- 1Biology Department, Woods Hole Oceanographic Institution, Woods Hole, MA, United States
- 2Hampshire College, Amherst, MA, United States
- 3Sea Mammal Research Unit, Scottish Oceans Institute, University of St Andrews, Fife, United Kingdom
- 4Department of Biology, Syracuse University, Syracuse, NY, United States
- 5Inter-American Tropical Tuna Commission, La Jolla, CA, United States
- 6Chicago Zoological Society’s Sarasota Dolphin Research Program, c/o Mote Marine Laboratory, Sarasota, FL, United States
Common bottlenose dolphins (Tursiops truncatus) produce individually distinctive signature whistles that are learned early in life and that help animals recognize and maintain contact with conspecifics. Signature whistles are the predominant whistle type produced when animals are isolated from conspecifics. Health assessments of dolphins in Sarasota, Florida (USA) provide a unique opportunity to record signature whistles, as dolphins are briefly separated from conspecifics. Recordings were first made in the mid 1970’s, and then nearly annually since 1984. The Sarasota Dolphin Whistle Database (SDWD) now contains 926 recording sessions of 293 individual dolphins, most of known age, sex, and matrilineal relatedness. The longest time span over which an individual has been recorded is 43 years, and 85 individuals have been recorded over a decade or more. Here we describe insights about signature whistle structure revealed by this unique and expansive dataset. Signature whistles of different dolphins show great variety in their fundamental frequency contours. Signature whistle types (with ‘whistle type’ defined as all whistles visually categorized as sharing a particular frequency modulation pattern) can consist of a single stereotyped element, or loop (single-loop whistles), or of multiple stereotyped loops with or without gaps (multi-loop whistles). Multi-loop signature whistle types can also show extensive variation in both number and contour of loops. In addition, fundamental frequency contours of all signature whistle types can be truncated (deletions) or embellished (additions), and other features are also occasionally incorporated. However, even with these variable features, signature whistle types tend to be highly stereotyped and easily distinguishable due to the extensive variability in contours among individuals. In an effort to quantify this individual distinctiveness, and to compare it to other species, we calculated Beecher’s Information Statistic and found it to be higher than for any other animal signal studied so far. Thus, signature whistles have an unusually high capacity to convey information on individual identity. We briefly review the large range of research projects that the SDWD has enabled thus far, and look ahead to its potential to answer a broad suite of questions about dolphin communication.
1 Introduction
Common bottlenose dolphins (Tursiops truncatus) produce a variety of sound types. Those used for communication include click-based “burst-pulse” sounds and narrow-band tonal whistles. The best studied of these sounds are signature whistles, which were first described by Melba and David Caldwell in the 1960’s (Caldwell and Caldwell, 1965, and see a more thorough description in Caldwell et al., 1990). They found that isolated dolphins primarily produced stereotyped whistle contours (patterns of frequency change over time) that were unique to each individual. The operational definition of signature whistles is that they are the predominant whistle type produced when animals are isolated from conspecifics (e.g., Janik and Sayigh, 2013). Signature whistles are unique among animal signals, in their role as “designed individual signatures” (Boughman and Moss, 2003). In contrast, most animals rely on “byproduct distinctiveness” (Boughman and Moss, 2003), or voice cues, for individual recognition (Sayigh et al., 2017). Signature whistles function in some ways like human names, in that they are learned, and function to help animals recognize and maintain contact with conspecifics (Sayigh et al., 1990; Sayigh et al., 1995; Janik and Slater, 1998; Sayigh et al., 1999).
After the Caldwells’ pioneering work, little research was carried out on dolphin signature whistles for almost two decades. This was due in no small part to the fact that dolphins do not reliably provide any visible cues associated with vocalization. Thus, identifying which individual is producing a sound, which is essential to studying any animal communication system, presents a major hurdle when studying dolphins. However, here we describe a powerful longitudinal dataset that consists of hundreds of recording sessions of known individual dolphins. We were able to identify which animals were vocalizing by recording individuals while they were being briefly handled. In Sarasota Bay, Florida, USA, brief catch-and-release events for tagging took place in the mid 1970’s (Irvine et al., 1981), allowing in-air recordings to be made (by MDS and Paul Graycar; Graycar, 1977). In the 1980’s, one of us (PLT) devised hydrophones with suction cups that could be attached directly onto the dolphin melon to more effectively identify the whistling animal (Tyack, 1985; Tyack, 1986; Figure 1). These hydrophones were first used in Sarasota in 1984, when animals were briefly held for life history research. Since that time, we have recorded dolphins with suction cup hydrophones during 45 life history and/or health assessments over 35 years. In these catch-and-release events, small groups of dolphins are encircled by a long net in shallow water and examined and sampled by veterinarians and biologists before being released on-site (Wells et al., 2004). This setting provides an ideal circumstance to record signature whistles, because dolphins typically whistle at high rates when separated from conspecifics (Watwood et al., 2005; Esch et al., 2009a). When combined with background information from decades of longitudinal monitoring through observational studies of the long-term resident members of the Sarasota Bay dolphin community (Scott et al., 1990; Wells, 2009; Wells, 2020), we have been able to compile a collection of high-quality recordings of signature whistles of animals of known age, sex, matrilineal relationships and patterns of social association.
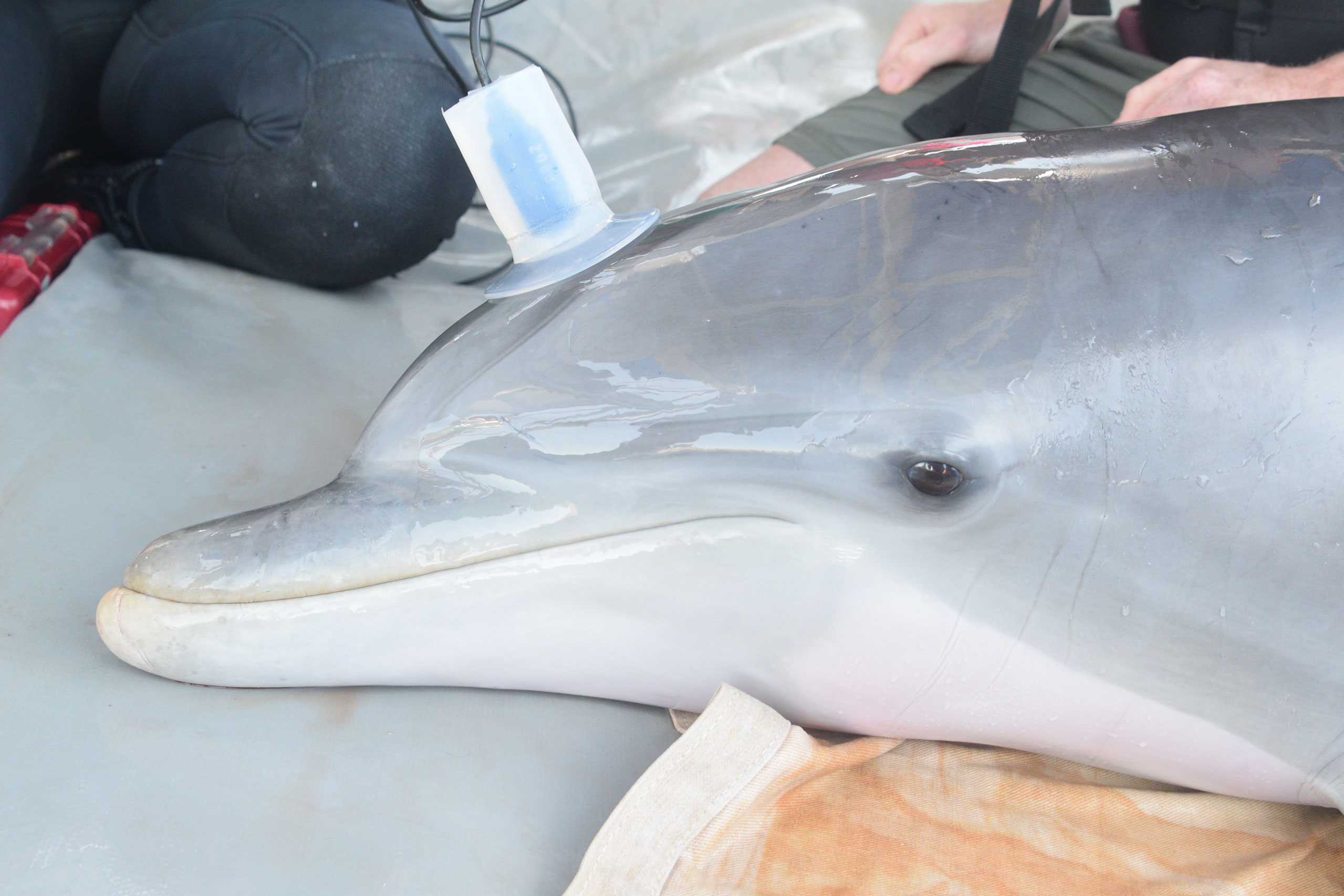
Figure 1 Common bottlenose dolphin being recorded with a suction-cup hydrophone directly on the melon, while being temporarily held during health assessments in Sarasota Bay, Florida. Photo taken by the Chicago Zoological Society’s Sarasota Dolphin Research Program under NOAA/National Marine Fisheries Service Scientific Research Permit.
Here we review the methods used over the years to record dolphins during catch-and release efforts, as well as to store and analyze data. We then describe some of the major features of this expansive whistle dataset (the Sarasota Dolphin Whistle Database). For these analyses, we defined the signature whistle as the predominant whistle type produced by an animal in a given recording session. (Dolphins do produce a large variety of other whistles, which are called non-signature whistles; these are discussed in section 4.1.6.) As is the standard for studies of dolphin whistles, we focus on visual categorizations of the contour, or pattern of changes over time, of the fundamental frequency, which can be identified in a spectrogram as the lowest frequency contour (higher frequency multiples of the fundamental frequency are called harmonics). The fundamental frequency contour alone has been shown to be sufficient to convey identity information (Janik et al., 2006). A ‘whistle type’ is defined as all whistles visually categorized as sharing a particular frequency modulation pattern (Sayigh et al., 2007; Kriesell et al., 2014); here we use the term ‘signature whistle type’ to describe a whistle type that has been identified as a signature whistle of a specific individual dolphin. Examples of several signature whistle types are shown in Figure 2. One of the most distinctive aspects of many bottlenose dolphin signature whistles is the presence of often-repetitive elements, called “loops” by the Caldwells (Caldwell et al., 1990). Whistles that consist of just a single, non-repeated element are referred to as “single-loop” whistles (Figure 2A). Whistles with multiple elements are termed “multi-loop” whistles; these loops can either all be alike or some can vary in contour shape (with introductory and/or terminal loops often different from central loops; Figures 2B, C). Loops can be either disconnected (Figure 2B) or connected (Figure 2C); if disconnected, they are typically separated by intervals of no more than 0.25 s (Esch et al., 2009b). Signature whistles are often produced in bouts, in which separate renditions of a given whistle, whether single or multi-loop, are typically separated by 1-10 s (Janik et al., 2013).
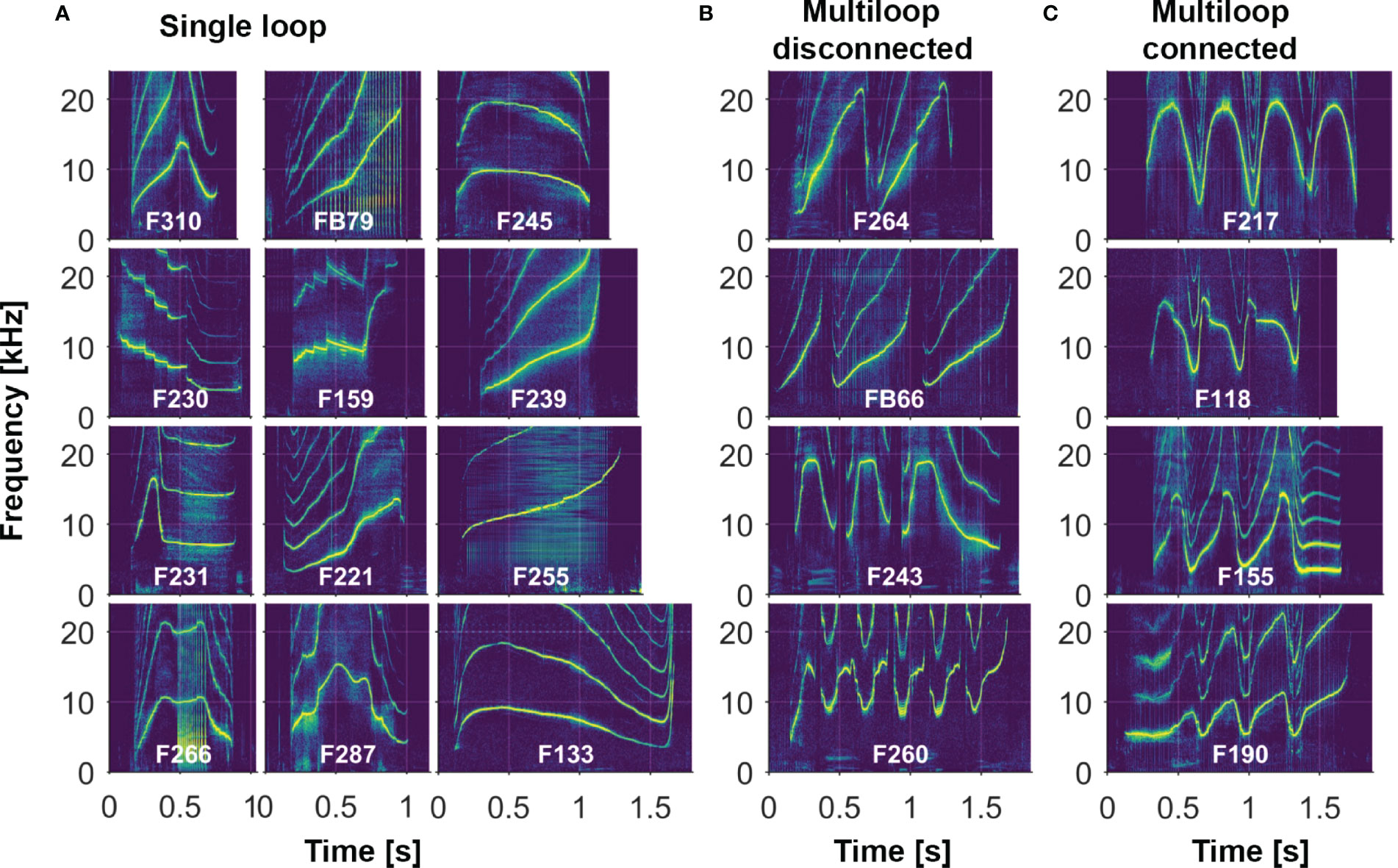
Figure 2 Examples of different whistle contour types from a subset of Sarasota dolphins (see text for more detail; labels represent animal identification numbers): (A) Single-loop whistles consist of a single, non-repeated element. (B, C) Multi-loop whistles consist of variable numbers of repetitive elements, which can be disconnected (B) or connected (C). Shown here are examples of multi-loop whistles with identical loops (F264, F217), different introductory loop (FB66, F118), different terminal loop (F243, F155), and different introductory and terminal loops (F260, F190). [Note also the presence of steps in the contours of F230 and F159].
