- 1Food Technology Division, School of Industrial Technology, Universiti Sains Malaysia, Gelugor, Malaysia
- 2Geography Division, School of Humanities, Universiti Sains Malaysia, Gelugor, Malaysia
Aquaculture plays a huge role in supporting the needs and demands of fish and other aquatic organisms globally. Being a vital contributor in the fisheries sector, aquatic sources derived from the aquaculture industry are well-known for their high nutritive values that are compromised with high-quality protein, essential omega-3 fatty acids, vitamins, and minerals. Aquaculture has also widened fish availability to regions and countries with limited access to farmed species, leading to improved nutrition and food security. However, the presence of dangerous contaminants, notably heavy metals, endocrine disrupting compounds, polycyclic aromatic hydrocarbons, microplastics, as well as antimicrobial drug residues, has been detected in the aquaculture products. In conjunction, the risk–benefit assessment (RBA) approach has been proposed to be used as a meaningful tool for evaluating the health impacts of the consumption of aquatic sources on the targeted population. This narrative review outlines a compilation of aquaculture products as a nutritional source for human consumption. The aquaculture industry is well-appreciated, especially in low-income countries, for achieving sustainable food security. The organoleptics and preferences of aquaculture products as means of dietary variations were demonstrated. The damaging contaminants contained in the commercially available aquaculture products were quantitatively outlined. Furthermore, the adaptation of the risk–benefit approach underlying its potent impacts on public health concerns, with its current challenges and limitations, is elucidated.
Introduction
To date, tremendous increase in the global population and extended life expectancy have alarmed the issue of food security. As such, securing a sustainable food supply chain is the key determinant for achieving the net balance of food demand and food consumption (Azra et al., 2021). Aquaculture is one of the essential sectors to contribute fishery products as a primary source of protein for human consumption (FAO, 2020). It was estimated that about 950 million people worldwide obtained their nutritious protein from fish sources, and in fewer than 50 years, the fish consumption trends per world capita had doubled (WFC, 2002). On a global basis, aquatic resources represent 17% of total amino acids supplied for dietary consumption, and half of the total global fish production is contributed by the aquaculture industry (FAO, 2020). In view of the negative environmental impact derived from the wild-caught fisheries sector, it is evident that aquaculture activity will become the key supporter to fulfill the global demand (Burgess et al., 2013; Golden et al., 2017). The increase in aquaculture practice for manipulating culturally relevant diet intake and nutrient fortification has marked another advantage for this sector (Rosenlund et al., 2010).
Aquatic animal foods offer numerous beneficial impacts on human health, particularly the presence of high omega-3 polyunsaturated fatty acids (PUFAs) in the dietary lipids of the fish, which are low in calories and high in minerals and vitamins (Eckhard and Leonor, 2018). The authentic quality proteins derived from the aquatic animals are also remarkable with enhanced biological functions such as antioxidants, cholesterol reductions, and anti-hypertensive effects (Heffernan et al., 2021). Notably, the presence of eicosapentaenoic acid (EPA) and docosahexaenoic acid (DHA) is the quintessential criterion for assessing the nutritional value (Guo et al., 2015). The beneficial effects of EPA and DHA are widely acknowledged, particularly for the reduction of cardiovascular, inflammatory, and neurological disease risks (Zhang et al., 2019). Additionally, the presence of multivitamins in the aquaculture products, such as the vitamin A, vitamin D, vitamin E, vitamin B12, folic acid, choline, and coenzyme-Q10 is important for the modulation of physiological activities, and aid in the improvement of visual, cognitive function and neurodevelopment in infants and children (Kennedy, 2016).
Despite their high nutritional values, aquatic products remain vulnerable to a range of safety risks attributed to their practices and environmental hazards (Hussan and Gon, 2016). The bioaccumulation of contaminants is a safety concern as it can be introduced at any value point. The adverse health effects of contaminant exposure on human will be effective following long-term dietary ingestion (Ling and Liao, 2007). The safety issues associated with aquaculture activities could be broadly classified into chemical, microbial, and environmental origins. These contaminants may originate from environmental pollution, socio-cultural habits prevailing in various regions, or malpractice in the cultivation process. Meanwhile, inappropriate cultural behavior and practices also lead to eutrophication and environmental degradation (Hussan and Gon, 2016). The concern of aggressive use of antibiotics in the aquaculture farms could be verdict with the incidence of the antimicrobial drug residue in the cultivated species (Gibson et al., 2020). Such antimicrobial residues could be directed at humans through the food chain and lead to the emergence of multi-drug resistance (Okocha et al., 2018).
In accordance with the scenario, several studies have been conducted to evaluate the complications of aquaculture product consumption on human health using the risk–benefit assessment (RBA) approach. Such an approach aims to develop useful dietary guidelines and recommended intake levels for the general and sub-group populations (Thomsen et al., 2021). The assessments were conducted based on specific farmed species, with detailed investigations on the health impacts of different consumption scenarios and exposure to the targeted sub-groups. In the same vein, scientific modeling, including hazard quotient, net integrated, and in vitro bio-accessibility, has been demonstrated to be an effective tool for predicting aquatic food consumption risk in humans (Thomsen et al., 2021).
With the aforementioned, the aim of this narrative review is to provide an overview of aquaculture practices as important role players in achieving sustainable food security from a global viewpoint. The nutritional compositions, organoleptic preferences, and global farmed aquaculture products for human consumption are summarized. This study postulates an initial platform to demonstrate the unique consequences of hazardous contaminants in aquaculture products for the concern of consumers. This work also provides information to reporting the application of the risk–benefit approach as a viable tool for hazard estimation in aquaculture.
The Global Production Trends of Aquaculture
Aquaculture production is constantly increasing yearly, while capture production has almost stabilized in the last 30 years. As such, aquaculture represents a huge potential to supplement the quantity of the catch while alleviating the pressure on natural stocks (Freitas et al., 2020). The global production of aquaculture is more than seventh in live-weight volume from 17 million in 1990 to 125 million in 2020 (Figure 1) and attained a total farm gate sale value of USD 281.5 billion (FAO, 2022). Today, aquaculture is more diverse, with 40% of fish, shellfish, aquatic plants, and algae species cultivated in various water systems globally (i.e., brackish, marine, and freshwater) (Metian et al., 2020). The main species groups that contributed to the top 60% of aquaculture production in 2020 included seaweed, crustaceans, carps, tilapia, salmon, and bivalve species (Figure 2). Meanwhile, up to 70% of global edible aquaculture volume is accounted for by freshwater fish, reflecting the favorable conversion from life to edible weight compared to bivalves and crustaceans (high shell weight) (Edwards et al., 2019).
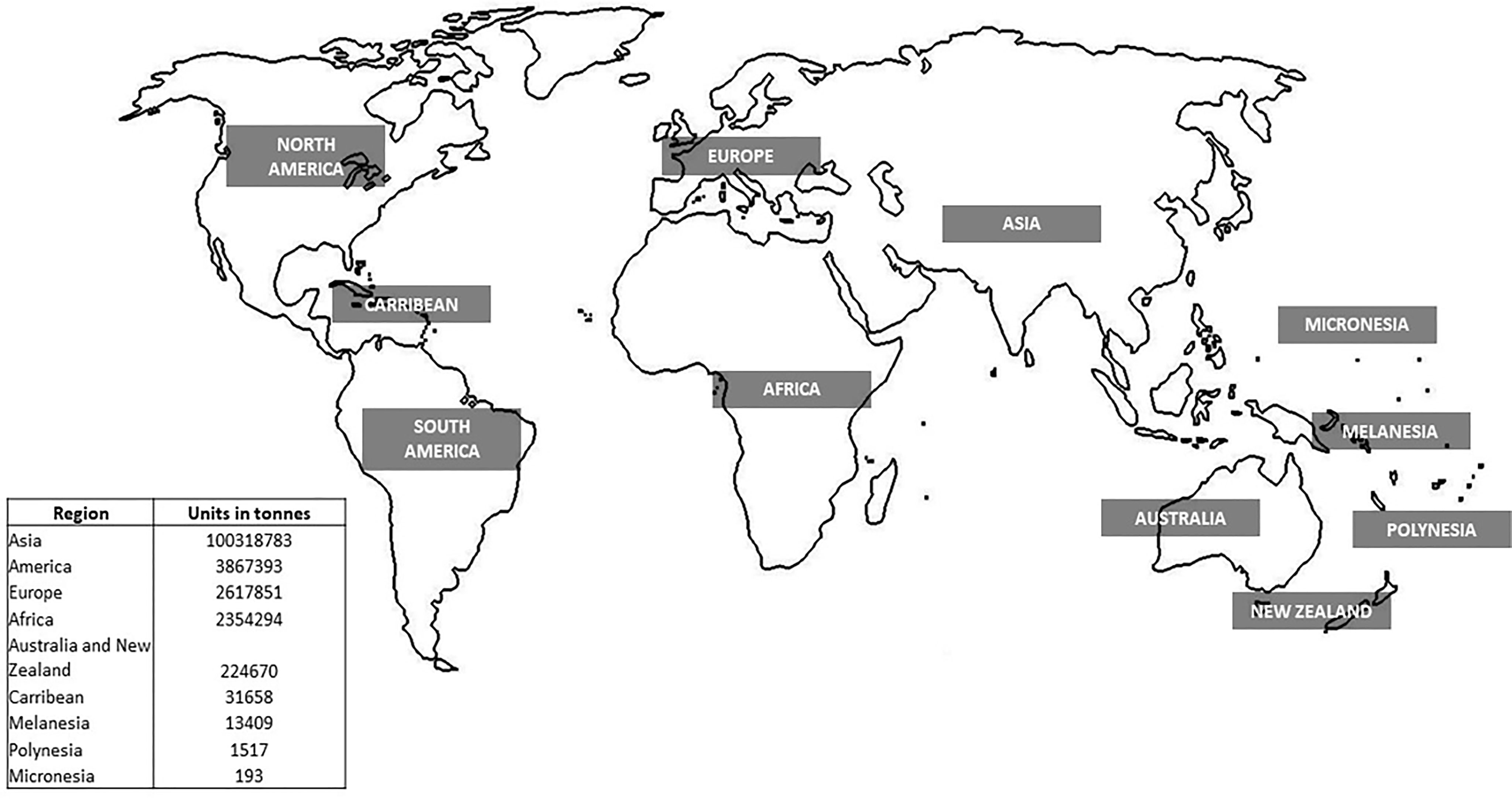
Figure 1 Global aquaculture production in tons from 1990 to 2020 (FAO, 2020).
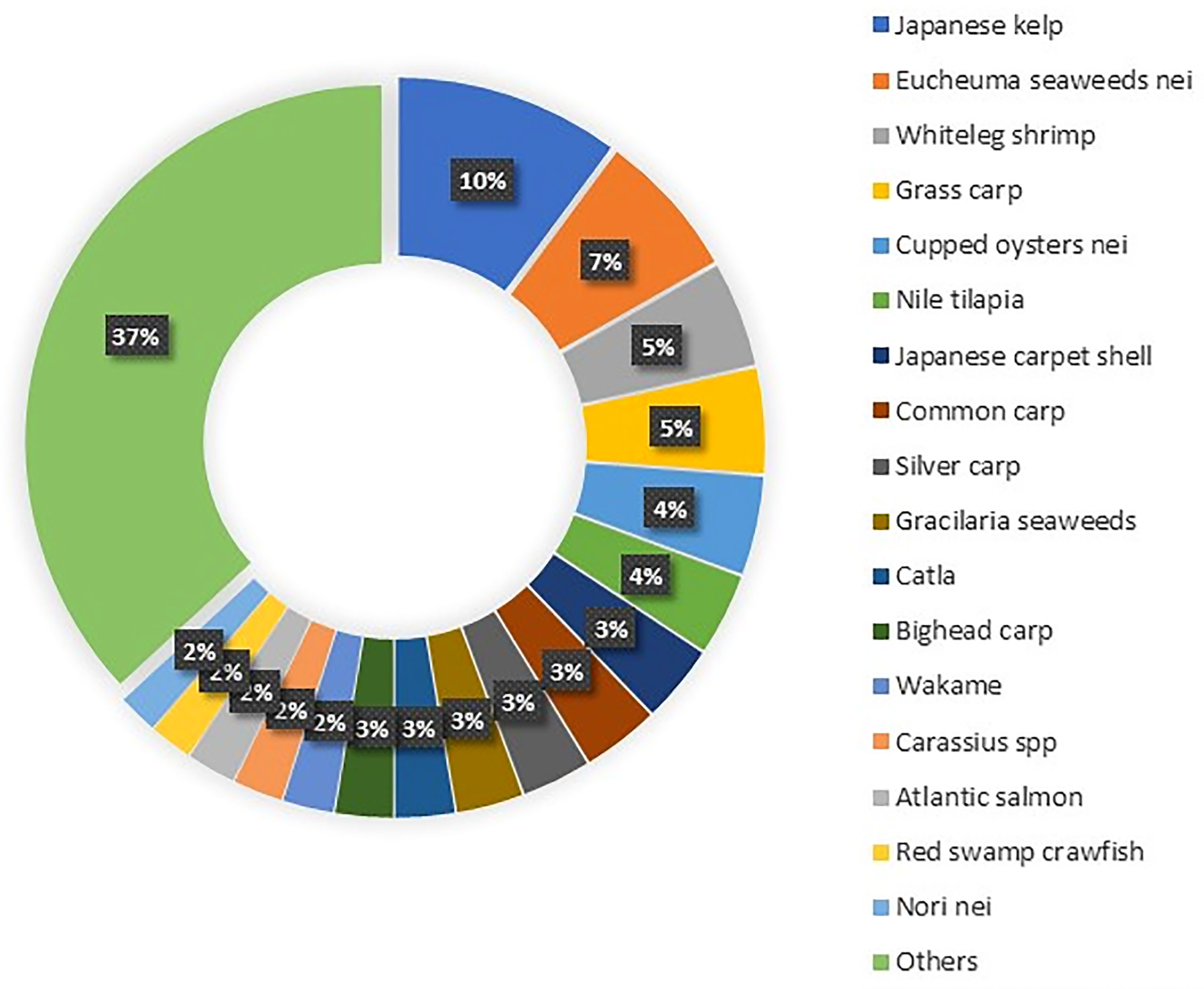
Figure 2 Major cultivated aquaculture species (FAO, 2022).
