- 1Department of Biology, Baylor University, Waco, TX, United States
- 2Antarctic Ecosystem Research Division, Southwest Fisheries Science Center, National Oceanic and Atmospheric Administration (NOAA) Fisheries, La Jolla, CA, United States
- 3Department of Ecology and Evolutionary Biology, University of California Santa Cruz, Santa Cruz, CA, United States
- 4Marine Mammal Commission, Bethesda, MD, United States
- 5Center of Applied Ecology and Sustainability (CAPES), Department of Ecology, Pontificia Universidad Católica de Chile, Santiago, Chile
- 6Instituto Antártico Chileno (INACH), Punta Arenas, Chile
- 7Department of Biology, Colorado State University, Fort Collins, CO, United States
- 8Department of Biology, Sonoma State University, Rohnert Park, CA, United States
Animals that display plasticity in behavioral, ecological, and morphological traits are better poised to cope with environmental disturbances. Here, we examined individual plasticity and intraspecific variation in the morphometrics, movement patterns, and dive behavior of an enigmatic apex predator, the leopard seal (Hydrurga leptonyx). Satellite/GPS tags and time-depth recorders were deployed on 22 leopard seals off the Western Antarctic Peninsula. Adult female leopard seals were significantly larger (454±59 kg) and longer (302±11 cm) than adult males (302±22 kg, 276±11 cm). As females were 50% larger than their male counterparts, leopard seals are therefore one of the most extreme examples of female-biased sexual size dimorphism in marine mammals. Female leopard seals also spent more time hauled-out on land and ice than males. In the austral spring/summer, three adult female leopard seals hauled-out on ice for 10+ days, which likely represent the first satellite tracks of parturition and lactation for the species. While we found sex-based differences in morphometrics and haul-out durations, other variables, including maximum distance traveled and dive parameters, did not vary by sex. Regardless of sex, some leopard seals remained in near-shore habitats, traveling less than 50 kilometers, while other leopard seals traveled up to 1,700 kilometers away from the tagging location. Overall, leopard seals were short (3.0±0.7 min) and shallow (29±8 m) divers. However, within this general pattern, some individual leopard seals primarily used short, shallow dives, while others switched between short, shallow dives and long, deep dives. We also recorded the single deepest and longest dive made by any leopard seal—1, 256 meters for 25 minutes. Together, our results showcased high plasticity among leopard seals tagged in a single location. These flexible behaviors and traits may offer leopard seals, an ice-associated apex predator, resilience to the rapidly changing Southern Ocean.
Introduction
Polar predators are particularly sensitive to rapid changes in their habitats (Estes et al., 1998; Williams et al., 2004; Costa et al., 2010; Estes et al., 2011). Further, apex polar predators, like polar bears and killer whales, play a disproportionately large role in shaping ecosystem function because of their position at the top of the food chain (Baum and Worm, 2009; Pagano et al., 2018; Hammerschlag et al., 2022). Consequently, changes to their populations can have widespread and cascading effects throughout entire food webs (Baum and Worm, 2009; Hunter et al., 2015; Hammerschlag et al., 2022). Species that exhibit plasticity in behavioral, ecological, and morphological traits are better poised to cope with habitat changes/disturbances (Charmantier et al., 2008; Dawson et al., 2011). Therefore, assessing individual plasticity and intraspecific variation in space use and life history traits of poorly studied polar predators is important for understanding how species operate in their environment, as well as their ability to cope with environmental change (Baum and Worm, 2009; Hays et al., 2016).
Leopard seals (Hydrurga leptonyx) are a quintessential Southern Ocean apex predator (Wilson, 1905; Hiruki et al., 1999; Southwell et al., 2008; Schwarz et al., 2013; Krause et al., 2015). Leopard seals are generalist predators, consuming a wide range of prey that includes krill, fish, cephalopods, seabirds, and even other pinnipeds (Penney and Lowry, 1967; Boveng et al., 1998; Walker et al., 1998; Hall-Aspland and Rogers, 2004; Casaux et al., 2009; Krause et al., 2015; Krause et al., 2020). This diverse diet makes leopard seals unique as one of only three marine mammals that feed on both endothermic and ectothermic prey (Werth, 2000). Within this broad dietary niche, individual leopard seals may switch prey types opportunistically (e.g., Casaux et al., 2009; Hocking et al., 2013; Krause et al., 2020). As a result of this dietary versatility, leopard seals directly influence Antarctic food web dynamics across multiple trophic levels, as well as exert top-down control on other pinnipeds (e.g., Antarctic fur seals, Arctocephalus gazella; Boveng et al., 1998; Schwarz et al., 2013; Krause et al., 2022).
It currently remains unclear how variability in diet and trophic position translates to other aspects of leopard seals’ biology, including their movement patterns, dive behavior, and morphometrics. A handful of studies in the past 15-20 years have provided the first insights into the at-sea movement patterns and dive behavior for the species (Rogers et al., 2005; Kuhn et al., 2006; Nordøy and Blix, 2009; Krause et al., 2015; Meade et al., 2015; Krause et al., 2016; Staniland et al., 2018). Some satellite tracked leopard seals, for example, traveled relatively short distances (<150 km; Rogers et al., 2005), while others traveled much farther (>800 km; Staniland et al., 2018). Time-depth recorders revealed that leopard seals made relatively short (2 min), shallow dives (<25 m; Kuhn et al., 2006; Krause et al., 2015; Krause et al., 2016). However, previous research was predominately carried out only during the austral summer (November – February; 80% of studies; Rogers et al., 2005; Kuhn et al., 2006; Nordøy and Blix, 2009; Krause et al., 2015; Meade et al., 2015; Krause et al., 2016; Staniland et al., 2018). Furthermore, the majority of studies only acquired tracking data for leopard seals, with tracking durations ranging from <1 to 466 days and sample sizes ranging from one to 12 seals (Rogers et al., 2005; Kuhn et al., 2006; Nordøy and Blix, 2009; Krause et al., 2015; Meade et al., 2015; Krause et al., 2016; Staniland et al., 2018). Previous studies have found that leopard seals were ~400 kg in mass and 290 cm in length (Hamilton, 1939; Laws, 1957; Rogers et al., 2005; van den Hoff et al., 2005; Kuhn et al., 2006; Nordøy and Blix, 2009; Krause et al., 2015; Meade et al., 2015; Staniland et al., 2018; Krause et al., 2020), with some data suggesting that body size varied regionally (van den Hoff et al., 2005). However, these measurements were primarily from adult females, and few morphometrics were paired with movement and/or dive data.
Therefore, the objective of this study was to assess the morphometrics, movement patterns, and dive behavior of leopard seals off the Western Antarctic Peninsula (WAP). We expand upon early studies by investigating the space use of leopard seals from the austral autumn to spring (April – November). We also examined plasticity and intraspecific variability in the horizontal and vertical space use patterns of leopard seals in the WAP. To do this, we deployed biologging instruments that transmitted data on the movement patterns and dive behavior of 22 leopard seals over the course of two field seasons from Cape Shirreff, Livingston Island. We paired tracking and dive behavior data with life history and morphological data to provide an integrated assessment of leopard seal biology.
Materials and methods
Study site and animal handling
Field work was conducted at the U.S. Antarctic Marine Living Resources (AMLR) Program research station on Cape Shirreff, Livingston Island, Antarctic Peninsula (62.47°S, 60.77°W; Figure 1). Cape Shirreff is the site of a long-term Convention for the Conservation of Antarctic Marine Living Resources (CCAMLR) monitoring program started in 1997/98 by the U.S. AMLR program. Leopard seals have been using this site to haul-out on land, and their population has grown due to the availability of abundant, seasonally-available, endothermic prey (Krause et al., 2015; Krause et al., 2020). Cape Shirreff is also notable for recently becoming ice-free year-round (since before the turn of the century; Goebel, pers. obs.). Here, we captured and chemically immobilized 22 leopard seals during the 2018 and 2019 field seasons (Table 1). While we had funding and logistic support for a third field season, it was cancelled due to travel constraints associated with the COVID-19 pandemic.
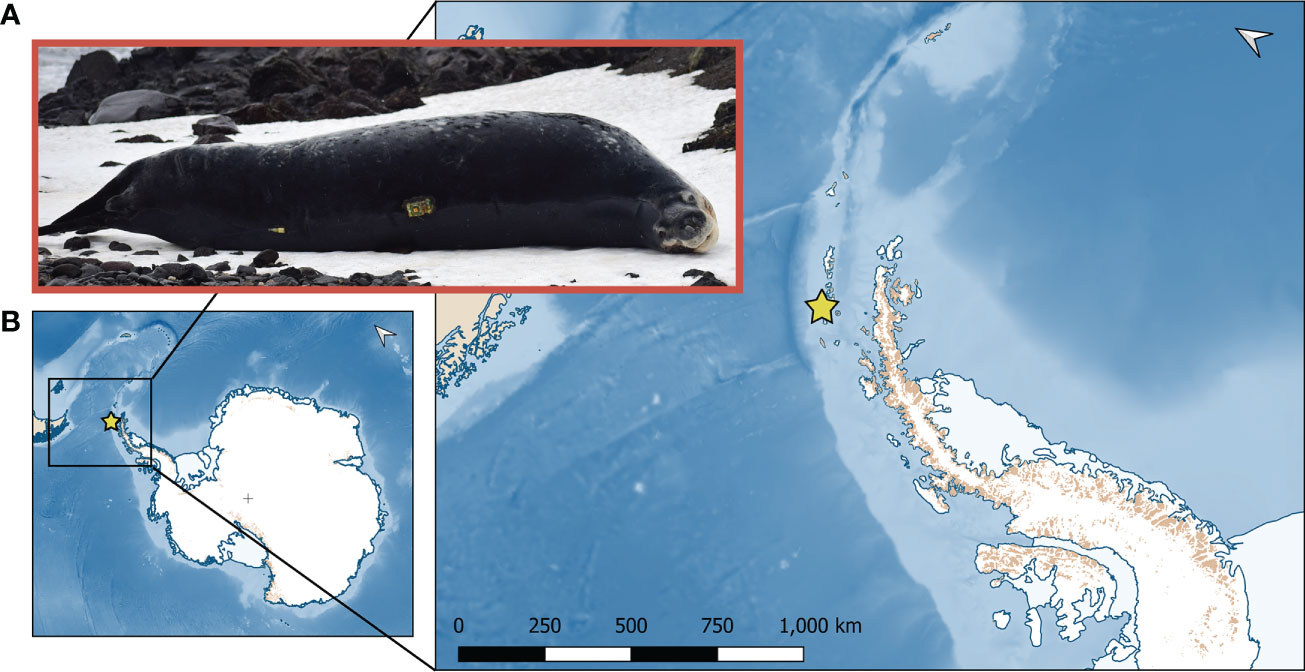
Figure 1 Leopard seal study site in the Western Antarctic Peninsula. (A) A representative adult female (#128) leopard seal with a mass of 491 kg (photo credit: S. Kienle) with a satellite/GPS biologger attached to the mid-back. (B) Animal handling and instrument deployment locations of leopard seals instrumented at Cape Shirreff (yellow star) in the South Shetland Islands off the Western Antarctic Peninsula of Antarctica in 2018 and 2019. Inset shows a zoomed-in view of the South Shetland Islands.
Specifically, eight adult females, three adult males, and one juvenile female were instrumented during the austral summer and fall of 2018, and nine adult females and one adult male were instrumented in the austral fall of 2019. One seal (female #397) was instrumented in both 2018 and 2019. We followed a butorphanol-midazolam protocol for chemical sedation; sedatives were administered with a jab stick following Pussini and Goebel, 2015. While leopard seals were sedated, we attached biologging instruments and collected morphometric data (e.g., mass, lengths, girths; Pussini and Goebel, 2015). At the end of each capture, we administered naltrexone, the chemical sedative-reversal agent for butorphanol following a slight modification to the protocol established by Pussini and Goebel, 2015; specifically, we did not reverse the midazolam using Flumazenil. We then monitored each individual’s recovery until the animal was fully mobile and alert.
