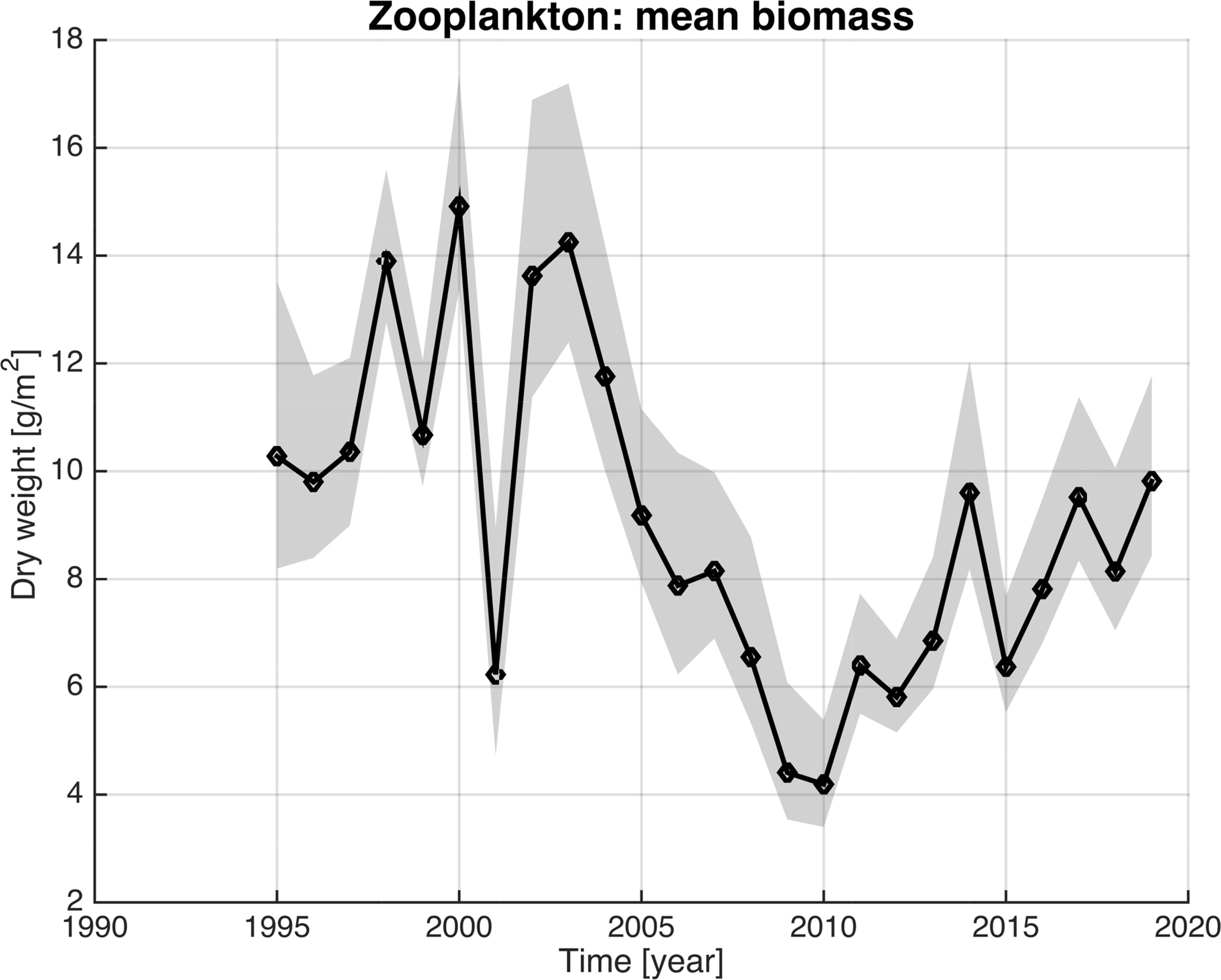
Figure 8 Median zooplankton biomass in the Norwegian Basin in May. The mean number of observations per year is 93. Solid line shows the median and the gray band represents the 95% confidence interval.
