- 1CAS Key Laboratory of Tropical Marine Bio-resources and Ecology, Guangdong Provincial Key Laboratory of Applied Marine Biology, South China Sea Institute of Oceanology (SCSIO), Chinese Academy of Sciences (CAS), Guangzhou, China
- 2Department of Ocean Science and Hong Kong Branch of The Southern Marine Science and Engineering Guangdong Laboratory (Guangzhou), The Hong Kong University of Science and Technology (HKUST), Kowloon, Hong Kong SAR, China
- 3Southern Marine Science and Engineering Guangdong Laboratory (Guangzhou), Guangzhou, China
- 4Chinese Academy of Sciences-The Hong Kong University of Science and Technology (CAS-HKUST) Sanya Joint Laboratory of Marine Science Research, Key Laboratory of Tropical Marine Biotechnology of Hainan Province, Sanya Institute of Oceanology, South China Sea Institute of Oceanology (SCSIO), Sanya, China
- 5Sanya National Marine Ecosystem Research Station, Tropical Marine Biological Research Station in Hainan, Chinese Academy of Sciences, Sanya, China
- 6University of Chinese Academy of Sciences, Beijing, China
Gregarious larval settlement represents an important window for chimera formation in reef corals, yet it remains largely unknown how aggregated settlement and early chimerism could modify the performance and responses of coral recruits under elevated temperature and pCO2. In this study, single and aggregated recruits of the broadcast spawning coral Acropora austera were exposed to contrasts of two temperatures (28 versus 30.5°C) and pCO2 levels (~500 versus 1000 μatm) for two weeks, and algal symbiont infection success, survivorship and growth were assessed. Results showed that symbiont infection success was mainly affected by temperature and recruit type, with reduced symbiont infection at increased temperature and consistently higher infection success in chimeric recruits compared to single recruits. Furthermore, although chimeric recruits with larger areal size had significantly higher survivorship in all treatments, the polyp-specific growth rates were considerably lower in chimeric entities than individual recruits. More importantly, the recruit type significantly influenced the responses of recruit polyp-specific growth rates to elevated temperature, with chimeras exhibiting lowered skeletal lateral growth under elevated temperature. These results demonstrate the benefits and costs associated with gregarious larval settlement for juvenile corals under ocean warming and acidification, and highlight the ecological role of larval settlement behavior in mediating the responses of coral recruits to climate change stressors.
Introduction
Larvae of sessile colonial marine organisms tend to settle in a gregarious manner and fuse into chimeric entities that simultaneously consist of at least two genetically distinct cells lineages (Keough, 1984). This phenomenon is widely documented in marine organisms including sponges, cnidarians, bryozoans and ascidians, and has been observed both in the laboratory settings and field (Keough, 1984; Santelices, 2004; Ben-Shlomo et al., 2008; Westerman et al., 2009; Puill-Stephan et al., 2012; Shefy et al., 2022). Chimerism could endow the newly settled recruits with an assortment of advantages and benefits, including larger size, greater genetic repertoires and better competitive ability, hence facilitating survivorship of chimeric juveniles and enhancing their capacity to cope with adverse environmental conditions. On the other hand, chimerism may also incur increased intra-specific competition, thus bearing some costs, such as reduced growth, tissue separation and even partial mortality (Buss, 1990; Yael et al., 2002; Santelices, 2004; Amar et al., 2008).
The importance of chimerism in sustaining and accelerating the adaptability and resilience of reef corals in the context of global climate change has been recognized recently, particularly given its potential role as an evolutionary rescue mechanism and active tool in mitigation of climate change impacts (Rinkevich, 2019a; Rinkevich, 2019b; Randall et al., 2020). Coral chimerism can be established at several specific stages during early ontogeny, either through aggregations of embryos and larvae (Yael et al., 2002; Puill-Stephan et al., 2012; Mizrahi et al., 2014; Jiang et al., 2015a; Sampayo et al., 2020), or contact and fusion between adjacent recruits via growth (Rinkevich, 2004; Nozawa and Hirose, 2011; Guerrini et al., 2021). Chimerism through early fusion can persist for very long time and into adult stages in reef corals (Puill-Stephan et al., 2009; Puill-Stephan et al., 2012; Schweinsberg et al., 2015; Guerrini et al., 2021). The allogenic fusion could lead to an immediate increase in areal size and genotypic diversity, hence rendering a potentially wider range of physiological qualities and enhancing the growth and survival likelihood of chimeric recruits compared to the individual and homogeneous ones (Raymundo and Maypa, 2004; Rinkevich, 2004; Rinkevich, 2019b).
Coral recruits are of vast importance to maintain adult populations and aid reef recovery, while they are extremely vulnerable and subject to high mortality rates (Ritson-Williams et al., 2009; Harrison, 2011), and this situation may be further compounded by the ongoing ocean warming and acidification driven by climate change (Anlauf et al., 2011; Foster et al., 2015; Jiang et al., 2018; Sun et al., 2020; Jiang et al., 2021). Despite the widely-accepted advantages and benefits with regard to coral chimerism, few studies have directly addressed the ecological consequences of coral chimerism in these susceptible early life history stages under future oceanic conditions.
Recently, evidence that chimeric corals are more resistant to climate change stressors than the nonchimeric has emerged. For instance, fused aggregations in larvae of the broadcast spawning coral Montipora capitata led to higher survivorship and thermal tolerance but lower growth rate in chimeric colonies compared to the solitary juveniles (Hancock et al., 2021). Likewise, tissue fusion between recruits of the brooding coral Pocillopora acuta significantly increased the survival likelihood under high temperature stress (Huffmyer et al., 2021). Moreover, one recent study found that the larger and chimeric recruits of the coral Colpophyllia natans exhibited reduced susceptibility to infectious agents than the solitary recruits (Williamson et al., 2022). These pioneer studies suggest that early aggregation and fusion in corals could boost the resilience and success of juveniles under environmental stress, in spite of the potential costs and tradeoffs (Rinkevich, 2019b).
Most reef corals are broadcast spawning corals, with a high synchrony in gamete release and larval development, thus providing probability for larval gregariousness upon settlement and the potential for chimera formation (Harrison, 2011; Puill-Stephan et al., 2012; Jiang et al., 2015a). Besides, settlement space on reef substrata is predicted to become increasingly fragmented, and under this circumstance, coral larvae will be more likely to settle gregariously and in aggregations, thus leading to the formation of chimeras (Sampayo et al., 2020). Therefore, a better understanding of how aggregated larval settlement in spawning corals may alter the responses of new recruits to environmental stress, particularly ocean warming and acidification, is needed, which could shed light upon the sexual propagation of corals for restoration under future climate change (Randall et al., 2020).
In this study, we investigated the effects of gregarious larval settlement on the early development in the recruits of a spawning coral Acropora austera under future warming and acidification conditions. This important reef builder in the Indo-Pacific region releases eggs without algal symbionts, and therefore newly settled recruits must capture symbionts from water column to establish symbiosis. We exposed chimeric and single recruits of A. austera to the combinations of two temperatures and two pCO2 levels, and measured the differences in survivorship, algal infection and growth rates between two types of recruits to uncover the role of chimerism in shaping the response of coral recruits to climate change stressors.