In addition to describing overall contour structures, we report basic parameters of the fundamental frequency contour from all unique signature whistle types recorded from this population. We also describe other whistle features, many of which have been defined in various publications. These include deletions (when a portion of the signature whistle is omitted; Tyack, 1986), additions (when atypical components are added to the signature whistle; similar to “embellishments” in pilot whale calls; see Zwamborn and Whitehead, 2017), sidebands indicative of amplitude modulation in portions of the whistle (Tyack, 1986; Tyack, 1991), biphonic whistle production (two distinct, not harmonically related tonal sounds produced simultaneously; e.g., Papale et al., 2015), breaks (abrupt changes in frequency, see Wang, 1995; Marley et al., 2017), stereotyped simultaneous pulsed components (Kaplan et al., 2018), steps (Oswald et al., 2007), and nonlinear phenomena such as subharmonics and chaotic or noisy components (see descriptions for right whale calls (Tyson et al., 2007), manatee calls (Mann et al., 2006), and humpback calls (Cazau et al., 2016)).
The Sarasota Dolphin Whistle Database has enabled a large variety of research projects. One that we present here is a comparison to other animal signals of the potential of signature whistle contours for acoustic individual identification (Linhart et al., 2019; Linhart et al., 2022). To examine this, we use a sample of signature whistles to calculate Beecher’s Information Statistic (Hs) as a measure of the amount of information that is theoretically available for differentiating individuals (Beecher, 1989). We then review past studies, which have focused on, among other things, whistle classification, contexts of whistle production, whistle development and stability, whistle copying, non-signature whistles, and playback studies of whistle function and perception. We lastly highlight some ongoing and planned research projects utilizing this unique and valuable resource.
2 Materials and methods
2.1 Recording methods
Whistle recordings are obtained during periodic catch and release sessions for life history studies and health assessments, where small groups of selected dolphins are encircled with a 500-m-long, 4-m-deep seine net in shallow water (< 2-m-deep). Each individual is brought aboard a specialized veterinary examination vessel, one at a time, where sex is determined, and it is weighed, measured for a standard suite of lengths and girths, measured ultrasonically for blubber thickness, examined by veterinarians externally and through ultrasonography, sampled, marked if necessary, photographed, and then released (Wells et al., 2004; Wells, 2009; Loughlin et al., 2010; Barratclough et al., 2019). Each animal is typically aboard the veterinary boat for less than one hour. Additional procedures, including acoustic playbacks, sometimes occur while the animal is restrained in the water. Acoustic recordings are made throughout the operations. Total time from encirclement to release is typically 2-4 hours, depending on numbers of animals and procedures performed.
Different recording methods have been used during the 47-year time span over which we have been recording dolphins during catch-and-release sessions. More detail about recording methods can be found in Graycar (1977), Sayigh et al. (1990) and Sayigh et al. (2007); only a brief summary is given here. In 1975-76 recordings were made in air with a microphone near the dolphin’s head while it was held in the water in a floating stretcher or on deck. The microphone was fed into an Uher Report L or Report IC reel-to reel tape recorder with a flat frequency response of 40-20,000 Hz. During the 1980s, animals were usually placed in a raft for processing, and subsequently they have been brought onto the deck of a specially designed boat. Recordings were typically made with suction-cup hydrophones on the melon for the entire duration the dolphin was held (30-90 min) on the raft or boat (Figure 1). Suction-cup hydrophones were also attached to the melon of animals being held in the water whenever possible; if for any reason this was not possible, we would hold a hydrophone nearby in the water. We have used hydrophones both custom-made and manufactured by High Tech Inc. (Gulfport, MS), typically with frequency responses extending at least to 30,000 Hz. During 1984-1989, recordings were made on cassette tapes with Sony or Marantz stereo recorders, with frequency responses extending to approximately 20,000 Hz. From 1990-2005, recordings were made on hifi VHS tapes with Panasonic video cassette recorders, with frequency responses extending to approximately 32,000 Hz. Since 2006, recordings have been made using either Sound Devices 744T or Tascam DR-680 digital recorders, with a typical sample rate of 96,000 Hz.
2.2 Data storage and management
Over the years we have worked opportunistically to organize and preserve these recordings. Grant support in 2004 allowed us to start digitizing all of the analog recordings collected over the previous decades, a process that has now been completed. Data storage has been a continual challenge, until the costs of both physical and cloud-based storage decreased substantially relatively recently. Thanks to recent support from several sources, we are finally in a position to quality check and back up all original recordings in digital format. However, long-term curation of this digital database remains a challenge that needs to be addressed.
2.3 Data extraction and signature whistle identification
Due to the complex organization of data collected over 4+ decades, and lack of funding for a centralized database, individual researchers have developed their own protocols for extracting data from source files and hand-written notes for different research projects. In addition to data extraction for individual research projects, efforts have been made to extract small samples (10-20 exemplars) of signature whistles from most recording sessions over the past decades, with the help of numerous students and volunteers. The fundamental aspect of this process has always been to visually identify (using spectrographic analysis) the predominant whistle type produced by a given individual during a recording session, and to assign this as the signature whistle. We set 10 exemplars as the minimum number that a dolphin produces in a single recording session in order to label it as a signature whistle. Illustrating the overwhelming prevalence of signature whistles in these recordings, we recently visually classified 34,029 (85%) of 39,840 high quality whistles as signature whistles in a subset of recordings of 110 dolphins. In most cases, this process of visual classification is straightforward and inter-observer reliability is high (Janik, 1999; Sayigh et al., 2007). Often these assignments can be verified by comparing whistles recorded from the same individual in different years. Through this process, we have built a catalog of signature whistles of most dolphins in the Sarasota community.
Different programs have been used for spectrographic analysis; these range from the analog Kay Sona-Graph prior to circa 1990, to a digital Kay DSP 5500 in the early 1990’s, to more cost-effective and PC-based programs such as SIGNAL, Avisoft SASLab Pro, Adobe Audition, Matlab, and Raven from the mid 1990’s up to today. All analyses presented here were carried out in Raven Pro 1.6 (K. Lisa Yang Center for Conservation Bioacoustics, Cornell, NY, USA) and Matlab R2020b (Mathworks, Natick, MA, USA).
2.4 Data analysis
2.4.1 Whistle contour categorization and description of other whistle features
To illustrate the range of signature whistle contour types in the Sarasota dolphin population, we selected a single representative example of each of 269 signature whistle types to include in Figure 3, and from which to obtain basic measurements of fundamental contour frequencies (24 of the 293 dolphins recorded were not included because we were not able to confidently assign a signature whistle contour, due to low whistle rates). Examples of other whistle features (defined above) were also noted in the process of selecting a single exemplar for each dolphin. We then inspected multiple signature whistles from each dolphin in the Sarasota Dolphin Whistle Database in order to assign them to particular contour types based on their typical structure. These include: 1) single-loop whistles; 2) multi-loop whistles with disconnected loops; 3) multi-loop whistles with intermittent loop connections (i.e., sometimes connected, sometimes not); and 4) multi-loop whistles with connected loops (Figure 2). As noted above, if disconnected, loops are typically separated by intervals of no more than 0.25 s (Esch et al., 2009b), whereas separate renditions of a given whistle, whether single or multi-loop, are typically separated by more than 1s (Janik et al., 2013). Multi-loop whistles were further categorized into those with different introductory loops, different terminal loops, or different introductory and terminal loops.
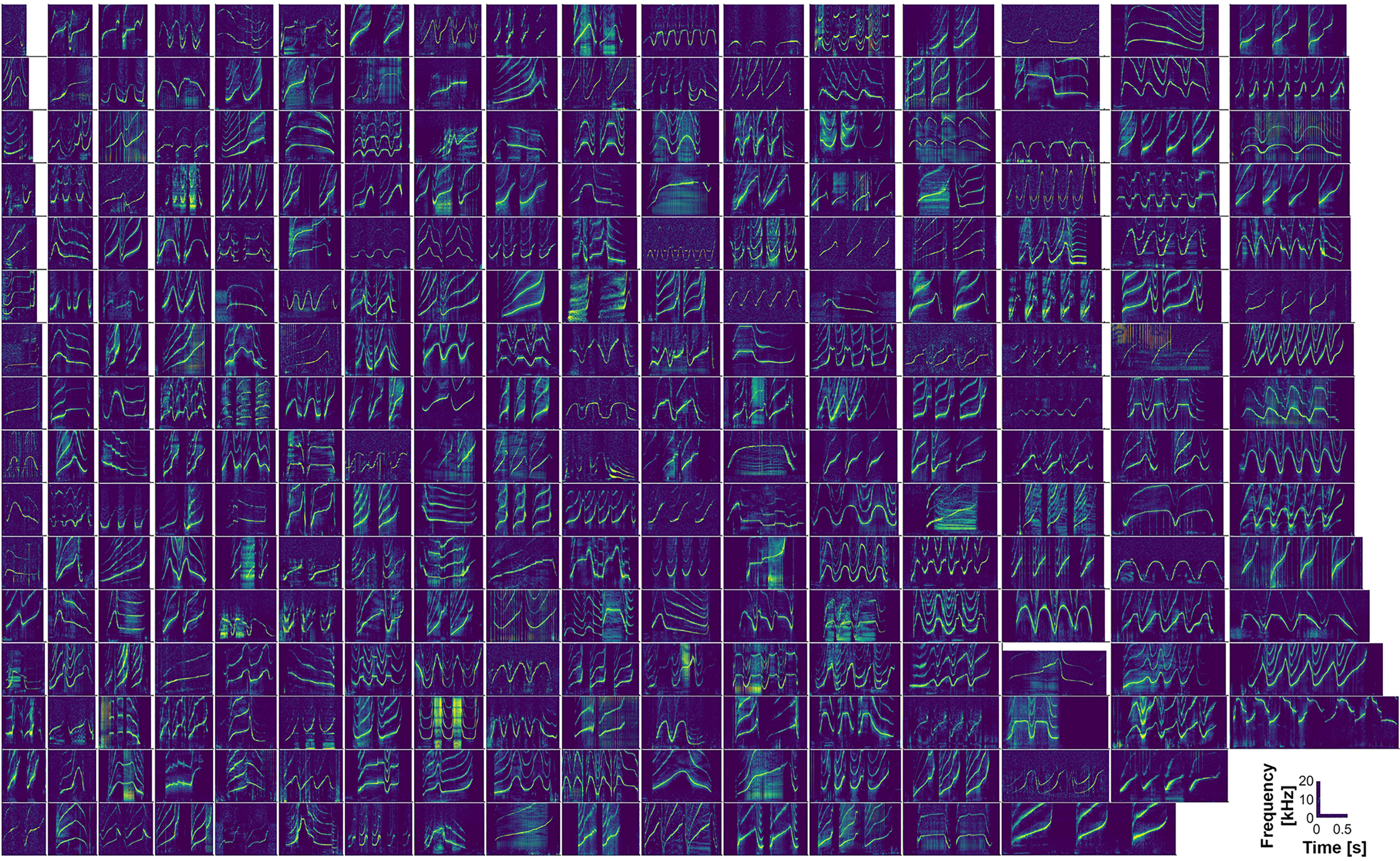
Figure 3 A single example spectrogram (48 kHz sample rate, 1024 pt Hanning window, 75% overlap) is plotted for each of 269 individual bottlenose dolphins. Whistles are sorted in columns based on increasing duration, and time and frequency axes are standardized.
2.4.2 Whistle frequency measurements
To assess the frequency range that signature whistles from the Sarasota population typically occupy, we extracted the fundamental frequency contour of each of the 269 different signature whistle exemplars. We used a supervised contour tracker to extract fundamental frequency with a 5 ms temporal resolution (King et al., 2018). The contour tracker first calculated a spectrogram with a fixed block size of 10 ms, a 5 ms overlap between adjacent blocks, and FFT size of 2048. To select the whistle contour, analysts marked time-frequency regions in the spectrogram within which the software automatically extracted the frequency with the highest power spectral density. This local spectral peak finder thus allowed analysts to extract the contour frequency in a semi-supervised manner rather than a completely manual approach. From the distribution of frequencies making up each contour, the 5th percentile, 50th percentile (median) and 95th percentile were extracted, and contour frequency range was estimated as the difference between the 95th and 5th percentile contour frequency. This analysis was intended to give a broad overview of signature whistle frequency parameters across individuals rather than variability within individuals; more in-depth analysis of individual variability in these parameters has been presented elsewhere (Esch et al., 2009b). Duration was not included in these measurements, as it is highly influenced by number of loops in multi-loop whistles, and was included in the analysis by Esch et al. (2009b). Frequency range was plotted against both maximum and minimum frequency, and the strength of these correlations were calculated.
