- 1State Key Laboratory of Estuarine and Coastal Research, Institute of Eco-Chongming, East China Normal University, Shanghai, China
- 2East China Sea Environmental Monitoring Center, State Oceanic Administration, Shanghai, China
- 3Key Laboratory of Marine Ecological Monitoring and Restoration Technologies, Ministry of Natural Resources, Shanghai, China
- 4Yangtze Delta Estuarine Wetland Ecosystem Observation and Research Station, Ministry of Education and Shanghai Science and Technology Committee, Shanghai, China
Saltmarshes are valued as key buffering ecosystems against global climate change and sea level rise. However, the knowledge deficit regarding links between colonization of saltmarsh fringes by plants and mud cracking in the lateral dimension considerably limits our understanding of marsh resilience. Here, the role of mud cracks in colonization by saltmarsh plants was investigated. A combination of field experiments, remote sensing, and experimental results revealed that: (1) potential mud cracking zones were formed at the seaward edge of saltmarshes under the influence of tide-induced wetting–drying cycles, where mud cracks were extensively distributed and colonized by new seedlings. (2) The seedling density in the mud cracks was higher than that in the patches, and seedlings in the mud cracks sprouted earlier than those in the patches. The results implied that mud cracking enhanced colonization by saltmarsh plants, rather than being a water stressor. (3) The two main ecological functions of mud cracks in saltmarsh colonization were acting as “seed traps” and “seedling growth promoters.” (4) Mud cracking could be a key factor influencing saltmarsh resilience, especially by promoting the colonization and dispersal of saltmarsh plants. Rapid colonization of potential zones with mud cracks could occur as soon as seeds are available. Our results could facilitate the development of appropriate saltmarsh rehabilitation strategies.
1 Introduction
Coastal wetlands such as saltmarshes are among the most valuable and productive ecosystems on the globe (Costanza et al., 1997), which perform key ecosystem functions such as wave attenuation (Bouma et al., 2010; Möller et al., 2014), shoreline stabilization (Temmerman et al., 2013; Bouma et al., 2014), carbon sequestration, nutrient retention (Li et al., 2014), and biodiversity conservation (Costanza et al., 2014; Kelleway et al., 2017). Thus, saltmarshes are crucial ecosystems that naturally buffer the effects of global climate change and sea level rise (SLR) (Duarte et al., 2013; Zhu et al., 2020). However, several saltmarshes are extensively eroded at the edges (Deegan et al., 2012; Silliman et al., 2012; Leonardi et al., 2016), and vegetation recovery forms the Achilles’ heel of saltmarsh resilience to SLR (Zhu et al., 2020). Consequently, effective and rapid efforts to restore vegetation at saltmarsh edges are essential.
The most notable characteristic of saltmarshes is the alternating wetting–drying periods with semimonthly inundation-exposure cycles, which leave the areas with high elevation and weak tides not submerged in seawater except during the spring tides. Consequently, extensive mud cracks have occurred on intertidal mudflats (Han, 2017). Elevation and tidal regime are two principle factors affecting mud cracking processes. Coastal wetlands in many areas are gradually expanding due to sediment-induced vertical accretion, such as in the Oosterschelde estuary in the Netherlands (Ma et al., 2014) and Yangtze River estuary (Yang et al., 2003; Ge et al., 2015a). As these are elevated features relative to the low-lying bare mudflats, saltmarshes are no longer subjected to inundation during each tide, but they become exposed to desiccation and evaporation, which are strongly associated with semilunar tidal cycles (Gardel et al., 2009). According to Fiot and Gratiot (2006), mud cracks appear if desiccation and evaporation processes last sufficiently at a given elevation (>2.6 m above hydrographic zero), and five consecutive days of emersion (CDOE) are sufficient for mud cracking to occur. Moreover, elevation-induced wetting-drying cycles vary considerably at the highest and seaward edges of a saltmarsh, which modifies the physical characteristics of mud surfaces in different ways. The study sites in Chongming Dongtan wetland still exhibit healed cracks that are a manifestation of previous wetting–drying cycles in the low-lying saltmarsh. Wetting cycles caused by tides do not shift cracks but heal them, thereby leaving scars on the mud surface (Figure 1B). Previous studies have only investigated the mud cracking process and factors influencing the process, such as moisture conditions, confining pressures (Morris et al., 1992; Mitchell, 1993), pore water pressure or suction (Fredlund and Rahardjo, 1993; Yesiller et al., 2000), and meteorological parameters (Gardel et al., 2009); however, ecological roles, such as the trapping of floating propagules during the formation of mud cracks, remain unknown.
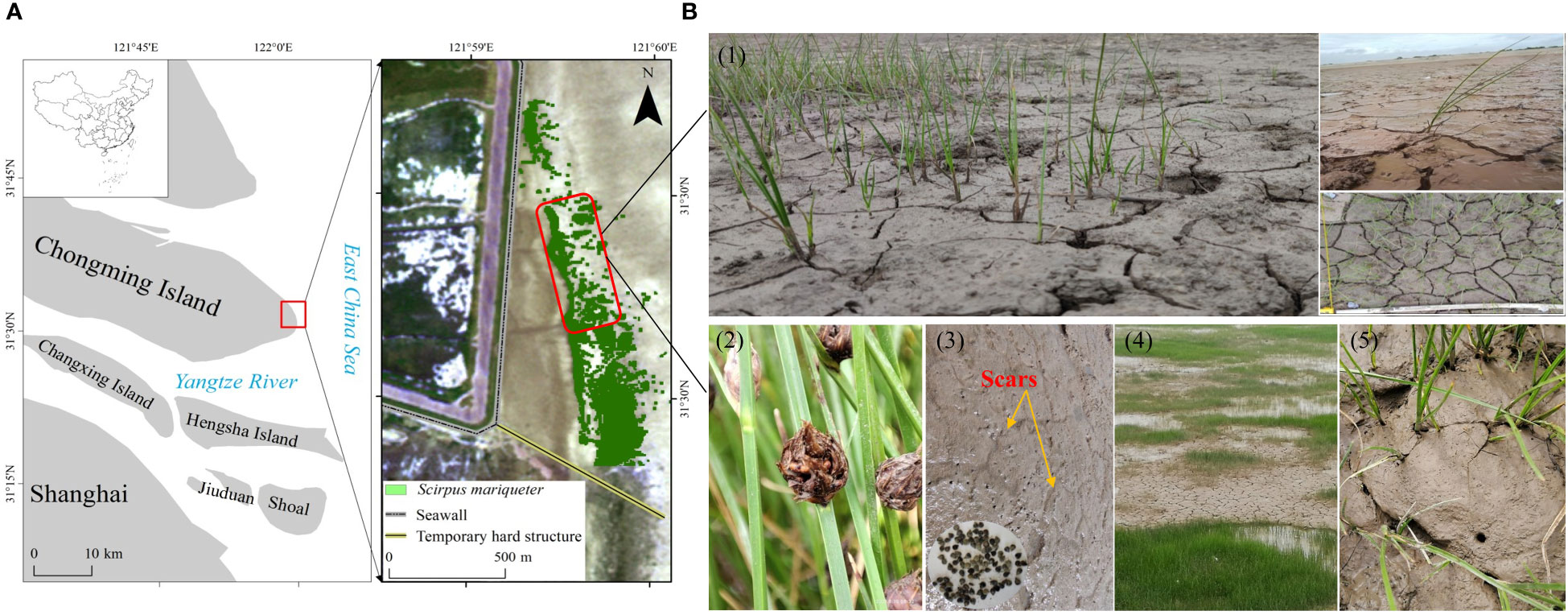
Figure 1 Study area and field site. (A) Location of the Chongming Dongtan wetland and distribution of S. mariqueter. (B1) Extensive formation of mud cracks on mud surfaces at the saltmarsh edge. (B2) Mature seeds and seed retention on mud surfaces and cracks in August 2021. (B3) Healed scars of mud cracks as an indicator of previous wetting–drying cycles on bare mudflats and S. mariqueter seeds trapped in mud cracks. (B4, B5) Seedlings in mud cracks and patches.
Mud cracks, which are affected by wetting–drying cycles induced by tides, are widely distributed in coastal wetlands, especially at saltmarsh edges. Mud cracks have previously been considered water stress indicators that reflect desiccation, which is potentially harmful to plant growth (Clarke and Myerscough, 1993; McKee et al., 2004). However, the conclusion has raised concerns among researchers. Fiot and Gratiot (2006) demonstrated that colonization of mudflats by vegetation was caused by mud dewatering and consolidation, which were dependent on the local topography (Anthony et al., 2008), including tide submersion frequency and wetting–drying cycles on intertidal mudflats. Gardel et al. (2009) also suggested that mud cracks are a key factor influencing mangrove resilience. In addition, vegetation recovery in tidal mudflats adjacent to a saltmarsh edge is essential for revegetation in relation to SLR (Zhu et al., 2020). Extensive mud cracks at the highest and seaward edge affect microtopography, as well as plant colonization and growth. The zones at the saltmarsh edges are substantially influenced by wave-induced erosion dynamics on tidal flats (Leonardi and Fagherazzi, 2014; Bouma et al., 2016; Wang et al., 2017), resulting in cliff erosion or seedling dislodgement. Therefore, the interplay between mud surface microtopography and the colonization of saltmarsh edges by plant seedlings should be investigated (Xie et al., 2019). Current studies on mud cracks have only focused on mud bar colonization and associated processes (Fiot and Gratiot, 2006; Gardel et al., 2009; Proisy et al., 2009). The knowledge deficit regarding links between colonization of saltmarsh fringes by plants and mud cracking in the lateral dimension substantially limits our understanding of marsh resilience to SLR.