Materials and methods
Coral sampling and preparation of new recruits
Five mature colonies of A. austera were collected from Luhuitou fringing reef (18°12′N, 109°28′E) and they spawned around 20:00 on 7th May, 2016. Egg-sperm bundles were mixed and gently agitated for cross fertilization. Fertilized embryos were raised in 0.5-μm-filtered seawater (FSW). Seven-day-old larvae were introduced to 6-well culture plates to prepare the solitary and chimeric recruits. Briefly, one small chip (3×3×2 mm) of crustose coralline algae (CCA) Hydrolithon onkodes, 20 larvae and 10 mL FSW were added into each well. Twenty-four hours later at the room temperature of 27°C, unsettled larvae and CCA chips were discarded. Dishes with settled larvae were carefully checked under a dissecting microscope and each recruit was scored as solitary or chimeric, and then marked with a number on the plates. There was an average of 22 single recruits (± 3, SD, n = 24, range: 17−26, Figure 1A) and seven aggregated recruits (± 1, SD, n = 24, range: 6−9, each with 2−7 polyps, Figures 1B, C) in each culture plate, respectively. Three culture plates were assigned to each experimental tank, with a total of six replicate plates for each treatment.
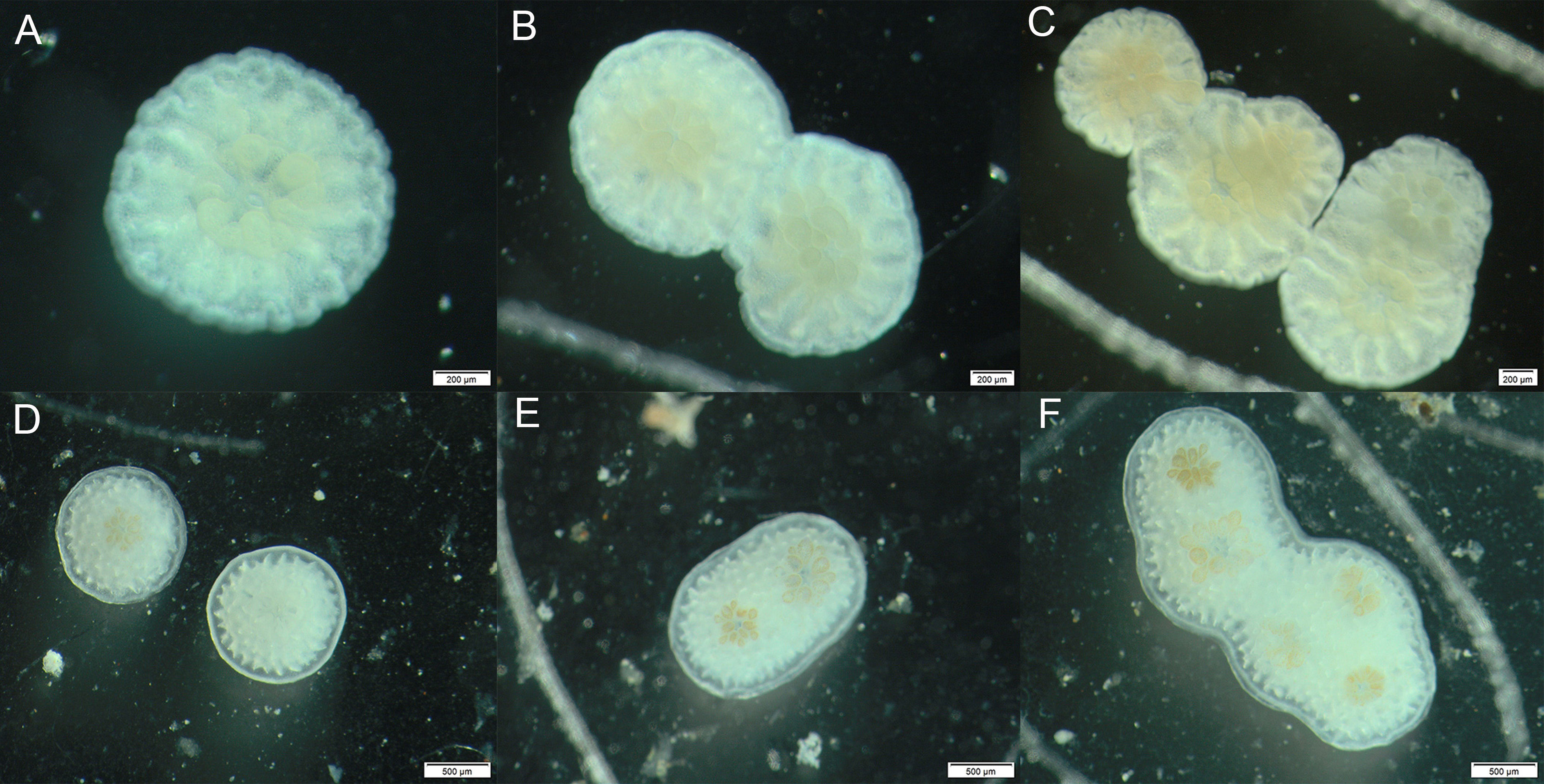
Figure 1 Newly settled single recruit (A) and aggregated recruits with two (B) or five (C) polyps that have fused into chimeras; successful algal infection in single (D) and chimeric recruits (E, F).
Experimental setup
Experimental treatments consisted of two temperatures and two pCO2 levels in a full factorial design as follows: (1) 28°C, ambient pCO2 (ATAC), (2) 28°C, high pCO2 (ATHC), (3) 30.5°C, ambient pCO2 (HTAC), (4) 30.5°C, high pCO2 (HTHC). The 28°C approximated the ambient seawater temperature during the spawning of A. austera at the study site. The ambient pCO2 treatment (500 µatm) was representative of the present-day pCO2 on Luhuitou fringing reef (Zhang et al., 2013). The future scenario treatments achieved a temperature increase of 2.5°C and pH decline of 0.3 units, which were close to the projections for the northern South China Sea by 2100 (Bopp et al., 2013; Gattuso et al., 2015).
Treatments were created in duplicate 75 L tanks receiving 0.5 μm-filtered and UV-sterilized seawater at a flow rate of 0.7 L min−1. Water temperature was controlled by temperature controllers and titanium heaters (Weipro, China). The carbonate chemistry within high pCO2 tanks was manipulated with pH regulators (Weipro, China) via a solenoid valve to control the bubbling of pure CO2 from compressed CO2 tanks. Submerged pumps (600 L h−1) were used to ensure well mixing of seawater within tanks. Each tank was illuminated from 07:00 to 19:00 with a series of full spectrum, T5 fluorescent bulbs (Giesemann), which provided a light intensity of 200 µmol photons m−2 s−1 at the tank bottom.
Seawater temperatures were continuously recorded using Hobo Pendant loggers at 15 min intervals. Salinity and pH were measured three times per day using Mettler-Toledo Seven Go. Seawater samples (100 ml) were taken every 3 days from each tank and preserved with mercuric chloride. Total alkalinity was measured with a Gran titrator (Apollo SciTech Model AS-ALK2). Aragonite saturation state (ΩArag) and pCO2 were calculated from the measured temperature, salinity, pH and alkalinity using CO2SYS (Lewis et al., 1998), with dissociation constants for carbonate determined by Dickson and Millero (1987) and the Dickson constant for the HSO− 4 (Dickson, 1990). Mean physical and chemical parameters are presented in Table 1.

Table 1 Summary of (men ± SD) physical and chemical conditions for each treatment during the experiment.
