- 1Tjärnö Marine Laboratory, Department of Marine Sciences, University of Gothenburg, Strömstad, Sweden
- 2Division of Food and Nutrition Science, Department of Biology and Biological Engineering, Chalmers University of Technology, Gothenburg, Sweden
- 3Swedish Food Agency, Uppsala, Sweden
- 4Department of Food and Meal Science, Kristianstad University, Kristianstad, Sweden
- 5Department of Food Science, Food Design and Consumer Behaviour, University of Copenhagen, Copenhagen, Denmark
Seaweed aquaculture can provide the growing human population with a sustainable source of proteins. Sea-based cultivation is an effective method for farming seaweeds on a large scale and can yield high biomass output. However, the quality and biochemical composition of the biomass is seasonally dependent, which limits the harvests to certain periods of the year. Here we show the possibility to extend the sea-based cultivation season of Ulva fenestrata when aiming for high protein levels, by post-harvest treatment in herring production process waters. We harvested U. fenestrata at an optimal period in terms of yield, but suboptimal in terms of protein content. We then cultivated the seaweed in onshore tank systems with the nutrient-rich process waters for 14 days. We monitored biomass yield, crude protein content, amino acid composition, and content of the health concerning metals arsenic, mercury, lead, and cadmium, as well as the sensory properties of the dried biomass. After cultivation in the process waters, biomass yields were 30 - 40% higher (210 – 230 g fresh weight) compared to in seawater (160 g fresh weight). Also, the crude protein and amino acid content increased three to five times in the process waters, reaching 12 - 17 and 15 – 21% dry weight, respectively. The protein enriched biomass followed food graded standards for heavy metal content, and consumption of the biomass does not exceed health based reference points. Additionally, no sensory attributes regarded as negative were found. This rapid, post-harvest treatment can help extend the cultivation season of sea-based seaweed farms, maximizing their output of sustainable proteins.
Introduction
In order to produce food that is sustainable for the growing world population, we need alternative future protein sources (Godfray et al., 2010; Olsen, 2015). Seaweed aquaculture is a promising source of sustainable food as it has a net uptake of nitrogen and phosphorus, and can capture greenhouse gas (Buschmann et al., 2017; Hasselstrom et al., 2018; Gephart et al., 2021). The worldwide production of seaweed is increasing exponentially, with over 32 million tonnes of seaweed produced annually at a value of USD 13.3 billion (FAO, 2018; FAO, 2020; Naylor et al., 2021). The distinct umami flavor has in recent years facilitated seaweeds as a delicious and interesting food ingredient in Western cuisine, especially in gastronomy and high-end restaurants (Mouritsen et al., 2018; Moerdijk-Poortvliet et al., 2022). Generally, the sensory quality of a food product, such as its appearance, odor, flavor, taste, and texture, plays a significant role in the public acceptance of novel food (Lawless, 2010; Moerdijk-Poortvliet et al., 2022). The consumer attitudes towards seaweed as a food has been shown to be very positive (Wendin and Undeland, 2020). However, to increase the understanding and recognition of seaweeds as a protein source and its gastronomic potential, its sensory attributes need to be further investigated. Special emphasis should be put on the avoidance of for example high intensity of bitterness, or other attributes that may occur in seaweed and are perceived by consumers as negative (Pérez-Lloréns et al., 2020).
Sea-based cultivation of seaweeds in coastal nearshore or offshore areas offer a practical and cost-efficient method of farming at a large scale (Araújo et al., 2021; Steinhagen et al., 2021). However, the systems are seasonally dependent, and environmental factors can significantly affect the growth and biochemical profile of the seaweeds (Venolia et al., 2020; Araújo et al., 2021; Steinhagen et al., 2021; Steinhagen et al., 2022). It is therefore important to optimize the harvest depending on its downstream application, to periods when the quality of the produced biomass can be ensured (Araújo et al., 2021; Steinhagen et al., 2022).
The green seaweed genus Ulva (sea lettuce) has gained attention by the aquaculture sector due to its many beneficial traits for large-scale cultivation (Buchholz et al., 2012; Lawton et al., 2013; Bolton et al., 2016). Some Ulva representatives have both high productivity and environmental tolerance (Bolton et al., 2016; Nardelli et al., 2019), and can efficiently bioremediate nutrients (Sode et al., 2013; Al‐Hafedh et al., 2015). The biomass has many nutritional properties interesting for consumers such as its protein content (5 - 22% dry weight (dw)) (Shuuluka et al., 2013; Jansen et al., 2022; Steinhagen et al., 2022), its content of all essential amino acids (Fleurence, 2004; Machado et al., 2020), and its relative abundance of minerals, antioxidants, vitamins, and fatty acids (Holdt and Kraan, 2011).
The nutritional and biochemical profile of Ulva changes depending on environmental conditions and nutrient availability (Bolton et al., 2016; Toth et al., 2020). The biomass quality of the Scandinavian Ulva fenestrata Postels and Ruprecht cultivated in an offshore seafarm in the Northern Hemisphere is highly dependent on the harvest season. For instance, fatty acids and protein content are highest in early spring (April), while biomass yield and carbohydrates are highest later in the season (May-June) (Steinhagen et al., 2022). Therefore, maximizing yield by harvesting late in the season results in a biomass with low protein content. However, by cultivating U. fenestrata in land-based settings it is possible to control the production cycle and biomass composition of the seaweeds independent of the season (Hafting et al., 2012; Hafting et al., 2015). We recently showed that U. fenestrata increases its growth, crude protein content and amino acid content when cultivated in herring production process waters (HPPWs) for 7 - 28 days (Stedt et al., 2022b), and cultivation of Ulva in other nutrient-rich waters show similar results (Nielsen et al., 2012; Nardelli et al., 2019).
The aim of this study was to determine if HPPWs can be used as a short-term, post-harvest treatment to boost the protein content of U. fenestrata harvested at an optimal harvest period (early summer) in terms of yield, but suboptimal in terms of protein content. The content of some prioritized toxic elements, commonly grouped as “heavy metals” were determined in the U. fenestrata biomass (arsenic, mercury, lead, and cadmium), and a simple exposure assessment was performed (US EPA, 2007). Furthermore, we wanted to assess if the sensory attributes (appearance, odor, taste, flavor, and texture) of the U. fenestrata biomass were affected by cultivation in HPPWs. We tested this by running a 14-day post-harvest experiment where we cultivated U. fenestrata, harvested from a nearby Swedish sea-based seaweed farm, in a land-based setting with two different HPPWs and a seawater control. We measured growth, crude protein content, amino acid composition, heavy metal content and conducted a sensory analysis on the biomass.
Materials and methods
Algal material and taxonomic identification
Algal material was collected from a sea-based seaweed farm (2 ha, 100 x 200 m) located in the Koster archipelago (Skagerrak), Sweden (58°51’34.0”N, 11°04′06.2”E). For details on the seafarm and cultivation conditions see Steinhagen et al. (2021). Individuals of U. fenestrata were collected from the seaweed farm at early-summer conditions on the 24th of May 2021 and had a mean crude protein content of 3.76 ± 0.22% dw (mean ± SEM, n = 3). Molecular identification of the algal material used in the present study has been described in detail by Toth et al. (2020) (GenBank accession numbers: MN240309, MN240310, MN240311).
Herring production process waters
The two herring production process waters (HPPWs) used in the experiments were collected in a food grade state in May 2021. The HPPWs were: (i) tub water (TUB) from in-house pre-processing storage of whole herring in 3% NaCl at a primary processor (Sweden Pelagic AB, Ellös, Sweden), and (ii) saturated salt brine (SAL) from maturation of herring fillets at a secondary processor (Klädesholmen Seafood AB, Rönnäng, Sweden). A detailed explanation of the HPPWs can be found in Stedt et al. (2022a). After collection, coarse particles (> 300 μm) were removed by filtration and the HPPWs were then stored at -60°C. The total ammonia, nitrate and nitrite were quantified using commercial enzymatic kits as described by Stedt et al. (2022a), while the inorganic phosphorus/orthophosphate content was measured with a standard curve made with monopotassium phosphate, as reported by Qvirist et al. (2015). Characterization of undiluted HPPWs is presented in Table 1. The HPPWs were diluted with filtered (0.2 μm + UV-light treated) deep-sea (40 m) seawater to 25 μM NH4+.

Table 1 Characterization of the seawater (SW) that was used as the control and to dilute the herring production process waters (HPPWs), as well as the undiluted HPPWs SAL and TUB (mean ± SEM, n = 3) collected in May 2021.
Experimental setup
The experiments included the two diluted HPPWs TUB and SAL, as well as a seawater control (SW), each set up in triplicates (Table 1). The seaweeds harvested from the seaweed farm were placed in 14 L tanks filled to 10 L at 14°C, under 16:8 h (L:D) light cycle, and at an irradiance of 100 μmol photons m-2 s-1 (light source: INDY66 LED 60 W 4000 K 6000 lm). The tanks were cleaned and the respective waters were renewed every second day to avoid nutrient depletion and microbial spoilage. Both salinity (WTW MultiLine 3420, Xylem Analytics) and pH (Mettler Toledo FiveEasy bench meter) remained stable throughout the experiment in all treatments (33.0 ± 1.0 ppt and 8.2 ± 0.2 respectively, mean ± SD).
