- 1Marine College, Shandong University, Weihai, Weihai, China
- 2School of Municipal and Environmental Engineering, Shandong Jianzhu University, Jinan, China
- 3Fisheries Development Service Department, Weihai Ocean Development Research Institute, Weihai, China
- 4Jihongtan Reservoir Management Station, Shandong Water Diversion Project Operation and Maintenance Center, Qingdao, China
Summer hypoxia and harmful algal bloom occurred sometimes in the nearshore of the northern Yellow Sea in recent years. Based on seven multidisciplinary investigations conducted from March to November 2016, except for April and October, the phytoplankton community and its association with ambient seawater physicochemical parameters in coastal waters of the northern Yellow Sea were comprehensively examined. In total, 39 taxa belonging to 4 phyla and 24 genera were identified. Diatoms and dinoflagellates were the dominant groups, which accounted for 64.1% and 30.8% of total species, respectively. An algal blooming event dominated by the diatom (Thalassiosira pacifica) occurred in March, which affects the shifting of diatom–dinoflagellate dominance. A notable dinoflagellate dominance occurred especially in surface water throughout the whole summer but changed to diatom dominance again from September. Hypoxic zones (<2 mg l-1)were observed in the bottom water in August, with minimum dissolved oxygen (DO) of 1.30 mg l-1. This low DO zone in August was clearly associated with the diatom blooming event (Thalassiosira pacifica) in March, as diatoms in surface waters sank into bottom waters and decomposed by the microbial community resulting in oxygen consumption. After the early-spring diatom bloom, thermohaline stratification occurred and prevented exchanges of dissolved oxygen, which eventually led to hypoxia in bottom waters. The effects of algal blooms on phytoplankton composition and hypoxia could have a cascaded effect on the fishery sustainability and aquaculture in nearshore waters of the northern Yellow Sea.
Introduction
Coastal waters are regions that are influenced by strong land–ocean interactions (Frigstad et al., 2020); they account for only a small portion of the ocean surface area (7%–8%) but are responsible for 25% of the global ocean primary production (Smith and Hollibaugh, 1993). Compared with open sea ecosystems, coastal waters are under substantial pressure and have become ecologically fragile regions in recent decades (Regnier et al., 2013; Fennel and Testa, 2019). Under the combined influences of climate change and human activities, coastal areas are more vulnerable to eutrophication, harmful algal blooms, and hypoxia (Bianchi and Allison, 2009; He and Silliman, 2019; Wei et al., 2020). As a feedback, hypoxia can influence fundamental biological and biochemical processes (Turner et al., 2008; Breitburg et al., 2018) and affect pelagic organisms from plankton to fish (O’Connor and Whitall, 2007; Breitburg et al., 2009; Roman et al., 2012; Bausch et al., 2019), which would destroy the potential functioning of the fragile coastal ecosystem. Phytoplankton is the main source of primary production and plays a key role in estuary ecosystems, which could be reflected by the variation of composition diversity and abundance (Falkowski and Woodhead, 1992). Previous studies have shown that the spring bloom is the period with the highest annual primary production and sinking of organic matter to the sediment; thereafter, the fate of this organic matter is a key driver for material fluxes, affecting ecosystem functioning and eutrophication feedback loops (Spilling et al., 2018). The potential functioning of the coastal ecosystem strongly depends on the quantity and quality of phytoplankton.
The Yantai nearshore ecosystem is located in the western portion of the northern Yellow Sea, where it is close to the Yantai-Weihai fishing ground and affected by typical anthropogenic activities such as sewage discharge and aquaculture. Similar to other coastal waters that are severely impacted by anthropogenic activities, our study area has also been suffering from serious eutrophication, jellyfish blooms, and hypoxia (Dong et al., 2012; Wang et al., 2012; Sun et al., 2015; Zhang et al., 2018; Yang et al., 2020). Since 2013, hypoxia was reported to cover approximately 54.0 km2 and cause mass mortality of the benthos species, such as sea cucumbers, abalones, and scallops, thus leading to huge economic and ecological losses. Bottom-layer hypoxia was also observed in the summers of most of the subsequent years (Yang et al., 2018; Zhang et al., 2018) in this studied area. Previous studies showed that phytoplankton blooms and their subsequent decomposition are important biological factors leading to oxygen depletion and hypoxia in Changjiang estuary and its adjacent waters (Wang, 2009; Chi et al., 2021). The diatom-dominated community sediments rapidly, having higher sinking rates, and contributes more carbon flux to the bottom waters during algal blooms (Guo et al., 2016; Li et al., 2018). Thus, it is important to understand the phytoplankton community composition and spatial and temporal changes, to assess algal bloom effects on shifting of diatom–dinoflagellate dominance, and on subsequent deoxygenation in coastal waters of northern Yellow Sea, China.
From March to November 2016, seven multidisciplinary investigations were conducted in the northern Shandong Peninsula, North Yellow Sea, China. The main goal of this study is to identify phytoplankton species (populations) associated with environmental factors to analyze the potential effects of spring diatom bloom on subsequent dinoflagellate dominance and formation of hypoxia. It is hypothesized that degradation of organic matter derived from diatom blooms is the cause of the formation of hypoxia.
2 Materials and Methods
2.1 Study Area and Cruise
The Yantai coast in the northern Yellow Sea covers an area of 423 km2 with a 118-km-long coastline, including Muping marine ranching (MR), Yantai–Weihai fishing ground (FG), and Sishili bay (SB). SB is a typical half-closed coastal bay affected by anthropogenic activities, such as harbor pollution and wastewater discharge from domestic, industrial, and agriculture areas (Wang et al., 2012). MR is an important aquaculture breeding base. The hydrological conditions of temperature, salinity, and water column stratification exhibit a seasonal cycle, leading to complex spatial–temporal heterogeneity in the phytoplankton community in the study area (Yang et al., 2020). Therefore, seven cruises were carried out from March to November 2016, except for April and October, in coastal waters of the northern Yellow Sea (121.51°E–122.03°E, 37.46°N–37.63°N). Thirty-five stations, with a sampling depth from 11 to 25 m, were established to include all representative areas within the domain. The locations of sampling stations are presented in Figure 1 and Table 1.

