- Center for Corrosion and Biofouling Control, Florida Institute of Technology, Melbourne, FL, United States
The majority of ships are coated with antifouling paint. These coatings can fail to completely protect from fouling due to mismatches between paint type and duty cycle, the presence of biocide tolerant fouling organisms, improperly applied, old or damaged paint, etc. Grooming antifouling coatings can provide a solution. Five commercially available antifouling coatings were applied to panels. Half of the replicates were groomed weekly, the other half were immersed and allowed to freely foul, undisturbed. Photographs were taken and panels were visually assessed monthly. Over the period of two years, all the undisturbed panels became fouled with a diverse community of macrofouling organisms including encrusting and arborescent bryozoans, barnacles, tube worms, oysters, tunicates and more. The groomed panels remained clean of macrofouling for an extended period of time, up to two years depending on the coating. Cover of biofilm was also low on groomed panels. Grooming was effective at maintaining different antifouling paints clear of macrofouling and decreasing cover of biofilms for up to two years of immersion.
Introduction
Grooming is the gentle, proactive, habitual, mechanical maintenance of the submerged portion of ship hulls to maintain them free of the majority of fouling and debris (Tribou & Swain, 2010; Hearin et al., 2015; Hunsucker et al., 2018a; Hunsucker et al., 2018b; Hunsucker et al., 2019; Swain et al., 2022). Fouling increases drag, fuel costs, maintenance costs and wear and tear on ships, greenhouse gas emissions and increases the risk of entrainment and transfer of non-indigenous species (NIS) (Dafforn et al., 2011; Schultz et al., 2011; McClay et al., 2015; Davidson et al., 2016; Hunsucker et al., 2018b; Hunsucker et al., 2019). Typically fouling on ships is controlled using coatings, the majority of which contain antifouling biocides (Martin and Ingle, 2009; Dafforn et al., 2011; Tribou and Swain, 2017). Antifouling coatings can fail to completely protect from fouling due to mismatches between paint type and duty cycle, the presence of biocide tolerant fouling organisms, improperly applied, old or damaged paint and many others (Davidson et al., 2009; Dafforn et al., 2011; IMO, 2011; Sylvester et al., 2011; Davidson et al., 2016).
Between dry docking, the solution to a fouled ship hull has been to clean the hull. Hull cleaning is reactive, requires a diver operated device, which introduces health and safety risks, and can damage coatings and introduce non-indigenous species (NIS; IMO, 2011; McClay et al., 2015; Hearin et al., 2016; Hunsucker et al., 2018b; Scianni and Georgiades, 2019). Despite these drawbacks, cleaning can increase the life of the hull coating and return ships to near clean conditions (Schultz et al., 2011; McClay et al., 2015; Georgiades et al., 2018; Hunsucker et al., 2018b; Hunsucker et al., 2019). Grooming has been proven to proactively provide the benefits of cleaning, without the risk of coating damage. Additionally, the ship is maintained free of fouling and does not show loss of performance associated with fouling (Hearin et al., 2015; Hearin et al., 2016; Tribou and Swain, 2017; Hunsucker et al., 2018b; Scianni and Georgiades, 2019).
Most ships have coatings containing copper (Martin and Ingle, 2009; Dafforn et al., 2011; Tribou and Swain, 2017). Due to concerns with copper accumulation in many ports, new biocides have been introduced to the market. Different biocides are more effective against some organisms, as organisms can become tolerant to biocides. Some examples include Amphibalanus amphitrite, Watersipora subtorquata and Bugula neritina which have all been shown to be tolerant to copper biocides (Weiss, 1947; Johnston and Keough, 2002; Piola and Johnston, 2006a; Piola and Johnston, 2006b; McKenzie et al., 2011; McElroy et al., 2017). Biocidal antifouling coatings use different modes of action to release their biocides. Ablative coatings work by a dissolution of the matrix, which slowly releases the biocide at a decreasing level over time as a leached layer builds up. Self polishing coatings work by hydrolysis of the paint matrix, resulting in a slow consistent release of biocide as long as there is sufficient movement of water over the hull (Lewis, 1998; Chambers et al., 2006; Almeida et al., 2007; Dafforn et al., 2011; Georgiades et al., 2018). Grooming may make antifouling coatings more efficient by minimizing the leached layer and maintaining high efficiency of biocide release (Tribou and Swain, 2017).
The International Maritime Organization has started working on a framework to decrease the transfer of invasive species on ship hulls. The framework highlights how practices that control and manage biofouling can reduce the risk of transferring non-indigenous species, improve hydrodynamic performance and may enhance energy efficiency and reduce ship emissions (IMO, 2011). Many countries are beginning to develop laws concerning hull condition of ships entering their territorial waters. The best practice is maintaining the ship hull as free of fouling as is practical (IMO, 2011; McClay et al., 2015; Scianni and Georgiades, 2019; Georgiades et al., 2020). Grooming has been shown to maintain a standard ablative copper antifouling coating free of macrofouling for up to 12 months and to decrease the amount of biofilm (Hearin et al., 2015; Hearin et al., 2016; Tribou and Swain, 2017; Hunsucker et al., 2018a; Hunsucker et al., 2019). The purpose of this study was to determine the ability of grooming to maintain five different commercial antifouling coatings free of fouling over long periods of time.
