- 1Escuela de Ciencias del Mar, Pontificia Universidad Catoílica de Valparaíso, Valparaíso, Chile
- 2Norwegian Research Center, Climate & Environment, Bergen, Norway
- 3Marine Aquaculture Station, Institute of Oceanography, Federal University of Rio Grande – FURG, Rio Grande, Brazil
Shrimp production in biofloc systems generates excess organic matter that must be removed from the system. Due to its ability to consume natural productivity, the integration of tilapia in shrimp culture could help to reduce the levels of total suspended solids in the biofloc system. The present study aimed to evaluate two stocking densities of tilapia in an integrated culture with shrimp Litopenaeus vannamei reared in a pilot-scale biofloc system. Two stocking densities of tilapia were tested, 35 and 65 fish m-3 in a recirculating system with 10 m3 tanks for shrimp culture and 4 m3 for tilapia culture with water recirculation of 965.66 ± 92.83 L h-1 during 78 days. The initial weight of shrimp was 0.9±0.1 g and of tilapia was 7.1±3.2 g. Shrimps were fed according to the feeding table and fish were underfed to stimulate bioflocs consumption. Selected water quality parameters were monitored during the trial. Tilapia densities did not affect shrimp growth (11.5±1.9 g and 10.1±0.7 g for 35 and 65 fish m-3 treatments, respectively). The tilapia presented a FCR lower than 1, proving that bioflocs were consumed by fish. The clarification time was shorter when compared to other studies with shrimp monoculture. Between the treatments, a reduction of 10 hours in the system clarification occurred when lower fish stocking density was used. The results demonstrate the feasibility of integrated shrimp and tilapia culture on a pilot scale, without compromising shrimp productivity.
1 Introduction
One of the major problems related to super intensive aquaculture is the generation of effluents with high organic loads. Feeding in intensive monocultures increment the discharge effluents with a high pollution potential, with a high concentration of nitrogen and phosphorus (Briggs and Funge-Smith, 1994). These problems have raised concerns in many countries due to the likely environmental impacts of this activity (Boyd et al., 2020).
The Integrated Multi-Trophic Aquaculture (IMTA) aims at the integration of species with different trophic levels in the culture system, in order to take advantage of the residues from the production of one species for the culture of other species (Troell et al., 2009). Even organisms that occupy the same trophic level in the system can be produced at IMTA if they make use of different food webs in that system (Boyd et al., 2020). This variety in the combination of organic and inorganic extractive species gives IMTA advantages in terms of biomitigation and economic gain, consequently more sustainability, when compared to monocultures (Biswas et al., 2020).
The biofloc system is another culture technology that has been adopted in shrimp culture. This system gives a new direction for the shrimp monoculture in regard to aquaculture sustainability. Due to a better maintenance of water quality, this system can reduce water use by up to 90% compared to traditional systems (Krummenauer et al., 2014), allowing culture at high stocking densities (Wasielesky et al., 2013), reducing the use of land (Liu et al., 2017) and optimizing the use of artificial food (Santhana Kumar et al., 2018). This is possible through the increase of the C: N ratio in water, with the use of organic carbon sources and constant aeration, stimulating the production of microorganisms that will both work on the metabolization of nitrogenous compounds and serve as natural food for reared animals (Krummenauer et al., 2011; Lara et al., 2017; Khanjani et al., 2023).
Shrimp production in the biofloc system has numerous economic advantages (Rego et al., 2017). Nevertheless, there are still environmental constraints such as the accumulation of organic matter during production in a system with minimal water exchange and high densities. This accumulation produces an excess of suspended solids, which increases the biological oxygen demand due to the high concentration of aerobic microorganisms present in bioflocs (Ray et al., 2010). Therefore, it is recommended that excess total suspended solids (TSS) in the biofloc system should be removed trough clarification processes (Gaona et al., 2017).
Sedimentation (removal of excess biofloc particles by gravity) efficiently removes excess solids suspended in the biofloc system (Gaona et al., 2016) that are normally discarded as effluents. Therefore, the rationale for integrating species in the biofloc system is to take advantage of this excess of microbial protein in the form of suspended solids, to feed other species and thus transform effluent into animal protein, making the system even more sustainable.
Tilapia (Oreochromis niloticus) presents some characteristics that make it an ideal species for culture in an IMTA system based on the biofloc system (Azim and Little, 2008; Monroy-Dosta et al., 2013; Poli et al., 2019). This species has a high tolerance for suspended solids, moderate dissolved oxygen, and high stocking densities (Azim and Little, 2008; Simão et al., 2013; Malpartida Pasco et al., 2018; Khanjani and Sharifinia, 2021). In addition, it is an omnivorous species, presenting filtering structures and a digestive system that allows it to be an organic extractive in potential, feeding on natural productivity of the biofloc system and removing the excess of total suspended solids. It can be used as a bioremediation agent in IMTA systems (Azim et al., 2003; Ekasari et al., 2014; Poli et al., 2019).
Commercial expansion of IMTA has been troublesome. Although the biological and environmental advantages of this practice are generally accepted by producers and society, some economic-related issues have yet to be overcome for its adoption (Ridler et al., 2007). Thus, pilot-scale studies aimed at integrating superintensive production of Pacific white shrimp (Litopenaeus vannamei) and Nile tilapia in the biofloc system are sorely needed for the development of large-scale integrated multitrophic cultures.
There are some studies reporting the integrated culture of shrimp and tilapia, though in low production densities and in clear water systems (Tendencia et al., 2004; Tendencia et al., 2006; Muangkeow et al., 2007; Cruz et al., 2008; Yuan et al., 2010; Muangkeow et al., 2011; Simão et al., 2013). More recently, Poli et al. (2019) studied the integrated culture of shrimp and tilapia in an experimental scale, and this work represents a first step towards a future IMTA using biofloc technology. Nevertheless, until now, there is no work with integrated superintensive production of shrimps and tilapia in a BFT system, on a pilot scale. The objective of this study was to evaluate the effect of different fish stocking densities in the integrated superintensive culture of white shrimp L. vannamei and Nile tilapia O. niloticus reared in biofloc system to promote the maintenance of TSS at the appropriate levels for shrimp culture, through the consumption of the excess of biofloc by the fish.
2 Material and methods
2.1 Experimental design
The experiment lasted 78 days and was performed in two treatments with three replicates: 1) T35 – integrated culture of shrimp (550 shrimp m-3) and tilapia at a stocking density of 35 fish m -3; and 2) T65 - integrated culture of shrimp (550 shrimp m-3) and tilapia at a stocking density of 65 fish m -3).
2.2 Culture conditions
The study was carried out at the Marine Station of Aquaculture, Federal University of Rio Grande (EMA-FURG), Southern Brazil (32°12’16S, 52°10’38W) in a greenhouse used for IMTA studies.
Nauplii of L. vannamei were purchased from the company Aquatec (Aquatec®, Canguaretama; Rio Grande do Norte, Brazil), and larval development was carried out in the Carcinoculture laboratory at EMA-FURG. The fish were purchased from a commercial farm in the municipality of Camaquã, Rio Grande do Sul, Brazil.
Sea water previously diluted in fresh water provided by the local supply company was used to formulate water with salinity of 15 g L-1. The salinity was kept at 15 with chlorinated fresh water and neutralized with vitamin C (Roselet et al., 2013). This experiment was approved by the Ethics and Animal Welfare Committee of the Federal University of Rio Grande - FURG (Case number 23116.005895/2016-42).
