- 1Southern Marine Science and Engineering Guangdong Laboratory (Guangzhou), Guangzhou, China
- 2Department of Estuarine and Delta Systems, Royal Netherlands Institute for Sea Research, Utrecht University, Yerseke, Netherlands
- 3School of Ecology, Environment and Resources, Guangdong University of Technology, Guangzhou, China
- 4Southern Marine Science and Engineering Guangdong Laboratory (Zhuhai), School of Marine Sciences, Sun Yat-Sen University, Zhuhai, China
- 5National Engineering Laboratory for Port Hydraulic Construction Technology, Tianjin Research Institute for Water Transport Engineering, Tianjin, China
- 6Department of Physical Geography, Faculty of Geosciences, Utrecht University, Utrecht, Netherlands
Identify critical factors driving seedling establishment is essential for saltmarsh restoration. Recent studies highlight drainage can facilitate seedling establishment for different saltmarsh species, yet the underling mechanism remains largely unknown. By a manipulative mesocosm experiment with contrasting sediment drainage treatments, we investigated the impacts of drainage on Spartina anglica seedlings survival, growth and stability. Surprisingly, neither S. anglica seedling survival nor seedling growth were observed to be directly promoted by well drained treatment. However, the critical erosion depth (maximum erosion tolerance) of S. anglica seedlings was found to positively correlate with seedling root length. Measurements on the sediment properties revealed that drainage increased the critical shear strength of sediments with lower water content and higher dry bulk density. These findings indicate that, by dewatering, drainage aids to sediment consolidation and critical shear strength, and thereby facilitate seedling establishment in tempering sediment dynamics. The latter is supported by a field study, in which we found that the magnitude of the sediment dynamics on two tidal flats were positively related to water content of sediment. Overall, present study suggests that drainage could be a key factor that determines seedling stability during the critical windows of opportunity for seedling establishment, and that it could be integrated into future designs for saltmarsh restoration to enhance establishment success.
Introduction
Saltmarshes are world-widely valued for their ecological services in blue carbon sequestration (Kirwan and Mudd, 2012) and coastal defence (Möller et al., 2014; Zhu et al., 2020a). Yet, saltmarshes are declining globally (Gedan et al., 2009; Silliman et al., 2009; Shepard et al., 2011) under pressures from sea level rise and human impacts (Craft et al., 2008; Lin et al., 2012; Kirwan and Megonigal, 2013). In reversing this, sufficient knowledge on saltmarsh plant establishment is required to provide science-based strategies to (re)create new marshes (Temmerman et al., 2013; Bouma et al., 2014; Hu et al., 2021).
Whereas it is widely recognized that saltmarsh plants can colonize bare intertidal mudflats both vegetatively via clonal growth and generatively by seedlings (Angelini and Silliman, 2012; Friess et al., 2012), seedling establishment is the crucial mechanism for marsh expansion in many coastal regions, such as Western Europe and Eastern Asia (Zhu et al., 2020b; Zhao et al., 2021). By driving rapid recruitment over extensive area, seedling establishment can be particularly important for many restoration desired sites, where tidal flats lack source vegetation or often are disconnected from existing vegetation by retreating marsh cliffs (Bouma et al., 2016; Zhu et al., 2020b). This render saltmarsh seedling establishment a key process determining restoration potentials (Hu et al., 2021).
Occurring in the dynamic intertidal environments, saltmarsh seedling establishment is a consequence of interactions between ecological, physical, and biogeochemical processes (Friess et al., 2012; Balke et al., 2014; Hu et al., 2015b). Based on experiments and modelling, studies on saltmarsh establishment strongly suggest that successful seedling establishment requires for a sequence of low-disturbance periods (referred to as windows of opportunity, WoO) following seedling dispersal (Balke et al., 2014; Hu et al., 2015b; Hu et al., 2021). During these critical periods, seedlings are expectants in gaining stability by rooting growth to resist subsequent erosion. However, energetic intertidal condition can impose multiple pressures on initial seedling establishment (Friess et al., 2012), the stochastic availability of WoO for a seedling may depend on the minimum duration of a WoO. That is, the shorter the required disturbance-free period, the more WoO will be available in time (Balke et al., 2014). As a consequence, saltmarsh seedling establishment is driven by a balance between seedling growth in rooting and sediment dynamics (Hu et al., 2021). Hence, knowledge of the critical factor and its control on seedling growth and stability is an important step determining the success of saltmarsh restoration.
Recent studies highlight that enhanced drainage condition can facilitate seedling establishment for different saltmarsh species (Fivash et al., 2020; Cao et al., 2021). Drainage is common features in saltmarsh, which can be generally caused by surface sheet flow towards channels during ebbing when the tidal area become exposed (Fagherazzi et al., 2004; Perillo et al., 2009). This tidally sheet flow will led to markedly difference in surface and subsurface hydrological characteristics, which may further develop a pressure head in sediment to induce interstitial porewater to drain vertically from the sediment (Fagherazzi et al., 2004; Winterwerp and Van Kesteren, 2004). That is, drainage of saltmarshes, that three-dimensionally linked to topography heterogeneity (e.g. channel networks, hollow-flat hummock micro-topography, Schwarz et al., 2014; Xie et al., 2018; Fivash et al., 2020) and soil type (e.g. sediment with different hydraulic resistance and capillary forces, Crooks and Pye, 2000; Winterwerp and Van Kesteren, 2004), constitute basic pathways for water recirculation in marshes sediments (Xin et al., 2022). By water recirculation, drainage is able to inject oxygen-rich water into anoxic sediments via porewater flow in them by a pressure head developing at low tide (Jansen et al., 2009), and thereby alleviate anoxic saline conditions (Rabouille et al., 2003; Lamers et al., 2013) that are toxic to salt marsh root layers (Redelstein et al., 2018). This process is suggested to be vital for the establishment of early-stage saltmarsh seedlings (Fivash et al., 2020; Cao et al., 2021), whose aerenchyma is underdeveloped (Burdick and Mendelssohn, 1987; Jung et al., 2008). Nevertheless, it is largely unknown to what extent drainage may affect saltmarsh seedling growth and stability, and whether these effects would dictate the availability of WoOs in determining the ultimate seedling establishment.
In this study, we hypothesize that drainage would facilitate saltmarsh seedling establishment 1) directly by promoting seedling survival and stabilizing root growth, and 2) indirectly by enhancing the sediment stability thereby reducing the chance of seedling loss due to sediment dynamics. To verify hypothesis 1, we tested the effects of drainage on seedling survival and growth in a manipulative mesocosm experiment. To verify hypothesis 2, we measured in the manipulative mesocosm experiment the critical erosion depth of seedlings (maximum erosion a seedling can tolerance) and critical shear strength (sediment strength in resist to erosion) of the sediment under different drainage treatments. This was followed by a field study, in which we related sediment dynamics to sediment water content on two tidal flats. By using a globally distributed foundation marsh species Spartina anglica (Strong and Ayres, 2013; Cao et al., 2018) and sediments from different tidal flats, we aim to extent current fundamental understanding on seedling establishment in the context of saltmarsh restoration.
Materials and Methods
Mesocosm Experiment
Study Sites and Sediment Collection
Sediment samples used in the mesocosm experiment were collected from two locations in the Scheldt estuary in the SW Netherlands (Figure 1). The estuary was semi-diurnal dominated, with tidal ranges from 380 cm near the mouth to 550 cm upstream (Baeyens et al., 1998). The pioneer vegetation of the estuary is mainly Spartina anglica, which was introduced to the area since 1920s and has formed monoculture marshes in the seaward part with elevation ranges from 60 to 200 cm NAP (Normal Amsterdam Peil, which is Dutch Ordance Level that approximately equal to mean high water level in the Scheldet estuary) (Groenendijk, 1986; van der Wal et al., 2008). The Scheldt estuary was composed of two main water bodies called Western Scheldt estuary and Eastern Scheldt estuary, wherein two large areas were realigned to recreate valuable saltmarshes recently:
(i) Rammegors
Rammegors (midpoint: 51°36’31”N, 4° 10’56”E) used to be an embanked polder in the Eastern Scheldt estuary. Due to the embankment, Rammegors has been refreshed by the rainwater for many years. Since 2016, regular tide has been introduced to Rammegors by a tide inlet. The average low and high tide in the Rammegors were 33 cm NAP and 136 cm NAP. After the opening of the inlet, fresh water species (dominated by Phragmites australis) has been largely degraded but still remained. Historically, Rammegors has been used as silt depot for construction reasons, and therefore no patterns of drainage gullies or creeks could be found for most pasts of the area. Field monitoring between 2015 and 2018 implied that elevation did not change in most parts of Rammegors, and soil in Rammegors is sandy and less clayey (Rijkswaterstaat, 2015).
(ii) Perkpolder
Perkpolder (midpoint: 51°23’27”N, 4° 1’25”E) used to be an agricultural land in the Weastern Scheldt estuary. In 2015, the dike preventing water from entering Perkpolder was breached, and sea water from Western Scheldt estuary was introduced to the area (Rijkswaterstaat, 2015). After the breach, a soft mud layer has quickly evolved to equilibrium on top of the former agriculture land (Bruneta et al., 2019). Measurements from 2016 to 2018 indicated that the bed-level elevation of tidal flat in Perkpolder had slightly increased to almost nothing. The tidal flat elevation in Perkpolder ranged between - 80 cm to +110 cm NAP (van de Lageweg et al., 2019). There was no natural vegetation establishment on the tidal flats, and the deposited sediment still consisted of soft, water saturated muddy sediment.
