- 1State Key Laboratory of Tropical Oceanography, South China Sea Institute of Oceanology, Chinese Academy of Sciences, Guangzhou, China
- 2Southern Marine Science and Engineering Guangdong Laboratory, Guangzhou, China
- 3Innovation Academy of South China Sea Ecology and Environmental Engineering, Chinese Academy of Sciences, Guangzhou, China
- 4College of Earth and Planetary Science, University of Chinese Academy of Sciences, Beijing, China
- 5Daya Bay Marine Biology Research Station, Chinese Academy of Sciences, Shenzhen, China
- 6Guangdong Zhihuan Innovative Environmental Technology Co. Ltd., Guangzhou, China
- 7Guangdong Marine Development Planning Research Center, Guangzhou, China
- 8Guangzhou Chemical Reagent Factory, Guangzhou, China
Mangroves are unique forest communities with an abundance of species, high productivity and high ecological, social and economic value. Evaluation of the stress resistance of mangrove plants has mainly focused on the effects of high salinity, heavy metals and flooding, with fewer studies evaluating resistance to upwelling stress. Mangrove species of Avicennia marina, Aegiceras corniculatum and Kandelia obovata were submitted to three temperature upwelling (5, 10 and 15°C) and several physiological and biochemical parameters were measured at six time points (0, 6, 12, 24, 72 and 168 h). The data demonstrated: a certain amount of damage occurred to mangrove plants in the face of prolonged upwelling; different mangrove plants have different response strategies to upwelling; mangrove plants are not sensitive to different upwelling temperatures; the resistance of mangrove plants to upwelling stress was in the following order: A. marina< K. obovata< A. corniculatum. Markers of damage such as relative electrical conductivity (REC), malondialdehyde (MDA) and reactive oxygen species (ROS) among all mangrove species were significantly higher with prolonged upwelling stress. The contents of photosynthetic pigments in all three mangrove species also increased. Superoxide dismutase activity (SOD) was maintained at a high level in both control and treatment groupss. By contrary, the change of peroxidase activity (POD) of A. marina and K. obovata was larger than that of A. corniculatum. Catalase activity (CAT) in A. marina and K. obovata significantly increased under upwelling at both 5 and 10°C while there was no obvious variation of CAT in A. corniculatum. Soluble protein and Soluble sugar contents showed no clear variation but stayed at fairly high levels. However, proline content in A. corniculatum significantly increased under long-term upwelling stress while this was not the case in the other two species. High correlation could be observed between A. marina and MDA, O2- and POD in PCA while A. corniculatum showed association with proline and soluble sugar. In conclusion, the ability of A. corniculatum to tolerate upwelling stress might be due mainly to increases in the activities of SOD and the inducing of proline biosynthesis, while, A marina and K. obovata tolerated upwelling stress by adjusting activity levels of SOD, POD and CAT. Segregation in both principal component analysis (PCA) and hierarchical cluster analysis (HCA) further indicated different tolerances and resistances to upwelling between the three species. Our study provides new insights into the stress response of mangroves to upwelling.
1 Introduction
Mangroves are trees or large shrubs, including ferns and a single species of palm, which normally grow in or adjacent to the intertidal zone and which have developed special adaptations for this environment (Mark Spalding, 2010). Known as “the Earth’s Tropical Coast Guard”, mangroves play several ecological roles including providing habitats for wildlife, stabilizing sediment, protecting shorelines and sequestering carbon (Walters et al, 2008; Simpson et al, 2019). Distributed in regions of warm temperatures, mangroves are susceptible to temperature variation. Studies of mangrove resistance to low temperatures have been widely reported (Peereman et al, 2021; Lu et al, 2021). The development of mangroves in Qinzhou Bay over the past 3000 years showed that low air temperature causes mangrove degradation by impacting their diversity, productivity and area (Zhang et al, 2021). It was also reported that cold event resulted in decreases in seedling growth rates and net ecosystem CO2 uptake in mangroves (Song et al., 2020). However, most studies of mangrove stressors have focused on low air temperatures induced by extreme climate events; studies on low water temperatures induced by ocean currents are rare. As a coastal ecosystem, mangroves are substantially affected by changing ocean currents such as upwelling activity (Ximenes et al, 2021). Upwelling is an oceanic process which transports cold and saline water to the surface (Wang et al, 2015). Exposure to water at low temperatures can harm the formation of mangrove propagules (Rachel Collin, 2016). Through harming the development of mangrove propagules, upwelling affected the distribution of mangrove plants (Ximenes et al., 2018). Low-temperature seawater caused by upwelling poses a threat to mangrove plants. In our previous study, Kandelia obovata (a mangrove plant) showed oxidative damage under upwelling stress with higher contents of hydrogen peroxide (H2O2) and malondialdehyde (MDA) (Li et al, 2022). Previous studies have also reported that low temperatures could impair water and nutrient intake, stimulate reactive oxygen species (ROS) production and disturb normal photosynthesis and metabolism (Ensminger et al, 2006; Viswanathan et al, 2007; Wang et al, 2019).
Plants mediate the adverse effects of temperature downshifts through stress tolerance defense mechanisms. The primary mechanisms involved in low temperature stress are related to the activation of ROS scavenger systems, alterations in protein and sugar synthesis, proline accumulations and photosynthesis changes (Theochairs et al, 2012). Under chilling, olives exhibited increased catalase (CAT), superoxide dismutase (SOD) and peroxidase (POD) activity to protect and stabilize cell membranes and structures, adapting to the environment (Petruccelli et al., 2022). The accumulation of osmoregulatory substances (e.g., soluble sugar, soluble protein, and proline) significantly increased cytosol concentration and prevented excessive protoplasmic dehydration, which enhanced cold tolerance (Jiang et al., 2022). Moreover, increasing chlorophyll contents to produce more carbon-based defensive chemicals could alleviate low temperature stress (Agathokleous et al, 2020).
Avicennia marina, Aegiceras corniculatum and Kandelia obovata are dominant mangrove species in South China, and it has been reported that these three species show different resistances to low temperature (Lin, 1997; Geng et al, 2021). The leaves of Avicennia marina suffered more serious damages than Aegiceras corniculatum and Kandelia obovata under 2008 chilling event in southern China (Chen et al, 2017). Besides, the distribution of mangroves on tidal zone reflects their adaptation to environmental changes (Ariel E. Lugo, 1974). Thus, low-tide zone mangroves (A. marina and A. corniculatum) and middle-tide zone mangrove (K. obovata) were chosen. In the present study, we investigated the physiological and biochemical characteristics of these three mangrove species to upwelling stress. This research focused on investigating the response mechanism of different mangrove species to upwelling, aiding understanding about the stress resistance of mangroves under upwelling, and providing a theoretical basis for mangrove protection. In detail, the objectives of the study were to:
(1) investigate indexes of injury including the membrane damage indicator (relative electrical conductivity and malonaldehyde content) and ROS production (superoxide anion radical and hydrogen peroxide) of three mangrove species under upwelling stresses of 5, 10 and 15°C at time points of 0, 6, 12, 24, 72 and 168 h of exposure.
(2) investigate indexes of protection including photosynthetic pigments (chlorophyll a, chlorophyll b, and carotenoid), antioxidant enzyme activities (superoxide dismutase, peroxidase, and catalase) and osmotic regulation substances (proline, soluble sugar and soluble protein) of three mangrove species during upwelling at water temperatures of 5, 10 and 15°C at time points of 0, 6, 12, 24, 72 and 168 h.
(3) employ principal component analysis (PCA) and hierarchical cluster analysis (HCA) to compare different responses to upwelling stress among different mangrove species.
2 Materials and methods
2.1 Plant materials and treatments
Healthy propagules of A. marina, A. corniculatum and K. obovata were collected form the nursey of Qi’ao Island Mangrove Nature Reserve in Guangdong. Seedlings were planted in clean sand and fertilized with 1/2 Hoagland’s solution. Three months later, the seedlings were subjected to upwelling stress. The upwelling stress conditions were as follow: water temperature (5, 10 and 15°C), measured at multiple time points (0, 6, 12, 24, 72 and 168 h), in each case at the same air temperature (25°C) and water salinity (20 psu), 20,000 light densities regularly, and 70% humidity. Among that, 0h is the blank control of the experiment. The experimental conditions were set based on the actual situation in upwelling zone and appropriate modifications were made in order to obtain significant changes in indoor simulation (Jianyu Hu, 2016; Burchard et al, 2017). Fresh leaves were collected for determination of physiological and biochemical indexes and each index had three repetitions.
2.2 Determination method
2.2.1 Determination of pigments
Chlorophyll a (Chl a), chlorophyll b (Chl b) and carotenoid (Caro) contents were detected by spectrophotometry (UV-1780, SHIMADZU, Japan). Leaf samples (0.2 g) were cut into 2-mm pieces and transferred to tubes with 20 ml solution of 95% ethanol for 24 h until the tissue faded. The content of photosynthetic pigments was determined at wavelengths of 665, 649 and 470 nm (Li et al, 2021).
2.2.2 Relative electrical conductivity
REC was measured using an electrical conductivity meter (DDB-303A, INESA, China), following (Zhang L. X. et al, 2021) with a slight modification. Leaf samples were washed with distilled water and then placed in tubes with 10 ml of distilled water. After 3 hours kept at room temperature, the initial electrical conductivity (R1) was measured. Then the samples were boiled for 20 min at 100°C. After cooling, the electrical conductivity was measured again (R2). REC was calculated as the percentage ratio of R1/R2.
2.2.3 Determination of superoxide anion radical
The content of O2- was measured using the hydroxylamine oxidation method with an assay kit (for plants) purchased from YaJi Biological Institute (Shanghai, China). The content was calculated from the reaction of O2- with hydroxylamine solution under acidic conditions (Yang et al, 2020).
