- 1Shandong Key Laboratory of Eco-Environmental Science for the Yellow River Delta, Binzhou University, Binzhou, China
- 2CAS Key laboratory of Coastal Environmental Processes and Ecological Remediation, Yantai Institute of Coastal Zone Research (YIC), Chinese Academy of Sciences (CAS), Shandong Key Laboratory of Coastal Environmental Processes, YICCAS, Yantai, China
Coastal wetlands are considered as important “blue carbon” sink, and mowing management induced by anthropogenic activities is anticipated to profoundly affect soil carbon stocks in coastal wetlands. However, the impacts of mowing management on soil organic carbon (SOC) and enzyme activities and the mechanisms responsible for associated changes in Phragmites australis wetland remain uncertain. We conducted a field mowing manipulation experiment [control (CK), mowing and returning straw in December (12MS), mowing and removing straw in December (12MR), mowing and returning straw in March (3MS), and mowing and removing straw in March (3MR)] in P. australis wetland of the Yellow River Delta and quantified their impacts on soil quality, SOC, SOC fractions, and enzyme activities. Results showed that mowing treatments led to overall increases in soil nutrients [total carbon (TC), total nitrogen (TN), total phosphorus (TP), NH4+, and NO3−] and decreases in soil C/N ratio. The effects of mowing treatments on soil nutrient content were pronounced on topsoil than deep soil, and the maximum value of TC, TN, and TP reached in the 12MR treatment. Compared with CK, the 12MS, 12MR, 3MS, and 3MR treatments at 0–10 cm depth significantly enhanced SOC content by 8.78%, 32.9%, 16.5%, and 30.1%, respectively, but only the 3MS treatment enhanced SOC by 16.5% at 10–20 cm depth. Mowing treatments increased dissolved organic carbon (DOC), microbial biomass carbon (MBC), particulate organic carbon (POC), and labile organic carbon (LOC) and the contents of DOC, MBC, POC, and LOC decreased with soil depth. Mowing treatments stimulated the activities of sucrase and urease in topsoil, but only the 3MR treatment improved alkaline phosphatase activity in topsoil. Path analysis indicated that mowing management dominantly modulates SOC by changing sucrase activity, alkaline phosphatase activity, TN, TP, NH4+, NO3−, DOC, and LOC in 0–10 cm depth. However, SOC was significantly controlled by sucrase activity, urease activity, TC, TN, TP, LOC, and POC in 10–20 cm depth. Collectively, our results indicate that the continuous mowing management is beneficial to enhance soil quality and carbon storage capacity of P. australis wetlands, which will contribute to enhance carbon sequestration and sink capacity of coastal wetlands.
Introduction
Coastal wetlands are among the most carbon-rich ecosystems on Earth due to high primary productivity, high carbon accumulation rate, and low organic matter decomposition rate (Osland et al., 2018; Chu et al., 2019). As a rough estimate, global coastal wetlands have the capacity to bury 116 Tg C/year, which are 10–100 times of carbon (C) burial higher than terrestrial forests ecosystems (Mcleod et al., 2011; Kirwan and Mudd, 2012). The “blue carbon” sinks in coastal wetlands have greater potential than terrestrial C sinks in reducing atmospheric carbon fluxes and mitigating climate change over longer periods (Han et al., 2018; Wang et al., 2019). In recent decades, anthropogenic activities have reduced coastal wetlands area and declined C sink function, leading to the release of CO2 emission newly increase by 0.45 Pg/Ca per year (Pendleton et al., 2012). Phragmites australis, a common perennial wetland grass with high carbon sequestration ability, is widespread distributed in coastal wetlands in China (Han et al., 2013). P. australis mowing is the most used management approaches in wetland system that may change soil microclimate, community structure, plant productivity, and soil carbon storage (Köbbing et al., 2013; Tanaka et al., 2017; Chai et al., 2019). Given that mowing management approaches may impact soil C with great uncertainty, it is essential to identify the response of soil C dynamics and storage to human activities to precisely predict soil C balance.
SOC plays a significant role in the ecosystem C cycle, and its content and quality are influenced by plant species composition, land use patterns, and management practices (Chai et al., 2019). Active SOC is the fraction of soil C with a high activity that shows different sensitivities to environmental changes (Cheng et al., 2008), which can be categorized into dissolved organic carbon (DOC), microbial biomass carbon (MBC), particulate organic carbon (POC), and labile organic carbon (LOC) (Xu et al., 2011). Removing plant aboveground tissue by mowing is anticipated to impact SOC and SOC fractions by changing biogeochemical processes such as soil microclimate and microorganisms, plant growth, and community composition (Tuffa et al., 2017; Zong and Shi, 2019). Mowing may have considerable effects on C fixation capacity of plants because it alters community diversity and primarily productivity, influencing allocation pattern of the aboveground and root biomass (Xu et al., 2014; Erktan et al., 2018). The change of root biomass and decomposition of root turnover may change root input of SOC and nutrient into soil, resulting in the alteration of soil C and active SOC (Xu et al., 2012). Previous studies showed that reasonable mowing could increase the content of SOC, MBC, and DOC, whereas excessive mowing could decrease the content of SOC, MBC, and total C, further resulting in the loss of soil carbon and nutrients (Shahzad et al., 2012; Chai et al., 2019). Soil enzyme activity, an indicator of soil quality and SOC change in response to ecosystem interference, can also be influenced by plant community composition and soil status (Acosta-Martinez et al., 2018; Song et al., 2019). Mowing can increase, decrease, and have no effect on soil enzyme activities and differed by different mowing measures (Yu et al., 2017; Jing et al., 2022; Song et al., 2022). Thus, understanding the response of active SOC and enzyme activities to mowing management is fundamentally important to broaden our knowledge of SOC allocation and enzyme activities dynamics to human activities in coastal wetlands.
Appropriate straw returning plays an important role in promoting soil quality and plant growth because of the favorable effects on soil physicochemical and biological characteristics and fertility (Xiu et al., 2019; Bu et al., 2020). The plant straw decomposition can provide a great number of soil organic matter and offer nutrient supply needed by plant (Wang et al., 2015). Further, the incorporation of straw may stimulate the nutrient use efficiency and nutrient cycling, which converts soil nutrients into the slowly source of nutrient supply (Zhang et al., 2016). Several studies have showed that the incorporation of straw into soil can increase SOC content, active SOC fractions, and microbial activities through improving carbon input (Wang et al., 2015; Song et al., 2019). For example, Chen et al. (2017) found that short-term rice straw return increased soil SOC, DOC, and MBC in rice-wheat cropping soil, whereas Hao et al. (2019) demonstrated that long-term addition of straw return enhanced soil SOC and MBC in farmland soil. Straw returning to the field often accelerate soil C pool and sequestration, further contributing to higher SOC by elevating SOC fractions (Li et al., 2016). Furthermore, straw mulching has confirmed to enhance soil enzyme activities and thereafter favored higher SOC pool and fractions (Zhang et al., 2016; Xiu et al., 2019). Hence, it is imperative to explore the effects of straw incorporation on SOC fractions and enzyme activities in coastal wetlands.
The coastal wetlands of the Yellow River Delta (YRD) possess high vegetation productivity and C sequestration capacity (Han et al., 2018), whereas it has degraded and shrunk by human activities, resulting in the decrease of C storage by 10.2% in the past decades (Ma et al., 2019). The area of P. australis in the YRD has reached 2,600 hm2, and P. australis mowing is a common human interference in this area (Guan et al., 2014). Mowing can modulate P. australis growth characteristics, improve plant diversity, and optimize wetlands ecological environment in coastal wetlands (Tanaka et al., 2017). Mowing can cause changes in soil water and salinity by changing microclimate, which profoundly affect the ecological characteristics and growth of P. australis community and thus affect the mineralization of SOC and the allocation of active SOC fractions (Setia et al., 2011; Guan et al., 2014; Zhao et al., 2018). In addition, mowing management measures also cause changes in SOC pool and C sequestration due to the alteration of the root resource allocation and aboveground regrowth (Asaeda et al., 2006; Song et al., 2015). However, the lack of knowledge about the effects of mowing management on SOC content, SOC fractions, and enzyme activities impedes efforts to establish a clear trajectory of C budget response to human interference in coastal wetlands. We hypothesized that the responses of SOC to mowing management were regulated by mowing-related changes, largely through soil nutrient, SOC fractions, and enzyme activities. To test our hypothesis, we conducted a field experiment that simulated different mowing measures in P. australis wetland of the YRD to elucidate the effects of mowing on soil quality, SOC content and fractions, and enzyme activities. Our main objectives were (i) to investigate the response of soil nutrient, SOC, and SOC fractions to mowing treatments; (ii) to understand how enzyme activities respond to changes in mowing management; and (iii) to illustrate the potential linkages between SOC and soil nutrient, SOC fractions, and enzyme activities under different mowing treatments.