2.4.3 Whistle stability
We visually compared signature whistles of several dolphins recorded over multiple years, and present examples of contours that are both highly stable and more labile over time. We did not attempt to quantify occurrences of these different trajectories, but this is a focus of ongoing research.
2.4.4 Quantification of individuality
A variety of metrics have been proposed over the years to quantify individual identity information (individuality) in animal signals. Recently, Linhart and colleagues (Linhart et al., 2019; Linhart et al., 2022) proposed that Beecher’s Information Statistic (Beecher, 1989) can serve as a robust and flexible metric for comparing individuality across animal communication systems.
To understand how individuality in bottlenose dolphin signature whistles compares to other animal communication systems, we calculated Beecher’s Information Statistic for a subset of dolphin signature whistles. We used largely the same whistle sample described by Sayigh et al. (2007), which was a random sample from the Sarasota Dolphin Whistle Database of 20 whistles from each of 20 individuals (totaling 400 whistles). However, because that dataset was constructed based on randomly selected whistles, we identified non-signature whistles visually (total of 7 whistles or <2% of full dataset: 1 non-signature from each of 3 animals, and 4 non-signatures from one animal) and replaced them with randomly selected signature whistles to obtain a curated dataset with 20 signature whistles for each of the 20 individual dolphins.
For each whistle, the fundamental frequency contour was extracted as above. Since whistles were mostly multi-loop in structure (as described in the introduction, and in section 3.2 below), a marker was inserted manually at the gap between separate loops, or at the obvious transition point between connected loops (the point that best separates repeating loops, often either at the minimum or maximum frequency). To estimate information content in whistles, 20 equally spaced fundamental frequency values as well as total duration were extracted from each loop following previous methods (e.g., Linhart and Šálek, 2017). This approach likely does not reflect all information available in the signature whistle (i.e. it does not capture non-contour features, features that are modulated more rapidly than can be sampled using 20 values per whistle, subtle or sequential features), so it is at best a conservative estimate of information content; however, it provides a standardized metric for comparisons to other species (Linhart et al., 2022). After calculating these 21 features (20 contour points and 1 duration) for each loop, measurements were averaged across all loops in a whistle. A principal components analysis was used to remove correlation between frequency measurements. Beecher’s information statistic (Beecher, 1989) was then calculated using a Matlab implementation of calc_HS from IDmeasurer (Linhart et al., 2019), using the number of samples (signature whistles) per individual animal as the sample size (20 in this case). The Matlab implementation was compared to the original R-implementation in IDmeasurer to ensure results were consistent.
3 Results
3.1 Data summary
Our recording library consists of 926 recording sessions of 293 individual dolphins, 147 male and 146 female. Of these, 26 were made in the 1970’s using a microphone in air near the dolphin; the remainder were made with suction-cup hydrophones since 1984. Most animals (58%) have been recorded more than once (up to a maximum of 18 times), with an overall mean of 3.1 times, and a mean of 4.7 for those recorded more than once. The longest time span over which an individual has been recorded is 43 years; 85 individuals have been recorded over a decade or more.
3.2 Whistle contour categorization
Signature whistles of Sarasota Bay dolphins can take a great variety of forms. A single example from each of 269 dolphins is shown in Figure 3 (as noted above, 24 of the 293 dolphins recorded were not included due to not being able to assign signature whistle contours to them). Whistle contour categorizations are summarized in Table 1, and examples of whistle types, as previously described, are shown in Figure 2. Overall, single-loops accounted for around a quarter of all signature whistle types (Figure 2, left side). Upsweep whistle types can occur as either single or multiple (disconnected) loops; (see FB66, FB79, F221, F239, F255 in Figure 2 as examples). Signature whistles that consisted only of a downsweep were extremely uncommon, with only two occurrences as single loop whistle types, and no occurrences where they comprised all loops of a multi-loop whistle type; Figure 3). Multi-loop whistle types were the dominant form of Sarasota signature whistles, comprising 74% of all signature whistle types. Half of these had disconnected loops (including multi-loop upsweeps; Figure 2B), and 44% had connected loops (Figure 2C). Some also had intermittent loop connections (6.5% of all multi-loop signature whistle types; Table 1). We further categorized multi-loop whistles into those with loops of the same contour shape (e.g. F264 and F217 in Figure 2), and those with introductory and/or terminal loops that were different from others in the whistle (e.g. FB66, F118, F243, F155, F260 and F190 in Figure 2). Proportions and sample sizes of these whistle types are listed in Table 1. However, note that loop contour shapes can be somewhat malleable; while not all renditions necessarily show exactly the same pattern, human observers are still highly reliable at grouping them as the same type (e.g., Sayigh et al., 2007).
3.3 Other whistle features
In addition to the variety of both single- and multi-loop structures that occur among Sarasota Bay bottlenose dolphin signature whistles, a wide range of other acoustic features are also evident. Examples of several of these features (steps, deletions, additions, biphonation, sidebands due to rapid amplitude modulation, simultaneous pulsed components, subharmonics) are shown in Figures 2–6. Figure 6 also shows examples of whistle types with variable numbers of loops. Additional examples of these as well as other features can be seen in Figure 3.
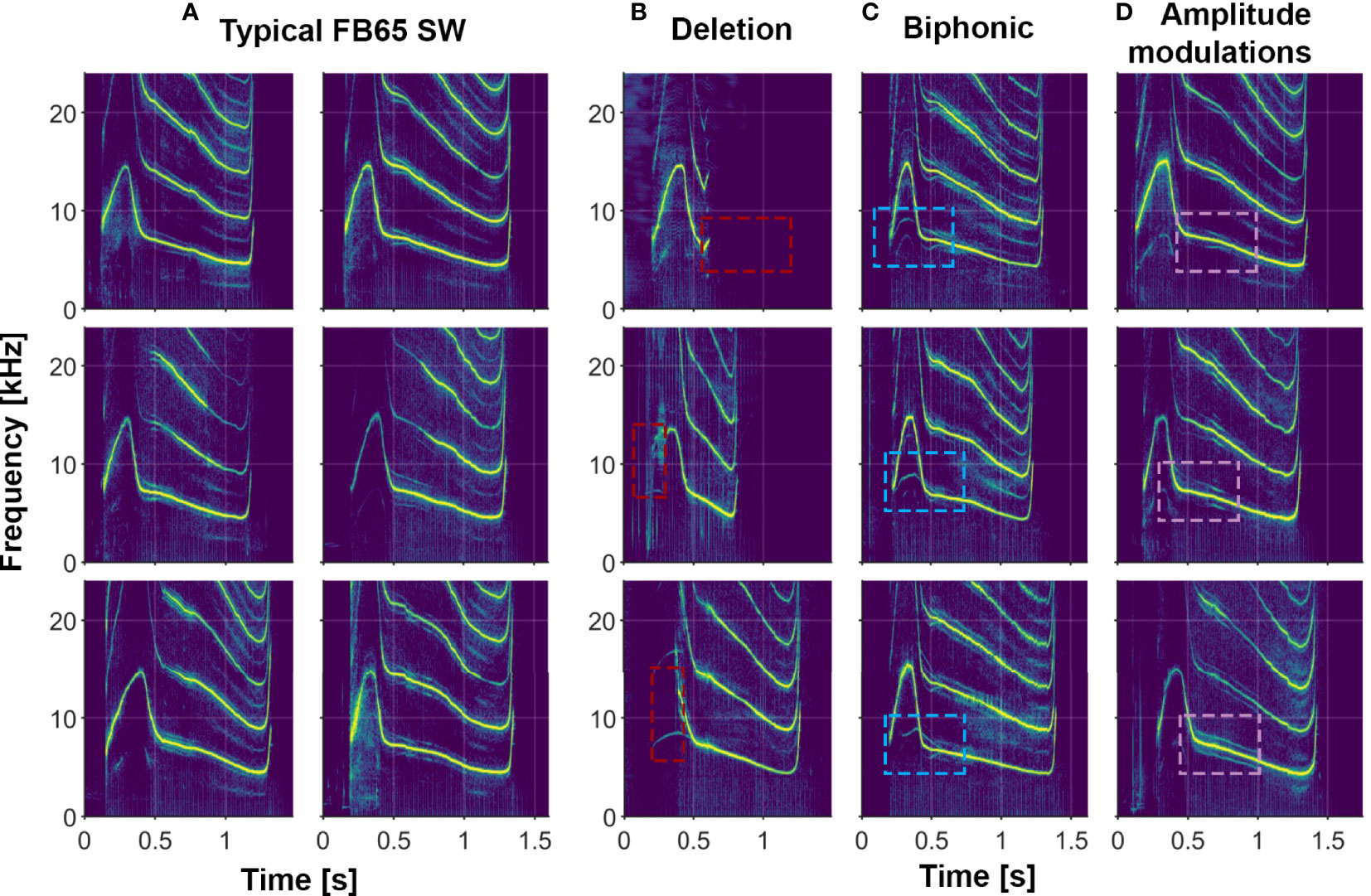
Figure 4 Examples of several whistle features in the signature whistles of a single animal (FB65). (A) Typical signature whistles; (B) Deletions where part of the signature whistle is omitted (marked by red boxes); (C) Biphonic vocalizations where a second independent contour is produced (marked by blue boxes); (D) Amplitude modulations that result in subtle spectral sidebands on either side of the fundamental frequency contour (marked by purple boxes).
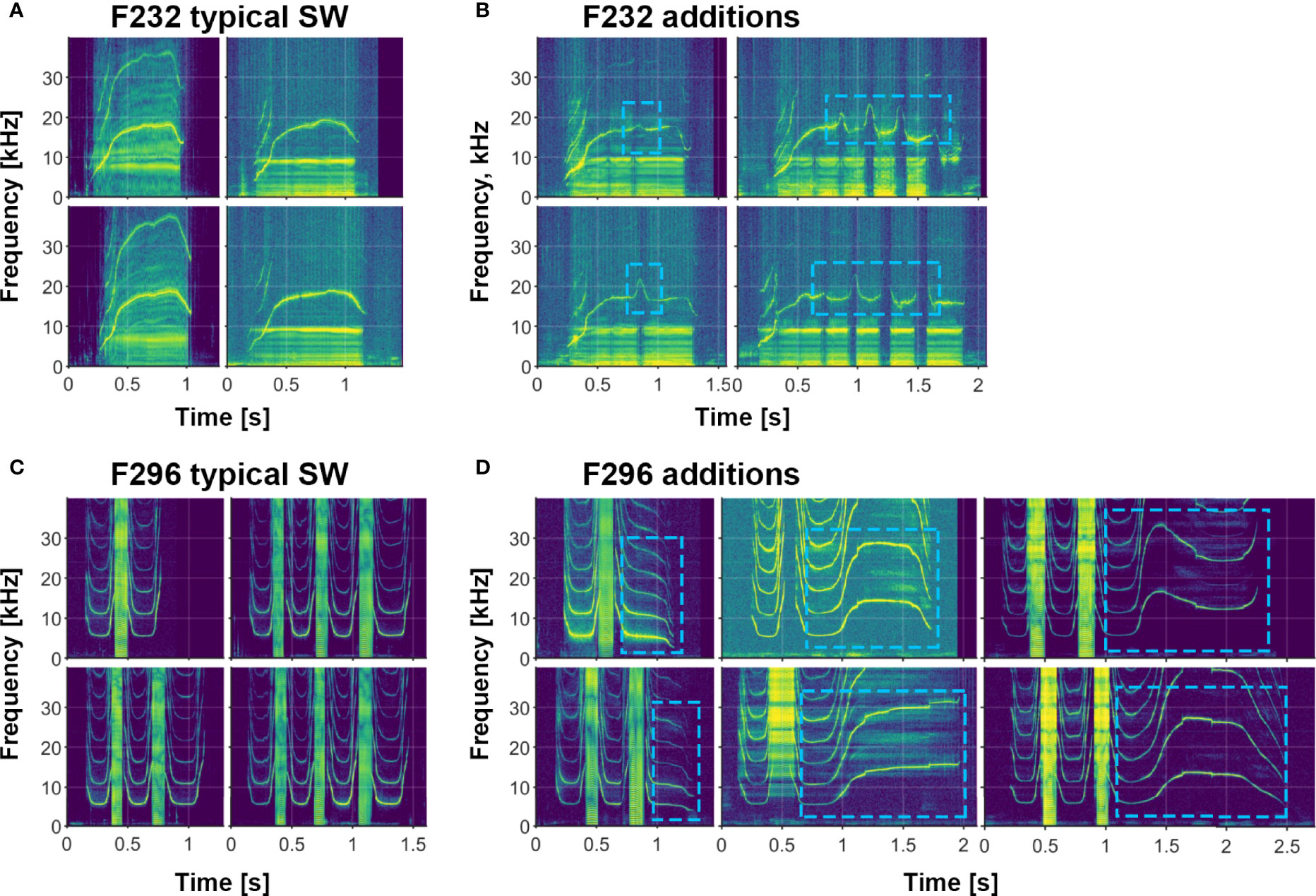
Figure 5 Examples of signature whistle additions and simultaneous burst-pulse components in two animals (F232 and F296). (A) Typical F232 signature whistle; note simultaneous whistle and burst-pulse components. (B) Signature whistle additions ranging from 1-4 inflections, all concurrent with gaps in burst-pulse component (marked by blue boxes). (C) Typical F296 signature whistle; note again concurrent burst-pulses. (D) Various additions (marked by blue boxes) that differ from the typical terminal loop.
