- 1Department of Biology, Portland State University, Portland, OR, United States
- 2Chicago Zoological Society’s Sarasota Dolphin Research Program, c/o Mote Marine Laboratory, Sarasota, FL, United States
Genetic analyses, initiated in 1984, have played a major role in our understanding of the structure and social relationships of the long-term resident community of common bottlenose dolphins (Tursiops truncatus) in Sarasota Bay, Florida (SBDC). One component of our ongoing study of the community’s social system involves using blood samples from periodic catch-and-release sampling for life history and health assessment studies, as well as skin from biopsy dart sampling studies and strandings, to investigate paternity and mating strategies in this community. These analyses, covering a span of four generations of calves, were originally based on chromosomes and protein electrophoresis, but with the advent of microsatellite DNA technology, the latter has become our analysis of choice. We have performed paternity analyses on 204 known mother-calf pairs. For 151 of the calves (74%), sires were identified within the SBDC, but for the remaining 26% of the calves all sampled males associated with the community were excluded and it is likely that a substantial contribution of paternity to the SBDC comes from outside the community. Of the SBDC males, only 52 males of the more than 200 potential sires were sires. The age of sires at time of conception of a calf ranged from 10 to 43 years, averaging 24 years old. These males have sired 1-7 calves during a documented period of reproductive tenure of as much as 24 years (average = 21.4 years). Four males have sired 6-7 calves each, these males siring calves in the community for more than 20 years. Another 19 males have each sired 3-5 calves and the remaining 30 males were identified as sires of 1-2 calves. Paired males sired 75% of the calves while 25% of the calves were sired by unpaired males. Females with multiple offspring generally had multiple sires for these offspring. These paternity analyses support the hypothesis that a major avenue of gene flow in the SBDC is through the males given their greater ranges, with occasional exchange via visits from males from other areas, as well as the occasional brief movements of females from outside the community range into the community.
Introduction
Being able to track the reproductive contribution of males is of major importance for understanding population social structure and defining mating systems. This involves being able to establish paternity to evaluate male reproductive contribution, understand social associations with other males and to investigate the contribution of males to gene flow between populations. Reproductive contribution and sex-related dispersal have been important motivators for a number of field studies on bottlenose dolphins with a wide global representation of populations, both coastal and offshore. These include population studies from the North Atlantic and U.S. waters (Wells et al., 1987; Duffield and Wells, 1991; Hoelzel et al., 1998; Duffield and Wells, 2002; Owen et al., 2002; Parsons et al., 2003; Natoli et al., 2005; Sellas et al., 2005; Quérouil et al., 2007; Rosel et al., 2009; Urian et al., 2009; Fernández et al., 2011; Mirimin et al., 2011; Louis et al., 2014; Barragán-Barrera et al., 2017) and Australia, Indo-Pacific and Melanesia (Connor et al., 1992; Connor et al., 2000a; Connor et al., 2001; Krützen et al., 2003; Möller and Beheregaray, 2004; Krützen et al., 2004a; Chabanne et al., 2021). The longest-running field studies have been those in Sarasota Bay on the west coast of Florida in the U.S. and in Shark Bay in western Australia. The Sarasota Bay study on Tursiops truncatus started in 1970 (Irvine and Wells, 1972; Scott et al., 1990; Wells, 1991) and the Shark Bay study on Tursiops aduncus began in 1982 (Connor and Smolker, 1985). Taken together, these two long-term studies on the social structure of wild bottlenose dolphin populations allow us to contrast male mating strategies and reproductive exchange in two very discrete coastal areas. This paper details the reproductive contribution of males and the mating system of bottlenose dolphins in the Sarasota Bay Bottlenose Dolphin Community (SBDC). The unique long-term nature of this dataset provides an unusual opportunity to add to the understanding of the social structure of this resident community.
Genetic work on the SBDC formally started in 1984 with our initial objectives of defining population structure and investigating paternity of the calves in this resident community. As of this summary, we have sampled more than 474 dolphins and this has provided us with nearly four decades of an unprecedented snapshot of paternities in this community. It includes calves sired during 1979-2017 and is an on-going study. The paternity assignments have been possible because of the unique combination of years of observational data on this community combined with catch-and-release and biopsy dart sampling programs, as well as collaborations with the local marine mammal stranding network members.
Over the decades we have used a diverse array of genetic tools for the investigation of this community (Duffield and Wells, 1991; Duffield and Wells, 2002), initially using chromosome heteromorphisms, protein electrophoresis, mtDNA and DNA fingerprinting (Duffield and Wells, 1991), moving to DNA microsatellites as that technique became available. Microsatellites, because they represent specific genetic loci, were more powerful for establishing individual genotypes that could then be used to determine relationships and paternity. To-date, we have developed DNA microsatellite profiles for the 474 bottlenose dolphins sampled from the Sarasota Bay area. We have used the information gained from these techniques to concentrate on observed patterns of paternity in this community. This has allowed us to address a number of questions regarding the mating system in this community, such as the number of resident males contributing to paternities, the ages of males at successful mating, the reproductive tenure of individual males, the relative contributions of paired males vs. unpaired males, and the possibility of gene flow with neighboring communities.
Materials and methods
Field studies
The bottlenose dolphins of Sarasota Bay, Florida, and vicinity have been studied since 1970, with new field and analytical techniques being incorporated as they have become available (Scott et al., 1990; Wells, 2009; Wells, 2020). Field efforts at the core of this paper have occurred along much of the central west coast of Florida, but have been focused in the region from southern Tampa Bay, southward to Venice Inlet, and offshore in the Gulf of Mexico to within several kilometers of the barrier island shorelines, defined as the home range of the long-term resident Sarasota Bay bottlenose dolphin community (Wells, 2014; Figure 1). “Community” is defined as a regional society of animals sharing ranges and social associates, but exhibiting genetic exchange with other social units (Wells et al., 1999). A community is distinguished from the similar concept of a “population” by the fact that the latter is typically defined as a closed reproductive unit.
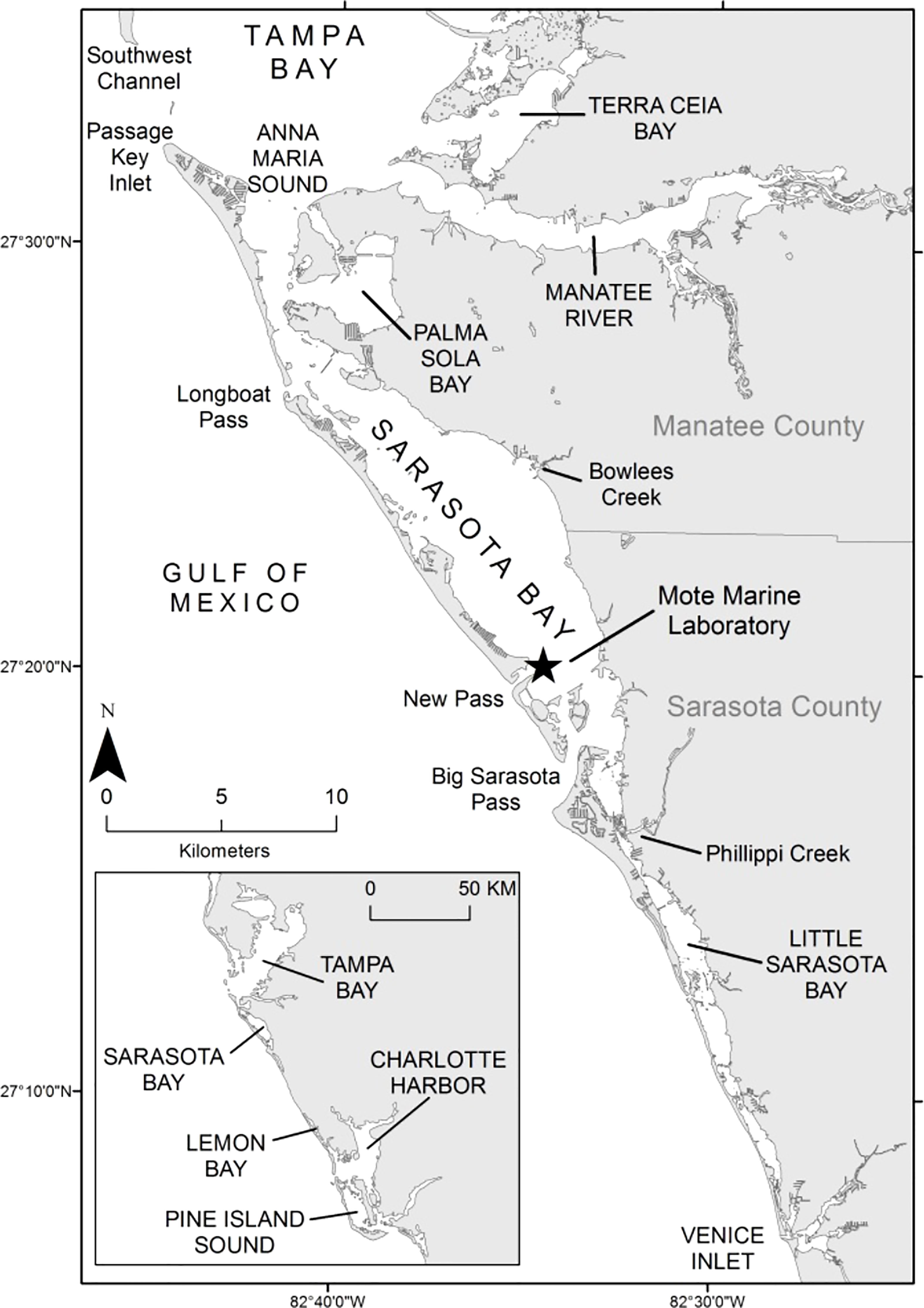
Figure 1 Study area of the Sarasota Dolphin Research Program along the west coast of Florida. The home range of the Sarasota Bay dolphin community extends southward from southern Tampa Bay to Venice Inlet. Other dolphin communities live in adjoining waters.