Survival, algal symbiont inoculation and growth
Throughout the experiment, all recruits were inspected under a dissecting microscope every 2−3 days to check their survival status based on the presence of polyp tissue and exposed bare skeleton. On the 5th day of the experiment, coral recruits were inoculated with freshly isolated algal symbionts from adult branches. Coral tissue was air-brushed from a 10-cm adult branch, after which the tissue slurry was homogenized, filtered through 100μm mesh and then centrifuged (3000 rpm for 3 minutes) for three times to remove coral host tissue debris. Symbiont cells were suspended in filtered seawater to prepare a stock solution at the density of 1.0×106 cells mL−1. Ten mL seawater from the corresponding tank, together with a 100 μL aliquot of symbiont stock solution, was added to each well of the culture plates to reach at a final symbiont concentration of approximately 1.0×104 cells mL−1. The culture plates were securely covered with lids to minimize seawater evaporation and to prevent gas exchange and were then partially submerged in experimental tanks. Recruits within each well were left to uptake symbionts for 8 hours and then seawater within each well was discarded and plates were immersed in seawater. One week later, recruits were inspected under the dissecting microscope to characterize their symbiotic status and symbiont infection success. Recruits were scored as symbiotic following Abrego et al. (2012) according to the criteria that brown symbiont cells and pigmentation were visible within tentacles and oral disc (Figures 1D, F). Algal infection rate was calculated as the ratio between the number of pigmented recruits and the total number of recruits in each plate. It is important to mentioned that a chimeric recruit was defined as symbiotic only when all polyps within this entity were infected with algal symbiont cells. At the last day of the experiment, each recruit was photographed using the cellSens software under the dissecting microscope. The skeletal planar area of recruits was determined from the photographs with a scale bar using ImageJ software, and the polyp-specific lateral growth rates were calculated as the rate of change in skeletal area over time and then standardized to the number of polyps within each recruit (Albright et al., 2008; Jiang et al., 2018).
Statistical analysis
Prior to analysis, data were checked for normality and homogeneity of variance using Shapiro-Wilk’s test and Levene’s test, respectively. When normality and homogeneity assumptions were violated, data were square root- or arctan-transformed. Three-way nested ANOVAs were used to examine the impact of temperature, pCO2 and recruit type on symbiont infection success, areal size and skeletal lateral growth, with temperature, pCO2 and recruit type as the fixed factors and tank as a random factor nested within temperature and pCO2. The random tank factor was dropped from the model to enhance the power of statistical analysis when the nested tank effect was insignificant (Quinn and Keough, 2002). Differences in recruit survivorship were compared among recruit type and treatments using a Kaplan–Meier analysis with log-rank tests.
Results
Algal pigmentation in recruits was first observed two days after inoculation, with brown symbiont cells clearly present in tentacles and oral discs (Figure 1). Generally, algal infection rates were significantly affected by recruit type, temperature, pCO2 and the interaction between temperature and pCO2 (Table 2). One week after inoculation, algal infection rates were significantly higher in chimeric recruits than the single recruits regardless of treatment (Table 2). Approximately 70% and 90% of single and chimeric recruits had successfully acquired symbionts at ATAC, and these ratios were reduced in other three treatments. While the proportions of pigmented recruits were reduced by about 50% at 30.5°C, high pCO2 did not affect symbiont infection at 30.5°C (HTHC; Figure 2A).
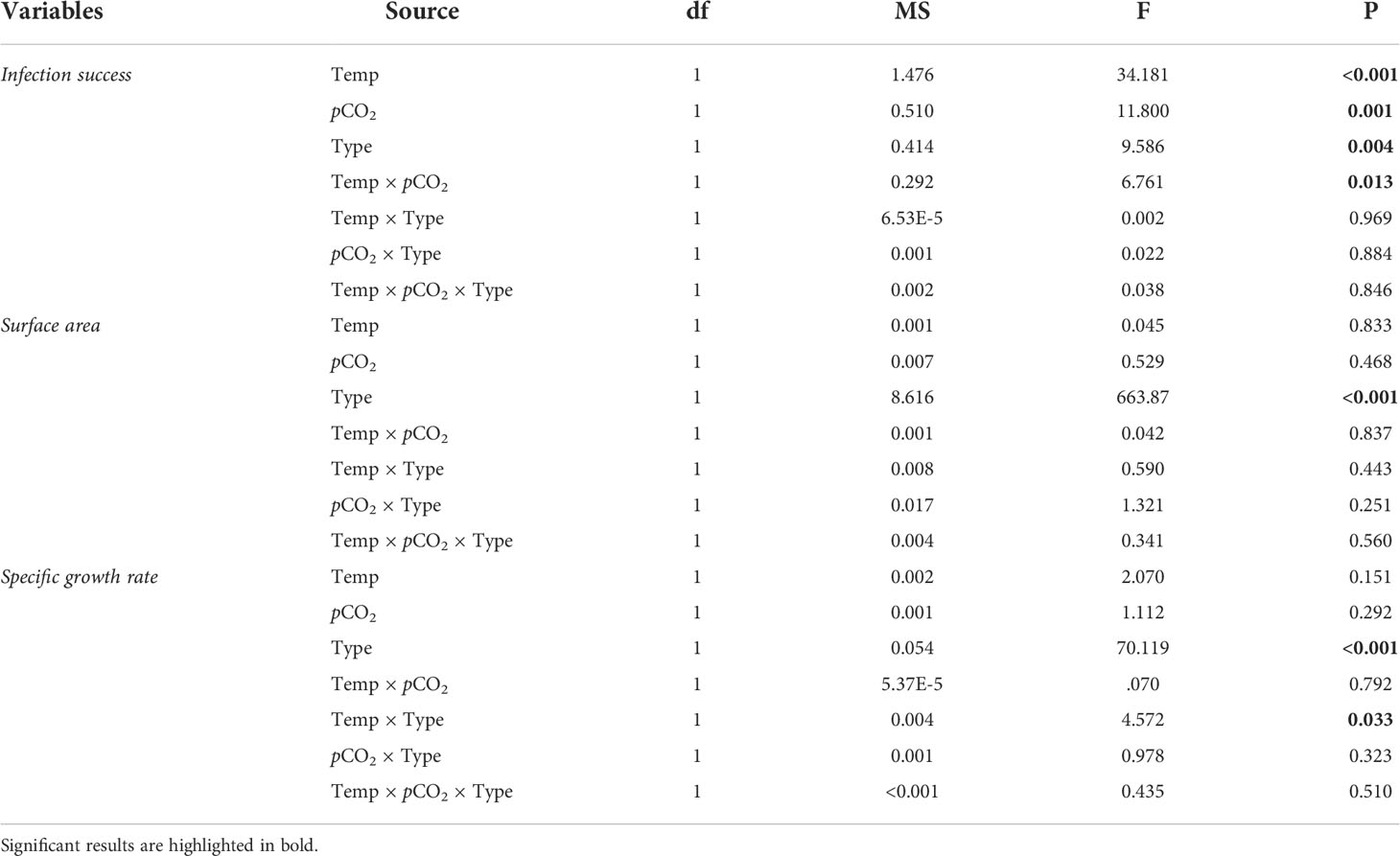
Table 2 Statistical results of three-way ANOVAs on the effects of temperature (28 vs. 30.5°C), pCO2 (~500 vs. ~1000 μatm) and recruit type (chimera vs. single) on the physiological responses of Acropora austera recruits.