To comprehensively elucidate the spatial synchronization of desiccation and propagule availability, there is a need for additional insights into the temporal synchronization of mud crack formation and seed availability. Therefore, the aim of this study was to investigate the mud cracking process and its ecological function in the establishment of saltmarsh plants. Our results revealed that mud cracks in coastal wetlands were not a stressor for plant activity, although they were a vital factor in the dispersal and colonization of saltmarsh fringes by plants. Furthermore, our study contributed to the promotion of vegetation establishment in mud crack zones in future saltmarsh restoration activities.
2 Materials and methods
2.1 Study site and field experiments
The study site was located at the east headland of Chongming Island (31°25′–31°38′N, 121°50′–122°05′E; Figure 1A), which is a part of the Chongming Dongtan National Nature Reserve and the largest alluvial island (total area of 1,267 km2) in the Yangtze Estuary, China (Zhao et al., 2019). The climate is subtropical monsoon, with an annual average temperature of 15.5 and being located on an intertidal mudflat, which is influenced by irregular semidiurnal tides and two maximum and minimum tides (15-day intervals) occurring monthly. The maximum and average tide heights range from 4.62 to 5.95 m and from 1.96 to 3.08 m, respectively (Ge et al., 2008; Li et al., 2014; Jiang et al., 2022). Under normal weather conditions, the mean current velocity in the saltmarsh is approximately 0.1 m s−1, while that in the low-lying mudflats is 0.3 m s−1 (Yang et al., 2020).
Our field investigation began in August 2021. The study area was in a low-lying saltmarsh, where the dominant pioneer plant species, Scirpus mariqueter, has colonized under frequent wetting–drying cycles. Narrow areas of mud cracks were distributed along the seaward edge of the saltmarsh, and extensive mud cracks were observed in the inner saltmarsh, where tidal flat elevation was relatively high (Figure 1). The saltmarsh plants are dispersed in a northwest and seaward direction owing to suitable elevation and hydrodynamics (Jiang et al., 2022). Propagules can be easily washed away at sites exposed to waves due to strong hydrodynamics. However, numerous mature seeds and new seedlings were observed in the mud cracks (Figures 1B1, B3, B5). To compare the differences in seedling densities between mud cracks and patches, 10 sample squares (50 cm × 50 cm) were randomly selected, and parameters such as plant density and the number of mud cracks and patches were recorded.
2.2 Tidal regime and water levels
To determine the effects of the tidal regime on mud cracking and plant establishment, we obtained tidal data between April and August 2021. Tide information was obtained from Sheshan station and tide tables published by the National Marine Data and Information Service (http://www.nmdis.gov.cn).
In this study, low-cloud Sentinel-2 remote sensing images from January to August 2021 were selected. The binary system of Sentinel-2A and Sentinel-2B guarantees a revisit period of five days and a spatial resolution of up to 10 m in the visible near-infrared band. Therefore, the high spatiotemporal resolution overcomes the adverse effects of cloudiness in estuarine and coastal regions to a certain extent and considerably enhances data availability.
The images (level 2A) used in this study, which covered seven periods, were downloaded from the European Space Agency (http://scihub.copernicus.eu/dhus/#/home); that is, the images were subjected to geometric and atmospheric (bottom-of-atmosphere reflectance) corrections. After the initial cropping of images, the water levels at the moment the satellite transited the study area were extracted based on a visual interpretation of each period. Based on the tidal level data obtained from Sheshan station (approximately 20 km east of Chongming Dongtan), the water levels were assumed to be the contour lines, and their elevation was the tidal height at the corresponding time (Fan et al., 2018). Because the multi-period images covered different tidal conditions, the highest and lowest water levels were considered the highest and lowest tide levels, respectively. The area between the highest and lowest tide levels was the potential mud cracking zone affected by wetting–drying cycles from periodic tides.
2.3 Manipulative experiments
2.3.1 Seed collection and experimental setup
Manipulative experiments were conducted to elucidate the phenomenon that mud cracks at the seaward edge promote colonization by S. mariqueter (Figure 2). Mature S. mariqueter seeds were randomly collected, and the mud used for the experiment was dug up in the Chongming Dongtan wetland in August 2021 (Figure 1A). All seeds were air-dried in the laboratory until absolutely dry (Xiao et al., 2016). The mud was transported to the laboratory and transferred into high-density polyethylene containers (65 cm × 41 cm × 15.5 cm, Figure 2). The properties of mud samples collected from the study site are presented in Table 1. Nine repeated treatments were set up, and 4,300 seeds (seed density of approximately 1.6 seeds/cm2) were evenly buried at a depth of 10 cm from the bottom of each container and covered with 3 cm of mud (Figure 2).
To avoid disturbance, all containers were placed at a fixed location until the experiment ended. The experiment was conducted for 1 month (August 2021) at an average daily temperature of 28°C. To simulate the effects of the tidal regime on mud crack formation and plant colonization, immersion–emersion cycles were conducted by draining seawater at 6:00 am and adding seawater at 18:00 pm for the first two days. Afterward, sediment moisture was used to maintain plant survival until day 15, and the procedure was repeated again.
2.3.2 Dynamic monitoring of mud cracks and plant growth
Mud cracking and seedling growth were dynamically monitored using a camera throughout the experimental period. The camera was kept at a fixed height for orthophotography at each monitored time (Figure 2). The number of CDOE, which is the probability of mud cracks occurring at a given elevation (Fiot and Gratiot, 2006), was recorded daily until no new cracks appeared. The number of mud cracks was computed based on new mud crack pixels appearing between two successive images. The crack intensity factor (CIF) was introduced as a descriptor of crack surficial dimensions (Miller et al., 1998; Yesiller et al., 2000; Gardel et al., 2009). CIF is defined as the ratio of crack area (Ac) to the total surface area (At) of a dry soil mass. In this study, CIF was used to quantify the number of mud cracks at the study site. Individual seedlings were also counted after germinating.
2.4 Data analysis
All statistical analyses were performed using IBM SPSS Statistics 23.0 (IBM Corporation, Armonk, NY, USA) and OriginPro 2021 (OriginLab Corporation, Northampton, MA, USA). A one-way ANOVA was used to test for significant differences in seedling densities between mud cracks and patches. The level of statistical significance was set at p <0.05.
Additionally, we monitored and recorded the evolution of mud cracks and seedling germination and growth during the experimental period using a camera. Parameters such as the lengths and areas of mud cracks and individual seedlings were analyzed using ArcGIS 10.7.1 (ESRI Inc., Redlands, CA, USA).
3 Results
3.1 Variations in tidal regimes
Tidal regimes influenced the alternating wetting–drying cycles on the mud surfaces, which potentially affected the distribution of mud cracks and the colonization of tidal flats by saltmarsh plants. The variations in tidal heights between April and August 2021 at Chongming Dongtan wetland were not significant, and the average tidal heights in April and August 2021 were 2.2 ± 0.9 m and 2.47 ± 0.9 m (0.54–3.99 m and 0.59–4.5 m), respectively (Figure 3A). The average interval of daily high tide was 11 h and low tide was 5.2 h. In addition, tidal water levels were computed according to tidal regimes at the Chongming Dongtan wetland from January to August 2021 (Figure 3B). The results showed that the waterline ranged from the seaward edge of the low-lying saltmarsh to the bare mudflats. Furthermore, the tidal occurrence zones, that is, from spring tide (high tide) to neap tide (low tide), coincided with the mud cracking zones in the low-lying saltmarsh, which were considered potential mud cracking zones as well as an ecological zone for plant dispersal and colonization (Figure 3B). The potential mud cracking zone was a dynamic area determined by the tidal flat elevation and tidal regime.
