- 1Graduate School of Agriculture, Kyoto University, Kyoto, Japan
- 2Osaka Museum of Natural History and C/O Institute for East China Sea, Nagasaki University, Nagasaki, Japan
- 3Graduate School of Fisheries Science, Hokkaido University, Hakodate, Hokkaido, Japan
- 4Graduate School of Bioresources, Mie University, Tsu, Japan
In situ emergence of the centric diatoms from the surface sediment, along with the occurrence of the vegetative cells in the water column, were monitored monthly in a shallow embayment, Ago Bay, of central Japan, where light penetrated to the seafloor. The in situ emergence flux (cells m-2 day-1) was measured by experiments using a ‘plankton emergence trap/chamber (PET chamber)’. During the study period from July 2006 to May 2008, germinating and rejuvenating cells of centric diatoms were successfully collected by the PET chamber. Furthermore, vegetative cells forming long-chain colonies, including the species which have not been known to form resting stage cells, were also found, indicating that these cells already inhabited the surface sediment prior to the start of the PET chamber experiments. The vegetative cells could be cells that grew after germination/rejuvenation and/or cells deposited from the upper layer in the water column. When comparing emergence flux in the PET chamber and the integrated abundance of the vegetative cells in the water column for the diatoms frequently observed, significant positive relationships were found for some diatom taxa. However, even for these taxa that showed a clear relationship, the magnitude of the vegetative population in the water column did not necessarily correlate with that of the emergence flux. These observations indicate that the magnitude of the vegetative population was not regulated directly by the emergence flux. The magnitude of the vegetative population could be dependent on the vegetative growth itself. This implies that the presence of vegetative cells in the water column is important at the time when environmental conditions become suitable for vegetative growth. In this context, the presence of various types of cells, such as germinating, rejuvenating, and vegetative cells, in the sediment is essential as seeds waiting for recruitment into the water column. Consequently, the seafloor in Ago Bay may act as a ‘refuge and nursery’ for centric diatoms. Based on the above, we demonstrated various patterns of life cycle strategies of the diatoms in a shallow coastal water/embayment.
Introduction
Among phytoplankton, diatoms and dinoflagellates are the major primary producers in coastal areas. Diatoms and dinoflagellates show species successions in response to drastic changes in the coastal environments (Margalef, 1958; Smayda, 1980; Reynolds, 1984). Many species of both diatoms and dinoflagellates can produce dormant benthic cells possessing the ability to survive adverse environments for vegetative growth (Dale, 1983; Hargraves and French, 1983; Belmonte and Rubino, 2019).
Dormant benthic cells for most species of dinoflagellates are known to be produced through sexual reproduction (von Stosch, 1967; Dale, 1983; Pfiester and Anderson, 1987) and for most species of centric diatoms through asexual reproduction (Hargraves and French, 1983; Hasle and Sims, 1985; Rines and Hargraves, 1988; Hargraves, 1990). Such dormant benthic cells are named ‘cysts’ for dinoflagellates, and ‘resting spores’ or ‘resting cells’ for diatoms, depending on their morphological/physiological features. The resting spore, characterized by a different shape from its vegetative cell and by a thick cell wall, is formed in the frustule of a vegetative cell and possesses high tolerance to adverse conditions for the vegetative growth. In contrast, the resting cell is one in which the physiological status of the vegetative cell becomes dormant without any morphological differences from the vegetative cell (McQuoid and Hobson, 1996). These dormant benthic cells are collectively called ‘resting stage cells’ but the reproduction modes of these two types of cells are separately referred to as ‘germination’ for resting spores and ‘rejuvenation’ for resting cells (Sicko-Goad, 1986; McQuoid and Hobson, 1996).
The life cycle of such resting stage cell forming centric diatoms has generally been explained as a conceptual diagram shown in Figure 1, which was drawn with reference to the reviews (McQuoid and Hobson, 1996; Belmonte and Rubino, 2019). The resting stage cells are formed under unfavorable conditions, such as nutrient depletion or low light conditions, for vegetative growth. When the benthic environmental conditions become favorable, the cells germinate (from resting spores) and rejuvenate (from resting cells), forming the vegetative population in the water column (McQuoid and Hobson, 1996; Itakura et al., 1997). Therefore, it is widely accepted that the resting stage cells act as ‘seed populations’ in their population dynamics (Hargraves and French, 1983; Pitcher, 1990), as is the case of dinoflagellates(Wall, 1971; Steidinger, 1975).
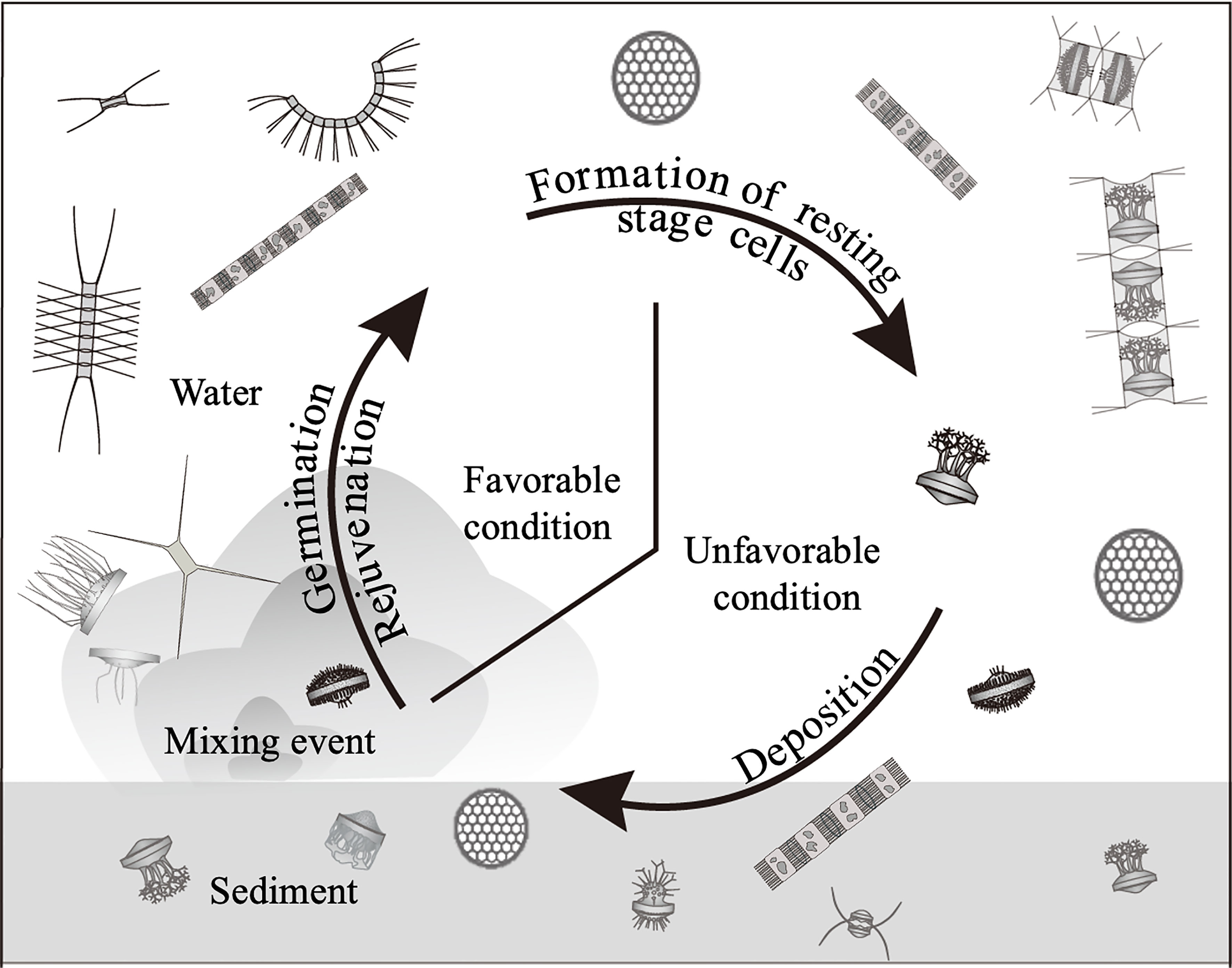
Figure 1 Conceptual diagram of life cycle of the centric diatoms forming the resting stage cells (resting spores and resting cells).
Accordingly, to understand the population dynamics of diatoms, the biology and ecology, with reference to germination/rejuvenation, of the resting stage cells have been studied under laboratory conditions (e.g., Imai et al., 1996; Itakura, 2000; Ueno and Ishikawa, 2009; Montresor et al., 2013). However, the conditions of the in situ environment are extremely complex and impossible to be mimicked in the laboratory. Therefore, to get a better understanding of the population dynamics in the natural environment, it is necessary to investigate the “germination” of the resting stage cells in situ.
Given the above circumstances, the ‘plankton emergence trap/chamber (PET chamber)’ has been developed to collect germinating cells (the vegetative cells just after germination) from the microalgal cysts in the surface sediments (Ishikawa et al., 2007). Subsequent study using the PET chamber successfully elucidated the mechanism of population dynamics of a toxic dinoflagellate of Alexandrium catenella (present name A. pacificum) (Ishikawa et al., 2014). However, there are no reported investigations that applied the PET chamber to diatoms or other microalgae that form resting stage cells/cysts.
In this study, we investigated the germination/rejuvenation of centric diatoms by using the PET chamber in order to elucidate the mechanisms of their population dynamics in a temperate shallow embayment. Based on the results of this investigation, we discuss the life cycle strategies of diatoms in such shallow coastal waters.
Materials and Methods
For this study, we selected Ago Bay, located on the southeast coast of Kii Peninsula, central Japan, as a model area of a typical coastal environment in the temperate zone (Figure 2). This bay is semi-enclosed with an area of 25 km2 and is connected to the Pacific Ocean through a narrow bay mouth of ca. 2 km width. There are no major rivers in the bay, so freshwater inflow is limited. The average water depth of the bay is shallow at about 10 m, implying that light penetrates to the seafloor throughout the year. Sampling was conducted at a site (34° 16.30’ N; 136° 48.40’ E), located at the southern extent of the bay at a frequency of once a month for about two years from July 2006 to May 2008 (Figure 2). The average depth at the site is 11 m. This is the same site where the study of A. catenella was conducted before, by using the PET chamber (Ishikawa et al., 2014).
