- 1Oxford Seascape Ecology Lab, School of Geography and the Environment, University of Oxford, Oxford, United Kingdom
- 2Seascape Analytics, Plymouth, United Kingdom
- 3Seascape Solutions, Mililani, HI, United States
- 4Emirates Nature - World Wide Fund for Nature, Dubai, United Arab Emirates
- 5Environment Agency-Abu Dhabi, Abu Dhabi, United Arab Emirates
- 6International Center for Biosaline Agriculture, Dubai, United Arab Emirates
- 7Departamento de Ecología e Hidrología, Universidad de Murcia, Murcia, Spain
Coastal seascapes are productive and diverse land-sea systems that provide many valuable benefits yet are increasingly threatened by human activity. Scaling up of nature-based solutions (NbS) to effectively protect, sustainably manage, and restore coastal seascapes is urgently required for mitigation of climate change and biodiversity loss while also providing socio-economic benefits. Evidence-based site selection is an important first step to improve the outcomes and avoid negative impacts when prioritizing NbS investments at national level. We developed a spatially explicit, integrative and culturally relevant ecosystem-based site selection process to identify a portfolio of seascapes for NbS consideration in the United Arab Emirates (UAE). The primary goal was to rank planning units based on potential for climate change mitigation action, positive impact to biodiversity and socio-economic benefits to people. The multi-criteria site-selection framework provided a rapid, transparent, repeatable and scalable tool. The highest weightings were assigned to blue carbon storage value, biodiversity conservation features, and local stakeholder preferred areas. Spatial proxies for benefits to people were represented by population density and accessibility to coastal seascapes, relative tourism and recreation potential, and importance of fish habitat and fishing grounds for food security. Participatory mapping of local knowledge and review of existing data ensured that both qualitative and quantitative criteria were reliable, up-to-date and locally relevant. Two distinct clusters of high suitability planning units were identified in the Abu Dhabi region and four along the north-western coast of the UAE. Most high suitability sites were located outside of existing marine protected areas. Alternative spatial scenarios without stakeholder bias underscored the suitability of sites identified through participatory mapping and highlighted additional priority sites for future scaling-up of NbS. A spatial corridor of medium and high suitability planning units across the region offers potential for designing well-connected NbS investments to accelerate and boost synergistic outcomes and increase resilience. The site selection framework provides a rapid tool that integrates local and global open access data at a range of scales with great potential for transferability to other regions worldwide.
1 Introduction
Effective and well-placed management actions to protect and restore coastal seascapes are urgently needed for climate change mitigation and to prevent further biodiversity loss, as well as securing ecosystem services that underpin social-ecological wellbeing (Waltham et al., 2020; Seddon et al., 2021). Ecosystems services are ecological structures and functions that actively or passively benefit humans and are increasingly at risk from human actions (Rocha et al., 2015; Halpern et al., 2019). The information and knowledge necessary to manage healthy and resilient coastal ecosystems are multifaceted, making the integration, analyses and translation of information into actionable knowledge a complex challenge (Abelson et al., 2020; Sheaves et al., 2021a). In the past decade, the umbrella term ‘nature-based solutions’ (NbS) has risen to prominence for reframing actionable policy on sustainable development, biodiversity conservation and climate mitigation and adaptation (Cohen-Shacham et al., 2016; Nesshöver et al., 2017). NbS are defined by the International Union for Conservation of Nature (IUCN) as actions to protect, sustainably manage and restore ecosystems, that address societal challenges effectively and adaptively, simultaneously providing human well-being and biodiversity benefits (Cohen-Shacham et al., 2019). The NbS actions are problem-focused and can include protection, restoration, habitat creation, and more recently have included nature-based enterprises such as ecotourism (Seddon et al., 2021).
Generally, the NbS approach seeks to provide multiple economic, social, and environmental benefits in a resource-efficient and adaptable way where it is intended that the benefits will form the steps along a transition pathway to a sustainable economy (Maes and Jacobs, 2017). For example, protecting, restoring or expanding mangroves would increase carbon sequestration and storage, maintain or increase biodiversity, protect human coastal communities from storm surges, provide food security through enhanced fish habitat supporting fisheries productivity, and generate opportunities for tourism and recreation (Su et al., 2021; Rahman et al., 2021).
Siting and scaling up NbS investments, however, is challenging and requires a pragmatic, pluralistic, and objective-driven approach that also has consistency in data use and analyses. Knowledge pluralism, a socio-political understanding of place, and the embedding of ecosystem services within a NbS site selection framework is part of a social movement toward more inclusive and localized decision making in sustainable development with potential for positive outcomes (Arkema et al., 2015; Giakoumi et al., 2018; Bennett, 2018). Lessons from global case studies show that successful projects usually involve local stakeholders and consider alternative livelihoods (Wylie et al., 2016). The collaborative process is also more likely to increase efficiency for limited management resources, reduce the risk of misdirected investment, link directly to policy, and focus attention on the key aspects that constrain and determine outcomes (Di Franco et al., 2020; Sheaves et al., 2021a). Multi-criteria spatial decision-support frameworks (Metzger et al., 2017; Adem Esmail and Geneletti, 2018; Pittman et al., 2018) that integrate social and ecological data, including local knowledge and stakeholder preferences, offer an appropriate tool for NbS site selection. Co-development of the entire NbS process assists in considering multiple competing priorities early in the process and increases the selection of locally relevant criteria and associated trade-offs, as well as increasing methodological transparency and the social acceptance of the project (Giordano et al., 2020).
Coastal ecosystem restoration is increasingly recognized as a scientifically credible NbS approach capable of supporting the delivery of multiple benefits. A growing body of evidence demonstrates that restoration interventions can be effective over large expanses (1,000s–100,000s ha), persist for decades, rapidly expand in size, be cost-effective, and generate social and economic benefits (Abelson et al., 2020; Bayraktarov et al., 2020; Saunders et al., 2020). Models indicate that restoration should produce greater outcomes when complemented by protected area management but can also be a cost-effective investment as an alternative to expanding protection (Possingham et al., 2015). It is becoming clear, however, that the success of restoration actions is context-dependent and, therefore, seascape context (e.g., surrounding socio-political and ecological variables) must be considered to avoid inappropriate site selection and for effective scaling up of NbS actions (Gilby et al., 2018; Bradley et al., 2020; Sheaves et al., 2021b).
Ecological connectivity is increasingly recognized as a critical process in the long term resilience of coastal ecosystems to disturbance and the flow of diverse benefits and biodiversity-positive outcomes (Barbier, 2018; Pittman et al., 2021; Chamberlain et al., 2022). In the global tropics, coastal seascapes often exist as spatially complex mosaics of interconnected habitat types (mangroves, seagrasses, algal flats, saltmarshes and coral reefs) where ecosystem services are modulated by the interactions between seascape patterns and ecological processes including animal movements and nutrient cycling (Carlson et al., 2021). Many of the key ecosystem services emerge from the synergistic interactions between combinations of habitat types (e.g., coral reefs, seagrasses, mangroves) (Moberg and Folke 1999; Sanchirico and Springborn, 2011) (Figure 1).
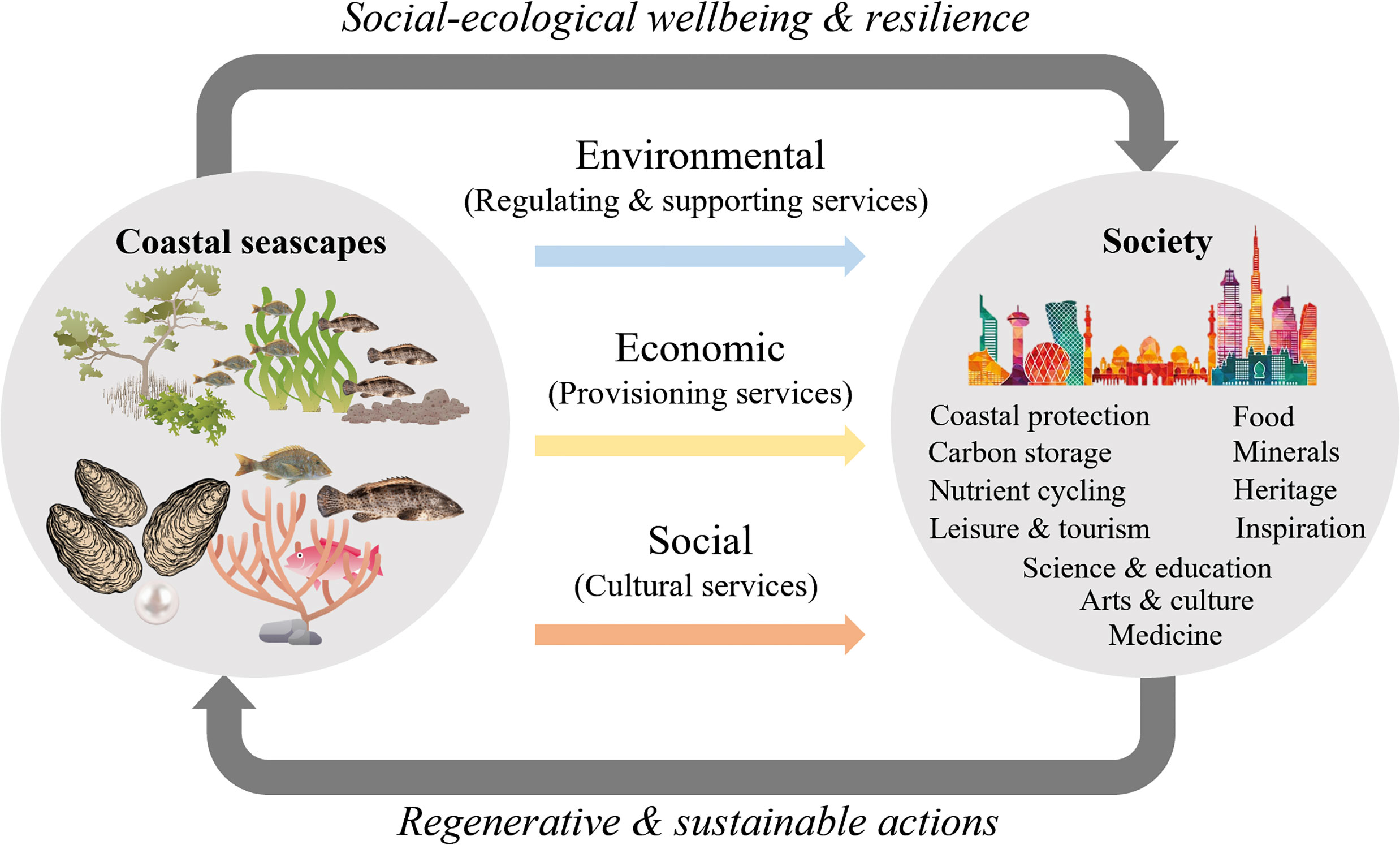
Figure 1 Mosaics of interconnected coastal habitat types underpin diverse ecosystem services that benefit human wellbeing that can be maintained by NbS for sustainability goals and ecosystem regeneration.
Seascape ecology has demonstrated that it is important to consider the ecological connectivity between coastal habitat types when valuing and managing seascapes for ecosystem services (Olds et al., 2016; Pittman et al., 2021). For example, the economic value of coastal protection from storms is greater when mangroves, seagrasses, and coral reefs co-occur (Guannel et al., 2016; Barbier, 2018; Carlson et al., 2021). Research indicates that in some places offshore fisheries catch is ecologically connected to the structure and function of nearshore vegetated seascapes (Hutchison et al., 2014; zu Ermgassen et al., 2021). To maintain and enhance benefits from synergistic interactions across seascapes requires that NbS strategies ensure seascape connectivity is recognized, protected and restored for sustainable and regenerative outcomes (Olds et al., 2016; Hilty et al., 2019), yet rarely is seascape composition (patch types and variety) and structural connectivity (spatial arrangement of patches) integrated into site selection frameworks (Pittman et al., 2018; Daigle et al., 2020). Rapid broad-scale active restoration with insufficient ecological and social consideration (e.g., local knowledge) could result in poor performance, negative (disservices) and unintended outcomes (Wodehouse and Rayment, 2019; Roman et al., 2021). Seascape ecology research suggests that to optimize performance of interventions, such as restoration, it is necessary to plan the design and placement of actions with a view to maximizing the potential for synergistic effects on animal populations and ecological functions (Gilby et al., 2021).
In the United Arab Emirates (UAE), and globally, increasing attention is being given to the potential for coastal NbS to enhance carbon storage capacity (i.e., blue carbon), benefit biodiversity and deliver multiple cultural and economic co-benefits (monetary and non-monetary) to people. For instance, in addition to generating revenue, activities such as tourism and recreation also contribute to the quality of life, sense of place, social connection, physical and mental well-being, learning, and other cultural ecosystem services. Public access to coastal ecosystems is instrumental to the flow and transformation of benefits from potential to realized (Ala-Hulkko et al., 2016). These are important components of national and local economies globally and in the UAE, and the neighboring Gulf States, are increasingly valued in forward looking strategies to diversify the economy away from fossil fuels (Shadab, 2018). Since the 1970s, UAE has been expanding mangrove forest extent and has ambitious new targets for mangrove protection and planting as part of the Nationally Determined Contributions (UAE, 2020) and commitment to the Glasgow Climate Pact (Decision CP.26). The political momentum for scaling up NbS is unprecedented, yet the investment pipeline is insufficiently populated with credible opportunities for accelerating investment, therefore, forming a barrier to strategic scaling up of NbS.
The primary goal of site selection was to identify and prioritize coastal seascapes with blue carbon potential and important biodiversity conservation features, and where NbS interventions can conserve, restore, and promote resilient coastal ecosystems that deliver multiple socio-economic benefits. For the Arabian Gulf coast of the UAE, we developed a spatially explicit and integrative site selection process by applying simple heuristic rules where sites were scored and ranked according to a set of stakeholder-derived criteria and weightings. The project, led by Emirates Nature-WWF, recognized the need to tackle the climate and biodiversity crises together where positive impacts to climate mitigation and biodiversity are desired equally (Pörtner et al., 2021) and socio-economic ecosystem services (i.e., tourism, recreation, and fisheries) are considered as co-benefits.
A rapid framework for site selection was required to align with management timelines and to be reliable, geographically comprehensive and sufficiently relevant to the local ecology, socio-political context and the key performance indicators. The key performance indicators target ecosystem services that are provided by coastal seascapes and recognized as high priorities by local and global sustainability policies. To support decision making, we developed and applied a spatial multi-criteria decision framework to identify and rank suitable sites for immediate (within 1 to 3 years) and future NbS investment in the UAE. The proposed NbS actions under consideration included oyster reef creation, mangrove restoration/afforestation, planting of edible saltmarsh plants, and enhanced area-based protection.
It was also important that the framework could be scaled up and transferable to other locations. To provide an alternate portfolio of sites without social bias, we also conducted the prioritization analysis with equal weighted criteria and by excluding stakeholder preferred areas. Although demonstrated in the UAE, the framework which couples open access global datasets and tools with local data is equally suitable for application elsewhere where there is a need to accelerate NbS strategies to meet a range of national and global policy goals (e.g., Global Climate Action, Aichi Biodiversity Targets, SDGs, post-2020 Global Biodiversity Framework) requiring a highly collaborative generation of solutions.
