- 1Conservation Biology, National Marine Mammal Foundation, San Diego, CA, United States
- 2Earth Resources Technology Inc., National Oceanic and Atmospheric Administration (NOAA) Affiliate, St. Petersburg, FL, United States
- 3National Oceanic and Atmospheric Administration (NOAA) Fisheries, Southeast Regional Office, St. Petersburg, FL, United States
- 4United States Navy Marine Mammal Program, Naval Information Warfare Center Pacific, San Diego, CA, United States
The use of auditory evoked potentials has been promoted as a means by which to collect audiometric information from odontocete cetaceans that are rarely encountered in stranding situations. This article presents the results of auditory evoked potential hearing tests collected from stranded odontocetes over nearly a decade. For six species, no audiograms previously existed – the dwarf sperm whale (Kogia sima), pygmy sperm whale (Kogia breviceps), northern right whale dolphin (Lissodelphis borealis), melon-headed whale (Peponocephala electra), long-beaked common dolphin (Delphinus capensis), and Atlantic spotted dolphin (Stenella frontalis). Additional hearing information was gathered for the pygmy killer whale (Feresa attenuata), a species for which only two prior audiograms had been collected. Audiograms for the delphinids demonstrated a typical dolphin-like form with upper-frequency limits of hearing > 149 kHz, except for the pygmy killer whales whose upper-frequency limit was between 103-107 kHz. The kogiid audiograms had a narrower region of increased sensitivity (80-128 kHz) closely aligned with their narrowband, high-frequency echolocation signals. Distinctions between kogiids and delphinids existed in the latencies of peaks of click-evoked auditory brainstem responses, with longer interwave intervals between P4 and N5 in the kogiids (mean of 0.60 ms vs. a mean of 0.37 ms in the delphinids). Modulation rate transfer functions collected in three of the species, suggested group-wide similarities in temporal processing capabilities.
1 Introduction
Auditory evoked potential (AEP) methods for testing hearing in odontocetes (toothed whales) are now regularly used in both research and clinical settings (e.g. Houser and Finneran, 2006b; Mooney et al., 2006; Popov et al., 2006; Houser et al., 2008; Finneran et al., 2013; Nachtigall and Supin, 2013; Finneran, 2020). The methods, which have become standardized in recent years (American National Standards Institute (ANSI), 2018), permit rapid acquisition of audiometric information without need for animal training and can be used in moribund and inattentive animals. The miniaturization and portability of AEP systems has further enabled rapid response to and data collection from stranded and rehabilitating odontocetes (Taylor et al., 2007; Finneran, 2009). With the exception of wild odontocete captures (Nachtigall et al., 2008; Mooney et al., 2009; Mooney et al., 2018; Wang et al., 2020), the opportunity to test odontocetes that are not under human care (e.g. bottlenose dolphins, belugas) is generally limited to stranding and rehabilitation events. These events provide unique opportunities to acquire information on odontocete species for which little or no audiometric information exists (Nachtigall et al., 2005; Cook et al., 2006; Finneran et al., 2009; Mann et al., 2010; Pacini et al., 2010; Pacini et al., 2011; Houser et al., 2018; Smith et al., 2018), and they have become an important element in addressing data-gaps related to the impact of anthropogenic noise on marine mammals (National Research Council (NRC), 2005; Southall et al., 2019).
A portable AEP system with custom software designed for cetacean testing [Evoked Response Study Tool (EVREST)] was created over a decade ago and has been made available for use by stranding networks (Finneran, 2009; Houser et al., 2018). The system has been used to test the hearing of stranded animals (Finneran et al., 2009; Houser et al., 2018) and animals held at marine mammal facilities and aquaria (Houser and Finneran, 2006b; Finneran et al., 2008; Houser et al., 2008; Strobel et al., 2017). In this article, we report on the use of EVREST to study the hearing of novel and seldom-tested odontocetes that stranded and underwent rehabilitation at different facilities within the United States. In each instance, an audiogram determined using sinusoidally amplitude modulated (SAM) tones and click-evoked responses were obtained. In some instances, a modulation rate transfer function (MRTF) was measured to determine the optimal relationship between the rate of SAM tone amplitude modulation and the amplitude of the auditory steady state response (ASSR) elicited by the SAM tone (Supin and Popov, 1995b; Finneran et al., 2007b). Where time or logistics did not permit an MRTF to be measured, the MRTF was estimated from the spectra of the click-evoked response since the spectral amplitudes of the click-evoked response have been shown to correlate well with the shape of the MRTF (Supin and Popov, 1995b; Finneran et al., 2007b). The audiograms and other auditory information presented herein are the first for some species (dwarf sperm whale (Kogia sima), pygmy sperm whale (Kogia breviceps), northern right-whale dolphin (Lissodelphis borealis), melon-headed whale (Peponocephala electra), long-beaked common dolphin (Delphinus capensis), Atlantic spotted dolphin (Stenella frontalis)) and add to the body of hearing data for another seldom-tested species (pygmy killer whale (Feresa attenuata)).
2 Materials and methods
2.1 Test coordination
Coordination with rehabilitation facilities and stranding response networks was made prior to contact about specific testing opportunities. Between 2012 and 2020, rehabilitation facilities or personnel at the National Oceanic and Atmospheric Administration’s Marine Mammal Health and Stranding Response Program (MMHSRP) contacted the first author when opportunities arose for testing recently stranded odontocetes and those that had entered rehabilitation. Hearing tests and other auditory assessments were performed under National Marine Fisheries research permits #16599 and #21026 or upon request of either the rehabilitation facility or the MMHSRP. Requests from the rehabilitation facility or MMHRSP were to fulfill pre-release health assessments associated with potential animal release determinations.
2.2 Subjects
Novel species that were tested included a pygmy sperm whale, a dwarf sperm whale, a northern right whale dolphin, a melon-headed whale, a long-beaked common dolphin, and an Atlantic spotted dolphin. Two pygmy killer whales were also tested, although this species had previously been the subject of AEP hearing tests (Montie et al., 2011).
A male dwarf sperm whale (MMPL2001) stranded on St. Pete Beach in Pinellas County, FL on 16 January 2020. The stranding was initially responded to by the Clearwater Marine Aquarium (CMA). The animal was subsequently moved from the beach to a CMA transport truck. The animal was given 10 mg midazolam IM prior to audiometric tests. As with other benzodiazepines, midazolam has no effect on the outcome of the hearing test; benzodiazepines can alter the wave latencies of the auditory brainstem response (ABR), but they do not affect wave amplitude, which is the critical characteristic for threshold testing (Adams et al., 1985; Hotz et al., 2000). The animal was euthanized later the same day.
A sub-adult, male melon-headed whale (47IMMS) stranded at Fort Morgan, AL and was initially responded to by the Dauphin Island Sea Lab, Alabama Marine Mammal Stranding Network on 2 September 2017. The animal was subsequently moved to the rehabilitation facilities at the Institute for Marine Mammal Studies (IMMS) in Gulfport, MS. Audiometric tests were performed on the animal on 3 October 2017. The animal was subsequently deemed releasable and was released to the Gulf of Mexico on 14 December 2017.
Two male pygmy killer whales (30IMMS and 31IMMS) stranded near Hancock, MS on 1 September 2015 and were transported to IMMS for rehabilitation. The two animals had audiometric tests performed at different times based upon their health status. The first of the animals (31IMMS) was tested on 13 October 2015 and the second (30IMMS) on 5 January 2016. Both animals were subsequently deemed releasable and were released into the Gulf of Mexico on 11 July 2016.
An adolescent, female northern right whale dolphin (Lb1701B) stranded in Ocean Beach of San Diego, CA on 5 May 2017. The dolphin was subsequently brought to the rehabilitation facility of SeaWorld San Diego and audiometric tests were performed on the same day. The attending veterinarian administered ceftiofur and danofloxacin intramuscularly prior to testing but neither drug is believed to have significant ototoxicity nor the time to have affected cochlear function before performance of the hearing test. The animal died the following day on 6 May 2017.
A neonate, female pygmy sperm whale (SWCKb1701b) stranded on La Jolla Shores Beach in San Diego, CA on 28 July 2017. The animal was subsequently transported to the rehabilitation facilities at SeaWorld San Diego. Audiometric tests were performed the following day. Due to the animal’s poor health status upon admission to the facility, the attending veterinarian administered ceftiofur, amikacin and enrofloxacin intramuscularly. Amikacin has known ototoxicity (Griffin, 1988; Tange, 1998), but it is unlikely that the drug would have had sufficient time to affect cochlear function prior to the time of testing. The animal died on 23 August 2017.
A young (1-2 yrs), male long-beaked common dolphin (Dc1304b) stranded on a beach in San Diego, CA on 14 May 2013. The dolphin was subsequently transported to the rehabilitation facilities at SeaWorld San Diego and audiometric testing was performed on the same day. The animal was subsequently released back into the wild on 8 October 2013. Another young (~3 yrs), male long-beaked common dolphin (Dc1601b) stranded on a beach in Oceanside, CA. The dolphin was also transported to the rehabilitation facilities at SeaWorld San Diego. A hearing test was performed on 19 June 2016 after the dolphin received a small dose of diazepam. It died three days later on 22 June.
An Atlantic spotted dolphin (SF1201) stranded in a bay at St. Andrew State Park, FL on 24 January 2012. The stranding was initially responded to by Gulf World Marine Park, but the dolphin was subsequently transported to the rehabilitation facility at SeaWorld Orlando on 25 January 2012. Hearing tests were performed on the adult female on 26 February 2012. The animal died on 23 April 2012.
2.3 Test procedures
Methods for performing audiometric tests varied somewhat over the course of the 8-yr period reported here, primarily due to changes in equipment used and the support capabilities available at the different rehabilitation facilities or site of stranding response. Hearing test methods were generally consistent with ANSI/ASA S3/SC1.6 (2018), but as most of the data were collected prior to publication of the standard, there were several notable exceptions: 1) the stimulus calibration method for tonal stimuli was based on the rms sound pressure level (SPL, dB re 1 µPa) vice the peak-to-peak equivalent sound pressure level (peSPL) recommended by the standard; and 2) the hearing test frequencies recommended by the standard were only used in one animal, which was tested after the standard was published (see dwarf sperm whale in Table 1). Below, common procedural details are provided. Additional details for each specific test situation follow the common procedures and are summarized in Table 1.