Measurements of Environmental Conditions
Water temperature and salinity were measured at three depths of 0, 4, and 1 m above the bottom (hereafter: b-1 m) at the sampling site, using a Multi-probe (Hach-Hydrolab, QUANTA). In addition, dissolved oxygen (DO) concentration and light intensity (PAR) at 0.3 m above the bottom (hereafter: b-0.3 m) were recorded every 20 minutes throughout the study period, using a compact memory oxygen meter (JFE Advantec, COMPACT-DOW) and a light quantum memory meter (JFE Advantec, COMPACT- LW), respectively. However, during the monitoring of the light, recording of its data occasionally failed due to the breakdown of the sensor.
Water Sampling and Treatments
Water samples were collected at different depths in the water column, using a Van-Dorn sampler. Samples (ca. 1,000 mL) collected from each depth were used to analyze nutrients and to count vegetative cells of centric diatoms.
A 100 mL aliquot of each water sample collected at 0 and b-1 m was filtered through a glass fiber filter (Whatman, GF/F) and the filtrate was frozen at -30°C until analysis of the following nutrients: dissolved inorganic nitrogen (NO3-N + NO2-N + NH4-N: DIN), phosphate (PO4-P; DIP), and silicate (SiO4-Si). The concentrations of these nutrients were measured with an autoanalyzer (BL TEC, swAAt) by the method of Strickland and Parsons (1972). Dissolved silicate was measured starting on a later date, February 2007.
To count the vegetative cells of the diatoms, 500 mL water samples collected at 0, 2, 4, 6, 8 and b-1 m were immediately fixed by adding borax-buffered formaldehyde at a final concentration of 1% (v/v). After allowing the preserved samples to settle for at least 24 h in the laboratory, the supernatant was removed by siphoning and concentrated to 10 mL. From this concentrated sample, a 1 mL of aliquot was spread onto a Sedgewick-Rafter chamber, and all of the vegetative cells of diatoms that appeared were identified and counted usually at 200 X magnification (400 or 600 X when needed) under an inverted epifluorescence microscope (Nikon, ECLIPSE TE200), along with confirming the viability of cells. The identification of the species was mainly referred to Rines and Hargraves (1988); Hasle and Syvertsen (1997) and Jensen and Moestrup (1998). In this microscopy, the counting was conducted until the total number of the vegetative cells was more than 200 cells. All cell counts were made in triplicate and the cell abundance was obtained as the average value (cells L-1). These cell abundances were finally integrated to obtain the total abundance of vegetative cells in the water column per m2 (cells m-2) by plotting the abundances (cells L-1) against their respective depths.
Sediment Sampling and PET Chamber Experiment
The sediment core samples were collected without disturbance using the method of Yokoyama and Ueda (1997), in which an acrylic core tube (6.4 cm diameter, 23 cm length) was set in the bucket of an Ekman grab (see Ishikawa et al., 2007). A total of six sediment core samples were obtained randomly within a 10 m radius at the sampling site. Among them, five core samples were used for the PET chamber experiments to collect in situ germinating/rejuvenating cells from the surface sediment and the remaining one for measuring the temperature of the sediment at a depth of 1 cm with a mercury thermometer immediately after the core sample was obtained.
A schematic diagram of the PET chamber used in the present study is shown in Figure 3. The chamber consists of a top cylinder and a bottom cylinder. The top cylinder is designed to contain filtered seawater and the bottom cylinder to contain sediments collected at the sampling site. In every experiment, we employed five such units and set them all together on a stainless-steel incubation platform (Figure 3). In addition to the above units, one unit was placed on the platform as a control chamber filled with filtered seawater at the sampling site but without sediment inside of the bottom cylinder, in order to check the contamination (i.e., invasion) of diatoms from outside the cylinder (i.e., ambient seawater). The six PET chambers were set at a bottom depth of ~11 m at the sampling site. After 24 h, the chambers were retrieved and the seawater in the top cylinder of the chamber was carefully collected. All of the above handling procedures using PET chambers were described in Ishikawa et al. (2007).
The water samples (ca. 500 mL) collected from the top cylinder were immediately fixed with borax-buffered formaldehyde at a final concentration of 1% (v/v). The fixed samples were brought back to the laboratory and allowed to settle for at least 24 h. Thereafter, the supernatant in the sample was removed by siphoning and concentrated to 100 mL. This concentrated sample was finally poured into the “combined plate chamber” (see Hasle, 1978) and allowed to settle again for at least 24 h before microscopy. All of the vegetative cells that appeared in the sample of a PET chamber were identified and counted at usually 200 X magnification (400 or 600 X when needed) using an inverted epifluorescence microscope (Nikon, ECLIPSE TE200) under blue light excitation, making it possible to detect the viable vegetative cells easily by observing their autofluorescence, even though the samples occasionally contained sediment silt and clay particles which were derived from resuspension of the surface sediment at the time when the water sample in the top cylinder of the PET chamber was collected. The vegetative cell numbers in the five PET chambers were first averaged and finally converted to daily ‘emergence flux’ per m2 (i.e., cells m-2 day-1) by taking the sediment surface exposed area on the base plate of the PET chamber (i.e., 32.2 cm2, see Ishikawa et al., 2007). It was assumed that grazing by zooplankton on the emerged cells during the PET chamber experiment was negligible, since large zooplankton were rarely observed in the samples, as was the case in a previous study of the dinoflagellate A. catenella conducted at this same site (Ishikawa et al., 2014).
In order to clarify the relationship between the emergence flux (cells m-2 day-1) and the abundance of vegetative cells in the water column (cells m-2), Spearman’s rank test was conducted using the software SPSS (ver. 22).
After the above series of studies, sediment cores were additionally collected without disturbance by the same method using Ekman grab as described above at the same sampling site in July 2020. The water sample containing fine sediment particles in the flocculent layer (sediment/water interface) was obtained by sucking with a dropper. The contents in the sample were directly observed under the microscope.
Results
Environmental Conditions
The water column was thermally stratified in the summer from July to September in 2006 and from June to September in 2007. During these periods, the temperatures at the surface (0 m) and the bottom (b-1 m) were in the range of 24.6 – 27.1°C and 19.1 – 23.4°C, respectively (Figure 4A). In contrast, the water column in other periods was vertically mixed, except in January 2007. The water temperatures in the water column were generally lower than 15°C from December to March in both 2006/2007 and 2007/2008, with minima of 9.0°C at 0 m and 8.7°C at b-1 m in January 2008. The temporal change in temperature of the sediment showed a similar trend to that in the water column, with a maximum of 25.2°C in July 2007 and a minimum of 10.0°C in January 2008.
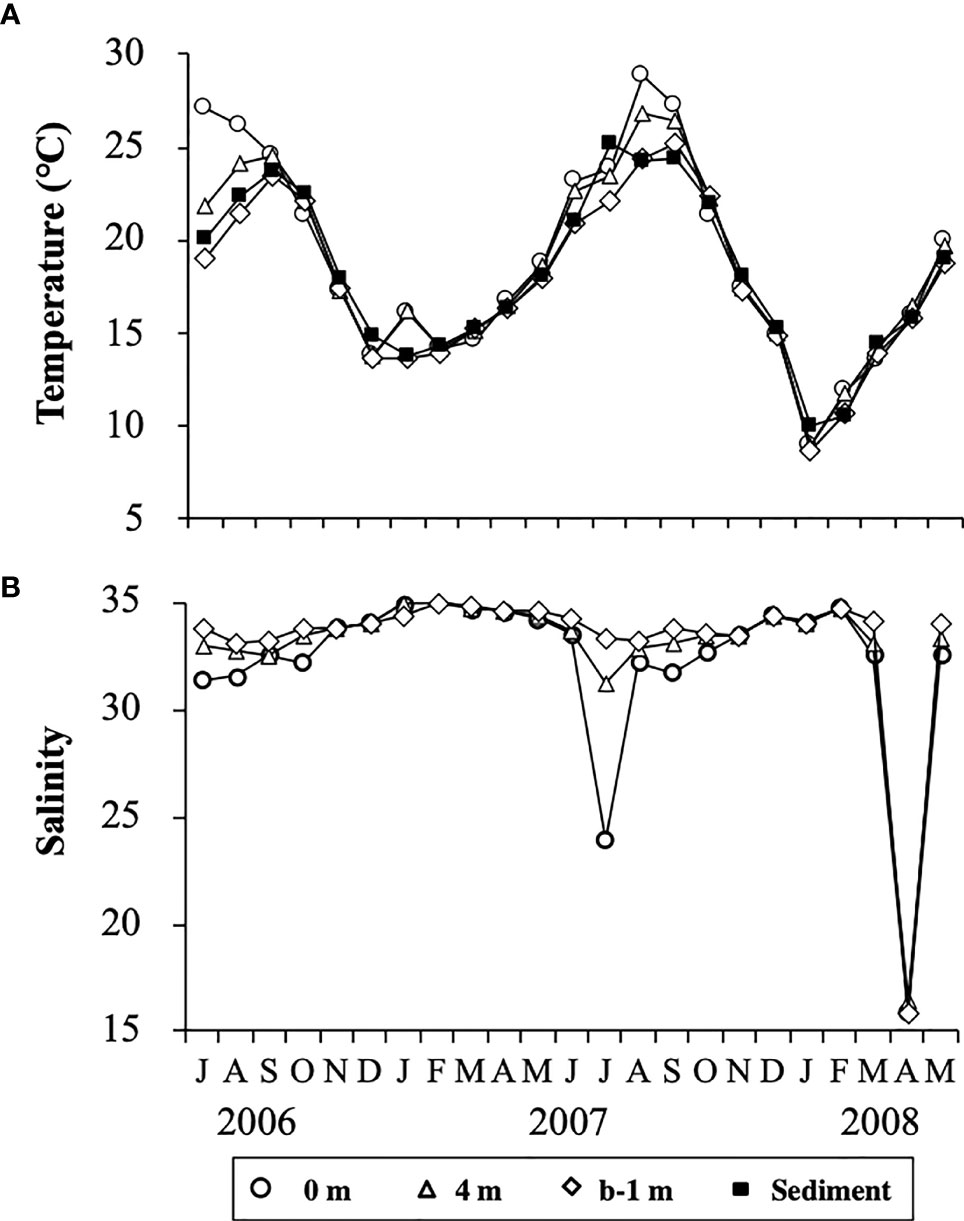
Figure 4 Temporal changes in temperature (A) at different depths in the water column and at 1 cm depth in the sediment, and salinity (B) at different depths in the water column at the sampling site in Ago Bay. b-1 m denotes the depth at 1 m above the bottom.