2.2.4 Determination of soluble protein, soluble sugar and proline
The SP content of the mangrove leaves was determined in accordance with the spectrophotometry method of (M.Bradford, 1976) using bovine serum albumin as the calibration standard. SS content was measured using anthrone colorimetry (Feng et al, 2019). The concentration of proline was determined according to ninhydrin colorimetry (Khoma et al, 2021).
2.2.5 Extraction and assay of lipid peroxidation, hydrogen peroxide and antioxidant enzymes
Fresh leaf samples (roughly 1.0 g) were ground into powder in liquid nitrogen and then homogenized with 0.1 mol/L Tris hydrochloride buffer (PH 7.8). Subsequently, the homogenate was subjected to centrifugation at 3500 rpm at 4°C for 20 min, and then the supernatant was cryopreserved for determination. The level of lipid peroxidation in mangrove leaves was expressed as malondialdehyde (MDA) content, according to the thiobarbituric acid (TBA) method (Md. Jahirul Islam, 2020).
The hydrogen peroxide (H2O2) content was determined using the absorption of titanium peroxide complex at 405nm. (Wang et al, 2020). Superoxide dismutase (SOD) activity was measured at 560 nm with the nitro blue tetrazolium (NBT) photoreduction method (Wu et al, 2020). Peroxidase (POD) activity was estimated based on the absorbance of guaiacol oxidation at 470 nm while catalase (CAT) activity was measured as the decrease rate in the absorbance of H2O2 (Li et al, 2013).
2.3 Statistical analyses
One-way analysis of variance (ANOVA) and post hoc multiple mean comparisons (Tukey’s test) were carried out to determine the significance of differences in each parameter, using SPSS (version 23). Data were presented as mean ± standard deviation (SD). P<0.05 was interpreted as indicating a significant difference between the control and treatment groupss. The responses of three mangrove plants to upwelling stress were profiled through hierarchical cluster analysis (HCA) and principal component analysis (PCA). PCA was conducted in Origin (version 2021) and HCA was performed in R (3.4.4).
3. Results
3.1 Effect of upwelling stress on the content of chlorophylls and carotenoids
As demonstrated in Figure 1, pigment increases were observed in all three mangrove species under upwelling stress. The contents of chl a, chl b and caro in leaves of A. marina and A. corniculatum slightly decreased at first and then increased. The contents of pigments in A. marina changed significantly under short term upwelling stress (6–24 h) (p<0.05). For A. corniculatum, chlorophyll and carotenoid contents changed significantly in a short period of time under 5°C upwelling while under 10 and 15°C they changed significantly in the later period (after 72h) (p<0.05). At 5°C, the pigment contents of K. obovata significantly increased during 6 h of exposure (p<0.05) while there was no significant difference in pigment contents during later time periods. By contrast, under 10 and 15°C upwellings, in K. obovata the pigment contents fluctuated.
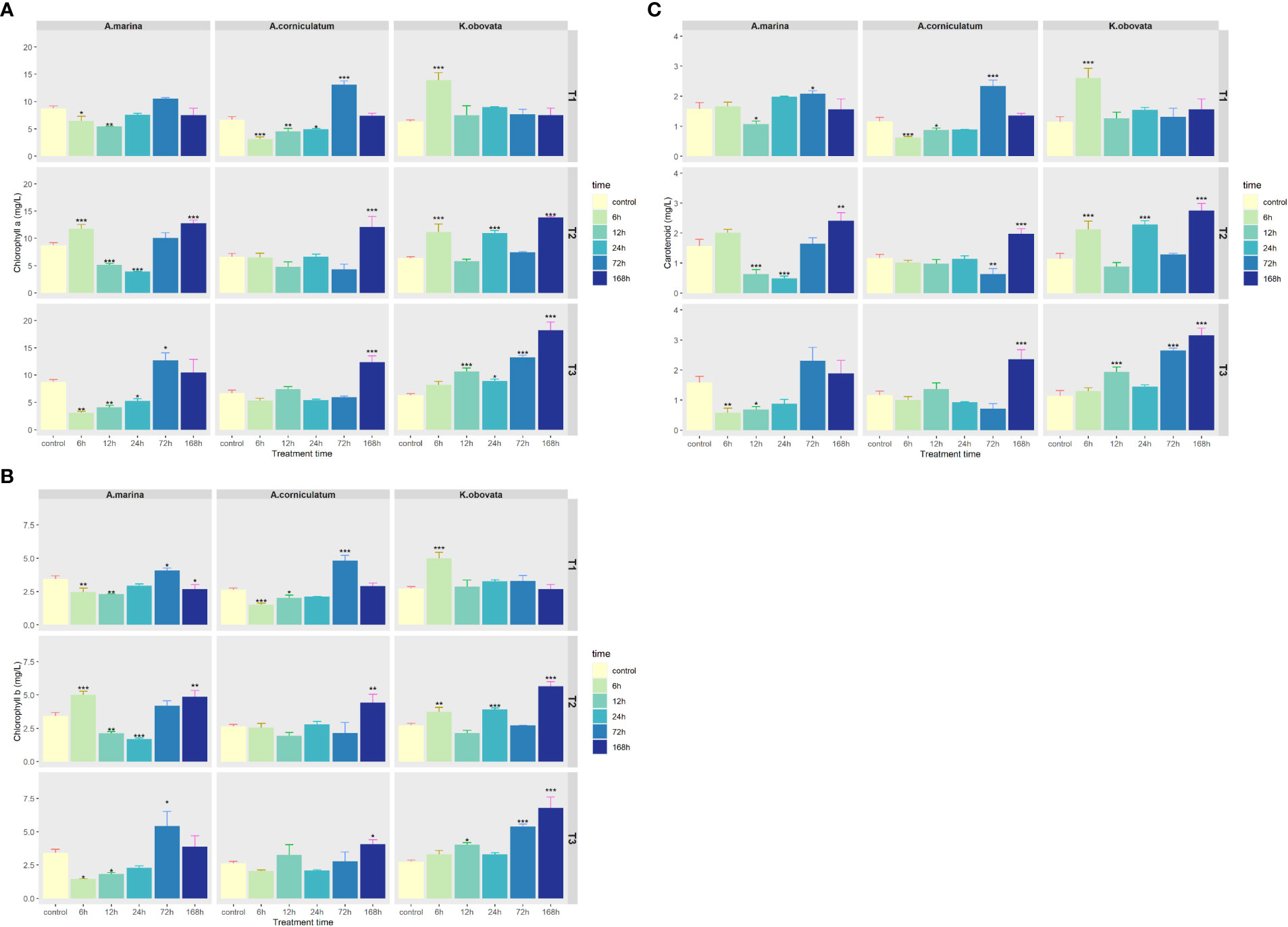
Figure 1 The changes of (A) Chlorophyll a, (B) Chlorophyll b and (C) Carotenoid contents under different upwelling stresses. * indicates that there are significant differences between control and treatment groups (p<0.05(*), p<0.01(**), p<0.001(***)). “T1” means 5°C upwelling stress, “T2” means 10°C upwelling stress and “T3” means 15°C upwelling stress.
3.2 Effect of upwelling stress on membrane systems
As shown in Figure 2A, RECs of A. marina first decreased and then increased over treatment time. RECs of A. corniculatum and K. obovata increased with increasing treatment time.
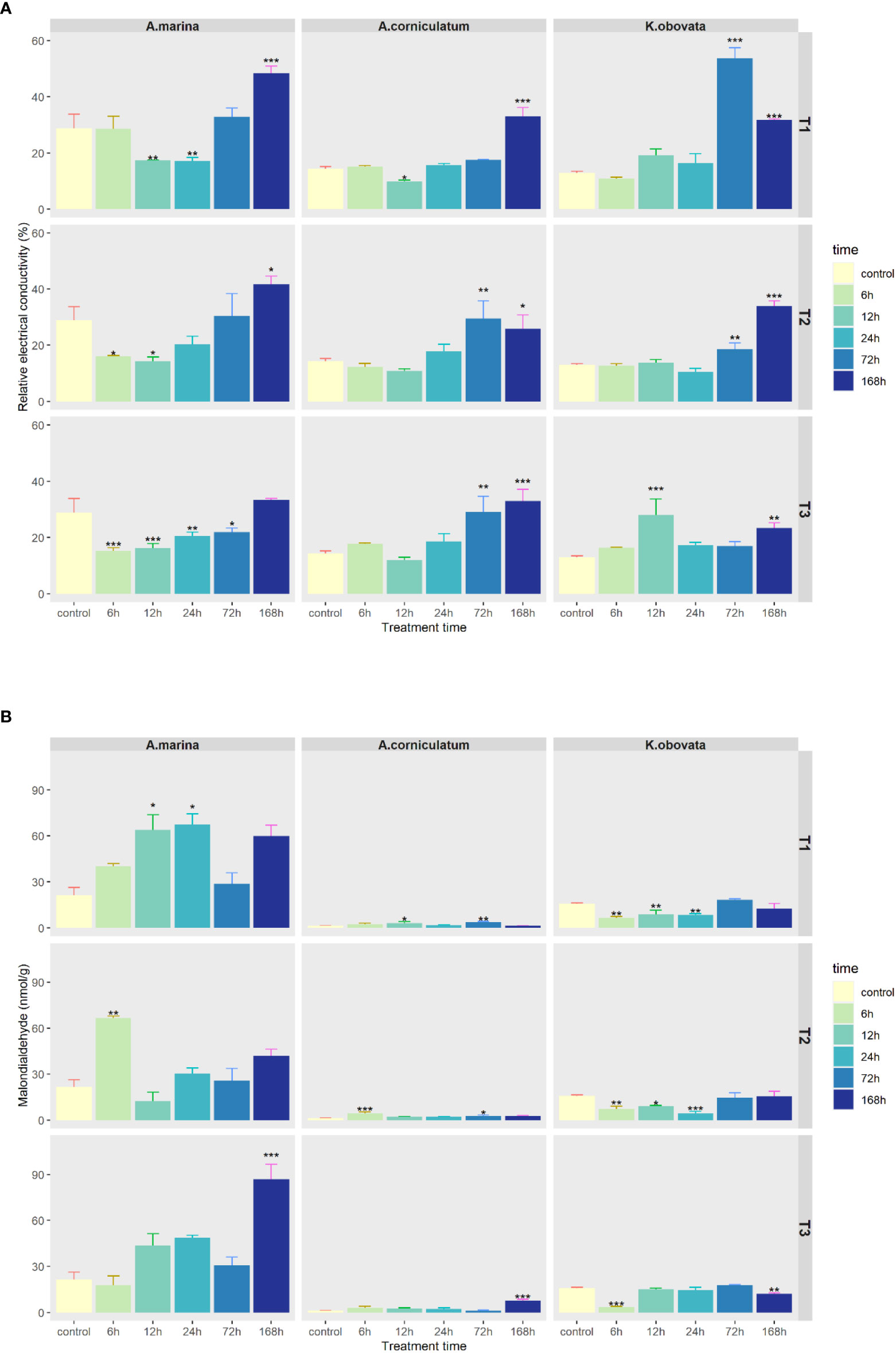
Figure 2 Changes of (A) Relative electrical conductivity and (B) Malondialdehyde content under upwelling stress. * indicates that there are significant differences between control and treatment groups (p<0.05(*), p<0.01(**), p<0.001(***)). “T1” means 5°C upwelling stress, “T2” means 10°C upwelling stress and “T3” means 15°C upwelling stress.