Methods
All coatings were applied according to manufacturer’s instructions as a complete system. Coatings consisted of one ablative copper (ACA), two self-polishing copper formulations (SPC1 & SPC2) which were supplied by Naval Surface Warfare Center – Carderock Division (NSWC – Carderock) as part of the intersite calibration project. The intersite calibration project is a regularly repeated test, performed at various test sites around the world (regularly Florida, California, Hawaii, Singapore, etc.). The same set of coatings undergo static immersion and are compared in terms of biofouling accumulation, community structure and fouling adhesion (Swain et al., 2000). Also included were two copper free formulations (CF1 & CF2) which were coated at Florida Institute of Technology (FIT). All coatings had three replicates that were immersed at the FIT static immersion test platform at Port Canaveral, Fl. A set of epoxy panels was included as a negative control. An additional set of three replicates of the antifouling coatings were randomized and placed on a backing plate which was installed on the FIT grooming test platform adjacent to the static immersion test platform. These coatings were groomed weekly using the robotic grooming robot described in (Hearin et al., 2015; Hearin et al., 2016; Hunsucker et al., 2018b; Hunsucker et al., 2019). No epoxy was included in the grooming because it has been shown to foul too quickly for grooming to be effective (Tribou and Swain, 2010) and could damage the grooming tool/brushes. The ACA coating is a standard which has been extensively tested (Tribou and Swain, 2015; Hearin et al., 2015; Hearin et al., 2016; Hunsucker et al., 2019).
Panels were assessed monthly over the span of two years. The static panels were removed from the water, photographed and visually assessed for percent cover (ASTM D6990, 2020). There are two data gaps (8/15 & 1/16) for the ungroomed CF coatings and the EPX where data was not able to be collected. The grooming panels were photographed in situ by a diver with a camera fitted with a water box. This enabled clear pictures regardless of water clarity and maintained the pictures at a set standoff distance and image size. Photographs were visually assessed in the lab following the same method as the static panels. Results are reported in terms of macrofouling cover and cover of specific taxonomic groups (i.e.: hydroid, encrusting bryozoan, barnacle, etc.). To compare fouling at the community level, a principal component analysis was run on the monthly average cover data. A series of one way ANOVAs was used to compare macrofouling cover and fouling cover by specific taxonomic groups.
Results
Macrofouling cover was significantly higher on the ungroomed than the groomed surfaces (One-way Anova, p<0.05; Figure 1). Groomed surfaces did not accumulate macrofouling until nearly two years immersion. Ungroomed surfaces began to accumulate macrofouling after only a few months, despite biocides. Epoxy was typically 95% covered with macrofouling and greater, showing that fouling pressure was diverse and high throughout the experiment. The only exception was CF2. This copper-free coating began to accumulate macrofouling on the groomed surfaces after 11 months. Upon closer examination, the groomed replicates had begun to wear through, as shown by the appearance of light colored epoxy barrier coat. Despite this, the groomed surfaces were able to be maintained in a clean condition at least 6 months longer than the ungroomed surfaces.
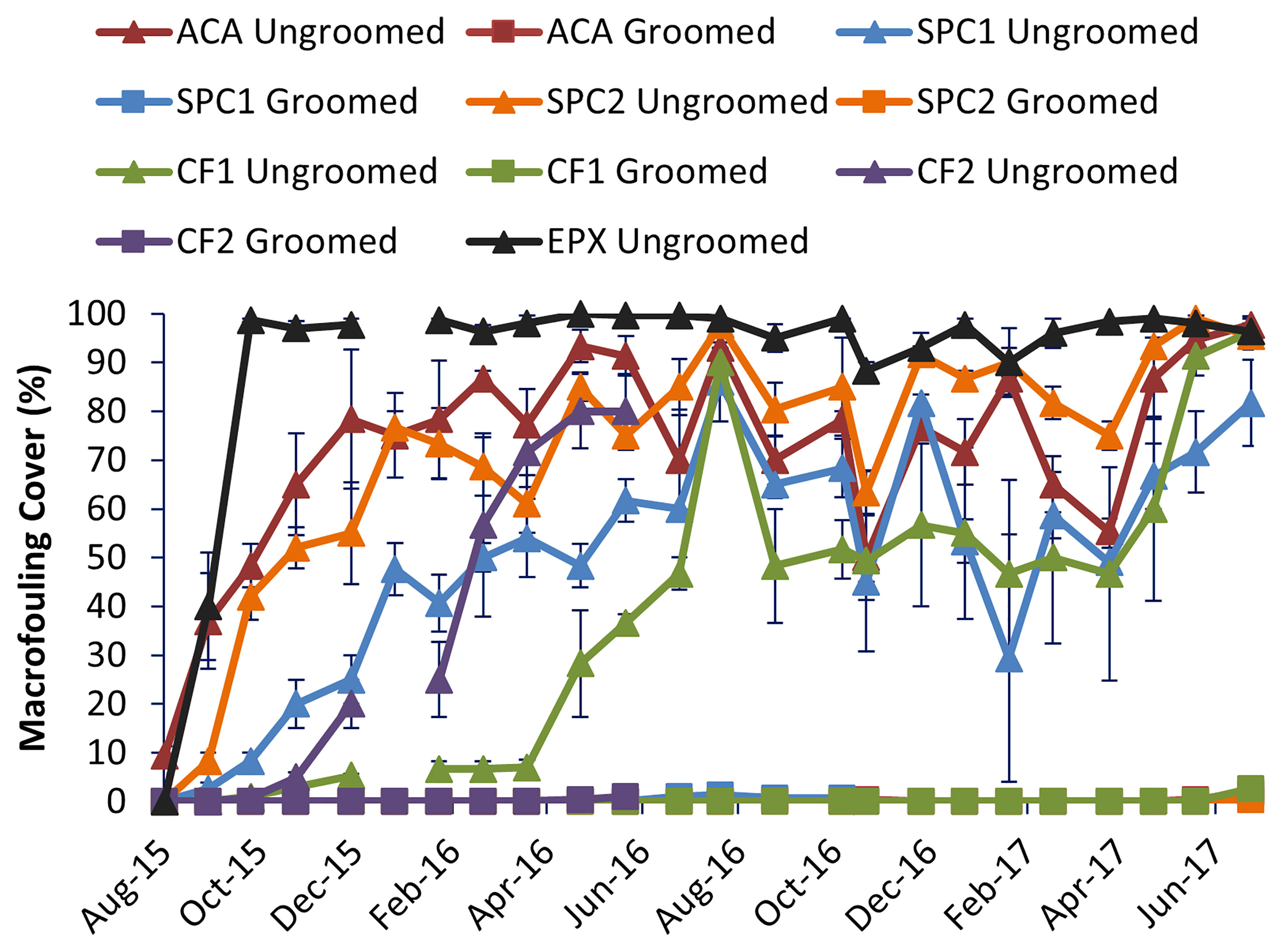
Figure 1 Comparison of macrofouling cover among the different coatings. Error bars represent one standard error, n=3 replicate panels for each coating and grooming condition.