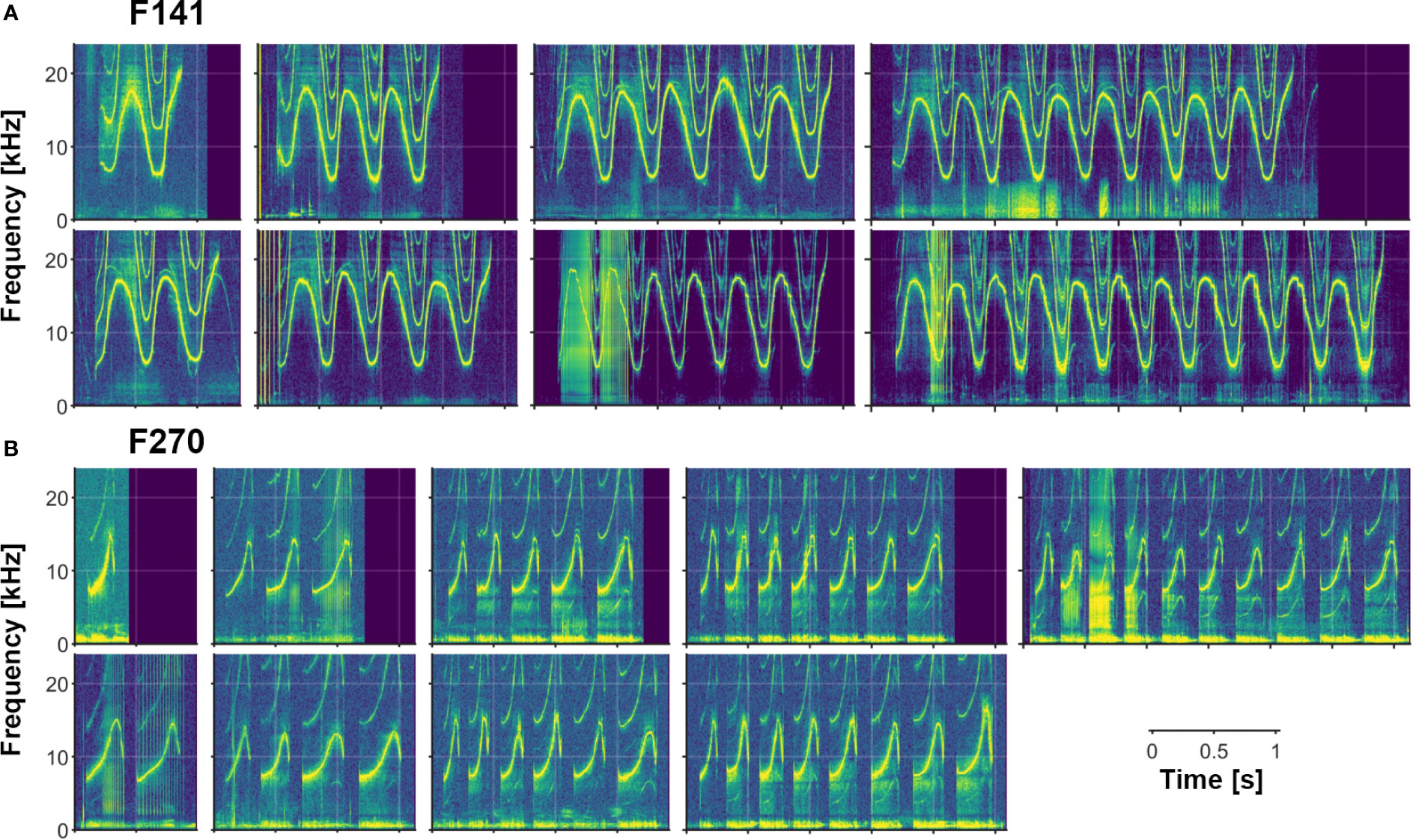
Figure 6 Examples of varying numbers of loops in multi-loop signature whistles. (A) F141 produces a connected multi-loop whistle containing 1 to at least 11 loops. (B) F270 produces a disconnected multi-loop whistle containing 1 to at least 10 loops (separated by typical inter-loop intervals; see text). [Note also subharmonics in many of F270’s whistles.].
3.4 Whistle frequency measurements
Frequency parameters can differ greatly between signature whistle types. To characterize the parameter space bottlenose dolphins use for their signature whistles in general we measured the frequency minimum, maximum and range for each of the 269 signature whistle exemplars shown in Figure 3. Minimum (5th percentile) contour frequency ranged from 1,781 to 14,766 Hz (mean ± SD of 5,807 ± 1,604), maximum (95th percentile) contour frequency ranged from 6,656 to 26,918 Hz (mean 15,380 ± 3,515) and median frequency ranged from 4,758 to 20,430 Hz (mean 9,976 ± 2,552 Hz). Total whistle frequency range, or “90% bandwidth” (assessed as the difference between 5th and 95th percentile contour frequencies) was from 1,439 - 21,907 Hz (mean 9,573 ± 3,385 Hz) and mainly depended on maximum frequency (R2 = 0.795) rather than minimum frequency (R2 = 0.016) (Figure 7). Note that values for maximum frequency and frequency range might be slightly underestimated as our early recording systems were not capable of recording frequencies above 20 kHz.
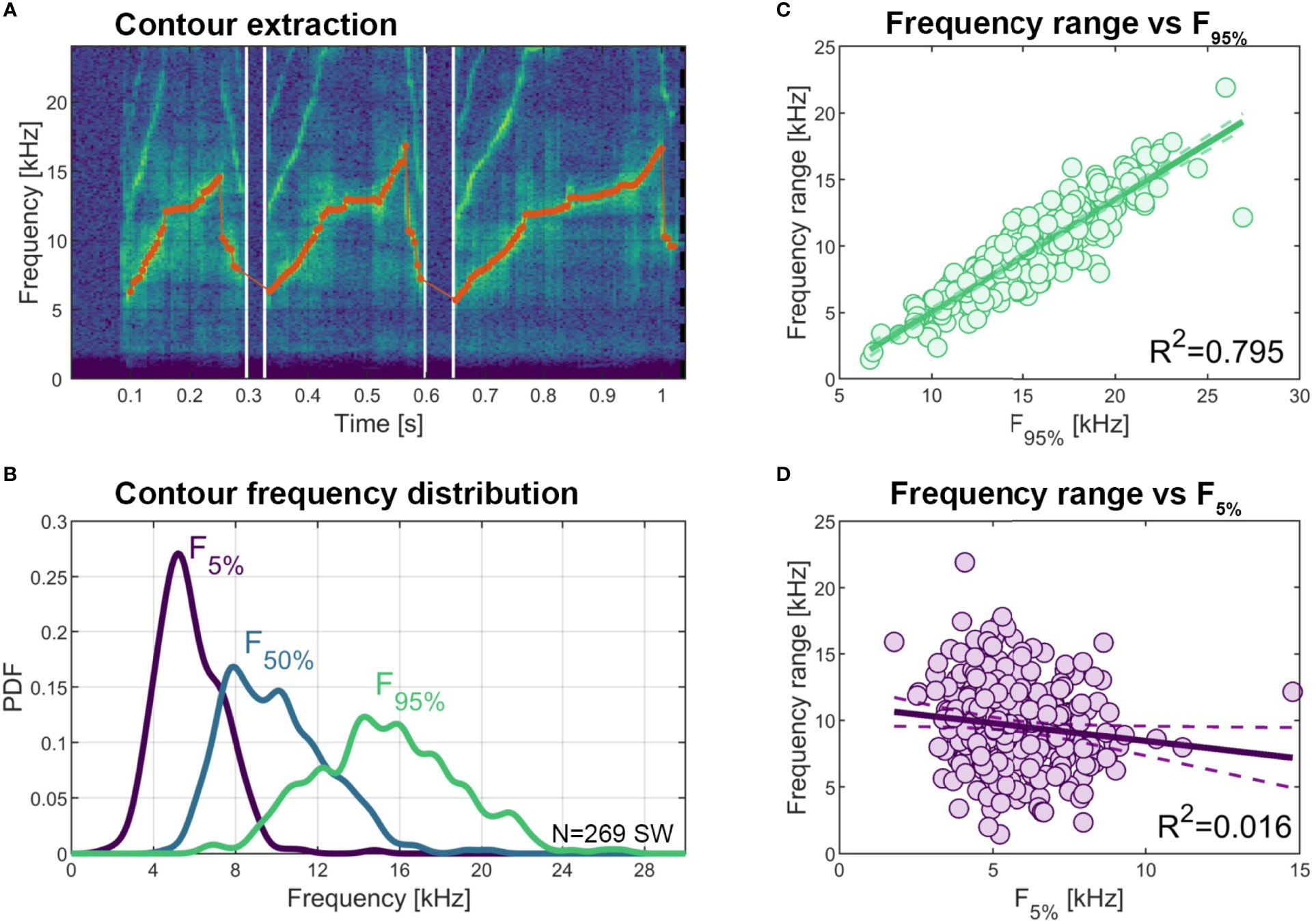
Figure 7 Variation in signature whistle contour frequency parameters. (A) Example spectrogram of a signature whistle with extracted fundamental frequency contour overlaid (red dots, 5 ms resolution). (B) Distribution (PDF: power density function) of lower 5th percentile, 50th percentile (median), and upper 95th percentile contour frequency across all 269 signature whistles. (C) Correlation between upper 95th percentile contour frequency and contour frequency range (calculated as the difference between 95th and 5th percentiles). (D) Correlation between lower 5th percentile contour frequency and contour frequency range.
3.5 Whistle stability
Visual comparisons of whistles of dolphins recorded over multiple years indicate that most whistles are highly stable (e.g., see Sayigh et al., 1990, Sayigh et al., 2007, Figure 8), with the longest recorded stability lasting 43 years. However, there are examples of whistles changing over time, some in subtle ways, and others more dramatic (see examples in Figure 9). We also observed rare examples of individuals apparently producing two different signature whistles in the same recording session (i.e., both were produced in approximately equal amounts), a phenomenon described by Caldwell and Caldwell (1968) for a common dolphin (Delphinus delphis) and by Caldwell et al. (1990) for 2 of the 120 bottlenose dolphins in their sample.
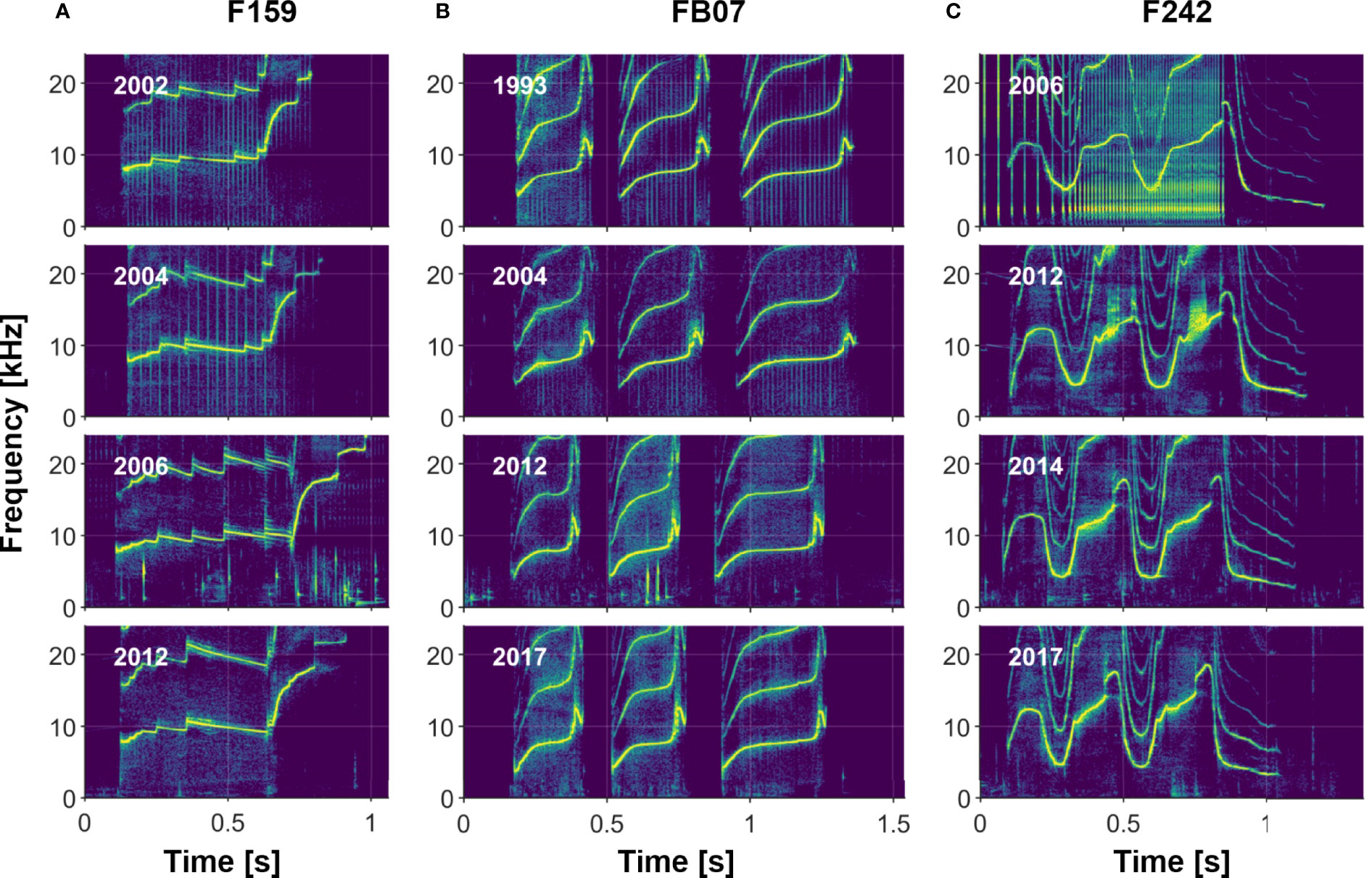
Figure 8 Three examples of individuals recorded across 10 or more years, illustrating signature whistle stability. (A) Female F159, who produces a single-loop whistle; (B) Female FB07, who produces a multi-loop disconnected whistle; and (C) Male F242, who produces a multi-loop connected whistle.
