- 1Key Laboratory for Ecological Environment in Coastal Areas, National Marine Environmental Monitoring Center, Dalian, China
- 2Department of Marine Chemistry, Marine Debris and Microplastic Research Center, National Marine Environmental Monitoring Center, Dalian, China
- 3Key Laboratory of Marine Bio-Resource Restoration and Habitat Reparation in Liaoning Province, Dalian Ocean University, Dalian, China
Heavy metal (HM) pollution in the marine environment has been of concern for decades. The potential impact of HMs carried by emerging marine pollutants such as microplastics (MPs) has attracted attention only in recent years. In this study, we investigated the single and combined chronic toxic effects (growth, burrowing behavior, and histopathology) of cadmium (Cd, three concentrations of low, medium, and high) and polystyrene (PS) microspheres (1 μm, 10 μg/L) on the coastal polychaete Perinereis aibuhitensis. Cd bioaccumulation under two exposure scenarios was also explored. Our results showed that Cd and PS did not affect worm growth for single or combined exposure, while 13.08 μg/L of Cd (CdL) alone significantly decreased the burrowing time of P. aibuhitensis in sediment. The presence of PS mitigated the hormetic effect of Cd on worm burrowing behavior, and this influence was Cd concentration-related. Cd body burdens in worms exposed to the medium and high Cd concentrations (CdM and CdH) were significantly greater than those of control worms with or without the presence of PS. In addition, Cd bioaccumulation was significantly higher with the coexistence of PS than those of Cd alone at the CdL and CdM groups. Histopathological analyses demonstrated a trend of epidermal and intestinal damages for single Cd/PS and their combined groups, and the contribution of PS-MPs should not be ignored. Our results indicate that the toxic effects and bioaccumulation pattern of Cd could be altered with the presence of PS-MPs for P. aibuhitensis, especially at environmentally relevant concentrations.
Introduction
As an emerging environmental contaminant, microplastics (MPs, < 5 mm in size) (Law and Thompson, 2014) are small fragments of plastic debris that have been found in both marine water and sediment environments worldwide. The bioaccumulation and toxicological consequence of MPs in marine ecosystem have received considerable concern for their bioavailability and potential impacts on marine biota. MPs were found to cause mechanical and physical injures such as behavior alteration and clogging of the digestive tract, or their effects could be physiological and biochemical, including growth and reproduction disturbance, neurotoxicity, oxidative damage, energy-related changes, immune dysfunction, histopathological, and ultrastructural alteration (Lu et al., 2016; Auta et al., 2017; Barboza et al., 2018a; Barboza et al., 2018b; Wang et al., 2019).Currently, it has also been widely recognized that MPs not only pose direct impacts on marine biota, but also act as vectors for several chemicals including hydrophobic organic chemicals such as polycyclic aromatic hydrocarbons (PAHs) (Liu et al., 2019b; Pannetier et al., 2019), metal ions (Holmes et al., 2012; Holmes et al., 2014; Brennecke et al., 2016), and antibiotics (Li et al., 2018), of which the adsorption of various heavy metals (HMs) by the MPs in the marine environment was highlighted (Brennecke et al., 2016), which may, in turn, alter the bioavailability and toxicity of HMs. For instance, Barboza et al. (2018a) found that fluorescence red polymer microspheres (1–5 μm diameter) likely sorbed mercury (Hg) from water and influenced Hg bioaccumulation in the tissues of European seabass, Dicentrarchus labrax, and a significant interaction between Hg and MPs was found. In addition, when a marine mysid (Neomysis awatschensis) was exposed to HMs (arsenic/As, cadmium/Cd, copper/Cu, lead/Pb, and zinc/Zn) premixed with MPs, it demonstrated increasing metal body burdens and thus aggravated oxidative damage and neurotoxicity (Eom et al., 2021). Likewise, the study of Yan et al. (2020) showed that the presence of MPs caused higher bioaccumulation of HMs (Cd, Pb, and Zn) in the gut of marine medaka (Oryzias melastigma), and co-exposure triggered more significant changes of specific bacterial species and gut function in male fishes. Nevertheless, in some circumstances, the toxicity of MPs themselves also plays an important role when combined with exposure to HMs. For example, Wen et al. (2018) reported that single or co-exposure of MPs and Cd could all induce oxidative stress and stimulate innate immunity in the juvenile discus fish (Symphysodon aequifasciatus); however, the presence of MPs led to reduced Cd accumulation, which highlighted the critical role of MPs themselves other than their “carrier effect” of increasing metal bioavailability in their combined toxicity.Although several model animals have been adopted to evaluate the combined effects of HMs with MPs, most of which focus on freshwater/marine fishes, bivalves, or zooplanktons, the performance of marine benthic organisms after combined exposure to HMs and MPs is rarely known. Moreover, there are inconsistent conclusions regarding the bioavailability or bioaccumulation of HMs with or without the presence of MPs among different test organisms. This process probably determines the toxicities of HMs and the potential of MPs as a carrier of HMs, which should be clarified using different model organisms which are representative of the marine environment.As a nonessential metal element, Cd is one of the most frequently detectable metal contaminants in the marine environment and organisms along coasts of China (Qiu, 2015; Zhang et al., 2015; Hao et al., 2019). Polystyrene (PS) MPs are among the most produced worldwide plastics, and the low reuse rate of these polymers results in their widespread presence in the aquatic environment (Eriksen et al., 2014), which were most frequently observed as a spherical form (Carpenter et al., 1972; Mani et al., 2015; Zhang et al., 2017). The polychaete Perinereis aibuhitensis is a commonly distributed and ecologically important infauna species that inhabited coastal sediments in China. Due to its essential role in influencing fluxes of organic matter, nutrients, and contaminants in sediments and across the sediment–water interface through bioturbation, it has been increasingly used as a bioindicator for metals (Cd, Pb, etc.) (Tian et al., 2014; Liu et al., 2019a) and organic compounds (petroleum hydrocarbons) (Sun et al., 2019) in ecotoxicological studies. Recently, the indicator role of ragworm (Nereididae) for MPs in the Haizhou Bay intertidal zone in China has been confirmed (Wang et al., 2021). In this study, we select P. aibuhitensis as the test organism and polystyrene (PS) and Cd as representatives of MPs and HMs with aims to (1) determine the single and combined effects of PS and Cd on the growth and burrowing behavior of worms; (2) quantify and compare the bioaccumulation of Cd in worms with or without the presence of PS; and (3) characterize the histopathological alterations in different tissues of worms caused by single or combined compounds under a 7-day seawater exposure pathway.
Materials and methods
Animal obtaining and culturing
Adult P. aibuhitensis, with lengths of approximately 8 to 12 cm, were purchased from Ruian aquaculture base, Taizhou, China. After transport to the lab, healthy and uninjured worms were selected and placed in several 10-L aquaria each containing approximately 8 cm depth of natural sediment that was collected from Yuan Island, the Yellow Sea (38°40′25″N, 122°09′47″E, sieved ≤ 1 mm) to acclimatize for 7 days at 10 ± 1°C with aerated filtered artificial seawater (FASW, < 0.45 μm, salinity 16.6 ± 0.1, pH 8.0 ± 0.1, DO 7.99 ± 0.2 mg/L) as overlying water. The overlying FASW was replaced once, and worms were fed with organic matter in natural sieved sediment without additional food supply during acclimation. One day before exposure, all worms were carefully picked out of the sediment and placed in clean FASW to empty their guts overnight.
Characterization of polystyrene microspheres
Commercial PS microspheres (Cat.# 4010A, nominal size of 1 μm, 1.0% solids) were purchased from Thermo Fisher Scientific Corporation, USA. The primary morphology and particle size were identified using a Hitachi transmission electron microscope (TEM, operating at 80 kV) system. The chemical composition of polystyrene microspheres was confirmed by micro-Fourier transform infrared spectroscopy (μ-FTIR) on a Nicolet iS5 spectrometer (Thermo Fisher Scientific, USA). A sample of PS microspheres was pressed onto a diamond crystal and their spectra were obtained at 8 cm−1 resolution and 8 scans.