Instrumentation and life history
Adult seals were instrumented with SPLASH 10-F tags (Wildlife Computers, Redmond, WA) using 5-minute epoxy (Loctite Quickset epoxy; Henkel Corporation, Rocky Hill, CT; Figure 1B). SPLASH tags were equipped with FastLoc GPS (hereafter “GPS”), 0.5W Argos satellite transmitters, and time-depth recorders. The single juvenile seal was instrumented with a Wildlife Computers SPOT6 tag, which only provided Argos satellite tracking data. The majority of tag attachments (n=18, 81%) were placed on the upper back of the seals to ensure that the antenna would break the surface when the seal was swimming at the surface and to combat the potential effects of rapid head movements and accelerations on tag functionality. In 2018, two tag attachments had to be placed on the newly grown fur on the head of two adult male seals (#140, #141) because the fur on their backs was molting (Table 1). We also attached the SPOT6 tag to the head of the juvenile (#145) due to the uncertainty regarding its swimming behavior and whether the tag would be exposed above water regularly if placed on the back. Seven leopard seals (n=7) had previously been equipped with flipper tags from long-term demographic studies conducted by the U.S. AMLR Program (Krause et al., 2015; Krause et al., 2016; Krause et al., 2020; Goebel and Krause, pers. comm.). The remaining leopard seals (n=13) were equipped with a flipper tag that was inserted into the webbing of one hindflipper while chemically sedated.
Animal sampling
Body mass (kg) was measured during instrument deployment using a sling, a digital scale (DynaLink Measurement Systems International, Rice Lake, WI), hand winch, and tripod. Standard length and girth measurements were taken along the seal’s body with the seal lying on its belly. All seals were in excellent body condition based on qualitative assessment of the visibility of bony protrusions following Hupman et al. (2020). We measured seals’ head length as the straight-line distance from the tip of the nose to the caudal end of the skull and mouth length from the tip of the nose to the caudal border of the lips. We took scaled photographs of fore- and hindflippers to compare size differences in flipper morphology. Head length, mouth length, and flipper lengths were estimated from scaled photographs in ImageJ v. 1.53K. Sex was determined visually based on the genitalia. Age was estimated based on the relationship between standard length and age established by Laws, 1957; specifically, a seal was classified as a juvenile if standard length was less than 260 cm (n=1) or as an adult if standard length exceeded 260 cm (n=20). Research was approved by the Institutional Animal Care and Use Committee at the University of California, Santa Cruz and conducted under federal authorizations for marine mammal research under National Marine Fisheries Service permit #19439 and an Antarctic Conservation Act permit #2018-016.
Tag set up and processing
SPLASH tags were programmed to ensure coverage throughout the year and maximize transmissions of hourly GPS locations, dive data, and histogram data, resulting in 450-500 transmissions/day. Tags were programmed to transmit information on haul-out periods, dive shape, maximum dive depth, and duration of individual dives, and histograms of depth and duration binned into 6-hour periods. For a dive to be recorded, the tag had to reach or exceed two meters in depth for one minute. Dive shapes were categorized through on-tag behavioral processing as: 1) square-shaped, where bottom time exceeded 50% of the total dive duration, 2) U-shaped, where bottom time was between 20-50% of the total dive duration, or 3) V-shaped, where bottom time was less than 20% of the total dive duration; any dives that could not be characterized were labeled as ‘unknown’ (Schreer and Testa, 1996; Schreer et al., 2001). Tags were considered ‘dry’ if the wet/dry sensor was dry for 30 seconds of any minute. A haul-out period began after the wet/dry sensor was dry for 20 consecutive minutes and ended when the sensor was immersed in saltwater. At the end of the transmission period, decoded data were download from the Wildlife Computers data portal for analysis.
Data and statistical analyses
We conducted analyses in R v. 4.1.2 (R Core Team, 2021), except where noted. All values are reported as mean ± standard deviation (s.d.). Each seal’s initial transmitted track was a combination of pre-filtered Argos System and GPS data. The pre-filtered track data did not include Argos location class “Z” or GPS locations with less the four satellites. Track data were first filtered to remove any obvious erroneous locations (e.g., incorrect hemisphere due to testing). GPS locations did not contain ellipse error (location error) information, but they were expected to have an error radius of approximately 50 meters when locations are fixed with four or more satellites (Dujon et al., 2014). Therefore, we assigned 50 meters to the semi-major axis, semi-minor axis, and error ellipse orientation for each GPS location, and removed any Argos locations without error ellipse values. Because the Argos locations contained near duplicate records (same date-time but differing location and spatial error information), we adjusted the date-time value for one of the near duplicate records by 10 seconds using the make.time.unique function from the xts package (Ryan et al., 2020). This allowed all Argos location and ellipse error information to inform the track interpolation. The track data were next filtered with a course-speed filter, sdafilter function from the argofilter package (Freitas, 2012), to remove points which indicated that the seal traveled greater than 3 m/s between locations. To interpolate each seal’s track, we fit a continuous-time correlated random walk model, informed by the ellipse error information, to the telemetry data using the crwMLE function from the crawl package (Johnson and London, 2018) and predicted locations at hourly time steps (Costa et al., 2010; McHuron et al., 2018).
GPS data were assessed separately to determine behavioral states. Each satellite location was assigned an at-sea trip number based on the haul-out status transmitted from the wet/dry sensor; a trip began when the seal left the haul-out location and included the location on land immediately following the seal’s return to land (Matsuoka et al., 2021). We visually examined the final trip classifications in QGIS version 3.20.3 (QGIS Development Team, 2021) using the detailed basemap from Quantarctica (Matsuoka et al., 2021) to ensure that locations classified as ‘hauled-out’ were on land/ice in relative proximity to haul-out locations based on areas where clusters of on-land locations occurred We also downloaded monthly ice extent data from the National Snow and Ice Data Center (https://nsidc.org/) to validate long haul-out periods on ice. We calculated maximum distance traveled (km) as the great circle (Haversine) distance from their tagging location to the farthest point on the track.
Dive depth and duration data were visually inspected for outliers, which were defined as dives exceeding 500 meters in depth or 30 minutes in duration based on all previously reported dive metrics for leopard seals (Kuhn et al., 2006; Krause et al., 2015; Krause et al., 2016). Outliers were inspected to ensure that dive durations were biologically meaningful and that these data were associated with high-quality GPS and/or Argos transmissions. Additionally, internal data-processing errors associated with dive durations were removed by assessing the internal drift sensor data. If the internal dive sensor exceeded a dive threshold of two meters, those data were excluded, providing a highly conservative approach for estimating dive duration summary statistics. We examined the variability in dive depth and duration of each seal through respective coefficients of variation (CV=standard deviation/mean). A low CV (values closer to 0) signifies that data are centered around the mean value and indicates stereotypy or consistency of behavior, while a high CV (values closer or exceeding 1) signifies that data are dispersed around the mean and indicates high variability in the recorded behavior (Gerhardt, 1991; Wainwright et al., 2008).
We calculated the ratio of fore- and hind-flipper (cm) to standard length (cm) for each seal to standardize for body size differences. We examined the morphological, movement, and dive data for deviations from normality using density and Q-Q plots and a Shapiro-Wilks test (R Core Team, 2021). Log transformations were performed when data were not normally distributed. We then assessed variance with F-tests.
We ran linear regression models to determine relationship between continuous variables. We used Pearson’s correlation to measure the strength of the relationship and then plotted and visualized these relationships (ggplot2, ggpubr, tidyverse, RColorBrewer; Neuwirth, 2014; Wickham, 2016; Wickham et al., 2019; Kassambara, 2020). We also ran linear mixed effects models to examine sex-specific differences among adults in seal morphometrics, movement patterns, and dive behavior. Linear mixed effects models had a Gaussian distribution and were run using the lme4 package (Bates et al., 2015). For morphometric variables, sex was included as the fixed effect, and individual was the random effect. For the movement pattern and dive behavior variables, the full model included both sex and body mass as fixed effects, and we included an interaction between the two; individual was included as the random effect. We ran all possible combinations of the model that included sex and/or body mass as the explanatory variable. Similarly, we compared differences in mean dive depth and dive duration between the three dive types (square, U, or V-shaped) using linear mixed effects models with dive shape as the fixed effect and individual as the random effect. In all cases, each candidate model was compared to a null model (intercept only) using likelihood ratio tests of null and residual deviances. We ranked models using the Akaike information criterion corrected for small sample sizes (AICc) using the aictab function in the AICcmodavg package (Mazerolle, 2020). We then evaluated the fit of the best fit model using an ANOVA in the car package (Type 2, Wald’s test; Fox and Weisberg, 2019). For the dive shape models, we estimated marginal means to perform post hoc pairwise contrasts between each dive shape; Tukey’s method was then used to adjust the p-value for multiple comparisons in the emmeans package (Lenth, 2021).
Results
Morphometrics
We obtained tracking, dive, and morphometric data from all 21 adult leopard seals (females: n=17; 81%; males: n=4, 19%) and tracking and morphometric data from one juvenile (n=1; female) at Cape Shirreff (N=22; Table 1). Leopard seal body masses ranged from 147-540 kg, standard lengths ranged from 213-320 cm, and axillary girths ranged from 125-239 cm. Mass and standard length were positively correlated among age classes (R2 = 0.64, F1,17 = 29.64, p<0.001) and among adults only (R2 = 0.43, F1,16 = 11.87, p=0.003). The linear regression equation between standard length and body mass that included all age classes was used to estimate body mass (n=2) and standard length (n=1) for the three females with missing values.
Adult females were significantly larger (454±59 kg) and longer (302±11 cm) than males (302±22 kg, χ2(1,21)=25.91, p<0.001; 276±11 cm; χ2(1,21)=21.94, p<0.001; Figure 2). Adult females also had greater axillary girths (196±21 cm) than adult males (156±8 cm; χ2(1,21)=15.01, p<0.001). The single juvenile had a mass of 147 kg, standard length of 213 cm, and axillary girth of 125 cm. Proportionally, adult leopard seal fore- and hindflippers were the same length when standardized for body length; fore- and hindflippers were each 23±2% of leopard seals’ total length. Adult leopard seal heads were 45±3 cm in length, and their mouths were 21±2 cm in length. Mouth length accounted for 47±4% of adult seal’s total head length and did not differ between the sexes.