2 Materials And Methods
2.1 Study Area
The geographical focus for NbS was on the coastal seascapes of Abu Dhabi emirate and the Northern Emirates (Ajman, Ras Al Khaimah, Sharjah, and Umm Al Quwain) along the Arabian Gulf coast of the United Arab Emirates (Figure 2). The focal area (53,680 km2) was divided into hexagonal planning units of 4 km2 each. Relatively high spatial resolution of the planning units was possible because of the detailed coastal habitat maps produced by Emirates Nature-WWF (Mateos-Molina et al., 2020; Mateos-Molina et al., 2021a) and the Environment Agency Abu Dhabi (EAD, 2018) and a national systematic conservation planning project to identify and map Areas of Particular Importance to Marine Biodiversity (APIMBs) (Ben Lamine et al., 2020). Dubai and UAE’s coastline in the Gulf of Oman were outside of the geographical scope due to key spatial data gaps on biodiversity that precluded the application of several essential criteria. Emirates Nature-WWF initiated and coordinated the project alongside government and academic partners.
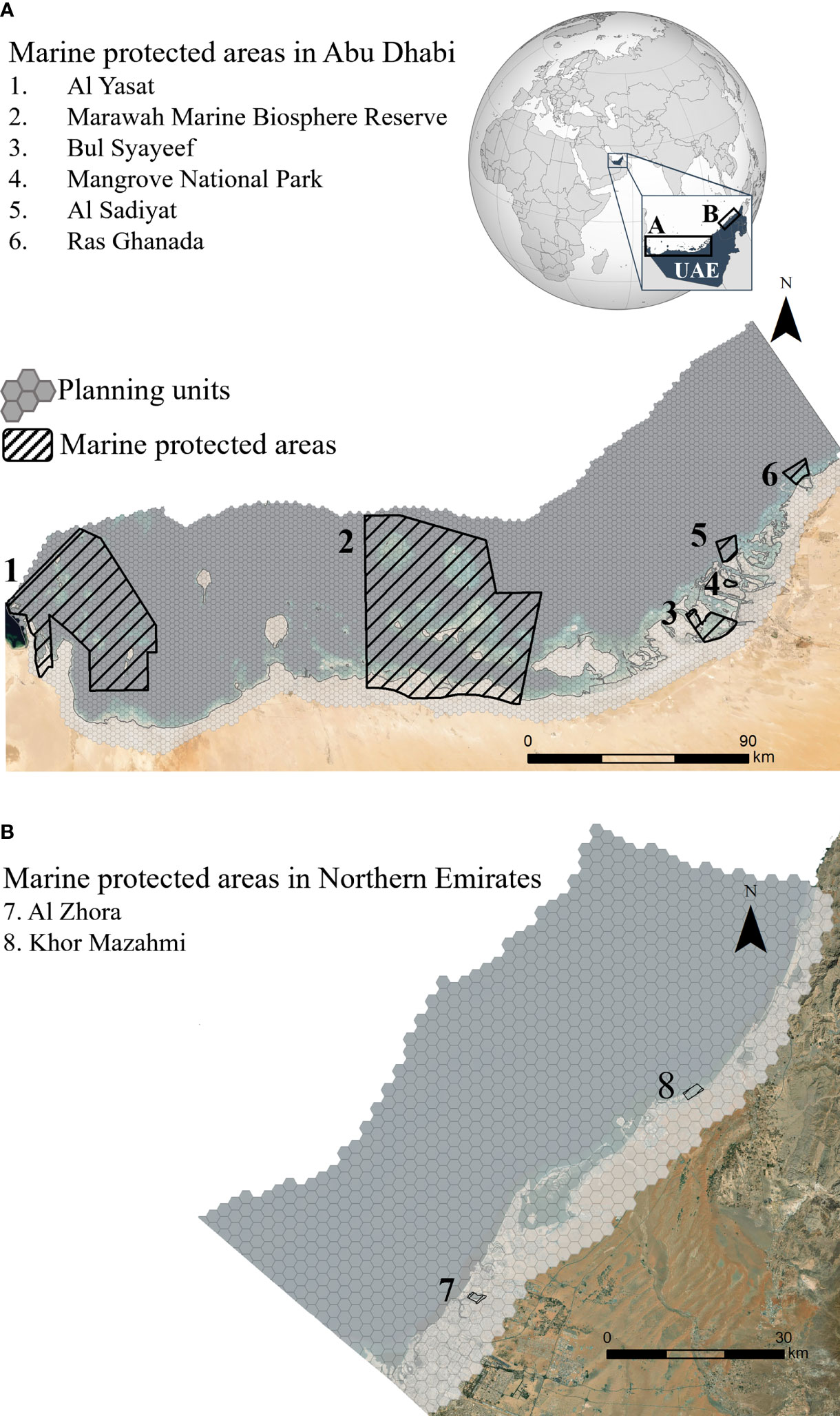
Figure 2 Map of the two study areas. (A) Abu Dhabi, and (B) Northern Emirates (Ajman, Ras Al-Khaimah, Sharjah and Umm Al-Quwain) in the United Arab Emirates (UAE). Planning units of the analytical grid were arranged in a spatial grid of 4 km2 hexagonal cells.
The population of the UAE is approximately 10 million people, mostly living in a rapidly growing urban seascape that has experienced widespread coastal habitat modification (Burt and Bartholomew, 2019). The UAE has several high-level and far-reaching policies aimed at diversifying its economy away from fossil fuels, growing the tourism sector and achieving sustainable living (e.g., Vision 2021 and the Green Growth Strategy) and is actively aligning government ministries to address the UN Sustainable Development Goals and UNFCCC (i.e., Nationally Determined Contributions, Net Zero by 2050, Climate Pact). The UAE’s tourism-led economic growth is forecast to rise to 12.4% of Gross Domestic Product by 2027, employing 6% of the working population (Shadab, 2018). Fish and fisheries (recreational, subsistence and commercial) in the UAE are important for food security and are an integral part of the cultural heritage. Archaeological evidence indicates that fisheries have been a significant subsistence activity for the people of the region since the 5th millennium BC (Lidour et al., 2020). Today, several commonly targeted fish species are considered overexploited (UAE National Framework Statement for Sustainable Fisheries 2019-2030). Climate change models predict an estimated drop in future fisheries catch potential by more than 40% by 2090 for the Arabian Gulf coast of the UAE, threatening food security and human health in the region (Golden et al., 2016; Wabnitz et al., 2018).
2.2 Site Selection Framework
A seven-step site selection framework was co-designed with stakeholders to identify and evaluate criteria, review the outcomes from the site selection models and select a subset of candidate seascapes for NbS interventions from the highest scoring sites (Figure 3). Our approach was structured, consultative, and spatially explicit and therefore fits the broad methodological approach of systematic conservation planning where ecosystem services are now being integrated (Villarreal-Rosas et al., 2020). Subsequent to the rapid site selection, a sub-seascape site selection process will provide a more detailed ecosystem services assessment a to inform the optimal placement and design of specific NbS at demonstration sites.
2.3 Integrating Stakeholder Knowledge and Preferences
The multi-stakeholder engagement was central to our way of working and aligned with Goal 2 (to consider local knowledge) and Goal 5 (to enhance cooperation and coordination with competent authorities) of the UAE’s National Biodiversity Strategy and Action Plan (2015-2021) and the 2030 Agenda for Sustainable Development. Recognizing coastal seascapes as complex social-ecological systems, the project was co-developed with management practitioners and government scientists where a participatory process that integrates stakeholders’ local ecological and cultural knowledge was crucial to produce relevant, realistic and locally accurate outputs (Elliott, 2013). Throughout the site selection process, the project provided opportunities for stakeholder opinions and preferences on sites to be voiced, documented and mapped through participatory mapping. Participatory mapping is a direct method for knowledge co-production and is an effective way to address knowledge gaps and reveal and integrate intangible and qualitative local cultural information and current opinions (Kenter et al., 2016; Burdon et al., 2019). In Workshop 1, stakeholders agreed to share local knowledge on the following criteria:
1) Preferred sites for current NbS investments based on local knowledge of land use and feasibility of NbS implementation.
2) Unsuitable sites for current NbS investments based on local knowledge of land use and feasibility of NbS implementation.
3) Locations where NbS related (e.g., mangrove restoration/afforestation) activities have occurred; and
4) Locally important fishing grounds (artisanal and subsistence fishing).
Considering locations of historical restoration actions recognizes that some investors may want to add value to existing investments while others may want to avoid existing investments. The task of mapping preferred sites for NbS investments can be interpreted as stakeholder opinions on sites with high potential for success based on perceived feasibility that included consideration of potential barriers and opportunities (e.g., field logistics, political acceptance, historical knowledge, compatible land ownership, confidential knowledge of future land use, achieving key project objectives).
Due to Covid-19 restrictions all engagement was coordinated through remote workshop participation using Microsoft® Teams software with participatory mapping conducted using Mural™ software. Intermittent correspondence was also conducted via email. Using Mural™ software, an online digital workspace for visual collaboration, workshop participants were provided with coastal maps of the UAE and asked to draw polygons over locations that, in their opinion, represented the criteria. In addition, they were asked to add notes to provide more information on why each area was delineated. Access to the maps remained open for three days after the workshop to allow participants time to reflect on the task and to consult colleagues.
2.4 Selecting and Weighting the Importance of Criteria
Where criteria-weighting methods are applied to drive the site prioritization scenarios it is important that the selection and weightings of criteria undergo rigorous discussion and review. The site selection process included three rounds of review and detailed discussion until the final criteria and weightings had reached unanimous agreement among the project team (Figure 3). The initial scoping and selection of criteria and weightings took place at the first project meeting. In Workshop 1, existing spatial data from EN-WWF, project partners and other sources were quality checked and presented as an initial set of candidate criteria data layers for review through an online survey and were then the focus of discussion. After Workshop 1, shortlisted criteria were evaluated and refined. At Workshop 2, the first model results were presented to stakeholders for review and discussion before running the final model. The stakeholder consensus was for a high weighting to be applied to planning units with blue carbon storage value, high biodiversity value and the stakeholder preferred areas delineated through the participatory mapping process (Table 1). A medium weighting was assigned to potential co-benefits for people (access, tourism and recreation, food security associated with fisheries). Other datasets considered but not used in the site selection model because of high uncertainty associated with outdated information, or geographical gaps included: coastal vulnerability to climate change, historical coastal habitat change, construction and dredging activity, land ownership and critical infrastructure.
2.5 Spatial Data Layers as Spatial Proxies for Criteria
Spatial proxies for individual criterion were developed and grouped by key performance indicators: 1) climate change mitigation impact (blue carbon storage); 2) positive biodiversity impact; 3) financing and feasibility, 4) other benefits to people achievable through accessibility, tourism and recreation potential, and 5) fisheries value of coastal seascapes. The spatial data sources representing the multi-criteria indicators included maps of seascape patterns, outcomes from biodiversity conservation prioritization, physical infrastructure, participatory mapping, and spatial proxies for variables that can influence the potential for benefits to people (Table S1, Supplementary Material). Because the focus was on a rapid site-selection process, a full data quality assessment was not conducted. However, the habitat maps and APIMBs and their associated input data underwent an extensive development process that included stakeholder input and validation (Ben Lamine et al., 2020; Mateos-Molina et al., 2020; Mateos-Molina et al., 2021a). Elsewhere we relied primarily on authoritative global datasets and stakeholder knowledge to fill remaining data gaps.
2.5.1 Climate Change Mitigation Impact (Blue Carbon Storage Capacity)
A spatial proxy for carbon storage value was developed by applying mean carbon stock values measured in discrete habitat types in the UAE (Schile et al., 2017) to coastal habitat maps of the two study areas (Mateos-Molina et al., 2020; Mateos-Molina et al., 2021a). Four data sources of mapped coastal habitat were merged to create the most up-to-date unified map of vegetated blue carbon habitat for the study area (Table S1). Vegetated coastal blue carbon habitats (seagrass, algal mats, mangroves, salt marsh, and coastal supratidal mudflats known locally as coastal sabkha) were attributed to the planning units. Total carbon stock (Mg C) was calculated for each planning unit by multiplying the area (ha) of each blue carbon habitat by the mean carbon stock values (Mg C ha-1) from Schile et al. (2017) and summing the result (Figure 4).
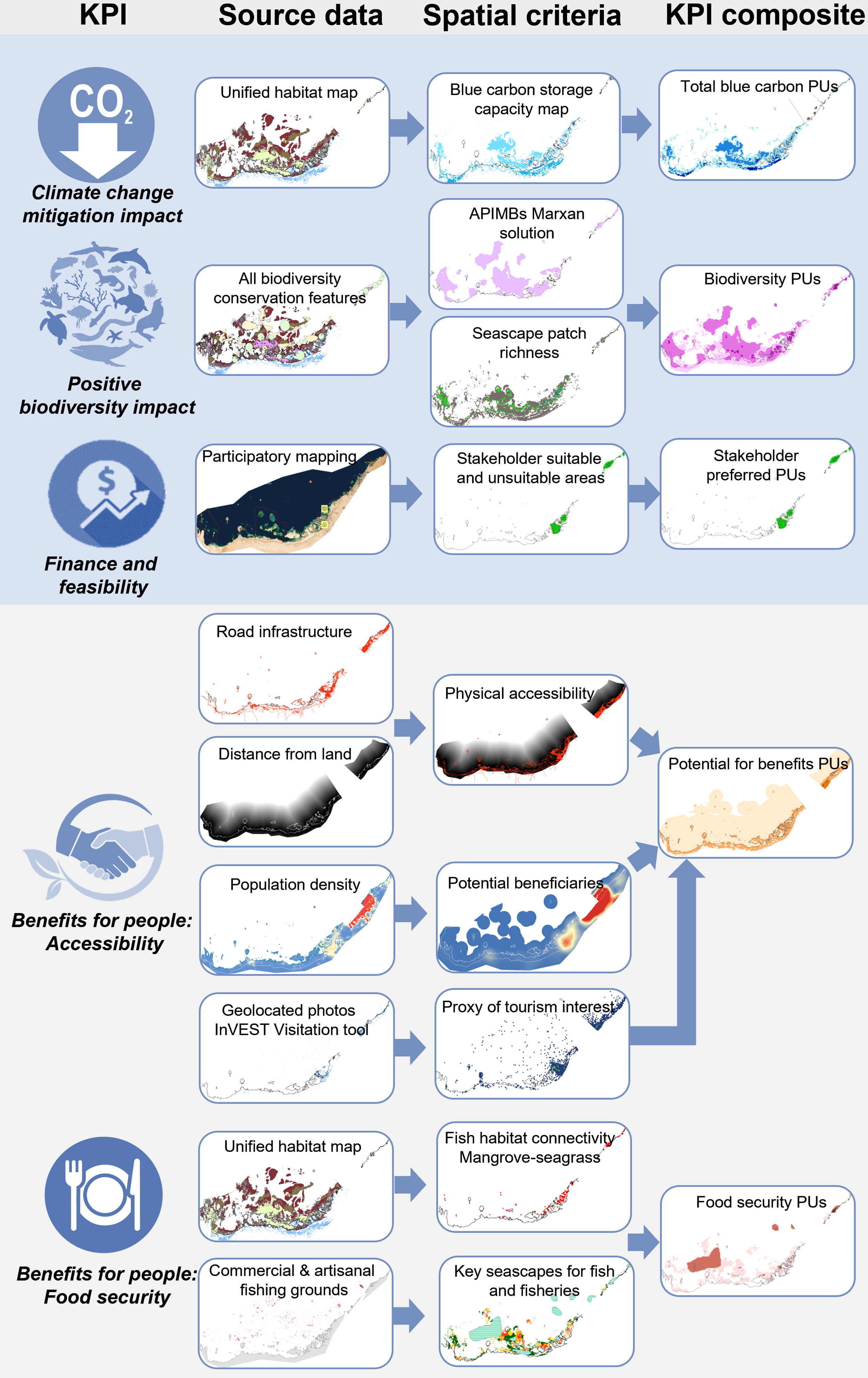
Figure 4 Development of spatial proxies to represent criteria to identify sites suitable for delivering key performance indicators (KPI). PUs, planning units.