Materials and methods
Study site
This research was conducted at the Research Station of Coastal Wetland in the Yellow River Delta (37°45′50″N, 118°59′24″E), which attaches to Yantai Institute of Coastal Zone Research, Chinese Academy of Sciences (Figure 1). This experimental site is located at supratidal wetlands of the Yellow River Delta. This area occupies a warm-temperate and continental monsoon climate with average annual temperature and average annual precipitation being 12.9°C and 560 mm, respectively. Monthly average temperature ranges from −2.8°C in January to 26.7°C in July and approximately 70% of the annual precipitation is occurred in the growing season. The groundwater table is shallow with an average depth of 1.14 m, and the periodic surface ponding is often happened after extreme rainfall. The hydrology of this area is influenced by the tidal process and the Yellow River runoff. The soil type mainly consists of wet and saline soil, and soil texture is mainly sandy clay loam. The dominant vegetation in our study site is Phragmites australis, with other species mainly including Suaeda salsa, Inperata cylindrica, and Tripoliun vulgare. The highest canopy height of the growing season is 1.7 m, and the canopy index closure range is between 0.3 and 0.8 (Han et al., 2018).
Experimental design and soil sampling
This filed experiment was installed in 2016 with 20 quadrats (6 × 6 m) in a P. australis–dominated flat (Figure 1). Five treatments were established as follows in this experiment: control (CK), mowing and returning straw in December (12MS), mowing and removing straw in December (12MR), mowing and returning straw in March (3MS), and mowing and removing straw in March (3MR). The reason for choosing these two mowing months is that December is the traditional harvest time in this area, whereas March is chosen to test whether mowing stimulates the germination of P. australis. Each treatment was replicated four times, and each quadrat was separated by cement barriers at a 40 cm distance. At the beginning of this experiment, P. australis covered nearly >95% in all treatments. The P. australis stems were removed at the height of 4–6 cm in the mowing plots once a year in December and March. The P. australis stems were removed out of the plots in 12MR and 3MR treatments, and the harvested P. australis stems were applied soil surface evenly in 12MS and 3MS treatments. Mowing treatments started in December 2016 and continued indefinitely according to the experimental design.
Soil samples were collected by mixing three soil cores (7.5 cm diameter) randomly from the topsoil (0–10 cm) and the subsoil (10–20 cm) of each plot in August 2020. After removing debris such as stones and plant and animal residues, the soil samples were sealed and transported to the laboratory. At each plot, soils with the same soil layer were mixed, homogenized, and then divided into two parts. One part was air dried and passed through a 2-mm sieve for analysis of SOC, POC, LOC, sucrase, urease, and alkaline phosphatase activity. The other part was stored at 4°C for analysis of MBC, DOC, NH4+, and NO3−.
Soil analysis
SOC was determined using the K dichromate volumetric method and then measured by the elemental analyzer. MBC was measured with the chloroform fumigation-direct extraction method after saturating the soil with water to minimize errors associated with variable soil moisture content between plots (Vance et al., 1987). DOC was extracted by deionized water (soil:water, 1:5) and filtrated by 0.45-μm membrane and then analyzed by the total organic carbon analyzer (Elementar, Germany). POC was determined by the sodium hexametaphosphate dispersion method (5 g/L) (Lee, 2013), and LOC was measured by the 333 mM KMnO4 digestion method (Xu et al., 2011). NH4+ and NO3− were determined by an AA3 automated flow injection analysis (SEAL Analytical GmbH, Norderstedt, Germany). Soil sucrase activity was measured by 5-dinitrylsalicylate colorimetrically and quantified at 508 nm using a spectrophotometer (722, Jingke, Shanghai). Soil urease activity was determined by sodium phenoxide and colorimetrically and measured at 578 nm with a UV spectrophotometer. Soil alkaline phosphatase activity was measured as described in Tan et al. (2014) and expressed as the amount of phenol released from the soil using a spectrophotometer at 510 nm.
Data analysis
One-way ANOVA based on the least significant difference (LSD) (p < 0.05) was used to determine the effects of mowing treatments on soil quality, SOC, SOC fractions, and enzyme activities. Pearson’s correlation coefficient analysis was conducted to evaluate the relationship between soil quality, SOC, SOC fractions, and enzyme activities. R3.6.1 statistical software and the Corrplot package were used for correlation analysis. Path analysis was performed to ascertain hypothetical pathways by which soil quality, SOC fractions, and enzyme activities might explain SOC. The data were fit to the model using maximum likelihood estimation. Path analysis was conducted using the AMOS 21.0 version. SPSS 22.0 was used to the statistical analysis. Origin Pro 2018 and ArcGIS 10.5 software were used for graphing.
Results
Variation in soil properties
Soil pH in the 0- to 10-cm and 10- to 20-cm soil layers showed little change after 4 years of mowing management (Table 1). All the mowing treatments increased soil salinity, although not significantly (Table 1). Four years of mowing treatments significantly increased soil total carbon (TC) (P < 0.05), with the highest enhancement of 17.5% and 16.8% under the 12MS treatment in the 0- to 10-cm and 10- to 20-cm soil layers, respectively. Soil total nitrogen (TN) levels under the 12MS and 3MR treatments in the 0- to 10-cm layer were significantly 68.8% and 78.4% higher than the CK treatment (P < 0.05), respectively. All treatments significantly increased soil TN by 44.9%–91.2% at the 10- to 20-cm layer. Soil total phosphorus (TP) levels in the 12MR, 12MS, 3MR, and 3MS treatments were 1.17, 1.45, 1.07, and 1.21 g/kg, respectively, at the 0- to 10-cm soil layer, significantly higher than that in the CK treatment (P < 0.05). However, soil TP did not differ with mowing management in the 10- to 20-cm soil layer. Across the two soil depths, all treatments significantly decreased soil C:N ratio (P < 0.05), and soil C:N ratio at the 0- to 10-cm and 10- to 20-cm soil layers reached to 21.3–25.6 and 23.1–27.9, respectively. Mowing treatments significantly increased soil NH4+ at the 0- to 10-cm soil sample, although only the 12MR treatment significantly enhanced soil NH4+ at the 10- to 20-cm soil sample. The 12MS, 3MR, and 3MS treatments significantly increased soil NO3− at the 0- to 10-cm soil layer by 110.2%, 63.9%, and 164%, respectively, whereas the 12MR, 3MR, and 3MS treatments significantly elevated soil NO3− at the 10- to 20-cm soil layer by 139.9%, 68.6%, and 114.1%, respectively (P < 0.05).
Variation in soil organic carbon and active fractions
Compared with the CK treatment, the 12MS, 12MR, 3MS, and 3MR treatments at the 0- to 10-cm soil layer significantly stimulated SOC content by 8.78%, 32.9%, 16.5%, and 30.1%, respectively, whereas only the 3MS treatment at the 10- to 20-cm soil layer significantly stimulated SOC content by 16.5% (P < 0.05, Figure 2A). Only the 12MR and 3MR treatments were found to be significant than the CK treatment (P < 0.05, Figure 2B), with the increased MBC content by 41.0% and 35.5% in the 0- to 10-cm soil layer, and 23.1% and 21.2% in the 10- to 20-cm soil layer, respectively. In the 0- to 10-cm soil layer, DOC content under four mowing treatments were 103.6%, 152.4%, 62.12%, and 163.7% higher than that under the CK treatment, respectively (P < 0.05, Figure 3C). Compared with CK, the 12MR, 12MS, 3MR, and 3MS treatments in the 10- to 20-cm soil layer significantly increased DOC content by 39.55%, 14.92%, 22.16%, and 43.65%, respectively (P < 0.05, Figure 2C). The 12MR and 12MS treatments in the 0- to 10-cm soil layer obtained significantly higher LOC content than the CK treatment, with average increases of 24.76% and 26.27%, respectively (P < 0.05, Figure 2D). In the 10- to 20-cm soil layer, only the 12MS treatment significantly enhanced LOC by 70.45% (P < 0.05, Figure 2D). Compared with that under the CK treatment, POC content in the 0- to 10-cm soil layer elevated significantly under the 12MR, 12MS, 3MR, and 3MS treatments by 30.00%, 35.54%, 27.95%, and 57.27%, whereas POC content in the 10- to 20-cm soil layer increased significantly under four treatments by 33.60%, 32.36%, 39.40%, and 49.04%, respectively (P < 0.05, Figure 2E).