Table 1 The sampling date and station numbers of environmental and biological parameters during each cruise.
2.2 Sampling and Processing
The profiles of temperature, salinity, and DO were recorded by the CTD device (RBRmaestro, Ottawa, Canada; RBR temperature and salinity probes, and a Model AADI DO optode). Discrete water samples were collected from standard depths, i.e., surface, 5 m, 10 m, 20 m, and 2 m above bottom, using 12-l Niskin bottles (KC Denmark Ltd., Silkeborg, Denmark) at each station. For analysis of phytoplankton assemblage, aliquots of 250-ml seawater samples were preserved with buffered formalin (2% final concentration). Following the methodology described by Utermöhl (1958), 10–25 ml of the subsamples was settled in a Hydro-bios chamber for 24 h, then identified and enumerated under an inverted light microscope (IX71, Olympus, Japan) at ×200 or ×400 magnification (Tomas, 1997). The lower size limit of resolution for this analysis was ~5μm.
2.3 Data Analysis
The diversity of phytoplankton assemblages was demonstrated by the Shannon–Weiner diversity index (H′, log base 2) (Sun and Liu, 2003). The dominant phytoplankton species were determined according to the McNaughton index (Y), which is calculated as follows:
where ni is the total abundance of species i, N is the total abundance of all species, and fi is the frequency of species i in all samples. Values of Y < 0.02, indicate that the species is not dominant.
The DO saturation of each sample was calculated based on the Weiss equation (Pytkowicx, 1971).
In the equations, DO′ is the equilibrium saturation concentration in water under certain environmental conditions and DO is the measured concentration.
2.4 Statistical Analysis
One-way analysis of variance (ANOVA) was applied to compare the parameters in different sampling sites, with the significant level of p set at 0.05. Pearson correlation analysis was conducted to access the relationships between phytoplankton abundance and environmental factors. A Kruskal–Wallis test was performed to reveal differences of phytoplankton in non-hypoxia and hypoxia zones. All statistical tests were conducted with the SPSS (Version 20.0 for Windows, SPSS Inc., La Jolla, CA, USA). The horizontal distributions of each parameter were plotted using Surfer 16 (Golden Software LLC, Golden, CO, USA). The variations in the phytoplankton abundance were conducted with OriginPro 2021 software.
3 Results
3.1 Environmental Factors
3.1.1 Temporal Variation of Temperature and Salinity
Water temperature showed strong monthly variation in the study area, and variations of water temperature in bottom were consistent with the surface layer (Figure 2). Water temperature was highest in August, with the average of 25.38 ± 0.91°C, and lowest in March, with the average of 3.38 ± 0.51°C in the surface layer. There were small differences in water temperature of the surface layer between June and July, with the average of 19.23 ± 0.99°C and 20.84 ± 1.65°C, respectively. The averaged salinity was lower in summer than the other seasons, and salinity in the surface layer was lower than that in the bottom layer especially from March to August (Figure 3). In the surface layer, the lowest salinity occurred in August with the average of 31.31 ± 0.15. On the whole, the mean temperature in surface water was higher than that in bottom water from March to August (p < 0.001). Similarly, surface salinity was lower than bottom salinity from March to August (p < 0.05 in March, p < 0.001 in other months). No differences in surface salinity and bottom salinity were detected in September and November (p > 0.05).
3.1.2 Spatial Distribution of Temperature and Salinity
As a typical half-closed coastal bay, the study area was affected by wastewater discharge from domestic, open sea waters and melting snow, which together leads to the different variations of salinity from coastal to offshore waters. In March, the temperature and salinity were lower in the coastal water than those in the outer part (Figure 2, 3). In May, the temperature and salinity increased from coastal to offshore waters. The relatively higher salinity and lower temperature distributed offshore in June and remained until August especially in bottom water. The temperature and salinity were homogeneous from September in both surface and bottom waters. However, the relatively lower temperature and higher salinity occurred from the inner bay (the west of studied area) in November.
3.2 Phytoplankton Assemblage
3.2.1 Species Composition
During the seven cruises, a total of 39 taxa belonging to 4 phyla and 24 genera were identified. Diatoms and dinoflagellates were the dominant groups, which accounted for 64.1% and 30.8% of total species, respectively. In general, diatoms were dominant in March, most of which were chain-forming diatoms such as T. pacifica and Paralia sulcata. Dinoflagellates became dominant from May to August in surface water (Figure 4), while the species amounts of Chrysophyta and Euglenophyta were low in all cruises.