2.5.2 Positive Biodiversity Impact
Two spatial criteria represented the regional spatial patterns of biodiversity: 1) Areas of Particular Importance for Marine Biodiversity (APIMBs), and 2) marine habitat diversity calculated as the number of critical habitat types in each planning unit. APIMBs were previously identified and mapped through an expert stakeholder-informed systematic conservation planning process using Marxan software (Ben Lamine et al., 2020; Mateos-Molina et al., 2021b). This analysis incorporated dredging as one of the most impactful and widespread pressures on marine species and ecosystems in the UAE due to its association with multiple activities in the Arabian Gulf, such as land reclamation and creation of transport channels for urban development. Planning units that overlapped APIMBs were identified as a priority space for biodiversity for this project (Figure 4).
Habitat diversity (number of patch types) has been linked positively to ecosystem multifunctionality from microbial assemblages to fish and beyond (Lefcheck et al., 2015; Alsterberg et al., 2017), offering a potentially useful spatial proxy for local biodiversity patterns. In addition to APIMBs, habitat diversity was calculated as the number of mapped critical habitats represented in the most recent marine habitat maps for Abu Dhabi and the Northern Emirates. Nine classes of benthic habitat types were included: algal mat, coral reef, halophytes, oyster reef, mangrove, mud flat, coastal sabkha, saltmarsh and seagrass. In the case of coral reefs all classes with coral were subsumed into a single class referred to generically as coral reefs. The total number of critical habitats occurring within 500 m of each critical habitat unit was calculated and attributed to each planning unit (Figure 4).
2.5.3 Finance and Feasibility of Implementation
Through participatory mapping and targeted discussions with stakeholders’ specific geographical areas were identified as being: 1.) most suitable for current NbS investments, 2.) unsuitable for current NbS investments, and 3.) areas with current or historical restoration or habitat creation such as mangrove planting. These areas were mapped by participants during Workshop 1 using a digital visual collaboration software (Mural™). Selection of suitable areas for current NbS investments were informed by collective local knowledge of site feasibility (field logistics, political acceptance, historical knowledge, confidential knowledge of future land use, potential for achieving project objectives). The proportion of mapped suitable areas within each planning unit was calculated (Figure 4). A rapid site visit to suitable areas took place after the participatory mapping to confirm if these areas were still suitable locations for demonstrating NbS.
In contrast, unsuitable sites were identified as private land, sensitive critical infrastructures and areas designated for future urban developments. Areas unsuitable for immediate NbS investments and areas of active restoration were not included as a criteria in the site selection model. Instead, these mapped areas remained as informative spatial overlays for decision makers to reference for future potential NbS.
2.5.4 Benefits for People: Site Accessibility and the Potential for Tourism and Recreation
The flow of benefits to people from NbS investments will be mediated by population proximity and physical access to nature (White et al., 2013; Pittman et al., 2019). Greater distance and low accessibility associated with local transport infrastructure and cost can reduce the frequency of human-seascape interactions. The benefits for people key performance indicator focused on physical accessibility and the potential for nature-based enterprise and recreational opportunities associated with coastal seascapes. Spatial proxies were created to represent the following criteria: 1) historical patterns of coastal tourism and recreation indicative of visitation patterns; 2) proximity to human populations as a proxy for the potential for ecosystem service delivery; 3) physical accessibility to coastal locations via road infrastructure; and 4) distance to land as a proxy for costs of public visitation and project feasibility associated with project field costs (i.e., higher costs for working in offshore areas).
2.5.4.1 Accessibility of Coastal Seascapes via Roads
Transportation infrastructure data for the UAE was acquired from OpenStreetMap (http://download.geofabrik.de/asia.html). Roads were categorized into four types relating to ease/speed of travel: 1) paths (unsuitable for cars), 2) small roads/tracks, 3) minor roads/highway links, and 4) major roads and highways. Planning units were assigned road accessibility scores (1-4) based on the most accessible (highest score) road category (Figure 4).
2.5.4.2 Accessibility Based on Distance From Land
The Euclidean distance from coastline was calculated for each pixel of a 100 m2 resolution grid spanning the study area to a maximum distance of 65 km offshore, equivalent to the seaward boundary of the Marawah Biosphere Reserve. This was agreed by stakeholders during Workshop 2 to be the maximum distance from shore that it was logistically feasible to implement any NbS project. Mean distance from coast was calculated for each planning unit (Figure 4).
2.5.4.3 Population Proximity to Coastal Seascapes
To incorporate the spatial patterns of human population density across the study area, a map of the 2020 population density of UAE was obtained from WorldPop (https://www.worldpop.org/) at 100 m2 resolution. Total population within 15 km of each grid cell was calculated and assigned to each corresponding 100 m2 cell and the mean of all cells was calculated for each 4 km2 hexagonal planning unit (Figure 4).
2.5.4.4 Tourism and Recreation Interest
Geotagged user-generated images shared on social media provides a pragmatic and rapid tool to map the patterns of visitation to coastal seascapes where no other relevant geospatial information is available (Wood et al., 2013; Arkema et al., 2021). The InVEST version 3.9 Recreation model (The Natural Capital Project) was used to calculate average annual photo-user-days (PUD) using the metadata from geotagged photographs available on the online photo sharing platform Flickr. Photo user days are defined as the number of days for which each unique user submitted photos in each location (i.e., planning unit) as identified by a unique ID, the timestamp, and geotag of each photo. Average annual PUD for the years 2005 to 2017 combined were mapped for each planning unit in this project (Figure 4).
2.5.5 Food Security Through NbS Focus on Fish Habitat
In practice, identifying and linking fishing grounds to coastal seascapes is often challenging due to the lack of reliable data on spatial patterns of fishing effort and the habitat use patterns of fished species (Sheaves et al., 2020). In the absence of reliable fisheries data, we created a simple linear distance-based spatial proxy to identify coastal seascapes with potential value to fisheries by identifying coastal seascapes proximal to fishing grounds.
Participatory mapping with stakeholders identified locally important nearshore fishing grounds (artisanal, recreational, and subsistence) and the proportion of identified areas within each planning unit was calculated. Commercial offshore fishing grounds were identified using nighttime satellite vessel detection data (2017-2020 inclusive) (Earth Observation Group). This method uses VIIRS (Visible Infrared Imaging Radiometer Suite) satellite data from a polar-orbiting satellite operated by NASA (US National Aeronautics and Space Administration) and NOAA (National Oceanic and Atmospheric Administration) to detect fishing boats at night from their deck lights. While it does not identify fishing vessels exclusively, fishing is known to occur during the nighttime in the UAE and this layer was used to identify areas where fishing boats are likely to operate as a subset of all vessels visible at night.
The sum of average vessel detections within 5 km of intertidal and subtidal coastal fish habitat types (all hard-bottom habitats, seagrasses, and mangroves) was calculated for each planning unit (Figure 4).
In the absence of reliable information on the spatial scale of fish movements, a scale of 5 km was selected to encompass the approximate scales of movement for fish known to be of commercial value in the Arabian Gulf (Green et al., 2015). Many of these species are known to use nearshore seascape mosaics as nurseries (Nagelkerken et al., 2015) before moving offshore where they are exposed to fisheries. At least 20% of fish species associated with coral reef ecosystems also rely on non-reef coastal habitat types (i.e., macroalgal beds, seagrasses, mangroves) and more than half of these are target species for local fisheries (Sambrook et al., 2019).
2.5.5.1 Seascape Connectivity
To consider the potential for positive synergistic effects from the proximity of critical habitat types, areas where seagrass and mangrove co-occurred within 500 m of one another (1 km diameter buffer) were mapped using the unified coastal habitat map. The proportion of co-occurring seagrass and mangrove habitats was calculated for each planning unit (Figure 4).
2.6 Integrating Criteria to Address the Objectives
Climate change mitigation impact (enhancing blue carbon) and finance and feasibility were each represented by a single criterion layer (Figure 5) and other key performance indicators were represented by multi-criterion composite layers grouped and merged by indicators as follows: 1) Positive biodiversity impact integrated APIMBs and critical habitat richness; 2) Benefits for people integrated tourism and recreation visitation (PUD), proximity to people (population density), and accessibility (distance to coast and proximity to roads); 3) Food security integrated local fishing grounds, coastal seascapes within 5 km of commercial fishing boats, and connected mangrove and seagrass habitats (Figure 5). Although each criterion layer showed different spatial patterns, a correlation analysis indicated that only climate change impact and the biodiversity criteria were significantly correlated (Pearson r = 0.4) suggesting that no redundancy occurred among criteria.
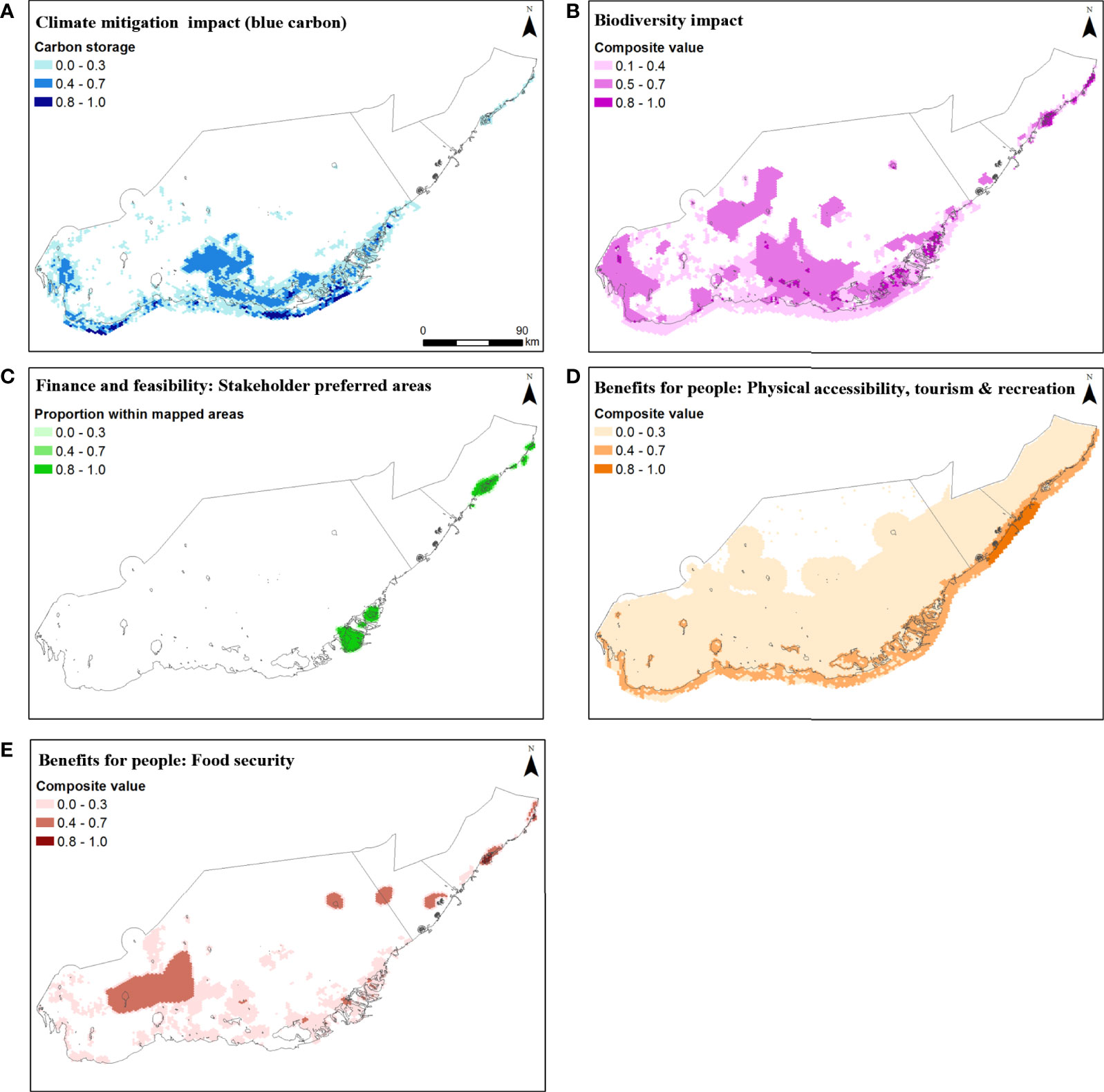
Figure 5 Composite spatial criteria representing key performance indicators including: (A) climate change mitigation impact (blue carbon storage), (B) positive biodiversity impact, (C) finance and feasibility (stakeholder preferred areas), and benefits for people via (D) accessibility, and (E) food security.
Criteria data layers were summed by key performance indicator and re-scaled between 0-1. Next, the five indicator composite data layers were summed by planning unit with highly weighted criteria of climate change mitigation impact (blue carbon), positive biodiversity impact, and stakeholder determined finance and feasibility each multiplied by two prior to summation. The weighted criteria final scores were scaled between 0-10 and each planning unit was classified using equal intervals into either a high (6.6-10), medium (3.4-6.5), or low (0-3.3) suitability class across the study area. To determine the contributions of the mapped composite indicator criteria to high suitability planning units we calculated the average score of all high suitability planning units by indicator and plotted the results in bar charts. At the request of stakeholders, the marine and coastal areas farther than 65 km from the coastline were excluded due to challenges in data availability, access, and related logistical considerations. MPAs and areas deemed by stakeholders to be not suitable for immediate NbS investment and areas with existing restoration action were not included in the model are instead presented as overlay spatial data for consideration.
2.7 Alternative Models With Different Criteria and Weightings
Two alternative multi-criteria site selection models were computed to explore the influence of removing stakeholder determined weightings and stakeholder preferred areas as used in Scenario 1 (Figure 6). Alternative Scenario 2 applied equal weighting for all criteria, including stakeholder preferred areas, and Alternative Scenario 3 applied equal weighting to all criteria and excluded stakeholder preferred areas.