In contrast to C. finmarchicus, C. hyperboreus does not reproduce in the Norwegian Basin (Wiborg, 1954), and its relative abundance can therefore be used as indicator of advection of Arctic waters from the Greenland and Iceland Seas. We here show that the previously demonstrated water mass, MEIW, and C. hyperboreus are advected to the Section N (Kristiansen et al., 2019), and extend further east to the Svinøy Section (Figure 9). Further more, the increased influx of water from the west during the recent Fresh period (Figure 5A) coincided with increased abundances of C. hyperboreus, at both sections, although not to the levels of the first decade. The variability of C. hyperboreus thus tends to follow that of the overall biomass (Figure 8) with maximum values in the Atlantic period, followed by low values and some years virtually absent in the Atlantic period, and finally a relative increase in the most recent Fresh period.
Discussion
Our analysis showed that the Norwegian Basin the three defined multi-annual periods turned out to have distinct differences in nutrients, and for both zooplankton biomass and species. In the Arctic period, 1995 to 2005, the Atlantic inflow was relative fresh and cold, and the observations show a pronounced layer of AIW, relative high nutrients and maximum values for zooplankton biomass and C. hyperboreus abundance. During the subsequent Atlantic period, 2006-2016, the Atlantic waters were relatively warm and saline, the layer of AIW almost disappeared, the level of nutrients was relatively low, and there was significant decrease in both in C. hyperboreus and zooplankton biomass. During the most recent Fresh period, 2017-2019, the Atlantic Water became less saline but still relative warm. There was still little evidence of AIW but increased presence of MEIW. During this period the nutrients remained at the level of the Atlantic period, but there was a relative increase in zooplankton biomass and C. hyperboreus. We first discuss the cause of the observed changes and proceed with potential effects on the ecosystem.
A major change during the study period (1995-2019) is the decrease of the AIW, defined with salinity less than 34.9 and temperatures between -0.5 and +1.0 °C (Swift and Aagaard, 1981; Blindheim, 1990; Jeansson et al., 2017), from the Arctic to the Atlantic period (Figures 3–6). A possible cause of this reduction is the increasing salinity of the Norwegian Atlantic Current into the early 2000s. This water partly turns southward in the Fram Strait as Return Atlantic Water, causing increase in the surface salinity in the Greenland Sea, leading to more saline and hence deeper winter convection (Lauvset et al., 2018). A possible interpretation could be that a more saline AIW vintage, that does not meet previous TS definition replaced the classical AIW type. However, the volumetric plots show that the AIW layer is largely reduced during the two latter periods and that this insulating layer between the Atlantic Water and the NSDW basically vanished. Another prominent feature is the MEIW that is transported with the East Icelandic Current into the southwestern Norwegian Basin and then follows the general cyclonic circulation (Meincke, 1978; Blindheim, 1990; Søiland et al., 2008; Semper et al., 2020; Hátún et al., 2021). According to the spatial maps of MEIW (Figure 5A), there is a tongue of the 34.9 isohaline that extend anomalous eastward in the southern Norwegian Sea during the period Fresh.
Related to the changes in the Arctic Water two partially related mechanisms are at play. First, the fueling of zooplankton via the EIC (Wiborg, 1954; Kristiansen et al., 2022). Kristiansen et al. (2019) found a coinciding decrease of MEIW and C. hyperboreus abundance at Section N in the early 2000s but an increase of C. hyperboreus since 2017 within the southwestern Norwegian Sea (Kristiansen et al., 2022). This paper shows that the increase in C. hyperboreus abundance with increasing Arctic Waters can also be traced to the eastern part of the Norwegian Basin (Figure 9). This mechanism is likely important during the Arctic and Fresh periods, but less so during the Atlantic period. Second, the generally higher level of C. hyperboreus during the Arctic period indicate advection from regions of higher concentration. Such areas are found further north in the Iceland and Greeland Seas (Wiborg, 1954; Hirche, 1997). Thus, the elevated abundance of C. hyperborus during the Arctic period is in accordance with increased inflow of AIW from the Greenland and Iceland Seas. This also applies to the overall mesozooplankton biomass within the Norwegian Basin since the abundance of C. hyperboreus and mesozooplankton biomass co-vary, which suggests that other zooplankton species are also advected eastwards with the Arctic waters.
However, our results show a significant reduction in the amount of winter nutrients contrasting the Arctic to the subsequent Atlantic and Fresh periods. This can be interpreted as a reduction in the potential for new production that correspond to the major changes in ocean climate. Note that the elevated levels of nutrients extend to intermediate depths, thus annual upper ocean winter refueling of nutrients could simply be explained by local enhanced winter mixing.