Before the experiment starts, an inoculum of 20% of the total volume of the shrimp tank, with mature bioflocs (with the complete nitrification process, without detection of ammonia and nitrite, and with detection of nitrate), was used in all treatments. The inoculum was taken from a 60-day culture of L. vannamei with a density of 400 m−3 in 35 m3 tanks located in a greenhouse. Sugar cane molasses, containing 37% of organic carbon, was used as a carbon source in the initial phases of cultivation for ammonia control. The inoculum’s initial concentration was TSS ± 400 mg L−1 and ± 65 mg L−1 of nitrate, indicating that the nitrification process was taking place in this matrix tank. Organic fertilization was carried out by manipulating the C: N ratio to 15:1 (Ebeling et al., 2006; Khanjani and Sharifinia, 2022).
During the study, shrimps were fed twice a day (9 a.m. and 5 p.m.) with species-specific commercial feed, containing 38% crude protein (Poty Active 38, 1.6 mm, Guabi®, Campinas, SP, Brazil), following the methodology described by Jory et al. (2001). Fish were fed twice a day (9 a.m. and 5 p.m.) with Guabitech Mirim QS commercial feed (1.0 mm) at the beginning of the experiment, adjusting the feed for Guabitech omnivorous QS (2-3 mm/5-5 mm), throughout the experimental period, as fish grew. Fish were underfed to stimulate the consumption of biofloc. In the first half of the experiment the feed was offered at a rate of 2% of the total fish biomass in each tank. This value was adjusted in the second half of the experiment, when feed was offered at a rate of 1%. Shrimps were stocked in the experimental tanks with an initial weight of 0.96 ± 0.1g. Tilapia, with an initial weight of 7.17 ± 3.15g, were previously acclimated to the salinity of the experiment and later stored in the experimental units.
2.3 Culture system
The biofloc-IMTA recirculation system includes a circular tank of 10 m3, where shrimps were stored, and another similar tank of 4 m³ of useful volume was stocked with tilapia. The water was pumped from the shrimp tank to the tilapia tank through PVC pipes (40mm in diameter) using submerged pumps (SB 2700, Sarlo better, Brazil), and returned to the shrimp tank by gravity, through tubes (40mm) installed 20 cm from the surface of the tank. The recirculation system operated for 24 hours, with an average flow of 965.6 ± 92.8 L h-1. Aeration was provided by a 4HP blower connected to the air distribution system by means of microperforated hoses. Each recirculation system contained a cylindrical-conical clarifier made of fiberglass with useful volume of 150 L. A shade cloth was installed on the greenhouse ceiling to attenuate 70% of the luminosity to avoid phytoplankton blooms and favor the heterotrophic nature of the system.
2.4 Water quality monitoring
The temperature (°C) and dissolved oxygen (mg L-1) in the tanks were monitored twice a day using a digital oximeter (model HI 9146-04, Hanna® instruments), the pH was measured daily, with a bench pH meter (Mettler Toledo, Five-Easy model). Salinity was checked weekly with an optical refractometer (Atago).
Total ammonia (UNESCO, 1983) and nitrite (Bendschneider and Robinson, 1952) were monitored daily. Nitrate (UNESCO, 1983), phosphate (Strickland and Parsons, 1972), alkalinity (APHA, 2017) and total suspended solids (Strickland and Parsons, 1972) were measured weekly.
Total suspended solids (TSS) were kept at 500 mg L-1 according to (Gaona et al., 2017). When the TSS value exceeded this limit, physical clarification was performed in the systems by removing suspended solids in all treatments (Gaona et al., 2011). The pH corrections were made to keep the values above 7.2 by the addition of hydrated lime - Ca (OH)2 - according to (Furtado et al., 2011). Alkalinity was corrected to maintain concentrations above 150 mg L - 1, following the same protocol.
2.5 Growth performance
Shrimp (n = 40) and fish (n = 20) growth was monitored through weekly and biweekly biometrics, respectively, using a digital scale with accuracy of 0.01g (Mars - Model AD 2000). Anesthetics were used to manipulate fish during weighing (benzocaine hydrochloride, 50 mg L-1). At the end of the experiment, all shrimp and fish remaining in the tanks were counted to determine the survival and growth. The parameters analyzed were: Survival (%) = (final shrimp number/initial shrimp number) × 100; Final average weight (g): final weight of live animals (g)/total number of animals; Total biomass (g): final weight of all live animals (g); Weekly growth rate (g week−1): weight gain (g)/number of weeks; Feed conversion ratio (FCR) = offered feed (g)/(final biomass (g) - initial biomass (g)); Productivity (kg m-3): [(final biomass (kg) - initial biomass (kg)) x 1000]/useful tank volume (L).
The parameters analyzed for the IMTA system as a whole (shrimp+fish) were: system productivity (Kg m-3): (FBs + FBf) - (IBs + IBf)/Total useful volume (m³), where FBs = final shrimp biomass; FBf = final fish biomass; IBs = Initial biomass of shrimps; IBf = Initial biomass of fish and Total useful volume = sum of the useful volume of the shrimp and fish tanks; FCR: (Fs + Ff)/(FBs + FBf) - (IBs - IBf), where Fs = feed offered for shrimp and Ff = feed offered for fish.
2.6 Statistical analysis
After verifying the normality and homoscedasticity of the data, the parameters of water quality and growth performance were submitted to the t-test for comparison of means. The survival data were transformed (arcsine x0.5) before analysis (Zar, 2010).
3 Results
3.1 Water quality
The main water quality parameters registered in the present study are showed in Table 1. The mean temperature was maintained above 28°C, dissolved oxygen above 6.0 mg L-1 and the pH above 7.5 and did not present significant differences between treatments (p > 0.05). Alkalinity (Figure 1) showed significantly higher (p<0.05) mean values (143.6 ± 35.2 mg L-1 of CaCO3) in treatment T35 than in treatment T65 (134.9 ± 33.2 mg L-1 of CaCO3). Salinity values fluctuated between 13 and 15 throughout the experiment, however, differences between treatments were non-significant (p > 0.05).
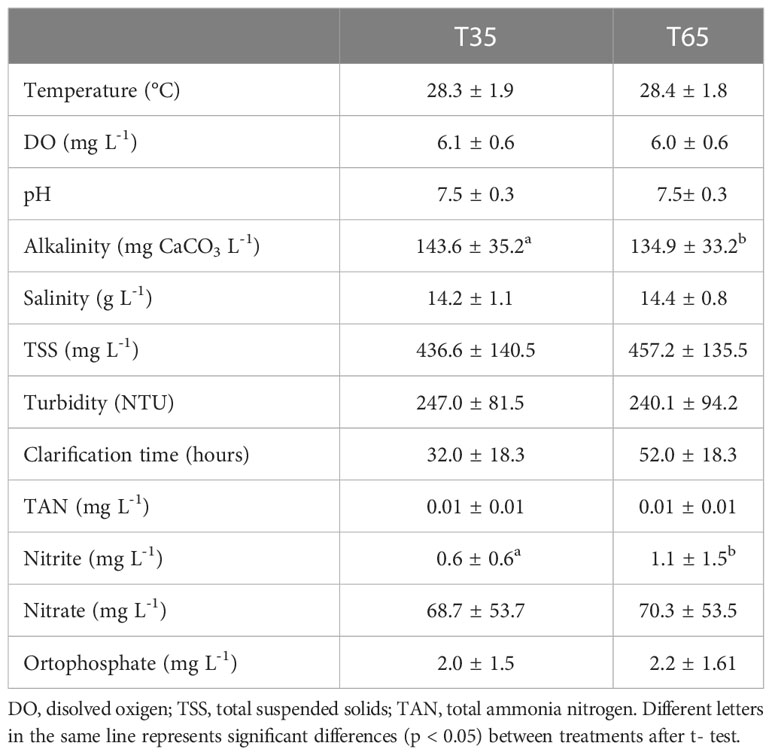
Table 1 Water quality parameters in treatments T35 (540 shrimp m-3 + 35 fish m-3) and T65 (540 shrimp m-3 + 65 fish m-3) throughout the experimental period (mean values ± standard deviation).