Chronic seawater exposure
A 7-day seawater exposure was conducted to investigate the single and combined effects of PS and Cd to P. aibuhitensis. One PS concentration (10 μg/L, ~1.8×104 particles/ml) and three Cd (low, medium, and high) concentrations were adopted in chronic experiments. The PS concentration used here was within the environmental concentration range (Wang et al., 2019). The lowest Cd concentration was determined based on the real environmental concentration of Cd in the Liaodong Bay reported by Guo et al. (2022). The highest Cd concentration was selected approximately 10% of the 96 h-LC50 of Cd for P. aibuhitensis (Liu et al., 2019a). Considering the potential impact of additive (NaN3) in PS suspension, a total of nine treatments were set up: 0 (blank control), NaN3 (additive control group), CdL (13.08 μg/L), CdM (123.50 μg/L), CdH (1563.33 μg/L), PS (10 μg/L), CdL (12.45 μg/L) + PS, CdM (128.63 μg/L) + PS, and CdH (1637.33 μg/L) + PS. The measured exposure concentrations of Cd in single and combined treatments were determined by inductively coupled plasma-mass spectrometry (ICP-MS, Agilent 7500ce, Agilent Technologies Co. Ltd., USA). The stock polystyrene microsphere suspension was freshly prepared by diluting the original PS suspension with ultrapure water (18.2 Ω, Millipore). Both the original and stock PS suspensions were sonicated in a water bath for 5 min before use to allow fully suspending the microspheres. A 1 mg/ml of Cd solution was prepared in ultrapure water as stock solution and then was diluted by FASW to a series of experimental concentrations.
Following overnight gut emptying in seawater, worms were randomly assigned to 500-ml plastic beakers (a total of 54 beakers), with each beaker containing one worm and 250 ml of exposure solutions/suspensions to start the 7 days of exposure. The exposure parameters (temperature, salinity, pH, and DO) were set in accordance with the conditions during acclimatization. Each beaker contained one worm (one replicate) and each treatment contained six replicates. The replicate numbers used in growth and burrowing tests were based on Cong et al. (2011), Cong et al. (2014) and Wang et al. (2015). The experimental seawater was replaced once and worms were fed with commercial fish food flakes every other day. Natural photoperiod in the laboratory (14-h light:10-h dark) was used. All beakers were inspected daily for oxygen supply and worm condition. Mortality and growth in all treatments were recorded after 7 days of exposure.
Burrowing test
The burrowing behavior of P. aibuhitensis was assessed following Bonnard et al. (2009) and Cong et al. (2014), with some modifications. After 7 days of seawater exposure, worms were carefully picked out of their beakers and placed individually in beakers containing approximately 5 cm of clean natural wet sediment (≤1 mm) to start the burrowing test. Worm burrowing positions were observed and recorded every 2 min for 1 h, and the time that it took each worm to become fully buried was recorded (n = 6). After the burrowing test, all worms were carefully picked out of the sediment and transferred to clean FASW to depurate their guts overnight.
Cd bioaccumulation
After the burrowing test and gut depuration overnight, worms were frozen at −80°C until use. Each worm was weighed and added with 1.42 g/ml HNO3 and 30% H2O2 (v:v = 4:1) to start the digestive process. Afterwards, the samples were transferred to a microwave digestion system and heated to 120°C within 6 min and holding for 6 min, followed by heating to 180°C within 6 min and holding for 15 min. All digested samples were then heated to evaporate HNO3 and diluted with ultrapure water for Cd determination using ICP-MS. Marine seaweed (GBW08517) was used as a certified reference for Cd determination to ensure internal quality assurance/quality control (QA/QC) practices. The recovery ranged from 96.5% to 103.5% (n = 3) for Cd.
Histological analyses
After depuration overnight, the fresh middle piece from 2 individual worms for each treatment was dissected within 3 min and fixed with Bouin’s solution for approximately 24 h. Afterwards, the tissues were removed from the fixed solution, trimmed, dehydrated with gradient ethanol, and embedded with paraffin as follows: 75% ethanol for 4 h, 85% ethanol for 2 h, 90% ethanol for 2 h, 95% ethanol for 1 h, absolute ethanol for 30 min two times, ethanol benzene for 5–10 min, xylene for 5–10 min two times, and 65°C melting paraffin for 1 h three times. Then, the paraffin-infiltrated tissues were embedded by melting paraffin in the embedding machine (JB-P5, Wuhan), and cooled on a freezing table at −20°C. After that, each paraffin block was cut with Slicer Pathology (RM2016, Leica, Shanghai) into slices of 4 μm thickness, and each slice was flattened on water at 40°C, picked up by a glass slide, and dried in an oven at 60°C. The slides were preserved at room temperature and histological images of worm transverse sections were photographed by a panoramic slice scanner (3DHISTECH, PANNORAMIC DESK/MIDI/250/1000, Hungary).
Statistical analyses
Statistical analyses were performed using SPSS 26.0 software (SPSS, Chicago, IL, USA). The normality of data and the homogeneity of variances were verified using the Kolmogorov–Smirnov test and Levene’s test, respectively. A one-way ANOVA analysis or nonparametric test (Kruskal–Wallis) was applied to determine the significant differences between the treatments and the controls depending on the assumptions of parametric tests fulfilled or not. Similarly, an independent sample t-test or a nonparametric test (Mann–Whitney) was used to analyze the statistical differences of exposure concentrations between the single and combined exposure groups. Differences were considered significant at p < 0.05.
Results
Characterization of PS microspheres
TEM images showed that PS-MPs were spherical shaped with the diameter approximately 1 μm (Figures 1A, B). FTIR analysis confirmed that the chemical composition of plastic microspheres used in this study was polystyrene, a common polymer, whose spectrum is shown in Figure 1C.
Mortality and growth
No worm was found dead after 7 days of seawater exposure. However, all worms but one (in CdM + PS group) tended to lose weight, ranging from 6.19% to 11.45% after exposure (Figure 2). Growth in the additive control group did not differ significantly with that in the blank control or the PS alone group, nor was a concentration-dependent growth effect observed (Figure 2). Also, there were no significant differences (p < 0.05) in weight loss between single/combined exposure groups and the control groups or between the Cd alone and combined groups.
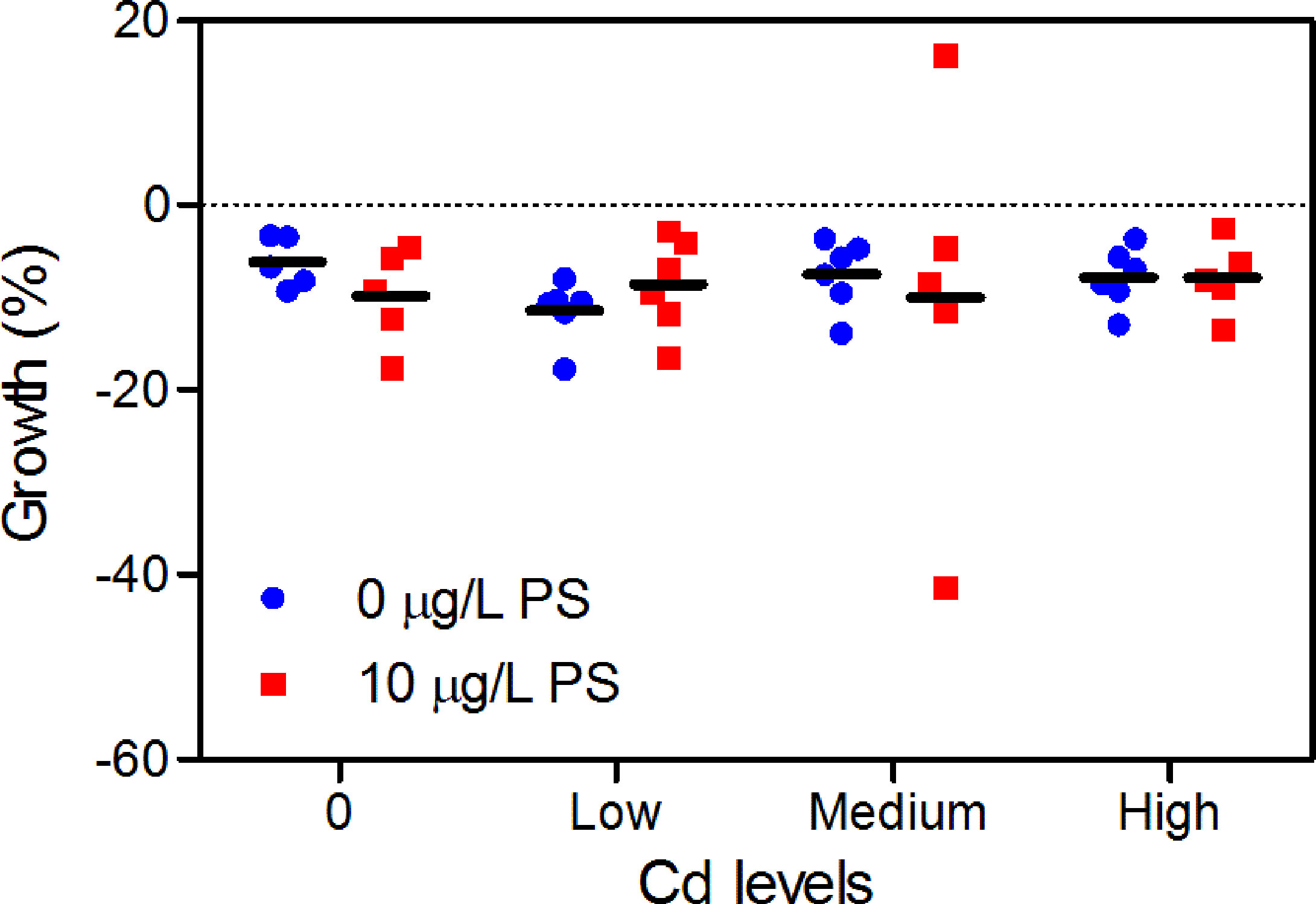
Figure 2 Growth (weight change percent) of P. aibuhitensis after 7 days of exposure to Cd alone and Cd combined with 10 μg/L of PS. Results are presented as scatter plot (n = 5–6) and mean (black bar).