Biofilm cover was uniformly low to absent on groomed panels after grooming (Figure 2). On ungroomed surfaces, the entire surface not covered with macrofouling was typically covered with biofilm. That biofilm was usually thick and silty. On groomed surfaces, the biofilms that remained after grooming were thin and lacked surface debris. The biofilm on groomed surfaces often resembled a surface stain, with no visible thickness (Figure 5).
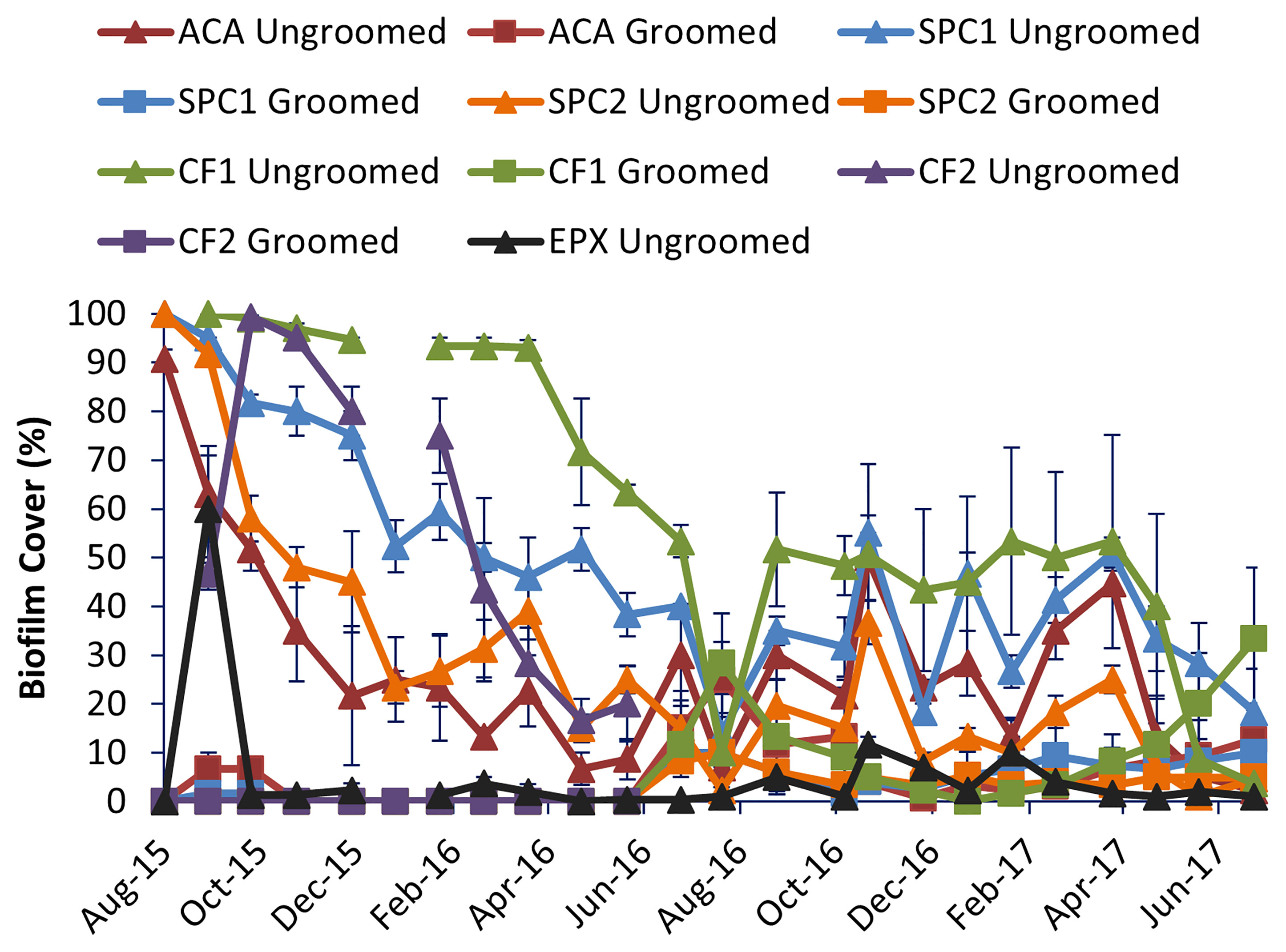
Figure 2 Comparison of biofilm cover among the different coatings. Error bars represent one standard error, n = 3 replicate panels for each coating and grooming condition.