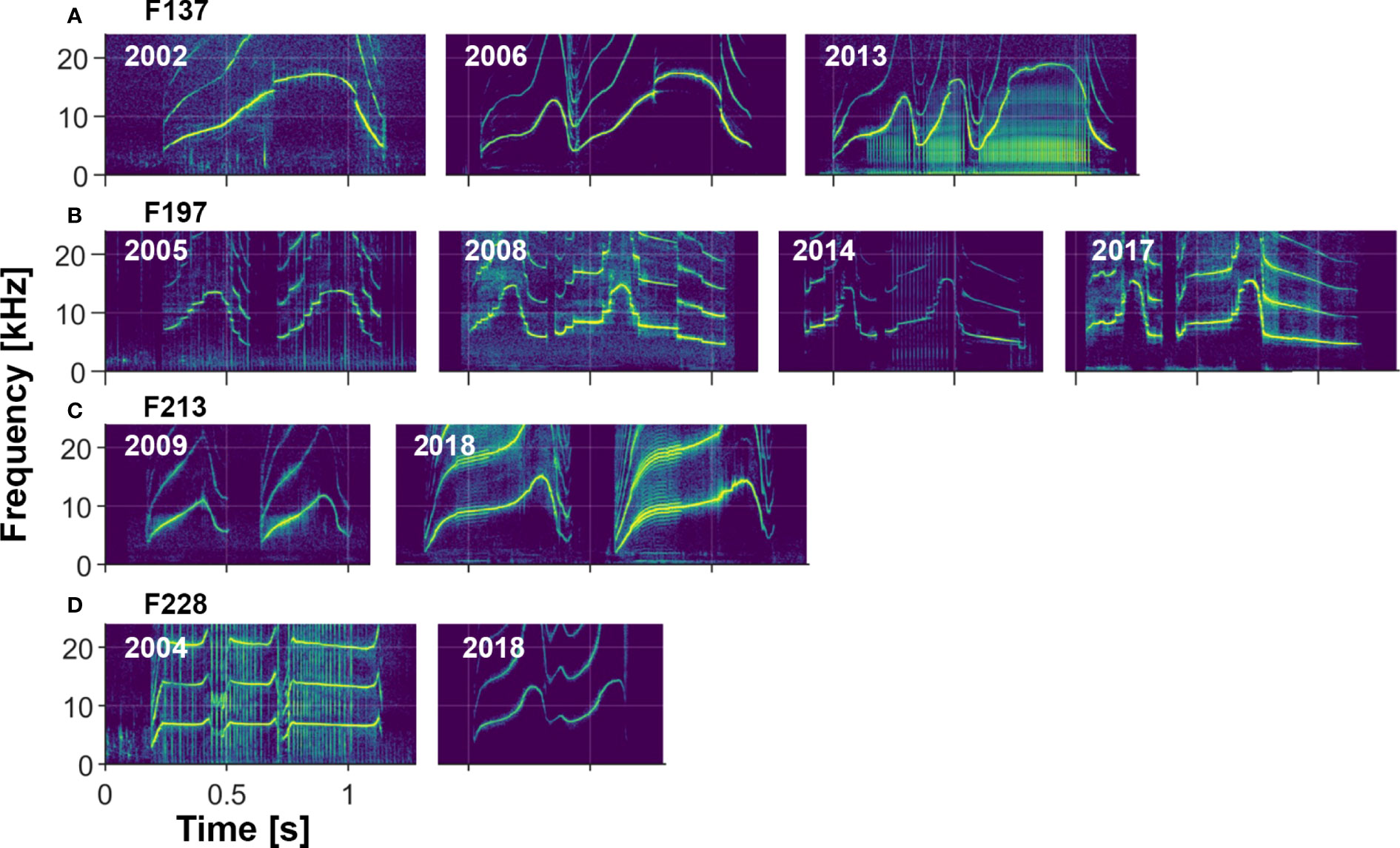
Figure 9 Four examples of changes to signature whistles over time. (A) F137 changed her whistle from a single-loop to a multi-loop form sometime between 2002 and 2006. Two loop versions still occurred in 2013 but the three loop version was more common. (B) F197 changed her whistle between 2005 and 2008, after which it remained fairly stable. (C) F213 changed her whistle between 2009 and 2018, increasing the frequency range and modulation of the contour. Sidebands indicative of amplitude modulation are evident in her 2018 whistle. (D) F228 made a drastic change to his whistle contour between 2004 (when he was with his mother) and 2018 (when he was with a male alliance partner).
3.6 Quantification of individuality
We estimated Beecher’s information statistic (Hs) at 13.7 bits of information based solely on fundamental frequency variation and loop duration, resulting in a theoretical cap of more than 13,000 different individual signatures that can be encoded given the inter-individual variability and intra-individual stereotypy in these parameters. Beecher’s Information Statistic compares within-individual variability to between-individual variability across a range of signal features, so is higher when signals show very high within-individual stereotypy and/or large between-individual differences. While the theoretical estimate of how many different signature whistles can be encoded within this communication system is far larger than any dolphin population and not practically relevant, it is a direct product of the highly stereotyped nature of signature whistle contours produced by one dolphin compared to the large variation observed between signature whistle contours from different dolphins.
4 Discussion
The Sarasota Dolphin Whistle Database, with its large number of recordings of different individuals, many recorded repeatedly over long time periods, has provided an unprecedented window into the range of variability of bottlenose dolphin signature whistles. Our summary of whistle contour types shows a great variety of forms, with a majority having multiple, repeated elements, called “loops.” Loop number is a highly variable feature, with single whistles containing anywhere from 1 to at least 11 loops. (These values are based on qualitative observations; quantifying this range for different individuals is the focus of ongoing research). In addition to the variety of both multi- and single-loop structures that occur among Sarasota Bay bottlenose dolphin signature whistles, a wide range of other acoustic features are also evident. These include phenomena previously described in bottlenose dolphin whistles such as deletions (Tyack, 1986), rapid amplitude modulation leading to sidebands in portions of the whistle spectrogram (Tyack, 1986; Tyack, 1991), biphonic whistle production (Papale et al., 2015), steps (Oswald et al., 2007), and breaks (Wang, 1995). In addition, we observed other features including additions, subharmonics, chaotic or noisy nonlinear components (e.g., Mann et al., 2006; Tyson et al., 2007; Cazau et al., 2016), and stereotyped simultaneous pulsed components (Kaplan et al., 2018). While tonal calls in the dolphin repertoire are called “whistles,” they are not produced the way humans whistle in air (pressure changes in vortices created by air flow over a sharp edge) but by vibration of phonic lips (Madsen et al., 2012). Dolphins have two pairs of phonic lips; they tend to click with their right pair and whistle with the left (Madsen et al., 2013). This explains how dolphins are able to whistle and click simultaneously, but production mechanisms of other phenomena reported here need further study.
Information content of Sarasota dolphin signature whistles, as calculated using Beecher’s Information Statistic (Hs), is higher than for any other animal signal studied so far (Linhart et al., 2022). This is not surprising when considering that most non-human animals use voice cues, called “byproduct distinctiveness” by Boughman and Moss (2003), but that bottlenose dolphins use vocal production learning to develop “designed individual signatures” (as defined by Boughman and Moss, 2003). These findings demonstrate the extensive differences among signature whistle types and highlight the importance of whistle diversity in the bottlenose dolphin communication system.
4.1 How has the Sarasota Dolphin Whistle Database expanded our understanding of dolphin communication?
The Sarasota Dolphin Whistle Database has provided the basis for a variety of studies of dolphin communication over the years. In the following sections, we will briefly describe some of these studies, as well as highlight areas for future research. [Note that the following review focuses only on studies that have involved the Sarasota Dolphin Whistle Database.] We will start with one of the thorniest issues facing studies of dolphin communication: whistle classification.
4.1.1 Whistle classification
Whistle classification is central to the study of dolphin signature whistles: in order to make sense of how and why whistles are being used by dolphins, we must have a reliable way of categorizing them. There have been several attempts to develop computerized whistle classification methods over the past several decades (e.g., Buck and Tyack, 1993; McCowan and Reiss, 1995; Kershenbaum et al., 2013), but none are as accurate as visual categorizations in grouping externally validated categories of whistles (see Janik, 1999; Sayigh et al., 2007). That is, human visual judgements are more accurate than any computerized method developed to date at grouping together signature whistles known to be produced by the same individual. Here we discuss several types of challenges that have faced researchers trying to develop computerized whistle classification methods: 1) subtle whistle features; 2) multi-loop whistles; and 3) variability in acoustic features.
4.1.1.1 Subtle whistle features
Nearly one quarter (24%) of all signature whistle types in the Sarasota community are in the form of upsweeps, either as repeated loops (19%, or 50 of 269) or single loops (5%, or 14 of 269, Table 1 and Figure 3). Computerized whistle classification techniques tend to emphasize the overall shape of the whistle contour, while smoothing subtle inflections. Thus, these techniques would tend to group many of these upsweep whistles into one or very few categories. However, we know from having recorded thousands of whistles from individual animals over the years that these subtle inflections are highly preserved across renditions of an animal’s signature whistle, and thus it is likely that they serve to distinguish one upsweep type signature whistle from another. This idea has in fact been supported by playback experiments (Sayigh et al., 1999; Janik et al., 2006), in which dolphins discriminated between upsweep whistles from different individuals. Deecke and Janik (2006) developed a machine learning technique for whistle classification that can distinguish between different upsweep whistles and preserves subtle modulation patterns (Oswald et al., 2021), but it needs further testing to compare it to human observer classification.
Pitfalls of computerized classification methods are seen most easily in upsweep whistles, but subtle features are also important in multi-loop whistles. Figure 10 shows an example of a subtle feature being preserved in a signature whistle copy (more on whistle copies in section 4.1.5 below), which provides further evidence of the importance of these features.
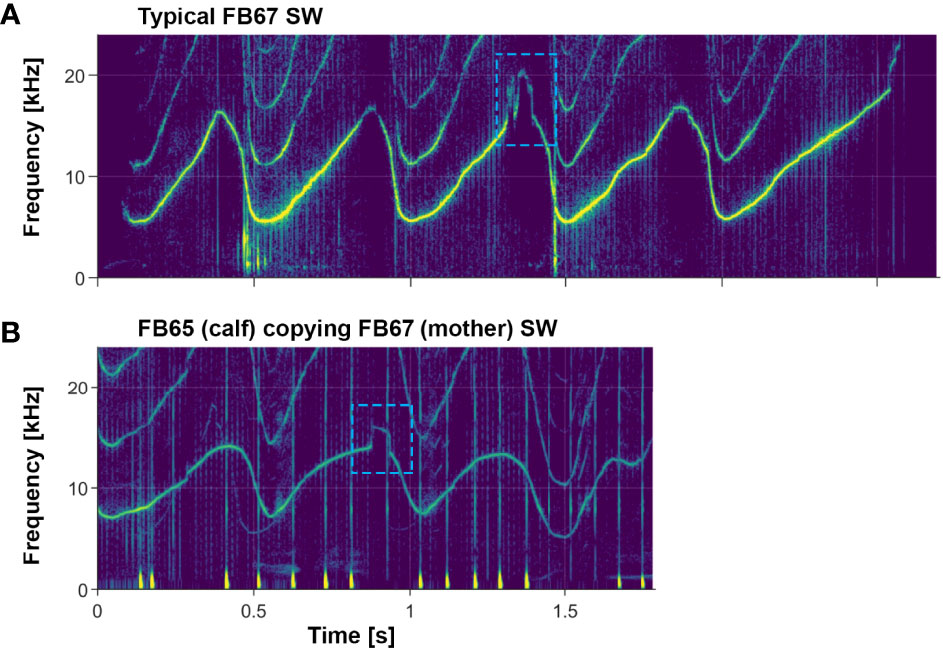
Figure 10 Example of a subtle whistle feature being preserved in a signature whistle copy. (A) Signature whistle of FB67, an adult female; she sometimes includes a subtle feature as seen in the 3rd loop of her whistle (blue dashed box). (B) Copy of FB67’s signature whistle emitted by her daughter FB65. Note the preservation of the subtle feature (blue dashed box) in the 2nd loop of the signature whistle copy. The faint whistle in the background is FB67 emitting her own whistle. Examples of FB65’s own signature whistle can be seen in Figure 4.
4.1.1.2 Multi-loop whistles
The majority of Sarasota Bay signature whistles are multi-looped, which can be connected, disconnected, or both. Janik and Slater (1998) defined an interval of 0.5s as the criterion for calling a whistle element a loop vs. a separate whistle, although Esch et al. (2009b) found that this interval is typically shorter, rarely exceeding 0.25s, and with a mean of 0.10s (from a sample of multi-loop whistles from 16 dolphins). These intervals tend to be highly stereotyped for a given dolphin, and Esch et al. (2009b) concluded that inter-loop intervals are likely an important component of signature whistle stereotypy.