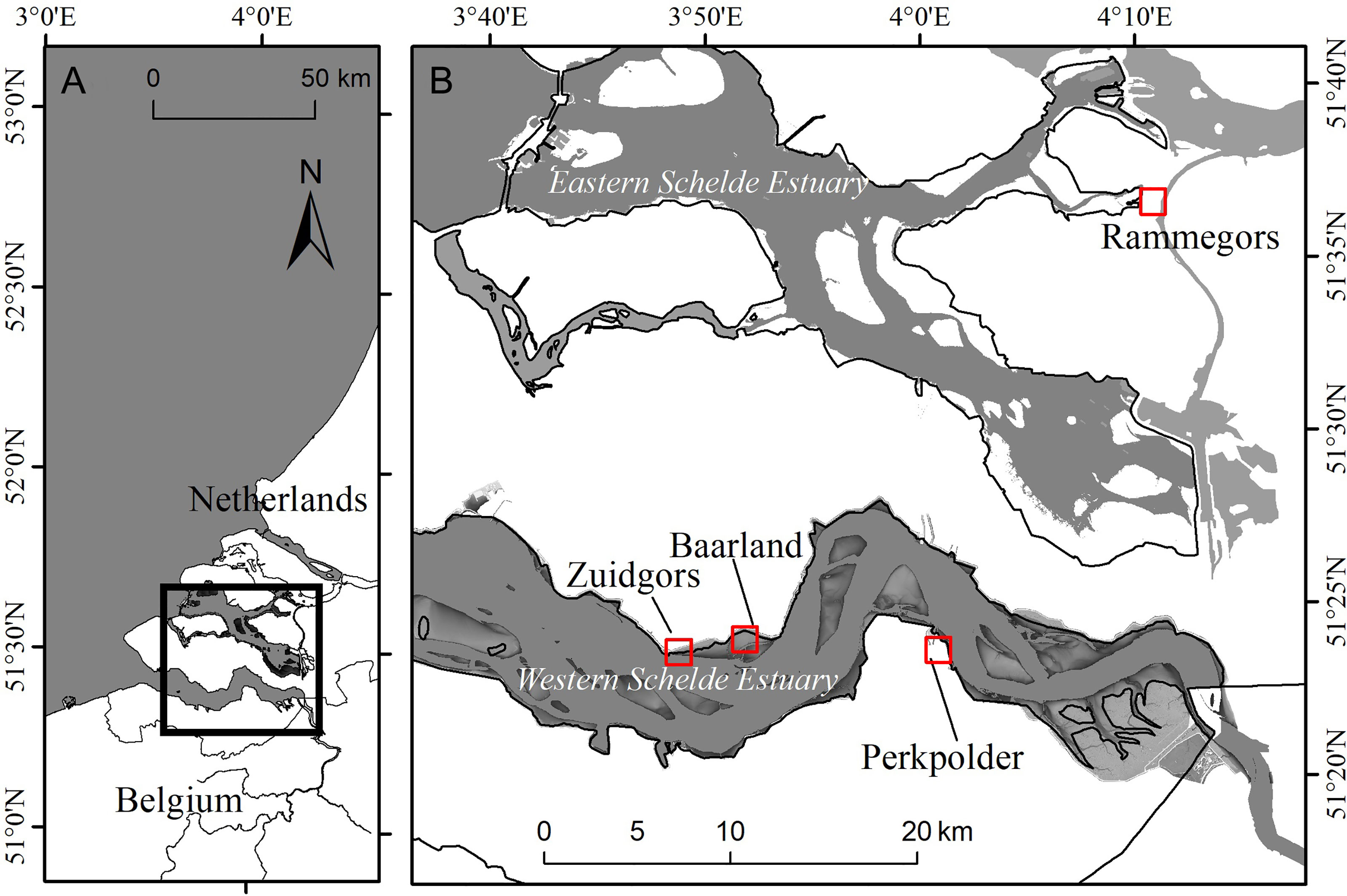
Figure 1 (A) Location of the Scheldt Estuary, and (B) Location of Rammegors (Eastern Schelde Estuary) and Perkpolder (Western scheldt) for sediment collection in the mesocosm experiment, and field study site of and Baarland and Zuidgors (both in the Western Scheldt estuary) for measuring sediment dynamics.
Both Rammegors and Perkpolder could be covered by water and dried every semi-diurnal tide cycle, and the wave actions in both sites were negligible because they were almost completely surrounded by sea-walls. Given that these two areas were lack for efficient drainage system but diffident in sediment texture, Rammegors and Perkpolder were ideal sites for sediment collection to study the effects of drainage on saltmarsh seedling establishment and to test the potential influence of different sediment types.
In Rammegors, due to the higher heterogeneity of vegetation canopy, two kinds of sediment was sampled, i.e. sediment from reed (Phragmites australis) covered marsh, and sediment from grass (Limonium sinense) covered marsh. In Perkpolder, where remains unvegetated, we collected the third kind of sediment. This resulted in three different kinds of sediments to be used in our mesocosm experiment: Rammegors grass (Ram. grass), Rammegors reed (Ram. reed), and Perkpolder (Perkpolder) with different sediment properties (Table 1). For each kind of sediment, we collected 8 replicate samples.
During sediment collection, experimental pots that consist of PVC pipes (with a dimension of 12 cm in width and 12.5 cm in height each) were used. These pots were individually hammered into the ground, after which the pots were carefully dug out again with sediment. To prevent sediment layers from mixing, both ends of the sediment pots were closed with a cap when transport to the institute. All sediment samples were then defaunated in one big tank under airtight and water (Eastern Scheldt sea water with an average salinity of 28ppt) sealed condition for two weeks. After defaunation, each sediment core was gently taken out of the pot, lined by a plastic bag, and put back to the same pot again. The plastic bag in each pot was used to prevent sediment being washed away, and to create different drainage treatments.
Seedling Growth Condition and Mesocosm Treatments
Seedlings of Spartina anglica were geminated from cool-stored seeds (at 4°C) that were collected from the Eastern Scheldt estuary, the Netherlands. The germination were performed in a container under an alternating temperature condition (30°C during the day and 25°C during night respectively). Seeds that visibly with a germ were selected as seedlings to be transplanted. To obtain similar seedling size, the germination were accomplished one week before above sediments were prepared.
Seedlings of S. anglica were transplanted at a depth of 1 cm below the sediment surface, with three seedlings each sediment pot. All sediment pots with seedlings were cultivated in a mesocosms in a climate room of a research institute (NIOZ, Yerseke, the Netherlands), where the temperature was set alternatively (same as seedling germination, see above) and the light was illuminated 12 h d−1 (550 μmol m−2 s−1 PAR) during the whole experiment time.
In order to investigate the effects of drainage on seedling establishment in different sediment types, we manipulated a mesocosm experiment with six paralleled treatments (2 drainage conditions × 3 sediments). For each treatment, four sediment pots (with 3 seedlings each, see above) were replicated, resulting in a total of 12 seedling per treatment (Table 2).
The two drainage condition were created by either making 10 small holes (with 1 cm diameter each) for dewatering at the bottom of the plastic bag that lined in the sediment pot (well drained treatment), or by making no holes at all (poorly drained treatment). To simulate a regular semi-diurnal tidal inundation of the pioneer tidal flat in the Scheldt Estuary, all sediment pots with seedlings were placed in a tidal mesocosm with a 3hrs/12hrs inundation (i.e., 3hrs flooding followed by 9hrs ebb every 12hrs). The tidal mesocosm was set up using a double tank design (for protocol details see Cao et al., 2018; van de Vijsel et al., 2020) that was able to provide seedlings with controllable tidal inundation regime. The water supplied to the tidal mesocosm was filtered Eastern Scheldt water (with an average salinity of 28ppt). In the mesocosm, replicate sediment pots with seedlings that under the same treatment were randomly distributed.
Measurements on Seedling Growth and Stability
After 20 days cultivating in the mesocosm, all seedlings were surveyed for their status of survived, toppled or dead. Survived seedlings were recorded when seedling visibly healthy with at least a vertical live shoot. Subsequently, the Critical Erosion Depth (CED) that a seedling could tolerate was tested for each survived seedling under all treatments. CED is defined as the minimum depth of sediment that needs to be removed before seedling toppling or dislodgment occurs. The CED test was quantified by applying step-wisely erosion from the sediment top, until seedling toppled or dislodged. This was carried out by the following procedure: first insert a disc with thickness of 1.5 mm underneath sediment pot and gently remove the pushed-up top sediment using a water spray, then individual sediment pot was exposed to a constant 30 cm/s current for 2 minutes in a flume. This disc adding and current flushing procedure was repeatedly applied until seedling toppled or dislodged (Cao et al., 2018). Thereafter, each survived seedling was measured for shoot and root length. When multiple shoots or roots were presented, the longest shoot or root was recorded.
Measurements on Sediment Properties
In examining the effects of drainage on sediment erodibility in the mesocosm experiment, we measured critical shear strength of sediments under different drainage treatments. Critical Shear Strength (CSS) was measured for all the three sediment types under both well drained and poor drained treatments. The CSS is a geotechnical characteristic that defines the erodibility of a sediment, and is widely used for many studies in demonstrating sedimentary mechanisms in the intertidal environments (Hu et al., 2015a). Generally, the lower the CSS is, the higher the erodibility of the sediment would perform. We measured the CSS with a shear vane device (Eijkelkamp Agrisearch Equipment, 2012). When using the device, we pressed the vane onto the sediment top and turning it to the right until the sediment starts moving. A value was read from the device for each test, which then been used to calculate the CSS at a resolution of 0.2186 kg/cm2.