Residency to the Sarasota community was assigned based on consideration of ranging patterns, social association patterns, and genetics (Wells et al., 1987; Sellas et al., 2005; Urian et al., 2009; Wells, 2014). Geographic and physiographic features help to define the community range. More than 89% of the resident sightings occurred inshore of the barrier island chain bounding Sarasota Bay to the west and south, and south of an extensive shallow sandbank that delineates Tampa Bay from Sarasota Bay (Wells, 2014). A year-round resident community of about 100-170 individuals, resident across decades, monitored across six generations, spanning as many as five concurrent generations and including individuals up to 67 years of age, has been identified for Sarasota Bay (Wells, 2009; Wells, 2014; Wells, 2020).
Findings from supplemental research in surrounding waters to the north in Tampa Bay (Urian et al., 2009), to the south in Charlotte Harbor and Pine Island Sound (Bassos-Hull et al., 2013), and offshore in the Gulf of Mexico (Sellas et al., 2005; Fazioli et al., 2006) provided additional perspective. Samples for genetic analyses and associated data have been obtained through a variety of techniques, including photographic identification, catch-and-release, remote biopsy dart sampling, and stranding response, as described below.
Photographic identification and long-term monitoring
Selection of dolphins for genetic sampling was based in large part on findings from individual identification from distinctive dorsal fin markings (Wells, 2018). Identifications of individuals were confirmed from rigorous standardized techniques for examination of high quality photographs (Rosel et al., 2011; Urian et al., 2015). Long-term monitoring of dolphins in the Sarasota Bay area since 1970 has included seasonal photographic identification surveys before 1992 and monthly surveys thereafter. The Sarasota Dolphin Research Program (SDRP) began year-round operations from a base established in Sarasota in 1992. Prior to 1992, surveys were conducted by researchers traveling to Sarasota specifically for field research sessions, typically during 2-4 months in the summer, and for several weeks in winter and spring. The photo-identification surveys involve teams of researchers in small (~7m long) boats photographing all dolphins encountered over survey routes through the entire study area (Wells, 2009). Sighting records are maintained in a Microsoft Access database, FinBase (Adams et al., 2006), designed to facilitate data entry and analyses, expedite matching and cataloging processes, and reduce errors associated with manual image final management. The SDRP FinBase contains more than 55,000 dolphin groups documented since 1970, involving more than 5,600 identifiable individuals from the west coast of Florida, and including more than 168,000 identifications. Some individuals have been documented more than 1,500 times over more than four decades. Relationships between mothers and calves were identified from repeated observations of close association, and confirmed when possible from genetic analyses.
Catch-and-release
Catch-and-release provided one of three opportunities for collection of samples for genetic analyses. Small groups of dolphins were encircled by a fast boat operated by a local commercial fisherman, deploying a 500-m-long by 4-m-deep seine net in shallow waters, typically less than 2 m deep (Loughlin et al., 2010). Individual dolphins were restrained by trained handlers under veterinary supervision, and moved to a specially designed veterinary examination vessel. One-by-one, dolphins were brought aboard the vessel, weighed, and placed on a shaded and padded deck, where they were examined and sampled (Wells et al., 2004; Barratclough et al., 2019). Blood for genetic analyses was drawn from the fluke vasculature, and kept cool until it arrived at Portland State University for analysis (Duffield and Wells, 1991; Duffield and Wells, 2002). Upon completion of examination, sampling, and other tests, each individual was released on-site.
Biopsy dart sampling
A second option for collection of samples for genetic analyses involved remote biopsy dart sampling. The body region below the dorsal fin, from several cms cranial to the anterior insertion to several cms caudal to the posterior insertion, was targeted for this sampling. Small samples of skin/blubber were obtained from a small boat paralleling a selected dolphin, and deploying a sampling dart from a specially designed rifle or a crossbow (Sellas et al., 2005). The sampling head at the end of the dart obtains a 10-mm-diameter sample, with a depth of about 15-20 mm. Skin samples were stored in DMSO and shipped to Portland State University for analyses.
Stranding response
A third opportunity for genetic sample collection comes from stranding response. About one third of the long-term resident dolphins that disappear from Sarasota Bay are eventually recovered as a carcass, typically by Mote Marine Laboratory’s Stranding Investigations Program (Wells et al., 2015). Samples for genetic analyses are obtained during necropsies as possible. Sampled tissues varied depending on carcass condition.
Age determination
An individual’s age is an important consideration for paternity assignments. Most of the dolphins of the Sarasota Bay dolphin community are of known age because they have been observed since their birth to a well-known resident mother. The ages of other dolphins are known from examination of growth layer groups in teeth extracted under local anesthesia during catch-and-release health assessments, and from stranded carcasses (Hohn et al., 1989). For the few remaining individuals for which ages are not known, they have been estimated as minimums based on the date of the earliest sighting record.
Genetic analyses
Evaluation of paternity was based on known mother-calf relationships as determined by the long-term observational work available for the Sarasota Bay bottlenose dolphin community. All known Sarasota Bay community males sampled up to the time of a paternity evaluation were included in each analysis. By 2019, this included 240 resident males; their DNA profiles were run against all mother-calf pairs. Overall, only 6.85% of the 482 identifiable dolphins considered to be residents of the SBDC at some point during 1993-2019 were of unknown sex and potentially >10 yrs old, providing an upper bound of no more than 33 unsampled potential resident sires. This does not include unidentifiable dolphins, which make up about 4% of those seen on any given dolphin survey, and which tend to be smaller, likely immature dolphins that had yet to acquire distinctive markings (Wells, 2014).
The initial techniques used for paternity were chromosome heteromorphism analysis, protein electrophoresis, mtDNA analysis and DNA fingerprinting (Duffield and Wells, 1991). These were used in concert to exclude males from paternity for a particular calf or to exclude all but a single male included as sire by more than one type of analysis. By 2005, DNA microsatellite analysis was the method of choice for paternity determination. Several of the microsatellite loci we used in this study have also been used in other studies of bottenose dolphin populations, especially the studies in Shark Bay (Krützen et al., 2001; Krützen et al., 2004b). Microsatellite profiles for the dolphins sampled from the Sarasota Bay community were established by analysis of eight microsatellite loci: EV37, EV14, and EV1 (Valsecchi and Amos, 1996); MK5, MK6, MK8 (Krützen et al., 2001); and KWM2a, KWM12a (Hoelzel et al., 1998). Fragment analysis was performed on an ABI 3100 or 3770 automated sequencer (Applied Biosystems, Foster City, CA). Amplification reactions used pure Taq™ Ready-To-Go™ PCR beads (Amersham Biosciences, Piscataway, NJ), and followed protocols provided by Valsecchi and Amos (1996). Sizing of allele fragments was done in the earlier years with Genescan analysis 2.1.1 (Applied Biosystems) and more recently with GeneMapper v 4.1 (Applied Biosystems, Waltham, MA). Alleles were scored by hand and recorded in an Excel database. Individual microsatellite profiles were used to evaluate relationships and paternities. Linkage disequilbrium was not observed for these loci and there was no evidence of null alleles (Owen et al. unpublished data). Size ranges and the number of allele sizes (based on ABI 3100 allele sizing) for each of the eight microsatellite loci are given in Table 1. The average number of alleles/loci was 11.12 (range: 7-25).