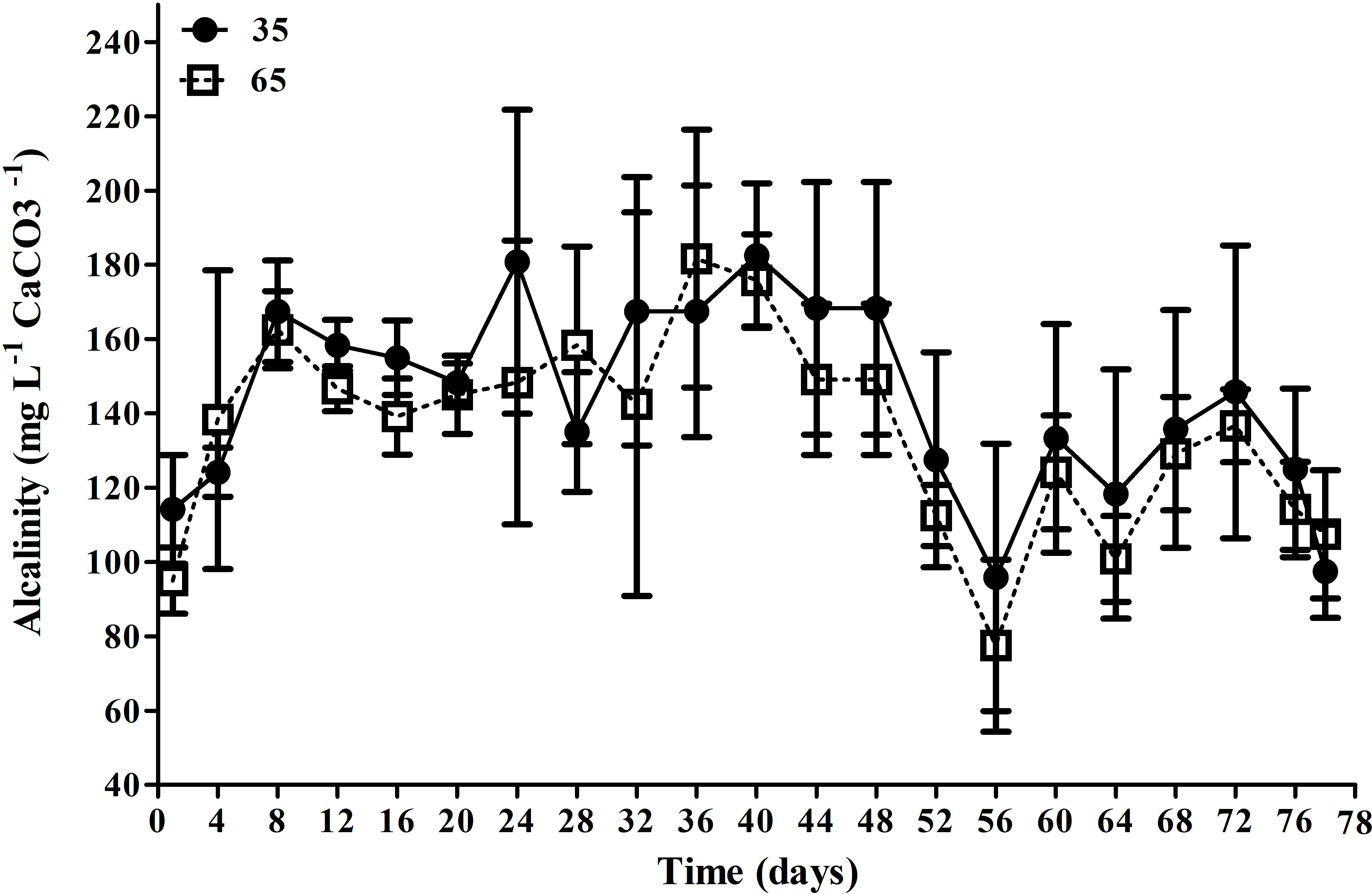
Figure 1 Mean alkalinity concentrations (mg L-1 of CaCO3) in the T35 and T65 treatments over the 78 day experimental period. Data are presented as mean ± standard deviation.
The concentrations of total ammoniacal nitrogen, nitrate and orthophosphate (Figure 2) did not show significant differences (p > 0.05) between treatments. The mean values of nitrite, on the other hand, were significantly higher (p < 0.05) in T65 (1.1 ± 1.5 mg L-1) than T35 treatment (0.6 ± 0.6 mg L-1).