At the end of mesocosm experiment, sediment redox potential was measured during ebbing by a banded ORP electrode that inserted at 1 cm depth of the sediment for each pot to compare the oxidizing capacity under different treatments. A sediment core was taken per replicate pot (for all three kind of sediment under both well drained and poorly drained treatments). Each sediment core was taken by a stander sized syringe (inner diameter = 2.8 cm, depth= 3 cm), then stored in a container and weighed for wet weight. All sediment samples were freeze dried for 72 hours and weighed again to calculate water content and dry bulk density:
Field Survey
To further determine the effect of drainage on sediment dynamics, we tested the relationship between sediment surface elevation change and sediment water content in the field. In the northern bank of Western Scheldt estuary, the Netherlands, we monitored sediment surface elevation change on two tidal flats in Baarland and Zuidgors (Figure 1). These two tidal flats were close in distance with similar elevation (0.30 NAP in Baarland, 0.45m NAP in Zuidgors, NAP is Normal Amsterdam Peil, which is Dutch Ordance Level that approximately equal to mean high water level in the Scheldet estuary), but contrast in wind exposure: Zuidgors was exposed to prevailing southwesterly wind, Baarland was sheltered by a seaward shoal (Callaghan et al., 2010).
At both sites, we monitored the surface elevation change for a month in Nov. 2014. For monitoring, Surface-Elevation-Dynamic (SED) sensors were used. The SED sensor is a stand-alone device that can continuously monitor surface elevation dynamics of intertidal flat with a high vertical resolution of 2 mm (for details in using the SED sensor, see Hu et al., 2015a). Due to the different cross-shore distance of the tidal flat between the two field sites, we inserted three and eight SED sensors along the elevation gradient in Baarland and Zuidgors, respectively. Close to each SED sensor, a pressure sensor (Ocean Sensor System. INC.) was deployed to measure wave condition. The presser sensor was placed 5 cm on top of the tidal flat and set at a measuring interval of 15 min with a frequency of 5 Hz. The significant wave height (Hs) data was derived from the presser sensors for analysis (Hu et al., 2015a). At each data point, we collected a sediment core close to each SED sensor. Afterwards, each sediment core was measured for sediment grain size (using Malvern P2000 machine) and water content (protocol same as in the mesocosm experiment, see above).
Statistics
Due to the binominal distribution of the seedling survival data, a generalized linear model (GLM) using the family “binominal” was performed to test the effect of drainage and sediment on seedling survival. Two-way ANOVAs was performed to examine effects of drainage and sediment on seedling shoot length, root length and CED. During this course, only those survived seedlings (visibly healthy with at least a vertical live shoot) were account for at the time of harvest. ANCOVA was used to test for correlations between the shoot length, root length and the CED within all treatments.
The critical shear strength and sediment properties was also analyzed with two-way ANOVAs to include the effects of drainage and sediment types. Student’s t-tests were applied to all seedling growth traits and sediment properties to compare the effect of drainage in individual treatment. In the field study, the obtained surface elevation dynamics at two contrasting sites were interpreted in relation to sediment water content and significant wave height. All the analyses were done in R (https://www.r-project.org) applying a significance level of 5%.
Results
Mesocosm Experiment
Effects of Drainage on Seedling Establishment
In the mesocosm experiment, the GLM analysis indicated that seedling survival was not affected by drainage (Figure 2A, p=0.295, Table 3), sediment (Figure 2A, p=0.745, Table 3) and their interactions (Figure 2A, p=0.202, Table 3). Similarly, effects of drainage, sediment and their interaction were not observed for either seedling shoot length or root length (Figure 2B, p>0.05, Table 4). Seedling length (for both shoot and root) in each sediment did not differed between well drained treatment and poorly drained treatment either.
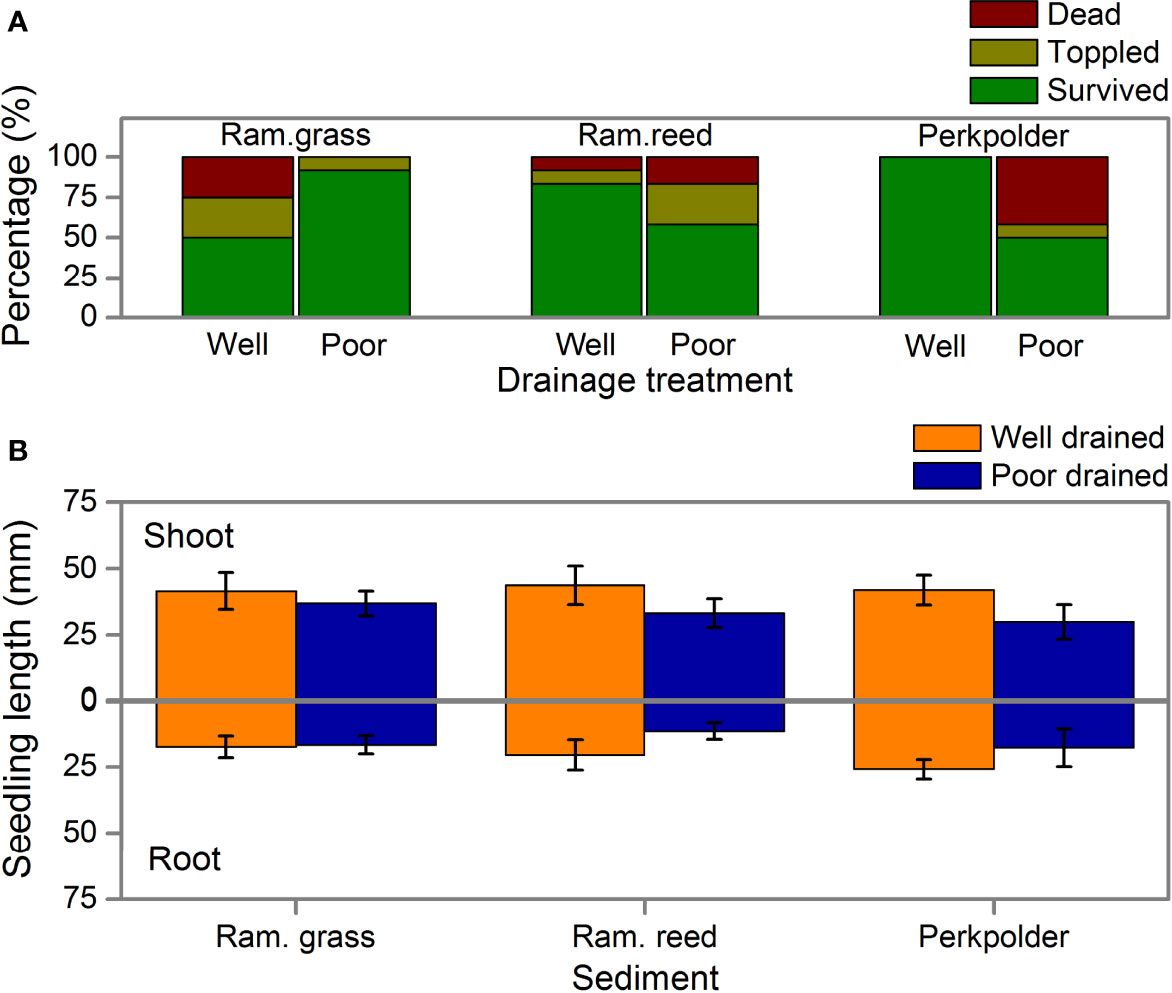
Figure 2 (A) Percentage of survived (dark green), toppled (dark yellow), and dead seedlings (dark red) at harvest in the mesocosm experiment, well drained and poor drained treatments are indicated at the bottom of the panel; (B) seedling length under 6 treatments after 20 days cultivating in the mesocosms. In (B), the well-drained and poor drained treatments were presented in orange and blue respectively. The three kinds of sediments (Ram.grass, Ram.reed, and Perkpolder) were shown for each column. (Data is presented as mean + se.).
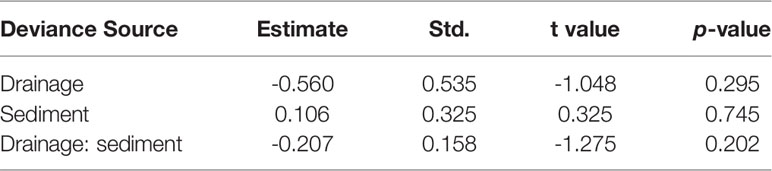
Table 3 Confidents of the generalized linear model (GLM) for examining the effects of drainage and sediment on seedling survival in the mesocosm experiment.
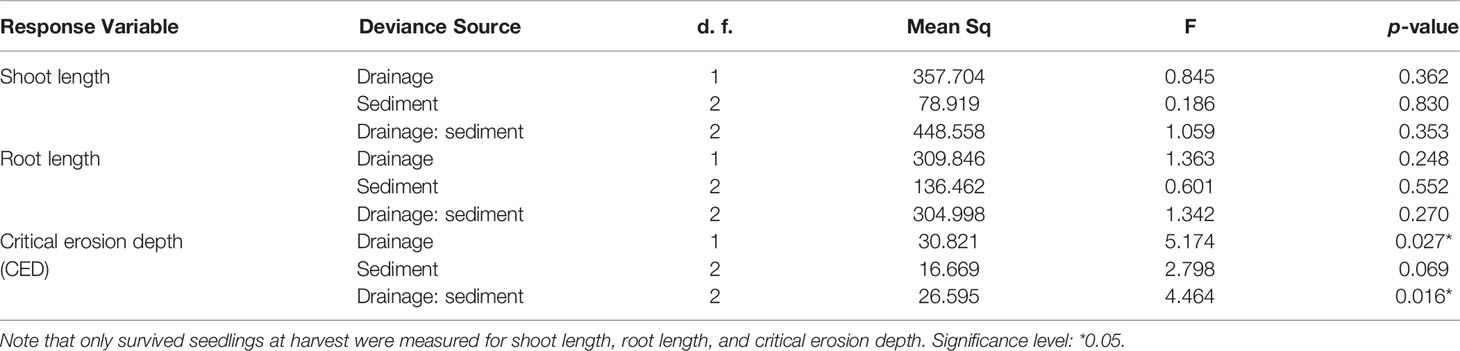
Table 4 Two-way ANOVAs table show effects of drainage, sediment, and their interactions on S. anglica seedling growth traits in the mesocosm experiment.
