- 1Co-Innovation Center of Jiangsu Marine Bio-industry Technology, Jiangsu Key Laboratory of Marine Bioresources and Environment, Jiangsu Ocean University, Lianyungang, China
- 2School of Marine Science and Fisheries, Jiangsu Key Laboratory of Marine Biotechnology, Jiangsu Ocean University, Lianyungang, China
Fucoidan and laminarin are polymolecular compounds that extracted from seaweed. As functional polysaccharides, they possess various biological functions such as immunomodulatory, anti-inflammatory, antiviral and antioxidant bioactivities. Fucoidan and laminarin modulate host immune responses by activating immune cells (macrophages, neutrophils, T lymphocytes) and inducing the expression of cytokines (TNFα, IL-1β, IL-6, IL-8, NF-κB). Intelectin is a novel lectin that plays important roles in bacterial agglutination and bactericidal effects by recognizing the polysaccharide components on the cell surface of pathogens, which can also enhance the phagocytosis of macrophages. The present study intends to explore the immunomodulatory effects of fucoidan, laminarin and intelectin on the macrophages of Megalobrama amblycephala. The results showed that the incubation of both fucoidan and laminarin at the concentration of 100 μg/mL for 4 h could enhance the phagocytic capacity of M. amblycephala macrophages, which also showed remodeled cytoskeleton with extended pseudopodia. In addition, fucoidan and laminarin could induce the expression of M. amblycephala intelectin (MaINTL) in the primary hepatocytes and macrophages, and the recombinant MaINTL (rMaINTL) could bind with fucoidan and laminarin. Furthermore, fucoidan and laminarin induced the expression of cytoskeletal remodeling related genes in the primary M. amblycephala macrophages, such as Rac1 and WASP, which should contribute to the enhancement of seaweed polysaccharides (fucoidan and laminarin) and MaINTL on the phagocytosis of M. amblycephala macrophages. However, even though fucoidan and laminarin could induced the expression of CXCL8, while the chemotaxis of M. amblycephala macrophages on neutrophils was not affected by them. These findings suggested that fucoidan and laminarin enhanced the phagocytic capacity of M. amblycephala macrophages via activation of MaINTL and up-regulation of the expression of downstream cytoskeletal remodeling related genes Rac1 and WASP. Therefore, fucoidan and laminarin could be used as immune enhancers to improve host immunity and disease resistance in M. amblycephala, which provided novel insights into the prevention and protection of fish bacterial diseases.
1 Introduction
Seaweed polysaccharides show immunomodulatory, antioxidant, anti-allergic, anti-tumor, anti-viral, anti-inflammatory and hepatoprotective effects in medical researches. Dietary supplementation of seaweed polysaccharides can promote the growth of aquatic animals, improve gut health and antioxidant capacity, and increase their resistance to bacterial and viral infections (Abdel-Latif et al., 2022). Specifically the immunomodulatory effects of seaweed polysaccharides are manifested as activating the proliferation and differentiation of immune cells (such as macrophages, NK cells and lymphocytes), and inducing their secretion of TNFa, IL-1β, IL-6, IL-10, and other cytokines. Fucoidan and laminarin are vital functional seaweed polysaccharides, which has greater potential for application in animal feed and medicine due to their benefits of various biological functions, wide range of sources, environmentally friendly.
Fucoidan are mainly sulphated polysaccharides consisting of fucose and sulfate groups. The structural diversity of lithoglycans is mainly species related. Typically, alpha (1→3) and/or (1→4) glycosidic bonds form the polysaccharide backbone in the presence of sulphate groups at the C-2, C-4 and/or C-3 positions. Biological functions with immunomodulatory, anti-obesity, anti-viral, anti-tumour, anti-diabetic and antioxidant properties (Li et al., 2021). It has obviously regulatory effects on the morphology and functions of immune cells and can induce the expression of cytokines. Studies have shown that fucoidan can promote the adhesion and migration of monocytes (Sapharikas et al., 2015), enhance the chemotaxis of neutrophils (Park et al., 2017), and regulate the morphology and phagocytosis of macrophages (Li et al., 2017). Moreover, fucoidan can regulate host inflammatory response by inducing the expression of inflammatory factors, such as TNFα, IL-6, IL-8, IL-4, IL-10, and GM-CSF (Jin and Yu, 2015; Peng et al., 2019).
Laminarans are water-soluble polysaccharides extracted from brown algae and have a molecular weight of mostly 3 to 10 kDa. The laminarans are polysaccharides built from residues of β-d-glucose, linked 1, 3- and 1, 6-glycosidic bonds according to their chemical properties. However, the ratio of 1, 3:1, 6 in laminarans varies according to species, developmental stage, season, and origin. This has led to differences in the biological functions of laminarans, which are now shown to have immunomodulatory, anti-cancer, anti-inflammatory, anti-bacterial, anti-oxidant and intestinal metabolism promoting functions (Usoltseva et al., 2020; Chen et al., 2021; Li et al., 2021). Similarly, laminarin can also promote the proliferation of mouse macrophages and induce the expression of inflammatory mediators, cytokines and transcription factors (Lee et al., 2012). In addition, laminarin can be absorbed in the small intestine, alleviates lipopolysaccharide (LPS) stimulated cellular inflammation, and increase the expression of tight junction-related proteins, thereby protect and maintain the intestinal barrier structures (Li et al., 2018; Yang et al., 2019). Fucoidan exhibits similar role as an intestinal modulator of improving gut health by regulating gut microbiota (Shang et al., 2016). These results suggest that laminarin and fucoidan are excellent candidates of immune enhancers.
Intelectin (INTL) is a secreted glycoprotein that first discovered in Xenopus lavis. As pathogen recognition molecule, INTL recognizes furan-ring-containing galactose in bacterial cell walls in the presence of Ca2+, thereby agglutinating pathogens and activating nonspecific immune responses (Tsuji et al., 2001; Wesener et al., 2017). Fish intelectins are able to bind with LPS and peptidoglycan (PGN), while the binding ability varies among different species (Yan et al., 2013). In addition, pathogenic microbial infection can generally induce the expression level of INTL, and INTL in turn recognizes bacterial polysaccharide components and then agglutinates pathogenic microorganisms (Nagata, 2018). Our previous studies also have found that Aeromonas hydrophila infection up-regulates the expression of Megalobrama amblycephala intelectin (MaINTL), and recombinant MaINTL protein can not only bind and agglutinate A. hydrophila, but also enhance the phagocytosis, bactericidal effect and chemotaxis of M. amblycephala macrophages (Ding et al., 2017; Ding et al., 2019; Zheng et al., 2021). Therefore, MaINTL plays immunomodulatory effects in multiple processes of immune response upon bacterial infection and is an excellent immune enhancer.
Macrophage is one of the most important immune cells that possess excellent phagocytosis and killing abilities (Hanington et al., 2009). Pattern recognition receptors (PRRs) are receptors on the surface of macrophages that directly recognize pathogen-associated molecular patterns (PAMPs), which can initiate phagocytosis by activating related signaling pathways to remodel macrophage cytoskeleton, then finally phagocytize and digest pathogens (Uribe-Querol and Rosales, 2020; Mylvaganam et al., 2021). More efficient phagocytosis of mouse macrophages is found when the medium containing mouse intelectin-1 protein, suggesting its contribution to the phagocytosis and clearance of microorganisms (Tsuji et al., 2009). The phagocytosis of M. amblycephala intelectin treated A. hydrophila by macrophages was also significantly enhanced (Ding et al., 2019). These studies suggest that intelectin has an efficiently regulatory effect on phagocytosis of macrophages.
Multiple mechanisms exist for activated macrophages to contribute to the inflammatory response. For instance, producing inflammatory mediators like IL-1, TNFα, IL-6 and ROS, or secreting chemokines like MCP-1, MIP-2 and CXCL8, which activate and recruit effector cells like neutrophils (Malik et al., 2010). Due to their high sensitivity to stimuli, huge numbers of neutrophils can be recruited within minutes to the sites of injury or inflammation. Chemotaxis will asymmetrically redistribute intracellular proteins and lipids, affecting the cellular morphology of neutrophils and causing polarization. The cell membrane folds where cytoskeletal elements like actin assemble create an anterior pole that migrates along a gradient of chemoattractant concentrations and a posterior pole that continuously retracts pseudopodia (Petri and Sanz, 2018). Chemokines secreted by macrophages can act as chemotactic agents for neutrophils and induce the chemotaxis of neutrophils. Studies have shown that virus-infected macrophages promote the chemotaxis of neutrophils by releasing Mip2/Cxcl2 (Mohanty et al., 2010).
Due to the deterioration of the aquaculture environment and the degradation of germplasm resources, the disease resistance of the M. amblycephala has gradually declined and the incidence rate has continued to increase. Among them, bacterial sepsis caused by A. hydrophila infection is the most serious, and has become a restriction to the aquaculture of M. amblycephala. Long-term use of antibiotics will not only cause drug resistance in cultured fish, but also affect the quality of aquatic products and cause food safety problems. In view of this, the development of efficient vaccines and immune enhancers with biosafety advantages has become a research hotspot and developmental trend for disease control in aquaculture. Seaweed polysaccharides have been used in food and pharmaceutical applications due to their wide source, sustainability and diverse biological functions. In aquaculture, seaweed polysaccharides have been added to diets as dietary supplements for their excellent immunomodulatory functions and have effectively promoted the growth, intestinal health and disease resistance of aquatic organisms, but their molecular mechanisms are still unclear. Therefore, it is important to study the immunomodulatory effects of seaweed polysaccharides on aquatic organisms at the molecular level. To explore the immunomodulatory effects of fucoidan and laminarin as immune enhancers on the macrophages and neutrophils of M. amblycephala, which lays a theoretical foundation for the development of environmentally friendly immunological agents, promoting the healthy and sustainable development of M. amblycephala aquaculture.
2 Materials and methods
2.1 Ethics statement
This study was approved by the Animal Care and Use Committee of Jiangsu Ocean University (protocol no. 2020-37; approval date: September 1, 2019). All procedures involving animals were performed in accordance with guidelines for the Care and Use of Laboratory Animals in China.