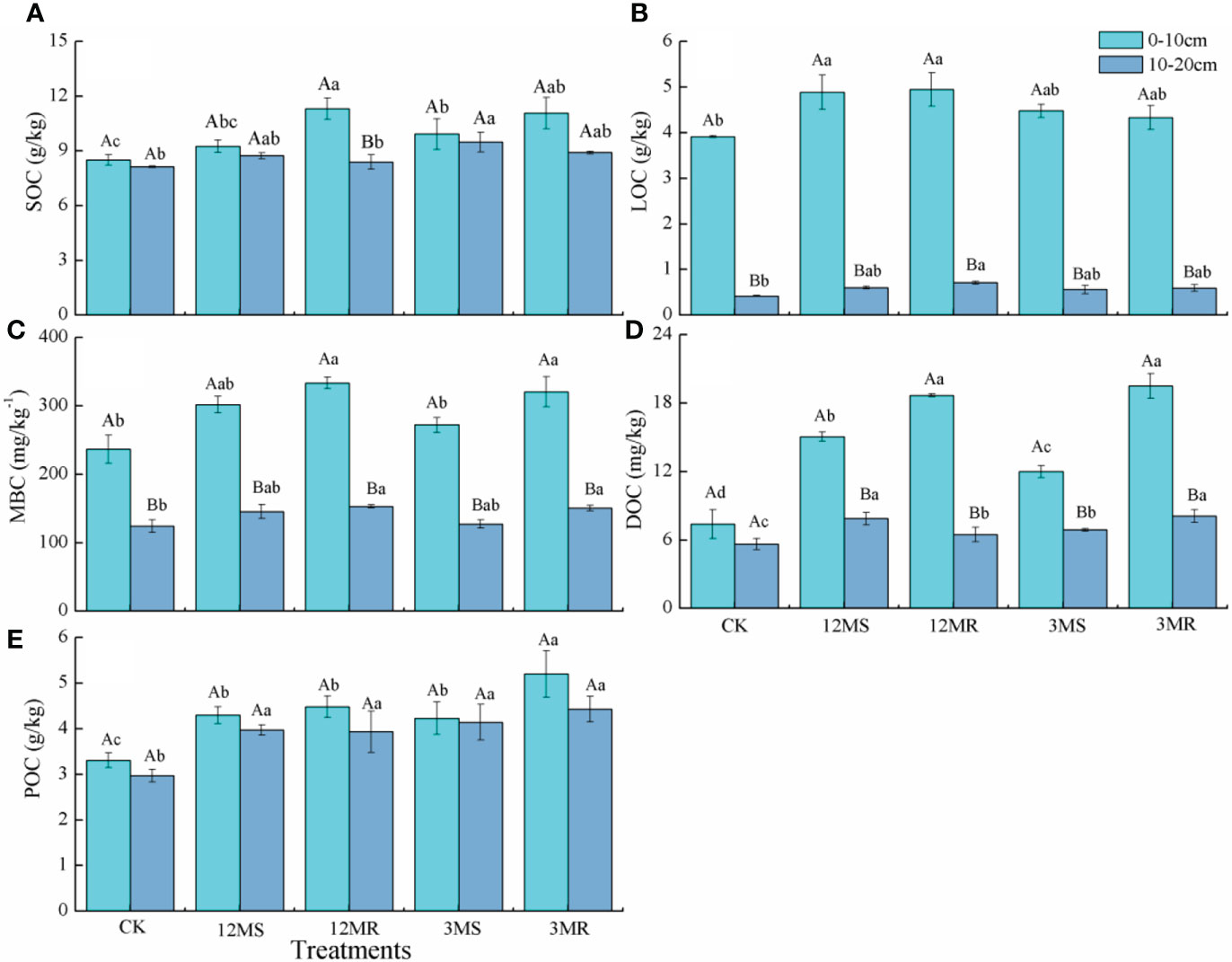
Figure 2 Soil organic carbon (SOC, A), labile organic carbon (LOC, B), microbial organic carbon (MBC, C), dissolved organic carbon (DOC, D), and particulate organic carbon (POC, E) under different treatments. Data are mean ± SD (n = 4). Different lowercase letters represent significant differences under different treatments. Different capital letters indicate significance between 0- to 10-cm and 10- to 20-cm soil layers of each treatment.
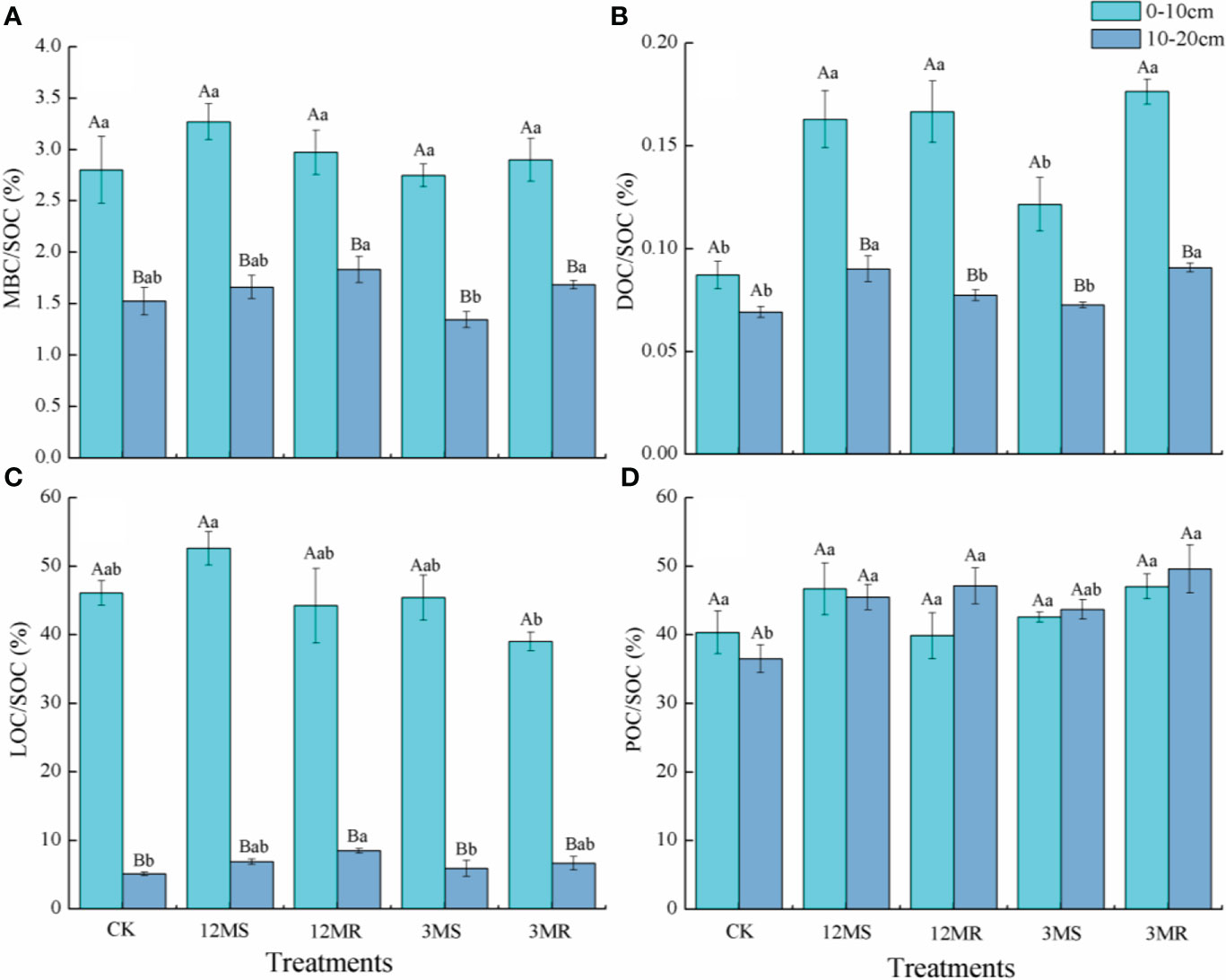
Figure 3 MBC/SOC (A), DOC/SOC (B), LOC/SOC (C), and POC/SOC (D) under different treatments. Data are mean ± SD (n = 4). Different lowercase letters represent significant differences under different treatments. Different capital letters indicate significance between 0- to 10-cm and 10- to 20-cm soil layers of each treatment.
Variation on allocation proportion of soil organic carbon fractions
Mowing management did not significantly affect MBC/SOC in the 0- to 10-cm and 10- to 20-cm soil layers (Figure 3A). Compared with the CK treatment, the 12MS, 12MR, and 3MR treatments in the 0- to 10-cm soil layer significantly enhanced DOC/SOC by 86.95%, 91.18%, and 102.34%, whereas the 12MS and 3MR treatments in the 10- to 20-cm soil layer significantly increased DOC/SOC by 30.27% and 31.16%, respectively (P < 0.05, Figure 3B). Among all the treatments, the 12MS and 3MR treatments at the 0- to 10-cm soil layer had the highest and lowest ratio of LOC/SOC, and these four treatments showed no significant difference compared with the CK treatment. However, the 12MR treatment at the 10- to 20-cm soil layer significantly improved LOC/SOC by 65.53% compared with the CK treatment (P < 0.05, Figure 3C). With respect to the POC/SOC ratio, the 12MS, 12MR, and 3MR treatments at the 10- to 20-cm soil layer were 24.5%, 29.09%, and 35.89% higher under the CK treatment, respectively (P < 0.05, Figure 3D).