Burrowing time
In all treatments, all except one worm (in CdL group) completely burrowed into the clean sediment (category 0) within 1 h upon transfer from the exposure media. The lack of burrowing for the worm was considered probably in poor condition, and it was therefore removed from further analysis. For Cd alone exposure, burrowing time in the CdL group was significantly lower than that in the blank control group, while burrowing in all concentrations of Cd + PS treatment was comparable to that of the 10 μg/L PS treatment (Figure 3). The significant differences in burrowing behavior among groups were only observed in CdL-alone-treated worms compared to those of CdL + PS and CdH treatments (Figure 3). Neither the PS alone nor the additive group affected worm burrowing behavior compared to the blank control.
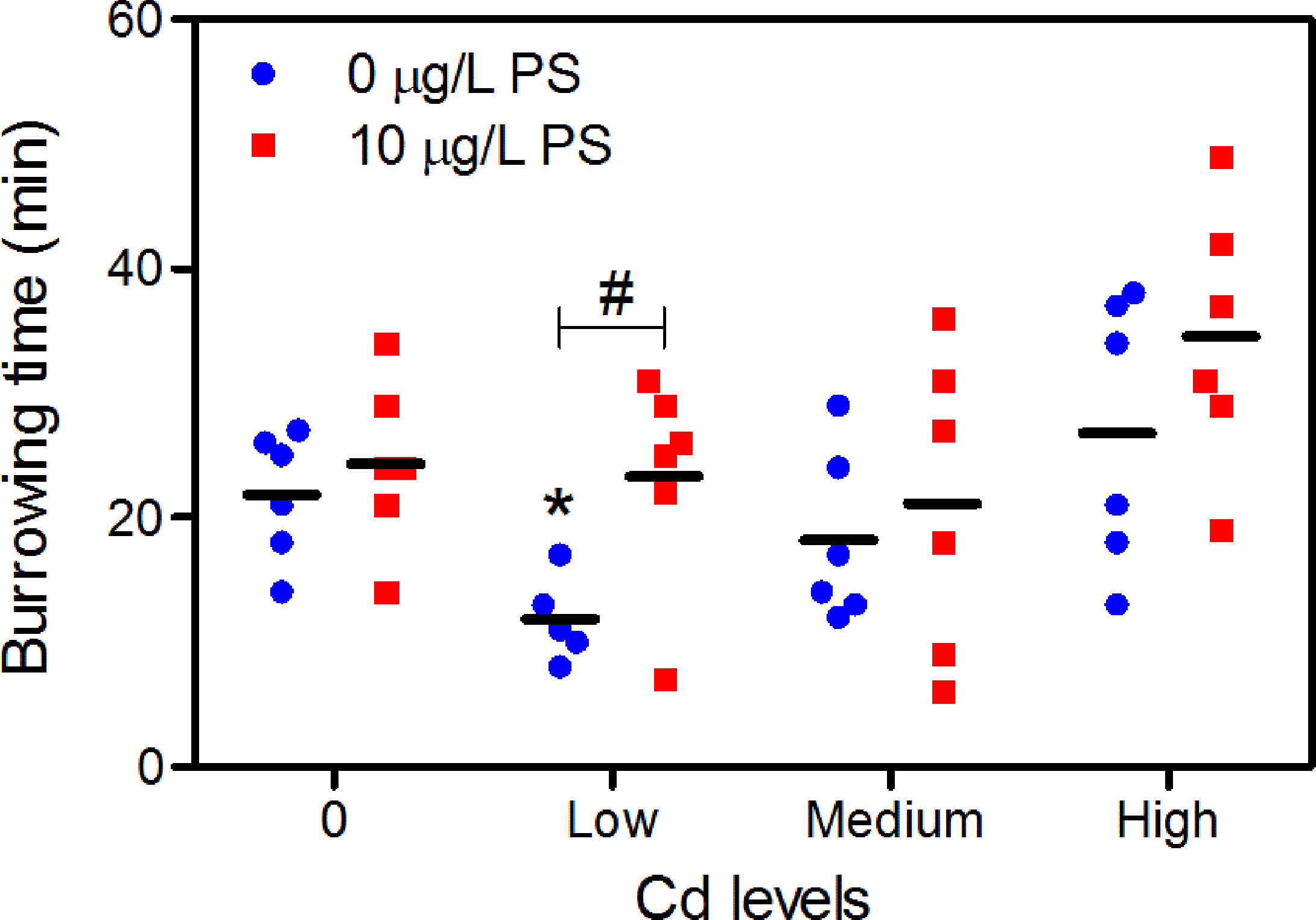
Figure 3 Burrowing time of P. aibuhitensis after 7 days of exposure to Cd alone and Cd combined with 10 μg/L of PS. Results are presented as scatter plot (n = 5–6) and mean (black bar). Significant difference against controls is shown as *(p < 0.05), while the difference between Cd and Cd + PS exposure is shown as #(p < 0.05).
Cd body burden
Cadmium was bioavailable in Cd/Cd + PS treatments and was accumulated in P. aibuhitensis. For both groups, Cd body burdens of worms increased significantly with increasing exposure concentrations (Figure 4). Cd body burdens at three exposure levels were 0.039 ± 0.008, 0.118 ± 0.033, and 2.47 ± 0.462 μg/g ww (mean ± SD) for worms in the Cd-alone treatment, and 0.055 ± 0.002, 0.203 ± 0.037, and 2.38 ± 0.581 μg/g ww for worms in the Cd + PS treatment, respectively. Cd body burdens in worms exposed to CdM and CdH were significantly greater than those of control worms with or without the presence of 10 μg/L PS (Figure 4). In addition, at low- and medium-exposure concentrations of Cd, Cd body burdens with the presence of 10 μg/L PS were significantly higher than those of Cd-alone exposures (Figure 4).