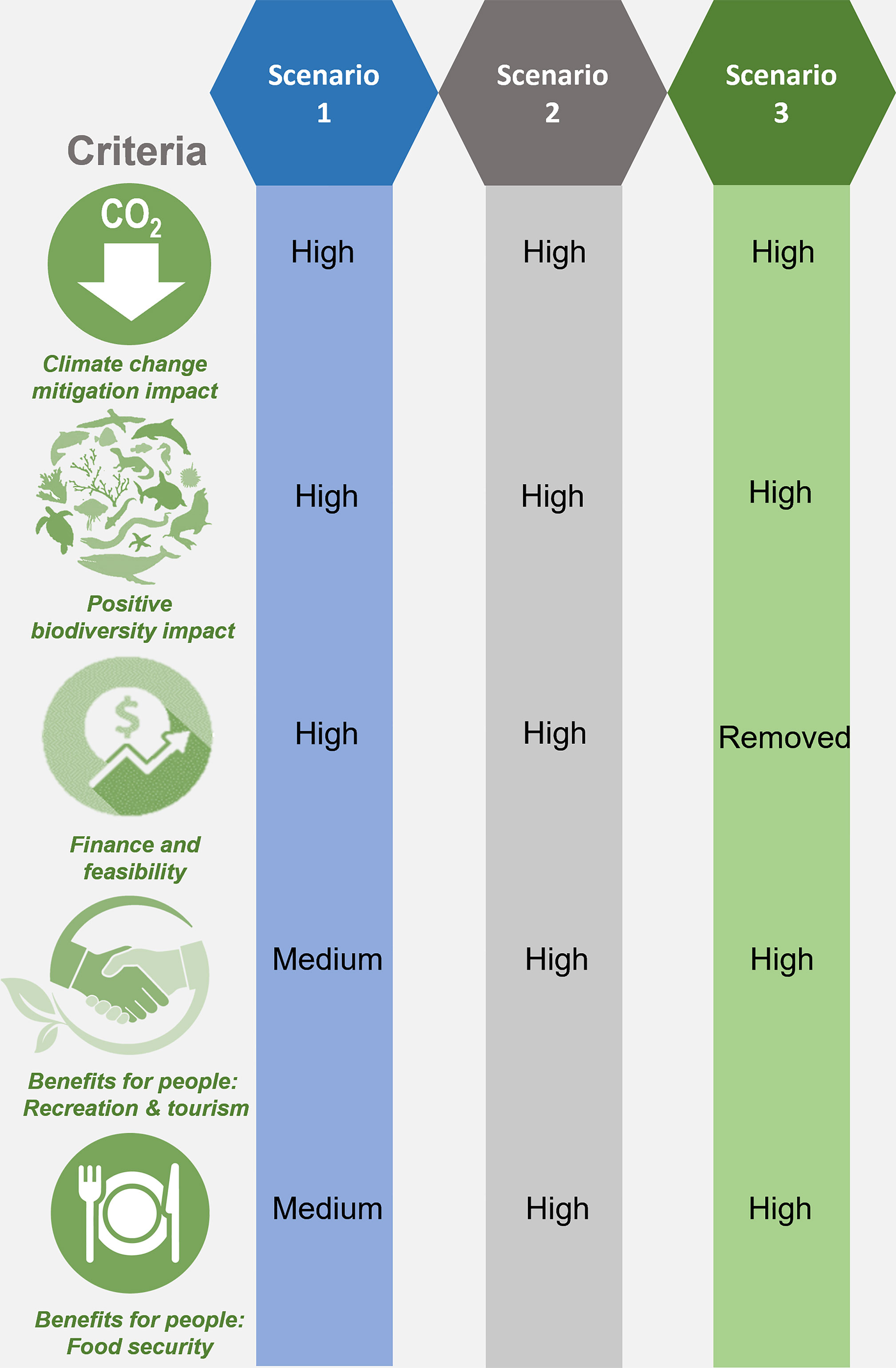
Figure 6 Three site selection model scenarios computed: Scenario 1 stakeholder-defined weightings and preferred areas for immediate investments; Scenario 2 equal weightings and stakeholder preferred areas for immediate investments, and Scenario 3 equal weightings and excluding stakeholder preferred areas.
2.8 Delineating Candidate Seascapes for NbS Actions
Clusters of high-scoring planning units were used to delineate candidate seascapes as operational units for implementing NbS. Seascapes were delineated based on visually distinct coastal geomorphology and hydrological patterns that appear to contain structurally coherent ecological patterns with potential for important ecological connectivity. For coastal lagoons, the terrestrial boundaries and the seaward openings guided the delineation. Although only provisional, the delineations were intended to encourage a whole-site approach that encompasses mosaics of interconnected habitat types and sufficient surrounding context to assess local factors that may promote or hinder NbS performance.
3 Results
The initial model scenario (Scenario 1) using stakeholder-informed weightings and preferred areas for immediate investment resulted in two large clusters of high scoring planning units (Khor Faridah and Bul Syayeef) and one high scoring planning unit (Mangrove National Park) in Abu Dhabi and four geographically distinct locations in the Northern Emirates. High priority areas identified in the initial model (Figures 7A, 8A) aligned well with stakeholder preferred areas identified through participatory mapping (Figure 5C). Spatial overlay of ‘unsuitable sites’ showed that none of the areas identified by stakeholders as currently unsuitable for NbS investment coincided with the highest suitability planning units in the model (Figures 7A, 8A). Participatory mapping data on known restoration activities revealed that two of the Abu Dhabi high suitability clusters (Figure 7A) and all four in the Northern Emirates have undergone some active restoration (Figure 8A). The two areas with the highest number of high scoring planning units were Khor Faridah, a large coastal lagoon seascape close to Abu Dhabi city, and Khor al Beidah, a large coastal lagoon seascape close to the city of Umm Al Quwain, Northern Emirates (Figures 9B, 11). These results were consistent across all three models, including those excluding stakeholder weightings and preferred areas. These two sites (Khor Faridah and Khor Beidah) have been confirmed as the project’s initial demonstration sites for NbS.
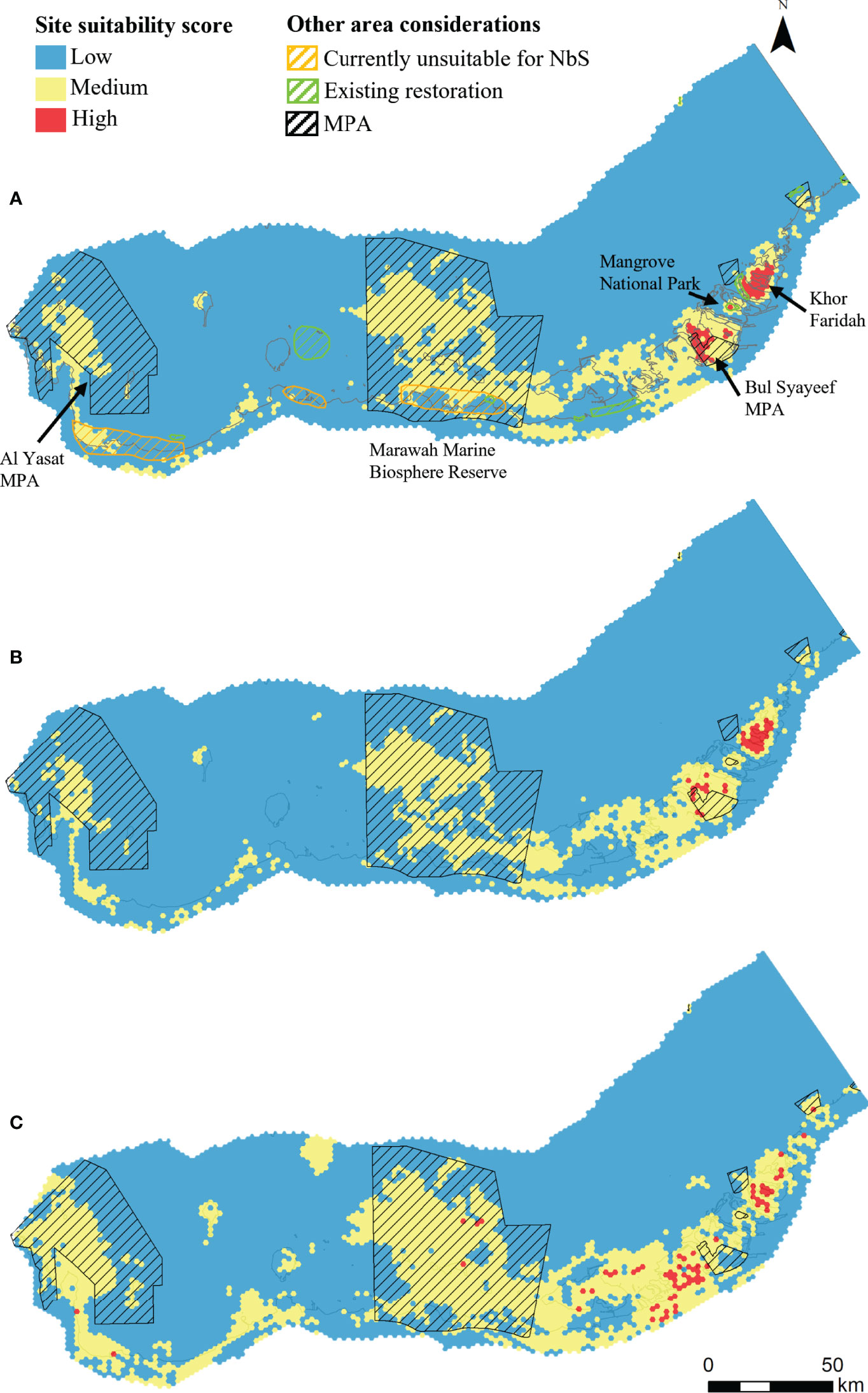
Figure 7 Site suitability scores for Abu Dhabi where the model uses (A) Scenario 1: Stakeholder defined weightings and preferred areas; (B) Scenario 2: Equal weighting for all criteria including stakeholder preferred areas; and (C). Scenario 3: Equal weighting for all criteria and stakeholder preferred areas excluded. Scores are classified into equal intervals of high (red), medium (yellow) and low (blue) suitability.
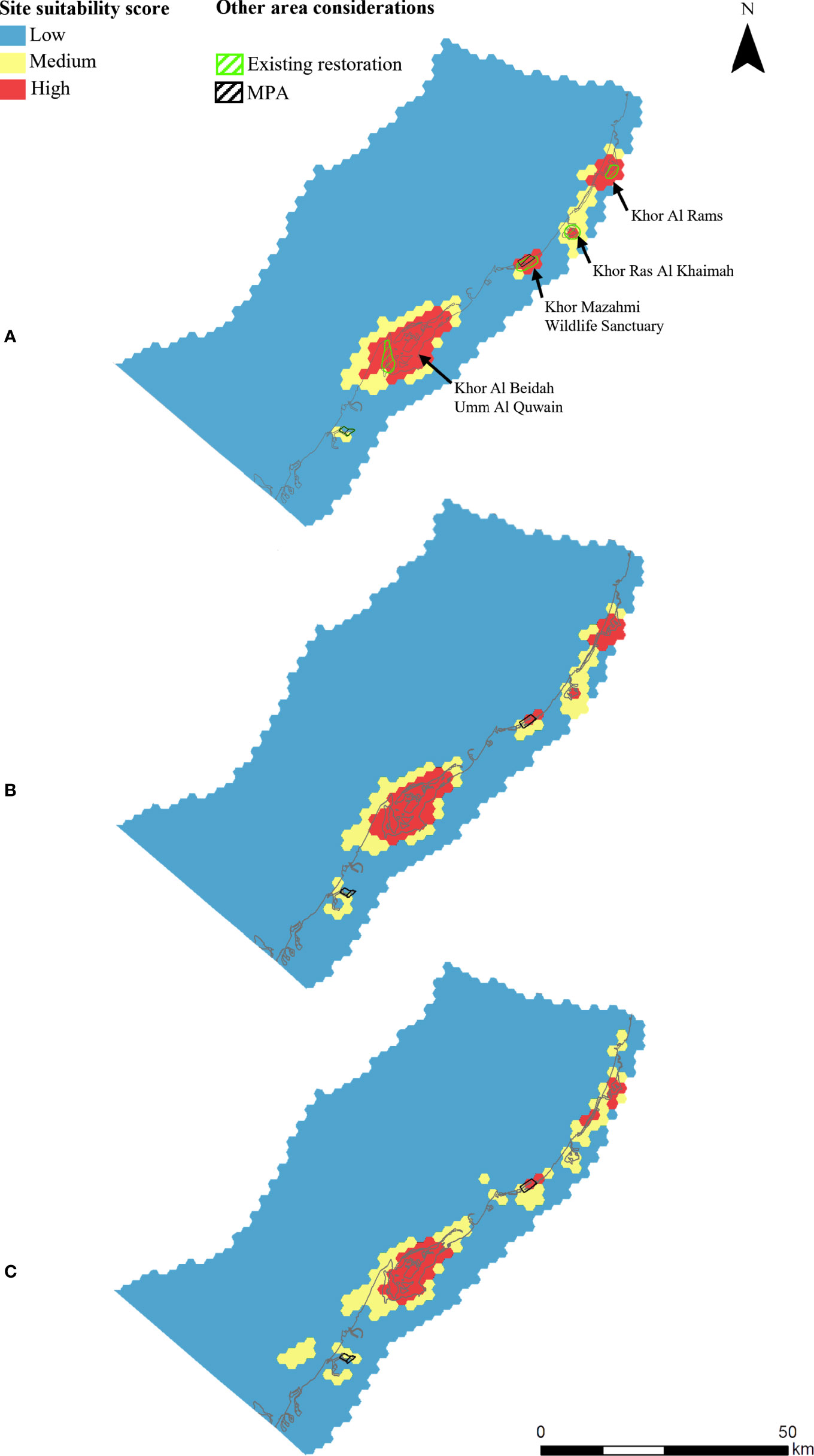
Figure 8 Site suitability scores for Northern Emirates where the model uses: (A) Scenario 1: Stakeholder defined weightings and preferred areas; (B) Scenario 2: Equal weighting for all key criteria including stakeholder preferred areas; and (C) Scenario 3: Equal weighting for all criteria and stakeholder preferred areas excluded. Scores are classified into equal intervals of high (red), medium (yellow) and low (blue) suitability.