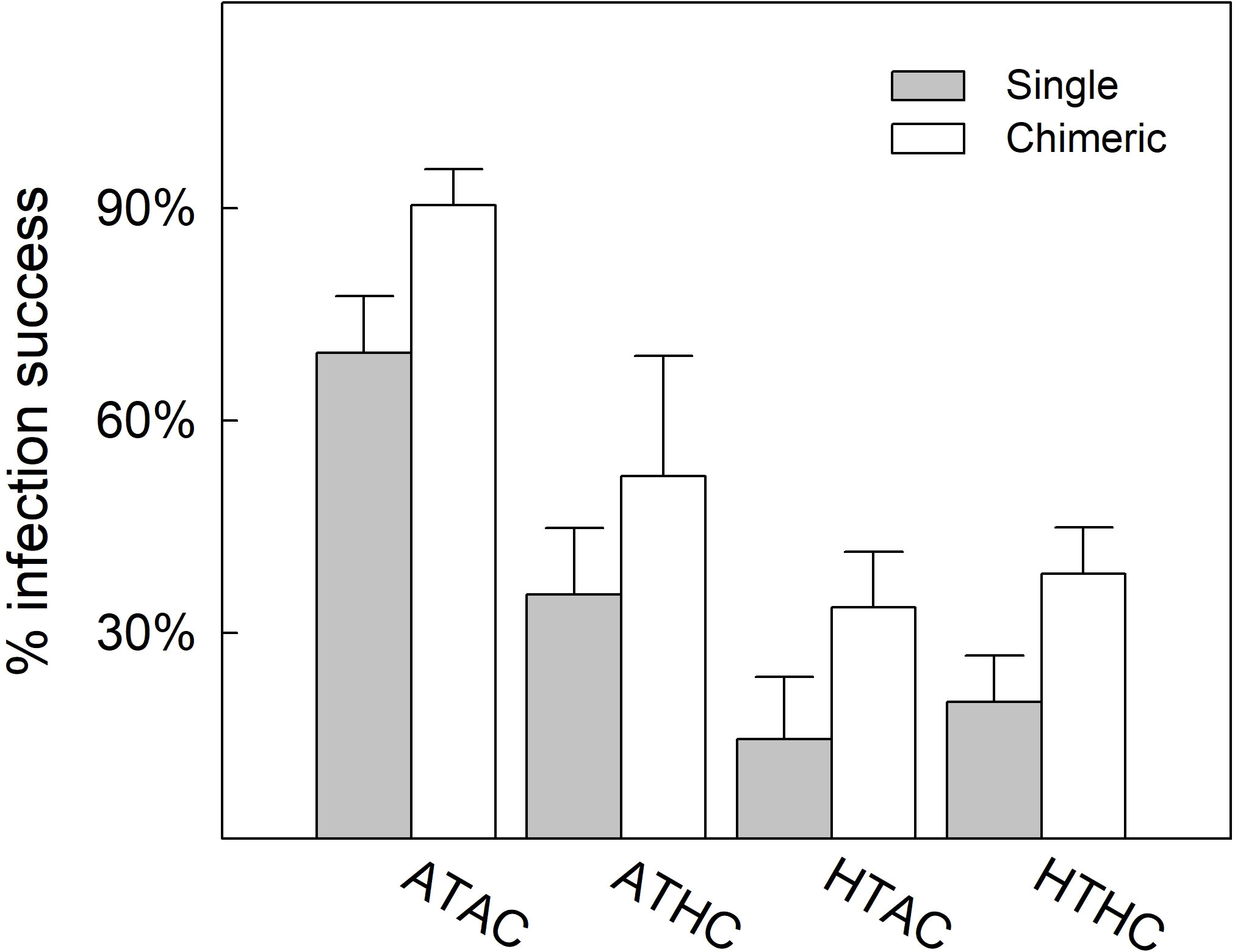
Figure 2 Algal infection rate of single and chimeric Acropora austera recruits under combinations of temperature (28°C, 30.5°C) and pCO2 (~500 μatm, ~1000 μatm). Data are presented as mean ± SE (n = 6). Experimental treatments: ambient temperature–ambient pCO2 (ATAC), ambient temperature–high pCO2 (ATHC), high temperature–ambient pCO2 (HTAC) and high temperature–high pCO2 (HTHC).
Regardless of recruit type, survival was similar among the four treatments (Figure 3A). Likewise, both the survival of single and chimeric recruits was unaffected by the treatment (Figures 3B, C). Irrespective of treatments, survivorship of chimeric recruits was significantly higher than that of single recruits (Figure 3D). Furthermore, in the four respective treatments, survivorship was consistently significantly higher in chimeric recruits compared to single recruits (Figures 3E–H).
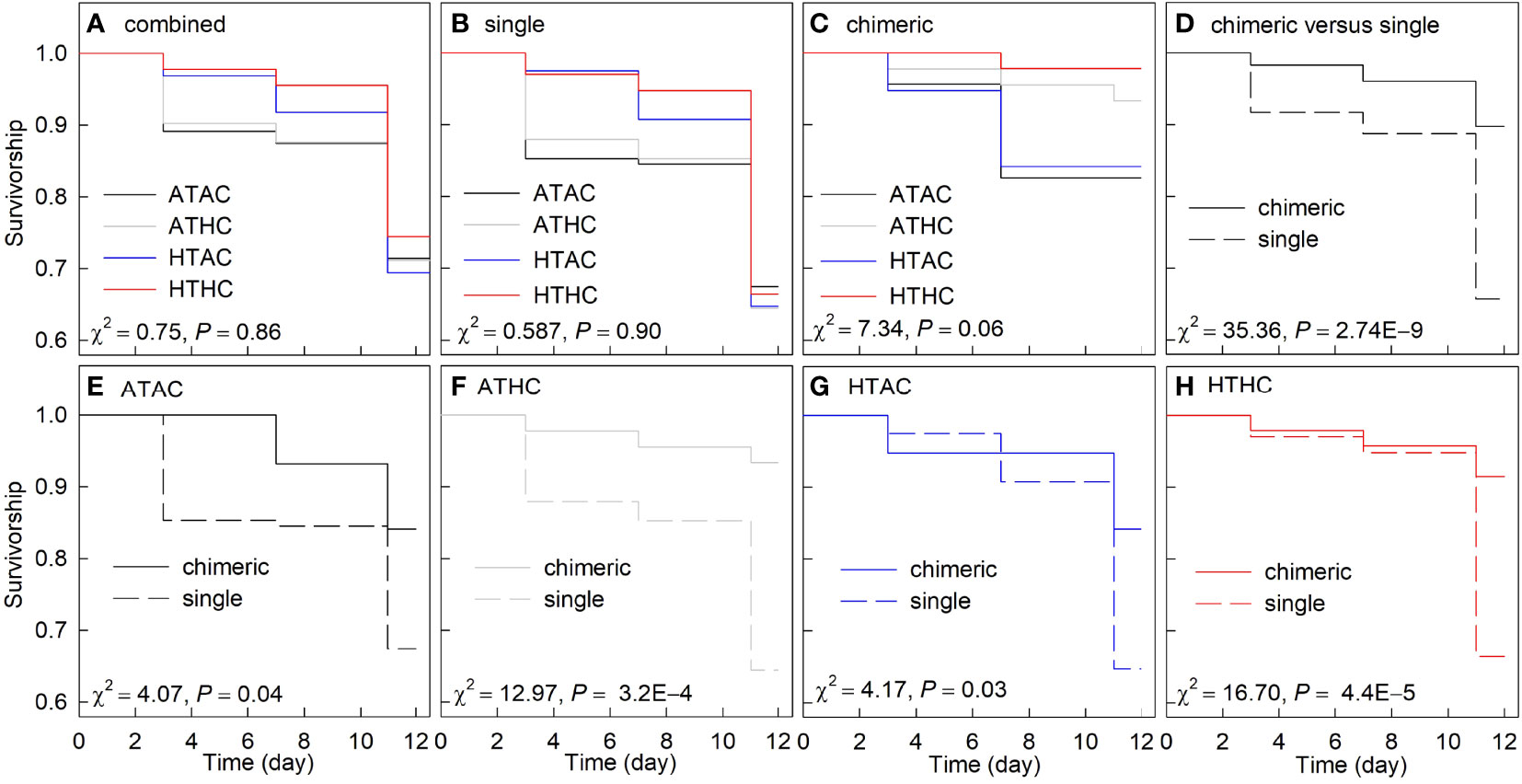
Figure 3 Proportional survival curves among treatments in all (A), (B) single, and (C) chimeric recruits; survival curves of single and chimeric recruits across all treatments (D) and the four respective treatments (E–G). Experimental treatments: ambient temperature–ambient pCO2 (ATAC, 28°C-500 μatm), ambient temperature–high pCO2 (ATHC, 28°C-1000 μatm), high temperature–ambient pCO2 (HTAC, 30.5°C-500 μatm) and high temperature–high pCO2 (HTHC, 30.5°C-1000 μatm). Differences in survival among the treatments were compared using the Kaplan–Meier (K–M) analysis and the statistical results were shown at each panel.