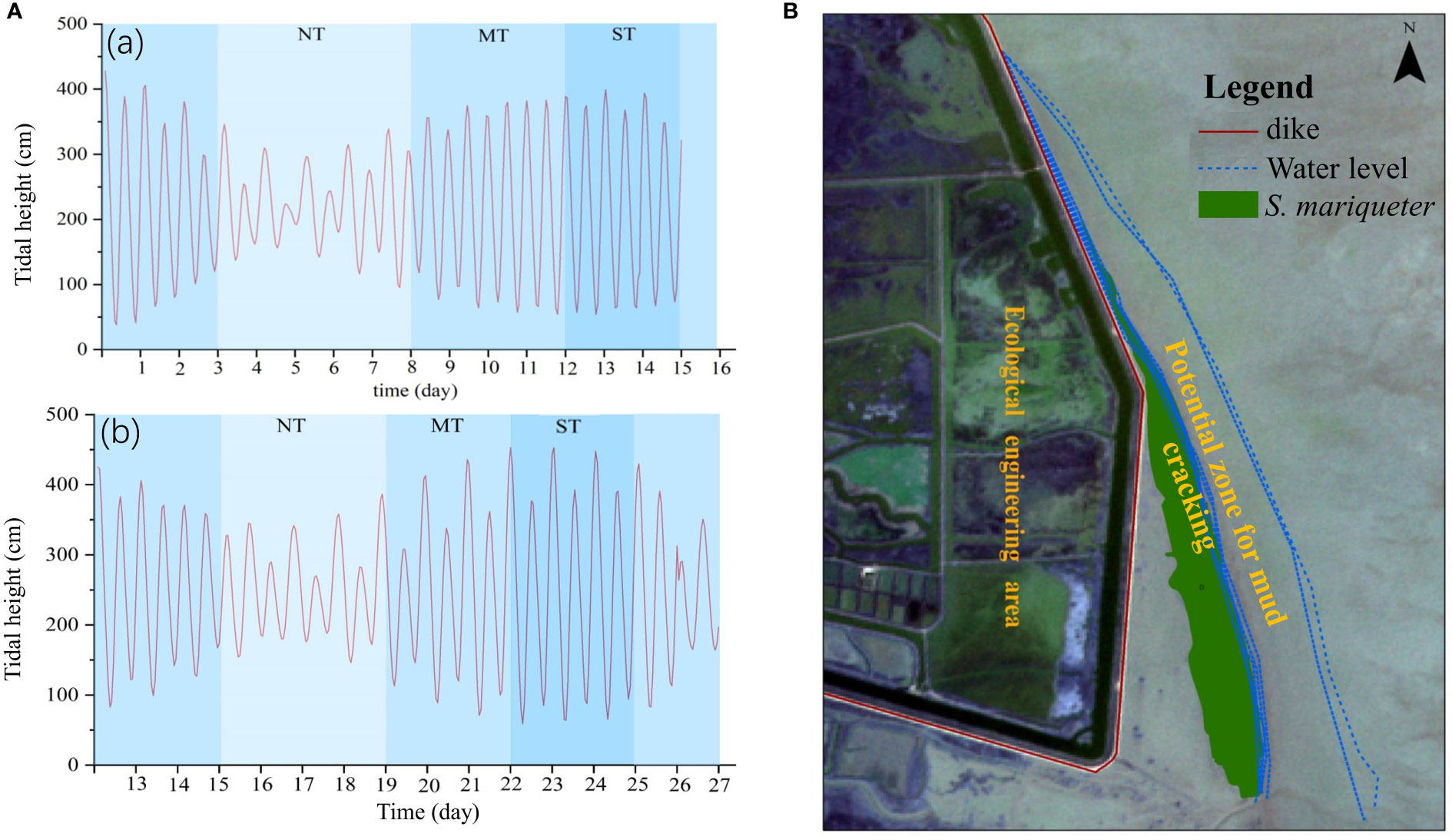
Figure 3 Tidal heights and tidal regimes in the Chongming Dongtan wetland. (A) Variations in tidal heights in April 2021 (a) and August 2021 (b); NT, MT, and ST are neap tide, middle tide, and spring tide, respectively. (B) Variations in tidal regimes from January to August 2021. The zone between water levels is defined as the potential mud cracking zone and a potential ecological restoration zone for plant dispersal and colonization.
3.2 Plant distribution between mud cracks and patches in the study site
According to our findings from the field survey, the mud cracks were irregularly distributed in polygons or lines on the tidal flats, and individual plants disproportionately colonized the mud cracks and patches. The results showed that most individual seedlings in the patches were established near mud cracks (Figure 4A). In addition, the number of seedlings in the mud cracks was significantly higher than that in the patches (p <0.01), and the mean seedling densities in the mud cracks and patches were 291 ± 13.91 individuals/m2 and 196 ± 7.92 individuals/m2, respectively (Figure 4B).
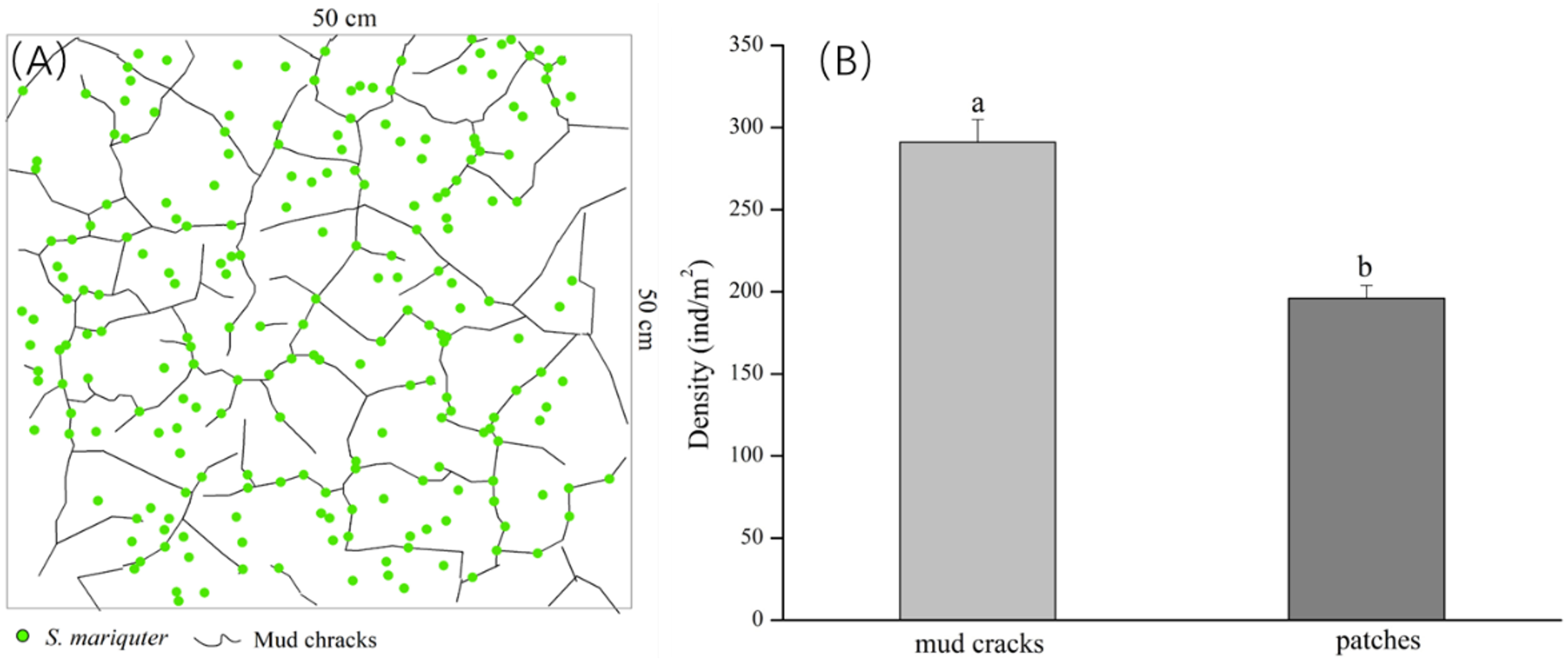
Figure 4 Distribution of S. mariqueter in mud cracks and patches in the study site. (A) An illustration of the distribution patterns of individual plants, and mud cracks: green dots are plants and black lines are mud cracks. (B) Bar graphs showing plant densities in the mud cracks and patches.
3.3 Mud crack formation and colonization by plants under laboratory conditions
3.3.1 Mud crack formation
Figure 5 illustrates the evolution of mud cracks during the experimental period, derived from mud crack images captured by the camera. The results showed that mud crack formation progressed gradually on the mud surface at the beginning of the first wetting–drying cycle and then developed rapidly after three days (Figure 5). Two mud cracks appeared on the mud surface on the second CDOE (Figure 5A), and a new crack appeared on the third CDOE (Figure 5B). The mud cracks were linearly distributed in the containers before new cracks appeared. The number of new mud cracks increased significantly, and the mud cracks gradually transformed into regular polygonal shapes on the fifth CDOE (Figure 5C). The mud cracks completely exhibited polygonal shapes (i.e., quadrilateral) on the seventh CDOE and formed a basic network of mud cracks (Figure 5D). No new mud cracks appeared after the seventh CDOE. Generally, all mud cracks in each container were formed within a period of 5–7 days (CDOE), and their formation decreased after the fifth CDOE, with no significant differences observed in subsequent wetting–drying cycles.