2.2 Experimental reagents
Fucoidan was purchased from Macklin Inc (Shanghai, China) and is sourced from brown algae with a purity of 98% and a molecular weight of 242.24 kDa. Laminarin was purchased from Xagelinbio Co., Ltd (Xi’an, China), which was derived from Laminaria japonica with 98% purity and a molecular weight of 504.4 kDa. RAC1 inhibitor (NSC 23766 trihydrochloride) was purchased from MedChem Express (Monmouth Junction, NJ, USA). WASP inhibitor (ab141085) was purchased from Abcam (Cambridge, MA, USA).
2.3 Preparation of the rMaINTL protein
The pET-32a-MaINTL protein was prepared as previously described (Zheng et al., 2021). Briefly, the encoding region of the MaINTL gene was amplified and inserted into the pre-digested pET-32a vector. The recombinant plasmids were transferred into BL21 competent cells (TransGen Biotech, Beijing, China), and induced by isopropyl-β-D-thiogalactopyranoside (IPTG) with a final concentration of 0.05 mM at 37°C for 6 h. Then, the rMaINTL protein were purified using His-tag Protein Purification Kit (Cowin Bio, Beijing, China) following the manufacturer’s instructions, and all procedures were performed at 4°C.
2.4 Isolation and treatment of macrophages
Isolation and purification of M. amblycephala primary macrophages as previously described (Ding et al., 2019). Briefly, 3-aminobenzoic acid ethyl ester methanesulfonate (MS-222, 100 mg/L) was used to anesthetize healthy adult M. amblycephala, and the head kidney was removed to prepare cell suspension. Then, 3.6 mL 45% percoll and 4 mL 31% percoll was added into a 10 mL centrifuge tube, respectively. The prepared cell suspension was carefully plated on the top of 31% percoll and centrifuged at 400 g for 30 min at 4°C. Then, the monocyte/macrophages cell layer between two layers of percoll were isolated. A small amount of residual red blood cells in the isolated monocyte/macrophages were lysed with red blood cell lysis buffer. After washing with PBS (phosphate buffered saline), the monocyte/macrophages were re-suspended using complete medium (L-15 medium with 5% FBS, 1% penicillin-streptomycin-gentamicin sulfate) and cultured at 28°C.
The well-grown macrophages were divided into three groups: the control group (treated with PBS), the fucoidan (100 μg/mL) treated group, the laminarin (100 μg/mL) treatment group, then incubated for 4 h at 28°C. After treatment, the total RNA and total proteins of macrophages from 3 groups were prepared to detect the expression of MaINTL and macrophage-derived cytokines.
2.5 Isolation and treatment of hepatocytes
The isolation and purification of the primary M. amblycephala hepatocytes was performed as previously described with some modifications (Berry et al., 1997). MS-222 was used to anesthetize healthy adult M. amblycephala, and the hepatopancreas was removed and cut into 1 mm3 pieces, then placed in sterilization solution (L-15 medium with 2% penicillin-streptomycin-gentamicin sulfate) for 20 min. The tissue was digested with 0.125% trypsin for 10 min and terminated with solution of L-15 medium with 10% FBS. The cell suspension was collected by centrifugation at 100 g for 3 min, and washed twice with PBS, then incubated in a 28°C cell incubator with complete medium (L-15 medium with 5% FBS, 1% penicillin-streptomycin-gentamicin sulfate). After adherence, the hepatocytes were divided into five groups, including the control group, fucoidan-50 (50 μg/mL), fucoidan-100 (100 μg/mL), laminarin-50 (50 μg/mL), and laminarin-100 (100 μg/mL) treated groups, with three replicates per group. The cells were treated with seaweed polysaccharides for 4 h and then the RNA and proteins were extracted and stored at -20°C.
2.6 cDNA preparation
Total RNA of macrophages and hepatocytes was extracted using the RNA Easy Fast Tissue Kit (TIANGEN, Beijing, China) according to the manufacturer’s instructions. The quality and concentration of total RNA were determined by agarose gel electrophoresis and NanoDrop 2000 (Thermo Fisher Scientific, Wilmington, DE, USA). In addition, the cDNA was synthesized using the PrimeScript® RT reagent Kit with gDNA Eraser (TaKaRa, Dalian, China) following the manufacturer’s protocol and stored at -20°C for real-time quantitative reverse transcription polymerase chain reaction (RT-qPCR) assay.
2.7 RT-qPCR analysis
The expression patterns of target genes were analyzed using RT-qPCR assay as previously reported (Zheng et al., 2021). Briefly, RT-qPCR was performed on an ABI StepOne Plus real-time PCR system (PerkinElmer Applied Biosystems, CA, USA) using QuantiNova™ SYBR® Green PCR Kit (TaKaRa) according to the manufacturer’s protocol. Relative expression levels of target genes were measured in terms of threshold cycle value (Ct) by the 2-ΔΔCt method (Livak and Schmittgen, 2001), and GAPDH (Glyceraldehyde-3-phosphate dehydrogenase) was selected as the internal reference (Ding et al., 2022). All reactions were performed in triplicate and the primers were listed in Table 1. Gene expression levels in the control group were set as 1, and the relative expression levels of experimental groups were presented as fold-change.
2.8 Western blotting
Western blotting assay was performed as previously described (Cui et al., 2022). Protein was separated on a 4-20% SDS-PAGE gel and transferred onto the polyvinylidene difluoride (PVDF) membrane using a Trans-Blot apparatus (BioRad, California, USA) for 2 h at 80 V. Non-specific reactivity was blocked with 5% (w/v) skim milk powder in TBS (tris buffered saline; 150 mM NaCl, 20 mM Tris-base, pH 7.4) for 1 h at 30°C. The PVDF membrane was incubated with anti-MaINTL polyclonal antibody (1: 3000) overnight at 4°C, then washed 3 times, and incubated with HRP-conjugated goat anti-rabbit IgG (Beyotime, Shanghai, China; 1: 2000 dilution) for 1 h at 30°C. Finally, the DAB Horseradish Peroxidase Color Development Kit (Beyotime) was used to detect the immunoreactive bands.
2.9 The quantitative binding of MaINTL with fucoidan and laminarin
The binding activity of MaINTL with fucoidan or laminarin was quantitatively measured following the previous description with some modifications (Yan et al., 2012). The 96-well high absorption capacity polystyrene microtiter plates were coated with 100 μg/mL of fucoidan or laminarin per well in 200 μL TBS, and an equal amount of TBS alone as negative controls. After incubation at 4°C overnight, the coated wells were washed with TBST solution (0.05% Tween-20 in TBS) and then blocked with 200 μL of blocking solution (5% (w/v) BSA in TBST) per well. The purified MaINTL protein (50 μg/mL) that diluted in blocking solution was added to the wells and incubated at 37°C for 2 h. The bound MaINTL was visualized with anti-MaINTL antibody (1: 1000 dilution) at 37°C for 1 h, then the plates were incubated with 100 μL peroxidase-conjugated goat anti-rabbit IgG (1: 2000 dilution) at 37°C for 1 h. The absorbance at OD450 (nm) was determined after adding DAB chromogenic solution, and the experiments were performed in triplicate.
2.10 Effects of fucoidan and laminarin on the phagocytosis of macrophages
A. hydrophila was cultured in LB medium at 37°C to the exponential growth phase, which was centrifuged and washed with PBS. Subsequently, A. hydrophila were heat inactivated at 70°C for 1 h and fluorescently labeled with FITC (Solarbio, Beijing, China) at 30°C for 1 h on a horizontal shaker. Finally, A. hydrophila was washed with PBS for 2 times and diluted to a concentration of 1×108 CFU/mL.
The phagocytic assay was performed as previously described (Ding et al., 2019). The primary M. amblycephala macrophages seeded onto an 24-well flat-bottomed microtiter plate (1 × 106 cells per well) were divided into 4 groups: the control group (DMSO), seaweed polysaccharide (100 μg/mL) treated group, seaweed polysaccharide (100 μg/mL) and RAC1 inhibitor (40 μM) treated group, seaweed polysaccharide (100 μg/mL) and WASP inhibitor (2.5 μM) treated group, which were treated at 28°C for 2 h, respectively. Then, the heat-killed FITC-labeled A. hydrophila was added into each well and phagocytized for 30 min. Then, all groups were washed for 3 times with pre-cooling PBS to remove the non-binding and non-internalized FITC-A. hydrophila. Subsequently, trypan blue was used to quench the fluorescence from the remaining non-internalized FITC-A. hydrophila (Loike and Silverstein, 1983; Lu et al., 2017). Thereafter, all samples were fixed with 4% paraformaldehyde for 10 min, stained with DAPI (Thermo Fisher Scientific) for 2 min, and washed with PBS for 3 times, then imaged using a fluorescence microscope (Olympus, Tokyo, Japan).
2.11 Regulation of fucoidan and laminarin on the cytoskeleton of macrophages
The primary M. amblycephala macrophages seeded onto an 24-well plate (1 × 106 cells per well) were incubated with PBS (control group), 100 μg/mL fucoidan (fucoidan-treated group) and 100 μg/mL laminarin (laminarin-treated group) at 28°C for 2 h, respectively. After washing with PBS, the cells were fixed with 4% paraformaldehyde (Beyotime) and permeabilized with 0.1% TritonX-100 (Beyotime) at 30°C for 10 min, respectively. Then, Actin-Tracker Green-488 (Beyotime) was stained for 40 min at 30°C in the dark, followed by staining with DAPI (Thermo Fisher Scientific) for 2 min. Finally, the cytoskeleton of macrophages were observed using a fluorescence microscope (Olympus, Tokyo, Japan).