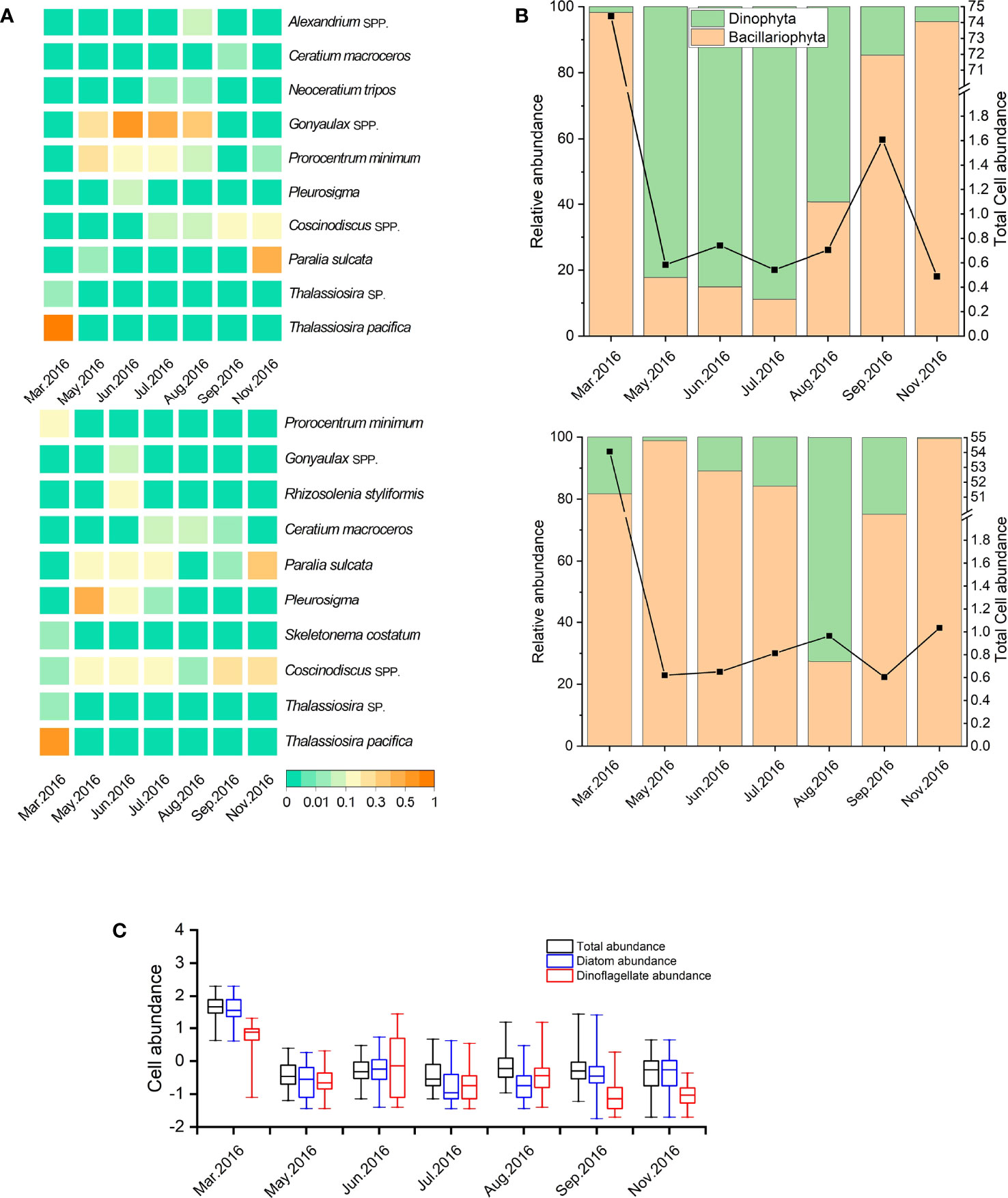
Figure 4 Variation in the dominance (Y) of phytoplankton species (or genera) in the study area (A); variation in relative abundance of diatoms and dinoflagellates in study area (B). The upper and bottom capture represent surface and bottom water, respectively. Variation in cell abundance of total phytoplankters, diatoms, and dinoflagellates (all data were transformed logarithmically [log10 (x)] before analyses; the bottom and top of the box represent the lower and upper quartiles, respectively. The band near the middle of the box represents the medium; the ends of the whisker represent the maximum and minimum values, respectively) (C).
3.2.2 Dominant Species
The top 10 dominant phytoplankton species (or genera) are listed in Figure 4A, including six diatom and four dinoflagellate species during the study period. Chain-forming diatoms, such as T. pacifica, P. sulcate, and Skeletonema costatum, were the most common dominant species in the study area. In March, T. pacifica showed exclusive dominance (Y = 0.91). P. sulcata was another dominant diatom in this nearshore area except in March and August, and its highest dominance occurred in November in the whole water. Coscinodiscus spp. were the dominant diatoms in bottom water and higher than in surface water from March to June. Prorocentrum minimum presented higher dominance in surface water from May to August and was the only dominant dinoflagellate in March in bottom water. Gonyaulax spp. maintained the highest dominance from June to August in surface water (Y = 0.35–0.66). Neoceratium tripos was dominant in both surface and bottom waters in July and August and was the dinoflagellate species with the highest dominance in August, whereas Alexandrium spp. showed a relatively high dominance (Y = 0.07) only in August in surface water.
3.2.3 Temporal and Spatial Distribution of Phytoplankton
The relative abundance of Bacillariophyta and Dinophyta is shown in Figure 4B. The ratio of diatom peaked in March, but the ratio of dinoflagellates in bottom water exhibited a higher value than that in surface water. The ratio of dinoflagellates increased greatly from May to June, with the maximum of 89.97%, and maintained a relatively high abundance until August in surface water. From September, the ratio of diatoms began to increase in both surface and bottom waters, with high ratios more than 96.77% in November. The total abundance of phytoplankton, diatoms, and dinoflagellates is shown in Figure 4B, and the abundance transformed logarithmically [log10 (x)] is shown in Figure 4C. After a relatively high abundance in March, the total of phytoplankton abundance showed low from May to August in both surface and bottom waters and then increased to a weaker peak in September in surface water.
The spatial distribution of total phytoplankton is shown in Figure 5. The phytoplankton abundance was relatively higher at station 6 (maximum at 2.01 × 105 cells l-1 in surface water) in March, with the diatom blooming event dominated by T. pacifica (this blooming threshold was determined based on “People’s Republic of China Marine Industry Standard: HY-T069-2005_Technical specification for red tide monitoring”). In May~June, the phytoplankton presented patches with higher abundance from northern offshore areas to coastal waters. In July, the phytoplankton cell abundance remained high in northern areas but decreased in August. In September, the phytoplankton cell abundance concentrated in the northwest and thereafter phytoplankton maintained low abundance but only concentrated in the east part of the study area in November.