Macronutrient concentrations, and silicate in particular, are generally higher in Arctic surface waters (Figure 7). New production is determined as the seasonal drop in NO3 concentrations, from a uniform depth profile of concentrations in winter to unexploitable levels below detection in summer. Therefore, we propose that if the observed lower original winter concentrations continue its downward trend (Hátún et al., 2017; Gundersen et al., 2021) the region will generate less seasonal new production overall. Additionally, diatom growth is only competitive to other phytoplankton at silicate concentrations >2 umol/L (Furnas, 1990; Egge and Aksnes, 1992). Diatoms will therefore lose their competitive edge at lower silicate concentrations in surface waters in developing spring blooms. Diatoms are considered one of the main staples for dominant zooplankton groups in the Norwegian Sea, and zooplankton growth is highly dependent on the timing and magnitude of the spring bloom (Melle et al., 2004; Eiane and Tande, 2009). Also, clearance and ingestion rates for, C. finmarchicus are at a peak during diatom spring blooms in the Norwegian Sea (Meyer-Harms et al., 1999). Therefore, silicate concentrations in surface waters, which showed a decline in the last number of decades (Rey, 2012; Hátún et al., 2017; Gundersen et al., 2021) may have impacted the amounts of diatoms available for major groups of zooplankton in these waters. Juvenile fish of commercial stocks in the Norwegian Sea (e.g. herring and cod) are strongly dependent on successful zooplankton growth and reproduction each year (Skjoldal, 2004; Gjøsæther, 2009). Therefore, lower initial silicate concentrations at the onset of the spring bloom season may lead to weaker diatom blooms and hence, less successful growth and survival of zooplankton and fish larvae in these oceans. However, zooplankton diets are diverse (Meyer-Harms et al., 1999) and hence, not solely dependent on diatom growth and development. Therefore, we propose that the magnitude and longevity of the spring bloom, determined by the total amount of available nitrate in surface waters at the onset of the spring bloom, may play an equally important role in determining the seasonal magnitude of new production available for zooplankton growth and development in these waters.
Changes in temperature can affect the growth rates (Campbell et al., 2001), length of growth season (Strand et al., 2020), and distribution of C. finmarchicus, and thus potentially influence on the zooplankton biomass and production. An extension of suitable habitats for C. finmarchicus have been observed in Arctic areas and related to temperature and retreat of the ice-edge (Aarflot et al., 2018; Moller and Nilsen, 2020; Tarling et al., 2022). The ability of C. finmarchicus to complete its life cycle is found to increase with temperature at the entrance to the Arctic (Tarling et al., 2022). The optimum temperature window for older copepodite stages of C. finmarchicus (CV and adults) based on distribution and abundance data is 6–11°C (Helaouet and Beaugrand, 2007). In the Norwegian Sea, C. finmarchicus occupy waters with surface temperatures from 0 to 13°C, with the highest abundance occurring between 5 and 9°C (Strand et al., 2020). Since the upper-ocean temperature during the three considered periods are all basically within these optimal windows, temperature may not be a major driver of ecosystem change in the Norwegian Basin during the present climate conditions.
While beyond the scope of this work, recent studies indicate that the here discussed contrasting periods in the Norwegian Basin have also affected fish (Eliasen et al., 2021; Kristiansen et al., 2022; Utne et al., 2022). The reduction of Arctic Water in the Norwegian Sea in the early 2000s resulted in reduced feeding of post-smolts of salmon (Utne et al., 2022), drop in marine growth of Norwegian salmon (Vollset et al., 2022), and in a marked shift of the herring feeding migration towards East Icelandic Current (Eliasen et al., 2021; Kristiansen et al., 2022). These results are in accordance with the results of this paper linking changes in the ocean climate to plankton production and species composition in the Norwegian Sea, and thereby changed productivity of the ecosystem. We acknowledge that we in this paper have not included data of phytoplankton which are needed to bridge proposed link from nutrients to zooplankton. Inclusion of such data would, however, add new uncertainties and for further details on primary production we refer to the paper of Silva et al. (this issue).