Salinity in the water column showed no clear temporal changes, being almost always in the range of 30 – 35 during the study period, although it occasionally decreased to 23.9 at 0 m in July 2007 and about 16 throughout the water column in April 2008 (Figure 4B). Such lower values of salinity were due to heavy rain fall (Japan Meteorological Agency, data available at https://www.data.jma.go.jp/gmd/risk/obsdl/).
Concentrations of DO at b-0.3 m showed a clear temporal change, with relatively low and high values when the water column was stratified or vertically mixed, respectively (Figure 5A). DO was often lower than 2 mg L-1 in the warmer seasons (from July to September) but generally higher than 4 mg L-1 in other seasons.
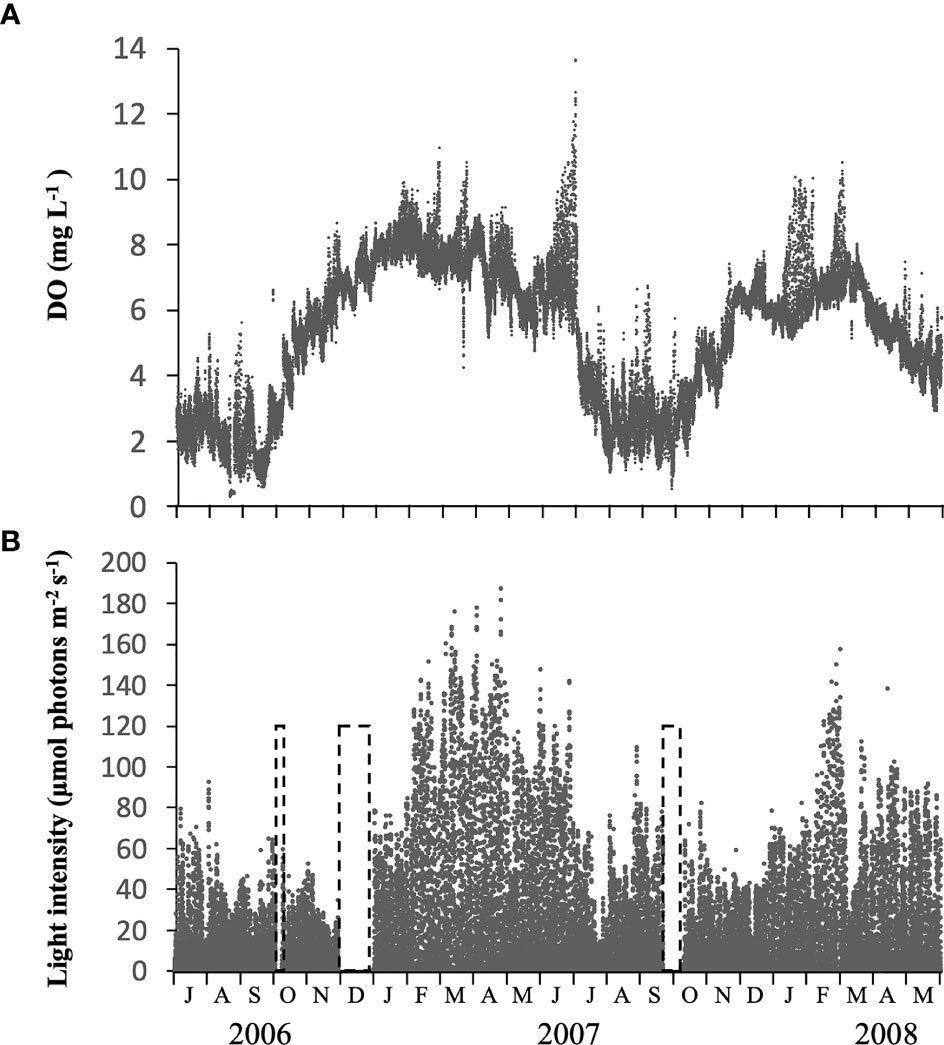
Figure 5 Temporal changes in concentration of dissolved oxygen (DO) (A) and light intensity (B) measured at 0.3 m above the bottom, using memory meters, at the sampling site in Ago Bay. The periods in the dashed frame indicate that no data was recorded.
The light intensity at b-0.3 m during daytime changed notably, not only seasonally but also daily, in the range of 1.3 – 187.0 µmol photons m-2 s-1 (Figure 5B). Throughout the study period, the light almost always penetrated to the bottom at intensities > 10 µmol photons m-2 s-1, and although there were cases when intensity was < 10 µmol photons m-2 s-1, it did not continue for more than 2 successive days. The average light intensity over the periods when the PET chamber experiment was conducted was 27.3 µmol photons m-2 s-1 (data not shown).
Concentrations of DIN (Figure 6A) and DIP (Figure 6B) changed temporally in a similar manner, being generally high at both depths (0 m and b-1 m) in the warm seasons from June/July to September/October in both years, 2006 and 2007, and low in cold seasons (January to March). During the study, the concentration of DIN fluctuated in the range of 1.4 -7.3 µM and 1.1 – 13.0 µM at 0 m and b-1 m, respectively, and DIP fluctuated in the range of 0.1-0.6 µM and 0.1-1.2 µM at 0 m and b-1 m, respectively. The concentration of SiO4 (Figure 6C) changed over a wider range between 1.7 and 32.2 µM in the water column, although no obvious temporal change was observed in the concentrations.
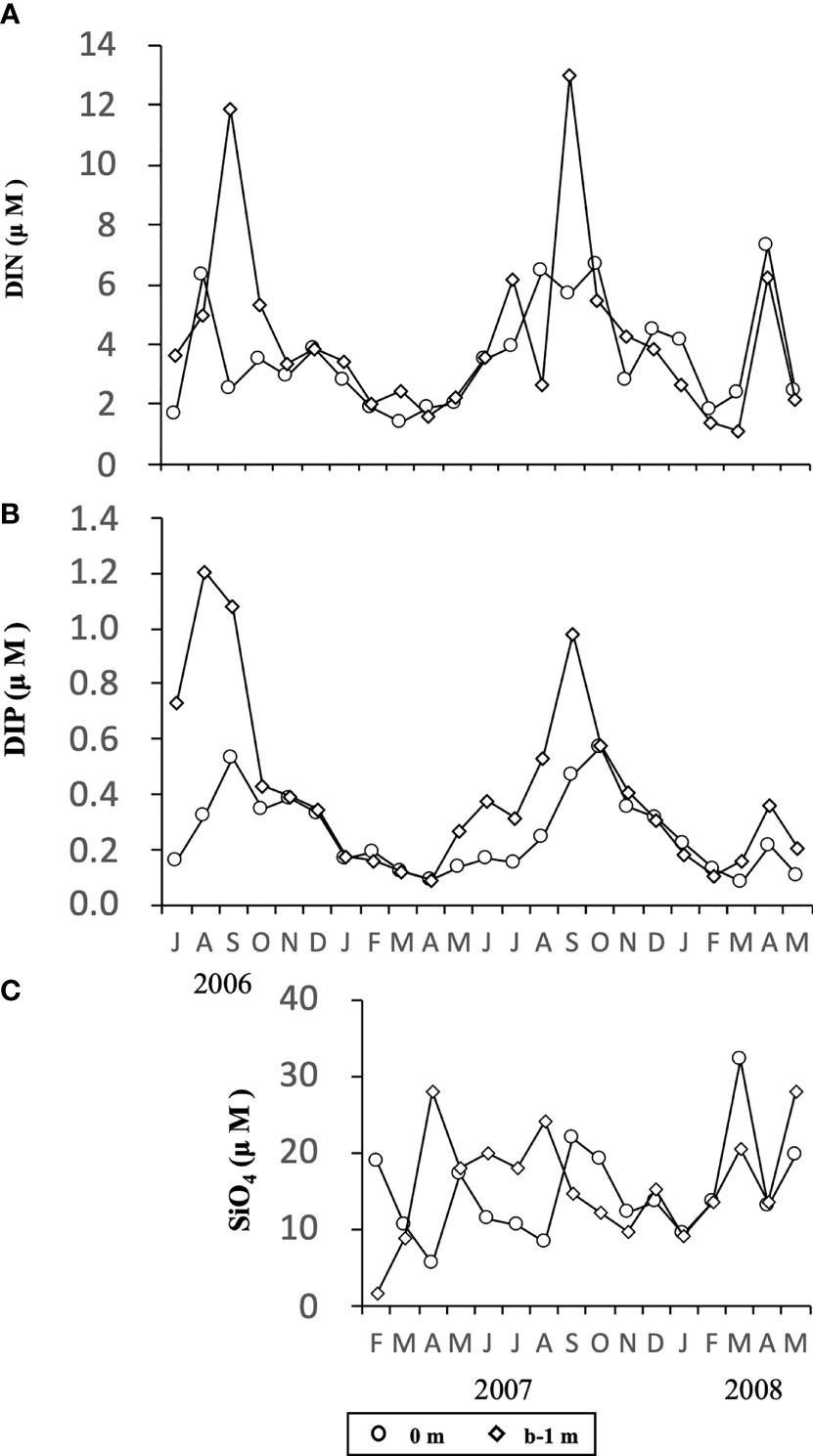
Figure 6 Temporal changes in concentrations of DIN (A), DIP (B) and SiO4 (C) at the depth of 0 m and b-1 m at the sampling site in Ago Bay. b-1 m denotes the depth at 1 m above the bottom.
As a whole, the environmental conditions measured at the sampling site tended to show changes with the seasons, like those in a typical temperate embayment.
Diatoms Observed in Water Column and PET Chamber
Over the course of this study, from July 2006 to May 2008, more than 79 species grouped in 34 genera, including unidentified species, and more than 55 species grouped in 28 genera were observed in samples representing the water column and the PET chamber, respectively (Table 1): all of the 55 species in the PET chamber appeared in the water column.