There was no significant effect of temperature, pCO2 or their interaction on areal size of recruits (Table 2), while chimeric recruits were significantly larger and more than twice the size of single recruits (Table 2; Figure 4A). At the polyp level, lateral growth rates of single recruits were significantly higher than chimeric recruits (Table 2). Moreover, there was a significant interaction between recruit type and temperature on the polyp-specific growth rate (Table 2), with growth rate being significantly reduced by temperature in chimeric recruits (Figure 4B).
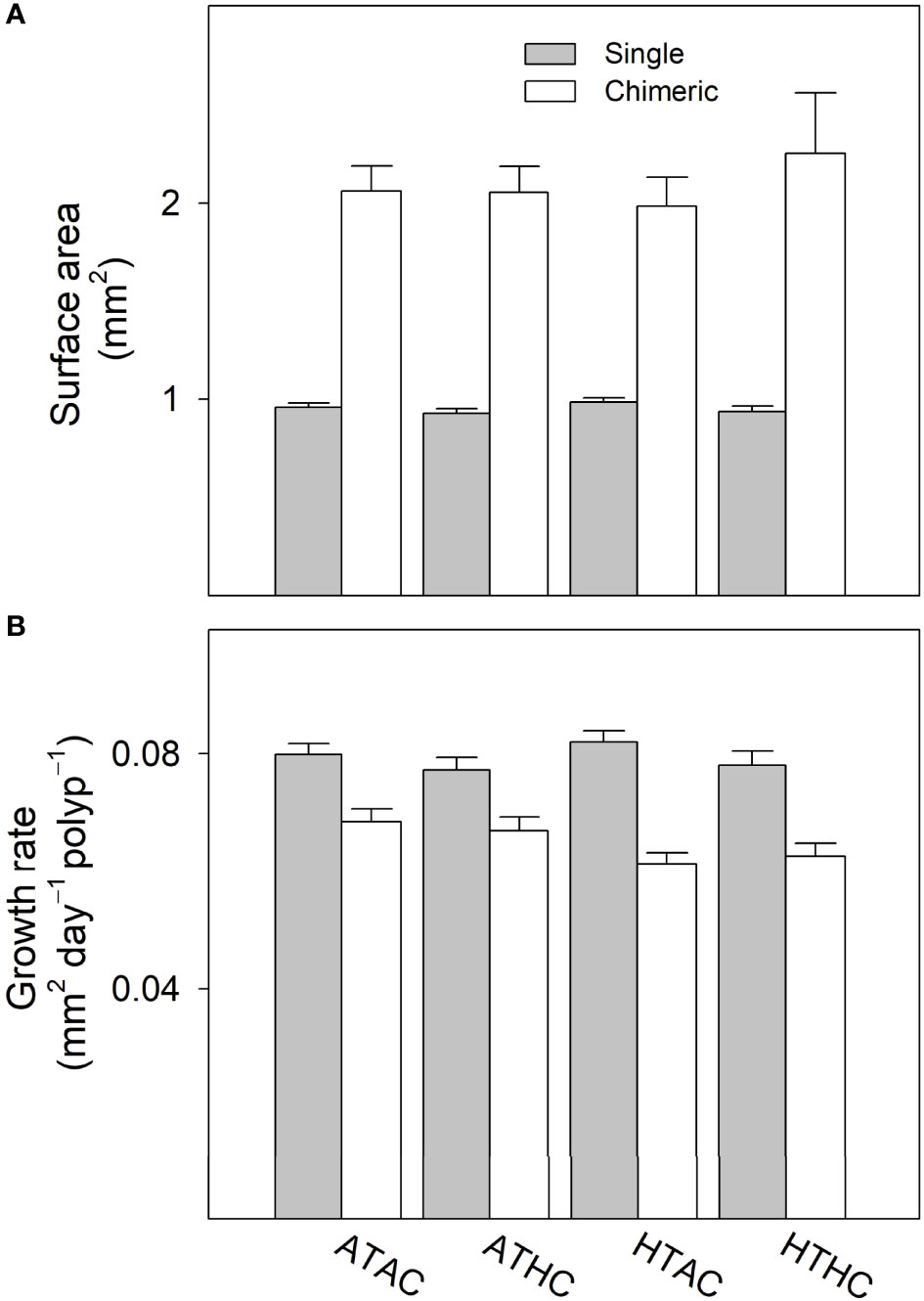
Figure 4 (A) Surface area and (B) growth rate of single and chimeric Acropora austera recruits under combinations of temperature (28°C, 30.5°C) and pCO2 (~500 μatm, ~1000 μatm). Data are presented as mean ± SE (n = 60 and 30 for single and chimeric recruits, respectively). Experimental treatments: ambient temperature–ambient pCO2 (ATAC), ambient temperature–high pCO2 (ATHC), high temperature–ambient pCO2 (HTAC) and high temperature–high pCO2 (HTHC).
Discussion
This study demonstrates that, under experimental conditions that mimic future projections, aggregated settlement of larvae in the reef coral A. austera could promote the survival and algal uptake success of new recruits within the first two weeks after settlement. Moreover, there were significant interactive effects between gregarious settlement and elevated temperature or pCO2 on recruit survival and growth. Specifically, chimeric recruits resulted from larval fusion had higher survivorship under high pCO2 and lower skeletal growth rates at increased temperature. These results suggest the complex role of larval aggregation in shaping post-settlement performance of corals in response to ocean warming and acidification, and provide direct evidence for the benefits and tradeoff associated with coral chimerism in determining the recruitment success under future climate change.