Asia remains the largest aquaculture producer, accounting for 92% (approximately 1 billion tons) of farmed aquatic animals and plants, followed by the United States of America (4%), Europe (2%), and Africa (2%). Among the top major producers of aquaculture countries, China plays an oversized role in nearly all fields of aquaculture production, with values of USD 167 million (FAO, 2022). The country has stabilized its role as the largest producer, processor, and trader of most aquatic animals for the past 20 years and has emerged as a leading consumer (Garlock et al., 2020). The slow-paced rate of aquaculture production in western countries is likely to reflect the restrictive and cumbersome regulatory structure, high coastal property values, the prioritization of commercial fishing, and tourism over aquaculture, in parallel with high labor costs (Garlock et al., 2020). In the Western hemisphere, single or dual-species production systems have been developed, which account for the remaining 1–2% of the global production. These advanced systems showed advantages toward improving the genetic and nutrition of the species. However, it remains vulnerable due to market volatility and pandemics such as COVID-19 (Naylor et al., 2021).
Nutrition and Human Consumption of Aquaculture Products
The annual global consumption of seafood products per capita has doubled over the last 50 years, and more than 3.1 billion people consumed at least 20% of their animal protein via seafood (FAO, 2016). Seafood protein is an important nutritional component in many countries, particularly in low-income countries (Guillen et al., 2019). Thus, the aquaculture industry contributes significantly to food security not only as a direct source of protein, micronutrients, and essential fatty acids, but also indirectly improves employment income for food purchase (Guillen et al., 2019). In 2018, 52% of total global fisheries consumption was attributed to aquaculture, and it is expected that the number will continue to increase in the future (FAO, 2020). China, the leading producer of aquaculture products, ranked first (56%) for farmed aquaculture product consumption (Guillen et al., 2019).
Essentially, Garlock et al. (2022) reported a positive correlation between aquatic product consumption and aquatic production on a national scale. Countries with developed aquaculture industries (i.e., production of more than 10,000 million tons) showed higher aquatic food consumption per capita. Similar trends were depicted in all global regions except North America, South America, and the Caribbean. Hence, aquaculture is definitely playing a critical role for the transition to a more environmentally sustainable diet, while attaining food security goals. Aquatic food production in fisheries is stagnant and distributional shifts in fish stocks due to climate change will reduce the capacity for fisheries to contribute to future aquatic food security in tropical, low-income nations (Lotze et al., 2019). Improving the facilities and technologies in regions with high rates of food insecurity and malnutrition, such as Africa, where aquaculture is limited, is crucial for stabilizing and improving access to aquatic food (Golden et al., 2016; Chan et al., 2019; Koehn et al., 2022).
The Role of Aquaculture in Food Security and Sustainability
Because of the expeditious growth of the human population, food from the aquatic environment is expected to be in high demand globally to fulfill the needs and preferences of consumers (Pradeepkiran, 2019). The median projection predicts the global population will grow to over 9.7 billion by 2050, and food demand is expected to rise even faster due to the emergence of a larger proportion of high-income societies who typically consume more animal protein than low-income societies (Kharas, 2010). In parallel, food security has become an utmost importance to third-world countries, where a large percentage of their population is low in income and a high share of total household expenditure is devoted to food (Jennings et al., 2016). Aquaculture plays an increasing role in aquatic food security. As in Africa, fish provide 19% of animal protein intake and play a unique role in providing various essential fatty acids and micronutrients that are commonly absent in land-based animals. Additionally, the fishing sector has supported the livelihoods of almost 12.3 million people (a quarter of the numbers are female), who are often considered among the poorest and most marginalized communities in Africa (Chan et al., 2019). In India, the second largest producer of fish in the world, the aquaculture sector contributes about 68% of fish in India. The sector supports the livelihoods of more than 28 million people in India, especially the marginalized and vulnerable communities. This scenario has validated the importance of this sector not only to promote socioeconomic growth but also to alleviate the poverty of this continent.
Aquaculture contributes to food security when the system is safe, efficient, sufficient in supply, and sustainable (Jennings et al., 2016). The use of modern land- and water-based technologies on aquaculture farms has increased the resource-efficiency of the system. The current Fish In–Fish Out (FIFO) ratio is reported to be decreased with values of 0.10 resulting from the innovation of fish aquafeed and modern technologies (McNally, 2021). This sustainable trend can predicate the improvement of the economic, environmental, and social sustainability of the production, processing methods, and supply chain of aquaculture products (Jennings et al., 2016). Thus, the sustainability of aquaculture should be the key to the solution in providing healthy, sustainable protein and employment opportunities to future generations (McNally, 2021).
Organoleptic Properties, Preferences and Perceptions Towards Aquaculture Products
The development of organoleptic research much focuses on giving better understanding of how the senses react of the food intake and is frequently used as the support of fundamental for the decision-making strategies as well as the consumer preferences. Sensory descriptive analysis is normally implemented in studies related to aquaculture because of its precise results that are easily associated with the physiochemical parameters. Among the most popular aquaculture fish species, gilthead sea bream, trout, and salmon are the most well-studied laboratory subjects (Calanche et al., 2020). In particular, the functional and sensory properties of the final products after dietary modification, substitution, or supplementation with different compounds were evaluated (Alexi et al., 2017; Badiola et al., 2017; Trullàs et al., 2017). Preliminarily, Gai et al. (2016) examined the use of pea protein concentrate and bacteria protein concentrate as dietary protein sources to feed rainbow trout. This study suggests that high preference was paid to trout fillets fed with a combination of alternative protein sources. Later, a scale of quality for the body shape of gilthead sea bream was developed (Fragkoulis et al., 2017). Therefore, it is essential to expand the knowledge of organoleptic properties in aquaculture, in addition to achieving adequate information for farmed fish quality recognition.
For perception analysis, consumers tend to value wild capture more than cultivated fish (Claret et al., 2014; Bacher, 2015; López-Mas et al., 2021). In a blinding test, Claret et al. (2014) discovered that consumers preferred farmed fish from different species over the wild option, but they favored the wild option once they knew the origin of the fish. The finding suggests that the choice of the consumer is not merely based on the sensory properties but is rather influenced by the psychological interpretation of the product details (López-Mas et al., 2021). In fact, the factors that influence the negative perception of aquaculture are based on preconceived ideas, attitudes, and beliefs (Bacher, 2015). The information provided to consumers and their cognitive processing, which involves beliefs, might bias the analysis of information by individuals and drive their preferences (Underwood, 2008). Although consumers from European regions are more concerned about the environmental or human health issues related to farmed and wild fish, they favorably prefer seafood products that are more natural (Schlag and Ystgaard, 2013).
Nutritional Benefits of Aquaculture Products
Nutrient deficiency is one of the global concerns occurring throughout the world (Tacon and Metian, 2013). Compared to terrestrial meat products (bovine meat, poultry meat, and pig meat), aquatic animals offer many benefits for human health. It is well documented that aquatic animals contribute to high levels of omega-3 fatty acids as the sources of dietary lipid and provide low calories but high-quality essential proteins (Eckhard and Leonor, 2018). Pioneer epidemiological evidence from a fishing village in Japan has shown that the consumption of aquatic animal products could effectively reduce the risk of cardiovascular disease (CVD) (Ebihara et al., 1982).
The role of the aquaculture sector in producing aquatic foods is quintessential not only for securing the global food supply but also for providing sufficient nutritional benefits, particularly for underdeveloped countries susceptible to malnutrition (Bogard et al., 2017). The rich nutrients in the aquatic foods may contribute to the fulfillment of human nutrient requirement, at the same time act as the active ingredients for developing nutraceutical products (Kim, 2013).
Protein and Amino Acids
Proteins are one of the essential macromolecules required by living organisms to carry out the functionality of the biological process. This versatile macromolecule is an initial substance for the enzyme, which functions as the catalyst for the hemoglobin and contributes to most of the functionalities of the body (i.e., immune system, growth, and movement). Aquatic animals are well known for their rich source of protein. The variability of the species, habitat, and cultivation characteristics are the key determinants in predicting the protein composition of the aquaculture organisms (Table 1).
Protein Content
Protein derived from aquatic sources can be categorized into 3 groups, namely, myofibrillar, structural protein, and sarcoplasmic proteins. As reported by Hernández-Ledesma and Herrero (2013), these proteins are rich in all EAAs, which are majorly dominated by lysine, valine, and leucine. These EAAs are important for determining the protein quality in food. Interestingly, the total EAA values of cultured aquatic organisms (Table 1) approach the recommended dietary values by FAO/WHO. The relatively high digestibility value (90%) indicates that the protein in aquaculture sources is highly nutritive (Tacon and Metian, 2013; Weichselbaum et al., 2013).
Generally, the protein content in cultivated organisms is directly correlated with the dietary feed. Commercial feeds are developed according to the type and maturity stage of the culture species (Akiyama, 1993). For example, the commercial feed of Nile Tilapia in Asia is formulated with fish meal (5–15%), fish oil (2–5%), soybean meals (20–60%), and vitamin and mineral premixes (0.1%). The total crude protein of Nile Tilapia accounted for between 30 and 40%, depending on the maturity stage (Creswell, 2005). The highlighted core nutrient in commercial feed is total crude protein, which promotes the growth of culture species (Ayuba and Iorkohol, 2012). As observed by Watanabe et al. (1990), the production of red tilapia increased because of the higher quantity of crude protein in the fish diet. The addition of crude protein into the feed meal is also important to achieve the balanced EAA ratio while also increasing the desirable nutrient load.
Protein Hydrolysate
The composition of high nutritive amino acids in cultured aquatic sources has garnered considerable interest recently with its potential applications in food supplements and pharmaceuticals. The evidence of physiological benefits of protein hydrolysate, including its antioxidants, anti-hypertensive, anti-inflammatory, antimicrobial, and hypocholesterolemia effects (Heffernan et al., 2021). The collagen hydrolysate of Pangasius pangasius (Pangas catfish) demonstrated anti-inflammatory properties through the activation of the residents and inflammatory tissue macrophages to secrete potent mediators, specifically pro-inflammatory cytokines implicated in the pathogenesis of tissue damage (Sivaraman and Shanthi, 2021). A study by Kaewsahnguan et al. (2021) reported that the salmon bone protein peptide consisted of phenylalanine, cystine, tyrosine, leucine, alanine, arginine, and glutamine, and these amino acids showed the greatest angiotensin-converting enzyme (ACE) inhibitory activity in ameliorating high blood pressure.
Recently, the role of fish-waste based protein hydrolysates for enhancing feed palatability and biological nutrients in the culture organisms has been discovered (Siddik et al., 2021). The inclusion of protein hydrolysate into the culture organism feed also showed greater growth performance, antioxidant activity, and immune response (Zheng et al., 2013; Wei et al., 2016). Most importantly, the culture organism fed with the fish protein hydrolysate could increase immunity and resistance to several specific viral, bacterial, and parasitic infections (Siddik et al., 2019; Chaklader et al., 2020).
Fat and Lipid Profile
Fats or oils are long polymer chains of hydrocarbons that consist of saturated, monounsaturated, and polyunsaturated fatty acids. Polyunsaturated fatty acids are essential lipids that cannot be synthesized naturally by mammalian organisms, and they can only be consumed from external sources (Hamilton et al., 2005). While aquaculture has increasingly become the major source of EPA and DHA for the burgeoning human population, it has also become the greatest consumer of the available supply of EPA and DHA (Tocher et al., 2019).
Omega-3 and Omega-6 PUFAs
Aquatic animals are major sources of long-chain fatty acids, including the famous omega-3 series (20:5n−3 EPA and 22:6n−3 DHA), as well as omega-6 arachidonic acid (20:4n−6 ARA). The composition of omega-3 and omega-6 PUFAs in the aquaculture products was in the range of 0.1 to 25.5% (Table 2). It is worth noting that the consumption of n−3 PUFAs could reduce the risk of coronary heart disease (Psota et al., 2006), cancer (Sasazuki et al., 2011), and aid in the development of cognitive and neurological functions (McNamara et al., 2015). According to the International Society of Fatty Acids and Lipids (ISSFAL), the ideal recommended dietary intake level of this fatty acid group is 500 mg per day for healthy adults. The action mode of omega-3 PUFAs is attributed to their ability to activate the pharmacological compounds, such as prostaglandins, as both EPA and DHA are the precursors of eicosanoids. Eicosanoids derived from omega-3 PUFAs showed excellent anti-inflammatory properties via the reduction of nuclear factor-κB activation and inhibition of the production of pro-inflammatory cytokines (Calder, 2015). Akbar et al. (2021) have inferred the effectiveness of PUFAs in preventing various physiological disorders, particularly the metabolic syndrome, cancer, and inflammation.
Subsequently, Oomen et al. (2000) suggested that individuals with frequent fatty fish intake were able to reduce the risk of getting CVD by 34%, and the consumption of fish up to 35 g/d would decrease CVD mortality. Recently, Zhang et al. (2020a) also reported that the incidence of cardiovascular heart disease decreased by 4% following a 20 g fish intake on a daily basis.
Muscle Color and Lean vs Fatty Fish
The fat content of fish is highly dependent on the muscle color. The white fish muscle (demersal fish) contains lower lipid content compared to pelagic fish, which consists of a higher proportion of dark colored muscle. Fish can also be classified based on their fat contents: (1) lean fish such as cod (<2%); and (2) fatty fish such as mackerel and salmon (>8%) (Venugopal, 2018). However, shellfish species, such as crustaceans and bivalves, are generally low in lipid content. Depending on the habitat, marine water fish species contain a higher content of omega-3 PUFAs than freshwater species, and fatty fish have been reported to contain the highest amount of EPA/DHA (Huss et al., 2004).
Fish Meal and Fish Oil Substitute
Traditionally, fish oil is used as a supplement to enhance essential fatty acids in aquaculture activities (Yun et al., 2013). Vergara et al. (1999) reported that the dietary lipid content of gilthead sea bream increased after the ingestion of good quality fish meal. The fish oil substitution also improved the lipid profiles of the rainbow trout (Yıldız et al., 2018; Gesto et al., 2021), gilthead sea bream (Ofori-Mensah et al., 2020), and red tilapia (Yu et al., 2021a). However, the limited source of fish oil has led to the substitute use of vegetable oils and microalgae as alternatives to the dietary lipid.
Recently, in China, Yu et al. (2021a) investigated the nutritional values and production performance of red tilapia using camelina seed oil, chia seed oil, cotton seed oil, canola oil, and a rapeseed oil-containing diet between the duration of 84 and 94 days at commercial scale. The most profound result from the use of a vegetable oil diet was the non-significant change in the proximate composition of the fish as compared to the fish oil diet, but there were significant reductions in the ratio of EPA/DHA, total DHA, and ARA, respectively. Surprisingly, the implementation of Nannochloropsis oculate (algae) in the Brachinos plicatilis muller (rotifer) diet showed improvement in the PUFA value (Eryalçın, 2019). In Mexico, a similar finding was documented by Legarda et al. (2021), where the supplementation of Ulva fasciata (seaweed) increased the DHA composition in the muscle portions of California yellowtail.