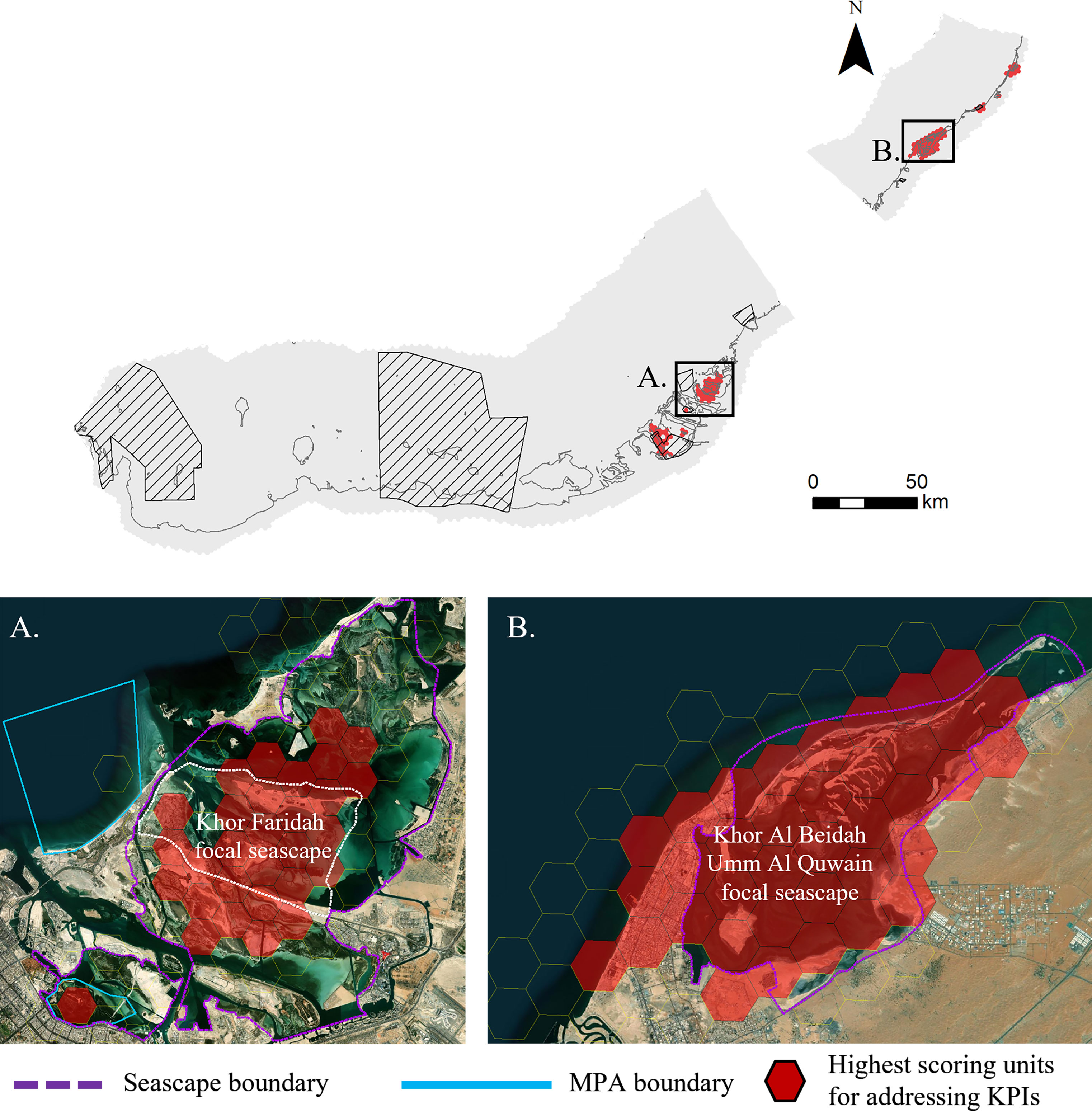
Figure 9 The high scoring planning units from Scenario 1 using stakeholder defined weightings and stakeholder preferred areas. Insets show the two largest high scoring clusters and the boundaries defining the operational ‘seascape’ units for implementing NbS: (A) Khor Faridah in Abu Dhabi, and (B) Khor Al Beidah in Umm Al Quwain.
3.1 Abu Dhabi’s High Suitability Seascapes for Immediate NbS Investment (Scenario 1)
A large cluster of high suitability planning units occur in the Khor Faridah region (Figures 7A, 9A). The overlaying of previous NbS investments shows extensive historical mangrove restoration/afforestation action within the southern portion of Khor Faridah and within the Mangrove National Park. Another high suitability cluster overlaps with the Bul Syayeef MPA and a single high suitability planning overlaps the Mangrove National Park. A larger number of planning units received a medium suitability score. These medium scoring planning units (yellow in the map) form a belt from east to west through Abu Dhabi coastal waters and occupy ~50% of the area within Marawah Marine Biosphere Reserve (Figure 7A). Another large cluster of medium scoring planning units exist in the western part of Abu Dhabi overlapping with Al Yasat MPA (Figure 7A).
Examination of mean values quantified for each objective (key performance indicator) across each candidate seascape revealed that Bul Syayeef and Marawah Marine Biosphere Reserve seascapes received the highest average score for climate change mitigation impact resulting from a high blue carbon storage value (Figure 10 and Figure S3). Khor Faridah and Marawah Marine Biosphere Reserve scored highest for the potential to achieve a positive biodiversity impact, even though in the initial stakeholder-driven model Marawah Marine Biosphere Reserve did not receive any of the high scoring planning units in Scenario 1. For finance and feasibility, Bul Syayeef received the highest score in Abu Dhabi followed by Mangrove National Park primarily due to selection by stakeholders. Mangrove National Park also received the highest score for benefits for people and food security (Figure 10 and Figure S3).
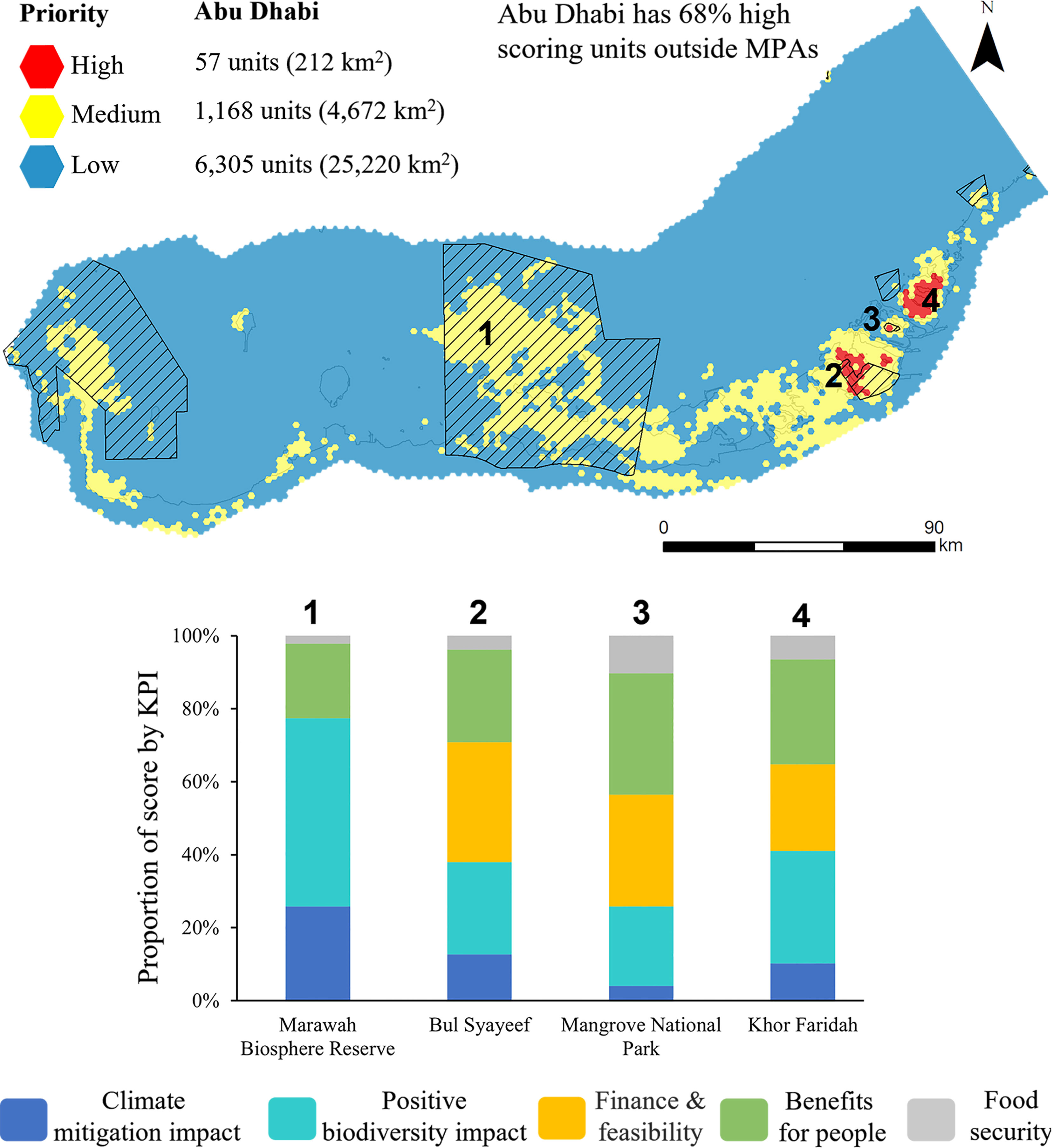
Figure 10 Summary of results from Scenario 1 showing the number of planning units in each suitability class for Abu Dhabi and the proportion of highest suitability units inside MPAs. Bar charts show the proportion of mean scores by key performance indicator just for the high suitability planning units at each seascape.
3.2 Northern Emirates’ High Suitability Seascapes for Immediate NbS Investment (Scenario 1)
Four spatially discrete high suitability clusters occur in the Northern Emirates (Figure 8A). The largest cluster of high suitability planning units occurs in the Umm Al Quwain (Figure 9B). Other discrete clusters occur at Khor Mazahmi Wildlife Sanctuary, Ras Al Khaimah and the lagoon at Al Rams (Figures 8A). All four of these high priority clusters overlap with existing mangrove plantations identified by stakeholders during Workshop 1. Mangrove planting has occurred on the western shore of Khor Al Beidah, within Khor Mazahmi Wildlife Sanctuary and Khor Ras Al Khaimah, while there are oyster farms at Al Rams.
In Scenario 1, the finance and feasibility criteria and positive biodiversity impact criteria contributed most to the high scoring planning units (Figure 12A and Figure S3). Although the climate change mitigation impact (blue carbon) criterion received a high weighting, the more spatially heterogeneous distribution of this criterion resulted in a lower contribution to the final scores. At the scale of candidate seascapes, Umm Al Quwain received the greatest number of high scoring planning units and the highest total score of all seascapes in the study area. Umm Al Quwain received the highest scores for finance and feasibility, as well as food security, highlighting its potential significance for NbS investment in this project. All high scoring seascapes in the Northern Emirates received uniformly high scores for benefits to people based on their proximity to population centers. Among these, Khor Mazahmi Wildlife Sanctuary (south Ras Al Khaimah) received the highest scores and Khor Ras Al Khaimah the lowest (Figure 11 and Figure S3). The candidate seascapes of the Northern Emirates received lower scores for climate mitigation than the Abu Dhabi seascapes, but higher scores for positive biodiversity impact, finance and feasibility, and benefits for people.
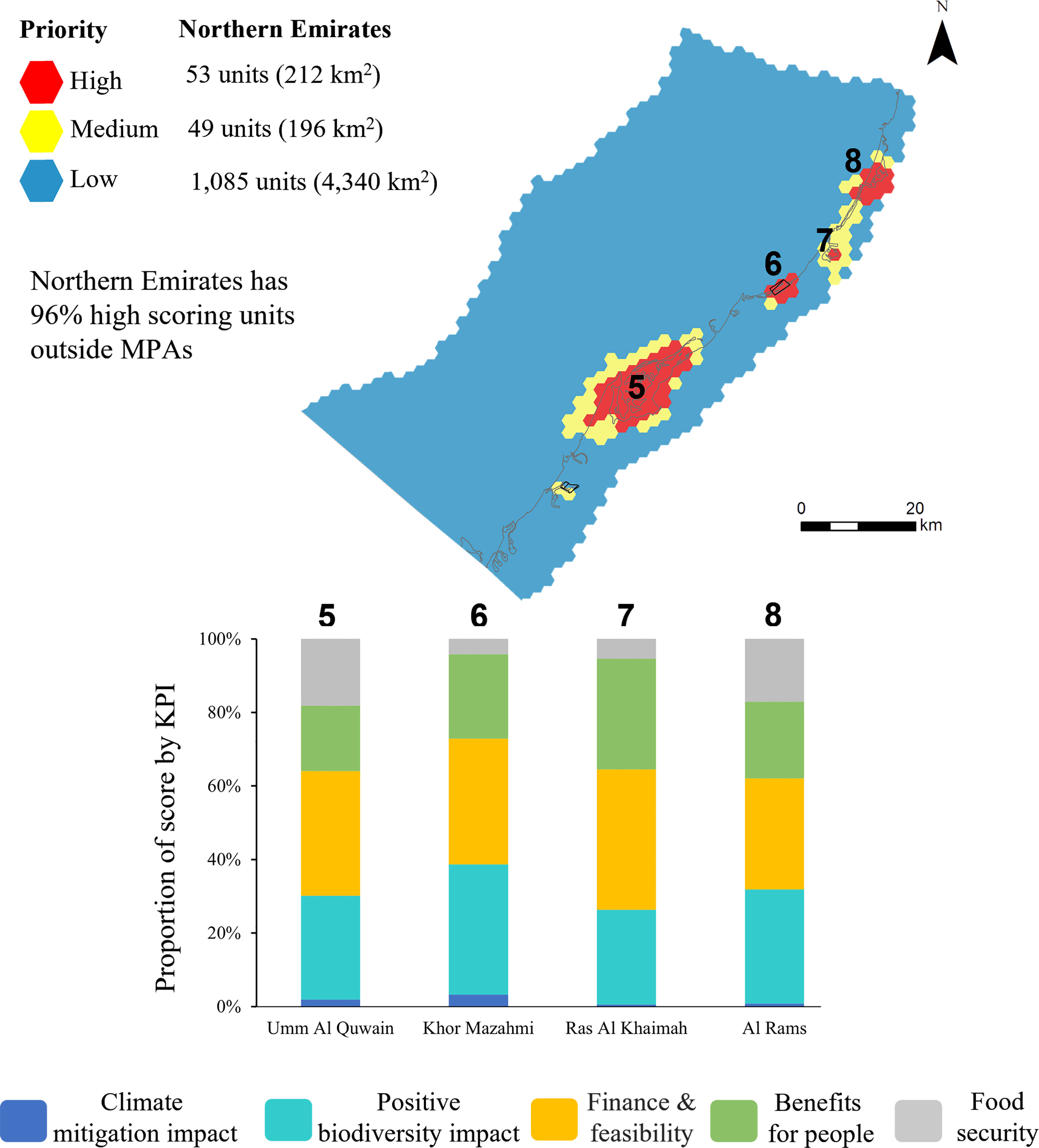
Figure 11 Summary of results from Scenario 1 showing the number of planning units in each suitability class for Northern Emirates and the proportion of highest suitability units inside MPAs. Bar charts show the proportion of mean scores by key performance indicator just for the high suitability planning units within each seascape.