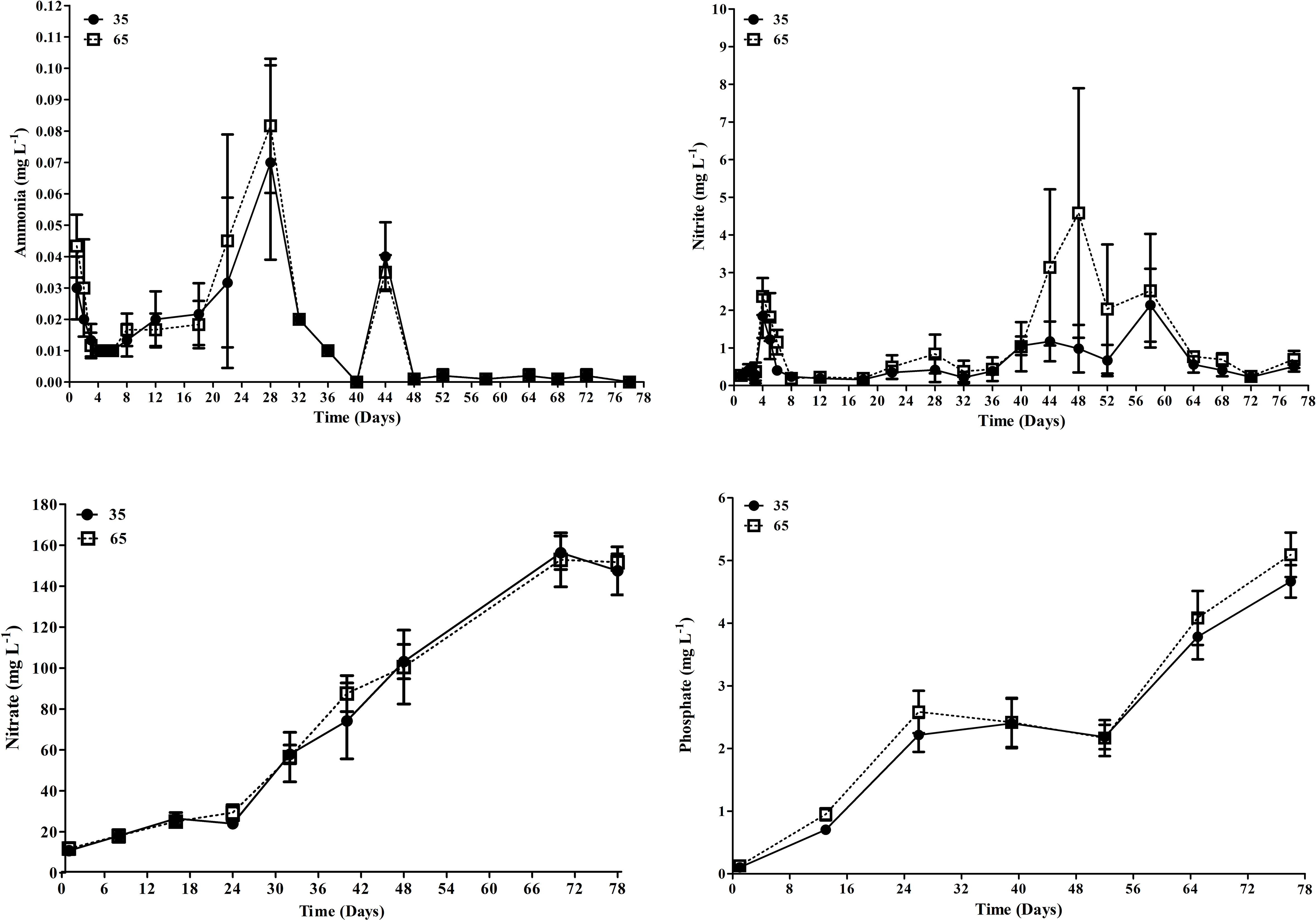
Figure 2 Mean concentrations of ammonia, nitrite, nitrate and phosphate (mg L-1) over the 78 day experimental period in T35 and T65 treatments. Data are presented as mean ± standard deviation.
3.2 TSS dynamics
The TSS concentrations and turbidity (Figure 3) did not show statistical differences (p > 0.05) between treatments. The management of total suspended solids (clarification) was necessary in both treatments in order to maintain the TSS values close to 500 mg L-1. The clarification was performed during 52 ± 18.3 h in T65 and for 32 ± 18.3h in T35, along all the experimental period (Table 1).
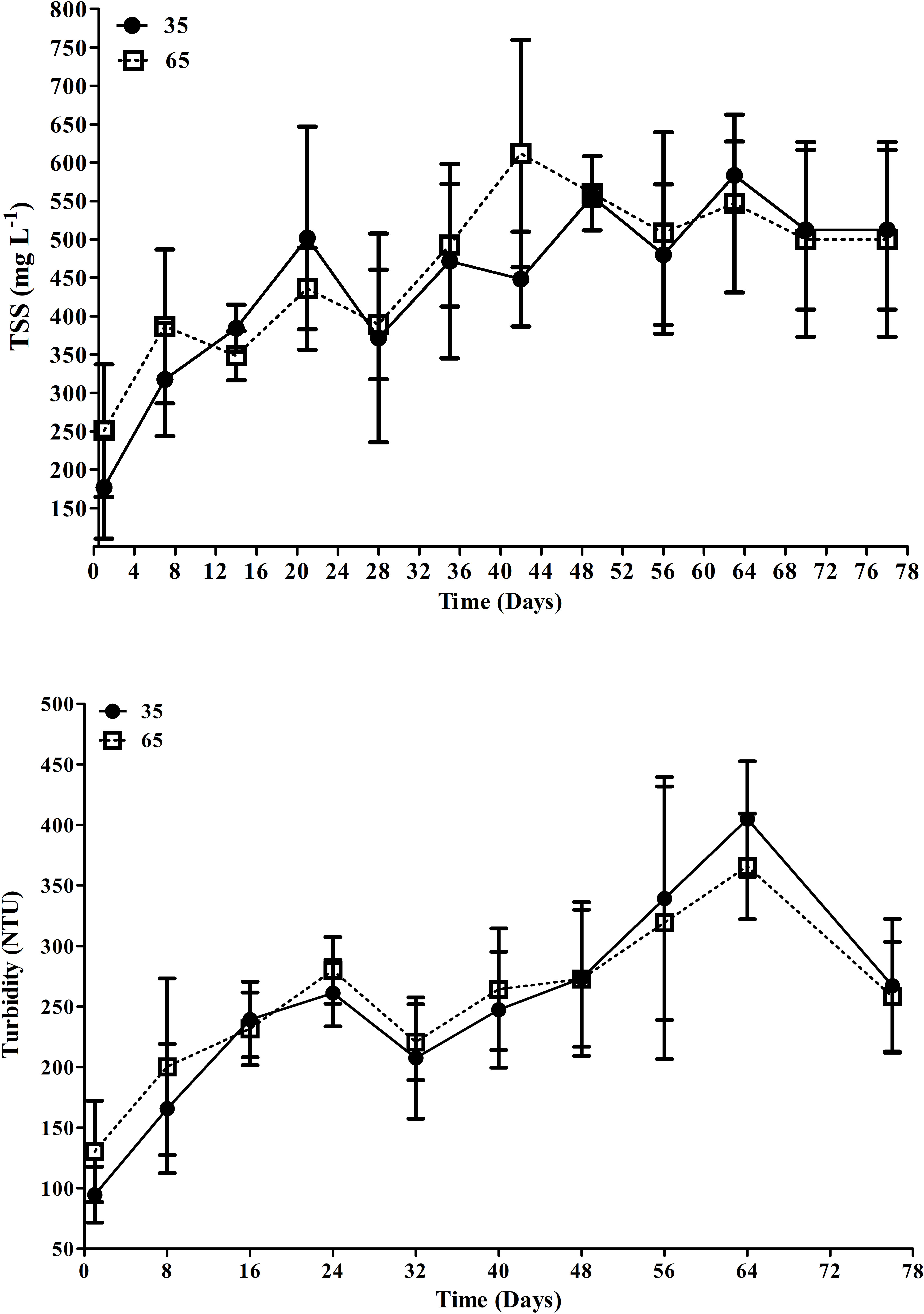
Figure 3 Mean TSS (total suspended solids) concentrations (mg L-1) and turbidity (NTU) in T35 and T65 treatments during the 78 days of experiment. Data are presented as mean ± standard deviation.
3.3 Growth performance
Shrimps showed similar growth in both treatments (p > 0.05) during the experiment, reaching a final weight of 10.1 ± 0.7 g and 11.5 ± 1.9 g in treatments T65 and T35, respectively. The results of growth performance in terms of final mean weight, survival, daily growth rate, feed conversion rate and productivity showed no statistical differences (p > 0.05) between treatments (Table 2).