The suggested link between the relative amount of Arctic to Atlantic Water masses, lateral influx of nutrients and arctic zooplankton, and zooplankton likely growth provide a potential for making ecosystem predictions. While atmospheric forcing which drive regional circulation and water mass modification, are basically unpredictable, the memory in terms of advected water masses may provide a forecast horizon on the order of several years.
Concluding Remarks
We investigate the major oceanographic changes of the Norwegian Basin over the period 1995 to 2019 and potential impacts on marine ecosystems. Limited evidence supports that temperature drive ecosystem changes, while changing nutrients levels in connection to water masses may play a stronger role. We find that nutrient concentrations are generally higher in Arctic waters, compared to Atlantic waters, and that during the Arctic period (1995-2005) nutrients were significantly higher compared to the subsequent Atlantic period (2006-2016), and Fresh period, (2017-2019). We have not investigated primary production per se but note that high level of nutrients and zooplankton abundance tend to be synchronized in the Norwegian Basin. During the Arctic period, and partly during the Fresh period, the observations indicate increased amount of arctic zooplankton that are advected from the Iceland – and Greenland seas into the Norwegian Basin. Since these large and nutritious arctic zooplankton are preferred food for large fish stock (e.g. Norwegian spring spawning herring), these shifts likely have major impacts on fish stock dynamics, and therefore also on the fisheries and economies.
Data Availability Statement
The raw data supporting the conclusions of this article will be made available by the authors, without undue reservation.
Author Contributions
All authors contributed to the article and approved the submitted version.
Funding
This study was carried out as part of the project “Sustainable multispecies harvest from the Norwegian Sea and adjacent ecosystems” funded by the Research Council of Norway (project number 299554). Data collection along Section N received support from the European Framework Programs under grant agreement numbers GA212643 (THOR), and 308299 (NACLIM) and from the Danish Ministry of Climate, Energy and Utilities through its climate support program to the Arctic (FARMON projects). The Argo data were collected and made freely available by the International Argo Program and the national programs that contribute to it. (https://argo.ucsd.edu, https://www.ocean-ops.org). The Argo Program is part of the Global Ocean Observing System. This study was supported by the Institute of Marine Research project “Monitoring of the environment and plankton condition in the Norwegian Sea” and the Research Council of Norway (NorArgo2, #269753).
Conflict of Interest
The authors declare that the research was conducted in the absence of any commercial or financial relationships that could be construed as a potential conflict of interest.
Publisher’s Note
All claims expressed in this article are solely those of the authors and do not necessarily represent those of their affiliated organizations, or those of the publisher, the editors and the reviewers. Any product that may be evaluated in this article, or claim that may be made by its manufacturer, is not guaranteed or endorsed by the publisher.
Acknowledgments
We thank all Captains and crew, the Plankton Chemistry and the Zooplankton Laboratories at IMR, the Environmental Department and the Pelagic Department at FAMRI, the Zooplankton Laboratory at MFRI (Marine and Freshwater Institute Iceland) for all shipboard collections and sample analysis over the years.
References
Aarflot J. M., Skjoldal H. R., Dalpadado P., Skern-Mauritzen M. (2018). Contribution of Calanus Species to the Mesozooplankton Biomass in the Barents Sea. ICES J. Marine Sci. 75, 2342–2354. doi: 10.1093/icesjms/fsx221