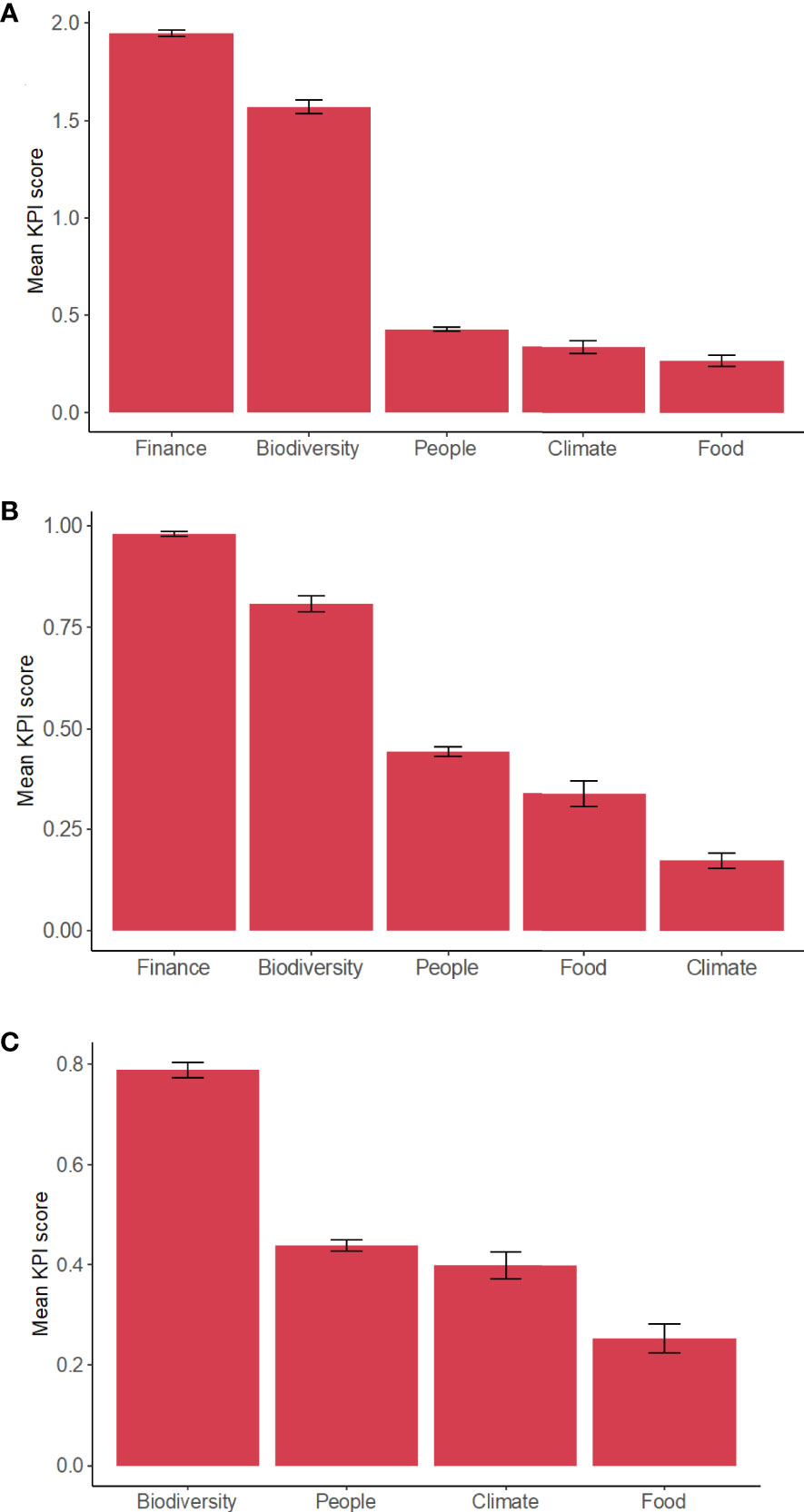
Figure 12 The relative contribution of key performance indicator (KPI score) composite criteria to the highest scoring (high) planning units for: (A) Scenario 1: Stakeholder weightings and preferred areas; (B) Scenario 2: Equal weighting for all criteria; and (C) Scenario 3: Equal weighting for all criteria and stakeholder preferred areas excluded. Error bars represent standard error of the mean.
3.3 Alternative Model Results
3.3.1 Scenario 2. Equal Criteria Weighting Including Stakeholder Preferred Areas
When equal weights were applied to each criterion, the same general areas in Abu Dhabi were identified as high suitability (Figure 7B) when compared with the stakeholder-driven model (Figure 7A). The most noticeable difference was fewer high and medium suitability planning units overall, particularly fewer high suitability units inside Bul Syayeef MPA and fewer medium suitability units inside Marawah Marine Biosphere Reserve. In addition, Mangrove National Park moved from a high to medium score. For the Northern Emirates, very little difference occurred with Scenario 2 using equal weighted criteria with the four high scoring clusters remaining distinct (Figure 8B). Khor Mahzami MPA received fewer high planning units on the landward side, and several of the units around Al Zhora MPA were reclassified from low to medium suitability. Khor Beidah at Umm Al Quwain remained stable as a high scoring area. The contributions to high scoring planning units were very similar to the stakeholder-driven model apart from food security (fisheries) which surpassed climate mitigation as a greater contributor to high scoring units (Figure 12B).
3.3.2 Scenario 3. Equal Criteria Weighting Without Stakeholder Preferred Areas
When the stakeholder preferred areas for immediate investment were excluded from the model, a larger proportion of the study area received a medium score forming a broad (yellow) band across Abu Dhabi waters including some offshore areas (Figure 7C). While high scoring planning units were found in some of the similar areas in the Northern Emirates (Figure 8C), they were much more dispersed in Abu Dhabi in comparison with models with stakeholder-driven models. High scoring planning units were still found at Khor Faridah, yet only one remained near Bul Syayeef MPA, with several clusters appearing to the west of this region (Figure 7C). In addition, several high scoring planning units appeared in the Marawah Marine Biosphere Reserve, as well as along the western coast of Abu Dhabi. The key performance indicators contributing most to the high suitability scores shifted with positive biodiversity impact contributing most followed by co-benefits for people (accessibility, recreation & tourism) and climate change mitigation impact (Figure 12C). Without stakeholder preferred areas, the prioritization analysis was driven to a greater extent by habitat features with high scores across multiple criteria. The naturally heterogeneous and patchy nature of these habitat features led to more disbursed clusters of high suitability planning units. This occurred most notably for the coastal area of Abu Dhabi, which features expansive and diverse mosaics of coastal and marine habitats, while in the Northern Emirates, areas of diverse coastal habitat are limited to several discrete inshore locations.
3.4 Suitable Sites for Scaling Up NbS With Future Investments
The stakeholder-driven model highlighted ample candidate seascapes for evaluation for immediate investments biased toward current project specific objectives and information available. To explore additional areas with potential for future scaling up, we removed the social bias by removing the highly weighted stakeholder preferred areas and applying equal weighting among all criteria. In comparison with Scenario 1 and 2, Scenario 3 produced smaller clusters of the highest scoring planning units with additional high suitability sites identified in Abu Dhabi west of Bul Syayeef MPA. Several high scoring planning units also occurred inside the Marawah Marine Biosphere Reserve and south of Al Yasat MPA. Khor Faridah again received a large cluster of high scoring units, but less tightly clustered than in the initial model. For the Northern Emirates, the results were similar to the initial model (Figure 7C) with clusters of high scoring planning units at Umm Al Quwain, Khor Mazahmi, Ras Al Khaimah and Al Rams (Figure 8C). In contrast to the initial model, positive biodiversity impact contributed most to the high scoring planning units in Scenario 3. These sites provide a rapid geographical scoping that identifies a spatial configuration of medium and high suitability areas with potential for scaling up and connecting NbS activities across the study area.
4 Discussion
Integrated social-ecological approaches are required to design durable NbS for multi-functional coastal seascapes where the objective is to promote the delivery of bundles of ecosystem services (Seddon et al., 2021). NbS are predicated on the capacity for healthy and restored ecosystems to make cost-effective and enduring contributions to addressing climate change, biodiversity loss, food security, and sustainable economic and social development (Nesshöver et al., 2017; Seddon et al., 2021). Integration of local cultural, ecological, and logistical perspectives and spatially explicit knowledge from stakeholders is critical for a pragmatic framework that generates locally relevant outcomes.
Here we co-developed a rapid, systematic, and transparent site selection framework with local stakeholders to help identify sites for immediate NbS interventions intended to demonstrate the NbS approach at a few pilot locations. Secondarily, alternative planning scenarios with reduced contemporary social bias were developed to provide a broader portfolio of candidate sites for scaling up actions in the future. The simplicity and transparency of the prioritization framework and the use of widely accessible data allow for the process to be replicated for the UAE or applied elsewhere in the world. The underlying objectives were based on broad and globally relevant key performance indicators and therefore offer flexibility in the data requirements that enable the geographically diverse application. Our framework used a multi-criteria approach and incorporated seascape spatial context to identify sites with potential for NbS actions to address the project’s key performance indicators with resilient outcomes. By incorporating ecological and social information the framework demonstrates an ecosystem-based approach for NbS (Cohen-Shacham et al., 2016).
Ultimately, at the scale of individual sites or candidate seascapes, the available ecological knowledge and socio-political conditions must be considered more deeply to design specific interventions. The context-specific design of interventions such as active restoration must be informed with knowledge of the trajectories and drivers of change (past and present), the ecosystem structure and function, the range of potential outcome scenarios, and the potential futures envisioned (Sheaves et al., 2021a). Where multiple candidate sites are available, comparative analyses can be conducted to consider past, present, and predicted future conditions using spatially explicit assessments that measure risks to ecosystem function and the potential benefits connected to a wide range of ecosystem services. For example, although the focal seascapes have been delineated based on visually distinct marine geomorphological and hydrological patterns these seascapes should not be managed as marine spaces isolated from surrounding landscapes. Although the positioning of NbS investments at locations where ecosystem services will most benefit people is important, the proximity to cities requires careful consideration because of the potential for impacts to project outcomes. Consequently, the quantification of supply, demand and the impact on benefits can further inform trade-off analyses and priority setting (Watson et al., 2019).
Local expert knowledge provided reliable, up-to-date, and locally relevant information on the status and trends of coastal ecosystems, coastal development plans, and insights into the risks from potential threats. Local knowledge of institutional socio-cultural context ensured that the sites and potential NbS interventions are supported by key institutional political and economic decision-makers. Where possible, a broad range of stakeholders could participate in the co-creation of criteria using participatory mapping with the potential to include human use patterns, including more detailed information on tourist and recreational visitation, historical coastal habitat change, wildlife observations, commercial opportunities, and investor interest, etc. Furthermore, incorporating predictions of climate change vulnerability and habitat suitability under sea-level change scenarios will add additional forecasting capacity to site selection.
Our approach combined both quantitative and qualitative datasets in the prioritization analysis. Participatory mapping was used to capture stakeholder preferences and fill data gaps where no quantitative spatial data was available. In this implementation we incorporated stakeholder preference directly in the prioritization process as one of the input criteria. An alternate approach for future implementations (assuming the data were available) would be to use only quantitative datasets in the prioritization, then have stakeholders select among identified areas. In data-poor situations, participatory mapping can be used to fill data gaps as well as capture stakeholder preference. In data-rich situations, participatory mapping can be used to capture stakeholder preferences during and/or after the prioritization procedure.
To identify a potential portfolio of sites beyond the highly suitable sites for immediate consideration in this project, a scenario was computed with similar criteria weightings and without the current stakeholders preferred areas. Even in the absence of stakeholder weightings, two of the key performance criteria, positive biodiversity impact, and finance/feasibility dominated the prioritization scores. This is likely because the underlying criteria; APIMB areas and stakeholder preferred NbS areas, respectively, differed from most of the quantitative criteria used because they are effectively presence/absence and consisted primarily of scores of 1 or 0. Since all criteria are scaled from 0-1, these criteria have a larger influence where they occur compared to the quantitative layers with a range of values. This should be considered when applying this framework and weightings adjusted accordingly. These criteria were weighted highly by stakeholders, though adding additional weight did little to change the outcome. If not weighted highly, perhaps these presence/absence criteria could be down-weighted to better match other criteria. Rather than scoring 1 for present, another approach could be to apply the average scaled score of all quantitative criteria. The only other presence/absence criterion used in the analyses was stakeholder mapped local fishing areas. This criterion did not have a large influence on site suitability scores, likely because it was combined with two other layers for the food security criterion and many of the mapped areas were located offshore where they did not overlap with other criteria.
A region-wide portfolio of diverse sites of different sizes, conservation features and conditions provide a forward look to scaling up while recognizing that criteria may differ at different scales and that design of effective actions requires knowledge at local site scales (tens of meters) to optimize benefits and reduce socio-economic costs (Gilby et al., 2021). Marine NbS are generally more expensive than terrestrial counterparts, and from an investor’s perspective, this places even greater emphasis on selecting locations and actions that will optimize returns on investments through high success rates and the magnitude of benefits flowing from ecosystem services (Lester et al., 2020). Integrated management of NbS can be designed to optimize multiple co-benefits through analysis of synergies and trade-offs while safeguarding ecosystem function through risk reduction strategies (Martín et al., 2020).
In addition to considering the multi-criteria prioritization framework, scaling up should consider potential ecological connectivity between high and medium scoring planning units. Identifying opportunities to connect or reconnect functional connectivity through restoration and creation of green and blue corridors could accelerate the provision of ecosystem services and increase the resilience of outcomes from investments (Hilty et al., 2019). A regional portfolio of actions that restores or enhances connectivity through corridors could have synergistic effects that together across a network of NbS actions generate whole region benefits that are greater than the sum of the individual NbS actions.
Techniques such as connectivity modeling (often but not always requiring species information) (Liu et al., 2018; Krost et al., 2018) can help to identify potential corridors and highlight places where NbS actions can be designed to enhance connectivity. Low scoring planning units should not be exempt from opportunities for successful NbS since the rapid site selection framework utilized only a simple set of spatial criteria and in some areas data gaps may have resulted in underscoring some planning units. Furthermore, when scaling up, it will be prudent to consider all coastal ecosystems as having potential for investment of different types and size. This geographically inclusive ‘no seascape left behind’ perspective will include even the most heavily modified and urbanized seascapes and may create linkages across the land-sea boundary between urban landscapes and city seascapes.
4.1 Climate Change
The priority areas are multi-functional seascapes with a variety of existing human uses and potential future human benefits. Site suitability for NbS investment must take place with consideration of human activity patterns and ecosystem vulnerabilities through human impact mapping. Coastal vulnerability to climate change processes especially sea level rise and the future impact on coastal habitats and species and habitat suitability is an important consideration for planning NbS that will influence feasibility and the sustainability of projects. Climate change models project that sea level will rise leading inevitably to a landward migration of some coastal fish habitat, a phenomenon known as shifting mosaics (Lauchlan and Nagelkerken, 2020). To optimize outcomes from NbS, adaptation strategies will need to account for changing habitat suitability for coastal vegetation with sea level rise, including identifying opportunities for future managed retreat/realignment (Spencer et al., 2016). Urbanized coastlines can sometimes create a phenomenon called ‘coastal squeeze’ where habitat or function is lost when engineered structures or elevation prevent habitats from transgressing landward in response to sea level rise (Pontee, 2013; Smart et al., 2021). Adaptation strategies can be modelled and evaluated to inform cost-benefit analyses and the design of effective management actions (Moritsch et al., 2021).
4.2 Marine Protected Areas
MPAs were initially considered as potential spatial proxies for low-risk areas but were not used as such in the analyses due to high perceived variability in management effectiveness among MPAs. In principle, the investment in NbS inside MPAs should offer investors the greater assurance of lower risk to investments from human impacts. However, the majority of high suitable planning units were located outside of the current network of MPAs. In the Northern Emirates almost all (98%) of the high suitability planning units were outside of MPAs, and in Abu Dhabi, 68% were outside MPAs. The importance of MPAs in NbS outcomes will depend on the type of NbS and the activities allowed within the MPAs, which vary depending on the objective of the designation (Gill et al., 2017; Giakoumi et al., 2018). Further research into the regulatory framework and effectiveness of each MPA in the project area is required to refine MPAs as a weighted criterion to integrate relative risks and benefits to NbS outcomes.