Variations in soil enzyme activities
Most soil enzyme activities increased after 4 years of mowing management treatments. In the 0- to 10-cm soil layer, the 12MR, 3MS, and 3MR treatments significantly improved soil sucrase activity by 42.04%, 28.42%, and 41.47%, respectively (P < 0.05, Figure 4A). However, only 12MR significantly improved soil sucrase activity at 10- to 20-cm soil layer by 44.13% (P < 0.05, Figure 4A). In treatments of 12MS, 3MS, and 3MR, soil urease activity at 0- to 10-cm soil layer showed increases of 24.45%, 14.52%, and 17.54%, respectively, compared with the CK (P < 0.05, Figure 4B). In comparison to the CK treatment, the 12MS, 12MR, 3MS, and 3MR treatments at 10- to 20-cm soil depth significantly increased soil urease activity by 17.51%, 28.42%, 31.26%, and 48.30%, respectively (P < 0.05, Figure 4B). Soil alkaline phosphatase activity at 0- to 10-cm soil layer under the 12MR, 3MS, and 3MR treatments were 27.89%, 22.65%, and 43.30% higher than the CK, respectively (P < 0.05, Figure 4C). Compared with the CK, the 12MS, 3MS, and 3MR treatments in the 10–20 cm depth significantly increased soil alkaline phosphatase activity by 18.88%, 22.88%, and 27.04%, respectively (P < 0.05, Figure 4C).
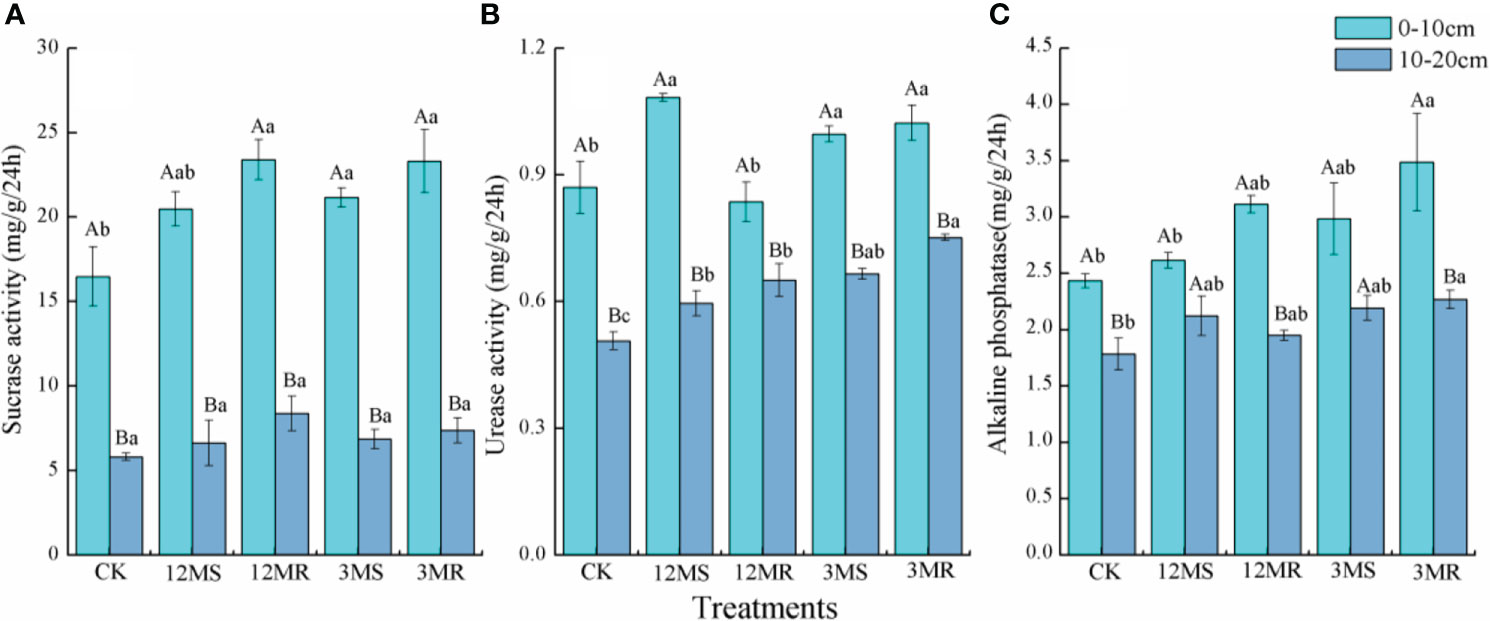
Figure 4 Sucrase activity (A), urease activity (B), and alkaline phosphatase activity (C) under different treatments. Data are mean ± SD (n = 4). Different lowercase letters represent significant differences under different treatments. Different capital letters indicate significance between 0- to 10-cm and 10- to 20-cm soil layers of each treatment.
Relationships between soil organic carbon fractions, soil enzyme activities, and soil nutrients
Pearson correlation analysis revealed significant positive correlations between soil quality, SOC, SOC fractions, and enzyme activities. For example, a highly significant positive correlation was observed between the SOC and TP, MBC, DOC, POC, NH4+, NO3−, and sucrase and alkaline phosphatase activity in the 0- to 10-cm soil layer (Figure 5A). MBC was significantly correlated with TP, DOC, POC, NH4+, NO3−, and sucrase activity. DOC was significantly correlated with TP, SOC, MBC, POC, NH4+, NO3−, and sucrase and alkaline phosphatase activity. In the 10- to 20-cm soil layer, SOC had significant positive relationships with TP, POC, and alkaline phosphatase activity (Figure 5B). Path analysis was performed to explore the underlying mechanisms of mowing management, soil enzyme activity, soil quality, SOC fractions, and SOC (Figure 6). The SOC in the 0- to 10-cm and 10- to 20-cm soil layers were explained by 89% and 93% of the variation using the path analysis model, respectively. Mowing treatments enhanced soil sucrase and alkaline phosphatase activities, then positively promoted TN, TP, NH4+, NO3−, DOC, and LOC, and hence improved SOC in the 0- to 10-cm soil depth (Figure 6A). With regard to the 10- to 20-cm soil layer, mowing management improved sucrase and urease activities, then positively influenced TC, TN, TP, LOC, and POC, and finally stimulated the content of SOC (Figure 6B).
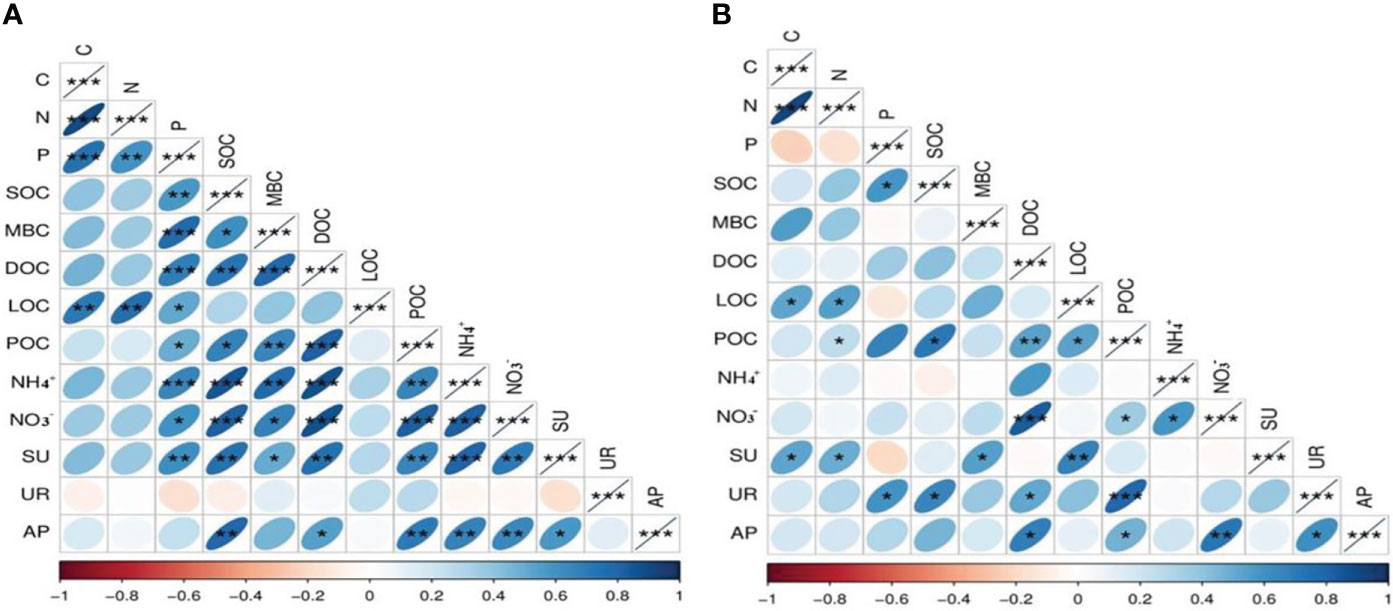
Figure 5 Pearson correlations between soil nutrient, SOC, SOC fractions, and soil enzyme activities under 0–10 cm depth (A) and 10–20 cm depth (B). The symbols *, **, and *** indicate significant correlations at the 0.05, 0.01, and 0.001 levels, respectively. The bottom axis is the legend, on which correlation coefficient values are represented by different colors.