The maximum erosion depth that S. anglica seedlings can withstand before dislodgment (Critical erosion depth, CED) was significantly affected by drainage and its interaction with sediment (Figure 3A, p<0.05, Table 4). For individual sediment, significant higher CED were observed in Ram. reed sediment (Figure 3A, p<0.05, student’s t test) and Perkpolder sediment (Figure 3A, p<0.05, student’s t test). A close to, but not significant effect of sediment on CED was observed (p=0.069, Table 4).
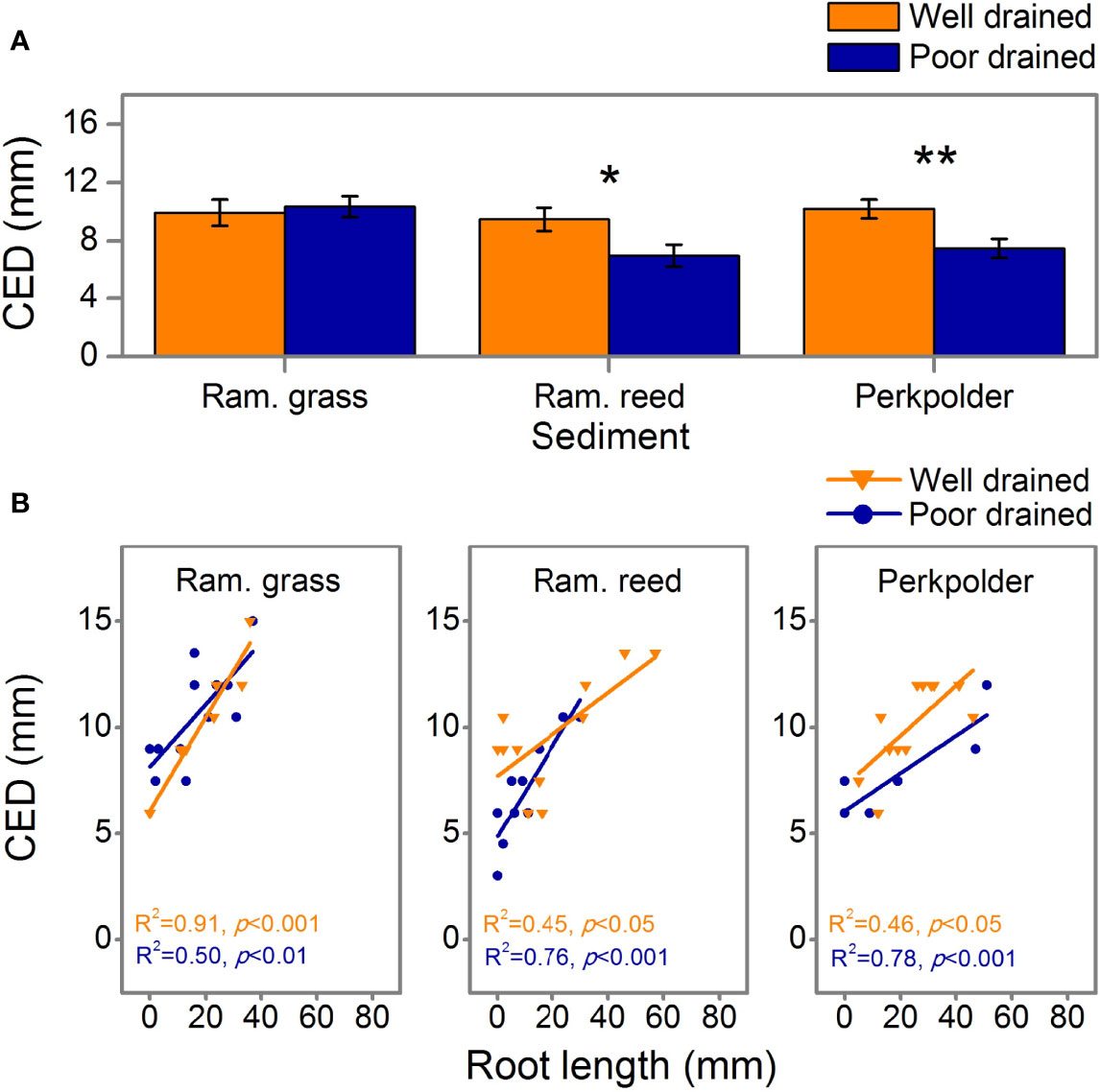
Figure 3 (A) Critical erosion depth (CED) of survived seedling in the mesocosm experiment (data is presented as mean + se. Significance level: *0.05, **0.01). (B) The relationship between CED and seedling root length, R2 and p values are presented at the bottom of each column. Note that data points are not equally plot due to different seedling survival. Colors indicate the same as Figure 2B.
Interestingly, we found that CED was linearly correlated with root length for all three kinds of sediments in our experiment, regardless the sediment was drained or not (Figure 3B; p<0.05). That is, in all sediment types, under both well drained and poorly drained sediment, the longer the roots of seedlings grown, the higher CED the seedlings could with stand when exposed to erosion disturbances.
Effects of Drainage on Sediment Characteristics
In our mesocosm experiment, both water content and bulk density were significantly affected by drainage and sediment (Figures 4A, B, p<0.01, Table 5). As expected, for all sediment types, water content was significantly lower in well drained treatment than in poorly drained treatment (Figure 4A, p= 0.014 for Ram. grass sediment, p=0.001 for Ram. reed sediment, p=0.043 for Perkpolder sediment, student’s t tests). The bulk density of Perkpolder sediment was significantly higher in compared to Ram. grass and Ram. reed (Figure 4B, p<0.001, Post-Hoc test) presumably because both of the later sediments were collected in vegetated areas in Rammegors with a significant higher organic content (Table 1).
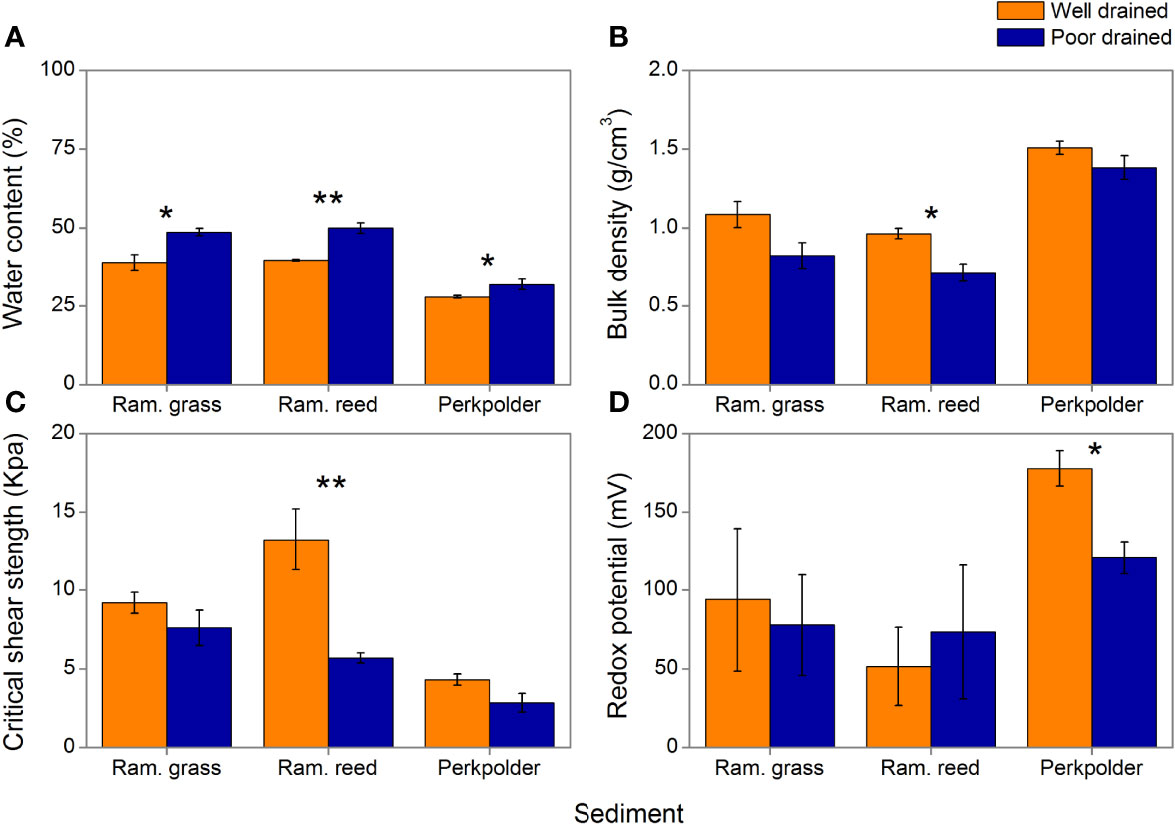
Figure 4 Sediment properties and critical shear stress measured in the mesocosm experiment: (A) water content, (B) bulk density, (C) critical shear stress, (D) redox potential. Colors indicate the same as Figure 2B. (Data is presented as mean + se. Significance level: *0.05, **0.01).