3.3 Low DO and Hypoxia
The DO concentration of the study area exhibited both spatial variation and seasonal fluctuation (Figure 6). The DO concentration in surface water was significantly higher than that in bottom water from March to August (p < 0.05 in July, p < 0.001 in other months), but there was no significant difference in September and November. In March and May, the DO concentrations in surface water waters were relatively high, ranging from 6.48 to 11.89 mg l-1, while a relatively low DO zone (minimum at 4.86 mg l-1) appeared in bottom water in May, which centered in the west part of the coastal area. The DO saturation ranged from 75.1% to 94.0% in March and 61.7% to 93.5% in May. The averaged DO increased slightly in June especially in surface water, while the low DO zones in bottom water expanded, with a DO concentration decreased to 4.10 mg l-1 in the center. The low DO zone expanded continuously from the west to east parts in July, with the lowest DO (<4.0 mg l-1) formed around stations 4–7 and a lower DO (< 6.0 mg l-1) distributed in most coastal areas in bottom water. In August, waters with low DO (<4.0 mg l-1) almost covered all parts of the study area in bottom water and the DO concentrations of five coastal stations (stations 6–8 and stations 12–13) reached the hypoxia level (1.31–1.77 mg l-1). The minimum of DO saturation was 16.2% at station 7. In September, the hypoxia zone was relieved and low DO (minimum to 3.54 mg l-1) was only observed in the offshore area. The low DO zone disappeared in November, and the DO concentration in the bottom water increased to saturation in the west part of the study area. Overall, the low DO zone in the bottom water from June to August distributed at the same stations where diatom abundance was high in March (stations 6 and 7).
4 Discussion
Summer oxygen depletion and even hypoxia in the northern mariculture areas of the Shandong Peninsula have been observed since 2013 (Liu et al., 2014; Zhai et al., 2014). In the present study, low DO (<3 mg l-1) or hypoxic zones (<2 mg l-1) were observed in bottom waters in August 2016. The low DO zone almost covered the whole of the survey area, with DO reaching its minimum of 1.31 mg l-1. The low DO zone in bottom water in August was clearly associated with the high phytoplankton cell abundance of surface waters in March. Previous studies have indicated that the formation of the hypoxic zone is closely related to the oxygen utilization by the particulate organic matter (Rabouille et al., 2008; Li et al., 2011), and the photosynthesis of surface phytoplankton is the main source of organic matter (Wei et al., 2007). In this study, a diatom bloom dominated by T. pacifica broke out in March, which could provide an organic carbon source. When algal mass sank to the bottom and decomposed by microbial community eventually, low DO and even hypoxia formed in bottom waters (Li et al., 2018; Chi et al., 2021).
4.1 The Correlation Between Algal Blooms and Low DO
Hypoxia has been widely observed in various estuaries and coastal and gulf regions, including the Gulf of Mexico, Chesapeake Bay, the Baltic Sea, and the Changjiang (Yangtze River) Estuary (Chen et al., 2007; Breitburg et al., 2009; Conley et al., 2011; Carstensen et al., 2014). Previous studies have shown that phytoplankton communities dominated by diatoms with higher sinking rates contribute more particulate organic matter to the bottom waters during algal blooms and may thus be more closely associated with bottom hypoxia in the Changjiang Estuary (Yangtze River) and its adjacent waters (Guo et al., 2016; Li et al., 2018). In addition, studies have shown that the spring diatom bloom could be followed by seasonal DO reduction in coastal areas (Egge and Aksnes, 1992). Besides, 40%–60% of annual carbon fixation takes place during the spring bloom in the most eutrophicated region of the Baltic Sea. When the fixed carbon sinks to the bottom, seasonal hypoxia occurred (Spilling et al., 2018).
In this study, the relationships between the numerical abundance of dinoflagellates and diatoms in surface and bottom water and the environmental factors in the corresponding layer from May to November are shown in Table 2. Results showed the significant negative relationship between surface diatom abundance and surface DO concentration (p < 0.05, r2 = -0.86), similar with the result between bottom dinoflagellate abundance and bottom DO concentration (p < 0.01, r2 = -0.92). It is worth noting that the relatively high Chl a values in the surface water appeared right in the region with low DO in the bottom water (Yang et al., 2020). Particularly, the spatial distribution of the hypoxic area in August was consistent with the zone of diatom blooms in March, which provided a clue of DO consumption resulting from organic matter degradation during an algal bloom period. Phytoplankton bloom could be the primary contribution to dissolved organic matter (DOM) and dissolved organic carbon (DOC) (Wei et al., 2007; Fan et al., 2018; Zhang et al., 2018). The origin and dynamics of DOM were studied in the same cruise in the study area. The results showed that DOM mainly produced in situ, indicating that in situ diatom blooms were the resource of DOM (Zhang et al., 2018).

Table 2 Pearson’s correlation coefficient of the relationship between abundance of diatoms or dinoflagellates and environmental parameters in surface (S) and bottom (B) water from May to November.
Degradation of organic matter is known to be a major factor contributing to the development of hypoxia (Wang et al., 2016; Su et al., 2017). In approximately the same study area, the microbial diversity in bottom water was significantly higher in summer, and Bacteroidetes was higher in August, which was the important organic-aggregate-associated bacteria during phytoplankton bloom (Wang et al., 2022). This may be due to that planktonic algae multiplied in spring and then sank to the bottom, increasing the influx of organic matter to the seabed in August. Besides, bacterial oxidation of sulfur compounds is known to occur extensively in the sediments under oxygen minimum zones and sulfate-reducing bacteria could indirectly promote phosphate release through organic matter mineralization (Diaz and Rosenberg, 2008; Sinkko et al., 2011). As one of sulfate-reducing bacteria, Pseudomonas could cause phosphate release. In the study area, Pseudomonas with higher abundance was found in the bottom in August, which indirectly proves that microbial decomposition accelerates the oxygen consumption in summer (Wang et al., 2022). Moreover, studies in dissolved inorganic nutrients in the same cruise also showed that dissolved silicon (DSi) was mainly generated by sediment release, accounting for 94.2% in this area, and DSi increased from June onward, reaching a maximum in August (Yang et al., 2020). The results suggested that variation of DSi associated with DO consumption in the bottom, indicating that the mineralization of the settled diatom could contribute to deoxygenation. Therefore, the formation of hypoxia was related to the quick sinking and subsequent mineralization of diatom blooms in the study area.