As shown in Figure 2B, the MDA contents in mangrove species were in the following decreasing order: A. marina (21.43–86.75 nmol/g), K. obovata (3.40–18.18 nmol/g), A. corniculatum (1.33–7.54 nmol/g). The MDA content of A. marina significantly increased under upwelling (p<0.05). Moreover, the MDA of A. marina in upwellings at 5 and 10°C reached a peak at 6–24 h of treatment, while at 15°C, the peak showed at 168 h. Accumulation of MDA in A. corniculatum occurred with a prolonged period of stress, while MDA decreased over time in K. obovata.
3.3 Effect of upwelling stress on the production of ROS
Changes of ROS in three mangrove plants under upwelling treatments are shown in Figure 3. The amount of O2- in each plant was in the following descending order: A. marina (2439.04–4810.27 μm/g), K. obovata (74.78–784.06 μm/g), A. corniculatum (38.79–674.09 μm/g) (Figure 3A). Compared to the control, O2- content significantly increased under upwelling in A. marina (p<0.05). The content of O2- in K. obovata decreased at first and then increased. The O2- content of A. corniculatum only significantly increased at 6 h under 5°C upwelling stress (p<0.05), while under 10 and 15°C there was no apparent change.
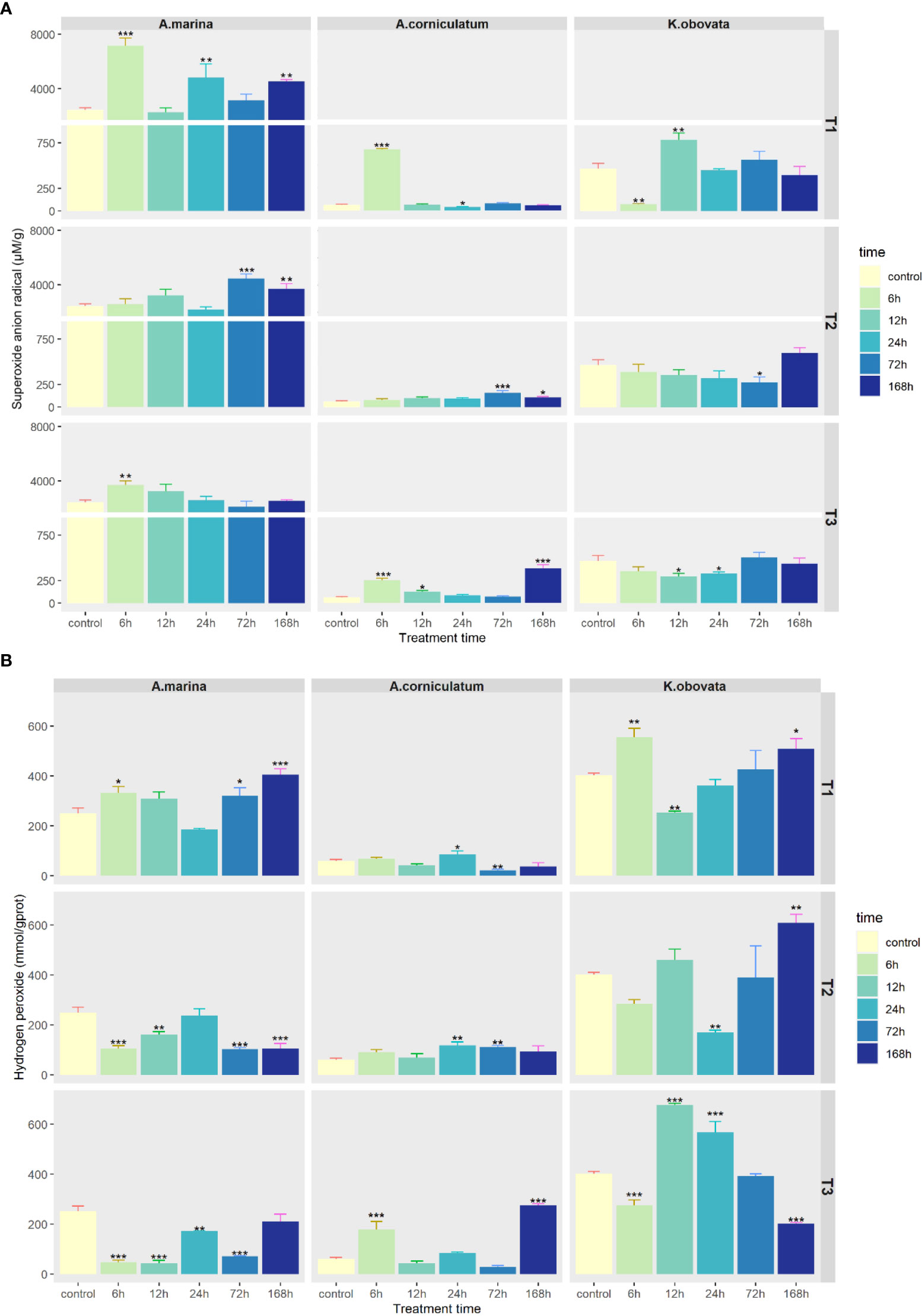
Figure 3 Changes of (A) Superoxide anion radical and (B) Hydrogen peroxide contents under upwelling stress. * indicates that there are significant differences between control and treatment groups [p<0.05(*), p<0.01(**), p<0.001(***)]. “T1” means 5°C upwelling stress, “T2” means 10°C upwelling stress and “T3” means 15°C upwelling stress.
Responses of H2O2 content to upwelling are shown in Figure 3B. H2O2 contents in A. marina under 5°C upwelling significantly increased, while under 10 and 15°C, they were significantly lower than the control (p<0.05). H2O2 content of A. corniculatum significantly decreased in the later period of the 5°C-upwelling treatment and significantly increased in the later period of the 10°C and 15°C upwelling periods (p<0.05). The H2O2 content of K. obovata first reduced and then generally rose under the 5 and 10°C upwellings. Under the 15°C upwelling, the H2O2 content of K. obovata ultimately decreased, and was lower than that observed in the control.
3.4 Effect of upwelling stress on the activity of antioxidant enzymes
The activities of some antioxidant enzymes (CAT, POD, SOD) during upwelling were investigated and are shown in Figure 4. The SOD activity of three mangrove species is basically the same (Figure 4A). There is no significant change in SOD under 5°C upwelling for A. marina. Under 10 and 15°C upwelling, the SOD of A. marina first decreased, and then significantly increased after 72 h (p<0.05). Exposed to upwelling, the activity of SOD in A. corniculatum and K. obovata declined slightly. Changes of POD were most obvious in A. marina, followed by K. obovata and A. corniculatum (Figure 4B). The POD of A. marina significantly increased with duration of upwelling (p<0.05). The POD of A. corniculatum significantly rosed for 6 and 72 h under a 5°C upwelling while showing a declining trend under 10 and 15°C upwellings (p<0.05). The POD of K. obovata first decreased and then significantly increased for 168 h under a 5°C upwelling (p<0.05). Under 10 and 15°C treatments, changes of POD showed a trend of increasing first and then decreasing.