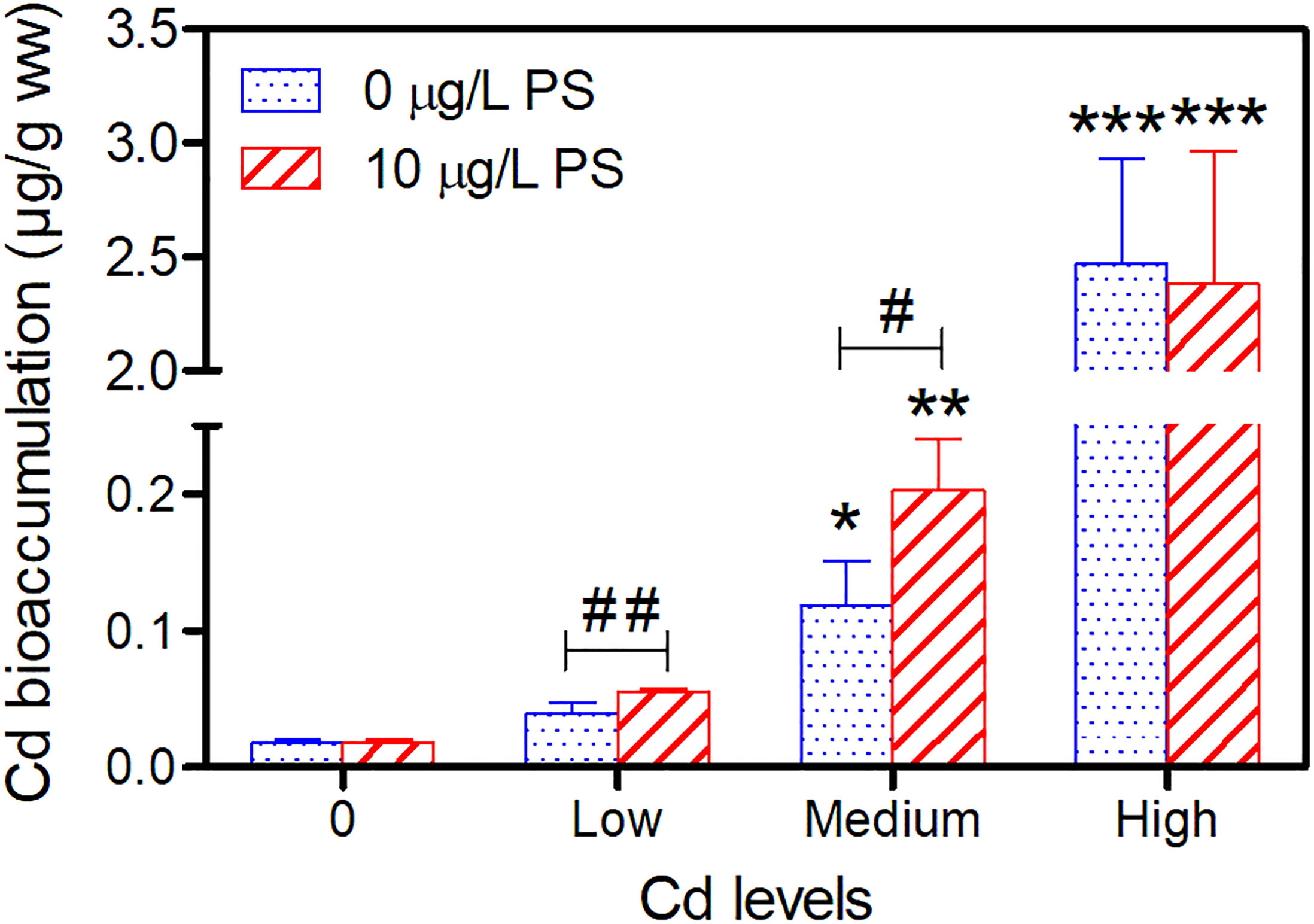
Figure 4 Cd bioaccumulation in P. aibuhitensis after 7 days of exposure to Cd alone and Cd combined with 10 μg/L of PS. Results are presented as means ± SD (n = 4). Significant differences against controls are shown as *(p < 0.05), **(p < 0.05), and ***(p < 0.001), while differences between Cd and Cd + PS exposure are shown as #(p < 0.05) and ##(p < 0.01).
Histopathological analyses of epidermis and intestinal canal
In the control group, the dorsal epidermis of worms showed normal histology with an intact cuticle, regular single-layer columnar epithelia cells, and discontinuously circular muscle from the outside to the inside, respectively (Figure 5A). However, worms exposed to Cd alone showed pathological alterations including increased numbers of gland cells, cytoplasmic loose, and vacuolation (Figures 5C, E, G), while worms exposed to PS alone mainly demonstrated increased numbers of gland cells and swelling of these cells (Figure 5B). When exposed to Cd and PS, worms showed major changes of epidermis characterized by the abundant number of gland cells (Figures 5D, F, H) and increased number and volume of vacuolation (Figure 5D).
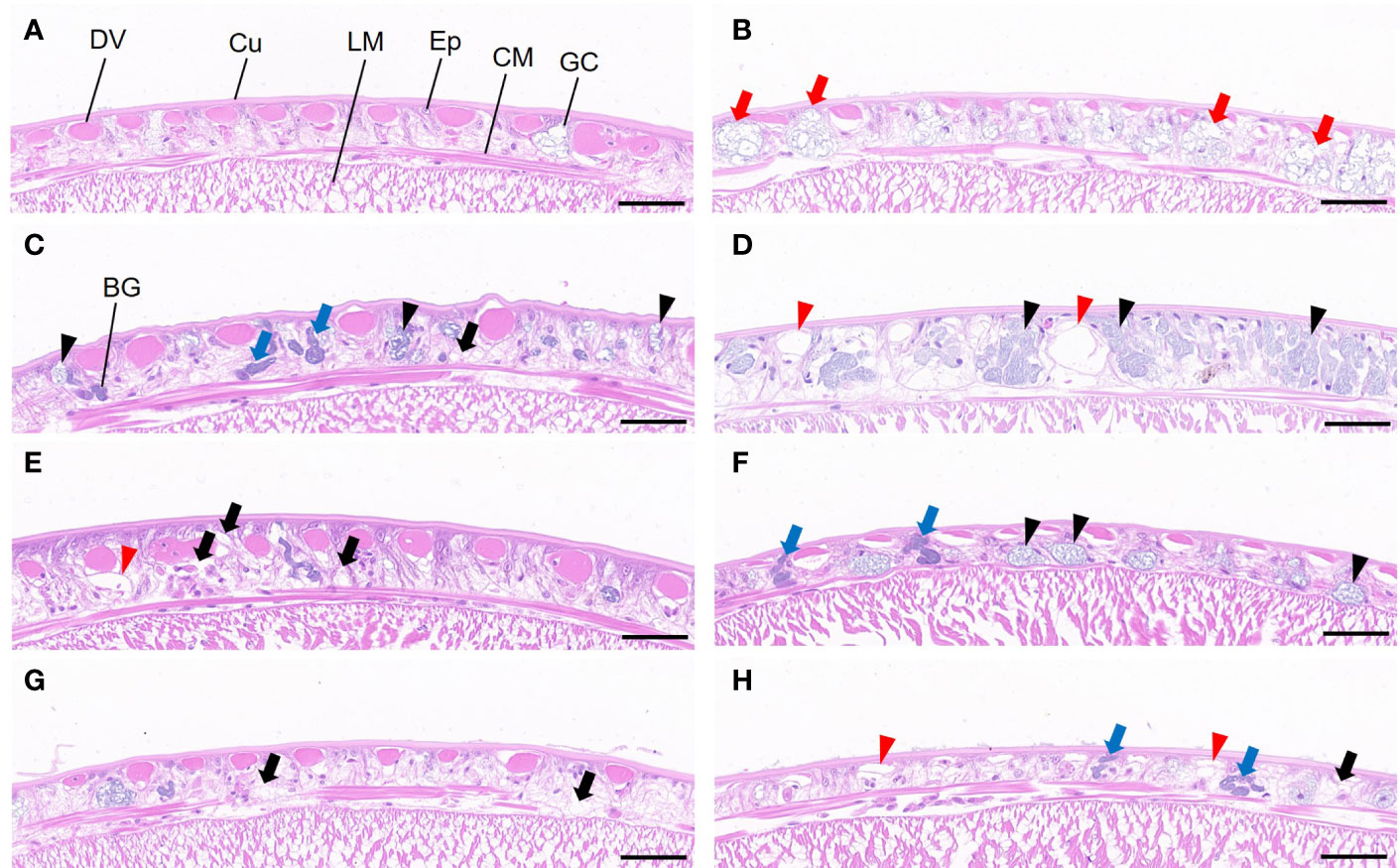
Figure 5 Histology (transverse section) of P. aibuhitensis dorsal body wall after 7 days of exposure to (A) Control, (B) 10 μg/L PS, (C) CdL, (D) CdL + PS, (E) CdM, (F) CdM + PS, (G) CdH, and (H) CdH + PS. Cu, cuticle; Ep, epithelial layer; DV, dorsal vessel; GC, gland cell; CM, circular muscle; LM, longitudinal muscle; BG, basophilic granule. The different symbols indicate cell swelling (red arrow), increase of basophilic granules (blue arrow), cytoplasmic loose (black arrow), cytosis of gland cells (black triangle), and cell vacuolation (red triangle). Scale bars are 50 μm.