The experiment started on the 24th of May 2021 and lasted 14 days. The seaweeds were cleaned and divided into tanks at a density of 12 g fresh weight (fw) L-1, i.e. 120 g fw tank-1. The total fw and crude protein content of the seaweed in each tank was measured on day 1, 3, 7, and 14. Samples for amino acid composition, heavy metal content, and sensory properties from U. fenestrata were analyzed at the end of the experiment.
Growth and crude protein content
The fresh weight in each tank was determined in a standardized way by weighing the U. fenestrata on a lab-scale (Sartorius TE1502S, Göttingen, Germany), after removing the excess water with a salad spinner. From each tank, random tissue samples were collected, frozen, freeze-dried and homogenized to a fine powder before further analysis.
The total nitrogen content was determined by combustion using a GSL elemental analyzer coupled to an isotope-ratio mass spectrometer (EA-IRMS, 20 - 22, Sercon Ltd., Crewe UK). The crude protein content was estimated based on the nitrogen-to-protein conversion factor of five for seaweeds (Angell et al., 2016).
Amino acid composition
Total amino acids (TAA) of U. fenestrata were analyzed following the procedure described in Trigo et al. (2021), with minor modifications. All analyzes were made in triplicates. To 100 mg of the freeze-dried, homogenized seaweed tissue sample, 4 mL of 6 M HCl was mixed in screw-cap glass tubes, after which all air in the tubes was replaced with nitrogen. The samples were hydrolyzed at 110°C for 24 h using a heat block, followed by dilution with 0.2 M acetic acid and filtering (0.22 μm; Fisher Scientific). Two μL of each sample were run in a LC/MS (Agilent 1100 HPLC) with a Phenomenex column (C18 250 μm x 4.6 μm x 3 μm), coupled to an Agilent 6210 quadrupole in the SIM positive mode (Agilent Technologies). Separation was conducted at 0.7 mL min-1 for 40 min using different ratios of mobile phase A (3% methanol, 0.2% formic acid and 0.01% acetic acid) and mobile phase B (50% methanol, 0.2% formic acid and 0.01% acetic acid). For the calibration curve, a mix consisting of 17 amino acids (Thermo Scientific) was used. Due to the use of acidic hydrolysis, tryptophan and cysteine could not be recovered, and glutamine and asparagine were co-determined with glutamic and aspartic acid, respectively. Data were analyzed using MassHunter Quantitative Analysis software (version B.09.00, Agilent Technologies).
Heavy metal content and exposure assessment
Randomly selected, freeze-dried, and homogenized tissue samples (0.5 g dw) from two tanks per treatment were analyzed for total arsenic (tAs), mercury (Hg), lead (Pb), and cadmium (Cd) at the Swedish Food Agency, Uppsala, Sweden. The analytical method follows the standard procedures of NMKL no. 186 and EN 15763:2009, which includes total microwave acid digestion of the samples using a mixture of concentrated nitric acid and hydrochloric acid (6 + 1 mL) before determination with inductively coupled plasma mass spectrometry (ICP-MS, Agilent 7700x). The inorganic arsenic (iAs) was analyzed at the Swedish Food Agency, Uppsala, Sweden, according to the European Standard EN 16802:2016. The method is based on extraction with dilute nitric acid and hydrogen peroxide in hot water bath, followed by analysis with strong anion exchange HPLC-ICP-MS (Agilent 1260 Infinity Quaternary LC and Agilent 7700x ICP-MS). Both methods are further described in (Kollander et al., 2019). As there are no regulations on the maximum levels of these heavy metals in seaweeds for food purposes other than for food supplements, the heavy metal contents were compared to the maximum allowed levels (MLs) in general foodstuff set by the European Union’s Commission Regulation (EC) No. 1881/2006 (version 03/05/2022) (European Commission, 2006).
In addition, the following equations were used to estimate the exposure to these heavy metals when consuming biomass of seaweeds cultivated in the different treatments:
Eq. 1
Eq. 2
Where Ci is the content of heavy metal i in dried U. fenestrata biomass (μg g dw-1), Di is the daily average intake of dried seaweeds in China (5.2 g day-1) adapted from Chen et al. (2018), bw is the reference body weight for adults (63.3 kg) as established by the EFSA (2017), and RfDi is the reference dose for heavy metal i (μg kg bw-1 day-1) as set by US EPA (2007). Because iAs is the most toxic species of arsenic, RfD values are only established for this form of As (US EPA, 2007). THQ is used in several seaweed studies (Chen et al., 2018; Filippini et al., 2021; Peng et al., 2022), and a THQ < 1 indicates that there are no health risks associated with the consumption of the dried U. fenestrata biomass concerning element i. The Di of 5.2 g day-1 was adopted as it is higher than the consumption in Japan (4 g day-1), but lower than in South Korea (8.5 g day-1) (Chen et al., 2018). Together with using a low reference body weight in comparison to the population in North America, Australia, and Europe (> 75 kg) (worlddata.info, 2022), this makes the exposure assessment rather conservative. The US EPA RfD was chosen over the EU Tolerable daily intake (TDI) in order to simplify comparisons with other publications in the area.
Sensory analysis
Sensory attributes of the biomass from cultivation in the control (SW) and the two HPPWs (TUB and SAL) were analyzed at the Kristianstad University, Sweden. To analyze the seaweed in a food like product, 2.5 g of pulverized seaweed tissue were blended into 150 g water. To keep the seaweed powder evenly distributed in the liquid, 2.0 g soy lecithin (Sosa ingredients, Spain) and 1.3 g xanthan gum (Guzmán Gastronomia SL, Spain) were added. Sensory attributes were analyzed by quantitative descriptive analysis with a trained analytical sensory panel, following ISO 13299:2016. The software Eye Question (version 4.10, the Netherlands) was used for sensory data collection. Each assessor gave their informed consent for participation after being informed about the products and the terms of participation: voluntary participation, freedom to leave the test without giving a reason, and the right to decline to answer specific questions.
Four assessors participated in the analytical sensory panel, which were all selected and trained according to ISO 8586:2012. During training reference samples were presented and the sensory attributes defined (Table 2). The panel was further trained on how to rate attribute on a numerical intensity scale ranging from 0 to 100. The assessments were performed in duplicate, in randomized order.
Statistical analysis
All statistical analyses, except for the sensory data, were performed in RStudio (v. 1.2.5001). All data were visually checked for homogeneity and normality with diagnostic plots (density-, normality- and QQ-plots). Furthermore, normality was checked with the Shapiro Wilk test for each combination of factor levels, and Levene’s test was used to test for homogeneity of variance at each level of time. Growth and crude protein content were analyzed using a two-way mixed model ANOVA with day as within (repeated measures) and treatment as between subject factors. Comparisons between treatment and day were analyzed post-hoc with pairwise paired t-test (Bonferroni corrected). Amino acids were analyzed with one-way ANOVA followed by Tukey’s post-hoc test for pairwise comparison.
The sensory data were subjected to two-way ANOVA with treatments and assessments as fixed effects. Significant differences (p < 0.05) between the treatments were calculated with Tukey’s post-hoc pairwise comparison test (IBM SPSS, version 26). Principal Component Analysis (PCA) was performed to give an overview of the data (Panel Check, version 1.4.2, Nofima, Norway).
Results
Growth and crude protein content
After 14 days, the biomass yield had increased from 120.00 g fw per tank to 228.67 ± 0.88, 213.33 ± 4.26, and 162.67 ± 7.84 g fw in SAL, TUB, and SW, respectively (mean ± SEM) (Figure 1A), resulting in 30 - 40% higher biomass yield in the HPPWs compared to in SW. There was a statistically significant interaction between treatment group and time in explaining growth (Table 3). The effect of treatment was significant at day 1, 3, 7, and 14 (p < 0.05), and the pairwise comparisons show that the mean fw was significantly higher in SAL and TUB compared to in SW on day 1, 3, 7, and 14 (p < 0.05).