2.12 Effects of fucoidan and laminarin on the recruitment of neutrophils by macrophages
The primary M. amblycephala neutrophils were prepared by dextran (Macklin, Shanghai, China) sedimentation and Ficoll (Solarbio, Beijing, China) density gradient centrifugation as previously described with some modifications (Chu et al., 2014). Briefly, 2 mL fresh blood was diluted with the same volume of PBS and immediately added to 5 mL 3% dextran solution, then mixed and settled at 30°C for 30-60 min, and waited for erythrocytes sedimentation. Then, 1 mL leukocyte-rich supernatant was isolated and gently added to the top of 3 mL Ficoll. The neutrophils were separated from the Ficoll solution after centrifugation, and the residual erythrocytes were lysed using red blood cell lysis buffer (Biomass, Beijing, China). The purified neutrophils were washed with PBS and elution medium (RPMI1640 medium with 2% FBS, 1% penicillin-streptomycin-gentamicin sulfate). Finally, the neutrophils were re-suspended using complete medium (RPMI1640 plus 5% FBS, 1% penicillin-streptomycin), and cultured at 28°C with 5% CO2.
The macrophages in the 24-well plates were divided into three groups, and were incubated with PBS (control group), 100 μg/mL fucoidan (fucoidan-treated group) and 100 μg/mL laminarin (laminarin-treated group) at 28°C for 4 h, respectively. the culture medium of macrophages was separately collected and the supernatant was prepared by centrifuging at 1000 g for 10 min. The chemotaxis assay of neutrophils was performed in triplicate using 24-well transwell chambers (Jet Bio-Filtration, Guangzhou, China). Then, 600 μL culture medium supernatant of macrophages was added in the lower chamber, and 100 μL neutrophils were plated in the upper chamber and incubated at 28°C for 4 h, of which migrated into the lower chamber were counted using light microscopy (Nikon, Tokyo, Japan).
2.13 Statistical analysis
In the present study, data were presented as mean ± SE. The statistical significance was assessed by one-way analysis of variance (ANOVA), and multiple comparison was performed using the Turkey method of SPSS (version 25.0, Chicago, USA). The P-value of 0.05 was considered as statistically significant difference.
3 Results
3.1 Fucoidan and laminarin enhanced the phagocytosis of M. amblycephala macrophages
In order to assess the regulatory effects of fucoidan and laminarin on the phagocytosis of primary M. amblycephala macrophages, the phagocytic assay was performed. As shown in Figure 1, A. hydrophila was labeled with FITC in green, and the nuclei of macrophages was stained with DAPI in blue. The experimental results showed that increased amount of A. hydrophila phagocytized by macrophages after treated with fucoidan (100 μg/mL) and laminarin (100 μg/mL), which indicating the immunopotentiation of these seaweed polysaccharides on M. amblycephala macrophages.
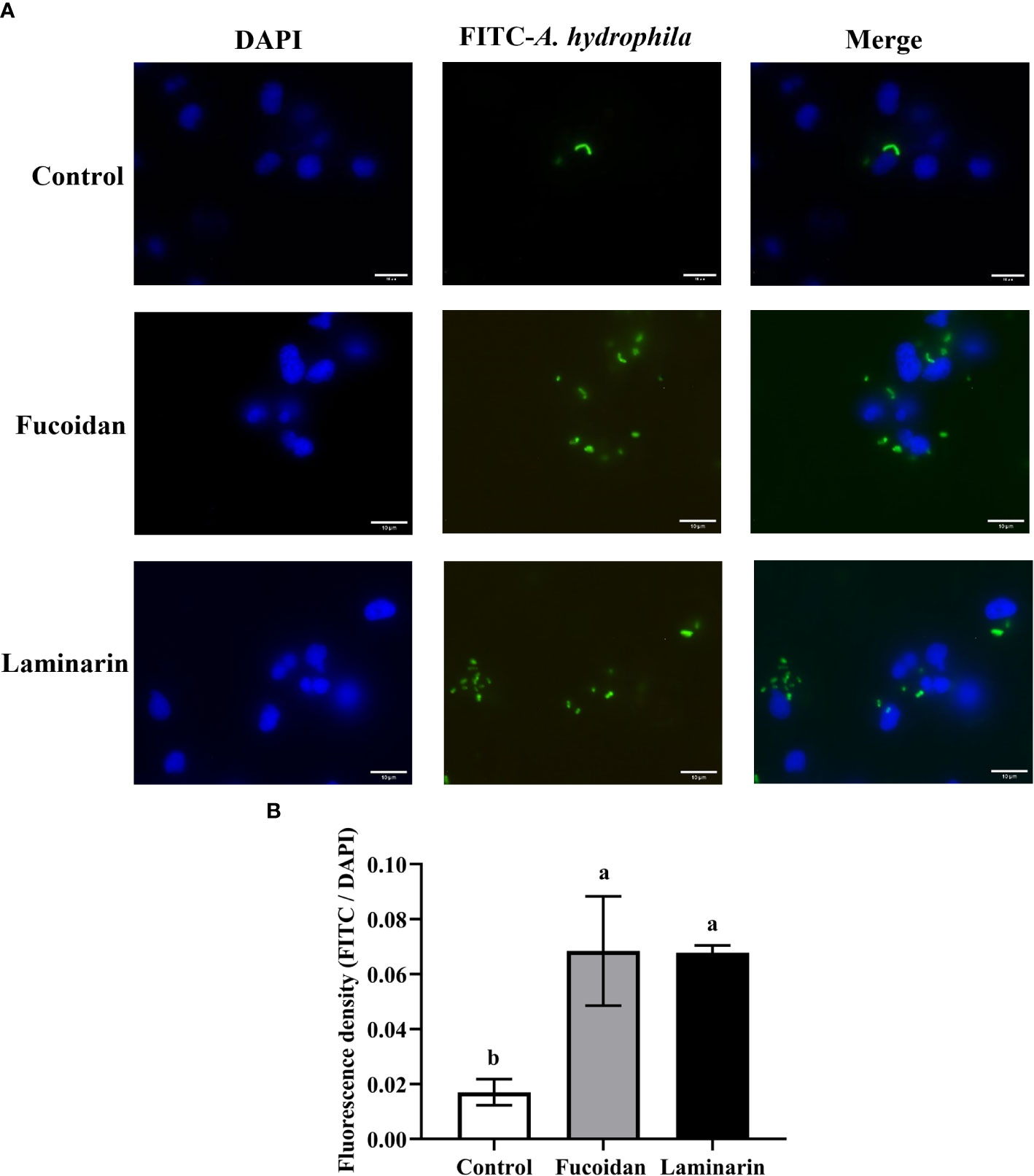
Figure 1 Fucoidan and laminarin increase the phagocytic capacity of macrophages against FITC-labeled A. hydrophilus. (A) For visual analysis, cells were fixed with 4% paraformaldehyde for 10 min and the nuclei were stained with DAPI, then imaged using a fluorescence microscope. The 3 columns of images represented the nuclei of macrophage stained with DAPI (blue), phagocytized FITC-labeled A. hydrophila (green), and the merged images, respectively. (B) Quantification of seaweed polysaccharides-induced phagocytosis, different alphabets indicate significant differences (P > 0.05). Scale bars = 10 μm.
3.2 Fucoidan and laminarin regulated the cytoskeleton of M. amblycephala macrophages
As the essence of phagocytosis is the remodeling of the cytoskeleton, thus the effects of seaweed polysaccharides (fucoidan and laminarin) on the cytoskeleton of macrophages was analyzed by fluorescent staining. Specifically, the cytoskeleton of M. amblycephala macrophages was stained with Actin-Tracker Green-488 in green, and the nuclei was stained with DAPI in blue. As shown in Figure 2, compared with the regular cytoskeletal structures of macrophages in the control group, the seaweed polysaccharides (fucoidan and laminarin) treated groups showed affected cytoskeleton, exhibiting extended pseudopodia and the deformation of macrophages. Moreover, laminarin showed more significant effects on the cytoskeletal structures of macrophage than that of fucoidan.
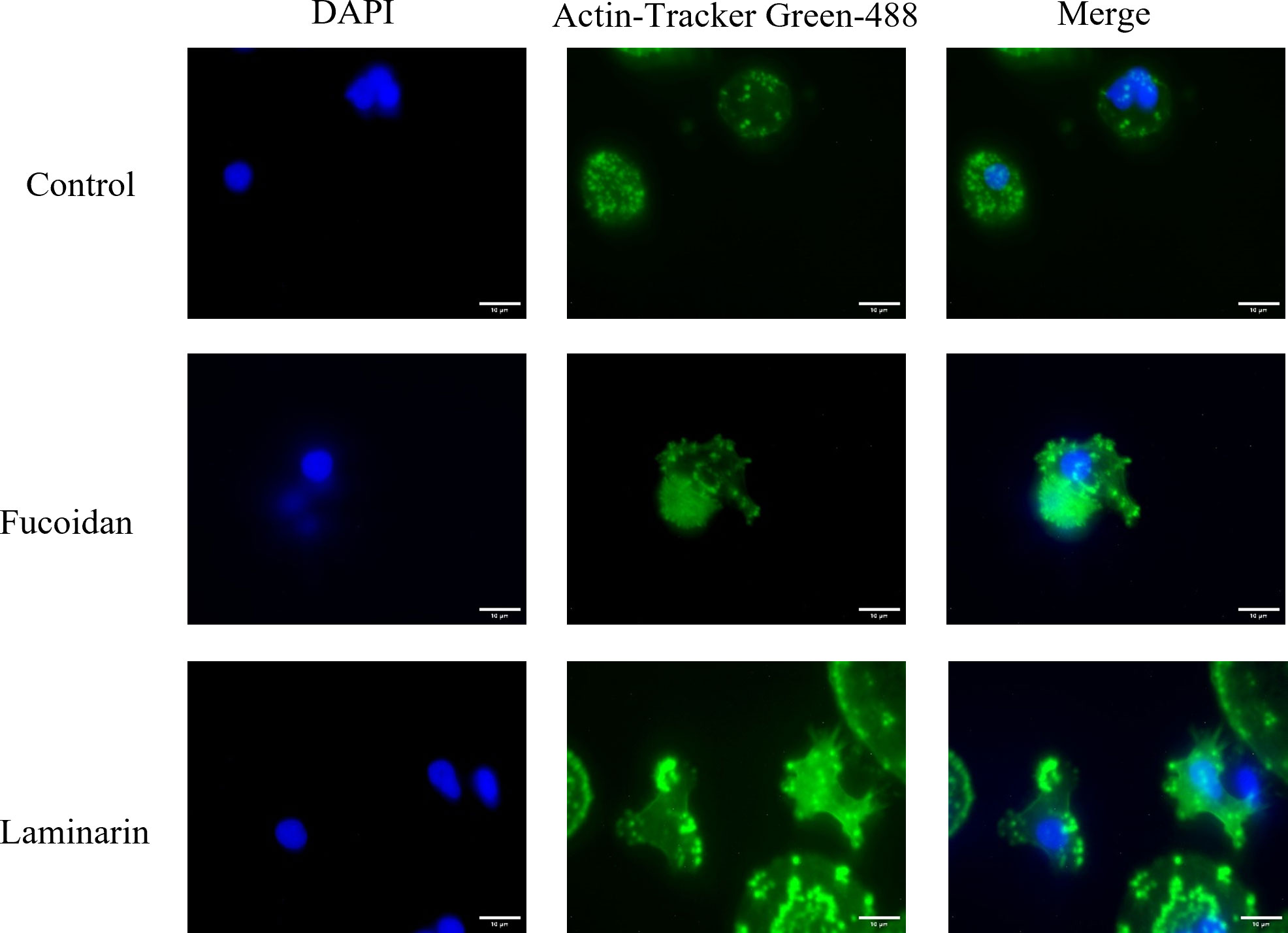
Figure 2 Fucoidan and laminarin induce skeletal remodeling of macrophages. The nuclei and skeleton of macrophages were stained with DAPI and Actin-Tracker Green-488, respectively, and then recorded under a fluorescent microscope. Image J was used to merge two fluorescent photographs with the same field of view to show the overall cell morphology. Scale bars = 10 μm.
