- Department of Marine Life Sciences, Jeju National University, Jeju, South Korea
Sargassum horneri (SH) is a brown macroalgal species commonly found along the coast of Japan, China, and Korea. SH possesses valuable bioactive compounds that can be developed as functional food ingredients as well as pharmaceutical agents for both humans and animals. In this study, SH was tested for its potential prebiotic effect. Several solvent-assisted extracts of SH were tested on the growth of three species of probiotics (LAB) (Lactobacillus plantarum, Lactobacillus pentosus, Lactobacillus brevis) and fish pathogen bacteria (Streptococcus iniae, Streptococcus parauberis, Edwersiella tarda) both in vitro and in vivo. According to the in vitro results, Celluclast extract (SHC) and crude polysaccharide extract (SHCPs) of SH showed outstanding growth enhancing activity in all LAB species and excellent antibacterial activity against pathogenic bacteria dose-dependently. Both SHC and SHCPs induced the production of secondary metabolites from LAB. The secondary metabolites successfully reduced pathogenic bacterial growth. Furthermore, in vivo experiments revealed that co-treatment with LAB and SHC/SHCPs diminished the mortality of Streptococcus parauberis infected zebrafish by modulating iNOS, COX-2 expressions. Similarly, SH act as an anti-inflammatory agent against S. parauberis infection by hindering NF-κB pathway activation. Conclusively, the results achieved from the study suggest that S. horneri has the potential to be used as a prebiotic dietary supplement and possesses a protective effect against S. parauberis infections in the aquaculture industry.
1 Introduction
Aquaculture, the rapidly growing food sector plays a dynamic role in providing food, employment, and income in South Korea. Aquaculture products are considered a high-quality protein source for human consumption therefore, demand for such products increases continuously. Over the past few years, aquaculture food production and demand have been severely affected by outbreaks of infectious viral, bacterial, and parasitic diseases (Lee et al., 2016). Bacterial diseases have been the most important causative factor of major losses in aquaculture food productions, mainly due to the ability of bacteria to survive in environments independently from their host, as well as the retention of bacteria in packed seafood products (Fernández-No et al., 2012; Woo and Park, 2013). Gram-negative bacteria such as Aeromonas, Edwardsiella, Pseudomonas, and Vibrio, as well as gram-positive bacteria such as Lactococcus and Streptococcus, have been identified as the common disease-causing bacteria in the aquaculture (Pridgeon and Klesius, 2012). Streptococcus parauberis is a non-motile, gram-positive, and alpha-hemolytic bacterium belonging to the family Streptococcaceae which was initially identified as a causative agent of Bovine mastitis. S. parauberis has been reported causing streptococcosis in most aquaculture fish species, and it was reported as a major causative agent in an outbreak of olive flounder Paralichthys olivaceus, which is a popular seafood source in Jeju Island, South Korea (Baeck et al., 2006).
Bacterial infections have been controlled using the crude application of antibiotics such as tetracycline, amoxicillin, and cefazolin, as well as chemicals such as formalin, hydrogen peroxide, and sodium chloride (Harikrishnan et al., 2010). Recently, the application of antibiotics has been reduced due to the detrimental effects on human health and the environment, as well as the development and transfer of resistance in other bacterial species (Cabello, 2006). In a study conducted on olive flounder, an antibiotic-resistant S. parauberis strain was isolated. Therefore, new alternative approaches are required to overcome these types of resistance in the pathogenic bacteria (Schmidt et al., 2000; Park et al., 2009). Probiotics have emerged as an alternative antibiotic source and play a significant role in antimicrobial applications in fisheries. It is well known that the gut microbiota is essential for supporting host health and well-being against pathogenic bacteria. The incorporation of probiotics with feed provides a number of advantages, such as increased feed value, inhibition of resistant pathogens, promotion of growth, and increased immunity (Wang et al., 2005; Yanbo and Zirong, 2006). In the last decade, lactic acid bacteria have gained recognition as probiotics and dietary supplements for the prevention and management of diseases. Probiotic activity is enhanced by prebiotics, non-active food constituents that have the ability to enhance the growth and production of secondary metabolites (Gibson et al., 2017). Prebiotics shift to the colon and is selectively fermented and become accessible to the probiotics. Seaweeds are underexploited plants with prebiotic activities. They provide a source of rich and valuable bioactive compounds, which have been proven to have prebiotic effects on several probiotic bacteria in both humans and animals (O’Sullivan et al., 2010).
According to previous reports, feeding fish with seaweed extracts improved the growth and reduced the mortality rate of pathogen-infected fish species (Eom et al., 2015; Lee et al., 2016). However, there is little information on the prebiotic role of brown seaweed Sargassum horneri (SH) on the effect of probiotics and its pathogen inhibition activity. Hence, this study was carried out to investigate the prebiotic potential of the products extracted from SH on lactic acid bacteria, pathogenic bacterial growth, and the mechanism of anti-inflammatory activity against S. paruberis infections in fish.
2 Materials and Methodology
2.1 Materials
S. horneri samples were collected along the coast of Jeju Island (33° 27’ 30.6” N, 126° 56’ 31.2” E) in South Korea in February 2020 and washed with fresh water to remove salt, epiphytes, sand, and other external contaminants, that had been attached to the surface of the sample. The samples were then dried using a Hybrid hot water Goodle dryer (Nagahawatta et al., 2021) and pulverized using a multifunctional grinder (Hanil electronics, model 12339938004). Analytical grade solvents were used to prepare crude samples (Dae-Jung Chemicals & Metals, Seoul, Korea). Carbohydrate-degrading enzymes Termamyl, Viscozyme, Ultraflo, Amyloglucosidase, and Celluclast) were purchased from Novo Nordisk (Bagsvaerd, Denmark).
2.2 Preparation of Distilled Water Extract (SHD), Ethanol Extract (SHE), Crude Polysaccharide Extract, and Five Types of Enzymatic Extract From Sargassum horneri
Dried seaweed powder was extracted using ethanol and distilled water, as previously described (Lee et al., 2016). Briefly, powdered SH was homogenized with solvents and evaporated using a rotary evaporator. Obtained samples were freeze-dried and used for further experiments. Five carbohydrases; Termamyl (Term), Viscozyme (Visco), Ultraflo (Ultra), Amyloglucosidase (AMG), and Celluclast were used for the enzymatic extraction of SH (Charoensiddhi et al., 2015). In short, 1 g of freeze-dried SH powder was dispersed in 100 mL distilled water and enzymes were separately introduced. pH was adjusted according to the optimal pH of each enzyme with the addition of 1 M HCl or 1 M NaOH. Enzymatic hydrolysis was performed for 24 h under optimum temperatures for each enzyme. Optimum pH and temperature for each enzyme are as follows: Viscozyme (pH 4.5, 50°C), Celluclast (pH 4.5, 50°C), Ultraflo (pH 7, 60°C), Amyloglucosidase (pH 4.5, 60°C) and Termamyl (pH 6, 60°C) (Shanura Fernando et al., 2018). Enzymes were heat-inactivated by heating in a boiling water bath for 10 min and hydrolysates were filtered, and pH was readjusted for 7. Crude polysaccharides were obtained from the Celluclast enzymatic extract of SH. Ethyl alcohol (1:3, v/v) was used to facilitate the precipitation of crude polysaccharides.
2.3 Culturing of LAB and Pathogenic Bacteria
The potential probiotic bacteria Lactobacillus brevis (KCCM 40399), Lactobacillus plantarum (KCCM 12116), Lactobacillus pentosus (KCCM 40997), and pathogenic bacteria such as Streptococcus iniae (KCTC 3657), Streptococcus parauberis (KCTC 3651), and Edwersiella tarda (KCTC 12267) were purchased from the Korean Culture Center of Microorganisms (KCCM) and KCTC (Korean Collection for Type Culture, Daejeon, Korea) respectively. LAB were cultured and incubated at 30°C in De%%Man, Rogosa, and Sharpe (MRS) broth and MRS agar medium (Difco Laboratories, Detroit, MI, USA), whereas pathogenic bacteria were cultured in brain heart infusion medium (BHIF) broth, and BHIF agar medium (Difco Laboratories, Detroit, MI, USA) at 30°C until needed.
2.4 Effects of Different SH Extracts on the Growth of LAB and Pathogenic Bacteria
The extracted SH samples were screened with LAB using a colony counting assay (Lee et al., 2016). LAB was cultured in MRS broth containing 100 μL of extracted samples and incubated for 24h at 30°C. Each bacterial suspension was diluted and incubated on MRS agar under the same incubation conditions. The number of colony-forming units (CFU) was counted, and growth rates were calculated. In a previous study, conducted to identify the prebiotic role of Ecklonia cava, Celluclast extract of E. cava (ECC) showed the highest growth effect in LAB (Lee et al., 2016). Therefore, ECC was used as a positive control in the experiment.
The antibacterial effect of the SH enzymatic extracts (SH-Visco, SH-Celluclast, SH-AMG, SH-Ultra and SH-Term) on three species of pathogenic bacteria, as well as the growth enhancement effect of the enzymatic extracts on LAB, were assessed using colony counting assays as mentioned above. Freeze-dried samples of extracts were added to bacteria-inoculated broth media at concentrations of 0.01, 0.1, 1, and 10 mg/mL, and the relative growth rate was calculated as a percentage value. In the above experiment, Celluclast extract (SHC) and crude polysaccharide extract (SHCPs) of SH showed superior activity compared to other extracts. Therefore, SHC and SHCPs were selected for further experiments. The chemical composition of the SHC and SHCPs were measured and analyzed in our previous study (Sanjeewa et al., 2019).
2.5 Effects of SHC and SHCPs on LAB Growth
For the identification of the influence of SHC and SHCPs on LAB growth, a colony counting assay was performed as described in section 2.4, with concentrations of 0.5, 1, 5, and 10 mg/mL. The relative growth rate was calculated as a percentage value.
2.6 Recovery of Secondary Metabolites From L. plantarum and L. pentosus Incubated With SHC and SHCPS
L. plantarum and L. pentosus were cultured in MRS broth medium with or without SHC (Lee et al., 2016). In brief, 100 µL of SHC was added to the MRS broth and autoclaved. L. plantarum and L. pentosus (109 CFU/mL) were inoculated into the MRS broth with or without containing SHC and incubated for 24h at 30°C. Each culture medium was then centrifuged three times at 11200 g for 30 min and supernatants were filtered using 0.45 μm filters and freeze-dried. The weight of the supernatant containing secondary metabolites were measured. Supernatants containing metabolites were used in the following experiments.
2.7 Effect of Secondary Metabolites of L. plantarum and L. pentosus on Pathogen Bacterial Growth
S. iniae, S. parauberia, and E. tarda (103 CFUs) were cultured in BHIF broth medium containing 100 μL of secondary metabolites (0.5, 1, 5, and 10 mg/mL) obtained from L. plantarum and L. pentosus cultured with or without SHC and incubated for 24h. After 24h, the bacteria were diluted and incubated on BHIF agar plates for another 24h. The number of CFUs were counted, and growth rates were calculated as percentages based on the number of bacteria in the BHIF broth medium only.