In addition, stratification of the water column has also been shown be an important factor contributing to hypoxia (Wiseman et al., 1997; Murphy et al., 2011; Hamidi et al., 2015). In the study area, temperature and salinity stratification appeared from June to September. In November, the temperature and salinity distributed vertical homogeneity with adequate water exchange. Water column stratification influences the replenishment of oxygen by restricting exchanges between near-bottom waters and surface waters. Moreover, the organic matters derived from diatom blooms sinking below the pycnocline led to more oxygen depletion in near-bottom waters in summer. Therefore, both the diatom bloom as a biological factor and the thermohaline stratification as a hydrological factor could affect the formation of hypoxia. To further reveal the contribution of phytoplankton blooms sinking to the organic carbon and DO reduction in the hypoxia zone, sediment traps together with the evaluation of carbon flux could be conducted in further investigations.
4.2 Shifting of Diatom-Dinoflagellate Dominance After Early-Spring Bloom
An increasing trend in dinoflagellate abundance has been detected throughout the Baltic Sea in recent decades, and Peridiniella catenata (syn. Gonyaulax catenata) dominated in the phytoplankton community during phytoplankton bloom in the end of April (Lignell et al., 1993; Wasmund and Uhlig, 2003; Klais et al., 2011; Hajdu et al., 2000; Stoecker et al., 2017). In this study, the relative abundance of dinoflagellate reached the highest value in May and remained in high abundance until July, after the early-spring diatom bloom (Figure 4). In particular, the dominance of Gonyaulax spp. was 0.66 in June, while the diatom species even had no dominance in May (Y < 0.02).
During the investigation, the spatial distribution of surface nutrients exhibited an obvious seaward gradient, and the nutrients were higher in the inshore zones than in the offshore zones, especially DIN and DIP (Sun et al., 2021), leading to the higher phytoplankton abundance off coastal waters in March. However, terrestrial input was not the primary source of DSi to the study area (Liu et al., 2006), as sediment was the main source of DSi (Sun et al., 2021). In the study area, DSi increased in June with the degradation of settled diatom and reached to a maximum in August (Yang et al., 2020). This variation of DSi was consistent with shifting of diatoms–dinoflagellates, which decreased after the diatom blooming but reached maximum supporting diatom dominance to the first in September. Therefore, early-spring diatom bloom could result in dinoflagellate dominance in subsequent months and restoration of diatom dominance with release of DSi after sedimentation.
In addition to the effects of nutrients, many other factors could contribute to dinoflagellate dominance in the nearshore area of the northern Yellow Sea. Changes in mixing conditions, stratification, and wind-induced cyst resuspension have all been invoked to explain the dinoflagellate increase in the Baltic Sea (Kremp, 2001; Klais et al., 2011; Lips et al., 2011; Sildever et al., 2017). As cysts of the dominant dinoflagellates, Gonyaulax spp. and Alexandrium spp. have been shown to be dominant in Yellow Sea sediments (Liu et al., 2012; Wu et al., 2018); the potential effects of cyst germination on dinoflagellate dominance especially during the deoxygenation period should be focused in future to explain the shifting of diatom–dinoflagellate in nearshore water of the northern Yellow Sea.
5 Conclusions
Diatoms and dinoflagellates are the dominant groups, and diatom blooms occurred nearshore (station 6) in March. Hypoxic zones (<2 mg l-1) were observed in bottom waters in August. The low DO zone in bottom waters in August was clearly associated with the high phytoplankton cell abundance of surface waters in March. Degradation of organic matter derived from diatom blooms is essential to the formation of low DO and shifting of diatom–dinoflagellate dominance. Moreover, water-column stratification, which occurred from May to August, could also be an important factor contributing to hypoxia. Moreover, water-column stratification, which occurred from May to August, could also be an important factor contributing to DO decrease and dinoflagellate dominance. Therefore, further in situ studies are needed for a better understanding of hypoxia and algal blooms in this typical nearshore area, to assure the health of aquaculture in Muping marine ranching and sustainability in fishery resource zones nearby.
Data Availability Statement
The original contributions presented in the study are included in the article/supplementary material. Further inquiries can be directed to the corresponding authors.
Author Contributions
XS contributed in conceptualization, methodology, and writing. ZL contributed in the application of statistical and writing of the draft. XD contributed in conducting the research and investigation process. GJ contributed in conducting the research and investigation process. LW contributed in project administration. XG contributed in project administration. QC contributed in data curation. LZ contributed in application of statistical to analyze the study data. All authors contributed to the article and approved the submitted version.
Funding
This study was financially supported by the National Key Research and Development Program of China (Grant No. 2018YFC1407501); the National Natural Science Foundation of China (NSFC) (Grant No. 41806152); Science Foundation of Shandong Jianzhu University (XNBS1937).
Conflict of Interest
The authors declare that the research was conducted in the absence of any commercial or financial relationships that could be construed as a potential conflict of interest.
Publisher’s Note
All claims expressed in this article are solely those of the authors and do not necessarily represent those of their affiliated organizations, or those of the publisher, the editors and the reviewers. Any product that may be evaluated in this article, or claim that may be made by its manufacturer, is not guaranteed or endorsed by the publisher.
Acknowledgments
The authors are grateful to Professor Jianmin Zhao, for sharing his program cruise to us, and Zhang Fan, student at Shandong University, for participation in the cruises and in counting phytoplankton samples.