3.3 Fucoidan and laminarin induced MaINTL expression in macrophages and hepatocytes
To evaluate the effects of seaweed polysaccharides on the expression of MaINTL, western blot and RT-qPCR assays were used to determine MaINTL expression patterns after fucoidan and laminarin treatment. As shown in Figures 3A, B, relative low expression levels of MaINTL were found in the control hepatocytes, the mainly synthetic and secretory cells of MaINTL, which were significantly up-regulated after treated with fucoidan and laminarin, indicating that seaweed polysaccharides induced the expression of MaINTL. Similar expression patterns of MaINTL were also found in macrophages, and the expression of MaINTL were also significantly up-regulated after fucoidan and laminarin treatment (Figures 3C, D). These results suggested that fucoidan and laminarin can increase the expression of MaINTL both in the M. amblycephala macrophages and hepatocytes.
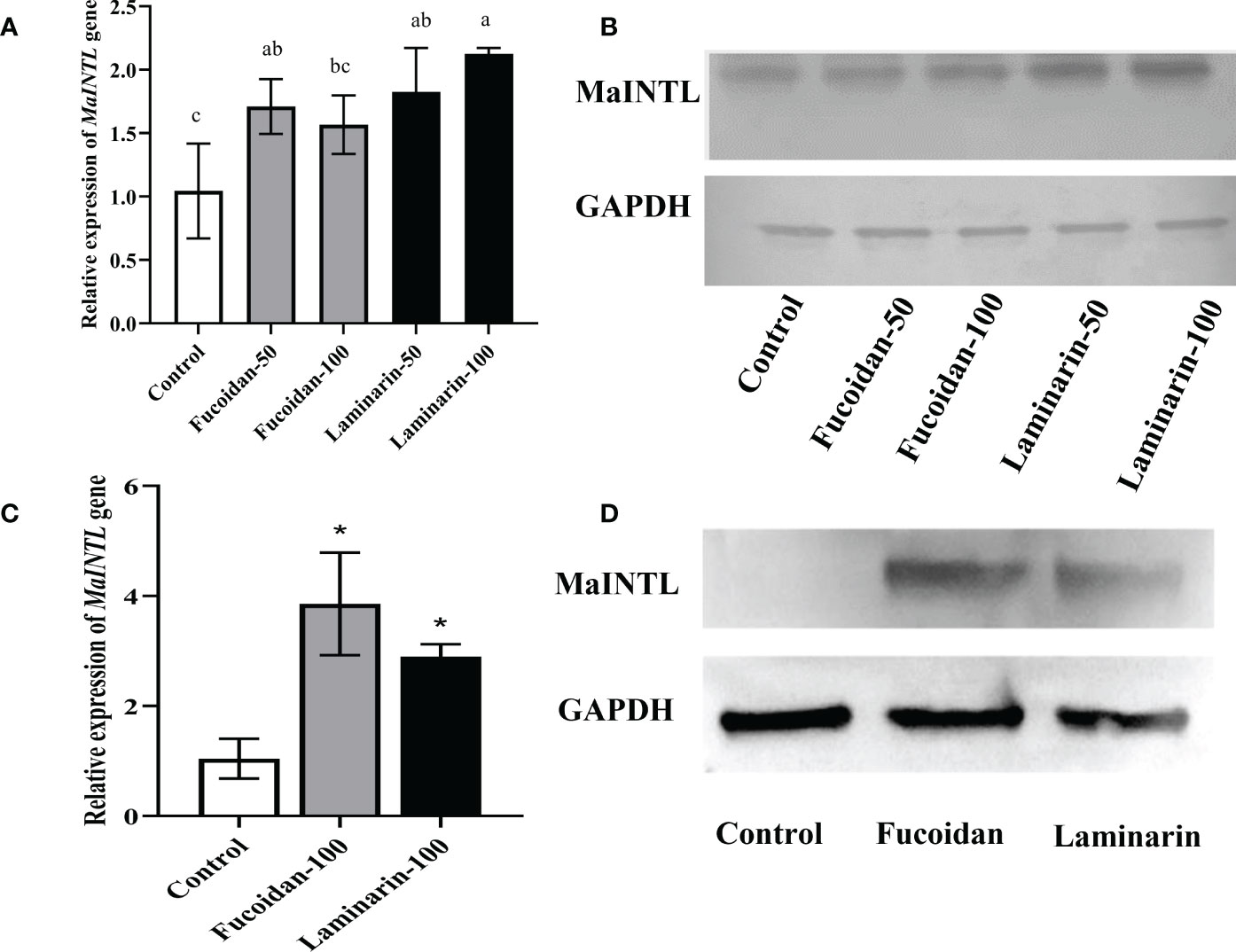
Figure 3 Induction of MaINTL expression by fucoidan and laminarin in macrophages and hepatocytes. (A) Induced expression of MaINTL by fucoidan and laminarin in hepatocytes detected by RT-qPCR, asterisks indicated notably statistical difference (P < 0.05). (B) Western blotting was performed to detect the induction of MaINTL expression by fucoidan and laminarin in hepatocytes. (C) Induced expression of MaINTL by fucoidan and laminarin in macrophages detected by RT-qPCR, asterisks indicated notably statistical difference (P < 0.05). (D) Western blotting was performed to detect the induction of MaINTL expression by fucoidan and laminarin in macrophages.
3.4 Fucoidan and laminarin bound with MaINTL and activated phagocytosis related signaling molecules
The rMaINTL protein was prepared with biological activity (Supplemental Fig. 1), and were used for the binding assay of rMaINTL with seaweed polysaccharides. Both seaweed polysaccharides and MaINTL can enhance host immunity by modulating the phagocytosis of macrophages, and INTL is a lectin that can bind with bacterial polysaccharide components. To identify the interaction of fucoidan and laminarin with MaINTL, the binding assay was carried out. As shown in Figure 4A, the absorbance at OD450 (nm) of laminarin and fucoidan groups was significantly higher than that of the control group, especially that of the fucoidan group, indicating that both fucoidan and laminarin can bind with MaINTL. Therefore, it could be speculated that seaweed polysaccharides (fucoidan and laminarin) can bind with MaINTL, thereby activated the downstream signaling pathways and enhanced the phagocytosis of M. amblycephala macrophages.
On account of the affected cytoskeleton of M. amblycephala macrophages post fucoidan and laminarin treatment, the expression levels of related signaling factors (CDC42, Rac1, WASP and ARPC) were detected. The results showed that the expression of Rac1 and WASP were significantly up-regulated post fucoidan and laminarin treatment (P < 0.05), while the expression of CDC42 and Arp2/3 complexes was not significantly affected (Figure 4B), suggesting that fucoidan and laminarin might regulate the cytoskeletal structures and phagocytosis of M. amblycephala macrophages by inducing the expression of MaINTL-Rac1-WASP axis. To verify the involvement of RAC1 and WASP in the enhanced phagocytosis process of macrophages that induced by seaweed polysaccharides, macrophages were pretreated with RAC1 and WASP inhibitors for 1 h, respectively, and then macrophages were treated with seaweed polysaccharides. The results showed that seaweed polysaccharides enhanced the phagocytosis of macrophages, while the inhibitors of RAC1 and WASP attenuated the enhanced phagocytosis (Figures 5A–D), indicating that seaweed polysaccharides enhanced the phagocytosis of M. amblycephala macrophages by upregulating the expression of RAC1 and WASP.