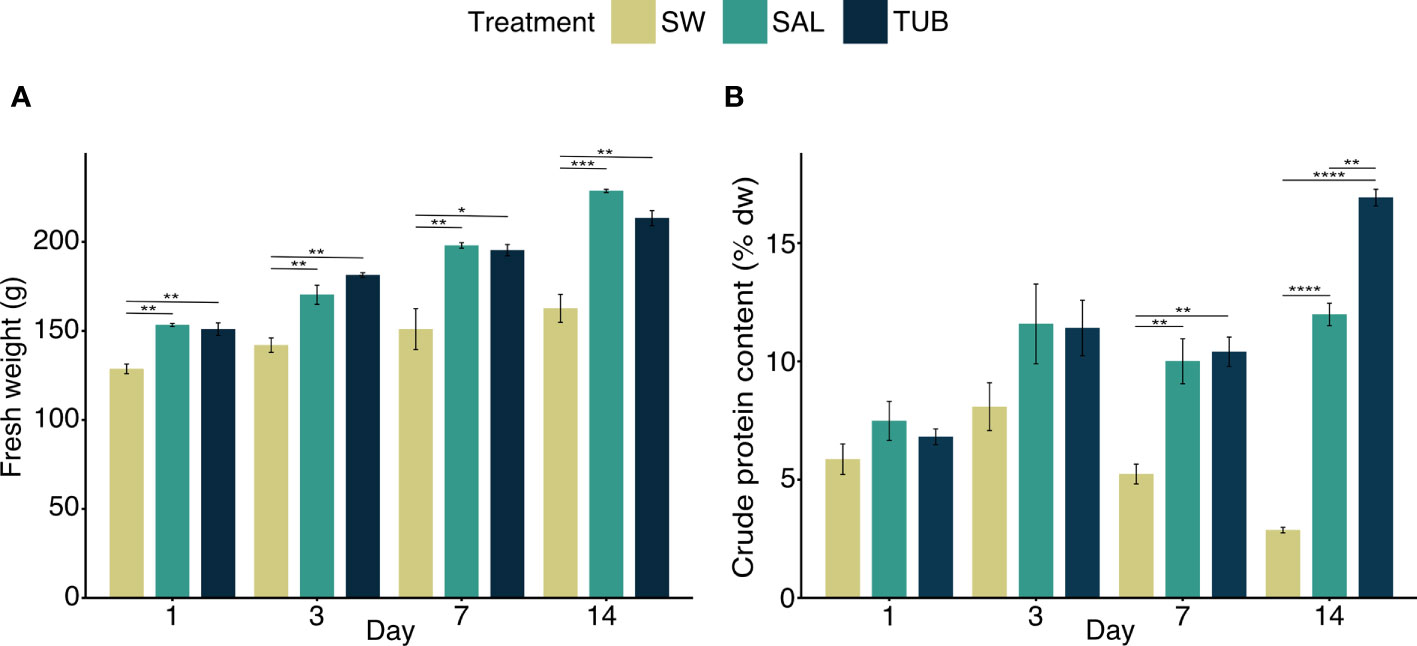
Figure 1 (A) Fresh weight (g) and (B) crude protein content (% dw) of Ulva fenestrata cultivated in seawater control (SW) and the two herring production process waters salt brine (SAL) and tub water (TUB) (mean ± SEM, n = 3). Significant differences from the pairwise paired t-test are denoted by asterisks, * < 0.05, ** < 0.01, *** < 0.001, and *** < 0.0001.
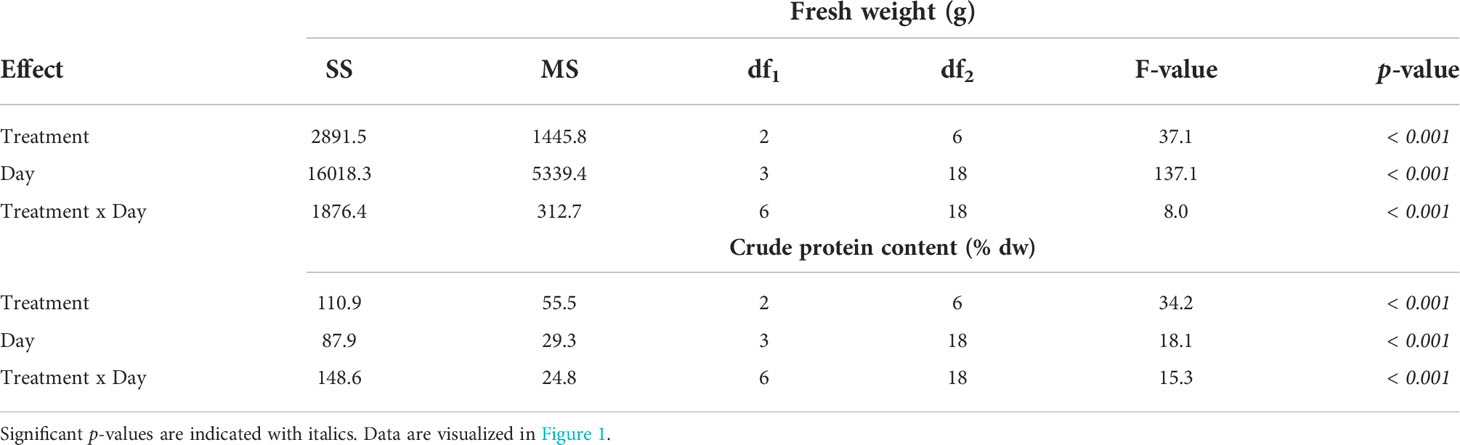
Table 3 Two-way mixed model ANOVA (Type III) of fresh weight (g) and crude protein content (% dw) of Ulva fenestrata cultivated under two different herring production process waters (SAL and TUB), as well as a seawater control (SW).
After 14 days, the crude protein content had increased from 3.76 ± 0.22% dw (day 0) to 11.98 ± 0.47 and 16.92 ± 0.35% dw in SAL and TUB, respectively, while it decreased to 2.87 ± 0.11% dw in SW (mean ± SEM) (Figure 1B). There was a statistically significant interaction between treatment group and time for crude protein content (Table 3), and treatment was significant at day 7 and 14 (p < 0.05). Seaweeds cultivated in TUB had higher crude protein content compared to seaweeds cultivated in SAL (p < 0.005), but the HPPWs generated biomass with four and six times higher crude protein content compared to SW.
Amino acid composition
The amino acid profile of the dried U. fenestrata biomass is presented in Table 4. The total amino acid (TAA) content in SAL (15.30 ± 1.10% dw) and TUB (20.69 ± 0.32% dw) were significantly higher compared to in SW (4.21 ± 0.26% dw) (p < 0.001), and all TAA were higher compared to the crude protein content estimated by the nitrogen-to-protein conversion factor of five (11.98 ± 0.47, 16.92 ± 0.35, and 2.87 ± 0.11% dw in SAL, TUB, and SW, respectively). As a result of the high content of the non-essential amino acid (NEAA) glutamic acid in SAL (19.35 ± 0.25% of TAA) and TUB (22.24 ± 0.19% of TAA) compared to in SW (13.13 ± 0.06% of TAA) the total essential amino acids (TEAA) in SAL (36.35 ± 0.28%) and TUB (33.20 ± 0.60%) were significantly lower compared to in SW (40.61 ± 0.47%) (p < 0.001) (mean ± SEM, n = 3). Based on the WHO/FAO/UNU (2007) requirements, lysine was the limiting amino acid in the control biomass, whereas histidine was the limiting amino acid in biomass cultivated in both HPPWs (Table 4).
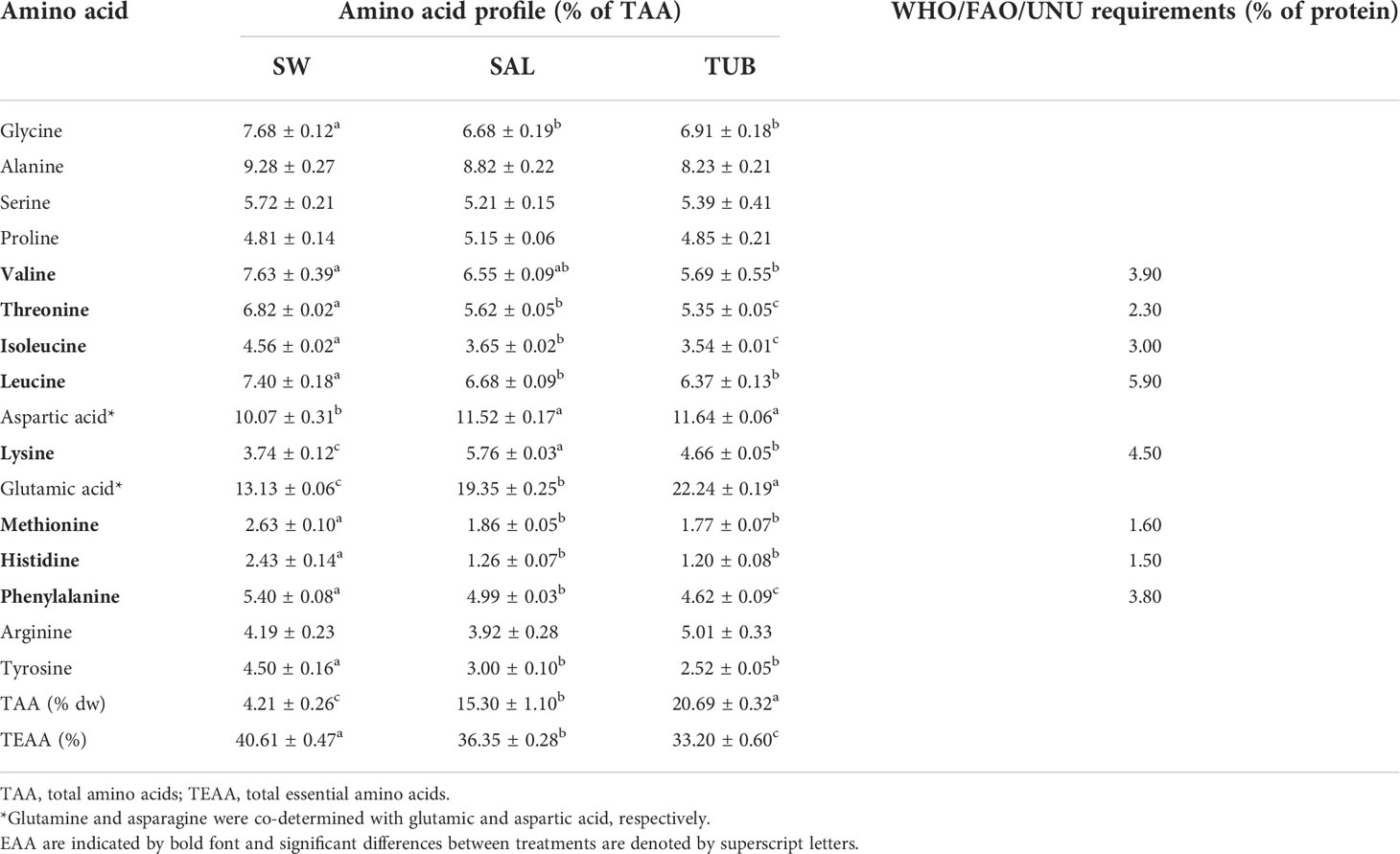
Table 4 Amino acid profile (% of TAA) of dried biomass of Ulva fenestrata cultivated in seawater control (SW) and the two herring production process waters salt brine (SAL) and tub water (TUB) (mean ± SEM, n = 3), as well as the amino acid profiles (% of protein) recommended by WHO/FAO/UNU (2007).