Vitamin
Fat Soluble Vitamins
Fat-soluble vitamins, the vitamins A, D, E, and K, are predominantly concentrated in the liver, viscera, and eyes of the fish. Fatty fish species contain higher vitamin A levels than lean fish, with reported differences of values between 75 and 1,450 IU (Venugopal, 2018). Cod, halibut, and sardine contain high levels of vitamin A, particularly in the liver (Kundam et al., 2018). In shrimps and oysters, vitamin A in the form of retinol has been discovered, whereas freshwater fish species are mainly enriched with vitamin A in the form of 3,4-dehydroretinol. Vitamin A is important for preventing xerophthalmia (eye inflammation that can lead to blindness), lingual cell keratinization, and epithelial intestinal tissue deterioration (Petricorena, 2015).
Vitamin D is predominantly present in the form of ergocalciferol (vitamin D2) or cholecalciferol (vitamin D3). Mackerel, herring flounder, marine trout, and shrimp demonstrated 0.06 to 300 μg of vitamin D (Bogard et al., 2015). This vitamin is important for regulating immune system of the body, and its deficiency may be associated with the occurrence of allergic asthma, osteoporosis, and multiple sclerosis (James et al., 2017).
Fatty fish is rich in vitamin E, which could be further categorized into four types: the α-, β-, γ-, and δ-tocopherol. The α-tocopherol is well-established for its function in enhancing dermatological health, owing to its vital role in the prevention of sunburn cell formation, lipid peroxidation, and inhibition of the action of nitric oxide and prostaglandins, such as prostaglandin E2 (PGE2) (Keen and Hassan, 2016). Meanwhile, vitamin K is generally known for its role in blood coagulation, and it is naturally occurring in two forms: the phytylmenaquinone (vitamin K1) and multiprenylmenaquinone (vitamin K2). A detailed quantitative study has been conducted to measure the micronutrient composition of 35 fish consumed as food from India. In the investigation, Amblypharyngodon mola, Puntius sophore, and Epinephelus spp. contained the highest amounts of vitamin K, ranging between 402.9 and 786.9 IU per 100 g of flesh (Mohanty et al., 2016). This highlights the significance of micronutrient composition in human nutrition, and such results will enhance the utility of fish at both the community level and clinical settings in the future.
Water Soluble Vitamins
Water-soluble vitamins, including thiamine (vitamin B1), riboflavin (vitamin B2), niacin (vitamin B3), pantothenic acid (vitamin B5), pyridoxine (vitamin B6), biotin (vitamin B7), folate (vitamin B9), and cobalamin (vitamin B12), are generally distributed in the flesh of aquatic organisms (Petricorena, 2015). B vitamins are essential in the formation of hemoglobin, the major component in red blood cells for the delivery of oxygen supply to the muscle cells (Williams, 2004). These vitamins are also involved in the energy production mechanism, particularly during carbohydrate and fat processing (Baj and Sieniawska, 2017).
Vitamin B1 (thiamine), an important co-enzyme, is essential for carbohydrate metabolism and modulation of the heart and nervous systems. Indications of thiamine deficiency are beri-beri and Wernicke Korsakoff syndrome (Thomson, 2000; Carpenter, 2012). This vitamin is most representative in dark-muscle fish (i.e., canned herring, mackerel, and pilchard) and shellfish (i.e., oysters), with an average content ranging between 0.1 and 1 mg/100 g of flesh (Petricorena, 2015). Vitamin B2, the riboflavin, is fundamental to supplying energy via fatty acid and amino acid degradations during energy production. Deficiency of riboflavin could lead to anemia and migraine prophylaxis (Baj and Sieniawska, 2017). In aquatic organisms, anchovies and oysters have been discovered to contain high amounts of riboflavin, approximately 2.33 to 2.63 mg per kg of flesh (Caprioli et al., 2018).
Fish flesh is a modest source of pyridoxine (vitamin B6), which accounted for 100 to 1,200 mg/100 g of flesh (Petricorena, 2015). Vitamin B6 is vital for the activation of energy production via amino acid conversion, ARA formation through the conversion of linoleic acid, blood glucose regulation, and hemoglobin synthesis (Petricorena, 2015). Cobalamin (Vitamin B12) is the most documented vitamin in fish and shellfish, with a concentration of up to 159 μg 100 g−1 (Venugopal, 2018). Bacteria, particularly Pseudomonas denitrificans, Bacillus megaterium, and Propionibacterium freudenreichii, are involved in the biosynthesis of cobalamin, and subsequently bioaccumulated through the natural food chain system (Gu and Li, 2016). Cobalamin is vital for the preservation and functioning of the neurological system, and its deficiency may cause demyelination of central and peripheral nerves, thus resulting in dementia (Rizzo and Laganà, 2019).
Minerals
Macrominerals such as calcium (Ca), magnesium (Mg), sodium (Na), potassium (K), iron (Fe), and phosphate (P) are essential for the growth and overall development of all living organisms. Aquatic organisms are one of the richest sources of mineral components, and these elements could accumulate in the body either through ingestion or absorption from the environment. Among the aquatic organisms, fish contain the highest amounts of macroelements (Na, Ca, and P) in the range of 100 to 250 μg g−1 (Venugopal, 2018).
Recently, mineral-based compounds derived from fish bone have gained attention due to their potential as mineral fortified foods. Sultanbawa and Aksnes (2006) have justified the potential use of tuna bone powders, which contain a proper ratio of Ca and P, as a food supplement. Additionally, minerals were isolated from the scales of Catla catla fish as sources of Ca, P, and Mg (Paul et al., 2017). Moreover, Ca and P were extracted from the bone of Lates calcarifer (sea bass) for the formulation of non-cytotoxic hydroxyapatite using heat treatment technology (Pal et al., 2017). Similarly, nanohydroxyapatite derived from Sardinella longiceps (sardine) has been reported to be promising for use in biomedical applications due to its excellent morphological structure, chemical group functionality, and cell viability (Surya et al., 2021).
Consumption Hazards of Aquaculture Products
Annually, approximately 1.8 million deaths are associated with food and drink contamination (WHO, 2015). In accordance with the public health issue, the food chain approach encompasses the entire food chain system, ranging from primary production to the final stage of human consumption, to ensure the safety, health, and nutritious elements of the products. Risk analysis is one of the important aspects of food safety evaluation under the food chain approach. This analysis involves scientific hazard identification, hazard characterization, and exposure assessment. In the aquaculture industry, risk analysis is implemented to avoid the incursion of microbial pathogens, chemicals, and other ecological impacts (Karunasagar, 2008).
Epidemiological studies define risk as a probability (without reference to the potential size of the adverse health impact), while hazard is interpreted as the presence of material or conditions that have the potential to cause harm or loss (Rout and Sikdar, 2017). There are 3 major broad categories of hazards that interact and impact the seafood supply chain from aquaculture subsectors: (1) chemical hazards derived from either natural or anthropogenic sources that may affect the survival rate of animals for human consumption; (2) human pathogen hazards that interact with aquatic products; and (3) animal pathogen hazards that may affect the quality of life of the products for use as seafood. The aquatic animals and plants have a particularly intricate relationship with their environment, that prone them to easy exposure and bioaccumulating to the diverse chemical and pathogen hazards present in water, sediment, and their feeds (Stentiford et al., 2022).
Three specific terms have been commonly used for evaluating toxicology perspectives in aquatic fields: the terminologies of bioconcentration, bioamplification, and bioaccumulation. Bioconcentration is a term that corresponds to a comparison of hazard component concentration between the living organism and its environmental surroundings (Sanou et al., 2021). The higher the bioconcentration factor, the higher the risk of product contamination. Bioaccumulation in aquatic farming is generally expressed by comparing the ratio of hazard component concentration to the sediment. This factor is effective in predicting the speed of absorption and excretion of living organisms (Sanou et al., 2021). Generally, bioaccumulation occurs in a living aquatic organism if the bioaccumulation factor is greater than 1 (Szefer et al., 1999). Meanwhile, bioamplification occurs when the rate of hazard concentration in muscle tissue excretion of aquatic species is slower than the fugacity capacity, thus resulting in both body mass and nutrition losses (Daley et al., 2009). This situation is expected to occur when the organism experiences a bioenergetic bottleneck or nutritional constraint related to metamorphosis, diet shifts, or reproduction (Drouillard, 2008). In this review, emphasis is placed on looking into the studies reported on the bioconcentration and bioaccumulation perspectives of hazardous materials in aquaculture products. Figure 3 illustrates the correlation between risk and benefit assessment in aquaculture practice.
Endocrine Disrupting Compounds
Endocrine disrupting compounds (EDCs) are mixtures of organic contaminants, that have the potential to elicit adverse effects on the hormonal systems of organisms (Deblonde et al., 2011; Bayen et al., 2013). These harmful contaminants could reduce the production and quality of sperm (Esteban et al., 2014; Giulivo et al., 2016), modify homeostasis (Giulivo et al., 2016), lower the growth rate (Giulivo et al., 2016), and cause the distortion of hormonal processes that lead to mental problems and obesity (Legler et al., 2015).
The origin of EDCs can be categorized into either natural or artificial compounds, such as hormones, secondary metabolite compounds (i.e., phthalates and alkylphenols), bisphenol A (BPA), polyhalogenated compounds, pharmaceuticals, and pesticides (Arditsoglou and Voutsa, 2008; Aris et al., 2014). The use of pesticides, antibiotics, and fertilizers for preventing parasitic and bacterial-borne diseases is one of the introduced sources of EDCs in the aquaculture system (Ali et al., 2016). The bioaccumulation of EDCs in fish is high due to its lipophilic properties and easily concentrated with the fat composition in the organisms (Waring and Harris, 2005). Long-term exposure of these substances will potentially generate adverse effects on human health as they diffuse into the food chain system.
The detection of EDCs, such as insecticides, pesticides, organophosphates, pharmaceutical drugs, steroid hormones, and phenolic compounds, has been reported recently in several cultured organisms (Table 3A). Generally, EDCs originate from the wastewater discharge, predominantly from residential, industrial, and agricultural plantations (Ismail et al., 2020; Olatoye et al., 2021). The presence of steroid hormone residues in the ecosystem is attributed to the less efficient sewage discharge from the residential area (Pessoa et al., 2014). In Malaysia, BPA is dominant at the mariculture cage site, indicating the widespread use of the chemical in aquaculture farms (Ismail et al., 2018). This statement was further justified via detailed laboratory work, where BPA was detected in golden pomfret, snapper, and sea bass, with the concentrations ranging between 0.078 and 0.0322 ng/g. Even though the detected level is considered safe for adults, it might pose some potential risks to children (Lee et al., 2015). Additionally, the content of polychlorinated biphenyl (PCB) was observed in the farmed yellow perch of all life stages. The mechanism can subsequently propagate through the food web and allow the fugacities of chemicals to increase through the successive trophic steps in the ecosystem (Daley et al., 2009). Meanwhile, exposure to herbicides and pesticides is correlated with the locally formulated fish feed. Plant-based formulated feeds are more likely to be the main source of lipophilic pollutants. The aggressive use of chemical spray in the agriculture farms also transferred the contaminations to the raw ingredient of the feeds, thus resulting in the bioaccumulation of the pollutants in the organism (Olatoye et al., 2021).
Heavy Metals
Over the past decades, the issue of toxic metal bioaccumulation in aquaculture organisms has become a hot topic in the scientific community. The release of metal elements into the surrounding environment does not occurs naturally nor anthropogenically, and significantly, this metal toxicity differs from that which is biologically essential to humans (Low et al., 2015). The adverse effects might impact humans in a long-term manner as dietary fish consumption (Ling and Liao, 2007). Heavy metal accumulation in the human body is associated with various health implications, including cancers (Saher and Kanwal, 2019), CVD (Sevim et al., 2020), hypertension (Martins et al., 2021), diabetes (Javaid et al., 2021), brain damage (Paithankar et al., 2021), disruption of immune function and impaired gastrointestinal system (Yu et al., 2021b), and anemia (Ashley-Martin et al., 2021). More importantly, pregnant women who were exposed to these toxic metals might experience miscarriage, stillbirth, premature birth, low birth weight, and fetal malformations (WHO, 2016).
Over the past decades, the presence of arsenic (As), cadmium (Cd), copper (Cu), cobalt (Co), iron (Fe), mercury (Hg), lead (Pb), manganese (Mn), selenium (Se), vanadium (V), and zinc (Zn) in aquaculture species has been extensively studied (Table 3B). The metal elements, As, Cd, Hg, and Pb, are known as the non-essential metals, but they can be toxic even in trace amounts (Munoz-Olivas, 2001). Evidence of heavy metal detection in aquaculture species was documented by Ju et al. (2017), where As, Cd, and Hg were reported in cultured tilapia. The research also proposed that sediment and water bodies from the culture pond greatly contributed to the metal bioaccumulation in the fish muscles. Mahat et al. (2018) also reported that the metal contamination occurred in the mussel culture pond. The detected concentrations of Pb and Cd in the mussel tissues exceeding the maximun permitable level. Additionally, crayfish from rice co-culture farming were found to be contaminated with As, Cd, and Pb. Since the detected levels were lower than the national permitable threshold and the reported HQ value was less than 1, the species was considered safe to be consumed (Tan et al., 2021).
The metal contaminants in the aquaculture cultivation areas are mainly derived from land urbanization, land reclamation, and petrochemical industries (Ju et al., 2017; Mahat et al., 2018). The release of environmental waste from a mine, either directly or indirectly, is one of the largest contributors to the metals discharged into the water stream (Rodriguez-Freire et al., 2016). Once it has been introduced into the water systems, the metal will be absorbed by the sediments, thus affecting the aquatic organisms for decades (MacDonald et al., 2016). Apart from that, the use of metal-enriched pellets and fish oil in the commercial diet also partly contributes to the contamination (Liang et al., 2016; Rodríguez-Hernández et al., 2017). Recently, Sanou et al. (2021) also discovered the presence of As, Cd, and Hg in farmed tilapia. The bioconcentration factor of the studies indicated that metals were transferred from bodies of water, and the zero value inferred that there was limited interaction of metal accumulation from sediments.