Argo (2000). Argo Float Data and Metadata From Global Data Assembly Centre (Argo GDAC) (SEANOE). doi: 10.17882/42182
Asbjørnsen H., Årthun M., Skagseth Ø., Eldevik T. (2019). Mechanisms of Ocean Heat Anomalies in the Norwegian Sea. J. Geophys. Res. Oceans 124, 3908–2923. doi: 10.1029/2018JC014649
Astthorsson O. S., Gislason A. (2003). Seasonal Variations in Abundance, Development and Vertical Distribution of Calanus finmarchicus, C. hyperboreus and C. glacialis in the East Icelandic Current. J. Plankton Res. 25, 843–854. doi: 10.1093/plankt/25.7.843
Bagøien E., Melle W., Kaartvedt S. (2012). Seasonal Development of Mixed Layer Depths, Nutrients, Chlorophyll and Calanus finmarchicus in the Norwegian Sea – A Basin-Scale Habitat Comparison. Progr. Oceanography 103, 58–79. doi: 10.1016/j.pocean.2012.04.014
Blindheim J. (1990). Arctic Intermediate Water in the Norwegian Sea. Deep Sea Res. 37 (9), 1475–1489. doi: 10.1016/0198-0149(90)90138-L
Blindheim J., Borovkov V., Hansen B., Malmberg S. A., Turrell W. R., Østerhus S. (2000). Upper Layer Cooling and Freshening in the Norwegian Sea in Relation to Atmospheric Forcing. Deep Sea Res. I 47, 655–680. doi: 10.1016/S0967-0637(99)00070-9
Broms C., Melle W. (2007). Seasonal Development of Calanus Finmarchicus in Relation to Phytoplankton Bloom Dynamics in the Norwegian Sea. Deep Sea Res. Part II 54, 2760–2775. doi: 10.1016/j.dsr2.2007.08.021
Broms C., Melle W., Kaartvedt S. (2009). Oceanic Distribution and Life Cycle of Calanus Species in the Norwegian Sea and Adjacent Waters. Deep Sea Res. Part II-Top. Stud. Oceanogr. 56, 1910–1921. doi: 10.1016/j.dsr2.2008.11.005
Broomé S., Nilsson J. (2018). Shear Dispersion and Delayed Propagation of Temperature Anomalies Along the Norwegian Atlantic Slope Current. Tellus 70A, 1–13. doi: 10.1080/16000870.2018.1453215
Campbell R. G., Wagner M. M., Teegarden G. J., Boudreau C. A., Durbin E. G. (2001). Growth and Development Rates of the Copepod Calanus Finmarchicus Reared in the Laboratory. Marine Ecol. Prog. Ser. 221, 161–183. doi: 10.3354/meps221161
Chafik L., Nilsson J., Skagseth Ø., Lundberg P. (2015). On the Flow of Atlantic Water and Temperature Anomalies in the Nordic Seas Toward the Arctic Ocean. J. Geophys. Res. Oceans 120, 7897–7918. doi: 10.1002/2015JC011012
Dickson R. R., Meincke J., Malmberg S.-A., Lee A. J. (1988). The “Great Salinity Anomaly” in the Northern North Atlantic 1968-82. Prog. Oceanogr. 20, 103–151. doi: 10.1016/0079-6611(88)90049-3
Eden C., Willebrand J. (2001). Mechanism of Interannual to Decadal Variability of the North Atlantic Circulation. J. Clim. 14, 2266–2280. doi: 10.1175/1520-0442(2001)014<2266:MOITDV>2.0.CO;2
Egge J., Aksnes D. (1992). Silicate as Regulating Nutrient in Phytoplankton Competition. Mar. Ecol. Prog. Ser. 83, 281–289. doi: 10.3354/meps083281
Eiane K., Tande K. S. (2009). “Meso and Microzooplankton,” in Ecosystem Barents Sea (Trondheim: Tapir Academic Press), vol. 587 . Eds. Sakshaug E., Johnsen G., Kovac K., pp, 209–234.
Eliasen S. K., Homrum E.Í., Jacobsen J. A., Kristiansen I., Óskarsson G. J., Salthaug A., et al. (2021). Spatial Distribution of Different Age Groups of Herring in Norwegian Sea, May 1996-2020. Front. Mar. Sci. 8. doi: 10.3389/fmars.2021.778725
Furevik T. (2001). Annual and Interannual Variability of Atlantic Water Temperatures in the Norwegian and Barents Seas: 1980–1996. Deep Sea Res. I 48 (2), 383–404. doi: 10.1016/S0967-0637(00)00050-9
Furnas M. J. (1990). In Situ Growth Rates of Marine Phytoplankton: Approaches to Measurement, Community and Species Growth Rate. J. Plankton Res. 12, 1117–1151. doi: 10.1093/plankt/12.6.1117
Gislason A. (2018). Life Cycles and Seasonal Vertical Distribution of Copepods in the Iceland Sea. Polar Biol. 41, 2575–2589. doi: 10.1007/s00300-018-2392-4
Gjøsæther H. (2009). “Commercial Fisheries (Fish, Seafood and Marine Mammals),” in Ecosystem Barents Sea (Trondheim: Tapir Academic Press), vol. 587 . Eds. Sakshaug E., Johnsen G., Kovac K., 373–414.
