- 1School of Biological Sciences, The University of Adelaide, Adelaide, SA, Australia
- 2Environment Institute, The University of Adelaide, Adelaide, SA, Australia
- 3School of Social Sciences, The University of Adelaide, Adelaide, SA, Australia
- 4College of Science and Engineering, Flinders University, Adelaide, SA, Australia
- 5SARDI Aquatic Sciences, Henley Beach, SA, Australia
Marine ecosystem restoration is fast becoming the primary tool for repairing the socio-ecological functions and economic benefits of coastal ecosystems. Healthy seascapes are characterized by many interacting species and intermingled habitats (e.g., seagrass, kelp, shellfish, sedimentary) that co-create ecological functions of substantial socio-economic value. These co-created functions not only build stability and resilience at seascape scales, but synergistically combine to enhance ecological productivity that is greater than the sum of the individual habitats. Yet, restoration practice is dominated by single-habitat approaches underpinned by single-species monocultures, potentially limiting the range of benefits that restoration can provide. We propose that for ecosystem restoration to meet its full potential in delivering socio-ecological benefits that are resilient to environmental change, restoration practices should plan beyond single-species and single-habitats to a multi-habitat seascape. Where multiple habitats are co-restored, their positive interactions mutually benefit each other to stabilize and even accelerate ecosystem recovery; such as co-restored shellfish and kelp forests on constructed reefs, which combine to stabilize sediment for seagrass recovery. As fisheries scientists and managers, food and social scientists, and ecologists and oceanographers, we describe multi-habitat marine restoration activities that are readily achievable and provide a vision for the diverse socio-ecological, economic, and culture benefits that may emerge from future seascape-level repair.
Introduction
To meet the socio-ecological goals of the United Nation’s Decade on Ecosystem Restoration (2021-2030), a central focus should be bringing restoration practice up to speed with current ecological theory. It is well-known that ecological complexity– the number of components (e.g., species, habitat types) in a system and the connections among them (e.g., species interactions, habitat connectivity, energy flows; Bullock et al., 2022) – is an essential part of nature; key to the productivity, function, and stability of ecosystems (Brown et al., 2001; Angelini et al., 2011; Isbell et al., 2015; Soliveres et al., 2016). Decades of research have demonstrated the productivity benefits and positive interactions of intermingled species (Bertness and Callaway, 1994; Tilman et al., 1996). Yet, restoration practice remains fixated on single-habitat approaches underpinned by single-species monocultures (Silliman et al., 2015) that rely upon inherently uncertain successional processes to recover complexity. Joining calls from terrestrial ecologists to diversify plantings to ensure restorations are multi-functional and resilient (Messier et al., 2021, Bullock et al., 2022), marine researchers have increasingly demonstrated the benefits of co-seeding multiple species to enhance and accelerate restorations (e.g., Angelini et al., 2015; Derksen-Hooijberg et al., 2018; Thomsen et al., 2022). These typically small-scale marine efforts provide evidence to embrace a multi-species, multi-habitat restoration approach, but it is now time to work at larger scales (McAfee et al., 2021a).
Coastal marine habitat-forming species (e.g., seagrass meadows, kelp forests, shellfish reefs, mangrove forests, saltmarsh) have experienced considerable and ongoing losses (Jackson et al., 2001; Beck et al., 2011; Goldberg et al., 2020; Dunic et al., 2021). These losses manifest in the exponential decay of the marine goods and services on which society depends (Worm et al., 2006), undermining our goals for a sustainable socio-ecological future (e.g., United Nation’s SDG 14). But recognition of ecosystem losses provide contemporary opportunities for widespread ecological repair. In many cases, the ecological foundations for rapid ecosystem recovery still exist (Duarte et al., 2020). And many of these lost habitats naturally co-occur (e.g., seagrass and bivalves, saltmarshes and mangroves) to provide divergent functions that synergistically benefit each habitat (Angelini et al., 2011; Thomsen et al., 2018; Gagnon et al., 2020). Consequently, restoration practices that prioritise the natural synergy of co-occurring habitats may enhance the ecological complexity and function that augments ecosystem services (Benayas et al., 2009; Renzi et al., 2019, Bullock et al., 2022).
In this perspective, we advocate for multi-habitat seascape restoration by describing its practical, ecological, and potential socio-economic benefits. We describe multi-habitat restorations that aim to restore mosaics of functionally-diverse habitats over tens of hectares, and provide a visionary perspective of the socio-ecological benefits that may emerge from successful multi-habitat restorations. Our restoration case-studies from South Australia, show how constructed boulder reefs provide opportunities to co-restore multiple habitats (shellfish, kelp, seagrass) that facilitate and synergise each other’s recovery (e.g., McAfee et al., 2021b). Similarly, we describe how re-introducing tidal flows into tidally-restricted areas (e.g., via tidal gates, sea walls) provides opportunity to restore mosaics of connected intertidal habitats (seagrass, mangrove, saltmarsh). From our experiences, we suggest that a multi-habitat approach may be particularly achievable where restorations involve new infrastructure (e.g., boulder reefs) or removal of obstructive infrastructure (e.g., tidal barriers). Such restoration activities are naturally collaborative, requiring diverse expertise across research, management, regulatory, and conservation organisations. We propose that a multi-habitat restoration approach is readily achievable, and may help ecosystem restoration meet its potential in delivering socio-ecological benefits that are resilient to environmental change.
Multi-habitat reef restoration: a case-study
A multi-habitat restoration is underway along South Australia’s most urbanised coastline (Adelaide’s metropolitan coast). Over the last 200 years, this coastline’s seafloor has experienced considerable re-structuring (Tanner, 2005); overfishing eradicated oyster reefs from 1,500 km of coastline (Alleway and Connell, 2015), and extensive seagrass meadows (6,200 ha) and kelp forests (70 km) were lost due to anthropogenic nutrient inputs (Connell et al., 2008; Tanner et al., 2014). In recent decades, water quality improvements have resulted in net recovery of 11,000 ha of seagrass in water deeper than 10 m (Fernandes et al., 2022). However, little-to-no near-shore seagrass (<10 m depth) has recovered as hysteresis effects mean that once seagrasses are lost, they cannot recover in hydrodynamically active environments due to sediment mobility (Cabaço et al., 2008; Infantes et al., 2009). Similarly, no oyster reefs have recovered due to the loss of the hard substratum (shell) required for their natural recovery (McAfee and Connell, 2020). To kickstart recovery in the hydrodynamically active nearshore, restoration efforts have focused on providing stable substrate for oysters (boulder reefs) and seagrasses (hessian bags; Tanner et al., 2014). These restoration efforts are now being combined with kelp transplants to facilitate positive interactions for their multi-habitat recovery.
Since 2017, boulder reefs have been constructed to restore South Australia’s lost oyster reefs (Ostrea angasi; 193 boulder reefs [L × W × H: <30 × 14 × 1 m] over 30 ha to date). Bringing diverse research and management expertise together to inform these reef restorations (McAfee et al., 2022) helped realise the opportunities for recovering multiple lost habitats. For example, these reefs provide suitable substrata to also recover lost kelp forests (Ecklonia radiata; Connell et al., 2008), and they reduce hydrodynamic energy and stabilize sediment around the reef base, providing conditions for seagrass recovery. Such hydrodynamic dampening likely underpins the positive interaction between seagrass and oyster reefs commonly observed at restorations elsewhere (e.g., Milbrandt et al., 2015; Sharma et al., 2016; Figure 1).