Heavy metal content and exposure assessment
The heavy metal content was similar between all treatments, and were all below the EU maximum levels (MLs) in foodstuff (Table 5). The Hg content was below the method’s quantification limit (< 0.036 μg g dw-1) for SW and TUB. Inorganic arsenic only contributed 0.45, 0.72, and 0.39% to the tAs in Ulva fenestrata biomass cultivated in SW, SAL, and TUB, respectively.
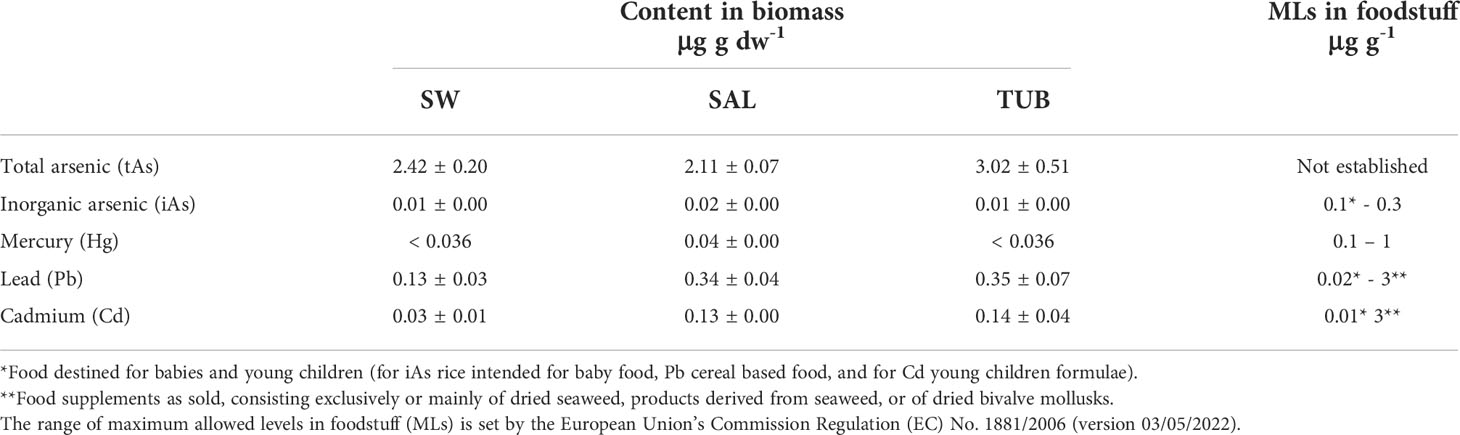
Table 5 Heavy metal content (μg g dw-1) in Ulva fenestrata biomass cultivated for 14 days in the seawater control (SW), and the two herring production process waters salt brine (SAL) and tub water (TUB) (mean ± SEM, n = 2).
The simple exposure assessment showed that the exposure dose from consuming 5.2 g dw day-1 of the U. fenestrata from any treatment is between 0.10 – 1.17% of the heavy metals respectively RfD values (Table 6). Consequently, the THQ is well below 1 for all the heavy metals, indicating no expected health risks concerning these heavy metals.
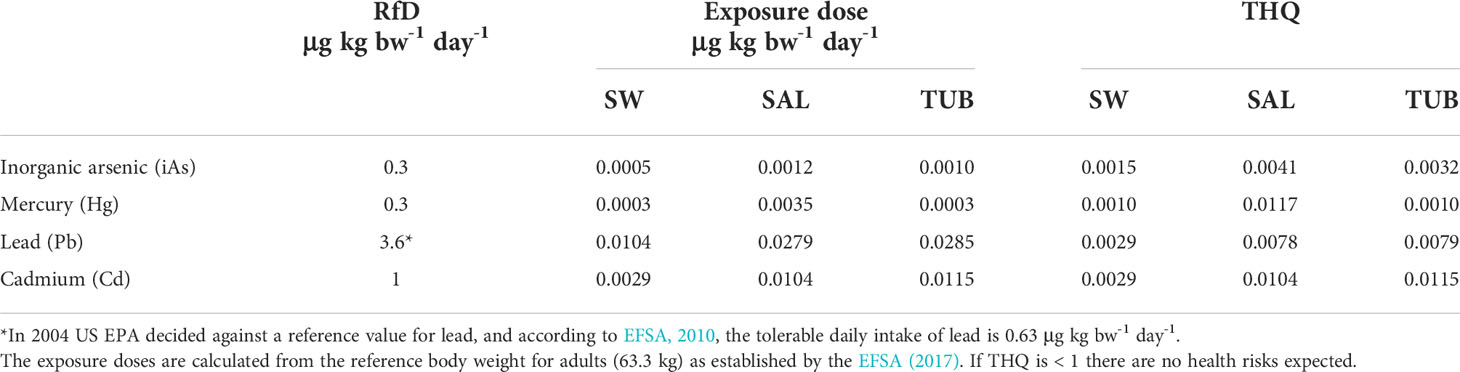
Table 6 Estimated exposure dose and target hazard quotient (THQ) when consuming 5.2 g dw of Ulva fenestrata cultivated for 14 days in the seawater control (SW), and the two herring production process waters salt brine (SAL) and tub water (TUB).
Sensory analysis
Ulva fenestrata cultivated in HPPWs were similar in most sensory attributes but differed from the control biomass with respect to a few attributes (Figure 2 and Table 7). The appearance of the U. fenestrata together with their total odor intensity were the sensory attributes contributing to the largest differences between the treatments. There was a significant difference between the SW and the HPPWs in appearance, where SW had a yellow-green color and the HPPWs a blue-green color (p < 0.001). The control was also significantly different from the HPPWs by having a less intense total odor (p < 0.001). The control was significantly different from TUB regarding seaweed odor (p < 0.05), while the difference was not significant compared to SAL (p = 0.06). Furthermore, the odor of fresh fish was not significantly different between the treatments. For taste, there was a significant difference between the treatments in saltiness, where SAL was experienced to be lower in saltiness compared to the other treatments. There was also a significant difference between TUB and the other two treatments in the perception of adhesiveness, where TUB was more adhesive than the others. However, there was no significant difference in flavor between the treatments, and they were all perceived equally in the texture attribute oiliness.