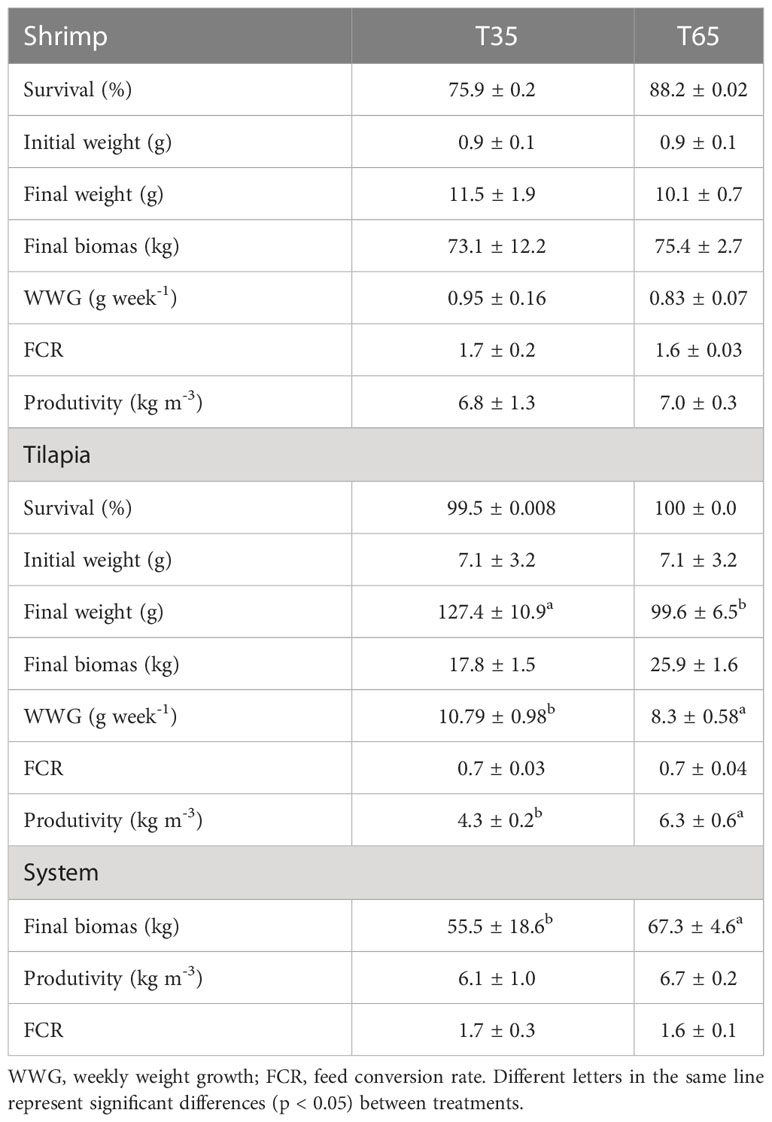
Table 2 Performance of L. vannamei and O. niloticus (mean values ± standard deviation) during the experimental period.
Fish growth was significantly higher (p < 0.05) in T35 than in T65 treatments. The productivity was higher in the T65 treatment. Survival and FCR, were similar in both treatments (Table 2).
For the IMTA system (shrimp + fish), the total final biomass was statistically higher in the T65 treatment. The total productivity of the system as a whole and the FCR showed no statistical differences between treatments (Table 2).
4 Discussion
Throughout the experimental period, the physical and chemical parameters of water quality were at suitable levels both for L. vannamei (Samocha and Prangnell, 2019) and for tilapia growth (Atwood et al., 2001; Azim and Little, 2008). Even with the culture of two species, the dissolved oxygen, a limiting factor for cultured organisms, mainly in a biofloc system, remained above 6 mg L-1, values above those recommended for reared species (Wasielesky et al., 2006; Tran-Duy et al., 2008).
The alkalinity showed a statistical difference between the treatments during the experimental period, however, the average values registered were within the recommended for L. vannamei (Furtado et al., 2014) and for tilapia (O. niloticus) (Cavalcante et al., 2009). The T65 treatment showed a lower mean alkalinity value than T35, which in turn, presented lower mean nitrite values (0.6 ± 0.6 mg L-1) than T65 (1.1 ± 1.1 mg L-1). In T65 was registered a peak of nitrite at 21 days of the study, reaching 4.5 ± 3.31 mg L-1 and, this possibly happened due to the presence of fish in the system at elevated densities, although with the addition of mature biofloc inoculum. The influence of fish density and the imbalance in the nitrification process are reported by Holanda et al. (2020) in a study integrating shrimp and mullets (Mugil liza). In addition, it is possible that the swimming of a great biomass of fish may have altered the size and shape of bioflocs, which affects the nitrification dynamics in the biofloc systems (Carvalho et al., 2006; Delatolla et al., 2009).
The presence of tilapia integrated in the system did not negatively affect the performance of the shrimp, independently of the fish densities used. The productivity of the shrimp was high, approximately 7.0 kg m-3, already expected in cultures in biofloc system and above the reported productivity values observed in studies evaluating the biofloc monoculture of L. vannamei in pilot scale (Krummenauer et al., 2011; Gaona et al., 2017). These authors obtained yields close to 4.1 kg m-3.
Even underfed tilapia showed zootechnical performance similar to that reported for tilapia culture in biofloc system. Zaki et al. (2020) showed an average final weight of 115 g for tilapia at a density of 60 fish m-³ for 84 days, with an initial weight of 50 grams. Malpartida Pasco et al. (2018), on the other hand, observed productivity of 21 kg m-3 in tanks of 10m³ growing tilapia at the density of 70 fish m-3 for 56 days in a biofloc system.
The FCR obtained for shrimp was similar to other studies with monoculture of L. vannamei in biofloc systems (Lara et al., 2017; Reis et al., 2019). In this present study, it is worth noting the low FCR values of tilapia, which were below 1.0 in both T65 (0.7 ± 0.04) and T35 (0.7 ± 0.03) treatments. This indicates that tilapias consumed the natural microbiota present in bioflocs, using it as a substantial part of their diet. A low feed rate was provided to tilapias (1% of biomass), which favored their consumption of bioflocs without impairing their growth despite the low amount of feed provided. A standard feed rate for tilapia in a biofloc system, with an approximate weight of 50 grams, is 3% of wet body weight (Zaki et al., 2020). The values obtained in the present study are not only beneficial from an environmental point of view, but also economically advantageous as it can decrease production costs due to the reduction of food used (a major cost in terms of production), producing two species at the same time. Environmentally, using less feed implies the generation of effluents with less polluting potential, making aquaculture environmentally friendly and socially acceptable (Tacon and Metian, 2008; Naylor et al., 2009). Ekasari et al. (2014) showed the consumption of bioflocs by red tilapia, regardless of the size of the fish. The same study also showed that the absorption capacity is 39 to 117 g of TSS/kg of fish. Poli et al. (2019) also found low FCR values (around 0.2) for Nile tilapia in integrated cultivation with L. vannamei, proving the tilapia’s potential to consume the excess solids produced by the shrimp. These results indicate that tilapias can feed on bioflocs and the integration of the two species in an IMTA system could be implemented to warrant a more sustainable culture system.