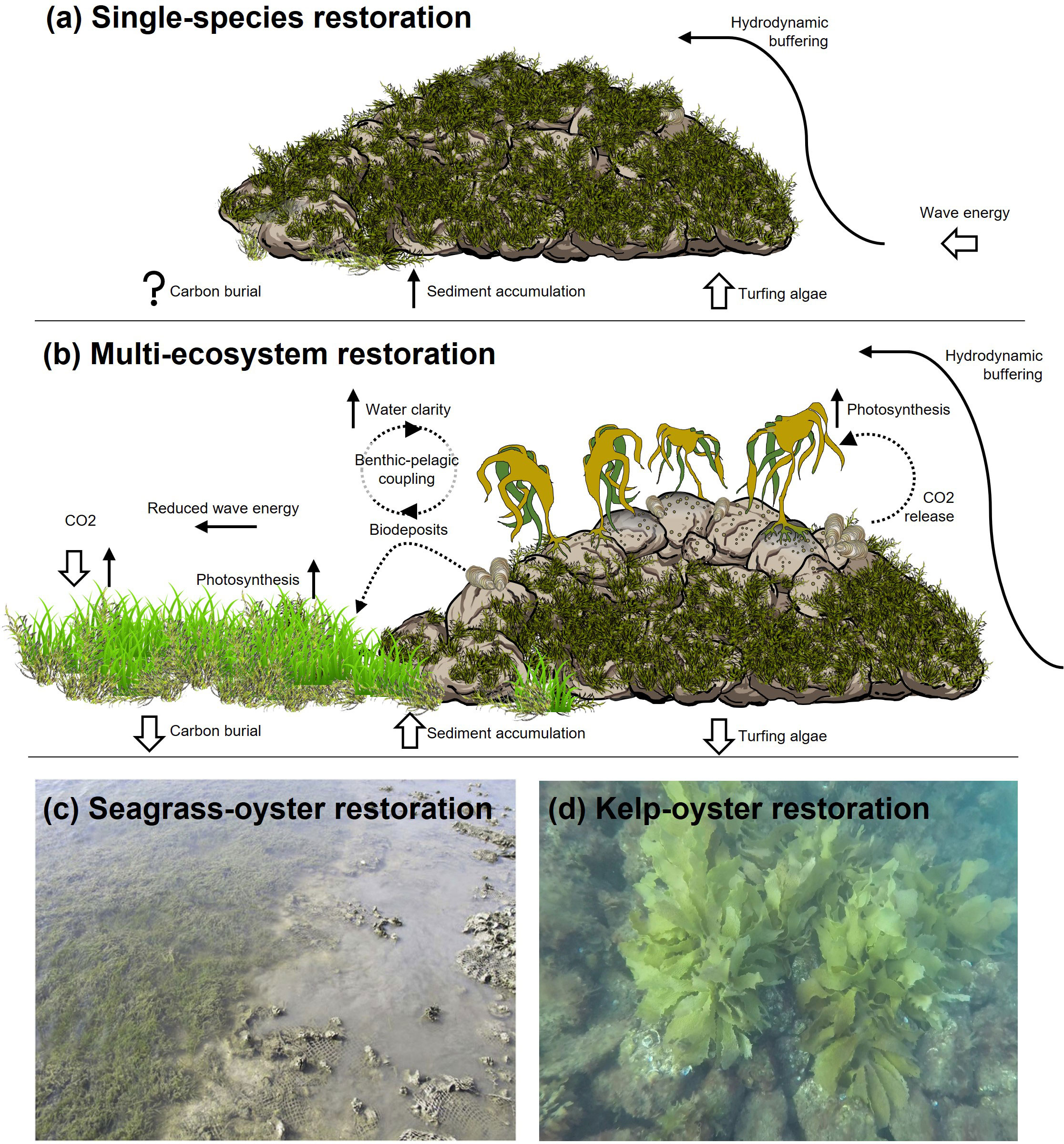
Figure 1 Restoration practice commonly focuses on (a) single-species approaches to habitat restoration, such as constructing reefs to restore oysters, whereas a (b) multi-habitat approach could utilise constructed reefs to recover multiple lost habitat-types (oysters, seagrass, kelp). Examples of multi-habitat restoration in practice demonstrate the facilitative benefits of co-restoration, including (c) constructed oyster reefs that buffer hydrodynamics and stabilise sediments to promote seagrass recovery (adapted from Milbrandt et al., 2015 with permission from Elsevier), and (d) kelp transplants atop constructed reefs that maintain substrata free of turfing algae to facilitate understory oyster recruitment (South Australia).
To co-restore seagrass among the constructed reefs, we placed hessians bags leeward of reefs to provide attachment substrata for naturally recruiting seagrass seedlings (Amphibolis antarctica). Hessian bags are a cost-effective technique that can be used for large-scale restoration (Tanner et al., 2014), provided hydrodynamic energy is sufficiently low for seedling attachment and to avoid resuspension. Despite the high energy of our study area’s near-shore environment, A.antarctica seedlings successfully recruited to the bags in the hydrological shadow of the reefs, where high rates of sediment depositation (relative to no-reef controls) indicate conditions for restoration success (McGlathery et al., 2012). It is hoped that, like previous restorations using hessian bags (Tanner et al., 2014), these A. antarctica patches will facilitate other seagrasses (Posidonia australis) and coalesce to create resilient meadows that eventually expand beyond the reef footprint. Although it is too early to measure the synergies of co-restored oysters and seagrass at these new restorations (constructed 2020 and 2021), evidence suggests the seagrass will benefit from oyster filter-feeding (improved water clarity, nutrient-rich deposits; Wall et al., 2008; Peterson and Hick, 2001), while the oysters may benefit from oxygen production by seagrass (Gagnon et al., 2020; Figure 1).
Ecological synergies are also anticipated from co-restoring oysters and kelp. Co-culturing oysters and kelp can create water chemistry that mutually benefits each other’s growth (Han et al., 2017), and they may also maintain conditions favourable for each other’s recruitment. For example, kelp canopies maintain exposed rocky substrate by shading and physically abrading their understory habitat (Connell, 2003; Irving and Connell, 2006), keeping it free of turf-forming algae that otherwise competitively excludes kelp and oyster recruits (Gorman and Connell, 2009; Figure 1). We utilised this function of kelp by transplanting kelp from healthy donor populations to form forests (8 plants/m2) atop our restoration reefs, attaching transplanted individuals to reef boulders (following Layton et al., 2021). Beneath our kelp transplants, oyster recruitment has increased by orders of magnitude (McAfee et al., 2021b), likely a function of the suppressed turf algae, as witnessed elsewhere (Shelamoff et al., 2019). Conversely, as the oyster habitat grows, the provision of shell substratum may facilitate kelp attachment (Lang and Buschbaum, 2010). Overall, multi-habitat restoration allows practitioners to radily generate these kinds of positive feedbacks between foundation species as restorations commence. This may be invaluable for steering restorations on the desired trajectory of recovery, especially during the rapid succession of multiple species witnessed on newly constructed reefs.
Prioritising ecological synergies in restoration practice
The scientific literature abounds with examples of interspecific foundation species combining to boost productivity, biodiversity, habitat growth, and to alleviate physical stressors and predation pressure (e.g., reviews by Renzi et al., 2019; Gagnon et al., 2020; Zabin et al., 2022). The co-produced functions and complexity created by interacting foundation species can help enhance the recovery (Lang’at et al., 2013), stability (Angelini et al., 2015) and resilience of multi-habitat ecosystems (Zabin et al., 2022), with productivity that far exceeds single-species habitats (Thomsen et al., 2018; Thomsen et al., 2022). Consequently, calls are increasing for restoration practice to utilise positive species interactions to restore seagrass meadows (Gagnon et al., 2020; Zhang et al., 2021), kelp forests (Eger et al., 2020), oyster reefs (Reeves et al., 2020, McAfee et al., 2021b), mangroves (Gedan and Silliman, 2009; Renzi et al., 2019) and saltmarsh (Derksen-Hooijberg et al., 2018).
It is our view that we should extend the benefits of co-species plantings to a multi-habitat approach focused on restoring mosaics of interacting habitats (Figure 1). In time, at scales beyond present capacity, this could involve designs to recover interconnected habitats across the land-to-seascape continuum, re-establishing energy flows between saltmarsh and mangrove communities to near- and off-shore seagrass meadows, kelp forests, and coral and shellfish reefs. Such a vision may not yet be economically realistic, but we can now embrace multi-habitat restoration to create localised mosaics as building blocks for widespread seascape connectivity. Similar to our case-study, multi-habitat restorations have involved localised plantings of adjacent patches of interacting oyster reefs, saltmarsh, and mangroves (Milbrandt et al., 2015; Donnelly et al., 2017; Walters et al., 2017). Alternatively, planted foundation species have been associated with hard substrata provided to facilitate associated communities (e.g., artificial reefs for oyster recruitment; Sharma et al., 2016; McAfee et al., 2021b; Pinnell et al., 2021). Such restorations create ‘living shorelines’ where co-occurring species generate structural and biological feedbacks that reduce hydrodynamic and shear stress, thus promoting sediment accretion and propagules to settle (Smith et al., 2020; Figure 1). Additionally, multi-habitat approaches are shown to enhance the resilience of restored plots to natural disturbances (Zabin et al., 2022) and to accelerate natural succession processes beyond those of single-species restorations (McAfee et al., 2021b; Pinnell et al., 2021).