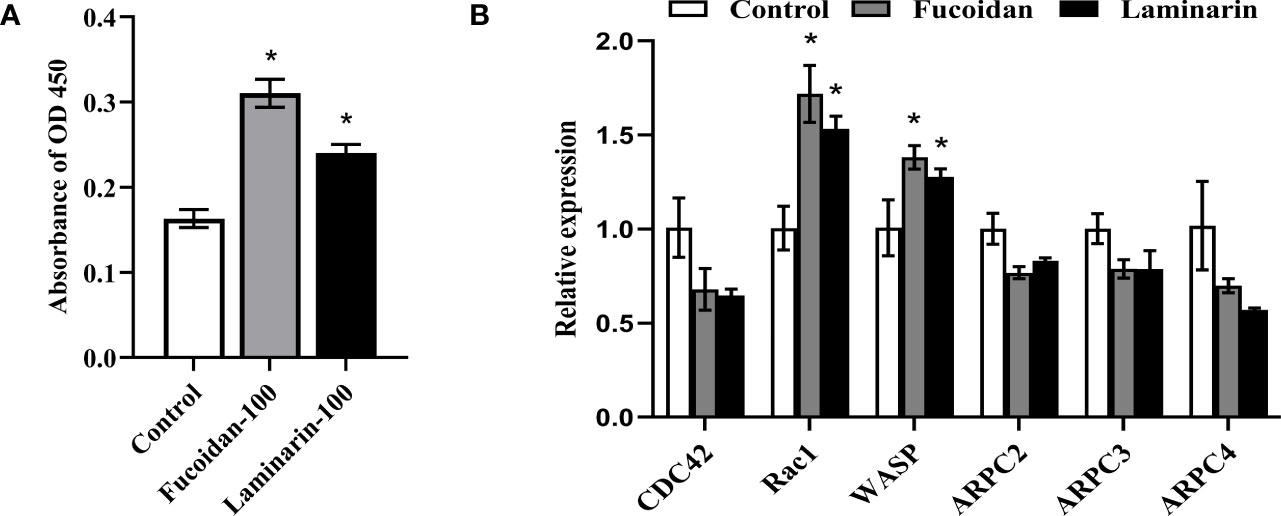
Figure 4 Fucoidan and laminarin bind with MaINTL and regulate the expression of candidate signaling molecules involved in the phagocytosis of macrophages. (A) 96-well plates were pre-coated with fucoidan (100 mg/mL) and laminarin (100 mg/mL), and their binding with MaINTL was detected by the absorbance at OD450 nm. Asterisks indicate significant variations (P < 0.05). (B) Fucoidan (100 mg/mL) and laminarin (100 mg/mL) stimulate the expression of signaling molecules that might be involved in the phagocytosis. Asterisks denoted significant differences (P < 0.05).
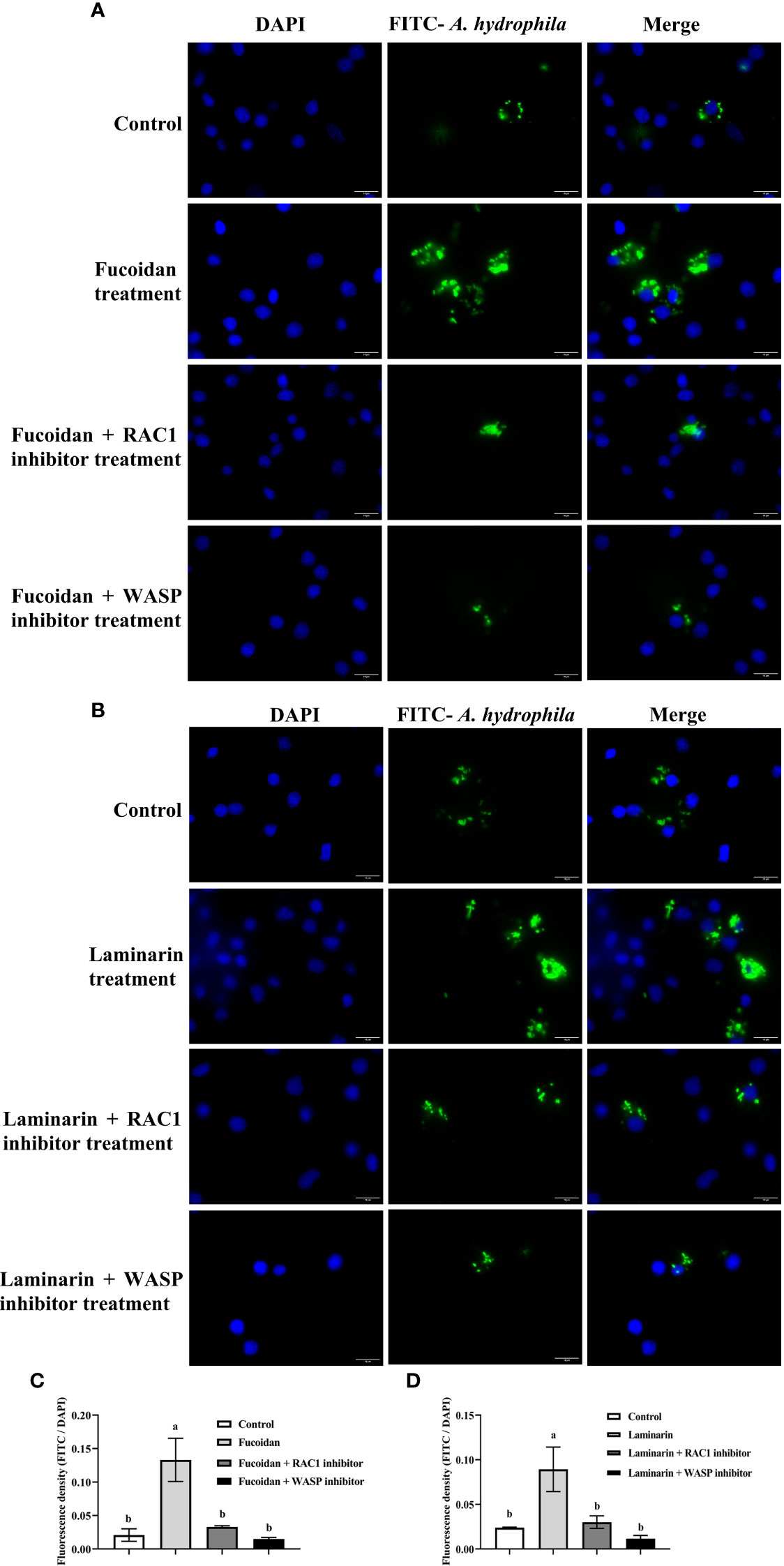
Figure 5 Effects of RAC-1/WASP on the phagocytosis of macrophages that induced by seaweed polysaccharides. (A) Blocking effects of RAC-1/WASP inhibitors on fucoidan-induced phagocytosis; (B) Blocking effects of RAC-1/WASP inhibitors on laminarin-induced phagocytosis; (C, D) Quantification of the blocking effects RAC-1/WASP inhibitors on seaweed polysaccharides-induced phagocytosis, different alphabets indicate significant differences (P < 0.05). Scale bars = 10 μm.
3.5 Effects of fucoidan and laminarin on the chemotaxis of neutrophils
The effects of fucoidan and laminarin on the expression of macrophage-derived cytokines were detected by RT-qPCR, and the results showed that the expression of CXCL8 and IL-1β was significantly up-regulated post fucoidan and laminarin treatment (P < 0.05; Figure 6A), indicating that fucoidan and laminarin might enhance the bactericidal activities of M. amblycephala macrophages and recruitment of neutrophils. CXCL8 is an important chemokine that can recruit neutrophils from blood vessels to the inflammatory sites by its chemotactic activity, thereby transwell migration assay was performed to detect the recruitment of neutrophils by fucoidan and laminarin treated macrophages. Specifically, the culture medium supernatant of fucoidan and laminarin treated macrophages was added in the lower chamber of 24-well transwell chamber, and primary neutrophils were plated in the upper chamber, of which the neutrophils that migrated into the lower chamber were counted. However, the results showed that the chemotaxis of neutrophils was not significantly different among 3 groups (Figures 6B, C), which might be due to the protein levels of induced CXCL8 by fucoidan and laminarin were not that sufficient.
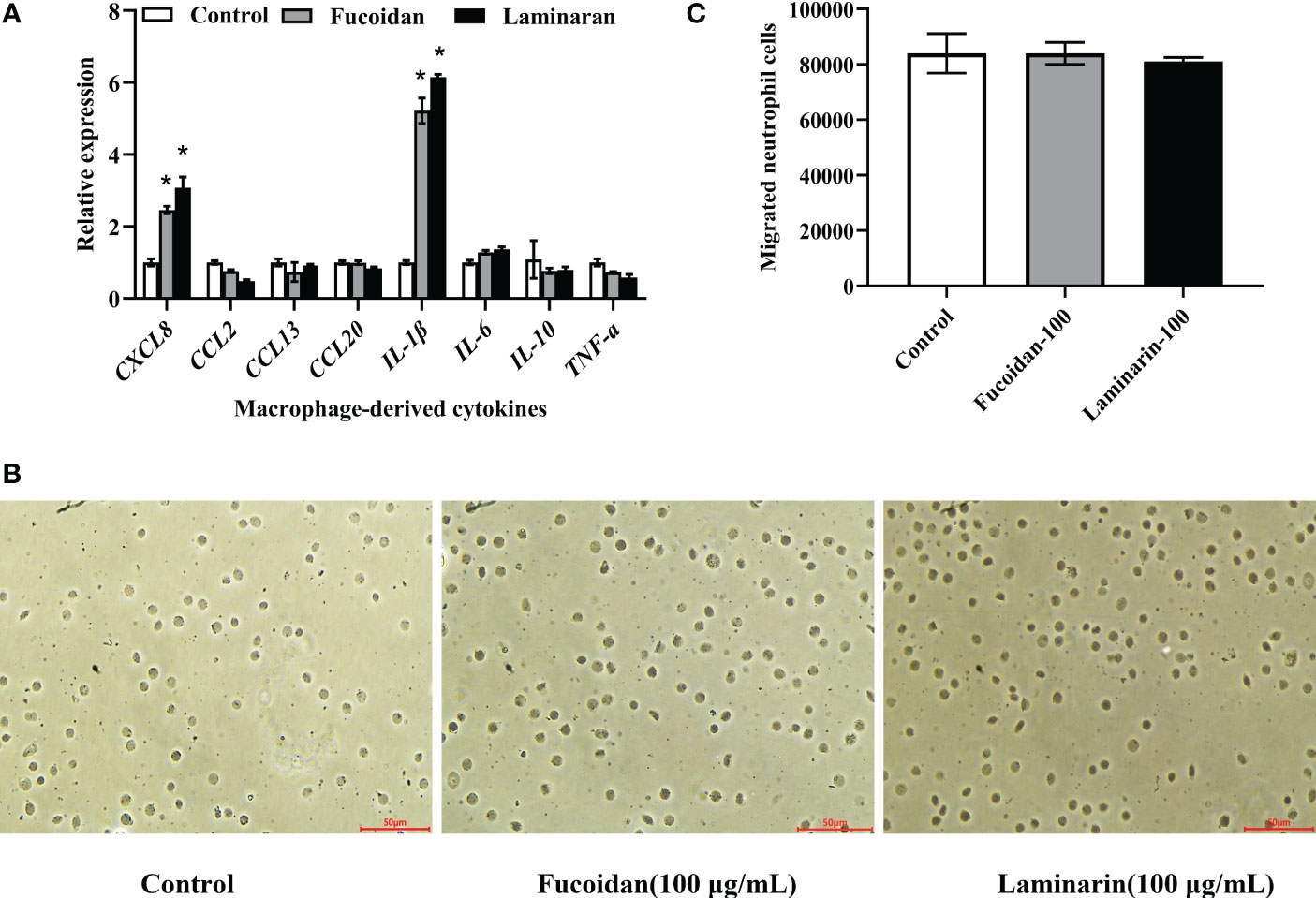
Figure 6 Effects of fucoidan and laminarin on the cytokines expression in macrophages and the chemotaxis of neutrophils. (A) Regulation of fucoidan and laminarin on the expression of cytokines in macrophages, and the asterisk indicated significant difference (P < 0.05). (B) Effects of fucoidan and laminarin cultured macrophages supernatant on the chemotaxis of neutrophils. Scale bars =50 μm. (C) Count statistics of chemotactic neutrophils.