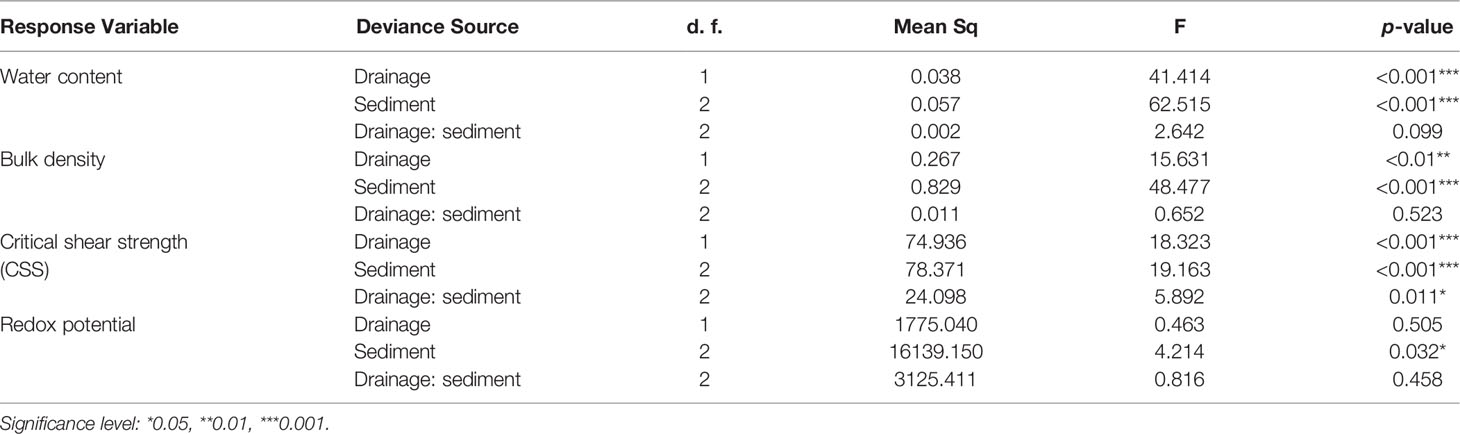
Table 5 Two-way ANOVAs table show effects of drainage, sediment, and their interactions on sediment properties in the mesocosm experiment.
Critical shear strength (CSS) was also detected to have been influenced by drainage and sediment (Figure 4C, p<0.001, Table 5). That is, the overall CSS was significantly lower in well drained sediments in compare to poorly drained sediments. For different sediment types, CSS of Perkpolder sediment was observed to be lower in compare to the other two sediment (Figure 4B, p<0.001, Post-Hoc test). Specially, in Ram. reed sediment, CSS was significantly higher in well drained treatments than in poorly drained treatments (Figure 4C, p<0.01, Student’s t test).
Drainage did not influence the redox potential (Figure 4D, p=0.505, Table 5), as only significant sediment effects was found (Figure 4D, p<0.5, Table 5). However, redox potential was significantly higher in Perkpolder sediment than in Ram. grass and Ram. reed (Figure 4D, p<0.05, Post-Hoc test). For Perkpolder sediment alone, redox potential was significantly increased under well drained sediment in compare to under poorly drained sediment (Figure 4D, p<0.05, Student’s t test).
Field Survey
Our field measurements showed that, sediment water content in Baarland and Zuidgors was positively correlated to sediment grain size (Figure 5A; R2 = 0.40, p<0.01). Sediment dynamics measured by SED sensors was positively correlated with the water content of the sediment across tidal flats (Figure 5B; R2 = 0.47, p<0.05). This means that, in the field, sediment drainage was strongly depend on sediment nature, and the higher water the sediment contains, the more dynamics it would exposed to under wave conditions, thereby reducing the likelihood of seedling establishment.
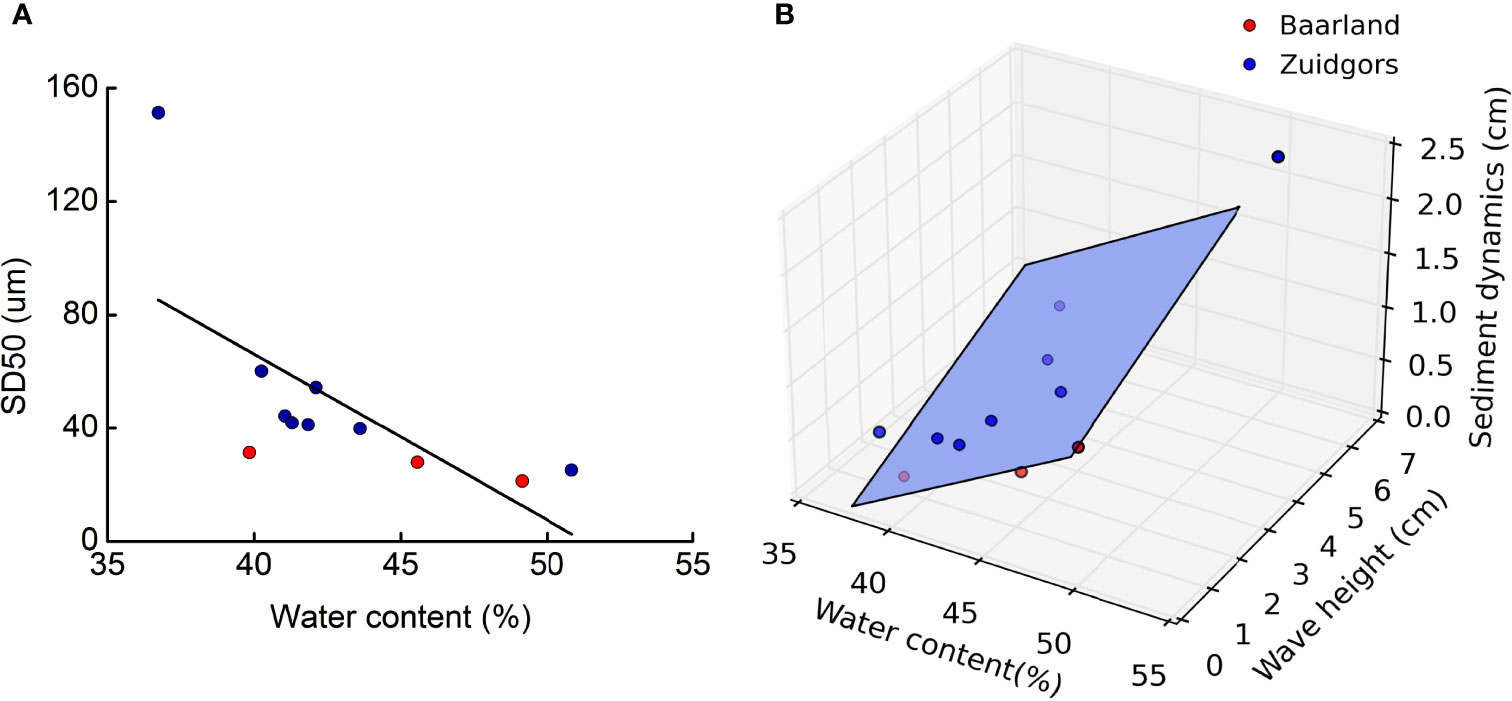
Figure 5 Field measurements in Baarland and Zuidgors showing (A) sediment water content was positively correlated to sediment grain size (R2 = 0.40, p<0.01), and (B) sediment dynamics depends on a combination of sediment water content and wave height (R2 = 0.47, p<0.05).
Discussion
Seedling establishment is a crucial phase that determining the success of saltmarsh restoration attempts (Friess et al., 2012; Balke et al., 2014; Hu et al., 2021). Whereas recent studies highlight drainage is a critical factor in facilitating saltmarsh seedling establishment (Fivash et al., 2020; Cao et al., 2021), much less is known on the underlining mechanisms for the effects of drainage. Using Spartina anglica as an example, present study demonstrates that drainage could enhance saltmarsh seedling stability indirectly by improving sediment strength during the windows of opportunity for seedling establishment.
Drainage Effects on Seedling Survival and Growth
In our mesocosm experiment, neither drainage nor sediment were observed to affect seedling growth and survival. This is surprising, because drainage in earlier studies was found to facilitate saltmarsh seedling survival and growth by relieving anoxic toxicity to root layers (Redelstein et al., 2018; Fivash et al., 2020; Cao et al., 2021). Although there were certain differences in soil water content showing that our drainage treatments were effective in dewatering all sediments, we note that drainage had no overall effects on redox potential of sediments. This discrepancy could possibly attribute to the fact that fast surface dynamics of pore water chemistry in intertidal flats during inundation (Jansen et al., 2009), and our measurements for redox potential during ebbing may not have captured the extreme event. Moreover, it is noteworthy that the dewatering of sediment in our experiment was via holes from the sediment bottom (internal drainage through the sediment), which is in stark contrast to the surface heterogeneity related drainage (e.g. channel networks, hollow-flat-hummock topography induced surface sheet flow on sediment top) that previous studies worked on (Fivash et al., 2020; Cao et al., 2021). While the dewatering of superficial sediment accompanied by evaporation could have significant influence on the exchange of metabolically gases, internal drainage, which depends on sediment nature, is more reliable for the resupply of sulfate and the removal of soluble end products of sulfate reduction (Bradley and Morris, 1990). The complexity in our redox potential measurement indicates that there could be other mechanisms associated with drainage in affecting saltmarsh seedling survival and growth.