Even with the integration of the fish in the system, it was necessary to remove the excess of total suspended solids through sedimentation process. The sedimentation time was different in the two treatments, with T65 being clarified for longer time, probably due to the high total biomass in this treatment. Gaona et al. (2016) performed clarification during 58 ± 12.2h in a monoculture of L. vannamei with a density of 350 shrimp m-2, with an initial weight of 0.18 ± 0.06g for 17 weeks. These data highlight that tilapias could contribute to the management of TSS, since the stocking density of shrimp used in the present study were higher than the reported previously by the authors. In the present study, the lower density of tilapia in T35 required less clarification time (36 ± 12 h), which corroborates that higher stocking densities of fish could contribute with the excess of organic matter in the water, hence increasing the TSS. In addition, the clarification time in T35 was shorter than that reported by Gaona et al. (2016) for L. vannamei monoculture at a lower density than the present study. In this study, it was observed that in treatment T65, where there was a greater biomass of fish, longer time of clarification were needed, which indicates that the system produced more TSS compared to treatment T35. Similar results were observed by Zaki et al. (2020) in a monoculture of tilapia in biofloc systems, showing that the increase in biofloc volume was directly proportional to the increase in stocking density.
Azim and Little (2008) reported a difficulty in maintaining TSS levels at 500 mg L-1 even using a clarifier and often the level reached exceeded 1000 mg L-1 TSS, growing tilapia from 80 to 120 grams and density of 12 kg m-3. The consumption of bioflocs by fish depends on the species cultivated, the feeding habits of the fish, and the size and density of bioflocs. It is possible that the consumption of bioflocs by fish also depends on the presence and rate of feed added to the tank. Therefore, new studies with different biomass ratios of shrimp and fish should be encouraged.
Most studies of IMTA cultures of L. vannamei and tilapia have worked with low shrimp densities (10 to 120 shrimp m-2) (Yuan et al., 2010; Muangkeow et al., 2011; Simão et al., 2013) and few are the studies of IMTA cultures in super-intensive densities. Poli et al. (2019) observed shrimp yields of up to 4 kg m-3 in the integrated culture of shrimp, Nile tilapia and Sarcocornia ambigua on a laboratory scale. Our work provides useful information for implementing biofloc cultures with shrimp and fish in integrated farming systems, diversifying production without loss in performance of the target species, which in the present study are the shrimp. From the productive perspective, the data presented here encourage the development of integrated shrimp and tilapia harvests in a biofloc system, in tanks on land.
5 Conclusion
The results obtained in the present study lead to the conclusion that the integrated culture of L. vannamei and O. niloticus in super-intensive systems using biofloc is possible, allowing the reduction of fish feeding rates without negatively influencing their growth. When higher densities of tilapia are used (65 fish m3), there is an increase in the total suspended solids concentrations in the water, also increasing the clarification time required to keep these concentrations within the levels suitable for the species. This study, on a pilot scale, proves that the IMTA systems of tilapia with L. vannamei based on bioflocs diversifies production without compromising the productivity of shrimp.
Data availability statement
The original contributions presented in the study are included in the article/supplementary material. Further inquiries can be directed to the corresponding author.
Ethics statement
This experiment was approved by the Ethics and Animal Welfare Committee of the Federal University of Rio Grande - FURG (Case number 23116.005895/2016-42).
Author contributions
Conceptualization: LP and MH. Data curation: LP and MH. Formal analysis: LP, MH and WW. Methodology: All authors. Project administration: ER and LP. Supervision: LP and WW. Writing—original draft: All authors. Writing—review and editing: LP, WW and ER. All authors contributed to the article and approved the submitted version.
Funding
The authors are grateful for the financial support provided by the European Community (ASTRAL Project – H2020 – Grant Agreement 863034). National Council for Scientific and Technological Development (CNPq) and Co- ordination for the Improvement of Higher- Level Personnel (CAPES). WW and LP are research fellows of CNPq.
Acknowledgments
Special thanks to GUABI Animal Health and Nutrition SA for donating the commercial diets and Hellyjúnyor Brandão for the assistance with the final corrections.
Conflict of interest
The authors declare that the research was conducted in the absence of any commercial or financial relationships that could be construed as a potential conflict of interest.
Publisher’s note
All claims expressed in this article are solely those of the authors and do not necessarily represent those of their affiliated organizations, or those of the publisher, the editors and the reviewers. Any product that may be evaluated in this article, or claim that may be made by its manufacturer, is not guaranteed or endorsed by the publisher.
References
APHA, A. W. W. A, W. P. C. A (2017). Standard methods for the examination of water and wastewater. 23rd edn (Washington DC: W. E. F. American Public Health Association, American Water Works Association. Pharmabooks).
Atwood H. L., Fontenot Q. C., Tomasso J. R., Isely J. J.. (2001). Toxicity of nitrite to Nile tilapia: Effect of fish size and environmental chloride. North Am. J. Aquacult. 63 (1), 49–51. doi: 10.1577/1548-8454(2001)063<0049:TONTNT>2.0.CO;2
Azim M. E., Verdegem M. C. J., Mantingh I., Van Dam A.A., Beveridge M. C. M.. (2003). Ingestion and utilization of periphyton grown on artificial substrates by Nile tilapia, oreochromis niloticus l. Aquacult. Res. 34 (1), 85–92. doi: 10.1046/j.1365-2109.2003.00802.x
Azim M. E., Little D. C. (2008). The biofloc technology (BFT) in indoor tanks: Water quality, biofloc composition, and growth and welfare of Nile tilapia (Oreochromis niloticus). Aquaculture 283 (1–4), 29–35. doi: 10.1016/j.aquaculture.2008.06.036
Bendschneider K., Robinson R. J. (1952). A new spectrophotometric method for the determination of nitrite in Sea water. J. Mar. Res. 11 (8), 87–96.