The intestinal epithelia of control worms are densely arranged with regular morphology and intact cell structure (Figure 6A). In contrast, the intestinal epithelia of worms exposed to the PS alone showed extensive epithelia cytolysis and vacuolation, and the epithelia layer was thinner than that of the control group (Figure 6B). The main pathological alterations of worms in Cd-alone treatments included disordered arrangement of intestinal epithelia (Figures 6C, G), partial epithelia cytolysis (Figures 6C, G), enlargement of cell space (Figure 6E), and partial villi exfoliation (Figures 6C, E, G), while the intestine epithelial changes in combined exposed worms mainly included cell vacuolation (Figure 6D), enlarged cell space (Figure 6F), extensive cytolysis (Figures 6D, H), and villi exfoliation (Figures 6F, H).
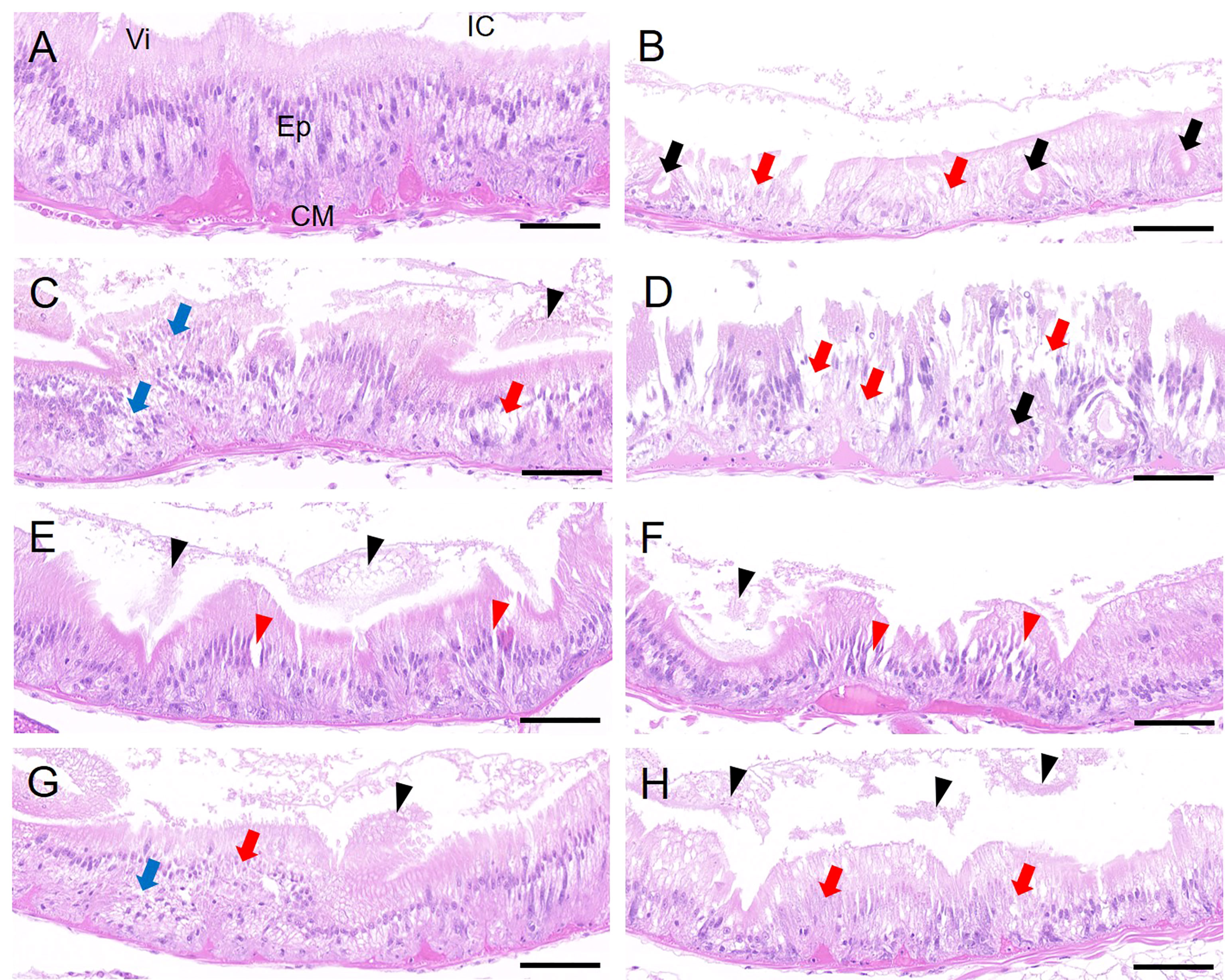
Figure 6 Histology (transverse section) of P. aibuhitensis intestinal canal after 7 days of exposure to (A) Control, (B) 10 μg/L PS, (C) CdL, (D) CdL + PS, (E) CdM, (F) CdM + PS, (G) CdH, and (H) CdH + PS. Vi, intestinal villi; Ep, intestinal epithelia; CM, circular muscle; IC, intestinal cavity. The different symbols indicate cytolysis (red arrow), vacuolation (black arrow), disordered arrangement (blue arrow), villi exfoliation (black triangle), and enlarged cell space (red triangle). Scale bars are 50 μm.
Discussion
Cd bioaccumulation
Bioaccumulation of HMs in marine organisms with the presence of MPs is an important issue that has received relatively less attention than other toxicological endpoints. Cadmium is ubiquitous in the marine environment and has been found to be readily adsorbing on the surface of PS-MPs (Zhou et al., 2020). The specific surface area and total pore volume were closely correlated with the adsorption capacity of the MPs, and the π–π interaction, electrostatic interaction, and oxygen-containing functional groups played crucial roles in the adsorption of Cd(II) onto the MPs (Zhou et al., 2020). It should also be noted that a higher desorption rate of Cd(II) from the surface of PS-MPs was observed in the simulated gut environment (Zhou et al., 2020). Therefore, there is potential for PS-MPs to alter the bioavailability of Cd through adsorption and desorption processes. Our study demonstrated that PS-MPs carrying Cd can be bioaccumulated in worms. Moreover, at low and medium Cd concentrations, the coexistence of PS significantly increased Cd body burdens in worms, while Cd bioaccumulation was comparable with or without the presence of PS at the highest Cd concentration (Figure 4). These findings highlighted that more attention should be paid to the interactions between Cd and PS at lower concentrations. Our results are in accordance with that of Yan et al. (2020), in which the combined treatment of PS (2.5 μm, 100 μg/L) and Cd (10 μg/L) caused higher Cd body burdens in the gut of marine medaka, O. melastigma. Such increase in accumulation of HMs mixed with MPs was also reported in European seabass (D. labrax) (Barboza et al., 2018a), marine mysid (N. awatschensis) (Eom et al., 2021), zebrafish (Danio rerio) (Lu et al., 2018), and Chinese mitten crab (Eriocheir sinensis) (Yang et al., 2022). As the adsorption of Cd by PS had been proven to occur upon mixing, we inferred that the absorbed Cd(II) from the surface of PS-MPs was readily released after ingestion under an acidic environment of worm intestine, and resulted in a faster bioaccumulation of Cd compared to the scenario of CdL- or CdM-alone exposure. These results provide evidence supporting the role of PS-MPs, which are involved in making Cd more bioavailable to benthic organisms in the marine environment. Also, the ingestion of Cd carried out by PS-MPs possibly poses a higher ecological risk to macroinvertebrates, especially at environmentally relevant concentrations as shown in this study.
Nevertheless, the increasing Cd body burden with the presence of PS-MPs was not always the case in our study. That is, at the highest Cd concentration, the accumulation of Cd in combined exposure groups was comparable to that of worms treated with Cd alone. This may be explained by the fact that PS surfaces are saturated by Cd, leading to similar Cd burdens independently of the presence of PS, which suggested the potential influence of metal concentration on the interaction between HMs and MPs. A similar result was also observed by Roda et al. (2020), in which the presence of PS-MPs (10–90 μm in diameter, 20 μg/L) did not significantly affect the Cu body burden in freshwater fish, Prochilodus lineatus. In summary, the existing bioaccumulation data suggest substantial variation in metal body burdens when combined with MPs, which is likely not only due to differences in metal type, concentration, and media conditions among studies, but also due to differences within and among species, and the properties of MPs, such as size and concentration, are also responsible for the observed bioavailability of HMs.