Uptake of algal symbionts and symbiosis establishment are of paramount importance to the early success and resilience of broadcast spawning corals (Suzuki et al., 2013; Yuyama and Higuchi, 2014; Hazraty-Kari et al., 2022), while this process is particularly susceptible to the ongoing ocean warming (Abrego et al., 2012; Yuyama et al., 2016; Yorifuji et al., 2017; Sun et al., 2020; Jiang et al., 2021; Williamson et al., 2021). Consistent with these aforementioned studies, our results also showed detrimental effects of heat stress on symbiont infection process, with symbiont infection rates being reduced by 50% at 30.5°C. It is important to note that the experimental inoculation method used here may differ from what would happen on natural reefs with a high diversity of free-living Symbiodiniaceae, and this could greatly influence the symbiont selection and acquisition in early stages of corals (Williamson et al., 2021; Matsuda et al., 2022). Furthermore, we found a significant interaction between increased temperature and pCO2 on algal uptake. Specifically, high pCO2 reduced the symbiont infection success at ambient temperature. Similarly, Suwa et al. (2010) observed that elevated pCO2 delayed symbiont acquisition in primary polyps of A. digitifera. Nevertheless, two prior studies also reported negligible effects of high pCO2 on symbiont uptake in recruits of the reef coral A. intermedia and Platygyra daedalea (Sun et al., 2020; Jiang et al., 2021). Therefore, this disparity suggests that the responses of symbiont acquisition in coral recruits to ocean acidification may be highly species-specific. However, the high pCO2 exhibited no effect on algal infection at elevated temperature herein, and it is possible that the influence of elevated temperature could be strong enough to mask the effect of high pCO2.
Interestingly, gregarious settlement and fusion between larvae provided clear advantage for symbiont infection success in chimeric recruits. This may be due to the fact that chimeric recruits had more polyps, hence increasing the probability of algae contact, attachment and capture. Likewise, one previous study reported that the smaller primary polyp of A. digitifera had reduced ability to capture algal symbionts, possibly due to its fewer number of ciliated gastrodermal cells and thus the weakened capacity to produce water currents in the coelenteron (Suwa et al., 2010). Alternatively, it has been demonstrated that the nutritional flux and resource sharing could occur between polyps within chimeric coral colonies in the coral Stylophora pistillata (Rinkevich and Weissman, 1987), and this raises the possibility that if one polyp within a chimeric entity had acquired algal symbionts, it could translocate the proliferated algae to the adjacent polyps. However, given the restricted algal pigmentation pattern in tentacle and oral disc region of coral recruits, the symbiont transfer and sharing between polyps within chimeric recruits seems unlikely here.
The fusion of multi-polyp chimeras resulted in larger areal size than the single recruits, and this immediate increases in size achieved through gregarious settlement led to significantly higher survivorship across treatments as compared to the single polyps. Theory posits that mortality risk in corals is inversely proportional to juvenile colony size, and the rapid increase in recruit size via aggregated settlement and fusion could overcome size-specific mortality thresholds. Our finding here aligned well with several studies showing the considerably increased likelihood of survival due to recruit fusion in corals (Raymundo and Maypa, 2004; Amar et al., 2008; Huffmyer et al., 2021). In particular, chimeric recruits tended to have higher survivorship under high pCO2, suggesting a favorable role of chimerism in sustaining the coral recruits under acidified conditions, however, reasons for such a phenomenon remains unclear. Similarly, larval and recruit fusion could result in higher thermal tolerance and survivorship as well as increased disease tolerance in several coral species (Hancock et al., 2021; Huffmyer et al., 2021; Williamson et al., 2022), plausibly as a result of the increased recruit size together with the greater genotypic diversity and the resultant diversity in stress response pathways and wider ranges of physiological qualities (Rinkevich, 2019b).
Noteworthy, growth rates of the single recruits were unaffected by high temperature and/or pCO2, and this contrasts to the majority of prior studies reporting the reduced lateral growth and calcification in juvenile corals under elevated temperature and especially high pCO2 (Albright et al., 2008; Albright et al., 2010; Suwa et al., 2010; Albright and Langdon, 2011; Foster et al., 2015; Jiang et al., 2015b). This discrepancy may be due to two major reasons. First, the short-term exposure duration and the relatively lower symbiont density after inoculation could have made recruits less susceptible to the sublethal thermal stress used here. Second, the coral A. austera in our study site showed higher calcification rates under ocean acidification, because of its capacity to upregulate calcium transporters (Yuan et al., 2019). Therefore, this inherent ability of this coral species to tolerate ocean acidification may also enable their recruits insensitive to high pCO2 conditions, and suggests that A. austera might be a good target in future restoration practice.
Although the areal size of these chimeric recruits was almost twice that of the single recruits, their specific growth rates per polyp were significantly lower than their solitary counterparts, indicating the cost and trade-off associated with chimerism. This observation is in agreement with previous studies showing that the aggregation behavior at settlement in corals could induce a cost, that is, reduced growth rate of each polyp within the chimeric entities (Amar et al., 2008; Hancock et al., 2021). However, Huffmyer et al. (2021) found that tissue fusion between Pocillopora acuta recruits did not provoke any apparent cost to polyp expansion under both ambient and elevated temperatures. Hence, the trade-off of chimerism in coral recruits could be taxa-dependent and manifested on differential aspects of early growth and development. Intriguingly, the recruit type exerted a significant effect on the specific growth rate in response to elevated temperature, with depressed growth rates at 30.5°C particularly in chimeric recruits. This clearly suggest increased competition between polyps in chimeras under elevated temperature and higher energy demand to maintain tissue fusion and compatibility, thereby compromising the investment in skeletal expansion. Therefore, despite the initial survival advantage provided by aggregated settlement and fusion, this trade-off and cost observed here may present an obstacle to the long-term growth performance of the whole entities.
Overall, our study demonstrated that gregarious larval settlement and chimerism in the spawning coral A. austera could provide advantages to the early recruits to favor algal infection and survival under future climatic conditions. However, the increased competition and the depressed growth rate at the polyp level within chimeric entities, especially under high temperature, strongly suggests a potential trade-off. Hence, this study illustrates the benefits and costs with regard to chimerism and larval settlement behavior. Given the critical window for early survival, our study provides additional support and a promising way to boost the adaptability of juvenile corals via chimerism and increase the chances of surviving and resilience in early stages in spawning corals. Although our short-term study did not observe signs of rejection, overgrowth and borderline formation within chimeras, further experimental studies are needed to characterize the implications of gregarious larval settlement and early chimerism in mitigating climate change stressors over much longer and ecologically relevant duration. Only with such information, combining with the development of techniques and protocols to optimize the fusion of gregariously settling larvae and chimera formation through manipulating larval settlement densities, can we fully harness this key early life-history traits to improve future sexual restoration success in reef corals.
Data availability statement
The original contributions presented in the study are included in the article/supplementary material. Further inquiries can be directed to the corresponding authors.
Author contributions
LJ, TY, and HH conceived and designed the study. LJ conducted the experiment, performed the laboratory and data analyses, and drafted the manuscript. Y-YZ, C-YL, L-TH, H-YT, G-WZ, M-LG, TY, and HH contributed to lab analysis and interpretation of the results. All authors contributed to the article and approved the submitted version.
Funding
This work was supported by the National Key Research and Development Program (2021YFC3100504 and 2021YFF0502804), Natural Science Foundation of Guangdong province (2018A0303130173), Key Special Project for Introduced Talent Teams of Southern Marine Science and Engineering Guangdong Laboratory (Guangzhou) (GML2019ZD409, GML2019ZD0402 and GML 2019ZD0404) and Science and Technology Planning Project of Guangdong Province, China (2020B1212060058). LJ was supported by Southern Marine Science and Engineering Guangdong Laboratory (Guangzhou) (SMSEGL20SC01) as a visiting scholar in HKB, HKUST.
Conflict of interest
The authors declare that the research was conducted in the absence of any commercial or financial relationships that could be construed as a potential conflict of interest.
Publisher’s note
All claims expressed in this article are solely those of the authors and do not necessarily represent those of their affiliated organizations, or those of the publisher, the editors and the reviewers. Any product that may be evaluated in this article, or claim that may be made by its manufacturer, is not guaranteed or endorsed by the publisher.