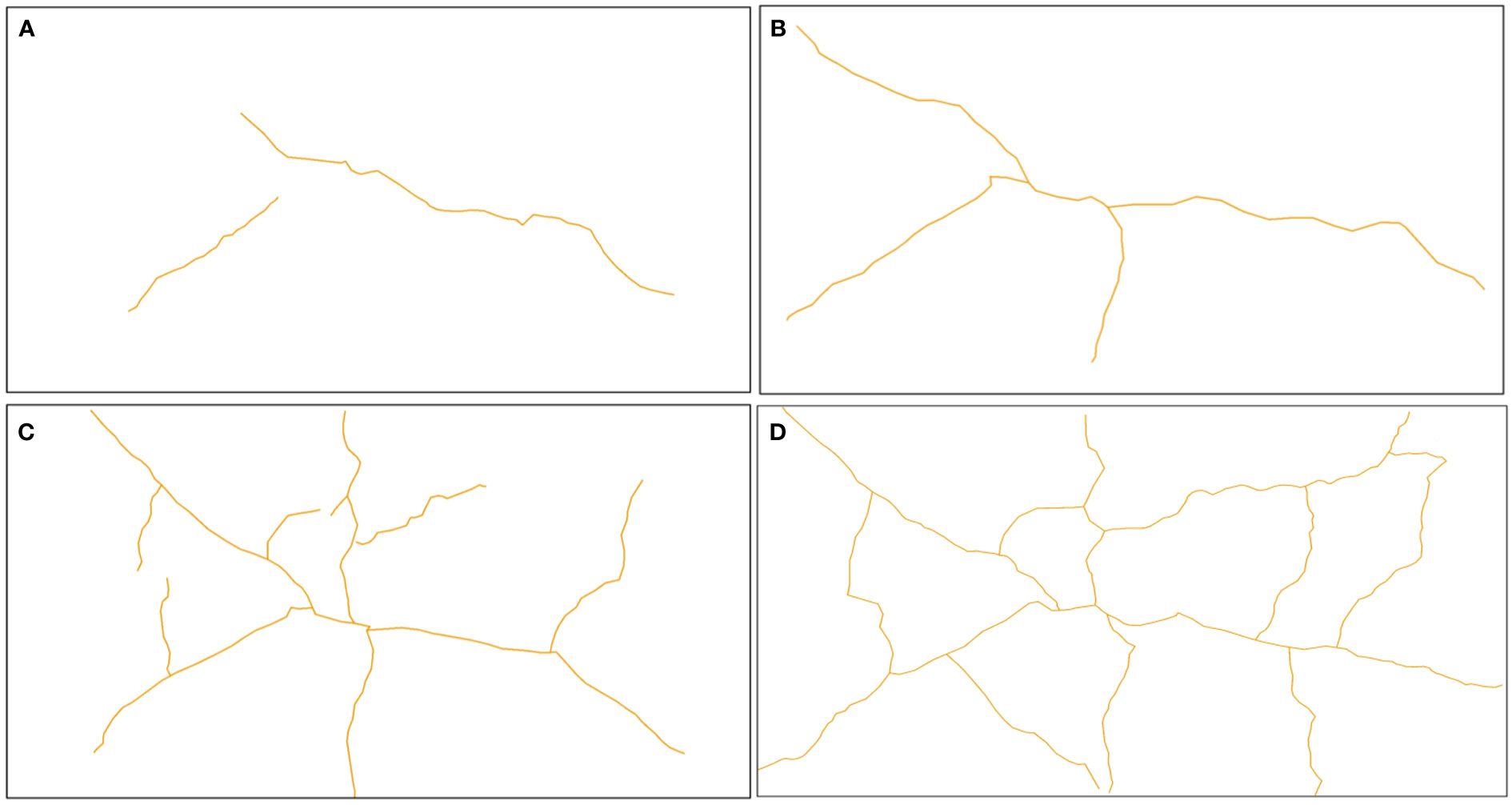
Figure 5 Evolution of mud cracks during the experimental period. (A) Mud cracks at the end of the second CDOE, (B) at the end of the third CDOE, (C) at the end of the fifth CDOE, (D) and at the end of the seventh CDOE. CDOE is consecutive days of emersion.
3.3.2 Plant colonization between mud cracks and patches
3.3.2.1 Plant colonization process
According to our results, seedlings emerged from the mud cracks into the patches (Figure 6), which is consistent with field observations. Seedlings colonized the mud cracks after the seventh CDOE (Figure 6B). Moreover, seedlings in the mud cracks germinated earlier than those in the patches (Figures 6A, B). “Seedling circles” based on the morphology of the mud cracks were left on the mud surface at the end of the experimental period, with the seedlings densely distributed in the mud cracks and sparsely distributed in the patches (Figures 6C, D). The number of individual seedlings in the mud cracks (453 seedlings) was higher than that in the patches (311 seedlings) and accounted for 59.3% of the total seedlings in each container.
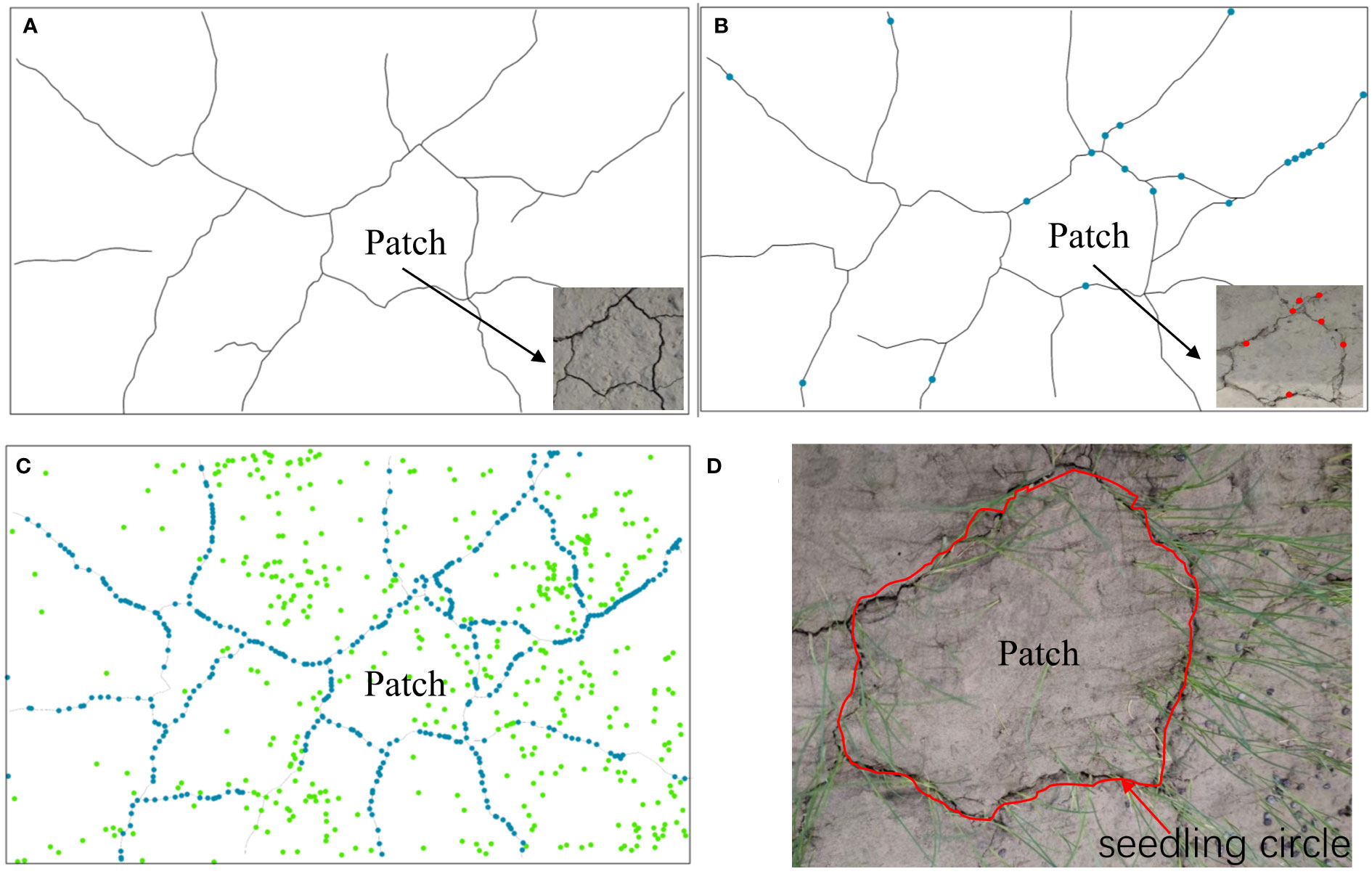
Figure 6 An illustration of the seedling colonization process. (A) Formation of mud cracks and patches. (B) Gradual colonization of mudflats along the mud cracks by individual seedlings. (C) Individual seedlings in mud cracks and patches. Green dots represent seedlings in the patches, and blue dots represent seedlings in the mud cracks. (D) A “seedling circle” formed at the end of the experimental period.
3.3.2.2 Variations in plant densities between mud cracks and patches
The one-month-long experimental results revealed that the average germination rate of S. mariqueter was approximately 23%. Detailed information on seedling properties, such as seedling heights, is presented in Table 2. Mud cracks evolved into irregular polygonal or linear cracks. The average length and width of mud cracks were 2.84 ± 0.31 m and 0.5 ± 0.37 cm, respectively. Generally, 15 ± 3 patches were developed in each container (Figure 7 and Table 2).