Coatings differed in terms of macrofouling composition (One-way Anova, p<0.05; Figures 3–5). On ungroomed copper coatings, dominant macrofouling primarily consisted of encrusting bryozoans in the genus Watersipora and the barnacle Amphibalanus amphitrite. On the copper free coatings, dominant macrofouling primarily consisted of the arborescent bryozoan Bugula neritina and tube worms in the genus Hydroides. Composition on epoxy consisted of hydroids, encrusting and arborescent bryozoans, barnacles, tube worms, oysters, sponges, tunicates and sea anemones (Figures 3, 5). The PCA (Figure 4) shows that most of the groomed surfaces cluster relatively closely together and are distinct from the ungroomed surfaces. The ungroomed surfaces are less closely grouped, but still make up one group, distinct from the groomed surfaces. The differences between groomed and ungroomed surfaces are primarily due to the cover of macrofouling and biofilms, with a lesser contribution from barnacles and tube worms. The first Principal Component is Macrofouling and accounted for 60% of the variability. The second split was Biofouling (24.6%) and the final split (8.8%) consisted of barnacles and tube worms combined.
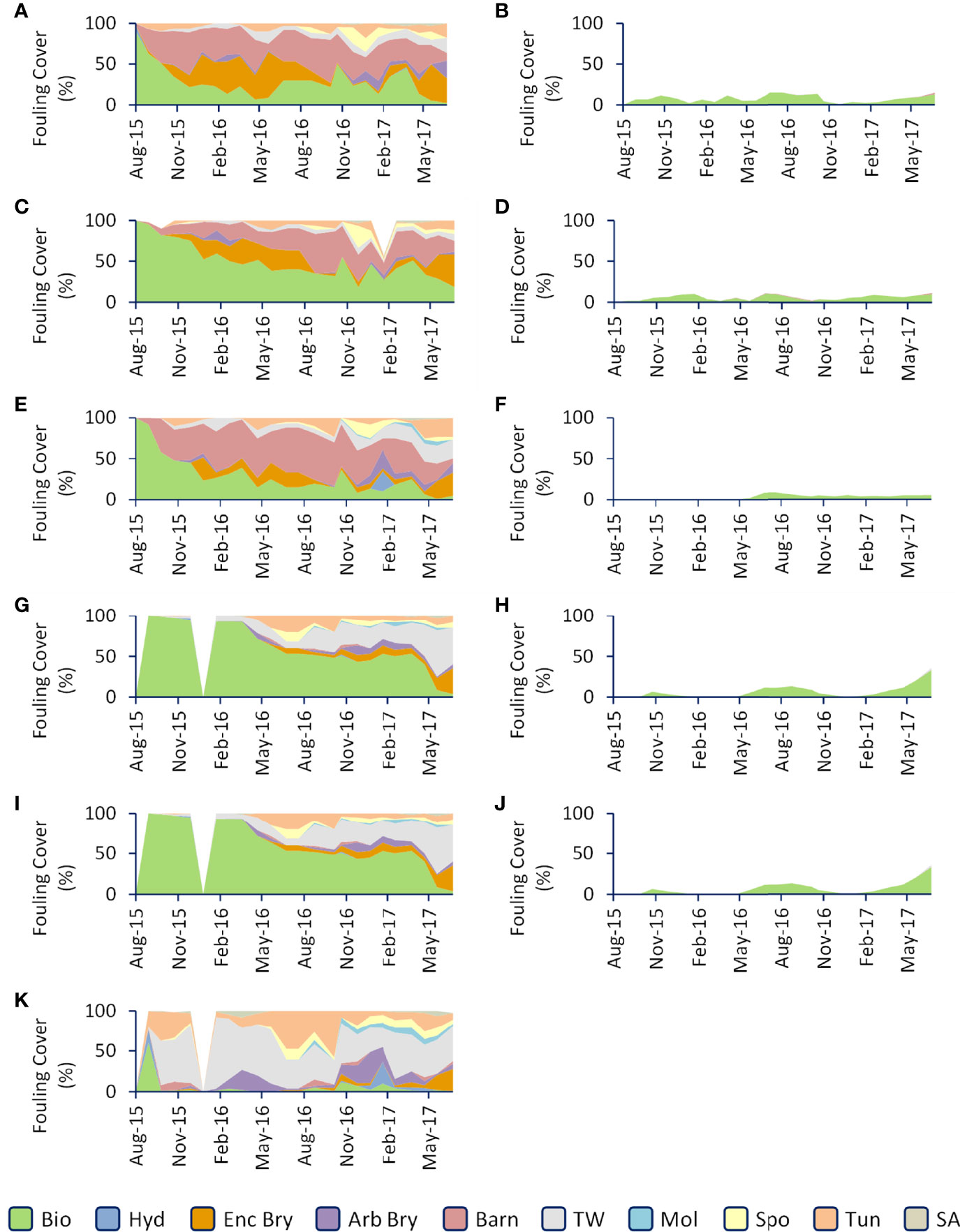
Figure 3 Comparison of fouling composition among the coatings. The left graphs (A, C, E, G, I, K) represent ungroomed surfaces, the right graphs (B, D, F, H, J) represent groomed surfaces. Coating order is as follows: ACA (A, B), SPC1 (C, D), SPC2 (E, F), CF1 (G, H), CF2 (I, J) and EPX (K). Bio, Biofilm; Hyd, Hydroid; Enc Bry, Encrusting Bryozoan; Arb Bry, Arborescent Bryozoan; Barn, Barnacle; TW, Tube Worm; Mol, Mollusc; Spo, Sponge; Tun, Tunicate; SA, Sea Anemone.