Microplastics
Microplastics (MPs) (fibers and films) are defined as synthetic plastic particulates with a diameter size of 100 nm–5 mm. It is commonly detected in the aquatic ecosystem, particularly the coastal, estuarine, and aquaculture water systems (Lusher, 2015). Two types of microplastic sources have been introduced into the aquaculture environment: the primary microplastic (commercial applications) (Zitko and Hanlon, 1991) and the secondary microplastic (degraded naturally in the environment through wave action, temperature change, and UV-radiation) (Wright et al., 2013) (Table 3C). Microplastic is pernicious and persistent, and it acts as a pathogen carrier in the water environment (Rochman et al., 2016). The number of MPs in the aquaculture water body system depends on its type, morphological size, residence size, and the circulating flow (Dong et al., 2021). Exposure to plastic aquaculture gear and tanks (Law and Thompson, 2014; Reinold et al., 2021), including the contaminated feeds, leads to microplastic contamination in commercial fish meal and cultured common carp (Cyprinus carpio) in Iran (Hanachi et al., 2019). The easy accessibility of MPs in the adsorption of contaminants will cause infinite harmful impacts on human beings (Xiang et al., 2021; Zhu, 2019). Typically, dysbacteriosis in the intestine will have immunity reduction in the human body system (Zhang et al., 2020b).
Antimicrobials Drugs
Recently, the use of antimicrobial drugs for preventing diseases as well as promoting the growth of aquaculture species has raised ascertainable concern (Çanak and Timur, 2020) (Table 3D). Lulijwa et al. (2020) revealed that up to 67 types of antibiotic compounds have been used among the top major aquaculture producing countries, notably in Bangladesh, China, and Vietnam. Antibiotics, particularly sulfonamide and tetracylines, are used in aquaculture practice to prevent bacterial infections, which are closely associated with the stress conditions (i.e., hypoxia, high fish density, and mass concentration of ammonia).
With utmost attention, the practical use of chloramphenicol, dimetridazole, ipronidazole, nitromidazoles, furazolidone, nitrofurazone, malachite green, and fluoroquinolones has been terminated in the aquaculture sector (FDA, 2020). Leumachite green was detected in the farmed seafood cultured in Montreal, Canada (Dinh et al., 2020). The worrying scenario related to the excessive use of antibiotics will not only cause an environmental threat but will also result in the antimicrobial resistance to living organisms (Han et al., 2021). There were approximately 170 incidences of antimicrobial resistance reported in the period from 2008 to 2018, when China ranked as the top country in the Asia region (Lulijwa et al., 2020). It should be noted that the main administration route of antimicrobial drugs is medicated either through the feed or through direct application to the water body (Preena et al., 2020).
Additionally, the undigested meal and feces excretion from the culture organism will indirectly retain the antimicrobial drug residue, therefore creating an antibiotic force fluctuation for the bacteria for survival and adaptation through different mechanisms, with the emergence of the resistance gene (Burridge et al., 2010). As reported by Sing et al. (2016), catfish from different culture environments showed an elevation and multiple antibiotic indices towards Salmonella spp., indicating the combined use of several antibiotics in the culture system. The ability of pathogen-resistant bacteria to transmit from aquaculture species to human beings is a major concern with respect to consumer health. The transmission can occur as long-term exposure to the antibiotic at a low level, which could attributed to the transfer of resistance gene with human pathogens via conjugation (Tomova et al., 2015; Scott et al., 2016).
Polycyclic Aromatic Hydrocarbon
Polycyclic aromatic hydrocarbons (PAHs) are lipophilic contaminants derived from either natural or anthropogenic origins. The sources of PAHs can be categorized into two: the petrogenic and pyrogenic origins (Zakaria et al., 2002). Environmental pollution from petrochemical industries, accidental oil spills from marine transportation, as well as effluent discharge from urban activities are the typical examples of petrogenic sources. While, pyrogenic sources include the partial combustion of organic matters, the release of exhausted gas and solid wastes from land transportation and biomass burning (Zakaria et al., 2002; Liu et al., 2009). The carcinogenic, mutagenic, and endocrine disruptor properties possessed by PAHs have made these substances an interesting scientific subject (Retnam et al., 2013; Shrivastava et al., 2017).
The bioaccumulation of PAHs in aquatic organisms occurs through the ingestion of suspended particles or direct uptake from the contaminated water through the skin or gills (Van der Oost et al., 2003). These substances can be biomagnified in organisms at the highest tropic level depending on their lipophilicity properties and metabolism resistance (Broman et al., 1992). These effects could enhance the risk of exposure to PAHs through the consumption of aquatic products (Pacini et al., 2013). Fish muscle and fish oils contain PAHs (Ohiozebau et al., 2017). Gadelha et al. (2019) also verified the presence of PAHs in bivalve species sampled from aquaculture farms located in Portugal. The study concluded that the spillage of diesel fuel from marine transport into the sea could be one of the possible root factors correlated with the presence of PAHs. PAHs have been found in fish and crustaceans too, due to petrogenic activity surrounding the aquaculture sites in Malaysia (Nasher et al., 2016).
The Evaluation of Risk–Benefit Correlation in Aquaculture Practice
Risk–benefit assessment (RBA) is a practical process for evaluating the potential for adverse and beneficial health impacts relative to food intake, components, and diet (Figure 3). This possibility trade of risk and benefit of an aquaculture organism through the quantification assessment could suggest an advisory in the consumption pattern to confer the health impacts (Cohen et al., 2005). The European Food Safety Authority outlines four steps for the implementation of RBA: (1) the identification of both hazard/benefit compounds; (2) the characterization and identification of both hazard/benefit compounds related to the reversibility, severity, and single-dose relationships; (3) the probability and exposure assessment of each identified compound for both hazard and benefit compounds in a population group; and (4) the risk and benefit assessment. Globally, there are three approaches to evaluating the RBA in aquaculture practice, namely, the hazard quotient assessment, net-integrated risk and benefit evaluation, and in vitro bioaccesibility evaluation (EFSA, 2010) (Table 4).
Hazard Quotient Assessment
The common approach to elucidating the RBA is closely related to the traditional risk assessment, by comparing the nutrient intake to the contaminants with dietary reference values. The comparison of contaminants can be made on the scale of exposure, through the division of exposure into reference values. The United States Environmental Protection Agency (USEPA, 2000) is the referred legislation for the monitoring of hazard risk assessment toward human health (EC—European Commission Regulation, 2001; Food Act 1983 and Food Regulations 1985 (1985); USEPA, 2000). Specifically, Hazard Quotient (HQ) and Hazard Index (HI) have been adopted as the reference guidelines for assessing potential health risks associated with the exposure of metal contaminants via fish consumption (USEPA, 2000). HQ is defined as the ratio of potential exposure to a substance and the level to which no adverse effects are expected, and HI is the sum of HQ for toxics that affect the organ system (USEPA, 2019). The measurements of HQ and HI are estimated using both experimental data and modeling assumptions. Generally, if the estimation value for HQ is beyond 0.2 or when the HI value exceeds 1, the occurrence probability of the chronic effect is high (Castilhos et al., 2006).
The aforementioned steps have been demonstrated by Fan et al. (2021), where the risk of cadmium exposure against the omega-3 PUFA intake was observed using the farmed Chinese mitten crab. The research team used the HQ formula to calculate the correlation between benefit and risk in an adult population. The study suggested that the daily intake benefit of Chinese mitten crab (0.02 to 0.07) had outweighed the risk, with the HQ value less than 1.
Net Integrated Risk and Benefit Evaluation
Another model equation approach, which adopts the concept of net integration between the risk and benefit, has been used to predict high-risk or lifestyle-related diseases affecting adults, mothers, and infants. The model applies an estimate through the subtraction of the net benefit from the net risk. A positive–negative outcome is used as an indicator to show whether the risk is outweighing the benefit, or vice versa. This modeling equation has been demonstrated in recent research conducted by Jing et al. (2021), where the benefits of omega 3-PUFAs in eutrophic reserved farmed fish culture against the risk of methylmercury exposure were studied among adults with coronary heart disease and visual recognition memory capability of infants. The study showed that the benefit of farmed fish has outweighed the risk as the integrated net-benefit value (0.4 to 1.2) was positive. Similarly, Yabanli and Tay (2021) have implemented the same formula for evaluating net integration of selenium against methylmercury ingestion using cultured sea bream in Turkey. The study revealed that the molar ratio between selenium and methylmercury was less than one, and the positive net-integrated value indicated little harmful risk in consuming the culture species.
In Vitro Bioaccessibility Evaluation
In vitro bioaccessibility evaluation is an experimental and modeling approach created by Afonso et al. (2015), where the risk and benefit were evaluated based on the number of ingested nutrients and contaminants. Traditionally, bioaccesibility has been applied to investigate the absorbable fraction from a food matrix after being consumed by a human being (Intawongse and Dean, 2006). Intawongse and Dean (2006) define bioaccesibility as the maximum fraction of a substance that can be released in the gastrointestinal digestion tract from its original food matrix (Intawongse and Dean, 2006). This process requires the functions of the digestive organs (stomach and intestine), including a series of enzymes for releasing the nutrients and contaminants prior to absorption into the circulatory system or elimination from the body. Conjunctively, the module has been adopted as a reliable tool as it is easy and rapid to handle. The assessment of benefits and risks with this technique was first studied using tuna (Thunnus spp.) (Afonso et al., 2015). Results revealed that the molar selenium over methylmercury ratios were higher than one (between 10 and 74). A probabilistic assessment of methylmercury risk versus selenium benefit showed that while a weekly meal of canned tuna presents a very low risk, raw, boiled, and grilled tuna consumption should not exceed a monthly meal, at least for pregnant and nursing women. The research tool was improved by utilizing the cutting-edge mathematical technique for a better balancing of the probabilistic results, which brought the assessment to a more accurate and realistic level (Afonso et al., 2018).
Challenges and Limitations of RBA
For the past decades, aesthetic attention has been paid towards the use of RBA in aquatic organisms, with the most frequently studied beneficial compounds being EPA, DHA, and selenium. Oppositely, methylmercury and dioxin are the most studied hazardous components in aquaculture practice. In the child-bearing woman, RBA suggested that this unique sub-group should limit the consumption of highly contaminated aquatic sources. Contradictorily, one to four servings of aquatic sources on a weekly basis are expected to be beneficial for the general population (Thomsen et al., 2021). It should be noted that the nutrient and risk content of the aquatic sources vary depending on the demographic, geographic, species, and cultural characteristics of country. Additionally, there is an urgent need to revise the RBA models that can aid in the decision-making process for both current and alternative consumption scenarios. To better reflect both scenarios, the heterogeneity of individual food consumption as well as the level of components in the food must be considered in the model. Also, the inclusion of other aspects, such as the environmental impact on RBA modeling, is vital for decision making. Such inclusion would require other advanced approaches with different elements to be created in the future (Thomsen et al., 2021).
Conclusion
Aquaculture is seen as an essential food producing sector for improving global food security and nutrition indices. Culture characteristics and feeding formulations are likely to influence the nutritional composition of the farmed species. With proper diets and feed formulation, the culture species could achieve a satisfactory quality of protein and lipid profiles, indirectly offering an alternative solution to overcome food insecurity. However, the evidence of contaminant accumulation in the aquaculture species is found to be a worrying scenario. The discharge of effluents from industrial areas and household residencies is found to be the main reason for the presence of chemical contaminants, notably EDC, PAHs, heavy metals, antimicrobial drugs, and MPs. It should be noted that all compounds might accumulate in the tissue of the culture organism and possess the possibility to transfer the hazard risk to human beings as part of the routine diet. The RBA approach is a trending and useful tool for evaluating the safety of aquatic organism consumption. Several model equations have been developed to offer convincing guidelines on the food intake and exposure of individuals. Nonetheless, the limited data of RBA in aquaculture practice is also found to be a challenging issue, and this needs to be frequently regulated to achieve a sustainable aquaculture industry.
Author Contributions
Conceptualization, NA, NS, and L.K. Lee. Methodology, NA. Writing—original draft preparation, NA. Writing—review & editing, NA and L.K. Lee. Supervision, NS, and L.K. Lee. Funding acquisition, NS. All authors listed have made a substantial, direct, and intellectual contribution to the work and approved it for publication.
Conflict of Interest
The authors declare that the research was conducted in the absence of any commercial or financial relationships that could be construed as a potential conflict of interest.
Publisher’s Note
All claims expressed in this article are solely those of the authors and do not necessarily represent those of their affiliated organizations, or those of the publisher, the editors and the reviewers. Any product that may be evaluated in this article, or claim that may be made by its manufacturer, is not guaranteed or endorsed by the publisher.
Acknowledgments
Authors are grateful to the Ministry of Higher Education Malaysia for the funding of Long-Term Research Grant Scheme (LRGS/1/2018/USM/01/1/5) (203/PHUMANITI/67215003).
References
Afonso C., Costa S., Cardoso C., Coelho I., Castanheira H., Lourenço, et al. (2018). Bioaccessibility in Risk-Benefit Analysis of Raw and Cooked Seabream Consumption. J. Food Compos. Anal. 68, 118–127. doi: 10.1016/j.jfca.2016.10.003
Afonso C., Costa S., Cardoso C., Olievia R., Lourenco H. M., Viula A., et al. (2015). Benefits and Risks Associated With Consumption of Raw, Cooked, and Canned Tuna (Thunnus Spp.) Based on the Bioaccessibility of Selenium and Methylmercury. Environ. Res. 143, 130–137. doi: 10.1016/j.envres.2015.04.019
Akbar S., Bhatti M. Z., Saeed R. F., Qazi A. S. (2021). Polyunsaturated Fatty Acids: Impact on Health and Disease Status. Life Sci. 2, 71–82. doi: 10.37185/lns.1.1.85
Akiyama T. (1993). Handbook of Nutrient Requirements of Finfish. Aquaculture 114, 204. doi: 10.1016/0044-8486(93)90313-n
Alexi N., Fountoulaki E., Grigorakis K. (2017). Quality of Reared Gilthead Sea Bream (Sparus Aurata) During Ice Storage, as Affected by Dietary Fish Oil Substitution; an Instrumental and Sensory Designation Approach. Aquac. Res. 48, 3817–3828. doi: 10.1111/are.13208
Ali H., Rico A., Murshed-e-Jahan K., Belton B. (2016). An Assessment of Chemical and Biological Product Use in Aquaculture in Bangladesh. Aquaculture 454, 199–209. doi: 10.1016/j.aquaculture.2015.12.025
Arditsoglou A., Voutsa D. (2008). Determination of Phenolic and Steroid Endocrine Disrupting Compounds in Environmental Matrices. Environ. Sci. pollut. Res. 15, 228–236. doi: 10.1065/espr2007.12.459
Aris A. Z., Shamsuddin A. S., Praveena S. M. (2014). Occurrence of 17α-Ethynylestradiol (EE2) in the Environment and Effect on Exposed Biota: A Review. Environ. Int. 69, 104–119. doi: 10.1016/j.envint.2014.04.011
Ashley-Martin J., Iannotti L., Lesorogol C., Hilton C. E., Olungah C. O., Zava T., et al. (2021). Heavy Metal Blood Concentrations in Association With Sociocultural Characteristics, Anthropometry and Anemia Among Kenyan Adolescents. Int. J. Environ. Health Res. 2, 1–15. doi: 10.1080/09603123.2021.1929871
Ayuba V. O., Iorkohol E. K. (2012). Proximate Composition of Some Commercial Fish Feeds Sold in Nigeria. J. Fish. Aquat. Sci. 8, 248–252. doi:10.3923/jfas.2013.248.252
Azra M. N., Okomoda V. T., Tabatabaei M., Hassan M., Ikhwanuddin M. (2021). The Contributions of Shellfish Aquaculture to Global Food Security: Assessing its Characteristics From a Future Food Perspective. Front. Mar. Sci. 8. doi: 10.3389/fmars.2021.654897
Azpeitia K., Ríos Y., Garcia I., Pagaldai J., Mendiola D.(2017). A sensory and nutritional validation of open ocean mussels (Mytilus galloprovincialis Lmk.) cultured in SE Bay of Biscay (Basque Country) compared to their commercial counterparts from Galician Rías (Spain). Int. Aquat. Res. 9, 89–106. doi: 10.1007/s40071-017-0159-0
Bacher K. (2015). Perceptions and Misconceptions of Aquaculture (FAO, Rome: Food and Agriculture Organization of United Nation).