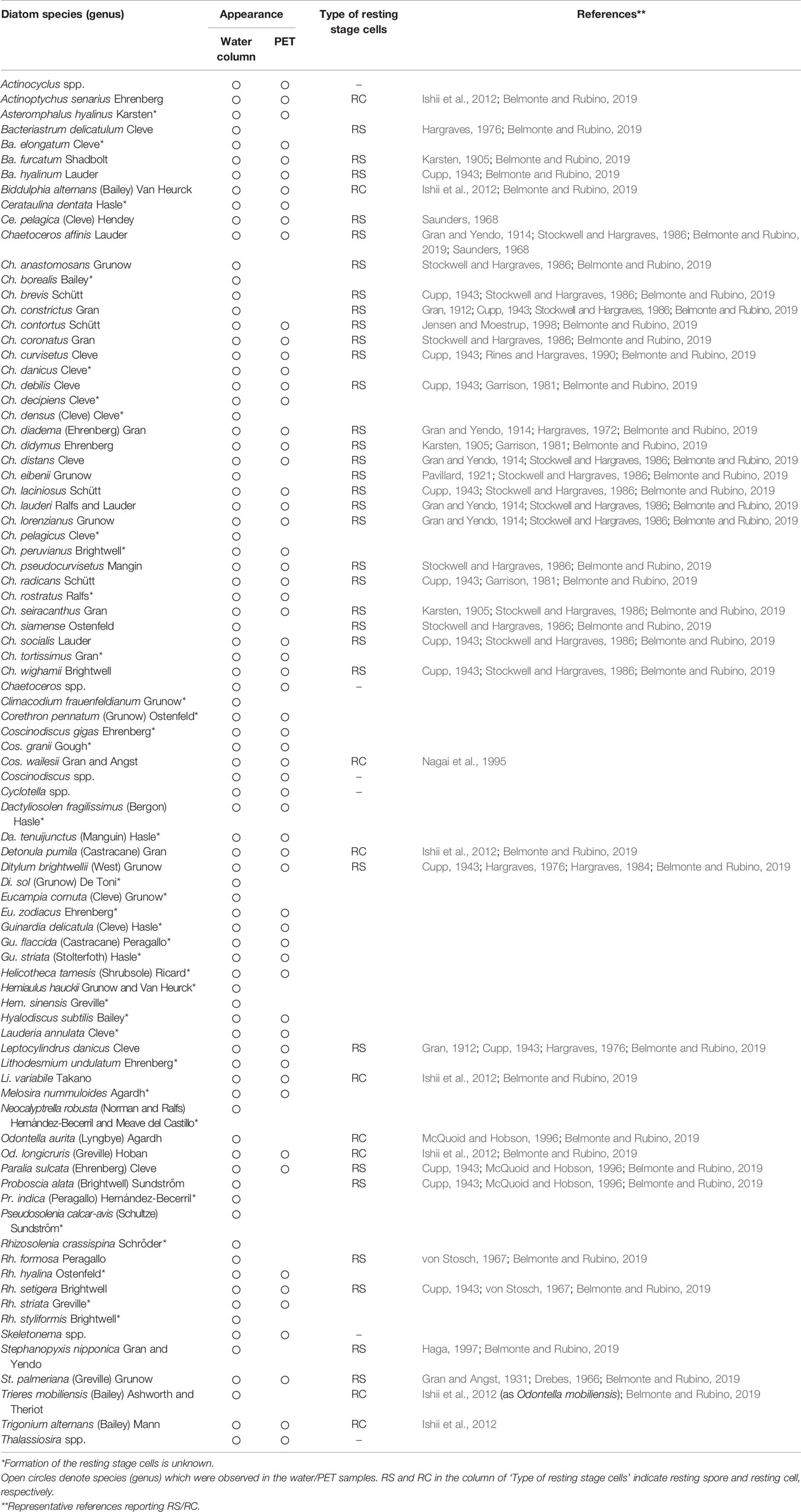
Table 1 Compiled list of species (genus) of centric diatoms observed in the water column and PET chamber at the sampling site in Ago Bay, during the period from July 2006 to May 2008.
Vegetative cells that frequently emerged in the PET chamber are shown in Figure 7. Among these vegetative cells, different types were found as follows. Vegetative cells with chloroplasts were observed beside a valve which was shed from a resting spore (Figure 7G). In addition, long chains of vegetative cells containing distinct chloroplasts were often observed for species of the genera Chaetoceros (e.g., Figures 7J, M), Skeletonema (Figure 7Ab) and Thalassiosira (Figure 7Af). Among the 55 species recognized in the PET chambers, 24 species have not been known to form the resting stage cells so far (Table 1).
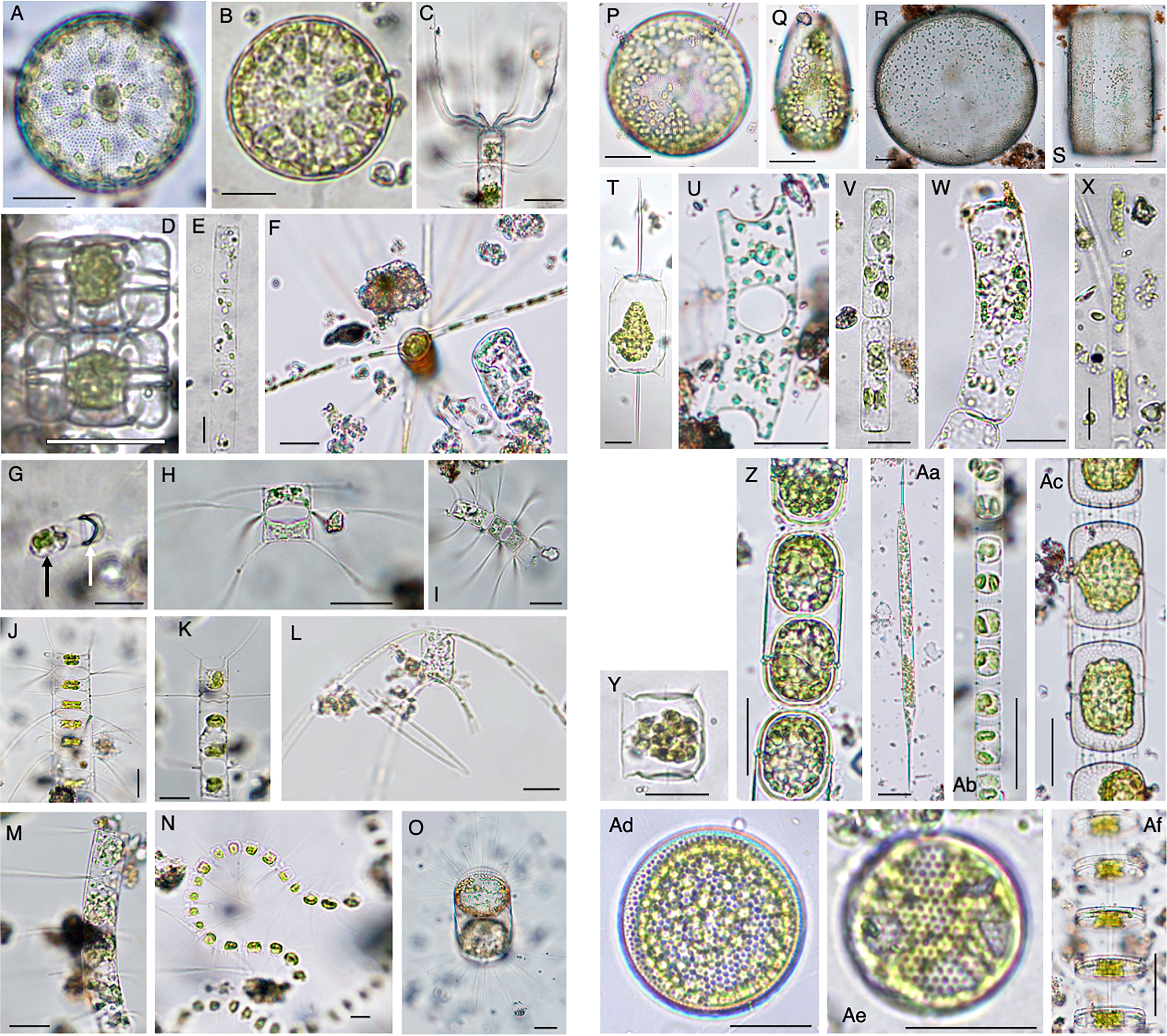
Figure 7 Photomicrographs showing representative centric diatoms that emerged in the PET chamber. Actinocyclus sp. (A), Actinoptychus senarius (B), Bacteriastrum elongatum (C), Biddulphia alternans (D), Cerataulina pelagica (E), Chaetoceros danicus (F), Chaetoceros debilis (G–I), Chaetoceros didymus (J), Chaetoceros distans (K), Chaetoceros peruvianus (L), Chaetoceros pseudocurvisetus (M), Chaetoceros socialis (N), Corethron pennatum (O), Coscinodiscus granii (P, Q), Coscinodiscus wailesii (R, S), Ditylum brightwellii (T), Eucampia zodiacus (U), Guinardia delicatula (V), Guinardia striata (W), Leptocylindrus danicus (X), Lithodesmium variabile (Y), Melosira nummuloides (Z), Rhizosolenia setigera (Aa), Skeletonema sp. (Ab), Stephanopyxis palmeriana (Ac), Thalassiosira spp. (Ad–Af). In (G), black and white arrows indicate a vegetative cell just after germination and a valve of the resting spore, respectively. Scale bars: 50 µm.
The top five of the most frequently detected species were common in both the water column and the PET chamber. Those species were Cerataulina pelagica, Chaetoceros debilis, Ch. didymus, Ch. distans and Leptocylindrus danicus. Furthermore, the genus Skeletonema also occurred quite frequently. Since identification of species of the genus Skeletonema is very difficult under an optical microscope in a routine observation (see Sarno et al., 2005), they are collectively referred to as Skeletonema spp. in this study. No obvious trend of temporal change in either the abundance of the vegetative cells or the emergence flux of the frequently observed diatoms was found during the study (Figure 8). This was also true for other diatoms detected in this study (data not shown). The maximum abundance of the vegetative cells in the water column reached between 109- 1010 cells m-2 for Ch. debilis and Skeletonema spp., between 108 – 109 cells m-2 for Ch. didymus and between 107 – 108 cells m-2 for Ce. Pelagica, Ch. distans, and Le. danicus. In the PET chamber, the emergence flux exceeded 1.0×105 cells m-2 day-1 for Ch. debilis and Skeletonema spp. For other species, the maximum flux was in the range between 102-104 cells m-2 day-1. The abundance of the vegetative cells in the water column and the emergence flux in the PET chamber for each of these five species and Skeletonema spp. varied notably during the study period, but no clear relationships were found in the temporal changes between either of them (the abundance of the vegetative cells or the emergence flux) and any of the environmental parameters, such as water temperature, salinity, DO, light intensity and concentrations of nutrients.