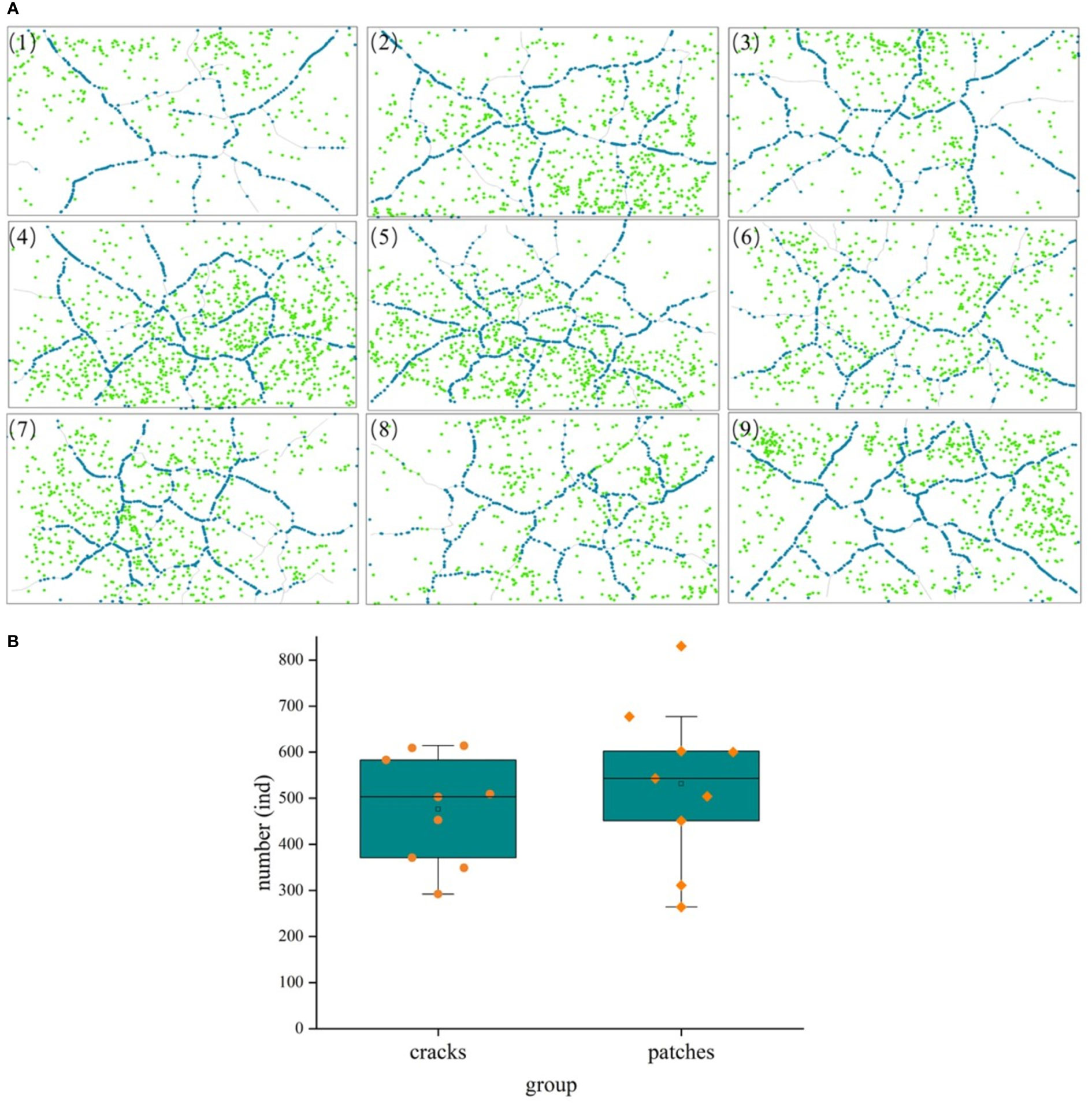
Figure 7 A comparison of seedling distribution in mud cracks and patches during the experimental period. (A) Distribution of mud cracks and seedlings in nine parallel samples (1 - 9), blue dots represent individual seedlings in mud cracks, whereas green dots represent seedlings in patches. (B) A comparison of seedling number in mud cracks and patches.
3.3.2.3 Variations in seedling density per unit area of mud cracks and patches
Individual seedlings in mud cracks and patches were compared per unit area (cm2) to assess the effects of mud cracks on saltmarsh colonization (Figure 8). Our results revealed that the total area of mud cracks was significantly smaller than that of patches; however, the mud cracks had more seedlings per unit area than the patches, which contributed to the successful colonization by the seedlings. For example, the length and area of mud cracks in sample 3 were 276.2 cm and 137.0 cm2, respectively, and the CIF value at the end of the experiment was 5.1%. Similarly, the seedling density in the mud cracks was 3.31 individuals/cm2 and 0.12 individuals/cm2 in the patches. The number of seedlings in the mud cracks accounted for 59.3% of the total seedling count in 5.1% of the area in sample 3 (Figure 8).
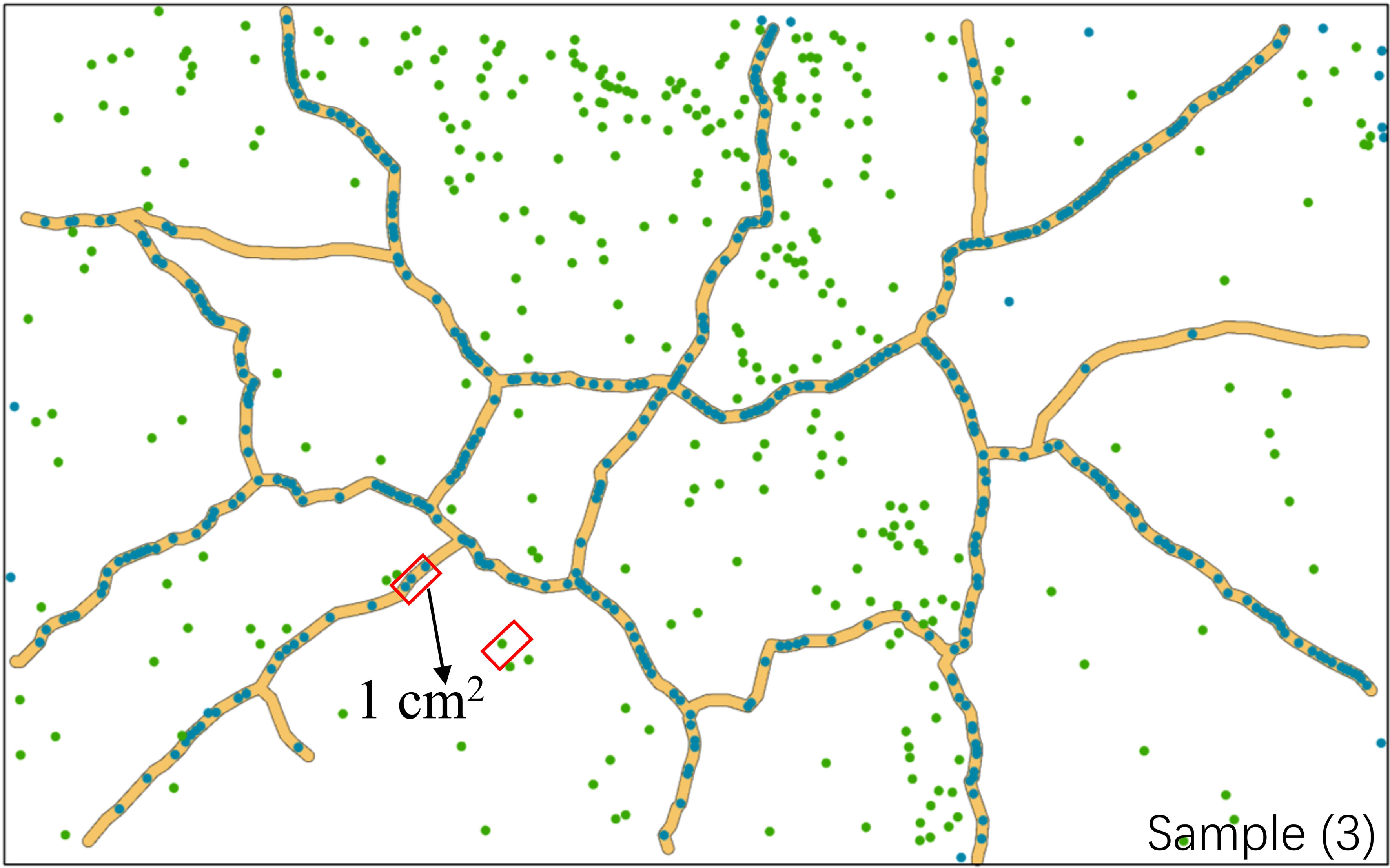
Figure 8 Variations in seedling densities between mud cracks and patches. The area marked in red represents one square unit (1 cm2). The image is from parallel sample 3.
Specific details regarding variations in seedling densities between mud cracks and patches are presented in Table 3. Generally, the number of individual seedlings in mud cracks (3.4 individuals/cm2) was higher than that in patches (0.2 individuals/cm2). The CIF data are presented in Table 3. Although the mean CIF value was 5.3%, it accounted for 48% of the total number of individual seedlings, indicating that mud cracks promote seedling colonization.