Badiola M., Gartzia I., Basurko O. C., Mendiola D. (2017). Land-Based Growth of Atlantic Salmon (Salmo Salar) and Consumers’ Acceptance. Aquac. Res. 48, 4666–4683. doi: 10.1111/are.13289
Baj T., Sieniawska E. (2017). Vitamins ( Elsevier Inc:London, United Kingdom), 281–292. doi: 10.1016/B978-0-12-802104-0.00013-5
Bayen S., Zhang H., Desai M. M., Ooi S. K., Kelly B. C. (2013). Occurrence and Distribution of Pharmaceutically Active and Endocrine Disrupting Compounds in Singapore’s Marine Environment: Influence of Hydrodynamics and Physical-Chemical Properties. Environ. pollut. 182, 1–18. doi: 10.1016/j.envpol.2013.06.028
Bhavan P. S., Radhakrishnan S., Seenivasan C., Shanthi R., Poongodi R., Kannan S. (2010). Proximate Composition and Profiles of Amino Acids and Fatty Acids in the Muscle of Adult Males and Females of Commercially Viable Prawn Species Macrobrachium Rosenbergii Collected from Natural Culture Environments. Int. J. Biol. 2, 107–119. doi: 10.5539/ijb.v2n2p107
Bogard J. R., Farook S., Marks G. C., Jillian W., Ben B., Masum A., et al. (2017). Higher Fish But Lower Micronutrient Intakes: Temporal Changes in Fish Consumption From Capture Fisheries and Aquaculture in Bangladesh. PLos One 12, 1–14. doi: 10.1371/journal.pone.0175098
Bogard J. R., Thilsted S. H., Marks G. C., Wahab M. A., Hossain M. A. R., Jakobsen J., et al. (2015). Nutrient Composition of Important Fish Species in Bangladesh and Potential Contribution to Recommended Nutrient Intakes. J. Food Compos. Anal. 42, 120–133. doi: 10.1016/j.jfca.2015.03.002
Broman D., Rolff C., Näf C., Zebühr Y., Fry B., Hobbie J. (1992). Using Ratios of Stable Nitrogen Isotopes to Estimate Bioaccumulation and Flux of Polychlorinated Dibenzo-P-Dioxins (PCDDs) and Dibenzofurans (PCDFs) in Two Food Chains From the Northern Baltic. Environ. Toxicol. Chem. 11, 331–345. doi: 10.1002/etc.5620110307
Burgess M. G., Polasky S., Tilman D. (2013). Predicting Overfishing and Extinction Threats in Multispecies Fisheries. Proc. Natl. Acad. Sci.U. S. A. 110, 15943–25948. doi: 10.1073/pnas.1314472110
Burridge L., Weis J. S., Cabello F., Pizzaro J., Bostick K. (2010). Chemical Use in Salmon Aquaculture: A Review of Current Practices and Possible Environmental Effects. Aquaculture 1, 7–23. doi: 10.1016/j.aquaculture.2010.05.020
Calanche J. B., Beltrán J. A., Hernández Arias A. J. (2020). Aquaculture and Sensometrics: The Need to Evaluate Sensory Attributes and the Consumers’ Preferences. Rev. Aquac. 12, 805–821. doi: 10.1111/raq.12351
Calder P. C. (2015). Marine Omega-3 Fatty Acids and Inflammatory Processes: Effects, Mechanisms and Clinical Relevance. Biochim. Biophys. Acta 1851, 469–484. doi: 10.1016/j.bbalip.2014.08.010
Çanak Ö., Timur G. (2020). An Initial Survey on the Occurrence of Staphylococcal Infections in Turkish Marine Aquacultur –1014). J. Appl. Ichthyol. 36, 932–941. doi: 10.1111/jai.14141
Capo X., Rubio M., Solomando A., Alomar C., Compa M., Sureda A., et al. (2021). Microplastic Intake and Enzymatic Responses in Mytilus Galloprovincialis Reared at the Vicinities of an Aquaculture Station. Chemosphere 280, 130575. doi: 10.1016/j.chemosphere.2021.130575.
Caprioli G., Sagratini G., Vittori S., Torregiani E. (2018). Optimization of an Extraction Procedure for the Simultaneous Quantification of Riboflavin, Nicotinamide and Nicotinic Acid in Anchovies (Engraulis Enrasicolus) by High-Performance Liquid Chromatography–Tandem Mass Spectrometry. J. Food Compos. Anal. 66, 23–29. doi: 10.1016/j.jfca.2017.11.004
Carpenter K. J. (2012). The Discovery of Thiamin. Ann. Nutr. Metab. 61, 219–223. doi: 10.1159/000343109
Castilhos Z. C., Rodrigues-Filho S., Rodrigues A. P. C., Roberto C. V., Shefa S., Marcello M. V., et al. (2006). Mercury Contamination in Fish From Gold Mining Areas in Indonesia and Human Health Risk Assessment. Sci. Total Environ. 1, 320–325. doi: 10.1016/j.scitotenv.2006.01.039
Chaklader M. R., Fotedar R., Howieson J., Siddik M. A. B., Foysal M. J. (2020). The Ameliorative Effects of Various Fish Protein Hydrolysates in Poultry by-Product Meal Based Diets on Muscle Quality, Serum Biochemistry and Immunity in Juvenile Barramundi, Lates Calcarifer. Fish. Shellfish Immunol. 104, 567–578. doi: 10.1016/j.fsi.2020.06.014
Chan C. Y., Tran N., Pethiyagoda S., Crissman C. C., Sulser T. B., Phillips M. J. (2019). Prospects and Challenges of Fish for Food Security in Africa. Glob. Food Sec. 20, 17–25. doi: 10.1016/j.gfs.2018.12.002
Chasanah E., Fithriani D., Poernomo A., Jeinie M. H., Huda N. (2021). The Nutritional Profile of Indonesian Salmon Van Java Mahseer T. Soro Species. Potravin. Slovak J. Food Sci. 15, 566–574. doi: 10.5219/1552.
Chen M., Zhou J., Lin J., Tang H., Shan Y., Chang A. K., et al. (2020). Changes in Oxidative Stress Biomarkers in Sinonovacula Constricta in Response to Toxic Metal Accumulation During Growth in an Aquaculture Farm. Chemosphere 248, 125974. doi: 10.1016/j.chemosphere.2020.125974
Claret A., Guerrero L., Ginés R., Grau A., Hernández M. D., Aguirre E., et al. (2014). Consumer Beliefs Regarding Farmed Versus Wild Fish. Appetite 79, 25–31. doi: 10.1016/j.appet.2014.03.031
Cohen J. T., Bellinger D. C., Connor W. E., Kriss-Etherton P. M., Lawrence P. M., Savitz D. A., et al. (2005). A Quantitative Risk-Benefit Analysis of Changes in Population Fish Consumption. Am. J. Prev. Med. 29, 325–334. doi: 10.1016/j.amepre.2005.07.003
Creswell D. (2005). The Feeding and Nutrition of the Tilapia. AQUA Cult. Asia Pacific Magazine, 32–33.
Daley J. M., Leadley T. A., Drouillard K. G. (2009). Evidence for Bioamplification of Nine Polychlorinated Biphenyl (PCB) Congeners in Yellow Perch (Perca Flavascens) Eggs During Incubation. Chemosphere 75, 1500–1505. doi: 10.1016/j.chemosphere.2009.02.013
Deblonde T., Cossu-Leguille C., Hartemann P. (2011). Emerging Pollutants in Wastewater: A Review of the Literature. Int. J. Hyg. Environ. Health 214, 442–448. doi: 10.1016/j.ijheh.2011.08.002
Dinh Q. T., Munoz G., Vo D. S., Do D. T., Bayen S., Sauve S. (2020). Analysis of Sulfonamides, Fluoroquinolones, Tetracyclines, Triphenylmethane Dyes and Other Veterinary Drug Residues in Cultured and Wild Seafood Sold in Montreal, Canada. J. Food Compos. Anal. 94, 103630. doi: 10.1016/j.jfca.2020.103630
Dong H., Chen Y., Wang J., Zhang Y., Zhang P., Li X., et al. (2021). Interactions of Microplastics and Antibiotic Resistance Genes and Their Effects on the Aquaculture Environments. J. Hazard. Mater. 403, 123961. doi: 10.1016/j.jhazmat.2020.123961
Drouillard K. G. (2008). “Biomagnification,” in Ecotoxicology Vol. 1 of Encyclopaedia of Ecology, vol. 5 . Eds. Jorgensen S. E., Fath B. D. (Oxford: Elsevier), 441–448.
Ebihara A., Suzuki M., Miyatake T., Hamamoto T., Goto K., Motonaga E., et al. (1982). Eicosapolyenoic Acids of Serum Lipids of Japanese Islanders With Low Incidence of Cardiovascular Diseases. J. Nutr. Sci.Vitaminol (Tokyo) 28, 441–453. doi: 10.3177/jnsv.28.441
Eckhard W. P., Leonor C. M. (2018). What Aquaculture Does for Taxonomy, Evo-Devo, Palaeontology, Biomechanics and Biomedical Research. J. Appl. Ichthyol. 34, 429–430. doi: 10.1111/jai.13670
Edwards P., Zhang W., Belton B., Little D. C. (2019). Misunderstandings, Myths and Mantras in Aquaculture: Its Contribution to World Food Supplies has Been Systematically Over Reported. Mar. Policy 106, 103547. doi: 10.1016/j.marpol.2019.103547
EFSA Scientific Committee (2010). Guidance on Human Health Risk-Benefit Assessment of Food. EFSA J. 8, 1673. doi: 10.2093/j.efsa.2010.1673
Eryalçın K. M. (2019). Nutritional Value and Production Performance of the Rotifer Brachionus Plicatilis Müller 1786 Cultured With Different Feeds at Commercial Scale. Aquac. Int. 27, 875–890. doi: 10.1007/s10499-019-00375-5
Esteban S., Gorga M., González-Alonso S., Petrovic M., Barceló D., Valcárcel Y. (2014). Monitoring Endocrine Disrupting Compounds and Estrogenic Activity in Tap Water From Central Spain. Environ. Sci. pollut. Res. 21, 9297–92310. doi: 10.1007/s11356-014-2847-2
European Commission Regulation (2001). Commission Regulation (EC) No 466/2001: Setting Maximum Levels for Certain Contaminants in Foodstuffs. Off J. Eur. Communities. 1–25.
Fan Z., Zou J., Wang Q., Qiu L., Hu G., Song C., et al. (2021). Quantitative Benefit and Risk Assessment of Cadmium and Nutrient Levels in Chinese Mitten Crab (Eriocheir Sinensis). Environ. Sci. pollut. Res. 28, 7322–7331. doi: 10.1007/s11356-020-10977-9
FAO (2016). The State of World Fisheries and Aquaculture. Contributing to Food Security and Nutrition for All( FAO, Rome).
FAO (2020). The State of World Fisheries and Aquaculture 2020. Sustainability in Action ( FAO: Rome). doi: 10.4060/ca9229en
FAO (2022). “Fishery and Aquaculture Statistics,” in Global Fisheries Commodities Production and Trade 1976-2018 (FishstatJ) ( Fishery and Aquaculture Statistics. Global Fisheries commodities production and trade,Rome1976–2018 (FishstatJ).
FDA (2020) Approved Aquaculture Drugs. Available at: https://www.fda.gov/animal-veterinary/aquaculture/approved-aquaculture-drugs (Accessed 2 Feb 2022).
Food Act 1983 and Food Regulations 1985. (1985) Available at: https://extranet. who.int/nutrition/gina/sites/default/filesstore/MYS%201985%20Food%20 Regulations_0.pdf (Accessed 24 Feb 2022).