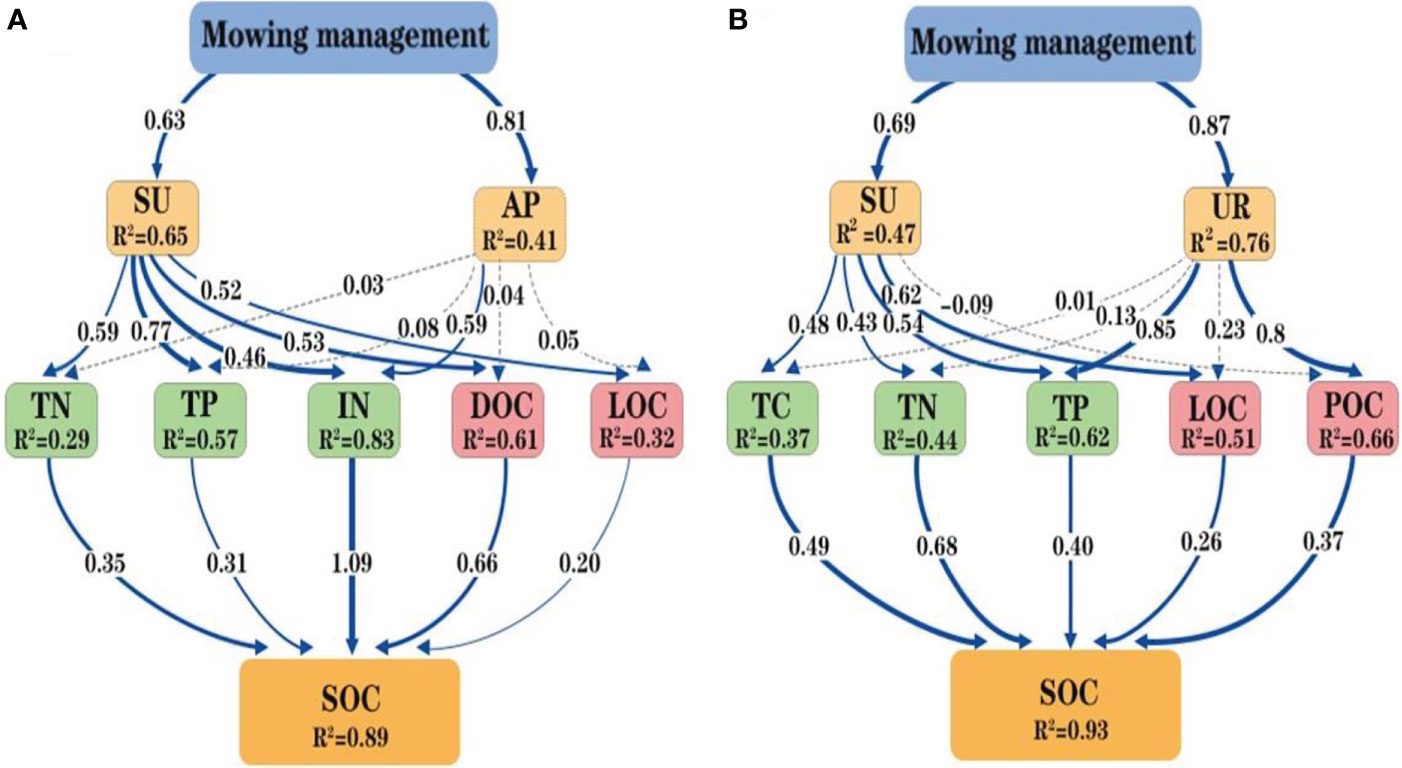
Figure 6 The structure equation model describes the plausible pathways by which mowing management treatment influenced SOC content at 0–10 cm depth (A) and 10–20 cm depth (B). Soil enzyme activities are shown in yellow, soil nutrients in green, SOC fractions in red, and SOC in orange. Blue solid arrows from thick to thin indicate positive pathways (p < 0.001, p < 0.01, and p < 0.05), and light dashed gray arrows indicate non-significant relationships (p > 0.05). The numbers next to arrows are standardized path coefficients, and the arrow width represents the strength of the relationship. The proportion of the variation (R2) values occurs aside each response variable in the model. SU, sucrase activity; UR, urease activity; AP, alkaline phosphatase activity; TC, total carbon; TN, total nitrogen; TP, total phosphatase; IN, NH4+ and NO3−; DOC, dissolved organic carbon; LOC, labile organic carbon; POC, particulate organic carbon; SOC, soil organic carbon.
Discussion
Effects of mowing treatments on soil properties
Soil salinity increased after 4 years of mowing management, especially in the 12MR and 3MR treatments (Table 1). We considered that mowing and removing reed straw treatment can cause an increase in bare ground and soil evaporation, decreasing soil moisture and increasing soil salinity (Marschner et al., 2015). Moreover, improved plant transpiration and more water absorb by root with increased plant growth may elevate soil salinity (Chen et al., 2019). Mowing is an important management practice on grassland that has been proved to improve ecosystem carbon stocks (Koncz et al., 2017). We found that mowing management increased soil TC and TN and decreased soil C/N ratio (Table 1), which is similar with the previous studies (Wei et al., 2016). Mowing disturbs the equilibrium between soil and plant by removing aboveground biomass and decreases water and nutrient supply requirements for aboveground parts, resulting in more reallocation of resources to roots (Xu et al., 2012; Tuffa et al., 2017). Accordingly, a more favorable energy environment induced by persistent mowing is helpful for facilitating plant productivity, root biomass, and root exudates, which may increase soil C and N stocks (Cong et al., 2014; Li et al., 2017; Yu et al., 2017). In addition, the incorporation of reed straw into the soil may improve soil physical, chemical, and microbial condition, thereby providing more energy for the accumulation of soil C and N (Zhang et al., 2016; Bu et al., 2020). Mowing management also decreased soil C/N ratio, indicating that mowing could stimulate the release of more nitrogen for use by plants. Mowing management treatments increased the concentrations of soil TP and NH4+ in 0- to 10-cm soil layer but had significant impact on NO3− concentration in both two soil layers (Table 1). The increased soil TP, NH4+, and NO3− were mainly related to enhanced plant biomass and root vitality induced by mowing, which led to more efficient recycling of P and N (Tanaka et al., 2017; Kotas et al., 2017). Positive correlations between soil TP, NH4+, NO3-, TC, TN, SOC, and SOC fractions provide evidence for the stimulation of soil quality and SOC among the different mowing management treatments.
Effects of mowing treatments on SOC
Our results suggested that 4 years of mowing led to a significant increase in SOC contents at 0- to 10-cm and 10- to 20-cm layers, indicating that the mowing management measures elevated SOC pool in P. australis wetland. These results were in line with previous studies (Ziter and MacDougall, 2013; Poeplau et al., 2016; Li et al., 2017), in which mowing enhanced SOC accumulation and changed SOC composition in grassland ecosystem. Moderate mowing improved the plant productivity by stimulating the dominance of efficiently regrowing plant species through the favorable energy environment for plant (Erktan et al., 2018; Zong and Shi, 2019). Our results supported these ideas and found that mowing regime improved P. australis vitality, dominance, and productivity (Cui et al., unpublished data), which agreed with previous studies (Asaeda et al., 2006; Köbbing et al., 2013). Four mowing management treatments can improve illumination and ventilation conditions, thereby promoting the germination rate and photosynthetic efficiency of P. australis for the improvement of reed density and primary productivity (Chai et al., 2019). SOC distribution is regulated by plant productivity and root biomass, because the root biomass and their exudates were closely correlated with SOC accumulation (Keller et al., 2015; Poeplau et al., 2015). Thus, higher SOC in the soil of mowing treatments than control could be partly ascribed to the improved P. australis productivity and C sequestration capacity via increased C input and N retention, as the P. australis biomass in four mowing treatments is higher than those in control plot (Cong et al., 2014; Li et al., 2017). Interestingly, we observed that SOC content in the 12MR and 3MR treatments were higher than the 12MS and 3MS treatments. After harvesting and returning the P. australis straw into the soil under the 12MS and 3MS treatments, the P. australis straw decomposed very slowly in the flooded anerobic environment, especially after experiencing 4 years of straw addition. The low SOC content mainly be attributed to the low decomposition rate of reed straw, restricting the P. australis germination and growth, as supported by our observation that P. australis density and biomass in the 12MR and 3MR treatments are lower than that in the 12MS and 3MS treatments (Cui et al., unpublished data).