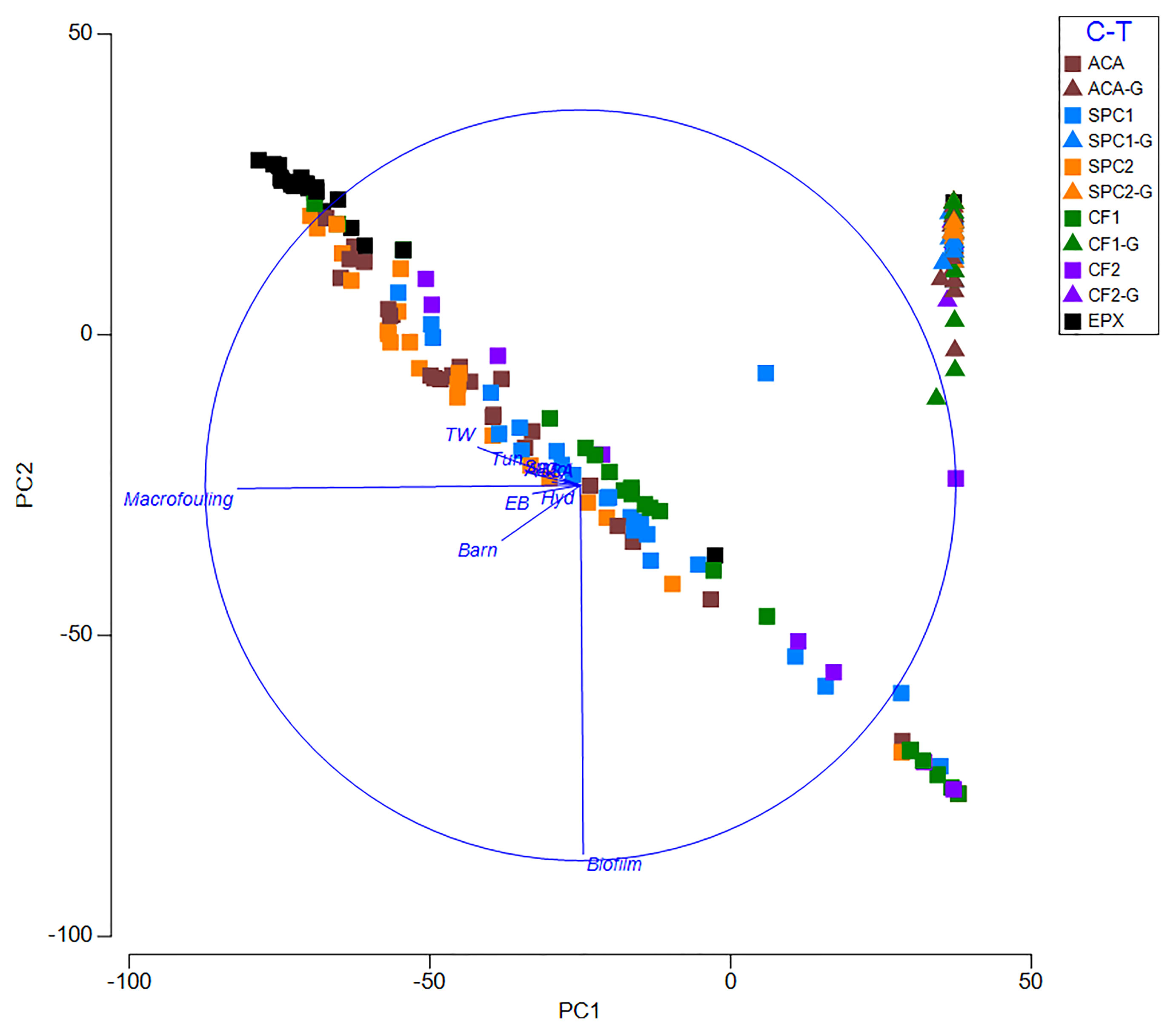
Figure 4 PCA comparing the fouling communities on groomed (triangles) and ungroomed (squares) coatings.
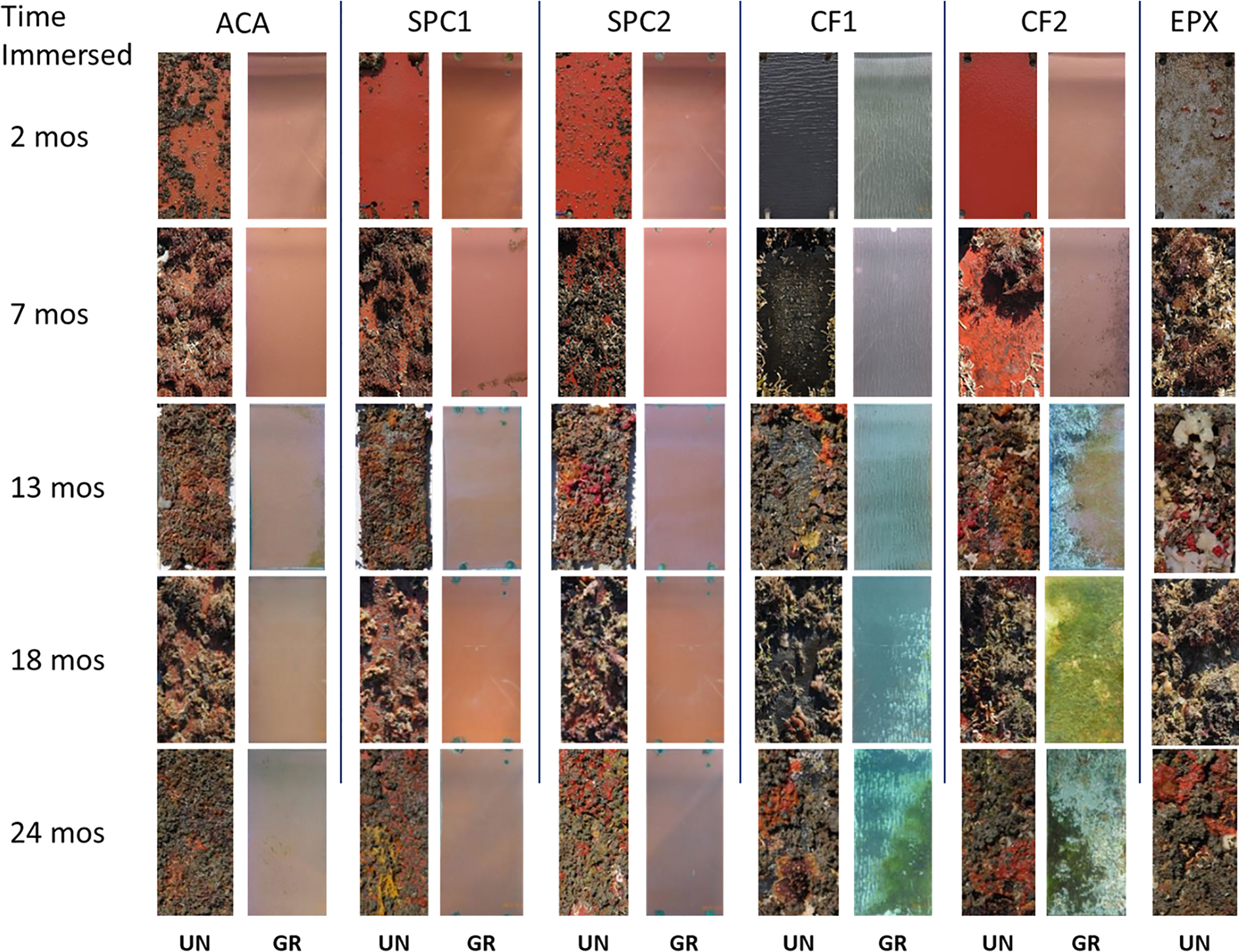
Figure 5 Representative images of the panels over the duration of the experiment. UN is ungroomed, GR is groomed. Panels measured 15x30.5 cm (6x12”).
Discussion
Grooming has proven time and again to be an effective way to maintain ship hull coatings free of macrofouling. Previous experiments have focused on an ablative copper coating found on 90% of US Navy Ships, and have successfully kept macrofouling off the surfaces for up to 12 months (Martin and Ingle, 2009; Hearin et al., 2015; Tribou and Swain, 2017). This experiment looked at the efficacy of grooming on five commercially available, biocide containing, antifouling coatings over two years. These coatings included the previously tested ablative copper coating, two self-polishing copper coatings and two copper-free formulations. All coatings were able to be maintained free of macrofouling for 11 to 24 months using weekly grooming. Ungroomed coatings began to accumulate macrofouling after one to two months immersion, with greater than 50% cover in as little as four and as much as 12 months.
Organisms may have different tolerance to biocides. This is suggested by the different dominant organisms on the copper and copper-free coatings. Ungroomed copper coatings were dominated by Amphibalanus amphitrite and the encrusting bryozoan Watersipora species complex. Both taxa are reported to be tolerant of copper and both are NIS at this site (Weiss, 1947; Piola and Johnston, 2006a; McKenzie et al., 2011; McElroy et al., 2017; Tribou and Swain, 2017). Ungroomed copper-free coatings were dominated by Hydroides tube worms and Bugula neritina (NIS). There are no reports in the literature of organism tolerance to these alternative biocides, however, B neritina and serpulid polychaetes have been reported to be copper and pollution tolerant (Johnston and Keough, 2002; Piola and Johnston, 2006a; Piola and Johnston, 2006b). Grooming was effective at preventing macrofouling accumulation, regardless of growth form. Groomed surfaces were free of low growing forms (tube worms and encrusting bryozoans), higher growth forms (barnacles) and softer growth forms (tunicates and arborescent bryozoans).