2.8 Analysis of Secondary Metabolites From L. plantarum Incubated With SHC
An Agilent Head Space-Gas Chromatography-Tandem Mass Spectrometer system (Agilent Technologies, CA, US) was used to conduct GC/MS analysis to identify secondary metabolites produced by L. plantarum when cultured in MRS medium containing SHC (Lee et al., 2016). Data analysis was done with the help of Wiley version 8.0 and NIST version 5.0 mass spectral libraries. An HP-5ms capillary column coated with (5%-phenyl)- methylpolysiloxane was used, whereas helium was used as the carrier gas at 1.5 mL/min constant flow rate. A flow rate of 1.5 mL/min was maintained in the injector with an injection temperature of 280°C. The initial column temperature was maintained at 44°C and was raised to 170°C at a rate of 3°C/min after sample injection.
2.9 In Vivo Experiments
Animal experiments were conducted in accordance with the experimental animal guidelines provided by Jeju National University Animal center and were authorized by the Animal Care Use Committee (IACUC) of Jeju National University (protocol 2020-0049)
2.9.1 Maintenance of Zebrafish
Zebrafish were purchased from Seoul Aquarium, Korea. Fish were separated into tanks (260 x 155 x 160 mm and volume of 3.5 L) (15 fish per tank) under controlled conditions (29°C with 14/10h light and dark cycle) and acclimated for two weeks with temperature 28.5 ± 1°C, salinity 0 g/L and a flow rate of 700 mL/min. They were fed twice daily with tetrabits flakes (Tetrabits GmbH D-49324 Melle, Germany) with a feeding rate of 3% of body weight in feed per day.
2.9.2 Experimental Diet Preparation With L. plantarum, SHC, and SHCPs
The formulated commercial fish feed (Tetrabit Gmbh D49304, 47.5% crude protein, 6.5% crude fat, 2.0% crude fiber, 10.5% crude ash) was mixed with 1% pure culture of L. plantarum (w/w) and SH extracts (1%, 3%, and 5% (w/w)). Briefly, L. plantarum cells were collected by centrifuging the incubated MRS medium and freeze-dried to obtain L. plantarum powder. Freeze-dried bacterial samples and freeze-dried SH extract (SHC or SHCPs) were dissolved in autoclaved distilled water and mixed with commercial feed. The extruded fish feed was freeze-dried and made into a powder. The fish were fed twice a day at 9.00 and 19.00 with the experimental diet for four weeks.
Fish were separated into 11 groups according to the experimental dietsas blank, positive control, 1% L. plantarum only, 1% SHC only, 3% SHC only, 5% SHC only, 1% SHCPs only, 1% L. plantarum + 1% SHC, 1% L. plantarum + 3% SHC, 1% L. plantarum + 5% SHC, and 1% L. plantarum +1% SHCPs. Fish, only fed with commercial fish feed was kept as a negative control to determine the normal mortality rate during the study period. A total of 330 zebrafish were used for the in vivo experiment.
2.9.3 Measurement of Body Weight of Zebrafish
The bodyweight of zebrafish was batch weighed every week from the start of the experimental diet (Ohaus Pioneer PX4201/E Precision scale, Seoul, South Korea). Growth performance was analyzed using fish weight gain following the method given in a previous study (Kesbiç and Yigit, 2019; Kesbiç et al., 2020).
2.9.4 Mortality Measurement of S. parauberis - Infected Fish
Fish were challenged with S. parauberis intraperitoneally with 10 μL PBS containing 1.1x 108 cells/μL in the fourth week of the experimental diet. The number of dead fish was recorded daily for 17 days.
2.9.5 Measurement of Inflammatory Responses in Pathogen Challenged Zebrafish
Sampling was done 48 h post-infection with S. parauberis. Four fish were selected from each tank randomly and anesthetized with 50 mg/L MS-222 (Tricaine Methanesulfonate) (Sigma Aldrich Inc. Louis USA). Muscles of S. parauberis-infected zebrafish were extracted, and protein lysates were prepared. Protein concentrations were measured using the BCA protein assay kit. Cyclooxygenase 2 (COX-2), inducible nitric oxide synthase (iNOS), and NF-κB pathway protein expression levels were analyzed by Western blot technique (Fernando et al., 2017). Proteins were subjected to SDS-PAGE, and protein bands were transferred to nitrocellulose membranes (Bio-Rad, Hercules, CA, USA). Membranes were blocked with 5% skimmed milk and incubated with primary and secondary antibodies. Enhanced chemiluminescence reagents (iNtRON, Sungnam, Korea) were used to develop the blots, and a FUSION SOLO Vilber Lourment system was used to photograph the developed blots. ImageJ software was aided in quantifying band intensities.
2.9.6 Hematoxylin and Eosin (H&E) Staining of S. parauberis Infected Tissues
Tissue samples were obtained from pathogen-infected fish at 48h post-infection. Samples were subjected to H&E staining (Paul, 2017). In short, samples were fixed in 10% buffered formalin and dehydrated using alcohol to remove water from the tissue. The tissues were then cleared using xylene or chloroform and impregnated with paraffin wax. Sections were de-paraffinized using xylene and rehydrated using ethanol and distilled water. H&E staining was used to stain the slides. Stained slides were dehydrated, mounted with coverslips, and examined with a microscope under a magnification of 40 to 1000X (Olympus, Tokyo, Japan).
2.10 Statistical Analysis
Data were analyzed using the GraphPad Prism 7 statistical analysis package (version 5.01; GraphPad Software Inc. San Diego, CA, USA). All data have been analyzed a minimum of three times and expressed as mean ± standard error (SE). Significant values were evaluated using one-way ANOVA test and Dunnett’s multiple range tests.
3 Results
3.1 SHC and SHCPs Showed the Highest Growth Rate in All Three LAB Species
A colony counting assay was performed to evaluate the effects of different solvent-assisted extracts of SH namely ethanol extract (SHE), distilled water extract (SHD), Celluclast extract (SHC), and crude polysaccharide extract of SH (SHCPs). According to the calculated CFU, SHC and SHCPs showed a comparative peak effect on the growth of all three types of LAB compared to the untreated controls (Figure 1A). Moreover, L. plantarum showed a significantly high growth rate in SHC (177.38 ± 8.46%) and SHCPs (192.37 ± 11.03%) groups compared to L. pentosus and L. brevis.
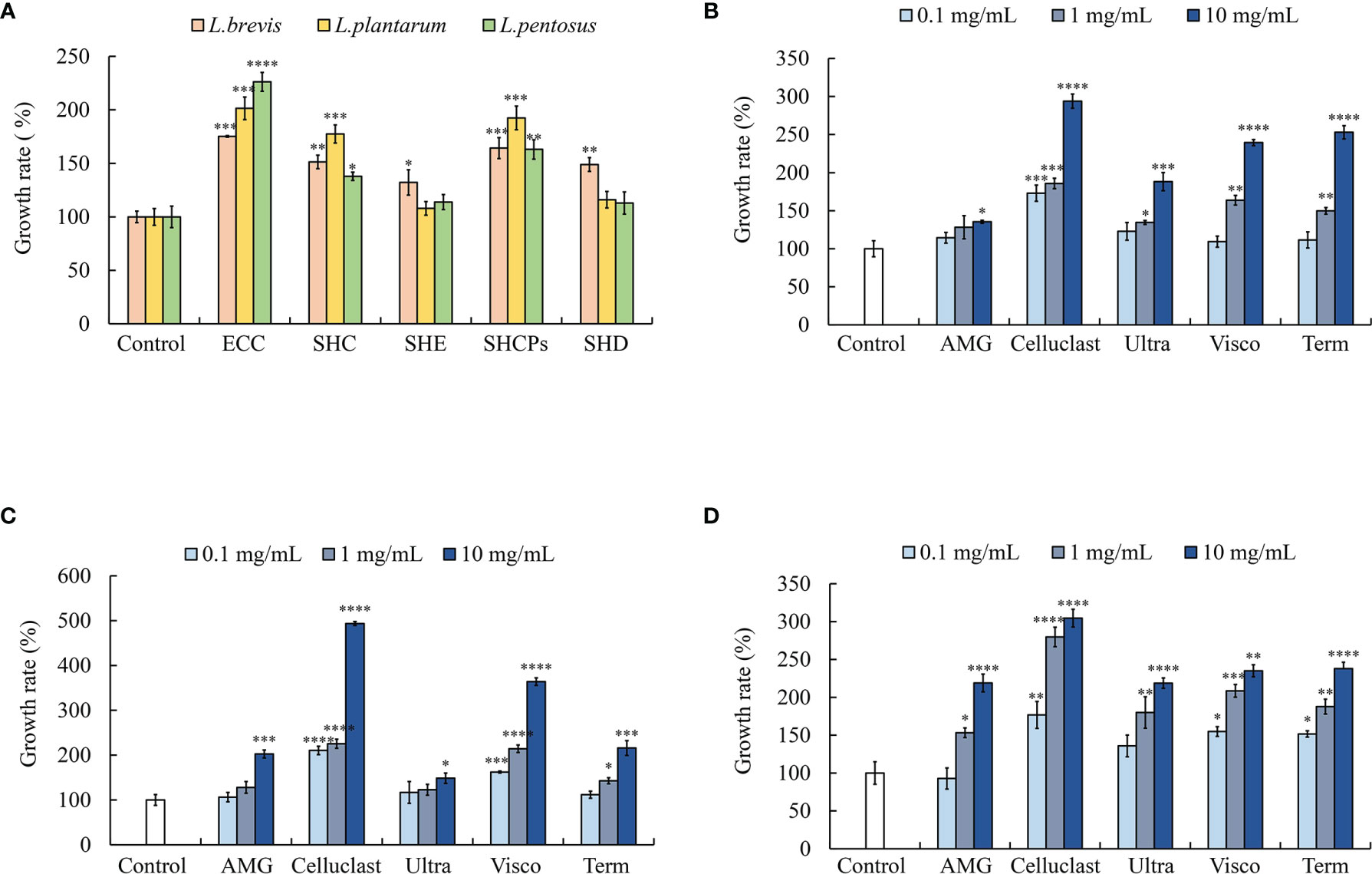
Figure 1 Effect of SH extracts on lactic acid bacterial (LAB) growth. (A) Growth of LAB in different solvent extracts of SH. Growth of (B) L. plantarum, (C) L. pentosus, and (D) L. brevis in different enzymatic extracts of SH. Experiment was triplicated and statistical evaluation was performed by comparing experimental and control groups. Values are significantly different from the control group at *p<0.05, **p<0.01, ***p<0.005 and ****p<0.0001. (SHE, S. horneri ethanol extract; SHC, S. horneri Celluclast extract; SHCPs, S. horneri crude polysaccharide extract; SHD, S. horneri distilled water extract; ECC, Ecklonia cava Celluclast extract; AMG, S. horneri AMG enzymatic extract; Celluclast, S. horneri Celluclast extract; Ultra, S. horneri Ultraflo enzymatic extract; Visco, S. horneri Viscozyme enzymatic extract and Term, S. horneri Termamyl enzymatic extract).