Biswas G., Kumar P., Ghoshal T. K., Kailasam M., De D., Bera A., et al. (2020). Integrated multi-trophic aquaculture (IMTA) outperforms conventional polyculture with respect to environmental remediation, productivity and economic return in brackishwater ponds. Aquaculture 516, 734626. doi: 10.1016/j.aquaculture.2019.734626
Boyd C. E., D’Abramo L. R., Glencross B. D., Huyben D. C., Juarez L. M., Lockwood G. S., et al. (2020). Achieving sustainable aquaculture: Historical and current perspectives and future needs and challenges. J. World Aquacult. Soc. 51 (3), 578–633. doi: 10.1111/jwas.12714
Briggs M. R. P., Funge-Smith S. J. (1994). A nutrient budget of some intensive marine shrimp ponds in Thailand. Aquacult. Fish. Manageme 25 (8), 789–811. doi: 10.1111/j.1365-2109.1994.tb00744.x
Carvalho G., Meyer R. L., Yuan Z., Keller J. (2006). Differential distribution of ammonia- and nitrite-oxidising bacteria in flocs and granules from a nitrifying/denitrifying sequencing batch reactor. Enzyme Microbial. Technol. 39 (7), 1392–1398. doi: 10.1016/j.enzmictec.2006.03.024
Cavalcante D. D. H., Poliato A. D. S., Ribeiro D. C., Magalhães F. B., Sá M. V.. (2009). Effects of CaCO3 liming on water quality and growth performance of fingerlings of Nile tilapia, oreochromis niloticus. Acta Scientiarum. Anim. Sci. 31 (3), 327–333. doi: 10.4025/actascianimsci.v31i3.6263
Cruz P. S., Andalecio M. N., Bolivar R. B., Fitzsimmons K.. (2008). Tilapia-shrimp polyculture in negros island, Philippines: A review. J. World Aquacult. Soc. 39 (6), 713–725. doi: 10.1111/j.1749-7345.2008.00207.x
Delatolla R., Tufenkji N., Comeau Y., Lamarre D., Gadbois A., Berk D.. (2009). In situ characterization of nitrifying biofilm: Minimizing biomass loss and preserving perspective. Water Res. 43 (6), 1775–1787. doi: 10.1016/j.watres.2009.01.009
Ebeling J. M., Timmons M. B., Bisogni J. J. (2006). Engineering analysis of the stoichiometry of photoautotrophic, autotrophic, and heterotrophic removal of ammonia-nitrogen in aquaculture systems. Aquaculture 257 (1–4), 346–358. doi: 10.1016/j.aquaculture.2006.03.019
Ekasari J., Angela D., Waluyo S. H., Bachtiar T., Surawidjaja E. H., Bossier P., et al. (2014). The size of biofloc determines the nutritional composition and the nitrogen recovery by aquaculture animals. Aquaculture 426–427 (April), 105–111. doi: 10.1016/j.aquaculture.2014.01.023
Furtado P. S., Poersch L. H., Wasielesky W. (2011). Effect of calcium hydroxide, carbonate and sodium bicarbonate on water quality and zootechnical performance of shrimp litopenaeus vannamei reared in bio-flocs technology (BFT) systems. Aquaculture 321 (1–2), 130–135. doi: 10.1016/j.aquaculture.2011.08.034
Furtado P. S., Poersch L. H., Wasielesky W. (2014). The effect of different alkalinity levels on litopenaeus vannamei reared with biofloc technology (BFT). Aquacult. Int. 23 (1), 345–358. doi: 10.1007/s10499-014-9819-x
Gaona C. A. P., et al. (2011). The effect of solids removal on water quality, growth and survival of litopenaeus vannamei in a biofloc technology culture system. Int. J. Recirculating Aquacult. 12 (1), 54–73. doi: 10.21061/ijra.v12i1.1354
Gaona C. A. P., Serra da F. P., Furtado P. S., Poersch L. H., Wasielesky W.. (2016). Biofloc management with different flow rates for solids removal in the litopenaeus vannamei BFT culture system. Aquacult. Int. 24 (5), 1263–1275. doi: 10.1007/s10499-016-9983-2
Gaona C. A. P., Souza de Almeida M., Viau V., Poersch L. H., Wasielesky W.. (2017). Effect of different total suspended solids levels on a litopenaeus vannamei (Boone 1931) BFT culture system during biofloc formation. Aquacult. Res. 48 (3), 1070–1079. doi: 10.1111/are.12949
Holanda M., Santana G., Furtado P., Vieira R., Ronzani V., Andr L., et al. (2020). Evidence of total suspended solids control by mugil liza reared in an integrated system with pacific white shrimp litopenaeus vannamei using biofloc technology. Aquacult. Rep. 18 (October 2019), 1–7. doi: 10.1016/j.aqrep.2020.100479
Jory D. E., Cabrera T. R., Dugger D. M., Fegan D., Lee P. G., Lawrence L., et al. (2001). A’ global review of shrimp feed Management : Status and perspectives. Aquaculture, 104–152.
Khanjani M. H., Mozanzadeh M. T., Sharifinia M., Emerenciano M. G. C.. (2023). Biofloc: A sustainable dietary supplement, nutritional value and functional properties. Aquaculture 562 (August 2022), 738757. doi: 10.1016/j.aquaculture.2022.738757
Khanjani M. H., Sharifinia M. (2021). Production of Nile tilapia oreochromis niloticus reared in a limited water exchange system: The effect of different light levels. Aquaculture 542 (January), 736912. doi: 10.1016/j.aquaculture.2021.736912
Khanjani M. H., Sharifinia M. (2022). Biofloc technology with addition molasses as carbon sources applied to litopenaeus vannamei juvenile production under the effects of different C/N ratios. Aquacult. Int. Springer Int. Publ. 30 (1), 383–397. doi: 10.1007/s10499-021-00803-5
Krummenauer D., Peixoto S., Cavalli R. O., Poersch L. H., Wasielesky W.. (2011). Superintensive culture of white shrimp, litopenaeus vannamei, in a biofloc technology system in southern Brazil at different stocking densities. J. World Aquacult. Soc. 42 (5), 726–733. doi: 10.1111/j.1749-7345.2011.00507.x
Krummenauer D., Samocha T., Poersch L., Lara G., Wasielesky W.. (2014). The reuse of water on the culture of pacific white shrimp, litopenaeus vannamei, in BFT system. J. World Aquacult. Soc. 45 (1), 3–14. doi: 10.1111/jwas.12093
Lara G., Honda M., Poersch L., Wasielesky W.. (2017). The use of biofilm and different feeding rates in biofloc culture system: the effects in shrimp growth parameters. Aquacult. Int. 25 (5), 1959–1970. doi: 10.1007/s10499-017-0151-0
Liu W., Luo G., Li L., Wang X., Wang J., Ma N., et al. (2017). Nitrogen dynamics and biofloc composition using biofloc technology to treat aquaculture solid waste mixed with distillery spent wash. North Am. J. Aquacult. 79 (1), 27–35. doi: 10.1080/15222055.2016.1223769
Malpartida Pasco J. J., Carvalho Filho J. W., de Espirito Santo C. M., Vinatea L.. (2018). Production of Nile tilapia oreochromis niloticus grown in BFT using two aeration systems. Aquacult. Res. 49 (1), 222–231. doi: 10.1111/are.13451
Monroy-Dosta M.d. C., de Lara R.A., Castro-Mejía J., Castro-Mejía G., Coelho-Emerenciano M. G.. (2013). Composición y abundancia de comunidades microbianas asociados al biofloc en un cultivo de tilapia. Rev. Biol. Marina y Oceanogr. 48 (3), 511–520. doi: 10.4067/S0718-19572013000300009
Muangkeow B., Ikejima K., Powtongsook S., Gallardo W.. (2007). Effects of white shrimp, litopenaeus vannamei (Boone), and Nile tilapia, oreochromis niloticus l., stocking density on growth, nutrient conversion rate and economic return in integrated closed recirculation system. Aquaculture 269 (1–4), 363–376. doi: 10.1016/j.aquaculture.2007.04.002
Muangkeow B., Ikejima K., Powtongsook S., Yi Y.. (2011). Growth and nutrient conversion of white shrimp litopenaeus vannamei (Boone) and Nile tilapia oreochromis niloticus l. in an integrated closed recirculating system. Aquacult. Res. 42 (9), 1246–1260. doi: 10.1111/j.1365-2109.2010.02713.x