Our results from correlation and path analysis suggested that the influencing factors that affected SOC were different under the 0- to 10-cm and 10- to 20-cm soil layers, whereas the factors would change depend on the alteration of soil properties and carbon fraction dynamics. Path analysis showed that SOC content in the 0- to 10-cm soil layer was directly controlled by TN, TP, NH4+, NO3−, DOC, and LOC and indirectly controlled by sucrase and alkaline phosphatase activity (Figure 6A). However, SOC content in the 10- to 20-cm soil layer was directly dominated by TC, TN, TP, POC, and LOC and indirectly regulated by the sucrase and urease activity (Figure 6B). A significant relationship was found between SOC and TN content in two soil layers (Wang et al., 2015; Assefa et al., 2017), which suggested that the increased TN content would be benefit for the accumulation of SOC in this N-deficient coastal wetlands (Zhao et al., 2018). Significant positive correlation between SOC and TP was exhibited in two soil layers, indicating that increased soil nutrient may improve the input of soil organic matter. DOC, POC, and LOC were most strongly correlated with SOC, and these SOC fractions would serve as the indicators of SOC content and soil quality, in line with findings obtained from the previous studies (Wang et al., 2015). Thus, mowing management accelerated more SOC storage by elevating soil nutrient and active SOC fractions in P. australis wetlands.
Effects of mowing treatments on SOC fractions
MBC, as an active form of SOC, has been regarded as an indicator of soil quality and function (Xu et al., 2011). The significantly higher MBC contents found in the 12MR and 3MR plots than the control plots could due to the higher root biomass and materials (Figure 2), which provide adequate nutrients and substrates for microorganisms to improve microbial growth and populations (Sheng et al., 2015). Mowing and removing reed straw can improve P. australis photosynthesis and increase distribution of C to soil, causing the enhancement of microbial C sources (Li et al., 2017). MBC significantly correlated with TP, SOC, DOC, POC, NH4+, NO3−, and sucrase activity, suggesting that mowing and removing reed straw increased soil nutrient availability and provided more favorable environment for the development and activity of microbial. The P. australis straw addition to the seasonally flooded wetlands is difficult to be decomposed, which limits oxygen exchange and restricts the growth of microorganisms, leading to lower MBC in the 12MS and 3MS treatments (Zhao et al., 2018). In addition, given that above- and belowground biomass input more nutrients on top soil, MBC concentration in the 0- to 10-cm depth was higher than in the 10- to 20-cm depth.
DOC is mainly derived from microbial decomposition of plant residues and soil organic matter and plays an important role in soil nutrient transformation and cycling. Our results showed that DOC contents in four mowing management treatments were significantly higher than in the control (Figure 2). The effects of mowing management on soil DOC content are likely driven by two mechanisms. First, mowing management increased input of aboveground biomass and accumulation of root exudation, resulting in the enhancement in DOC. In addition, mowing and straw returning caused greater DOC input through root exudation of labile carbon and decomposition of straw residues (Xie et al., 2013; Wang et al., 2015). Second, these results of correlation analysis confirmed that soil DOC content promoted by soil TP, MBC, POC, NH4+, NO3−, and sucrase activity. Mowing treatments also enhanced the activity of soil microorganisms and the water-soluble metabolites in soil (Yu et al., 2020). Notably, the increased DOC effect was stronger in the 12MR and 3MR treatments than that of the 12MS and 3MS treatments. This finding could be because the continuous reed straw returning to the seasonally flooded wetlands decomposed very slowly in anaerobic environment and inhibited the P. australis regrowth, thereby suppressing the input of new labile C.
LOC is the component with the fastest turnover rate of SOC, and the changes of LOC can reflect the availability and timeliness of soil organic matter in various degrees (Xu et al., 2011). Our results showed that the 12MS and 12MR treatments significantly increased LOC content compared with the CK (Figure 2), indicating that mowing in December can stimulate activity of soil microorganism and promote bio-accumulation of LOC in soil (Chen et al., 2016). In addition, close correlations among LOC, TC, and TN in both two soil layers were observed, which suggested that TC and TN were driving force of LOC turnover in this region. This was because soil TC and TN promoted the decomposition and utilization rate of microbial, resulting in the enhancement of soil organic matter input into the soil, thus improving the LOC content (Chen et al., 2012). POC is mainly derived from undecomposed or partially decomposed plant residues and microbial biomass and is very sensitive to land use and management practice (Xu et al., 2011; Sharma et al., 2014). The improved content of POC was also attributed to the higher plant residues and root biomass compared with the CK, which might create more beneficial environment to POC accumulation by providing litter and root exudates for soil microorganisms (Sharma et al., 2014). The significant positive correlation between POC and other SOC fractions also supported the above explanations. Mowing practices enhanced more organic matter input and did not damage soil aggregates, thus making POC enriched in soil aggregates.
Effects of mowing treatments on enzyme activities
The critical roles that enzymes play in biochemical processes and nutrient cycling have been demonstrated in wetlands (Acosta-Martinez et al., 2018). Sucrase, urease, and alkaline phosphatase are considered as important indices of soil C, N, and P cycling, respectively. In our study, the activities of sucrase, urease, and alkaline phosphatase were the highest in the surface layer and decreased with the soil depth. This is mainly due to the abundant nutrient in surface soil that was driven by the greater inputs from plant litter, residues, and root exudates. We found that the 12MR, 3MS, and 3MR treatments only significantly increased the sucrase activity in the 0- to 10-cm soil depth (Figure 4), which is consistent with the results of Song et al. (2022), who found mowing removal or return straw treatments significantly enhanced the sucrase activity in surface soil of reed wetlands. Mowing management could improve soil quality by increasing aboveground and root biomass input in this study. The sustained nutrient input from plant or root to soils favors the growth and activity of microorganisms, accelerating the synthesis of sucrase enzyme (Finzi et al., 2015). Regression analysis showed that sucrase enzyme activity was controlled by soil C, N, P, SOC, SOC fractions, NH4+, and NO3−, which supported these above explanations. Moreover, SOC and its fractions can also adhere to soil enzymes to form humus–protein complexes to protect enzymes from decomposition (Mohammadi et al., 2017). Our results revealed that mowing management enhanced urease activity, and only the 3MR treatment increased alkaline phosphatase activity in both two soil layers (Figure 4). Similar results were also observed in steppe, where the urease and alkaline phosphatase activities increased significantly after nine years of mowing (Jing et al., 2022). Mowing management effectively improved urease enzyme activity, indicating that mowing accelerated the conversion efficiency of soil N. Increase in urease enzyme activity from mowing was may attributed to improved root activity and exudates, which boosted soil microbial metabolism and activity. In addition, the differences in microclimates under different mowing management treatments also might affect soil enzyme activities.
Conclusion
This study explicitly illustrated the responses of soil properties, SOC, SOC fractions, and enzyme activities to different mowing management treatments in P. australis wetland of the YRD, China. Results showed that mowing management treatments significantly increased soil TC, TN, and TP and decreased soil C/N ratio. Four years of mowing treatments stimulated SOC, SOC fractions, and soil enzyme activities in reed wetlands, and the increase effect was pronounced in the 0- to 10-cm soil layer. Moreover, SOC in mowing and removing straw treatments showed the higher value than mowing and returning straw treatments. Mowing management improved SOC content by changing soil nutrient, SOC fractions, and enzyme activities. Therefore, we suggest that mowing and removing P. australis straw in December and March in coastal wetlands of the YRD can be considered an effective strategy that benefits soil quality, enzyme activities, and carbon sequestration and optimizes the coastal wetlands function.
Data availability statement
The original contributions presented in the study are included in the article/supplementary material. Further inquiries can be directed to the corresponding authors.
Author contributions
QC: data curation, formal analysis, methodology, and writing—original draft. HY: data curation and supervision. GW: visualization, methodology, and investigation. JM: conceptualization and formal analysis. LF: review and editing. JL: statistical analysis. All authors reviewed, edited, and approved the manuscript.
Funding
This study was financially supported by the National Natural Science Foundation of China (41901118 and 41971126), the Youth Innovation Support Program of Shandong Universities (2021KJ081), and the Natural Science Foundation of Shandong Province (ZR2020MD005 and ZR2020MD007).
Conflict of interest
The authors declare that the research was conducted in the absence of any commercial or financial relationships that could be construed as a potential conflict of interest.
Publisher’s note
All claims expressed in this article are solely those of the authors and do not necessarily represent those of their affiliated organizations, or those of the publisher, the editors and the reviewers. Any product that may be evaluated in this article, or claim that may be made by its manufacturer, is not guaranteed or endorsed by the publisher.