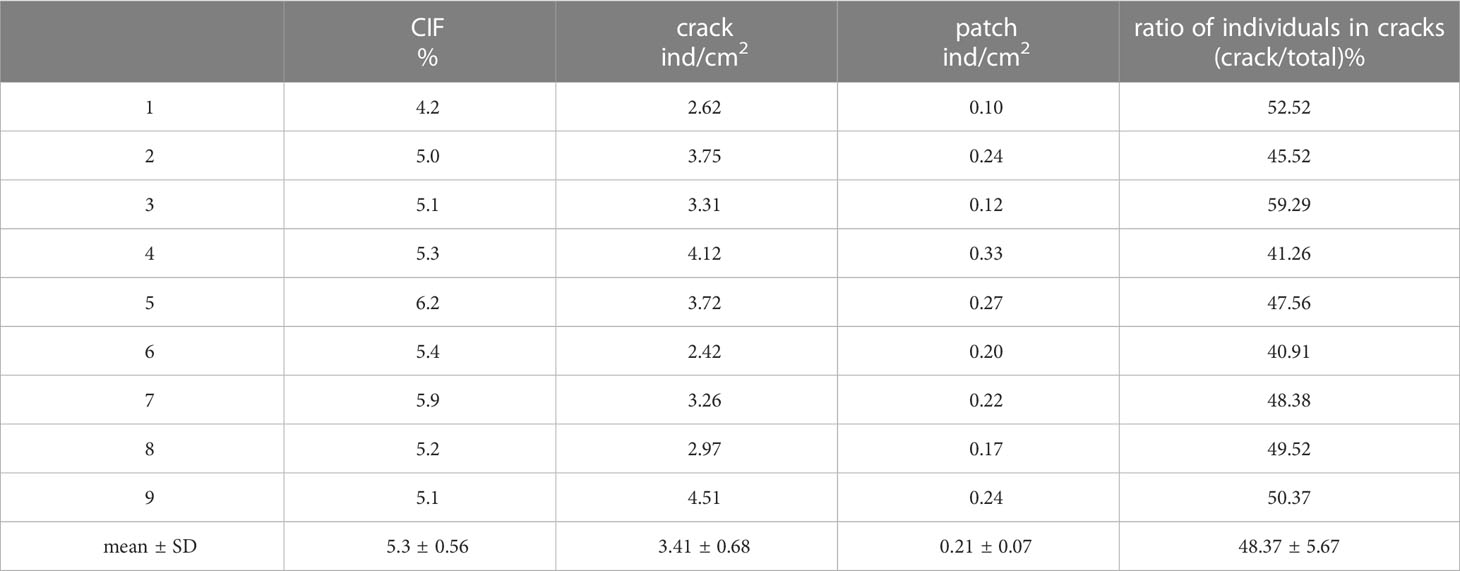
Table 3 Variations in seedling densities between mud cracks and patches in the nine experimental containers.
4 Discussion
4.1 Mud crack formation
The present study contributed to the work of Fiot and Gratiot (2006), which investigated the probabilities of mud cracking and confirmed the vital role of mud cracks in promoting S. mariqueter propagule establishment. According to our results, the mud cracking zones, which are determined by water levels, were mainly located at low-lying saltmarsh edges. Below such zones, the mud remained soft because frequent inundations prevented extensive dewatering. The establishment of propagules in the zones is difficult because they are easily resuspended by tidal currents (Figure 3). Some studies have investigated mud crack occurrence on mudflats, such as at an elevation of 2.67 m above hydrographic zero (Clarke and Myerscough, 1993; Fiot and Gratiot, 2006; Proisy et al., 2009); however, the ecological functions of mud cracks have not been investigated. Therefore, future field restoration efforts should focus on critical zones under elevation–tidal interrelationships to determine the precise areas for mud cracking, such as the mean water level, mean high water level, and mean neap tide high water level on coastal wetlands, to precisely delineate the potential zones for plant colonization.
In the controlled experiment, the mud cracks were well-formed within a short period; mud cracks emerged on the second CDOE and became stable on the seventh CDOE (CDOE was approximately 7 d). This observation was consistent with the results of a previous laboratory experiment on different soils showing a short and intensive mud crack formation episode during drying after a wetting event (Yesiller et al., 2000). Moreover, our finding was consistent with the results of a field experiment conducted during the 2008 equinoctial spring tide in French Guiana, where mud cracks formed within 1.5 days (Gardel et al., 2009). However, the present study only considered the mud cracking process based on CDOE and mud crack morphology, such as crack length and width. We did not consider their depth, as it cannot be estimated from photographs.
Factors influencing tidal flats, such as abiotic and biotic processes, enhance mud cracking. Previous studies have shown that wetting–drying cycles enhance mud crack formation (Yesiller et al., 2000; Gardel et al., 2009). The first drying episode causes shrinkage, which structurally rearranges soil particles and potentially breaks soil particle bonds, thereby weakening the soil (Young, 2012). The subsequent wetting cycle is believed to further weaken and soften the soil and reduce cohesion through water supply. Therefore, a new drying cycle substantially activates the mud cracking process at the weakest part of the soil, where cohesion is low (Gardel et al., 2009). In addition, biological activities (bioturbation by burrowing and root penetration) increase the drainage capacity of soils, and they considerably enhance desiccation and mud crack formation (Gardel et al., 2009). Other processes, such as desiccation and shrinkage, freezing and thawing, and syneresis, also influence the development of mud cracks (Yesiller et al., 2000).
Further studies involving the technical measurement of mud cracks are required. Our study was restricted to small areas and could not be repeated at the same site due to difficulties in accessing such soft, muddy, and flooded environments (Proisy et al., 2009). According to Lefebvre et al. (2004), elevation profiles of several hectometers are the most that can be feasibly achieved during a ground survey. Rosso et al. (2006) and Anthony et al. (2008) demonstrated that emerging remote sensing techniques based on lidar (light detection and ranging) may be beneficial for studying intertidal muddy habitats. Moreover, future studies should focus on enhancing our understanding of the relationship between seasonality and the mud cracking process, as well as the role of neap and spring tides in field consolidation processes. The present study recommends interdisciplinary gap-bridging involving sedimentology, geomorphology, hydrology, saltmarsh ecology, and remote sensing.
4.2 Influence of mud cracking zones on saltmarsh colonization
Our results revealed that mud cracking varied with water level and was often accompanied by the establishment of saltmarsh plants. A previous study has shown that a specific functional range for the growth of the genus Avicennia in French Guiana is between mean water level and mean high water level (Proisy et al., 2009), which is like the intertidal range for Avicennia marina in southeastern Australia (Clarke and Myerscough, 1993). Notably, the extensive mud cracks observed in this study occurred in such intertidal areas. The present study has demonstrated that the outline of mud cracking zones, located at intertidal zones, can act as an indicator for. Therefore, such coastal wetland zones require restoration and more precise and long-term monitoring, considering their key ecological functions. In addition, owing to the disturbances associated with hydrodynamics caused by waves and currents, it is imperative to understand the influence of sediment dynamics on the formation and stabilization of mud cracks and on saltmarsh plant establishment. Quantifying the total area covered by mud cracks in saltmarshes is essential for determining the role of mud cracks in saltmarsh plant establishment.
4.3 Ecological functions of mud cracks in saltmarshes
Saltmarsh colonization and dispersal cannot increase rapidly on a large scale without the influence of a typical and visible event that strongly enhances seed trapping. We speculate that it is not a single biological event but rather a physical event associated with the mud surface, such as extensive mud cracking. The main ecological functions of mud cracks in saltmarsh colonization are as follows:
(1) Mud cracks act as “seed traps.” The number of seeds, floating rate, and germination rate are crucial for saltmarsh plant establishment and dispersal. S. mariqueter establishment and dispersal are fast in summer, with high seed density (8,400 seeds/m2), a high floating rate (up to 80%), and a low germination rate (28%), being observed (Jiang et al., 2022). The high number of seeds produced ensures survival in each reproductive period (Delgado et al., 2001). Furthermore, the open mud cracks acted as cages for floating saltmarsh plant seeds, especially under weak hydrodynamic conditions. The soaked S. mariqueter seeds were washed away in August 2021, transported by tides, and then randomly trapped in mud cracks. Notably, the high germination rate of S. mariqueter seeds (seven days) and two days for anchorage in summer considerably favor seedling establishment (Jiang et al., 2022). This confirmed the rapid and transient process between seed germination and mud cracking (approximately 7 d). Finally, a large amount of suspended sediment carried by weak tides gradually settled and was retained in mud cracks on tidal flats due to the interaction between sediment viscosity and burial, thereby making it more difficult for seeds trapped in mud cracks to be washed away or easily resuspended. The mud cracks filled with fine sediment particles exhibited multiple linear crack scars on the tidal flat surfaces (Figure 1B). The Chongming Dongtan saltmarsh exhibited continuous sedimentation from April to October, and the accumulated accretion exceeded 20 cm (Li and Yang, 2007). Therefore, the geomorphology of tidal flats changed gradually, which facilitated seed burial.
Our study showed that seedling densities in the mud cracks were significantly higher than those in the patches in field observations, which was consistent with laboratory results. The results obtained in the laboratory could be because the seeds were fixed without hydrodynamic transportation and sediment burial. In addition, mud cracks lost their function as seed traps.