We acknowledge that present study manipulated only two drainage treatments, under one pioneer tidal inundation regime, and thus represents a limitation. In natural saltmarshes, drainage often exhibit strong heterogeneity and inter-correlated with other environmental factors (for a review see Xin et al., 2022). Generally, a hydraulic driven drainage gradient is readily present to impact saltmarsh colonization along elevation (Chapman, 1960; Davy et al., 2011), with increasing distances to creeks (Sanderson et al., 2000; Hughes et al., 2012), or raising and lowering of topography (Ward et al., 2016; Xie et al., 2018; Mossman et al., 2019). Superimposed on this, is drainage condition has substantial influences on other environmental stressors such as salinity, which can induce ion toxicity and inhibit saltmarsh seedling emergence if tolerance threshold been surpassed (Munns and Tester, 2008; Nichols et al., 2009). For example, hypersalinity has been reported to hamper seedling establishment in the supratidal uplands as a consequence of severe droughts (He et al., 2017; Xie et al., 2019). However, such hampering effects may not be a problem at lower elevations, where higher moisture content and more stable salinity level can be expected under frequent tidal inundation (van Katwijk and Wijgergangs, 2004). Therefore, our results that no difference in S. anglica seedlings survival and growth could consequent from that soil water content and salinity in our mesocosm experiment fit within the tolerance threshold of S. anglica (Suding and Hobbs, 2009; Xie et al., 2019). The genus of the latter, Spartina spp., is known as a primary colonizer that has high tolerance for salinity (Xie et al., 2019) and poorly drained, waterlogged sediments in low tidal frame (Sullivan et al., 2018). Further exploration to disentangle independent effect of drainage and salinity on saltmarsh seedling establishment along a gradient would be valuable.
Drainage Effects on Seedling Stability
The critical erosion depth (CED) of seedlings in our experiment was shown to be positively correlated to seedling root length. Given that seedling establishment requires short-term bed erosion to be always lower than the CED (Hu et al., 2021), the antagonistic relationship indicates that, for a given seedling root length, CED could be more easily exceeded in sediment with a lower critical erosion threshold. The likelihood to exceed CED can be easier in sediment that lack drainage, because drainage is a determinant of sediment water content, which may affect consolidation and the resistance of sediment to erosion (Crooks et al., 2002). In our case, there were demonstrable effects of drainage on sediment water content, bulk density, and consequently on the critical shear strength. Hence, present results suggest that drainage could be a general determinant factor that seedling stability depend up on.
The effects of drainage in determining seedling stability can further be supported by our field data, in which we found that short-term sediment erosion increased with water content on two tidal flats with different wave exposure. Although there might be nonlinear relationship between drainage and sediment water content in the field, the effects of drainage are manifested primary as changes in the water content of sediments, and consequently on the interactions between particles and surrounding porewater (Crooks et al., 2002). Previous works have shown that the erosion of sediment occurs when repulsive forces between sediment particles exceed the attractive forces, and is thus strongly dependent on the capillary forces in porewater and the cohesion behaviour of the sediment (Crooks and Pye, 2000). That is, the increasing trend of sediment erosion with water content in our field survey could be related, at least in part, to local drainage condition.
In macro intertidal systems, even though wave forcing appears to drive landscape erosion (Pye, 2000; Callaghan et al., 2010; Leonardi et al., 2016), the erodibility of the sediment itself could determine the erosion rate at the local scale (Feagin et al., 2009; Lo et al., 2017; Wang et al., 2017). The spatially and temporally varying bed shear stress of tidal flats could also affect the short-term sediment dynamic patterns that may dictate fronting saltmarsh colonization (Hu et al., 2015b; Hu et al., 2015c). Such short-term sediment dynamics can be especially important in early seedling establishment (Bouma et al., 2016), since experiments have shown that CED is influenced by the cumulative sediment dynamic regime a seedling experienced (Cao et al., 2018). This means that drainage can also externally contribute to seedling tolerance to sediment erosion in the long-term on an intertidal flat that is often reworked by hydrodynamics.
Implications for WoO in Seedling Establishment and Saltmarsh Restoration
To be able to successfully establish, saltmarsh seedling needs to develop sufficient root anchoring during disturbance-free period (referred to as windows of opportunity, WoO) to improve resistance to withstand subsequent disturbances (Friess et al., 2012; Balke et al., 2014). This makes the minimum required WoO length for a seedling to successfully establishment variable depending on seedling root growth rate and local sediment erosion (Hu et al., 2015b; Hu et al., 2021). However, although several saltmarsh pioneer species (e.g., Salicornia spp., Spartina spp., Elytrigia spp.) were shown to exhibit rapid root emergence and development (Pestrong, 1969; Friess et al., 2012; Redelstein et al., 2018), marsh seedling size is generally small in the first several weeks after germination (within tens of mms in our case) in compare to other wetland species, such as mangroves (Balke et al., 2011; Friess et al., 2012). Sediment erosion is therefore expected to be the main mechanism causing initial saltmarsh seedling establishment failure (Hu et al., 2015b; Cao et al., 2018). In this regard, well drained sediments that with higher critical shear strength could be essential for enhancing initial marsh seedling establishment chances. Hence, present study suggests that drainage could play a key indirect role in seedling establishment when WoO opens for initial seedling establishment. That is, by dewatering, drainage aids to sediment consolidation and strength, and thus facilitates seedling establishment by reducing sediment dynamics.
In the last decades, saltmarsh restoration has been increasingly appreciated to compensate for the loss of valuable coastal habitats. However, restored saltmarshes often failed to be equivalent to natural marshes because of frequently waterlogged conditions under poor drainage (Masselink et al., 2017; Sullivan et al., 2018). For instance, a comparison between nineteen restored sites and natural marshes of the UK showed that restored saltmarshes are commonly lack for topographic heterogeneity and with greater potential for water accumulation (Lawrence et al., 2018). As a consequence, the top sediment layer for saltmarsh seedlings to anchor is generally low in shear strength and susceptible to erosion (Crooks and Pye, 2000). The latter case could especially true in many relatively young realigned marsh landscapes, where high rates of sedimentation frequently occurred with a smoothing topography (Garbutt et al., 2006; Mazik et al., 2010). Therefore, present results have important implication for saltmarsh restoration in that, by management measures to well-conceive local drainage conditions, sediment strength associated seedling stability could be enhanced. Restoration projects may be able to improve drainage with limited extra cost. For example, by taking advantage of local channel networks or existing agriculture farm ditches (Gerdan et al., 2009), or by manipulating of a hummock micro-topography (Xie et al., 2018; Mossman et al., 2019; Fivash et al., 2020), one could ameliorate drainage cost-effectively.
Conclusion
Understanding saltmarsh seedling establishment has attracted a major of interests in marine science from the standpoint of coastal restoration. Present study highlights that drainage-improved sediment strength could enhances saltmarsh seedling stability in balancing sediment dynamics. This adds to previous works on windows of opportunity (WoO) for seedling establishment in that, common physical factor on the tidal flat, such as drainage, may dictate the route of seedling establishment when WoO opens. Future restoration are suggested to ameliorate drainage in a cost-effective way to improve the primary establishment of saltmarshes.
Data Availability Statement
The raw data supporting the conclusions of this article will be made available by the authors, without undue reservation.
Author Contributions
The experiment in this research is designed based on the ideas of ZZ and TB; HC, ZH, and ZZ collected and analysed the data; HC led the writing of the manuscript. All authors contributed to the manuscript and approved to submit to Frontiers in Marine science.
Funding
We acknowledge the financial support from the Program for Guangdong Introducing Innovative and Entrepreneurial Teams (2019ZT08L213) and the Key Special Project for Introduced Talents Team of Southern Marine Science and Engineering Guangdong Laboratory (Guangzhou) (GML2019ZD0403) and the National Natural Science Foundation of China (Grants: 41901126; 42006153). We acknowledge the RWS-funded Perkpolder and Rammegors projects, and the Zuidgors project, which was funded by the Province of Zeeland. These three projects aim to obtain a better understanding of saltmarsh establishment, to thereby improve the nature management of the Scheldt estuary.
Conflict of Interest
The authors declare that the research was conducted in the absence of any commercial or financial relationships that could be construed as a potential conflict of interest.
Publisher’s Note
All claims expressed in this article are solely those of the authors and do not necessarily represent those of their affiliated organizations, or those of the publisher, the editors and the reviewers. Any product that may be evaluated in this article, or claim that may be made by its manufacturer, is not guaranteed or endorsed by the publisher.
Acknowledgments
We highly appreciate MsC student Annick van der Laan from the Utrecht University for her energetic help in data collecting during the mesocosm experiment.
References
Angelini C., Silliman B. R. (2012). Patch Size-Dependent Community Recovery After Massive Disturbance. Ecology 93, 101–110. doi: 10.1890/11-0557.1
Baeyens W., van Eck B., Lambert C., Wollast R., Goeyens L. (1998). Generaldescription of the Scheldt Estuary. Hydrobiologia 366, 1–14. doi: 10.1007/978-94-017-3573-5_1
Balke T., Bouma T. J., Horstman E. M., Webb E. L., Erftemeijer P. L. A., Herman P. M. J. (2011). Windows of Opportunity: Thresholds to Mangrove Seedling Establishment on Tidal Flats. Mar. Ecol. Prog. Ser. 440, 1–9. doi: 10.3354/meps09364
Balke T., Herman P. M., Bouma T. J. (2014). Critical Transitions in Disturbance-Driven Ecosystems: Identifying Windows of Opportunity for Recovery. J. Ecol. 102, 700–708. doi: 10.1111/1365-2745.12241
Bouma T. J., van Belzen J., Balke T., van Dalen J., Klaassen P., Hartog A. M., et al. (2016). Short-Term Mudflat Dynamics Drive Long-Term Cyclic Salt Marsh Dynamics. Limnol. Oceanogr. 61, 2261–2275. doi: 10.1002/lno.10374
Bouma T. J., van Belzen J., Balke T., Zhu Z., Airoldi L., Blight A. J., et al. (2014). Identifying Knowledge Gaps Hampering Application of Intertidal Habitats in Coastal Protection: Opportunities and Steps to Take. Coast. Eng. 87, 147–157. doi: 10.1016/j.coastaleng.2013.11.014
Bradley P. M., Morris J. T. (1990). Physical Characteristics of Salt Marsh Sediments: Ecological Implications. Mar. Ecol. Prog. Ser. 61, 245–252. doi: 10.3354/meps061245
Bruneta R., de Paiva J. S., Ciavola P. (2019). Morphological Evolution of an Intertidal Area Following a Set-Back Scheme: A Case Study From the Perkpolder Basin (Netherlands). Front. Earth Sci. 7. doi: 10.3389/feart.2019.00228
Burdick D. M., Mendelssohn I. A. (1987). Waterlogging Responses in Dune, Swale and Marsh Populations of Spartina Patens Under Field Conditions. Oecologia 74 (3), 321–329. doi: 10.1007/BF00378924
Callaghan D. P., Bouma T. J., Klaassen P., van der Wal D., Stive M. J. F., Herman P. M. J. (2010). Hydrodynamic Forcing on Salt-Marsh Development: Distinguishing the Relative Importance of Waves and Tidal Flows. Estuar. Coast. Shelf. Sci. 89, 73–88. doi: 10.1016/j.ecss.2010.05.013
Cao H., Zhu Z., Balke T., Zhang L., Bouma T. J. (2018). Effects of Sediment Disturbance Regimes on Spartina Seedling Establishment: Implication for Salt Marsh Creation and Restoration. Limnol. Oceanogr. 63, 647–659. doi: 10.1002/lno.10657
Cao H., Zhu Z., van Belzen J., Gourgue O., van de Koppel J., Temmerman S., et al. (2021). Salt Marsh Establishment in Poorly Consolidated Muddy Systems: Effects of Surface Drainage, Elevation and Plant Age. Ecosphere 12 (9), e03755. doi: 10.1002/ecs2.3755
Chapman V. J. (1960). “The Plant Ecology of Scolt Head Island,” in Scolt Head Island. Ed. Steers J. A. (Cambridge, UK: W. Heffer and Sons), (pp. 85–163).