Fragkoulis S., Christou M., Karo R., Ritas C., Tzokas C., Batargias C., et al. (2017). Scaling of Body-Shape Quality in Reared Gilthead Seabream Sparus Aurata L. Consumer Preference Assessment, Wild Standard and Variability in Reared Phenotype. Aquac. Res. 48, 2402–2410. doi: 10.1111/are.13076
Freitas J., Vaz-Pires P., Câmara J. S. (2020). From Aquaculture Production to Consumption: Freshness, Safety, Traceability and Authentication, the Four Pillars of Quality. Aquaculture 518, 734857. doi: 10.1016/j.aquaculture.2019.734857
Gadelha J. R., Rocha A. C., Camacho C., Eljaral E., Peris A., Amminot Y., et al. (2019). Persistent and Emerging Pollutants Assessment on Aquaculture Oysters (Crassostrea Gigas) From NW Portuguese Coast (Ria De Aveiro). Sci. Total Environ. 666, 731–742. doi: 10.1016/j.scitotenv.2019.02.280
Gai F., Peiretti P. G., Brugiapaglia A., Gasco L. (2016). Effects of Dietary Protein Source and Feeding Regime on Growth Performance, Nutrient Digestibility, Fatty Acids, and Quality Characteristics of Rainbow Trout, Oncorhynchus Mykiss, Fillets. J. World Aquac. Soc 47, 496–507. doi: 10.1111/jwas.12294
Garlock T., Asche F., Anderson J., Bjørndal T., Kumar G., Lorenzen K., et al. (2020). A Global Blue Revolution: Aquaculture Growth Across Regions, Species, and Countries. Rev. Fish. Sci. Aquac. 28, 1–10. doi: 10.1080/23308249.2019.1678111
Garlock T., Asche F., Anderson J., Ceballos-Concha A., Love D. C., Osmundsen T. C., et al. (2022). Aquaculture: The Missing Contributor in the Food Security Agenda. Glob. Food Sec. 32, 100620. doi: 10.1016/j.gfs.2022.100620
Gesto M., Madsen L., Andersen N. R., Kertaouid N. K., Kestemontd P., Jokumsenet A., et al. (2021). Early Performance, Stress- and Disease-Sensitivity in Rainbow Trout Fry (Oncorhynchus Mykiss) After Total Dietary Replacement of Fish Oil With Rapeseed Oil. Effects EPA DHA supplement. Aquacult. 536, 736446. doi: 10.1016/j.aquaculture.2021.736446
Gibson J. S., Wai H., Ooi S. S. M. L., Hmwe E. M. M., Wai S. S., Hthun L. L., et al. (2020). Antimicrobials Use and Resistance on Integrated Poultry-Fish Farming Systems in the Ayeyarwady Delta of Myanmar. Sci. Rep. 10, 16149. doi: 10.1038/s41598-020-73076-2
Girolametti F., Annibaldi A., Carnevali O., Pignalosa P., Illuminati S., Truzzi C. (2021). Potential Toxic Elements (PTEs) in Wild and Farmed Atlantic Bluefin Tuna (Thunnus Thynnus) From Mediterranean Sea: Risks and Benefits for Human Consumption. Food Control 125, 108012. doi: 10.1016/j.foodcont.2021.108012
Giulivo M., Lopez de Alda M., Capri E., Barceló D. (2016). Human Exposure to Endocrine Disrupting Compounds: Their Role in Reproductive Systems, Metabolic Syndrome and Breast Cancer. A Review. Environ. Res. 151, 251–264. doi: 10.1016/j.envres.2016.07.011
Golden C. D., Allison E. H., Cheung W. W. L., Dey M. M., Halpern B. S., McCauley D. J., et al. (2016). Nutrition: Fall in Fish Catch Threatens Human Health. Nature 534, 317–320. doi: 10.1038/534317a
Golden C. D., Seto K. L. S., Dey M. M., Chen O. L., Gephart J. A., Myers S. S., et al. (2017). Does Aquaculture Support the Needs of Nutritionally Vulnerable Nations? Front. Mar. Sci. 4. doi: 10.3389/fmars.2017.00159
Guillen J., Natale F., Carvalho N., Casey J., Hofherr J., Druon J. N., et al. (2019). Global Seafood Consumption Footprint. Ambio 48, 111–122. doi: 10.1007/s13280-018-1060-9
Gu Q., Li P. (2016). “Biosynthesis of Vitamins by Probiotic Bacteria,” in Probiotics and Prebiotics in Human Nutrition and Health. Available at: https://www.intechopen.com/chapters/50488. ( IntechOpen,London).
Gunalan B, Nina Tabitha S., Soundarapandian P., Anand T (2013). Nutritive value of cultured white leg shrimp Litopenaeus vannamei. IJFA 5, 166–171. doi: 10.5897/IJFA2013.0333
Guo Y. R., Gu S. Q., Wang X. C., Zhuang K. J., Wang S., Shi J. (2015). Nutrients and non-Volatile Taste Compounds in Chinese Mitten Crab by-Products. Fish. Sci. 81, 193–203. doi: 10.1007/s12562-014-0816-9
Hamilton M. C., Hites R. A., Schwager S. J., Foran J. A., Kruth B. A., Carpenter D. O. (2005). Lipid Composition and Contaminants in Farmed and Wild Salmon. Environ. Sci. Technol. 39, 8622–8629. doi: 10.1021/es050898y
Hanachi P., Karbalaei S., Walker T. R., Cole M., Hosseini S. V. (2019). Abundance and Properties of Microplastics Found in Commercial Fish Meal and Cultured Common Carp (Cyprinus Carpio). Environ. Sci. Pollut. Res. 26, 23777–23787. doi: 10.1007/s11356-019-05637-6
Han Q. F., Zhao S., Zhang X. R., Wang X. L., Song C., Wang S. G. (2020). Distribution, Combined Pollution and Risk Assessment of Antibiotics in Typical Marine Aquaculture Farms Surrounding the Yellow Sea, North China. Environ. Int. 138, 105551. doi: 10.1016/j.envint.2020.105551.
Han Q. F., Zhang X. R., Xu X. Y., Ding Z. J., Zhao S., Wang S. J. (2021). Antibiotics in Marine Aquaculture Farms Surrounding Laizhou Bay, Bohai Sea: Distribution Characteristics Considering Various Culture Modes and Organism Species. Sci. Total Environ. 760, 143863. doi: 10.1016/j.scitotenv.2020.143863
Heffernan S., Giblin L., O’Brien N. (2021). Assessment of the Biological Activity of Fish Muscle Protein Hydrolysates Using In Vitro Model Systems. Food Chem. 359, 129852. doi: 10.1016/j.foodchem.2021.129852
Hernández-Ledesma B., Herrero M. (2013). Bioactive Compounds From Marine Foods: Plant and Animal Sources. United States: Wiley-Blackwell.
Hu B., Zhou J., Qiu H., Lai X., Li J., Wu D., et al. (2021). Comparison of Nutritional Quality and Volatile Flavor Compounds Among Bighead Carp from Three Aquaculture Systems.Saudi J. Biol. Sci.28,4291–4299. doi: 10.1016/j.sjbs.2021.03.079
Huss H. H., Ababouch L., Gram L., Huss H. H., Ababouch L., Gram L. (2004) Assessment and Management of Seafood Safety and Quality. Available at: https://seafood.oregonstate.edu/sites/agscid7/files/snic/assessment-and-management-of-seafood-safety-and-quality.pdf.
Hussan A., Gon T. (2016). Common Problems in Aquaculture and Their Preventive Measures. Aquac. Times J. 2, 6–9.
Intawongse M., Dean J. R. (2006). In-Vitro Testing for Assessing Oral Bioaccessibility of Trace Metals in Soil and Food Samples. TrAC - Trends Anal. Chem. 25, 876–886. doi: 10.1016/j.trac.2006.03.010
Ismail N. A. H., Wee S. Y., Aris A. Z. (2018). Bisphenol A and Alkylphenols Concentrations in Selected Mariculture Fish Species From Pulau Kukup, Johor, Malaysia. Mar. pollut. Bull. 127, 536–540. doi: 10.1016/j.marpolbul.2017.12.043
Ismail N. A. H., Wee S. Y., Haron D. E. M., Kamarulzaman N. H., Aris A. Z. (2020). Occurrence of Endocrine Disrupting Compounds in Mariculture Sediment of Pulau Kukup, Johor, Malaysia. Mar. pollut. Bull. 150, 110735. doi: 10.1016/j.marpolbul.2019.110735
Ismail N. A. H, Aris A. Z., Wee S. Y., Nasir H. M., Razak M. R., Kamarulzaman N. H., et al. (2021). Occurrence and Distribution of Endocrine-Disrupting Chemicals in Mariculture Fish and the Human Health Implications. Food Chem. 345, 128806. doi: 10.1016/j.foodchem.2020.128806.
James J., Weaver V., Cantorna M. T. (2017). Control of Circulating IgE by the Vitamin D Receptor In Vivo Involves B Cell Intrinsic and Extrinsic Mechanisms. J. Immunol. 198, 1164–1171. doi: 10.4049/jimmunol.1601213
Javaid A., Akbar I., Javed H., Khan U., Iftikhar H., Zahra D., et al. (2021). Role of Heavy Metals in Diabetes: Mechanisms and Treatment Strategies. Crit. Rev. Eukaryot. Gene Expr. 31, 65–80. doi: 10.1615/CRITREVEUKARYOTGENEEXPR.2021037971
Jennings S., Stentiford G. D., Leocadio A. M., Jeffery K. R., Metcalfe J. D., Katsiadaki I., et al. (2016). Aquatic Food Security: Insights Into Challenges and Solutions From an Analysis of Interactions Between Fisheries, Aquaculture, Food Safety, Human Health, Fish and Human Welfare, Economy and Environment. Fish. Fish. 17, 893–938. doi: 10.1111/faf.12152
Jing M., Lin D., Lin J., Li Q., Yan H., Feng X. (2021). Mercury, Microcystins and Omega-3 Polyunsaturated Fatty Acids in Farmed Fish in Eutrophic Reservoir: Risk and Benefit Assessment. Environ. pollut. 270, 116047. doi: 10.1016/j.envpol.2020.116047
Ju Y. R., Chen C. W., Chen C. F., Chuang X. Y., Dong C. D. (2017). Assessment of Heavy Metals in Aquaculture Fishes Collected From Southwest Coast of Taiwan and Human Consumption Risk. Int. Biodeterior. Biodegrad. 124, 314–325. doi: 10.1016/j.ibiod.2017.04.003
Kaewsahnguan T., Noitang S., Sangtanoo P., Srimongkol P., Saisavoey T., Reamtong O., et al. (2021). A Novel Angiotensin I-Converting Enzyme Inhibitory Peptide Derived From the Trypsin Hydrolysates of Salmon Bone Proteins. PLos One 16, e0256595. doi: 10.1371/journal.pone.0256595
Karunasagar I. (2008). “Food Safety and Public Health Risks Associated With Products of Aquaculture,” in Understanding and Applying Risk Analysis in Aquaculture. Eds. Bondad-Reantaso M. G., Arthur J. R., Subasinghe R. P. (Rome: FAO), 9–25.
Keen M., Hassan I. (2016). Vitamin E in Dermatology. Indian Dermatol. Online J. 7, 311–315. doi: 10.4103/2229-5178.185494
Kennedy D. O. (2016). B Vitamins and the Brain: Mechanisms, Dose and Efficacy—A Review. Nutrients 8, 68–73. doi: 10.3390/nu8020068
Kharas H. (2010). The Emerging Middle Class in Developing CountriesParis, France: OECD Publishing, 61 pp.
Kim S. K. (2013). Marine Nutraceuticals: Prospects and Perspectives. 1st Ed (England: Taylor and Francis).
Koehn J. Z., Allison E. H., Villeda K., Chen Z., Nixon M., Crigler E., et al. (2022). Fishing for Health: Do the World’s National Policies for Fisheries and Aquaculture Align With Those for Nutrition? Fish. Fish. 23, 125–142. doi: 10.1111/faf.12603
Kundam D. N., Acham I. O., Girgih A. T. (2018). Bioactive Compounds in Fish and Their Health Benefits. Asian Food Sci. J. 4, 1–14. doi: 10.9734/afsj/2018/41803
Law K. L., Thompson R. C. (2014). Microplastics in the Seas. Science 345, 144–145. doi: 10.1126/science.1254065
Lee C. C., Jiang L. Y., Kuo Y. L., Chen S. Y., Hsieh C. Y., Hung C. F., et al. (2015). Characteristics of Nonylphenol and Bisphenol A Accumulation by Fish and Implications for Ecological and Human Health. Sci. Total Environ. 502, 417–425. doi: 10.1016/j.scitotenv.2014.09.042
Legarda E. C., Viana M. T., Zaragoza O. B. D. R., Skrzynska A. K., Braga A., Lorenzo M. A., et al. (2021). Effects on Fatty Acids Profile of Seriola Dorsalis Muscle Tissue Fed Diets Supplemented With Different Levels of Ulva Fasciata From an Integration Multi-Trophic Aquaculture System. Aquaculture 535, 736414. doi: 10.1016/j.aquaculture.2021.736414
Legler J., Fletcher T., Govarts E., Porta M., Blumberg B., Heindel J. J., et al. (2015). Obesity, Diabetes, and Associated Costs of Exposure to Endocrine-Disrupting Chemicals in the European Union. J. Clin. Endocrinol. Metab. 100, 1278–1288. doi: 10.1210/jc.2014-4326
Liang P., Wu S. C., Zhang J., Chao Y., Yu S., Wong M. H. (2016). The Effects of Mariculture on Heavy Metal Distribution in Sediments and Cultured Fish Around the Pearl River Delta Region, South China. Chemosphere 148, 171–178. doi: 10.1016/j.chemosphere.2015.10.110
Ling M. P., Liao C. M. (2007). Risk Characterization and Exposure Assessmentin Arseniasis-Endemic Areas of Taiwan. Environ. Int. 33, 98–107. doi: 10.1016/j.envint.2006.08.006
Liu Y., Chen L., Huang Q., Li W. Y., Tang Y. J., Zhao J. F. (2009). Source Apportionment of Polycyclic Aromatic Hydrocarbons (PAHs) in Surface Sediments of the Huangpu River, Shanghai, China. Sci. Total Environ. 407, 2931–2938. doi: 10.1016/j.scitotenv.2008.12.046
López-Mas L., Claret A., Reinders M. J., Banovic M., Krystallis A., Guerrero L. (2021). Farmed or Wild Fish? Segmenting European Consumers Based on Their Beliefs. Aquaculture 532, 735992. doi: 10.1016/j.aquaculture.2020.735992
Lotze H. K., Tittensor D. P., Bryndum-Buchholz A., Eddy T. D., Cheung W. W. L., Galbraith E. D., et al. (2019). Global Ensemble Projections Reveal Trophic Amplification of Ocean Biomass Declines With Climate Change. Proc. Natl. Acad. Sci.U. S .A. 116, 12907–12912. doi: 10.1073/pnas.1900194116
Low K. H., Zain S. M., Abas M. R., Salleh K. M., Teo Y. Y. (2015). Distribution and Health Risk Assessment of Trace Metals in Freshwater Tilapia From Three Different Aquaculture Sites in Jelebu Region (Malaysia). Food Chem. 177, 390–396. doi: 10.1016/j.foodchem.2015.01.059
Lulijwa R., Rupia E. J., Alfaro A. C. (2020). Antibiotic Use in Aquaculture, Policies and Regulation, Health and Environmental Risks: A Review of the Top 15 Major Producers. Rev. Aquac. 12, 640–663. doi: 10.1111/raq.12344
Lusher A. (2015). Microplastics in the Marine Environment: Distribution, Interactions and Effects. Mar. Anthr. Litter. 157, 245–307. doi: 10.1007/978-3-319-16510-310
MacDonald L. A., Wiklund J. A., Elmes M. C., Wolfe B. B., Hall R. I. (2016). Paleolimnological Assessment of Riverine and Atmospheric Pathways and Sources of Metal Deposition at a Floodplain Lake (Slave River Delta, Northwest Territories, Canada). Sci. Total Environ. 544, 812–823. doi: 10.1016/j.scitotenv.2015.11.173
Mahat N. A., Muktar N. K., Ismail R., Razak F. I. A. B., Wahab R. A., Keyon A. S. A. (2018). Toxic Metals in Perna Viridis Mussel and Surface Seawater in Pasir Gudang Coastal Area, Malaysia, and its Health Implications. Environ. Sci. pollut. Res. 25, 30224–30235. doi: 10.1007/s11356-018-3033-8
Martins A. C., Almeida L. A. C. B., Urbano M. R., Carvalvo M. F. H., Silva A. M. R., Tinkov A. A., et al. (2021). An Updated Systematic Review on the Association Between Cd Exposure, Blood Pressure and Hypertension. Ecotoxicol. Environ. Saf. 208, 111636. doi: 10.1016/j.ecoenv.2020.111636
McNally N. (2021) The Importance of Modern Aquaculture for Food Security. Alltech. Retrieved 24th May 2022. Available at: https://www.alltech.com/blog/importance-modern-aquaculture-food-security.