4 Discussion
Seaweed polysaccharides have been studied and applied as immunostimulants in the animal feed for their immunomodulatory effects, among which fucoidan and laminarin are vital functional seaweed polysaccharides. The regulation of fucoidan and laminarin on the proliferation, migration and phagocytosis of macrophages have been reported previously (Lee et al., 2012; Li et al., 2017), as well as their promotion on the expression of inflammatory factors (Jin and Yu, 2015; Peng et al., 2019). Similarly, the present study found that fucoidan and laminarin promoted the remodeling of M. amblycephala macrophages cytoskeleton and enhanced their phagocytic ability, which might be attributed to their activation and binding with MaINTL, so as induced the expression of downstream phagocytosis related signaling factors such as Rac1 and WASP. And the macrophage phagocytosis induced by seaweed polysaccharide was diminished after blocked by RAC1 and WASP inhibitors, indicating that RAC1 and WASP are involved in the signaling pathway of macrophage phagocytosis induced by seaweed polysaccharide. These results indicated the immunoregulatory effects of fucoidan and laminarin on M. amblycephala macrophages via activation of MaINTL.
4.1 Fucoidan and laminarin enhance macrophage phagocytosis
Macrophages are important immune cells in host immune system that are involved in mediating inflammatory responses, repairing tissue damage, and identifying and phagocytizing pathogens. There are a variety of receptors on the surface of macrophages, among which the receptors related to polysaccharide recognition mainly including toll-like receptors (TLRs), mannose receptor (MR), dectin-1, scavenger receptor (SR), complement receptor 3 (CR3) (Taylor et al., 2005). The present study speculated that fucoidan and laminarin could bind with polysaccharide receptors on the surface of macrophages, thereby activating the Rac1-WASP signaling pathways in macrophages, inducing the changes of macrophages cytoskeleton, and then enhancing the phagocytic ability of macrophages. As an important member of Ras GTPase family, Rac1 is a key molecule that can affect cytoskeletal reorganization by inducing actin polymerization and F-actin formation (Wells et al., 2004), which is a necessary step for the phagocytosis of macrophages.
In addition, Gracilaria fisheri polysaccharides have been shown to bind with the dectin-1 receptor on the surface of macrophages, then induce the phagocytosis of macrophages and release cytokines (TNFα, IL-1β and IL-6) (Khongthong et al., 2021). Alginate promotes the phagocytosis by up-regulating the expression of TLR4 and stimulating Akt/NF-κB and p38 MAPK signaling pathways (Bi et al., 2017). In a study of osteosarcoma, fucoidan impairs the dynamic remodeling of cytoskeleton by inhibiting the activation of the Rac1/PAK1/LIMK1/cofilin signaling axis and significantly inhibiting the migratory capacity of U2OS cells (Zhang et al., 2020). Fucoidan-Sargassum decreases the expression of CDC42/WASP and inhibits the migration of cancer cells (Pan et al., 2019). These results suggested that seaweed polysaccharides could alter the cytoskeleton and promote the phagocytosis and migration by regulating the expression of Rac1/CDC42 and WASP, which was similar to the results of present study.
4.2 Interaction of fucoidan and laminarin with MaINTL
Intelectin is a kind of novel soluble and secretory lectin with conserved fibrinogen-related domain (FReD), and the D5 region of the intelectin domain is a new type of carbohydrate-recognition domain (CRD domain), which can recognize and bind with pathogen-specific carbohydrate in a Ca2+-dependent manner (Chen et al., 2020). Interestingly, MaINTL does not possess a CRD domain, but MaINTL can not only recognize LPS, a bacterial cell wall component, and also fucoidan and laminarin. These results suggested the presence of specific glycan binding sites in MaINTL protein, although they remain to be investigated. It has been revealed that the CRD domain is not present in amphioxus INTL, while its domain 5 (203-302 aa) is able to bind with LPS or PGN and agglutinate bacteria as efficient as the full-length INTL protein (Yan et al., 2018). Lectins are usually covalently bound to polysaccharides, which bind to asparagine (N-glycan) and serine or threonine (O-glycan) in proteins to form protein-polysaccharide complexes that perform biological functions (Valbuena et al., 2016). For example, mannose and serum C-type lectin are covalently bound to form mannose-binding lectins, which play an important role in intrinsic immunity, complement activation, immunomodulation, and phagocytosis (Vasta et al., 1999). However, the specific binding mode of MaINTL and seaweed polysaccharides need to be further investigated.
Previously, we have revealed that MaINTL was most highly expressed in the liver of M. amblycephala and could promote the phagocytosis of macrophages (Ding et al., 2017; Ding et al., 2019). Therefore, we verified the effects of fucoidan and laminarin on the expression of MaINTL in both macrophages and hepatocytes. Binding of seaweed polysaccharides with receptors on the surface of macrophages should induce MaINTL expression in the macrophages and hepatocytes.
4.3 Regulation of fucoidan and laminarin on the expression of cytokines
Seaweed polysaccharides are involved in the immune response by activating immune cells and inducing the secretion of cytokines. The present study showed that fucoidan and laminarin promoted the expression of CXCL8 and IL-1β in macrophages, while the expressions of IL-6, IL-10 and TNF-α did not significantly affected. The activation of NF-κB and MAPKs signaling pathways of RAW264.7 murine macrophages and natural killer cells by fucoidan from Nizamuddinia zanardinii has been reported, with increased secretion of TNF-α, IL‐1β, IL‐6 and INF‐γ (Tabarsa et al., 2020). Laminarin increases the phagocytic capacity of rainbow trout macrophages and significantly up-regulated the expression of TNF-α and CXCL8 (Morales-Lange et al., 2015). These results indicate that different seaweed polysaccharides can regulate the expression of different inflammatory cytokines in different cellular models. In addition, even for the same seaweed polysaccharide, the composition of the polysaccharide mixture affected the expression of different cytokines. For example, fucoidan from Undaria increases the expression of INF-γ, but has no significant regulatory effect on the expression of IL-4, IL-6 and TNF-α (Maruyama et al., 2015).
Activated macrophages secrete a large number of cytokines and chemokines, such as IL-6, CXCL8, IL-10, TNF-α, CCL2, CCL3, CCL4, CCL5, thereby participating in the process of host non-specific immunity and specific immunity (Fantuzzi et al., 2003; Kiguchi et al., 2009; Schulz et al., 2021). Macrophages induce the expression of chemokines CXCL1/CXCL2, thereby promoting the chemotaxis of neutrophils (De Filippo et al., 2013). Macrophages can also release IL-1β, a cytokine critical for neutrophils recruitment (Sil et al., 2017). These evidences suggest that macrophage can secrete chemokines that mediating the recruitment of neutrophils. Neutrophils and macrophages cooperate closely in the host immune response, which is involved in the prevention of pathogenic microbial invasion, pathogens clearance, and tissue repair. Receptors on the surface of neutrophils recognize chemotactic agents and sense their concentrations, leading to the polarization and motility of neutrophils through the activation of related signaling pathways (Petri and Sanz, 2018). CXCL8 is an important chemokine that can recruit neutrophils from blood vessels to the inflammatory sites by its chemotactic activity (Matsushima et al., 2022), which is an vital link between macrophages and neutrophils. Therefore, transwell migration assay was performed to detect the recruitment of neutrophils by fucoidan and laminarin treated macrophages. The present study found that the chemotaxis of neutrophils by the supernatant of macrophages treated with seaweed polysaccharides was not enhanced, despite the expression of neutrophils-specific chemokine CXCL8 was induced upon treatment. This may be because the protein levels of induced CXCL8 by fucoidan and laminarin are insufficient.
5 Conclusions
In brief, fucoidan and laminarin affected the cytoskeletal structures of macrophages and enhanced the phagocytosis, which should attributed to their activation and binding with MaINTL, so as the induced expression levels of Rac1/WASP, and after blockade by RAC1 and WASP inhibitors, seaweed polysaccharide-induced phagocytosis by macrophages was diminished. Moreover, even though the expression of chemokines CXCL8 in macrophages is up-regulated after the treatment of fucoidan and laminarin, while the chemotaxis of neutrophils are not significantly affected, which might due to the limited protein levels of CXCL8. The results provided novel insights into the immune regulatory effects of seaweed polysaccharides, and also suggested that fucoidan and laminarin are excellent candidates for fishery immune enhancers. The addition of seaweed polysaccharides as immune enhancers may increase the resistance of M. amblycephala to pathogenic microorganisms, thus promoting the healthy and sustainable development of M. amblycephala aquaculture.
Data availability statement
The original contributions presented in the study are included in the article/Supplementary material. Further inquiries can be directed to the corresponding authors.
Ethics statement
This study was approved by the Animal Care and Use Committee of Jiangsu Ocean University (protocol no. 2020-37; approval date: September 1, 2019). All procedures involving animals were performed in accordance with guidelines for the Care and Use of Laboratory Animals in China.