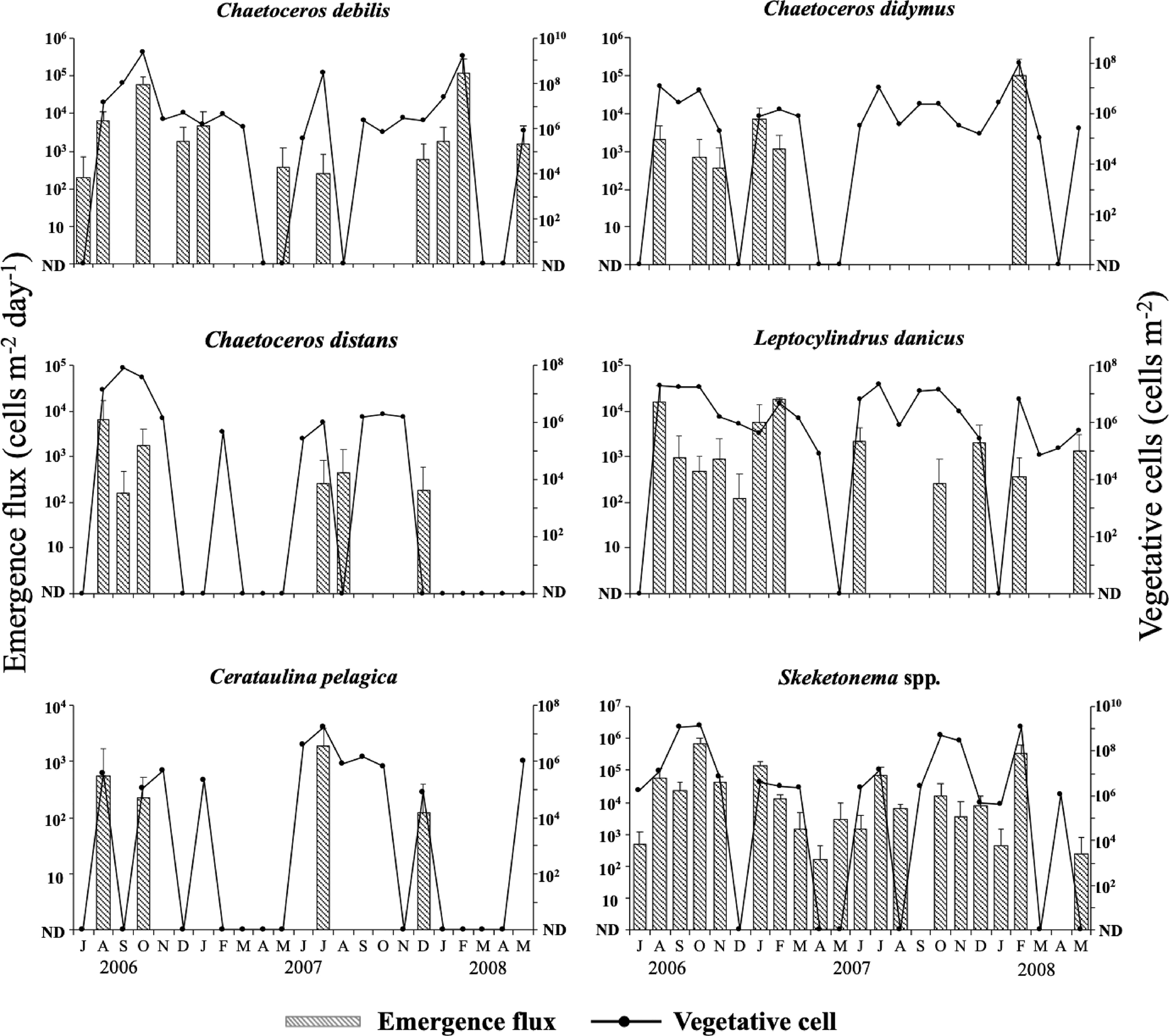
Figure 8 Temporal changes in the integrated abundance of the vegetative cells in the water column and the emergence flux in the PET chamber for six diatom taxa at the sampling site in Ago Bay. ND denotes that the cells were not detected. Bars represent standard deviations (+SD).
For these diatom taxa, in many cases their vegetative cells occurred in the water column when their cells emerged in the PET chamber. Analysis of the relationship between the abundance of the vegetative cells and the emergence flux by Spearman’s rank test revealed a significant positive relationship for Ch. debilis (r = 0.50, n = 23, p < 0.05), Ch. didymus (r = 0.47, n = 23, p < 0.05) and Skeletonema spp. (r = 0.76, n = 23, p < 0.01), but not for Ch. distans (r = 0.41, n = 23, p > 0.05), Le. danicus (r = 0.38, n = 23, p > 0.05) and Ce. pelagica (r = 0.36, n = 23, p > 0.05) (Table 2).
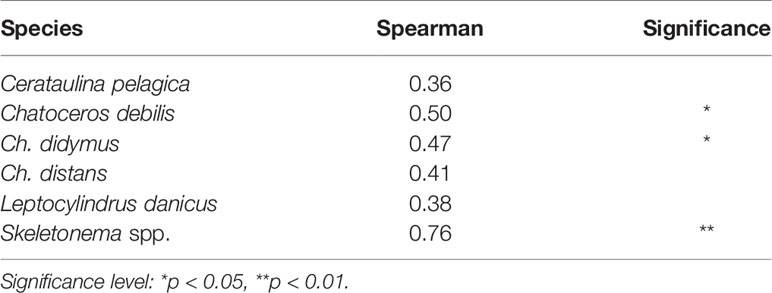
Table 2 Correlations between the integrated abundance of the vegetative cells (cells m-2) in the water column and the emergence flux (cells m-2 day-1) revealed by the Spearman’s rank test.
Throughout the PET chamber experiments, vegetative cells were not observed in the chamber employed as a control, confirming that there was no contamination from outside the PET chamber.
In the water samples of the flocculent layer obtained in July 2020, many diatom species forming long chains of vegetative cells with distinct chloroplasts in each cell were observed. Chaetoceros species dominated, but other genera (Bacteriastrum and Skeletonema) were also observed (Figure 9).
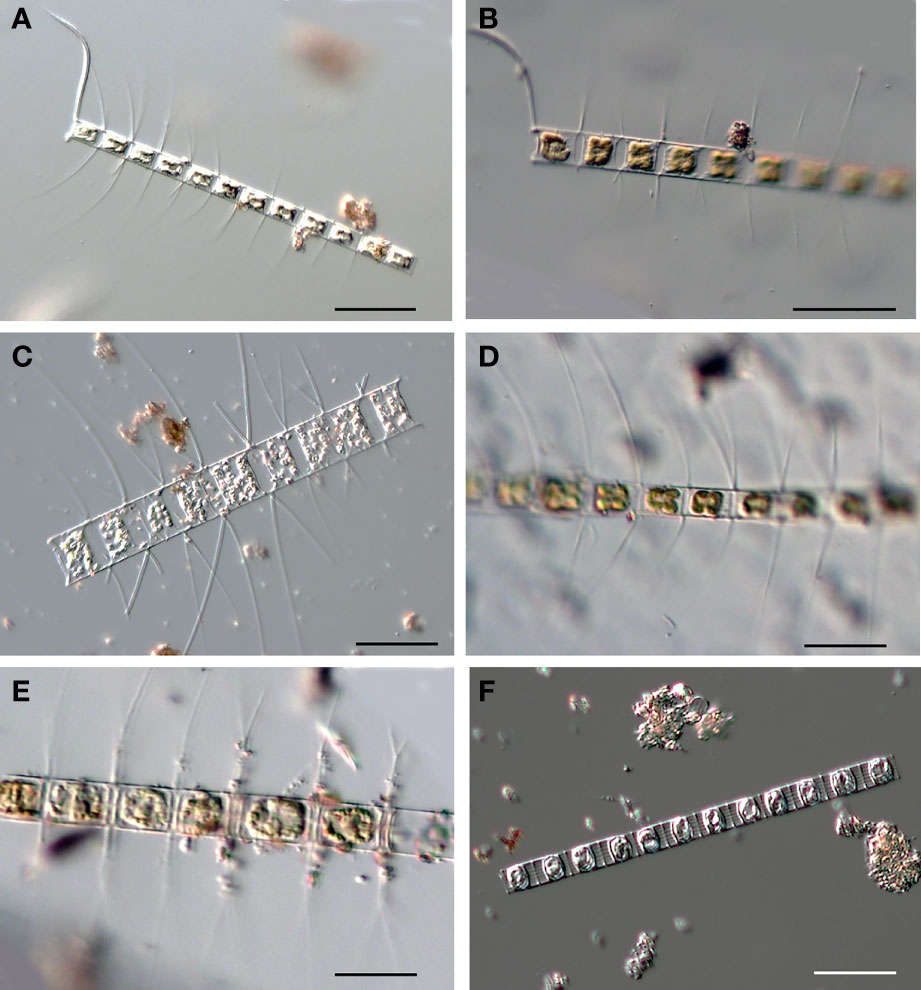
Figure 9 Photomicrographs, as examples, of the diatoms forming long chains observed in the flocculent layer of the sediment core sample collected at the PET chamber experiment site in Ago Bay. Chaetoceros affinis (A, B), Chaetoceros lorenzianus (C), Chaetoceros compressus (D), Bacteriastrum sp. (E), Skeletonema sp. (F). Scale bars: 50 µm.