Generally, trace metals (such as Cd, Cu, Zn, and Ag) accumulated in aquatic invertebrates are typically detoxified by sequestration to intracellular ligands (Ng et al., 2008). In the polychaete N. diversicolor, for example, the induction of physiological detoxification mechanisms can be triggered to control its body concentration of certain metals, the process of which is involved in the formation of metal-containing extracellular granules, mineralized lysosomes, excretion of metals by exocytosis via coelomocytes, and synthesis and turnover of metal binding proteins (metallothioneins, etc.) (Cong et al., 2011). Given the physiological detoxification mechanisms of HMs by worms, it could well be that the above processes were changed to some degree with the presence of MPs. Therefore, future studies should be directed to understand the metal distribution patterns in different intracellular ligands (cytosolic proteins and insoluble deposits) and detoxification processes during metal accumulation with or without the presence of MPs.
Burrowing behavior
Infauna species such as P. aibuhitensis are involved in the biogeochemical cycling of nutrients and contaminants in coastal environment, the process of which is closely related to its burrowing activity. Previous studies showed that burrowing behavior had been identified as a sensitive indicator of estuarine health status assessment for the polychaete, Hedisite diversicolor (Métais et al., 2019), which is mainly distributed in European and North American coasts. In addition, the altered burrowing pattern of this species was influenced by various environmental pollutants including HMs, metal and plastic nanoparticles, antibiotics, and environmental factors such as pH and temperature (Bonnard et al., 2009; Cong et al., 2014; Nogueira and Nunes, 2020; Silva et al., 2020; Bhuiyan et al., 2021). The main alterations after exposure to the above pollutants and environmental factors include prolonged time taken for the individuals to start the burrowing process and to completely bury them in sediment, as well as increased proportion of unborrowed individuals. Interestingly, in this study, we found that the burrowing behavior of P. aibuhitensis was significantly excited at the lowest Cd (13.08 μg/L)-alone exposure as shown by the shorter burrowing time compared to that of the control. Afterwards, burrowing time gradually increased with increasing Cd concentrations. In other words, the hormetic effect of Cd on worm burrowing activity was observed, demonstrated by a typical U-shaped response profile, and this mode of action by Cd was also reported in the study of Liu et al. (2019a). Nevertheless, we did not observe a significant impact of PS MPs on the burrowing of P. aibuhitensis in this study, under either single or combined circumstance. This is in contrast with the finding of Silva et al. (2020), in which the burrowing time of H. diversicolor was significantly prolonged after 28 days of exposure to PS nanoplastics (100 nm) within a concentration range of 5 to 500 μg/L. This discrepancy should be attributed to the different sizes and concentrations of PS used, different exposure durations, and, in consequence, different species performances. Nonetheless, we found that the burrowing performance of worms was significantly different at the lowest Cd concentration with the coexistence of PS compared to that of Cd alone. Again, this emphasized the importance of testing single and combined impacts of the above two compounds at low concentrations, that is, at environmentally relevant concentrations.
Behavioral alterations may be the result of the integration of effects in several physiological systems such as neurological, sensorial, hormonal, and metabolic (Cong et al., 2011; Silva et al., 2020). Therefore, the exploration of these multiple factors will help interpret the behavioral alterations and discrepancy induced by HMs and MPs. In this study, at two other higher Cd concentrations, although no significant difference of burrowing behavior between the combined groups and the single Cd groups was observed, the worms in combined treatments did have longer burrowing time than those exposed to Cd alone. From an ecological perspective, this hypoactivity of burrowing with the presence of MPs suggested an altered fitness of worms that may lead to a higher susceptibility to predators, and a reduction in sediment oxygenation, which may consequently impact other organisms and affect nutrients’ cycle (Scott and Sloman, 2004; Fonseca et al., 2017; Silva et al., 2020).
Histopathological alterations
The middle cross sections of P. aibuhitensis were analyzed for histopathological alterations after 7 days of exposure. We found that the epidermis and intestinal epithelium layer of P. aibuhitensis demonstrated histopathological alterations after single Cd/PS or combined exposure, whereas muscles did not show obvious lesion. Previous studies have confirmed that both HMs and MPs can cause pathological changes of different tissues (gill, hepatopancreas, intestine, etc.) in different organisms, such as shrimp (Litopenaeus vannamei) (Hsieh et al., 2021), medaka (Oryzias latipes) (Rochman et al., 2013), zebrafish (D. rerio) (Lei et al., 2018), and earthworms (Eisenia fetida) (Jiang et al., 2020). However, to the best of our knowledge, this study is the first time that histopathological influences of Cd and PS, either individual or combined, were demonstrated in the ecologically and economically important species, P. aibuhitensis.
We found that the main alterations in dorsal epidermis of worms exposed to Cd alone were shown as the increased numbers of gland cells and basophilic granules (Figure 6C), cytoplasmic loose (Figures 6C, E, G), and a little vacuolation (Figure 6E), indicating the occurrence of cell degradation and structural damage of epidermis. In contrast, when exposed to the PS alone, the major characteristic of worm epidermis was the increasing numbers of gland cells and swelling of these cells. In addition, the coexistence of Cd and PS was inclined to aggravate the situation of vacuolation and gland cell proliferation in epidermis compared to those of single Cd exposure (Figures 6D, F, H), especially at the lowest Cd combined group. The main function of epidermal gland cells is to secrete mucus, which forms a barrier on the body wall to resist the invasion of bacteria and exogenous substances, and facilitate the physiological behavior of organisms such as burrowing of sediment-dwelling organisms. The above abnormalities suggested that the defense function of worm epidermis was possibly damaged and its exacerbation by the contribution of PS should not be ignored.
The intestinal canal is the most commonly analyzed part for lesions following ingestion of pollutants. Our results demonstrated partial cytolysis, cell disordered arrangement, cell space enlargement, and villi exfoliation of intestines in Cd-alone-treated worms. In particular, these changes tended to be more apparent in combined exposed worms mainly characterized by large areas of cytolysis and villi exfoliation. Similarly, this consequence could be attributed to the presence of PS, which alone resulted in extensive cytolysis, vacuolation, and a thinner layer of intestine epithelia of worms (Figure 6B). Similar histopathological changes of intestines induced by HMs and MPs were also reported in other aquatic organisms. A marked loss of all the digestive gland structure, cell autolysis, and loss of basophilia were observed in the digestive gland of Antarctic limpets (Nacella concinna) after 72 h of exposure to a concentration of 0.5 mg/L Cd (Najle et al., 2000). Jiang et al. (2020) showed that intestines of earthworms (E. fetida) exposed to PS-MPs (1.3 μm, 1,000 μg/kg) exerted enlarged intestinal cells with irregular shapes and altered size of cell nuclei, and intestinal cell lysis was also often observed. Lu et al. (2018) found that 2,000 μg/L of PS-MPs (5 μm and 20 μm) induced inflammation in zebrafish gut. These findings highlighted that intestinal epithelia were one of the main target organs potentially affected by HMs and MPs, whose process probably impaired the nutrient status, energy supply, and defense capability of organisms as a consequence.
Previous studies have pointed out that the histopathological responses that occurred in organisms can vary depending on factors such as animal biology (age, condition factor, etc.), exposure concentrations, exposure time, exposure pathway, size of particles, the presence of other contaminants, surface charge of particles, and carrying out of depuration period (Lu et al., 2016). In this study, the coexistence of PS and Cd tended to generate a more severe damage of epidermis and intestine epithelia. Nevertheless, it should be noted that the histopathological analyses in our study mainly focus on the qualitative analyses, and quantitative results such as the percentage of tissue damaged worms and the percentage of main histopathological characteristics under single and combined exposure conditions still need further investigation using more replicates. In addition, the potential biochemical processes involved in these alterations should also be clarified in future studies.