Biofilms that remain after grooming were thin, patchy and lacked adhered sediment. On ungroomed surfaces, biofilms tended to cover all open space, were thick and had a fluffy appearance due to attached sediment and flocculated material. This was previously reported and quantified by Hunsucker et al. (2018a) who found that groomed biofilms were significantly thinner, with a different composition and had lower drag than ungroomed ones. The thin, adherent biofilm left behind after grooming has been labeled tenacious biofilm, to differentiate it from standard biofilms accumulated during static immersion (Hearin et al., 2015; Hearin et al., 2016; Hunsucker et al., 2018a).
The length of effect of grooming varied among the coatings. The copper coatings remained macrofouling free and with very low biofilm cover, consisting primarily of low-form, tenacious biofilms for the full two years. SPC2 was especially effective, with only low cover of biofilm over the entire experiment. The copper-free coatings were more variable. CF2 was ended early because of excessive coating loss, as observed in the wear through of the coating. By the end of the experiment, CF1 was also starting to show coating loss. No estimate of coating loss on the ungroomed surfaces could be made because of the high cover of macrofouling hiding the surface of the coatings. The copper coatings did not have visible coating loss and maintained high efficacy. This has been shown in previous experiments, where coating loss was similar among groomed and ungroomed ablative copper coatings over a six year study (Tribou and Swain, 2017). Coating thickness could not be measured in this study because base panel material was not metallic and destructive methods could not be performed on the static panels, which were part of a larger experiment. However, observationally, copper coatings did not show evidence of the loss of even the top layer of coating. Additionally, no plume of paint was observed during grooming as is often observed during cleaning.