Discussion
Vegetative Cells Emerged in the PET Chamber
In one of the samples of the PET chamber, a single vegetative cell with chloroplasts was observed just beside the valve of the resting spore (Figure 7G). Following the identification criteria for the species of the resting spores of diatoms presented by Ishii et al. (2011), the valve was morphologically identified as that of Ch. debilis, indicating that this single cell should have been a cell that germinated from a resting spore of the species during the 24 h of the PET chamber experiment. Similar cells were also observed for other species (photographs not shown). In addition, no contaminations of vegetative cells were observed in the PET chamber used as a control. These support that the monitoring of germinated/rejuvenated cells in the in situ sediment using the PET chamber was successful in this study.
Apart from the germinating cells, a large number of vegetative cells of diatom species which have not been known to form resting stage cells were observed in the PET chamber. The number of such species was 24, constituting about half of the total species that emerged in the PET chamber during the study period (Table 1). However, as suggested by Lewis et al. (1999) and Ueno and Ishikawa (2009), many unknown species of diatoms that form resting stage cells might exist. In this context, it is noteworthy that the resting stage cells of six species (Actinoptycus senarius, Biddulphia alternans, Detonula pumila, Lithodesmium variabile, Odontella longicruris and O. mobiliensis) were reported in the sediments collected in Ago Bay (Ishii et al., 2012) and the vegetative cells of all of these species emerged in the PET chamber in this study (Table 1). Therefore, it is possible that some additional species that emerged in the PET chamber can form resting stage cells.
It is intriguing that many long chains consisting of 4 or more cells were observed in the samples of the PET chamber (e.g., Figures 7J, Ab, Af). Considering that the cell division rate of diatoms is known to generally be one to two times per day, even for the fastest dividing species (Eppley, 1977; Frunas, 1990; Itakura et al., 1993), it is unlikely that those chains were formed after germination/rejuvenation of the resting stage cells within 24 h in the PET chamber experiments, suggesting that the vegetative cells consisting of the long chains had existed originally on the surface sediment before the experiment of the PET chamber started. Indeed, in the samples of the flocculent layer, many diatom species forming long chains of vegetative cells with distinct chloroplasts in each cell were observed (Figure 9). Vegetative cells were also found in the water of the benthic boundary layer (flocculent layer) off Oregon, U.S.A. (Wetz et al., 2004).
Vegetative cells could grow at light intensities > 9.4 µmol photons m-2 s-1 for Skeletonema costatum (sensu lato) (Itakura, 2000) and > 10 µmol photons m-2 s-1 for Coscinodiscus wailesii (Nishikawa and Yamaguchi, 2006) and Eucampia zodiacus (Nishikawa and Yamaguchi, 2008). This suggests that the two types of the living diatoms forming long chains in the flocculent layer of the surface sediment at the sampling site, where light almost always penetrated to the bottom (average depth 11 m) at intensities of > 10 µmol photons m-2 s-1 (Figure 5B), were present, although they could not be differentiated, as follows:
(1) the vegetative cells reproduced in the flocculent layer after germination/rejuvenation prior to the PET chamber experiment
(2) the vegetative cells had been deposited from the water column into the flocculent layer prior to the PET chamber experiment
In this study, the presence of diatom cells forming long chains in the flocculent layer was revealed by the PET chamber experiment. This is the reason why we used the term ‘emergence’ for the flux measured by the PET chamber rather than ‘germination/rejuvenation’.
Relationship Between Emergence of the Vegetative Cells and Environmental Factors
Throughout the study period, the emergence flux of the diatoms fluctuated largely but no clear trends of temporal changes appeared (Figure 8). Itakura (2000) reported, on the basis of laboratory culture experiments, that the resting stage cells of diatoms (Sk. costatum (sl), Chaetoceros spp. and Thalassiosira spp.) could germinate/rejuvenate from the sediments collected at Hiroshima Bay, Japan, at temperatures from 10 – 28°C and suggested that temperature is not a limiting factor on the germination/rejuvenation of their resting stage cells. Meanwhile, the vegetative cells that emerged in the PET chamber included not only the vegetative cells that germinated/rejuvenated from the sediment but also two types of cells forming long chains, as described above. The vegetative cells of the diatoms generally grow at a wide range of temperatures in laboratory experiments (Eppley, 1977). Furthermore, it has been reported that no obvious trends of temporal changes in the occurrence of vegetative cells in the water column were observed (Itakura et al., 1997; Itakura, 2000). These findings indicate that the temporal change of emergence flux could not be determined by the temporal change of temperature. In addition, there seemed to be no clear relationships between any environmental condition (salinity, DO, light intensity, or nutrients) and emergence flux in this study. On the other hand, it is well known that light is a primary factor to induce germination/rejuvenation of diatom resting stage cells (Hollibaugh et al., 1981; Imai et al., 1996). Hollibaugh et al. (1981) reported that resting stage cells of three species of the genus Chaetoceros did not germinate at a light intensity of 1.3 µmol photons m-2 s-1 or less. Itakura (2000) also reported that resting stage cells of Ch. didymus could not germinate under a low light intensity of 0.25 µmol photons m-2 s-1 or less, but could actively do so at a light intensity of 12 µmol photons m-2 s-1 or over. Furthermore, Imai et al. (1996) revealed that resting stage cells of Sk. costatum (sl) and Chaetoceros spp. needed a light intensity of about 4 µmol photons m-2 s-1 or over for germination/rejuvenation. Since the light during daytime almost always penetrated to the bottom at intensities > 10 µmol photons m-2 s-1 throughout the study period at the present study site and its average intensity during the period of PET chamber experiments was 27.3 µmol photons m-2 s-1, it should not be considered that light was a limiting factor on the germination/rejuvenation of resting stage cells of the diatoms that emerged in the PET chamber and on the maintenance of the two types of vegetative cells mentioned above. Therefore, unlike in deeper seas with lower light intensity, such as the Seto Inland Sea of Japan (Imai et al., 1996) and the East China Sea (Ishikawa and Furuya, 2004), where the resting stage cells in the sediment cannot germinate/rejuvenate unless those cells reach the euphotic layer as a result of mixing of the water column, the seafloor in a shallow embayment such as Ago Bay could provide an advantageous environment for diatoms to maintain their vegetative cells in the flocculent layer on the surface sediment even if there were no mixing of the water column.
Quantitative Relationships Between Both the Vegetative Cells in the Water Column and Those in the PET Chamber
Since the cells that emerged in the PET chamber consisted of various types of vegetative cells, careful interpretation is needed to quantitatively compare the relationships between the abundance of the vegetative cells in the water column and emergence flux. However, even if it is assumed that all the vegetative cells in the PET chamber were resuspended into the water column at the same time, the level of statistical significance varied depending on the diatom taxa (Table 2). Furthermore, several cases of disagreement were found for the relationships between the abundance of the vegetative cells and the emergence flux, even for the species (i.e., Ch. debilis and Ch. didymus) that showed a significant positive relationship. Namely, there were some cases when a large population of the vegetative cells occurred in the water column despite small emergence flux in the PET chamber (e.g., Ch. debilis in July 2007, Ch. didymus in October 2006 and February 2007). This was true for Skeletonema spp. (e.g., in October and November 2007), although it is necessary to pay attention to the statistical interpretation of the significant result due to the possibility of the content of multiple species. As pointed out for the case of dinoflagellates whose germination rate/flux was monitored (Ishikawa and Taniguchi, 1996; Ishikawa et al., 2014), these above considerations demonstrated that the magnitude of the emergence flux does not determine that of the vegetative populations in the water column, since the growth of the vegetative cells is largely dependent on complex factors, such as water temperature, light intensity, concentration of nutrients, competition with other phytoplankton species and predation by zooplankton. Namely, like to cyst-forming dinoflagellates (e.g., Ishikawa and Taniguchi, 1996; Ishikawa et al., 2014), the presence of vegetative cells, even in small numbers, in the water column by recruitment of the vegetative cells on the surface sediment (flocculent layer) as ‘seeds’ is important for proliferation of the diatoms at the time when environmental conditions become favorable for vegetative growth.
On the seafloor in a shallow embayment, such as Ago Bay, where the light always penetrates, various types of the vegetative cells demonstrated above wait for recruitment into the water column as seeds. This means that the flocculent layer on the seafloor acts as a ‘refuge and nursery’ for the vegetative population in the water column.