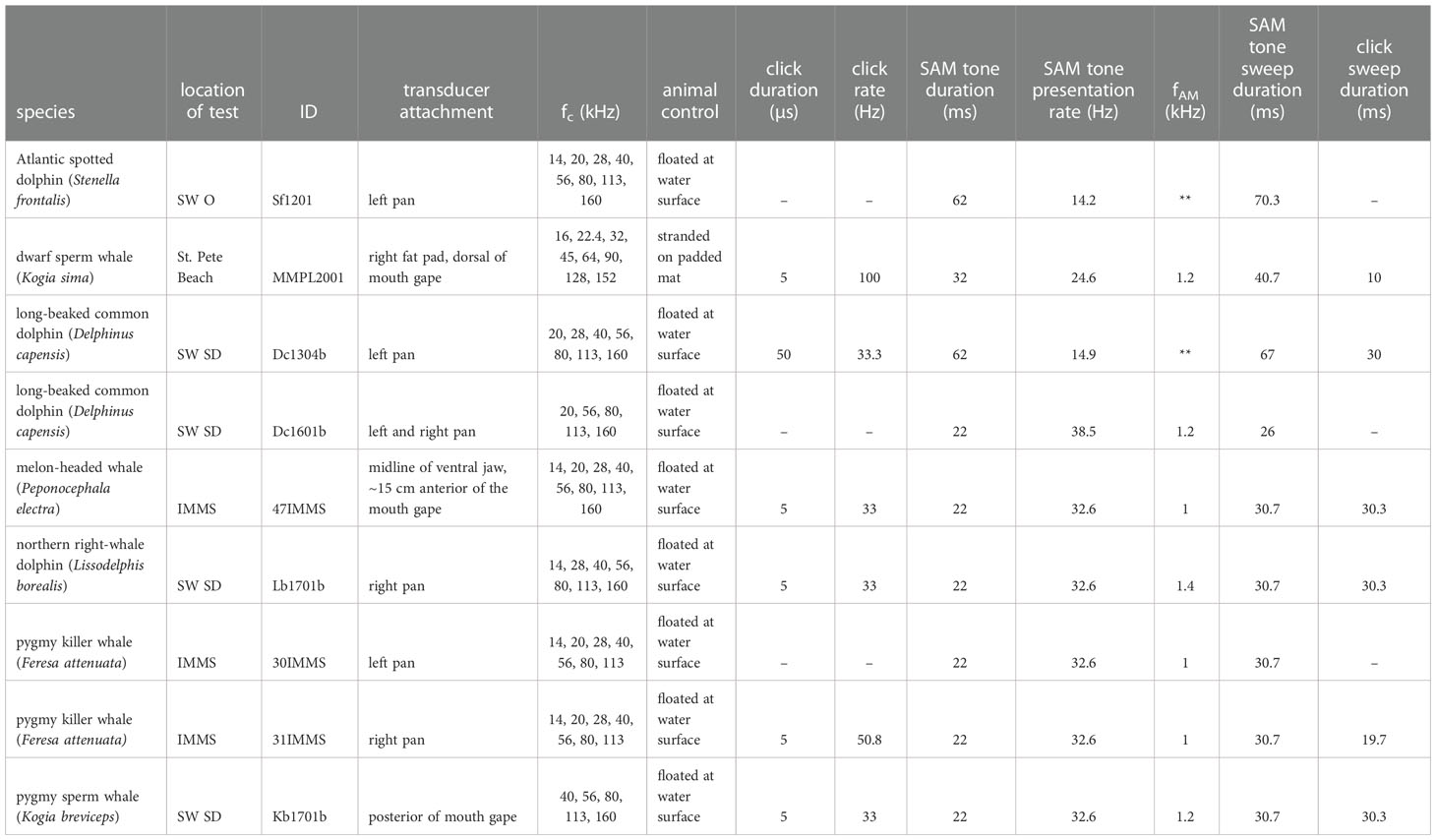
Table 1 Species tested, animal ID, location of test (SW O, SeaWorld Orlando; SW SD, SeaWorld San Diego; IMMS, Institute for Marine Mammal Studies), and animal control during testing. Test parameters include: transducer attachment location, center frequency (fc) of test stimuli, click duration and presentation rate, SAM tone duration, rate of presentation, and amplitude modulation rate (fAM), and the sweep duration used for evoked response AEP recordings.
Tonal stimuli for all hearing tests except those in the Atlantic spotted dolphin and the first long-beaked common dolphin tested consisted of individual SAM tones with durations from 22-32 ms. Amplitude modulation frequencies (fAM, 100% depth) ranged from 1 to 1.4 kHz and were based either on prior experience with the species, an evaluation of click-evoked spectra collected prior to the hearing test, or the outcome of an MRTF test performed prior to the hearing test. SAM tone carrier frequencies (fc) varied from 10 to 160 kHz. For the two animals with the earliest test dates, multiple simultaneous SAM tone stimuli were utilized for the hearing test (Finneran et al., 2007c; Finneran and Houser, 2007; Finneran et al., 2008; Branstetter et al., 2008). Each multiple SAM tone stimulus consisted of a combination of SAM tones containing unique fc and fAM. All fc were separated by an octave and carrier fAM were separated by 50 Hz centered around the known or presumed optimal rate of fAM for the species. For the Atlantic spotted dolphin, 4-SAM and 5-SAM configurations were used (4-SAM: fc =14, 28, 56, and 113 kHz; fAM =900, 950, 1000, and 1050 Hz/5-SAM: fc =10, 20, 40, 80 and 160 kHz; fAM =900, 950, 1000, 1050 and 1100 Hz). For the first long-beaked common dolphin tested, 3-SAM and 4-SAM configurations were used (3-SAM: fc =28, 56, and 113 kHz; fAM = 950, 1000, and 1050 Hz/4-SAM: fc =20, 40, 80 and 160 kHz; fAM =950, 1000, 1050 and 1100 Hz). Click stimuli were produced by applying a positive rectangular pulse to the sound projector with a duration of 50 or 5 µs. This produced a broadband stimulus waveform suited for the generation of a large auditory brainstem response (ABR). (Note* - Reductions in the duration of the click stimulus were made early in the study to eliminate a double-pulse produced by the transducer when using the 50-µs stimulus.)
The generation of acoustic stimuli and the recording of evoked responses were performed using EVREST software (Finneran, 2008; Finneran, 2009). All stimuli were projected from a rugged notebook computer with a PCI or PCIe expansion chassis that contained a multifunction data acquisition board (National Instruments NI PCI-6251 or PCIe-6251). Stimuli were digitally synthesized and converted to analog at a 1 MHz update rate and 16-bit resolution, low-pass filtered at 200 kHz (eight-pole Butterworth, Krohn-Hite 3C series), and attenuated (custom, 0–70 dB range) before being applied to the stimulus projector. A piezoelectric transducer embedded in a silicon suction cup (termed ‘‘jawphone’’) was used as the stimulus projector and was attached to the acoustically receptive area of the posterior mandible (“pan” region) for all of the delphinids (Moore et al., 1995; Møhl et al., 1999; Brill et al., 2001), except the melon-headed whale (see Table 1). For the dwarf sperm whale, placement corresponded to the location of the lateral fat pad just dorsal of the mouth gape. Placement was slightly different for the pygmy sperm whale because a quick evaluation of the click-evoked ABR as a function of transducer placement suggested the optimal location was posterior of the mouth gape. Animals were either held at the water surface by animal care staff or held out of water on a padded mat. The sound projector was either an ITC 1042 (International Transducer Corp., Santa Barbara, CA) or a TC 4013 (Reson, Slangerup, Denmark) transducer. The ITC 1042 was used on all animals except one of the long-beaked common dolphins (Dc1304b), which required the use of the TC 4013. The jawphones were calibrated multiple times over the 8-yr period, as previously described (Finneran and Houser, 2006; Houser and Finneran, 2006a). Calibration was performed by measuring the underwater SPL (SAM tones) or peak-peak SPL (clicks) produced at a distance of 15 cm from the surface of the jawphone face. The distance of the receiving hydrophone was derived from anatomical measurements in bottlenose dolphins (Tursiops truncatus). This approach has produced reasonable agreement between AEP thresholds collected with jawphones – both in air and underwater – and those behaviorally measured underwater (Finneran and Houser, 2006; Houser and Finneran, 2006a). Approximation to the species tested and reported here are probably similar for delphinid adults since their masses and sizes are similar to that of adult bottlenose dolphins. However, received level estimates for the smaller kogiids and delphinid neonates and adolescents are likely to be underestimated because of the smaller distance between the jawphone contact location and the auditory bullae.
Evoked potentials were measured using three 10-mm gold cup surface electrodes embedded in silicon suction cups and placed on the head and back. For adult delphinids, a noninverting electrode was located on the dorsal midline approximately 8 cm posterior to the blowhole. For neonate/adolescent delphinids, the placement of the noninverting electrode was scaled to the animal’s size (relative to that of an adult). For the pygmy sperm whale, the optimal location of the noninverting electrode (~8 cm behind the blowhole) was determined by measuring the peak-peak amplitude of the click-evoked ABR at different distances posterior to the blowhole. For the dwarf sperm whale, insufficient time was available for determining the optimal placement, so a distance of ~8 cm posterior to the blowhole was utilized. In all cases, a ground electrode was placed on the animal’s back near the dorsal fin and an inverting electrode was located midway between the noninverting and ground electrodes. Electrodes were coupled to the skin surface using conductive paste and electrode signals were passed to a biopotential amplifier (Grass ICP-511) which amplified (x105) and filtered (0.3–3 kHz) the voltage between the noninverting and inverting electrodes. The biopotential amplifier output was digitized with 16-bit resolution at a rate of 20-40 kHz for SAM tone stimuli, 100-200 kHz for click stimuli, and 1 MHz for multiple SAM tone stimuli. The digitized signals were then divided into a number of “sweeps”, each temporally aligned with a stimulus onset (see Table 1 for sweep duration), and the sweeps were synchronously averaged. Sweeps with peak instantaneous voltage above 12–50 µV were excluded from averaging; generally, reject voltages ranged from 12-20 µV.
Click-evoked potentials were averaged over 1024 sweeps. A maximum of 1024 sweeps were also collected for SAM tone stimuli, however, the presence or absence of an evoked response was determined after integral multiples of 256 sweeps. If a response was detected, the measurement was completed; otherwise, an additional 256 sweeps were collected and the process repeated until a maximum of 1024 sweeps was collected. At each integral multiple of 256 sweeps, the sweeps were averaged using a weighted averaging technique (Elberling and Wahlgreen, 1985) and the presence or absence of the ASSR determined by comparing the magnitude squared coherence (MSC) statistic, based on 16 “subaverages” (Dobie and Wilson, 1996; Finneran et al., 2007a), to a critical value at the level of α=0.01. Threshold testing began at SPLs ranging from 100 to 120 dB re 1 µPa. The SPL was subsequently adjusted according to a modified up/down staircase procedure after each measurement. If a response was detected, the SPL was reduced by the step size ΔL; if a response was not detected, the SPL was increased by ΔL. The initial ΔL varied from 10 to 30 dB. After each change from detection to non-detection or vice versa, the step size was reduced according to the rules:
Lk+1 = 0.4ΔLk (for reversals following detections),
ΔLk+1 = 0.45ΔLk (for reversals following non-detections),
where ΔLk is the step size for the kth measurement. The staircase was terminated when the step size for the next measurement was <3 dB. The threshold was defined as the SPL of the lowest detected ASSR (American National Standards Institute (ANSI), 2018). The procedure for determining threshold was the same when using multiple SAM tone stimuli, except that each component tone (i.e., unique carrier frequency) was evaluated and adjusted independently from all other components in the stimulus. When possible, an upper-frequency limit (UFL) of hearing was determined for each whale as the highest frequency corresponding to an interpolated threshold of 120 dB re 1 µPa.