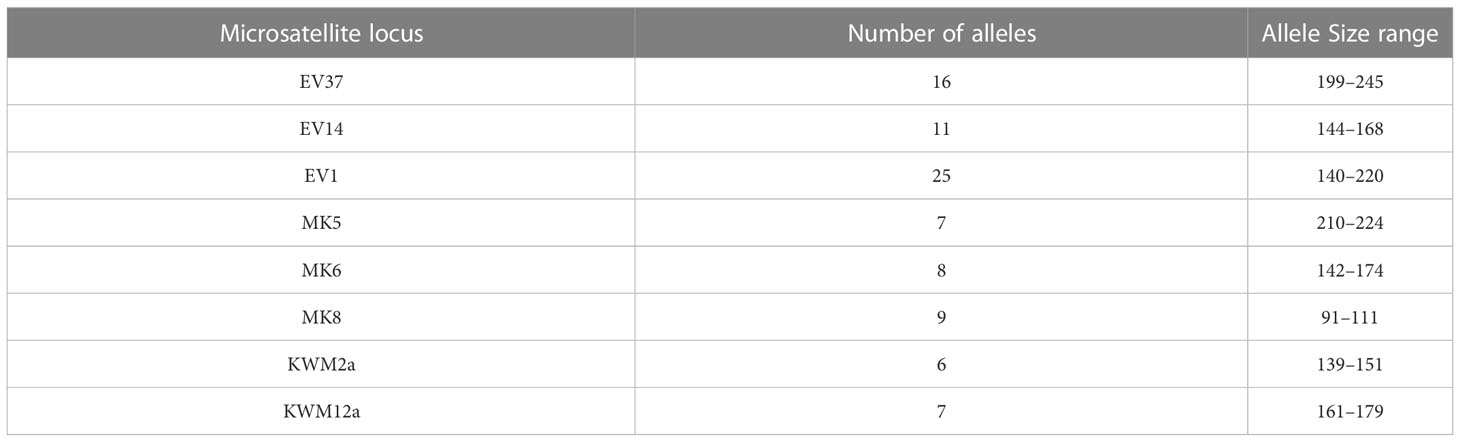
Table 1 Size range and number of alleles of the various microsatellite loci in the Sarasota Bay Dolphin Community.
Features of Sarasota Bay bottlenose dolphin sires
We examined adult male ages, morphometrics and social association patterns from the long-term datasets of the SDRP to try to identify features that might distinguish breeding from non-breeding males (Wells, 2009). Sighting records from photographic identification survey data provided information on the timing of births to long-term resident females, and on social association patterns. Age data were obtained from observations from the year of birth, or from examination of growth layer groups in teeth (Hohn et al., 1989), with adult males classified as those at least 10 years old. Morphometric data (mass and total length) were obtained from catch-and-release sessions designed to collect life history and/or health data (Wells et al., 2004).
The ages, sizes and social behavior of adult dolphins around the estimated time of conception were examined for cases in which only a single sire was identified from genetic analyses, and when a relatively narrow period of female receptivity/conception could be identified. The estimated time of conception was back-calculated from the estimated birth date of a calf, when no more than 30 days passed from the last observation of a mother alone and her first observation with a new calf. In total, 60 cases of births met these criteria. The mid-point of this time span was assigned as the estimated birth date. Based on the typical gestation period of bottlenose dolphins (O’Brien and Robeck, 2012), the estimated mid-point of the period of receptivity/conception was calculated as occurring 12.5 months earlier. All adult males seen with the female during a 30-day period centered on the estimated conception date were considered for social association analyses. Historical social associations were measured as half-weight coefficients of association between the female and each associated male (COA; Cairns and Schwager, 1987; Wells et al., 1987), as obtained through queries of FinBase. Each COA was calculated over the entire period from the first sighting record of the female through the estimated conception date, including hundreds of sighting records for most females. Within the 30-day period centered on the estimated conception date, adult male associations were also measured by the number of sightings with the female and the span of days over which these sightings occurred. Morphometric data were included when they were available from catch-and-release sessions within a span of one year before to one year after the estimated conception year.
Statistical comparisons of sires vs. non-sires involved 2-tailed t-tests, with equal variances for mass, length, and age, and Mann-Whitney U tests were used for COAs, with statistical significance assigned when p < 0.05.
Results
Paternity
Of the more than 450 mother-calf pairs in the Sarasota Bay dolphin community identified to date (see Wells et al., in prep.), we have evaluated 204 calves from known dams. For 151 of these calves (74%), sires were identified within the community and for the remaining 53 calves (26%), all sampled males associated with the community were excluded. Of the calves with sires identified in the community, 133 calves (88%) had a single sire assignment with all other sampled males excluded. The remaining 18 calves had two to three possible sires in the Sarasota Bay community, and these were excluded from further analyses.
There have been 52 males identified as sires out of more than 200 males in the community (Table 2). Two males as young as 12-13 yrs of age sired calves and the youngest male was 10 yrs. Data on testis sizes from ultrasonic measurements during catch-and-release health assessments, along with testosterone concentrations in blood, were obtained for seven males sampled within one year of their first documented siring event. Testis length ranged from 22 to 33 cm, with diameters from 5.7 to 8.3 cm, and testosterone ranged from 24.6 to 72.5 ng/ml (Wells et al. in prep.). These males ranged in total body length from 262 to 277 cm. On average, males were 263.5 cm (+14.9 cm sd, n = 26) long by the time they sired calves, and the shortest sire was 218 cm at 15 yrs of age (Wells et al. in prep.). The oldest male to sire a calf was 43 yrs of age before he was not seen again in the community, but the next oldest male sired a calf at 40 and was observed in the community for another 10 years after siring the last of 7 documented calves.
The number of years (observed reproductive tenure) over which an individual male was documented to sire calves ranged up to 24 years (Table 2), with a mean of 10.7 years, but with a median of 3 years. Looking at males that sired more than one calf, the average number of years over which a given male sired calves was 9.1 years (2-24) and the median reproductive tenure was 7 years. Three males have sired a minimum of 7 calves each, over an observed reproductive tenure of 13-20 years and with age ranges at the time of conception from 14-43 yrs. Another 19 males have sired 3-6 calves each, spanning 3-24 years, with the remaining 30 identified as sire of 1-2 calves. Overall, 37% of the 52 males have sired only one calf over the time period of this study, 19% sired two calves, 15% sired 3 calves, 15% sired 4 calves, 6% sired 5 calves, 4% sired 6 calves and 4% sired 7 calves. Generally, males sired calves from their mid-teens into their mid-forties and the average age at which males sired calves was 21.4 years (10-43) and median age was 25 yrs. The average age at conception for males that only sired one calf in the 38 years of recorded paternities was 23.4 years old (15-38); 6 of these were in their teens, 9 were 22-38 yrs. Most of the calves born to a given female were sired by different males. There were instances where a given female had two calves by a given male (2 of 2 calves, 2 of four calves, 2 of 5 calves, 2 of 7 calves) and one case in which 3 of 6 calves were assigned to the same male.
Patterns of reproductive tenure
Across the four decades of this study, a large percentage of the calves were sired by a limited number of males (Table 3). For example, during 1979-1989, 15 of the 24 calves (63%) were sired by just 4 males. During 1990-1999, 13 of 32 calves (41%) were sired by 4 males. During 2000-2009, 21 of 35 calves (60%) were sired by 6 males, while during 2010-2018, 25 of 45 calves (56%) were sired by 7 males.
The specific males siring calves changed over the decades and a variety of siring patterns were seen in this sampling of calves. Some males were identified as sires for only a year or two, others had longer reproductive tenures. For example, one male, FB28, sired calves across three decades (1979-2003). Three males, FB06, FB10 and FB60, sired calves over two decades until their death or disappearance (Table 2). However, some males, such as FB48 and FB28 were observed in the community for another ten to 12 years after their last known siring events. FB26 and FB36 sired calves in Sarasota Bay in the 1980’s and then not again until the 2000’s. Similar patterns were seen through the last 2 decades (2000-2018): F162 (1995-2008), F110 (2001-2012), F128 (2009-2017), F138 (2007-2017) and F142 (2006-2016) sired calves across two decades. F162 was observed in the community until 2016, although his last documented calf was conceived in 2008. For calves sired from 2010-2018, 16 males not previously identified as sires were sires of 1-4 calves. The average age at conception of the first calf for these males was 19 yrs (range 12->24), similar to the age range of the previously identified sires. Although it is possible that previous calves of these males have not yet been sampled (e.g., calves sired outside of Sarasota), given the comprehensive sampling of the calves especially in the most recent decades it seems more likely that this represents the movement of these males into the ranks of currently active sires. Continued evaluation of calves in this community will help to explore this further.