3.2 SHC Showed the Highest Effect on LAB Growth and Highest Inhibition of Pathogenic Bacterial Growth Among the SH Enzymatic Extracts
The five types of SH enzymatic extracts significantly induced LAB growth in a dose-dependent manner. Among the enzymatic extracts, Celluclast extract treatment (SHC) showed excellent and significant dose-dependent growth enhancement in all LAB species. The highest growth of L. plantarum was observed in Celluclast extract treated group at the concentration of 10 mg/mL (293.81± 9.32%). AMG treatment showed no significant differences in L. plantarum growth compared to untreated control in 0.1 and 1 mg/mL concentrations (Figure 1B). The growth of L. pentosus was significantly induced with treatment of Celluclast and Viscozyme enzymatic extracts under all concentrations (0.1, 1 and 10 mg/mL) but all the other enzymatic extracts showed no significant increase in growth at 0.1 mg/mL concentration (Figure 1C). In contrast to L. plantarum and L. pentosus, L. brevis showed significant increase in growth in all the enzymatic extracts treated growth medium (Figure 1D). These results indicate that the enzymatic extracts of SH have the potential to stimulate beneficial probiotic bacterial growth.
To assess the protective action of the above-mentioned enzymatic extracts against S. parauberis, S. iniae, and E. tarda, a colony counting assay was carried out. As shown inFigure 2, the enzymatic extracts of SH actively inhibited the growth of pathogenic bacteria dose dependently. Results indicated that Celluclast extract treatment led to a higher growth inhibition on all pathogenic bacterial species compared to the non-treated groups, especially, the highest growth inhibition was observed in S. iniae compared to the untreated control group (14.34 ± 7.06%) (Figure 2C). These results indicate that SH possesses antibacterial effects against pathogenic bacteria.
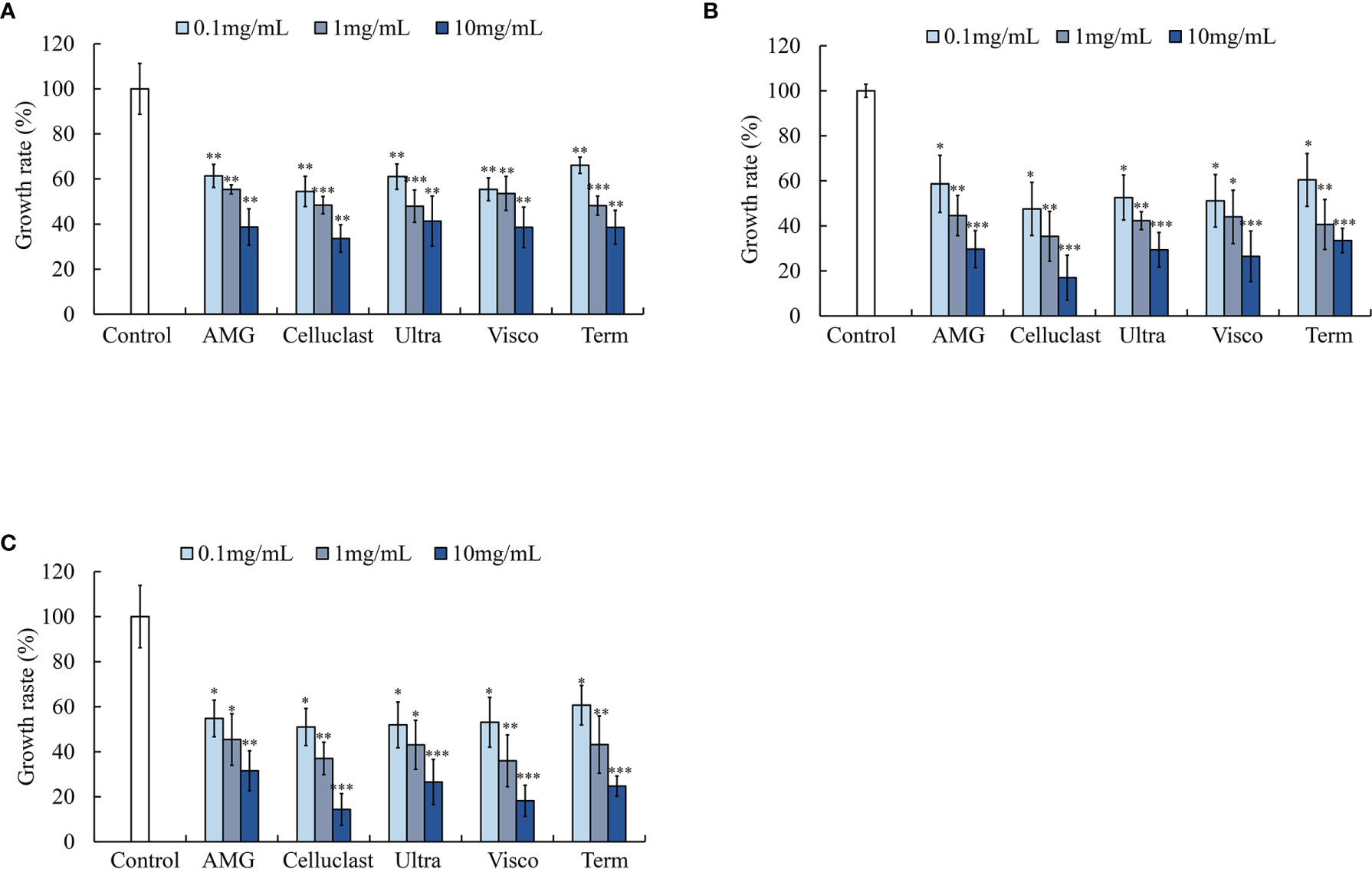
Figure 2 Effect of various enzymatic extracts of SH on pathogenic bacterial growth (A) S. parauberis, (B) E. tarda and (C) S. iniae growth. Experiment was triplicated and statistical evaluation was done to compare the experimental and control groups. Values are significantly different from the control group at *p<0.05, **p<0.01, and ***p<0.005 (AMG: S. horneri AMG enzymatic extract; Celluclast: S. horneri Celluclast extract; Ultra: S. horneri Ultraflo enzymatic extract; Visco: S. horneri Viscozyme enzymatic extract and Term: S. horneri Termamyl enzymatic extract). The samples were treated to the bacterial culture at different concentrations as 0.1,1 and 10 mg/mL.
3.3 SHCPs Was More Effective in Stimulating the Growth of LAB
The proximate composition analysis of SHC and SHCPs was reported in our earlier study (Sanjeewa et al., 2019). According to the results, the polysaccharide contents of SHC and SHCPs were 57.94%, and 65.01% respectively whereas the sulfate content of SHCPs was 12.5%. Therefore, to analyze the effect of polysaccharide content on the prebiotic activity of SH extracts, the growth rates of all three species of LAB corresponding to SHC and SHCPs were tested and shown in Figure 3. Both samples displayed potent growth-increasing ability in LAB, and SHCPs were proven to be more effective for the growth of all three LAB species. The treatment of SHCPs caused a significant increase in L. brevis growth when at 5 and 10 mg/mL concentrations, while a significant change in growth in SHC was only observed at 10 mg/mL (Figure 3A). L. pentosus showed significant growth enhancement in all concentrations of SHCPs and in 1,5 and 10 mg/mL concentrations in SHC (Figure 3B). Figure 3C shows that the treatment of SHCPs and SHC resulted in a gradual increase in growth of L. plantarum in a dose increasing manner. Therefore, the samples showed dose-dependent growth improvement in LAB species.
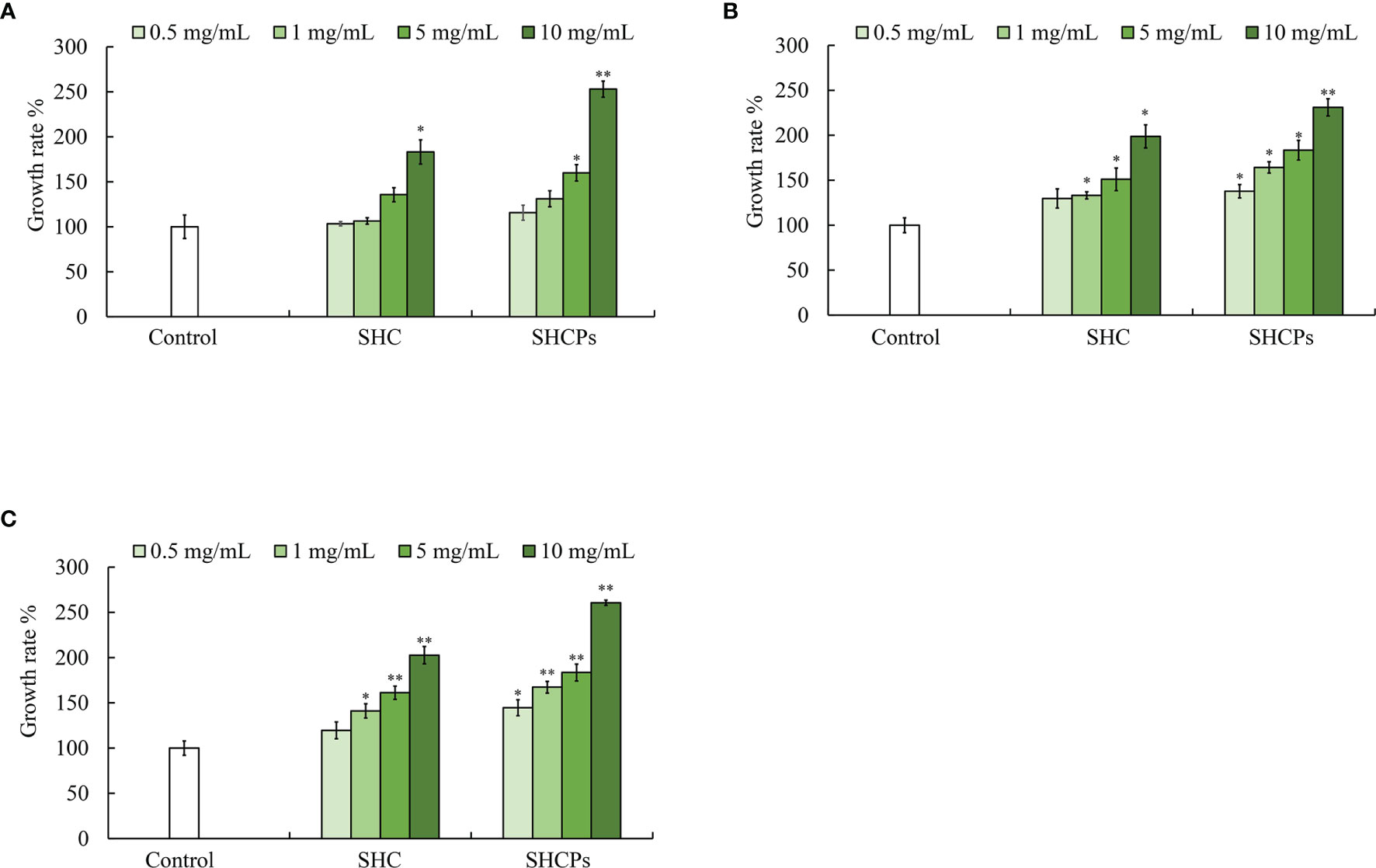
Figure 3 Effect of SHC and SHCPs on probiotic bacterial growth Growth of (A) L. brevis, (B) L. pentosus and (C) L. plantarum. Experiment was triplicated and statistical evaluation was performed to compare the experimental and control groups. Values are significantly different from control group at *p<0.05, **p<0.01 (SHCPs: S. horneri crude polysaccharide extract; SHC: S. horneri Celluclast extract). The samples were treated to bacterial culture with different concentrations as 0.5,1,5 and 10 mg/mL.