Life Cycle Strategies of Diatoms in a Shallow Coastal Waters
It has generally been suggested that the resting stage cells require resuspension, for their germination/rejuvenation, into the euphotic layer from the surface sediment by water mixing, as summarized in Figure 1. However, resuspension might be a dispensable condition for the cells to germinate/rejuvenate in a shallow embayment, such as Ago Bay, since the light penetrates to the seafloor. In addition, even the vegetative cells germinated/rejuvenated from the surface sediment and/or deposited from the water column could be alive on the surface sediment, as mentioned above. Based on this point of view, we propose three patterns of life cycle strategies for the diatoms in the bay (Figure 10);
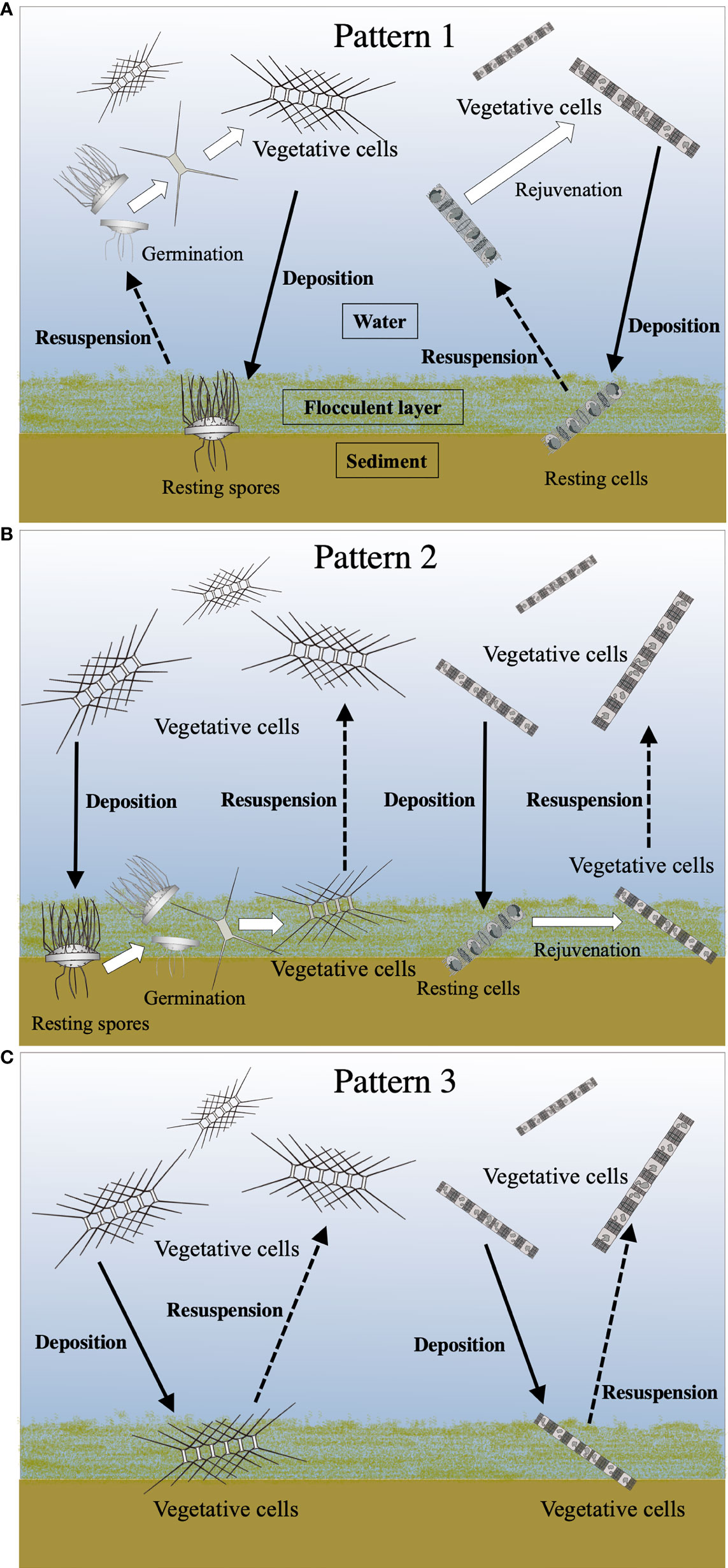
Figure 10 Conceptual diagrams of various life cycle patterns of the centric diatoms. See the text for details of Pattern 1 (A), Pattern 2 (B) and Pattern 3 (C). Solid and dotted lines indicate the movement (deposition and resuspension, respectively) of the cells between the water column and the surface sediment. White arrows denote transformations of the cell stage.
Pattern 1: the resting stage cells, which are resuspended from the surface sediment into the upper layer where the light condition is more favorable for their vegetative growth, germinate/rejuvenate (Figure 10A).
Pattern 2: the resting stage cells germinated/rejuvenated on the surface sediment remain as the forms of the vegetative cells in the flocculent layer of the surface sediment, waiting for the opportunity to be resuspended into the upper layer (Figure 10B).
Pattern 3: the vegetative cells once deposited from the water column in the flocculent layer of the surface sediment remain there until being resuspended into the upper layer (Figure 10C).
Consequently, the seafloor in a shallow embayment, where the light penetrates, enables the diatoms to possess not only a simple life cycle strategy that proceeds from the resting stage cells to the vegetative cells, but also unique strategies that proceed from the vegetative cells to the vegetative cells. These links between the planktonic forms and the benthic forms might be recognized as complex life cycle strategies of the diatoms. In conclusion, such complex life cycle strategies could promote the thriving of the diatoms in the temperate coastal waters/embayment where the environmental conditions vary widely throughout the seasons.
Further investigations of the emergence of the diatoms using the PET chamber are needed to get a better understanding of their population dynamics in different locations, including deep sea areas.
Data Availability Statement
The raw data supporting the conclusions of this article will be made available by the authors, without undue reservation.
Author Contributions
AI and KI designed and conducted the research, and collected the data. All authors contributed to data interpretation. KI wrote the first version of the manuscript and AI edited it. KM and II reviewed the manuscript. All authors approved the submitted version.
Funding
This study was supported by a Grant-in-Aid for Scientific Research (C) (18580180) from the Japan Society for the Promotion of Science.
Conflict of Interest
The authors declare that the research was conducted in the absence of any commercial or financial relationships that could be construed as a potential conflict of interest.
Publisher’s Note
All claims expressed in this article are solely those of the authors and do not necessarily represent those of their affiliated organizations, or those of the publisher, the editors and the reviewers. Any product that may be evaluated in this article, or claim that may be made by its manufacturer, is not guaranteed or endorsed by the publisher.
Acknowledgments
We are grateful to Dr. Keigo Yamamoto of the Research Institute of Environment, Agriculture and Fisheries, Osaka Prefecture, for his kind cooperation in nutrient analysis. We also express our sincere thanks to Dr. Taisuke Otsuka of the Lake Biwa Museum, Shiga Prefecture, for his kind guidance in statistical analysis of the data. Our special thanks are extended to the staff members of the Fisheries Research Laboratory of Mie University and our colleagues at the university for their help in the field work.
References
Belmonte G., Rubino F. (2019). “Resting cysts from coastal marine plankton”, in Oceanography and Marine Biology, An Annual Review,. Eds. Hawkins S. J., Allcock A. L., Bates A. E., Firth L. B., Smith I. P., Swearer S. E., Todd P. A. (Boca Raton: CRC Press), 57, 1–88.
Cupp E. E. (1943). Marine Plankton Diatoms of the West Coast of North America (Berkeley: University of California Press).
Dale B. (1983). “Dinoflagellate Resting Cysts: Benthic Plankton”, in Survival Strategies of the Algae. Ed. Frexell G. A. (Cambridge: Cambridge University Press), 69–136.
Drebes G. (1966). On the Life History of the Marine Plankton Diatom Stephanopyxis Palmeriana. Helgol. Wiss. Meeresunters 13, 101–114. doi: 10.1007/BF01612659
Eppley R. W. (1977). “The Growth and Culture of Diatoms”, in The Biology of Diatom. Ed. Werner D. (Oxford: Blackwell Scientific Publications), 24–64.
Furnas M. J. (1990). In situ growth rate of marine phytoplankton: approached to measurement, community and species growth rates. J. Plankton Res. 12, 1117–1151. doi: 10.1093/plankt/12.6.1117
Garrison D. L. (1981). Monterey Bay Phytoplankton. II. Resting Spore Cycles in Coastal Diatom Populations. J. Plankton Res. 3, 137–156. doi: 10.1093/plankt/3.1.137
Gran H. H. (1912). “Pelagic Plant Life”, in The Depths of the Ocean. Eds. Murray J., Hijort J. (London: Macmillan & Co., Ltd), 307–386.
Gran H. H., Angst E. C. (1931). Plankton Diatoms of Puget Sound. Publ. Puget Sound Biol. Sta. Univ. Wash. 7, 417–519.
Gran H. H., Yendo K. (1914). Japanese Diatoms. I. On Chaetoceras. II. On Stephanopyxia (Videnskapsselskapets Skrifter. I. Mat.-Naturu. Klasse. 1913. No. 8) (Christiania: Utgit for Friftjof Nansens Fond).
Haga M. (1997). Morphology of Vegetative and Resting Spore Valves of. Stephanopyxis Nipponica. Diatom Res. 12, 217–228. doi: 10.1080/0269249X.1997.9705416
Hargraves P. E. (1972). Studies on Marine Plankton Diatom. I. Chaetoceros Diadema (Ehr.) Gran: Life Cycle, Structural Morphology, and Regional Distribution. Phycologia 11, 247–257. doi: 10.2216/i0031-8884-11-3-247.1
Hargraves P. E. (1976). Studies on Marine Plankton Diatoms.II. Resting Spore Morphology. J. Phycol. 12, 118–128. doi: 10.1111/j.1529-8817.1976.tb02838.x
Hargraves P. E. (1984). “Resting Spore Formation in the Marine Diatom Ditylum Brightwellii (West) Gran”, in Proceedings of the Seventh International Diatom Symposium. Ed. Mann D. G. (Koenigstein: O. Koeltz Science Publishers), 33–46.
Hargraves P. E. (1990). Studies on Marine Plankton Diatoms. V. Morphology and Distribution on Leptocylindrus Minimus Gran. Beihefte zur Nova Hedwigia 100, 47–60.
Hargraves P. E., French F. W. (1983). “Diatom Resting Spores: Significance and Strategies”, in Survival Strategies of the Algae. Ed. Fryxell G. A. (New York: Cambridge University Press), 49–68.
Hasle G. R. (1978). “The Inverted-Microscope Method”, in Phytoplankton Manual. Ed. Sournia A. (Paris: UNESCO), 88–96.
Hasle G. R., Sims P. A. (1985). The Morphology of the Diatom Resting Spores Syringidium Bicorne and Syringidium Simplex. Bri. Phycol. J. 20, 219–225. doi: 10.1080/00071618500650231
Hasle G. R., Syvertsen E. E. (1997). “Marine Diatoms”, in Identifying Marine Phytoplankton. Ed. Tomas C. R. (San Diego: Academic press), 5–385.
Hollibaugh J. T., Seibert D. L. R., Thomas W. H. (1981). Observations on the Survival and Germination of Resting Spores of Three Chaetoceros (Bacillariophyceae) Species. J. Phycol. 17, 1–9. doi: 10.1111/j.1529-8817.1981.tb00812.x
Imai I., Itakura S., Yamaguchi M., Honjo T. (1996). “Selective Germination of Heterosigma Akashiwo (Raphidophyceae) Cysts in Bottom Sediments Under Low Light Condition: A Possible Mechanism of Red Tide Initiation”, in Harmful and Toxic Algal Blooms. Eds. Yasumoto T., Oshima Y., Fukuyo Y. (Paris: UNESCO), 197–200.