Promoting productivity through connectivity
Mosaics of interacting habitats are a defining feature of healthy coastal seascapes that connect habitats from intertidal (e.g., saltmarsh, mangroves) to subtidal settings (e.g., kelp forests, seagrass, shellfish reefs). Across the land-to-sea continuum, habitats are hydrologically and functionally connected by ontogenetic movements of animals, fluxes of nutrients and organic matter, and predator-prey interactions that support many economically important species (Nagelkerken et al., 2015; Sheaves et al., 2015). Habitat mosaics provide conditions for enhanced growth and survival (e.g., abundant food and shelter from predators) of numerous fish, crab, and other invertebrates species (Nagelkerken, 2009), and are well recognized nursery areas where juveniles mature before moving to adult populations to replenish fisheries (Reis-Santos et al., 2013, Nagelkerken et al., 2015; Lefcheck et al., 2019). This nursery function is strongly influenced by the quality and spatial setting of the surrounding seascape (Olson et al., 2019), as many fish use different habitats during their larval, juvenile, and adult life stages. Therefore, to safeguard nursery function, restorations should aim to promote linkages among fragmented seascapes and maximise the synergy of multiple interacting habitats (Crook et al., 2015; Gilby et al., 2018). In this context, single-species restorations may limit our ability to repair the connectivity of diverse habitats.
Multi-habitat restoration may allow us to replicate naturally co-occurring coastal habitats in planned mosaics (Milbrandt et al., 2015), enhancing the network of available habitats and the overall seascape carrying capacity (Gilby et al., 2018). Creating localised habitat mosaics increases the probability that diverse species will recruit (e.g., those preferentially settling on reef, in kelp, or seagrass), enhancing the diversity and abundance of fish assemblages and other fisheries species. Importantly, this enhances the abundance of harvestable species through gains in fisheries productivity, rather than from attraction processes (Gilby et al., 2021). Attraction processes readily boost fish abundance and diversity on constructed reefs, as witnessed on our new reef restorations (unpublished data). As these restorations mature, it will be important to disentangle the boosted productivity attributable to multi-habitat restorations. Such knowledge would inform spatial and temporal frameworks for optimising multi-habitat restorations to support increased biodiversity and functional connectivity that extend beyond the fringes of restored habitats (Olds et al., 2016; Pittman et al., 2021).
Supporting quality seafood
Restored nursery habitats can enhance fish productivity and diversity (e.g., oyster reefs; Zu Ermgassen et al., 2016). Yet, few species utilise just one nursery habitat (Bostrom et al., 2011) and productive seascape nurseries involve functionally-connected habitat mosaics (Nagelkerken et al., 2015). We believe that once mature, multi-habitat restorations could benefit human health with more productive fisheries that may foster new market opportunities. Seafood is rich in essential nutrients (e.g., iron, zinc, vitamin A) and omega-3 fatty acids that are essential to human health (Thilsted et al., 2016; Hicks et al., 2021). One million premature deaths are annually linked to deficiencies in these essential nutrients (Calder, 2015; Haddad et al., 2016), so it is no wonder that sustainable fisheries are key to promoting global human health (HLPE, 2014). Fish obtain their omega-3 content from diet, particularly from primary producers (phytoplankton, macrophytes) whose nutritional content reflects the quality of their surrounding environment (Galloway and Winder, 2015). Whereas ecological degradation threatens nutritional quality (Robinson et al., 2022), restoration offers potential to improve the conditions underlying seafood quality through repair of multi-habitat mosaics. However, quantifying linkages between seafood quality and multi-habitat restorations will require decadal-scale monitoring (e.g., >15 years) that far exceeds typical monitoring programs (Bayraktarov et al., 2016).
Where restoration repairs functional seascapes, it could enhance access to nutritional seafood through two mechanisms: (i) through higher nutritional content of the primary producers that can propagate up food webs to harvestable fish (Galloway and Winder, 2015), and (ii) by increasing species richness to provide access to more diverse diets that provide more nutritional benefit (i.e., diverse micronutrient profiles; Bernhardt and O’Connor, 2021; Robinson et al., 2022). Technological advances allow increasingly accurate tracing of the geographic origin (seafood provenance) and nutritional quality of seafood, helping create new seafood markets and maximising the socio-economic benefits of restored seascapes (Gopi et al., 2019). Validating seafood provenance to healthy habitats helps promote the certification of sustainably sourced high-quality seafood, and empowers managers, authorities, and consumers to champion sustainable production. Our vision is that where restored seascapes bolster productivity and fisheries, seafood provenancing could certify production to create local added value products that connect consumers and sustainable commerical fisheries to restoration programs.
Blue carbon opportunities
Conservation and restoration of blue carbon habitats (seagrass, mangroves, saltmarsh) are considered key nature-based solutions to provide climate mitigation through carbon sequestration (Barbier, 2019; Macreadie et al., 2021). Habitat connectivity is critical to the carbon sequestration function of blue carbon ecosystems because a large proportion of carbon is externally derived and deposited by tides, waves, or terrestrial run-off (Geraldi et al., 2019). Therefore, the successful restoration of blue carbon abatement functions depends on connectivity between organic carbon sources and sink habitats (Hyndes et al., 2014; Smale et al., 2018). Single-species restorations can sequester carbon (e.g., seagrass; Greiner et al., 2013) and be used to repair connectivity among existing habitats. Yet, we see the benefit of a multi-habitat approach in generating close connectivity between carbon source and sink habitats (e.g., adjacent kelp forests and seagrass, respectively), such as in the near-shore environment of our case-study. Furthermore, our case-study shows that a multi-habitat approach can introduce blue carbon benefits into restorations that may not otherwise draw down carbon, such as the restoration of oyster reefs in isolation (Fodrie et al., 2017).
Restoring multiple, interconnected coastal habitats that resemble natural spatial mosaics is likely to enhance organic carbon transfer and burial, maximising the carbon sequestration potential of restoration activities (Smale et al., 2018). Other than active plantings, this may be achieved by focusing actions on restoring the hydrodynamic conditions required to support tidal wetland habitat complexes. For example, re-introducing tidal flows to previously cut-off/reclaimed coastal land, which is the focus of Australia’s recently announced blue carbon crediting method for the national Emissions Reduction Fund (Lovelock et al., 2022). In South Australia, case-studies re-introducing tidal flows have demonstrated successful restoration of wetland habitat mosaics (intermingled saltmarsh, mangrove, and intertidal seagrass) that resemble those of undisturbed environments (Dittmann et al., 2019). In time, this approach could re-connect intertidal habitats to the broader seascape through combination with near-shore subtidal restorations (e.g., shellfish reefs, seagrass) that are connected to deeper water habitats (e.g., kelp forests). Such projects could restore multiple, interconnected coastal habitats concurrently, enhancing carbon abatement functions by connecting donor and receiver habitats (Smale et al., 2018).
Cultural seascapes
Coastlines are cultural places of significance to community well-being, both in terms of the spiritual connections and economic livelihoods they support (McNiven, 2004, Clarke et al., 2013, Nardini, 2019). Coasts are places to socialise, recreate, experience nature, and make a living, and therefore embody a range of different values and personal relationships people have with the sea (Grice et al., 2012; Kobryn et al., 2018). Encouragingly, this socio-cultural complexity is increasingly incorporated into restoration planning, as social engagement provides a foundation for project success (DeAngelis et al., 2020; Clarke et al., 2021; McAfee et al., 2021c). Incorporating the diversity of socio-cultural values and uses of coastlines can encourage cultural stewardship (Lyver et al., 2016) and may assist policy makers to identify potential sources of conflict, as well as the socio-cultural compatability of suggested restorations (Brown and Raymond, 2014). We believe that over the coming decades, marine restoration has the potential to play an even greater role in shaping people’s perspectives and stewardship of the sea. Contemporary marine restoration is a young concept, but recent momentum (i.e. UN Decade on Ecosystem Restoration) suggests restoration efforts will continue to grow and engage people in coastal stewardship. Here, we provide a brief vision of the potential socio-ecological and economic benefits (Figure 2) that could emerge from successful seascape restorations, benefits that are not limited to multi-habitat restorations.