3.4 SHC and SHCPs Improved the Secondary Metabolite Production of LAB in MRS Medium
Secondary metabolites are considered auxiliary compounds produced by microbes that are not required for normal cell growth. Nonetheless, they have other beneficial biological activities, such as antibacterial effects. Therefore, the effects of SHC and SHCPs on secondary metabolite production from LAB were tested in this study. Co-culturing SHC and SHCPs with MRS medium was demonstrated to induce the production of secondary metabolites by L. plantarum, L. pentosus, and L. brevis (Table 1). However, L. plantarum cultured in SHC and SHCPs containing MRS media showed a significant increase in the production of secondary metabolites (718.70 ± 10.0 mg and 574.17 ± 5.0 mg respectively) compared to L. pentosus and L. brevis when given the same culture conditions. Moreover, it was observed that L. plantarum cultured in SHC treated medium produced higher amount of secondary metabolites than L. plantarum cultured in SHCPs containing medium.
3.5 Secondary Metabolites of L. plantarum and L. pentosus Reduced the Growth of Pathogenic Bacteria
To identify the potential functions of secondary metabolites produced by L. plantarum and L. pentosus, we tested their antibacterial activity (Table 2). Secondary metabolites produced by both L. plantarum and L. pentosus cultured with or without SHC containing media inhibited the growth of pathogenic bacteria in a significant manner. Secondary metabolites obtained from the LAB cultured in an SHC-containing medium noticeably inhibited pathogen growth (S. parauberis, S. iniae, and E. tarda) compared to the control groups. In this experiment, SHCPs was not used due to the lower secondary metabolite production by both L. plantarum and L. pentosus in SHCPs containing medium compared to SHC containing medium. Similarly, L. brevis was not used due to the slower growth rate, as well as the lower production of secondary metabolites.
Obtained results showed that SH is a potential prebiotic for beneficial bacteria, with SHC and SHCPs showing superior activity over other enzymatic extracts. Hence, further experiments were planned with SHC and SHCPs, while L. plantarum was used as the model probiotic bacteria in this study due to its popularity as a starter probiotic as well as its abundance in the gastrointestinal tract in both humans and fish (Arasu et al., 2016). S. parauberis was used as the pathogenic bacteria in vivo experiments due to its high infectivity and its popularity as a common disease-causing bacterium in aquaculture.
3.6 Feeding of Prebiotics Improved the Growth Performance and Reduced the Mortality of S. parauberis Infected Zebrafish Significantly
The experimental diet was fed to zebrafish for 30 days and the weight gain was recorded each week. The effects of diet on growth performance are shown in Figure 4A. The results showed that co-treatment of L. plantarum with SHC and SHCPs had a direct effect on zebrafish growth. After 30 days of feeding the diet, the final biomass of the fish had improved in all groups compared to the group fed with commercial feed only. However, there was no significant difference between the experimental groups.
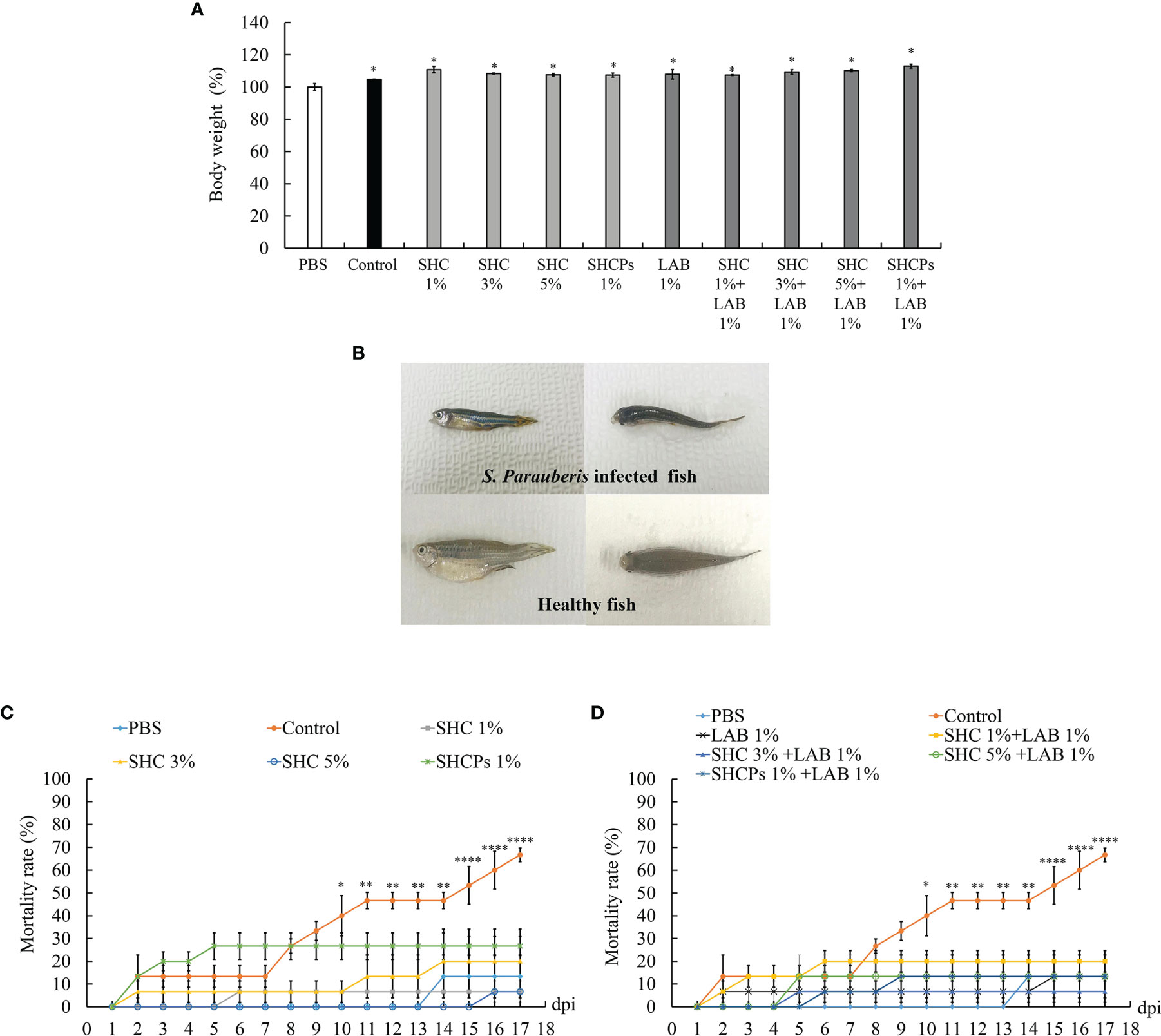
Figure 4 Effect of experimental diet on S. parauberis infected zebrafish. (A) growth performance of zebrafish, (B) morphology, (C) accumulated mortality (diet containing SH extracts only), and (D) accumulated mortality (diet containing LAB and SH extracts). Statistical evaluation was performed to compare the experimental and control groups. Values are significantly different from PBS group at *p<0.05, **p<0.01, and ****p<0.0001.
For the assessment of the role of SH as a prebiotic, zebrafish were injected intraperitoneally with 1.18 x 108 cells/µL of S. parauberis after four weeks of experimental diets, and the number of dead fish was counted. The results showed that infected fish displayed symptoms especially, darkened body coloration, cachexia (Figure 4B), and abnormal swimming patterns. During this period, the number of dead fish in the infected groups increased significantly compared to that in the non-infected group. However, supplementation with L. plantarum and SH extracts (SHC and SHCPs) led to a decline in mortality rates effectively (Figures 4C, D). The mortality rate of the group of infected fish fed with the control diet (commercial feed) only reached 66.67%, whereas the mortality rates of other treated groups were lower than 30%. The lowest mortality rate of pathogen-infected fish was observed in the groups co-treated with SH and L. plantarum.
3.7 Supplementation of SHC Reduced the Expressions Levels of iNOS and COX-2 Proteins in S. parauberis Infected Zebrafish
The anti-inflammatory effect of the SH diet on inflammatory mediators such as inducible nitric oxide synthase (iNOS) and cyclooxygenase-2 (COX-2) protein levels, in S. parauberis-infected fish, were assessed via Western blot analysis (Figures 5A, B). The protein expression results for iNOS and COX-2 are shown in Figures 5C, D. The results indicated that the expression of iNOS and COX-2 was significantly increased in pathogen-infected zebrafish. Meanwhile, the expression of proteins was downregulated following the supplementation of experimental diet. Significant reduction of iNOS and COX-2 expressions in the SHCPs treated group was observed compared to the control group and 5% SHC treated group showed the highest inhibition of iNOS expression in infected fish (Figure 5C). The overall highest inhibition of iNOS and COX-2 expression was observed in 1% L. plantarum + 5% SHC treated group (0.1591 ± 0.0014, 0.1385 ± 0.0003 respectively) (Figure 5D). Overall, the results show that SHC amended the mortality of S. parauberis-infected zebrafish via obstructing the inflammatory mediator expression.