The age of males at conception of their last documented calf was particularly interesting. While some males were identified as sires up to their death, for others there were many years between the last known calf and the death or disappearance of the male. This could reflect the fact that we have not been able to obtain samples from all the calves, however, the observation that there are several males that have >10 years following their last known Sarasota Bay calf suggests that this may be a real pattern. The oldest male known to sire a calf was 43 yrs of age at conception – the oldest male at disappearance and presumed death was 52 yrs of age. The only calf recorded for one male, FB78, was sired when he was 31 yrs old but he was sighted in the community for another 12 years before he died. However, he also suffered from severe scoliosis throughout his life, and his dorsal fin was mutilated from a boat propeller strike at age 11. Looking at males (N= 18) with five or more years past their last identified calf, the average age of the males at their last identified calf was 27.1 yrs (15-40 yrs) and at death or disappearance was 36.9 yrs (21-52 yrs).
Male pairs
Adult males often live in strongly bonded pairs (e.g., Wells et al., 1987; Owen et al., 2002). Over the course of our study, knowledge of the sex and age composition of the Sarasota community has increased, such that by the early 1990s most of the adult males were known. Owen et al. (2002) reported that on average, for the years 1994-2000, 67% of adult males were seen with sufficient frequency to allow them to be assigned status as paired or unpaired. Of these, 57% were paired, on average, during any given year, although the numbers of paired vs. unpaired adult males during years prior to 1994 and for sires from outside of the Sarasota area cannot be determined. Male pairs generally persisted for decades, however, males could form new pair associations, be unpaired or go between being paired one year and unpaired another. We looked at the paired/unpaired status of the sires at the time of conception of a calf to evaluate the effect of pairing on reproductive success in the SBDC.
We had paired vs. unpaired male paternity data for 130 calves; 97 calves (74.6%) were sired by Sarasota Bay males that were paired at the time of conception, 33 (25.4%) were sired by unpaired males. With respect to the pairs of males that sired these 97 calves, for twelve of these pairs, both males sired calves throughout the span of their reproductive tenure, which could be as long as 15-16 years, and these pairs were responsible for 59 of the calves. For nine of the pairs, the two males were together for the entire time that we scored calves from them, producing from 2 calves (1 to each male) to 7 calves to the pair. Calves were either conceived in the same year or to one or the other of the males over a period of up to 15 years. For many of the pairs there were spaces between calves of up to 7 years, possibly a reflection of their being in another part of their range or that they had sired calves that we have not yet been able to test. There were instances where a given male had subsequent partners over time. For example, FB10 was paired with FB46 together siring 8 calves during 1995-2003. FB46 was not seen after 2003. FB10 then partnered with FB36 and together they sired 6 calves during 2004-2009. FB36 had been paired with FB38 and together they had sired 5 calves during 1985-1999, until FB38 died. There were also cases where paired males had also sired calves when unpaired (single). There were 13 additional pairs in which only one of the males sired calves, ranging from 1-5 calves for a total of 38 calves. Some of these pairs had been observed together for 15 years and some of these males also sired calves when not paired.
Fifteen males were unpaired when they conceived a calf. Single males sired from 1 to 6 calves each. Ten of these males also sired calves when paired, but there were examples where all the calves they sired were conceived when the males were unpaired: for example, two unpaired males sired 6 calves each over 10-14 years. Five males sired 3 calves each while unpaired, and were subsequently observed in pair associations where they sired additional calves.
Features of Sarasota Bay bottlenose dolphin sires
Presumed receptive females were typically seen with a variety of adult males during their estimated period of conception – no single or paired males monopolized females during this period. Only 40.4% of sires vs. non-sires were with the mothers over a longer span of time during the estimated conception period, 36.8% of sires were seen with the mothers more frequently than non-sires.
On average, sires and non-sires were not significantly different in total body length (Table 4), but 61.3% of sires were significantly heavier than non-sires (n = 31 pair-wise comparisons). Overall, 51.0% of sires were older than non-sires (n = 192 pair-wise comparisons) and on average, sires were significantly older than non-sires (Table 4). Overall COAs between adult males and adult females tended to be low, and a female’s lifetime associations with sires and non-sires were not significantly different (Table 4). On average, individual males within pairs were remarkably closely matched for age, length, mass, and COA with the females.
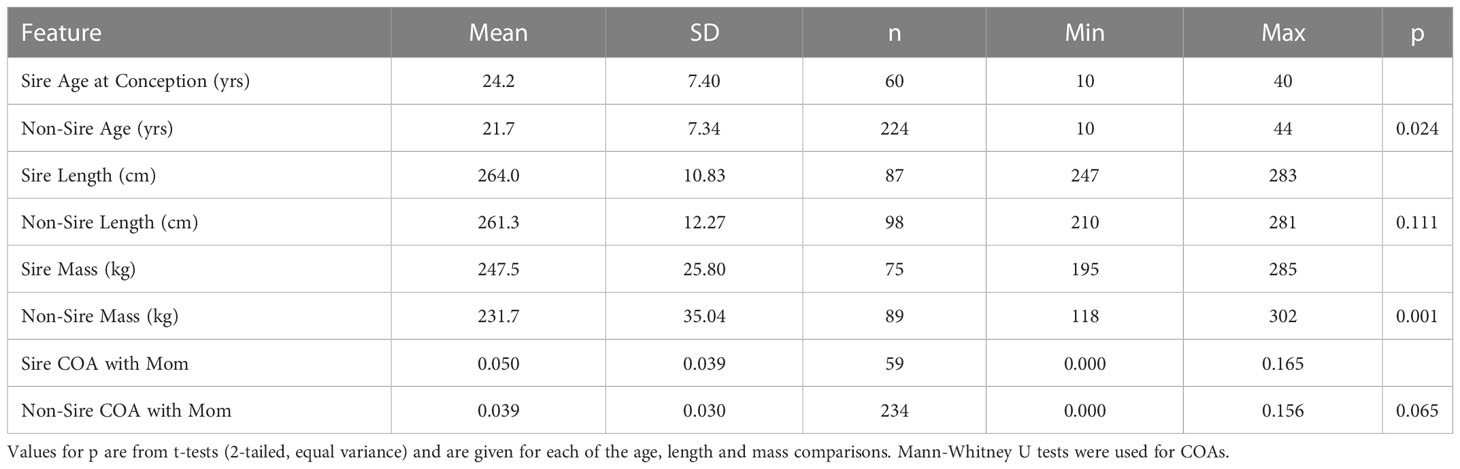
Table 4 Comparisons of features of known sires and non-sire adult male associates of presumed receptive females during the month surrounding their estimated date of conception.
Discussion
Paternity in the Sarasota Bay dolphin community
The age, sex and genetic relationships are known for >90% of the SBDC (Wells, 2003). The ability to make single sire assignments in the SBDC has been possible due in part to the allelic diversity seen in the microsatellites, especially in EV37, EV14 and EV1 (Table 1). Even more importantly, this has been possible because of the existence of detailed information on nearly every individual in the resident community, including known mother-calf relationships, as well as birth and death years, allowing us to narrow paternal assignments. With the synergism between the genetic data and long-term behavioral and life history data, we have assigned single paternities of males in the SBDC to 133 calves, with an additional 18 calves for which two to three males could have been sires. Bearing in mind that there are a limited number of Sarasota residents for which sex is not yet confirmed, the majority of successful sires were 20-43 yrs old at conception of their calves (Table 2), although some males in their early teens sired calves. There were 30+ year-old males that sired only one calf in the Sarasota Bay community during this 38-year record of paternity. There were also males that sired multiple calves relatively consistently until they died, as well as males that sired a number of calves, but were still observed in the community for a number of years after their last documented calf. Sires and dams for all the assigned calves were unrelated and the calves born to a given female were most often sired by different males.