References
Abrego D., Willis B. L., Oppen M. J. H. V. (2012). Impact of light and temperature on the uptake of algal symbionts by coral juveniles. PloS One 7, e50311. doi: 10.1371/journal.pone.0050311
Albright R., Langdon C. (2011). Ocean acidification impacts multiple early life history processes of the Caribbean coral Porites astreoides. Global Change Biol. 17, 2478–2487. doi: 10.1111/j.1365-2486.2011.02404.x
Albright R., Mason B., Langdon C. (2008). Effect of aragonite saturation state on settlement and post-settlement growth of Porites astreoides larvae. Coral. Reefs. 27, 485–490. doi: 10.1007/s00338-008-0392-5
Albright R., Mason B., Miller M., Langdon C. (2010). Ocean acidification compromises recruitment success of the threatened Caribbean coral Acropora palmata. Proc. Natl. Acad. Sci. U.S.A. 107, 20400–20404. doi: 10.1073/pnas.1007273107
Amar K.-O., Chadwick N. E., Rinkevich B. (2008). Coral kin aggregations exhibit mixed allogeneic reactions and enhanced fitness during early ontogeny. BMC Evolution. Biol. 8, 126. doi: 10.1186/1471-2148-8-126
Anlauf H., D’Croz L., O’Dea A. (2011). A corrosive concoction: The combined effects of ocean warming and acidification on the early growth of a stony coral are multiplicative. J. Exp. Mar. Bio Ecol. 397, 13–20. doi: 10.1016/j.jembe.2010.11.009
Ben-Shlomo R., Motro U., Paz G., Rinkevich B. (2008). Pattern of settlement and natural chimerism in the colonial urochordate botryllus schlosseri. Genetica 132, 51–58. doi: 10.1007/s10709-007-9148-3
Bopp L., Resplandy L., Orr J. C., Doney S. C. (2013). Multiple stressors of ocean ecosystems in the 21st century: projections with CMIP5 models. Biogeosciences 10, 6225–6245. doi: 10.5194/bg-10-6225-2013
Buss L. W. (1990). Competition within and between encrusting clonal invertebrates. Trends Ecol. Evol. 5, 352–356. doi: 10.1016/0169-5347(90)90093-S
Dickson A. G. (1990). Standard potential of the reaction: AgCl(s) + 12H2(g) = ag(s) + HCl(aq), and and the standard acidity constant of the ion HSO4– in synthetic sea water from 273.15 to 318.15 K. J. Chem. Thermodyn. 22, 113–127. doi: 10.1016/0021-9614(90)90074-Z
Dickson A. G., Millero F. J. (1987). A comparison of the equilibrium constants for the dissociation of carbonic acid in seawater media. Deep. Sea. Res. Part A. Oceanogr. Res. Papers. 34, 1733–1743. doi: 10.1016/0198-0149(87)90021-5
Foster T., Gilmour J. P., Chua C. M., Falter J. L., Mcculloch M. T. (2015). Effect of ocean warming and acidification on the early life stages of subtropical Acropora spicifera. Coral. Reefs. 34, 1217–1226. doi: 10.1007/s00338-015-1342-7
Gattuso J.-P., Magnan A., Billé R., Cheung W. W. L., Howes E. L., Joos F., et al. (2015). Contrasting futures for ocean and society from different anthropogenic CO2 emissions scenarios. Science 349. doi: 10.1126/science.aac4722
Guerrini G., Shefy D., Douek J., Shashar N., Goulet T. L., Rinkevich B. (2021). Spatial distribution of conspecific genotypes within chimeras of the branching coral stylophora pistillata. Sci. Rep. 11, 22554. doi: 10.1038/s41598-021-00981-5
Hancock J. R., Barrows A. R., Roome T. C., Huffmyer A. S., Matsuda S. B., Munk N. J., et al. (2021). Coral husbandry for ocean futures: leveraging abiotic factors to increase survivorship, growth, and resilience in juvenile montipora capitata. Mar. Ecol. Prog. Ser. 657, 123–133. doi: 10.3354/meps13534
Harrison P. L. (2011). Sexual reproduction of scleractinian corals coral reefs: an ecosystem in transition (Dordrecht, Netherlands: Springer), p59–p85.
Hazraty-Kari S., Masaya M., Kawachi M., Harii S. (2022). The early acquisition of symbiotic algae benefits larval survival and juvenile growth in the coral acropora tenuis. J. Exp. Zool. Part A.: Ecol. Integr. Physiol. 337, 559–565. doi: 10.1002/jez.2589
Huffmyer A. S., Drury C., Majerová E., Lemus J. D., Gates R. D. (2021). Tissue fusion and enhanced genotypic diversity support the survival of pocillopora acuta coral recruits under thermal stress. Coral. Reefs. 40, 447–458. doi: 10.1007/s00338-021-02074-1
Jiang L., Huang H., Yuan X. C., Yuan T., Zhang Y. Y., Wen K. C., et al. (2015b). Effects of elevated pCO2 on the post-settlement development of Pocillopora damicornis. J. Exp. Mar. Bio Ecol. 473, 169–175. doi: 10.1016/j.jembe.2015.09.004
Jiang L., Lei X., Liu S., Huang H. (2015a). Fused embryos and pre-metamorphic conjoined larvae in a broadcast spawning reef coral. F1000Research 4, 1–11. doi: 10.12688/f1000research.6136.1
Jiang L., Zhang F., Guo M.-L., Guo Y.-J., Zhang Y.-Y., Zhou G.-W., et al. (2018). Increased temperature mitigates the effects of ocean acidification on the calcification of juvenile Pocillopora damicornis, but at a cost. Coral. Reefs. 37, 71–79. doi: 10.1007/s00338-017-1634-1
Jiang L., Zhou G.-W., Zhang Y.-Y., Lei X.-M., Yuan T., Guo M.-L, et al. (2021). Plasticity of symbiont acquisition in new recruits of the massive coral platygyra daedalea under ocean warming and acidification. Coral. Reefs 40, 1563–1576. doi: 10.1007/s00338-021-02151-5
Keough M. J. (1984). Kin-recognition and the spatial distribution of larvae of the bryozoan bugula neritina (L.). Evolution 38, 142–147. doi: 10.1111/j.1558-5646.1984.tb00267.x
Lewis E., Wallace D., Allison L. J. (1998). Program developed for CO2 system calculations (Tennessee: Carbon Dioxide Information Analysis Center, Oak Ridge National Laboratory, US Department of Energy).
Matsuda S. B., Chakravarti L. J., Cunning R., Huffmyer A. S., Nelson C. E., Gates R. D., et al. (2022). Temperature-mediated acquisition of rare heterologous symbionts promotes survival of coral larvae under ocean warming. Global Change Biol. 28, 2006–2025. doi: 10.1111/gcb.16057
Mizrahi D., Navarrete S. A., Flores A. A. V. (2014). Groups travel further: pelagic metamorphosis and polyp clustering allow higher dispersal potential in sun coral propagules. Coral. Reefs. 33, 443–448. doi: 10.1007/s00338-014-1135-4
Nozawa Y., Hirose M. (2011). When does the window close?: the onset of allogeneic fusion 2-3 years post-settlement in the scleractinian coral, echinophyllia aspera. Zool. Stud. 50, 396–396.