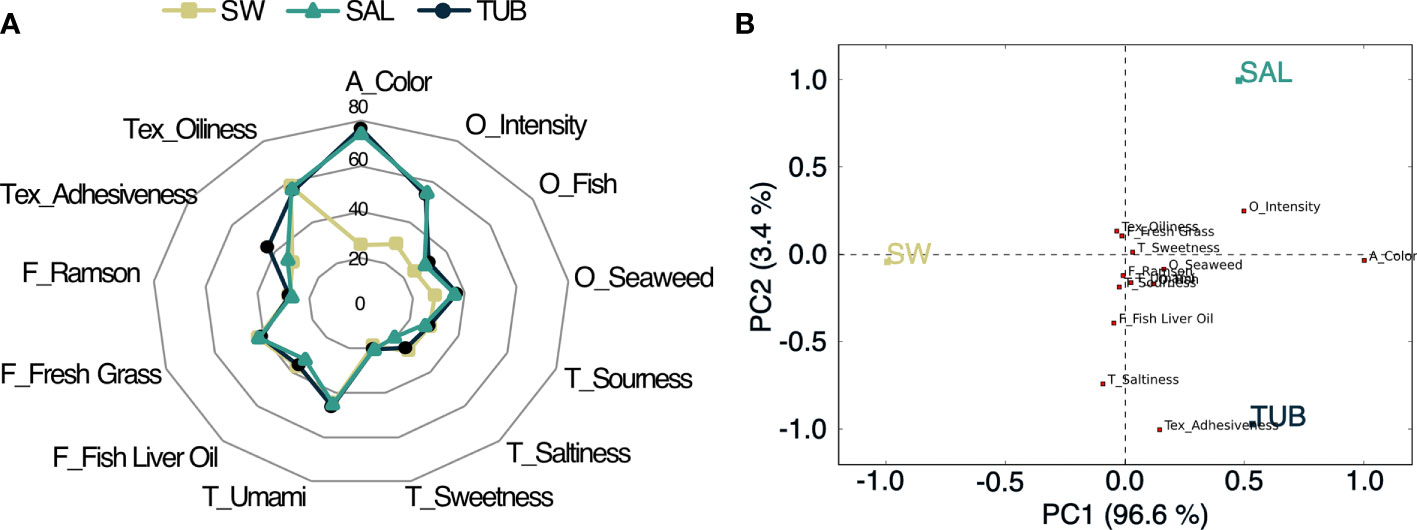
Figure 2 (A) Sensory profile showing the mean values, and (B) Principle Component Analysis (PCA) of the sensory attributes for Ulva fenestrata cultivated in seawater control (SW) and the two herring production process waters salt brine (SAL) and tub water (TUB). In the PCA, the first main component (PC1) accounts for 96.6% of the data variation and the second main component (PC2) accounts for 3.4%.
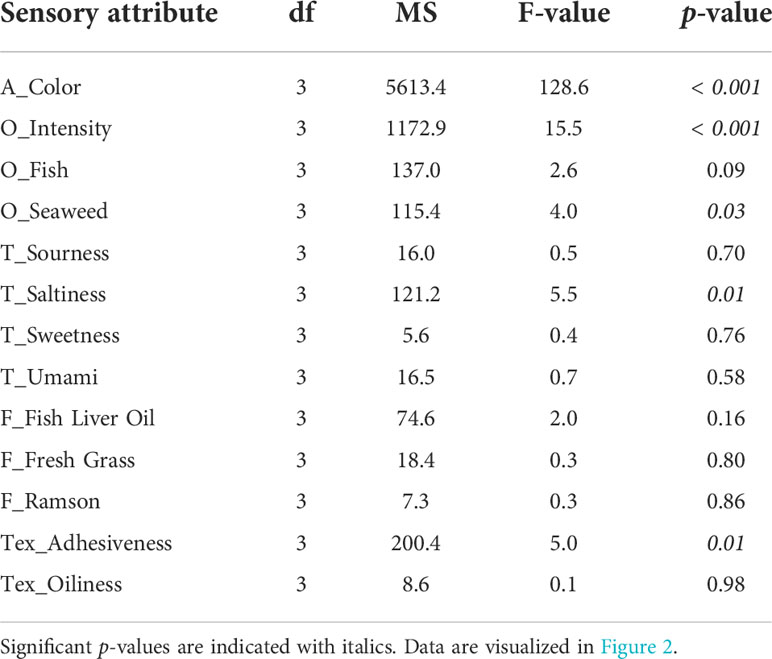
Table 7 Two-way ANOVA of the sensory attributes for Ulva fenestrata cultivated under two different herring production process waters (SAL and TUB), as well as a seawater control (SW).
Discussion
Biomass composition and quality of farmed U. fenestrata are dependent on seasonal conditions (Steinhagen et al., 2022). Consequently, the harvest period of sea-based cultivated biomass is limited to certain seasons of the year depending on the desired biochemical properties of the biomass and downstream application. Here, we show the prospects of using herring production process waters (HPPWs) as a post-harvest treatment for U. fenestrata cultivated for food purposes. In only 7 to 14 days, the biomass harvested at the suboptimal harvest period in regards to its biomass protein content, almost doubled in biomass yield and increased in crude protein content over four times, when cultivated in the HPPWs. We show that around 450 g dw day-1 can be consumed (based on the THQ) regarding heavy metals (iAs, Hg, Pb, Cd), which is much higher than the average consumption in Japan (4.0 g dw day-1), China (5.2 g dw day-1), and South Korea (8.5 g dw day-1) (Chen et al., 2018). Furthermore, the sensory attributes of the biomass were not perceived as negatively affected by the HPPWs.
Seaweed growth is generally limited by nutrients, and increasing the accessibility to nitrogen and phosphate often results in higher growth (Hurd et al., 2014). In our experiment, U. fenestrata grew more in the HPPWs compared to in the SW already after the first day of cultivation, and after 14 days the biomass yields were 30 – 40% higher in the HPPWs compared to in the SW. These results are in agreement with previously reported studies, which show increased growth when seaweeds are cultivated in association with waters emerging from the aquaculture sector (Bolton et al., 2009; Korzen et al., 2016; Ashkenazi et al., 2019), or liquid pig manure (Nielsen et al., 2012). The biomass harvested from the seafarm had a crude protein content of 3.76% dw, and by cultivating the U. fenestrata for only 14 days in the HPPWs the crude protein content increased three times in SAL (11.98% dw), and over four times in TUB (16.92% dw). We recently showed similar increases for U. fenestrata when cultivated in HPPWs (Stedt et al., 2022b). However, to the best of our knowledge, such rapid increases in crude protein content have not been reported elsewhere. To compete with soy and animal derived proteins, it is important to achieve such high protein levels, as this will allow the production of more pure protein from extractions and counteract its relatively low digestibility (Harrysson et al., 2018; Harrysson et al., 2019; Trigo et al., 2021).
After 7 days, the crude protein content in the U. fenestrata cultivated in the seawater control decreased. This may be explained by sporulation events occurring during the cultivation period. Changing environmental conditions can trigger spore release in Ulva fenestrata (Balar and Mantri, 2019), but interestingly, sporulation events were not observed within the HPPWs treatments. If the HPPWs contain some unidentified sporulation inhibitors these would be highly valuable to identify, as sporulation events can be detrimental to commercial cultivations of Ulva. The decrease in crude protein content of the U. fenestrata in the seawater control was also reflected in their lower intensity of green color of the blades at the end of the experiment, while the green color intensified in U. fenestrata cultivated in the HPPWs. The intensified color is probably an effect of accumulation of N-containing photosynthetic pigments such as chlorophyll in the biomass and protein bound chlorophyll (Robertson-Andersson et al., 2009; Aryee et al., 2018; Stedt et al., 2022a).
The total amino acid composition showed that the nitrogen-to-protein conversion factor of five underestimated the protein content in the U. fenestrata biomass in this experiment. Based on our TAA data, a more accurate conversion factor for our samples would be, on average, 6.6. Apart from tryptophan, which could not be recovered due to the acid hydrolysis, all the essential amino acids were found in the U. fenestrata biomass. Of particular interest is the relatively high content of lysine in the biomass cultivated in the HPPWs, as this specific EAA is often considered the limiting amino acid in plant-based proteins (Sá et al., 2020; Thiviya et al., 2022). Overall, the results indicate that biomass of U. fenestrata cultivated in the HPPWs result in a high-quality protein source (Friedman, 1996; Sá et al., 2020). To reach the recommended daily intake of all EAA, an adult with 63.3 kg body weight (EFSA, 2017) needs to consume 1209 g dw of U. fenestrata cultivated in SW (limited by lysine), and 330 and 255 g dw of U. fenestrata cultivated in SAL and TUB (limited by histidine), respectively (data not shown). The results from this study indicate that the different treatments influence the AA composition, and that the treatments have a high impact on the daily intake needed to reach the recommendations set by the WHO/FAO/UNU (2007).