During 2010-2018, a large number of males not previously identified as sires sired calves, more than was normally seen as new sires across the earlier decades. They sired from 1-4 calves in this interval, along with males previously identified to have sired calves in previous decades (Table 2). The reason for this change is not clear, but since we were able to examine a similar proportion of the calves born in each of these years, it does not seem to be related to having identified more calf-sire pairs. Possible explanations may include the death of older sires, older sires becoming reproductively senescent or unable to compete with the younger sires, or an inability of the oldest sires to reproduce based on life-time accumulation of environmental contaminants such as PCBs that can interfere with reproduction (Wells et al., 2005). The increase in new sires may also be related to the observed decline and leveling off of environmental contaminants such as PCBs in Sarasota Bay dolphins (Kucklick et al., 2022) with concomitant changes in the age structure of the males of the SBDC showing males to live longer.
Given that in our sampling of paternity in the Sarasota Bay community for the 204 calves that we have analyzed to-date paternity was assigned to only 52 of the 200 males in the community, it is apparent that not all adult males were siring calves, so we looked at characteristics of the males that would correlate with their breeding success (Table 4). Age appeared to be a feature characterizing breeding males. In spite of the fact that females can still produce calves at 48 years of age, and very few males live to that age (Wells, 2000; Lacy et al., 2021), 77% of sires were older than the mothers, on average exceeding their age by 5.1 years (+ 10.89 yrs, sd, n = 57). Body condition, in the form of mass, also appeared to be a factor, as successful sires were significantly heavier than non-sires. In addition, familiarity with the female may play a role in the determination of breeding status for males since COA’s were higher for successful sires. These observations would support the hypothesis that in the mating system in this community, some form of male-to-male competition and male dominance is operating. Therefore, the presence of older males remaining in the community for some years after siring calves may also be indicative of a change in their dominance, while other males continued to sire calves throughout their lifetime.
Mating system
Wells et al. (1987) hypothesized a promiscuous mating system for the Sarasota community based on the brevity of male-female associations, low level of paternal investment, large testes, high concentration of sperm in ejaculate (possibly reflecting sperm competition) and scarring from agonistic conspecific interactions. Certainly, in the SBDC, both males and females mate with multiple partners during a breeding season and our observations support dominance among males in the SBDC mating system. However, there is also a possibility for female choice. Cryptic female choice was identified in the late 1990’s (Eberhard 1985, Birkhead and Meller, 1998) and has been related to mechanisms as varied as morphology and behavior. With respect to cetaceans, it has been suggested that one of the functions of the complex system of folds that comprise the female “pseudocervix” in cetaceans could be related to post-copulatory sexual selection (Orbach et al., 2017) making it possible for female choice to contribute to breeding success by controlling semen deposition. Observations from a bottlenose dolphin breeding program where multiple copulations with multiple males were seen for a number of females, but paternity was consistently assigned to a single male (Duffield pers obs.) offer support for the possibility of a role for female choice. In the SBDC, where a number of males are known to consort with a female at the time of estrus and both members of a male pair are offered opportunities for mating, the possibility of female choice is supported both by the increased familiarity of the successful sires and by the observation that for some of the male pairs consistently only one of the males was successful over a number of calves. Whether this is achieved by behavioral mechanisms or by the mechanical manipulation of the pseudocervix is unknown, but would be an interesting avenue for further research. Promiscuity has also been proposed for the IndoPacific bottlenose dolphin population in Shark Bay where females were also observed consorting with a number of males (Connor et al., 1996), similar to the SBDC.
Male pairs and alliances
A large repertoire of mating strategies has been reported for male bottlenose dolphins, ranging from single roving males to pairs and occasional trios as in the SBDC (Wells et al., 1987; Wells, 2000) to the complex alliances seen in Tursiops aduncus in Shark Bay (Connor et al., 2000b; Connor et al., 2001; Krützen et al., 2003; Connor and Krützen, 2015; Connor et al., 2017). Of the calves sired by SBDC, 74.6% were conceived by males that were paired at the time of conception of the calf and 24.4% were conceived by unpaired males. For the paired males where both sired calves throughout the period of reproductive tenure, some of the calves were sired in the same year, others were spread throughout the period of reproductive tenure for the pair. There were examples of the members of the pair staying together over the 15 years during which they sired calves. There were also examples of males that lost a partner to death or disappearance who then formed another alliance in which they sired calves. But there were also males that sired calves as singles, including males that seemed to be between pair alliances and males that were never seen to have paired. It was apparent that, although the unpaired male strategy was less common, the males while unpaired also had extended reproductive tenures and sired multiple calves. This contrasts with the nature of the male-male bonds observed in the long-term study of Indopacific bottlenose dolphins in Shark Bay, Australia (Möller et al., 2001; Connor and Krützen, 2015) where not only are there strong associations (first-order alliances) between pairs and trios of males that can last up to 20 yrs (Connor et al., 1992; Connor et al., 2000b; Krützen et al., 2003), but these groups also form second-order alliances and labile super-alliances with other groups of males (Connor et al., 1999; Connor et al., 2011; Connor and Krützen, 2015). Possibly the muted sexual dimorphism of T. aduncus makes it necessary for males to work together in larger associations for access to, and control of, the females than seen in the SBDC, where both paired and single males successfully sire calves.
In addition, in the SBDC pairs are not kin (Wells et al., 1987; Duffield and Wells, 2002, this chapter), while in the Shark Bay population kinship may be a factor in long-term pair alliances (Krützen et al., 2003; Parsons et al., 2003). Observations of bottlenose dolphins under human care have shown that both males in a pair may copulate with a particular receptive female (McBride, 1940; Tayler and Saayman, 1972), so males may not be able to know if they are the sire of a particular calf. In the absence of an individual male’s ability to assess its own reproductive success, the overall greater reproductive success of males in pairs as compared to alone would drive a continued tendency toward pair formation, even in the absence of kinship.
Movement between communities
The SBDC is one of a mosaic of adjacent communities along Florida’s central west coast (Irvine and Wells, 1972; Wells et al., 1980; Irvine et al., 1981; Wells, 1986; Wells et al., 1987; Sellas et al., 2005; Fazioli et al., 2006; Urian et al., 2009; Bassos-Hull et al., 2013) and although these communities have discrete home ranges built around resident females, their calves and associates, low rates of immigration and emigration have been documented. Males in particular seem to move between these communities and to have larger home ranges than the females based on patterns of social affiliation and long-term site fidelity (Urian et al., 2009). Male pairs have larger ranging areas and core areas than unpaired males (Owen et al., 2002) and an adult male from Sarasota was observed escorting a known estrous female of a neighboring area (Wells, 1991). Of the 52 males known to be part of the SBDC and exclusively assigned as sires for 1 to 7 calves, 16 were themselves known to have been born to females in the SBDC. However, the rest were not previously identified as calves during the more than 50 years of this long-term study. Many of the males that sired several calves did so relatively consistently throughout their time of reproductive tenure (Table 2). For other males, both paired and unpaired, there were periods ranging from 5 to 13 years between siring events. The gaps in siring calves may represent times when Sarasota Bay males moved between communities as previously shown. Movements of males from nearby resident communities, possibly extending to males from Gulf populations moving past the coastal areas, may also account for the 26% of the calves for which all the sampled Sarasota resident males had been excluded from paternity. It would be reasonable to think that SBDC males moving between local communities could explain the gaps in reproductive tenure seen with the Sarasota Bay males. Although “unknown sires” were expected in the early years of the study before more of the males had been sampled, calves with unknown sires have consistently been found throughout the decades of this study and we still see the exclusion of all known males sampled in the Sarasota Bay community for a number of the current calves. These observations strongly support that these communities are not closed demographic units that would meet the definition of populations, but are neighboring local communities with genetic exchange across their observed geographic boundaries (Wells, 1986; Duffield and Wells, 1991).
A variety of dispersal patterns exist among different bottlenose dolphin species or populations, possibly related at least in part to their use of different habitats (Natoli et al., 2005). For example, Shark Bay males disperse more than females (Krützen et al., 2004a), although still showing locational philopatry (Tsai and Mann, 2012), supporting potential male-mediated gene flow between resident and presumably transient groups. Sellas et al. (2005) compared four resident inshore bottlenose dolphin stocks (including the SBDC) and Gulf of Mexico coastal dolphins. They demonstrated significant philopatry and population differentiation, but that the individuals within each group were not highly related. This also suggests that, despite the philopatry and differentiation, there may be some level of genetic exchange among these populations, although whether via the males or females was not investigated. Population studies provide differing evidence of female philopatry and male-mediated dispersal in bottlenose dolphins. Rosel et al. (2009) and Louis et al. (2014), looking at populations along the Northwest and Northeast Atlantic coasts, saw significant differentiation among coastal populations, with little or no evidence of significant sex-biased dispersal. In contrast, fine-scale studies of genetic structure of bottlenose dolphins around the Iberian Peninsula (Fernández et al., 2011) provided no evidence of sex-biased dispersal, but did see evidence of gene flow between resident and immediately neighboring populations. There were high levels of gene flow between oceanic and coastal populations in Azores-Madeira and Portugal (Quérouil et al., 2007), although no evidence was found for sex-biased dispersal. In other areas, a wider dispersal of males than females was noted with females being more philopatric (Möller and Beheregaray, 2004; Krützen et al., 2004a). On the other hand, in the Eastern Mediterranean Sea it was found that the gene flow was mediated through females (Gaspari et al., 2015).