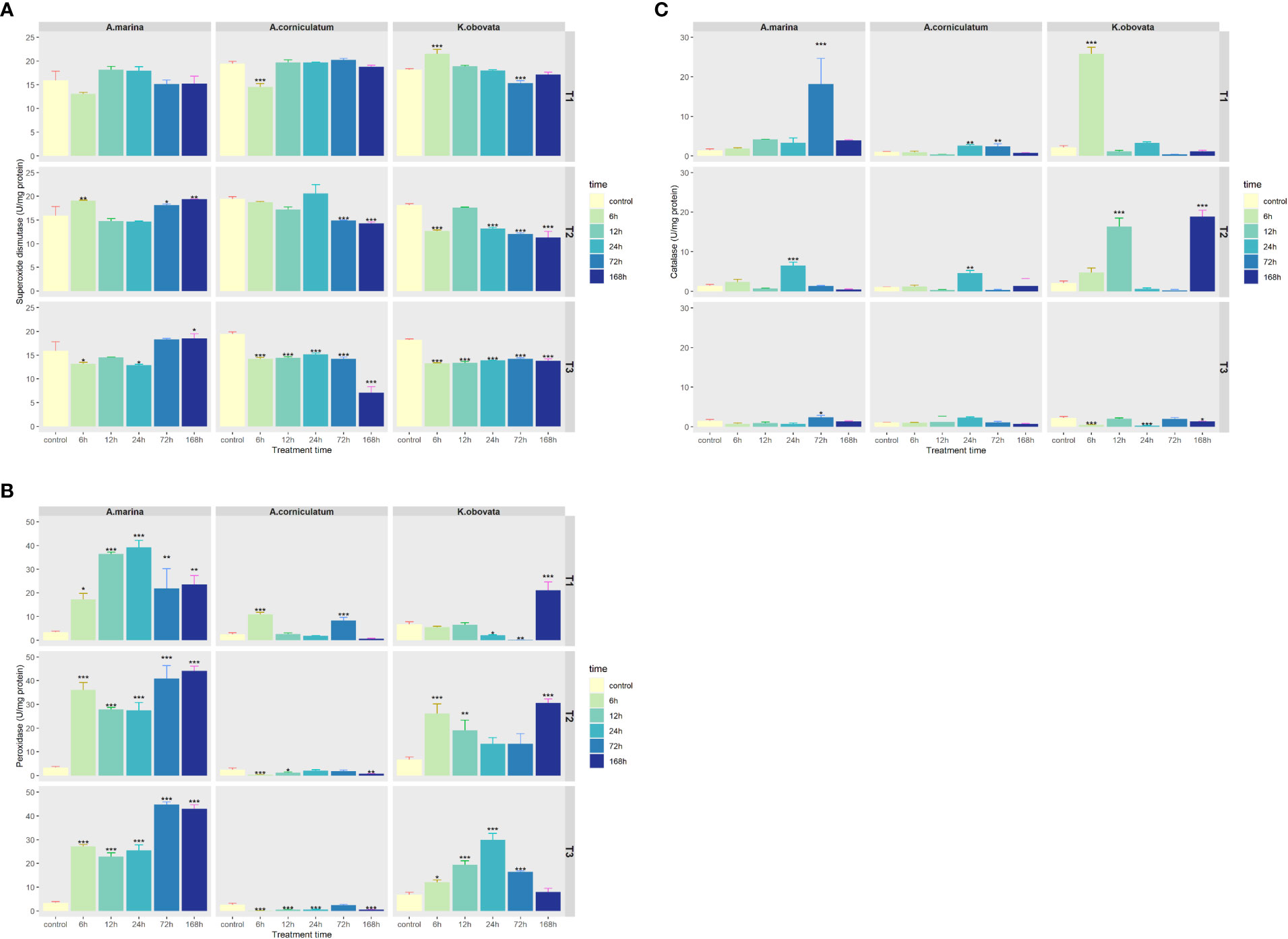
Figure 4 Changes of (A) Superoxide dismutase, (B) Peroxidase and (C) Catalase activities under upwelling stress. * indicates that there are significant differences between control and treatment groups (p<0.05(*), p<0.01(**), p<0.001(***)). “T1” means 5°C upwelling stress, “T2” means 10°C upwelling stress and “T3” means 15°C upwelling stress.
Under 5 and 10°C upwellings, the activities of CAT in the three mangrove plants changed more than at 15°C (Figure 4C). The CAT of A. marina and A. corniculatum reached its peak mainly at 24 and 72 h while K. obovata showed a different trend. Under 5°C, the maximum CAT of K. obovata appeared at 6 h and then declined. In K. obovata, at 10°C CAT reached a peak at 12 and 168 h.
3.5 Effect of upwelling stress on the content of osmotic adjusting substances
The variation in levels of proline contents in A. marina and K. obovata was different to that of A. corniculatum (Figure 5A). Changes of proline content in A. marina and K. obovata were comparatively small, ranging from 16.84 to 121.71μg/g and 21.53 to 174.00 μg/g, respectively. Interestingly, 72 and 168 h upwelling stress induced a tremendous increase in the proline content of A. corniculatum (p<0.05).
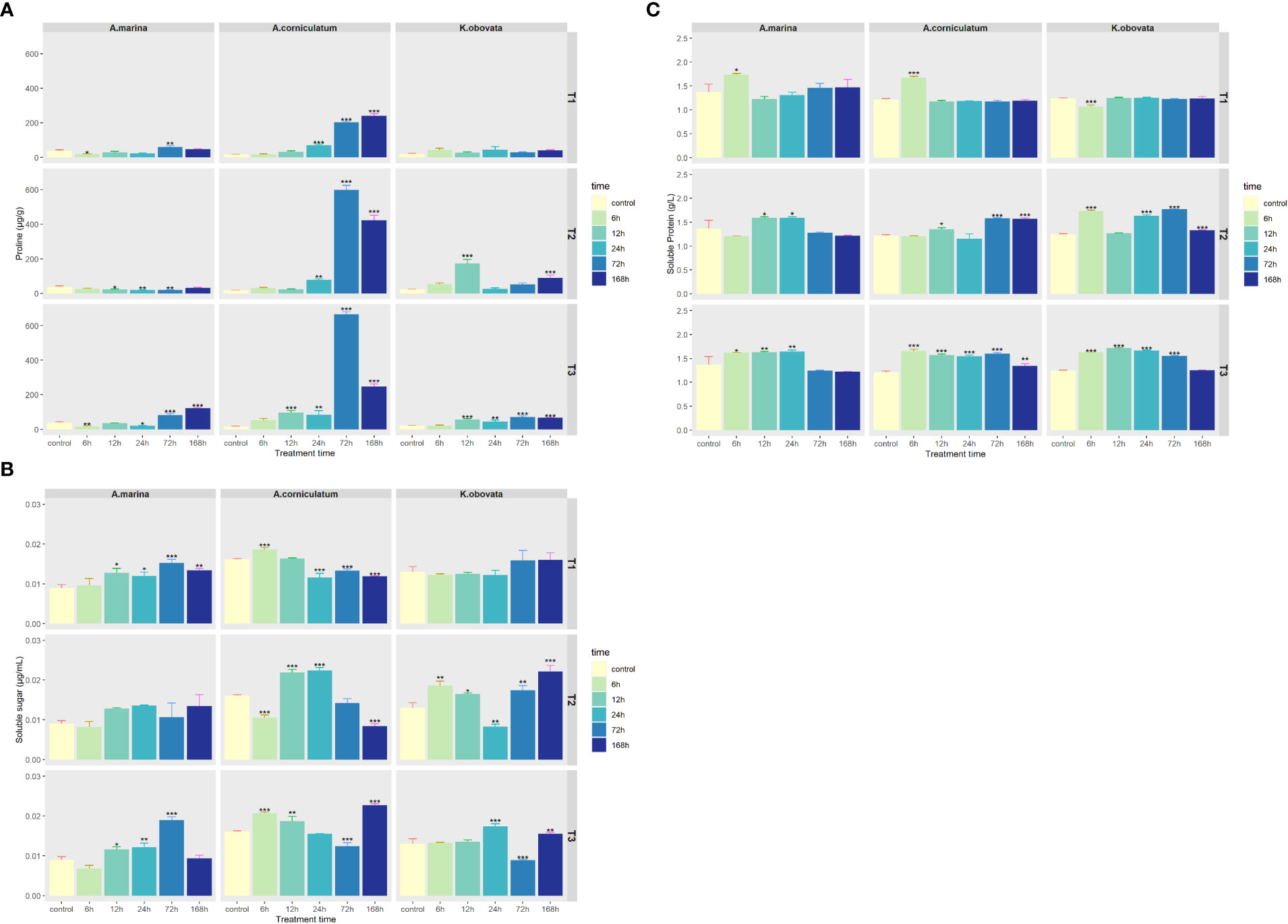
Figure 5 Changes of (A) proline, (B) Soluble sugar and (C) Soluble protein contents under upwelling stress. * indicates that there are significant differences between control and treatment groups [p<0.05(*), p<0.01(**), p<0.001(***)]. “T1” means 5℃ upwelling stress, “T2” means 10℃ upwelling stress and “T3” means 15℃ upwelling stress.
SS contents in A. marina showed an increase over treatment time (Figure 5B). The SS contents in A. corniculatum first increased and then decreased under stress. There were no significant differences in K. obovata in a 5°C upwelling. The minimum SS of K. obovata occurred after 24 h at 10°C, and after 72 h at 15°C. Under upwelling stress, SP contents in three mangrove plants were essentially consistent (Figure 5C). At 5°C, the SP contents of the three mangrove plants stabilized at about the same level except after 6 h of stress. At 10 and 15°C, the SP contents of the three mangrove plants significantly increased for 12–72 h (p<0.05).
3.6 Principal component analysis
The biplots of PC1 and PC2 based on the analysis of physiological and biochemical traits in three mangrove species under different temperature upwellings were shown in Figures 6 and 7. The first two principal components explained 49.4% of the total variance. The percentage variance and eigenvalues for the first component (PC1) and the second principal component (PC2) were presented in Table 1. PC1 explained 28.6% of the variation. Pigments (chl a, chl b and caro), H2O2, REC, MDA and CAT showed a high positive association in PC1 while SOD showed a negative association (loading coefficient >0.2214, Table 1). PC2 explained 20.8% of the variation. While there was a high positive correlation between MDA, O2- and POD parameters with PC2, proline and SS were negatively correlated with PC2 (loading coefficient >0.2022, Table 1). In addition, a correlation was observed in A. marina between O2-, MDA and POD. Proline and SS were associated with A. corniculatum. Furthermore, a clear distinction between mangrove species under upwelling stress was observed. However, different upwelling temperatures did create an obvious separation in the biplot (Figure 7).