Naylor R. L., Hardy R. W., Bureau D. P., Chiu A., Elliott M., Farrell A. P., et al. (2009). Feeding aquaculture in an era of finite resources. Proc. Natl. Acad. Sci. 106 (36), 15103–15110. doi: 10.1073/pnas.0905235106
Poli M. A., Legarda E. C., de Lorenzo M. A., Martins M. A., do Nascimento Vieira F.. (2019). Pacific white shrimp and Nile tilapia integrated in a biofloc system under different fish-stocking densities. Aquaculture 498 (August 2018), 83–89. doi: 10.1016/j.aquaculture.2018.08.045
Poli M. A., Legarda E. C., de Lorenzo M. A., Martins M. A., Seiffert W. Q., do Nascimento Vieira F., et al. (2019). Integrated multitrophic aquaculture applied to shrimp rearing in a biofloc system. Aquaculture 511, 1–6. doi: 10.1016/j.aquaculture.2019.734274
Ray A. J., Lewis B. L., Browdy C. L., Leffler J. W., et al. (2010). Suspended solids removal to improve shrimp (Litopenaeus vannamei) production and an evaluation of a plant-based feed in minimal-exchange, superintensive culture systems. Aquaculture 299 (1–4), 89–98. doi: 10.1016/j.aquaculture.2009.11.021
Rego M. A. S., Sabbag O. J., Soares R., Peixoto S.. (2017). Financial viability of inserting the biofloc technology in a marine shrimp litopenaeus vannamei farm: a case study in the state of pernambuco, Brazil. Aquacult. Int. Springer Int. Publ. 25 (1), 473–483. doi: 10.1007/s10499-016-0044-7
Reis W. G., Wasielesky W., Abreu P. C., Brandão H., Krummenauer D.. (2019). Rearing of the pacific white shrimp litopenaeus vannamei (Boone 1931)in BFT system with different photoperiods: Effects on the microbial community, water quality and zootechnical performance. Aquaculture 508 (February), 19–29. doi: 10.1016/j.aquaculture.2019.04.067
Ridler N., Wowchuk M., Robinson B., Barrington K., Chopin T., Robinson S., et al. (2007). Integrated multi-trophic aquaculture (IMTA): A potential strategic choice for farmers. Aquacult. Econ. Manage. 11 (1), 99–110. doi: 10.1080/13657300701202767
Roselet F., Maicá P., Martins T., Abreu P. C.. (2013). Comparison of open-air and semi-enclosed cultivation system for massive microalgae production in sub-tropical and temperate latitudes. Biomass Bioenergy 59, 418–424. doi: 10.1016/j.biombioe.2013.09.014
Samocha T. M., Prangnell D. I. (2019). System treatment and preparation. Sustain. Biofloc. Syst. Mar. Shrimp, 119–131. doi: 10.1016/b978-0-12-818040-2.00006-x
Santhana Kumar V., Pandey P. K., Anand T., Bhuvaneswari G. R., Dhinakaran A., Kumar S.. (2018). Biofloc improves water, effluent quality and growth parameters of penaeus vannamei in an intensive culture system. J. Environ. Management. 215, 206–215. doi: 10.1016/j.jenvman.2018.03.015
Simão B. R., et al. (2013). Stocking densities and feeding strategies in shrimp and tilapia polyculture in tanks. Pesquisa Agropecuaria Bras. 48 (8), 1088–1095. doi: 10.1590/S0100-204X2013000800039
Strickland J., Parsons T. (1972). A practical handbook of seawater analysis. 2nd edn (Ottawa: Fisheries Research Board of Canada). doi: 10.1007/978-1-4615-5439-4_19
Tacon A. G. J., Metian M. (2008). Global overview on the use of fish meal and fish oil in industrially compounded aquafeeds: Trends and future prospects. Aquaculture 285 (1–4), 146–158. doi: 10.1016/j.aquaculture.2008.08.015
Tendencia E. A., Dela Peña M. R., Choresca C. H. (2006). Effect of shrimp biomass and feeding on the anti-vibrio harveyi activity of tilapia sp. in a simulated shrimp-tilapia polyculture system. Aquaculture 253 (1–4), 154–162. doi: 10.1016/j.aquaculture.2005.08.004
Tendencia E. A., Dela Peña M. R., Fermin A. C., Lio-Po G., Choresca C. H., Inui Y.. (2004). Antibacterial activity of tilapia tilapia hornorum against vibrio harveyi. Aquaculture 232 (1–4), 145–152. doi: 10.1016/S0044-8486(03)00531-3
Tran-Duy A., Schrama J. W., van Dam A. A., Verreth J. A. J.. (2008). Effects of oxygen concentration and body weight on maximum feed intake, growth and hematological parameters of Nile tilapia, oreochromis niloticus. Aquaculture 275 (1–4), 152–162. doi: 10.1016/j.aquaculture.2007.12.024
Troell M., Joyce A., Chopin T., Neori A., Buschmann A. H., Fang J. G.. (2009). Ecological engineering in aquaculture - potential for integrated multi-trophic aquaculture (IMTA) in marine offshore systems. Aquaculture 297 (1–4), 1–9. doi: 10.1016/j.aquaculture.2009.09.010
Wasielesky W., Atwood H., Stokes A., Browdy C. L.. (2006). Effect of natural production in a zero exchange suspended microbial floc based super-intensive culture system for white shrimp litopenaeus vannamei. Aquaculture 258 (1–4), 396–403. doi: 10.1016/j.aquaculture.2006.04.030
Wasielesky W., Froes C., Fóes G., Krummenauer D., Lara G., Poersch L.. (2013). Nursery of litopenaeus vannamei reared in a biofloc system: The effect of stocking densities and compensatory growth. J. Shellfish Res. 32 (3), 799–806. doi: 10.2983/035.032.0323
Yuan D., Yi Y., Yakupitiyage A., Fitzimmons K., Diana J. S. (2010). Effects of addition of red tilapia (Oreochromis spp.) at different densities and sizes on production, water quality and nutrient recovery of intensive culture of white shrimp (Litopenaeus vannamei) in cement tanks. Aquaculture 298 (3–4), 226–238. doi: 10.1016/j.aquaculture.2009.11.011
Zaki M. A. A., Alabssawy A. N., Nour A. E. A. M., El Basuini M. F., Dawood M. A. O., Alkahtani S., et al. (2020). The impact of stocking density and dietary carbon sources on the growth, oxidative status and stress markers of Nile tilapia (Oreochromis niloticus) reared under biofloc conditions. Aquacult. Rep. 16 (January), 100282. doi: 10.1016/j.aqrep.2020.100282
Keywords: integrated culture, Litopenaeus vannamei, Oreochromis niloticus, total suspended solids, zero water exchange
Citation: Holanda M, Ravagnan E, Lara G, Santana G, Furtado P, Cardozo A, Wasielesky W Jr and Poersch LH (2023) Integrated multitrophic culture of shrimp Litopenaeus vannamei and tilapia Oreochromis niloticus in biofloc system: A pilot scale study. Front. Mar. Sci. 10:1060846. doi: 10.3389/fmars.2023.1060846
Received: 03 October 2022; Accepted: 20 February 2023;
Published: 22 March 2023.
Edited by:
Francisco Vargas-Albores, National Council of Science and Technology (CONACYT), MexicoReviewed by:
Mansour Torfi Mozanzadeh, South Iran Aquaculture Research Center, IranMoslem Sharifinia, Iranian Fisheries Science Research Institute, Iran
Copyright © 2023 Holanda, Ravagnan, Lara, Santana, Furtado, Cardozo, Wasielesky and Poersch. This is an open-access article distributed under the terms of the Creative Commons Attribution License (CC BY). The use, distribution or reproduction in other forums is permitted, provided the original author(s) and the copyright owner(s) are credited and that the original publication in this journal is cited, in accordance with accepted academic practice. No use, distribution or reproduction is permitted which does not comply with these terms.
*Correspondence: Luis Henrique Poersch, bHBvZXJzY2hAZ21haWwuY29t