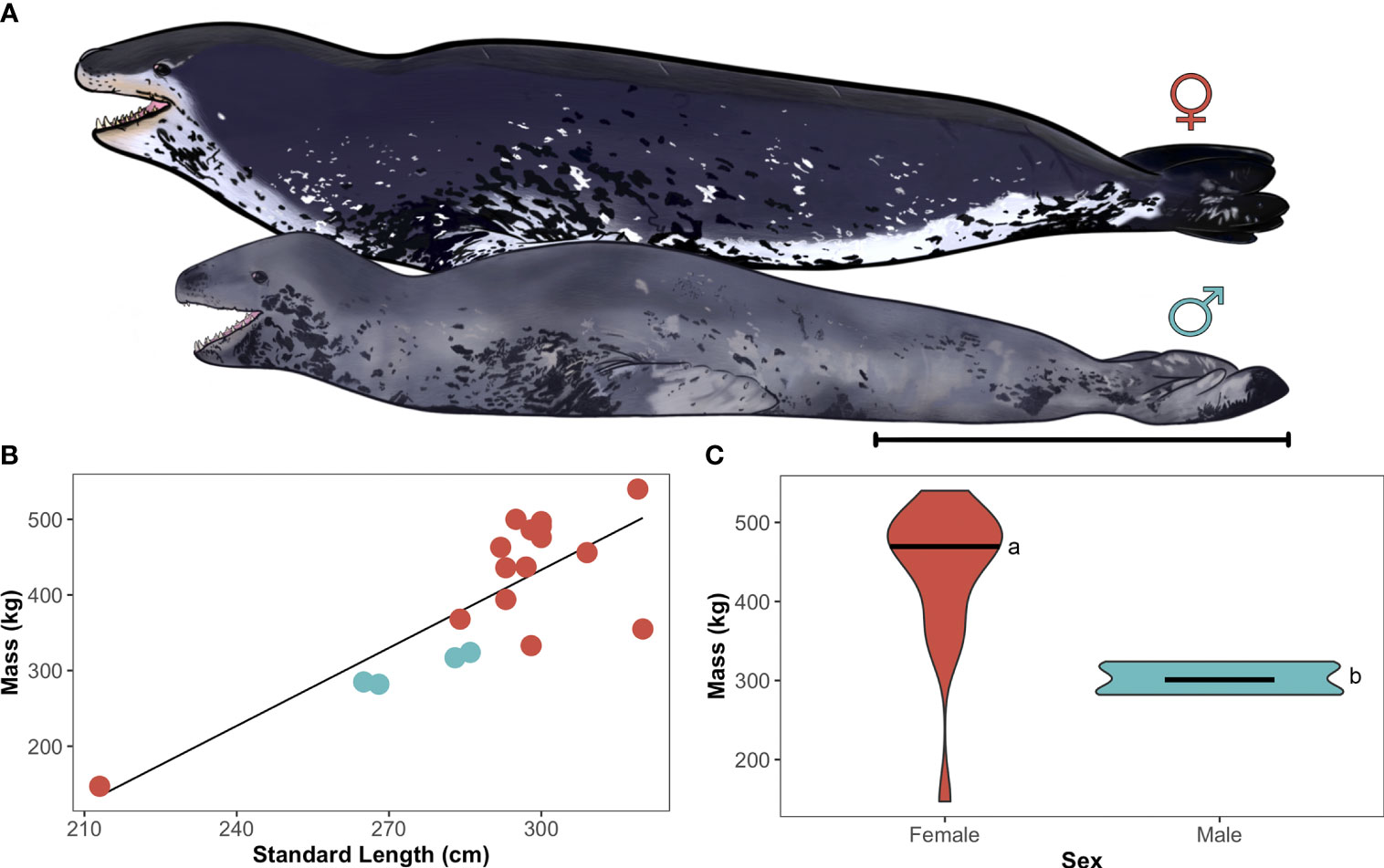
Figure 2 Female-biased sexual size dimorphism in adult leopard seals. (A) Illustration of scaled body morphometric differences between adult female (top) and male (bottom) leopard seals. Art by Aaron Kirkpatrick. Scale bar represents 1 m. (B) Linear regression of standard length (cm) and mass (kg) for adult leopard seals (females: red-orange, males: gray-blue; y=-600+3.4x, R2 = 0.80, p<0.001). Each point represents an individual seal. (C) Violin plot comparing sex-specific differences in body mass (kg), where females are significantly larger than males (a, b; t14.4 = 8.49, p<0.001). Horizontal bars represent the 25th, 50th (median), and 75th quartiles.
We documented the presence and absence of scars on 20 of the 22 leopard seals. All but one leopard seal had one to 17 visible wounds and/or scars on their body. Two leopard seals showed evidence of broken and re-modeled flipper bones (male #144, female #159). Five leopard seals showed evidence of recently acquired injuries, which included cuts on the cheeks, flippers, and hindquarters. One of these five (female #12) also had a recent cut on her eyelid and seven skin lesions. Female leopard seals had more injuries (6±6 wounds, range: 0-17) than males (2±1 wounds, range: 1-2).
Movement patterns
Satellite tags transmitted data for approximately 5 months (139±71 days; Table 1); tracking durations lasted 19 to 290 days. Leopard seals ranged from South Georgia Island to Adelaide Island (Figure 3). Leopard seals traveled a mean distance of 556±558 km from Cape Shirreff between January and December 2018 and April to December 2019. There was a variety of maximum distances traveled, from 46 km (female #128) to just over 1,669 km (female #57). Maximum distance traveled was not driven by sex, age class, body mass, or number of transmission days (Figures 3B, C). For example, the leopard seal tracked for most days (288 days; female #100) traveled a maximum distance of 139 km, while the leopard seal tracked for the fewest days (19 days; female #157) traveled 204 km. The single repeat individual (female #397) was tracked for 160 days in 2018 and traveled 512 km; she was subsequently tracked for 192 days in 2019 and traveled 878 km.
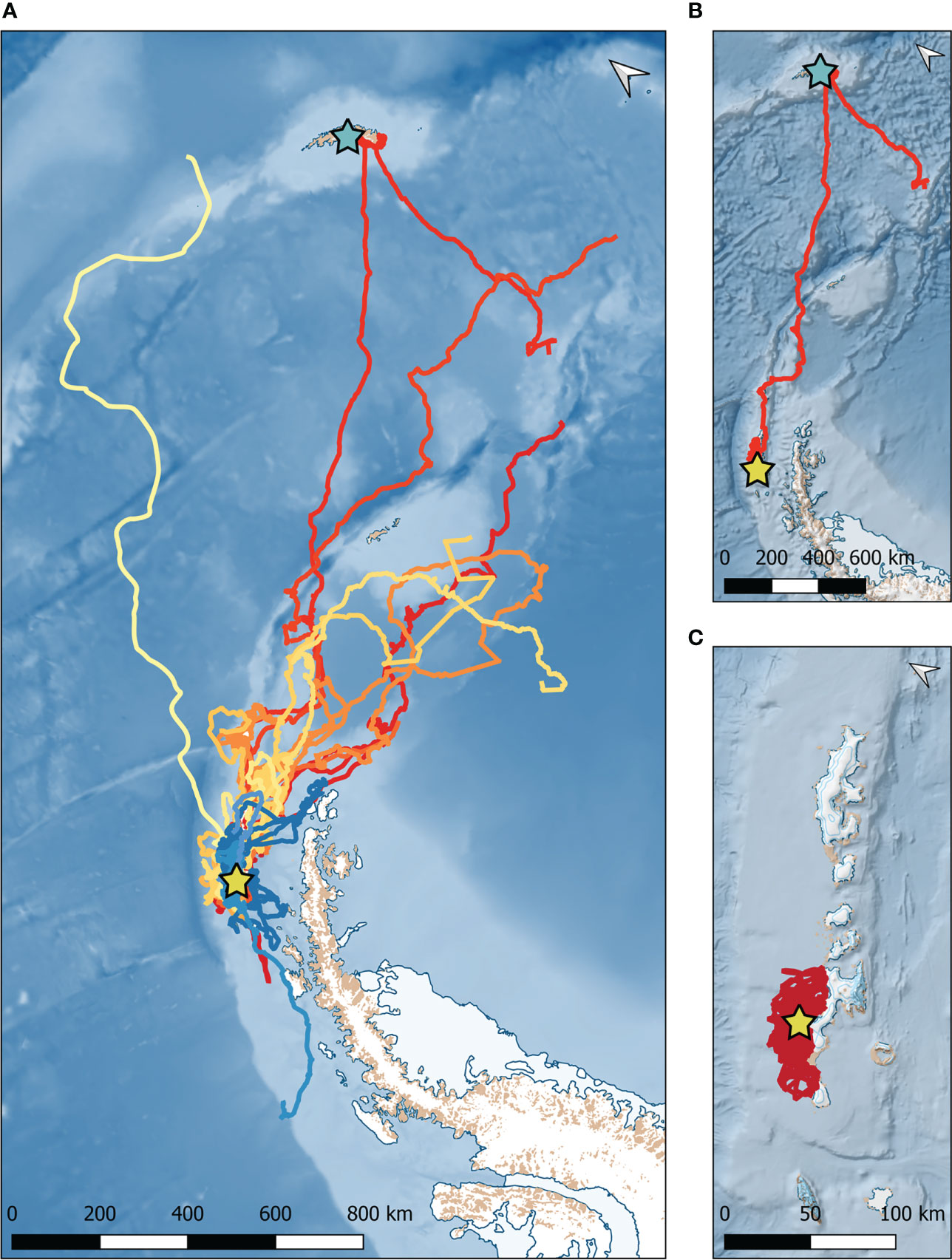
Figure 3 Comparison of movement patterns of leopard seals instrumented at Cape Shirreff. (A) GPS tracks of 22 leopard seals that transmitted for 139 ± 71 (mean ± standard deviation) days. Each color represents a different seal. Warm colors (reds, oranges, yellows) represent female seals. Cooler colors (blues) represent male seals. The yellow star represents Cape Shirreff. (B) GPS track of the seal (adult female #57) that traveled the farthest maximum distance (1,669 km) from Cape Shirreff to South Georgia (represented by the aqua star). (C) GPS track of the seal (adult female #128) that traveled the shortest maximum distance (46 km) from Cape Shirreff.
During the tracking period, leopard seals spent an average of 82±8% of their time at-sea and 18±8% hauled-out (Table 2). The shortest percentage of time a leopard seal spent at-sea was 59% (female #153), and the longest percentage of time at-sea was 96% (female #161). Females spent a greater percentage of time hauled-out (19±9%) compared to males (11±4%; χ2(1,20)=4.06, p=0.04; Figure 4). Females’ mean haul-out time was 15±4 h compared to 12±4 h for males. Similarly, the mean time females spent on at-sea trips was 74±48 h, compared to 92±42 h for males, although time spent at-sea did not significantly differ between the sexes. The longest continuous period a leopard seal spent in the water was 35 days (832 h; male #141).
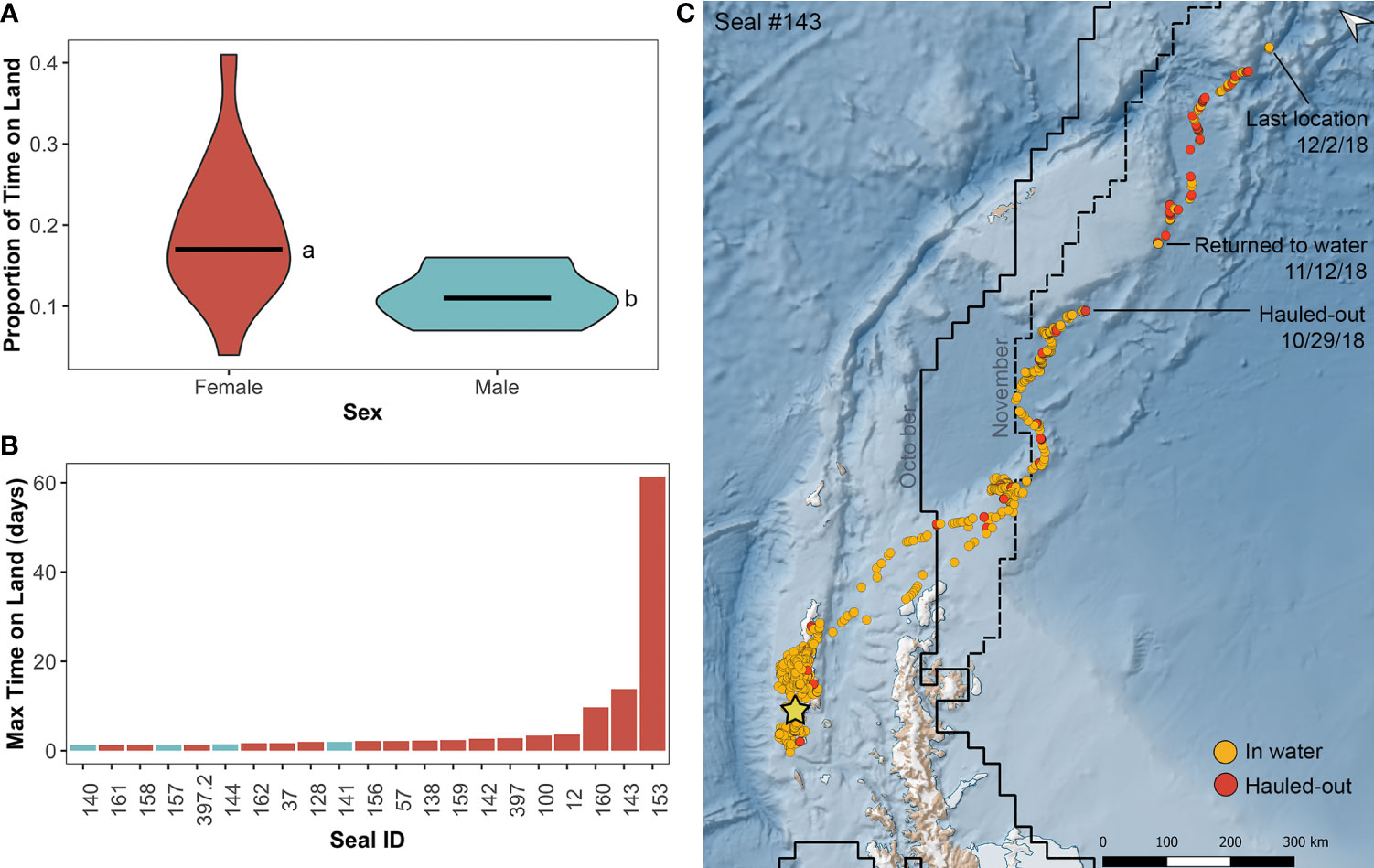
Figure 4 Haul-out behavior of leopard seals instrumented at Cape Shirreff. (A) Violin plot comparing sex-specific differences in proportion of time spent on land, where females (red-orange) spend significantly more time hauled-out than males (blue-gray; a, b; t11.4 = 2.87, p=0.015). Horizontal bars represent the 25th, 50th (median), and 75th quartiles. (B) Bar graph of maximum time spent on land (days) for each seal color coded by sex. (C) Satellite track of the adult female seal (#143) that hauled-out for 14 days between October 29 and November 12, 2018. Orange circles represent at-sea locations, and red points represent hauled-out locations. The straight black line shows the mean ice extent for October 2018, and the dashed line shows the mean ice extent for November 2018; data were downloaded from the National Snow and Ice Data Center.