4.3 Local Threats to Coastal Seascapes
Threats and stressors were not modelled explicitly for this rapid site selection process due to insufficient information available. Instead, consideration was given to threats through collective stakeholder local knowledge which informed site suitability. Future applications could, however, make use of land cover data as a proxy for landscape development and land cover change to identify losses and gains to coastal seascapes and help assess the current condition and ecological trajectory of the seascape. In addition, information on future land use from strategic urban plans may reveal potential for contested space. The condition of the landscape or watershed of adjacent coastal ecosystems can influence the suitability of a site for active restoration (Saunders et al., 2017). Accounting for the climate vulnerability of coastal seascapes and associated communities is a significant and complex challenge. At the scale of candidate seascapes, it will be important to model key hydrodynamic dynamic variables such as sea-level rise, salinity and thermal stress that can influence the future opportunities and viability of NbS. At this stage, input from a broader range of stakeholders including landowners, developers and industry will be important.
4.4 Fish Habitat and Fisheries
Food provisioning and economic activity through fisheries are key ecosystem services provided by marine and coastal ecosystems in the UAE. While we did collect information on recreational/subsistence/artisanal fishing activities through participatory mapping, we were not able to obtain commercial fishing data from the UAE government agencies and looked instead to a global proxy to fill this data gap. We used satellite nighttime vessel detection (VIIRS – Visible Infrared Imaging Radiometer Suite) data to identify potential fishing areas and level of effort based on the location and number of fishing vessels as a subset of all vessels visible at night. While this data is not specific to fishing vessels, we assumed that the number of fishing vessels is relative to the total number of vessels. Furthermore, we attribute this proxy value for commercial fishing pressure to coastal habitats within 5 km to identify potential nursery habitats. While there is a rather high level of uncertainty in this approach, it was necessary to fill an important data gap.
4.5 Spatial Variability in Blue Carbon Storage
In this project, we assigned a single habitat-type specific mean carbon storage value uniformly to each mapped habitat type using the best available data. Studies of the spatial variability of carbon storage indicate that carbon storage is highly variable across a seascape mosaic and can be influenced by terrestrial input and seascape composition and spatial configuration (Gullström et al., 2018; Huxham et al., 2018). For example, mangroves in proximity to seagrass beds or mudflats increase the carbon storage capacity of the connected seagrasses and mudflats (Asplund et al., 2021). While data on carbon storage were available for intertidal plants and sediments in the UAE, the role of subtidal unvegetated sediments in nutrient cycling and carbon storage remains an important knowledge gap. Strategic sampling of carbon across a land-sea gradient and across structural features such as edges between adjacent patch types and in places influenced by different hydrodynamic forces, vegetation and sediment types could reveal where carbon storage potential can be optimized through NbS.
Scaling up of NbS is accelerating globally as a viable approach to addressing multiple crises and is encouraged by global and national policy, international conservation organizations, grassroots community initiatives and increasingly funded by philanthropists and the commercial sector. This progress must be part of an integrated cross-sectoral sustainability strategy and be ecologically informed, aligned with local perspectives, strive for inclusivity and the consideration of intangible and non-monetary values. With this study, we present a transferable framework for rapid NbS site selection that incorporates these considerations and can be implemented in data poor regions to address this unprecedented and timely momentum for scaling-up implementation of NbS.
Data Availability Statement
The raw data supporting the conclusions of this article will be made available by the authors, without undue reservation.
Author Contributions
SP led the research and wrote the first draft of the paper through a contract with EN-WWF. DM-M and MA conceived and led the project. KS and SP conducted the spatial analyses. All other authors provided guidance throughout the project, supplied data, and contributed edits to refine subsequent versions of the manuscript.
Funding
This project was funded by HSBC through the Nature Based Solutions Accelerator, a global initiative of the HSBC Climate Solutions Partnership. The funder had no role in study design, data collection and analysis, decision to publish, or preparation of the manuscript.
Conflict of Interest
SP is employed by Seascape Analytics Ltd. KS and JD are employed by Seascape Solutions LLC.
The remaining authors declare that the research was conducted in the absence of any commercial or financial relationships that could be construed as a potential conflict of interest.
Publisher’s Note
All claims expressed in this article are solely those of the authors and do not necessarily represent those of their affiliated organizations, or those of the publisher, the editors and the reviewers. Any product that may be evaluated in this article, or claim that may be made by its manufacturer, is not guaranteed or endorsed by the publisher.
Acknowledgments
We thank our UAE partners and data providers, as well as HSBC MENAT and PLC for providing philanthropic funding through the global Climate Solutions Partnership. The funder had no role in study design, data collection and analysis, decision to publish, or preparation of the manuscript. EN-WWF are grateful to Her Excellency Dr. Shaikha Salem Al Dhaheri, Secretary General of the Environment Agency of Abu Dhabi and Ahmed Al Hashmi, Acting Executive Director of Terrestrial and Marine Biodiversity at EAD, for their advice throughout this study. We thank Anil Kumar and the EAD GIS department for sharing key data layers to support this study. We are grateful to Rashyd Zaaboul, Dr Ali Elbattay, Amna Al Mansoori, Hind Al Ameri, Maitha Al Hameli, Hammad Abubaker, Ibrahim Bugla, Mona Moller, Dionysia Angeliki for their input to the project design and workshops.
Supplementary Material
The Supplementary Material for this article can be found online at: https://www.frontiersin.org/articles/10.3389/fmars.2022.832480/full#supplementary-material
References
Abelson A., Reed D. C., Edgar G. J., Smith C. S., Kendrick G. A., Orth R. J., et al. (2020). Challenges for Restoration of Coastal Marine Ecosystems in the Anthropocene. Front. Mar. Sci. 7. doi: 10.3389/fmars.2020.544105
Adem Esmail B., Geneletti D. (2018). Multi-Criteria Decision Analysis for Nature Conservation: A Review of 20 Years of Applications. Methods Ecol. Evol. 9 (1), 42–53. doi: 10.1111/2041-210X.12899
Ala-Hulkko T., Kotavaara O., Alahuhta J., Helle P., Hjort J. (2016). Introducing Accessibility Analysis in Mapping Cultural Ecosystem Services. Ecol. Indic. 66, 416–427. doi: 10.1016/j.ecolind.2016.02.013
Alsterberg C., Roger F., Sundbäck K., Juhanson J., Hulth S., Hallin S., et al. (2017). Habitat Diversity and Ecosystem Multifunctionality: The Importance of Direct and Indirect Effects. Sci. Adv. 3 (2), e1601475. doi: 10.1126/sciadv.1601475
Arkema K. K., Verutes G. M., Wood S. A., Clarke-Samuels C., Rosado S., Canto M., et al (2015). Embedding Ecosystem Services in Coastal Planning Leads to Better Outcomes for People and Nature. P Natl. Acad. Sci. U. S. A. 112 (24), 7390–7395. doi: 10.1073/pnas.1406483112
Arkema K. K., Fisher D. M., Wyatt K., Wood S. A., Payne H. J. (2021). Advancing Sustainable Development and Protected Area Management With Social Media-Based Tourism Data. Sustainability 13 (5), 2427. doi: 10.3390/su13052427
Asplund M. E., Dahl M., Ismail R. O., Arias-Ortiz A., Deyanova D., Franco J. N., et al. (2021). Dynamics and Fate of Blue Carbon in a Mangrove–Seagrass Seascape: Influence of Landscape Configuration and Land-Use Change. Land. Ecol. 36 (5), 1489–1509. doi: 10.1007/s10980-021-01216-8
Barbier E. B. (2018). “Seascape Economics: Valuing Ecosystem Services Across the Seascape,” in Seascape Ecology, 1st Edition. Ed. Pittman S. J. (Hoboken, New Jersey:Wiley).
Bayraktarov E., Brisbane S., Hagger V., Smith C. S., Wilson K. A., Lovelock C. E., et al. (2020). Priorities and Motivations of Marine Coastal Restoration Research. Front. Mar. Sci. 7. doi: 10.3389/fmars.2020.00484
Ben Lamine E., Mateos-Molina D., Antonopoulou M., Burt J. A., Das H. S., Javed S., et al. (2020). Identifying Coastal and Marine Priority Areas for Conservation in the United Arab Emirates. Biodivers. Conserv. 29 (9), 2967–2983. doi: 10.1007/s10531-020-02007-4
Bennett N. J. (2018). Navigating a Just and Inclusive Path Towards Sustainable Oceans. Mar Policy 97, 139–146. doi: 10.1016/j.marpol.2018.06.001
Bradley M., Nagelkerken I., Baker R., Sheaves M. (2020). Context Dependence: A Conceptual Approach for Understanding the Habitat Relationships of Coastal Marine Fauna. BioScience 70 (11), 986–1004. doi: 10.1093/biosci/biaa100
Burdon D., Potts T., McKinley E., Lew S., Shilland R., Gormley K., et al. (2019). Expanding the Role of Participatory Mapping to Assess Ecosystem Service Provision in Local Coastal Environments. Ecosyst. Serv. 39, 101009. doi: 10.1016/j.ecoser.2019.101009
Burt J. A., Bartholomew A. (2019). Towards More Sustainable Coastal Development in the Arabian Gulf: Opportunities for Ecological Engineering in an Urbanized Seascape. Mar. Pollut. Bull. 142, 93–102. doi: 10.1016/j.marpolbul.2019.03.024
Carlson R. R., Evans L. J., Foo S. A., Grady B. W., Li J., Seeley M., et al. (2021). Synergistic Benefits of Conserving Land-Sea Ecosystems. Global Ecol. Conserv. 28, e01684. doi: 10.1016/j.gecco.2021.e01684
Chamberlain D. A., Possingham H. P., Phinn S. R. (2022). Decision-Making With Ecological Process for Coastal and Marine Planning: Current Literature and Future Directions. Aquat. Ecol. 56, 1–9. doi: 10.1007/s10452-021-09896-9
Cohen-Shacham E., Andrade A., Dalton J., Dudley N., Jones M., Kumar C., et al. (2019). Core Principles for Successfully Implementing and Upscaling Nature-Based Solutions. Environ. Sci. Policy 98, 20–29. doi: 10.1016/j.envsci.2019.04.014
Cohen-Shacham E., Walters G., Janzen C., Maginnis S. (Eds.) (2016). Nature-Based Solutions to Address Global Societal Challenges (Gland, Switzerland: IUCN), xiii + 97pp.
Daigle R. M., Metaxas A., Balbar A. C., McGowan J., Treml E. A., Kuempel C. D., et al. (2020). Operationalizing Ecological Connectivity in Spatial Conservation Planning With Marxan Connect. Methods Ecol. Evol. 11 (4), 570–579. doi: 10.1111/2041-210X.13349
Di Franco A., Hogg K. E., Calò A., Bennett N. J., Sévin-Allouet M. A., Alaminos O. E., et al. (2020). Improving Marine Protected Area Governance Through Collaboration and Co-Production. J. Environ. Manage 269, 110757. doi: 10.1016/j.jenvman.2020.110757
EAD (2015) EnviroPortal, Environment Agency Abu Dhabi. Abu Dhabi, United Arab Emirates Available at: https://enviroportal.ead.ae/map/.
EAD (2018). Mangrove Habitat Satellite Imagery Project (Abu Dhabi, United Arab Emirates:Environment Agency Abu Dhabi).
Elliott M. (2013). The 10-Tenets for Integrated, Successful and Sustainable Marine Management. Mar Pollut. Bull. 74 (1), 1–5. doi: 10.1016/j.marpolbul.2013.08.001
Giakoumi S., McGowan J., Mills M., Beger M., Bustamante R. H., Charles A., et al. (2018). Revisiting “Success” and “Failure”"" Of Marine Protected Areas: A Conservation Scientist Perspective. Front. Mar Sci. 5. doi: 10.3389/fmars.2018.00223
Gilby B. L., Olds A. D., Brown C. J., Connolly R. M., Henderson C. J., Maxwell P. S., et al. (2021). Applying Systematic Conservation Planning to Improve the Allocation of Restoration Actions at Multiple Spatial Scales. Restor. Ecol. 29 (5), e13403. doi: 10.1111/rec.13403
Gilby B. L., Olds A. D., Connolly R. M., Henderson C. J., Schlacher T. A. (2018). Spatial Restoration Ecology: Placing Restoration in a Landscape Context. Bioscience 68 (12), 1007–1019. doi: 10.1093/biosci/biy126
Gill D. A., Mascia M. B., Ahmadia G. N., Glew L., Lester S. E., Barnes M., et al. (2017). Capacity Shortfalls Hinder the Performance of Marine Protected Areas Globally. Nature 543 (7647), 665–669. doi: 10.1038/nature21708
Giordano R., Pluchinotta I., Pagano A., Scrieciu A., Nanu F. (2020). Enhancing Nature-Based Solutions Acceptance Through Stakeholders’ Engagement in Co-Benefits Identification and Trade-Offs Analysis. Sci. Total Environ. 713, 136552. doi: 10.1016/j.scitotenv.2020.136552
Golden C. D., Allison E. H., Cheung W. W., Dey M. M., Halpern B. S., McCauley D. J., et al. (2016). Nutrition: Fall in Fish Catch Threatens Human Health. Nat. News 534 (7607), 317. doi: 10.1038/534317a
Green A. L., Maypa A. P., Almany G. R., Rhodes K. L., Weeks R., Abesamis R. A., et al. (2015). Larval Dispersal and Movement Patterns of Coral Reef Fishes, and Implications for Marine Reserve Network Design. Biol. Rev. 90 (4), 1215–1247. doi: 10.1111/brv.12155
Guannel G., Arkema K., Ruggiero P., Verutes G. (2016). The Power of Three: Coral Reefs, Seagrasses and Mangroves Protect Coastal Regions and Increase Their Resilience. PloS One 11 (7), e0158094. doi: 10.1371/journal.pone.0158094
Gullström M., Lyimo L. D., Dahl M., Samuelsson G. S., Eggertsen M., Anderberg E., et al. (2018). Blue Carbon Storage in Tropical Seagrass Meadows Relates to Carbonate Stock Dynamics, Plant–Sediment Processes, and Landscape Context: Insights From the Western Indian Ocean. Ecosystems 21 (3), 551–566. doi: 10.1007/s10021-017-0170-8
Halpern B. S., Frazier M., Afflerbach J., Lowndes J. S., Micheli F., O'Hara C., et al. (2019). Recent Pace of Change in Human Impact on the World’s Ocean. Sci. Rep. 9, 1160. doi: 10.1038/s41598-019-47201-9
Hilty J. A., Keeley A. T., Merenlender A. M., Lidicker W. Z. Jr. (2019). Corridor Ecology: Linking Landscapes for Biodiversity Conservation and Climate Adaptation (Washington DC: Island Press).