An MRTF was collected from the pygmy sperm whale, melon-headed whale, and northern right whale dolphin. The MRTF measurements used an 80-kHz carrier in all tests, but the range of fAM varied between subjects. For the melon headed whale and pygmy sperm whale, fAM varied from 0.4 to 2 kHz in 50 Hz steps; for the northern right whale dolphin, fAM varied from 0.1 to 2 kHz in 100 Hz steps. SPLs for the MRTF stimuli were 110 dB. ASSR amplitudes were subsequently corrected for the biopotential amplifier frequency response prior to plotting ASSR amplitude as a function of fAM for the MRTF measurements.
3 Results
3.1 Dwarf sperm whale
Figure 1 shows the (top) click-evoked ABR from a single click series, (middle) frequency spectrum of the click-evoked ABR, and (bottom) the audiogram of the dwarf sperm whale. As with all of the species described in this paper, the click-evoked ABR waveform was qualitatively similar to that of bottlenose dolphins (Tursiops truncatus) and other odontocete species (Supin et al., 2001), and the waveform peaks were labeled as previously described (Popov and Supin, 1990). (Note – the N2 complex was not labeled, as bifurcation of the peak made it difficult to label the peaks with confidence.) Latencies of the ABR peaks were 1.15 (P1), 3.08 (P3), 3.68 (P4), and 4.3 ms (N5). The peak-peak amplitude of the ABR was 10.1 µV. The click-evoked spectral content spread upward to ~2-3 kHz, showed peaks at 600 Hz and 1.2 kHz, and had a notch between the peaks at ~900 Hz.
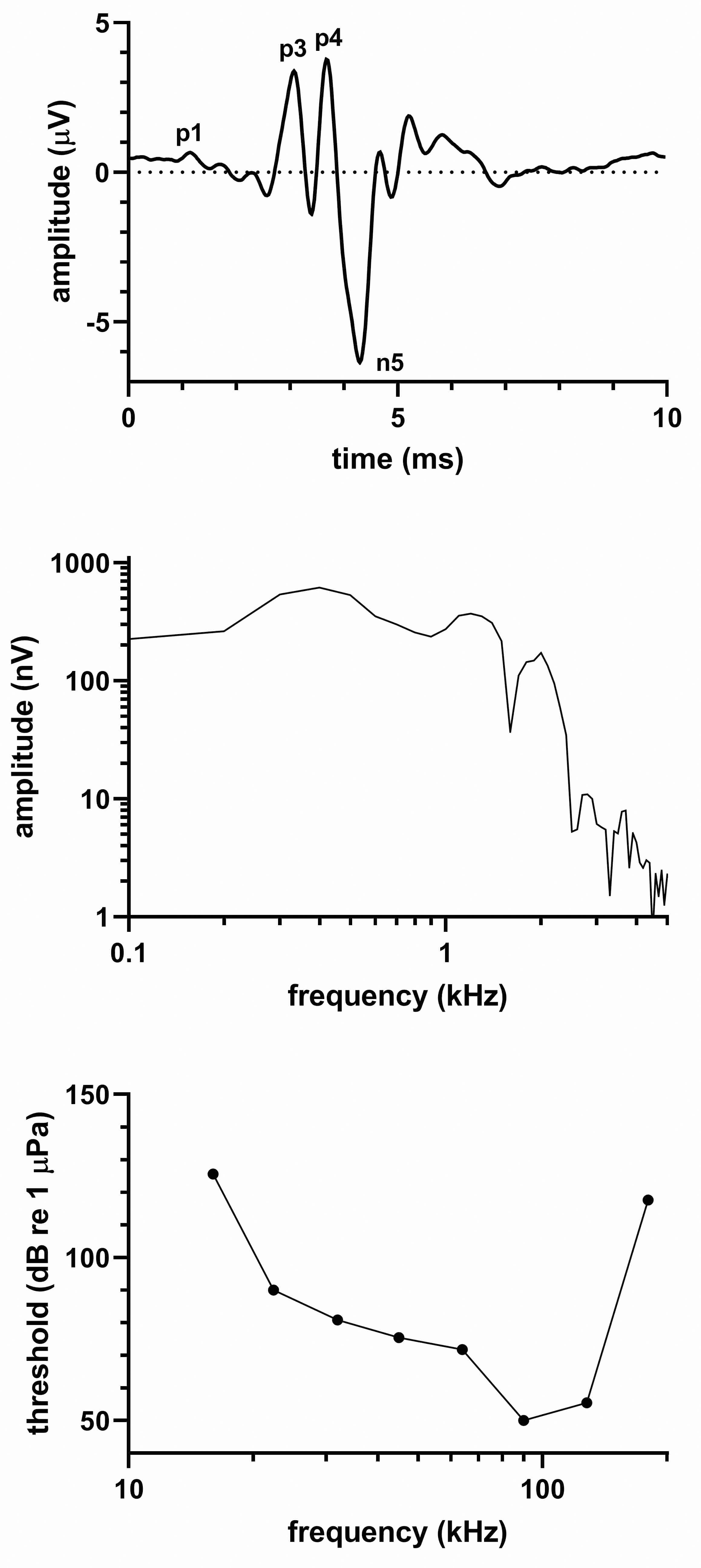
Figure 1 (top) Click-evoked ABR, (middle) frequency spectrum of the ABR waveform, and (bottom) audiogram for the dwarf sperm whale. The click-evoked ABR waveform is labeled as previously described (Popov and Supin, 1990).
The audiogram had a bandwidth typical of very high frequency hearing specialists (Southall et al., 2019). Good sensitivity (defined here as a threshold < 80 dB re 1 µPa) occurred between 45-128 kHz and the UFL was found to exceed the highest tested frequency of 180 kHz. Unlike a typical delphinid audiogram, a dip in the sensitivity curve (i.e. increased sensitivity) occurred at tested frequencies of 90 and 128 kHz where the animal had thresholds < 55 dB re 1 µPa.
3.2 Pygmy sperm whale
Figure 2 shows (top) click-evoked ABRs from three identical click series, (middle) frequency spectra of the respective click-evoked ABRs, and (bottom) the audiogram of the pygmy sperm whale. Latencies of the ABR peaks were 2.13 (P1), 2.93 (P3), 3.45 (P4), and 4.03 ms (N5), and the peak-peak amplitude of the averaged ABR was 8 µV. The click-evoked spectra frequency content spread upward to ~2 kHz, showed peaks at 600-700 Hz and 1.2 kHz, and a notch between the peaks at 1 kHz. The MRTF followed patterns observed in the click-evoked ABR spectra, showing peaks at 600 Hz and 1.2 kHz and a high-frequency cut-off between 2-3 kHz.
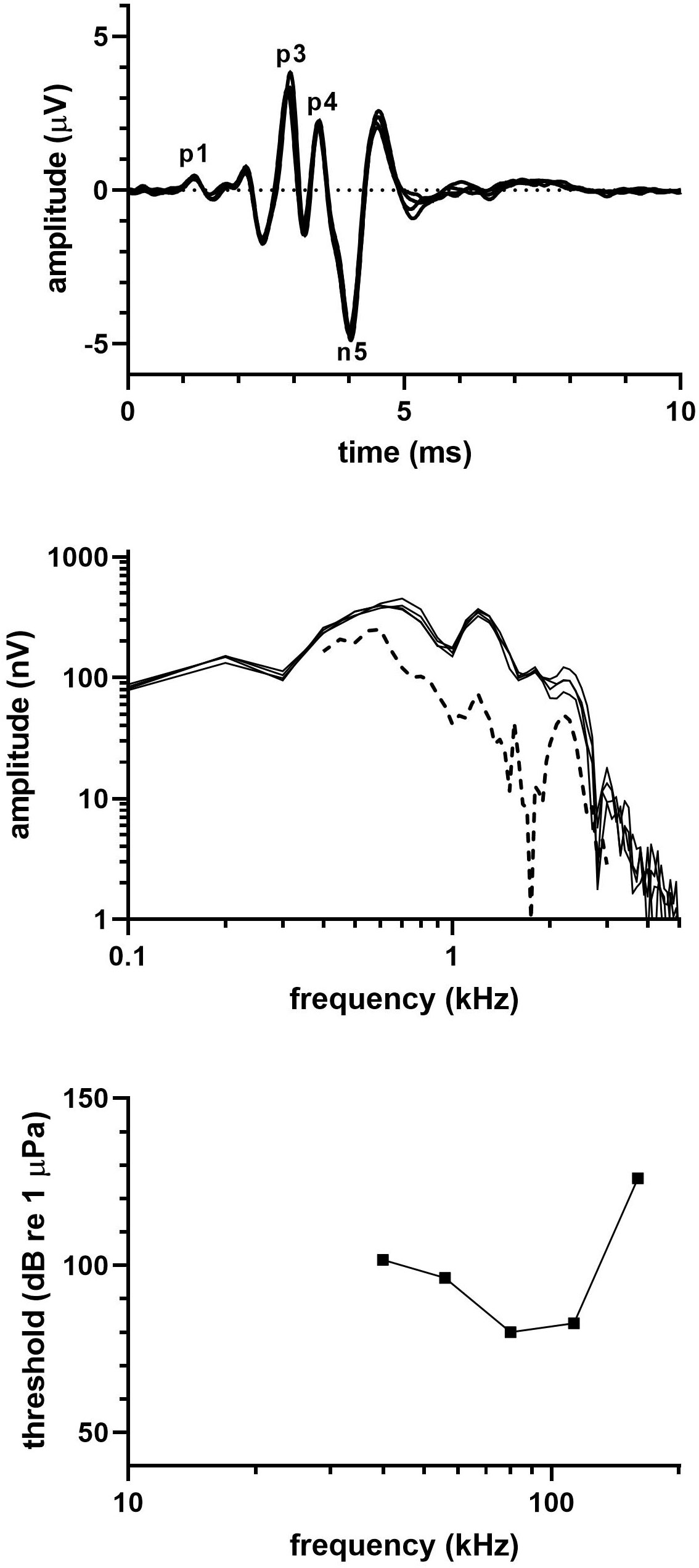
Figure 2 (top) Click-evoked ABR, (middle) frequency spectrum of the ABR waveform [solid line] and MRTF [dashed line], (bottom) and audiogram for the pygmy sperm whale.