Given that rates of permanent immigration and emigration are low in SBDC, gene flow is largely meditated through temporary movements of males and/or females into and out of the community range. Our observation and paternity work in Sarasota Bay suggests that gene flow in the SBDC is largely through the males given their greater ranges and their overlap with neighboring communities, while the females are more philopatric. However, there are also possibilities for exchange via visits from males from other areas, as well as the occasional brief movements of females among communities. The patterns of gene flow we have described would seem to provide the localized communities with a level of resilience in the event of a localized unusual mortality event or other environmental catastrophe. Continued monitoring and sampling of this community and those in surrounding waters will help to illuminate the social structures of these complex societies, and mechanisms that may impact their conservation.
Conclusion
Studies that have been on-going since 1970 allowed a multi-decade synthesis of extensive genetic and observational data to establish the patterns of paternity in the Sarasota Bay Dolphin Community (SBDC). Based on 204 known mother-calf pairs, we made the following observations:
* Only 52 of the 200 known males in the SBDC have sired calves, indicating that a large percentage of the calves were sired by a limited number of males.
* The average age at which males sired calves was 21 years, but males of ages 10-43 years have sired calves.
* Four males have sired 6-7 calves each, one of these males siring calves in the community for more than 20 years. Another 19 males have each sired 3-5 calves and the remaining 30 males were identified as sires of 1-2 calves.
* A number of older males were still present in the community for more than 10 years after their last identified calf.
* Both males known to be in strongly bonded pairs and males that were unpaired sired calves: 74.6% of the calves were sired by paired males, but 25.4% of the calves were sired by males that were single and not paired when they sired the calf.
* Successful sires were on average older than the mothers and significantly heavier than non-sires and had greater prior familiarity with the females.
* A promiscuous mating system is characteristic of the SBDC, with suggestions both of male dominance and the possibility of cryptic female choice.
* Sires were identified within the community for 152 of the calves (74%), but for the remaining 26% of the calves, all sampled males associated with the community were excluded. It seems likely that a contribution of paternity to the Sarasota Bay Dolphin Community comes from outside that community and that there is reproductive exchange among bottlenose dolphin communities.
Data availability statement
The original contributions presented in the study are included in the article/supplementary material. Further inquiries can be directed to the corresponding author.
Ethics statement
Ethical review and approval was not required for the animal study because the animals were wild.
Author contributions
RW: Field observations and manuscript preparation. DD: Genetic analysis and manuscript preparation. All authors contributed to the article and approved the submitted version.
Funding
Support for the research has come from the Charles and Margery Barancik Foundation, Earthwatch Institute, Dolphin Quest, Disney, NOAA, Mote Scientific Foundation, the U.S. Environmental Protection Agency, the International Whaling Commission, the Office of Naval Research, the Gulf of Mexico Research Initiative, Harbor Branch Oceanographic Institute ProtectWild Dolphins Program, and Fundacion Oceanografic, among others.
Acknowledgments
Research was conducted under a series of Scientific Research Permits issued by the National Marine Fisheries Service, renewed every five years, and Institutional Animal Care and Use Committee approvals through Mote Marine Laboratory, renewed annually. Special thanks to Dalin D’Alessandro and Amy Dolan for their help with the DNA database. This work would not have been possible without the dedicated efforts over decades by a large team of Sarasota Dolphin Research Program staff, students, volunteers, veterinarians, and collaborators from around the world. In particular, the vision and curiosity of Blair Irvine gave the research its start in 1970, and continuing interest by Blair and Michael Scott led to the development of the large team effort that has continued the research through its first 50 years. Jay Sweeney initiated sampling for genetics in 1984, as a part of life history and health assessment research. We thank Aleta Hohn for age estimates from analyses of teeth, and Rae Stone and National Marine Mammal Foundation veterinarians for ultrasonic testes measurements. Biopsy dart sampling supplementing health assessments was accomplished primarily by Anna Sellas, Aaron Barleycorn, Jason Allen, and Brian Balmer. We thank personnel associated with Mote Marine Laboratory’s Stranding Investigations Program for samples from stranded dolphins. Primary staff involved in photo-ID data collection and/or analyses through 2019 included Suzanne Hofmann, Kim Urian, Kim Bassos Hull, Kristi Fazioli, Edward Owen, Caryn Owen, Stephanie Nowacek, Jason Allen, Aaron Barleycorn, Katie McHugh, Shauna McBride, Sunnie Brenneman, Robin Perrtree, and Allison Honaker.
Conflict of interest
The authors declare that the research was conducted in the absence of any commercial or financial relationships that could be construed as a potential conflict of interest.
Research was conducted under a series of Scientific Research Permits issued by the National Marine Fisheries Service, renewed every five years, and Institutional Animal Care and Use Committee approvals through Mote Marine Laboratory, renewed annually.
Publisher’s note
All claims expressed in this article are solely those of the authors and do not necessarily represent those of their affiliated organizations, or those of the publisher, the editors and the reviewers. Any product that may be evaluated in this article, or claim that may be made by its manufacturer, is not guaranteed or endorsed by the publisher.
References
Adams J. D., Speakman T., Zolman E., Schwacke L. H. (2006). Automating image matching, cataloging, and analysis for photo-identification research. Aquat. Mammals 32 (3), 374–384. doi: 10.1578/AM.32.3.2006.374
Barragán-Barrera D. C., May-Collado L. J., Tezanos-Pinto G., Islas-Villaneuva V., Correa-Cárdenas C. A., Caballero S. (2017). High genetic structure and low mitochondrial diversity in bottlenose dolphins of the archipelago of Bocas del Toro, Panama: A population at risk? PLoS 12, e0189370. doi: 10.1371/journal.pone.0189370
Barratclough A., Wells R. S., Schwacke L. H., Rowles T. K., Gomez F. M., Fauquier D. A., et al. (2019). Health assessments of common bottlenose dolphins (Tursiops truncatus): Past, present, and potential conservation applications. Front. Vet. Sci 6. doi: 10.3389/fvets.2019.00444
Bassos-Hull K., Perrtree R., Shepard C., Schilling S., Barleycorn A., Allen J., et al. (2013). Long-term site fidelity and seasonal abundance estimates of common bottlenose dolphins (Tursiops truncatus) along the southwest coast of Florida and responses to natural perturbations. J. Cetacean Res. Manage. 13, 19–30.
Birkhead T. R., Meller A. P. (1998). Sperm competition and sexual selection (Cambridge, MA: Academic Press).
Cairns S. J., Schwager S. (1987). A comparison of association indices. Anim. Behav. 35, 1455–1469. doi: 10.1016/S0003-3472(87)80018-0
Chabanne D. B. H., Allen S. J., Sherwin W. B., Finn H., Krützen M. (2021). Inconsistency between socio-spatial and genetic structure in a coastal dolphin population. Front. Mar. Sci. 7, e617540. doi: 10.3389/mars.2020.61/540
Connor R. C., Cloffi W. R., Randic S., Allen S. J., Watcon-Capps J., Krützen M. (2017). Male Alliance behaviour and mating access varies with habitat in a dolphin social network. Nat. Sci. Repts., 46354. doi: 10.1038/srep46354
Connor R. C., Heithaus M. R., Barre L. M. (1999). Super-alliance of bottlenose dolphins. Nature, 371–571-572. doi: 10.1038/17501
Connor R. C., Heithaus M. R., Barre L. M. (2001). Complex social structure, alliance stability and mating access in a bottlenose dolphin ‘super-alliance’. Proc. R. Soc B. 268, 263–267. doi: 10.1098/rsbl.2000.1357
Connor R. C., Krützen M. (2015). Male Dolphin alliances in Shark Bay: Changing perspectives in a 30-year study. Anim. Behav. 103, 223–235. doi: 10.1016/j.anbehav.2015.02.019
Connor R. C., Read A. J., Wrangham R. W. (2000b). Male Reproductive strategies and social bonds. in cetacean societies, field studies of dolphins and whales. Eds. Mann J., Conner R. C., Tyack P. L., Whitehead H. (Chicago, IL: The University of Chicago Press), 247–269.