Craft C., Clough J., Ehman J., Joye S., Park R., Pennings S., et al. (2008). Forecasting the Effects of Accelerated Sea-Level Rise on Tidal Marsh Ecosystem Services. Front. Ecol. Environ. 7 (2), 73–78. doi: 10.1890/070219
Crooks S., Pye K. (2000). “Sedimentological Controls on the Erosion and Morphology of Saltmarshes: Implications for Flood Defense and Habitat Recreation,” in Coastal and Estuarine Environments: Sedimentology, Geomorphology and Geoarchaeology. Eds. Pye K., Allen J. R. L. (London, UK; Geological Society Publishing House), (pp. 207–222).
Crooks S., Schutten J., Sheern G. D., Pye K., Davy A. J. (2002). Drainage and Elevation as Factors in the Restoration of Salt Marsh in Britain. Ecol. Restor. 10 (3), 591–602. doi: 10.1046/j.1526-100X.2002.t01-1-02036.x
Davy A. J., Brown M. J. H., Mossman H. L., Grant A. (2011). Colonization of a Newly Developing Salt Marsh: Disentangling Independent Effects of Elevation and Redox Potential on Halophytes. J. Ecol. 99, 1350–1357. doi: 10.1111/j.1365-2745.2011.01870.x
Eijkelkamp Agrisearch Equipment (2012) Operating Instructions: Pocket Vane Tester, Giesberg, the Netherlands. Available at: https://en.eijkelkamp.com/products/field-measurement-equipment/pocket-vane-tester.html.
Fagherazzi S., Gabet E. J., Furbish D. J. (2004). The Effect of Bidirectional Flow on Tidal Planforms. Earth Surf. Process. Landf. 29, 295–309. doi: 10.1002/esp.1016
Feagin R. A., Lozada-Bernard S. M., Ravens T. M., Möller I., Yeager K. M., Baird A. H. (2009). Does Vegetation Prevent Wave Erosion of Salt Marsh Edges. Proc. Natl. Acad. Sci. U. S. A. 106 (25), 10,109–10,113. doi: 10.1073/pnas.0901297106
Fivash G., van Belzen J., Temmink R. J. M., Didderen K., Lengkeek W., van der Heide T., et al. (2020). Elevated Micro-Topography Boosts Growth Rates in Salicornia Procumbens by Amplifying a Tidally Driven Oxygen Pump: Mplications for Natural Recruitment and Restoration. Ann. Bot. 125, 353–364. doi: 10.1093/aob/mcz137
Friess D. A., Krauss K. W., Horstman E. M., Balke T., Bouma T. J., Galli D., et al. (2012). Are All Intertidal Wetlands Naturally Created Equal? Bottlenecks, Thresholds and Knowledge Gaps to Mangrove and Saltmarsh Ecosystems. Biol. Rev. 87, 346–366. doi: 10.1111/j.1469-185x.2011.00198.x
Garbutt R. A., Reading C. J., Wolters M., Gray A. J., Rothery P. (2006). Monitoring the Development of Intertidal Habitats on Former Agricultural Land After the Managed Realignment of Coastal Defences at Tollesbury, Essex, UK. Mar. Pollut. Bull. 53, 155–164. doi: 10.1016/j.marpolbul.2005.09.015
Gedan K. B., Silliman B. R., Bertness M. D. (2009). Centuries of Human-Driven Change in Salt Marsh Ecosystems. Ann. Rev. Mar. Sci. 1, 117–141. doi: 10.1146/annurev.marine.010908.163930
Groenendijk A. M. (1986). Establishment of a Spartina-Anglica Population on a Tidal Mudflat - A Field Experiment. J. Environ. Manage. 22, 1–12.
He Q., Silliman B. R., Cui B. (2017). Incorporating Thresholds Into Understanding Salinity Tolerance: A Study Using Salt-Tolerant Plants in Salt Marshes. Ecol. Evol. 7, 6326–6333. doi: 10.1002/ece3.3209
Hu Z., Belzen J., Wal D., Balke T., Wang Z. B., Stive M., et al. (2015b). Windows of Opportunity for Salt Marsh Vegetation Establishment on Bare Tidal Flats: The Importance of Temporal and Spatial Variability in Hydrodynamic Forcing. J. Geophys. Res. Biogeosci. 120, 1450–1469. doi: 10.1002/2014jg002870
Hu Z., Borsje B., van Belzen J., Willemsen P., Wang H., Peng Y., et al. (2021). Mechanistic Modeling of Marsh Seedling Establishment Provides a Positive Outlook for Coastal Wetland Restoration Under Global Climate Change. Geophys. Res. Lett. 48, e2021GL095596. doi: 10.1029/2021GL095596
Hughes A. L. H., Wilson A. M., Morris J. T. (2012). Hydrologic Variability in a Salt Marsh: Assessing the Links Between Drought and Acute Marsh Dieback. Estuar. Coast. Shelf. Sci. 111, 95–106. doi: 10.1016/j.ecss.2012.06.016
Hu Z., Lenting W., Bouma T. J. (2015a). Continuous Monitoring Bed-Level Dynamics on an Intertidal Flat: Introducing Novel, Stand-Alone High-Resolution SED-Sensors. Geomorphology 245, 223–230. doi: 10.1016/j.geomorph.2015.05.027
Hu Z., Wang Z. B., Zitman T. J., Stive M. J., Bouma T. J. (2015c). Predicting Long-Term and Short-Term Tidal Flat Morphodynamics Using a Dynamic Equilibrium Theory. J. Geophys. Res. Earth 120, 1803–1823. doi: 10.1002/2015JF003486
Jansen S., Walpersdorf E., Werner U., Billerbeck M., Böttcher M. E., De Beer D. (2009). Functioning of Intertidal Flats Inferred From Temporal and Spatial Dynamics of O2, H2S and pH in Their Surface Sediment. Ocean. Dyn. 59, 317–332. doi: 10.1007/s10236-009-0179-4
Jung J., Lee S. C., Choi H. K. (2008). Anatomical Patterns of Aerenchyma in Aquatic and Wetland Plants. J. Plant Biol. 51 (6), 428–439. doi: 10.1007/BF03036065
Kirwan M. L., Megonigal J. P. (2013). Tidal Wetland Stability in the Face of Human Impacts and Sea-Level Rise. Nature 504, 53–60. doi: 10.1038/nature12856
Kirwan M. L., Mudd S. M. (2012). Response of Salt-Marsh Carbon Accumulation to Climate Change. Nature 489, 550–553. doi: 10.1038/nature11440
Lamers L. P. M., Govers L. L., Janssen I. C. J. M., Jeroen J. M., van der welle M. E. W., Van Katwijk M. M., et al. (2013). Sulfide as a Soil Phytotoxin – a Review. Front. Plant Sci. 4. doi: 10.3389/fpls.2013.00268
Lawrence P. J., Smith G., Sullivan M. J. P., Mossman H. L. (2018). Restored Saltmarshes Lack the Topographic Diversity Found in Natural Habitat. Ecol. Eng. 115, 58–66. doi: 10.1016/j.ecoleng.2018.02.007
Leonardi N., Ganju N. K., Fagherazzi S. (2016). A Linear Relationship Between Wave Power and Erosion Determines Saltmarsh Resilience to Violent Storms and Hurricanes. Proc. Natl. Acad. Sci. U. S. A. 113, 64–68. doi: 10.1073/pnas.1510095112
Lin N., Emanuel K., Oppenheimer M., Vanmarcke E. (2012). Physically Based Assessment of Hurricane Surge Threat Under Climate Change. Nat. Clim. Chang. 2, 462–467. doi: 10.1038/nclimate1389
Lo V. B., Bouma T. J., van Belzen J., Van Colen C., Airodi L. (2017). Interactive Effects of Vegetation and Sediment Properties on Erosion of Salt Marshes in the Northern Adriatic Sea. Mar. Environ. 131, 32–42. doi: 10.1016/j.marenvres.2017.09.006
Masselink G., Hanley M. E., Halwyn A. C., Blake W., Kingston K., Newton T., et al. (2017). Evaluation of Salt Marsh Restoration by Means of Self-Regulating Tidal Gate–Avon Estuary, South Devon, UK. Ecol. Eng. 106, 174–190. doi: 10.1016/j.ecoleng.2017.05.038
Mazik K., Musk W., Dawes O., Solyanko K., Brown S., Mander L., et al. (2010). Managed Realignment as Compensation for the Loss of Intertidal Mudflat: A Short Term Solution to a Long Term Problem? Estuar. Coast. Shelf. Sci. 90, 11–20. doi: 10.1016/j.ecss.2010.07.009
Möller I., Kudella M., Rupprecht F., Spencer T., Paul M., van Wesenbeeck B. K., et al. (2014). Wave Attenuation Over Coastal Salt Marshes Under Storm Surge Conditions. Nat. Geosci. 7, 727–731. doi: 10.1038/ngeo2251
Mossman H. L., Grant A., Davy A. J. (2019). Manipulating Saltmarsh Microtopography Modulates the Effects of Elevation on Sediment Redox Potential and Halophyte Distribution. J. Ecol. 108, 1–13. doi: 10.1111/1365–2745.13229
Munns R., Tester M. (2008). Mechanisms of Salinity Tolerance. Annu. Rev. Plant Biol. 59, 651–681. doi: 10.1146/annurev.arplant.59.032607.092911
Nichols P. G. H., Malik A. I., Stockdale M., Colmer T. D. (2009). Salt Tolerance and Avoidance Mechanisms at Germination of Annual Pasture Legumes: Importance for Adaptation to Saline Environments. Plant Soil 315, 241–255. doi: 10.1007/s11104-008-9747-5
Perillo G. M. E., Wolanski E., Cahoon D. R. (2009). Coastal Wetlands: An Integrated Ecosystem Approach (Amsterdam: Elsevier Science).