References
Acosta-Martinez V., Cano A., Johnson J. (2018). Simultaneous determination of multiple soil enzyme activities for soil health-biogeochemical indices. Appl. Soil Ecol. 126, 121–128. doi: 10.1016/j.apsoil.2017.11.024
Asaeda T., Rajapakse L., Manatunge J., Sahara N. (2006). The effect of summer harvesting of Phragmites australis on growth characteristics and rhizome resource storage. Hydrobiologia 553, 327–335. doi: 10.1007/s10750-005-1157-6
Assefa D., Rewald B., Sandén H., Rosinger C., Abiyu A., Yitaferu B., et al. (2017). Deforestation and land use strongly effect soil organic carbon and nitrogen stock in northwest ethiopia. Catena 153, 89–99. doi: 10.1016/j.catena.2017.02.003
Bu R. Y., Ren T., Lei M. J., Liu B., Li X. K., Cong R. H., et al. (2020). Tillage and straw-returning practices effect on soil dissolved organic matter, aggregate fraction and bacteria community under rice-rice-rapeseed rotation system. Agric. Ecosyst. Environ. 287, 106681. doi: 10.1016/j.agee.2019.106681
Chai Q. L., Ma Z. Y., Chang X. F., Wu G. L., Zheng J. Y., Li Z. W., et al. (2019). Optimizing management to conserve plant diversity and soil carbon stock of semi-arid grasslands on the loess plateau. Catena 172, 781–788. doi: 10.1016/j.catena.2018.09.034
Chen X. L., Chen H. Y. H., Chen X., Wang J., Chen B., Wang D., et al. (2016). Soil labile organic carbon and carbon-cycle enzyme activities under different thinning intensities in Chinese fir plantations. Appl. Soil Ecol. 107, 162–169. doi: 10.1016/j.apsoil.2016.05.016
Cheng X. L., Chen J. Q., Luo Y. Q., Henderson R., An S. Q., Zhang Q. F., et al. (2008). Assessing the effects of short-term Spartina alterniflora invasion on labile and recalcitrant c and n pools by means of soil fractionation and stable c and n isotopes. Geoderma 145, 177–184. doi: 10.1016/j.geoderma.2008.02.013
Chen X., Hao B. H., Jing X., He J. S., Ma W. H., Zhu B. (2019). Minor responses of soil microbial biomass, community structure and enzyme activities to nitrogen and phosphorus addition in three grassland ecosystems. Plant Soil 444, 21–37. doi: 10.1007/s11104-019-04250-3
Chen X. M., Liu J. X., Deng Q., Yan J. H., Zhang D. Q. (2012). Effects of elevated CO2 and nitrogen addition on soil organic fractions in a subtropical forest. Plant Soil 357 (1/2), 25–34. doi: 10.1007/s11104-012-1145-3
Chen Z. M., Wang H. Y., Liu X. W., Zhao X. L., Lu D. J., Zhou J. M., et al. (2017). Changes in soil microbial community and organic carbon fractions under short-term straw return in a rice-wheat cropping system. Soil Tillage Res. 165, 121–127. doi: 10.1016/j.still.2016.07.018
Chu X. J., Han G. X., Xing Q. F., Xia J. Y., Sun B. Y., Li X. G., et al. (2019). Changes in plant biomass induced by soil moisture variability drive interannual variation in the net ecosystem CO2 exchange over a reclaimed coastal wetland. Agr. For. Meteoral. 264, 138–148. doi: 10.1016/j.agrformet.2018.09.013
Cong W. F., van Ruijven J., Mommer L., De Deyn G. B., Berendse F., Hoffland E. (2014). Plant species richness promotes soil carbon and nitrogen stocks in grasslands without legumes. J. Ecol. 102, 1163–1170. doi: 10.1111/1365-2745.12280
Erktan A., Roumet C., Bouchet D., Stokes A., Pailler F., Munoz F. (2018). Two dimensions define the variation of fine root traits across plant communities under the joint influence of ecological succession and annual mowing. J. Ecol. 106 (5), 2031–2042. doi: 10.1111/1365-2745.12953
Finzi A. C., Abramoff R. Z., Spiller K. S., Brzostek E. R., Darby B. A., Kramer M. A., et al. (2015). Rhizosphere processes are quantitatively important components of terrestrial carbon and nutrient cycles. Glob. Change Biol. 21 (5), 2082–2094. doi: 10.1111/gcb.12816
Guan B., Li Y. Z., Xia J. B., Dong H. H., Lu Z. B., Yu J. B. (2014). Ecological characteristics of Phragmites australis vegetation at different water table levels and their relation to environmental factors in the yellow river delta. Chin. J. Eco. 33 (10), 2633–2639. doi: 10.13292/j.1000-4890.2014.0224
Han G. X., Sun B. Y., Chu X. J., Xing Q. H., Song W. M., Xia J. Y. (2018). Precipitation events reduce soil respiration in a coastal wetland based on four-year continuous field measurements. Agr. For. Meteoral. 256, 292–303. doi: 10.1016/j.agrformet.2018.03.018
Han G. X., Yang L. Q., Yu J. B., Wang G. M., Mao P. L., Gao Y. J. (2013). Environmental controls on net ecosystem CO2 exchange over a reed (Phragmites australis) wetland in the yellow river delta, China. Estuar. Coast. 36 (2), 401–413. doi: 10.1007/s12237-012-9572-1
Hao M., Hu H., Liu Z., Dong Q., Sun K., Feng Y., et al. (2019). Shifts in microbial community and carbon sequestration in farmland soil under long term conservation tillage and straw returning. Appl. Soil Ecol. 136, 43–54. doi: 10.1016/j.apsoil.2018.12.016
Jing J. Q., Sarenqilimoge, Qin J., Zhang H. F., Li M., Yan D. L. (2022). Effects of utilization methods on soil microbial community structure and soil enzyme activity in Stipa baicalensis steppe. Chi. J. Grassl. 4 (02), 33–40. doi: 10.16742/j.zgcdxb.20210130
Keller J., Anthony T., Clark D., Gabriel K., Gamalath D., Kabala R., et al. (2015). Soil organic carbon and nitrogen storage in two southern California salt marshes: the role of prerestoration vegetation. Bull. South. Calif. Acad. Sci. 114 (1), 22–23. doi: 10.3160/0038-3872-114.1.22
Kirwan M. L., Mudd S. M. (2012). Response of salt-marsh carbon accumulation to climate change. Nature 489 (7417), 550–553. doi: 10.1038/nature11440
Köbbing J. F., Thevs N., Zerbe S. (2013). The utilisation of reed (Phragmites australis): a review. Mires. Peat. 13, 1–14. doi: 10.1017/9780521391689.034
Koncz P., Pintér K., Balogh J., Papp M., Hidy D., Csintalan Z., et al. (2017). Extensive grazing in contrast to mowing is climate-friendly based on the farm-scale greenhouse gas balance. Agric. Ecosyst. Environ. 240, 121–134. doi: 10.1016/j.agee.2017.02.022
Kotas P., Choma M., Šantrůčková H., Lepš J., Tříska J., Kaštovská E. (2017). Linking above- and belowground responses to 16 years of fertilization, mowing, and removal of the dominant species in a temperate grassland. Ecosystems 20, 354–367. doi: 10.1007/s10021-016-0031-x
Lee J. H. (2013). An overview of phytoremediation as a potentially promising technology for environmental pollution control. Biotechnol. Bioproc. E. 18 (3), 431–439. doi: 10.1007/s12257-013-0193-8
Li S. Y., Gu X., Zhuang J., An T. T., Pei J. B., Xie H. T., et al. (2016). Distribution and storage of crop residue carbon in aggregates and its contribution to organic carbon of soil with low fertility. Soil Tillage Res. 155, 199–206. doi: 10.1016/j.still.2015.08.009
Li J. Y., Zhang Q. C., Li Y., Liu Y. M., Xu J. M., Di H. J. (2017). Effects of long-term mowing on the fractions and chemical composition of soil organic matter in a semiarid grassland. Biogeosciences 14 (10), 2685–2696. doi: 10.5194/bg-14-2685-2017
Ma T. T., Li X. W., Bai J. H., Ding S. Y., Zhou F. W., Cui B. S. (2019). Four decades' dynamics of coastal blue carbon storage driven by land use/land cover transformation under natural and anthropogenic processes in the yellow river delta, China. Sci. Total Environ. 655, 741–750. doi: 10.1016/j.scitotenv.2018.11.287
Marschner P., Hatam Z., Cavagnaro T. R. (2015). Soil respiration, microbial biomass and nutrient availability after the second amendment are influenced by legacy effects of prior residue addition. Soil Biol. Biochem. 88, 169–177. doi: 10.1016/j.soilbio.2015.05.023
Mcleod E., Chmura G. L., Bouillon S., Salm R., Björk M., Duarte C. M. (2011). A blueprint for blue carbon: toward an improved understanding of the role of vegetated coastal habitats in sequestering CO2. Front. Ecol. Environ. 9 (10), 552–560. doi: 10.1890/110004