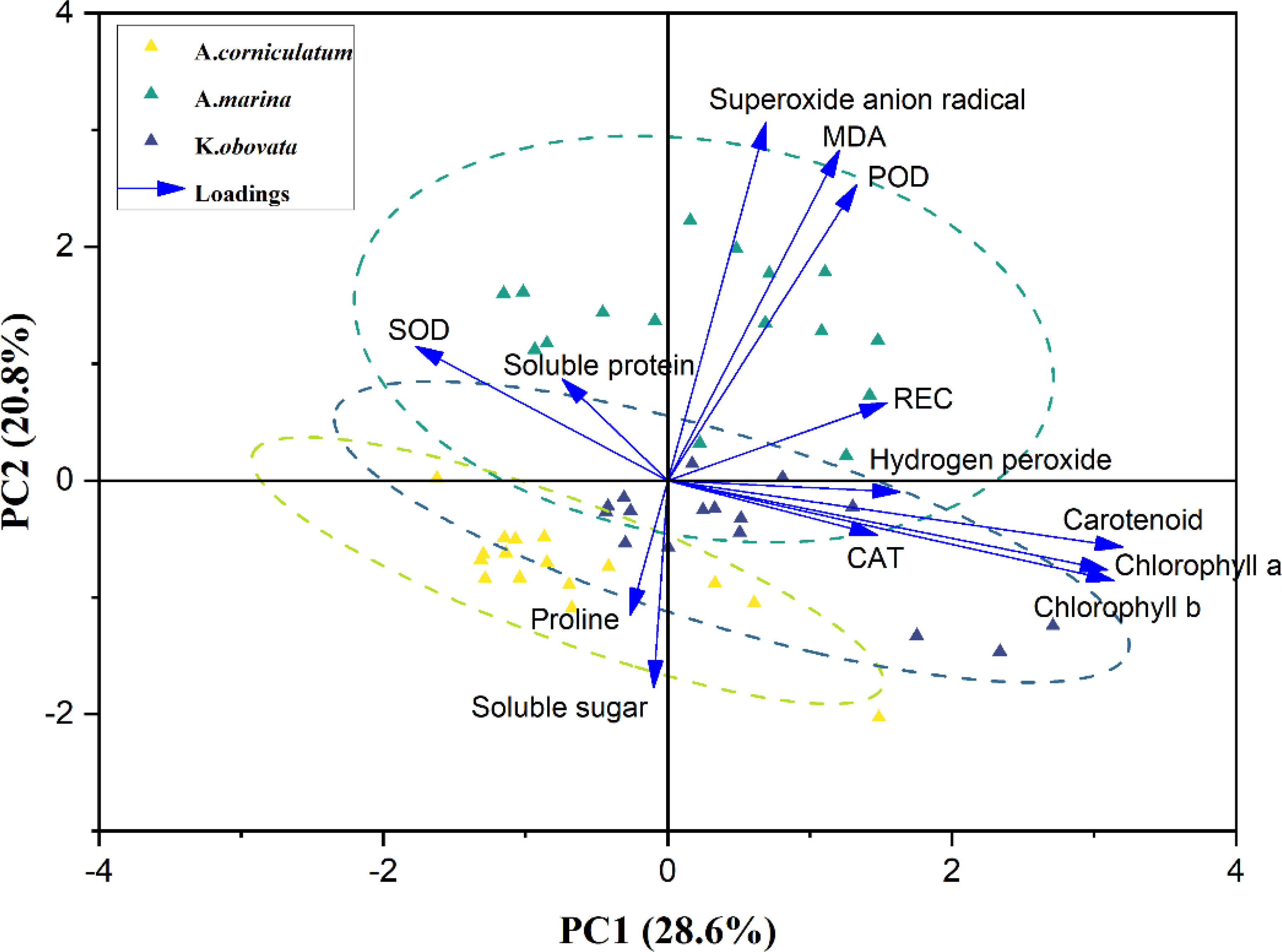
Figure 6 Biplot for the first two principal components was analyzed using the principal component analysis (PCA) for 13 traits among 3 mangrove species possessing different treatment. Yellow, green and gray triangles are representing three different mangrove plants. Arrows represented traits while its length is based on the contribution of each trait to separate the accessions. Relative electrical conductivity (REC), malondialdehyde (MDA), superoxide dismutase (SOD), peroxidase (POD) and catalase (CAT).
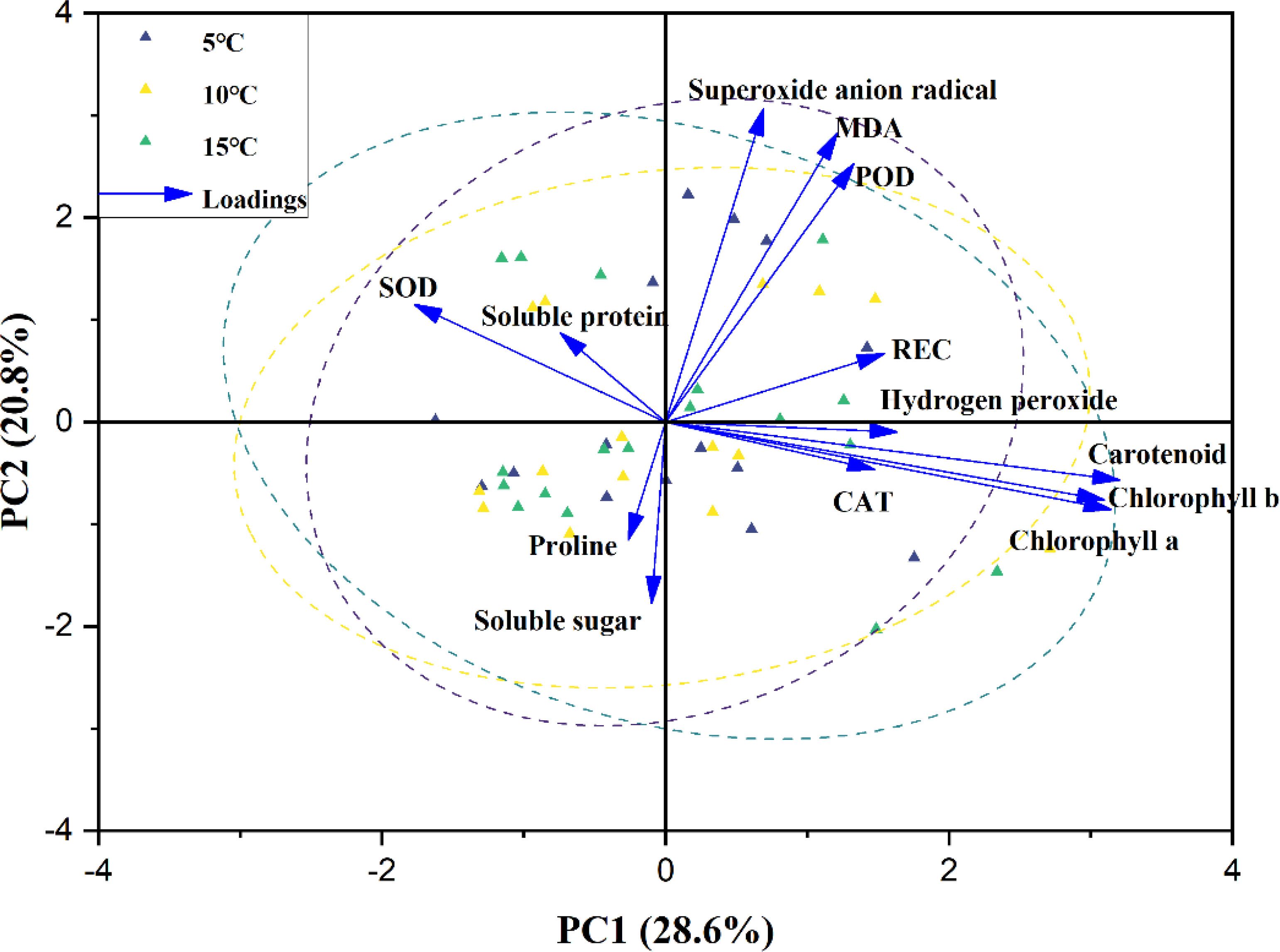
Figure 7 Biplot for the first two principal components was analyzed using the principal component analysis (PCA) for 13 traits among 54 samples under 3 different upwelling temperature. Yellow, green and gray triangles are representing three different upwelling temperature. Arrows represented traits while its length is based on the contribution of each trait to separate the accessions. Relative electrical conductivity (REC), malondialdehyde (MDA), superoxide dismutase (SOD), peroxidase (POD) and catalase (CAT).