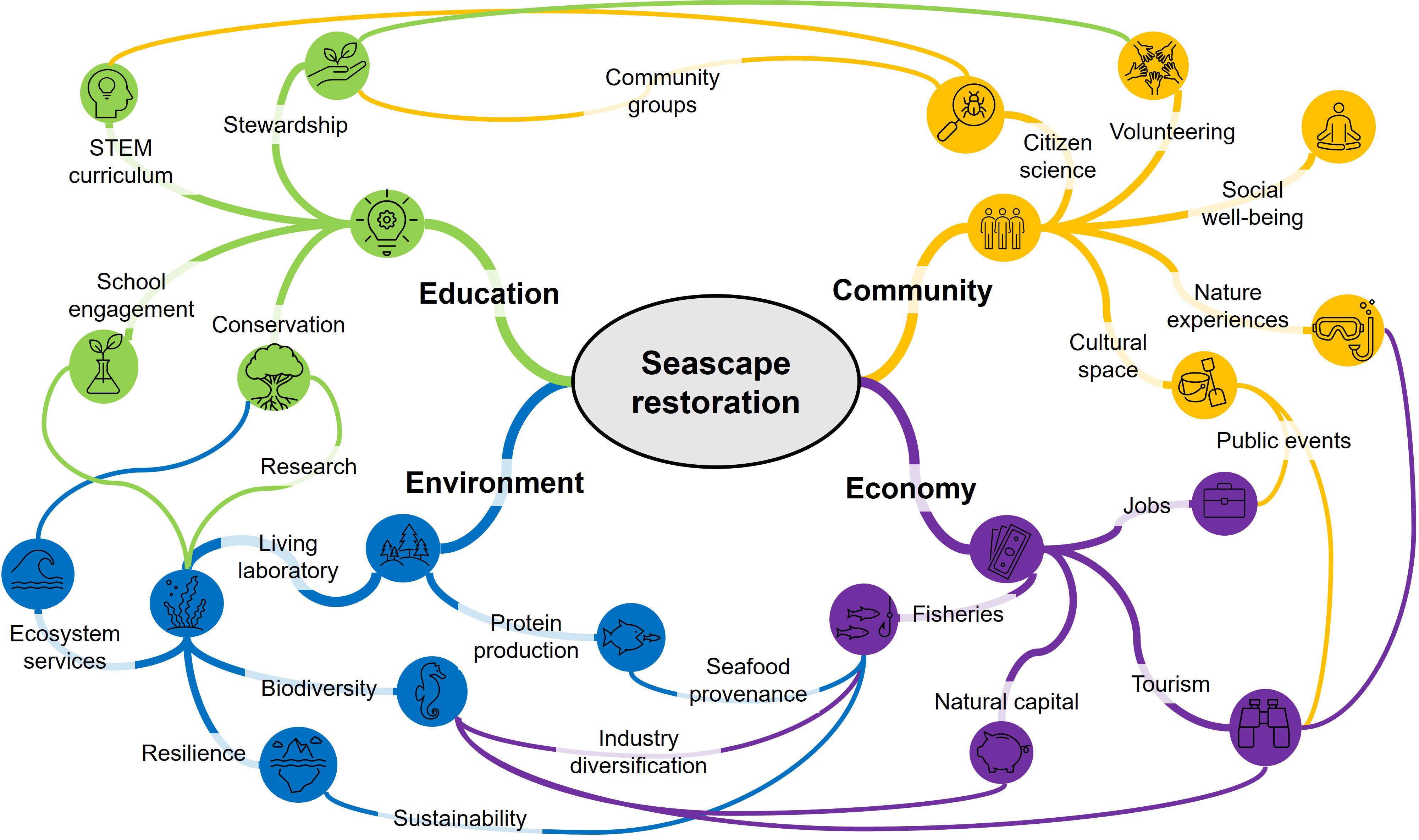
Figure 2 A vision of the broad socio-ecological and economic benefits that could emerge where restorations support healthy functional seascapes. Note that all nodes within each of the four sectors are related, and that many more linkages between sector nodes are likely.
The restoration and conservation of functional seascapes has the potential to generate considerable socio-cultural benefits beyond their long discussed ecological services (e.g., Costanza et al., 1997). Restorations can repair the elements of nature that provide value to people either directly or indirectly (i.e., natural capital; Blignaut et al., 2014), and can therefore support human well-being in a variety of ways (Smith et al., 2017). For example, restorations provide diverse opportunities for hands-on public participation, such as volunteer-led planting or monitoring, collecting or growing propagules, or bagging oyster shell (Tanner et al., 2014, Walters et al., 2017). Such stewardship can provide important well-being benefits through increased interaction and sense of attachment to nature, and by fostering community agency to protect nature (Kibler et al., 2018). Once restored, enhanced cultural services from multi-habitat mosaics may include more aesthetic appeal (ecological diversity; Smith et al., 2017) and enriched recreation experiences, including improved fishing and eco-tourism (e.g., kayaking, SCUBA diving, wildlife viewing; Ruiz-Frau et al., 2013). Other cultural services could include new education (e.g., living laboratories, school engagement) and tourism opportunities that support local livelihoods (Brancalion et al., 2014; Figure 2). Additionally, engagement with cultural knowledge, including Indigenous knowledge about the coastal restoration sites, can enrich historical narratives and assist in site and species selection for restoration (Thurstan et al., 2020; Reyes-García et al. 2018). Such engagement with Indigenous interests and input provides opportunities to build Indigenous agency and social justice into restoration projects (Kamelamela et al., 2022).
Discussion
Theory suggests that multi-habitat restorations will achieve ecological functions, productivity, and resilience that are greater than the sum of their individual parts. Multi-habitat restoration provides opportunities to create ecologically complex habitat mosaics, thus augmenting the recruitment of biodiverse communities, and habitat connectivity that supports seascape-level functionality. Prioritising ecological heterogeneity has been likened to the risk management of investment portfolios, whereby diversifying the ecological assets of restorations makes them more resilient to change and sudden impacts (e.g., extreme climate events; Zabin et al., 2022). Consequently, a multi-habitat approach provides a means of incorporating ecological resilience and adaptive capacity into restoration efforts (e.g., Donaher et al., 2021), while generating the valuable cultural services and opportunities for public participation that stem from restoration and conservation initiatives (Figure 2).
Our multi-habitat restoration case-studies were enabled by bringing together and leveraging the diverse restoration expertise in the State of South Australia. In bringing practitioners, researchers, and regulators together across multiple institutes, the project accessed diverse expertise in reef building, oceanography, restoration ecology, and coastal management (McAfee et al., 2021a). This increased the operational capacity and socio-ecological vision of the restoration, transitioning it from a shellfish restoration to a multi-habitat approach with expanded goals (e.g., carbon sequestration) and stakeholders. In time, it is hoped that this expanded vision will increase the project’s return on investment, which will require long-term monitoring. But in the short term, our multi-habitat approach has boosted the local ‘restoration economy’ by feeding diverse material supply chains (BenDor et al., 2015) and generated opportunities for public participation through seagrass seedling collection and oyster shell cleaning. Such socio-economic benefits are likely important for maintaining local support and social licence for future restorations. Otherwise, marine restorations may be ‘out of sight, out of mind’, risking the legitimacy for governments to invest (McAfee et al., 2020). There is little doubt that to leave a positive environmental legacy, ongoing public engagement is needed to ensure the cultural benefits (Figure 2) and sustainability of restoration activities.