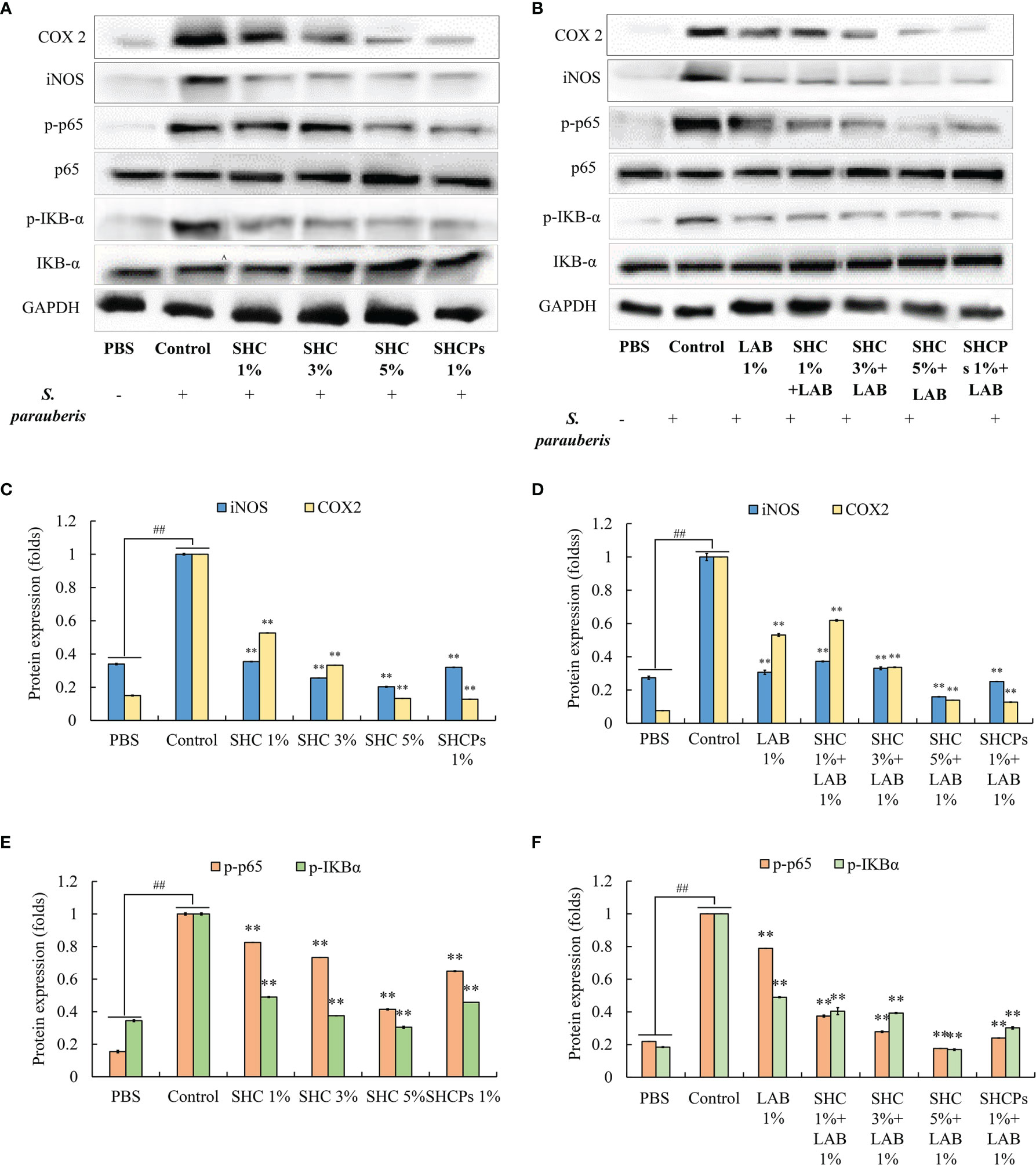
Figure 5 Effect of experimental diet on expression levels of inflammatory mediators and NF-κB pathway related proteins. (A, B) Protein expression inhibition by SH extracts, (C, D) iNOS and COX-2 expression (E, F) NF-κB pathway protein expression. Statistical evaluation was done to compare the experimental and control groups. Values are significantly different from control group at **p<0.01 or ##p < 0.01 compared to non-treated group (PBS group).
3.8 SHC Significantly Inhibited the Activation of NF-κB Signaling Pathway
To explore whether the anti-inflammatory activity of SH extracts in S. parauberis-infected zebrafish is mediated by NF-κB pathway-related protein expression, the levels of NF-κB protein expression were determined by Western blot. Thus, we evaluated the expression of p65 and IKB-α in zebrafish infected with S. parauberis and treated with SH extracts. As shown in Figures 5E, F, the results revealed that compared to the non-infected group, expression levels of p65 and IKBα in zebrafish were increased due to S. parauberis infection. Moreover, supplementation with the experimental diet containing SH extracts and L. plantarum actively inhibited the phosphorylation of the above-mentioned proteins. As indicated in Figure 5F, the highest inhibition of p-p65 and p-IKBα was observed in fish fed with 1% L. plantarum + 5% SHC group (0.1763 ± 0.0003 and 0.1691 ± 0.0050 respectively). These results suggest that SH possesses anti-inflammatory activity via inhibiting the activation of NF-κB signaling pathway.
3.9 Pathological Features of Zebrafish Infected With S. parauberis
The infection with S. parauberis leads to serious injuries in zebrafish, especially in the gastrointestinal (GI) tract. Histological sections of tissues from the GI tract demonstrated the presence of bacteria in the intestinal lumen of infected fish. We also observed an increase in the number of goblet cells involved in the intestinal mucosal formation and immunity of epithelial cells in the experimental diet-supplemented fish compared to infected fish fed with the control diet. According to the H&E staining results, fish in the 5% SHC+1% L. plantarum supplemented group had the highest increase in goblet cell density. Similarly, we observed extensive necrosis in epithelial cells in the intestine caused by pathogenic bacterial infection, which was lessened with the supplementation of SHC (Figure 6).
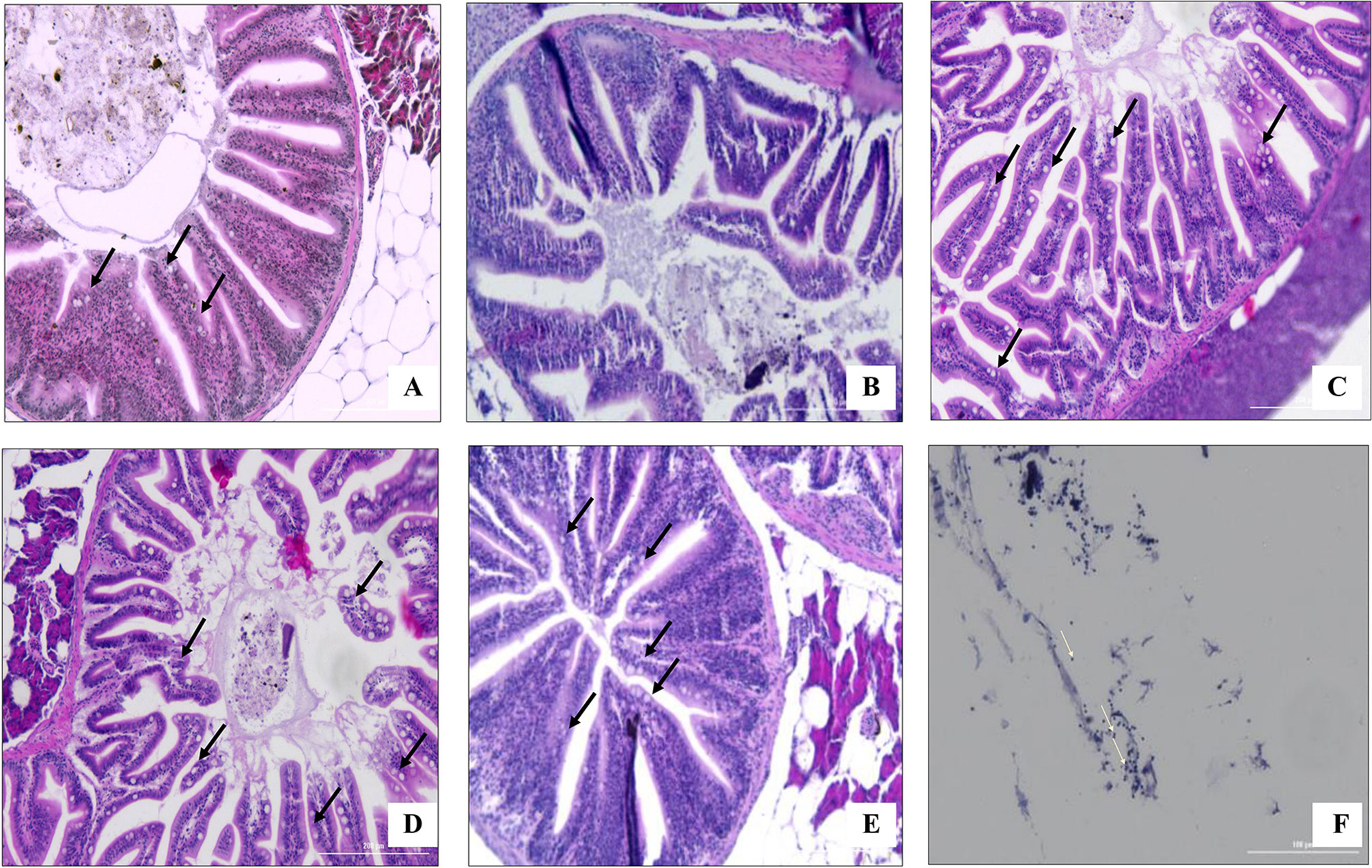
Figure 6 Histological analysis of the goblet cells (black arrow) in the intestine of zebrafish challenged with S. parauberis. (A) non-infected group with control diet (B) infected group fed with control diet, (C) infected group fed with 5% SHC supplemented diet, (D) infected group fed with 1% L. plantarum supplemented diet, (E) infected group fed with 1% L. plantarum + 5% SHC supplemented diet and (F) bacterial cells in the intestinal lumen (white arrow). (SHC, S. horneri Celluclast extract).
3.10 Incubation of L. plantarum With SHC Produced Several Secondary Metabolites Compounds That May Affect Antibacterial Activity
According to the results obtained from in vivo experiments, SHC showed higher activity in inhibiting S. parauberis infection when co-treated with L. plantarum. Therefore, for the identification and analysis of secondary metabolites produced by L. plantarum in an SHC-containing growth medium, GC/MS analysis was performed. For the purpose of providing a negative control, GC/MS analysis was also performed with MRS broth without SHC treatment. Results of the analysis of secondary metabolites in SHC-treated broth are shown in Figure 7. According to the results, the MRS broth containing L. plantarum contained compounds with similar peaks to those of in SHC-treated broth, such as L-Lactic acid, N-methoxy-N-methyl acetamide, diethyl phthalate, and propanoic acid.