Conclusions
Overall, this study compared the chronic toxicities and bioaccumulation of Cd with or without the presence of PS, through a 7-day seawater exposure pathway, using the estuary polychaete P. aibuhitensis as the test organism. The burrowing time of P. aibuhitensis only showed a significant decrease in the CdL-alone treatment, suggesting a hormetic effect of Cd. The coexistence of PS significantly prolonged worm burrowing time in the CdL group, and increased worm body burden in CdL and CdM groups, indicating the potential influence of metal concentration on the interaction between HMs and MPs. Histopathological analyses demonstrated mainly epidermal and intestinal alterations by Cd, PS, and their combined treatments. Also, the presence of PS tended to aggravate the pathological changes of these tissues. Our results emphasized the importance of exploring interactions and toxicities of HMs and MPs at environmentally relevant concentrations. Future studies should explore the underlying mechanisms involved in the above processes to help understand the interactions between HMs and MPs and their joint risk in the marine environment.
Data availability statement
The original contributions presented in the study are included in the article. Further inquiries can be directed to the corresponding authors.
Author contributions
YC: experiment operation, statistical analyses, and original draft writing. YL: experiment preparation and partial operation. HZ: histopathological analyses. ZL: conceptualization and writing–review. MZ: experiment preparation. FJ: conceptualization and experiment preparation. YW and JW: supervision, writing–review, and editing. All authors contributed to the manuscript revision and approved the submitted version.
Funding
This research was supported by grants from the National Natural Science Foundation of China (No. 41706117), the Liaoning Revitalization Talents Program (XLYC2007013), and the National Key Research and Development Program of China (2016YFC1402204).
Conflict of interest
The authors declare that the research was conducted in the absence of any commercial or financial relationships that could be construed as a potential conflict of interest.
Publisher’s note
All claims expressed in this article are solely those of the authors and do not necessarily represent those of their affiliated organizations, or those of the publisher, the editors and the reviewers. Any product that may be evaluated in this article, or claim that may be made by its manufacturer, is not guaranteed or endorsed by the publisher.
References
Auta H. S., Emenike C. U., Fauziah S. H. (2017). Distribution and importance of microplastics in the marine environment: a review of the sources, fate, effects, and potential solutions. Environ. Int. 102, 165–176. doi: 10.1016/j.envint.2017.02.013
Barboza L. G. A., Vieira L. R., Branco V., Figueiredo N., Carvalho F., Carvalho C., et al. (2018a). Microplastics cause neurotoxicity, oxidative damage and energy-related changes and interact with the bioaccumulation of mercury in the European seabass, Dicentrarchus labrax (Linnaeus 1758). Aquat. Toxicol. 195, 49–57. doi: 10.1016/j.aquatox.2017.12.008
Barboza L. G. A., Vieira L. R., Guilhermino L. (2018b). Single and combined effects of microplastics and mercury on juveniles of the European seabass (Dicentrarchus labrax): Changes in behavioural responses and reduction of swimming velocity and resistance time. Environ. Pollut. 236, 1014–1019. doi: 10.1016/j.envpol.2017.12.082
Bhuiyan K. A., Rodríguez B. M., Pires A., Riba I., Dellvals Á., Freitas R., et al. (2021). Experimental evidence of uncertain future of the keystone ragworm Hediste diversicolor (O.F. müller 1776) under climate change conditions. Sci. Total Environ. 750, 142031. doi: 10.1016/j.scitotenv.2020.142031
Bonnard M., Roméo M., Amiard-Triquet C. (2009). Effects of copper on the burrowing behavior of estuarine and coastal invertebrates the polychaete Nereis diversicolor and the bivalve Scrobicularia plana. Hum. Ecol. Risk Assess. 15, 11–26. doi: 10.1080/10807030802614934
Brennecke D., Duarte B., Paiva F., Cacador I., Canning-Clode J. (2016). Microplastics as vector for heavy metal contamination from the marine environment. Estuar. Coast. Shelf. Sci. 178, 189–195. doi: 10.1016/j.ecss.2015.12.003
Carpenter E. J., Anderson S. J., Harvey G. R., Miklas H. P., Peck B. B. (1972). Polystyrene spherules in coastal waters. Science 178 (4062), 749–750. doi: 10.1126/science.178.4062.749
Cong Y., Banta G. T., Selck H., Berhanu D., Valsami-Jones E., Forbes V. E. (2011). Toxic effects and bioaccumulation of nano-, micron- and ionic-Ag in the polychaete, Nereis diversicolor. Aquat. Toxicol. 105 (3-4), 403–411. doi: 10.1016/j.aquatox.2011.07.014
Cong Y., Banta T. G., Selck H., Berhanu D., Valsami-Jones E., Forbes E. V. (2014). Cellular toxicity and bioaccumulation of sediment-associated silver nanoparticles in the estuarine polychaete, Nereis (Hediste) diversicolor. Aquat. Toxicol. 156, 106–115. doi: 10.1016/j.aquatox.2014.08.001
Eom H. J., Haque M. N., Lee S., Rhee J. S. (2021). Exposure to metals premixed with microplastics increases toxicity through bioconcentration and impairs antioxidant defense and cholinergic response in a marine mysid. Comp. Biochem. Physiol. C Toxicol. Pharmacol. 249, 109142. doi: 10.1016/j.cbpc.2021.109142
Eriksen M., Lebreton L., Carson H., Thiel M., Moore C., Borerro J., et al. (2014). Plastic pollution in the world’s oceans: more than 5 trillion plastic pieces weighing over 250,000 tons afloat at Sea. PLoS One 9, 12. doi: 10.1371/journal.pone.0111913
Fonseca T. G., Morais M. B., Rocha T., Abessa D. M. S., Aureliano M., Bebianno M. J. (2017). Ecotoxicological assessment of the anticancer drug cisplatin in the polychaete Nereis diversicolor. Sci. Total Environ. 575, 162–172. doi: 10.1016/j.scitotenv.2016.09.185
Guo W., Zou J., Liu S., Chen X., Kong X., Zhang H., et al. (2022). Seasonal and spatial variation in dissolved heavy metals in liaodong bay, China. Int. J. Environ. Res. Public Health 19 (1), 608. doi: 10.3390/ijerph19010608
Hao Z., Chen L., Wang C., Zou X., Zheng F., Feng W., et al. (2019). Heavy metal distribution and bioaccumulation ability in marine organisms from coastal regions of hainan and zhoushan, China. Chemosphere 226, 340–350. doi: 10.1016/j.chemosphere.2019.03.132
Holmes L. A., Turner A., Thompson R. C. (2012). Adsorption of trace metals to plastic resin pellets in the marine environment. Environ. Pollut. 160, 42–48. doi: 10.1016/j.envpol.2011.08.052
Holmes L. A., Turner A., Thompson R. C. (2014). Interactions between trace metals and plastic production pellets under estuarine conditions. Mar. Chem. 167, 25–32. doi: 10.1016/j.marchem.2014.06.001
Hsieh S. L., Wu Y. C., Xu R. Q., Chen Y. T., Chen C. W., Singhania R. R., et al. (2021). Effect of polyethylene microplastics on oxidative stress and histopathology damages in Litopenaeus vannamei. Environ. Pollut. 288, 117800. doi: 10.1016/j.envpol.2021.117800
Jiang X., Chang Y., Zhang T., Qiao Y., Klobučar G., Li M. (2020). Toxicological effects of polystyrene microplastics on earthworm (Eisenia fetida). Environ. Pollut. 259, 113896. doi: 10.1016/j.envpol.2019.113896
Law K. L., Thompson R. C.. (2014). Microplastics in the seas. Sci. 345(6193), 144–145. doi: 10.1126/science.1254065
Lei L., Wu S., Lu S., Liu M., Song Y., Fu Z., et al. (2018). Microplastic particles cause intestinal damage and other adverse effects in zebrafish Danio rerio and nematode Caenorhabditis elegans. Sci. Total Environ. 619–620, 1–8. doi: 10.1016/j.scitotenv.2017.11.103