Pestrong R. (1969). The Shear Strength of Tidalmarsh Sediments. J. Sediment.Petrol. 39, 322–396. doi: 10.1306/74D71C44-2B21-11D7-8648000102C1865D
Pye K. (2000). “Saltmarsh Erosion in Southeast England: Mechanisms, Causes and Implications,” in British Saltmarshes (Yorkshire: Westbury Publishing), 359–396.
Rabouille C., Denis L., Dedieu K., Stora G., Lansard B., Grenz C. (2003). Oxygen Demand in Coastal Marine Sediments: Comparing in Situ Microelectrodes and Laboratory Core Incubations. J. Exp. Mar. Biol. Ecol. 285–286, 49–69. doi: 10.1016/S0022-0981(02)00519-1
Redelstein R., Zote G., Balke T. (2018). Seedling Stability in Waterlogged Sediments: An Experiment With Saltmarsh Plants. Mar. Ecol. Prog. Ser. 590, 95–108. doi: 10.3354/meps12463
Rijkswaterstaat. (2015). Nieuwe Buitendijkse Natuur Perkpolder Van Dichtbij Te Bewonderen. Available at: https://www.rijkswaterstaat.nl/over-ons/nieuws/nieuwsarchief/p2015/12/nieuwe-buitendijkse-natuur-perkpolder-van-dichtbij-te-bewonderen.aspx.
Sanderson E. W., Ustin S. L., Foin T. C. (2000). The Influence of Tidal Channels on the Distribution of Salt Marsh Plant Species in Petaluma Marsh, CA, USA. Plant Ecol. 146 (1), 29–41. doi: 10.1023/A:1009882110988
Schwarz C., Ye Q. H., van der Wal D., Zhang L. Q., Bouma T. J., Ysebaert T., et al. (2014). Impacts of Salt Marsh Plants on Tidal Channel Initiation and Inheritance. J. Geophys. Res. 119 (2), 385–400. doi: 10.1002/2013JF002900
Shepard C. C., Crain C. M., Beck M. W. (2011). The Protective Role of Coastal Marshes: A Systematic Review and Meta-Analysis. PLoS One 6, e27374. doi: 10.1371/journal.pone.0027374
Silliman B. R., Grosholz T., Bertness M. D. (2009). Human Impacts on Salt Marshes: A Global Perspective (Berkeley, CA: University of California Press).
Strong D. R., Ayres D. R. (2013). Ecological and Evolutionary Misadventures of Spartina. Annu. Rev. Ecol. Evol. Syst. 44, 389–410. doi: 10.1146/annurevecolsys-110512-135803
Suding K. N., Hobbs R. J. (2009). Threshold Models in Restoration and Conservation: A Developing Framework. Trends Ecol. Evol. 24, 271–279. doi: 10.1016/j.tree.2008.11.012
Sullivan M. J. P., Davy A. J., Grant A., Mossman H. L. (2018). Is Saltmarsh Restoration Success Constrained by Matching Natural Environments or Altered Succession? A Test Using Niche Models. J. Appl. Ecol. 55, 1207–1217. doi: 10.1111/1365-2664.13033
Temmerman S., Meire P., Bouma T. J., Herman P. M. J., Ysebaert T., De Vriend H. J. (2013). Ecosystem-Based Coastal Defence in the Face of Global Change. Nature 504, 79–83. doi: 10.1038/nature12859
van de Lageweg W. I., de Paiva J. N. S., De Vet P. L. M., van der Werf J. J., de Louw P. G. B., Walles B., et al. (2019) Perkpolder Tidal Restauration: Final Report. Center of Expertise Delta Technology, the Netherlands. Available at: https://www.zeeweringenwiki.nl/images/a/a4/Perkpolder_Eindrapportage.pdf.
van der Wal D., Wielemaker-Van den Dool A., Herman P. M. J. (2008). Spatial Patterns, Rates and Mechanisms of Saltmarsh Cycles (Westerschelde, The Netherlands). Estuar. Coast. Shelf. Sci. 76, 357–368. doi: 10.1016/j.ecss.2007.07.017
van de Vijsel R. C., van Belzen J., Bouma T. J., van der Wal D., Cusseddu V., Purkis S. J., et al. (2020). Estuarine Biofilm Patterns: Modern Analogues for Precambrian Self-Organization. Earth Surf. Process. Landf. 45 (5), 1141–1154. doi: 10.25850/nioz/7b.b.m
van Katwijk M. M., Wijgergangs L. J. M. (2004). Effects of Locally Varying Exposure, Sediment Type and Low-Tide Water Cover on Zostera Marina Recruitment From Seed. Aquat. Bot. 80, 1–12. doi: 10.1016/j.aquabot.2004.04.003
Wang H., van der Wal D., Li X., Van Belzen J., Herman P. M. J., Hu Z., et al. (2017). Zooming in and Out: Scale Dependence of Extrinsic and Intrinsic Factors Affecting Salt Marsh Erosion. J. Geophys. Res. Earth Surf. 122 (7), 1455–1470. doi: 10.1002/2016JF004193
Ward R. D., Burnside N. G., Joyce C. B., Sepp K. (2016). Importance of Microtopographyin Determining Plant Community Distribution in Baltic Coastal Wetlands. J. Coast. Res. 32 (5), 1062–1070. doi: 10.2112/JCOASTRES-D-15-00065.1
Winterwerp J. C., Van Kesteren W. G. M. (2004). Introduction to the Physics of Cohesive Sediment in the Marine Environment (Amsterdam: Elsevier).
Xie T., Cui B., Li S., Bai J. (2018). Topography Regulates Edaphic Suitability for Seedling Establishment Associated With Tidal Elevation in Coastal Salt Marshes. Geoderma 337, 1258–1266. doi: 10.1016/j.geoderma.2018.07.053
Xie T., Cui B., Li S., Zhang S. (2019). Management of Soil Thresholds for Seedling Emergence to Re-Establish Plant Species on Bare Flats in Coastal Salt Marshes. Hydrobiologia 827, 51–63. doi: 10.1007/s10750-018-3589-9
Xin P., Wilson A., Shen C., Ge Z., Moffett K. B., Santos I. R., et al. (2022). Surface Water and Groundwater Interactions in Salt Marshes and Their Impact on Plant Ecology and Coastal Biogeochemistry. Rev. Geophys. 60, e2021RG000740. doi: 10.1029/2021RG000740
Zhao Z., Zhang L., Li X., Yuan L., Bouma T. J. (2021). The Onset of Secondary Seed Dispersal is Controlled by Germination-Features: A Neglected Process in Sudden Saltmarsh Establishment. Limnol. Oceanogr. 66, 3070–3084. doi: 10.1002/lno.11860
Zhu Z., van Belzen J., Zhu Q., van de Koppel J., Bouma T. J. (2020b). Vegetation Recovery on Neighboring Tidal Flats Forms an Achilles’ Heel of Saltmarsh Resilience to Sea Level Rise. Limnol. Oceanogr. 65, 51–62. doi: 10.1002/lno.11249
Keywords: saltmarsh, restoration, seedling, establishment, stability, drainage, windows of opportunity
Citation: Cao H, Zhu Z, Hu Z, Wang H and Bouma TJ (2022) Drainage-Improved Sediment Strength Enhances Saltmarsh Seedling Establishment Chance. Front. Mar. Sci. 9:874680. doi: 10.3389/fmars.2022.874680
Received: 12 February 2022; Accepted: 09 May 2022;
Published: 02 June 2022.
Edited by:
Dongdong Shao, Beijing Normal University, ChinaReviewed by:
Tian Xie, Beijing Normal University, ChinaMan Qi, Central China Normal University, China
Copyright © 2022 Cao, Zhu, Hu, Wang and Bouma. This is an open-access article distributed under the terms of the Creative Commons Attribution License (CC BY). The use, distribution or reproduction in other forums is permitted, provided the original author(s) and the copyright owner(s) are credited and that the original publication in this journal is cited, in accordance with accepted academic practice. No use, distribution or reproduction is permitted which does not comply with these terms.
*Correspondence: Zhenchang Zhu, emhlbmNoYW5nLnpodUBnZHV0LmVkdS5jbg==