A full audiogram was not obtained for the pygmy sperm whale. However, the partial audiogram appeared similar in shape to that of the dwarf sperm whale; although thresholds were higher than in the dwarf sperm whale, the region of best hearing sensitivity was similar (occurring at measured frequencies of 80 and 113 kHz). The estimated UFL was 153 kHz, although this was possibly an underestimate given that the thresholds appeared to be higher than expected for an animal of this age and size.
3.3 Melon-headed whale
Figure 3 shows (top) click-evoked ABRs from three click series, (middle) frequency spectra of the respective click-evoked ABRs, and (bottom) the audiogram of the melon headed whale. Latencies of the ABR peaks as determined from the averaged time-waveform were 1.50 (P1), 2.59 (P3), 3.27 (P4), and 3.64 ms (N5), and the peak-peak amplitude of the averaged ABR was 6.9 µV. The click-evoked ABR frequency content spread upward to ~2 kHz, showed peaks at 500 Hz and 1.2 kHz, and a notch between the peaks at 900 Hz. The MRTF showed amplitude peaks at 550 Hz and 1.05 kHz, and a high-frequency cut-off above ~2 kHz.
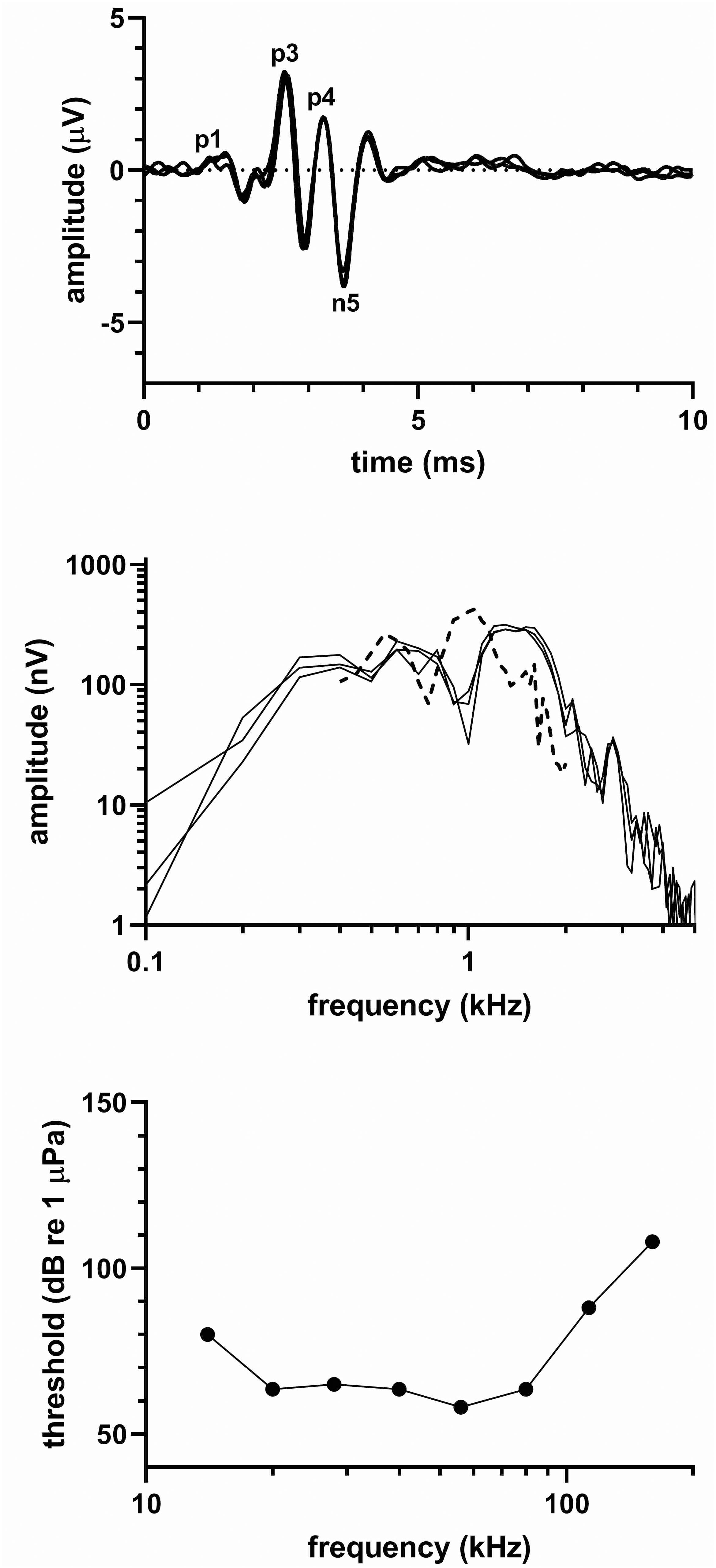
Figure 3 (top) Click-evoked ABR, (middle) frequency spectrum of the ABR waveform [solid line] and MRTF [dashed line], (bottom) and audiogram for the melon-headed whale.
The audiogram was delphinid in nature with thresholds <65 dB re 1 µPa across a two-octave range (20-80 kHz). The UFL exceeded 160 kHz, which was the highest frequency tested and which had a threshold of 108 dB re 1 µPa.
3.4 Northern right whale dolphin
Figure 4 shows (top) click-evoked ABRs from two click series, (middle) frequency spectra of the respective click-evoked ABRs, and (bottom) the audiogram of the northern right whale dolphin. Latencies of the ABR peaks as determined from the averaged time-waveform were 1.63 (P1), 2.30 (P3), 2.90 (P4), and 3.27 ms (N5), and the peak-peak amplitude of the averaged ABR was 11.5 µV. The click-evoked spectra frequency content spread upward to ~2-3 kHz, showed peaks at 700 Hz and 1.3 kHz, and a notch between the peaks at 1 kHz. The MRTF showed amplitude peaks at 600 Hz and 1.1 kHz and a high-frequency cut-off ~2 kHz.
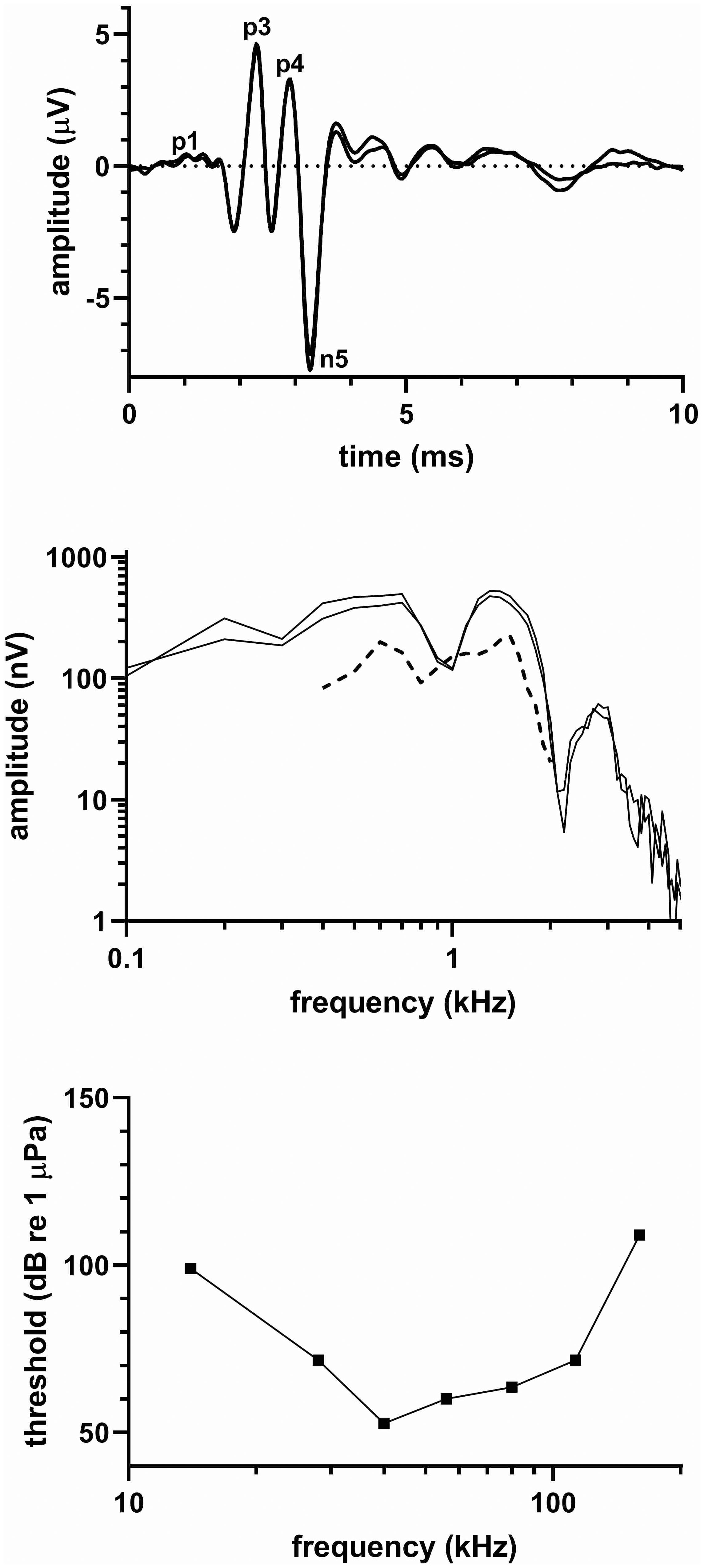
Figure 4 (top) Click-evoked ABR, (middle) frequency spectrum of the ABR waveform [solid line] and MRTF [dashed line], (bottom) and audiogram for the northern right whale dolphin.
The audiogram was delphinid in nature with thresholds <65 dB re 1 µPa from 40-80 kHz. The UFL exceeded 160 kHz, which was the highest frequency tested and which had a threshold of 109 dB re 1 µPa.
3.5 Long-beaked common dolphin
Figure 5 shows a (top left) click-evoked ABR from a single click series, (top right) the frequency spectrum of the click-evoked ABR, and (bottom left) the audiogram for one long-beaked common dolphin (Dc1304b); (bottom right) shows an audiogram from the additional long-beaked common dolphin (Dc1601b). Latencies of the ABR peaks were 1.28 (P1), 2.20 (P3), 2.77 (P4), and 3.12 ms (N5), and the peak-peak amplitude of the ABR was 12.5 µV. The click-evoked spectrum extended to ~2 kHz, showed peaks at 600 Hz and 1.3 kHz, and a notch between the peaks at 1 kHz.