References
Bausch A. R., Juhl A. R., Donaher N. A., Cockshutt A. M. (2019). Combined Effects of Simulated Acidification and Hypoxia on the Harmful Dinoflagellate Amphidinium Carterae. Mar. Biol. 166 (80), 1–19. doi: 10.1007/s00227-019-3528-y
Bianchi T. S., Allison M. A. (2009). Large-River Delta-Front Estuaries as Natural “Recorders” of Global Environmental Change. Proc. Natl. Acad. Sci. 106 (20), 8085.e8092. doi: 10.1073/pnas.0812878106
Breitburg D., Hondorp D. W., Davias L. A., Diaz R. J. (2009). Hypoxia, Nitrogen, and Fisheries: Integrating Effects Across Local and Global Landscapes. Annu. Rev. Mar. Sci. 1, 329–349. doi: 10.1146/annurev.marine.010908.163754
Breitburg D., Levin L. A., Oschlies A., Gregoire M., Chavez F. P., Conley D. J., et al. (2018). Declining Oxygen in the Global Ocean and Coastal Waters. Science. 359 (6371), 46. doi: 10.1126/science.aam7240
Carstensen J., Andersen J. H., Gustafsson B. G., Conley D. J. (2014). Deoxygenation of the Baltic Sea During the Last Century. Proc. Natl. Acad. Sci. U.S.A. 111 (15), 5628–5633. doi: 10.1073/pnas.1323156111
Chen C. C., Gong G. C., Shiah F. K. (2007). Hypoxia in the East China Sea: One of the Largest Coastal Low-Oxygen Areas in the World. Mar. Environ. Res. 64, 399–408. doi: 10.1016/j.marenvres.2007.01.007
Chi L., Song X., Ding Y., Yuan Y., Wang W., Cao X., et al. (2021). Heterogeneity of the Sediment Oxygen Demand and its Contribution to the Hypoxia Off the Changjiang Estuary and Its Adjacent Waters. Mar. Pollut. Bull. 172, 112920. doi: 10.1016/j.marpolbul.2021.112920
Conley D. J., Carstensen J., Aigars J., Axe P., Bonsdorff E., Eremina T., et al. (2011). Hypoxia Is Increasing in the Coastal Zone of the Baltic Sea. Environ. Sci. Technol. 45 (16), 6777–6783. doi: 10.1021/es201212r
Diaz R. J., Rosenberg R. (2008). Spreading Dead Zones and Consequences for Marine Ecosystems. Science. 321, 926–929. doi: 10.1126/science.1156401
Dong Z., Liu D., Wang Y., Di B., Song X., Shi Y., et al. (2012). A Report on a Moon Jellyfish Aurelia Aurita Bloom in Sishili Bay, Northern Yellow Sea of China in 2009. Aquat. Ecosyst. Health 15 (2), 161–167. doi: 10.1080/14634988.2012.689583
Egge J. K., Aksnes D. L. (1992). Silicate as Regulating Nutrient in Phytoplankton Competition. Mar. Ecol. Prog. Ser. 83 (2-3), 281–289. doi: 10.3354/meps083281
Falkowski P. G., Woodhead A. D. (1992). Primary Productivity and Biogeochemical Cycles in the Sea. Plenum. Press. 43. doi: 10.1007/978-1-4899-0762-2
Fan X., Cheng F., Yu Z., Song X. (2018). The Environmental Implication of Diatom Fossils in the Surface Sediment of the Changjiang River Estuary (CRE) and Its Adjacent Area. J. Oceanol. Limnol. 37 (2), 552–567. doi: 10.1007/s00343-019-8037-9
Fennel K., Testa J. M. (2019). Biogeochemical Controls on Coastal Hypoxia. Annu. Rev. Mar. Sci. 11, 105–130. doi: 10.1146/annurev-marine-010318-095138
Frigstad H., Kaste Y., Deininger A., Kvalsund K., Christensen G., Bellerby R. G. J., et al. (2020). Influence of Riverine Input on Norwegian Coastal Systems. Front. Mar. Sci. 7. doi: 10.3389/fmars.2020.00332
Guo S., Sun J., Zhao Q., Feng Y., Huang D., Liu S., et al. (2016). Sinking Rates of Phytoplankton in the Changjiang (Yangtze River) Estuary: A Comparative Study Between Prorocentrum Dentatum and Skeletonema Dorhnii Bloom. J. Mar. Syst. 154, 5–14. doi: 10.1016/j.jmarsys.2015.07.003
Hajdu S., Edler L., Olenina I., Witek B.. (2000). Spreading and Establishment of the Potentially Toxic Dinoflagellate Prorocentrum Minimum in the Baltic Sea. Int. Rev. Hydrobiol. 85 (5-6), 561–575. doi: 10.1002/1522-2632(200011)85:5/6<561
Hamidi S. A., Bravo H. R., Klump J. V., Waples J. T. (2015). The Role of Circulation and Heat Fluxes in the Formation of Stratification Leading to Hypoxia in Green Bay, Lake Michigan. J. Great. Lake. Res. 41 (4), 1024–1036. doi: 10.1016/j.jglr.2015.08.007
He Q., Silliman B. R. (2019). Climate Change, Human Impacts, and Coastal Ecosystems in the Anthropocene. Curr. Biol. 29 (19), R1021–R1035. doi: 10.1016/j.cub.2019.08.042
Klais R., Tamminen T., Kremp A., Spilling K., Olli K. (2011). Decadal-Scale Changes of Dinoflagellates and Diatoms in the Anomalous Baltic Sea Spring Bloom. PloS One 6 (6), e21567. doi: 10.1371/journal.pone.0021567
Kremp A. (2001). Effects of Cyst Resuspension on Germination and Seeding of Two Bloom-Forming Dinoflagellates in the Baltic Sea. Mar. Ecol. Prog. Ser. 216, 57–66. doi: 10.3354/meps216057
Lignell R., Heiskanen A. S., Kuosa H., Gundersen K., Kuuppoleinikki P., Pajuniemi R., et al. (1993). Fate of a Phytoplankton Spring Bloom: Sedimentation and Carbon Flow in the Planktonic Food Web in the Northern Baltic. Mar. Ecol. Prog. Ser. 94 (3), 239–239. doi: 10.3354/meps094239