Seaweeds can accumulate heavy metals from its surrounding environment (Gaudry et al., 2007; Jarvis and Bielmyer-Fraser, 2015), which may be a concern for food applications. Of particular focus have been the inorganic form of arsenic, as it is cancerogenic (Duinker et al., 2020; Blikra et al., 2021). Our results show that the iAs in the U. fenestrata biomass was between 0.39 and 0.75% of the tAs, which corresponds with the general assumption that the fraction of iAs in seaweeds is around 1% of the tAs (Duinker et al., 2020; Blikra et al., 2021). Both the iAs and the other heavy metals analyzed were well below the MLs for regulated foodstuff (European Commission, 2006; European Commission, 2018), indicating that the biomass can safely be consumed regarding these elements. However, it is important to note that the MLs are not permanent, but constantly evaluated and adjusted to revised standards. MLs for new foodstuff are continuously added, and as seaweeds are increasingly being consumed by the European population, the European Commission have requested the European Food Safety Authority (EFSA) for a scientific report on the dietary exposure of metals from seaweeds (European Commission, 2022). Our health risk assessment showed that if a person with a body weight of 63.3 kg consumes 5.2 g dw day-1 [average consumption in China (Chen et al., 2018)] from any of the treatments, they would be exposed to 0.1 - 1.2% of the heavy metals’ RfD. Using the more restrictive tolerable daily intake values for Pb (0.63 μg kg bw-1 day-1) and Cd (0.36 μg kg bw-1 day-1) set by EFSA (EFSA, 2009; EFSA, 2010) exposes the consumer to maximum 4.5% and 3.2% of the RfD for Pb and Cd, respectively. The contents of some heavy metals can be reduced by post-processing of the biomass, such as blanching, washing, and boiling (Hanaoka et al., 2001; Stévant et al., 2018; Ownsworth et al., 2019; Blikra et al., 2021), which would further decrease the possible health risks associated with consumption of the biomass. Furthermore, the bioavailability of the heavy metals needs to be investigated to establish how readily they are absorbed to the bloodstream during digestion (Domínguez-González et al., 2010; Intawongse et al., 2018).
The sensory analysis supported the effect of intensified color when the biomass was cultivated in the HPPWs. Sensory analysis further showed significant differences between the HPPW treatments and the control in the intensity of overall odor and seaweed odor. Marine aromas can be correlated to several compounds belonging to groups of hydrocarbons, alcohols, carboxylic acids, aldehydes and ketones, esters, fatty acids, and halogenated or sulfur compounds (Sánchez-García et al., 2021). Among others, species, handling, and geography have great impact on the presence of odors and odor intensity in seaweeds (Sánchez-García et al., 2019). Earlier studies have shown positive consumer attitudes towards seaweeds as food, where the most preferred forms of consuming seaweeds were as snacks, bread and in various dishes (Wendin and Undeland, 2020). For example, adding seaweed to seafood dishes seems to be an example without any negative taste experiences (Chapman et al., 2015). Our results showed that the sensory attributes of the biomass were not considered to be negatively affected after cultivation in HPPWs. With suitable marketing, seaweed can become a natural, sustainable and innovative food resource with the potential to play an important role towards a more green and sustainable future for the planet (Pérez-Lloréns et al., 2020).
Conclusion
This study addresses the “Farm to Fork Strategy” emphasized by the EU’s Green Deal by providing insights into different steps surrounding seaweed cultivation; from enhancing the biomass composition of the crop, to ensuring a safe food product and evaluating its sensorial aspects. In conclusion, we show that the generally low protein content of late season U. fenestrata biomass can be boosted through short-term, post-harvest treatments with herring production process waters (HPPWs). Generally, harvesting U. fenestrata later in the season will allow for higher biomass yields. After 14 days in the HPPWs the protein content of the U. fenestrata was at similar levels as those observed early in the season, when the crude protein content is at its peak (20.79% dw) (Steinhagen et al., 2022). Furthermore, we show that the biomass can be consumed as food based on our documented levels of heavy metals, and that there are no effects regarded as negative from the cultivation in HPPWs on the sensory attributes of the biomass.
Data availability statement
The original contributions presented in the study are included in the article/supplementary material. Further inquiries can be directed to the corresponding author.
Ethics statement
Ethical review and approval was not required for the study on human participants in accordance with the local legislation and institutional requirements. The patients/participants provided their written informed consent to participate in this study.
Author contributions
KS: conceptualization, data curation, formal analysis, investigation, methodology, project administration, validation, visualization, writing – original draft & review & editing. SS: conceptualization, investigation, methodology, resources, writing – review & editing. JT: investigation, data curation, writing – review & editing. BK: funding acquisition, resources, writing – review & editing. IU: funding acquisition, resources, writing – review & editing. GT: supervision, validation, writing – review & editing. KW: investigation, resources, writing – review & editing. HP: funding acquisition, resources, supervision, validation, writing – review & editing. All authors read and agreed to the published version of the manuscript.
Funding
This study was supported by the Swedish Research Council Formas (CirkAlg-project, Grant no. 2018-01839).
Acknowledgments
The authors would like to thank Benedikt Schrofner-Brunner at the University of Gothenburg for valuable input on statistics.
Conflict of interest
The authors declare that the research was conducted in the absence of any commercial or financial relationships that could be construed as a potential conflict of interest.
Publisher’s note
All claims expressed in this article are solely those of the authors and do not necessarily represent those of their affiliated organizations, or those of the publisher, the editors and the reviewers. Any product that may be evaluated in this article, or claim that may be made by its manufacturer, is not guaranteed or endorsed by the publisher.
Abbreviations
HPPW(s), herring production process water(s); TUB, tub water from in-house pre-processing storage of whole herring at a primary processor; SAL, salt brine from maturation of herring fillets at a secondary processor; SW, seawater control; US EPA, United States Environmental Protection Agency; EFSA, European Food Safety Authority; RfD, reference doses for heavy metals set by US EPA; MLs, maximum allowed levels of heavy metals in foodstuff set by the European Union’s Commission Regulation (EC) No. 1881/2006 (version 03/05/2022); THQ, Target Hazard Quotient; THQ, < 1 indicates that there are no health risks concerning element i.
References
Al-Hafedh Y. S., Alam A., Buschmann A. H. (2015). Bioremediation potential, growth and biomass yield of the green seaweed Ulva lactuca in an integrated marine aquaculture system at the red Sea coast of Saudi Arabia at different stocking densities and effluent flow rates. Rev. Aquaculture 7 (3), 161–171. doi: 10.1111/raq.12060
Angell A. R., Mata L., de Nys R., Paul N. A. (2016). The protein content of seaweeds: a universal nitrogen-to-protein conversion factor of five. J. Appl. Phycol 28 (1), 511–524. doi: 10.1007/s10811-015-0650-1
Araújo R., Vázquez Calderón F., Sánchez López J., Azevedo I. C., Bruhn A., Fluch S., et al. (2021). Current status of the algae production industry in europe: an emerging sector of the blue bioeconomy. Front. Mar. Sci. 7 (1247). doi: 10.3389/fmars.2020.626389
Aryee A. N. A., Agyei D., Akanbi T. O. (2018). Recovery and utilization of seaweed pigments in food processing. Curr. Opin. Food Sci. 19, 113–119. doi: 10.1016/j.cofs.2018.03.013
Ashkenazi D. Y., Israel A., Abelson A. (2019). A novel two-stage seaweed integrated multi-trophic aquaculture. Rev. Aquaculture 11 (1), 246–262. doi: 10.1111/raq.12238
Balar N. B., Mantri V. A. (2019). Insights into life cycle patterns, spore formation, induction of reproduction, biochemical and molecular aspects of sporulation in green algal genus Ulva: implications for commercial cultivation. J. Appl. Phycol 32, 473–484. doi: 10.1007/s10811-019-01959-7
Blikra M. J., Wang X., Philip J., Dagbjørn S. (2021). Saccharina latissima cultivated in northern Norway: reduction of potentially toxic elements during processing in relation to cultivation depth. Foods 10 (6), 1290. doi: 10.3390/foods10061290
Bolton J. J., Cyrus M. D., Brand M. J., Joubert M., Macey B. M. (2016). Why grow Ulva? its potential role in the future of aquaculture. Perspect. Phycol 3 (3), 113–120. doi: 10.1127/pip/2016/0058
Bolton J. J., Robertson-Andersson D. V., Shuuluka D., Kandjengo L. (2009). Growing Ulva (Chlorophyta) in integrated systems as a commercial crop for abalone feed in south Africa: a SWOT analysis. J. Appl. Phycol 21 (5), 575–583. doi: 10.1007/s10811-008-9385-6
Buchholz C. M., Krause G., Buck B. H. (2012). “Seaweed and man,” in Seaweed biology: Novel insights into ecophysiology, ecology and utilization. Eds. Wiencke C., Bischof K. (Berlin, Heidelberg: Springer Berlin Heidelberg), 471–493.
Buschmann A. H., Camus C., Infante J., Neori A., Israel Á., Hernández-González M. C., et al. (2017). Seaweed production: overview of the global state of exploitation, farming and emerging research activity. Eur. J. Phycol 52 (4), 391–406. doi: 10.1080/09670262.2017.1365175
Chapman A. S., Stévant P., Larssen W. E. (2015). Food or fad? challenges and opportunities for including seaweeds in a Nordic diet. Botanica Marina 58 (6), 423–433. doi: 10.1515/bot-2015-0044
Chen Q., Pan X.-D., Huang B.-F., Han J.-L. (2018). Distribution of metals and metalloids in dried seaweeds and health risk to population in southeastern China. Sci. Rep. 8 (1), 3578. doi: 10.1038/s41598-018-21732-z
Domínguez-González R., Romarís-Hortas V., García-Sartal C., Moreda-Piñeiro A., Barciela-Alonso M., Bermejo-Barrera P. (2010). Evaluation of an in vitro method to estimate trace elements bioavailability in edible seaweeds. Talanta 82 (5), 1668–1673. doi: 10.1016/j.talanta.2010.07.043
Duinker A., Kleppe M., Fjære E., Biancarosa I., Heldal H. E., Dahl L., et al. (2020). “Knowledge update on macroalgae food and safety: based on data generated in the period 2014-2019 by the institute of marine research, Norway,” (Havsforskningsinstitutet).