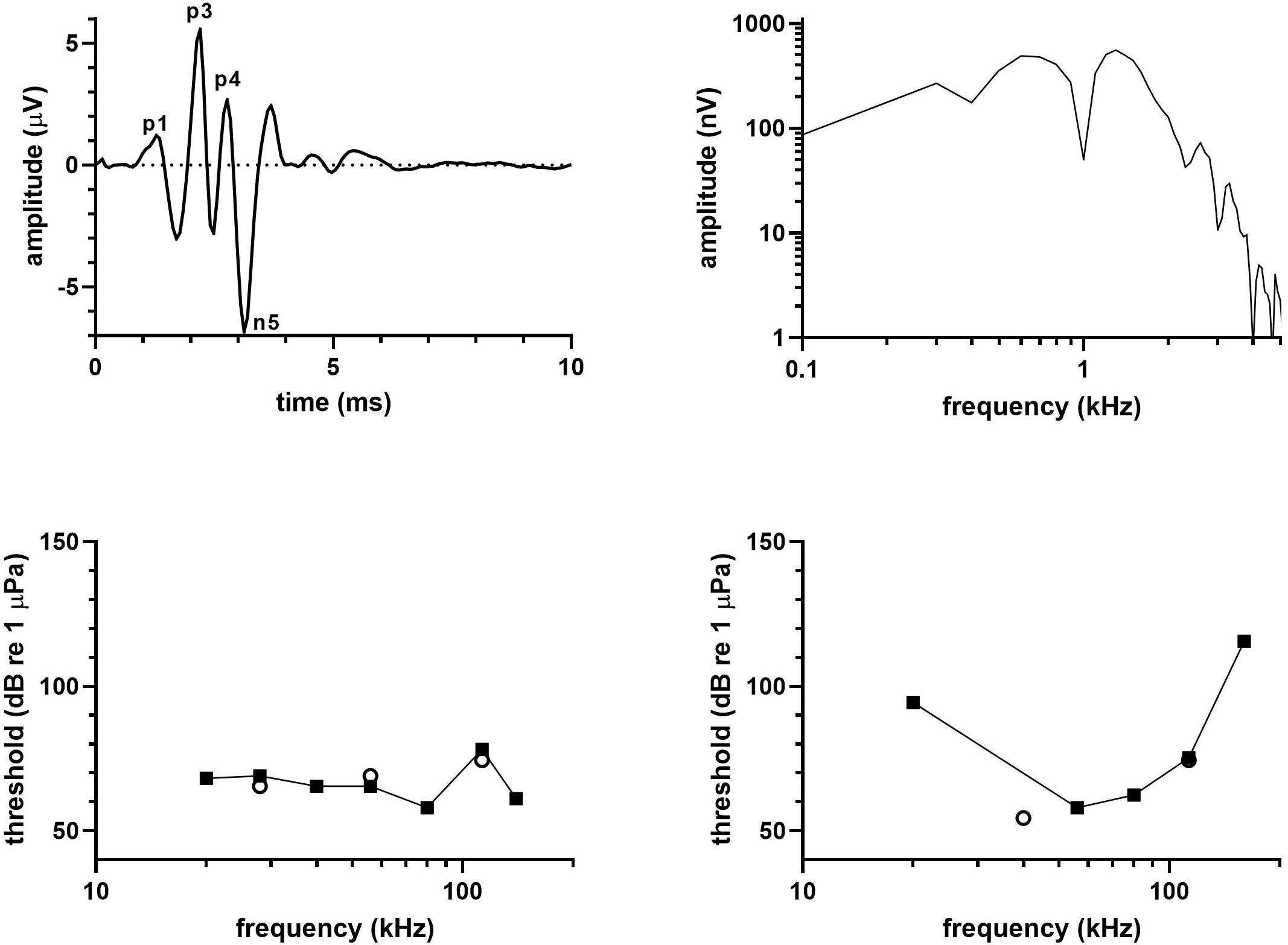
Figure 5 (top left) Click-evoked ABR, (top right) frequency spectrum of the ABR waveform, and (bottom left) audiogram for a long-beaked common dolphin (Dc1304b). (bottom right) Audiogram for an additional long-beaked common dolphin (Dc1601b) for which click-evoked ABRs were not obtained. In both bottom panels, open circles correspond to spot-checks of threshold with the contact transducer placed on the opposite side of the mandible.
The audiogram for the 3 yr-old long-beaked common dolphin (Dc1601b) was delphinid in nature with thresholds <65 dB re 1 µPa from 40-80 kHz. The UFL exceeded 160 kHz, which was the highest frequency tested and which had a threshold of 116 dB re 1 µPa. The audiogram exhibited the typical steep reduction in sensitivity as it approached the UFL, but this was not observed in the younger (1-2 yr-old) long-beaked common dolphin (Dc1304b). No UFL was identified in this animal either and thresholds <70 dB re 1 µPa were found across most of the range of hearing. Thresholds at several frequencies in the youngest animal and one frequency in the older animal were verified by placing the contact transducer on the pan opposite that used for the primary threshold determinations.
3.6 Atlantic spotted dolphin
Due to time constraints on sampling, no click-evoked data were obtained from the Atlantic spotted dolphin and the audiogram was determined through using both 4-SAM and 5-SAM ASSR methods. Figure 6 shows the audiogram from the Atlantic spotted dolphin. Lowest thresholds (67-70 dB re 1 µPa) were observed from 28 to 80 kHz, although a peculiar notch in hearing sensitivity was observed at 56 kHz (threshold=86 dB re 1 µPa). The UFL was estimated at 149 kHz.
3.7 Pygmy killer whale
Figure 7 shows the (a) click-evoked ABR from a single click series and (b) the frequency spectrum of the click-evoked ABR for the pygmy killer whale (31IMMS). The bottom panel of Figure 7 shows the audiogram from both rehabilitated pygmy killer whales plus the audiograms of two pygmy killer whales previously tested (Montie et al., 2011). Latencies of the ABR peaks were 1.43 (P1), 2.45 (P3), 3.10 (P4), and 3.48 ms (N5), and the peak-peak amplitude of the ABR was 11.5 µV. The click-evoked ABR frequency content spread upward to ~2 kHz, showed peaks at 400 Hz and 1.2 kHz, and a notch between the peaks at 900 Hz.
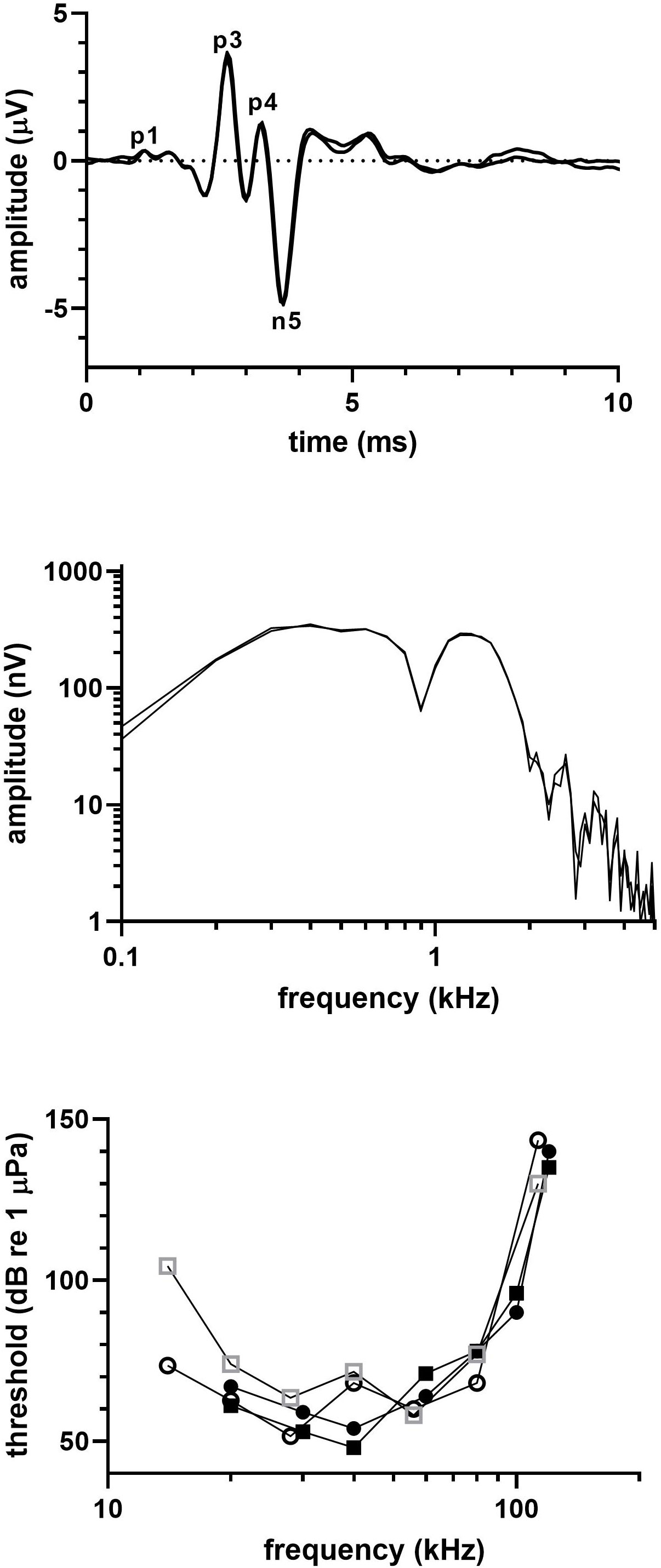
Figure 7 (top) Click-evoked ABR and (middle) frequency spectrum of the ABR waveform for a pygmy killer whale. (bottom) Audiograms for the two pygmy killer whales tested in this study (open square=30IMMS, open circle=31IMMS), as well as the audiograms of two pygmy killer whales previously tested (filled symbols; Montie et al., 2011).
The audiograms were typical of delphinid audiograms. Thresholds were <70 dB re 1 µPa from 20-80 kHz in one animal (31IMMS), but somewhat less sensitive in the other (30IMMS) with thresholds <80 dB re 1 µPa across the same range. The estimated UFL was 103 kHz in 30IMMS and 107 kHz in 31 IMMS, which was at least ½ octave lower than the other species tested.
4 Discussion
This paper presents evoked potential audiograms for six cetacean species for which no prior audiograms existed: the dwarf sperm whale, pygmy sperm whale, northern right whale dolphin, melon-headed whale, Atlantic spotted dolphin, and the long-beaked common dolphin. Audiograms for the pygmy killer whale complemented the only two other audiograms that exist for the species. The audiograms of the different delphinid species were generally consistent with respect to frequency range, shape of the audiogram, and UFL, with few exceptions, although the kogiids demonstrated some distinguishing characteristics potentially unique to their genus.