The push to replace highly toxic tributyl tin (TBT) led to an increase in copper based ship hull coatings and increased interest in developing alternative biocides. Recently, attention has turned to controlling invasive species transport on ship hulls. IMO developed guidelines to manage biofouling to manage introduction of non indigenous species (IMO, 2011). This standard recommends that ship hulls be maintained as free of fouling as is practical (IMO, 2011). In response many countries, including New Zealand, Australia and the United States, have begun to develop or have developed hull biofouling standards for ships to enter territorial waters with a clean hull (Cunningham et al., 2019; Scianni and Georgiades, 2019; Georgiades et al., 2020). Grooming was shown, in this experiment, to maintain five antifouling coatings clean of macrofouling for extended periods of time. A ship could fulfill “clean hull” requirements in a hull management plan through the use of hull grooming.
Conclusions
Grooming provides long term control of macrofouling on five different antifouling coatings. All copper coatings remained essentially free of macrofouling and with reduced biofilms for two years. The copper-free coatings worked well with grooming, until the coatings began to wear through. This provides many potential benefits including lower fuel costs and less wear and tear on engines due to reduced drag, less green house gas emissions and a reduced chance of transporting invasive species due to reduction of macrofouling on the hull.
Data Availability Statement
The datasets presented in this article are not readily available. Requests to access the datasets should be directed toZXJhbHN0b25AZml0LmVkdQ==.
Author Contributions
ER was the primary author. KH was lead researcher on the static immersion panels. HG operated the grooming robot. GS is the director of the research group. All authors contributed to earlier drafts of the manuscript. All authors contributed to the article and approved the submitted version.
Conflict of Interest
The authors declare that the research was conducted in the absence of any commercial or financial relationships that could be construed as a potential conflict of interest.
Publisher’s Note
All claims expressed in this article are solely those of the authors and do not necessarily represent those of their affiliated organizations, or those of the publisher, the editors and the reviewers. Any product that may be evaluated in this article, or claim that may be made by its manufacturer, is not guaranteed or endorsed by the publisher.
Acknowledgments
We would like to acknowledge the generous funding from the Office of Naval Research (Grants N00014-10-1-0919; N00014-11-1-0915). We would also like to thank the program chairs Dr. Stephen McElvany and Dr. Paul Armistead, as well as Inter-site Calibration Project support from Dr. Eric Holm. We thank our colleagues in CCBC who provided support in the field and lab to get this work done.
References
Almeida E., Diamantino T., Sousa O. (2007). Marine Paints: The Particular Case of Antifouling Paints. Prog. Org. Coat. 59, 2–20. doi: 10.1016/j.porgcoat.2007.01.017
ASTM D6990 (2020). Standard Practice for Evaluating Biofouling Resistance and Physical Performance of Marine Coating Systems (West Conshohocken, PA: ASTM International), 2003. doi: 10.1520/D6990-20
Chambers L., Stokes K., Walsh F., Wood R. (2006). Modern Approaches to Marine Antifouling Coatings. Surf. Coat. Tech. 201, 3642–3652. doi: 10.1016/j.surfcoat.2006.08.129
Cunningham S., Teirney L., Brunton J., McLeod R., Bowman R., Richards D., et al. (2019). Mitigating the Threat of Invasive Marine Species to Fiordland: New Zealand’s First Management Plan. Manage. Biol. Invas. 10, 690–708. doi: 10.3391/mbi.2019.10.4.07
Dafforn K., Lewis J., Johnston E. (2011). Antifouling Strategies: History and Regulation, Ecological Impacts and Mitigation. Mar. Poll. Bull. 62, 453–465. doi: 10.1016/j.marpolbul.2011.01.012
Davidson I., Brown C., Sytsma M., Ruiz G. (2009). The Role of Containerships as Transfer Mechanisms of Marine Biofouling Species. Biofouling 25, 645–655. doi: 10.1080/08927010903046268
Davidson I., Scianni C., Hewitt C., Everett R., Holm E., Tamburri M., et al. (2016). Assessing the Drivers of Ship Biofouling Management – Aligning Industry and Biosecurity Goals. Biofouling 32, 411–428. doi: 10.1080/08927014.2016.1149572
Georgiades E., Growcott A., Kluza D. (2018). “Technical Guidance on Biofouling Management for Vessels Arriving to New Zealand,” in MPI Technical Paper No: 2018/07. WELLINGTON, MPI.
Georgiades E., Kluza D., Bates T., Lubarsky K., Brunton J., Growcott A., et al. (2020). Regulating Vessel Biofouling to Support New Zealand’s Marine Biosecurity System – A Blue Print for Evidence-Based Decision Making. Front. Mar. Sci. 19. doi: 10.3389/fmars.2020.00390
Hearin J., Hunsucker K., Swain G., Gardner H., Stephens A., Lieberman K. (2016). Analysis of Mechanical Grooming at Various Frequencies on a Large Scale Test Panel Coated With a Fouling-Release Coating. Biofouling 32, 561–569. doi: 10.1080/08927014.2016.1167880
Hearin J., Hunsucker K., Swain G., Stephens A., Gardner H., Lieberman K., et al. (2015). Analysis of Long-Term Mechanical Grooming on Large-Scale Test Panels Coated With an Antifouling and a Fouling-Release Coating. Biofouling 31, 625–638. doi: 10.1080/08927014.2015.1081687
Hunsucker K., Braga C., Erdogan C., Gardner H., Hearin J., Ralston E., et al. (2018b). “The Advantages of Proactive in-Water Hull Grooming From a Biologist’s Perspective,” in Hullpic’ 18. 3rd Hull Performance & Insight Conference, Redworth, HullPIC. pp 210–pp 222.