Liu F., Lu Z., Wu H., Ji C. (2019a). Dose-dependent effects induced by cadmium in polychaete Perinereis aibuhitensis. Ecotoxicol. Environ. Saf. 169, 714–721. doi: 10.1016/j.ecoenv.2018.11.098
Liu G., Zhu Z., Yang Y., Sun Y., Yu F., Ma J. (2019b). Sorption behavior and mechanism of hydrophilic organic chemicals to virgin and aged microplastics in freshwater and seawater. Environ. Pollut. 246, 26–33. doi: 10.1016/j.envpol.2018.11.100
Li J., Zhang K., Zhang H. (2018). Adsorption of antibiotics on microplastics. Environ. Pollut. 237, 460–467. doi: 10.1016/j.envpol.2018.02.050
Lu K., Qiao R., An H., Zhang Y. (2018). Influence of microplastics on the accumulation and chronic toxic effects of cadmium in zebrafish (Danio rerio). Chemosphere 202, 514–520. doi: 10.1016/j.chemosphere.2018.03.145
Lu Y., Zhang Y., Deng Y., Jiang W., Zhao Y., Geng J., et al. (2016). Uptake and accumulation of polystyrene microplastics in zebrafish (Danio rerio) and toxic effects in liver. Environ. Sci. Technol. 50, 4054–4060. doi: 10.1021/acs.est.6b00183
Mani T., Hauk A., Walter U., Burkhardt-Holm P. (2015). Microplastics profile along the Rhine river. Sci. Rep. 5, 17988. doi: 10.1038/srep17988
Métais I., Châtel A., Mouloud M., Perrein-Ettajani H., Bruneau M., Gillet P., et al. (2019). Is there a link between acetylcholinesterase, behaviour and density populations of the ragworm Hediste diversicolor? Mar. Pollut. Bull. 142, 178–182. doi: 10.1016/j.marpolbul.2019.03.026
Najle R., Elissondo M., Gentile S., Gentile M., Vacarezza G., Solana H. (2000). Histopathology of the digestive gland of an Antarctic limpet exposed to cadmium. Sci. Total Environ. 247 (2-3), 263–268. doi: 10.1016/s0048-9697(99)00495-7
Ng T. Y. T., Rainbow P. S., Amiard-Triquet C., Amiard J. C., Wang W. X. (2008). Decoupling of cadmium biokinetics and metallothionein turnover in a marine polychaete after metal exposure. Aquat. Toxicol. 89, 47–54. doi: 10.1016/j.aquatox.2008.06.001
Nogueira A. F., Nunes B. (2020). Effects of low levels of the antibiotic ciprofloxacin on the polychaete Hediste diversicolor: biochemical and behavioural effects. Environ. Toxicol. Pharmacol. 80, 103505. doi: 10.1016/j.etap.2020.103505
Pannetier P., Morin B., Clerandeau C., Laurent J., Chapelle C., Cachot J. (2019). Toxicity assessment of pollutants sorbed on environmental microplastics collected on beaches: part II-adverse effects on Japanese medaka early life stages. Environ. Pollut. 248, 1098–1107. doi: 10.1016/j.envpol.2018.12.091
Qiu Y. W. (2015). Bioaccumulation of heavy metals both in wild and mariculture food chains in daya bay, south China. Estuar. Coast. Shelf. S163, 7–14. doi: 10.1016/j.ecss.2015.05.036
Rochman C. M., Hoh E., Kurobe T., Teh S. J. (2013). Ingested plastic transfers hazardous chemicals to fish and induces hepatic stress. Sci. Rep. 3, 3263. doi: 10.1038/srep03263
Roda J. F. B., Lauer M. M., Risso W. E., Bueno Dos Reis Martinez C. (2020). Microplastics and copper effects on the neotropical teleost Prochilodus lineatus: Is there any interaction? Comp. Biochem. Physiol. A Mol. Integr. Physiol. 242, 110659. doi: 10.1016/j.cbpa.2020.110659
Scott G. R., Sloman K. A. (2004). The effects of environmental pollutants on complex fish behaviour: integrating behavioural and physiological indicators of toxicity. Aquat. Toxicol. 68, 369–392. doi: 10.1016/j.aquatox.2004.03.016
Silva M. S. S., Oliveira M., Valente P., Figueira E., Martins M., Pires A. (2020). Behavior and biochemical responses of the polychaeta Hediste diversicolor to polystyrene nanoplastics. Sci. Total Environ. 707, 134434. doi: 10.1016/j.scitotenv.2019.134434
Sun F., Zhou Q., Wang M., An J. (2019). Joint stress of copper and petroleum hydrocarbons on the polychaete Perinereis aibuhitensis at biochemical levels. Ecotoxicol. Environ. Saf. 72, 1887–1892. doi: 10.1016/j.ecoenv.2009.04.017
Tian Y., Liu H., Wang Q., Zhou H., Tang X. (2014). Acute and chronic toxic effects of Pb2+ on polychaete Perinereis aibuhitensis: morphological changes and responses of the antioxidant system. J. Environ. Sci. 26, 1681–1688. doi: 10.1016/j.jes.2014.06.008
Wang F., Qi H. X., You J. (2015). Joint toxicity of sediment-associated DDT and copper to a polychaete, Nereis succinea. Ecotoxicology 24 (2), 424–432. doi: 10.1007/s10646-014-1391-7
Wang J. X., Song K. X., Sun Y. X., Fang T., Li J. Z., Zhang T., et al. (2021). Indicator function of ragworm (Nereididae) on sediment microplastic in haizhou bay intertidal zone. Huan Jing Ke Xue 42 (9), 4341–4349. doi: 10.13227/j.hjkx.202012069
Wang Y., Zhang D., Zhang M., Mu J., Ding G., Mao Z., et al. (2019). Effects of ingested polystyrene microplastics on brine shrimp, Artemia parthenogenetica. Environ. Pollut. 244, 715–722. doi: 10.1016/j.envpol.2018.10.024
Wen B., Jin S. R., Chen Z. Z., Gao J. Z., Liu Y. N., Liu J. H., et al. (2018). Single and combined effects of microplastics and cadmium on the cadmium accumulation, antioxidant defence and innate immunity of the discus fish (Symphysodon aequifasciatus). Environ. Pollut. 243, 462–471. doi: 10.1016/j.envpol.2018.09.029
Yang Z., Zhu L., Liu J., Cheng Y., Waiho K., Chen A., et al. (2022). Polystyrene microplastics increase Pb bioaccumulation and health damage in the Chinese mitten crab Eriocheir sinensis. Sci. Total Environ. 829, 154586. doi: 10.1016/j.scitotenv.2022.154586
Yan W., Hamid N., Deng S., Jia P. P., Pei D. S. (2020). Individual and combined toxicogenetic effects of microplastics and heavy metals (Cd, Pb, and zn) perturb gut microbiota homeostasis and gonadal development in marine medaka (Oryzias melastigma). J. Hazard Mater. 397, 122795. doi: 10.1016/j.jhazmat.2020.122795
Zhang L., Shi Z., Jiang Z., Zhang J., Wang F., Huang X. (2015). Distribution and bioaccumulation of heavy metals in marine organisms in east and west guangdong coastal regions, south China. Mar. Pollut. Bull. 101, 930–937. doi: 10.1016/j.marpolbul.2015.10.041
Zhang W., Zhang S., Wang J., Wang Y., Mu J., Wang P., et al. (2017). Microplastic pollution in the surface waters of the bohai Sea, China. Environ. Pollut. 231 (Pt 1), 541–548. doi: 10.1016/j.envpol.2017.08.058
Keywords: heavy metal, microplastics, benthic organism, behavior, body burden, histopathology
Citation: Cong Y, Lou Y, Zhao H, Li Z, Zhang M, Jin F, Wang Y and Wang J (2022) Polystyrene microplastics alter bioaccumulation, and physiological and histopathological toxicities of cadmium in the polychaete Perinereis aibuhitensis. Front. Mar. Sci. 9:939530. doi: 10.3389/fmars.2022.939530
Received: 09 May 2022; Accepted: 30 June 2022;
Published: 25 July 2022.
Edited by:
Sílvia C. Gonçalves, Polytechnic Institute of Leiria, PortugalReviewed by:
Youssef Lahbib, Tunis University, TunisiaGianfranco Santovito, University of Padua, Italy
Copyright © 2022 Cong, Lou, Zhao, Li, Zhang, Jin, Wang and Wang. This is an open-access article distributed under the terms of the Creative Commons Attribution License (CC BY). The use, distribution or reproduction in other forums is permitted, provided the original author(s) and the copyright owner(s) are credited and that the original publication in this journal is cited, in accordance with accepted academic practice. No use, distribution or reproduction is permitted which does not comply with these terms.
*Correspondence: Ying Wang, wangying@nmemc.org.cn; Juying Wang, jywang@nmemc.org.cn