EFSA (2009). Scientific opinion of the panel on contaminants in the food chain on a request from the European commission on cadmium in food. EFSA J. 980 (980), 1–139. doi: 10.2903/j.efsa.2009.980
EFSA (2010). Panel on contaminants in the food chain (CONTAM); scientific opinion on lead in food. EFSA J. 8 (4), 1570(151). doi: 10.2903/j.efsa.2010.1570
EFSA (European Food Safety Authority) (2017). Dietary reference values for nutrients summary report. (EFSA Supporting Publications) 14 (12), e15121.98. doi: 10.2903/sp.efsa.2017.e15121
European Commission (2006). Commission regulation (EC) no 1881/2006 of 19 December 2006 setting maximum levels for certain contaminants in foodstuffs. Off. J. Eur. Union France: Union Strasbourg 364/5, 5–24. Available at: http://data.europa.eu/eli/reg/2006/1881/oj
European Commission (2018). Commission recommendation (EU) 2018/464 of 19 march 2018 on the monitoring of metals and iodine in seaweed, halophytes and products based on seaweed. Off. J. Eur. Union. L78/16, 16–18. Available at: http://data.europa.eu/eli/reco/2018/464/oj
European Commission (2022). “Annex: request from the European commision to EFSA for an EFSA scientific report on dietary exposure to metals and iodine in seaweed and halophytes in the European population,” (European Union). Ref. Ares(2022)612869 - 26/01/2022.
FAO (2018). “The global status of seaweed production, trade and utilization,” in Globefish research programme (Rome: Food and Agriculture Organization of the United Nations).
FAO (2020). “The state of world fisheries and aquaculture 2020,” in Sustainability in action (Rome: Food and Agriculture Organization of the United Nations).
Filippini M., Baldisserotto A., Menotta S., Fedrizzi G., Rubini S., Gigliotti D., et al. (2021). Heavy metals and potential risks in edible seaweed on the market in Italy. Chemosphere 263, 127983. doi: 10.1016/j.chemosphere.2020.127983
Fleurence J. (2004). “Seaweed proteins,” in Proteins in food processing. Ed. Yada R. Y. (Cambridge, UK: Food Science, Technology and Nutrition: Woodhead Publishing Limited), 197–211.
Friedman M. (1996). Nutritional value of proteins from different food sources. a review. J. Agric. Food Chem. 44 (1), 6–29. doi: 10.1021/jf9400167
Gaudry A., Zeroual S., Gaie-Levrel F., Moskura M., Boujrhal F. Z., El Moursli R. C., et al. (2007). Heavy metals pollution of the atlantic marine environment by the Moroccan phosphate industry, as observed through their bioaccumulation in Ulva lactuca. Water Air Soil pollut. 178 (1), 267–285. doi: 10.1007/s11270-006-9196-9
Gephart J. A., Henriksson P. J. G., Parker R. W. R., Shepon A., Gorospe K. D., Bergman K., et al. (2021). Environmental performance of blue foods. Nature 597 (7876), 360–365. doi: 10.1038/s41586-021-03889-2
Godfray H. C. J., Beddington J. R., Crute I. R., Haddad L., Lawrence D., Muir J. F., et al. (2010). Food security: the challenge of feeding 9 billion people. Science 327 (5967), 812–818. doi: 10.1126/science.1185383
Hafting J. T., Craigie J. S., Stengel D. B., Loureiro R. R., Buschmann A. H., Yarish C., et al. (2015). Prospects and challenges for industrial production of seaweed bioactives. J. Phycol 51 (5), 821–837. doi: 10.1111/jpy.12326
Hafting J. T., Critchley A. T., Cornish M. L., Hubley S. A., Archibald A. F. (2012). On-land cultivation of functional seaweed products for human usage. J. Appl. Phycol 24 (3), 385–392. doi: 10.1007/s10811-011-9720-1
Hanaoka K.i., Yosida K., Tamano M., Kuroiwa T., Kaise T., Maeda S. (2001). Arsenic in the prepared edible brown alga hijiki, Hizikia fusiforme. Appl. organometallic Chem. 15 (6), 561–565. doi: 10.1002/aoc.195
Harrysson H., Hayes M., Eimer F., Carlsson N. G., Toth G. B., Undeland I. (2018). Production of protein extracts from Swedish red, green, and brown seaweeds, Porphyra umbilicalis kutzing, Ulva lactuca Linnaeus, and Saccharina latissima (Linnaeus) j. v. lamouroux using three different methods. J. Appl. Phycol 30 (6), 3565–3580. doi: 10.1007/s10811-018-1481-7
Harrysson H., Konasani V. R., Toth G. B., Pavia H., Albers E., Undeland I. (2019). Strategies for improving the protein yield in pH-shift processing of Ulva lactuca Linnaeus: effects of ulvan lyases, pH-exposure time, and temperature. ACS Sustain. Chem. Eng. 7 (15), 12688–12691. doi: 10.1021/acssuschemeng.9b02781
Hasselstrom L., Visch W., Grondahl F., Nylund G. M., Pavia H. (2018). The impact of seaweed cultivation on ecosystem services - a case study from the west coast of Sweden. Mar. pollut. Bull. 133, 53–64. doi: 10.1016/j.marpolbul.2018.05.005
Holdt S. L., Kraan S. (2011). Bioactive compounds in seaweed: functional food applications and legislation. J. Appl. Phycol 23 (3), 543–597. doi: 10.1007/s10811-010-9632-5
Hurd C. L., Lobban C. S., Bischof K., Harrison P. J. (2014). “Nutrients,” in Seaweed ecology and physiology. Eds. Hurd C. L., Lobban C. S., Bischof K., Harrison P. J. (Cambridge: Cambridge University Press), 238–293.
Intawongse M., Kongchouy N., Dean J. R. (2018). Bioaccessibility of heavy metals in the seaweed Caulerpa racemosa var. corynephora: Human health risk from consumption. Instrumentation Sci. Technol. 46 (6), 628–644. doi: 10.1080/10739149.2018.1427105
Jansen H. M., Bernard M. S., Nederlof M. A. J., van der Meer I. M., van der Werf A. (2022). Seasonal variation in productivity, chemical composition and nutrient uptake of Ulva spp. (Chlorophyta) strains. J. Appl. Phycol 34, 1649–1660. doi: 10.1007/s10811-022-02708-z
Jarvis T. A., Bielmyer-Fraser G. K. (2015). Accumulation and effects of metal mixtures in two seaweed species. Comp. Biochem. Physiol. C Toxicol. Pharmacol. 171, 28–33. doi: 10.1016/j.cbpc.2015.03.005
Kollander B., Sand S., Almerud P., Ankarberg E. H., Concha G., Barregård L., et al. (2019). Inorganic arsenic in food products on the Swedish market and a risk-based intake assessment. Sci. Total Environ. 672, 525–535. doi: 10.1016/j.scitotenv.2019.03.289
Korzen L., Abelson A., Israel A. (2016). Growth, protein and carbohydrate contents in Ulva rigida and Gracilaria bursa-pastoris integrated with an offshore fish farm. J. Appl. Phycol 28 (3), 1835–1845. doi: 10.1007/s10811-015-0691-5
Lawless H. T. (2010). Sensory evaluation of food: principles and practices (New York: New York: Springer).