Gundersen K., Lien V. S., Møgster J. S., Nilsen J.E.Ø., Vindenes H. (2021). “Declining Silicate and Nitrate Concentrations in the Northern North Atlantic,” in Copernicus Marine Service Ocean State Report, Issue 5, Journal of Operational Oceanography, vol. 14:sup1, s23–s31. doi: 10.1080/1755876X.2021.1946240
Häkkinen S., Rhines P. B. (2004). Decline of Subpolar North Atlantic Circulation During the 1990s. Science 304, 555–559. doi: 10.1126/science.1094917
Hansen B., Larsen K. M. H., Hátún H., Kristiansen R., Mortensen E., Østerhus S. (2015). Transport of Volume, Heat, and Salt Towards the Arctic in the Faroe Current 1993–2013. Ocean Sci. 11, 743–757. doi: 10.5194/os-11-743-2015
Hátún H., Azetsu-Scott K., Somavilla R., Rey F., Johnson C., Mathis M., et al. (2017). The Subpolar Gyre Regulates Silicate Concentrations in the North Atlantic. Sci. Rep. 7. doi: 10.1038/s41598-017-14837-4
Hátún H., Chafik L., Larsen K. M. H. (2021). The Norwegian Sea Gyre - A Regulator of Iceland-Scotland Ridge Exchanges. Front. Mar. Sci. doi: 10.3389/fmars.2021.694614
Hátún H., Sandø A. B., Drange H., Hansen B., Valdimarsson H. (2005). Influence of the Atlantic Subpolar Gyre on the Thermohaline Circulation. Science 309, 1841–1844. doi: 10.1126/science.1114777
Helaouet P., Beaugrand G. (2007). Macroecology of Calanus Finmarchicus and C. Helgolandicus in the North Atlantic Ocean and Adjacent Seas. Mar. Ecol. Progr. Ser. 345, 147–165. doi: 10.3354/meps06775
Helland-Hansen B., Nansen F. (1909). The Norwegian Sea: Its Physical Oceanography Based Upon the Norwegian Researches 1900–1904. (Bergen: A/S John Griegs Boktrykkeri) 390 pp.
Hirche H.-J. (1997). “Life Cycle of the Copepod Calanus Hyperboreus at the Greenland Sea,” in Mar. Biol, vol. 128. , 607–618.
Holliday N. P., Bersch M., Berx B., Chafik L., Cunningham S., Florindo-López C., et al. (2020). Ocean Circulation Causes the Largest Freshening Event for 120 Years in Eastern Subpolar North Atlantic. Nat. Com. 11, 585. doi: 10.1038/s41467-020-14474-y
Holliday N. P., Hughes S. L., Bacon S., Beszczynska-Möller A., Hansen B., Lavin A., et al. (2008). Reversal of the 1960s to 1990s Freshening Trend in the Northeast North Atlantic and Nordic Seas. Geophys. Res. Lett. 35, L03614. doi: 10.1029/2007GL032675
ICES (2021a). Working Group of International Pelagic Surveys (WGIPS). ICES Sci. Rep. 3 (40), 481. doi: 10.17895/ices.pub.8055
ICES (2021b). Working Group on the Integrated Assessments of the Norwegian Sea (WGINOR; Outputs From 2020 Meeting). ICES Sci. Rep. 3 (35), 114. doi: 10.17895/ices.pub.8021
Jeansson E., Olsen A., Jutterstrøm S. (2017). Arctic Intermediate Water in the Nordic Sea–2009. Deep Sea Res. Part1 128, 82–97. doi: 10.1016/j.dsr.2017.08.013
Krahman G., Visbeck M., Reverdin G. (2001). Formation and Propagation of Temperature Anomalies Along the North Atlantic Current. J. Phys. Oceanogr. 31, 1287–1303. doi: 10.1175/1520-0485(2001)031<1287:FAPOTA>2.0.CO;2
Kristiansen I., Gaard E., Hátún H., Jonasdottir S., Ferreira A. S. A. (2016). Persistent Shift of Calanus Spp. In the Southwestern Norwegian Sea Since 2003, Linked to Ocean Climate. ICES J. Mar. Sci. 73, 1319–1329. doi: 10.1093/icesjms/fsv222
Kristiansen I., Hátún H., Jacobsen J. A., Eliasen S. K., Petursdottir H., Gaard E. (2022). Spatial Variability of the Feeding Conditions for the Norwegian Spring Spawning Herring in May. Front. Mar. Sci. doi: 10.3389/fmars.2022.823006