The longest haul-out period for a leopard seal was for 61 days and occurred from October 23 to December 24, 2019 (1,473 h; female #153). The second longest haul-out period was for 14 days from October 29 to November 12, 2018 (331 hours; female #143), and the third longest haul-out period was for 10 days from August 20 to August 30, 2019 (233 hours; female #160). For these three long haul-outs, all three tags continuously transmitted as ‘dry’ and therefore entered haul-out mode during these periods. At the end of the 14-day haul-out, female #143 re-entered the water, which activated the tag’s ‘wet/dry’ switch (indicated by change from ‘dry’ to ‘wet’ transmission status messages), and the seal resumed diving. At the end of the 61-day haul-out, female #153 tag’s wet/dry switch was activated, signaling that the tag got wet at the end of this period; however, there was not clear evidence that the seal began diving from the transmitted data. In comparison, the 10-day haul-out by female #160 ended when the instrumented stopped transmitting entirely.
Leopard seals went on 47±29 at-sea trips during the tagging period, where an at-sea trip is defined as the period spent at-sea between two haul-out periods. The number of at-sea trips was positively related to the number of days that the instrumented transmitted (F1,19 = 68.31, p<0.001). Number of at-sea trips varied between three foraging trips (male #157, tracked 19 days; female #161, tracked 31 days) to 108 days (female #162, tracked 217 days).
Dive behavior
Leopard seals dove to a mean depth of 29±8 m (range: 17-48 m) and had a mean dive duration of 3.0 ± 0.7 min (range: 2-5 min; Table 3). Dive depth was positively related to dive duration (F1,19 = 11.84, p=0.002; Figure 5A). Dive depth was not related to sex or body mass; however, larger seals (i.e., seals with larger body mass) dove deeper than smaller seals (χ2(1,20)=5.98, p=0.015). The maximum depth and duration recorded for a dive was from an adult male (#141) that dove to 1,256 m for 25 min.
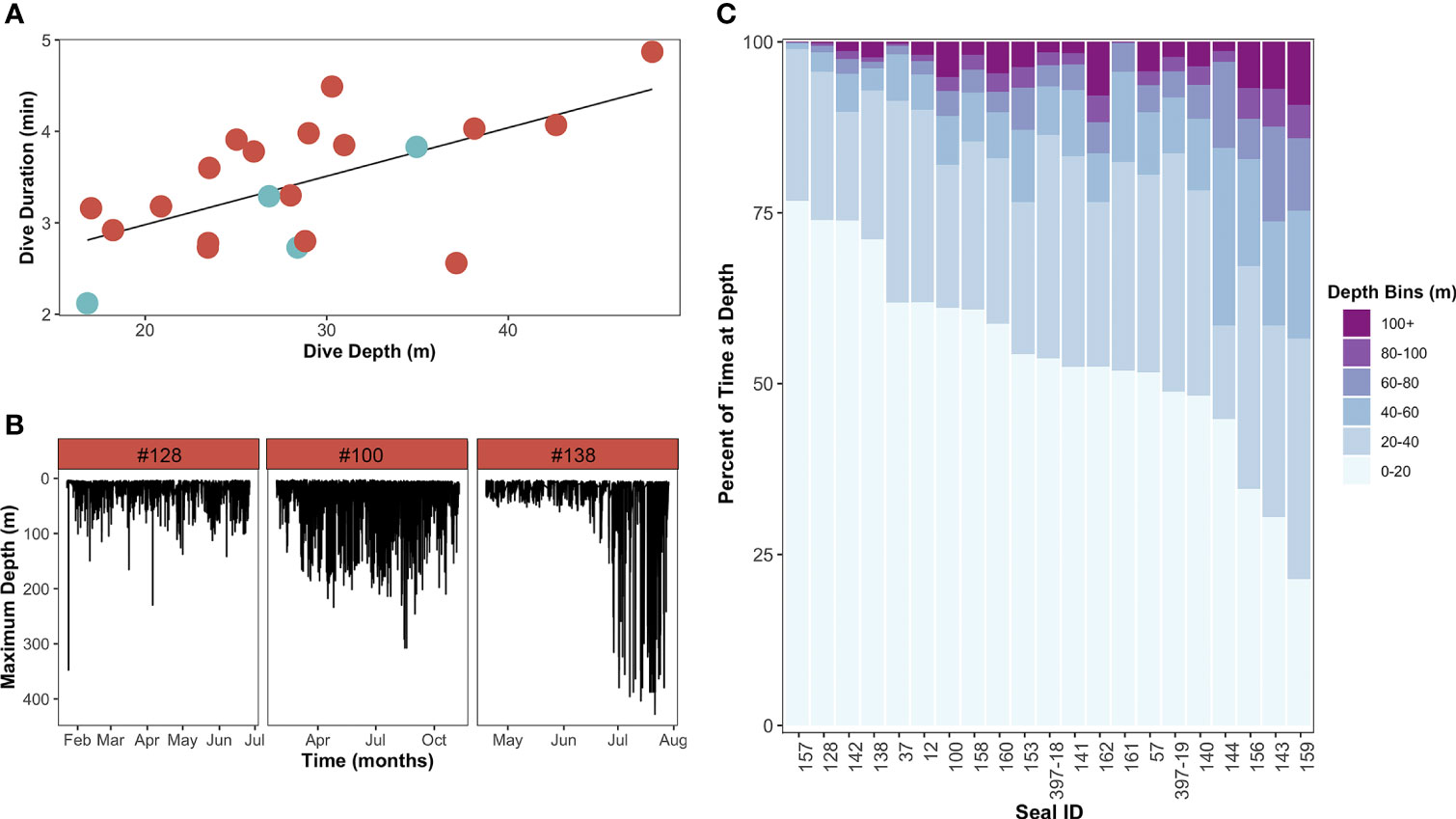
Figure 5 Dive behavior of leopard seals instrumented at Cape Shirreff. (A) Linear regression of the relationship between dive depth and dive duration (y=1.9+0.053x, R2 = 0.62, p=0.003). Each individual is represented by a circle and colored by sex (red-orange circles are females, blue-gray circles are males). (B) Representative dive profiles of three representative adult female leopard seals demonstrating variability in dive depth over time, including a shallow diver [left; #128; mean depth: 17 ± 13 m; coefficient of variation (CV): 0.79], a deep diver (middle; #100; mean depth: 29 ± 33 m; CV: 1.12), and a seasonally variable diver (right; #138, 24 ± 40 m; CV: 1.71). (C) Histogram demonstrating the percentage of time each seal spent in different depth bins. Depth bins are sorted from deepest (100+ m; top) to shallowest (0-20 m; bottom).
The greatest proportion of leopard seal dives occurred between 0-40 m and lasted between 0-5 min. Leopard seals showed variability in the proportion of time spent at different dive depths and durations (Figure 5B). While some seals barely exceeded 60 m in depth and did not have dive durations longer than 9 min (e.g., male #157, female #161), others (e.g., female #162) spent nearly 10% of their time at depths greater than 100 m and had dive durations in excess of 9 minutes. Overall, dive depth was more variable (CV=1.0±0.3; range: 0.5-1.7) than dive duration (CV=0.5±0.1; range: 0.3-0.6; Table 3). Specifically, some seals were stereotypic divers that primarily used shallow or deep dives throughout the tagging period; other seals were flexible and switched (regularly or seasonally) between shallow and (relatively) deep dives (Figure 5C).
Most leopard seal dives were square-shaped (68±13%). Leopard seals also used U-shaped (28±12%) and V-shaped (5±3%) dives, albeit less frequently. There was no relationship between the proportion of square or U-shaped dives with sex and/or body mass. However, the proportion of V-shaped dives significantly decreased as a function of sex (χ2(1,20)=8.24, p=0.004) and body mass (χ2(1,20)=8.85, p=0.003). Among all leopard seals, U-shaped dives were deeper (38±12 m) than square (24±8 m) or V-shaped dives (32±9 m; χ2(2,62)=66.68, p<0.001). In contrast, V-shaped dives were longer (4.0±0.9) than U- (3.3±0.7 min) or square-shaped dives (3.5±0.7 min; χ2(2,62)=75.87, p<0.001).
Discussion
Leopard seals are sexually dimorphic marine predators that have variable space use around the Antarctic Peninsula and subantarctic islands. This study is the largest dataset on the morphometrics, movement patterns, and dive behavior of leopard seals to date. The results expand our understanding of leopard seals’ life history, spatial ecology, and diving behavior and showcase high intraspecific variation among seals from a single location.
Morphometric patterns
Adult female leopard seals from Cape Shirreff were larger than males, with some females reaching nearly twice the size of their male counterparts. The largest animal sampled in our study was an adult female (540 kg), while the smallest adult was a male (282 kg). Furthermore, the smallest adult female in this study was nine kilograms heavier than the largest male. Female-biased sexual size dimorphism in leopard seals appears to extend to other phenotypic traits—females are also longer and have a larger girth than males.
As we were interested in understanding how representative these patterns were across leopard seals more broadly, we analyzed all published morphometric data for adults in this species. We acquired data from 158 leopard seals (this study; Hamilton, 1939; Laws, 1957; Rogers et al., 2005; Kuhn et al., 2006; Nordøy and Blix, 2009; Krause et al., 2015; Meade et al., 2015; Staniland et al., 2018; Krause et al., 2020). We were not able to include data from the only published study of sexual dimorphism as it did not report individual or sex-specific values (van den Hoff et al., 2005). This extended dataset suggests that female-biased sexual dimorphism is a general characteristic of leopard seals. Females were significantly larger (430±62 kg; n=52) and longer (304±20 cm, n=60) than males (292±29 kg, n=11; 280±18 cm, n=29), supporting the ‘reverse’ sexual dimorphism first noted by Murdoch and Bruce (1894).