Puill-Stephan E., van Oppen M. J. H., Pichavant-Rafini K., Willis B. L. (2012). High potential for formation and persistence of chimeras following aggregated larval settlement in the broadcast spawning coral, acropora millepora. Proc. R. Soc. B.: Biol. Sci. 279, 699–708. doi: 10.1098/rspb.2011.1035
Puill-Stephan E., Willis B. L., van Herwerden L., van Oppen M. J. (2009). Chimerism in wild adult populations of the broadcast spawning coral acropora millepora on the great barrier reef. PloS One 4, e7751. doi: 10.1371/journal.pone.0007751
Quinn G., Keough M. (2002). Experimental design and data analysis for biologists (Melbourne, Australia: Cambridge University Press).
Randall C. J., Negri A. P., Quigley K. M., Foster T., Ricardo G. F., Webster N. S., et al. (2020). Sexual production of corals for reef restoration in the anthropocene. Mar. Ecol. Prog. Ser. 635, 203–232. doi: 10.3354/meps13206
Raymundo L. J., Maypa A. P. (2004). Getting bigger faster: Mediation of size-specific mortality via fusion in juvenile coral transplants. Ecol. Appl. 14, 281–295. doi: 10.1890/02-5373
Rinkevich B. (2004). Allorecognition and xenorecognition in reef corals: a decade of interactions. Hydrobiologia 530, 443–450. doi: 10.1007/s10750-004-2686-0
Rinkevich B. (2019a). The active reef restoration toolbox is a vehicle for coral resilience and adaptation in a changing world. J. Mar. Sci. Eng. 7, 201. doi: 10.3390/jmse7070201
Rinkevich B. (2019b). Coral chimerism as an evolutionary rescue mechanism to mitigate global climate change impacts. Global Change Biol. 25, 1198–1206. doi: 10.1111/gcb.14576
Rinkevich B., Weissman I. (1987). Chimeras in colonial invertebrates: A synergistic symbiosis or somatic-and germ-cell parasitism? Symbiosis 4, 117–134.
Ritson-Williams R., Arnold S. N., Fogarty N. D., Steneck R. S., Vermeij M. J. A., Paul V. J. (2009). New perspectives on ecological mechanisms affecting coral recruitment on reefs. Smithson. Contrib. to. Mar. Sci. 38, 437–457. doi: 10.5479/si.01960768.38.437
Sampayo E. M., Roff G., Sims C. A., Rachello-Dolmen P. G., Pandolfi J. M. (2020). Patch size drives settlement success and spatial distribution of coral larvae under space limitation. Coral. Reefs. 39, 387–396. doi: 10.1007/s00338-020-01901-1
Santelices B. (2004). Mosaicism and chimerism as components of intraorganismal genetic heterogeneity. J. Evolution. Biol. 17, 1187–1188. doi: 10.1111/j.1420-9101.2004.00813.x
Schweinsberg M., Weiss L. C., Striewski S., Tollrian R., Lampert K. P. (2015). More than one genotype: how common is intracolonial genetic variability in scleractinian corals? Mol. Ecol. 24, 2673–2685. doi: 10.1111/mec.13200
Shefy D., Guerrini G., Marom N., Shashar N., Rinkevich B. (2022). Settling in aggregation: Spatial planning consideration for brooding coral transplants. Mar. Environ. Res. 176, 105612. doi: 10.1016/j.marenvres.2022.105612
Sun Y.-F., Jiang L., Gong S.-Q., Guo M.-L., Yuan X.-C., Zhou G.-W., et al. (2020). Impact of ocean warming and acidification on symbiosis establishment and gene expression profiles in recruits of reef coral Acropora intermedia. Front. Microbiol. 11, 532447. doi: 10.3389/fmicb.2020.532447
Suwa R., Nakamura M., Morita M., Shimada K., Iguchi A., Sakai K., et al. (2010). Effects of acidified seawater on early life stages of scleractinian corals (Genus Acropora). Fisher. Sci. 76, 93–99. doi: 10.1007/s12562-009-0189-7
Suzuki G., Yamashita H., Kai S., Hayashibara T., Suzuki K., Iehisa Y., et al. (2013). Early uptake of specific symbionts enhances the post-settlement survival of Acropora corals. Mar. Ecol. Prog. Ser. 494, 149–158. doi: 10.3354/meps10548
Westerman E. L., Dijkstra J. A., Harris L. G. (2009). High natural fusion rates in a botryllid ascidian. Mar. Biol. 156, 2613–2619. doi: 10.1007/s00227-009-1287-x
Williamson O. M., Allen C. E., Williams D. E., Johnson M. W., Miller M. W., Baker A. C. (2021). Neighboring colonies influence uptake of thermotolerant endosymbionts in threatened Caribbean coral recruits. Coral. Reefs 40, 867–879. doi: 10.1007/s00338-021-02090-1
Williamson O. M., Dennison C. E., O’Neil K. L., Baker A. C. (2022). Susceptibility of Caribbean brain coral recruits to stony coral tissue loss disease (SCTLD). Front. Mar. Sci. 9. doi: 10.3389/fmars.2022.821165
Yael B., GÂo D., Dan G., Baruch R. (2002). Soft-coral natural chimerism: a window in ontogeny allows the creation of entities comprised of incongruous parts. Mar. Ecol. Prog. Ser. 231, 91–99. doi: 10.3354/meps231091
Yorifuji M., Harii S., Nakamura R., Fudo M. (2017). Shift of symbiont communities in Acropora tenuis juveniles under heat stress. Peerj 5, e4055. doi: 10.7717/peerj.4055
Yuan X., Huang H., Zhou W., Guo Y., Yuan T., Liu S. (2019). Gene expression profiles of two coral species with varied resistance to ocean acidification. Mar. Biotechnol. 21, 151–160. doi: 10.1007/s10126-018-9864-y
Yuyama I., Higuchi T. (2014). Comparing the effects of symbiotic algae (Symbiodinium) clades C1 and d on early growth stages of Acropora tenuis. PloS One 9, e98999. doi: 10.1371/journal.pone.0098999
Yuyama I., Nakamura T., Higuchi T., Hidaka M. (2016). Different stress tolerances of juveniles of the coral Acropora tenuis associated with clades C1 and d Symbiodinium. Zool. Stud. 55, 19–19. doi: 10.6620/ZS.2016.55-19
Keywords: gregarious settlement, chimerism, coral recruit, early development, climate change
Citation: Jiang L, Zhang Y-Y, Liu C-Y, Huang L-T, Tong H-Y, Zhou G-W, Guo M-L, Yuan T and Huang H (2022) Gregarious larval settlement mediates the responses of new recruits of the reef coral Acropora austera to ocean warming and acidification. Front. Mar. Sci. 9:964803. doi: 10.3389/fmars.2022.964803
Received: 09 June 2022; Accepted: 29 August 2022;
Published: 15 September 2022.
Edited by:
Wei Jiang, Guangxi University, ChinaReviewed by:
Sheila A. Kitchen, California Institute of Technology, United StatesYi Guan, Guangdong Ocean University, China
Copyright © 2022 Jiang, Zhang, Liu, Huang, Tong, Zhou, Guo, Yuan and Huang. This is an open-access article distributed under the terms of the Creative Commons Attribution License (CC BY). The use, distribution or reproduction in other forums is permitted, provided the original author(s) and the copyright owner(s) are credited and that the original publication in this journal is cited, in accordance with accepted academic practice. No use, distribution or reproduction is permitted which does not comply with these terms.
*Correspondence: Tao Yuan, eXVhbnRhb0BzY3Npby5hYy5jbg==; Hui Huang, aHVhbmdodWlAc2NzaW8uYWMuY24=