(2) Mud cracks acted as “seedling growth promoters.” The network formed by mud cracks influenced water content and water circulation in the tidal sediment. Mud cracks functioned like well-developed miniature tidal creeks on tidal surfaces: at high tide, mud cracks became a channel of seawater and were rapidly filled with seawater, whereas at ebb tide, seawater on tidal flats flowed into the mud cracks and was gradually drained. The phenomenon was vital for saltmarsh plant growth as it provided sufficient water during the early growth stage, especially for young root development. In addition, it alleviated stress caused by waterlogging due to the morphology of low tidal zones, such as pits. For example, the sprouted young seedlings can die due to the exposure of roots to hypoxic conditions caused by prolonged flooding. Therefore, mud cracks can create pathways for seawater transportation on tidal flats and substantially increase the hydraulic conductivity of sediments (Yesiller et al., 2000), which promotes water uptake by plants during the early growth stages. Furthermore, local sediment properties, such as mud compaction and pore water, vary considerably with mud cracking (Fiot and Gratiot, 2006). Mud cracks alter the oxygen content of sediment, increase aeration, and in turn enhanced respiration in saltmarsh plants. Saltmarsh plants consume oxygen and release carbon dioxide during respiration, resulting in a low oxygen content in sediment. Numerous mud cracks in tidal flats allow oxygen to penetrate the sediment from the atmosphere and allow continuous release of carbon dioxide from the sediment into the atmosphere, which in turn enhances the total respiration of saltmarsh plants. The traits of S. mariqueter (plant height, root length, and root diameter) in mud cracks and patches were significantly different (Table 2). Mud cracks increase nutrient availability in tidal flat sediments. Both organic and mineral nutrients in sediments must be decomposed by soil microorganisms before they are absorbed and used by plants. Insufficient oxygen in sediments, or anaerobic status, reduces microbial activity, especially for aerobic microorganisms, and inhibits complete organic matter decomposition and the release of soil nutrients (Marschner, 2021). The occurrence of mud cracks improved the oxygen status of the saltmarsh, thereby increasing microbial activity due to sufficient oxygen, which in turn, decomposed organic matter and released large quantities of soil nutrients, as well as improving the content and utilization of nutrients required for plant growth.
The seedling density in the mud cracks at the study site was significantly higher than that in patches, which was consistent with the results of manipulative experiments (CIF = 5.3%). Mud cracks accounted for approximately 48.37% of the total seed germination in 5.3% of the study area (Figure 8 and Table 3). The high density of seedlings that colonized the mud cracks formed a “seedling circle” or “seedling lines” (Figures 6D, 7), which may protect seedlings in the patches in the field from hydrodynamic disturbances, dislodgement, or uprooting.
A strong correlation has been observed between seedling recruitment and mud cracking (Gardel et al., 2009; Proisy et al., 2009). A previous field study revealed that the trapping of Avicennia germinans propagules in ephemeral mud cracks accounted for 95% of the sprouting on the coastal fringe. Desiccation was identified as a major mechanism of colonization by the species (Fiot and Gratiot, 2006). According to Proisy et al. (2009), nearly instantaneous mangrove germination over tens of hectares was observed in repeated testing at the same sites. Mud cracks may be sighted across colonized areas whenever seedlings emerge. Similarly, patches of S. mariqueter were established simultaneously at the saltmarsh edges within a short period (Jiang et al., 2022), where mud cracking occurred. Nevertheless, the mechanisms associated with the ecological role of mud cracks in saltmarsh colonization remain unclear. Therefore, future studies should focus on aspects such as seed arrival, seed availability, and seed retention rates. In addition, they should focus variation on propagule dispersal between flooding and dewatering periods.
4.4 Implications for saltmarsh restoration
It is likely that a future pioneer saltmarsh restoration could benefit from the undetermined role of mud cracks highlighted in our study. Suitable restoration measures should be adopted based on the different tidal zones or elevations of saltmarshes. For example, by marking patches ready to be colonized in low-lying saltmarshes, mud cracks can be directly targeted as restoration zones, where the chances of successful replanting are the highest. An efficient approach is sowing large quantities of seeds in mud crack zones during the ebb (neap) tide to promote seed germination. Mud cracks in high-lying saltmarshes may not be conducive to high-water-demanding plants due to desiccation induced by high elevation. Therefore, plants with considerable water-tolerance capacities, such as Phragmites australis, could be suitable for such areas.
5 Conclusion
Mud cracks are often considered stressors for plant growth, which may be different in saltmarsh ecosystems. This study demonstrated that mud cracks facilitated colonization by saltmarsh plants. Our key findings were as follows: (1) Mud cracks were easily formed in the narrow zone from the seaward edge of the saltmarsh to the bare mudflats owing to tide-induced wetting–drying cycles, and young seedlings colonized thereafter. (2) The seedling density in the mud cracks was higher than that in the patches; mud cracks were formed within seven days (CDOE: approximately 7 d), and no significant changes were observed in subsequent wetting–drying cycles; seedlings in the mud cracks sprouted earlier than those in the patches. (3) The mud cracks acted as “seed traps” and “seedling growth promoters” for saltmarsh colonization. The results suggest that mud cracks in coastal wetlands can considerably enhance colonization by saltmarsh plants. Our results could facilitate the development of appropriate saltmarsh rehabilitation strategies and enhance our understanding of the interaction between topography and vegetation from the perspective of mud cracks in saltmarshes.
Data availability statement
The original contributions presented in the study are included in the article/supplementary material. Further inquiries can be directed to the corresponding author.
Author contributions
CJ designed the study, analyzed data, and wrote the manuscript. CJ and XL revised the manuscript together. WZ, LX, and LS contributed significantly to data collection and manuscript preparation. All authors contributed to the article and approved the submitted version.
Funding
This work was financially supported by the National Natural Science Foundation of China (Grant Nos. 42176164 and 42141016).
Acknowledgments
The authors would like to thank Bin Yang and Shiwei Lin for helping with experimental setup.
Conflict of interest
The authors declare that the research was conducted in the absence of any commercial or financial relationships that could be construed as a potential conflict of interest.
Publisher’s note
All claims expressed in this article are solely those of the authors and do not necessarily represent those of their affiliated organizations, or those of the publisher, the editors and the reviewers. Any product that may be evaluated in this article, or claim that may be made by its manufacturer, is not guaranteed or endorsed by the publisher.
References
Anthony E. J., Dolique F., Gardel A., Gratiot N., Proisy C., Polidori L. (2008). Nearshore intertidal topography and topographic-forcing mechanisms of an Amazon-derived mud bank in French Guiana. Continental Shelf Res. 28 (6), 813–822. doi: 10.1016/j.csr.2008.01.003
Bouma T. J., De Vries M. B., Herman P. M. J. (2010). Comparing ecosystem engineering efficiency of two plant species with contrasting growth strategies. Ecology 91 (9), 2696–2704. doi: 10.1890/09-0690.1
Bouma T. J., van Belzen J., Balke T., Zhu Z. C., Airoldi L., Blight A. J., et al. (2014). Identifying knowledge gaps hampering application of intertidal habitats in coastal protection: opportunities & steps to take. Coast. Eng. 87, 147–157. doi: 10.1016/j.coastaleng.2013.11.014
Bouma T. J., Van Belzen J., Balke T., Van Dalen J., Klaassen P., Hartog A. M., et al. (2016). Short-term mudflat dynamics drive long-term cyclic salt marsh dynamics. Limnology Oceanography. 61 (6), 2261–2275. doi: 10.1002/lno.10374
Clarke P. J., Myerscough P. J. (1993). The intertidal distribution of the grey mangrove (Avicennia marina) in southeastern Australia: the effects of physical conditions, interspecific competition, and predation on propagule establishment and survival. Aust. J. Ecol. 18 (3), 307–315. doi: 10.1111/j.1442-9993.1993.tb00458.x
Costanza R., d'Arge R., De Groot R., Farber S., Grasso M., Hannon B., et al. (1997). The value of the world’s ecosystem services and natural capital. Nature 387 (6630), 253–260. doi: 10.1038/387253a0
Costanza R., de Groot R., Sutton P., Van der Ploeg S., Anderson S. J., Kubiszewski L., et al. (2014). Changes in the global value of ecosystem services. Global Environ. Change 26, 152–158. doi: 10.1016/j.gloenvcha.2014.04.002
Deegan L. A., Johnson D. S., Warren R. S., Peterson B. J., Fleeger J. W., Fagherazzi S., et al. (2012). Coastal eutrophication as a driver of salt marsh loss. Nature 490 (7420), 388–392. doi: 10.1038/nature11533
Delgado P., Hensel P. F., Jime´nez J. A., Day J. W. (2001). The importance of propagule establishment and physical factors in mangrove distributional patterns in a Costa Rican estuary. Aquat. Bot. 71 (3), 157–178. doi: 10.1016/S0304-3770(01)00188-7
Duarte C. M., Losada I. J., Hendriks I. E., Mazarrasa I., Marbà N. (2013). The role of coastal plant communities for climate change mitigation and adaptation. Nat. Climate Change 3 (11), 961–968. doi: 10.1038/nclimate1970
Fan Y. S., Chen S. L., Zhao B., Pan S. Q., Jiang C., Ji H. Y. (2018). Shoreline dynamics of the active yellow river delta since the implementation of water-sediment regulation scheme: a remote-sensing and statistics-based approach. Estuarine Coast. Shelf Sci. 200, 406–419. doi: 10.1016/j.ecss.2017.11.035
Fiot J., Gratiot N. (2006). Structural effects of tidal exposures on mudflats along the French Guiana coast. Mar. Geology 228 (1-4), 25–37. doi: 10.1016/j.margeo.2005.12.009
Gardel A., Proisy C., Lesourd S., Philippe S., Caillaud J., Gontharet S., et al. (2009). A better understanding of mud cracking processes gained from in situ measurements on an intertidal mudflat in French Guiana. J. Coast. Res. 56, 424–428. doi: 10.2307/25737611
Ge Z. M., Cao H. B., Cui L. F., Zhao B., Zhang L. Q. (2015a). Future vegetation patterns and primary production in the coastal wetlands of East China under sea level rise, sediment reduction, and saltwater intrusion. J. Geophysical Research: Biogeosciences 120 (10), 1923–1940. doi: 10.1002/2015JG003014
Ge Z. M., Wang T. H., Wang K. Y., Wang X. M. (2008). Characteristics of coastal wetland ecosystem of the Yangtze estuary and conservation for key communities (Beijing, China: Science Press), 189.