Female-biased sexual dimorphism is common among animals but uncommon in mammals (Fairbairn et al., 2007; Swanson et al., 2013). It is only observed in a few disparate mammalian taxa, including rodents (e.g., some species of chinchillas, chipmunks, voles) and carnivorans (e.g., Kalahari meerkats, spotted hyenas; (Schulte-Hostedde et al., 2001; Clutton-Brock, 2007; Swanson et al., 2013; Kilanowski and Koprowski, 2017). Among marine mammals, baleen whales are the only clade to show widespread female-biased sexual dimorphism; females are up to 5% longer than males in all 14 extant species (Brownell and Ralls, 1986; Dines et al., 2014; Mesnick and Ralls, 2018). Among pinnipeds, female-biased dimorphism is mostly absent (although male-biased dimorphism is quite common). Female-biased sexual dimorphism has only been documented in a couple of species: Weddell (Leptonychotes weddellii) and ringed seals (Pusa hispida; Bryden et al., 1984; Staniland et al., 2005; Proffitt et al., 2007; Mellish et al., 2011; Langley et al., 2018; Shaughnessy and Southwell, 2019; Ferguson et al., 2019). In Weddell seals, differences between females and males are small (6% or less; Proffitt et al., 2007; Mellish et al., 2011; Langley et al., 2018). In ringed seals, interestingly, the degree of female-biased size dimorphism varies latitudinally, with females ranging from 19% to 37% larger than males (Ferguson et al., 2019). In comparison to pinniped and other marine mammals, the degree of size dimorphism between leopard seal sexes is exceptional–females are 50% larger than their male counterparts.
The evolution of female-biased sexual size dimorphism is often attributed to reduced male-male competition, where a relaxation of selective pressure for body size leads to males becoming smaller than females (Ralls, 1976; Isaac, 2005). Alternatively, some studies have found relationships between polyandrous mating systems, female-biased operational sex ratios, intense female reproductive competition, and/or greater development of secondarily selected sexual characteristics (Clutton-Brock, 2007). In marine mammals, female-biased sexual dimorphism is thought to be energetically necessary to facilitate the capital breeding strategy employed by many phocids and mysticetes, where females lactate while fasting, relying on internal energy stores acquired prior to parturition (Costa and Maresh, 2022).
Spotted hyenas are the only large terrestrial carnivore to exhibit female-biased sexual dimorphism (Swanson et al., 2013). Spotted hyenas are an extreme example of sexual dimorphism, as adult females exhibit many behavioral, ecological, and morphological traits that differ from adult males (Boydston et al., 2001; Boydston et al., 2005; Swanson et al., 2013). For example, adult female spotted hyenas are larger and more aggressive than adult males, have preferential access to food resources, and have uniquely adapted ‘male-like’ genitalia (Swanson et al., 2013). Adult female spotted hyenas showed sexual size dimorphism in traits associated with mass, length, and girth (Swanson et al., 2013), similar to what was documented here in leopard seals. In spotted hyenas, female-biased sexual size dimorphism is hypothesized to be associated with competition for food, dominance status, and reproductive success (Boydston et al., 2001; Isaac, 2005; Holekamp et al., 2012; Swanson et al., 2013; Ilany et al., 2021).
Similar to spotted hyenas, resource acquisition may play an important role in driving and/or maintaining sexual dimorphism in leopard seals. At Cape Shirreff, for example, large adult female leopard seals are observed more frequently than males, small females, or juveniles (Goebel and Krause, pers. obs.). The increased frequency of large adult females overlaps with the seasonal increase in available endothermic prey—notably, Gentoo and chinstrap penguin chicks and Antarctic fur seal pups (Krause et al., 2015; Krause et al., 2020). Recent stable isotope analyses also show that adult female leopard seals at Cape Shirreff undergo a rapid dietary switch from lower to higher trophic level prey items, likely representing the transition from small ectothermic prey to large endothermic prey; this dietary switch also coincides temporally with the birth of Antarctic fur seal pups and penguin chicks (Krause et al., 2020). Interestingly, this large trophic level dietary switch is only documented in adult females, not males. Males at Cape Shirreff consistently feed at lower trophic levels than females, and, anecdotally, only one male has only ever been observed hunting near fur seal beaches (Krause et al., 2020). Further, Hiruki et al. (1999) documented that only adult female leopard seals, not males, targeted Antarctic fur seals around Seal Island (a nearby island in the South Shetland Island chain). Animal-borne video cameras deployed on adult female leopard seals at Cape Shirreff also document kleptoparasitism, where some female leopard seals will steal (or attempt to steal) prey caught by other individuals (Krause et al., 2015). While the sample size was small (n=7 observed kleptoparasitism events), larger females were always successful at defending or stealing prey compared to smaller females (Krause et al., 2015). We also found that adult females had four times more injuries and scars compared to males. Similarly, Hamilton (1939) observed that the skulls of large adult females had more broken teeth compared to other age classes, potentially as the result of “combat”. While our sample size is small, we hypothesize that adult females acquire more injuries than males as a result of competition and resource defense with conspecifics, as well as from targeting large, highly mobile, aggressive endothermic prey.
Together, these data suggest that females, especially large bodied females, are better at both acquiring large endothermic prey and defending prey compared to smaller individuals of both sexes. However, we also note that males also consume endothermic prey (Kooyman, 1965; Walker et al., 1998; Hall-Aspland and Rogers, 2004) in other regions, suggesting endothermic prey is an important prey resource for both sexes. We therefore suggest that large body size in females offers a selective advantage in resource acquisition and defense, potentially leading to foraging territoriality in this sexually dimorphic species. However, data on the mating behavior and increased sampling across the species range are needed to test this hypothesis and better elucidate the selective pressures driving the evolution of sexual size dimorphism in this species.
Movement patterns
Leopard seals tagged at Cape Shirreff ranged from Adelaide Island to South Georgia Island. Leopard seals traveled ~560 kilometers from their tagging location, but individuals were highly variable in maximum distance traveled. One seal, for example, traveled only 46 kilometers from Cape Shirreff, while another traveled nearly 1,700 km to South Georgia. Similarly, previous studies documented leopard seals traveling short (<150 km) or long (800-1,950 km) distances from their tagging location (Rogers et al., 2005; Kuhn et al., 2006; Krause et al., 2015; Staniland et al., 2018). Regardless of distance traveled, leopard seals spent ~80% of their time in the water, similar to previous reports (Kuhn et al., 2006; Staniland et al., 2018). Leopard seals have been described as both ‘uncommon’ and ‘cryptic’, and it is likely due to this combination of variable movement patterns and large portions of time spent in the water (as opposed to hauled-out; Southwell et al., 2008).
AMLR has monitored the residency patterns of individual leopard seals at Cape Shirreff from 2011 to present. Thirty-six percent (n=8) of leopard seals in this study were seasonal (summer) residents around the South Shetlands and had previously been observed at Cape Shirreff (Goebel and Krause, pers. obs.). Notably, all the summer residents were adult females. Our tracking data show that these summer residents primarily used nearshore habitats around Cape Shirreff and surrounding islands during the austral fall and winter. In contrast to the summer residents, 64% (n=14) of leopard seals in this study had not been identified during annual summer surveys conducted by AMLR (Goebel and Krause, pers. obs.). Rather, these ‘transient’ leopard seals were only observed during the deployment period and have not been observed at the Cape since (this study; Goebel and Krause, pers. obs.).
In both years of the study, all leopard seals, both summer residents and transients, left the South Shetland Islands by October, which likely coincides with the breeding season. Pinnipeds require solid substrate—land or ice—for parturition and lactation (Costa and McHuron, 2022). Lobodontines, like leopard seals, are considered ice-obligate breeders that travel to breeding habitats during the austral spring and early summer (Siniff, 1991; Southwell et al., 2003). Opportunistic observations of leopard seal adult-pup pairs have occurred from the beginning of October to the end of December, supporting an austral spring to summer breeding season for the species (Brown, 1952; Tikhomirov, 1975; Rogers et al., 1996; Southwell et al., 2003; Acevedo et al., 2017; Bester et al., 2021; Joiris, 1991). However, data on the exact timing and duration of breeding season are lacking. Prior to this study, the longest haul-out period recorded for a leopard seal was for three days (Staniland et al., 2018).
Here, three adult female leopard seals hauled-out on ice for ten or more days between August and December. One female (#143) hauled-out on ice at the edge of the Weddell Sea for two weeks from the end of October to mid-November. At the end of this extended haul-out, the female returned to the water and resumed diving. In pinnipeds, lactation duration varies widely, ranging from four days to over a year, and ice-associated pinnipeds often have shorter lactation periods due to their unstable ice habitat (Bowen et al., 1985; Schulz and Don Bowen, 2005; Costa and Maresh, 2022). We therefore hypothesize that the 14-day haul-out period for this adult female documented the entire parturition and lactation period in leopard seals. A two-week lactation duration is consistent with that of other pack ice seals, especially those that fast during the haul-out period (Robinson and Pomeroy, 2022). Similarly, the timing of this haul-out period at the end of October to early November corresponds with other studies observations of leopard seal adult-pup pairs and newborn pups during this time period (Brown, 1952; Joiris, 1991; Southwell et al., 2003; Acevedo et al., 2017; Bester et al., 2021).
Another female seal (#160) hauled-out on ice near Elephant Island off the Antarctic Peninsula for 10 days at the end of August; however, the instrument stopped transmitting, so it is unknown how long the total haul-out period was for this seal. A third female (#153) had the longest recorded haul-out of 61 days on ice off the Antarctic Peninsula; however, it was unclear whether the seal resumed diving at the end of the haul-out. It is possible, for example, that the tag fell off on the ice or the seal died, and the tag continued to transmit as dry until the wet/dry sensor was activated as a result of the ice break up and/or the seal’s body entering the water. The 61-day haul-out, greatly exceeds the 28-to-49-day lactation interval observed in Weddell seals, the sister taxa of leopard seals, a species that goes into the water and feeds during lactation (Wheatley et al., 2006; Wheatley et al., 2008). It is also considerably longer than 35 days, which is the longest documented lactation for a pinniped that does not enter the water to feed during lactation (e.g., Hawaiian monk seals; Johanos et al., 1994).
Regardless of length, the timing of all three of these extended female haul-outs in the austral spring and summer matches the predicted breeding season for leopard seals, as well as lobodontines more broadly (Skinner and Klages, 1994; Southwell et al., 2003; Wheatley et al., 2006; Wheatley et al., 2008; Shaughnessy and Southwell, 2019). Our data, along with previously reported observations of adult-pup pairs, suggests plasticity in the timing of breeding season for leopard seals, with breeding occurring throughout a three-month window during the austral spring to summer. Together, the tracking and haul-out data demonstrate that adult female leopard seals haul-out for parturition and lactation on pack ice. Interestingly, the most recent observations of adult-pup pairs (n=3) have been in southern Chile; similar to the seals in this study, all three births occurred on ice floes near large glaciers, suggesting that ice may be the preferred (or required) breeding substrate for this species (this study; Acevedo and Martinez, 2013; Acevedo et al., 2017).
Dive behavior
Despite their large body size, leopard seals are overwhelmingly short (mean duration range: 2-5 min) and shallow (mean depth range: 17-48 m) divers. Although limited comparable data exist, this finding is consistent with all previous work (Kuhn et al., 2006; Nordøy and Blix, 2009; Krause et al., 2015), supporting the species’ classification as unimpressive divers. In many air-breathing marine vertebrates, dive depth and duration increase with body mass, as animals with larger body sizes have greater oxygen storage capabilities that enable longer dives (Schreer et al., 2001; Costa, 2007). Leopard seals are an exception to this pattern, similar to the benthic-diving walrus (Odobenus rosmarus) and shallow-diving blue whales (Balaenoptera musculus; Schreer et al., 2001; Costa, 2007). One explanation for why leopard seals do not take long, deep dives is simply that they do not need to do so. Leopard seals are often observed at the surface of the water when hunting large endothermic prey; if/when leopard seal prey occur in shallow waters, there is no reason for leopard seals to dive to their maximum capacity (Penney and Lowry, 1967; Hiruki et al., 1999; Costa, 2007; Krause et al., 2015; Krause et al., 2016; Krause and Rogers, 2019).
The vast majority (96%) of leopard seal dives are square-shaped (68%) or U-shaped (28%); both dive types are characterized by spending extended periods at the bottom of the dive (square shaped: >50%; U-shaped: 20-50%). These two dive types are, by far, the most common type of dive used by many diverse groups of marine vertebrates, including cetaceans, fish, pinnipeds, seabirds, and turtles, and are associated with benthic and pelagic foraging (Schreer and Testa, 1996; Schreer et al., 2001; Seminoff et al., 2006; Queiroz et al., 2017; Fortune et al., 2020; Lassauce et al., 2020). Square and U-shaped dives often occur in bouts and have relatively uniform depths (Schreer and Testa, 1996; Schreer et al., 2001). Because of the extended bottom time, square and U-shaped dives are often associated with targeting aggregated prey at depth (Schreer and Testa, 1996; Schreer et al., 2001; Queiroz et al., 2017). In line with optimal foraging theory, these diving marine vertebrates maximize the amount of time spent at the bottom of a dive in profitable prey patches (Queiroz et al., 2017).