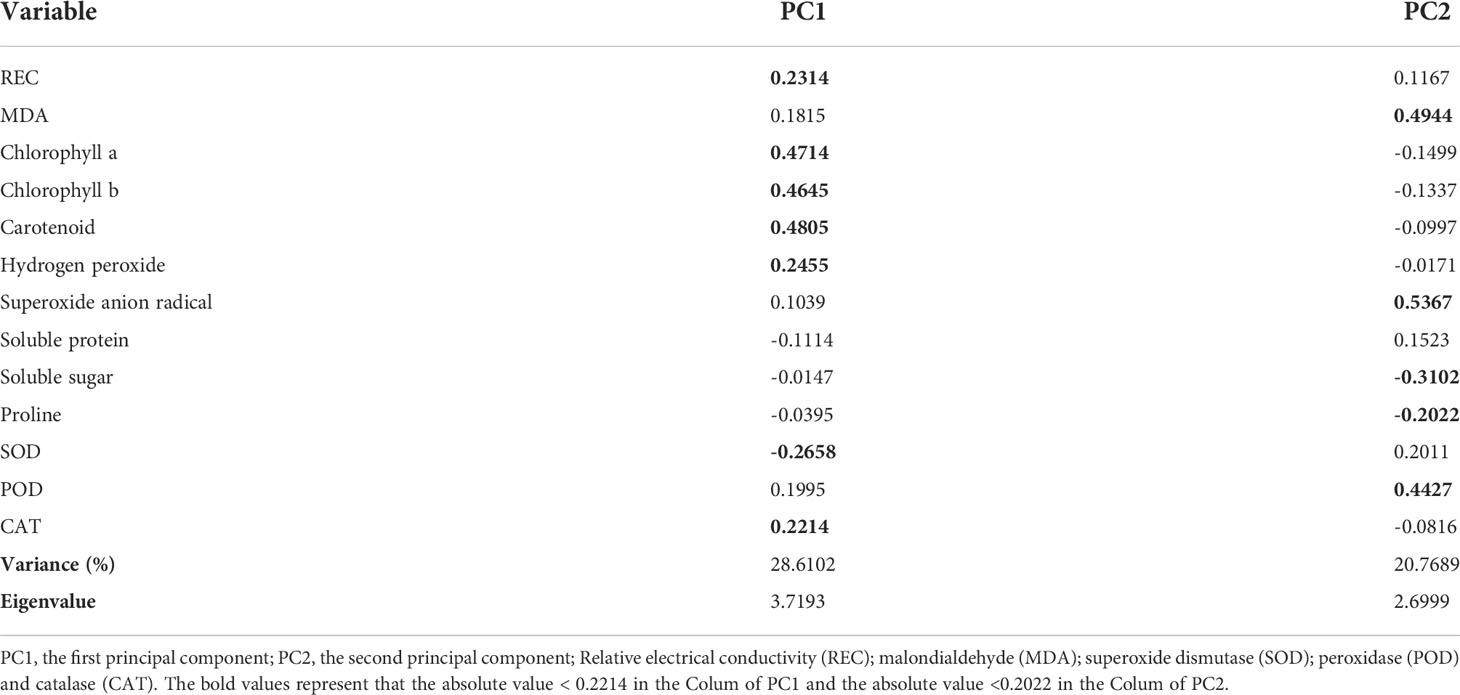
Table 1 Variable loading, percentage variation and eigenvalue derived from PCA for the measured characteristics in three mangrove species under all upwelling treatments.
3.7 Hierarchical clustering analysis
Physiological and biochemical parameters were subjected to hierarchical cluster analysis using a Euclidean distance matrix via Ward’s method of agglomeration (Figure 8). Based on the dendrogram result, three major clusters were observed which were consistent with PCA. Each cluster was basically composed of one mangrove species. Accordingly, leaf samples of A. marina under different upwelling treatments were placed in cluster 1, samples of A. corniculatum were placed in cluster 2, and samples of K. obovata, and two of A. corniculatum, were placed in cluster 3.
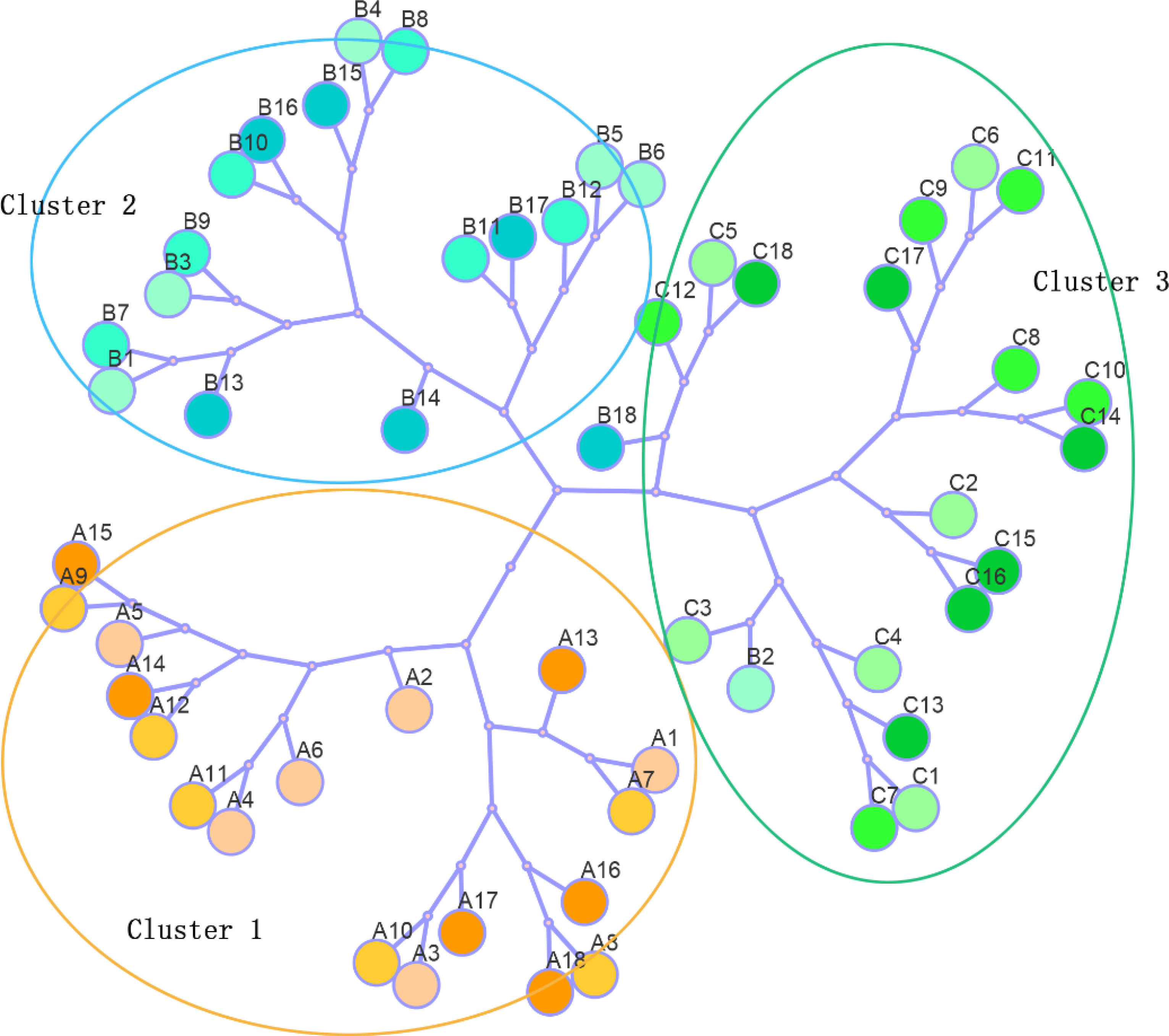
Figure 8 Dendrogram was exhibited by cluster analysis of A. marina, A. corniculatum and K. obovata under different upwelling treatment based on the Euclidean distance using physiological and biochemical traits. A represented A. marina (yellow circles), B represented A. corniculatum (blue circles), C represented K. obovata (green circles). Numbers 1-18 represented 5°C upwelling stress-0h, 6h, 12h, 24h,72h and 168h; 10°Cupwelling stress-0h, 6h, 12h, 24h, 72h and 168h; 15°C upwelling stress-0h, 6h, 12h, 24h, 72h and 168h.
4. Discussion
As a result of global climate change, upwelling activity becomes more frequent. Air-water temperature differences caused by upwellings may affect the development, growth and distribution of mangroves. Thus, A. marina, A. corniculatum and K. obovata were selected for a comprehensive investigation into the regulatory mechanisms underlying upwelling stress.
4.1 Damages of mangrove plans under upwelling stress
Low temperature dysregulates active oxygen metabolism in plants, which leads to oxidative stress, cell membrane lipid peroxidation, protein denaturation and nucleotide damage (Jingjing Yu, 2020). Accumulations of O2- and H2O2 in three mangrove species were observed under 5°C upwelling stress (Figure 3). The O2- content of A. marina was much higher than that of A. corniculatum and K. obovata, indicating that A. marina was more sensitive to upwelling stress. Moreover, in A. marina the lower the temperature, the higher the ROS contents. Similar results were reported in silage corn under chilling stress (Jiaxu Wu, 2022). However, there was no such trend in A. corniculatum and K. obovata, indicating that they were less sensitive to upwelling temperature.
REC and MDA are usually selected as indicators of increased membrane leakage and decreased membrane fluidity. Membrane systems are particularly sensitive to low temperature and ROS-induced oxidation of membrane lipid is a reflection of stress-induced damage at the cellular level (Zheng et al, 2021). In the present study, REC increased in the three mangrove species under upwelling stress (Figure 2A), indicating that upwelling stress induced membrane injury. MDA content in A. marina significantly increased while it remained stable in A. corniculatum and K. obovata (Figure 2B). Moreover, the MDA of A. marina was much higher than that of A. corniculatum and K. obovata, which could be explained by the accumulation of O2-.
4.2 Adjustment mechanism of mangrove plants under upwelling stress
Photosynthetic systems are sensitive to temperature variation, and reduced chlorophyll content usually occurs in plants at low temperatures because chlorophyll biosynthetic enzymes are affected and so biosynthesis progresses slowly (Matej Vosnjak, 2021). In our study, reductions in pigment contents could be observed in A. marina and A. corniculatum under short-term upwelling stress (6–24 h) which indicates that short-term upwelling caused a degree of damage to mangroves (Figure 1). With increased stress time, pigment contents increased and by significantly more than that of the control group (p<0.05), which could be attributed to the protection of protective pigments and other antioxidants in the leaves. Plants are also able to construct a defense system that actively increases their chlorophyll contents and prevents decreases in photosynthesis and energy production (Zhang et al, 2019). In our study, mangroves showed a certain tolerance to long-term upwelling stress because this stress induced increases in pigment contents (Agathokleous et al, 2020). Photosynthesis results in a large increase in the production of ROS (Foyer, 2018). The three mangrove species maintained relatively stable and high chlorophyll contents under long-term upwelling stress, which might be another reason for the high ROS production.