Han G. X. (2017). Effect of tidal action and drying-wetting cycles on carbon exchange in a salt marsh: progress and prospects. Acta Ecol. Sin. 37 (24), 8170–8178. doi: 10.5846/stxb201611182347
Jiang C., Li X. Z., Xue L. M., Yan Z. Z., Liang X., Chen X. C. (2022). Pioneer salt marsh species Scirpus mariqueter disperses quicker in summer with seed contribution from current and last year. Estuarine Coast. Shelf Sci. 264, 107682. doi: 10.1016/j.ecss.2021.107682
Kelleway J. J., Cavanaugh K., Rogers K., Feller L. C., Ens E., Doughty C., et al. (2017). Review of the ecosystem service implications of mangrove encroachment into salt marshes. Global Change Biol. 23 (10), 3967–3983. doi: 10.1111/gcb.13727
Lefebvre J. P., Dolique F., Gratiot N. (2004). Geomorphic evolution of a coastal mudflat under oceanic influences: an example from the dynamic shoreline of French Guiana. Mar. Geology 208 (2-4), 191–205. doi: 10.1016/j.margeo.2004.04.008
Leonardi N., Fagherazzi S. (2014). How waves shape salt marshes. Geology 42 (10), 887–890. doi: 10.1130/G35751.1
Leonardi N., Ganju N. K., Fagherazzi S. (2016). A linear relationship between wave power and erosion determines saltmarsh resilience to violent storms and hurricanes. Proc. Natl. Acad. Sci. 113 (1), 64–68. doi: 10.1073/pnas.1510095112
Li X. Z., Ren L. J., Liu Y., Craft C., Mander Ü., Yang S. L. (2014). The impact of the change in vegetation structure on the ecological functions of salt marshes: the example of the Yangtze estuary. Regional Environ. Change 14 (2), 623–632. doi: 10.1007/s10113-013-0520-9
Li H., Yang S. L. (2007). A review of influence of saltmarsh vegetation on physical processes in intertidal wetland. Advance Earth Sci. 22 (16), 583–591.
Ma Z., Ysebaert T., Wal D. V. D., Jong D. J. D., Li X. Z., Herman P. M. J. (2014). Long-term salt marsh vertical accretion in a tidal bay with reduced sediment supply. Estuarine Coast. Shelf Science. 146, 14–23. doi: 10.1016/j.ecss.2014.05.001
Marschner P. (2021). Processes in submerged soils–linking redox potential, soil organic matter turnover and plants to nutrient cycling. Plant Soil 464 (1-2), 1–12. doi: 10.1007/s11104-021-05040-6
McKee K. L., Mendelssohn I. A. D., Materne M. (2004). Acute salt marsh dieback in the Mississippi river deltaic plain: a drought-induced phenomenon? Global Ecol. Biogeography 13 (1), 65–73. doi: 10.1111/j.1466-882X.2004.00075.x
Miller C. J., Mi H., Yesiller N. (1998). Experimental analysis of desiccation crack propagation in clay liners. J. Am. Water Resour. Association AWRA 34 (3), 677–686. doi: 10.1111/j.1752-1688.1998.tb00964.x
Möller I., Kudella M., Rupprecht F., Spencer T., Paul M., Wesenbeeck B. K. V., et al. (2014). Wave attenuation over coastal salt marshes under storm surge conditions. Nat. Geosci. 7 (10), 727–731. doi: 10.1038/ngeo2251
Morris P. H., Graham J., Williams D. J. (1992). Cracking in drying soils. Can. Geotechnical J. 29 (2), 263–277. doi: 10.1139/t92-030
Proisy C., Gratiot N., Anthony E. J., Gardel A., Fromard F., Heuret P. (2009). Mud bank colonization by opportunistic mangroves: a case study from French Guiana using lidar data. Continental Shelf Res. 29 (3), 632–641. doi: 10.1016/j.csr.2008.09.017
Rosso P. H., Ustin S. L., Hastings A. (2006). Use of lidar to study changes associated with spartina invasion in San Francisco bay marshes. Remote Sens. Environ. 100 (3), 295–306. doi: 10.1016/j.rse.2005.10.012
Silliman B. R., van de Koppel J., McCoy M. W., Diller J., Kasozi G. N., Earl K., et al. (2012). Degradation and resilience in Louisiana salt marshes after the BP-Deepwater horizon oil spill. Proc. Natl. Acad. Sci. 109 (28), 11234–11239. doi: 10.1073/pnas.1204922109
Temmerman S., Meire P., Bouma T. J., Herman P. M. J., Ysebaert T., Vriend H. J. D. (2013). Ecosystem-based coastal defence in the face of global change. Nature 504 (7478), 79–83. doi: 10.1038/nature12859
Wang H., van der Wal D., Li X. Y., van Belzen J., Herman P. M. J., Hu Z., et al. (2017). Zooming in and out: scale dependence of extrinsic and intrinsic factors affecting salt marsh erosion. J. Geophysical Research: Earth Surface 122 (7), 1455–1470. doi: 10.1002/2016JF004193
Xiao D. R., Zhang C., Zhang L. Q., Zhu Z. C., Tian K., Gao W. (2016). Seed dispersal capacity and post-dispersal fate of the invasive Spartina alterniflora in saltmarshes of the Yangtze Estuary. Estuar Coast Shelf Sci. 169, 158–163. doi: 10.1016/j.ecss.2015.11.032
Xie T., Cui B., Li S., Bai J. (2019). Topography regulates edaphic suitability for seedling establishment associated with tidal elevation in coastal salt marshes. Geoderma 337, 1258–1266. doi: 10.1016/j.geoderma.2018.07.053
Yang S. L., Belkin I. M., Belkina A. I., Zhao Q. Y., Zhu J., Ding P. X. (2003). Delta response to decline in sediment supply from the Yangtze river: evidence of the recent four decades and expectations for the next half-century. Estuarine Coast. Shelf Sci. 57 (4), 689–699. doi: 10.1016/S0272-7714(02)00409-2
Yang S. L., Luo X. X., Temmerman S., Kirwan M., Bouma T. J., Xu K. H., et al. (2020). Role of delta-front erosion in sustaining salt marshes under sea-level rise and fluvial sediment decline. Limnology Oceanography 65 (9), 1990–2009. doi: 10.1002/lno.11432
Yesiller N., Miller C. J., Inci G., Yaldo K. (2000). Desiccation and cracking behavior of three compacted landfill liner soils. Eng. Geology 57 (1-2), 105–121. doi: 10.1016/S0013-7952(00)00022-3
Young R. N. (2012). Soil properties and behaviour (Amsterdam: Elsevier Scientific Publishing Company).
Zhao L. X., Xu C., Ge Z. M., van de Koppel J., Liu Q. X. (2019). The shaping role of self-organization: linking vegetation patterning, plant traits and ecosystem functioning. Proceeding R. Soc. B: Biol. Sci. 286 (1900), 20182859. doi: 10.1098/rspb.2018.2859
Keywords: mud cracks, colonization, tidal regime, saltmarsh, restoration
Citation: Jiang C, Li X, Zhao W, Xue L and Su L (2023) Mud cracks promote colonization by pioneer saltmarsh plants. Front. Mar. Sci. 10:1081353. doi: 10.3389/fmars.2023.1081353
Received: 27 October 2022; Accepted: 04 April 2023;
Published: 25 April 2023.
Edited by:
Zeng Zhou, Hohai University, ChinaReviewed by:
Tian Xie, Beijing Normal University, ChinaZhenchang Zhu, Guangdong University of Technology, China
Steven G. Sandi, Deakin University, Australia
Copyright © 2023 Jiang, Li, Zhao, Xue and Su. This is an open-access article distributed under the terms of the Creative Commons Attribution License (CC BY). The use, distribution or reproduction in other forums is permitted, provided the original author(s) and the copyright owner(s) are credited and that the original publication in this journal is cited, in accordance with accepted academic practice. No use, distribution or reproduction is permitted which does not comply with these terms.
*Correspondence: Xiuzhen Li, eHpsaUBza2xlYy5lY251LmVkdS5jbg==