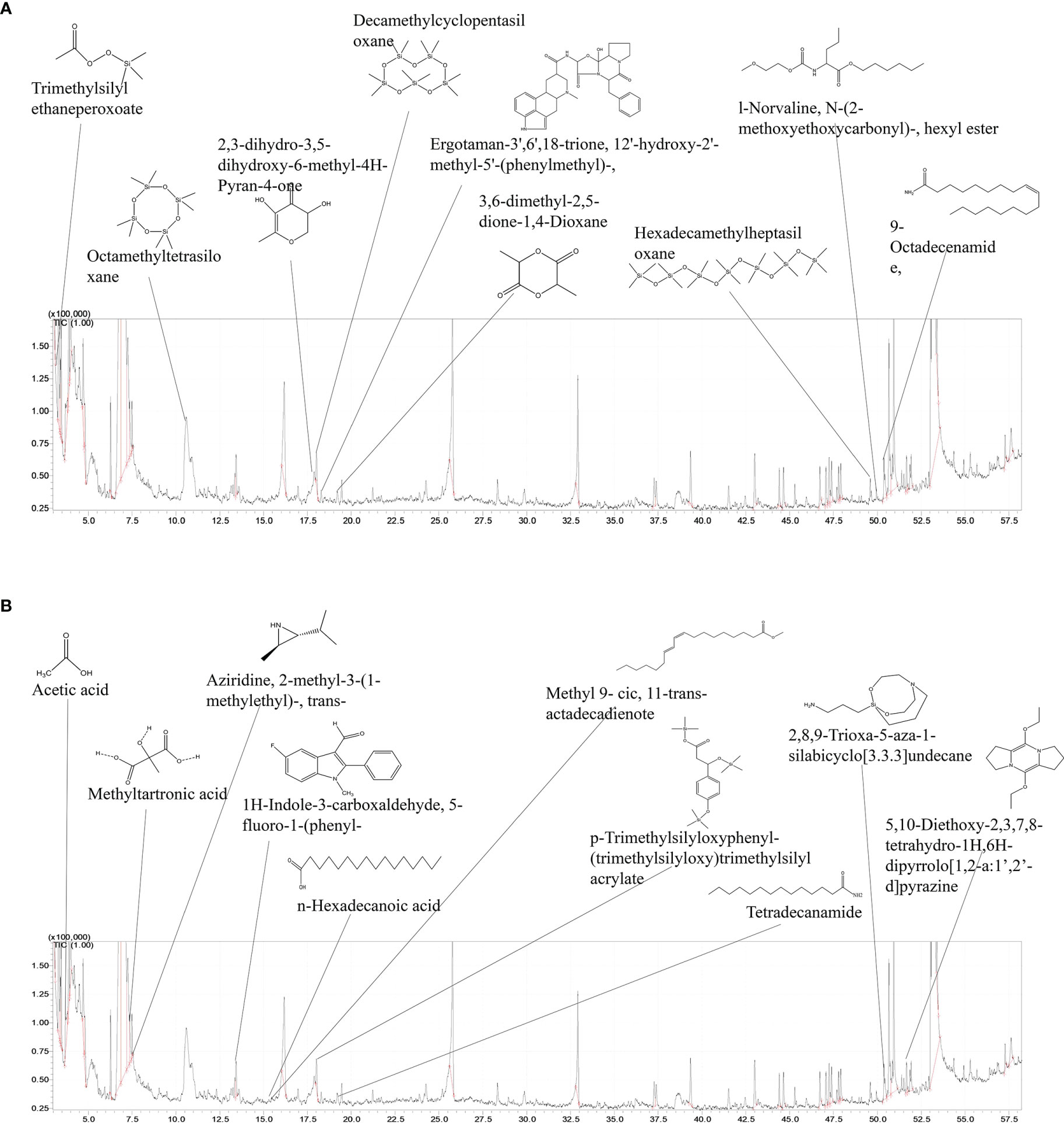
Figure 7 GC/MS analysis of secondary metabolites obtained from L. plantarum incubated with SHC. (A) GC/MS spectrum of secondary metabolites produced in only MRS containing medium only (B) GC/MS spectrum of secondary metabolites.
Interestingly, tetradecanamide, 2,8,9-Trioxa-5-aza-1-silabicyclo [3.3.3] undecane, 5, 10-Diethoxy-2, 3, 7, 8-tetrahydro- 1H,6H-dipyrrolo[1,2-a:1’,2’-d] pyrazine,1H-Indole-3- carboxaldehyde, and 5- fluoro-1-phenyl were only observed in MRS medium supplemented with SHC. Such compounds were identified by comparing their spectra to those in the mass spectra library. Compounds identified from L. plantarum secondary metabolites were aromatic and heterocyclic, which are suspected to possess the antibacterial effects on pathogenic bacteria used in this study.
4 Discussion
Due to its high polysaccharide content, seaweed can be used as a prebiotic ingredient for both human and animal health applications (O’Sullivan et al., 2010). The notable effect of prebiotics is by modulating the intestinal microbiota population, which results in inhibition of pathogenic bacteria by limiting their proliferation. This study demonstrated the potential of SH to enhance beneficial probiotic bacterial growth and secondary metabolite production, thereby resulting in the reduction of pathogenic bacterial infections in fish. Likewise, supplementation with SH was also shown to result in increased body weight in zebrafish. Probiotics such as lactic acid bacteria (LAB) are beneficial microorganisms having numerous health benefits. LAB are a group of gram-positive, non-spore-forming anaerobic or facultative aerobic cocci or rods (Hayek and Ibrahim, 2013). They metabolize growth medium components and produce bacterial secondary metabolites that are important for the health and nutrition of other organisms (Ustyugova et al., 2012). LAB are widely used as probiotics in poultry and swine management practices (Patterson and Burkholder, 2003) and have also been recently used as an additive in fish feed, resulting in improved growth and management of diseases in the aquaculture (Ringo and Gatesoupe, 1998).
The use of seaweed as prebiotics is an important alternative approach for controlling diseases in fish through a variety of mechanisms. LAB breakdown components found in seaweed and produce bioactive compounds having antibacterial activity. Further, microbial fermentation of seaweed results in new bioactive complexes with antioxidant properties and other activities, as occurs, for example, through fermentation of Ekclonia cava by Candida utilis (Wijesinghe and Jeon, 2012). Another study showed that fermentation of Hizikia fusiforme by L. brevis enhanced the antioxidant and anti-inflammatory activities of its water extract (Song et al., 2011). Also, fermentation of Sargassum siliquanstrum with LAB was proven to enhance its anti-inflammatory property (Lee et al., 2016). Such antioxidant properties and anti-inflammatory properties reduce the oxidative stress and inflammation caused by external factors such as pathogens in fish.
Co-culturing of LAB with seaweed extracts resulted in increased growth of LAB.
In particular, the growth of L. plantarum was enhanced significantly when treated with SHC and SHCPs compared to non-treated groups. In addition, compared to SHC, SHCPs led to a higher increase in lactic acid bacterial growth. This is likely due to the increased polysaccharide content in the extract (Sanjeewa et al., 2017), as an earlier study reported that a high polysaccharide content was found to result in a good prebiotic activity (O’Sullivan et al., 2010). A study conducted on several Indian seaweeds, has also proven that the high dietary fiber content and polysaccharide content in E. compressa results in higher growth of probiotics (Ajanth Praveen et al., 2019). Thus, prebiotics are able to boost the growth of beneficial probiotic bacteria and thereby promote the production of secondary metabolites that are beneficial to host health. The results from previous studies agreed with the results obtained from the present study. Apart from its prebiotic activity, seaweeds are also proven to have antibacterial activity against various infectious bacteria. In an early study, several cultivated seaweeds were screened for their antibacterial activity and verified the possible use of seaweeds as an antimicrobial source (Bansemir et al., 2006). Similarly, we observed that SH enzymatic extracts, especially SHC acted as a protective agent against fish pathogenic bacteria such as E. tarda, S. parauberis and S. iniae, independently from its prebiotic action on LAB. This was confirmed by culturing pathogenic bacteria in SH enzymatic extracts-containing culture medium and observing their growth compared to the non-treated control groups. Moreover, both SHC and SHCPs significantly improved the production of secondary metabolites by all three species of LAB compared to LAB cultured in MRS culture medium only. Furthermore, secondary metabolites produced from both L. plantarum and L. pentosus were proven to possess antibacterial action against the common fish pathogens used in this study. Based on GC/MS results, we identified that culturing of L. plantarum in S. horneri-containing medium produced tetradecanamide, 2,8,9-Trioxa-5-aza-1-silabicyclo [3,3,3] undecane, 5,10-Diethoxy-2,3,7,8-tetrahydro-1G, 6H-dipyrrolo[1,2-a:1,2-d] pyrazine, as secondary metabolites, all of which are suspected of having potential antibacterial activity against all three pathogenic bacterial species. This may be the reason for the higher antibacterial activity of secondary metabolites from L. plantarum against S. parauberis. However, further investigations are required to characterize its antibacterial activity. A previous, similar study reported that L.plantarum produced 3-ethyl-6-pentofuranosyl imidazo (4,5-c) pyrazole-1(6H)-carbaldehyde which has antibacterial properties when grown in MRS medium containing Ecklonia cava extract (Lee et al., 2016). Moreover, it has been reported that secondary metabolites, such as oleic acid, octanoic acid, butanamide, and decanoic acid derivatives produced by L. plantarum have antifungal activities (Poornachandra Rao et al., 2019).
Probiotics and prebiotics like immune-stimulants, act as alternatives to antibiotics and chemotherapeutics which have negative effects such as resistance development and mutations in pathogens (Lopez et al., 2003). Because of such benefits, probiotics and prebiotics have received substantial consideration for incorporation into fish feed. A study conducted on groupers (Epinephelus coioides) showed that feeding on L. plantarum, enhanced their growth and disease resistance in a significant manner (Son et al., 2009). In early studies, SH was proven to increase the growth performance of aquaculture fish such as black sea bream and turbot (Shi et al., 2019; Wang et al., 2019). Correspondingly, our study revealed that feeding on prebiotics resulted in comparatively higher weight gain in zebrafish compared to the non-treated group. The increase in body weight may be due to the supplementation of the experimental diet, which helps in augmenting the digestive activity via vitamin and enzyme synthesis and improving the microbial flora in the gut (Son et al., 2009; Ngamkala et al., 2010).
The pathogenic bacteria used in this study are responsible for the disease streptococcosis, which is related to acute and chronic mortality in many aquaculture fish species. The results of this study suggested that the co-application of L. plantarum and SH improved the survival rate of zebrafish infected with S. parauberis. Infected fish showed darkened skin, inflammation, and cachexia, symptoms which were relieved following the co-treatment with L. plantarum and SH. Similar findings were obtained from studies conducted on the prebiotic potential of E. cava in E. tarda -infected zebrafish (Lee et al., 2016) and olive flounder (Lee et al., 2016; Vidhya Hindu et al., 2018), indicating that the incorporation of seaweed in fish feed results in improved growth and decreased mortality of infected fish. Histological studies showed that co-treatment with L. plantarum and SH improved goblet cell density in infected fish. Goblet cells are specialized epithelial cells that play an important role in innate defense in the gut, especially by exerting a protective mechanism against exogenous conditions, including protection from pathogenic bacteria (Kim and Ho, 2010). Similar results were obtained in previous studies conducted on the effects of U. pinnatifida on intestinal health of gibel carp and the effect of chitosan silver nanoparticles on immunomodulation in zebrafish (Udayangani et al., 2017; Cui et al., 2020).