Our study dramatically increases the maximum dive depth and duration reported for the species. Previously, the deepest dive for a leopard seal was 425 m (Kuhn et al., 2006). Four of our study seals exceeded that previous record, with one leopard seal diving to 1,256 meters. On the 1,256 m dive, this seal also had the longest reported dive duration of 25 minutes (Kuhn et al., 2006; Nordøy and Blix, 2009; Krause et al., 2015). However, seals that made deep dives did not show a pattern of deep-diving across their at-sea trips. Leopard seals, therefore, have the physiological capacity for longer, deeper dives even though they generally don’t use it, similar to other shallow-diving but large bodied marine mammals (Costa, 2007; Garde et al., 2018).
Leopard seals in this study range from stereotyped shallow divers to variable divers that regularly switch between shallow and (relatively) deep dives. Additionally, some leopard seals appear to switch between short and deeper dives seasonally. This is consistent with a recent study by Krause et al. (2020) that found intraspecific variation in leopard seal diets based on stable isotope analyses. In particular, Krause et al. (2020) hypothesized that leopard seals in the WAP rely on lower trophic level prey (e.g., fish and krill) during the spring when larger endothermic prey are less available. However, leopard seal diets change quickly and seasonally (Krause et al., 2020). Adult female leopard seals, for example, transitioned to feeding at higher trophic levels in the austral summer as large endothermic prey (e.g., Antarctic fur seals, penguins) were highly concentrated and available (Hiruki et al., 1999; Krause et al., 2020). The ability to switch between different dive types based on prey abundance and distribution is highly advantageous in generalist marine predators, especially those in rapidly changing environments (Beever et al., 2017; Kienle et al., 2019).
Intraspecific variation and plasticity
Leopard seals show high intraspecific variation in morphometrics, movement patterns, and dive behavior; this pattern is true both for leopard seals instrumented at Cape Shirreff and, more broadly, for leopard seals around Antarctica (Rogers et al., 2005; van den Hoff et al., 2005; Kuhn et al., 2006; Nordøy and Blix, 2009; Krause et al., 2015; Meade et al., 2015; Krause et al., 2016; Staniland et al., 2018; Krause et al., 2020). One life history trait that affects intraspecific variation in leopard seals is sex. Females are larger, have longer haul-out periods, exhibit summer residency patterns at Cape Shirreff, and seasonally specialize on higher tropic-level prey than males (Krause et al., 2015; Krause et al., 2020). However, sex-specific differences do not explain all the variability in leopard seal movement patterns and dive behavior.
Leopard seals exhibit high variability in space use across sexes and age classes. Leopard seals travel short and long distances and are both seasonal residents and transients (Rogers et al., 2005; Kuhn et al., 2006; Nordøy and Blix, 2009; Meade et al., 2015; Staniland et al., 2018). Leopard seals are short, shallow divers, which differs from mass-specific predictions of diving capabilities (Kuhn et al., 2006; Nordøy and Blix, 2009; Krause et al., 2015; Krause et al., 2016). Leopard seals also show individual and seasonal variation in dive behavior consistent with dietary studies showing seasonal variability in diet (Krause et al., 2015; Guerrero et al., 2021).
The one repeat leopard seal in this study showed flexibility in movement patterns between years. For example, in 2019, this female seal traveled >300 km farther from Cape Shirreff and went on 2.5 times more at-sea trips; however, there was between-year differences in satellite tracking durations, which may explain these differences. Between years, however, this repeat female also shows stereotyped movement patterns and dive behavior. Specifically, the repeat female showed similar diving patterns (depth, duration, dive type) across both years of the study. We also found evidence of variability in juvenile leopard seal movement patterns and dive behavior. Only one other juvenile seal has ever been instrumented, and it traveled a maximum distance of 40 km from its tagging location over 21 days (Kuhn et al., 2006). In contrast, the juvenile in this study traveled 1,582 km from its tagging location over 71 days.
Future studies that sample across the species range with a broader representation of life history stages are necessary to tease apart the factors that drive intraspecific variation in this apex predator. Regardless, the variability observed in this study suggests that leopard seals may have the plasticity to persist in one of the most rapidly changing habitats on the planet (Thurman et al., 2020). Furthermore, leopard seals may have more flexible habitat requirements than previously thought based on the sustained presence of leopard seals in subpolar habitats in subantarctic islands, Chile, and New Zealand (Gwynn, 1953; Walker et al., 1998; Acevedo and Martinez, 2013; Aguayo-Lobo et al., 2011; Acevedo et al., 2017; Hupman et al., 2020).
Leopard seals’ unique morphology, movement patterns, and dive behavior work in tandem and allow the species to be a flexible apex predator that feed at the top and bottom of the Southern Ocean food web. As an apex predator feeding on the top of the Southern Ocean food chain, they exert top-down control on pinniped and penguin populations (Hiruki et al., 1999; Schwarz et al., 2013; Krause et al., 2015; Krause et al., 2020; Krause et al., 2022). For example, leopard seals are responsible for the local population collapse of Antarctic fur seals in the South Shetland Islands (Boveng et al., 1998; Schwarz et al., 2013; Krause et al., 2022). However, leopard seals’ dependence on resources from the bottom of the food web makes the species vulnerable to the predicted changes in krill populations as a result of climate change and overfishing (Friedlaender et al., 2011; Forcada et al., 2012). Thus, leopard seals are both indirectly competing for krill as a resource but also directly impacting other krill consumers as a predator. As both krill predators and apex predators, leopard seals have an oversized impact on ecosystem structure and functioning, and changes in their abundance and distribution have the potential to dramatically restructure polar and subpolar ecosystem (Pagano et al., 2018; Laidre et al., 2020; Cloyed et al., 2021; Hammerschlag et al., 2022). Therefore, understanding phenotypic plasticity of this poorly studied apex predator should be of the utmost importance moving forward.
Data availability statement
The original contributions presented in the study are included in the article/supplementary material. Further inquiries can be directed to the corresponding author.
Ethics statement
The animal study was reviewed and approved by Institutional Animal Care and Use Committee at the University of California, Santa Cruz, federal authorizations for marine mammal research under National Marine Fisheries Service permit #19439, and an Antarctic Conservation Act permit #2018-016.
Author contributions
Conceptualization – DEC, DPC, MEG, SBK, SSK, and SJT; Funding, project administration – DEC, DPC, MEG, SBK, SSK, and SJT; Methodology – DEC, DPC, RB-C, MEG, SBK, SSK, and SJT; Data curation – DPC, SSK, and EL; Formal analysis – SSK and EL; Visualization – RB-C, SSK, and EL; Writing (original) – SSK; Writing (editing) – RB-C, DEC, DPC, MEG, SBK, SSK, EL, and SJT. All authors contributed to the article and approved the submitted version.
Acknowledgments
We gratefully acknowledge the following individuals and organizations for their invaluable help in completing this project, including the entire crew of the Lawrence M. Gould, NOAA Antarctic Marine Living Resources Program, M. L. Benton, P. Cunningham, A. Kirkpatrick, A. Leahy, E. Sperou, and the U.S. Antarctic Program, with special thanks to C. Ferrier, D. Krause, and G. Watters. The authors also appreciate the helpful reviews and comments by D. Jacoby, M. Bester, and D. Ainley that improved the manuscript. We also thank The Chilean Antarctic Institute (INACH) for their support at Cape Shirreff and the ANID PIA/BASAL FB0002. This work was funded by NSF #1644256.
Conflict of interest
The authors declare that the research was conducted in the absence of any commercial or financial relationships that could be construed as a potential conflict of interest.
Publisher’s note
All claims expressed in this article are solely those of the authors and do not necessarily represent those of their affiliated organizations, or those of the publisher, the editors and the reviewers. Any product that may be evaluated in this article, or claim that may be made by its manufacturer, is not guaranteed or endorsed by the publisher.
References
Acevedo J., González A., Garthe S., González I., Gómez R., Aguayo-Lobo A. (2017). Births of leopard seals Hydrurga leptonyx in southern Chile. Polar. Biol. 40, 713–717. doi: 10.1007/s00300-016-1968-0
Acevedo J., Martinez F. (2013). Residence of the leopard seal in the Magellan strait: A potential sub-Antarctic population inhabiting the waters of southern Chile? Polar. Biol. 36, 453–456. doi: 10.1007/s00300-012-1275-3
Aguayo-Lobo A., Jorge Acevedo R., Brito J. L., Paola Acuña G., Bassoi M., Secchi E. R., et al. (2011). Presence of the leopard seal, Hydrurga leptonyx (De blainville, 1820), on the coast of Chile: An example of the Antarctica - South America connection in the marine environment. Oecologia Australis 15, 69–85. doi: 10.4257/oeco.2011.1501.07
Bates D., Maechler M., Bolker B., Walker S. (2015). Fitting linear mixed-effects models using lme4. J. Stat. Software 67, 1–48. doi: 10.18637/jss.v067.i01
Baum J. K., Worm B. (2009). Cascading top-down effects of changing oceanic predator abundances. J. Anim. Ecol. 78, 699–714. doi: 10.1111/j.1365-2656.2009.01531.x
Beever E. A., Hall L. E., Varner J., Loosen A. E., Dunham J. B., Gahl M. K., et al. (2017). Behavioral flexibility as a mechanism for coping with climate change. Front. Ecol. Environ. 15, 299–308. doi: 10.1002/fee.1502
Bester M. N., Lübcker N., Haddad W., Bornemann H., Wege M. (2021). Antarctic Pack ice seal observations during spring across the lazarev Sea. Polar. Rec. 57, 1–6. doi: 10.1017/S003224742100005X
Boveng P. L., Hiruki L. M., Schwartz M. K., Bengtson J. L. (1998). Population growth of Antarctic fur seals: Limitation by a top predator, the leopard seal? Ecology 79, 2863–2877. doi: 10.1890/0012-9658(1998)079[2863:PGOAFS]2.0.CO;2
Bowen W. D., Oftedal O. T., Boness D. J. (1985). Birth to weaning in 4 days: remarkable growth in the hooded seal, Cystophora cristata. Can. J. Zoology 63, 2841–2846. doi: 10.1139/z85-424
Boydston E. E., Kapheim K. M., van Horn R. C., Smale L., Holekamp K. E. (2005). Sexually dimorphic patterns of space use throughout ontogeny in the spotted hyena (Crocuta crocuta). J. Zoology 267, 271–281. doi: 10.1017/S0952836905007478
Boydston E. E., Morelli T. L., Holekamp Boydston K. E., Morelli E. E., Holekamp T. L. (2001). Sex differences in territorial behavior exhibited by the spotted hyena (Hyaenidae, Crocuta crocuta). Ethology 107, 369–385. doi: 10.1046/j.1439-0310.2001.00672.x
Brown K. G. (1952). Observations on the newly born leopard seal. Nature 170, 982–983. doi: 10.1038/170982a0
Brownell R. L., Ralls K. (1986). Potential for sperm competition in baleen whales. Rep. Int. Whaling Comm. 8, 97–112.