Ishii K., Ishikawa A., Imai I. (2012). Newly Identified Resting Stage Cells of Diatoms From Sediments Collected in Ago Bay, Central Part of Japan. Plankton Benthos Res. 7, 1–7. doi: 10.3800/pbr.7.1
Ishii K., Iwataki M., Matsuoka K., Imai I. (2011). Proposal of Identification Criteria for Resting Spores of Chaetoceros Species (Bacillariophyceae) From a Temperate Coastal Sea. Phycologia 50, 351–362. doi: 10.2216/10-36.1
Ishikawa A., Furuya K. (2004). The Role of Diatom Resting Stages in the Onset of the Spring Bloom in the East China Sea. Mar. Biol. 145, 633–639. doi: 10.1007/s00227-004-1331-9
Ishikawa A., Hattori M., Imai I. (2007). Development of the “Plankton Emergence Trap/Chamber (PET Chamber)”, A New Sampling Device to Collect in Situ Germinating Cells From Cysts of Microalgae in Surface Sediments of Coastal Waters. Harmful Algae 6, 301–307. doi: 10.1016/j.hal.2006.04.005
Ishikawa A., Hattori M., Ishii K., Kulis D. M., Anderson D. M., Imai I. (2014). In Situ Dynamics of Cyst and Vegetative Cell Population of the Toxic Dinoflagellate Alexandrium Catenella in Ago Bay, Central Japan. J. Plankton Res. 36, 1333–1343. doi: 10.1093/plankt/fbu048
Ishikawa A., Taniguchi A. (1996). Contribution of Benthic Cysts to the Population Dynamics of Scrippsiella Spp. (Dinophyceae) in Onagawa Bay, Northeast Japan. Mar. Ecol. Prog. Ser. 140, 169–178. doi: 10.3354/meps140169
Itakura S. (2000). Physiological Ecology of the Resting Stage Cells of Coastal Planktonic Diatoms. Bull. Fish. Environ. Inland Sea 2, 67–130.
Itakura S., Imai I., Itoh K. (1997). “Seed Bank” of Coastal Planktonic Diatoms in Bottom Sediments of Hiroshima Bay, Seto Inland Sea, Japan. Mar. Biol. 128, 497–508. doi: 10.1007/s002270050116
Itakura S., Yamaguchi M., Imai I. (1993). Resting Spore Formation and Germination of Chaetoceros Didymus Var. Protuberans (Bacillariophyceae) in Clonal Culture. Nippon Suisan Gakkaishi 59, 807–813. doi: 10.2331/suisan.59.807
Japan Meteorological Agency (2008) Precipitation. Available at: https://www.data.jma.go.jp/gmd/risk/obsdl/ (Accessed on August 2021).
Jensen K. G., Moestrup Ø. (1998). The Genus Chaetoceros (Bacillariophyceae) in Inner Danish Coastal Waters. Opera Bot. 133, 5–68. doi: 10.1111/j.1756-1051.1998.tb01103.x
Karsten G. (1905). Das Phytoplankton Des Antarktischen Meeres Nach Dem Material Der Deutschen Tiefsee-Expedition 1898-1899. Dtsch. Tiefsee Exped. 2, 1–136.
Lewis J., Haris A. S. D., Jones K. J., Edmonds R. L. (1999). Long-Term Survival of Marine Planktonic Diatoms and Dinoflagellates in Stored Sediment Samples. J. Plankton Res. 21, 343–354. doi: 10.1093/plankt/21.2.343
Margalef R. (1958). “Temporal Succession and Spatial Heterogeneity in Natural Phytoplankton”, in Perspectives in Marine Biology, Ed. Buzzati-Traverso A. A. (Barkley: University of California Press), 323–349.
McQuoid M. R., Hobson L. A. (1996). Diatom Resting Stages. J. Phycol. 32, 889–902. doi: 10.1111/j.0022-3646.1996.00889.x
Montresor M., Di Prisco C., Sarno D., Margiotta F., Zingone A. (2013). Diversity and Germination Patterns of Diatom Resting Stages at a Coastal Mediterranean Site. Mar. Ecol. Prog. Ser. 484, 79–95. doi: 10.3354/meps10236
Nagai S., Hori Y., Manabe T., Imai I. (1995). Morphology and Rejuvenation of Coscinodiscus Wailesii Gran (Bacillariophyceae) Resting Cells Found in Bottom Sediments of Harima-Nada, Seto Inland Sea, Japan. Nippon Suisan Gakkaishi 61, 179–185. doi: 10.2331/suisan.61.179
Nishikawa T., Yamaguchi M. (2006). Effect of Temperature on Light-Limited Growth of the Harmful Diatom Coscinodiscus Wailesii, a Causative Organism in the Bleaching of Aqua-Cultured Porphyra Thalli. Harmful Algae 7, 561–566. doi: 10.1016/j.hal.2007.12.021
Nishikawa T., Yamaguchi M. (2008). Effect of Temperature on Light-Limited Growth of the Harmful Diatom Eucampia Zodiacus Ehrenberg, a Causative Organism in the Discoloration of Porphyra Thalli. Harmful Algae 5, 141–147. doi: 10.1016/j.hal.2005.06.007
Pavillard J. (1921). Sur La Reproduction Du Chaetoceros Eibenii Meunier. Compt. Rend. Séanc. l’Acad. Sci. 172, 469–471.
Pfiester L. A., Anderson D. M. (1987). “Dinoflagellate Reproduction”, in The Biology of Dinoflagellates. Ed. Taylor F. J. R. (Oxford: Blackwell), 611–648.
Pitcher G. C. (1990). Phytoplankton Seed Populations of the Cape Peninsula Upwelling Plume, With Particular Reference to Resting Spores of Chaetoceros (Bacillariophyceae) and Their Role in Seeding Upwelling Waters. Estuar. Coast. Shelf Sci. 31, 283–301. doi: 10.1016/0272-7714(90)90105-Z
Reynolds C. S. (1984). Phytoplankton Periodicity: The Interactions of Form, Function and Environmental Variability. Freshw. Biol. 14, 111–142. doi: 10.1111/j.1365-2427.1984.tb00027.x
Rines J. E. B., Hargraves P. E. (1988). The Chaetoceros Ehrenberg (Bacillariophyceae) Flora of Narragansett Bay, Rhode Island, U.S.A. Bibl. Psychiat. 79, 1–196.
Rines J. E. B., Hargraves P. E. (1990). Morphology and Taxonomy of Chaetoceros Compressus Lauder Var. Hirtisetus Var. Nova, With Preliminary Consideration of Closely Related Taxa. Diatom Res. 5, 113–127. doi: 10.1080/0269249X.1990.9705097
Sarno D., Kooistra W. H., Medlin L. K., Percopo I., Zingone A. (2005). Diversity in the Genus Skeletonema (Bacillariophyceae). II. An Assessment of the Taxonomy of S. Costatum-Like Species With the Description of Four New Species. J. Phycol. 41, 151–176. doi: 10.1111/j.1529-8817.2005.04067.x
Saunders R. P. (1968). Cerataulina Pelagica (Cleve) Hendey. Florida Board Conserv. Leaflet 1 (Pt. 2, No. 5), 1–11.
Sicko-Goad L. (1986). Rejuvenation of Melosira Granulata (Bacillariophyceae) Resting Cells From the Anoxic Sediments of Douglas Lake, Michigan. II. Electron Microscopy. J. Phycol. 22, 28–35. doi: 10.1111/j.1529-8817.1986.tb02510.x
Smayda T. J. (1980). “Phytoplankton Species Succession”, in The Physiological Ecology of Phytoplakton. Ed. Morris I. (Oxford: Blackwell), 493–570.
Stedinger K. A. (1975). “Basic Factors Influencing Red Tides”, in Proceedings of the First International Conference on Toxic Dinoflagellate Blooms. Ed. Lo-Cicero V. R. (Wakefield: Mass Sci Tech Found), 153–162.
Stockwell D. A., Hargraves P. E. (1986). “Morphological Variability Within Resting Spores of the Marine Diatom Genus Chaetoceros Ehrenberg”, in Proceedings of the Eighth International Diatom Symposium. Ed. Richard M. (Koenigstein: Koeltz Scientific Books), 81–95.
Strickland J. D. H., Parsons T. R. (1972). A Practical Handbook of Seawater Analysis. Fish. Res. Bd. Can. Bull. 167, 1–310.
Ueno R., Ishikawa A. (2009). Evaluation of Functionality as a Seed Population of Resting Stage Cells of Centric Diatoms in Surface Sediments of Ago Bay, Central Part of Japan. Bull. Plankton Soc. Jpn. 56, 1–12.
von Stosch H. A. (1967). “Diatomeen”, in Vegetative Fortpflanzung, Parthenogense Und Apogamie Bei Algen, Handbuch Der Pflanzenphysiologie. Ed. Rhuland W. (Berlin: Springer-Verlag), 657–681.
Wall D. (1971). Biological Problems Concerning Fossilizable Dinoflagellates. Geosci. Man. 3, 1–15. doi: 10.1080/00721395.1971.9989704
Wetz M. S., Wheeler P. A., Letelier R. M. (2004). Light-Induced Growth of Phytoplankton Collected During the Winter From the Benthic Boundary Layer Off Oregon, USA. Mar. Ecol. Prog. Ser. 280, 95–104. doi: 10.3354/meps280095
Keywords: diatom, resting stage cell, vegetative cell, emergence, PET chamber, shallow embayment, life cycle strategy
Citation: Ishii K-I, Matsuoka K, Imai I and Ishikawa A (2022) Life Cycle Strategies of the Centric Diatoms in a Shallow Embayment Revealed by the Plankton Emergence Trap/Chamber (PET Chamber) Experiments. Front. Mar. Sci. 9:889633. doi: 10.3389/fmars.2022.889633
Received: 04 March 2022; Accepted: 21 April 2022;
Published: 18 July 2022.
Edited by:
Tamara Cibic, Istituto Nazionale di Oceanografia e di Geofisica Sperimentale, ItalyReviewed by:
David U. Hernández-Becerril, National Autonomous University of Mexico, MexicoGenuario Belmonte, University of Salento, Italy
Copyright © 2022 Ishii, Matsuoka, Imai and Ishikawa. This is an open-access article distributed under the terms of the Creative Commons Attribution License (CC BY). The use, distribution or reproduction in other forums is permitted, provided the original author(s) and the copyright owner(s) are credited and that the original publication in this journal is cited, in accordance with accepted academic practice. No use, distribution or reproduction is permitted which does not comply with these terms.
*Correspondence: Akira Ishikawa, aXNoaWthd2FAYmlvLm1pZS11LmFjLmpw; Ken-Ichiro Ishii, cmVzdGluZ3Nwb3JlQGdtYWlsLmNvbQ==
†Present address: Ken-Ichiro Ishii, SeedBank Co. Ltd., Kyoto, Japan