Hutchison J., Spalding M., zu Ermgassen P. (2014). “The Role of Mangroves in Fisheries Enhancement,” in The Nature Conservancy and Wetlands International. Wetlands International, Cambridge. pp. 54. Available at: https://www.wetlands.org/publications/the-role-of-mangroves-in-fisheries-enhancement/
Huxham M., Whitlock D., Githaiga M., Dencer-Brown A. (2018). Carbon in the Coastal Seascape: How Interactions Between Mangrove Forests, Seagrass Meadows and Tidal Marshes Influence Carbon Storage. Curr. Forestry Rep. 4 (2), 101–110. doi: 10.1007/s40725-018-0077-4
Kenter J. O., Jobstvogt N., Watson V., Irvine K. N., Christie M., Bryce R. (2016). The Impact of Information, Value-Deliberation and Group-Based Decision-Making on Values for Ecosystem Services: Integrating Deliberative Monetary Valuation and Storytelling. Ecosyst. Serv. 21, 270–290. doi: 10.1016/j.ecoser.2016.06.006
Krost P., Goerres M., Sandow V. (2018). Wildlife Corridors Under Water: An Approach to Preserve Marine Biodiversity in Heavily Modified Water Bodies. J. Coast Conserv. 22 (1), 87–104. doi: 10.1007/s11852-017-0554-0
Lauchlan S. S., Nagelkerken I. (2020). Species Range Shifts Along Multistressor Mosaics in Estuarine Environments Under Future Climate. Fish Fisheries 21 (1), 32–46. doi: 10.1111/faf.12412
Lefcheck J. S., Byrnes J. E., Isbell F., Gamfeldt L., Griffin J. N., Eisenhauer N., et al. (2015). Biodiversity Enhances Ecosystem Multifunctionality Across Trophic Levels and Habitats. Nat. Commun. 6 (1), 6936. doi: 10.1038/ncomms7936
Lester S. E., Dubel A. K., Hernán G., McHenry J., Rassweiler A. (2020). Spatial Planning Principles for Marine Ecosystem Restoration. Front. Mar. Sci. 7. doi: 10.3389/fmars.2020.00328
Lidour K., Béarez P., Méry S. (2020). Analysis of Archaeological Fish Remains Provides New Insights Into Seasonal Mobility in Eastern Arabia During the Mid-Holocene. The Subsistence Fisheries of UAQ36, Umm Al-Quwain Lagoon (United Arab Emirates). J. Archaeol Sci: Rep. 30, 102223. doi: 10.1016/j.jasrep.2020.102223
Liu C., Newell G., White M., Bennett A. F. (2018). Identifying Wildlife Corridors for the Restoration of Regional Habitat Connectivity: A Multispecies Approach and Comparison of Resistance Surfaces. PloS One 13 (11), e0206071. doi: 10.1371/journal.pone.0206071
Maes J., Jacobs S. (2017). Nature-Based Solutions for Europe’s Sustainable Development. Conserv Lett. 10 (1), 121–124. doi: 10.1111/conl.12216
Martín E. G., Giordano R., Pagano A., van der Keur P., Costa M. M. (2020). Using a System Thinking Approach to Assess the Contribution of Nature-Based Solutions to Sustainable Development Goals. Sci. Total Environ. 738, 139693. doi: 10.1016/j.scitotenv.2020.139693
Mateos-Molina D., Antonopoulou M., Baldwin R., Bejarano I., Burt J. A., García-Charton J. A., et al. (2020). Applying an Integrated Approach to Coastal Marine Habitat Mapping in the North-Western United Arab Emirates. Mar. Environ. Res. 161, 105095. doi: 10.1016/j.marenvres.2020.105095
Mateos-Molina D., Lamine E. B., Antonopoulou M., Burt J. A., Das H. S., Javed S., et al. (2021a). Synthesis and Evaluation of Coastal and Marine Biodiversity Spatial Information in the United Arab Emirates for Ecosystem-Based Management. Mar. Pollut. Bull. 167, 112319. doi: 10.1016/j.marpolbul.2021.112319
Mateos-Molina D., Pittman S. J., Antonopoulou M., Baldwin R., Chakraborty A., García-Charton J. A., et al. (2021b). An Integrative and Participatory Coastal Habitat Mapping Framework for Sustainable Development Actions in the United Arab Emirates. Appl. Geogr 136, 102568. doi: 10.1016/j.apgeog.2021.102568
Metzger J. P., Esler K., Krug C., Arias M., Tambosi L., Crouzeilles R., et al. (2017). Best Practice for the Use of Scenarios for Restoration Planning. Curr. Opin. Env. Sust 29, 14–25. doi: 10.1016/j.cosust.2017.10.004
Moberg F., Folke C. (1999). Ecological Goods and Services of Coral Reef Ecosystems. Ecol. Econ. 29(2), 215–233. doi: 10.1016/S0921-8009(99)00009-9
Moritsch M. M., Young M., Carnell P., Macreadie P. I., Lovelock C., Nicholson E., et al. (2021). Estimating Blue Carbon Sequestration Under Coastal Management Scenarios. Sci. Total Environ. 777, 145962. doi: 10.1016/j.scitotenv.2021.145962
Nagelkerken I., Sheaves M., Baker R., Connolly R. M. (2015). The Seascape Nursery: A Novel Spatial Approach to Identify and Manage Nurseries for Coastal Marine Fauna. Fish Fisheries 16 (2), 362–371. doi: 10.1111/faf.12057
Nesshöver C., Assmuth T., Irvine K. N., Rusch G. M., Waylen K. A., Delbaere B., et al. (2017). The Science, Policy and Practice of Nature-Based Solutions: An Interdisciplinary Perspective. Sci. Total Environ. 579, 1215–1227. doi: 10.1016/j.scitotenv.2016.11.106
Olds A. D., Connolly R. M., Pitt K. A., Pittman S. J., Maxwell P. S., Huijbers C. M., et al. (2016). Quantifying the Conservation Value of Seascape Connectivity: A Global Synthesis. Global Ecol. Biogeogr 25 (1), 3–15. doi: 10.1111/geb.12388
Pittman S. J., Poti M., Jeffrey C. F., Kracker L. M., Mabrouk A. (2018). Decision Support Framework for the Prioritization of Coral Reefs in the US Virgin Islands. Ecol. Inform 47, 26–34. doi: 10.1016/j.ecoinf.2017.09.008
Pittman S. J., Rodwell L. D., Shellock R. J., Williams M., Attrill M. J., Bedford J., et al. (2019). Marine Parks for Coastal Cities: A Concept for Enhanced Community Well-Being, Prosperity and Sustainable City Living. Mar Policy 103, 160–171. doi: 10.1016/j.marpol.2019.02.012
Pittman S. J., Yates K. L., Bouchet P. J., Alvarez-Berastegui D., Andréfouët S., Bell S. S., et al. (2021). Seascape Ecology: Identifying Research Priorities for an Emerging Ocean Sustainability Science. Mar. Ecol. Prog. Ser. 663, 1–29. doi: 10.3354/meps13661
Pontee N. (2013). Defining Coastal Squeeze: A Discussion. Ocean Coast Manage 84, 204–207. doi: 10.1016/j.ocecoaman.2013.07.010
Pörtner H. O., Scholes R. J., Agard J., Archer E., Arneth A., Bai X., et al. (2021). IPBES-IPCC Co-Sponsored Workshop Report on Biodiversity and Climate Change. Intergovernmental Science-Policy Platform on Biodiversity and Ecosystem Services (IPBES) and Intergovernmental Panel on Climate Change (IPCC). doi: 10.5281/zenodo.5101125
Possingham H. P., Bode M., Klein C. J. (2015). Optimal Conservation Outcomes Require Both Restoration and Protection. PloS Biol. 13 (1), e1002052. doi: 10.1371/journal.pbio.1002052
Rahman M. M., Zimmer M., Ahmed I., Donato D., Kanzaki M., Xu M. (2021). Co-Benefits of Protecting Mangroves for Biodiversity Conservation and Carbon Storage. Nat. Commun. 12 (1), 1–9. doi: 10.1038/s41467-021-24207-4
Rocha J., Yletyinen J., Biggs R., Blenckner T., Peterson G. (2015). Marine Regime Shifts: Drivers and Impacts on Ecosystems Services. Philos. T Roy Soc. B 370 (1659), 20130273. doi: 10.1098/rstb.2013.0273
Roman L. A., Conway T. M., Eisenman T. S., Koeser A. K., Barona C. O., Locke D. H., et al. (2021). Beyond’trees are Good’: Disservices, Management Costs, and Tradeoffs in Urban Forestry. Ambio 50 (3), 615–630. doi: 10.1007/s13280-020-01396-8
Sambrook K., Hoey A. S., Andréfouët S., Cumming G. S., Duce S., Bonin M. C. (2019). Beyond the Reef: The Widespread Use of non-Reef Habitats by Coral Reef Fishes. Fish Fisheries 20 (5), 903–920. doi: 10.1111/faf.12383
Sanchirico J. N., Springborn M. (2011). How to Get There From Here: Ecological and Economic Dynamics of Ecosystem Service Provision. Environ. Res. Econ 48 (2), 243–267. doi: 10.1007/s10640-010-9410-5
Saunders M. I., Bode M., Atkinson S., Klein C. J., Metaxas A., Beher J., et al. (2017). Simple Rules can Guide Whether Land-or Ocean-Based Conservation Will Best Benefit Marine Ecosystems. PloS Biol. 15 (9), e2001886. doi: 10.1371/journal.pbio.2001886
Saunders M. I., Doropoulos C., Bayraktarov E., Babcock R. C., Gorman D., Eger A. M., et al. (2020). Bright Spots in Coastal Marine Ecosystem Restoration. Curr. Biol. 30 (24), R1500–R1510. doi: 10.1016/j.cub.2020.10.056
Schile L. M., Kauffman J. B., Crooks S., Fourqurean J. W., Glavan J., Megonigal J. P. (2017). Limits on Carbon Sequestration in Arid Blue Carbon Ecosystems. Ecol. Appl. 27 (3), 859–874. doi: 10.1002/eap.1489
Seddon N., Smith A., Smith P., Key I., Chausson A., Girardin C., et al. (2021). Getting the Message Right on Nature-Based Solutions to Climate Change. Global Change Biol. 27 (8), 1518–1546. doi: 10.1111/gcb.15513
Shadab S. (2018). Tourism and Economic Growth in the United Arab Emirates: A Granger Causality Approach. IOSR J. Business Manage 20 (4), 1–6. doi: 10.9790/487X-2004030106
Sheaves M., Abrantes K., Barnett A., Benham C., Dale P., Mattone C., et al. (2020). The Consequences of Paradigm Change and Poorly Validated Science: The Example of the Value of Mangroves to Fisheries. Fish Fisheries 21 (5), 1067–1075. doi: 10.1111/faf.12479
Sheaves M., Mattone C., Connolly R. M., Hernandez S., Nagelkerken I., Murray N., et al. (2021b). Ecological Constraint Mapping: Understanding Outcome-Limiting Bottlenecks for Improved Environmental Decision-Making in Marine and Coastal Environments. Front. Mar Sci., 1133. doi: 10.3389/fmars.2021.717448
Sheaves M., Waltham N. J., Benham C., Bradley M., Mattone C., Diedrich A., et al. (2021a). Restoration of Marine Ecosystems: Understanding Possible Futures for Optimal Outcomes. Sci. Total Environ. 796, 148845. doi: 10.1016/j.scitotenv.2021.148845
Smart L. S., Vukomanovic J., Sills E. O., Sanchez G. (2021). Cultural Ecosystem Services Caught in a ’Coastal Squeeze’ Between Sea Level Rise and Urban Expansion. Global Environ. Change 66, 102209. doi: 10.1016/j.gloenvcha.2020.102209
Spencer T., Schuerch M., Nicholls R. J., Hinkel J., Lincke D., Vafeidis A. T., et al. (2016). Global Coastal Wetland Change Under Sea-Level Rise and Related Stresses: The DIVA Wetland Change Model. Global Planet Change 139, 15–30. doi: 10.1016/j.gloplacha.2015.12.018
Su J., Friess D. A., Gasparatos A. (2021). A Meta-Analysis of the Ecological and Economic Outcomes of Mangrove Restoration. Nat. Commun. 12 (1), 1–13. doi: 10.1038/s41467-021-25349-1
TIES (2015). Available at: https://ecotourism.org/what-is-ecotourism/.
UAE (2020) Second Nationally Determined Contribution of the United Arab Emirates. December 2020. UAE Government Submission to UNFCCC. Available at: https://tinyurl.com/9yya2z9h.
Villarreal-Rosas J., Sonter L. J., Runting R. K., López-Cubillos S., Dade M. C., Possingham H. P., et al. (2020). Advancing Systematic Conservation Planning for Ecosystem Services. Trends Ecol. Evol. 35 (12), 1129–1139. doi: 10.1016/j.tree.2020.08.016
Wabnitz C. C., Lam V. W., Reygondeau G., Teh L. C., Al-Abdulrazzak D., Khalfallah M., et al. (2018). Climate Change Impacts on Marine Biodiversity, Fisheries and Society in the Arabian Gulf. PloS One 13 (5), e0194537. doi: 10.1371/journal.pone.0194537
Waltham N. J., Elliott M., Lee S. Y., Lovelock C., Duarte C. M., Buelow C., et al. (2020). UN Decade on Ecosystem Restoration 2021–2030—What Chance for Success in Restoring Coastal Ecosystems? Front. Mar Sci. 7. doi: 10.3389/fmars.2020.00071
Watson K. B., Galford G. L., Sonter L. J., Koh I., Ricketts T. H. (2019). Effects of Human Demand on Conservation Planning for Biodiversity and Ecosystem Services. Conserv Biol. 33 (4), 942–952. doi: 10.1111/cobi.13276
White M. P., Alcock I., Wheeler B. W., Depledge M. H. (2013). Coastal Proximity, Health and Well-Being: Results From a Longitudinal Panel Survey. Health Place 23, 97–103. doi: 10.1016/j.healthplace.2013.05.006
Wodehouse D. C., Rayment M. B. (2019). Mangrove Area and Propagule Number Planting Targets Produce Sub-Optimal Rehabilitation and Afforestation Outcomes. Est Coast Shelf Sci. 222, 91–102. doi: 10.1016/j.ecss.2019.04.003
Wood S. A., Guerry A. D., Silver J. M., Lacayo M. (2013). Using Social Media to Quantify Nature-Based Tourism and Recreation. Sci. Rep. 3, 2976. doi: 10.1038/srep02976
Wylie L., Sutton-Grier A. E., Moore A. (2016). Keys to Successful Blue Carbon Projects: Lessons Learned From Global Case Studies. Mar Policy 65, 76–84. doi: 10.1016/j.marpol.2015.12.020
Keywords: prioritization, participatory mapping, climate action, blue carbon, biodiversity, seascape restoration, Arabian Gulf
Citation: Pittman SJ, Stamoulis KA, Antonopoulou M, Das HS, Shahid M, Delevaux JMS, Wedding LM and Mateos-Molina D (2022) Rapid Site Selection to Prioritize Coastal Seascapes for Nature-Based Solutions With Multiple Benefits. Front. Mar. Sci. 9:832480. doi: 10.3389/fmars.2022.832480
Received: 09 December 2021; Accepted: 28 March 2022;
Published: 29 April 2022.
Edited by:
Carolyn J. Lundquist, National Institute of Water and Atmospheric Research (NIWA), New ZealandReviewed by:
Elina A. Virtanen, Finnish Environment Institute (SYKE), FinlandNathan John Waltham, James Cook University, Australia
Katherine Dafforn, Macquarie University, Australia
Phoebe Jan Stewart-Sinclair, The University of Queensland, Australia
Copyright © 2022 Pittman, Stamoulis, Antonopoulou, Das, Shahid, Delevaux, Wedding and Mateos-Molina. This is an open-access article distributed under the terms of the Creative Commons Attribution License (CC BY). The use, distribution or reproduction in other forums is permitted, provided the original author(s) and the copyright owner(s) are credited and that the original publication in this journal is cited, in accordance with accepted academic practice. No use, distribution or reproduction is permitted which does not comply with these terms.
*Correspondence: Daniel Mateos-Molina, dmateos@enwwf.ae