Plants have evolved an efficient enzyme antioxidant mechanism to prevent the oxidative damages caused by ROS (Popov et al, 2020). Among the enzymatic systems, SOD is able to rapidly eliminate O2- and convert it into H2O2, and the generated H2O2 is then converted to water and dioxygen by POD and CAT (Honglin Huang, 2019). Stable and high levels of SOD activity were observed in the three mangrove species when exposed to an upwelling stress (Figure 4A) and this might indicate the essential involvement of SOD in scavenging ROS. Compared to the other mangrove plants, POD activity of A. marina also showed strong activity (Figure 4B). However, the ROS contents of A. marina remained high indicating that the plants’ ROS scavenging machinery was not able to defend against oxidative injury. The same results occurred in K. obovata with its accumulation of ROS and rather high levels of SOD and POD activity. Under upwelling stress, CAT activity was low in all three mangrove plants (Figure 4C) indicating their “weak affinity” to H2O2; this result was also observed in barley under conditions of drought and low temperatures (Zhanassova et al., 2021). Changes in SOD, POD and CAT activity and ROS accumulation in response to upwelling were different in the three mangrove species, indicating that different mangrove plants use different enzyme response mechanisms under these circumstances.
Increases in osmosis-regulating substances (including proline, soluble sugar and soluble protein) can effectively promote the ability of osmotic regulation, enzyme and protein stabilization, ROS detoxification, and the integrity of protective membranes (M. L. Cai, 2020). Proline contents of A. marina and K. obovata showed no obvious variation under upwelling stress, and proline content of A. corniculatum significantly increased under long-term stress (Figure 5A). This showed that long-term upwelling stimulated proline production in A. corniculatum to alleviate stress, and proline played an important role in ROS scavenging. Both SS and SP contents among three mangrove species showed slight changes under upwelling stress (Figure 5B, Figure 5C). These results showed that osmotic adjustment substances might not function as the main defense against the stresses of upwelling.
4.3 Species effect and temperature effect under upwelling stress
PCA was performed to detect the relationships among parameters, temperature and mangrove species, during upwelling stress (Figures 6, 7). Pigment contents, ROS, REC, MDA, CAT and POD were observed in the same quadrant, and ANOVA results showed an increasing trend under upwelling stress suggesting some homogeneity in the regulatory mechanism of these traits in the face of upwelling stress for three mangrove species (Behrooz Sarabi, 2022). Previous studies showed that plants respond differently to different temperatures under chilling stress (Ok et al, 2020; Wang et al, 2021). In our research, none of the mangrove species exhibited markedly different responses to variations in upwelling water temperatures, indicating that mangroves are not sensitive to temperature variations in upwelling stress compared to low air or water temperatures.
However, PCA results revealed substantial differences in the dynamics of the responses by A. marina, A. corniculatum and K. obovata to upwelling stress, and these results were further characterized by the results of HCA (Figure 8). Both the PCA and HCA results showed the well separation of different mangrove species during upwelling, which revealed that different mangrove species show distinct sensitivities and tolerances to upwelling stress. The sensitivity of mangrove species to upwelling was preliminarily characterized as A. corniculatum< K. obovata< A. marina which was consistent with their sensitivity to low air temperature (Chen et al, 2017). The aggregation of species and indicators could well explain the tolerance of different species to upwelling. Correlations between A. marina and MDA, O2- and POD in PCA revealed that A. marina might be the most intolerant species to upwelling stress with its high activities of SOD and POD but high accumulation of MDA and ROS. A. corniculatum did not show damage during short-term upwelling and proline played a major role in alleviating stress during long-term upwelling. According to the PCA and ANOVA results, K. obovata alleviated and adapted to upwelling stress by adjusting antioxidant enzyme activity.
5 Conclusion
The changes of A. marina, A. corniculatum and K. obovata under three upwelling temperatures over different exposure durations were comprehensively analyzed. The results showed that upwelling stress causes varying degrees of damage to mangrove plants, and species separation in response was observed, although there was no distinct difference in response of each species to upwelling temperature. Upwelling stress triggered accumulation in ROS resulting in increases of REC and MDA. In accordance with REC, MDA and ROS accumulation, the amount of resistance to upwelling was preliminarily characterized as A. marina< K. obovata< A. corniculatum, and PCA and HCA results strongly supported this conclusion. Upwelling stress also induced increases in plant pigments to synthesize more energy in order to resist adversity. Further, the response mechanisms of each mangrove species to upwelling were different. A. marina rapidly activated antioxidant enzyme systems though not enough to scavenge the over-produced ROS. Increased osmotic adjustment substances might be an effective means for A. corniculatum to resist long-term upwelling stress. As for K. obovata, an antioxidant enzyme system played an important role in resisting upwelling stress. These findings may provide a theoretical basis for further analysis of the physiological and biochemical mechanism of stress tolerance of mangrove plants.
Data availability statement
The original contributions presented in the study are included in the Article/supplementary material, further inquiries can be directed to the corresponding author.
Author contributions
MW and XL contributed to conception and design of the study. XL organized the database, performed the statistical analysis and wrote the first draft of the manuscript. YW, YZ, ZY, JX and FG contributed to review and edit the manuscript. All authors contributed to the article and approved the submitted version.
Funding
This research was supported by the National Natural Science Foundation of China (NO. 31971480), Key Special Project for Introduced Talents Team of Southern Marine Science and Engineering Guangdong Laboratory (Guangzhou) (Nos. 241GML2019ZD0303 and GML2019ZD0303) and the Strategic Priority Research Program of the Chinese Academy of Sciences (No. XDA19060204).
Conflict of interest
Author JX is employed by Guangdong Zhihuan Innovative Environmental Technology Co. Ltd, China. Author FG is employed by Guangzhou Chemical Reagent Factory, China.
The remaining authors declare that the research was conducted in the absence of any commercial or financial relationships that could be construed as a potential conflict of interest.
Publisher’s note
All claims expressed in this article are solely those of the authors and do not necessarily represent those of their affiliated organizations, or those of the publisher, the editors and the reviewers. Any product that may be evaluated in this article, or claim that may be made by its manufacturer, is not guaranteed or endorsed by the publisher.
References
Agathokleous E., Feng Z., Penuelas J. (2020). Chlorophyll hormesis: Are chlorophylls major components of stress biology in higher plants? Sci. Total. Environ. 726, 138637. doi: 10.1016/j.scitotenv.2020.138637
Ariel E. Lugo S. C. S. (1974). The ecology of mangroves. Annu. Rev. Ecol. Evolution. Systematics. 5, 39–64. doi: 10.1146/annurev.es.05.110174.000351
Behrooz Sarabi J. G. (2022). Evaluating the physiological and biochemical responses of melon plants to NaCl salinity stress using supervised and unsupervised statistical analysis. Plant Stress 4, 100067. doi: 10.1016/j.stress.2022.100067
Bradford M. M. (1976). A rapid and sensitive method for the quantitation of microgram quantities of protein utilizing the principle of protein-dye binding.Anal.Biochem. Analytical. Biochem. 72 (1-2), 248–254. doi: 10.1006/abio.1976.9999
Burchard H., Basdurak N. B., Gräwe U., Knoll M., Mohrholz V., Müller S. (2017). Salinity inversions in the thermocline under upwelling favorable winds. Geophysical. Res. Lett. 44 (3), 1422–1428. doi: 10.1002/2016gl072101
Cai M. L., Zhang Q. L., Zheng X. T., Zhai J. J., Peng C. L. (2020). Comparison of leaves and stems of paederia scandens (Lour.) merr. in tolerance to low temperature. Photosynthetica 58 (3), 846–852. doi: 10.32615/ps.2020.034
Chen L., Wang W., Li Q. Q., Zhang Y., Yang S., Osland M. J., et al. (2017). Mangrove species responses to winter air temperature extremes in China. Ecosphere 8 (6), e01865. doi: 10.1002/ecs2.1865
Chinnusamy V., Zhu J., Zhu J. (2007). Cold stress regulation of gene expression in plants. Trends Plant Sci. 12 (10), 444–451. doi: 10.1016/j.tplants.2007.07.002
Ensminger I., Busch F., Huner N. P. A. (2006). Photostasis and cold acclimation sensing low temperature through photosynthesis. Physiologia. Plantarum. 126, 28–44. doi: 10.1111/j.1399-3054.2005.00627.x
Feng J., Dong P., Li R-M., Li C-L., Xie X-B., Shi Q-S. (2019). Effects of wood fiber properties on mold resistance of wood polypropylene composites. International Biodeterioration & Biodegradation 140, 152–159. doi: 10.1016/j.ibiod.2019.04.005