The first line of the defense system in fish is the innate immune system (Harikrishnan et al., 2010). Probiotic activation in the gut induces the activation of the innate immune system and induction of pro-inflammatory cytokines when invaded by foreign pathogens. Bacterial infections result in the production of inflammatory responses and production of NO, a free radical with numerous pathological functions. Increased generation of NO leads to the activation of iNOS and COX (Wang et al., 2007). The inducible form of COX, called COX-2, is produced extensively by macrophages at the site of inflammation (Wang et al., 1995). Previous work showed the expression of iNOS and COX-2 to be up-regulated in S. parauberis-infected zebrafish in the non-treated group compared to the L. plantarum and SH treated groups. According to our study, co-application of L. plantarum significantly downregulated the expression of iNOS and COX-2. The reduction in inflammatory mediators was reported to decrease the effects of pathogenic bacteria in fish (Vojtech et al., 2009). Numerous studies have demonstrated the ability of SH to reduce iNOS and COX-2 expression during inflammation in in vitro and in in vivo models (Acheson, 1969; Sanjeewa et al., 2018). Due to the reduction of iNOS and COX-2, pain, swelling, and stiffness are decreased. The anti-inflammatory activity of SH was further tested by analyzing its effects on signal transduction pathways in S. parauberis-infected zebrafish.
It has been reported that inflammatory responses are due to the activation of complex signaling pathways, such as NF-κB. p65 and IKB-α are fundamental proteins in the NF-κB pathway, which are triggered by pathogen infection and eventually phosphorylated into the nucleus to bind with DNA, resulting in the regulation of mRNA expression of genes associated with inflammation including iNOS and COX-2 (Kim, 2014). The study showed that infection with S. parauberis resulted in an increase in phosphorylation of the above-mentioned proteins. However, treatment with SH extracts significantly suppressed the phosphorylation of NF-κB proteins, resulting in downregulation of NF-κB signaling cascade. These results collectively suggest that SH has anti-inflammatory activity against pathogenic bacteria-induced inflammation.
Conclusion
In agreement with the results obtained from our study, S. horneri (SH) efficiently improved the growth of probiotic bacteria such as L.plantarum, L. pentosus, and L. brevis, whereas it inhibited the growth of pathogenic bacteria such as S. iniae, St. parauberis, and E. tarda. Similarly, SH reduced the mortality of S. parauberis-infected zebrafish. These findings are also supported by the downregulation of several inflammatory mediators in the NF-κB pathway and the antibacterial activity against the pathogenic bacteria. Hence, these results strongly suggest that SH extracts possess potential prebiotic activity, which can be utilized in disease management.
Data Availability Statement
The original contributions presented in the study are included in the article/supplementary material. Further inquiries can be directed to the corresponding author.
Ethics Statement
The animal study was reviewed and approved by Animal Care Use Committee (IACUC) of Jeju National University (protocol 2020-0049).
Author Contributions
NL: Conceptualization, Methodology, Software, Validation, Formal analysis, Investigation, Writing- Original draft preparation; Y-SK: Resources, Data Curation, Supervision; DN: Software, Resources; TJ: Resources; HJi: Resources; H-WY: Resources; HJa: Resources; Y-JJ: Conceptualization, Supervision, Writing- Review and Editing, Project administration, Funding acquisition. All authors contributed to the article and approved the submitted version.
Funding
This research was supported by the Basic Science Research Program through the National Research Foundation of Korea (NRF), funded by the Ministry of Education (2019R1A6A1A03033553).
Conflict of Interest
The authors declare that the research was conducted in the absence of any commercial or financial relationships that could be construed as a potential conflict of interest.
Publisher’s Note
All claims expressed in this article are solely those of the authors and do not necessarily represent those of their affiliated organizations, or those of the publisher, the editors and the reviewers. Any product that may be evaluated in this article, or claim that may be made by its manufacturer, is not guaranteed or endorsed by the publisher.
References
Acheson E. D. (1969). [Personal Record Linkage]. Methods Inf. Med. Suppl. 4, 233–244. doi: 10.1016/j.ijbiomac.2016.02.025
Ajanth Praveen M., Karthika Parvathy K. R., Jayabalan R., Balasubramanian P. (2019). Dietary Fiber From Indian Edible Seaweeds and its in-Vitro Prebiotic Effect on the Gut Microbiota. Food Hydrocolloids 96, 343–353. doi: 10.1016/j.foodhyd.2019.05.031
Arasu M. V., Al-Dhabi N. A., Ilavenil S., Choi K. C., Srigopalram S. (2016). In Vitro Importance of Probiotic Lactobacillus Plantarum Related to Medical Field. Saudi J. Biol. Sci. 23 (), S6–S10. doi: 10.1016/j.sjbs.2015.09.022
Baeck G. W., Kim J. H., Gomez D. K., Park S. C. (2006). Isolation and Characterization of Streptococcus Sp. From Diseased Flounder (Paralichthys Olivaceus) in Jeju Island. J. Vet. Sci. 7 (1), 53–58. doi: 10.4142/jvs.2006.7.1.53
Bansemir A., Blume M., Schröder S., Lindequist U. (2006). Screening of Cultivated Seaweeds for Antibacterial Activity Against Fish Pathogenic Bacteria. Aquaculture 252 (1), 79–84. doi: 10.1016/j.aquaculture.2005.11.051
Cabello F. C. (2006). Heavy Use of Prophylactic Antibiotics in Aquaculture: A Growing Problem for Human and Animal Health and for the Environment. Environ. Microbiol. 8 (7), 1137–1144. doi: 10.1111/j.1462-2920.2006.01054.x
Charoensiddhi S., Franco C., Su P., Zhang W. (2015). Improved Antioxidant Activities of Brown Seaweed Ecklonia Radiata Extracts Prepared by Microwave-Assisted Enzymatic Extraction. J. Appl. Phycol. 27 (5), 2049–2058. doi: 10.1007/s10811-014-0476-2
Cui H, Wang Z, Liu J, Wang Y, Wang Z, Fu J, et al.(2020) Effects of a highly purified fucoidan from Undaria pinnatifida on growth performance and intestine health status of gibel carp Carassius auratus gibelio. Aquaculture Nutrition. 26,1,47-59. doi: 10.1111/anu.12966
Eom S.-H., Santos J. A., Kim J.-H., Jung W.-K., Kim D.-H., Kim Y.-M. (2015). In Vitro Antibacterial and Synergistic Activity of an Ecklonia Cava Extract Against Anti Biotic-Resistant Streptococcus Parauberis. Fish. Aquat. Sci. 18 (3), 241–247. doi: 10.5657/fas.2015.0241
Fernández-No I. C., Böhme K., Calo-Mata P., Cañas B., Gallardo J. M., Barros-Velázquez J. (2012). Isolation and Characterization of Streptococcus Parauberis From Vacuum-Packaging Refrigerated Seafood Products. Food Microbiol. 30 (1), 91–97. doi: 10.1016/j.fm.2011.10.012
Fernando I. P. S., Sanjeewa K. K. A., Samarakoon K. W., Lee W. W., Kim H.-S., Kang N., et al. (2017). A Fucoidan Fraction Purified From Chnoospora Minima; a Potential Inhibitor of LPS-Induced Inflammatory Responses. Int. J. Biol. Macromol. 104, 1185–1193. doi: 10.1016/j.ijbiomac.2017.07.031
Gibson G. R., Hutkins R., Sanders M. E., Prescott S. L., Reimer R. A., Salminen S. J., et al. (2017). Expert Consensus Document: The International Scientific Association for Probiotics and Prebiotics (ISAPP) Consensus Statement on the Definition and Scope of Prebiotics. Nat. Rev. Gastroenterol. Hepatol. 14 (8), 491–502. doi: 10.1038/nrgastro.2017.75
Harikrishnan R., Balasundaram C., Heo M. S. (2010). Effect of Probiotics Enriched Diet on Paralichthys Olivaceus Infected With Lymphocystis Disease Virus (LCDV). Fish Shellfish Immunol. 29 (5), 868–874. doi: 10.1016/j.fsi.2010.07.031
Harikrishnan R., Jin C. N., Kim M. C., Kim J. S., Balasundaram C., Heo M. S. (2010). Effectiveness and Immunomodulation of Chemotherapeutants Against Scuticociliate Philasterides Dicentrarchi in Olive Flounder. Exp. Parasitol. 124 (3), 306–314. doi: 10.1016/j.exppara.2009.11.005
Hayek S. A., Ibrahim S. A. (2013). Current Limitations and Challenges With Lactic Acid Bacteria: A Review. Food Nutr. Sci. 04 (11), 73–87. doi: 10.4236/fns.2013.411A010
Kesbiç O. S., Acar Ü, Yilmaz S., Aydin ÖD. (2020). Effects of Bergamot (Citrus Bergamia) Peel Oil-Supplemented Diets on Growth Performance, Haematology and Serum Biochemical Parameters of Nile Tilapia (Oreochromis Niloticus). Fish Physiol. Biochem. 46 (1), 103–110. doi: 10.1007/s10695-019-00700-y
Kesbiç O. S., Yigit M. (2019). Structural and Chemical Changes of Grape Seed Extract After Thermal Processing and its Use in Rainbow Trout (Oncorhynchus Mykiss) Diets as an Organic Feed Supplement. Aquaculture 503, 275–281. doi: 10.1016/j.aquaculture.2019.01.021
Kim H. K. (2014). Role of ERK/MAPK Signalling Pathway in Anti-Inflammatory Effects of Ecklonia Cava in Activated Human Mast Cell Line-1 Cells. Asian Pac J. Trop. Med. 7 (9), 703–708. doi: 10.1016/S1995-7645(14)60120-6
Kim Y. S., Ho S. B. (2010). Intestinal Goblet Cells and Mucins in Health and Disease: Recent Insights and Progress. Curr. Gastroenterol. Rep. 12 (5), 319–330. doi: 10.1007/s11894-010-0131-2