4.1 Pygmy and dwarf sperm whale
Dwarf and pygmy sperm whales are narrow-band high-frequency (NBHF) species with echolocation click center frequencies recorded between 120-130 kHz, root mean squared bandwidths of ~3 kHz, and a half-power beamwidth of ~9 degrees (Marten, 2000; Malinka et al., 2021b). Though deep-diving species, the echolocation behavior of the kogiids is more closely aligned with that of NBHF porpoise and dolphin species that inhabit nearshore, cluttered environments, possibly as an adaptation to avoid detection by predatory killer whales or to capitalize on low ocean noise levels above 100 kHz (Madsen et al., 2005). The audiograms of the kogiids diverge from the delphinids measured here in that the region of best sensitivity ranged from 80-128 kHz, which is consistent with sensitivity at the frequencies at which NBHF echolocation occurs. Unfortunately, only a partial audiogram was obtained from the pygmy sperm whale and the thresholds were higher than expected based on experience with similar sized delphinids and the results obtained from the dwarf sperm whale. Thus, it cannot be ruled out that the pygmy sperm whale might have had some underlying pathology affecting hearing, or that the optimal placement of the contact transducer was not achieved, especially considering the unusual jaw morphologies in these species and that the jawphones were placed in different locations.
Ridgway and Carder (2001) previously attempted to collect tone-pip evoked ABRs from a pygmy sperm whale. The waveforms obtained were noisy and difficult to replicate, but the overall latencies of major waves, likely P4 and N5, were similar to the latencies observed in the current study. The latencies of waves P3, P4 and N5 observed in this study were longer than those observed in the delphinids, for what are probably homologous waves. The increased latency was most pronounced between P4 and N5; the average P4-N5 inter-wave latency in the kogiids was 0.60 + 0.02 ms, while in the delphinids from this study it was 0.37 + 0.01 ms. Measurements derived from data available for other species, or reported in the literature, include: harbor porpoise (Phocoena phocoena; n=1) = 0.34 ms (Mulsow et al., 2018); common dolphin (Delphinus delphis, n=14) = 0.34 + 0.05; killer whale (Orcinus orca; n=4) = 0.74 + 0.07 ms (Lucke et al., 2016); short-finned pilot whale (Globicephala macrorhynchus; n=1) = 0.6 ms (Schlundt et al., 2011); and Gervais’ beaked whale (Mesoplodon europaeus, n=1) 0.5 ms (Finneran et al., 2009). The longer P4-N5 inter-wave latency in the Gervais’ beaked whale (725 kg), pilot whale (823 kg), and killer whales (1500-3500 kg) might be explained by the larger mass of the animals and the concomitant changes in the size of the brain. However, in the absence of pathology, the longer inter-wave latencies in the kogiids relative to comparably sized or larger delphinids/phocoenids suggests a normal yet slower neurological transmission through the midbrain (e.g. lateral lemniscus and inferior colliculus). We hypothesize that this finding might be related to the echolocation behavior of the kogiids (and possibly other deep diving species). As a group, the deep diving beaked whales and kogiids feed at depths of hundreds of meters and demonstrate long inter-click intervals (ICIs; 200-400 ms) indicative of tracking prey at greater ranges than is typical of shallow-diving delphinids and porpoises (Johnson et al., 2004a; Zimmer et al., 2005; Johnson et al., 2006; Malinka et al., 2021b). It is feasible that the differences in the P4-N5 inter-wave latency reflects some unknown adaptation toward regular echolocation in the deep ocean environment, potentially enabling the encoding of time differences between click production and echo reception across durations not typically observed in shallow-diving odontocetes. “Regular” echolocation can be defined as that in which the ICI exceeds the acoustic two-way travel time between the echolocator and its target of interest, presumably to avoid range ambiguity in target range estimation (Ladegaard et al., 2019). In the bottlenose dolphin (Tursiops truncatus), an odontocete that typically exploits shallower coastal environments, regular echolocation changes into a mode of click “packet” production once the ICI exceeds ~100-150 ms (Finneran, 2013; Ladegaard et al., 2019). In other words, the bottlenose dolphin cannot maintain an ICI beyond some critical time interval while generating regular echolocation clicks, while the kogiids and beaked whales do so regularly. Unfortunately, there are few available published click-evoked ABR waveforms available for deep-diving species and the concomitant increase in the mass of most deep-diving species could be a confounding factor in demonstrating any relationship between mid-brain latencies and echolocation behavior.
4.2 The delphinids
The audiograms of the remaining odontocetes, all of which were delphinids, were generally similar given the expected variability in threshold measurements obtained with previous AEP measurements (Finneran and Houser, 2006; Houser and Finneran, 2006a). Excluding the pygmy killer whales (and one of the long-beaked common dolphins for which the UFL was not defined), the audiograms of the non-kogiid odontocetes all showed the typical delphinid pattern with high UFLs (defined as an AEP threshold of 120 dB) at frequencies > ~150 kHz. In contrast, the pygmy killer whales had slightly lower UFLs; animals in this study had UFLs of 103 and 107 kHz, whereas previously tested animals had estimated UFLs of 112 kHz (Montie et al., 2011). The UFLs are similar to the UFLs of killer whales (Branstetter et al., 2017), long-finned and short-finned pilot whales (Globicephala melas and G. mactorhynchus, Pacini et al., 2010; Schlundt et al., 2011), and a Gervais’ beaked whale (Finneran et al., 2009). It has been hypothesized that the hearing range of odontocetes might scale inversely with odontocete mass (Schlundt et al., 2011). However, if this is the case, the pygmy killer whales deviate from the trend as they have a mass typical of mid-sized odontocetes (e.g., similar to bottlenose dolphins).
4.3 MRTFs and click-evoked spectra
An electrophysiological MRTF describes the relationship between the spectral amplitude of an auditory steady state response and the rate of amplitude modulation. It has been collected in numerous odontocete cetaceans (Dolphin et al., 1995; Supin and Popov, 1995a; Klishin et al., 2000; Mooney et al., 2006; Finneran et al., 2007b; Mooney et al., 2009; Smith et al., 2018), and the relationship between the ASSR and the amplitude modulation rate has been proposed as means of investigating the resolution of temporal processing (Viemeister, 1979; Viemeister and Plack, 1993; Supin and Popov, 1995b). MRTFs were obtained for three novel species in this study – the pygmy sperm whale, melon-headed whale, and northern right whale dolphin. Each MRTF had a characteristic low-pass filter shape with a low-pass cutoff between 2-3 kHz, similar to what has been observed in other odontocetes (Dolphin, 1995; Dolphin et al., 1995; Popov and Klishin, 1998; Klishin et al., 2000; Cook et al., 2006; Mooney et al., 2006; Finneran et al., 2009; Mooney et al., 2009; Linnenschmidt et al., 2012; Smith et al., 2018). (Note, however, that 2 kHz was the maximum modulation rate tested in the pygmy sperm whale and northern right whale dolphin).
In instances where time is of the essence and an MRTF cannot be collected, it is possible to use the spectrum of the click-evoked ABR to approximate the MRTF (Supin and Popov, 1995b; Finneran et al., 2007b), albeit with some caveats. Specifically, the spectrum of the click-evoked response does not necessarily exhibit amplitude peaks at the same frequencies of the MRTF, although they are similar. In this study, the amplitude peaks of both the MRTF and the spectrum of the click-evoked response were similar in the northern right whale dolphin (700 Hz and 1.3 kHz) and the pygmy sperm whale (600 Hz and 1.2 kHz), but were offset for the melon-headed whale. Amplitude peaks in the melon-headed whale MRTF were 550 Hz and 1.05 kHz, whereas the amplitude peaks of the click-evoked spectra were 600 Hz and 1.3 kHz. Differences between the functions are possibly a result of different frequency resolutions (the MRTF had a 50-Hz frequency resolution, whereas the click-evoked spectra had a frequency resolution of 100 Hz), but might also exist due to the carrier frequency used for the MRTF or the level of the click used to elicit the click-evoked response. Differences in the location of MRTF amplitude peaks have been observed to vary slightly as a function of the carrier frequency, although the overall low-pass filter shape remains intact (Finneran et al., 2007b). Similarly, clicks emitted at stimulus levels closer to the threshold of detection appear to produce ABRs with spectra closer to that obtained with SAM tone-derived MRTFs (Supin and Popov, 1995b).
All of the species tested in this study demonstrated peaks in the click-evoked ABR spectrum at frequencies between 400-600 Hz and 1.2-1.3 kHz. As an estimate of the MRTF, this shows a broad commonality across a diverse group of cetacean species, all of which echolocate. It has been presumed that the rapid temporal processing observed in these toothed whales is related to the ability to echolocate (e.g., Mooney et al., 2006), although no assessment of temporal processing has yet been performed in a non-echolocating cetacean (ie., baleen whale) for comparison. Nevertheless, the commonality among the odontocetes suggests an auditory system adapted to rapid temporal processing that supports regular echolocation under differing ecological conditions (e.g., short-range (<100 m) echolocation in shallow-water and cluttered environments, and long-range (hundreds of meters) echolocation in deep-water environments), as well as the terminal buzz observed prior to prey capture or in interactions with targets during echolocation research tasks (Johnson et al., 2004b; Johnson et al., 2006; Wisniewska et al., 2014; Wisniewska et al., 2015; Malinka et al., 2021a).
4.4 Caveats
The thresholds measured in the AEP hearing tests presented here are likely biased to some extent by the calibration procedure. The calibration distance of 15-cm, which was established based on adult bottlenose dolphin anatomy (Finneran and Houser, 2006; Houser and Finneran, 2006a), likely results in an error of the true threshold when applied to smaller species or neonates of species with adult morphology comparable to that of the adult bottlenose dolphin. This would occur as a result of a shorter distance between the transmitter and receiver (i.e. the auditory bulla), as well as the relationship between the brain and body mass of a species (i.e. far-field potentials decrease inversely with the distance from the dipole source, Supin et al., 2001). The degree to which these two phenomena affect threshold estimates will vary as a function of subject species and age; nevertheless, threshold estimates should be sufficiently close to the true threshold of the subject such that species hearing characteristics can be determined in small to mid-sized odontocetes.
5 Conclusions
The use of AEP methods to test the hearing of small cetaceans has long been promoted as a means of acquiring data on cetacean species for which no data exist. These species are not maintained under human care and are generally only accessible as a result of stranding. The data presented here are a realization of the idea that portable AEP systems could be used to rapidly test the hearing of stranded or rehabilitating toothed whales. Implemented in partnership with the national stranding network and rehabilitation facilities, six novel odontocete species were subject to AEP hearing tests enabling the first audiograms for these species to be obtained. Additional data collected on the pygmy killer whale further bolstered confidence in earlier hearing tests performed in this species. Information from the click-evoked ABR, and in some species the MRTF, provided additional insight into the temporal processing capabilities of toothed whales and how they might be impacted by ecological constraints.