Of course, practitioners will need to evaluate their restoration goals against the pros and cons of adopting a multi-habitat approach, many of which are yet to be quantified. Depending on the environmental context and goals of the project, a multi-habitat approach may be unnecessary (e.g., where single-species restorations can leverage existing connectivity), or even detrimental; where a multi-habitat approach could limit funding dedicated to individual habitats, potentially reducing the area and monitoring of restorations. Additionally, introducing habitat connectivity does not always yield positive or clear outcomes (Geraldi et al., 2009; Gilby et al., 2019) as organismal responses are highly complex and challenging to monitor. Funding for long-term monitoring is a major challenge for marine restorations (Bayraktarov et al., 2016), and the ecological and social benefits of a multi-habitat approach may not emerge for some time and require expensive and logistically challenging long-term monitoring programs.
Conclusion
Over a quarter-century ago, Naveh (1994) wrote on the vital role of restoration in creating a human-nature symbiosis to urgently repair the environment. To achieve this, Naveh (1994) warned that restorationists must “broaden their spatial and conceptual scales from the restoration of small, degraded nature islands to the restoration of large, open landscapes with their natural and cultural patterns and processes”. This Decade on Ecosystem Restoration provides new opportunity to embrace larger scales and new methodologies to advance restoration practice, including multi-habitat approaches. Evidence abounds on the natural synergises created by co-occurring habitats and the critical role of habitat connectivity to ensure that seascapes functionally benefit society, regulate climate, and boost ecological stability. Now is the time to bring people together to plan for such large-scale repair.
Data availability statement
The original contributions presented in the study are included in the article/supplementary material. Further inquiries can be directed to the corresponding author.
Author contributions
DM lead the writing of the manuscript. All authors contributed to the writing and editing of the manuscript, and approved its submission.
Conflict of interest
The authors declare that the research was conducted in the absence of any commercial or financial relationships that could be construed as a potential conflict of interest.
Publisher’s note
All claims expressed in this article are solely those of the authors and do not necessarily represent those of their affiliated organizations, or those of the publisher, the editors and the reviewers. Any product that may be evaluated in this article, or claim that may be made by its manufacturer, is not guaranteed or endorsed by the publisher.
References
Alleway H. K., Connell S. D. (2015). Loss of an ecological baseline through the eradication of oyster reefs from coastal ecosystems and human memory. Conserv. Biol. 29 (3), 795–804. doi: 10.1111/cobi.12452
Angelini C., Altieri A. H., Silliman B. R., Bertness M. D. (2011). Interactions among foundation species and their consequences for community organization, biodiversity, and conservation. BioScience 61 (10), 782–789. doi: 10.1525/bio.2011.61.10.8
Angelini C., van der Heide T., Griffin J. N., Morton J. P., Derksen-Hooijberg M., Lamers L. P., Smolders A. J, et al. (2015). Foundation species' overlap enhances biodiversity and multifunctionality from the patch to landscape scale in southeastern united states salt marshes. Proc. R. Soc. B: Biol. Sci. 282 (1811), 20150421. doi: 10.1098/rspb.2015.0421
Barbier E. B. (2019). “The value of coastal wetland ecosystem services,” in Coastal Wetlands: An Integrated Ecosystem Approach. (Amsterdam, Netherlands: Elsevier), pp. 947–964.
Bayraktarov E., Saunders M. I., Abdullah S., Mills M., Beher J., Possingham H. P., et al. (2016). The cost and feasibility of marine coastal restoration. Ecol. Appl. 26 (4), 1055–1074. doi: 10.1890/15-1077
Beck M. W., Brumbaugh R. D., Airoldi L., Carranza A., Coen L. D., Crawford C., et al. (2011). Oyster reefs at risk and recommendations for conservation, restoration, and management. Bioscience 61 (2), 107–116. doi: 10.1525/bio.2011.61.2.5
Benayas J. M. R., Newton A. C., Diaz A., Bullock J. M. (2009). Enhancement of biodiversity and ecosystem services by ecological restoration: a meta-analysis. Science 325 (5944), 1121–1124. doi: 10.1126/science.1172460
BenDor T. K., Livengood A., Lester T. W., Davis A., Yonavjak L. (2015). Defining and evaluating the ecological restoration economy. Restor. Ecol. 23 (3), 209–219. doi: 10.1111/rec.12206
Bernhardt J. R., O’Connor M. I. (2021). Aquatic biodiversity enhances multiple nutritional benefits to humans. Proc. Natl. Acad. Sci. 118 (15), e1917487118 . doi: 10.1073/pnas.1917487118
Bertness M. D., Callaway R. (1994). Positive interactions in communities. Trends Ecol. Evol. 9 (5), 191–193. doi: 10.1016/0169-5347(94)90088-4
Blignaut J., Aronson J., de Groot R. (2014). Restoration of natural capital: A key strategy on the path to sustainability. Ecol. Eng. 65, 54–61. doi: 10.1016/j.ecoleng.2013.09.003
Bostrom C., Pittman S. J., Simenstad C., Kneib R. T. (2011). Seascape ecology of coastal biogenic habitats: advances, gaps, and challenges. Mar. Ecol. Prog. Ser. 427, 191–217. doi: 10.3354/meps09051
Brancalion P. H., Cardozo I. V., Camatta A., Aronson J., Rodrigues R. R. (2014). Cultural ecosystem services and popular perceptions of the benefits of an ecological restoration project in the Brazilian Atlantic forest. Restor. Ecol. 22 (1), 65–71. doi: 10.1111/rec.12025
Brown G., Raymond C. M. (2014). Methods for identifying land use conflict potential using participatory mapping. Landscape Urban Plann. 122, 196–208. doi: 10.1016/j.landurbplan.2013.11.007
Brown J. H., Whitham T. G., Morgan Ernest S. K., Gehring C. A. (2001). Complex species interactions and the dynamics of ecological systems: long-term experiments. Science 293 (5530), 643–650. doi: 10.1126/science.293.5530.643
Bullock J. M., Fuentes‐Montemayor E., McCarthy B., Park K., Hails R. S., Woodcock B. A., et al. (2022). Future restoration should enhance ecological complexity and emergent properties at multiple scales. Ecography, 2022 (4).
Cabaço S., Santos R., Duarte C. M. (2008). The impact of sediment burial and erosion on seagrasses: a review. Estuarine Coast. Shelf Sci. 79, 354–366. doi: 10.1016/j.ecss.2008.04.021
Calder P. C. (2015). Marine omega-3 fatty acids and inflammatory processes: Effects, mechanisms and clinical relevance. Biochim. Biophys. Acta (BBA)-Molecular Cell Biol. Lipids 1851 (4), 469–484. doi: 10.1016/j.bbalip.2014.08.010
Clarke B., Stocker L., Coffey B., Leith P., Harvey N., Baldwin C., et al. (2013). Enhancing the knowledge–governance interface: Coasts, climate and collaboration. Ocean Coast. Manage. 86, 88–99. doi: 10.1016/j.ocecoaman.2013.02.009
Clarke B., Thet A. K., Sandhu H., Dittmann S. (2021). Integrating cultural ecosystem services valuation into coastal wetlands restoration: A case study from south Australia. Environ. Sci. Policy 116, 220–229. doi: 10.1016/j.envsci.2020.11.014
Connell S. D. (2003). The monopolization of understorey habitat by subtidal encrusting coralline algae: a test of the combined effects of canopy-mediated light and sedimentation. Mar. Biol. 142 (6), 1065–1071. doi: 10.1007/s00227-003-1021-z
Connell S. D., Russell B. D., Turner D. J., Shepherd S.A., Kildea T., Miller D., et al. (2008). Recovering a lost baseline: missing kelp forests from a metropolitan coast. Mar. Ecol. Prog. Ser. 360, 63–72. doi: 10.3354/meps07526
Costanza R., d'Arge R., De Groot R., Farber S., Grasso M., Hannon B., et al (1997). The value of the world's ecosystem services and natural capital. Nature 387 (6630), 253–260.