Lee W., Ahn G., Oh J. Y., Kim S. M., Kang N., Kim E. A., et al. (2016). A Prebiotic Effect of Ecklonia Cava on the Growth and Mortality of Olive Flounder Infected With Pathogenic Bacteria. Fish Shellfish Immunol. 51, 313–320. doi: 10.1016/j.fsi.2016.02.030
Lee S.-J., Lee D.-G., Kim M., Kong C.-S., Yu K.-H., Kim Y.-Y., et al. (2016). Enhancement of Anti-Inflammatory Activity by Fermentation of. Sargassum Siliquanstrum. 26 (3), 318–324. doi: 10.5352/JLS.2016.26.3.318
Lee W., Oh J. Y., Kim E. A., Kang N., Kim K. N., Ahn G., et al. (2016). A Prebiotic Role of Ecklonia Cava Improves the Mortality of Edwardsiella Tarda-Infected Zebrafish Models via Regulating the Growth of Lactic Acid Bacteria and Pathogen Bacteria. Fish Shellfish Immunol. 54, 620–628. doi: 10.1016/j.fsi.2016.05.018
Lopez N., Cuzon G., Gaxiola G., Taboada G., Valenzuela M., Pascual C., et al. (2003). Physiological, Nutritional, and Immunological Role of Dietary Beta 1-3 Glucan and Ascorbic Acid 2-Monophosphate in Litopenaeus Vannamei Juveniles. Aquaculture 224 (1-4), 223–243. doi: 10.1016/S0044-8486(03)00214-X
Nagahawatta D. P., Sanjeewa K. K. A., Jayawardena T. U., Kim H.-S., Yang H.-W., Jiang Y., et al. (2021). Drying Seaweeds Using Hybrid Hot Water Goodle Dryer (HHGD): Comparison With Freeze-Dryer in Chemical Composition and Antioxidant Activity. Fish. Aquat. Sci. 24 (1), 19–31. doi: 10.47853/FAS.2021.e3
Ngamkala S., Futami K., Endo M., Maita M., Katagiri T. (2010). Immunological Effects of Glucan and Lactobacillus Rhamnosus GG, a Probiotic Bacterium, on Nile Tilapia Oreochromis Niloticus Intestine With Oral Aeromonas Challenges. Fish. Sci. 76 (5), 833–840. doi: 10.1007/s12562-010-0280-0
O’Sullivan L., Murphy B., McLoughlin P., Duggan P., Lawlor P. G., Hughes H., et al. (2010). Prebiotics From Marine Macroalgae for Human and Animal Health Applications. Mar. Drugs 8 (7), 2038–2064. doi: 10.3390/md8072038
Park Y. K., Nho S. W., Shin G. W., Park S. B., Jang H. B., Cha I. S., et al. (2009). Antibiotic Susceptibility and Resistance of Streptococcus Iniae and Streptococcus Parauberis Isolated From Olive Flounder (Paralichthys Olivaceus). Vet. Microbiol. 136 (1-2), 76–81. doi: 10.1016/j.vetmic.2008.10.002
Patterson J. A., Burkholder K. M. (2003). Application of Prebiotics and Probiotics in Poultry Production. Poult Sci. 82 (4), 627–631. doi: 10.1093/ps/82.4.627
Paul M. (2017).Histological slide preparation of fish tissues (paraffin method). Calcutta. India: University of Calcutta Calcutta, IND; doi: 10.13140/RG.2.2.15130.34243
Poornachandra Rao K., Deepthi B. V., Rakesh S., Ganesh T., Achar P., Sreenivasa M. Y. (2019). Antiaflatoxigenic Potential of Cell-Free Supernatant From Lactobacillus Plantarum MYS44 Against Aspergillus Parasiticus. Probiotics Antimicrob. Proteins 11 (1), 55–64. doi: 10.1007/s12602-017-9338-y
Pridgeon J. W., Klesius PHJASR. (2012). Major Bacterial Diseases in Aquaculture and Their Vaccine Development 7, 1–16. doi: 10.1079/PAVSNNR20127048
Ringo E., Gatesoupe F. J. (1998). Lactic Acid Bacteria in Fish: A Review. Aquaculture 160 (3-4), 177–203. doi: 10.1016/S0044-8486(97)00299-8
Sanjeewa K. K., Fernando I. P., Kim E. A., Ahn G., Jee Y., Jeon Y. J. (2017). Anti-Inflammatory Activity of a Sulfated Polysaccharide Isolated From an Enzymatic Digest of Brown Seaweed Sargassum Horneri in RAW 264. 7 Cells Nutr. Res. Pract. 11 (1), 3–10. doi: 10.4162/nrp.2017.11.1.3
Sanjeewa K. K. A., Fernando I. P. S., Kim S. Y., Kim H. S., Ahn G., Jee Y., et al. (2018). In Vitro and In Vivo Anti-Inflammatory Activities of High Molecular Weight Sulfated Polysaccharide; Containing Fucose Separated From Sargassum Horneri: Short Communication. Int. J. Biol. Macromol. 107 (Pt A), 803–807. doi: 10.1016/j.ijbiomac.2017.09.050
Sanjeewa K. K. A., Jayawardena T. U., Kim S.-Y., Kim H.-S., Ahn G., Kim J., et al. (2019). Fucoidan Isolated From Invasive Sargassum Horneri Inhibit LPS-Induced Inflammation via Blocking NF-κb and MAPK Pathways. Algal. Res. 41, 101561. doi: 10.1016/j.algal.2019.101561
Schmidt A. S., Bruun M. S., Dalsgaard I., Pedersen K., Larsen J. L. (2000). Occurrence of Antimicrobial Resistance in Fish-Pathogenic and Environmental Bacteria Associated With Four Danish Rainbow Trout Farms. Appl. Environ. Microbiol. 66 (11), 4908–4915. doi: 10.1128/AEM.66.11.4908-4915.2000
Shanura Fernando I. P., Asanka Sanjeewa K. K., Samarakoon K. W., Lee W. W., Kim H.-S., Ranasinghe P., et al. (2018). Antioxidant and Anti-Inflammatory Functionality of Ten Sri Lankan Seaweed Extracts Obtained by Carbohydrase Assisted Extraction. Food Sci. Biotechnol. 27 (6), 1761–1769. doi: 10.1007/s10068-018-0406-1
Shi Q., Rong H., Hao M., Zhu D., Aweya J. J., Li S., et al. (2019). Effects of Dietary Sargassum Horneri on Growth Performance, Serum Biochemical Parameters, Hepatic Antioxidant Status, and Immune Responses of Juvenile Black Sea Bream Acanthopagrus Schlegelii. J. Appl. Phycol. 31 (3), 2103–2113. doi: 10.1007/s10811-018-1719-4
Son V. M., Chang C. C., Wu M. C., Guu Y. K., Chiu C. H., Cheng W. (2009). Dietary Administration of the Probiotic, Lactobacillus Plantarum, Enhanced the Growth, Innate Immune Responses, and Disease Resistance of the Grouper Epinephelus Coioides. Fish Shellfish Immunol. 26 (5), 691–698. doi: 10.1016/j.fsi.2009.02.018
Song H-S, Eom S-H, Kang Y-M, Choi J-D, Kim Y-MJKJoF, Sciences A. (2011)Enhancement of the antioxidant and anti-inflammatory activity of Hizikia fusiforme water extract by lactic acid bacteria fermentation. Korean Journal of Fisheries and Aquatic Sciences. 44,2,111-7. doi: 10.5657/KFAS.2011.44.2.111
Udayangani R. M. C., Dananjaya S. H. S., Nikapitiya C., Heo G. J., Lee J., De Zoysa M. (2017). Metagenomics Analysis of Gut Microbiota and Immune Modulation in Zebrafish (Danio Rerio) Fed Chitosan Silver Nanocomposites. Fish Shellfish Immunol. 66, 173–184. doi: 10.1016/j.fsi.2017.05.018
Ustyugova E. A., Timofeeva A. V., Stoyanova L. G., Netrusov A. I., Katrukha G. S. (2012). Characteristics and Identification of Bacteriocins Produced by Lactococcus Lactis Subsp Lactis 194-K. Appl. Biochem. Micro+. 48 (6), 557–563. doi: 10.1134/S0003683812060105
Vidhya Hindu S., Chandrasekaran N., Mukherjee A., Thomas J. (2018). A Review on the Impact of Seaweed Polysaccharide on the Growth of Probiotic Bacteria and its Application in Aquaculture. Aquacult. Int. 27 (1), 227–238. doi: 10.1007/s10499-018-0318-3
Vojtech L. N., Sanders G. E., Conway C., Ostland V., Hansen J. D. (2009). Host Immune Response and Acute Disease in a Zebrafish Model of Francisella Pathogenesis. Infect. Immun. 77 (2), 914–925. doi: 10.1128/IAI.01201-08
Wang M. T., Honn K. V., Nie D. (2007). Cyclooxygenases, Prostanoids, and Tumor Progression. Cancer Metastasis Rev. 26 (3-4), 525–534. doi: 10.1007/s10555-007-9096-5
Wang C., Hu W., Wang L., Qiao H., Wu H., Xu Z. (2019). Effects of Dietary Supplementation With Sargassum Horneri Meal on Growth Performance, Body Composition, and Immune Response of Juvenile Turbot. J. Appl. Phycol. 31 (1), 771–778. doi: 10.1007/s10811-018-1590-3
Wang R., Neumann N. F., Shen Q., Belosevic M. (1995). Establishment and Characterization of a Macrophage Cell-Line From the Goldfish. Fish Shellfish Immun. 5 (5), 329–346. doi: 10.1006/fsim.1995.0032
Wang Y. B., Xu Z. R., Xia M. S. (2005). The Effectiveness of Commercial Probiotics in Northern White Shrimp Penaeus Vannamei Ponds. Fish. Sci. 71 (5), 1036–1041. doi: 10.1111/j.1444-2906.2005.01061.x
Wijesinghe W. A. J. P., Jeon Y.-J. (2012). Biological Activities and Potential Industrial Applications of Fucose Rich Sulfated Polysaccharides and Fucoidans Isolated From Brown Seaweeds: A Review. Carbohydr. Polymers 88 (1), 13–20. doi: 10.1016/j.carbpol.2011.12.029
Woo S. H., Park S. I. (2013). Streptococcous Parauberis Infection in Starry Flounder, Platichthys Stellatus: Characterization of Innate Immune Responses Following Experimental Infection. Fish Shellfish Immunol. 35 (2), 413–420. doi: 10.1016/j.fsi.2013.04.047
Keywords: Sargassum horneri, prebiotics, Streptococcus parauberis, secondary metabolites, Diet
Citation: Liyanage NM, Kim Y-S, Nagahawatta DP, Jin H, Yang H-W, Jayawardhana HHACK, Jayawardena TU and Jeon Y-J (2022) Sargassum horneri as a Prebiotic Dietary Supplement for Immunity Development in Streptococcus parauberis Infected Zebrafish Model. Front. Mar. Sci. 9:901676. doi: 10.3389/fmars.2022.901676
Received: 22 March 2022; Accepted: 31 May 2022;
Published: 08 July 2022.
Edited by:
Hüseyin Sevgili, Applied Sciences University of Isparta, TurkeyReviewed by:
Santhiyagu Prakash, Tamil Nadu Fisheries University, IndiaMohammed Fouad El Basuini, Tanta University, Egypt
Osman Sabri Kesbic, Kastamonu University, Turkey
Copyright © 2022 Liyanage, Kim, Nagahawatta, Jin, Yang, Jayawardhana, Jayawardena and Jeon. This is an open-access article distributed under the terms of the Creative Commons Attribution License (CC BY). The use, distribution or reproduction in other forums is permitted, provided the original author(s) and the copyright owner(s) are credited and that the original publication in this journal is cited, in accordance with accepted academic practice. No use, distribution or reproduction is permitted which does not comply with these terms.
*Correspondence: You-Jin Jeon, eW91amluMjAxNEBnbWFpbC5jb20=
†These authors have contributed equally to this work