Mohammadi M. F., Jalali S. G., Kooch Y., Said-Pullicino D. (2017). The effect of landform on soil microbial activity and biomass in a hyrcanian oriental beech stand. Catena 149, 309–317. doi: 10.1016/j.catena.2016.10.006
Osland M. J., Gabler C. A., Grace J. B., Day R. H., McCoy M. L., McLeod J. L., et al. (2018). Climate and plant controls on soil organic matter in coastal wetlands. Glob. Chang. Biol. 24 (11), 5361–5379. doi: 10.1111/gcb.14376
Pendleton L., Donato D. C., Murray B. C., Crooks S., Jenkins W. A., Sifleet S., et al. (2012). Estimating global”blue carbon” emissions from conversion and degradation of vegetated coastal ecosystems. PloS One 7 (9), e43542. doi: 10.1371/journal.pone.0043542
Poeplau C., Aronsson H., Myrbeck Å., Kätterer T. (2015). Effect of perennial ryegrass cover crop on soil organic carbon stocks in southern Sweden. Geoderma Reg. 4, 126–133. doi: 10.1016/j.geodrs.2015.01.004
Poeplau C., Marstorp H., Thored K., Kätterer T. (2016). Effect of grassland cutting frequency on soil carbon storage-a case study on public lawns in three Swedish cities. Soil 2 (2), 175–184. doi: 10.5194/soil-2-175-2016
Setia R., Marschner P., Baldock J., Chittleborough D., Verma V. (2011). Relationships between carbon dioxide emission and soil properties in salt-affected landscapes. Soil Biol. Biochem. 43 (3), 667–674. doi: 10.1016/j.soilbio.2010.12.004
Shahzad T., Chenu C., Repinçay C., Mougin C., Ollier J. L., Fontaine S. (2012). Plant clipping decelerates the mineralization of recalcitrant soil organic matter under multiple grassland species. Soil Biol. Biochem. 51, 73–80. doi: 10.1016/j.soilbio.2012.04.014
Sharma V., Hussain S., Sharma K. R., Arya V. M. (2014). Labile carbon pools and soil organic carbon stocks in the foothill Himalayas under different land use systems. Geoderma 232, 81–87. doi: 10.1016/j.geoderma.2014.04.039
Sheng H., Zhou P., Zhang Y. Z., Kuzyakov Y., Zhou Q., Ge T., et al. (2015). Loss of labile organic carbon from subsoil due to land-use changes in subtropical China. Soil Biol. Biochem. 88, 148–157. doi: 10.1016/j.soilbio.2015.05.015
Song Y., Burgess P., Han H. R., Huang B. R. (2015). Carbon balance of turfgrass systems in response to seasonal temperature changes under different mowing heights. J. Am. Soc. Hortic. Sci. 140 (4), 317–322. doi: 10.21273/JASHS.140.4.317
Song T. H., Chen J. X., Wang L. D., Wu Q. T., Wu M. D., Han G. X., et al. (2022). Effects of mowing management on soil ezyme activities and physicochemical factors in coastal reed wetland. Acta Agric. Jiangxi. 34 (02), 100–107. doi: 10.19386/j.cnki.jxnyxb.2022.02.016
Song X. Y., Li Y., Yue X., Hussain Q., Zhang J. J., Liu Q., et al. (2019). Effect of cotton straw-derived materials on native soil organic carbon. Sci. Total Environ. 663, 38–44. doi: 10.1016/j.scitotenv.2019.01.311
Tanaka T. S., Irbis C., Kumagai H., Wang P., Li K., Inamura T. (2017). Effect of Phragmites japonicus harvest frequency and timing on dry matter yield and nutritive value. J. Environ. Manage. 187, 436–443. doi: 10.1016/j.jenvman.2016.11.008
Tan X., Kong L., Yan H., Wang Z., He W., Wei G. (2014). ). influence of soil factors on the soil enzyme inhibition by cd. Acta Agric. Scand. Sect. B Soil Plant Sci. 64, 666–674. doi: 10.1080/09064710.2014.953985
Tuffa S., Hoag D., Treydte A. C. (2017). Clipping and irrigation enhance grass biomass and nutrients: Implications for rangeland management. Acta Oecol. 81, 32–39. doi: 10.1016/j.actao.2017.05.001
Vance E. D., Brookes P. C., Jenkinson D. S. (1987). An extraction method for measuring soil microbial biomass c. Soil. Biol. Biochem. 19, 703–707. doi: 10.1016/0038-0717(87)90052-6
Wang W., Lai D. Y. F., Wang C., Pan T., Zeng C. (2015). Effects of rice straw incorporation on active soil organic carbon pools in a subtropical paddy field. Soil Tillage Res. 152, 8–16. doi: 10.1016/j.still.2015.03.011
Wang F., Lu X., Sanders C. J., Tang J. (2019). Tidal wetland resilience to sea level rise increases their carbon sequestration capacity in united states. Nat. Commun. 10, 1–11. doi: 10.1038/s41467-019-13294-z
Wei L., Liu J., Su J. S., Jing G. H., Zhao J., Cheng J. M., et al. (2016). Effect of clipping on soil respiration components in temperate grassland of loess plateau. Eur. J. Soil Sci. 75, 157–167. doi: 10.1016/j.ejsobi.2016.06.003
Xie Z. B., Xu Y. P., Liu G., Liu Q., Zhu J. G., Tu C., et al. (2013). Impact of biochar application on nitrogen nutrition of rice, greenhousegas emissions and soil organic carbon dynamics in two paddy soils of China. Plant Soil 370, 527–540. doi: 10.1007/s11104-013-1636-x
Xiu L., Zhang W. M., Sun Y. Y., Wu D., Meng J., Chen W. F. (2019). Effects of biochar and straw returning on the key cultivation limitations of albic soil and soybean growth over 2 years. Catena 173, 481–493. doi: 10.1016/j.catena.2018.10.041
Xu M. G., Lou Y. L., Sun X. L., Wang W., Baniyamuddin M., Zhao K. (2011). Soil organic carbon active fractions as early indicators for total carbon change under straw incorporation. Biol. Fertil. Soils 47, 745–752. doi: 10.007/s00374-011-0579-8
Xu X., Luo Y. Q., Shi Z., Zhou X. H., Li D. J. (2014). Consistent proportional increments in responses of belowground net primary productivity to long-term warming and clipping at various soil depths in a tallgrass prairie. Oecologia 174 (3), 1045–1054. doi: 10.1007/s00442-013-2828-z
Xu X., Niu S., Sherry R. A., Zhou X. H., Zhou J. Z., Luo Y. Q. (2012). Interannual variability in responses of belowground net primary productivity (NPP) and NPP partitioning to long-term warming and clipping in a tallgrass prairie. Glob. Chang. Biol. 18 (5), 1648–1656. doi: 10.1111/j.1365-2486
Yu Q. G., Hu X., Ma J. W., Ye J., Sun W. C., Wang Q., et al. (2020). Effects of long-term organic material applications on soil carbon and nitrogen fractions in paddy fields. Soil Tillage Res. 2020, 104483. doi: 10.1016/j.still.2019.104483
Yu P. J., Liu S. W., Han K. X., Guan S. C., Zhou D. W. (2017). Conversion of cropland to forage land and grassland increases soil labile carbon and enzyme activities in northeastern China. Agric. Ecosyst. Environ. 245, 83–91. doi: 10.1016/j.agee.2017.05.013
Zhang P., Chen X. L., Wei T., Yang Z., Jia Z. K., Yang B., et al. (2016). Effects of straw incorporation on the soil nutrient contents, enzyme activities, and crop yield in a semiarid region of China. Soil Tillage Res. 160, 65–72. doi: 10.1016/j.still.2016.02.006
Zhao Q. Q., Bai J. H., Zhang G. L., Jia J., Wang W., Wang X. (2018). Effects of water and salinity regulation measures on soil carbon sequestration in coastal wetlands of the yellow river delta. Geoderma 319, 219–229. doi: 10.1016/j.geoderma.2017.10.058
Ziter C., MacDougall A. S. (2013). Nutrients and defoliation increase soil carbon inputs in grassland. Ecology 94, 106–116. doi: 10.1890/11-2070.1
Keywords: coastal wetlands, mowing treatments, soil organic carbon, soil organic carbon fractions, soil enzyme activities
Citation: Cui Q, Yang HJ, Wang GM, Ma JZ, Feng L and Liu JT (2022) Response of soil carbon fractions and enzyme activities to mowing management on in a coastal wetland of the yellow river delta. Front. Mar. Sci. 9:993181. doi: 10.3389/fmars.2022.993181
Received: 13 July 2022; Accepted: 25 July 2022;
Published: 17 August 2022.
Edited by:
Junhong Bai, Beijing Normal University, ChinaCopyright © 2022 Cui, Yang, Wang, Ma, Feng and Liu. This is an open-access article distributed under the terms of the Creative Commons Attribution License (CC BY). The use, distribution or reproduction in other forums is permitted, provided the original author(s) and the copyright owner(s) are credited and that the original publication in this journal is cited, in accordance with accepted academic practice. No use, distribution or reproduction is permitted which does not comply with these terms.
*Correspondence: Qian Cui, Y3VpcWlhbi4xOTg5QDE2My5jb20=; Guangmei Wang, Z213YW5nQHlpYy5hYy5jbg==