Hunsucker K., Ralston E., Gardner H., Swain G. (2019). “Specialized Grooming as a Mechanical Method to Prevent Marine Invasive Species Recruitment and Transport on Ship Hulls,” in Impacts of Invasive Species on Coastal Environments: Coasts in Crisis. Eds. Makowski C., Finkl C. (Cham, Switzerland: Springer), 247–265.
Hunsucker K., Vora G., Hunsucker J., Gardner H., Leary D., Kim S., et al. (2018a). Biofilm Community Structure and the Associated Drag Penalties of a Groomed Fouling Release Ship Hull Coating. Biofouling 34, 162–172. doi: 10.1080/08927014.2017.1417395
IMO (2011) 2011 Guidelines for the Control and Management of Ships’ Biofouling to Minimize the Transfer of Invasive Aquatic Species. MEPC 62/24/Add.1. Available at: https://wwwcdn.imo.org/localresources/en/KnowledgeCentre/IndexofIMOResolutions/MEPCDocuments/MEPC.207(62).pdf.
Johnston E., Keough M. (2002). Direct and Indirect Effects of Repeated Pollution Events on Marine Hard-Substrate Assemblages. Ecol. Appl. 12, 1212–1228. doi: 10.2307/3061047
Martin F., Ingle M. (2009). Shipboard Coatings Developments and Emerging Surface Technologies. Presented at ASETSDefense 2009 (Westminster, CO: Sustainable Surface Engineering for Aerospace and Defense Workshop).
McClay T., Zabin C., Davidson I., Young R., Elam D. (2015). Vessel Biofouling Prevention and Management Options Report (London: United States Coast Guard).
McElroy D., Hochuli D., Doblin M., Murphy R., Blackburn R., Coleman R. (2017). Effect of Copper on Multiple Successional Stages of a Marine Fouling Assemblage. Biofouling 33, 904–916. doi: 10.1080/08927014.2017.1384468
McKenzie L., Brooks R., Johnston E. (2011). Heritable Pollution Tolerance in a Marine Invader. Envi. Res. 111, 926–932. doi: 10.1016/j.envres.2010.12.007
Piola R., Johnston E. (2006a). Differential Resistance to Extended Copper Exposure in Four Introduced Bryozoans. Mar. Ecol. Prog. Ser. 311, 103–114. doi: 10.3354/meps311103
Piola R., Johnston E. (2006b). Differential Tolerance to Metals Among Populations of the Introduced Bryozoan Bugula Neritina. Mar. Biol. 148, 997–1010. doi: 10.1007/s00227-005-0156-5
Schultz M., Bendick J., Holm E., Hertel W. (2011). Economic Impact of Biofouling on a Naval Surface Ship. Biofouling 27, 87–98. doi: 10.1080/08927014.2010.542809
Scianni C., Georgiades E. (2019). Vessel in-Water Cleaning or Treatment: Identification of Environmental Risks and Science Needs for Evidence-Based Decision Making. Front. Mar. Sci. 26. doi: 10.3389/fmars.2019.00467
Swain G., Anil A., Baier R., Chia F., Conte E., Cook A., et al. (2000). Biofouling and Barnacle Adhesion Data for Fouling-Release Coatings Subjected to Static Immersion at Seven Marine Sites. Biofouling 16, 331–344. doi: 10.1080/08927010009378456
Swain G., Caglar E., Lauren F., Harrison G., Michael H., John H., et al. (2022). Proactive In-Water Ship Hull Grooming as a Method to Reduce the Environmental Footprint of Ships. Front. Mar. Sci. 8.
Sylvester F., Kalaci O., Leung B., Lacoursiere-Roussel A., Murray C., Choi F., et al. (2011). Hull Fouling as an Invasion Vector: Can Simple Models Explain a Complex Problem? J. Appl. Ecol. 48, 415–423. doi: 10.1111/j.1365-2664.2011.01957.x
Tribou M., Swain G. (2010). The Use of Proactive in-Water Grooming to Improve the Performance of Ship Hull Antifouling Coatings. Biofouling 26, 47–56. doi: 10.1080/08927010903290973
Tribou M., Swain G. (2015). Grooming Using Rotating Brushes as a Proactive Method to Control Ship Hull Fouling. Biofouling 31, 309–319. doi: 10.1080/08927014.2015.1041021
Tribou M., Swain G. (2017). The Effects of Grooming on a Copper Ablative Coating: A Six Year Study. Biofouling 33, 494–504. doi: 10.1080/08927014.2017.1328596
Keywords: grooming, antifouling, copper, copper-free, non-indigenous species
Citation: Ralston E, Gardner H, Hunsucker KZ and Swain G (2022) The Effect of Grooming on Five Commercial Antifouling Coatings. Front. Mar. Sci. 9:836555. doi: 10.3389/fmars.2022.836555
Received: 15 December 2021; Accepted: 28 April 2022;
Published: 30 May 2022.
Edited by:
Gary H. Dickinson, The College of New Jersey, United StatesReviewed by:
Shiguo Li, Research Center for Eco-environmental Sciences (CAS), ChinaChristopher M. Spillmann, United States Naval Research Laboratory, United States
Copyright © 2022 Ralston, Gardner, Hunsucker and Swain. This is an open-access article distributed under the terms of the Creative Commons Attribution License (CC BY). The use, distribution or reproduction in other forums is permitted, provided the original author(s) and the copyright owner(s) are credited and that the original publication in this journal is cited, in accordance with accepted academic practice. No use, distribution or reproduction is permitted which does not comply with these terms.
*Correspondence: Emily Ralston, ZXJhbHN0b25AZml0LmVkdQ==