Crook D. A., Lowe W. H., Allendorf F. W., Erős T., Finn D. S., Gillanders B. M., et al. (2015). Human effects on ecological connectivity in aquatic ecosystems: integrating scientific approaches to support management and mitigation. Sci. Total Environ. 534, 52–64. doi: 10.1016/j.scitotenv.2015.04.034
DeAngelis B. M., Sutton-Grier A. E., Colden A., Arkema K. K., Baillie C. J., Bennett R. O., et al. (2020). Social factors key to landscape-scale coastal restoration: lessons learned from three US case studies. Sustainability 12 (3), 869. doi: 10.3390/su12030869
Derksen-Hooijberg M., Angelini C., Lamers L. P., Borst A., Smolders A., Hoogveld J. R., et al. (2018). Mutualistic interactions amplify saltmarsh restoration success. J. Appl. Ecol. 55 (1), 405–414. doi: 10.1111/1365-2664.12960
Dittmann S., Mosley L., Clanahan M., Quinn J., Crooks S., Emmer I., et al. (2019). “Proof of concept for tidal re-connection as a blue carbon offset project,” in Goyder institute for water research technical report series no. 19/29. (Adelaide, SA: Goyder Institute)
Donaher S. E., Baillie C. J., Smith C. S., Zhang Y. S., Albright A., Trackenberg S. N., et al. (2021). Bivalve facilitation mediates seagrass recovery from physical disturbance in a temperate estuary. Ecosphere 12 (11), e03804. doi: 10.1002/ecs2.3804
Donnelly M., Shaffer M., Connor S., Sacks P., Walters L. (2017). Using mangroves to stabilize coastal historic sites: deployment success versus natural recruitment. Hydrobiologia 803 (1), 389–401. doi: 10.1007/s10750-017-3155-x
Duarte C. M., Agusti S., Barbier E., Britten G. L., Castilla J. C., Gattuso J. P., et al. (2020). Rebuilding marine life. Nature 580 (7801), 39–51. doi: 10.1038/s41586-020-2146-7
Dunic J. C., Brown C. J., Connolly R. M., Turschwell M. P., Côté I. M. (2021). Long-term declines and recovery of meadow area across the world’s seagrass bioregions. Global Change Biol. 27 (17), 4096–4109. doi: 10.1111/gcb.15684
Eger A. M., Marzinelli E., Gribben P., Johnson C. R., Layton C., Steinberg P. D., et al. (2020). Playing to the positives: using synergies to enhance kelp forest restoration. Front. Mar. Sci., 544. doi: 10.3389/fmars.2020.00544
Fernandes M. B., Hennessy A., Law W. B., Daly R., Gaylard S., Lewis M., et al. (2022). Landsat historical records reveal large-scale dynamics and enduring recovery of seagrasses in an impacted seascape. Sci. Total Environ. 813, 152646. doi: 10.1016/j.scitotenv.2021.152646
Fodrie F. J., Rodriguez A. B., Gittman R. K., Grabowski J. H., Lindquist N. L., Peterson C. H., et al. (2017). Oyster reefs as carbon sources and sinks. Proc. R. Soc. B: Biol. Sci. 284 (1859), 20170891. doi: 10.1098/rspb.2017.0891
Gagnon K., Rinde E., Bengil E. G., Carugati L., Christianen M. J., Danovaro R., et al. (2020). Facilitating foundation species: The potential for plant–bivalve interactions to improve habitat restoration success. J. Appl. Ecol. 57 (6), 1161–1179. doi: 10.1111/1365-2664.13605
Galloway A. W. E., Winder M. (2015). Partitioning the relative importance of phylogeny and environmental conditions on phytoplankton fatty acids. PloS One 10, e0130053. doi: 10.1371/journal.pone.0130053
Gedan K. B., Silliman B. R. (2009). Using facilitation theory to enhance mangrove restoration. AMBIO: A J. Hum. Environ. 38 (2), 109–109. doi: 10.1579/0044-7447-38.2.109
Geraldi N. R., Ortega A., Serrano O., Macreadie P. I., Lovelock C. E., Krause-Jensen D., et al. (2019). Fingerprinting blue carbon: rationale and tools to determine the source of organic carbon in marine depositional environments. Front. Mar. Sci. 263. doi: 10.3389/fmars.2019.00263
Geraldi N. R., Powers S. P., Heck K. L., Cebrian J. (2009). Can habitat restoration be redundant? Response of mobile fishes and crustaceans to oyster reef restoration in marsh tidal creeks. Marine Ecology Progress Series 389, 171–180.
Gilby B. L., Olds A. D., Chapman S., Goodridge Gaines L. A., Henderson C. J., Ortodossi N. L., et al. (2021). Attraction versus production in restoration: spatial and habitat effects of shellfish reefs for fish in coastal seascapes. Restor. Ecol. 29 (7), e13413. doi: 10.1111/rec.13413
Gilby B. L., Olds A. D., Henderson C. J., Ortodossi N. L., Connolly R. M., Schlacher T. A. (2019). Seascape context modifies how fish respond to restored oyster reef structures. ICES Journal of Marine Science 76 (4), 1131–1139.
Gilby B. L., Olds A. D., Peterson C. H., Connolly R. M., Voss C. M., Bishop M. J., et al. (2018). Maximizing the benefits of oyster reef restoration for finfish and their fisheries. Fish Fisheries 19 (5), 931–947. doi: 10.1111/faf.12301
Goldberg L., Lagomasino D., Thomas N., Fatoyinbo T. (2020). Global declines in human-driven mangrove loss. Global Change Biol. 26 (10), 5844–5855. doi: 10.1111/gcb.15275
Gopi K., Mazumder D., Sammut J., Saintilan N. (2019). Determining the provenance and authenticity of seafood: A review of current methodologies. Trends Food Sci. Technol. 91, 294–304. doi: 10.1016/j.tifs.2019.07.010
Gorman D., Connell S. D. (2009). Recovering subtidal forests in human-dominated landscapes. J. Appl. Ecol. 46 (6), 1258–1265. doi: 10.1111/j.1365-2664.2009.01711.x
Greiner J. T., McGlathery K. J., Gunnell J., McKee B. A. (2013). Seagrass restoration enhances “blue carbon”. sequestration Coast. waters. PloS One 8 (8), e72469. doi: 10.1371/journal.pone.0072469
Grice A. C., Cassady J., Nicholas D. M. (2012). Indigenous and non-indigenous knowledge and values combine to support management of nywaigi lands in the Queensland coastal tropics. Ecol. Manage. Restor. 13, 93–97. doi: 10.1111/j.1442-8903.2011.00621.x
Haddad L., Hawkes C., Webb P., Thomas S., Beddington J., Waage J., et al. (2016). A new global research agenda for food. Nature 540 (7631), 30–32. doi: 10.1038/540030a
Han T., Shi R., Qi Z., Huang H., Liang Q., Liu H.. (2017). Interactive effects of oyster and seaweed on seawater dissolved inorganic carbon systems: implications for integrated multi-trophic aquaculture. Aquaculture Environ. Interact. 9, 469–478. doi: 10.3354/aei00246
Hicks C. C., Graham N. A., Maire E., Robinson J. P. (2021). Secure local aquatic food systems in the face of declining coral reefs. One Earth 4 (9), 1214–1216. doi: 10.1016/j.oneear.2021.08.023
HLPE (2014). Sustainable fisheries and aquaculture for food security and nutrition (Rome: A report by the High Level Panel of Experts on Food Security and Nutrition of the Committee on World Food Security).