Kristiansen I., Hátún H., Petursdottir H., Gislason A., Broms C., Melle W., et al. (2019). Decreased Influx of Calanus Spp. Into the South-Western Norwegian Sea Since 2003. Deep Sea Res. Part I: Oceanographic Res. Papers 149, 103048. doi: 10.1016/j.dsr.2019.05.008
Larsen K. M. H., Hátún H., Hansen B., Kristiansen R. (2012). Atlantic Water in The Faroe Area: Sources and Variability. ICES J. Marine Sci. 69, 802–808. doi: 10.1093/icesjms/fss028
Lauvset S. K., Brakstad A., Våge K., Olsen A., Jeansson E., Mork K. A. (2018). Continued Warming, Salinification and Oxygenation of the Greenland Sea Gyre. Tellus 70A, 1–9. doi: 10.1080/16000870.2018.1476434
Møller E. F., Nielsen T. G. (2020). Borealization of Arctic Zooplankton—Smaller and Less Fat Zooplankton Species in Disko Bay, Western Greenland. Limnol. Oceanogr. 65, 1175–1188. doi: 10.1002/lno.11380
Macrander A., Valdimarsson H., Jónsson S. (2014). Improved Transport Estimate of the East Icelandic Current 2002–2012. J. Geophys. Res. Oceans 119, 3407–3424. doi: 10.1002/2013JC009517
Marshall J., Kushner Y., Battisti D., Chang P., Czaja A., Dickson R., et al. (2001). North Atlantic Climate Variability: Phenomena, Impacts and Mechanisms. Int. J. Climatol. 21, 1863–1898. doi: 10.1002/joc.693
Meincke J. (1978). On the Distribution of Low Salinity Intermediate Waters Around the Faroes. Dtsch. Hydrogr. Zeitschrift 31, 50–64. doi: 10.1007/BF02226000
Melle W., Ellertsen B., Skjodal H. R. (2004). “Zooplankton: The Link to Higher Trophic Levels,” in The Norwegian Sea Ecosystem Trondheim: Tapir Academic Press. Ed. Skjoldal H. R. (Tapir, Trondheim, Norway: Tapir Academic Press), 209–234.
Melle W., Runge J., Head E., Plourde S., Castellani C., Licandro P., et al. (2014). The North Atlantic Ocean as Habitat for Calanus finmarchicus: Environmental Factors and Life History Traits. Prog. Oceanogr. 129, 244–284. doi: 10.1016/j.pocean.2014.04.026
Meyer-Harms B., Irigoien X., Head R., Harris R. (1999). Selective Feeding on Natural Phytoplankton by Calanus Finmarchicus Before, During, and After the 1997 Spring Bloom in the Norwegian Sea. Limnol. Oceanogr. 44, 154–165. doi: 10.4319/lo.1999.44.1.0154
Mork K. A., Blindheim J. (2000). Variations in the Atlantic Inflow to the Nordic Sea–1996. Deep Sea Res. I 47, 1035–1057. doi: 10.1016/S0967-0637(99)00091-6
Mork K. A., Skagseth Ø., Ivshin V., Ozhigin V., Hughes S. L., Valdimarsson H. (2014). Advective and Atmospheric Forced Changes in Heat and Fresh Water Content in the Norwegian Se–2010. Geophys. Res. Lett. 41, 6221–6228. doi: 10.1002/2014GL061038
Mork K. A., Skagseth Ø., Søiland H. (2019). Recent Warming and Freshening of the Norwegian Sea Observed by Argo Data. J. Clim 32 (12), 3695–3705. doi: 10.1175/JCLI-D-18-0591.1
Read J. F., Pollard R. T. (1992). Water Masses in the Region of the Iceland Faeroes Front. J. Phys. Oceanogr. 22, 1365–1378. doi: 10.1175/1520-0485(1992)
Rey F. (2012). Declining Silicate Concentrations in the Norwegian and Barents Seas. – ICES J. Mar. Sci. 69, 208–212. doi: 10.1093/icesjms/fss007
Rossby T., Ozhigin V., Ivshin V., Bacon S. (2009). An Isopycnal View of the Nordic Seas Hydrography With Focus on Properties of the Lofoten Basin. Deep Sea Res. Part I 56 (11), 1955–1971. doi: 10.1016/j.dsr.2009.07.005