Data availability statement
The raw data supporting the conclusions of this article will be made available by the authors, without undue reservation.
Ethics statement
The animal study was reviewed and approved by Institutional Animal Care and Use Committee of the National Marine Mammal Foundation.
Author contributions
DH obtained funding to support the project, collected and analysed data, and was the primary author of the manuscript. LN, EF, JM and JF collected data and contributed to the writing of the article. All authors contributed to the article and approved the submitted version.
Funding
This work was funded in part under a contract from the U.S. Navy Living Marine Resources (contract #N39430-15-R-1667). Publication charges were paid for through the generous support of the Jeanette Fuller Ridgway Scientific Writing Fund.
Acknowledgments
The authors wish to give special thanks to all of the facilities involved with the rehabilitation and testing of the animals reported in this paper. Specifically, the authors wish to thank Dr. Moby Solangi and Dr. Deborah Moore of the Institute for Marine Mammal Studies (Gulfport, MS), Jon Peterson and Dr. Michelle Davis of SeaWorld Orlando (Orlando, FL), and Dr. Elsburgh Clarke, Dr. Hendrik Nollens, Dr. Todd Schmitt and Jody Westberg of SeaWorld San Diego (San Diego, CA). Additional thanks are given to all of the support personnel that supported the animals during the hearing tests. Thanks, are also given to Dr. Teri Rowles of NOAA for her support and assistance in enabling this research effort. This is scientific contribution #343 of the National Marine Mammal Foundation.
Conflict of interest
Author LN was employed by Earth Resources Technology Inc.
The remaining authors declare that the research was conducted in the absence of any commercial or financial relationships that could be construed as a potential conflict of interest.
Publisher’s note
All claims expressed in this article are solely those of the authors and do not necessarily represent those of their affiliated organizations, or those of the publisher, the editors and the reviewers. Any product that may be evaluated in this article, or claim that may be made by its manufacturer, is not guaranteed or endorsed by the publisher.
References
Adams D. A., Mcclelland R. J., Houston H. G., Gamble W. G. (1985). The effects of diazepam on the auditory brain stem responses. Br. J. Audiol. 19, 277–280. doi: 10.3109/03005368509078984
American National Standards Institute (ANSI) (2018). “Procedure for determining audiograms in toothed whales through evoked potential methods,” in Accredited standards committee S3/SC 1, animal bioacoustics (New York, NY: Acoustical Society of America).
Branstetter K., Finneran J. J., Houser D. S. (2008). Frequency and level dependent masking of the multiple auditory steady-state response in the bottlenose dolphin (Tursiops truncatus). J. Acoustical Soc. America 123, 2928–2935. doi: 10.1121/1.2896753
Branstetter B. K., St. Leger J., Acton D., Stewart J., Houser D., Finneran J. J., et al. (2017). Killer whale (Orcinus orca) behavioral audiograms. J. Acoustical Soc. America 141, 2387–2398. doi: 10.1121/1.4979116
Brill R. L., Moore P. W. B., Dankiewicz L. A. (2001). Assessment of dolphin (Tursiops truncatus) auditory sensitivity and hearing loss using jawphones. J. Acoustical Soc. America 109, 1717–1722. doi: 10.1121/1.1356704
Cook M. L. H., Varela R. A., Goldstein J. D., Mcculloch S. D., Bossart G. D., Finneran J. J., et al. (2006). Beaked whale auditory evoked potential hearing measurements. J. Comp. Physiol. A. 192, 489–495. doi: 10.1007/s00359-005-0086-1
Dobie R. A., Wilson M. J. (1996). A comparison of t test, F test, and coherence methods of detecting steady-state auditory-evoked potentials, distortion-product otoacoustic emissions, or other sinusoids. J. Acoustical Soc. America 100, 2236–2246. doi: 10.1121/1.417933
Dolphin W. F. (1995). “Steady-state auditory evoked potentials in three cetacean species elicited using amplitude-modulated stimuli,” in Sensory systems of aquatic mammals. Eds. Kastelein R. A., Thomas J. A., Nachtigall P. E. (Woerden, The Netherlands: De Spil Publishers).
Dolphin W. F., Au W. W. L., Nachtigall P. E., Pawloski J. (1995). Modulation rate transfer functions to low-frequency carriers in three species of cetaceans. J. Comp. Physiol. A. 177, 235–245. doi: 10.1007/BF00225102
Elberling C., Wahlgreen O. (1985). Estimation of auditory brainstem response, ABR, by means of Bayesian inference. Scandinavian Audiol. 14, 89–96. doi: 10.3109/01050398509045928
Finneran J. J. (2009). Evoked response study tool: a portable, rugged system for single and multiple auditory evoked potential measurements. J. Acoustical Soc. America 126, 491–500. doi: 10.1121/1.3148214
Finneran J. J. (2013). Dolphin ‘packet’ use during long-range echolocation tasks. J. Acoustical Soc. America 133, 1796–1810. doi: 10.1121/1.4788997
Finneran J. J. (2020). Conditioned attenuation of dolphin monaural and binaural auditory evoked potentials after preferential stimulation of one ear. J. Acoustical Soc. America 147, 2302–2313. doi: 10.1121/10.0001033
Finneran J. J., Houser D. S. (2006). Comparison of in-air evoked potential and underwater behavioral hearing thresholds in four bottlenose dolphins (Tursiops truncatus). J. Acoustical Soc. America 119, 3181–3192. doi: 10.1121/1.2180208
Finneran J. J., Houser D. S. (2007). Bottlenose dolphin (Tursiops truncatus) steady-state evoked responses to multiple simultaneous sinusoidal amplitude modulated tones. J. Acoustical Soc. America 121, 1775–1782. doi: 10.1121/1.2431330
Finneran J. J., Houser D. S., Blasko D., Hicks C., Hudson J., Osborn M. (2008). Estimating bottlenose dolphin (Tursiops truncatus) hearing thresholds from single and multiple simultaneous auditory evoked potentials. J. Acoustical Soc. America 123, 542–551. doi: 10.1121/1.2812595
Finneran J. J., Houser D. S., Mase-Guthrie B., Ewing R. Y., Lingenfelser R. G. (2009). Auditory evoked potentials in a stranded gervais’ beaked whale (Mesoplodon europaeus). J. Acoustical Soc. America 126, 484–490. doi: 10.1121/1.3133241
Finneran J. J., Houser D. S., Schlundt C. E. (2007a). Objective detection of bottlenose dolphin (Tursiops truncatus) steady-state auditory evoked potentials in response to AM/FM tones. Aquat. Mammals 33, 43–54. doi: 10.1578/AM.33.1.2007.43
Finneran J. J., London H. R., Houser D. S. (2007b). Modulation rate transfer functions in bottlenose dolphins (Tursiops truncatus) with normal hearing and high-frequency hearing loss. J. Comp. Physiol. A. 193, 835–843. doi: 10.1007/s00359-007-0238-6
Finneran J. J., Mulsow J., Houser D. S. (2013). Auditory evoked potentials in a bottlenose dolphin during moderate-range echolocation tasks. J. Acoustical Soc. America 134, 4532–4547. doi: 10.1121/1.4826179
Finneran J. J., Schlundt C. E., Branstetter B., Dear R. L. (2007c). Assessing temporary threshold shift in a bottlenose dolphin (Tursiops truncatus) using multiple simultaneous auditory evoked potentials. J. Acoustical Soc. America 122, 1249–1264. doi: 10.1121/1.2749447
Griffin J. P. (1988). Drug-induced ototoxicity. Br. J. Audiol. 22, 195–210. doi: 10.3109/03005368809076453
Hotz M. A., Ritz R., Linder L., Scollo-Lavizzari G., Haefeli W. E. (2000). Auditory and electroencephalographic effects of midazolam and α-hydroxy-midazolam in healthy subjects. Br. J. Clin. Pharmacol. 49, 72–79. doi: 10.1046/j.1365-2125.2000.00104.x
Houser D. S., Finneran J. J. (2006a). A comparison of underwater hearing sensitivity in bottlenose dolphins (Tursiops truncatus) determined by electrophysiological and behavioral methods. J. Acoustical Soc. America 120, 1713–1722. doi: 10.1121/1.2229286
Houser D. S., Finneran J. J. (2006b). Variation in the hearing sensitivity of a dolphin population obtained through the use of evoked potential audiometry. J. Acoustical Soc. America 120, 4090–4099. doi: 10.1121/1.2357993
Houser D. S., Gomez-Rubio A., Finneran J. J. (2008). Evoked potential audiometry of 13 pacific bottlenose dolphins (Tursiops truncatus gilli). Mar. Mammal Sci. 24, 28–41. doi: 10.1111/j.1748-7692.2007.00148.x
Houser D. S., Moore K. M. T., Sharp S. M., Hoppe J. M., Finneran J. J. (2018). Cetacean evoked potential audiometry by stranding networks enables more rapid accumulation of hearing information in stranded odontocetes. J. Cetacean Res. Manage. 18, 93–101.
Johnson M., Madsen P. T., Zimmer W. M. X., Aguilar De Soto N., Tyack P. L. (2004a). Beaked whales echolocate on prey. Proc. R. Soc. London B. 271, S383–S386. doi: 10.1098/rsbl.2004.0208
Johnson M., Madsen P. T., Zimmer W. M. X., Aguilar De Soto N., Tyack P. (2006). Foraging blainville’s beaked whales (Mesoplodon densitorstris) produce distinct click types matched to different phases of echolocation. J. Exp. Biol. 209, 5038–5050. doi: 10.1242/jeb.02596
Johnson M., Madsen P. T., Zimmer W. M. X., De Soto N. A., Tyack P. L. (2004b). Beaked whales echolocate on prey. Proc. R. Soc. B.: Biol. Sci. 271 Suppl 6, S383–S386. doi: 10.1098/rsbl.2004.0208
Klishin V. O., Popov V. V., Supin A. Y. (2000). Hearing capabilities of a beluga whale, Delphinapterus leucas. Aquat. Mammals 26, 212–228.