A typical approach in computerized methods aimed at classifying multi-loop whistles has been to select a single loop as the basis of comparison (e.g., Fripp et al., 2005; Watwood et al., 2005; King et al., 2018). Depending on the question, this can preserve sufficient contrast between whistles, but it can be problematic for signature whistle types where individual loops are not all the same. As discussed earlier, almost half (43.5%) of multi-loop whistles in Sarasota have a stereotyped introductory and/or terminal loop that differs from the central loops. Isolating single loops, in addition to eliminating temporal features, therefore ignores some of the structure present in these whistles, which is likely an important aspect of their individual distinctiveness. Likewise, when connected loops are separated from each other and analyzed individually, some of the distinctiveness of the whistle is lost, and this is especially true, as described above, when all loops do not have a consistent shape. However, methods other than visual comparisons for comparing multi-loop whistles with different numbers of loops are fraught with difficulties (e.g., Sayigh et al., 2007). This is not surprising when one considers that most methods involve some form of superimposition of whistle contours over one another, albeit with corrections for different durations such as dynamic time warping (e.g., Buck and Tyack, 1993; Deecke and Janik, 2006).
4.1.1.3 Variability in acoustic features among signature whistles from the same individual
Although the fundamental frequency contour is typically consistent enough to be used as a reliable cue in differentiating among different signature whistle types (Janik et al., 2006), there can be variability in acoustic features across signature whistles from the same individual. As mentioned previously, multi-loop whistles with different introductory and/or terminal loops are sometimes produced without those loops, and even those with consistent loops can sometimes vary the structure of one or more loops. Connections between loops may or may not be present in different whistle renditions, and loop number can vary greatly. Whistle features such as noisy or chaotic components, subharmonics, biphonation, or simultaneous pulses, may or may not be consistently present. Deletions and additions can vary greatly in structure when they occur. Finally, individual whistle parameters can vary within whistle types and encode additional contextual information (Janik et al., 1994). Thus, there is a diversity of features that may add to individuality in a whistle or carry additional information and these can vary across signature whistle renditions. In addition, a small sample may not represent the full range of variability in a given whistle, rendering classifications of some exemplars inaccurate.
4.1.1.4 Visual classification and future directions
Based on the issues described here, we believe that visual classification remains one of the best ways to categorize dolphin signature whistles. The human eye is exceptional at discerning shapes and patterns, even features that are very subtle. Visual classifications have been referred to in the literature as “subjective” or “qualitative” (e.g., McCowan and Reiss, 2001; Bazúa-Durán and Au, 2002; Ansmann, 2005; Camargo et al., 2006). However, if similarity scales and multiple judges are utilized, these issues can be addressed. And, of course, computerized methods are designed and implemented by humans, so by necessity include subjective aspects of what features should or should not be emphasized (e.g., smoothing of subtle features, or focus only on single loops). In the end, the true test for classification is studying how dolphins perceive and categorize these calls. But we do know that visual classification of spectrograms is more accurate at assigning signature whistles to the dolphin producing them than are several computerized methods that have been tested (Janik, 1999; Sayigh et al., 2007; Kershenbaum et al., 2013).
However, visual classifications involving multiple judges are time consuming and this motivates the pursuit of other methodologies. Approaches that involve artificial intelligence (including especially spectrogram-based deep learning) have potential to learn to discriminate signature whistles based on the same spectrogram features that human observers currently make decisions on, including subtle features, variable numbers of loops, and variable loop features (e.g., Deecke and Janik, 2006). We are currently collaborating on efforts to develop machine learning methods for whistle classification. The Sarasota Dolphin Whistle Database, with its large number of identified whistles from known individuals, is the ideal data set with which to pursue these methods. Toward this aim, we are currently developing a systematic, verified, curated database of dolphin signature whistles, which should provide the raw data needed to develop methods for accurate and flexible whistle classification.
4.1.2 Signature whistle production in free-swimming dolphins
Cook et al. (2004) found that individual dolphins produced the same signature whistle types during brief catch-and-release events and when swimming freely. This finding enabled studies of signature whistle production in free-swimming Sarasota dolphins, through comparisons to recordings made during health assessments. Using this method, three studies (Buckstaff, 2004; Cook et al., 2004, and Watwood et al., 2005) found signature whistles comprised 38-70% of total whistles produced by free-swimming dolphins. These values are undoubtedly influenced by context. Esch et al. (2009a) found that dolphins produced signature whistles at lower rates while free-swimming than during brief catch-and-release events. Watwood et al. (2005) found that adult allied males were more likely to produce their signature whistles when voluntarily separated from their partner than when together with their partner. Cook et al. (2004) found that signature whistle rates were highest during socializing, and lowest during travelling, when compared to other activity states (feeding, milling, and resting); these same trends were found in overall whistle rates (signature plus non-signature) both by Cook et al. (2004) and Jones and Sayigh (2002). Buckstaff (2004) found that overall whistle rates were significantly higher at the onset vs. during or after vessel approaches. These studies highlight the importance of both documenting recording context as well as sampling a range of different contexts when studying whistle rates.
Janik et al. (2013) studied temporal patterns of signature whistles in recordings made during focal animal behavioral observations, or follows, of dolphins in Sarasota. They found that signature whistles tended to occur in bouts separated by 1-10 sec, whereas non-signature whistles of the same type tended to be separated by intervals of less than 1 sec or more than 10 sec. They thus devised a method called SIGID that utilizes this distinctive bout structure to identify signature whistles in recordings where there is no accompanying information about individual signature whistles (as would be the case in most areas other than Sarasota Bay). This method has been used in numerous study sites in the past decade (Quick and Janik, 2012; King and Janik, 2013; Gridley et al., 2014; Kriesell et al., 2014; Luís et al., 2016; Hiley et al., 2017; Fearey et al., 2019; La Manna et al., 2020; Longden et al., 2020).
4.1.3 Signature whistle development
Caldwell and Caldwell (1979) studied signature whistle development in dolphin calves in human care and found that the whistle typically forms during the first few months of life, with strong evidence for learning from other dolphins in the same tank. Since then, several studies have focused on signature whistle development in Sarasota dolphins. Sayigh et al. (1990) compared whistles of a small sample of mothers and calves recorded during health assessments, and discovered an apparent sex difference, whereby male calves were more likely to produce whistles similar to those of their mothers, and female calves more likely to produce whistles highly distinct from those of their mothers. A later study (Sayigh et al., 1995) found the same effect with a larger sample size of 42 calves. Sayigh (1992) conducted focal animal behavioral observations of several calves during their first few months of life, which Caldwell and Caldwell (1979) had found was the typical time period of whistle development. Sayigh (1992) found evidence suggestive of the number of associates influencing a calf’s tendency to develop a whistle similar to or different from that of the mother (see also Tyack and Sayigh, 1997; Tyack, 1997). Fripp et al. (2005) found that Sarasota calves developed whistles more similar, in a general sense, to those of members of the Sarasota community vs. a neighboring community, and suggested that calves modeled their signature whistles on those of community members. Miksis et al. (2002) compared whistles of 10 Sarasota dolphins to those of 10 dolphins under human care and found that the latter were significantly more likely to include “flat” or constant frequency components in their whistles. The “bridge” whistle produced by trainers to reinforce dolphins is typically a constant frequency, and Miksis et al. (2002) suggested that some calves may have incorporated this sound into their signature whistles. These studies provide evidence that signature whistles are learned, placing dolphins into a select group of mammals (including elephants, bats and whales, as well as humans) that are capable of vocal production learning (Janik and Slater, 2000).
In recent years we have rekindled the study of vocal development in Sarasota dolphins by examining the large sample size of calves in the Sarasota Dolphin Whistle Database. Although this work is currently ongoing, we have found in a sample of 166 mother-calf pairs (82 male and 84 female) that about one third (32%) produced whistles very similar to those of their mothers (as judged by human observers), but the sex difference seen by Sayigh et al. (1990; 1995) was no longer evident. Strong similarities among sibling whistles were observed in several cases, including some where calves were not producing whistles similar to their mother. Anecdotal suggestions of similarities are also evident from the Sarasota Dolphin Whistle Database. In one case, two females born in the same year (1978) have similar whistles. Although some similarities are to be expected by chance, it is tempting to speculate that some are the result of learning from associates (as also suggested by Fripp et al. 2005). These findings suggest that we need to delve more deeply into association patterns of calves during the whistle development period, and this is the goal of ongoing work.
4.1.4 Signature whistle stability
Our earlier work on mother-calf whistle comparisons (Sayigh et al., 1990; Sayigh et al., 1995) led us to believe that signature whistles were highly stable, and in fact they are for most adult females (see examples of F159 and FB07 in Figure 8). However, Watwood et al. (2004) found that closely allied males (alliances) tended to have similar signature whistles, and speculated that this might be a learned vocal convergence that signaled social affiliation. Our ongoing work with the Sarasota Dolphin Whistle Database has revealed that changes in signature whistles over time, both gradual and dramatic, can occur in both males and females, as illustrated in Figure 9. The topic of signature whistle stability is addressed in more detail in Leon-Lopez et al. (in prep); however, additional studies are needed to determine what factors dictate whether an individual’s whistle remains stable or changes over time.
4.1.5 Signature whistle copying
Sayigh et al. (1990) and Sayigh (1992) observed instances of apparent signature whistle copying in early health assessment recordings. This phenomenon was then studied in detail by King et al. (2013), with a continued focus on health assessment recordings in which two dolphins were simultaneously recorded with suction-cup hydrophones (copying cannot be discerned with single hydrophone recordings unless whistles overlap in time). King et al. (2013) found that signature whistle copying occurs between close associates (mother-calf pairs and male alliances), suggesting an affiliative function. Tyack (1991) and King et al. (2013) further suggested that copies were recognizable as such due to consistent modifications of acoustic parameters. We have since found evidence of signature whistle copying in recordings made with digital acoustic tags (DTAGs) and a goal of ongoing work is to quantify its occurrence in free-swimming dolphins. Although further study is needed to determine how signature whistle copies function in dolphin communication, it appears likely that they may serve as a way to initiate contact with a specific individual, as found in another population (e.g., King and Janik, 2013).
4.1.6 Non-signature whistles
Even after decades of research on signature whistles, surprisingly little is known about non-signature whistles. (These whistles were called “variant” whistles in the earlier literature, although we have since moved away from that terminology.) Studies that have quantified signature whistle production in free-swimming dolphins, as described above, have yielded numbers ranging from about 40-70% of whistles (Buckstaff, 2004; Cook et al., 2004; Watwood et al., 2005), meaning that non-signatures are an important component of the whistle repertoire. Sayigh et al. (1990) suggested that male dolphins produced more non-signatures than females, based on a small sample of recordings made during health assessments. This finding seems accurate based on more recent and ongoing studies that have found evidence for shared non-signature whistle production by males; for example, Sayigh et al. (2017) found that several males produced a shared non-signature whistle type in response to playbacks of non-signature whistles. Research is currently ongoing to determine the role of this and other non-signature whistles in the dolphin vocal repertoire, and to quantify the apparent sex difference in non-signature whistle production.
4.1.7 Playback experiments to study signature whistle function and perception
The Sarasota Dolphin Whistle Database has been the source of stimuli for a variety of playback experiments over the years; only a brief description will be presented here. Sayigh et al. (1999) played back whistles of close relatives and familiar associates, matched as much as possible for level of association, and found that dolphins responded more strongly to whistles of relatives. Since familiarity was controlled for, these results were interpreted as evidence that signature whistles functioned in individual recognition. Later experiments were designed to determine which cues dolphins used for recognition. Two non-mutually exclusive possibilities were the distinctive frequency modulation pattern, or contour, of whistles, and “voice cues” that result from the morphology of the vocal apparatus. Voice cues, which have been referred to as “byproduct distinctiveness” (Boughman and Moss, 2003), are the typical means of individual recognition for non-human terrestrial mammals. Janik et al. (2006) followed the same playback protocol as Sayigh et al. (1999), but played back synthetic whistles, with all potential voice cues removed. They found the same results as Sayigh et al. (1999), indicating that the contour alone was sufficient for individual recognition. To determine if voice cues might also be used, Sayigh et al. (2017) played back non-signature whistles, again using the same protocol as Sayigh et al. (1999), but this time did not find that dolphins discriminated between relatives and familiar associates. Given that sounds may be filtered by gas-filled structures in the upper respiratory tract that could potentially change shape with depth, it was perhaps not surprising to find that voice cues might not provide reliable information on individual identity (Tyack, 2000; Sayigh et al., 2017).
These studies (Sayigh et al., 1999; Janik et al., 2006; Sayigh et al., 2017) were carried out with temporarily restrained dolphins; more recently we have begun carrying out playbacks with free-swimming dolphins while recording video from drones. The goals of these experiments are multi-fold, and include looking at functions of signature whistles, non-signature whistles, signature whistle copies, and non-whistle sounds, as well as how noise impacts dolphin communication through the phenomenon known as communication masking.
4.1.8 Tag studies
We have been deploying non-invasive, suction-cup-attached DTAGs (Johnson and Tyack, 2003) on Sarasota dolphins since 2001. These tags give rare insights into communication among known individuals in the wild, especially when multiple animals can be tagged simultaneously to facilitate identification of the calling individual. The Sarasota Dolphin Whistle Database has proven critical to interpreting these recordings, enabling us to unequivocally differentiate between signature and non-signature whistles of tagged individuals as well as some of the conspecifics with whom they are interacting. This information was used by Kragh et al. (2019), who found that signature whistles tended to be emitted at higher output levels (resulting in longer detection ranges) than non-signature whistles, and that dolphins only partially compensated for increasing noise by increasing vocal output through the Lombard response.