Søiland H., Prater M. D., Rossby T. (2008). Rigid Topographic Control of Currents in the Nordic Seas. Geophys. Res. Lett. 35, 1–5. doi: 10.1029/2008GL034846
Semper S., Pickart R. S., Våge K., Larsen K. M., Hátún H., Hansen B. (2020). The Iceland-Faroe Slope Jet: A Conduit for Dense Water Toward the Faroe Bank Channel Overflow. Nat. Com. 11, 5390. doi: 10.1038/s41467-020-19049-5
Skagseth Ø., Mork K. A. (2012). Heat Content in the Norwegian Se–2010. ICES J. Mar. Sci. 69, 826–832. doi: 10.1093/icesjms/fss026
Strand E., Bagøien E., Edwards M., Broms C., Klevjer T. (2020). Spatial Distributions and Seasonality of Four Calanus Species in the Northeast Atlantic. Prog. Oceanogr 185, 102344. doi: 10.1016/j.pocean.2020.102344
Swift J. H., Aagaard K. (1981). Seasonal Transitions and Water Mass Formation in the Iceland and Greenland Seas. Deep Sea Res. 28, 1107–1129. doi: 10.1016/0198-0149(81)90050-9
Tarling G. A., Freer J. J., Banas N. S., Belcher A., Blackwell M., Castellani C., et al. (2022). Can a Key Boreal Calanus Copepod Species Now Complete its Life-Cycle in the Arctic? Evidence and Implications for Arctic Food-Webs. Ambio 51 (2), 333–344. doi: 10.1007/s13280-021-01667-y
Utne K. R., Skagseth Ø., Wennevik V., Broms C. T., Melle W., Thorstad E. B. (2022). Impacts of a Changing Ecosystem on the Feeding and Feeding Conditions for Atlantic Salmon During the First Months at Sea. Front. Mar. Sci. 9, 824614. doi: 10.3389/fmars.2022.824614
Voet G., Quadfasel D., Mork K. A., Søiland H. (2010). The Mid-Depth Circulation of the Nordic Seas Derived From Profiling Float Observations. Tellus 62A, 516–529. doi: 10.1111/j.1600-0870.2010.00444.x
Vollset K. W., Urdal K., Utne K. R., Thorstad E. B., Sægrov H., Raunsgard A., et al (2022). Ecological Regime Shift in the North-East Atlantic Revealed From the Unprecedented Reduction in Marine Growth of Atlantic Salmon. Sci. Adv. 8, eabk2542.
Keywords: Arctic water, Atlantic water, nutrients variations, zooplankton, Norwegain basin, water masses
Citation: Skagseth Ø, Broms C, Gundersen K, Hátún H, Kristiansen I, Larsen KMH, Mork KA, Petursdottir H and Søiland H (2022) Arctic and Atlantic Waters in the Norwegian Basin, Between Year Variability and Potential Ecosystem Implications. Front. Mar. Sci. 9:831739. doi: 10.3389/fmars.2022.831739
Received: 08 December 2021; Accepted: 22 March 2022;
Published: 02 May 2022.
Edited by:
Gilles Reverdin, Centre National de la Recherche Scientifique (CNRS), FranceReviewed by:
Terry Whitledge, Retired, United StatesJan Marcin Weslawski, Polish Academy of Sciences, Poland
Naomi Penny Holliday, University of Southampton, United Kingdom
Copyright © 2022 Skagseth, Broms, Gundersen, Hátún, Kristiansen, Larsen, Mork, Petursdottir and Søiland. This is an open-access article distributed under the terms of the Creative Commons Attribution License (CC BY). The use, distribution or reproduction in other forums is permitted, provided the original author(s) and the copyright owner(s) are credited and that the original publication in this journal is cited, in accordance with accepted academic practice. No use, distribution or reproduction is permitted which does not comply with these terms.
*Correspondence: Øystein Skagseth, b3lzdGVpbi5za2Fnc2V0aEBoaS5ubw==