Connor R. C., Richards A. F., Smolker R. A., Mann J. (1996). Patterns of female attractiveness in Indian ocean bottlenose dolphins. Behav 133, 37–69. doi: 10.1163/156853996X00026
Connor R. C., Smolker R. S. (1985). Habituated dolphins (Tursiops sp.) in Western Australia. J. Mamm. 66 (2), 398–400. doi: 10.2307/1381260
Connor R. C., Smolker R. A., Richards A. F. (1992). Two levels of alliance formation among male bottlenose dolphins (Tursiops sp.). Proc. Natl. Acad. Sci. U.S.A. 89, 987–990. doi: 10.1073/pnas.89.3.987
Connor R. C., Watson-Capps J. J., Sherwin W. B., Krützen M. (2011). A new level of complexity in the male alliance networks of Indian ocean bottlenose dolphins (Tursiops sp.). Biol. Letters. 7, 623–626. doi: 10.1098/rsbl.2010.0852
Connor R. C., Wells R. S., Mann J., Read A. J. (2000a). “The bottlenose dolphin, social relationship in a fission-fusion society,” in Cetacean societies, field studies of dolphins and whales. Eds. Mann J., Conner R. C., Tyack P. L., Whitehead H. (Chicago, IL: The University of Chicago Press), 91–126.
Duffield D. A., Wells R. S. (1991). “The combined application of chromosome, protein and molecular data in the determination of social unit structure and dynamics in Tursiops truncatus,” in Genetic ecology of whales and dolphins, vol. 13 . Ed. Hoelzel A. R. (Cambridge, U.K.; International Whaling Comm Special Issue), 155–169.
Duffield D. A., Wells R. S. (2002). “Molecular profile of a resident community of bottlenose dolphins, Tursiops truncatus,” in Cell and molecular biology of marine mammals. Ed. Pfeiffer C. J. (Malabar, FL: Krieger Publ. Co.), 3–11.
Eberhard W. G. (1985). Sexual selection and animal genitalia (Cambridge, MA: Harvard Univ. Press). doi: 10.4159/harvard.9780674330702
Fazioli K. L., Hofmann S., Wells R. S. (2006). Use of coastal gulf of Mexico waters by distinct assemblages of bottlenose dolphins. Tursiops truncatus. Aquat. Mamm. 32, 212–222. doi: 10.1578/AM.32.2.2006.212
Fernández R., Santos M. B., Pierce G. J., Llavona Á., López A., Silva M. A., et al. (2011). Fine-scale genetic structure of bottlenose dolphins, Tursiops truncatus, in Atlantic coastal waters of the Iberian peninsula. Hydrobiologia 670, 111–125. doi: 10.1007/s10750-011-0669-5
Gaspari S., Scheinin A., Holcer D., Fortuna C., Natali C., Genov T., et al. (2015). Drivers of population structure of the bottlenose dolphin (Tursiops truncatus) in the Mediterranean Sea. Evol. Biol. 42, 177–190. doi: 10.07/s11692-015-9309-8
Hoelzel A. R., Potter C. W., Best P. B. (1998). Genetic differentiation between parapatric ‘nearshore’ and ‘offshore’ populations of the bottlenose dolphin. Proc. R. Soc. B. 265, 1177–1183. doi: 10.1098/rspb.1998.0416
Hohn A. A., Scott M. D., Wells R. S., Sweeney J. C., Irvine A. B. (1989). Growth layers in teeth from known-age, free-ranging bottlenose dolphins. Mar. Mamm. Sci. 5 (4), 315–342. doi: 10.1111/j.1748-7692.1989.tb00346.x
Irvine A. B., Scott M. D., Wells R. S., Kaufmann J. H. (1981). Movements and activities of the Atlantic bottlenose dolphin, Tursiops truncatus, near Sarasota, Florida. Fish. Bull. U.S. 79, 671–688.
Irvine B., Wells R. S. (1972). Results of attempts to tag Atlantic bottlenosed dolphins (Tursiops truncatus). Cetology 13, 1–5.
Krützen M., Barre L. M., Connor R. C., Mann J., Sherwin W. B. (2004a). ‘O father: where are thou?’ – paternity assessment in an open fission-fusion society of wild bottlenose dolphins (Tursiops sp.) in Shark Bay, Western Australia. Mol. Ecol. 13, 1975–1990. doi: 10.1111/j.1365-294X.2004.02192.x
Krützen M., Sherwin W. B., Berggren P., Gales N. J. (2004b). Population structure in an inshore cetacean revealed by microsatellite and mtDNA analysis: Bottlenose dolphins (Tursiops sp.) in Shark Bay, Western Australia. Mar. Mamm. Sci. 20, 28–47. doi: 10.1111/j.1748-7692.2004.tb01139.x
Krützen M., Sherwin W. B., Connor R. C., Barré L. M., Van de Casteele T., Mann J., et al. (2003). Contrasting relatedness patterns in bottlenose dolphins (Tursiops sp.) with difference alliance strategies. Proc. R. Soc B. 270, 497–502. doi: 10.1098/rspb.2002.2229
Krützen M., Valsecchi E., Connor R. C., Sherwin W. B. (2001). Characterization of microsatellite loci in Tursiops aduncus. Mol. Ecol. Notes 1, 170–172. doi: 10.1046/j.1471-8278.2001.00065.x
Kucklick J., Boggs A., Huncik K., Moors A., Davis E., Ylitalo G., et al. (2022). Temporal tends of persistent organic pollutants in Sarasota bay common bottlenose dolphins (Tursiops truncatus). Front.in Mar. Sci. 9. doi: 10.3389/fmars.2022.763918
Lacy R. C., Wells R. S., Scott M. D., Allen J. B., Barleycorn A. A., Urian K. W., et al. (2021). Assessing the viability of the Sarasota bay community of bottlenose dolphins. Front. Mar. Sci. 8. doi: 10.3389/fmars.2021.788086
Loughlin T., Cunningham L., Gales N., Wells R. S., Boyd I. (2010). “Marking and capturing,” in Marine mammal ecology and conservation: A handbook of techniques. Eds. Boyd I., Bowen D., Iverson S. (Oxford, UK; Oxford University Press), 16–41.
Louis M., Viricel A., Lucas T., Peltier H., Alfonsi E., Barrow S., et al. (2014). Habitat-driven population structure of bottlenose dolphins, Tursiops truncatus, in the north-east Atlantic. Mol. Ecol. 23, 857–874. doi: 10.1111/mec.12653
Mirimin L., Miller R., Dillane E., Berrow S. D., Ingram S., Cross T. F., et al. (2011). Fine-scale population genetic structuring of bottlenose dolphins in Irish coastal waters. Anim. Conserv. 14, 342–353. doi: 10.1111/j.1469-1795-2010.00432.x
Möller L. M., Beheregaray L. B. (2004). Genetic evidence for sex-biased dispersal in resident bottlenose dolphins (Tursiops aduncus). Mol. Ecol. 13, 1607–1612. doi: 10.1111/j.1365-294X.2004.02137.x
Möller L. M., Beheregaray L. B., Harcourt R., Krützen M. (2001). Alliance membership and kinship in wild male bottlenose dolphins (Tursiops aduncus) of southeastern Australia. Proc. R. Soc B. 268, 1941–1947. doi: 10.1098/rspb.2001.1756
Natoli A., Birkun A., Aguilar A., Lopez A., Hoelzel A. R. (2005). Habitat structure and the dispersal of male and female bottlenose dolphins (Tursiops truncatus). Proc. R. Soc B 272, 1217–1226. doi: 10.1098/rspb.2005.3076
O’Brien J. K., Robeck T. R. (2012). The relationship of maternal characteristics and circulating progesterone concentrations with reproductive outcome in the bottlenose dolphin (Tursiops truncatus) after artificial insemination, with and without ovulation induction, and natural breeding. Theriogenology 78 (3), 469–482. doi: 10.1016/j.theriogenology.2012.02.011
Orbach D. N., Marshall C. D., Mesnick S. L., Würsig B. (2017). Patterns of cetacean vaginal folds yield insights into functionality. PloS One 12 (3), e0175037. doi: 10.137/journal.pone.0175037
Owen E. C. G., Wells R. S., Hofmann S. (2002). Ranging and social association patterns of paired and unpaired adult male Atlantic bottlenose dolphins, Tursiops truncatus, in Sarasota, Florida, provide no evidence for alternative male strategies. Can. J. Zool. 80, 2072–2089. doi: 10.1139/Z02-195
Parsons K. M., Durban J. W., Claridge D. E., Balcomb J. C., Noble L. R., Thompson P. M. (2003). Kinship as a basis for alliance formation between male bottlenose dolphins, Tursiops truncatus, in the Bahamas. Anim. Behav. 66, 185–194. doi: 10.1006/anbe.2003.2186
Quérouil S., Silva M. A., Freitas L., Prieto R., Magalhães S., Dinis A., et al. (2007). High gene flow in oceanic bottlenose dolphins (Tursiops truncatus) of the north Atlantic. Conserv. Genet. 8, 1405–1419. doi: 10.1007/s10592-007-9291-5
Rosel P. E., Hansen L., Hohn A. A. (2009). Restricted dispersal in a continuously distributed marine species: Common bottlenose dolphinis Tursiops truncatus in coastal waters of the western north Atlantic. Mol. Ecol. 18, 5030–5045. doi: 10.1111/j.1365-294X.2009.04413.x
Rosel P. E., Mullin K. D., Garrison L., Schwacke L., Adams J., Balmer B., et al. (2011). Photo-identification Capture-Mark-Recapture techniques for estimating abundance of bay, sound and estuary populations of bottlenose dolphins along the U.S. East coast and gulf of Mexico: A workshop report. NOAA Tech. Memorandum, 30.