Lips U., Lips I., Liblik T., Kikas V., Altoja K., Buhhalko N., et al. (2011). Vertical Dynamics of Summer Phytoplankton in a Stratified Estuary (Gulf of Finland, Baltic Sea). Ocean. Dynam. 61 (7), 903–915. doi: 10.1007/s10236-011-0421-8
Li Z., Song S., Li C., Yu Z. (2018). The Sinking of the Phytoplankton Community and Its Contribution to Seasonal Hypoxia in the Changjiang (Yangtze River) Estuary and Its Adjacent Waters. Estuar. Coast. Shelf. Sci. 208, 170–179. doi: 10.1016/j.ecss.2018.05.007
Liu G., Cai X., Tong F., Wang L., Zhang X. (2014). Investigation of Massive Death of Sea Cucumber in Artificial Reef Zone of Shuangdao Bay, Weihai. Fish. Inform. Strateg. 29, 122–129. doi: 10.13233/j.cnki.fishis.2014.02.020
Liu Y. H., Liu X. J., Xing Y. H., Jin Y., Ma Y. Q., Liu X. B. (2006). Analysis of the Water Quality in the Sea Area of Sishili Bay of Yantai in 2003. Trans. Oceanol. Limnol. 3, 93–97. doi: 10.13984/j.cnki.cn37-1141.2006.03.014
Liu D., Shi Y., Di B., Sun Q., Wang Y., Dong Z., et al. (2012). The Impact of Different Pollution Sources on Modern Dinoflagellate Cysts in Sishili Bay, Yellow Sea, China. Mar. Micropaleontol. 84-85, 1–13. doi: 10.1016/j.marmicro.2011.11.001
Li X., Yu Z., Song X., Cao X., Yuan Y. (2011). The Seasonal Characteristics of Dissolved Oxygen Distribution and Hypoxia in the Changjiang Estuary. J. Coast. Res. 27, 52–62. doi: 10.2112/JCOASTRES-D-11-00013.1
Murphy R. R., Kemp W. M., Ball W. P. (2011). Long-Term Trends in Chesapeake Bay Seasonal Hypoxia, Stratification, and Nutrient Loading. Estuar. Coast. 34 (6), 1293–1309. doi: 10.1007/s12237-011-9413-7
O’Connor T., Whitall D. (2007). Linking Hypoxia to Shrimp Catch in the Northern Gulf of Mexico. Mar. Pollut. Bull. 54 (4), 460–463. doi: 10.1016/j.marpolbul.2007.01.017
Pytkowicx R. M. (1971). On the Apparent Oxygen Utilization and the Preformed Phosphate in the Oceans. Limnol. Oceanogr. 16 (1), 39–42. doi: 10.4319/lo.1971.16.1.0039
Rabouille C., Conley D. J., Dai M. H., Cai W. J., Chen C., Lansard B., et al. (2008). Comparison of Hypoxia Among Four River-Dominated Ocean Margins: The Changjiang (Yangtze), Mississippi, Pearl, and Rhone Rivers. Cont. Shelf. Res. 28, 1527–1537. doi: 10.1016/j.csr.2008.01.020
Regnier P., Friedlingstein P., Ciais P., Mackenzie F. T., Gruber N., Janssens I. A., et al. (2013). Anthropogenic Perturbation of the Carbon Fluxes From Land to Ocean. Nat. Geosci. 6 (8), 597–607. doi: 10.1038/NGEO1830
Roman M. R., Pierson J. J., Kimmel D. G., Boicourt W. C., Zhang X. (2012). Impacts of Hypoxia on Zooplankton Spatial Distributions in the Northern Gulf of Mexico. Estuar. Coast. 35 (5), 1261–1269. doi: 10.1007/s12237-012-9531-x
Sildever S., Kremp A., Enke A., Buschmann F., Maljutenko I., Lips I. (2017). Spring Bloom Dinoflagellate Cyst Dynamics in Three Eastern Sub-Basins of the Baltic Sea. Cont. Shelf. Res. 137, 46–55. doi: 10.1016/j.csr.2016.11.012
Sinkko H., Lukkari K., Jama A. S., Sihvonen L. M., Sivonen K., Leivuori M., et al. (2011). Phosphorus Chemistry and Bacterial Community Composition Interact in Brackish Sediments Receiving Agricultural Discharges. PloS One 6, e21555. doi: 10.1371/journal.pone.0021555
Smith S. V., Hollibaugh J. T. (1993). Coastal Metabolism and the Oceanic Organic Carbon Balance. Rev. Geophys. 31, 75–89. doi: 10.1029/92RG02584
Spilling K., Olli K., Lehtoranta J., Kremp A., Tedesco L., Tamelander T., et al. (2018). Shifting Diatom-Dinoflagellate Dominance During Spring Bloom in the Baltic Sea and its Potential Effects on Biogeochemical Cycling. Front. Mar. Sci. 5. doi: 10.3389/fmars.2018.00327
Stoecker D. K., Hansen P. J., Caron D. A., Mitra A. (2017). Mixotrophy in the Marine Plankton. Annu. Rev. Mar. Sci. 9, 311–335. doi: 10.1146/annurev-marine-010816-060617
Su J., Dai M., He B., Wang L., Gan J., Guo X., et al. (2017). Tracing the Origin of the Oxygen-Consuming Organic Matter in the Hypoxic Zone in a Large Eutrophic Estuary: The Lower Reach of the Pearl River Estuary, China. Biogeosciences 14 (18), 4085–4099. doi: 10.5194/bg-14-4085-2017
Sun X., Dong Z., Zhang W., Sun X., Hou C., Liu Y., et al. (2021). Seasonal and Spatial Variations in Nutrients Under the Influence of Natural and Anthropogenic Factors in Coastal Waters of the Northern Yellow Sea, China. Mar. Pollut. Bull. 175, 113171. doi: 10.1016/j.marpolbul.2021.113171
Sun J., Liu D. (2003). The Application of Diversity Indices in Marine Phytoplankton Studies. Acta Oceanol. Sin. 26, 62–75. doi: 10.3321/j.issn:0253-4193.2004.01.007