4.2 Future directions
Our current focus on developing a systematic, verified database of dolphin whistles promises to greatly expand the reach of the Sarasota Dolphin Whistle Database. Our current protocol involves labelling all whistles in our recordings made during health assessments. This extensive data set preserves information on sequential whistle production, as well as on proportions of signature whistles, non-signature whistles, and signature whistle copies produced by different individuals. We are now in a position to study aspects of whistle production such as the range of variability in signature whistles, production of shared non-signature whistles, signature whistle development, and much more. We are actively collaborating with researchers who are using our unique dataset to develop machine learning tools for classifying signature whistles. This research direction ties in extremely well with the Sarasota Bay Listening Network, a network of hydrophones that are continuously recording in various locations throughout the Sarasota Bay study area (e.g., Rycyk et al., 2020). These recordings promise to expand our understanding of how signature whistles are used in the daily lives of dolphins, and to provide insights into the comparability of visual and acoustic data when studying association patterns and habitat usage. Also, by testing and ground-truthing these whistle classification methods in Sarasota, where we have extensive data on signature whistles, we can tailor methods for study sites that do not have such extensive background data. Such methods could potentially be used for acoustic mark-recapture studies and long-term monitoring in areas where populations are poorly known or at risk.
4.3 Conclusions
The Sarasota Dolphin Whistle Database provides unique insights into the range of variability that can occur in dolphin signature whistles from one population. Whistle parameters such as frequencies, number of loops (and thus number of inflection points and duration), and presence of non-linear features vary widely. This large data set thus provides a foundation against which we should critically evaluate studies that are based on smaller sample sizes of whistles. For example, can we compare variability across different populations or even species without knowing whether the full range of variability has been sampled? Assessments of whistle characteristics based on small sample sizes may be biased toward vocalizations of a few individuals who were whistling at high rates at the time of recording. Can we assess the importance of features such as number of inflection points, which has been used as a measure of “complexity,” without knowledge of context? For example, Esch et al. (2009a) found that dolphins produced whistles with more loops (which are correlated with inflection points) when under stress, and produced fewer loops during undisturbed conditions. To our knowledge, this is the only study that has examined variation in loop number according to context, and the findings contradict the idea that loop number (i.e., number of inflection points) is correlated with “complexity.” We urge caution in drawing conclusions about whistle features when limited data are available about context and range of variability.
The Sarasota Dolphin Research Program provides a unique natural laboratory for studies of dolphin communication. Nowhere else is there the combined knowledge about so many aspects of the biology of individual dolphins: sex, age, matrilineal relatedness, social association patterns, ranges, health and reproductive status, and, of course, signature whistles. Over the years, the Sarasota Dolphin Whistle Database has facilitated numerous research projects at several institutions and enabled breakthroughs on dolphin communication and cognition. As we continue to develop this resource into a systematic whistle database, we are positioned to ask and answer a broad suite of questions about dolphin communication.
Data availability statement
Requests for the raw data supporting the conclusions of this article will be considered by the authors.
Ethics statement
Research described in this study was reviewed and approved by Institutional Animal Care and Use Committees at both the University of North Carolina Wilmington and the Woods Hole Oceanographic Institution.
Author contributions
LS and VJ contributed to data collection, data analysis, and writing; FJ contributed to data analysis, data visualization and writing, MS, PT and RW contributed to data collection and writing. All authors contributed to the article and approved the submitted version.
Funding
Funding for data collection and analysis over the years has been provided by the National Science Foundation, The Royal Society of London, Dolphin Quest, Adelaide M. and Charles B. Link Foundation, Marine Mammal Commission, National Oceanic and Atmospheric Administration, Earthwatch Institute, Protect Wild Dolphins Fund of the Harbor Branch Oceanographic Institute, Grossman Family Foundation, WHOI Ocean Life Institute, Vulcan Machine Learning Center for Impact, and the Allen Institute for Artificial Intelligence. Current support for PT’s involvement is provided by the Office of Naval Research Grants N00014-18-1-2062 and N00014-20-1-2709 through a subaward from Carnegie Mellon University. Current support for LS’s involvement is provided by the Adelaide M. & Charles B. Link Foundation and Dolphin Quest.
Acknowledgments
Many, many people have contributed to this multi-decadal effort, both in the field and in the lab. Without the initial efforts of Blair Irvine starting in 1970, and ongoing efforts of the staff, students, volunteers, and collaborators of the Sarasota Dolphin Research Program, none of the whistle recordings or background information on the recorded dolphins would exist. It would be difficult to name all of the people who assisted in the catch-and-release operations and playbacks in the field, but without catcher Larry Fulford, and the Net Rats, as they are collectively called, these studies would have been impossible. We also thank all the people on the data analysis side, whose efforts made many of our studies and this synthesis possible. This includes many former students of LS while she was at the University of North Carolina Wilmington (UNCW), but most notably Lynne Williams Hodge, who created the backbone of the database that we continue to use today. Others who made substantial contributions include Mandy Cook, Carter Esch, Guen Jones, Kim Fleming, Jessica Maher, and Nikki Vollmer. More recently, Maia Austin, Gemma Bekki, Gracie Gavazzi, Nicole el Haddad, Kristi Kaleel, Evan Morrison, Campbell van Horn, and Jessica Veo contributed to our efforts at WHOI. Many thanks also to Renae Brodie, Beth Jakob, Lisa Mangiamele, Sarah Partan, and three reviewers for their insightful comments.
Conflict of interest
The authors declare that the research was conducted in the absence of any commercial or financial relationships that could be construed as a potential conflict of interest.
Publisher’s note
All claims expressed in this article are solely those of the authors and do not necessarily represent those of their affiliated organizations, or those of the publisher, the editors and the reviewers. Any product that may be evaluated in this article, or claim that may be made by its manufacturer, is not guaranteed or endorsed by the publisher.
References
Ansmann I. C. (2005). The Whistle Repertoire and Acoustic Behaviour of Short-Beaked Common Dolphins, Delphinus delphis, Around the British Isles, With Applications for Acoustic Surveying (University of Wales Bangor).
Barratclough A., Wells R. S., Schwacke L. H., Rowles T. K., Gomez F. M., Fauquier D. A., et al. (2019). Health Assessments of Common Bottlenose Dolphins (Tursiops truncatus): Past, Present, and Potential Conservation Applications. Front. Vet. Sci 6. doi: 10.3389/fvets.2019.00444
Bazúa-Durán C., Au W. W. (2002). The Whistles of Hawaiian Spinner Dolphins. J. Acoust. Soc. Am. 112, 3064–3072. doi: 10.1121/1.1508785
Beecher M. D. (1989). Signalling Systems for Individual Recognition: An Information Theory Approach. Anim. Behav. 38, 248–261. doi: 10.1016/S0003-3472(89)80087-9
Boughman J. W., Moss C. F. (2003). “Social Sounds: Vocal Learning and Development of Mammal and Bird Calls,” in Acoustic Communication, Springer Handbook of Auditory Research. Eds. Simmons A. M., Fay R. R., Popper A. N. (New York, NY: Springer), 138–224. doi: 10.1007/0-387-22762-8_4
Buckstaff K. C. (2004). Effects of Watercraft Noise on the Acoustic Behavior of Bottlenose Dolphins, Tursiops truncatus, in Sarasota Bay, Florida. Mar. Mamm. Sci. 20, 709–725. doi: 10.1111/j.1748-7692.2004.tb01189.x
Buck J. R., Tyack P. L. (1993). A Quantitative Measure of Similarity for Tursiops truncatus Signature Whistles. J. Acoust. Soc. Am. 94, 2497–2506. doi: 10.1121/1.407385
Caldwell M. C., Caldwell D. K. (1965). Individualized Whistle Contours in Bottle-Nosed Dolphins (Tursiops truncatus). Nature 207, 434–435. doi: 10.1038/207434a0
Caldwell M. C., Caldwell D. K. (1968). Vocalization of Naive Captive Dolphins in Small Groups. Science 159, 1121–1123. doi: 10.1126/science.159.3819.1121
Caldwell M. C., Caldwell D. K. (1979). “The Whistle of the Atlantic Bottlenosed Dolphin (Tursiops truncatus)—Ontogeny,” in Behavior of Marine Animals (Boston, MA: Springer), 369–401.
Caldwell M. C., Caldwell D. K., Tyack P. L. (1990). “Review of the Signature-Whistle Hypothesis for the Atlantic Bottlenose Dolphin,” in Biology of Marine Mamm. Eds. Leatherwood J. E., Reeves R. R. (San Diego: Academic Press), 199–234.
Camargo F. S., Rollo M. M. Jr., Giampaoli V., Bellini C. (2006). Whistle Variability in South Atlantic Spinner Dolphins From the Fernando De Noronha Archipelago Off Brazil. J. Acoust. Soc. Am. 120, 4071–4079. doi: 10.1121/1.2359704
Cazau D., Adam O., Aubin T., Laitman J. T., Reidenberg J. S. (2016). A Study of Vocal Nonlinearities in Humpback Whale Songs: From Production Mechanisms to Acoustic Analysis. Sci. Rep. 6, 1–12. doi: 10.1038/srep31660
Cook M. L. H., Sayigh L. S., Blum J. E., Wells R. S. (2004). Signature–whistle Production in Undisturbed Free–Ranging Bottlenose Dolphins (Tursiops truncatus). Proc. R. Soc. London Ser. B: Biol. Sci. 271, 1043–1049. doi: 10.1098/rspb.2003.2610
Deecke V. B., Janik V. M. (2006). Automated Categorization of Bioacoustic Signals: Avoiding Perceptual Pitfalls. J. Acoust. Soc. Am. 119, 645–653. doi: 10.1121/1.2139067
Esch H. C., Sayigh L. S., Blum J. E., Wells R. S. (2009a). Whistles as Potential Indicators of Stress in Bottlenose Dolphins (Tursiops truncatus). J. Mamm. 90, 638–650. doi: 10.1644/08-MAMM-A-069R.1
Esch H. C., Sayigh L. S., Wells R. S. (2009b). Quantifying Parameters of Bottlenose Dolphin Signature Whistles. Mar. Mamm. Sci. 25, 976–986. doi: 10.1111/j.1748-7692.2009.00289.x
Fearey J., Elwen S. H., James B. S., Gridley T. (2019). Identification of Potential Signature Whistles From Free-Ranging Common Dolphins (Delphinus delphis) in South Africa. Anim. Cogn. 22, 777–789. doi: 10.1007/s10071-019-01274-1
Fripp D., Owen C., Quintana-Rizzo E., Shapiro A., Buckstaff K., Jankowski K., et al. (2005). Bottlenose Dolphin (Tursiops truncatus) Calves Appear to Model Their Signature Whistles on the Signature Whistles of Community Members. Anim. Cogn. 8, 17–26. doi: 10.1007/s10071-004-0225-z
Graycar P. J. (1977). Whistle Dialects of the Atlantic Bottlenosed Dolphin, Tursiops truncatus (US: ProQuest Information & Learning).
Gridley T., Cockcroft V. G., Hawkins E. R., Blewitt M. L., Morisaka T., Janik V. M. (2014). Signature Whistles in Free-Ranging Populations of Indo-Pacific Bottlenose Dolphins, Tursiops aduncus. Mar. Mamm. Sci. 30, 512–527. doi: 10.1111/mms.12054
Hiley H. M., Perry S., Hartley S., King S. L. (2017). What’s Occurring? Ultrasonic Signature Whistle Use in Welsh Bottlenose Dolphins (Tursiops truncatus). Bioacoustics 26, 25–35. doi: 10.1080/09524622.2016.1174885
Irvine A. B., Scott M. D., Wells R. S., Kaufmann J. H. (1981). Movements and Activities of the Atlantic Bottlenose Dolphin, Tursiops truncatus, Near Sarasota, Florida. Fish Bull. U.S. 79, 671–688.