Author contributions
Conceptualization, ZD and XZ; methodology, YZ; software, XW; validation, JX; formal analysis, MZ; investigation, HL and YL; resources, XC; data curation, YT; writing—original draft preparation, HL; writing—review and editing, ZD and XZ; visualization, HL and YL; supervision, ZD and XZ; project administration, XZ; funding acquisition, ZD, XZ, and HC. All authors contributed to the article and approved the submitted version.
Funding
This work was supported by Chinese Postdoctoral Science Foundation (No. 2020M671386), Jiangsu Agricultural Science and Technology Innovation Fund (No. CX(20)3110), the Postdoctoral Science Foundation of Jiangsu Province (2020Z157) and Lianyungang City (China), and the Priority Academic Program Development of Jiangsu Higher Education Institutions (Innovation project No. 2022JSPAPD017).
Conflict of interest
The authors declare that the research was conducted in the absence of any commercial or financial relationships that could be construed as a potential conflict of interest.
Publisher’s note
All claims expressed in this article are solely those of the authors and do not necessarily represent those of their affiliated organizations, or those of the publisher, the editors and the reviewers. Any product that may be evaluated in this article, or claim that may be made by its manufacturer, is not guaranteed or endorsed by the publisher.
Supplementary material
The Supplementary Material for this article can be found online at: https://www.frontiersin.org/articles/10.3389/fmars.2023.1124880/full#supplementary-material
Abbreviations
Arpc2, recombinant actin related protein 2/3 complex subunit 2; CCL2/13/20, C-C motif chemokine ligand 2/13/20; CDC42, cell division cycle 42; CR3, complement receptor 3; CRD, carbohydrate-recognition domain; Ct, cycle value; CXCL1/8, C-X-C motif chemokine ligand 1/8; DAPI, 4',6-diamidino-2-phenylindole; DMSO, dimethyl sulfoxide; EF1ɑ, elongation Factor 1; FITC, fluorescein isothiocyanate isomer; FReD, fibrinogen-related domain; GAPDH, glyceraldehyde-3-phosphate dehydrogenase; GM-CSF, granulocyte-macrophage colony stimulating Factor; HRP, horse radish peroxidase; IgG, immunoglobulin G; IL-1β/4/6/8/10, interleukin-1β/4/6/8/10; INF‐γ, interferon‐γ; INTL, intelectin; IPTG, isopropyl-b-D-thiogalactopyranoside; LB, lysogeny broth; LIMK1, LIM domain kinase 1; MAPK, mitogen-activated protein kinase; MCP-1, monocyte chemotactic protein-1; MIP-2, macrophage inflammatory protein 2; MR, mannose receptor; MS-222, 3-aminobenzoic acid ethyl ester methane sulfonate; NF-kB, nuclear factor kappa B; PAK1, p21 (RAC1) activated kinase 1; PAMPs, pathogen-associated molecular patterns; PBS, phosphate buffered saline; PGN, peptidoglycan; PRRs, pattern recognition receptors; PVDF, polyvinylidene difluoride; RAC1, Rac family small GTPase 1; ROS, reactive oxygen species; RT-qPCR, real-time quantitative reverse transcription polymerase chain reaction; SDS-PAGE, polyacrylamide gel electrophoresis; SR, scavenger receptor; TBS, tris buffered saline; TBST, tris buffered saline with Tween-20; TLRs, toll-like receptors; TNFɑ, tumour necrosis factor α; WASP, Wiskott–Aldrich syndrome protein.
References
Abdel-Latif H. M. R., Dawood M. A. O., Alagawany M., Faggio C., Nowosad J., Kucharczyk D. (2022). Health benefits and potential applications of fucoidan (FCD) extracted from brown seaweeds in aquaculture: An updated review. Fish Shellfish Immunol. 122, 115–130. doi: 10.1016/j.fsi.2022.01.039
Berry M. N., Grivell A. R., Grivell M. B., Phillips J. W. (1997). Isolated hepatocytes–past, present and future. Cell Biol. Toxicol. 13 (4-5), 223–233. doi: 10.1023/a:1007402505482
Bi D., Zhou R., Cai N., Lai Q., Han Q., Peng Y., et al. (2017). Alginate enhances toll-like receptor 4-mediated phagocytosis by murine RAW264.7 macrophages. Int. J. Biol. Macromol. 105, 1446–1454. doi: 10.1016/j.ijbiomac.2017.07.129
Chen L., Li J., Yang G. (2020). A comparative review of intelectins. Scand. J. Immunol. 92 (1), e12882. doi: 10.1111/sji.12882
Chen J., Yang J., Du H., Aslam M., Wang W., Chen W., et al. (2021). Laminarin, a major polysaccharide in stramenopiles. Mar. Drugs 19 (10), 576. doi: 10.3390/md19100576
Chu C. Q., Lu X. J., Li C. H., Chen J. (2014). Molecular characterization of a CXCL8-like protein from ayu and its effect on chemotaxis of neutrophils and monocytes/macrophages. Gene 548, 48–55. doi: 10.1016/j.gene.2014.07.006
Cui H., Shen X., Zheng Y., Guo P., Gu Z., Gao Y., et al. (2022). Identification, expression patterns, evolutionary characteristics and recombinant protein activities analysis of CD209 gene from megalobrama amblycephala. Fish Shellfish Immunol. 126, 47–56. doi: 10.1016/j.fsi.2022.04.043
De Filippo K., Dudeck A., Hasenberg M., Nye E., van Rooijen N., Hartmann K., et al. (2013). Mast cell and macrophage chemokines CXCL1/CXCL2 control the early stage of neutrophil recruitment during tissue inflammation. Blood 121 (24), 4930–4937. doi: 10.1182/blood-2013-02-486217
Ding Z., Wang X., Liu Y., Zheng Y., Li H., Zhang M., et al. (2022). Dietary mannan oligosaccharides enhance the non-specific immunity, intestinal health, and resistance capacity of juvenile blunt snout bream (Megalobrama amblycephala) against aeromonas hydrophila. Front. Immunol. 13. doi: 10.3389/fimmu.2022.863657
Ding Z., Zhao X., Wang J., Zhang F., Wang W., Liu H. (2019). Intelectin mediated phagocytosis and killing activity of macrophages in blunt snout bream (Megalobrama amblycephala). Fish Shellfish Immunol. 87, 129–135. doi: 10.1016/j.fsi.2019.01.001
Ding Z., Zhao X., Zhan Q., Cui L., Sun Q., Lin L., et al. (2017). Characterization and expression analysis of an intelectin gene from Megalobrama amblycephala with strong response to bacterial infection and excellent bacterial binding and agglutination activity. Fish Shellfish Immunol. 61, 100–110. doi: 10.1016/j.fsi.2016.12.023
Fantuzzi L., Belardelli F., Gessani S. (2003). Monocyte/macrophage-derived CC chemokines and their modulation by HIV-1 and cytokines: A complex network of interactions influencing viral replication and AIDS pathogenesis. J. Leukoc. Biol. 74 (5), 719–725. doi: 10.1189/jlb.0403175
Hanington P. C., Tam J., Katzenback B. A., Hitchen S. J., Barreda D. R., Belosevic M. (2009). Development of macrophages of cyprinid fish. Dev. Comp. Immunol. 33 (4), 411–429. doi: 10.1016/j.dci.2008.11.004
Jin J. O., Yu Q. (2015). Fucoidan delays apoptosis and induces pro-inflammatory cytokine production in human neutrophils. Int. J. Biol. Macromol. 73, 65–71. doi: 10.1016/j.ijbiomac.2014.10.059
Khongthong S., Theapparat Y., Roekngam N., Tantisuwanno C., Otto M., Piewngam P. (2021). Characterization and immunomodulatory activity of sulfated galactan from the red seaweed gracilaria fisheri. Int. J. Biol. Macromol 189, 705–714. doi: 10.1016/j.ijbiomac.2021.08.182
Kiguchi N., Maeda T., Kobayashi Y., Fukazawa Y., Kishioka S. (2009). Leptin enhances CC-chemokine ligand expression in cultured murine macrophage. Biochem. Biophys. Res. Commun. 384 (3), 311–315. doi: 10.1016/j.bbrc.2009.04.121
Lee J. Y., Kim Y. J., Kim H. J., Kim Y. S., Park W. (2012). Immunostimulatory effect of laminarin on RAW 264. 7 Mouse macrophages. Molecules 17 (5), 5404–5411. doi: 10.3390/molecules17055404
Li Q. M., Teng H., Zha X. Q., Pan L. H., Luo J. P. (2018). Sulfated laminaria japonica polysaccharides inhibit macrophage foam cell formation. Int. J. Biol. Macromol. 111, 857–861. doi: 10.1016/j.ijbiomac.2018.01.103
Li P., Wang H., Shao Q., Kong B., Qu X. (2017). Fucoidan modulates cytokine production and migration of THP-1-derived macrophages via colony-stimulating factor-1. Mol. Med. Rep. 15 (4), 2325–2332. doi: 10.3892/mmr.2017.6228
Li Y., Zheng Y., Zhang Y., Yang Y., Wang P., Imre B., et al. (2021). Brown algae carbohydrates: Structures, pharmaceutical properties, and research challenges. Mar. Drugs 19 (11), 620. doi: 10.3390/md19110620
Livak K. J., Schmittgen T. D. (2001). Analysis of relative gene expression data using real-time quantitative PCR and the 2(-delta delta C(T)) method. Methods 25 (4), 402–408. doi: 10.1006/meth.2001.1262
Loike J. D., Silverstein S. C. (1983). A fluorescence quenching technique using trypan blue to differentiate between attached and ingested glutaraldehyde-fixed red blood cells in phagocytosing murine macrophages. J. Immunol. Methods 57 (1-3), 373–379. doi: 10.1016/0022-1759(83)90097-2