Sun S., Zhang F., Li C., Wang S., Wang M., Tao Z., et al. (2015). Breeding Places, Population Dynamics, and Distribution of the Giant Jellyfish Nemopilema Nomurai (Scyphozoa: Rhizostomeae) in the Yellow Sea and the East China Sea. Hydrobiologia 754 (1), 59–74. doi: 10.1007/s10750-015-2266-5
Turner R. E., Rabalais N. N., Justic D. (2008). Gulf of Mexico Hypoxia: Alternate States and a Legacy. Environ. Sci. Technol. 42 (7), 2323–2327. doi: 10.1021/es071617k
Utermöhl H. (1958). ). Zur Vervolkommung Der Quantitative Phytoplankton-Methodik. Mitt. Int. Ver. Theor. Angew. Limnol. 9, 1–38. doi: 10.1080/05384680.1958.11904091
Wang B. (2009). Hydromorphological Mechanisms Leading to Hypoxia Off the Changjiang Estuary. Mar. Environ. Res. 67 (1), 53–58. doi: 10.1016/j.marenvres.2008.11.001
Wang H., Dai M., Liu J., Kao S., Zhang C., Cai W., et al. (2016). Eutrophication-Driven Hypoxia in the East China Sea Off the Changjiang Estuary. Environ. Sci. Technol. 50 (5), 2255–2263. doi: 10.1021/acs.est.5b06211
Wang L., Liang Z., Guo Z., Cong W., Song M., Wang Y., et al. (2022). Response Mechanism of Microbial Community to Seasonal Hypoxia in Marine Ranching. Sci. Tot. Environ. 811, 152387. doi: 10.1016/j.scitotenv.2021.152387
Wang Y., Liu D., Dong Z., Di B., Shen X. (2012). Temporal and Spatial Distributions of Nutrients Under the Influence of Human Activities in Sishili Bay, Northern Yellow Sea of China. Mar. Pollut. Bull. 64 (12), 2708–2719. doi: 10.1016/j.marpolbul.2012.09.024
Wasmund N., Uhlig S. (2003). Phytoplankton Trends in the Baltic Sea. ICES. J. Mar. Sci. 60 (2), 177–186. doi: 10.1016/S1054-3139(02)00280-1
Wei H., He Y., Li Q., Liu Z., Wang H. (2007). Summer Hypoxia Adjacent to the Changjiang Estuary. J. Mar. Syst. 67, 292–303. doi: 10.1016/j.jmarsys.2006.04.014
Wei Q., Wang B., Zhang X., Ran X., Fu M., Sun X., et al. (2020). Contribution of the Offshore Detached Changjiang (Yangtze River) Diluted Water to the Formation of Hypoxia in Summer. Sci. Tot. Environ. 764, 142838. doi: 10.1016/j.scitotenv.2020.142838
Wiseman W., Rabalais N. N., Turner R. E., Dinnel S. P., MacNaughton A. (1997). Seasonal and Interannual Variability Within the Louisiana Coastal Current: Stratification and Hypoxia. J. Mar. Syst. 12 (1-4), 237–248. doi: 10.1016/S0924-7963(96)00100-5
Wu H., Luan Q., Guo M., Gu H., Zhai Y., Tan Z. (2018). Phycotoxins in Scallops (Patinopecten Yessoensis) in Relation to Source, Composition and Temporal Variation of Phytoplankton and Cysts in North Yellow Sea, China. Mar. Poll. Bull. 135, 1198–1204. doi: 10.1016/j.marpolbul.2018.08.045
Yang B., Gao X., Xing Q. (2018). Geochemistry of Organic Carbon in Surface Sediments of a Summer Hypoxic Region in the Coastal Waters of Northern Shandong Peninsula. Cont. Shelf. Res. 171, 113–125. doi: 10.1016/j.csr.2018.10.015
Yang B., Gao X., Zhao J., Lu Y., Gao T. (2020). Biogeochemistry of Dissolved Inorganic Nutrients in an Oligotrophic Coastal Mariculture Region of the Northern Shandong Peninsula, North Yellow Sea. Mar. Poll. Bull. 150, 110693. doi: 10.1016/j.marpolbul.2019.110693
Zhai W. D., Zheng N., Huo C., Xu Y., Zhao H. D., Li Y. W., et al. (2014). Subsurface pH and Carbonate Saturation State of Aragonite on the Chinese Side of the North Yellow Sea: Seasonal Variations and Controls. Biogeosciences 11, 1103–1123. doi: 10.5194/bg-11-1103-2014
Keywords: phytoplankton, diatom, dinoflagellate, algal bloom, hypoxia
Citation: Sun X, Li Z, Ding X, Ji G, Wang L, Gao X, Chang Q and Zhu L (2022) Effects of Algal Blooms on Phytoplankton Composition and Hypoxia in Coastal Waters of the Northern Yellow Sea, China. Front. Mar. Sci. 9:897418. doi: 10.3389/fmars.2022.897418
Received: 22 March 2022; Accepted: 21 April 2022;
Published: 20 May 2022.
Edited by:
Zhangxi Hu, Chinese Academy of Sciences (CAS), ChinaReviewed by:
Duncan A. Purdie, University of Southampton, United KingdomYuqiu Wei, Chinese Academy of Fishery Sciences (CAFS), China
Copyright © 2022 Sun, Li, Ding, Ji, Wang, Gao, Chang and Zhu. This is an open-access article distributed under the terms of the Creative Commons Attribution License (CC BY). The use, distribution or reproduction in other forums is permitted, provided the original author(s) and the copyright owner(s) are credited and that the original publication in this journal is cited, in accordance with accepted academic practice. No use, distribution or reproduction is permitted which does not comply with these terms.
*Correspondence: Xiaohong Sun, c3VueGlhb2hvbmdAc2R1LmVkdS5jbg==; Zhao Li, bGl6aGFvMTlAc2RqenUuZWR1LmNu