Lawton R. J., Mata L., de Nys R., Paul N. A. (2013). Algal bioremediation of waste waters from land-based aquaculture using Ulva: Selecting target species and strains. PLoS One 8 (10), e77344. doi: 10.1371/journal.pone.0077344
Machado M., Machado S., Pimentel F. B., Freitas V., Alves R. C., Oliveira M. (2020). Amino acid profile and protein quality assessment of macroalgae produced in an integrated multi-trophic aquaculture system. Foods 9 (10), 1–15. doi: 10.3390/foods9101382
Moerdijk-Poortvliet T. C. W., de Jong D. L. C., Fremouw R., de Reu S., de Winter J. M., Timmermans K., et al. (2022). Extraction and analysis of free amino acids and 5′-nucleotides, the key contributors to the umami taste of seaweed. Food Chem. 370, 131352. doi: 10.1016/j.foodchem.2021.131352
Mouritsen O. G., Rhatigan P., Pérez-Lloréns J. L. (2018). World cuisine of seaweeds: science meets gastronomy. Int. J. Gastronomy Food Sci. 14, 55–65. doi: 10.1016/j.ijgfs.2018.09.002
Nardelli A. E., Chiozzini V. G., Braga E. S., Chow F. (2019). Integrated multi-trophic farming system between the green seaweed Ulva lactuca, mussel, and fish: a production and bioremediation solution. J. Appl. Phycol 31 (2), 847–856. doi: 10.1007/s10811-018-1581-4
Naylor R. L., Hardy R. W., Buschmann A. H., Bush S. R., Cao L., Klinger D. H., et al. (2021). A 20-year retrospective review of global aquaculture. Nature 591 (7851), 551–563. doi: 10.1038/s41586-021-03308-6
Nielsen M. M., Bruhn A., Rasmussen M. B., Olesen B., Larsen M. M., Moller H. B. (2012). Cultivation of Ulva lactuca with manure for simultaneous bioremediation and biomass production. J. Appl. Phycol 24 (3), 449–458. doi: 10.1007/s10811-011-9767-z
Olsen Y. (2015). How can mariculture better help feed humanity? Front. Mar. Sci. 2 (46). doi: 10.3389/fmars.2015.00046
Ownsworth E., Selby D., Ottley C. J., Unsworth E., Raab A., Feldmann J., et al. (2019). Tracing the natural and anthropogenic influence on the trace elemental chemistry of estuarine macroalgae and the implications for human consumption. Sci. Total Environ. 685, 259–272. doi: 10.1016/j.scitotenv.2019.05.263
Peng Z., Guo Z., Wang Z., Zhang R., Wu Q., Gao H., et al. (2022). Species-specific bioaccumulation and health risk assessment of heavy metal in seaweeds in tropic coasts of south China Sea. Sci. Total Environ. 832, 155031. doi: 10.1016/j.scitotenv.2022.155031
Pérez-Lloréns J. L., Mouritsen O. G., Rhatigan P., Cornish M. L., Critchley A. T. (2020). Seaweeds in mythology, folklore, poetry, and life. J. Appl. Phycol 32 (5), 3157–3182. doi: 10.1007/s10811-020-02133-0
Qvirist L., Carlsson N.-G., Andlid T. (2015). Assessing phytase activity–methods, definitions and pitfalls. J. Biol. Methods 2 (1), e16. doi: 10.14440/jbm.2015.58
Robertson-Andersson D. V., Wilson D. T., Bolton J. J., Anderson R. J., Maneveldt G. W. (2009). Rapid assessment of tissue nitrogen in cultivated Gracilaria gracilis (Rhodophyta) and Ulva lactuca (Chlorophyta). Afr. J. Aquat. Sci. 34 (2), 169–172. doi: 10.2989/ajas.2009.34.2.7.894
Sá A. G. A., Moreno Y. M. F., Carciofi B. A. M. (2020). Plant proteins as high-quality nutritional source for human diet. Trends Food Sci. Technol. 97, 170–184. doi: 10.1016/j.tifs.2020.01.011
Sánchez-García F., Mirzayeva A., Roldán A., Castro R., Palacios V., et al. (2021). Effect of different cooking methods on sea lettuce (Ulva rigida) volatile compounds and sensory properties. J. Sci. Food Agric. 101 (3), 970–980. doi: 10.1002/jsfa.10705
Sánchez-García F., Mirzayeva A., Roldán A., Castro R., Palacios V., García-Barroso C., et al. (2019). Evolution of volatile compounds and sensory characteristics of edible green seaweed (Ulva rigida) during storage at different temperatures. J. Sci. Food Agric. 99 (12), 5475–5482. doi: 10.1002/jsfa.9808
Shuuluka D., Bolton J. J., Anderson R. J. (2013). Protein content, amino acid composition and nitrogen-to-protein conversion factors of Ulva rigida and Ulva capensis from natural populations and Ulva lactuca from an aquaculture system, in south Africa. J. Appl. Phycol 25 (2), 677–685. doi: 10.1007/s10811-012-9902-5
Sode S., Bruhn A., Balsby T. J. S., Larsen M. M., Gotfredsen A., Rasmussen M. B. (2013). Bioremediation of reject water from anaerobically digested waste water sludge with macroalgae (Ulva lactuca, chlorophyta). Bioresource Technol. 146, 426–435. doi: 10.1016/j.biortech.2013.06.062
Stedt K., Trigo J. P., Steinhagen S., Nylund G. M., Forghani B., Pavia H., et al. (2022a). Cultivation of seaweeds in food production process waters: evaluation of growth and crude protein content. Algal Res. 63, 102647. doi: 10.1016/j.algal.2022.102647
Stedt K., Gustavsson O., Kollander B., Undeland I., Toth G. B., Pavia H. (2022b). Cultivation of Ulva fenestrata using herring production process waters increases biomass yield and protein content. Front. Mar. Sci., 1762. doi: 10.3389/fmars.2022.988523
Steinhagen S., Enge S., Cervin G., Larsson K., Edlund U., Schmidt A. E. M., et al. (2022). Harvest time can affect the optimal yield and quality of sea lettuce (Ulva fenestrata) in a sustainable sea-based cultivation. Front. Mar. Sci. 9. doi: 10.3389/fmars.2022.816890
Steinhagen S., Enge S., Larsson K., Olsson J., Nylund G. M., Albers E., et al. (2021). Sustainable large-scale aquaculture of the northern hemisphere sea lettuce, Ulva fenestrata, in an off-shore seafarm. J. Mar. Sci. Eng. 9 (6), 615. doi: 10.3390/jmse9060615
Stévant P., Marfaing H., Duinker A., Fleurence J., Rustad T., Sandbakken I., et al. (2018). Biomass soaking treatments to reduce potentially undesirable compounds in the edible seaweeds sugar kelp (Saccharina latissima) and winged kelp (Alaria esculenta) and health risk estimation for human consumption. J. Appl. Phycol 30 (3), 2047–2060. doi: 10.1007/s10811-017-1343-8
Thiviya P., Gamage A., Gama-Arachchige N. S., Merah O., Madhujith T. (2022). Seaweeds as a source of functional proteins. Phycology 2 (2), 216–243. doi: 10.3390/phycology2020012
Toth G. B., Harrysson H., Wahlstrom N., Olsson J., Oerbekke A., Steinhagen S., et al. (2020). Effects of irradiance, temperature, nutrients, and pCO(2) on the growth and biochemical composition of cultivated Ulva fenestrata. J. Appl. Phycol 32, 3243–3254. doi: 10.1007/s10811-020-02155-8
Trigo J. P., Engström N., Steinhagen S., Juul L., Harrysson H., Toth G. B., et al. (2021). In vitro digestibility and caco-2 cell bioavailability of sea lettuce (Ulva fenestrata) proteins extracted using pH-shift processing. Food Chem. 356, 129683. doi: 10.1016/j.foodchem.2021.129683
US EPA (2007). Concepts, methods, and data sources for cumulative health risk assessment of multiple chemicals, exposures and effects: a resource document (Washingtong, DC: US Environmental Protection Agency).
Venolia C. T., Lavaud R., Green-Gavrielidis L. A., Thornber C., Humphries A. T. (2020). Modeling the growth of sugar kelp (Saccharina latissima) in aquaculture systems using dynamic energy budget theory. Ecol. Model. 430, 109151. doi: 10.1016/j.ecolmodel.2020.109151
Wendin K., Undeland I. (2020). Seaweed as food – attitudes and preferences among Swedish consumers. a pilot study. Int. J. Gastronomy Food Sci. 22, 100265. doi: 10.1016/j.ijgfs.2020.100265
WHO/FAO/UNU (2007). “Protein and amino acid requirements in human nutrition,” in WHO technical report series 935. report of joint WHO/FAO/UNU expert consultation. Geneva, Switzerland: World Health Organization.
worlddata.info (2022) Average sizes of men and women. Available at: https://www.worlddata.info/average-bodyheight.php (Accessed 5 July 2022).
Keywords: macroalgae, ulva, food application, proteins, arsenic, heavy metals, exposure assessment, wastewater
Citation: Stedt K, Steinhagen S, Trigo JP, Kollander B, Undeland I, Toth GB, Wendin K and Pavia H (2022) Post-harvest cultivation with seafood process waters improves protein levels of Ulva fenestrata while retaining important food sensory attributes. Front. Mar. Sci. 9:991359. doi: 10.3389/fmars.2022.991359
Received: 11 July 2022; Accepted: 06 September 2022;
Published: 26 September 2022.
Edited by:
Mercedes González-Wangüemert, University of Algarve, PortugalReviewed by:
Leonel Pereira, University of Coimbra, PortugalJosé M. Rico, University of Oviedo, Spain
Copyright © 2022 Stedt, Steinhagen, Trigo, Kollander, Undeland, Toth, Wendin and Pavia. This is an open-access article distributed under the terms of the Creative Commons Attribution License (CC BY). The use, distribution or reproduction in other forums is permitted, provided the original author(s) and the copyright owner(s) are credited and that the original publication in this journal is cited, in accordance with accepted academic practice. No use, distribution or reproduction is permitted which does not comply with these terms.
*Correspondence: Kristoffer Stedt, a3Jpc3RvZmZlci5zdGVkdEBndS5zZQ==