Hyndes G. A., Nagelkerken I., McLeod R. J., Connolly R. M., Lavery P. S., Vanderklift M. A. (2014). Mechanisms and ecological role of carbon transfer within coastal seascapes. Biol. Rev. 89 (1), 232–254. doi: 10.1111/brv.12055
Infantes E., Terrados J., Orfila A., Cañellas B., Alvarez-Ellacuria A. (2009). Wave energy and the upper depth limit distribution of posidonia oceanica. Botanica Marina 52 (5), 419–427. doi: 10.1515/BOT.2009.050
Irving A. D., Connell S. D. (2006). Physical disturbance by kelp abrades erect algae from the understorey. Mar. Ecol. Prog. Ser. 324, 127–137. doi: 10.3354/meps324127
Isbell F., Craven D., Connolly J., Loreau M., Schmid B., Beierkuhnlein C., et al. (2015). Biodiversity increases the resistance of ecosystem productivity to climate extremes. Nature 526 (7574), 574–577. doi: 10.1038/nature15374
Jackson J. B., Kirby M. X., Berger W. H., Bjorndal K. A., Botsford L. W., Bourque B. J., et al. (2001). Historical overfishing and the recent collapse of coastal ecosystems. Science 293 (5530), 629–637. doi: 10.1126/science.1059199
Kamelamela K. L., Springer H. K., Keakealani R. K.U., Ching M. U., Ticktin T., Ohara R. D., et al. (2022). Kōkua aku, kōkua mai: An indigenous consensus-driven and place-based approach to community led dryland restoration and stewardship. For. Ecol. Manage. 506, 119949. doi: 10.1016/j.foreco.2021.119949
Kibler K., Cook G., Chambers L., Donnelly M., Hawthorne T., Rivera F., et al. (2018). Integrating sense of place into ecosystem restoration: a novel approach to achieve synergistic social-ecological impact. Ecol. Soc. 23 (4), 25. doi: 10.5751/ES-10542-230425
Kobryn H. T., Brown G., Munro J., Moore S. A. (2018). Cultural ecosystem values of the Kimberley coastline: An empirical analysis with implications for coastal and marine policy. Ocean Coast. Manage. 162, 71–84. doi: 10.1016/j.ocecoaman.2017.09.002
Lang’at J. K., Kirui B. K., Skov M. W., Kairo J. G., Mencuccini M., Huxham M., et al. (2013). Species mixing boosts root yield in mangrove trees. Oecologia 172 (1), 271–278. doi: 10.1007/s00442-012-2490-x
Lang A. C., Buschbaum C. (2010). Facilitative effects of introduced pacific oysters on native macroalgae are limited by a secondary invader, the seaweed sargassum muticum. J. Sea Res. 63, 119–128. doi: 10.1016/j.seares.2009.11.002
Layton C., Cameron M. J., Shelamoff V., Tatsumi M., Wright J. T., Johnson C. R. (2021). A successful method of transplanting adult ecklonia radiata kelp, and relevance to other habitat-forming macroalgae. Restor. Ecol. 29 (5), e13412. doi: 10.1111/rec.13412
Lefcheck J. S., Hughes B. B., Johnson A. J., Pfirrmann B. W., Rasher D. B., Smyth A. R., et al. (2019). Are coastal habitats important nurseries? A meta-analysis. Conserv. Lett. 12 (4), e12645. doi: 10.1111/conl.12645
Lovelock C. E., Adame M. F., Bradley J., Dittmann S., Hagger V., Hickey S. M., et al. (2022). An Australian blue carbon method to estimate climate change mitigation benefits of coastal wetland restoration. Restor. Ecol., e13739. doi: 10.1111/rec.13739
Lyver P. O. B., Akins A., Phipps H., Kahui V., Towns D. R., Moller H., et al. (2016). Key biocultural values to guide restoration action and planning in New Zealand. Restor. Ecol. 24 (3), 314–323. doi: 10.1111/rec.12318
Macreadie P. I., Costa M. D., Atwood T. B., Friess D. A., Kelleway J. J., Kennedy H., et al. (2021). Blue carbon as a natural climate solution. Nat. Rev. Earth Environ. 2 (12), 826–839. doi: 10.1038/s43017-021-00224-1
McAfee D., Alleway H. K., Connell S. D. (2020). Environmental solutions sparked by environmental history. Conserv. Biol. 34 (2), 386–394. doi: 10.1111/cobi.13403
McAfee D., Connell S. D. (2020). Cuing oyster recruitment with shell and rock: Implications for timing reef restoration. Restor. Ecol. 28, 506–511. doi: 10.1111/rec.13134
McAfee D., Costanza R., Connell S. D. (2021a). Valuing marine restoration beyond the ‘too small and too expensive’. Trends Ecol. Evol. 36 (11), 968–971. doi: 10.1016/j.tree.2021.08.002
McAfee D., Larkin C., Connell S. D. (2021b). Multi-species restoration accelerates recovery of extinguished oyster reefs. J. Appl. Ecol. 58 (2), 286–294. doi: 10.1111/1365-2664.13719
McAfee D., McLeod I. M., Alleway H. K., Bishop M. J., Branigan S., Connell S. D., et al. (2022). Turning a lost reef ecosystem into a national restoration program. Conserv. Biol. doi: 10.1111/cobi.13958
McAfee D., Reinhold S. L., Alleway H. K., Connell S. D. (2021c). Environmental solutions fast-tracked: Reversing public scepticism to public engagement. Biol. Conserv. 253, 108899. doi: 10.1016/j.biocon.2020.108899
McGlathery K. J., Reynolds L. K., Cole L. W., Orth R. J., Marion S. R., Schwarzschild A. (2012). Recovery trajectories during state change from bare sediment to eelgrass dominance. Mar. Ecol. Prog. Ser. 448, 209–221. doi: 10.3354/meps09574
McNiven I. (2004). Saltwater people: spiritscapes, maritime rituals and the archaeology of Australian indigenous seascapes. World Archaeology 35 (3), 329–349. doi: 10.1080/0043824042000185757
Messier C., Bauhus J., Sousa-Silva R., Auge H., Baeten L., Barsoum N., et al. (2021). For the sake of resilience and multifunctionality, let's diversify planted forests! Conserv. Lett. 15 (1), e12829. doi: 10.1111/conl.12829
Milbrandt E. C., Thompson M., Coen L. D., Grizzle R. E., Ward K. (2015). A multiple habitat restoration strategy in a semi-enclosed Florida embayment, combining hydrologic restoration, mangrove propagule plantings and oyster substrate additions. Ecol. Eng. 83, 394–404. doi: 10.1016/j.ecoleng.2015.06.043
Nagelkerken I. (2009). “Evaluation of nursery function of mangroves and seagrass beds for tropical decapods and reef fishes: patterns and underlying mechanisms,” in Ecological connectivity among tropical coastal ecosystems. Ed. Nagelkerken I. (Dordrecht, the Netherlands: Springer Science and Business Media), 357–399.
Nagelkerken I., Sheaves M., Baker R., Connolly R. M. (2015). The seascape nursery: a novel spatial approach to identify and manage nurseries for coastal marine fauna. Fish Fisheries 16 (2), 362–371. doi: 10.1111/faf.12057
Nardini D. (2019). “Living the dream”: Surfing as cultural heritage on australia’s gold coast. Int. J. History Sport 36 (6), 592–610. doi: 10.1080/09523367.2019.1664475
Naveh Z. (1994). From biodiversity to ecodiversity: a landscape-ecology approach to conservation and restoration. Restor. Ecol. 2 (3), 180–189. doi: 10.1111/j.1526-100X.1994.tb00065.x
Olds A. D., Connolly R. M., Pitt K. A., Pittman S. J., Maxwell P. S., Huijbers C. M., et al. (2016). Quantifying the conservation value of seascape connectivity: a global synthesis. Global Ecol. Biogeography 25 (1), 3–15. doi: 10.1111/geb.12388
Olson A. M., Hessing-Lewis M., Haggarty D., Juanes F. (2019). Nearshore seascape connectivity enhances seagrass meadow nursery function. Ecol. Appl. 29 (5), e01897. doi: 10.1002/eap.1897
Peterson B. J., Heck K. L. Jr. (2001). Positive interactions between suspension-feeding bivalves and seagrass a facultative mutualism. Mar. Ecol. Prog. Ser. 213, 143–155. doi: 10.3354/meps213143
Pinnell C. M., Ayala G. S., Patten M. V., Boyer K. E. (2021). Seagrass and oyster reef restoration in living shorelines: effects of habitat configuration on invertebrate community assembly. Diversity 13 (6), 246. doi: 10.3390/d13060246
Pittman S. J., Yates K. L., Bouchet P. J., Alvarez-Berastegui D., Andréfouët S., Bell S. S., et al. (2021). Seascape ecology: identifying research priorities for an emerging ocean sustainability science. Mar. Ecol. Prog. Ser. 663, 1–29. doi: 10.3354/meps13661
Reeves S. E., Renzi J. J., Fobert E. K., Silliman B. R., Hancock B., Gillies C. L. (2020). Facilitating better outcomes: how positive species interactions can improve oyster reef restoration. Frontiers in Marine Science, 7, 656.