McNamara R. K., Vannest J. V., Valentine C. J. (2015). Role of Perinatal Long-Chain Omega-3 Fatty Acids in Cortical Circuit Maturation: Mechanisms and Implications for Psychopathology. World J. Psychiatry 5, 15–34. doi: 10.5498/wjp.v5.i1.15
Metian M., Troell M., Christensen V., Steenbeek J., Pouil S. (2020). Mapping Diversity of Species in Global Aquaculture. Rev. Aquac. 12, 1090–1100. doi: 10.1111/raq.12374
Minhaz T. M., Sarker J., Khan M. N. A., Khatoon H., Alim M. A., Khalequzzaman S. M., et al. (2020). Data on Growth Performance, Proximate Composition, and Fatty Acid Content of Edible Oyster (Crassostrea spp.), Farmed on Shell String Along Cox’s Bazar Coast. Data Br. 26, 106450. doi: 10.1016/j.dib.2020.106450.
Mohanty B. P., Sankar T. V., Ganguly S., Mahanty A., Anadan R., Chakraborty R., et al. (2016). Micronutrient Composition of 35 Food Fishes From India and Their Significance in Human Nutrition. Biol. Trace Elem. Res. 174, 448–458. doi: 10.1007/s12011-016-0714-3
Monteiro J. P., Melo T., Skjermo J., Forbord S., Broch O. J., Domingues P., et al. (2021). Effect of Harvesting Month and Proximity to Fish Farm Sea Cages on the Lipid Profile of Cultivated Saccharina Latissima. Algal Res. 54, 102201. doi: 10.1016/j.algal.2021.102201
Munoz-Olivas C. C. (2001). “Speciation Related to Human Health,” in Trace Element Speciation for Environment, Food and Health, 331–353. doi: 10.1039/9781847552204-00331. ( RCS Publishing: Milan).
Nasher E., Heng L. Y., Zakaria Z., Surif S. (2016). Health Risk Assessment of Polycyclic Aromatic Hydrocarbons Through Aquaculture Fish Consumption, Malaysia. Environ. Forensics 17, 97–106. doi: 10.1080/15275922.2015.1133733
Naylor R. L., Hardy R. W., Buschmann A. H., Bush S. R., Cao L., Klinger D. H., et al. (2021). A 20-Year Retrospective Review of Global Aquaculture. Nature 591, 551–563. doi: 10.1038/s41586-021-03308-6
Nölle N., Genschick S., Schwadorf K., Hrenn H., Brandner S., Biesalski H. K. (2020). Fish as a Source of (Micro)Nutrients to Combat Hidden Hunger in Zambia. Food Secur. 12, 1385–1406. doi: 10.1007/s12571-020-01060-9
Noordin W. N., Ibrahim S., Nawawi R. A., Sari M. D., Huda N. (2019). Nutritional Composition, Sensory Evaluation and Halal Perspective African Catfish (Clarias gariepinus) Fed with Diets Containing Najasa (Impurities). Curr. Res. Nutr. Food Sci. 7, 436–448. doi: 10.12944/CRNFSJ.7.2.13
Ocharoen Y., Boonphakdee C., Boonphakdee T., Shinn A. P., Moonmangmee S. (2018). High levels of the Endocrine Disruptors Bisphenol-A and 17βestradiol Detected in Populations of Green Mussel, Perna Viridis, Cultured in the Gulf of Thailand. Aquaculture 497, 348–356. doi: 10.1016/j.aquaculture.2018.07.057
Ofori-Mensah S., Yıldız M., Arslan M., Eldem V. (2020). Fish Oil Replacement With Different Vegetable Oils in Gilthead Seabream, Sparus Aurata Diets: Effects on Fatty Acid Metabolism Based on Whole-Body Fatty Acid Balance Method and Genes Expression. Aquaculture 529, 735609. doi: 10.1016/j.aquaculture.2020.735609
Ohiozebau E., Tendler B., Codling G., Kelly E., Giesy J. P., Johnson P. D., et al. (2017). Potential Health Risks Posed by Polycyclic Aromatic Hydrocarbons in Muscle Tissues of Fishes From the Athabasca and Slave Rivers, Canada. Environ. Geochem. Health 39, 139–160. doi: 10.1007/s10653-016-9815-3
Okocha R. C., Olatoye I. O., Adedeji O. B. (2018). Food Safety Impacts of Antimicrobial Use and Their Residues in Aquaculture. Public Health Rev. 39, 21. doi: 10.1186/s40985-018-0099-2
Olatoye I. O., Okocha R. C., Oridupa O. A., Nwishienyi C. N., Tiamiyu A. M., Adedeji O. B. (2021). Atrazine in Fish Feed and African Catfish (Clarias Gariepinus) From Aquaculture Farms in Southwestern Nigeria. Heliyon 7, e06076. doi: 10.1016/j.heliyon.2021.e06076
Oliveira A. R., Sardinha-Silva A., Andrews P. L. R., Green D., Cooke G. M., Hall S., et al. (2020a). Microplastics Presence in Cultured and Wild-Caught Cuttlefish, Sepia Officinalis. Mar. Pollut. Bull. 160, 111553. doi: 10.1016/j.marpolbul.2020.111553
Oliveira M., De Gasperi Portella C., Ramalhosa M. J., Delerue-Matos C., Sant’Ana L. S., Morais S. (2020b). Polycyclic Aromatic Hydrocarbons in Wild and Farmed Whitemouth Croaker and Meagre from Different Atlantic Ocean fishing areas: Concentrations and Human Health Risk Assessment. Food Chem. Toxicol. 146, 111797. doi: 10.1016/j.fct.2020.111797.
Oomen C. M., Feskens E. J. M., Räsänen L., Fidanza F., Nissinen A. M., Menotti A., et al. (2000). Fish Consumption and Coronary Heart Disease Mortality in Finland, Italy, and the Netherlands. Am. J. Epidemiol. 151, 999–1006. doi: 10.1093/oxfordjournals.aje.a010144
Pacini N., Abate V., Brambilla G., Felip E. D., Filippis S. P. D., Luca S. D., et al. (2013). Polychlorinated Dibenzodioxins, Dibenzofurans, and Biphenyls in Fresh Water Fish From Campania Region, Southern Italy. Chemosphere 9, 80–89. doi: 10.1016/j.chemosphere.2012.07.039
Paithankar J. G., Saini S., Dwivedi S., Sharma A., Chowdhuri D. K. (2021). Heavy Metal Associated Health Hazards: An Interplay of Oxidative Stress and Signal Transduction. Chemosphere 262, 128350. doi: 10.1016/j.chemosphere.2020.128350
Pal A., Paul S., Choudhury A. R., Krishna V., Dasc B. M., Sinha A. (2017). Synthesis of Hydroxyapatite From Lates Calcarifer Fish Bone for Biomedical Applications. Mater. Lett. 203, 01588. doi: 10.1016/j.matlet.2017.05.103
Paul S., Pal A., Choudhury A. R., Bodhakc S., Ballac S. K., Sinhaa A., et al. (2017). Effect of Trace Elements on the Sintering Effect of Fish Scale Derived Hydroxyapatite and its Bioactivity. Ceram. Int. 43, 15678–15684. doi: 10.1016/j.ceramint.2017.08.127
Pessoa G. P., Souza N. C., Vidal C. B., Alves J. A. C., Paulo I. M. F., Nascimento R. F., et al. (2014). Occurrence and Removal of Estrogens in Brazilian Wastewater Treatment Plants. Sci. Total Environ. 490, 288–295. doi: 10.1016/j.scitotenv.2014.05.008
Petricorena Z. C. (2015). “Chemical Composition of Fish and Fishery Products,” in Handbook of Food Chemistry, vol. 1. , 28. doi: 10.1007/978-3-642-41609-5_12-1 ( Springer:German)
Pleadin J., Lešić T., Krešić G., Barić R., Bogdanović T., Oraić D., et al. (2017). Nutritional Quality of Different Fish Species Farmed in the Adriatic Sea. Ital. J. Food Sci. 29, 537–549. doi: 10.14674/IJFS-706
Pradeepkiran J. A. (2019). Aquaculture Role in Global Food Security With Nutritional Value: A Review. Transl. Anim. Sci. 3, 903–910. doi: 10.1093/tas/txz012
Preena P. G., Swaminathan T. R., Kumar V. J. R., Singh I. S. B. (2020). Antimicrobial Resistance in Aquaculture: A Crisis for Concern. Biologia. (Bratisl). 75, 1497–1517. doi: 10.2478/s11756-020-00456-4
Psota T., Gebauer S. K., Kris-Etherton P. (2006). Dietary Omega-3 Fatty Acid Intake and Cardiovascular Risk. Am. J. Cardiol. 98, 3i–18i. doi: 10.1016/j.amjcard.2005.12.022
Reinold S., Herrera A., Saliu F., Gonzálezc C. H., Lasagni I. M. M., Lagsini M., et al. (2021). Evidence of Microplastic Ingestion by Cultured European Sea Bass (Dicentrarchus Labrax). Mar. pollut. Bull. 168, 112450. doi: 10.1016/j.marpolbul.2021.112450
Retnam A., Zakaria M. P., Juahir H., Aris A. Z., Zali M. A., Kasim M. F. (2013). Chemometric Techniques in Distribution, Characterisation and Source Apportionment of Polycyclic Aromatic Hydrocarbons (PAHS) in Aquaculture Sediments in Malaysia. Mar. pollut. Bull. 69, 55–66. doi: 10.1016/j.marpolbul.2013.01.009
Rizzo G., Laganà A. S. (2019). “A Review of Vitamin B12,” in Molecular Nutrition: Vit 2020, 105–156. doi: 10.1016/B978-0-12-811907-5.00005-1 ( Academic Press: United States).
Rochman C. M., Browne M. A., Underwood A. J., Franeker J. A., Thompson R. C., Linda A., et al. (2016). The Ecological Impacts of Marine Debris: Unraveling the Demonstrated Evidence From What is Perceived. Ecology 97, 302–312. doi: 10.1890/14-2070.1
Rodriguez-Freire L., Avasarala S., Ali A. M. S., Agnew D., Hoover J. H., Artyushkova K., et al. (2016). Post Gold King Mine Spill Investigation of Metal Stability in Water and Sediments of the Animas River Watershed. Environ. Sci. Technol. 50, 11539–11548. doi: 10.1021/acs.est.6b03092
Rodríguez-Hernández Á., Camacho M., Henríquez-Hernández L. A., Boada L. D., Valerón P. F., Zaccaroni A., et al. (2017). Comparative Study of the Intake of Toxic Persistent and Semi Persistent Pollutants Through the Consumption of Fish and Seafood From Two Modes of Production (Wild-Caught and Farmed). Sci. Total Environ. 575, 919–931. doi: 10.1016/j.scitotenv.2016.09.142
Rosenlund G., Corraze G., Izquierdo M., Torstensen B. (2010). “The Effects of Fish Oil Replacement on Nutritional and Organoleptic Qualities of Farmed Fish,” in Fish Oil Replacement and Alternative Lipid Sources in Aquaculture Feeds, 487–522. doi: 10.1201/9781439808634-c15 ( Taylor and Francis Group: United Kingdom).
Rout B. K., Sikdar B. K. (2017). Hazard Identification, Risk Assessment, and Control Measures as an Effective Tool of Occupational Health Assessment of Hazardous Process in an Iron Ore Pelletizing Industry. Indian J. Occup. Environ. Med. 21, 56–71. doi: 10.4103/ijoem.IJOEM_19_16
Saher N. U., Kanwal N. (2019). Assessment of Some Heavy Metal Accumulation and Nutritional Quality of Shellfish With Reference to Human Health and Cancer Risk Assessment: A Seafood Safety Approach. Environ. Sci. pollut. Res. 26, 5189–5201. doi: 10.1007/s11356-018-3764-6
Sanou A., Coulibaly S., Coulibaly M., N’Goran N’Dri S., Atse B. C. (2021). Assessment of Heavy Metal Contamination of Fish From a Fish Farm by Bioconcentration and Bioaccumulation Factors. Egypt. J. Aquat. Biol. Fish. 25, 821–841. doi: 10.21608/EJABF.2021.151995
Santána L. S., Iwamoto A. A., Crepaldi D. V., Boaventura T. P., Teixeira L. V., Teixeira E. A., et al. (2019). Comparative study of the chemical composition, fatty acid profile, and nutritional quality of Lophiosilurus alexandri (Siluriformes: Pseudopimelodidae), a Brazilian carnivorous freshwater fish, grown in lotic, lentic, and aquaculture environments. Neotrop. Ichthyol. 17, e190050. doi: 10.1590/1982-0224-20190050.