Bryden M. M., Smith M. S. R., Tedman R. A., Featherston D. W. (1984). Growth of the Weddell seal, Leptonychotes weddellii (Pinnipedia). Aust. J. Zoology 32, 33–41. doi: 10.1071/ZO9840033
Casaux R., Baroni A., Ramón A., Carlini A., Bertolin M., DiPrinzio C. Y. (2009). Diet of the leopard seal Hydrurga leptonyx at the Danco Coast, Antarctic Peninsula. Polar. Biol. 32, 307–310. doi: 10.1007/s00300-008-0567-0
Charmantier A., Mccleery R. H., Cole L. R., Perrins C., Kruuk L. E. B., Sheldon B. C. (2008). Adaptive phenotypic plasticity in response to climate change in a wild bird population. Science 320, 800–803. doi: 10.1126/science.1157174
Cloyed C. S., Wilson R. M., Balmer B. C., Hohn A. A., Schwacke L. H., Zolman E. S., et al. (2021). Specialization of a mobile, apex predator affects trophic coupling among adjacent habitats. Sci. Rep. 11, 19611. doi: 10.1038/s41598-021-99017-1
Clutton-Brock T. (2007). Sexual selection in males and females. Science 318, 1882–1885. doi: 10.1126/science.1133311
Costa D. P. (2007). “Diving physiology of marine vertebrates,” in Encyclopedia of Life Sciences (Hoboken, New Jersey, USA: Wiley), 1–7. doi: 10.1002/9780470015902.a0004230
Costa D. P., Huckstadt L. A., Crocker D. E., McDonald B. I., Goebel M. E., Fedak M. A. (2010). Approaches to studying climatic change and its role on the habitat selection of Antarctic pinnipeds. Integr. Comp. Biol. 50, 1018–1030. doi: 10.1093/icb/icq054
Costa D. P., Maresh J. L. (2022). “Reproductive energetics of phocids,” in Ethology and Behavioral Ecology of Phocids. Eds. Costa D. P., McHuron E. A. (Switzerland: Springer Cham), 281–309.
Costa D. P., McHuron E. A. (2022). Ethology and behavioral ecology of phocids (Switzerland: Springer Cham). Available at: https://link.springer.com/bookseries/15983.
Dawson T. P., Jackson S. T., House J. I., Prentice I. C., Mace G. M. (2011). Beyond predictions: Biodiversity conservation in a changing climate. Science 332, 53–58. doi: 10.1126/science.1200303
Dines J. P., Otárola-Castillo E., Ralph P., Alas J., Daley T., Smith A. D., et al. (2014). Sexual selection targets cetacean pelvic bones. Evolution 68, 3296–3306. doi: 10.1111/evo.12516
Dujon A. M., Lindstrom R. T., Hays G. C. (2014). The accuracy of fastloc-GPS locations and implications for animal tracking. Methods Ecol. Evol. 5, 1162–1169. doi: 10.1111/2041-210X.12286
Estes J. A., Terborgh J., Brashares J. S., Power M. E., Berger J., Bond W. J., et al. (2011). Trophic downgrading of planet earth. Science 333, 301–306. doi: 10.1126/science.1205106
Estes J. A., Tinker M. T., Williams T. M., Doak D. F. (1998). Killer whale predation on sea otters linking oceanic and nearshore ecosystems. Science 282, 473–476. doi: 10.1126/science.282.5388.473
Fairbairn D. J., Blanckenhorn W. U., Székely T. (2007). Sex, size and gender roles: evolutionary studies of sexual size dimorphism (New York: Oxford University Press).
Ferguson S. H., Yurkowski D. J., Young B. G., Willing C., Zhu X., Muir D. C., et al. (2019). Do intraspecific life history patterns follow interspecific predictions? A test using latitudinal variation ringed seals. Population Ecol. 61, 371–382. doi: 10.1002/1438-390X.12008
Forcada J., Trathan P. N., Boveng P. L., Boyd I. L., Burns J. M., Costa D. P., et al. (2012). Responses of Antarctic pack-ice seals to environmental change and increasing krill fishing. Biol. Conserv. 149, 40–50. doi: 10.1016/j.biocon.2012.02.002
Fortune S. M. E., Ferguson S. H., Trites A. W., Hudson J. M., Baumgartner M. F. (2020). Bowhead whales use two foraging strategies in response to fine-scale differences in zooplankton vertical distribution. Sci. Rep. 10, 1–18. doi: 10.1038/s41598-020-76071-9
Fox J., Weisberg S. (2019). An {R} companion to applied regression. 3rd ed. (Thousand Oaks CA: Sage. URL). Available at: https://socialsciences.mcmaster.ca/jfox/Books/Companion/.
Freitas C. (2012) Argosfilter: Argos locations filter. r package version 0.63. Available at: https://CRAN.R-project.org/package=argosfilter.
Friedlaender A. S., Johnston D. W., Fraser W. R., Burns J., Patrick N. H., Costa D. P. (2011). Ecological niche modeling of sympatric krill predators around Marguerite bay, Western Antarctic Peninsula. Deep-Sea Res. Part II: Topical Stud. Oceanog. 58, 1729–1740. doi: 10.1016/j.dsr2.2010.11.018
Garde E., Jung-Madsen S., Ditlevsen S., Hansen R. G., Zinglersen K. B., Heide-Jørgensen M. P. (2018). Diving behavior of the Atlantic walrus in high Arctic Greenland and Canada. J. Exp. Mar. Biol. Ecol. 500, 89–99. doi: 10.1016/j.jembe.2017.12.009
Gerhardt H. C. (1991). Female mate choice in treefrogs static and dynamic acoustic criteria. Anim. Behav. 42, 615–634. doi: 10.1016/S0003-3472(05)80245-3
Guerrero A. I., Pinnock A., Negrete J., Rogers T. L. (2021). Complementary use of stable isotopes and fatty acids for quantitative diet estimation of sympatric predators, the Antarctic pack-ice seals. Oecologia 197, 729–742. doi: 10.1007/s00442-021-05045-z
Gwynn A. M. (1953). The status of leopard seals at heard island and macquarie island, 1948–1950. ANARE Interim. Rep. 3, 1–33.
Hall-Aspland S. A., Rogers T. L. (2004). Summer diet of leopard seals (Hydrurga leptonyx) in prydz bay, Eastern Antarctica. Polar. Biol. 27, 729–734. doi: 10.1007/s00300-004-0662-9
Hammerschlag N., Fallows C., Meÿer M., Seakamela S. M., Orndorff S., Kirkman S., et al. (2022). Loss of an apex predator in the wild induces physiological and behavioural changes in prey. Biol. Lett. 18, 20210476. doi: 10.1098/rsbl.2021.0476
Hays G. C., Ferreira L. C., Sequeira A. M. M., Meekan M. G., Duarte C. M., Bailey H., et al. (2016). Key questions in marine megafauna movement ecology. Trends Ecol. Evol. 31, 463–475. doi: 10.1016/j.tree.2016.02.015
Hiruki L. M., Schwartz M. K., Boveng P. L. (1999). Hunting and social behaviour of leopard seals (Hydrurga leptonyx) at seal island, south Shetland islands, Antarctica. J. Zoology 249, 97–109. doi: 10.1111/j.1469-7998.1999.tb01063.x
Hocking D. P., Evans A. R., Fitzgerald E. M. G. (2013). leopard seals (Hydrurga leptonyx) use suction and filter feeding when hunting small prey underwater. Polar. Biol. 36, 211–222. doi: 10.1007/s00300-012-1253-9
Holekamp K. E., Smith J. E., Strelioff C. C., van Horn R. C., Watts H. E. (2012). Society, demography and genetic structure in the spotted hyena. Mol. Ecol. 21, 613–632. doi: 10.1111/j.1365-294X.2011.05240.x
Hunter D. O., Britz T., Jones M., Letnic M. (2015). Reintroduction of Tasmanian devils to mainland Australia can restore top-down control in ecosystems where dingoes have been extirpated. Biol. Conserv. 191, 428–435. doi: 10.1016/j.biocon.2015.07.030
Hupman K., Visser I. N., Fyfe J., Cawthorn M., Forbes G., Grabham A. A., et al. (2020). From vagrant to resident: occurrence, residency and births of leopard seals (Hydrurga leptonyx) in new Zealand waters. New Z. J. Mar. Freshw. Res. 54, 1–23. doi: 10.1080/00288330.2019.1619598
Ilany A., Holekamp K. E., Akçay E. (2021). Rank-dependent social inheritance determines social network structure in spotted hyenas. Science 373, 348–352. doi: 10.1126/science.abc1966
Isaac J. L. (2005). Potential causes and life-history consequences of sexual size dimorphism in mammals. Mammal Rev. 35, 101–115. doi: 10.1111/j.1365-2907.2005.00045.x
Johanos T. C., Beckerm B. L., Ragen T. J. (1994). Annual reproductive-cycle of the female Hawaiian monk seal (Monachus schauinslandi). Mar. Mammal Sci. 10, 13–30. doi: 10.1111/j.1748-7692.1994.tb00386.x
Johnson D. S., London J. M. (2018). Crawl: an r package for fitting continuous-time correlated random walk models to animal movement data (Zenodo). doi: 10.5281/zenodo.596464
Joiris C. R. (1991). Spring distribution and ecological role of seabirds and marine mammals in the Weddell Sea, Antarctica. Polar. Biol. 11, 415–424. doi: 10.1007/BF00233076
Kassambara A. (2020) Ggpubr: 'ggplot2' based publication ready plots. r package version 0.4.0. Available at: https://CRAN.R-project.org/package=ggpubr.
Kienle S. S., Cacanindin A., Kendall T., Richter B., Ribeiro-French C., Castle L., et al. (2019). Hawaiian Monk seals exhibit behavioral flexibility when targeting prey of different size and shape. J. Exp. Biol. 222, jeb194985. doi: 10.1242/jeb.194985
Kilanowski A. L., Koprowski J. L. (2017). Female-biased sexual size dimorphism: Ontogeny, seasonality, and fecundity of the cliff chipmunk (Tamias dorsalis). J. Mammal. 98, 204–210. doi: 10.1093/jmammal/gyw172
Krause D. J., Bonin C. A., Goebel M. E., Reiss C. S., Watters G. M. (2022). The rapid population collapse of a key marine predator in the northern Antarctic Peninsula endangers genetic diversity and resilience to climate change. Front. Mar. Sci. 8. doi: 10.3389/fmars.2021.796488
Krause D. J., Goebel M. E., Kurle C. M. (2020). Leopard seal diets in a rapidly warming polar region vary by year, season, sex, and body size. BMC Ecol. 20, 1–15. doi: 10.1186/s12898-020-00300-y
Krause D. J., Goebel M. E., Marshall G. J., Abernathy K. (2015). Novel foraging strategies observed in a growing leopard seal (Hydrurga leptonyx) population at Livingston island, Antarctic peninsula. Anim. Biotelemetry 3, 1–14. doi: 10.1186/s40317-015-0059-2
Krause D. J., Goebel M. E., Marshall G. J., Abernathy K. (2016). ). Summer diving and haul-out behavior of leopard seals (Hydrurga leptonyx) near mesopredator breeding colonies at Livingston Island, Antarctic Peninsula. Mar. Mammal Sci. 32, 839–867. doi: 10.1111/mms.12309
Krause D. J., Rogers T. L. (2019). Food caching by a marine apex predator, the leopard seal (Hydrurga leptonyx). Can. J. Zoology 97, 573–578. doi: 10.1139/cjz-2018-0203
Kuhn C. E., McDonald B. I., Shaffer S. A., Barnes J., Crocker D. E., Burns J., et al. (2006). Diving physiology and winter foraging behavior of a juvenile leopard seal (Hydrurga leptonyx). Polar. Biol. 29, 303–307. doi: 10.1007/s00300-005-0053-x
Laidre K. L., Atkinson S., Regehr E. V., Stern H. L., Born E. W., Wiig Ø., et al. (2020). Interrelated ecological impacts of climate change on an apex predator. Ecol. Appl. 30, e02071. doi: 10.1002/eap.2071
Langley I., Fedak M., Nicholls K., Boehme L. (2018). Sex-related differences in the postmolt distribution of Weddell seals (Leptonychotes weddellii) in the southern Weddell Sea. Mar. Mammal Sci. 34, 403–419. doi: 10.1111/mms.12461
Lassauce H., Chateau O., Erdmann M., Wantiez L. (2020). Diving behavior of the reef manta ray (Mobula alfredi) in new Caledonia: More frequent and deeper night-time diving to 672 meters. PloS One 15, 15. doi: 10.1371/journal.pone.0228815
Laws R. (1957). On the growth rate of the leopard seal Hydrurga leptonyx (de blainville, 1820). Saugetierkundliche Mitt. 5, 49–55.