Reis-Santos P., Tanner S. E., Vasconcelos R. P., Elsdon T. S., Cabral H. N., Gillanders B. M. (2013). Connectivity between estuarine and coastal fish populations: contributions of estuaries are not consistent over time. Mar. Ecol. Prog. Ser. 491, 177–186. doi: 10.3354/meps10458
Renzi J. J., He Q., Silliman B. R. (2019). Harnessing positive species interactions to enhance coastal wetland restoration. Front. Ecol. Evol. 131. doi: 10.3389/fevo.2019.00131
Reyes-García V., Fernández-Llamazares Á., McElwee P., Molnár Z., Öllerer K., Wilson S. J., et al. (2018). The contributions of indigenous peoples and local communities to ecological restoration. Restor. Ecol. 27 (1), 3–8. doi: 10.1111/rec.12894
Robinson J. P., Maire E., Bodin N., Hempson T. N., Graham N. A., Wilson S. K., et al. (2022). Climate-induced increases in micronutrient availability for coral reef fisheries. One Earth 5 (1), 98–108. doi: 10.1016/j.oneear.2021.12.005
Ruiz-Frau A., Hinz H., Edwards-Jones G., Kaiser M. J. (2013). Spatially explicit economic assessment of cultural ecosystem services: Non-extractive recreational uses of the coastal environment related to marine biodiversity. Mar. Policy 38, 90–98. doi: 10.1016/j.marpol.2012.05.023
Sharma S., Goff J., Moody R. M., Byron D., Heck K. L. Jr., Powers S. P., et al. (2016). Do restored oyster reefs benefit seagrasses? Exp. study Northern Gulf Mexico. Restor. Ecol. 24 (3), 306–313. doi: 10.1111/rec.12329
Sheaves M., Baker R., Nagelkerken I., Connolly R. M. (2015). True value of estuarine and coastal nurseries for fish: incorporating complexity and dynamics. Estuaries Coasts 38, 401–414. doi: 10.1007/s12237-014-9846-x
Shelamoff V., Layton C., Tatsumi M., Cameron M. J., Wright J. T., Johnson C. R. (2019). Ecosystem engineering by a canopy-forming kelp facilitates the recruitment of native oysters. Restor. Ecol. 27 (6), 1442–1451. doi: 10.1111/rec.13019
Silliman B. R., Schrack E., He Q., Cope R., Santoni A., van der Heide T., et al. (2015). Facilitation shifts paradigms and can amplify coastal restoration efforts. Proc. Natl. Acad. Sci. 112 (46), 14295–14300. doi: 10.1073/pnas.1515297112
Smale D. A., Moore P. J., Queirós A., Higgs S., Burrows M. T. (2018). Appreciating interconnectivity between habitats is key to blue carbon management. Front. Ecol. Environ. 16 (2), 71–73. doi: 10.1002/fee.1765
Smith A. C., Harrison P. A., Soba M. P., Archaux F., Blicharska M., Egoh B. N., et al. (2017). How natural capital delivers ecosystem services: A typology derived from a systematic review. Ecosystem Serv. 26, 111–126. doi: 10.1016/j.ecoser.2017.06.006
Smith C. S., Rudd M. E., Gittman R. K., et al. (2020). Coming to terms with living shorelines: a scoping review of novel restoration strategies for shoreline protection. Front. Mar. Sci. 7,434. doi: 10.3389/fmars.2020.00434
Soliveres S., van der Plas F., Manning P., Prati D., Gossner M. M., Renner S. C., et al. (2016). Biodiversity at multiple trophic levels is needed for ecosystem multifunctionality. Nature 536 (7617), 456–459. doi: 10.1038/nature19092
Tanner J. E. (2005). “Three decades of habitat change in gulf st. Vincent, south Australia,” in Habitat modification and its influence on prawn and crab fisheries, 199. (Adelaide: Transactions of the Royal Society of South Australia)
Tanner J. E., Irving A. D., Fernandes M., Fotheringham D., McArdle A., Murray‐Jones S. (2014). Seagrass rehabilitation off metropolitan Adelaide: a case study of loss, action, failure and success. Ecol. Manage. Restor. 15 (3), 168–179. doi: 10.1111/emr.12133
Thilsted S. H., Thorne-Lyman A., Webb P., Bogard J. R., Subasinghe R., Phillips M. J., et al. (2016). Sustaining healthy diets: The role of capture fisheries and aquaculture for improving nutrition in the post-2015 era. Food Policy 61, 126–131. doi: 10.1016/j.foodpol.2016.02.005
Thomsen M. S., Altieri A. H., Angelini C., Bishop M. J., Gribben P. E., Lear G., et al. (2018). Secondary foundation species enhance biodiversity. Nat. Ecol. Evol. 2 (4), 634–639. doi: 10.1038/s41559-018-0487-5
Thomsen M. S., Altieri A. H., Angelini C., Bishop M. J., Bulleri F., Farhan R., et al. (2022). Heterogeneity within and among co-occurring foundation species increases biodiversity. Nat. Commun. 13 (1), 1–9. doi: 10.1038/s41467-022-28194-y
Thurstan R. H., Diggles B. K., Gillies C. L., Strong M. K., Kerkhove R., Buckley S. M., et al. (2020). Charting two centuries of transformation in a coastal social-ecological system: A mixed methods approach. Global Environ. Change 61, 102058. doi: 10.1016/j.gloenvcha.2020.102058
Tilman D., Wedin D., Knops J. (1996). Productivity and sustainability influenced by biodiversity in grassland ecosystems. Nature 379 (6567), 718–720. doi: 10.1038/379718a0
Wall C. C., Peterson B. J., Gobler C. J. (2008). Facilitation of seagrass zostera marina productivity by suspension-feeding bivalves. Mar. Ecol. Prog. Ser. 357, 165–174. doi: 10.3354/meps07289
Walters L., Donnelly M., Sacks P., Campbell D. (2017). “Lessons learned from living shoreline stabilization in popular tourist areas: boat wakes, volunteer support, and protecting historic structures,” in In living shorelines (Boca Raton, Florida: CRC Press), pp. 235–248.
Worm B., Barbier E. B., Beaumont N., Duffy J. E., Folke C., Halpern B. S., et al. (2006). Impacts of biodiversity loss on ocean ecosystem services. Science 314 (5800), 787–790. doi: 10.1126/science.1132294
Zabin C. J., Jurgens L. J., Bible J. M., Patten M. V., Chang A. L., Grosholz E. D., et al. (2022). Increasing the resilience of ecological restoration to extreme climatic events. Front. Ecol. Environ. doi: 10.1002/fee.2471
Zhang Y. S., Gittman R. K., Donaher S. E., Trackenberg S.N., Van der Heide T., Silliman B. R. (2021). Inclusion of intra-and interspecific facilitation expands the theoretical framework for seagrass restoration. Front. Mar. Sci 8, 645673. doi: 10.3389/fmars.2021.645673
Keywords: habitat connectivity, landscape ecology, positive interactions, public engagement, socio-economics, ecosystem services, multi-species restoration
Citation: McAfee D, Reis-Santos P, Jones AR, Gillanders BM, Mellin C, Nagelkerken I, Nursey-Bray MJ, Baring R, da Silva GM, Tanner JE and Connell SD (2022) Multi-habitat seascape restoration: optimising marine restoration for coastal repair and social benefit. Front. Mar. Sci. 9:910467. doi: 10.3389/fmars.2022.910467
Received: 01 April 2022; Accepted: 05 July 2022;
Published: 04 August 2022.
Edited by:
Elisabeth Marijke Anne Strain, University of Tasmania, AustraliaReviewed by:
Patrick Smallhorn-west, James Cook University, AustraliaCopyright © 2022 McAfee, Reis-Santos, Jones, Gillanders, Mellin, Nagelkerken, Nursey-Bray, Baring, da Silva, Tanner and Connell. This is an open-access article distributed under the terms of the Creative Commons Attribution License (CC BY). The use, distribution or reproduction in other forums is permitted, provided the original author(s) and the copyright owner(s) are credited and that the original publication in this journal is cited, in accordance with accepted academic practice. No use, distribution or reproduction is permitted which does not comply with these terms.
*Correspondence: Dominic McAfee, ZG9taW5pYy5tY2FmZWVAYWRlbGFpZGUuZWR1LmF1