Sasazuki S., Inoue M., Iwasaki M., Sawada N., Shimazu T., Yamaji T., et al. (2011). Intake of N-3 and N-6 Polyunsaturated Fatty Acids and Development of Colorectal Cancer by Subsite: Japan Public Health Center-Based Prospective Study. Int. J. Cancer. 29, 1718–1729. doi: 10.1002/ijc.25802
Schlag A. K., Ystgaard K. (2013). Europeans and Aquaculture: Perceived Differences Between Wild and Farmed Fish. Br. Food J. 115, 209–222. doi: 10.1108/00070701311302195
Scott G. I., Porter D. E., Norman R. S., Scott C. H., Uyaguari-Diaz M. I., Maruya K. A., et al. (2016). Antibiotics as CECs: An Overview of the Hazards Posed by Antibiotics and Antibiotic Resistance. Front. Mar. Sci. 3. doi: 10.3389/fmars.2016.00024
Sevim Ç., Doğan E., Comakli S. (2020). Cardiovascular Disease and Toxic Metals. Curr. Opin. Toxicol. 6, 251–259. doi: 10.1016/j.cdtm.2020.02.005
Shrivastava M., Lou S., Zelenyuk A., Tao S. (2017). Global Long-Range Transport and Lung Cancer Risk From Polycyclic Aromatic Hydrocarbons Shielded by Coatings of Organic Aerosol. Proc. Natl. Acad. Sci.U. S. A. 114, 1246–1251. doi: 10.1073/pnas.1618475114
Siddik M. A. B., Chungu P., Fotedar R., Howieson J. (2019). Bioprocessed Poultry by-Product Meals on Growth, Gut Health and Fatty Acid Synthesis of Juvenile Barramundi, Lates Calcarifer (Bloch). PLos One 14, 1–18. doi: 10.1371/journal.pone.0215025
Siddik M. A. B., Howieson J., Fotedar R., Partridge G. J. (2021). Enzymatic Fish Protein Hydrolysates in Finfish Aquaculture: A Review. Rev. Aquac. 13, 406–430. doi: 10.1111/raq.12481
Sing C. K., Khan M. Z. I., Mohd D. H. H., Aziz A. R. (2016). Prevalence of Salmonella Sp. In African Catfish (Clarias Gariepinus) Obtained From Farms and Wet Markets in Kelantan, Malaysia and Their Antibiotic Resistance. Sains Malaysiana 45, 1597–1602.
Sivaraman K., Shanthi C. (2021). Role of Fish Collagen Hydrolysate in Attenuating Inflammation—An In Vitro Study. J. Food Biochem. 45, e13876. doi: 10.1111/jfbc.13876.
Sriket P., Benjakul S., Visessanguan W., Kijroongrojana K. (2007). Comparative Studies on Chemical Composition and Thermal Properties of Black Tiger Shrimp (Penaeus monodon) and white shrimp (Penaeus Vannamei) Meats. Food Chem. 103, 1199–1207. doi: 10.1016/j.foodchem.2006.10.039
Stentiford G. D., Peeler E. J., Tyler C. R., Bickley L. K., Holt C. C., Bass D., et al. (2022). A Seafood Risk Tool for Assessing and Mitigating Chemical and Pathogen Hazards in the Aquaculture Supply Chain. Nat. Food 3, 169–178. doi: 10.1038/s43016-022-00465-3
Sultanbawa Y., Aksnes A. (2006). Tuna Process Waste – an Unexploited Resource. INFOFISH Inter. 3, 37–40.
Surya P., Nithin A., Sundaramanickam A., Sathish M. (2021). Synthesis and Characterization of Nano-Hydroxyapatite From Sardinella Longiceps Fish Bone and its Effects on Human Osteoblast Bone Cells. J. Mech. Behav. Biomed. Mater. 119, 104501. doi: 10.1016/j.jmbbm.2021.104501
Szefer P., Ali A. A., Ba-Haroon A. A., Rajeh A. A., Geldon J., Nabrzyski M. (1999). Distribution and Relationships of Selected Trace Metals in Molluscs and Associated Sediments From the Gulf of Aden, Yemen. Environ. Pollut. 106, 299–314. doi: 10.1016/S0269-7491(99)00108-6
Szlinder-Richert J., Usydus Z., Malesa-Ciećwierz M., Polak-Juszczak L., Ruczyńska W. (2011). Marine and Farmed Fish on the Polish Market: Comparison of the Nutritive Value and Human Exposure to PCDD/Fs and Other Contaminants. Chemosphere 85, 1725–1733. doi: 10.1016/j.chemosphere.2011.09.019
Tacon A. G. J., Metian M. (2013). Fish Matters: Importance of Aquatic Foods in Human Nutrition and Global Food Supply. Rev. Fish. Sci. 21, 22–38. doi: 10.1080/10641262.2012.753405
Tan Y., Peng B., Wu Y., Xiong L., Sun J., Peng G., et al. (2021). Human Health Risk Assessment of Toxic Heavy Metal and Metalloid Intake via Consumption of Red Swamp Crayfish (Procambarus Clarkii) From Rice-Crayfish Co-Culture Fields in China. Food Control 128, 108181. doi: 10.1016/j.foodcont.2021.108181
Thomsen S. T., Assunção R., Afonso C., Boué G., Cardoso C., Cubadda F., et al. (2021). Human Health Risk–Benefit Assessment of Fish and Other Seafood: A Scoping Review. Crit. Rev. Food Sci. Nutr. 6, 1–22. doi: 10.1080/10408398.2021.1915240
Thomson A. D. (2000). Mechanisms of Vitamin Deficiency in Chronic Alcohol Misusers and the Development of the Wernicke-Korsakoff Syndrome. Alcohol Alcohol Suppl. 35, 2–7. doi: 10.1093/alcalc/35.supplement1.2
Tocher D. R., Betancor M. B., Sprague M., Olsen R. E., Napier J. A. (2019). Omega-3 Long-Chain Polyunsaturated Fatty Acids, EPA and DHA: Bridging the Gap Between Supply and Demand. Nutrients 11, 89. doi: 10.3390/nu11010089
Tomova A., Ivanova L., Buschmann A. H., Rioseco M. R., Kalsi R. K., Godfrey H. P., et al. (2015). Antimicrobial Resistance Genes in Marine Bacteria and Human Uropathogenic Escherichia Coli From a Region of Intensive Aquaculture. Environ. Microbiol. Rep. 7, 803–809. doi: 10.1111/1758-2229.12327
Trullàs C., Tres A., Saldo J., Fontanillas R., Sala R. (2017). Quality Characteristics of Fillets of Rainbow Trout Fed Acid or Re-Esterified Rapeseed Oils as Dietary Fat Sources. Aquaculture 480, 22–31. doi: 10.1016/j.aquaculture.2017.07.040
Underwood C. (2008).Belief and Attitude Change in the Context of Human Development. Available at: https://www.eolss.net/sample-chapters/c11/E6-60-03-07.pdf (Accessed 13 Feb 2022).
USEPA (2019). National-Scale Air Toxics Assessment Glossary of Terms. (Washington, USA). U.S. Environmental Protection Agency, National Risk Management Research Laboratory, Office of Research and Development. Available at: https://www.epa.gov/sites/default/files/2020-02/documents/hq_2019_year_in_review.pdf (Accessed 15 Feb 2022).
USEPA (2000). Guidance for Assessing Chemical Contaminant Data for Use in fish Advisories (Washington, DC: United States Environ Prot Agency).
Van der Oost R., Beyer J., Vermeulen N. P. E. (2003). Fish Bioaccumulation and Biomarkers in Environmental Risk Assessment: A Review. Environ. Toxicol. Pharmacol. 13, 57–149. doi: 10.1016/S1382-6689(02)00126-6
Venugopal V. (2018). Nutrients and Nutraceuticals From Seafood. Bio. Mol. Food, 1–45. doi: 10.1007/978-3-319-54528-8_36-2
Vergara J. M., López-Calero G., Robaina L., Izquierdo M. S., Aksnes A. (1999). Growth, Feed Utilization and Body Lipid Content of Gilthead Seabream (Sparus Aurata) Fed Increasing Lipid Levels and Fish Meals of Different Quality. Aquaculture 179, 35–44. doi: 10.1016/S0044-8486(99)00150-7
Waring R. H., Harris R. M. (2005). Endocrine Disrupters: A Human Risk? Mol. Cell Endocrinol. 244, 2–9. doi: 10.1016/j.mce.2005.02.007
Watanabe W. O., Clark J. H., Dunham J. B., Wickhlund R. B., Olla B. L. (1990). Culture of Florida Red Tilapia in Marine Cages: The Effect of Stocking Density and Dietary Protein on Growth. Aquaculture 90, 123–134. doi: 10.1016/0044-8486(90)90336-L
Weichselbaum E., Coe S., Buttriss J., Stanner S. (2013). Fish in the Diet: A Review. Nutr. Bull. 38, 128–177. doi: 10.1111/nbu.12021
Wei Y., Liang M., Mu Y., Zheng K., Xu H. (2016). The Effect of Ultrafiltered Fish Protein Hydrolysate Level on Growth Performance, Protein Digestibility and mRNA Expression of PepT1 in Juvenile Turbot (Scophthalmus Maximus L.). Aquac. Nutr. 22, 1006–1017. doi: 10.1111/anu.12319
WFC (2002) Fish for All: An Issue for Everyone. A Concept ( World Fish Center). Available at: https://hdl.handle.net/20.500.12348/2262 (Accessed 10 Feb 2022).
WHO (2015) Food Safety ( WHO). Available at: https://www.who.int/health-topics/food-safety (Accessed 19 May 2022).
WHO (2016) Lead Poisoning and Health. Available at: https://www.who.int/news-room/fact-sheets/detail/lead-poisoning-and health#:~:text=Lead%20exposure%20can%20have%20serious,intellectual%20disability%20and%20behavioural%20disorders (Accessed 16 Feb 2022).
Williams M. H. (2004). Dietary Supplements and Sports Performance: Introduction and Vitamins. J. Int. Soc Sports Nutr. 1, 1–6. doi: 10.1186/1550-2783-1-2-1
Wright S. L., Thompson R. C., Galloway T. S. (2013). The Physical Impacts of Microplastics on Marine Organisms: A Review. Environ. Pollut. 178, 483–492. doi: 10.1016/j.envpol.2013.02.031
Wu F., Wang Y., Leung J. Y. S., Huang W., Zeng J., Tang Y., et al. (2020). Accumulation of Microplastics in Typical Commercial Aquatic Species: A case Study at a Productive Aquaculture Site in China. Sci. Total Environ. 708, 135432. doi: 10.1016/j.scitotenv.2019.135432
Xiang S., Xie Y., Sun X., Du H., Wang J. (2021). Identification and Quantification of Microplastics in Aquaculture Environment. Front. Mar. Sci. 8. doi: 10.3389/fmars.2021.804208
Yabanli M., Tay S. (2021). Selenium and Mercury Balance in Sea Bream Obtained From Different Living Environments in Turkey: A Risk Assessment for the Consumer Health. Environ. Sci. pollut. Res. 28, 36069–36075. doi: 10.1007/s11356-021-13366-y
Yıldız M., Eroldoğan T. O., Ofori-Mensah S., Engin M., Baltac M. A. (2018). The Effects of Fish Oil Replacement by Vegetable Oils on Growth Performance and Fatty Acid Profile of Rainbow Trout: Re-Feeding With Fish Oil Finishing Diet Improved the Fatty Acid Composition. Aquaculture 488, 123–133. doi: 10.1016/j.aquaculture.2017.12.030
Yun B., Xue M., Wang J., Fan Z. Y., Wu X. F., Zheng Y. H., et al. (2013). Effects of Lipid Sources and Lipid Peroxidation on Feed Intake, Growth, and Tissue Fatty Acid Compositions of Largemouth Bass (Micropterus Salmoides). Aquac. Int. 21, 101013. doi: 10.1007/s10499-012-9538-0
Yu J., Wen X., You C., Chen C., Li Y. (2021a). Comparison of the Growth Performance and Long-Chain Polyunsaturated Fatty Acids (LC-PUFA) Biosynthetic Ability of Red Tilapia (O. Mossambicus and O. Niloticus) Fed Fish Oil or Vegetable Oil Diet at Different Salinities. Aquaculture 542, 374–378. doi: 10.1016/j.aquaculture.2021.736899
Yu L., Yu Y., Xiao Y., Tian F., Narbad A., Zhai Q., et al. (2021b). Lead-Induced Gut Injuries and the Dietary Protective Strategies: A Review. J. Funct. Foods 83, 104528. doi: 10.1016/j.jff.2021.104528
Zakaria M. P., Takada H., Tsutsumi S., Ohno K., Yamada J., Kouno E., et al. (2002). Distribution of Polycyclic Aromatic Hydrocarbons (PAHs) in Rivers and Estuaries in Malaysia: A Widespread Input of Petrogenic PAHs. Environ. Sci. Technol. 36, 1907–1918. doi: 10.1021/es011278
Zhang Y., Min J., Zhang L. (2019). Anti-Inflammatory and Immunomodulatory Effects of Marine N-3 Polyunsaturated Fatty Acids on Human Health and Diseases. J. Ocean. Univ. China 18, 481–492. doi: 10.1007/s11802-019-3620-4
Zhang B., Xiong K., Cai J., Ma A. (2020a). Fish Consumption and Coronary Heart Disease: A Meta-Analysis. Nutrients 12, 1–22. doi: 10.3390/nu12082278
Zhang N., Zhou Y. W., Bai Y. (2020b). Research Progress in Effect of Microplastics on Intestinal Flora. China Plast. 34, 103–110.
Zheng K., Liang M., Yao H., Wang J., Chang Q. (2013). Effect of Size-Fractionated Fish Protein Hydrolysate on Growth and Feed Utilization of Turbot (Scophthalmus Maximus L.). Aquac. Res. 44, 895–902. doi: 10.1111/j.1365-2109.2012.03094.x
Zhu Z. L. (2019). The Joint Toxicity and Adsorption Behavior Between Typical Microplastics and PPCPS in Aquatic Environment (in Chinese) ( Shan Dong University,China).
Keywords: aquaculture, food security, heavy metals, nutrient, hazard, sustainability
Citation: Arshad N, Samat N and Lee LK (2022) Insight Into the Relation Between Nutritional Benefits of Aquaculture Products and its Consumption Hazards: A Global Viewpoint. Front. Mar. Sci. 9:925463. doi: 10.3389/fmars.2022.925463
Received: 21 April 2022; Accepted: 23 June 2022;
Published: 22 July 2022.
Edited by:
Mohammad Mahfujul Haque, Bangladesh Agricultural University, BangladeshReviewed by:
Omid Safari, Ferdowsi University of Mashhad, IranSairatul Dahlianis Ishak, Universiti Malaysia, Terengganu, Malaysia
Copyright © 2022 Arshad, Samat and Lee. This is an open-access article distributed under the terms of the Creative Commons Attribution License (CC BY). The use, distribution or reproduction in other forums is permitted, provided the original author(s) and the copyright owner(s) are credited and that the original publication in this journal is cited, in accordance with accepted academic practice. No use, distribution or reproduction is permitted which does not comply with these terms.
*Correspondence: Lai Kuan Lee, bC5rLmxlZUB1c20ubXk=