Ladegaard M., Mulsow J., Houser D. S., Jensen F. H., Johnson M., Madsen P. T., et al. (2019). Dolphin echolocation behaviour during active long-range target approaches. J. Exp. Biol. 222, jeb189217. doi: 10.1242/jeb.189217
Linnenschmidt M., Wahlberg M., Hansen J. D. (2012). The modulation rate transfer function of a harbour porpoise (Phocoena phocoena). J. Comp. Physiol. A. 199, 115–126. doi: 10.1007/s00359-012-0772-8
Lucke K., Finneran J. J., Almunia J., Houser D. S. (2016). Variability in click-evoked potentials in killer whales (Orcinus orca) and identification of a hearing impaired whale. Aquat. Mammals 42, 184–192. doi: 10.1578/AM.42.2.2016.184
Møhl B., Au W. W. L., Pawloski J., Nachtigall P. E. (1999). Dolphin hearing: Relative sensitivity as a function of point of application of a contact sound source in the jaw and head region. J. Acoustical Soc. America 105, 3421–3424. doi: 10.1121/1.426959
Madsen P. T., Carder D. A., Bedholm K., Ridgway S. H. (2005). Porpoise clicks from a sperm whale nose – convergent evolution of 130 kHz pulses in toothed whale sonars? Bioacoustics 15, 195–206. doi: 10.1080/09524622.2005.9753547
Malinka C. E., Rojano-Doñate L., Madsen P. T. (2021a). Directional biosonar beams allow echolocating harbour porpoises to actively discriminate and intercept closely-spaced targets. J. Exp. Biol. doi: 10.1242/jeb.242779
Malinka C. E., Tønnesen P., Dunn C. A., Claridge D. E., Gridley T., Elwen S. H., et al. (2021b). Echolocation click parameters and biosonar behaviour of the dwarf sperm whale (Kogia sima). J. Exp. Biol. 224. doi: 10.1242/jeb.240689
Mann D., Hill-Cook M., Manire C., Greenhow D., Montie E., Powell J., et al. (2010). Hearing loss in stranded odontocete dolphins and whales. PloS One 5, e13824. doi: 10.1371/journal.pone.0013824
Marten K. (2000). Ultrasonic analysis of pygmy sperm whale (Kogia breviceps) and hubbs’ beaked whale (Mesoplodon carlhubbsi) clicks. Aquat. Mammals 26, 45–48.
Montie E. W., Manire C. A., Mann D. A. (2011). Live CT imaging of sound reception anatomy and hearing measurements in the pygmy killer whale, Fresa attenuata. J. Exp. Biol. 214, 945–955. doi: 10.1242/jeb.051599
Mooney T. A., Castellote M., Quakenbush L., Hobbs R., Gaglione E., Goertz C. (2018). Variation in hearing within a wild population of beluga whales (Delphinapterus leucas). J. Exp. Biol. 221, 1–13. doi: 10.1242/jeb.171959
Mooney T. A., Nachtigall P. E., Taylor K. A., Rasmussen M. H., Miller L. A. (2009). Auditory temporal resolution of a wild white-beaked dolphin (Lagenorhynchus albirostris). J. Comp. Physiol. A. 195, 375–384. doi: 10.1007/s00359-009-0415-x
Mooney T. A., Nachtigall P. E., Yuen M. M. L. (2006). Temporal resolution of the risso’s dolphin, grampus griseus, auditory system. J. Comp. Physiol. A. 192, 373–380. doi: 10.1007/s00359-005-0075-4
Moore P. W. B., Pawloski D. A., Dankiewicz L. (1995). “Interaural time and intensity difference thresholds in the bottlenose dolphin (Tursiops truncatus),” in Sensory systems of aquatic mammals. Eds. Kastelein R. A., Thomas J. A., Nachtigall P. E. (Woerden, The Netherlands: De Spil Publishers).
Mulsow J., Finneran J. J., Houser D. S., Nordstrom C., Barrett-Lennard L. G., Burkard R. F. (2018). Click reception in the harbor porpoise (Phocoena phocoena): Effects of electrode and contact transducer location on the auditory brainstem response. J. Acoustical Soc. Am. 143, 2076–2084. doi: 10.1121/1.5030921
Nachtigall P. E., Mooney T. A., Taylor K. A., Miller L. A., Rasmussen M. H., Akamatsu T., et al. (2008). Shipboard measurements of the hearing of the white-beaked dolphin Lagenorhynchus albirostris. J. Exp. Biol. 211, 642–647. doi: 10.1242/jeb.014118
Nachtigall P. E., Supin A. Y. (2013). A false killer whale reduces its hearing sensitivity when a loud sound is preceded by a warning. J. Exp. Biol. 216, 3062–3070. doi: 10.1242/jeb.085068
Nachtigall P. E., Yuen M. M. L., Mooney T. A., Taylor K. A. (2005). Hearing measurements from a stranded infant risso’s dolphin, Grampus griseus. J. Exp. Biol. 208, 4181–4188. doi: 10.1242/jeb.01876
National Research Council (NRC) (2005). Marine mammal populations and ocean noise (Washington, DC: National Academies Press).
Pacini A. F., Nachtigall P. E., Kloepper L. N., Linnenschmidt M., Sogorb A., Matias S. (2010). Audiogram of a formerly stranded long-finned pilot whale (Globicephala melas) measured using auditory evoked potentials. J. Exp. Biol. 213, 3138–3143. doi: 10.1242/jeb.044636
Pacini A. F., Nachtigall P. E., Quintos C. T., Schofield T. D., Look D. A., Levine G. A., et al. (2011). Audiogram of a stranded blainville’s beaked whale (Mesoplodon densirostris) measured during auditory evoked potentials. J. Exp. Biol. 214, 2409–2415. doi: 10.1242/jeb.054338
Popov V. V., Klishin V. O. (1998). EEG Study of hearing in the common dolphin, Delphinus delphis. Aquat. Mammals 24, 13–20.
Popov V., Supin A. Y. (1990). “Electrophysiological studies of hearing in some cetaceans and a manatee,” in Sensory abilities in cetaceans. Eds. Thomas J. A., Kastelein R. A. (New York: Plenum Press).
Popov V. V., Supin A. Y., Wang D., Wang K. (2006). Nonconstant quality of auditory filters in the porpoises, Phocoena phocoena and Neophocaena phocaenoides (Cetacea, phocoenidae). J. Acoustical Soc. America 119, 3173–3180. doi: 10.1121/1.2184290
Ridgway S. H., Carder D. A. (2001). Assessing hearing and sound production in cetaceans not available for behavioral audiograms: Experiences with sperm, pygmy sperm, and gray whales. Aquat. Mammals 27, 267–276.
Schlundt C. E., Dear R. L., Houser D. S., Bowles A. E., Reidarson T., Finneran J. J. (2011). Auditory evoked potentials in two short-finned pilot whales (Globicephala macrorhynchus). J. Acoustical Soc. America 129, 1111–1116. doi: 10.1121/1.3531875
Smith A. B., Pacini A. F., Nachtigall P. E. (2018). Modulation rate transfer functions from four species of stranded odontocete (Stenella longirostris, feresa attenuata, globicephala melas, and mesoplodon densirostris). J. Comp. Physiol. A. 204, 377–389. doi: 10.1007/s00359-018-1246-4
Southall B. L., Finneran J. J., Reichmuth C., Nachtigall P. E., Ketten D. R., Bowles A. E., et al. (2019). Marine mammal noise exposure criteria: Auditory weighting functions and TTS/PTS onset. Aquat. Mammals 45, 125–232. doi: 10.1578/AM.45.2.2019.125
Strobel M. M., Houser D. S., Moore K. T., Clough P. L., Davis M. R., Staggs L. A., et al. (2017). Auditory evoked potentials and behavioral considerations with hearing loss in small cetaceans: Application as a standard diagnostic test in health assessment. J. Zoo Wildife Med. 48, 979–986. doi: 10.1638/2017-0045R.1
Supin A. Y., Popov V. (1995a). “Frequency tuning and temporal resolution in dolphins,” in Sensory systems of aquatic mammals. Eds. Kastelein R. A., Thomas J. A., Nachtigall P. E. (Woerden, The Netherlands: De Spil Publishers).
Supin A. Y., Popov V. V. (1995b). Envelope-following response and modulation transfer function in the dolphin’s auditory system. Hearing Res. 92, 38–46. doi: 10.1016/0378-5955(95)00194-8
Supin A. Y., Popov V. V., Mass A. M. (2001). The sensory physiology of aquatic mammals (Boston: Kluwer Academic Publishers).
Taylor K. A., Nachtigall P. E., Mooney T. A., Supin A. Y., Yuen M. M. L. (2007). A portable system for the evaluation of the auditory capabilities of marine mammals. Aquat. Mammals 33, 93–99. doi: 10.1578/AM.33.1.2007.93
Viemeister N. F. (1979). Temporal modulation transfer functions based upon modulation thresholds. J. Acoustical Soc. America 66, 1364–1380. doi: 10.1121/1.383531
Viemeister N. F., Plack C. J. (1993). “Time analysis,” in Human psychophysics. Eds. Yost W. A., Popper A. N., Fay R. R. (New York: Springer-Verlag).
Wang Z.-T., Li J., Duan P.-X., Mei Z.-G., Niu F.-Q., Akamatsu T., et al. (2020). Evoked-potential audiogram variability in a group of wild Yangtze finless porpoises (Neophocaena asiaeorientalis asiaeorientalis). J. Comp. Physiol. A. 206, 527–541. doi: 10.1007/s00359-020-01426-6
Wisniewska D. M., Johnson M., Nachtigall P. E., Madsen P. T. (2014). Buzzing during biosonar-based interception of prey in the delphinids Tursiops truncatus and Pseudorca crassidens. J. Exp. Biol. 217, 4279–4282. doi: 10.1242/jeb.113415
Wisniewska D. M., Ratcliffe J. M., Beedholm K., Christensen C. B., Johnson M., Koblitz J. C., et al. (2015). Range-dependent flexibility in the acoustic field of view of echolocating porpoises (Phocoena phocoena). Elife 4, e05651. doi: 10.7554/eLife.05651.016
Keywords: hearing, odontocete, toothed whale, auditory evoked potential, audiogram
Citation: Houser DS, Noble L, Fougeres E, Mulsow J and Finneran JJ (2022) Audiograms and click spectra of seven novel and seldom-tested odontocetes. Front. Mar. Sci. 9:984333. doi: 10.3389/fmars.2022.984333
Received: 01 July 2022; Accepted: 08 December 2022;
Published: 21 December 2022.
Edited by:
Mark Meekan, Australian Institute of Marine Science (AIMS), AustraliaReviewed by:
Aude Pacini, University of Hawaii at Manoa, United StatesDmitry Nechaev, Severtsov Institute of Ecology and Evolution (RAS), Russia
Copyright © 2022 Houser, Noble, Fougeres, Mulsow and Finneran. This is an open-access article distributed under the terms of the Creative Commons Attribution License (CC BY). The use, distribution or reproduction in other forums is permitted, provided the original author(s) and the copyright owner(s) are credited and that the original publication in this journal is cited, in accordance with accepted academic practice. No use, distribution or reproduction is permitted which does not comply with these terms.
*Correspondence: Dorian S. Houser, dorian.houser@nmmf.org