Lu X. J., Chen Q., Chen J., Chen J. (2017). Molecular identification and functional analysis of KLF2 in Plecoglossus altivelis (ayu): It’s regulatory role in monocyte/macrophage activation. Fish Shellfish Immunol. 62, 257–264. doi: 10.1016/j.fsi.2017.01.035
Malik I. A., Moriconi F., Sheikh N., Naz N., Khan S., Dudas J., et al. (2010). Single-dose gamma-irradiation induces up-regulation of chemokine gene expression and recruitment of granulocytes into the portal area but not into other regions of rat hepatic tissue. Am. J. Pathol. 176 (4), 1801–1815. doi: 10.2353/ajpath.2010.090505
Maruyama H., Tamauchi H., Kawakami F., Yoshinaga K., Nakano T. (2015). Suppressive effect of dietary fucoidan on proinflammatory immune response and MMP-1 expression in UVB-irradiated mouse skin. Planta Med. 81 (15), 1370–1374. doi: 10.1055/s-0035-1557821
Matsushima K., Yang D., Oppenheim J. J. (2022). Interleukin-8: An evolving chemokine. Cytokine 153, 155828. doi: 10.1016/j.cyto.2022.155828
Mohanty S. K., Ivantes C. A., Mourya R., Pacheco C., Bezerra J. A. (2010). Macrophages are targeted by rotavirus in experimental biliary atresia and induce neutrophil chemotaxis by Mip2/Cxcl2. Pediatr. Res. 67 (4), 345–351. doi: 10.1203/PDR.0b013e3181d22a73
Morales-Lange B., Bethke J., Schmitt P., Mercado L. (2015). Phenotypical parameters as a tool to evaluate the immunostimulatory effects of laminarin in oncorhynchus mykiss. Aquac Res. 46 (11), 2707–2715. doi: 10.1111/are.12426
Mylvaganam S., Freeman S. A., Grinstein S. (2021). The cytoskeleton in phagocytosis and macropinocytosis. Curr. Biol. 31 (10), R619–R632. doi: 10.1016/j.cub.2021.01.036
Nagata S. (2018). Xenopus laevis macrophage-like cells produce XCL-1, an intelectin family serum lectin that recognizes bacteria. Immunol. Cell Biol. 96 (8), 872–878. doi: 10.1111/imcb.12048
Pan T. J., Li L. X., Zhang J. W., Yang Z. S., Shi D. M., Yang Y. K., et al. (2019). Antimetastatic effect of fucoidan-sargassum against liver cancer cell invadopodia formation via targeting integrin αVβ3 and mediating αVβ3/Src/E2F1 signaling. J. Cancer. 10 (20), 4777–4792. doi: 10.7150/jca.26740
Park J., Cha J. D., Choi K. M., Lee K. Y., Han K. M., Jang Y. S. (2017). Fucoidan inhibits LPS-induced inflammation in vitro and during the acute response in vivo. Int. Immunopharmacol 43, 91–98. doi: 10.1016/j.intimp.2016.12.006
Peng Y., Song Y., Wang Q., Hu Y., He Y., Ren D., et al. (2019). In vitro and in vivo immunomodulatory effects of fucoidan compound agents. Int. J. Biol. Macromol. 127, 48–56. doi: 10.1016/j.ijbiomac.2018.12.197
Petri B., Sanz M. J. (2018). Neutrophil chemotaxis. Cell Tissue Res. 371 (3), 425–436. doi: 10.1007/s00441-017-2776-8
Sapharikas E., Lokajczyk A., Fischer A. M., Boisson-Vidal C. (2015). Fucoidan stimulates monocyte migration via ERK/p38 signaling pathways and MMP9 secretion. Mar. Drugs 13 (7), 4156–4170. doi: 10.3390/md13074156
Schulz C., Petzold T., Ishikawa-Ankerhold H. (2021). Macrophage regulation of granulopoiesis and neutrophil functions. Antioxid Redox Sign. 35 (3), 182–191. doi: 10.1089/ars.2020.8203
Shang Q., Shan X., Cai C., Hao J., Li G., Yu G. (2016). Dietary fucoidan modulates the gut microbiota in mice by increasing the abundance of lactobacillus and ruminococcaceae. Food Funct. 7 (7), 3224–3232. doi: 10.1039/c6fo00309e
Sil P., Wicklum H., Surell C., Rada B. (2017). Macrophage-derived IL-1β enhances monosodium urate crystal-triggered NET formation. Inflammation Res. 66 (3), 227–237. doi: 10.1007/s00011-016-1008-0
Tabarsa M., Dabaghian E. H., You S., Yelithao K., Cao R., Rezaei M., et al. (2020). The activation of NF-κB and MAPKs signaling pathways of RAW264.7 murine macrophages and natural killer cells by fucoidan from Nizamuddinia zanardinii. Int. J. Biol. Macromol 148, 56–67. doi: 10.1016/j.ijbiomac.2020.01.125
Taylor P. R., Martinez-Pomares L., Stacey M., Lin H. H., Brown G. D., Gordon S. (2005). Macrophage receptors and immune recognition. Annu. Rev. Immunol. 23, 901–944. doi: 10.1146/annurev.immunol.23.021704.115816
Tsuji S., Uehori J., Matsumoto M., Suzuki Y., Matsuhisa A., Toyoshima K., et al. (2001). Human intelectin is a novel soluble lectin that recognizes galactofuranose in carbohydrate chains of bacterial cell wall. J. Biol. Chem. 276 (26), 23456–23463. doi: 10.1074/jbc.M103162200
Tsuji S., Yamashita M., Hoffman D. R., Nishiyama A., Shinohara T., Ohtsu T., et al. (2009). Capture of heat-killed Mycobacterium bovis bacillus calmette-guérin by intelectin-1 deposited on cell surfaces. Glycobiology 19 (5), 518–526. doi: 10.1074/jbc.M103162200
Uribe-Querol E., Rosales C. (2020). Phagocytosis: Our current understanding of a universal biological process. Front. Immunol. 11. doi: 10.3389/fimmu.2020.01066
Usoltseva R. V., Belik A. A., Kusaykin M. I., Malyarenko O. S., Zvyagintsevа T. N., Ermakova S. P. (2020). Laminarans and 1,3-β-D-glucanases. Int. J. Biol. Macromol. 163, 1010–1025. doi: 10.1016/j.ijbiomac.2020.07.034
Valbuena G., Madrid J. F., Martínez de Ubago M., Gómez-Santos L., Alonso E., Díaz-Flores L., et al. (2016). N-glycans in Xenopus laevis testis characterised by lectin histochemistry. Reprod. Fertil Dev. 28 (3), 337–348. doi: 10.1071/RD14077
Vasta G. R., Quesenberry M., Ahmed H., O’Leary N. (1999). C-type lectins and galectins mediate innate and adaptive immune functions: their roles in the complement activation pathway. Dev. Comp. Immunol. 23 (4-5), 401–420. doi: 10.1016/s0145-305x(99)00020-8
Wells C. M., Walmsley M., Ooi S., Tybulewicz V., Ridley A. J. (2004). Rac1-deficient macrophages exhibit defects in cell spreading and membrane ruffling but not migration. J. Cell Sci. 117 (7), 1259–1268. doi: 10.1242/jcs.00997
Wesener D. A., Dugan A., Kiessling L. L. (2017). Recognition of microbial glycans by soluble human lectins. Curr. Opin. Struct. Biol. 44, 168–178. doi: 10.1016/j.sbi.2017.04.002
Yan J., Chen L., Liu Z., Chen Y., Sun Y., Han J., et al. (2018). The D5 region of the intelectin domain is a new type of carbohydrate recognition domain in the intelectin gene family. Dev. Comp. Immunol. 85, 150–160. doi: 10.1016/j.dci.2018.02.021
Yan J., Wang J., Zhao Y., Zhang J., Bai C., Zhang C., et al. (2012). Identification of an amphioxus intelectin homolog that preferably agglutinates gram-positive over gram-negative bacteria likely due to different binding capacity to LPS and PGN. Fish Shellfish Immunol. 33 (1), 11–20. doi: 10.1016/j.fsi.2012.03.023
Yan J., Zhang C., Zhang Y., Li K., Xu L., Guo L., et al. (2013). Characterization and comparative analyses of two amphioxus intelectins involved in the innate immune response. Fish Shellfish Immunol. 34 (5), 1139–1146. doi: 10.1016/j.fsi.2013.01.017
Yang H. S., Haj F. G., Lee M., Kang I., Zhang G., Lee Y. (2019). Laminaria japonica extract enhances intestinal barrier function by altering inflammatory response and tight junction-related protein in lipopolysaccharide-stimulated caco-2 cells. Nutrients 11 (5), 1001. doi: 10.3390/nu11051001
Zhang M., Chen L., Liu Y., Chen M., Zhang S., Kong D. (2020). Sea Cucumber (Cucumaria frondosa) fucoidan inhibits osteosarcoma adhesion and migration by regulating cytoskeleton remodeling. Oncol. Rep. 44 (2), 469–476. doi: 10.3892/or.2020.7614
Keywords: fucoidan, laminarin, intelectin, macrophages, phagocytosis
Citation: Li H, Liu Y, Teng Y, Zheng Y, Zhang M, Wang X, Cheng H, Xu J, Chen X, Zhao X and Ding Z (2023) Enhancement of seaweed polysaccharides (fucoidan and laminarin) on the phagocytosis of macrophages via activation of intelectin in blunt snout bream (Megalobrama amblycephala). Front. Mar. Sci. 10:1124880. doi: 10.3389/fmars.2023.1124880
Received: 15 December 2022; Accepted: 27 February 2023;
Published: 10 March 2023.
Edited by:
Noora Barzkar, King Mongkut’s University of Technology North Bangkok, ThailandCopyright © 2023 Li, Liu, Teng, Zheng, Zhang, Wang, Cheng, Xu, Chen, Zhao and Ding. This is an open-access article distributed under the terms of the Creative Commons Attribution License (CC BY). The use, distribution or reproduction in other forums is permitted, provided the original author(s) and the copyright owner(s) are credited and that the original publication in this journal is cited, in accordance with accepted academic practice. No use, distribution or reproduction is permitted which does not comply with these terms.
*Correspondence: Xiaoheng Zhao, emhhb3hpYW9oZW5nQGpvdS5lZHUuY24=; Zhujin Ding, ZGluZ3podWppbkBqb3UuZWR1LmNu
†These authors have contributed equally to this work