Janik V. M. (1999). Pitfalls in the Categorization of Behaviour: A Comparison of Dolphin Whistle Classification Methods. Anim. Behav. 57, 133–143. doi: 10.1006/anbe.1998.0923
Janik V. M., King S. L., Sayigh L. S., Wells R. S. (2013). Identifying Signature Whistles From Recordings of Groups of Unrestrained Bottlenose Dolphins (Tursiops truncatus). Mar. Mamm. Sci. 29, 109–122. doi: 10.1111/j.1748-7692.2011.00549.x
Janik V. M., Sayigh L. S. (2013). Communication in Bottlenose Dolphins: 50 Years of Signature Whistle Research. J. Comp. Physiol. A 199, 479–489. doi: 10.1007/s00359-013-0817-7
Janik V. M., Sayigh L. S., Wells R. S. (2006). Signature Whistle Shape Conveys Identity Information to Bottlenose Dolphins. Proc. Natl. Acad. Sci. 103, 8293–8297. doi: 10.1073/pnas.0509918103
Janik V. M., Slater P. J. (1998). Context-Specific Use Suggests That Bottlenose Dolphin Signature Whistles are Cohesion Calls. Anim. Behav. 56, 829–838. doi: 10.1006/anbe.1998.0881
Janik V. M., Slater P. J. (2000). The Different Roles of Social Learning in Vocal Communication. Anim. Behav. 60, 1–11. doi: 10.1006/anbe.2000.1410
Janik V. M., Todt D., Dehnhardt G. (1994). Signature Whistle Variations in a Bottlenosed Dolphin, Tursiops truncatus. Behav. Ecol. Sociobiol. 35, 243–248. doi: 10.1007/BF00170704
Johnson M. P., Tyack P. L. (2003). A Digital Acoustic Recording Tag for Measuring the Response of Wild Marine Mammals to Sound. IEEE J. Oceanic Eng. 28, 3–12. doi: 10.1109/JOE.2002.808212
Jones G. J., Sayigh L. S. (2002). Geographic Variation in Rates of Vocal Production of Free-Ranging Bottlenose Dolphins. Mar. Mamm. Sci. 18, 374–393. doi: 10.1111/j.1748-7692.2002.tb01044.x
Kaplan J. D., Melillo-Sweeting K., Reiss D. (2018). Biphonal Calls in Atlantic Spotted Dolphins (Stenella frontalis): Bitonal and Burst-Pulse Whistles. Bioacoustics 27, 145–164. doi: 10.1080/09524622.2017.1300105
Kershenbaum A., Sayigh L. S., Janik V. M. (2013). The Encoding of Individual Identity in Dolphin Signature Whistles: How Much Information is Needed? PLoS One 8, e77671. doi: 10.1371/journal.pone.0077671
King S. L., Friedman W. R., Allen S. J., Gerber L., Jensen F. H., Wittwer S., et al. (2018). Bottlenose Dolphins Retain Individual Vocal Labels in Multi-Level Alliances. Curr. Biol. 28, 1993–1999. doi: 10.1016/j.cub.2018.05.013
King S. L., Janik V. M. (2013). Bottlenose Dolphins can Use Learned Vocal Labels to Address Each Other. Proc. Natl. Acad. Sci. 110, 13216–13221. doi: 10.1073/pnas.1304459110
King S. L., Sayigh L. S., Wells R. S., Fellner W., Janik V. M. (2013). Vocal Copying of Individually Distinctive Signature Whistles in Bottlenose Dolphins. Proc. R. Soc. B: Biol. Sci. 280, 20130053. doi: 10.1098/rspb.2013.0053
Kragh I. M., McHugh K., Wells R. S., Sayigh L. S., Janik V. M., Tyack P. L., et al. (2019). Signal-Specific Amplitude Adjustment to Noise in Common Bottlenose Dolphins (Tursiops truncatus). J. Exp. Biol. 222 (23), jeb216606. doi: 10.1242/jeb.216606
Kriesell H. J., Elwen S. H., Nastasi A., Gridley T. (2014). Identification and Characteristics of Signature Whistles in Wild Bottlenose Dolphins (Tursiops truncatus) From Namibia. PLoS One 9, e106317. doi: 10.1371/journal.pone.0106317
La Manna G., Rako-Gospić N., Sarà G., Gatti F., Bonizzoni S., Ceccherelli G. (2020). Whistle Variation in Mediterranean Common Bottlenose Dolphin: The Role of Geographical, Anthropogenic, Social, and Behavioral Factors. Ecol. Evol. 10, 1971–1987. doi: 10.1002/ece3.6029
Leon-Lopez B., Sayigh L., Scott M., Tyack P., Wells R., Janik V. In preparation. Life-long stability in signature whistles of bottlenose dolphins (Tursiops truncatus).
Linhart P., Mahamoud-Issa M., Stowell D., Blumstein D. T. (2022). The Potential for Acoustic Individual Identification in Mammals. Mamm. Biol., 102:1–17. doi: 10.1007/s42991-021-00222-2
Linhart P., Osiejuk T. S., Budka M., Šálek M., Špinka M., Policht R., et al. (2019). Measuring Individual Identity Information in Animal Signals: Overview and Performance of Available Identity Metrics. Methods Ecol. Evol. 10, 1558–1570. doi: 10.1111/2041-210X.13238
Linhart P., Šálek M. (2017). The Assessment of Biases in the Acoustic Discrimination of Individuals. PLoS One 12, e0177206. doi: 10.1371/journal.pone.0177206
Longden E. G., Elwen S. H., McGovern B., James B. S., Embling C. B., Gridley T. (2020). Mark–recapture of Individually Distinctive Calls—a Case Study With Signature Whistles of Bottlenose Dolphins (Tursiops truncatus). J. Mamm. 101, 1289–1301. doi: 10.1093/jmammal/gyaa081
Loughlin T., Cunningham L., Gales N., Wells R. S., Boyd I. (2010). “Marking and Capturing,” in Marine Mamm. Ecology and Conservation: A Handbook of Techniques. Eds. Boyd I., Bowen D., Iverson S. (New York:Oxford University Press), 16–41.
Luís A. R., Couchinho M. N., Dos Santos M. E. (2016). Signature Whistles in Wild Bottlenose Dolphins: Long-Term Stability and Emission Rates. Acta Ethologica 19, 113–122. doi: 10.1007/s10211-015-0230-z
Madsen P. T., Jensen F. H., Carder D., Ridgway S. (2012). Dolphin Whistles: A Functional Misnomer Revealed by Heliox Breathing. Biol. Lett. 8, 211–213. doi: 10.1098/rsbl.2011.0701
Madsen P. T., Lammers M., Wisniewska D., Beedholm K. (2013). Nasal Sound Production in Echolocating Delphinids (Tursiops truncatus and Pseudorca crassidens) is Dynamic, But Unilateral: Clicking on the Right Side and Whistling on the Left Side. J. Exp. Biol. 216, 4091–4102. doi: 10.1242/jeb.091306
Mann D. A., O’Shea T. J., Nowacek D. P. (2006). Nonlinear Dynamics in Manatee Vocalizations. Mar. Mammal. Sci. 22, 548–555. doi: 10.1111/j.1748-7692.2006.00036.x
Marley S. A., Erbe C., Kent C. P. S. (2017). Underwater Recordings of the Whistles of Bottlenose Dolphins in Fremantle Inner Harbour, Western Australia. Sci. Data 4, 170126. doi: 10.1038/sdata.2017.126
McCowan B., Reiss D. (1995). Quantitative Comparison of Whistle Repertoires From Captive Adult Bottlenose Dolphins (Delphinidae, Tursiops truncatus): A Re-Evaluation of the Signature Whistle Hypothesis. Ethology 100, 194–209. doi: 10.1111/j.1439-0310.1995.tb00325.x
McCowan B., Reiss D. (2001). The Fallacy of ‘Signature Whistles’ in Bottlenose Dolphins: A Comparative Perspective of ‘Signature Information’in Animal Vocalizations. Anim. Behav. 62, 1151–1162. doi: 10.1006/anbe.2001.1846
Miksis J. L., Tyack P. L., Buck J. R. (2002). Captive Dolphins, Tursiops truncatus, Develop Signature Whistles That Match Acoustic Features of Human-Made Model Sounds. J. Acoust. Soc. Am. 112, 728–739. doi: 10.1121/1.1496079
Oswald J. N., Rankin S., Barlow J., Lammers M. O. (2007). A Tool for Real-Time Acoustic Species Identification of Delphinid Whistles. J. Acoust. Soc. Am. 122, 587–595. doi: 10.1121/1.2743157
Oswald J. N., Walmsley S. F., Casey C., Fregosi S., Southall B., Janik V. M. (2021). Species Information in Whistle Frequency Modulation Patterns of Common Dolphins. Philos. Trans. R. Soc. B 376, 20210046. doi: 10.1098/rstb.2021.0046
Papale E., Buffa G., Filiciotto F., Maccarrone V., Mazzola S., Ceraulo M., et al. (2015). Biphonic Calls as Signature Whistles in a Free-Ranging Bottlenose Dolphin. Bioacoustics 24, 223–231. doi: 10.1080/09524622.2015.1041158
Quick N. J., Janik V. M. (2012). Bottlenose Dolphins Exchange Signature Whistles When Meeting at Sea. Proc. R. Soc. B: Biol. Sci. 279, 2539–2545. doi: 10.1098/rspb.2011.2537
Rycyk A. M., Moore R. B. T., Wells R. S., McHugh K. A., McCabe E. J. B., Mann D. A. (2020). Passive Acoustic Listening Stations (PALS) Show Rapid Onset of Ecological Effects of Harmful Algal Blooms in Real Time. Sci. Rep. 10, 1–12. doi: 10.1038/s41598-020-74647-z
Sayigh L. S. (1992). Development and Functions of Signature Whistles of Free-Ranging Bottlenose Dolphins, Tursiops truncatus (Massachusetts Institute of Technology/Woods Hole Oceanographic Institution).
Sayigh L. S., Esch H. C., Wells R. S., Janik V. M. (2007). Facts About Signature Whistles of Bottlenose Dolphins, Tursiops truncatus. Anim. Behav. 74, 1631–1642. doi: 10.1016/j.anbehav.2007.02.018
Sayigh L. S., Tyack P. L., Wells R. S., Scott M. D. (1990). Signature Whistles of Free-Ranging Bottlenose Dolphins Tursiops truncatus: Stability and Mother-Offspring Comparisons. Behav. Ecol. Sociobiol. 26, 247–260. doi: 10.1007/BF00178318
Sayigh L. S., Tyack P. L., Wells R. S., Scott M. D., Irvine A. B. (1995). Sex Difference in Signature Whistle Production of Free-Ranging Bottlenose Dolphins, Tursiops truncatus. Behav. Ecol. Sociobiol. 36, 171–177. doi: 10.1007/BF00177793
Sayigh L. S., Tyack P. L., Wells R. S., Solow A. R., Scott M. D., Irvine A. B. (1999). Individual Recognition in Wild Bottlenose Dolphins: A Field Test Using Playback Experiments. Anim. Behav. 57, 41–50. doi: 10.1006/anbe.1998.0961
Sayigh L. S., Wells R. S., Janik V. M. (2017). What’s in a Voice? Dolphins do Not Use Voice Cues for Individual Recognition. Anim. Cogn. 20, 1067–1079. doi: 10.1007/s10071-017-1123-5
Scott M. D., Wells R. S., Irvine A. B. (1990). “A Long-Term Study of Bottlenose Dolphins on the West Coast of Florida,” in The Bottlenose Dolphin. Eds. Leatherwood S., Reeves R. R. (San Diego: Academic Press), 235–244.
Tyack P. (1985). An Optical Telemetry Device to Identify Which Dolphin Produces a Sound. J. Acoust. Soc. Am. 78, 1892–1895. doi: 10.1121/1.392777
Tyack P. (1986). Whistle Repertoires of Two Bottlenosed Dolphins, Tursiops truncatus : Mimicry of Signature Whistles? Behav. Ecol. Sociobiol. 18, 251–257. doi: 10.1007/BF00300001
Tyack P. (1991). “Use of a Telemetry Device to Identify Which Dolphin Produces a Sound,” in Dolphin Societies: Discoveries and Puzzles. Eds. Pryor K., Norris K. (Berkeley and Los Angeles, California, and London, England:University of California Press), 319–340.
Tyack P. L. (1997). Development and Social Functions of Signature Whistles in Bottlenose Dolphins Tursiops truncatus. Bioacoustics 8, 21–46. doi: 10.1080/09524622.1997.9753352
Tyack P. L. (2000). Dolphins Whistle a Signature Tune. Science 289, 1310–1311. doi: 10.1126/science.289.5483.1310
Tyack P. L., Sayigh L. S. (1997). Vocal Learning in Cetaceans, in: Social Influences on Vocal Development (New York, NY, US: Cambridge University Press), 208–233. doi: 10.1017/CBO9780511758843.011
Tyson R. B., Nowacek D. P., Miller P. J. (2007). Nonlinear Phenomena in the Vocalizations of North Atlantic Right Whales (Eubalaena glacialis) and Killer Whales (Orcinus orca). J. Acoust. Soc. Am. 122, 1365–1373. doi: 10.1121/1.2756263
Wang D. W. (1995). Whistles of Bottlenose Dolphins: Comparisons Among Populations. Aquat. Mamm. 21, 65–77.
Watwood S. L., Owen E. C., Tyack P. L., Wells R. S. (2005). Signature Whistle Use by Temporarily Restrained and Free-Swimming Bottlenose Dolphins, Tursiops truncatus. Anim. Behav. 69, 1373–1386. doi: 10.1016/j.anbehav.2004.08.019
Watwood S. L., Tyack P. L., Wells R. S. (2004). Whistle Sharing in Paired Male Bottlenose Dolphins, Tursiops truncatus. Behav. Ecol. Sociobiol. 55, 531–543. doi: 10.1007/s00265-003-0724-y
Wells R. S. (2009). Learning From Nature: Bottlenose Dolphin Care and Husbandry. Zoo Biol. 28, 1–17. doi: 10.1002/zoo.20252
Wells R. S. (2020). The Sarasota Dolphin Research Program in 2020: Celebrating 50 Years of Research, Conservation, and Education. Aquat. Mamm. 46, 502–504. doi: 10.1578/AM.46.5.2020.502
Wells R. S., Rhinehart H. L., Hansen L. J., Sweeney J. C., Townsend F. I., Stone R., et al. (2004). Bottlenose Dolphins as Marine Ecosystem Sentinels: Developing a Health Monitoring System. EcoHealth 1, 246–254. doi: 10.1007/s10393-004-0094-6
Keywords: signature whistle, communication, cognition, database, individual identity
Citation: Sayigh LS, Janik VM, Jensen FH, Scott MD, Tyack PL and Wells RS (2022) The Sarasota Dolphin Whistle Database: A unique long-term resource for understanding dolphin communication. Front. Mar. Sci. 9:923046. doi: 10.3389/fmars.2022.923046
Received: 18 April 2022; Accepted: 27 May 2022;
Published: 04 August 2022.
Edited by:
Rebecca Dunlop, The University of Queensland, AustraliaReviewed by:
Tess Gridley, Sea Search Research and Conservation, South AfricaSalvatore Siciliano, Fundação Oswaldo Cruz (Fiocruz), Brazil
Bruno Díaz López, Bottlenose Dolphin Research Institute (BDRI), Spain
Copyright © 2022 Sayigh, Janik, Jensen, Scott, Tyack and Wells. This is an open-access article distributed under the terms of the Creative Commons Attribution License (CC BY). The use, distribution or reproduction in other forums is permitted, provided the original author(s) and the copyright owner(s) are credited and that the original publication in this journal is cited, in accordance with accepted academic practice. No use, distribution or reproduction is permitted which does not comply with these terms.
*Correspondence: Laela S. Sayigh, lsayigh@whoi.edu