Scott M. D., Wells R. S., Irvine A. B. (1990). “A long-term study of bottlenose dolphins on the west coast of Florida,” in The bottlenose dolphin. Eds. Leatherwood S., Reeves R. R. (San Diego: Academic Press), 235–244.
Sellas A. B., Wells R. S., Rosel P. E. (2005). Mitochondrial and nuclear DNA analyses reveal fine scale geographic structure in bottlenose dolphins (Tursiops truncatus) in the gulf of Mexico. Conserv. Genet. 6, 715–728. doi: 10.1007/s10592-005-9031-7
Tayler C. K., Saayman G. S. (1972). The social organization of dolphins, (Tursiopsaduncus) and baboons (Papio ursinus): Some comparisons and assessments. Ann. Cape Prov. Mus. Nat. Hist. 9, 11–49.
Tsai Y. J., Mann J. (2012). Dispersal, philopatry, and the role of fission-fusion dynamics in bottlenose dolphins. Mar. Mamm. Sci. 29 (2), 261–279. doi: 10.1111/j.1748-7692.2011.00559.x
Urian K., Gorgone A., Read A., Balmer B., Wells R., Berggren P., et al. (2015). Recommendations for photo-identification methods used in capture-recapture models with cetaceans. Mar. Mammal Sci. 31 (1), 298–321. doi: 10.1111/mms.12141
Urian K. W., Hofmann S., Wells R. S., Read A. J. (2009). Fine-scale population structure of bottlenose dolphins, Tursiops truncatus, in Tampa bay, Florida. Mar. Mamm. Sci. 25, 619–638. doi: 10.1111/j.1748-7692.2009.00284.x
Valsecchi E., Amos W. (1996). Microsatellite markers for the study of cetacean populations. Mol. Ecol. 5, 151–156. doi: 10.1111/j.1365-294x.1996.tb00301.x
Wells R. S. (1986). Population structure of bottlenose dolphins: Behavioral studies of bottlenose dolphins along the central west coast of Florida (Santa Cruz, CA; National Marine Fisheries Service, Southeast Fisheries Center), 70.
Wells R. S. (1991). “The role of long-term study in understanding the social structure of a bottlenose dolphin community,” in Dolphin societies: Discoveries and puzzles. Eds. Pryor K., Norris K. S. (Berkeley: Univ. of California Press), 199–225.
Wells R. S. (2000). “Reproduction in wild bottlenose dolphins: Overview of patterns observed during a long-term study,” in Bottlenose dolphin reproduction workshop report. Eds. Duffield D., Robeck T. (Silver Springs, MD: AZA Mar. Mammal Taxon Advisory Group), 57–74.
Wells R. S. (2003). “Dolphin social complexity: Lessons from long-term study and life history,” in Animal social complexity: Intelligence, culture and individualized societies. Eds. de Waal F. B. M., Tyack P. L. (Cambridge, MA: Harvard University Press), 32–56.
Wells R. S. (2009). Learning from nature: Bottlenose dolphin care and husbandry. Zoo Biol. 28, 1–17. doi: 10.1002/zoo.20252
Wells R. S. (2014). “Social structure and life history of common bottlenose dolphins near Sarasota bay, Florida: Insights from four decades and five generations,” in Primates and cetaceans: Field research and conservation of complex mammalian societies, primatology monographs. Eds. Yamagiwa J., Karczmarski L. (Tokyo, Japan: Springer), 149–172. doi: 10.1007/978-4-431-54523-1_8
Wells R. S. (2018). “Identification methods,” in Encyclopedia of marine mammals, 3rd Ed. Eds. Würsig B., Thewissen J. G. M., Kovacs K. (San Diego, CA: Academic Press/Elsevier), 503–509.
Wells R. S. (2020). The Sarasota dolphin research program in 2020: Celebrating 50 years of research, conservation, and education. Aquat. Mammals 25 (5), 502–503. doi: 10.1578/AM.46.5.2020.502
Wells R. S., Allen J. B., Lovewell G. N., Gorzelany J., DeLynn R. E., Fauquier D. A., et al. (2015). Carcass-recovery rates for resident bottlenose dolphins in Sarasota bay, Florida. Mar. Mammal Sci. 31, 355–368. doi: 10.1111/mms.12142
Wells R. S., Boness D. J., Rathbun G. B. (1999). “Behavior,” in Biology of marine mammals. Eds. Reynolds J. E. III, Rommel S. A. (Washington, DC: Smithsonian Institution Press), 578.
Wells R. S., Irvine A. B., Scott M. D. (1980). “The social ecology of inshore odontocetes,” in Cetacean behavior: Mechanisms and functions. Ed. Herman L. M. (New York: J. Wiley & Sons), 263–317, 463.
Wells R. S., Rhinehart H. L., Hansen L. J., Sweeney J. C., Townsend F. I., Stone R., et al. (2004). Bottlenose dolphins as marine ecosystem sentinels: Developing a health monitoring system. EcoHealth 1, 246–254. doi: 10.1007/s10393-004-0094-6
Wells R. S., Scott M. D., Irvine A. B. (1987). “The social structure of free-ranging bottlenose dolphins,” in Curr. mamm. Ed. Genoways H. H. (New York, NY: Plenum), 247–305. doi: 10.1007/978-1-4757-9909-5_7
Wells R. S., Tornero V., Borrell A., Aguilar A., Rowles T. K., Rhinehart H. L., et al. (2005). Integrating life history and reproductive success data to examine potential relationships with organochlorine compounds for bottlenose dolphins (Tursiops truncatus) in Sarasota bay, Florida. Sci. Total Environ. 349, 106–119. doi: 10.1016/j.scitotenv.2005.01.010
Keywords: Sarasota Bay dolphin community, DNA microsatellites, male contribution, social structure, mating patterns, reproductive success
Citation: Duffield D and Wells R (2023) Paternity patterns in a long-term resident bottlenose dolphin community. Front. Mar. Sci. 10:1076715. doi: 10.3389/fmars.2023.1076715
Received: 21 October 2022; Accepted: 20 January 2023;
Published: 02 February 2023.
Edited by:
James Scott Maki, Marquette University, United StatesReviewed by:
Stephanie Plön, Stellenbosch University, South AfricaCindy R. Elliser, Pacific Mammal Research (PacMam), United States
Ewa Krzyszczyk, Bangor University, United Kingdom
Keith Hernandez, Oregon State University, United States
Copyright © 2023 Duffield and Wells. This is an open-access article distributed under the terms of the Creative Commons Attribution License (CC BY). The use, distribution or reproduction in other forums is permitted, provided the original author(s) and the copyright owner(s) are credited and that the original publication in this journal is cited, in accordance with accepted academic practice. No use, distribution or reproduction is permitted which does not comply with these terms.
*Correspondence: Debbie Duffield, ZHVmZmllbGRkQHBkeC5lZHU=