Lenth R. V. (2021). Emmeans: Estimated marginal means, aka least-squares means. r package version 1.6.3. Available at: https://CRAN.R-project.org/package=emmeans.
Matsuoka K., Skoglund A., Roth G., de Pomereu J., Griffiths H., Headland R., et al (2021). Quantarctica, an integrated mapping environment for Antarctica, the Southern Ocean, and sub-Antarctic islands. Environmental Modelling & Software 140, 105015. doi: 10.1016/j.envsoft.2021.105015
Mazerolle M. J. (2020). AICcmodavg: Model selection and multimodel inference based on (Q)AIC(c). r package version 2.3-1. Available at: https://cran.r-project.org/package=AICcmodavg.
McHuron E. A., Block B. A., Costa D. P. (2018). Movements and dive behavior of juvenile California sea lions from año nuevo island. Mar. Mammal Sci. 34, 238–249. doi: 10.1111/mms.12449
Meade J., Ciaglia M. B., Slip D. J., Negrete J., Márquez M. E. I., Mennucci J., et al. (2015). Spatial patterns in activity of leopard seals Hydrurga leptonyx in relation to sea ice. Mar. Ecol. Prog. Ser. 521, 265–275. doi: 10.3354/meps11120
Mellish J. E., Hindle A. G., Horning M. (2011). Health and condition in the adult weddell seal of McMurdo sound, Antarctica. Zoology 114, 177–183. doi: 10.1016/j.zool.2010.11.007
Mesnick S., Ralls K. (2018). “Sexual dimorphism,” in Encyclopedia of marine mammals (San Diego: Academic Press), 848–853.
Murdoch W. G. B., Bruce W. S. (1894). From Edinburgh to the Antarctic: An artist's notes and sketches during the Dundee Antarctic expedition of 1892-93 (London: Longmans, Green, and Co).
Neuwirth E. (2014) RColorBrewer: ColorBrewer palettes. r package version 1.1-2. Available at: https://CRAN.R-project.org/package=RColorBrewer.
Nordøy E. S., Blix A. S. (2009). Movements and dive behaviour of two leopard seals (Hydrurga leptonyx) off Queen Maud Land, Antarctica. Polar. Biol. 32, 263–270. doi: 10.1007/s00300-008-0527-8
Pagano A. M., Durner G. M., Rode K. D., Atwood T. C., Atkinson S. N., Peacock E., et al. (2018). High-energy, high-fat lifestyle challenges an Arctic apex predator, the polar bear. Science 359, 568–572. doi: 10.1126/science.aan8677
Penney R. L., Lowry G. (1967). Leopard seal predation of Adelie penguins. Ecology 48, 878–882. doi: 10.2307/1933751
Proffitt K. M., Garrott R. A., Rotella J. J., Wheatley K. E. (2007). Environmental and senescent related variations in Weddell seal body mass: implications for age-specific reproductive performance. Oikos 116, 1683–1690. doi: 10.1111/j.0030-1299.2007.16139.x
Pussini N., Goebel M. E. (2015). A safer protocol for field immobilization of leopard seals (Hydrurga leptonyx). Mar. Mammal Sci. 31, 1549–1558. doi: 10.1111/mms.12232
QGIS Development Team (2021). QGIS geographic information system (Open Source Geospatial Foundation). Available at: http://qgis.org.
Queiroz N., Vila-Pouca C., Couto A., Southall E. J., Mucientes G., Humphries N. E., et al. (2017). Convergent foraging tactics of marine predators with different feeding strategies across heterogeneous ocean environments. Front. Mar. Sci. 4. doi: 10.3389/fmars.2017.00239
Ralls K. (1976). Mammals in which females are larger than males. Q. Rev. Biol. 51, 245–276. doi: 10.1086/409310
R Core Team (2021). R: A language and environment for statistical computing (Vienna, Austria: R Foundation for Statistical Computing). Available at: https://www.R-project.org/.
Robinson K. J., Pomeroy P. P. (2022). “Breeding behavior,” in Ethology and behavioral ecology of phocids. Eds. Costa D. P., McHuron E. A. (Switzerland: Springer Cham), 229–279.
Rogers T. L., Cato D. H., Bryden M. M. (1996). Behavioral significance of underwater vocalizations of captive leopard seals Hydrurga leptonyx. Mar. Mammal Sci. 12, 414–427. doi: 10.1111/j.1748-7692.1996.tb00593.x
Rogers T. L., Hogg C. J., Irvine A. (2005). Spatial movement of adult leopard seals (Hydrurga leptonyx) in prydz bay, Eastern Antarctica. Polar. Biol. 28, 456–463. doi: 10.1007/s00300-004-0703-4
Ryan J. A., Ulrich J. M., Bennett R., Joy C. (2020) Xts: eXtensible time series. r package version 0.12.1. Available at: https://CRAN.R-project.org/package=xts.
Schreer J. F., Kovacs K. M., Hines H. (2001). Comparative diving patterns of pinnipeds and seabirds. Ecol. Monogr. 71, 137–162. doi: 10.1890/0012-9615(2001)071[0137:CDPOPA]2.0.CO;2
Schreer J. F., Testa J. W. (1996). Classification of Weddell seal diving behavior. Mar. Mammal Sci. 12, 227–250. doi: 10.1111/j.1748-7692.1996.tb00573.x
Schulte-Hostedde A. I., Millar J. S., Hickling G. J. (2001). Sexual dimorphism in body composition of small mammals. Can. J. Zoology 79, 1016–1020. doi: 10.1139/cjz-79-6-1016
Schulz T. M., Don Bowen W. (2005). The evolution of lactation strategies in pinnipeds: a phylogenetic analysis. Ecol. Monogr. 75, 159–177. doi: 10.1890/04-0319
Schwarz L. K., Goebel M. E., Costa D. P., Kilpatrick A. M. (2013). Top-down and bottom-up influences on demographic rates of Antarctic fur seals Arctocephalus gazella. J. Anim. Ecol. 82, 903–911. doi: 10.1111/1365-2656.12059
Seminoff J. A., Todd Jones T., Marshall G. J. (2006). Underwater behaviour of green turtles monitored with video-time-depth recorders: what’s missing from dive profiles? Mar. Ecol. Prog. Ser. 322, 269–280. doi: 10.3354/meps322269
Shaughnessy P. D., Southwell C. (2019). Are crabeater seals, Lobodon carcinophaga, sexually dimorphic for size during the breeding season? Mar. Mammal Sci. 32, 677–683. doi: 10.1111/mms.12549
Siniff D. B. (1991). An overview of the ecology of Antarctic seals. Am. Zoologist 31, 143–149. doi: 10.1093/icb/31.1.143
Skinner N. T. W., Klages J. (1994). On some aspects of the biology of the Ross seal Ommatophoca rossii from king haakon VII Sea, Antarctica. Polar. Biol. 14, 467–472. doi: 10.1007/BF00239051
Southwell C., Kerry K., Ensor P., Woehler E. J., Rogers T. (2003). The timing of pupping by pack-ice seals in East Antarctica. Polar. Biol. 26, 648–652. doi: 10.1007/s00300-003-0534-8
Southwell C., Paxton C. G. M., Borchers D., Boveng P., Rogers T., de la Mare W. K. (2008). Uncommon or cryptic? challenges in estimating leopard seal abundance by conventional but state-of-the-art methods. Deep-Sea Res. Part I: Oceanog. Res. Papers 55, 519–531. doi: 10.1016/j.dsr.2008.01.005
Staniland I. J., Ratcliffe N., Trathan P. N., Forcada J. (2018). Long term movements and activity patterns of an Antarctic marine apex predator: The leopard seal. PloS One 13, e0197767. doi: 10.1371/journal.pone.0197767
Staniland I., Ruckstuhl K. E., Clutton-Brock T. H. (2005). “Sexual segregation in seals,” in Sexual segregation in vertebrates: ecology of the two sexes. Eds. Ruckstuhl K. E., Neuhaus P. (New York: Cambridge University Press), 53–73.
Swanson E. M., McElhinny T. L., Dworkin I., Weldele M. L., Glickman S. E., Holekamp K. E. (2013). Ontogeny of sexual size dimorphism in the spotted hyena (Crocuta crocuta). J. Mammal. 94, 1298–1310. doi: 10.1644/12-MAMM-A-277.1
Thurman L. L., Stein B. A., Beever E. A., Foden W., Geange S. R., Green N., et al. (2020). Persist in place or shift in space? evaluating the adaptive capacity of species to climate change. Front. Ecol. Environ. 18, 520–528. doi: 10.1002/fee.2253
Tikhomirov E. (1975). The biology of the ice forms of seals in the pacific section of the Antarctic. Rapports process-verbauz Des. reunions 169, 409–412.
van den Hoff J., Fraccaro R., Mitchell P., Field I., Mcmahon C., Burton H., et al. (2005). Estimating body mass and condition of leopard seals by allometrics. J. Wildlife Manage. 69, 1015–1023. doi: 10.2193/0022-541X(2005)069[1015:EBMACO]2.0.CO;2
Wainwright P. C., Mehta R. S., Higham T. E. (2008). Stereotypy, flexibility and coordination: Key concepts in behavioral functional morphology. J. Exp. Biol. 211, 3523–3528. doi: 10.1242/jeb.007187
Walker T. R., Boyd I. L., McCafferty D. J., Huin N., Taylor R. I., Reid K. (1998). Seasonal occurrence and diet of leopard seals (Hydrurga leptonyx) at Bird Island, South Georgia. Antarctic. Sci. 10, 75–81. doi: 10.1017/s0954102098000108
Werth A. (2000). “Feeding in marine mammals,” in Feeding: Form, function, and evolution of tetrapod verbrates. Ed. Schwenk K. (Orlando: Academic Press), 475–514. doi: 10.1016/b978-012632590-4/50017-4
Wheatley K. E., Bradshaw C. J. A., Davis L. S., Harcourt R. G., Hindell M. A. (2006). Influence of maternal mass and condition on energy transfer in Weddell seals. J. Anim. Ecol. 75, 724–733. doi: 10.1111/j.1365-2656.2006.01093.x
Wheatley K. E., Bradshaw C. J. A., Harcourt R. G., Hindell M. A. (2008). Feast or famine: Evidence for mixed capital-income breeding strategies in Weddell seals. Oecologia 155, 11–20. doi: 10.1007/s00442-007-0888-7
Wickham H., Averick M., Bryan J., Chang W., D'Agostino McGowan L., François R., et al. (2019). Welcome to the tidyverse. J. Open Source Software 4, 1686. doi: 10.21105/joss.01686
Williams T. M., Estes J. A., Doak D. F., Springer A. M. (2004). Killer appetites: Assessing the role of predators in ecological communities. Ecology 85, 3373–3384. doi: 10.1890/03-0696
Keywords: apex predator, dive behavior, flexibility, intraspecific variation, leopard seal, morphometrics, movement patterns, sexual dimorphism
Citation: Kienle SS, Goebel ME, LaBrecque E, Borras-Chavez R, Trumble SJ, Kanatous SB, Crocker DE and Costa DP (2022) Plasticity in the morphometrics and movements of an Antarctic apex predator, the leopard seal. Front. Mar. Sci. 9:976019. doi: 10.3389/fmars.2022.976019
Received: 22 June 2022; Accepted: 26 July 2022;
Published: 17 August 2022.
Edited by:
David M. P. Jacoby, University of Lancaster, United KingdomReviewed by:
Marthan Bester, University of Pretoria, South AfricaDavid Ainley, H.T. Harvey & Associates, United States
Copyright © 2022 Kienle, Goebel, LaBrecque, Borras-Chavez, Trumble, Kanatous, Crocker and Costa. This is an open-access article distributed under the terms of the Creative Commons Attribution License (CC BY). The use, distribution or reproduction in other forums is permitted, provided the original author(s) and the copyright owner(s) are credited and that the original publication in this journal is cited, in accordance with accepted academic practice. No use, distribution or reproduction is permitted which does not comply with these terms.
*Correspondence: Sarah S. Kienle, U2FyYWhfS2llbmxlQGJheWxvci5lZHU=