Foyer C. H. (2018). Reactive oxygen species, oxidative signaling and the regulation of photosynthesis. Environ. Exp. Bot. 154, 134–142. doi: 10.1016/j.envexpbot.2018.05.003
Geng Q., Wang Z., Tao J., Kimura M. K., Liu H., Hogetsu T., et al. (2021). Ocean currents drove genetic structure of seven dominant mangrove species along the coastlines of southern China. Front. Genet. 12, 615911. doi: 10.3389/fgene.2021.615911
Huang H., Ullah F., Zhou D., Yi M., Zhao Yu (2019). Mechanisms of ROS regulation of plant development and stress responses. Front. Plant Sci. 10. doi: 10.3389/fpls.2019.00800
Islam GMd. J., Kim J. W., Begum M. K., Sohel Md. A. T., Lim Y.S. (2020). Physiological and biochemical changes in sugar beet seedlings to confer stress adaptability under drought condition. Plants (Basel). 9 (11), 1511. doi: 10.3390/plants9111511
Jiang G., Hassan M. A., Muhammad N., Arshad M., Chen X., Xu Y., et al. (2022). Comparative physiology and transcriptome analysis of young spikes in response to late spring coldness in wheat (Triticum aestivum l.). Front. Plant Sci. 13. doi: 10.3389/fpls.2022.811884
Jianyu Hu X. W. (2016). Progress on upwelling studies in the China seas. Rev. Geophysics. 54 (3), 653–673. doi: 10.1002/2015rg000505
Jingjing Yu D. S., Yang D., Dong T., Tang Z., Li H., Han Y., et al. (2020). Chilling and heat stress-induced physiological changes and MicroRNA-related mechanism in sweetpotato (Ipomoea batatas l.). Front. Plant Sci. 11. doi: 10.3389/fpls.2020.00687
Khoma Y. A., Nesterenko O. G., Kutsokon N. K., Khudolieieva L. V., Shevchenko V. V., Rashydov N. M. (2021). Proline content in the leaves of poplar and willow under water deficit. Regul. Mech. Biosyst. 12 (3), 519–522. doi: 10.15421/022171
Lei Wang J. Z., Liu C. (2021). Arash arabmarkade). antioxidant activity of potato seedlings at different storage temperatures. Int. J. Chem. Eng., 1–11. doi: 10.1155/2021/5573644
Li M., Wang Z., Chen L. Q., Wang J. J., Li H. Y., Han Y. H., et al. (2021). The relationship between the photosynthetic pigments, carotenoids and yield of broomcorn millet (Panicum miliaceum; poaceae). Appl. Ecol. Environ. Res. 19 (1), 191–203. doi: 10.15666/aeer/1901_191203
Li X-M., Wang Y-S., Dong J-D., Wu M-L. (2022). Physiological and biochemical response of kandelia obovata to upwelling stress. Water 14 (6), 899. doi: 10.3390/w14060899
Li H.X., Xiao Y., Cao L.L., Yan Xu, Li C., Shi H.-Y., et al. (2013). Cerebroside c increases tolerance to chilling injury and alters lipid composition in wheat roots. PloS One 8 (9), e73380. doi: 10.1371/journal.pone.0073380
Lu W.X., Zhang B.H., Zhang Y.Y., Yang S.C. (2021). Differentiation of cold tolerance in an artificial population of a mangrove species, Kandelia obovata, is associated with geographic origins. Front. Plant Sci. 12, 695746. doi: 10.3389/fpls.2021.695746
Ok J., Kim S. H., Ma K.B., Kim D., Jeong H. S., Shin H. (2020) Seaonal fluctuation of freezing tolerance and soluble sugar content in three sweet persimmon cultivars Hortic. Sci. technol 39 (3), 305–313. doi: 10.7235/HORT.20210027
Peereman J., Hogan J. A., Lin T.-C. (2021). Cold wave-induced reductions in NDII and ChlRE for North-Western pacific mangroves varies with latitude and climate history. Remote Sens. 13 (14), 2732. doi: 10.3390/rs13142732
Petruccelli R., Bartolini G., Ganino T., Zelasco S., Lombardo L., Perri E., et al. (2022). Cold stress, freezing adaptation, varietal susceptibility of Olea europaea l.: A review. Plants (Basel). 11 (10), 1367. doi: 10.3390/plants11101367
Popov V. N., Naraikina N. V. (2020). Change of antioxidant enzyme activity during low-temperature hardening of Nicotiana tabacum l. and Secale cereale l. Russian J. Plant Physiol. 67 (5), 898–905. doi: 10.1134/s1021443720050118
Rachel Collin I. O. (2016). Influence of seasonal environmental variation on the reproduction of four tropical marine gastropods. Mar. Ecol. Prog. Ser. 555, 125–139. doi: 10.3354/meps11815
Simpson L. T., Stein C. M., Osborne T. Z., Feller I. C. (2019). Mangroves dramatically increase carbon storage after 3 years of encroachment. Hydrobiologia 834 (1), 13–26. doi: 10.1007/s10750-019-3905-z
Song W., Feng J., Krauss K. W., Zhao Y., Wang Z., Lin G. (2020). Non-freezing cold event stresses can cause significant damage to mangrove seedlings: assessing the role of warming and nitrogen enrichment in a mesocosm study. Environ. Res. Commun. 2 (3). doi: 10.1088/2515-7620/ab7a77
Theochairs A., Clément C., Barka E. A. (2012). Physiological and molecular changes in plants grown at low temperatures. Planta 235 (6), 1091–1105. doi: 10.1007/s00425-012-1641-y
Vosnjak M., Šircelj H., Hudina M., Usenik V. (2021). Response of chloroplast pigments, sugars and phenolics of sweet cherry leaves to chilling. Sci. Rep. 11 (1), 7210. doi: 10.1038/s41598-021-86732-y
Walters B. B., Ronnback Patrik , Kovacs J. M., Crona B., Hussain S. A., Badola R., et al. (2008). Ethnobiology, socio-economics and management of mangrove forests: A review. Aquat. Bot. 89 (2), 220–236. doi: 10.1016/j.aquabot.2008.02.009
Wang D., Gouhier T. C., Menge B. A., Ganguly A. R. (2015)7539. Intensification and spatial homogenization of coastal upwelling under climate change. Nature 518, 390–394. doi: 10.1038/nature14235
Wang L., Zhang J.L., Liu C., Arabmarkadeh A.. (2021). Antioxidant Activity of Potato Seedlings at Different Storage Temperatures. Int. J. Chem. Engineering, 1–11. doi: 10.1155/2021/5573644
Wang R.R., Huang J., Liang A.C., Wang Y., Mur Alejandro Jose Mur, Wang M., et al. (2020). Zinc and copper enhance cucumber tolerance to fusaric acid by mediating its distribution and toxicity and modifying the antioxidant system. Int. J. Mol. Sci. 21 (9). doi: 10.3390/ijms21093370
Wang Z., Yu D., Zheng C., Wang Y., Cai Lu, Guo J., et al. (2019). Ecophysiological analysis of mangrove seedlings Kandelia obovata exposed to natural low temperature at near 30°N. J. Mar. Sci. Eng. 7 (9), 292. doi: 10.3390/jmse7090292
Wu L.L., Deng Z., Cao L.F., Meng Li (2020). Effect of plant density on yield and quality of perilla sprouts. Sci. Rep. 10 (1), 9937. doi: 10.1038/s41598-020-67106-2
Wu J., Nadeem M., Galagedara L., Thomas R., Cheema M. (2022). Effects of chilling stress on morphological, physiological, and biochemical attributes of silage corn genotypes during seedling establishment. Plants (Basel). 11 (9), 1217. doi: 10.3390/plants11091217
Ximenes A. C., Ponsoni L., Lira C. F., Dahdouh-Guebas F., Koedam N. (2021). Seasonal atmospheric and oceanographic factors influencing poleward mangrove expansion in the southeastern American coast. Estuarine. Coast. Shelf. Sci. 262, 107607. doi: 10.1016/j.ecss.2021.107607
Ximenes A. C., Ponsoni L., Lira C. F., Koedam N., Dahdouh-Guebas F. (2018). Does Sea surface temperature contribute to determining range limits and expansion of mangroves in Eastern south America (Brazil)? Remote Sens. 10 (11), 1787. doi: 10.3390/rs10111787
Yang J., Cao Y., Zhang N. (2020). Spectrophotometric method for superoxide anion radical detection in a visible light (400-780 nm) system. Spectrochim. Acta A. Mol. Biomol. Spectrosc. 239, 118556. doi: 10.1016/j.saa.2020.118556
Zhanassova K., Kurmanbayeva A., Gadilgereyeva B., Yermukhambetova R., Iksat N., Amanbayeva U., et al. (2021). ROS status and antioxidant enzyme activities in response to combined temperature and drought stresses in barley. Acta Physiologiae. Plantarum. 43 (8), 114. doi: 10.1007/s11738-021-03281-7
Zhang L. X., Chang Q. S., Hou X. G., Chen S. D., Zhang Q. M., Wang J. Z., et al. (2021). Biochemical and photosystem characteristics of wild-type and chl b-deficient mutant in tree peony (Paeonia suffruticosa). Photosynthetica 59 (2), 256–265. doi: 10.32615/ps.2021.019
Zhang F.H., Lu K., Gu Y., Zhang L., Li W.Y., Li Ze (2019). Effects of low-temperature stress and brassinolide application on the photosynthesis and leaf structure of tung tree seedlings. Front. Plant Sci. 10. doi: 10.3389/fpls.2019.01767
Zhang Y., Meng X., Xia P., Li Z. (2021). Response of mangrove development to air temperature variation over the past 3000 years in qinzhou bay, tropical China. Front. Earth Sci. 9. doi: 10.3389/feart.2021.678189
Keywords: upwelling, mangrove, photosynthetic pigments, membrane permeability, reactive oxygen species, osmoregulatory system, antioxidant enzyme
Citation: Li X, Wang Y, Zhang Y, Xiang J, Yang Z, Gu F and Wu M (2022) Evaluating the physiological and biochemical responses of different mangrove species to upwelling. Front. Mar. Sci. 9:989055. doi: 10.3389/fmars.2022.989055
Received: 08 July 2022; Accepted: 25 July 2022;
Published: 16 August 2022.
Edited by:
Fajin Chen, Guangdong Ocean University, ChinaReviewed by:
Peng Zhao, Hainan University, ChinaWenzhe Xu, Tianjin University of Science and Technology, China
Senthilnathan Lakshmanan, Amet University, India
Copyright © 2022 Li, Wang, Zhang, Xiang, Yang, Gu and Wu. This is an open-access article distributed under the terms of the Creative Commons Attribution License (CC BY). The use, distribution or reproduction in other forums is permitted, provided the original author(s) and the copyright owner(s) are credited and that the original publication in this journal is cited, in accordance with accepted academic practice. No use, distribution or reproduction is permitted which does not comply with these terms.
*Correspondence: Meilin Wu, bWx3dUBzY3Npby5hYy5jbg==